- 1Department of Neurosciences, Psychology, Drug Research and Child Health (NEUROFARBA), University of Florence, Florence, Italy
- 2Division of Hematology, Careggi University Hospital, Florence, Italy
- 3Meyer University Children’s Hospital, University of Florence, Florence, Italy
- 4Centre of Excellence, Division of Pediatric Oncology/Hematology, Meyer University Children’s Hospital, Florence, Italy
- 5Division of Pediatric Oncology/Hematology, University Hospital of Pisa, Pisa, Italy
- 6Division of Pediatric Oncology/Hematology, University Hospital of Ospedali Riuniti, Ancona, Italy
Inborn errors of immunity (IEI) are genetic disorders characterized by a wide spectrum of clinical manifestations, ranging from increased susceptibility to infections to significant immune dysregulation. Among these, primary immune regulatory disorders (PIRDs) are mainly presenting with autoimmune manifestations, and autoimmune cytopenias (AICs) can be the first clinical sign. Significantly, AICs in patients with IEI often fail to respond to first-line therapy. In pediatric patients, autoimmune cytopenias can be red flags for IEI. However, for these cases precise indicators or parameters useful to suspect and screen for a hidden congenital immune defect are lacking. Therefore, we focused on chronic/refractory AIC patients to perform an extensive clinical evaluation and multiparametric flow cytometry analysis to select patients in whom PIRD was strongly suspected as candidates for genetic analysis. Key IEI-associated alterations causative of STAT3 GOF disease, IKAROS haploinsufficiency, activated PI3Kδ syndrome (APDS), Kabuki syndrome and autoimmune lymphoproliferative syndrome (ALPS) were identified. In this scenario, a dysregulated immunophenotype acted as a potential screening tool for an early IEI diagnosis, pivotal for appropriate clinical management and for the identification of new therapeutic targets.
Introduction
Inborn errors of immunity (IEI) are an expanding universe of disorders, not only characterized by an infectious diathesis but also displaying a wide variety of other clinical features (1). Primary Immune Regulatory Disorders (PIRDs) are a relevant subgroup of IEI that is particularly characterized by autoimmune manifestations (2, 3). The number of genetic defects belonging to this category has strikingly expanded over time (4), and atypical manifestations of known PIRDs have progressively been unveiled (5).
In this dynamic setting, target organs of the autoimmune process may be diverse, but autoimmune cytopenias (AICs) undoubtedly play a leading role (6–8). Indeed, the relative risk of AIC appears to be at least 120 times higher in patients with IEI, compared to the general population, and increases up to 830 times if we consider autoimmune hemolytic anemia (AIHA) alone (6). Moreover, the combination of AIHA and immune thrombocytopenia (ITP) is often the clinical presentation of a PIRD (9–11), and potentially bears a genetic explanation in 65% of cases (12). Indeed, some specific immunological alterations, if accompanied with AIHA, ITP, autoimmune neutropenia (AIN), or their combinations (Evans syndrome, ES) could be significant red flags for an associated IEI (13). These include both humoral and cell-mediated immune defects, like reduced serum immunoglobulin levels and low T cell counts (3, 12, 14, 15), while only scant evidence regarding deeper immunological studies in AICs is available (16).
Regarding treatment, AICs in patients with IEI often fail to respond to first-line therapy, and the best management for refractory AICs still needs to be fully elucidated (17–20). Intravenous immunoglobulins (IVIG) and immunosuppressants are, in some cases, effective (17–19); interestingly, immunomodulatory drugs may significantly attenuate immunological alterations in PIRDs – as seen in ALPS (21, 22) – while rituximab can lead to a persistent hypogammaglobulinemia and potentially unmask an underlying genetic defect (23). Importantly, attaining a definitive molecular diagnosis might open new targeted therapeutic options, as seen in LRBA and CTLA-4 deficiencies as well as in other PIRDs (20, 24–26).
In this context, we sought to investigate the immunological and genetic background of pediatric patients affected by refractory mono- or multi-lineage AICs, eventually associated with additional signs of immune dysregulation. We applied extensive multiparametric flow cytometry, an already established tool in detecting and monitoring IEI (27, 28), to lymphocyte phenotyping on AIC background, in order to select patients in whom PIRD was suspected, and to direct next-generation sequencing (NGS) analysis. Immune phenotyping acted as a potential screening tool for an underlying IEI, thus permitting an early molecular diagnosis and a specific treatment.
Methods
Patient Selection and Data Collection
This prospective study included 30 pediatric and adolescent - young adult (AYA) patients (median age 8,5 years, range 1-24 years) referred to A. Meyer Children Hospital Oncology-Hematology Department for mono- or multilineage AIC, defined by immunological evaluation and/or differential diagnosis with other hematologic causes (i.e. bone marrow failure or malignancies). We recruited patients presenting with: chronic refractory ITP and/or AIHA (>12 months) requiring at least a second-line treatment; and/or AIN not self-resolving (>12 months).
According to the European Hematology Association (EHA) and the Intercontinental Childhood ITP Study (ICIS) working group criteria, ITP was defined by blood platelet count <100x109/l on two separate measurements (29); AIHA by Hb level <11 g/dl and at least one hemolysis criteria among the following: reticulocytosis >120x109/l, total bilirubin >1 mg/dl, and haptoglobin <10 mg/dl (30). AIN was defined as neutrophil counts <1,5x109/l on two separate measurements, after excluding other secondary causes (31). Patients’ classification into two groups, isolated AIC (AIC-alone) and AIC with strong suspicion of IEI (AIC-sIEI), was based on clinical signs of immune dysregulation and/or immunodeficiency, defined as: hypogammaglobulinemia, auto/hyper-inflammation, organ-specific autoimmunity, splenomegaly, lymphadenopathy, lymphoid malignancies and/or recurrent or opportunistic infections. Eventual family history of immune disorders was considered as inclusion criteria.
First-line therapy for cytopenias included intravenous immunoglobulin (IVIG) and/or corticosteroids according to local practice; therefore, refractory ITP and AIHA were treated with second- and third-line therapy (mycophenolate mofetil, MMF; sirolimus; rituximab; eltrombopag). Due to the severity of their clinical condition at time of referral, a few patients directly underwent second- or third-line therapy. To assess response to conventional therapy, the following criteria were used: for ITP, a platelet count >30x109/l with at least a two-fold increase of the pre-treatment count (29). For AIHA, Hb level ≥10 g/dl with an increase of at least 2 g/dl from baseline was considered. A response as defined above lasting at least 2 months would classify a patient as a responder (32).
The study was reviewed and approved by Meyer Hospital Pediatric Ethics Committee, and written informed consent was obtained of all included patients or their parents, according to their age. Data related to patients’ clinical and family history was collected (Supplementary Table 1). Patients’ peripheral blood was analyzed at different time points to perform immunophenotyping (Figure 1), whereas genetic analysis was conducted on both patients and their parents in order to better interpret the genetic results.
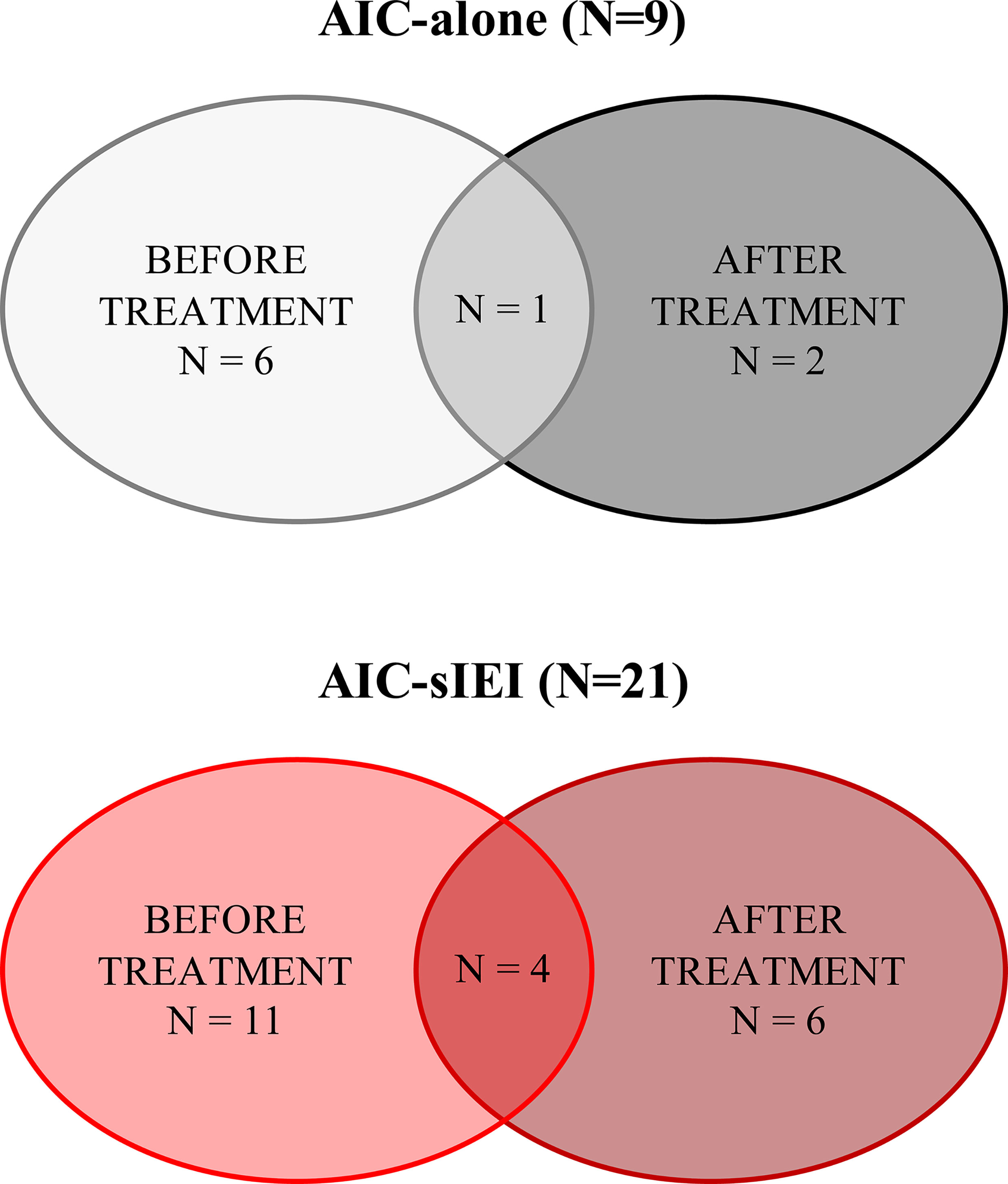
Figure 1 Summary of patients’ sample collection. Patients’ samples were collected before 2nd-line treatment (IVIG and/or corticosteroids) and/or after 2nd- or 3rd-line treatment, which included MMF, sirolimus, rituximab, and eltrombopag. N, count.
