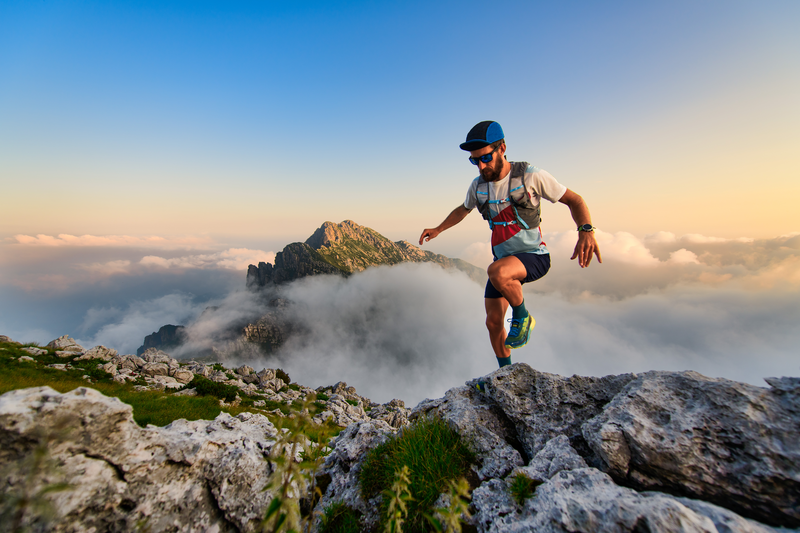
95% of researchers rate our articles as excellent or good
Learn more about the work of our research integrity team to safeguard the quality of each article we publish.
Find out more
REVIEW article
Front. Immunol. , 22 November 2021
Sec. Vaccines and Molecular Therapeutics
Volume 12 - 2021 | https://doi.org/10.3389/fimmu.2021.771242
Coronavirus disease 2019 (COVID-19) pandemic is a serious threat to global public health and social and economic development. Various vaccine platforms have been developed rapidly and unprecedentedly, and at least 16 vaccines receive emergency use authorization (EUA). However, the causative pathogen severe acute respiratory syndrome coronavirus-2 (SARS-CoV-2) has continued to evolve and mutate, emerging lots of viral variants. Several variants have successfully become the predominant strains and spread all over the world because of their ability to evade the pre-existing immunity obtained after previous infections with prototype strain or immunizations. Here, we summarized the prevalence and biological structure of these variants and the efficacy of currently used vaccines against the SARS-CoV-2 variants to provide guidance on how to design vaccines more rationally against the variants.
Severe acute respiratory syndrome coronavirus-2 (SARS-CoV-2), the causative pathogen of coronavirus disease 2019 (COVID-19), was first reported in early December 2019 in Wuhan, China (1). As of 18, October 2021, the COVID-19 pandemic has resulted in more than 240 million confirmed cases worldwide, with at least 4.9 million deaths (2). To deal with the ongoing COVID-19 pandemic, a variety of vaccines have been developed rapidly and unprecedentedly. Various vaccine platforms are available for the development of SARS-CoV-2 vaccines, such as protein or peptide subunit, recombinant viral vectors, nucleic acid (DNA or mRNA)-based vaccines, live attenuated virus, inactivated whole virus, and virus-like particle, etc. There are 126 vaccines currently in clinical development and 194 vaccine candidates at the pre-clinical developmental stage (3). More importantly, at least 16 vaccines have now gained EUA from their National Regulators Authorities (NRAs) of different countries. Globally, as of 18, October 2021, a cumulative number of 6 billion vaccine doses have been administered (2). Interestingly, clinical trial results showed that all of them could provide good efficacies to protect immunized individuals against infection with SARS-CoV-2 prototypical, wild-type SARS-CoV-2 Wuhan strain, especially to arrest the disease progression and severity in real and natural environments.
However, with the number of infected individuals increasing and the epidemic continuing, SARS-CoV-2 has been found to continue to evolve and mutate, with many viral variants emerging in different regions of countries. A few variants have successfully evolved and become the predominant strains to replace the prototype strain under selective pressure and then spread out across the world with the pandemic (4). Correspondingly, a key issue has been raised whether the currently available emergency vaccines could effectively protect against these emerging epidemic variants.
The SARS-CoV-2 uses the angiotensin-converting enzyme 2 (ACE2), the same receptor as SARS-CoV (5), to infect humans. The spike (S) protein on the viral envelope of SARS-CoV-2 with a total length of 1,273 amino acids recognizes and binds to the ACE2 receptor on the host cell. S protein contains five functional domains. N-terminal domain (NTD, 12–306 residues) and receptor-binding domain (RBD, 328-533 residues) on S1 subunit are responsible for receptor binding. The fusion peptide (FP, 815-826 residues), heptapeptide repeat sequence 1 (HR1, 912–984 residues), and HR2 (1163–1213 residues) on S2 subunit are involved in the process of membrane fusion (6). The earliest reported SARS-CoV-2 variant had a D614G mutant on the S protein in April 2020 (7). Since then, the emergency of SARS-CoV-2 variants has attracted significant attention. If SARS-CoV-2 variants show an effective adaptation to a new host, they might have positive genetic mutations that affect receptor binding and enhance multiplication and propagation of the virus in infected people (8). Up to now, more than 1200 amino acid sites of the S protein have been found capable to produce mutations with the genome of Wuhan-Hu-1 (GenBank: NC_045512) as a reference sequence (Figure 1A). Different countries have reported a large of SARS-CoV-2 new variants. These mutations were mainly concentrated on RBD, NTD, and HR1 of the S protein. To deeply understand the impact of these mutations on the transmission capacity and virulence of SARS-CoV-2, we elaborated on mutations and epidemiology of SARS-CoV-2 variants and their evasive immune effects to different emergency used vaccines. Our works provide a scientific background for designing and developing more effective vaccines against SARS-CoV-2 variants.
Figure 1 Global mutation landscape of spike and mutation prevalence across lineages in variants of concern or interest. (A) Global mutation landscape of spike. Based on a spike alignment containing 3,105,458 sequences around the world (https://cov.lanl.gov, accessed 3 October 2021.), the frequencies of mutations on the different positions of the spike protein of SARS-COV-2 were analyzed and shown in the figure. (B) Mutation prevalence across lineages in variants of concern or interest. We select the mutations with > 95% prevalence in at least one lineage to product the mutation prevalence across lineages of concern or interest, using outbreak lineage comparison (https://outbreak.info/compare-lineages, accessed 13 October 2021) in 4,204,861 sequences. The color of blue means the higher mutation frequency. The grey color means no mutations detected.
For unification and standardization, WHO, recently, reclassified SARS-CoV-2 variants as variants of concern (VOC) and variants of interest (VOI) emerging around the world, based on their prevalence, transmissibility, disease severity, and immune escape ability (Table 1) (15). VOC consist of the following variants. Alpha as known as B.1.1.7 and new Q sub-lineages in Pango lineage (9), 20I (V1) in next strain clade, GRY (formerly GR/501Y.V1) in GISAID clade, was first identified in United Kingdom, in September 2020. Beta, known as B.1.351 in Pango lineage (10), 20H (V2) in nextstrain clade, GH/501Y.V2 in GISAID clade, was first identified in South Africa, in May 2020. Gamma, known as P.1 in Pango lineage (11), 20J (V3) in nextstrain clade, GR/501Y.V3 in GISAID clade, was first identified in Brazil, in November 2020. Delta, known as B.1.617.2 and new AY sub-lineages in Pango lineage (12), 21A in next strain clade, G/478K.V1 in GISAID clade, was first identified in India, in October 2020.
VOI contain Lambda (a.k.a., C.37) from Peru (13) and Mu (a.k.a., B.1.621) from Colombia (14). Lambda with an emerging mutation(F490S) in RBD and a deletion (at positions 247/253) in NTD has been detected in at least 33 countries (Figure 2A) (16). Mu with an emerging mutation (R346K) in RBD and a P681H mutation in the furin cleavage site has been detected in at least 49 countries (Figure 2B) (17). In addition, several variants such as B.1.427/429, B.1.525, B.1.526, and B.1.617.1, previously designated as VOI have finally proven to no longer pose a significant risk to global public health (15).
Figure 2 Frequencies of VOC and VOI in relevant positions. We collect and analyze the frequencies for the spike mutations in VOI (A, B) and VOC (C–F) worldwide from December 24, 2019 to October 13, 2021, using the Analyze Align (AA) tool at cov.lanl.gov (https://cov.lanl.gov/content/index). The log on the vertical axis indicates the amino acid frequency in the data set and the horizontal axis shows the mutation sites in the S protein, with NC_045512 as a reference. The gray box indicates deletion.
Interestingly, we observed there were 3,121,316 sequences containing the G614 mutation in a total of 3,148,973 spike sequences (ratio, 0.99) from cov.lanl.gov (https://cov.lanl.gov/content/index) and found that the first discovered mutation D614G existed in almost all variants. This mutation benefits the open state of the homologous S protein trimer, which can help the S protein to bind closely with the receptor and thus promoting infection and transmission (18). Moreover, there are many combinations of mutations in the RBD among VOC. These usually occur in several key positions across different VOC lineages, such as K417, L452, T478, E484, and N501, P681 etc. (Figure 1B). Importantly, convergent evolution of mutations confers to the stronger ability of VOC for either transmission or immune evasion (19).
Since April 2021, VOC have accounted for more than 70% of all widespread variants globally. Among these, B.1.1.7 has attributed nearly half of all detected strains. Another prevalent strain belongs to B.1.351 lineage, which remained stable at around 7% of all detected strains. The epidemic variants B.1.617.2, identified Indian strain and P.1, earlier reported Brazilian strain have been increasing rapidly. The detected proportion of B.1.617.2 has rose to over 80% in August 2021 (Figure 3).
