- 1Department of Pediatrics, Emory University School of Medicine, Atlanta, GA, United States
- 2Center for Childhood Infections and Vaccines of Children’s Healthcare of Atlanta and Emory University, Atlanta, GA, United States
Despite the significant progress that has been made to eliminate vertical HIV infection, more than 150,000 children were infected with HIV in 2019, emphasizing the continued need for sustainable HIV treatment strategies and ideally a cure for children. Mother-to-child-transmission (MTCT) remains the most important route of pediatric HIV acquisition and, in absence of prevention measures, transmission rates range from 15% to 45% via three distinct routes: in utero, intrapartum, and in the postnatal period through breastfeeding. The exact mechanisms and biological basis of these different routes of transmission are not yet fully understood. Some infants escape infection despite significant virus exposure, while others do not, suggesting possible maternal or fetal immune protective factors including the presence of HIV-specific antibodies. Here we summarize the unique aspects of HIV MTCT including the immunopathogenesis of the different routes of transmission, and how transmission in the antenatal or postnatal periods may affect early life immune responses and HIV persistence. A more refined understanding of the complex interaction between viral, maternal, and fetal/infant factors may enhance the pursuit of strategies to achieve an HIV cure for pediatric populations.
Introduction
Pediatric AIDS was first described in 1982, shortly after the first adult cases were reported (1). Of the estimated 37.5 million people currently living with HIV-1 as of 2020, 1.7 million of those individuals are children (2). While heterosexual transmission is the major transmission mode in adults, the majority of pediatric infections occur through mother-to-child transmission (MTCT) during pregnancy, labor and delivery, and postpartum through breastfeeding. Tremendous progress has been made in both prevention and treatment of HIV in children. However, despite the implementation of prevention of mother-to-child transmission (PMTCT) measures that decrease the risk of vertical HIV transmission to less than 5% (3, 4), approximately 150,000 children were newly infected with HIV in 2020, the majority of whom live in sub-Saharan Africa (5). As with HIV infection in adults, the primary targets for infection are activated CD4+ T cells expressing the CCR5 co-receptor. Risk of MTCT transmission is influenced by geography, maternal viral load, co-infections, delivery mode, and breast-feeding (6), among other factors. The precise mechanisms of MTCT and how its timing influences establishment of HIV infection and persistence have not been fully elucidated. In this review, we aim to summarize what is known about HIV MTCT, the immunologic and virologic factors involved in HIV transmission in the fetal and neonatal/infant periods, and provide implications for HIV cure strategies targeting perinatally infected children.
Routes and Mechanisms of Vertical Transmission
Mother-to-child transmission (MTCT) remains the most important route of pediatric HIV acquisition and, in the absence of preventative measures, transmission rates range from 15% to 45% via three distinct routes: in utero, intrapartum, and in the postnatal period through breastfeeding. Overall, it is estimated that 20-25%, 35-50% and 25-45% of perinatal HIV transmissions occur in utero, intrapartum, and through breastfeeding, respectively (7–9). It is important to note that despite the prolonged exposure to HIV during fetal development, delivery, and breastfeeding, MTCT of HIV is relatively inefficient, and many children born to women with HIV do not become infected, even in absence of preventative services. Here, we will discuss the biological mechanisms of HIV MTCT and with emphasis on the factors influencing transmission. While this is still an active area of research, further elucidating these mechanisms of transmission is likely to inform cure approaches that should be considered for the almost 2 million children living with HIV.
In Utero Transmission
Transmission of HIV in utero is the least efficient route of MTCT, accounting for an estimated absolute rate of 5-10% of MTCT for women not receiving antiretroviral therapy (ART) (7, 8). The major risk factors for in utero transmission are high maternal viral loads and placental inflammation (8, 10, 11). The mechanisms of in utero HIV transmission are incompletely understood, but some have been proposed. Early studies suggested that in utero transmission may occur though HIV in the amniotic fluid coming into contact with fetal mucosal surfaces (12). Although the fetal gastrointestinal tract is populated with targets for HIV infection (e.g., CD4+CCR5+ T cells) (13), more recent studies have found that even in women with detectable virus in the plasma, HIV is not detected in the amniotic fluid (14–16). Another study found that amniotic fluid has innate inhibitory activity against the replication of HIV (17). Consequently, it is more likely that in utero transmission occurs primarily through the placenta. The placenta is a highly effective barrier that successfully inhibits most pathogens from reaching fetal circulation through various protective mechanisms. The placenta is composed of fetal-derived trophoblast progenitor cells that differentiate into specialized cell layers. These specialized trophoblasts have broad antiviral activity in addition to acting as a physical barrier to pathogens (18). Maternal blood comes into direct contact with the placenta by 10-12 weeks of gestation when trophoblasts form the placental villi and mediate the exchange of gases, nutrients, and waste products between maternal and fetal tissue (18).
While in utero transmission of HIV has been documented to occur as early as 8 weeks gestation (19), the vast majority of transmissions via this mode occur in the third trimester (20, 21). There are two mechanisms of transplacental transmission: infection of the trophoblasts and transcytosis across the trophoblastic layer. Although trophoblasts have low to no expression of CD4 receptors, multiple studies have detected HIV in these cells (22–24). More recent evidence suggests that transmission occurs primarily through cell-associated virus, as trophoblasts appear to be naturally nonpermissive to cell-free HIV (25). In a study designed to mimic the complex cellular architecture of the placenta, transcytosis and infection of the trophoblastic layer were only accomplished by cell-associated virus (26). New evidence suggests that cell-associated HIV enters trophoblasts via fusion with maternal lymphocytes (27). This cell-fusion mediated spread of HIV may be less sensitive to ART than cell-free infection, causing the placenta to become a potential reservoir for the virus during pregnancy (27). It is important to note that even when HIV successfully traverses the placenta, transmission to the fetus is not guaranteed. Studies have shown that both infected and uninfected infants had maternal cells with HIV DNA in their dried cord blood, and there was no significant difference between the rate of infection and the presence of HIV-infected cells in cord blood (28, 29). These findings suggest that there are likely more factors, some of which we will discuss in later sections of this paper, governing in utero transmission of HIV.
Intrapartum Transmission
The most common route of HIV MTCT occurs during labor and delivery, accounting for an absolute rate of 10-20% of infections in children born to women not receiving ART (7, 8). A major risk factor for intrapartum transmission is again maternal viral load (30–32), and while in the United States the current standard of care is delivery by Caesarian section unless women are on ART with low viral loads, most women deliver vaginally elsewhere in the world (33). Although multiple mechanisms of intrapartum transmission have been proposed, most of the evidence supports infection via exposure of infant mucosal surfaces to maternal secretions and blood during birth. The fetal intestines are populated with high levels CD4+CCR5+ T cells (13), and evidence suggests that higher viral loads in the birth canal correlate with increased transmission rates (10). Additionally, factors that would increase contact of fetal mucosal surfaces with maternal viral secretions also increase transmission rates. For example, genital ulcers have been shown to cause an increase in intrapartum transmission rates (34). This association remained significant even after adjusting for plasma HIV viral load. Several possible mechanisms are thought to explain this association including increased recruitment of HIV-infected CD4+ T cells to the mucosal surface of the genital lesions resulting in increased viral load in the genital tract (35, 36). Finally, the protective effect of elective Caesarian section against MTCT of HIV is attributed to the reduction of fetal contact with the birth canal (37–39).
Another proposed mechanism of intrapartum transmission is maternal-fetal microtransfusion (also called placental microtransfusion) causing fetal exposure to maternal blood containing both cell-free HIV RNA and cell-associated HIV DNA. Although the cause is unknown, microtransfusions occur at or near time of delivery when there is a disruption of the placental barrier and are thought to increase when uterine contractions intensify causing membranes to rupture. One study done using placental alkaline phosphatase (PLAP) as a marker for microtransfusions found that in women who had vaginal deliveries, high levels of PLAP were associated with higher intrapartum transmission risk (40). However, there was no significant association between PLAP (microtransfusion) levels and perinatal transmission as a whole. As described above, further studies designed to detect maternal cells in dried fetal cord blood as a proxy for microtransfusion also found no correlation (28, 29). Maternal-fetal microtransfusions are still relatively understudied, however.
Postpartum Transmission
Breastfeeding contributes to a considerable proportion of pediatric HIV infections accounting for an estimated absolute rate of 5-15% of MTCT for women not receiving ART (8). Premastication, where an adult chews foods before feeding it to the child, is also a risk factor for MTCT and this risk is associated with predisposing oral conditions that lead to the presence of blood in the mouth (41). It was recognized early on that breastfeeding was a potential mechanism for HIV transmission; however, the World Health Organization recommends that women in sub-Saharan Africa (with high HIV prevalence) breastfeed their children due to lack of consistent access to a safe water supply (42). Other factors such as the cost of formula and the stigma associated with not breastfeeding play into this decision (7). In order for MTCT to occur during breast feeding, the virus must first pass through the mammary epithelium, remain infectious within breast milk, traverse the infant mucosal barriers and establish infection. Studies in ex vivo organ tissue model systems and in vivo animal models have provided evidence that transmission can occur via the oral route (43, 44). Oral inoculations of simian immunodeficiency virus (SIV) have been shown to cause infection in infant rhesus macaques, providing proof of concept, although often these experimental inoculums contained higher doses of virus than would be seen in normal levels in breast milk (45–47). Cord blood and neonatal blood contain low levels of CD4+ T cells expressing CCR5, but, as described above, CD4+CCR5+ T cells populating the fetal intestines are abundant (13), a finding that has also been described in infant rhesus macaques (48–50). The mammary epithelium, like the placenta, however, successfully inhibits many pathogens from entering the breast milk through various protective mechanisms. Additionally, there is evidence that breast milk and saliva have innate inhibitory activity that restricts HIV transmission (51–54). The protective mechanisms of the maternal and fetal immune responses that limit breast milk transmission will be discussed in more detail below.
One major risk factor for MTCT during breastfeeding is viral load in the breast milk (10), and both cell-free and cell-associated HIV are thought to contribute to transmission. HIV RNA levels in breast milk correlate with plasma levels but are generally 100-fold lower (8, 55). Conditions that increase breast milk viral shedding are associated with higher transmission rates. Nonexclusive breastfeeding and infrequent emptying of the breast can lead to breast inflammation secondary to milk stasis, which in turn increases the viral load and has a strong association with increased MTCT (56–61). However, conflicting research found that nonexclusive breast feeding and mastitis do not significantly increase viral loads in breast milk (56). Still, HIV transmission was increased in breastfed infants who also received solid foods when compared to exclusively breastfed children (58). This mixed feeding is thought to cause disruption of the infant’s gut mucosal lining secondary to the introduction of non-breast-milk foods and early introduction of pathogens or foreign antigens, leading to immune activation and increased susceptibility to infection (62–64). Microbiome differences between mixed fed and exclusively breastfed infants may also impact transmission (65). Multiple studies suggested that cell-associated viruses also have a role in transmission during breastfeeding (66, 67). Understanding these biological mechanisms of breast milk viral spread is an important first step in characterizing the establishment of HIV reservoirs during this period. Further, it should be recognized that breastfeeding transmission can occur outside of the neonatal period and infants may be diagnosed after more time has elapsed since infection than occurs with in utero and intrapartum transmission. This later diagnosis, coupled with rapid immune system changes in early life, likely leads to a unique immunovirologic environment defining HIV persistence in children infected through the breastfeeding route.
Factors Influencing Vertical Transmission
MTCT is multifactorial, with different viral, maternal, and fetal/infant factors at play that influence the risk of HIV transmission (Figure 1). Knowledge of these risk factors and understanding of their roles in MTCT have been crucial to develop preventative measures and here we postulate that their consideration should also inform the investigation into curative approaches.
Viral Factors Impacting MTCT
Viral Burden
Maternal viral load has been shown to be the strongest predictor of perinatal HIV transmission. Many studies have demonstrated increased risk of transmission with high levels of maternal viremia and high p24 antigenemia (68–71). Acute infection during pregnancy is associated with increased risk of perinatal transmission and is likely related to the high viral loads in plasma and the genital tract (72). Consequently, maternal viral suppression via use of ART during pregnancy has been proven to lower the risk of perinatal transmission. This was first supported by the 076 trial which showed that administration of zidovudine during pregnancy, delivery, and to the newborn for the first 6 months of life reduced transmission by nearly 70% in women with HIV who did not breast-feed (73). Subsequent studies have indicated that transmission is reduced even further with triple-drug regimens (74). Several studies have tried to establish a threshold of maternal viral load below which transmission does not occur (75); however, it has been demonstrated that transmission can occur in some cases where women have low levels of HIV RNA in the blood, indicating the presence of other factors influencing the transmission process. In one study that included 320 women from 18 different centers in France showed that perinatal transmission occurred in 12% of the women at less than 1000 copies/ml compared to 29% in women with more than 10,000 copies/ml (76). In contrast, in Bangkok 281 pregnant women were followed and no transmission occurred in those with virus load less than 2,000 copies/ml (77).
Several behavioral practices have been associated with increased risk of maternal to infant HIV transmission due to resultant increases in viral load. These behavioral factors include illicit drug use (IDU), cigarette smoking, and alcohol use. IDU is associated with increased risk of MTCT (78–81). Alcohol and drug use like heroin, amphetamine and cocaine, may be associated with failure to control viral load in the presence of ART, hence increased transmission rate (82–85). Additionally, transmission may be increased through drug interactions with ART and placental injury (86). Some have demonstrated an association between IDU and lack of adherence to ART which can lead to increased viral load (83, 85–87).
In addition to plasma viral load, viral levels in cervicovaginal fluid and breast milk are also thought to influence transmission risk in the intrapartum and postnatal periods (56, 88–90). As referenced above, levels of HIV DNA in breast milk cells (ie, CD4+ T cells) are positively correlated with postnatal transmission, and this intracellular HIV DNA is not significantly reduced by maternal ART. Risk of transmission is higher during early lactation due to the increased cellular content of colostrum (91). Both maternal ART and extended infant prophylaxis (at least with a single agent) during breastfeeding significantly reduces but does not eliminate MTCT, an observation that likely reflects virus persistence in CD4+ T cells (92–97).
Viral Genotype and Phenotype
Several studies of viral variants in mothers and infants attempted to characterize HIV quasispecies associated with MTCT and have yielded conflicting results. Major, minor, and multiple variant transmission events have been described (98–100). Most evidence suggests that a single or restricted subset of maternal viral variants establish infection in the infant suggesting selective pressure during the transmission process. However, the basis and the factors controlling MTCT bottleneck are not yet fully understood. Different properties of virus populations have been reported in studies analyzing vertical transmission stratified by timing, with transmission during the intrapartum period found to be primarily associated with the transmission of minor maternal variants while in utero transmission was more likely to occur with single or multiple HIV variants (100–104). Maternal antiretroviral drug resistance is associated with transmission during breastfeeding, but not during the in utero/intrapartum periods (105).
