- Department of Rheumatology and Immunology, Ruijin Hospital, Shanghai Jiao Tong University School of Medicine, Shanghai, China
Adult-onset immunodeficiency syndrome due to anti-interferon (IFN)-γ autoantibodies has attracted much attention in recent years. It usually occurs in previously healthy people and usually presents as chronic, recurrent, and hard-to-control infections that can be effectively treated with aggressive antibiotic therapy. Adult-onset immunodeficiency syndrome is also referred to as AIDS-like syndrome. Anti-type I IFN (IFN-I) autoantibodies have been reported to play a significant role in the pathogenesis of coronavirus disease 2019 (COVID-19) and preexisting anti-IFN-I autoantibodies are associated with an increased risk of severe COVID-19. This review summarizes the effects of anti-IFN autoantibodies on the susceptibility and severity of various infectious diseases, including SARS-CoV-2 infection. In addition, we discuss the role of anti-IFN autoantibodies in the pathogenesis of autoimmune diseases that are characterized by recurrent infections.
Introduction
In recent years, life-threatening diseases caused by anti-cytokine autoantibodies have received widespread attention, especially those in previously healthy adults. Patients usually demonstrate a unique infectious syndrome associated with high-titer neutralizing autoantibodies that target a specific cytokine. The autoantibodies may block the activity of the corresponding cytokine, which enhances susceptibility to one or more pathogenic bacteria. For example, granulocyte-macrophage colony-stimulating factor (GM-CSF) is required for surfactant degradation in the pulmonary alveoli and boosts innate immunity (1–3). Neutralizing anti-GM-CSF autoantibodies were discovered in patients with acquired pulmonary alveolar proteinosis (PAP), a respiratory disease in which surfactant protein accumulates in the lung (4, 5). Patients with acquired PAP are vulnerable to typical respiratory pathogens as well as opportunistic infections caused by pathogens such as Nocardia (6), nontuberculous mycobacteria (NTM) (7), Histoplasma (8), and Cryptococcus (9). Interleukin (IL)-17A, IL-17F, and IL-22 are considered to be important in mucosal immunity. They work together to produce proinflammatory cytokines that are important in neutrophil recruitment, granulopoiesis, and the generation of antimicrobial peptides such as S100 protein and defensins (10, 11). Chronic mucocutaneous candidiasis (CMC) has been observed in individuals with anti-IL-17A, anti-IL-17F, and anti-IL-22 autoantibodies, resulting from these autoantibodies blocking the Th-17 immunity (12). Furthermore, these autoantibodies and CMC are also linked to autoimmune polyendocrinopathy-candidiasis-ectodermal dystrophy syndrome (APECED) and thymoma (13). Other anti-cytokine autoantibodies, such as anti-IL-2 autoantibodies, have been found in human immunodeficiency virus (HIV)-infected patients (14); anti-G-CSF autoantibodies were identified in Felty’s syndrome (FS), in which neutropenia is associated with bacterial infections (15); and anti-IL-6 autoantibodies have been confirmed to be associated with severe bacterial infections, including Escherichia coli, Streptococcus intermedius, and Staphylococcus aureusinfections (14). In summary, anti-cytokine autoantibodies have been considered as an important etiology of immunodeficiency, though there are also many immunodeficiencies that do not rely on anti-cytokine autoantibodies, like GATA2 deficiency, one of the most prevalent causes of hereditary bone marrow failure. Its phenotype is distinguished by immunodeficiency, which results in invasive viral, mycobacterial, and fungal infections (16). In addition, there are other autoimmune manifestations observed with considerable frequency in patients with primary antibody deficiencies, including common variable immunodeficiency (CVID) and selective IgA deficiency (sIgAD), but also in patients with combined immunodeficiency disorders (CID) (17).
Interferons (IFNs) play a pivotal role in the immune system. They form the first line of defense in humans in response to pathogen invasion, especially against viral infection (18, 19). IFNs can be divided into three families, type I IFN (mostly IFN-α and IFN-β, several other subtypes also included), type II IFN (IFN-γ), and type III IFN (IFN-λ). All types of IFNs work through the JAK/STAT pathway where they bind receptors and sequentially active Janus family kinases (JAK and TYK) and signal transducer and activator of transcription (STAT), which leads to transcriptional activation of interferon stimulating genes (20).
Adult-onset immunodeficiency syndrome (AOID) differs from primary immunodeficiency disease (PID). Patients with AOID always have high titers of anti-IFN-γ autoantibodies, which is strongly associated with higher mortality (21–23). Since the coronavirus disease 2019 pneumonia (COVID-19) outbreak, it has been discovered in several studies that preexisting autoantibodies against type I IFNs (anti-IFN-I autoantibodies) are linked to the severity of COVID-19, which has aroused great interest in academic circles (24–26). Both AOID and COVID-19 are infectious diseases that usually lead to severe conditions in patients; therefore, the present study considers how anti-IFN autoantibodies are closely related to infection, particularly severe infectious diseases. This review summarizes the effects of anti-IFN autoantibodies on the susceptibility and severity of different infectious diseases. The role of anti-IFN autoantibodies in autoimmune diseases complicated with recurrent infection is also discussed.
IFNs and Their Abs
IFN, first described by A. Isaacs and J. Lindenmann in 1957 (27), was thought to be an antiviral substance. The mechanism by which interferon acts against viruses is to stimulate the production of cytokines and the upregulation of antiviral effector proteins (28). Host cell death is an intrinsic cell defense mechanism during infection. Several studies have conclusively demonstrated that the necroptosis and pyroptotic cell death pathways are crucial for the release and action of inflammatory cytokines (29–31). Th1 cells, a separate subset of CD4+ Th cells, is also critical for protection against pathogens (32).
IFNs are the first cytokines discovered and provide the basis for understanding the structure, pathways, evolution, and functions of other cytokines and their receptors. They are also the first cytokines used in therapy (33). IFNs are used to treat autoimmune diseases including multiple sclerosis (34), tumors such as lymphoma (35), infectious diseases such as disseminated nocardiosis (36), and hepatitis C (37). Recently, it is even considered as a potential therapeutic target for COVID-19.
Type I IFN
Type I IFNs, including IFN-α, IFN-β, IFN-ω, IFN-ϵ, IFN-κ, IFN-δ, and IFN-τ (33), are potent antiviral molecules, acting in both innate immunity (in particular via their secretion by plasmacytoid dendritic cells) and cell-intrinsic immunity (in most if not all cell types) (27). All these IFNs mentioned above bind to IFN-α receptor (IFNαR), which is composed of two subunits, IFNαR1 and IFNαR2. Once the type I IFNs bind to the receptor, the binding causes the phosphorylation of TYK2 and JAK1, which are associated with IFNαR1 and IFNαR2 separately; the phosphorylation of TYK2 and JAK1 subsequently leads to the phosphorylation of STAT1/STAT2. Activated STATs dimerize, translocate to the nucleus, and regulate type I IFN-inducible gene expression so as to stimulate the production of cytokines and the upregulation of antiviral effector proteins (28, 38). Duncan et al. reviewed monogenic lesions of type I IFN signaling pathways and informed our understanding of the type I IFN system within the concerted antiviral response (39).
Anti-IFN-I autoantibodies can be found in patients treated with IFN-a or IFN-β (40) and exist in almost all patients with autoimmune polyendocrine syndrome type-1 (APS-1) (41). They are also observed in patients with systemic lupus erythematosus (SLE) (42). Lately, they have been shown to affect life-threatening COVID-19 (24). Moreover, it is reported that inborn errors are more common in patients under the age of 60 years, whereas autoantibodies are more common in patients over the age of 70 years in COVID-19 (43).
Type II IFN
Type II IFNs, also called IFN-γ, are generated mainly by natural killer (NK) cells, and NKT cells are one of the crucial cytokines for host defense against intracellular pathogens associated with antigen presentation, macrophage differentiation and activation, production of proinflammatory cytokines, cell death, tumor immunity, and autoimmunity (44). Cytokine-induced NK cell activation is regulated by IL-12. In the early stages of infection, phagocytes secrete IL-12 after binding with its receptor on NK cells, which results in activation of STAT4 and eventual production of IFN-γ. NK cell-mediated IFN-γ production promotes the activation of phagocytes, leads to increased secretion of IL-12 by phagocytes, and finally establishes a positive feedback loop (38, 45).
IFN-γ receptor (IFNγR) is presented in nearly every cell type, except for mature erythrocytes (46). The receptor is made up of IFNR1 and IFNR2 and belongs to the class II cytokine receptor family. Similar to type I IFNs, after binding to the IFNγR, IFN-γ activates the JAK-STAT pathway and mediates various biological responses (46, 47).
