- 1Department of Biotherapy, Cancer Center, West China Hospital, Sichuan University, Chengdu, China
- 2Department of Neurosurgery, West China Hospital, Sichuan University, Chengdu, China
Immune checkpoint inhibitors (ICIs), as one of the innovative types of immunotherapies, including programmed cell death-1 (PD-1), programmed cell death-ligand 1 (PD-L1), and cytotoxic T lymphocyte antigen 4 (CTLA-4) inhibitors, have obtained unprecedented benefit in multiple malignancies. However, the immune response activation in the body organs could arise immune-related adverse events (irAEs). Checkpoint inhibitor colitis (CIC) is the most widely reported irAEs. However, some obscure problems, such as the mechanism concerning gut microbiota, the confusing differential diagnosis with inflammatory bowel disease (IBD), the optimal steroid schedule, the reintroduction of ICIs, and the controversial prognosis features, influence the deep understanding and precise diagnosis and management of CIC. Herein, we based on these problems and comprehensively summarized the relevant studies of CIC in patients with NSCLC, further discussing the future research direction of this specific pattern of irAEs.
Highlights
● Gut microbiota, including Faecalibacterium prausnitzii, Bacteroides fragilis, and Lactobacillus reuteri, plays a critical role in the development of immune checkpoint-related colitis.
● Endoscopic biopsy is the gold standard of diagnosis, while endoscopic findings were not significantly associated with the severity of immune checkpoint-related colitis.
● Current management is based on symptomatic and steroid therapy. The utilization of biological agents according to the severity of immune checkpoint-related colitis grade is reasonable and recommended.
● Longer survival was found in patients who had a deteriorated or recurrent CIC instead of improved or resolved CIC. The influence of CIC outcome on overall survival requires to be explored further.
Introduction
Lung cancer is the leading cause of death among all cancers. In 2021, an estimated 131,880 deaths from lung cancer were recorded in the United States, with the death rate at 22% (1). As the most common type of lung cancer, non-small cell lung cancer (NSCLC) faces difficulties that over 50% of patients are diagnosed with advanced disease initially, for which the 5-year survival is nearly 5% (2). Moreover, the recommended standard treatment, chemotherapy and/or radiotherapy, offers generally modest efficacy for metastatic NSCLC patients. The 5-year survival varies from 6% to 30% when patients are treated with chemotherapy and/or radiotherapy (3, 4). Besides, target therapies, such as EGFR, ALK, and ROS1 tyrosine kinase inhibitors (TKIs), have contributed to unprecedented improvements for survival in NSCLC patients, with more moderate toxicity response than chemotherapy (5).
Recently, the emergence of immunotherapy fosters hopes for the treatment of multiple malignancies. Immune checkpoint inhibition, one of the innovative classes of immunotherapy, serves to restrain suppressive T-cell co-stimulatory signals, primarily cytotoxic CD8+ T cells, by inhibiting programmed death protein-1 (PD-1), PD-ligand 1 (PD-L1), and cytotoxic T-lymphocyte associated protein-4 (CTLA-4) (6, 7). PD-1 inherently presents in T cells, B cells, tumor-infiltrating lymphocytes, monocytes, and dendritic cells (DCs). PD-L1 exists on the surface of both antigen-presenting cells (APCs) and tumor cells (8–10), resulting in self-tolerance promotion and autoimmunity attenuation after interacting with PD-1. Similarly, CTLA-4 is typically expressed on CD4+/CD8+ T cells, B-cell subsets, and thymocytes, which is associated with the suppression of T-cell activity (11). Immune checkpoint inhibitors (ICIs) have the ability of strengthening the immune attack and promoting tumor killing (12, 13), thus improving the survival of cancer patients.
To date, PD-1 inhibitors (nivolumab, pembrolizumab), PD-L1 inhibitors (atezolizumab, durvalumab), and CTLA-4 inhibitor (ipilimumab) have been approved by the U.S. Food and Drug Administration (FDA) and the European Medicines Agency (EMA) for the treatment of advanced NSCLC (14). The combination of nivolumab and ipilimumab contributed to prolonged overall survival (OS) and progression-free survival (PFS) in patients with NSCLC (15–17).
However, the eliminated immunoregulatory control could cause unrestrained immune response activation in body organs (18), like the gastrointestinal tract, liver, lung, heart, skin, and arthrosis, which leads to immune-related adverse events (irAEs) ultimately (19, 20). Among clinically reported irAEs, gastrointestinal toxicity is the most common irAEs that results in ICI discontinuation (21–23). Because of the similar symptoms with inflammatory bowel disease (IBD) and/or intestinal infection, the diagnosis of checkpoint inhibitor colitis (CIC) might be confused. As a result, precise diagnosis and optimized therapy are essential for recognizing this specific pattern of irAEs.
Prior studies have demonstrated the incidence, risk factors, diagnosis, and management of CIC (24–29). Nonetheless, they have variable concentrations and orientations, which are complementary but not deep and comprehensive. This review comprehensively summarized the relevant research studies and advanced progress of ICI-related colitis, especially the aspects less mentioned before, like the mechanism, evidence-based management schedules, rechallenge of ICIs, and prognosis of CIC. Besides, we provided a summary of cases and case series regarding CIC in NSCLC, aiming at identifying the clinicopathological and endoscopic features of these typical patients.
Incidence and Onset
Nearly 20%–30% of patients would develop diarrhea after ICI therapy, while no more than 5% of patients have colitis (30). Patients treated with CTLA-4 inhibitors tend to experience three times higher CIC frequency than those with PD-1/PD-L1 inhibitors (19, 31, 32). It has been proposed that CTLA-4 blockade induces CD4+ T-cell activation, resulting in a generalized immune response. In contrast, PD-1/PD-L1 blockades aim at late T-cell proliferation and, therefore, cause a more localized immune reaction (33). According to previous meta-analysis and systematic reviews (30, 33, 34), the overall incidence of diarrhea was 30.2%–35.4% for CTLA-4 inhibitors and 12.1%–13.7% for PD-1/PD-L1 inhibitors. The all-grade incidence of colitis was 5.7%–39.1% for CTLA-4 inhibitors and 0.7%–31.6% for PD-1/PD-L1 inhibitors. In lung cancer, the rates of all-grade and high-grade diarrhea were 9.1%–11.0% and 0.6%–1.1% for PD-1/PD-L1 inhibitors, respectively (34). All-grade and high-grade colitis caused by PD-1/PD-L1 inhibitors separately occurred at 0.4%–0.9% and 0.1%–0.6%. The combination of PD-1/PD-L1 inhibitors and CTLA inhibitors could reach 40.4% in all-grade diarrhea/colitis. We searched PubMed and Web of Science from 2017 to October 10, 2021, and included 32 occurrences of CIC in NSCLC patients. The search term we utilized were “non-small cell lung cancer*” AND “colitis*” AND “immunotherapy*” OR “immune checkpoint inhibitors*.” All related case reports were included (Table 1). In our analysis, over 70% of patients were PD-1 inhibitor-related colitis, and patients treated with PD-1+ CTLA inhibitors were prone to suffer grade 3 CIC (Figure 1A).
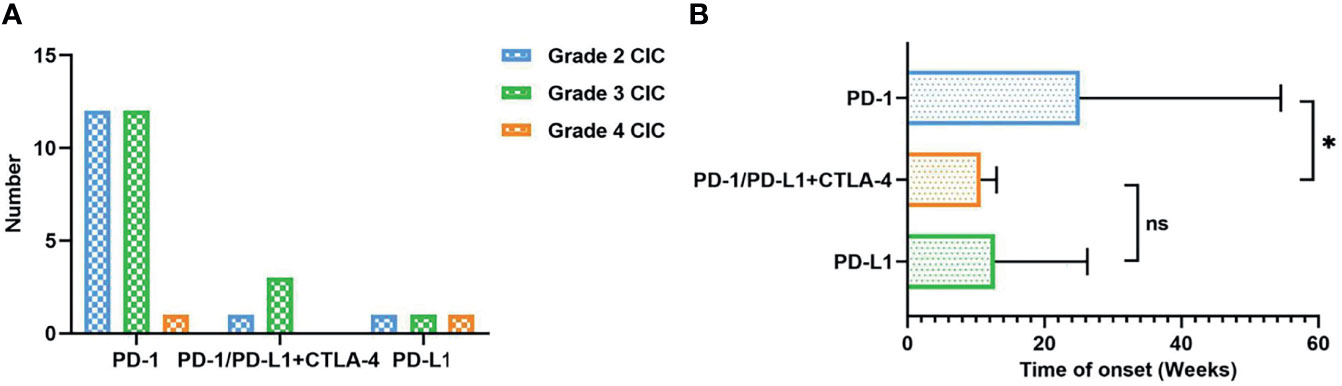
Figure 1 (A) The number of CIC patients according to different ICI therapies and severity grade. (B) Time of onset (weeks) classified by different ICI therapies. CIC, checkpoint inhibitor colitis; ICIs, immune checkpoint inhibitors. *P < 0.05; ns, no significant.
ICI-mediated colitis could appear at any time, such as during treatment initiation, the steroid tapering period, or after treatment termination (59). The overall onset of CIC spanned from 0 to 6.3 months (25, 53, 60–63). Prior research studies suggested that CIC onset might vary from different ICI types. Colitis induced by CTLA-4 inhibitors seemed to happen later than that caused by PD-1/PD-L1 inhibitors. The median time to the onset of ipilimumab-induced colitis was approximately 6 to 7 weeks, with an average after two to three infusions (64–68). The range of the median onset from the last injection to diarrhea was 0 to 2 months (67, 69). As for PD-1/PD-L1 inhibitors, symptomatic colitis was less predictable with the onset from nearly 1 week to 2 years (19, 70, 71). Besides, the onset of CIC seemed to be significantly earlier for patients who received combined ICIs (70). Our analysis also showed that CIC patients with PD-1/PD-L1 and CTLA-4 therapy had earlier onset than those with PD-1 inhibitors (10 vs. 25 weeks, P < 0.05) (Figure 1B). Moreover, it could also be affected by other therapies, including previous anticancer treatment, antibiotics, and non-steroidal anti-inflammatory drugs (NSAIDs) (72). The development of CIC was of great difficulty to be predicted before symptom onset (73). Lord et al. (74) suggested that the resolution time of CIC could exceed 130 days, over 10 times than the half-life period of ipilimumab. This implies the long-term influence of immunotherapy on the immune system instead of simply checkpoint blockade at the moment of treatment in the immune cascade. Therefore, we should keep continuous vigilance during the period of ICI utilization.
Mechanism
The underlying mechanism of ICI-related colitis still remains obscure. However, extensive research studies have suggested that the proposed mechanisms included the hyperactivation of effector T cell (Teff cell), the infiltration of lymphocytes, and the increase of circulating memory T cells, thus causing proinflammatory status and the emergence of autoimmune-type presentation (25, 75–77). Besides, the gut microbiome also plays a critical role in the regulation of intestinal mucosal homeostasis (Figure 2) (78, 79).
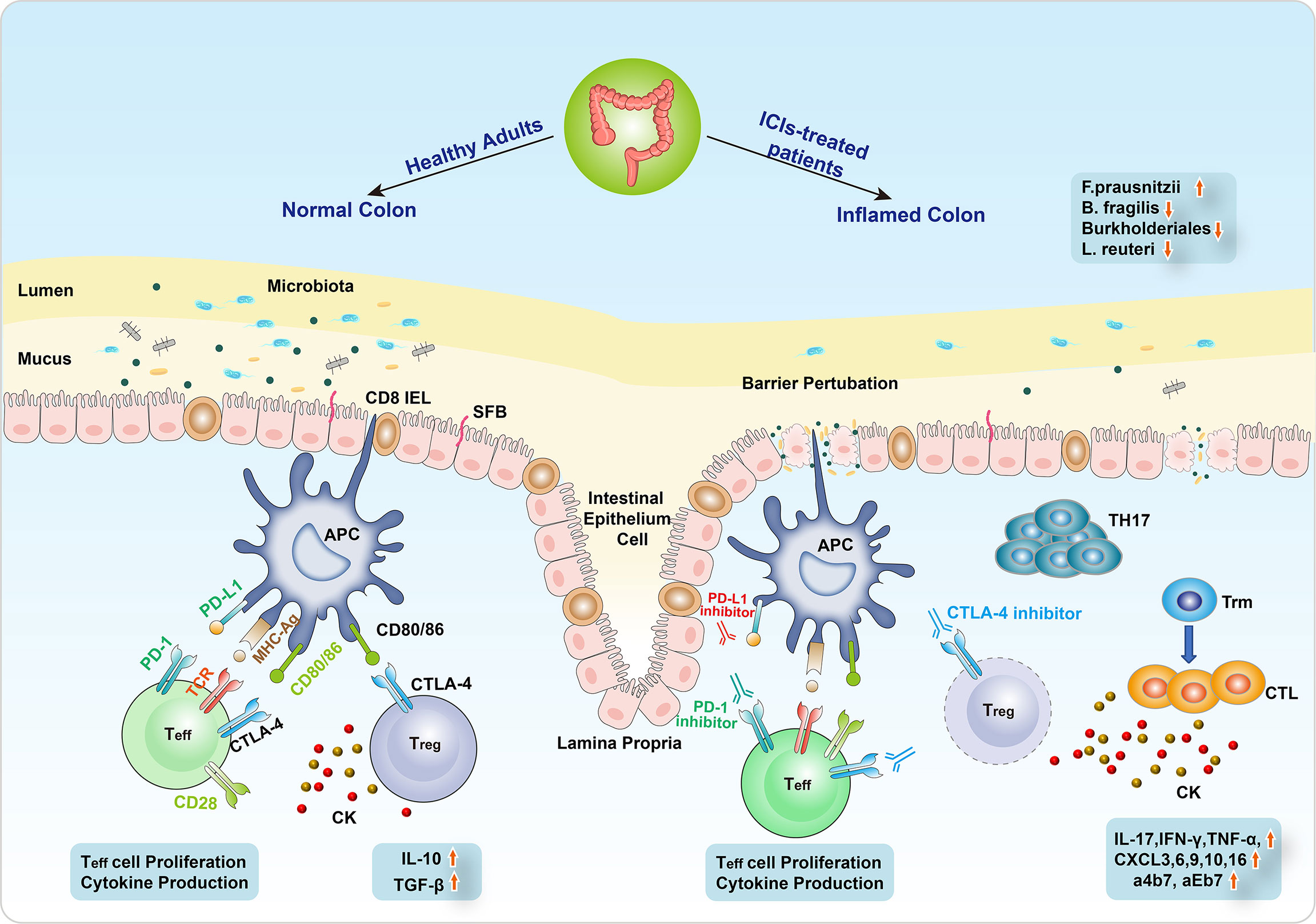
Figure 2 The mechanism of ICI-related colitis. The normal intestinal epithelial barrier is a highly organized mucosal surface that prevents microbiota from entering into the lamina propria. The epithelium is composed of a single layer of intestinal epithelial cells (IECs) covered by a mucus layer. Segmented filamentous bacteria (SFB) seldom breach the barrier of mucus and contact with the IECs. Immune checkpoint inhibitors (ICIs) modulate the microbiota–intestinal barrier equilibrium by inducing IEC apoptosis, resulting in barrier perturbation. The alterations of intestinal flora include the decrease of Bacteroides fragilis, Burkholderiales, and Lactobacillus reuteri and the increase of Faecalibacterium prausnitzii. Barrier perturbation is further aggravated possibly due to pathogenic Th17 cells, leading to severe intestinal toxicity resembling an early sign of colitis. Besides, the differentiation from CD8+ tissue-resident memory T (Trm) cells to cytotoxic T lymphocytes (CTLs) induced cytokines (IL-17, IFN-γ, TNF-α) to participate in the CIC emergence. In addition, CXCR3, 6, 9/10, 16, and a4b7/aEb7 receptors are also involved in the development of CIC.
Mechanism of irAEs
To target and eliminate tumor cells, T cells need two stimulating signals to be activated (80–82). One is the direct antigen stimulation mediated by the T-cell receptor (TCR) and major histocompatibility complex (MHC) class II molecule on the APCs. The other depends on the co-stimulation between CD28 receptor on T cells and CD80/86 (B7) on APCs. T-cell activation results in its proliferation, cytokine production, and CTLA4 and PD1 expression (83). The role of CTLA-4, which is constitutively located on the surface of FOXP3 regulatory CD4+ T cells, is to terminate this co-stimulation. As a co-inhibitory ligand, CTLA-4 harbors higher affinity than CD28, contributing to competitively bind CD80/86, thus blunting the immune response. In addition to the CTLA-4-mediated inhibitory interaction between T cells and APCs, immunosuppressive cytokines produced by regulatory T cells (Treg cells) include IL-10 and TGF-β and also inhibit T-cell activation and proliferation (84, 85). Conversely, PD-1 with PD-L1 does not restrain co-stimulation but suppresses the TCR downstream signaling, which results in the reduction of transcription factors and cytokines like TBET and GATA3, as well as the enhanced inhibitory effect of Treg cells (86, 87). Both CTLA and PD-1/PD-L1 inhibitors could strengthen the activation and proliferation of Teff cells, abrogate Treg cell function, induce inflammatory cytokines, probably boost humoral autoimmunity (88), and cause a range of autoimmunity-related adverse effects in multiple organs (Figure 2).
Mechanism of CIC
Several animal models with the absence of immune checkpoints have been utilized to simulate the immunological effects of CIC. Mice knocked out CTLA-4 presented diffuse immune cells infiltration in various organs and fatal colitis caused by increased T-cell activity (85, 89, 90). The PD-1 axis is of great importance in the development of innate lymphoid cells (ILCs), which have been regarded as critical effective cells at gastrointestinal mucosal surfaces (91–93). The severity of CIC was correlated to the growing mucosal number of group 3 ILCs (ILC3s) (94). Moreover, a high-level infiltration of CD4+ T cells and CD8+ T cells was reported in patients with severe colitis following immunotherapy, of which the former was more prevalent in those who underwent anti-CTLA-4 therapy and the latter usually occurred in anti-PD-1-induced colitis (95–97). Recently, a comprehensive single-cell analysis of CIC illustrated the differentiation from CD8+ tissue-resident memory T (Trm) cells to cytotoxic T lymphocytes (CTLs) (97). It was hypothesized that the abundant CD8+ Trm cells in the normal colon exerted a vital effect on CIC, and their activation prompted the subsequent assembly of CD4+ and CD8+ T cells. In addition, cytokines may participate in the emergence of CIC. The preclinical model of CIC has observed the rise of IL-17 (11, 98). The inducible gene and expression of IFN-γ and TNF-α, which induced cell death, showed a substantial increase in ICI-related colitis patients (97, 99). TNF-like cytokine 1A (TL1A) and its receptor DR3 were also upregulated in ICI-mediated colitis (100). Besides, CXCR3 and CXCR6 chemokine receptor (CXCR9/10 and CXCR16, respectively) genes presented high-level expression on colitis-related T-cell population, upregulating T-cell activity (101). Besides, three genes (ITGB7, ITGA4, and ITGAE), encoding a4b7 and aEb7 integrin receptors, were expressed in colitis-associated T-cell clusters. This might lead to lymphocyte retention in the intestinal mucosa (Figure 2) (102).