Immunophenotyping
Peripheral blood (PB) obtained from patients was processed within 24h after collection to perform immunophenotyping. Upon red blood cell lysis with ammonium chloride, cells were stained to identify T, B and NK cell subsets using the monoclonal antibodies listed in Supplementary Table 2. Flow cytometry data were collected using a MACSQuant Analyzer 10 flow cytometer (Miltenyi Biotec, Bergisch Gladbach, Germany), and analyzed with Flowlogic Software (v. 7.3, Inivai Technologies, Victoria, Australia). The expression of CD3, CD4, CD8, CD27, CD45RA, CD31 was used to identify recent thymic emigrants (RTE, CD45RA+CD31+), naïve (CD27+CD45RA+), central memory (CM, CD27+CD45RA-), effector memory (EM, CD27-CD45RA-) and terminally differentiated effector memory T cells (EMRA, CD27-CD45RA+). Treg cells were identified by CD25 and CD127 expression (CD4+CD25+CD127low), and distinguished in naïve (CD45RA+) and memory (CD45RA-) Treg. Double negative T cells (DNT) were identified by CD4 and CD8 expression within the TCRαβ T subset (TCRαβ+CD4-CD8-). The CD19+ B cell subpopulations were defined based on the differential expression of CD27 and IgD into naïve (CD27-IgD+), pre-switched memory (CD27+IgD+) and switched memory (CD27+IgD-). Plasmablasts (IgM-CD38++) and transitional B cells (CD24+CD38+) were evaluated. NK cells were defined based on CD56 expression (CD3-CD56+). A minimum of 20000 events within the lymphocyte population gate were collected, and gating strategy is shown in Supplementary Table 3. Absolute cell count was calculated from total lymphocyte numbers obtained by differential blood count.
Genetic Analysis
Genetic testing was performed on the AIC-sIEI group, as patients presenting with isolated AIC (AIC-alone) did not meet the clinical and immunological criteria necessary to suspect an immunodeficit. Genomic DNA (gDNA) was extracted from peripheral blood obtained from patients and their parents using the BioRobot EZ1 Workstation (Qiagen, Milan, Italy) and quantified. Sequencing analysis was performed through target resequencing of 58 immune dysregulation-associated genes (Supplementary Table 4) using MiSeq Illumina platform (Illumina, San Diego, USA), or through whole-exome sequencing (WES) according to the protocols indicated. Sequence reads were aligned to the NCBI38/hg38 reference genome using a pipeline based on BWA and Picard, and variants were called using the GATK toolkit. Variants annotation (ANNOVAR tool) and prioritization was performed according to the standard guidelines of the American College of Medical Genetics and Genomics (ACMG) (33), by using a combination of prediction programs (SIFT, PolyPhen, pMUT, Mutation taster, FATHMM score, CADD score) to distinguish potentially damaging variants from those predicted to have neutral effect. Variants that were called less than 5X, off-target, synonymous, or with minor allele frequency (MAF) >1% in the Exome Aggregation Consortium (ExAC, Cambridge, MA http://exac.broadinstitute.org) were eliminated. For WES, data were filtered for a panel of >400 genes published by the International Union of Immunological Societies (IUIS) expert committee on IEI (34).
Statistical Analysis
Analysis of lymphocyte main populations (total lymphocytes, CD3 T cells, CD4 T cells, CD8 T cells, CD19 B cells and CD56 NK cells) count (x109/l) was performed using Microsoft Excel (v. 365, Microsoft Corporation, Redmond, USA), and comparisons between the two groups were made using the Student t-test (two-tailed). GraphPad Prism (v. 8.0, San Diego, USA) was used for univariate analysis of CD4, CD8, Treg and B cell subpopulation frequencies, by applying the nonparametric Mann-Whitney test (two-tailed). P values <0.05 were considered significant. Multivariate analysis on T lymphocyte subsets was performed by Principal Component Analysis (PCA), PAleontological STatistics (PAST, v. 4.03, University of Oslo). PCA is a technique for reducing the dimensionality of large datasets, minimizing information loss and increasing interpretability. The majority of the variation of flow cytometric datasets is captured by the 2 most dominant principal components (Component 1 and 2), representing a Cartesian space in which each sample (patient) is allocated. Samples are plotted to visualize similarities and differences. The overlay of the 2D (2 Dimensional) plot of the scores (patients) with the 2D plot of the loadings (combination of cell subsets) allows the identification of the variables that most contribute to the characterization of the single patient.
Results
Patients’ Clinical Presentation
We enrolled 30 patients, 21 males (70%) and 9 females (30%) and classified them into two groups: isolated AIC (AIC-alone) and AIC with strong suspicion of IEI (AIC-sIEI) based on the associated other clinical signs of immunodeficiency beyond AIC. Cohort clinical and laboratory features are shown in Table 1 and Supplementary Table 1. The most represented cytopenia lineages are ITP and AIHA, the latter peculiar to patients with signs of immune defect. In the AIC-sIEI group, splenomegaly and hypogammaglobulinemia are the most frequent clinical signs, and almost all patients with lymphadenopathy (6/7) also presented with splenomegaly.
Imbalance of Naïve and Memory T Lymphocyte Compartments in AIC Patients With Signs of Immune Dysregulation
As we aimed at defining possible congenital immune defects, causative of a wide spectrum of manifestations other than the cytopenia, we performed an extended immunophenotyping on lymphocyte subsets. Regardless of the diagnostic group, 22 patients were investigated before 2nd-line treatment (Figure 1). No significant differences concerning the absolute counts of the main immune cell populations were identified by groups comparison (Table 2). Absolute count of T, B and NK cells are also available for each patient (Supplementary Table 5).
Analysis performed on CD4+ T cell subsets showed significantly lower frequency of recent thymic emigrants (RTE) and naïve T cells in AIC-sIEI patients compared to AIC, with an increase of T CD4+ central memory (CM) compartment (Figures 2A–E). The same imbalance between naïve and memory compartments was observed for cytotoxic CD8+ T cells (Figures 2F–I). As Treg cells play a pivotal role in peripheral homeostasis, we also evaluated their total frequency, as well as the fraction of naïve and memory Tregs. Patients with AIC-sIEI presented a heterogeneous distribution of Treg subpopulations when compared to the AIC-only group. We also observed a reduction of total (P<0.05) and naïve Tregs, and an increase of memory Treg compartment, as detected for the other T cell lineages (Figures 2J–L).