Phylogenetic analyses of HIV env in infected infants showed that they have a more homogenous virus population when compared to their mothers (106, 107). Studies have also shown that macrophage-tropic and non-syncytium-inducing (NSI) or CCR5-utilizing HIV viral strains are selectively transmitted (108). Another characteristic found in transmitted viruses was shorter variable loops and fewer putative N-linked glycosylation (PNG) sites encoded in env (70, 109–111). Different HIV subtypes may also have distinct MTCT rates. Work conducted in Tanzania showed that subtype C is preferentially transmitted from mother to child compared to subtype A and D (100). In another study from Kenya, the MTCT rate appeared to be higher among mothers infected with subtype D compared with subtype A (112). However, no such differences have been observed in other cohorts (100, 112–115).
Host Factors Impacting MTCT
Genetic Factors
Genetic polymorphisms in the coding and regulatory regions of HIV receptors and their ligands influence the risk of HIV acquisition. Infants with a single nucleotide polymorphism (SNP) in the CD4 gene at position C868T that may modify the tertiary structure of CD4 were more likely to acquire HIV compared to infants with wild type CD4 (116). In the setting of MTCT, most of the transmitted viruses use CCR5 as a coreceptor (117). As has been shown for horizontal infection in adults, the presence of a 32-bp deletion in the coding region of the CCR5 gene (CCR5-Δ32) in the homozygous state in infants results in non-functional coreceptors and confers protection from vertical infection (118, 119). Heterozygosity also exerts a protective effect when carried by mothers due to lower maternal viral burden (107). Conversely, polymorphisms in the CCR5 promoter region at positions 59029 and 59353T increase the expression of CCR5 leading to increased risk of MTCT when carried by infants (120–123).
Increased risk of vertical transmission was also seen with genetic polymorphisms resulting in decreased expression of the natural ligands for HIV coreceptors (CCL3, CCL4, and CCL5) (124).
Genetic variations affecting innate immunity may also influence MTCT. Defensins are antimicrobial peptides that are expressed by epithelial cells, known for innate mucosal defense and antiviral activities. Defensins inhibit HIV infection via different mechanisms including direct binding to virions as well as disturbing intracellular signaling by modulation of host cell surface receptors. Three SNPs in the 5′ untranslated region of β-defensin-1 (DEFB1) gene were reported to modulate risk of MTCT: −52G/A, -20(G/A) and −44C/G (125–127). Braida et al. described an association between -44(C/G) and HIV infection in Italian pediatric population (125). Another study found a lower copy number of DEFB104 among HIV-infected children when compared to HIV-exposed children and healthy controls, suggesting that DEFB104 may have a potential protective role against vertical transmission (128).
Multiple studies have investigated the influence of HLA concordance between mother and infant on vertical transmission and as well as risk of disease progression in infected infants (129–131). Specific maternal HLA polymorphisms, including B4901, B5301, A2/6802 and B18, have also been associated with decreased risk of MTCT (132–134). HLA-G, a non-classical class I MHC gene highly expressed in placental trophoblasts, has several SNPs found to be associated with decreased risk of vertical transmission (135, 136). Certain class II MHC alleles have also been reported to influence MTCT among certain ethnicities such as DQB1*0604, DR3, DR13, DRB1*1501 (137–139).
Gender specific differences in MTCT have been reported in several cohorts (140, 141). Female infants are reported to have two- to three-fold increased risk of infection at birth compared to male infants (7, 140, 141). This increased susceptibility has been linked to subversion of innate immunity, with female fetuses acquiring maternal variants resistant to type I interferons (142). Sex-specific differences in in utero infection have also been attributed to the fact that in utero mortality rates of HIV-infected male infants are disproportionately higher and thus more HIV-infected female infants are liveborn. It is also proposed that a minor histocompatibility reaction between infant male Y chromosome-derived antigens and maternal lymphocytes reduce the risk of MTCT to boys (140).
Maternal Co-Infections
Several coexisting maternal infections have been found to be associated with increased MTCT of HIV. Multiple studies demonstrated an increased risk of vertical transmission in the setting of chorioamnionitis (143–145), primarily related to disruption of the placental barrier and entrance of HIV-infected cells into the amniotic fluid (143, 144, 146). Chorioamnionitis complications including preterm labor and premature rupture of membranes can also lead to increased risk of MTCT related to immaturity of the skin and mucosal membranes as well as the premature fetal immune system. In a multicenter prospective cohort, clinical and biologic factors that contributed to MTCT were studied. Histologic chorioamnionitis was found to be a major risk factor for transmitting HIV (147).
Adachi and colleagues evaluated the effect of sexually transmitted infections (STIs) on risk of MTCT in a large cohort of HIV-infected pregnant women and found increased rates of HIV transmission in the presence of a another sexually transmitted disease (148). In general, inflammation of the maternal genital tract mucosa has been shown to increase rate of vertical transmission independent of maternal plasma HIV load (59). Infections resulting in genital ulcer disease such as HSV-2 are also associated with increased genital shedding of HIV (35, 149, 150). Conflicting data exist regarding maternal syphilis infection and MTCT of HIV. A study in Zimbabwe showed that active maternal syphilis at the time of delivery was not associated with intra-partum MTCT risk while several other groups have demonstrated an increased risk of vertical HIV acquisition in the setting of maternal syphilis (146, 151). HIV and Hepatitis B virus (HBV) co-infection are associated with increased HBV, but not HIV transmission to the infant. Maternal Hepatitis C virus (HCV) co-infection has been linked to higher rates of vertical HIV transmission (152–160).
Unprotected sexual intercourse during pregnancy itself is thought to be associated with increased risk of MTCT (161, 162). Bulterys et al. conducted a prospective cohort study in Rwanda that showed that unprotected sexual intercourse with multiple partners before and during pregnancy in a population with high HIV-1 seroprevalence may increase risk of transmission from infected mother to infants (161). Another study by Burns and colleagues also found higher frequency of intercourse during pregnancy among women transmitting HIV to their infants (163). Potential mechanisms include increased HIV strain diversity (i.e., superinfection) (164, 165) and vaginal or cervical inflammation due to microabrasions or STIs that result in chorioamnionitis and/or increased viral shedding in genital fluids (164).
Tuberculosis (TB) is one of the most important causes of mortality and morbidity in HIV infection, especially in women residing in TB endemic areas. Active TB infection increases HIV viral load which is a known risk factor for perinatal transmission (166–168). Gupta et al., found a 2.5-fold increase in the odds of MTCT of HIV in pregnant women with TB/HIV coinfection, after adjusting for maternal and infant factors (169). Similarly, malaria and HIV coinfection is associated with an increased risk of adverse outcomes in pregnant women (170) as well as increased HIV viral load (171). As such, a few studies have suggested an increased risk of perinatal transmission with maternal malaria (172–174), yet others failed to demonstrate this relationship (144, 175, 176). Finally, other common viral infections, such as Cytomegalovirus (CMV), may play a critical role in influencing MTCT. One described mechanism has been through enhancement of placental susceptibility to HIV infection (177).
Maternal Immunological Factors
There are multiple maternal immunological factors associated with increased risk of perinatal transmission, including low CD4+ T cell count, CD4+ T cell percentage, and CD4/CD8 ratio (178). Shivakoti et al. showed that high maternal soluble CD14 concentration during the peripartum period was associated with increased risk of MTCT, independent of maternal viral load, CD4+ T cell count and ART exposure (179), implicating immune activation in transmission.
Adaptive immune responses play an important role in transmission from mother to infant. MTCT is a unique setting where HIV-1 acquisition occurs in the presence of naturally elicited HIV-specific antibodies that are passively transferred prior to birth. Several studies have examined the impact of maternal antibodies on MTCT; however, there have been conflicting results and their role in protecting infants against HIV-1 transmission remains unclear. These contradicting results may be explained by small cohort sizes, lack of control for the other known risk factors for HIV transmission, timing, and methods used in infant diagnosis, and potential clade-specific differences in virus–antibody interactions. Initial studies demonstrated that higher levels of maternal HIV-1 envelope (Env)-specific IgG antibody responses were associated with reduced transmission risk (180, 181).This association was not observed in subsequent studies and research focus was shifted to examine the effect of neutralizing antibodies (nAbs) and their role in modulating the risk of transmission (182, 183). Work conducted in nonhuman primates showed that passive immunization of infants with a cocktail of HIV-1-neutralizing antibodies provided partial protection against oral simian-human immunodeficiency virus transmission (184). Subsequent human studies showed that high levels of maternal nAbs were correlated with reduced MTCT rates while others failed to confirm this association (185–188). However, founder viruses in infants are generally more resistant to neutralization by maternal antibodies (189, 190), suggesting that the transmitted variants are able to escape nAbs and supportive of the idea that maternal antibodies may confer partial protection.
In a large cohort of non-breastfeeding HIV-1 infected women enrolled in the pre-ART era Women and Infant Transmission Study (WITS), Permar and her group found that maternal V3-specific IgG binding responses and CD4 binding site-blocking responses correlated and were independently predictive of reduced MTCT risk (191). They also found that both binding and neutralizing responses targeting the C-terminal region of HIV envelope (Env) were associated with decreased risk of transmission (191). Antibody effector functions beyond neutralization have also been examined for their contribution to MTCT. Maternal antibody-dependent cellular cytotoxicity (ADCC) is thought to have a role in protection against HIV-1 transmission. In one such investigation, plasma and breast milk obtained soon after delivery from 9 transmitting and 10 non-transmitting women in Kenya demonstrated that breast milk Env-specific IgG responses with ADCC activity were associated with decreased MTCT risk (192). However, Pollara et al. found no association of ADCC-mediating responses and MTCT risk in HIV clade C breastfeeding women in Malawi (193). In addition to antibodies and antibody effector functions, Lohman-Payne et al. also reported breast milk HIV gag-specific IFNg responses to be associated with protection from MTCT via breastfeeding in a Kenyan cohort (194).
Infant Immunological Factors
Most infants born to HIV-1 infected mothers do not become infected despite having an immature immune system and repeated exposures to HIV-1 throughout the peripartum and breastfeeding periods. To survive to term, the developing fetus must avoid generating an inflammatory response to the many foreign maternal antigens to which it is exposed during development resulting in a predominantly tolerogenic immune system in the fetus and newborn (195). The tolerogenic environment is facilitated by high levels of anti-inflammatory cytokines such as TGFb and IL-10 (196), which likely subvert immune activation and establishment of infection upon HIV exposure. TGFb also directs naïve CD4+ T cell to differentiate to Tregs resulting in a larger Treg pool in infants, representing 15% of fetal blood T cells compared to 5% of adult blood T cells. These Tregs promote immune tolerance and are long-lived (196, 197). Much of these regulatory cells reside in intestinal tissue and are critical for mucosal immune homeostasis. In addition to higher levels of Tregs, the CD4+ effector cells of infants are predominantly of the Th17 and Th2 phenotype (rather than Th1 as in adults) (198, 199). Th17 cells are critical to maintaining the integrity of the intestinal mucosal barrier and may thus restrict dissemination of infection. Conversely, Tugizov and colleagues have reported lower levels of innate proteins that restrict infection, such as defensins, in infant compared to adult oral epithelia (43).
In the mother-child transmission pair, the infant may be disadvantaged in that the transmitted virus in has already adapted to evade a genetically similar immune system. Anti-HIV-1 antibodies and T cells transferred to the child either in utero or through breastmilk have pre-adapted to the transmitted virus and may be ineffective. Additionally, because of shared HLA alleles the transmitted virus may be preadapted to escape CD8+ T cells targeting HIV epitopes restricted by HLA alleles inherited from the mother further complicating pediatric HIV-1 infection (200, 201). HIV-specific cellular immune responses are detected in exposed uninfected infants, but their role in influencing virus acquisition is uncertain (202, 203).
Implications for Cure Approaches
The World Health Organization recommendations state that all infants and children under two years of age with confirmed HIV-1 begin ART immediately at the time of diagnosis irrespective of CD4+ T cell counts (204). Despite this recommendation, HIV-1-infected children are one third less likely to receive ART than infected adults (205). Reasons for this include fewer drugs available for use by children, higher treatment cost, and dependence on a caregiver to provide ART (206). These factors can complicate the design and implementation of cure studies in children.
A major barrier to HIV cure is the establishment of a reservoir of HIV-infected cells that persists despite suppressive ART and can give rise to rebound viremia if ART is interrupted. In adults, it is well documented that viral reservoirs are established during early stages of HIV infection (207) and this finding is supported by the SIV/macaque model in which rapid seeding of the viral reservoir within 3 days of infection has been described (208). Furthermore, rebound of viremia is almost always seen after ART discontinuation in both horizontally infected adults and perinatally-infected children (209–211). However, little is known about the exact timing of reservoir seeding in different MTCT settings and whether there are differences in reservoir establishment in relation to time of infection and mode of transmission. Increased understanding of these factors may aid in the development of approaches to eradicate viral reservoirs and/or induce viral remission (sustained viral suppression in absence of ART) in children.
The uniqueness of the in-utero transmission window allows for rapid detection and treatment as children born to women with HIV can be screened at birth. Such early detection is not generally feasible in adult transmissions. Infants diagnosed at birth via rapid point-of-care testing can begin ART within the first few hours of life (212). Infections arising from intra- or post-partum transmission will not be detected at the time of birth, so follow-up care and repeated testing are required to monitor these transmission modes. In these instances, early ART initiation is not as practical in resource limited settings, although current United States guidelines recommend triple drug prophylaxis (given at therapeutic dosing) for neonates with high risk of acquiring HIV infection.
Contemporary studies of HIV reservoirs in perinatally infected infants and children have focused on in utero transmission, following the description of the ‘Mississippi child’. This child received ART between 30 hours and 18 months of age, and then remained persistently aviremic for 27 months after discontinuation of ART before rebound (209, 213). In utero infection was documented, but the child did not develop HIV-specific antibody or T cell responses, thought due to the very early initiation of ART. Despite the eventual rebound, this partial remission led to several clinical trials of very early ART (typically considered to be within 48 hours) to limit the size of the HIV reservoir and investigate the possibility of achieving post-treatment control after ART interruption. Results from the Early Infant Treatment (EIT) study in Botswana have demonstrated that very early ART leads to an exceptionally small reservoir of intact proviral HIV DNA as well as an improved innate and adaptive antiviral immune response compared to delayed ART (214). As of this writing, results from the ART interruption phase of the very early ART trials, including EIT and IMPAACT P1115 (215), have not been published. Kuhn and colleagues describe challenges in meeting predetermined virologic and immunologic criteria for ART interruption in the LEOPARD trial of very early/early ART conducted in South Africa (216).
The South African Children with HIV Early antiRetroviral therapy (CHER) trial (217), that included children with in utero and intrapartum HIV infection, has led to a number of key findings regarding persistent HIV reservoirs. One report demonstrated rare intact proviral sequences in children after 6-9 years of ART initiated after 2 months of age but within the first year of life using near full-length proviral amplification and sequencing (218). This group further identified clones of infected cells shortly after birth that could also be detected after almost a decade on ART, suggesting that clonal expansion of reservoir cells maintains HIV persistence in children as it does for adults (219). Two additional case reports of long-term HIV remission have emerged, one from the CHER trial, with unknown timing of infection (in utero vs intrapartum) (220) and the other from a French cohort, with presumed intrapartum transmission (221), both of whom started ART at 2-3 months of life.
