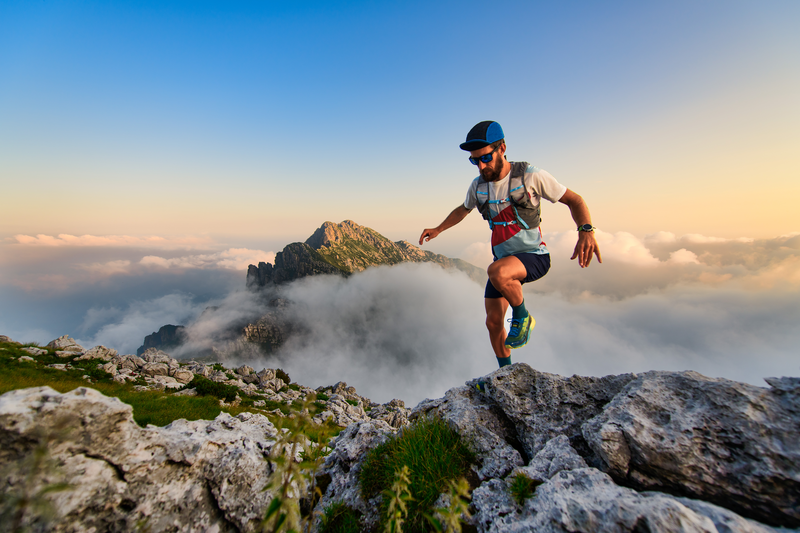
95% of researchers rate our articles as excellent or good
Learn more about the work of our research integrity team to safeguard the quality of each article we publish.
Find out more
REVIEW article
Front. Immunol. , 15 September 2021
Sec. Nutritional Immunology
Volume 12 - 2021 | https://doi.org/10.3389/fimmu.2021.743022
This article is part of the Research Topic Breast Milk and Passive Immunity during the COVID-19 Pandemic View all 11 articles
A correction has been applied to this article in:
Corrigendum: The effects of COVID-19 on the placenta during pregnancy
Coronavirus disease 2019 (COVID-19) caused by the severe acute respiratory syndrome coronavirus 2 (SARS-CoV-2) has caused a global pandemic. The virus primarily affects the lungs where it induces respiratory distress syndrome ranging from mild to acute, however, there is a growing body of evidence supporting its negative effects on other system organs that also carry the ACE2 receptor, such as the placenta. The majority of newborns delivered from SARS-CoV-2 positive mothers test negative following delivery, suggesting that there are protective mechanisms within the placenta. There appears to be a higher incidence of pregnancy-related complications in SARS-CoV-2 positive mothers, such as miscarriage, restricted fetal growth, or still-birth. In this review, we discuss the pathobiology of COVID-19 maternal infection and the potential adverse effects associated with viral infection, and the possibility of transplacental transmission.
The World Health Organization (WHO) declared a global pandemic of coronavirus disease 2019 (COVID-19) in March 2020, caused by the Severe Acute Respiratory Syndrome Coronavirus 2 (SARS-CoV-2) (1). As of August 2021, the number of total cases surpassed 200 million and resulted in more than 4 million deaths. There is an ongoing effort to understand transmission, incidence, disease pathogenesis and the short- and long-term impacts following infection. In particular, the impact of SARS-CoV-2 infection on mothers and their babies (2). Evidence suggests that pregnant women with COVID-19 are more susceptible to severe disease with a higher risk of preterm birth (3–5), as well as higher risk of maternal and/or fetal death (6, 7). These findings are reminiscent of the dire outcomes from other similar respiratory viral infections, such as influenza A/H1N1 (8–11), severe acute respiratory syndrome (SARS) (12), and Middle East Respiratory Syndrome (MERS) (13, 14), where infected pregnant women are at increased risk of severe morbidity and mortality to both themselves and their infants (2). While most neonates born to SARS-CoV-2 positive mothers test negative and do not present with virus-induced disease, there have been some cases of newborns testing positive and presenting with early-onset symptoms (15). Whether this is due to the trans-placental transmission of SARS-CoV-2, or infection following delivery is still not well understood (16–18). Examination of the placentas from SARS-CoV-2 positive mothers have mixed reports on viral positivity, and not all neonates born from mothers with a SARS-CoV-2 positive placenta test positive for the virus (19). This suggests that there is a protective mechanism/barrier within the placenta, where its success may rely on the presence or absence of certain receptors/pathways. Fortunately, SARS-CoV-2 positive neonates are yet to present with any congenital defects (20). In this review, we provide an overview of the literature of SARS-CoV-2 infection during pregnancy, as well as the pathobiology of the placenta which may protect the growing fetus.
The immune system changes during pregnancy in such a way that it adapts to the growth of a semi-allogeneic fetus in the body of the mother, resulting in a distinct immune response to different infections during pregnancy (21–23). It has been well documented that in patients with COVID-19, particularly those with severe disease, have profound immune dysregulation (24). Studies have revealed an increase in blood leukocytes (leukocytosis), which was characterized by a decrease in lymphocytes (lymphopenia) and an increase in neutrophil-to-lymphocyte ratio (NLR) (25, 26). Using immunophenotyping analyses, researchers discovered that patients with severe COVID-19 had fewer natural killer (NK), CD3+, CD4+, and CD8+ T cells than those with the non-severe disease (27). NK cells were also found to be functionally exhausted during SARS-CoV-2 infection (28–30). Moreover, a reduction in circulating NK cell population has been reported during gestation (31). NK cells have key roles in the innate immune response by killing transformed cells, as consequence of viral infections or oncogenesis; NK cells are also major sources of pro-inflammatory cytokines such as granulocyte-macrophage colony-stimulating factor (GM-CSF) and interferon gamma (IFN-γ), which can restore or activate the antiviral property of the myeloid compartment; thus, any decrease in these cell populations may alter the ability to clear viruses (32). Evidence has shown that lymphopenia and enhanced NLR can be further amplified by COVID-19 disease severity (33). Compared to patients with moderate COVID-19, individuals with severe disease had lower numbers of cytotoxic T lymphocytes (CTLs) (33). Studies investigating COVID-19 patients’ lung tissue and bronchoalveolar lavage fluid (BALF) samples found T cell hyperactivation and/or upregulation of pro-apoptotic factors, including first apoptosis signal receptor (FAS), TNF‐related apoptosis‐inducing ligand (TRAIL), and caspase 3, as the main causes of T cell depletion (34–36). Alterations in CD4+ T cell population toward T helper-2 (Th)-2 phenotypes rather than Th1 phenotypes have been found during pregnancy, which contributes to the promotion of humoral immune responses over cellular immune responses (37). There is also a balance between regulatory T cell (Treg) and Th17 cells during pregnancy; with a shift towards Tregs to ensure fetal-maternal immune tolerance and to prevent fetal allograft rejection (38). In terms of innate immune cells, evidence suggests that, while absolute peripheral blood monocyte counts are not significantly different between patients with severe COVID-19 and those with moderate disease, the activation status of the monocyte/macrophage system is significantly altered (39). It was shown that monocyte/macrophage alterations caused by SARS-CoV-2 infection are similar to a condition known as familial hemophagocytic lymphohistiocytosis (HLH), a systemic inflammatory disorder involving cytokine production and cytopenia (40–42). HLH can be triggered either by abnormalities in genes regulating NK and cytotoxic CD8+ T cell degranulation or by conditions such as autoimmune disease, malignancy, and viral infection (40, 41). It was found that patients with H1N1 influenza who experienced the ‘cytokine storm,’ characterized by the extreme and excessive immune and inflammatory response (43), had mutations in genes associated with HLH (44). Many studies, however, do not support the link between HLH and COVID-19 (45–47). Wood et al., found that only three of 40 COVID-19 patients had Hscores >169, the cut-off used to identify HLH (47). Several studies have reported widespread infiltration of monocytes/macrophages in the lung tissue samples taken from COVID-19 patients (35, 48, 49). Single-cell studies revealed that monocyte-derived FCN1+ macrophages were the most abundant macrophage subset found in BALF samples from severe COVID-19 patients (35). Furthermore, it was discovered that peripheral monocyte trafficking and subsequent differentiation into macrophages in the lungs of COVID-19 patients contributes to pro-inflammatory responses and further activation of innate immune cells (49). Changes in the innate immune system during pregnancy, also, involve the pattern recognition receptors Toll-like receptors (TLRs), in particular TLR4 (50, 51). There are three different levels of TLR4 activation during pregnancy. First, TLR4 activation and the inflammatory response rise during the first trimester, allowing blastocyst implantation. Following that, a decrease in TLR4 activation happens during the second trimester in order to create an anti-inflammatory response for fetal growth. Eventually, TLR4 activation and the inflammatory response increase again in the third trimester to support labor and delivery (52). Infection with COVID-19 leads to pyroptosis of host cells and the release of danger associated molecular patterns (DAMPs) that can act as ligands for TLR molecules and trigger a greater inflammatory response (31). Studies are needed to determine whether such changes in the immune system result in higher susceptibility or are protective against COVID-19 during pregnancy (31).