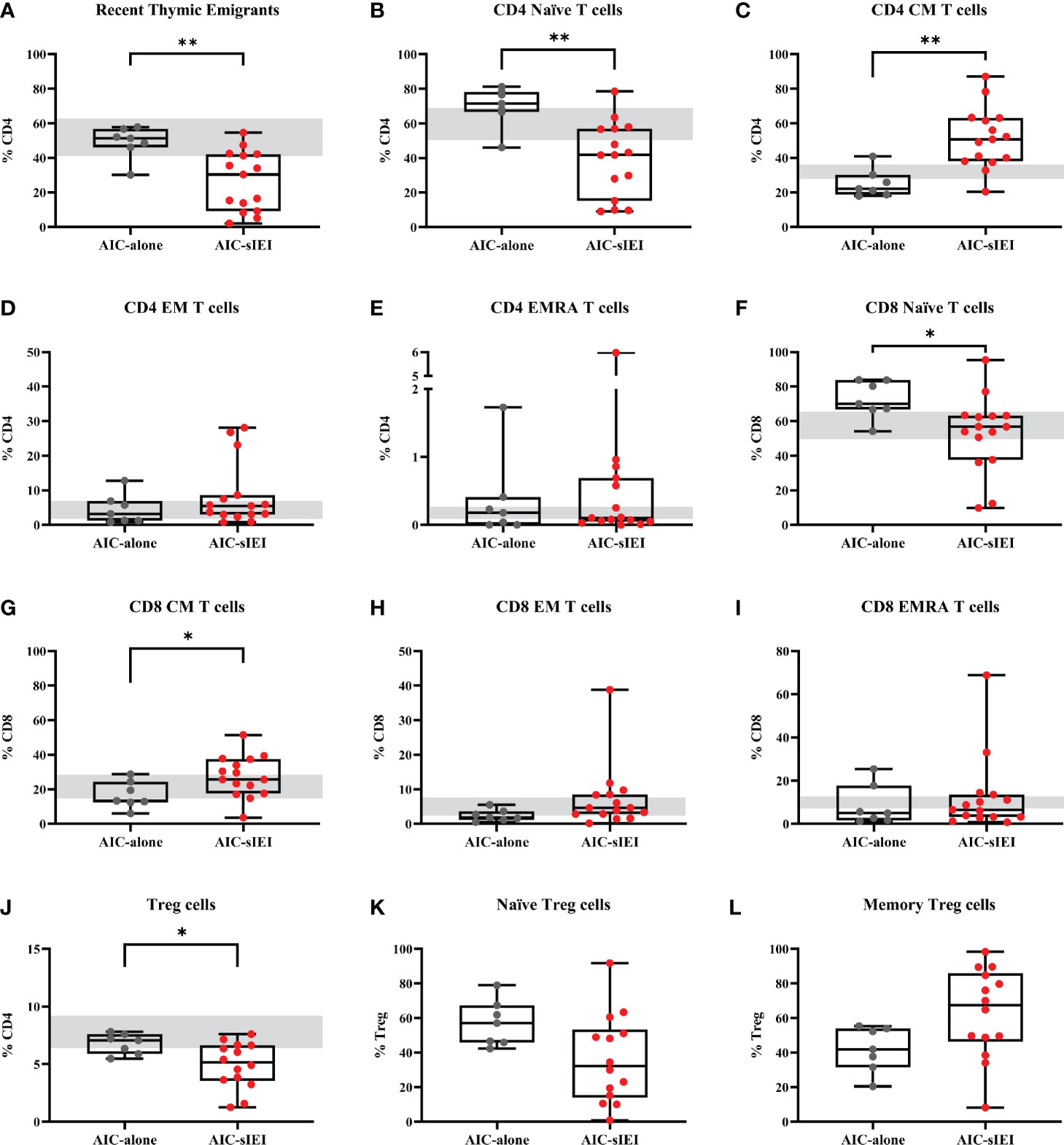
Figure 2 T cell subpopulations immunophenotyping analysis before 2nd- and 3rd-line treatment. Lymphocyte frequencies data (%) of AIC-alone (N=7) and AIC-sIEI (N=15) patients relative to (A–E) helper CD4+ T cells, (F–I) cytotoxic CD8+ T cells and (J–L) Treg subpopulations. (L) Treg subsets were not available for P11. Box plots show the 25th percentile (bottom edge), 50th percentile (median) and 75th percentile (top edge); vertical lines at the top and bottom indicate minimum and maximum values. Grey bars indicate control range, based on age-matched median values (35–37). p-values <0.05 (*) or <0.01 (**) are indicated. CM, central memory T cells; EM, effector memory T cells; EMRA, terminally differentiated effector memory T cells.
T cell subsets frequencies were then analyzed by PCA. Notably, among the AIC-sIEI group (red triangles), 11 patients out of 15 defined a specific subgroup, uniformly distributed in an area far from the AIC-only group that skewed towards CD4+ and CD8+ memory T subsets, while other 4 patients lay inside the AIC-only group area (grey dots) (Figure 3).
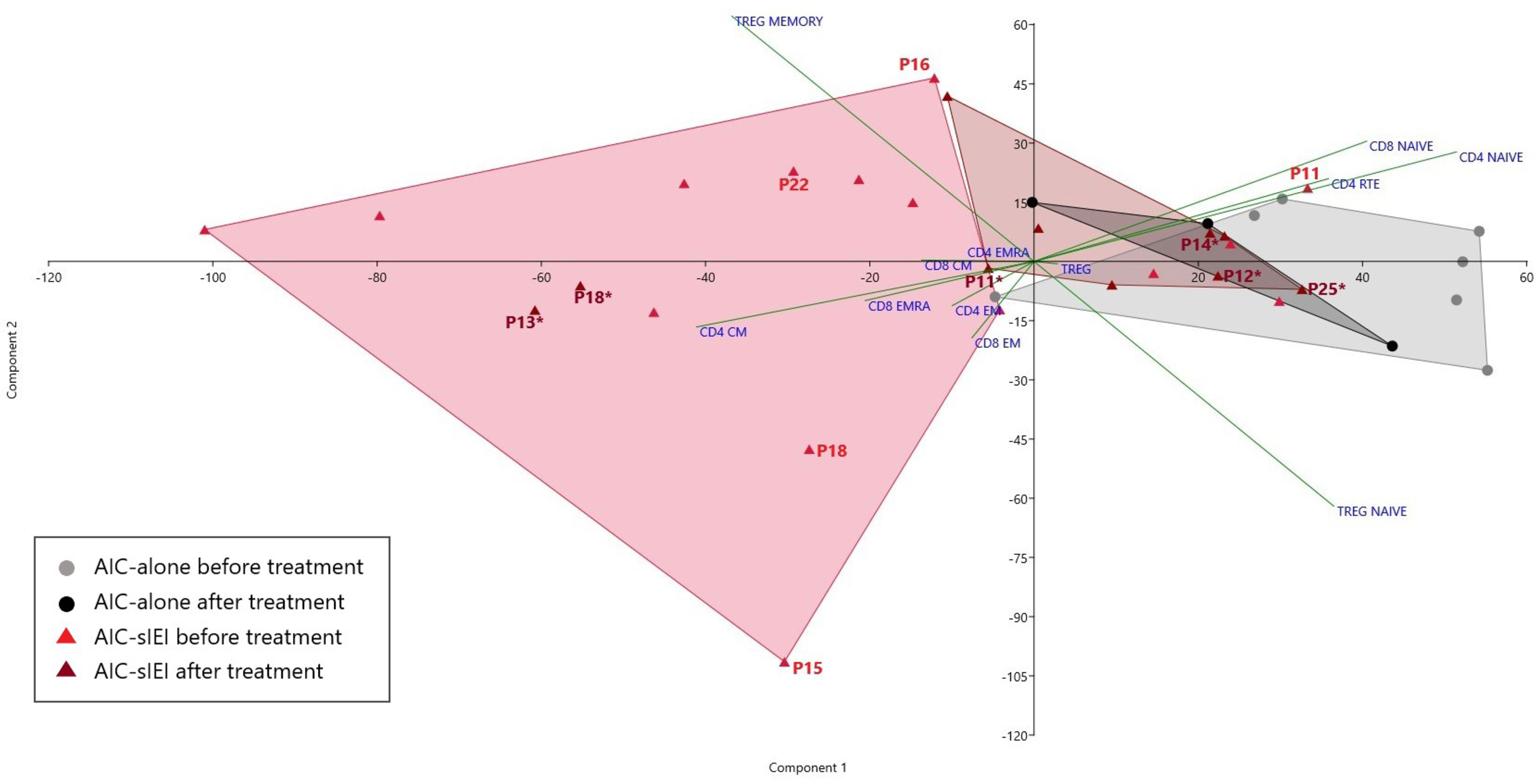
Figure 3 PCA of T cell subsets frequencies before and after 2nd- and/or 3rd-line treatment. Scatter plot displaying the distribution of T cell subsets frequency for AIC-alone and AIC-sIEI patients pre- and post-immunosuppressive (2nd- and/or 3rd-line) treatment (AIC-alone: N=7 before treatment, N=3 after treatment; AIC-sIEI: N=15 before treatment, N=10 after treatment). Grey and red areas indicate patients’ clusterization. AIC-sIEI patients who underwent genetic analysis are indicated for both time points (P, before treatment; P*, after treatment).
Concerning TCRαβ double negative T cell (DNT) evaluation before treatment, two patients (P14 and P18) were found to be in ALPS-range (i.e., >6% of CD3+TCRαβ+ T cells) (38), while other patients displayed borderline DNTs (Supplementary Table 1).
Surprisingly, we did not detect any significant difference within B cell subsets, including CD21 low B cells, by univariate (Supplementary Figure 1) and PCA analysis (data not shown). However, we observed very low switched memory B cell frequencies in patients with hypogammaglobulinemia, as previously described (39).
Effects of Immunomodulatory Treatment on T Cell Subsets
Patients presenting with chronic/refractory AIC require a differential clinical management than patients with acute, transient AIC, which may need to be further adapted in presence of additional signs of immune dysregulation (13). In particular, in our cohort a higher proportion of AIC-sIEI patients underwent 2nd- and 3rd-line treatment, and for 6 patients the severity of their clinical status led to the choice of HSCT as definitive therapy. Conversely, none of the patients only presenting with AIC required HSCT, and those cases with isolated neutropenia needed no treatment (N=3) (Table 3).
In order to assess the treatment effect on T lymphocyte subsets, we compared immunophenotypic data obtained from AIC-alone and AIC-sIEI groups both before and after treatment with immunomodulatory agents (MMF and/or sirolimus) by PCA (Figures 1 and 3). Upon therapy, patients with isolated AIC did not significantly change their position in the PCA plot. On the other hand, AIC-sIEI subjects shifted towards the naïve area of the diagram, with the exception of P13 and P18 who segregated independently, suggesting a different clinical response to treatment (Figure 3).
Identification of Variants in IEI-Associated Genes
Based on immunophenotyping results, we performed genetic analysis in patients with family history of immune disorders and/or signs of IEI, in order to identify the molecular bases of the observed immunological defect. Due to the advances made in sequencing technology, more than half of the patients underwent targeted NGS panel sequencing (14/21, Supplementary Table 4), comprising 58 genes, while in the remaining ones (7/21) WES analysis was performed (34).
