- 1Department of Integrated Traditional Chinese and Western Medicine, Tongji Hospital, Tongji Medical College of Huazhong University of Science and Technology, Wuhan, China
- 2Department of Nephrology, Zhongnan Hospital of Wuhan University, Wuhan, China
The morbidity and mortality of autoimmune diseases (Ads) have been increasing worldwide, and the identification of novel therapeutic strategies for prevention and treatment is urgently needed. Sirtuin 1 (SIRT1), a member of the class III family of nicotinamide adenine dinucleotide (NAD+)-dependent histone deacetylases, has been reported to participate in the progression of several diseases. SIRT1 also regulates inflammation, oxidative stress, mitochondrial function, immune responses, cellular differentiation, proliferation and metabolism, and its altered functions are likely involved in Ads. Several inhibitors and activators have been shown to affect the development of Ads. SIRT1 may represent a novel therapeutic target in these diseases, and small molecules or natural products that modulate the functions of SIRT1 are potential therapeutic agents. In the present review, we summarize current studies of the biological functions of SIRT1 and its role in the pathogenesis and treatment of Ads.
Introduction
Autoimmune diseases (Ads) are characterized by the dysregulation of the immune system, which results in the overproduction of autoantibodies, an imbalance in tolerance to self-antigens, and immune-mediated end-organ damage (1). Multiple types of Ads seriously affect the quality of life and labour ability and impose a substantial economic and psychological burden on society and families. To date, the etiology and pathogenesis of these diseases have remained invariably unknown, and complex and diverse elements associated with the environment, genetic risk factors, mental factors, and infection, which can cause an imbalance in autoimmune processes and immunological tolerance (2–4).
Sirtuin 1 (SIRT1), a nicotinamide adenine dinucleotide (NAD+)-dependent histone deacetylase, has been reported to participate in regulating various biological processes, such as energetic homeostasis (5), inflammation, oxidative stress (6), mitochondrial biogenesis (7), cell apoptosis (8), and autophagy (9). There have been preclinical and clinical studies indicating the significance of SIRT1 in the pathogenesis of Ads, including rheumatoid arthritis (RA), systemic lupus erythematosus (SLE), inflammatory bowel disease (IBD), multiple sclerosis (MS), etc. In women Hashimoto’s disease patients with type 1 diabetes mellitus, SIRT1 contributed to the pathogenesis of early cardiac dysfunction (10). Agathe et al. performed microarray experiments to identify SIRT1 as a relevant gene candidate associated with pathological angiogenesis in autoimmune arthritis mice (11). The deletion of SIRT1 in endothelial promoted a proliferative, proapoptotic and activated state of endothelial cells through the acetylation of p53 and p65, and resulted in the progress of proangiogenic capacities. The deficiency of SIRT1 in endothelial cells delayed the resolution of experimental arthritis. DNA hypomethylation was the first epigenetic pattern determined in SLE patients. The overexpression of SIRT1 was found in CD4+ T cells of a murine lupus model (12), however, SIRT1-null mice showed with immunoglobulin deposition in the kidneys and a high level of serum antinuclear antibody (13). The activator of SIRT1 plays a protective role in pristane-induced lupus mice, with the alleviation of proteinuria and decreased deposition of immune globulin in the kidneys (14). SIRT1 also may represent a biomarker of relapses and a potential target for therapeutic intervention in MS (15). The decreased expression of SIRT 1 has been found to increase the levels of proinflammatory cytokines that are being involved in the pathogenesis of IBD. On the contrary, the reduced expression of SIRT1 1 maintains the gastrointestinal barrier in IBD (16).
SIRT1 is a promising candidate molecule in the treatment due to different physiological and pathological processes in Ads. In the present manuscript, we will summarize and discuss the functions of SIRT1 in Ads and the therapeutic potential of targeting SIRT1 (Table 1).
Sirtuins and SIRT1
Acetylation, an evolutionarily conserved posttranslational modification of lysine residues, mainly facilitates chromatin formation and gene transcription. Protein deacetylases eliminate the effect of protein acetyltransferases by removing the acetyl groups added to the lysine residues (40). These enzymes are called histone deacetylases (HDACs), which are divided into four classes in mammals (41). Class III HDACs or silent information regulator 2 (Sir2) are NAD+-dependent HDACs that modify histone proteins and nonhistone proteins via deacetylation (42). Sirtuins, a family of highly conserved NAD+-dependent HDACs, share homology with Sir2 of the yeast Saccharomyces cerevisiae and show no sequence similarity to the other HDACs (43). According to existing studies, humans have 7 sirtuin paralogues, SIRT1–7, characterized by different binding targets, tissue specificities, functions, and localization (44, 45). SIRT1, SIRT6, and SIRT7 are mainly distributed in the nucleus (46, 47). SIRT2 is mainly found in the cytoplasm. SIRT1 and SIRT2 also share nuclear-cytoplasmic shuttling (48). SIRT3, SIRT4, and SIRT5 are located in mitochondria, and SIRT3 is also expressed in the nucleus under normal conditions (49–51). SIRT1, the protein with the largest molecular mass (120 kDa) and member of the sirtuin family with the highest amino acid sequence homology with yeast SIR2 (52), has been most widely studied (53).
SIRT1, located on chromosome 10q21.3, consists of 8 introns and 11 exons. The structure of the SIRT1 protein contains 747 amino acid residues and a catalytic core region flanked by variable NH2- and COOH-terminal domains consisting of approximately 250 amino acids (54). The variety of terminal domains is associated with the diversity of sirtuin functions. This domain forms the hairpin structure that compliments with the β sheet of the NAD+-binding domain, and the NH2-terminal domain potentiates the catalytic activity (55). In addition, extensions of the NH2- and COOH-termini influence the functions of SIRT1, which are the targets of posttranslational modifications (56). Through the action of NAD+, SIRT1 removes the acetyl moieties of ϵ-acetyl-lysine residues of histones and other target proteins, thereby producing 2’-O-acetyl-ADP-ribose, nicotinamide, and the deacetylated substrate (57, 58). SIRT1 not only deacetylates lysine residues of histones, such as lysine 16 of H4, lysine 26 of H1, and lysine 9 of H3, but also regulates the activity of a number of transcription factors via deacetylation (59). SIRT1 epigenetically silences these target proteins at the transcriptional or posttranslational level, such as forkhead box class O (FoxOs), p53, nuclear factor-κB (NF-κB), nuclear factor E2-related factor 2 (Nrf2), HIF1α, AMP-activated protein kinase (AMPK), β-catenin, mitochondrial peroxisome proliferator-activated receptor γ coactivator 1 alpha (PCG-1α), proliferator-activated receptor gamma (PPARγ), and Notch. SIRT1 participates in a series of pathological and physiological processes, including cell metabolism and DNA repair. The regulation of diverse physiological signalling pathways and targets by SIRT1 makes it a promising therapeutic target. However, the specific mechanism of SIRT1 in Ads is unknown.
Immunological Functions of SIRT1
Due to continuous exposure to a variety of pathogenic agents, such as bacteria, viruses, and fungi, the body defends against these potentially fatal infections through a series of highly regulated responses known as innate and adaptive immunity. The targets of SIRT1 can affect immune cells and immune responses to modulate the progression of chronic autoimmune and inflammatory diseases (Table 2).
Innate Immunity
Circulating monocytes from the blood enter the tissue and then develop into macrophages after differentiation and maturation. These phagocytes affect the immunopathogenesis of Ads. In SIRT1-deficient macrophages, hyperacetylation of NF-κB p65 results in increased levels of proinflammatory cytokines, such as tumour necrosis factor (TNF)-α and interleukin (IL)-1β, compared to the control (60). Furthermore, mice lacking SIRT1 in macrophages have high levels of activated macrophages in the liver and adipose tissues, which promote insulin resistance and metabolic syndrome. Clearly, p50/p65 is located in the cytoplasm mainly through an interaction with the inhibitor protein κB (IκB), and the activation of macrophages causes the degeneration of IκB, transferring NF-kB to the nucleus and finally promoting the expression of inflammatory genes (74). In RAW264.7 macrophages, siRNA-mediated knockdown of SIRT1 increased the expression of activated NF-κB and inflammatory factors and cytokines (61). Imperatore et al. reported an essential role for SIRT1 in the self-renewal of macrophages through the regulation of the cell cycle and longevity pathways (62). Overexpression of SIRT1 in bone marrow-derived macrophages increases their proliferative capacity. Silencing and deleting the SIRT1 gene restricts the self-renewal of macrophages. Moreover, SIRT1 inhibition negatively regulates the G1/S transition, cell cycle progression, and renewal, which are associated with the inhibition of E2F1 and Myc and the activation of FoxO1. Activator protein-1 (AP-1), which is composed of c-Fos and c-Jun, is also regulated by SIRT1 to affect inflammatory gene transcription. SIRT1 binds to c-Fos and c-Jun and deacetylates c-Jun, thereby inhibiting the transcriptional activity of AP-1 in peritoneal macrophages and reducing AP-1-associated expression of inflammatory mediators, including cyclooxygenase-2 (COX2) and prostaglandin E2 (63).
