- 1Beijing Key Laboratory of Emerging Infectious Diseases, Institute of Infectious Diseases, Beijing Ditan Hospital, Capital Medical University, Beijing, China
- 2Beijing Ditan Hospital, Capital Medical University, Beijing, China
- 3Intensive Care Medicine, Beijing Ditan Hospital, Capital Medical University, Beijing, China
- 4Clinical and Research Center of Infectious Diseases, Beijing Ditan Hospital, Capital Medical University, Beijing, China
Background: The global outbreak of coronavirus disease 2019 (COVID-19) has turned into a worldwide public health crisis and caused more than 100,000,000 severe cases. Progressive lymphopenia, especially in T cells, was a prominent clinical feature of severe COVID-19. Activated HLA-DR+CD38+ CD8+ T cells were enriched over a prolonged period from the lymphopenia patients who died from Ebola and influenza infection and in severe patients infected with SARS-CoV-2. However, the CD38+HLA-DR+ CD8+ T population was reported to play contradictory roles in SARS-CoV-2 infection.
Methods: A total of 42 COVID-19 patients, including 32 mild or moderate and 10 severe or critical cases, who received care at Beijing Ditan Hospital were recruited into this retrospective study. Blood samples were first collected within 3 days of the hospital admission and once every 3–7 days during hospitalization. The longitudinal flow cytometric data were examined during hospitalization. Moreover, we evaluated serum levels of 45 cytokines/chemokines/growth factors and 14 soluble checkpoints using Luminex multiplex assay longitudinally.
Results: We revealed that the HLA-DR+CD38+ CD8+ T population was heterogeneous, and could be divided into two subsets with distinct characteristics: HLA-DR+CD38dim and HLA-DR+CD38hi. We observed a persistent accumulation of HLA-DR+CD38hi CD8+ T cells in severe COVID-19 patients. These HLA-DR+CD38hi CD8+ T cells were in a state of overactivation and consequent dysregulation manifested by expression of multiple inhibitory and stimulatory checkpoints, higher apoptotic sensitivity, impaired killing potential, and more exhausted transcriptional regulation compared to HLA-DR+CD38dim CD8+ T cells. Moreover, the clinical and laboratory data supported that only HLA-DR+CD38hi CD8+ T cells were associated with systemic inflammation, tissue injury, and immune disorders of severe COVID-19 patients.
Conclusions: Our findings indicated that HLA-DR+CD38hi CD8+ T cells were correlated with disease severity of COVID-19 rather than HLA-DR+CD38dim population.
Introduction
Coronavirus disease 2019 (COVID-19) started as an epidemic in Wuhan in 2019 and has become a pandemic (1–3). It rapidly triggered a worldwide public health crisis. As of June, 28, 2021, a total of 180,654,652 cases were identified to be infected by severe acute respiratory syndrome coronavirus 2 (SARS-CoV-2), with 3,920,463 fatal cases, according to the data from WHO. Although the earliest vaccines are already being rolled out in a host of countries, herd immunity to COVID-19 might be very difficult to achieve with current vaccines (4–7). In this case, the spread of SARS-CoV-2 infection would be kept out of control.
Consistent with other respiratory viral infections, adaptive immune responses, particularly cytotoxic T cells, play a vital role in SARS- CoV-2 infection (8–10). It remains a puzzle whether T cell responses in COVID-19 patients are moderate, excessive, or dysfunctional, with evidences provided for all ends of the spectrum. Numerous studies indicated that progressive lymphopenia, especially in T cells, might be highly involved in the pathological process of SARS-CoV-2 infection (11–14). Co-expression of Human Leukocyte Antigen DR (HLA-DR) and CD38 associated with activation of CD8+ T cells was reported to accumulate over a prolonged period from the lymphopenia patients who died from Ebola and influenza infection (15–19). These activated HLA-DR+CD38+ CD8+T cells were also noted in mild/moderate and severe cases of COVID-19 patients and displayed a tight correlation with severity of COVID-19 (20–22). However, several studies indicated that CD38+HLA-DR+CD8+ T cells could play a recovery role of activating immunity and eliminating the virus (23, 24). It seemed that the effects of CD38+HLA-DR+ CD8+ T cells in COVID-19 patients varied widely in different studies. Nevertheless, little is known about phenotype and function of CD38+HLA-DR+ CD8+ T cells and association with clinical outcome in COVID-19 patients.
Here, we revealed that the HLA-DR+CD38+ CD8+ T population is heterogeneous of two subpopulations, HLA-DR+CD38dim and HLA-DR+CD38hi with distinct characteristics.
Furthermore, we found that elevated fraction of HLA-DR+CD38hi rather than HLA-DR+CD38+ CD8+ T cells were persistently accumulated in COVID-19 patients, especially in severe and critical cases. These HLA-DR+CD38hi CD8+ T cells existed in an overactivated and consequently immune disordered state, with high expression of several coinhibitory and costimulatory molecules. This population displayed increased apoptotic sensitivity, impaired killing potential, and more exhausted phenotype and transcriptional regulation, compared to HLA-DR+CD38dim CD8+ T cells. Of note, the clinical and laboratory data support the notion that HLA-DR+CD38hi CD8+ T cells were correlated with disease severity of COVID-19 rather than HLA-DR+CD38dim population.
Materials and Methods
Patients
A total of 42 COVID-19 patients in this retrospective cohort study were enrolled from Beijing Ditan Hospital from March 13, 2020 to April 25, 2020. All enrolled patients were confirmed to be infected with SARS-CoV-2 by RT-PCR assays. This study was approved by the Committee of Ethics at Beijing Ditan Hospital, Capital Medical University. M/M patients were mild and moderate patients, and S/C patients were severe and critical patients, according to the guidelines on the diagnosis and treatment of new coronavirus pneumonia (version 7) by the National Health Commission of China issued on March 3, 2020. These 42 patients included 32 mild or moderate (M/M) patients and 10 severe or critical (S/C) patients. All baseline medical record information including demographic data and clinical characteristics were obtained within the first day after hospital admission (Table S1). Blood samples were first collected within 3 days of the hospital admission and once every 3–7 days during hospitalization. The median age of the patients was 37 years (range 20–75) with 50% men and 50% women. Among these 42 patients, the most common were hypertension (five cases), diabetes (three cases), chronic pulmonary disease (three cases), chronic kidney disease (one case), cardiovascular disease (one case). Other clinical details are shown in Table S1.
Ethics
This study was approved by Committee of Ethics at Beijing Ditan Hospital, Capital Medical University [NO. JDLKZ (2020) D (036)-01] with informed consents acquired from all enrolled patients. This study complied with all relevant ethical regulations for work with human participants, and informed consent was obtained. Samples were collected from patients who provided informed consent to participate in the study.
Peripheral Blood Mononuclear Cells and Serum Isolation
The PBMCs were collected in EDTA at the indicated time points. PBMCs were separated by density gradient centrifugation with lymphocyte separation solution. Serum samples were collected in serum separation tube. The blood was centrifuged at 2,000 rpm for 10 min at 20°C, and the serum was stored at −80°C and thawed at the time of assays. All samples were processed and analyzed within 24 h of collection.