Type III IFN
The type III IFN (refers to IFN-λ), first described in 2003, is the most recently recognized type of IFNs (48). IFN-λ is secreted by most cells but acts mainly on epithelial surfaces because of the restricted receptor expression (49).
In simple terms, no matter what type of interferons, anti-IFN autoantibodies with high titers in serum interrupt the activation of the downstream responsive pathway by blocking the combination between IFNs and their receptor and the consequence is increased infection rates (Figures 1, 2) (50).
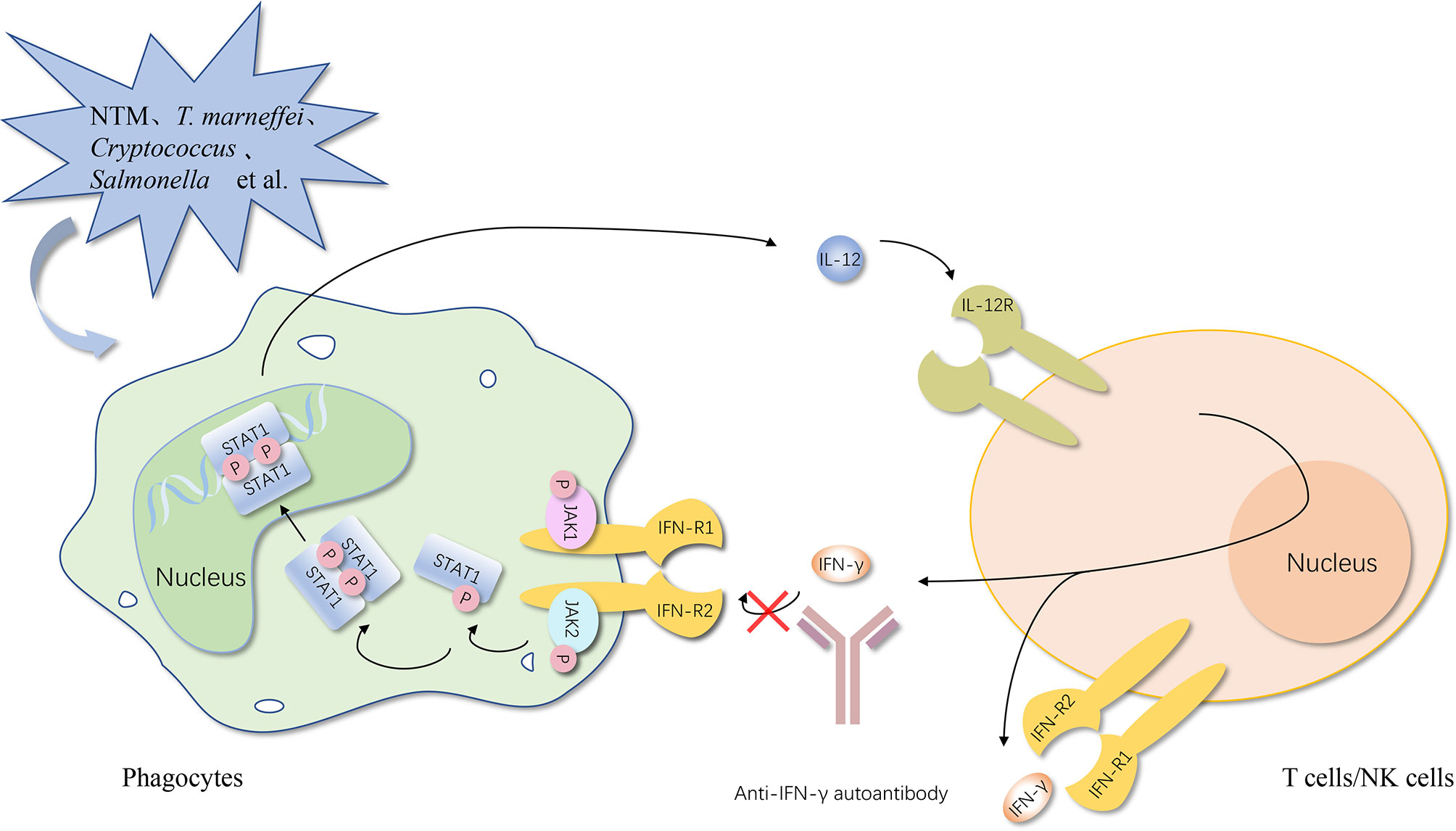
Figure 1 Anti-IFN-γ autoantibody increases the susceptibility to opportunistic pathogens via the IFN-γ/IL-12 axis. Once the opportunistic pathogens invade, activated phagocytes produce IL-12. After binding with its receptor on NK cells and NKT cells, IL-12 induces the secretion of IFN-γ. IFN-γ can bind to the IFN-γ receptor on phagocytes and promote the activation of phagocytes, thereby further increasing the production of anti-microbial proteins to control opportunistic pathogens and IL-12, establishing a positive feedback loop finally. Anti-IFN-γ autoantibody causes opportunistic infections by impairing the binding of type I IFNs to their receptor and the activation of the downstream responsive pathway. IFN, interferon; IFN-R, interferon-receptor; IL-12, interleukin-12; STAT, signal transducer and activator of transcription; P, phosphorylation; JAK, Janus kinase. The red cross represents the pathway blocked by the antibody.
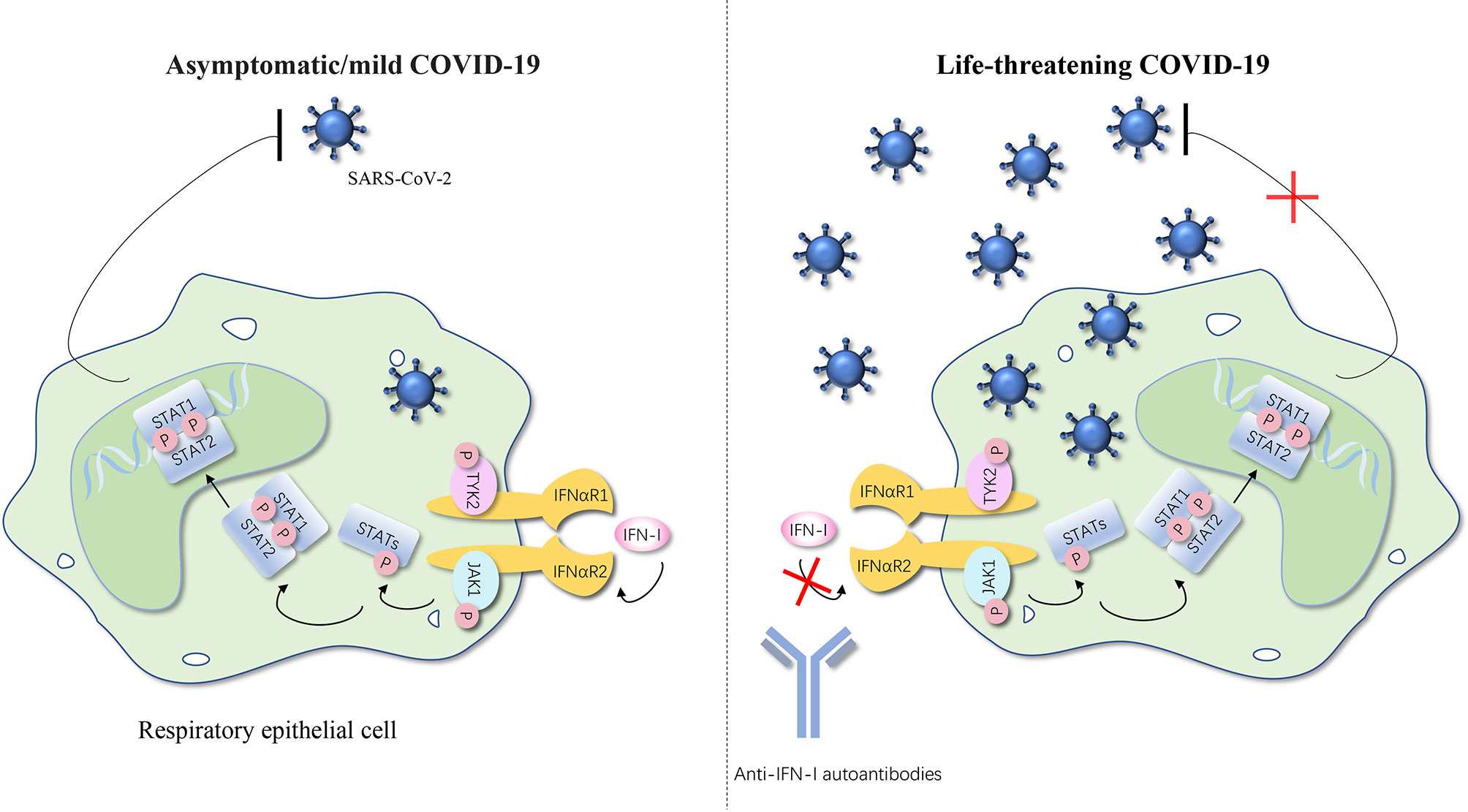
Figure 2 Anti-IFN-I autoantibodies underlie life-threatening COVID-19. IFN-I can bind to the IFNαR and cause the phosphorylation of TYK2 and JAK1, thereby further activating STATs. STATs translocate to the nucleus and regulate type I IFN–inducible gene expression to achieve the anti-SARS-CoV-2 activity. Autoantibodies against type I IFNs may underlie critical COVID-19 by blocking the binding of type I IFNs to their receptor and impairing type I IFN immunity. The number of SARS-CoV-2 represents the severity of the COVID-19. IFN, interferon; IFNαR, interferon-α receptor; STAT, signal transducer and activator of transcription; P, phosphorylation; JAK, Janus kinase; TYK, tyrosine kinase; SARS-CoV-2, severe acute respiratory syndrome coronavirus 2; COVID-19, coronavirus disease 2019 pneumonia. The red cross represents the pathway blocked by the autoantibodies.
