- 1Department of Colorectal Surgery, First Affiliated Hospital, School of Medicine, Zhejiang University, Hangzhou, China
- 2Department of Medical Oncology, First Affiliated Hospital, School of Medicine, Zhejiang University, Hangzhou, China
- 3Department of Anorectal Surgery, Haiyan People’s Hospital, Jiaxing, China
Colorectal cancer (CRC) is one of the most common cancers worldwide. However, the treatment outcomes of immunotherapy in microsatellite-stable (MSS) CRC remain unsatisfactory. As the majority of CRC cases display a molecular MSS/mismatch repair-proficient (pMMR) profile, it is particularly meaningful to explore the clinical applications of adaptive immune therapy in MSS CRC patients. In this review, we summarized the therapeutic approaches of adoptive immune therapies, including cytokines, therapeutic cancer vaccines, adoptive T-cell therapy, and immune checkpoint inhibitors, in the treatment of MSS CRCs.
Introduction
Colorectal cancer (CRC) has a high incidence and is the third leading cause of cancer-related death worldwide (1). In recent years, despite the decrease in the overall incidence of CRC patients (2), the incidence of CRC in young patients has nearly doubled (3). Unfortunately, therapeutic options for colorectal cancer patients are still limited despite current systemic chemotherapy, targeted therapy, and local therapy tools. Recently, immunotherapy has become increasingly popular in the field of cancer therapy. The adaptive immune response plays an important role in the response to immunotherapy. The adaptive immune response always presents as specific and long-term memory, which result in durable responses. It has been confirmed that the presence of tumor-infiltrating lymphocytes (TILs) is associated with improved overall survival (4, 5), and the success of TILs in one patient with KRAS G12D mutation indicates the promise of adaptive immunotherapy in CRC patients (6). In addition, immune checkpoint inhibitors (ICIs) have shown great success in cancers such as melanoma and lung cancer (7–9). However, microsatellite-stable (MSS) metastatic colorectal cancer (mCRC) is usually considered to be a typical “cold” cancer that presents a poor response to immunotherapy. Indeed, MSS mCRC accounts for approximately 95% of all mCRC cases (10). It is important to explore how these patients can benefit from adaptive immunotherapy. This review highlights the current role of adaptive immune therapies, including cytokines, vaccines, cell therapies, and ICIs and their potential clinical applications in patients with MSS mCRC (Figure 1).
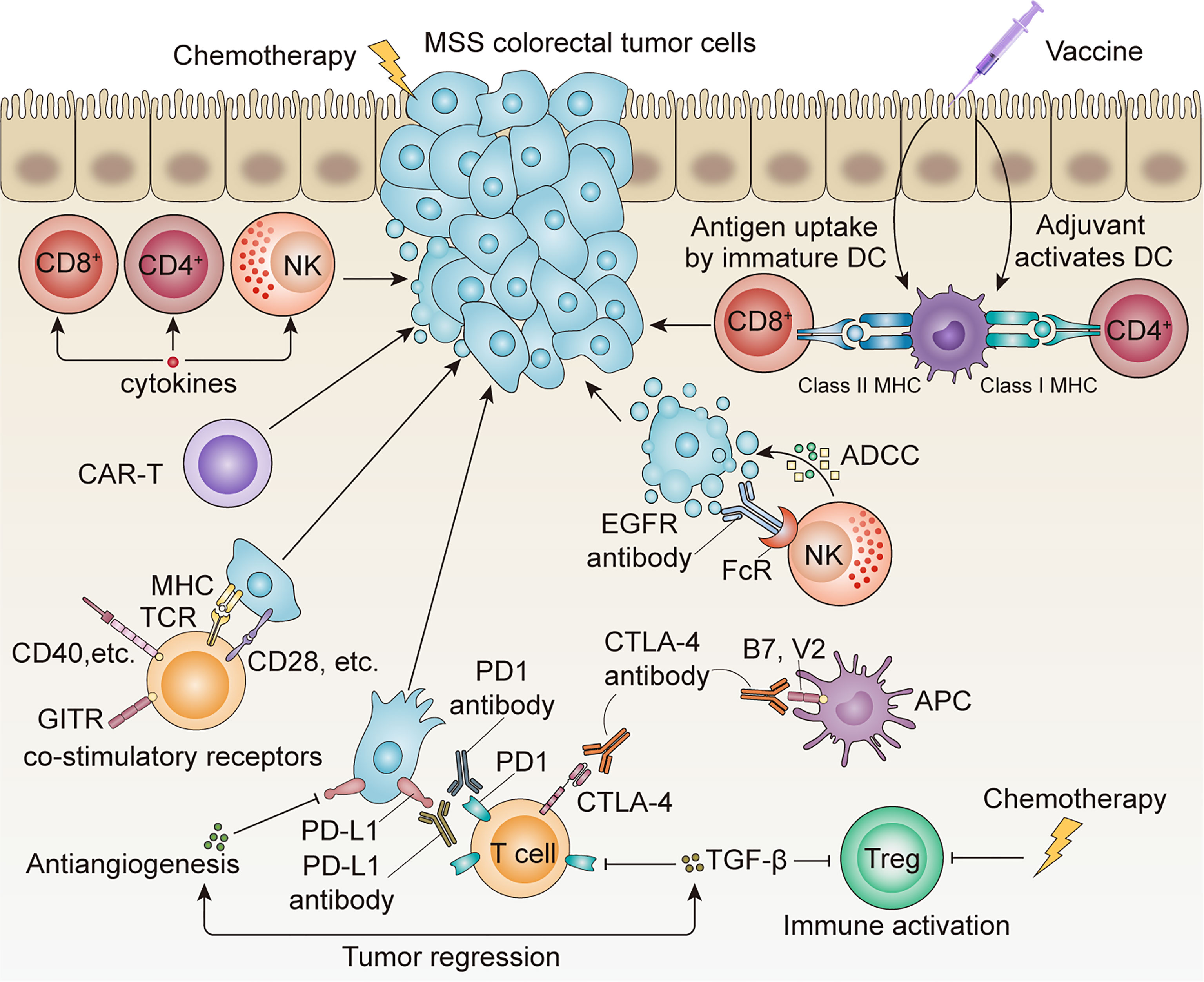
Figure 1 Application of multiple adaptive immunotherapies in MSS CRC. ICI monotherapy is often ineffective, and the combination of chemotherapy, antiangiogenesis therapy, anti-EGFR antibody, and other systemic therapies is expected to achieve better therapeutic outcomes.