Dendritic cells (DCs) play a key protective role in promoting antigen-specific responses by adaptive immune cells and in producing a variety of chemokines and cytokines that recruit immune cells into the target tissues following the invasion of pathogenic microorganisms (75). SIRT1 regulates the generation of cytokines, such as IL-12 and TGFβ-1, by DCs through HIF1α modulation, which potentially regulates the formation of helper T (Th)-1 cells and regulatory T cells (Tregs) and the function of DCs (64, 76). The inhibition of SIRT1 in DCs promotes the differentiation of Th1 cells while restricting the differentiation of Tregs. In a mouse colitis model, transferring naïve CD4+ T cells into mice with DCs with a specific deletion of SIRT1 aggravated colonic inflammation and promoted weight loss. In cocultures of activated DCs with CD4+ T cells, pharmacological SIRT1 inhibition increases the Th1/Treg ratio and the expression of IFN-γ and IL-12 and reduces the expression of TGFβ1 (77). In a mouse asthma model, pharmacological inhibition of SIRT1 increases the activity of PPARγ and inhibits Th2 cell responses in allergic airway inflammation, which are associated with an imbalance in the maturation and migration of lung DCs (65). The loss of SIRT1 limits DC transfer into the draining lymph nodes, leading to disrupted Th2 cell differentiation. In a mouse OVA-induced airway inflammation model with DC-specific deletion of SIRT1 (66), the maturation and migration of DCs are reduced. Coculture of activated SIRT1-deficient DCs with CD4+ T cells inhibits the differentiation of Th17 cells, which is reversed by anti-IL-27 and anti-IFN-β antibodies. In respiratory syncytial virus infection, SIRT1 promotes the activation of DCs to produce efficient antiviral immune responses (78).
Adaptive Immunity
The role of SIRT1 in the adaptive immune response was mainly identified to be a negative regulator of T cell function. The levels of SIRT1 are increased in activated T cells and anergic T cells compared to mature naïve T cells (67). Compared with wild-type littermates, CD4+ T cells from SIRT1-deficient mice appear to exhibit greater proliferation and cytokine production (67). However, mice with a SIRT1 deletion do not present abnormal T or B cells, suggesting that SIRT1 is unlikely to be a key factor contributing to the activation of T or B cells. SIRT1 deficiency is still an important factor associated with a higher risk of Ads (79).
The hyperactivation of T cells with a specific deletion of SIRT1 is likely to be associated with the loss of inhibition of NF-κB and AP-1 activity. SIRT1 inhibits the AP-1 signalling pathway mainly through the deacetylation of c-Jun, thereby inhibiting T cell activation and proliferation. In addition to directly regulating the activity of transcription factors, SIRT1 regulates related genes that affect T cell proliferation and function. Bclaf1, initially identified as a Bcl-2-binding protein, is considered required for T cell activation (80). SIRT1 is a suppressor of Bclaf1 transcription that inhibits the activity of NF-κB and deacetylates histone lysine residues. The differences in Bclaf1 levels and Bclaf1 locus histone deacetylation between wild-type animals and mice with systemic knockout of SIRT1 are not significant in naïve, un-activated T cells (81). In the presence of IL-6, IL-23, and TGFβ, naïve T cells cultured with antigen-presenting cells induce the generation of Th17 cells, which have been proven to participate in the immunopathogenesis of certain Ads (68). SIRT1 is expressed at high levels in Th17 cells and plays an essential role in Th17 cell formation. SIRT1 binds to and deacetylates the transcription factor retinoid acid receptor-related orphan receptor gamma (RORγt), promoting the differentiation of Th17 cells by activating IL-17 and repressing the IL-2 promoter (82). Mice with a targeted deletion of SIRT1 in T cells showed a reduction in Th17 differentiation through the suppression of IL-17 expression and induction of IL-2 expression. Additionally, SIRT1 also limits Th17 differentiation by deacetylating signal transducer and activator of transcription (STAT)-3, which is required for RORγt transcription (69). Several studies found that increasing rather than suppressing SIRT1 may inhibit Th17 differentiation (70, 83). More evidence is needed to prove the direct effect of SIRT1 on Th17 development. SIRT1 also functions as a negative regulator in the differentiation of IL-9-secreting effector cells and Th9 effector cells, which have been shown to possess antitumour and antiallergic activities (84). Targeted deletion of SIRT1 in mouse CD4+ T cells or silencing of SIRT1 in mouse or human T cells promotes the differentiation of Th9 cells and IL-9 production, whereas ectopic expression of SIRT1 inhibits IL-9 production and Th9 differentiation (85). Additionally, IL-9 produced by SIRT1-deficient T cells protects against tumours and increases the levels of allergic pulmonary inflammation.
IL-2 plays an important role in the proliferation of activated T cells and in preventing their apoptosis induced by the high-affinity IL-2 receptor CD25 through the phosphoinositide-3- kinase (PI3K)/Akt pathway to suppress FoxO1, 3, and 4 (71). SIRT1 is a very important deacetylase of FoxO family members. Normally, SIRT1 is likely to regulate the activation of T cells via the deacetylation of FoxO proteins and the inhibition of FoxO apoptotic signalling and the IL-2 signalling pathway.
SIRT1 regulates the acetylation and stability of FoxP3, a crucial transcription factor involved in the differentiation of Tregs (86). Hyperacetylation of FoxP3 diminishes its polyubiquitination and increases its stability. The inhibition of sirtuins downregulates the acetylation of FoxP3 and promotes the ubiquitination and degeneration of FoxP3. In addition, the Notch receptor in Tregs plays an essential role in the survival of Tregs and is associated with the antiapoptotic effect of the Notch1 intracellular domain. SIRT1 was shown to stabilize the Notch1 intracellular domain proximal to the membrane to promote Treg survival (87). However, in contrast, SIRT1 inhibition promotes the formation of FoxP3+ Tregs with elevated immunosuppressive activity (88). Beier et al. reported that conventional CD4+FoxP3- T cell-specific deletion of SIRT1 in mice rarely affected the numbers of T cells and their activation and proliferation but increased the expression of FoxP3 and suppressive activity of Tregs in vitro and in vivo (72). Compared to wild-type mice, mice with specific deletions of SIRT1 in FoxP3+ Tregs survived significantly longer with mismatched heart allografts. Wild-type mice treated with SIRT1 selective inhibitors (splitomicin and EX-527) showed similar results. Another study reported that mice with a SIRT1 deletion and mismatched renal allografts experienced longer survival than wild-type mice (89). CD4+ T cells isolated from a cervical heterotopic heart transplantation mouse model treated with sirtinol (sirtuin inhibitor) showed significantly lower expression of IL-17A and RORγt and higher expression of FoxP3 (90). In vivo, sirtinol reduces the differentiation of Th17 cells and increases the proportion of Treg cells among splenocytes. Additionally, co-transfection of SIRT1 with FoxP3 increases FoxP3 proteasomal degeneration, while SIRT1 inhibition increases FOXP3 transcriptional activity in human Treg (91). SIRT1 inhibition might increase FoxP3 acetylation to promote the production and functions of FoxP3+ Tregs and inhibit the acetylation of RORγt and the differentiation of Th17 cells, thereby affecting the Th17/Treg ratio (92, 93). Nonetheless, SIRT1-/- mice develop spontaneous and severe Ads (67) but not mice with SIRT1-deficient CD4+ T cells. A potential explanation for this finding is alterations in thymic T cell selection in SIRT1-/- mice and the expression of autoimmune regulator (AIRE), which is required for the former. SIRT1 also plays a key role in regulating the expression of AIRE (79).
The function of SIRT1 in another group of lymphocytes has rarely been studied. SIRT1 regulates the activation of B cells through CD38 and NAD (94). In another study, SIRT1 regulated antibody maturation in B cells (95). The activation of B cells resulted in the inhibition of SIRT1 and the upregulation of activation-induced cytidine deaminase (AICDA). B cells obtained from mice with B cell-specific deletion of SIRT1 showed reduced deacetylation in activated B cells, increased AICDA levels, and the induction of antibody maturation. More studies are needed to clarify the role of SIRT1 in the activation and differentiation of B cells.
SIRT1 in Autoimmune Diseases
SLE
SLE is a multisystemic and chronic inflammatory disorder characterized by autoantibody production, immune complex deposition, inflammation, and damage to multiple tissues and organs. Levels of the SIRT1 mRNA and protein are significantly increased in patients with active lupus nephritis (LN) compared with those in remission or healthy patients (73). Moreover, histological features of LN biopsies were related to increased SIRT1 expression in proliferative forms. SIRT1 expression showed a strong power to discriminate kidney damage in patients with SLE. Hu et al. found that the administration of SIRT1-siRNA to MRL/lpr mice significantly increases levels of acetylated H3 and H4 in CD4+ T cells and reduces serum anti-dsDNA antibody levels and renal pathological scores, particularly tubulointerstitial scores (12). The results suggested that the overexpression of SIRT1 in vivo was associated with lupus pathogenesis and that SIRT1 inhibition mitigated the damage induced by lupus in MRL/lpr mice. Consiglio et al. analysed genomic DNA from the peripheral blood of 367 patients with SLE and 290 healthy controls in a Brazilian population and found that SIRT1 promoter variant rs3758391 modifies SLE morbidity, with the rs3758391 T allele serving as a risk factor for nephritis and a higher systemic lupus erythematosus disease activity index (SLEDAI) (96). Nevertheless, researchers have not yet elucidated how the SIRT1 rs3758391 variant functionally affects SLE severity. In another study, the activity of DNA methyltransferase 1 (DNMT1) was inhibited in CD4+ T cells isolated from 22 patients with active SLE transfected with si-SIRT1. Ultraviolet B radiation suppressed SIRT1 mRNA and protein expression by activating aryl hydrocarbon receptor (AhR) and downregulated the activity of DNMT1 in CD4+ T cells by binding to the SIRT1 promoter (97). B cell hyperactivity is a major characteristic of SLE and is involved in the progression of SLE. Wang et al. (17) transfected mouse B cells BaF3 with a SIRT1 vector or shRNA targeting SIRT1, and the results showed that SIRT1 overexpression promotes BaF3 cell proliferation and increases the expression of proinflammatory cytokines (IL-6 and TNF-α). In addition, p65 was significantly activated and phosphorylated, and the expression of B cell CLL/lymphoma 3 (Bcl-3) was increased. SIRT1 might be a potential risk factor for the development of SLE.