Figure 3 Emergence and prevalence of several important SARS-CoV-2 variants around the world. (A) The maximum-likelihood phylogenetic tree of SARS-CoV-2. The bootstrap values were shown at the corresponding nodes. The branches with dots at the ends represented randomly selected sequences. (B) The prevalence of several important variants from February to August, 2021. The ordinate represented the proportion of different linages. The strains were shown by different colors that marked on the map.
Korber et al. first reported that the variant with the D614G mutation on the S protein emerged during early COVID19 pandemic (20). Since then, it has rapidly become the predominant circulating variant. At the same time, a study showed that D614G variant infections resulted in a higher level of viral load and became more infectious or transmissible than D614 wild type virus did, although no evidence supported that D614G variant was associated with high mortality or disease severity (21). This finding was consistent with the results from animal challenge experiments (22). Interestingly, antibodies induced by either G614 variant or the prototype strain infections have cross-neutralization activity (21, 22).
B.1.1.7 lineage, also known as VOC-202012/01, or 20B/501Y.V1, first emerged in September 2020 (23, 24) and became the predominant strain in UK quickly. It has been detected in at least 180 countries (25). B.1.1.7 contains 7 amino acid substitutions in the S protein (Figure 2C), a N501Y mutation in the RBD (i.e., an amino acid substitution of asparagine to tyrosine at position 501) and 3 amino acids deletions in the viral NTD (i.e., the deletions of histidine, valine and tyrosine at positions 69-70 and 144/145, respectively) (26). Another mutation P681H is adjacent to the furin cleavage site which plays an essential role in viral transmission and infection (27). Thus, B.1.1.7 may be more transmissible and possibly stronger pathogenicity than other SARS-CoV-2 variants (28). Even so, neutralization antibody (nAb) titers of sera from immunized individuals or convalescent patients against B.1.1.7 lineage only slightly decreased, based on the pseudovirus neutralization assay (29).
B.1.351 lineage (a.k.a., Beta or 20H/501Y.V2) first identified in South Africa has been detected in at least 114 countries and 51 states of USA (30). During December 2020 to March 2021, the average daily prevalence of B.1.351 in South African reached up to 80% (30). B.1.351 lineage contains 9 amino acid substitutions in the S protein, such as K417N, E484K, and N501Y in the viral RBD, and 3 amino acids deleted at positions 242-244 in the viral NTD (10). The combination of three mutations K417N/E484K/N501Y in the RBD region resulted in a significant decrease of anti-RBD nAb titers. Of these mutations, E484K plays a major role (31). K417N reduces the neutralizing activity through attenuating its polar contact with the complementary determination region (31). The 242-244 deletion reduces the neutralizing activity of anti-NTD nAb via changing the conformation of the NTD antigen supersite (Figure 2D) (32, 33). More importantly, B.1.351 variants showed significant resistance to the nAb in the sera of recovered patients and immunized population (34). Interestingly, the serum of convalescent patients infected with the B.1.351 variant showed effective cross-neutralization against other variants (35).
P.1 lineage (a.k.a., Gamma) was first identified in Brazil with several mutations of biological significance such as E484K, N501Y and K417T in the RBD and five mutations in the NTD (Figure 2E) (32, 33). Currently, P.1 has been detected in at least 75 countries. From April to June 2021, 80% daily infected individuals in Brazil were caused by P.1 (36). The study demonstrated that the infectivity of P.1 might be 1.7 to 2.4 times higher than non-P.1 infections (37). Moreover, the sera from immunized individuals or convalescent plasma showed a more significant drop of neutralizing activity against P.1 (38).
B.1.617.2 (a.k.a., Delta), reclassified by WHO as VOC due to its high transmissibility and pathogenicity, has been detected in at least 157 countries since its outbreak in April 2021. More than 90% cases per day were caused by B.1.617.2 in India (39). It contains 8 amino acid substitutions in the spike protein and 2 amino deletions, i.e., L452R and T478K mutations in RBD along with a P681R in the furin cleavage site (Figure 2F) (40). These mutations not only enhance the ability of B.1.617.2 to bind with the ACE2 receptor, but also improve the cutting efficiency between S1 and S2 units. Eventually, the variant is highly contagious, with a stronger transmission and a higher risk of hospitalization, when compared to B.1.1.7 (41). It is noteworthy that seropositive individuals vaccinated current available vaccines could not provide sufficient protection to prevent B.1.617.2 infections (42, 43).
Here, we have examined all the vaccines having received EUA from RNAs (Table 2) and their protective efficacies on the prototype strain of SARS-CoV-2 and its various variants (Table 3).
Since December 2, 2020, BNT162b2 (Pfizer – BioNTech – Fosun Pharma) was used as an emergency vaccine in the UK for the first time to prevent people over 16 years of age against the infection with COVID-19 (84). Much later, the U.S. FDA approved the emergency use of the Pfizer vaccine (85). BNT162b2 was constructed based on an mRNA modified by nucleosides with substitution of two prolines encoding the complete S protein (S-2P) of the prototype strain SARS-CoV-2 and encapsulated in a nanoparticle lipid (LNP) which aims to protect mRNA from the degradation (44). A phase 2/3 clinical trial result showed that BNT162b2 conferred almost 95% protection against SARS-CoV-2 infection after the second dose (61). Other studies showed that the vaccine efficacies were approximately 88%, 93%, and 75.0% against the Delta, Alpha, and Beta variants, respectively (41, 62). Skowronski et al. conducted a clinical study in British Columbia and Canada, and found that the vaccine was 61% effective against Gamma variants at ≥21 days after individuals received the first dose of the vaccine (63). BNT162b2 vaccination effectively reduced the risk of hospitalization of patients infected with SARS-CoV-2 prototype strain, as well as the Delta strain (42). Although the protection against variant B.1.351 significantly reduced, antibodies induced by BNT162b2 showed effective against variants B.1.1.7, COH.20G/677H Columbus Ohio, and 20A.EU2 Europe (86). Interestingly, the effect of vaccination might be affected by several factors. Among these, age might be a key factor. Collier et al. showed a significant drop of serum nAb titers with age in immunized individuals, and the protection against variants B.1.1.7, B.1.351, and P.1 also decreased. Moreover, the number of SARS-CoV-2 specific memory B cells, IFN-γ+ and/or IL-2+ T cells was lower in vaccinated people over 80 years old than in younger people (87). Besides, BNT162b2 vaccine indicated high efficacy in pregnant women and immunized adolescents aged 12 to 15 years, and the efficacy was similar to that in the general population (88, 89). Interestingly, in a study in the UK, antibody responses to BNT162b2 showed that women and the individuals young and previously infected with SARS-CoV-2 had higher IgG levels afterward vaccination (90). However, whether gender is the factor to affect the immunization remains controversial (90, 91). In immunocompromised individuals receiving immunosuppressive drugs, Hadjadj et al. observed that antibody responses had partial neutralizing activity against Alpha, and even weaker against Delta stains, and their T cell responses were also altered (92). Therefore, immunosuppressive drugs for specific diseases might affect the vaccine-induced immunological effects. However, in patients with solid malignancies, the nAb (seroconversion: 79% in a vaccine, 84% in control) and polyfunctional T cell responses could be observed six months after vaccination (93).
Another mRNA-based vaccine, known as mRNA-1273, was developed by Moderna and the National Institute of Allergy and Infectious Diseases. It was prepared by using mRNA, which encoded the prefusion stabilized S-2P protein, then encapsulated in a lipid nanoparticle (45). The mRNA-1273 vaccine was approved for emergency use by the FDA on December 18, 2020 (94). A phase 3 clinical trial demonstrated 94.1% efficacy of mRNA-1273 vaccine against COVID-19 illnesses, including serious illnesses, in participants aged ≥18 years (64). Also, the mRNA-1273 showed highly protective efficacies against variants B.1.1.7 and B.1.351 infection in all cases of COVID-19, i.e., 88.1% and 61.3% ≥ 14 days post-one dose, 100% and 96.4% post-two doses (65). Furthermore, the mRNA-1273 vaccine remained highly effective against Gamma and emerging Delta and Mu variants , and the vaccine efficacies against these variants were 95.5%, 86.7% and 90.4%, respectively. However, the efficacies against these variants gradually decreased with the duration of the post-vaccination time (66). On the other hand, the vaccine-induced antibodies showed neutralizing activity against four variants, including nCoV/USA_WA1/2020 (line A.1), EHC-083E (line B.1), B.1.1.7, and N501Y (95), but the neutralization activity against B.1.351 variant was significantly lost (96). Remarkably, S protein-specific IFN-γ+ T lymphocyte responses against B.1.1.7 and B.1.351 variants were induced after immunization despite reduced nAb titers (97). Unlike another mRNA vaccine, BNT162b2, similar levels of nAb were observed in mRNA-1273 vaccinated adolescents aged 12 to 15 years, in people aged 56 years and older, and in pregnant women (98, 99). Besides, the immune status of the body also affected vaccine efficacy. A study of immunocompromised populations showed seropositivity rates of 98.1% for healthcare workers, 37.2% for solid organ transplants, 83.8% for autoimmune diseases, 54.7% for hematologic malignancies and 82.4% for solid tumors (100). In addition to the above, Moderna developed another vaccine against the B.1.351 and other variants, called mRNA-1273.351, based on cross-neutralizing activity (101).
