- 1Department of Gastroenterology, Qilu Hospital, Cheeloo College of Medicine, Shandong University, Jinan, China
- 2Laboratory of Translational Gastroenterology, Qilu Hospital, Cheeloo College of Medicine, Shandong University, Jinan, China
- 3Advanced Medical Research Institute, Shandong University, Jinan, China
- 4Robot Engineering Laboratory for Precise Diagnosis and Therapy of GI Tumor, Qilu Hospital, Cheeloo College of Medicine, Shandong University, Jinan, China
- 5Key Laboratory for Experimental Teratology of Ministry of Education, Shandong University, Jinan, China
Levels of type 2 cytokines are elevated in the blood and intestinal tissues of ulcerative colitis (UC) patients in the active phase; this phenomenon indicates the participation of type 2 immune response in UC progression. The beneficial effects of melatonin in dextran sodium sulfate (DSS) and 2,4,6-trinitrobenzene sulfonic acid (TNBS) colitis models have been illustrated, but its role in the oxazolone (Oxa)-induced colitis model (driven by type 2 immune response) remains relatively unknown. We investigated the relationship between melatonin concentration and the severity of UC, revealing a significantly negative correlation. Subsequently, we investigated the effects of melatonin in Oxa-induced colitis mice and the potential underlying mechanisms. Administration of melatonin significantly counteracted body weight loss, colon shortening, and neutrophil infiltration in Oxa-induced colitis mice. Melatonin treatment mitigated Oxa-induced colitis by suppressing type 2 immune response. In addition, melatonin attenuated intestinal permeability by enhancing the expression of ZO-1 and occludin in colitis mice. Interestingly, the protective effect of melatonin was abolished when the mice were co-housed, indicating that the regulation of gut microbiota by melatonin was critical in alleviating Oxa-induced colitis. Subsequently, 16S rRNA sequencing was performed to explore the microbiota composition. Decreased richness and diversity of intestinal microbiota at the operational taxonomic unit (OTU) level resulted from melatonin treatment. Melatonin also elevated the abundance of Bifidobacterium, a well-known probiotic, and reduced proportions of several harmful bacterial genera, such as Desulfovibrio, Peptococcaceae, and Lachnospiraceae. Fecal microbiota transplantation (FMT) was used to explore the role of microbiota in the function of melatonin in Oxa-induced colitis. Microbiota transplantation from melatonin-treated mice alleviated Oxa-induced colitis, suggesting that the microbiome participates in the relief of Oxa-induced colitis by melatonin. Our findings demonstrate that melatonin ameliorates Oxa-induced colitis in a microbiota-dependent manner, suggesting the therapeutic potential of melatonin in treating type 2 immunity-associated UC.
Introduction
Inflammatory bowel disease (IBD) is a chronic disease of the gastrointestinal (GI) tract that leads to frequent diarrhea, abdominal pain, weight loss, and impaired GI motility (1). IBD is classified into ulcerative colitis (UC) and Crohn’s disease (CD) based on clinical symptoms (2, 3). The former is the predominant pathological subtype of IBD (4). However, the critical factors for the initiation and development of UC remain elusive (5, 6). Multiple risk factors might contribute to the UC progression, including mutations in some genes such as Nod2 and Card9, abnormal activation of immune cells, and disorders of gut microflora (7–10). Concentrations of type 2 cytokines, such as IL-5 and IL-13, increase in the blood and intestinal tissues of UC patients in the active phase, indicating that type 2 immune response may contribute to UC progression (11, 12). In contrast, secretion of amphiregulin (Areg) by group 2 innate lymphoid cells (ILC2s) is indispensable for tissue repair in colitis, suggesting the complicated roles of type 2 immunity in different phases of UC (13). Oxazolone (Oxa) induces colitis in mice (involving type 2 cytokines), which recapitulates a similar immune response in the active stage of UC patients (14, 15).
Melatonin (N-acetyl-5-methoxytryptamine) is mainly secreted by the pineal gland and participates in several physiological processes, such as circadian rhythm management and immune modulation (16–18). In addition to the brain, the GI tract contains melatonin, whose concentration is higher in the GI tract than that in the circulation by 10 to 400 times (19). The secretion of melatonin in the GI tract is controlled by dietary rather than circadian rhythms (20–22). Melatonin in the GI tract is principally produced by enterochromaffin cells (EC), certain types of immune cells, and intestinal commensals (19, 23–25). The liver degrades virtually all of melatonin, suggesting that GI melatonin is confined to the gut–liver axis, instead of circulating into other organs, and that it functions locally (26).
There are a variety of therapeutic options for UC, including immunosuppressive hormones such as glucocorticoids or their analogs, and monoclonal antibodies targeting inflammatory molecules (4). However, adverse effects and low responsiveness limit the utility of these strategies. Therefore, safe and effective treatment of UC requires substantial further investigation. Melatonin affects the permeability, motility, and barrier function of the intestine as an anti-inflammatory agent and antioxidant (27–29). Melatonin ameliorates the inflammatory reaction and colonic injury in experimental colitis induced by both dextran sodium sulfate (DSS) and 2,4,6-trinitrobenzene sulfonic acid (TNBS) in mice and rats (28, 30–32). Clinically, melatonin treatment relieves abdominal pain and diarrhea (27). Notably, melatonin can also change the composition of the gut microbiota (33). In addition, melatonin increases the abundance of Firmicutes and Bacteroides and reduces the proportion of harmful bacteria in mice (34, 35). However, it remains to be explored whether melatonin affects the microbiota to influence the pathological consequences in UC.
In this study, we demonstrated a negative correlation between the melatonin concentration in colonic tissues and UC progression. We also determined that the levels of key enzymes for melatonin synthesis were diminished in UC patients. Melatonin relieved Oxa-induced colitis in mice, concomitantly suppressing ILC2s in a microbiota-dependent manner. Our findings provide new evidence for the beneficial role of melatonin in type 2 immunity-related colitis.
Materials and Methods
Study Patients
This study was approved by the Clinical Ethical Committee of Shandong University (ECSBMSSDU2020-1-035). Each participant signed a written informed consent form prior to participation. The basic patient information is shown in Table 1. Eleven patients with UC who underwent colonoscopy for disease surveillance were enrolled. The diagnosis was based on well-established clinical, endoscopic, and histopathological criteria. The healthy controls (HCs) included 17 healthy volunteers who underwent colonoscopy for physical examination. During the colonoscopy, inflamed intestinal mucosal biopsies of UC patients were obtained, as well as normal areas in HCs in the descending colon or sigmoid colon. Collected biopsies were placed in 4% formalin solution or stored at –80°C.
Quantification of Melatonin in the Human Colon
Total proteins from colonic biopsies were extracted and centrifuged at 12,000 rpm for 15 min at room temperature. Supernatants were collected, and melatonin concentration was assessed using a human melatonin ELISA kit (CUSABIO, CSB-E08132h) per the manufacturer’s instructions.
Fixation and Histology
The tissue was then fixed for 24 h at room temperature. Subsequently, a standard dehydration procedure was performed, followed by paraffin embedding. Intestinal tissue was sectioned into 4-µm thin sections for staining. The slides were stained with H&E and scored in a blinded manner, according to a previously published scoring system (36). Briefly, each section was scored based on the following criteria: inflammation, dysplasia, hyperplasia, edema, crypt degeneration, and epithelial damage. The total lesion scores reflect the sum of individual scores.
RNA Extraction, Reverse Transcription, and qPCR
Total RNA from colonic biopsies was isolated using RNA isolation reagent (Vazyme, R401-01), and the concentration was measured. Total RNA (1 μg) was reverse transcribed into cDNA using the HiScript III RT SuperMix for qPCR (+gDNA wiper) kit (Vazyme, R323-01). Subsequently, cDNA was amplified using the StepOnePlus™ Real-Time PCR System (Thermo Fisher; 4376600). The PCR mixture containing 10 μl of 2× AceQ®Universal SYBR qPCR Master Mix (Vazyme, Q511-02), 1 μl of cDNA, forward and reverse primers (0.8 μl each), and diethyl pyrocarbonate (DEPC) water (7.4 μl) was prepared. The primers used for qPCR are listed in Table 2. GAPDH was used as the internal standard to normalize target gene expression. For analysis, the 2−ΔΔCt method was used to calculate the mRNA levels of the target genes.
Mouse Colitis Model
All animal experiments were carried out in compliance with and approved by the Shandong University Specific Pathogen Free (SPF) animal center. All experimental animal procedures were approved by the Animal Care and Animal Experiments Committee of Shandong University (ECSBMSSDU2020-2-057). Animal studies were conducted in a gender- and age-matched manner. Male C57BL/6 mice aged 6–8 weeks were obtained from Nanjing GemPharmatech Animal Center and maintained in SPF facilities at Shandong University. For the induction of Oxa-induced colitis, mice were anesthetized with 3.5 μl/g of 2% sodium pentobarbital. Oxa (4-ethoxymethylene-2-phenyl-2-oxazolin-5-one) (Sigma Chemical Co., St. Louis, MO) was dissolved in a 1:1 H2O/ethanol mixture (50% ethanol). Then, 4.5 μl/g of 3% Oxa solution was administered via a 3.5-F catheter. The catheter was inserted 4 cm proximal to the anal verge, and the Oxa was injected. To ensure the distribution of Oxa within the entire colon, mice were held in a vertical position for 5 min after the injection. For melatonin treatment, mice were administered 50 mg/kg of melatonin (experimental group) or solvent (control group) daily (via gavage) for 1 week before induction of colitis according to published articles (37–39). Melatonin was purchased from MedChemExpress (CAS Number: 73-31-4) and dissolved in dimethyl sulfoxide (DMSO).
Residential Model of Mice
Separated mice were obtained from Nanjing GemPharmatech Animal Center, fed in separate cages, and handled separately. Co-housed mice were kept together in one cage for at least 7 days before melatonin treatment.
Antibiotic Treatment and Fecal Microbiota Transplantation
All the mice used in the fecal microbiota transplantation (FMT) study came from the same batch and were kept together at least 7 days before the experiment. During FMT, the housing environment, the same as before, was strictly controlled to ensure consistency. Mice were administered daily (via gavage) with 200 μl of autoclaved water supplemented with antibiotics (ampicillin 1 g/L, gentamicin 1 g/L, metronidazole 1 g/L, neomycin 1 g/L, and vancomycin 0.5 g/L). After 1 week, antibiotic treatment was stopped, and the mice were recolonized by FMT, receiving stools from melatonin-treated mice and controls. Mice received stools daily for 1 week by oral gavage before Oxa colitis was induced.