Anti-IFN-γ Autoantibodies in AOID
Infection can be regarded as a normal event in anyone’s lifetime. However, if someone experiences chronic, recurrent, hard-to-control, or unusual serious infections of common pathogens compared with the normal population, then a potential deficiency of the immune defense can be considered.
Since 2004, some infectious diseases caused by opportunistic pathogens have been reported in adults without immunodeficiency (51–55). They were not infected with HIV but, similar to AIDS, showed clinical manifestations due to extremely low immunity. Browne et al. first came up with the term “adult-onset immunodeficiency syndrome” to describe this new type of disease because its major patient population is composed of adults (56). To date, a few studies regarding AOID have been conducted and published. The cumulative reports confirmed the critical role of anti-IFN-γ autoantibody in the pathogenesis of AOID (21, 57).
As we know, genetic deficiencies of the innate immune pathway lead to severe intracellular pathogen infections, which is known as Mendelian susceptibility to mycobacterial disease (MSMD), also called PID (58). PID are more common in children although both children and adults can be affected (58). However, for most reported AOID cases, infection occurred in adults aged around 50 years (18–78 years). Interestingly, almost all of the patients with AOID come from Asia, or live elsewhere but were born in Asia, which means that there is a distinct racial predisposition in the population (59). Accumulated reports strongly suggested that the AOID is highly associated with the expression of human leukocyte antigen (HLA). Chi et al. presented that HLA-DRB1*16:02 and HLA-DQB1*05:02 may account for the pathogenesis of NTM and varicella-zoster virus infection (57). HLA-DQB1*05:01 and HLA-DQB1*05:02 were also found in patients with a very high risk of critical AOID (60).
In addition, it is reported that both genetic factors and environmental exposure contribute to AOID. Moreover, autoimmunity caused by the anti-IFN-γ autoantibody plays an important role as well (21). Browne et al. showed that neutralizing anti-IFN-γ autoantibody was detectable in 88% of adults with multiple opportunistic infections in Asia, and up to 41 cytokine autoantibodies were detected in 97 opportunistic infection patients in Taiwan and Thailand. The results indicate that only anti-IFN-γ autoantibodies are closely associated with opportunistic infections in patients (56).
In patients with AOID, the clinical presentation, site of infection, and related pathogens may vary from one race to another. Thereinto, Nontuberculous mycobacteria (NTM) is the most common pathogenic microorganism (61). Rapidly growing mycobacteria (RGM), such as Mycobacteria abscess, are the most common NTM species isolated from patients in Thailand, China, and the Philippines while Mycobacterium avium complex (MAC) is mainly present in Japanese and non-Asian patients (59, 62). Lymph nodes are the most usual organ involved in patients with RGM infection, while bone and lung infections are the predominant apparatuses in patients with MAC infection (59). NTM are ubiquitous, low pathogenic environmental bacteria that widely exist in water, soil, and man-made environments (63, 64). The incidence of various infections caused by weakly virulent organisms increases year by year and has become more common than those caused by Mycobacterium tuberculosis (61). Disseminated NTM infections mainly occur in individuals with obvious immunodeficiencies, such as hairy cell leukemia patients, post-transplant patients, advanced HIV infection patients, individuals taking glucocorticoid or immunosuppressive therapies, and those with Mendelian defects in the IL-12/IFN-γ axis (65). Recently, an increasing number of disseminated NTM infections have been observed in HIV-negative individuals with no obvious immunosuppression, and the anti-IFN-γ autoantibody was identified as an important risk factor (64, 66, 67).
Talaromyces marneffei (T. marneffei) is another extremely important pathogen in AOID. T. marneffei infection in humans is supposed to be caused by inhalation of T. marneffei conidia in the environment (68). Upon entering the human body, it can replicate in yeast form in macrophages to cause infection, ranging from local infection in lungs and skin to disseminated systemic infection (69). The same as NTM infection, T. marneffei infection usually occurs in immunocompromised individuals with impaired cell-mediated immunity, including secondary immunodeficiency due to HIV infection, cancer, and immunosuppressive therapy. Among the patients with T. marneffei infection, the lungs and skin were the dominant organs affected (70). However, the patients with anti-IFN-γ autoantibody are more likely to have pleural effusion and multiple organ involvement (23). Chen et al. confirmed that patients who tested positive for anti-IFN-γ autoantibody usually have more frequently exhibited disseminated systemic infections with severe pleural effusion, have stronger inflammatory responses, are more likely to be infected by other intracellular pathogen, and have worse disease outcomes despite aggressive antimicrobial therapy (71).
In addition, other microorganisms including Salmonella species, Cryptococcus neoformans, Histoplasmosis capsulate, Burkholderia pseudomallei, Listeria, Varicella–zoster virus, Cytomegalovirus, and Epstein–Barr virus are also frequently identified as opportunistic pathogens in this patient group (56, 59, 72). Co-infection with at least 2 pathogens is more pinpoint to diagnose AOID.
Abs Against Type I IFNs in Life-Threatening COVID-19
COVID-19, which is caused by severe acute respiratory syndrome coronavirus 2 (SARS-CoV-2), has become a serious public health emergency of international concern (73, 74). Up to September 1, 2021, it has infected over 200 million people around the world (https://coronavirus.jhu.edu/map.html). It is reported that most people infected with COVID-19 are asymptomatic or have just mild symptoms and about 20% may present with life-threatening complications, including multisystem organ failure, acute respiratory distress syndrome, and ultimately death (73, 75).
Risk factors for disease severity include older age, male sex, ethnicity, increased BMI, and pre-existing comorbidities such as chronic lung disease, cardiovascular disease, hypertension, diabetes, and cancer (76). Additionally, host genetic factors are also relevant to COVID-19 susceptibility and severity (77, 78). Pathogen recognition receptors of the innate immune system, e.g., toll-like receptors (TLRs), recognize pathogen-associated molecular patterns and activate IFN-related immune responses. They stimulate the production of proinflammatory cytokines via NF-κB activation and activate the interferon regulatory factors (IRFs), which induce type I IFN expression (79). IFNAR1 and IFNAR2 bind type I IFNs and are involved in signal transduction by activating the JAK-STAT pathway. Efficient IFN-I-mediated antiviral responses are critical for viral clearance. Zhang et al. reported that at least 3.5% of patients with life-threatening COVID-19 had known (AR IRF7 and IFNAR1 deficiencies or AD TLR3, TICAM1, TBK1, and IRF3 deficiencies) or new (AD UNC93B1, IRF7, IFNAR1, and IFNAR2 deficiencies) genetic defects at eight of the 13 candidate loci involved in the TLR3- and IRF7-dependent induction and amplification of type I IFNs (80). Chiara et al. found TLR7 deleterious variants in 2.1% of severely affected males and none of the asymptomatic participants (81). Autoimmune polyendocrine syndrome type 1 (APS-1), also known as APECED, is a monogenic inborn error of immunity typically caused by biallelic deleterious variants of the autoimmune regulator (AIRE) gene (82). Bastard et al. presented in their study that virtually all patients with APS-1 produce autoantibodies against type I IFNs, and 86% of them were hospitalized for COVID-19 pneumonia, including 68% who were admitted to an intensive care unit, 50% of whom required mechanical ventilation, and 18% of whom died (83). Moreover, other GWASs identified the SNP rs11385942 on chromosome 3, situated at 3p21.31 and around the genes SLC6A20, LZTFL1, CCR9, FYCO1, CXCR6, and XCR1, as a risk variant (84–86). Simply speaking, COVID-19 is characterized by excessive activation of both the innate and adaptive immune systems, giving rise to hyperinflammatory cytokine storms that mainly result in organ damage (87).
