- 1Department of Medical Sciences, University of Ferrara, Ferrara, Italy
- 2Center for Studies on Gender Medicine, Department of Medical Sciences, University of Ferrara, Ferrara, Italy
- 3Laboratory for Technologies of Advanced Therapies (LTTA), University of Ferrara, Ferrara, Italy
Merkel cell polyomavirus (MCPyV) is the main causative agent of Merkel cell carcinoma (MCC), a rare but aggressive skin tumor with a typical presentation age >60 years. MCPyV is ubiquitous in humans. After an early-age primary infection, MCPyV establishes a clinically asymptomatic lifelong infection. In immunocompromised patients/individuals, including elders, MCC can arise following an increase in MCPyV replication events. Elders are prone to develop immunesenescence and therefore represent an important group to investigate. In addition, detailed information on MCPyV serology in elders has been debated. These findings cumulatively indicate the need for new research verifying the impact of MCPyV infection in elderly subjects (ES). Herein, sera from 226 ES, aged 66–100 years, were analyzed for anti-MCPyV IgGs with an indirect ELISA using peptides mimicking epitopes from the MCPyV capsid proteins VP1-2. Immunological data from sera belonging to a cohort of healthy subjects (HS) (n = 548) aged 18–65 years, reported in our previous study, were also included for comparisons. Age-/gender-specific seroprevalence and serological profiles were investigated. MCPyV seroprevalence in ES was 63.7% (144/226). Age-specific MCPyV seroprevalence resulted as 62.5% (25/40), 71.7% (33/46), 64.9% (37/57), 63.8% (30/47), and 52.8% (19/36) in ES aged 66–70, 71–75, 76–80, 81–85, and 86–100 years, respectively (p > 0.05). MCPyV seroprevalence was 67% (71/106) and 61% (73/120) in ES males and females, respectively (p > 0.05). Lack of age-/gender-related variations in terms of MCPyV serological profiles was found in ES (p > 0.05). Notably, serological profile analyses indicated lower optical densities (ODs) in ES compared with HS (p < 0.05), while lower ODs were also determined in ES males compared with HS males (p < 0.05). Our data cumulatively suggest that oncogenic MCPyV circulates in elders asymptomatically at a relatively high prevalence, while immunesenescence might be responsible for a decreased IgG antibody response to MCPyV, thereby potentially leading to an increase in MCPyV replication levels. In the worse scenario, alongside other factors, MCPyV might drive MCC carcinogenesis, as described in elders with over 60 years of age.
Introduction
Merkel cell polyomavirus (MCPyV) is a small DNA virus with oncogenic potential (1). MCPyV is the main causative factor of Merkel cell carcinoma (MCC), a rare but aggressive skin tumor with a presentation age above 60 years (2). DNA sequences belonging to this polyomavirus (PyV) have been reported in ~80% of MCC tissues (1).
MCPyV genome is made up of a circular double-stranded DNA of ~5,400 base pairs containing three functional domains (3). These domains encompass a non-coding control region and early and late coding regions (4, 5), which regulate the early and late gene expression, respectively (6). The early region is involved in MCPyV replication initiation, whereas it comprises genes encoding for transcripts generated by alternative splicing, including the two proteins with oncogenic potential named large T (LT) and small (sT) antigens. The continuous expression of both LT and sT, alongside viral DNA integration into host genome, is required for the MCC onset and development (7–9). Additionally, LT/sT expression also appears to be linked to improper DNA methylation (10), an epigenetic process regulating gene expression (11). The late region encodes for MCPyV capsid proteins, known as major capsid protein 1 (VP1) and minor capsid protein 2 (VP2) (7). Differently from other PyVs, MCPyV does not express the VP3 protein (4).
Previous serological studies aimed to detect antibodies against MCPyV VPs have reported conflicting data on MCPyV prevalence in adulthood and middle/advanced age, with a seroprevalence ranging between 46% and 87% being described in healthy adults (3, 12–26) and between 58% and 95% in the elderly (12–15, 21–23, 25–29). Despite these conflicting rates, previous studies concordantly imply that MCPyV appears to be almost ubiquitous in the general population, which is asymptomatically infected (18, 27, 30, 31). After primary infection, which occurs during childhood (28, 32, 33), MCPyV establishes a lifelong but inoffensive infection in healthy individuals (32, 34). However, in certain circumstances, such as immune system impairment in the host, increased viral activity can occur. MCPyV may induce the dysregulated expression of host cell genes, which ultimately increase the MCC occurrence. Immunosuppression plays an important role in MCC onset (9, 35–37). Indeed, a higher occurrence of MCC has been observed in patients with immunocompromising conditions, including human immunodeficiency virus (HIV) infection (38–41), leukemia, and other hematologic malignancies (42, 43), as well as patients being pharmacologically treated for autoimmune diseases, solid organ transplantation, and cancers of other types (7, 9, 44–46). Notably, as only 10% of MCC patients have overt immunodeficiency, it is likely that in most patients cancer development is promoted by a loss of antiviral/cancer immune surveillance during advanced age (38, 47). Physiological immune impairment as a result of aging, or immunosenescence, could therefore favor high levels of MCPyV replication, ultimately leading to MCC onset. It has been reported that MCPyV viral load increases among elders, suggesting an age-related association of viral replication in the host (34, 48). Considering these aspects, assessing the impact of MCPyV infection in various immunocompromised groups, including elders, can improve early identification of individuals at risk of developing MCC.
In a previous study, we developed and validated a specific and sensitive indirect ELISA method with two linear synthetic peptides/mimotopes that mimic the MCPyV VP1 and VP2 antigens to detect circulating IgGs against MCPyV immunogenic antigens (18). Herein, we aimed to evaluate the impact of MCPyV infection in elders by taking advantage from our recently developed immunoassay. Specifically, the prevalence of serum IgGs against MCPyV in healthy elderly subjects (ES) aged from 66 to 100 years was investigated. MCPyV seroprevalence and serological profiles were therefore determined in age- and gender-stratified ES. It is worth noting that MCPyV infection in ES is of paramount importance for identifying elderly individuals potentially at risk of developing MCC in healthy populations.
Materials and Methods
Human Sera
Serum samples were obtained from n = 226 (mean age ± standard deviation of mean [SD], 78 ± [7] years) healthy ES. Samples were stored at −80°C until testing. Analyses were conducted in age-stratified ES, i.e., 66–70 (n = 40), 71–75 (n = 46), 76–80 (n = 57), 81–85 (n = 47), and 86–100 (n = 36) year-old groups. In addition, data from a set of sera belonging to a cohort of healthy subjects (HS) (n = 548, mean age ± SD, 42 ± [13] years) aged 18–65 years, reported in our previous study (18), were included for comparisons. Specifically, HS were stratified herein according to age in 18–25 (n = 78), 26–30 (n = 52), 31–35 (n = 59), 36–40 (n = 61), 41–45 (n = 76), 46–50 (n = 65), 51–55 (n = 50), 56–60 (n = 55), and 61–65 (n = 52) year-old groups. Serum samples were collected at the Clinical Laboratory Analysis, University Hospital of Ferrara, Ferrara, Italy, from discarded laboratory analysis samples, after routine analyses, before their destruction by incineration. The hospital records indicated these samples as belonging to healthy subjects. Indeed, blood analysis parameters were all in the normal index range. In addition, sera were collected anonymously and coded with indications of age and gender. Written informed consent was obtained from all subjects. The County Ethical Committee, Ferrara, Italy, approved the study (ID: 151078).
Merkel Cell Polyomavirus VP1 and VP2 Linear Synthetic Peptides
A recently developed and validated indirect ELISA was employed to detect specific IgGs against MCPyV in sera from ES, as described (18). The indirect ELISA provides the use of two linear synthetic peptides/mimotopes, known as MCPyV VP1 S and VP2 F (S and F peptides), which have previously been designed and employed as mimotopes for detecting circulating IgGs against MCPyV in a group of HS (18). The synthetic peptides were synthesized using standard procedures and purchased from UFPeptides s.r.l., Ferrara, Italy.
Amino acid (a.a.) sequences of VP1 S (24 a.a. residues) and VP2 F (25 a.a. residues) peptides, are as follows:VP1 S: NH2-NSPDLPTTSNWYTYTYDLQPKGSS-COOH,VP2 F: NH2-SLSPTSRLQIQSNLVNLILNSRWVF-COOH.
Indirect ELISA
Indirect ELISA was performed as reported (18). Immunological plates (Nunc-immuno plate PolySorp, Thermo Fisher Scientific, Milan, Italy) were coated with 5 μg of S and/or F peptide for each well and diluted in 100 μl of Coating Buffer 1×, pH 9.6 (Candor Bioscience, Wangen, Germany). The plates coated with peptides were incubated at 4°C for 16 h. Subsequently, immunological plates were washed three times with Washing Buffer (Candor Bioscience, Wangen, Germany), to remove the unbound peptides. During the blocking phase, 200 μl/well of blocking solution containing the casein and Tween detergent (Candor Bioscience, Wangen, Germany) was added to each well and incubated at 37°C for 90 min. Plates were rinsed three times with Washing Buffer, before adding serum samples. Each well was covered with 100 μl containing the serum samples diluted 1:20 in low cross-buffer (Candor Bioscience, Wangen, Germany). Sera in each plate comprise the following: i) positive controls, represented by immune human sera derived from patients with MCPyV-positive MCC (18); ii) negative controls, represented by three human MCPyV-negative sera (18); and iii) sera from ES under analysis. Immunological plates with sera were incubated at 37°C for 90 min. Each sample was analyzed in three replicates. Wells were washed three times, and the secondary antibody was added to each sample. This solution consists of a goat anti-human IgG heavy (H) and light (L) chain specific peroxidase conjugate (Calbiochem-Merck, Darmstadt, Germany) diluted 1:10,000 in low cross-buffer. The solution was added to each well, and plates were incubated at room temperature (RT) for 90 min. After 90 min of incubation, plates were washed three times, and then 100 μl of 2,2′-azino-bis-3-ethylbenzthiazoline-6-sulfonic acid (ABTS) solution (Sigma-Aldrich, Milan, Italy) was added to each well. Plates were incubated at RT for 45 min. ABTS reacted with the peroxidase enzyme to yield the color reaction. Finally, the plate was read spectrophotometrically (Thermo Electron Corp., model Multiskan EX, Vantaa, Finland) at a wavelength (λ) of 405 nm. Color intensity in wells was determined by optical density (OD) reading. The OD readings correspond to the amount of immune complexes formed by the specific antibodies bound to S and F synthetic peptides/mimotopes.