Gut Microbiome and CIC
Immunotherapy could modulate microbiota–gut barrier equilibrium by intraepithelial lymphocyte (IEL)-mediated apoptosis of intestinal epithelial cells (IECs), leading to barrier perturbation (103). The role of dysbiosis has been proposed to initiate irAEs, in which the product exposure derived by the microbiome could induce innate immune responses, probably activating self-reactive immune cells (104). Several species of gut bacteria have been indicated to potentiate Treg cell induction, which maintained intestinal tolerance (105). CD4+ T helper cells induced by IL-17 (Th17), located in the lamina propria to focus specifically on intestinal-resident segmented filamentous bacteria (SFB), could deteriorate systemic autoimmune diseases under some circumstances (Figure 2) (106, 107).
Currently, accumulating evidence has demonstrated that gut microbiome features may be linked with the development of CIC and tumor response to ICI therapy (Figure 2) (77, 108–111). Chaput et al. (112) conducted a prospective study to evaluate the intestinal microbiome characteristics at baseline and the time of gastrointestinal toxicity in 26 patients who received ipilimumab. The results showed that ipilimumab could not influence the composition of the microbiome. Nonetheless, decreased microbiota diversity was found in ICI-related colitis, especially some genera like Faecalibacterium prausnitzii (F. prausnitzii) and Bacteroides fragilis (B. fragilis) in the Firmicutes phylum.
Enrichment of F. prausnitzii might be associated with the occurrence of colitis (112). As an obligate anaerobe, F. prausnitzii has the normal function of keeping colonic mucosa integrity (111). The increase of F. prausnitzii was linked to T-cell proliferation in the gut mucosa and facilitated the recruitment of Treg cells and a4b7 T cells. In addition, it could enhance CTL concentration in the tumor microenvironment, prolonging the OS and PFS (113). The strong and beneficial response would be presented in patients with the abundance of F. prausnitzii receiving anti-CTLA-4 and/or anti-PD-1 inhibitors, possibly with the cost of immunotherapy-induced colitis.
Fragilis increase is considered to be the protective factor of CIC (81, 108). It acts to play an anti-inflammatory role in the gastrointestinal tract (114). The CTLA-4 pathway was involved in the immunoregulatory process, in which B. fragilis produced polysaccharide A to upregulate the level of IL-10, thus alleviating inflammation (115). Moreover, the anti-CTLA-4 blockade has been verified to favor B. fragilis outgrowth in the colon (116). Although B. fragilis frequency keeps stable after anti-CTLA-4 therapy (81), the elevated number of B. fragilis could further decrease the size of tumor in patients treated with ipilimumab. Besides, anti-CTLA-4 antibodies could also promote dysbiosis by reducing Burkholderiales and increasing Clostridiales bacteria presentation (103, 116, 117). The combination of Burkholderia cepacia (B. cepacia) and B. fragilis or the administration of Bacteroides thetaiotaomicron (B. thetaiotaomicron) allowed germ-free (GF) mice to decrease the gut toxicity and elicit anticancer immune response presented in normal microbiota mice (116, 118). This might be attributed to the accumulation and maturation of plasmacytoid DCs, which produced IL-12 subsequently to stimulate ICOS+ Treg cells and thus promote antitumor immunity, induced by these bacteria in the gastrointestinal lamina propria. Furthermore, the response of anti-CTLA-4 inhibitors required microbiota-dependent T-cell activation. The therapeutic efficacy of the anti-CTLA-4 inhibitor could be restored by adoptively transferring B. fragilis-specific T cells into GF mice (117). Similar experiments conducted with PD-L1 inhibitors showed that Bifidobacterium species could mediate antitumor efficacy (119). This bacterium could attenuate bowel inflammation mediated by Treg cells without detriments to the antitumor response (120). Wang et al. (94) also established ICI-related colitis models to find the change of gut microbiota. The microbiota profiling showed that Lactobacillus presented lower abundance in the ICI-treated mice stool than in the control group. Similarly, a trinitrobenzene sulfonic acid (TNBS)-built mouse model showed that intestinal inflammation could be suppressed by specific Lactobacillus reuteri strains (121). In addition, L. reuteri could augment DC function to improve antitumor response after blocking PD-L1.
Therefore, the species and relative abundance of gut microbiota could influence the development of CIC. Some specific microbial species might orchestrate the inflammation initiation, while other subsets might help perpetuate CIC. More efforts should be dedicated to further learn the complex symbiosis between bacteria and ICI-induced colitis, with the ultimate aim to manipulate the microbiota for the balance of tumor response and irAEs.
Risk Factors
The current risk factors for CIC cover past medical history and tumor type, therapy management, and molecular markers. Specifically, these included medication history, preexisting autoimmune diseases, tumor type, combined ICI therapy, and inflammatory cell levels.
Past Medical History and Tumor Type
The utilization of NSAIDs has been regarded to increase the risk of CIC (77). Patients with CIC (31%) were more common to use NSAIDs than those without CIC (5%) (P = 0.003), which is similar with IBD, and there is a correlation between NSAIDs and increased IBD flare risk (122). Conversely, vitamin D use was found to reduce the risk for ICI-related colitis (123, 124). In addition, the risk of CIC of patients who had previous irAEs is controversial. Some studies suggested that patients who had irAEs with the first ICI initiation would experience a higher risk of irAEs following alternate ICI therapy (125, 126). However, other studies showed that patients did not develop recurrent severe diarrhea or CIC when they changed to subsequent PD-1/PD-L1 inhibitor therapy after ipilimumab (127, 128). Moreover, the occurrence of CIC might relate to malignancy types. Melanoma seemed to have a higher risk of CIC compared with renal carcinoma and NSCLC (33). However, the reason behind the discrepancy between the rates from these two cancer types remains obscure.
Autoimmune disorders, including IBD, were not always considered in ICI clinical trial criterion, since the disease might worsen by non-specific T-cell activation of ICIs. Nearly 27% of patients have underlying disease exacerbation after ICI therapy, which means increased or recurrent prior manifestations (129). Few studies demonstrated that patients with preexisting autoimmune diseases would experience a higher incidence of CIC (129, 130). The occurrence of CIC in underlying IBD patients who received ipilimumab was reported at 30%. Besides, Menzies et al. (126) reported that five cases with prior IBD history who received PD-1 blockade had great tolerance without disease flare. Therefore, PD-1 inhibitors are more recommended for IBD patients than ipilimumab. Currently, a prospective clinical protocol is recruiting IBD patients who have ICI indications (AIM-NIVO, NCT03816345). This would bring critical instruction for ICI therapy of IBD patients in evaluating risk and developing decision-making.
Therapy Management
The combination of ICI therapy is reported to present a higher occurrence of ICI-related colitis. A recent meta-analysis included 27 prospective studies reporting the risk of CIC in patients with lung cancer who received ICI therapy (31, 131). The results showed that the combination of anti-CTLA-4 and anti-PD-1 therapy had a higher risk of all grades of CIC than anti-PD-1 monotherapy (RR: 1.61, 95% CI: 1.14–2.29). The combined ICIs, such as ipilimumab and pembrolizumab/nivolumab (132) and durvalumab and tremelimumab (133, 134), obtained above 10% occurrence of colitis (79, 135). Moreover, the sequential administration of ipilimumab before nivolumab showed higher rates of grade 3 colitis than nivolumab before ipilimumab (16% vs. 10%) (136). No particular toxicities were found in the combination therapy (137). The same class of ICI therapy had a similar incidence of all grades of CIC. However, the combination of chemotherapy/radiotherapy and immunotherapy could not increase the incidence of colitis but could promote tumor response rates, PFS, and OS in NSCLC (138, 139).
Molecular Markers
Several potential molecular markers, such as increased IL-17, neutrophil, eosinophil, and WBC levels, have merged to predict the development of ICI-mediated colitis (31, 140–142). Decreased baseline granulocyte colony-stimulating factor (GCSF) level was found in CIC patients (143, 144). Moreover, the association between some cell surface markers and GI-irAEs was also investigated. Shahabi et al. (145) explored the gene expression profiling of whole blood in 162 patients who were treated with ipilimumab. They found that pretreatment elevated the baseline levels of genes involved in cell cycle (WDR1, FP36L2, PCGF1, BAT1, BANF1, and SPTAN1), vesicle trafficking-correlated genes (VAMP3, SNAP23, and PICALM), and immune-related genes (RAC2, IL-32, IL2RG, CD37, CD4, and CD3E), and these were associated with developing GI-irAEs. After 3 weeks of treatment, the biomarker which distinguished GI-irAEs and non-GI-irAEs was CD177, a specific neutrophil surface biomarker that exerts effects in activating neutrophils and recruiting inflammatory cells. However, it could not be utilized alone to predict irAE occurrence because of large individual variability and its low sensitivity. Besides, CEA-associated cell adhesion molecule (CEACAM), as the essential adherent mediator in the migration of neutrophils, also showed a significant increase in the ICI-related GI toxicity group. Therefore, the changes in CEACAM and CD177 expression might become more sensitive markers than the absolute neutrophil level in the peripheral blood, and these activation steps happen in the early neutrophil recruitment.
Manifestations
Clinical Manifestations
The majority of clinical trials discriminate diarrhea from colitis, although they overlap in the most practical cases. Diarrhea is evaluated by stool increase per day. Colitis is evaluated according to clinical symptoms (blood or mucus in stool, abdominal pain) or the diagnostic findings on the imaging and endoscopic observations (146). The grading of diarrhea/colitis was according to the Common Terminology Criteria for Adverse Events v5.0 (147).
Similar to IBD, diarrhea is the most common clinical symptom of CIC (Figure 3A). It is also characterized by abdominal pain, hematochezia, fever, vomiting, nausea, and loss of appetite (77). Intestinal perforation and weight loss are less common (148). In our analysis, almost all patients developed diarrhea, ranging from 4 to 30 times. Additionally, other common clinical manifestations also include abdominal pain (25%), loss of appetite (18.75%), and hematochezia (12.5%). Obstipation, tachycardia, and malnutrition were also reported in our patients with CIC.
Imaging Manifestations
CT and/or MRI is the major radiology examination for CIC. However, CT presents a relatively low negative predictive value, with 53%–85% sensitivity and 75%–78% specificity (22, 149, 150). Colonic mucosal hyperenhancement is the most common CT sign of CIC, followed by mesenteric vessel engorgement, bowel wall thickening, fluid-filled distended colon, pericolic fat stranding, and bowel wall edema (151). In our analysis, the most common features of CT in our analysis were bowel wall thickening (10/32) (Figure 3B). Universally, the patterns of CIC could be divided into pancolitis, segmental colitis associated with diverticulosis (SCAD) enterocolitis, and enteritis (152–154). It is reported that the different CT patterns of CIC are related to corresponding clinical features. For example, patients with diffuse colitis usually have watery diarrhea, while patients with SCAD pattern have mixed watery and bloody diarrhea and cramping pain (154). When severe colitis was complicated with bowel perforation and toxic megacolon, CT would be an effective tool for diagnosis (155). The sign of non-dependent extraluminal air close to the intestine might be conducive to bowel perforation. PET-CT was also applied in CIC patients with increased 2-deoxy-2-(18F) fluoro-D-glucose (FDG) uptake (50, 150). One of our cases showed colon inflammatory activity when PET/CT was performed (45).
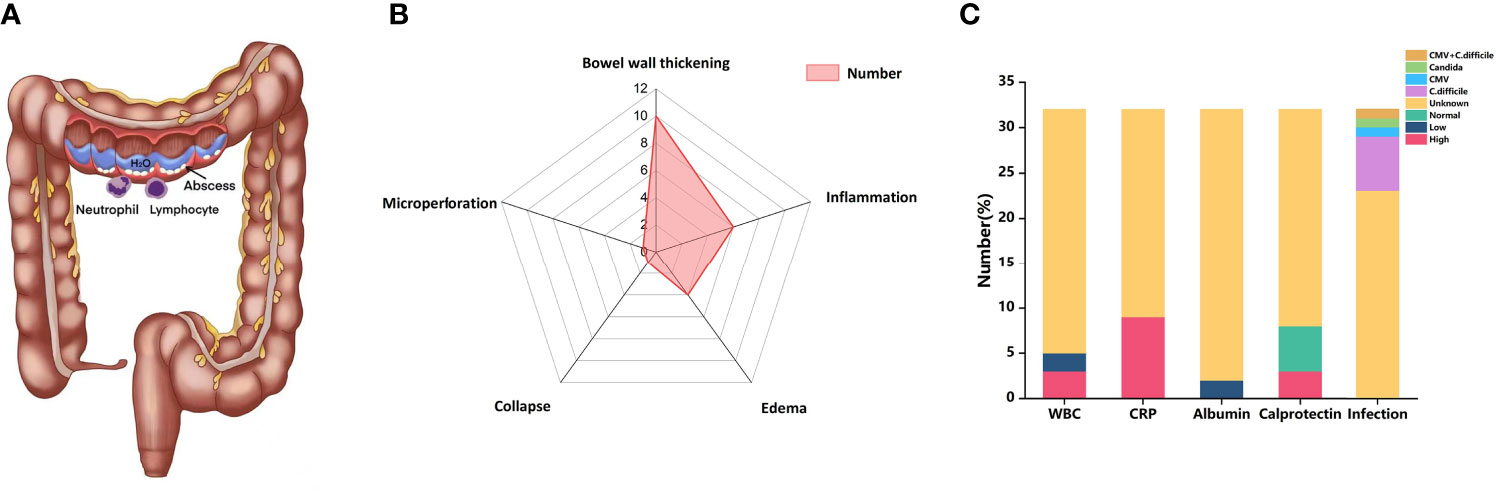
Figure 3 (A) CIC physically presented more similarly with colitis than IBD, involving lymphocyte and neutrophil infiltration. (B) Radar chart of CT features of CIC. (C) Bar chart of laboratory features of CIC. CIC, checkpoint inhibitor colitis; ICIs, immune checkpoint inhibitors; WBC, while blood cells; CRP, C-reactive protein; CMV, cytomegalovirus; C. difficile, Clostridium difficile.
Laboratory Features
The serological findings of patients with CIC are not specific. Some literature has reported that markers such as C-reactive protein (CRP) or low serum albumin level counts increased in the serum of IMC patients, but this may be influenced by systemic inflammation. In a retrospective study by Abolhassani et al., 88 patients with advanced melanoma were diagnosed irAEs after receiving ICIs, and 42% of them had a high CRP level (156). In our analysis, 9 of 32 patients had an increased level of CRP, and two patients showed low albumin levels (Figure 3C). Other serum findings may also be related to CIC, such as increased IL-17 (157), eosinophil count (158), and neutrophil–lymphocyte ratio. Some patients with CIC may show anemia on serological findings because of chronic bleeding in the digestive tract (77). In our analysis, three patients showed a decreased level of WBC (Figure 3C). Besides, elevations were found in serum leucine-rich α2-glycoprotein (LRG) and creatinine (42). LRG was reported to distinguish different colitis forms like drug-related and Cytomegalovirus (CMV) colitis (42).
Fecal calprotectin is often elevated in the stool specimens of patients developing IMC, which is a predictor of disease activity and prognosis. We found two patients with CIC had elevated fecal calprotectin (Figure 3C). Zou et al. (159) retrospectively analyzed the fecal calprotectin of patients with CIC and found that it was increased at the onset of IMC while decreased after treatment. In terms of infectious pathogens, Clostridium difficile (C. difficile) was the most common pathogen. CMV and Candida were also reported in our included patients (Figure 3C).
Endoscopic Features
Early endoscopy examination is encouraged for diagnosis and prognostic assessment if CIC is suspected (22). Left-sided colitis (31%–43%) is the most commonly reported in CIC cases, followed with pancolitis (involvement of ≥3 segments) (23%–40%) and ileitis (11%–14%) (160, 161). Endoscopic inflammatory findings usually present with mucosal ulceration and non-ulcerative inflammation with similar incidence. Non-ulcerative inflammation is characterized by erythema, exudate, erosion, friability, loss of vascular pattern, and edematous or granular mucosa (58, 72, 79). Among these, erythema (14/32) and edema (11/32) were the most common endoscopic features in our CIC patients (Figure 4). Most inflammation changes are predominantly diffuse instead of patchy (73). However, half of the diarrhea patients were observed to have patchy distribution endoscopically (77), which resembles the presentation of IBD (162), especially ulcerative colitis (UC) (53, 58). Fewer individuals with CIC (35%) were found to have segmental performance, as what often appeared in Crohn’s disease (77). There is no particular grading standard for the endoscopic manifestations in CIC. Nonetheless, the Mayo score, the van der Heide score for UC, or Simple Endoscopic Score (SES) for Crohn’s disease could be utilized (61, 163, 164).
Several studies illustrated that clinical symptoms were not significantly associated with endoscopic manifestations (22, 165). We also conducted a correlation analysis between CIC grade and endoscopic (P = 0.581)/histological features (P = 0.821), with no significant difference found (Table 2). However, the endoscopic findings, such as ulceration and pancolitis, are the indicative factors for steroid-refractory colitis (61). The findings in the endoscope could be categorized as low risk and high risk for steroid response. High-risk presentations were defined as either ulceration larger than 1 cm and/or deeper than 2 mm or extensive colitis from the proximity to the splenic flexure of the colon (166). High-risk patients need frequent utilization of biologics (vedolizumab or infliximab). Besides, they were significantly correlated with longer hospital stays, more disease recurrence, and more requirements for the repeat endoscopy (167). Furthermore, CIC patients who received colonoscopy within 1 week of onset would experience a significantly shorter period of symptoms and steroid therapy (166).