Western Blotting
Total proteins of colon tissue from Oxa-colitis mice were extracted. Proteins were separated by 10% sodium dodecyl sulfate–polyacrylamide gel electrophoresis and transferred onto a polyvinylidene fluoride membrane. The membrane was incubated with the primary antibody at 4°C overnight and then incubated with the secondary antibody for 60 min at room temperature. Immunoblots were detected using an enhanced chemiluminescent substrate (Millipore). Antibodies: anti-ZO-1 antibody (1:1,000; Thermo Fisher), anti-occludin antibody (1:1,000; Thermo Fisher), anti-GAPDH antibody (1:5000; ProteinTech, USA), anti-claudin-1 antibody (1:1,000; Thermo Fisher), anti-claudin-5 antibody (1:1,000; Abcam), and goat anti-rabbit IgG (1:5,000; Zhongshan Gold Bridge, Beijing, China).
Metagenomic Analysis of Microbiome
A pellet of mouse feces was collected after melatonin treatment and stored at –80°C until further use. 16S rRNA sequencing was performed by Shanghai Majorbio, and the data were analyzed on the Majorbio cloud platform. DNA was isolated using the E.Z.N.A.® soil kit (Omega Bio-Tek, Norcross, GA, USA), and V3–V4 was amplified by PCR. The primers were 338F (5′-ACTCCTACGGGAGGCAGCAG-3′) and 806R (5′-GGACTACHVGGGTWTCTAAT-3′). PCR products were then sequenced using the Illumina MiSeq platform (Illumina, San Diego, USA) and analyzed using UPARSE software (version 7.1).
Isolation of Lamina Propria Lymphocytes (LPLs) and Flow Cytometry
Isolation of intestinal lamina propria cells and flow cytometry were conducted as previously described (40). To isolate lymphocytes from the lamina propria, the intestinal segment (approximately 0.5 cm) was digested in a complete Roswell Park Memorial Institute (RPMI) medium containing DNase I (150 μg/ml, Sigma) and collagenase VIII (300 U/ml, Sigma) at 37°C in a 5% CO2 incubator for 1.5 h. The digested segments were ground and filtered through a 100-μm cell strainer. The cells were collected from the interphase of an 80% and 40% Percoll gradient after spinning at 2,500 rpm for 15 min at room temperature. The CD16/32 antibody (eBioscience) was used to block the Fc receptors before surface staining. Lymphocytes isolated from the intestinal lamina propria were stained with antibodies against the following markers: GATA3 (PB), RORγt (PE), IL-13 (Alexa Fluor 488), IL-5 (APC), IL-22 (APC), CD45.2 (AF700), CD127 (PE), KLRG1 (PerCP-Cy5.5), CD11b (APC-Cy7), and Ly6G (FITC). Lin comprised APC-Cy7 or APC-eFluor 780-CD3, CD5, CD19, B220, Ly6G, FcϵR1, CD11c, CD11b, Ter119, NK1.1, and CD16/CD32. For cytokine staining, cells were stimulated with 50 ng/ml of phorbol myristate acetate (PMA) and 500 ng/ml of ionomycin for 2 h, and Brefeldin A (2 µg/ml) was added 2 h before cells were harvested. Live and dead cells were identified using the Live and Dead Violet Viability Kit or Zombie Aqua Fixable Viability Kit (BioLegend).
Sorting, Culture, and Treatment of ILC2s In Vitro
ILC2s (Lin−KLRG1+CD127+) were sorted by flow cytometry (Beckman, Moflo Astrios EQ). Sorted ILC2s were cultured in IMDM medium containing 10% fetal bovine serum (FBS), 10 ng/ml of IL-2, 10 ng/ml of IL-7, 10 ng/ml of IL-25, and 10 ng/ml of IL-33. Purified ILC2s were treated with melatonin for 4 or 16 h, and cytokine production by ILC2s was assessed by flow cytometry.
Statistical Analysis
Unless otherwise noted, statistical analyses were performed using the unpaired two-tailed Student’s t-test with GraphPad Prism. Results are expressed as mean ± SD (*p < 0.05, **p < 0.01, ***p < 0.001, ****p < 0.0001).
Results
Melatonin in the Colon Reduces and Negatively Correlates With Disease Severity in Ulcerative Colitis Patients
To investigate the role of melatonin in UC, we measured the concentration of melatonin in the descending and sigmoid colon of UC patients and HCs. Compared with those in HCs, the levels of melatonin decreased drastically in UC patients (Figure 1A). H&E staining indicated that the pathological consequence of UC was more severe than that of HCs (Figure 1B). Notably, a negative association emerged between melatonin concentration and histologic severity in all 11 patients with UC and 4 random HCs (Figure 1C). In addition, the mRNA levels of AANAT and HIOMT, the key enzymes in the melatonin biosynthesis pathway, were significantly less in patients with UC than in HCs (Figures 1D, E). Taken together, these data suggest that the level of melatonin decreases in UC patients and correlates negatively with disease severity, indicating that melatonin may be involved in UC progression.
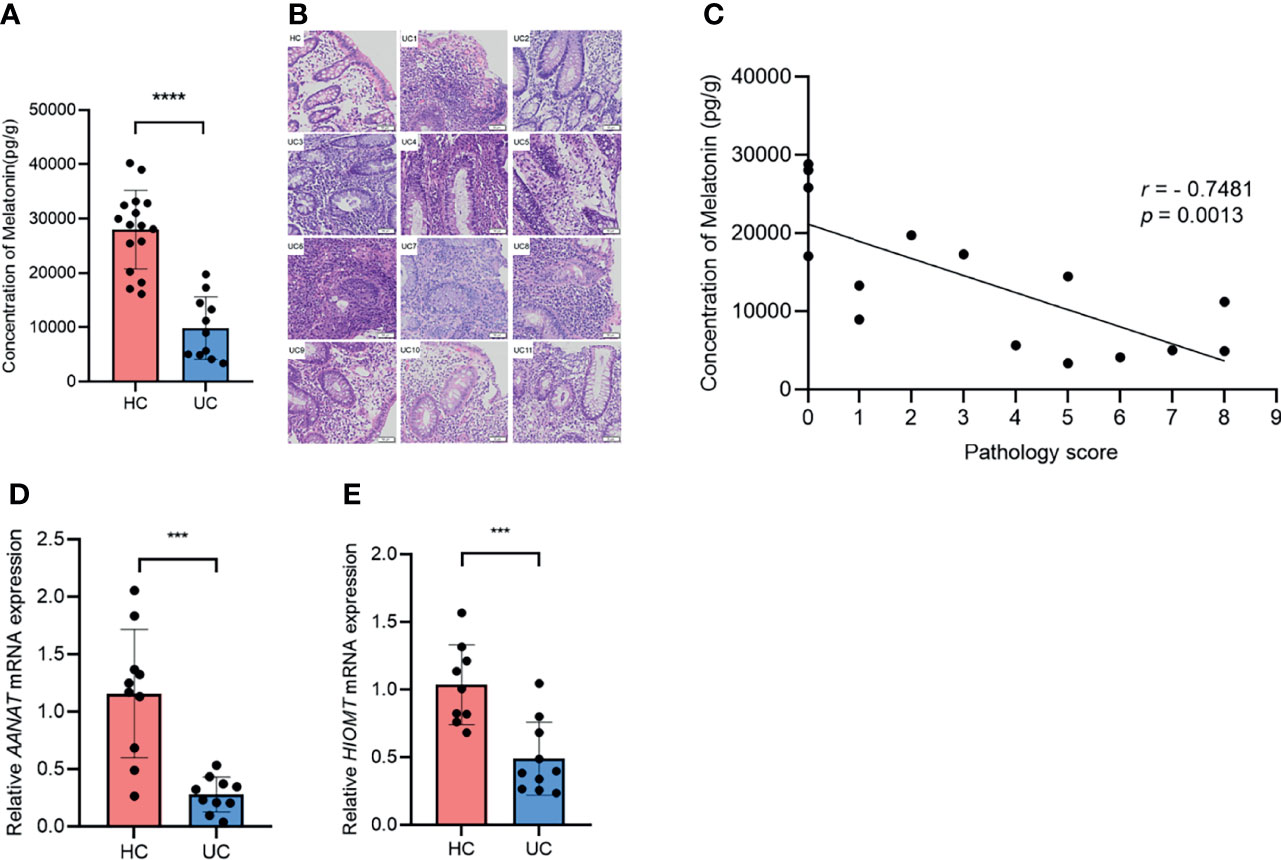
Figure 1 Melatonin in the colon decreases and negatively associates with disease severity in ulcerative colitis (UC) patients. (A) Melatonin concentrations in healthy control (HC) and UC patients were detected by ELISA. (B) Representative H&E staining of colonic sections (×20). (C) Correlation of melatonin concentration and histological severity scores of all 11 UC patients and 4 random HCs. (D, E) AANAT and HIOMT mRNA expression levels in HCs and UC patients (all values were normalized to the HC group). ***p < 0.001; ****p < 0.0001.
Melatonin Mitigates Oxazolone-Induced Colitis
Oxa-induced colitis, an experimental animal colitis model, is mediated by the type 2 immune response. The experimental model shows pathological similarity to UC in humans, particularly regarding the elevated levels of type 2 cytokines. To explore the effect of melatonin on colitis in vivo, Oxa was administered to mice by enema after anesthesia. Compared with HC, Oxa-induced colitis mice showed significant inflammation after enema (Supplementary Figures 1A–D). Melatonin treatment at a daily dose of 50 mg/kg for 7 days (MT50) markedly ameliorated Oxa-induced colitis in mice, in terms of less body weight loss and longer colon length (Figures 2A–D). H&E staining revealed that melatonin treatment prevented intestinal inflammation, and the pathology score of the colon in the MT50 group was lower than that in the MT0 group (Figures 2E–G). Next, we examined inflammatory cell infiltration by flow cytometry and the production of inflammatory cytokines by qPCR. Melatonin treatment resulted in a significant reduction of CD11b+Ly6G+ neutrophils compared with the control group (MT0), and a decreased production of inflammatory cytokines, such as Tnfa and Il-1β (Figures 2H–K). Accordingly, lower expression levels of tight junction-associated proteins (e.g., occludin and ZO-1) correlate with higher levels of inflammation and reflect worsened disease; these proteins were significantly upregulated in the MT50 group (Figures 2L–N). These results indicate that melatonin alleviates Oxa-induced colitis, as indicated by a decreased inflammatory response and elevated intestinal integrity.