The cutoff for each S and F synthetic peptide was determined in each indirect ELISA run, as the mean of the OD readings of n = 3 negative control sera plus three standard deviations of mean (mean +3 SDs) (18, 49, 50), as described previously for other ELISA methods (51, 52). Immune serum samples were considered MCPyV-positive when reacting to both S and F synthetic peptides, in three replica ELISA experiments carried out by three independent operators, without data variability.
Indirect ELISA dilutional linearity (accuracy) was assessed by testing n = 15 of 10-fold diluted samples, from 1:20 to 1:2,560 (53). Sera with high (n = 5), medium (n = 5), and low (n = 5) OD values were selected and tested in triplicate (18).
Statistical Analysis
MCPyV seroprevalence rates were statistically analyzed applying the two-sided chi-square test for comparing ES and HS groups as well as age-/gender-stratified ES and HS cohorts (54, 55). OD values from the same ES and HS groups/cohorts were analyzed with the D’Agostino–Pearson normality test, and then parametric and non-parametric tests were applied according to normal and non-normal variables, respectively, as reported (53, 56). In detail, ODs from age-stratified ES and HS cohorts were analyzed with one-way ANOVA and Kruskal–Wallis multiple comparison tests, according to normal and non-normal variables, respectively (OD medians, 95% CI). ODs from the entire ES and HS groups as well as gender-stratified ES and HS cohorts were analyzed with Mann–Whitney U test. Spearman’s correlation coefficient r was used to evaluate the OD concordance between S and F peptides. Linear regression R2 coefficient was used to evaluate the agreement between sample dilutions and ODs, for both S and F peptides. Statistical analyses were carried out using Graph Pad Prism version 8.0 for Windows (Graph Pad, La Jolla, USA) (57). p-Values <0.05 were considered statistically significant (58).
Results
Indirect ELISA Reliability Assessment
In the first phase of our investigation, the accuracy of the indirect ELISA was determined by performing serial dilutions, from 1:20 to 1:2,560, on three sets of MCPyV-seropositive samples (n = 15) from our previous study (18), with known high (n = 5), medium (n = 5), and low (n = 5) OD values. For each peptide, dilutions were assayed in triplicate. Subsequently, ODs and dilution values were compared. The assay had a high correlation between ODs and sample dilutions when the S peptide was employed, with an R2 of 0.9786 (p < 0.0001), 0.9959 (p < 0.0001), and 0.9768 (p < 0.0001) for samples with high, medium, and low ODs, respectively (Figure 1A). The assay also showed a high correlation between ODs and sample dilutions when F peptide was used, with an R2 of 0.9773 (p < 0.0001), 0.9784 (p < 0.0001), and 0.9846 (p < 0.0001) for samples with known high, medium, and low ODs (Figure 1A).
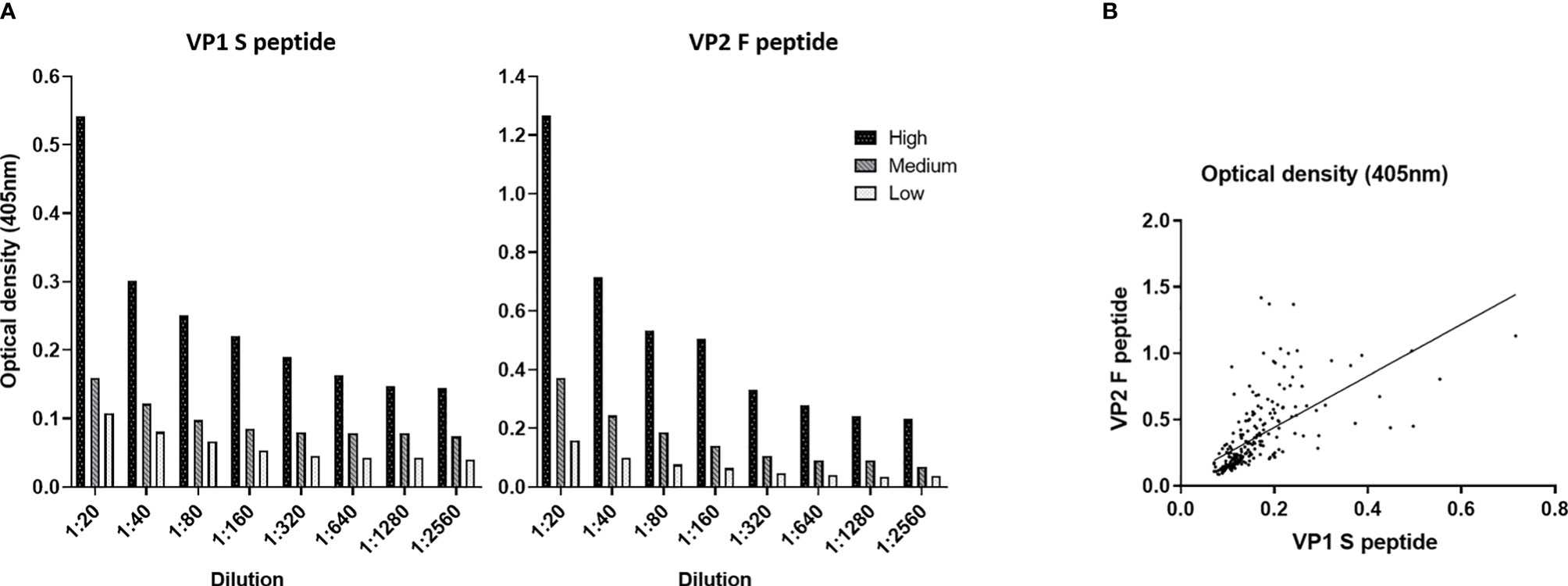
Figure 1 Dilution linearity and correlation of optical density (OD) values obtained using Merkel cell polyomavirus (MCPyV) VP1 S and VP2 F peptides. (A) OD response to serial dilutions (1:20, 1:80, 1:160, 1:320, 1:640, 1:1,280, and 1:2,560) was evaluated on n = 15 MCPyV-seropositive sera with known high (n = 5), medium (n = 5), and low (n = 5) ODs. Each dilution was assayed in triplicate for each MCPyV VP1 S and VP2 F peptide; and OD values and sera dilutions were compared by linear regression analysis. High correlation between ODs and dilutions was found for VP1 S peptide with an R2 of 0.9786 (p < 0.0001), 0.9959 (p < 0.0001), and 0.9768 (p < 0.0001) for samples with high, medium, and low ODs, respectively. Good correlation between ODs and dilutions was found when VP2 F peptide was employed, with an R2 of 0.9773 (p < 0.0001) for sample with known high ODs, 0.9784 (p < 0.0001) for samples with medium ODs, and 0.9846 (p < 0.0001) for samples with low ODs. (B) The concordance in ODs between the VP1 S and VP2 F peptides was evaluated on the entire set of sera from elderly subjects (ES) (n = 226) using Spearman’s correlation analysis. A good correlation between S peptide and F peptide was found, with an r of 0.7991 and a p < 0.0001.
Thereafter, OD concordance between MCPyV VP1 S and VP2 F peptides for the whole set of ES sera (n = 226) was evaluated by Spearman’s correlation analysis. Results indicate a good degree of correlation between ODs for S and F peptides, with a Spearman’s coefficient r of 0.7991 (p < 0.0001) (Figure 1B).
Detection of Serum IgG Antibodies Against Merkel Cell Polyomavirus by Indirect ELISA in Elderly Subjects
In order to assess the distribution of MCPyV infection in elders, sera from ES (n = 226) were tested by indirect ELISA for IgG Abs reactivity to MCPyV VP1 S and VP2 F peptides/mimotopes. A similar overall prevalence of 67.7% (153/226) and 71.2% (161/226) was obtained in sera from ES reacting to S and F peptides, respectively (p > 0.05) (Table 1).
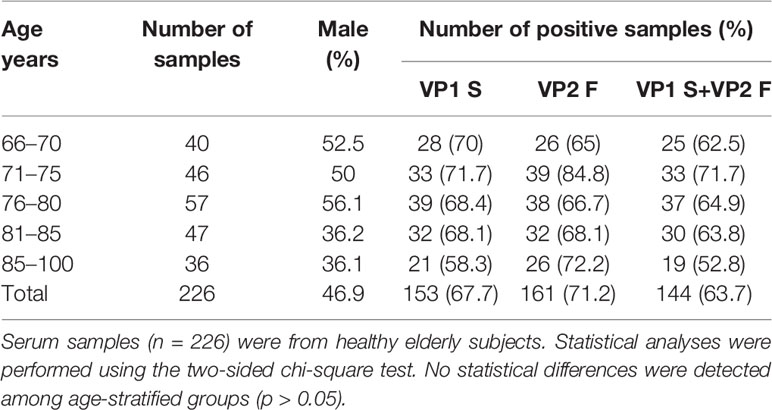
Table 1 Seroprevalence of IgG antibodies reacting with Merkel cell polyomavirus VP1 S and VP2 F peptides in healthy elderly subjects.
