- 1Provincial-Level Key Laboratory for Molecular Medicine of Major Diseases and The Prevention and Treatment with Traditional Chinese Medicine Research in Gansu Colleges and Universities, Gansu University of Chinese Medicine, Lanzhou, China
- 2Gansu Institute of Cardiovascular Diseases, The First People’s Hospital of Lanzhou City, Lanzhou, China
- 3Key Laboratory of Space Radiobiology of Gansu Province & Key Laboratory of Heavy Ion Radiation Biology and Medicine of Chinese Academy of Sciences Institute of Modern Physics, Chinese Academy of Sciences, Lanzhou, China
- 4Key Laboratory of Dunhuang Medicine and Transformation at Provincial and Ministerial Level, Gansu University of Chinese Medicine, Lanzhou, China
The tumor microenvironment is essential for the formation and development of tumors. Cytokines in the microenvironment may affect the growth, metastasis and prognosis of tumors, and play different roles in different stages of tumors, of which transforming growth factor β (TGF-β) and tumor necrosis factor α (TNF-α) are critical. The two have synergistic and antagonistic effect on tumor regulation. The inhibition of TGF-β can promote the formation rate of tumor, while TGF-β can promote the malignancy of tumor. TNF-α was initially determined to be a natural immune serum mediator that can induce tumor hemorrhagic necrosis, it has a wide range of biological activities and can be used clinically as a target to immune diseases as well as tumors. However, there are few reports on the interaction between the two in the tumor microenvironment. This paper combs the biological effect of the two in different aspects of different tumors. We summarized the changes and clinical medication rules of the two in different tissue cells, hoping to provide a new idea for the clinical application of the two cytokines.
Introduction
The process of tumor formation is complex and changeable and closely related to changes in its microenvironment. As an important part of the tumor microenvironment, cytokines have a two-way relationship with cancer. On the one hand, cytokines can directly affect carcinogenesis and metastasis by changing the phenotype of tumors. On the other hand, cytokines can also play a role through host immune system to produce specific responses against tumors (1). The two most mentioned cytokines in the tumor microenvironment are TGF-β and TNF-α. Interestingly, these two cytokines play a two-way role in the process of tumor formation, that is they can both inhibit tumor formation and promote tumor development (2). However, the interaction between TGF-β and TNF-α during tumor formation is still unclear. This article reviews the two sides of the interaction between TGF-β and TNF-α during tumor formation.
Role of TGF-β in the Process of Tumor Formation
TGF-β was originally thought to induce the proliferation of rat renal fibroblasts (3). In normal environment, TGF-β, as an anti-inflammatory factor, has been proved to inhibit the production and function of effector T cells and antigen-presenting dendritic cells, and can regulate natural killer cells, macrophages, dendritic cells and granulocytes to inhibit inflammation (4, 5). Interestingly, in tumors, its effects are multifaceted, depending on the stage of the tumor. In the early stage, TGF-β, as an effective growth inhibitor, can inhibit the epithelial cell cycle and promote cell apoptosis, thus inhibiting the occurrence and development of tumors (6). In the middle and late stages, TGF-β can induce and promote epithelial-mesenchymal transformation (EMT), increase the activity and invasiveness of tumor cells and participate in tumor malignant progression and angiogenesis (7). In view of the above phenomenon, some literatures have proposed explanations. In the early stage, TGF-β is involved in the inhibition and apoptosis of tumor cells as a major tumor suppressor factor. In the middle and late stages, tumor cells develop resistance to TGF-β or are reinterpreted by tumor cells to promote tumor growth (8). Therefore, TGF-β plays an important role in tumor formation and development.
Role of TNF-α in the Process of Tumor Formation
Tumor necrosis factor (TNF) family is an important class of cytokines, which play an important role in the regulation of a series of physiological and pathological reactions such as cell proliferation, differentiation, apoptosis, immune responses and inflammation (9). TNF-α is produced by monocytes and macrophages, it can not only regulate the immune function and cause necrosis of some tumor cells, but also mediate pathophysiological reactions such as inflammatory processes, tissue injury and shock (10). The proinflammatory effect of TNF-α can promote the formation of tumors. Studies have shown that TNF-α can lead to highly invasive diseases in many malignant tumors, and effectively increase the transcriptional levels of different inflammatory factors and chemokines, and also increase the metastatic phenotype of cancer cells to promote the progression of cancer, so it plays an important role in regulating the proliferation, migration and invasion of various types of cancer cells (11–13). The bidirectional regulatory effect of TNF-α on tumors also plays a key role in cancer progression.
Interaction Between TNF-α and TGF-β
TNF-α is recognized as a strong pro-inflammatory factor and an important mediator of immune protection. But the role of TGF-β is complicated. Although it has a pro-inflammatory effect, it is more regarded as an effective immunosuppressive cytokine. Both of them are important cytokines involved in the process of tumor formation, and their interaction relationship mainly depends on the influence of the microenvironment.
TNF-α and TGF-β can promote each other’s production, and TNF-α has been shown to affect TGF-β expression in many cells and tissues, such as endothelial cells, lung epithelial cells, macrophages, as well as subcutaneous adipose tissue (14). A correlation was also found between TNF-α mRNA expression and TGF-β mRNA expression in fibroblasts as well as in thyroid cells (15–17). Similarly, TGF-β can also activate the expression of TNF-α in in vitro and in vivo experiments and plays an important role in tissue injury repair, inflammation and tumor growth (18–21). In addition to direct mutual stimulation of the two, TNF-α has an effect on TGF-β receptors, and TNF-α can increase TGF-β type I and type II receptor expression and stimulate Smad3 phosphorylation in rat fibroblasts (22). The mutual stimulation of the two is also closely related to their respective signaling pathways, and TNF-α can lead to increased TGF-β1 mRNA and promote the expression of TGF-β1 by activating the extracellular regulated kinase (ERK) specific pathway in fibroblasts (23). Subsequent in-depth studies revealed that TNF-α can induce the expression of activator protein 1 (AP-1) and allow it to bind to DNA, which leads to increased transcription of the TGF-β1 gene (24). There are also relevant reports pointing out that up-regulation of TGF-β1 expression induced by TNF-α may be associated with activation of the NF-κB pathway (25–27). TGF-β1 and TNF-α have mutually stimulating effect, and their mutual induction and crosstalk in their respective pathways leads to non-destructive tissue remodeling, ultimately leading to myocardial fibrosis, dysfunction and heart failure (28).
TNF-α and TGF-β can also inhibit each other, and increased TNF-α can inhibit TGF-β-mediated gene or signaling pathway conduction in the previous literature (29–31). It has also been shown that elevated TGF-β content can also inhibit the expression of TNF-α and its receptor in different cell lines, TGF-β can be produced by autocrine means in microglia, and can inhibit TNF-α production and prevent oxidative stress response, a phenomenon that contributes to the survival of phagocytic microglia (32). TGF-β inhibited the induction of TNF-α expression at both protein and mRNA levels in rat astrocytes (33). In phagocytes, TGF-β inhibited TNF-α production by mediating the expression of the major antigen and adhesion-promoting protein (BAD1) in type B dermatitis (34). In human venous endothelial cells (EC), TGF-β can down-regulate TNF-α receptors, thus exerting an immunosuppressive effect (35). TNF-α and TGF-β can interact differently in different microenvironments of different cell lines, which may be the result of self-regulation by the body in order to maintain the homeostasis of the normal microenvironment. However, the mechanism of the interaction between the two on tumorigenesis is still unclear. There are ten hallmarks which have been published about the process of normal cells that transforming into tumor cells, including self-sufficient growth signals, insensitivity to growth signals, avoidance of apoptosis, limit potential replication, sustained angiogenesis, tissue invasion and metastasis, avoidance of immune destruction, promotion of tumor inflammation, deregulating cellular energetics and genome instability and mutation (36). However, it has been investigated that the interaction between TNF-α and TGF-β is also two-sided during tumor formation. The authors reviewed the dual roles of proliferation, apoptosis, inflammatory response, immune regulation, EMT, tissue invasion and metastasis, and genomic instability in the process of tumor formation.
Role of TNF-α and TGF-β in Cell Proliferation
The first step in the transformation of normal cells into cancer is often abnormal changes in proliferation ability, and TNF-α and TGF-β play multiple roles in the regulation of cell proliferation, as shown in Table 1. First of all the two have a two-way effect in the coordinated regulation of proliferation, which can synergistically enhance the proliferation ability of tumor cells and increase the characteristics of tumor stem cell in pancreatic cancer cells (MiaPaCa-2) (37). In rheumatoid synovial fibroblasts (RSF), TNF-α and TGF-β can synergistically stimulate the proliferation of RSF, which is related to the activated RAS gene (38). It is found in myofibroblasts (MFBIC) that although TGF-β has a slight inhibitory effect on the cell growth, when used in combination with TNF-α, TGF-β can promote cell proliferation and stimulate the formation of liver fibrosis (39). In addition to the synergistic promotion, there is also a synergistic inhibitory effect. In human promyelocytic leukemia cells (HL-60), both inhibit cell growth, which is associated with down-regulation of c-myc expression (40). In hematopoietic stem cells, hematopoietic progenitor cells and cord blood megakaryocytes (MK), TNF-α and TGF-β can inhibit cell proliferation and colony-forming ability (41, 42). It has also been found that in mouse hepatocytes, TGF-β1 can inhibit the production of hepatic growth factor (HGF), while TNF-α is positively correlated with the production of TGF-β, and inhibiting the level of TNF-α can inhibit the production of TGF-β1, thereby enhancing liver tissue regeneration (43). TNF-α and TGF-β also have antagonistic effect on proliferation. In human fibroblasts and nasal epithelial cells (HNECs), TGF-β dose-dependently inhibits TNF-α-stimulated cell proliferation (44, 45). In the normal central nervous system, increased TGF-β1 inhibits the proliferation of brain endothelial cells (BEC), whereas in cerebral ischemia or other neurotic processes, activated microglia increase TNF-α production, which can promote BEC proliferation (46).
In summary, in pancreatic cancer, rheumatoid synovial fibroblasts and myofibroblasts, TNF-α and TGF-β can promote cell proliferation, while in human promyelocytic leukemia cells and hematopoietic cells, the two can inhibit cell proliferation. In fibroblasts, nasal epithelial cell and neurological diseases, TGF-β can restrain the pro-proliferative effect of TNF-α.
Role of TNF-α and TGF-β in Apoptosis
In addition to abnormal proliferation, avoiding cell apoptosis is also an important manifestation in the process of cell carcinogenesis, as shown in Table 2 and Figure 1. For Schwann cells (SC), oligodendrocytes (OLG), human umbilical vein endothelial cells (HUVEC) and malignant glioma cells (SMA-560), both cytokines have a significant synergistic effect on promoting apoptosis (54–58), and they can also induce the expression of apoptosis-related proteins and play a synergistic effect. In HL-60 cells, TGF-β1 and TNF-α can induce apoptosis by down-regulating the expression of the anti-apoptotic protein Bcl-2 (47). In gastric cancer cells (SNU620), both synergistically promote up-regulation of the transcription level of the pro-apoptotic protein Bim, thereby promoting apoptosis (48). However, in hepatic stellate cells (HSCs), TGF-β and TNF-α can synergistically inhibit apoptosis levels not only by reducing the expression of the spontaneously apoptotic CD95L gene but also by inducing NF-κB activation and up-regulating the anti-apoptotic protein Bcl-xL (49).