Cytokines and Costimulatory Receptors
Extracellular cytokine components dynamically regulate the tumor microenvironment. The aberrant expression of cytokines is strongly correlated with the pathogenesis and progression of CRC (11). Cytokines have direct antitumor effects and have been used as cancer immunotherapies for decades, especially in metastatic melanoma and metastatic renal cell carcinoma (12, 13). Regarding CRC, evidence has shown that IL-6 can activate autophagy through the IL-6/JAK2/BECN1 pathway and promote chemotherapy resistance in CRC (14). One recent study showed that combined treatment with IL-2 and Akkermansia muciniphila leads to stronger antitumor efficacy in CRC patient-derived tumor tissue and proposed this novel therapeutic strategy with prospecting application in the future (15). Another study showed that IL-2 combined with TNFSF14 results in an increase in CD8+ central memory cells and a decrease in tumor size in preclinical exploration (16). Several clinical studies have proven that cytokines are tolerable safe, and more clinical trials are underway to explore their efficiency (11).
Another immunotherapy type is costimulatory receptors; among them, OX40 and 4-1BB are members of the TNF receptor and have the ability to activate stimulatory receptors and have shown promising antitumor effects in preclinical studies (17, 18). However, OX40 antibodies only show strong tumor suppressive effects when the tumor load is low, and further studies indicate that costimulatory receptors can improve treatment efficiency when combined with ICIs (19). A clinical trial (NCT 02179918) of multiple solid tumors was conducted on the combined treatment of a 4-1BB agonist plus a PD-1 inhibitor.
Therapeutic Cancer Vaccines
Therapeutic cancer vaccines are diverse and include cell-based vaccines, protein/peptide vaccines, and genetic vaccines. Cancer vaccines are divided into tumor-associated antigen (TAA) vaccines and tumor-specific antigen vaccines. Currently, no significant breakthrough has been found in CRC vaccines, and no such vaccines have been approved for clinical treatment. However, as research continues, vaccines can present more potent antigens and activate both CD4+ and CD8+ T cells.
The most mature TAA vaccines in CRC are carcinoembryonic antigen (CEA) vaccines, melanoma-associated antigen (MAGE) vaccines, and intestinal protein guanylyl cyclase 2C (GUCY2C) vaccines. CEA is regularly overexpressed in CRC, but it is not cancer specific and is also expressed by normal epithelial cells (20). One of the first studies of CEA vaccines showed preliminary clinical benefit: two of 12 patients experienced dramatic tumor regression (21). Subsequently, studies suggested that different CEA peptide or mRNA vaccines can generate or boost specific T-cell responses and may provide clinical benefit in CRC patients (22, 23). However, the treatment outcome of CEA vaccines is not satisfactory because the clinical response rate does not exceed 17% (24) and consequently autoimmunity occurs. MAGE vaccines have the ability to induce an orchestrated CD4+ and CD8+ immune response. However, only one case report showed that MAGE-A4-H/K-HELP could induce Th1-dependent cells and decrease tumor growth and CEA tumor markers in colon cancer patients (25). Due to the poor response to a single vaccine, more combination treatment regimens of vaccines and personalized peptide vaccines have been studied and investigated in clinical trials. Most vaccines in these studies were combined with chemotherapies, such as 5-fluorouracil. Sato et al. (26) found that this combination therapy could actually maintain or augment immunological responses, but there was no confirmed decrease in tumor burden. GUCY2C vaccines focus on minimum residual disease in colon cancer patients and aim to reduce the recurrence rate (27). A preliminary phase I study suggested that the GUCY2C vaccine was safe and effective at inducing the CD8+ T-cell response in early-stage patients (28).
Regarding tumor-specific antigen vaccines, the number and quality of neoantigens are high (29). Tumor-specific vaccines have the advantage of recognizing cancer cells from normal cells and minimizing the risk of vaccination-reduced severe adverse events. However, MSS CRC usually shows fewer gene alterations than CRC with microsatellite instability-high (MSI-H) status or POLE mutations. In addition, heterogeneity exists and remains a challenge for developing a tumor-specific antigen vaccine. Neoantigens caused by KRAS are common in CRC, and peptides derived from mutated KRAS show certain anticancer activity in vaccination trials. In one case report, CRC lung metastasis patients quickly exhibited tumor regression after treatment with activated T cells recognizing G12D KRAS (6). Another clinical trial found that two out of seven patients were responsive after vaccine infusion (30).
Vaccines are able to activate cytotoxic T lymphocytes (CTLs), and the correlation between infiltrating lymphocytes and overall survival is significant in patients with MSS disease (31, 32). Therefore, a combination of ICIs and vaccines could be a new treatment strategy in the future. Preliminary animal studies have shown encouraging results (33), and several clinical trials are ongoing (Table 1).