SARS-CoV-2 enters the body through the nasal passage and infects pulmonary cells by binding to the receptor angiotensin-converting enzyme 2 (ACE2) (31, 53–55). It has been found that ACE2 expresses in respiratory and intestinal track, placenta, ovaries, vagina, and uterus (56). Cell entry is further facilitated by viral spike (S) protein priming induced by trans-membrane serine protease 2 (TMPRSS2) (53–55). Cells co-expressing both ACE2 and TMPRSS2 have been found to have a higher susceptibility to SARS-CoV-2 entry (57) (Figure 1). In addition, furin, trypsin, and cathepsins B and L have been reported to be capable of cleaving the spike glycoprotein binding at the S1/S2 site, allowing the virus to enter (53, 58, 59). ACE2 has been shown to be expressed by fetal kidney, ilium, and rectal cells from as early as 15 weeks, barely detectable at 15 weeks in the lungs with undetected expression thereafter, and undetectable in the cerebral ependymal, parenchymal and cardiac cells (60). It has been found that only a proportion of cells which are located in the fetal adrenal gland and the kidney co-expressed ACE2 and TMPRSS2. It was discovered that placental cytotrophoblasts and syncytiotrophoblasts (STBs) express ACE2 from 7 weeks onward, suggesting that SARS-CoV-2 could cross into the placenta at any gestational age (60). Investigation of ACE2 and TMPRSS2 co-expression in the developing embryo up to day 14 (from surplus IVF human embryos) has revealed the co-localization of these genes, raising concern to increased susceptibility to SARS-CoV-2 fetal infection in the early stages of embryonic development (61). To date, cohort studies of SARS-CoV-2 positive mothers with mild symptoms or asymptomatic, have reported no adverse effects to the mother or neonate regardless of the timing of the infection (i.e. first versus third trimester) (62, 63). However, women with severe SARS-CoV-2 infection that required critical care had higher odds of complications, particularly a higher incidence of iatrogenic pre-term delivery mostly due to fear of sudden maternal decompensation (64).
The placenta offers a protective barrier that does not allow the fetus to become exposed to maternal infections (31). The human placenta primarily consists of a number of specific fetal-derived cells called trophoblasts, of which there are three main types. These include terminally differentiated multinuclear syncytiotrophoblast cells, which are in direct contact with the maternal blood and line the villus tree, progenitor villous cytotrophoblast cells, which underlie the syncytiotrophoblast, and invasive extravillous trophoblast (EVT) cells, which anchor the chorionic villi to the uterus and modify its vasculature (Figure 2) (31). Various potential causes may play a role in the vertical transmission of the virus from the mother to the fetus. These include direct damage to the villous tree with a break in the protective syncytiotrophoblast layer, which could be caused by virus-induced apoptosis and vascular damage in the placenta, spread through the virus-infected maternal endothelium to the extravillous trophoblast, trafficking of infected maternal immune cells throughout the syncytiotrophoblast, paracellular or transcellular transport (for example, immunoglobulin-mediated transcytosis) into fetal capillaries, transmission via swallowed or aspirated amniotic fluid (65, 66), as well as ascending infection from the vagina (Figure 3) (31). To define the possibility of vertical transmission of SARS-CoV-2 infection in different studies, a classification system has been proposed by a multidisciplinary team of the WHO (68). Given the timing of vertical transmission, in utero, intrapartum, and early postnatal period, four possibilities exist: confirmed, possible, unlikely, and indeterminate (68). Vertical transmission is considered “possible” if evidence suggests it but cannot confirm infection. However, if there is poor support of diagnosis, but vertical transmission cannot be completely ruled out, this is considered as “unlikely”. The “indeterminate” possibility is when the tests required to define the classification have not been performed (68). Recent findings confirming the presence of SARS-CoV-2 mRNA or virions in syncytiotrophoblasts have strongly suggested transplacental infection caused by the SARS-CoV-2 (69, 70). Nonetheless, given that the presence of SARS-CoV-2 in the blood sample of COVID-19 patients is reported to be around 1%, therefore the likelihood of SARS-CoV-2 being able to directly infect syncytiotrophoblasts is low (71). Another alternative way of transmitting SARS-CoV-2 infection to the neonate is through the vagina during childbirth (72, 73).
Figure 3 Possible mechanisms of transplacental transmission. There are several potential mechanisms involved in the virus’s vertical transmission from mother to fetus. (1) Infection caused by direct villous tree damage. (2) Infection through the maternal endothelium to the extravillous trophoblast. (3) Infection caused by maternal immune cell trafficking and transcellular transport. (4) Infection through the vagina. Adapted from (67).
Whilst the possibility of transmitting SARS-CoV-2 from mother to fetus during pregnancy is suggested, the role of the placenta in infection with the virus has not yet been fully understood. However, evidence suggests that pathogens can overcome this barrier, infect the fetus, and even cause serious complications in newborns, such as microcephaly and ocular abnormalities (74). Such pathogens include Cytomegalovirus (CMV), herpes simplex virus (HSV), varicella-zoster virus, and Zika virus (ZIKV) (20, 75–77). It is currently unclear whether neonates who tested positive for SARS-CoV-2 have been infected with the virus from their mothers during pregnancy or have been infected during labor or after birth. (Table 1). Evidence based on infant antibody tests suggests vertical transmission of the virus may be possible. It was discovered that infants born to women infected with SARS-CoV-2 had higher immunoglobulin (Ig)G and IgM levels for SARS-CoV-2 (31, 89, 90). The presence of IgG in the fetus may indicate the transfer of this immunoglobulin from the mother to the fetus during pregnancy, but the presence of IgM indicates that the fetus has produced and secreted this immunoglobulin in response to viral infection because in contrast to IgG, IgM is unable to cross the placenta due to its higher molecular weight (89, 90).