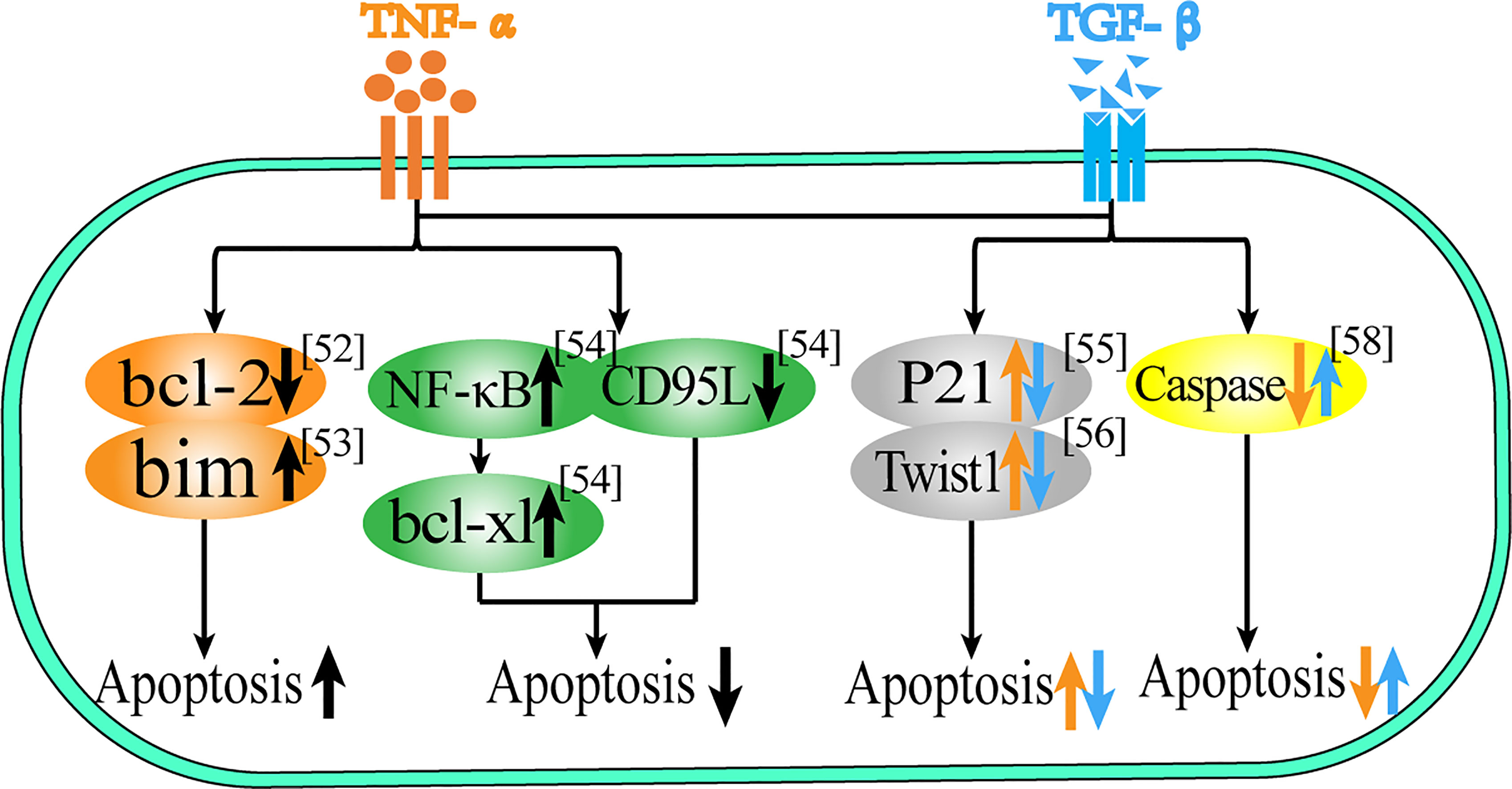
Figure 1 Role of TNF-α and TGF-β in apoptosis. This figure summarizes the regulatory effect of TNF-α and TGF-β on different proteins in terms of apoptosis. The black arrow represents the common regulatory role of TNF-α and TGF-β, and the orange arrow represents the effect of TNF-α, the blue arrow represents the effect of TGF-β.
In terms of apoptosis, there is not only a synergistic effect but also a mutual antagonism between the two. In epithelial cells, TGF-β1 can induce epithelial cell apoptosis, while TNF-α can induce the anti-apoptotic protein P21 to regulate the level of apoptosis (50). In melanoma cells, TGF-β1 can induce cell death, while TNF-α can reduce the relative cell death number, a phenomenon that may be associated with both regulating Twist1 protein levels (51). In splenocytes, TGF-β has an anti-apoptotic effect, while TNF-α can exacerbate the apoptotic process (52). In osteoblasts (MC3T3-E1), TGF-β1 can attenuate TNF-α-induced caspase gene expression to reduce TNF-α-induced apoptosis in murine osteoblasts (53). Thus, TNF-α and TGF-β have multiple roles in regulating cell proliferation and apoptosis, and the release of these cytokines by the body indirectly determines the survival fate of cells.
Therefore, both of them can promote apoptosis in SC, OLG, HOVEC, SMA-560, HL-60 and gastric cancer cells, while in hepatic stellate cells, both of them can inhibit apoptosis. In epithelial cells and melanoma cells, TGF-β induces apoptosis while TNF-α is anti-apoptotic, and in splenocytes and osteoblasts, TGF-β is anti-apoptotic while TNF-α induces apoptosis.
Role of TNF-α and TGF-β in Inflammation and Immunomodulation
Inflammation is an important intermediate process of carcinogenesis, and immune regulation disorders can induce the formation of cancer microenvironment. TNF-α and TGF-β are two important cytokines involved in immune regulation and inflammatory response. TNF-α and TGF-β also have two-way functions in immune regulation and participation in inflammation, as shown in Table 3 and Figure 2. These functions may vary depending on the microenvironment.
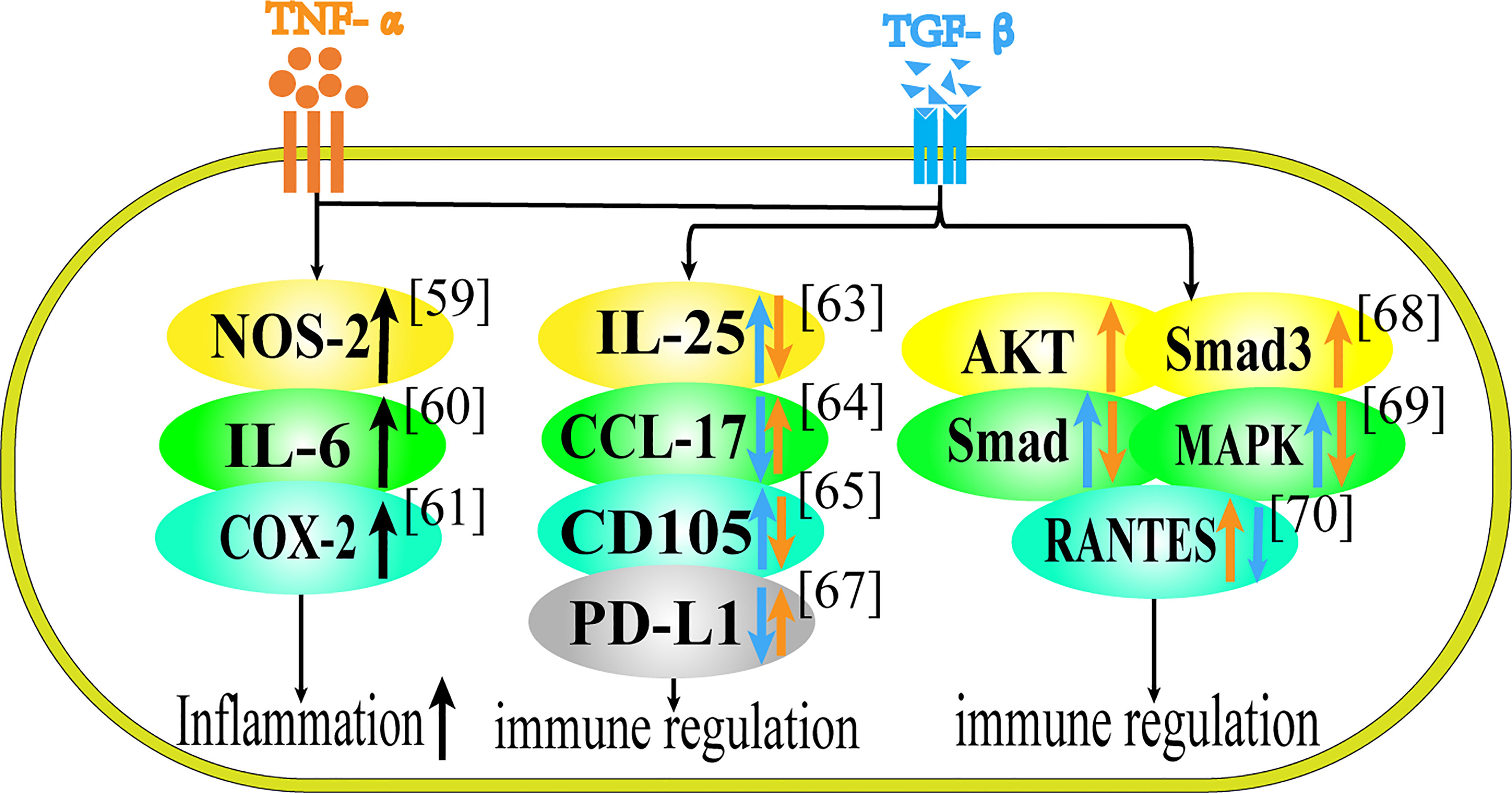
Figure 2 Role of TNF-α and TGF-β in Inflammation and Immune Regulation. This figure summarizes the regulatory effect of TNF-α and TGF-β on different proteins in terms of immune regulation. Black arrows represent the common regulatory effect of TNF-α and TGF-β. Orange arrows represent the effect of TNF-α, and blue arrows represent the effect of TGF-β.
The synergistic effect of TNF-α and TGF-β is mostly manifested in jointly promoting the release of inflammatory factors. In astrocytes, the co-stimulation of TNF-α and TGF-β1 increased the expression of NOS-2 compared with cytokine stimulation alone (59). TNF-α and TGF-β1 can synergistically increase IL-6 secretion in IEC-6 cells (60). In mesenchymal stem cells TNF-α and TGF-β can increase the release of proinflammatory factors such as COX-2, that is to say, in the presence of TNF-α, TGF-β1 is converted into pro-inflammatory cytokines, and when they act together on MSCs, they can play a synergistic effect in promoting inflammation (61). Besides, TGF-β can reverse the inhibitory effect of MSCs on T cell proliferation, and cooperate with TNF-α to promote immune response (69).
Through literature research, we found that the antagonistic effect of TNF-α and TGF-β have been reported in terms of immune regulation and inflammatory response. Regarding the antagonism of the two cytokines, studies have shown that in inflammatory bowel disease (IBD), TNF-α can inhibit the synthesis of IL-25, while TGF-β1 can stimulate the up-regulation of IL-25 in colon tissue (62). TGF-β1 can inhibit the production of CCL-17 in human epidermal cells induced by TNF-α, suggesting that TGF-β1 may have a certain effect on the treatment of atopic dermatitis (63). TNF-α can down-regulate the expression of CD105, while TGF-β1 can up-regulate it in vascular endothelial cells and this differential expression can regulate the damage repair of endothelial cells (64). TGF-β secreted by human umbilical cord mesenchymal stem cells can inhibit TNF-α and relieve atopic dermatitis (70). In patients with systemic lupus erythematosus (SLE), exogenous TNF-α can restore the expression of PD-L1 in lupus cells, while TGF-β, on the contrary, can inhibit the expression of PD-L1 in lupus cells (65).
