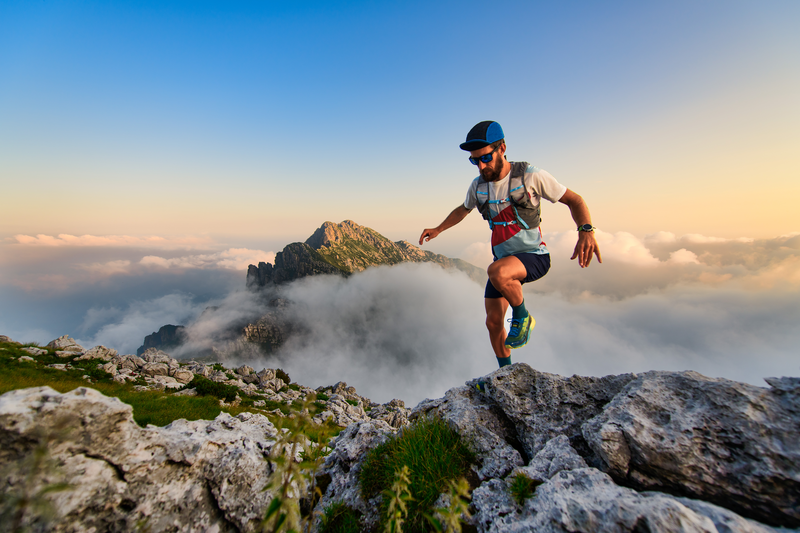
94% of researchers rate our articles as excellent or good
Learn more about the work of our research integrity team to safeguard the quality of each article we publish.
Find out more
SYSTEMATIC REVIEW article
Front. Immunol. , 03 January 2022
Sec. Alloimmunity and Transplantation
Volume 12 - 2021 | https://doi.org/10.3389/fimmu.2021.792098
Background: Multiple preclinical studies have demonstrated that bone‐marrow derived mesenchymal stromal (stem) cells [MSC(M)] positively influence the severity of sepsis symptoms and mortality in rodent models. However, this remains an inconclusive finding.
Objective: To review the effect of naïve MSC(M) in rodent models of sepsis.
Methods: The PubMed, EMBASE, and Web of Science databases were searched up to August 31, 2021. Inclusion criteria according to PICOS criteria were as follows: (1) population: rodents; (2) intervention: unmodified MSC(M); (3) comparison: not specified; (4) primary outcome: the effects of MSC(M) cell therapy on the mortality of rodent models of sepsis and endotoxemia; (5) study: experimental studies. Multiple prespecified subgroup and meta-regression analysis were conducted. Following quality assessment, random effects models were used for this meta-analysis.The inverse variance method of the fixed effects model was used to calculate the pooled odds ratios (ORs) and their 95% confidence intervals (CIs).
Results: twenty-four animal studies met the inclusion criteria. Our results revealed an overall OR difference between animals treated with naïve MSC(M) and controls for mortality rate was 0.34(95% confidence interval: 0.27-0.44; P < 0.0001). Significant heterogeneity among studies was observed.
Conclusions: The findings of this meta-analysis suggest that naïve MSC(M) therapy decreased mortality in rodent models of sepsis. Additionally, we identified several key knowledge gaps, including the lack of large animal studies and uncertainty regarding the optimal dose of MSC(M) transplantation in sepsis. Before MSC(M) treatment can advance to clinical trials, these knowledge gaps must be addressed.
A potentially lethal disease involving multiple systems, sepsis is caused by an abnormal balance between the host’s proinflammatory response and antiinflammatory response to infection (1, 2). Approximately 40% of deaths in the intensive care unit (ICU) are caused by sepsis, one of the principal causes of mortality worldwide (3, 4). The development of treatments for sepsis requires substantial advances in understanding of the diagnostic mechanisms and regulatory mechanisms that modulate the host’s response to infecting organisms. Despite substantial progress in understanding, there remain significant challenges in translating these advances into clinically effective therapies (5, 6). Presently, no specific treatment is available for sepsis and septic shock. Only symptomatic management is available, which includes the infusion of antibiotics and catecholamines (7). It appears that cellular therapy may offer great potential for the treatment of sepsis and septic shock. There are a number of different source tissues that can be used to treat sepsis, such as human bone marrow, adipose, olfactory mucosa, placenta, umbilical cord, and umbilical cord blood (8). Among the different kinds of mesenchymal stem cells that have been studied in preclinical studies, bone marrow derived mesenchymal stem cells have been thoroughly tested.
Bone marrow mesenchymal stromal cells (MSC(M), also known as bone marrow stem cells, or bone marrow-derived stem and progenitor cells) were firstly described by Freidenstein et al. in 1968 (9). They were adherent, fibroblast-like clonogenic cells with a high capability of replicating and differentiating into osteoblasts and adipocytes (10, 11). Cells in the bone marrow comprise less than 0.1% of mesenchymal stromal cells. However, MSC(M) can be harvested and expanded easily in culture, which makes them attractive for research. Additionally, allogeneic therapy is also available. It is because of these characteristics that MSCs are attractive for clinical trials. The feasibility and efficacy of mesenchymal stem-cell-based therapy has been examined in a large number of clinical trials (12, 13). It is estimated that over 2000 patients have benefited from over 400 clinical trials using MSCs to treat graft-versus-host disease, diabetes, hematological malignancies, cardiovascular disease, neurologic diseases, and autoimmune diseases.
