- 1Department of General Surgery, Qilu Hospital, Cheeloo College of Medicine, Shandong University, Jinan, China
- 2Department of Colorectal Surgery, National Cancer Center/National Clinical Research Center for Cancer/Cancer Hospital, Chinese Academy of Medical Sciences and Peking Union Medical College, Beijing, China
- 3Institute of Basic Medical Sciences, Qilu Hospital, Cheeloo College of Medicine, Shandong University, Jinan, China
- 4Department of Urology, National Cancer Center/National Clinical Research Center for Cancer/Cancer Hospital, Chinese Academy of Medical Sciences and Peking Union Medical College, Beijing, China
- 5Department of Obstetrics and Gynecology, Quanzhou First Hospital Affiliated to Fujian Medical University, Quanzhou, China
- 6Department of Pathology, Qilu Hospital, Cheeloo College of Medicine, Shandong University, Jinan, China
- 7Department of Ultrasound, Qilu Hospital, Cheeloo College of Medicine, Shandong University, Jinan, China
Background: Macrophage extracellular traps (METs) and tumor-infiltrating macrophages contribute to the progression of several diseases. But the role of METs and tumor-infiltrating macrophages in colon cancer (CC) has not been illuminated. In this study, we aimed to clarify the prognostic value of METs for CC patients and to explore the interaction between CC cells and METs in vitro and in vivo.
Methods: A training cohort consisting of 116 patients and a validation cohort of 94 patients were enrolled in this study. Immunofluorescence (IF) staining was conducted to determine METs formation in CC patients. Cox regression was used to perform prognostic analysis and screen out the best prognostic model. A nomogram was established to predict 5-year overall survival (OS). The correlation between METs with clinicopathological features and inflammatory markers was analyzed. The formation of METs in vitro was detected by SYTOX® green and IF staining, and the effect of METs on CC cells was detected by transwell assays. PAD2-IN-1, a selective inhibitor of peptidylarginine deiminase 2 (PAD2), was introduced to destroy the crosstalk between CC cells and METs in vitro and in vivo.
Results: METs levels were higher in CC tissues and were an independent prognostic factor for CC patients. The prognostic model consisting of age, tumors local invasion, lymph node metastasis and METs were confirmed to be consistent and accurate for predicting the 5-year OS of CC patients. Besides, METs were correlated with distant metastasis and inflammation. Through in vitro experiments, we confirmed that there was a positive feedback loop between CC cells and METs, in that METs promoted the invasion of CC cells and CC cells enhanced the production of METs, in turn. This interaction could be blocked by PAD2-IN-1 inhibitors. More importantly, animal experiments revealed that PAD2-IN-1 inhibited METs formation and CC liver metastasis in vivo.
Conclusions: METs were the potential biomarker of CC patient prognosis. PAD2-IN-1 inhibited the crosstalk between CC cells and METs in vitro and in vivo, which should be emphasized in CC therapy.
Introduction
Colorectal cancer (CRC) is one of the most common malignancies, which can be further divided into colon cancer (CC) and rectal cancer (RC). According to the global cancer statistics in 2020, the incidence of CRC ranks third, and the mortality rate ranks second among all cancers (1, 2). With continuous improvement of early screening and treatment strategies for CRC, the prognosis of patients with orthotopic CRC has significantly improved. However, the prognosis of patients with metastatic CRC (mCRC) who have received postoperative adjuvant chemoradiotherapy and radiofrequency therapy is still poor, with a 5-year overall survival (OS) rate less than 15%, which is the main challenge for CRC treatment at present (2).
With the development of molecular biology technology, new therapeutic methods such as molecular targeted therapy have been proposed for CRC patients. But improvement of the survival rate and therapeutic effects are still unsatisfactory for patients with advanced CRC, especially those with distant metastasis (3). One of the primary reasons is that the drugs used to treat CRC are mainly targeted at tumor cells. However, tumors are not only composed of a group of homologous malignant cells, but can be considered a special ‘organ’, which is composed of tumor cells and other heterogeneous cells (4). Non-malignant cells such as stromal cells, immune cells, and other cells infiltrate the tumor, and the dynamic interaction network among the different cell sub-classes regulates the biological behavior of tumor cells and ultimately determines tumor progression, the prognosis of tumor patients, and the response to the onco-therapy. Immune cells constitute a very important non-malignant cell population in tumors (5, 6). CRC is an immune sensitive tumor, with numerous immune cells infiltrating the tumor microenvironment (7). Therefore, the targeted treatment of immune cells in CRC should be emphasized and studies on more effective predictors related to immune cells are necessary, in order to greatly improve the survival rate of CRC patients, especially those with distant metastasis.
Macrophages exist in and around many solid tumors and together with other important immune cells, such as NK cells, T cells, and neutrophils, constitute up to 80% of tumor infiltrating immune cells. Macrophages differentiate from peripheral blood monocytes when recruited to tissues and are the largest proportion of myeloid immune cells in tumor tissues. The main function of macrophages is to recognize and phagocytize antigens and present them to T cells, as well as regulate the activity of the acquired immune response. Macrophages are thus considered the bridge between innate immunity and acquired immune response (8, 9). Moreover, macrophages produce different tumor-promoting factors, such as reactive oxygen species, growth factors, and angiogenic factors, which promote tumor invasion and metastasis (10).
Plasticity is a widely accepted feature of bone marrow cells, especially the monocyte-macrophage system (11). With more thorough research, scientists have found that macrophages are involved in the pathological process of many diseases in the form of extracellular traps (ETs), also referred to as macrophage extracellular traps (METs). This discovery has gone beyond the traditional theory that macrophages can polarize into M1 (classical macrophages) and M2 (alternative activated macrophages) phenotypes under different environmental stimuli (12, 13). The formation of ETs is a type of cell death that is different from apoptosis or necrosis. It is characterized by the release of depolymerized chromatin from immune cells and the formation of a reticular structure after being activated by some stimulating factors (13). Besides the DNA skeleton, ETs also contain histone, granule protein, and other proteins (14). At present, research on ETs mainly focuses on neutrophil extracellular traps (NETs), which promote the progress of a variety of tumors and correlate with poor patient prognosis (15–19). METs have been reported to be involved in the response process of bacteria and their toxins, the lipid-rich lesions in atherosclerosis, and acute kidney injury (20–23). However, the research about METs in the field of cancer is limited to nonfunctional pancreatic neuroendocrine tumors, and there is little relevant research in CRC (24).
About 70% of CC patients present with macrophage infiltration, but its role in the progression of CC is still controversial. Some reports suggest that tumor infiltrating macrophages promote tumor progression and metastasis. Other reports show that intratumoral macrophages have the advantage of prolonged survival (7, 25). In CC, the role of METs, a special cell death form of macrophages, in the progression and metastasis of CC needs to be further elucidated. In this study, we first described the correlation between METs with the prognosis of patients with CC, and confirmed that presence of METs was an independent risk factor for the prognosis of CC. Additionally, we elucidated the interaction between METs and CC cells in vitro, showing that METs promoted the invasion of CC cells and CC cells also promoted the formation of METs. More importantly, we found that PAD2-IN-1, a selective inhibitor of PAD2, destroyed the interaction between METs and CC cells in vitro and inhibited METs formation and colon cancer liver metastasis in vivo. Our study provided a new target for the treatment of CC patients, especially those with distant metastasis, and also identified a new idea for CC immunotherapy.
Methods and Materials
Patients and Specimens
The training cohort consisting of 116 patients was selected following the criteria: (i) patients who underwent radical resection with a clear surgical margin; (ii) patients with available formalin-fixed tumor tissues, follow-up information and complete medical records; (iii) patients with a postsurgical survival time of more than 1 month; and (iv) patients with no history of other malignancies. The validation cohort of 94 patients was purchased from Shanghai Outdo Biotech Company. The tumors were classified and staged according to the 8th AJCC/UICC TNM classification system. Informed consent was obtained from all patients. All experiments were approved and supervised by the Ethics Committee of Qilu Hospital of Shandong University.
The clinical features of all the patients are described in Supplemental Table 1.
Immunofluorescence Staining of Paraffin-Embedded Tissues
To visualize METs in patients’ tissue samples, paraffin-embedded tissue sections and TMA were subjected to immunofluorescence (IF) staining. METs were specifically detected by mouse anti-CD68 (ab955, 1:200, Abcam) and rabbit anti-Citrullinated histone H3 (H3Cit, ab5103, 1:200, Abcam) antibodies. Tris-EDTA was used for antigen retrieval and 5% bovine serum albumin (BSA) was used for nonspecific antigen blocking at room temperature. The sections were incubated with anti-CD68 and anti-H3Cit primary antibodies at 4°C overnight. After that, the sections were washed with PBS and incubated with CY3-conjugated goat anti-mouse (1:200, Servicebio) and Alexa Fluor® 488-conjugated goat anti-rabbit (1:200, Servicebio) secondary antibodies at room temperature for 2 hours. Finally, the sections were mounted in Anti-fade Mounting Medium with DAPI (Beyotime Biotechnology).