Speaking of the antagonism of the two cytokines in their respective pathways, the increase of TNF-α can lead to the activation of AKT, and activated AKT can interact with Smad3, which leads to the inhibition of TGF-β pathway in regulatory T cells, so TNF-α can weaken the differentiation and function of Treg cells induced by TGF-β in autoimmune diseases through AKT and Smad3 signaling pathways (66). In fibroblasts, TNF-α can inhibit TGF-β1-induced activation of Smad2/3 and p38 MAPK pathway and terminate nerve growth factor (NGF) expression, which inhibits the regeneration of neurons (67). In rheumatoid synovial fibroblasts, TGF-β1 inhibits the expression of RANTES induced by TNF-α in a dose-dependent manner, possibly due to the decreased binding of NF-κB to the RANTES promoter (68).
In summary, TNF-α and TGF-β can increase the release of inflammatory factors and play pro-inflammatory or anti-inflammatory effect in different cells and tissues. In epidermal cells and patients with systemic lupus erythematosus, TNF-α is proinflammatory and TGF-β is anti-inflammatory. In vascular endothelial cells, inflammatory bowel disease, T cells and fibroblasts, TNF-α is anti-inflammatory and TGF-β is pro-inflammatory.
Role of TNF-α and TGF-β in EMT and Tissue Invasion and Metastasis
EMT is an important process of cancer invasion and changes in cell-extracellular matrix (ECM) interactions also contribute to these pathological conditions (71–73). TNF-α and TGF-β play important roles in EMT and tumor formation, and they play different regulatory roles in different cell lines and different microenvironments, as shown in Table 4 and Figure 3. In previous studies, a large number of literatures reported that the two play a synergistic role in fibrosis and EMT.
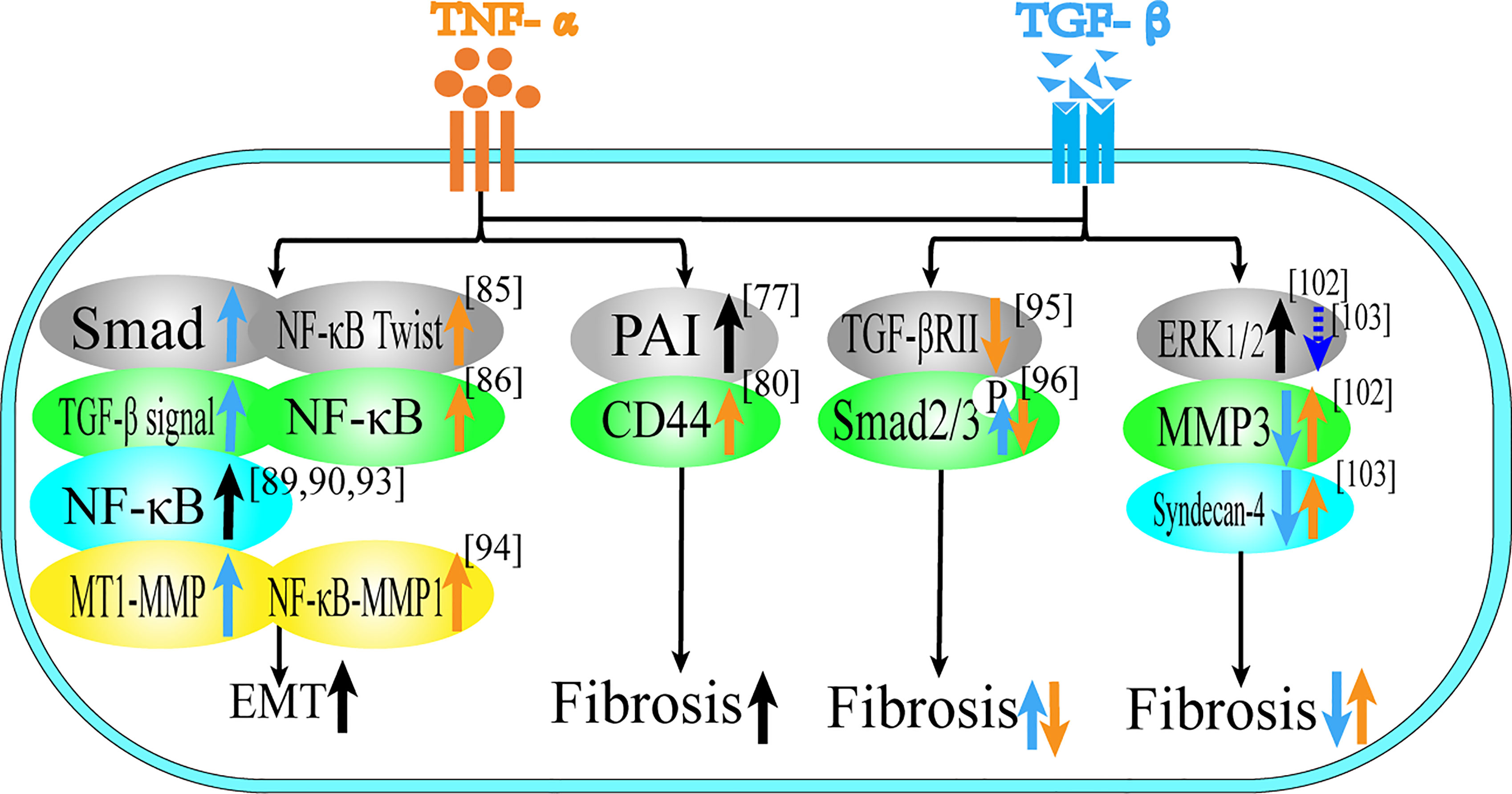
Figure 3 Role of TNF-α and TGF-β in EMT and tissue invasion and metastasis. This figure summarizes the regulatory effect of TNF-α and TGF-β on different proteins in terms of tissue invasion and metastasis, black arrows represent the common regulatory effect of TNF-α and TGF-β, reflecting the common regulatory effect of the two, orange arrows represent the effect of TNF-α, and blue arrows represent the effect of TGF-β.
For fibrosis, both of them play a synergistic role in different tissues. In rat kidney and lung tissues, TNF-α and TGF-β cooperatively induce fibrosis through their respective pathways (74–76), which is related to the expression of Plasminogen activator inhibitor-1 (PAI-1) (95). In the retina, TNF-α activates TGF-β signal transduction to induce the formation of fibrotic lesions in retinal pigment epithelium cells (77), which is related to the promotion of the expression of CD44 and MMP9 (96).
In EMT, TNF-α and TGF-β can stimulate intercellular transformation of bronchial epithelial cells and promote cell migration in combination (78). In addition, TNF-α can also enhance the endothelial mesenchymal transition induced by TGF-β (97, 98). Most of the literature focuses on the synergistic promotion of EMT in tumor cells. In terms of breast cancer, TGF-β and TNF-α can not only induce EMT, but also form a stable breast cancer stem cell phenotype (79, 80). EMT of breast cancer cells is regulated by TGF-β/Smad-dependent pathway and activated by TNF-α/NF-κB/Twist, which both promote tumor metastasis and synergistically promote breast cancer cell migration (99, 100). Activation of gene transcription programs associated with poor prognosis and increased malignancy in breast cancer is associated with activation of the NF-κB signaling pathway (101, 102). In cervical cancer and ovarian cancer, TNF-α and TGF-β induce EMT and cancer stem cell-like properties through the NF-κB/Twist axis (81, 82). In lung cancer, TNF-α enhances TGF-β1-induced EMT and enhances TGF-β1-induced cell contraction (103). In A549 cells, TGF-β1 induces EMT in a Smad-dependent manner, while TNF-α accelerates this process and may be involved in the regulation of miR-23a (83). Combined use of TNF-α and TGF-β can promote the dryness of H460 lung cancer through NF-κB and FoxM1 pathways (84). Moreover, studies have shown that the release of TNF-α and TGF-β1 from macrophages can synergistically increase the migration rate and persistence of 3D cancer cells. TGF-β1 mainly acts via the MT1-MMP pathway, while TNF-α acts mainly through the NFκB-MMP1 pathway (85). Therefore, TNF-α and TGF-β play a mutually promoting role in the regulation of fibrosis and EMT, and they complement each other and are two essential factors in the process of tumor formation.
TNF-α and TGF-β not only have synergistic effects on EMT and fibrosis, but also have antagonistic effects in some microenvironments. This antagonism is mainly reflected in fibroblasts, the regulation of type I collagen, nucleus pulposus cells and liver cancer. TGF-β can stimulate the production of extracellular matrix of fibroblasts, while TNF-α has anti-fibrotic activity and TNF-α can down-regulate the level of TGF-βRII protein through proteolysis in human skin fibroblasts, thereby attenuating the effect of extracellular matrix response to TGF-β (86). TNF-α inhibits phosphorylation of Smad2/3 by TGF-β1, thereby inhibiting the differentiation of myofibroblasts (87). In addition, high levels of TNF-α may inhibit fibroblast migration by elevating the level of Smad7 and reducing the phosphorylation of Smad2 (88). TGF-β can enhance the expression of type I collagen gene, while TNF-α has an antagonistic effect on the expression of type I collagen gene, which may be related to the bidirectional regulation of MAPK pathway (89–92). TGF-β1 can reverse the TNF-α-induced increase of MMP3 in nucleus pulposus cells (NP), which may be due to the activation of ERK1/2 signaling pathway, and treatment with ERK1/2 inhibitors (PD98059 and U0126) can abolish the antagonistic effect of TGF-β1 on TNF-α-mediated catabolic response (93). TGF-β1 antagonizes TNF-α -mediated up-regulation of syndecan-4 in NP cells. Treatment with ERK1/2 and NF-κB inhibitors can reduce TNF-α up-regulation of syndecan-4. This suggests that TGF-β1 exerts an anabolic effect on intervertebral discs by inhibiting syndecan-4 expression (94). In terms of liver cancer, TGF-β treatment can induce Huh7 in liver cancer cells to up-regulate autophagy gene expression, strongly activate autophagy and induce EMT, while TNF-α inhibits TGF-β-induced EMT levels by inhibiting autophagy (104).
In kidney, lung tissue and retinal pigment epithelial cells, the two together induce fibrosis; in breast cancer, ovarian cancer, cervical cancer, lung cancer and bronchial epithelial cells, the two together induce EMT. In fibroblasts, TGF-β promotes fibrosis, while TNF-α is anti-fibrotic; in liver cancer Huh7 cells, TGF-β induces EMT, while TNF-α resists EMT.
Role of TNF-α and TGF-β in Genomic Instability and Mutation
Mitotic abnormalities in normal cells frequently lead to missegregation of chromosomes, which produces genomic instability that triggers the development and progression of tumors (105). TNF-α and TGF-β have dual roles in causing genomic instability and mutations, as shown in Table 5. Studies have showed that there is a positive correlation between TNF-α and TGF-β and TP53 mutations (106, 109). Aberrant expression of androgen receptor (AR) -dependent transcriptional programs is a decisive pathology in the development of prostate cancer, and studies have shown that TNF-α and TGF-β can be involved in mediating AR-dependent gene transcription (107). Radiation can induce persistent genomic instability of bone marrow cells in mice, which is associated with high expression of TNF-α with TGF-β (110).TGF-β can up-regulate the activity of the pseudoxanthoma elasticum genesis gene ABCC6 promoter in HepG2 cells, while TNF-α has the opposite effect (108).
In summary, in monocytes and macrophages and cholangiocarcinoma, the two are positively correlated with TP53 mutant gene; in fibroblasts, the two jointly inhibit gene mutation. In addition, they can also affect the development of prostate cancer by regulating AR-dependent gene transcription. In the treatment of malaria parasites and liver cancer, the two are antagonistic.
