- 1Hunan Collaborative Innovation Center for Utilization of Botanical Functional Ingredients, College of Animal Science and Technology, Hunan Agricultural University, Changsha, China
- 2Department of Food Science and Biotechnology, Faculty of Agriculture, Kagoshima University, Kagoshima, Japan
- 3Pig Breeding Research Insititute, Hunan Xinguang’an Agricultural Husbandry Co., Ltd., Changsha, China
- 4State Key Laboratory of Animal Nutrition, Institute of Animal Sciences, Chinese Academy of Agricultural Sciences, Beijing, China
As the precursor of vitamin A, β-carotene has a positive effect on reproductive performance. Our previous study has shown that β-carotene can increase antioxidant enzyme activity potentially through regulating gut microbiota in pregnant sows. This study aimed to clarify the effect of β-carotene on reproductive performance and postpartum uterine recovery from the aspect of inflammation and gut microbiota by using a mouse model. Twenty-seven 6 weeks old female Kunming mice were randomly assigned into 3 groups (n=9), and fed with a diet containing 0, 30 or 90 mg/kg β-carotene, respectively. The results showed that dietary supplementation of β-carotene reduced postpartum uterine hyperemia and uterine mass index (P<0.05), improved intestinal villus height and villus height to crypt depth ratio, decreased serum TNF-α and IL-4 concentration (P<0.05), while no differences were observed in litter size and litter weight among three treatments. Characterization of gut microbiota revealed that β-carotene up-regulated the relative abundance of genera Akkermansia, Candidatus Stoquefichus and Faecalibaculum, but down-regulated the relative abundance of Alloprevotella and Helicobacter. Correlation analysis revealed that Akkermansia was negatively correlated with the IL-4 concentration, while Candidatus Stoquefichus and Faecalibaculum had a negative linear correlation with both TNF-α and IL-4 concentration. On the other hand, Alloprevotella was positively correlated with the TNF-α, and Helicobacter had a positive correlation with both TNF-α and IL-4 concentration. These data demonstrated that dietary supplementation of β-carotene contributes to postpartum uterine recovery by decreasing postpartum uterine hemorrhage and inhibiting the production of inflammatory cytokines potentially through modulating gut microbiota.
Introduction
Regular consumption of fruits and vegetables are believed to decrease the incidence of chronic disease (1), and the beneficial health effects may attribute to secondary plant compounds such as carotenoids (2, 3). β-carotene is one of the main dietary carotenoids, and it can be converted to vitamin A in cells of intestinal mucosa, liver, uterus, and ovaries (4), although the mechanisms are not clear (5). β-carotene metabolism plays an important role during mammalian embryonic development (6). Recent studies have suggested that dietary β-carotene supplementation during late pregnancy has benefit to the birth weight of offspring in sows (7, 8), and injection of β-carotene can increase the litter size (9). Moreover, β-carotene has been reported to suppress inflammatory responses in macrophages by inhibiting NF-κB translocation (10), which can induce the expression of pro-inflammatory genes (11) and promote inflammatory diseases (12). The uterus is an important reproductive organ with fibromuscular characteristics (13). During childbirth, sustain stretching muscle tissue, resulting tissue damage and adverse outcomes (14), promote postpartum recovery of uterus, which is beneficial to the mother. A previous study has shown that β-carotene can reduce the incidence of retained placenta and metritis in cats (15), and uterine involution may have been more complete and that uterine inflammation may have been reduced in cows which received the β-carotene (16). The above results suggested that β-carotene may contribute to postpartum recovery.
The diverse collection of microorganisms that inhabit the gastrointestinal tract, collectively called the gut microbiota, profoundly influences many aspects of host physiology, including nutrient metabolism, resistance to infection and immune system development (17). Previous studies have suggested that pregnancy is associated with a significant alteration of the gut microbiota, which can affect the physiological state and metabolism of host (18). As a “metabolic organ,” gut microbiota undergo adaptive changes during pregnancy, especially in the third trimester (19). Gut microbiota can influence intestinal permeability, thereby promoting translocation of bacterial products to induce inflammation (20), which occurs throughout pregnancy (21). Our previous studies have also revealed that gut microbiota is closely correlated with inflammation and intestinal mucosal barrier function (22), and dietary supplementation of β-carotene can regulate host gut microbiota (8).
On the basis of previous studies, this study aimed to investigate the effect of β-carotene on the reproductive performance and postpartum uterine recovery, and challenged to elucidate the possible mechanism from the perspective of gut microbiota by using a mouse model.
Materials and Methods
Animals, Diets and Experimental Design
All animal protocols were performed in accordance with guidelines of the Animal Care and Use Committee of Hunan Agricultural University (Permission No. 2020041). Twenty-seven female and twenty-seven male Kunming mice aged 6 weeks were purchased from Hunan SJA Laboratory Animal Co, Ltd. (Changsha, Hunan, China). Each mouse was housed individually in cages with wood shavings bedding under controlled light (12-h light/day) and temperature (23°C), and free access to water and feed. After acclimatization for 1 week, female mice were randomly assigned to 3 groups (n=9), and fed with a basal diet (control group, CLT), a diet containing 30 mg/kg of β-carotene (β-carotene low dose group, CARL), or a diet containing 90 mg/kg of β-carotene (β-carotene high dose group, CARH), based on our previous study, respectively. Male mice were put in female cages from 8:00 PM to 8:00 AM until the copulatory plug was observed, and recorded the date.
Parturition Record and Sample Collection
Food intake was recorded every three days during pregnancy. On the day of delivery, the mice were anesthetized and sacrificed, and the date, uterine mass, as well as the number of mice pups born live and the birth weight were recorded. Ileum and uterine samples were fixed in 4% para-formaldehyde solution immediately, liver, the rest of ileum and uterine organs and sample of cecum contents were collected, immediately put them in liquid nitrogen for quick freezing, and collected blood samples, after standing for 30 minutes, centrifuged at 1500 g for 10 minutes to obtain serum and store at -80°C.
Histology
For hematoxylin and eosin (H&E): Ileum and uterine tissues were fixed in formalin overnight, dehydrated by titrating in ethanol (50%), and submitted to Wuhan Servicebio Technology Co., Ltd (Wuhan, Hubei, China) for paraffin embedding, sectioning, then put the slices into xylene I for 20 min, xylene II for 20 min, absolute ethanol I for 5 min, absolute ethanol II for 5min, 75% alcohol for 5 min, and wash with tap water. The slices were stained with hematoxylin dye solution for 3-5 min, washed with tap water, differentiated with differentiation solution, washed with tap water, hematoxylin-eosin stain returned to blue, and rinsed with running water. The slices were dehydrated with 85% and 95% gradient alcohol for 5 min, and stained with eosin for 5 min. Put absolute ethanol I for 5 min, absolute ethanol II for 5 min, absolute ethanol III for 5 min, dimethyl I for 5 min, xylene II for 5 min, transparent and sealed with neutral gum. The microscopes, image acquisition and length measurement were performed using MShot biological microscope ML31.
Measurement of Antioxidant Indexes of Liver
Liver samples were accurately weighed, normal saline was added according to mass: volume = 1:9 and homogenized, then centrifuged at 4000 rpm for 10 min at 4°C. Total antioxidant capacity (T-AOC) and the level of MDA reflected by thiobarbituric acid reactive substances (TBARS) were measured using respective assay kits (Nanjing jiancheng Bioengineering Institute, Nanjing, China). Protein content was determined by using a BCA protein assay kit (Nanjing jiancheng Bioengineering Institute, Nanjing, China). All values were normalized by the protein content of the same sample.