IF assessments were performed by two independent experienced pathologists. METs were defined as DNA-, CD68- and H3Cit-positive MET fibers exceeding 20 μm in length (23), and macrophages were defined as co-localized CD68 and DNA without H3Cit. 5 random fields under HPF were selected over the whole section for the quantification of macrophages or METs in tumor and para-tumor tissues. Extracellular structures exceeding 20 μm in length with DNA-, CD68- and H3Cit-positive staining were calculated, manually. The mean value was regarded as the final score of macrophages infiltration or METs formation in the tumor of each patient, as described (24).
Cell Lines and Agents
Human colon cancer cell lines HCT116 and SW480 were purchased from the Cell Bank of Chinese Academy of Sciences. HCT116 and SW480 were cultured in Dulbecco’s modified Eagle’s medium (DMEM, Hyclone) with 10% fetal bovine serum (FBS, Gibco Life Technologies), 100 U/ml penicillin, and 100 μg/ml streptomycin at 37°C under 95% air and 5% CO2. All cell lines were authenticated using short tandem repeat (STR) analysis, and the databases of the Chinese Academy of Sciences and American Type Culture Collection were used as references.
The PAD2 inhibitor PAD2-IN-1 was purchased from MedChemExpress (MCE, HY-136557). DNase I was purchased from ThermoFisher Scientific.
Monocytes Isolation and Macrophages Culture
Monocytes isolation and macrophages culture were performed as previously described (26). Briefly, human monocytes were isolated from the peripheral blood of healthy donors using Ficoll density gradient centrifugation. Under sterile conditions, monocytes were resuspended in serum-free RPMI-1640 medium and seeded into 12-well culture plate in the density of 5 × 105/ml. After 2 hours incubation at 37°C under 95% air and 5% CO2, monocytes will adhere to the culture plate. The cell medium was then replaced with complete RPMI-1640 culture medium containing 10% (v/v) pooled human serum and 20 mM L-glutamine and cultured for 8 days.
In Vitro METs Formation Assay
To prepare the METs inducing medium, phorbol 12-myristate 13-acetate (PMA) was added to complete RPMI-1640 medium and the final concentration was adjusted to 5 μM. Under sterile conditions, the medium was removed from the culture plate wells and the wells were washed with PBS three times. Approximately 1 mL of METs inducing medium was added to each well containing macrophages. After 12 hours of incubation, METs were measured using SYTOX® Green (S7020, Thermo Fisher Scientific) and IF staining.
For IF staining, cells were fixed with 4% paraformaldehyde at 4°C for 15 minutes and the nonspecific antigen was blocked with 5% goat serum at 37°C for 1 hours. Primary mouse anti-CD68 (ab955, 1:200, Abcam) and rabbit anti-H3Cit (ab5103, 1:200, Abcam) antibodies were applied and incubated at 4°C overnight. CY3-conjugated goat anti-mouse (1:200, Servicebio) and Alexa Fluor® 488-conjugated goat anti-rabbit (1:200, Servicebio) secondary antibodies were used for 2 hours at room temperature. The percentage of the field of view positive for the green signal (H3Cit) was regarded as the level of METs formation and was measured using Image J (NIH).
SYTOX® Green was also introduced to detect the extracellular DNA chains. SYTOX® Green was diluted to 2 μM with RPMI-1640 and was added into each well. After 30 minutes of incubation at 37°C, the green signal was determined using a fluorescence microscope (Olympus IX70).
Conditioned Medium Preparation
The preparation of conditioned medium (CM) was performed as described (27). Briefly, HCT-116 cells were cultured in DMEM medium with 10% FBS until the cell density became 40%-50%. Then replace the complete medium with serum-free medium and incubate the cells for 48 hours. The supernatant of the medium was collected and centrifuged for 1000g to discard the pellets. The supernatant was ten times concentrated with Amicon Ultra 15ml filters at 4000 g.
To determine whether colon cancer cells could induce METs, macrophages were stimulated with the CM from HCT116 cells for 12 hours at 37°C. METs were then visualized and assessment through SYTOX® Green and IF staining as above described.
Transwell Assays
Transwell assays were performed using 24 plates with transwell chambers (8 µm pore diameter, Corning). After METs were induced in the lower chamber, 5 × 104 HCT-116 cells or 2 × 104 SW480 cells were seeded to the upper chambers with matrigel coated (diluted at 1:6 with DMEM; Corning). After 36 hours, the cells attached to the bottom of the chamber were fixed with methanol for 20 minutes and then stained with 0.5% crystal violet (Beyotime) for 30 minutes at room temperature. Images of 5 random visual fields of microscopy at ×200 magnification were exported to Image J (NIH) for cell counting.
Western Blotting
Western blotting assay was performed as described (27, 28). Briefly, total protein was extracted using RIPA lysis buffer (Beyotime) with 1% PMSF (Beyotime) and 1% phosphatase inhibitor (Solarbio). Protein concentration was determined using a bicinchoninic acid (BCA) Assay Kit (Beyotime). Protein extracts were resolved through 10% SDS-PAGE after denaturation, transferred to PVDF membranes (0.22µm, Millipore) and probed with rabbit anti-H3Cit (ab5103, 1:1000, Abcam) and rabbit anti-H3 (ab1791, 1:5000, Abcam) primary antibodies at 4°C overnight. The membranes were then incubated in the second antibody for 1 hour at room temperature. An enhanced chemiluminescence (Millipore) were used for HRP detection. Quantitative analysis of Western blotting bands was performed using Image J (NIH).
In Vivo Experiments
Rat anti–mouse Ly6G antibody (BP0075-1, BioXcell) was used for neutrophil depletion (100 μg/mice, i.p.) as described (23). 5×105 MC-38 cells were injected into the caudal vein of each BALB/c mouse, in the presence or absence of PAD2-IN-1 (20 mg/kg, i.p.). The presence of METs was detected by IF staining, as above described. The weights of livers were measured to assess the actual tumor burden. The number of nodules on the livers was counted and confirmed by HE staining. All animal experiments were approved by the Medical Ethics Committee of Shandong University.
Statistical Methods
SPSS 17.0 and GraphPad Prism 5.0 software were used for statistical analysis and chart generation. The survival curves were plotted using the Kaplan-Meier method, and the log-rank test was conducted to determine the statistical significance. The Harrell’s concordance index (C-index) was calculated using “survival” of R package by RStudio software. The independent prognostic factors were analyzed in Cox proportional hazards regression model. The nomogram was established to predict 5-year OS, and the calibration plot was used to display the accuracy and predictive value of the prognostic models. Student’s t test was used for the comparison of two independent groups. Paired t test was used for the comparison of two paired groups. p values < 0.05 was considered statistically significant.
Results
Patient Characteristics
In total, 116 patients with CC were included in the training cohort and 94 patients with CC were included in the validation cohort. Variables of clinicopathological characteristics of patients are shown in Supplemental Table 1.
In the training cohort, the median age of 116 patients was 64 years old (ranging from 30 to 91), and 33.6% of these patients were female. A total of 102 patients (87.9%) had T3/T4 tumors, while 45 patients (38.8%) had lymph node metastasis (LNM) and 11 patients (9.5%) had distant metastasis, and all of them were in stage III/IV.
In the validation cohort, the median age of 94 patients was 65 years old (ranging from 27 to 90), and 48.9% of these patients were female. In total, 83 patients (88.3%) had T3/T4 tumor, 34 patients (36.2%) had LNM, and 5 patients (5.32%) had distant metastasis, and all of them were in stage III/IV.
Prognostic Value of METs for CC Patients
To determine the levels of macrophage infiltration and METs formation in CC, we performed immunofluorescence (IF) staining on a total of 116 patients as the training cohort (Figure 1A). Both macrophage infiltration and METs presented with significantly higher levels in CC tissues than those in para-tumor tissues (Figure 1B and Supplemental Figure 1A). Univariate Cox regression analysis was performed to analyze the prognostic value of macrophage infiltration, METs, and other clinicopathological factors. Results showed that in CC, METs (p=0.007) exhibited a better prognostic value than macrophage infiltration (p=0.117) (Figure 1C). There was no correlation between macrophage infiltration and METs formation (Figure 1D and Supplemental Figures 1B, C). The tumor local invasion (T stage, p=0.020), positive lymph node metastasis (N stage, p<0.001), distant metastasis (M stage, p<0.001), and advanced TNM stage (p<0.001) were also strongly correlated with the poor prognosis (Table 1).