The inactivated SARS-CoV-2 (lnCoV) vaccine was produced by the Sinopharm-Beijing Bio-Institute of Biological Products (BIBP) and the Sinopharm-Wuhan Institute of Biological Products (WIBP) received the EUA. Aluminum hydroxide adjuvanted vaccine was prepared by culturing viruses in infected Vero cell lines, then inactivating viruses (HB02 and WIV04) with β-propiolactone and chromatography purification (50, 51). Phase 3 clinical studies reported 72.8% and 78.1% vaccine efficacies and 94.5 and 156.0 geometric mean titers (GMTs) at day 14 after the second dose for Sinopharm-WBIP and Sinopharm-BBIP, respectively, that were effective against prototype SARS-CoV-2 infection (51). Besides, Li et al. showed that the overall efficacy after vaccination was 59.0% against SARS-CoV-2, 70.2% against COVID -19 moderate and nearly 100% against severe disease during the Delta variant outbreak in Guangzhou city, China (67). Although, sera from recipients BBIBP-CorV vaccine showed neutralizing activity against the variant B.1.1.7 and the prototype strain, most sera samples showed complete or partial loss of the neutralization against B.1.351 lineage (102, 103). Remarkably, similar to BNT162b2, the influence of gender on immune response is also controversial. One study showed significantly higher vaccine efficacy in women than in men during the Delta variant epidemic (67), but another study showed gender had no effect on IgM and IgG antibodies responses (104). However, age can affect the IgG antibody response, and the antibody level of elderly recipients (≥42 years old) is significantly lower than that of younger (104).
Another inactivated vaccine against SARS-CoV-2 was developed by Sinovac Biotech Company via a bioreactor (52). This inactivated vaccine was cleared for emergency use in July 2020 and subsequently approved by the WHO (105). A phase 3 clinical trial reported that the vaccine efficacies were 50.7% in Brazil (68), 83.5% in Turkey (69), and 65.9% in Chile (70), respectively. However, it was highly effective against severe forms, hospitalizations and deaths of COVID-19 (106). In particular, vaccine-induced serum nAb remained effective against D614G, B.1.1.7 and B.1.429 variants (107), but that was significantly less effective against B.1.351 (107), P.1 and P.2 variants (68). Interestingly, although an IFN-γ-secreted T-cell response and a B-cell response were significantly induced in a Chilean study, no differences were observed between the age of 18-59 and over 60 (70). Nevertheless, CoronaVac was able to induce a strong nAb response (GMTs: 86.4-142.2) in children and adolescents aged from 3 to 17 years (108). In order to study the response of specific immune populations to the CoronaVac vaccine, Sinovac conducted a phase 4 clinical trial, which observed a reduction more than 15% in seroconversion rates and nAb titers after the second dose compared to controls (109).
The University of Oxford, in collaboration with the British company AstraZeneca and the Serum Institute of India, developed the AZD1222 vaccine, which was prepared using a chimpanzee adenovirus deficient in the replication ChAdOx1 to express the S protein of SARS -CoV-2 (46). The WHO has approved the AZD-1222 vaccine for the prevention of COVID-19 (110). However, several side effects, such as disseminated intravascular coagulation and brain blood vessels have been reported in people who received this vaccine (111). Some EU countries suspended the use of this vaccine. After carefully evaluating the available data generated by the vaccine, WHO recommended continuing vaccination in a bulletin issued on March 17, 2021 (112). Approximately 14 days after the second dose, the AZD-1222 vaccine showed 66.7% efficacy against the SARS-CoV-2 prototype stain (71), 70.4% against Alpha (72), 10.4% against Beta (73), 66% against Delta (42). Although the number of cases to analyze was limited, ChAdOx1 nCoV-19 showed 64% efficacy against Gamma variant infection (74). These results indicated that the vaccine lost its protective effect against the Beta variant, but still was effective against other VOC. On the other hand, a study that vaccination was more effective in individuals aged 18 to 34 than in those aged 35 to 64 years, also in those showing signs of the prior SARS-CoV-2 infection than in those no infection. These results were confirmed by a study in populations vaccinated with ChAdOx1 or BNT162b2 SARS-CoV-2 vaccine (90, 113). However, only 28.6% of immunosuppressed patients had detectable immune responses in humoral or T-cell responses after the first administration of ChAdOx1 nCoV-19 vaccine (114).
Ad26.COV2.S was a vaccine-based recombinant non-replicating adenoviral vector type 26 (rAd26) expressing a stabilized SARS-CoV-2 S-2P protein with a furin cleavage site mutation, developed by the Center for Virology and Vaccine Research, Harvard Medical School, collaborating with Janssen Vaccines & Prevention, Leiden (47). Studies conducted by Sadoff et al. followed by Johnson & Johnson, reported that approximately 28 days after administration, the overall vaccine efficacies were 66.1% and 85.4% for the prevention of moderate to severe and severe COVID19 diseases, respectively (75, 76). The similar vaccine efficacy was observed for variant B.1.351, i.e., 64.0% for moderate to severe COVID-19 cases and 81.7% for severe critical COVID-19 cases (75). Also, phase 3 clinical trial study showed the efficacies of Ad26.COV2.S were 68% against moderate to severe COVID-19 and 88% against severe or critical COVID-19 in the real world of Brazil, however, this effectiveness was lower in older participants than younger participants (77). Remarkably, the humoral and cellular immunity levels peaked 71 days after vaccination and could be maintained for at least 8 months. Moreover, as the time after vaccination increases, Ad26.COV2.S showed higher and broader neutralizing ability against all VOC (115). It was also noteworthy that vaccine-induced T cell responses largely retained the ability to control SARS-CoV-2 variants, although Ad26.COV2.S-induced nAb titers were reduced against B.1.351 and P.1 variants (77).
Sputnik V was a combined vaccine of a rAd26 and a recombinant adenoviral vector type 5 (rAd5) expressing an S protein (Russian Gamaleya Institute). A heterologous prime-boost regimen was utilized for the vaccine consisting of the first dose (rAd26) and the second dose (rAd5) at an interval of 21 days. An interim analysis in phase 3 clinical trial showed that the Sputnik V vaccine could induce the efficacies of 91.6% against COVID-19 and 100% against severe COVID-19 at 21 days after the first dose (48). The high levels of vaccine-induced humoral and cellular responses were similar between different age groups and between males and females, and were not affected by previous SARS-CoV-2 infections (48). In addition, in a randomized controlled study in Argentina, the participant’s sera demonstrated neutralizing activity against the SARS-CoV-2 prototype strain and B.1.1.7 variant, although a significant reduction in the GMTs was observed against the variant containing the E484K mutation such as B.1.351 variant (116).
Ad5-nCoV was a vaccine, rAd5-based vaccine that expresses an un-modified S protein, which developed by the CanSinoBIO-Beijing Institute of Biotechnology (49). According to the CanSino report, the overall efficacies were 65% to 69% and 90% to 96% for critical COVID-19 at 14 to 28 days after the first dose of Ad5-nCoV, respectively (78). The antibody response induced by Ad5-nCoV vaccine peaked on day 28 post-vaccination and the specific T-cell response peaked on 14 days post-vaccination in adults aged between 18 and 60 years (117). Based on this promising result from the first human trials, CanSino conducted further investigations in a broad range of age groups, in which showed that administration with prime-boost regimen in children and adolescents aged 6-17 years (GMTs: 1037.5) produced higher levels of immune responses than adults aged 18-55 years (GMTs: 647.2) and those aged 56 years and older (GMTs: 338.0) (118). Consistent with this study, the level of immune response to the vaccine declined significantly with age in adults aged 18 years and older, and that was severely affected by the pre-existing high anti-Ad5 immunity (119).
ZF2001, a RBD-based protein subunit vaccine was developed by Chinese Academy of Sciences and Anhui Zhifei Longcom Biopharmaceutical Co. Ltd. and received its EUA. In clinical trial phase 2, the ZF2001 showed high levels of nAb titers (GMTs: 94.5 for 25 µg and 117.8 for 50 µg) and complete seroconversion rates (100%) after three doses of vaccination (54). Also, a balanced immune response of Th1 and Th2 cells was observed (54). ZF2001 vaccine-elicited nAb had higher neutralizing activity against the B.1.351 variant compared to convalescents and individuals vaccinated CoronaVac, although the neutralizing activity was reduced compared to the D614G variant (103, 120). Recently, Zhifei Longcom released the latest data from phase 3 clinical trials. Indeed, this study showed that the efficacies of the ZF2001 vaccine were 77.54% and 92.93% against Delta and Alpha infections, respectively. In addition, the efficacies of all cases of COVID-19 (81.76%) and severe forms (100%) were also high (79).
EpiVacCorona was an aluminum hydroxide adjuvanted peptide vaccine which was covalently bound to the N protein (Vector State Research Centre of Virology and Biotechnology). The peptides were selected from the most conserved region of protein S. The EpiVacCorona vaccine provided protective immunity and eliminated antibody-dependent enhancement (ADE), which could be induced by antibodies specific for NTDs after infection. In a phase 1/2 clinical trial, Ryzhikov et al. observed a seroconversion rate of 100% and nAb titers ranging from 1:20 to 1: 160 on day 42 after the first vaccination (58).
COVAXIN (a.k.a BBV152) was an inactivated SARS-CoV-2 strain (NIV-2020-770) adjuvant aluminum hydroxide (Algel) -Imidazoquinoline (IMDG) or Algel developed by Bharat Biotech (53), and was approved by the Drug Controller General of India (DCGI) for emergency use. In phase 1 trial, BBV152 showed good safety and tolerance, as well as, demonstrated humoral and Th1-responsive cellular immunity (121), that persisted during the three-month follow-up (122). Moreover, the vaccine induced elevated T-cell memory responses (122). A two-dose regimen of inactivated virus adjuvant Algel-IMDG could confer protection of 65.2% against B.1.617.2 variant, followed by an overall efficacy of 77.8% (80), and that efficacy against B.1.617.2 variant was not significant differences in immune responses between the age groups under 60 and over 60 (80). BBV152 was also effectively to neutralize the Alpha variant but weakly neutralize the Beta and Delta variants (123, 124).