MSC(M) may hold the key for sepsis and are therefore an appealing reparative therapy. There is now a growing body of preclinical evidence investigating the efficacy of MSC(M) therapy (14–36); however, there are often conflicting results in the literature. Güldner et al. found that the survival rate of mice did not differ among treated with mouse MSC(M), human MSC(M),and the untreated animals (CLP) (26). A similar result was also published by Silva et al. In their study there was no statistical difference in survival rates between the MSC(M) and CLP groups (34). Prior meta-analysis either took a broader approach to mesenchymal stem cells therapy or database was only until October 1, 2017 (37, 38). Neither have offered a meta-analysis of the relevant study to investigate mortality rate of MSC(M) transplantation for sepsis. Our aim was to perform a meta-analysis to review published animal studies employing the use of naïve MSC(M) therapy following sepsis, and to provide information for the future clinical translation of MSC(M) to the bedside.
In accordance with Preferred Reporting Items for Systematic Reviews and Meta-Analyses (PRISMA) (39), a meta-analysis has been conducted. Analyzing data that is available in published articles does not require ethical approval or consent from the patient. The article provides all supporting data and the online supplement provides additional information
The researchers conducted a systematic literature search using 3 databases, including MEDLINE, EMBASE, and Web of Science, to screen for targeted studies on the efficacy of BMSCs in treating sepsis. The detailed search strategy is shown in Additional file 1: Table S1. The last search was updated on August 31, 2021. The publication language was limited to English. We also searched the reference lists of eligible studies.
According to the PICOS scheme (population, intervention, control, outcome, and study design), the studies’ eligibility criteria were formulated (40). This meta-analysis included studies that met the following criteria: (i) They tested the efficacy of MSC(M) treatment in animal models of sepsis (all types of animals and both genders). (ii) The research centered on animal models of sepsis or endotoxemia. (iii) The study included mortality as part of its evaluation index. When two or more articles contained overlapping data, the most recent or informative article was used. (iv) Present experimental studies in original research papers. (v) The study was published in English.
The exclusion criteria were as follows: (i) Researches that only evaluated the efficacy of transfected or modified cell transplantation. (ii) Studies that only tested stem cells other than MSC(M). (iii) MSC(M) administered before sepsis model.
All published articles were independently reviewed by two investigators after the removal of duplicates. After the two investigators reached an agreement, irrelevant studies were excluded. For a comprehensive review, all relevant articles were retrieved, and two researchers independently evaluated each article using the above selection criteria. Any disagreements or uncertainties were resolved by consensus, and if necessary by a third investigator.
The following information were abstracted by two investigators independently and entered electronically: author, publishing year, study country, animal characteristics (species, gender, sample size, and model), intervention characteristics (origin, dose, route, and timing of the MSC(M) treatment), follow-up (the longest observation time of outcomes after MSC(M) administration), and our primary measures related to secondary outcomes. If only graphs were available, values were calculated from images using GetData Graph Digitizer software. Analyzing the data involved averaging the two researchers’ readings. In the absence of the standard deviation, the standard error was converted into a standard deviation by multiplying it by the square root of the group size. When multiple experimental groups that were distinguished by a variety of factors such as cell dose or delivery route and timing were contrasted with the control group, these groups were considered separate and independent studies. When results were evaluated over a range of follow-up periods, only the longest interval was selected.
Study authors assessed independently the risk of bias for each study included using the method developed by the Systematic Review Center for Laboratory Animal Experimentatio(SYRCLE) (41). This tool evaluates selection bias, performance bias, detection bias, attribution bias, and reporting bias and grades them according to “Yes, No, or Unclear.” Arguments were resolved through discussions with additional authors.
The primary outcome of this meta-analysis was mortality. OR was calculated between the MSC(M) treated group and the control group to determine the combined effect size. All statistical analyses and graphs were performed by R (version 4.0.2), using a random-effects model and the Hedges calculation (42). Typically, an effect size of 0.2 represents a small effect, 0.5 represents a medium effect, and 0.8 represents a large effect (43). A P-value <0.05 was considered statistically significant. The I2 statistic was used to analyze heterogeneity, and it was divided into three categories, low (25–50%), moderate (50–75%), and high (>75%) (44).
We used six clinical characteristics to group the effect size of outcome: Gender (male, female); sepsis model (CLP, non-CLP Roteneone); MSC(M) species (Allogeneic, Syngeneic, or Xenogeneic); MSC(M) dose (<1×106, ≥1×106 and <1×107, ≥1×107); follow-up duration (≤6hours, >6 hours); delivery route (intravenous or intraperitoneal). In order to examine the possible associations between the outcomes and the above clinical characteristics, subgroup analyses and meta-regression analyses were performed (45). Sensitivity analyses were performed by omitting one study at a time to evaluate whether the results were affected by a single study. Publication bias was evaluated using funnel plots (46), and the symmetry of funnel plots was performed with Egger regression (47). If necessary, any non-negligible bias would be corrected using the trim-and-fill approach (48).
A total of 1551 records were identified following the search procedure shown in Figure 1, including 184 articles in PubMed, 538 articles in EMBASE, and 784 articles in Web of Science. After excluding duplicates, 432 studies were screened by title and abstract and 364 studies were subsequently excluded. Full-text screening for eligibility was performed on 68 studies and, based on the inclusion and exclusion criteria, 24 studies were included in this systematic review, No new records met our inclusion criteria, and thus, all were excluded.