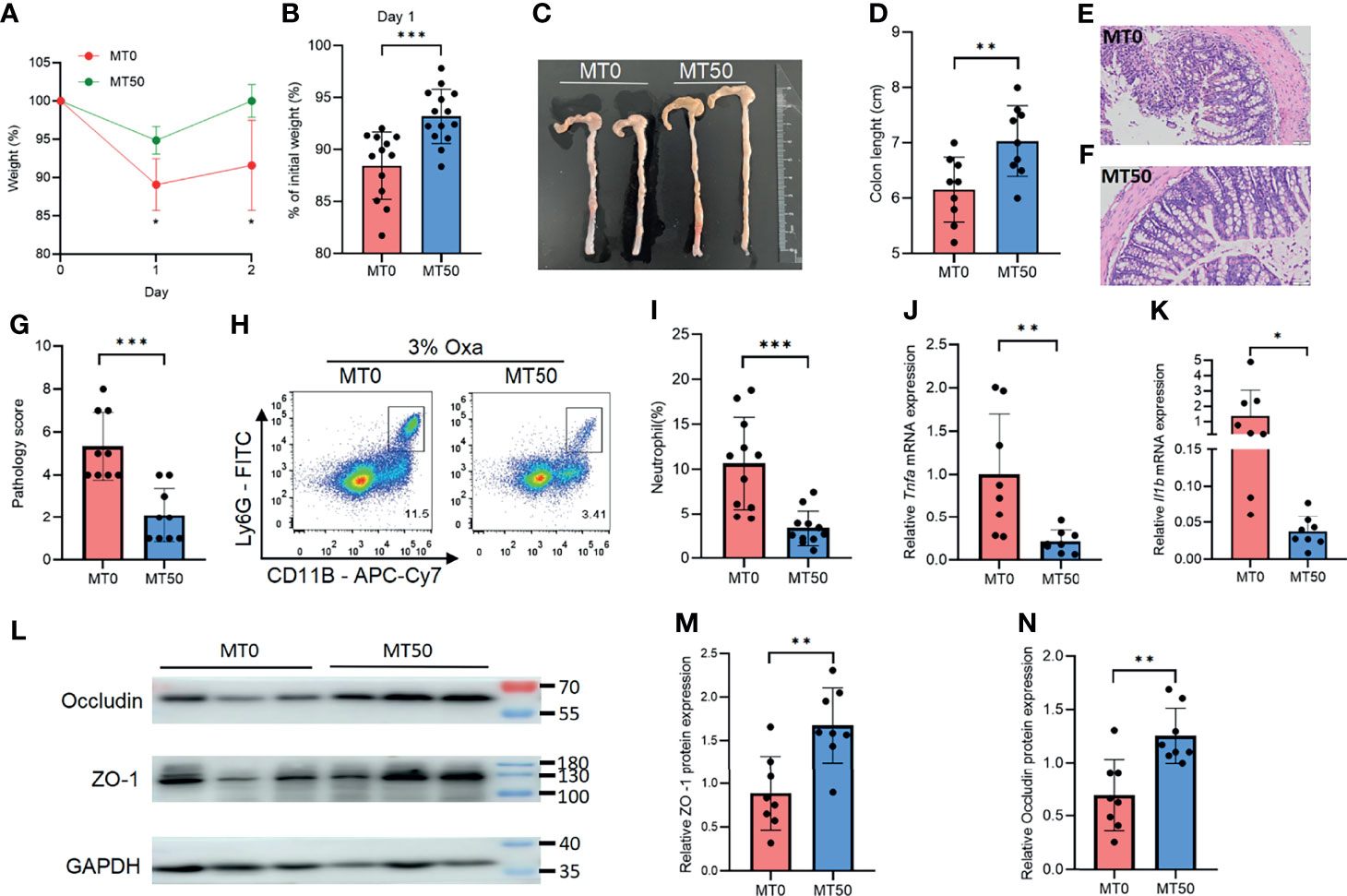
Figure 2 Melatonin mitigates oxazolone (Oxa)-induced colitis. (A) Body weight changes after 3% Oxa-treated (day 0). (B) Weight changes at day 1. (C, D) Colon length at day 1. (E–G) Representative H&E staining (×20) and pathological score of the inflamed gut epithelium. (H, I) Flow cytometry analysis of colonic CD45+CD11b+Ly6G+ neutrophil subsets in melatonin-treated and melatonin-untreated mice at day 1 after 3% oxazolone enema. (J, K) qPCR of Tnfa and Il-1b mRNA in colonic tissues in melatonin-treated and melatonin-untreated mice at day 1 after 3% oxazolone enema (all values were normalized to the melatonin-untreated group). (L) Immunoblot for ZO-1 and occludin in colonic tissue and (M, N) the densitometric analysis of ZO-1 and occludin immunoblot of melatonin-treated and melatonin-untreated mice. Data are representative of at least two independent experiments. Data are shown as mean ± SD. *p < 0.05; **p < 0.01; ***p < 0.001.
Melatonin Inhibits Type 2 Immunity in Oxazolone-Induced Colitis
Owing to Oxa-induced colitis being mediated by type 2 immunity, the effect of melatonin on the secretion of type 2 inflammatory cytokines was assessed, and melatonin treatment markedly suppressed the production of IL-5 and IL-13 in the colon of the MT50 group (Figures 3A, B and Supplementary Figure 2A). Additionally, the mRNA expression of IL-5 and IL-13 was also inhibited by melatonin (Figures 3C, D). Notably, IL-5 and IL-13 were mainly secreted by CD45+Lin−GATA3+ cells in the colon of Oxa-induced colitis (Supplementary Figure 2B), suggesting that ILC2s were the main producers of type 2 cytokines rather than Th2 cells. Melatonin significantly decreased the production of IL-5 and IL-13 in a dose- and time-dependent manner in both large and small intestinal ILC2s isolated from the lamina propria in vitro (Figures 3E, F and Supplementary Figures 2C–F). In contrast, ILC3s, another subset of ILC2s, were unaffected by melatonin (Supplementary Figures 2G, H). To eliminate the possibility that other compartments of LPLs mediate the effect of melatonin, the sorted ILC2s from the mouse large intestine were treated with melatonin, showing that the secretion of IL-5 and IL-13 by ILC2s was significantly suppressed by melatonin (Figures 3G, H). Collectively, these results suggest that melatonin directly inhibits ILC2s, which might be responsible for its role in improving Oxa-induced colitis.
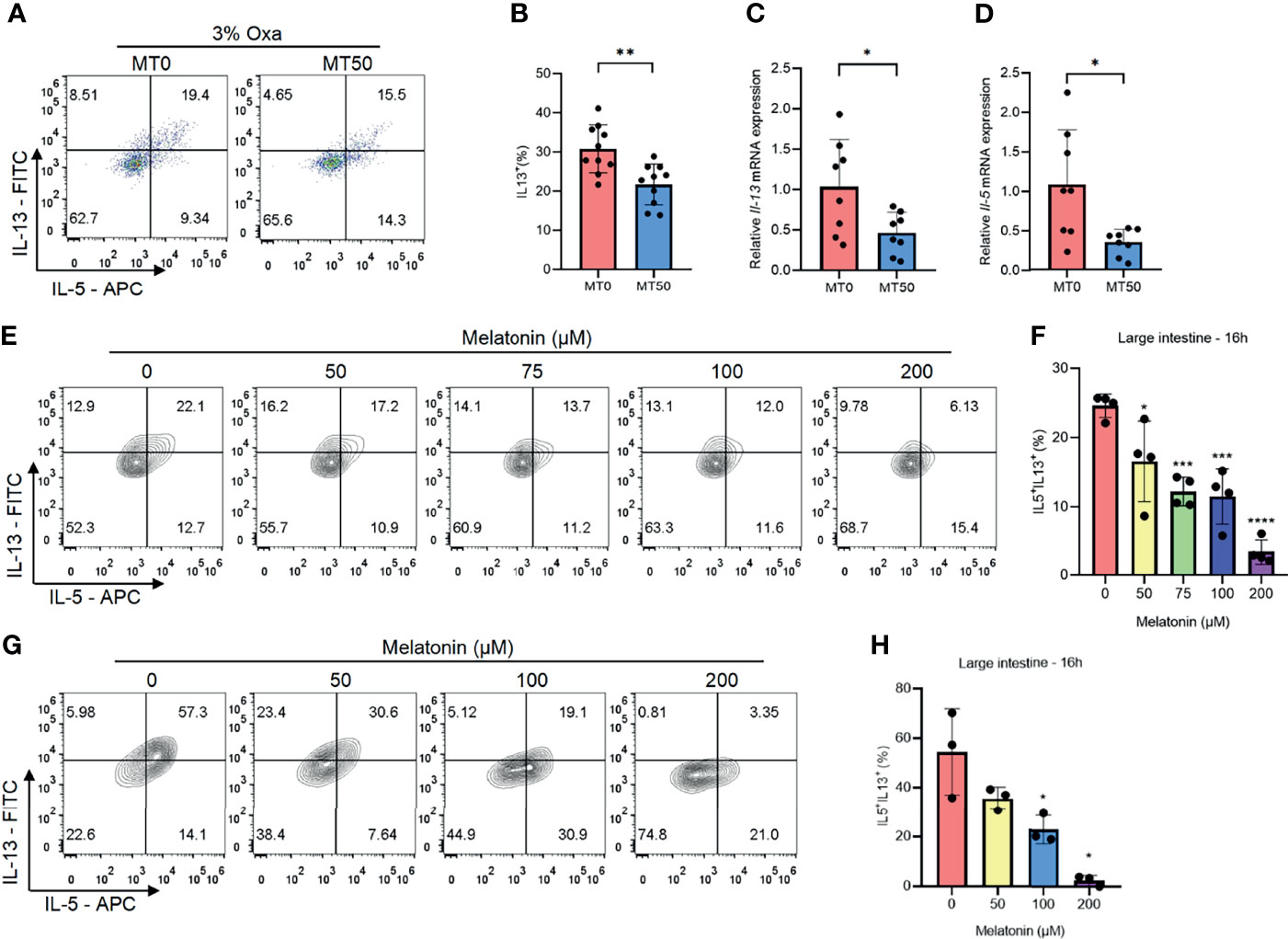
Figure 3 Melatonin inhibits type 2 immunity in oxazolone (Oxa)-induced colitis. (A, B) Flow cytometry analysis of IL-5 and IL-13 in colonic CD45+Lin−GATA3+ ILC2s and quantification of IL-13 in colonic ILC2s in melatonin-treated and melatonin-untreated mice at day 1 after 3% oxazolone enema. (C, D) qPCR of Il-5 and Il-13 mRNA of colonic tissue in melatonin-treated and melatonin-untreated mice at day 1 after 3% oxazolone enema (all values were normalized to the melatonin-untreated group). (E, F) Representative flow cytometry plots and quantification of large intestinal CD45+Lin−GATA3+ ILC2 function treated with melatonin for 16 h in LPLs in vitro. (G, H) Representative flow cytometry plots and quantification of purified large intestinal CD45+Lin−CD127+KLRG1+ ILC2 function treated with melatonin for 16 h in vitro. Data are representative of at least two independent experiments. Data are shown as mean ± SD. *p < 0.05; **p < 0.01; ***p < 0.001; ****p < 0.0001.