Adoptive T-Cell Therapy
The patient’s T cells can target a specific antigen that is expressed on the surface of tumor cells. Thus, several types of T cells have been used for adoptive T-cell therapy, including TILs, chimeric antigen receptor (CAR)-engineered T cells and cancer-specific T-cell receptor-engineered T cells. These modified cells are reintroduced into the human body to fight against cancer cells and have achieved great success in hematological malignancies (34). However, the role of adoptive T-cell therapy in CRC is still unclear, and the treatment efficacy and safety remain to be verified in clinical studies.
CAR T-cell therapy has become increasingly popular in the last decade, and we are currently in the fourth generation of CAR T-cell immunotherapy (35). In the course of treatment, the patient’s immune cells are first harvested and cultured in vitro, and then CARs are artificially integrated into the T cells. Finally, the CAR T cells are infused back into the patient. The complexity of the entire process leads to the high cost of CAR T-cell therapy and high requirements for companies. Another significant problem with CAR T-cell therapy is toxicity. Cytokine release syndrome (CRS), which is not observed with traditional chemotherapy, is the main side effect and is caused by the release of inflammatory cytokines by continuously proliferating CAR T cells.
Multiple ongoing clinical trials of various potential targets of CAR T-cell therapy for colorectal cancer, including solid tumor targets such as GUCY2C, CEA, and TAG-72 and circulating tumor cell targets such as EpCAM, are shown in Table 1.
Different clinical studies have also employed different administration methods for CAR T cells, such as intravenous infusion, intraperitoneal infusion, and direct hepatic artery infusion (36). One of the first human CAR T-cell trials on metastatic colorectal cancer reported by Hege et al. (37) found that CART72 showed good safety and tolerance despite quick clearance from the blood. Another preclinical study (38) focusing on GUCY2C found certain effectiveness against mCRC in mouse models and in xenograft models of human CRC, and further human clinical trials are currently being recruited in China. Preliminary antitumor activity is also reflected in CAR T-133 cells, EpCAM CAR T cells, NKG2D CAR T cells, and HER2 CAR T cells (36, 39–42). Ongoing clinical trials of CAR T-cell therapy in the treatment of mCRC are shown in Table 2.
To date, there have been no particularly encouraging results about CAR T-cell therapy in mCRC. Although several promising CAR T-cell therapies have shown success in preclinical models, additional human data are still needed.
Immune Checkpoint Inhibitors
The most well-studied immune checkpoints include PD-1, PD-L1, CTLA-4, LAG-3, and TIM-3. Despite the great success of the KEYNOTE 177 study (43) on MSI-H CRC, the majority of clinical trials of ICIs in MSS CRC have ended in failure. ICI monotherapy did not obtain positive results in MSS CRC patients in the KEYNOTE 016 and phase 2 CheckMate 142 trials. ICIs also failed as maintenance therapy in the MODUL study (44). PD-1 and CTLA-4 antibody combination therapy is a third-line treatment compared with best supportive care (45). The lack of mutant neoantigens, immune evasion, and neoangiogenesis are responsible for poor responses (46–49). In addition to treatment regimen exploration, the effect of metastatic sites on the efficacy of immunotherapy is also worthy of further study. Several studies have found that patients with liver metastasis often have a poor response to immunotherapy (50, 51). To overcome these problems, several new combination regimens have been explored. The rationale for combination therapy is that chemotherapy or targeted therapy can alter the tumor microenvironment (TME). Although different factors, such as low neoantigen burden and alterations in JAK/STAT pathways, may influence the treatment efficiency of ICIs, it is believed that the insensitivity of MSS CRC to immunotherapy could be reversed by altering the TME. The TME is a dynamic environment and is composed of cancer cells, extracellular matrix, immune cells, nutrients, and other components (52, 53).
Regarding combined checkpoint inhibitors, a phase I study found manageable safety and promising antitumor activity with the antilymphocyte activation gene (LAG-3) antibody plus pembrolizumab in MSS mCRC (54). Anti-TIM-3 antibody combined with anti-PD-1 antibody showed preliminary signs of antitumor activity in solid tumors (55).
Combination With Chemotherapy
Chemotherapeutic drugs seem to reduce the levels of Tregs and increase the proliferation of homeostatic T cells (56). Oxaliplatin was proven to feasibly and substantially expand the response to ICIs in lung adenocarcinoma mouse models (57). Therefore, the phase II study POCHI (NCT 04262687) of the combination of oxaliplatin, capecitabine, bevacizumab, and pembrolizumab in CRC patients is ongoing.
Another study found that 5-fluorouracil could increase tumor visibility to immune cells, and the treatment response improved with sequential administration of 5-fluorouracil and ICIs compared with concurrent administration of ICIs with 5-fluorouracil (58).
Combination With Radiotherapy
Radiotherapy can release neoantigens and inflammatory cytokines during treatment (59) and influence nonirradiated sites by activating the immune response (60), which is called the abscopal effect. However, the abscopal effect was observed in melanoma when ipilimumab was combined with localized radiotherapy (61). Regarding CRC, a phase II study enrolled 22 patients with MSS/mismatch repair-proficient (pMMR) CRC, and only one patient experienced regression at nonirradiated sites (62). There are a growing number of clinical trials exploring the combined efficiency of radiotherapy and immunotherapy. A phase II clinical trial of short-course radiotherapy combined with mFOLFOX6 chemotherapy and avelumab immunotherapy in Lebanon and Jordan enrolled 13 patients with MSS rectal cancer. One patient had preoperative progression, three patients achieved pCR, and three achieved near pCR (TRG1 score). That is, half of the patients achieved very significant tumor regression.