The characteristics of the 24 studies are summarized in Table 1. All studies were carried out in rodents (rats and mice). Intervention included MSCs obtained from mice, rat, or human bone marrow. The most common sepsis models were the cecal ligation and puncture (CLP) and intraperitoneal injection of lipopolysaccharide (LPS). Following induction of sepsis, MSC(M) were injected within a few hours of the induction of the sepsis animal models. The mean follow-up ranged from 8 days to 20 weeks. The most common delivery routes used for MSC(M) were intravenous route or intraperitoneal route. The total dose of MSC(M) administered ranged from 1×105 to 1×107 cells.
The RoB assessment results of included studies is summarized in Table 2. There was no study considered to have a low RoB. There was no significant difference in the baseline characteristics between the experimental and control groups, reducing the chance of selection bias based on animal characteristics. No study has explicitly described how random sequences are generated despite the random allocation of experimental and control subjects. Accordingly, the RoB was deemed to be “unclear” in the sequence generation domain of all studies included. However, no study attempted to describe the method of concealed allocation. An evaluation of randomized outcomes was reported, the intervention received by each animal was blinded to researchers and, in one study (22), the evaluator’s blindness was noted. Based on the provided signal questions, all of the included studies showed a low risk of attrition bias and reporting bias. In one study that had a high risk of bias, the decrease in animal numbers was not accounted for between methods and results (15). In one study (16), there were other problems that caused a high risk of bias, such as pollution, experimental design, etc. Furthermore, we did not identify any additional sources of bias or bias tools in relation to systematic risks that were not included.
We conducted a sensitivity analysis to evaluate the stability of the results by sequential omission of each study if heterogeneity between the studies existed. The rate of mortality outcome was not significantly affected by any study (Figure 2).
A total of 24 animal studies involving 1278 animals were used in this meta-analysis and reported animal mortality rates. Heterogeneity test results showed I2 = 9% and P = 0.34, indicating that the heterogeneity between the studies was low; thus, a fixed effects model was used. As shown in Figure 3, the pooled results demonstrated that the mortality of the animals after MSC(M) treatment was significantly reduced (OR 0.34, 95% CI 0.27-0.44, P<0.0001).
Figure 3 Forest plot summarizing the effects of mesenchymal stem cell therapy on the mortality of preclinical models of sepsis and endotoxemia.
Subgroup analysis was performed based on the animal species and model, as well as the model gender, species, Sepsis model, and MSC(M) dose, injection time, source, and injection route. In general, significant efficacy of MSC(M) transplantation was observed in most subgroups. In animal models with female participants, MSC(M) for females (OR=0.26, 95% CI 0.13-0.49, P <0.001) had a greater effect than MSC(M) for male participants (OR=0.27, 95% CI 0.19-0.30, P<0.001) and not reported group (OR=0.69, 95% CI 0.42-1.13, P<0.001) (Figure S1). Furthermore, MSC(M) administered to non-CLP-induced sepsis animals (OR=0.26, 95% CI 0.16-0.41, P<0.001) were more beneficial than those administered to CLP-induced sepsis animals (OR=0.39, 95% CI 0.28-0.52, P<0.001) (Figure S2), and MSC(M) administered to rats (OR=0.23, 95% CI 0.10-0.55, P<0.001) were more beneficial than those administered to mice (OR= 0.36, 95% CI 0.27-0.45, P<0.001) (Figure S3). A significant decrease in mortality rate is observed in both doses of ≥1×106 and <1×107 MSC(M) (OR=0.21, 95% CI 0.14-0.32, P<0.001) and ≥1×107 MSC(M) (OR=0.32, 95% CI 0.06-1.85, P<0.001), as compared to doses of <1×106 MSC(M) (OR=0.50, 95% CI 0.35-0.70, P<0.001) (Figure S4). Additionally, injection times of ≤6h (OR=0.26, 95% CI 0.19-0.37, P<0.001) and better than >6h (OR=0.48, 95% CI 0.33-0.70, P<0.001) after sepsis induction were noted (Figure S5). Further, xenogenic MSC(M) (OR=0.30, 95% CI 0.19-0.47, P<0.001) significantly reduced mortality compared to syngeneic MSC(M) (OR=0.33, 95% CI 0.21-0.51, P < 0.001) and allogenic MSC(M) (OR=0.40, 95% CI 0.20-0.62, P<0.001) (Figure S6). As well, intraperitoneal administration (OR=0.04, 95% CI 0.00-0.37, P<0.001) is superior to intravenous administration (OR=0.36, 95% CI 0.28-0.46, P<0.001) after sepsis induction in animal models (Figure S7).
We assessed publication bias by funnel plots (Figure 4). No evident publication bias was observed by visual inspection. The Egger’s test presented publication bias for mortality rates (P = 0.004). After adopting trim-and-fill correction for mortality rates outcomes, the estimated value remained unchanged.
Figure 4 Funnel plot for the mortality rate. Each dot in the figure represents a study, with the y-axis signifying study quality and the x-axis showing the study results. SMD, standardized mean difference.