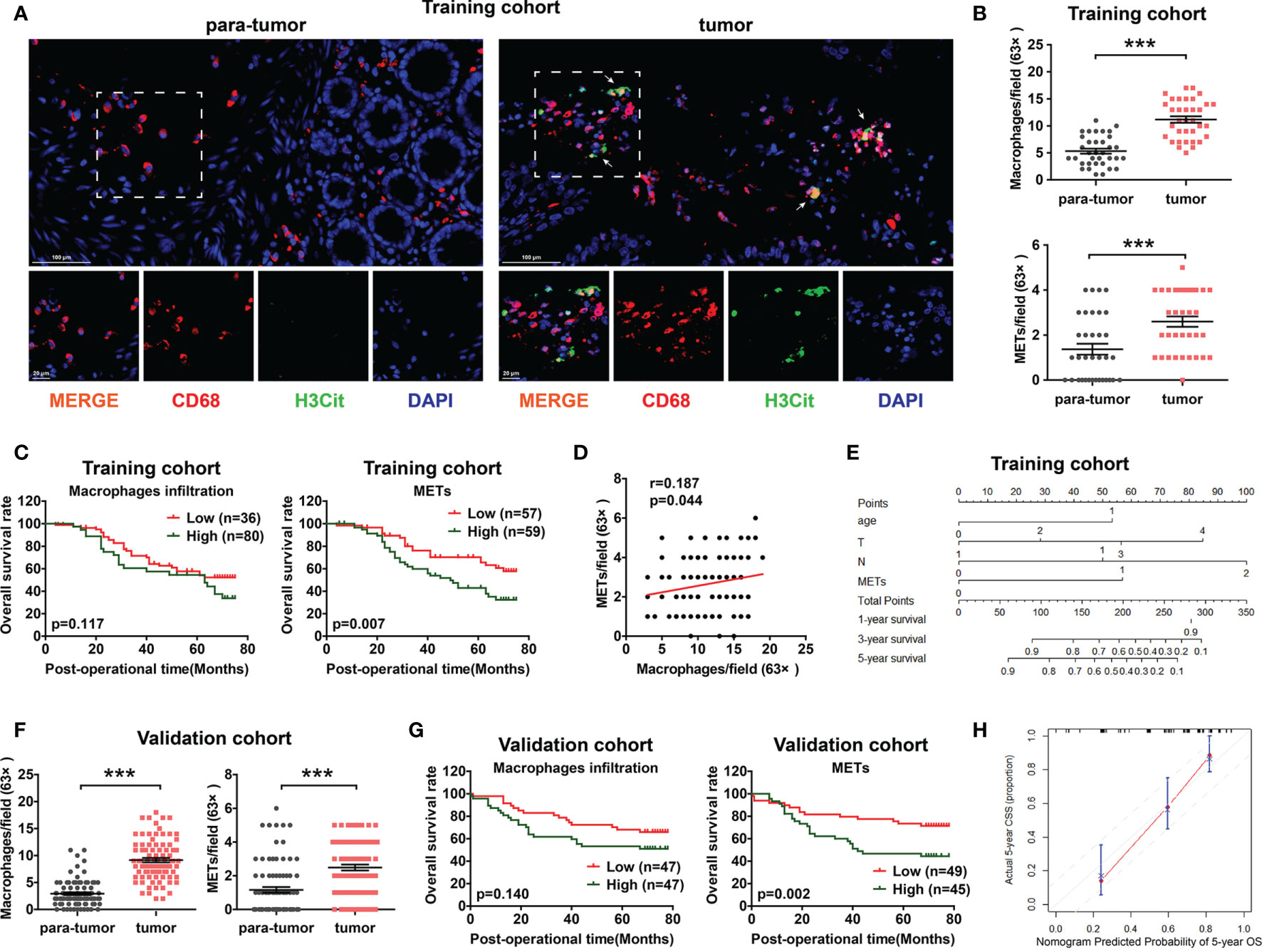
Figure 1 The prognostic value of METs for CC patients in training cohort and validation cohort. (A) Representative images of immunofluorescence staining of METs in CC patients. Arrows indicated the presence of METs. (B) In the training cohort, macrophage infiltration (upper panel) and METs (lower panel) were higher expressed in CC tissues than in para-tumor tissues. (C) High METs levels were correlated with poor prognosis of CC patients in training cohort (right panel), while macrophage infiltration had no prognostic value (left panel). (D) No significant correlation was observed between macrophage infiltration and METs formation in training cohort. (E) A nomogram for 5-year OS predictive probability. (F) In the validation cohort, macrophage infiltration (left panel) and METs (right panel) were higher expressed in CC tissues than those in para-tumor tissues. (G) The level of METs rather than macrophage infiltration correlated with poor prognosis of CC patients in validation cohort. (H) Calibration plot for 5-year overall survival. *** represents p<0.001. In (B, F), data were calculated by Paired t test. In (C, G), data were calculated by long-rank test. In (D), data were calculated by Pearson correlation test.
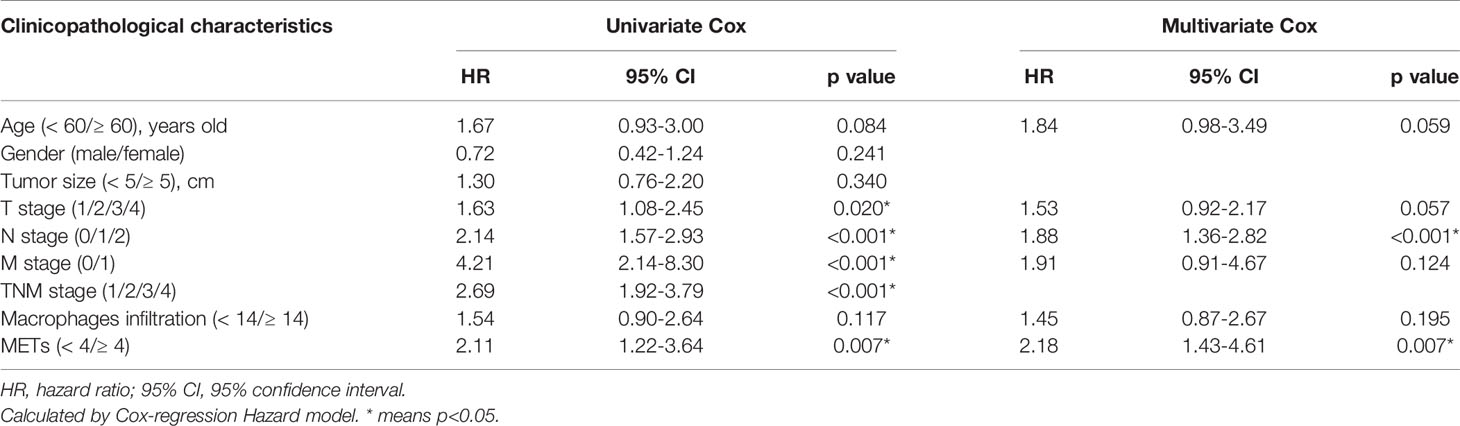
Table 1 Univariate and multivariate Cox regression analysis of prognostic factors for overall survival in CC.
Multivariate Cox regression analysis was further performed to identify the independent prognostic factors. All factors with p value ≤0.20 in univariate analysis were included in multivariate analysis, except TNM stage because of its natural interaction with T, N, or M stage. We found that presence of METs was an independent prognostic factor in CC (p=0.007). Patients with higher METs had 2.18 fold higher risk of cancer-caused death than those with lower METs (Table 1). In addition, we chose prognostic factors with p value ≤0.10 in multivariate analysis to establish a prognostic model including age, T stage, N stage, and METs. Concordance index (C-index) was used in our study to assess the consistency and accuracy of predictive models. This model showed the highest C-index (0.730), surpassing TNM stage and any single clinicopathological factors (Table 2). Thus, METs combined with age, T stage, and N stage were selected for the establishment of a nomogram to predict the 5-year overall survival rate (Figure 1E).
Moreover, we determined the presence of METs through IF staining in 94 CC patients as the validation cohort. Consistent with the results of the training cohort, presence of METs was higher in CC tissues and was a significant prognostic indicator for CC patients in the validation cohort (Figures 1F–G and Supplemental Figure 1D). The prognostic model including age, T stage, N stage, and METs also showed the highest C-index (0.737) (Table 2). The calibration plot presented excellent prognostic values and centralized mainly in the 10% margin of error for 5-year predictive overall survival rate (Figure 1H).
Correlation Between METs With Clinicopathological Features and Inflammatory Markers
We then analyzed the correlation between METs formation and clinicopathological features in the training cohort and validation cohort. High METs levels were significantly associated with distant metastasis, but not tumor local invasion and lymph node metastasis (Figures 2A, B). Meanwhile, considering the close relationship between METs with inflammation, we analyzed the correlation between METs formation and the systemic markers of inflammation, including procalcitonin (PCT), neutrophil-to-lymphocyte ratio (NLR) and platelet-to-lymphocyte ratio (PLR). Patients with abnormally elevated PCT and NLR levels showed the higher levels of METs (Figure 2C).