There have been relatively fewer studies of reservoir characteristics in children infected postpartum through breastfeeding. However, presently over half of new infections occur postnatally through breast milk (9). As this route has become predominant in perinatal HIV infection, a nonhuman primate model has been established to better understand virologic and immunologic features of lentivirus infection following postpartum transmission, more precisely define anatomic sites of virus persistence, and test strategies to promote reservoir eradication or remission (46, 47, 222–224). These and other ongoing studies may inform the design of future cure-directed clinical trials in children with HIV infection acquired through breast milk.
Regardless of the mode of transmission earlier ART is generally associated with a smaller reservoir during viremia suppression (210, 214, 225–237), although starting ART within 14 days of life may not lead to significantly greater HIV DNA persistence compared to within 48 hours of life (238). ART initiation within 8 days has also been associated with a faster HIV DNA decay compared to ART started at 5 months (239). Female sex and maternal acute HIV infection during pregnancy have been associated with higher levels of persistent HIV DNA (240).
While protocol-specified ART interruption has not occurred in recent clinical trials, studies of intermittent viremia vs. sustained viral suppression can be informative to identify biomarkers that may be predictive of viral rebound dynamics. These findings are by nature complicated by behavioral factors, including adherence to ART regimens. Millar and colleagues assessed factors associated with intermittent viremia in in utero infected infants started on early ART (in the first 3 weeks of life) (240). A smaller reservoir size as measured by total HIV DNA in PBMCs was not correlated with maintained viral suppression whereas a longer time to initial viral suppression was, implicating immunologic contributions to aviremia, although this finding may have been influenced by ART nonadherence. In children in the EIT study who started on ART at < 7 days of life, sustained HIV RNA suppression was associated with negative HIV serostatus and negative qualitative HIV DNA PCR at ~20 months (225). HIV seronegativity has also been described in other studies of early or very early ART and has been proposed as an estimate for reservoir size (227, 228, 241–244).
Identification of cure strategies that target latently infected cells, allow immune recognition and clearance of the reservoir, and/or promote viral remission are currently considered extremely high priority for the field. Unlike in adults, few cure strategies have been tested in children to date, with the exception of very early ART that it is now understood is insufficient to lead to cure in the majority of individuals. However, there are several approaches that may provide benefit in the setting of perinatal HIV transmission, and their study should be carefully considered for certain populations (Figure 2). The design of such studies should take into consideration not only the timing of ART initiation, but also the duration of ART (and therefore age of the trial participant), as well as the known or presumed mode of HIV transmission (in utero, intrapartum, or postpartum). For example, a neonate infected in utero and initiating very early ART is likely to be vastly different both virologically and immunologically from an adolescent who acquired HIV through breastfeeding with delayed ART initiation and periods of unsuppressed viremia over years. In the former case, adding the cure directed therapy at the time of early ART initiation may lead to an extremely small reservoir size (or even prevent reservoir establishment in the best-case scenario). This cure-directed therapy might include one or multiple broadly neutralizing antibodies delivered by passive (as is being tested in IMPAACT 2008 (215) or active immunization along with ART. A novel proposed strategy, termed “surge and purge,” combines very early ART, passive antibody administration, and immune stimulation to destabilize reservoir establishment (245). Infants and children with very small reservoirs may also be good candidates for approaches designed to silence HIV expression (“block and lock”). For the school aged or adolescent child with a larger reservoir size, the “kick and kill” approach may be more effective, with a latency reversal agent used to reactivate virus expression followed by immune-based clearance of infected cells. Studies in nonhuman primates support the notion that pre-existing reservoir size as well as age-related factors may influence susceptibility to latency reversal (246, 247). Therapeutic HIV vaccines designed to boost antiviral T cell responses may have benefit in kick and kick strategies targeting established infection (223, 248) and possibly in limiting reservoir size early in infection, although in both settings the impact may depend on the child’s age at vaccination, the timing of ART, and the degree of immune exhaustion present. Results from the HVRRICANE trial are forthcoming, in which a prime-boost vaccine strategy will be tested in combination with a TLR4 adjuvant (hypothesized to reverse latency) in HIV-1-infected children with history of early ART or previous immunization with one of the vaccine components (215).
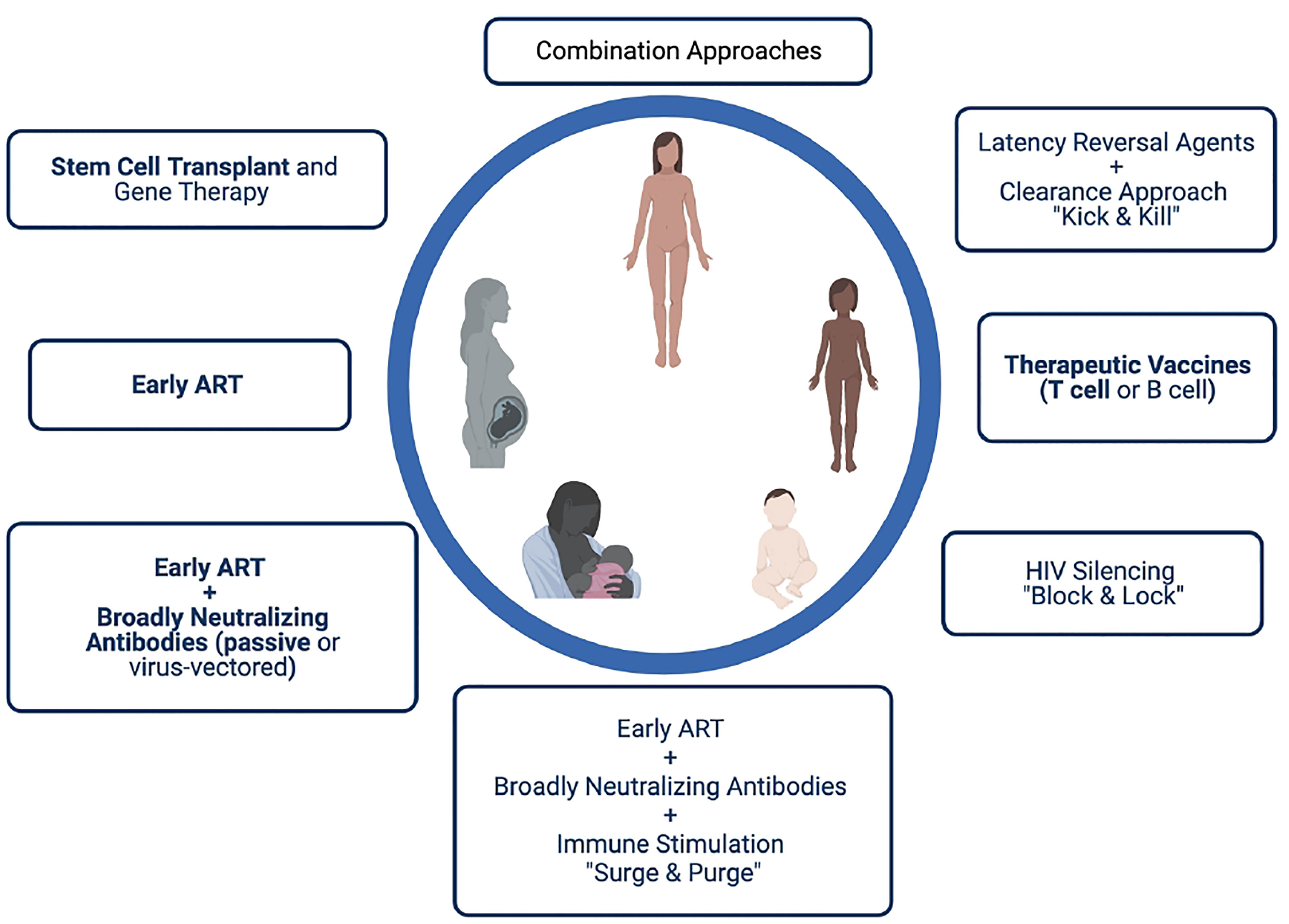
Figure 2 Possible HIV cure approaches for perinatally infected infants, children and adolescents. Strategies in bold have been or are being studied in pediatric clinical trials.
In summary, HIV MTCT is promoted by distinct immunopathogenesis for the three different routes of transmission that may also affect HIV persistence. Consideration of the complex interaction between viral, maternal, and fetal/infant factors may enhance the pursuit of strategies to achieve an HIV cure for pediatric populations. Future preclinical and clinical trials from the neonatal period through adolescence will further elucidate how MTCT influences the potential for HIV cure.
Author Contributions
OA is the first author, the rest of the coauthors AC, KB, JP contributed to the manuscript. All authors reviewed the final manuscript. All authors contributed to the article and approved the submitted version.
Funding
AC would like to acknowledge funding support from the NIH (R01 AI133706, P01 AI131276, R37 AI157862, and UM1 AI164566).
Conflict of Interest
The authors declare that the research was conducted in the absence of any commercial or financial relationships that could be construed as a potential conflict of interest.
Publisher’s Note
All claims expressed in this article are solely those of the authors and do not necessarily represent those of their affiliated organizations, or those of the publisher, the editors and the reviewers. Any product that may be evaluated in this article, or claim that may be made by its manufacturer, is not guaranteed or endorsed by the publisher.
References
1. Centers for Disease C. Unexplained Immunodeficiency and Opportunistic Infections in Infants–New York, New Jersey, California. MMWR Morb Mortal Wkly Rep (1982) 31(49):665–7.
2. UNAIDS. Global HIV & AIDS Statistics - Fact Sheet. UNAIDS (2011). Available at: https://www.unaids.org/en/resources/fact-sheet (Accessed 10/06/2020).
3. WHO. Antiretroviral Drugs for Treatment Pregnant Women and Preventing HIV Infection in Infants: Recommendations for a Public Health Approach. Geneva: WHO (2010).
4. HIV/AIDS) UJUNPo. Press Release: “World Leaders Launch Plan to Eliminate New HIV Infections Among Children by 2015”. Geneva: UNAIDS (2011).
6. Goulder PJ, Lewin SR, Leitman EM. Paediatric HIV Infection: The Potential for Cure. Nat Rev Immunol (2016) 16(4):259–71. doi: 10.1038/nri.2016.19
7. Lehman DA, Farquhar C. Biological Mechanisms of Vertical Human Immunodeficiency Virus (HIV-1) Transmission. Rev Med Virol (2007) 17(6):381–403. doi: 10.1002/rmv.543
8. Tobin NH, Aldrovandi GM. Immunology of Pediatric HIV Infection. Immunol Rev (2013) 254(1):143–69. doi: 10.1111/imr.12074
9. Luzuriaga K, Mofenson LM. Challenges in the Elimination of Pediatric HIV-1 Infection. N Engl J Med (2016) 374(8):761–70. doi: 10.1056/NEJMra1505256
10. John GC, Nduati RW, Mbori-Ngacha DA, Richardson BA, Panteleeff D, Mwatha A, et al. Correlates of Mother-to-Child Human Immunodeficiency Virus Type 1 (HIV-1) Transmission: Association With Maternal Plasma HIV-1 RNA Load, Genital HIV-1 DNA Shedding, and Breast Infections. J Infect Dis (2001) 183(2):206–12. doi: 10.1086/317918
11. Kumar SB, Rice CE, Milner DA Jr., Ramirez NC, Ackerman WE 4th, Mwapasa V, et al. Elevated Cytokine and Chemokine Levels in the Placenta Are Associated With in-Utero HIV-1 Mother-to-Child Transmission. AIDS (2012) 26(6):685–94. doi: 10.1097/QAD.0b013e3283519b00
12. Fazely F, Sharma PL, Fratazzi C, Greene MF, Wyand MS, Memon MA, et al. Simian Immunodeficiency Virus Infection via Amniotic Fluid: A Model to Study Fetal Immunopathogenesis and Prophylaxis. J Acquir Immune Defic Syndr (1988) (1993) 6(2):107–14.