The use of MSC(M) therapy in sepsis has not been implemented in the clinical management of patients with sepsis despite numerous preclinical studies showing that MSC(M) could decrease the mortality rate of sepsis and improve sepsis. For MSC(M), several issues remain unclear with respect to delivery timing, routes of administration, and dosage. Since animal experiments serve as a basis for designing clinical trials, it is important to examine the combined effects of preclinical and clinical studies. How are stem cell therapies indicated for acute or chronic sepsis, what doses of MSC(M) are optimal, how are MSC(M) delivered, how long do MSC(M) survive in the hostile environment of the disease, and how can sepsis mortality be further improved? (Figure 5). It has been reported that previous meta-analyses either considered multiple different mesenchymal stem cell types or that the data was only available until October 1, 2017. However, neither has provided a meta-analysis of the relevant study to examine the mortality rate following MSC(M) transplantation for sepsis. In this study, we demonstrate that MSC(M) can potentially reduce mortality rates of sepsis in animal models. This provides insight into the potential therapeutic applications of MSC(M) in preclinical studies of sepsis. According to our knowledge, two clinical phases 1 trials (Table 3) have been conducted to test the safety and feasibility of MSC(M) therapy for patients with septic shock and sepsis. Based on these studies, there were no serious clinical or physiological safety signals, drawing the conclusion that MSC(M) treatment was safe and well-tolerated in critical patients experiencing a septic shock.
Figure 5 Key issues that the settlement of which would facilitate the transition of BMSCs research in sepsis from bench to bedside.
According to the results of this meta-analysis: (1) MSC(M) therapy significantly reduced the mortality rate of sepsis animal models, supporting the possibility of using MSC(M) therapy for preclinical studies of sepsis. (2) The administration time was correlated with mortality. MSC(M) therapy initiated within 6 hours of the onset of sepsis exhibited the greatest efficacy. (3) For the mortality rate, intraperitoneal administration injection seems to show the greatest efficacy, followed by intravenous administration, however, this finding should be interpreted cautiously. (4) Xenogenic MSC(M) significantly reduced the mortality rate of sepsis in the animal models, compared to Syngeneic and allogeneic MSC(M). (5) It is unclear from the included studies what is the appropriate dosage of MSC(M). In contrast to the previously published meta-analysis (38), which indicated that less than or equal to 1.0 × 106 MSCs was the ideal dose, the conclusion of our analysis was that more than or equal to 1.0 ×106 MSC(M) is the ideal dose. Therefore, it will be necessary to conduct additional research to determine the ideal dose of MSC(M) for the treatment of sepsis. As a general rule, the above various subgroup analyses are only able to generate hypotheses rather than confirm them. As a result of the subgroup analysis, the results are speculative because they are based on the reanalysis of published data rather than the results of a well-constructed randomized controlled trial. Thus, despite the fact that the subgroup analysis provided updated evidence, the results of this analysis should be considered cautiously.
Numerous preclinical studies have demonstrated the benefit of MSC(M) in animal models of sepsis. The precise mechanism by which MSC(M) may exert beneficial effects in sepsis is still being elucidated, but it appears that multiple mechanisms may contribute. Besides mesenchymal stem cells’ homing properties, progress has also been made with understanding the mechanisms of benefit, including paracrine factors, mitochondrial transfer, and the development of extracellular vesicles and microvesicles. (Figure 6).
Firstly, MSC(M) may be most helpful in alleviating sepsis due to their anti-inflammatory properties. The MSC(M) can promote the repolarization of monocytes and/or macrophages from a type 1 to a type 2 phenotype, characterized by high levels of intracellular IL-10 secretion, increased phagocytosis, low levels of TNF‐α and interferon‐γ production (49). Reprogrammed type 2 monocytes produced by MSC(M) produce large amounts of IL-10, which inhibits neutrophil influx into injured tissue and prevents further damage. Additionally, MSC(M) can also interfere with the differentiation, maturation, and function of dendritic cells, causing them to adopt a regulatory phenotype (50, 51). The MSC(M) can modulate natural killer cells, which are involved in eradicating virus-infected and damaged cells, as well as releasing a range of proinflammatory cytokines, such as interferon‐γ. It has been demonstrated in a number of studies that MSC(M), in co-culture with natural killer cells, impair their cytotoxic activity, their cytokine production, and their ability to release granzyme B (52). Moreover, MSC(M) have also been shown to have antimicrobial effects in animals. MSC(M) contributed to improved bacterial clearance by secreting antibacterial proteins/peptides such as LL-37 (53),lipocalin-2 (54), and so on. Secondly, several studies have demonstrated that MSC(M) have beneficial effects on metabolomics through mitochondrial transfer using extracellular vesicles. In a model of lipopolysaccharide-induced ALI, mitochondrial transfer through connexin-43 was partially responsible for restoring ATP levels (55). The therapeutic effects of MSC(M) in LPS-induced ALI were abrogated when mutant MSC(M) with defective gap junctions or MSC(M) with dysfunctional mitochondria were used. In addition, a model of E.coli pneumonia has shown that mitochondria transfer from MSC(M) to macrophages is necessary for enhancing macrophage bioenergetics and phagocytosis, as well as the antimicrobial effects of MSC(M) in vivo (56). Finally, extracellular vesicles (EVs) derived from MSC(M) may contribute to the paracrine effect. These MSC(M)-EVs contribute molecules (such as proteins, peptides, mRNA, microRNA, and lipids) with immunoregulatory properties. MSC(M)-EVs have been found to mimic MSC(M) in alleviating sepsis in healthy people and may serve as an alternative to whole-cell therapy. In contrast to MSC(M), MSC(M)-EVs may offer specific advantages due to their low immunogenicity and high safety profile. Even though several studies have demonstrated the benefits of MSC(M)-EVs in sepsis, the underlying mechanisms of MSC(M)-EVs remain unclear. In order to further understand the mechanism by which beneficial effects are mediated, we need to increase our understanding of paracrine factors and the transfer of mitochondria and microcapsules.