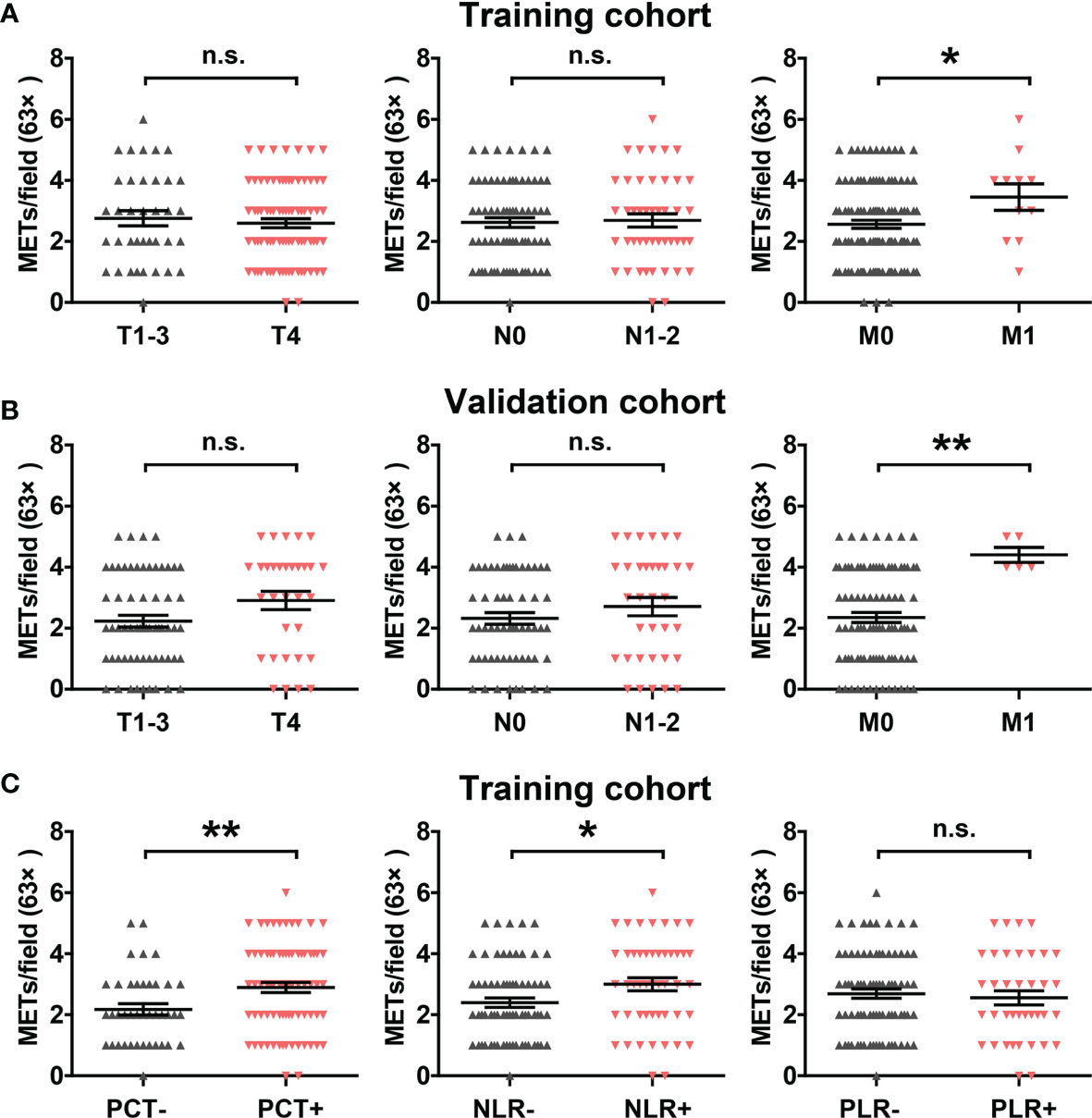
Figure 2 Correlation between METs with clinicopathological features and inflammatory markers. (A) Correlation between METs levels with T stage, N stage and M stage in the training cohort. (B) Correlation between METs levels with T stage, N stage and M stage in the validation cohort. (C) Correlation between METs levels with inflammatory markers PCT, NLR and PLR in the training cohort. * represents p<0.05, ** represents p<0.01. In (A–C), data were calculated by student’s t test. "n.s." means nonsense.
Crosstalk Between CC Cells and METs In Vitro
Given that the presence of METs in the two cohorts was significantly related to the distant metastasis and poor prognosis of CC patients, we tried to investigate whether METs promoted the metastatic ability of colon cancer in vitro. We first detected METs formation induced by PMA stimulation, through SYTOX® green (Figure 3A) and IF staining (Figure 3B). Results showed that 12 hours of stimulation with 5 μM PMA induced the formation of METs (Figure 3C). Then, we used transwell chambers to co-culture CC cells HCT-116 and SW480 (upper chamber) with macrophages or METs (lower chamber). DNase I was introduced to inhibit METs. As expected, METs significantly promoted the invasion of HCT116 and SW480 cells, while DNase I treatment reversed it (Figure 3D).
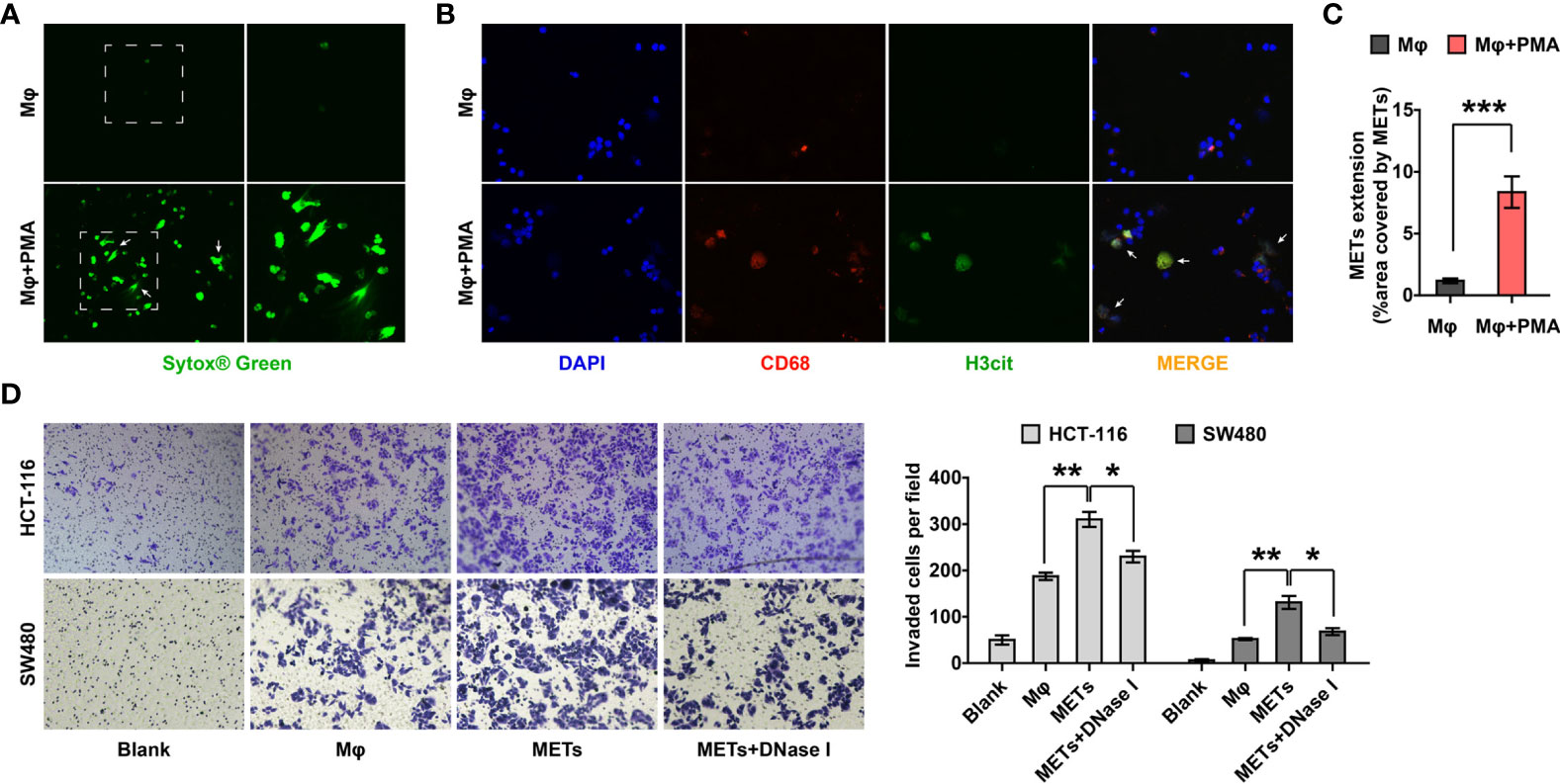
Figure 3 METs promoted the invasion of CC cells. (A, B) Representative images of SYTOX® green (A) and IF (B) staining of METs formation. Arrows indicated the presence of METs. (C) Quantification of METs proved that PMA stimulation induced the formation of METs. (D) Invasion of HCT-116 and SW480 cells were detected with transwell assay after co-culturing with macrophages or METs, with or without DNase I treatment. * represents p<0.05, ** represents p<0.01, *** represents p<0.001. In (C, D), data were calculated by student’s t test.