Measurement of Inflammatory Cytokines
Serum tumor necrosis factor-α (TNF-α) and interleukin-4 (IL-4) concentration were detected using ELISA kits (Nanjing jiancheng Bioengineering Institute, Nanjing, China) according to the manufacturer’s manual.
Characterization of Gut Microbiota
Gut microbiota was characterized by 16S rRNA gene sequencing as described previously (8). Briefly, Total DNA was extracted from cecum contents by using a DNA Isolation Kit (MoBio Laboratories, Carlsbad, CA, USA) following the manufacturer’ s manual. The V3–4 hypervariable region of the bacterial 16S rRNA gene was amplified with the primers 338F (5′-ACTCCTACGGGAGGCAGCA-3′) and 806R (5′-GGACTACHVGGGTWTCTAAT-3′). The PCR was carried out on a Mastercycler Gradient (Eppendorf, Germany) using 25 µL reaction volumes, containing 12.5 µL KAPA 2G Robust Hot Start Ready Mix, 1 µL Forward Primer (5 µmol/L), 1 µL Reverse Primer (5 µmol/L), 5 µL DNA (total template quantity is 30 ng), and 5.5 µL H2O. Cycling parameters were 95°C for 5min, followed by 28 cycles of 95°C for 45 s, 55°C for 50 s, and 72°C for 45 s with a final extension at 72°C for 10min. The PCR products were purified using a QIAquick Gel Extraction Kit (QIAGEN, Germany), and quantified using Real Time PCR, and sequenced on Miseq platform at Allwegene Technology Inc., Beijing, China. Qualified reads were separated using the sample-specific barcode sequences and trimmed with Illumina Analysis Pipeline Version 2.6, and then the dataset was analyzed using QIIME (Version 1.8.0).
Statistical Analysis
Results were expressed as means ± SD. The significant differences between groups were analyzed by one-way ANOVA tests, followed by Fisher’ s least significant difference (LSD) tests with the SPSS statistical software (IBM SPSS Statistics, version 19). Probabilities that were <0.05 were regarded as significant. Bivariate associations between serum TNF-α concentration and the abundance of microbiota, bivariate associations between serum IL-4 concentration and the abundance of microbiota were assessed by Spearman rank correlations, respectively. Then the linear relationship between the abundance of genus regulated significantly by β-carotene and inflammatory factors were analyzed using linear regression method. Principal component analysis (PCA) can reflect the difference and distance between samples by analyzing the composition of OUT (97% similarity) of different samples. Variance decomposition is used to reflect the difference of multiple groups of data on the two-dimensional coordinate map, and the coordinate axis takes two eigenvalues that can reflect the maximum variance value. If the sample composition is more similar, the closer the distance reflected in the PCA diagram.
Results
The Effect of β-Carotene on Litter Performance of Mice
As shown in Table 1, there was no significant difference in average daily food intake during pregnancy in mice, and no significant difference in litter size, weight and offspring average weight between CTL, CARL and CARH group.
The Effect of β-Carotene on Uterine Morphology and Inflammation
As shown in Figure 1, there is more intrauterine blood stasis in CTL group than that in the CARL and CARH group (A). Meanwhile, β-carotene significantly decreased the uterine mass index (P<0.05) (B), serum TNF-α (P<0.05) (C) and IL-4 concentration (P<0.05) (D), as compared with CTL group. However, there was no difference on T-AOC (E) and MDA level (F) in liver among the three groups.
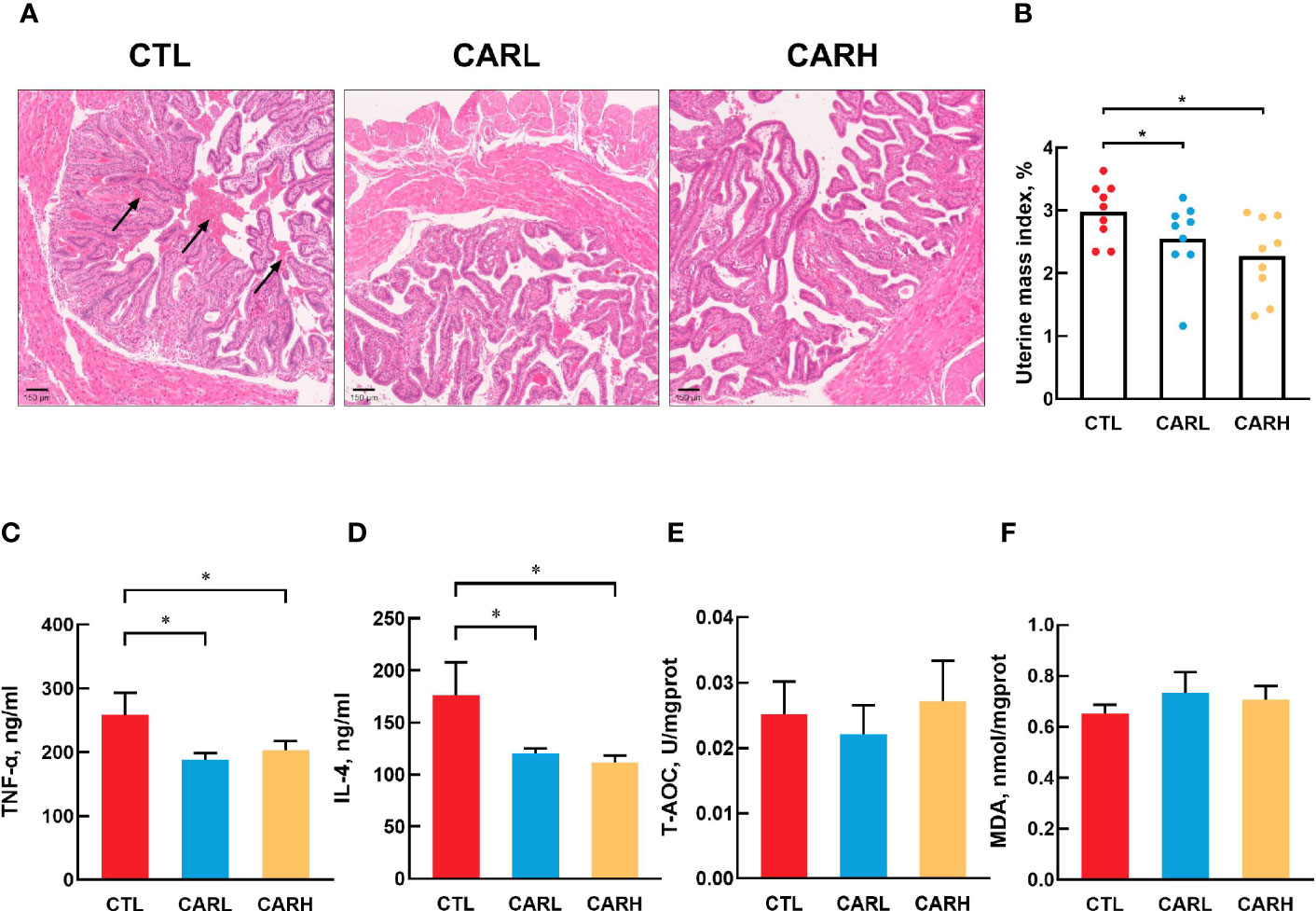
Figure 1 Effect of β-carotene on uterine morphology and serum biomarkers. Representative images of hematoxylin and eosin (H&E) stained uterine section (A), uterine mass index=uterine mass/body weight (B), serum TNF-α (C), IL-4 (D) concentration, total antioxidant capacity of liver (E), MDA content (F). CTL, a basal diet; CARL, a basal diet containing 30 mg/kg β-carotene; CARH, a basal diet containing 90 mg/kg β-carotene. Data were shown as means ± SD (n = 9). *P < 0.05.