Effect of TNF-α and TGF-β in Other Aspects
In other respects, TNF-α and TGF-β also interact, as shown in Table 6. TGF-β1 can alleviate TNF-α-induced intestinal epithelial barrier disorders, and TGF-β1 reduction significantly alleviated TNF-α-induced changes in proteins ZO-1 and occludin, these results showed that TGF-β1 could protect intestinal integrity after challenge with TNF-α (111). TGF-β1 can inhibit the synthesis of prolactin (PRL) in a dose-dependent manner, while TNF-α can stimulate the synthesis and release of prolactin, and the two cytokines antagonize each other, indicating that their relative concentrations can determine whether PRL synthesis is upregulated or downregulated (112). In terms of osteoclast formation, TGF-β enhances the formation of o TNF-α-induced osteoclasts, which may be reflected that TGF-β can induce the expression of SOCS (113–115). In addition to their different relative roles in different tissues or cells, the two also have different changes in different time periods. For example, in 1-5 months after radiotherapy of A549 cells, TGF-β did not change significantly (slightly more in the first month but not statistically significant), but TNF-α showed a significant trend to increase (116).
The Application of TNF-α and TGF-β in Tumor Treatment
Some progress has been made in animal tumor model experiments. For example, in vivo studies in mice and rats demonstrated that low-dose tail vein injection of TNF-α enhanced the anti-tumor activity of pegylated liposomal doxorubicin (117). There are also experiments to enhance the activity of local TNF-α by removing soluble TNF-α receptors in vitro, thus taking advantage of the strong antitumor activity of TNF-α (118). Clinical studies have proved that the hemorrhagic necrosis of the tumor can be quickly observed after the combined use of TNF-α and melphalan (119). The results of clinical trials have shown that the use of the TNF-αantagonist etanercept can alleviate the condition of some patients with ovarian cancer (120), but it has not achieved clinical remission in patients with advanced breast cancer (121).
In related experiments with TGF-β, different inhibitors have achieved satisfactory results in cell culture and animal models. TGF-β blocking peptide P114 can significantly reduce tumor growth (122). However, in clinical trials of cancer, the results are poor or inconsistent with animal experiments, which may be due to the differences between tumor-bearing tissues and naturally formed tumor tissues. However, there are few clinical reports on the combination of the two in the treatment of diseases, and it is unknown whether the combination of the two can achieve better efficacy. The following are some drugs we can currently learn about TNF-α and TGF-β. The FDA-approved inhibitors of TNF-α are infliximab, adalimumab, etanercept, golimumab and certolizumab. The role of TGF-β in tumors is complicated, so many inhibitors are in clinical trials. Inhibitors of TGF-β are mainly classified into four categories, TGF-β receptor kinase small molecule inhibitors, monoclonal antibodies that prevent TGF-β binding to receptor complexes, ligand traps, and TGF-β activation inhibitors (123). Several commonly used drugs related to TNF and TGF are shown in Table 7.
Drugs targeting TNF-α or TGF-β have limitations and side effects. For example, TNF-α inhibitors can cause opportunistic infections, invasive fungal infections, autoimmune diseases and the development of lymphoma, and increase skin derived incidence of solid tumors (127). The side effects of TGF-β are minor, but long-term systemic inhibition of TGF-β therapy can affect wound healing, tissue repair, and anti-inflammatory effects (128). At present, there have been experiments to develop drugs from the perspective of ligand receptors, hoping to improve clinical efficacy. However, TNF-α and TGF-β are in the upstream position of the cellular pathway, and simple blocking of both will affect many downstream protein abnormalities, so it is necessary to specifically block downstream target proteins that cause a pathological feature may be better.
Traditional Chinese medicine (TCM) is a multi-target in the treatment of diseases, and each target can synergize with each other with few side effect, which provides many possibilities for the combination of traditional Chinese and western medicine. The therapeutic effects of Chinese herbs on TNF-α and TGF-β have been reported. Guizhi Fuling capsule can down-regulate the expression of proinflammatory cytokines IL-1β and TNF-α (129). Dachaihu Decoction can reduce TNF-α and TGF-β in nonalcoholic fatty liver model rat (130). Psoralen, can reduce the levels of TGF-β and TNF-α in bleomycin-induced pulmonary fibrosis (131), and it can also inhibit TNF-α-induced inflammation of synovial cells by down-regulating the synthesis of IL-1β protein (132).
According to different purposes, different intervention measures can be taken for TGF-β and TNF-α. For example, in the treatment of tumors, if the two play a synergistic role in promoting tissue or cell proliferation, inflammation, EMT and inhibiting apoptosis, they can be blocked at the same time. However, if the two play a role in inhibiting tissue or cell proliferation, inflammation, EMT and promoting apoptosis, the expression of both can be promoted. Similarly, when two cytokines exert an antagonistic effect, they need to be blocked or promoted according to their current effects on the tumor. At different time points of tumor development, drug intervention can be given according to the changes of the two at different time points. For instance, as mentioned above, it can promote the production of TGF-β in the early stage to achieve the purpose of anti-tumor, but in the middle and late stages of the tumor, it is necessary to inhibit TGF-β to resist tumor angiogenesis. TNF-α also seems to have this logic, that is, it can regulate tumor apoptosis in the early stage, and it can increase tumor invasion and metastasis in the late stage. In most reports, it is described that the two should be used to effectively kill cancer in the early stage (133, 134), and the two should be targeted for treatment in the late stage (135, 136). However, we have proposed that the two play more antagonistic effects in the early stage and evolution of the tumor, and synergistic effects occur in the late stage of the tumor, and whether targeted therapy is needed or not needs to be distinguished according to different tissues and cells.
According to the above five effects of TGF-β and TNF-α on tumor genesis and development, we made the following generalizations. In proliferation, the antagonistic effect of the two was mainly in epidermis, and the synergistic effect was mainly in hematopoietic system. In terms of apoptosis, the antagonistic effect of the two was mainly in skin and bone, and the synergistic effect was mainly in glial cells. In terms of inflammatory response, the antagonistic effect is mostly skin and intestinal tract, and the synergistic effect is mostly in glial cells and stem cells. In EMT and fibrosis, the antagonistic effect is mostly in normal tissues, while the synergistic effect is mostly in epithelium and cancer. For genomic instability, the antagonistic effect of the two was mainly in hepatocytes, while the synergistic effect was mainly in fibroblasts and macrophages. So we guess that the co-action of the two is mostly in the endoderm, while the antagonistic action is mostly in the ectoderm. This corresponds to the theory of Yin and Yang and the theory of exterior and interior in Traditional Chinese medicine, as concluded in Figure 4.
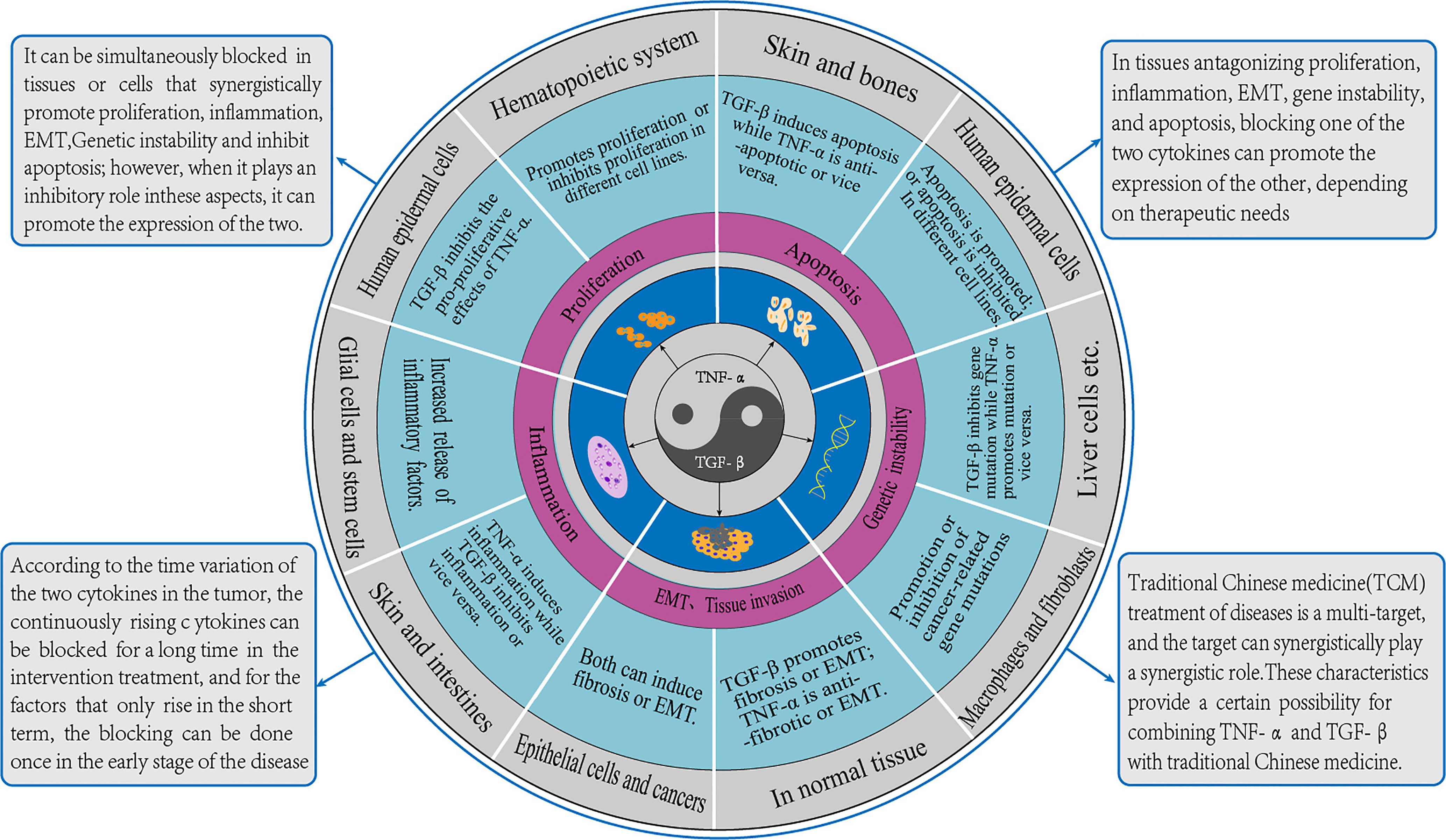
Figure 4 TGF-β and TNF-α action diagram. The crosstalk effect of TGF-β and TNF-α is similar to the relationship between Yin and Yang in Traditional Chinese medicine, which restricts and balances each other. This figure summarizes the roles of the two and their corresponding cell types described in the paper. The methods of synergism, antagonism and time-varying were put forward, and the prospects of integrated traditional Chinese and western medicine were also discussed.
Conclusion
In summary, based on the bifacial nature of TNF-α and TGF-β in the body microenvironment, this article describes the interaction between the two in the process of tumor formation from five aspects: proliferation, apoptosis, inflammation, EMT and genomic instability. Summarizing the different functional roles of the two in different microenvironments helps to speculate and control the evolution of cancer. For decades, researchers have been committed to the study of cytokines to treat cancer-related diseases. When designing relevant treatment methods, the direct effects of cytokines and the mutual regulation of multiple factors in immunity must be considered. In addition, traditional Chinese medicine has the advantages of multiple points of marked effect, synergy, and less side effects, which can supplement the inadequate treatment of modern medicine, and it also provides many possibilities for the combined application of traditional Chinese medicine with TNF-α and TGF-β.
Author Contributions
Z-wL and Y-mZ are responsible for the literature review and article writing. L-yZ and TZ are responsible for the guidance and article modification. Y-yL, G-cZ, Z-mM, and MS are responsible for the collection and sorting of materials. J-pH and ND are responsible for the guidance of ideas. Y-qL provide thought guidance and drafts. All authors contributed to the article and approved the submitted version.
Funding
National Natural Science Foundation of China (No. 81973595); National Natural Science Foundation of China (No. 82004094); Support Program for Longyuan Youth and Fundamental Research Funds for the Universities of Gansu Province. (No. GZTZ[2021]17-1); Provincial university industry support project in Gansu (No. 2020C-15).
