- 1Department of Gynaecology and Obstetrics, The Second Affiliated Hospital of Fujian Medical University, Quanzhou, China
- 2Department of Gynaecology and Obstetrics, Shenzhen Hospital of University of Hong Kong, Shenzhen, China
- 3Centre of Neurological and Metabolic Research, The Second Affiliated Hospital of Fujian Medical University, Quanzhou, China
- 4Diabetes and Metabolism Division, Garvan Institute of Medical Research, Sydney, NSW, Australia
Intrauterine adhesion (IUA) is an endometrial fibrosis disease caused by repeated operations of the uterus and is a common cause of female infertility. In recent years, treatment using mesenchymal stem cells (MSCs) has been proposed by many researchers and is now widely used in clinics because of the low immunogenicity of MSCs. It is believed that allogeneic MSCs can be used to treat IUA because MSCs express only low levels of MHC class I molecules and no MHC class II or co-stimulatory molecules. However, many scholars still believe that the use of allogeneic MSCs to treat IUA may lead to immune rejection. Compared with allogeneic MSCs, autologous MSCs are safer, more ethical, and can better adapt to the body. Here, we review recently published articles on the immunomodulation of allogeneic and autologous MSCs in IUA therapy, with the aim of proving that the use of autologous MSCs can reduce the possibility of immune rejection in the treatment of IUAs.
Introduction
Intrauterine adhesion (IUA), also known as Asherman syndrome, is condition involving endometrial fibrosis caused by damage to the basal layer of the uterus, leading to partial or full adhesion of the uterine cavity (1, 2). IUA is usually accompanied by decreased menstrual flow, and in severe cases, secondary amenorrhea. Moreover, IUA affects embryo implantation and development as a result of reduction or even complete disappearance of intrauterine volume, leading to female infertility and recurrent miscarriage (3). However, normal endometrium is not scarred during repair. Under the influence of uterine manipulation and inflammatory cytokines, the endometrium participates in hypoxia, reduces new blood vessels, and controls the expression of adhesion-related cytokines (4).
At present, hysteroscopic endometrial adhesion decomposition combined with various methods, such as insertion of an intrauterine balloon (5), administration of hyaluronic acid gel (6) and polyethylene oxide-sodium carboxymethylcellulose gel (2), etc., can be used to treat IUA. However, the common adhesion release can only improve the problem of uterine cavity stenosis and cannot completely reverse endometrial fibrosis. Therefore, researchers have proposed the use of stem cells to treat IUA because of their regenerative ability (7).
Recently, Cao et al. (8) reported that the use of umbilical cord MSCs in allogeneic cell therapy could improve the treatment of recurrent uterine adhesions, and subsequently conducted a phase I clinical trial. Since umbilical cord MSCs have low immunogenicity and low tumorigenicity, they have great advantages in the application of IUA. On the one hand, clinical trials showed that endometrial thickening and IUA score after treatment were lower than those before treatment. On the other hand, DNA short tandem repeat (STR) analysis showed the regenerated endometrium only containing patient DNA, so that umbilical cord MSCs exhibit good safety. Mare (9) also confirmed that allogeneic MSCs can regulate the protein pattern and increase the proliferation of glandular epithelial cells, thereby exerting an anti-scarring effect. Although the immunogenicity of MSCs is lower than that of other stem cells, immune rejection of allogeneic MSCs can still occur. Ankrum et al. (10) pointed out that antibodies and immune rejection may be produced during the treatment of allogeneic donor MSCs. In other words, MSCs may not have immune privileges.
Currently, the mechanism by which allogeneic mesenchymal stem cells induce immune rejection remains unclear. Most researchers believe that the human leukocyte antigen (HLA) of allogeneic MSCs does not match the receptor type (11). Others have suggested that immune rejection caused by allogeneic MSCs is also related to immune cells (12), immunoactive substances (3, 13) and immune organs (14). Currently, there is no good method to control immune rejection. A prior study suggested (10) that the immune persistence of MSCs could be improved, and immune tolerance could be enhanced. More importantly, others have suggested (11) that knockout of beta-2 microglobulin could be performed to enhance repair. The most direct approach is the use of autologous MSCs. Kim et al. believe (15) that the combination of MSC spheres and autologous composite sheets could be used to avoid immune rejection and improve the effectiveness of stem cell therapy. This article reviews the comparison between allogeneic and autologous MSCs. In addition, we will summarize the mechanism by which allogeneic mesenchymal stem cell therapy induces immunoregulation. Furthermore, we will discuss the therapeutic prospects of autologous MSCs.
Intrauterine Adhesion
IUA often progresses as a consequence of uterine cavity operation. Deans et al. conducted (16) a retrospective analysis of 1856 patients with intrauterine adhesions and found that 67% of curettage was induced or spontaneous, while 22% was due to postpartum bleeding. Christina et al. (17) considered that antagonistic effects occur due to increased prolactin levels and decreased estrogen levels in the postpartum period; therefore, the endometrium is more prone to atrophy. Additionally, IUA is associated with inflammatory cytokines. The experimental results of Mo et al. (18) demonstrated that preoperative inflammation in IUA patients was significantly higher than that in non-IUA patients. At present, the only confirmed infectious factor that causes IUA is genital tuberculosis (4). Research has shown (19) that Mycobacterium tuberculosis infection of the uterine cavity can lead to focal ulceration, necrosis, or bleeding of the endometrial tissue, while destruction of the endometrium can cause partial or complete IUA. Another important reason is the insufficient endometrial blood perfusion (20). For example, uterine artery embolism, B-Lynch suture, hysteroscopic myomectomy, etc. (21). Due to the long-term insufficient blood supply to the endometrium, it is difficult to regenerate, which increases the possibility of IUA (Figure 1).
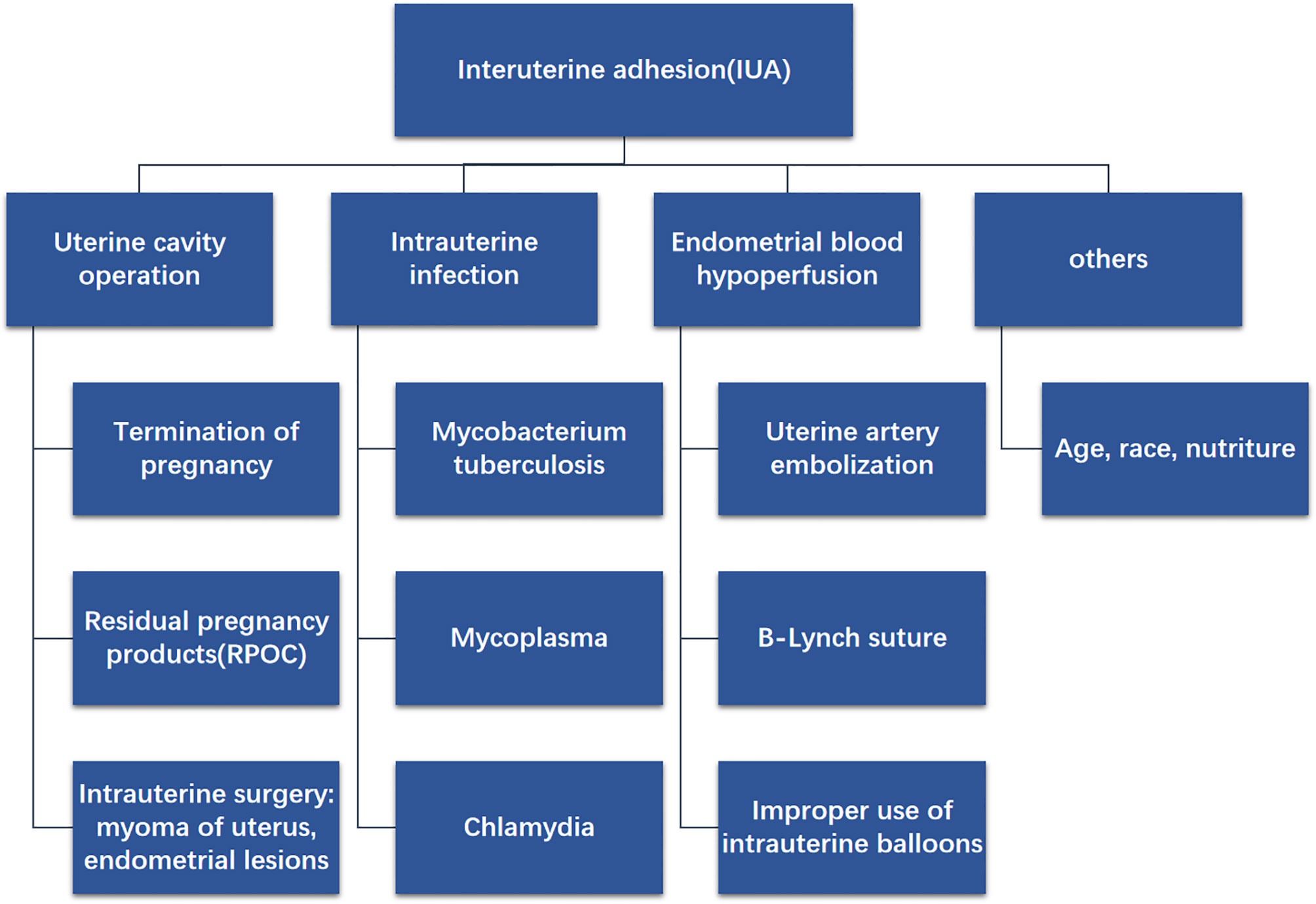
Figure 1 Diagram shows the possible causes of IUA, for example uterine cavity operation, intrauterine infection, endometrial blood hypoperfusion and others.
At present, the immune mechanism of intrauterine adhesions is not clear, Zhao et al. (22) believe that it is related to the imbalance of vaginal flora and microecology. The causes of microecological imbalance may be related to the long-term use of antibiotics, frequent sexual intercourse, vaginal flushing, and decreased estrogen. These factors greatly increase the incidence of uterine inflammation. When the endometrium is normal, it can resist the invasion of these inflammatory cytokines. However, once the endometrium is damaged due to uterine operation, the surface barrier is destroyed. On the one hand, bacteria invade the endometrium, causing local inflammatory reactions, and the pro-inflammatory cytokines IL-6 and IFN-γ increase. Negative feedback reduced the activity of matrix metalloproteinase (MMP) and promoted the generation of endometrial fibrosis. On the other hand, damaged epithelial cells (23) release IL-25, IL-33, TSLP, other cells can directly or indirectly promote Th2 immune response to promote fibrosis. What’s more, NF-κB (24) transcription cytokine promotes the expression of intrauterine adhesion inflammatory cytokines and plays a central role in inflammatory diseases. It also closely intersects with the pathogenic cytokines of intrauterine adhesion such as TGF-β, TNF-α, IL-1 and IL-18. Wang et al. (25) found that the expression of NF-κB in endometrium from patients with endometrial adhesion was significantly higher than that in normal endometrium (Figure 2).