Effect of Melatonin on Oxazolone-Induced Colitis Depends on Gut Microbiota
Accumulating evidence indicates that there is a link between gut microbiota and intestinal inflammation in UC, in line with the crucial function of microbial colonization of the immune system. We noticed that the protective effect of melatonin on weight loss and colonic shortening in Oxa-induced colitis was abolished in co-housed mice, suggesting that the microbiome is the key target of melatonin in controlling gut inflammation (Figures 4A–C). There was no significant difference in the histological severity of colitis and neutrophil infiltration between the MT0 and MT50 groups from co-housed mice (Figures 4D–H). Accordingly, microbiota communication also abolished the effect of melatonin on the expression of inflammatory cytokines and tight junction-associated proteins (Figures 4I–M). Together, the regulation of gut microbiota by melatonin is critical for alleviating Oxa-induced colitis.
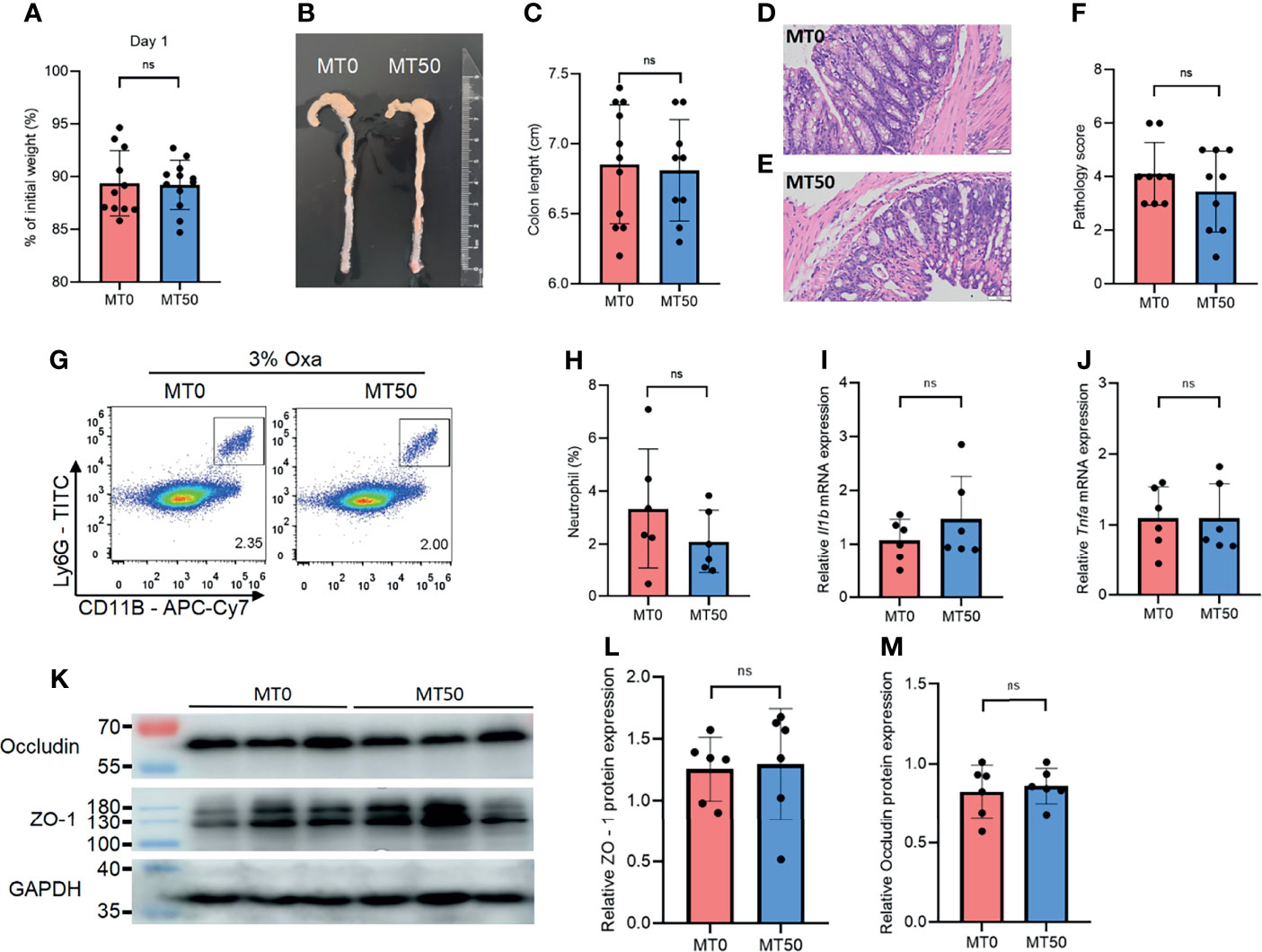
Figure 4 Effect of melatonin on oxazolone (Oxa)-induced colitis depends on gut microbiota. (A) Weight changes and colon length (B, C) in co-housed mice at day 1 after 3% oxazolone enema. (D–F) Representative H&E staining of colonic sections (×20) and pathological score of inflamed colonic tissue. (G, H) Flow cytometry analysis of colonic CD45+CD11b+Ly6G+ neutrophil subsets in co-housed mice at day 1 after 3% oxazolone enema. (I, J) qPCR of Tnfa and Il-1b mRNA in colonic tissues in co-housed mice at day 1 after 3% oxazolone enema (all values were normalized to the melatonin-untreated group). (K–M) Immunoblot and densitometric analysis of ZO-1 and occludin in co-housed mice. Data are representative of at least two independent experiments. Data are shown as mean ± SD. ns, no significance.
Melatonin Alters Microbiota Composition
Given that the effect of melatonin on colitis induced by Oxa is mediated by gut microbiota, we collected feces of separately housed or co-housed mice treated or untreated with melatonin, and 16S rRNA gene high-throughput pyrosequencing was performed. Principal coordinate analysis (PCoA) was used to assess the overall microbiome composition (beta diversity), which showed significant differences in clustering between vehicle- and melatonin-treated groups of separately housed mice, while there was no significant difference between co-housed mice treated or untreated with melatonin (Figures 5A–C). The richness and diversity of flora in melatonin-treated mice from separately housed mice were significantly lower than those in the melatonin-treated group according to the Ace, Chao, and Shannon indices of operational taxonomic unit (OTU) level (Figures 5D–F). There was no significant difference in the richness and diversity between melatonin-untreated separately housed mice and co-housed mice. The abundance of Verrucomicrobiota and Actinobacteria in the melatonin-treated separately housed group was greater than that in the other groups at the phylum level (Supplementary Figure 3A). At the genus level, the melatonin-treated group, compared with the melatonin-untreated group, showed significant suppression of proportions of Desulfovibrio, Lachnospiraceae, and Peptococcaceae and increased abundance of Bifidobacterium (Figures 6A–E and Supplementary Figure 3B). Additionally, the significant difference of the main genus between the melatonin-treated and melatonin-untreated groups is shown as a circos plot (Supplementary Figure 3C). Taken together, melatonin treatment causes significant changes in the intestinal microbiota, some of which are erased by co-housing.
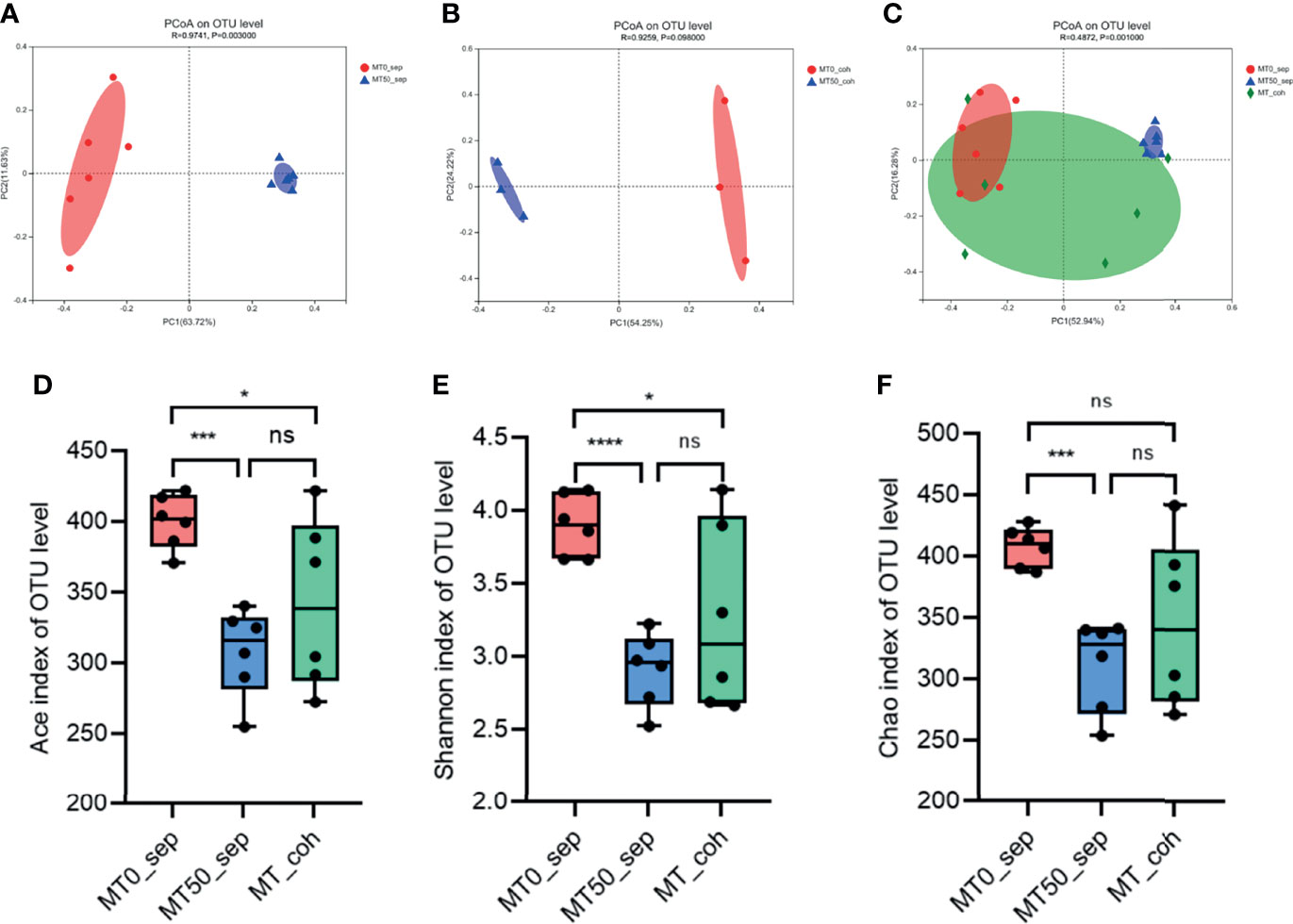
Figure 5 Melatonin alters microbiota composition in alpha and beta diversity. (A) Principal coordinate analysis (PCoA) of fecal microbiota in separately housed mice treated or untreated with melatonin for 7 days. (B) PCoA of fecal microbiota in co-housed mice treated or untreated with melatonin for 7 days. (C) PCoA of fecal microbiota among separately housed melatonin-treated mice, separately housed melatonin-untreated mice, and co-housed mice. PCoA is based on binary Jaccard distance. ANOSIM and 999 times permutation tests were utilized. (D–F) Shannon, Chao, and Ace indices of operational taxonomic unit (OTU) levels. Data are expressed as mean ± SD; statistical significance was determined by a two-sided Student’s t-test or one-way ANOVA. *p < 0.05; ***p < 0.001; ****p < 0.0001. ns, no significance.
