- 1College of Veterinary Medicine, Huazhong Agricultural University, Wuhan, China
- 2College of Food Science and Technology, Huazhong Agricultural University, Wuhan, China
Antibiotics are widely used for infectious diseases and feed additives for animal health and growth. Antibiotic resistant caused by overuse of antibiotics poses a global health threat. It is urgent to choose safe and environment-friendly alternatives to antibiotics to promote the ecological sustainable development of the pig industry. Phytochemicals are characterized by little residue, no resistance, and minimal side effects and have been reported to improve animal health and growth performance in pigs, which may become a promising additive in pig production. This paper summarizes the biological functions of recent studies of phytochemicals on growth performance, metabolism, antioxidative capacity, gut microbiota, intestinal mucosa barrier, antiviral, antimicrobial, immunomodulatory, detoxification of mycotoxins, as well as their action mechanisms in pig production. The review may provide the theoretical basis for the application of phytochemicals functioning as alternative antibiotic additives in the pig industry.
Introduction
Antibiotics are natural products or derivatives of natural products, and widely used for infectious diseases and feed additives for animal health and growth over the past decades (1). Antibiotics may lose effectiveness against a growing number of bacterial pathogens, reducing competition and expanding the resources available to resistant bacteria, which results in antibiotic resistance (AR) (2, 3). AR is spreading rapidly because once a resistance gene evolves in one bacterium, it can spread to other cells and other bacterial species (3, 4). AR accounts for hundreds of thousands of deaths annually, and its wider implications present us with a growing global health threat (5). Development of AR and measures to combat AR have become important issues that are not counterbalanced by the development of new therapeutic agents (6, 7).
Pork is considered as a good and cheap alternative source of animal protein and a rich source of B complex vitamins (8). The development of hog production does not only meet the demand of pork and its products, but also contribute a lot to the farmers’ income increase, rural labor employment, transformation of grains, and stimulating the development of related industries. However, pig breeding is endangered by a variety of infectious diseases. Pork and its products not only infect people with bacterium disease, but also viral diseases, parasitic diseases and spiral diseases (9, 10). The unnecessary use of antibiotics and concomitant rapid growth of AR is a widely acknowledged threat to the development, and sustainability of pig production. It is reported that antibiotic consumption in food animal production was conservatively estimated at 93,309 tons in 2017. Globally, sales are expected to increase by 11.5% in 2030 to 104,079 tons (11). Overuse of antibiotics results in increasing levels of AR and food residues in the pig industry, and the arsenal of effective antibiotics is diminishing, which lead to severe challenges in food safety, animal health, and environmental protection (12, 13). World Health Organization (WHO) has declared it a risk for both human and animal health (14). Many countries have approved the withdrawal of colistin as a feed additive in animals, including Brazil (November, 2016), Thailand (February, 2017), China (April, 2017), Japan (July, 2018), Malaysia (January, 2019), Argentina (February, 2019), and India (July, 2019) (15, 16). Therefore, alternative measures need to be sought to maintain swine health and performance. Some alternatives including plant extracts, essential oils, probiotics, prebiotics, symbiotic, dietary fiber and enzymes, antimicrobial peptides, and functional amino acids have been used as feed alternatives to improve health and growth performance in pig industry (17–19).
Phytochemicals have been used for many years to treat various ailments. Some of the compounds of plant origin such as phenolic compounds (apigenin, quercetin, curcumin and resveratrol), organosulfur compounds (allicin), terpenes (eugenol, thymol, carvacrol, capsaicin, and artemisinin) and aldehydes (cinnamaldehyde and vanillin) have different properties such as antimicrobial (including antibacterial, antifungal, antiviral and antiprotozoal), antioxidant, immunomodulatory, and detoxification of mycotoxins, as well as the improvement of intestinal morphology and integrity of the intestinal mucosa (20–22). Phytochemicals are characterized by little residue, no resistance, and minimal side effects and serve as powerful therapeutics against pathogenic bacteria or act as functional additives, which have been reported to improve animal health and growth performance in pigs. Phytochemicals have great potential as substitutes for antibiotics, and may become a promising additive in pig production. The development of new antibiotics around proven natural scaffolds is the best short-term solution to the rising crisis of antibiotic resistance (23). In this article, we discuss the pharmacological applications of phytochemicals as alternatives to conventional antibiotics. The potential action mechanisms of phytochemicals in swine production are also reviewed. Some thoughts for future research and application are put forward to improve swine health.
Pharmacological Applications of Phytochemicals in Pig Production
Phytochemicals are widely used in the animal production industry. The diet supplemented with phytochemicals can improve meat quality, increase the amino acid concentration of muscle, increase the villus height: crypt depth ratio, and induce positive effects on serum biochemical parameters and immune function in swine (24). For instance, coix seed (scientific name Coix lacryma-jobi), from a grain-bearing perennial tropical plant (25), has been used to fortify the spleen and inhibit dampness, significantly enhance the average weight gain, and reduce the feed/meat ratio in post-weaning pigs. coix seed administration decreases the pH value of gastric juice and elevates the density and length of gastrointestinal villi. Coix seed exerts a good potential growth performance effect in weaned pigs, which is associated with increased amounts of Lactobacillus and Bacteroides and the enrichment of microbial metabolic pathways. (26). Additionally, wolfberry is well known for its health benefits in Asian countries and has a positive effect on boar semen quality (27). Moreover, the active and safe compounds isolated from plants can additionally serve as drug leading for therapeutic purposes (28). Phytochemicals are volatile lipophilic compounds, which are constituted of a complex mixture of terpenoids and phenols, which function as antioxidant, antimicrobial, immunomodulatory effects, as well as improving intestinal morphology and integrity of the intestinal mucosa (29, 30).
Effect of Phytochemicals on Pig Growth Performance
Due to the concerns regarding AR and antibiotic residues, antibiotic growth promoters have completely or partially banned to use in pig products in several countries. AR and residues are important factors related to animal growth and animal product quality. Natural bioactive compounds can prevent the animal growth check and enhance gastrointestinal health. Phytogenic feed additives have been widely used in view of the plant-derived properties and growth-promoting effect, which suggests that phytogenic feed additives will be a promising alternative to antibiotic growth promoters (21, 31). It is shown that the 300 ppm Laminarin group has a higher ADFI (average daily feed intake) and a higher ADG (average daily gain) than the basal group (32). Laminarin supplementation elevates villus height in the duodenum and jejunum, improves performance, and prevents post-weaning intestinal dysfunction. Laminarin exerts a positive influence on intestinal health through alterations in the gastrointestinal microbiome (32, 33). The results from research evaluating phytogenic additives as growth performance enhancers are variable (34). YGF251, extracted from herbs including Phlomisumbrosa Turez, Cynancumwilfordii Hemsley, Zingiberofficinale Rosc, and Platycodi Radix, positively promotes growth performance, nutrient digestibility, immune function, and fecal gas emission in pigs. However, Dietary supplementation with 0.05% herbal extract YGF251 in low protein diets does not effectively improve growth performance (31). Studies show that supplementation of combined Chestnut (Ch) and Quebracho (Qu) increase the serum concentration of albumin and albumin/globulin ratio, but decreased creatinine, and did not influence growth performance (35). However, tannins extracted from Ch and Qu have been applied on intensive swine farms due to their ability to improve animal performance and health. These positive and prominent effects are frequently associated with the anti-inflammatory activity and antioxidant activities in weaning and post-weaned piglets (36, 37). Particularly, 500 and 1,000 mg/kg of microencapsulated tannic acid (TA) is safe to be included in the swine diet and that 1,000 mg/kg of microencapsulated TA has beneficial effects on intestinal morphology, intestinal nutrient transporter, and intestinal microbiota in weaning piglets. Microencapsulated TA functions as a suitable alternative to antibiotics for improving growth performance in weaning piglets (38). It is demonstrated that 0.3 g/kg Eucommia ulmoides leaf extracts (ELE) increase the average daily gain compared with basal diet and alkaline phosphatase (AKP) levels. Total antioxidant capacity (T-AOC) is elevated in serum and liver, accompanied with a higher villus height of the duodenum and jejunum (39). Besides, dietary supplementation of artificial sweetener and capsicum oleoresin can mitigate the negative consequences of heat stress on pig performance (40). Supplementation with B. papyrifera leaf extract can increase the growth performance and antioxidant capacity of weaned piglets (41). The effect of phytochemicals on growth performance elevation may associate with its antioxidant capacity or anti-inflammatory activity. Natural phytochemicals represent a promising non-antibiotic tool to allow better intestinal health, nutrient digestibility, and general health status, thereby leading to improve growth performance.
Effect of Phytochemicals on Metabolism
Phytochemicals improve metabolism by regulating the expression of metabolism-related proteins and antioxidant stress-related proteins. Phytochemicals may exert beneficial health effects by regulating energy metabolism and activating enzymatic mechanisms, and may substitute dietary antibiotics. A paper reports that cinnamon bark extract contributes to improve glucose metabolism and lipid profile in fructose-fed rats (42). Also, oral supplementation with food-derived phospholipids has been associated with beneficial effects on lipid and lipoprotein metabolism (43).
It can be concluded that supplemented with 2% of inulin extract from chicory root or 4% of dried chicory root for 40 d significantly modulates the expression of liver proteins associated with energetic metabolism, particularly those involved in cholesterol and TG metabolism in growing pigs. Additionally, both dietary additives increase the expression of proteins related to hepatocyte protection against oxidative stress (44). Diet supplementation with dried Chicory root or inulin causes significant changes in the expression of liver cytoskeletal proteins, and alters the expression of kidney proteins engaged in energy metabolism and stress response in pigs (45). Moreover, Chicory root supplements have a potential ability to improve the liver mineral content oxidoreductive homeostasis in growing pigs (46). Particularly, a proteomic study shows that inulin-type fructans can enhance the anti-inflammatory properties by downregulation of hepatic acute phase protein CK18 in growing pigs (44). Sows fed with Garcinol (200 or 600 mg garcinol per kg) in late gestation and lactation (from the 90th day of pregnancy to day 21 postpartum) improve the maternal health and antioxidative status, milk protein content, acid–base balance in the umbilical cord blood, and growth performance in piglets (47). Reductions of the fat percentage in milk on day 7 and day 14 are found in sows supplemented with Oregano (OEO) treatment. Milk from sows supplemented with OEO during lactation has the greatest number of T lymphocytes, and a trend for greater milk intake is observed in piglets (48). Moreover, dietary supplementation with OEO can be effective in reducing the performance loss due to the outdoor-rearing system without modifying most of the peculiar traits of the meat, and improve growth rate and antioxidative status in outdoor-reared (49). Black pepper extract (BPE) supplementation has positively enhanced the growth performance, nutrient digestibility, fecal microbial, fecal gas emission, and meat quality of finishing pigs (50). Betaine 1250 mg/kg (Low Betaine) or 2500 mg/kg (High Betaine) may promote muscle fatty acid uptake via up-regulating genes related to fatty acid transporters including FAT/CD36, FATP1 and FABP3. On the other hand, Betaine activates AMPK and up-regulates genes related to fatty acid oxidation including PPARα and CPT1 in a lasting 42-days feeding experiment (51). 3.5% linseed oil (LSO) supplementation increases immunoglobulins, modifying the fatty acid composition. The concentration of 18: 3n-3 fatty acids is higher in the milk of LSO sows (gestation of day 107 to the lactation of 28th day) and n-3 polyunsaturated fatty acid (PUFA) in the tissues of piglets weaned on day 21 is increased. And the genes of D5D (Δ5-desaturase) and D6D (Δ6-desaturase) expression of piglets are affected (52). Flaxseed oil may have a positive effect on alleviating muscle protein loss and carbohydrate oxidation impairment induced by LPS challenge through regulation of TLR4/NOD and Akt/FOXO signaling pathways (53). Murtilla or murta (Ugni molinae Turcz), a plant of the Myrtaceae family, is rich in phenolic components. Murtilla extract (MT-ex) exerts antioxidant activity, and anti-inflammatory (54). However, MT-ex administration generates an important decrease in sperm metabolism, especially ATP production, which might affect the fertilization potential due to the reduction in sperm motility. Nevertheless, the metabolic decrease would allow greater efficiency in the refrigeration of boar semen at 17°C (8). Therefore, phytochemicals may play important roles in the metabolic regulation of proteins or genes associated with energetic metabolism, hepatocyte protection, fatty acid transporters, and oxidation. The metabolic effect of phytochemicals might be the key to explain the diverse pharmacological effects described in practical applications, which would contribute to a better understanding of its various functional properties in pig production.
Effect of Phytochemicals on Antioxidative Capacity
Oxidant and oxidative stress accelerate the free radical generation resulting in cell, DNA, protein, and lipid damage and loss of biological function, which are linked to many diseases (55–57). Free radical mediated oxidative stress may play a decisive role in the pathogenesis and progression of chronic metabolic diseases. Anti-oxidative therapy has been proposed as a promising and effective strategy for preventing metabolic diseases (58, 59).
Antioxidant activity of phytochemicals is one of their most intensively investigated properties. Oxidation of many biological substances causes cellular damage and metabolic diseases (60). The decreased antioxidant capacity and reactive oxygen species (ROS) level elevation are involved in weaning-induced intestinal dysfunction, resulting in intestinal oxidative stress (61), which is frequently associated with inflammation, elevation of interleukin 1β (IL-1β), tumor necrosis factor α (TNF-α), and interleukin 6 (IL-6), decreasing the abundance of tight junction proteins, and undermining the integrity of the intestinal barrier. Excessive ROS exerts adverse effects on cells by reacting with phospholipids and oxidizing sulfhydryl groups in enzymes, proteins, and DNA.
It has been reported that OEO exerts antimicrobial and antioxidative ability to improve intestinal barrier function and growth in pigs. OEO supplementation to maternal rations during late gestation and lactation can lead to improvements in progeny health and performance with a reduced incidence of mortality and lower need for medication (62). The main active compounds carvacrol and thymol in OEO possess ROS-scavenging activity in vitro. Dietary supplementation with 100 mg/kg carvacrol–thymol (1:1) decreases the intestinal oxidative stress and influences selected microbial populations without changing the biomarkers of the intestinal barrier in weaning piglets (63). Another study reports that thymol (510 mg/kg feed) contributes to regulating the integrity of the intestinal mucosa because of their anti-inflammatory and antioxidant properties (64). Plant extract resveratrol attenuates oxidative stress-induced intestinal barrier injury through PI3K/Akt-mediated Nrf2 signaling pathway (65). However, due to the labile nature, volatiles, and being rapidly absorbed in the upper gastrointestinal tract, the stability of EO during feed processing is often questionable to result in a challenge. Here, a report demonstrates that lipid matrix microparticles are able to maintain the stability of thymol and allow a slow and progressive intestinal release of thymol in weaned pigs (66).