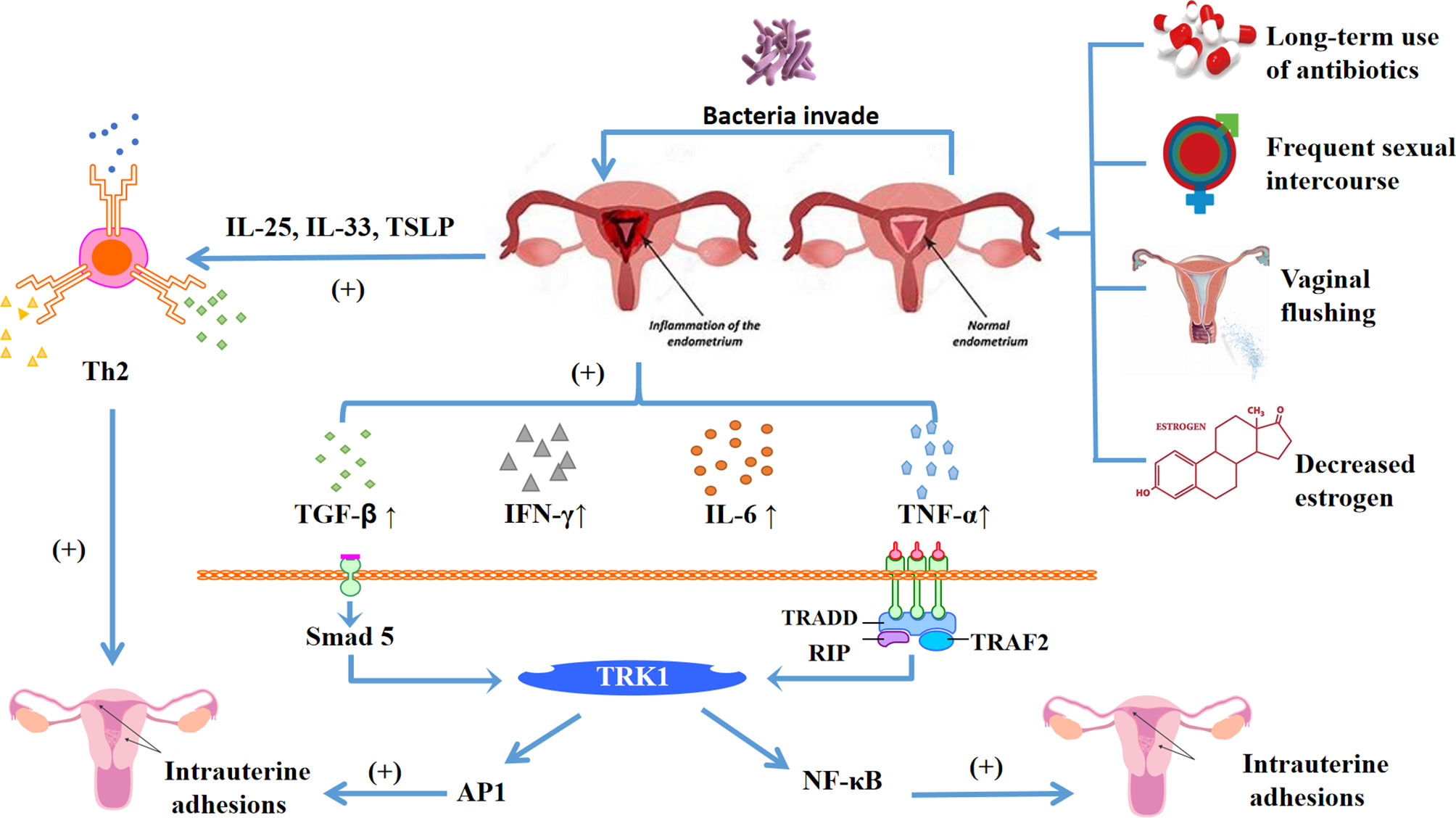
Figure 2 Diagram shows the main mechanism of intrauterine adhesions, the damaged endometrium is affected by long-term antibiotic treatment, frequent sexual intercourse, vaginal flushing, decreased estrogen and other factors, which lead to the homeostasis of the internal environment changing. Broken rings in the microenvironment make bacteria easier to invade, and the damaged endometrium has an inflammatory response. On the one hand, it can release inflammatory cytokines IL-25, IL-33, TSLP, activate helper Th2 cells, and promote endometrial fibrosis. On the other hand, it can also release inflammatory cytokines TGF-β, IFN-γ, IL-6, TNF-α, etc. Among them, TGF-β can be through TGF-β-Smad5 pathway, and TNF-α and NF-κB jointly promote endometrial fibrosis, thereby forming intrauterine adhesions.
Currently, the commonly used clinical treatment method is transcervical resection of adhesions (TCRA). To improve the efficacy of the IUA, an intrauterine contraceptive device and intrauterine balloon can be inserted first (26). Intrauterine devices and balloons can expand the narrow uterine cavity to improve the long-term prognosis of patients. In addition, we can also increase biological barriers, such as new cross-linked hyaluronan gel (27), auto-cross-linked hyaluronan gel (28), and estrogen gel (29). However, the prognosis of IUA is still not ideal. Some researchers have postulated (21) that the degree of recurrence of IUA is related to the degree of adhesion. In addition, Hanstede et al. (30) compared the degree of IUA and the recurrence rate of patients with hysteroscopic adhesiolysis. The results indicated that the recurrence rate of first-degree IUA was 3.8% (n=24), that of second-degree IUA was 33.1% (n=211), and that of third-degree IUA was 35.4% (n=226).
Furthermore, the release of adhesions also needs to promote the regeneration and repair of endometrial fibrosis. Due to the regenerative ability of stem cells, researchers are considering the use of stem cells for treatment. Studies have shown (31) that collagen scaffolds loaded with human umbilical cord mesenchymal stem cells can promote endometrial structural reconstruction and functional recovery (Table 1).
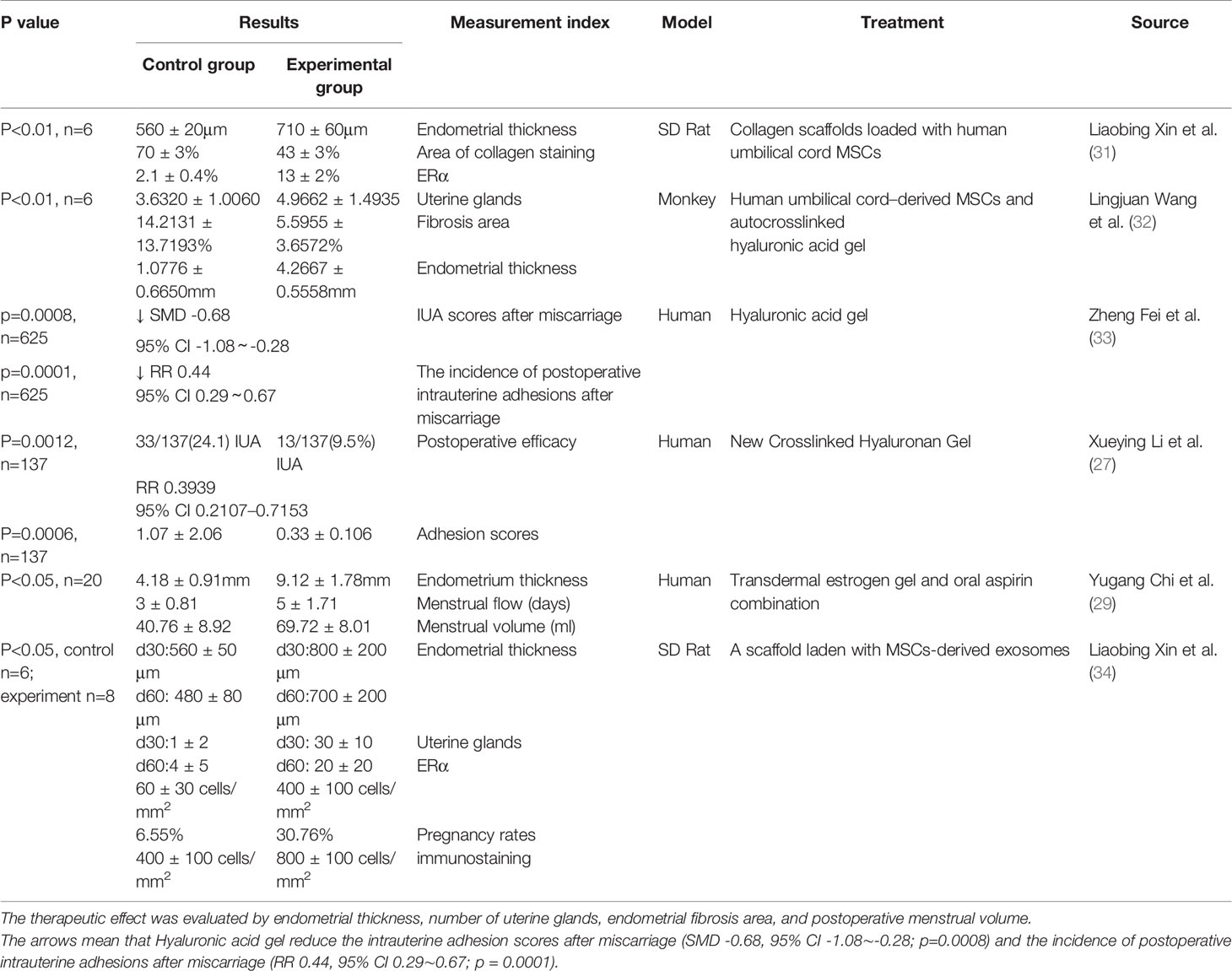
Table 1 This table clearly summarizes some treatment methods for IUA, including hyaluronic acid, mesenchymal stem cells and their combined application.
Mesenchymal Stem Cells
Background of Mesenchymal Stem Cells
Mesenchymal stem cells (MSCs) are pluripotent stem cells which can attach to the wall where they grow and replicate (35). The sources of MSCs are relatively abundant; for example, they can be extracted from the bone marrow, umbilical cord, adipose tissue, peripheral blood, and so on (36). MSCs have become a research hotspot for their diverse functions, which include cell differentiation and proliferation, regulation of inflammatory processes, control of oxidative stress, and angiogenesis (37).
Types of Mesenchymal Stem Cells
Currently, there are several classifications of MSCs; donor MSCs can be classified as either allogeneic or autologous. Allogeneic MSCs are derived from other donors for implantation into the recipient patient. Because they are extracted from a foreign body, the number of allogeneic MSCs can be quite large. However, since allogeneic MSCs are equivalent to foreign matter, they are not ethically recognized in humans (38). In contrast, autologous MSCs are cells that are extracted from the patient’s own tissues, induced and differentiated, and then re-implanted for treatment. Therefore, they adapt better to the patient’s body, and are less likely to suffer from immune rejection. In the past decade (39), genetic modification of autologous hematopoietic stem cells has been used for the treatment of single-gene diseases. Moreover, they are more in line with people’s ethical requirements and are recognized by more people. At present, autologous MSCs have been used to treat several diseases, including multiple sclerosis (40), post-burn scar treatment (41), refractory rectovaginal Crohn’s fistulas (42), and patients with advanced tumors (43), among others (Table 2).
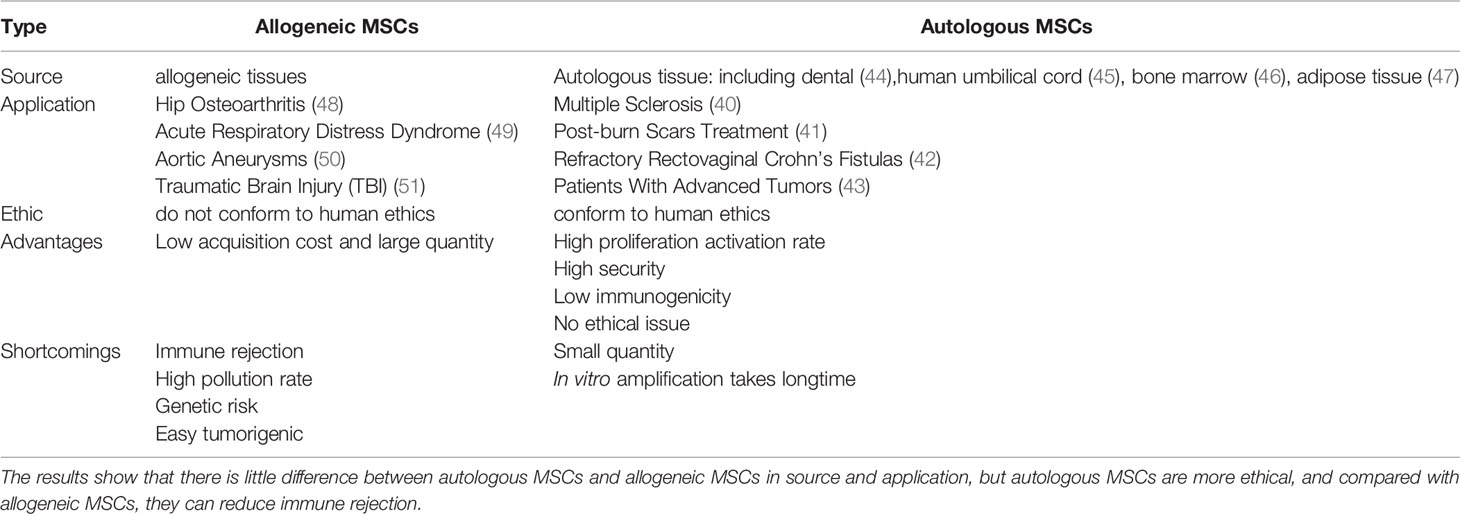
Table 2 This table mainly compares the differences between autologous MSCs and allogeneic MSCs from five aspects: source, application, ethics, advantages and disadvantages.
In addition, MSCs can be classified by source, as either dental pulp MSCs (44), human umbilical cord MSCs (45), bone marrow MSCs (46), and adipose tissue-derived MSCs (47). In addition, if they are classified by developmental potency, they can be divided into totipotent stem cells (TSCs), pluripotent stem cells, and unipotent stem cells.