In contrast, the activation of SIRT1 by resveratrol, a SIRT1 activator, attenuates proteinuria, glomerulonephritis, and the serum levels of IgG1 and IgG2a in mice with pristane-induced lupus (14). Furthermore, resveratrol also suppresses CD69 and CD71 expression on CD4+ T cells, as well as CD4+ T cell proliferation, induced CD4+ T cell apoptosis, and decreased the number of CD4 IFNγ+ Th1 cells, the proliferation of B cells, and the ratio of Th1/Th2 cells in vitro (as shown in Figure 1). Resveratrol protects against lupus-induced tissue damage and may represent a potential therapeutic agent for the treatment of SLE. Moreover, SIRT1 knockout mice show higher levels of anti-dsDNA and anti-nuclear antigen IgG and IgM immunoglobulin than wild-type mice (13). A potential explanation is that histone modification dysregulation is related to the incidence of SLE (98). The deletion of SIRT1 in activated B cells results in the production of autoantibodies targeting nuclear antigens, dsDNA, and ribonucleoprotein (95). Compared to healthy controls, B cells obtained from mice and patients with SLE exhibit increased expression of AICDA, which is related to decreased SIRT1 expression. However, the occurrence of SLE is also related to the increase in SIRT1 levels, which may be due to the overall imbalance of transcription and hyperacetylation.
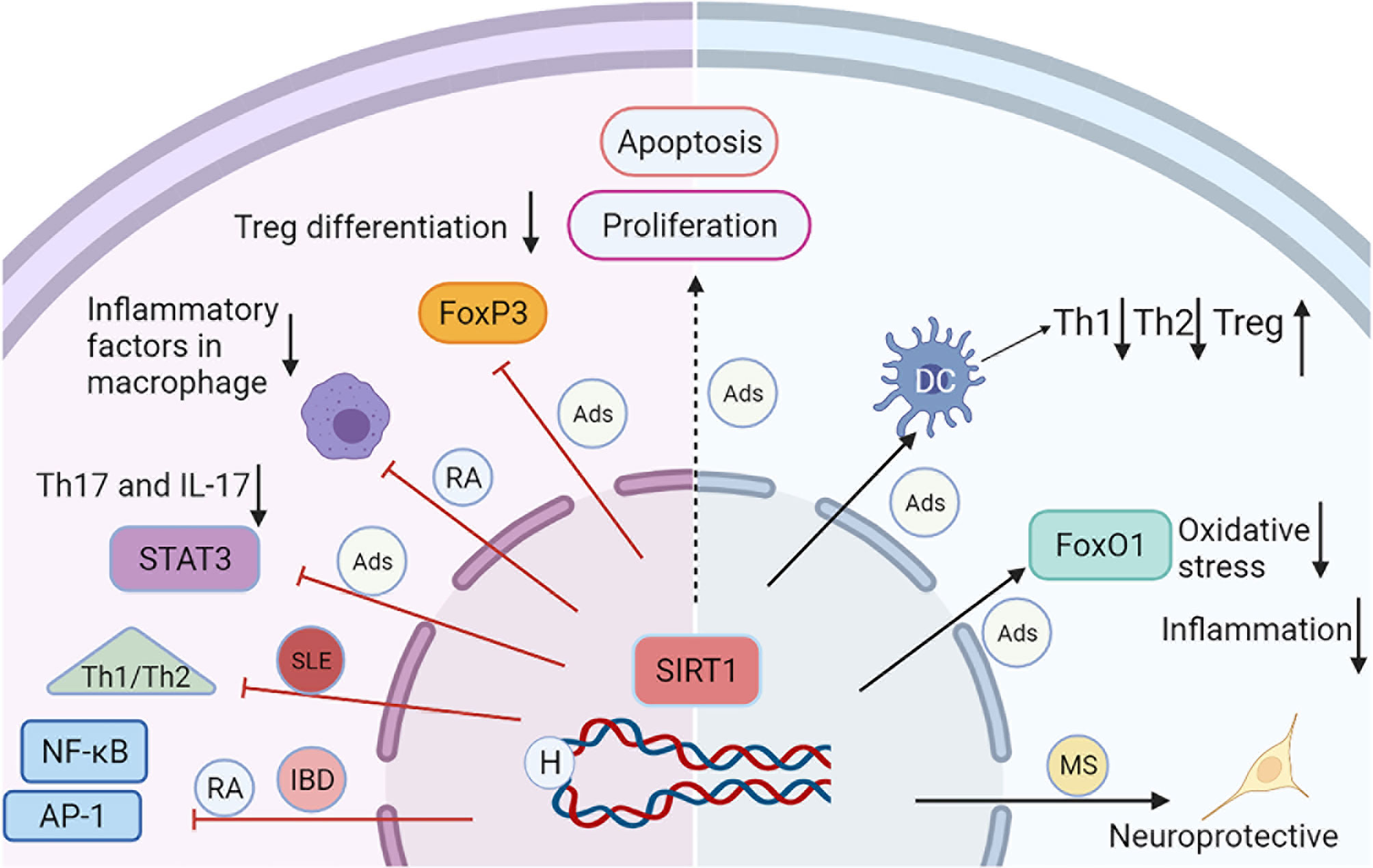
Figure 1 A schematic diagram illustrating the major mechanisms of SIRT1 in selected autoimmune diseases. The activation of SIRT1 reduces the acetylation and activation of transcription factors, such as NF-kB, STAT3, AP-1, and FoxP3, leading to decreased inflammation, and apoptosis. SIRT1 can inhibit inflammation and oxidative stress through the deacetylation of FoxO1. SIRT1 also regulates DC-mediated Th1, Th2, and Treg immune responses. All these processes interact each other and contribute to the progression of Ads.
RA
RA is one of the most prevalent chronic disorders defined as a systemic breakdown of self-tolerance and immune-mediated inflammation characterized by cartilage/bone destruction and joint dysfunction (99). The inflammatory proliferation of RA fibroblast-like synoviocytes (FLSs) is the most important pathological feature of RA, which generates invasive synovial pannus and a series of pro-inflammatory cytokines and produces proteases that directly destroy bone and cartilage. Therefore, an effective method to slow the progression of RA is to promote apoptosis and inhibit the proliferation, invasion, and migration of FLSs, which suppress synovial inflammation and alleviate joint deformation. SIRT1 activation induces the apoptosis of FLSs through the activation of caspase-3 and the PI3K/Akt signalling pathway. Engler et al. found that SIRT1 silencing promotes the proliferation and adhesion of FLSs (100). SIRT1 overexpression not only reduces the production of proinflammatory cytokines but also inhibits the proliferation, invasion, and migration of FLSs, thereby effectively alleviating RA synovial inflammation (18, 19). These effects are associated with the activation of NF-κB (as shown in Figure 1). The upregulation of SIRT1 reduces COX2 levels in RA-FLSs by inhibiting the activation of AP-1 and NF-κB (101). The DNA binding activity of AP-1 is significantly increased in the synovial tissues of patients with RA and positively correlates with the disease activity of RA. Inhibition of AP-1 activity helps alleviate the disease (102).
SIRT1 activation by resveratrol reduces the activation of T cells, the production of TNF-α and IL-12, and the expression of CD28 and CD80 and upregulates CTLA4 expression in collagen-induced arthritis (CIA) mice and T cells. SIRT1 inhibition in T cells suppresses the resveratrol-induced inhibition of T cells and increases the acetylation of c-Jun and the incidence and severity of CIA (20). Resveratrol inhibits the activation of the MAPK signalling pathway and the expression of IL-1β in synovial tissues of CIA rats and exerts a positive regulatory effect on the development of arthritis (21). In mice with myeloid cell-specific deletion of SIRT1, an arthritis model (serum transfer from K/BxN arthritis mice) showed more severe inflammatory responses and pathological changes, including increases in IL-1β and TNF-α levels, as well as increases in the number of TRAP+ osteoclasts in the ankles (22). Compared to wild-type mice, macrophages obtained from mice with selective deletion of SRIT1 show increased migration, polarization, and proinflammatory cytokine production, which are associated with hyperacetylation of p65 and activation of NF-κB. Based on these results, SIRT1 suppresses the activation of innate immune cells, leading to less joint inflammation and damage. In contrast, Woo et al. (23) observed less joint inflammation and damage in myeloid cell-specific SIRT1 knockout arthritis mice than in wild-type mice with CIA. SIRT1 inhibition in CIA mice also reduces the production of inflammatory cytokines, MMPs and RORγt and decreases the proliferation of Th1, Th17, and DCs. In addition, impaired DC maturation and a reduction in the Th1/Th17 immune response are observed in these mice. The explanation for these differences among different studies may be attributed to the different pathogeneses in different arthritis models. The serum transfer model from K/BxN arthritis mice does not require the participation of T, B, and innate immune cells, which are required to produce autoantibodies in the CIA model.