Flow Cytometric Analysis
PBMCs were incubated with directly conjugated antibodies for 30 min at 4°C. The cells were then washed before flow cytometric analysis. Antibodies used were anti-human CD3-BUV737, CD4-BUV395, PD-1-BV711, CD38-FITC, GITR-BV605 (BD Biosciences, San Diego, CA, USA), CD8-BV510, CTLA-4-BV786, OX40-APC-Fire750, 4-1BB-BV421, HLA-DR-AF700 (BioLegend, San Diego, CA, USA), TIGIT- PE-Cy7, LAG-3-APC, ICOS-PE, (Ebioscience, San Diego, CA, USA), and the corresponding isotype controls. Data acquisition was performed on an LSR Fortessa flow cytometer (BD Biosciences), and data analysis was performed using FlowJo Software (Tree Star, Ashland, OR, USA).
Intracellular Staining
PBMCs, isolated as described above, were resuspended to 1×106 cells/ml in PBS. The cells were surface-stained with CD3-BV786, CD38-BUV737, HLA-DR-PE, CCR7-BV421, CD45RA-AF700, CD71-APC-H7 (BD), CD4-APC-Fire750, CD8-BV510 (BioLegend) for 30 min in the dark at 4°C, followed by fixation and permeabilization. After permeabilization, cells were stained with ki67-FITC, Granzyme B-AF700, T-bet-BV421, BAX-FITC, Bcl2-PE (BD Biosciences), Eomes-PE-Cy7 (Ebioscience), perforin-APC (BioLegend) antibodies for 30 min in the dark at room temperature. Following staining, cells were washed and acquired on an LSRFortessa.
45 Cytokines/Chemokines/Growth Factors and 14 Soluble Checkpoints Multiplex Assay
The serum of 27 COVID-19 patients were assayed for the two multiplexed bead immunoassays. First, we tested 45 ProcartaPlex Human Cytokine/Chemokine/Growth Factor Panel (Invitrogen, Calsbad, CA, USA), including BDNF, Eotaxin/CCL11, EGF, FGF-2, GM-CSF, GROα/CXCL1, HGF, NGFβ, LIF, IFNα, IFNγ, IL-1β, IL-1α, IL-1Rα, IL-2, IL-4, IL-5, IL-6, IL-7, IL-8/CXCL8, IL-9, IL-10, IL-12p70, IL-13, IL-15, IL-17α, IL-18, IL-21, IL-22, IL-23, IL-27, IL-31, IP-10/CXCL10, MCP-1/CCL2, MIP-1α/CCL3, MIP1β/CCL4, RANTES/CCL5, SDF-1α/CXCL12, TNFα, TNFβ/LTA, PDGF-BB, PLGF, SCF, VEGF-A, and VEGF-D. Second, we tested the 14 ProcartaPlex Human ImmunoOncology Checkpoint Panel (Invitrogen), including BTLA, GITR, HVEM, IDO, LAG-3, PD-1, PD-L1, PD-L2, TIM-3, CD28, CD80, 4-1BB, CD27, and CD152. All data were acquired according to the manufacturer’s protocol using Luminex MAGPIX® instrument (Luminex Co., Austin, TX, USA) and analyzed using ProcartaPlex Analyst 1.0 software (Invitrogen).
Statistical Analysis
GraphPad5 (GraphPad Software, La Jolla, CA, USA) or SPSS (IBM Corporation, New York, NY, USA) was used for statistical calculations. Data are expressed as the mean ± standard deviation (SD) and percentage (frequency), and the normality of each variable was evaluated using the Kolmogorov–Smirnov test. In cases of two normally distributed data, the comparison of variables was respectively performed using unpaired or paired two-tailed Student’s t tests. One-way ANOVA test followed by Tukey’s multiple comparisons test or Holm-Sidak’s multiple comparisons test was performed for comparing two more independent or matched samples. When the data were not normally distributed, the comparison of variables was performed with a Mann–Whitney U test or a Wilcoxon matched-pairs signed-rank test for unpaired and paired data, respectively. For comparing two more samples, a Kruskal–Wallis test or Friedman test followed by Dunn’s multiple comparisons test was applied for independent and matched samples. Comparisons of patient characteristics were analyzed using Fisher’s exact test (categorical variables) or Kruskal–Wallis test (continuous variables). P and correlation coefficient values were obtained using the Spearman’s correlation test. For all analyses, P values <0.05 were considered statistically significant.
Results
COVID−19 Cohort
We recruited 42 confirmed COVID-19 patients who received care at Beijing Ditan Hospital. The clinical courses of these cases included 32 mild or moderate (M/M) and 10 severe or critical (S/C) cases have been described in Table S1. Twenty age- and gender-matched healthy donors (HDs) were enrolled as controls. Blood samples were first collected within 3 days of the hospital admission and once every 3–7 days during hospitalization. The study was approved by the Committee of Ethics at Beijing Ditan Hospital, Capital Medical University, Beijing, China.
Persistent Elevated HLA-DR+CD38hi CD8+ T Cells in S/C Group COVID-19 Cases
To determine the activated status of CD8+ T cells, we first analyzed co-expression of HLA-DR and CD38, which are key markers of CD8+ T cell activation during viral infection. Based on expression of CD38, three subpopulations were defined among activated HLA-DR+CD8+ T cells: HLA-DR+CD38− (fraction I), HLA-DR+CD38dim (fraction II), HLA-DR+CD38hi (fraction III), as shown in Figure 1A. Patients of S/C group were found to have an obvious peak in HLA-DR+CD38hi CD8+ T cell (fraction III) within 2–3 weeks post onset (Figures S1A, B). We then observed a significantly higher percentage of HLA-DR+CD38hi CD8+ T cells in all patients including M/M and S/C groups at the peak point, compared to healthy controls. Moreover, the percentage of HLA-DR+CD38hi CD8+ T cells was dramatically higher in S/C patients than M/M patients (23.48 vs. 3.203%, Figure 1B). HLA-DR+CD38dim CD8+ T cells showed a similar trend but less increase. Consequently, the ratio of HLA-DR+CD38dim to HLA-DR+CD38hi CD8+ T cells was significantly higher in M/M than S/C COVID-19 patients in CD8 T cells (Figure S2). In contrast, no significant difference was found in HLA-DR+CD38− subset among three groups.