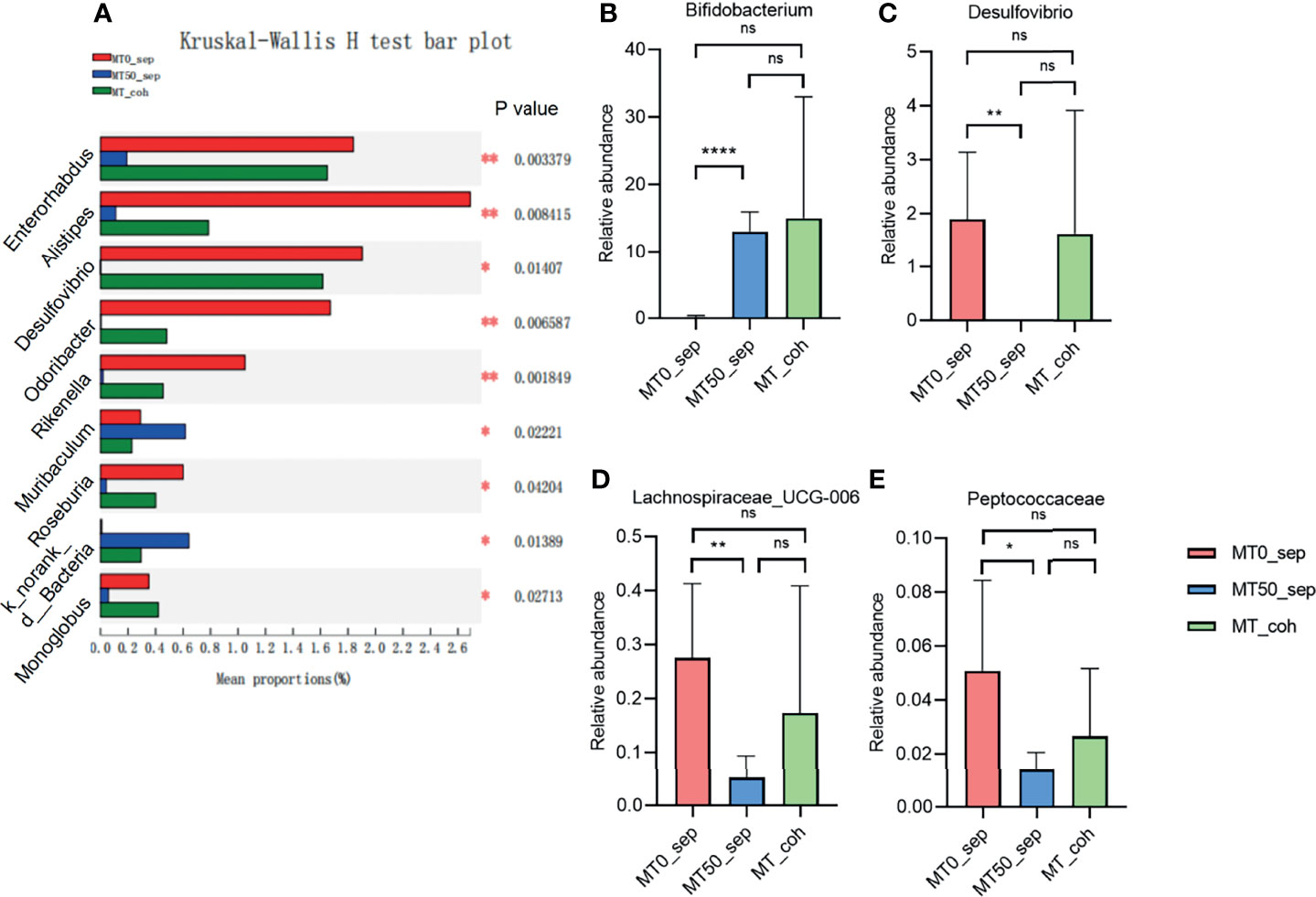
Figure 6 Melatonin regulates certain microbiota on genus level. (A) Kruskal–Wallis H test for differences between separated and co-housed mice for 7-day melatonin treatment on genus levels. (B–E) Relative abundance of Bifidobacterium, Desulfovibrio, Lachnospiraceae_UCG-006, and Peptococcaceae on genus levels. Data are expressed as mean ± SD. *p < 0.05; **p < 0.01; ****p < 0.0001. ns, no significance.
Microbiota Transplantation From Melatonin-Treated Mice Alleviates Oxazolone-Induced Colitis
As melatonin significantly changed the composition of the bacterial community, we sought to explore whether FMT from melatonin-treated mice could alleviate Oxa-induced colitis. Fecal samples collected from melatonin-treated or untreated mice were transplanted into antibiotic-treated mice, and Oxa was administered to the mice with fecal transplantation to induce colitis (Figure 7A). Antibiotic-treated mice receiving melatonin-treated feces showed marked relief of Oxa-induced colitis, including weight loss, colonic shortening, and histological severity (Figures 7B–G), which coincided with a decrease in the level of the inflammatory cytokine TNFα, but not in the level of IL-1β, in the colon (Figures 7H, I). Accordingly, the expression of tight junction-associated proteins, occludin and ZO-1, was markedly upregulated in mice transplanted with microbiota from melatonin-treated mice (Figures 7J–L), while there was no significant difference of type 2 inflammatory cytokines between the two groups (Figures 7M, N). These results indicate that melatonin alleviates Oxa-induced colitis by regulating microbiota.
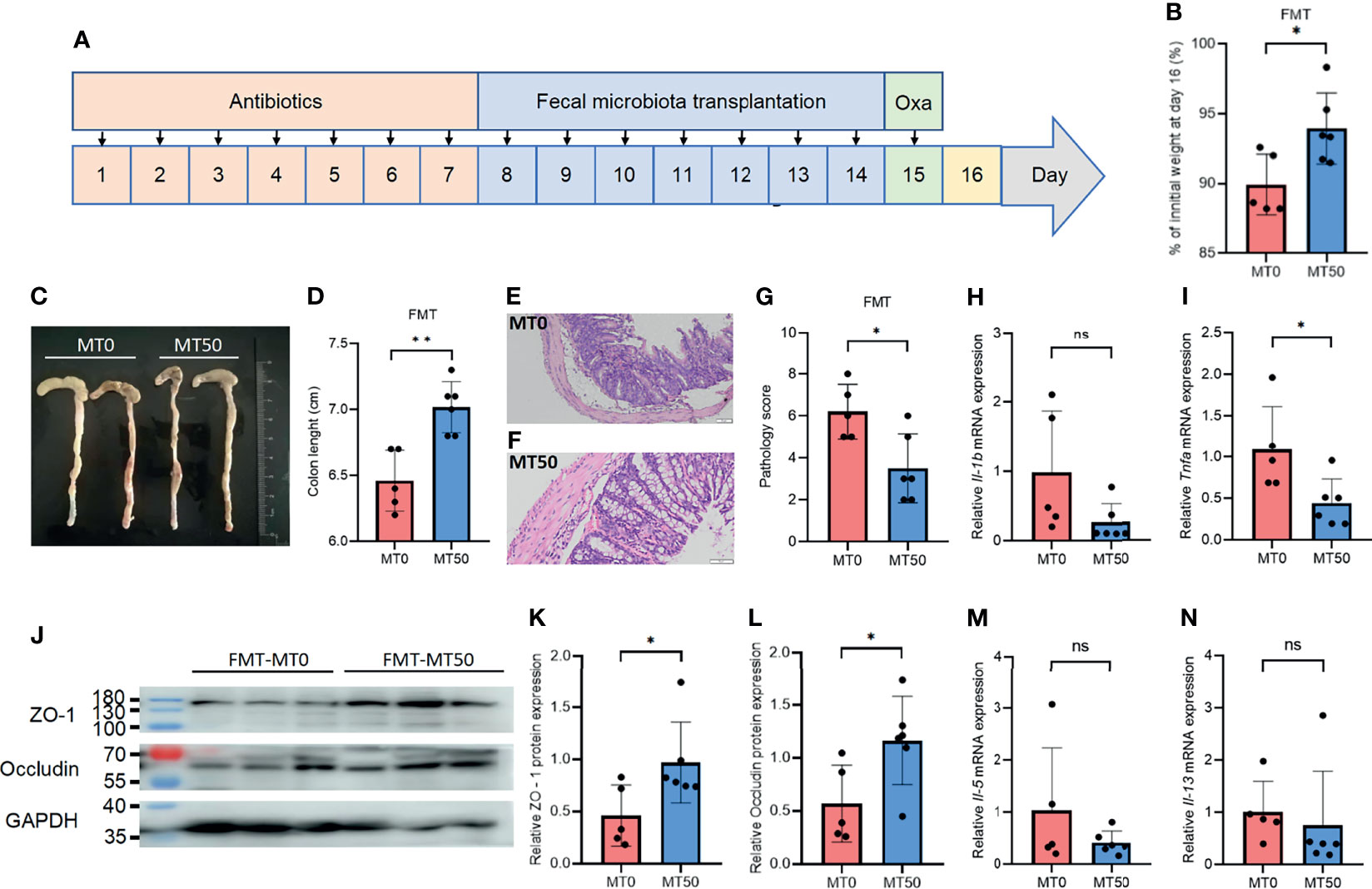
Figure 7 Microbiota transplantation from melatonin-treated mice alleviates oxazolone (Oxa)-induced colitis. (A) The process of fecal microbiota transplantation. The mice were treated with antibiotics for 7 days to clear the gut microbiota (day 1 to day 7) and transplanted feces from 7-day melatonin-treated or melatonin-untreated mice (day 8 to day 14) before 3% oxazolone enema (day 15). Mice were sacrificed on day 16. (B) Body weight changes in fecal microbiota transplantation (FMT) mice at day 16. (C, D) Colon length in FMT mice at day 16. (E–G) Representative H&E staining (×20) and pathological score of the inflamed gut epithelium. (H, I) qPCR of Tnfa and Il-1b mRNA in colonic tissues in FMT mice at day 16 after 3% oxazolone enema (all values were normalized to MT0 group). (J–L) Immunoblot and densitometric analyses of ZO-1 and occludin in FMT mice at day 16 after 3% oxazolone enema. (M, N) qPCR of Il-5 and Il-13 mRNA in colonic tissues in FMT mice at day 16 after 3% oxazolone enema (all values were normalized to MT0 group). Data are representative of two independent experiments. Data are shown as mean ± SD. *p < 0.05; **p < 0.01; ***p < 0.001. ns, no significance.