Pathological Features
The pathological features of CIC present from focal active colon injury with patchy crypt abscess to diffuse mucous inflammation (95). We found that inflammatory cell infiltration (50%), cryptitis, and/or crypt abscesses (33.3%) were the most frequently reported pathological features (Figure 4). These presentations might precede the onset of clinical symptoms. Coutzac et al. (95) included asymptomatic patients who received colonoscopy 1 to 2 weeks after treatment with ipilimumab and found that colon inflammation existed before the symptom development, although most of the symptoms of the patients occurred 3 weeks later. When they ultimately presented with symptomatic diarrhea or colitis, the histological observations exhibited more severe lamina propria infiltration. CIC pathological patterns could be summarized as follows: active colitis, chronic active colitis, microscopic (collagenous and lymphocytic) colitis, ischemic colitis, increased apoptosis, and non-specific inflammatory reactive changes (168–170). Patterns overlapping might exist in the microscopic presentation of CIC.
Active colitis is the most frequent pattern of histopathologic finding in ICI-mediated colitis. The typical manifestations of active colitis are lamina propria neutrophilic infiltrate, crypt abscesses, and neutrophilic cryptitis, with or without crypt dropout/atrophy (171, 172). The atrophic crypt epithelium is attenuated, which admixes apoptotic debris and inflammatory cell (173), resembling mycophenolate-caused colitis. Granulomas accompanied with crypt rupture have also been found (32, 174). Intraepithelial lymphocytes and increased apoptosis might or might not appear.
Microscopic colitis means lymphocytic and collagenous colitis (95), which has been found in 12% of CIC cases (66, 79, 175). Usually, lymphocytic colitis has the histological characteristics of a normal crypt structure, with increased lymphoplasmacytic inflammation in the lamina propria and intraepithelial lymphocyte infiltration, but no significant acute inflammatory presentation (176, 177). Collagenous colitis is an infrequent manifestation of CIC, which has increased intraepithelial lymphocytes and thickened subepithelial collagen (66). However, the atypical feature of microscopic colitis has also been reported, like crypt abscesses and neutrophilic cryptitis of active IBD (178). Gallo et al. (41) reported a patient with lung cancer who received atezolizumab presented collagenous colitis along with acute neutrophilic inflammation. Besides, Choi et al. (179) showed that patients with microscopic colitis induced by ICIs would have higher hospitalization rates and more aggressive course of disease and need more treatments like steroids and/or immunosuppressants than those without exposure to ICIs.
Chronic active colitis, one of the pathological patterns of IBD, has also been reported in ICI-mediated colon inflammation, particularly in patients with recurrent ICI therapy. This type is characterized by increased basal plasmacytosis and lymphoplasmacytic infiltration in the lamina propria, along with acute inflammation presentation of crypt abscess/cryptitis (172). The chronic manifestations, Paneth cell or pseudo-pyloric metaplasia and crypt structural distortion, constantly appear (63). The chronic feature could appear simultaneously with active colitis or develop gradually based on it. Yamauchi et al. (53) reported that three cases with colitis induced by nivolumab exhibited similar endoscopic and histopathologic features with ulcerative colitis at the initiation of diagnosis, and therapy for ulcerative colitis was applied successfully on these patients. Besides, one study (172) found that nearly half of patients who appeared with active colitis initially developed chronic features mentioned above. Therefore, ICI-related colitis has been considered as a specific IBD form (77). Approximately 60% of patients could have chronic inflammatory presence in the pathological section of ICI-induced colonic injury (22, 32, 180).
Increased crypt epithelial apoptosis always occurs in concurrence with other CIC patterns, including lymphocytic colitis, active colitis, and chronic active colitis. Isolated increased apoptosis with the absence of active and/or chronic inflammatory manifestation, imitating graft-versus-host disease, is less common (169). Ischemic colitis is also a rare pattern of CIC, presenting with atrophic crypts, reactive epithelial changes, and fibrosis in the lamina propria (32). In addition, non-specific inflammatory reactive changes, including lymphoplasmacytic infiltrate increase in the lamina propria along with epithelial attenuation and reactive changes and depleted mucin, are also presented in the colon injury pattern.
Diagnosis
New emerging abdominal symptoms after immunotherapy for NSCLC, especially acute diarrhea, need consideration of CIC (181). However, few patients only present with abdominal pain instead of diarrhea (50). Typically, the diagnosis of CIC is made by exclusion of infection, IBD, and tumor metastasis. Fecal etiology helps to rule out intestinal microbial infection. Endoscopy with biopsy, contributing to evaluate inflammatory severity, has been considered as the gold standard criteria for the diagnosis of ICI-related colitis. In addition, imaging examination like CT also assists in diagnosing serious complications such as toxic megacolon and intestinal perforation.
The most common differential diagnoses include infectious intestinal diseases and IBD. The infectious pathogens, such as CMV, Salmonella, C. difficile, and parasites, should be examined foremost for the diagnosis of CIC. It is worthy to notice that CIC could develop with bowel infection at the same time. One of our cases developed CIC that coexisted with CMV and Salmonella (46). Therefore, in addition to etiology detection, endoscopic biopsy is essential for accurate diagnosis. Moreover, tumor immunity could be triggered by blocking the pathways of PD-1/PD-L1/CTLA-4, while the immunity to infection could be inhibited. Therefore, several cases were infected with C. difficile after CIC (43). Under this condition, CIC would get worse, and it is necessary to add antibiotics in time. Besides, the pathogenesis and endoscopic manifestations of CIC resemble those of IBD (162, 166). However, there still exist differences in pathological appearance between CIC and IBD. Histologically, CIC is characterized with active colitis, such as neutrophilic infiltration, crypt microabscesses, and prominent crypt epithelial cell apoptosis, while IBD usually shows chronic inflammation signs, including basal lymphocytosis, crypt architectural irregularity, and Paneth cell metaplasia (173). In addition, compared with UC, CIC showed less basal plasmacytosis (14% vs. 92%), less crypt distortion (23% vs. 75%), and more apoptotic bodies ((17.6 ± 15.3 vs. 8.2 ± 4.2) (182). It is noteworthy that the clinical presentations could distinguish these different clinical entities when the histologic findings overlap, including the onset of symptoms soon after ICI initiation and other concurrent irAEs (25). Moreover, recent research illustrated distinct immunological features of colonoscopy from CIC and IBD patients, with predominantly CD4+ T cells and Treg cells in the anti-CTLA-4-induced colitis and IBD patients, respectively (95).
In summary, the precise diagnosis of CIC requires multidisciplinary discussion and persistent monitoring, which also plays a critical role in grade assessment.
Management
At present, minimal prospective trials have been designed to evaluate the optimal therapeutic modality for CIC. Consequently, current management guidelines are on the strength of expert opinion and retrospective studies, recommending systemic corticosteroids as the first-line therapy uniformly (19, 71, 183). The treatment is ideally initiated not exceeding 5 days of symptom appearance (23). The specific management is mainly based on severity degree. Pretreatment examinations include infectious etiology (stool parasitism and calprotectin), blood testing (C-reactive protein, celiac disease serology, comprehensive metabolic panel, complete blood count, etc.), hepatitis serology, and tuberculosis testing, which are beneficial for monitoring treatment response and preparing for biologics if corticosteroids do not work (184).
Steroid
Systemic high-dose glucocorticoids are typically effective for CIC cases, equaled to prednisone of 1–2 mg/kg/day, obtaining nearly 90% efficacy (185).
Steroid Therapy Classified by Grade
For grade 1 diarrhea/colitis, supportive treatments, such as anti-motility agents (atropine/diphenoxylate, loperamide), adequate hydration, and dietary adjustments (low-fiber, bland diet), are recommended (186). Budesonide has been proposed to reduce symptoms in patients with ICI-mediated colitis (19). ICIs could continue to be utilized without delay or interruption in the management of colitis.
For grade 2 diarrhea/colitis, ruling out the infectious factors, systemic corticosteroids (prednisone/intravenous methyl-prednisolone of 1–2 mg/kg/day) should be initiated until symptom improves to grade 1 or less. After that, the taper of steroids is recommended for at least 4 to 6 weeks (186). Endoscopy and abdominal CT should be considered strongly. In terms of ICIs, anti-CTLA-4 agents should be discontinued permanently, while anti-PD-L1 agents could be continuously held. Besides, PD-L1 inhibitors also could be resumed as monotherapy when symptoms have improved/resolved, or prednisone is reduced to 10 mg/day or less (19, 183).
For grade 3 to 4 diarrhea/colitis, the discontinuation of ICIs should be immediate and permanent (19, 71, 183, 187). Patients are usually admitted to the hospital for urgent evaluation and continuous monitoring. Intravenous methylprednisolone of 1–2 mg/kg is the prompted initial therapy (24, 79). It is also necessary to keep electrolyte balance and implement aggressive fluid resuscitation for grade 3–4 CIC patients. The corticosteroid response for grade 2–4 toxicity should be assessed early after 2–3 days. Non-responders are recommended for escalation to a biological agent.
We also investigated the therapeutic features stratified by CIC grade (Table 3) and depicted the steroid duration and taper (Figure 5). We unified different steroid types to methylprednisolone (MP) equivalents and further classified them into high-dose, intermediate-dose, and low-dose groups, based on the initial administered dose. Since we observed that not all cases pointed out the weight of the patients, two distinct steroid dose specifications (mg/kg/day and mg/day) were established to describe the utilization of steroid. Our data showed that the median steroid initial dose was nearly 60 mg/day or 1 mg/kg/day (MP), with no significant difference found in grade 2 and 3–4 CIC patients (Table 3). In terms of dose group, low-dose steroid was more frequently used in grade 3–4 CIC patients, while high-dose steroid was more common in grade 2 CIC patients, although no significant discrepancy existed (Table 3). Extensive sample size studies with more specific steroid data are required to illustrate steroid use.
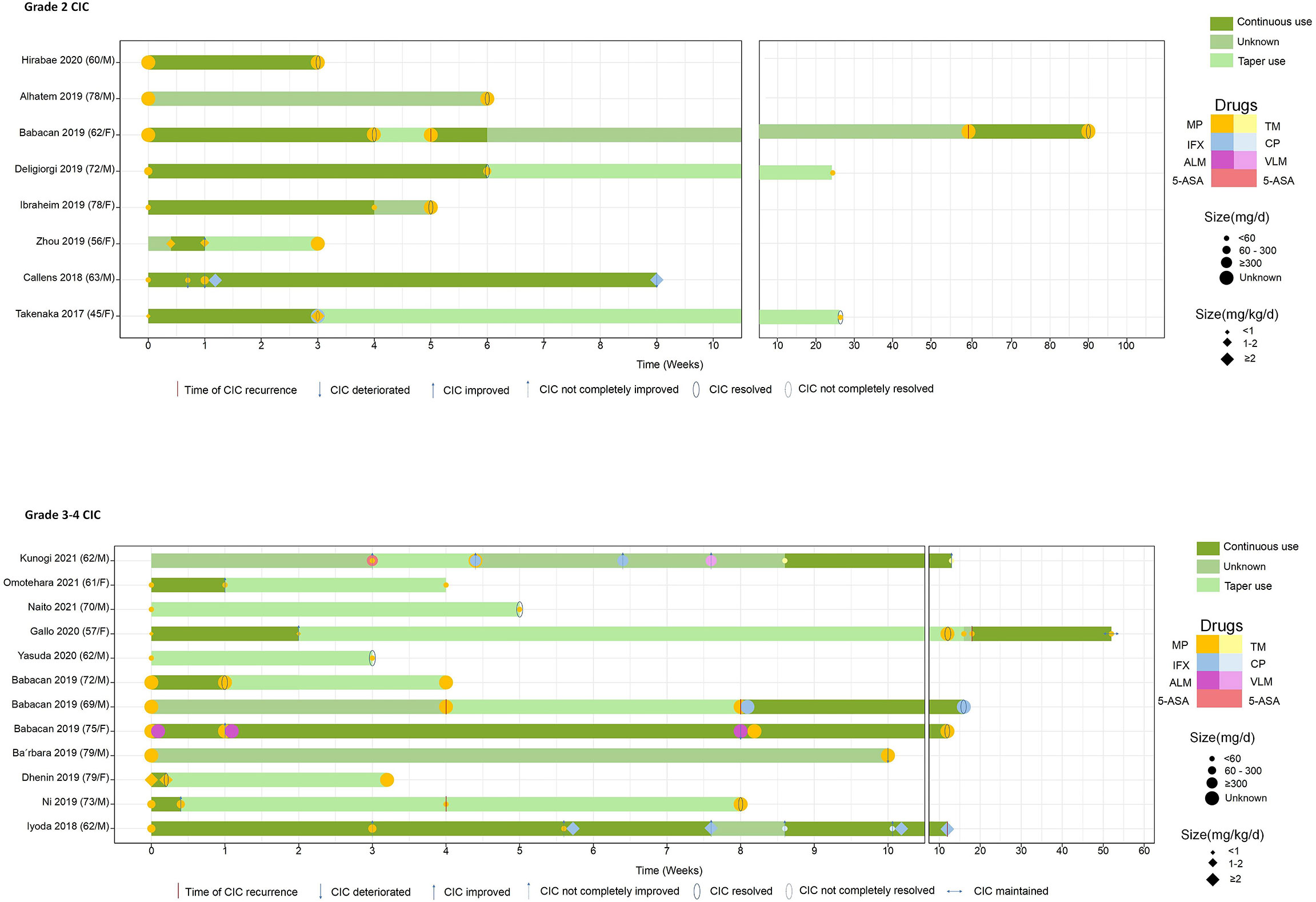
Figure 5 The drugs that every case utilized and the definite continuous and taper time. All the steroid doses were converted to equivalent MP. MP, methylprednisolone; TM, tacrolimus; IFX, infliximab; CP, cyclosporine; ALM, adalimumab; VLM, vedolizumab; 5-ASA, 5-aminosalicylic acid.
The Quantifying, Taper, and Maintenance of Steroid
Limited data underscore the quantifying of steroids in treating ICI-related diarrhea/colitis. A retrospective study (188) included 90 patients with ICI-induced gastroenterocolitis and divided them into three groups based on steroid dose: “limited or none,” prednisone equivalents <7.5 mg/day on average for over 2 months; “moderate,” prednisone equivalents >7.5 mg/day on average for over 2 months; and “high,” prednisone equivalents ≥1 mg/kg/day for over 1 week (189, 190). They found that the high-dose steroid group had decreased PFS (HR = 2.54, 95% CI: 1.11–5.80, P = 0.03), while OS was not influenced by systemic steroid treatment for gastroenterocolitis mediated by ICIs, after the adjustment of covariates. Another study focused on the maintenance of steroid management (191). The results showed that grade 3 CIC has a median average steroid duration of 58 days. Overall grade steroid duration was 94 days, twice than grade 3 or 4 CIC steroid duration. This placed quite a few patients at risk of iatrogenic complications such as mood change and infection. Therefore, continuous supportive care during the utilization of steroid should be reinforced as well (192). The recommended taper time was 4 to 8 weeks (193, 194). The cases we included had a median steroid taper duration at nearly 5 weeks, with grade 2 and grade 3–4 CIC at about 10 and 5 weeks, respectively (P = 0.041) (Table 3). In 20 CIC patients with exact steroid data, 9 of them chose the steroid decrement after the improvement or resolution of CIC, and the duration of continuous steroid use varied from 3 days to 12 weeks (Figure 5).
Biological Agents
Approximately 30%–60% of ICI-reduced diarrhea/colitis patients appear recalcitrant to first-line glucocorticoid, showing no response to high-dose steroid within 72 h of onset or no complete response in 1 week (61). They should be considered for second-line immunosuppressants. Besides, patients who suffer relapses during the steroid decrement or after finishing the steroid course also require additional immunosuppression (28), with the recurrent incidence of 44% for CTLA-4 inhibitors and 34% for PD-1/PD-L1 inhibitors (195). Similarly, our analysis also suggested that patients were more likely to receive biological agents (42.86% vs. 6.25%, P = 0.018) if they suffered from a deteriorated or recurrent CIC outcome rather than an improved or resolved outcome (Table 4). Additionally, colon ulceration, pancolitis, and high van der Heide or Mayo score are identified as the predictive factors correlated to the utilization for secondary immunosuppression (22, 61, 167). In addition, the biopsy specimens of CIC patients who required infliximab showed higher CD68 scores and CD8/FoxP3 ratios than those who responded to steroid (196).
Current studies demonstrate that infliximab (IFX) and vedolizumab are the primary therapeutic approaches for steroid-refractory CIC patients (31, 71). Vedolizumab could be utilized in cases when infliximab is contraindicated or ineffective (186).
IFX, a TNF-α antagonist, has been verified to promote tumor immunity and relieve resistance to PD-1 inhibitors significantly (195, 197). Few prospective studies are conducted to illustrate the dose of IFX, while different guidelines showed almost universal recommendations that the initial dose is at 5 mg/kg (q2w) until symptoms improve (19, 71, 183, 198). Seventy-two percent of patients recover with one IFX dose, though the requirement for two or three doses is common (19, 77, 196, 197). However, IFX is reported to induce a rare type of hepatitis (199) and is limited in patients with hepatitis B virus or latent tuberculosis history (200, 201). Moreover, IFX utilization is notably correlated with lymphomas and skin cancer in young adults, while this correlation might be confounded by overall increased malignancy risk and previous thiopurine utilization (202). Golimumab, certolizumab, adalimumab, and etanercept could be alternatives to IFX if paradoxical adverse events occur in CIC patients (203).
Vedolizumab, an antagonist of α4β7 on CD4+ T cells, prevents T cells from aggregating and homing into the inflammatory intestinal mucosa. The unique gut selective mechanism could avoid systemic immunosuppression without substantially changing antitumor response to chemotherapy (28). It is administered to treat steroid-refractory cases with 300 mg infusions at 0, 2, and 6 weeks or until laboratory or clinical improvement (204, 205). Bergqvist et al. (206) reported seven patients with steroid-refractory or steroid-dependent CIC who recovered by using vedolizumab. CIC with microscopic colitis on histopathology also has a response to vedolizumab (205). The potential risk of tumor progression in patients with lymph nodes metastasis might be minimized by vedolizumab since its gut selectivity (207). In addition, utilizing vedolizumab, which has the same potential as IFX, could not increase secondary tumor risk in vulnerable individuals.