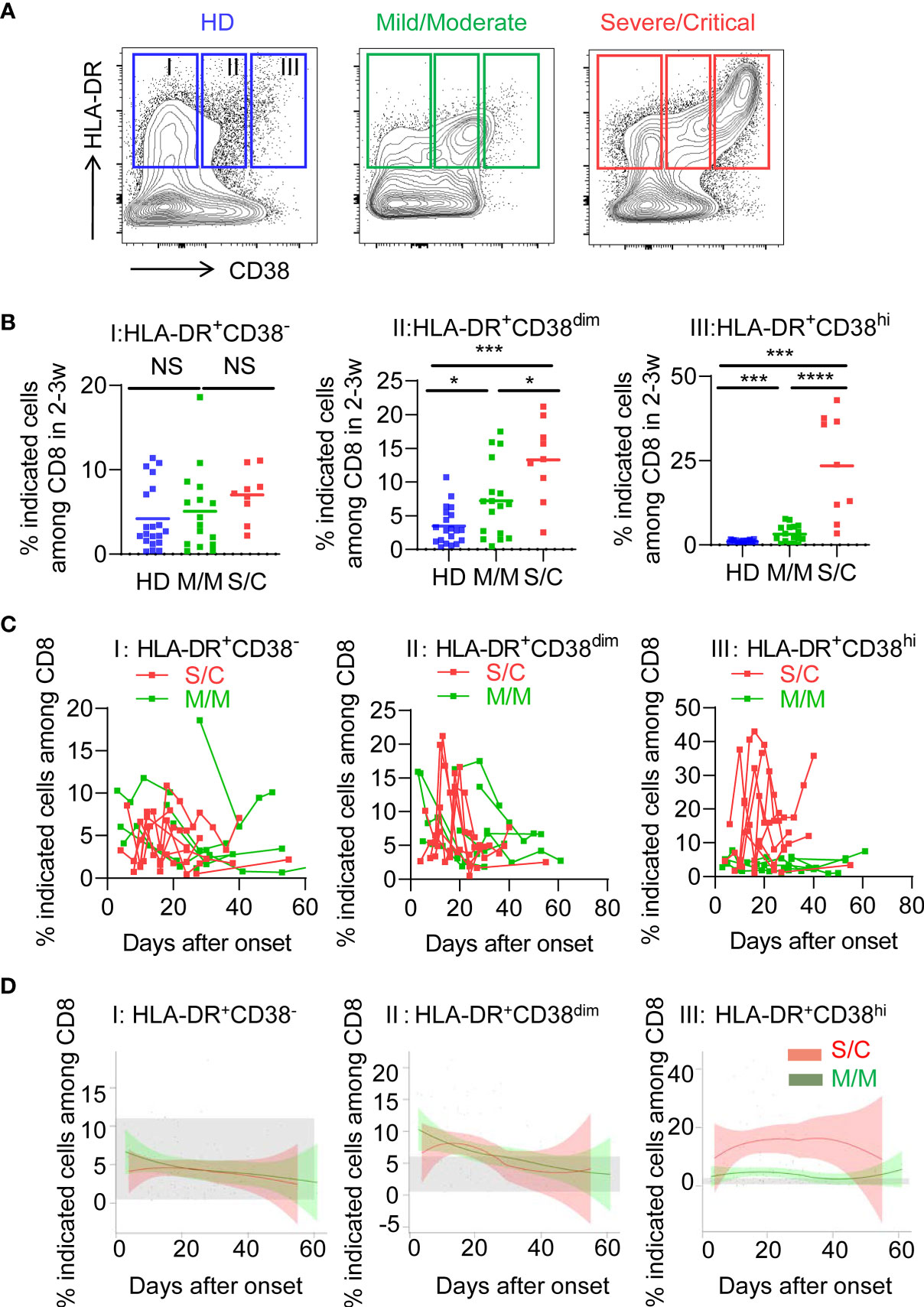
Figure 1 Elevated HLA-DR+CD38hi CD8+ T cells during acute infection of COVID-19. Flow cytometry analysis of HLA-DR and CD38 expression was performed on PBMCs collected from healthy donors, M/M and S/C patients with COVID-19 infection. (A) Representative FACS contour plots showed three subpopulations of HLA-DR+ CD8+ T cells from healthy donor and COVID-19 patients: HLA-DR+CD38− (I), HLA-DR+CD38dim (II), HLA-DR+CD38hi (III). (B) Scatter dot plots of the three percentages of HLA-DR+ CD8+ T cells from healthy donors and COVID-19 patients within 2–3 weeks post onset (n = 9–20 each group). P Values were obtained by unpaired two-tailed Student’s t tests and Mann–Whitney U test and repeated measures by one-way ANOVA or Kruskal-Wallis test followed by Tukey’s or Dunn’s multiple comparisons test. *P < .05, **P < .01, ***P < .001, ****P < .0001. (C) Longitudinal data of three subpopulations were graphed for eight S/C and seven M/M patients with three time points at least. (D) Temporal changes of three subpopulations in M/M (n =32) and S/C (n = 10) groups during hospitalization were shown. The 95% confidence interval indicated by colored areas. The normal range of each population was gray shaded region.
We further examined the longitudinal flow cytometric data in eight S/C and eight M/M cases. S/C patients developed elevated HLA-DR+CD38hi CD8+ T cells early in the infection and displayed persistently high percentage of this population (peak 43%) during the whole course of hospitalization. In contrast, percentage of HLA-DR+CD38hi CD8+ T cell in M/M cases increased slightly and transiently in the course of illness. As expected, there were no differences in kinetics of HLA-DR+CD38− and HLA-DR+CD38dim CD8+ T cells between S/C and M/M patients (Figure 1C). Furthermore, we combined all flow data of each patient (32 M/M and 10 S/C cases) and plotted their fluctuation patterns against the time point post onset. Consistently, these aggregating data showed that percentage of HLA-DR+CD38hi CD8+ T cells rather than the other two subsets was persistently higher in S/C patients than in M/M cases during hospitalization (Figure 1D). Meanwhile, we observed no significant changes of HLA-DR+CD38hi CD4+ T cells in S/C and M/M patients with COVID-19 infection (Figure S3). Overall, our data showed that persistent accumulation of HLA-DR+CD38hi CD8+ T cells was associated with severity of COVID-19.
Elevation of HLA-DR+CD38hi CD8+ T Cells Correlated With Immune Disorders and Tissue Injury in COVID-19 Patients
Next, we applied the longitudinal data of all patients and analyzed the correlation between dynamic changes of circulating HLA-DR+CD38hi CD8+ T cells and laboratory parameters (Table 1). We found percentage of HLA-DR+CD38hi CD8+ T cells was negatively correlated with absolute counts of lymphocytes, total T cells, CD4, CD8 T cells, B cells, and NK cells, but not neutrophil and monocyte counts. We also observed significant negative correlations between percentage of HLA-DR+CD38hi CD8+ T cells and hemoglobin (R=−0.546, P<0.0001). Additionally, coagulation-related parameters including platelet count, D-dimer, prothrombin time (PT), and activated partial thromboplastin time (APTT) were detected. We showed that percentage of HLA-DR+CD38hi CD8+ T cells was significantly associated with D-dimer, which was indicated to correlate with COVID-19 severity (R=0.452, P=0.0003).
We further found positive correlations between HLA-DR+CD38hi CD8+ T cells and levels of C-response protein (CRP) and serum amyloid A (SAA), suggesting systemic inflammation (R= 0.475, P<0.0001; R=0.565, P<0.0001). Moreover, the percentage of HLA-DR+CD38hi CD8+ T cells was found to have positive correlations with aspartate transaminase (AST) and total bilirubin (TB) in COVID-19 patients (R=0.397, P<0.0001; R=0.398, P<0.0001), and a strong negative correlation with albumin (R=−0.481, P<0.0001). These data suggested that high percentage of HLA-DR+CD38hi CD8+ T cells was involved in liver injury induced by COVID-19. Consistently, levels of lactate dehydrogenase (LDH) and creatinine (CRE) were correlated with the percentage of HLA-DR+CD38hi CD8+ T cells, respectively (R=0.643, P<0.0001; R=0.354, P=0.0003), indicating myocardial and renal injury (Table 1). HLA-DR+CD38dim and HLA-DR+CD38− CD8 T cells showed no correlation with the clinical characteristics above. Collectively, these results suggested the involvement of HLA-DR+CD38hi CD8+ T cells in immune disorders and tissue injury in COVID-19 patients.
Phenotypic and Functional Characterization of HLA-DR+CD38hi CD8+ T Cells
To assess the phenotypic status of HLA-DR+CD38hi CD8+ T cells from COVID-19 patients, we performed additional stains on selected 20 samples from 18 patients. We determined the developmental stage of HLA-DR+CD38hi CD8+ T cells through dissecting T cells into naïve (TN: CD45RA+, CD27+, CCR7+), central memory (TCM: CD45RA−, CD27+, CCR7+), transitional memory (TTM: CD45RA−, CD27+, CCR7−), effector memory (TEM: CD45RA−, CD27−, CCR7−), and effector T cells (TE: CD45RA+, CD27−, CCR7−). HLA-DR+CD38hi CD8+ T cells consisted of enhanced percentage of TTM, constant proportion of TCM and TEM, and decreased TN and TE (Figure 2).