Discussion
UC is a common chronic GI disease with histopathological features of tissue damage, lymphocyte infiltration, epithelial destruction, and ulcer formation (41). UC is associated with recurrent episodes and is often associated with mental disorders such as anxiety and depression (42). Melatonin improves emotional states in patients; thus, it is often used as an auxiliary medication in the treatment of many diseases. No severe adverse effects are observed (18, 25, 43). The concentration of melatonin in venous blood is significantly reduced in patients with UC (44). Notably, urinary excretion of 6-sulfatoxymelatonin, a metabolite of melatonin in the liver, is greater in UC patients than in HC, suggesting an increase in intestinal melatonin level (44, 45). In this study, we used biopsy specimens from human descending or sigmoid colon to assess the concentration of melatonin by ELISA, which clarified the fact that melatonin levels in UC patients gradually decreased with the severity of inflammation, revealing a regulatory potential of melatonin during UC.
Melatonin is highly enriched in the digestive tract, especially in the intestine, where its concentration is greater than that in the circularity system by a factor of 10–400 (19). A major amount of intestinal melatonin is reported to be secreted by EC, which are neuroendocrine-immune cells that are critical for intestinal homeostasis (31, 46). EC is capable of sensing bacterial metabolites or neurotransmitters in the intestine and responds accordingly. Due to the drastic alteration of the intestinal microenvironment during UC, EC showed a significant reduction in sensory function, but enhanced secretion, such as 5-HT and antimicrobial peptides (47). Since EC is the major producer of melatonin in the gut, decreased melatonin levels in UC patients highlight the role of EC in the context of colitis, indicating that comprehensive and in-depth research on the function of EC remains to be investigated.
There are three main experimental animal models of colitis: the DSS, TNBS, and Oxa models (30, 48, 49). Melatonin relieves DSS colitis through epithelial components, such as goblet cells or stem cells, and affects the intestinal microbiota to improve oxidative stress in DSS colitis (50, 51). In addition, melatonin attenuates TNBS-induced colitis through a melatonin receptor-independent pathway in mice and relieves TNBS colitis through the TLR4/MyD88/NF-κB signaling pathway in rats (30). Nevertheless, the effect of melatonin on type 2 immunity-dependent colitis needs to be documented, as levels of type 2 cytokines, including IL-5 and IL-13, increase in UC patients (11, 12). Oxa-induced colitis is a type 2 immunity-based disease with increased expression levels of IL-4, IL-5, and IL-13 (14). Our data show that melatonin significantly mitigates Oxa-induced colitis, providing new evidence on the beneficial function of melatonin in the field of experimental colitis. Because of the rapid progression of Oxa-induced colitis and a longer melatonin duration, only pretreatment with melatonin was used in vivo during this report, which was a limitation of this study.
Melatonin regulates a variety of immune cells, such as T helper cells, Treg cells, B cells, macrophages, and mast cells, but it remains unclear whether melatonin affects ILCs (25). Our results reveal that ILC2-mediated type 2 immunity functions as a pro-inflammatory cell compartment in Oxa-induced colitis, and melatonin inhibits ILC2s in the disease. Intriguingly, melatonin inhibits ILC2s but not ILC3s, indicating a specific regulation of ILC2s by melatonin. Melatonin acts on target cells via three types of receptors located in the cell membrane or nucleus, including the classical membrane receptor MT1/MT2, the nuclear receptor superfamily RZR/RORα, and quinone reductase 2 (Nqo2, also called MT3) (25, 52, 53). The expression of melatonin receptors MT1 and MT2 in ILC2s is relatively small, and RZR/RORα is a positive regulator of ILC2s (13, 54). Therefore, MT3 might be responsible for the regulation of ILC2s by melatonin. The mechanism by which melatonin controls ILC2s requires further investigation. Notably, co-housing of mice abolished the relief of Oxa-induced colitis by melatonin, indicating that the microbiota overwhelms other parts in mediating the beneficial effects of melatonin.
The effects of melatonin on the microbiota have been studied in different contexts. The abundance of Akkermansia muciniphila increased and the abundance of Bacteroides massiliensis decreased in stressed mice after melatonin administration (55). Oral melatonin supplementation alleviated lipid accumulation and reversed gut microbiota dysbiosis in high-fat diet-fed mice, along with an altered abundance of Bacteroides and Alistipes (56). Melatonin controls the gut microbiota of mice via Reg3β (57). Notably, different methods (gavage or intraperitoneal injection) and various doses of melatonin lead to different changes in the gut flora. In this study, we observed that melatonin reduced the amount of certain harmful bacterial genera such as Desulfovibrio, Peptococcaceae, and Lachnospiraceae and increased the abundance of beneficial genera such as Bifidobacterium. In accordance, Bifidobacterium, a well-known probiotic, colonizes the intestinal mucus layer and can modulate mucus production by goblet cells. It adheres to intestinal mucus and secretes metabolites that upregulate the major mucin MUC2 to maintain intestinal integrity (58). As an over-the-counter (OTC) medicine, Bifidobacterium improves UC clinically and pathologically (59, 60). This study found that melatonin was able to stimulate Bifidobacterium in Oxa-induced colitis, which may be an important pathway on its anti-colitic effect. In contrast, Desulfovibrio and Peptococcaceae are opportunistic pathogens, which are positively related to gut inflammation (59). The severity of colitis can be mitigated by reducing them (60, 61), which provides us another potential mechanism for the treatment of colitis by melatonin. Additionally, Lachnospiraceae_UCG-006 is related to short-chain fatty acid metabolism, which may participate in the process of colitis and liver inflammation (62, 63). Accordingly, the transplantation of feces from melatonin-treated mice mitigates Oxa-induced colitis, validating that melatonin alleviates type 2 immunity-related colitis in a microbiota-dependent manner.
In conclusion, our study demonstrates that melatonin mitigates type 2 immune response-induced colitis and uncovers the indispensable role of microbiota in this process. Further studies are needed to elucidate how melatonin shapes gut microflora and controls ILC2s mechanistically, as well as how to use melatonin against UC clinically. The identification of melatonin-regulated commensals could provide new therapeutic strategies for the treatment of UC.
Conclusion
Our findings demonstrate that melatonin ameliorates Oxa-induced colitis in a microbiota-dependent manner, suggesting the therapeutic potential of melatonin in UC by targeting type 2 immune response.
Data Availability Statement
The datasets presented in this study can be found in online repositories. The names of the repository/repositories and accession number(s) can be found below: https://www.ncbi.nlm.nih.gov/, PRJNA752219.
Ethics Statement
The studies involving human participants were reviewed and approved by the Clinical Ethical Committee of Shandong University (ECSBMSSDU2020-1-035). The patients/participants provided their written informed consent to participate in this study.
Author Contributions
Z-xZ, S-yL, and Y-qL designed and performed the experiments, analyzed the data, and wrote the paper. Y-yC wrote the paper. XY, G-jK, and JL performed the experiments and analyzed the results. M-qZ, JS, Y-jZ, R-cZ, and B-yJ contributed to the concept and technical support. B-cF and X-lZ supervised the project. All authors participated in revising the manuscript.
Funding
This study was supported by the National Key R&D Program of China (2020YFA0804400), the National Natural Science Foundation of China (81873550, 82070552, 81800462, and 82071854), and the Youth Interdiscipline Innovative Research Group of Shandong University (2020QNQT009). This study is also supported by the Taishan Scholars Program of Shandong Province and the National Clinical Research Center for Digestive Diseases supporting technology project (2015BAI13B07).
Conflict of Interest
The authors declare that the research was conducted in the absence of any commercial or financial relationships that could be construed as a potential conflict of interest.
Publisher’s Note
All claims expressed in this article are solely those of the authors and do not necessarily represent those of their affiliated organizations, or those of the publisher, the editors and the reviewers. Any product that may be evaluated in this article, or claim that may be made by its manufacturer, is not guaranteed or endorsed by the publisher.
Acknowledgments
The authors thank the technical assistance from the FACS facility of Advanced Medical Research Institute of Shandong University. They would like to thank Editage (www.editage.cn) for the English-language editing.
Supplementary Material
The Supplementary Material for this article can be found online at: https://www.frontiersin.org/articles/10.3389/fimmu.2021.783806/full#supplementary-material
Supplementary Figure 1 | (Related to Figure 2) Inflammation presents in oxazolone-induced colitis mice compared with healthy mice. (A, B) Representative HE staining (10×) and pathological score of healthy and inflamed gut epithelium. (C, D) qPCR of Tnfa and Il-1b mRNA in colonic tissues in healthy controls and Oxa-induced colitis (all values were normalized to healthy controls).
Supplementary Figure 2 | (Related toto Figure 3) Melatonin inhibits ILC2s but not ILC3s in LPLs in vitro. (A) Flow cytometry analysis of IL-5 and IL-13 in colonic CD45+Lin—GATA3+ ILC2s (secrete IL-5 and IL-13 mainly) and CD45+Lin—GATA3— ILC1s & ILC3s (IL-5 and IL-13 are not secreted) in Oxa-induced colitis. That indicated the right gating in our gating strategy. (B) Representative flow cytometry analysis of IL-13 secretion at day 1 after 3% Oxa-treated. (C, D) Representative flow cytometry plots and quantification of large intestinal and (E, F) Small intestinal CD45+Lin—GATA3+ ILC2s function treated by melatonin for 4 hours in LPLs in vitro. (G, H) Quantification of small and large intestinal CD45+Lin—RORγt+ ILC3s function treated by melatonin for 16 hours in LPLs in vitro. Data are representative of at least two independent experiments. Data are shown as mean ± SD. * p < 0.05; ** p < 0.01; *** p < 0.001.