In terms of reproductive performance, cWGRE (cultured wild ginseng root extract) has positive effects on male reproductive function via suppression of ROS production (67). Supplementation with astragalus polysaccharides (APS), as well as murtilla extract (MT-ex), can effectively preserve sperm motility, acrosome integrity and mitochondrial membrane potential through inhibiting the protein dephosphorylation caused by ROS via cAMP-PKA signaling pathway. The compound can eliminate the excessive mitochondrial ROS, improve antioxidant capacities and enhance ATP levels, which is an advantage in extending the useful life of boar sperm (8). Besides, OEO dietary supplementation increases the retention of α-tocopherol and antioxidant capacity in spermatozoa of boars fed with a fish oil-fortified diet. Moreover, a feeding diet containing 500 mg/kg OEO greatly attenuates sperm membrane and DNA oxidative damage by reducing ROS production (68). Grape seed procyanidin B2 (GSPB2) inhibits oxidative stress-induced apoptosis of porcine ovarian granulosa cells through the increased let-7a (69). In conclusion, phytochemicals may activate a series of signal transduction to clear ROS, reduce apoptosis levels, suppress the expression level of inflammatory factors, maintain intestinal mucosal integrity and change oxidative stress levels, to maintain normal cell and body functions.
Effect of Phytochemicals on Intestinal Mucosa Barrier
The gut is hypothesized to be the “motor” of critical illness and the intestinal epithelium absorbs nutrients, which may be as the first-line protection against pathogenic microbes and as the central coordinator of mucosal immunity to play roles (70, 71). The gut plays a dynamic role in organ integrity, immunity, and body defense against harmful antigens, toxins, and pathogens.
Weaning is a major critical period in pig husbandry, which involves complex dietary, social, and environmental stresses that interfere with gut development. Significant efforts are being made to identify natural alternatives to support homeostasis in the piglet gut, in particular during the weaning period (72).
Supplement with Cynara scolymus extract (CSE) for 15 days can be considered as a nutritional strategy to prevent enteric disorders and improve intestinal health in post-weaned piglets (73). 100 mg/kg Forsythia suspensa extract (FSE) or 160 mg/kg chito-oligosaccharide (COS) can increase performance by modulating intestinal permeability, antioxidant status and immune function in younger pigs for lasting 28 days (74). Plant essential oil (PEO) improves the growth performance and intestinal mucosa growth in weaned pigs, contributing to mediate the improvement in intestinal integrity and function (75). Capsicum oleoresin (CAP) and garlic botanical (GAR) are natural products and widely used in foods and drugs. Feeding CAP and GAR increases the expression of genes related to the integrity of membranes in infected weaning pigs and enhances gut mucosa health, which results in ameliorating the diarrhea and clinical immune responses in infected pigs fed plant extracts (76). Supplementation of essential oil from Brazilian red pepper or chlorohydroxyquinoline in weanling pig diets affects gut microbiota and histology but not affecting performance and organ weight, and high doses of essential oil can reduce the incidence of diarrhea (77). Piglets fed the Sangrovit (SAG) diet have an average lower value for crypt depth of the jejunum and greater values for villus height in the ileum and ratios of villus height to crypt depth in the jejunum and in the ileum. SAG can potentially improve the intestinal morphology and modify the intestinal luminal environment (78). Rotavirus (RV) impairs intestinal morphology, antioxidant capacity, and microbiota, and increases apoptosis of jejunal epithelial cells of in piglets. Dietary Lentinan (LNT) supplementation is found to improve intestinal morphology, permeability, and apoptosis of jejunal epithelial cells in piglets (79). OEO can induce a higher glycoconjugate production in the gut, creating a physical barrier against microorganisms. OAE (an aqueous extract of oregano) supplementation improves the production of glycoconjugates, enhances the protection of intestinal mucosa in pig gut (80). Rosemary (Rosmarinus officinalis L.) extract (RE) has multiple pharmacological and biological activities, and has been used as a food additive. Piglet supplementation with RE shows longer villus height and villus height/crypt depth in the jejunum and ileum, in addition to a lesser crypt depth in the jejunum and ileum, which can improve growth performance, nutrient digestibility, and intestinal morphology in weaned piglets (81). Cactus (O. ficus indica) intake does not affect piglet development in lactating sows, but a higher length of intestinal villi in the jejunum and transverse portion to improve live weight in post-weaning piglets (82).
The OEO-treated pigs show decreased endotoxin levels in serum and increased villus height and the expression of Occludin and Zonula occludens-1 (ZO-1) in the jejunum (83). The main active compounds carvacrol and thymol in OEO contribute to have antimicrobial and antioxidative capacity to improve intestinal barrier function and growth in pigs (62). In addition, supplementation of AGP or microencapsulated organic acids (OA) and EO does not significantly attenuate the induced inflammation, reduce digestive enzyme activities, and elevate gut permeability in piglets infected with Escherichia coli F4 (ETEC F4) in weaned piglets (84). However, the herbal extract mixture (HEM), a mixture of golden-and-silver honeysuckle (Lonicera japonica Thunb), huangqi (Astragalus menbranaceus), duzhong leaves (Eucommia folium) and dangshen (Codonopsis pilosula), had no effects on growth performance and organ weight of weaned pigs. However, compared with the control group, HEM can improve intestinal morphology and elevate the expression of nutrient transporters in the ileum (85). Practices have focused on supplementing in weaning piglets with phytochemicals to alleviate weaning complications. Some benefits are observed with supplementation of phytochemicals, and further research explored the potential of maternal supplementation during fetal and postnatal life to support homeostasis in the piglet gut.
Effect of Phytochemicals on Gut Microbiota
Intestinal health is related to transform of the intestinal microbiome composition, leakage of the mucosal barrier, and/or inflammation, which is determined by host, nutritional, microbial, and environmental factors (86). The dynamic gut microbiota of mammals contributes to regulating immune responses, engaging in the metabolism of dietary nutrients, and constituting a resistance line against pathogenic bacteria infection (87). Gut microbiota shifts or dysbiosis are accompanied with colitis and infectious diseases (88, 89).
Gut microbiota has a crucial role in intestinal absorption and digestion, intestinal immune regulation, and intestinal health of weaned piglets (38). Gut bacterial dysbiosis usually results in post-weaning diarrhea (18, 90, 91), which lead to intestinal barrier injury, reduce the size of the intestinal villi, result in atrophy of the enterocytes and lower digestive capacity, and decrease the weight gain of the piglet (82, 92). Intestinal tract is an important target of nutritional regulation. Strategies that modulate the microbiota and intestinal integrity of the pig in a favorable way should be sought. It is demonstrated that Firmicutes and Bacteroidetes are the most abundant phyla in the fecal microbiota of piglets, accounting for more than 90% of the fecal bacterial community at both pre-weaning and post-weaning periods (72). Coix seed supplement significantly increases the abundance of phylum Bacteroidetes and genus Lactobacillus, and reduces the abundance of phylum Prevotella in the gut microbiota, which could be associated with growth performance in weaned piglets (26). Dietary supplementation with tannic acid (TA) elevates the expression of nutrient transporter genes such as solute carrier family 15, member 1 (SLC15A1) and solute carrier family 6, member 19 (SLC6A19) of the ileum in weaning piglets. The relative abundances of Firmicutes at the phylum level are deceased. However, TA elevates the relative abundances of Bacteroidetes at the phylum level, Bacteroidia at the class level, and Bacteroidales at the order level (38). Herb of purple loosestrife (Lythrum salicaria L. from Lythraceae family) (LSH) has no significant influence on microbiota diversity and metabolic activity, but is able to modulate the gut microbiota composition, which could be applied as therapy or prevention of post-weaning diarrhea in piglets (93). Cortex Phellodendri Extract (CPE) also has a positive effect on diarrhea in weaning piglets. CPE can inhibit the growth of harmful intestinal bacteria including Escherichia and Shigella. Meanwhile, the composition of gut microbiota, the diversity, and evenness of gut microflora have been elevated (94). Dietary oleum cinnamomi (OCM) and marine macroalgal extracts supplementation modulate the intestinal microbiota and improve intestinal function in weaned piglets (72, 95). Grape seed proanthocyanidins (GSPs) with half-dose colistin are equivalent to antibiotic treatment and assist weaned animals in resisting intestinal oxidative stress by increasing diversity and improving the balance of gut microbes. Dietary GSPs result in increasing concentrations of propionic acid and butyric acid to activate GPR41, which may in turn contribute to improve the intestinal mucosa barrier, decrease intestinal permeability, improve intestinal morphology, reduce the occurrence of diarrhea (96, 97).
Besides the weaned piglets, the gut microbiota modulated by phytochemicals also contributes to pigs at other growth stages. Addition of 1.5% bamboo vinegar powder promotes the growth and development of growing-finishing pigs, increases the abundance of Firmicute/Bacteroidetes, enhances the ability of the host to absorb food energy and store more body fat in 37-days experiments. Additionally, bamboo vinegar powder has positive effects on promoting the abundance of Lactobacillus and Thalassospira and on inhibiting Streptococcus and Prevotella growth (98). 1% Wakame seaweed powder maybe alter intestinal microflora preferentially, namely, which are observed that an increase in Lactobacillus and a decrease of Escherichia coli to improve the gut health and immunity of pigs (99). Feeding the diet supplemented with dried Jerusalem artichoke (DJA) tubers, as a source of inulin-type fructans, affects the microbiota activity in the large intestine by decreasing proteolytic fermentation and detrimental bacterial enzyme activity. 4% of DJA tubers modifies the microbiota ecology in the large intestine of young pigs to a greater extent than 2% of inulin from chicory root and the applied probiotics do not enhance the effects of prebiotics (100). Additionally, supplementing with OEO during lactation increases the relative abundance of Lactobacillaceae family and Fibrobacteriaceae and Akkermansiaceae in sows. However, analysis of piglet microbiota reveals a relative decrease in Enterobacteriaceae, nevertheless butyrate producers (Lachnospiraceae family) are increased at two weeks and four weeks of age (62). Together, phytochemicals can increase the abundance of beneficial bacteria such as lactic acid bacteria, reduce the abundance of harmful bacteria such as Escherichia coli and Streptococcus, maintain the stability and balance of intestinal flora, regulate immune response, improve intestinal structure and nutrient metabolism, thus maintaining intestinal health and growth and development of pigs.
Effect of Phytochemicals on Antiviral
It has been well documented that phytochemicals possess strong antiviral action against several DNA and RNA viruses (60, 101). The effective and efficient properties of pure natural products are a novel source of rich antiviral drugs, which exerts antiviral strategy in animal infectious disease.
Phytochemicals differently regulate the expression of genes related to immunity in alveolar macrophages of PRRSV (porcine reproductive and respiratory syndrome virus)-infected pigs (102). Curcumin can block PRRSV internalization and virus-mediated cell fusion in vitro (103), and Xanthohumol inhibits PRRSV proliferation and alleviates oxidative stress induced by PRRSV (104). Sugar cane extract (SCE)-treated pigs show a significant enhancement of natural killer cytotoxicity, lymphocyte proliferation, phagocytic function of monocytes, and interferon-gamma (IFN-γ) production of CD4+ and gammadelta T cells compared with the controls after pseudorabies virus (PRV) infection, which may be extensively applied in field for the prevention of infections (105). A study investigates that different concentrations of Radix isatidis polysaccharide can inhibit pseudorabies virus (PRV) replication by 14.674-30.840%, prevent infection at rates of 6.668-14.923%, and kill this virus at rates of 32.214-67.422% (106). Porcine epidemic diarrhea virus (PEDV) causes lethal diarrhea in suckling piglets. Even commercial vaccines do not guarantee effective protection against PEDV. It is urgent to find alternatives to antibiotics to prevent diarrhea in the context of anti-drug prohibition. Isoflavonoid is the major component of Puerarin (PR), which is isolated from the Chinese herb Gegen and possesses anti-inflammatory and antiviral activities. PR (0.5 mg/kg body weight between days 5 and 9) plays antiviral and anti-inflammatory roles in piglets infected with PEDV (104.5 TCID50 per pig on day 9) via regulating the interferon and NF-κB signaling pathways both in vitro and in vivo. In addition, Tomatidine inhibits PEDV replication mainly by targeting 3CL protease (107, 108). However, formic acid has no function in any anti-PEDV activity in the early steps of the viral cycle with the only exception of a slight effect of viral inactivation at the lowest PEDV MOI. Formic acid at a dose of only 1,200 ppm can effectively inhibit PEDV replication in Vero cells (109). However, the combination of essential oil and benzoic acid enhances the degradation of PEDV RNA (110). Rotavirus (RV) impairs intestinal morphology, antioxidant capacity, and microbiota, and increases apoptosis of jejunal epithelial cells of in piglets. It is reported that lentinan (LNT) relieves RV-induced diarrhea in piglets, which can be due to the increase in antioxidant capacity, reduction in apoptosis and improvement of the microbiota-increased gut barrier (79). Porcine circovirus type 2 (PCV2) often causes multiple system failure in nursery pigs. Cepharanthine and Curcumin can inhibit mitochondrial apoptosis induced by PCV2 by through increasing Bcl-2, reducing Bax, caspase-3, ROS, and decreasing mitochondrial membrane potential (MMP) (111). Selenizing astragalus polysaccharides (sAPS) attenuates oxidative stress-induced PCV2 replication promotion through inhibition of autophagy via the increased phosphorylation of Akt and mTOR (112). Additionally, PCV2 induced immunosuppression and total flavonoids of Spatholobus suberectus Dunn (TFSD) might be able to protect animals from virus infection via regulation of immune function and inhibition of oxidative stress (113). Additionally, an essential oil blend of three oils, E. globulus, P. sylvestris, and L. latifolia, can enhance the pig humoral immune system by enhancing IgG levels and reducing IgM levels, suggesting that this novel formulation functions as a potential agent to minimize African swine fever virus (ASFV) transmission in an in vivo trial in swine (114). To sum up, inhibition of virus replication in different ways may be the main advantage of phytochemicals and can be supplemented by alleviating oxidative stress, regulating interferon expression, reducing cell apoptosis and improving the intestinal barrier. Therefore, these results demonstrate that phytochemicals exert promise as a new antiviral drug in the future.
Effect of Phytochemicals on Antimicrobial Activity
Phytochemicals possess strong potential as antimicrobial agents. The antimicrobial action of essential oils not only depends on phytochemical constituents but also interactions among different components leading to synergistic or antagonistic activities (60). The plant extracts containing nonpolar compounds such as α-amyrin, friedelan-3-one, lupeol, and β-sitosterol, damage the internal and external anatomy of the cytoplasmic membrane and inner structure, and lead to an increased influx of propidium iodide into treated bacterial cells to exert antimicrobial and anti-inflammatory properties (28).