The Effect of β-Carotene on Mucosal Morphology
Mucosal crypt-villus axis affects the metabolic and inflammatory response (23), and the damage of intestinal cause to increase the intestinal permeability and inflammatory responses (24), thus the mucosal morphology of mice was observed. As shown in Figure 2, dietary supplementation of β-carotene improved the integrity of mucosa (A), and significantly increased villus height (P<0.05) (B) and ratio of villus height to crypt depth (P<0.05) (C).
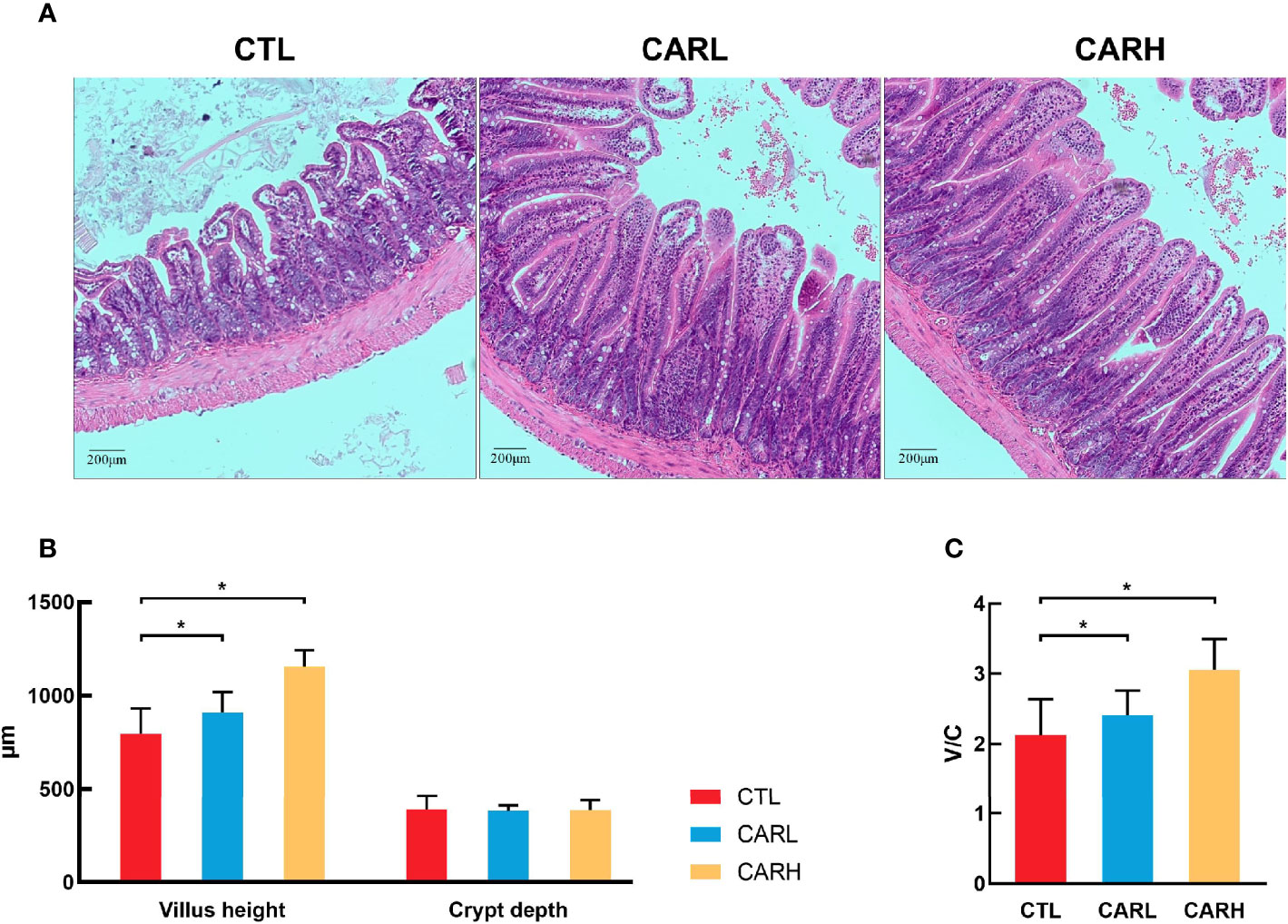
Figure 2 The effect of β-carotene on gut morphology. Representative images of ileum mucosa section stained by hematoxylin and eosin (H&E) (A), villus height and crypt depth of ileum (B), ratio of villus height to crypt depth (C) were analyzed. CTL, a basal diet; CARL, a basal diet containing 30 mg/kg β-carotene; CARH, a basal diet containing 90 mg/kg β-carotene. Data were shown as means ± SD (n = 9). *P < 0.05.
The Effect of β-Carotene on Gut Microbiota
The result of principal component analysis (PCA) of gut microbiota has been shown in Figure 3A, β-carotene had a significant effect on the structure of gut microbiota (Anosim: R=0.135, P=0.049). β-carotene had limited effect on the Shannon index (Figure 3B), however, the Chao 1 index (Figure 3C), Observed species index (Figure 3D) and PD whole tree index (Figure 3E) of CARH group were significantly higher than that of CTL group (P<0.05).