Supplementary Figure 3 | (Related to Figure 5) Melatonin alters microbiota composition. (A) Community bar plot analysis of fecal microbiota from separated and co-housed mice for 7-day-melatonin-treatment on Phylum levels. (B) Heatmap of different genus between separated and co-housed mice for 7-day-melatonin-treatment. (C) Contribution of fecal microbiota from separated and co-housed mice for 7-day-melatonin-treatment on genus levels in Circos plot.
References
1. Knights D, Lassen KG, Xavier RJ. Advances in Inflammatory Bowel Disease Pathogenesis: Linking Host Genetics and the Microbiome. Gut (2013) 62:1505–10. doi: 10.1136/gutjnl-2012-303954
2. Pascal V, Marta P, Natalia B, Francesc C, David C, Alba S, et al. A Microbial Signature for Crohn’s Disease. Gut (2017) 66:813–22. doi: 10.1136/gutjnl-2016-313235
3. Lee T, Thomas C, Kirill S, Annemarie S, Ilias L, Alesia W, et al. Oral Versus Intravenous Iron Replacement Therapy Distinctly Alters the Gut Microbiota and Metabolome in Patients With IBD. Gut (2017) 66:863–71. doi: 10.1136/gutjnl-2015-309940
4. Lamb CA, Kennedy NA, Raine T, Hendy PA, Smith PJ, Limdi JK, et al. British Society of Gastroenterology Consensus Guidelines on the Management of Inflammatory Bowel Disease in Adults. Gut (2019) 68:s1–s106. doi: 10.1136/gutjnl-2019-318484
5. Ananthakrishnan AN. Epidemiology and Risk Factors for IBD. Nat Rev Gastroenterol Hepatol (2015) 12:205–17. doi: 10.1038/nrgastro.2015.34
6. Mak WY, Zhao M, Ng SC, Burisch J. The Epidemiology of Inflammatory Bowel Disease: East Meets West. J Gastroenterol Hepatol (2020) 35:380–9. doi: 10.1111/jgh.14872
7. Larabi A, Barnich N, Nguyen HTT. New Insights Into the Interplay Between Autophagy, Gut Microbiota and Inflammatory Responses in IBD. Autophagy (2020) 16:38–51. doi: 10.1080/15548627.2019.1635384
8. Yamamoto-Furusho JK, Fonseca-Camarillo G, Furuzawa-Carballeda J, Sarmiento-Aguilar A, Barreto-Zuñiga R, Martínez-Benitez B, et al. Caspase Recruitment Domain (CARD) Family (CARD9, CARD10, CARD11, CARD14 and CARD15) are Increased During Active Inflammation in Patients With Inflammatory Bowel Disease. J Inflammation (Lond) (2018) 15:13. doi: 10.1186/s12950-018-0189-4
9. Mitsialis V, Wall S, Liu P, Ordovas-Montanes J, Parmet T, Vukovic M, et al. Single-Cell Analyses of Colon and Blood Reveal Distinct Immune Cell Signatures of Ulcerative Colitis and Crohn’s Disease. Gastroenterology (2020) 159:591–608 e510. doi: 10.1053/j.gastro.2020.04.074
10. Geremia A, Biancheri P, Allan P, Corazza GR, Di Sabatino A. Innate and Adaptive Immunity in Inflammatory Bowel Disease. Autoimmun Rev (2014) 13:3–10. doi: 10.1016/j.autrev.2013.06.004
11. Butera A, Di Paola M, Vitali F, De Nitto D, Covotta F, Borrini F, et al. IL-13 Mrna Tissue Content Identifies Two Subsets of Adult Ulcerative Colitis Patients With Different Clinical and Mucosa-Associated Microbiota Profiles. J Crohns Colitis (2020) 14:369–80. doi: 10.1093/ecco-jcc/jjz154
12. Heller F, Florian P, Bojarski C, Richter J, Christ M, Hillenbrand B, et al. Interleukin-13 is the Key Effector Th2 Cytokine in Ulcerative Colitis That Affects Epithelial Tight Junctions, Apoptosis, and Cell Restitution. Gastroenterology (2005) 129:550–64. doi: 10.1016/j.gastro.2005.05.002
13. Vivier E, Artis D, Colonna M, Diefenbach A, Di Santo JP, Eberl G, et al. Innate Lymphoid Cells: 10 Years on. Cell (2018) 174:1054–66. doi: 10.1016/j.cell.2018.07.017
14. Heller F, Fuss IJ, Nieuwenhuis EE, Blumberg RS, Strober W. Oxazolone Colitis, a Th2 Colitis Model Resembling Ulcerative Colitis, is Mediated by IL-13-Producing NK-T Cells. Immunity (2002) 17:629–38. doi: 10.1016/s1074-7613(02)00453-3
15. Zhang L, Cao N, Wang Y, Wang Y, Wu C, Cheng X, et al. Improvement of Oxazolone-Induced Ulcerative Colitis in Rats Using Andrographolide. Molecules (2019) 25(1):76. doi: 10.3390/molecules25010076
16. Cajochen C, Krauchi K, Wirz-Justice A. Role of Melatonin in the Regulation of Human Circadian Rhythms and Sleep. J Neuroendocrinol (2003) 15:432–7. doi: 10.1046/j.1365-2826.2003.00989.x
17. Tordjman S, Chokron S, Delorme R, Charrier A, Bellissant E, Jaafari N, et al. Melatonin: Pharmacology, Functions and Therapeutic Benefits. Curr Neuropharmacol (2017) 15:434–43. doi: 10.2174/1570159X14666161228122115
18. Cipolla-Neto J, Amaral FGD. Melatonin as a Hormone: New Physiological and Clinical Insights. Endocr Rev (2018) 39:990–1028. doi: 10.1210/er.2018-00084
19. Acuna-Castroviejo D, Escames G, Venegas C, Díaz-Casado ME, Lima-Cabello E, López LC, et al. Extrapineal Melatonin: Sources, Regulation, and Potential Functions. Cell Mol Life Sci (2014) 71:2997–3025. doi: 10.1007/s00018-014-1579-2
20. Challet E. The Circadian Regulation of Food Intake. Nat Rev Endocrinol (2019) 15:393–405. doi: 10.1038/s41574-019-0210-x
21. Kvetnoy IM, Ingel IE, Kvetnaia TV, Malinovskaya NK, Rapoport SI, Raikhlin NT, et al. Gastrointestinal Melatonin: Cellular Identification and Biological Role. Neuro Endocrinol Lett (2002) 23:121–32.
22. Bubenik GA. Localization, Physiological Significance and Possible Clinical Implication of Gastrointestinal Melatonin. Biol Signals Recept (2001) 10:350–66. doi: 10.1159/000046903
23. Raikhlin NT, Kvetnoy IM, Tolkachev VN. Melatonin may be Synthesised in Enterochromaffin Cells. Nature (1975) 255:344–5. doi: 10.1038/255344a0
24. Gao J, Xu K, Liu H, Liu G, Bai M, Peng C, et al. Impact of the Gut Microbiota on Intestinal Immunity Mediated by Tryptophan Metabolism. Front Cell Infect Microbiol (2018) 8:13. doi: 10.3389/fcimb.2018.00013
25. Ma N, Zhang J, Reiter RJ, Ma X. Melatonin Mediates Mucosal Immune Cells, Microbial Metabolism, and Rhythm Crosstalk: A Therapeutic Target to Reduce Intestinal Inflammation. Med Res Rev (2020) 40:606–32. doi: 10.1002/med.21628
26. Munoz-Perez JL, López-Patiño MA, Álvarez-Otero R, Gesto M, Soengas JL, Míguez JM. Characterization of Melatonin Synthesis in the Gastrointestinal Tract of Rainbow Trout (Oncorhynchus Mykiss): Distribution, Relation With Serotonin, Daily Rhythms and Photoperiod Regulation. J Comp Physiol B (2016) 186:471–84. doi: 10.1007/s00360-016-0966-4
27. Mannino G, Caradonna F, Cruciata I, Lauria A, Perrone A, Gentile C. Melatonin Reduces Inflammatory Response in Human Intestinal Epithelial Cells Stimulated by Interleukin-1beta. J Pineal Res (2019) 67:e12598. doi: 10.1111/jpi.12598
28. Li JH, Yu JP, Yu HG, Xu XM, Yu LL, Liu J, et al. Melatonin Reduces Inflammatory Injury Through Inhibiting NF-Kappab Activation in Rats With Colitis. Mediators Inflammation (2005) 2005:185–93. doi: 10.1155/MI.2005.185
29. Tan DX, Manchester LC, Esteban-Zubero E, Zhou Z, Reiter RJ. Melatonin as a Potent and Inducible Endogenous Antioxidant: Synthesis and Metabolism. Molecules (2015) 20:18886–906. doi: 10.3390/molecules201018886
30. Chamanara M, Rashidian A, Mehr SE, Dehpour AR, Shirkohi R, Akbarian R, et al. Melatonin Ameliorates TNBS-Induced Colitis in Rats Through the Melatonin Receptors: Involvement of TLR4/Myd88/NF-Kappab Signalling Pathway. Inflammopharmacology (2019) 27:361–71. doi: 10.1007/s10787-018-0523-8
31. Esteban-Zubero E, López-Pingarrón L, Alatorre-Jiménez MA, Ochoa-Moneo P, Buisac-Ramón C, Rivas-Jiménez M, et al. Melatonin’s Role as a Co-Adjuvant Treatment in Colonic Diseases: A Review. Life Sci (2017) 170:72–81. doi: 10.1016/j.lfs.2016.11.031
32. Gao T, Wang T, Wang Z, Cao J, Dong Y, Chen Y. Melatonin-Mediated MT2 Attenuates Colitis Induced by Dextran Sodium Sulfate via PI3K/AKT/Nrf2/SIRT1/Roralpha/NF-Kappab Signaling Pathways. Int Immunopharmacol (2021) 96:107779. doi: 10.1016/j.intimp.2021.107779
33. Park YS, Kim SH, Park JW, Kho Y, Seok PR, Shin JH, et al. Melatonin in the Colon Modulates Intestinal Microbiota in Response to Stress and Sleep Deprivation. Intest Res (2020) 18:325–36. doi: 10.5217/ir.2019.00093
34. Bai YF, Wang SW, Wang XX, Weng YY, Fan XY, Sheng H, et al. The Flavonoid-Rich Quzhou Fructus Aurantii Extract Modulates Gut Microbiota and Prevents Obesity in High-Fat Diet-Fed Mice. Nutr Diabetes (2019) 9:30. doi: 10.1038/s41387-019-0097-6
