- Department of Infectious Diseases and Microbiology, University of Lübeck, Lübeck, Germany
Although macrophages are considered for host cells for the multiplication of Leishmania, recent studies indicate the important role of neutrophil granulocytes as host cells for these intracellular parasites. Neutrophils have been shown to be massively and rapidly recruited to the site of Leishmania infection where they represent the first cells to encounter the parasites. Exposure to ATP and UTP have been shown to enhance anti-Leishmania activity of macrophages and intralesional injection of UTP led to strongly reduced parasite load in vivo. Since the in vivo anti-leishmanial effect of extracellular UTP correlated with enhanced neutrophil recruitment and enhanced ROS production at the site of Leishmania infection we hypothesized that exposure to extracellular nucleotides can directly enhance the killing of Leishmania by neutrophils. Since purinergic signaling is an essential mechanism of neutrophil activation the aim of the present study was to assess whether purinergic exposure results in the activation of anti-leishmanial neutrophil functions and, therefore, represent an essential component of enhanced anti-leishmanial defense in leishmaniasis. We could show that exposure to ATP and UTP led to activation and enhanced CD11b expression of primary human neutrophils in vitro. Leishmania-induced ROS production was strongly enhanced by extracellular ATP and UTP. Importantly, exposure to ATP and UTP resulted in enhanced killing of Leishmania donovani by neutrophils. In addition, ATP strongly enhanced the secretion of IL-8 and IL-1β by Leishmania-exposed neutrophils. Our results suggest that signaling via the P2 receptor and phosphorylation of Erk1/2, Akt and p38 are involved in the purinergic enhancement of anti-leishmanial functions of neutrophils.
Introduction
Leishmaniases comprise vector-borne parasitic diseases with multiple clinical manifestations caused by the unicellular protozoon parasites of the genus Lesihmania. Visceral leishmaniasis (VL) is the most serious form of leishmaniasis and has an incidence of estimated 400 000 cases per year (source: Centers for Disease Control and Prevention). In the mammalian host Leishmania parasites are obligatory intracellular pathogens. Since Leishmania parasites cannot actively penetrate and invade host cells, Leishmania depend on the phagocytic activity for their entry into their host cells. Although classically macrophages are considered for host cells for the multiplication of Leishmania, recent studies indicate the important role of neutrophil granulocytes as host cells for these intracellular parasites. Neutrophils have been shown to be massively and rapidly recruited to the site of Leishmania infection where they represent the first cells to encounter the parasites (1, 2). Neutrophils can play both protective as well as disease promoting roles in leishmaniasis. On the one hand neutrophils participate in the elimination of parasites through the production of reactive oxygen species (ROS), the release of azurophilic granules that contain antimicrobial proteins such as neutrophil elastase (NE) and myeloperoxidase (MPO) (3, 4). In addition, neutrophil extracellular traps (NETs) have been described to kill some Leishmania spp (5). On the other hand, neutrophils can also play a detrimental role in leishmaniasis (6, 7). Neutrophils can provide a transient intracellular niche for the intracellular pathogen and function as “Trojan horses” to transfer the parasites to macrophages (8, 9).
Adenosine triphosphate (ATP) and uridine triphosphate (UTP) are intracellular nucleotides that are essential for nucleic acid synthesis as well as source of energy. However, extracellular ATP and UTP represent damage associated molecular patterns (DAMPs) that modulate inflammatory and immune responses against infectious agents (10). The level of extracellular ATP can reach hundreds of micromolar concentration (11). Extracellular ATP and UTP act on leukocytes upon binding to surface P2 receptors. P2 receptors include the P2X and P2Y receptors. P2X receptors function as ligand-gated ion channels. ATP is a native agonist for all seven P2X receptors (12, 13). P2Y receptors are G-protein coupled receptors (GPCR). Preferred agonists of P2Y receptors are the purines ATP and ADP, the pyrimidines UTP, UDP and UDP-sugars (12–14). ATP can bind to all eight P2Y receptors except to the P2Y6 receptor. UTP is a native agonist of P2Y2, P2Y4 and P2Y11 (12, 13).
Exposure to ATP and UTP have been shown to enhance anti-Leishmania activity of macrophages (15). Parasite load in L. amazonensis infected macrophages was reduced in vitro after treatment with ATP (16). In an experimental model of Leishmania infection intralesional injection of UTP led to strongly reduced parasite load in vivo (17). Since the in vivo anti-leishmanial effect of extracellular UTP correlated with enhanced neutrophil recruitment and enhanced ROS production at the site of Leishmania infection (17) we hypothesized that exposure to extracellular nucleotides can directly enhance the killing of Leishmania by neutrophils and this effect play a substantial role in the anti-leishmanial effect of extracellular nucleotide treatment in Leishmania infection.
Neutrophils express several P2X and P2Y receptors and signaling via purinergic receptors has been shown to represent a fundamental mechanism of neutrophils activation (18). Purinergic signaling control PMN chemotaxis, modulate life span, and activate neutrophil functions such as phagocytosis and ROS release and were reported to be required for activation of PMN by a wide variety of stimuli (18, 19). Since purinergic signaling is an essential mechanism of neutrophil activation (20) the aim of the present study was to assess whether purinergic exposure results in the activation of anti-leishmanial neutrophils functions and, therefore, represent an essential component of enhanced anti-leishmanial defense in leishmaniasis. We could show that exposure to ATP and UTP strongly enhance anti-leishmanial functions of primary human neutrophils in vitro. Our results suggest that signaling via the P2 receptor and phosphorylation of Erk1/2, Akt and p38 are involved in the purinergic enhancement of anti-leishmanial functions of neutrophils.
Materials and Methods
Isolation of Human Neutrophil Granulocytes
Primary human neutrophils were isolated from peripheral blood of healthy volunteers by Histopaque and Percoll gradient centrifugation as described elsewhere (21). The blood collection was conducted with the understanding and written consent of each participant and was approved by the ethical committee of the Medical Faculty of the University of Lübeck (18-187). Blood was layered on a two-layer density gradient consisting of lymphocyte separation medium 1077 (upper layer, PAA, Pasching, Austria) and Histopaque® 1119 (bottom layer, Sigma-Aldrich, Deisenhofen, Germany) and centrifuged for 5 min at 300 × g followed by 20 min at 800 × g. Cells from the upper layer consisting mainly of lymphocytes and monocytes were discarded. The granulocyte-rich lower layer was collected leaving the erythrocyte pellet at the bottom of the tube. Granulocytes were washed once in PBS, resuspended in complete medium (RPMI 1640 medium, Sigma) supplemented with 50 µM 2-mercaptoethanol, 2 mM L-glutamine, 10 mM HEPES (all from Biochrom, Berlin, Germany) and 10% fetal calf serum (FCS, Gibco, Karlsruhe, Germany) and further fractionated on a discontinuous Percoll® (Amersham Biosciences, Uppsala, Sweden) gradient consisting of layers with densities of 1.105 g/ml (85%), 1.100 g/ml (80%), 1.093 g/ml (75%), 1.087 g/ml (70%), and 1.081 g/ml (65%). After centrifugation for 20 min at 800 × g, the interface between the 80 and 70% Percoll® layers was collected, washed once in PBS and resuspended in complete medium. All procedures were conducted at room temperature. The cell preparations contained > 99.9% granulocytes as determined by morphological examination of > 1,000 cells on Giemsa stained cytocentrifuge (Shandon, Pittsburgh, PA) slides. Cell viability was > 99%, as determined by trypan blue exclusion.
Flow Cytometry Analysis of Cell Surface Expression of Activation Markers
Neutrophils (500.000 cells/sample) were resuspended in FACS buffer [1x PBS (Thermo Fisher) supplemented with 1% bovine serum albumin and 1% human serum] in a V-bottom plate. After washing once with FACS buffer the cells were stained with FITC-conjugated mAb to human CD62L (clone DREG-56, IgG1, BD Biosciences) and PE-conjugated mAb to human CD11b (clone 2LPM19c, IgG1, Dako, Waldbronn, Germany) for 30 min at 4°C protected from light. After two wash steps with FACS buffer at 4°C the cells were resuspended in FACS buffer and measured with a BD FACS Canto II (BD) flow cytometer.
Assessment of Neutrophil Apoptosis and Viability
Apoptosis of neutrophils was determined by Annexin-V FLUOS binding as Annexin-V exhibits calcium-dependent binding to phosphatidylserine (PS) expressed in the outer leaflet of the cell membrane of apoptotic neutrophils. Assessment of membrane integrity and identification of necrotic cells was performed by counterstaining with propidium iodide (PI). For staining, 5×105 cells/100 µl were transferred to a U-tube and 10 µl Annexin-V buffer (10 mM HEPES + 140 mM NaCl, pH 7.4), 1 µl 1 M CaCl2, 1 µl PI (Sigma-Aldrich) and 1 µl Annexin-V FLUOS (Sigma-Aldrich) were added. Samples were incubated 10 min at 4°C protected from light. Analysis was carried out by flow cytometry.
Leishmania donovani Culture and Staining With CFSE
Virulent L. donovani promastigotes (strain MHOM/IN/82/Patna 1) were cultivated in Schneider’s Drosophila Medium with L-glutamine (Genaxxon, Ulm, Germany) supplemented with 10% FCS, 100 U/ml penicillin, 100 μg/ml streptomycin, and 2% sterile filtered human urine at 27°C in a humidified air atmosphere containing 5% CO2. For seeding of cultures, a parasite density of 1 × 106 cells/ml was used. The parasite counting was conducted in a hemocytometer with a chamber depth of 0.02 mm (0.0025 mm2, depth 0.02 mm, VWR). The culture was considered to be in the stationary phase 72 h after seeding. Serial passaging was conducted until passage 10.