Conflict of Interest
The authors declare that the research was conducted in the absence of any commercial or financial relationships that could be construed as a potential conflict of interest.
Publisher’s Note
All claims expressed in this article are solely those of the authors and do not necessarily represent those of their affiliated organizations, or those of the publisher, the editors and the reviewers. Any product that may be evaluated in this article, or claim that may be made by its manufacturer, is not guaranteed or endorsed by the publisher.
References
1. Amedei A, Prisco D, D' Elios MM. The Use of Cytokines and Chemokines in the Cancer Immunotherapy. Recent Pat Anticancer Drug Discov (2013) 8(2):126–42. doi: 10.2174/1574892811308020002
2. Balkwill F. TNF-Alpha in Promotion and Progression of Cancer. Cancer Metastasis Rev (2006) 25(3):409–16. doi: 10.1007/s10555-006-9005-3
3. Roberts AB, Anzano MA, Lamb LC, Smith JM, Sporn MB. New Class of Transforming Growth Factors Potentiated by Epidermal Growth Factor: Isolation From Non-Neoplastic Tissues. Proc Natl Acad Sci USA (1981) 78(9):5339–43. doi: 10.1073/pnas.78.9.5339
4. Sanjabi S, Oh SA, Li MO. Regulation of the Immune Response by TGF-β: From Conception to Autoimmunity and Infection. Cold Spring Harb Perspect Biol (2017) 9(6):a022236. doi: 10.1101/cshperspect.a022236
5. Wrzesinski SH, Wan YY, Flavell RA. Transforming Growth Factor-Beta and the Immune Response: Implications for Anticancer Therapy. Clin Cancer Res (2007) 13(18 Pt 1):5262–70. doi: 10.1158/1078-0432.CCR-07-1157
6. Bierie B, Moses HL. Transforming Growth Factor Beta (TGF-Beta) and Inflammation in Cancer. Cytokine Growth Factor Rev (2010) 21(1):49–59. doi: 10.1016/j.cytogfr.2009.11.008
7. Hu Q, Hisamatsu T, Haemmerle M, Cho MS, Pradeep S, Afshar-Kharghan V. Role of Platelet-Derived Tgfβ1 in the Progression of Ovarian Cancer. Clin Cancer Res (2017) 23(18):5611–21. doi: 10.1158/1078-0432.CCR-16-3272
8. Batlle E, Massagué J. Transforming Growth Factor-β Signaling in Immunity and Cancer. Immunity (2019) 50(4):924–40. doi: 10.1016/j.immuni.2019.03.024
9. Smith CA, Farrah T, Goodwin RG. The TNF Receptor Superfamily of Cellular and Viral Proteins: Activation, Costimulation, and Death. Cell (1994) 76(6):959–62. doi: 10.1016/0092-8674(94)90372-7
10. Zhang W, Chen Z, Li F, Kamencic H, Juurlink B, Gordon JR, et al. Tumour Necrosis Factor-Alpha (TNF-Alpha) Transgene-Expressing Dendritic Cells (DCs) Undergo Augmented Cellular Maturation and Induce More Robust T-Cell Activation and Anti-Tumour Immunity Than DCs Generated in Recombinant TNF-Alpha. Immunology (2003) 108(2):177–88. doi: 10.1046/j.1365-2567.2003.01489.x
11. Akiyama M, Hideshima T, Hayashi T, Tai YT, Tai YT, Mitsiades CS, Mitsiades N, et al. Nuclear factor-kappaB P65 Mediates Tumor Necrosis Factor Alpha-Induced Nuclear Translocation of Telomerase Reverse Transcriptase Protein. Cancer Res (2003) 63(1):18–21.
12. Shen Z, Zhou R, Liu C, Wang Y, Zhan W, Shao Z, et al. MicroRNA-105 Is Involved in TNF-α-Related Tumor Microenvironment Enhanced Colorectal Cancer Progression. Cell Death Dis (2017) 8(12):3213. doi: 10.1038/s41419-017-0048-x
13. Si H, Lu H, Yang X, Mattox A, Jang M, Bian Y, et al. TNF-α Modulates Genome-Wide Redistribution of Δnp63α/TAp73 and NF-κb cREL Interactive Binding on TP53 and AP-1 Motifs to Promote an Oncogenic Gene Program in Squamous Cancer. Oncogene (2016) 35(44):5781–94. doi: 10.1038/onc.2016.112
14. de Souza Dantas Oliveira SH, de Souza Aarão TL, da Silva Barbosa L, Souza Lisbôa PG, Tavares Dutra CD, Margalho Sousa L, et al. Immunohistochemical Analysis of the Expression of TNF-Alpha, TGF-Beta, and Caspase-3 in Subcutaneous Tissue of Patients With HIV Lipodystrophy Syndrome. Microb Pathog (2014) 67-68:41–7. doi: 10.1016/j.micpath.2014.02.004
15. Helmig S, Aliahmadi N, Stephan P, Döhrel J, Schneider J. TNF-α-308 Genotypes Are Associated With TNF-α and TGF-β1 mRNA Expression in Blood Leucocytes of Humans. Cytokine (2011) 53(3):306–10. doi: 10.1016/j.cyto.2010.11.018
16. Helmig S, Stephan P, Döhrel J, Schneider J. TNF-α mRNA Expression Correlates With TGF-β mRNA Expression In Vivo. Inflammation (2011) 34(4):255–9. doi: 10.1007/s10753-010-9231-2
17. Pekary AE, Berg L, Wang J, Lee P, Dubinett SM, Hershman JM. TNF-Alpha, TSH, and Aging Regulate TGF-Beta Synthesis and Secretion in FRTL-5 Rat Thyroid Cells. Am J Physiol (1995) 268(3 Pt 2):R808–15. doi: 10.1152/ajpregu.1995.268.3.R808
18. Wiseman DM, Polverini PJ, Kamp DW, Leibovich SJ. Transforming Growth Factor-Beta (TGF Beta) Is Chemotactic for Human Monocytes and Induces Their Expression of Angiogenic Activity. Biochem Biophys Res Commun (1988) 157(2):793–800. doi: 10.1016/S0006-291X(88)80319-X
19. Chen Y, Kam CS, Liu FQ, Liu Y, Lui VC, Lamb JR, et al. LPS-Induced Up-Regulation of TGF-Beta Receptor 1 Is Associated With TNF-Alpha Expression in Human Monocyte-Derived Macrophages. J Leukoc Biol (2008) 83(5):1165–73. doi: 10.1189/jlb.0807521
20. Wang X, Ma W, Han S, Meng Z, Zhao L, Yin Y, et al. TGF-β Participates Choroid Neovascularization Through Smad2/3-VEGF/TNF-α Signaling in Mice With Laser-Induced Wet Age-Related Macular Degeneration. Sci Rep (2017) 7(1):9672. doi: 10.1038/s41598-017-10124-4
21. Paduch R, Niedziela P. TGF-Beta1 Influence on TNF-Alpha Production and sTNF-Rs Shedding in a Coculture of Colon Carcinoma Cell Spheroids With Normal Cells. In Vitro Cell Dev Biol Anim (2009) 45(7):371–7. doi: 10.1007/s11626-009-9190-9
22. Voloshenyuk TG, Hart AD, Khoutorova E, Gardner JD. TNF-α Increases Cardiac Fibroblast Lysyl Oxidase Expression Through TGF-β and PI3Kinase Signaling Pathways. Biochem Biophys Res Commun (2011) 413(2):370–5. doi: 10.1016/j.bbrc.2011.08.109
23. Sullivan DE, Ferris MB, Pociask D, Brody AR. Tumor Necrosis Factor Induces Transforming Growth Factor1 Expression in Lung Fibroblasts Through the Extracellular Signal-Regulated Kinase Pathway. Am J Respir Cell Mol Biol (2005) 32(4):342–9. doi: 10.1165/rcmb.2004-0288OC
24. Sullivan DE, Ferris M, Nguyen H, Abboud E, Brody AR. TNF-Alpha Induces TGF-Beta1 Expression in Lung Fibroblasts at the Transcriptional Level via AP-1 Activation. J Cell Mol Med (2009) 13(8B):1866–76. doi: 10.1111/j.1582-4934.2008.00647.x
25. Zhao Y, Tian B, Sadygov RG, Zhang Y, Brasier AR. Integrative Proteomic Analysis Reveals Reprograming Tumor Necrosis Factor Signaling in Epithelial Mesenchymal Transition. J Proteomics (2016) 148:126–38. doi: 10.1016/j.jprot.2016.07.014
26. Della Latta V, Cecchettini A, Del Ry S, Morales MA. Bleomycin in the Setting of Lung Fibrosis Induction: From Biological Mechanisms to Counteractions. Pharmacol Res (2015) 97:122–30. doi: 10.1016/j.phrs.2015.04.012
27. Kabel AM, Omar MS, Elmaaboud MAA. Amelioration of Bleomycin-Induced Lung Fibrosis in Rats by Valproic Acid and Butyrate: Role of Nuclear Factor Kappa-B, Proinflammatory Cytokines and Oxidative Stress. Int Immunopharmacol (2016) 39:335–42. doi: 10.1016/j.intimp.2016.08.008
28. Kassiri Z, Defamie V, Hariri M, Oudit GY, Anthwal S, Dawood F, et al. Simultaneous Transforming Growth Factor Beta-Tumor Necrosis Factor Activation and Cross-Talk Cause Aberrant Remodeling Response and Myocardial Fibrosis in Timp3-Deficient Heart. J Biol Chem (2009) 284(43):29893–904. doi: 10.1074/jbc.M109.028449
29. Kähäri VM, Chen YQ, Bashir MM, Rosenbloom J, Uitto J. Tumor Necrosis Factor-Alpha Down-Regulates Human Elastin Gene Expression. Evidence for the Role of AP-1 in the Suppression of Promoter Activity. J Biol Chem (1992) 267(36):26134–41. doi: 10.1016/S0021-9258(18)35727-2
30. Kähäri VM, Chen YQ, Su MW, Ramirez F, Uitto J. Tumor Necrosis Factor-Alpha and Interferon-Gamma Suppress the Activation of Human Type I Collagen Gene Expression by Transforming Growth Factor-Beta 1. Evidence for Two Distinct Mechanisms of Inhibition at the Transcriptional and Posttranscriptional Levels. J Clin Invest (1990) 86(5):1489–95. doi: 10.1172/JCI114866
31. Verrecchia F, Pessah M, Atfi A, Mauviel A. Tumor Necrosis Factor-Alpha Inhibits Transforming Growth Factor-Beta/Smad Signaling in Human Dermal Fibroblasts via AP-1 Activation. J Biol Chem (2000) 275(39):30226–31. doi: 10.1074/jbc.M005310200