35. Ghareghani M, Reiter RJ, Zibara K, Farhadi N. Latitude, Vitamin D, Melatonin, and Gut Microbiota Act in Concert to Initiate Multiple Sclerosis: A New Mechanistic Pathway. Front Immunol (2018) 9:2484. doi: 10.3389/fimmu.2018.02484
36. Dieleman LA, Palmen MJ, Akol H, Bloemena E, Peña AS, Meuwissen SG, et al. Chronic Experimental Colitis Induced by Dextran Sulphate Sodium (DSS) is Characterized by Th1 and Th2 Cytokines. Clin Exp Immunol (1998) 114:385–91. doi: 10.1046/j.1365-2249.1998.00728.x
37. Galley HF, McCormick B, Wilson KL, Lowes DA, Colvin L, Torsney C. Melatonin Limits Paclitaxel-Induced Mitochondrial Dysfunction in Vitro and Protects Against Paclitaxel-Induced Neuropathic Pain in the Rat. J Pineal Res (2017) 63(4):e12444. doi: 10.1111/jpi.12444
38. Laothong U, Pinlaor P, Boonsiri P, Pairojkul C, Priprem A, Johns NP, et al. Melatonin Inhibits Cholangiocarcinoma and Reduces Liver Injury in Opisthorchis Viverrini-Infected and N-Nitrosodimethylamine-Treated Hamsters. J Pineal Res (2013) 55:257–66. doi: 10.1111/jpi.12068
39. Guo Y, Chen H, Wang QJ, Qi X, Li Q, Fu W, et al. Prolonged Melatonin Treatment Promote Testicular Recovery by Enhancing RAC1-Mediated Apoptotic Cell Clearance and Cell Junction-Dependent Spermatogensis After Heat Stress. Theriogenology (2021) 162:22–31. doi: 10.1016/j.theriogenology.2020.12.015
40. Li S, Bostick JW, Ye J, Qiu J, Zhang B, Urban JF Jr, et al. Aryl Hydrocarbon Receptor Signaling Cell Intrinsically Inhibits Intestinal Group 2 Innate Lymphoid Cell Function. Immunity (2018) 49:915–928 e915. doi: 10.1016/j.immuni.2018.09.015
41. Ordás I, Eckmann L, Talamini M, Baumgart DC, Sandborn WJ. Ulcerative Colitis. Lancet (2012) 380:1606–19. doi: 10.1016/s0140-6736(12)60150-0
42. Neuendorf R, Harding A, Stello N, Hanes D, Wahbeh H. Depression and Anxiety in Patients With Inflammatory Bowel Disease: A Systematic Review. J Psychosom Res (2016) 87:70–80. doi: 10.1016/j.jpsychores.2016.06.001
43. Garcia IG, Rodriguez-Rubio M, Mariblanca AR, de Soto LM, García LD, Villatoro JM, et al. A Randomized Multicenter Clinical Trial to Evaluate the Efficacy of Melatonin in the Prophylaxis of SARS-Cov-2 Infection in High-Risk Contacts (Mecovid Trial): A Structured Summary of a Study Protocol for a Randomised Controlled Trial. Trials (2020) 21:466. doi: 10.1186/s13063-020-04436-6
44. Chojnacki C, Wiśniewska-Jarosińska M, Kulig G, Majsterek I, Reiter RJ, Chojnacki J. Evaluation of Enterochromaffin Cells and Melatonin Secretion Exponents in Ulcerative Colitis. World J Gastroenterol (2013) 19:3602–7. doi: 10.3748/wjg.v19.i23.3602
45. Chen M, Mei Q, Xu J, Lu C, Fang H, Liu X. Detection of Melatonin and Homocysteine Simultaneously in Ulcerative Colitis. Clin Chim Acta (2012) 413:30–3. doi: 10.1016/j.cca.2011.06.036
46. Konturek SJ, Konturek PC, Brzozowska I, Pawlik M, Sliwowski Z, Cześnikiewicz-Guzik M, et al. Localization and Biological Activities of Melatonin in Intact and Diseased Gastrointestinal Tract (GIT). J Physiol Pharmacol (2007) 58:381–405.
47. Qin HY, Xavier Wong HL, Zang KH, Li X, Bian ZX. Enterochromaffin Cell Hyperplasia in the Gut: Factors, Mechanism and Therapeutic Clues. Life Sci (2019) 239:116886. doi: 10.1016/j.lfs.2019.116886
48. MacEachern SJ, Keenan CM, Papakonstantinou E, Sharkey KA, Patel BA. Alterations in Melatonin and 5-HT Signalling in the Colonic Mucosa of Mice With Dextran-Sodium Sulfate-Induced Colitis. Br J Pharmacol (2018) 175:1535–47. doi: 10.1111/bph.14163
49. Terry PD, Villinger F, Bubenik GA, Sitaraman SV. Melatonin and Ulcerative Colitis: Evidence, Biological Mechanisms, and Future Research. Inflammation Bowel Dis (2009) 15:134–40. doi: 10.1002/ibd.20527
50. Kim SW, Kim S, Son M, Cheon JH, Park YS. Melatonin Controls Microbiota in Colitis by Goblet Cell Differentiation and Antimicrobial Peptide Production Through Toll-Like Receptor 4 Signalling. Sci Rep (2020) 10:2232. doi: 10.1038/s41598-020-59314-7
51. Liu XW, Wang CD. Melatonin Alleviates Circadian Rhythm Disruption Exacerbating DSS-Induced Colitis by Inhibiting the Distribution of HMGB1 in Intestinal Tissues. Int Immunopharmacol (2019) 73:108–17. doi: 10.1016/j.intimp.2019.05.005
52. Singh M, Jadhav HR. Melatonin: Functions and Ligands. Drug Discovery Today (2014) 19:1410–8. doi: 10.1016/j.drudis.2014.04.014
53. Carlberg C, Wiesenberg I. The Orphan Receptor Family RZR/ROR, Melatonin and 5-Lipoxygenase: An Unexpected Relationship. J Pineal Res (1995) 18:171–8. doi: 10.1111/j.1600-079x.1995.tb00157.x
54. Liu Z, Yang X, Liu X, Mu Y, Wang L, Song X, et al. Analysis of Expression of ILC2 Cells in Nasal Mucosa Based on Animal Model of Allergic Bacterial Infection Rhinitis. J Infect Public Health (2021) 14:77–83. doi: 10.1016/j.jiph.2019.09.010
55. Gao T, Wang Z, Dong Y, Cao J, Lin R, Wang X, et al. Role of Melatonin in Sleep Deprivation-Induced Intestinal Barrier Dysfunction in Mice. J Pineal Res (2019) 67:e12574. doi: 10.1111/jpi.12574
56. Yin J, Li Y, Han H, Chen S, Gao J, Liu G, et al. Melatonin Reprogramming of Gut Microbiota Improves Lipid Dysmetabolism in High-Fat Diet-Fed Mice. J Pineal Res (2018) 65:e12524. doi: 10.1111/jpi.12524
57. Bajic D, Niemann A, Hillmer AK, Mejias-Luque R, Bluemel S, Docampo M, et al. Gut Microbiota-Derived Propionate Regulates the Expression of Reg3 Mucosal Lectins and Ameliorates Experimental Colitis in Mice. J Crohns Colitis (2020) 14:1462–72. doi: 10.1093/ecco-jcc/jjaa065
58. Engevik MA, Luk B, Chang-Graham AL, Hall A, Herrmann B, Ruan W, et al. Bifidobacterium Dentium Fortifies the Intestinal Mucus Layer via Autophagy and Calcium Signaling Pathways. mBio (2019) 10(3):e01087–19. doi: 10.1128/mBio.01087-19
59. Rowan F, Docherty NG, Murphy M, Murphy B, Calvin Coffey J, O'Connell PR. Desulfovibrio Bacterial Species are Increased in Ulcerative Colitis. Dis Colon Rectum (2010) 53:1530–6. doi: 10.1007/DCR.0b013e3181f1e620
60. Cui H, Cai Y, Wang L, Jia B, Li J, Zhao S, et al. Berberine Regulates Treg/Th17 Balance to Treat Ulcerative Colitis Through Modulating the Gut Microbiota in the Colon. Front Pharmacol (2018) 9:571. doi: 10.3389/fphar.2018.00571
61. Monk JM, Lepp D, Zhang CP, Wu W, Zarepoor L, Lu JT, et al. Diets Enriched With Cranberry Beans Alter the Microbiota and Mitigate Colitis Severity and Associated Inflammation. J Nutr Biochem (2016) 28:129–39. doi: 10.1016/j.jnutbio.2015.10.014
62. Guo W, Xiang Q, Mao B, Tang X, Cui S, Li X, et al. Protective Effects of Microbiome-Derived Inosine on Lipopolysaccharide-Induced Acute Liver Damage and Inflammation in Mice via Mediating the TLR4/NF-Kappab Pathway. J Agric Food Chem (2021) 69(27):7619–28. doi: 10.1021/acs.jafc.1c01781
Keywords: inflammatory bowel disease, ulcerative colitis, melatonin, ILC2, microbiota
Citation: Zhao Z-x, Yuan X, Cui Y-y, Liu J, Shen J, Jin B-y, Feng B-c, Zhai Y-j, Zheng M-q, Kou G-j, Zhou R-c, Li L-x, Zuo X-l, Li S-y and Li Y-q (2022) Melatonin Mitigates Oxazolone-Induced Colitis in Microbiota-Dependent Manner. Front. Immunol. 12:783806. doi: 10.3389/fimmu.2021.783806
Received: 27 September 2021; Accepted: 23 December 2021;
Published: 18 January 2022.
Edited by:
Axel Kornerup Hansen, University of Copenhagen, DenmarkReviewed by:
Philip Brandon Busbee, University of South Carolina, United StatesJose Garrido-Mesa, King’s College London, United Kingdom
Copyright © 2022 Zhao, Yuan, Cui, Liu, Shen, Jin, Feng, Zhai, Zheng, Kou, Zhou, Li, Zuo, Li and Li. This is an open-access article distributed under the terms of the Creative Commons Attribution License (CC BY). The use, distribution or reproduction in other forums is permitted, provided the original author(s) and the copyright owner(s) are credited and that the original publication in this journal is cited, in accordance with accepted academic practice. No use, distribution or reproduction is permitted which does not comply with these terms.
*Correspondence: Yan-qing Li, Liyanqing@sdu.edu.cn; Shi-yang Li, lishiyang@sdu.edu.cn