13. Bunders MJ, van der Loos CM, Klarenbeek PL, van Hamme JL, Boer K, Wilde JCH, et al. Memory CD4(+)CCR5(+) T Cells Are Abundantly Present in the Gut of Newborn Infants to Facilitate Mother-to-Child Transmission of HIV-1. Blood (2012) 120(22):4383–90. doi: 10.1182/blood-2012-06-437566
14. Lobato AC, Aguiar RA, Aleixo AW, Andrade BA, Cavallo IK, Kakehasi FM, et al. HIV-1 RNA Detection in the Amniotic Fluid of HIV-Infected Pregnant Women. Mem Inst Oswaldo Cruz (2010) 105(5):720–1. doi: 10.1590/S0074-02762010000500021
15. Maiques V, García-Tejedor A, Perales A, Córdoba J, Esteban RJ. HIV Detection in Amniotic Fluid Samples. Amniocentesis Can Be Performed in HIV Pregnant Women? Eur J Obstet Gynecol Reprod Biol (2003) 108(2):137–41. doi: 10.1016/S0301-2115(02)00405-0
16. Mohlala BK, Tucker TJ, Besser MJ, Williamson C, Yeats J, Smit L, et al. Investigation of HIV in Amniotic Fluid From HIV-Infected Pregnant Women at Full Term. J Infect Dis (2005) 192(3):488–91. doi: 10.1086/431604
17. Farzin A, Boyer P, Ank B, Nielsen-Saines K, Bryson Y. Amniotic Fluid Exhibits an Innate Inhibitory Activity Against HIV Type 1 Replication In Vitro. AIDS Res Hum Retroviruses (2013) 29(1):77–83. doi: 10.1089/aid.2011.0355
18. Arora N, Sadovsky Y, Dermody TS, Coyne CB. Microbial Vertical Transmission During Human Pregnancy. Cell Host Microbe (2017) 21(5):561–7. doi: 10.1016/j.chom.2017.04.007
19. Lewis SH, Reynolds-Kohler C, Fox HE, Nelson JA. HIV-1 in Trophoblastic and Villous Hofbauer Cells, and Haematological Precursors in Eight-Week Fetuses. Lancet (1990) 335(8689):565–8. doi: 10.1016/0140-6736(90)90349-A
20. Rouzioux C, Costagliola D, Burgard M, Blanche S, Mayaux MJ, Griscelli C, et al. Estimated Timing of Mother-To-Child Human Immunodeficiency Virus Type 1 (HIV-1) Transmission by Use of a Markov Model. Am J Epidemiol (1995) 142(12):1330–7. doi: 10.1093/oxfordjournals.aje.a117601
21. Chouquet C, Richardson S, Burgard M, Blanche S, Mayaux MJ, Rouzioux C, et al. Timing of Human Immunodeficiency Virus Type 1 (HIV-1) Transmission From Mother to Child: Bayesian Estimation Using a Mixture. Stat Med (1999) 18(7):815–33. doi: 10.1002/(SICI)1097-0258(19990415)18:7<815::AID-SIM74>3.0.CO;2-G
22. Menu E, Mbopi-Keou FX, Lagaye S, Pissard S, Mauclère P, Scarlatti G, et al. Selection of Maternal Human Immunodeficiency Virus Type 1 Variants in Human Placenta. European Network for In Utero Transmission of HIV-1. J Infect Dis (1999) 179(1):44–51. doi: 10.1086/314542
23. Zachar V, Zacharova V, Fink T, Thomas RA, King BR, Ebbesen P, et al. Genetic Analysis Reveals Ongoing HIV Type 1 Evolution in Infected Human Placental Trophoblast. AIDS Res Hum Retroviruses (1999) 15(18):1673–83. doi: 10.1089/088922299309711
24. De Andreis C, Simoni G, Rossella F, Castagna C, Pesenti E, Porta G, et al. HIV-1 Proviral DNA Polymerase Chain Reaction Detection in Chorionic Villi After Exclusion of Maternal Contamination by Variable Number of Tandem Repeats Analysis. AIDS (1996) 10(7):711–5. doi: 10.1097/00002030-199606001-00004
25. Ross AL, Cannou C, Barré-Sinoussi F, Menu E. Proteasome-Independent Degradation of HIV-1 in Naturally Non-Permissive Human Placental Trophoblast Cells. Retrovirology (2009) 6:46. doi: 10.1186/1742-4690-6-46
26. Lagaye S, Derrien M, Menu E, Coïto C, Tresoldi E, Mauclère P, et al. Cell-To-Cell Contact Results in a Selective Translocation of Maternal Human Immunodeficiency Virus Type 1 Quasispecies Across a Trophoblastic Barrier by Both Transcytosis and Infection. J Virol (2001) 75(10):4780–91. doi: 10.1128/JVI.75.10.4780-4791.2001
27. Tang Y, Woodward BO, Pastor L, George AM, Petrechko O, Nouvet FJ, et al. Endogenous Retroviral Envelope Syncytin Induces HIV-1 Spreading and Establishes HIV Reservoirs in Placenta. Cell Rep (2020) 30(13):4528–39.e4. doi: 10.1016/j.celrep.2020.03.016
28. Biggar RJ, Lee TH, Wen L, Broadhead R, Kumwenda N, Taha TE, et al. The Role of Transplacental Microtransfusions of Maternal Lymphocytes in HIV Transmission to Newborns. AIDS (2008) 22(17):2251–6. doi: 10.1097/QAD.0b013e328314e36b
29. Lee TH, Chafets DM, Biggar RJ, McCune JM, Busch MP. The Role of Transplacental Microtransfusions of Maternal Lymphocytes in In Utero HIV Transmission. J Acquir Immune Defic Syndr (2010) 55(2):143–7. doi: 10.1097/QAI.0b013e3181eb301e
30. Magder LS, Mofenson L, Paul ME, Zorrilla CD, Blattner WA, Tuomala RE, et al. Risk Factors for In Utero and Intrapartum Transmission of HIV. J Acquir Immune Defic Syndr (2005) 38(1):87–95. doi: 10.1097/00126334-200501010-00016
31. Fawzi W, Msamanga G, Renjifo B, Spiegelman D, Urassa E, Hashemi L, et al. Predictors of Intrauterine and Intrapartum Transmission of HIV-1 Among Tanzanian Women. AIDS (2001) 15(9):1157–65. doi: 10.1097/00002030-200106150-00011
32. Mofenson LM, Lambert JS, Stiehm ER, Bethel J, Meyer WA, Whitehouse J, et al. Risk Factors for Perinatal Transmission of Human Immunodeficiency Virus Type 1 in Women Treated With Zidovudine. Pediatric AIDS Clinical Trials Group Study 185 Team. N Engl J Med (1999) 341(6):385–93. doi: 10.1056/NEJM199908053410601
33. Briand N, Jasseron C, Sibiude J, Azria E, Pollet J, Hammou Y, et al. Cesarean Section for HIV-Infected Women in the Combination Antiretroviral Therapies Era, 2000-2010. Am J Obstet Gynecol (2013) 209(4):335.e1–12. doi: 10.1016/j.ajog.2013.06.021
34. Drake AL, John-Stewart GC, Wald A, Mbori-Ngacha DA, Bosire R, Wamalwa DC, et al. Herpes Simplex Virus Type 2 and Risk of Intrapartum Human Immunodeficiency Virus Transmission. Obstet Gynecol (2007) 109(2 Pt 1):403–9. doi: 10.1097/01.AOG.0000251511.27725.5c
35. Mbopi-Kéou FX, Legoff J, Grésenguet G, Si-Mohamed A, Matta M, Mayaud P, et al. Genital Shedding of Herpes Simplex Virus-2 DNA and HIV-1 RNA and Proviral DNA in HIV-1- and Herpes Simplex Virus-2-Coinfected African Women. J Acquir Immune Defic Syndr (2003) 33(2):121–4. doi: 10.1097/00126334-200306010-00001
36. McClelland RS, Wang CC, Overbaugh J, Richardson BA, Corey L, Ashley RL, et al. Association Between Cervical Shedding of Herpes Simplex Virus and HIV-1. AIDS (2002) 16(18):2425–30. doi: 10.1097/00002030-200212060-00007
37. Parazzini F, Ricci E, Chiaffarino F, Di Cintio E, Di Cintio E, Pardi G, et al.Elective Caesarean-Section Versus Vaginal Delivery in Prevention of Vertical HIV-1 Transmission: A Randomised Clinical Trial. Lancet (1999) 353(9158):1035–9. doi: 10.1016/s0140-6736(98)08084-2
38. Andiman W, Bryson Y, de Martino M, Bryson Y, Farley J, Fowler H, et al. The Mode of Delivery and the Risk of Vertical Transmission of Human Immunodeficiency Virus Type 1–A Meta-Analysis of 15 Prospective Cohort Studies. N Engl J Med (1999) 340(13):977–87. doi: 10.1056/NEJM199904013401301
39. Kennedy CE, Yeh PT, Pandey S, Betran AP, Narasimhan M. Elective Cesarean Section for Women Living With HIV: A Systematic Review of Risks and Benefits. AIDS (2017) 31(11):1579–91. doi: 10.1097/QAD.0000000000001535
40. Kwiek JJ, Mwapasa V, Milner DA Jr, Alker AP, Miller WC, Tadesse E, et al. Maternal-Fetal Microtransfusions and HIV-1 Mother-to-Child Transmission in Malawi. PloS Med (2006) 3(1):e10. doi: 10.1371/journal.pmed.0030010
41. Ivy WI, Dominguez KL, Rakhmanina NY, Iuliano AD, Danner SP, Borkowf CB, et al. Premastication as a Route of Pediatric HIV Transmission: Case–Control and Cross-Sectional Investigations. JAIDS J Acquired Immune Defic Syndr (2012) 59(2):207–12. doi: 10.1097/QAI.0b013e31823b4554
43. Tugizov SM, Herrera R, Veluppillai P, Greenspan D, Soros V, Greene WC, et al. Differential Transmission of HIV Traversing Fetal Oral/Intestinal Epithelia and Adult Oral Epithelia. J Virol (2012) 86(5):2556–70. doi: 10.1128/JVI.06578-11
44. Milush JM, Kosub D, Marthas M, Schmidt K, Scott F, Wozniakowski A, et al. Rapid Dissemination of SIV Following Oral Inoculation. AIDS (2004) 18(18):2371–80.
45. Van Rompay KK, Abel K, Lawson JR, Singh RP, Schmidt KA, Evans T, et al. Attenuated Poxvirus-Based Simian Immunodeficiency Virus (SIV) Vaccines Given in Infancy Partially Protect Infant and Juvenile Macaques Against Repeated Oral Challenge With Virulent SIV. J Acquir Immune Defic Syndr (2005) 38(2):124–34. doi: 10.1097/00126334-200502010-00002
46. Obregon-Perko V, Bricker KM, Mensah G, Uddin F, Kumar MR, Fray EJ, et al. Simian-Human Immunodeficiency Virus SHIV.C.CH505 Persistence in ART-Suppressed Infant Macaques Is Characterized by Elevated SHIV RNA in the Gut and a High Abundance of Intact SHIV DNA in Naive CD4+ T Cells. J Virol (2020) 95(2):e01669–20. doi: 10.1128/JVI.01669-20
47. Mavigner M, Habib J, Deleage C, Rosen E, Mattingly C, Bricker K, et al. Simian Immunodeficiency Virus Persistence in Cellular and Anatomic Reservoirs in Antiretroviral Therapy-Suppressed Infant Rhesus Macaques. J Virol (2018) 92(18):e00562–18. doi: 10.1128/JVI.00562-18
48. Chahroudi A, Cartwright E, Lee ST, Mavigner M, Carnathan DG, Lawson B, et al. Target Cell Availability, Rather Than Breast Milk Factors, Dictates Mother-To-Infant Transmission of SIV in Sooty Mangabeys and Rhesus Macaques. PloS Pathog (2014) 10(3):e1003958. doi: 10.1371/journal.ppat.1003958
49. Veazey RS, Lifson JD, Pandrea I, Purcell J, Piatak M, Lackner AA. Simian Immunodeficiency Virus Infection in Neonatal Macaques. J Virol (2003) 77(16):8783–92. doi: 10.1128/jvi.77.16.8783-8792.2003
50. Wang X, Xu H, Pahar B, Alvarez X, Green LC, Dufour J, et al. Simian Immunodeficiency Virus Selectively Infects Proliferating CD4+ T Cells in Neonatal Rhesus Macaques. Blood (2010) 116(20):4168–74. doi: 10.1182/blood-2010-03-273482
51. Lyimo MA, Howell AL, Balandya E, Eszterhas SK, Connor RI. Innate Factors in Human Breast Milk Inhibit Cell-Free HIV-1 But Not Cell-Associated HIV-1 Infection of CD4+ Cells. J Acquir Immune Defic Syndr (2009) 51(2):117–24. doi: 10.1097/QAI.0b013e3181a3908d
52. Fouda GG, Jaeger FH, Amos JD, Ho C, Kunz EL, Anasti K, et al. Tenascin-C Is an Innate Broad-Spectrum, HIV-1-Neutralizing Protein in Breast Milk. Proc Natl Acad Sci USA (2013) 110(45):18220–5. doi: 10.1073/pnas.1307336110
53. Mall AS, Habte H, Mthembu Y, Peacocke J, de Beer C. Mucus and Mucins: Do They Have a Role in the Inhibition of the Human Immunodeficiency Virus? Virol J (2017) 14(1):192. doi: 10.1186/s12985-017-0855-9
54. Kazmi SH, Naglik JR, Sweet SP, Evans RW, O'Shea S, Banatvala JE, et al. Comparison of Human Immunodeficiency Virus Type 1-Specific Inhibitory Activities in Saliva and Other Human Mucosal Fluids. Clin Vaccine Immunol (2006) 13(10):1111–8. doi: 10.1128/CDLI.00426-05
55. Milligan C, Overbaugh J. The Role of Cell-Associated Virus in Mother-To-Child HIV Transmission. J Infect Dis (2014) 210(suppl_3):S631–40. doi: 10.1093/infdis/jiu344
56. Lunney KM, Iliff P, Mutasa K, Ntozini R, Magder LS, Moulton LH, et al. Associations Between Breast Milk Viral Load, Mastitis, Exclusive Breast-Feeding, and Postnatal Transmission of HIV. Clin Infect Dis (2010) 50(5):762–9. doi: 10.1086/650535
57. Kuhn L, Sinkala M, Kankasa C, Semrau K, Kasonde P, Scott N, et al. High Uptake of Exclusive Breastfeeding and Reduced Early Post-Natal HIV Transmission. PloS One (2007) 2(12):e1363. doi: 10.1371/journal.pone.0001363
58. Coovadia HM, Rollins NC, Bland RM, Little K, Coutsoudis A, Bennish ML, et al. Mother-To-Child Transmission of HIV-1 Infection During Exclusive Breastfeeding in the First 6 Months of Life: An Intervention Cohort Study. Lancet (2007) 369(9567):1107–16. doi: 10.1016/S0140-6736(07)60283-9
59. Coutsoudis A, Dabis F, Fawzi W, Gaillard P, Haverkamp G, Harris DR, et al. Late Postnatal Transmission of HIV-1 in Breast-Fed Children: An Individual Patient Data Meta-Analysis. J Infect Dis (2004) 189(12):2154–66. doi: 10.1086/420834
60. Gejo NG, Weldearegay HG, W/tinsaie KT, Mekango DE, Woldemichael ES, Buda AS, et al. Exclusive Breastfeeding and Associated Factors Among HIV Positive Mothers in Northern Ethiopia. PloS One (2019) 14(1):e0210782. doi: 10.1371/journal.pone.0210782
61. Coutsoudis A, Pillay K, Spooner E, Kuhn L, Coovadia HM. Influence of Infant-Feeding Patterns on Early Mother-to-Child Transmission of HIV-1 in Durban, South Africa: A Prospective Cohort Study. South African Vitamin A Study Group. Lancet (1999) 354(9177):471–6. doi: 10.1016/S0140-6736(99)01101-0
62. Semrau K, Kuhn L, Brooks DR, Cabral H, Sinkala M, Kankasa C, et al. Exclusive Breastfeeding, Maternal HIV Disease, and the Risk of Clinical Breast Pathology in HIV-Infected, Breastfeeding Women. Am J Obstet Gynecol (2011) 205(4):344.e1–8. doi: 10.1016/j.ajog.2011.06.021
63. Semba RD, Kumwenda N, Hoover DR, Taha TE, Quinn TC, Mtimavalye L, et al. Human Immunodeficiency Virus Load in Breast Milk, Mastitis, and Mother-to-Child Transmission of Human Immunodeficiency Virus Type 1. J Infect Dis (1999) 180(1):93–8. doi: 10.1086/314854
64. Embree JE, Njenga S, Datta P, Nagelkerke NJD, Ndinya-Achola JO, Mohammed Z, et al. Risk Factors for Postnatal Mother-Child Transmission of HIV-1. AIDS (2000) 14(16):2535–41. doi: 10.1097/00002030-200011100-00016
65. Wood LF, Brown BP, Lennard K, Karaoz U, Havyarimana E, Passmore J-AS, et al. Feeding-Related Gut Microbial Composition Associates With Peripheral T-Cell Activation and Mucosal Gene Expression in African Infants. Clin Infect Dis (2018) 67(8):1237–46. doi: 10.1093/cid/ciy265
66. Rousseau CM, Nduati RM, Richardson BA, John-Stewart GC, Mbori-Ngacha DA, Kreiss JK, et al. Association of Levels of HIV-1–Infected Breast Milk Cells and Risk of Mother-To-Child Transmission. J Infect Dis (2004) 190(10):1880–8. doi: 10.1086/425076
67. Ndirangu J, Viljoen J, Bland RM, Danaviah S, Thorne C, Vande Perre P, et al. Cell-Free (RNA) and Cell-Associated (DNA) HIV-1 and Postnatal Transmission Through Breastfeeding. PloS One (2012) 7(12):e51493. doi: 10.1371/journal.pone.0051493
68. Thea DM, Steketee RW, Pliner V, Bornschlegel K, Brown T, Orloff S, et al. The Effect of Maternal Viral Load on the Risk of Perinatal Transmission of HIV-1. AIDS (1997) 11(4):437–44. doi: 10.1097/00002030-199704000-00006
69. Cao Y, Krogstad P, Korber BT, Koup RA, Muldoon M, Macken C, et al. Maternal HIV-1 Viral Load and Vertical Transmission of Infection: The Ariel Project for the Prevention of HIV Transmission From Mother to Infant. Nat Med (1997) 3(5):549–52. doi: 10.1038/nm0597-549
70. Scarlatti G, Hodara V, Rossi P, Muggiasca L, Bucceri A, Albert J, et al. Transmission of Human Immunodeficiency Virus Type 1 (HIV-1) From Mother to Child Correlates With Viral Phenotype. Virology (1993) 197(2):624–9. doi: 10.1006/viro.1993.1637
71. O’Shea S, Newell M-L, Dunn DT, Garcia-Rodriguez M-C, Bates I, Mullen J, et al. Maternal Viral Load, CD4 Cell Count and Vertical Transmission of HIV-1. J Med Virol (1998) 54(2):113–7. doi: 10.1002/(SICI)1096-9071(199802)54:2<113::AID-JMV8>3.0.CO;2-9
72. Morrison CS, Demers K, Kwok C, Bulime S, Rinaldi A, Munjoma M, et al. Plasma and Cervical Viral Loads Among Ugandan and Zimbabwean Women During Acute and Early HIV-1 Infection. AIDS (2010) 24(4):573–82. doi: 10.1097/QAD.0b013e32833433df
73. Connor EM, Sperling RS, Gelber R, Kiselev P, Scott G, O'Sullivan MJ, et al. Reduction of Maternal-Infant Transmission of Human Immunodeficiency Virus Type 1 With Zidovudine Treatment. Pediatric AIDS Clinical Trials Group Protocol 076 Study Group. N Engl J Med (1994) 331(18):1173–80. doi: 10.1056/NEJM199411033311801
74. Cooper ER, Charurat M, Mofenson L, Hanson CI, Pitt J, Diaz C, et al. Combination Antiretroviral Strategies for the Treatment of Pregnant HIV-1-Infected Women and Prevention of Perinatal HIV-1 Transmission. J Acquir Immune Defic Syndr (2002) 29(5):484–94. doi: 10.1097/00042560-200204150-00009
75. Dickover RE, Garratty EM, Herman SA, Sim MS, Plaeger S, Boyer PJ, et al. Identification of Levels of Maternal HIV-1 RNA Associated With Risk of Perinatal Transmission: Effect of Maternal Zidovudine Treatment on Viral Load. JAMA (1996) 275(8):599–605. doi: 10.1001/jama.1996.03530320023029
76. Mayaux MJ, Dussaix E, Isopet J, Rekacewicz C, Mandelbrot L, Ciraru-Vigneron N, et al. Maternal Virus Load During Pregnancy and Mother-To-Child Transmission of Human Immunodeficiency Virus Type 1: The French Perinatal Cohort Studies. J Infect Dis (1997) 175(1):172–5. doi: 10.1093/infdis/175.1.172
77. Shaffer N, Roongpisuthipong A, Siriwasin W, Chotpitayasunondh T, Chearskul S, Young NL, et al. Maternal Virus Load and Perinatal Human Immunodeficiency Virus Type 1 Subtype E Transmission, Thailand. J Infect Dis (1999) 179(3):590–9. doi: 10.1086/314641
78. Burns DN, Landesman S, Muenz LR, Nugent RP, Goedert JJ, Minkoff H, et al. Cigarette Smoking, Premature Rupture of Membranes, and Vertical Transmission of HIV-1 Among Women With Low CD4+ Levels. J Acquir Immune Defic Syndr (1988) (1994) 7(7):718–26.