Strikingly, genetic analysis of 12/21 AIC-sIEI subjects was inconclusive or needed further investigation, which is currently underway. Of note, two of these (P20 and P29) had clinical and immunological features of common variable immunodeficiency (CVID). Conversely, 9/21 patients presenting with clinical features of immune dysregulation displayed disease-associated variants in the following genes (Figure 4 and Table 4): FAS, UNC13D, STAT3, CARD11, PIK3CD, KMT2D, IKZF1, and AIRE.
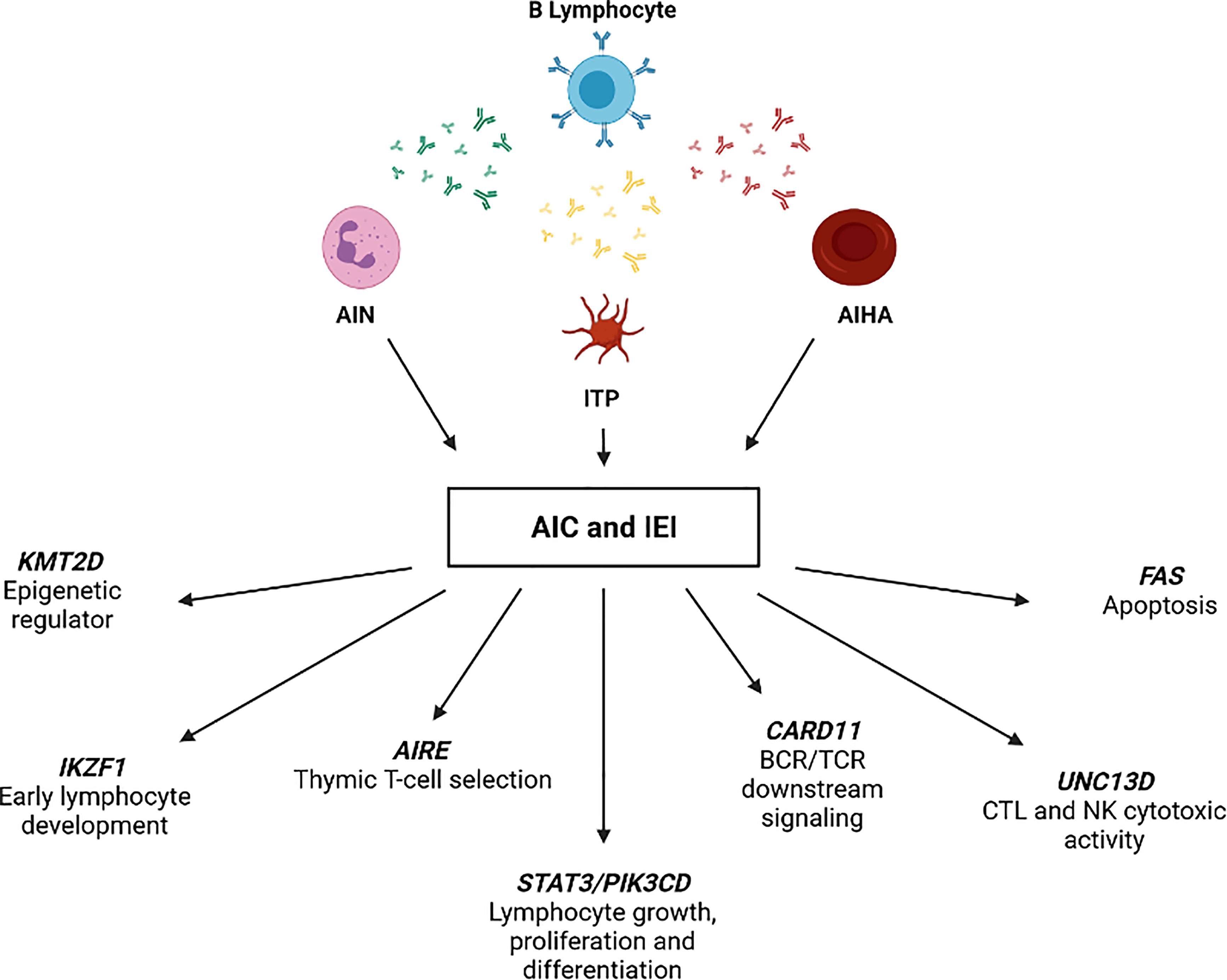
Figure 4 IEI-associated gene variants identified in patients with AIC and signs of immune defect (AIC-sIEI).
We identified a T158fs FAS mutation in P14, presenting with both a family history and clinical signs of autoimmune lymphoproliferative syndrome (ALPS), including high DNT frequency (21.75%) and AIHA. Coherently, he also displayed a reduced FAS expression in T cell subsets. Since other family members carried the same mutation, despite a less profound impact on protein expression, we hypothesize that P14 may also present a somatic loss of heterozygosity (sLOH) in the DNT population (40). Sanger sequencing of DNA extracted from sorted DNTs is currently ongoing.
Two loss-of-function (LOF) mutations in UNC13D (I848L and A995P, in cis) were found in P12, who clinically displayed chronic ITP and lymphoproliferation. These findings are in accordance with a previous report that considered the same UNC13D variants as predisposing to ALPS development (41). Interestingly, a novel variant in UNC13D gene (R1075W) was detected in P22, who presented a CVID-like clinical phenotype with bilineage autoimmune cytopenia (AIN+ITP) and hypogammaglobulinemia.
A de novo heterozygous germline STAT3 P715L mutation previously described (42–44) was identified in P18, presenting with life-threatening AIHA and other clinical findings associated with STAT3 gain-of-function (GOF) (44, 45).
Molecular investigations performed on P16, presenting with AIHA, family history of autoimmunity, celiac disease and splenomegaly led to identification of the I544L gene variant. The variant was previously reported as benign, although autoimmune features - including cytopenias - have already been associated with hypomorphic CARD11 mutations (46).
A known E525A PIK3CD mutation was detected in P15, who presented with lymphadenopathy, splenomegaly and AIHA. Based on these genetic and clinical findings, Activated PI3Kδ Syndrome (APDS) was diagnosed (47, 48).
Kabuki syndrome (KS), a rare multisystemic immune disorder, was diagnosed in P13 carrying the novel heterozygous E1738* mutation in KMT2D gene (49). The patient displayed typical dysmorphic features, chronic ITP and recurrent infections, which have been previously reported in other KS patients (50, 51).
A heterozygous R502L mutation in IKZF1 gene was identified in P25, who came to our attention for Burkitt lymphoma, and subsequently developed AIN and ITP. Functional studies revealed that this genotype leads to reduced protein stability and to impaired IKAROS homo- and heterodimerization by haploinsufficiency (52).
We found the AIRE V301M heterozygous mutation in P11, displaying acute and persistent AIN and ITP. Homozygous AIRE mutations cause Autoimmune Polyendocrinopathy Candidiasis Ectodermal Dystrophy (APECED). Cytopenias have rarely been reported in APECED, even though P11 lacks other typical features and disease-specific autoantibodies (53, 54). However, heterozygous AIRE mutations - including V301M - may hide behind common autoimmune disorders, and lead to variable clinical manifestations among family members (55, 56).
Discussion
This study confirms the strong relationship between AICs and IEI (13, 16), focusing on the potential role of extensive multiparametric flow cytometry and PCA as screening tools for an underlying genetic disorder. T cell phenotypes analyzed before 2nd- or 3rd-line treatment revealed an imbalanced T CD4+ and CD8+ profile in patients with AIC-sIEI. In particular, we observed a significant predominance of the mature/memory T cell compartment, counterbalanced by a reduction of T naïve and RTE subsets. Moreover, a reduced Treg frequency was detected in the AIC-sIEI group. These findings suggest the presence of an underlying immune dysregulation that skews the T cell-mediated response towards an activated status. In a clinical context, this corresponds to autoimmune features with or without lymphoproliferation, which are typically associated with PIRDs (57).
The heterogeneity of lymphocyte frequency data is in line with the high variability of IEIs that may clinically display autoimmune cytopenias (8, 13). These include CVID, which typically bears abnormal B cell subsets including a reduction in switched memory B cells (CD19+CD27+IgD-) frequency (58), especially in patients with autoimmune features (59). The scant number of CVID cases in our cohort (P20 and P29 only) may justify the lack of statistical significance in the frequencies of CD19+CD27+IgD- cells between the AIC and AIC-sIEI groups, as well as for other B cell subsets (e.g., CD21low). Interestingly, evidence suggests that the risk of autoimmunity in CVID is particularly increased in patients bearing a reduction in naïve CD4 cells, RTEs, naïve CD8 and Treg counts (60–62). These findings are surprisingly superimposable to our immunophenotyping results in the AIC-sIEI group, implying that such imbalanced T cell profile clinically correlates with autoimmunity not only in CVID but also in the entire PIRDs galaxy.
Moreover, immunophenotyping revealed elevated levels of DNT cells (38) in two patients: one affected by ALPS-FAS (P14) and the other bearing a STAT3 GOF mutation (P18), which has recently been depicted as a possible cause of ALPS-Undetermined (ALPS-U) (63). Other patients in both groups displayed borderline DNTs, consistent with recent findings in other autoimmune contexts (64, 65). Indeed, our immunophenotypic results actually agree with an approach based on clinical and family history to select patients that should undergo molecular testing.