Moreover, with a few exceptions, sera testing negative for S peptide did not react to F peptide. In detail, 7.5% (17/226) of sera resulting negative for S peptide were positive for F peptide, while 3.9% (9/226) of sera were negative for F, while being positive for S peptide. The combined overall prevalence, for both S and F peptides, in ES sera resulted as 63.7% (144/226) (Table 1). Serum reactivity to MCPyV was then determined in age-stratified ES, and rates were compared. A total of 62.5% (25/40), 71.7% (33/46), 64.9% (37/57), 63.8% (30/47), and 52.8% (19/36) of serum samples were found to be positive for IgG Abs reacting to MCPyV in ES cohorts aged 66–70, 71–75, 76–80, 81–85, and 86–100 years, respectively (Table 1). No statistically significant differences in MCPyV seroreactivity were determined among groups (p > 0.05).
In order to evaluate an association between MCPyV infection and gender, MCPyV seroprevalence was investigated in gender-stratified ES. To this purpose, the presence of IgG Abs against MCPyV was determined in sera from male (n = 106) and female (n = 120) ES, and rates were compared. The prevalence of serum Abs reacting to MCPyV resulted as 67% (71/106) and 61% (73/120) in male and female groups, respectively, with no differences between the two gender groupings (p > 0.05).
Serological Profiles of IgG Reacting to Merkel Cell Polyomavirus in Elderly Subjects
Serological profiles of IgG Abs reactivity to S and F peptides, both for single peptides and in combination, were analyzed. Immunological data were taken from the entire ES cohort (n = 226), and results are reported as OD readings at λ 405 nm. The median [interquartile range (IQR)] OD values for S peptide and F peptide in ES sera were 0.14 (0.10–0.18) and 0.25 (0.18–0.49), respectively, while for combined S and F peptides, these resulted as 0.18 (0.13–0.30). The median (IQR) ODs for S peptide and F peptide, both for single mimotopes and in combination, were then determined in age-stratified ES, and values were compared. The median (IQR) OD values for S peptide resulted as 0.14 (0.10–0.17), 0.15 (0.11–0.21), 0.13 (0.11–0.17), 0.13 (0.11–0.20), and 0.13 OD (0.10–0.19) in age-stratified ES groups aged 66–70, 71–75, 76–80, 81–85, 86–100 years, respectively (Figure 2A). The median (IQR) OD values for F peptide resulted as 0.25 (0.17–0.53), 0.25 (0.18–0.53), 0.24 (0.16–0.42), 0.29 (0.18–0.50), and 0.28 (0.20–0.46) in age-stratified ES groups aged 66–70, 71–75, 76–80, 81–85, and 86–100 years, respectively (Figure 2B). The median (IQR) ODs for combined S and F peptides resulted as 0.17 (0.12–0.26), 0.19 (0.13–0.37), 0.16 (0.12–0.26), 0.19 (0.12–0.31), and 0.19 (0.12–0.32) in age-stratified ES groups aged 66–70, 71–75, 76–80, 81–85, and 86–100 years, respectively (Figure 2C). No statistically significant differences in OD values for peptides S and F, both for single peptides and in combination, were determined among age-stratified ES groups (p > 0.05). Similarly, Spearman’s correlation analysis showed a lack of correlation between age and OD levels, for peptides S and F, in the entire cohort of ES (p > 0.05).
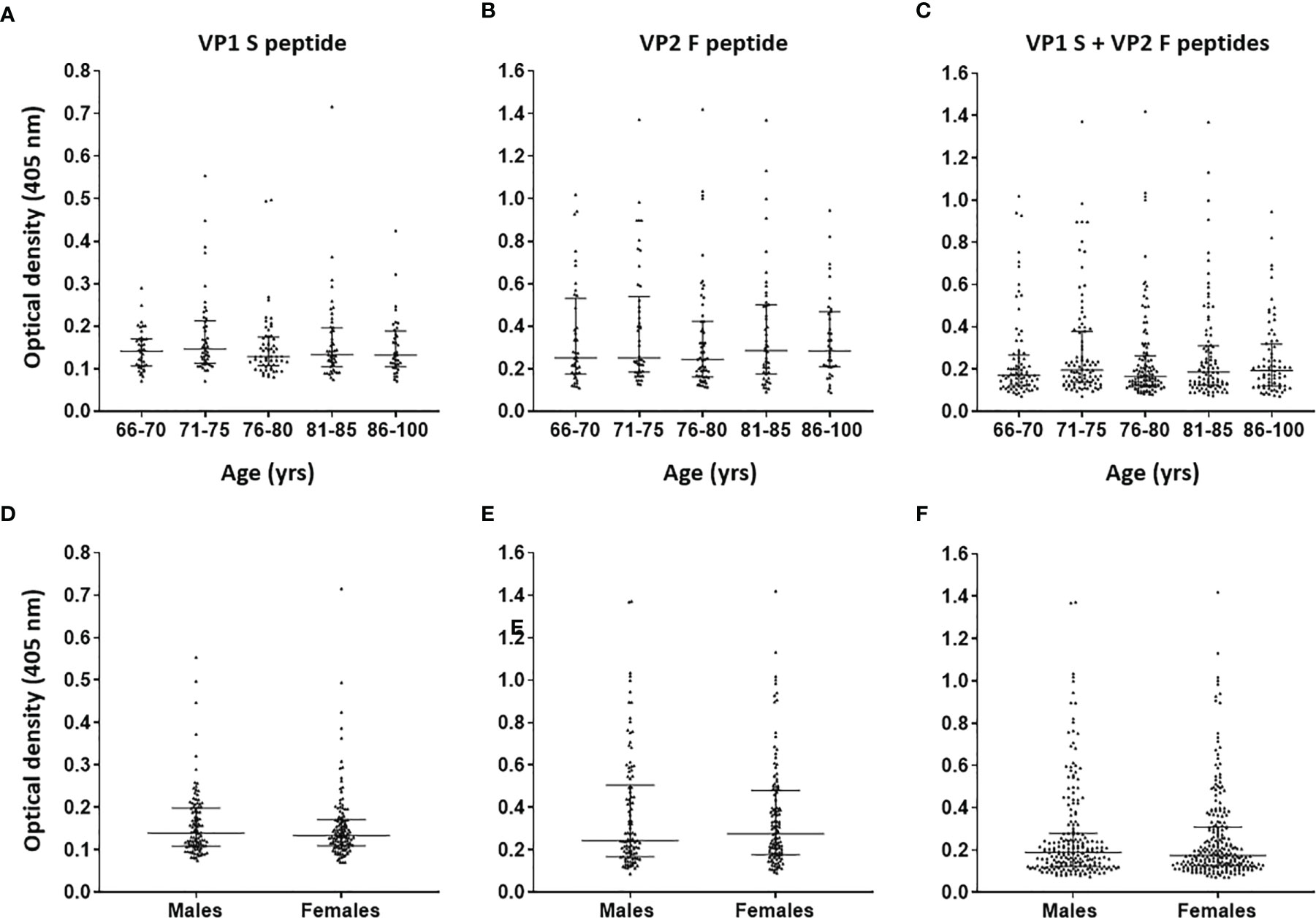
Figure 2 Serological profiles of serum antibody reactivity to Merkel cell polyomavirus (MCPyV) VP1 S (A, D), VP2 F (B, E) peptides and for combined S and F peptides (C, F) in age- and gender-stratified elderly subjects (ES). Immunologic data are from age- and gender-stratified ES, and results are reported as optical density (OD) value readings at λ 405 nm for serum samples assayed in indirect ELISA. In the scatter dot plot, each dot represents the dispersion of ODs for each sample. The median is indicated by the line inside the scatter plot with the interquartile range (IQR) in age-stratified ES, i.e., 66–70 (n = 40), 71–75 (n = 46), 76–80 (n = 57), 81–85 (n = 47), and 86–100 (n = 36) years and in gender-stratified ES, i.e., males (n = 106) and females (n = 120). No statistically significant differences in MCPyV seroreactivity were determined among age-/gender-stratified groups (p > 0.05).
The median (IQR) ODs for S peptide and F peptide, both for single peptides and in combination, were then determined in ES males (n = 106) and females (n = 120), whereas values were compared. The ODs for S and F peptides in the male group were 0.14 (0.11–0.2) and 0.24 (0.17–0.50), respectively, while these resulted as 0.13 (0.11–0.17) and 0.28 (0.18–0.49), respectively, in the female group (Figures 2D, E). Moreover, the median (IQR) ODs for combined S and F peptides were 0.19 (0.12–0.28) in males and 0.17 (0.13–0.30) in females (Figure 2F). No statistically significant differences in ODs for peptides S and F, both for single peptides and in combination, were determined between males and females (p > 0.05).