79. Turner BJ, Hauck WW, Fanning TR, Markson LE. Cigarette Smoking and Maternal-Child HIV Transmission. J Acquir Immune Defic Syndr Hum Retrovirol (1997) 14(4):327–37. doi: 10.1097/00042560-199704010-00004
80. Matheson PB, Schoenbaum E, Greenberg B, Pliner V. Association of Maternal Drug Use During Pregnancy With Mother-to-Child HIV Transmission. New York City Perinatal HIV Transmission Collaborative Study. AIDS (1997) 11(7):941–2.
81. Rodriguez EM, Mofenson LM, Chang BH, Rich KC, Fowler MG, Smeriglio V, et al. Association of Maternal Drug Use During Pregnancy With Maternal HIV Culture Positivity and Perinatal HIV Transmission. AIDS (1996) 10(3):273–82. doi: 10.1097/00002030-199603000-00006
82. Carrico AW, Johnson MO, Moskowitz JT, Neilands TB, Morin SF, Charlebois ED, et al. Affect Regulation, Stimulant Use, and Viral Load Among HIV-Positive Persons on Anti-Retroviral Therapy. Psychosom Med (2007) 69(8):785–92. doi: 10.1097/PSY.0b013e318157b142
83. Baum MK, Rafie C, Lai S, Sales S, Page B, Campa A. Crack-Cocaine Use Accelerates HIV Disease Progression in a Cohort of HIV-Positive Drug Users. J Acquir Immune Defic Syndr (2009) 50(1):93–9. doi: 10.1097/QAI.0b013e3181900129
84. Ellis RJ, Childers ME, Cherner M, Lazzaretto D, Letendre S, Grant I. Increased Human Immunodeficiency Virus Loads in Active Methamphetamine Users Are Explained by Reduced Effectiveness of Antiretroviral Therapy. J Infect Dis (2003) 188(12):1820–6. doi: 10.1086/379894
85. Chander G, Lau B, Moore RD. Hazardous Alcohol Use: A Risk Factor for Non-Adherence and Lack of Suppression in HIV Infection. J Acquir Immune Defic Syndr (2006) 43(4):411–7. doi: 10.1097/01.qai.0000243121.44659.a4
86. Purohit V, Rapaka RS, Schnur P, Shurtleff D. Potential Impact of Drugs of Abuse on Mother-to-Child Transmission (MTCT) of HIV in the Era of Highly Active Antiretroviral Therapy (HAART). Life Sci (2011) 88(21-22):909–16. doi: 10.1016/j.lfs.2011.03.022
87. Arnsten JH, Demas PA, Grant RW, Gourevitch MN, Farzadegan H, Howard AA. Impact of Active Drug Use on Antiretroviral Therapy Adherence and Viral Suppression in HIV-Infected Drug Users. J Gen Intern Med (2002) 17(5):377–81. doi: 10.1007/s11606-002-0044-3
88. John GC, Nduati RW, Mbori-Ngacha D, Overbaugh J, Welch M, Richardson BA, et al. Genital Shedding of Human Immunodeficiency Virus Type 1 DNA During Pregnancy: Association With Immunosuppression, Abnormal Cervical or Vaginal Discharge, and Severe Vitamin A Deficiency. J Infect Dis (1997) 175(1):57–62. doi: 10.1093/infdis/175.1.57
89. Clemetson DBA. Detection of HIV DNA in Cervical and Vaginal Secretions. JAMA (1993) 269(22):2860. doi: 10.1001/jama.1993.03500220046024
90. Loussert-Ajaka I, Mandelbrot L, Delmas MC, Bastian H, Benifla JL, Farfara I, et al. HIV-1 Detection in Cervicovaginal Secretions During Pregnancy. AIDS (1997) 11(13):1575–81. doi: 10.1097/00002030-199713000-00005
91. Rousseau CM, Nduati RM, Richardson BA, Steele MS, John-Stewart GC, Mbori-Ngacha DA, et al. Longitudinal Analysis of Human Immunodeficiency Virus Type 1 RNA in Breast Milk and of Its Relationship to Infant Infection and Maternal Disease. J Infect Dis (2003) 187(5):741–7. doi: 10.1086/374273
92. Nagot N, Kankasa C, Tumwine JK, Meda N, Hofmeyr GJ, Vallo R, et al. Extended Pre-Exposure Prophylaxis With Lopinavir–Ritonavir Versus Lamivudine to Prevent HIV-1 Transmission Through Breastfeeding Up to 50 Weeks in Infants in Africa (ANRS 12174): A Randomised Controlled Trial. Lancet (2016) 387(10018):566–73. doi: 10.1016/S0140-6736(15)00984-8
93. Flynn PM, Taha TE, Cababasay M, Fowler MG, Mofenson LM, Owor M, et al. Prevention of HIV-1 Transmission Through Breastfeeding: Efficacy and Safety of Maternal Antiretroviral Therapy Versus Infant Nevirapine Prophylaxis for Duration of Breastfeeding in HIV-1-Infected Women With High CD4 Cell Count (IMPAACT PROMISE): A Randomi. JAIDS J Acquir Immune Defic Syndr (2018) 77(4):383–92. doi: 10.1097/QAI.0000000000001612
94. Flynn PM, Taha TE, Cababasay M, Butler K, Fowler MG, Mofenson LM, et al. Association of Maternal Viral Load and CD4 Count With Perinatal HIV-1 Transmission Risk During Breastfeeding in the PROMISE Postpartum Component. JAIDS J Acquired Immune Deficiency Syndromes (2021) 88(2):206–13. doi: 10.1097/QAI.0000000000002744
95. Shapiro RL, Hughes MD, Ogwu A, Kitch D, Lockman S, Moffat C, et al. Antiretroviral Regimens in Pregnancy and Breast-Feeding in Botswana. New Engl J Med (2010) 362(24):2282–94. doi: 10.1056/NEJMoa0907736
96. Luoga E, Vanobberghen F, Bircher R, Nyuri A, Ntamatungiro AJ, Mnzava D, et al. Brief Report: No HIV Transmission From Virally Suppressed Mothers During Breastfeeding in Rural Tanzania. J Acquir Immune Defic Syndr (2018) 79(1):e17–20. doi: 10.1097/QAI.0000000000001758
97. Giuliano M, Andreotti M, Liotta G, Jere H, Sagno J-B, Maulidi M, et al. Maternal Antiretroviral Therapy for the Prevention of Mother-To-Child Transmission of HIV in Malawi: Maternal and Infant Outcomes Two Years After Delivery. PloS One (2013) 8(7):e68950. doi: 10.1371/journal.pone.0068950
98. Kliks S, Contag CH, Corliss H, Learn G, Rodrigo A, Wara D, et al. Genetic Analysis of Viral Variants Selected in Transmission of Human Immunodeficiency Viruses to Newborns. AIDS Res Hum Retroviruses (2000) 16(13):1223–33. doi: 10.1089/08892220050116998
99. Zhang H, Tully DC, Hoffmann FG, He J, Kankasa C, Wood C. Phylogenetic and Phenotypic Analysis of HIV Type 1 Env Gp120 in Cases of Subtype C Mother-to-Child Transmission. AIDS Res Hum Retroviruses (2002) 18(18):1415–23. doi: 10.1089/088922202320935492
100. Renjifo B, Chung M, Gilbert P, Mwakagile D, Msamanga G, Fawzi W, et al. In-Utero Transmission of Quasispecies Among Human Immunodeficiency Virus Type 1 Genotypes. Virology (2003) 307(2):278–82. doi: 10.1016/S0042-6822(02)00066-1
101. Dickover RE, Garratty EM, Plaeger S, Bryson YJ. Perinatal Transmission of Major, Minor, and Multiple Maternal Human Immunodeficiency Virus Type 1 Variants In Utero and Intrapartum. J Virol (2001) 75(5):2194–203. doi: 10.1128/JVI.75.5.2194-2203.2001
102. Kwiek JJ, Russell ES, Dang KK, Burch CL, Mwapasa V, Meshnick SR, et al. The Molecular Epidemiology of HIV-1 Envelope Diversity During HIV-1 Subtype C Vertical Transmission in Malawian Mother–Infant Pairs. AIDS (2008) 22(7):863–71. doi: 10.1097/QAD.0b013e3282f51ea0
103. Ahmad N, Baroudy BM, Baker RC, Chappey C. Genetic Analysis of Human Immunodeficiency Virus Type 1 Envelope V3 Region Isolates From Mothers and Infants After Perinatal Transmission. J Virol (1995) 69(2):1001–12. doi: 10.1128/jvi.69.2.1001-1012.1995
104. Wolinsky S, Wike C, Korber B, Hutto C, Parks WP, Rosenblum L, et al. Selective Transmission of Human Immunodeficiency Virus Type-1 Variants From Mothers to Infants. Science (1992) 255(5048):1134–7. doi: 10.1126/science.1546316
105. Boyce CL, Sils T, Ko D, Wong-On-Wing A, Beck IA, Styrchak SM, et al. Maternal HIV Drug Resistance Is Associated With Vertical Transmission and Is Prevalent in Infected Infants. Clin Infect Dis (2021). doi: 10.1093/cid/ciab744
106. Baan E, de Ronde A, Luchters S, Vyankandondera J, Lange JM, Pollakis G, et al. HIV Type 1 Mother-to-Child Transmission Facilitated by Distinctive Glycosylation Sites in the Gp120 Envelope Glycoprotein. AIDS Res Hum Retroviruses (2012) 28(7):715–24. doi: 10.1089/aid.2011.0023
107. Ometto L, Zanchetta M, Mainardi M, De Salvo GL, Garcia-Rodriguez MC, Gray L, et al. Co-Receptor Usage of HIV-1 Primary Isolates, Viral Burden, and CCR5 Genotype in Mother-to-Child HIV-1 Transmission. AIDS (2000) 14(12):1721–9. doi: 10.1097/00002030-200008180-00006
108. Derdeyn CA, Hunter E. Viral Characteristics of Transmitted HIV. Curr Opin HIV AIDS (2008) 3(1):16–21. doi: 10.1097/COH.0b013e3282f2982c
109. John GC, Kreiss J. Mother-To-Child Transmission of Human Immunodeficiency Virus Type 1. Epidemiologic Rev (1996) 18(2):149–57. doi: 10.1093/oxfordjournals.epirev.a017922
110. Bryson YJ. Perinatal HIV-1 Transmission: Recent Advances and Therapeutic Interventions. AIDS (1996) 10 Suppl 3:S33–42.