Thymol activity is related to alterations in the bacteria cell wall, but cinnamaldehyde activity is associated with the cell wall of both enterobacteria and protein and fatty acid changes for C. perfringens (115). In addition, an additive effect is shown for carvacrol-oregano essential oil for Escherichia coli, formic acid-carvacrol and formic acid-thymol for Salmonella spp. and carvacrol-cinamaldehyde for C. perfringens (29). Ginseng polysaccharides (GPS) (200 mg/kg) supplementation improves immunity-related biomolecular levels in sow serum and milk during late pregnancy and lactation, which may be further beneficial to piglet health and growth through biological transmission effects (116). In a lasting 28-days experiment, water extract of Artemisia ordosica (WEAO) supplementation improves the apparent nutrient digestibility of piglets in a linear or quadratic dose‐dependent manner. In addition, dietary WEAO quadratically increases serum concentrations of IL‐1, IL‐4, TNF‐α, soluble surface antigen CD8 (sCD8), immunoglobulins (Ig)‐A and linearly increased serum concentrations of IL‐2, IL‐6, IgG, IgM. Furthermore, dietary WEAO linearly or quadratically decreases serum concentrations of malondialdehyde but quadratically increases the activities of antioxidant enzymes and total antioxidative capacity (117).
Enterotoxigenic Escherichia coli is considered one of the main causes of diarrhea in weaning piglets. 2% (20 g/kg) chestnut extract (CE) can represent a promising alternative to antibiotics immediately after weaning for improving growth performance and reducing post-weaning diarrhea (PWD) caused by ETEC F4 (118). Anethole (300 mg/kg) coated with corn starch can improve the growth performance of weaned piglets infected by Escherichia coli K88 through decreasing the expression of TLR5, TLR9, MyD88, IL-1β, TNF-α, IL-6, and IL-10 in the jejunum, attenuating intestinal barrier disruption and enriching the abundance of beneficial flora in the intestines of the piglets (119). Acetone crude leaf extracts of Syzygium and Eugenia (Myrtaceae) have good antimicrobial activity as well as a protective role on intestinal epithelial cells against enterotoxigenic E.coli bacterial adhesion (120). Plant-derived natural steroid compound phytosterol (PS) supplementation exerts similar effects on growth, anti-inflammation and intestinal microorganisms as supplementation with polymyxin E in piglets. Despite of no significant effect on growth, 0.2 g/kg PS supplementation activates CD4+ T cells and results in a Th2 shift, which suggests that PS can improve immunity and anti-inflammatory activity and ameliorate diarrhea in weaned piglets (121). Baicalin–aluminum complex (BBA) administration alters the structure of the gut microbiomes. Then the diarrhea rate reduces significantly after treatment with BBA in piglets (122). Scutellaria baicalensis extracts (SBE) supplementation can potently attenuate diarrhea in weaning piglets and decrease inflammatory cytokine expression through inhibiting the NF-κB and P38 signaling pathways, attenuating Escherichia coli K88-induced acute intestinal injury in weaned piglets (123).
H. parasuis infection provokes the expression of cytokines and pathways activation, and induces the release of high-mobility group box 1 (HMGB1). HMGB1 protein is related to the pathogenesis of various infectious pathogens. Baicalin displays important anti-inflammatory and anti-microbial activities. Baicalin significantly reduces the release of HMGB1 in peripheral blood monocytes induced by H. parasuis (124). Tea polyphenols can inhibit H. parasuis growth in a dose-dependent manner and attenuate the biofilm formation of H. parasuis. In addition, tea polyphenols inhibit the expression of H. parasuis virulence-related factors. Moreover, tea polyphenols can confer protection against a lethal dose of H. parasuis and reduce pathological tissue damage induced by H. parasuis (125). Together, some plants are known as nutraceuticals because they exhibit antimicrobial properties for susceptible in pig production. These activities of phytochemicals may be achieved by rebuilding the structure of bacterial cell membranes and cell walls, inhibiting the expression of bacterial virulence factors, regulating the expression levels of inflammatory factors, and improving antioxidant enzyme activity.
Effect of Phytochemicals on Immunomodulatory
Phytochemicals are hopeful alternatives to chemotherapeutics in animal production owing to their immunostimulant. Phytochemicals may activate the immunity of the host either by directly stimulating the innate immune system or through commensal microorganisms sustenance and pathogens inhibition (126, 127). The dietary administration of phytochemicals can modulate the following biological processes related to innate immune effector cells, namely “leukocyte activation”, “leukocyte activation involved in immune response”, “neutrophil activation”, and “neutrophil degranulation” (127). Neutrophils are rapidly recruited to infected tissues and can engulf bacteria directly or produce toxic antimicrobial mediators, which have always been considered as uncomplicated front-line troopers of the innate immune system equipped with limited proinflammatory duties (128).
Plants containing immunostimulatory properties improve the activity of lymphocytes, macrophages, and NK cells and thereby increase phagocytosis and stimulate interferon synthesis (129). Medicinal plants propose an alternate to conventional therapeutic strategies for numerous ailments, particularly when suppression of inflammation is expected (130, 131). It has been reported that dietary supplementation of phytochemicals increases the number of white blood cells and other immune parameters and possesses significant anti-inflammatory activity (132). Chinese medicinal herbs (CMH) supplementation composed of Panax ginseng, Dioscoreaceae opposite, Atractylodes macrocephala, Glycyrrhiza uralensis, Ziziphus jujube and Platycodon grandiflorum can enhance the immune activities of polymorphonuclear leucocytes (PMNs), and reduce diarrhea frequency in weanling pigs. (133). ORE treatment alters the lymphocyte proportion and the ratio of CD4+ and CD8+ T-cells in the serum of non-stimulated and in LPS-stimulated piglets (134). NF-κB is downregulated on peripheral blood mononuclear cells (PBMCs) in outdoor reared pigs when fed the ORE diet (135). Leukocyte populations in blood reveal that the percentages of Th lymphocytes, γδ T lymphocytes, and B lymphocytes are more affected by body weight in weanling piglets. The percentage of this leukocyte population is higher in high birth weight (HBW) piglets receiving CKTL + COL diet [a cocktail of feed additives containing cranberry extract, encapsulated carvacrol, yeast-derived products, and extra vitamins A, D, E, and B complex (CKTL); CKTL diet with bovine colostrum in replacement of plasma proteins (CKTL + COL)] when compared with low birth weight (LBW) piglets of the same group (136). The E. coli challenge tends to increase the number of total WBC on d 5 and increase it on d 11 post-infection compared with the sham group, but the plant extracts (10 ppm of capsicum oleoresin, 10 ppm of garlic botanical, or 10 ppm of turmeric oleoresin) treatments in the E. coli–challenged group decrease total WBC and the number of neutrophils (137), and feeding each of the plant extracts has effects on expression of several genes that are counter to the effects of E. coli (76). Additionally, Agrimonia procera (AP) increases the immune response in LPS-treated piglets (138). SBE supplementation can potently attenuate diarrhea in weaning piglets and decrease inflammatory cytokine expression through inhibiting the NF-κB and p38 pathways (123). Moreover, dietary supplementation with isoquinoline alkaloids extracted from Macleaya cordata R. Br. boosts the immune system, regulates the metabolic process and finally promotes growth and development in swine (139). The immunomodulatory effects of plant-derived compounds are increasingly attracting people’s interest. Studies that applied plant extracts with known components, or single constituents, and de facto measured parameters with the relevant to immune function as outcome measures are included (140). Several studies proving to have effects on weaned piglet immune regulation apply a ready-to-use plant product. The immunomodulatory effects are mainly inspected on a cellular (monocytes/macrophages, neutrophil granulocytes, NK cells, and T and B lymphocytes) and molecular level (cytokines, immunoglobulins), data on the potential molecular genetical mechanisms accounting such immunological effects in the literature is limited (140).
Effect of Phytochemicals on Detoxification of Mycotoxins
Deoxynivalenol (DON), zearalenone (ZEN), and aflatoxin B1 (AFB1) are the main mycotoxins that frequently contaminate maize and grain cereals which are the main feedstuff in pig diets (141). The effects of toxic include immune modulation, disruption of intestinal barrier function, and cytotoxicity leading to cell death, which all result in impaired pig performance and impose risks to the health of both humans and animals (142, 143). It is reported that the genera Lactobacillus (particularly in DON) and Bacteroides dominate the bacterial flora in both the DON and ZEN dietary treatments, and there may be potential opportunities to isolate and characterize useful probiotics that decrease the level of mycotoxins (143). However, phytochemicals may potentially reduce tissue damage mediated by mycotoxins by regulating immune function, improving the abundance of intestinal flora, and decreasing oxidative stress.
Liver is the earliest target for AFB1 toxicity in both humans and animals (144). Grape pomace is able to reduce the gastrointestinal absorption of mycotoxins (145). Dietary grape seed meal (GSM) can mitigate the AFB1-induced toxicity in pigs (146). Diet containing 8% GSM (grape seed meal) by-products ameliorates histological liver injury and oxidative stress in the liver of the pig after weaning exposed to AFB1 through the contaminated feed for 28 days, accompanied by decreasing MAPK (mitogen-activated protein kinase) signaling pathway and inhibition of NF-κB signaling pathway by AFB1 diet (144). After 30 days of experiment, GSM (8% inclusion in the diet) and AFB1 (320 g/kg feed) increase the relative abundance of phylum Bacteroidetes and Proteobacteria. However, compared to the individual treatments, the Firmicutes abundance is decreased in a synergic manner. An additive or synergistic action of the two treatments were identified for Lactobacillus, Prevotella, and Campylobacter. On the contrary, a rather antagonistic effect is observed on Lachnospira (147). Additionally, Dihydromyricetin (DHM) alleviates cell injury induced by DON and it is possibly through its antioxidant activity, anti-inflammatory activity, or ability to regulate metabolic pathways (148). Astragalus polysaccharides (APS) can attenuate the immune stress induced by ochratoxin A (OTA) in vitro and in vivo via activation of AMPK/SIRT-1 signaling pathway (149). Plant derivatives can be used to diminish the detrimental effects caused by mycotoxins in pigs, more specifically in the gastrointestinal tract (141). Phytobiotics, such as plant extracts, typically include bioactive extracts and characterize with antioxidative and anti-inflammatory properties (150). Phytobiotics are often included in mycotoxin-detoxifying agent formulas to mitigate the negative effects of multiple mycotoxins in diets fed to pigs, which is directly related to the gut health and gut microflora of pigs (151). In summary, the effects of phytochemicals on mycotoxin poisoning can be achieved by regulating signaling pathways, improving histological damage, oxidative stress, flora abundance, and regulating anti-inflammatory activity and metabolic activity.
The Action Mechanisms of Phytochemicals
Phytochemicals exhibit antimicrobial activity, antioxidative capacity, anti-inflammatory activity, and other properties against pathogenic microorganisms (bacteria, fungi, and viruses) or risks. Although the chemical composition and function attributes of phytochemicals have been studied previously, the comparison of their chemical constituents using different extraction methods as well as the linked mechanisms still remain unexplored (152). A number of mechanisms have been proposed and proven. The studies have focused on enzymatic activity, endocrine disruption, plasma membrane homeostasis, singling pathway. In this manuscript, the antimicrobial, antivirus, antioxidant, and immune regulation mechanisms of phytochemicals are mainly discussed.
Antimicrobial and Antivirus Mechanism of Phytochemicals in Pig Production
It is demonstrated that numerous antimicrobial phytochemicals have been discovered or better characterized (22, 153). Wang et al. summarizs the antimicrobial mechanisms of ginseng, one of a phytochemicals (a) inhibit the microbial motility and quorum-sensing ability; (b) affect the formation of biofilms and destroy the mature biofilms, which can weaken the infection ability of the microbes; (c) perturb membrane lipid bilayers, thus causing the formation of pores, leakages of cell constituents and eventually cell death; (d) active of the host immune system and attenuate microbes induced apoptosis, inflammation, and DNA damages, which can protect or help the host fight against microbial infections; and (e) inhibit the efflux of antibiotics that can descend the drug resistance of the microbial (154). Besides ginseng, cinnamon extracts have been reported to inhibit bacteria by damaging cell membranes, altering the lipid profile, inhibiting ATPases, cell division, and biofilm formation (155). Carvacrol can cross the bacterial plasma membrane and bind molecules such as ATP or monovalent cations such as K+ and transport them out of the bacterial cell depending on a phenolic OH group, seriously altering the membrane potential and homeostasis (22, 156). Thymol has the ability to incorporate into the polar-head group region of the lipid bilayer, altering the structural integrity and fluidity of the membrane through hydrogen bonding and hydrophobic interactions exerting antimicrobial activity (157, 158). Cortex Phellodendri Extract (CPE) has a positive effect on diarrhea in weaning piglets. The mechanism behind these effects is that CPE can diminish the adhesion of ETEC to intestinal epithelial cells by suppressing the expression of the bacterial flagellum genes (94). Furthermore, Cranberry extract (20 μg or 100 μg/mL) inhibits in vitro adhesion of F4+ and F18+ E. coli to pig intestinal epithelium and reduces in vivo excretion of pigs orally challenged with F18+ verotoxigenic E. coli (159).
Many plant-derived natural substances are potential keys to designing antiviral therapies for inhibiting viral replication and infection (160, 161). The main active ingredients of Chinese medicines or phytochemicals such as quercetin, kaempferol, luteolin, isorhamnetin, baicalein, naringenin, and wogonin could target on AEC2 and 3CL protein of COVID-19. They exert the antivirus, inhibiting inflammatory mediators, regulating immunity, and eliminating free radical properties through the signaling pathways of IL-17, arachidonic acid, HIF-1, NF-κB, Ras, and TNF (162). Pterodontic acid is isolated from L. pterodonta and shows selective antiviral activity of influenza A virus, which is most probably associated with inhibiting the replication of influenza A virus by blocking nuclear export of viral RNP complexes, and attenuating the inflammatory response by inhibiting activation of the NF-κB pathway (163). Isoflavonoid is the major component of Puerarin (PR), which is isolated from the Chinese herb Gegen and possesses anti-inflammatory and antiviral activities. PR supplementation inhibits PEDV-induced NF-κB activation, demonstrating that PR has the potential to be an effective antiviral feed additive (108). PRRSV is one of the major swine pathogens. Tea seed saponins (TS) inhibit PRRSV-induced cell apoptosis and effectively suppress PRRSV replication by reducing the expression of the host cellular gene PABP, and significantly restrain virus N gene/protein expression (164). Xanthohumol, a prenylated flavonoid found in hops, significantly suppresses PRRSV proliferation by activating the Nrf2-HMOX1 pathway, which may be helpful for developing a novel prophylactic and therapeutic strategy against PRRSV infection (104). Studies showed that APS attenuated PCV2 infection by inhibiting oxidative stress and endoplasmic reticulum stress and by blocking NF-κB pathway (165, 166), and selenizing astragalus polysaccharide (sAPS) attenuates PCV2 replication promotion through autophagy inhibition via PI3K/AKT activation relying on Akt and mTOR phosphorylation (112). Aloe (Ae) extract can hamper completely the proliferation of PEDV in Vero and IPEC-J2 cells in vitro, and can reduce virus load and pathological changes in the intestinal tract of pig challenge with highly pathogenic PEDV variant GDS01 infection. However, Ae could not degrade S1 protein in vitro, indicating that Ae may directly inactivate the virus infectivity, but no effect on virus nucleic and virus invasion (167). Virus-infected cells release signals to recruit and activate immune cells. These immune cells secrete a variety of cytokines and chemokines to recruit more immune cells to the lesion site (168). Phytochemicals can be beneficial for animal health and considered as an alternative agent for antimicrobial and antivirus therapy. Although, potential targets and pathways of phytochemicals prescriptions were predicted through network pharmacological analysis. Antimicrobial and antivirus mechanisms of phytochemicals in pig production need further to be investigated.