Serum IgG Reactivity to Merkel Cell Polyomavirus in Senior Elderly Subjects Versus Younger Healthy Subjects
In the second phase, MCPyV seroprevalence and serologic profiles in ES were compared with those obtained in sera from HS, which had previously been investigated in our laboratory (18). The prevalence of serum anti-MCPyV IgGs, combining S and F peptide reactivity, in HS was 62.9% (345/548) (18). No statistical differences in MCPyV seroprevalence were found either between the two entire cohorts of ES and HS (p > 0.05) or in MCPyV seroprevalence and serologic profiles among age-stratified ES and HS (p > 0.05). Notably, serologic profile analysis indicated that S peptide ODs resulted as lower in the ES group (n = 226, median [IQR], 0.13, 0.10–0.19) than in HS (n = 548, 0.15, 0.11–0.21, p < 0.05) (Figure 3A). Contrariwise, F peptide ODs were similar in ES (0.25, 0.18–0.49) and HS groups (0.27, 0.2–0.42) (p > 0.05) (Figure 3B). Lower ODs for combined S and F peptides were detected in ES group (0.18, 0.13–0.29) compared with HS (0.2, 0.14–0.3) (p < 0.05) (Figure 3C).
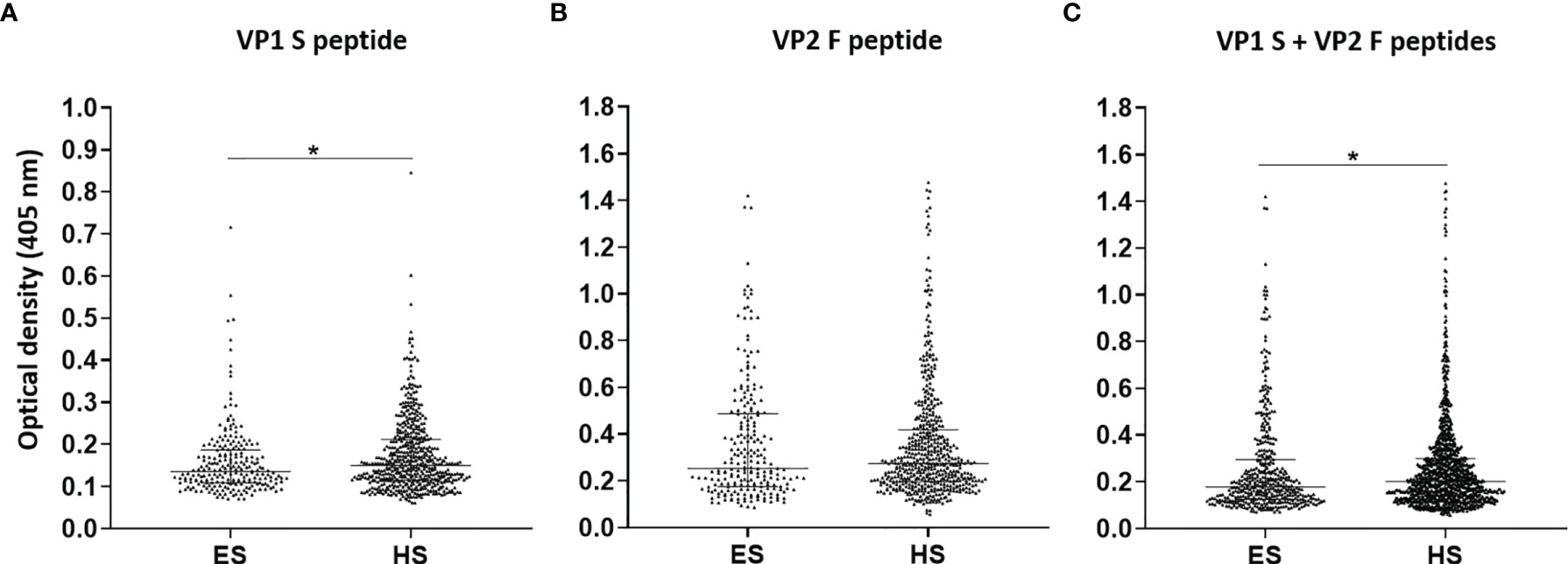
Figure 3 Serologic profiles of serum antibody reactivity to Merkel cell polyomavirus (MCPyV) VP1 S (A) and VP2 F (B) peptides and for combined S and F peptides (C) evaluated in elderly subjects (ES) compared with healthy subjects (HS). Immunologic data are from ES (n = 226) and HS (n = 548), and results are reported as optical density (OD) value readings at λ 405 nm for sera analyzed in indirect ELISA. In the scatter dot plot, each dot represents the dispersion of ODs for each sample. The median is indicated by the line inside the scatter plot with the interquartile range (IQR) in ES and HS cohorts. (A) *p < 0.05; (C) *p < 0.05.
Afterwards, MCPyV seroprevalence was compared in ES (n = 106) and HS (n = 210) males, as well as in ES (n = 120) and HS (n = 338) females (18). A total of 65.2% (137/210) HS males and 61.5% (208/338) HS females presented IgGs against MCPyV (18). No statistically significant differences were determined among groups (p > 0.05). As regards the serologic profiles, ODs for S peptide resulted as lower in ES males (0.14, 0.11–0.2) than in HS males (0.16, 0.13–0.21, p < 0.01) (Figure 4A), while no statistical differences in F peptide ODs were determined in ES (0.24, 0.16–0.5) and HS males (0.29, 0.21–0.43) (p > 0.05) (Figure 4B). Furthermore, lower ODs for combined S and F peptides were detected in ES males (0.19, 0.12–0.28) when compared with HS males (0.21, 0.15–0.31, p < 0.01) (Figure 4C). The ODs for S peptide in ES females (0.13, 0.11–0.17) were similar when compared with those in HS females (0.14, 0.1–0.21, p > 0.05) (Figure 4D). Similar ODs for F peptide were also found in ES (0.28, 0.18–0.48) and HS females (0.25, 0.18–0.41, p > 0.05) (Figure 4E). No differences in ODs for combined S and F peptides were detected in ES (0.17, 0.13–0.31) and HS females (0.2, 0.13–0.3, p > 0.05) (Figure 4F).
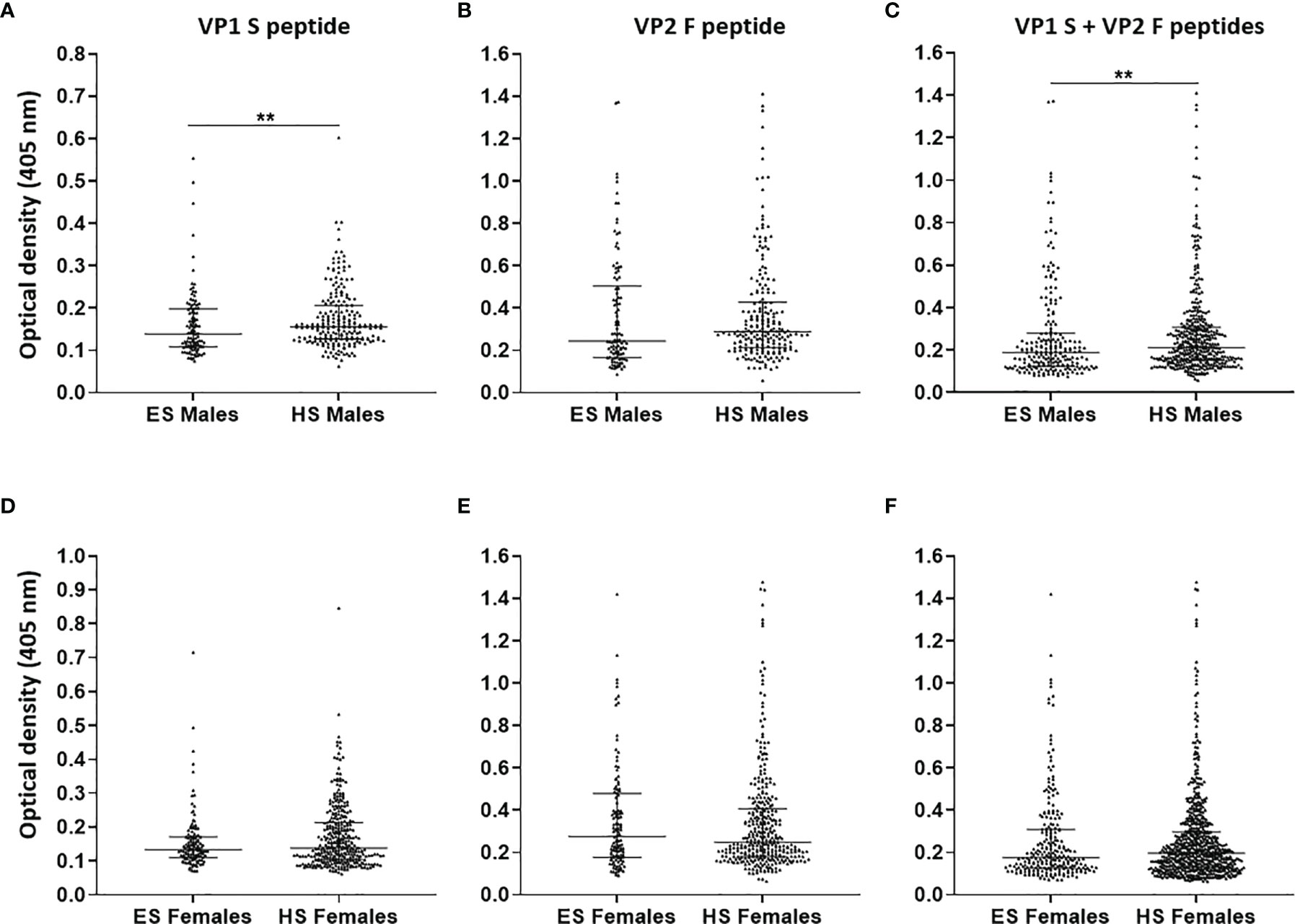
Figure 4 Serologic profiles of serum antibody reactivity to Merkel cell polyomavirus (MCPyV) VP1 S (A, D) and VP2 F (B, E) peptides and for combined S and F peptides (C, F) evaluated in gender-stratified elderly subjects (ES) compared with gender-stratified healthy subjects (HS). Immunologic data are from gender-stratified ES (n = 106 males and n = 120 females) and HS (n = 210 males and n = 338 females). Results are expressed as optical density (OD) value readings at λ 405 nm for serum samples assayed in indirect ELISA. In the scatter dot plot, each dot represents the dispersion of ODs for each sample. The median is indicated by the line inside the scatter plot with the interquartile range (IQR) for each group of subjects analyzed. (A) **p < 0.01; (C) **p < 0.01.