Several studies have employed single cell RNA sequencing (scRNA-seq) to gain an understanding of the molecular features of SARS-CoV-2 infection (91–95). In a study by Lu et al., which compared ACE2 and TMPRSS2 gene expression between fetal, placental tissues and adult tissues, a small proportion of trophoblast cells, as well as various fetal organs such as the heart, kidney, stomach, and adrenal glands, had ACE2 expression. The study showed that only the kidney and adrenal gland expressed TMPRSS2 (96). Pique-Regi et al. discovered that very few cells during any of the three trimesters expressed both ACE2 and TMPRSS2. Using single-nuclear RNAseq (snRNA-seq), it has been shown that the placenta is unlikely to express ACE2 and TMPRSS2, and thus be infected by SARS-CoV-2 (59). Using scRNA-seq data, Ashary et al., identified only a small proportion of STB in the first trimester and EVT in the second trimester had ACE2 and TMPRSS2 expression. The ACE2+TMPRSS2+STBs were highly differentiated and expressed genes engaged in mitochondrial metabolism and glucose transport. In addition, the ACE2+TMPRSS2+EVTs were found to have endovascular trophoblast markers. The researchers found that these cells could be the targets of SARS-CoV-2 entry (97). Moreover, robust immune responses at the maternal-fetal interface of SARS-CoV-2-infected women was discovered (98). Researchers found overexpression of interferon-related genes, and increased activation of NK cells and T cells (98–100). Also, it was found that there was an association between SARS-CoV-2 infection and local immune responses at the maternal-fetal interface (98). in a study by Nagy et al, the impact of mutations in SARS-CoV-2 viral genes on clinical outcomes was explored. The study found that mutations in the nucleocapsid phosphoprotein-N, nonstructural proteins-4 (NSP4), NSP6, Open Reading Frame-3a (ORF3a), and ORF8 were associated with mild outcome, while mutations in NSP7 were linked to severe disease (101).
The identification of new biomarkers and prevention strategies requires the fundamental understanding and control of how SARS-CoV-2 spreads to the lungs and elicits a multi-organ inflammatory response. (Table 2). These infection processes rely on their location and spatial context: which cells in which tissue locations are most susceptible to infection (105), infected cell-to-uninfected cell associations, and biochemical factor release of different cell types in response to infection (106). These spatiotemporal relationships in the inflammatory cascade give rise to positive or negative prognoses, and their understanding can triage patients at greater or lesser risks of infection, of response to infection, and inform new therapeutics and treatment regimens (107). Spatial immunoprofiling is rapidly advancing due to several recent technologies: advanced instrumentation, molecular barcoding and immunolabelling, providing a much richer portrait of the immune landscape (108), and recent approaches in biostatics and theoretical biology are incorporating imaging data to deconstruct the relationships between cells and disease within their tissue context (109–111). Spatial resolved transcriptomics are changing the ways in which we interrogate complex tissues and were voted the ‘Method of the Year 2020’ by the journal Nature Methods (112). These technologies combine the benefits in advancements in microscopy and advanced imaging, with simultaneous read out of transcript and proteomic data, thereby alleviating the challenges associated with single cell or bulk profiling. The maintenance of spatial context is key in understanding the underlying cellular profiles, biology, specialization and tissue organization and has begun shedding light into consortia studies such as the Human Cell Atlas. A number of technologies currently exist for RNA applications: Nanostring GeoMX Digital Spatial Profiler (DSP), 10x Genomics Visium, MERFISH and proteomic: Nanostring GeoMX DSP, Akoya Biosciences CODEX, Imaging Mass spectrometry (IMC) (113). Recent application of these methodologies to COVID-19 autopsy tissue studies from lungs, kidney, liver and heart tissue has provided deep insights into cell types and genes implicated with severe COVID-19 disease severity (114).
Once region- or cell-specific spatial information is derived from histology sections, statistical relationships between cells and tissues and mathematical predictions of their future behavior with or without treatment are often sought. There exist numerous tools to detect and segment single cell locations from this spatial information. While open-source ImageJ, developed in 1987, remains popular for microscopic image analysis (115–117), more recent software such as CellProfiler, Icy, ilastik, and QuPath provide user-friendly interfaces for the development of bioimage analysis macroscripts (118, 119). Once single-cell data can be derived, spatial relationships can be determined. The most common of which is intercellular clustering or associations, often calculated as cell density within concentric circles away from each cell’s center and averaged across all imaged cells (120). For instance, to characterize the distribution of SARS-CoV-2 bodies from macrophages or monocytes or tissue structures to estimate inflammatory progression (121).
Taking into account the changing physiology and immune responses during gestation, pregnant women are more susceptible to developing severe COVID-19, which can lead to pregnancy-related complications. There is limited information for the association of COVID-19 and its direct complications to the growing fetus during pregnancy. These may include preterm birth, stillbirth, or long-term complications for the newborn (122). A study conducted on 827 pregnant women, who have been given the COVID-19 mRNA vaccine, found that the proportion of adverse pregnancy and neonatal outcomes were similar to incidence reported in similar studies conducted prior to the pandemic (123). Furthermore, vaccination of pregnant women has been shown to result in maternal IgG production 5 days after the first dose of vaccination, as well as the transplacental transfer of IgG 16 days after the first dose of vaccination (124). However, longitudinal follow-up is needed to monitor those who are vaccinated, especially during the first trimester, in order to be informed about maternal, pregnancy, and neonatal outcomes. Another important consideration with COVID-19 infection during pregnancy is that current diagnostic tests such as X-ray and CT scans cannot be performed in pregnant women due to potential risks to the growing fetus (125). These factors may therefore delay the diagnosis and treatment of pregnant women, particularly those with more severe symptoms.
These factors may therefore delay the diagnosis and treatment of pregnant women, particularly those with more severe symptoms. Screening tests may be helpful in this respect because of the possibility of transmitting the virus from the mother to the fetus. Understanding the disease progression and its relationship to manifestation severity is necessary to therapeutically intervene and reduce the associated morbidity.
All authors listed have made a substantial, direct, and intellectual contribution to the work, and approved it for publication.
This project is supported by the Queensland University of Technology (QUT) ECR grant to AK, MA, NS, and JR. NS is supported by a US Department of Defense Prostate Cancer Early Investigator Award Fellowship (PC190533). MA is supported by an Advance Queensland Fellowship (AQIRF1312018). FSFG is funded by a UQ Diamantina Institute laboratory start-up package, Australian and New Zealand Sarcoma Association – Sarcoma Research Grant, a priority-driven collaborative cancer research scheme grant co-funded by Cancer Australia and Cure Cancer (#1158085), and a US Department of Defense – Breast Cancer Research Program – breakthrough award level 1 (#BC200025). AK is supported by an NHMRC Fellowship (APP1157741) and Cure Cancer (APP1182179).
FSFG is a consultant and has a funded research agreement with Biotheus Inc.
The remaining authors declare that the research was conducted in the absence of any commercial or financial relationships that could be construed as a potential conflict of interest.
All claims expressed in this article are solely those of the authors and do not necessarily represent those of their affiliated organizations, or those of the publisher, the editors and the reviewers. Any product that may be evaluated in this article, or claim that may be made by its manufacturer, is not guaranteed or endorsed by the publisher.