Stationary phase L. donovani promastigotes were labeled with CFSE (Cell Trace CFSE, Invitrogen, Thermo Fischer Scientific) by incubating the parasites in a volume of 1 ml at a concentration of 1x108/ml with 5 µM CFSE in PBS for 15 min. with shaking. 500 µl complete medium was then added and incubated for another 5 min with shaking. Cell were then centrifuged at 1300 x g and resuspended in complete medium.
In Vitro Infection of Neutrophils With Leishmania donovani Promastigotes
Neutrophils and L. donovani promastigotes were co-incubated in complete medium at a parasite-neutrophil ratio of 10:1 and a neutrophil density of 5 × 106 cells/ml for 3 h at 37°C in a humidified air atmosphere containing 5% CO2. Subsequently, the infection rate was assessed on Giemsa-stained cytocentrifuged samples. The cells were washed six times in 1 × PBS at 400 × g for 10 min to remove extracellular Leishmania. Infected cells were again adjusted to 5 × 106 cells/ml.
ROS Assay
The luminol-based chemiluminescence assay was used to detect the sum of intra- and extracellular ROS. 4×105 neutrophils (2x106 cells/ml) were seeded per well in a 96-well LUMITRAC™ 600 plates (Greiner Bio-One, Frickenhausen Germany) and mixed with a final concentration of 60 µM luminol (Sigma-Aldrich). Neutrophils were exposed to ATP (500 µM), UTP (500 µM), or LPS (100 ng/ml) or fMLP (1 µM). ROS-dependent luminol chemiluminescence was assessed using an infinite 200 reader and the Tecan i-control 1.7 Software (Tecan, Crailsheim, Germany). ROS release was monitored for 2 h every 2 min at 37°C. For statistical analysis, the area under the curve (AUC) value of each sample was calculated.
To assess the effect of ATP and UTP on the ROS production of Leishmania-exposed neutrophils, neutrophils were co-incubated with L. donovani promastigotes in the presence or absence of ATP or UTP and the ROS production was assessed for 60 min by using the luminol based chemiluminescence assay.
Assessment of Intracellular Ca2+-Concentration
Intracellular Ca2+-concentration was determined by using a flow cytometry-based method with the fluorescent Ca2+-sensitive indicator Fluo-4 AM as described (22). Briefly, neutrophils, 1x107/ml, were labeled with 10 µM Fluo-4 AM (AAT Bioquest, Sunnyvale, CA) for 30 min in the dark. After washing with PBS cells were resuspended in complete medium at a concentration of 5x106/ml. Neutrophils were infected with Leishmania by co-incubating neutrophils with L. donovani promastigotes at a neutrophil to Leishmania ratio of 1:10 for 2h at 37°C. ATP (500 µM) or UTP (500 µM) was added to the infected neutrophils. Fluorescence intensity was assessed for 3 min in FITC channel by using a BD FACS Canto II flow cytometer (BD). The stimuli were added at the time point 30 sec of the flow cytometry analysis. Fluo-4-labeled non-infected cells were used as negative controls, Fluo-4-labeled non-infected cells exposed to ionomycin (10 µM) were used as positive control.
Western Blot Analysis
Neutrophils (5×106 cells/ml) were exposed to ATP (500 µM), to Leishmania donovani promastigotes (Leishmania to neutrophil ratio 10:1) or a combination of ATP with Leishmania donovani promastigotes for 15 min at 37°C. In samples with the P2-antagonist PPADS (Tocris, Bio-Techne, Wiesbaden, Germany) the neutrophils were pretreated with the inhibitor (30 µM) for 15 min. Whole cell lysates were prepared as described (23). Western blot analysis was carried out by using antibodies against human phospho Akt (Thr308), phospho-p44/42 MAPK (ERK1/2, thr202/Tyr204), phospho p38 MAPK (Thr180/Tyr182) and beta-actin (all from Cell Signaling Technology) and probed with HRP-conjugated anti-rabbit IgG secondary antibody (New England Biolabs, USA). The signals were detected by using Immobilon Western Chemiluminescence HRP substrate (Millipore, USA) with a Fusion Fxt Chemiluminescence reader (Vilber Loumat, Germany). Signals of pAkt, pERK1/2, or pp38 were related to beta-actin signals on the same blots or of the same sample by using ImageJ software (NIH, USA). Data were then normalized by sum of all data point in a replicate as described (24).
Phagocytosis Assays
Phagocytosis of Latex Microspheres
Neutrophils (5×105 cells/100 μl) were preincubated for 30 min with ATP (500 µM), UTP (500 µM), LPS (100 ng/ml) or a combination of LPS with ATP or UTP. Subsequently, FluoSphere carboxylate-modified latex microspheres (Invitrogen™) with a diameter of 1 μm at a final concentration of 0.015% (v/v) were added and the co-culture was incubated for further 30 min. Cultures were placed on ice to stop phagocytosis and cells were washed to remove non-ingested beads. Phagocytosis was assessed by flow cytometry using a FACS Canto II flow cytometer (BD).
Phagocytosis of L. donovani Promastigotes
Neutrophils (5×105 cells/100 μl) were preincubated for 30 min with ATP (500 µM), UTP (500 µM) or left untreated. Subsequently, neutrophils were co-incubated with CFSE-labeled L. donovani promastigotes in 200 µl complete medium in flat-bottom 96-well plate at a parasite-neutrophil ratio of 5:1 and a neutrophil density of 5 × 106 cells/ml for 120 min at 37°C in a humidified air atmosphere containing 5% CO2. L. donovani promastigotes were labeled with Cell Trace CFSE (Invitrogen) according to the manufacturer’s protocol. Phagocytosis was assessed by flow cytometry using a BD FACS Canto II flow cytometer (BD). The infection rate was also checked microscopically on Giemsa-stained cytocentrifuged samples in order to control whether the CFSE-labeled promastigotes were inside the neutrophils rather than attached to the outer surface.
Cytokine Determination
Neutrophils (1x106 cells/100 µl) were exposed to ATP (500 µM), UTP (500 µM), L. donovani promastigotes or a combination of ATP and UTP with L. donovani promastigotes or medium alone for 18 h at 37°C, 5% CO2. Cultures were centrifuged at 300 x g for 10 minutes to separate cytokine-containing supernatants from cells. Detection of IL-8 and IL-1β in the supernatants was conducted by using Duo-Set ELISAs (R&D Systems) according to the manufacturer’s instructions.
Assessment of Killing of Leishmania by Neutrophils
Leishmania-infected neutrophils were exposed to ATP (500 µM), UTP (500 µM) or LPS (100 ng/ml) as well as to the combination of LPS with ATP and UTP for 18 h at 37°C in a humidified air atmosphere containing 5% CO2. A limiting dilution culture assay was then used to detect viable L. donovani parasites in neutrophils and survival of L. donovani parasites as described previously (25). Briefly, serial 1.5-fold dilutions of L. donovani-infected neutrophil suspensions (5 × 106 cells/ml) were plated in three replicates in 96-well flat-bottom microtiter plates containing Schneider’s Drosophila Medium. The plates were incubated at 27°C in humidified air atmosphere containing 5% CO2 for 7-10 days. The growth of L. donovani promastigotes was detected microscopically. The last dilution resulting in a growth of parasites in > 50% of the wells is given as a quantitative measure of the parasite load in the neutrophil cell suspension.
Statistical Analysis
If not stated differently, the presented data were collected/generated from minimum of three independent experiments with neutrophils isolated from different blood donors. Statistical analysis was performed with the GraphPad Prism software 6 using the ordinary one-way ANOVA followed by Turkey’s multiple comparison. A p-value ≤0.05 was considered statistically significant.
Results
Effect of Extracellular ATP and UTP on CD62L Shedding and CD11b Expression on Neutrophil Granulocytes
Since activation of PMN leads to shedding of L-selectin (CD62L) from the cell surface (26), analysis of CD62L expression is a widely used method to distinguish CD62Lneg/low activated cells from CD62Lhigh non-activated neutrophils (27). To obtain information regarding the activation status of neutrophils upon exposure to ATP and UTP the expression of L-selectin (CD62L) was assessed via flow cytometry. As compared to untreated cells, a marked shedding of CD62L was observed in neutrophils upon a 30 min exposure to ATP and UTP (Figure 1A).
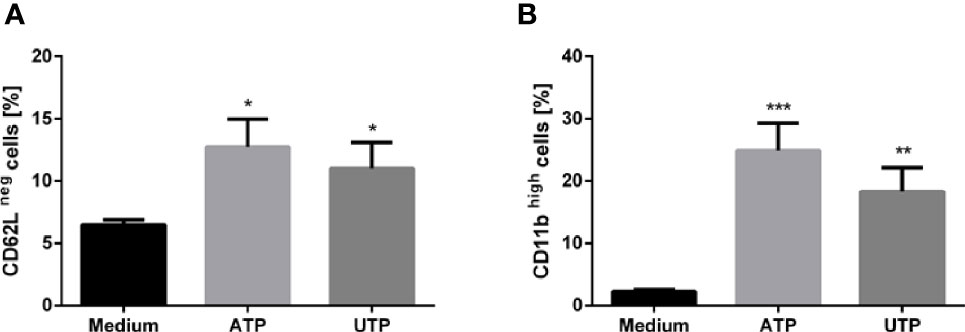
Figure 1 The effect of extracellular ATP and UTP on the cell surface expression of CD62L and CD11b on neutrophils. Primary human neutrophils were incubated for 30 in the presence of ATP (500 µM) or UTP (100 µM). The expression of CD62L (A) and CD11b (B) was assessed by using flow cytometry. Bar diagrams show % ± SD (n=3). *p < 0.05, **p < 0.01, ***p < 0.001.
Granule exocytosis is an important function of activated neutrophils. During this process, the granule membrane incorporates into the cell membrane. Consequently, increasing expression of granule-membrane markers such as CD11b (28) can be observed on the cell surface of activated PMN. To investigate whether exposure to ATP and UTP affects granule exocytosis the cell surface expression of the degranulation marker integrin alpha M (CD11b) was assessed by using flow cytometry. The expression of CD11b was significantly enhanced on ATP- and UTP-treated neutrophils. (Figure 1B). These data indicate an activated state and granule exocytosis of neutrophils upon exposure to extracellular ATP and UTP.