Combination With Anti-EGFR Antibody
The combination of immunotherapy and EGFR antibodies can also enhance the immune response due to antibody-dependent cell-mediated cytotoxicity (ADCC) (63). One phase Ib/II trial of cetuximab combined with ICIs in nine mCRC patients showed that 67% of patients achieved stable disease (64). Another phase II trial of panitumumab plus ipilimumab and nivolumab in 56 refractory mCRC patients achieved a 35% 12-week response rate and 5.7-month progression-free survival (PFS) time (65).
Combination With Antiangiogenesis Therapy
Immunotherapy is most commonly combined with VEGF inhibitors. Patients treated with antiangiogenic therapy had higher infiltration of CD4+FOXP3+ regulatory T cells and enhanced expression of checkpoint ligand programmed death-ligand 1 (PD-L1) in renal cell carcinoma specimens than the corresponding control (66). This phenomenon may be related to improved nutrient supply and oxygen demand with antiangiogenic therapy. However, an increasing number of studies have found that antiangiogenic drugs can modulate the TME, independent of their antiangiogenic effects. Regorafenib was found to have the ability to inhibit JAK1/2-STAT1 and MAPK signaling by targeting the RET-Src axis and to induce PD-L1 and IDO1 expression in melanoma (67). Another study found that regorafenib could reverse M2 macrophage polarization and the regorafenib dosage may be halved when combined with ICIs (68).
The REGNIVO study (69) showed a 36% overall response in 25 MSS CRC patients treated with regorafenib and nivolumab. However, among 48 patients in the REGOMUM study, no patient showed a partial or complete response. Therefore, this combination regimen still needs validation in a phase III study, and many clinical trials are ongoing.
Combination With TGF-β Antibody
TGF-β upregulation plays an important role in the immune evasion of MSS mCRC. It has been reported that TGF-β can promote T-cell exclusion and block the acquisition of the Th1 effector phenotype (70). TGF-β inhibitors can render tumors susceptible to ICIs. Preclinical studies support the potential use of anti-PD-L1/TGF-β trap fusion proteins in clinical use, and related clinical trials are ongoing. Src homology 2 domain-containing tyrosine phosphatase 2 (Shp2) (71) and prostaglandin E2 (PGE2) receptor 4 (EP4) (72) are both potential strategies for enhancing the efficacy of immunotherapy for MSS mCRC by regulating immunosuppressive myeloid cells.
Conclusion
Immunotherapy has a confirmed survival benefit for MSI-H mCRC. For MSS CRC, trials of monotherapy with ICIs and ICIs combined with other treatments, such as MEK inhibitors, have failed in the past decade. However, explorations of adaptive immune therapy in MSS CRC are ongoing. Clinical trials of cytokines, costimulatory receptors, CAR T cells and different combinations of anti-VEGF or anti-EGFR agents plus ICIs are ongoing. Preliminary data have indicated the great promise of combined adaptive immune therapy in MSS mCRC, and this type of treatment is expected to transform cold tumors into hot tumors.
Author Contributions
All authors contributed to the study conception and design. Material preparation, data collection, and analysis were performed by DW, TX, and GW. The first draft of the manuscript was written by HZ, and all authors commented on previous versions of the manuscript. All authors read and approved the final manuscript.
Conflict of Interest
The authors declare that the research was conducted in the absence of any commercial or financial relationships that could be construed as a potential conflict of interest.
Publisher’s Note
All claims expressed in this article are solely those of the authors and do not necessarily represent those of their affiliated organizations, or those of the publisher, the editors and the reviewers. Any product that may be evaluated in this article, or claim that may be made by its manufacturer, is not guaranteed or endorsed by the publisher.
References
1. Siegel RL, Miller KD, Fuchs HE, Jemal A. Cancer Statistics, 2021. CA Cancer J Clin (2021) 71(1):7–33. doi: 10.3322/caac.21654
2. Done JZ, Fang SH. Young-Onset Colorectal Cancer: A Review. World J Gastrointest Oncol (2021) 13(8):856–66. doi: 10.4251/wjgo.v13.i8.856