Characteristics of MSCs
MSCs have many notable characteristics. First, MSCs cannot differentiate and self-renew (52). Hsiao et al. (53) proposed that treatment with undifferentiated MSCs can improve insulin resistance in diabetic rats, rebalance inflammation, and improve blood sugar levels. Regarding the differentiation ability of MSCs, researchers have suggested that MSCs can differentiate into adipocytes, osteoblasts, and chondrocytes. In addition, detection of MSC surface antigens indicated that the expression of markers such as CD166, CD44, CD29, CD73, CD90, and CD105 increased significantly with the increase of transmission algebra (20). Moreover, MSCs also express CD11, CD14, CD45, and CD34. However, as the transmission algebra increases, these markers are reduced or lost. Therefore, MSCs can be detected using the above-mentioned markers.
Mechanism and Application of MSCs in the Treatment of IUA
MSC Immunization
Current research indicates that application of bone marrow MSCs can promote endometrial regeneration by suppressing the innate and adaptive immune systems (54). On the one hand, in the innate immune system, MSCs can inhibit the activation, proliferation and cytotoxicity of natural killer cells (NK cells) (55). Conversely, in adaptive immunity, MSCs (56) can inhibit the proliferation of B and T cells, and further prevent the proliferation and differentiation of T cells into pro-inflammatory TH1 and TH17 helper T cells. In addition, MSCs can promote the differentiation of T cells into tolerant Treg cells (57), and can regulate the action of monocytes, dendritic cells, and NK cells by secreting chemokines (58), such as IGF-1, TGF-β, bFGF, HGF, IL-6, SDF-1, M-CSF, VEGF, PIGF, and MCP-1 (55).
Toll-like receptors (TLR) are also involved in the immunotherapy of MSCs. When faced with a danger signal, TLRs recognize danger signals and are activated to trigger a cellular response, mobilizing innate and adaptive immune cells (59). The results showed that MSCs combined with TLR4 promoted the secretion of pro-inflammatory cytokines. In contrast, if combined with TLR3, MSCs promote the secretion of anti-inflammatory cytokines (60). When microorganisms are infected, exogenous danger signals such as endotoxins or lipopolysaccharides (LPS) disappear. Meanwhile, abnormal or damaged cells overflow into the circulatory system, resulting in the expression of heat shock proteins, an endogenous danger signal. Get rid of the “danger signal” to activate the sentinel innate immune cells (for example, dendritic cells) TLR. Various immune cells are recruited to the site of endometrial damage (61).
Moreover, researchers have found that the concentration of macrophages in the endometrium of patients with IUA decreased, which may be related to a decrease in CSF-1 (62). Otherwise, another study indicated (63) that transplantation of Human amniotic epithelial cells (HAECs) can be performed through autophagy-induced recovery of damaged endometrium. VEGF expression in the menstrual and proliferative phases can promote the reconstruction of endometrial tissue. HAECs can increase the expression of VEGF, promote angiogenesis, and promote the recovery of endometrial fibrosis.
Differentiation Therapy of MSCs
Mesenchymal stem cells have the capability to differentiate After MSCs reach the tissue, they secrete stem cell cytokines such as SCF and M-CSF to reactivate the differentiation potential of the endogenous stem cells of the damaged tissue, thus promoting the generation of new tissue cells to replace damaged cells, resulting in tissue recovery; this is termed stem cell differentiation therapy (64). At present, MSC differentiation has been applied in various fields. A set of clinical treatment data of Ashman syndrome showed (65) that patients receiving autologous CD9 +, CD44 +, CD90 + bone marrow MSC transplantation experienced improved endometrial vascularization and an increase in endometrial thickness. One such patient subsequently underwent in vitro fertilization and embryo transfer, and successfully carried a fetus to term.
Differentiation therapy of MSCs can also be applied to other aspects, such as the treatment of Parkinson’s disease, in which EDSCs are promoted to differentiate into dopamine-producing cells (66). Studies have shown (67) that CD90, CD146, and PDGFR-β in HEDSCs can differentiate into neuron-like cells. Moreover, they can also differentiate into cholinergic neuron-like cells (68), oligodendrocyte-like cells (69), insulin-producing cells (70), cardiomyocyte-like cells (71), megakaryocyte-like cells (72), and urothelial cells (73).
Stem Cell Paracrine Therapy
MSCs coordinate tissue recovery by releasing soluble paracrine cytokines (74). Treatment of IUA through the paracrine pathway is currently the most popular research method as IUA is partially affected by inflammatory cytokines. One of the subgroups of inflammatory cytokines is IL-1ra in the acute inflammatory paracrine effect of MSCs. IL-1ra inhibits cytokine stimulation of the helper T lymphocyte system, as well as the production of the inflammatory cytokines TNF-α in macrophages in an IL-1ra-dependent manner (74).
Furthermore, because exosomes can make mesenchymal stem cells specialize in the treatment of certain diseases, they are now an active field. Exosomes are small, single-membrane secretory organelles with a diameter of 30–200 nm (75). Recent studies have shown (76) that the function, targeting, and mechanism-driven accumulation of specific cellular components in exosomes indicate that they play a role in regulating intercellular communication.
At present, exosomes are also used in the IUA. Yao et al. (77) demonstrated that exosomes from MSCs can reverse the epithelial to mesenchymal transition (EMT) through action of the TGF-β1/Smad pathway and promote the repair of damaged endometrium in 64 female rabbits. Liao et al. (78) also summarized the manner by which different exosomes could be used to treat female reproductive disorders, and concluded that MSCs can improve female fertility in in vitro and in vivo models. Therapeutic mechanisms include angiogenesis, immune regulation, anti-fibrosis, and anti-oxidative stress. In addition, after subcutaneous injection of a mixture of matrix gel and MSCs in mice, the number of hemoglobin or CD31+ cells increased, indicating that MSCs can promote the formation of functional capillaries (79). Furthermore, Zhao et al. (80) demonstrated that ADSC-ex promotes endometrial regeneration and fertility recovery. Moreover, Tao et al. (81) found through experiments that Mir-29a in bone marrow MSC exosomes can inhibit fibrosis in the process of endometrial adhesion repair.
Homing Characteristics of Stem Cells
The homing function of stem cells functions to recruit stem cells by disrupting the tissue-secreted chemokine system (82). It is well known that chemokine SDF1 and its special receptor CXCR4 play a key role in the homing of BMSCs (83). Moreover, studies have shown that ERα promotes the proliferation and migration of BMSCs through SDF1/CXCR4 (84). Furthermore, the CXCL12/CXCR4 protein ligand is a chemokine receptor complex that can transmit stem cells to the uterus (85), and thus promote endometrial repair. In addition, G-CSF has been shown to mobilize hematopoietic stem cells from the bone marrow into the blood, thereby reducing migration to the endometrium (86). G-CSF can also enhance the expression of cytokeratin, vimentin, integrin, and leukemia inhibitory cytokine (LIF) and regulate endometrial function (7).
Immunoregulation of Allogeneic MSCs
It is well known that the immunoregulation and regeneration characteristics of MSCs are the reason why they are used to treat many diseases. Because bone marrow MSCs are considered to have low immunogenicity, their implantation does not trigger histocompatibility disorders or predict possible immune rejection (87). The low immunity of MSCs is due to the lack of expression of major histocompatibility complex II (MHCII) and the low expression of costimulatory molecules such as MHCI, CD80, and CD86 (88).
Studies have shown that under certain conditions, bone marrow MSCs can secrete proinflammatory cytokines and act as antigen-presenting cells to promote immune responses (89). Bone marrow MSCs can effectively reduce phagocytosis and antigen presentation of monocytes/macrophages, and promote the expression of immunosuppressive molecules such as interleukin IL-10 and programmed cell death 1 ligand 1 in these cells (90). In addition, they can effectively inhibit the maturation of dendritic cells and their ability to produce pro-inflammatory cytokines, in addition to stimulating strong T-cell responses (90). Furthermore, MSCs can inhibit the generation and pro-inflammatory properties of CD4 + T helper cells 1 (Th1) and Th17 cells, and promotes the proliferation and inhibition of regulatory T cells. Bone marrow MSCs can also damage the expansion, cytokine secretion, and cytotoxic activity of pro-inflammatory CD8 + T cells (90).
Allogeneic MSCs and Monocytes/Macrophages
Immunomodulatory macrophages can be categorized as either pro-inflammatory M1 and anti-inflammatory M2 macrophages (91). Some scholars have pointed out that MSCs can effectively promote the polarization of macrophages into the M2 type. This process is mediated by a variety of soluble molecules, including PGE2, IDO, HGF, IL-1RA, TSG6, TGF-β, etc. (90). In addition, MSCs induce M2 polarization, the anti-inflammatory cytokines IL-10, arginase-1, and TGF-β increase, and the pro-inflammatory cytokines TNF-α, IL-12, and IL-1 decrease, inhibit T cell response, and induce the production of regulatory T cells. Finally, this leads to further immunosuppression, which is conducive to the treatment of allogeneic MSCs (92).
Furthermore, when human umbilical cord-derived MSCs (UC-MSCs) are co-cultured with monocytes and macrophages, the expression of HLA-DR/DP/DQ and CD86 is reduced, and the phagocytosis and antigen presentation ability of monocytes and macrophages is decreased (93). This indicates that, compared with ordinary stem cells, UC-MSCs can reduce the chance immune rejection caused by allogeneic transplantation. In addition, US-MSCs can induce CD14+, CD16+, and CD206+ in mice after being phagocytosed by monocytes, leading to an increase in the anti-inflammatory cytokines IL-10 and PD-L1 (94) (Figure 3).
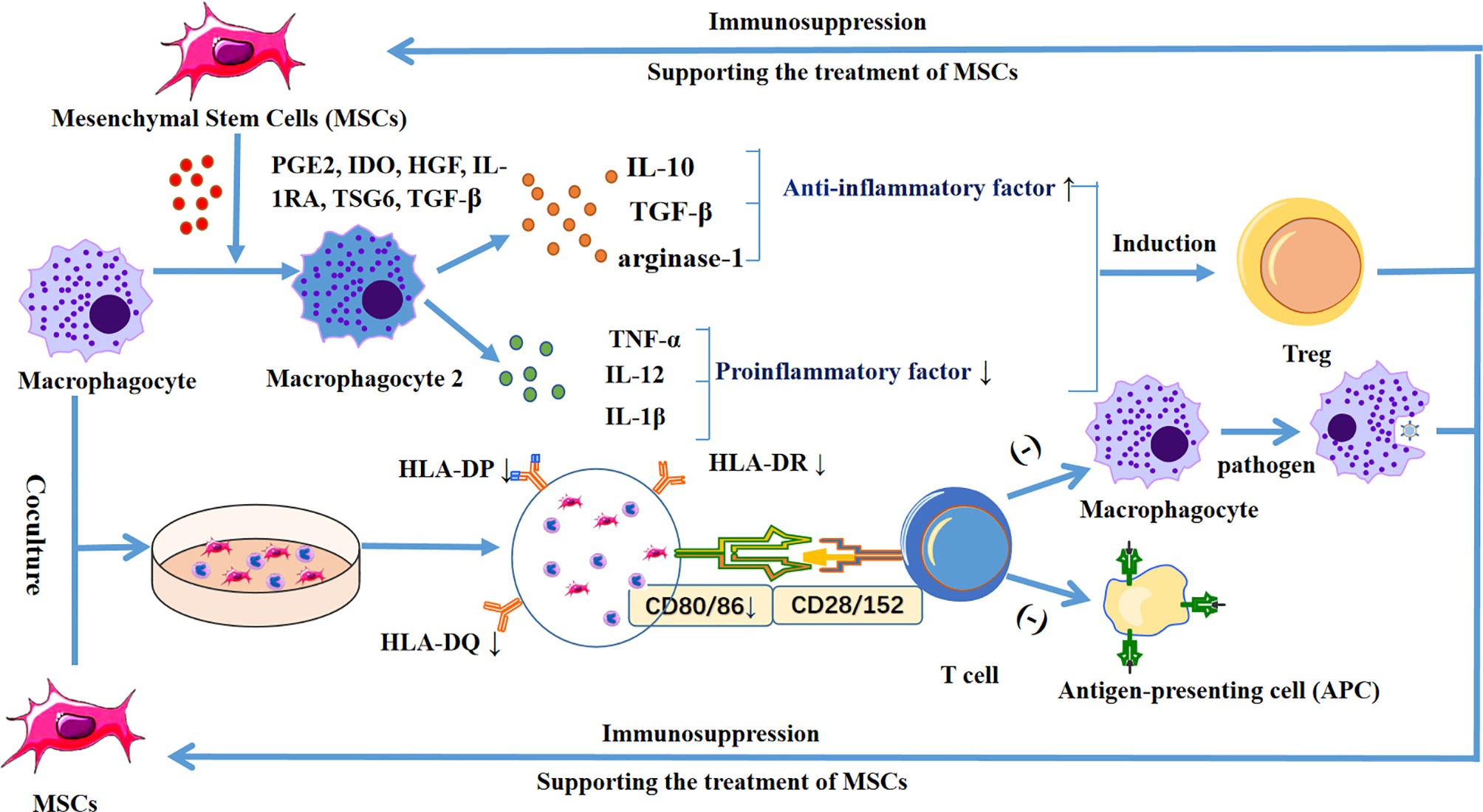
Figure 3 Diagram shows the release of PGE2, IDO, HGF, IL-1RA, TSG6, and TGF-β by MSCs, which stimulates macrophages to differentiate into M2 macrophages, increases anti-inflammatory cytokines IL-10, TGF-β, and arginase-1, and decreases the pro-inflammatory cytokines TNF-α, IL-12, and IL-1β, inhibiting the T cell response, and inducing regulatory T cells. In addition, when MSCs were co-cultured with macrophages, the expression levels of HLA-DR/DP/DQ and CD86 were decreased, thereby reducing the phagocytic ability and antigen presentation ability of macrophages, leading to further immunosuppression and supporting the therapeutic effect of MSCs.