Effect of Extracellular ATP and UTP on the Production of ROS by Neutrophils
The production of reactive oxygen species (ROS), the oxidative burst, is one of the most important mechanisms of neutrophil granulocytes to kill phagocytosed microbes. The ability to induce the production of ROS is characteristic for many neutrophil-activating agents. Therefore, we investigated the effect of ATP and UTP on the ROS production by human neutrophils. The production of MPO-derived ROS and extracellular superoxide was assessed by the luminol-based chemiluminescence assay. PMN were incubated in medium alone as negative control or in the presence of ATP (500 µM) or UTP (500 µM). Exposure to ATP and UTP did not induce a significant level of ROS production (Figures 2A–F).
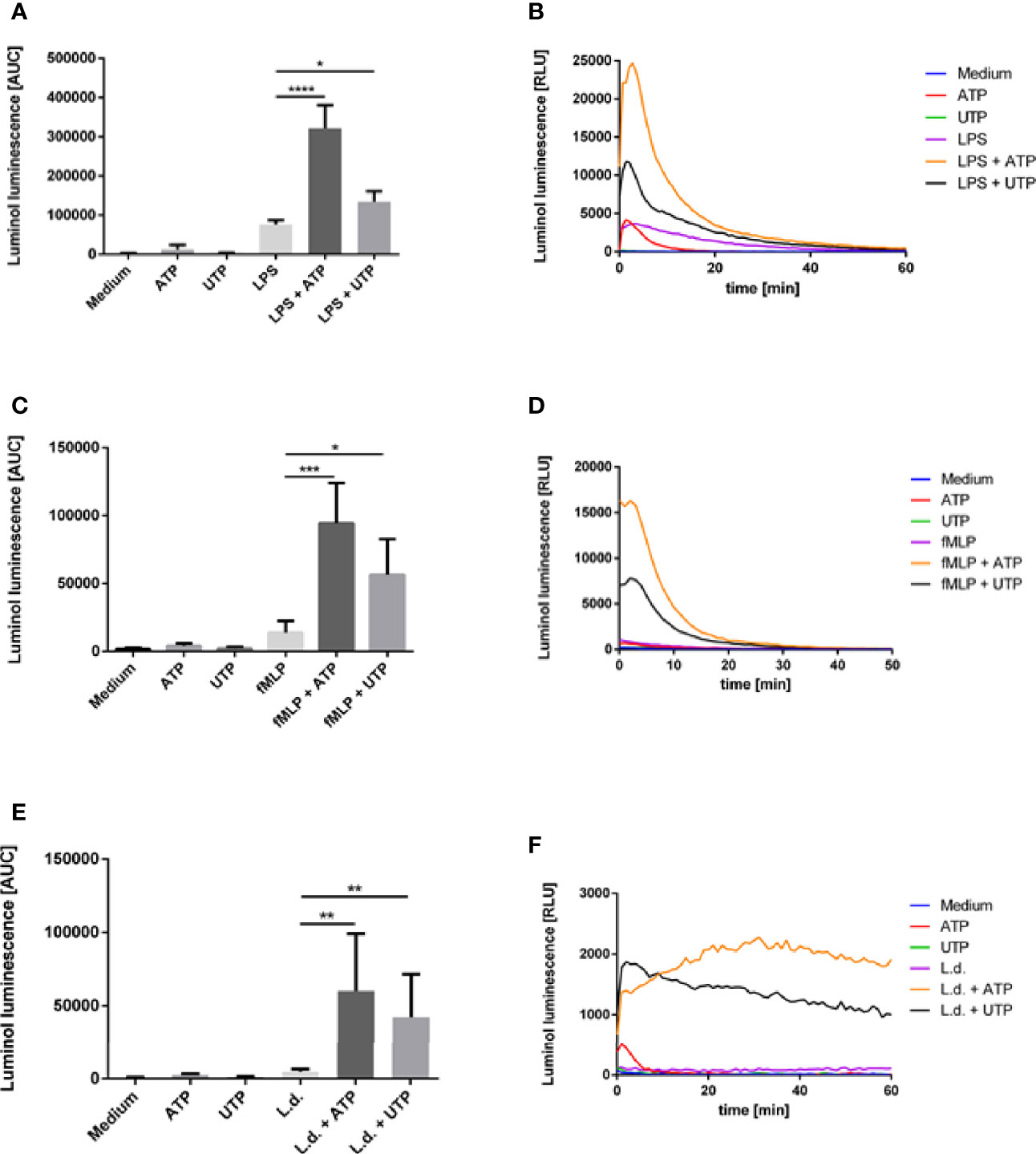
Figure 2 The effect of extracellular ATP and UTP on the ROS production by neutrophils. Neutrophils were treated with (A, B) ATP, UTP, LPS and the combination of LPS with ATP and UTP; (C, D) ATP, UTP, fMLP and the combination of fMLP with ATP and UTP or (E, F) ATP, UTP, coincubated with L. donovani promastigotes or co-incubated with L. donovani promastigotes in the presence of ATP or UTP. Production of total ROS was measured by luminol luminescence. (B, D, F) Representative kinetic curves of ROS production by neutrophils. (A, C, E) ROS production shown by calculating the area under the curve (AUC) values. Statistical analysis by ordinary one-way ANOVA with a post hoc Turkey’s test. Asterisks above the bars indicate significance compared to untreated cells. n=3, *p ≤ 0,05, **p ≤ 0,01, ***p ≤ 0,001, ****p ≤ 0,0001.
A characteristic feature of low-level PMN activation is the priming, i.e., the amplification of the neutrophil’s functional response to other stimuli. We have investigated whether ATP and UTP can amplify LPS- or fMLP-induced ROS production of neutrophils. Treatment with LPS (Figures 2A, B) and fMLP (Figures 2C, D) resulted in ROS production. Importantly, exposure of LPS- and fMLP-preincubated cells to ATP and UTP markedly and significantly enhanced the production of ROS (Figures 2A–D). These data show that ATP and UTP alone do not induce significant level of ROS production but enhance LPS- and fMLP-induced ROS production in a synergistic manner.
Since ROS production is regarded as an essential mechanism of killing of Leishmania by neutrophils (29) next we investigated the effect of ATP and UTP on the ROS production of neutrophils in response to L. donovani. Coincubation of neutrophils with L. donovani promastigotes induced a low and statistically not significant level of ROS production (Figures 2E, F). Simultaneous exposure of neutrophils to L. donovani and to ATP or UTP, however, led to strong ROS production (Figures 2E, F). These data show that ATP and UTP enhance Leishmania-induced ROS production in a synergistic manner.
Phagocytic Activity of Neutrophils Is Enhanced by Exposure to ATP
Next, we investigated whether ATP and UTP, in addition to their effects on ROS production, also activate other antimicrobial effector functions of neutrophils. Since purinergic signaling was recently shown to enhance phagocytic capacity of macrophages (30) we hypothesized that ATP and UTP can exert an activating effect on phagocytosis by neutrophils. Neutrophils were exposed to ATP and UTP and subsequently phagocytosis of fluorescence-labeled latex beads was analyzed by flow cytometry. Exposure of neutrophils to ATP and UTP only slightly enhanced the phagocytosis of latex beads by neutrophils (Figure 3A). Exposure to LPS enhanced both the ratio of phagocytosing cells (Figure 3A) and the number of phagocytosed particles per neutrophil, shown as mean fluorescence intensity (MFI) (Figure 3B). Simultaneous exposure of LPS-stimulated neutrophils to ATP and UTP strongly and synergistically enhanced the LPS-induced phagocytic capacity of neutrophils (Figures 3A, B).