3. Miller KD, Nogueira L, Mariotto AB, Rowland JH, Yabroff KR, Alfano CM, et al. Cancer Treatment and Survivorship Statistics, 2019. CA Cancer J Clin (2019) 69(5):363–85. doi: 10.3322/caac.21565
4. Zhang L, Conejo-Garcia JR, Katsaros D, Gimotty M, Massobrio G, Regnani A, et al. Intratumoral T Cells, Recurrence, and Survival in Epithelial Ovarian Cancer. N Engl J Med (2003) 348(3):203–13. doi: 10.1056/NEJMoa020177
5. Galon J, Costes A, Sanchez-Cabo F, Kirilovsky B, Mlecnik C, Lagorce-Pages M, et al. Type, Density, and Location of Immune Cells Within Human Colorectal Tumors Predict Clinical Outcome. Science (2006) 313(5795):1960–4. doi: 10.1126/science.1129139
6. Tran E, Robbins PF, Lu YC, Prickett TD, Gartner JJ, Jia L, et al. T-Cell Transfer Therapy Targeting Mutant KRAS in Cancer. N Engl J Med (2016) 375(23):2255–62. doi: 10.1056/NEJMoa1609279
7. Tartarone A, Roviello G, Lerose R, Roudi R, Aieta M, Zoppoli P. Anti-PD-1 Versus Anti-PD-L1 Therapy in Patients With Pretreated Advanced Non-Small-Cell Lung Cancer: A Meta-Analysis. Future Oncol (2019) 15(20):2423–33. doi: 10.2217/fon-2018-0868
8. Petrelli F, Ferrara R, Signorelli D, Ghidini C, Proto R, Roudi MN, et al. Immune Checkpoint Inhibitors and Chemotherapy in First-Line NSCLC: A Meta-Analysis. Immunotherapy (2021) 13(7):621–31. doi: 10.2217/imt-2020-0224
9. Cuevas LM, Daud AI. Immunotherapy for Melanoma. Semin Cutan Med Surg (2018) 37(2):127–31. doi: 10.12788/j.sder.2018.028
10. Overman MJ, Ernstoff MS, Morse MA. Where We Stand With Immunotherapy in Colorectal Cancer: Deficient Mismatch Repair, Proficient Mismatch Repair, and Toxicity Management. Am Soc Clin Oncol Educ Book (2018) 38:239–47. doi: 10.1200/EDBK_200821
11. Li J, Huang L, Zhao H, Yan Y, Lu J. The Role of Interleukins in Colorectal Cancer. Int J Biol Sci (2020) 16(13):2323–39. doi: 10.7150/ijbs.46651
12. Steininger J, Gellrich FF, Schulz A, Westphal D, Beissert S, Meier F. Systemic Therapy of Metastatic Melanoma: On the Road to Cure. Cancers (Basel). (2021) 13(6). doi: 10.3390/cancers13061430
13. Wrangle JM, Patterson A, Johnson CB, Neitzke DJ, Mehrotra S, Denlinger CE, et al. IL-2 and Beyond in Cancer Immunotherapy. J Interferon Cytokine Res (2018) 38(2):45–68. doi: 10.1089/jir.2017.0101
14. Fuqing Hu DS, Yumeng Y, Changsheng H, Chentao S, Jingqin L, Yaqi C, et al. IL-6 Regulates Autophagy and Chemotherapy Resistance by Promoting BECN1 Phosphorylation. Nat. Commun (2021) 12(1):3651. doi: 10.1038/s41467-021-23923-1
15. Shi L, Sheng J, Chen G, Zhu P, Shi C, Li B, et al. Combining IL-2-Based Immunotherapy With Commensal Probiotics Produces Enhanced Antitumor Immune Response and Tumor Clearance. J Immunother Cancer (2020) 8(2). doi: 10.1136/jitc-2020-000973
16. Fernandez MF, Qiao G, Tulla K, Prabhakar BS, Maker AV. Combination Immunotherapy With LIGHT and Interleukin-2 Increases CD8 Central Memory T-Cells In Vivo. J Surg Res (2021) 263:44–52. doi: 10.1016/j.jss.2021.01.010
17. Lynch DH. The Promise of 4-1BB (CD137)-Mediated Immunomodulation and the Immunotherapy of Cancer. Immunol Rev (2008) 222:277–86. doi: 10.1111/j.1600-065X.2008.00621.x
18. Jensen SM, Maston LD, Gough MJ, Ruby CE, Redmond WL, Crittenden M, et al. Signaling Through OX40 Enhances Antitumor Immunity. Semin Oncol (2010) 37(5):524–32. doi: 10.1053/j.seminoncol.2010.09.013
19. Yan LH, Liu XL, Mo SS, Zhang D, Mo XW, Tang WZ. OX40 as a Novel Target for the Reversal of Immune Escape in Colorectal Cancer. Am J Transl Res (2021) 13(3):923–34.
20. Gold P, Freedman SO. Demonstration of Tumor-Specific Antigens in Human Colonic Carcinomata by Immunological Tolerance and Absorption Techniques. J Exp Med (1965) 121:439–62. doi: 10.1084/jem.121.3.439
21. Fong L, Hou Y, Rivas A, Benike C, Yuen A, Fisher GA, et al. Altered Peptide Ligand Vaccination With Flt3 Ligand Expanded Dendritic Cells for Tumor Immunotherapy. Proc Natl Acad Sci USA (2001) 98(15):8809–14. doi: 10.1073/pnas.141226398
22. Lesterhuis WJ, De Vries IJ, Schreibelt G, Schuurhuis DH, Aarntzen EH, De Boer A, et al. Immunogenicity of Dendritic Cells Pulsed With CEA Peptide or Transfected With CEA mRNA for Vaccination of Colorectal Cancer Patients. Anticancer Res (2010) 30(12):5091–7.