Allogeneic MSCs and Dendritic Cells
Dendritic cells (DCs) are antigen-presenting cells that can induce T cells to produce an immune response. MSCs can inhibit the maturation of dendritic cells by regulating the expression of HLA-DR, CD40, OX40L, CD80, and CD83 (95), and upregulating the expression of PD-L1 (96), thereby reducing its ability to activate T cells. In addition, when DCs are co-cultured with MSCs, the pro-inflammatory cytokines secreted by CD1c+ DCs are reduced, and the anti-inflammatory cytokine IL-10 secreted by plasmacytoid DCs is increased (97). Moreover, studies have shown that exosomes released by adipose-derived mesenchymal stem cells can inhibit IL-6 and increase the expression of the anti-inflammatory cytokines IL-10 and TGF-β (98). Furthermore, treatment of mouse dendritic cells with MSC-derived exosomes did not stimulate T cell proliferation after LPS activation (98)
Allogeneic MSCs and T Cells
In the T cell family, CD4 + helper T cells and CD8 + cytotoxic T cells play important roles in the immune regulation of MSCs. On the one hand, CD4 + T cells can bind to CD40 and CD40 ligands on their cell surface, thereby enhancing the ability of dendritic cells to induce CTL. On the other hand, CD40 can maintain CTL activity by triggering the secretion of IL-2 (99). However, MSCs inhibit the proliferation of these two tesla cells through paracrine and cell contact, thereby reducing the release of the pro-inflammatory cytokines TNF-α and IFN-γ, and reducing the immune rejection between allogeneic MSC receptors (100).
In addition, exosomes extracted from mouse MSCs can inhibit T cell proliferation by upregulating cyclin-dependent kinase inhibitor 1 B and downregulating cyclin-dependent kinase 2 (101). Bone marrow mesenchymal stem cells can transform Th1 to Th2 mediated by dendritic cells and induce Tregs (56), reduce pro-inflammatory cytokines IFN-α, IL-17, and IL-6, and increase the anti-inflammatory cytokines IL-4 and IL-10 (102). Moreover, exosomes extracted from umbilical cord-derived mesenchymal stem cells can inhibit CD8 + T cells and Th1 cells, reduce the secretion of pro-inflammatory cytokines IFN-γ and TNF-α, induce Tregs, and promote the secretion of the anti-inflammatory cytokines IL-10 (103) (Figure 4).
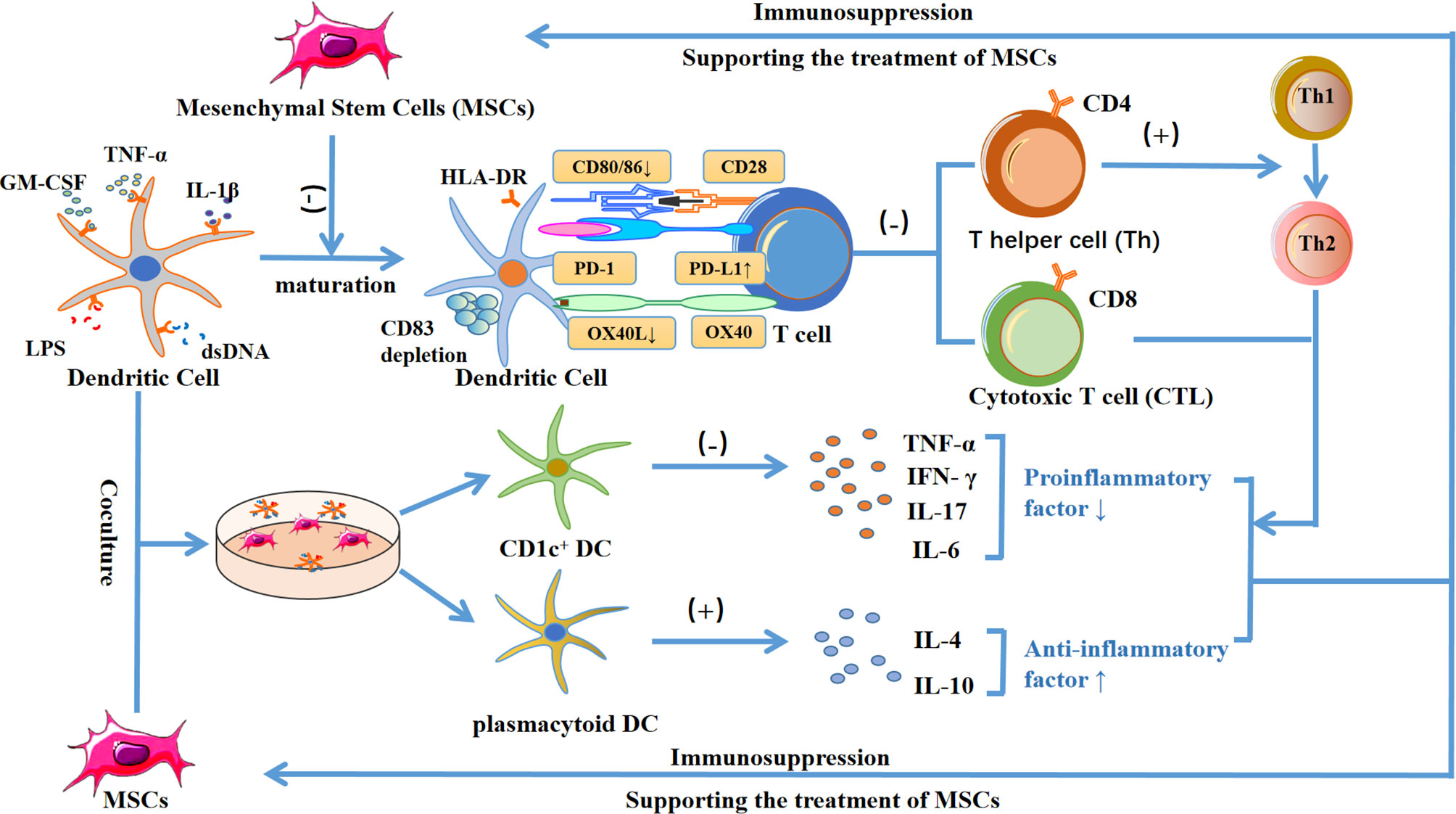
Figure 4 Diagram showing the mechanism by which MSCs inhibit the maturation of dendritic cells, reduce the ability to activate T cells, and decrease HLA-DR, CD40, OX40L, CD80, CD83, CD86 expression, while increasing PD-L1 expression. This process causes the inhibition of CD4+ and CD8+ T cells by paracrine or cell contact. On the one hand, inhibiting CD4+ helper T cells can inhibit the binding of CD40 ligands with CD40 on the surface of dendritic cells, thereby reducing the transformation to CD8+ T cells. In addition, inhibiting CD4+ helper T cells can reduce the release of IL-2 and formation of CD8 + T cells, and ultimately reduce the release of pro-inflammatory cytokines TNF-α and IFN-γ. On the other hand, MSCs can induce the differentiation of regulatory T cells and trigger dendritic cells to promote the transformation of Th1 to Th2, resulting in a decrease of the pro-inflammatory cytokines TNF-α, IFN-γ, IL-17 and IL-6, and an increase in the levels of the anti-inflammatory cytokines IL-4 and IL-10, which contributes to the treatment of MSCs. In addition, when dendritic cells were co-cultured with MSCs, the secretion of pro-inflammatory cytokines by CD1c+ DC decreased, and the secretion of anti-inflammatory cytokine IL-10 by plasmacytoid DC increased, which further led to immunosuppression and supported the treatment of MSCs.
Immune Rejection Reaction Between Allogeneic and Autologous Mesenchymal Stem Cells
Chronic rejection is the most serious major adverse reaction after allogeneic transplantation, and is also the main obstacle affecting patient survival (104). Due to the presence of alloreactive T cells in the allograft, the allograft recipient usually experiences an acute graft-versus-host response (GVHR) (105–108). Possible mechanisms of this response include host tissue injury, increased secretion of pro-inflammatory cytokines (TNF-α, IFN-γ, IL-1, IL-2, IL-12), and activation of dendritic cells (DCs), macrophages, NK cells, and cytotoxic T cells (109). Because of the low immunogenicity of human mesenchymal stem cells (HMSCs), some scholars have proposed (97) that BMSCs do not express the costimulatory molecules B7-1, B7-2, CD40, and CD40 ligands, and thus may not activate allogeneic reactive T cells.
However, in recent years, some scholars have proposed that although the immunogenicity of mesenchymal stem cells is low, thus fact alone does not explain why mesenchymal stem cells have “immune privileges” (10). Allogeneic MSCs transplanted into the body can still trigger immune rejection; however, at present, it is unclear whether this rejection has a major impact on the treatment effect. This article provides a detailed description of the above-mentioned factors that may affect host immune rejection.
Host Tissue Injury
In the treatment of osteoarthritis, Djouad et al. (110) initially proposed that although allogeneic mouse MSCs can be transplanted into mice with strong immunity to form bone, lymphocyte infiltration can be seen around newly formed tissues. Fischer et al. (111) also found more severe graft-versus-host disease (GVHD) in mice receiving allogeneic hematopoietic stem cell transplantation than in wild-type mice in clinical models. The main reason explaining this is that during allogeneic hematopoietic stem cell transplantation, damaged or dead epithelial cells release endogenous risk signals and are perceived by pattern recognition receptors on antigen-presenting cells, triggering the release of proinflammatory cytokines and T cells from major donor sources, which attack and destroy host cells and tissues, causing graft-versus-host disease (112).
In addition, studies have shown that the survival rate of allogeneic mesenchymal stem cells is limited when transplanted into the body. Liesz et al. proposed that even in uninjured adult brains, transplanted MSCs can be rejected within 14 days (113). This indicates that after allogeneic MSCs are transplanted into the body, the survival rate is still not guaranteed and some cannot coexist with the host. There are also reports that pigs (114), rats (115) and baboons (116) have dissolved allogeneic antibodies after inoculating allogeneic mesenchymal stem cells, which further confirmed the possibility of immune rejection between allogeneic and autologous MSCs.
Increased Secretion of Pro-Inflammatory Cytokines and Activation of Immunocytes
Barnhoorn et al. (117) showed that the titer of anti-donor immunoglobulin G increased significantly 7 days after the subcutaneous injection of IFN-γ-activated allogeneic mesenchymal stem cells in pigs. Furthermore, in the use of MSCs to treat traumatic brain injury, it has been proposed (54) that allogeneic mesenchymal stem cells can be actively rejected by the host immune response, mainly due to the cytotoxic CD8+ T cell-mediated response, which limits the therapeutic effect. Melissa et al. (51) released Fas ligand (FasL) through agarose gel to induce the apoptosis of cytotoxic CD8+ T cells, thereby reducing immune rejection induced by allogeneic mesenchymal stem cells during transplantation.
In addition, CD4+ Tm cells have been shown to induce allograft rejection by activating cytotoxic CD8+ T cells and helping B cells produce allogeneic antibodies (118, 119). Moreover, some scholars have found (120) that activated CD4 + T cells secrete IL-2 and IFN-γ, thereby damaging the structure of the extracellular matrix, precipitating extracellular collagen, promoting the proliferation of fibroblasts, and ultimately leading to immune rejection. It has also been suggested (121) that CD4+ helper T cells and monocytes can be recruited into the neointima and secrete IL-1, IL-6, and TNF-α, which enables smooth muscle cells to migrate and proliferate in the elastic layer of the endometrium, ultimately leading to graft-versus-host reaction (GVHR).