Increasing research studies support the early introduction of biological agents in the therapy algorithm (60, 197, 208). Wang et al. (60) pointed out that a long period of steroid monotherapy could increase the infectious risk in contrast to a short period of steroid such as IFX, which suggests that the early utilization of steroid-sparing therapy might improve the prognosis. Abu-Sbeih et al. (208) recruited 179 CIC patients who received biologic therapy (IFX and vedolizumab) to explore their clinical outcomes. They found that over half of the patients significantly experienced lower hospitalization, shorter symptom duration, shorter steroid taper/taper attempt, and higher success rate of steroid taper. Decreased fecal calprotectin levels and the promoted histologic regression rate were found in patients treated with over three infusions of biologics. Usually, the biologics withdrawal happens in the endoscopy finding of mucosal healing, if there is an attempt to resume ICI therapy. However, no prospective studies have emerged to verify the effectiveness of biologics as first-line therapy. ASCO guidelines stated that individualized therapeutic decisions should be recommended all along (79).
Besides, there are also other potential biologics for refractory ICI-related colitis (203). Anti-IL-1 blockade (anakinra), anti-IL-17 blockade (ixekizumab), anti-IL-23 and anti-IL-12 blockade (ustekinumab) (209), mycophenolate mofetil (MMF) (210, 211), and ciclosporin (54), aiming at blocking critical inflammatory components participating in the pathophysiological process of irAEs, are reported to exert considerable effects in treating severe CIC. Besides, Janus kinase (JAK) inhibitors (tofacitinib) have shown efficacy in CIC patients (212). More studies are warranted to evaluate the usefulness of these biologics in ICI-induced colitis.
Fecal Transplants
The intestinal microbiome has been hypothesized to play an essential role in the occurrence of CIC. Various gut microbiomes are correlated to induce or alleviate ICI-mediated colitis. Fecal transplantation, contributing to gut microbiome reconstitution and regulatory T cells relatively increasing within the colon mucosa, has been proposed as an optional therapeutic approach for patients with severe or refractory CIC. However, available guidance comes from limited case reports. Wang et al. (213) reported two cases with non-healing CIC who completely recovered after receiving fecal transplantation. Another case that was reported by Fasanello et al. had CIC healed successfully with fecal transplantation (214). Convincing prospective studies are required to verify these observations.
Surgery
Patients with CIC could deteriorate to colonic perforation, although with the estimated incidence of 1% to 1.5% (71). Emergency colectomy is recommended to be the most effective treatment. Specifically, subtotal colectomy is preferred since the colon lesions are extensive. Segmental colectomy is usually utilized in the remaining severe colonic inflammation in the postoperative period (77). We also included two NSCLC cases with colonic perforation after receiving ICI therapy (50). One recovered after surgery and rechallenge ICIs with ongoing partial regression (50), while the other ultimately succumbed to infectious complications (55). The predictive risk factors for colon perforation are limited. Smith et al. (215) found that CIC patients who received ipilimumab followed with high-dose IL-2 treatment were prone to present perforation than patients who received one of these therapies alone. Heightened vigilance and timely surgery to this severe complication can improve the clinical outcomes effectively.
Prognosis
Several speculations arise from the association between CIC outcome and survival, whose evidence is rare and conflicting. Two retrospective studies reported that patients who developed ICI-related diarrhea/colitis had a superior OS than those without gastrointestinal toxicity (60, 216). In addition, two simultaneous or sequential irAEs may herald better clinical outcomes. Patients with both colitis and rash caused by immune therapy had a significantly better OS (28.6 vs. 19.9 months, P = 0.018) and PFS (16.1 vs. 3.2 months, P = 0.001), in contrast to patients with colitis but no rash (217). Obviously, irAEs indicated encouraging tumor response and clinical benefit in patients, which meant longer PFS and OS (216–219). Similarly, our analysis showed that CIC patients with deteriorated or recurrent outcomes had longer OS than those with improved or resolved outcomes (28.5 vs. 13 months, P = 0.015) (Table 4). Besides, the CIC course was shorter in CIC patients with resolution or improvement, compared with those with deterioration and recurrence (0.84 vs. 6.25 months, P < 0.001) (Table 4). Moreover, we found that over 60% of patients with grade 2 CIC experienced resolved or improved CIC outcomes, while nearly half of patients with grade 3–4 CIC experienced deteriorated or recurrent CIC outcome (Table 3). Generally, higher grade irAEs were reported to suffer inferior prognosis (220). However, several studies showed that no significant correlation was observed between CIC and survival (221, 222). Therefore, the correlation between irAEs and survival needs further verification, and the involved mechanisms are warranted to be clarified.
Predictors for CIC are still on exploration. Several serum markers like CRP, LDH, and fecal calcitonin and their association with CIC prognosis have been investigated. Justine et al. revealed that patients with elevated CRP level had a higher risk of irAE recurrence (223). LDH ≥618 IU/L was related to a poor prognosis in CIC patients (60). Conversely, the decrease of fecal calcitonin after treatment occurred more significantly in patients with CIC remission under endoscopy, which could be used as a non-invasive tool for monitoring and evaluating the response of CIC (159). Nevertheless, the value of fecal calcitonin was limited by its 54% specificity in predicting the endoscopic remission of CIC (166). Furthermore, CIC with immuno-suppressive therapy might be associated with improved OS and PFS compared with CIC without immunosuppressive treatment (25, 60). Higher grade diarrhea could predict great prognosis (216). In addition, the application of antibiotics at any time was reported to decrease the incidence of CIC recrudescence, and patients in our analysis who experienced poor CIC prognosis (P = 0.025) or had higher CIC grade (P = 0.025) were more frequently utilized antibiotics (Tables 3 and 4). However, it could increase the frequency of hospitalization and intensive care unit hospitalization and exacerbate CIC severity (224).
Rechallenge and Recurrence
A great number of meta-analyses and retrospective studies showed that the resumption after irAEs was potentially effective and relatively safe in selected individuals with careful monitoring (225–229). Universally, patients who improved to grade 1 or less diarrhea/colitis could resume ICI therapy. Any grade 4 irAEs, especially cardiac and neurologic toxicities, are not allowed to continue or reuse ICI (225). The patterns of ICI reintroduction included the same ICI utilized before, the same type of ICIs, and the switching of ICIs of distinct types. Kitagawa et al. (230) showed the safety and efficacy of rechallenging ICIs in patients with NSCLC reported in prior studies. They found that switching ICI class therapy obtained a superior outcome, with over 50% of patients experiencing stable or partial regression disease. Gobbini et al. (231) demonstrated that NSCLC patients discontinuing for irAEs had more prolonged survival than those discontinuing for disease progression. After ICI rechallenge, the recurrence rate of irAEs varied from 37% to 78% (227, 232–234). Among these recurrent irAEs, colitis showed significantly higher incidence than other irAEs (227). Nearly 20%–30% of patients would experience recurrent grade 3 CIC (195, 233). In our analysis, 12.5% (4/32) of patients (36, 43, 50, 54) were retreated with the same ICI therapy used before, one of which experienced the recurrence of grade 3 enterocolitis (54). All of these patients who were reintroduced to ICI therapy harbored deteriorated/recurrent CIC outcomes (P = 0.037) (Table 4). Furthermore, a multicenter retrospective study compared the clinical outcomes in ICI retreatment and discontinuation group patients (229). The results showed that retreatment patients had fewer steroid requirements and less hospitalizations (P = 0.007). Besides, the outcomes of recurrent irAEs were found to be more severe if patients were treated with immunosuppressants before, and thus, they need more intensive immunosuppressive treatment. A retrospective study (205) investigated a patient subset of reinitiation ICI therapy. They found that most of the patients need steroid, while a minority of them required IFX or vedolizumab, regardless of whether in the initial or recurrent episode. Our analysis showed that 25% (8/32) of patients (36, 41, 43, 46, 48, 54, 57) had recurrent ICI-mediated colitis without ICI retreatment, which received subsequent therapy involving probiotics, antidiarrheal drugs, antiviral drugs, steroid, and immunosuppressants. Four cases with CIC finally did not improve or deteriorate after the second treatment (36, 41, 48, 54). The risk factors associated with CIC recurrence included early irAE development, long duration of the initial CIC, initial application of immunosuppressants, pneumonitis, hepatitis, age over 65, advanced tumor stage, CTLA-4 inhibitors use (227), and less antibiotic therapy (205, 224). In addition, the factors—good performance status at ICI resumption, the duration and response of the initial ICI course, longer ICI-free interval, and high PD-L1 expression—were considered so far as correlated to clinical benefit from ICI retreatment (235, 236). Actually, with the increasing interest in immunotherapy reintroduction in recent years, we still need further ad-hoc-designed and prospective studies to assess the efficacy and safety of the diverse immunotherapy strategies.
Summary
ICI-mediated colitis is the most common irAEs. The incidence varied with different types of ICIs, with combined ICI therapy obtaining the highest occurrence and severity. Gut microbiota plays a critical role in the development of CIC. Endoscopic biopsy is the gold standard of diagnosis, and the consideration combined with medication history is necessary. Current management is based on symptomatic and steroid therapy. The utilization of biological agents according to the severity of CIC grade is reasonable and recommended. The association between CIC and survival is obscure and requires to be explored further. Rechallenge of ICIs is on heated debate. On the condition of symptom control, the reintroduction of ICI is worthy of consideration, with persistent monitoring and evaluation certainly.
Strengths and Limitations
This review summarized the latest studies to demonstrate the comprehensive characteristics of ICI-related colitis, especially the mechanisms and treatment. We also collected the CIC cases with NSCLC as an example to analyze the differences between different CIC grades and outcomes. The association between endoscopic findings and the severity of ICIs-related colitis has been evaluated as well. We hoped that our comprehensive review could bring some inspiration in clinical practice. In addition, we explored the correlation between initial steroid dose and CIC outcome, but no significant results were found due to the small sample size and limited accurate data. Although we focused on the NSCLC cases with ICI-mediated colitis, the understanding of irAEs is regardless of cancer species. Moreover, we are conducting real-world studies in our center and we hope that large sample data could present more reliable results.
Future Direction
In the future, early prediction and detection and timely and effective treatment are the two main aspects we need to investigate continuously.
Most studies have focused on the predictive factors for survival outcomes or treatment response (237, 238), while comparatively limited research studies could find biomarkers characterizing irAEs (239, 240). Lim et al. established the CYTOX score covering 11 proinflammatory cytokines and validated its efficacy in predicting severe irAEs which need high-dose corticosteroid therapy (241). However, in view of the extensive organ specificity or systemic presentations in irAEs and the distinct potentially inflammatory mechanisms concerning the adverse events, it is still difficult to translate relevant results to clinical practice. Besides, specific biomarkers are warranted to be explored with a large number of verified preclinical models.
As for the treatment, the optimal steroid dose and continuous/taper time remain inconclusive. Besides, steroid utilization for treating irAEs has become a field with growing controversy. Quite a bit of evidence suggested that the antitumor efficacy of ICI could be weakened by the wide range of immunosuppressive function of steroid (242–244). However, a single-center study (245) recruited 372 patients according to the different indications (irAEs and others) for steroid. They found that using systemic steroid for irAEs could not influence tumor response and OS, while for other indications (usually correlated to poor prognosis), it could. In addition, we also know little about the immune microenvironment effect by systemic corticosteroids administration. A few case reports implied a temporal correlation between steroid therapy, decreased cytokine expression, and irAE resolution (96). Notably, Tyan et al. (143) found that steroid could disrupt the specific harmonization pattern in irAE patients, which suggested the elimination of activated immune states and thus verified the worse outcome in patients without irAEs or in patients treated with steroid. More subgroup analysis is required to associate irAEs and steroid utilization with survival. Except for steroid treatment, current clinical trials, concerning the use of IFX and vedolizumab (NCT04407247) for CIC patients and tofacitinib (NCT04768504) for refractory CIC patients, are looking forward to presenting profound results. Clinical studies on fecal transplant involved in the gut microbiome have also been in the phase of recruiting participants (NCT03819296 and NCT04038619).
Numerous scientists have been persistently working to investigate the development of irAEs. We hope that one day in the future, irAEs could be specifically predicted and its risk could be assessed by simple and convenient detection biomarkers. The cooperation among multiple disciplines should be strengthened for the continuous monitoring of CIC. Novel treatment strategies are expected with a few side effects. Furthermore, we also need more research studies about the clinicopathological and biological characteristics of CIC to help us better understand this specific subset of irAEs.
Statistical Analysis
We analyzed the differences of CIC onset in different ICI therapy groups by Mann–Whitney U test. The descriptive analysis was conducted to describe the baseline features and the intergroup discrepancies in distinct CIC grade and CIC outcome groups. Fisher’s exact or chi-square test and Kruskal–Wallis test were performed to analyze categorical and continuous variables, respectively. Categorical variables were expressed as counts and proportions. Continuous variables were delineated as means and standard deviations. We utilized the statistical software packages R and EmpowerStats (X&Y Solutions Inc., Boston, MA, USA) to perform all the statistical analyses. The statistical significance was presented as two-sided P-values less than 0.05.
Author Contributions
LT and JW searched the literature and wrote the manuscript. NL and YZ helped collect the literature and participated in the discussion. LT and WH performed the statistical analysis. JL and XM examined and verified the study. All authors contributed to the article and approved the submitted version.
Conflict of Interest
The authors declare that the research was conducted in the absence of any commercial or financial relationships that could be construed as a potential conflict of interest.
Publisher’s Note
All claims expressed in this article are solely those of the authors and do not necessarily represent those of their affiliated organizations, or those of the publisher, the editors and the reviewers. Any product that may be evaluated in this article, or claim that may be made by its manufacturer, is not guaranteed or endorsed by the publisher.