23. Liu KJ, Wang CC, Chen LT, Cheng AL, Lin DT, Wu YC, et al. Generation of Carcinoembryonic Antigen (CEA)-Specific T-Cell Responses in HLA-A*0201 and HLA-A*2402 Late-Stage Colorectal Cancer Patients After Vaccination With Dendritic Cells Loaded With CEA Peptides. Clin Cancer Res (2004) 10(8):2645–51. doi: 10.1158/1078-0432.ccr-03-0430
24. Wagner S, Mullins CS, Linnebacher M. Colorectal Cancer Vaccines: Tumor-Associated Antigens vs Neoantigens. World J Gastroenterol (2018) 24(48):5418–32. doi: 10.3748/wjg.v24.i48.5418
25. Takahashi N, Ohkuri T, Homma S, Ohtake J, Wakita D, Togashi Y, et al. First Clinical Trial of Cancer Vaccine Therapy With Artificially Synthesized Helper/ Killer-Hybrid Epitope Long Peptide of MAGE-A4 Cancer Antigen. Cancer Sci (2012) 103(1):150–3. doi: 10.1111/j.1349-7006.2011.02106.x
26. Sato Y, Fujiwara T, Mine T, Mine H, Shomura S, Homma Y, et al. Immunological Evaluation of Personalized Peptide Vaccination in Combination With a 5-Fluorouracil Derivative (TS-1) for Advanced Gastric or Colorectal Carcinoma Patients. Cancer Sci (2007) 98(7):1113–9. doi: 10.1111/j.1349-7006.2007.00498.x
27. Lisby AN, Flickinger JC Jr., Bashir B, Weindorfer M, Shelukar S, Crutcher M, et al. GUCY2C as a Biomarker to Target Precision Therapies for Patients With Colorectal Cancer. Expert Rev Precis Med Drug Dev (2021) 6(2):117–29. doi: 10.1080/23808993.2021.1876518
28. Snook AE, Baybutt TR, Xiang B, Abraham TS, Flickinger JC Jr, Hyslop T, et al. Split Tolerance Permits Safe Ad5-GUCY2C-PADRE Vaccine-Induced T-Cell Responses in Colon Cancer Patients. J Immunother Cancer (2019) 7(1):104. doi: 10.1186/s40425-019-0576-2
29. Rooney MS, Shukla SA, Wu CJ, Getz G, Hacohen N. Molecular and Genetic Properties of Tumors Associated With Local Immune Cytolytic Activity. Cell (2015) 160(1-2):48–61. doi: 10.1016/j.cell.2014.12.033
30. Toubaji A, Achtar M, Provenzano M, Herrin VE, Behrens R, Hamilton M, et al. Pilot Study of Mutant Ras Peptide-Based Vaccine as an Adjuvant Treatment in Pancreatic and Colorectal Cancers. Cancer Immunol Immunother (2008) 57(9):1413–20. doi: 10.1007/s00262-008-0477-6
31. Baker K, Zlobec I, Tornillo L, Terracciano L, Jass JR, Lugli A. Differential Significance of Tumour Infiltrating Lymphocytes in Sporadic Mismatch Repair Deficient Versus Proficient Colorectal Cancers: A Potential Role for Dysregulation of the Transforming Growth Factor-Beta Pathway. Eur J Cancer (2007) 43(3):624–31. doi: 10.1016/j.ejca.2006.11.012
32. Deschoolmeester V, Baay M, Van Marck E, Van Marck J, Weyler P, Vermeulen F, et al. Tumor Infiltrating Lymphocytes: An Intriguing Player in the Survival of Colorectal Cancer Patients. BMC Immunol (2010) 11:19. doi: 10.1186/1471-2172-11-19
33. Liu C, Lu Z, Xie Y, Guo Q, Geng F, Sun B, et al. Soluble PD-1-Based Vaccine Targeting MUC1 VNTR and Survivin Improves Anti-Tumor Effect. Immunol Lett (2018) 200:33–42. doi: 10.1016/j.imlet.2018.06.004
34. Sur D, Havasi A, Cainap C, Samasca G, Burz C, Balacescu O, et al. Chimeric Antigen Receptor T-Cell Therapy for Colorectal Cancer. J Clin Med (2020) 9(1). doi: 10.3390/jcm9010182
35. Yeku OO, Brentjens RJ. Armored CAR T-Cells: Utilizing Cytokines and Pro-Inflammatory Ligands to Enhance CAR T-Cell Anti-Tumour Efficacy. Biochem Soc Trans (2016) 44(2):412–8. doi: 10.1042/BST20150291
36. Ang WX, Li Z, Chi Z, Du SH, Chen C, Tay JC, et al. Intraperitoneal Immunotherapy With T Cells Stably and Transiently Expressing Anti-EpCAM CAR in Xenograft Models of Peritoneal Carcinomatosis. Oncotarget (2017) 8(8):13545–59. doi: 10.18632/oncotarget.14592
37. Hege KM, Bergsland EK, Fisher GA, Nemunaitis JJ, Warren RS, McArthur JG, et al. Safety, Tumor Trafficking and Immunogenicity of Chimeric Antigen Receptor (CAR)-T Cells Specific for TAG-72 in Colorectal Cancer. J Immunother Cancer (2017) 5:22. doi: 10.1186/s40425-017-0222-9
38. Magee MS, Abraham TS, Baybutt TR, Flickinger JC Jr, Ridge NA, Marszalowicz GP, et al. Human GUCY2C-Targeted Chimeric Antigen Receptor (CAR)-Expressing T Cells Eliminate Colorectal Cancer Metastases. Cancer Immunol Res (2018) 6(5):509–16. doi: 10.1158/2326-6066.CIR-16-0362
39. Zhang C, Wang Z, Yang Z, Wang M, Li S, Li Y, et al. Phase I Escalating-Dose Trial of CAR-T Therapy Targeting CEA(+) Metastatic Colorectal Cancers. Mol Ther (2017) 25(5):1248–58. doi: 10.1016/j.ymthe.2017.03.010
40. Deng X, Gao F, Li N, Li Q, Zhou Y, Yang T, et al. Antitumor Activity of NKG2D CAR-T Cells Against Human Colorectal Cancer Cells In Vitro and In Vivo. Am J Cancer Res (2019) 9(5):945–58.