Anti-IFN-I autoantibodies account for life-threatening COVID-19 in at least 10.2% of patients. To be specific, it is detected in at least 2.6% of women and 12.5% of men with a life-threatening disease. As a control, it is undetectable in all 663 patients with asymptomatic or mild SARS-CoV-2 infection, and detectable in only 0.3% of 1227 healthy individuals (24). Furthermore, both in vivo and in vitro, these autoantibodies against type I IFNs showed their neutralizing activity, including the ability to block SARS-CoV-2 infection (24). Collectively, these data provide compelling evidence that neutralizing anti-IFN-I autoantibodies are important to the elimination of SARS-CoV-2 infection and can even explain the severity of the disease (24).
Neutralizing anti-IFN-I autoantibodies may impair the binding of type I IFNs to IFNαR and the activation of the downstream pathway, block the antiviral effect of type I IFNs, and result in life-threatening COVID-19. Bastard et al. also constructed a two-step model to explain the pathogenesis of life-threatening COVID-19 with insufficient type I IFN immunity during the first few days of infection resulting in underlying viral growth and dissemination and leading to the unleashing of damaging secondary, excessive pulmonary, and systemic inflammation (24–26).
Therapy Strategies of Diseases Associated With Anti-IFN Abs
Standardized antimicrobial therapy is the most important treatment but it is still difficult to cure just by using antibiotics in AOID patients with anti-IFN-γ autoantibody (88). Decreasing the titer of anti-IFN-γ autoantibody is considered the key to curing patients. Likewise, as a substitution to ectogenous type I IFNs, treatments including plasmapheresis to deplete monoclonal antibodies, some specific interventions to inhibit the production of reactive B cells, and plasmablast depletion were confirmed to be beneficial in COVID-19 patients with autoantibodies against type I IFNs (24, 83, 89)
Cyclophosphamide
Cyclophosphamide is a nitrogen mustard analog, which exerts its effects through inhibition of DNA replication. The drug acts by activating capable of preventing protein synthesis through DNA and RNA crosslinking and eventually inhibits cell division (90, 91). Cyclophosphamide has been used to treat several neoplastic diseases such as lymphomas, leukemia, ovary, and breast cancer, and is also identified as an immunosuppressant (91). Chetchotisakd et al. reported the use of pulse intravenous cyclophosphamide in 7 patients who had refractory, progressive Mycobacterium abscessus infection. Finally, 5 patients had favorable outcomes without hospitalization while 2 patients were hospitalized due to relapse. Even more to the point, the anti-IFN-γ autoantibody titers among all the 7 patients significantly decreased during treatment, and the degrees of reduction among satisfactory prognosis vs. poor prognosis were significantly different (92).
Rituximab
Rituximab is a monoclonal antibody binding to the CD20 antigen and used to treat types of cancer and certain autoimmune diseases (93). Browne et al. used rituximab in 4 previously healthy women with a high-titer anti-IFN-γ autoantibody and disseminated NTM who had progressive disease despite aggressive antimicrobial therapy. After receiving differing amounts of rituximab, all patients achieved clinical remission along with a reduction in antibody titer and relief of inhibition in the IFN-γ pathway (94). Carlos et al. presented in the literature the first case of clinical remission in a patient who had prolonged COVID-19 symptoms by using monoclonal neutralizing antibodies (95).
Daratumumab
Daratumumab is an IgGκ monoclonal antibody targeting CD38, which is highly expressed in plasma cells, plasmablasts, B cells in early maturation stages, and myeloma cells (96). Prior studies have shown that CD38 is an effective treatment for multiple myeloma patients with a striking reduction of RF, ANCA, and ANA titer (97). Sebastian et al. described the first use of daratumumab in a patient with no prior history of immunodeficiency disease who had a high titer of neutralizing anti-IFN-γ autoantibody. The patient’s disease was not under control despite receiving various antibiotics and multiple cycles of rituximab and bortezomib. However, treatment with daratumumab eventually resulted in clinical and radiographic improvement (98).
Exogenous IFN
Exogenous recombinant IFN binds to circulating antibodies, resulting in inhibition of their pathogenicity. Here, timing is of the essence. In COVID-19, early application of IFN was beneficial to decrease viral load and improved the prognosis of disease, whereas delayed IFN treatment was of no benefit compared with the placebo group (99). Concerning AOID, Harada et al. presented a case of a 65-year-old Japanese man who was infected with NTM. In this case, the antibiotics treatment did not function well enough and exogenous subcutaneous injection of IFN-γ therapy had a good response (100).
Plasma Exchange
Therapeutic plasma exchange is a plasma purification treatment capable of eliminating large molecular weight substances from blood (101). It is the fastest way to remove circulating autoantibodies. Nicolas et al. reported four patients with life-threatening COVID-19 who were treated with plasma exchange as a rescue therapy (89).
Other Treatments
Transfusion of convalescent plasma to infected patients has emerged as an effective therapy for severe COVID-19. It attenuates the severe inflammatory response by providing cytokines and other factors regulating the immune response against SARS-CoV-2 (75).
In conclusion, these reports suggest that patients with anti-IFNs autoantibodies always have a chronic, persistent infection, and we should pay attention to long-term treatment. Cyclophosphamide, autoantibody depletion with plasma exchange, B-cell depletion with anti-CD20 antibody, and supplementation of recombinant IFN have been successfully used as adjuvant therapies combined with antimicrobial therapy in a small percentage of patients. Among patients treated with antibiotics alone, antibiotics and rituximab, and antibiotics and cyclophosphamide, the median time to infection clearance was 3, 4, and 5 years, respectively (59).
Prospect of Anti-IFNs Autoantibodies in Autoimmune Diseases
In autoimmune diseases, autoantibodies against one cytokine or more cytokines have been reported, but their spectrum and clinical effect remain largely unknown (38, 42). In different circumstances, they may be beneficial or harmful, depending not only on the activity of the autoantibodies themselves but also on the intrinsic role of the target cytokines. It is noteworthy that autoantibodies against type I and type II IFN in serum were detected in up to 21.6% of patients with SLE (42). Their effect on IFN gene expression characteristics and pathogenesis of SLE is unknown, but they influence IFN signaling, disease activity, and response to biologic therapy (102). On the one hand, their existence may help to classify patients with SLE or other autoimmune diseases currently diagnosed with similar pathological features. Gupta et al. used the luciferase immunoprecipitation system method to detect and quantify 24 different anti-cytokine autoantibodies and evaluated a total of 498 patients diagnosed with SLE, primary Sjogren’s syndrome, or rheumatoid arthritis. Eventually, the increased SLEDAI score and abnormal laboratory tests in SLE patients were almost entirely attributable to the presence of anti-IFN-γ autoantibody (42). On the other hand, despite the continuous progress in the treatment of SLE in recent years, infection is still the primary cause of morbidity and death of SLE patients (103). We noted that some patients with SLE often present with recurrent and refractory infections clinically, which are still difficult to control and prone to recurrence despite being treated with antibiotics aggressively. Among them, cytomegalovirus, Epstein–Barr virus, and varicella-zoster virus are the most common pathogenic agent, and Klebsiella pneumoniae, Acinetobacter baumannii, and Cryptococcus neoformans are not rare. These pathogens are also involved in AOID.
Thus, we believe that the level of neutralizing anti-IFN autoantibodies in patients with SLE and other autoimmune diseases complicated with repeated infection deserves further investigation. It may have important suggestive significance for the prognosis and outcome of the disease, and may also have important value in guiding the treatment of this kind of disease.
Conclusion
To date, cumulative reports and studies suggest that anti-IFN-I autoantibodies play an important role in life-threatening COVID-19. Meanwhile, anti-IFN-γ autoantibodies are crucial to AOID. Anti-cytokine autoantibodies can have severe consequences as well as highly varied manifestations. It is necessary to detect serum anti-IFN autoantibody levels for patients with clinical manifestations of multiple opportunistic pathogen infections or refractory infections. At present, no standard treatment guidelines have been established for anti-IFN autoantibody-related infections, and control of infection combined with immunotherapy is a feasible method. In autoimmune diseases, the growing importance of cytokines and cytokine autoantibodies is starting to emerge, especially since we are now in an era of targeted therapy using biological preparations. A better understanding of the resistant properties and biological functions of cytokine autoantibodies in autoimmune disease patients may help to design future treatment. Further research to study key questions regarding the pathogenicity of anti-IFN autoantibodies in autoimmune disease patients infected with opportunistic pathogens will become feasible.