32. Ryu KY, Cho GS, Piao HZ, Kim WK. Role of TGF-β in Survival of Phagocytizing Microglia: Autocrine Suppression of TNF-α Production and Oxidative Stress. Exp Neurobiol (2012) 21(4):151–7. doi: 10.5607/en.2012.21.4.151
33. Benveniste EN, Tang LP, Law RM. Differential Regulation of Astrocyte TNF-Alpha Expression by the Cytokines TGF-Beta, IL-6 and IL-10. Int J Dev Neurosci (1995) 13(3-4):341–9. doi: 10.1016/0736-5748(94)00061-7
34. Finkel-Jimenez B, Wüthrich M, Klein BS. BAD1, an Essential Virulence Factor of Blastomyces Dermatitidis, Suppresses Host TNF-Alpha Production Through TGF-Beta-Dependent and -Independent Mechanisms. J Immunol (2002) 168(11):5746–55. doi: 10.4049/jimmunol.168.11.5746
35. Weiss JM, Cuff CA, Berman JW. TGF-Beta Downmodulates Cytokine-Induced Monocyte Chemoattractant Protein (MCP)-1 Expression in Human Endothelial Cells. A Putative Role for TGF-Beta in the Modulation of TNF Receptor Expression. Endothelium (1999) 6(4):291–302. doi: 10.3109/10623329909078496
36. Hanahan D, Weinberg RA. Hallmarks of Cancer: The Next Generation. Cell (2011) 144(5):646–74. doi: 10.1016/j.cell.2011.02.013
37. Kali A, Ostapchuk YO, Belyaev NN. Tnfα and Tgfβ-1 Synergistically Increase the Cancer Stem Cell Properties of MiaPaCa-2 Cells. Oncol Lett (2017) 14(4):4647–58. doi: 10.3892/ol.2017.6810
38. Kitasato H, Noda M, Akahoshi T, Okamoto R, Koshino T, Murakami Y, et al. Activated Ras Modifies the Proliferative Response of Rheumatoid Synovial Cells to TNF-Alpha and TGF-Alpha. Inflammation Res (2001) 50(12):592–7. doi: 10.1007/PL00000239
39. Bachem MG, Sell KM, Melchior R, Kropf J, Eller T, Gressner AM. Tumor Necrosis Factor Alpha (TNF Alpha) and Transforming Growth Factor Beta 1 (TGF Beta 1) Stimulate Fibronectin Synthesis and the Transdifferentiation of Fat-Storing Cells in the Rat Liver Into Myofibroblasts. Virchows Arch B Cell Pathol Incl Mol Pathol (1993) 63(2):123–30. doi: 10.1007/BF02899251
40. Robinson-Benion C, Salhany KE, Hann SR, Holt JT. Antisense Inhibition of C-Myc Expression Reveals Common and Distinct Mechanisms of Growth Inhibition by TGF Beta and TNF Alpha. J Cell Biochem (1991) 45(2):188–95. doi: 10.1002/jcb.240450210
41. Wang L, Xiao Q, Wang CH, Li X, Luo SQ, Tang CW. Vasoactive Intestinal Polypeptide Suppresses Proliferation of Human Cord Blood-Derived Hematopoietic Progenitor Cells by Increasing TNF-α and TGF-β Production in the Liver. Genet Mol Res (2014) 13(4):9032–43. doi: 10.4238/2014.October.31.18
42. Lu L, Wang LS, Cooper RJ, Liu HJ, Turner K, Weich N, et al. Suppressive Effects of TNF-Alpha, TGF-Beta1, and Chemokines on Megakaryocytic Colony Formation in CD34+ Cells Derived From Umbilical Cord Blood Compared With Mobilized Peripheral Blood and Bone Marrow. J Hematother Stem Cell Res (2000) 9(2):195–204. doi: 10.1089/152581600319405
43. Martino RB, Coelho AM, Kubrusly MS, Leitão R, Sampietre SN, Machado MC, et al. Pentoxifylline Improves Liver Regeneration Through Down-Regulation of TNF-α Synthesis and TGF-β1 Gene Expression. World J Gastrointest Surg (2012) 4(6):146–51. doi: 10.4240/wjgs.v4.i6.146
44. Kamijo R, Takeda K, Nagumo M, Konno K. Suppression of TNF-Stimulated Proliferation of Diploid Fibroblasts and TNF-Induced Cytotoxicity Against Transformed Fibroblasts by TGF-Beta. Biochem Biophys Res Commun (1989) 158(1):155–62. doi: 10.1016/S0006-291X(89)80191-3
45. Min YG, Rhee CS, Kwon SH, Lee KS, Yun JB. Effects of IL-1 Beta, TNF-Alpha, and TGF-Beta on Proliferation of Human Nasal Epithelial Cells In Vitro. Am J Rhinol (1998) 12(4):279–82. doi: 10.2500/105065898781390064
46. Welser JV, Li L, Milner R. Microglial Activation State Exerts a Biphasic Influence on Brain Endothelial Cell Proliferation by Regulating the Balance of TNF and TGF-β1. J Neuroinflamm (2010) 7:89. doi: 10.1186/1742-2094-7-89
47. Chen YZ, Wu Y, Huang MJ, Lu LH. Effect of Endogenous TGF-Beta1 and TNF-Alpha on the As(2)O(3) Inducing Apoptosis of HL-60 Cells. Zhonghua Xue Ye Xue Za Zhi (2003) 24(5):231–4.
48. Ha Thi HT, Lim HS, Kim J, Kim YM, Kim HY, Hong S. Transcriptional and Post-Translational Regulation of Bim Is Essential for TGF-β and TNF-α-Induced Apoptosis of Gastric Cancer Cell. Biochim Biophys Acta (2013) 1830(6):3584–92. doi: 10.1016/j.bbagen.2013.03.006
49. Saile B, Matthes N, El Armouche H, Neubauer K, Ramadori G. The Bcl, NFkappaB and P53/P21waf1 Systems Are Involved in Spontaneous Apoptosis and in the Anti-Apoptotic Effect of TGF-Beta or TNF-Alpha on Activated Hepatic Stellate Cells. Eur J Cell Biol (2001) 80(8):554–61. doi: 10.1078/0171-9335-00182
50. Yamasaki M, Kang HR, Homer RJ, Chapoval SP, Cho SJ, Lee BJ, et al. P21 Regulates TGF-Beta1-Induced Pulmonary Responses via a TNF-Alpha-Signaling Pathway. Am J Respir Cell Mol Biol (2008) 38(3):346–53. doi: 10.1165/rcmb.2007-0276OC
51. Menon DR, Wels C, Bonyadi Rad E, Joshi S, Knausz H, Lade-Keller J, et al. TGF-β1 and TNF-α Differentially Regulate Twist1 Mediated Resistance Towards BRAF/MEK Inhibition in Melanoma. Pigment Cell Melanoma Res (2013) 26(6):912–6. doi: 10.1111/pcmr.12139
52. Keswani T, Bhattacharyya A. Splenocyte Apoptosis in Plasmodium Berghei ANKA Infection: Possible Role of TNF-α and TGF-β. Parasite Immunol (2013) 35(2):73–90. doi: 10.1111/pim.12005
53. Chua CC, Chua BH, Chen Z, Landy C, Hamdy RC. TGF-Beta1 Inhibits Multiple Caspases Induced by TNF-Alpha in Murine Osteoblastic MC3T3-E1 Cells. Biochim Biophys Acta (2002) 1593(1):1–8. doi: 10.1016/S0167-4889(02)00257-4
54. Skoff AM, Lisak RP, Bealmear B, Benjamins JA. TNF-Alpha and TGF-Beta Act Synergistically to Kill Schwann Cells. J Neurosci Res (1998) 53(6):747–56. doi: 10.1002/(SICI)1097-4547(19980915)53:6<747::AID-JNR12>3.0.CO;2-V
55. Yu C, Takeda M, Soliven B. Regulation of Cell Cycle Proteins by TNF-Alpha and TGF-Beta in Cells of Oligodendroglial Lineage. J Neuroimmunol (2000) 108(1-2):2–10. doi: 10.1016/S0165-5728(99)00278-7
56. Emmanuel C, Foo E, Medbury HJ, Matthews J, Comis A, Zoellner H. Synergistic Induction of Apoptosis in Human Endothelial Cells by Tumour Necrosis Factor-Alpha and Transforming Growth Factor-Beta. Cytokine (2002) 18(5):237–41. doi: 10.1006/cyto.2002.1042
57. Emmanuel C, Huynh M, Matthews J, Kelly E, Zoellner H. TNF-α and TGF-β Synergistically Stimulate Elongation of Human Endothelial Cells Without Transdifferentiation to Smooth Muscle Cell Phenotype. Cytokine (2013) 61(1):38–40. doi: 10.1016/j.cyto.2012.09.017
58. Ashley DM, Sampson JH, Archer GE, Hale LP, Bigner DD. Local Production of TGF Beta1 Inhibits Cerebral Edema, Enhances TNF-Alpha Induced Apoptosis and Improves Survival in a Murine Glioma Model. J Neuroimmunol (1998) 86(1):46–52. doi: 10.1016/S0165-5728(98)00017-4
59. Hamby ME, Gragnolati AR, Hewett SJ, Hewett JA. TGF Beta 1 and TNF Alpha Potentiate Nitric Oxide Production in Astrocyte Cultures by Recruiting Distinct Subpopulations of Cells to Express NOS-2. Neurochem Int (2008) 52(6):962–71. doi: 10.1016/j.neuint.2007.10.010
60. McGee DW, Bamberg T, Vitkus SJ, McGhee JR. A Synergistic Relationship Between TNF-Alpha, IL-1 Beta, and TGF-Beta 1 on IL-6 Secretion by the IEC-6 Intestinal Epithelial Cell Line. Immunology (1995) 86(1):6–11.
61. Lerrer S, Liubomirski Y, Bott A, Abnaof K, Oren N, Yousaf A, et al. Co-Inflammatory Roles of Tgfβ1 in the Presence of Tnfα Drive a Pro-Inflammatory Fate in Mesenchymal Stem Cells. Front Immunol (2017) 8:479. doi: 10.3389/fimmu.2017.00479
62. Fina D, Franzè E, Rovedatti L, Corazza GR, Biancone L, Sileri PP, et al. Interleukin-25 Production is Differently Regulated by TNF-α and TGF-β1 in the Human Gut. Mucosal Immunol (2011) 4(2):239–44. doi: 10.1038/mi.2010.68
63. Sumiyoshi K, Nakao A, Setoguchi Y, Tsuboi R, Okumura K, Ogawa H. TGF-Beta/Smad Signaling Inhibits IFN-Gamma and TNF-Alpha-Induced TARC (CCL17) Production in HaCaT Cells. J Dermatol Sci (2003) 31(1):53–8. doi: 10.1016/S0923-1811(02)00141-X
64. Li C, Guo B, Ding S, Rius C, Langa C, Kumar P, et al. TNF Alpha Down-Regulates CD105 Expression in Vascular Endothelial Cells: A Comparative Study With TGF Beta 1. Anticancer Res (2003) 23(2B):1189–96.