Patients with RA suffer from multiple cartilage/bone erosion events and osteoporosis in the middle and late stages, a common clinical complication that can lead to joint deformity, severe dysfunction, and disability. SIRT1 heterozygous female mice show a significant decrease in bone density, suggesting that SIRT1 plays a role in regulating bone metabolism (103). Osteoblast-specific deletion of SIRT1 in mice significantly reduces osteoblast differentiation and bone mass and promotes the activation of the NF-κB signalling pathway and the differentiation and maturity of osteoclasts (24). Activated SIRT1 deacetylates NF-κB and p53 and reduces IL-1β, iNOS, and IL-6 levels and the inflammation and apoptosis of articular chondrocytes (25). Therefore, SIRT1 modulates the erosive destruction of articular cartilage and bone by regulating the differentiation, maturation, and apoptosis of osteoblasts, osteoclasts, and chondrocytes, reducing the disability rate of individuals with RA.
IBD
IBD is characterized by chronic relapsing intestinal inflammation and gastrointestinal bleeding, and includes two main types: Crohn’s disease and ulcerative colitis. IBD has received increasing attention in recent decades due to its increasing incidence rate worldwide (more than 2 million individuals have been diagnosed with IBD), especially in China. Although the exact pathogenesis of IBD is not fully understood, genetics, intestinal microbiota, and environmental factors have been considered as main regulators. The identification of new targets and definitive methods for IBD treatment is urgently needed. Several studies have reported that decreases in SIRT1 expression are critical for the development of IBD.
Sharma et al. (26) used dextran sulfate sodium-induced (DSS) IBD mice, which are the most commonly used IBD model because they share many manifestations and pathological characteristics with human disease, to explore the role of SIRT1 in the development of colonic inflammation. Treatment with resveratrol significantly improves DSS-induced colitis and restores the SIRT1 mRNA levels. Ren et al. (27) found that the SIRT1 activator SRT1720 decreases the disease activity index, inflammatory cytokine levels, and colon histological score in mice with DSS-induced colitis, whereas nicotinamide (SIRT1 inhibitor) administration exerts the opposite effects. Resveratrol suppresses the activation of the NLRP-3 inflammasome and alleviates bowel inflammation in mice with radiation-induced IBD (104). SIRT1 also participates in the development of chronic spontaneous colitis in an IL-10-deficient mouse model (105). Talero et al. found that IL-10-deficient mice are characterized by increased levels of cytokines and decreased SIRT1 mRNA levels in the colonic mucosa, which are associated with the upregulation of the autophagy pathway, promoting inflammation and dysplasia in mice. Caruso et al. (106) detected reduced levels of the SIRT1 mRNA and protein in colon tissues from mice with 2,4,6-trinitrobenzenesulfonic acid-induced colitis. The administration of a SIRT1 antagonist, EX-527, to mice increased the disease severity and infiltration of CD3+ T cells in the colon. Cay10591, a SIRT1 agonist, decreased the generation of proinflammatory cytokines. Moreover, the expression of the SIRT1 mRNA and protein was decreased in lamina propria mononuclear cells from patients with IBD, while treatment with a SIRT1 activator inhibited the activation of NF-κB and the generation of proinflammatory cytokines. Taken together, SIRT1 activation attenuates colitis, and SIRT1 may represent a promising target for treating IBD.
In contrast, several studies also found that SIRT1 may stimulate the pathogenesis of IBD. In one study, intestinal-specific deletion of SIRT1 protected mice from the development of colitis (28). DSS did not induce colitis successfully in SIRT1-deficient mice with decreased expression of inflammatory genes. Thus, the deletion of SIRT1 in the intestine exerts a positive effect on the development of IBD. In another study, Akimova et al. primarily determined the role of SIRT1-targeted T cells in the development and pathogenesis of chronic colitis in mice (29). The authors reported that adoptive transfer of CD4+ CD25- Foxp3- T effector (TE) cells from wild-type mice into B6/Rag1-/-mice induced chronic colitis, which was related to the expansion of disease-producing Th1 effector cells that promoted increases in weight loss and the infiltration of T cells into the colon. Moreover, the adoptive transfer of TE cells from SIRT1-deficient mice into B6/Rag1-/-mice resulted in lower colitis disease activity and reduced weight loss, as well as a 2.8-fold increase in the formation of iTregs, compared with mice receiving wild-type T cells. Therefore, naïve T cells tend to differentiate into iTregs in the absence of SIRT1. In a second mouse model, treatment with a SIRT1 inhibitor, EX-527, reduced weight loss and colonic inflammation and increased iTreg differentiation. The deletion of SIRT1 may inhibit the development of colitis through the induction of Tregs.
Both protective and deleterious effects of SIRT1 have been reported on individuals with IBD. The protective actions of SIRT1 are associated with decreased acetylation of NF-κB, which results in increased expression of proinflammatory cytokines. In contrast, the deletion or silencing of SIRT1 inhibits colitis through the induction of Tregs, which are essential for the maintenance of gastrointestinal homeostasis.
MS
MS is a chronic neuroinflammatory and demyelinating disease. Genetic and environmental factors may influence the susceptibility to and progression of MS. Several recent studies have provided evidence supporting the beneficial effects of SIRT1 on demyelinating and inflammatory diseases, such as MS. Compared with patients with MS who are in remission and healthy controls, SIRT1 activity is significantly decreased in patients with active MS (30). In addition, SIRT1 colocalizes with CD4+, CD68+, oligodendrocytes (OLGs), and glial fibrillary acidic protein-positive cells in MS lesions. Although a wide distribution of cells expresses SIRT1, higher SIRT1 expression was detected in the MS lesions than in the area adjacent to the MS lesions. Moreover, peripheral blood mononuclear cells (PBMCs) from patients with active MS expressed higher levels of the SIRT1 mRNA and protein than those from patients in remission and healthy controls. Based on these results, SIRT1 may represent a biomarker of relapse (15). Ciriello and Hewes et al. (31, 32) found that phosphorylated SIRT1 (p-SIRT1) and H3K9me3 are possible biomarkers for MS relapse, and SIRT1 and H3K9me3 potentially predict the response to glatiramer acetate (GA, a widely used drug in patients with MS) therapy. Higher SIRT1 mRNA and H3K9me2 levels are detected in responders to GA treatment than in nonresponders.
Shindler et al. tested whether activators of SIRT1, namely, SRT647 and SRT501, prevent neuronal loss caused by optic neuritis in an SJL/J model of experimental autoimmune encephalitis (EAE) (107). Activated SIRT1 inhibits retinal ganglion cell loss in a dose-dependent manner. In contrast, the inhibition of SIRT1 with sirtinol blocks the neuroprotective effects. In addition, activated SIRT1 increases the axonal density, protecting against neuronal damage and long-term neurological dysfunction. However, treatment with an activator does not reduce the disease index of EAE or attenuate optic nerve inflammation, indicating that neuroprotection is not associated with immunosuppression (as shown in Figure 1). In another study, SIRT1 activation attenuated optic neuritis induced by a neurotropic strain of hepatitis virus and MHV-A59 and reduced ROS levels (33). In addition, the activation of SIRT1 by oral resveratrol reduced disease severity in a mouse model of chronic EAE (108) and decreased neuronal loss and paralysis (109). SIRT1 protein expression was upregulated in the nuclei of NG2+ or PDGFRα+ oligodendrocyte progenitor cells in demyelinated brain lesions, which may inhibit the regeneration of functionally competent oligodendrocytes (110). McDougald et al. investigated the neuroprotective potential of SIRT1 using adeno-associated virus vector gene transfer in an EAE mouse model. Vector-SIRT1 improved the optokinetic response and protected retinal ganglion cells compared to Vector-eGFP controls (111). Treatment with resveratrol also reduces the production of proinflammatory cytokines, such as IL-6 and IL-12/23 p40, in EAE mice (34). SIRT1 overexpression in EAE mice significantly decreases the clinical score, inflammation, and myelin loss and improves axon preservation and neuronal survival (35). The neuroprotective effects appear to be associated with upregulated levels of NAD+ and brain-derived neurotrophic factors. Treatment with NAD+ for EAE in C57BL/6 mice alleviates the severity of EAE and activates SIRT1 (36). T cells are involved in regulating SIRT1 expression and the pathogenesis of most autoimmune syndromes, including MS. However, the regulatory effects of T cells on MS are not fully understood. Zhang et al. reported that adiponectin inhibits Th17 cell-mediated mouse autoimmune CNS inflammation. This process might be associated with increases in SIRT1 and PPARγ levels and the inhibition of RORγt and Th17 cell differentiation (37). Wang et al. reported that methylene blue reduces the clinical indices of mouse EAE models and attenuates pathological injuries in the spinal cord. The protective effects are associated with activation of SIRT1 and the Th17/Treg balance (38). SIRT1 inhibition increases the expression of FasL and promotes the apoptosis of CD4+ and CD8+ cells from patients with MS (39).