Author Contributions
LC constructed the figures and wrote the manuscript. CY designed the review’s structure and revised the manuscript. XC designed the figures and revised the manuscript. All authors contributed to the review and approved the submitted version.
Funding
This work was supported by the National Natural Science Foundation of China (81801596).
Conflict of Interest
The authors declare that the research was conducted in the absence of any commercial or financial relationships that could be construed as a potential conflict of interest.
Publisher’s Note
All claims expressed in this article are solely those of the authors and do not necessarily represent those of their affiliated organizations, or those of the publisher, the editors and the reviewers. Any product that may be evaluated in this article, or claim that may be made by its manufacturer, is not guaranteed or endorsed by the publisher.
References
1. Akagawa KS, Kamoshita K TT. Effects of Granulocyte-Macrophage Colony-Stimulating Factor and Colony-Stimulating Factor-1 on the Proliferation and Differentiation of Murine Alveolar Macrophages. J Immunol (1988)141 3383–90.
2. Shibata Y, Berclaz PY, Chroneos ZC, Yoshida M, Whitsett JA, Trapnell BC. GM-CSF Regulates Alveolar Macrophage Differentiation and Innate Immunity in the Lung Through PU.1. Immunity (2001) 15:557–67. doi: 10.1016/s1074-7613(01)00218-7
3. Bhattacharya P, Thiruppathi M, Elshabrawy HA, Alharshawi K, Kumar P, Prabhakar BS. GM-CSF: An Immune Modulatory Cytokine That can Suppress Autoimmunity. Cytokine (2015) 75(2):261–71. doi: 10.1016/j.cyto.2015.05.030
4. Kitamura T, Tanaka N, Watanabe J, Uchida, Kanegasaki S, Yamada Y, et al. Idiopathic Pulmonary Alveolar Proteinosis as an Autoimmune Disease With Neutralizing Antibody Against Granulocyte/Macrophage Colony-Stimulating Factor. J Exp Med (1999) 190:875–80. doi: 10.1084/jem.190.6.875
5. Piccoli L, Campo I, Fregni CS, Rodriguez BM, Minola A, Sallusto F, et al. Neutralization and Clearance of GM-CSF by Autoantibodies in Pulmonary Alveolar Proteinosis. Nat Commun (2015) 6:7375. doi: 10.1038/ncomms8375
6. Abdul Rahman JA, Moodley YP MJP. Pulmonary Alveolar Proteinosis Associated With Psoriasis and Complicated by Mycobacterial Infection: Successful Treatment With Granulocyte-Macrophage Colony Stimulating Factor After a Partial Response to Whole Lung Lavage. Respirology (2004) 9:419–22. doi: 10.1111/j.1440-1843.2004.00594.x
7. Wu XK LQ. Pulmonary Alveolar Proteinosis Complicated With Nocardiosis: A Case Report and Review of the Literature. World J Clin cases (2021) 9:2874–83. doi: 10.12998/wjcc.v9.i12.2874
8. Garrido L, Mata-Essayag S, Hartung de Capriles C, Eugenia Landaeta M, Pacheco I, Fuentes Z. Pulmonary Histoplasmosis: Unusual Histopathologic Findings. Pathol Res Pract (2006) 202(5):373–8. doi: 10.1016/j.prp.2005.10.012
9. Kuo CY, Wang SY, Shih HP, Tu KH, Huang WC, Ding JY, et al. Disseminated Cryptococcosis Due to Anti-Granulocyte-Macrophage Colony-Stimulating Factor Autoantibodies in the Absence of Pulmonary Alveolar Proteinosis. J Clin Immunol (2017) 37(2):143–52. doi: 10.1007/s10875-016-0364-4
10. Xu S, Cao X. Interleukin-17 and its Expanding Biological Functions. Cell Mol Immunol (2010) 7(3):164–74. doi: 10.1038/cmi.2010.21
11. Lindahl H, Olsson T. Interleukin-22 Influences the Th1/Th17 Axis. Front Immunol (2021) 12:618110. doi: 10.3389/fimmu.2021.618110
12. Puel A, Doffinger R, Natividad A, Chrabieh M, Barcenas-Morales G, Picard C, et al. Autoantibodies Against IL-17A, IL-17F, and IL-22 in Patients With Chronic Mucocutaneous Candidiasis and Autoimmune Polyendocrine Syndrome Type I. J Exp Med (2010) 207(2):291–7. doi: 10.1084/jem.20091983
13. Kisand K, Boe Wolff AS, Podkrajsek KT, Tserel L, Link M, Kisand KV, et al. Chronic Mucocutaneous Candidiasis in APECED or Thymoma Patients Correlates With Autoimmunity to Th17-Associated Cytokines. J Exp Med (2010) 207(2):299–308. doi: 10.1084/jem.20091669
14. Nanki T, Onoue I, Nagasaka K, Takayasu A, Ebisawa M, Hosoya T, et al. Suppression of Elevations in Serum C Reactive Protein Levels by Anti-IL-6 Autoantibodies in Two Patients With Severe Bacterial Infections. Ann Rheum Dis (2013) 72:1100–2. doi: 10.1136/annrheumdis-2012-202768
15. Hellmich B, Csernok E, Schatz H, Gross WL, Schnabel A. Autoantibodies Against Granulocyte Colony-Stimulating Factor in Felty’s Syndrome and Neutropenic Systemic Lupus Erythematosus. Arthritis Rheum (2002) 46(9):2384–91. doi: 10.1002/art.10497
16. Fabozzi F, Strocchio L, Mastronuzzi A, Merli P. GATA2 and Marrow Failure. Best Pract Res Clin Haematol (2021) 34(2):101278. doi: 10.1016/j.beha.2021.101278
17. Allenspach E, Torgerson TR. Autoimmunity and Primary Immunodeficiency Disorders. J Clin Immunol (2016) 36 Suppl 1:57–67. doi: 10.1007/s10875-016-0294-1
18. Plotnikova M, Lozhkov A, Romanovskaya-Romanko E, Baranovskaya I, Sergeeva M, Kаа K, et al. IFN-Lambda1 Displays Various Levels of Antiviral Activity In Vitro in a Select Panel of RNA Viruses. Viruses (2021) 13(8):1602–15. doi: 10.3390/v13081602
19. Banos-Lara Mdel R, Harvey L, Mendoza A, Simms D, Chouljenko VN, Wakamatsu N, et al. Impact and Regulation of Lambda Interferon Response in Human Metapneumovirus Infection. J Virol (2015) 89(1):730–42. doi: 10.1128/JVI.02897-14
20. Chow KT, Gale M Jr. Snapshot: Interferon Signaling. Cell (2015) 163(7):1808– e1. doi: 10.1016/j.cell.2015.12.008
21. Guo J, Ning XQ, Ding JY, Zheng YQ, Shi NN, Wu FY, et al. Anti-IFN-Gamma Autoantibodies Underlie Disseminated Talaromyces Marneffei Infections. J Exp Med (2020) 217(12):e20190502. doi: 10.1084/jem.20190502
22. Kampitak T, Suwanpimolkul G, Browne S, Suankratay C. Anti-Interferon-Gamma Autoantibody and Opportunistic Infections: Case Series and Review of the Literature. Infection (2011) 39(1):65–71. doi: 10.1007/s15010-010-0067-3
23. Browne SK. Anticytokine Autoantibody-Associated Immunodeficiency. Annu Rev Immunol (2014) 32:635–57. doi: 10.1146/annurev-immunol-032713-120222
24. Bastard P, Rosen LB, Zhang Q, Michailidis E, Hoffmann HH, Zhang Y, et al. Autoantibodies Against Type I Ifns in Patients With Life-Threatening COVID-19. Science (2020) 370(6515):eabd4585. doi: 10.1126/science.abd4585
25. Zhang Q, Bastard P, Liu Z, Le Pen J, Moncada-Velez M, Chen J, et al. Inborn Errors of Type I IFN Immunity in Patients With Life-Threatening COVID-19. Science (2020) 370(6515):eabd4570. doi: 10.1126/science.abd4570