ZyCoV-D was the first DNA vaccine developed by Zydus Research Center and Vaccine Technology Center, and Cadila Healthcare Ltd, and received the EUA to be approved for use in humans on August 20, 2021 (59). ZyCoV-D comprised an optimized plasmid DNA coding spike protein along with an IgE signal sequence and was administered on the skin by the PharmaJet® Tropis® Needle-Free Injection System (NFIS) (60). In phase 1 study, ZyCoV-D has shown good safety and tolerability. Besides, the cellular and humoral immune responses were observed after three doses of 2 mg vaccine administered 28 days apart, and these immune responses were maintained till day 70 (125). The Indian government reported 67% vaccine efficacy against symptomatic COVID-19 in a phase 3 clinical trial (59).
Soberana and Abdala were two vaccines constructed from recombinant RBD conjugated to tetanus toxoid or aluminum (55). These two vaccines developed in Cuba has been approved by the Cuban National Control Center for Drugs and Medical Devices (CECMED) to prevent COVID-19. CECMED reported that the Abdala and Soberana vaccines were 92.28% and 91.2% effective against COVID-19, respectively (81). A regimen three-dose, i.e., two-dose Soberana02 followed by one-dose Soberana Plus showed great protective efficacy against the Delta variant. The Instituto Finlay de Vacunas reported 91.7% protective efficacy against Delta variant after three doses (56).
NVX-CoV2373 was a recombinant protein subunit vaccine, composed of trimeric full-length S protein and Matrix-M1 adjuvant can induce Th1 phenotype CD4+ T cell response (57), which obtained its EUA on November 1 from Indonesia. However, NVX-CoV2373 demonstrated the promising results of a phase 3 clinical trial, in which the efficacies of 89.7% against SARS-CoV-2 prototype strain and 85.6% against B.1.1.7 and 51% against B.1.351 were observed after individuals received two doses of the vaccine (82, 83). Previously, Shen et al. observed that the sera of individuals who had received NVX-CoV2373 still contained high levels of nAb against the B.1.1.7 variant (126), but these nAb levels were significantly reduced 14.5-fold against B.1.315 (127). In addition, although the NVX-CoV2373 vaccine was found to induce a weak humoral immune response in the elderly, however, seroconversion occurred almost completely, with anti-spike protein IgG seroconversion rate of 97%, and nAb seroconversion rate of 100% (128).
With the COVID-19 pandemic, SARS-CoV-2 evolves persistently and the number of variants increases significantly. Except for alpha, which is only highly transmissible, the other VOC are highly transmissible, with high mortality and severity of clinical cases. Among all VOC, Beta is the most infectious and pathogenic. These variants are more prevalent among young people, but are less severe. All vaccines currently used for preventing COVID-19 are based on the SARS-CoV-2 prototype strain. Although these vaccines still have great efficacy against the earliest D614 G variant and Alpha variant, they show different efficacies to other VOC and VOI. The levels of cellular and humoral immunity induced by vaccines are influenced significantly by age, the use of immunosuppressive drugs, and a history of previously infected with SARS-CoV-2. The existing vaccine platforms can also be used to rapidly prepare new vaccines against these variants. Cross-neutralizing activities and screening key epitopes among variants may be promising strategies for developing more effective vaccines that provide a comprehensive immune response against all variants.
XF contributed to the concept, and revised the manuscript. YZ did literature retrieval, collected and analyzed data, and wrote the manuscript. J-LB did literature retrieval and wrote the manuscript. MG and XL did literature retrieval. All authors contributed to the article and approved the submitted version.
This work was supported by grants from the Applied Basic Research Key Project of Wuhan Municipal Bureau of Science and Technology (2020020601012218) and the Fundamental Research Funds for the Central Universities (HUST COVID-19 Rapid Response Call No. 2020kfyXGYJ040).
The authors declare that the research was conducted in the absence of any commercial or financial relationships that could be construed as a potential conflict of interest.
All claims expressed in this article are solely those of the authors and do not necessarily represent those of their affiliated organizations, or those of the publisher, the editors and the reviewers. Any product that may be evaluated in this article, or claim that may be made by its manufacturer, is not guaranteed or endorsed by the publisher.
We gratefully acknowledge Sheffield COVID-19 Genomics Group and all members to provide the platform of COVID-19 Viral Genome Analysis Pipeline (https://cov.lanl.gov/content/index) for the tracking Changes in SARS-CoV-2 Spike. We thank all members from the Su, Wu, and Andersen labs at Scripps Research to unify COVID-19 and SARS-CoV-2 epidemiology and genomic data (https://outbreak.info/)
1. Zhu N, Zhang D, Wang W, Li X, Yang B, Song J, et al. China Novel Coronavirus Investigating and Research Team. A Novel Coronavirus From Patients With Pneumonia in China, 2019. N Engl J Med (2020) 382(8):727–33. doi: 10.1056/NEJMoa2001017
2. World Health Organization. WHO Coronavirus (COVID-19) Dashboard: Global Situation (2021). Available at: https://covid19.who.int/ (Accessed 18 October 2021).
3. World Health Organization. COVID-19 Vaccines: WHO’s Landscape of COVID-19 Vaccine Candidates (2021). Available at: https://www.who.int/publications/m/item/draft-landscape-of-covid-19-candidate-vaccines (Accessed 15 October 2021).
4. Abdool Karim SS, de Oliveira T. New SARS-CoV-2 Variants - Clinical, Public Health, and Vaccine Implications. N Engl J Med (2021) 384(19):1866–8. doi: 10.1056/NEJMc2100362
5. Zhou P, Yang XL, Wang XG, Hu B, Zhang L, Zhang W, et al. A Pneumonia Outbreak Associated With a New Coronavirus of Probable Bat Origin. Nature (2020) 579(7798):270–3. doi: 10.1038/s41586-020-2012-7
6. Barnes CO, West AP Jr, Huey-Tubman KE, Hoffmann MAG, Sharaf NG, Hoffman PR, et al. Structures of Human Antibodies Bound to SARS-CoV-2 Spike Reveal Common Epitopes and Recurrent Features of Antibodies. Cell (2020) 182(4):828–42.e16. doi: 10.1016/j.cell.2020.06.025
7. Plante JA, Liu Y, Liu J, Xia H, Johnson BA, Lokugamage KG, et al. Spike Mutation D614G Alters SARS-CoV-2 Fitness. Nature (2021) 592(7852):116–21. doi: 10.1038/s41586-020-2895-3
8. Holmes KV. Adaptation of SARS Coronavirus to Humans. Science (2005) 309(5742):1822–3. doi: 10.1126/science.1118817
9. COVID-19 Genomics Consortium UK (CoG-UK). Preliminary Genomic Characterisation of an Emergent SARS-CoV-2 Lineage in the UK Defined by a Novel Set of Spike Mutations (2020). Available at: https://virological.org/t/563.
10. Tegally H, Wilkinson E, Giovanetti M, Iranzadeh A, Fonseca V, Giandhari J, et al. Emergence and Rapid Spread of a New Severe Acute Respiratory Syndrome-Related Coronavirus 2 (SARS-CoV-2) Lineage With Multiple Spike Mutations in South Africa. MedRxiv [Preprint] (2021) 2020.12.21.20248640. doi: 10.1101/2020.12.21.20248640
11. Faria NR, Mellan TA, Whittaker C, Claro IM, Candido DDS, Mishra S, et al. Genomics and Epidemiology of the P.1 SARS-CoV-2 Lineage in Manaus, Brazil. Science (2021) 372(6544):815–21. doi: 10.1126/science.abh2644
12. Center for Disease Control and Prevention. SARS-CoV-2 Variant Classifications and Definitions. Available at: https://www.cdc.gov/coronavirus/2019-ncov/variants/variant-info.html#Interest (Accessed 13 October 2021).
13. Kimura I, Kosugi Y, Wu J, Yamasoba D, Butlertanaka EP, Tanaka YL, et al. SARS-CoV-2 Lambda Variant Exhibits Higher Infectivity and Immune Resistance. BioRxiv [Preprint] (2021) 2021.07.28.454085. doi: 10.1101/2021.07.28.454085
14. Laiton-Donato K, Franco-Muñoz C, Álvarez-Díaz DA, Ruiz-Moreno HA, Usme-Ciro JA, Prada DA, et al. Characterization of the Emerging B.1.621 Variant of Interest of SARS-CoV-2. Infect Genet Evol (2021) 95:105038. doi: 10.1016/j.meegid.2021.105038
15. World Health Organization. COVID-19 Vaccines: Tracking SARS-CoV-2 Variants (2021). Available at: https://www.who.int/en/activities/tracking-SARS-CoV-2-variants/ (Accessed 15 October 2021).
16. Latif AA, Mullen JL, Alkuzweny M, Tsueng J, Cano M, the Center for Viral Systems Biology, et al. C.37 Lineage Report . Available at: https://outbreak.info/situation-reports?pango=C.37&loc=PER&selected=PER (Accessed 1 September 2021).
17. Latif AA, Mullen JL, Alkuzweny M, Tsueng J, Cano M, the Center for Viral Systems Biology, et al. B.1.621 Lineage Report. Available at: https://outbreak.info/situation-reports?pango=B.1.621&loc=COL&loc=GBR&selected=COL (Accessed 18 October 2021).