Conclusions
In recent years, the function of SIRT1 has expanded far beyond its initial impression as a prominent NAD+-dependent class III HDAC of the sirtuin family. SIRT1 participates in the complex coordination of the immune system and Ads. Although various articles have examined the roles of SIRT1 in suppressing the promotion of autoimmune diseases, many studies described in this review support the hypothesis that SIRT1 represents a possible biomarker of relapses and a potential target for therapeutic intervention in multiple Ads, including SLE, RA, IBD, MS, regardless of whether it functions as an activator or inhibitor. SIRT1 regulates the expression and activity of some transcription factors and genes, affecting immune cell activation, differentiation, and function.
SIRT1 not only affects histones deacetylation, but also deacetylation of various transcription factors, including p65, p53, FoxO family, STAT3, PGC1α, and PPARγ, leading to transcription repression. SIRT1 regulates the activity of p53 and FoxO3 through deacetylation and promotes cell survival via suppression of apoptosis and cell death in response to DNA damage and oxidative stress. The deficiency of SIRT1-mediated deacetylation of FoxO3a causes increased etoposide-induced apoptosis. On bone tissues, SIRT1 maintains its “self-renewal” ability through the inhibition of inflammation, oxidative stress, and senescence. SIRT1 also exerts anti-inflammatory effects through the inhibition of NF-kB, AP-1, and STAT3 pathways. SIRT1 causes the deacetylation and inactivation of STAT3 during caloric restriction. SIRT1 inhibits the NF-kB pathway through deacetylation of p65 and regulates cellular response to hypoxia via deacetylation of HIF-1α. However, loss of SIRT1 might lead to improved immune surveillance against pathogenic infection and nonself antigens, not all diseases benefit from the activation of SIRT1 or might even worsen Th2-mediated immune responses. All these highlight an important transcription modulatory function by SIRT1 activity, and the essential roles of SIRT1 in different Ads and different stages of the disease.
Although substantial progress has been achieved, the study of SIRT1 functions in the immune response is still in the early stage (Figure 1). In future studies, experiments are designed to understand how SIRT1 affects different cell types in a coordinated manner within the immune system and the different roles of SIRT1 in different subsets of T cells, B cells, and dendritic cells will be valuable. The precise function of SIRT1 in the development of Ads remains unclear, and future studies are also required to elucidate the molecular pathways and targets regulated by SIRT1 and their roles in treating Ads, which can be used to design precise and more efficient therapies with limited detrimental or unwanted effects. However, new models, methods, and techniques for investigating SIRT1 must be developed to promote its clinical application.
Author Contributions
PS and XD wrote the first draft of the manuscript. All authors participated in manuscript revision and have approved the submitted version of the manuscript.
Conflict of Interest
The authors declare that the research was conducted in the absence of any commercial or financial relationships that could be construed as a potential conflict of interest.
Publisher’s Note
All claims expressed in this article are solely those of the authors and do not necessarily represent those of their affiliated organizations, or those of the publisher, the editors and the reviewers. Any product that may be evaluated in this article, or claim that may be made by its manufacturer, is not guaranteed or endorsed by the publisher.
References
1. Kechida M. Update on Autoimmune Diseases Pathogenesis. Curr Pharm Des (2019) 25(27):2947–52. doi: 10.2174/1381612825666190709205421
2. Shen HH, Yang YX, Meng X, Luo XY, Li XM, Shuai ZW, et al. NLRP3: A Promising Therapeutic Target for Autoimmune Diseases. Autoimmun Rev (2018) 17(7):694–702. doi: 10.1016/j.autrev.2018.01.020
3. Bruserud Ø, Oftedal BE, Wolff AB, Husebye ES. AIRE-Mutations and Autoimmune Disease. Curr Opin Immunol (2016) 43:8–15. doi: 10.1016/j.coi.2016.07.003
4. Lee KH, Kronbichler A, Park DD, Park Y, Moon H, Kim H, et al. Neutrophil Extracellular Traps (NETs) in Autoimmune Diseases: A Comprehensive Review. Autoimmun Rev (2017) 16(11):1160–73. doi: 10.1016/j.autrev.2017.09.012
5. Rodgers JT, Lerin C, Haas W, Gygi SP, Spiegelman BM, Puigserver P. Nutrient Control of Glucose Homeostasis Through a Complex of PGC-1alpha and SIRT1. Nature (2005) 434(7029):113–8. doi: 10.1038/nature03354
6. Singh V, Ubaid S. Role of Silent Information Regulator 1 (SIRT1) in Regulating Oxidative Stress and Inflammation. Inflammation (2020) 43(5):1589–98. doi: 10.1007/s10753-020-01242-9
7. Zhao Q, Tian Z, Zhou G, Niu Q, Chen J, Li P, et al. SIRT1-Dependent Mitochondrial Biogenesis Supports Therapeutic Effects of Resveratrol Against Neurodevelopment Damage by Fluoride. Theranostics (2020) 10(11):4822–38. doi: 10.7150/thno.42387
8. Luo J, Nikolaev AY, Imai S, Chen D, Su F, Shiloh A, et al. Negative Control of P53 by Sir2alpha Promotes Cell Survival Under Stress. Cell (2001) 107(2):137–48. doi: 10.1016/S0092-8674(01)00524-4
9. Salminen A, Kaarniranta K. SIRT1: Regulation of Longevity via Autophagy. Cell Signal (2009) 21(9):1356–60. doi: 10.1016/j.cellsig.2009.02.014
10. Łukawska-Tatarczuk M, Franek E, Czupryniak L, Joniec-Maciejak I, Pawlak A, Wojnar E, et al. Sirtuin 1, Visfatin and IL-27 Serum Levels of Type 1 Diabetic Females in Relation to Cardiovascular Parameters and Autoimmune Thyroid Disease. Biomolecules (2021) 11(8):1108–10. doi: 10.3390/biom11081110
11. Leblond A, Pezet S, Cauvet A, Casas C, Pires Da Silva J, Hervé R, et al. Implication of the Deacetylase Sirtuin-1 on Synovial Angiogenesis and Persistence of Experimental Arthritis. Ann Rheum Dis (2020) 79(7):891–900. doi: 10.1136/annrheumdis-2020-217377
12. Hu N, Long H, Zhao M, Yin H, Lu Q. Aberrant Expression Pattern of Histone Acetylation Modifiers and Mitigation of Lupus by SIRT1-siRNA in MRL/lpr Mice. Scand J Rheumatol (2009) 38(6):464–71. doi: 10.3109/03009740902895750
13. Sequeira J, Boily G, Bazinet S, Saliba S, He X, Jardine K, et al. Sirt1-Null Mice Develop an Autoimmune-Like Condition. Exp Cell Res (2008) 314(16):3069–74. doi: 10.1016/j.yexcr.2008.07.011
14. Wang ZL, Luo XF, Li MT, Xu D, Zhou S, Chen HZ, et al. Resveratrol Possesses Protective Effects in a Pristane-Induced Lupus Mouse Model. PloS One (2014) 9(12):e114792. doi: 10.1371/journal.pone.0114792
15. Tegla CA, Azimzadeh P, Andrian-Albescu M, Martin A, Cudrici CD, Trippe R 3rd, et al. SIRT1 Is Decreased During Relapses in Patients With Multiple Sclerosis. Exp Mol Pathol (2014) 96(2):139–48. doi: 10.1016/j.yexmp.2013.12.010
16. Devi K, Singh N, Jaggi AS. Dual Role of Sirtuin 1 in Inflammatory Bowel Disease. Immunopharmacol Immunotoxicol (2020) 42(5):385–91. doi: 10.1080/08923973.2020.1790595
17. Wang Q, Yan C, Xin M, Han L, Zhang Y, Sun M. Sirtuin 1 (Sirt1) Overexpression in BaF3 Cells Contributes to Cell Proliferation Promotion, Apoptosis Resistance and Pro-Inflammatory Cytokine Production. Med Sci Monit (2017) 23:1477–82. doi: 10.12659/MSM.900754