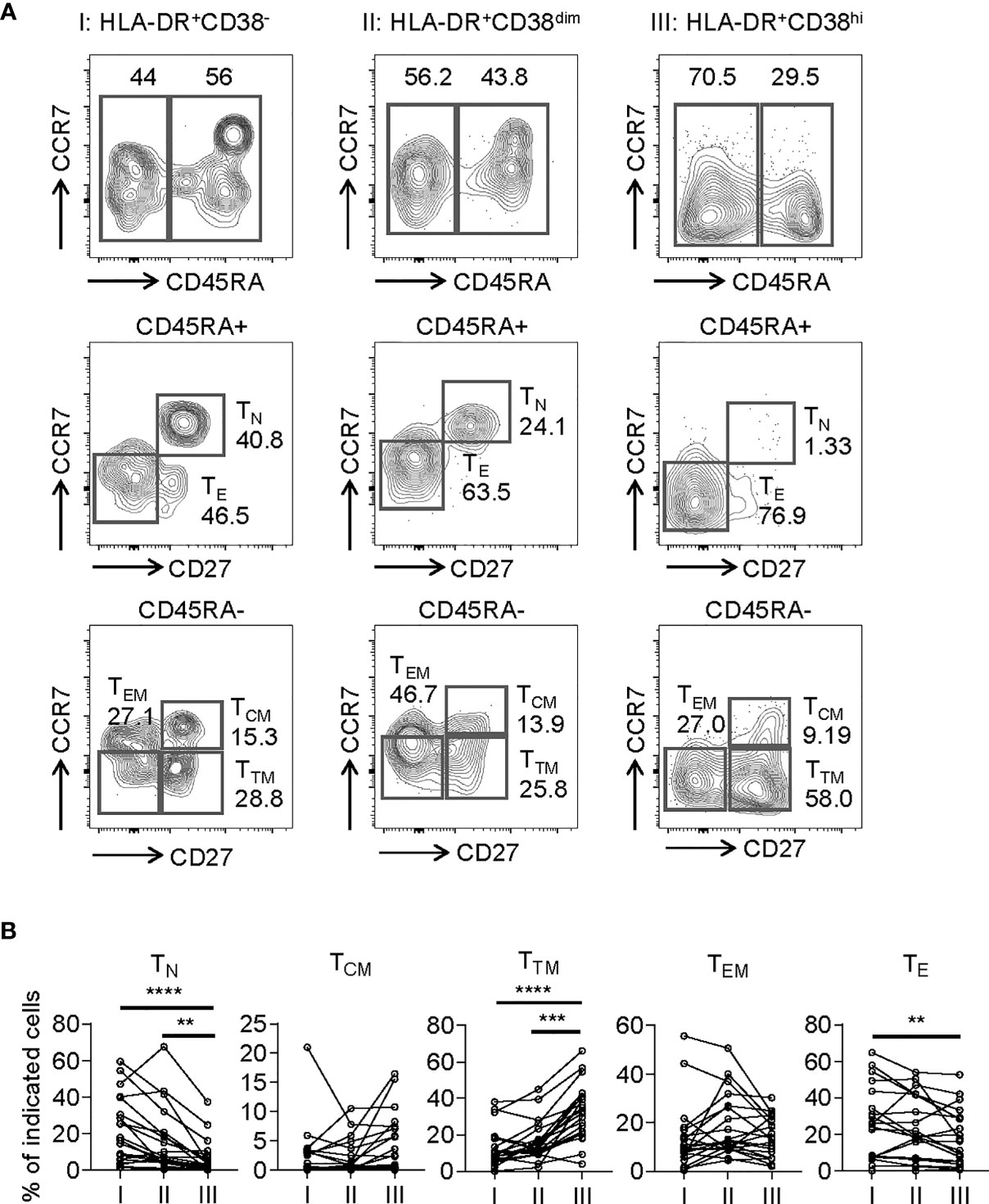
Figure 2 HLA-DR+CD38hi CD8+ T cells consisted of enhanced percentage of TTM and decreased TN and TE. Flow cytometry analysis of TN, TCM, TTM, TEM, and TE frequency was performed on PBMCs collected from patients with infection of COVID-19 (n = 20). (A) Gating strategy for TN, TCM, TTM, TEM, and TE in three CD8+ T populations. (B) The percentage of TN, TCM, TTM, TEM, and TE on each CD8+ T population (I, II, III). P Values were obtained by paired two-tailed Student’s t tests and Wilcoxon matched-pairs signed-rank test and repeated measures by one-way ANOVA or Friedman test followed by Holm-Sidak’s multiple comparisons or Dunn’s multiple comparisons test. **P < .01, ***P < .001, ****P < .0001.
Consistent with activation, HLA-DR+CD38hi CD8+ T cells (fraction III) displayed significantly higher levels of CD69, an early activation marker, compared with fractions I and II. We also evaluated expression of costimulatory molecules, including inducible T-cell costimulator (ICOS), OX40, TNF receptor superfamily member 9 (4-1BB), and glucocorticoid-induced tumor necrosis factor receptor (GITR). HLA-DR+CD38hi CD8+ T cells showed elevated expression of ICOS, OX40, 4-1BB, and GITR compared to fraction I. The level of OX40 in fraction III was higher than in fraction II, while fraction II expressed the highest levels of 4-1BB and GITR (Figure 3). Interestingly, we also found HLA-DR+CD38hi CD8+ T cells expressed higher levels of numerous coinhibitory molecules, including Programmed Death-1 receptor (PD-1), T cell immunoglobulin and mucin-domain containing-3 receptor (TIM-3), LAG-3 (Lymphocyte Activating 3), compared to fractions I and II. No significant difference was found in TIGIT expression of these three fractions. To investigate the intrinsic regulation of HLA-DR+CD38hi CD8+ T cells, we examined the expression of T-bet and Eomesodermin (Eomes), two key transcription factors governing CD8+ T cell exhaustion. We found that HLA-DR+CD38hi CD8+ T cells contained higher percentage of T-betdimEomeshi cells, which represented a terminal exhausted status, than fraction I and II. Meanwhile, these three fractions had comparable T-bethiEomesdim cells (Figure 4).