18. Yurkovetskiy L, Wang X, Pascal KE, Tomkins-Tinch C, Nyalile TP, Wang Y, et al. Structural and Functional Analysis of the D614G SARS-CoV-2 Spike Protein Variant. Cell (2020) 183(3):739–51.e8. doi: 10.1016/j.cell.2020.09.032
19. Winger A, Caspari T. The Spike of Concern-The Novel Variants of SARS-CoV-2. Viruses (2021) 13(6):1002. doi: 10.3390/v13061002
20. Korber B, Fischer WM, Gnanakaran S, Yoon H, Theiler J, Abfalterer W, et al. Tracking Changes in SARS-CoV-2 Spike: Evidence That D614G Increases Infectivity of the COVID-19 Virus. Cell (2020) 182(4):812–27.e19. doi: 10.1016/j.cell.2020.06.043
21. Hou YJ, Chiba S, Halfmann P, Ehre C, Kuroda M, Dinnon KH 3rd, et al. SARS-CoV-2 D614G Variant Exhibits Efficient Replication Ex Vivo and Transmission In Vivo. Science (2020) 370(6523):1464–8. doi: 10.1126/science.abe8499
22. Volz E, Hill V, McCrone JT, Price A, Jorgensen D, O’Toole Á, et al. Evaluating the Effects of SARS-CoV-2 Spike Mutation D614G on Transmissibility and Pathogenicity. Cell (2021) 184(1):64–75.e11. doi: 10.1016/j.cell.2020.11.020
23. Tang JW, Tambyah PA, Hui DS. Emergence of a New SARS-CoV-2 Variant in the UK. J Infect (2021) 82(4):e27–8. doi: 10.1016/j.jinf.2020.12.024
24. Kirby T. New Variant of SARS-CoV-2 in UK Causes Surge of COVID-19. Lancet Respir Med (2021) 9(2):e20–1. doi: 10.1016/S2213-2600(21)00005-9
25. Latif AA, Mullen JL, Alkuzweny M, Tsueng J, Cano M, the Center for Viral Systems Biology, et al. B.1.1.7 Lineage Report. Available at: https://outbreak.info/situation-reports?pango=B.1.1.7&loc=GBR&loc=USA&loc=USA_US-CA&selected=GBR (Accessed 18 October 2021).
26. McCallum M, De Marco A, Lempp FA, Tortorici MA, Pinto D, Walls AC, et al. N-Terminal Domain Antigenic Mapping Reveals a Site of Vulnerability for SARS-CoV-2. Cell (2021) 184(9):2332–47.e16. doi: 10.1016/j.cell.2021.03.028
27. Lubinski B, Tang T, Daniel S, Jaimes JA, Whittaker GR. Functional Evaluation of Proteolytic Activation for the SARS-CoV-2 Variant B.1.1.7: Role of the P681H Mutation. BioRxiv [Preprint] (2021) 2021.04.06.438731. doi: 10.1101/2021.04.06.438731
28. Davies NG, Jarvis CI, CMMID COVID-19 Working Group, Edmunds WJ, Jewell NP, Diaz-Ordaz K, et al. Increased Mortality in Community-Tested Cases of SARS-CoV-2 Lineage B.1.1.7. Nature (2021) 593(7858):270–4. doi: 10.1038/s41586-021-03426-1
29. Supasa P, Zhou D, Dejnirattisai W, Liu C, Mentzer AJ, Ginn HM, et al. Reduced Neutralization of SARS-CoV-2 B.1.1.7 Variant by Convalescent and Vaccine Sera. Cell (2021) 184(8):2201–11.e7. doi: 10.1016/j.cell.2021.02.033
30. Latif AA, Mullen JL, Alkuzweny M, Tsueng J, Cano M, the Center for Viral Systems Biology, et al. B.1.351 Lineage Report. Available at: https://outbreak.info/situation-reports?pango=B.1.351&loc=ZAF&loc=USA&loc=USA_US-CA&selected=ZAF (Accessed 18 October 2021).
31. Tegally H, Wilkinson E, Lessells RJ, Giandhari J, Pillay S, Msomi N, et al. Sixteen Novel Lineages of SARS-CoV-2 in South Africa. Nat Med (2021) 27(3):440–6. doi: 10.1038/s41591-021-01255-3
32. Yuan M, Huang D, Lee CD, Wu NC, Jackson AM, Zhu X, et al. Structural and Functional Ramifications of Antigenic Drift in Recent SARS-CoV-2 Variants. Science (2021) 373(6556):818–23. doi: 10.1126/science.abh1139
33. Gobeil SM, Janowska K, McDowell S, Mansouri K, Parks R, Stalls V, et al. Effect of Natural Mutations of SARS-CoV-2 on Spike Structure, Conformation, and Antigenicity. Science (2021) 373(6555):eabi6226. doi: 10.1126/science.abi6226
34. Zhou D, Dejnirattisai W, Supasa P, Liu C, Mentzer AJ, Ginn HM, et al. Evidence of Escape of SARS-CoV-2 Variant B.1.351 From Natural and Vaccine-Induced Sera. Cell (2021) 184(9):2348–61.e6. doi: 10.1016/j.cell.2021.02.037
35. Moyo-Gwete T, Madzivhandila M, Makhado Z, Ayres F, Mhlanga D, Oosthuysen B, et al. Cross-Reactive Neutralizing Antibody Responses Elicited by SARS-CoV-2 501y.V2 (B.1.351). N Engl J Med (2021) 384(22):2161–3. doi: 10.1056/NEJMc2104192
36. Latif AA, Mullen JL, Alkuzweny M, Tsueng J, Cano M, the Center for Viral Systems Biology, et al. P.1 Lineage Report. Available at: https://outbreak.info/situation-reports?pango=P.1&loc=BRA&loc=USA&loc=JPN&selected=BRA (Accessed 18 October 2021).
37. Chen X, Chen Z, Azman AS, Sun R, Lu W, Zheng N, et al. Comprehensive Mapping of Neutralizing Antibodies Against SARS-CoV-2 Variants Induced by Natural Infection or Vaccination. MedRxiv [Preprint] (2021) 2021.05.03.21256506. doi: 10.1101/2021.05.03.21256506
38. Dejnirattisai W, Zhou D, Supasa P, Liu C, Mentzer AJ, Ginn HM, et al. Antibody Evasion by the P.1 Strain of SARS-CoV-2. Cell (2021) 184(11):2939–54.e9. doi: 10.1016/j.cell.2021.03.055
39. Latif AA, Mullen JL, Alkuzweny M, Tsueng J, Cano M, the Center for Viral Systems Biology, et al. B.1.617.2 Lineage Report. Available at: https://outbreak.info/situation-reports?pango=B.1.617.2&loc=IND&loc=GBR&loc=USA&selected (Accessed 18 October 2021).
40. Cherian S, Potdar V, Jadhav S, Yadav P, Gupta N, Das M, et al. SARS-CoV-2 Spike Mutations, L452R, T478K, E484Q and P681R, in the Second Wave of COVID-19 in Maharashtra, India. Microorganisms (2021) 9(7):1542. doi: 10.3390/microorganisms9071542
41. Julia Stowe NACG, Thelwall ETNG, Amirthalingam MEMZ, Chand MRJL. Effectiveness of COVID-19 Vaccines Against Hospital Admission With the Delta (B.1.617.2) Variant. Public Health England (2021). Available at: https://khub.net/documents/135939561/479607266/Effectiveness+of+COVID-19+vaccines+against+hospital+admission+with+the+Delta+%28B.1.617.2%29+variant.pdf/1c213463-3997-ed16-2a6f-14e5deb0b997?t=1623689315431.