18. Li G, Xia Z, Liu Y, Meng F, Wu X, Fang Y, et al. SIRT1 Inhibits Rheumatoid Arthritis Fibroblast-Like Synoviocyte Aggressiveness and Inflammatory Response via Suppressing NF-κB Pathway. Biosci Rep (2018) 38(3):BSR20180541. doi: 10.1042/BSR20180541
19. Niederer F, Ospelt C, Brentano F, Hottiger MO, Gay RE, Gay S, et al. SIRT1 Overexpression in the Rheumatoid Arthritis Synovium Contributes to Proinflammatory Cytokine Production and Apoptosis Resistance. Ann Rheum Dis (2011) 70(10):1866–73. doi: 10.1136/ard.2010.148957
20. Zou T, Yang Y, Xia F, Huang A, Gao X, Fang D, et al. Resveratrol Inhibits CD4+ T Cell Activation by Enhancing the Expression and Activity of Sirt1. PloS One (2013) 8(9):e75139. doi: 10.1371/journal.pone.0075139
21. Yang G, Chang CC, Yang Y, Yuan L, Xu L, Ho CT, et al. Resveratrol Alleviates Rheumatoid Arthritis via Reducing ROS and Inflammation, Inhibiting MAPK Signaling Pathways, and Suppressing Angiogenesis. J Agric Food Chem (2018) 66(49):12953–60. doi: 10.1021/acs.jafc.8b05047
22. Hah YS, Cheon YH, Lim HS, Cho HY, Park BH, Ka SO, et al. Myeloid Deletion of SIRT1 Aggravates Serum Transfer Arthritis in Mice via Nuclear Factor-κB Activation. PloS One (2014) 9(2):e87733. doi: 10.1371/journal.pone.0087733
23. Woo SJ, Lee SM, Lim HS, Hah YS, Jung ID, Park YM, et al. Myeloid Deletion of SIRT1 Suppresses Collagen-Induced Arthritis in Mice by Modulating Dendritic Cell Maturation. Exp Mol Med (2016) 48(3):e221. doi: 10.1038/emm.2015.124
24. Edwards JR, Perrien DS, Fleming N, Nyman JS, Ono K, Connelly L, et al. Silent Information Regulator (Sir)T1 Inhibits NF-κB Signaling to Maintain Normal Skeletal Remodeling. J Bone Miner Res (2013) 28(4):960–9. doi: 10.1002/jbmr.1824
25. Lei M, Wang JG, Xiao DM, Fan M, Wang DP, Xiong JY, et al. Resveratrol Inhibits Interleukin 1β-Mediated Inducible Nitric Oxide Synthase Expression in Articular Chondrocytes by Activating SIRT1 and Thereby Suppressing Nuclear Factor-κb Activity. Eur J Pharmacol (2012) 674(2-3):73–9. doi: 10.1016/j.ejphar.2011.10.015
26. Sharma M, Mohapatra J, Wagh A, Patel HM, Pandey D, Kadam S, et al. Involvement of TACE in Colon Inflammation: A Novel Mechanism of Regulation via SIRT-1 Activation. Cytokine (2014) 66(1):30–9. doi: 10.1016/j.cyto.2013.12.010
27. Ren MT, Gu ML, Zhou XX, Yu MS, Pan HH, Ji F, et al. Sirtuin 1 Alleviates Endoplasmic Reticulum Stress-Mediated Apoptosis of Intestinal Epithelial Cells in Ulcerative Colitis. World J Gastroenterol (2019) 25(38):5800–13. doi: 10.3748/wjg.v25.i38.5800
28. Lo Sasso G, Ryu D, Mouchiroud L, Fernando SC, Anderson CL, Katsyuba E, et al. Loss of Sirt1 Function Improves Intestinal Anti-Bacterial Defense and Protects From Colitis-Induced Colorectal Cancer. PloS One (2014) 9(7):e102495. doi: 10.1371/journal.pone.0102495
29. Akimova T, Xiao H, Liu Y, Bhatti TR, Jiao J, Eruslanov E, et al. Targeting Sirtuin-1 Alleviates Experimental Autoimmune Colitis by Induction of Foxp3+ T-Regulatory Cells. Mucosal Immunol (2014) 7(5):1209–20. doi: 10.1038/mi.2014.10
30. Li R, Sun X, Shu Y, Wang Y, Xiao L, Wang Z, et al. Serum CCL20 and its Association With SIRT1 Activity in Multiple Sclerosis Patients. J Neuroimmunol (2017) 313:56–60. doi: 10.1016/j.jneuroim.2017.10.013
31. Ciriello J, Tatomir A, Hewes D, Boodhoo D, Anselmo F, Rus V, et al. Phosphorylated SIRT1 as a Biomarker of Relapse and Response to Treatment With Glatiramer Acetate in Multiple Sclerosis. Exp Mol Pathol (2018) 105(2):175–80. doi: 10.1016/j.yexmp.2018.07.008
32. Hewes D, Tatomir A, Kruszewski AM, Rao G, Tegla CA, Ciriello J, et al. SIRT1 as a Potential Biomarker of Response to Treatment With Glatiramer Acetate in Multiple Sclerosis. Exp Mol Pathol (2017) 102(2):191–7. doi: 10.1016/j.yexmp.2017.01.014
33. Khan RS, Dine K, Das Sarma J, Shindler KS. SIRT1 Activating Compounds Reduce Oxidative Stress Mediated Neuronal Loss in Viral Induced CNS Demyelinating Disease. Acta Neuropathol Commun (2014) 2:3. doi: 10.1186/2051-5960-2-3
34. Imler TJ Jr., Petro TM. Decreased Severity of Experimental Autoimmune Encephalomyelitis During Resveratrol Administration Is Associated With Increased IL-17+IL-10+ T Cells, CD4(-) IFN-Gamma+ Cells, and Decreased Macrophage IL-6 Expression. Int Immunopharmacol (2009) 9(1):134–43. doi: 10.1016/j.intimp.2008.10.015
35. Nimmagadda VK, Bever CT, Vattikunta NR, Talat S, Ahmad V, Nagalla NK, et al. Overexpression of SIRT1 Protein in Neurons Protects Against Experimental Autoimmune Encephalomyelitis Through Activation of Multiple SIRT1 Targets. J Immunol (Baltimore Md 1950) (2013) 190(9):4595–607. doi: 10.4049/jimmunol.1202584
36. Wang J, Zhao C, Kong P, Sun H, Sun Z, Bian G, et al. Treatment With NAD(+) Inhibited Experimental Autoimmune Encephalomyelitis by Activating AMPK/SIRT1 Signaling Pathway and Modulating Th1/Th17 Immune Responses in Mice. Int Immunopharmacol (2016) 39:287–94. doi: 10.1016/j.intimp.2016.07.036
37. Zhang K, Guo Y, Ge Z, Zhang Z, Da Y, Li W, et al. Adiponectin Suppresses T Helper 17 Cell Differentiation and Limits Autoimmune CNS Inflammation via the SIRT1/Pparγ/Rorγt Pathway. Mol Neurobiol (2017) 54(7):4908–20. doi: 10.1007/s12035-016-0036-7
38. Wang J, Zhao C, Kong P, Bian G, Sun Z, Sun Y, et al. Methylene Blue Alleviates Experimental Autoimmune Encephalomyelitis by Modulating AMPK/SIRT1 Signaling Pathway and Th17/Treg Immune Response. J Neuroimmunol (2016) 299:45–52. doi: 10.1016/j.jneuroim.2016.08.014
39. Martin A, Tegla CA, Cudrici CD, Kruszewski AM, Azimzadeh P, Boodhoo D, et al. Role of SIRT1 in Autoimmune Demyelination and Neurodegeneration. Immunol Res (2015) 61(3):187–97. doi: 10.1007/s12026-014-8557-5
40. Vega RB, Horton JL, Kelly DP. Maintaining Ancient Organelles: Mitochondrial Biogenesis and Maturation. Circ Res (2015) 116(11):1820–34. doi: 10.1161/CIRCRESAHA.116.305420
41. Yang XJ, Seto E. The Rpd3/Hda1 Family of Lysine Deacetylases: From Bacteria and Yeast to Mice and Men. Nat Rev Mol Cell Biol (2008) 9(3):206–18. doi: 10.1038/nrm2346
42. Hadden MJ, Advani A. Histone Deacetylase Inhibitors and Diabetic Kidney Disease. Int J Mol Sci (2018) 19(9):201–9. doi: 10.3390/ijms19092630
43. Finkel T, Deng CX, Mostoslavsky R. Recent Progress in the Biology and Physiology of Sirtuins. Nature (2009) 460(7255):587–91. doi: 10.1038/nature08197
44. Kitada M, Kume S, Kanasaki K, Takeda-Watanabe A, Koya D. Sirtuins as Possible Drug Targets in Type 2 Diabetes. Curr Drug Targets (2013) 14(6):622–36. doi: 10.2174/1389450111314060002
45. Sacconnay L, Carrupt PA, Nurisso A. Human Sirtuins: Structures and Flexibility. J Struct Biol (2016) 196(3):534–42. doi: 10.1016/j.jsb.2016.10.008
46. Morigi M, Perico L, Benigni A. Sirtuins in Renal Health and Disease. J Am Soc Nephrol (2018) 29(7):1799–809. doi: 10.1681/ASN.2017111218
47. Chang AR, Ferrer CM, Mostoslavsky R. SIRT6, a Mammalian Deacylase With Multitasking Abilities. Physiol Rev (2020) 100(1):145–69. doi: 10.1152/physrev.00030.2018
48. Sun L, Fang J. Macromolecular Crowding Effect Is Critical for Maintaining SIRT1's Nuclear Localization in Cancer Cells. Cell Cycle (Georgetown Tex) (2016) 15(19):2647–55. doi: 10.1080/15384101.2016.1211214
49. Salvatori I, Valle C, Ferri A, Carrì MT. SIRT3 and Mitochondrial Metabolism in Neurodegenerative Diseases. Neurochem Int (2017) 109:184–92. doi: 10.1016/j.neuint.2017.04.012