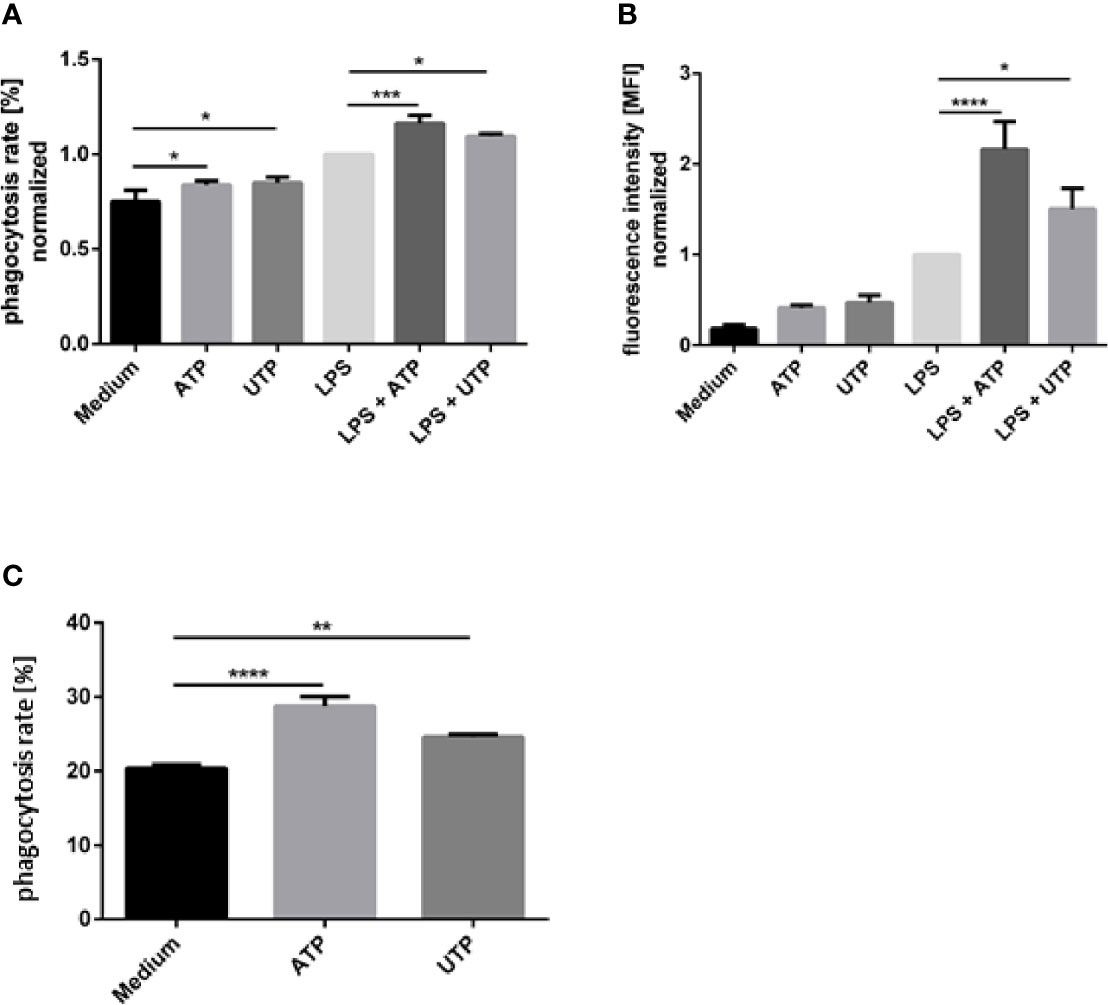
Figure 3 The effect of extracellular ATP and UTP on the phagocytic capacity of neutrophils. (A, B) Neutrophils were exposed to of ATP (500 µM) or UTP (500 µM) in the presence or absence of LPS (100 ng/ml) or left untreated. FluoSphere carboxylate-modified latex microspheres with a diameter of 1 μm were added and incubated for 30 min. Phagocytosis was assessed by flow cytometry by analyzing the ratio (%) of neutrophils with ingested fluorescent beads (A) and mean fluorescence intensity (MFI) of cells that phagocytosed beads (B). (C) Neutrophils were co-incubated with CFSE-labeled L. donovani promastigotes for 120 min at a neutrophil to Leishmania ratio of 1:5. Phagocytosis was assessed by flow cytometry by analyzing the ratio (%) of neutrophils with ingested CFSE-labeled Leishmania. Statistical analysis by ordinary one-way ANOVA with a post hoc Turkey’s test. n=3, *p ≤ 0,05, **p ≤ 0,01, ***p ≤ 0,001, ****p ≤ 0,0001.
Prerequisite of intracellular killing of Leishmania by neutrophils is the ingestion of the parasites. The effect of ATP and UTP on the ingestion of Leishmania parasites was assessed by using CFSE-labeled L. donovani promastigotes. Exposure to both ATP and UTP enhanced the phagocytosis of Leishmania by neutrophils (Figure 3C).
Exposure to ATP and UTP Leads to Enhanced Killing of Intracellular Leishmania by Neutrophils
Neutrophils can play both protective as well as disease promoting roles in leishmaniasis. On the one hand neutrophils can kill intracellular Leishmania. On the other hand, if intracellular Leishmania are not killed, neutrophils can provide an intracellular niche for the survival of these intracellular pathogens. Our finding, that exposure to extracellular ATP and UTP induces ROS production (Figure 2) suggests that neutrophils can kill Leishmania at an enhanced level. The enhanced ingestion of the parasites (Figure 3C), on the one hand may facilitate the killing of more parasites. However, on the other hand, if the parasites are not killed, enhanced ingestion of Leishmania may also lead to the survival of more parasites.
To test whether exposure to ATP and/or UTP can enhance antileishmanial activity of neutrophils Leishmania donovani-infected neutrophils were exposed to ATP and UTP and the intracellular killing of the pathogens was assessed. Exposure of neutrophils to ATP and UTP significantly and markedly increased the killing of L. donovani (Figure 4). Exposure of L. donovani promastigotes to ATP and UTP did not affect the viability of the parasites indicating that ATP and UTP do not directly kill L. donovani promastigotes (data not shown). Therefore, the increased killing of L. donovani is due to activation of neutrophil anti-leishmanial activity by ATP and UTP.
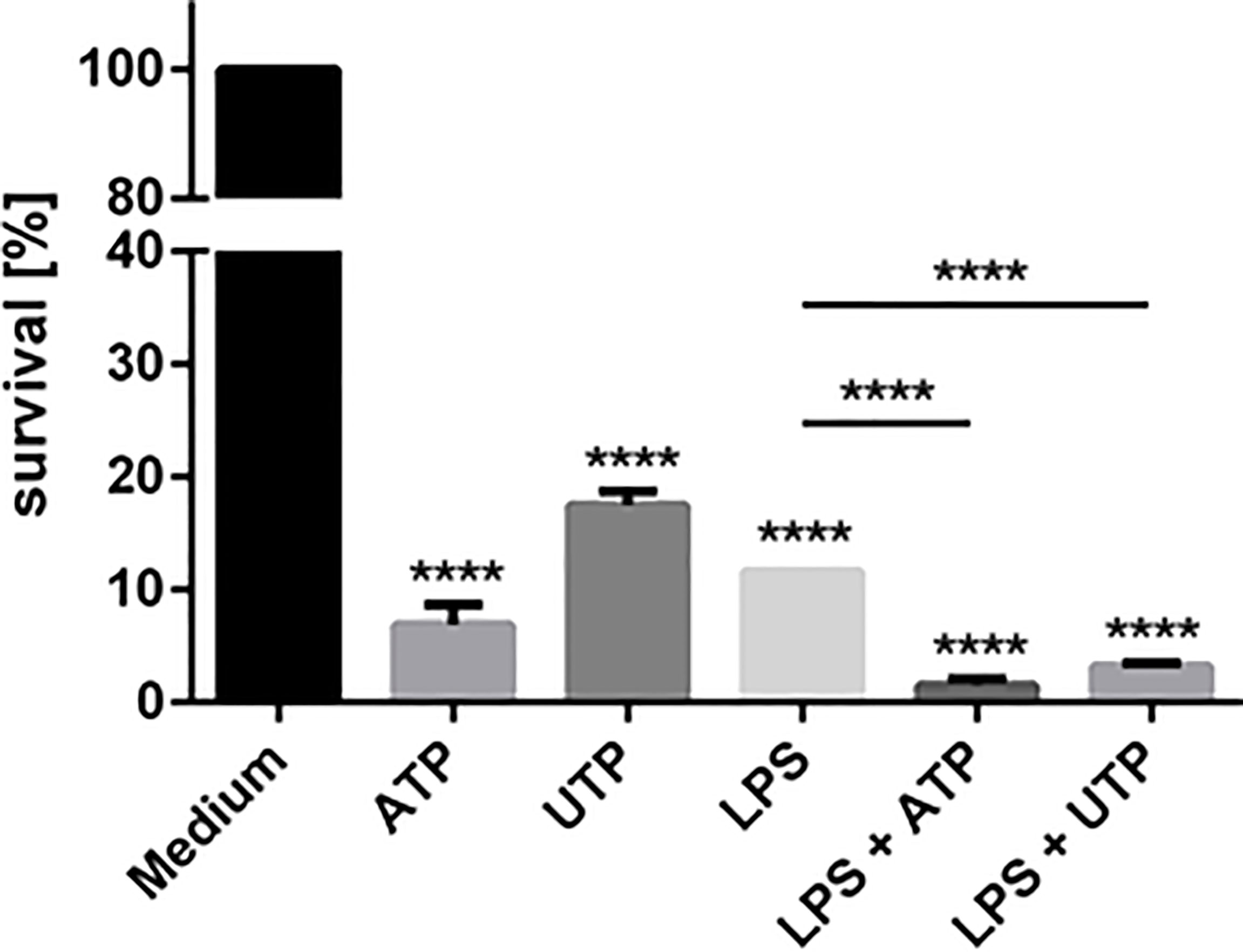
Figure 4 The effect of extracellular ATP and UTP on the intracellular killing of Leishmania donovani parasites by neutrophils. Primary human neutrophils were infected with L. donovani promastigotes (ratio 1:10) for 3 h at 37°C, 5% CO2. The infection rate was controlled on Giemsa-stained cytocentrifuge slides. After removing the free, non-ingested parasites the infected cells were treated with ATP, UTP, LPS and the combination of LPS with ATP or UTP or left untreated (medium). After 18 h of incubation at 37°C, 5% CO2 the survival of L. donovani parasites was determined by using a limiting dilution assay. The bars show % of viable Leishmania compared to untreated neutrophils ± SD (n=3). Statistical analysis by ordinary one-way ANOVA with a post hoc Turkey’s test. Asterisks above the bars indicate significance compared to untreated cells. ****p ≤ 0.0001.
As shown in Figure 2, ATP and UTP synergize with other activating stimuli such as LPS and fMLP. Since in preliminary studies we observed that exposure to LPS leads to enhanced anti-laishmanial activity of neutrophils (data not shown) we investigated whether the ATP and UTP exert a synergistic effect with LPS regarding the capacity of neutrophils to kill intracellular Leishmania. Simultaneous exposure of Leishmania-infected neutrophils to LPS and ATP or UTP enhanced significantly the killing of the parasites in a synergistic manner (Figure 4).
Exposure to ATP and UTP Results in Enhanced Production of Pro-Inflammatory Cytokines by Neutrophils
In addition to their antimicrobial effector functions neutrophils can regulate inflammatory and immune responses by releasing cytokines (31). After having seen that extracellular ATP and UTP can activate effector functions of neutrophils we investigated whether exposure to ATP and UTP can also influence the proinflammatory regulatory functions of neutrophils. Such a function can contribute to the antimicrobial effects of ATP and UTP in vivo.