Discussion
Detailed information on MCPyV serology in the healthy elderly population is still being debated. Herein, the impact of MCPyV infection in ES was determined using an innovative indirect ELISA with two linear synthetic peptides that mimic MCPyV VP antigens (18). MCPyV seroprevalence and serologic profile in ES according to age and gender were therefore determined.
In a first phase, the performance of the indirect ELISA was evaluated by i) testing assay dilutional linearity (53, 59–61), or accuracy, for MCPyV VP1 S and VP2 F peptides and by ii) comparing S and F peptide ODs. Linear regression analyses showed a remarkable goodness of fit between sample dilutions and ODs, with an R2 ranging from 0.09768 to 0.09959 (p < 0.0001) being found, for both peptides. Furthermore, Spearman’s analysis, indicated good concordance between S and F peptide ODs, with an r of 0.7991 (p < 0.0001). These data indicate that not only is this assay robust in detecting anti-MCPyV IgGs with high accuracy, a key prerequisite for reliable ELISAs (18, 62–66), but also S and F peptides can be exploited simultaneously, showing high concordance. These data indicate that this method is accurate, while providing high reliability in detecting anti-MCPyV IgGs (18, 67, 68).
Analysis was afterwards carried out on sera from ES aged from 66 to 100 years with unknown MCPyV serology. Sera were investigated for IgG reactivity to MCPyV antigens. Sera were considered MCPyV-positive when IgGs reacted to both S and F peptides (18).
The overall prevalence, obtained by combining MCPyV-positive ES sera, for both peptides, resulted as 63.7%, a proportion similar to 62.9%, which we previously obtained in HS aged 18–65 years (18). These data suggest that MCPyV circulates asymptomatically not only in the adult population but also in elders at a relatively high prevalence. Over the years, serological evidence of circulating MCPyV in adults has been described with highly variating rates ranging between 46% and 87%, according to the study considered (3, 12–16, 19–26). Distinct prevalence figures across studies have been described for individuals aged over 60 years, with rates grouping from about 58%–70% (13, 14, 21–23, 29) to approximately 73%–95% (12, 15, 25–28, 69). Differences in geographical region/study populations could reflect these variations across studies (25, 69). However, variating rates have been reported across studies conducted in the same geographical region (15, 20, 22, 23, 25, 28, 29, 69, 70). Therefore, the current data available do not allow to reach robust conclusions regarding the presence of an MCPyV seroprevalence pattern according to the geographical region. Multicenter studies based on large sample sizes should be performed to clarify this issue. Moreover, methodological differences in immunoassays, which may result in variating rates, could also be considered an explanation for these variations across studies (21, 25). It should be recalled that previous MCPyV-based immunological methods mainly employ VLPs as antigens for immunoreactions, which are in vitro-generated recombinant MCPyV VPs (3, 12, 14, 20, 27, 71, 72). VLP-based assays might be susceptible to overestimation/cross-reaction, as VLPs share common antigens with other PyVs (16, 27, 73), therefore potentially hampering the result (27, 73). Our immunoassay differs from previous methods since it is based on two synthetic linear peptides mimicking portions of MCPyV VPs. Indeed, the S and F peptide a.a. chains used herein present a very marginal identity with corresponding VPs from other PyVs, as has been demonstrated (18). In addition, VLP-based systems necessitate time-consuming/expensive protocols (27, 73), which might be susceptible to methodological flaws. Considering these aspects, our indirect ELISA exhibits high specificity in detecting anti-MCPyV IgGs, while cost/time efficiency and a simple procedure are also provided (18).
In terms of MCPyV seroprevalence, no variations with age were determined in ES (p > 0.05). Comparable rates were also obtained when comparing age-stratified ES cohorts with age-stratified HS cohorts from our previous study (p > 0.05) (18). This result suggests that MCPyV serology appears to not differ from adulthood to middle/advanced age, persisting lifelong without significant fluctuations. Despite previous studies being characterized by variating MCPyV seroprevalence rates (74), our results are in agreement with previous studies reporting a lack of/slight variations with age (12, 14, 21, 22, 24, 26, 27, 69). MCPyV establishes a lifelong asymptomatic infection in adults. In physiological conditions, this kind of infection may provide a long-lasting/lifetime source of viral antigens, which are permanently exposed to the host immune system, while continuous, consequent antigen stimulation is provoked (14, 24). An alternative scenario provides for the gradual production/exposure of MCPyV antigens to the immune system during adulthood, which leads to a gradual immune response increase in an age-dependent manner (75), as described for other viruses (76). As for other PyVs (33), the gradual production of antiviral IgGs might be prompted by an increase in MCPyV replication levels or reinfections with different strains throughout life (21, 25). Consistently, MCPyV seroreactivity has also been reported to increase with age (13, 23, 28, 70).
It is important to point out that lower OD levels were found in ES compared with HS from our previous study (p < 0.05) (18). In addition, despite the entire ES group having statistically similar MCPyV seroprevalence rates, the older cohort, aged 86–100 years, exhibited the relatively lowest rate, i.e., 53%, between 10% and 20% lower than the remaining cohorts aged below 85 years. These results, in agreement with previous findings, suggest a possible decrease in IgG levels against MCPyV during senility (15, 25, 26). Indeed, the immune system is prone to decline in old-aged subjects (77), potentially leading to a reduced response to MCPyV. At the same time, as high MCPyV DNA amounts have been described in elders, a link between age and an increase in viral replication levels has also been theorized (34). Physiological immune senescence could therefore favor higher levels of MCPyV replication, as a minor response to MCPyV may occur (29, 32, 34, 78), which in turn might lead to MCC carcinogenesis. This is understandable, as MCC mainly arises during advanced senility following MCPyV reactivation (35, 36). The impairment of antiviral/cancer immune surveillance in advanced age can therefore favor MCPyV-driven MCC carcinogenesis (38, 47). However, Spearman’s analysis and comparisons among, and within, age-stratified ES and HS cohorts showed a lack of OD variation with age. The relatively small fraction of serum samples available for immunoreactions characterizing the age-stratified ES cohorts could justify this result. Further investigations with larger study cohorts might clarify the presence of variations in MCPyV seroreactivity according to age.
In summary, the data in the present study, along with evidence reported previously (12, 14, 21, 22, 24, 26, 27, 69), cumulatively suggest that, after seroconversion early in life (22, 69), MCPyV infection seems to remain stable throughout adulthood by evoking a physiological immune response, which might be followed by a decrease in MCPyV seroreactivity during advanced senility. The decrease in antiviral/cancer immune surveillance might lead to higher MCPyV replication levels ultimately leading to MCC onset (38, 47).
No differences in terms of both MCPyV seroprevalence and ODs were determined between ES males and females (p > 0.05). These data, in agreement with those reported previously (18, 25–28, 69), support the view that MCPyV infection is homogenously distributed in humans without gender-based disparity. Notably, a decrease in ODs with age was observed in males, but not in females, with low levels being found in ES males compared with HS males from our previous study (p < 0.05) (18). This evidence suggests that males might be more predisposed to develop immune senescence than females, which in turn might possibly favor higher MCPyV replication levels. A gender dimorphism in immune response impairment with age has been described as more pronounced in males than females (79). Indeed, although some evidences indicate a lack of gender differences (80), MCC seems to occur more frequently in males than females (81–84).
As MCPyV LT/sT plays a role in MCC onset/development (7–9), investigating the presence of serum IgGs against these viral oncoproteins in the healthy population, to identify MCC-risk individuals, might present clinical relevance. Our ELISA method can potentially be extended by using different linear peptides/mimotopes mimicking portions of MCPyV LT/sT antigens, as performed previously (85). This methodological approach, which might allow a more comprehensive evaluation of the MCPyV infection in the healthy population, in immunosuppressed individuals and in MCC-risk patients, will be employed in further studies.
Our study presents some limitations. First, S and F peptides, used herein as mimotopes to detect circulating IgGs against MCPyV, were not tested for the detection of IgGs against MCPyV variants with mutations in the sequences corresponding to the used peptides. However, in silico analyses conducted previously to assess the theoretical reliability of the two peptides as mimotopes/antigens indicated that both peptides were able to detect IgGs to a variety of known MCPyV strains with high concordance (18). Second, a relatively small number of sera from ES have been tested. However, the sample size employed herein is statistically appropriate and significant.