111. Van’T Wout AB, Kootstra NA, Mulder-Kampinga GA, Albrecht-van Lent N, Scherpbier HJ, Veenstra J, et al. Macrophage-Tropic Variants Initiate Human Immunodeficiency Virus Type 1 Infection After Sexual, Parenteral, and Vertical Transmission. J Clin Invest (1994) 94(5):2060–7. doi: 10.1172/JCI117560
112. Yang C, Li M, Newman RD, Shi Y-P, Ayisi J, van Eijk AM, et al. Genetic Diversity of HIV-1 in Western Kenya: Subtype-Specific Differences in Mother-to-Child Transmission. AIDS (2003) 17(11):1667–74. doi: 10.1097/00002030-200307250-00011
113. Walter J, Kuhn L, Aldrovandi GM. Advances in Basic Science Understanding of Mother-to-Child HIV-1 Transmission. Curr Opin HIV AIDS (2008) 3(2):146–50. doi: 10.1097/COH.0b013e3282f50bb2
114. Koulinska IN, Villamor E, Chaplin B, Msamanga G, Fawzi W, Renjifo B, et al. Transmission of Cell-Free and Cell-Associated HIV-1 Through Breast-Feeding. J Acquir Immune Defic Syndr (1999) (2006) 41(1):93–9. doi: 10.1097/01.qai.0000179424.19413.24
115. Eshleman SH, Church JD, Chen S, Guay LA, Mwatha A, Fiscus SA, et al. Comparison of HIV-1 Mother-to-Child Transmission After Single-Dose Nevirapine Prophylaxis Among African Women With Subtypes A, C and D. J Acquir Immune Defic Syndr (2006) 42(4):518–21. doi: 10.1097/01.qai.0000221676.22069.b8
116. Choi RY, Farquhar C, Juno J, Mbori-Ngacha D, Lohman-Payne B, Vouriot F, et al. Infant CD4 C868T Polymorphism Is Associated With Increased Human Immunodeficiency Virus (HIV-1) Acquisition. Clin Exp Immunol (2010) 160(3):461–5. doi: 10.1111/j.1365-2249.2010.04096.x
117. Shearer WT, Kalish LA, Zimmerman PA. CCR5 HIV-1 Vertical Transmission. Women and Infants Transmission Study Group. J Acquir Immune Defic Syndr Hum Retrovirol (1998) 17(2):180–1. doi: 10.1097/00042560-199802010-00014
118. Mandl CW, Aberle SW, Henkel JH, Puchhammer-Stöckl E, Heinz FX. Possible Influence of the Mutant CCR5 Allele on Vertical Transmission of HIV-1. J Med Virol (1998) 55(1):51–5. doi: 10.1002/(SICI)1096-9071(199805)55:1<51::AID-JMV9>3.0.CO;2-N
119. Philpott S, Burger H, Charbonneau T, Grimson R, Vermund SH, Visosky A, et al. CCR5 Genotype and Resistance to Vertical Transmission of HIV-1. J Acquir Immune Defic Syndr (1999) 21(3):189–93. doi: 10.1097/00126334-199907010-00002
120. Kostrikis LG, Neumann AU, Thomson B, Korber BT, McHardy P, Karanicolas R, et al. A Polymorphism in the Regulatory Region of the CC-Chemokine Receptor 5 Gene Influences Perinatal Transmission of Human Immunodeficiency Virus Type 1 to African-American Infants. J Virol (1999) 73(12):10264–71. doi: 10.1128/JVI.73.12.10264-10271.1999
121. Pedersen BR, Kamwendo D, Blood M, Mwapasa V, Molyneux M, North K, et al. CCR5 Haplotypes and Mother-to-Child HIV Transmission in Malawi. PloS One (2007) 2(9):e838. doi: 10.1371/journal.pone.0000838
122. Singh KK, Hughes MD, Chen J, Phiri K, Rousseau C, Kuhn L, et al. Associations of Chemokine Receptor Polymorphisms With HIV-1 Mother-to-Child Transmission in Sub-Saharan Africa: Possible Modulation of Genetic Effects by Antiretrovirals. J Acquir Immune Defic Syndr (2008) 49(3):259–65. doi: 10.1097/QAI.0b013e318186eaa4
123. Salkowitz JR, Bruse SE, Meyerson H, Valdez H, Mosier DE, Harding CV, et al. CCR5 Promoter Polymorphism Determines Macrophage CCR5 Density and Magnitude of HIV-1 Propagation in vitro. Clin Immunol (2003) 108(3):234–40. doi: 10.1016/S1521-6616(03)00147-5
124. De Rossi A. Virus-Host Interactions in Paediatric HIV-1 Infection. Curr Opin HIV AIDS (2007) 2(5):399–404. doi: 10.1097/COH.0b013e3282ced163
125. Braida L, Boniotto M, Pontillo A, Tovo PA, Amoroso A, Crovella S. A Single-Nucleotide Polymorphism in the Human Beta-Defensin 1 Gene Is Associated With HIV-1 Infection in Italian Children. AIDS (2004) 18(11):1598–600. doi: 10.1097/01.aids.0000131363.82951.fb
126. Ricci E, Malacrida S, Zanchetta M, Montagna M, Giaquinto C, De Rossi A. Role of Beta-Defensin-1 Polymorphisms in Mother-to-Child Transmission of HIV-1. J Acquir Immune Defic Syndr (2009) 51(1):13–9. doi: 10.1097/QAI.0b013e31819df249
127. Milanese M, Segat L, Pontillo A, Arraes LC, de Lima Filho JL, Crovella S. DEFB1 Gene Polymorphisms and Increased Risk of HIV-1 Infection in Brazilian Children. AIDS (2006) 20(12):1673–5. doi: 10.1097/01.aids.0000238417.05819.40
128. Milanese M, Segat L, Arraes LC, Garzino-Demo A, Crovella S. Copy Number Variation of Defensin Genes and HIV Infection in Brazilian Children. J Acquir Immune Defic Syndr (2009) 50(3):331–3. doi: 10.1097/QAI.0b013e3181945f39
129. Polycarpou A, Ntais C, Korber BT, Elrich HA, Winchester R, Krogstad P, et al. Association Between Maternal and Infant Class I and II HLA Alleles and of Their Concordance With the Risk of Perinatal HIV Type 1 Transmission. AIDS Res Hum Retroviruses (2002) 18(11):741–6. doi: 10.1089/08892220260139477
130. Aikhionbare FO, Hodge T, Kuhn L, Bulterys M, Abrams EJ, Bond VC. Mother-To-Child Discordance in HLA-G Exon 2 Is Associated With a Reduced Risk of Perinatal HIV-1 Transmission. AIDS (2001) 15(16):2196–8. doi: 10.1097/00002030-200111090-00019
131. MacDonald KS, Embree J, Njenga S, Nagelkerke NJD, Ngatia I, Mohammed Z, et al. Mother-Child Class I HLA Concordance Increases Perinatal Human Immunodeficiency Virus Type 1 Transmission. J Infect Dis (1998) 177(3):551–6. doi: 10.1086/514243
132. MacDonald KS, Embree JE, Nagelkerke NJ, Castillo J, Ramhadin S, Njenga S, et al. The HLA A2/6802 Supertype Is Associated With Reduced Risk of Perinatal Human Immunodeficiency Virus Type 1 Transmission. J Infect Dis (2001) 183(3):503–6. doi: 10.1086/318092
133. Farquhar C, Rowland-Jones S, Mbori-Ngacha D, Redman M, Lohman B, Slyker J, et al. Human Leukocyte Antigen (HLA) B*18 and Protection Against Mother-to-Child HIV Type 1 Transmission. AIDS Res Hum Retroviruses (2004) 20(7):692–7. doi: 10.1089/0889222041524616
134. Mackelprang RD, John-Stewart G, Carrington M, Richardson B, Rowland-Jones S, Gao X, et al. Maternal HLA Homozygosity and Mother-Child HLA Concordance Increase the Risk of Vertical Transmission of HIV-1. J Infect Dis (2008) 197(8):1156–61. doi: 10.1086/529528
135. Fabris A, Catamo E, Segat L, Morgutti M, Arraes LC, de Lima-Filho JL, et al. Association Between HLA-G 3’UTR 14-Bp Polymorphism and HIV Vertical Transmission in Brazilian Children. AIDS (2009) 23(2):177–82. doi: 10.1097/QAD.0b013e32832027bf
136. Aikhionbare FO, Kumaresan K, Shamsa F, Bond VC. HLA-G DNA Sequence Variants and Risk of Perinatal HIV-1 Transmission. AIDS Res Ther (2006) 3:28. doi: 10.1186/1742-6405-3-28
137. Kilpatrick DC, Hague RA, Yap PL, Mok JY. HLA Antigen Frequencies in Children Born to HIV-Infected Mothers. Dis Markers (1991) 9(1):21–6.
138. Winchester R, Chen Y, Rose S, Selby J, Borkowsky W. Major Histocompatibility Complex Class II DR Alleles DRB1*1501 and Those Encoding HLA-DR13 Are Preferentially Associated With a Diminution in Maternally Transmitted Human Immunodeficiency Virus 1 Infection in Different Ethnic Groups: Determination by an Automated Sequence-Based Typing Method. Proc Natl Acad Sci USA (1995) 92(26):12374–8. doi: 10.1073/pnas.92.26.12374
139. Just JJ, Abrams E, Louie LG, Urbano R, Wara D, Nicholas SW, et al. Influence of Host Genotype on Progression to Acquired Immunodeficiency Syndrome Among Children Infected With Human Immunodeficiency Virus Type 1. J Pediatr (1995) 127(4):544–9. doi: 10.1016/S0022-3476(95)70110-9
140. Biggar RJ, Taha TE, Hoover DR, Yellin F, Kumwenda N, Broadhead R. Higher In Utero and Perinatal HIV Infection Risk in Girls Than Boys. J Acquir Immune Defic Syndr (2006) 41(4):509–13. doi: 10.1097/01.qai.0000191283.85578.46
141. Taha TE, Nour S, Kumwenda NI, Broadhead RL, Fiscus SA, Kafulafula G, et al. Gender Differences in Perinatal HIV Acquisition Among African Infants. Pediatrics (2005) 115(2):e167–72. doi: 10.1542/peds.2004-1590
142. Adland E, Millar J, Bengu N, Muenchhoff M, Fillis R, Sprenger K, et al. Author Correction: Sex-Specific Innate Immune Selection of HIV-1 In Utero Is Associated With Increased Female Susceptibility to Infection. Nat Commun (2020) 11(1). doi: 10.1038/s41467-020-16215-7
143. St Louis ME, Kamenga M, Brown C, Tarande Manzila AMNM, Batter V, Behets F, et al. Risk for Perinatal HIV-1 Transmission According to Maternal Immunologic, Virologic, and Placental Factors. Jama (1993) 269(22):2853–9. doi: 10.1001/jama.1993.03500220039023
144. Mwanyumba F, Gaillard P, Inion I, Verhofstede C, Claeys P, Chohan V, et al. Placental Inflammation and Perinatal Transmission of HIV-1. J Acquir Immune Defic Syndr (2002) 29(3):262–9. doi: 10.1097/00042560-200203010-00006
145. Temmerman M, Nyong’o AO, Bwayo J, Fransen K, Coppens M, Piot P. Risk Factors for Mother-to-Child Transmission of Human Immunodeficiency Virus-1 Infection. Am J Obstet Gynecol (1995) 172(2 Pt 1):700–5. doi: 10.1016/0002-9378(95)90597-9
146. Wabwire-Mangen F, Gray RH, Mmiro FA, Ndugwa C, Abramowsky C, Wabinga H, et al. Placental Membrane Inflammation and Risks of Maternal-to-Child Transmission of HIV-1 in Uganda. J Acquir Immune Defic Syndr (1999) 22(4):379–85. doi: 10.1097/00126334-199912010-00009
147. Van Dyke RB, Korber BT, Popek E, Macken C, Widmayer SM, Bardeguez A, et al. The Ariel Project: A Prospective Cohort Study of Maternal-Child Transmission of Human Immunodeficiency Virus Type 1 in the Era of Maternal Antiretroviral Therapy. J Infect Dis (1999) 179(2):319–28. doi: 10.1086/314580
148. Adachi K, Xu J, Yeganeh N, Camarca M, Morgado MG, Watts D, et al. Combined Evaluation of Sexually Transmitted Infections in HIV-Infected Pregnant Women and Infant HIV Transmission. PloS One (2018) 13(1):e0189851. doi: 10.1371/journal.pone.0189851
149. Hitti J, Watts DH, Burchett SK, Schacker T, Selke S, Brown ZA, et al. Herpes Simplex Virus Seropositivity and Reactivation at Delivery Among Pregnant Women Infected With Human Immunodeficiency Virus-1. Am J Obstet Gynecol (1997) 177(2):450–4. doi: 10.1016/S0002-9378(97)70214-X
150. McClelland RS, Wang CC, Richardson BA, Corey L, Ashley RL, Mandaliya K, et al. A Prospective Study of Hormonal Contraceptive Use and Cervical Shedding of Herpes Simplex Virus in Human Immunodeficiency Virus Type 1-Seropositive Women. J Infect Dis (2002) 185(12):1822–5. doi: 10.1086/340639
151. Gray RH, Wabwire-Mangen F, Kigozi G, Sewankambo NK, Serwadda D, Moulton LH, et al. Randomized Trial of Presumptive Sexually Transmitted Disease Therapy During Pregnancy in Rakai, Uganda. Am J Obstet Gynecol (2001) 185(5):1209–17. doi: 10.1067/mob.2001.118158
152. Menendez C, Sanchez-Tapias JM, Kahigwa E, Mshinda H, Costa J, Vidal J, et al. Prevalence and Mother-to-Infant Transmission of Hepatitis Viruses B, C, and E in Southern Tanzania. J Med Virol (1999) 58(3):215–20. doi: 10.1002/(SICI)1096-9071(199907)58:3<215::AID-JMV5>3.0.CO;2-K
153. Tess BH, Rodrigues LC, Newell ML, Dunn DT, Lago TD. Breastfeeding, Genetic, Obstetric and Other Risk Factors Associated With Mother-to-Child Transmission of HIV-1 in Sao Paulo State, Brazil. Sao Paulo Collaborative Study for Vertical Transmission of HIV-1. AIDS (1998) 12(5):513–20. doi: 10.1097/00002030-199805000-00013
154. England K, Thorne C, Newell ML. Vertically Acquired Paediatric Coinfection With HIV and Hepatitis C Virus. Lancet Infect Dis (2006) 6(2):83–90. doi: 10.1016/S1473-3099(06)70381-4
155. Giovannini M, Tagger A, Ribero ML, Zuccotti G, Pogliani L, Grossi A, et al. Maternal-Infant Transmission of Hepatitis C Virus and HIV Infections: A Possible Interaction. Lancet (1990) 335(8698):1166. doi: 10.1016/0140-6736(90)91174-9
156. Hershow RC, Riester KA, Lew J, Quinn TC, Mofenson LM, Davenny K, et al. Increased Vertical Transmission of Human Immunodeficiency Virus From Hepatitis C Virus-Coinfected Mothers. Women and Infants Transmission Study. J Infect Dis (1997) 176(2):414–20. doi: 10.1086/514058
157. Paccagnini S, Principi N, Massironi E, Tanzi E, Romanò L, Muggiasca ML, et al. Perinatal Transmission and Manifestation of Hepatitis C Virus Infection in a High Risk Population. Pediatr Infect Dis J (1995) 14(3):195–9. doi: 10.1097/00006454-199503000-00005
158. Papaevangelou V, Pollack H, Rochford G, Kokka R, Hou Z, Chernoff D, et al. Increased Transmission of Vertical Hepatitis C Virus (HCV) Infection to Human Immunodeficiency Virus (HIV)-Infected Infants of HIV- and HCV-Coinfected Women. J Infect Dis (1998) 178(4):1047–52. doi: 10.1086/515668
159. Thomas DL, Villano SA, Riester KA, Hershow R, Mofenson LM, Landesman SH, et al. Perinatal Transmission of Hepatitis C Virus From Human Immunodeficiency Virus Type 1-Infected Mothers. Women and Infants Transmission Study. J Infect Dis (1998) 177(6):1480–8. doi: 10.1086/515315
160. Tovo PA, Palomba E, Ferraris G, Principi N, Ruga E, Dallacasa P, et al. Increased Risk of Maternal-Infant Hepatitis C Virus Transmission for Women Coinfected With Human Immunodeficiency Virus Type 1. Italian Study Group for HCV Infection in Children. Clin Infect Dis (1997) 25(5):1121–4. doi: 10.1086/516102
161. Bulterys M, Landesman S, Burns DN, Rubinstein A, Goedert JJ. Sexual Behavior and Injection Drug Use During Pregnancy and Vertical Transmission of HIV-1. JAIDS J Acquired Immune Deficiency Syndromes (1997) 15(1):76–82. doi: 10.1097/00042560-199705010-00012
162. Matheson PB, Thomas PA, Abrams EJ, Pliner V, Lambert G, Bamji M, et al. Heterosexual Behavior During Pregnancy and Perinatal Transmission of HIV-1. New York City Perinatal HIV Transmission Collaborative Study Group. AIDS (1996) 10(11):1249–56. doi: 10.1097/00002030-199609000-00011
163. Burns DN, Landesman S, Wright DJ, Waters D, Mitchell RM, Rubinstein A, et al. Influence of Other Maternal Variables on the Relationship Between Maternal Virus Load and Mother-to-Infant Transmission of Human Immunodeficiency Virus Type 1. J Infect Dis (1997) 175(5):1206–10. doi: 10.1086/593569
164. Taha TE, Gray RH. Genital Tract Infections and Perinatal Transmission of HIV. Ann N Y Acad Sci (2000) 918:84–98. doi: 10.1111/j.1749-6632.2000.tb05477.x
165. Bulterys M, Chao A, Dushimimana A, Phocas NP, Kurawige J-B, Musanganire F, et al. Multiple Sexual Partners and Mother-to-Child Transmission of HIV-1. AIDS (1993) 7(12):1639–45. doi: 10.1097/00002030-199312000-00015
166. Day JH, Grant AD, Fielding KL, Morris L, Moloi V, Charalambous S, et al. Does Tuberculosis Increase HIV Load? J Infect Dis (2004) 190(9):1677–84. doi: 10.1086/424851
167. Goletti D, Weissman D, Jackson RW, Graham NM, Vlahov D, Klein RS, et al. Effect of Mycobacterium Tuberculosis on HIV Replication. Role of Immune Activation. J Immunol (1996) 157(3):1271–8.