26. Zhang Q, Bastard P, Bolze A, Jouanguy E, Zhang SY, Effort CHG, et al. Life-Threatening Covid-19: Defective Interferons Unleash Excessive Inflammation. Med (N Y) (2020) 1(1):14–20. doi: 10.1016/j.medj.2020.12.001
27. Isaacs A, Lindenmann J. Virus Interference. I. The Interferon. Proc R Soc Lond B Biol Sci (1957) 147(927):258–67. doi: 10.1098/rspb.1957.0048
28. Gokhale NS, Smith JR, Van Gelder RD, Savan R. RNA Regulatory Mechanisms That Control Antiviral Innate Immunity. Immunol Rev (2021) 304 77–96. doi: 10.1111/imr.13019
29. Chan FK, Luz NF, Moriwaki K. Programmed Necrosis in the Cross Talk of Cell Death and Inflammation. Annu Rev Immunol (2015) 33:79–106. doi: 10.1146/annurev-immunol-032414-112248
30. Bergsbaken T, Fink SL, Cookson BT. Pyroptosis: Host Cell Death and Inflammation. Nat Rev Microbiol (2009) 7(2):99–109. doi: 10.1038/nrmicro2070
31. Li Y, Guo X, Hu C, Du Y, Guo C, Di W, et al. Type I IFN Operates Pyroptosis and Necroptosis During Multidrug-Resistant a. Baumannii Infection. Cell Death Differ (2018) 25(7):1304–18. doi: 10.1038/s41418-017-0041-z
32. Weinstein JS, Laidlaw BJ, Lu Y, Wang JK, Schulz VP, Li N, et al. STAT4 and T-Bet Control Follicular Helper T Cell Development in Viral Infections. J Exp Med (2018) 215(1):337–55. doi: 10.1084/jem.20170457
33. Pestka S KC, Walter MR. Interferons, Interferon-like Cytokines, and their Receptors. Immunol Rev (2004) 202:8–32. doi: 10.1111/j.0105-2896.2004.00204.x
34. Cohan SL, Hendin BA, Reder AT, Smoot K, Avila R, Mendoza JP, et al. Interferons and Multiple Sclerosis: Lessons From 25 Years of Clinical and Real-World Experience With Intramuscular Interferon Beta-1a (Avonex). CNS Drugs (2021) 35(7):743–67. doi: 10.1007/s40263-021-00822-z
35. Lapenta C, Donati S, Spadaro F, Lattanzi L, Urbani F, Macchia I, et al. Lenalidomide Improves the Therapeutic Effect of an Interferon-Alpha-Dendritic Cell-Based Lymphoma Vaccine. Cancer Immunol Immunother (2019) 68(11):1791–804. doi: 10.1007/s00262-019-02411-y
36. Derungs T, Leo F, Loddenkemper C, Schneider T. Treatment of Disseminated Nocardiosis: A Host–Pathogen Approach With Adjuvant Interferon Gamma. Lancet Infect Dis (2021) 21(10):e334–40. doi: 10.1016/s1473-3099(20)30920-8
37. Huang Y, Qi M, Liao C, Xun J, Zou J, Huang H, et al. Analysis of the Efficacy and Safety of Pegylated Interferon-Alpha2b Treatment in Inactive Hepatitis B Surface Antigen Carriers. Infect Dis Ther (2021) 10:2323–31. doi: 10.1007/s40121-021-00511-w
38. Meager A, Wadhwa M, Dilger P, Bird C, Thorpe R, Newsom-Davis J, et al. Anti-Cytokine Autoantibodies in Autoimmunity: Preponderance of Neutralizing Autoantibodies Against Interferon-Alpha, Interferon-Omega and Interleukin-12 in Patients With Thymoma and/or Myasthenia Gravis. Clin Exp Immunol (2003) 132:128–36. doi: 10.1046/j.1365-2249.2003.02113.x
39. Duncan CJA, Randall RE, Hambleton S. Genetic Lesions of Type I Interferon Signalling in Human Antiviral Immunity. Trends Genet (2021) 37(1):46–58. doi: 10.1016/j.tig.2020.08.017
40. Vallbracht A, Treuner J, Flehmig B, Joester KE. Interferon-Neutralizing Antibodies in a Patient Treated With Human Fibroblast Interferon. Nature (1981) 289:496–7. doi: 10.1038/289496a0
41. Levin M. Anti-Interferon Auto-Antibodies in Autoimmune Polyendocrinopathy Syndrome Type 1. PloS Med (2006) 3(7):e292. doi: 10.1371/journal.pmed.0030292
42. Gupta S, Tatouli IP, Rosen LB, Hasni S, Alevizos I, Manna ZG, et al. Distinct Functions of Autoantibodies Against Interferon in Systemic Lupus Erythematosus: A Comprehensive Analysis of Anticytokine Autoantibodies in Common Rheumatic Diseases. Arthritis Rheumatol (2016) 68(7):1677–87. doi: 10.1002/art.39607
43. Bastard P, Gervais A, Le Voyer T, Rosain J, Philippot Q, Manry J, et al. Autoantibodies Neutralizing Type I Ifns are Present in ~4% of Uninfected Individuals Over 70 Years Old and Account for ~20% of COVID-19 Deaths. Sci Immunol (2021) 6, eabl4340. doi: 10.1126/sciimmunol.abl4340
44. Billiau A, Matthys P. Interferon-Gamma: A Historical Perspective. Cytokine Growth Factor Rev (2009) 20(2):97–113. doi: 10.1016/j.cytogfr.2009.02.004
45. Alspach E, Lussier DM, Schreiber RD. Interferon Gamma and its Important Roles in Promoting and Inhibiting Spontaneous and Therapeutic Cancer Immunity. Cold Spring Harb Perspect Biol (2019) 11(3):a028480. doi: 10.1101/cshperspect.a028480
46. Bach EA, Aguet M, Schreiber RD. The IFN Gamma Receptor: A Paradigm for Cytokine Receptor Signaling. Annu Rev Immunol (1997) 15:563–91. doi: 10.1146/annurev.immunol.15.1.563
47. Hibino Y, Mariano TM, Kumar CS, Kozak CA, Pestka S. Expression and Reconstitution of a Biologically Active Mouse Interferon Gamma Receptor in Hamster Cells. Chromosomal Location of an Accessory Factor. J Biol Chem (1991) 266(11):6948–51. doi: 10.1016/S0021-9258(20)89594-5
48. Kotenko SV, Gallagher G, Baurin VV, Lewis-Antes A, Shen M, Shah NK, et al. IFN-Lambdas Mediate Antiviral Protection Through a Distinct Class II Cytokine Receptor Complex. Nat Immunol (2003) 4(1):69–77. doi: 10.1038/ni875
49. Sommereyns C, Paul S, Staeheli P, Michiels T. IFN-Lambda (IFN-Lambda) Is Expressed in a Tissue-Dependent Fashion and Primarily Acts on Epithelial Cells In Vivo. PloS Pathog (2008) 4(3):e1000017. doi: 10.1371/journal.ppat.1000017
50. Shih HP, Ding JY, Yeh CF, Chi CY, Ku CL. Anti-Interferon-γ Autoantibody-Associated Immunodeficiency. Curr Opin Immunol (2021) 72:206–214. doi: 10.1016/j.coi.2021.05.007
51. Hoflich C, Sabat R, Rosseau S, Temmesfeld B, Slevogt H, Docke WD, et al. Naturally Occurring Anti-IFN-Gamma Autoantibody and Severe Infections With Mycobacterium Cheloneae and Burkholderia Cocovenenans. Blood (2004) 103(2):673–5. doi: 10.1182/blood-2003-04-1065
52. Hanitsch LG, Lobel M, Muller-Redetzky H, Schurmann M, Suttorp N, Unterwalder N, et al. Late-Onset Disseminated Mycobacterium Avium Intracellulare Complex Infection (MAC), Cerebral Toxoplasmosis and Salmonella Sepsis in a German Caucasian Patient With Unusual Anti-Interferon-Gamma Igg1 Autoantibodies. J Clin Immunol (2015) 35(4):361–5. doi: 10.1007/s10875-015-0161-5
53. Liew WK, Thoon KC, Chong CY, Tan NWH, Cheng DT, Chan BSW, et al. Juvenile-Onset Immunodeficiency Secondary to Anti-Interferon-Gamma Autoantibodies. J Clin Immunol (2019) 39(5):512–8. doi: 10.1007/s10875-019-00652-1
54. Otome O, O’Reilly M, Lim L. Disseminated Mycobacterium Haemophilum Skeletal Disease in a Patient With Interferon-Gamma Deficiency. Intern Med J (2015) 45(10):1073–6. doi: 10.1111/imj.12875