Antioxidant and Immune Regulation Mechanisms of Phytochemicals in Pig Production
The main antioxidant and anti-inflammatory mechanisms found in phytochemicals are largely attributed to the regulation of signaling pathways. The Nrf2-ARE signaling is an important mediator of cellular response during an oxidative stress condition. Nrf2 may be considered as a key factor that protects cells against oxidative damage and contributes to the survival of the cell (169). Tagetes erecta flowers essential oils exerting antioxidative stress, anti-apoptotic and anti-inflammatory effects may rely on Nrf2/HO-1 and NF-κB signaling pathways (170). Isoegomaketone (IK), an essential oil component of Perilla frutescens (L.) Britt, inhibits NO production through simultaneous induction of HO-1 and inhibition of the IFNβ-STAT-1 pathway possessing anti-inflammatory activity (171).
NF-κB is a critical regulator of immunity and responsible for the transcription of the gene encoding many pro-inflammatory cytokines and chemokines (172). NF-κB activation results in tissue changes characteristic of inflammation. Schisandra chinensis extract (SCE) and Schisandra chinensis lignans (SCL) can greatly inhibit the TLR4/NF-κB/IKKα signaling pathway, and indicate that Schisandra chinensis ameliorates depressive-like behaviors by regulating microbiota-gut-brain axis via its anti-inflammation activity (173). Astilbe Chinensis ethanol extracts (ACE) inhibit LPS or thioglycollate (TG)-induced inflammation by blocking the NF-κB signaling pathway in macrophages (174). Additionally, MAPK and protein kinase A (PKA) signaling pathways are involved in antioxidant and immune regulation (175).
OEO is generally attributed to its antimicrobial and anti-inflammatory effects and contributes to promoting intestinal barrier integrity in pigs. The protective effect of OEO on the intestine is associated with the decrease of intestinal E. coli population and the inactivation of the JNK, ERK1/2, Akt, and NF-κB signaling pathways (83). Illicium verum extracts (IVE) and probiotics with added glucose oxidase (PGO) promote antioxidant capacity through upregulating the hepatic and jejunal Nrf2/Keap1 pathway of weaned piglets. Therefore, IVE and PGO can be recommended as a new potential alternative to antibiotics in piglets’ diets (176). Ulva prolifera extracts contain multiple functional active substances, which relieves oxidative stress via Nrf2 signaling, but not through AMPK pathway in weaned piglets treated with hydrogen peroxide (177). It is shown that SBE supplementation can potently attenuate diarrhea in weaning piglets and decrease inflammatory cytokine expression through inhibiting the NF-κB and P38 signaling pathways (123). Additionally, KEGG Pathway enrichment analysis by RNA sequencing shows that PI3K-Akt, AMPK, Rap1, and peroxisome signaling pathways are enriched in Tibetan pig liver after Chinese wolfberry (Lycium barbarum) and Astragalus (Astragalus membranaceus) extract treatment, and the plant extracts exert antioxidant and anti-stress ability (178). In vitro, OEO induces SOD1 and GSH expression through Nrf2 activation and alleviates hydrogen peroxide-induced oxidative damage in IPEC-J2 Cells (179). A low dose of eugenol (100 μM) improves selectively permeable barrier function during LPS-induced inflammation and tends to increase TEER values in the IPEC-J2 cell line (180). The antioxidant and immune regulation effects of phytochemicals are partly related to the activation of signaling pathways. These facts justify the urgency for therapeutic alternatives in pig production, preferentially combining selective antioxidant and anti-inflammatory activities, which may be efficiently to promote growth performance. Although numerous antioxidant and anti-inflammatory phytochemicals have been discovered or better characterized, in most cases, their mechanisms of action still have been clarified (Figure 1).
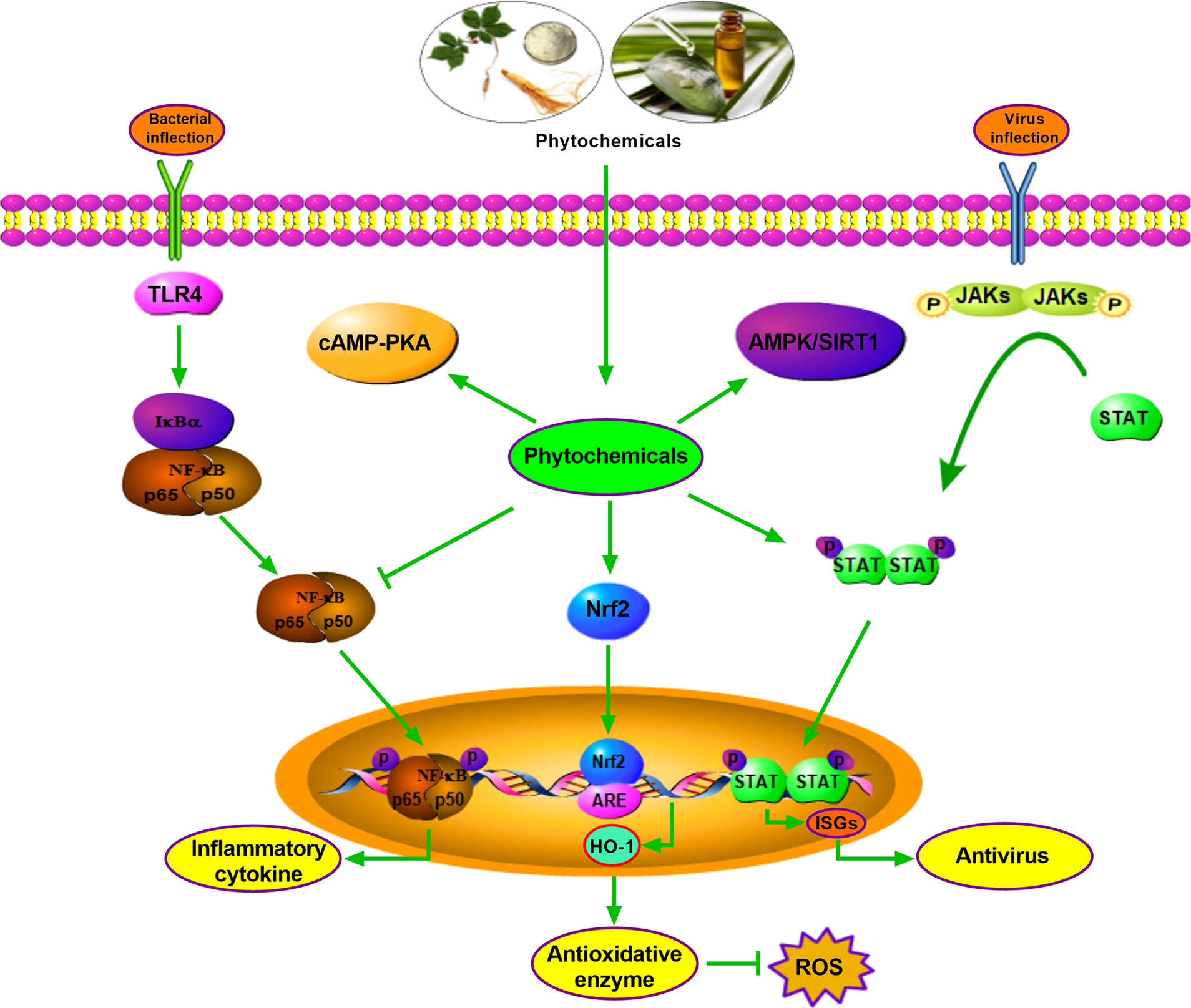
Figure 1 Antimicrobial, antivirus, antioxidant mechanism of parts phytochemicals. Phytochemicals exert antimicrobial effect by inhibiting NF-kB signaling, and function as antioxidant role by activating Nrf2-HO-1 pathway. Phytochemicals show antivirus function relying on STAT pathway. Other pathways such as cAMP-PKA and AMPK/SIRT1 are also involved in antimicrobial, antivirus or antioxidant effects.
Discussion and Prospect
Scientific feeding strategies need to be considered to achieve certain goals in pig production. It is imperative to develop by-products or feed additives with high nutritional value, low cost, and easy access, to solve the shortage of feed resources, alleviate the current situation of human and animal competition for food, reduce drug resistance and environmental pollution, and provide safe meat. In the application of feed additives to improve animal performance and health, the chemical composition of feed additives, the mechanism of action, the release efficiency of the target of action, the relationship between feed and additives, nontoxic side and no residue, are important factors that should be considered. Plants are the source of a large proportion of medicines. Phytochemicals are considered to have medicinal properties possessing a wide variety of bioactivities including antimicrobial, antiviral, antioxidant, anti-inflammatory (181). Some novel biological functions including medical and commercial benefits of some phytochemicals have been fully explored, which can be acted as an alternative to antibiotics (182, 183).
Antibiotics have played a crucial role in the growth and development of the swine industry. Their efficiency in increasing growth rate, improving feed utilization, and reducing mortality from clinical disease is well documented (184). However, antibiotic residues, drug resistance, and environmental pollution seriously threaten human health and the sustainable development of the pig industry. It is necessary to improve nutritional programs in swine diets using phytochemicals, which contributes to increasing production efficiency and profitability, and reducing the environmental impact of pork production. The prohibition of antibiotics in pig feed provides a good application prospect for the development of effective phytochemical additives (Table 1). The principles of choosing by-products or additives are that they do not require more time or effort to process (188). However, there is limited information available on the pharmacodynamics/kinetics of phytochemicals in pig production. Firstly, efficient extraction methods to obtain active ingredients may be a pending challenge for many phytochemicals. It is imperative to use modern technologies including supercritical fluid extraction, subcritical extraction, liquid or solvent-free microwave extraction to obtain the effective substances. Secondly, the morphological damage, toxicity, and effectiveness, as well as the dosage in animals need to develop new techniques and to evaluate. Furthermore, the potential pharmacological interactions of ingredients and action mechanisms need to be further investigated. Importantly, the quick growth rate, stress-load effects of combinational feed additives, and cost/benefit analysis can be conducted to understand the achievable application of phytochemicals in pig production. Besides, careful consideration of group size, sample size, and how these factors may influence study outcomes, protocol implementation, sample collection, and recording of important information will be beneficial to the research progress of effective alternatives of antibiotics (189). Some studies of phytochemicals in pig production indicate the fruitfulness but others are still unclear. Experiments are needed to evaluate its economic aspects and efficacy under clinical conditions, so that phytochemicals can be used as natural medicine alternatives to antibiotics in the pig industry.
Author Contributions
The idea for this article came from LL and HD. All authors contributed to structuring, drafting, writing, critically revising, and agreed to the published version of the manuscript.
Funding
This work was sponsored by the National Natural Science Foundation of China(Grant: 32172808).
Conflict of Interest
The authors declare that the research was conducted in the absence of any commercial or financial relationships that could be construed as a potential conflict of interest.
Publisher’s Note
All claims expressed in this article are solely those of the authors and do not necessarily represent those of their affiliated organizations, or those of the publisher, the editors and the reviewers. Any product that may be evaluated in this article, or claim that may be made by its manufacturer, is not guaranteed or endorsed by the publisher.