IL-8 (CXCL8) is a major chemotactic factor for neutrophils. However, neutrophils not only respond to but also secrete IL-8 (32). This autocrine mechanism is thought to serve as positive feedback for neutrophil recruitment. Because neutrophil recruitment is generally considered to be proinflammatory, we tested the effect of extracellular ATP and UTP on the IL-8 release by human neutrophils. Neutrophils were exposed to L. donovani, ATP or UTP or to the combination of L. donovani with ATP or UTP for 18 h and the IL-8 content of the supernatants was analyzed by ELISA. Exposure to ATP or UTP alone did not induce the release of IL-8 (Figure 5A). Co-incubation with L. donovani promastigotes resulted in the release of high IL-8 levels (Figure 5A). Importantly, extracellular ATP strongly enhanced the L. donovani-induced IL-8 release (Figure 5A). Exposure to UTP enhanced slightly the Leishmania-induced IL-8 release. However, this effect was not statistically significant (Figure 5A).
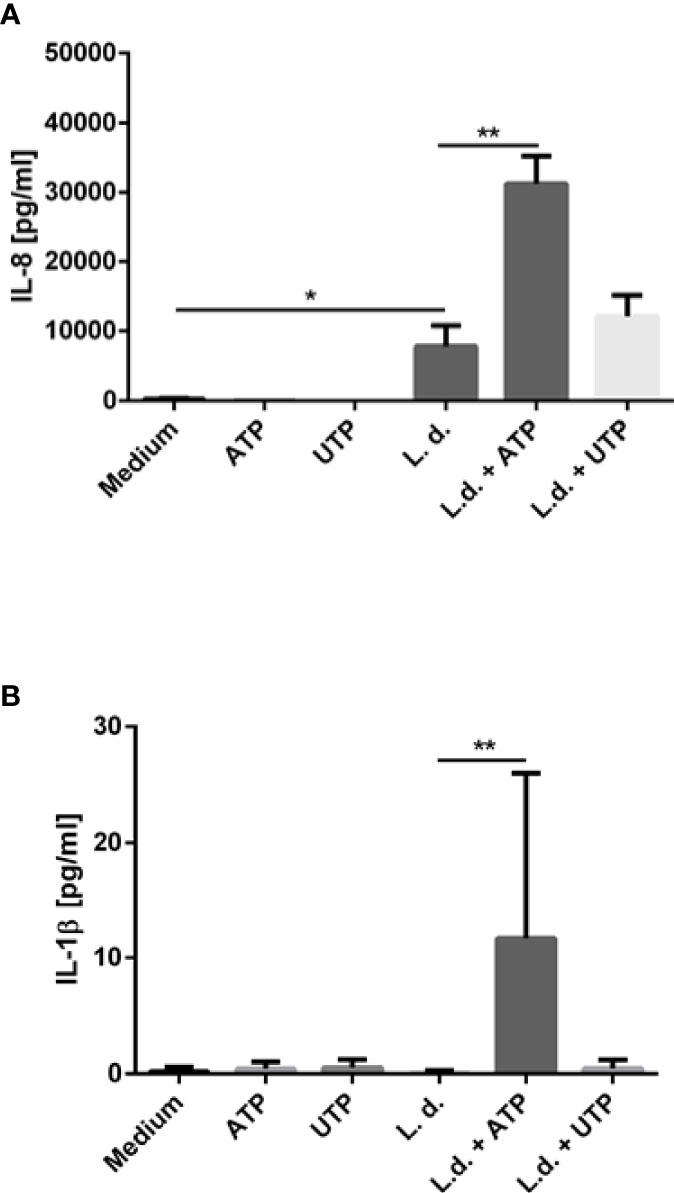
Figure 5 The effect of extracellular ATP and UTP on the Leishmania-induced cytokine production by neutrophils. Primary human neutrophils were infected with L. donovani promastigotes (ratio 1:10) for 3 h at 37°C, 5% CO2. After removing the free, non-ingested parasites the infected cells were treated with ATP, UTP or left untreated for 18 h. The IL-8 content (A) and the IL-1β (B) content of the supernatants was analyzed by ELISA. n=3, *p ≤ 0,05, **p ≤ 0,01.
Activation of purinergic receptors plays also a major role in the immune responses through the secretion of pro-inflammatory cytokines. Extracellular ATP was reported to activate the NALP3 inflammasome which initiates a cascade of proteolytic events commencing with the conversion of procaspase-1 to caspase-1 resulting in the processing and secretion of IL-1β (33). In our present study co-incubation of neutrophils with L. donovani did not induce the release of IL-1β (Figure 5B). Exposure to extracellular ATP or UTP also did not induce IL-1β release (Figure 5B). Simultaneous exposure to L. donovani and ATP, however, resulted in the release of IL-1β from primary human neutrophils (Figure 5B).
Exposure to ATP and UTP Results in Enhanced Intracellular Ca2+-Concentration
Purinergic signaling has been described to lead to Gαq-dependent activation of phospholipase C-β (PLCβ) resulting in the hydrolysis of PtdIns (4,5)P2 producing the second messengers Ins (1,4,5)P3 which then in turn mobilized intracellular Ca2+ (30). In order to investigate whether this pathway can be involved in the enhanced killing of Leishmania in neutrophils upon exposure to ATP and UTP we next assessed the effect of extracellular ATP and UTP on Ca2+ mobilization in Leishmania-infected neutrophils. A strong intracellular Ca2+ mobilization was observed in infected neutrophils upon treatment with ATP and UTP (Figure 6). Treatment with ionomycin was used in these experiments as positive control (Figure 6).
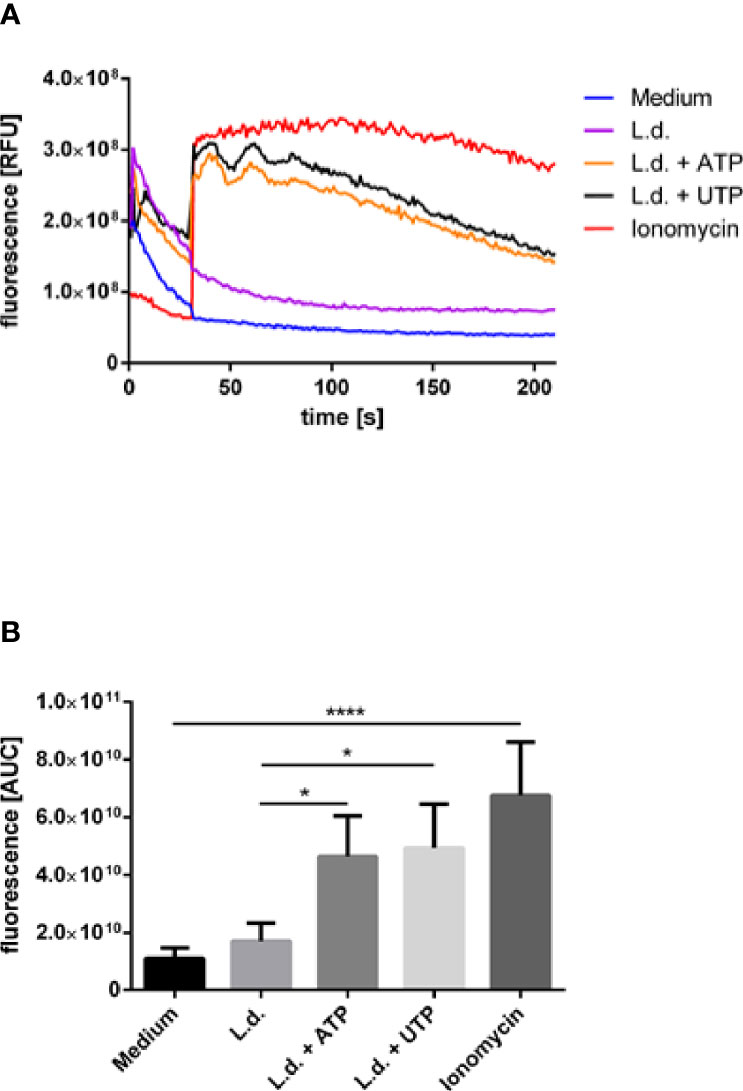
Figure 6 The effect of extracellular ATP and UTP on the intracellular Ca2+-concentration in L. donovani-infected neutrophils. Neutrophils were labeled with the fluorescent Ca2+-sensitive indicator Fluo-4 AM and infected with L. donovani promastigotes. Infected neutrophils were exposed to ATP (500 µM) or UTP (500 µM) and the fluorescence intensity was assessed for 3 min by using flow cytometry. The stimuli were added at the time point 30 s of the flow cytometry analysis. Fluo-4-labeled non-infected cells were used as negative controls, Fluo-4-labeled non-infected cells exposed to ionomycin (10 µM) were used as positive controls. (A) Representative kinetic curves of Fluo-4 AM fluorescence in neutrophils. (B) Fluo-4 AM fluorescence shown by calculating the area under the curve (AUC) values calculated between the time points 30 sec and 210 sec. Statistical analysis by ordinary one-way ANOVA with a post hoc Turkey’s test. Asterisks above the bars indicate significance compared to untreated cells. n=3, *p ≤ 0,05, ****p ≤ 0,0001.
Extracellular ATP Induces Phosphorylation of Akt, Erk1/2 and p38 MAPK in L. donovani-Infected Neutrophils
Purinergic signaling has been described to lead to Gαq-dependent activation of phospholipase C-β (PLCβ). Activation of PLCβ results in the hydrolysis of diacylglycerol (DAG) which activates protein kinase C (PKC) which then in turn activates the serine/threonine kinase ERK1/2 (34). Signaling events involving Akt, Erk1/2 and p38 MAPK are also involved in inflammasome activation and the subsequent processing of IL-1β. Having observed a synergistic effect of ATP and L. donovani on the IL-1β-release of human primary neutrophils (Figure 5) we next investigated whether phosphorylation of Akt, Erk1/2 and p38 MAPK is affected upon simultaneous contact of neutrophils to L. donovani and ATP. Western blot analysis reveled an upregulation of the phosphorylation of Akt, Erk1/2 and p38 MAPK upon exposure to extracellular ATP in L. donovani-infected neutrophils (Figure 7).