1. Senthil R, Kunchithapathan B, Ramalingam S, Manivannan P. COVID-19 Awareness and Its Impact in Rural and Urban Puducherry–a Community Based Cross Sectional Study. J Evol Med Dent Sci (2020) 9(51):3862–8. doi: 10.14260/jemds/2020/847
2. Knight M, Bunch K, Vousden N, Morris E, Simpson N, Gale C, et al. Characteristics and Outcomes of Pregnant Women Admitted to Hospital With Confirmed SARS-Cov-2 Infection in UK: National Population Based Cohort Study. BMJ (2020) 8:369. doi: 10.1136/bmj.m2107
3. Zambrano LD, Ellington S, Strid P, Galang RR, Oduyebo T, Tong VT, et al. Update: Characteristics of Symptomatic Women of Reproductive Age With Laboratory-Confirmed SARS-Cov-2 Infection by Pregnancy Status—United States, January 22–October 3, 2020. MMWR Morb Mortal Wkly Rep 69(44):1641–7. doi: 10.15585/mmwr.mm6944e3
4. Delahoy MJ, Whitaker M, O'Halloran A, Chai SJ, Kirley PD, Alden N, et al. Characteristics and Maternal and Birth Outcomes of Hospitalized Pregnant Women With Laboratory-Confirmed COVID-19—COVID-NET, 13 States, March 1–August 22, 2020. MMWR Morb Mortal Wkly Rep (2020) 69(38)1347–54. doi: 10.15585/mmwr.mm6938e1
5. Panagiotakopoulos L, Myers TR, Gee J, Lipkind HS, Kharbanda EO, Ryan DS, et al. SARS-Cov-2 Infection Among Hospitalized Pregnant Women: Reasons for Admission and Pregnancy Characteristics—Eight US Health Care Centers, March 1–May 30, 2020. MMWR Morb Mortal Wkly Rep 69. (2020) 69(38):1355–9. doi: 10.15585/mmwr.mm6938e2
6. Khalil A, von Dadelszen P, Draycott T, Ugwumadu A, O'Brien P, Magee L, et al. Change in the Incidence of Stillbirth and Preterm Delivery During the COVID-19 Pandemic. (2020) 324(7):705–6. doi: 10.1001/jama.2020.12746
7. Takemoto ML, Menezes MO, Andreucci CB, Nakamura-Pereira M, Amorim MMR, Katz L, et al. The Tragedy of COVID-19 in Brazil: 124 Maternal Deaths and Counting. (2020) 151(1):154–6. doi: 10.1002/ijgo.13300
8. BMJ AIIJ. ANZIC Influenza Investigators and Australasian Maternity Outcomes Surveillance System Critical Illness Due to 2009 a/H1N1 Influenza in Pregnant and Postpartum Women: Population Based Cohort Study. BMJ (2010) 340:c1279. doi: 10.1136/bmj.c1279
9. Jamiesan D, Honein M, Rasmussen S, Williams JL, Swerdlow DL, Biggerstaff MS, et al. Novel Influenza A (H1N1) Pregnancy Working Group. H1N1 2009 Influenza Virus Infection During Pregnancy in the USA. Lancet (2009) 374(9688):451–8. doi: 10.1016/S0140-6736(09)61304-0
10. Louie J, Acosta M, Jamieson DJ, Honein MA. California Pandemic (H1n1) Working Group. N Engl J Med (2010) 362(1):27–35. doi: 10.1056/NEJMoa0910444
11. Siston AM, Rasmussen SA, Honein MA, Fry AM, Seib K, Callaghan WM, et al. Pandemic 2009 Influenza a (H1N1) Virus Illness Among Pregnant Women in the United States. JAMA (2010) 303(15):1517–25. doi: 10.1001/jama.2010.479
12. Yamashita M, Yamate M, Li GM, Ikuta K. Susceptibility of Human and Rat Neural Cell Lines to Infection by SARS-Coronavirus. Biochem Biophys Res Commun (2005) 334(1):79–85. doi: 10.1016/j.bbrc.2005.06.061
13. Mullins E, Evans D, Viner RM, O'Brien P, Morris E. Coronavirus in Pregnancy and Delivery: Rapid Review. Ultrasound Obstet Gynecol (2020) 55(5):586–92. doi: 10.1101/2020.03.06.20032144
14. Alfaraj SH, Al-Tawfiq JA, Memish ZA. Middle East Respiratory Syndrome Coronavirus (MERS-CoV) Infection During Pregnancy: Report of Two Cases & Review of the Literature. J Microbiol Immunol Infect (2019) 52(3):501–3. doi: 10.1007/978-3-319-74365-3_49-1
15. Gajbhiye RK, Modi DN, Mahale SD. Pregnancy Outcomes, Newborn Complications and Maternal-Fetal Transmission of SARS-CoV-2 in Women With COVID-19: A Systematic Review of 441 Cases. MedRxiv (2020). doi: 10.1101/2020.04.11.20062356
16. Schwartz DA, Dhaliwal A. Infections in Pregnancy With COVID-19 and Other Respiratory RNA Virus Diseases are Rarely, If Ever, Transmitted to the Fetus: Experiences With Coronaviruses, Parainfluenza, Metapneumovirus Respiratory Syncytial Virus, and Influenza. Arch Pathol Lab Med (2020) 144(8):920–8. doi: 10.5858/arpa.2020-0211-SA
17. Zaigham M, Andersson O. Maternal and Perinatal Outcomes With COVID-19: A Systematic Review of 108 Pregnancies. Acta obstetricia gynecologica Scandinavica (2020) 99(7):823–9. doi: 10.1111/aogs.13867
18. Alzamora MC, Paredes T, Caceres D, Webb CM, Valdez LM, La Rosa M. Severe COVID-19 During Pregnancy and Possible Vertical Transmission. (2020) 37(8):861–865. doi: 10.1055/s-0040-1710050
19. Robbins JR, Bakardjiev AI. Pathogens and the Placental Fortress. Curr Opin Microbiol (2012) 15(1):36–43. doi: 10.1016/j.mib.2011.11.006
20. Auriti C, De Rose DU, Santisi A, Martini L, Piersigilli F, Bersani I, et al. Pregnancy and Viral Infections: Mechanisms of Fetal Damage, Diagnosis and Prevention of Neonatal Adverse Outcomes From Cytomegalovirus to SARS-CoV-2 and Zika Virus. Biochim Biophys Acta (BBA)-Molecular Basis Dis (2021) 1867(10):166198. doi: 10.1016/j.bbadis.2021.166198
21. Jamieson DJ, Theiler RN, Rasmussen SA. Emerging Infections and Pregnancy. Emerg Infect Dis (2006) 12(11):1638. doi: 10.3201/eid1211.060152
22. Schjenken JE, Tolosa JM, Paul JW, Clifton VL, Smith R. Mechanisms of Maternal Immune Tolerance During Pregnancy. (2012) 11:211–42.