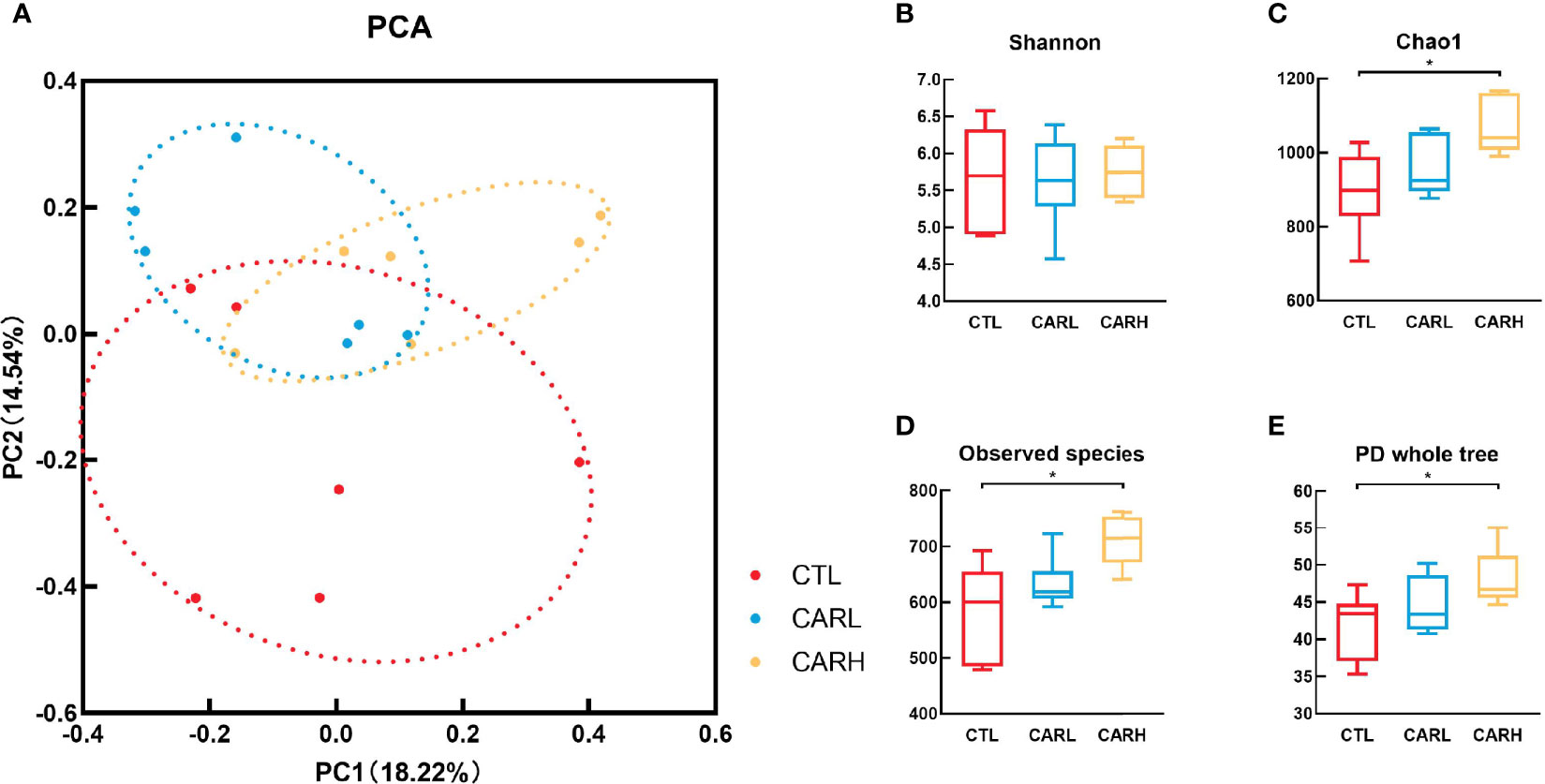
Figure 3 Effects of β -carotene on microbiota diversity. Effect of β-carotene on genus-based (n=6) PCA (A), Shannon index (B), Chao 1 index (C), Observed species index (D) and PD whole tree index (E). CTL, a basal diet; CARL, a basal diet containing 30 mg/kg β-carotene; CARH, a basal diet containing 90 mg/kg β-carotene. Data were shown as means ± SD (n = 6). Anosim: R = 0.135, P= 0.049, *P < 0.05.
The analysis of gut microbiota at the phyla level showed that β-carotene significantly down-regulated the relative abundance of Proteobacteria (P<0.05) (Figures 4A, B), while β-carotene significantly decreased the relative abundances of Helico-bacteraceae (Figure 4C) and Peptococcaceae (Figure 4D) at the family level (P<0.05), as compared with CTL group. At the genus level, β-carotene up-regulated the relative abundance of Akkermansia, Candidatus Stoquefichus and Faecalibaculum, and down-regulated the relative abundances of Alloprevotella and Helicobacter (P<0.05), which were selected from top 40 genera that affected by β-carotene (Figure 4E).
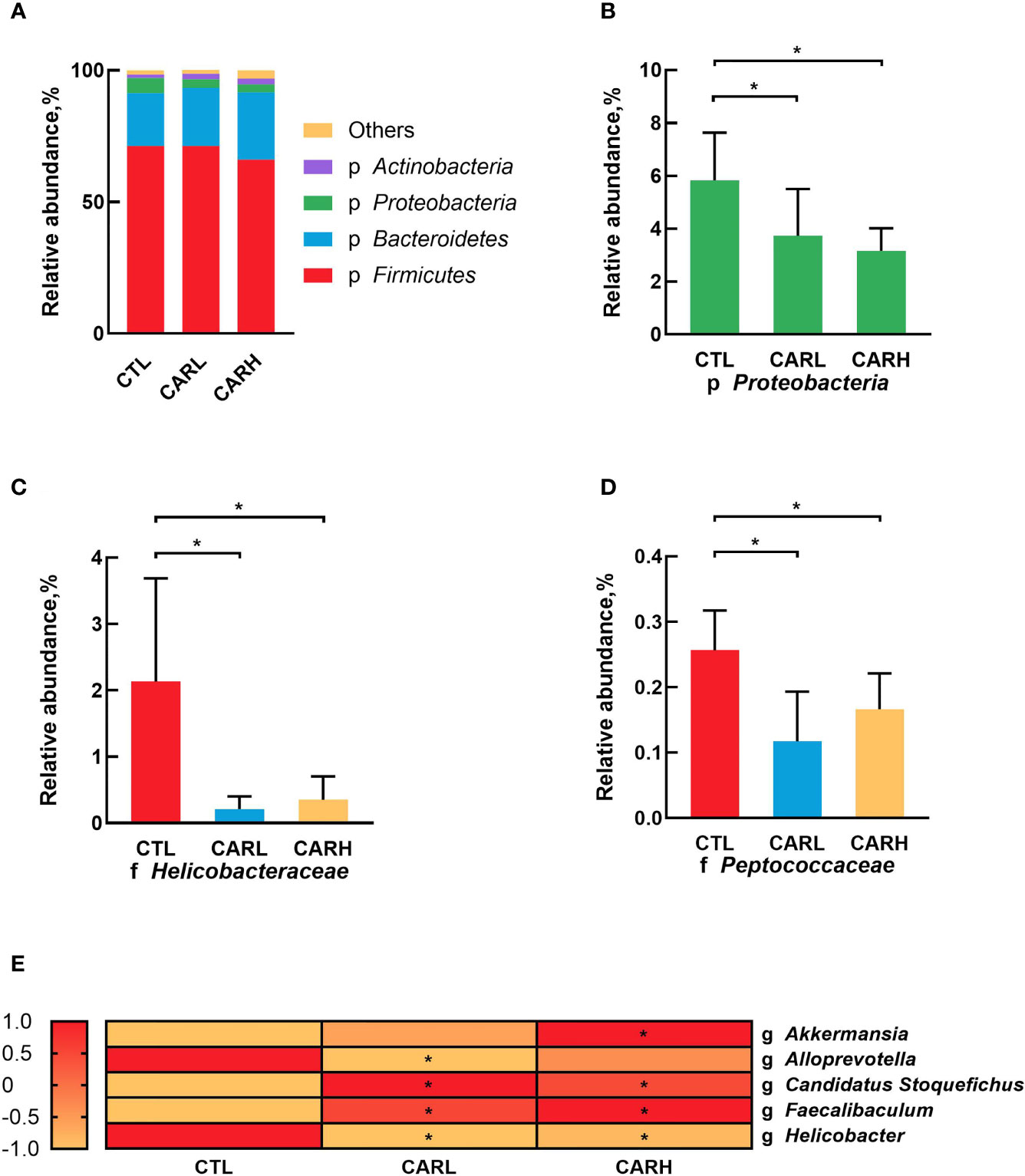
Figure 4 Modulation of gut microbiota by β-carotene. Effect of β-carotene on the relative abundance of main microbes at the phylum level (A), proteobacteria phylum (B), Helicobacteraceae family (C) and Peptococcaceae family (D), and main microbial genera (E) were analyzed by 16S rRNA gene sequencing. CTL, a basal diet; CARL, a basal diet containing 30 mg/kg β-carotene; CARH, a basal diet containing 90 mg/kg β-carotene. Data were shown as means ± SD (n = 6). *P < 0.05.