In conclusion, evaluating the impact of MCPyV infection in ES is of paramount importance for identifying individuals potentially at risk of MCC. Our indirect ELISA proved to be reliable in investigating IgGs reacting to MCPyV VP mimotopes in ES sera. Circulating anti-MCPyV IgGs were determined in sera from ES, while a lack of age-/gender-related variations in terms of MCPyV seroprevalence and serologic profile was found. The results of the present study suggest that oncogenic MCPyV circulates in the elderly asymptomatically without variations according to age and gender, at a relatively high prevalence. However, the entire ES group reported lower ODs compared with HS, while lower ODs were also found in ES males compared with HS males. This result may suggest that immunesenescence might be responsible for a decreased IgG antibody response to MCPyV, thereby potentially leading to an increase in MCPyV replication levels. In a few cases, alongside other risk factors, this phenomenon might prompt MCPyV-driven MCC onset, as described in elders aged over 60 years.
Data Availability Statement
The raw data supporting the conclusions of this article will be made available by the authors, without undue reservation.
Ethics Statement
The studies involving human participants were reviewed and approved by County Ethical Committee, Ferrara, Italy (ID:151078). The patients/participants provided their written informed consent to participate in this study.
Author Contributions
Conceptualization: MT and JR. Methodology: CM and JR. Software: CM and JR. Formal analysis: CM. Investigation: CM and GP. Resources: MG. Data curation and statistical analysis: CM. Writing—original draft preparation: CM and JR. Writing—review and editing: MT, FM, and MG. Visualization: CM, CL, and EM. Supervision: JR and MT. Funding acquisition: JR and MT. All authors contributed to the article and approved the submitted version.
Funding
This work was supported, in part, by grants IG 21956 (to JR), IG 21617 (to MT) from the Associazione Italiana per la Ricerca sul Cancro (AIRC), Milan, Italy, and University of Ferrara, FAR grants 2021 (FM and MT).
Conflict of Interest
Authors CM, CL, EM, FM, MT, and JR are holders of the Italian patent application number 102020000021235 (I0188839) BRE-mma, filed on September 8, 2020. Data of this work were enclosed, in part, in the aforementioned Italian patent.
The remaining authors declare that the research was conducted in the absence of any commercial or financial relationships that could be construed as a potential conflict of interest.
Publisher’s Note
All claims expressed in this article are solely those of the authors and do not necessarily represent those of their affiliated organizations, or those of the publisher, the editors and the reviewers. Any product that may be evaluated in this article, or claim that may be made by its manufacturer, is not guaranteed or endorsed by the publisher.
Acknowledgments
We thank Professor Georgia Emma Gili for revising the English text of the manuscript.
References
1. Feng H, Shuda M, Chang Y, Moore PS. Clonal Integration of a Polyomavirus in Human Merkel Cell Carcinoma. Science (2008) 319:1096–100. doi: 10.1126/science.1152586
2. Jin HT, Park SJ, Choi EK, Kim YS. The Frequency of Merkel Cell Polyomavirus in Whole Blood From Immunocompetent and Immunosuppressed Patients With Kidney Disease and Healthy Donors. Microb Pathog (2019) 131:75–80. doi: 10.1016/j.micpath.2019.03.020
3. Coursaget P, Samimi M, Nicol JTJ, Gardair C, Touzé A. Human Merkel Cell Polyomavirus: Virological Background and Clinical Implications. APMIS (2013) 121:755–69. doi: 10.1111/apm.12122
4. Schowalter R, Buck C. The Merkel Cell Polyomavirus Minor Capsid Protein. Plos Pathog (2013) 9:1003558. doi: 10.1371/journal.ppat.1003558
5. McIlroy D, Halary F, Bressollette-Bodin C. Intra-Patient Viral Evolution in Polyomavirus-Related Diseases. Philos Trans R Soc B Biol Sci (2019) 37(1773):20180301. doi: 10.1098/rstb.2018.0301
6. Prezioso C, Obregon F, Ambroselli D, Petrolo S, Checconi P, Rodio DM, et al. Merkel Cell Polyomavirus (MCPyV) in the Context of Immunosuppression: Genetic Analysis of Noncoding Control Region (NCCR) Variability Among a HIV-1-Positive Population. Viruses (2020) 12(5):507. doi: 10.3390/v12050507
7. Pietropaolo V, Prezioso C, Moens U. Merkel Cell Polyomavirus and Merkel Cell Carcinoma. Cancers (Basel) (2020) 12:1774. doi: 10.3390/cancers12071774
8. Del Marmol V, Lebbé C. New Perspectives in Merkel Cell Carcinoma. Curr Opin Oncol (2019) 31:72–83. doi: 10.1097/CCO.0000000000000508
9. Rotondo JC, Bononi I, Puozzo A, Govoni M, Foschi V, Lanza G, et al. Merkel Cell Carcinomas Arising in Autoimmune Disease Affected Patients Treated With Biologic Drugs Including Anti-TNF. Clin Cancer Res (2017) 23:3929–34. doi: 10.1158/1078-0432.CCR-16-2899
10. Improta G, Ritter C, Pettinato A, Vasta V, Schrama D, Fraggetta F, et al. MGMT Promoter Methylation Status in Merkel Cell Carcinoma: In Vitro Versus Invivo. J Cancer Res Clin Oncol (2017) 143:1489–97. doi: 10.1007/s00432-017-2413-7
11. Moore LD, Le T, Fan G. DNA Methylation and Its Basic Function. Neuropsychopharmacology (2013) 38:23–38. doi: 10.1038/npp.2012.112
12. Pastrana DV, Tolstov YL, Becker JC, Moore PS, Chang Y, Buck CB. Quantitation of Human Seroresponsiveness to Merkel Cell Polyomavirus. PloS Pathog (2009) 5:e1000578. doi: 10.1371/journal.ppat.1000578
13. Zhou X, Nakashima K, Ito M, Zhang X, Sakai S, Feng C, et al. Prevalence and Viral Loads of Polyomaviruses BKPyV, JCPyV, MCPyV, TSPyV and NJPyV and Hepatitis Viruses HBV, HCV and HEV in HIV-Infected Patients in China. Sci Rep (2020) 10(1):17066. doi: 10.1038/s41598-020-74244-0
14. Vahabpour R, Aghasadeghi MR, Salehi-Vaziri M, Mohajel N, Keyvani H, Nasimi M, et al. Prevalence of Merkel Cell Polyomavirus in Tehran: An Age-Specific Serological Study. Iran Red Crescent Med J (2016) 18:e26097. doi: 10.5812/ircmj.26097
15. Kamminga S, van der Meijden E, Feltkamp MCW, Zaaijer HL. Seroprevalence of Fourteen Human Polyomaviruses Determined in Blood Donors. PloS One (2018) 13:e0206273. doi: 10.1371/journal.pone.0206273
16. Šroller V, Hamšíková E, Ludvíková V, Vochozková P, Kojzarová M, Fraiberk M, et al. Seroprevalence Rates of BKV, JCV, and MCPyV Polyomaviruses in the General Czech Republic Population. J Med Virol (2014) 86:1560–8. doi: 10.1002/jmv.23841
17. Csoboz B, Rasheed K, Sveinbjørnsson B, Moens U. Merkel Cell Polyomavirus and non-Merkel Cell Carcinomas: Guilty or Circumstantial Evidence? APMIS (2020) 128(2):104–20. doi: 10.1111/apm.13019
18. Mazziotta C, Lanzillotti C, Torreggiani E, Oton-Gonzalez L, Iaquinta MR, Mazzoni E, et al. Serum Antibodies Against the Oncogenic Merkel Cell Polyomavirus Detected by an Innovative Immunological Assay With Mimotopes in Healthy Subjects. Front Immunol (2021) 12:676627. doi: 10.3389/fimmu.2021.676627
19. Faust H, Pastrana DV, Buck CB, Dillner J, Ekström J. Antibodies to Merkel Cell Polyomavirus Correlate to Presence of Viral DNA in the Skin. J Infect Dis (2011) 203:1096–100. doi: 10.1093/infdis/jiq173
20. Touzé A, Le Bidre E, Laude H, Fleury MJJ, Cazal R, Arnold F, et al. High Levels of Antibodies Against Merkel Cell Polyomavirus Identify a Subset of Patients With Merkel Cell Carcinoma With Better Clinical Outcome. J Clin Oncol (2011) 29:1612–9. doi: 10.1200/JCO.2010.31.1704
21. Zhang C, Liu F, He Z, Deng Q, Pan Y, Liu Y, et al. Seroprevalence of Merkel Cell Polyomavirus in the General Rural Population of Anyang, China. PloS One (2014) 9:e106430. doi: 10.1371/journal.pone.0106430
22. Carter JJ, Paulson KG, Wipf GC, Miranda D, Madeleine MM, Johnson LG, et al. Association of Merkel Cell Polyomavirus-Specific Antibodies With Merkel Cell Carcinoma. J Natl Cancer Inst (2009) 101:1510–22. doi: 10.1093/jnci/djp332
23. Kean JM, Rao S, Wang M, Garcea RL. Seroepidemiology of Human Polyomaviruses. PloS Pathog (2009) 5:e1000363. doi: 10.1371/journal.ppat.1000363
24. Tolstov YL, Knauer A, Chen JG, Kensler TW, Kingsley LA, Moore PS, et al. Asymptomatic Primary Merkel Cell Polyomavirus Infection Among Adults. Emerg Infect Dis (2011) 17:1371–80. doi: 10.3201/eid1708.110079
25. Viscidi RP, Rollison DE, Sondak VK, Silver B, Messina JL, Giuliano AR, et al. Age-Specific Seroprevalence of Merkel Cell Polyomavirus, BK Virus, and JC Virus. Clin Vaccine Immunol (2011) 18:1737–43. doi: 10.1128/CVI.05175-11
26. Van Der Meijden E, Bialasiewicz S, Rockett RJ, Tozer SJ, Sloots TP, Feltkamp MCW. Different Serologic Behavior of MCPyV, TSPyV, HPyV6, HPyV7 and HPyV9 Polyomaviruses Found on the Skin. PloS One (2013) 8:e81078. doi: 10.1371/journal.pone.0081078
27. Touzé A, Gaitan J, Arnold F, Cazal R, Fleury MJ, Combelas N, et al. Generation of Merkel Cell Polyomavirus (MCV)-Like Particles and Their Application to Detection of MCV Antibodies. J Clin Microbiol (2010) 48:1767–70. doi: 10.1128/JCM.01691-09
28. Martel-Jantin C, Pedergnana V, Nicol JÔTJ, Leblond V, Trégouët DA, Tortevoye P, et al. Merkel Cell Polyomavirus Infection Occurs During Early Childhood and Is Transmitted Between Siblings. J Clin Virol (2013) 58:288–91. doi: 10.1016/j.jcv.2013.06.004
29. Sadeghi M, Aronen M, Chen T, Jartti L, Jartti T, Ruuskanen O, et al. Merkel Cell Polyomavirus and Trichodysplasia Spinulosa-Associated Polyomavirus DNAs and Antibodies in Blood Among the Elderly. BMC Infect Dis (2012) 12:383. doi: 10.1186/1471-2334-12-383
30. U.S. Department of Health and Human Services. Report on Carcinogens Monograph on Merkel Cell Polyomavirus. National Toxic Program (2016) 1–83.