Current Treatment of Allogeneic MSCs Immune Rejection
At present, immune rejection of allogeneic mesenchymal stem cells still occurs. Some scholars have proposed that treatment can be refined by improving the persistence of mesenchymal stem cells and the immune tolerance of mesenchymal stem cells (10).
Improve the Persistence of MSCs
Recent advances have shown that solid organ transplantation can induce mixed hematopoietic chimerism in allogeneic MSC transplantation through donor hematopoietic stem cell transplantation (122). Moreover, the immunization isolation membrane device is used to temporarily prevent rejection by wrapping differentiated allogeneic cells in collagen or gelatin gels. Zanotti et al. have shown that subcutaneous injection of bone marrow MSCs wrapped in alginate can significantly improve their survival in GVHD mice (123).
In addition, in order to overcome the immune rejection caused by transplantation of allogeneic mesenchymal stem cells into the body and prolong the survival time of allogeneic mesenchymal stem cells, it is also necessary to ensure their persistence through host and MSC modification. For example, mesenchymal stem cells can be pre-treated or loaded with biological agents to increase the expression of surface receptors or the production of immunosuppressive cytokines (124).
Improving the Immune Tolerance of MSCs
In addition to improving immune tolerance, we can improve the efficacy of allogeneic MSCs by extending their survival time in vivo. On the one hand, the immune tolerance of allogeneic MSCs can be improved by treatment with exosomes or secreting nutritional and immunoregulatory cytokines to mediate hit-and-run mechanisms (125). However, it has also been proposed that the immune system can be reprogrammed through apoptotic bodies, thereby prolonging the duration of allogeneic MSCs after injection.
Induction Therapy
Induction therapy can be divided into T cell consumption and T cell non-consumption strategies. The former involves anti-thymocyte globulin (ATG), anti-CD3 antibody (OKT3), and alemtuzumab, which reduce the release of the proinflammatory cytokines TNF-α and IFN-γ by consuming T cells. While anti-IL-2 antibodies such as daclizumab, brazillicoxib, and anti-CD20 antibodies such as rituximab and anti-cytotoxin are considered non-depleting agents for T cells (126). IL-2, IL-6, and IL-1 are autophagy-related cytokines (63), and anti-IL-2 antibodies can reduce immune rejection by reducing autophagy induction.
New Direction of IUA Treatment: Autologous MSC
Autologous MSCs are safer to use because they use their own stem cells to avoid being attacked by B and T cells. In the treatment of IUA, in order not to affect subsequent fertility, the use of autologous MSCs is more in line with ethical requirements. Therefore, an increasing number of people are currently advocating the use of autologous MSCs for the treatment of female IUA. Such treatment reduces immune rejection, thereby reducing the recipient’s attack on the graft, while the female reproductive system avoids the controversy of potential genetic changes. In the treatment of intrauterine adhesions, this would also guarantee female fertility in later periods.
In 2011, a case study reported the implantation of autologous BMSCs into the uterine cavity in patients with severe IUA at 8 weeks of pregnancy (127). In 2014, a study found that the thickness of the endometrium slightly increased in patients with severe AS when they were injected with CD34+ or cultured autologous bone marrow stromal cells (128). In 2016, a case study reported the effect of injection of autologous peripheral blood CD133+ cells into the uterine spiral artery on endometrial reconstruction in 16 patients with refractory infertility (129). In the same year, 7 patients with severe IUA underwent autologous menstrual blood stromal cell transplantation, with two cases later successfully conceiving. In previous studies, transplantation of bone marrow/collagen complex into patients with severe IUA could help to achieve pregnancy and live birth after treatment (130). Our research group (131) recently proved that the combination of autologous adipose-derived mesenchymal stem cells (ADSCs) and gel can increase the endometrial thickness and reduce the fibrosis area through the BMP7-Smad5 pathway, resulting in successful conception.
Although transplantation with autologous MSCs is safer and more ethical than allogeneic MSCs, there are still some problems in the clinical applications. First, the number of extracted autologous MSCs is very limited as they can only be extracted from the host body (132). Second, after autologous MSCs are extracted, the in vitro culture cycle is long, which may not fully meet the needs of the body. Finally, there is a significant difference between the secretion and immune regulation of autologous MSCs (133).
Conclusion and Prospective
Immune rejection is very common in allogeneic transplantation and is one of the top five causes of death in patients undergoing allogeneic transplantation (134). To avoid adverse reactions, MSCs with low immunogenicity have become a research hotspot in recent years. However, some scholars have proposed that allogeneic mesenchymal stem cells can still trigger immune rejection. At present, the mechanism by which autologous MSCs avoid immune rejection is unclear. However, the experimental results showed that when autologous MSCs and allogeneic MSCs were injected into the body for treatment, autologous MSCs survived longer and there were clusters of immune cells around allogeneic MSCs (113).
In addition, IUA is an important factor affecting female infertility. The regenerative ability of MSCs can be harnessed repair and restore the function of the fibrotic endometrium. However, from an ethical point of view, it is still unclear whether allogeneic MSCs affect the development of offspring after implantation in the human body. Therefore, autologous MSCs are recommended for the treatment of IUA. At present, there is no effective method to solve the problem of extracting fewer stem cells from autologous MSCs, but the increase in exosomes may not only solve the problem of immune rejection of allogeneic MSCs, but also that of the low number of autologous MSCs.
Further studies to overcome the immune rejection caused by allogeneic MSCs during the treatment process are necessary. Owing to the many sources of allogeneic MSCs and the high efficiency of in vitro culture, the treatment of immune rejection caused by allogeneic MSCs is still receiving widespread attention (135). On the other hand, an obvious solution is to immediately use autologous MSCs as a ready-made product. In addition, new products such as acellular exosomes and MSCs derived from human pluripotent stem cells (hPSCs) are exciting developments that are attracting significant attention (136).
Author Contributions
J-mC, Q-yH, SL, and Q-yS contributed to the conception and design of the review. J-mC drafted and finalized the manuscript. SL and Q-yS contributed equally to writing the review. SL and Q-yS revised the manuscript and provided critical advice on the content of the manuscript. All authors contributed to the article and approved the submitted version.
Funding
This work was supported by the Science and Technology Bureau of Quanzhou (grant number 2020CT003).
Conflict of Interest
The authors declare that the research was conducted in the absence of any commercial or financial relationships that could be construed as a potential conflict of interest.
Publisher’s Note
All claims expressed in this article are solely those of the authors and do not necessarily represent those of their affiliated organizations, or those of the publisher, the editors and the reviewers. Any product that may be evaluated in this article, or claim that may be made by its manufacturer, is not guaranteed or endorsed by the publisher.
Acknowledgments
We are thankful to The Second Affiliated Hospital of Fujian Medical University for providing infrastructure facilities. What’s more, we would like to thank Editage (www.editage.cn) for English language editing.
References
1. Han Y, Li X, Zhang Y, Han Y, Chang F, Ding J. Mesenchymal Stem Cells for Regenerative Medicine. Cells (2019) 8:886. doi: 10.3390/cells8080886
2. Healy MW, Schexnayder B, Connell MT, Terry N, DeCherney AH, Csokmay JM, et al. Intrauterine Adhesion Prevention After Hysteroscopy: A Systematic Review and Meta-Analysis. Am J Obstet Gynecol (2016) 215:267–75.e7. doi: 10.1016/j.ajog.2016.05.001
3. Leung RK, Lin Y, Liu Y. Recent Advances in Understandings Towards Pathogenesis and Treatment for Intrauterine Adhesion and Disruptive Insights From Single-Cell Analysis. Reprod Sci (2021) 28:1812–26. doi: 10.1007/s43032-020-00343-y
4. Evans-Hoeker EA, Young SL. Endometrial Receptivity and Intrauterine Adhesive Disease. Semin Reprod Med (2014) 32:392–401. doi: 10.1055/s-0034-1376358
5. Amer MI, Abd-El-Maeboud KH, Abdelfatah I, Salama FA, Abdallah AS. Human Amnion as a Temporary Biologic Barrier After Hysteroscopic Lysis of Severe Intrauterine Adhesions: Pilot Study. J Minim Invasive Gynecol (2010) 17:605–11. doi: 10.1016/j.jmig.2010.03.019
6. Guida M, Acunzo G, Di Spiezio Sardo A, Bifulco G, Piccoli R, Pellicano M, et al. Effectiveness of Auto-Crosslinked Hyaluronic Acid Gel in the Prevention of Intrauterine Adhesions After Hysteroscopic Surgery: A Prospective, Randomized, Controlled Study. Hum Reprod (2004) 19:1461–4. doi: 10.1093/humrep/deh238
7. Azizi R, Aghebati-Maleki L, Nouri M, Marofi F, Negargar S, Yousefi M. Stem Cell Therapy in Asherman Syndrome and Thin Endometrium: Stem Cell- Based Therapy. BioMed Pharmacother (2018) 102:333–43. doi: 10.1016/j.biopha.2018.03.091
8. Cao Y, Sun H, Zhu H, Zhu X, Tang X, Yan G, et al. Allogeneic Cell Therapy Using Umbilical Cord MSCs on Collagen Scaffolds for Patients With Recurrent Uterine Adhesion: A Phase I Clinical Trial. Stem Cell Res Ther (2018) 9:192. doi: 10.1186/s13287-018-0904-3
9. Mambelli LI, Mattos RC, Winter GH, Madeiro DS, Morais BP, Malschitzky E, et al. Changes in Expression Pattern of Selected Endometrial Proteins Following Mesenchymal Stem Cells Infusion in Mares With Endometrosis. PloS One (2014) 9:e97889. doi: 10.1371/journal.pone.0097889
10. Ankrum JA, Ong JF, Karp JM. Mesenchymal Stem Cells: Immune Evasive, Not Immune Privileged. Nat Biotechnol (2014) 32:252–60. doi: 10.1038/nbt.2816
11. Shao L, Zhang Y, Pan X, Liu B, Liang C, Zhang Y, et al. Knockout of Beta-2 Microglobulin Enhances Cardiac Repair by Modulating Exosome Imprinting and Inhibiting Stem Cell-Induced Immune Rejection. Cell Mol Life Sci (2020) 77:937–52. doi: 10.1007/s00018-019-03220-3
12. Zangi L, Margalit R, Reich-Zeliger S, Bachar-Lustig E, Beilhack A, Negrin R, et al. Direct Imaging of Immune Rejection and Memory Induction by Allogeneic Mesenchymal Stromal Cells. Stem Cells (Dayton Ohio) (2009) 27:2865–74. doi: 10.1002/stem.217