Correlation Analysis of Gut Microbiota and Serum Inflammatory Cytokines
The correlation between the abundance of the top 40 genera and serum inflammatory cytokines concentration were presented in Figure 5A. Serum TNF-α concentration had a significantly positive correlation with the relative abundances of Alloprevotella (P<0.05), Candidatus Arthromitus (P<0.05), Helicobacter (P<0.05) and Lachnospiraceae UCG 006 (P<0.05), but had a negative correlation with the relative abundance of Candidatus Stoquefichus (P<0.05) and Faecalibaculum (P<0.05). Moreover, there was a significant positive correlation between serum IL-4 concentration and the relative abundance of Helicobacter (P<0.05), while serum IL-4 concentration was negatively associated with the relative abundances of Akkermansia (P<0.05), Candidatus Stoquefichus (P<0.05) and Faecalibaculum (P<0.05). The linear association of these microbial genera with serum inflammatory cytokines was shown in Figures 5B-F.
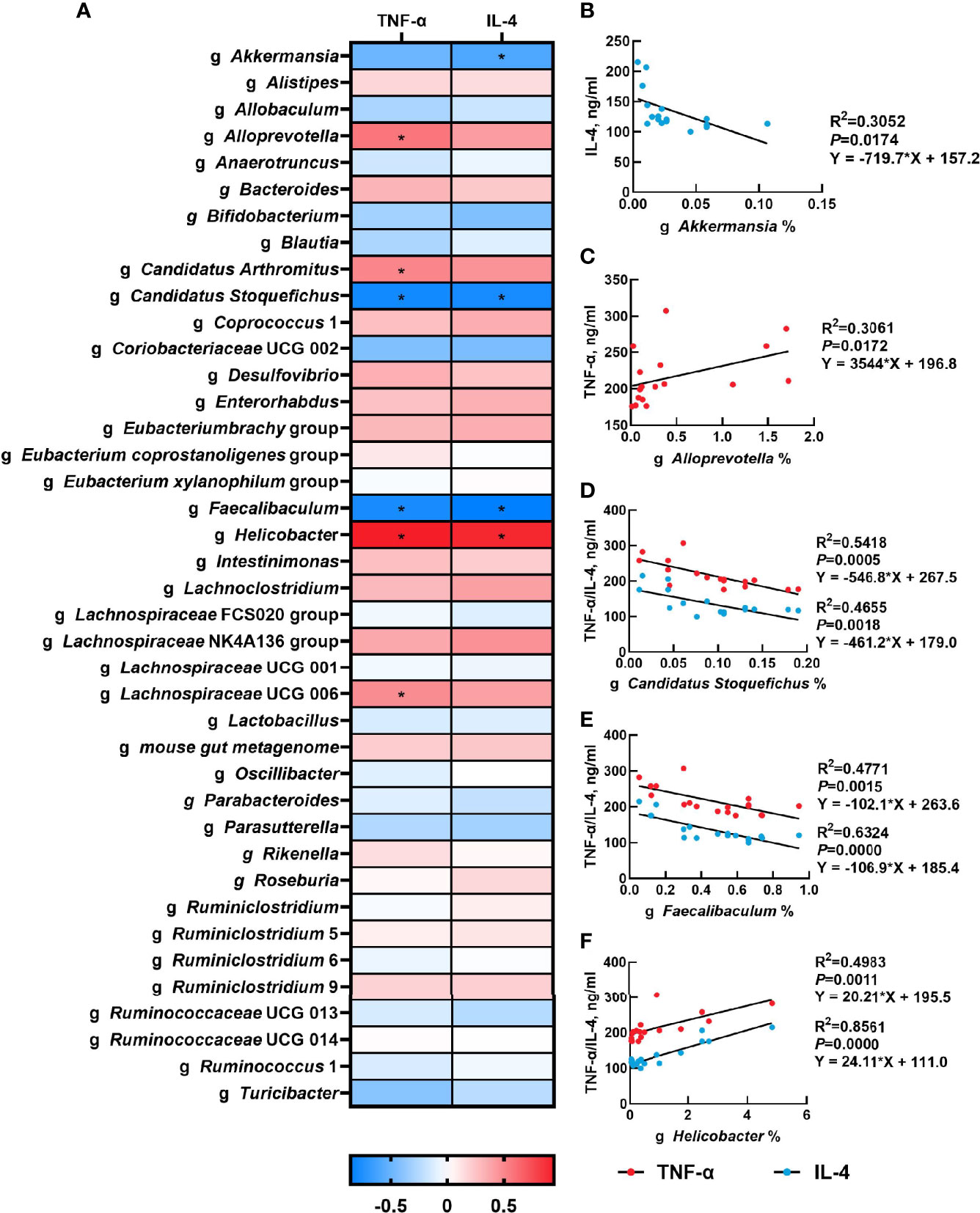
Figure 5 Correlation analysis of gut microbiota and serum inflammatory cytokines. The correlation analysis between the abundance of top 40 microbial genera and serum inflammatory cytokines concentration (A), and the linear correlation analysis between relative abundance of main microbial genera and inflammatory cytokines that significantly regulated by β-carotene (B-F) were analyzed by Spearman’s correlation analysis. CTL, a basal diet; CARL, a basal diet containing 30 mg/kg β-carotene; CARH, a basal diet containing 90 mg/kg β-carotene. (*P < 0.05).
Discussion
Multiple studies have focused on the effect of β-carotene on offspring, however, the impact of β-carotene on maternal and postpartum recovery is not clear. Our recent study has shown that dietary supplementation of β-carotene had a positive effect on the reproductive performance of sows (8), and in vitro inhibitory effect of β-carotene on cytokines was revealed by Lin et al. (25). Delivery or the release of the placenta often causes damage to the epithelium of the endometrium (26), and postpartum uterine hemorrhage is a common phenomenon (27). The present study showed that β-carotene could contribute to uterine recovery through reducing intrauterine blood stasis, with decreased uterine mass index and inflammation. Mild increase in inflammation has been considered as a normative part of healthy pregnancy, but abnormally elevated level of inflammation during pregnancy is associated with adverse birth outcomes (28). In this study, dietary supplementation of β-carotene decreased serum TNF-α and IL-4 concentration in mice after delivery. TNF-α plays a role in the mechanism of inflammation-induced preterm labor through increasing uterine smooth muscle cell collagen contractility (29, 30). TNF-α may directly promote tissue damage in pregnancy, as suggested by in vitro studies where TNF-α activated maternal monocytes bound to LFA-1 on placental syncytiotrophoblast and induced apoptosis (31). Immune regulation at the maternal-fetal interface is complex due to conflicting immunological objectives: protection of the fetus from maternal pathogens and prevention of immune-mediated rejection of the semi-allogeneic fetus and placenta (32). As an anti-inflammatory factor, IL-4 exerts immuno-suppressive effect by stimulating B-cells and T-cells (33). IL-4 is one of the pleiotropic anti-inflammatory cytokines that function mainly by suppressing the proinflammatory milieu (34). In this study, β-carotene decreased serum IL-4 concentration, but it has been reported that IL-4-knockout mice have normal pregnancies with respect to fetal growth and development, this would suggest that the role of an individual cytokine may not be crucial to the success of pregnancy (35). Oxidative stress can activate inflammatory cytokines (36), and our previous study demonstrated that improving antioxidant capacity can alleviate inflammation (37). Based on the relationship between inflammation and oxidation, we detected the antioxidant capacity of mice liver, but the results showed that β-carotene had no significant effect on the T-AOC and MDA content. Therefore, β-carotene exerted the anti-inflammatory effect in mice independent its antioxidant capacity. Inflammation is closely related to intestinal barrier function (38), β-carotene is mainly absorbed in the intestine, it has affinity with intestinal epithelial cells, enters the cytoplasm through the brush edge membrane, and then plays role after being catalyzed by enzymes (39). β-carotene robusts modulator of mucosal barriers (40), it improves villus height and villus height to crypt depth ratio and microbiota composition in this study, which has positive effect on the barrier function.