42. Callaway E. Delta Coronavirus Variant: Scientists Brace for Impact. Nature (2021) 595(7865):17–8. doi: 10.1038/d41586-021-01696-3
43. Sheikh A, McMenamin J, Taylor B, Robertson C. Public Health Scotland and the EAVE II Collaborators. SARS-CoV-2 Delta VOC in Scotland: Demographics, Risk of Hospital Admission, and Vaccine Effectiveness. Lancet (2021) 397(10293):2461–2. doi: 10.1016/S0140-6736(21)01358-1
44. Vogel AB, Kanevsky I, Che Y, Swanson KA, Muik A, Vormehr M, et al. BNT162b Vaccines Protect Rhesus Macaques From SARS-CoV-2. Nature (2021) 592(7853):283–9. doi: 10.1038/s41586-021-03275-y
45. Corbett KS, Edwards DK, Leist SR, Abiona OM, Boyoglu-Barnum S, Gillespie RA, et al. SARS-CoV-2 mRNA Vaccine Design Enabled by Prototype Pathogen Preparedness. Nature (2020) 586(7830):567–71. doi: 10.1038/s41586-020-2622-0
46. van Doremalen N, Lambe T, Spencer A, Belij-Rammerstorfer S, Purushotham JN, Port JR, et al. ChAdOx1 Ncov-19 Vaccine Prevents SARS-CoV-2 Pneumonia in Rhesus Macaques. Nature (2020) 586(7830):578–82. doi: 10.1038/s41586-020-2608-y
47. Bos R, Rutten L, van der Lubbe JEM, Bakkers MJG, Hardenberg G, Wegmann F, et al. Ad26 Vector-Based COVID-19 Vaccine Encoding a Prefusion-Stabilized SARS-CoV-2 Spike Immunogen Induces Potent Humoral and Cellular Immune Responses. NPJ Vaccines (2020) 5:91. doi: 10.1038/s41541-020-00243-x
48. Logunov DY, Dolzhikova IV, Shcheblyakov DV, Tukhvatulin AI, Zubkova OV, Dzharullaeva AS, et al. Safety and Efficacy of an Rad26 and Rad5 Vector-Based Heterologous Prime-Boost COVID-19 Vaccine: An Interim Analysis of a Randomised Controlled Phase 3 Trial in Russia. Lancet (2021) 397(10275):671–81. doi: 10.1016/S0140-6736(21)00234-8
49. Wu S, Zhong G, Zhang J, Shuai L, Zhang Z, Wen Z, et al. A Single Dose of an Adenovirus-Vectored Vaccine Provides Protection Against SARS-CoV-2 Challenge. Nat Commun (2020) 11(1):4081. doi: 10.1038/s41467-020-17972-1
50. Wang H, Zhang Y, Huang B, Deng W, Quan Y, Wang W, et al. Development of an Inactivated Vaccine Candidate, BBIBP-CorV, With Potent Protection Against SARS-CoV-2. Cell (2020) 182(3):713–21.e9. doi: 10.1016/j.cell.2020.06.008
51. Al Kaabi N, Zhang Y, Xia S, Yang Y, Al Qahtani MM, Abdulrazzaq N, et al. Effect of 2 Inactivated SARS-CoV-2 Vaccines on Symptomatic COVID-19 Infection in Adults: A Randomized Clinical Trial. JAMA (2021) 326(1):35–45. doi: 10.1001/jama.2021.8565
52. Gao Q, Bao L, Mao H, Wang L, Xu K, Yang M, et al. Development of an Inactivated Vaccine Candidate for SARS-CoV-2. Science (2020) 369(6499):77–81. doi: 10.1126/science.abc1932
53. Mohandas S, Yadav PD, Shete-Aich A, Abraham P, Vadrevu KM, Sapkal G, et al. Immunogenicity and Protective Efficacy of BBV152, Whole Virion Inactivated SARS- CoV-2 Vaccine Candidates in the Syrian Hamster Model. iScience (2021) 24(2):102054. doi: 10.1016/j.isci.2021.102054
54. Yang S, Li Y, Dai L, Wang J, He P, Li C, et al. Safety and Immunogenicity of a Recombinant Tandem-Repeat Dimeric RBD-Based Protein Subunit Vaccine (ZF2001) Against COVID-19 in Adults: Two Randomised, Double-Blind, Placebo-Controlled, Phase 1 and 2 Trials. Lancet Infect Dis (2021) 21(8):1107–19. doi: 10.1016/S1473-3099(21)00127-4
55. Valdes-Balbin Y, Santana-Mederos D, Quintero L, Fernández S, Rodriguez L, Sanchez Ramirez B, et al. SARS-CoV-2 RBD-Tetanus Toxoid Conjugate Vaccine Induces a Strong Neutralizing Immunity in Preclinical Studies. ACS Chem Biol (2021) 16(7):1223–33. doi: 10.1021/acschembio.1c00272
56. Instituto Finlay de Vacunas. Confirman Los Resultados De Ensayo Clínico Fase III Desarrollado Por El Instituto Pasteur De Irán Eficacia De Vacunas SOBERANA-02 Y SOBERANA Plus Frente a La Cepa DeltaFigure and Figure Legends . Available at: https://www.finlay.edu.cu/blog/confirman-los-resultados-de-ensayo-clinico-fase-iii-desarrollado-por-el-instituto-pasteur-de-iran-eficacia-de-vacunas-soberana-02-y-soberana-plus-frente-a-la-cepa-delta/.
57. Keech C, Albert G, Cho I, Robertson A, Reed P, Neal S, et al. Phase 1-2 Trial of a SARS-CoV-2 Recombinant Spike Protein Nanoparticle Vaccine. N Engl J Med (2020) 383(24):2320–32. doi: 10.1056/NEJMoa2026920
58. Ryzhikov AB, Ryzhikov EA, Bogryantseva MP, Usova SV, Danilenko ED, Nechaeva EA, et al. A Single Blind, Placebo-Controlled Randomized Study of the Safety, Reactogenicity and Immunogenicity of the “EpiVacCorona” Vaccine for the Prevention of COVID-19, in Volunteers Aged 18–60 Years (Phase I–II). Russian J Infect Immun (2021) 11(2):283–96. doi: 10.15789/2220-7619-ASB-1699
59. Ministry of Science & Technology. DBT-BIRAC Supported ZyCoV-D Developed by Zydus Cadila Receives Emergency Use Authorization . Available at: https://www.pib.gov.in/PressReleasePage.aspx?PRID=1747669.
60. Dey A, Chozhavel Rajanathan TM, Chandra H, Pericherla HPR, Kumar S, Choonia HS, et al. Immunogenic Potential of DNA Vaccine Candidate, ZyCoV-D Against SARS-CoV-2 in Animal Models. Vaccine (2021) 39(30):4108–16. doi: 10.1016/j.vaccine.2021.05.098
61. Polack FP, Thomas SJ, Kitchin N, Absalon J, Gurtman A, Lockhart S, et al. Safety and Efficacy of the BNT162b2 mRNA Covid-19 Vaccine. N Engl J Med (2020) 383(27):2603–15. doi: 10.1056/NEJMoa2034577
62. Abu-Raddad LJ, Chemaitelly H, Butt AA. National Study Group for COVID-19 Vaccination. Effectiveness of the BNT162b2 Covid-19 Vaccine Against the B.1.1.7 and B.1.351 Variants. N Engl J Med (2021) 385(2):187–9. doi: 10.1056/NEJMc2104974
63. Skowronski DM, Setayeshgar S, Zou M, Prystajecky N, Tyson JR, Galanis E, et al. Single-Dose mRNA Vaccine Effectiveness Against SARS-CoV-2, Including Alpha and Gamma Variants: A Test-Negative Design in Adults 70 Years and Older in British Columbia, Canada. Clin Infect Dis (2021) ciab616. doi: 10.1093/cid/ciab616
64. Baden LR, El Sahly HM, Essink B, Kotloff K, Frey S, Novak R, et al. Efficacy and Safety of the mRNA-1273 SARS-CoV-2 Vaccine. N Engl J Med (2021) 384(5):403–16. doi: 10.1056/NEJMoa2035389
65. Chemaitelly H, Yassine HM, Benslimane FM, Al Khatib HA, Tang P, Hasan MR, et al. mRNA-1273 COVID-19 Vaccine Effectiveness Against the B.1.1.7 and B.1.351 Variants and Severe COVID-19 Disease in Qatar. Nat Med (2021) 27(9):1614–21. doi: 10.1038/s41591-021-01446-y
66. Bruxvoort KJ, LS Sy, Qian L, BK A, Luo Y, GS L, et al. Effectiveness of mRNA-1273 Against Delta, Mu, and Other Emerging Variants. MedRxiv [Preprint] (2021) 2021.09.29.21264199. doi: 10.1101/2021.09.29.21264199
67. Li XN, Huang Y, Wang W, Jing QL, Zhang CH, Qin PZ, et al. Effectiveness of Inactivated SARS-CoV-2 Vaccines Against the Delta Variant Infection in Guangzhou: A Test-Negative Case-Control Real-World Study. Emerg Microbes Infect (2021) 10(1):1751–9. doi: 10.1080/22221751.2021.1969291
68. Moutinho S. “Chinese COVID-19 Vaccine Maintains Protection in Variant-Plagued Brazil”. In: New York: SCIENCEINSIDER (2021). Available at: https://www.science.org/news/2021/04/chinese-covid-19-vaccine-maintains-protection-variant-plagued-brazil.
69. Tanriover MD, Doğanay HL, Akova M, Güner HR, Azap A, Akhan S, et al. Efficacy and Safety of an Inactivated Whole-Virion SARS-CoV-2 Vaccine (CoronaVac): Interim Results of a Double-Blind, Randomised, Placebo-Controlled, Phase 3 Trial in Turkey. Lancet (2021) 398(10296):213–22. doi: 10.1016/S0140-6736(21)01429-X
70. Bueno SM, Abarca K, González PA, Gálvez NM, Soto JA, Duarte LF, et al. Interim Report: Safety and Immunogenicity of an Inactivated Vaccine Against SARS-CoV2 in Healthy Chilean Adults in a Phase 3 Clinical Trial. MedRxiv [Preprint] (2021), 2021.03.31.21254494. doi: 10.1101/2021.03.31.21254494
71. Voysey M, Costa Clemens SA, Madhi SA, Weckx LY, Folegatti PM, Aley PK, et al. Single-Dose Administration and the Influence of the Timing of the Booster Dose on Immunogenicity and Efficacy of ChAdOx1 Ncov-19 (AZD1222) Vaccine: A Pooled Analysis of Four Randomised Trials. Lancet (2021) 397(10277):881–91. doi: 10.1016/S0140-6736(21)00432-3
72. Emary KRW, Golubchik T, Aley PK, Ariani CV, Angus B, Bibi S, et al. Efficacy of ChAdOx1 Ncov-19 (AZD1222) Vaccine Against SARS-CoV-2 Variant of Concern 202012/01 (B.1.1.7): An Exploratory Analysis of a Randomised Controlled Trial. Lancet (2021) 397(10282):1351–62. doi: 10.1016/S0140-6736(21)00628-0
73. Madhi SA, Baillie V, Cutland CL, Voysey M, Koen AL, Fairlie L, et al. Efficacy of the ChAdOx1 Ncov-19 Covid-19 Vaccine Against the B.1.351 Variant. N Engl J Med (2021) 384(20):1885–98. doi: 10.1056/NEJMoa2102214
74. Clemens SAC, Folegatti PM, Emary KRW, Weckx LY, Ratcliff J, Bibi S, et al. Efficacy of ChAdOx1 Ncov-19 (AZD1222) Vaccine Against SARS-CoV-2 Lineages Circulating in Brazil. Nat Commun (2021) 12(1):5861. doi: 10.1038/s41467-021-25982-w
75. Sadoff J, Gray G, Vandebosch A, Cárdenas V, Shukarev G, Grinsztejn B, et al. Safety and Efficacy of Single-Dose Ad26.Cov2.S Vaccine Against Covid-19. N Engl J Med (2021) 384(23):2187–201. doi: 10.1056/NEJMoa2101544
76. Johnson & Johnson. Johnson & Johnson Announces Single-Shot Janssen COVID-19 Vaccine Candidate Met Primary Endpoints in Interim Analysis of its Phase 3 ENSEMBLE Trial (2021). Available at: https://www.jnj.com/johnson-and-johnson-announces-single-shot-janssen-covid-19-vaccine-candidate-met-primary-endpoints-in-interim-analysis-of-its-phase-3-ensemble-trial.