Tumor cells also promote the formation of ETs in vitro (18). In order to verify whether CC cells promoted METs formation, the conditioned medium (CM) of HCT-116 cells was collected to stimulate macrophages. Results showed that CM significantly promoted the formation of METs (Figures 4A–C). These results demonstrated that there might be an interaction network between CC cells and METs, that is, METs could promote the invasion of CC cells and CC cells could enhance the production of METs, in turn.
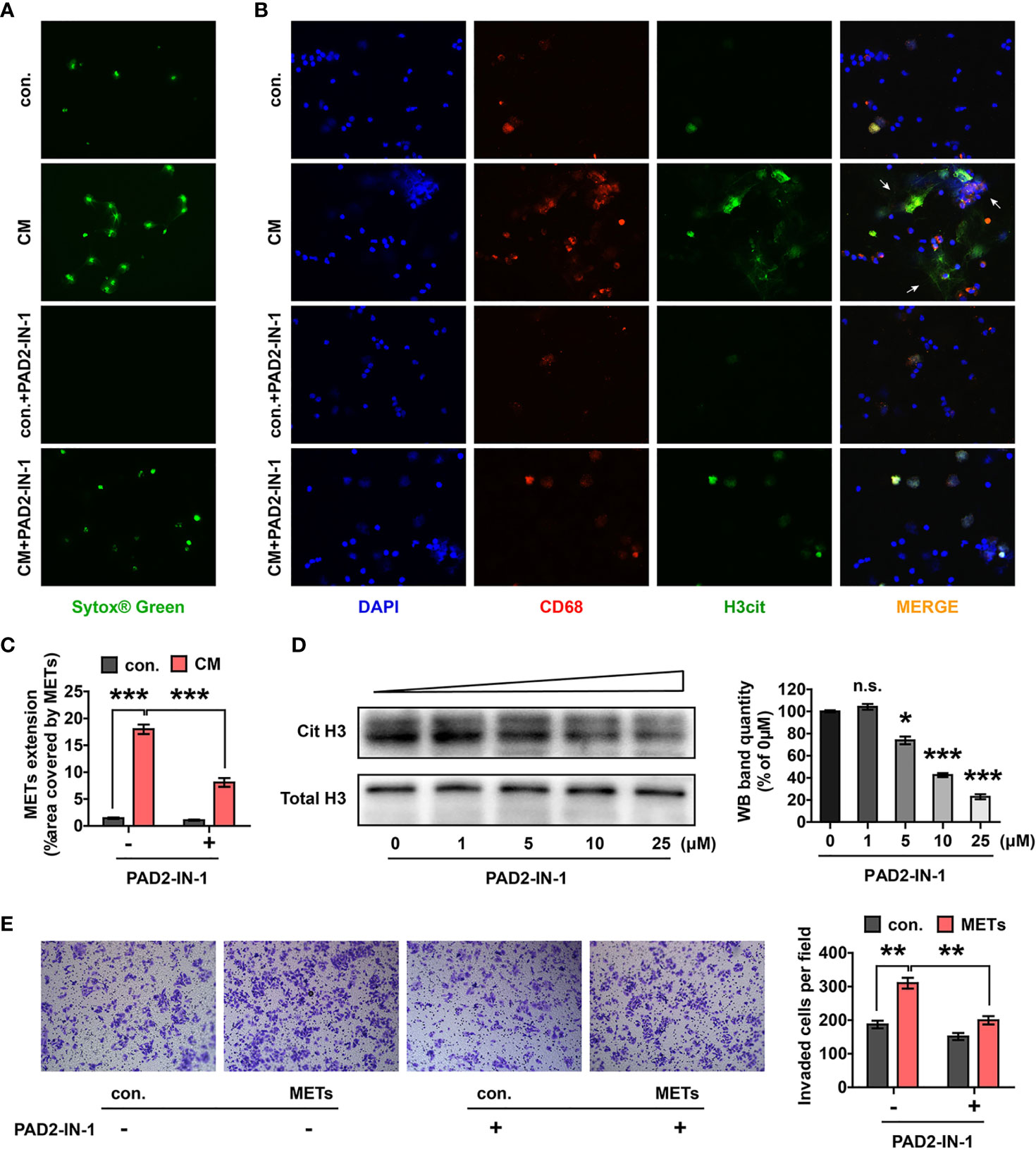
Figure 4 CM of CC cells induced METs formation and the crosstalk between CC cells and METs could be destroyed by PAD2-IN-1. (A, B) Representative images of SYTOX® green (A) and IF (B) staining of METs after the stimulation of HCT-116 cells CM with or without PAD2-IN-1. Arrows indicated the presence of METs. (C) Quantification of METs proved that CM stimulation induced the formation of METs, which could be destroyed by PAD2-IN-1. (D) Western blotting assay confirmed that PAD2-IN-1 inhibited histone H3 citrullination of macrophages. (E) Invasion of HCT-116 cells were detected with transwell assay after co-culturing with METs, with or without PAD2-IN-1. CM stimulation enhanced the invasive ability of HCT-116 cells, which could be destructed by PAD2-IN-1. * represents p<0.05, ** represents p<0.01. *** represents p<0.001. In (C–E), data were calculated by student’s t test. "n.s." means nonsense.
Crosstalk Between CC Cells and METs Could Be Destroyed by PAD2-IN-1
Peptidylarginine deiminases (PADs) have been reported to be widely involved in the formation of ETs, of which PAD2 is the only member confirmed to function in METs formation (29). PAD2-IN-1, a selective PAD2 inhibitor, has been confirmed to inhibit the histone H3 citrullination induced by PAD2 in vitro (30). We first stimulated macrophages with different concentrations of PAD2-IN-1 to select the optimal concentration of PAD2-IN-1 to inhibit histone H3 citrullination of macrophages. PAD2-IN-1 at 25 μM was determined as the best condition (Figure 4D). After that, HCT-116 cell CM with or without 25 μM PAD2-IN-1 was used to stimulate macrophages and the formation of METs was detected. We found that PAD2-IN-1 significantly inhibited METs formation induced by CM (Figures 4A–C). Moreover, we introduced PAD2-IN-1 to the co-culture system of METs and HCT-116 cells to investigate whether PAD2-IN-1 inhibited METs-induced invasion of CC cells. Results showed that the improvement of CC cells invasion induced by METs was significantly reversed by PAD2-IN-1 (Figure 4E). The above data supported that PAD2-IN-1 inhibited the crosstalk between CC cells and METs in vitro, which should be emphasized in CC therapy.
Therapeutic Effect of PAD2-IN-1 In Vivo
Experiments in vivo were further performed to verify the crosstalk between CC cells and METs and the therapeutic value of PAD2-IN-1 in vivo. To eliminate the interference of NETs, we first use the anti-mouse Ly6G antibody to deplete neutrophils as described (23). After that, we inoculated MC-38 cells into the tail vein of BALB/c mice and treated the mice with PAD2-IN-1. IF staining was performed to detect the presence of METs and H&E staining was performed to confirm that the nodules were formed by CC cells. We found that CC cells were mainly colonized around blood vessels and were surrounded by METs (Figures 5A, B). PAD2-IN-1 not only inhibited METs formation (Figure 5A) but also reduced the liver metastasis of CC cells (Figures 5B–D), which was consistent with results of in vitro experiments. Besides, our results also suggested that PAD2-IN-1 treatment suppressed macrophage infiltration in the mice livers. This finding was consistent with previous studies on the correlation of PAD2 with the infiltration of inflammatory cells and macrophage activation, which revealed the multiple roles of PAD2 in the regulation of tumor immune microenvironment (31, 32).