23. Silasi M, Cardenas I, Kwon JY, Racicot K, Aldo P, Mor G. Viral Infections During Pregnancy. (2015) 73(3):199–213. doi: 10.1111/aji.12355
24. Khosroshahi LM, Rokni M, Mokhtari T, Noorbakhsh F. Immunology, Immunopathogenesis and Immunotherapeutics of COVID-19; an Overview. Int Immunopharmacol (2021) 93:107364. doi: 10.1016/j.intimp.2020.107364
25. Huang C, Wang Y, Li X, Ren L, Zhao J, Hu Y, et al. Clinical Features of Patients Infected With 2019 Novel Coronavirus in Wuhan, China. Lancet (2020) 395(10223):497–506. doi: 10.1016/S0140-6736(20)30183-5
26. Liu J, Li S, Liu J, Liang B, Wang X, Wang H, et al. Longitudinal Characteristics of Lymphocyte Responses and Cytokine Profiles in the Peripheral Blood of SARS-CoV-2 Infected Patients. EBioMedicine (2020) 55:102763. doi: 10.1016/j.ebiom.2020.102763
27. Chen G, Wu D, Guo W, Cao y, Huang D, Wang H, et al. Clinical and Immunological Features of Severe and Moderate Coronavirus Disease 2019. J Clin Invest (2020) 130(5):2620–9. doi: 10.1172/JCI137244
28. Zheng M, Gao Y, Wang G, Song G, Liu S, Sun D, et al. Functional Exhaustion of Antiviral Lymphocytes in COVID-19 Patients. Cell Mol Immunol (2020) 17(5):533–5. doi: 10.1038/s41423-020-0402-2
29. Giamarellos-Bourboulis EJ, Netea MG, Rovina N, Akinosoglou K, Antoniadou A, Antonakos N, et al. Complex Immune Dysregulation in COVID-19 Patients With Severe Respiratory Failure. Cell Host Microbe (2020) 27(6):992–1000. e3. doi: 10.1016/j.chom.2020.04.009
30. Khosroshahi LM, Rezaei N. Dysregulation of the Immune Response in Coronavirus Disease 2019. Cell Biol Int (2021) 45(4):702–7. doi: 10.1002/cbin.11517
31. Wastnedge EAN, Reynolds RM, van Boeckel SR, Stock SJ, Denison FC, Maybin JA, et al. Pregnancy and COVID-19. Physiol Rev (2021) 101(1):303–18. doi: 10.1152/physrev.00024.2020
32. Björkström NK, Strunz B, Ljunggren H-G. Natural Killer Cells in Antiviral Immunity. Nat Rev Immunol (2021) p:1–12.
33. Chua RL, Lukassen S, Trump S, Hennig BP, Wendisch D, Pott F, et al. COVID-19 Severity Correlates With Airway Epithelium–Immune Cell Interactions Identified by Single-Cell Analysis. Nat Biotechnol (2020) 38(8):970–9. doi: 10.1038/s41587-020-0602-4
34. Mathew D, Giles JR, Baxter AE, Oldridge DA, Greenplate AR, Wu JE, et al. Deep Immune Profiling of COVID-19 Patients Reveals Distinct Immunotypes With Therapeutic Implications. Science (2020) 369(6508). doi: 10.1126/science.abc8511
35. Liao M, Liu Y, Yuan J, Wen Y, Xu G, Zhao J, et al. Single-Cell Landscape of Bronchoalveolar Immune Cells in Patients With COVID-19. Nat Med (2020) 26(6):842–4. doi: 10.1038/s41591-020-0901-9
36. Wichmann D, Sperhake JP, Lütgehetmann M, Steurer S, Edler C, Heinemann A, et al. Autopsy Findings and Venous Thromboembolism in Patients With COVID-19: A Prospective Cohort Study. Ann Internal Med (2020) 173(4):268–77. doi: 10.7326/L20-1206
37. Piccinni M-P, Romagnani S. Regulation of Fetal Allograft Survival by Hormone-Controlled Th1-and Th2-Type Cytokines. Immunol Res (1996) 15(2):141–50. doi: 10.1007/BF02918503
38. Muyayalo KP, Huang DH, Zhao SJ, Xie T, Mor G, Liao AH. COVID-19 and Treg/Th17 Imbalance: Potential Relationship to Pregnancy Outcomes. Am J Reprod Immunol (2020) 84(5):e13304. doi: 10.1111/aji.13304
39. Qin C, Zhou L, Hu Z, Zhang S, Yang S, Tao Y, et al. Dysregulation of Immune Response in Patients With COVID-19 in Wuhan, China; Clinical Infectious Diseases; Oxford Academic. Clin Infect Dis (2020) 71(15):762–768. doi: 10.1093/cid/ciaa248
40. Halyabar O, Chang MH, Schoettler ML, Schwartz MA, Baris EH, Benson LA, et al. Calm in the Midst of Cytokine Storm: A Collaborative Approach to the Diagnosis and Treatment of Hemophagocytic Lymphohistiocytosis and Macrophage Activation Syndrome. Pediatr Rheumatol (2019) 17(1):1–12. doi: 10.1186/s12969-019-0309-6
41. Henderson LA, Canna SW, Schulert GS, Volpi S, Lee PY, Kernan KF, et al. On the Alert for Cytokine Storm: Immunopathology in COVID-19. Arthritis Rheumatol (2020) 72(7):1059–63. doi: 10.1002/art.41285
42. Soy M, Atagündüz P, Atagündüz I, Sucak GT. Hemophagocytic Lymphohistiocytosis: A Review Inspired by the COVID-19 Pandemic. Rheumatol Int (2021) 41(1):7–18. doi: 10.1007/s00296-020-04636-y
43. Tisoncik JR, Korth MJ, Simmons CP, Farrar J, Martin TR, Katze MG. Into the Eye of the Cytokine Storm. Microbiol Mol Biol Rev (2012) 76(1):16–32. doi: 10.1128/MMBR.05015-11
44. Schulert GS, Zhang M, Fall N, Husami A, Kissell D, Hanosh A, et al. Whole-Exome Sequencing Reveals Mutations in Genes Linked to Hemophagocytic Lymphohistiocytosis and Macrophage Activation Syndrome in Fatal Cases of H1N1 Influenza. J Infect Dis (2016) 213(7):1180–8. doi: 10.1093/infdis/jiv550
45. Clark KEN, Nevin WD, Mahungu T, Lachmann H, Singh A. Assessment of the Hemophagocytic Lymphohistiocytosis Hscore in Patients With Coronavirus Disease 2019. Clin Infect Dis (2020) 28:ciaa1463. doi: 10.1093/cid/ciaa1463
46. Ruscitti P, Bruno F, Berardicurti O, Acanfora C, Pavlych V, Palumbo P, et al. Lung Involvement in Macrophage Activation Syndrome and Severe COVID-19: Results From a Cross-Sectional Study to Assess Clinical, Laboratory and Artificial Intelligence–Radiological Differences. Ann rheumatic Dis (2020) 79(9):1152–5. doi: 10.1136/annrheumdis-2020-218048
47. Wood H, Jones JR, Hui K, Mare T, Pirani T, Galloway J, et al. Secondary HLH is Uncommon in Severe COVID-19. Br J Haematol (2020) 190(5):e283–e285. doi: 10.1111/bjh.16934
48. Yao X, Li TY, He ZC, Ping YF, Liu HW, Yu SC, et al. A Pathological Report of Three COVID-19 Cases by Minimally Invasive Autopsies. Zhonghua bing li xue za zhi= Chin J Pathol (2020) 49:E009–9. doi: 10.3760/cma.j.cn112151-20200312-00193
49. Liao M, Liu Y, Yuan J, Wen Y, Xu G, Zhao J. The Landscape of Lung Bronchoalveolar Immune Cells in COVID-19 Revealed by Single-Cell RNA Sequencing. MedRxiv (2020). doi: 10.1101/2020.02.23.20026690
50. Amirchaghmaghi E, Taghavi SA, Shapouri F, Saeidi S, Rezaei A, Aflatoonian R. The Role of Toll Like Receptors in Pregnancy. (2013) 7(3):147.
51. Young BC, Stanic AK, Panda B, Rueda BR, Panda A. Longitudinal Expression of Toll-Like Receptors on Dendritic Cells in Uncomplicated Pregnancy and Postpartum. (2014) 210(5):445.e1–445.e6. doi: 10.1016/j.ajog.2013.11.037
52. Firmal P, Shah VK, Chattopadhyay S. Insight Into TLR4-Mediated Immunomodulation in Normal Pregnancy and Related Disorders. Front Immunol (2020) 11:807. doi: 10.3389/fimmu.2020.00807
53. Hoffmann M, Kleine-Weber H, Schroeder S, Krüger N, Herrler T, Erichsen S, et al. SARS-CoV-2 Cell Entry Depends on ACE2 and TMPRSS2 and Is Blocked by a Clinically Proven Protease Inhibitor. (2020) 181(2):271–80.e8. doi: 10.1016/j.cell.2020.02.052
54. Cascella M, Rajnik M, Aleem A, Dulebohn SC, Di Napoli R. Features, Evaluation and Treatment Coronavirus (COVID-19). In: StatPearls Treasure Island (FL):StatPearls Publishing. (2021).