References
1. Mohr KI. History of Antibiotics Research. Curr Top Microbiol Immunol (2016) 398:237–72. doi: 10.1007/82_2016_499
2. Conlan S, Thomas PJ, Deming C, Park M, Lau AF, Dekker JP, et al. Single-Molecule Sequencing to Track Plasmid Diversity of Hospital-Associated Carbapenemase-Producing Enterobacteriaceae. Sci Transl Med (2014) 6(254):254ra126. doi: 10.1126/scitranslmed.3009845
3. Lerminiaux NA, Cameron ADS. Horizontal Transfer of Antibiotic Resistance Genes in Clinical Environments. Can J Microbiol (2019) 65(1):34–44. doi: 10.1139/cjm-2018-0275
4. Klümper U, Riber L, Dechesne A, Sannazzarro A, Hansen LH, Sørensen SJ, et al. Broad Host Range Plasmids Can Invade an Unexpectedly Diverse Fraction of a Soil Bacterial Community. Isme J (2015) 9(4):934–45. doi: 10.1038/ismej.2014.191
5. Bengtsson-Palme J, Kristiansson E, Larsson DGJ. Environmental Factors Influencing the Development and Spread of Antibiotic Resistance. FEMS Microbiol Rev (2018) 42(1):fux053. doi: 10.1093/femsre/fux053
6. Plantinga NL, Wittekamp BH, van Duijn PJ, Bonten MJ. Fighting Antibiotic Resistance in the Intensive Care Unit Using Antibiotics. Future Microbiol (2015) 10(3):391–406. doi: 10.2217/fmb.14.146
7. Holmer I, Salomonsen CM, Jorsal SE, Astrup LB, Jensen VF, Høg BB, et al. Antibiotic Resistance in Porcine Pathogenic Bacteria and Relation to Antibiotic Usage. BMC Vet Res (2019) 15(1):449–9. doi: 10.1186/s12917-019-2162-8
8. Jofré I, Cuevas M, de Castro LS, de Agostini Losano JD, Torres MA, Alvear M, et al. Antioxidant Effect of a Polyphenol-Rich Murtilla (Ugni Molinae Turcz.) Extract and Its Effect on the Regulation of Metabolism in Refrigerated Boar Sperm. Oxid Med Cell Longev (2019) 2019:2917513. doi: 10.1155/2019/2917513
9. Farez S, Morley RS. Potential Animal Health Hazards of Pork and Pork Products. Rev Sci Tech (1997) 16(1):65–78. doi: 10.20506/rst.16.1.992
10. Montone AMI, De Sabato L, Suffredini E, Alise M, Zaccherini A, Volzone P, et al. Occurrence of HEV-RNA in Italian Regional Pork and Wild Boar Food Products. Food Environ Virol (2019) 11(4):420–6. doi: 10.1007/s12560-019-09403-2
11. Tiseo K, Huber L, Gilbert M, Robinson TP, Van Boeckel TP. Global Trends in Antimicrobial Use in Food Animals From 2017 to 2030. Antibiotics (Basel Switzerland) (2020) 9(12):918. doi: 10.3390/antibiotics9120918
12. Betts JW, Hornsey M, La Ragione RM. Novel Antibacterials: Alternatives to Traditional Antibiotics. Adv Microb Physiol (2018) 73:123–69. doi: 10.1016/bs.ampbs.2018.06.001
13. Silveira RF, Roque-Borda CA, Vicente EF. Antimicrobial Peptides as a Feed Additive Alternative to Animal Production, Food Safety and Public Health Implications: An Overview. Anim Nutr (2021) 7(3):896–904. doi: 10.1016/j.aninu.2021.01.004
14. Diana A, Boyle LA, Leonard FC, Carroll C, Sheehan E, Murphy D, et al. Removing Prophylactic Antibiotics From Pig Feed: How Does It Affect Their Performance and Health? BMC Vet Res (2019) 15(1):67–7. doi: 10.1186/s12917-019-1808-x
15. Shen Y, Zhou H, Xu J, Wang Y, Zhang Q, Walsh TR, et al. Anthropogenic and Environmental Factors Associated With High Incidence of Mcr-1 Carriage in Humans Across China. Nat Microbiol (2018) 3(9):1054–62. doi: 10.1038/s41564-018-0205-8
16. Wang Y, Xu C, Zhang R, Chen Y, Shen Y, Hu F, et al. Changes in Colistin Resistance and Mcr-1 Abundance in Escherichia Coli of Animal and Human Origins Following the Ban of Colistin-Positive Additives in China: An Epidemiological Comparative Study. Lancet Infect Dis (2020) 20(10):1161–71. doi: 10.1016/s1473-3099(20)30149-3
17. Kantas D, Papatsiros VG, Tassis PD, Giavasis I, Bouki P, Tzika ED. A Feed Additive Containing Bacillus Toyonensis (Toyocerin((R)) ) Protects Against Enteric Pathogens in Postweaning Piglets. J Appl Microbiol (2015) 118(3):727–38. doi: 10.1111/jam.12729
18. Jing Y, Liu H, Xu W, Yang Q. 4,4'-Diaponeurosporene-Producing Bacillus Subtilis Promotes the Development of the Mucosal Immune System of the Piglet Gut. Anat Rec (Hoboken) (2019) 302(10):1800–7. doi: 10.1002/ar.24102
19. Xu B, Fu J, Zhu L, Li Z, Jin M, Wang Y. Overall Assessment of Antibiotic Substitutes for Pigs: A Set of Meta-Analyses. J Anim Sci Biotechnol (2021) 12(1):3. doi: 10.1186/s40104-020-00534-2
20. Omonijo FA, Ni L, Gong J, Wang Q, Lahaye L, Yang C. Essential Oils as Alternatives to Antibiotics in Swine Production. Anim Nutr (2018) 4(2):126–36. doi: 10.1016/j.aninu.2017.09.001
21. Rossi B, Toschi A, Piva A, Grilli E. Single Components of Botanicals and Nature-Identical Compounds as a Non-Antibiotic Strategy to Ameliorate Health Status and Improve Performance in Poultry and Pigs. Nutr Res Rev (2020) 33(2):218–34. doi: 10.1017/s0954422420000013
22. Álvarez-Martínez FJ, Barrajón-Catalán E, Herranz-López M, Micol V. Antibacterial Plant Compounds, Extracts and Essential Oils: An Updated Review on Their Effects and Putative Mechanisms of Action. Phytomedicine (2021) 90:153626. doi: 10.1016/j.phymed.2021.153626
23. Rossiter SE, Fletcher MH, Wuest WM. Natural Products as Platforms To Overcome Antibiotic Resistance. Chem Rev (2017) 117(19):12415–74. doi: 10.1021/acs.chemrev.7b00283
24. Lin ZN, Ye L, Li ZW, Huang XS, Lu Z, Yang YQ, et al. Chinese Herb Feed Additives Improved the Growth Performance, Meat Quality, and Nutrient Digestibility Parameters of Pigs. Anim Model Exp Med (2020) 3(1):47–54. doi: 10.1002/ame2.12104
25. Liu S, Li F, Zhang X. Structural Modulation of Gut Microbiota Reveals Coix Seed Contributes to Weight Loss in Mice. Appl Microbiol Biotechnol (2019) 103(13):5311–21. doi: 10.1007/s00253-019-09786-z
26. Li Z, Lin Z, Lu Z, Feng Z, Chen Q, Deng S, et al. Coix Seed Improves Growth Performance and Productivity in Post-Weaning Pigs by Reducing Gut pH and Modulating Gut Microbiota. AMB Express (2019) 9(1):115–5. doi: 10.1186/s13568-019-0828-z
27. Yang Q, Xing Y, Qiao C, Liu W, Jiang H, Fu Q, et al. Semen Quality Improvement in Boars Fed With Supplemental Wolfberry (Lycium Barbarum). Anim Sci J (2019) 90(12):1517–22. doi: 10.1111/asj.13295
28. Famuyide IM, Fasina FO, Eloff JN, McGaw LJ. The Ultrastructural Damage Caused by Eugenia Zeyheri and Syzygium Legatii Acetone Leaf Extracts on Pathogenic Escherichia Coli. BMC Vet Res (2020) 16(1):326. doi: 10.1186/s12917-020-02547-5
29. Gomez-Garcia M, Sol C, de Nova PJG, Puyalto M, Mesas L, Puente H, et al. Antimicrobial Activity of a Selection of Organic Acids, Their Salts and Essential Oils Against Swine Enteropathogenic Bacteria. Porcine Health Manag (2019) 5:32. doi: 10.1186/s40813-019-0139-4
30. Lagha R, Ben Abdallah F, Al-Sarhan BO, Al-Sodany Y. Antibacterial and Biofilm Inhibitory Activity of Medicinal Plant Essential Oils Against Escherichia Coli Isolated From UTI Patients. Molecules (2019) 24(6):1161. doi: 10.3390/molecules24061161
31. Lei XJ, Lee SI, Kim IH. Effects of Different Levels of Dietary Protein With or Without Plant Extract YGF251 on Growth Performance, Nutrient Digestibility, Blood Profiles, Fecal Microbial Shedding, and Fecal Gas Emission in Growing Pigs. Anim Sci J (2019) 90(4):547–53. doi: 10.1111/asj.13162
32. Rattigan R, Sweeney T, Maher S, Thornton K, Rajauria G, O'Doherty JV. Laminarin-Rich Extract Improves Growth Performance, Small Intestinal Morphology, Gene Expression of Nutrient Transporters and the Large Intestinal Microbial Composition of Piglets During the Critical Post-Weaning Period. Br J Nutr (2020) 123(3):255–63. doi: 10.1017/s0007114519002678
33. Vigors S, O'Doherty J, Rattigan R, Sweeney T. Effect of Supplementing Seaweed Extracts to Pigs Until D35 Post-Weaning on Performance and Aspects of Intestinal Health. Mar Drugs (2021) 19(4):183. doi: 10.3390/md19040183
34. Silva Junior CD, Martins CCS, Dias FTF, Sitanaka NY, Ferracioli LB, Moraes JE, et al. The Use of an Alternative Feed Additive, Containing Benzoic Acid, Thymol, Eugenol, and Piperine, Improved Growth Performance, Nutrient and Energy Digestibility, and Gut Health in Weaned Piglets. J Anim Sci (2020) 98(5):skaa119. doi: 10.1093/jas/skaa119
35. Caprarulo V, Hejna M, Giromini C, Liu Y, Dell'Anno M, Sotira S, et al. Evaluation of Dietary Administration of Chestnut and Quebracho Tannins on Growth, Serum Metabolites and Fecal Parameters of Weaned Piglets. Anim (Basel) (2020) 10(11):1945. doi: 10.3390/ani10111945
36. Liu HW, Li K, Zhao JS, Deng W. Effects of Chestnut Tannins on Intestinal Morphology, Barrier Function, Pro-Inflammatory Cytokine Expression, Microflora and Antioxidant Capacity in Heat-Stressed Broilers. J Anim Physiol Anim Nutr (Berl) (2018) 102: (3):717–26. doi: 10.1111/jpn.12839
37. Caprarulo V, Giromini C, Rossi L. Review: Chestnut and Quebracho Tannins in Pig Nutrition: The Effects on Performance and Intestinal Health. Animal (2021) 15(1):100064. doi: 10.1016/j.animal.2020.100064
38. Wang M, Huang H, Hu Y, Huang J, Yang H, Wang L, et al. Effects of Dietary Microencapsulated Tannic Acid Supplementation on the Growth Performance, Intestinal Morphology, and Intestinal Microbiota in Weaning Piglets. J Anim Sci (2020) 98(5):skaa112. doi: 10.1093/jas/skaa112
39. Ding H, Cao A, Li H, Zhao Y, Feng J. Effects of Eucommia Ulmoides Leaf Extracts on Growth Performance, Antioxidant Capacity and Intestinal Function in Weaned Piglets. J Anim Physiol Anim Nutr (Berl) (2020) 104(4):1169–77. doi: 10.1111/jpn.13333
40. Biggs ME, Kroscher KA, Zhao LD, Zhang Z, Wall EH, Bravo DM, et al. Dietary Supplementation of Artificial Sweetener and Capsicum Oleoresin as a Strategy to Mitigate the Negative Consequences of Heat Stress on Pig Performance. J Anim Sci (2020) 98(5):skaa131. doi: 10.1093/jas/skaa131
41. Chen G, Shui S, Chai M, Wang D, Su Y, Wu H, et al. Effects of Paper Mulberry (Broussonetia Papyrifera) Leaf Extract on Growth Performance and Fecal Microflora of Weaned Piglets. BioMed Res Int (2020) 2020:6508494. doi: 10.1155/2020/6508494
42. Kannappan S, Jayaraman T, Rajasekar P, Ravichandran MK, Anuradha CV. Cinnamon Bark Extract Improves Glucose Metabolism and Lipid Profile in the Fructose-Fed Rat. Singapore Med J (2006) 47(10):858–63.
43. Robert C, Couëdelo L, Knibbe C, Fonseca L, Buisson C, Errazuriz-Cerda E, et al. Rapeseed Lecithin Increases Lymphatic Lipid Output and α-Linolenic Acid Bioavailability in Rats. J Nutr (2020) 150(11):2900–11. doi: 10.1093/jn/nxaa244
44. Lepczynski A, Herosimczyk A, Ozgo M, Marynowska M, Pawlikowska M, Barszcz M, et al. Dietary Chicory Root and Chicory Inulin Trigger Changes in Energetic Metabolism, Stress Prevention and Cytoskeletal Proteins in the Liver of Growing Pigs - a Proteomic Study. J Anim Physiol Anim Nutr (Berl) (2017) 101(5):e225–36. doi: 10.1111/jpn.12595
45. Robak P, Ożgo M, Lepczyński A, Herosimczyk A, Barszcz M, Taciak M, et al. Proteome Changes in Renal Cortex and Medulla Induced by Dietary Supplementation With Inulin-Type Fructans in Growing Pigs. J Anim Physiol Anim Nutr (Berl) (2019) 103(6):1837–47. doi: 10.1111/jpn.13170
46. Lepczyński A, Herosimczyk A, Barszcz M, Ożgo M, Michałek K, Grabowska M, et al. Diet Supplemented Either With Dried Chicory Root or Chicory Inulin Significantly Influence Kidney and Liver Mineral Content and Antioxidative Capacity in Growing Pigs. Animal (2021) 15(2):100129. doi: 10.1016/j.animal.2020.100129
47. Wang T, Yao W, Xia J, Li J, Shao Y, Huang F. Dietary Supplementation With Garcinol During Late Gestation and Lactation Facilitates Acid-Base Balance and Improves the Performance of Sows and Newborn Piglets1. J Anim Sci (2019) 97(11):4557–66. doi: 10.1093/jas/skz292
48. Ariza-Nieto C, Bandrick M, Baidoo SK, Anil L, Molitor TW, Hathaway MR. Effect of Dietary Supplementation of Oregano Essential Oils to Sows on Colostrum and Milk Composition, Growth Pattern and Immune Status of Suckling Pigs. J Anim Sci (2011) 89(4):1079–89. doi: 10.2527/jas.2010-3514