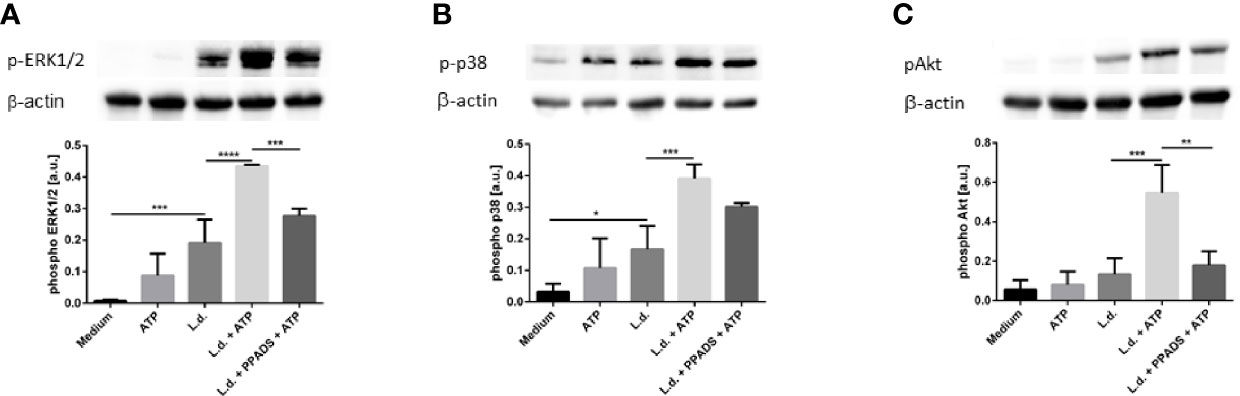
Figure 7 The effect of extracellular ATP and UTP on the phosphorylation of p38, Akt and ERK1/2 in neutrophils. Neutrophils were exposed to ATP (500 µM), UTP (500 µM), Leishmania donovani promastigotes (Leishmania to neutrophil ratio 10:1) or a combination of ATP or UTP with Leishmania donovani promastigotes for 15 min at 37°C. In samples with the P2-antagonist PPADS the neutrophils were pretreated with the inhibitor (30 µM) for 15 min. Whole cell lysates were prepared and phosphorylation of ERK1/2 (A), p38 (B) and Akt (C) were analyzed by Western Blot. Representative blots are shown in the upper parts of the panels. Phosphorylation of ERK1/2, p38 and Akt was quantified by densitometry analysis. Signals of pAkt, pERK1/2, or pp38 were related to β-actin signals on the same blots. n=4, *p ≤ 0,05, **p ≤ 0,01, ***p ≤ 0,001, ****p ≤ 0,0001.
In order to demonstrate that the observed phosphorylation events are induced by signaling via purinergic receptors the effect of the non-selective P2 purinergic receptor antagonist PPADS was investigated. Treatment with PPADS strongly and significantly reduced the phosphorylation of ERK1/2 and Akt in neutrophils exposed to L. donovani and ATP (Figures 7A, C). The inhibitory effect of PPADS on the phosphorylation of p38 MAPK was apparent, however, statistically not significant (Figure 7B).
Exposure to Extracellular ATP and UTP Does Not Compromise Viability of Neutrophils
Since in our experiments a relatively high concentration (500 µM) of ATP and UTP was applied to neutrophils for a time period varying between 15 min and over night exposure, we tested the effect of ATP and UTP treatment on the apoptosis and viability of neutrophils. Neutrophils are short lived cells, they undergo spontaneous apoptosis within hours both in vivo and in vitro (35, 36). Apoptosis and viability of neutrophils were assessed after 1h, 4h and 20h exposure to 500 µM of ATP and UTP. Extracellular ATP and UTP neither enhanced apoptosis nor exerted any toxic effects (Supplemental Figure 1). In contrary, exposure to ATP and UTP showed a tendency to reduce apoptosis and enhance viability. This effect was statistically significant after 20h exposure of neutrophils to ATP (Supplemental Figure 1).
Discussion
In the present study we demonstrated that extracellular ATP and UTP results in enhanced anti-leishmanial effector functions of neutrophil granulocytes in vitro. We could show that activation status, granule degranulation, ROS-production, phagocytic capacity of primary human neutrophils and intracellular Ca2+ concentration are enhanced upon exposure to ATP and UTP. Exposure of Leishmania-infected neutrophils to ATP and UTP resulted in a strongly enhanced ability of neutrophils to kill the intracellular parasites. Importantly, exposure to ATP resulted in the production and release of strongly enhanced levels of IL-8 and IL-1β by Leishmania-infected neutrophils. We could show that simultaneous exposure to Leishmania donovani promastigotes and ATP leads to the phosphorylation of ERK1/2, p38 MAPK and Akt and that P2 purinergic receptors are involved in these phosphorylation events.
At inflammatory foci the concentration of ATP and other purine and pyrimidine nucleotides can be very high, the ATP concentration was shown to reach hundreds of micromolar concentrations (11). These high concentrations have significant biological effects. In vitro studies with ATP showed significant biological effect of extracellular ATP in the concentration of 10-3 -10 -4 M (37). ATP (10-3 -10 -4 M) also leads to mobilization of intracellular Ca2+ (38). In an in vitro study ATP exerted an inhibitory effect on spontaneous neutrophil apoptosis in concentrations between 100 and 1000 µM (39). Based on these findings in our present study we used ATP and UTP at a concentration of 500 µM. In our study, exposure to ATP and UTP also showed a tendency to reduce apoptosis and enhance viability. This effect was statistically significant after 20h exposure of neutrophils to ATP.
Purinergic activation of macrophages by ATP and UTP was shown reduced parasite load in Leishmania infected macrophages (15). Parasite load in L. amazonensis infected macrophages was reduced in vitro after treatment with UTP (17). Enhanced elimination of intracellular Leishmania by macrophages has been described upon exposure to extracellular ATP (40) and UTP (41). By using the air pouch technique UTP treatment of Leishmania-infected mice was shown to induce neutrophil recruitment and enhanced ROS production. However, the source of ROS was not investigated in this study. We hypothesized that neutrophils were the likely source of ROS after UTP treatment. Since neutrophils were recently recognized as host cells for the intracellular parasite Leishmania our present study focused on the effect of extracellular ATP on UTP on the functions and, importantly, on the capacity of neutrophils to kill intracellular Leishmania.
Previous studies described the activating effect of purinergic regulation on selected individual function of neutrophils such as degranulation (37, 42), ROS production (42) and mobilization of intracellular Ca2+ (38) we here followed a more comprehensive approach to investigate neutrophil functions and investigate these functions in relation to infection with Leishmania donovani.
The production of reactive oxygen species (ROS) is a major antimicrobial effector functions of neutrophils. This function is regarded as essential mechanism to kill intracellular Leishmania. We observed that ATP or UTP alone did not induce the production of significant levels of ROS. Exposure to Leishmania also did not induce the production of significant ROS levels. Importantly, in the presence of ATP and UTP high ROS levels were produced upon exposure to Leishmania parasites. We observed similar synergistic effects of ATP and UTP with the microbial stimuli LPS and fMLP. Purinergic activation was shown to be closely connected to other activating signaling pathways. Extracellular ATP was shown to synergize with TNF-alpha for the activation of DCs (43). A good example for the connection between purinergic and other signaling pathways is our finding regarding the secretion of IL-1β upon exposure of Leishmania-infected neutrophils to ATP. It is generally accepted that the activation of NLRP3 inflammasome require two signals, i) a priming signal which results in the upregulation of NLRP3 and pro-IL-1β and ii) and a second signal leading to the assembly of the NLRP3 inflammasome complex (44). The first signal is provided by pattern recognition receptors recognizing microbial constituents such as toll like receptors (TLR) (45). The second signals include DAMPs such as extracellular nucleotides (46). Accordingly, ATP-induced release of IL-1β was shown to require a priming step by LPS (47).
Several pattern recognition receptors have been reported to be involved in the recognition of Leishmania parasites such as TLR2 (48), TLR4 (49), TLR9 (50) and the NLR (reviewed in 51). Importantly, these receptors are known to provide a signal for NLRP3 activation. NLRP3-IL-1β axis is important for the defense against Leishmania amazonensis. Leishmania-induced activation of NLRP3 inflammasome and subsequent IL-1β production facilitated host resistance to Leishmania infection in macrophages in vitro and in mice in vivo (52). Extracellular ATP at a concentration of 500 µM was reported to induce IL-1β signaling and control of L. amazonensis infection in macrophages in vitro (53). Extracellular ATP was shown to activate NLRP3 inflammasome in murine neutrophils resulting in IL-1β secretion (34). Here we showed that extracellular ATP acts as second signal in Leishmania-infected human neutrophils to induce IL-1β release.
Extracellular ATP was shown to be involved in the stimulation of neutrophils with fMLP but also through receptors for IL-8, C5a and LTB4 (20). The production of LTB4 has been reported to be involved in the ATP- and UTP-mediated killing of Leishmania by macrophages (15, 54). In a previous study we demonstrated that neutrophils infected with Leishmania release an increased amount of LTB4 (55). It is, therefore, tempting to hypothesize that neutrophil-derived LTB4, in an autocrine manner, is involved in the ATP- and UTP-mediated enhanced elimination of intracellular Leishmania by neutrophils.