The microbiota play a critical role in maintaining health (41), including nutrient acquisition, immune programming, and protection from pathogens. Our previous studies have revealed that β-carotene possess an effect on gut microbiota (8), which are closely related to inflammation (22). The maternal gut microbiota undergoes dramatic changes throughout the gestation period, in which α-diversity will gradually increase throughout the lactation period (42). During pregnancy, the natural homeostasis of the gut microbiota changes toward a more inflammatory state (43), which may associate with the maternal low-grade inflammation caused by reduced fetal immune rejection. Results of the present study showed that β-carotene can significantly increase chao 1 index, observed species index and PD whole tree index, which are often used to estimate the total number of species. Specifically, β-carotene down-regulated the relative abundance of Proteobacteria, the microbial phylum that has been reported to be associated with increased susceptibility to colitis (44). Moreover, dietary supplementation of β-carotene improves intestinal integrity with decreased serum IL-4 concentration, which was negatively associated with the relative abundances of Akkermansia, a microbe genus that has been reported to increase the number of goblet cells and augment barrier integrity (45), which suggesting that the decrease of IL-4 may be related to the occurrence of low-grade inflammation in normal pregnancy. Additionally, β-carotene increased the relative abundance of genus Candidatus Stoquefichus, which was negatively correlated with serum TNF-α and IL-4 concentration in the present study and results by Lu et al. (46). Supplementation of β-carotene also enriched the genus Faecalibaculum, which contributes to butyrate production along with a shift from lactate metabolism to increased short chain fatty acids (SCFAs) production and carbohydrate metabolism (47). SCFAs mediate the transmission of signals between the microbiome and the immune system and are responsible for maintaining balance in the anti-inflammatory reaction (48). On the other hand, β-carotene reduced the relative abundance of microbial genera including Alloprevotella and Helicobacter. Correlation analysis revealed that there was a significant positive correlation between the abundance of Alloprevotella and serum TNF-α level, while the abundance of Helicobacter was positively correlated with serum levels of TNF-α and IL-4. Alloprevotella has been reported as producing SCFAs, and has a potential negative correlation with obesity, diabetes and metabolic syndrome (49). Helicobacter has a potential correlation with enteritis (50), and is considered as the majority of colonized individuals developing chronic inflammation (51). Therefore, the beneficial effects of β-carotene on inflammation may depend on a combined impact on the gut microbiota.
Conclusions
In conclusion, dietary supplementation of β-carotene showed a positive effect on reproductive performance and postpartum recovery, with reduced postpartum hemorrhage and uterine mass index, improved villus height and villus height to crypt depth ratio, potentially by regulating inflammation-related gut microbiota. Analysis on gut microbiota revealed that β-carotene up-regulated the relative abundance of Akkermansia, Candidatus Stoquefichus and Faecalibaculum at the genus level, but down-regulated the relative abundance of Proteobacteria at the phylum level, Helicobacteraceae and Peptococcaceae at the family level, Alloprevotella and Helicobacter at the genus level. And there was a significant correlation between microbiota and serum levels of inflammatory factors.
Data Availability Statement
The original contributions presented in the study are publicly available. This data can be found here: https://www.ncbi.nlm.nih.gov/sra/PRJNA781130.
Ethics Statement
The animal study was reviewed and approved by Animal Care and Use Committee of Hunan Agricultural University (Permission No. 2020041).
Author Contributions
XZY, ZH and SW are the primary investigators in this study. RH, JY, QZ, BL and XPY participated in the animal experiments. HZ and JH revised the manuscript. SW designed this study and wrote the manuscript as corresponding author. All authors contributed to the article and approved the submitted version.
Funding
This work was partially supported by the funds from the National Natural Science Foundation of China (31741115), Fellowship of China Postdoctoral Science Foundation (2021T140715), and Hunan Provincial Natural Science Foundation for Distinguished Young Scholars (2019JJ30012).
Conflict of Interest
Author XPY was employed by the company Hunan Xinguang’an Agricultural Husbandry Co., Ltd.
The remaining authors declare that the research was conducted in the absence of any commercial or financial relationships that could be construed as a potential conflict of interest.
Publisher’s Note
All claims expressed in this article are solely those of the authors and do not necessarily represent those of their affiliated organizations, or those of the publisher, the editors and the reviewers. Any product that may be evaluated in this article, or claim that may be made by its manufacturer, is not guaranteed or endorsed by the publisher.