49. Forte C, Ranucci D, Beghelli D, Branciari R, Acuti G, Todini L, et al. Dietary Integration With Oregano (Origanum Vulgare L.) Essential Oil Improves Growth Rate and Oxidative Status in Outdoor-Reared, But Not Indoor-Reared, Pigs. J Anim Physiol Anim Nutr (Berl) (2017) 101(5):e352–61. doi: 10.1111/jpn.12612
50. Sampath V, Shanmugam S, Park JH, Kim IH. The Effect of Black Pepper (Piperine) Extract Supplementation on Growth Performance, Nutrient Digestibility, Fecal Microbial, Fecal Gas Emission, and Meat Quality of Finishing Pigs. Anim (Basel) (2020) 10(11):1965. doi: 10.3390/ani10111965
51. Li S, Wang H, Wang X, Wang Y, Feng J. Betaine Affects Muscle Lipid Metabolism via Regulating the Fatty Acid Uptake and Oxidation in Finishing Pig. J Anim Sci Biotechnol (2017) 8:72. doi: 10.1186/s40104-017-0200-6
52. Chen XL, Wang N, Tian ML, Wang L, Liu T, Zhang XW, et al. Dietary Linseed Oil in the Maternal Diet Affects Immunoglobulins, Tissue Fatty Acid Composition and Expression of Lipid Metabolism-Related Genes in Piglets. J Anim Physiol Anim Nutr (Berl) (2017) 101(5):e257–65. doi: 10.1111/jpn.12599
53. Kang P, Wang Y, Li X, Wan Z, Wang X, Zhu H, et al. Effect of Flaxseed Oil on Muscle Protein Loss and Carbohydrate Oxidation Impairment in a Pig Model After Lipopolysaccharide Challenge. Br J Nutr (2020) 123(8):859–69. doi: 10.1017/S0007114519002393
54. Aron PM, Kennedy JA. Flavan-3-Ols: Nature, Occurrence and Biological Activity. Mol Nutr Food Res (2008) 52(1):79–104. doi: 10.1002/mnfr.200700137
55. Dizdaroglu M, Jaruga P. Mechanisms of Free Radical-Induced Damage to DNA. Free Radic Res (2012) 46(4):382–419. doi: 10.3109/10715762.2011.653969
56. He Y, Sang Z, Zhuo Y, Wang X, Guo Z, He L, et al. Transport Stress Induces Pig Jejunum Tissue Oxidative Damage and Results in Autophagy/Mitophagy Activation. J Anim Physiol Anim Nutr (Berl) (2019) 103(5):1521–9. doi: 10.1111/jpn.13161
57. Chen X, Li X, Xu X, Li L, Liang N, Zhang L, et al. Ferroptosis and Cardiovascular Disease: Role of Free Radical-Induced Lipid Peroxidation. Free Radic Res (2021) 55(4):1–11. doi: 10.1080/10715762.2021.1876856
58. Li H, He Y, Zhang C, Ba T, Guo Z, Zhuo Y, et al. NOX1 Down-Regulation Attenuated the Autophagy and Oxidative Damage in Pig Intestinal Epithelial Cell Following Transcriptome Analysis of Transport Stress. Gene (2020) 763:145071. doi: 10.1016/j.gene.2020.145071
59. Zhang H, Xu Z, Zhao H, Wang X, Pang J, Li Q, et al. Anthocyanin Supplementation Improves Anti-Oxidative and Anti-Inflammatory Capacity in a Dose-Response Manner in Subjects With Dyslipidemia. Redox Biol (2020) 32:101474–4. doi: 10.1016/j.redox.2020.101474
60. Wani AR, Yadav K, Khursheed A, Rather MA. An Updated and Comprehensive Review of the Antiviral Potential of Essential Oils and Their Chemical Constituents With Special Focus on Their Mechanism of Action Against Various Influenza and Coronaviruses. Microb Pathog (2021) 152:104620. doi: 10.1016/j.micpath.2020.104620
61. Hu J, Nie Y, Chen S, Xie C, Fan Q, Wang Z, et al. Leucine Reduces Reactive Oxygen Species Levels via an Energy Metabolism Switch by Activation of the mTOR-HIF-1α Pathway in Porcine Intestinal Epithelial Cells. Int J Biochem Cell Biol (2017) 89:42–56. doi: 10.1016/j.biocel.2017.05.026
62. Hall HN, Wilkinson DJ, Le Bon M. Oregano Essential Oil Improves Piglet Health and Performance Through Maternal Feeding and Is Associated With Changes in the Gut Microbiota. Anim Microbiome (2021) 3(1):2. doi: 10.1186/s42523-020-00064-2
63. Wei HK, Xue HX, Zhou ZX, Peng J. A Carvacrol-Thymol Blend Decreased Intestinal Oxidative Stress and Influenced Selected Microbes Without Changing the Messenger RNA Levels of Tight Junction Proteins in Jejunal Mucosa of Weaning Piglets. Animal (2017) 11(2):193–201. doi: 10.1017/S1751731116001397
64. Toschi A, Tugnoli B, Rossi B, Piva A, Grilli E. Thymol Modulates the Endocannabinoid System and Gut Chemosensing of Weaning Pigs. BMC Vet Res (2020) 16(1):289. doi: 10.1186/s12917-020-02516-y
65. Zhuang Y, Wu H, Wang X, He J, He S, Yin Y. Resveratrol Attenuates Oxidative Stress-Induced Intestinal Barrier Injury Through PI3K/Akt-Mediated Nrf2 Signaling Pathway. Oxid Med Cell Longev (2019) 2019:7591840. doi: 10.1155/2019/7591840
66. Choi J, Wang L, Ammeter E, Lahaye L, Liu S, Nyachoti M, et al. Evaluation of Lipid Matrix Microencapsulation for Intestinal Delivery of Thymol in Weaned Pigs. Transl Anim Sci (2020) 4(1):411–22. doi: 10.1093/tas/txz176
67. Yun SJ, Bae GS, Park JH, Song TH, Choi A, Ryu BY, et al. Antioxidant Effects of Cultured Wild Ginseng Root Extracts on the Male Reproductive Function of Boars and Guinea Pigs. Anim Reprod Sci (2016) 170:51–60. doi: 10.1016/j.anireprosci.2016.04.002
68. Liu Q, Duan RJ, Zhou YF, Wei HK, Peng J, Li JL. Supplementing Oregano Essential Oil to Boar Diet With Strengthened Fish Oil: Effects on Semen Antioxidant Status and Semen Quality Parameters. Andrologia (2017) 49(10):e12764. doi: 10.1111/and.12764
69. Zhang JQ, Wang XW, Chen JF, Ren QL, Wang J, Gao BW, et al. Grape Seed Procyanidin B2 Protects Porcine Ovarian Granulosa Cells Against Oxidative Stress-Induced Apoptosis by Upregulating Let-7a Expression. Oxid Med Cell Longev (2019) 2019:1076512. doi: 10.1155/2019/1076512
70. Klingensmith NJ, Coopersmith CM. The Gut as the Motor of Multiple Organ Dysfunction in Critical Illness. Crit Care Clin (2016) 32(2):203–12. doi: 10.1016/j.ccc.2015.11.004
71. Otani S, Coopersmith CM. Gut Integrity in Critical Illness. J Intensive Care (2019) 7:17. doi: 10.1186/s40560-019-0372-6
72. Sweeney T, O'Doherty JV. Marine Macroalgal Extracts to Maintain Gut Homeostasis in the Weaning Piglet. Domest Anim Endocrinol (2016) 56:S84–9. doi: 10.1016/j.domaniend.2016.02.002
73. Martinez G, Dieguez SN, Fernandez Paggi MB, Riccio MB, Perez Gaudio DS, Rodriguez E, et al. Effect of Fosfomycin, Cynara Scolymus Extract, Deoxynivalenol and Their Combinations on Intestinal Health of Weaned Piglets. Anim Nutr (2019) 5(4):386–95. doi: 10.1016/j.aninu.2019.08.001
74. Zhao P, Piao X, Zeng Z, Li P, Xu X, Wang H. Effect of Forsythia Suspensa Extract and Chito-Oligosaccharide Alone or in Combination on Performance, Intestinal Barrier Function, Antioxidant Capacity and Immune Characteristics of Weaned Piglets. Anim Sci J (2017) 88(6):854–62. doi: 10.1111/asj.12656
75. Su G, Zhou X, Wang Y, Chen D, Chen G, Li Y, et al. Dietary Supplementation of Plant Essential Oil Improves Growth Performance, Intestinal Morphology and Health in Weaned Pigs. J Anim Physiol Anim Nutr (Berl) (2020) 104(2):579–89. doi: 10.1111/jpn.13271
76. Liu Y, Song M, Che TM, Lee JJ, Bravo D, Maddox CW, et al. Dietary Plant Extracts Modulate Gene Expression Profiles in Ileal Mucosa of Weaned Pigs After an Escherichia Coli Infection. J Anim Sci (2014) 92(5):2050–62. doi: 10.2527/jas.2013-6422
77. Cairo PLG, Gois FD, Sbardella M, Silveira H, de Oliveira RM, Allaman IB, et al. Effects of Dietary Supplementation of Red Pepper (Schinus Terebinthifolius Raddi) Essential Oil on Performance, Small Intestinal Morphology and Microbial Counts of Weanling Pigs. J Sci Food Agric (2018) 98(2):541–8. doi: 10.1002/jsfa.8494
78. Chen J, Kang B, Zhao Y, Yao K, Fu C. Effects of Natural Dietary Supplementation With Macleaya Cordata Extract Containing Sanguinarine on Growth Performance and Gut Health of Early-Weaned Piglets. J Anim Physiol Anim Nutr (Berl) (2018) 102(6):1666–74. doi: 10.1111/jpn.12976
79. Mao X, Hu H, Xiao X, Chen D, Yu B, He J, et al. Lentinan Administration Relieves Gut Barrier Dysfunction Induced by Rotavirus in a Weaned Piglet Model. Food Funct (2019) 10(4):2094–101. doi: 10.1039/c8fo01764f
80. Dall'Aglio C, Mercati F, Faeti V, Acuti G, Trabalza Marinucci M, De Felice E, et al. Immuno- and Glyco-Histochemistry as a Tool to Evaluate the Oregano Supplemented Feed Effects in Pig Gut. Eur J Histochem (2020) 64(1):3110. doi: 10.4081/ejh.2020.3110
81. Yang M, Yin Y, Wang F, Bao X, Long L, Tan B, et al. Effects of Dietary Rosemary Extract Supplementation on Growth Performance, Nutrient Digestibility, Antioxidant Capacity, Intestinal Morphology, and Microbiota of Weaning Pigs. J Anim Sci (2021) 99(9):skab237. doi: 10.1093/jas/skab237
82. Ortiz R, Orozco A, Ordaz G, Lopez M, Perez RE. Effect of the Addition of Cactus (O. Ficus-Indica) to the Lactating Sows' Diet on Piglet Development at Lactation and Post-Weaning. J Adv Vet Anim Res (2019) 6(3):290–9. doi: 10.5455/javar.2019.f347
83. Zou Y, Xiang Q, Wang J, Peng J, Wei H. Oregano Essential Oil Improves Intestinal Morphology and Expression of Tight Junction Proteins Associated With Modulation of Selected Intestinal Bacteria and Immune Status in a Pig Model. BioMed Res Int (2016) 2016:5436738–5436738. doi: 10.1155/2016/5436738
84. Choi J, Wang L, Liu S, Lu P, Zhao X, Liu H, et al. Effects of a Microencapsulated Formula of Organic Acids and Essential Oils on Nutrient Absorption, Immunity, Gut Barrier Function, and Abundance of Enterotoxigenic Escherichia Coli F4 in Weaned Piglets Challenged With E. Coli F4. J Anim Sci (2020) 98(9):skaa259. doi: 10.1093/jas/skaa259
85. Wang M, Huang H, Hu Y, Liu Y, Zeng X, Zhuang Y, et al. Effects of Dietary Supplementation With Herbal Extract Mixture on Growth Performance, Organ Weight and Intestinal Morphology in Weaning Piglets. J Anim Physiol Anim Nutr (Berl) (2020) 104(5):1462–70. doi: 10.1111/jpn.13422
86. Ducatelle R, Goossens E, De Meyer F, Eeckhaut V, Antonissen G, Haesebrouck F, et al. Biomarkers for Monitoring Intestinal Health in Poultry: Present Status and Future Perspectives. Vet Res (2018) 49(1):43. doi: 10.1186/s13567-018-0538-6
87. Xu B, Yan Y, Huang J, Yin B, Pan Y, Ma L. Cortex Phellodendri Extract's Anti-Diarrhea Effect in Mice Related to Its Modification of Gut Microbiota. BioMed Pharmacother (2020) 123:109720. doi: 10.1016/j.biopha.2019.109720
88. Kamada N, Seo SU, Chen GY, Núñez G. Role of the Gut Microbiota in Immunity and Inflammatory Disease. Nat Rev Immunol (2013) 13(5):321–35. doi: 10.1038/nri3430
89. Belkaid Y, Hand TW. Role of the Microbiota in Immunity and Inflammation. Cell (2014) 157(1):121–41. doi: 10.1016/j.cell.2014.03.011
90. Zou TD, Deng CX, Wang ZR, Ye YL, You JM. Dietary Alanyl-Glutamine Improves Growth Performance of Weaned Piglets Through Maintaining Intestinal Morphology and Digestion-Absorption Function. Animal (2019) 13(9):1826–33. doi: 10.1017/S1751731119000223
91. Tang W, Chen D, Yu B, He J, Huang Z, Zheng P, et al. Capsulized Faecal Microbiota Transplantation Ameliorates Post-Weaning Diarrhea by Modulating the Gut Microbiota in Piglets. Vet Res (2020) 51(1):55. doi: 10.1186/s13567-020-00779-9
92. Yin J, Li F, Kong X, Wen C, Guo Q, Zhang L, et al. Dietary Xylo-Oligosaccharide Improves Intestinal Functions in Weaned Piglets. Food Funct (2019) 10(5):2701–9. doi: 10.1039/c8fo02485e
93. Dadi TH, Vahjen W, Zentek J, Melzig MF, Granica S, Piwowarski JP. Lythrum Salicaria L. Herb and Gut Microbiota of Healthy Post-Weaning Piglets. Focus on Prebiotic Properties and Formation of Postbiotic Metabolites in Ex Vivo Cultures. J Ethnopharmacol (2020) 261:113073. doi: 10.1016/j.jep.2020.113073
94. Xu X, Pan Y, Xu B, Yan Y, Yin B, Wang Y, et al. Effects of Cortex Phellodendri Extract on Post-Weaning Piglets Diarrhea. Vet Med Sci (2020) 6(4):901–9. doi: 10.1002/vms3.304
95. Yi D, Fang Q, Hou Y, Wang L, Xu H, Wu T, et al. Dietary Supplementation With Oleum Cinnamomi Improves Intestinal Functions in Piglets. Int J Mol Sci (2018) 19(5):1284. doi: 10.3390/ijms19051284
96. Han M, Song P, Huang C, Rezaei A, Farrar S, Brown MA, et al. Dietary Grape Seed Proanthocyanidins (GSPs) Improve Weaned Intestinal Microbiota and Mucosal Barrier Using a Piglet Model. Oncotarget (2016) 7(49):80313–26. doi: 10.18632/oncotarget.13450
97. Wu Y, Ma N, Song P, He T, Levesque C, Bai Y, et al. Grape Seed Proanthocyanidin Affects Lipid Metabolism via Changing Gut Microflora and Enhancing Propionate Production in Weaned Pigs. J Nutr (2019) 149(9):1523–32. doi: 10.1093/jn/nxz102