13. Murphy N, Treacy O, Lynch K, Morcos M, Lohan P, Howard L, et al. TNF-Alpha/IL-1beta-Licensed Mesenchymal Stromal Cells Promote Corneal Allograft Survival via Myeloid Cell-Mediated Induction of Foxp3(+) Regulatory T Cells in the Lung. FASEB J (2019) 33:9404–21. doi: 10.1096/fj.201900047R
14. Xu LH, Fang JP, Hong DL, Wu YF. Application of Mesenchymal Stromal Cells in Bone Marrow Transplantation for Sensitized Recipients. Acta Haematol (2012) 127:105–9. doi: 10.1159/000333554
15. Kim S, Han YS, Lee JH, Lee SH. Combination of MSC Spheroids Wrapped Within Autologous Composite Sheet Dually Protects Against Immune Rejection and Enhances Stem Cell Transplantation Efficacy. Tissue Cell (2018) 53:93–103. doi: 10.1016/j.tice.2018.06.005
16. Deans R, Abbott J. Review of Intrauterine Adhesions. J Minim Invasive Gynecol (2010) 17:555–69. doi: 10.1016/j.jmig.2010.04.016
17. Salazar CA, Isaacson K, Morris S. A Comprehensive Review of Asherman’s Syndrome: Causes, Symptoms and Treatment Options. Curr Opin Obstet Gynecol (2017) 29:249–56. doi: 10.1097/GCO.0000000000000378
18. Moquin-Beaudry G, Colas C, Li Y, Bazin R, Guimond JV, Haddad E, et al. The Tumor-Immune Response Is Not Compromised by Mesenchymal Stromal Cells in Humanized Mice. J Immunol (Baltimore Md 1950) (2019) 203:2735–45. doi: 10.4049/jimmunol.1900807
19. Sharma JB, Sharma E, Sharma S, Dharmendra S. Female Genital Tuberculosis: Revisited. Indian J Med Res (2018) 148:S71–83. doi: 10.4103/ijmr.IJMR_648_18
20. Chen Y, Liu L, Luo Y, Chen M, Huan Y, Fang R. Prevalence and Impact of Chronic Endometritis in Patients With Intrauterine Adhesions: A Prospective Cohort Study. J Minim Invasive Gynecol (2017) 24:74–9. doi: 10.1016/j.jmig.2016.09.022
21. Dreisler E, Kjer JJ. Asherman’s Syndrome: Current Perspectives on Diagnosis and Management. Int J Womens Health (2019) 11:191–8. doi: 10.2147/IJWH.S165474
22. Zhao X, Zhao Q, Zhu X, Huang H, Wan X, Guo R, et al. Study on the Correlation Among Dysbacteriosis, Imbalance of Cytokine and the Formation of Intrauterine Adhesion. Ann Trans Med (2020) 8:52. doi: 10.21037/atm.2019.11.124
23. Placek K, Schultze JL, Aschenbrenner AC. Epigenetic Reprogramming of Immune Cells in Injury, Repair, and Resolution. J Clin Invest (2019) 129:2994–3005. doi: 10.1172/JCI124619
24. Yimam M, Lee YC, Moore B, Jiao P, Hong M, Nam JB, et al. Analgesic and Anti-Inflammatory Effects of UP1304, a Botanical Composite Containing Standardized Extracts of Curcuma Longa and Morus Alba. J Integr Med (2016) 14:60–8. doi: 10.1016/S2095-4964(16)60231-5
25. Wang X, Ma N, Sun Q, Huang C, Liu Y, Luo X. Elevated NF-kappaB Signaling in Asherman Syndrome Patients and Animal Models. Oncotarget (2017) 8:15399–406. doi: 10.18632/oncotarget.14853
26. Lin XN, Zhou F, Wei ML, Yang Y, Li Y, Li TC, et al. Randomized, Controlled Trial Comparing the Efficacy of Intrauterine Balloon and Intrauterine Contraceptive Device in the Prevention of Adhesion Reformation After Hysteroscopic Adhesiolysis. Fertil Steril (2015) 104:235–40. doi: 10.1016/j.fertnstert.2015.04.008
27. Moghaddam AS, Afshari JT, Esmaeili SA, Saburi E, Joneidi Z, Momtazi-Borojeni AA. Cardioprotective microRNAs: Lessons From Stem Cell-Derived Exosomal microRNAs to Treat Cardiovascular Disease. Atherosclerosis (2019) 285:1–9. doi: 10.1016/j.atherosclerosis.2019.03.016
28. Mais V, Cirronis MG, Peiretti M, Ferrucci G, Cossu E, Melis GB. Efficacy of Auto-Crosslinked Hyaluronan Gel for Adhesion Prevention in Laparoscopy and Hysteroscopy: A Systematic Review and Meta-Analysis of Randomized Controlled Trials. Eur J Obstet Gynecol Reprod Biol (2012) 160:1–5. doi: 10.1016/j.ejogrb.2011.08.002
29. Chi Y, He P, Lei L, Lan Y, Hu J, Meng Y, et al. Transdermal Estrogen Gel and Oral Aspirin Combination Therapy Improves Fertility Prognosis via the Promotion of Endometrial Receptivity in Moderate to Severe Intrauterine Adhesion. Mol Med Rep (2018) 17:6337–44. doi: 10.3892/mmr.2018.8685
30. Hanstede MM, van der Meij E, Goedemans L, Emanuel MH. Results of Centralized Asherman Surgery, 2003-2013. Fertil Steril (2015) 104:1561–8 e1. doi: 10.1016/j.fertnstert.2015.08.039
31. Xin L, Lin X, Pan Y, Zheng X, Shi L, Zhang Y, et al. A Collagen Scaffold Loaded With Human Umbilical Cord-Derived Mesenchymal Stem Cells Facilitates Endometrial Regeneration and Restores Fertility. Acta Biomater (2019) 92:160–71. doi: 10.1016/j.actbio.2019.05.012
32. Wang L, Yu C, Chang T, Zhang M, Song S, Xiong C, et al. In Situ Repair Abilities of Human Umbilical Cord-Derived Mesenchymal Stem Cells and Autocrosslinked Hyaluronic Acid Gel Complex in Rhesus Monkeys With Intrauterine Adhesion. Sci Adv (2020) 6:eaba6357. doi: 10.1126/sciadv.aba6357
33. Fei Z, Xin X, Fei H, Cui YC. Meta-Analysis of the Use of Hyaluronic Acid Gel to Prevent Intrauterine Adhesions After Miscarriage. Eur J Obstet Gyn R B (2020) 244:1–4. doi: 10.1016/j.ejogrb.2019.10.018
34. Xin LB, Lin XN, Zhou F, Li C, Wang XF, Yu HY, et al. A Scaffold Laden With Mesenchymal Stem Cell-Derived Exosomes for Promoting Endometrium Regeneration and Fertility Restoration Through Macrophage Immunomodulation. Acta Biomaterialia (2020) 113:252–66. doi: 10.1016/j.actbio.2020.06.029
35. Uccelli A, Moretta L, Pistoia V. Mesenchymal Stem Cells in Health and Disease. Nat Rev Immunol (2008) 8:726–36. doi: 10.1038/nri2395
36. Elahi KC, Klein G, Avci-Adali M, Sievert KD, MacNeil S, Aicher WK. Human Mesenchymal Stromal Cells From Different Sources Diverge in Their Expression of Cell Surface Proteins and Display Distinct Differentiation Patterns. Stem Cells Int (2016) 2016:5646384. doi: 10.1155/2016/5646384
37. Costa LA, Eiro N, Fraile M, Gonzalez LO, Saa J, Garcia-Portabella P, et al. Functional Heterogeneity of Mesenchymal Stem Cells From Natural Niches to Culture Conditions: Implications for Further Clinical Uses. Cell Mol Life Sci (2021) 78:447–67. doi: 10.1007/s00018-020-03600-0
38. Monti M, Perotti C, Del Fante C, Cervio M, Redi CA, Fondazione Irccs Policlinico San Matteo P. Stem Cells: Sources and Therapies. Biol Res (2012) 45:207–14. doi: 10.4067/S0716-97602012000300002
39. Khan-Farooqi H, Chodosh J. Autologous Limbal Stem Cell Transplantation: The Progression of Diagnosis and Treatment. Semin Ophthalmol (2016) 31:91–8. doi: 10.3109/08820538.2015.1114862
40. Petrou P, Kassis I, Levin N, Paul F, Backner Y, Benoliel T, et al. Beneficial Effects of Autologous Mesenchymal Stem Cell Transplantation in Active Progressive Multiple Sclerosis. Brain (2020) 143:3574–88. doi: 10.1093/brain/awaa333
41. Zahorec P, Sarkozyova N, Ferancikova N, Bukovcan P, Danisovic L, Bohac M, et al. Autologous Mesenchymal Stem Cells Application in Post-Burn Scars Treatment: A Preliminary Study. Cell Tissue Bank (2021) 22:39–46. doi: 10.1007/s10561-020-09862-z
42. Lightner AL, Dozois EJ, Dietz AB, Fletcher JG, Friton J, Butler G, et al. Matrix-Delivered Autologous Mesenchymal Stem Cell Therapy for Refractory Rectovaginal Crohn’s Fistulas. Inflamm Bowel Dis (2020) 26:670–7. doi: 10.1093/ibd/izz215
43. Ruano D, Lopez-Martin JA, Moreno L, Lassaletta A, Bautista F, Andion M, et al. First-In-Human, First-In-Child Trial of Autologous MSCs Carrying the Oncolytic Virus Icovir-5 in Patients With Advanced Tumors. Mol Ther (2020) 28:1033–42. doi: 10.1016/j.ymthe.2020.01.019
44. Sharpe PT. Dental Mesenchymal Stem Cells. Development (2016) 143:2273–80. doi: 10.1242/dev.134189
45. Ding DC, Chang YH, Shyu WC, Lin SZ. Human Umbilical Cord Mesenchymal Stem Cells: A New Era for Stem Cell Therapy. Cell Transplant (2015) 24:339–47. doi: 10.3727/096368915X686841
46. Baker N, Boyette LB, Tuan RS. Characterization of Bone Marrow-Derived Mesenchymal Stem Cells in Aging. Bone (2015) 70:37–47. doi: 10.1016/j.bone.2014.10.014
47. Bourin P, Bunnell BA, Casteilla L, Dominici M, Katz AJ, March KL, et al. Stromal Cells From the Adipose Tissue-Derived Stromal Vascular Fraction and Culture Expanded Adipose Tissue-Derived Stromal/Stem Cells: A Joint Statement of the International Federation for Adipose Therapeutics and Science (IFATS) and the International Society for Cellular Therapy (ISCT). Cytotherapy (2013) 15:641–8. doi: 10.1016/j.jcyt.2013.02.006
48. Maki CB, Beck A, Wallis CCC, Choo J, Ramos T, Tong R, et al. Intra-Articular Administration of Allogeneic Adipose Derived MSCs Reduces Pain and Lameness in Dogs With Hip Osteoarthritis: A Double Blinded, Randomized, Placebo Controlled Pilot Study. Front Vet Sci (2020) 7:570. doi: 10.3389/fvets.2020.00570
49. Zheng G, Huang L, Tong H, Shu Q, Hu Y, Ge M, et al. Treatment of Acute Respiratory Distress Syndrome With Allogeneic Adipose-Derived Mesenchymal Stem Cells: A Randomized, Placebo-Controlled Pilot Study. Respir Res (2014) 15:39. doi: 10.1186/1465-9921-15-39
50. Akita N, Narita Y, Yamawaki-Ogata A, Usui A, Komori K. Therapeutic Effect of Allogeneic Bone Marrow-Derived Mesenchymal Stromal Cells on Aortic Aneurysms. Cell Tissue Res (2021) 383:781–93. doi: 10.1007/s00441-020-03295-6
51. Alvarado-Velez M, Enam SF, Mehta N, Lyon JG, LaPlaca MC, Bellamkonda RV. Immuno-Suppressive Hydrogels Enhance Allogeneic MSC Survival After Transplantation in the Injured Brain. Biomaterials (2021) 266:120419. doi: 10.1016/j.biomaterials.2020.120419
52. Cheng YH, Dong JC, Bian Q. Small Molecules for Mesenchymal Stem Cell Fate Determination. World J Stem Cells (2019) 11:1084–103. doi: 10.4252/wjsc.v11.i12.1084
53. Hsiao CY, Chen TH, Huang BS, Chen PH, Su CH, Shyu JF, et al. Comparison Between the Therapeutic Effects of Differentiated and Undifferentiated Wharton’s Jelly Mesenchymal Stem Cells in Rats With Streptozotocin-Induced Diabetes. World J Stem Cells (2020) 12:139–51. doi: 10.4252/wjsc.v12.i2.139
54. Wang Y, Chen X, Cao W, Shi Y. Plasticity of Mesenchymal Stem Cells in Immunomodulation: Pathological and Therapeutic Implications. Nat Immunol (2014) 15:1009–16. doi: 10.1038/ni.3002
55. Mutlu L, Hufnagel D, Taylor HS. The Endometrium as a Source of Mesenchymal Stem Cells for Regenerative Medicine. Biol Reprod (2015) 92:138. doi: 10.1095/biolreprod.114.126771
56. Benor A, Gay S, DeCherney A. An Update on Stem Cell Therapy for Asherman Syndrome. J Assisted Reprod Genet (2020) 37:1511–29. doi: 10.1007/s10815-020-01801-x
57. Luz-Crawford P, Kurte M, Bravo-Alegria J, Contreras R, Nova-Lamperti E, Tejedor G, et al. Mesenchymal Stem Cells Generate a CD4+CD25+Foxp3+ Regulatory T Cell Population During the Differentiation Process of Th1 and Th17 Cells. Stem Cell Res Ther (2013) 4:65. doi: 10.1186/scrt216
58. Jiang Y, Zhang P, Zhang X, Lv L, Zhou Y. Advances in Mesenchymal Stem Cell Transplantation for the Treatment of Osteoporosis. Cell Prolif (2021) 54:e12956. doi: 10.1111/cpr.12956
59. Nemeth K, Mayer B, Mezey E. Modulation of Bone Marrow Stromal Cell Functions in Infectious Diseases by Toll-Like Receptor Ligands. J Mol Med (Berl) (2010) 88:5–10. doi: 10.1007/s00109-009-0523-7