77. Alter G, Yu J, Liu J, Chandrashekar A, Borducchi EN, Tostanoski LH, et al. Immunogenicity of Ad26.COV2.S Vaccine Against SARS-CoV-2 Variants in Humans. Nature (2021) 596(7871):268–72. doi: 10.1038/s41586-021-03681-2
78. CanSino. CanSino Announces Vaccine Efficacy of Convidecia in Its Phase 3 Trial (2021). Available at: http://www.cansinotech.com.cn/html/1/156/231/index.html.
79. Zhifei Longcom. The Delta Protection Rate Is 77.54%! Zhifei Releases the Most Internationally Competitive New Crown Vaccine Phase III Data. Available at: http://www.zflongkema.com/news/qyyw/2021-08-27/234.html.
80. Ella R, Reddy S, Blackwelder W, Potdar V, Yadav P, Sarangi V, et al. Efficacy, Safety, and Lot to Lot Immunogenicity of an Inactivated SARS-CoV-2 Vaccine (BBV152): a, Double-Blind, Randomised, Controlled Phase 3 Trial. MedRxiv [Preprint] (2021), 2021.06.30.21259439. doi: 10.1101/2021.06.30.21259439
81. Aguilar-Guerra TL, Fajardo-Díaz EM, Gorry C. Cuba’s National Regulatory Authority & COVID-19: Olga Lidia Jacobo-Casanueva MS Director, Center for State Control of Medicines and Medical Devices (CECMED). MEDICC Rev (2021) 23(3-4):9–14. doi: 10.37757/MR2021.V23.N3.3
82. Heath PT, Galiza EP, Baxter DN, Boffito M, Browne D, Burns F, et al. Safety and Efficacy of NVX-CoV2373 Covid-19 Vaccine. N Engl J Med (2021) 385(13):1172–83. doi: 10.1056/NEJMoa2107659
83. Shinde V, Bhikha S, Hoosain Z, Archary M, Bhorat Q, Fairlie L, et al. Efficacy of NVX-CoV2373 Covid-19 Vaccine Against the B.1.351 Variant. N Engl J Med (2021) 384(20):1899–909. doi: 10.1056/NEJMoa2103055
84. FDA Authorizes Pfizer-BioNTech COVID-19 Vaccine. Med Lett Drugs Ther (2021) 63(1615):1–2. Available at: https://secure.medicalletter.org/w1615a.
85. Tano E, San Martin S, Girgis S, Martinez-Fernandez Y, Sanchez Vegas C. Perimyocarditis in Adolescents After Pfizer-BioNTech COVID-19 Vaccine. J Pediatr Infect Dis Soc (2021) piab060. doi: 10.1093/jpids/piab060
86. Tada T, Dcosta BM, Samanovic MI, Herati RS, Cornelius A, Zhou H, et al. Convalescent-Phase Sera and Vaccine-Elicited Antibodies Largely Maintain Neutralizing Titers Against Global SARS-CoV-2 Variant Spikes. mBio (2021) 12(3):e0069621. doi: 10.1128/mBio.00696-21
87. Collier DA, Ferreira IATM, Kotagiri P, Datir RP, Lim EY, Touizer E, et al. Age-Related Immune Response Heterogeneity to SARS-CoV-2 Vaccine BNT162b2. Nature (2021) 596(7872):417–22. doi: 10.1038/s41586-021-03739-1
88. Dagan N, Barda N, Biron-Shental T, Makov-Assif M, Key C, Kohane IS, et al. Effectiveness of the BNT162b2 mRNA COVID-19 Vaccine in Pregnancy. Nat Med (2021) 27(10):1693–5. doi: 10.1038/s41591-021-01490-8
89. Frenck RW Jr, Klein NP, Kitchin N, Gurtman A, Absalon J, Lockhart S, et al. Safety, Immunogenicity, and Efficacy of the BNT162b2 Covid-19 Vaccine in Adolescents. N Engl J Med (2021) 385(3):239–50. doi: 10.1056/NEJMoa2107456
90. Wei J, Stoesser N, Matthews PC, Ayoubkhani D, Studley R, Bell I, et al. Antibody Responses to SARS-CoV-2 Vaccines in 45,965 Adults From the General Population of the United Kingdom. Nat Microbiol (2021) 6(9):1140–9. doi: 10.1038/s41564-021-00947-3
91. Eyre DW, Lumley SF, Wei J, Cox S, James T, Justice A, et al. Quantitative SARS-CoV-2 Anti-Spike Responses to Pfizer-BioNTech and Oxford-AstraZeneca Vaccines by Previous Infection Status. Clin Microbiol Infect (2021) 27(10):1516.e7–1516.e14. doi: 10.1016/j.cmi.2021.05.041
92. Hadjadj J, Planas D, Ouedrani A, Buffier S, Delage L, Nguyen Y, et al. Immunogenicity of BNT162b2 Vaccine Against the Alpha and Delta Variants in Immunocompromised Patients. MedRxiv [Preprint] (2021), 2021.08.08.21261766. doi: 10.1101/2021.08.08.21261766
93. Waldhorn I, Holland R, Goshen-Lago T, Shirman Y, Szwarcwort-Cohen M, Reiner-Benaim A, et al. Six-Month Efficacy and Toxicity Profile of BNT162b2 Vaccine in Cancer Patients With Solid Tumors. Cancer Discovery (2021) 11(10):2430–5. doi: 10.1158/2159-8290.CD-21-1072
94. Oliver SE, Gargano JW, Marin M, Wallace M, Curran KG, Chamberland M, et al. The Advisory Committee on Immunization Practices’ Interim Recommendation for Use of Moderna COVID-19 Vaccine - United States, December 2020. MMWR Morb Mortal Wkly Rep (2021) 69(5152):1653–6. doi: 10.15585/mmwr.mm695152e1
95. Edara VV, Hudson WH, Xie X, Ahmed R, Suthar MS. Neutralizing Antibodies Against SARS-CoV-2 Variants After Infection and Vaccination. JAMA (2021) 325(18):1896–8. doi: 10.1001/jama.2021.4388
96. Wang P, Nair MS, Liu L, Iketani S, Luo Y, Guo Y, et al. Antibody Resistance of SARS-CoV-2 Variants B.1.351 and B.1.1.7. Nature (2021) 593(7857):130–5. doi: 10.1038/s41586-021-03398-2
97. Collier AY, McMahan K, Yu J, Tostanoski LH, Aguayo R, Ansel J, et al. Immunogenicity of COVID-19 mRNA Vaccines in Pregnant and Lactating Women. JAMA (2021) 325(23):2370–80. doi: 10.1001/jama.2021.7563
98. Ali K, Berman G, Zhou H, Deng W, Faughnan V, Coronado-Voges M, et al. Evaluation of mRNA-1273 SARS-CoV-2 Vaccine in Adolescents. N Engl J Med (2021) NEJMoa2109522. doi: 10.1056/NEJMoa2109522
99. Anderson EJ, Rouphael NG, Widge AT, Jackson LA, Roberts PC, Makhene M, et al. Safety and Immunogenicity of SARS-CoV-2 mRNA-1273 Vaccine in Older Adults. N Engl J Med (2020) 383(25):2427–38. doi: 10.1056/NEJMoa2028436
100. Haidar G, Agha M, Lukanski A, Linstrum K, Troyan R, Bilderback A, et al. Immunogenicity of COVID-19 Vaccination in Immunocompromised Patients: An Observational, Prospective Cohort Study Interim Analysis. MedRxiv [Preprint] (2021), 2021.06.28.21259576. doi: 10.1101/2021.06.28.21259576
101. Wu K, Choi A, Koch M, Ma L, Hill A, Nunna N, et al. Preliminary Analysis of Safety and Immunogenicity of a SARS-CoV-2 Variant Vaccine Booster. MedRxiv [Preprint] (2021), 2021.05.05.21256716. doi: 10.1101/2021.05.05.21256716
102. Wang GL, Wang ZY, Duan LJ, Meng QC, Jiang MD, Cao J, et al. Susceptibility of Circulating SARS-CoV-2 Variants to Neutralization. N Engl J Med (2021) 384(24):2354–6. doi: 10.1056/NEJMc2103022
103. Huang B, Dai L, Wang H, Hu Z, Yang X, Tan W, et al. Serum Sample Neutralisation of BBIBP-CorV and ZF2001 Vaccines to SARS-CoV-2 501y.V2. Lancet Microbe (2021) 2(7):e285. doi: 10.1016/S2666-5247(21)00082-3
104. Banga Ndzouboukou JL, Zhang Y, Lei Q, Lin X, Yao Z, Fu H, et al. Human IgM and IgG Responses to an Inactivated SARS-CoV-2 Vaccine. Curr Med Sci (2021) 41(6):1–8. doi: 10.1007/s11596-021-2461-8
105. Mallapaty S. WHO Approval of Chinese CoronaVac COVID Vaccine Will be Crucial to Curbing Pandemic. Nature (2021) 594(7862):161–2. doi: 10.1038/d41586-021-01497-8
106. Jara A, Undurraga EA, González C, Paredes F, Fontecilla T, Jara G, et al. Effectiveness of an Inactivated SARS-CoV-2 Vaccine in Chile. N Engl J Med (2021) 385(10):875–84. doi: 10.1056/NEJMoa2107715
107. Chen Y, Shen H, Huang R, Tong X, Wu C. Serum Neutralising Activity Against SARS-CoV-2 Variants Elicited by CoronaVac. Lancet Infect Dis (2021) 21(8):1071–2. doi: 10.1016/S1473-3099(21)00287-5
108. Han B, Song Y, Li C, Yang W, Ma Q, Jiang Z, et al. Safety, Tolerability and Immunogenicity of an Inactivated SARS-CoV-2 Vaccine (CoronaVac) in Healthy Children and Adolescents: A Randomised, Double-Blind, and Placebo-Controlled, Phase 1/2 Clinical Trial . Available at: http://dx.doi.org/10.2139/ssrn.3820545.