References
1. Siegel RL, Miller KD, Fuchs HE, Jemal A. Cancer Statistics, 2021 (Vol 71, Pg 7, 2021). CA Cancer J Clin (2021) 71(4):359–9. doi: 10.3322/caac.21654
2. Cabezon-Gutierrez L, Custodio-Cabello S, Palka-Kotlowska M, Alonso-Viteri S, Khosravi-Shahi P. Biomarkers of Immune Checkpoint Inhibitors in Non-Small Cell Lung Cancer: Beyond PD-L1. Clin Lung Cancer (2021) 22(5):381–9. doi: 10.1016/j.cllc.2021.03.006
3. Ettinger DS, Wood DE, Aggarwal C, Aisner DL, Akerley W, Bauman JR, et al. Non-Small Cell Lung Cancer, Version 1.2020 Featured Updates to the NCCN Guidelines. J Natl Compr Cancer Netw (2019) 17(12):1464–72. doi: doi: 10.6004/jnccn.2019.0059
4. Bei Y, Chen X, Raturi VP, Liu K, Ye S, Xu Q, et al. Treatment Patterns and Outcomes Change in Early-Stage Non-Small Cell Lung Cancer in Octogenarians and Older: A SEER Database Analysis. Aging Clin Exp Res (2021) 33(1):147–56. doi: 10.1007/s40520-020-01517-z
5. Lamberti G, Andrini E, Sisi M, Rizzo A, Parisi C, Di Federico A, et al. Beyond EGFR, ALK and ROS1: Current Evidence and Future Perspectives on Newly Targetable Oncogenic Drivers in Lung Adenocarcinoma. Crit Rev Oncol Hematol (2020) 156:103119. doi: 10.1016/j.critrevonc.2020.103119
6. Li X, Shao C, Shi Y, Han W. Lessons Learned From the Blockade of Immune Checkpoints in Cancer Immunotherapy. J Hematol Oncol (2018) 11:31. doi: 10.1186/s13045-018-0578-4
7. Li Y, Li F, Jiang F, Lv X, Zhang R, Lu A, et al. A Mini-Review for Cancer Immunotherapy: Molecular Understanding of PD-1/PD-L1 Pathway & Translational Blockade of Immune Checkpoints. Int J Mol Sci (2016) 17(7):1151. doi: 10.3390/ijms17071151
8. Reddy HG, Schneider BJ, Tai AW. Immune Checkpoint Inhibitor-Associated Colitis and Hepatitis. Clin Trans Gastroenterol (2018) 9(11):206. doi: 10.1038/s41424-018-0049-9
9. Lin X, Lu X, Luo G, Xiang H. Progress in PD-1/PD-L1 Pathway Inhibitors: From Biomacromolecules to Small Molecules. Eur J Med Chem (2020) 186:111876. doi: 10.1016/j.ejmech.2019.111876
10. Hayashi H, Nakagawa K. Combination Therapy With PD-1 or PD-L1 Inhibitors for Cancer. Int J Clin Oncol (2020) 25(5):818–30. doi: 10.1007/s10147-019-01548-1
11. Postow MA, Sidlow R, Hellmann MD. Immune-Related Adverse Events Associated With Immune Checkpoint Blockade. N Engl J Med (2018) 378(2):158–68. doi: 10.1056/NEJMra1703481
12. Shannon VR. Pneumonitis Associated With Immune Checkpoint Inhibitors Among Patients With Non-Small Cell Lung Cancer. Curr Opin Pulm Med (2020) 26(4):326–40. doi: 10.1097/MCP.0000000000000689
13. Naidoo J, Nishino M, Patel SP, Shankar B, Rekhtman N, Illei P, et al. Immune-Related Pneumonitis After Chemoradiotherapy and Subsequent Immune Checkpoint Blockade in Unresectable Stage III Non-Small-Cell Lung Cancer. Clin Lung Cancer (2020) 21(5):E435–44. doi: 10.1016/j.cllc.2020.02.025
14. Remon J, Passiglia F, Ahn M-J, Barlesi F, Forde PM, Garon EB, et al. Immune Checkpoint Inhibitors in Thoracic Malignancies: Review of the Existing Evidence by an IASLC Expert Panel and Recommendations. J Thorac Oncol (2020) 15(6):914–47. doi: 10.1016/j.jtho.2020.03.006
15. Hellmann MD, Ciuleanu T-E, Pluzanski A, Lee JS, Otterson GA, Audigier-Valette C, et al. Nivolumab Plus Ipilimumab in Lung Cancer With a High Tumor Mutational Burden. N Engl J Med (2018) 378(22):2093–104. doi: 10.1056/NEJMoa1801946
16. Reck M, Borghaei H, O'Byrne KJ. Nivolumab Plus Ipilimumab in Non-Small-Cell Lung Cancer. Future Oncol (2019) 15(19):2287–302. doi: 10.2217/fon-2019-0031
17. Reck M, Ciuleanu T-E, Dols MC, Schenker M, Zurawski B, Menezes J, et al. Nivolumab (NIVO) Plus Ipilimumab (IPI)+2 Cycles of Platinum-Doublet Chemotherapy (Chemo) vs 4 Cycles Chemo as First-Line (1L) Treatment (Tx) for Stage IV/recurrent Non-Small Cell Lung Cancer (NSCLC): CheckMate 9la. J Clin Oncol (2020) 38(15):9501–9501. doi: 10.1200/JCO.2020.38.15_suppl.9501
18. Rizzo A, Brandi G. Biochemical Predictors of Response to Immune Checkpoint Inhibitors in Unresectable Hepatocellular Carcinoma. Cancer Treat Res Commun (2021) 27:100328. doi: 10.1016/j.ctarc.2021.100328
19. Puzanov I, Diab A, Abdallah K, Bingham CO III, Brogdon C, Dadu R, et al. Managing Toxicities Associated With Immune Checkpoint Inhibitors: Consensus Recommendations From the Society for Immunotherapy of Cancer (SITC) Toxicity Management Working Group. J Immunother Cancer (2017) 5:95. doi: 10.1186/s40425-017-0300-z
20. Haanen JBAG, Carbonnel F, Robert C, Kerr KM, Peters S, Larkin J, et al. Management of Toxicities From Immunotherapy: ESMO Clinical Practice Guidelines for Diagnosis, Treatment and Follow-Up (Vol 28, Pg 119, 2017). Ann Oncol (2018) 29:264–6. doi: 10.1093/annonc/mdy162
21. Abu-Sbeih H, Ali FS, Luo W, Raju G, Wang Y. The Importance of Endoscopic and Histological Evaluation in the Management of Immune Checkpoint Inhibitor-Induced Colitis. Am J Gastroenterol (2018) 113:S112–3. doi: 10.14309/00000434-201810001-00197
22. Wang Y, Abu-Sbeih H, Mao E, Ali N, Qiao W, Van Anh T, et al. Endoscopic and Histologic Features of Immune Checkpoint Inhibitor-Related Colitis. Inflamm Bowel Dis (2018) 24(8):1695–705. doi: 10.1093/ibd/izy104
23. Marin-Acevedo JA, Harris DM, Burton MC. Immunotherapy-Induced Colitis: An Emerging Problem for the Hospitalist. J Hosp Med (2018) 13(6):413–8. doi: 10.12788/jhm.2925
24. Kroner PT, Mody K, Farraye FA. Immune Checkpoint Inhibitor-Related Luminal GI Adverse Events. Gastrointest Endosc (2019) 90(6):881–92. doi: 10.1016/j.gie.2019.09.009
25. Som A, Mandaliya R, Alsaadi D, Farshidpour M, Charabaty A, Malhotra N, et al. Immune Checkpoint Inhibitor-Induced Colitis: A Comprehensive Review. World J Clin Cases (2019) 7(4):405–18. doi: 10.12998/wjcc.v7.i4.405
26. Menon T, Afzali A. Immune-Mediated Colitis. Curr Treat Options Gastroenterol (2019) 17(4):506–23. doi: 10.1007/s11938-019-00263-0
27. Sehgal K, Khanna S. Gut Microbiome and Checkpoint Inhibitor Colitis. Intest Res (2020) 19(4):360–4. doi: 10.5217/ir.2020.00116
28. Dougan M, Wang Y, Rubio-Tapia A, Lim JK. AGA Clinical Practice Update on Diagnosis and Management of Immune Checkpoint Inhibitor Colitis and Hepatitis: Expert Review. Gastroenterology (2021) 160(4):1384–93. doi: 10.1053/j.gastro.2020.08.063
29. Portenkirchner C, Kienle P, Horisberger K. Checkpoint Inhibitor-Induced Colitis-A Clinical Overview of Incidence, Prognostic Implications and Extension of Current Treatment Options. Pharmaceuticals (2021) 14(4):e1344805. doi: 10.3390/ph14040367
30. Wang DY, Ye F, Zhao S, Johnson DB. Incidence of Immune Checkpoint Inhibitor-Related Colitis in Solid Tumor Patients: A Systematic Review and Meta-Analysis. Oncoimmunology (2017) 6(10). doi: 10.1080/2162402X.2017.1344805
31. Soularue E, Lepage P, Colombel JF, Coutzac C, Faleck D, Marthey L, et al. Enterocolitis Due to Immune Checkpoint Inhibitors: A Systematic Review. Gut (2018) 67(11):2056–67. doi: 10.1136/gutjnl-2018-316948
32. Gonzalez RS, Salaria SN, Bohannon CD, Huber AR, Feely MM, Shi C. PD-1 Inhibitor Gastroenterocolitis: Case Series and Appraisal of 'Immunomodulatory Gastroenterocolitis'. Histopathology (2017) 70(4):558–67. doi: 10.1111/his.13118
33. Khoja L, Day D, Chen TW-W, Siu LL, Hansen AR. Tumour- and Class-Specific Patterns of Immune-Related Adverse Events of Immune Checkpoint Inhibitors: A Systematic Review. Ann Oncol (2017) 28(10):2377–85. doi: 10.1093/annonc/mdx286
34. Tandon P, Bourassa-Blanchette S, Bishay K, Parlow S, Laurie SA, McCurdy JD. The Risk of Diarrhea and Colitis in Patients With Advanced Melanoma Undergoing Immune Checkpoint Inhibitor Therapy: A Systematic Review and Meta-Analysis. J Immunother (2018) 41(3):101–8. doi: 10.1097/CJI.0000000000000213
35. Kunogi Y, Tominaga K, Abe K, Kanazawa M, Tanaka T, Watanabe S, et al. Refractory Immune Checkpoint Inhibitor-Induced Colitis Improved by Tacrolimus: A Case Report. Healthcare (2021) 9(4):418. doi: 10.3390/healthcare9040418
36. Mourad AP, De Robles MS. Chemoimmunotherapy-Related Enteritis Resulting in a Mechanical Small Bowel Obstruction - A Case Report. Int J Surg Case Rep (2021) 79:131–4. doi: 10.1016/j.ijscr.2020.12.096
37. Naito T, Nosaka T, Takahashi K, Ofuji K, Matsuda H, Ohtani M, et al. A Case of Immune Checkpoint Inhibitor-Related Colitis With a Distinctive Endoscopic Finding of Colonic Pseudolipomatosis. Clin J Gastroenterol (2021) 14(5):1431–6. doi: 10.1007/s12328-021-01459-7
38. Omotehara S, Nishida M, Yamanashi K, Sakurai K, Katsurada T, Komatsu Y, et al. A Case of Immune Checkpoint Inhibitor-Associated Gastroenteritis Detected by Ultrasonography. J Clin Ultrasound (2021) 49(6):605–9. doi: 10.1002/jcu.22975
39. Hirabae A, Ichihara E, Sunami R, Ota M, Iwamoto Y, Maeda Y, et al. Rapid Disease Progression of Advanced Non-Small Cell Lung Cancer Five Months After Cessation of Pembrolizumab. Acta Med Okayama (2020) 74(5):423–5. doi: 10.18926/AMO/60802
40. Grover S, Bond SA, Mansour MK, Friedman S. Management of Immunotherapy Colitis: Special Considerations in the COVID-19 Era. Cancer (2020) 126(21):4630–3. doi: 10.1002/cncr.33125
41. Gallo A, Talerico R, Novello L, Giustiniani MC, D'Argento E, Bria E, et al. Collagenous Colitis and Atezolizumab Therapy: An Atypical Case. Clin J Gastroenterol (2021) 14(1):165–9. doi: 10.1007/s12328-020-01276-4
42. Yoshimura K, Saku A, Karayama M, Inui N, Sugimura H, Suda T. Leucine-Rich α(2)-Glycoprotein as a Potential Biomarker for Immune-Related Colitis After Anti-PD-L1 Therapy: A Report of a Case Series. Clin Lung Cancer (2020) 21(6):e516–22. doi: 10.1016/j.cllc.2020.04.005
43. Babacan NA, Tanvetyanon T. Superimposed Clostridium Difficile Infection During Checkpoint Inhibitor Immunotherapy-Induced Colitis. J Immunother (2019) 42(9):350–3. doi: 10.1097/CJI.0000000000000270
44. Zhou C, Klionsky Y, Treasure ME, Bruno DS. Pembrolizumab-Induced Immune-Mediated Colitis in a Patient With Concurrent Clostridium Difficile Infection. Case Rep Oncol (2019) 12(1):164–70. doi: 10.1159/000497155
45. Deligiorgi MV, Panayiotidis MI, Trafalis DT. Parathyroid Hormone Related Protein (PTHrP)-Mediated Hypercalcemia in Malignancy Associated With Anti-PD-1 Immune Checkpoint Inhibitor Treatment and Related Inflammatory Reactions. Int Immunopharmacol (2019) 77:105942. doi: 10.1016/j.intimp.2019.105942
46. Ni J, Zhang X, Zhang L. Opportunistic Bowel Infection After Corticosteroid Dosage Tapering in a Stage IV Lung Cancer Patient With Tislelizumab-Related Colitis. Thorac Cancer (2020) 11(6):1699–702. doi: 10.1111/1759-7714.13401
47. Alhatem A, Patel K, Eriksen B, Bukhari S, Liu C. Nivolumab-Induced Concomitant Severe Upper and Lower Gastrointestinal Immune-Related Adverse Effects. ACG Case Rep J (2019) 6(11):e00249. doi: 10.14309/crj.0000000000000249
48. Cancela-Díez B, Gómez-De Rueda F, Antolinos Pérez MJ, Jiménez-Morales A, López-Hidalgo JL. Acute Coronary Syndrome and Recurrent Colitis as Immune-Related Adverse Events in a Lung Cancer Patient. J Oncol Pharm Pract (2020) 26(1):252–5. doi: 10.1177/1078155219865596
49. Dhenin A, Samartzi V, Lejeune S, Seront E. Cascade of Immunologic Adverse Events Related to Pembrolizumab Treatment. BMJ Case Rep (2019) 12(6):e229149. doi: 10.1136/bcr-2018-229149
50. Beck TN, Kudinov AE, Dulaimi E, Boumber Y. Case Report: Reinitiating Pembrolizumab Treatment After Small Bowel Perforation. BMC Cancer (2019) 19:379. doi: 10.1186/s12885-019-5577-5
51. Ibraheim H, Green M, Papa S, Powell N. Topical Beclometasone Dipropionate in the Management of Immune Checkpoint Inhibitor-Induced Microscopic Colitis. BMJ Case Rep (2019) 12(4):e226481. doi: 10.1136/bcr-2018-226481
52. Yasuda Y, Urata Y, Tohnai R, Ito S, Kawa Y, Kono Y, et al. Immune-Related Colitis Induced by the Long-Term Use of Nivolumab in a Patient With Non-Small Cell Lung Cancer. Internal Med (2018) 57(9):1269–72. doi: 10.2169/internalmedicine.9230-17
53. Yamauchi R, Araki T, Mitsuyama K, Tokito T, Ishii H, Yoshioka S, et al. The Characteristics of Nivolumab-Induced Colitis: An Evaluation of Three Cases and a Literature Review. BMC Gastroenterol (2018) 18(1):135. doi: 10.1186/s12876-018-0864-1
54. Iyoda T, Kurita N, Takada A, Watanabe H, Ando M. Resolution of Infliximab-Refractory Nivolumab-Induced Acute Severe Enterocolitis After Cyclosporine Treatment in a Patient With Non-Small Cell Lung Cancer. Am J Case Rep (2018) 19:360–4. doi: 10.12659/AJCR.908570
55. Callens R, Tamsin A, van Zandweghe L. Nivolumab-Induced Fulminant Immune-Related Colitis Despite Infliximab in a Patient With NSCLC. J Thorac Oncol (2019) 14(3):e49–50. doi: 10.1016/j.jtho.2018.11.014
56. Gonzalez-Vazquez S, de-la-Riva-Onandia S, Ignacio Echeveste J, Munoz-Navas M. Immune Mediated Colitis Caused by Lung Cancer Treatment With Atezolizumab. Rev Esp Enferm Dig (2017) 109(12):863–4. doi: 10.17235/reed.2017.5060/2017
57. Takenaka T, Yamazaki K, Miura N, Harada N, Takeo S. Osimertinib Reactivated Immune-Related Colitis After Treatment With Anti-PD1 Antibody for Non-Small Cell Lung Cancer. Invest New Drugs (2017) 35(6):848–50. doi: 10.1007/s10637-017-0481-9
58. Kubo K, Kato M, Mabe K. Nivolumab-Associated Colitis Mimicking Ulcerative Colitis. Clin Gastroenterol Hepatol (2017) 15(9):XXXV–XXXVI. doi: 10.1016/j.cgh.2017.03.026
59. Durrechou Q, Domblides C, Sionneau B, Lefort F, Quivy A, Ravaud A, et al. Management of Immune Checkpoint Inhibitor Toxicities. Cancer Manage Res (2020) 12:9139–57. doi: 10.2147/CMAR.S218756
60. Wang Y, Abu-Sbeih H, Mao E, Ali N, Ali FS, Qiao W, et al. Immune-Checkpoint Inhibitor-Induced Diarrhea and Colitis in Patients With Advanced Malignancies: Retrospective Review at MD Anderson. J Immunother Cancer (2018) 6. doi: 10.1186/s40425-018-0346-6
61. Foppen MHG, Rozeman EA, van Wilpe S, Postma C, Snaebjornsson P, van Thienen JV, et al. Immune Checkpoint Inhibition-Related Colitis: Symptoms, Endoscopic Features, Histology and Response to Management. ESMO Open (2018) 3(1):e000278. doi: 10.1136/esmoopen-2017-000278
62. Hayashi Y, Hosoe N, Takabayashi K, Limpias Kamiya KJL, Tsugaru K, Shimozaki K, et al. Clinical, Endoscopic, and Pathological Characteristics of Immune Checkpoint Inhibitor-Induced Gastroenterocolitis. Dig Dis Sci (2021) 66(6):2129–34. doi: 10.1007/s10620-020-06441-w
63. Assarzadegan N, Montgomery E, Anders RA. Immune Checkpoint Inhibitor Colitis: The Flip Side of the Wonder Drugs. Virchows Arch (2018) 472(1):125–33. doi: 10.1007/s00428-017-2267-z
64. Yanai S, Nakamura S, Matsumoto T. Nivolumab-Induced Colitis Treated by Infliximab. Clin Gastroenterol Hepatol (2017) 15(4):E80–1. doi: 10.1016/j.cgh.2016.09.017
65. Gondal B, Patel P, Gallan A, Hart J, Bissonnette M. Gastrointestinal Adverse Effects of Immunomodulation: Acute Colitis With Immune Check Point Inhibitor Nivolumab. Inflamm Bowel Dis (2016) 22:S12–3. doi: 10.1097/01.MIB.0000480057.88945.e3
66. Baroudjian B, Lourenco N, Pages C, Chami I, Maillet M, Bertheau P, et al. Anti-PD1-Induced Collagenous Colitis in a Melanoma Patient. Melanoma Res (2016) 26(3):308–11. doi: 10.1097/CMR.0000000000000252
67. Weber JS, Kaehler KC, Hauschild A. Management of Immune-Related Adverse Events and Kinetics of Response With Ipilimumab. J Clin Oncol (2012) 30(21):2691–7. doi: 10.1200/JCO.2012.41.6750
68. Hillock NT, Heard S, Kichenadasse G, Hill CL, Andrews J. Infliximab for Ipilimumab-Induced Colitis: A Series of 13 Patients. Asia Pac J Clin Oncol (2017) 13(5):E284–90. doi: 10.1111/ajco.12651
69. Boutros C, Tarhini A, Routier E, Lambotte O, Ladurie FL, Carbonnel F, et al. Safety Profiles of Anti-CTLA-4 and Anti-PD-1 Antibodies Alone and in Combination. Nat Rev Clin Oncol (2016) 13(8):473–86. doi: 10.1038/nrclinonc.2016.58
70. Wang DY, Mooradian MJ, Kim D, Shah NJ, Fenton SE, Conry RM, et al. Clinical Characterization of Colitis Arising From Anti-PD-1 Based Therapy. Oncoimmunology (2019) 8(1):e1524695. doi: 10.1080/2162402X.2018.1524695
71. Haanen JBAG, Carbonnel F, Robert C, Kerr KM, Peters S, Larkin J, et al. Management of Toxicities From Immunotherapy: ESMO Clinical Practice Guidelines for Diagnosis, Treatment and Follow-Up. Ann Oncol (2017) 28(suppl_4):iv119–42. doi: 10.1093/annonc/mdx225
72. Nishida T, Iijima H, Adachi S. Immune Checkpoint Inhibitor-Induced Diarrhea/Colitis: Endoscopic and Pathologic Findings. World J Gastrointest Pathophysiol (2019) 10(2):17–28. doi: 10.4291/wjgp.v10.i2.17
73. Berman D, Parker SM, Siegel J, Chasalow SD, Weber J, Galbraith S, et al. Blockade of Cytotoxic T-Lymphocyte Antigen-4 by Ipilimumab Results in Dysregulation of Gastrointestinal Immunity in Patients With Advanced Melanoma. Cancer Immun (2010) 10:11–1.