We have identified several limitations to our meta-analysis. Firstly, our approach can include only those studies that have been traditionally published in English. Unpublished data may influence our results. Furthermore, none of the included studies examined the safety of MSC(M) injection in sepsis models in animals. We are unable to evaluate the clinical safety of MSC(M). Finally, a good study requires a sample size calculation that is based on formal procedures. However, no study in the meta-analysis had conducted a sample calculation, indicating insufficient statistical power to determine the treatment effect.
The limited treatment options for sepsis call for new interventions. To date, preclinical studies in rodents have shown MSC(M) to be an effective treatment for sepsis using preclinical research. The study of the efficacy of MSC(M) transplantation for sepsis has identified important future directions that preclinical research needs to address before clinical trials begin. Research gaps identified include the inability to directly compare routes, doses, sources, and timings of MSC(M) administration, as well as the lack of standardization of clinically meaningful behavioral outcomes. The effectiveness of MSC(M) transplantation for sepsis must be evaluated in large animal studies and further investigation is required to determine whether immunosuppression is necessary.
The original contributions presented in the study are included in the article/Supplementary Material. Further inquiries can be directed to the corresponding author.
LZ supervised the project. LG, JZ, and HD analyzed the data. ZH and CC extracted the data. LG and LZ wrote the paper. All authors contributed to the article and approved the submitted version.
This work was supported by National Natural Science Foundation of China (No. 31670838, No.32000151, No.81371358, No.81974213), Key Project of Hunan Provincial Science and Technology Innovation (2020SK2102), Hunan Provincial Natural Science Foundation of China (2021JJ40830).
The authors declare that the research was conducted in the absence of any commercial or financial relationships that could be construed as a potential conflict of interest.
All claims expressed in this article are solely those of the authors and do not necessarily represent those of their affiliated organizations, or those of the publisher, the editors and the reviewers. Any product that may be evaluated in this article, or claim that may be made by its manufacturer, is not guaranteed or endorsed by the publisher.
The Supplementary Material for this article can be found online at: https://www.frontiersin.org/articles/10.3389/fimmu.2021.792098/full#supplementary-material
1. Liu V, Escobar GJ, Greene JD, Soule J, Whippy A, Angus DC, et al. Hospital Deaths in Patients With Sepsis From 2 Independent Cohorts. JAMA (2014) 312(1):90–2. doi: 10.1001/jama.2014.5804
2. Singer M, Deutschman CS, Seymour CW, Shankar-Hari M, Annane D, Bauer M, et al. The Third International Consensus Definitions for Sepsis and Septic Shock (Sepsis-3). JAMA (2016) 315(8):801–10. doi: 10.1001/jama.2016.0287
3. Fleischmann C, Scherag A, Adhikari NK, Hartog CS, Tsaganos T, Schlattmann P, et al. Assessment of Global Incidence and Mortality of Hospital-Treated Sepsis. Current Estimates and Limitations. Am J Respir Crit Care Med (2016) 193(3):259–72. doi: 10.1164/rccm.201504-0781OC
4. Kaukonen KM, Bailey M, Suzuki S, Pilcher D, Bellomo R. Mortality Related to Severe Sepsis and Septic Shock Among Critically Ill Patients in Australia and New Zealand, 2000-2012. JAMA (2014) 311(13):1308–16. doi: 10.1001/jama.2014.2637
5. Adhikari NK, Fowler RA, Bhagwanjee S, Rubenfeld GD. Critical Care and the Global Burden of Critical Illness in Adults. Lancet (2010) 376(9749):1339–46. doi: 10.1016/S0140-6736(10)60446-1
6. Gotts JE, Matthay MA. Sepsis: Pathophysiology and Clinical Management. BMJ (2016) 353:i1585. doi: 10.1136/bmj.i1585
7. Mehta S, Gill SE. Improving Clinical Outcomes in Sepsis and Multiple Organ Dysfunction Through Precision Medicine. J Thorac Dis (2019) 11(1):21–8. doi: 10.21037/jtd.2018.11.74