ATP and other nucleotides such as UTP are released from cells at sites of inflammation in high concentrations. Extracellular ATP and related purine and pyrimidine nucleotides exert their functions as danger signals via signaling through membrane-bound purinergic P2 receptors. P2 receptor signaling is mediated by pathways involving the phosphorylation of ERK1/2, Akt and p38 MAPK (34). Having seen the activating effects of extracellular nucleotides on neutrophil function we used an inhibitor approach to show that the observed effects were mediated by purinergic receptor(s). Extracellular ATP and UTP act on leukocytes upon binding to surface P2 receptors. ATP is an agonist for all seven P2X receptors and can bind to all eight P2Y receptors except to the P2Y6 receptor (12–14). UTP is an agonist of P2Y2, P2Y4 and P2Y11 (12, 13). To obtain a hint regarding the receptors involved in the observed affects the non-selective P2 receptors antagonist PPADS was used. By using PPADS we could show the involvement of P2-receptor(s) in the ATP-induced signaling events that involve the phosphorylation of ERK1/2, Akt and p38 MAPK.
Taking together our results show that extracellular ATP and UTP activate anti-leishmanial effector and also regulatory functions of human neutrophils. Therefore, therapy options using extracellular nucleotides can target Leishmania-infected neutrophils leading to enhanced elimination of parasite load. Moreover, since the presence of high numbers of neutrophils and high extracellular ATP and UTP-concentrations is characteristic for multiple inflammatory and infectious conditions, our results suggest that ATP- and UTP-induced activation of neutrophils has a major and clinically relevant pathophysiological impact. In one hand neutrophils activated by extracellular ATP and/or UTP exert an enhanced antimicrobial activity. On the other hand neutrophils activated by extracellular ATP and/or UTP may have the potential to exert a disease promoting effect in chronic or autoinflammatory conditions.
Data Availability Statement
The raw data supporting the conclusions of this article will be made available by the authors, without undue reservation.
Ethics Statement
The studies involving human participants were reviewed and approved by Ethical Committee of the Medical Faculty of the University of Lübeck. The patients/participants provided their written informed consent to participate in this study.
Author Contributions
SM conducted laboratory work, analysed data, and prepared the figures. TL designed the study, analysed data, and wrote the manuscript. All authors contributed to the article and approved the submitted version.
Conflict of Interest
The authors declare that the research was conducted in the absence of any commercial or financial relationships that could be construed as a potential conflict of interest.
Publisher’s Note
All claims expressed in this article are solely those of the authors and do not necessarily represent those of their affiliated organizations, or those of the publisher, the editors and the reviewers. Any product that may be evaluated in this article, or claim that may be made by its manufacturer, is not guaranteed or endorsed by the publisher.
Acknowledgments
The authors thank Mr. Daniel Dömer, M.Sc. for his assistance and advice regarding the assay for measuring intracellular Ca2+-concentration.
Supplementary Material
The Supplementary Material for this article can be found online at: https://www.frontiersin.org/articles/10.3389/fimmu.2021.747049/full#supplementary-material
References
1. Müller K, Zandbergen G, Hansen B, Laufs H, Jahnke N, Solbach W, et al. Chemokines, Natural Killer Cells and Granulocytes in the Early Course of Leishmania Major Infection in Mice. Med Microbiol Immunol (2001) 190:73–6. doi: 10.1007/s004300100084
2. Peters NC, Egen JG, Secundino N, Debrabant A, Kimblin N, Kamhawi S, et al. In Vivo Imaging Reveals an Essential Role for Neutrophils in Leishmaniasis Transmitted by Sand Flies. Science (2008) 321:970–4. doi: 10.1126/science.1159194
3. Segal AW. How Neutrophils Kill Microbes? Annu Rev Immunol (2005) 23:197–223. doi: 10.1146/annurev.immunol.23.021704.115653
4. Nauseef WM. How Human Neutrophils Kill and Degrade Microbes: An Integrated View. Immunol Rev (2007) 219:88–102. doi: 10.1111/j.1600-065X.2007.00550.x
5. Guimaraes-Costa AB, Nascimento MTC, Froment GS, Soares RPP, Morgado FN, Conceicao-Silva F, et al. Leishmania Amazonensis Promastigotes Induce and Are Killed by Neutrophil Extracellular Traps. Proc Natl Acad Sci (2009) 106:6748–53. doi: 10.1073/pnas.0900226106
6. Regli IB, Passelli K, Hurrell BP, Tacchini-Cottier F. Survival Mechanisms Used by Some Leishmania Species to Escape Neutrophil Killing. Front Immunol (2017) 8:1558. doi: 10.3389/fimmu.2017.01558
7. Carlsen ED, Liang Y, Shelite TR, Walker DH, Melby PC, Soong L. Permissive and Protective Roles for Neutrophils in Leishmaniasis: Complex Roles for Neutrophils in Leishmaniasis. Clin Exp Immunol (2015) 182:109–18. doi: 10.1111/cei.12674
8. van Zandbergen G, Klinger M, Mueller A, Dannenberg S, Gebert A, Solbach W, et al. Cutting Edge: Neutrophil Granulocyte Serves as a Vector for Leishmania Entry Into Macrophages. J Immunol (2004) 173:6521–5. doi: 10.4049/jimmunol.173.11.6521
9. Chaves MM, Lee SH, Kamenyeva O, Ghosh K, Peters NC, Sacks D. The Role of Dermis Resident Macrophages and Their Interaction With Neutrophils in the Early Establishment of Leishmania Major Infection Transmitted by Sand Fly Bite. PloS Pathog (2020) 16:e1008674. doi: 10.1371/journal.ppat.1008674
10. Coutinho-Silva R, Ojcius DM. Role of Extracellular Nucleotides in the Immune Response Against Intracellular Bacteria and Protozoan Parasites. Microbes Infect (2012) 14:1271–7. doi: 10.1016/j.micinf.2012.05.009
11. Morciano G, Sarti AC, Marchi S, Missiroli S, Falzoni S, Raffaghello L, et al. Use of Luciferase Probes to Measure ATP in Living Cells and Animals. Nat Protoc (2017) 12:1542–62. doi: 10.1038/nprot.2017.052
12. Lovászi M, Branco Haas C, Antonioli L, Pacher P, Haskó G. The Role of P2Y Receptors in Regulating Immunity and Metabolism. Biochem Pharmacol (2021) 187:114419. doi: 10.1016/j.bcp.2021.114419
13. Zimmermann H. Ectonucleoside Triphosphate Diphosphohydrolases and Ecto-5’-Nucleotidase in Purinergic Signaling: How the Field Developed and Where We Are Now. Purinergic Signal (2021) 17(1):117–25. doi: 10.1007/s11302-020-09755-6
14. Giuliani AL, Sarti AC, Di Virgilio F. Ectonucleotidases in Acute and Chronic Inflammation. Front Pharmacol (2021) 11:619458. doi: 10.3389/fphar.2020.619458
15. Thorstenberg ML, Martins MDA, Figliuolo V, Silva CLM, Savio LEB, Coutinho-Silva R. P2Y2 Receptor Induces L. Amazonensis Infection Control in a Mechanism Dependent on Caspase-1 Activation and IL-1β Secretion. Mediators Inflamm (2020) 2020:2545682. doi: 10.1155/2020/2545682
16. Marques-da-Silva C, Chaves MM, Rodrigues JC, Corte-Real S, Coutinho-Silva R, Persechini PM. Differential Modulation of ATP-Induced P2X7-Associated Permeabilities to Cations and Anions of Macrophages by Infection With Leishmania Amazonensis. PloS One (2011) 6:e25356. doi: 10.1371/journal.pone.0025356
17. Marques-da-Silva C, Chaves MM, Thorstenberg ML, Figliuol VR, Vieira FS, Chaves SP, et al. Intralesional Uridine-5’-Triphosphate (UTP) Treatment Induced Resistance to Leishmania Amazonensis Infection by Boosting Th1 Immune Responses and Reactive Oxygen Species Production. Purinergic Signal (2018) 14:201–11. doi: 10.1007/s11302-018-9606-7
18. Wang X, Chen D. Purinergic Regulation of Neutrophil Function. Front Immunol (2018) 9:399. doi: 10.3389/fimmu.2018.00399
19. Rubenich DS, de Souza PO, Omizzollo N, Lenz GS, Sevigny J, Braganhol E. Neutrophils: Fast and Furious-The Nucleotide Pathway. Purinergic Signal (2021) 17:371–83. doi: 10.1007/s11302-021-09786-7
20. Chen Y, Yao Y, Sumi Y, Li A, To UK, Elkhal A, et al. Purinergic Signaling: A Fundamental Mechanism in Neutrophil Activation. Sci Signal (2010) 125:ra45. doi: 10.1126/scisignal.2000549
21. Laufs H, Müller K, Fleischer J, Reiling N, Jahnke N, Jensenius JC, et al. Intracellular Survival of Leishmania Major in Neutrophil Granulocytes After Uptake in the Absence of Heat-Labile Serum Factors. Infect Immun (2002) 70:826–35. doi: 10.1128/IAI.70.2.826-835.2002
22. Karsten CM, Laumonnier Y, Köhl J. Functional Analysis of C5a Effector Responses In Vitro and In Vivo. In: Gadjeva M, editor. The Complement System: Methods and Protocols, Methods in Molecular Biology, vol. 1100. New York: Springer Science+Business Media (2014). doi: 10.1007/978-1-62703-724-2_23
23. Behnen M, Möller S, Brozek A, Klinger M, Laskay T. Extracellular Acidification Inhibits the ROS-Dependent Formation of Neutrophil Extracellular Traps. Front Immunol (2017) 8:184. doi: 10.3389/fimmu.2017.00184
24. Degasperi A, Birtwistle MR, Volinsky N, Rauch J, Kolch W, Kholodenko BN. Evaluating Strategies to Normalise Biological Replicates of Western Blot Data. PloS One (2014) 9:e87293. doi: 10.1371/journal.pone.0087293
25. Laskay T, Wittmann I, Diefenbach A, Röllinghoff M, Solbach W. Control of Leishmania Major Infection in BALB/c Mice by Inhibition of Early Lymphocyte Entry Into Peripheral Lymph Nodes. J Immunol (1997) 158:1246–53.