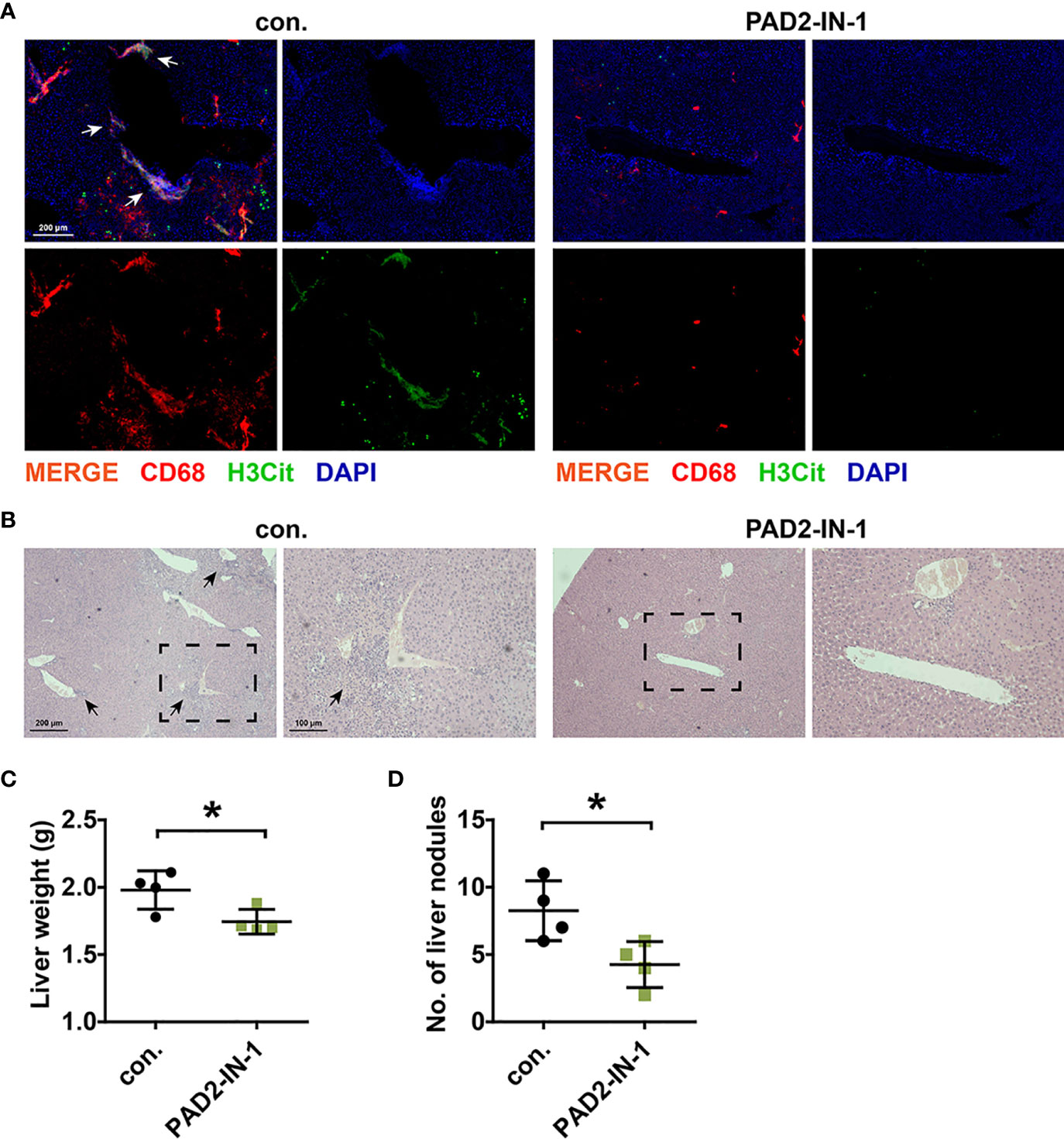
Figure 5 PAD2-IN-1 inhibited METs formation and colon cancer liver metastasis in vivo. (A) Representative images of IF staining of METs in metastasis lesions in liver. Arrows indicated the presence of METs. (B) Representative images of H&E staining of metastasis lesions in liver. Arrows indicated the metastasis lesions. (C) Livers were weighted to assess the tumor burden of liver metastatic foci. (D) Number of metastasis nodules in livers of mice. * represents p<0.05. In (C, D), data were calculated by student’s t test.
In conclusion, we found that numbers of METs were high in CC tissues and were correlated with poor prognosis and distant metastasis of CC patients. Through in vitro and in vivo experiments, we confirmed that there was a positive feedback loop between CC cells and METs, which could be blocked by PAD2-IN-1 inhibitors. This discovery filled the research gap of METs in the field of CC, identified a new mechanism of macrophages in the progression of CC, and provided new ideas and potential therapeutic targets for the immunotherapy of CC patients, especially patients with distant metastasis.
Discussion
The development of malignancies relies on a complex tissue environment referred to as the tumor microenvironment. This environment is critical for tumor growth, metastasis, and tumor-associated angiogenesis (33). Numerous tumor cells, mesenchymal cells, and immune cells as well as lymphatic vessels and blood vessels constitute the tumor microenvironment, which may be conducive to the occurrence and development of tumors (34). Studies have shown that inflammation has a close relationship with tumors. The tumor microenvironment composed of main inflammatory cells is a necessary participant and supporter of several tumor biological behaviors (35). Among these inflammatory cells, tumor-associated macrophages (TAMs) and tumor-associated neutrophils (TANs) account for a large proportion. TAMs are one of the major immune cells that infiltrate the tumor and are the main producers of multiple inflammation mediators (such as chemokines), which contribute to the activation and maintenance of chronic inflammatory processes (10). At present, increasing numbers of studies have confirmed that tumor-infiltrating macrophages and domestication of tumor microenvironment by macrophages are essential in tumor development. In the tumor microenvironment, macrophages can express pro- or anti-tumoral functions. This plasticity is a characteristic of the monocyte-macrophage system and reflects the particularity of these cells (11).
CC is a malignant tumor with abundant macrophage infiltration (36, 37). However, the role of macrophages in the progression of CC is still controversial. Some studies showed that high macrophage infiltration along the tumor front brings a high survival advantage for patients with CRC (38). However, more studies demonstrate that TAM promotes the growth and development of tumors (39, 40). These findings suggest that there may be other factors that function in the crosstalk of macrophages and colon cancer cells.
METs formation is a special death form of macrophages. The main components of METs, roughly the same as NETs, include extracellular fibers composed of DNA extending outside the cell boundary, which can be degraded by DNase I or micrococcal nuclease treatment. By staining the known ET components (such as citrullinated histone and elastase), the formation of METs is determined (13). METs can be induced by PMA, LPS, TNF-α, and other factors, and participate in the response to bacteria and toxins, as well as pathological processes such as acute kidney injury (20–23). In tumor research, METs have only been reported as closely related to the poor prognosis of patients with nonfunctional pancreatic neuroendocrine tumors. However, the clinical significance and molecular mechanisms of METs in CC are still lacking. Our study confirmed for the first time that presence of METs was involved in the progression of CC and affected the survival outcome of CC patients. Through immunofluorescence assays, we found that macrophage infiltration was not significantly related to the prognosis of patients, while METs formation was an independent prognostic of CC patients. High METs predicted the worst prognosis for CC patients. This result was consistent with a previous study showing that macrophages participate in the immune response of nonfunctional pancreatic neuroendocrine tumors in the form of METs (24). However, they did not confirm this breakthrough conclusion through in vivo and in vitro experiments.
The potential value of METs in the clinical diagnosis and treatment of CC patients may be huge. Given that we have confirmed the critical role of METs in CC, we conducted further in vitro experiments using CC cell lines. Through transwell assays, we found that the co-culture of PMA-induced METs with CC cells promoted the invasion of CC cells. Additionally, the CM of CC cells also enhanced the formation of METs. This finding confirmed that there was a positive feedback between METs and CC cells. They promoted each other and together led to tumor progression and poor prognosis of CC patients. Cytokines including Interferon γ (IFN-γ), tumor necrosis factor α (TNF-α), and interleukin 8 (IL-8) have been confirmed to induce METs formation (13, 41). IL-8 and TNF-α can be secreted by CC cells and correlate with CC progression, which may be the molecular basis for the formation of METs induced by CC cells (42, 43). Further studies are needed to elucidate the cytokines and the regulation mechanisms involved in the process of CC cells-mediated METs formation.
Peptidylarginine deiminases (PADs) catalyze the conversion of positively charged arginine residues to neutrally charged citrulline. The N-terminal tails of H3 and H4 histones are the main targets of PADs because of their arginine-richment (44). PADs family consists of 5 members: PAD1, PAD2, PAD3, PAD4 and PAD6. PAD2 and PAD4 have been clearly confirmed to regulate NETs release (44–46), but the role of PAD2 and PAD4 in METs is controversial. A study finds that PAD2 mRNA is strongly expressed while PAD4 expression is very low in RAW 264.7 mouse macrophages. PAD2 expresion is significantly related with METs formation (29). However, another study confirms the positive expression of PAD4 in mouse macrophages from lymphoid tissue, which promoted the formation of METs (47). Besides, some studies show that not all observed METs are composed of histones and are generated in a PAD-dependent manner. METs can also be released independent of PAD enzymes-mediated histone citrullination (41, 48). In this study, we mainly focused on PAD2 and confirmed for the first time that PAD2-IN-1, an inhibitor of PAD2, inhibited the interaction between METs and CC cells. More and more tumor-targeted drugs are not designed to destroy tumor cells, but to block the interaction between tumor cells and other cells, such as PD-1/PD-L1 monoclonal antibodies and anti-VEGF bevacizumab. Our research focused on the positive feedback between METs and CC cells. This discovery fills the research gap of METs in the field of CC, described a new mechanism of macrophages in the progression of CC, and provided new ideas and potential therapeutic targets for the immunotherapy of CC patients, especially patients with distant metastasis.