98. Qu H, Huang Y, Shi Y, Liu Y, Wu S, Bao W. Effect of Bamboo Vinegar Powder as an Antibiotic Alternative on the Digesta Bacteria Communities of Finishing Pigs. Can J Microbiol (2018) 64(10):732–43. doi: 10.1139/cjm-2018-0058
99. Shimazu T, Borjigin L, Katoh K, Roh SG, Kitazawa H, Abe K, et al. Addition of Wakame Seaweed (Undaria Pinnatifida) Stalk to Animal Feed Enhances Immune Response and Improves Intestinal Microflora in Pigs. Anim Sci J (2019) 90(9):1248–60. doi: 10.1111/asj.13274
100. Barszcz M, Taciak M, Skomial J. The Effects of Inulin, Dried Jerusalem Artichoke Tuber and a Multispecies Probiotic Preparation on Microbiota Ecology and Immune Status of the Large Intestine in Young Pigs. Arch Anim Nutr (2016) 70(4):278–92. doi: 10.1080/1745039X.2016.1184368
101. Allahverdiyev A, Duran N, Ozguven M, Koltas S. Antiviral Activity of the Volatile Oils of Melissa Officinalis L. Against Herpes Simplex Virus Type-2. Phytomedicine (2004) 11(7-8):657–61. doi: 10.1016/j.phymed.2003.07.014
102. Kim K, Ji P, Song M, Che TM, Bravo D, Pettigrew JE, et al. Dietary Plant Extracts Modulate Gene Expression Profiles in Alveolar Macrophages of Pigs Experimentally Infected With Porcine Reproductive and Respiratory Syndrome Virus. J Anim Sci Biotechnol (2020) 11:74. doi: 10.1186/s40104-020-00475-w
103. Du T, Shi Y, Xiao S, Li N, Zhao Q, Zhang A, et al. Curcumin is a Promising Inhibitor of Genotype 2 Porcine Reproductive and Respiratory Syndrome Virus Infection. BMC Vet Res (2017) 13(1):298. doi: 10.1186/s12917-017-1218-x
104. Liu X, Song Z, Bai J, Nauwynck H, Zhao Y, Jiang P. Xanthohumol Inhibits PRRSV Proliferation and Alleviates Oxidative Stress Induced by PRRSV via the Nrf2-HMOX1 Axis. Vet Res (2019) 50(1):61. doi: 10.1186/s13567-019-0679-2
105. Lo DY, Chien MS, Yeh KS, Koge K, Lin CC, Hsuan SL, et al. Effects of Sugar Cane Extract on Pseudorabies Virus Challenge of Pigs. J Vet Med Sci (2006) 68(3):219–25. doi: 10.1292/jvms.68.219
106. Tong C, Chen Z, Liu F, Qiao Y, Chen T, Wang X. Antiviral Activities of Radix Isatidis Polysaccharide Against Pseudorabies Virus in Swine Testicle Cells. BMC Complement Med Ther (2020) 20(1):48. doi: 10.1186/s12906-020-2838-4
107. Wang P, Bai J, Liu X, Wang M, Wang X, Jiang P. Tomatidine Inhibits Porcine Epidemic Diarrhea Virus Replication by Targeting 3CL Protease. Vet Res (2020) 51(1):136. doi: 10.1186/s13567-020-00865-y
108. Wu M, Zhang Q, Yi D, Wu T, Chen H, Guo S, et al. Quantitative Proteomic Analysis Reveals Antiviral and Anti-Inflammatory Effects of Puerarin in Piglets Infected With Porcine Epidemic Diarrhea Virus. Front Immunol (2020) 11:169. doi: 10.3389/fimmu.2020.00169
109. Gómez-García M, Puente H, Argüello H, Mencía-Ares Ó, Rubio P, Carvajal A. In Vitro Assessment of Antiviral Effect of Natural Compounds on Porcine Epidemic Diarrhea Coronavirus. Front Vet Sci (2021) 8:652000. doi: 10.3389/fvets.2021.652000
110. Gebhardt JT, Woodworth JC, Jones CK, Tokach MD, Gauger PC, Main RG, et al. Determining the Impact of Commercial Feed Additives as Potential Porcine Epidemic Diarrhea Virus Mitigation Strategies as Determined by Polymerase Chain Reaction Analysis and Bioassay. Trans Anim Sci (2018) 3(1):93–102. doi: 10.1093/tas/txy100
111. Xu Y, Zheng J, Sun P, Guo J, Zheng X, Sun Y, et al. Cepharanthine and Curcumin Inhibited Mitochondrial Apoptosis Induced by PCV2. BMC Vet Res (2020) 16(1):345. doi: 10.1186/s12917-020-02568-0
112. Liu D, Xu J, Qian G, Hamid M, Gan F, Chen X, et al. Selenizing Astragalus Polysaccharide Attenuates PCV2 Replication Promotion Caused by Oxidative Stress Through Autophagy Inhibition via PI3K/AKT Activation. Int J Biol Macromolecules (2018) 108:350–9. doi: 10.1016/j.ijbiomac.2017.12.010
113. Fu YF, Jiang LH, Zhao WD, Xi-Nan M, Huang SQ, Yang J, et al. Immunomodulatory and Antioxidant Effects of Total Flavonoids of Spatholobus Suberectus Dunn on PCV2 Infected Mice. Sci Rep (2017) 7(1):8676. doi: 10.1038/s41598-017-09340-9
114. Babikian HY, Jha RK, Truong QL, Nguyen LT, Babikyan Y, Nguyen HT, et al. Novel Formulation With Essential Oils as a Potential Agent to Minimize African Swine Fever Virus Transmission in an In Vivo Trial in Swine. Vet World (2021) 14(7):1853–66. doi: 10.14202/vetworld.2021.1853-1866
115. Gomez-Garcia M, Arguello H, Puente H, Mencia-Ares O, Gonzalez S, Miranda R, et al. In-Depth In Vitro Evaluation of the Activity and Mechanisms of Action of Organic Acids and Essential Oils Against Swine Enteropathogenic Bacteria. Front Vet Sci (2020) 7:572947. doi: 10.3389/fvets.2020.572947
116. Xi QY, Jiang Y, Zhao S, Zeng B, Wang F, Wang LN, et al. Effect of Ginseng Polysaccharides on the Immunity and Growth of Piglets by Dietary Supplementation During Late Pregnancy and Lactating Sows. Anim Sci J (2017) 88(6):863–72. doi: 10.1111/asj.12678
117. Xing Y, Wu Y, Mao C, Sun D, Guo S, Xu Y, et al. Water Extract of Artemisia Ordosica Enhances Antioxidant Capability and Immune Response Without Affecting Growth Performance in Weanling Piglets. J Anim Physiol Anim Nutr (Berl) (2019) 103(6):1848–56. doi: 10.1111/jpn.13171
118. Girard M, Hu D, Pradervand N, Neuenschwander S, Bee G. Chestnut Extract But Not Sodium Salicylate Decreases the Severity of Diarrhea and Enterotoxigenic Escherichia Coli F4 Shedding in Artificially Infected Piglets. PloS One (2020) 15(2):e0214267. doi: 10.1371/journal.pone.0214267
119. Yi Q, Liu J, Zhang Y, Qiao H, Chen F, Zhang S, et al. Anethole Attenuates Enterotoxigenic Escherichia Coli-Induced Intestinal Barrier Disruption and Intestinal Inflammation via Modification of TLR Signaling and Intestinal Microbiota. Front Microbiol (2021) 12:647242. doi: 10.3389/fmicb.2021.647242
120. Famuyide IM, Aro AO, Fasina FO, Eloff JN, McGaw LJ. Antibacterial Activity and Mode of Action of Acetone Crude Leaf Extracts of Under-Investigated Syzygium and Eugenia (Myrtaceae) Species on Multidrug Resistant Porcine Diarrheagenic Escherichia Coli. BMC Vet Res (2019) 15(1):162. doi: 10.1186/s12917-019-1914-9
121. Hu Q, Zhuo Z, Fang S, Zhang Y, Feng J. Phytosterols Improve Immunity and Exert Anti-Inflammatory Activity in Weaned Piglets. J Sci Food Agric (2017) 97(12):4103–9. doi: 10.1002/jsfa.8277
122. Fu S, Zhuang F, Guo L, Qiu Y, Xiong J, Ye C, et al. Effect of Baicalin-Aluminum Complexes on Fecal Microbiome in Piglets. Int J Mol Sci (2019) 20(10):2390. doi: 10.3390/ijms20102390
123. Huang C, Wang Y, He X, Jiao N, Zhang X, Qiu K, et al. The Involvement of NF-κb/P38 Pathways in Scutellaria Baicalensis Extracts Attenuating of Escherichia Coli K88-Induced Acute Intestinal Injury in Weaned Piglets. Br J Nutr (2019) 122(2):152–61. doi: 10.1017/s0007114519000928
124. Fu S, Liu H, Chen X, Qiu Y, Ye C, Liu Y, et al. Baicalin Inhibits Haemophilus Parasuis-Induced High-Mobility Group Box 1 Release During Inflammation. Int J Mol Sci (2018) 19(5):1307. doi: 10.3390/ijms19051307
125. Guo L, Guo J, Liu H, Zhang J, Chen X, Qiu Y, et al. Tea Polyphenols Suppress Growth and Virulence-Related Factors of Haemophilus Parasuis. J Vet Med Sci (2018) 80(7):1047–53. doi: 10.1292/jvms.18-0085
126. López Nadal A, Ikeda-Ohtsubo W, Sipkema D, Peggs D, McGurk C, Forlenza M, et al. Feed, Microbiota, and Gut Immunity: Using the Zebrafish Model to Understand Fish Health. Front Immunol (2020) 11:114. doi: 10.3389/fimmu.2020.00114
127. Firmino JP, Vallejos-Vidal E, Balebona MC, Ramayo-Caldas Y, Cerezo IM, Salomón R, et al. Diet, Immunity, and Microbiota Interactions: An Integrative Analysis of the Intestine Transcriptional Response and Microbiota Modulation in Gilthead Seabream (Sparus Aurata) Fed an Essential Oils-Based Functional Diet. Front Immunol (2021) 12:625297. doi: 10.3389/fimmu.2021.625297
128. Liew PX, Kubes P. The Neutrophil's Role During Health and Disease. Physiol Rev (2019) 99(2):1223–48. doi: 10.1152/physrev.00012.2018
129. Kuralkar P, Kuralkar SV. Role of Herbal Products in Animal Production - An Updated Review. J Ethnopharmacol (2021) 278:114246. doi: 10.1016/j.jep.2021.114246
130. Zhao H, Zhao N, Zheng P, Xu X, Liu M, Luo D, et al. Prevention and Treatment of Osteoporosis Using Chinese Medicinal Plants: Special Emphasis on Mechanisms of Immune Modulation. J Immunol Res (2018) 2018:6345857. doi: 10.1155/2018/6345857
131. Tasneem S, Liu B, Li B, Choudhary MI, Wang W. Molecular Pharmacology of Inflammation: Medicinal Plants as Anti-Inflammatory Agents. Pharmacol Res (2019) 139:126–40. doi: 10.1016/j.phrs.2018.11.001
132. Roome T, Dar A, Naqvi S, Ali S, Choudhary MI. Aegiceras Corniculatum Extract Suppresses Initial and Late Phases of Inflammation in Rat Paw and Attenuates the Production of Eicosanoids in Rat Neutrophils and Human Platelets. J Ethnopharmacol (2008) 120(2):248–54. doi: 10.1016/j.jep.2008.08.025
133. Huang CW, Lee TT, Shih YC, Yu B. Effects of Dietary Supplementation of Chinese Medicinal Herbs on Polymorphonuclear Neutrophil Immune Activity and Small Intestinal Morphology in Weanling Pigs. J Anim Physiol Anim Nutr (Berl) (2012) 96(2):285–94. doi: 10.1111/j.1439-0396.2011.01151.x
134. Stelter K, Frahm J, Paulsen J, Berk A, Kleinwächter M, Selmar D, et al. Effects of Oregano on Performance and Immunmodulating Factors in Weaned Piglets. Arch Anim Nutr (2013) 67(6):461–76. doi: 10.1080/1745039x.2013.858897
135. Cappelli K, Sabino M, Trabalza-Marinucci M, Acuti G, Capomaccio S, Menghini L, et al. Differential Effects of Dietary Oregano Essential Oil on the Inflammation Related Gene Expression in Peripheral Blood Mononuclear Cells From Outdoor and Indoor Reared Pigs. Front Vet Sci (2021) 8:602811. doi: 10.3389/fvets.2021.602811
136. Lo Verso L, Talbot G, Morissette B, Guay F, Matte JJ, Farmer C, et al. The Combination of Nutraceuticals and Functional Feeds as Additives Modulates Gut Microbiota and Blood Markers Associated With Immune Response and Health in Weanling Piglets. J Anim Sci (2020) 98(8):skaa208. doi: 10.1093/jas/skaa208
137. Liu Y, Song M, Che TM, Almeida JA, Lee JJ, Bravo D, et al. Dietary Plant Extracts Alleviate Diarrhea and Alter Immune Responses of Weaned Pigs Experimentally Infected With a Pathogenic Escherichia Coli. J Anim Sci (2013) 91(11):5294–306. doi: 10.2527/jas.2012-6194
138. Gräber T, Kluge H, Granica S, Horn G, Kalbitz J, Brandsch C, et al. Agrimonia Procera Exerts Antimicrobial Effects, Modulates the Expression of Defensins and Cytokines in Colonocytes and Increases the Immune Response in Lipopolysaccharide-Challenged Piglets. BMC Vet Res (2018) 14(1):346–6. doi: 10.1186/s12917-018-1680-0
139. Kantas D, Papatsiros VG, Tassis PD, Athanasiou LV, Tzika ED. Effect of a Natural Feed Additive (Macleaya Cordata), Containing Sanguinarine, on the Performance and Health Status of Weaning Pigs. Anim Sci J (2015) 86(1):92–8. doi: 10.1111/asj.12240
140. Peterfalvi A, Miko E, Nagy T, Reger B, Simon D, Miseta A, et al. Much More Than a Pleasant Scent: A Review on Essential Oils Supporting the Immune System. Molecules (2019) 24(24):4530. doi: 10.3390/molecules24244530
141. Holanda DM, Kim SW. Mycotoxin Occurrence, Toxicity, and Detoxifying Agents in Pig Production With an Emphasis on Deoxynivalenol. Toxins (Basel) (2021) 13(2):171. doi: 10.3390/toxins13020171
142. Marin S, Ramos AJ, Cano-Sancho G, Sanchis V. Mycotoxins: Occurrence, Toxicology, and Exposure Assessment. Food Chem Toxicol (2013) 60:218–37. doi: 10.1016/j.fct.2013.07.047
143. Reddy KE, Kim M, Kim KH, Ji SY, Baek Y, Chun JL, et al. Effect of Commercially Purified Deoxynivalenol and Zearalenone Mycotoxins on Microbial Diversity of Pig Cecum Contents. Anim Biosci (2021) 34(2):243–55. doi: 10.5713/ajas.20.0137
144. Taranu I, Hermenean A, Bulgaru C, Pistol GC, Ciceu A, Grosu IA, et al. Diet Containing Grape Seed Meal by-Product Counteracts AFB1 Toxicity in Liver of Pig After Weaning. Ecotoxicol Environ Saf (2020) 203:110899. doi: 10.1016/j.ecoenv.2020.110899