PCA performed on pre-treatment T cell immunophenotype showed that patients belonging to the AIC-sIEI group uniformly cluster in an area skewed towards the memory compartment (Figure 3), consistent with the presence of an underlying immune dysregulation. Such finding confirms the relevant role of PCA in classifying IEIs (28), and paves the way for its potential usefulness as a screening tool for patients with AICs deserving further genetic analyses. Interestingly, post-treatment PCA revealed a counter-shift of AIC-sIEI patients towards an equilibrium of naïve and memory T cell frequencies. On the other hand, treatment did not significantly impact on the position of the AIC-alone cluster in the PCA plot. This phenomenon highlights that immunomodulatory therapy (MMF and/or sirolimus) determines a partial rebalance of immune dysregulation in the AIC-sIEI subjects, consistent with a good clinical response. Therefore, these drugs might be an early treatment choice for patients with chronic/refractory AICs associated with signs of IEI, and their use should be considered according to the patient’s clinical status, as previously proposed (20). Further studies will be required to define the best therapeutic strategy for AIC patients carrying a still undiagnosed IEI.
Of note, two patients remained in the T memory area of the PCA plot after therapy: P13 and P18, respectively affected by KS and STAT3 GOF disease (49, 66). For P18, such lack of response was most likely due to the life-threatening clinical contingency that brought the patient directly to HSCT (44), without attempting targeted treatment with JAK-inhibitors and tocilizumab (26). The complex immunologic background of KS, due to an altered methylation of crucial transcription factors (51), may explain the persistence of T memory-skewed subsets in P13, which could possibly be reversed only by future applications of epigenome editing (67). Therefore, we may speculate that unbalanced immunophenotypes after immunomodulant therapy can act as a warning sign for the need, in highly selected patients, of additional treatment steps such as HSCT or - if available - targeted drugs. Further studies are needed in order to clarify this aspect.
Importantly, genetic analysis showed that IEI-causing mutations were detected in patients displaying suggestive clinical features or a positive family history (AIC-sIEI group, Table 1). This finding confirms previous results of a recent retrospective study (13), highlighting that associated clinical signs together with extended immunophenotyping (16) should guide physicians in the decision of performing genetic testing. Notably, 12/21 AIC-sIEI subjects had an inconclusive genetic analysis and are undergoing additional investigations, as well as P11 (AIRE), P16 (CARD11) and P22 (UNC13D) whose WES is currently being processed to rule out whether other mutations may cause the clinical phenotype. Given the increasing number of genes associated with IEI (1), especially within the PIRD microcosm (4), we cannot exclude that future reinterpretation of WES may unravel novel IEI-causing genotypes.
Overall, we identified several genetic causes of immune dysregulation, whose immunophenotypic behavior before and/or after immunomodulant therapy is potentially explainable. In ALPS (P14), for instance, sirolimus has already demonstrated to induce a partial normalization of biomarkers (22). On the other hand, ALPS-like disorders such as STAT3 GOF disease (P18) and APDS (P15) (68–70), as well as CARD11 loss-of-function mutations (P16) (46), distort intracellular signaling cascades, leading to the previously described altered immunophenotype. Interestingly, hyperactivation of PI3Kδ (P15) enhances mTOR signaling, skewing the differentiation of CD8+ T cells towards short-living effector cells and impairing the development of memory T and B cells (71). Such mechanism gives a possible explanation to the peripheral position of P15 in the pre-treatment PCA plot (Figure 3). PI3Kδ’s pathway ultimately leads to the suppression of FOXO1, a transcription factor supporting critical genes for lymphocyte development, including IKAROS (P25, heterozygous IKZF1 mutation) (48, 72). Nevertheless, P25’s immunophenotype after therapy shows adequate frequencies of naïve T cells - similarly to other patients with IKAROS dimerization haploinsufficiency (52). Finally, P11 (heterozygous AIRE mutation) displayed elevated T naïve frequencies compared to other AIC-sIEI patients, which normalized upon treatment (Figure 3). Interestingly, P11 presented a decrease in RTE frequencies, similar to previous reports in APECED (73, 74): such finding may potentially support the contribution of the V301M AIRE variant to the patient’s complex autoimmune phenotype.
Interestingly, we observed a lower frequency of AIC-alone patients in our cohort compared to recently published studies (13, 16), which could be ascribable to the different inclusion criteria and to the prospective nature of our work. Moreover, two AIC-sIEI patients presenting with multi-lineage cytopenia (P27 and P28) also displayed autoimmune hepatitis (AIH), which it is known to be associated with severe aplastic anemia (SAA). Nevertheless, an aplastic etiology was ruled out performing bone marrow aspirates and biopsies, which revealed a picture compatible with refractory cytopenia of childhood (RCC). Thus, refractory cytopenia was also recently reported as associated with autoimmune hepatitis (75). In light of their clinical behavior and immunophenotyping features, we initially interpreted these cytopenias as immune-mediated, although the clinical evolution revealed over time RCC. Therefore, we decided to include these patients in our study to raise awareness of possible overlapping hematological conditions at the time of clinical presentation.
Our real-life study has some limitations, mainly due to the restricted sample size and the scarce number of patients that underwent flow cytometry both before and after treatment. Moreover, immunophenotyping was mainly performed during acute clinical presentation, therefore we cannot exclude that these abnormalities are due to the concomitant inflammatory status, rather than the underlying immune dysregulation. However, a recent retrospective study pointed out similar immunologic alterations in patients affected by AICs with a known genetic etiology (16). Coherent findings in two differently designed studies potentially confirm that the immunologic imbalance detected in our AIC-sIEI population should not be ascribable to the concurrent inflammatory background.
In conclusion, the tight interconnection between hematology and immunology is particularly represented by AICs, which underlie an IEI in a not negligible proportion of cases (13). This study confirms that such relationship is particularly recognizable in PIRDs and further demonstrates the kaleidoscopic presentations of IEI (3), which undoubtedly need a multidisciplinary approach. While clinical signs and family history are paramount to suspect an underlying IEI, extended immunophenotyping and PCA may potentially act as screening tools to identify patients deserving genetic analyses. In our case, patients with a strong suspicion of IEI and those who actually received a molecular diagnosis presented with T lymphocyte subsets significantly skewed towards the memory and effector compartments. Our immunophenotypic results allowed us to build a speculative model explicating how the detected genotypes may impact on specific steps of T lymphocyte’s life-cycle (Figure 5). Moreover, this study highlights that performing immunophenotyping before and after immunomodulatory therapy may also act as a monitor for treatment response. Larger prospective investigations are needed to improve current knowledge on clinical warning signs of IEI. Achieving a prompt diagnosis may rapidly lead to target therapies (20, 76), or definitive treatments such as HSCT or gene editing (77, 78).
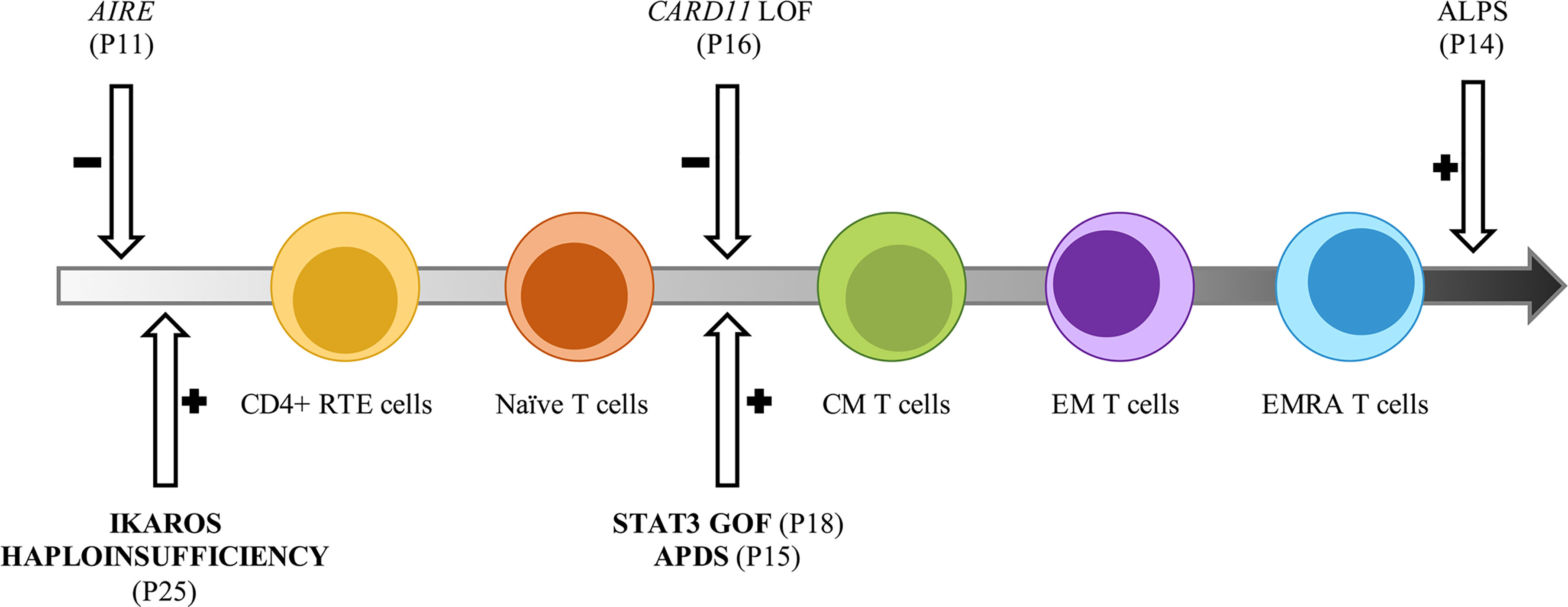
Figure 5 Potential impact of inborn errors of immunity on T cells development and function. Model representative of the T cell subsets alterations observed in the AIC-sIEI group. The gray arrow shows T cell populations skewed towards memory compartment and terminal effectors. Patients harboring a disease-associated (bold) or potentially relevant gene variant are indicated.