8. Laroye C, Gibot S, Reppel L, Bensoussan D. Concise Review: Mesenchymal Stromal/Stem Cells: A New Treatment for Sepsis and Septic Shock. Stem Cells (2017) 35(12):2331–9. doi: 10.1002/stem.2695
9. Friedenstein AJ, Petrakova KV, Kurolesova AI, Frolova GP. Heterotopic of Bone Marrow. Analysis of Precursor Cells for Osteogenic and Hematopoietic Tissues. Transplantation (1968) 6(2):230–47. doi: 10.1097/00007890-196803000-00009
10. Jiang Y, Jahagirdar BN, Reinhardt RL, Schwartz RE, Keene CD, Ortiz-Gonzalez XR, et al. Pluripotency of Mesenchymal Stem Cells Derived From Adult Marrow. Nature (2002) 418(6893):41–9. doi: 10.1038/nature00870
11. Pittenger MF, Mackay AM, Beck SC, Jaiswal RK, Douglas R, Mosca JD, et al. Multilineage Potential of Adult Human Mesenchymal Stem Cells. Science (1999) 284(5411):143–7. doi: 10.1126/science.284.5411.143
12. Ho MS, Mei SH, Stewart DJ. The Immunomodulatory and Therapeutic Effects of Mesenchymal Stromal Cells for Acute Lung Injury and Sepsis. J Cell Physiol (2015) 230(11):2606–17. doi: 10.1002/jcp.25028
13. Lombardo E, van der Poll T, DelaRosa O, Dalemans W. Mesenchymal Stem Cells as a Therapeutic Tool to Treat Sepsis. World J Stem Cells (2015) 7(2):368–79. doi: 10.4252/wjsc.v7.i2.368
14. Németh K, Leelahavanichkul A, Yuen PS, Mayer B, Parmelee A, Doi K, et al. Bone Marrow Stromal Cells Attenuate Sepsis via Prostaglandin E(2)-Dependent Reprogramming of Host Macrophages to Increase Their Interleukin-10 Production. Nat Med (2009) 15(1):42–9. doi: 10.1038/nm.1905
15. Bi L-K, Tang B, Zhu B, Xie C, Li S, Lin T, et al. Systemic Delivery of IL-10 by Bone Marrow Derived Stromal Cells has Therapeutic Benefits in Sepsis Therapy*. Prog Biochem Biophys Prog Biochem Biophys (2010) 37(60):678–85. doi: 10.3724/SP.J.1206.2010.00107
16. Mei SH, Haitsma JJ, Dos Santos CC, Deng Y, Lai PF, Slutsky AS, et al. Mesenchymal Stem Cells Reduce Inflammation While Enhancing Bacterial Clearance and Improving Survival in Sepsis. Am J Respir Crit Care Med (2010) 182(8):1047–57. doi: 10.1164/rccm.201001-0010OC
17. Liang ZX, Sun JP, Wang P, Tian Q, Yang Z, Chen LA. Bone Marrow-Derived Mesenchymal Stem Cells Protect Rats From Endotoxin-Induced Acute Lung Injury. Chin Med J (Engl) (2011) 124(17):2715–22.
18. Krasnodembskaya A, Samarani G, Song Y, Zhuo H, Su X, Lee JW, et al. Human Mesenchymal Stem Cells Reduce Mortality and Bacteremia in Gram-Negative Sepsis in Mice in Part by Enhancing the Phagocytic Activity of Blood Monocytes. Am J Physiol Lung Cell Mol Physiol (2012) 302(10):L1003–13. doi: 10.1152/ajplung.00180.2011
19. Hall SR, Tsoyi K, Ith B, Padera RF Jr, Lederer JA, Wang Z, et al. Mesenchymal Stromal Cells Improve Survival During Sepsis in the Absence of Heme Oxygenase-1: The Importance of Neutrophils. Stem Cells (2013) 31(2):397–407. doi: 10.1002/stem.1270
20. Zhao Y, Yang C, Wang H, Li H, Du J, Gu W, et al. Therapeutic Effects of Bone Marrow-Derived Mesenchymal Stem Cells on Pulmonary Impact Injury Complicated With Endotoxemia in Rats. Int Immunopharmacol (2013) 15(2):246–53. doi: 10.1016/j.intimp.2012.12.008
21. Chao YH, Wu HP, Wu KH, Tsai YG, Peng CT, Lin KC, et al. An Increase in CD3+CD4+CD25+ Regulatory T Cells After Administration of Umbilical Cord-Derived Mesenchymal Stem Cells During Sepsis. PloS One (2014) 9(10):e110338. doi: 10.1371/journal.pone.0110338
22. Kim H, Darwish I, Monroy MF, Prockop DJ, Liles WC, Kain KC. Mesenchymal Stromal (Stem) Cells Suppress Pro-Inflammatory Cytokine Production But Fail to Improve Survival in Experimental Staphylococcal Toxic Shock Syndrome. BMC Immunol (2014) 15:1. doi: 10.1186/1471-2172-15-1
23. Luo CJ, Zhang FJ, Zhang L, Geng YQ, Li QG, Hong Q, et al. Mesenchymal Stem Cells Ameliorate Sepsis-Associated Acute Kidney Injury in Mice. Shock (2014) 41(2):123–9. doi: 10.1097/SHK.0000000000000080
24. Sepúlveda JC, Tomé M, Fernández ME, Delgado M, Campisi J, Bernad A, et al. Cell Senescence Abrogates the Therapeutic Potential of Human Mesenchymal Stem Cells in the Lethal Endotoxemia Model. Stem Cells (2014) 32(7):1865–77. doi: 10.1002/stem.1654