Data Availability Statement
The original contributions presented in the study are included in the article/Supplementary Material. Further inquiries can be directed to the corresponding author.
Ethics Statement
The studies involving human participants were reviewed and approved by the Ethics Committee of Qilu Hospital of Shandong University. The patients/participants provided their written informed consent to participate in this study. The animal study was reviewed and approved by the Ethics Committee of Qilu Hospital of Shandong University.
Author Contributions
CL, XQ and TC contributed to conception and design of the study. TC and YW carried out our research. ZN and TZ collected the specimens and perform the follow-up. CL and JW participated in data analysis and interpretation. TC and CL wrote the manuscript. All authors contributed to manuscript revision, read, and approved the submitted version.
Funding
Our study was supported by National Natural Science Foundation of China (Grant No. 81372810).
Conflict of Interest
The authors declare that the research was conducted in the absence of any commercial or financial relationships that could be construed as a potential conflict of interest.
Publisher’s Note
All claims expressed in this article are solely those of the authors and do not necessarily represent those of their affiliated organizations, or those of the publisher, the editors and the reviewers. Any product that may be evaluated in this article, or claim that may be made by its manufacturer, is not guaranteed or endorsed by the publisher.
Acknowledgments
We appreciate the assistance from Prof. Daoxin Ma, Dr. Ruiqing Wang, and other teammates. We thank all enrolled patients for the support.
Supplementary Material
The Supplementary Material for this article can be found online at: https://www.frontiersin.org/articles/10.3389/fimmu.2021.779325/full#supplementary-material
Abbreviations
CRC, colorectal cancer; CC, colon cancer; RC, rectal cancer; mCRC, metastatic colorectal cancer; OS, overall survival; TAMs, tumor-associated macrophages; TANs, tumor-associated neutrophils; ETs, extracellular traps; METs, macrophage extracellular traps; NETs, neutrophil extracellular traps; PAD2, peptidylarginine deiminase 2; IF, immunofluorescence staining; H3Cit, Citrullinated histone H3; PMA, phorbol 12-myristate 13-acetate; CM, conditioned medium; C-index, concordance index; PCT, procalcitonin; NLR, neutrophil-to-lymphocyte ratio; PLR, platelet-to-lymphocyte ratio.
References
1. Sung H, Ferlay J, Siegel RL, Laversanne M, Soerjomataram I, Jemal A, et al. Global Cancer Statistics 2020: GLOBOCAN Estimates of Incidence and Mortality Worldwide for 36 Cancers in 185 Countries. CA Cancer J Clin (2021) 71(3):209–49. doi: 10.3322/caac.21660
2. Siegel RL, Miller KD, Goding Sauer A, Fedewa SA, Butterly LF, Anderson JC, et al. Colorectal Cancer Statistics, 2020. CA Cancer J Clin (2020) 70(3):145–64. doi: 10.3322/caac.21601
3. Kobayashi H, Mochizuki H, Sugihara K, Morita T, Kotake K, Teramoto T, et al. Characteristics of Recurrence and Surveillance Tools After Curative Resection for Colorectal Cancer: A Multicenter Study. Surgery (2007) 141(1):67–75. doi: 10.1016/j.surg.2006.07.020
4. Hanahan D, Weinberg RA. Hallmarks of Cancer: The Next Generation. Cell (2011) 144(5):646–74. doi: 10.1016/j.cell.2011.02.013
5. Galon J, Costes A, Sanchez-Cabo F, Kirilovsky A, Mlecnik B, Lagorce-Pagès C, et al. Type, Density, and Location of Immune Cells Within Human Colorectal Tumors Predict Clinical Outcome. Science (2006) 313(5795):1960–4. doi: 10.1126/science.1129139
6. Wang E, Miller LD, Ohnmacht GA, Mocellin S, Perez-Diez A, Petersen D, et al. Prospective Molecular Profiling of Melanoma Metastases Suggests Classifiers of Immune Responsiveness. Cancer Res (2002) 62(13):3581–6.
7. Nagtegaal ID, Marijnen CA, Kranenbarg EK, Mulder-Stapel A, Hermans J, van de Velde CJ, et al. Local and Distant Recurrences in Rectal Cancer Patients Are Predicted by the Nonspecific Immune Response; Specific Immune Response has Only a Systemic Effect–A Histopathological and Immunohistochemical Study. BMC Cancer (2001) 1:7. doi: 10.1186/1471-2407-1-7
8. Solinas G, Germano G, Mantovani A, Allavena P. Tumor-Associated Macrophages (TAM) as Major Players of the Cancer-Related Inflammation. J Leukoc Biol (2009) 86(5):1065–73. doi: 10.1189/jlb.0609385
9. Noy R, Pollard JW. Tumor-Associated Macrophages: From Mechanisms to Therapy. Immunity (2014) 41(1):49–61. doi: 10.1016/j.immuni.2014.06.010
10. Mantovani A, Bottazzi B, Colotta F, Sozzani S, Ruco L. The Origin and Function of Tumor-Associated Macrophages. Immunol Today (1992) 13(7):265–70. doi: 10.1016/0167-5699(92)90008-u
11. Sica A, Mantovani A. Macrophage Plasticity and Polarization In Vivo Veritas. J Clin Invest (2012) 122(3):787–95. doi: 10.1172/jci59643
12. Chow OA, von Köckritz-Blickwede M, Bright AT, Hensler ME, Zinkernagel AS, Cogen AL, et al. Statins Enhance Formation of Phagocyte Extracellular Traps. Cell Host Microbe (2010) 8(5):445–54. doi: 10.1016/j.chom.2010.10.005
13. Doster RS, Rogers LM, Gaddy JA, Aronoff DM. Macrophage Extracellular Traps: A Scoping Review. J Innate Immun (2018) 10(1):3–13. doi: 10.1159/000480373
14. Galluzzi L, Vitale I, Aaronson SA, Abrams JM, Adam D, Agostinis P, et al. Molecular Mechanisms of Cell Death: Recommendations of the Nomenclature Committee on Cell Death 2018. Cell Death Differentiation (2018) 25(3):486–541. doi: 10.1038/s41418-017-0012-4
15. Liu K, Sun E, Lei M, Li L, Gao J, Nian X, et al. BCG-Induced Formation of Neutrophil Extracellular Traps Play an Important Role in Bladder Cancer Treatment. Clin Immunol (2019) 201:4–14. doi: 10.1016/j.clim.2019.02.005
16. Cools-Lartigue J, Spicer J, Najmeh S, Ferri L. Neutrophil Extracellular Traps in Cancer Progression. Cell Mol Life Sci (2014) 71(21):4179–94. doi: 10.1007/s00018-014-1683-3
17. Takesue S, Ohuchida K, Shinkawa T, Otsubo Y, Matsumoto S, Sagara A, et al. Neutrophil Extracellular Traps Promote Liver Micrometastasis in Pancreatic Ductal Adenocarcinoma via the Activation of Cancer−Associated Fibroblasts. Int J Oncol (2020) 56(2):596–605. doi: 10.3892/ijo.2019.4951
18. Nie M, Yang L, Bi X, Wang Y, Sun P, Yang H, et al. Neutrophil Extracellular Traps Induced by IL8 Promote Diffuse Large B-Cell Lymphoma Progression via the TLR9 Signaling. Clin Cancer Res (2019) 25(6):1867–79. doi: 10.1158/1078-0432.Ccr-18-1226
19. Yang L, Liu Q, Zhang X, Liu X, Zhou B, Chen J, et al. DNA of Neutrophil Extracellular Traps Promotes Cancer Metastasis via CCDC25. Nature (2020) 583(7814):133–8. doi: 10.1038/s41586-020-2394-6
20. Doster RS, Sutton JA, Rogers LM, Aronoff DM, Gaddy JA. Streptococcus Agalactiae Induces Placental Macrophages To Release Extracellular Traps Loaded With Tissue Remodeling Enzymes via an Oxidative Burst-Dependent Mechanism. mBio (2018) 9(6). doi: 10.1128/mBio.02084-18
21. Pertiwi KR, de Boer OJ, Mackaaij C, Pabittei DR, de Winter RJ, Li X, et al. Extracellular Traps Derived From Macrophages, Mast Cells, Eosinophils and Neutrophils are Generated in a Time-Dependent Manner During Atherothrombosis. J Pathol (2019) 247(4):505–12. doi: 10.1002/path.5212
22. Allison SJ. Acute Kidney Injury: Macrophage Extracellular Traps in Rhabdomyolysis-Induced AKI. Nat Rev Nephrol (2018) 14(3):141. doi: 10.1038/nrneph.2018.5
23. Okubo K, Kurosawa M, Kamiya M, Urano Y, Suzuki A, Yamamoto K, et al. Macrophage Extracellular Trap Formation Promoted by Platelet Activation Is a Key Mediator of Rhabdomyolysis-Induced Acute Kidney Injury. Nat Med (2018) 24(2):232–8. doi: 10.1038/nm.4462
24. Xu SS, Li H, Li TJ, Li S, Xia HY, Long J, et al. Neutrophil Extracellular Traps and Macrophage Extracellular Traps Predict Postoperative Recurrence in Resectable Nonfunctional Pancreatic Neuroendocrine Tumors. Front Immunol (2021) 12:577517. doi: 10.3389/fimmu.2021.577517
25. Etoh T, Shibuta K, Barnard GF, Kitano S, Mori M. Angiogenin Expression in Human Colorectal Cancer: The Role of Focal Macrophage Infiltration. Clin Cancer Res (2000) 6(9):3545–51.