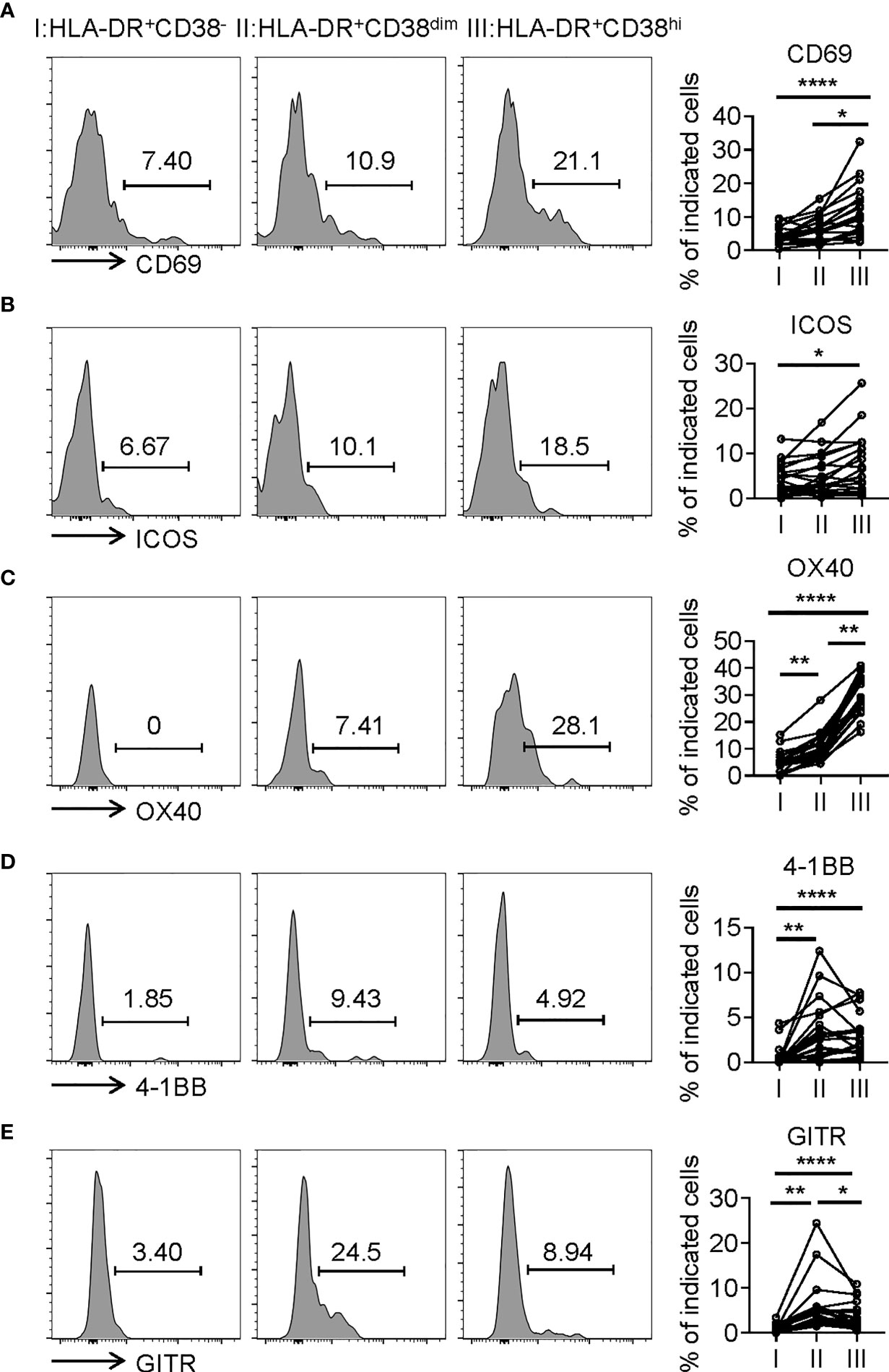
Figure 3 HLA-DR+CD38hi CD8+ T cells exhibited the phenotype of overactivation. Flow cytometry analysis of expression of CD69 (A), ICOS (B), OX40 (C), 4-1BB (D), and GITR (E) on three CD8+ T populations (I, II, III) from COVID-19 patients (n=20). Representative histograms (left) and plots (right) were shown. P Values were obtained by paired two-tailed Student’s t tests and repeated measures by one-way ANOVA test followed by Holm-Sidak’s multiple comparisons test. *P < .05, **P < .01, ****P < .0001.
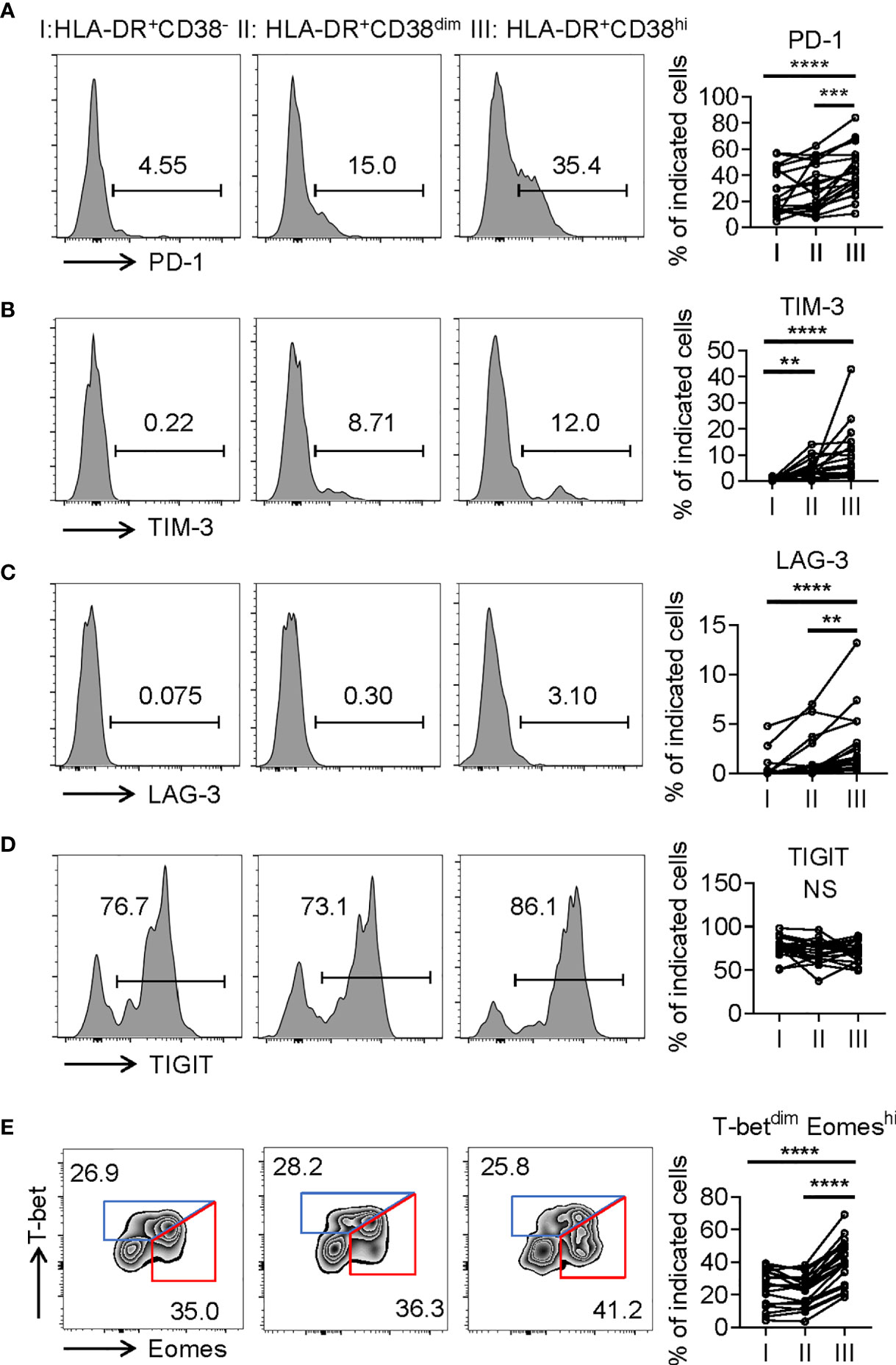
Figure 4 HLA-DR+CD38hi CD8+ T cells displayed phenotypic and transcriptional state of exhaustion. (A–D) Flow cytometry analysis of expression of PD-1 (A), TIM3 (B), LAG3 (C), and TIGIT (D) on the three CD8+ T population (I, II, III) from COVID-19 patients (n = 20). Representative histograms (left) and plots (right) were shown. (E) Representative flow data (left) and dot plots (right) of percentage of T-betdimEomeshi and T-bethiEomesdim cells among I, II, III from COVID-19 patients (n = 20). P Values were obtained by paired two-tailed Student’s t tests and repeated measures by one-way ANOVA test followed by Holm-Sidak’s multiple comparisons test. **p < .01, ***p < .001, ****p < .0001.