41. Teng R, Zhao J, Zhao Y, Gao J, Li H, Zhou S, et al. Chimeric Antigen Receptor-Modified T Cells Repressed Solid Tumors and Their Relapse in an Established Patient-Derived Colon Carcinoma Xenograft Model. J Immunother (2019) 42(2):33–42. doi: 10.1097/CJI.0000000000000251
42. Wang Y, Chen M, Wu Z, Tong C, Dai H, Guo Y, et al. CD133-Directed CAR T Cells for Advanced Metastasis Malignancies: A Phase I Trial. Oncoimmunology (2018) 7(7):e1440169. doi: 10.1080/2162402X.2018.1440169
43. Casak SJ, Marcus L, Fashoyin-Aje L, Mushti SL, Cheng J, Shen YL, et al. FDA Approval Summary: Pembrolizumab for the First-Line Treatment of Patients With MSI-H/dMMR Advanced Unresectable or Metastatic Colorectal Carcinoma. Clin Cancer Res (2021). doi: 10.1158/1078-0432.CCR-21-0557
44. Grothey A TJ, Arnold D, et al. LBA19 Fluoropyrimidine (FP)þ Bevaci- Zumab (BEV)þ Atezolizumab vs FP/BEV in BRAFwt Metastatic Colorectal can- Cer (mCRC): Findings From Cohort 2 of MODUL–a Multicentre, Randomized Trial of Biomarker-Driven Maintenance Treatment Following First-Line Induc- Tion Therapy. Ann Oncol (2018) 29(suppl 8):mdy424.020.
45. Chen EX, Jonker DJ, Loree JM, Kennecke HF, Berry SR, Couture F, et al. Effect of Combined Immune Checkpoint Inhibition vs Best Supportive Care Alone in Patients With Advanced Colorectal Cancer: The Canadian Cancer Trials Group CO.26 Study. JAMA Oncol (2020) 6(6):831–8. doi: 10.1001/jamaoncol.2020.0910
46. Le DT, Durham JN, Smith KN, Wang H, Bartlett BR, Aulakh LK, et al. Mismatch Repair Deficiency Predicts Response of Solid Tumors to PD-1 Blockade. Science (2017) 357(6349):409–13. doi: 10.1126/science.aan6733
47. Spranger S, Bao R, Gajewski TF. Melanoma-Intrinsic Beta-Catenin Signalling Prevents Anti-Tumour Immunity. Nature (2015) 523(7559):231–5. doi: 10.1038/nature14404
48. Luke JJ, Bao R, Sweis RF, Spranger S, Gajewski TF. WNT/beta-Catenin Pathway Activation Correlates With Immune Exclusion Across Human Cancers. Clin Cancer Res (2019) 25(10):3074–83. doi: 10.1158/1078-0432.CCR-18-1942
49. Ghiringhelli F, Fumet JD. Is There a Place for Immunotherapy for Metastatic Microsatellite Stable Colorectal Cancer? Front Immunol (2019) 10:1816. doi: 10.3389/fimmu.2019.01816
50. Wang C, Sandhu J, Ouyang C, Ye J, Lee PP, Fakih M. Clinical Response to Immunotherapy Targeting Programmed Cell Death Receptor 1/Programmed Cell Death Ligand 1 in Patients With Treatment-Resistant Microsatellite Stable Colorectal Cancer With and Without Liver Metastases. JAMA Netw Open (2021) 4(8):e2118416. doi: 10.1001/jamanetworkopen.2021.18416
51. Yu J, Green MD, Li S, Sun Y, Journey SN, Choi JE, et al. Liver Metastasis Restrains Immunotherapy Efficacy via Macrophage-Mediated T Cell Elimination. Nat Med (2021) 27(1):152–64. doi: 10.1038/s41591-020-1131-x
52. Hanahan D, Coussens LM. Accessories to the Crime: Functions of Cells Recruited to the Tumor Microenvironment. Cancer Cell (2012) 21(3):309–22. doi: 10.1016/j.ccr.2012.02.022
53. Duan Q, Zhang H, Zheng J, Zhang L. Turning Cold Into Hot: Firing Up the Tumor Microenvironment. Trends Cancer (2020) 6(7):605–18. doi: 10.1016/j.trecan.2020.02.022
54. Elena Garralda AS, et al. A Phase 1 First-in-Human Study of the Anti-LAG-3 Antibody MK4280 (Favezelimab) Plus Pembrolizumab in Previously Treated, Advanced Microsatellite Stable Colorectal Cancer. J Clin Oncol (2021) 39:(suppl 15; abstr 3584). doi: 10.1200/JCO.2021.39.15_suppl.3584
55. Curigliano G, Gelderblom H, Mach N, Doi T, Tai D, Forde PM, et al. Phase I/Ib Clinical Trial of Sabatolimab, an Anti-TIM-3 Antibody, Alone and in Combination With Spartalizumab, an Anti-PD-1 Antibody, in Advanced Solid Tumors. Clin Cancer Res (2021) 27(13):3620–9. doi: 10.1158/1078-0432.CCR-20-4746
56. Sun X, Suo J, Yan J. Immunotherapy in Human Colorectal Cancer: Challenges and Prospective. World J Gastroenterol (2016) 22(28):6362–72. doi: 10.3748/wjg.v22.i28.6362
57. Pfirschke C, Engblom C, Rickelt S, Cortez-Retamozo C, Garris F, Pucci T, et al. Immunogenic Chemotherapy Sensitizes Tumors to Checkpoint Blockade Therapy. Immunity (2016) 44(2):343–54. doi: 10.1016/j.immuni.2015.11.024
58. Zhao X, Kassaye B, Wangmo D, Lou E, Subramanian S. Chemotherapy But Not the Tumor Draining Lymph Nodes Determine the Immunotherapy Response in Secondary Tumors. iScience (2020) 23(5):101056. doi: 10.1016/j.isci.2020.101056
59. Weichselbaum RR, Ishwaran H, Yoon T, Nuyten DS, Baker SW, Khodarev N, et al. An Interferon-Related Gene Signature for DNA Damage Resistance Is a Predictive Marker for Chemotherapy and Radiation for Breast Cancer. Proc Natl Acad Sci USA (2008) 105(47):18490–5. doi: 10.1073/pnas.0809242105
60. Franke AJ, Skelton WP, Starr JS, Parekh JJ, Lee MJ, Overman C, et al. Immunotherapy for Colorectal Cancer: A Review of Current and Novel Therapeutic Approaches. J Natl Cancer Inst (2019) 111(11):1131–41. doi: 10.1093/jnci/djz093
61. Postow MA, Callahan MK, Barker CA, Yamada J, Yuan S, Kitano Y, et al. Immunologic Correlates of the Abscopal Effect in a Patient With Melanoma. N Engl J Med (2012) 366(10):925–31. doi: 10.1056/NEJMoa1112824
62. Segal NH KN, Cercek A, et al. Non-Randomized Phase II Study to as- Sess the Efficacy of Pembrolizumab (Pem) Plus Radiotherapy (RT) or Ablation in Mismatch Repair Proficient (pMMR) Metastatic Colorectal Cancer (mCRC) Patients. In: American Society of Clinical Oncology Annual Meeting. Chicago: American Society of Clinical Oncology (2016).