25. Ou H, Zhao S, Peng Y, Xiao X, Wang Q, Liu H, et al. Comparison of Bone Marrow Tissue- and Adipose Tissue-Derived Mesenchymal Stem Cells in the Treatment of Sepsis in a Murine Model of Lipopolysaccharide-Induced Sepsis. Mol Med Rep (2016) 14(4):3862–70. doi: 10.3892/mmr.2016.5694
26. Güldner A, Maron-Gutierrez T, Abreu SC, Xisto DG, Senegaglia AC, Barcelos PR, et al. Expanded Endothelial Progenitor Cells Mitigate Lung Injury in Septic Mice. Stem Cell Res Ther (2015) 6:230. doi: 10.1186/s13287-015-0226-7
27. Liu W, Gao Y, Li H, Wang H, Ye M, Jiang G, et al. Intravenous Transplantation of Mesenchymal Stromal Cells has Therapeutic Effects in a Sepsis Mouse Model Through Inhibition of Septic Natural Killer Cells. Int J Biochem Cell Biol (2016) 79:93-103. doi: 10.1016/j.biocel.2016.08.013
28. Xu S, Zhou Z, Li H, Liu Z, Pan X, Wang F, et al. BMSCs Ameliorate Septic Coagulopathy by Suppressing Inflammation in Cecal Ligation and Puncture-Induced Sepsis. J Cell Sci (2018) 131(3). doi: 10.1242/jcs.211151
29. Li S, Wu H, Han D, Ma S, Fan W, Wang Y, et al. A Novel Mechanism of Mesenchymal Stromal Cell-Mediated Protection Against Sepsis: Restricting Inflammasome Activation in Macrophages by Increasing Mitophagy and Decreasing Mitochondrial ROS. Oxid Med Cell Longev (2018) 2018:3537609. doi: 10.1155/2018/3537609
30. Saeedi P, Halabian R, Fooladi A. Antimicrobial Effects of Mesenchymal Stem Cells Primed by Modified LPS on Bacterial Clearance in Sepsis. J Cell Physiol (2019) 234(4):4970–86. doi: 10.1002/jcp.27298
31. Saeedi P, Halabian R, Fooladi A. Mesenchymal Stem Cells Preconditioned by Staphylococcal Enterotoxin B Enhance Survival and Bacterial Clearance in Murine Sepsis Model. Cytotherapy (2019) 21(1):41–53. doi: 10.1016/j.jcyt.2018.11.002
32. Laroye C, Boufenzer A, Jolly L, Cunat L, Alauzet C, Merlin JL, et al. Bone Marrow vs Wharton’s Jelly Mesenchymal Stem Cells in Experimental Sepsis: A Comparative Study. Stem Cell Res Ther (2019) 10(1):192. doi: 10.1186/s13287-019-1295-9
33. Luo F, Jiang W, Xu Y, Liu XM, Wang W, Zhang W, et al. The Mechanisms Involved in Mesenchymal Stem Cell Alleviation of Sepsis-Induced Acute Lung Injury in Mice: A Pilot Study. Curr Ther Res Clin Exp (2020) 93:100593. doi: 10.1016/j.curtheres.2020.100593
34. Silva A, Amorim ÉA, Barbosa-Silva MC, Lima MN, Oliveira HA, Granja MG, et al. Mesenchymal Stromal Cells Protect the Blood-Brain Barrier, Reduce Astrogliosis, and Prevent Cognitive and Behavioral Alterations in Surviving Septic Mice. Crit Care Med (2020) 48(4):e290–8. doi: 10.1097/CCM.0000000000004219
35. Varkouhi AK, He X, Teixeira Monteiro AP, Amatullah H, Tsoporis JN, Gupta S, et al. Immunophenotypic Characterization and Therapeutics Effects of Human Bone Marrow- and Umbilical Cord-Derived Mesenchymal Stromal Cells in an Experimental Model of Sepsis. Exp Cell Res (2021) 399(2):112473. doi: 10.1016/j.yexcr.2021.112473
36. Guo J, Wang R, Liu D. Bone Marrow-Derived Mesenchymal Stem Cells Ameliorate Sepsis-Induced Acute Kidney Injury by Promoting Mitophagy of Renal Tubular Epithelial Cells via the SIRT1/Parkin Axis. Front Endocrinol (Lausanne) (2021) 12:639165. doi: 10.3389/fendo.2021.639165
37. Lalu MM, Sullivan KJ, Mei SH, Moher D, Straus A, Fergusson DA, et al. Evaluating Mesenchymal Stem Cell Therapy for Sepsis With Preclinical Meta-Analyses Prior to Initiating a First-in-Human Trial. Elife (2016) 5. doi: 10.7554/eLife.17850
38. Sun XY, Ding XF, Liang HY, Zhang XJ, Liu SH, Bing-Han, et al. Efficacy of Mesenchymal Stem Cell Therapy for Sepsis: A Meta-Analysis of Preclinical Studies. Stem Cell Res Ther (2020) 11(1):214. doi: 10.1186/s13287-020-01730-7
39. Moher D, Liberati A, Tetzlaff J, Altman DG, PRISMA Group. Preferred Reporting Items for Systematic Reviews and Meta-Analyses: The PRISMA Statement. BMJ (2009) 339:b2535. doi: 10.1136/bmj.b2535
40. Riva JJ, Malik KM, Burnie SJ, Endicott AR, Busse JW. What is Your Research Question? An Introduction to the PICOT Format for Clinicians. J Can Chiropr Assoc (2012) 56(3):167–71.