145. Guindon-Kezis KA, Mulder JE, Massey TE. In Vivo Treatment With Aflatoxin B1 Increases DNA Oxidation, Base Excision Repair Activity and 8-Oxoguanine DNA Glycosylase 1 Levels in Mouse Lung. Toxicology (2014) 321:21–6. doi: 10.1016/j.tox.2014.03.004
146. Taranu I, Marin DE, Palade M, Pistol GC, Chedea VS, Gras MA, et al. Assessment of the Efficacy of a Grape Seed Waste in Counteracting the Changes Induced by Aflatoxin B1 Contaminated Diet on Performance, Plasma, Liver and Intestinal Tissues of Pigs After Weaning. Toxicon (2019) 162:24–31. doi: 10.1016/j.toxicon.2019.02.020
147. Grosu IA, Pistol GC, Taranu I, Marin DE. The Impact of Dietary Grape Seed Meal on Healthy and Aflatoxin B1 Afflicted Microbiota of Pigs After Weaning. Toxins (Basel) (2019) 11(1):25. doi: 10.3390/toxins11010025
148. Long H, Xin Z, Zhang F, Zhai Z, Ni X, Chen J, et al. The Cytoprotective Effects of Dihydromyricetin and Associated Metabolic Pathway Changes on Deoxynivalenol Treated IPEC-J2 Cells. Food Chem (2021) 338:128116. doi: 10.1016/j.foodchem.2020.128116
149. Liu D, Su J, Lin J, Qian G, Chen X, Song S, et al. Activation of AMPK-Dependent SIRT-1 by Astragalus Polysaccharide Protects Against Ochratoxin A-Induced Immune Stress In Vitro and In Vivo. Int J Biol Macromol (2018) 120(Pt A):683–92. doi: 10.1016/j.ijbiomac.2018.08.156
150. Kim SW, Fan MZ, Applegate TJ. Nonruminant Nutrition Symposium on Natural Phytobiotics for Health of Young Animals and Poultry: Mechanisms and Application. J Anim Sci (2008) 86(14 Suppl):E138–139. doi: 10.2527/jas.2007-0769
151. Holanda DM, Kim YI, Parnsen W, Kim SW. Phytobiotics With Adsorbent to Mitigate Toxicity of Multiple Mycotoxins on Health and Growth of Pigs. Toxins (2021) 13(7):442. doi: 10.3390/toxins13070442
152. Wang X, Shen Y, Thakur K, Han J, Zhang J-G, Hu F, et al. Antibacterial Activity and Mechanism of Ginger Essential Oil Against Escherichia Coli and Staphylococcus Aureus. Molecules (Basel Switzerland) (2020) 25(17):3955. doi: 10.3390/molecules25173955
153. Guglielmi P, Pontecorvi V, Rotondi G. Natural Compounds and Extracts as Novel Antimicrobial Agents. Expert Opin Ther Pat (2020) 30(12):949–62. doi: 10.1080/13543776.2020.1853101
154. Wang L, Huang Y, Yin G, Wang J, Wang P, Chen Z-Y, et al. Antimicrobial Activities of Asian Ginseng, American Ginseng, and Notoginseng. Phytother Res (2020) 34(6):1226–36. doi: 10.1002/ptr.6605
155. Vasconcelos NG, Croda J, Simionatto S. Antibacterial Mechanisms of Cinnamon and Its Constituents: A Review. Microbial Pathogen (2018) 120:198–203. doi: 10.1016/j.micpath.2018.04.036
156. Hemaiswarya S, Doble M. Synergistic Interaction of Eugenol With Antibiotics Against Gram Negative Bacteria. Phytomedicine (2009) 16(11):997–1005. doi: 10.1016/j.phymed.2009.04.006
157. Gill AO, Holley RA. Inhibition of Membrane Bound ATPases of Escherichia Coli and Listeria Monocytogenes by Plant Oil Aromatics. Int J Food Microbiol (2006) 111(2):170–4. doi: 10.1016/j.ijfoodmicro.2006.04.046
158. Di Pasqua R, Betts G, Hoskins N, Edwards M, Ercolini D, Mauriello G. Membrane Toxicity of Antimicrobial Compounds From Essential Oils. J Agric Food Chem (2007) 55(12):4863–70. doi: 10.1021/jf0636465
159. Coddens A, Loos M, Vanrompay D, Remon JP, Cox E. Cranberry Extract Inhibits In Vitro Adhesion of F4 and F18(+)Escherichia Coli to Pig Intestinal Epithelium and Reduces In Vivo Excretion of Pigs Orally Challenged With F18(+) Verotoxigenic E. Coli. Vet Microbiol (2017) 202:64–71. doi: 10.1016/j.vetmic.2017.01.019
160. Chojnacka K, Witek-Krowiak A, Skrzypczak D, Mikula K, Młynarz P. Phytochemicals Containing Biologically Active Polyphenols as an Effective Agent Against Covid-19-Inducing Coronavirus. J Funct Foods (2020) 73:104146. doi: 10.1016/j.jff.2020.104146
161. Yang Y, Islam MS, Wang J, Li Y, Chen X. Traditional Chinese Medicine in the Treatment of Patients Infected With 2019-New Coronavirus (SARS-CoV-2): A Review and Perspective. Int J Biol Sci (2020) 16(10):1708–17. doi: 10.7150/ijbs.45538
162. Huang YF, Bai C, He F, Xie Y, Zhou H. Review on the Potential Action Mechanisms of Chinese Medicines in Treating Coronavirus Disease 2019 (COVID-19). Pharmacol Res (2020) 158:104939. doi: 10.1016/j.phrs.2020.104939
163. Guan W, Li J, Chen Q, Jiang Z, Zhang R, Wang X, et al. Pterodontic Acid Isolated From Laggera Pterodonta Inhibits Viral Replication and Inflammation Induced by Influenza A Virus. Molecules (2017) 22(10):1738. doi: 10.3390/molecules22101738
164. Li E, Sun N, Zhao JX, Sun YG, Huang JG, Lei HM, et al. In Vitro Evaluation of Antiviral Activity of Tea Seed Saponins Against Porcine Reproductive and Respiratory Syndrome Virus. Antivir Ther (2015) 20(7):743–52. doi: 10.3851/imp2937
165. Xue H, Gan F, Zhang Z, Hu J, Chen X, Huang K. Astragalus Polysaccharides Inhibits PCV2 Replication by Inhibiting Oxidative Stress and Blocking NF-κb Pathway. Int J Biol Macromol (2015) 81:22–30. doi: 10.1016/j.ijbiomac.2015.07.050
166. Xue H, Gan F, Qian G, Hu J, Hao S, Xu J, et al. Astragalus Polysaccharides Attenuate PCV2 Infection by Inhibiting Endoplasmic Reticulum Stress In Vivo and In Vitro. Sci Rep (2017) 7:40440. doi: 10.1038/srep40440
167. Xu Z, Liu Y, Peng P, Liu Y, Huang M, Ma Y, et al. Aloe Extract Inhibits Porcine Epidemic Diarrhea Virus In Vitro and In Vivo. Vet Microbiol (2020) 249:108849–9. doi: 10.1016/j.vetmic.2020.108849
168. Dai YJ, Wan SY, Gong SS, Liu JC, Li F, Kou JP. Recent Advances of Traditional Chinese Medicine on the Prevention and Treatment of COVID-19. Chin J Nat Med (2020) 18(12):881–9. doi: 10.1016/s1875-5364(20)60031-0
169. Shaw P, Chattopadhyay A. Nrf2-ARE Signaling in Cellular Protection: Mechanism of Action and the Regulatory Mechanisms. J Cell Physiol (2020) 235(4):3119–30. doi: 10.1002/jcp.29219
170. Cui G, Wei F, Wei M, Xie L, Lin Z, Feng X. Modulatory Effect of Tagetes Erecta Flowers Essential Oils via Nrf2/HO-1/NF-κb/P65 Axis Mediated Suppression of N-Methyl-N'nitro-N-Nitroguanidine (MNNG) Induced Gastric Cancer in Rats. Mol Cell Biochem (2021) 476(3):1541–54. doi: 10.1007/s11010-020-04005-0
171. Jin CH, Lee HJ, Park YD, Choi DS, Kim DS, Kang SY, et al. Isoegomaketone Inhibits Lipopolysaccharide-Induced Nitric Oxide Production in RAW 264.7 Macrophages Through the Heme Oxygenase-1 Induction and Inhibition of the Interferon-Beta-STAT-1 Pathway. J Agric Food Chem (2010) 58(2):860–7. doi: 10.1021/jf9033333
172. Oeckinghaus A, Hayden MS, Ghosh S. Crosstalk in NF-κb Signaling Pathways. Nat Immunol (2011) 12(8):695–708. doi: 10.1038/ni.2065
173. Yan T, Wang N, Liu B, Wu B, Xiao F, He B, et al. Schisandra Chinensis Ameliorates Depressive-Like Behaviors by Regulating Microbiota-Gut-Brain Axis via Its Anti-Inflammation Activity. Phytother Res (2021) 35(1):289–96. doi: 10.1002/ptr.6799
174. Gil TY, Jin BR, Hong CH, Park JH, An HJ. Astilbe Chinensis Ethanol Extract Suppresses Inflammation in Macrophages via NF-κb Pathway. BMC Complement Med Ther (2020) 20(1):302. doi: 10.1186/s12906-020-03073-5
175. Huang HC, Ho YC, Lim JM, Chang TY, Ho CL, Chang TM. Investigation of the Anti-Melanogenic and Antioxidant Characteristics of Eucalyptus Camaldulensis Flower Essential Oil and Determination of Its Chemical Composition. Int J Mol Sci (2015) 16(5):10470–90. doi: 10.3390/ijms160510470
176. Zhang J, Liu Y, Yang Z, Yang W, Huang L, Xu C, et al. Illicium Verum Extracts and Probiotics With Added Glucose Oxidase Promote Antioxidant Capacity Through Upregulating Hepatic and Jejunal Nrf2/Keap1 of Weaned Piglets. J Anim Sci (2020) 98(3):skaa077. doi: 10.1093/jas/skaa077
177. Feng Y, An Z, Chen H, He X, Wang W, Li X, et al. Ulva Prolifera Extract Alleviates Intestinal Oxidative Stress via Nrf2 Signaling in Weaned Piglets Challenged With Hydrogen Peroxide. Front Immunol (2020) 11:599735. doi: 10.3389/fimmu.2020.599735
178. Hao Z, Li Z, Huo J, Li J, Liu F, Yin P. Effects of Chinese Wolfberry and Astragalus Extract on the Antioxidant Capacity of Tibetan Pig Liver. PloS One (2021) 16(1):e0245749–e0245749. doi: 10.1371/journal.pone.0245749
179. Zou Y, Wang J, Peng J, Wei H. Oregano Essential Oil Induces SOD1 and GSH Expression Through Nrf2 Activation and Alleviates Hydrogen Peroxide-Induced Oxidative Damage in IPEC-J2 Cells. Oxid Med Cell Longev (2016) 2016:5987183. doi: 10.1155/2016/5987183
180. Hui Q, Ammeter E, Liu S, Yang R, Lu P, Lahaye L, et al. Eugenol Attenuates Inflammatory Response and Enhances Barrier Function During Lipopolysaccharide-Induced Inflammation in the Porcine Intestinal Epithelial Cells. J Anim Sci (2020) 98(8):skaa245. doi: 10.1093/jas/skaa245
181. Huang Q, Liu X, Zhao G, Hu T, Wang Y. Potential and Challenges of Tannins as an Alternative to in-Feed Antibiotics for Farm Animal Production. Anim Nutr (Zhongguo xu mu shou yi xue hui) (2018) 4(2):137–50. doi: 10.1016/j.aninu.2017.09.004
182. Ács K, Balázs VL, Kocsis B, Bencsik T, Böszörményi A, Horváth G. Antibacterial Activity Evaluation of Selected Essential Oils in Liquid and Vapor Phase on Respiratory Tract Pathogens. BMC Complement Altern Med (2018) 18(1):227. doi: 10.1186/s12906-018-2291-9
183. Basavegowda N, Patra JK, Baek KH. Essential Oils and Mono/bi/tri-Metallic Nanocomposites as Alternative Sources of Antimicrobial Agents to Combat Multidrug-Resistant Pathogenic Microorganisms: An Overview. Molecules (2020) 25(5):1058. doi: 10.3390/molecules25051058
184. Cromwell GL. Why and How Antibiotics Are Used in Swine Production. Anim Biotechnol (2002) 13(1):7–27. doi: 10.1081/abio-120005767
185. Pasipanodya CN, Tekedza TT, Chatiza FP, Gororo E. Efficacy of Neem (Azadirachta Indica) Aqueous Fruit Extracts Against Sarcoptes Scabiei Var. Suis in Grower Pigs. Trop Anim Health Prod (2021) 53(1):135. doi: 10.1007/s11250-020-02545-7
186. Fu J, Yang Q, Li Y, Li P, Wang L, Li X. A Mechanism by Which Astragalus Polysaccharide Protects Against ROS Toxicity Through Inhibiting the Protein Dephosphorylation of Boar Sperm Preserved at 4 Degrees C. J Cell Physiol (2018) 233(7):5267–80. doi: 10.1002/jcp.26321
187. Zhang G, Zhao J, Dong W, Song X, Zang J, Ni S, et al. Effects of Tea Tree Oil Supplementation on Growth Performance, Antioxidant Capacity, Immune Status and Microbial Community in Weaned Pigs. Arch Anim Nutr (2021) 75(2):121–36. doi: 10.1080/1745039x.2021.1877074
188. Lin B, Yan J, Zhong Z, Zheng X. A Study on the Preparation of Microbial and Nonstarch Polysaccharide Enzyme Synergistic Fermented Maize Cob Feed and Its Feeding Efficiency in Finishing Pigs. BioMed Res Int (2020) 2020:8839148. doi: 10.1155/2020/8839148
189. Olsen KM, Gabler NK, Rademacher CJ, Schwartz KJ, Schweer WP, Gourley GG, et al. The Effects of Group Size and Subtherapeutic Antibiotic Alternatives on Growth Performance and Morbidity of Nursery Pigs: A Model for Feed Additive Evaluation. Transl Anim Sci (2018) 2(3):298–310. doi: 10.1093/tas/txy068
Keywords: pharmacological applications and action mechanisms, alternatives, antibiotics, pig production, phytochemicals
Citation: Li L, Sun X, Zhao D and Dai H (2021) Pharmacological Applications and Action Mechanisms of Phytochemicals as Alternatives to Antibiotics in Pig Production. Front. Immunol. 12:798553. doi: 10.3389/fimmu.2021.798553
Received: 20 October 2021; Accepted: 22 November 2021;
Published: 09 December 2021.
Edited by:
Yangchun Cao, Northwest A and F University, ChinaReviewed by:
Julio Plaza-Diaz, Children’s Hospital of Eastern Ontario (CHEO), CanadaXin Wu, Chinese Academy of Sciences (CAS), China
Copyright © 2021 Li, Sun, Zhao and Dai. This is an open-access article distributed under the terms of the Creative Commons Attribution License (CC BY). The use, distribution or reproduction in other forums is permitted, provided the original author(s) and the copyright owner(s) are credited and that the original publication in this journal is cited, in accordance with accepted academic practice. No use, distribution or reproduction is permitted which does not comply with these terms.
*Correspondence: Hanchuan Dai, ZGFpaGNoQDEyNi5jb20=