55. King YA, Hu CH, Lee YJ, Lin CF, Liu D, Wang KH. Disseminated Cutaneous Mycobacterium Kansasii Infection Presenting With Rosai-Dorfman Disease-Like Histological Features in a Patient Carrying Anti-Interferon-Gamma Autoantibodies. J Dermatol (2017) 44(12):1396–400. doi: 10.1111/1346-8138.13973
56. Browne SK, Burbelo PD, Chetchotisakd P, Suputtamongkol Y, Kiertiburanakul S, Shaw PA, et al. Adult-Onset Immunodeficiency in Thailand and Taiwan. N Engl J Med (2012) 367(8):725–34. doi: 10.1056/NEJMoa1111160
57. Chi CY, Chu CC, Liu JP, Lin CH, Ho MW, Lo WJ, et al. Anti-IFN-Gamma Autoantibodies in Adults With Disseminated Nontuberculous Mycobacterial Infections Are Associated With HLA-DRB1*16:02 and HLA-DQB1*05:02 and the Reactivation of Latent Varicella-Zoster Virus Infection. Blood (2013) 121(8):1357–66. doi: 10.1182/blood-2012-08-452482
58. Nekooie-Marnany N, Deswarte C, Ostadi V, Bagherpour B, Taleby E, Ganjalikhani-Hakemi M, et al. Impaired IL-12- and IL-23-Mediated Immunity Due to IL-12Rbeta1 Deficiency in Iranian Patients With Mendelian Susceptibility to Mycobacterial Disease. J Clin Immunol (2018) 38(7):787–93. doi: 10.1007/s10875-018-0548-1
59. Hong GH, Ortega-Villa AM, Hunsberger S, Chetchotisakd P, Anunnatsiri S, Mootsikapun P, et al. Natural History and Evolution of Anti-Interferon-Gamma Autoantibody-Associated Immunodeficiency Syndrome in Thailand and the United States. Clin Infect Dis (2020) 71(1):53–62. doi: 10.1093/cid/ciz786
60. Ku CL, Lin CH, Chang SW, Chu CC, Chan JF, Kong XF, et al. Anti-IFN-Gamma Autoantibodies Are Strongly Associated With HLA-DR*15:02/16:02 and HLA-DQ*05:01/05:02 Across Southeast Asia. J Allergy Clin Immunol (2016) 137(3):945–8 e8. doi: 10.1016/j.jaci.2015.09.018
61. Wu U-I, Holland SM. Host Susceptibility to Non-Tuberculous Mycobacterial Infections. Lancet Infect Dis (2015) 15(8):968–80. doi: 10.1016/s1473-3099(15)00089-4
62. Hase I, Morimoto K, Sakagami T, Ishii Y, van Ingen J. Patient Ethnicity and Causative Species Determine the Manifestations of Anti-Interferon-Gamma Autoantibody-Associated Nontuberculous Mycobacterial Disease: A Review. Diagn Microbiol Infect Dis (2017) 88(4):308–15. doi: 10.1016/j.diagmicrobio.2017.05.011
63. Sarro YDS, Butzler MA, Sanogo F, Kodio O, Tolofoudie M, Goumane MS, et al. Development and Clinical Evaluation of a New Multiplex PCR Assay for a Simultaneous Diagnosis of Tuberculous and Nontuberculous Mycobacteria. EBioMedicine (2021) 70:103527. doi: 10.1016/j.ebiom.2021.103527
64. Qiu Y, Huang J, Li Y, Zeng W, Pan M, Cen J, et al. Talaromyces Marneffei and Nontuberculous Mycobacteria Co-Infection in HIV-Negative Patients. Sci Rep (2021) 11(1):16177. doi: 10.1038/s41598-021-95686-0
65. Xie Y, Xie J, Meijer AH, Schaaf MJM. Glucocorticoid-Induced Exacerbation of Mycobacterial Infection Is Associated With a Reduced Phagocytic Capacity of Macrophages. Front Immunol (2021) 12:618569. doi: 10.3389/fimmu.2021.618569
66. Nagamura N, Imada T. Anti-Interferon-Gamma Autoantibody Related Disseminated Nontuberculous Mycobacteriosis With Pathological Features of Immunoglobulin G4-Related Disease. Immunol Med (2021) 41, 1–6. doi: 10.1080/25785826.2021.1914962
67. Nishimura-Tagui M, Hayama K, Fujita H, Miyamoto Y, Ishii N, Terui T. Disseminated Mycobacterium Massiliense Skin Infection in an Immunocompromised Patient Requiring Long-Term Treatment. J Dermatol (2021) 48(4):e201–e2. doi: 10.1111/1346-8138.15808
68. Wei W, Ning C, Huang J, Wang G, Lai J, Han J, et al. Talaromyces Marneffei Promotes M2-Like Polarization of Human Macrophages by Downregulating SOCS3 Expression and Activating the TLR9 Pathway. Virulence (2021) 12(1):1997–2012. doi: 10.1080/21505594.2021.1958470
69. Cao C, Xi L, Chaturvedi V. Talaromycosis (Penicilliosis) Due to Talaromyces (Penicillium) Marneffei: Insights Into the Clinical Trends of a Major Fungal Disease 60 Years After the Discovery of the Pathogen. Mycopathologia (2019) 184(6):709–20. doi: 10.1007/s11046-019-00410-2
70. Chan JF, Lau SK, Yuen KY, Woo PC. Talaromyces (Penicillium) Marneffei Infection in non-HIV-Infected Patients. Emerg Microbes Infect (2016) 5:e19. doi: 10.1038/emi.2016.18
71. Chen ZM, Li ZT, Li SQ, Guan WJ, Qiu Y, Lei ZY, et al. Clinical Findings of Talaromyces Marneffei Infection Among Patients With Anti-Interferon-Gamma Immunodeficiency: A Prospective Cohort Study. BMC Infect Dis (2021) 21(1):587. doi: 10.1186/s12879-021-06255-9
72. Chawansuntati K, Rattanathammethee K, Wipasa J. Minireview: Insights Into Anti-Interferon-Gamma Autoantibodies. Exp Biol Med (Maywood) (2021) 246(7):790–5. doi: 10.1177/1535370220981579
73. Berenguer J, Ryan P, Rodriguez-Bano J, Jarrin I, Carratala J, Pachon J, et al. Characteristics and Predictors of Death Among 4035 Consecutively Hospitalized Patients With COVID-19 in Spain. Clin Microbiol Infect (2020) 26(11):1525–36. doi: 10.1016/j.cmi.2020.07.024
74. Guan WJ, Ni ZY, Hu Y, Liang WH, Ou CQ, He JX, et al. Clinical Characteristics of Coronavirus Disease 2019 in China. N Engl J Med (2020) 382(18):1708–20. doi: 10.1056/NEJMoa2002032
75. Acosta-Ampudia Y, Monsalve DM, Rojas M, Rodriguez Y, Gallo JE, Salazar-Uribe JC, et al. COVID-19 Convalescent Plasma Composition and Immunological Effects in Severe Patients. J Autoimmun (2021) 118:102598. doi: 10.1016/j.jaut.2021.102598
76. Phua J, Weng L, Ling L, Egi M, Lim C-M, Divatia JV, et al. Intensive Care Management of Coronavirus Disease 2019 (COVID-19): Challenges and Recommendations. Lancet Respir Med (2020) 8(5):506–17. doi: 10.1016/s2213-2600(20)30161-2
77. Garg S, Kim L, Whitaker M, O’Halloran A, Cummings C, Holstein R PM, et al. Hospitalization Rates and Characteristics of Patients Hospitalized With Laboratory-Confirmed Coronavirus Disease 2019 - COVID-NET, 14 States, March 1-30,2020. MMWR Morb Mortal Wkly Rep (2020) 69:458–64. doi: 10.15585/mmwr.mm6915e3
78. Boutin S, Hildebrand D, Boulant S, Kreuter M, Ruter J, Pallerla SR, et al. Host Factors Facilitating SARS-Cov-2 Virus Infection and Replication in the Lungs. Cell Mol Life Sci (2021) 78(16):5953–76. doi: 10.1007/s00018-021-03889-5
79. Khanmohammadi S, Rezaei N. Role of Toll-Like Receptors in the Pathogenesis of COVID-19. J Med Virol (2021) 93(5):2735–9. doi: 10.1002/jmv.26826
80. Zhang Q, Bastard P, Liu Z, Le Pen J, Moncada-Velez M, Chen J, et al. Inborn Errors of Type I IFN Immunity in Patients With Life-Threatening COVID-19. Science (2020) 370, eabd4570. doi: 10.1126/science.abd4570
81. Fallerini C, Daga S, Mantovani S, Benetti E, Picchiotti N, Francisci D, et al. Association of Toll-Like Receptor 7 Variants With Life-Threatening COVID-19 Disease in Males: Findings From a Nested Case-Control Study. Elife (2021) 10:e67569. doi: 10.7554/eLife.67569