55. Tay MZ, Poh CM, Ré nia L, MacAry PA, Ng LFP. The Trinity of COVID-19: Immunity, Inflammation and Intervention. Nat Rev Immunol (2020) 20 (6):363–74. doi: 10.1038/s41577-020-0311-8
56. Auriti C, De Rose DU, Tzialla C, Caforio L, Ciccia M, Manzoni P, et al. Vertical Transmission of SARS-CoV-2 (COVID-19): Are Hypotheses More Than Evidences? Am J Perinatol (2020) 37(S02):S31–8. doi: 10.1055/s-0040-1714346
57. Rabi FA, Al Zoubi MS, Kasasbeh GA, Salameh DM, Al-Nasser AD. SARS-CoV-2 and Coronavirus Disease 2019: What We Know So Far. Pathogens (2020) 9(3):231. doi: 10.3390/pathogens9030231
58. Jaimes JA, Millet JK, Whittaker GRJI. Proteolytic Cleavage of the SARS-CoV-2 Spike Protein and the Role of the Novel S1/S2 Site. iScience (2020) 23 (6):101212. doi: 10.1016/j.isci.2020.101212
59. Pique-Regi R, Romero R, Tarca AL, Luca F, Xu Y, Alazizi A, et al. Does the Human Placenta Express the Canonical Cell Entry Mediators for SARS-CoV-2? Elife (2020) 9:e58716. doi: 10.7554/eLife.58716
60. Faure-Bardon V, Isnard P, Roux N, Leruez-Ville M, Molina T, Bessieres B, et al. Protein Expression of Angiotensin-Converting Enzyme 2, a SARS-CoV-2-Specific Receptor, in Fetal and Placental Tissues Throughout Gestation: New Insight for Perinatal Counseling. Ultrasound Obstetrics Gynecol (2021) 57(2):242–7. doi: 10.1002/uog.22178
61. Weatherbee BA, Glover DM, Zernicka-Goetz M. Expression of SARS-CoV-2 Receptor ACE2 and the Protease TMPRSS2 Suggests Susceptibility of the Human Embryo in the First Trimester. Open Biol (2020) 10(8):200162. doi: 10.1098/rsob.200162
62. Cosma S, Carosso AR, Cusato J, Borella F, Carosso M, Bovetti M, et al. Coronavirus Disease 2019 and First-Trimester Spontaneous Abortion: A Case-Control Study of 225 Pregnant Patients. Am J Obstetrics Gynecol (2021) 224(4):391.e1–e7. doi: 10.1016/j.ajog.2020.10.005
63. la Cour Freiesleben N, Egerup P, Hviid KVR, Severinsen ER, Kolte AM, Westergaard D, et al. SARS-CoV-2 in First Trimester Pregnancy: A Cohort Study. Hum Reprod (2021) 36(1):40–7. doi: 10.1093/humrep/deaa311
64. Khalil A, Kalafat E, Benlioglu C, O'Brien P, Morris E, Draycott T, et al. SARS-CoV-2 Infection in Pregnancy: A Systematic Review and Meta-Analysis of Clinical Features and Pregnancy Outcomes. EClinicalMedicine (2020) 25:100446. doi: 10.1016/j.eclinm.2020.100446
65. Blumberg DA, Underwood MA, Hedriana HL, Lakshminrusimha S. Vertical Transmission of SARS-CoV-2: What is the Optimal Definition? Am J Perinatol (2020) 37(08):769–72. doi: 10.1055/s-0040-1712457
66. Zamaniyan M, Ebadi A, Aghajanpoor S, Rahmani Z, Haghshenas M, Azizi S. Preterm Delivery in Pregnant Woman With Critical COVID-19 Pneumonia and Vertical Transmission. Prenat Diagn (2020) 40(13):1759–1761. doi: 10.1002/pd.5713
67. Komine-Aizawa S, Takada K, Hayakawa S. Placental barrier against COVID-19. Placenta (2020) 99:45–9. doi: 10.1016/j.placenta.2020.07.022
68. Auriti C, De Rose DU, Mondì V, Stolfi I, Tzialla C. Neonatal SARS-CoV-2 Infection: Practical Tips. Pathogens (2021) 10(5):611. doi: 10.3390/pathogens10050611
69. Patanè L, Morotti D, Giunta MR, Sigismondi C, Piccoli MG, Frigerio L, et al. Vertical Transmission of COVID-19: SARS-CoV-2 RNA on the Fetal Side of the Placenta in Pregnancies With COVID-19 Positive Mothers and Neonates at Birth. Am J Obstet Gynecol (2020) 2(3):100145. doi: 10.1016/j.ajogmf.2020.100145
70. Algarroba G, Rekawek P, Vahanian SA. Visualization of SARS-CoV-2 Virus Invading the Human Placenta Using Electron Microscopy. Am J Obstet Gynecol (2020) 223(2):275–78. doi: 10.1016/j.ajog.2020.05.023
71. Wang W, Xu Y, Gao R, Lu R, Han K, Wu G, et al. Detection of SARS-CoV-2 in Different Types of Clinical Specimens. JAMA (2020) 323(18):1843–4. doi: 10.1001/jama.2020.3786
72. Juan J, Gil MM, Rong Z, Zhang Y, Yang H, Poon LC. Effect of Coronavirus Disease 2019 (COVID-19) on Maternal, Perinatal and Neonatal Outcome: Systematic Review. J Obstet Gynecol (2020) 56(1):15–27. doi: 10.1002/uog.22088
73. Yang Z, Liu Y. Vertical Transmission of Severe Acute Respiratory Syndrome Coronavirus 2: A Systematic Review. Am J Perinatol (2020) 37(10):1055. doi: 10.1055/s-0040-1712161
74. Coyne CB, Lazear HM. Zika Virus—Reigniting the TORCH. Nat Rev Microbio (2016) 14(11):707–15. doi: 10.1038/nrmicro.2016.125
75. Stegmann B, Carey JC. TORCH Infections. Toxoplasmosis, Other (Syphilis, Varicella-Zoster, Parvovirus B19), Rubella, Cytomegalovirus (CMV), and Herpes Infections. Curr Womens Health Rep (2002) 2(4):253–8.