65. Ou JN, Wiedeman AE, Stevens AM. TNF-α and TGF-β Counter-Regulate PD-L1 Expression on Monocytes in Systemic Lupus Erythematosus. Sci Rep (2012) 2:295. doi: 10.1038/srep00295
66. Zhang Q, Cui F, Fang L, Hong J, Zheng B, Zhang JZ. TNF-α Impairs Differentiation and Function of TGF-β-Induced Treg Cells in Autoimmune Diseases Through Akt and Smad3 Signaling Pathway. J Mol Cell Biol (2013) 5(2):85–98. doi: 10.1093/jmcb/mjs063
67. Ohta M, Chosa N, Kyakumoto S, Yokota S, Okubo N, Nemoto A, et al. Il−1β and TNF−α Suppress TGF−β−Promoted NGF Expression in Periodontal Ligament−Derived Fibroblasts Through Inactivation of TGF−β−Induced Smad2/3−and P38 MAPK−mediated Signals. Int J Mol Med (2018) 42(3):1484–94. doi: 10.3892/ijmm.2018.3714
68. Cho ML, Min SY, Chang SH, Kim KW, Heo SB, Lee SH, et al. Transforming Growth Factor Beta 1(TGF-Beta1) Down-Regulates TNFalpha-Induced RANTES Production in Rheumatoid Synovial Fibroblasts Through NF-kappaB-Mediated Transcriptional Repression. Immunol Lett (2006) 105(2):159–66. doi: 10.1016/j.imlet.2006.02.003
69. Xu C, Yu P, Han X, Du L, Gan J, Wang Y, et al. TGF-β Promotes Immune Responses in the Presence of Mesenchymal Stem Cells. J Immunol (2014) 192(1):103–9. doi: 10.4049/jimmunol.1302164
70. Park HH, Lee S, Yu Y, Yoo SM, Baek SY, Jung N, et al. TGF-β Secreted by Human Umbilical Cord Blood-Derived Mesenchymal Stem Cells Ameliorates Atopic Dermatitis by Inhibiting Secretion of TNF-α and IgE. Stem Cells (2020) 38(7):904–16. doi: 10.1002/stem.3183
71. Thiery JP. Epithelial-Mesenchymal Transitions in Tumour Progression. Nat Rev Cancer (2002) 2(6):442–54. doi: 10.1038/nrc822
72. Noble PW, Jiang D. Matrix Regulation of Lung Injury, Inflammation, and Repair: The Role of Innate Immunity. Proc Am Thorac Soc (2006) 3(5):401–4. doi: 10.1513/pats.200604-097AW
73. Toole BP, Zoltan-Jones A, Misra S, Ghatak S. Hyaluronan: A Critical Component of Epithelial-Mesenchymal and Epithelial-Carcinoma Transitions. Cells Tissues Organs (2005) 179(1-2):66–72. doi: 10.1159/000084510
74. Guo NF, Cao YJ, Chen X, Zhang Y, Fan YP, Liu J, et al. Lixisenatide Protects Doxorubicin-Induced Renal Fibrosis by Activating wNF-κb/TNF-α and TGF-β/Smad Pathways. Eur Rev Med Pharmacol Sci (2019) 23(9):4017–26. doi: 10.26355/eurrev_201905_17832
75. Malik S, Suchal K, Khan SI, Bhatia J, Kishore K, Dinda AK, et al. Apigenin Ameliorates Streptozotocin-Induced Diabetic Nephropathy in Rats via MAPK-NF-κb-TNF-α and TGF-β1-MAPK-Fibronectin Pathways. Am J Physiol Renal Physiol (2017) 313(2):F414–22. doi: 10.1152/ajprenal.00393.2016
76. Huang C, Wu X, Wang S, Wang W, Guo F, Chen Y, et al. Combination of Salvia Miltiorrhiza and Ligustrazine Attenuates Bleomycin-Induced Pulmonary Fibrosis in Rats via Modulating TNF-α and TGF-β. Chin Med (2018) 13:36. doi: 10.1186/s13020-018-0194-9
77. Kamitani S, Yamauchi Y, Kawasaki S, Takami K, Takizawa H, Nagase T, et al. Simultaneous Stimulation With TGF-β1 and TNF-α Induces Epithelial Mesenchymal Transition in Bronchial Epithelial Cells. Int Arch Allergy Immunol (2011) 155(2):119–28. doi: 10.1159/000318854
78. Takahashi E, Nagano O, Ishimoto T, Yae T, Suzuki Y, Shinoda T, et al. Tumor Necrosis Factor-Alpha Regulates Transforming Growth Factor-Beta-Dependent Epithelial-Mesenchymal Transition by Promoting Hyaluronan-CD44-Moesin Interaction. J Biol Chem (2010) 285(6):4060–73. doi: 10.1074/jbc.M109.056523
79. Asiedu MK, Ingle JN, Behrens MD, Radisky DC, Knutson KL. TGFbeta/TNF(alpha)-Mediated Epithelial-Mesenchymal Transition Generates Breast Cancer Stem Cells With a Claudin-Low Phenotype. Cancer Res (2011) 71(13):4707–19. doi: 10.1158/0008-5472.CAN-10-4554
80. Liao SJ, Luo J, Li D, Zhou YH, Yan B, Wei JJ, et al. TGF-β1 and TNF-α Synergistically Induce Epithelial to Mesenchymal Transition of Breast Cancer Cells by Enhancing TAK1 Activation. J Cell Commun Signal (2019) 13(3):369–80. doi: 10.1007/s12079-019-00508-8
81. Dong W, Sun S, Cao X, Cui Y, Chen A, Li X, et al. Exposure to TNF-α Combined With TGF-β Induces Carcinogenesis In Vitro via NF-κb/Twist Axis. Oncol Rep (2017) 37(3):1873–82. doi: 10.3892/or.2017.5369
82. Li H, Chen A, Yuan Q, Chen W, Zhong H, Teng M, et al. NF-κb/Twist Axis Is Involved in Chysin Inhibition of Ovarian Cancer Stem Cell Features Induced by Co-Treatment of TNF-α and TGF-β. Int J Clin Exp Pathol (2019) 12(1):101–12.
83. Saito A, Suzuki HI, Horie M, Ohshima M, Morishita Y, Abiko Y, et al. An Integrated Expression Profiling Reveals Target Genes of TGF-β and TNF-α Possibly Mediated by MicroRNAs in Lung Cancer Cells. PLoS One (2013) 8(2):e56587. doi: 10.1371/journal.pone.0056587
84. Yuan Q, Wen M, Xu C, Chen A, Qiu YB, Cao JG, et al. 8-Bromo-7-Methoxychrysin Targets NF-κb and FoxM1 to Inhibit Lung Cancer Stem Cells Induced by Pro-Inflammatory Factors. J Cancer (2019) 10(21):5244–55. doi: 10.7150/jca.30143
85. Li R, Hebert JD, Lee TA, Xing H, Boussommier-Calleja A, Hynes RO, et al. Macrophage-Secreted Tnfα and Tgfβ1 Influence Migration Speed and Persistence of Cancer Cells in 3D Tissue Culture via Independent Pathways. Cancer Res (2017) 77(2):279–90. doi: 10.1158/0008-5472.CAN-16-0442
86. Yamane K, Ihn H, Asano Y, Tamaki K. Antagonistic Effects of TNF-Alpha on TGF-Beta Signaling Through Down-Regulation of TGF-Beta Receptor Type II in Human Dermal Fibroblasts. J Immunol (2003) 171(7):3855–62. doi: 10.4049/jimmunol.171.7.3855
87. Goldberg MT, Han YP, Yan C, et al. TNF-α Suppresses α-Smooth Muscle Actin Expression in Human Dermal Fibroblasts: An Implication for Abnormal Wound Healing. J Invest Dermatol (2007) 127(11):2645–55. doi: 10.1038/sj.jid.5700890
88. Al-Mulla F, Leibovich SJ, Francis IM, Bitar MS. Impaired TGF-β Signaling and a Defect in Resolution of Inflammation Contribute to Delayed Wound Healing in a Female Rat Model of Type 2 Diabetes. Mol Biosyst (2011) 7(11):3006–20. doi: 10.1039/c0mb00317d
89. Verrecchia F, Mauviel A. TGF-β and TNF-α: Antagonistic Cytokines Controlling Type I Collagen Gene Expression. Cell Signal (2004) 16(8):873–80. doi: 10.1016/j.cellsig.2004.02.007
90. Verrecchia F. Interactions Fonctionnelles Entre La Voie De Signalisation Du TGF-Beta Par Les Smads Et Le TNF-Alpha: Implications Dans La Régulation De L'expression Du Collagène De Type I [Functional Interactions Between the TGF-Beta Signaling Pathway via the Smads and TNF-Alpha: Implications for the Regulation of Type I Collagen Expression]. J Soc Biol (2005) 199(4):329–36. doi: 10.1051/jbio:2005034
91. Gosselin LE, Martinez DA. Impact of TNF-Alpha Blockade on TGF-Beta1 and Type I Collagen mRNA Expression in Dystrophic Muscle. Muscle Nerve (2004) 30(2):244–6. doi: 10.1002/mus.20056
92. Varela-Rey M, Montiel-Duarte C, Osés-Prieto JA, López-Zabalza MJ, Jaffrèzou JP, Rojkind M, et al. P38 MAPK Mediates the Regulation of Alpha1(I) Procollagen mRNA Levels by TNF-Alpha and TGF-Beta in a Cell Line of Rat Hepatic Stellate Cells (1). FEBS Lett (2002) 528(1-3):133–8. doi: 10.1016/S0014-5793(02)03276-3
93. Yang H, Gao F, Li X, Wang J, Liu H, Zheng Z. TGF-β1 Antagonizes TNF-α Induced Up-Regulation of Matrix Metalloproteinase 3 in Nucleus Pulposus Cells: Role of the ERK1/2 Pathway. Connect Tissue Res (2015) 56(6):461–8. doi: 10.3109/03008207.2015.1054030
94. Yang H, Liu H, Li X, Pan H, Li Z, Wang J, et al. TNF-α and TGF-β1 Regulate Syndecan-4 Expression in Nucleus Pulposus Cells: Role of the Mitogen-Activated Protein Kinase and NF-κb Pathways [Published Correction Appears in Connect Tissue Res. 2015 Nov;56(6):504]. Connect Tissue Res (2015) 56(4):281–7. doi: 10.3109/03008207.2014.996702
95. Yamada-Nomoto K, Yoshino O, Akiyama I, Iwase A, Ono Y, Nakamura T, et al. PAI-1 in Granulosa Cells Is Suppressed Directly by Statin and Indirectly by Suppressing TGF-β and TNF-α in Mononuclear Cells by Insulin-Sensitizing Drugs. Am J Reprod Immunol (2017) 78(1):1–20. doi: 10.1111/aji.12669
96. Hatanaka H, Mukai A, Ito E, Ueno M, Sotozono C, Kinoshita S, et al. Epigenetic Regulation of the Epithelial Mesenchymal Transition Induced by Synergistic Action of TNF-α and TGF-β in Retinal Pigment Epithelial Cells. Biochem Biophys Res Commun (2021) 544:31–7. doi: 10.1016/j.bbrc.2021.01.060
97. Yoshimatsu Y, Kimuro S, Pauty J, Takagaki K, Nomiyama S, Inagawa A, et al. TGF-Beta and TNF-Alpha Cooperatively Induce Mesenchymal Transition of Lymphatic Endothelial Cells via Activation of Activin Signals. PLoS One (2020) 15(5):e0232356. doi: 10.1371/journal.pone.0232356
98. Yoshimatsu Y, Wakabayashi I, Kimuro S, Takahashi N, Takahashi K, Kobayashi M, et al. TNF-α Enhances TGF-β-Induced Endothelial-to-Mesenchymal Transition via TGF-β Signal Augmentation. Cancer Sci (2020) 111(7):2385–99. doi: 10.1111/cas.14455
99. Li CW, Xia W, Huo L, Lim SO, Wu Y, Hsu JL, et al. Epithelial-Mesenchymal Transition Induced by TNF-α Requires NF-κb-Mediated Transcriptional Upregulation of Twist1. Cancer Res (2012) 72(5):1290–300. doi: 10.1158/0008-5472.CAN-11-3123
100. Liu P, He K, Song H, Ma Z, Yin W, Xu LX. Deferoxamine-Induced Increase in the Intracellular Iron Levels in Highly Aggressive Breast Cancer Cells Leads to Increased Cell Migration by Enhancing TNF-α-Dependent NF-κb Signaling and TGF-β Signaling. J Inorg Biochem (2016) 160:40–8. doi: 10.1016/j.jinorgbio.2016.04.014
101. Qi Y, Kai K, Creighton CJ, Eckhardt B, Saya H, Tripathy D, et al. Abstract 1078: TGF-β and TNF-α Activate Gene Transcription Programs Associated With Poor Breast Cancer Prognosis. Cancer Res (2015) 75(15 Supplement):1078–8. doi: 10.1158/1538-7445.AM2015-1078
102. Trivanović D, Jauković A, Krstić J, Nikolić S, Okić Djordjević I, Kukolj T, et al. Inflammatory Cytokines Prime Adipose Tissue Mesenchymal Stem Cells to Enhance Malignancy of MCF-7 Breast Cancer Cells via Transforming Growth Factor-β1. IUBMB Life (2016) 68(3):190–200. doi: 10.1002/iub.1473
103. Yamauchi Y, Kohyama T, Takizawa H, Kamitani S, Desaki M, Takami K, et al. Tumor Necrosis Factor-Alpha Enhances Both Epithelial-Mesenchymal Transition and Cell Contraction Induced in A549 Human Alveolar Epithelial Cells by Transforming Growth Factor-Beta1. Exp Lung Res (2010) 36(1):12–24. doi: 10.3109/01902140903042589
104. Dash S, Sarashetti PM, Rajashekar B, Chowdhury R, Mukherjee S. TGF-β2-Induced EMT Is Dampened by Inhibition of Autophagy and TNF-α Treatment. Oncotarget (2018) 9(5):6433–49. doi: 10.18632/oncotarget.23942
105. Lauriola A, Martello A, Fantini S, Marverti G, Zanocco-Marani T, Davalli P, et al. Depletion of Trichoplein (TpMs) Causes Chromosome Mis-Segregation, DNA Damage and Chromosome Instability in Cancer Cells. Cancers (Basel) (2020) 12(4):E993. doi: 10.3390/cancers12040993
106. Singh R, Dagar P, Pal S, Basu B, Shankar BS. Significant Alterations of the Novel 15 Gene Signature Identified From Macrophage-Tumor Interactions in Breast Cancer. Biochim Biophys Acta Gen Subj (2018) 1862(3):669–83. doi: 10.1016/j.bbagen.2017.12.004
107. Hsiao JJ, Ng BH, Smits MM, Martinez HD, Jasavala RJ, Hinkson IV, et al. Research Resource: Androgen Receptor Activity Is Regulated Through the Mobilization of Cell Surface Receptor Networks. Mol Endocrinol (2015) 29(8):1195–218. doi: 10.1210/me.2015-1021
108. Jiang Q, Matsuzaki Y, Li K, Uitto J. Transcriptional Regulation and Characterization of the Promoter Region of the Human ABCC6 Gene. J Invest Dermatol (2006) 126(2):325–35. doi: 10.1038/sj.jid.5700065
109. Al-Bahrani R, Abuetabh Y, Zeitouni N, Sergi C. Cholangiocarcinoma: Risk Factors, Environmental Influences and Oncogenesis. Ann Clin Lab Sci (2013) 43(2):195–210.