Data Availability Statement
The datasets presented in this article are not readily available because according to the protocol approved by Pediatric Ethics Committee for the current study, data sharing is limited to analysis results and not to raw datasets. Requests to access the datasets should be directed to ZWxlb25vcmEuZ2FtYmluZXJpQHVuaWZpLml0.
Ethics Statement
The studies involving human participants were reviewed and approved by the Pediatric Ethics Committee, Meyer University Children Hospital, Florence, Italy. Written informed consent to participate in this study was provided by the participants’ legal guardian/next of kin.
Author Contributions
ES, BM, EA, SCM, and MT performed data collection. ES, BM, and SCM performed immunophenotyping analysis. ES, BM, EA, and SCM analyzed data. MLC performed genetic analysis and analyzed data. EC, IF, LL, ID’A, MV, and EG were responsible for patient recruitment and supplied patient care. ES, BM, EA, FC, SCM, and EG wrote the original draft of the article. CF and EG supervised the work. ES and BM have contributed equally to this work and share first authorship. All authors contributed to the article and approved the submitted version.
Funding
This work was supported by the Ministry of Health grant (Ricerca Finalizzata 2016, Ministero Della Salute RF-2016-02362384), by the Jeffrey Modell Foundation Specific Defect Research Grant (Autoimmune Cytopenias as ‘New warning sign’ of Primary Immunodeficiency Disorders) and by Ente Cassa di Risparmio di Firenze (EG).
Conflict of Interest
The authors declare that the research was conducted in the absence of any commercial or financial relationships that could be construed as a potential conflict of interest.
Publisher’s Note
All claims expressed in this article are solely those of the authors and do not necessarily represent those of their affiliated organizations, or those of the publisher, the editors and the reviewers. Any product that may be evaluated in this article, or claim that may be made by its manufacturer, is not guaranteed or endorsed by the publisher.
Acknowledgments
We acknowledge all patients and their families for their support and cooperation; Rayan Goda and Maddalena Bagni for their valuable support in patients’ clinical evaluation and helpful discussions; Giulia Trippella and Serena Chiellino for their support in data collection. Figure 4 was created with BioRender.com and exported under a paid subscription.
Supplementary Material
The Supplementary Material for this article can be found online at: https://www.frontiersin.org/articles/10.3389/fimmu.2021.790455/full#supplementary-material
References
1. Notarangelo LD, Bacchetta R, Casanova JL, Su HC. Human Inborn Errors of Immunity: An Expanding Universe. Sci Immunol (2020) 5(49):eabb1662. doi: 10.1126/sciimmunol.abb1662
2. Chan AY, Torgerson TR. Primary Immune Regulatory Disorders: A Growing Universe of Immune Dysregulation. Curr Opin Allergy Clin Immunol (2020) 20(6):582–90. doi: 10.1097/ACI.0000000000000689
3. Thalhammer J, Kindle G, Nieters A, Rusch S, Seppänen MRJ, Fischer A, et al. Initial Presenting Manifestations in 16,486 Patients With Inborn Errors of Immunity Include Infections and Noninfectious Manifestations. J Allergy Clin Immunol (2021) 148(5):1332–41.e5. doi: 10.1016/j.jaci.2021.04.015
4. Tangye SG, Al-Herz W, Bousfiha A, Chatila T, Cunningham-Rundles C, Etzioni A, et al. Human Inborn Errors of Immunity: 2019 Update on the Classification From the International Union of Immunological Societies Expert Committee. J Clin Immunol (2020) 40(1):24–64. doi: 10.1007/s10875-019-00737-x
5. Consonni F, Ciullini Mannurita S, Gambineri E. Atypical Presentations of IPEX: Expect the Unexpected. Front Pediatr (2021) 9:643094. doi: 10.3389/fped.2021.643094
6. Fischer A, Provot J, Jais JP, Alcais A, Mahlaoui N, Adoue D, et al. Autoimmune and Inflammatory Manifestations Occur Frequently in Patients With Primary Immunodeficiencies. J Allergy Clin Immunol (2017) 140(5):1388–93.e8. doi: 10.1016/j.jaci.2016.12.978
7. Schmidt RE, Grimbacher B, Witte T. Autoimmunity and Primary Immunodeficiency: Two Sides of the Same Coin? Nat Rev Rheumatol (2017) 14(1):7–18. doi: 10.1038/nrrheum.2017.198
8. Seidel MG. Autoimmune and Other Cytopenias in Primary Immunodeficiencies: Pathomechanisms, Novel Differential Diagnoses, and Treatment. Blood (2014) 124(15):2337–44. doi: 10.1182/blood-2014-06-583260
9. Rivalta B, Zama D, Pancaldi G, Facchini E, Cantarini ME, Miniaci A, et al. Evans Syndrome in Childhood: Long Term Follow-Up and the Evolution in Primary Immunodeficiency or Rheumatological Disease. Front Pediatr (2019) 7:304. doi: 10.3389/fped.2019.00304
10. Besnard C, Levy E, Aladjidi N, Stolzenberg MC, Magerus-Chatinet A, Alibeu O, et al. Pediatric-Onset Evans Syndrome: Heterogeneous Presentation and High Frequency of Monogenic Disorders Including LRBA and CTLA4 Mutations. Clin Immunol (2018) 188:52–7. doi: 10.1016/j.clim.2017.12.009
11. Abraham RS. How to Evaluate for Immunodeficiency in Patients With Autoimmune Cytopenias: Laboratory Evaluation for the Diagnosis of Inborn Errors of Immunity Associated With Immune Dysregulation. Hematol (United States) (2020) 1):661–72. doi: 10.1182/hematology.2020000173
12. Hadjadj J, Aladjidi N, Fernandes H, Leverger G, Magérus-Chatinet A, Mazerolles F, et al. Pediatric Evans Syndrome Is Associated With a High Frequency of Potentially Damaging Variants in Immune Genes. Blood (2019) 134(1):9–21. doi: 10.1182/blood-2018-11-887141
13. Westermann-Clark E, Meehan CA, Meyer AK, Dasso JF, Amre D, Ellison M, et al. Primary Immunodeficiency in Children With Autoimmune Cytopenias: Retrospective 154-Patient Cohort. Front Immunol (2021) 12:649182. doi: 10.3389/fimmu.2021.649182
14. Al Ghaithi I, Wright NAM, Breakey VR, Cox K, Warias A, Wong T, et al. Combined Autoimmune Cytopenias Presenting in Childhood. Pediatr Blood Cancer (2016) 63(2):292–8. doi: 10.1002/pbc.25769
15. Grimes AB, Kim TO, Kirk SE, Flanagan J, Lambert MP, Grace RF, et al. Refractory Autoimmune Cytopenias in Pediatric Evans Syndrome With Underlying Systemic Immune Dysregulation. Eur J Haematol (2021) 106(6):783–7. doi: 10.1111/ejh.13600
16. Zama D, Conti F, Moratti M, Cantarini ME, Facchini E, Rivalta B, et al. Immune Cytopenias as a Continuum in Inborn Errors of Immunity: An in-Depth Clinical and Immunological Exploration. Immun Inflamm Dis (2021) 9(2):583–94. doi: 10.1002/iid3.420
17. Go RS, Winters JL, Kay NE. How I Treat Autoimmune Hemolytic Anemia. Blood (2017) 129(22):2971–9. doi: 10.1182/blood-2016-11-693689
18. Cuker A, Neunert CE. How I Treat Refractory Immune Thrombocytopenia. Blood (2016) 128(12):1547–54. doi: 10.1182/blood-2016-03-603365
19. Farruggia P, Dufour C. Diagnosis and Management of Primary Autoimmune Neutropenia in Children: Insights for Clinicians. Ther Adv Hematol (2015) 6(1):15–24. doi: 10.1177/2040620714556642
20. Seidel MG. Treatment of Immune-Mediated Cytopenias in Patients With Primary Immunodeficiencies and Immune Regulatory Disorders (PIRDs). Hematol (United States) (2020) 1:673–9. doi: 10.1182/hematology.2020000153
21. Teachey DT, Greiner R, Seif A, Attiyeh E, Bleesing J, Choi J, et al. Treatment With Sirolimus Results in Complete Responses in Patients With Autoimmune Lymphoproliferative Syndrome. Br J Haematol (2009) 145(1):101–6. doi: 10.1111/j.1365-2141.2009.07595.x
22. Klemann C, Esquivel M, Magerus-Chatinet A, Lorenz MR, Fuchs I, Neveux N, et al. Evolution of Disease Activity and Biomarkers on and Off Rapamycin in 28 Patients With Autoimmune Lymphoproliferative Syndrome. Haematologica (2017) 102(2):e52–6. doi: 10.3324/haematol.2016.153411
23. Ottaviano G, Marinoni M, Graziani S, Sibson K, Barzaghi F, Bertolini P, et al. Rituximab Unveils Hypogammaglobulinemia and Immunodeficiency in Children With Autoimmune Cytopenia. J Allergy Clin Immunol Pract (2020) 8(1):273–82. doi: 10.1016/j.jaip.2019.07.032
24. Lo B, Zhang K, Lu W, Zheng L, Zhang Q, Kanellopoulou C, et al. Patients With LRBA Deficiency Show CTLA4 Loss and Immune Dysregulation Responsive to Abatacept Therapy. Science (80-) (2015) 349(6246):436–40. doi: 10.1126/science.aaa1663
25. Lee S, Moon JS, Lee CR, Kim HE, Baek SM, Hwang S, et al. Abatacept Alleviates Severe Autoimmune Symptoms in a Patient Carrying a De Novo Variant in CTLA-4. J Allergy Clin Immunol (2016) 137(1):327–30. doi: 10.1016/j.jaci.2015.08.036
26. Forbes LR, Vogel TP, Cooper MA, Castro-Wagner J, Schussler E, Weinacht KG, et al. Jakinibs for the Treatment of Immune Dysregulation in Patients With Gain-of-Function Signal Transducer and Activator of Transcription 1 (STAT1) or STAT3 Mutations. J Allergy Clin Immunol (2018) 142(5):1665–9. doi: 10.1016/j.jaci.2018.07.020
27. van der Burg M, Kalina T, Perez-Andres M, Vlkova M, Lopez-Granados E, Blanco E, et al. The EuroFlow PID Orientation Tube for Flow Cytometric Diagnostic Screening of Primary Immunodeficiencies of the Lymphoid System. Front Immunol (2019) 10:246. doi: 10.3389/fimmu.2019.00246
28. Attardi E, Di Cesare S, Amodio D, Giancotta C, Cotugno N, Cifaldi C, et al. Phenotypical T Cell Differentiation Analysis: A Diagnostic and Predictive Tool in the Study of Primary Immunodeficiencies. Front Immunol (2019) 10:2735. doi: 10.3389/fimmu.2019.02735
29. Rodeghiero F, Stasi R, Gernsheimer T, Michel M, Provan D, Arnold DM, et al. Standardization of Terminology, Definitions and Outcome Criteria in Immune Thrombocytopenic Purpura of Adults and Children: Report From an International Working Group. Blood (2009) 113(11):2386–93. doi: 10.1182/blood-2008-07-162503
30. Aladjidi N, Leverger G, Leblanc T, Picat MQ, Michel G, Bertrand Y, et al. New Insights Into Childhood Autoimmune Hemolytic Anemia: A French National Observational Study of 265 Children. Haematologica (2011) 96(5):655–63. doi: 10.3324/haematol.2010.036053
31. Dinauer M. The Phagocyte System and Disorders of Granulopoiesis and Granulocyte Function. In: Nathan D, Orkin S, editors. Nathan and Oshi’s Hematology of Infancy and Childhood. Philadelphia, PA: WBS Saunders (2003). p. 923–1010.