82. Bruserud O, Oftedal BE, Landegren N, Erichsen MM, Bratland E, Lima K, et al. A Longitudinal Follow-Up of Autoimmune Polyendocrine Syndrome Type 1. J Clin Endocrinol Metab (2016) 101(8):2975–83. doi: 10.1210/jc.2016-1821
83. Bastard P, Orlova E, Sozaeva L, Levy R, James A, Schmitt MM, et al. Preexisting Autoantibodies to Type I Ifns Underlie Critical COVID-19 Pneumonia in Patients With APS-1. J Exp Med (2021) 218(7):e20210554. doi: 10.1084/jem.20210554
84. Pairo-Castineira E, Clohisey S, Klaric L, Bretherick AD, Rawlik K, Pasko D, et al. Genetic Mechanisms of Critical Illness in COVID-19. Nature (2021) 591(7848):92–8. doi: 10.1038/s41586-020-03065-y
85. Ellinghaus D, Degenhardt F, Bujanda L, Buti M, Albillos A, Invernizzi P, et al. Genomewide Association Study of Severe Covid-19 With Respiratory Failure. N Engl J Med (2020) 383(16):1522–34. doi: 10.1056/NEJMoa2020283
86. Shelton JF, Shastri AJ, Ye C, Weldon CH, Filshtein-Sonmez T, Coker D, et al. Trans-Ancestry Analysis Reveals Genetic and Nongenetic Associations With COVID-19 Susceptibility and Severity. Nat Genet (2021) 53(6):801–8. doi: 10.1038/s41588-021-00854-7
87. Rodriguez Y, Novelli L, Rojas M, De Santis M, Acosta-Ampudia Y, Monsalve DM, et al. Autoinflammatory and Autoimmune Conditions at the Crossroad of COVID-19. J Autoimmun (2020) 114:102506. doi: 10.1016/j.jaut.2020.102506
88. Aoki A, Sakagami T, Yoshizawa K, Shima K, Toyama M, Tanabe Y, et al. Clinical Significance of Interferon-Gamma Neutralizing Autoantibodies Against Disseminated Nontuberculous Mycobacterial Disease. Clin Infect Dis (2018) 66(8):1239–45. doi: 10.1093/cid/cix996
89. de Prost N, Bastard P, Arrestier R, Fourati S, Mahevas M, Burrel S, et al. Plasma Exchange to Rescue Patients With Autoantibodies Against Type I Interferons and Life-Threatening COVID-19 Pneumonia. J Clin Immunol (2021) 41(3):536–44. doi: 10.1007/s10875-021-00994-9
90. Li P, Chen F, Zheng J, Yang Y, Li Y, Wang Y, et al. Cyclophosphamide Abrogates the Expansion of CD4(+)Foxp3(+) Regulatory T Cells and Enhances the Efficacy of Bleomycin in the Treatment of Mouse B16-F10 Melanomas. Cancer Biol Med (2021) j.issn.2095-3941. doi: 10.20892/j.issn.2095-3941.2021.0027
91. Queiros V, Azeiteiro UM, Soares A, Freitas R. The Antineoplastic Drugs Cyclophosphamide and Cisplatin in the Aquatic Environment - Review. J Hazard Mater (2021) 412:125028. doi: 10.1016/j.jhazmat.2020.125028
92. Chetchotisakd P, Anunnatsiri S, Nanagara R, Nithichanon A, Lertmemongkolchai G. Intravenous Cyclophosphamide Therapy for Anti-IFN-Gamma Autoantibody-Associated Mycobacterium Abscessus Infection. J Immunol Res (2018) 2018:6473629. doi: 10.1155/2018/6473629
93. Muley SA, Jacobsen B, Parry G, Usman U, Ortega E, Walk D, et al. Rituximab in Refractory Chronic Inflammatory Demyelinating Polyneuropathy. Muscle Nerve (2020) 61(5):575–9. doi: 10.1002/mus.26804
94. Browne SK, Zaman R, Sampaio EP, Jutivorakool K, Rosen LB, Ding L, et al. Anti-CD20 (Rituximab) Therapy for Anti-IFN-Gamma Autoantibody-Associated Nontuberculous Mycobacterial Infection. Blood (2012) 119(17):3933–9. doi: 10.1182/blood-2011-12-395707
95. Rabascall CX, Lou BX, Navetta-Modrov B, Hahn SS. Effective Use of Monoclonal Antibodies for Treatment of Persistent COVID-19 Infection in a Patient on Rituximab. BMJ Case Rep (2021) 14(8):e243469. doi: 10.1136/bcr-2021-243469
96. Palumbo A, Chanan-Khan A, Weisel K, Nooka AK, Masszi T, Beksac M, et al. Daratumumab, Bortezomib, and Dexamethasone for Multiple Myeloma. N Engl J Med (2016) 375(8):754–66. doi: 10.1056/NEJMoa1606038
97. Frerichs KA, Verkleij CPM, Bosman PWC, Zweegman S, Otten H, van de Donk N. CD38-Targeted Therapy With Daratumumab Reduces Autoantibody Levels in Multiple Myeloma Patients. J Transl Autoimmun (2019) 2:100022. doi: 10.1016/j.jtauto.2019.100022
98. Ochoa S, Ding L, Kreuzburg S, Treat J, Holland SM, Zerbe CS. Daratumumab (Anti-CD38) for Treatment of Disseminated Nontuberculous Mycobacteria in a Patient With Anti-Interferon-Gamma Autoantibodies. Clin Infect Dis (2021) 72(12):2206–8. doi: 10.1093/cid/ciaa1086
99. Omrani AS, Saad MM, Baig K, Bahloul A, Abdul-Matin M, Alaidaroos AY, et al. Ribavirin and Interferon Alfa-2a for Severe Middle East Respiratory Syndrome Coronavirus Infection: A Retrospective Cohort Study. Lancet Infect Dis (2014) 14(11):1090–5. doi: 10.1016/s1473-3099(14)70920-x
100. Harada M, Furuhashi K, Karayama M, Suzuki Y, Hozumi H, Enomoto N, et al. Subcutaneous Injection of Interferon Gamma Therapy Could be Useful for Anti-IFN-Gamma Autoantibody Associated Disseminated Nontuberculous Mycobacterial Infection. J Infect Chemother (2021) 27(2):373–8. doi: 10.1016/j.jiac.2020.09.031
101. Elali I, Delasos L, Phachu D, Shah M, Mu J, Kaplan AA. Membrane-Based Therapeutic Plasma Exchange: Hemodynamics and Operational Characteristics Leading to Procedure Failure. J Clin Apher (2021) 36:841–48. doi: 10.1002/jca.21936
102. Morimoto AM, Flesher DT, Yang J, Wolslegel K, Wang X, Brady A, et al. Association of Endogenous Anti-Interferon-Alpha Autoantibodies With Decreased Interferon-Pathway and Disease Activity in Patients With Systemic Lupus Erythematosus. Arthritis Rheum (2011) 63(8):2407–15. doi: 10.1002/art.30399
Keywords: adult-onset immunodeficiency syndrome, coronavirus disease 2019 pneumonia, autoantibodies against IFNs, autoimmune disease, infectious disease
Citation: Chen L-F, Yang C-D and Cheng X-B (2021) Anti-Interferon Autoantibodies in Adult-Onset Immunodeficiency Syndrome and Severe COVID-19 Infection. Front. Immunol. 12:788368. doi: 10.3389/fimmu.2021.788368
Received: 02 October 2021; Accepted: 22 November 2021;
Published: 22 December 2021.
Edited by:
Raymond P. Donnelly, United States Food and Drug Administration, United StatesReviewed by:
Saul Oswaldo Lugo Reyes, National Institute of Pediatrics, MexicoGillian Inara Rice, The University of Manchester, United Kingdom
Copyright © 2021 Chen, Yang and Cheng. This is an open-access article distributed under the terms of the Creative Commons Attribution License (CC BY). The use, distribution or reproduction in other forums is permitted, provided the original author(s) and the copyright owner(s) are credited and that the original publication in this journal is cited, in accordance with accepted academic practice. No use, distribution or reproduction is permitted which does not comply with these terms.
*Correspondence: Xiao-Bing Cheng, Y3hiMTE5NTdAcmpoLmNvbS5jbg==