74. Lord JD, Hackman RC, Moklebust A, Thompson JA, Higano CS, Chielens D, et al. Refractory Colitis Following Anti-CTLA4 Antibody Therapy: Analysis of Mucosal FOXP3(+) T Cells. Dig Dis Sci (2010) 55(5):1396–405. doi: 10.1007/s10620-009-0839-8
75. Liu Y-H, Zang X-Y, Wang J-C, Huang S-S, Xu J, Zhang P. Diagnosis and Management of Immune Related Adverse Events (irAEs) in Cancer Immunotherapy. Biomed Pharmacother (2019) 120:109437. doi: 10.1016/j.biopha.2019.109437
76. Barnes MJ, Griseri T, Johnson AMF, Young W, Powrie F, Izcue A. CTLA-4 Promotes Foxp3 Induction and Regulatory T Cell Accumulation in the Intestinal Lamina Propria. Mucosal Immunol (2013) 6(2):324–34. doi: 10.1038/mi.2012.75
77. Marthey L, Mateus C, Mussini C, Nachury M, Nancey S, Grange F, et al. Cancer Immunotherapy With Anti-CTLA-4 Monoclonal Antibodies Induces an Inflammatory Bowel Disease. J Crohns Colitis (2016) 10(4):395–401. doi: 10.1093/ecco-jcc/jjv227
78. Siakavellas SI, Bamias G. Checkpoint Inhibitor Colitis: A New Model of Inflammatory Bowel Disease? Curr Opin Gastroenterol (2018) 34(6):377–83. doi: 10.1097/MOG.0000000000000482
79. Bellaguarda E, Hanauer S. Checkpoint Inhibitor-Induced Colitis. Am J Gastroenterol (2020) 115(2):202–10. doi: 10.14309/ajg.0000000000000497
80. Tadano H, Torigoe T. Immune-Related Adverse Events of Immune Checkpoint Inhibitors. Nihon Rinsho Meneki Gakkai Kaishi (2017) 40(2):102–8. doi: 10.2177/jsci.40.102
81. Miller PL, Carson TL. Mechanisms and Microbial Influences on CTLA-4 and PD-1-Based Immunotherapy in the Treatment of Cancer: A Narrative Review. Gut Pathog (2020) 12(1):43. doi: 10.1186/s13099-020-00381-6
82. Sharpe AH, Abbas AK. Focus on Research: T-Cell Costimulation - Biology, Therapeutic Potential, and Challenges. N Engl J Med (2006) 355(10):973–5. doi: 10.1056/NEJMp068087
83. Nagorsen D, Scheibenbogen C, Marincola FM, Letsch A, Keilholz U. Natural T Cell Immunity Against Cancer. Clin Cancer Res (2003) 9(12):4296–303.
84. Qureshi OS, Zheng Y, Nakamura K, Attridge K, Manzotti C, Schmidt EM, et al. Trans-Endocytosis of CD80 and CD86: A Molecular Basis for the Cell-Extrinsic Function of CTLA-4. Science (2011) 332(6029):600–3. doi: 10.1126/science.1202947
85. Wing K, Onishi Y, Prieto-Martin P, Yamaguchi T, Miyara M, Fehervari Z, et al. CTLA-4 Control Over Foxp3(+) Regulatory T Cell Function. Science (2008) 322(5899):271–5. doi: 10.1126/science.1160062
86. Patsoukis N, Bardhan K, Chatterjee P, Sari D, Liu B, Bell LN, et al. PD-1 Alters T-Cell Metabolic Reprogramming by Inhibiting Glycolysis and Promoting Lipolysis and Fatty Acid Oxidation. Nat Commun (2015) 6:6692. doi: 10.1038/ncomms7692
87. Patsoukis N, Li L, Sari D, Petkova V, Boussiotis VA. PD-1 Increases PTEN Phosphatase Activity While Decreasing PTEN Protein Stability by Inhibiting Casein Kinase 2. Mol Cell Biol (2013) 33(16):3091–8. doi: 10.1128/MCB.00319-13
88. Ramos-Casals M, Brahmer JR, Callahan MK, Flores-Chavez A, Keegan N, Khamashta MA, et al. Immune-Related Adverse Events of Checkpoint Inhibitors. Nat Rev Dis Primers (2020) 6(1):31. doi: 10.1038/s41572-020-0160-6
89. Tivol EA, Borriello F, Schweitzer AN, Lynch WP, Bluestone JA, Sharpe AH. Loss of CTLA-4 Leads to Massive Lymphoproliferation and Fatal Multiorgan Tissue Destruction, Revealing a Critical Negative Regulatory Role of CTLA-4. Immunity (1995) 3(5):541–7. doi: 10.1016/1074-7613(95)90125-6
90. Klocke K, Sakaguchi S, Holmdahl R, Wing K. Induction of Autoimmune Disease by Deletion of CTLA-4 in Mice in Adulthood. Proc Natl Acad Sci U S A (2016) 113(17):E2383–92. doi: 10.1073/pnas.1603892113
91. Beck KE, Blansfield JA, Tran KQ, Feldman AL, Hughes MS, Royal RE, et al. Enterocolitis in Patients With Cancer After Antibody Blockade of Cytotoxic T-Lymphocyte-Associated Antigen 4. J Clin Oncol (2006) 24(15):2283–9. doi: 10.1200/JCO.2005.04.5716
92. Guthery SL, Heubi JE, Filipovich A. Enteral Metronidazole for the Prevention of Graft Versus Host Disease in Pediatric Marrow Transplant Recipients: Results of a Pilot Study. Bone Marrow Transplant (2004) 33(12):1235–9. doi: 10.1038/sj.bmt.1704474
93. Kaehler KC, Piel S, Livingstone E, Schilling B, Hauschild A, Schadendorf D. Update on Immunologic Therapy With Anti-CTLA-4 Antibodies in Melanoma: Identification of Clinical and Biological Response Patterns, Immune-Related Adverse Events, and Their Management. Semin Oncol (2010) 37(5):485–98. doi: 10.1053/j.seminoncol.2010.09.003
94. Wang T, Zheng N, Luo Q, Jiang L, He B, Yuan X, et al. Probiotics Lactobacillus Reuteri Abrogates Immune Checkpoint Blockade-Associated Colitis by Inhibiting Group 3 Innate Lymphoid Cells. Front Immunol (2019) 10. doi: 10.3389/fimmu.2019.01235
95. Coutzac C, Adam J, Soularue E, Collins M, Racine A, Mussini C, et al. Colon Immune-Related Adverse Events: Anti-CTLA-4 and Anti-PD-1 Blockade Induce Distinct Immunopathological Entities. J Crohns Colitis (2017) 11(10):1238–46. doi: 10.1093/ecco-jcc/jjx081
96. Yoshino K, Nakayama T, Ito A, Sato E, Kitano S. Severe Colitis After PD-1 Blockade With Nivolumab in Advanced Melanoma Patients: Potential Role of Th1-Dominant Immune Response in Immune-Related Adverse Events: Two Case Reports. BMC Cancer (2019) 19(1):1019. doi: 10.1186/s12885-019-6138-7
97. Luoma AM, Suo S, Williams HL, Sharova T, Sullivan K, Manos M, et al. Molecular Pathways of Colon Inflammation Induced by Cancer Immunotherapy. Cell (2020) 182(3):655–+. doi: 10.1016/j.cell.2020.06.001
98. Song M-Y, Hong C-P, Park SJ, Kim J-H, Yang B-G, Park Y, et al. Protective Effects of Fc-Fused PD-L1 on Two Different Animal Models of Colitis. Gut (2015) 64(2):260–71. doi: 10.1136/gutjnl-2014-307311
99. Badran YR, Cohen JV, Brastianos PK, Parikh AR, Hong TS, Dougan M. Concurrent Therapy With Immune Checkpoint Inhibitors and TNF Alpha Blockade in Patients With Gastrointestinal Immune-Related Adverse Events. J Immunother Cancer (2019) 7(1):226. doi: 10.1186/s40425-019-0711-0
100. Bamias G, Delladetsima I, Perdiki M, Siakavellas SI, Goukos D, Papatheodoridis GV, et al. Immunological Characteristics of Colitis Associated With Anti-CTLA-4 Antibody Therapy. Cancer Invest (2017) 35(7):443–55. doi: 10.1080/07357907.2017.1324032
101. Tokunaga R, Zhang W, Naseem M, Puccini A, Berger MD, Soni S, et al. CXCL9, CXCL10, CXCL11/CXCR3 Axis for Immune Activation - A Target for Novel Cancer Therapy. Cancer Treat Rev (2018) 63:40–7. doi: 10.1016/j.ctrv.2017.11.007
102. Neurath MF. Targeting Immune Cell Circuits and Trafficking in Inflammatory Bowel Disease. Nat Immunol (2019) 20(8):970–9. doi: 10.1038/s41590-019-0415-0
103. Pitt JM, Vetizou M, Waldschmitt N, Kroemer G, Chamaillard M, Boneca IG, et al. Fine-Tuning Cancer Immunotherapy: Optimizing the Gut Microbiome. Cancer Res (2016) 76(16):4602–7. doi: 10.1158/0008-5472.CAN-16-0448
104. Khan Z, Hammer C, Guardino E, Chandler GS, Albert ML. Mechanisms of Immune-Related Adverse Events Associated With Immune Checkpoint Blockade: Using Germline Genetics to Develop a Personalized Approach. Genome Med (2019) 11(1):39. doi: 10.1186/s13073-019-0652-8
105. Belkaid Y, Hand TW. Role of the Microbiota in Immunity and Inflammation. Cell (2014) 157(1):121–41. doi: 10.1016/j.cell.2014.03.011
106. Yang Y, Torchinsky MB, Gobert M, Xiong H, Xu M, Linehan JL, et al. Focused Specificity of Intestinal T(H)17 Cells Towards Commensal Bacterial Antigens. Nature (2014) 510(7503):152–+. doi: 10.1038/nature13279
107. Anderson R, Theron AJ, Rapoport BL. Immunopathogenesis of Immune Checkpoint Inhibitor-Related Adverse Events: Roles of the Intestinal Microbiome and Th17 Cells. Front Immunol (2019) 10. doi: 10.3389/fimmu.2019.02254
108. Dubin K, Callahan MK, Ren B, Khanin R, Viale A, Ling L, et al. Intestinal Microbiome Analyses Identify Melanoma Patients at Risk for Checkpoint-Blockade-Induced Colitis. Nat Commun (2016) 7:10391. doi: 10.1038/ncomms10391
109. Derosa L, Routy B, Kroemer G, Zitvogel L. The Intestinal Microbiota Determines the Clinical Efficacy of Immune Checkpoint Blockers Targeting PD-1/PD-L1. Oncoimmunology (2018) 7(6):e1434468. doi: 10.1080/2162402X.2018.1434468
110. Goubet A-G, Daillere R, Routy B, Derosa L, Roberti PM, Zitvogel L. The Impact of the Intestinal Microbiota in Therapeutic Responses Against Cancer. C R Biol (2018) 341(5):284–9. doi: 10.1016/j.crvi.2018.03.004
111. Routy B, Le Chatelier E, Derosa L, Duong CPM, Alou MT, Daillere R, et al. Gut Microbiome Influences Efficacy of PD-1-Based Immunotherapy Against Epithelial Tumors. Science (2018) 359(6371):91–+. doi: 10.1126/science.aan3706
112. Chaput N, Lepage P, Coutzac C, Soularue E, Le Roux K, Monot C, et al. Baseline Gut Microbiota Predicts Clinical Response and Colitis in Metastatic Melanoma Patients Treated With Ipilimumab. Ann Oncol (2019) 30(12):2012–2. doi: 10.1093/annonc/mdz224
113. Gopalakrishnan V, Spencer CN, Nezi L, Reuben A, Andrews MC, Karpinets TV, et al. Gut Microbiome Modulates Response to Anti-PD-1 Immunotherapy in Melanoma Patients. Science (2018) 359(6371):97–103. doi: 10.1126/science.aan4236
114. Huang JY, Lee SM, Mazmanian SK. The Human Commensal Bacteroides Fragilis Binds Intestinal Mucin. Anaerobe (2011) 17(4):137–41. doi: 10.1016/j.anaerobe.2011.05.017
115. Mazmanian SK, Round JL, Kasper DL. A Microbial Symbiosis Factor Prevents Intestinal Inflammatory Disease. Nature (2008) 453(7195):620–5. doi: 10.1038/nature07008
116. Vetizou M, Pitt JM, Daillere R, Lepage P, Waldschmitt N, Flament C, et al. Anticancer Immunotherapy by CTLA-4 Blockade Relies on the Gut Microbiota. Science (2015) 350(6264):1079–+. doi: 10.1126/science.aad1329
117. Roy S, Trinchieri G. Microbiota: A Key Orchestrator of Cancer Therapy. Nat Rev Cancer (2017) 17(5):271–+. doi: 10.1038/nrc.2017.13
118. Dasgupta S, Erturk-Hasdemir D, Ochoa-Reparaz J, Reinecker H-C, Kasper DL. Plasmacytoid Dendritic Cells Mediate Anti-Inflammatory Responses to a Gut Commensal Molecule via Both Innate and Adaptive Mechanisms. Cell Host Microbe (2014) 15(4):413–23. doi: 10.1016/j.chom.2014.03.006
119. Sivan A, Corrales L, Hubert N, Williams JB, Aquino-Michaels K, Earley ZM, et al. Commensal Bifidobacterium Promotes Antitumor Immunity and Facilitates Anti-PD-L1 Efficacy. Science (2015) 350(6264):1084–9. doi: 10.1126/science.aac4255
120. Wang F, Yin Q, Chen L, Davis MM. Bifidobacterium can Mitigate Intestinal Immunopathology in the Context of CTLA-4 Blockade. Proc Natl Acad Sci U S A (2018) 115(1):157–61. doi: 10.1073/pnas.1712901115
121. Mu Q, Tavella VJ, Luo XM. Role of Lactobacillus Reuteri in Human Health and Diseases. Front Microbiol (2018) 9. doi: 10.3389/fmicb.2018.00757
122. Ananthakrishnan AN, Higuchi LM, Huang ES, Khalili H, Richter JM, Fuchs CS, et al. Aspirin, Nonsteroidal Anti-Inflammatory Drug Use, and Risk for Crohn Disease and Ulcerative Colitis: A Cohort Study. Ann Internal Med (2012) 156(5):350–9. doi: 10.7326/0003-4819-156-5-201203060-00007
123. Grover S, Dougan M, Tyan K, Giobbie-Hurder A, Blum SM, Ishizuka J, et al. Vitamin D Intake is Associated With Decreased Risk of Immune Checkpoint Inhibitor-Induced Colitis. Cancer (2020) 126(16):3758–67. doi: 10.1002/cncr.32966
124. Tyan K, Grover S, Dougan M, Sullivan RJ, Giobbie-Hurder A, Blum S, et al. Association of Vitamin D Intake With Decreased Risk of Immune Checkpoint Inhibitor-Induced Colitis. J Clin Oncol (2020) 38(5):S89. doi: 10.1200/JCO.2020.38.5_suppl.89
125. Bowyer S, Prithviraj P, Lorigan P, Larkin J, McArthur G, Atkinson V, et al. Efficacy and Toxicity of Treatment With the Anti-CTLA-4 Antibody Ipilimumab in Patients With Metastatic Melanoma After Prior Anti-PD-1 Therapy. Br J Cancer (2016) 114(10):1084–9. doi: 10.1038/bjc.2016.107
126. Menzies AM, Johnson DB, Ramanujam S, Atkinson VG, Wong ANM, Park JJ, et al. Anti-PD-1 Therapy in Patients With Advanced Melanoma and Preexisting Autoimmune Disorders or Major Toxicity With Ipilimumab. Ann Oncol (2017) 28(2):368–76. doi: 10.1093/annonc/mdw443
127. Wolchok JD, Neyns B, Linette G, Negrier S, Lutzky J, Thomas L, et al. Ipilimumab Monotherapy in Patients With Pretreated Advanced Melanoma: A Randomised, Double-Blind, Multicentre, Phase 2, Dose-Ranging Study. Lancet Oncol (2010) 11(2):155–64. doi: 10.1016/S1470-2045(09)70334-1
128. Kumar V, Chaudhary N, Garg M, Floudas CS, Soni P, Chandra AB. Current Diagnosis and Management of Immune Related Adverse Events (irAEs) Induced by Immune Checkpoint Inhibitor Therapy. Front Pharmacol (2017) 8. doi: 10.3389/fphar.2017.00049
129. Johnson DB, Sullivan RJ, Ott PA, Carlino MS, Khushalani NI, Ye F, et al. Ipilimumab Therapy in Patients With Advanced Melanoma and Preexisting Autoimmune Disorders. JAMA Oncol (2016) 2(2):234–40. doi: 10.1001/jamaoncol.2015.4368
130. Kaehler KC, Eigentler TK, Gesierich A, Heinzerling L, Loquai C, Meier F, et al. Ipilimumab in Metastatic Melanoma Patients With Pre-Existing Autoimmune Disorders. Cancer Immunol Immunother (2018) 67(5):825–34. doi: 10.1007/s00262-018-2134-z
131. Bishay K, Tandon P, Bourassa-Blanchette S, Laurie SA, McCurdy JD. The Risk of Diarrhea and Colitis in Patients With Lung Cancer Treated With Immune Checkpoint Inhibitors: A Systematic Review and Meta-Analysis. Curr Oncol (Toronto Ont) (2020) 27(5):486–94. doi: 10.3747/co.27.6251
132. Paz-Ares L, Ciuleanu T-E, Cobo M, Schenker M, Zurawski B, Menezes J, et al. First-Line Nivolumab Plus Ipilimumab Combined With Two Cycles of Chemotherapy in Patients With Non-Small-Cell Lung Cancer (CheckMate 9LA): An International, Randomised, Open-Label, Phase 3 Trial. Lancet Oncol (2021) 22(2):198–211. doi: 10.1016/S1470-2045(20)30641-0
133. Hellmann MD, Paz-Ares L, Bernabe Caro R, Zurawski B, Kim SW, Carcereny Costa E, et al. Nivolumab Plus Ipilimumab in Advanced Non-Small-Cell Lung Cancer. N Engl J Med (2019) 381(21):2020–31. doi: 10.1056/NEJMoa1910231
134. Antonia S, Goldberg SB, Balmanoukian A, Chaft JE, Sanborn RE, Gupta A, et al. Safety and Antitumour Activity of Durvalumab Plus Tremelimumab in Non-Small-Cell Lung Cancer: A Multicentre, Phase 1b Study. Lancet Oncol (2016) 17(3):299–308. doi: 10.1016/S1470-2045(15)00544-6
135. Rocco D, Gravara LD, Gridelli C. The New Immunotherapy Combinations in the Treatment of Advanced Non-Small Cell Lung Cancer: Reality and Perspectives Open Access Plus. Curr Clin Pharmacol (2020) 15(1):11–9. doi: 10.2174/1574884714666190809124555
136. Weber JS, Gibney G, Sullivan RJ, Sosman JA, Slingluff CL Jr., Lawrence DP, et al. Sequential Administration of Nivolumab and Ipilimumab With a Planned Switch in Patients With Advanced Melanoma (CheckMate 064): An Open-Label, Randomised, Phase 2 Trial. Lancet Oncol (2016) 17(7):943–55. doi: 10.1016/S1470-2045(16)30126-7
137. Sznol M, Ferrucci PF, Hogg D, Atkins MB, Wolter P, Guidoboni M, et al. Pooled Analysis Safety Profile of Nivolumab and Ipilimumab Combination Therapy in Patients With Advanced Melanoma. J Clin Oncol (2017) 35(34):3815–+. doi: 10.1200/JCO.2016.72.1167
138. Geng Y, Zhang Q, Feng S, Li C, Wang L, Zhao X, et al. Safety and Efficacy of Combination Therapy Using Programmed Cell Death Protein-1/Programmed Cell Death Ligand-1 Inhibitors and Radiotherapy in Patients With Non-Small-Cell Lung Cancer: A Systematic Review and Meta-Analysis. Cancer Med (2021) 10(4):1222–39. doi: 10.1002/cam4.3718
139. Wang M, Liang H, Wang W, Zhao S, Cai X, Zhao Y, et al. Immune-Related Adverse Events of a PD-(L)1 Inhibitor Plus Chemotherapy Versus a PD-(L)1 Inhibitor Alone in First-Line Treatment for Advanced Non-Small Cell Lung Cancer: A Meta-Analysis of Randomized Control Trials. Cancer (2021) 127(5):777–86. doi: 10.1002/cncr.33270
140. Fujisawa Y, Yoshino K, Otsuka A, Funakoshi T, Fujimura T, Yamamoto Y, et al. Fluctuations in Routine Blood Count Might Signal Severe Immune-Related Adverse Events in Melanoma Patients Treated With Nivolumab. J Dermatol Sci (2017) 88(2):225–31. doi: 10.1016/j.jdermsci.2017.07.007
141. Manson G, Norwood J, Marabelle A, Kohrt H, Houot R. Biomarkers Associated With Checkpoint Inhibitors. Ann Oncol (2016) 27(7):1199–206. doi: 10.1093/annonc/mdw181
142. Tarhini AA, Zahoor H, Lin Y, Malhotra U, Sander C, Butterfield LH, et al. Baseline Circulating IL-17 Predicts Toxicity While TGF-Beta 1 and IL-10 are Prognostic of Relapse in Ipilimumab Neoadjuvant Therapy of Melanoma. J Immunother Cancer (2015) 3:39. doi: 10.1186/s40425-015-0081-1
143. Tyan K, Baginska J, Brainard M, Giobbie-Hurder A, Severgnini M, Manos M, et al. Cytokine Changes During Immune-Related Adverse Events and Corticosteroid Treatment in Melanoma Patients Receiving Immune Checkpoint Inhibitors. Cancer Immunol Immunother (2021) 70(8):2209–21. doi: 10.1007/s00262-021-02855-1
144. Meshkibaf S, Martins AJ, Henry GT, Kim SO. Protective Role of G-CSF in Dextran Sulfate Sodium-Induced Acute Colitis Through Generating Gut-Homing Macrophages. Cytokine (2016) 78:69–78. doi: 10.1016/j.cyto.2015.11.025
145. Shahabi V, Berman D, Chasalow SD, Wang L, Tsuchihashi Z, Hu B, et al. Gene Expression Profiling of Whole Blood in Ipilimumab-Treated Patients for Identification of Potential Biomarkers of Immune-Related Gastrointestinal Adverse Events. J Trans Med (2013) 11:75. doi: 10.1186/1479-5876-11-75
146. Di Giacomo AM, Biagioli M, Maio M. The Emerging Toxicity Profiles of Anti-CTLA-4 Antibodies Across Clinical Indications. Semin Oncol (2010) 37(5):499–507. doi: 10.1053/j.seminoncol.2010.09.007
147. NCI Common Terminology Criteria for Adverse Events (CTCAE) Data Files . Available at: https://ctep.cancer.gov/protocolDevelopment/electronic_applications/ctc.htm#ctc_50.