32. Barcellini W, Fattizzo B, Zaninoni A, Radice T, Nichele I, Di Bona E, et al. Clinical Heterogeneity and Predictors of Outcome in Primary Autoimmune Hemolytic Anemia: A GIMEMA Study of 308 Patients. Blood (2014) 124(19):2930–6. doi: 10.1182/blood-2014-06-583021
33. Richards S, Aziz N, Bale S, Bick D, Das S, Gastier-Foster J, et al. Standards and Guidelines for the Interpretation of Sequence Variants: A Joint Consensus Recommendation of the American College of Medical Genetics and Genomics and the Association for Molecular Pathology. Genet Med (2015) 17(5):405–24. doi: 10.1038/gim.2015.30
34. Bousfiha A, Jeddane L, Picard C, Al-Herz W, Ailal F, Chatila T, et al. Human Inborn Errors of Immunity: 2019 Update of the IUIS Phenotypical Classification. J Clin Immunol (2020) 40(1):66–81. doi: 10.1007/s10875-020-00758-x
35. Schatorjé EJH, Gemen EFA, Driessen GJA, Leuvenink J, van Hout RWNM, de Vries E. Paediatric Reference Values for the Peripheral T Cell Compartment. Scand J Immunol (2012) 75(4):436–44. doi: 10.1111/j.1365-3083.2012.02671.x
36. Ding Y, Zhou L, Xia Y, Wang W, Wang Y, Li L, et al. Reference Values for Peripheral Blood Lymphocyte Subsets of Healthy Children in China. J Allergy Clin Immunol (2018) 142(3):970–3.e8. doi: 10.1016/j.jaci.2018.04.022
37. Garcia-Prat M, Álvarez-Sierra D, Aguiló-Cucurull A, Salgado-Perandrés S, Briongos-Sebastian S, Franco-Jarava C, et al. Extended Immunophenotyping Reference Values in a Healthy Pediatric Population. Cytom Part B - Clin Cytom (2019) 96(3):223–33. doi: 10.1002/cyto.b.21728
38. Abinun M, Albert M, Beaussant Cohen S, Buckland M, Bustamante J, Cant A, et al. ESID Registry 2019 - Working Definitions for Clinical Diagnosis of PID. European Society for Immunodeficiencies (2019). Available at: https://esid.org/Working-Parties/Registry-Working-Party/Diagnosis-criteria.
39. Eroglu FK, Aerts Kaya F, Cagdas D, Özgür TT, Yılmaz T, Tezcan İ, et al. B Lymphocyte Subsets and Outcomes in Patients With an Initial Diagnosis of Transient Hypogammaglobulinemia of Infancy. Scand J Immunol (2018) 88(4):e12709. doi: 10.1111/sji.12709
40. Magerus-Chatinet A, Neven B, Stolzenberg MC, Daussy C, Arkwright PD, Lanzarotti N, et al. Onset of Autoimmune Lymphoproliferative Syndrome (ALPS) in Humans as a Consequence of Genetic Defect Accumulation. J Clin Invest (2011) 121(1):106–12. doi: 10.1172/JCI43752
41. Aricò M, Boggio E, Cetica V, Melensi M, Orilieri E, Clemente N, et al. Variations of the UNC13D Gene in Patients With Autoimmune Lymphoproliferative Syndrome. PloS One (2013) 8(7):e68045. doi: 10.1371/journal.pone.0068045
42. Sediva H, Dusatkova P, Kanderova V, Obermannova B, Kayserova J, Sramkova L, et al. Short Stature in a Boy With Multiple Early-Onset Autoimmune Conditions Due to a STAT3 Activating Mutation: Could Intracellular Growth Hormone Signalling Be Compromised? Horm Res Paediatr (2017) 88(2):160–6. doi: 10.1159/000456544
43. Mauracher AA, Eekels JJM, Woytschak J, van Drogen A, Bosch A, Prader S, et al. Erythropoiesis Defect Observed in STAT3 GOF Patients With Severe Anemia. J Allergy Clin Immunol (2020) 145(4):1297–301. doi: 10.1016/j.jaci.2019.11.042
44. Ciullini Mannurita S, Goda R, Schiavo E, Coniglio ML, Azzali A, Fotzi I, et al. Case Report: Signal Transducer and Activator of Transcription 3 Gain-Of-Function and Spectrin Deficiency: A Life-Threatening Case of Severe Hemolytic Anemia. Front Immunol (2021) 11:620046. doi: 10.3389/fimmu.2020.620046
45. Milner JD, Vogel TP, Forbes L, Ma CA, Stray-Pedersen A, Niemela JE, et al. Early-Onset Lymphoproliferation and Autoimmunity Caused by Germline STAT3 Gain-of-Function Mutations. Blood (2015) 125(4):591–9. doi: 10.1182/blood-2014-09-602763
46. Dorjbal B, Stinson JR, Ma CA, Weinreich MA, Miraghazadeh B, Hartberger JM, et al. Hypomorphic Caspase Activation and Recruitment Domain 11 (CARD11) Mutations Associated With Diverse Immunologic Phenotypes With or Without Atopic Disease. J Allergy Clin Immunol (2019) 143(4):1482–95. doi: 10.1016/j.jaci.2018.08.013
47. Jamee M, Moniri S, Zaki-Dizaji M, Olbrich P, Yazdani R, Jadidi-Niaragh F, et al. Clinical, Immunological, and Genetic Features in Patients With Activated Pi3kδ Syndrome (APDS): A Systematic Review. Clin Rev Allergy Immunol (2020) 59(3):323–33. doi: 10.1007/s12016-019-08738-9
48. Nunes-Santos CJ, Uzel G, Rosenzweig SD. PI3K Pathway Defects Leading to Immunodeficiency and Immune Dysregulation. J Allergy Clin Immunol (2019) 143(5):1676–87. doi: 10.1016/j.jaci.2019.03.017
49. Adam MP, Banka S, Bjornsson HT, Bodamer O, Chudley AE, Harris J, et al. Kabuki Syndrome: International Consensus Diagnostic Criteria. J Med Genet (2019) 56(2):89–95. doi: 10.1136/jmedgenet-2018-105625
50. Giordano P, Lassandro G, Sangerardi M, Faienza MF, Valente F, Martire B. Autoimmune Haematological Disorders in Two Italian Children With Kabuki Syndrome. Ital J Pediatr (2014) 40:10. doi: 10.1186/1824-7288-40-10
51. Stagi S, Gulino AV, Lapi E, Rigante D. Epigenetic Control of the Immune System: A Lesson From Kabuki Syndrome. Immunol Res (2016) 64(2):345–59. doi: 10.1007/s12026-015-8707-4
52. Kuehn HS, Niemela JE, Stoddard J, Mannurita SC, Shahin T, Goel S, et al. Germline IKAROS Dimerization Haploinsufficiency Causes Hematologic Cytopenias and Malignancies. Blood (2021) 137(3):349–63. doi: 10.1182/blood.2020007292
53. Fierabracci A, Pellegrino M, Frasca F, Kilic SS, Betterle C. APECED in Turkey: A Case Report and Insights on Genetic and Phenotypic Variability. Clin Immunol (2018) 194:60–6. doi: 10.1016/j.clim.2018.06.012
54. Husebye ES, Anderson MS, Kämpe O. Autoimmune Polyendocrine Syndromes. N Engl J Med (2018) 378(12):1132–41. doi: 10.1056/NEJMra1713301
55. Bruserud Ø, Oftedal BE, Wolff AB, Husebye ES. AIRE-Mutations and Autoimmune Disease. Curr Opin Immunol (2016) 43:8–15. doi: 10.1016/j.coi.2016.07.003
56. Oftedal BE, Hellesen A, Erichsen MM, Bratland E, Vardi A, Perheentupa J, et al. Dominant Mutations in the Autoimmune Regulator AIRE Are Associated With Common Organ-Specific Autoimmune Diseases. Immunity (2015) 42(6):1185–96. doi: 10.1016/j.immuni.2015.04.021
57. Walter JE, Ayala IA, Milojevic D. Autoimmunity as a Continuum in Primary Immunodeficiency. Curr Opin Pediatr (2019) 31(6):851–62. doi: 10.1097/MOP.0000000000000833