Consistent with higher frequency of terminally differentiated cells, HLA-DR+CD38hi CD8+ T cells showed elevated BAX expression and decreased Bcl-2 expression compared with fraction I and II, indicative of high susceptibility to apoptosis. We next investigated killing potential of HLA-DR+CD38hi CD8+ T cells using intracellular staining of granzyme B and perforin, which are responsible for cytotoxic T lymphocytes to exert their killing function. Distinct from classical exhausted T cells, HLA-DR+CD38hi CD8+ T cells showed no significant changes of granzyme B and perforin intracellular staining compared with fraction I. Meanwhile, HLA-DR+CD38dim CD8+ T cells exhibited the highest levels of granzyme B and perforin. Subsequently, we further confirmed that HLA-DR+CD38hi CD8+ T cells are highly proliferative by expressing higher levels ki67 and CD71 (Figure 5). In all, these data suggested an overactivated and consequently disordered immune status of HLA-DR+CD38hi CD8+ T cells during acute COVID-19 infection.
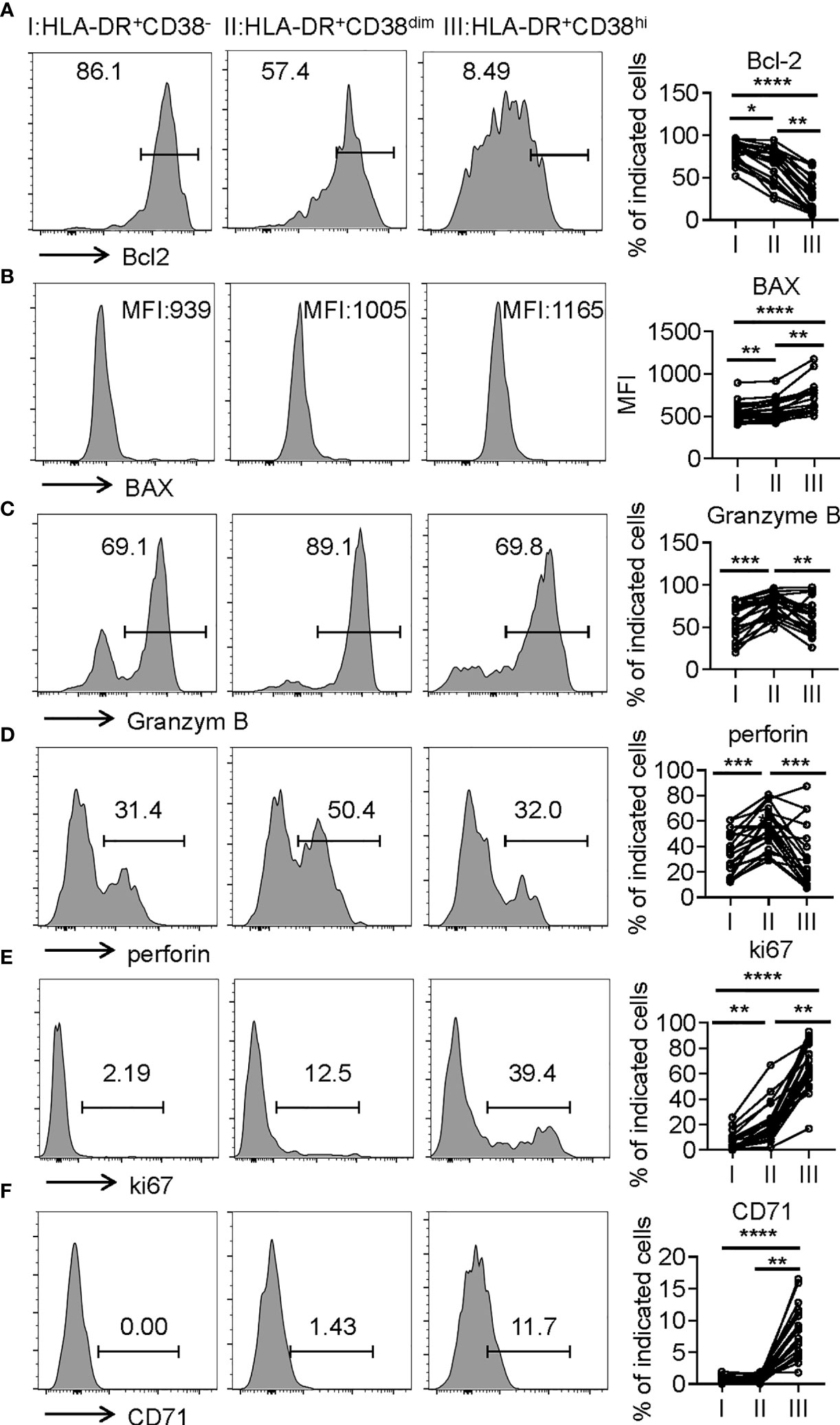
Figure 5 HLA-DR+CD38hi CD8+ T cells exhibited enhanced susceptibility to apoptosis and highly proliferative potential. Flow cytometry analysis of the expression of Bcl-2 (A), BAX (B), Granzyme B (C), perforin (D), ki67 (E), and CD71 (F) on the three CD8+ T population (I, II, III) from patients with infection of COVID-19 (n = 20). Representative histograms (left) and plots (right) display the expression of the above receptors on I, II, III. P Values were obtained by paired two-tailed Student’s t tests and repeated measures by one-way ANOVA test followed by Holm-Sidak’s multiple comparisons test. *p < .05, **p < .01, ***p < .001, ****p < .0001.
HLA-DR+CD38hi CD8+ T Cells Correlated With Storm of Cytokines and Soluble Checkpoint Molecules
In our previous study, high levels of cytokines and soluble checkpoint molecules were reported to correlate with S/C illness of COVID-19 (25, 26). Due to the disordered immune status of HLA-DR+CD38hi CD8+ T cells, we wondered the effects of these cells in the storms of cytokines and soluble checkpoint molecules. We evaluated serum levels of 45 cytokines/chemokines/growth factors and 14 soluble checkpoints using Luminex multiplex assay from 27 COVID-19 patients at different time points during hospitalization and collected longitudinal data. Levels of 17 factors such as HGF, IL-18, IL-1RA, MCP-1, RANTES, IL-10, and SCF showed significantly positive correlations with percentage of HLA-DR+CD38hi CD8+ T cells. Moreover, 10 serum soluble checkpoint molecules, such as TIM3, CD27, IDO, and LAG3, were positively correlated with percentage of HLA-DR+CD38hi CD8+ T cells. Moreover, two other populations HLA-DR+CD38− and HLA-DR+CD38dim showed no correlations to storm of cytokines and soluble checkpoint molecules (Table 2 and Figure S4). Thus, we hypothesized that elevated HLA-DR+CD38hi CD8+ T group might potentially contribute to the storm of cytokine and soluble checkpoint molecules occurring in COVID-19 patients.