50. Hershberger KA, Martin AS, Hirschey MD. Role of NAD(+) and Mitochondrial Sirtuins in Cardiac and Renal Diseases. Nat Rev Nephrol (2017) 13(4):213–25. doi: 10.1038/nrneph.2017.5
51. Wang T, Wang Y, Liu L, Jiang Z, Li X, Tong R, et al. Research Progress on Sirtuins Family Members and Cell Senescence. Eur J Med Chem (2020) 193:112207. doi: 10.1016/j.ejmech.2020.112207
52. Fang Y, Nicholl MB. Sirtuin 1 in Malignant Transformation: Friend or Foe? Cancer Lett (2011) 306(1):10–4. doi: 10.1016/j.canlet.2011.02.019
53. Yan T, Huang J, Nisar MF, Wan C, Huang W. The Beneficial Roles of SIRT1 in Drug-Induced Liver Injury. Oxid Med Cell Longev (2019) 2019:8506195. doi: 10.1155/2019/8506195
54. Kang H, Suh JY, Jung YS, Jung JW, Kim MK, Chung JH. Peptide Switch Is Essential for Sirt1 Deacetylase Activity. Mol Cell (2011) 44(2):203–13. doi: 10.1016/j.molcel.2011.07.038
55. Davenport AM, Huber FM, Hoelz A. Structural and Functional Analysis of Human SIRT1. J Mol Biol (2014) 426(3):526–41. doi: 10.1016/j.jmb.2013.10.009
56. Flick F, Lüscher B. Regulation of Sirtuin Function by Posttranslational Modifications. Front Pharmacol (2012) 3:29. doi: 10.3389/fphar.2012.00029
57. Fiorino E, Giudici M, Ferrari A, Mitro N, Caruso D, De Fabiani E, et al. The Sirtuin Class of Histone Deacetylases: Regulation and Roles in Lipid Metabolism. IUBMB Life (2014) 66(2):89–99. doi: 10.1002/iub.1246
58. Xie J, Zhang X, Zhang L. Negative Regulation of Inflammation by SIRT1. Pharmacol Res (2013) 67(1):60–7. doi: 10.1016/j.phrs.2012.10.010
59. Karbasforooshan H, Roohbakhsh A, Karimi G. SIRT1 and microRNAs: The Role in Breast, Lung and Prostate Cancers. Exp Cell Res (2018) 367(1):1–6. doi: 10.1016/j.yexcr.2018.03.023
60. Schug TT, Xu Q, Gao H, Peres-da-Silva A, Draper DW, Fessler MB, et al. Myeloid Deletion of SIRT1 Induces Inflammatory Signaling in Response to Environmental Stress. Mol Cell Biol (2010) 30(19):4712–21. doi: 10.1128/MCB.00657-10
61. Yoshizaki T, Schenk S, Imamura T, Babendure JL, Sonoda N, Bae EJ, et al. SIRT1 Inhibits Inflammatory Pathways in Macrophages and Modulates Insulin Sensitivity. Am J Physiol Endocrinol Metab (2010) 298(3):E419–428. doi: 10.1152/ajpendo.00417.2009
62. Imperatore F, Maurizio J, Vargas Aguilar S, Busch CJ, Favret J, Kowenz-Leutz E, et al. SIRT1 Regulates Macrophage Self-Renewal. EMBO J (2017) 36(16):2353–72. doi: 10.15252/embj.201695737
63. Zhang R, Chen HZ, Liu JJ, Jia YY, Zhang ZQ, Yang RF, et al. SIRT1 Suppresses Activator Protein-1 Transcriptional Activity and Cyclooxygenase-2 Expression in Macrophages. J Biol Chem (2010) 285(10):7097–110. doi: 10.1074/jbc.M109.038604
64. Liu G, Bi Y, Xue L, Zhang Y, Yang H, Chen X, et al. Dendritic Cell SIRT1-Hif1α Axis Programs the Differentiation of CD4+ T Cells Through IL-12 and TGF-β1. Proc Natl Acad Sci USA (2015) 112(9):E957–965. doi: 10.1073/pnas.1420419112
65. Legutko A, Marichal T, Fiévez L, Bedoret D, Mayer A, de Vries H, et al. Sirtuin 1 Promotes Th2 Responses and Airway Allergy by Repressing Peroxisome Proliferator-Activated Receptor-γ Activity in Dendritic Cells. J Immunol (Baltimore Md 1950) (2011) 187(9):4517–29. doi: 10.4049/jimmunol.1101493
66. Yang H, Lee SM, Gao B, Zhang J, Fang D. Histone Deacetylase Sirtuin 1 Deacetylates IRF1 Protein and Programs Dendritic Cells to Control Th17 Protein Differentiation During Autoimmune Inflammation. J Biol Chem (2013) 288(52):37256–66. doi: 10.1074/jbc.M113.527531
67. Zhang J, Lee SM, Shannon S, Gao B, Chen W, Chen A, et al. The Type III Histone Deacetylase Sirt1 is Essential for Maintenance of T Cell Tolerance in Mice. J Clin Invest (2009) 119(10):3048–58. doi: 10.1172/JCI38902
68. Omenetti S, Bussi C, Metidji A, Iseppon A, Lee S, Tolaini M, et al. The Intestine Harbors Functionally Distinct Homeostatic Tissue-Resident and Inflammatory Th17 Cells. Immunity (2019) 51(1):77–89.e76. doi: 10.1016/j.immuni.2019.05.004
69. Limagne E, Thibaudin M, Euvrard R, Berger H, Chalons P, Végan F, et al. Sirtuin-1 Activation Controls Tumor Growth by Impeding Th17 Differentiation via STAT3 Deacetylation. Cell Rep (2017) 19(4):746–59. doi: 10.1016/j.celrep.2017.04.004
70. Gardner PJ, Joshi L, Lee RW, Dick AD, Adamson P, Calder VL. SIRT1 Activation Protects Against Autoimmune T Cell-Driven Retinal Disease in Mice via Inhibition of IL-2/Stat5 Signaling. J Autoimmun (2013) 42:117–29. doi: 10.1016/j.jaut.2013.01.011
71. Stahl M, Dijkers PF, Kops GJ, Lens SM, Coffer PJ, Burgering BM, et al. The Forkhead Transcription Factor FoxO Regulates Transcription of p27Kip1 and Bim in Response to IL-2. J Immunol (Baltimore Md 1950) (2002) 168(10):5024–31. doi: 10.4049/jimmunol.168.10.5024
72. Beier UH, Wang L, Bhatti TR, Liu Y, Han R, Ge G, et al. Sirtuin-1 Targeting Promotes Foxp3+ T-Regulatory Cell Function and Prolongs Allograft Survival. Mol Cell Biol (2011) 31(5):1022–9. doi: 10.1128/MCB.01206-10
73. Olivares D, Perez-Hernandez J, Forner MJ, Perez-Soriano C, Tormos MC, Saez GT, et al. Urinary Levels of Sirtuin-1 Associated With Disease Activity in Lupus Nephritis. Clin Sci (London Engl 1979) (2018) 132(5):569–79. doi: 10.1042/CS20171410
74. Taniguchi K, Karin M. NF-κB, Inflammation, Immunity and Cancer: Coming of Age. Nat Rev Immunol (2018) 18(5):309–24. doi: 10.1038/nri.2017.142
75. Chen X, Lu Y, Zhang Z, Wang J, Yang H, Liu G. Intercellular Interplay Between Sirt1 Signalling and Cell Metabolism in Immune Cell Biology. Immunology (2015) 145(4):455–67. doi: 10.1111/imm.12473
76. O'Neill LA, Hardie DG. Metabolism of Inflammation Limited by AMPK and Pseudo-Starvation. Nature (2013) 493(7432):346–55. doi: 10.1038/nature11862
77. Rasha F, Mims BM, Castro-Piedras I, Barnes BJ, Grisham MB, Rahman RL, et al. The Versatility of Sirtuin-1 in Endocrinology and Immunology. Front Cell Dev Biol (2020) 8:589016. doi: 10.3389/fcell.2020.589016
78. Owczarczyk AB, Schaller MA, Reed M, Rasky AJ, Lombard DB, Lukacs NW. Sirtuin 1 Regulates Dendritic Cell Activation and Autophagy During Respiratory Syncytial Virus-Induced Immune Responses. J Immunol (Baltimore Md 1950) (2015) 195(4):1637–46. doi: 10.4049/jimmunol.1500326
79. Chuprin A, Avin A, Goldfarb Y, Herzig Y, Levi B, Jacob A, et al. The Deacetylase Sirt1 is an Essential Regulator of Aire-Mediated Induction of Central Immunological Tolerance. Nat Immunol (2015) 16(7):737–45. doi: 10.1038/ni.3194
80. McPherson JP, Sarras H, Lemmers B, Tamblyn L, Migon E, Matysiak-Zablocki E, et al. Essential Role for Bclaf1 in Lung Development and Immune System Function. Cell Death Differ (2009) 16(2):331–9. doi: 10.1038/cdd.2008.167
81. Kong S, Kim SJ, Sandal B, Lee SM, Gao B, Zhang DD, et al. The Type III Histone Deacetylase Sirt1 Protein Suppresses P300-Mediated Histone H3 Lysine 56 Acetylation at Bclaf1 Promoter to Inhibit T Cell Activation. J Biol Chem (2011) 286(19):16967–75. doi: 10.1074/jbc.M111.218206
82. Lim HW, Kang SG, Ryu JK, Schilling B, Fei M, Lee IS, et al. SIRT1 Deacetylates Rorγt and Enhances Th17 Cell Generation. J Exp Med (2015) 212(5):607–17. doi: 10.1084/jem.20132378
83. Gardner PJ, Yazid S, Chu CJ, Copland DA, Adamson P, Dick AD, et al. TNFα Regulates SIRT1 Cleavage During Ocular Autoimmune Disease. Am J Pathol (2015) 185(5):1324–33. doi: 10.1016/j.ajpath.2015.01.017