76. Arvin AM, Moffat JF, Sommer M, Oliver S, Che X, Vleck S, et al. Varicella-Zoster Virus T Cell Tropism and the Pathogenesis of Skin Infection. Curr Top Microbiol Immunol (2010) 342:189–209. doi: 10.1007/82_2010_29
77. Pereira L. Congenital Viral Infection: Traversing the Uterine-Placental Interface. Annu Rev Virol (2018) 5(1):273–99. doi: 10.1146/annurev-virology-092917-043236
78. Kotlyar AM, Grechukhina O, Chen A, Popkhadze S, Grimshaw A, Tal O, et al. Vertical Transmission of Coronavirus Disease 2019: A Systematic Review and Meta-Analysis. Am J Obstet Gynecol (2021) 224(1):35–53.e3. doi: 10.1016/j.ajog.2020.07.049
79. Yoon SH, Kang JM, Ahn JG. Clinical Outcomes of 201 Neonates Born to Mothers With COVID-19: A Systematic Review. Eur Rev Med Pharmacol Sci (2020) 24(14):7804–15. doi: 10.26355/eurrev_202007_22285
80. Novoa RH, Quintana W, Llancarí P, Urbina-Quispe K, Guevara-Ríos E, Ventura W. Maternal Clinical Characteristics and Perinatal Outcomes Among Pregnant Women With Coronavirus Disease 2019. A Systematic Review. Travel Med Infect Dis (2021) 39:101919. doi: 10.1016/j.tmaid.2020.101919
81. Chi J, Gong W, Gao Q. Clinical Characteristics and Outcomes of Pregnant Women With COVID-19 and the Risk of Vertical Transmission: A Systematic Review. Arch Gynecol Obstetrics (2020) p:1–9. doi: 10.1007/s00404-020-05889-5
82. Jafari M, Pormohammad A, Sheikh Neshin SA, Ghorbani S, Bose D, Alimohammadi S, et al. Clinical Characteristics and Outcomes of Pregnant Women With COVID-19 and Comparison With Control Patients: A Systematic Review and Meta-Analysis. Rev Med Virol (2021) p:e2208. doi: 10.1002/rmv.2208
83. Islam M, Poly TN, Walther BA, Yang HC, Wang CW, Hsieh WS, et al. Clinical Characteristics and Neonatal Outcomes of Pregnant Patients With COVID-19: A Systematic Review. Front Med (2020) 7:909. doi: 10.3389/fmed.2020.573468
84. Rodrigues C, Baía I, Domingues R, Barros H. Pregnancy and Breastfeeding During COVID-19 Pandemic: A Systematic Review of Published Pregnancy Cases. Front Public Health (2020) 8:806. doi: 10.3389/fpubh.2020.558144
85. Akhtar H, Patel C, Abuelgasim E, Harky A. COVID-19 (SARS-CoV-2) Infection in Pregnancy: A Systematic Review. Gynecol Obstetric Invest (2020) 85(4):295–306. doi: 10.1159/000509290
86. Trippella G, Ciarcià M, Ferrari M, Buzzatti C, Maccora I, Azzari C, et al. COVID-19 in Pregnant Women and Neonates: A Systematic Review of the Literature With Quality Assessment of the Studies. Pathogens (2020) 9(6):485. doi: 10.3390/pathogens9060485
87. Amaral WN, Moraes CL, Rodrigues APDS, Noll M, Arruda JT, Mendonça CR. Maternal Coronavirus Infections and Neonates Born to Mothers With SARS-CoV-2: A Systematic Review. Healthcare (2020) 8(4):511. Multidisciplinary Digital Publishing Institute. doi: 10.3390/healthcare8040511
88. Deniz M, Tezer H. Vertical Transmission of SARS CoV-2: A Systematic Review. J Maternal-Fetal Neonatal Med (2020) 21:1–8. doi: 10.1080/14767058.2020.1793322
89. Dong L, Tian J, He S, Zhu C, Wang J, Liu C, et al. Possible Vertical Transmission of SARS-CoV-2 From an Infected Mother to Her Newborn. (2020) 323(18):1846–8. doi: 10.1001/jama.2020.4621
90. Zeng H, Xu C, Fan J, Tang Y, Deng Q, Zhang W, et al. Antibodies in Infants Born to Mothers With COVID-19 Pneumonia. (2020) 323(18):1848–9. doi: 10.1001/jama.2020.4861
91. Colaco S, Chhabria K, Singh D, Bhide A, Singh N, Singh A, et al. Expression Map of Coronavirus Receptors and Associated Factors in Developing Human Embryos. J Assist Reprod Genet (2021) 38(7):1709–20. doi: 10.1007/s10815-021-02192-3
92. Lukassen S, Chua RL, Trefzer T, Kahn NC, Schneider MA, Muley T, et al. SARS-CoV-2 Receptor ACE2 and TMPRSS2 Are Primarily Expressed in Bronchial Transient Secretory Cells. EMBO J (2020) 39(10):e105114. doi: 10.15252/embj.20105114
93. Qi J, Zhou Y, Hua J, Zhang L, Bian J, Liu B, et al. The ScRNA-Seq Expression Profiling of the Receptor ACE2 and the Cellular Protease TMPRSS2 Reveals Human Organs Susceptible to SARS-CoV-2 Infection. Int J Environ Res Public Health (2021) 18(1):284. doi: 10.3390/ijerph18010284
94. Seow JJW, Pai R, Mishra A, Shepherdson E, Lim TKH, Goh BKP, et al. ScRNA-Seq Reveals ACE2 and TMPRSS2 Expression in TROP2+ Liver Progenitor Cells: Implications in COVID-19 Associated Liver Dysfunction. Front Med (Lausanne) (2021) 8:603374. doi: 10.3389/fmed.2021.603374
95. Singh M, Bansal V, Feschotte C. A Single-Cell RNA Expression Map of Human Coronavirus Entry Factors. Cell Rep (2020) 32(12):108175. doi: 10.1016/j.celrep.2020.108175
96. Lü M, Qiu L, Jia G, Guo R, Leng Q. Single-Cell Expression Profiles of ACE2 and TMPRSS2 Reveals Potential Vertical Transmission and Fetus Infection of SARS-CoV-2. Aging (Albany NY) (2020) 12(20):19880. doi: 10.18632/aging.104015
97. Ashary N, Bhide A, Chakraborty P, Colaco S, Mishra A, Chhabria K, et al. Single-Cell RNA-Seq Identifies Cell Subsets in Human Placenta That Highly Expresses Factors Driving Pathogenesis of SARS-CoV-2. Front Cell Dev Biol (2020) 8:783. doi: 10.3389/fcell.2020.00783
98. Lu-Culligan A, Chavan AR, Vijayakumar P, Irshaid L, Courchaine EM, Milano KM, et al. Maternal Respiratory SARS-CoV-2 Infection in Pregnancy is Associated With a Robust Inflammatory Response at the Maternal-Fetal Interface. Med (2021) 2(5):591–610. e10. doi: 10.1016/j.medj.2021.04.016
99. Maucourant C, Filipovic I, Ponzetta A, Aleman S, Cornillet M, Hertwig L, et al. Natural Killer Cell Immunotypes Related to COVID-19 Disease Severity. Sci Immunol (2020) 5(50):eabd6832. doi: 10.1126/sciimmunol.abd6832
100. Björkström NK, Ponzetta A. Natural Killer Cells and Unconventional T Cells in COVID-19. Curr Opin Virol (2021) 49:176–82. doi: 10.1016/j.coviro.2021.06.005
101. Nagy Á., Pongor S, Győrffy B. Different Mutations in SARS-CoV-2 Associate With Severe and Mild Outcome. Int J Antimicrobial Agents (2021) 57(2):106272. doi: 10.1016/j.ijantimicag.2020.106272
102. Mariappan V, Manoharan PS, R P, Shanmugam L, Rao SR, Pillai AB. Potential Biomarkers for the Early Prediction of SARS-COV-2 Disease Outcome. Microbial Pathogen (2021) p:105057. doi: 10.1016/j.micpath.2021.105057
103. Xiong TY, Redwood S, Prendergast B, Chen M. Coronaviruses and the Cardiovascular System: Acute and Long-Term Implications. Eur Heart J (2020) 41(19):1798–800. doi: 10.1093/eurheartj/ehaa231