31. Mazzoni E, Rotondo JC, Marracino L, Selvatici R, Bononi I, Torreggiani E, et al. Detection of Merkel Cell Polyomavirus DNA in Serum Samples of Healthy Blood Donors. Front Oncol (2017) 7:294. doi: 10.3389/fonc.2017.00294
32. Prezioso C, Lella FMD, DM R, Bitossi C, Trancassini M, Mele A, et al. Merkel Cell Polyomavirus DNA Detection in Respiratory Samples: Study of a Cohort of Patients Affected by Cystic Fibrosis. Viruses (2019) 11(6):571. doi: 10.3390/v11060571
33. Cason C, Monasta L, Zanotta N, Campisciano G, Maestri I, Tommasino M, et al. Antibody Response to Polyomavirus Primary Infection: High Seroprevalence of Merkel Cell Polyomavirus and Lymphoid Tissue Involvement. J Neurovirol (2018) 24:314–22. doi: 10.1007/s13365-017-0612-2
34. Hashida Y, Kamioka M, Tanaka M, Hosokawa S, Murakami M, Nakajima K, et al. Ecology of Merkel Cell Polyomavirus in Healthy Skin Among Individuals in an Asian Cohort. J Infect Dis (2016) 213:1708–16. doi: 10.1093/infdis/jiw040
35. Tabachnick-Cherny S, Pulliam T, Church C, Koelle DM, Nghiem P. Polyomavirus-Driven Merkel Cell Carcinoma: Prospects for Therapeutic Vaccine Development. Mol Carcinog (2020) 59:807–21. doi: 10.1002/mc.23190
36. Decaprio JA. Molecular Pathogenesis of Merkel Cell Carcinoma. Annu Rev Pathol Mech Dis (2021) 16:69–91. doi: 10.1146/annurev-pathmechdis-012419-032817
37. Ma J, Brewer JD. Merkel Cell Carcinoma in Immunosuppressed Patients. Cancers (Basel) (2014) 6:1328–50. doi: 10.3390/CANCERS6031328
38. Goldstein RH, DeCaprio JA. Merkel Cell Carcinoma in the HIV-1/AIDS Patient. Cancer Treat Res (2019) 177:211–29. doi: 10.1007/978-3-030-03502-0_8
39. Manganoni MA, Farisoglio C, Tucci G, Venturini M, Marocolo D, Aquilano MC, et al. Merkel Cell Carcinoma and HIV Infection: A Case Report and Review of the Literature. AIDS Patient Care STDS (2007) 21:447–51. doi: 10.1089/apc.2006.0152
40. Izikson L, Nornhold E, Iyer JG, Nghiem P, Zeitouni NC. Merkel Cell Carcinoma Associated With HIV: Review of 14 Patients. AIDS (2011) 25:119–21. doi: 10.1097/QAD.0b013e328340a19c
41. Vahabpour R, Nasimi M, Naderi N, Salehi-Vaziri M, Mohajel N, Sadeghi F, et al. Merkel Cell Polyomavirus IgG Antibody Levels Are Associated With Progression to AIDS Among HIV-Infected Individuals. Arch Virol (2017) 162:963–9. doi: 10.1007/s00705-016-3186-z
42. Zheng G, Chattopadhyay S, Sud A, Sundquist K, Sundquist J, Försti A, et al. Second Primary Cancers in Patients With Acute Lymphoblastic, Chronic Lymphocytic and Hairy Cell Leukaemia. Br J Haematol (2019) 185:232–9. doi: 10.1111/bjh.15777
43. Yu KK, Dasanu CA. Rapidly Fatal Dissemination of Merkel Cell Carcinoma in a Patient Treated With Alemtuzumab for Chronic Lymphocytic Leukemia. Conn Med (2016) 80:353–8.
44. Clarke CA, Robbins HA, Tatalovich Z, Lynch CF, Pawlish KS, Finch JL, et al. Risk of Merkel Cell Carcinoma After Solid Organ Transplantation. J Natl Cancer Inst (2015) 107(2):dju382. doi: 10.1093/jnci/dju382
45. Lanoy E, Engels EA. Skin Cancers Associated With Autoimmune Conditions Among Elderly Adults. Br J Cancer (2010) 103:112–4. doi: 10.1038/sj.bjc.6605733
46. Boldorini R, Allegrini S, Tognon M, Miglio U, Rossi D, Pawlita M, et al. Merkel Cell Carcinoma Arising in Inguinal Lymph Node in a Patient With Von Willebrand Disease After Multiple Blood Transfusions. J Clin Virol (2014) 60:73–5. doi: 10.1016/j.jcv.2014.02.007
47. Samimi M. Immune Checkpoint Inhibitors and Beyond: An Overview of Immune-Based Therapies in Merkel Cell Carcinoma. Am J Clin Dermatol (2019) 20:391–407. doi: 10.1007/s40257-019-00427-9
48. Shahzad N, Hussain I, Gilani US, Tayyeb A, Aslam MA, Khurshid M, et al. Merkel Cell Polyomavirus DNA Sequences in the Blood of Healthy Population of Pakistan. Future Microbiol (2019) 14:599–608. doi: 10.2217/fmb-2018-0314
49. Saraswati K, Phanichkrivalkosil M, Day NPJ, Blacksell SD. The Validity of Diagnostic Cut-Offs for Commercial and in-House Scrub Typhus IgM and IgG ELISAs: A Review of the Evidence. PloS Negl Trop Dis (2019) 13:e0007158. doi: 10.1371/journal.pntd.0007158
50. Classen DC, Morningstar JM, Shanley JD. Detection of Antibody to Murine Cytomegalovirus by Enzyme-Linked Immunosorbent and Indirect Immunofluorescence Assays. J Clin Microbiol (1987) 25:600–4. doi: 10.1128/jcm.25.4.600-604.1987
51. Lardeux F, Torrico G, Aliaga C. Calculation of the ELISA’s Cut-Off Based on the Change-Point Analysis Method for Detection of Trypanosoma Cruzi Infection in Bolivian Dogs in the Absence of Controls. Mem Inst Oswaldo Cruz (2016) 111:501–4. doi: 10.1590/0074-02760160119
52. Meyer P, Crowther JR. The ELISA Guidebook. In: Methods in Molecular Biology Vol. 149. Humana Press (2001). p. 421. doi: 10.1016/s0923-2508(00)01178-5
53. Rotondo JC, Oton-Gonzalez L, Mazziotta C, Lanzillotti C, Iaquinta MR, Tognon M, et al. Simultaneous Detection and Viral DNA Load Quantification of Different Human Papillomavirus Types in Clinical Specimens by the High Analytical Droplet Digital PCR Method. Front Microbiol (2020) 11:591452. doi: 10.3389/fmicb.2020.591452
54. Rotondo JC, Candian T, Selvatici R, Mazzoni E, Bonaccorsi G, Greco P, et al. Tracing Males From Different Continents by Genotyping JC Polyomavirus in DNA From Semen Samples. J Cell Physiol (2017) 232:982–5. doi: 10.1002/jcp.25686
55. Rotondo JC, Borghi A, Selvatici R, Magri E, Bianchini E, Montinari E, et al. Hypermethylation-Induced Inactivation of the IRF6 Gene as a Possible Early Event in Progression of Vulvar Squamous Cell Carcinoma Associated With Lichen Sclerosus. JAMA Dermatol (2016) 152(8):928–33. doi: 10.1001/jamadermatol.2016.1336
56. Nakagawa S, Schielzeth H. Repeatability for Gaussian and non-Gaussian Data: A Practical Guide for Biologists. Biol Rev (2010) 85:935–56. doi: 10.1111/j.1469-185X.2010.00141.x
57. Rotondo JC, Oton-Gonzalez L, Selvatici R, Rizzo P, Pavasini R, Campo GC, et al. SERPINA1 Gene Promoter Is Differentially Methylated in Peripheral Blood Mononuclear Cells of Pregnant Women. Front Cell Dev Biol (2020) 8:550543. doi: 10.3389/fcell.2020.550543
58. Mazzoni E, Pellegrinelli E, Mazziotta C, Lanzillotti C, Rotondo JC, Bononi I, et al. Mother-To-Child Transmission of Oncogenic Polyomaviruses BKPyV, JCPyV and SV40. J Infect (2020) 163:91–8. doi: 10.1016/j.jinf.2020.02.006
59. Huth SP, Relford R, Steiner JM, Strong-Townsend MI, Williams DA. ORIGINAL RESEARCH: Analytical Validation of an ELISA for Measurement of Canine Pancreas-Specific Lipase. Vet Clin Pathol (2010) 39:346–53. doi: 10.1111/j.1939-165X.2010.00245.x
60. Thomsson O, Ström-Holst B, Sjunnesson Y, Bergqvist AS. Validation of an Enzyme-Linked Immunosorbent Assay Developed for Measuring Cortisol Concentration in Human Saliva and Serum for its Applicability to Analyze Cortisol in Pig Saliva. Acta Vet Scand (2014) 56(1):55. doi: 10.1186/s13028-014-0055-1