168. Toossi Z, Mayanja-Kizza H, Hirsch CS, Edmonds KL, Spahlinger T, Hom DL, et al. Impact of Tuberculosis (TB) on HIV-1 Activity in Dually Infected Patients. Clin Exp Immunol (2001) 123(2):233–8. doi: 10.1046/j.1365-2249.2001.01401.x
169. Gupta A, Bhosale R, Kinikar A, Gupte N, Bharadwaj R, Kagal A, et al. Maternal Tuberculosis: A Risk Factor for Mother-to-Child Transmission of Human Immunodeficiency Virus. J Infect Dis (2011) 203(3):358–63. doi: 10.1093/jinfdis/jiq064
170. Taha TE, Canner JK, Dallabetta GA, Chiphangwi JD, Liomba G, Wangel AM, et al. Childhood Malaria Parasitaemia and Human Immunodeficiency Virus Infection in Malawi. Trans R Soc Trop Med Hyg (1994) 88(2):164–5. doi: 10.1016/0035-9203(94)90277-1
171. Hoffman IF, Jere CS, Taylor TE, Munthali P, Dyer JR, Wirima JJ, et al. The Effect of Plasmodium Falciparum Malaria on HIV-1 RNA Blood Plasma Concentration. AIDS (1999) 13(4):487–94. doi: 10.1097/00002030-199903110-00007
172. Brahmbhatt H, Sullivan D, Kigozi G, Askin F, Wabwire-Mangenm F, Serwadda D, et al. Association of HIV and Malaria With Mother-to-Child Transmission, Birth Outcomes, and Child Mortality. J Acquir Immune Defic Syndr (2008) 47(4):472–6. doi: 10.1097/QAI.0b013e318162afe0
173. Brahmbhatt H, Kigozi G, Wabwire-Mangen F, Serwadda D, Sewankambo N, Lutalo T, et al. The Effects of Placental Malaria on Mother-to-Child HIV Transmission in Rakai, Uganda. AIDS (2003) 17(17):2539–41. doi: 10.1097/00002030-200311210-00020
174. Ayouba A, Badaut C, Kfutwah A, Cannou C, Juillerat A, Gangnard S, et al. Specific Stimulation of HIV-1 Replication in Human Placental Trophoblasts by an Antigen of Plasmodium Falciparum. AIDS (2008) 22(6):785–7. doi: 10.1097/QAD.0b013e3282f560ee
175. Mwapasa V, Rogerson SJ, Molyneux ME, Abrams ET, Kamwendo DD, Lema VM, et al. The Effect of Plasmodium Falciparum Malaria on Peripheral and Placental HIV-1 RNA Concentrations in Pregnant Malawian Women. AIDS (2004) 18(7):1051–9. doi: 10.1097/00002030-200404300-00014
176. Msamanga GI, Taha TE, Young AM, Brown ER, Hoffman IF, Read JS, et al. Placental Malaria and Mother-to-Child Transmission of Human Immunodeficiency Virus-1. Am J Trop Med Hyg (2009) 80(4):508–15. doi: 10.4269/ajtmh.2009.80.508
177. Johnson EL, Chakraborty R. HIV-1 at the Placenta: Immune Correlates of Protection and Infection. Curr Opin Infect Dis (2016) 29(3):248–55. doi: 10.1097/QCO.0000000000000267
178. Fowler MG, Rogers MF. Overview of Perinatal HIV Infection. J Nutr (1996) 126(suppl_10):2602S–7S. doi: 10.1093/jn/126.suppl_10.2602S
179. Shivakoti R, Gupta A, Ray JC, Uprety P, Gupte N, Bhosale R, et al. Soluble CD14: An Independent Biomarker for the Risk of Mother-To-Child Transmission of HIV in a Setting of Preexposure and Postexposure Antiretroviral Prophylaxis. J Infect Dis (2016) 213(5):762–5. doi: 10.1093/infdis/jiv479
180. Rossi P, Moschese V, Broliden PA, Fundaró C, Quinti I, Plebani A, et al. Presence of Maternal Antibodies to Human Immunodeficiency Virus 1 Envelope Glycoprotein Gp120 Epitopes Correlates With the Uninfected Status of Children Born to Seropositive Mothers. Proc Natl Acad Sci (1989) 86(20):8055–8. doi: 10.1073/pnas.86.20.8055
181. Broliden PA, Moschese V, Ljunggren K, Rosen J, Fundaro C, Plebani A, et al. Diagnostic Implication of Specific Immunoglobulin G Patterns of Children Born to HIV-Infected Mothers. AIDS (London England) (1989) 3(9):577–82. doi: 10.1097/00002030-198909000-00004
182. Pancino G, Leste-Lasserre T, Burgard M, Costagliola D, Ivanoff S, Blanche S, et al. Apparent Enhancement of Perinatal Transmission of Human Immunodeficiency Virus Type 1 by High Maternal Anti-Gp160 Antibody Titer. J Infect Dis (1998) 177(6):1737–41. doi: 10.1086/517435
183. Guevara H, Casseb J, Zijenah LS, Mbizvo M, Oceguera LF 3rd, Hanson CV, et al. Maternal HIV-1 Antibody and Vertical Transmission in Subtype C Virus Infection. J Acquir Immune Defic Syndr (2002) 29(5):435–40. doi: 10.1097/00042560-200204150-00002
184. Hofmann-Lehmann R, Vlasak J, Rasmussen RA, Smith BA, Baba TW, Liska V, et al. Postnatal Passive Immunization of Neonatal Macaques With a Triple Combination of Human Monoclonal Antibodies Against Oral Simian-Human Immunodeficiency Virus Challenge. J Virol (2001) 75(16):7470–80. doi: 10.1128/JVI.75.16.7470-7480.2001
185. Dickover R, Garratty E, Yusim K, Miller C, Korber B, Bryson Y. Role of Maternal Autologous Neutralizing Antibody in Selective Perinatal Transmission of Human Immunodeficiency Virus Type 1 Escape Variants. J Virol (2006) 80(13):6525–33. doi: 10.1128/JVI.02658-05
186. Ugen KE, Goedert JJ, Boyer J, Refaeli Y, Frank I, Williams WV, et al. Vertical Transmission of Human Immunodeficiency Virus (HIV) Infection. Reactivity of Maternal Sera With Glycoprotein 120 and 41 Peptides From HIV Type 1. J Clin Invest (1992) 89(6):1923–30. doi: 10.1172/JCI115798
187. Broliden K, Sievers E, Tovo PA, Moschese V, Scarlatti G, Broliden PA, et al. Antibody-Dependent Cellular Cytotoxicity and Neutralizing Activity in Sera of HIV-1-Infected Mothers and Their Children. Clin Exp Immunol (2008) 93(1):56–64. doi: 10.1111/j.1365-2249.1993.tb06497.x
188. Omenda MM, Milligan C, Odem-Davis K, Nduati R, Richardson BA, Lynch J, et al. Evidence for Efficient Vertical Transfer of Maternal HIV-1 Envelope–Specific Neutralizing Antibodies But No Association of Such Antibodies With Reduced Infant Infection. JAIDS J Acquired Immune Deficiency Syndromes (2013) 64(2):163–6. doi: 10.1097/QAI.0b013e31829f6e41
189. Kliks SC. Features of HIV-1 That Could Influence Maternal-Child Transmission. JAMA: J Am Med Assoc (1994) 272(6):467.
190. Wu X, Parast AB, Richardson BA, Nduati R, John-Stewart G, Mbori-Ngacha D, et al. Neutralization Escape Variants of Human Immunodeficiency Virus Type 1 Are Transmitted From Mother to Infant. J Virol (2006) 80(2):835–44. doi: 10.1128/JVI.80.2.835-844.2006
191. Martinez DR, Vandergrift N, Douglas AO, McGuire E, Bainbridge J, Nicely NI, et al. Maternal Binding and Neutralizing IgG Responses Targeting the C-Terminal Region of the V3 Loop Are Predictive of Reduced Peripartum HIV-1 Transmission Risk. J Virol (2017) 91(9):e02422–16. doi: 10.1128/JVI.02422-16
192. Mabuka J, Nduati R, Odem-Davis K, Peterson D, Overbaugh J. HIV-Specific Antibodies Capable of ADCC Are Common in Breastmilk and Are Associated With Reduced Risk of Transmission in Women With High Viral Loads. PloS Pathog (2012) 8(6):e1002739. doi: 10.1371/journal.ppat.1002739
193. Pollara J, McGuire E, Fouda GG, Rountree W, Eudailey J, Overman RG, et al. Association of HIV-1 Envelope-Specific Breast Milk IgA Responses With Reduced Risk of Postnatal Mother-To-Child Transmission of HIV-1. J Virol (2015) 89(19):9952–61. doi: 10.1128/JVI.01560-15
194. Lohman-Payne B, Slyker JA, Moore S, Maleche-Obimboe E, Wamalwae DC, Richardson BA, et al. Breast Milk Cellular HIV-Specific Interferon γ Responses Are Associated With Protection From Peripartum HIV Transmission. AIDS (2012) 26(16):2007–16. doi: 10.1097/QAD.0b013e328359b7e0
195. Mor G, Aldo P, Alvero AB. The Unique Immunological and Microbial Aspects of Pregnancy. Nat Rev Immunol (2017) 17(8):469–82. doi: 10.1038/nri.2017.64
196. Mold JE, Michaelsson J, Burt TD, Muench MO, Beckerman KP, Busch MP, et al. Maternal Alloantigens Promote the Development of Tolerogenic Fetal Regulatory T Cells In Utero. Science (2008) 322(5907):1562–5. doi: 10.1126/science.1164511
197. Takahata Y, Nomura A, Takada H, Ohga S, Furuno K, Hikino S, et al. CD25+CD4+ T Cells in Human Cord Blood: An Immunoregulatory Subset With Naive Phenotype and Specific Expression of Forkhead Box P3 (Foxp3) Gene. Exp Hematol (2004) 32(7):622–9. doi: 10.1016/j.exphem.2004.03.012
198. Hebel K, Weinert S, Kuropka B, Knolle J, Kosak B, Jorch G, et al. CD4+ T Cells From Human Neonates and Infants Are Poised Spontaneously to Run a Nonclassical IL-4 Program. J Immunol (2014) 192(11):5160–70. doi: 10.4049/jimmunol.1302539
199. Siegrist CA. Neonatal and Early Life Vaccinology. Vaccine (2001) 19(25-26):3331–46. doi: 10.1016/S0264-410X(01)00028-7
200. Goulder PJ, Brander C, Tang Y, Tremblay C, Colbert RA, Addo MM, et al. Evolution and Transmission of Stable CTL Escape Mutations in HIV Infection. Nature (2001) 412(6844):334–8. doi: 10.1038/35085576
201. Wilson CC, Brown RC, Korber BT, Wilkes BM, Ruhl DJ, Sakamoto D, et al. Frequent Detection of Escape From Cytotoxic T-Lymphocyte Recognition in Perinatal Human Immunodeficiency Virus (HIV) Type 1 Transmission: The Ariel Project for the Prevention of Transmission of HIV From Mother to Infant. J Virol (1999) 73(5):3975–85. doi: 10.1128/JVI.73.5.3975-3985.1999
202. Liu AY, Lohman-Payne B, Chung MH, Kiarie J, Kinuthia J, Slyker J, et al. Maternal Plasma and Breastmilk Viral Loads Are Associated With HIV-1-Specific Cellular Immune Responses Among HIV-1-Exposed, Uninfected Infants in Kenya. Clin Exp Immunol (2015) 180(3):509–19. doi: 10.1111/cei.12599
203. Lohman-Payne B, Sandifer T, Ohainle M, et al. In-Uteroinfection With HIV-1 Associated With Suppressed Lymphoproliferative Responses at Birth. Clin Exp Immunol (2014) 178(1):86–93. doi: 10.1111/cei.12386
204. The Use of Antiretroviral Drugs for Treating and Preventing HIV Infection. Available at: http://apps.who.int/iris/bitstream/handle/10665/85321/9789241505727_eng.pdf?sequence=1 (Accessed 11/23/2018).
205. UNAIDS. The Gap Report. Available at: http://www.unaids.org/sites/default/files/media_asset/UNAIDS_Gap_report_en.pdf (Accessed 11/26/2018).
206. Organization GWH. WHO Recommendations on the Diagnosis of HIV Infection in Infants and Children (2010). Available at: http://whqlibdoc.who.int/publications/2010/9789241599085_eng.pdf?ua=1 (Accessed 11/26/2018).