60. Waterman RS, Tomchuck SL, Henkle SL, Betancourt AM. A New Mesenchymal Stem Cell (MSC) Paradigm: Polarization Into a Pro-Inflammatory MSC1 or an Immunosuppressive MSC2 Phenotype. PloS One (2010) 5:e10088. doi: 10.1371/journal.pone.0010088
61. Liotta F, Angeli R, Cosmi L, Fili L, Manuelli C, Frosali F, et al. Toll-Like Receptors 3 and 4 are Expressed by Human Bone Marrow-Derived Mesenchymal Stem Cells and can Inhibit Their T-Cell Modulatory Activity by Impairing Notch Signaling. Stem Cells (Dayton Ohio) (2008) 26:279–89. doi: 10.1634/stemcells.2007-0454
62. Liu D, Wang J, Zhao G, Jiang P, Song M, Ding H, et al. CSF1-Associated Decrease in Endometrial Macrophages may Contribute to Asherman’s Syndrome. Am J Reprod Immunol (2020) 83:e13191. doi: 10.1111/aji.13191
63. Li B, Zhang Q, Sun J, Lai D. Human Amniotic Epithelial Cells Improve Fertility in an Intrauterine Adhesion Mouse Model. Stem Cell Res Ther (2019) 10:257. doi: 10.1186/s13287-019-1368-9
64. Takano H, Ohtsuka M, Akazawa H, Toko H, Harada M, Hasegawa H, et al. Pleiotropic Effects of Cytokines on Acute Myocardial Infarction: G-CSF as a Novel Therapy for Acute Myocardial Infarction. Curr Pharm Des (2003) 9:1121–7. doi: 10.2174/1381612033455008
65. Nagori CB, Panchal SY, Patel H. Endometrial Regeneration Using Autologous Adult Stem Cells Followed by Conception by In Vitro Fertilization in a Patient of Severe Asherman’s Syndrome. J Hum Reprod Sci (2011) 4:43–8. doi: 10.4103/0974-1208.82360
66. Wolff EF, Mutlu L, Massasa EE, Elsworth JD, Eugene Redmond D Jr., Taylor HS. Endometrial Stem Cell Transplantation in MPTP- Exposed Primates: An Alternative Cell Source for Treatment of Parkinson’s Disease. J Cell Mol Med (2015) 19:249–56. doi: 10.1111/jcmm.12433
67. Wolff EF, Gao XB, Yao KV, Andrews ZB, Du H, Elsworth JD, et al. Endometrial Stem Cell Transplantation Restores Dopamine Production in a Parkinson’s Disease Model. J Cell Mol Med (2011) 15:747–55. doi: 10.1111/j.1582-4934.2010.01068.x
68. Noureddini M, Verdi J, Mortazavi-Tabatabaei SA, Sharif S, Azimi A, Keyhanvar P, et al. Human Endometrial Stem Cell Neurogenesis in Response to NGF and bFGF. Cell Biol Int (2012) 36:961–6. doi: 10.1042/CBI20110610
69. Ebrahimi-Barough S, Kouchesfahani HM, Ai J, Massumi M. Differentiation of Human Endometrial Stromal Cells Into Oligodendrocyte Progenitor Cells (OPCs). J Mol Neurosci (2013) 51:265–73. doi: 10.1007/s12031-013-9957-z
70. Santamaria X, Massasa EE, Feng Y, Wolff E, Taylor HS. Derivation of Insulin Producing Cells From Human Endometrial Stromal Stem Cells and Use in the Treatment of Murine Diabetes. Mol Ther (2011) 19:2065–71. doi: 10.1038/mt.2011.173
71. Hida N, Nishiyama N, Miyoshi S, Kira S, Segawa K, Uyama T, et al. Novel Cardiac Precursor-Like Cells From Human Menstrual Blood-Derived Mesenchymal Cells. Stem Cells (2008) 26:1695–704. doi: 10.1634/stemcells.2007-0826
72. Wang J, Chen S, Zhang C, Stegeman S, Pfaff-Amesse T, Zhang Y, et al. Human Endometrial Stromal Stem Cells Differentiate Into Megakaryocytes With the Ability to Produce Functional Platelets. PloS One (2012) 7:e44300. doi: 10.1371/journal.pone.0044300
73. Shoae-Hassani A, Mortazavi-Tabatabaei SA, Sharif S, Seifalian AM, Azimi A, Samadikuchaksaraei A, et al. Differentiation of Human Endometrial Stem Cells Into Urothelial Cells on a Three-Dimensional Nanofibrous Silk-Collagen Scaffold: An Autologous Cell Resource for Reconstruction of the Urinary Bladder Wall. J Tissue Eng Regener Med (2015) 9:1268–76. doi: 10.1002/term.1632
74. Walter J, Ware LB, Matthay MA. Mesenchymal Stem Cells: Mechanisms of Potential Therapeutic Benefit in ARDS and Sepsis. Lancet Respir Med (2014) 2:1016–26. doi: 10.1016/S2213-2600(14)70217-6
75. Pegtel DM, Gould SJ. Exosomes. Annu Rev Biochem (2019) 88:487–514. doi: 10.1146/annurev-biochem-013118-111902
76. Kalluri R, LeBleu VS. The Biology, Function, and Biomedical Applications of Exosomes. Science (2020) 367:eaau6977. doi: 10.1126/science.aau6977
77. Yao Y, Chen R, Wang G, Zhang Y, Liu F. Exosomes Derived From Mesenchymal Stem Cells Reverse EMT via TGF-Beta1/Smad Pathway and Promote Repair of Damaged Endometrium. Stem Cell Res Ther (2019) 10:225. doi: 10.1186/s13287-019-1332-8
78. Liao Z, Liu C, Wang L, Sui C, Zhang H. Therapeutic Role of Mesenchymal Stem Cell-Derived Extracellular Vesicles in Female Reproductive Diseases. Front Endocrinol (2021) 12:665645. doi: 10.3389/fendo.2021.665645
79. Liang X, Zhang L, Wang S, Han Q, Zhao RC. Exosomes Secreted by Mesenchymal Stem Cells Promote Endothelial Cell Angiogenesis by Transferring miR-125a. J Cell Sci (2016) 129:2182–9. doi: 10.1242/jcs.170373
80. Zhao S, Qi W, Zheng J, Tian Y, Qi X, Kong D, et al. Exosomes Derived From Adipose Mesenchymal Stem Cells Restore Functional Endometrium in a Rat Model of Intrauterine Adhesions. Reprod Sci (Thousand Oaks Calif) (2020) 27:1266–75. doi: 10.1007/s43032-019-00112-6
81. Tan Q, Xia D, Ying X. miR-29a in Exosomes From Bone Marrow Mesenchymal Stem Cells Inhibit Fibrosis During Endometrial Repair of Intrauterine Adhesion. Int J Stem Cells (2020) 13:414–23. doi: 10.15283/ijsc20049
82. Xiao L, Song Y, Huang W, Yang S, Fu J, Feng X, et al. Expression of SOX2, NANOG and OCT4 in a Mouse Model of Lipopolysaccharide-Induced Acute Uterine Injury and Intrauterine Adhesions. Reprod Biol Endocrinol (2017) 15:14. doi: 10.1186/s12958-017-0234-9
83. Wang X, Gao Y, Shi H, Liu N, Zhang W, Li H. Influence of the Intensity and Loading Time of Direct Current Electric Field on the Directional Migration of Rat Bone Marrow Mesenchymal Stem Cells. Front Med (2016) 10:286–96. doi: 10.1007/s11684-016-0456-9
84. Zhou Q, Wu X, Hu J, Yuan R. Abnormal Expression of Fibrosis Markers, Estrogen Receptor Alpha and Stromal Derived Factor1/Chemokine (CXC Motif) Receptor4 Axis in Intrauterine Adhesions. Int J Mol Med (2018) 42:81–90. doi: 10.3892/ijmm.2018.3586
85. Sahin Ersoy G, Zolbin MM, Cosar E, Moridi I, Mamillapalli R, Taylor HS. CXCL12 Promotes Stem Cell Recruitment and Uterine Repair After Injury in Asherman’s Syndrome. Mol Ther Methods Clin Dev (2017) 4:169–77. doi: 10.1016/j.omtm.2017.01.001
86. Du H, Naqvi H, Taylor HS. Ischemia/reperfusion Injury Promotes and Granulocyte-Colony Stimulating Factor Inhibits Migration of Bone Marrow-Derived Stem Cells to Endometrium. Stem Cells Dev (2012) 21:3324–31. doi: 10.1089/scd.2011.0193
87. Sanabria-de la Torre R, Quiñones-Vico MI, Fernández-González A, Sánchez-Díaz M, Montero-Vílchez T, Sierra-Sánchez Á, et al. Alloreactive Immune Response Associated to Human Mesenchymal Stromal Cells Treatment: A Systematic Review. J Clin Med (2021) 10:2991. doi: 10.3390/jcm10132991
88. Le Blanc K, Tammik C, Rosendahl K, Zetterberg E, Ringden O. HLA Expression and Immunologic Properties of Differentiated and Undifferentiated Mesenchymal Stem Cells. Exp Hematol (2003) 31:890–6. doi: 10.1016/S0301-472X(03)00110-3
89. Krampera M. Mesenchymal Stromal Cell ’Licensing’: A Multistep Process. Leukemia (2011) 25:1408–14. doi: 10.1038/leu.2011.108
90. Muller L, Tunger A, Wobus M, von Bonin M, Towers R, Bornhauser M, et al. Immunomodulatory Properties of Mesenchymal Stromal Cells: An Update. Front Cell Dev Biol (2021) 9:637725. doi: 10.3389/fcell.2021.637725
91. Shapouri-Moghaddam A, Mohammadian S, Vazini H, Taghadosi M, Esmaeili SA, Mardani F, et al. Macrophage Plasticity, Polarization, and Function in Health and Disease. J Cell Physiol (2018) 233:6425–40. doi: 10.1002/jcp.26429
92. Yang R, Gao H, Chen L, Fang N, Chen H, Song G, et al. Effect of Peripheral Blood-Derived Mesenchymal Stem Cells on Macrophage Polarization and Th17/Treg Balance In Vitro. Regener Ther (2020) 14:275–83. doi: 10.1016/j.reth.2020.03.008
93. Maqbool M, Algraittee SJR, Boroojerdi MH, Sarmadi VH, John CM, Vidyadaran S, et al. Human Mesenchymal Stem Cells Inhibit the Differentiation and Effector Functions of Monocytes. Innate Immun (2020) 26:424–34. doi: 10.1177/1753425919899132
94. de Witte SFH, Luk F, Sierra Parraga JM, Gargesha M, Merino A, Korevaar SS, et al. Immunomodulation By Therapeutic Mesenchymal Stromal Cells (MSC) Is Triggered Through Phagocytosis of MSC By Monocytic Cells. Stem Cells (Dayton Ohio) (2018) 36:602–15. doi: 10.1002/stem.2779
95. Dong L, Chen X, Shao H, Bai L, Li X, Zhang X. Mesenchymal Stem Cells Inhibited Dendritic Cells Via the Regulation of STAT1 and STAT6 Phosphorylation in Experimental Autoimmune Uveitis. Curr Mol Med (2018) 17:478–87. doi: 10.2174/1566524018666180207155614
96. Lu Z, Meng S, Chang W, Fan S, Xie J, Guo F, et al. Mesenchymal Stem Cells Activate Notch Signaling to Induce Regulatory Dendritic Cells in LPS-Induced Acute Lung Injury. J Trans Med (2020) 18:241. doi: 10.1186/s12967-020-02410-z
97. Aggarwal S, Pittenger MF. Human Mesenchymal Stem Cells Modulate Allogeneic Immune Cell Responses. Blood (2005) 105:1815–22. doi: 10.1182/blood-2004-04-1559
98. Shahir M, Mahmoud Hashemi S, Asadirad A, Varahram M, Kazempour-Dizaji M, Folkerts G, et al. Effect of Mesenchymal Stem Cell-Derived Exosomes on the Induction of Mouse Tolerogenic Dendritic Cells. J Cell Physiol (2020) 235:7043–55. doi: 10.1002/jcp.29601
99. Krampera M, Glennie S, Dyson J, Scott D, Laylor R, Simpson E, et al. Bone Marrow Mesenchymal Stem Cells Inhibit the Response of Naive and Memory Antigen-Specific T Cells to Their Cognate Peptide. Blood (2003) 101:3722–9. doi: 10.1182/blood-2002-07-2104
100. Krampera M, Cosmi L, Angeli R, Pasini A, Liotta F, Andreini A, et al. Role for Interferon-Gamma in the Immunomodulatory Activity of Human Bone Marrow Mesenchymal Stem Cells. Stem Cells (Dayton Ohio) (2006) 24:386–98. doi: 10.1634/stemcells.2005-0008
101. Lee S, Kim S, Chung H, Moon JH, Kang SJ, Park CG. Mesenchymal Stem Cell-Derived Exosomes Suppress Proliferation of T Cells by Inducing Cell Cycle Arrest Through P27kip1/Cdk2 Signaling. Immunol Lett (2020) 225:16–22. doi: 10.1016/j.imlet.2020.06.006
102. Ge W, Jiang J, Arp J, Liu W, Garcia B, Wang H. Regulatory T-Cell Generation and Kidney Allograft Tolerance Induced by Mesenchymal Stem Cells Associated With Indoleamine 2,3-Dioxygenase Expression. Transplantation (2010) 90:1312–20. doi: 10.1097/TP.0b013e3181fed001
103. Guo L, Lai P, Wang Y, Huang T, Chen X, Luo C, et al. Extracellular Vesicles From Mesenchymal Stem Cells Prevent Contact Hypersensitivity Through the Suppression of Tc1 and Th1 Cells and Expansion of Regulatory T Cells. Int Immunopharmacol (2019) 74:105663. doi: 10.1016/j.intimp.2019.05.048
104. Justiz Vaillant AA, Mohseni M. Chronic Transplantation Rejection. Treasure Island (FL: StatPearls (2021).
105. Elfenbein GJ, Sackstein R. Primed Marrow for Autologous and Allogeneic Transplantation: A Review Comparing Primed Marrow to Mobilized Blood and Steady-State Marrow. Exp Hematol (2004) 32:327–39. doi: 10.1016/j.exphem.2004.01.010
106. Tabbara IA, Kairouz S, Nahleh Z, Mihalcea AM. Current Concepts in Allogeneic Hematopoietic Stem Cell Transplantation. Anticancer Res (2003) 23:5055–67.