61. Bhadricha H, Khatkhatay MI, Desai M. Development of an in House ELISA for Human Intact Osteocalcin and Its Utility in Diagnosis and Management of Osteoporosis. Clin Chim Acta (2019) 489:117–23. doi: 10.1016/j.cca.2018.12.007
62. Cambron P, Jacquet JM, Hoet B, Lievens M. Development and Technical and Clinical Validation of a Quantitative Enzyme-Linked Immunosorbent Assay for the Detection of Human Antibodies to Hepatitis B Surface Antigen in Recipients of Recombinant Hepatitis B Virus Vaccine. Clin Vaccine Immunol (2009) 16:1236–46. doi: 10.1128/CVI.00431-08
63. Dehus O, Zimmer J, Döring S, Führer F, Hanschmann KM, Holzhauser T, et al. Development and in-House Validation of an Allergen-Specific ELISA for Quantification of Bet V 4 in Diagnostic and Therapeutic Birch Allergen Products. Anal Bioanal Chem (2015) 407:1673–. doi: 10.1007/s00216-014-8418-z
64. Hosamani M, Basagoudanavar SH, Tamil Selvan RP, Das V, Ngangom P, Sreenivasa BP, et al. Venkataramanan R. A Multi-Species Indirect ELISA for Detection of non-Structural Protein 3ABC Specific Antibodies to Foot-and-Mouth Disease Virus. Arch Virol (2015) 160:937–44. doi: 10.1007/s00705-015-2339-9
65. Hock BD, Stamp LK, Hayman MW, Keating PE, Helms ETJ, Barclay ML. Development of an ELISA-Based Competitive Binding Assay for the Analysis of Drug Concentration and Antidrug Antibody Levels in Patients Receiving Adalimumab or Infliximab. Ther Drug Monit (2016) 53:606–10. doi: 10.1097/FTD.0000000000000229
66. Leeming DJ, Willumsen N, Sand JMB, Holm Nielsen S, Dasgupta B, Brodmerkel C, et al. A Serological Marker of the N-Terminal Neoepitope Generated During LOXL2 Maturation is Elevated in Patients With Cancer or Idiopathic Pulmonary Fibrosis. Biochem Biophys Rep (2019) 17:38–43. doi: 10.1016/j.bbrep.2018.11.002
67. Cabán-Hernández K, Gaudier JF, Ruiz-Jiménez C, Espino AM. Development of Two Antibody Detection Enzyme-Linked Immunosorbent Assays for Serodiagnosis of Human Chronic Fascioliasis. J Clin Microbiol (2014) 52(3):766–72. doi: 10.1128/JCM.02875-13
68. Teimouri A, Hossein M, Modarressi, Shojaee S, Mohebali M, Rezaian M, et al. Development, Optimization, and Validation of an in-House Dot-ELISA Rapid Test Based on SAG1 and GRA7 Proteins for Serological Detection of Toxoplasma Gondii Infections. Infect Drug Resist (2019) 12:2657–69. doi: 10.2147/IDR.S219281
69. Nicol JTJ, Robinot R, Carpentier A, Carandina G, Mazzoni E, Tognon M, et al. Age-Specific Seroprevalences of Merkel Cell Polyomavirus, Human Polyomaviruses 6, 7, and 9, and Trichodysplasia Spinulosa-Associated Polyomavirus. Clin Vaccine Immunol (2013) 20:363–8. doi: 10.1128/CVI.00438-12
70. Tolstov YL, Pastrana DV, Feng H, Becker JC, Jenkins FJ, Moschos S, et al. Human Merkel Cell Polyomavirus Infection II. MCV is a Common Human Infection That Can be Detected by Conformational Capsid Epitope Immunoassays. Int J Cancer (2009) 125:1250–6. doi: 10.1002/ijc.24509
71. Li TC, Iwasaki K, Katano H, Kataoka M, Nagata N, Kobayashi K, et al. Characterization of Self-Assembled Virus-Like Particles of Merkel Cell Polyomavirus. PloS One (2015) 10:e0115646. doi: 10.1371/journal.pone.0115646
72. Fleury MJJ, Nicol JTJ, Samimi M, Arnold F, Cazal R, Ballaire R, et al. Identification of the Neutralizing Epitopes of Merkel Cell Polyomavirus Major Capsid Protein Within the BC and EF Surface Loops. PloS One (2015) 26:e0121751. doi: 10.1371/journal.pone.0121751
73. Samimi M, Molet L, Fleury M, Laude H, Carlotti A, Gardair C, et al. Prognostic Value of Antibodies to Merkel Cell Polyomavirus T Antigens and VP1 Protein in Patients With Merkel Cell Carcinoma. Br J Dermatol (2016) 174:813–22. doi: 10.1111/bjd.14313
74. Lunn RM, Jahnke GD, Rabkin CS. Tumour Virus Epidemiology. Philos Trans R Soc B Biol Sci (2017) 372(1732):20160266. doi: 10.1098/rstb.2016.0266
75. Mertz KD, Junt T, Schmid M, Pfaltz M, Kempf W. Inflammatory Monocytes Are a Reservoir for Merkel Cell Polyomavirus. J Invest Dermatol (2010) 130:1146–51. doi: 10.1038/jid.2009.392
76. Simon AK, Hollander GA, McMichael A. Evolution of the Immune System in Humans From Infancy to Old Age. Proc R Soc B Biol Sci (2015) 282:20143085. doi: 10.1098/rspb.2014.3085
77. Hazeldine J, Lord JM. Immunesenescence: A Predisposing Risk Factor for the Development of COVID-19? Front Immunol (2020) 11:573662. doi: 10.3389/fimmu.2020.573662
78. Liu W, MacDonald M, You J. Merkel Cell Polyomavirus Infection and Merkel Cell Carcinoma. Curr Opin Virol (2016) 20:20–7. doi: 10.1016/j.coviro.2016.07.011
79. Fan H, Dong G, Zhao G, Liu F, Yao G, Zhu Y, et al. Gender Differences of B Cell Signature in Healthy Subjects Underlie Disparities in Incidence and Course of SLE Related to Estrogen. J Immunol Res (2014) 2014:1–17. doi: 10.1155/2014/814598
80. Heli K, Böhling T, Koljonen V, Tukiainen E, Haglund C, Pokhrel A, et al. Merkel Cell Carcinoma - A Population-Based Epidemiological Study in Finland With a Clinical Series of 181 Cases. Eur J Cancer (2012) 48:737–42. doi: 10.1016/j.ejca.2011.06.001
82. Agelli M, Clegg LX, M A, LX C. Epidemiology of Primary Merkel Cell Carcinoma in the United States. J Am Acad Dermatol (2003) 49:832–41. doi: 10.1016/S0190-9622(03)02108-X
83. Stang A, Becker J, Nghiem P, Ferlay J. The Association Between Geographic Location and Incidence of Merkel Cell Carcinoma in Comparison to Melanoma: An International Assessment. Eur J Cancer (2018) 94:47–60. doi: 10.1016/J.EJCA.2018.02.003
84. Kieny A, Cribier B, Meyer N, Velten M, Jégu J, Lipsker D. Epidemiology of Merkel Cell Carcinoma. A Population-Based Study From 1985 to 2013, in Northeastern of France. Int J Cancer (2019) 144:741–5. doi: 10.1002/IJC.31860
Keywords: elderly individuals, Merkel cell polyomavirus, MCPyV, Merkel cell carcinoma, immunological assay, enzyme-linked immunosorbent assay, immune system, immunosenescence
Citation: Mazziotta C, Lanzillotti C, Govoni M, Pellielo G, Mazzoni E, Tognon M, Martini F and Rotondo JC (2021) Decreased IgG Antibody Response to Viral Protein Mimotopes of Oncogenic Merkel Cell Polyomavirus in Sera From Healthy Elderly Subjects. Front. Immunol. 12:738486. doi: 10.3389/fimmu.2021.738486
Received: 08 July 2021; Accepted: 22 September 2021;
Published: 18 October 2021.
Edited by:
Masakazu Kamata, University of Alabama at Birmingham, United StatesCopyright © 2021 Mazziotta, Lanzillotti, Govoni, Pellielo, Mazzoni, Tognon, Martini and Rotondo. This is an open-access article distributed under the terms of the Creative Commons Attribution License (CC BY). The use, distribution or reproduction in other forums is permitted, provided the original author(s) and the copyright owner(s) are credited and that the original publication in this journal is cited, in accordance with accepted academic practice. No use, distribution or reproduction is permitted which does not comply with these terms.
*Correspondence: John Charles Rotondo, cnRuam5jQHVuaWZlLml0; Fernanda Martini, bXJmQHVuaWZlLml0