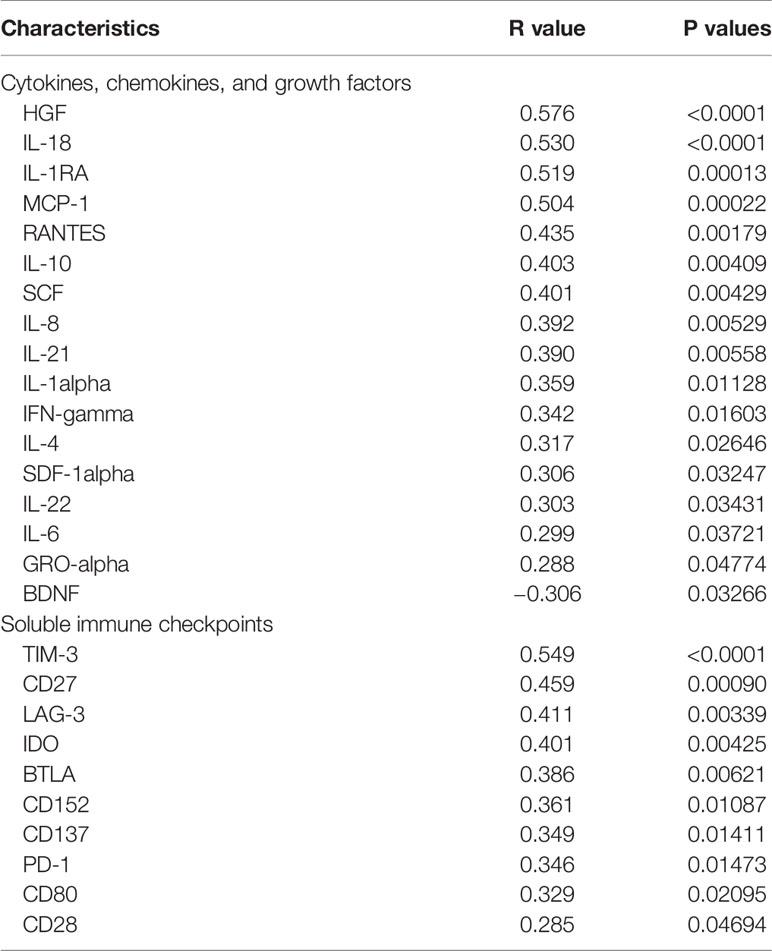
Table 2 Correlations between CD38hiHLA-DR+ percentage and soluble immune checkpoints, cytokines, chemokines, and growth factors in patients infected with SARS-CoV2.
Discussion
Previous studies noted both T cell activation and exhaustion during SARS-CoV-2 infection (10, 14, 27, 28). Although COVID-19 patients did develop severe lymphopenia in response to T cell exhaustion, an elevated proportion of HLA-DR+CD38+ CD8+ T cells suggests a potent adaptive immune response in these patients (29). HLA-DR and CD38 molecules, which are transmembrane glycoproteins, are present on immature T and B lymphocytes and are re-expressed during immune response. Thus, expression of HLA-DR and CD38 respectively on CD8+ T cells reflects immune activation. In particular, co-expression of CD38 and HLA-DR on CD8+ T cells was regarded as a better marker of immune activation during influenza, Dengue, Ebola, and HIV-1 viral infections (30–35). However, in SARS-CoV-2 infection, HLA-DR+CD38+ CD8+ T cells were reported to play contradictory roles. Severe COVID-19 patients showed a significant increase of HLA-DR+CD38+ CD8+ T cells compared to mild cases (20, 36). In a cohort of critical COVID-19 patients with hypertension, the percentage of CD38+HLA-DR+ fraction among CD8+ T cells was higher in the patients with fatal outcomes compared with the surviving patients (37). These studies suggested the involvement of CD38+HLA-DR+ CD8+ T cells in severe progression of COVID-19. In contrast, about 20% of patients had no increase in CD38+HLA-DR+ CD8+ T cells above the level found in HD (22). A study with a cohort of 6 severe and 11 mild COVID-19 patients found no significant differences of CD38+HLA-DR+ CD8+ T cells between mild and severe patients (36). Furthermore, Wang et al. observed that the number of CD38+HLA-DR+ CD8+ T cells was markedly higher in recovering group than severe persistence group among severe COVID-19 patients (23). Activated CD8+ T cells with CD38 signature contributed to the elimination of SARS-CoV-2 in the lungs, indicating a recovery role of these cells for boosting immune response and eliminating virus (24). These contradictory results of CD38+HLA-DR+ CD8+ T cells in COVID-19 patients implied the heterogeneity of this population, which was supported by our findings.
In the present study, we found that HLA-DR+CD38+ CD8+ T cells contained two distinct subpopulations, HLA-DR+CD38hi and HLA-DR+CD38dim. HLA-DR+CD38hi CD8+ T cells were demonstrated to only accumulate in COVID-19 patients, especially S/C cases. The proportion of HLA-DR+CD38hi CD8+ T cells was significantly higher in S/C than M/M group. Notably, a high frequency of HLA-DR+CD38hi CD8+ T cells strongly correlated with severe lymphopenia, systemic inflammation, and tissue injury, suggesting a predictive value of this cell population for disease progression in COVID-19 patients. Conversely, HLA-DR+CD38dim CD8+ T cells existed in both M/M and S/C patients, even healthy individuals. Additionally, S/C cases had transient elevation of HLA-DR+CD38dim CD8+ T cells, but a prolonged high percentage of HLA-DR+CD38hi fraction. Phenotypic analysis of these two subsets further demonstrated that HLA-DR+CD38+ CD8+ T cells were heterogeneous. It was revealed that HLA-DR+CD38dim CD8+ T cells expressed low levels of inhibitory checkpoints, high levels of 4-1BB and GITR, stronger killing potential, and weaker sensitivity to apoptosis. Meanwhile, HLA-DR+CD38hi CD8+ T cells were in a state of overactivation, or exhaustion, manifested by expression of multiple inhibitory and stimulatory checkpoints, more exhausted transcriptional regulation, higher apoptotic sensitivity, and impaired killing potential. Consistently, M/M patients showed a high ratio of HLA-DR+CD38dim to HLA-DR+CD38hi, implying activated immune responses and effective virus clearance, whereas much lower ratio of HLA-DR+CD38dim to HLA-DR+CD38hi was found in S/C group, representing immune exhaustion, systemic tissue injury, and subsequently poor outcome. Thus, a distinct ratio of these two subsets might contribute to different immune response and clinical outcome of HLA-DR+CD38+ CD8+ T cells in COVID-19 progression.
To our knowledge, HLA-DR+CD38hi CD8+ T cells were first reported to associate with a series of soluble immune checkpoint molecules, including sTIM3, sCD27, sLAG3, and sIDO. Considering the theory that soluble forms of checkpoint molecules are produced by cleavage of membrane-bound protein or by mRNA expression (38, 39), we supposed that HLA-DR+CD38hi CD8+ T cell with high expression of membrane-bound molecules contributed to the storm of soluble checkpoint molecules. Our previous study also demonstrated the same soluble molecules as predictive biomarkers for disease severity of COVID-19, further supporting the important role of HLA-DR+CD38hi CD8+ T cells (25). In addition, elevated levels of soluble checkpoints as well as membrane-bound forms on HLA-DR+CD38hi CD8+ T cells included stimulatory and inhibitory molecules, which boost potent immune response and maintain self-tolerance. Thus, the total effects of these HLA-DR+CD38hi CD8+ T cells and heterogeneous checkpoint molecules on immune response are difficultly computable in S/C cases of COVID-19, which reflected a broad and complicated dysregulation of T cell immunity. The severity of COVID-19 might represent a consequence from the imbalance between stimulatory and inhibitory checkpoints.