109. Medeiros-Ribeiro AC, Aikawa NE, Saad CGS, Yuki EFN, Pedrosa T, Fusco SRG, et al. Immunogenicity and Safety of the CoronaVac Inactivated Vaccine in Patients With Autoimmune Rheumatic Diseases: A Phase 4 Trial. Nat Med (2021) 27(10):1744–51. doi: 10.1038/s41591-021-01469-5
110. World Health Organization. WHO Recommendation AstraZeneca/SKBio - COVID-19 Vaccine (ChAdOx1-S [Recombinant]) (2021). Available at: https://extranet.who.int/pqweb/vaccines/covid-19-vaccine-chadox1-s-recombinant.
111. European Medicines Agency. COVID-19 Vaccine AstraZeneca: Benefits Still Outweigh the Risks Despite Possible Link to Rare Blood Clots With Low Blood Platelets (2021). Available at: https://www.ema.europa.eu/en/news/covid-19-vaccine-astrazeneca-benefits-still-outweigh-risks-despite-possible-link-rare-blood-clots.
112. World Health Organization. WHO Statement on AstraZeneca COVID-19 Vaccine Safety Signals (2021). Available at: https://www.who.int/news/item/17-03-2021-who-statement-on-astrazeneca-covid-19-vaccine-safety-signals.
113. Pouwels KB, Pritchard E, Matthews PC, Stoesser N, Eyre DW, Vihta KD, et al. Effect of Delta Variant on Viral Burden and Vaccine Effectiveness Against New SARS-CoV-2 Infections in the UK. Nat Med (2021). doi: 10.1038/s41591-021-01548-7
114. Prendecki M, Clarke C, Edwards H, McIntyre S, Mortimer P, Gleeson S, et al. Humoral and T-Cell Responses to SARS-CoV-2 Vaccination in Patients Receiving Immunosuppression. Ann Rheum Dis (2021) 80(10):1322–9. doi: 10.1136/annrheumdis-2021-220626
115. Barouch DH, Stephenson KE, Sadoff J, Yu J, Chang A, Gebre M, et al. Durable Humoral and Cellular Immune Responses 8 Months After Ad26.COV2.S Vaccination. N Engl J Med (2021) 385(10):951–3. doi: 10.1056/NEJMc2108829
116. Ikegame S, Siddiquey MNA, Hung C, Haas G, Brambilla L, Oguntuyo KY, et al. Neutralizing Activity of Sputnik V Vaccine Sera Against SARS-CoV-2 Variants. MedRxiv [Preprint] (2021), 2021.03.31.21254660. doi: 10.1101/2021.03.31.21254660
117. Zhu FC, Li YH, Guan XH, Hou LH, Wang WJ, Li JX, et al. Safety, Tolerability, and Immunogenicity of a Recombinant Adenovirus Type-5 Vectored COVID-19 Vaccine: A Dose-Escalation, Open-Label, Non-Randomised, First-in-Human Trial. Lancet (2020) 395(10240):1845–54. doi: 10.1016/S0140-6736(20)31208-3
118. Zhu F, Jin P, Zhu T, Wang W, Ye H, Pan H, et al. Safety and Immunogenicity of a Recombinant Adenovirus Type-5-Vectored COVID-19 Vaccine With a Homologous Prime-Boost Regimen in Healthy Participants Aged 6 Years and Above: A Randomised, Double-Blind, Placebo-Controlled, Phase 2b Trial. Clin Infect Dis (2021) ciab845. doi: 10.1093/cid/ciab845
119. Zhu FC, Guan XH, Li YH, Huang JY, Jiang T, Hou LH, et al. Immunogenicity and Safety of a Recombinant Adenovirus Type-5-Vectored COVID-19 Vaccine in Healthy Adults Aged 18 Years or Older: A Randomised, Double-Blind, Placebo-Controlled, Phase 2 Trial. Lancet (2020) 396(10249):479–88. doi: 10.1016/S0140-6736(20)31605-6
120. Cao Y, Yisimayi A, Bai Y, Huang W, Li X, Zhang Z, et al. Humoral Immune Response to Circulating SARS-CoV-2 Variants Elicited by Inactivated and RBD-Subunit Vaccines. Cell Res (2021) 31(7):732–41. doi: 10.1038/s41422-021-00514-9
121. Ella R, Vadrevu KM, Jogdand H, Prasad S, Reddy S, Sarangi V, et al. Safety and Immunogenicity of an Inactivated SARS-CoV-2 Vaccine, BBV152: A Double-Blind, Randomised, Phase 1 Trial. Lancet Infect Dis (2021) 21(5):637–46. doi: 10.1016/S1473-3099(20)30942-7
122. Ella R, Reddy S, Jogdand H, Sarangi V, Ganneru B, Prasad S, et al. Safety and Immunogenicity of an Inactivated SARS-CoV-2 Vaccine, BBV152: Interim Results From a Double-Blind, Randomised, Multicentre, Phase 2 Trial, and 3-Month Follow-Up of a Double-Blind, Randomised Phase 1 Trial. Lancet Infect Dis (2021) 21(7):950–61. doi: 10.1016/S1473-3099(21)00070-0
123. Sapkal GN, Yadav PD, Ella R, Deshpande GR, Sahay RR, Gupta N, et al. Inactivated COVID-19 Vaccine BBV152/COVAXIN Effectively Neutralizes Recently Emerged B.1.1.7 Variant of SARS-CoV-2. J Travel Med (2021) 28(4):taab051. doi: 10.1093/jtm/taab051
124. Yadav PD, Sapkal GN, Ella R, Sahay RR, Nyayanit DA, Patil DY, et al. Neutralization of Beta and Delta Variant With Sera of COVID-19 Recovered Cases and Vaccinees of Inactivated COVID-19 Vaccine BBV152/Covaxin. J Travel Med (2021) 28(7):taab104. doi: 10.1093/jtm/taab104
125. Momin T, Kansagra K, Patel H, Sharma S, Sharma B, Patel J, et al. Safety and Immunogenicity of a DNA SARS-CoV-2 Vaccine (ZyCoV-D): Results of an Open-Label, Non-Randomized Phase I Part of Phase I/II Clinical Study by Intradermal Route in Healthy Subjects in India. EClinicalMedicine (2021) 38:101020. doi: 10.1016/j.eclinm.2021.101020
126. Shen X, Tang H, McDanal C, Wagh K, Fischer W, Theiler J, et al. SARS-CoV-2 Variant B.1.1.7 Is Susceptible to Neutralizing Antibodies Elicited by Ancestral Spike Vaccines. Cell Host Microbe (2021) 29(4):529–39.e3. doi: 10.1016/j.chom.2021.03.002
127. Shen X, Tang H, Pajon R, Smith G, Glenn GM, Shi W, et al. Neutralization of SARS-CoV-2 Variants B.1.429 and B.1.351. N Engl J Med (2021) 384(24):2352–4. doi: 10.1056/NEJMc2103740
128. Formica N, Mallory R, Albert G, Robinson M, Plested JS, Cho I, et al. Different Dose Regimens of a SARS-CoV-2 Recombinant Spike Protein Vaccine (NVX-CoV2373) in Younger and Older Adults: A Phase 2 Randomized Placebo-Controlled Trial. PLoS Med (2021) 18(10):e1003769. doi: 10.1371/journal.pmed.1003769
Keywords: COVID-19, SARS-CoV-2 variants, vaccine efficacy, immune evasive, epidemiology
Citation: Zhang Y, Banga Ndzouboukou J-L, Gan M, Lin X and Fan X (2021) Immune Evasive Effects of SARS-CoV-2 Variants to COVID-19 Emergency Used Vaccines. Front. Immunol. 12:771242. doi: 10.3389/fimmu.2021.771242
Received: 06 September 2021; Accepted: 29 October 2021;
Published: 22 November 2021.
Edited by:
Sofia A Casares, Naval Medical Research Center, United StatesReviewed by:
Teodor Doru Brumeanu, Uniformed Services University of the Health Sciences, United StatesCopyright © 2021 Zhang, Banga Ndzouboukou, Gan, Lin and Fan. This is an open-access article distributed under the terms of the Creative Commons Attribution License (CC BY). The use, distribution or reproduction in other forums is permitted, provided the original author(s) and the copyright owner(s) are credited and that the original publication in this journal is cited, in accordance with accepted academic practice. No use, distribution or reproduction is permitted which does not comply with these terms.
*Correspondence: Xionglin Fan, eGxmYW5AaHVzdC5lZHUuY24=
Disclaimer: All claims expressed in this article are solely those of the authors and do not necessarily represent those of their affiliated organizations, or those of the publisher, the editors and the reviewers. Any product that may be evaluated in this article or claim that may be made by its manufacturer is not guaranteed or endorsed by the publisher.
Research integrity at Frontiers
Learn more about the work of our research integrity team to safeguard the quality of each article we publish.