84. Kaplan MH, Hufford MM, Olson MR. The Development and In Vivo Function of T Helper 9 Cells. Nat Rev Immunol (2015) 15(5):295–307. doi: 10.1038/nri3824
85. Wang Y, Bi Y, Chen X, Li C, Li Y, Zhang Z, et al. Histone Deacetylase SIRT1 Negatively Regulates the Differentiation of Interleukin-9-Producing CD4(+) T Cells. Immunity (2016) 44(6):1337–49. doi: 10.1016/j.immuni.2016.05.009
86. van Loosdregt J, Vercoulen Y, Guichelaar T, Gent YY, Beekman JM, van Beekum O, et al. Regulation of Treg Functionality by Acetylation-Mediated Foxp3 Protein Stabilization. Blood (2010) 115(5):965–74. doi: 10.1182/blood-2009-02-207118
87. Marcel N, Perumalsamy LR, Shukla SK, Sarin A. The Lysine Deacetylase Sirtuin 1 Modulates the Localization and Function of the Notch1 Receptor in Regulatory T Cells. Sci Signal (2017) 10(473):eaah4679. doi: 10.1126/scisignal.aah4679
88. Kwon HS, Lim HW, Wu J, Schnölzer M, Verdin E, Ott M. Three Novel Acetylation Sites in the Foxp3 Transcription Factor Regulate the Suppressive Activity of Regulatory T Cells. J Immunol (Baltimore Md 1950) (2012) 188(6):2712–21. doi: 10.4049/jimmunol.1100903
89. Levine MH, Wang Z, Xiao H, Jiao J, Wang L, Bhatti TR, et al. Targeting Sirtuin-1 Prolongs Murine Renal Allograft Survival and Function. Kidney Int (2016) 89(5):1016–26. doi: 10.1016/j.kint.2015.12.051
90. Ye Q, Zhang M, Wang Y, Fu S, Han S, Wang L, et al. Sirtinol Regulates the Balance of Th17/Treg to Prevent Allograft Rejection. Cell Biosci (2017) 7:55. doi: 10.1186/s13578-017-0182-2
91. van Loosdregt J, Brunen D, Fleskens V, Pals CE, Lam EW, Coffer PJ. Rapid Temporal Control of Foxp3 Protein Degradation by Sirtuin-1. PloS One (2011) 6(4):e19047. doi: 10.1371/journal.pone.0019047
92. Buhrmann C, Busch F, Shayan P, Shakibaei M. Sirtuin-1 (SIRT1) is Required for Promoting Chondrogenic Differentiation of Mesenchymal Stem Cells. J Biol Chem (2014) 289(32):22048–62. doi: 10.1074/jbc.M114.568790
93. Assadiasl S, Mooney N, Mohebbi B, Fatahi Y, Soleimanifar N. Sirtuin 1: A Dilemma in Transplantation. J Transplant (2020) 2020:9012980. doi: 10.1155/2020/9012980
94. Aksoy P, Escande C, White TA, Thompson M, Soares S, Benech JC, et al. Regulation of SIRT 1 Mediated NAD Dependent Deacetylation: A Novel Role for the Multifunctional Enzyme CD38. Biochem Biophys Res Commun (2006) 349(1):353–9. doi: 10.1016/j.bbrc.2006.08.066
95. Gan H, Shen T, Chupp DP, Taylor JR, Sanchez HN, Li X, et al. B Cell Sirt1 Deacetylates Histone and non-Histone Proteins for Epigenetic Modulation of AID Expression and the Antibody Response. Sci Adv (2020) 6(14):eaay2793. doi: 10.1126/sciadv.aay2793
96. Consiglio CR, Juliana da Silveira S, Monticielo OA, Xavier RM, Brenol JC, Chies JA. SIRT1 Promoter Polymorphisms as Clinical Modifiers on Systemic Lupus Erythematosus. Mol Biol Rep (2014) 41(7):4233–9. doi: 10.1007/s11033-014-3294-3
97. Wu Z, Mei X, Ying Z, Sun Y, Song J, Shi W. Ultraviolet B Inhibition of DNMT1 Activity via AhR Activation Dependent SIRT1 Suppression in CD4+ T Cells From Systemic Lupus Erythematosus Patients. J Dermatol Sci (2017) 86(3):230–7. doi: 10.1016/j.jdermsci.2017.03.006
98. Hu N, Qiu X, Luo Y, Yuan J, Li Y, Lei W, et al. Abnormal Histone Modification Patterns in Lupus CD4+ T Cells. J Rheumatol (2008) 35(5):804–10. doi: 10.1016/j.jbspin.2007.10.004
99. Weyand CM, Goronzy JJ. The Immunology of Rheumatoid Arthritis. Nat Immunol (2021) 22(1):10–8. doi: 10.1038/s41590-020-00816-x
100. Engler A, Tange C, Frank-Bertoncelj M, Gay RE, Gay S, Ospelt C. Regulation and Function of SIRT1 in Rheumatoid Arthritis Synovial Fibroblasts. J Mol Med (Berlin Germany) (2016) 94(2):173–82. doi: 10.1007/s00109-015-1332-9
101. Yang CM, Chen YW, Chi PL, Lin CC, Hsiao LD. Resveratrol Inhibits BK-Induced COX-2 Transcription by Suppressing Acetylation of AP-1 and NF-κB in Human Rheumatoid Arthritis Synovial Fibroblasts. Biochem Pharmacol (2017) 132:77–91. doi: 10.1016/j.bcp.2017.03.003
102. Asahara H, Fujisawa K, Kobata T, Hasunuma T, Maeda T, Asanuma M, et al. Direct Evidence of High DNA Binding Activity of Transcription Factor AP-1 in Rheumatoid Arthritis Synovium. Arthritis Rheum (1997) 40(5):912–8. doi: 10.1002/art.1780400520
103. Cohen-Kfir E, Artsi H, Levin A, Abramowitz E, Bajayo A, Gurt I, et al. Sirt1 Is a Regulator of Bone Mass and a Repressor of Sost Encoding for Sclerostin, a Bone Formation Inhibitor. Endocrinology (2011) 152(12):4514–24. doi: 10.1210/en.2011-1128
104. Sun H, Cai H, Fu Y, Wang Q, Ji K, Du L, et al. The Protection Effect of Resveratrol Against Radiation-Induced Inflammatory Bowel Disease via NLRP-3 Inflammasome Repression in Mice. Dose Response Publ Int Hormesis Soc (2020) 18(2):1559325820931292. doi: 10.1177/1559325820931292
105. Talero E, Alcaide A, Ávila-Román J, García-Mauriño S, Vendramini-Costa D, Motilva V. Expression Patterns of Sirtuin 1-AMPK-Autophagy Pathway in Chronic Colitis and Inflammation-Associated Colon Neoplasia in IL-10-Deficient Mice. Int Immunopharmacol (2016) 35:248–56. doi: 10.1016/j.intimp.2016.03.046
106. Caruso R, Marafini I, Franzè E, Stolfi C, Zorzi F, Monteleone I, et al. Defective Expression of SIRT1 Contributes to Sustain Inflammatory Pathways in the Gut. Mucosal Immunol (2014) 7(6):1467–79. doi: 10.1038/mi.2014.35
107. Shindler KS, Ventura E, Rex TS, Elliott P, Rostami A. SIRT1 Activation Confers Neuroprotection in Experimental Optic Neuritis. Invest Ophthalmol Visual Sci (2007) 48(8):3602–9. doi: 10.1167/iovs.07-0131
108. Shindler KS, Ventura E, Dutt M, Elliott P, Fitzgerald DC, Rostami A. Oral Resveratrol Reduces Neuronal Damage in a Model of Multiple Sclerosis. J Neuroophthalmol (2010) 30(4):328–39. doi: 10.1097/WNO.0b013e3181f7f833
109. Fonseca-Kelly Z, Nassrallah M, Uribe J, Khan RS, Dine K, Dutt M, et al. Resveratrol Neuroprotection in a Chronic Mouse Model of Multiple Sclerosis. Front Neurol (2012) 3:84. doi: 10.3389/fneur.2012.00084
110. Prozorovski T, Ingwersen J, Lukas D, Göttle P, Koop B, Graf J, et al. Regulation of Sirtuin Expression in Autoimmune Neuroinflammation: Induction of SIRT1 in Oligodendrocyte Progenitor Cells. Neurosci Lett (2019) 704:116–25. doi: 10.1016/j.neulet.2019.04.007
Keywords: autoimmune diseases, SIRT1, inflammation, rheumatoid arthritis, systemic lupus erythematosus
Citation: Shen P, Deng X, Chen Z, Ba X, Qin K, Huang Y, Huang Y, Li T, Yan J and Tu S (2021) SIRT1: A Potential Therapeutic Target in Autoimmune Diseases. Front. Immunol. 12:779177. doi: 10.3389/fimmu.2021.779177
Received: 20 September 2021; Accepted: 08 November 2021;
Published: 23 November 2021.
Edited by:
Li-Tung Huang, Kaohsiung Chang Gung Memorial Hospital, TaiwanReviewed by:
Manolo Sambucci, Santa Lucia Foundation (IRCCS), ItalyMario Galgani, University of Naples Federico II, Italy
Copyright © 2021 Shen, Deng, Chen, Ba, Qin, Huang, Huang, Li, Yan and Tu. This is an open-access article distributed under the terms of the Creative Commons Attribution License (CC BY). The use, distribution or reproduction in other forums is permitted, provided the original author(s) and the copyright owner(s) are credited and that the original publication in this journal is cited, in accordance with accepted academic practice. No use, distribution or reproduction is permitted which does not comply with these terms.
*Correspondence: Shenghao Tu, shtu@tjh.tjmu.edu.cn
†These authors have contributed equally to this work