63. Holubec L, Polivka J Jr., Safanda M, Karas M, Liska V. The Role of Cetuximab in the Induction of Anticancer Immune Response in Colorectal Cancer Treatment. Anticancer Res (2016) 36(9):4421–6. doi: 10.21873/anticanres.10985
64. Boland PM HA, Maguire O, et al. A Phase Ib/II Study of Cetuximab and Pembrolizumab in RAS-Wt mCRC. In: American Society of Clinical Oncology Annual Meeting. Chicago: American Society of Clinical Oncology (2018).
65. Sangmin Lee M, Loeher PJ, et al. Phase II Study of Ipilimumab, Nivolumab, and Panitumumab in Patients With KRAS/NRAS/BRAF Wild-Type (WT) Microsatellite Stable (MSS) Metastatic Colorectal Cancer (mCRC). In: American Society of Clinical Oncology Gastrointestinal Cancers Symposium. J Clin Oncol (2021) 39:(suppl 3; abstr 7). doi: 10.1200/JCO.2021.39.3_suppl.7
66. Liu XD, Hoang A, Zhou L, Kalra S, Yetil A, Sun M, et al. Resistance to Antiangiogenic Therapy Is Associated With an Immunosuppressive Tumor Microenvironment in Metastatic Renal Cell Carcinoma. Cancer Immunol Res (2015) 3(9):1017–29. doi: 10.1158/2326-6066.CIR-14-0244
67. Wu RY, Kong PF, Xia LP, Huang Y, Li ZL, Tang YY, et al. Regorafenib Promotes Antitumor Immunity via Inhibiting PD-L1 and IDO1 Expression in Melanoma. Clin Cancer Res (2019) 25(14):4530–41. doi: 10.1158/1078-0432.CCR-18-2840
68. Ou DL, Chen CW, Hsu CL, Chung CH, Feng ZR, Lee BS, et al. Regorafenib Enhances Antitumor Immunity via Inhibition of P38 Kinase/Creb1/Klf4 Axis in Tumor-Associated Macrophages. J Immunother Cancer (2021) 9(3). doi: 10.1136/jitc-2020-001657
69. Fukuoka S, Hara H, Takahashi N, Kojima T, Kawazoe A, Asayama N, et al. Regorafenib Plus Nivolumab in Patients With Advanced Gastric or Colorectal Cancer: An Open-Label, Dose-Escalation, and Dose-Expansion Phase Ib Trial (REGONIVO, Epoc1603). J Clin Oncol (2020) 38(18):2053–61. doi: 10.1200/JCO.19.03296
70. Tauriello DVF, Palomo-Ponce S, Stork D, Berenguer-Llergo A, Badia-Ramentol J, Iglesias M, et al. TGFbeta Drives Immune Evasion in Genetically Reconstituted Colon Cancer Metastasis. Nature (2018) 554(7693):538–43. doi: 10.1038/nature25492
71. Wang S, Yao Y, Li H, Zheng G, Lu S, Chen W. Tumor-Associated Macrophages (TAMs) Depend on Shp2 for Their Anti-Tumor Roles in Colorectal Cancer. Am J Cancer Res (2019) 9(9):1957–69.
Keywords: adaptive immune response, colorectal cancer, immune therapy, vaccines, CAR-T
Citation: Wang D, Zhang H, Xiang T and Wang G (2021) Clinical Application of Adaptive Immune Therapy in MSS Colorectal Cancer Patients. Front. Immunol. 12:762341. doi: 10.3389/fimmu.2021.762341
Received: 21 August 2021; Accepted: 17 September 2021;
Published: 13 October 2021.
Edited by:
Nurit Hollander, Tel Aviv University, IsraelReviewed by:
Raheleh Roudi, University of Minnesota, United StatesXianda Zhao, University of Minnesota, United States
Copyright © 2021 Wang, Zhang, Xiang and Wang. This is an open-access article distributed under the terms of the Creative Commons Attribution License (CC BY). The use, distribution or reproduction in other forums is permitted, provided the original author(s) and the copyright owner(s) are credited and that the original publication in this journal is cited, in accordance with accepted academic practice. No use, distribution or reproduction is permitted which does not comply with these terms.
*Correspondence: Gang Wang, anhoeXdnQDEyNi5jb20=
†These authors have contributed equally to this work