104. Ponti G, Maccaferri M, Ruini C, Tomasi A, Ozben T. Biomarkers Associated With COVID-19 Disease Progression. Crit Rev Clin Lab Sci (2020) 57(6):389–99. doi: 10.1080/10408363.2020.1770685
105. Gavriatopoulou M, Korompoki E, Fotiou D, Ntanasis-Stathopoulos I, Psaltopoulou T, Kastritis E, et al. Organ-Specific Manifestations of COVID-19 Infection. Clin Exp Med (2020) p:1–14. doi: 10.1007/s10238-020-00648-x
106. Chen LY, Quach TT. COVID-19 Cytokine Storm Syndrome: A Threshold Concept. Lancet Microbe (2021) 2(2):e49. doi: 10.1016/S2666-5247(20)30223-8
107. Rendeiro AF, Ravichandran H, Bram Y, Chandar V, Kim J, Meydan C, et al. The Spatial Landscape of Lung Pathology During COVID-19 Progression. Nature (2021) p:1–6. doi: 10.1038/s41586-021-03475-6
108. Kulasinghe A, Tan CW, dos Santos Miggiolaro AF, Monkman J, Bhuva D, Junior JD, et al. Spatial Profiling of Lung SARS-CoV-2 and Influenza Virus Infection Dissects Virus-Specific Host Responses and Gene Signatures. medRxiv (2020). doi: 10.1158/1557-3265.COVID-19-21-S03-04
109. King J, Eroumé KS, Truckenmüller R, Giselbrecht S, Cowan AE, Loew L, et al. Ten Steps to Investigate a Cellular System With Mathematical Modeling. PloS Comput Biol (2021) 17(5):e1008921. doi: 10.1371/journal.pcbi.1008921
110. Fletcher A, Osborne J. Seven Challenges in the Multiscale Modelling of Multicellular Tissues. WIREs Mechanisms of Disease (2020) 3:e1527. doi: 10.20944/preprints202007.0022.v1
111. Bravo RR, Baratchart E, West J, Schenck RO, Miller AK, Gallaher J, et al. Hybrid Automata Library: A Flexible Platform for Hybrid Modeling With Real-Time Visualization. PloS Comput Biol (2020) 16(3):e1007635. doi: 10.1371/journal.pcbi.1007635
112. Method of the Year 2020: Spatially Resolved Transcriptomics. Nat Methods (2021) 18(1):1–1. doi: 10.1038/s41592-020-01042-x
113. Sadeghi Rad H, Bazaz SR, Monkman J, Ebrahimi M, Warkiani N, Rezaei K, et al. The Evolving Landscape of Predictive Biomarkers in Immuno-Oncology With a Focus on Spatial Technologies. (2020) 9(11):e1215. doi: 10.1002/cti2.1215
114. Delorey TM, Ziegler CGK, Heimberg G, Normand R, Yang Y, Segerstolpe Å, et al. COVID-19 Tissue Atlases Reveal SARS-CoV-2 Pathology and Cellular Targets. Nature (2021) 595(7865):107–13. doi: 10.1038/s41586-021-03570-8
115. Schneider CA, Rasband WS, Eliceiri KW. NIH Image to Imagej: 25 Years of Image Analysis. Nat Methods (2012) 9(7):671–5. doi: 10.1038/nmeth.2089
116. Rueden CT, Schindelin J, Hiner MC, DeZonia BE, Walter AE, Arena ET, et al. Imagej2: Imagej for the Next Generation of Scientific Image Data. BMC Bioinf (2017) 18(1):1–26. doi: 10.1186/s12859-017-1934-z
117. Rueden CT, Eliceiri KW. The Imagej Ecosystem: An Open and Extensible Platform for Biomedical Image Analysis. Microscopy Microanalysis (2017) 23(S1):226–7. doi: 10.1017/S1431927617001817
118. McQuin C, Goodman A, Chernyshev V, Kamentsky L, Cimini BA, Karhohs KW, et al. Cellprofiler 3.0: Next-Generation Image Processing for Biology. PloS Biol (2018) 16(7):e2005970. doi: 10.1371/journal.pbio.2005970
119. Bankhead P, Loughrey MB, Fernández JA, Dombrowski Y, McArt DG, Dunne PD, et al. Qupath: Open Source Software for Digital Pathology Image Analysis. Sci Rep (2017) 7(1):1–7. doi: 10.1038/s41598-017-17204-5
120. Allenby MC, Misener R, Panoskaltsis N, Mantalaris A. A Quantitative Three-Dimensional Image Analysis Tool for Maximal Acquisition of Spatial Heterogeneity Data. Tissue Eng Part C: Methods (2017) 23(2):108–17. doi: 10.1089/ten.tec.2016.0413
121. Ferrando-Martinez S, Moysi E, Pegu A, Andrews S, Nganou Makamdop K, Ambrozak D, et al. Accumulation of Follicular CD8+ T Cells in Pathogenic SIV Infection. J Clin Invest (2018) 128(5):2089–103. doi: 10.1172/JCI96207
122. De Rose DU, Piersigilli F, Ronchetti MP, Santisi A, Bersani I, Dotta A, et al. Novel Coronavirus Disease (COVID-19) in Newborns and Infants: What We Know So Far. Ital J Pediatr (2020) 46(1):1–8. doi: 10.1186/s13052-020-0820-x
123. Shimabukuro TT, Kim SY, Myers TR, Moro PL, Oduyebo T, Panagiotakopoulos L, et al. Preliminary Findings of mRNA Covid-19 Vaccine Safety in Pregnant Persons. N Engl J Med (2021) 384(24):2273–82. doi: 10.1056/NEJMoa2104983
124. Prabhu M, Murphy EA, Sukhu AC, Yee J, Singh S, Eng D, et al. Antibody Response to Coronavirus Disease 2019 (COVID-19) Messenger RNA Vaccination in Pregnant Women and Transplacental Passage Into Cord Blood. Obstet Gynecol (2021) 138(2):278–80. doi: 10.1097/AOG.0000000000004438
Keywords: COVID-19, placenta, SARS-CoV-2, transplacental infection, pregnancy
Citation: Rad H S, Röhl J, Stylianou N, Allenby M C, Bazaz S R, Warkiani ME, Guimaraes FSF, Clifton VL and Kulasinghe A (2021) The Effects of COVID-19 on the Placenta During Pregnancy. Front. Immunol. 12:743022. doi: 10.3389/fimmu.2021.743022
Received: 17 July 2021; Accepted: 26 August 2021;
Published: 15 September 2021.
Edited by:
Veronique Demers-Mathieu, Medolac Laboratories, United StatesReviewed by:
Pavithra Vijayakumar, University of Rochester, United StatesCopyright © 2021 Rad, Röhl, Stylianou, Allenby, Bazaz, Warkiani, Guimaraes, Clifton and Kulasinghe. This is an open-access article distributed under the terms of the Creative Commons Attribution License (CC BY). The use, distribution or reproduction in other forums is permitted, provided the original author(s) and the copyright owner(s) are credited and that the original publication in this journal is cited, in accordance with accepted academic practice. No use, distribution or reproduction is permitted which does not comply with these terms.
*Correspondence: Arutha Kulasinghe, YXJ1dGhhLmt1bGFzaW5naGVAdXEuZWR1LmF1
Disclaimer: All claims expressed in this article are solely those of the authors and do not necessarily represent those of their affiliated organizations, or those of the publisher, the editors and the reviewers. Any product that may be evaluated in this article or claim that may be made by its manufacturer is not guaranteed or endorsed by the publisher.
Research integrity at Frontiers
Learn more about the work of our research integrity team to safeguard the quality of each article we publish.