41. Hooijmans CR, Rovers MM, de Vries RB, Leenaars M, Ritskes-Hoitinga M, Langendam MW. Syrcle’s Risk of Bias Tool for Animal Studies. BMC Med Res Methodol (2014) 14:43. doi: 10.1186/1471-2288-14-43
42. Durlak JA. How to Select, Calculate, and Interpret Effect Sizes. J Pediatr Psychol (2009) 34(9):917–28. doi: 10.1093/jpepsy/jsp004
43. Schulz KF, Chalmers I, Hayes RJ, Altman DG. Empirical Evidence of Bias. Dimensions of Methodological Quality Associated With Estimates of Treatment Effects in Controlled Trials. JAMA (1995) 273(5):408–12. doi: 10.1001/jama.273.5.408
44. Higgins JP, Thompson SG, Deeks JJ, Altman DG. Measuring Inconsistency in Meta-Analyses. BMJ (2003) 327(7414):557–60. doi: 10.1136/bmj.327.7414.557
45. Higgins JP, Thompson SG. Quantifying Heterogeneity in a Meta-Analysis. Stat Med (2002) 21(11):1539–58. doi: 10.1002/sim.1186
46. Lau J, Ioannidis JP, Terrin N, Schmid CH, Olkin I. The Case of the Misleading Funnel Plot. BMJ (2006) 333(7568):597–600. doi: 10.1136/bmj.333.7568.597
47. Egger M, Davey Smith G, Schneider M, Minder C. Bias in Meta-Analysis Detected by a Simple, Graphical Test. BMJ (1997) 315(7109):629–34. doi: 10.1136/bmj.315.7109.629
48. Sterne JA, Sutton AJ, Ioannidis JP, Terrin N, Jones DR, Lau J, et al. Recommendations for Examining and Interpreting Funnel Plot Asymmetry in Meta-Analyses of Randomised Controlled Trials. BMJ (2011) 343:d4002. doi: 10.1136/bmj.d4002
49. Maggini J, Mirkin G, Bognanni I, Holmberg J, Piazzón IM, Nepomnaschy I, et al. Mouse Bone Marrow-Derived Mesenchymal Stromal Cells Turn Activated Macrophages Into a Regulatory-Like Profile. PloS One (2010) 5(2):e9252. doi: 10.1371/journal.pone.0009252
50. Jiang XX, Zhang Y, Liu B, Zhang SX, Wu Y, Yu XD, et al. Human Mesenchymal Stem Cells Inhibit Differentiation and Function of Monocyte-Derived Dendritic Cells. Blood (2005) 105(10):4120–6. doi: 10.1182/blood-2004-02-0586
51. Nauta AJ, Kruisselbrink AB, Lurvink E, Willemze R, Fibbe WE. Mesenchymal Stem Cells Inhibit Generation and Function of Both CD34+-Derived and Monocyte-Derived Dendritic Cells. J Immunol (2006) 177(4):2080–7. doi: 10.4049/jimmunol.177.4.2080
52. Poggi A, Prevosto C, Massaro AM, Negrini S, Urbani S, Pierri I, et al. Interaction Between Human NK Cells and Bone Marrow Stromal Cells Induces NK Cell Triggering: Role of NKp30 and NKG2D Receptors. J Immunol (2005) 175(10):6352–60. doi: 10.4049/jimmunol.175.10.6352
53. Krasnodembskaya A, Song Y, Fang X, Gupta N, Serikov V, Lee JW, et al. Antibacterial Effect of Human Mesenchymal Stem Cells is Mediated in Part From Secretion of the Antimicrobial Peptide LL-37. Stem Cells (2010) 28(12):2229–38. doi: 10.1002/stem.544
54. Gupta N, Krasnodembskaya A, Kapetanaki M, Mouded M, Tan X, Serikov V, et al. Mesenchymal Stem Cells Enhance Survival and Bacterial Clearance in Murine Escherichia Coli Pneumonia. Thorax (2012) 67(6):533–9. doi: 10.1136/thoraxjnl-2011-201176
55. Islam MN, Das SR, Emin MT, Wei M, Sun L, Westphalen K, et al. Mitochondrial Transfer From Bone-Marrow-Derived Stromal Cells to Pulmonary Alveoli Protects Against Acute Lung Injury. Nat Med (2012) 18(5):759–65. doi: 10.1038/nm.2736
56. Jackson MV, Morrison TJ, Doherty DF, McAuley DF, Matthay MA, Kissenpfennig A, et al. Mitochondrial Transfer via Tunneling Nanotubes is an Important Mechanism by Which Mesenchymal Stem Cells Enhance Macrophage Phagocytosis in the In Vitro and In Vivo Models of ARDS. Stem Cells (2016) 34(8):2210–23. doi: 10.1002/stem.2372
Keywords: sepsis, meta-analysis, efficacy, animal experimentation, bone marrow stromal cell (BMSC)
Citation: Ge L, Zhao J, Deng H, Chen C, Hu Z and Zeng L (2022) Effect of Bone Marrow Mesenchymal Stromal Cell Therapies in Rodent Models of Sepsis: A Meta-Analysis. Front. Immunol. 12:792098. doi: 10.3389/fimmu.2021.792098
Received: 12 October 2021; Accepted: 08 December 2021;
Published: 03 January 2022.
Edited by:
Robert James Hayashi, Washington University School of Medicine in St. Louis, United StatesReviewed by:
Tatiana Maron-Gutierrez, Oswaldo Cruz Foundation (Fiocruz), BrazilCopyright © 2022 Ge, Zhao, Deng, Chen, Hu and Zeng. This is an open-access article distributed under the terms of the Creative Commons Attribution License (CC BY). The use, distribution or reproduction in other forums is permitted, provided the original author(s) and the copyright owner(s) are credited and that the original publication in this journal is cited, in accordance with accepted academic practice. No use, distribution or reproduction is permitted which does not comply with these terms.
*Correspondence: Liuwang Zeng, emVuZ2xpdXdhbmdAY3N1LmVkdS5jbg==
Disclaimer: All claims expressed in this article are solely those of the authors and do not necessarily represent those of their affiliated organizations, or those of the publisher, the editors and the reviewers. Any product that may be evaluated in this article or claim that may be made by its manufacturer is not guaranteed or endorsed by the publisher.
Research integrity at Frontiers
Learn more about the work of our research integrity team to safeguard the quality of each article we publish.