26. Ley K, Gaehtgens P, Fennie C, Singer MS, Lasky LA, Rosen SD. Lectin-Like Cell Adhesion Molecule 1 Mediates Leukocyte Rolling in Mesenteric Venules In Vivo. Blood (1991) 77:2553–5. doi: 10.1182/blood.V77.12.2553.2553
27. Neufert C, Pai RK, Noss EH, Berger M, Boom WH, Harding CV. Mycobacterium Tuberculosis 19-kDa Lipoprotein Promotes Neutrophil Activation. J Immunol (2001) 2001) 167:1542–9. doi: 10.4049/jimmunol.167.3.1542
28. Sengelov H, Kjeldsen L, Diamond MS, Springer TA, Borregaard N. Subcellular Localization and Dynamics of Mac-1 (Alpha M Beta 2) in Human Neutrophils. J Clin Invest (1993) 92:1467–76. doi: 10.1172/JCI116724
29. Hurrell BP, Regli IB, Tacchini-Cottier F. Different Leishmania Species Drive Distinct Neutrophil Functions. Trends Parasitol (2016) 32:392–401. doi: 10.1016/j.pt.2016.02.003
30. Zumerle S, Calì B, Munari F, Angioni R, Di Virgilio F, Molon B, et al. Intercellular Calcium Signaling Induced by ATP Potentiates Macrophage Phagocytosis. Cell Rep (2019) 27:1–10.e4. doi: 10.1016/j.celrep.2019.03.011
31. Tamassia N, Bianchetto-Aguilera F, Arruda-Silva F, Gardiman E, Gasperini S, Calzetti F, et al. Cytokine Production by Human Neutrophils: Revisiting the “Dark Side of the Moon”. Eur J Clin Invest (2018) 48 Suppl 2:e12952. doi: 10.1111/eci.12952
32. Cassatella MA. Neutrophil-Derived Proteins: Selling Cytokines by the Pound. Adv Immunol (1999) 73:369–509. doi: 10.1016/s0065-2776(08)60791-9
33. Karmakar M, Katsnelson MA, Dubyak GR, Pearlman E. Neutrophil P2X7 Receptors Mediate NLRP3 Inflammasome-Dependent IL-1β Secretion in Response to ATP. Nat Commun (2016) 7:10555. doi: 10.1038/ncomms10555
34. Erb L, Weisman GA. Coupling of P2Y Receptors to G Proteins and Other Signaling Pathways. Wiley Interdiscip Rev Membr Transp Signal (2012) 1:789–803. doi: 10.1002/wmts.62
35. Savill JS, Wyllie AH, Henson JE, Walport MJ, Henson PM, Haslett C. Macrophage Phagocytosis of Aging Neutrophils in Inflammation. Programmed Cell Death in the Neutrophil Leads to Its Recognition by Macrophages. J Clin Invest (1989) 83(3):865–75. doi: 10.1172/JCI113970
36. Payne CM, Glasser L, Tischler ME, Wyckoff D, Cromey D, Fiederlein R, et al. Programmed Cell Death of the Normal Human Neutrophil: An In Vitro Model of Senescence. Microsc Res Tech (1994) 28(4):327–44. doi: 10.1002/jemt.1070280408
37. Cockcroft S, Stutchfield J. ATP Stimulates Secretion in Human Neutrophils and HL60 Cells via a Pertussis Toxin-Sensitive Guanine Nucleotide-Binding Protein Coupled to Phospholipase C. FEBS Lett (1989) 245:25–9. doi: 10.1016/0014-5793(89)80184-x
38. Gasmi L, McLennan AG, Edwards SW. Diadenosine Polyphosphates Induce Intracellular Ca2+ Mobilization in Human Neutrophils via a Pertussis Toxin Sensitive G-Protein. Immunology (1997) 90:154–9. doi: 10.1046/j.1365-2567.1997.00123.x
39. Vaughan KR, Stokes L, Prince LR, Marriott HM, Meis S, Kassack MU, et al. Inhibition of Neutrophil Apoptosis by ATP Is Mediated by the P2Y11 Receptor. J Immunol (2007) 179:8544–53. doi: 10.4049/jimmunol.179.12.8544
40. Chaves SP, Torres-Santos EC, Marques C, Figliuolo VR, Persechini PM, Coutinho-Silva R, et al. Modulation of P2X(7) Purinergic Receptor in Macrophages by Leishmania Amazonensis and Its Role in Parasite Elimination. Microbes Infect (2009) 11:842–9. doi: 10.1016/j.micinf.2009.05.001
41. Marques-da-Silva C, Chaves MM, Chaves SP, Figliuolo VR, Meyer-Fernandes JR, Corte-Real S, et al. Infection With Leishmania Amazonensis Upregulates Purinergic Receptor Expression and Induces Host-Cell Susceptibility to UTP-Mediated Apoptosis. Cell Microbiol (2011) 13:1410–28. doi: 10.1111/j.1462-5822.2011.01630.x
42. Dichmann S, Idzko M, Zimpfer U, Hofmann C, Ferrari D, Luttmann W, et al. Adenosine Triphosphate-Induced Oxygen Radical Production and CD11b Up-Regulation: Ca(++) Mobilization and Actin Reorganization in Human Eosinophils. Blood (2000) 95:973–8. doi: 10.1182/blood.V95.3.973.003k47_973_978
43. Schnurr M, Then F, Galambos P, Scholz C, Siegmund B, Endres S, et al. Extracellular ATP and TNF-Alpha Synergize in the Activation and Maturation of Human Dendritic Cells. J Immunol (2000) 165:4704–9. doi: 10.4049/jimmunol.165.8.4704
44. Jo EK, Kim JK, Shin DM, Sasakawa C. Molecular Mechanisms Regulating NLRP3 Inflammasome Activation. Cell Mol Immunol (2016) 13:148–59. doi: 10.1038/cmi.2015.95
45. Franchi L, Muñoz-Planillo R, Núñez G. Sensing and Reacting to Microbes Through the Inflammasomes. Nat Immunol (2012) 13:325–32. doi: 10.1038/ni.2231
46. Mariathasan S, Weiss DS, Newton K, McBride J, O'Rourke K, Roose-Girma M, et al. Cryopyrin Activates the Inflammasome in Response to Toxins and ATP. Nature (2006) 440:228–32. doi: 10.1038/nature04515
47. Mehta VB, Hart J, Wewers MD. ATP-Stimulated Release of Interleukin (IL)-1beta and IL-18 Requires Priming by Lipopolysaccharide and Is Independent of Caspase-1 Cleavage. J Biol Chem (2001) 276:3820–6. doi: 10.1074/jbc.M006814200
48. Becker I, Salaiza N, Aguirre M, Delgado J, Carrillo-Carrasco N, Kobeh LG, et al. Leishmania Lipophosphoglycan (LPG) Activates NK Cells Through Toll-Like Receptor-2. Mol Biochem Parasitol (2003) 130:65–74. doi: 10.1016/s0166-6851(03)00160-9
49. Kropf P, Freudenberg MA, Modolell M, Price HP, Herath S, Antoniazi S, et al. Toll-Like Receptor 4 Contributes to Efficient Control of Infection With the Protozoan Parasite Leishmania Major. Infect Immun (2004) 72:1920–8. doi: 10.1128/IAI.72.4.1920-1928.2004
50. Liese J, Schleicher U, Bogdan C. TLR9 Signaling Is Essential for the Innate NK Cell Response in Murine Cutaneous Leishmaniasis. Eur J Immunol (2007) 37:3424–34. doi: 10.1002/eji.200737182
51. Gurung P, Kanneganti TD. Innate Immunity Against Leishmania Infections. Cell Microbiol (2015) 17:1286–94. doi: 10.1111/cmi.12484
52. Lima-Junior DS, Costa DL, Carregaro V, Cunha LD, Silva AL, Mineo TW, et al. Inflammasome-Derived IL-1β Production Induces Nitric Oxide-Mediated Resistance to Leishmania. Nat Med (2013) 19:909–15. doi: 10.1038/nm.3221
53. Chaves MM, Sinflorio DA, Thorstenberg ML, Martins MDA, Moreira-Souza ACA, Rangel TP, et al. Non-Canonical NLRP3 Inflammasome Activation and IL-1β Signaling Are Necessary to L. Amazonensis Control Mediated by P2X7 Receptor and Leukotriene B4. PloS Pathog (2019) 15:e1007887. doi: 10.1371/journal.ppat.1007887
54. Chaves MM, Marques-da-Silva C, Monteiro AP, Canetti C, Coutinho-Silva R. Leukotriene B4 Modulates P2X7 Receptor-Mediated Leishmania Amazonensis Elimination in Murine Macrophages. J Immunol (2014) 192:4765–73. doi: 10.4049/jimmunol.1301058
Keywords: Leishmania donovani, ATP, UTP, purinergic, intracellular killing, neutrophils
Citation: Möller S and Laskay T (2021) Purinergic Enhancement of Anti-Leishmanial Effector Functions of Neutrophil Granulocytes. Front. Immunol. 12:747049. doi: 10.3389/fimmu.2021.747049
Received: 25 July 2021; Accepted: 06 September 2021;
Published: 18 October 2021.
Edited by:
Wanderley De Souza, Federal University of Rio de Janeiro, BrazilReviewed by:
Robson Coutinho-Silva, Federal University of Rio de Janeiro, BrazilDominik Rückerl, The University of Manchester, United Kingdom
Copyright © 2021 Möller and Laskay. This is an open-access article distributed under the terms of the Creative Commons Attribution License (CC BY). The use, distribution or reproduction in other forums is permitted, provided the original author(s) and the copyright owner(s) are credited and that the original publication in this journal is cited, in accordance with accepted academic practice. No use, distribution or reproduction is permitted which does not comply with these terms.
*Correspondence: Tamás Laskay, VGFtYXMuTGFza2F5QHVrc2guZGU=