148. Martins F, Sofiya L, Sykiotis GP, Lamine F, Maillard M, Fraga M, et al. Adverse Effects of Immune-Checkpoint Inhibitors: Epidemiology, Management and Surveillance. Nat Rev Clin Oncol (2019) 16(9):563–80. doi: 10.1038/s41571-019-0218-0
149. Garcia-Neuer M, Marmarelis ME, Jangi SR, Luke JJ, Ibrahim N, Davis M, et al. Diagnostic Comparison of CT Scans and Colonoscopy for Immune-Related Colitis in Ipilimumab-Treated Advanced Melanoma Patients. Cancer Immunol Res (2017) 5(4):286–91. doi: 10.1158/2326-6066.CIR-16-0302
150. Barina AR, Bashir MR, Howard BA, Hanks BA, Salama AK, Jaffe TA. Isolated Recto-Sigmoid Colitis: A New Imaging Pattern of Ipilimumab-Associated Colitis. Abdom Radiol (2016) 41(2):207–14. doi: 10.1007/s00261-015-0560-3
151. Gupta A, De Felice KM, Loftus EV Jr., Khanna S. Systematic Review: Colitis Associated With Anti-CTLA-4 Therapy. Aliment Pharmacol Ther (2015) 42(4):406–17. doi: 10.1111/apt.13281
152. Mekki A, Dercle L, Lichtenstein P, Marabelle A, Michot J-M, Lambotte O, et al. Detection of Immune-Related Adverse Events by Medical Imaging in Patients Treated With Anti-Programmed Cell Death 1. Eur J Cancer (2018) 96:91–104. doi: 10.1016/j.ejca.2018.03.006
153. Tirumani SH, Ramaiya NH, Keraliya A, Bailey ND, Ott PA, Hodi FS, et al. Radiographic Profiling of Immune-Related Adverse Events in Advanced Melanoma Patients Treated With Ipilimumab. Cancer Immunol Res (2015) 3(10):1185–92. doi: 10.1158/2326-6066.CIR-15-0102
154. Kim KW, Ramaiya NH, Krajewski KM, Shinagare AB, Howard SA, Jagannathan JP, et al. Ipilimumab-Associated Colitis: CT Findings. Am J Roentgenol (2013) 200(5):W468–74. doi: 10.2214/AJR.12.9751
155. Mitchell KA, Kluger H, Sznol M, Hartman DJ. Ipilimumab-Induced Perforating Colitis. J Clin Gastroenterol (2013) 47(9):781–5. doi: 10.1097/MCG.0b013e31828f1d51
156. Abolhassani A-R, Schuler G, Kirchberger MC, Heinzerling L. C-Reactive Protein as an Early Marker of Immune-Related Adverse Events. J Cancer Res Clin Oncol (2019) 145(10):2625–31. doi: 10.1007/s00432-019-03002-1
157. Schindler K, Harmankaya K, Kuk D, Mangana J, Michielin O, Hoeller C, et al. Correlation of Absolute and Relative Eosinophil Counts With Immune-Related Adverse Events in Melanoma Patients Treated With Ipilimumab. J Clin Oncol (2014) 32(15):S9096. doi: 10.1200/jco.2014.32.15_suppl.9096
158. Nakamura Y, Tanaka R, Maruyama H, Ishitsuka Y, Okiyama N, Watanabe R, et al. Correlation Between Blood Cell Count and Outcome of Melanoma Patients Treated With Anti-PD-1 Antibodies. Jpn J Clin Oncol (2019) 49(5):431–7. doi: 10.1093/jjco/hyy201
159. Zou F, Wang X, Glitza IC, McQuade JL, Wang J, Zhang HC, et al. Fecal Calprotectin Concentration to Assess Endoscopic and Histologic Remission in Patients With Cancer With Immune-Mediated Diarrhea and Colitis. J Immunother Cancer (2021) 9(1):1. doi: 10.1136/jitc-2020-002058
160. Wright AP, Piper MS, Bishu S, Stidham RW. Systematic Review and Case Series: Flexible Sigmoidoscopy Identifies Most Cases of Checkpoint Inhibitor-Induced Colitis. Aliment Pharmacol Ther (2019) 49(12):1474–83. doi: 10.1111/apt.15263
161. Herlihy JD, Beasley S, Simmelink A, Maddukuri V, Amin A, Kamionek M, et al. Flexible Sigmoidoscopy Rather Than Colonoscopy Is Adequate for the Diagnosis of Ipilimumab-Associated Colitis. South Med J (2019) 112(3):154–8. doi: 10.14423/SMJ.0000000000000944
162. Dougan M. Checkpoint Blockade Toxicity and Immune Homeostasis in the Gastrointestinal Tract. Front Immunol (2017) 8. doi: 10.3389/fimmu.2017.01547
163. van der Heide H, van den Brandt-Gradel V, Tytgat GN, Endert E, Wiltink EH, Schipper ME, et al. Comparison of Beclomethasone Dipropionate and Prednisolone 21-Phosphate Enemas in the Treatment of Ulcerative Proctitis. J Clin Gastroenterol (1988) 10(2):169–72. doi: 10.1097/00004836-198804000-00013
164. Verschuren EC, van den Eertwegh AJ, Wonders J, Slangen RM, van Delft F, van Bodegraven A, et al. Clinical, Endoscopic, and Histologic Characteristics of Ipilimumab-Associated Colitis. Clin Gastroenterol Hepatol (2016) 14(6):836–42. doi: 10.1016/j.cgh.2015.12.028
165. Hughes MS, Molina GE, Chen ST, Zheng H, Deshpande V, Fadden R, et al. Budesonide Treatment for Microscopic Colitis From Immune Checkpoint Inhibitors. J Immunother Cancer (2019) 7(1):292. doi: 10.1186/s40425-019-0756-0
166. Abu-Sbeih H, Ali FS, Luo W, Qiao W, Raju GS, Wang Y. Importance of Endoscopic and Histological Evaluation in the Management of Immune Checkpoint Inhibitor-Induced Colitis. J Immunother Cancer (2018) 6(1):95. doi: 10.1186/s40425-018-0411-1
167. Jain A, Lipson EJ, Sharfman WH, Brant SR, Lazarev MG. Colonic Ulcerations may Predict Steroid-Refractory Course in Patients With Ipilimumab-Mediated Enterocolitis. World J Gastroenterol (2017) 23(11):2023–8. doi: 10.3748/wjg.v23.i11.2023
168. Patil PA, Zhang X. Pathologic Manifestations of Gastrointestinal and Hepatobiliary Injury in Immune Checkpoint Inhibitor Therapy. Arch Pathol Lab Med (2021) 145(5):571–82. doi: 10.5858/arpa.2020-0070-RA
169. Zhang ML, Neyaz A, Patil D, Chen J, Dougan M, Deshpande V. Immune-Related Adverse Events in the Gastrointestinal Tract: Diagnostic Utility of Upper Gastrointestinal Biopsies. Histopathology (2020) 76(2):233–43. doi: 10.1111/his.13963
170. Shivaj UN, Jeffery L, Gui X, Smith SCL, Ahmad OF, Akbar A, et al. Immune Checkpoint Inhibitor-Associated Gastrointestinal and Hepatic Adverse Events and Their Management. Ther Adv Gastroenterol (2019) 12:1756284819884196. doi: 10.1177/1756284819884196
171. Karamchandani DM, Chetty R. Immune Checkpoint Inhibitor-Induced Gastrointestinal and Hepatic Injury: Pathologists' Perspective. J Clin Pathol (2018) 71(8):665–71. doi: 10.1136/jclinpath-2018-205143
172. Chen JH, Pezhouh MK, Lauwers GY, Masia R. Histopathologic Features of Colitis Due to Immunotherapy With Anti-PD-1 Antibodies. Am J Surg Pathol (2017) 41(5):643–54. doi: 10.1097/PAS.0000000000000829
173. Star KV, Ho VT, Wang HH, Odze RD. Histologic Features in Colon Biopsies Can Discriminate Mycophenolate From GVHD-Induced Colitis. Am J Surg Pathol (2013) 37(9):1319–28. doi: 10.1097/PAS.0b013e31829ab1ef
174. Sakellariou S, Zouki DN, Ziogas DC, Pouloudi D, Gogas H, Delladetsima I. Granulomatous Colitis in a Patient With Metastatic Melanoma Under Immunotherapy: A Case Report and Literature Review. BMC Gastroenterol (2021) 21(1):227. doi: 10.1186/s12876-021-01812-7
175. Bavi P, Butler M, Serra S, Chetty R. Immune Modulator-Induced Changes in the Gastrointestinal Tract. Histopathology (2017) 71(3):494–6. doi: 10.1111/his.13224
176. Garcia-Varona A, Odze RD, Makrauer F. Lymphocytic Colitis Secondary to Ipilimumab Treatment. Inflamm Bowel Dis (2013) 19(2):E15–6. doi: 10.1002/ibd.22846
177. St Romain PE, Salama AKS, Ferguson NL, Burbridge RA. Ipilimumab-Associated Lymphocytic Colitis: A Case Report. Trans Gastrointest Cancer (2016) 5(1):44–6. doi: 10.3978/j.issn.2224-4778
178. Ayata G, Ithamukkala S, Sapp H, Shaz BH, Brien TP, Wang HH, et al. Prevalence and Significance of Inflammatory Bowel Disease-Like Morphologic Features in Collagenous and Lymphocytic Colitis. Am J Surg Pathol (2002) 26(11):1414–23. doi: 10.1097/00000478-200211000-00003
179. Choi K, Abu-Sbeih H, Samdani R, Gonzalez GN, Raju GS, Richards DM, et al. Can Immune Checkpoint Inhibitors Induce Microscopic Colitis or a Brand New Entity? Inflamm Bowel Dis (2019) 25(2):385–93. doi: 10.1093/ibd/izy240
180. Du-Thanh A, Pallure V, Girard C, Dereure O, Guillot B. Clostridium Difficile Infection may Loom Behind Ipilimumab-Induced Auto-Immune Colitis. Eur J Dermatol (2015) 25(4):344–4. doi: 10.1684/ejd.2015.2561
181. Ledezma B, Binder S, Hamid O. Atypical Clinical Response Patterns to Ipilimumab: Four Case Studies of Advanced Melanoma. Clin J Oncol Nurs (2011) 15(4):393–403. doi: 10.1188/11.CJON.393-403
182. Adler BL, Pezhouh MK, Kim A, Luan L, Zhu Q, Gani F, et al. Histopathological and Immunophenotypic Features of Ipilimumab-Associated Colitis Compared to Ulcerative Colitis. J Internal Med (2018) 283(6):568–77. doi: 10.1111/joim.12744
183. Brahmer JR, Lacchetti C, Schneider BJ, Atkins MB, Brassil KJ, Caterino JM, et al. Management of Immune-Related Adverse Events in Patients Treated With Immune Checkpoint Inhibitor Therapy: American Society of Clinical Oncology Clinical Practice Guideline. J Clin Oncol (2018) 36(17):1714–+. doi: 10.1200/JCO.2017.77.6385
184. Friedman CF, Proverbs-Singh TA, Postow MA. Treatment of the Immune-Related Adverse Effects of Immune Checkpoint Inhibitors: A Review. JAMA Oncol (2016) 2(10):1346–53. doi: 10.1001/jamaoncol.2016.1051
185. Collins M, Michot JM, Danlos FX, Mussini C, Soularue E, Mateus C, et al. Inflammatory Gastrointestinal Diseases Associated With PD-1 Blockade Antibodies. Ann Oncol (2017) 28(11):2860–5. doi: 10.1093/annonc/mdx403
186. Thompson JA, Schneider BJ, Brahmer J, Andrews S, Armand P, Bhatia S, et al. Management of Immunotherapy-Related Toxicities. Version 1.2019. J Natl Compr Cancer Netw (2019) 17(3):255–88. doi: 10.6004/jnccn.2019.0013
187. Grover S, Rahma OE, Hashemi N, Lim RM. Gastrointestinal and Hepatic Toxicities of Checkpoint Inhibitors: Algorithms for Management. Am Soc Clin Oncol Educ Book (2018) 38:13–9. doi: 10.1200/EDBK_100013
188. Thompson LL, Katznelson E, Leet DE, Durbin SM, Yoon J, Reynolds KL, et al. Impact of Systemic Corticosteroids on Survival Outcomes in Immune Checkpoint Inhibitor-Induced Gastroenterocolitis. Eur J Cancer (Oxford Engl 1990) (2021) 142:143–6. doi: 10.1016/j.ejca.2020.09.022
189. Faje AT, Lawrence D, Flaherty K, Freedman C, Fadden R, Rubin K, et al. High-Dose Glucocorticoids for the Treatment of Ipilimumab-Induced Hypophysitis is Associated With Reduced Survival in Patients With Melanoma. Cancer (2018) 124(18):3706–14. doi: 10.1002/cncr.31629
190. Horvat TZ, Adel NG, Thu-Oanh D, Momtaz P, Postow MA, Callahan MK, et al. Immune-Related Adverse Events, Need for Systemic Immunosuppression, and Effects on Survival and Time to Treatment Failure in Patients With Melanoma Treated With Ipilimumab at Memorial Sloan Kettering Cancer Center. J Clin Oncol (2015) 33(28):3193–+. doi: 10.1200/JCO.2015.60.8448
191. Favara DM, Spain L, Au L, Clark J, Daniels E, Diem S, et al. Five-Year Review of Corticosteroid Duration and Complications in the Management of Immune Checkpoint Inhibitor-Related Diarrhoea and Colitis in Advanced Melanoma. ESMO Open (2020) 5(4):e000585. doi: 10.1136/esmoopen-2019-000585
192. Baden LR, Swaminathan S, Angarone M, Blouin G, Camins BC, Casper C, et al. Prevention and Treatment of Cancer-Related Infections, Version 2.2016. J Natl Compr Cancer Netw (2016) 14(7):882–913. doi: 10.6004/jnccn.2016.0093
193. Pernot S, Ramtohul T, Taieb J. Checkpoint Inhibitors and Gastrointestinal Immune-Related Adverse Events. Curr Opin Oncol (2016) 28(4):264–8. doi: 10.1097/CCO.0000000000000292
194. Kähler KC, Hassel JC, Heinzerling L, Loquai C, Mössner R, Ugurel S, et al. Management of Side Effects of Immune Checkpoint Blockade by Anti-CTLA-4 and Anti-PD-1 Antibodies in Metastatic Melanoma. J Dtsch Dermatol Ges (2016) 14(7):662–81. doi: 10.1111/ddg.13047
195. Abu-Sbeih H, Ali FS, Naqash AR, Owen DH, Patel S, Otterson GA, et al. Resumption of Immune Checkpoint Inhibitor Therapy After Immune-Mediated Colitis. J Clin Oncol (2019) 37(30):2738–45. doi: 10.1200/JCO.19.00320
196. Lo YC, Price C, Blenman K, Patil P, Zhang X, Robert ME. Checkpoint Inhibitor Colitis Shows Drug-Specific Differences in Immune Cell Reaction That Overlap With Inflammatory Bowel Disease and Predict Response to Colitis Therapy. Am J Clin Pathol (2021) 156(2):214–28. doi: 10.1093/ajcp/aqaa217
197. Johnson DH, Zobniw CM, Trinh VA, Ma J, Bassett RL Jr., Abdel-Wahab N, et al. Infliximab Associated With Faster Symptom Resolution Compared With Corticosteroids Alone for the Management of Immune-Related Enterocolitis. J Immunother Cancer (2018) 6(1):103. doi: 10.1186/s40425-018-0412-0
198. Johnston RL, Lutzky J, Chodhry A, Barkin JS. Cytotoxic T-Lymphocyte-Associated Antigen 4 Antibody-Induced Colitis and its Management With Infliximab. Dig Dis Sci (2009) 54(11):2538–40. doi: 10.1007/s10620-008-0641-z
199. Zhang HC, Luo W, Wang Y. Acute Liver Injury in the Context of Immune Checkpoint Inhibitor-Related Colitis Treated With Infliximab. J Immunother Cancer (2019) 7(1):47. doi: 10.1186/s40425-019-0532-1
200. Robert C, Long GV, Brady B, Dutriaux C, Maio M, Mortier L, et al. Nivolumab in Previously Untreated Melanoma Without BRAF Mutation. N Engl J Med (2015) 372(4):320–30. doi: 10.1056/NEJMoa1412082
201. Rastogi P, Sultan M, Charabaty AJ, Atkins MB, Mattar MC. Ipilimumab Associated Colitis: An IpiColitis Case Series at MedStar Georgetown University Hospital. World J Gastroenterol (2015) 21(14):4373–8. doi: 10.3748/wjg.v21.i14.4373