References
1. Kaulmann A, Bohn T. Carotenoids, Inflammation, and Oxidative Stress–Implications of Cellular Signaling Pathways and Relation to Chronic Disease Prevention. Nutr Res (2014) 34(11):907–29. doi: 10.1016/j.nutres.2014.07.010
2. Xue C, Li Y, Lv H, Zhang L, Bi C, Dong N, et al. Oleanolic Acid Targets the Gut-Liver Axis to Alleviate Metabolic Disorders and Hepatic Steatosis. J Agric Food Chem (2021) 69(28):7884–97. doi: 10.1021/acs.jafc.1c02257
3. Hamer M, Chida Y. Intake of Fruit, Vegetables, and Antioxidants and Risk of Type 2 Diabetes: Systematic Review and Meta-Analysis. J Hypertens (2007) 25(12):2361–9. doi: 10.1097/HJH.0b013e3282efc214
4. Oliveira RC, Guerreiro BM, Morais Junior NN, Araujo RL, Pereira RA, Pereira MN. Supplementation of Prepartum Dairy Cows With Beta-Carotene. J Dairy Sci (2015) 98(9):6304–14. doi: 10.3168/jds.2014-9037
5. Schweigert FJ, Buchholz I, Schuhmacher A, Gropp J. Effect of Dietary Beta-Carotene on the Accumulation of Beta-Carotene and Vitamin A in Plasma and Tissues of Gilts. Reproduction Nutrition Dev (2001) 41(1):47–55. doi: 10.1051/rnd:2001111
6. Wassef L, Shete V, Hong A, Spiegler E, Quadro L. Beta-Carotene Supplementation Decreases Placental Transcription of LDL Receptor-Related Protein 1 in Wild-Type Mice and Stimulates Placental Beta-Carotene Uptake in Marginally Vitamin A-Deficient Mice. J Nutr (2012) 142(8):1456–62. doi: 10.3945/jn.112.162677
7. Chen J, Chen J, Zhang Y, Lv Y, Qiao H, Tian M, et al. Effects of Maternal Supplementation With Fully Oxidised Beta-Carotene on the Reproductive Performance and Immune Response of Sows, as Well as the Growth Performance of Nursing Piglets. Br J Nutr (2020) 125(1):62–70. doi: 10.1017/S0007114520002652
8. Yuan X, Yan J, Hu R, Li Y, Wang Y, Chen H, et al. Modulation of Gut Microbiota and Oxidative Status by β-Carotene in Late Pregnant Sows. Front Nutr (2020) 7:612875. doi: 10.3389/fnut.2020.612875
9. Krammer G, Aurich J. Effect of Intramuscularly Administered Beta-Carotene on Reproductive Performance in Sows. Berliner Und Munchener Tierarztliche Wochenschrift (2010) 123(11-12):496–9. doi: 10.2376/0005-9366-123-496
10. Bai S-K, Lee S-J, Na H-J, Ha K-S, Han J-A, Lee H, et al. Beta-Carotene Inhibits Inflammatory Gene Expression in Lipopolysaccharide-Stimulated Macrophages by Suppressing Redox-Based NF-kappaB Activation. Exp Mol Med (2005) 37(4):323–34. doi: 10.1038/emm.2005.42
11. Gilroy DW, Lawrence T, Perretti M, Rossi AG. Inflammatory Resolution: New Opportunities for Drug Discovery. Nat Rev Drug Discovery (2004) 3(5):401–16. doi: 10.1038/nrd1383
12. Makarov SS. NF-kappaB as a Therapeutic Target in Chronic Inflammation: Recent Advances. Mol Med Today (2000) 6(11):441–8. doi: 10.1016/S1357-4310(00)01814-1
13. Calcagno M, Pastore M, Scotto di Palumbo V. Laparoendoscopic Single-Site Assisted Vaginal Hysterectomy. Int J Gynaecol Obstet (2012) 116(3):264–5. doi: 10.1016/j.ijgo.2011.10.012
14. Vila Pouca MCP, Ferreira JPS, Oliveira DA, Parente MPL, Mascarenhas MT, Natal Jorge RM. Simulation of the Uterine Contractions and Foetus Expulsion Using a Chemo-Mechanical Constitutive Model. Biomech Model Mechanobiol (2019) 18(3):829–43. doi: 10.1007/s10237-019-01117-5
15. Chew BP, Weng BB, Kim HW, Wong TS, Park JS, Lepine AJ. Uptake of Beta-Carotene by Ovarian and Uterine Tissues and Effects on Steroidogenesis During the Estrous Cycle in Cats. Am J Veterinary Res (2001) 62(7):1063–7. doi: 10.2460/ajvr.2001.62.1063
16. Kaewlamun W, Okouyi M, Humblot P, Techakumphu M, Ponter AA. Does Supplementing Dairy Cows With Beta-Carotene During the Dry Period Affect Postpartum Ovarian Activity, Progesterone, and Cervical and Uterine Involution? Theriogenology (2011) 75(6):1029–38. doi: 10.1016/j.theriogenology.2010.11.010
17. Fung TC, Olson CA, Hsiao EY. Interactions Between the Microbiota, Immune and Nervous Systems in Health and Disease. Nat Neurosci (2017) 20(2):145–55. doi: 10.1038/nn.4476
18. Koren O, Goodrich JK, Cullender TC, Spor A, Laitinen K, Backhed HK, et al. Host Remodeling of the Gut Microbiome and Metabolic Changes During Pregnancy. (Cell) (2012) 150(3):470–80. doi: 10.1016/j.cell.2012.07.008
19. Li G, Yin P, Chu S, Gao W, Cui S, Guo S, et al. Correlation Analysis Between GDM and Gut Microbial Composition in Late Pregnancy. J Diabetes Res (2021) 2021:8892849. doi: 10.1155/2021/8892849
20. Verdam FJ, Fuentes S, de Jonge C, Zoetendal EG, Erbil R, Greve JW, et al. Human Intestinal Microbiota Composition is Associated With Local and Systemic Inflammation in Obesity. Obes (Silver Spring) (2013) 21(12):E607–15. doi: 10.1002/oby.20466
21. de Castro J, Sevillano J, Marciniak J, Rodriguez R, Gonzalez-Martin C, Viana M, et al. Implication of Low Level Inflammation in the Insulin Resistance of Adipose Tissue at Late Pregnancy. Endocrinology (2011) 152(11):4094–105. doi: 10.1210/en.2011-0068
22. Hu R, He Z, Liu M, Tan J, Zhang H, Hou DX, et al. Dietary Protocatechuic Acid Ameliorates Inflammation and Up-Regulates Intestinal Tight Junction Proteins by Modulating Gut Microbiota in LPS-Challenged Piglets. J Anim Sci Biotechnol (2020) 11:92. doi: 10.1186/s40104-020-00492-9
23. Xiong X, Yang H, Tan B, Yang C, Wu M, Liu G, et al. Differential Expression of Proteins Involved in Energy Production Along the Crypt-Villus Axis in Early-Weaning Pig Small Intestine. Am J Physiol Gastrointest Liver Physiol (2015) 309(4):G229–37. doi: 10.1152/ajpgi.00095.2015
24. Yu H, Shang L, Zeng X, Li N, Liu H, Cai S, et al. Risks Related to High-Dosage Recombinant Antimicrobial Peptide Microcin J25 in Mice Model: Intestinal Microbiota, Intestinal Barrier Function, and Immune Regulation. J Agric Food Chem (2018) 66(43):11301–10. doi: 10.1021/acs.jafc.8b03405
25. Lin HW, Chang TJ, Yang DJ, Chen YC, Wang M, Chang YY. Regulation of Virus-Induced Inflammatory Response by Beta-Carotene in RAW264. 7 Cells Food Chem (2012) 134(4):2169–75. doi: 10.1016/j.foodchem.2012.04.024
26. Sheldon IM, Cronin JG, Bromfield JJ. Tolerance and Innate Immunity Shape the Development of Postpartum Uterine Disease and the Impact of Endometritis in Dairy Cattle. Annu Rev Anim Biosci (2019) 7:361–84. doi: 10.1146/annurev-animal-020518-115227
27. Zhang Q, Li T, Xu Y, Hu Y. The New Clinical Application of Bilateral-Contralateral Cervix Clamp in Postpartum Hemorrhage: A Retrospective Cohort Study. BMC Pregnancy Childbirth (2021) 21(1):53. doi: 10.1186/s12884-020-03518-2
28. McDade TW, Borja JB, Largado F, Adair LS, Kuzawa CW. Adiposity and Chronic Inflammation in Young Women Predict Inflammation During Normal Pregnancy in the Philippines. J Nutr (2016) 146(2):353–7. doi: 10.3945/jn.115.224279