110. Irons SL, Serra V, Bowler D, Chapman K, Militi S, Lyng F, et al. The Effect of Genetic Background and Dose on Non-Targeted Effects of Radiation. Int J Radiat Biol (2012) 88(10):735–42. doi: 10.3109/09553002.2012.715793
111. Xiao K, Cao S, Jiao L, Song Z, Lu J, Hu C. TGF-β1 Protects Intestinal Integrity and Influences Smads and MAPK Signal Pathways in IPEC-J2 After TNF-α Challenge. Innate Immun (2017) 23(3):276–84. doi: 10.1177/1753425917690815
112. Lemini M, Ruiz-Herrera X, Ledesma-Colunga MG, Díaz-Lezama N, De Los Ríos EA, López-Barrera F, et al. Prolactin Anterior Pituitary Expression and Circulating Levels are Reduced in Obese and Diabetic Rats: Role of TGF-β and TNF-α. Am J Physiol Regul Integr Comp Physiol (2015) 308(9):R792–9. doi: 10.1152/ajpregu.00327.2014
113. Fox SW, Fuller K, Bayley KE, Lean JM, Chambers TJ. TGF-Beta 1 and IFN-Gamma Direct Macrophage Activation by TNF-Alpha to Osteoclastic or Cytocidal Phenotype. J Immunol (2000) 165(9):4957–63. doi: 10.4049/jimmunol.165.9.4957
114. de Matos FR, de Moraes M, Nonaka CF, de Souza LB, de Almeida Freitas R. Immunoexpression of TNF-α and TGF-β in Central and Peripheral Giant Cell Lesions of the Jaws. J Oral Pathol Med (2012) 41(2):194–9. doi: 10.1111/j.1600-0714.2011.01075.x
115. Lovibond AC, Haque SJ, Chambers TJ, Fox SW. TGF-Beta-Induced SOCS3 Expression Augments TNF-Alpha-Induced Osteoclast Formation. Biochem Biophys Res Commun (2003) 309(4):762–7. doi: 10.1016/j.bbrc.2003.08.068
116. Brouckaert P, Takahashi N, van Tiel ST, Hostens J, Eggermont AM, Seynhaeve AL, et al. Tumor Necrosis Factor-Alpha Augmented Tumor Response in B16BL6 Melanoma-Bearing Mice Treated With Stealth Liposomal Doxorubicin (Doxil) Correlates With Altered Doxil Pharmacokinetics. Int J Cancer (2004) 109(3):442–8. doi: 10.1002/ijc.11703
117. Josephs SF, Ichim TE, Prince SM, Kesari S, Marincola FM, Escobedo AR, et al. Unleashing Endogenous TNF-Alpha as a Cancer Immunotherapeutic. J Transl Med (2018) 16(1):242. doi: 10.1186/s12967-018-1611-7
118. Selinsky CL, Howell MD. Soluble Tumor Necrosis Factor Receptor Type I Enhances Tumor Development and Persistence In Vivo. Cell Immunol (2000) 200:81–7. doi: 10.1006/cimm.2000.1622
119. Madhusudan S, Muthuramalingam SR, Braybrooke J, Wilner S, Kaur K, Han C, et al. A Phase Ii Study of Etanercept (Enbrel), a Tumour Necrosis Factor-Alpha Inhibitor in Recurrent Ovarian Cancer. Int J Gynecol Cancer (2004) 14(Suppl 1):61–2.
120. Madhusudan S, Foster M, Muthuramalingam SR, Braybrooke JP, Wilner S, Kaur K, et al. A Phase II Study of Etanercept (Enbrel), a Tumor Necrosis Factor Alpha Inhibitor in Patients With Metastatic Breast Cancer. Clin Cancer Res (2004) 10(19):6528–34. doi: 10.1158/1078-0432.CCR-04-0730
121. Díaz-Valdés N, Basagoiti M, Dotor J, Aranda F, Monreal I, Monreal I, Riezu-Boj JI, et al. Induction of Monocyte Chemoattractant Protein-1 and Interleukin-10 by TGFbeta1 in Melanoma Enhances Tumor Infiltration and Immunosuppression. Cancer Res (2011) 71(3):812–21. doi: 10.1158/0008-5472.CAN-10-2698
122. Derynck R, Turley SJ, Akhurst RJ. Tgfβ Biology in Cancer Progression and Immunotherapy. Nat Rev Clin Oncol (2021) 18(1):9–34. doi: 10.1038/s41571-020-0403-1
123. Tolcher AW, Berlin JD, Cosaert J, Kauh J, Chan E, Piha-Paul SA, et al. A Phase 1 Study of Anti-Tgfβ Receptor Type-II Monoclonal Antibody LY3022859 in Patients With Advanced Solid Tumors. Cancer Chemother Pharmacol (2017) 79(4):673–80. doi: 10.1007/s00280-017-3245-5
124. Teixeira AF, Ten Dijke P, Zhu HJ. On-Target Anti-TGF-β Therapies Are Not Succeeding in Clinical Cancer Treatments: What Are Remaining Challenges? Front Cell Dev Biol (2020) 8:605. doi: 10.3389/fcell.2020.00605
125. Dutta A, Li J, Fedele C, Sayeed A, Singh A, Violette SM, et al. αvβ6 Integrin Is Required for Tgfβ1-Mediated Matrix Metalloproteinase2 Expression. Biochem J (2015) 466(3):525–36. doi: 10.1042/BJ20140698
126. Trcey D, Klareskog L, Sasso EH, Salfeld JG, Tak PP. Tumor Necrosis Factor Antagonist Mechanisms of Action: A Comprehensive Review. Pharmacol Ther (2008) 117(2):244–79. doi: 10.1016/j.pharmthera.2007.10.001
127. Isaka Y. Targeting TGF-β Signaling in Kidney Fibrosis. Int J Mol Sci (2018) 19(9):2532. doi: 10.3390/ijms19092532
128. Li TJ, Qiu Y, Mao JQ, Yang PY, Rui YC, Chen WS. Protective Effects of Guizhi-Fuling-Capsules on Rat Brain Ischemia/Reperfusion Injury. J Pharmacol Sci (2007) 105(1):34–40. doi: 10.1254/jphs.FP0070450
129. Yang JM, Sun Y, Wang M, Zhang XL, Zhang SJ, Gao YS, et al. Regulatory Effect of a Chinese Herbal Medicine Formula on Non-Alcoholic Fatty Liver Disease. World J Gastroenterol (2019) 25(34):5105–19. doi: 10.3748/wjg.v25.i34.5105
130. Du MY, Duan JX, Zhang CY, Yang HH, Guan XX, Zhong WJ, et al. Psoralen Attenuates Bleomycin-Induced Pulmonary Fibrosis in Mice Through Inhibiting Myofibroblast Activation and Collagen Deposition. Cell Biol Int (2019) 44(1):98–107. doi: 10.1002/cbin.11205
131. Wang C, Al-Ani MK, Sha Y, Chi Q, Dong N, Yang L, et al. Psoralen Protects Chondrocytes, Exhibits Anti-Inflammatory Effects on Synoviocytes, and Attenuates Monosodium Iodoacetate-Induced Osteoarthritis. Int J Biol Sci (2019) 15(1):229–38. doi: 10.7150/ijbs.28830
132. Wei J, Xu H, Liu Y, Li B, Zhou F. Effect of Captopril on Radiation-Induced TGF-β1 Secretion in EA. Hy926 Human Umbilical Vein Endothelial Cells. Oncotarget (2017) 8(13):20842–50. doi: 10.18632/oncotarget.15356
133. Diaz Arguello OA, Haisma HJ. Apoptosis-Inducing TNF Superfamily Ligands for Cancer Therapy. Cancers (Basel) (2021) 13(7):1543. doi: 10.3390/cancers13071543
134. Strait AA, Wang XJ. Setting Up Clinical Trials for Success: Applying Preclinical Advances in Combined Tgfβ/PD-L1 Inhibition to Ongoing Clinical Studies. Mol Carcinog (2021), 1–4. doi: 10.1002/mc.23373
135. Kasprzak A. The Role of Tumor Microenvironment Cells in Colorectal Cancer (CRC) Cachexia. Int J Mol Sci (2021) 22(4):1565. doi: 10.3390/ijms22041565
Keywords: TGF-β, TNF-α, proliferation, apoptosis, inflammation, genomic instability, epithelial-mesenchymal transition
Citation: Liu Z-w, Zhang Y-m, Zhang L-y, Zhou T, Li Y-y, Zhou G-c, Miao Z-m, Shang M, He J-p, Ding N- and Liu Y-q (2022) Duality of Interactions Between TGF-β and TNF-α During Tumor Formation. Front. Immunol. 12:810286. doi: 10.3389/fimmu.2021.810286
Received: 06 November 2021; Accepted: 09 December 2021;
Published: 05 January 2022.
Edited by:
Jun Wang, Dalhousie University, CanadaReviewed by:
Watcharin Loilome, Khon Kaen University, ThailandMaya Gulubova, Trakia University, Bulgaria
Copyright © 2022 Liu, Zhang, Zhang, Zhou, Li, Zhou, Miao, Shang, He, Ding and Liu. This is an open-access article distributed under the terms of the Creative Commons Attribution License (CC BY). The use, distribution or reproduction in other forums is permitted, provided the original author(s) and the copyright owner(s) are credited and that the original publication in this journal is cited, in accordance with accepted academic practice. No use, distribution or reproduction is permitted which does not comply with these terms.
*Correspondence: Yong-qi Liu, bGl1eW9uZ3FpNzNAMTYzLmNvbQ==
†These authors share first authorship