207. Takata H, Buranapraditkun S, Kessing C, Fletcher JLK, Muir R, Tardif V, et al. Delayed Differentiation of Potent Effector CD8+T Cells Reducing Viremia and Reservoir Seeding in Acute HIV Infection. Sci Trans Med (2017) 9(377):eaag1809. doi: 10.1126/scitranslmed.aag1809
208. Whitney JB, Hill AL, Sanisetty S, Penaloza-MacMaster P, Liu J, Shetty M, et al. Rapid Seeding of the Viral Reservoir Prior to SIV Viraemia in Rhesus Monkeys. Nature (2014) 512(7512):74–7. doi: 10.1038/nature13594
209. Luzuriaga K, Gay H, Ziemniak C, Sanborn KB, Somasundaran M, Rainwater-Lovett K, et al. Viremic Relapse After HIV-1 Remission in a Perinatally Infected Child. New Engl J Med (2015) 372(8):786–8. doi: 10.1056/NEJMc1413931
210. Bitnun A, Samson L, Chun TW, Kakkar F, Brophy J, Murray D, et al. Early Initiation of Combination Antiretroviral Therapy in HIV-1-Infected Newborns Can Achieve Sustained Virologic Suppression With Low Frequency of CD4+ T Cells Carrying HIV in Peripheral Blood. Clin Infect Dis (2014) 59(7):1012–9. doi: 10.1093/cid/ciu432
211. Giacomet V, Trabattoni D, Zanchetta N, Biasin M, Gismondo M, Clerici M, et al. No Cure of HIV Infection in a Child Despite Early Treatment and Apparent Viral Clearance. Lancet (2014) 384(950):1320. doi: 10.1016/S0140-6736(14)61405-7
212. Jani IV, Meggi B, Mabunda N, Vubil A, Sitoe NE, Tobaiwa O, et al. Accurate Early Infant HIV Diagnosis in Primary Health Clinics Using a Point-of-Care Nucleic Acid Test. J Acquir Immune Defic Syndr (2014) 67(1):e1–4. doi: 10.1097/QAI.0000000000000250
213. Persaud D, Gay H, Ziemniak C, Chen YH, Piatak M Jr, Chun T-W, et al. Absence of Detectable HIV-1 Viremia After Treatment Cessation in an Infant. N Engl J Med (2013) 369(19):1828–35. doi: 10.1056/NEJMoa1302976
214. Garcia-Broncano P, Maddali S, Einkauf KB, Jiang C, Gao C, Chevalier J, et al. Early Antiretroviral Therapy in Neonates With HIV-1 Infection Restricts Viral Reservoir Size and Induces a Distinct Innate Immune Profile. Sci Trans Med (2019) 11(520):eaax7350. doi: 10.1126/scitranslmed.aax7350
215. Safety and Effects of Using Prime-boost HIVIS DNA and MVA-CMDR Vaccine Regimens With or Without Toll-like Receptor 4 Agonist on HIV Reservoirs in Perinatally HIV Infected Children and Youth (HVRRICANE). Available at: https://clinicaltrials.gov/ct2/show/NCT04301154.
216. Kuhn L, Strehlau R, Shiau S, Patelc F, Shena Y, Technauc K-G, et al. Early Antiretroviral Treatment of Infants to Attain HIV Remission. EClinicalMedicine (2020) 18:100241. doi: 10.1016/j.eclinm.2019.100241
217. Violari A, Cotton MF, Gibb DM, Babiker AG, Steyn J, Madhi SA, et al. Early Antiretroviral Therapy and Mortality Among HIV-Infected Infants. New Engl J Med (2008) 359(21):2233–44. doi: 10.1056/NEJMoa0800971
218. Katusiime MG, Halvas EK, Wright I, Joseph K, Bale MJ, Kirby-McCullough B, et al. Intact HIV Proviruses Persist in Children Seven to Nine Years After Initiation of Antiretroviral Therapy in the First Year of Life. J Virol (2020) 94(4):e01519–19. doi: 10.1128/JVI.01519-19
219. Bale M, Katusiime M-G, Wells D, Wu X, Spindler J, Halvas EK, et al. Early Emergence and Long-Term Persistence of HIV-Infected T-Cell Clones in Children. mBio (2021) 12:e00568–21. doi: 10.1128/mBio.00568-21
220. Violari A, Cotton MF, Kuhn L, Schramm DB, Paximadis M, Loubser S, et al. A Child With Perinatal HIV Infection and Long-Term Sustained Virological Control Following Antiretroviral Treatment Cessation. Nat Commun (2019) 10(1):412–23. doi: 10.1038/s41467-019-08311-0
221. Frange P, Faye A, Avettand-Fenoël V, Bellaton E, Descamps D, Angin M, et al. HIV-1 Virological Remission Lasting More Than 12 Years After Interruption of Early Antiretroviral Therapy in a Perinatally Infected Teenager Enrolled in the French ANRS EPF-CO10 Paediatric Cohort: A Case Report. Lancet HIV (2016) 3(1):e49–54. doi: 10.1016/S2352-3018(15)00232-5
222. Goswami R, Nelson AN, Tu JJ, Dennis M, Feng L, Kumar A, et al. Analytical Treatment Interruption After Short-Term Antiretroviral Therapy in a Postnatally Simian-Human Immunodeficiency Virus-Infected Infant Rhesus Macaque Model. mBio (2019) 10(5):e01971–19. doi: 10.1128/mBio.01971-19
223. Bricker KM, Obregon-Perko V, Uddin F, Williams B, Uffman E, Garrido C, et al. Therapeutic Vaccination of SIV-Infected, ART-Treated Infant Rhesus Macaques Using Ad48/MVA in Combination With TLR-7 Stimulation. PloS Pathog (2020) 16(10):e1008954. doi: 10.1371/journal.ppat.1008954
224. Obregon-Perko V, Bricker K, Chahroudi A. The Brain Retains: Nonhuman Primate Models for Pediatric HIV-1 in the CNS. Curr HIV/AIDS Rep (2020) 17(4):343–53. doi: 10.1007/s11904-020-00503-4
225. Ajibola G, Garcia-Broncano P, Maswabi K, Bennett K, Hughes MD, Moyo S, et al. Viral Reservoir in Early-Treated Human Immunodeficiency Virus-Infected Children and Markers for Sustained Viral Suppression. Clin Infect Dis (2021) 22:e997–1003. doi: 10.1093/cid/ciab143
226. Martínez-Bonet M, Puertas MC, Fortuny C, Ouchi D, Mellado MJ, Rojo P, et al. Establishment and Replenishment of the Viral Reservoir in Perinatally HIV-1-Infected Children Initiating Very Early Antiretroviral Therapy. Clin Infect Dis (2015) 61(7):1169–78. doi: 10.1093/cid/civ456
227. Persaud D, Patel K, Karalius B, Rainwater-Lovett K, Ziemniak C, Ellis A, et al. Influence of Age at Virologic Control on Peripheral Blood Human Immunodeficiency Virus Reservoir Size and Serostatus in Perinatally Infected Adolescents. JAMA Pediatr (2014) 168(12):1138. doi: 10.1001/jamapediatrics.2014.1560
228. Rocca S, Zangari P, Cotugno N, Ferns B, Foster C, De Rossi A, et al. Human Immunodeficiency Virus (HIV)-Antibody Repertoire Estimates Reservoir Size and Time of Antiretroviral Therapy Initiation in Virally Suppressed Perinatally HIV-Infected Children. J Pediatr Infect Dis Soc (2019) 8(5):433–8. doi: 10.1093/jpids/piy080
229. Tagarro A, Chan M, Zangari P, Ferns B, Foster C, De Rossi A, et al. Early and Highly Suppressive Antiretroviral Therapy Are Main Factors Associated With Low Viral Reservoir in European Perinatally HIV-Infected Children. J Acquired Immune Deficiency Syndromes (1999) (2018) 79(2):269–76. doi: 10.1097/QAI.0000000000001789
230. Kuhn L, Paximadis M, Da Costa Dias B, Loubser S, Strehlau R, Patel F, et al. Age at Antiretroviral Therapy Initiation and Cell-Associated HIV-1 DNA Levels in HIV-1-Infected Children. PloS One (2018) 13(4):e0195514. doi: 10.1371/journal.pone.0195514
231. Ananworanich J, Avihingsanon A. HIV and Noncommunicable Diseases: The Asian Perspective. J Acquir Immune Defic Syndr (2014) 67 Suppl 1:S99–103. doi: 10.1097/QAI.0000000000000262
232. Uprety P, Chadwick EG, Rainwater-Lovett K, Ziemniak C, Luzuriaga K, Capparelli EV, et al. Cell-Associated HIV-1 DNA and RNA Decay Dynamics During Early Combination Antiretroviral Therapy in HIV-1-Infected Infants. Clin Infect Dis (2015) 61(12):1862–70. doi: 10.1093/cid/civ688
233. Luzuriaga K, Tabak B, Garber M, Chen YH, Ziemniak C, McManus MM, et al. HIV Type 1 (HIV-1) Proviral Reservoirs Decay Continuously Under Sustained Virologic Control in HIV-1–Infected Children Who Received Early Treatment. J Infect Dis (2014) 210(10):1529–38. doi: 10.1093/infdis/jiu297
234. Massanella M, Puthanakit T, Leyre L, Jupimai T, Sawangsinth P, de Souza M, et al. Continuous Prophylactic ARV/ART Since Birth Reduces Seeding and Persistence of the Viral Reservoir in Vertically HIV-Infected Children. Clin Infect Dis (2020) 73:427–38. doi: 10.1093/cid/ciaa718
235. Foster C, Domínguez-Rodríguez S, Tagarro A, Gkouleli T, Heaney J, Watters S, et al. The CARMA Study: Early Infant Antiretroviral Therapy—Timing Impacts on Total HIV-1 DNA Quantitation 12 Years Later. J Pediatr Infect Dis Soc (2021) 10(3):295–301. doi: 10.1093/jpids/piaa071
236. Van Zyl GU, Bedison MA, Van Rensburg AJ, Laughton B, Cotton MF, Mellors JW. Early Antiretroviral Therapy in South African Children Reduces HIV-1-Infected Cells and Cell-Associated HIV-1 RNA in Blood Mononuclear Cells. J Infect Dis (2015) 212(1):39–43. doi: 10.1093/infdis/jiu827
237. Avettand-Fenoel V, Lechenadec J, Diallo MS, Fillion M, Melard A, Samri A, et al. Initiating Antiretroviral Treatment Early in Infancy Has Long-Term Benefits on the Human Immunodeficiency Virus Reservoir in Late Childhood and Adolescence. Clin Infect Dis (2021). doi: 10.1093/cid/ciaa1931
238. Kuhn L. Early Infant Treatment: Still a Long Way to Go to Reach Human Immunodeficiency Virus Remission. Clin Infect Dis (2021) 72(3):394–5. doi: 10.1093/cid/ciaa033
239. Veldsman KA, Rensburg A, Isaacs S, Naidoo S, Laughton B, Lombard C, et al. HIV-1 DNA Decay Is Faster in Children Who Initiate ART Shortly After Birth Than Later. J Int AIDS Soc (2019) 22(8):e25368. doi: 10.1002/jia2.25368
240. Millar JR, Bengu N, Vieira VA, Adland E, Roider J, Muenchhoff M, et al. Early Initiation of Antiretroviral Therapy Following In Utero HIV Infection Is Associated With Low Viral Reservoirs But Other Factors Determine Subsequent Plasma Viral Rebound. J Infect Dis (2021). doi: 10.1093/infdis/jiab223
241. Veldsman KA, Laughton B, Janse van Rensburg A, Zuidewind P, Dobbels E, Barnabas S, et al. Viral Suppression Is Associated With HIV-Antibody Level and HIV-1 DNA Detectability in Early Treated Children at 2 Years of Age. AIDS (2021) 35(8):1247–52. doi: 10.1097/QAD.0000000000002861.
242. McManus WR, Bale MJ, Spindler J, Wiegand A, Musick A, Patro SC, et al. HIV-1 in Lymph Nodes Is Maintained by Cellular Proliferation During Antiretroviral Therapy. J Clin Invest (2019) 129(11):4629–42. doi: 10.1172/JCI126714
243. Bitnun A, Ransy DG, Brophy J, Kakkar F, Hawkes M, Samson L, et al. Clinical Correlates of Human Immunodeficiency Virus–1 (HIV-1) DNA and Inducible HIV-1 RNA Reservoirs in Peripheral Blood in Children With Perinatally Acquired HIV-1 Infection With Sustained Virologic Suppression for at Least 5 Years. Clin Infect Dis (2020) 70(5):859–66. doi: 10.1093/cid/ciz251
244. Palma P, McManus M, Cotugno N, Rocca S, Rossi P, Luzuriaga K. The HIV-1 Antibody Response: A Footprint of the Viral Reservoir in Children Vertically Infected With HIV. Lancet HIV (2020) 7(5):e359–65. doi: 10.1016/S2352-3018(20)30100-4
245. Singh V, Dashti A, Mavigner M, Chahroudi A. Latency Reversal 2.0: Giving the Immune System a Seat at the Table. Curr HIV/AIDS Rep (2021) 18(2):117–27. doi: 10.1007/s11904-020-00540-z
246. Nixon CC, Mavigner M, Sampey GC, Brooks AD, Spagnuolo RA, Irlbeck DM, et al. Systemic HIV and SIV Latency Reversal via Non-Canonical NF-κb Signalling In Vivo. Nature (2020) 578(7793):160–5. doi: 10.1038/s41586-020-1951-3
247. Dashti A, Waller C, Mavigner M, Schoof N, Bar KJ, Shaw GM, et al. SMAC Mimetic Plus Triple-Combination Bispecific HIVxCD3 Retargeting Molecules in SHIV.C.CH505-Infected, Antiretroviral Therapy-Suppressed Rhesus Macaques. J Virol (2020) 94(21):e00793–20. doi: 10.1128/JVI.00793-20
Keywords: HIV, MTCT, cure, pediatric, shock and kill, antiretroviral, breast milk, reservoir
Citation: Amin O, Powers J, Bricker KM and Chahroudi A (2021) Understanding Viral and Immune Interplay During Vertical Transmission of HIV: Implications for Cure. Front. Immunol. 12:757400. doi: 10.3389/fimmu.2021.757400
Received: 12 August 2021; Accepted: 27 September 2021;
Published: 21 October 2021.
Edited by:
Ashley L. St John, Duke-NUS Medical School, SingaporeCopyright © 2021 Amin, Powers, Bricker and Chahroudi. This is an open-access article distributed under the terms of the Creative Commons Attribution License (CC BY). The use, distribution or reproduction in other forums is permitted, provided the original author(s) and the copyright owner(s) are credited and that the original publication in this journal is cited, in accordance with accepted academic practice. No use, distribution or reproduction is permitted which does not comply with these terms.
*Correspondence: Ann Chahroudi, YW5uLm0uY2hhaHJvdWRpQGVtb3J5LmVkdQ==