29. Alexander HA, Sooranna SR, Myatt L, Johnson MR. Myometrial Tumor Necrosis Factor-Alpha Receptors Increase With Gestation and Labor and Modulate Gene Expression Through Mitogen-Activated Kinase and Nuclear Factor-Kappab. Reprod Sci (2012) 19(1):43–54. doi: 10.1177/1933719111413297
30. Fitzgibbon J, Morrison JJ, Smith TJ, O’Brien M. Modulation of Human Uterine Smooth Muscle Cell Collagen Contractility by Thrombin, Y-27632, TNF Alpha and Indomethacin. Reprod Biol Endocrinol (2009) 7:2. doi: 10.1186/1477-7827-7-2
31. Romanowska-Prochnicka K, Felis-Giemza A, Olesinska M, Wojdasiewicz P, Paradowska-Gorycka A, Szukiewicz D. The Role of TNF-Alpha and Anti-TNF-Alpha Agents During Preconception, Pregnancy, and Breastfeeding. Int J Mol Sci (2021) 22(6):2922. doi: 10.3390/ijms22062922
32. Casazza RL, Lazear HM, Miner JJ. Protective and Pathogenic Effects of Interferon Signaling During Pregnancy. Viral Immunol (2020) 33(1):3–11. doi: 10.1089/vim.2019.0076
33. West GA, Matsuura T, Levine AD, Klein JS, Fiocchi C. Interleukin 4 in Inflammatory Bowel Disease and Mucosal Immune Reactivity. Gastroenterology (1996) 110(6):1683–95. doi: 10.1053/gast.1996.v110.pm8964392
34. Chatterjee P, Chiasson VL, Bounds KR, Mitchell BM. Regulation of the Anti-Inflammatory Cytokines Interleukin-4 and Interleukin-10 During Pregnancy. Front Immunol (2014) 5:253. doi: 10.3389/fimmu.2014.00253
35. Svensson L, Arvola M, Sallstrom MA, Holmdahl R, Mattsson R. The Th2 Cytokines IL-4 and IL-10 are Not Crucial for the Completion of Allogeneic Pregnancy in Mice. J Reprod Immunol (2001) 51(1):3–7. doi: 10.1016/S0165-0378(01)00065-1
36. Reuter S, Gupta SC, Chaturvedi MM, Aggarwal BB. Oxidative Stress, Inflammation, and Cancer: How are They Linked? Free Radic Biol Med (2010) 49(11):1603–16. doi: 10.09.006
37. Wu S, Yano S, Chen J, Hisanaga A, Sakao K, He X, et al. Polyphenols From Lonicera Caerulea L. Berry Inhibit LPS-Induced Inflammation Through Dual Modulation of Inflammatory and Antioxidant Mediators. J Agric Food Chem (2017) 65(25):5133–41. doi: 10.1021/acs.jafc.7b01599
38. Pastorelli L, De Salvo C, Mercado JR, Vecchi M, Pizarro TT. Central Role of the Gut Epithelial Barrier in the Pathogenesis of Chronic Intestinal Inflammation: Lessons Learned From Animal Models and Human Genetics. Front Immunol (2013) 4:280. doi: 10.3389/fimmu.2013.00280
39. Nagao A, Maeda M, Lim BP, Kobayashi H, Terao J. Inhibition of Beta-Carotene-15,15’-Dioxygenase Activity by Dietary Flavonoids. J Nutr Biochem (2000) 11(6):348–55. doi: 10.1016/S0955-2863(00)00090-5
40. Hui J, Li L, Li R, Wu M, Yang Y, Wang J, et al. Effects of Supplementation With Beta-Carotene on the Growth Performance and Intestinal Mucosal Barriers in Layer-Type Cockerels. Anim Sci J (2020) 91(1):e13344. doi: 10.1111/asj.13344
41. Flint HJ, Scott KP, Louis P, Duncan SH. The Role of the Gut Microbiota in Nutrition and Health. Nat Rev Gastroenterol Hepatol (2012) 9(10):577–89. doi: 10.1038/nrgastro.2012.156
42. Ji YJ, Li H, Xie PF, Li ZH, Li HW, Yin YL, et al. Stages of Pregnancy and Weaning Influence the Gut Microbiota Diversity and Function in Sows. J Appl Microbiol (2019) 127(3):867–79. doi: 10.1111/jam.14344
43. Gomez-Arango LF, Barrett HL, Wilkinson SA, Callaway LK, McIntyre HD, Morrison M, et al. Low Dietary Fiber Intake Increases Collinsella Abundance in the Gut Microbiota of Overweight and Obese Pregnant Women. Gut Microbes (2018) 9(3):189–201. doi: 10.1080/19490976.2017.1406584
44. Shin NR, Whon TW, Bae JW. Proteobacteria: Microbial Signature of Dysbiosis in Gut Microbiota. Trends Biotechnol (2015) 33(9):496–503. doi: 10.1016/j.tibtech.2015.06.011
45. Shin NR, Lee JC, Lee HY, Kim MS, Whon TW, Lee MS, et al. An Increase in the Akkermansia Spp. Population Induced by Metformin Treatment Improves Glucose Homeostasis in Diet-Induced Obese Mice. Gut (2014) 63(5):727–35. doi: 10.1136/gutjnl-2012-303839
46. Lu Y-X, He C-Z, Wang Y-X, Ai Z-S, Liang P, Yang C-Q. Effect of Entecavir on the Intestinal Microflora in Patients With Chronic Hepatitis B: A Controlled Cross-Sectional and Longitudinal Real-World Study. Infect Dis Ther (2020) 10(1):241–52. doi: 10.1007/s40121-020-00355-w
47. Ke X, Walker A, Haange SB, Lagkouvardos I, Liu Y, Schmitt-Kopplin P, et al. Synbiotic-Driven Improvement of Metabolic Disturbances is Associated With Changes in the Gut Microbiome in Diet-Induced Obese Mice. Mol Metab (2019) 22:96–109. doi: 10.1016/j.molmet.2019.01.012
48. Szczuko M, Kikut J, Maciejewska D, Kulpa D, Celewicz Z, Zietek M. The Associations of SCFA With Anthropometric Parameters and Carbohydrate Metabolism in Pregnant Women. Int J Mol Sci (2020) 21(23). doi: 10.3390/ijms21239212
49. Shang Q, Song G, Zhang M, Shi J, Xu C, Hao J, et al. Dietary Fucoidan Improves Metabolic Syndrome in Association With Increased Akkermansia Population in the Gut Microbiota of High-Fat Diet-Fed Mice. J Funct Foods (2017) 28:138–46. doi: 10.1016/j.jff.2016.11.002
50. Belkaid Y, Hand TW. Role of the Microbiota in Immunity and Inflammation. Cell (2014) 157(1):121–41. doi: 10.1016/j.cell.2014.03.011
Keywords: β-carotene, postpartum uterine recovery, gut microbiota, inflammation, reproductive performance
Citation: Yang X, He Z, Hu R, Yan J, Zhang Q, Li B, Yuan X, Zhang H, He J and Wu S (2021) Dietary β-Carotene on Postpartum Uterine Recovery in Mice: Crosstalk Between Gut Microbiota and Inflammation. Front. Immunol. 12:744425. doi: 10.3389/fimmu.2021.744425
Received: 20 July 2021; Accepted: 01 November 2021;
Published: 24 November 2021.
Edited by:
Xia Xiong, Institute of Subtropical Agriculture (CAS), ChinaReviewed by:
Lijun Zou, Hunan First Normal University, ChinaHongkui Wei, Huazhong Agricultural University, China
Jinming You, Jiangxi Agricultural University, China
Copyright © 2021 Yang, He, Hu, Yan, Zhang, Li, Yuan, Zhang, He and Wu. This is an open-access article distributed under the terms of the Creative Commons Attribution License (CC BY). The use, distribution or reproduction in other forums is permitted, provided the original author(s) and the copyright owner(s) are credited and that the original publication in this journal is cited, in accordance with accepted academic practice. No use, distribution or reproduction is permitted which does not comply with these terms.
*Correspondence: Jianhua He, jianhuahy@hunau.net; Shusong Wu, wush688@hunau.edu.cn
†These authors have contributed equally to this work and share first authorship