107. Zeng D, Lan F, Hoffmann P, Strober S. Suppression of Graft-Versus-Host Disease by Naturally Occurring Regulatory T Cells. Transplantation (2004) 77:S9–S11. doi: 10.1097/01.TP.0000106475.38978.11
108. Slavin S. Graft-Versus-Host Disease, the Graft-Versus-Leukemia Effect, and Mixed Chimerism Following Nonmyeloablative Stem Cell Transplantation. Int J Hematol (2003) 78:195–207. doi: 10.1007/BF02983795
109. van den Brink MR, Burakoff SJ. Cytolytic Pathways in Haematopoietic Stem-Cell Transplantation. Nat Rev Immunol (2002) 2:273–81. doi: 10.1038/nri775
110. Djouad F, Plence P, Bony C, Tropel P, Apparailly F, Sany J, et al. Immunosuppressive Effect of Mesenchymal Stem Cells Favors Tumor Growth in Allogeneic Animals. Blood (2003) 102:3837–44. doi: 10.1182/blood-2003-04-1193
111. Fischer JC, Bscheider M, Eisenkolb G, Lin CC, Wintges A, Otten V, et al. RIG-I/MAVS and STING Signaling Promote Gut Integrity During Irradiation- and Immune-Mediated Tissue Injury. Sci Transl Med (2017) 9:eaag2513. doi: 10.1126/scitranslmed.aag2513
112. Heidegger S, van den Brink MR, Haas T, Poeck H. The Role of Pattern-Recognition Receptors in Graft-Versus-Host Disease and Graft-Versus-Leukemia After Allogeneic Stem Cell Transplantation. Front Immunol (2014) 5:337. doi: 10.3389/fimmu.2014.00337
113. Liesz A, Dalpke A, Mracsko E, Antoine DJ, Roth S, Zhou W, et al. DAMP Signaling Is a Key Pathway Inducing Immune Modulation After Brain Injury. J Neurosci (2015) 35:583–98. doi: 10.1523/JNEUROSCI.2439-14.2015
114. Cho PS, Messina DJ, Hirsh EL, Chi N, Goldman SN, Lo DP, et al. Immunogenicity of Umbilical Cord Tissue Derived Cells. Blood (2008) 111:430–8. doi: 10.1182/blood-2007-03-078774
115. Schu S, Nosov M, O’Flynn L, Shaw G, Treacy O, Barry F, et al. Immunogenicity of Allogeneic Mesenchymal Stem Cells. J Cell Mol Med (2012) 16:2094–103. doi: 10.1111/j.1582-4934.2011.01509.x
116. Beggs KJ, Lyubimov A, Borneman JN, Bartholomew A, Moseley A, Dodds R, et al. Immunologic Consequences of Multiple, High-Dose Administration of Allogeneic Mesenchymal Stem Cells to Baboons. Cell Transplant (2006) 15:711–21. doi: 10.3727/000000006783981503
117. Barnhoorn MC, Van Halteren AGS, Van Pel M, Molendijk I, Struijk AC, Jansen PM, et al. Lymphoproliferative Disease in the Rectum 4 Years After Local Mesenchymal Stromal Cell Therapy for Refractory Perianal Crohn’s Fistulas: A Case Report. J Crohns Colitis (2019) 13:807–11. doi: 10.1093/ecco-jcc/jjy220
118. Zhang QW, Rabant M, Schenk A, Valujskikh A. ICOS-Dependent and -Independent Functions of Memory CD4 T Cells in Allograft Rejection. Am J Transplant Off J Am Soc Transplant Am Soc Transplant Surgeons (2008) 8:497–506. doi: 10.1111/j.1600-6143.2007.02096.x
119. Chen Y, Heeger PS, Valujskikh A. In Vivo Helper Functions of Alloreactive Memory CD4+ T Cells Remain Intact Despite Donor-Specific Transfusion and Anti-CD40 Ligand Therapy. J Immunol (Baltimore Md 1950) (2004) 172:5456–66. doi: 10.4049/jimmunol.172.9.5456
120. Pichler M, Rainer PP, Schauer S, Hoefler G. Cardiac Fibrosis in Human Transplanted Hearts is Mainly Driven by Cells of Intracardiac Origin. J Am Coll Cardiol (2012) 59:1008–16. doi: 10.1016/j.jacc.2011.11.036
121. Chih S, Chong AY, Mielniczuk LM, Bhatt DL, Beanlands RS. Allograft Vasculopathy: The Achilles’ Heel of Heart Transplantation. J Am Coll Cardiol (2016) 68:80–91. doi: 10.1016/j.jacc.2016.04.033
122. Leonard DA, Cetrulo CL Jr., McGrouther DA, Sachs DH. Induction of Tolerance of Vascularized Composite Allografts. Transplantation (2013) 95:403–9. doi: 10.1097/TP.0b013e31826d886d
123. Zanotti L, Sarukhan A, Dander E, Castor M, Cibella J, Soldani C, et al. Encapsulated Mesenchymal Stem Cells for In Vivo Immunomodulation. Leukemia (2013) 27:500–3. doi: 10.1038/leu.2012.202
124. Ankrum JA, Miranda OR, Ng KS, Sarkar D, Xu C, Karp JM. Engineering Cells With Intracellular Agent-Loaded Microparticles to Control Cell Phenotype. Nat Protoc (2014) 9:233–45. doi: 10.1038/nprot.2014.002
125. Prockop DJ. Concise Review: Two Negative Feedback Loops Place Mesenchymal Stem/Stromal Cells at the Center of Early Regulators of Inflammation. Stem Cells (Dayton Ohio) (2013) 31:2042–6. doi: 10.1002/stem.1400
126. Zhao L, Hu C, Han F, Chen D, Cheng J, Wu J, et al. Induction Therapy With Mesenchymal Stromal Cells in Kidney Transplantation: A Meta-Analysis. Stem Cell Res Ther (2021) 12:158. doi: 10.1186/s13287-021-02219-7
127. Wang H, Jin P, Sabatino M, Ren J, Civini S, Bogin V, et al. Comparison of Endometrial Regenerative Cells and Bone Marrow Stromal Cells. J Trans Med (2012) 10:207. doi: 10.1186/1479-5876-10-207
128. Singh N, Mohanty S, Seth T, Shankar M, Bhaskaran S, Dharmendra S. Autologous Stem Cell Transplantation in Refractory Asherman’s Syndrome: A Novel Cell Based Therapy. J Hum Reprod Sci (2014) 7:93–8. doi: 10.4103/0974-1208.138864
129. Santamaria X, Cabanillas S, Cervelló I, Arbona C, Raga F, Ferro J, et al. Autologous Cell Therapy With CD133+ Bone Marrow-Derived Stem Cells for Refractory Asherman’s Syndrome and Endometrial Atrophy: A Pilot Cohort Study. Hum Reprod (Oxford England) (2016) 31:1087–96. doi: 10.1093/humrep/dew042
130. Zhao G, Cao Y, Zhu X, Tang X, Ding L, Sun H, et al. Transplantation of Collagen Scaffold With Autologous Bone Marrow Mononuclear Cells Promotes Functional Endometrium Reconstruction via Downregulating DeltaNp63 Expression in Asherman’s Syndrome. Sci China Life Sci (2017) 60:404–16. doi: 10.1007/s11427-016-0328-y
131. Zhao YX, Chen SR, Huang QY, Chen WC, Xia T, Shi YC, et al. Repair Abilities of Mouse Autologous Adipose-Derived Stem Cells and ShakeGel3D Complex Local Injection With Intrauterine Adhesion by BMP7-Smad5 Signaling Pathway Activation. Stem Cell Res Ther (2021) 12:191. doi: 10.1186/s13287-021-02258-0
132. Bravery CA, Carmen J, Fong T, Oprea W, Hoogendoorn KH, Woda J, et al. Potency Assay Development for Cellular Therapy Products: An ISCT Review of the Requirements and Experiences in the Industry. Cytotherapy (2013) 15:9–19. doi: 10.1016/j.jcyt.2012.10.008
133. Melief SM, Zwaginga JJ, Fibbe WE, Roelofs H. Adipose Tissue-Derived Multipotent Stromal Cells Have a Higher Immunomodulatory Capacity Than Their Bone Marrow-Derived Counterparts. Stem Cells Trans Med (2013) 2:455–63. doi: 10.5966/sctm.2012-0184
134. Chan S, Hawley CM, Campbell KL, Morrison M, Campbell SB, Isbel NM, et al. Transplant Associated Infections-The Role of the Gastrointestinal Microbiota and Potential Therapeutic Options. Nephrol (Carlton) (2020) 25:5–13. doi: 10.1111/nep.13670
135. Reinders ME, de Fijter JW, Roelofs H, Bajema IM, de Vries DK, Schaapherder AF, et al. Autologous Bone Marrow-Derived Mesenchymal Stromal Cells for the Treatment of Allograft Rejection After Renal Transplantation: Results of a Phase I Study. Stem Cells Trans Med (2013) 2:107–11. doi: 10.5966/sctm.2012-0114
136. Wang LT, Liu KJ, Sytwu HK, Yen ML, Yen BL. Advances in Mesenchymal Stem Cell Therapy for Immune and Inflammatory Diseases: Use of Cell-Free Products and Human Pluripotent Stem Cell-Derived Mesenchymal Stem Cells. Stem Cells Trans Med (2021) 10:1288–303. doi: 10.1002/sctm.21-0021
Glossary
Keywords: immunoregulation, allogeneic MSCs, autologous MSC, intrauterine adhesion, rejection reaction
Citation: Chen J-m, Huang Q-y, Zhao Y-x, Chen W-h, Lin S and Shi Q-y (2021) The Latest Developments in Immunomodulation of Mesenchymal Stem Cells in the Treatment of Intrauterine Adhesions, Both Allogeneic and Autologous. Front. Immunol. 12:785717. doi: 10.3389/fimmu.2021.785717
Received: 29 September 2021; Accepted: 01 November 2021;
Published: 15 November 2021.
Edited by:
Ursula Grohmann, University of Perugia, ItalyReviewed by:
Bojana Simovic Markovic, University of Kragujevac, SerbiaElba Mónica Vermeulen, Consejo Nacional de Investigaciones Científicas y Técnicas (CONICET) Laboratorio de Células Presentadoras de Antígeno y Respuesta Inflamatoria, Instituto de Biología y Medicina Experimental, Argentina
Copyright © 2021 Chen, Huang, Zhao, Chen, Lin and Shi. This is an open-access article distributed under the terms of the Creative Commons Attribution License (CC BY). The use, distribution or reproduction in other forums is permitted, provided the original author(s) and the copyright owner(s) are credited and that the original publication in this journal is cited, in accordance with accepted academic practice. No use, distribution or reproduction is permitted which does not comply with these terms.
*Correspondence: Qi-yang Shi, d3NxeTIxNEAxNjMuY29t; Shu Lin, c2h1bGluMTk1NkAxMjYuY29t