26. Zhang Y, Rayner BS, Jensen M, Hawkins CL. In Vitro Stimulation and Visualization of Extracellular Trap Release in Differentiated Human Monocyte-Derived Macrophages. J Vis Exp (2019) 153). doi: 10.3791/60541
27. Chen T, Liu H, Liu Z, Li K, Qin R, Wang Y, et al. FGF19 and FGFR4 Promotes the Progression of Gallbladder Carcinoma in an Autocrine Pathway Dependent on GPBAR1-cAMP-EGR1 Axis. Oncogene (2021) 40(30):4941–53. doi: 10.1038/s41388-021-01850-1
28. Chen T, Li K, Liu Z, Liu J, Wang Y, Sun R, et al. WDR5 Facilitates EMT and Metastasis of CCA by Increasing HIF-1α Accumulation in Myc-Dependent and Independent Pathways. Mol Ther (2021) 29(6):2134–50. doi: 10.1016/j.ymthe.2021.02.017
29. Mohanan S, Horibata S, McElwee JL, Dannenberg AJ, Coonrod SA. Identification of Macrophage Extracellular Trap-Like Structures in Mammary Gland Adipose Tissue: A Preliminary Study. Front Immunol (2013) 4:67. doi: 10.3389/fimmu.2013.00067
30. Muth A, Subramanian V, Beaumont E, Nagar M, Kerry P, McEwan P, et al. Development of a Selective Inhibitor of Protein Arginine Deiminase 2. J Med Chem (2017) 60(7):3198–211. doi: 10.1021/acs.jmedchem.7b00274
31. Foulquier C, Sebbag M, Clavel C, Chapuy-Regaud S, Al Badine R, Méchin MC, et al. Peptidyl Arginine Deiminase Type 2 (PAD-2) and PAD-4 But Not PAD-1, PAD-3, and PAD-6 Are Expressed in Rheumatoid Arthritis Synovium in Close Association With Tissue Inflammation. Arthritis Rheum (2007) 56(11):3541–53. doi: 10.1002/art.22983
32. Musse AA, Li Z, Ackerley CA, Bienzle D, Lei H, Poma R, et al. Peptidylarginine Deiminase 2 (PAD2) Overexpression in Transgenic Mice Leads to Myelin Loss in the Central Nervous System. Dis Model Mech (2008) 1(4-5):229–40. doi: 10.1242/dmm.000729
33. Quail DF, Joyce JA. Microenvironmental Regulation of Tumor Progression and Metastasis. Nat Med (2013) 19(11):1423–37. doi: 10.1038/nm.3394
34. Mukhtar RA, Nseyo O, Campbell MJ, Esserman LJ. Tumor-Associated Macrophages in Breast Cancer as Potential Biomarkers for New Treatments and Diagnostics. Expert Rev Mol Diagn (2011) 11(1):91–100. doi: 10.1586/erm.10.97
35. Coussens LM, Werb Z. Inflammation and Cancer. Nature (2002) 420(6917):860–7. doi: 10.1038/nature01322
36. Wang H, Tian T, Zhang J. Tumor-Associated Macrophages (TAMs) in Colorectal Cancer (CRC): From Mechanism to Therapy and Prognosis. Int J Mol Sci (2021) 22(16):8470. doi: 10.3390/ijms22168470
37. Fan L, Xu C, Ge Q, Lin Y, Wong CC, Qi Y, et al. A. Muciniphila Suppresses Colorectal Tumorigenesis by Inducing TLR2/NLRP3-Mediated M1-Like TAMs. Cancer Immunol Res (2021) 9(10):1111–24. doi: 10.1158/2326-6066.Cir-20-1019
38. Forssell J, Oberg A, Henriksson ML, Stenling R, Jung A, Palmqvist R. High Macrophage Infiltration Along the Tumor Front Correlates With Improved Survival in Colon Cancer. Clin Cancer Res (2007) 13(5):1472–9. doi: 10.1158/1078-0432.Ccr-06-2073
39. Zhang Y, Zhao Y, Li Q, Wang Y. Macrophages, as a Promising Strategy to Targeted Treatment for Colorectal Cancer Metastasis in Tumor Immune Microenvironment. Front Immunol (2021) 12:685978. doi: 10.3389/fimmu.2021.685978
40. Väyrynen JP, Haruki K, Lau MC, Väyrynen SA, Zhong R, Dias Costa A, et al. The Prognostic Role of Macrophage Polarization in the Colorectal Cancer Microenvironment. Cancer Immunol Res (2021) 9(1):8–19. doi: 10.1158/2326-6066.Cir-20-0527
41. Rayner BS, Zhang Y, Brown BE, Reyes L, Cogger VC, Hawkins CL. Role of Hypochlorous Acid (HOCl) and Other Inflammatory Mediators in the Induction of Macrophage Extracellular Trap Formation. Free Radical Biol Med (2018) 129:25–34. doi: 10.1016/j.freeradbiomed.2018.09.001
42. Hwang WL, Yang MH, Tsai ML, Lan HY, Su SH, Chang SC, et al. SNAIL Regulates Interleukin-8 Expression, Stem Cell-Like Activity, and Tumorigenicity of Human Colorectal Carcinoma Cells. Gastroenterology (2011) 141(1):279–91. doi: 10.1053/j.gastro.2011.04.008
43. Hsu HH, Cheng SF, Chen LM, Liu JY, Chu CH, Weng YJ, et al. Over-Expressed Estrogen Receptor-Alpha Up-Regulates hTNF-Alpha Gene Expression and Down-Regulates Beta-Catenin Signaling Activity to Induce the Apoptosis and Inhibit Proliferation of LoVo Colon Cancer Cells. Mol Cell Biochem (2006) 289(1-2):101–9. doi: 10.1007/s11010-006-9153-3
44. Wang Y, Li M, Stadler S, Correll S, Li P, Wang D, et al. Histone Hypercitrullination Mediates Chromatin Decondensation and Neutrophil Extracellular Trap Formation. J Cell Biol (2009) 184(2):205–13. doi: 10.1083/jcb.200806072
45. Wu Z, Deng Q, Pan B, Alam HB, Tian Y, Bhatti UF, et al. Inhibition of PAD2 Improves Survival in a Mouse Model of Lethal LPS-Induced Endotoxic Shock. Inflammation (2020) 43(4):1436–45. doi: 10.1007/s10753-020-01221-0
46. Leshner M, Wang S, Lewis C, Zheng H, Chen XA, Santy L, et al. PAD4 Mediated Histone Hypercitrullination Induces Heterochromatin Decondensation and Chromatin Unfolding to Form Neutrophil Extracellular Trap-Like Structures. Front Immunol (2012) 3:307. doi: 10.3389/fimmu.2012.00307
47. El Shikh MEM, El Sayed R, Nerviani A, Goldmann K, John CR, Hands R, et al. Extracellular Traps and PAD4 Released by Macrophages Induce Citrullination and Auto-Antibody Production in Autoimmune Arthritis. J Autoimmun (2019) 105:102297. doi: 10.1016/j.jaut.2019.06.008
Keywords: colon cancer, macrophage extracellular traps (METs), prognosis, PAD2, PAD2-IN-1
Citation: Chen T, Wang Y, Nan Z, Wu J, Li A, Zhang T, Qu X and Li C (2021) Interaction Between Macrophage Extracellular Traps and Colon Cancer Cells Promotes Colon Cancer Invasion and Correlates With Unfavorable Prognosis. Front. Immunol. 12:779325. doi: 10.3389/fimmu.2021.779325
Received: 18 September 2021; Accepted: 15 November 2021;
Published: 01 December 2021.
Edited by:
Franz Rödel, University Hospital Frankfurt, GermanyReviewed by:
Jennifer Angeline Gaddy, Vanderbilt University Medical Center, United StatesJonathan Cools-Lartigue, Montreal General Hospital, Canada
Clare Hawkins, University of Copenhagen, Denmark
Copyright © 2021 Chen, Wang, Nan, Wu, Li, Zhang, Qu and Li. This is an open-access article distributed under the terms of the Creative Commons Attribution License (CC BY). The use, distribution or reproduction in other forums is permitted, provided the original author(s) and the copyright owner(s) are credited and that the original publication in this journal is cited, in accordance with accepted academic practice. No use, distribution or reproduction is permitted which does not comply with these terms.
*Correspondence: Chen Li, lichensdu0809@163.com