58. Seidel MG, Kindle G, Gathmann B, Quinti I, Buckland M, van Montfrans J, et al. The European Society for Immunodeficiencies (ESID) Registry Working Definitions for the Clinical Diagnosis of Inborn Errors of Immunity. J Allergy Clin Immunol Pract (2019) 7(6):1763–70. doi: 10.1016/j.jaip.2019.02.004
59. Alachkar H, Taubenheim N, Haeney MR, Durandy A, Arkwright PD. Memory Switched B Cell Percentage and Not Serum Immunoglobulin Concentration Is Associated With Clinical Complications in Children and Adults With Specific Antibody Deficiency and Common Variable Immunodeficiency. Clin Immunol (2006) 120(3):310–8. doi: 10.1016/j.clim.2006.05.003
60. Gereige JD, Maglione PJ. Current Understanding and Recent Developments in Common Variable Immunodeficiency Associated Autoimmunity. Front Immunol (2019) 10:2753. doi: 10.3389/fimmu.2019.02753
61. Bateman EAL, Ayers L, Sadler R, Lucas M, Roberts C, Woods A, et al. T Cell Phenotypes in Patients With Common Variable Immunodeficiency Disorders: Associations With Clinical Phenotypes in Comparison With Other Groups With Recurrent Infections. Clin Exp Immunol (2012) 170:202–11. doi: 10.1111/j.1365-2249.2012.04643.x
62. Arumugakani G, Wood PMD, Carter CRD. Frequency of Treg Cells Is Reduced in CVID Patients With Autoimmunity and Splenomegaly and Is Associated With Expanded CD21lo B Lymphocytes. J Clin Immunol (2010) 30(2):292–300. doi: 10.1007/s10875-009-9351-3
63. Molnár E, Radwan N, Kovács G, Andrikovics H, Henriquez F, Zarafov A, et al. Key Diagnostic Markers for Autoimmune Lymphoproliferative Syndrome With Molecular Genetic Diagnosis. Blood (2020) 136(17):1933–45. doi: 10.1182/blood.2020005486
64. Tarbox JA, Keppel MP, Topcagic N, Mackin C, Ben Abdallah M, Baszis KW, et al. Elevated Double Negative T Cells in Pediatric Autoimmunity. J Clin Immunol (2014) 34(5):594–9. doi: 10.1007/s10875-014-0038-z
65. Brandt D, Hedrich CM. Tcrαβ+CD3+CD4–CD8– (Double Negative) T Cells in Autoimmunity. Autoimmun Rev (2018) 17(4):422–30. doi: 10.1016/j.autrev.2018.02.001
66. Consonni F, Dotta L, Todaro F, Vairo D, Badolato R. Signal Transducer and Activator of Transcription Gain-of-Function Primary Immunodeficiency/Immunodysregulation Disorders. Curr Opin Pediatr (2017) 29(6):711–7. doi: 10.1097/MOP.0000000000000551
67. Lee J, Bayarsaikhan D, Bayarsaikhan G, Kim J-S, Schwarzbach E, Lee B. Recent Advances in Genome Editing of Stem Cells for Drug Discovery and Therapeutic Application. Pharmacol Ther (2020) 209:107501. doi: 10.1016/j.pharmthera.2020.107501
68. Bride K, Teachey D. Autoimmune Lymphoproliferative Syndrome: More Than a FAScinating Disease. F1000Research (2017) 6:1928. doi: 10.12688/f1000research.11545.1
69. Todaro F, Tamassia N, Pinelli M, Moratto D, Dotta L, Grassi A, et al. Multisystem Autoimmune Disease Caused by Increased STAT3 Phosphorylation and Dysregulated Gene Expression. Haematologica (2019) 104(7):e322–5. doi: 10.3324/haematol.2018.202374
70. Hafezi N, Zaki-Dizaji M, Nirouei M, Asadi G, Sharifinejad N, Jamee M, et al. Clinical, Immunological, and Genetic Features in 780 Patients With Autoimmune Lymphoproliferative Syndrome (ALPS) and ALPS-Like Diseases: A Systematic Review. Pediatr Allergy Immunol (2021) 32(7):1519–32. doi: 10.1111/pai.13535
71. Lucas CL, Zhang Y, Venida A, Wang Y, Hughes J, McElwee J, et al. Heterozygous Splice Mutation in PIK3R1 Causes Human Immunodeficiency With Lymphoproliferation Due to Dominant Activation of PI3K. J Exp Med (2014) 211(13):2537–47. doi: 10.1084/jem.20141759
72. Okkenhaug K. Signaling by the Phosphoinositide 3-Kinase Family in Immune Cells. Annu Rev Immunol (2013) 31:675–704. doi: 10.1146/annurev-immunol-032712-095946
73. Heikkilä N, Laakso SM, Mannerström H, Kekäläinen E, Saavalainen P, Jarva H, et al. Expanded CD4+ Effector/Memory T Cell Subset in APECED Produces Predominantly Interferon Gamma. J Clin Immunol (2016) 36(6):555–63. doi: 10.1007/s10875-016-0302-5
74. Peterson P, Husebye E. Polyendocrine Syndromes. In: Rose N, Mackay I, editors. The Autoimmune Diseases. Cambridge, MA: Academic Press (2020). p. 731–48.
75. Rasmussen LK, Stenbøg EV, Kerndrup GB, Hasle H. Autoimmune Hepatitis and Seronegative Hepatitis Associated With Myelodysplastic Syndrome in Children. J Pediatr Hematol Oncol (2016) 38(8):e274–7. doi: 10.1097/MPH.0000000000000651
76. Delmonte OM, Castagnoli R, Calzoni E, Notarangelo LD. Inborn Errors of Immunity With Immune Dysregulation: From Bench to Bedside. Front Pediatr (2019) 7:353. doi: 10.3389/fped.2019.00353
77. Castagnoli R, Delmonte OM, Calzoni E, Notarangelo LD. Hematopoietic Stem Cell Transplantation in Primary Immunodeficiency Diseases: Current Status and Future Perspectives. Front Pediatr (2019) 7:295. doi: 10.3389/fped.2019.00295
Keywords: autoimmune cytopenia, autoimmune thrombocytopenia, autoimmune hemolytic anemia, autoimmune neutropenia, Evans syndrome, immunophenotyping, primary immune regulatory disorder (PIRD), inborn errors of immunity (IEIs)
Citation: Schiavo E, Martini B, Attardi E, Consonni F, Ciullini Mannurita S, Coniglio ML, Tellini M, Chiocca E, Fotzi I, Luti L, D’Alba I, Veltroni M, Favre C and Gambineri E (2022) Autoimmune Cytopenias and Dysregulated Immunophenotype Act as Warning Signs of Inborn Errors of Immunity: Results From a Prospective Study. Front. Immunol. 12:790455. doi: 10.3389/fimmu.2021.790455
Received: 06 October 2021; Accepted: 22 November 2021;
Published: 04 January 2022.
Edited by:
Markus G. Seidel, Medical University of Graz, AustriaReviewed by:
Sujal Ghosh, Heinrich Heine University of Düsseldorf, GermanyRoshini Sarah Abraham, Nationwide Children’s Hospital, United States
Emma Westermann-Clark, University of South Florida, United States
Copyright © 2022 Schiavo, Martini, Attardi, Consonni, Ciullini Mannurita, Coniglio, Tellini, Chiocca, Fotzi, Luti, D’Alba, Veltroni, Favre and Gambineri. This is an open-access article distributed under the terms of the Creative Commons Attribution License (CC BY). The use, distribution or reproduction in other forums is permitted, provided the original author(s) and the copyright owner(s) are credited and that the original publication in this journal is cited, in accordance with accepted academic practice. No use, distribution or reproduction is permitted which does not comply with these terms.
*Correspondence: Eleonora Gambineri, ZWxlb25vcmEuZ2FtYmluZXJpQHVuaWZpLml0; ZS5nYW1iaW5lcmlAbWV5ZXIuaXQ=
†These authors have contributed equally to this work and share first authorship