202. Cohn HM, Dave M, Loftus EV Jr. Understanding the Cautions and Contraindications of Immunomodulator and Biologic Therapies for Use in Inflammatory Bowel Disease. Inflamm Bowel Dis (2017) 23(8):301–15. doi: 10.1097/MIB.0000000000001199
203. Martins F, Sykiotis GP, Maillard M, Fraga M, Ribi C, Kuntzer T, et al. New Therapeutic Perspectives to Manage Refractory Immune Checkpoint-Related Toxicities. Lancet Oncol (2019) 20(1):e54–64. doi: 10.1016/S1470-2045(18)30828-3
204. Onuki T, Morita E, Sakamoto N, Nagai Y, Sata M, Hagiwara K. Severe Upper Gastrointestinal Disorders in Pembrolizumab-Treated Non-Small Cell Lung Cancer Patient. Respirol Case Rep (2018) 6(6):e00334. doi: 10.1002/rcr2.334
205. Abu-Sbeih H, Ali FS, Alsaadi D, Jennings J, Luo W, Gong Z, et al. Outcomes of Vedolizumab Therapy in Patients With Immune Checkpoint Inhibitor-Induced Colitis: A Multi-Center Study. J Immunother Cancer (2018) 6(1):142. doi: 10.1186/s40425-018-0461-4
206. Bergqvist V, Hertervig E, Gedeon P, Kopljar M, Griph H, Kinhult S, et al. Vedolizumab Treatment for Immune Checkpoint Inhibitor-Induced Enterocolitis. Cancer Immunol Immunother (2017) 66(5):581–92. doi: 10.1007/s00262-017-1962-6
207. Hsieh AH, Ferman M, Brown MP, Andrews JM. Vedolizumab: A Novel Treatment for Ipilimumab-Induced Colitis. BMJ Case Rep (2016) 2016:bcr2016216641. doi: 10.1136/bcr-2016-216641
208. Abu-Sbeih H, Ali FS, Wang X, Mallepally N, Chen E, Altan M, et al. Early Introduction of Selective Immunosuppressive Therapy Associated With Favorable Clinical Outcomes in Patients With Immune Checkpoint Inhibitor-Induced Colitis. J Immunother Cancer (2019) 7(1):93. doi: 10.1186/s40425-019-0577-1
209. Thomas AS, Ma W, Wang Y. Ustekinumab for Refractory Colitis Associated With Immune Checkpoint Inhibitors. N Engl J Med (2021) 384(6):581–3. doi: 10.1056/NEJMc2031717
210. Mir R, Shaw HM, Nathan PD. Mycophenolate Mofetil Alongside High-Dose Corticosteroids: Optimizing the Management of Combination Immune Checkpoint Inhibitor-Induced Colitis. Melanoma Res (2019) 29(1):102–6. doi: 10.1097/CMR.0000000000000543
211. Alcantar D, Al-Jaashaami L, Giron F. A Case of Immune Checkpoint Inhibitor Refractory Colitis Treated With Mycophenolate and High-Dose Steroids. Cureus (2019) 11(12):e6392. doi: 10.7759/cureus.6392
212. Bishu S, Melia J, Sharfman W, Lao CD, Fecher LA, Higgins PDR. Efficacy and Outcome of Tofacitinib in Immune Checkpoint Inhibitor Colitis. Gastroenterology (2021) 160(3):932–4.e933. doi: 10.1053/j.gastro.2020.10.029
213. Wang Y, Wiesnoski DH, Helmink BA, Gopalakrishnan V, Choi K, DuPont HL, et al. Fecal Microbiota Transplantation for Refractory Immune Checkpoint Inhibitor-Associated Colitis. Nat Med (2018) 24(12):1804–8. doi: 10.1038/s41591-018-0238-9
214. Fasanello MK, Robillard KT, Boland PM, Bain AJ, Kanehira K. Use of Fecal Microbial Transplantation for Immune Checkpoint Inhibitor Colitis. ACG Case Rep J (2020) 7(4):e00360. doi: 10.14309/crj.0000000000000360
215. Smith FO, Goff SL, Klapper JA, Levy C, Allen T, Mavroukakis SA, et al. Risk of Bowel Perforation in Patients Receiving Interleukin-2 After Therapy With Anti-CTLA 4 Monoclonal Antibody. J Immunother (2007) 30(1):130–0. doi: 10.1097/01.cji.0000211334.06762.89
216. Abu-Sbeih H, Ali FS, Qiao W, Lu Y, Patel S, Diab A, et al. Immune Checkpoint Inhibitor-Induced Colitis as a Predictor of Survival in Metastatic Melanoma. Cancer Immunol Immunother (2019) 68(4):553–61. doi: 10.1007/s00262-019-02303-1
217. Molina GE, Allen IM, Hughes MS, Zubiri L, Lee H, Mooradian MJ, et al. Prognostic Implications of Co-Occurring Dermatologic and Gastrointestinal Toxicity From Immune Checkpoint Inhibition Therapy for Advanced Malignancies: A Retrospective Cohort Study. J Am Acad Dermatol (2020) 82(3):743–6. doi: 10.1016/j.jaad.2019.07.049
218. Ezponda Casajus A, Calvo Imirizaldu M, de Torres Tajes JP, Garcia-Baizan A, Castanon Alvarez E, Cano Rafart D, et al. Immune-Related Adverse Events as Predictors of Response in Cancer Patients Undergoing Immunotherapy. Radiologia (2020) 62(2):131–8. doi: 10.1016/j.rxeng.2019.10.006
219. Masuda K, Shoji H, Nagashima K, Yamamoto S, Ishikawa M, Imazeki H, et al. Correlation Between Immune-Related Adverse Events and Prognosis in Patients With Gastric Cancer Treated With Nivolumab. BMC Cancer (2019) 19(1):974. doi: 10.1186/s12885-019-6150-y
220. Pollack MH, Betof A, Dearden H, Rapazzo K, Valentine I, Brohl AS, et al. Safety of Resuming Anti-PD-1 in Patients With Immune-Related Adverse Events (irAEs) During Combined Anti-CTLA-4 and Anti-PD1 in Metastatic Melanoma. Ann Oncol (2018) 29(1):250–5. doi: 10.1093/annonc/mdx642
221. Freeman-Keller M, Kim Y, Cronin H, Richards A, Gibney G, Weber JS. Nivolumab in Resected and Unresectable Metastatic Melanoma: Characteristics of Immune-Related Adverse Events and Association With Outcomes. Clin Cancer Res (2016) 22(4):886–94. doi: 10.1158/1078-0432.CCR-15-1136
222. Weber JS, Hodi FS, Wolchok JD, Topalian SL, Schadendorf D, Larkin J, et al. Safety Profile of Nivolumab Monotherapy: A Pooled Analysis of Patients With Advanced Melanoma. J Clin Oncol (2017) 35(7):785–+. doi: 10.1200/JCO.2015.66.1389
223. Lauwyck J, Beckwee A, Santens A, Schwarze JK, Awada G, Vandersleyen V, et al. C-Reactive Protein as a Biomarker for Immune-Related Adverse Events in Melanoma Patients Treated With Immune Checkpoint Inhibitors in the Adjuvant Setting. Melanoma Res (2021) 31(4):371–7. doi: 10.1097/CMR.0000000000000748
224. Abu-Sbeih H, Herrera LN, Tang T, Altan M, Chaftari AP, Okhuysen PC, et al. Impact of Antibiotic Therapy on the Development and Response to Treatment of Immune Checkpoint Inhibitor-Mediated Diarrhea and Colitis. J Immunother Cancer (2019) 7(1):242. doi: 10.1186/s40425-019-0714-x
225. Simonaggio A, Michot JM, Voisin AL, Le Pavec J, Collins M, Lallart A, et al. Evaluation of Readministration of Immune Checkpoint Inhibitors After Immune-Related Adverse Events in Patients With Cancer. JAMA Oncol (2019) 5(9):1310–7. doi: 10.1001/jamaoncol.2019.1022
226. Haanen J, Ernstoff M, Wang Y, Menzies A, Puzanov I, Grivas P, et al. Rechallenge Patients With Immune Checkpoint Inhibitors Following Severe Immune-Related Adverse Events: Review of the Literature and Suggested Prophylactic Strategy. J Immunother Cancer (2020) 8(1):e000604. doi: 10.1136/jitc-2020-000604
227. Dolladille C, Ederhy S, Sassier M, Cautela J, Thuny F, Cohen AA, et al. Immune Checkpoint Inhibitor Rechallenge After Immune-Related Adverse Events in Patients With Cancer. JAMA Oncol (2020) 6(6):865–71. doi: 10.1001/jamaoncol.2020.0726
228. Park R, Lopes L, Saeed A. Outcomes Following Immunotherapy Re-Challenge After Immune-Related Adverse Event: Systematic Review and Meta-Analysis. Immunotherapy (2020) 12(16):1183–93. doi: 10.2217/imt-2020-0103
229. Abou Alaiwi S, Xie W, Nassar AH, Dudani S, Martini D, Bakouny Z, et al. Safety and Efficacy of Restarting Immune Checkpoint Inhibitors After Clinically Significant Immune-Related Adverse Events in Metastatic Renal Cell Carcinoma. J Immunother Cancer (2020) 8(1):e000144. doi: 10.1136/jitc-2019-000144
230. Kitagawa S, Hakozaki T, Kitadai R, Hosomi Y. Switching Administration of Anti-PD-1 and Anti-PD-L1 Antibodies as Immune Checkpoint Inhibitor Rechallenge in Individuals With Advanced Non-Small Cell Lung Cancer: Case Series and Literature Review. Thorac Cancer (2020) 11(7):1927–33. doi: 10.1111/1759-7714.13483
231. Gobbini E, Toffart AC, Perol M, Assie J-B, Duruisseaux M, Coupez D, et al. Immune Checkpoint Inhibitors Rechallenge Efficacy in Non-Small-Cell Lung Cancer Patients. Clin Lung Cancer (2020) 21(5):E497–510. doi: 10.1016/j.cllc.2020.04.013
232. Kartolo A, Holstead R, Khalid S, Emack J, Hopman W, Baetz T. Safety of Immunotherapy Rechallenge After Immune-Related Adverse Events in Patients With Advanced Cancer. J Immunother (2021) 44(1):41–8. doi: 10.1097/CJI.0000000000000337
233. Allouchery M, Lombard T, Martin M, Rouby F, Sassier M, Bertin C, et al. Safety of Immune Checkpoint Inhibitor Rechallenge After Discontinuation for Grade >= 2 Immune-Related Adverse Events in Patients With Cancer. J Immunother Cancer (2020) 8(2):1. doi: 10.1136/jitc-2020-001622
234. Santini FC, Rizvi H, Plodkowski AJ, Ni A, Lacouture ME, Gambarin-Gelwan M, et al. Et Al: Safety and Efficacy of Re-Treating With Immunotherapy After Immune-Related Adverse Events in Patients With NSCLC. Cancer Immunol Res (2018) 6(9):1093–9. doi: 10.1158/2326-6066.CIR-17-0755
235. Levra MG, Cotte F-E, Corre R, Calvet C, Gaudin A-F, Penrod JR, et al. Immunotherapy Rechallenge After Nivolumab Treatment in Advanced Non Small Cell Lung Cancer in the Real-World Setting: A National Data Base Analysis. Lung Cancer (Amsterdam Netherlands) (2020) 140:99–106. doi: 10.1016/j.lungcan.2019.12.017
236. Ito K, Oguri T, Takeda N, Fukumitsu K, Fukuda S, Kanemitsu Y, et al. A Case of Non-Small Cell Lung Cancer With Long-Term Response After Re-Challenge With Nivolumab. Respir Med Case Rep (2020) 29:100979. doi: 10.1016/j.rmcr.2019.100979
237. Kaehler KC, Hassel JC, Heinzerling L, Loquai C, Moessner R, Ugurel S, et al. Management of Side Effects of Immune Checkpoint Blockade by Anti-CTLA-4 and Anti-PD-1 Antibodies in Metastatic Melanoma. J Dtsch Dermatol Ges (2016) 14(7):662–81. doi: 10.1111/ddg.13047
238. Bridge JA, Lee JC, Daud A, Wells JW, Bluestone JA. Cytokines, Chemokines, and Other Biomarkers of Response for Checkpoint Inhibitor Therapy in Skin Cancer. Front Med (2018) 5. doi: 10.3389/fmed.2018.00351
239. Khan S, Khan SA, Luo X, Fattah FJ, Saltarski J, Gloria-McCutchen Y, et al. Immune Dysregulation in Cancer Patients Developing Immune-Related Adverse Events. Br J Cancer (2019) 120(1):63–8. doi: 10.1038/s41416-018-0155-1
240. Head L, Gorden N, Van Gulick R, Amato CM, Frazer-Abel A, Robinson W, et al. Biomarkers to Predict Immune-Related Adverse Events With Checkpoint Inhibitors. J Clin Oncol (2019) 37(8):S131. doi: 10.1200/JCO.2019.37.8_suppl.131
241. Lim SY, Lee JH, Gide TN, Menzies AM, Guminski A, Carlino MS, et al. Circulating Cytokines Predict Immune-Related Toxicity in Melanoma Patients Receiving Anti-PD-1-Based Immunotherapy. Clin Cancer Res (2019) 25(5):1557–63. doi: 10.1158/1078-0432.CCR-18-2795
242. Scott SC, Pennell NA. Early Use of Systemic Corticosteroids in Patients With Advanced NSCLC Treated With Nivolumab. J Thorac Oncol (2018) 13(11):1771–5. doi: 10.1016/j.jtho.2018.06.004
243. Fuca G, Galli G, Poggi M, Lo Russo G, Proto C, Imbimbo M, et al. Modulation of Peripheral Blood Immune Cells by Early Use of Steroids and its Association With Clinical Outcomes in Patients With Metastatic Non-Small Cell Lung Cancer Treated With Immune Checkpoint Inhibitors. ESMO Open (2019) 4(1):e000457. doi: 10.1136/esmoopen-2018-000457
244. Jove M, Vilarino N, Nadal E. Impact of Baseline Steroids on Efficacy of Programmed Cell Death-1 (PD-1) and Programmed Death-Ligand 1 (PD-L1) Blockade in Patients With Advanced Non-Small Cell Lung Cancer. Trans Lung Cancer Res (2019) 8:S364–8. doi: 10.21037/tlcr.2019.06.06
245. Gaucher L, Adda L, Sejourne A, Joachim C, Chaby G, Poulet C, et al. Impact of the Corticosteroid Indication and Administration Route on Overall Survival and the Tumor Response After Immune Checkpoint Inhibitor Initiation. Ther Adv Med Oncol (2021) 13:1758835921996656. doi: 10.1177/1758835921996656
Keywords: immune checkpoint inhibitor, colitis, diarrhoea, mechanism, diagnosis, management, prognosis
Citation: Tang L, Wang J, Lin N, Zhou Y, He W, Liu J and Ma X (2021) Immune Checkpoint Inhibitor-Associated Colitis: From Mechanism to Management. Front. Immunol. 12:800879. doi: 10.3389/fimmu.2021.800879
Received: 24 October 2021; Accepted: 29 November 2021;
Published: 21 December 2021.
Edited by:
Hamid Reza Mirzaei, Tehran University of Medical Sciences, IranReviewed by:
Xuchen Zhang, Yale University, United StatesAlessandro Rizzo, Sant’Orsola-Malpighi Polyclinic, Italy
Copyright © 2021 Tang, Wang, Lin, Zhou, He, Liu and Ma. This is an open-access article distributed under the terms of the Creative Commons Attribution License (CC BY). The use, distribution or reproduction in other forums is permitted, provided the original author(s) and the copyright owner(s) are credited and that the original publication in this journal is cited, in accordance with accepted academic practice. No use, distribution or reproduction is permitted which does not comply with these terms.
*Correspondence: Jiyan Liu, bGl1aml5YW4xOTcyQDE2My5jb20=; Xuelei Ma, ZHJtYXh1ZWxlaUBnbWFpbC5jb20=
†These authors have contributed equally to this work