Both progressive lymphopenia and cytokine release syndrome were prominent clinical features of S/C COVID-19 in addition to dyspnea, hypoxemia, and acute respiratory distress (40–42). As expected, these HLA-DR+CD38hi CD8+ T cells were positively correlated to numerous inflammatory cytokines, IL-18, IL-10, IL-21, IL-1α, IFN-γ, IL-4, IL-22, and IL-6, implying a dysregulated state of these cells. It was in agreement with the characteristics of these cells. Notably, a few chemokines, including MCP-1, RANTES, and IL-8, showed significantly positive correlations with HLA-DR+CD38hi CD8+ T cells. Consistently, CD8+ T cells were identified in lung and liver tissues from COVID-19 patients by postmortem biopsy in previous studies (24, 43–45). Thus, we speculated that these cells might accumulate in target organs towards chemokines and could be a potential culprit of tissue injury. The notion was supported by a close correlation between the proportion of these cells and clinical parameters of systemic inflammation and tissue injury.
COVID-19 patients including S/C and M/M cases showed elevated percentages of HLA-DR+CD38hiCD8+ T cells up to 60 d after symptom onset. This finding is distinct from the responses of activated CD8+ T cells that were found in other acute viral infections, in which the activated T cells returned to baseline much faster (46–48). This implies the persistence of viral antigen continually stimulating these responses. Previous studies demonstrated that the median duration of viral shedding was 20 days in survivors, but SARS-CoV-2 was detectable until death in non-survivors (3). This finding was consistent with the persistently high percentage of HLA-DR+CD38hi CD8+ T cells from a death in the present study. Surprisingly, despite a respiratory virus, SARS-CoV-2 RNA in rectal samples was found to remain for a long period, with a higher positive rate and higher viral load than the paired respiratory samples. It is worth noting that the longest duration observed was 43 days, much longer than the usual 3–5 weeks from symptom onset to discharge for most patients (49). However, M/M COVID-19 patients showed low but prolonged activated CD8+ T cells, which could be explained by the fact that gastrointestinal viral reservoir of SARS-CoV-2 exists persistently even in mild and asymptomatic patients.
Taken together, we found accumulation of a novel HLA-DR+CD38hi population instead of heterogeneous HLA-DR+CD38+ CD8+ T cells during SARS-CoV-2 infection, especially in severe and critical cases. These HLA-DR+CD38hi CD8+ T cells existed in an overactivated and consequently immune disordered state, with high expression of multiple coinhibitory and costimulatory molecules (22, 50). Of note, a high frequency of HLA-DR+CD38hi CD8+ T cells strongly correlated with severe lymphopenia, systemic inflammation, and storm of cytokines and soluble checkpoint molecules, indicating a predictive value of this cell population for disease progression in COVID-19 patients.
Our study has several limitations, including small sample size, unmatched ages between groups, and variable sampling interval for each patient. More importantly, due to lack of functional data, it is difficult to determine the precise functional characteristics of HLA-DR+CD38hi and HLA-DR+CD38dim CD8+ T cells. Therefore, more evidences are urgently needed to investigate whether these two subsets play distinct roles in the pathogenesis and severity of COVID-19.
Data Availability Statement
The datasets presented in this study can be found in online repositories. The names of the repository/repositories and accession number(s) can be found in the article/Supplementary Material.
Ethics Statement
The studies involving human participants were reviewed and approved by the Committee of Ethics at Beijing Ditan Hospital. The patients/participants provided their written informed consent to participate in this study.
Author Contributions
JD performed the experiments, analyzed the data, and wrote the manuscript. LW, GL, and MH collected samples and performed the experiments. DW collected clinical data. KH, YY, and CS collected samples. YS and RS recruited patients. HZ participated in the critical review of the manuscript. JH designed and performed the experiments. JL conducted the study and recruited patients. YK conceived the study, performed the experiments, and wrote the manuscript. All authors contributed to the article and approved the submitted version.
Funding
This work was supported by the National Natural Science Foundation of China (81971307), Beijing Natural Science Foundation (M21007), Beijing Municipal Science & Technology Commission (Z201100007920017), National Key Sci-Tech Special Project of China (2018ZX10302207), and National Key Research and Development Program of China (2020YFC0846200).
Conflict of Interest
The authors declare that the research was conducted in the absence of any commercial or financial relationships that could be construed as a potential conflict of interest.
Publisher’s Note
All claims expressed in this article are solely those of the authors and do not necessarily represent those of their affiliated organizations, or those of the publisher, the editors and the reviewers. Any product that may be evaluated in this article, or claim that may be made by its manufacturer, is not guaranteed or endorsed by the publisher.
Acknowledgments
We thank all patients involved in the study and all medical staff involved in the diagnosis and treatment of patients with COVID-19 in Beijing Ditan Hospital. We sincerely thank all the patients and healthy donors included in this study.
Supplementary Material
The Supplementary Material for this article can be found online at: https://www.frontiersin.org/articles/10.3389/fimmu.2021.735125/full#supplementary-material
References
1. Huang C, Wang Y, Li X, Ren L, Zhao J, Hu Y, et al. Clinical Features of Patients Infected With 2019 Novel Coronavirus in Wuhan, China. Lancet (2020) 395(10223):497–506. doi: 10.1016/S0140-6736(20)30183-5
2. Du Y, Tu L, Zhu P, Mu M, Wang R, Yang P, et al. Clinical Features of 85 Fatal Cases of COVID-19 From Wuhan. A Retrospective Observational Study. Am J Respir Crit Care Med (2020) 201(11):1372–9. doi: 10.1164/rccm.202003-0543OC
3. Zhou F, Yu T, Du R, Fan G, Liu Y, Liu Z, et al. Clinical Course and Risk Factors for Mortality of Adult Inpatients With COVID-19 in Wuhan, China: A Retrospective Cohort Study. Lancet (2020) 395(10229):1054–62. doi: 10.1016/S0140-6736(20)30566-3
4. Wouters OJ, Shadlen KC, Salcher-Konrad M, Pollard AJ, Larson HJ, Teerawattananon Y, et al. Challenges in Ensuring Global Access to COVID-19 Vaccines: Production, Affordability, Allocation, and Deployment. Lancet (2021) 397(10278):1023–34. doi: 10.1016/S0140-6736(21)00306-8
5. Dai L, Gao GF. Viral Targets for Vaccines Against COVID-19. Nat Rev Immunol (2021) 21(2):73–82. doi: 10.1038/s41577-020-00480-0
6. Forni G, Mantovani A. COVID-19 Vaccines: Where We Stand and Challenges Ahead. Cell Death Differ (2021) 28(2):626–39. doi: 10.1038/s41418-020-00720-9
7. Su S, Du L, Jiang S. Learning From the Past: Development of Safe and Effective COVID-19 Vaccines. Nat Rev Microbiol (2021) 19(3):211–9. doi: 10.1038/s41579-020-00462-y
8. Shi Y, Wang Y, Shao C, Huang J, Gan J, Huang X, et al. COVID-19 Infection: The Perspectives on Immune Responses. Cell Death Differ (2020) 27(5):1451–4. doi: 10.1038/s41418-020-0530-3
9. Chen G, Wu D, Guo W, Cao Y, Huang D, Wang H, et al. Clinical and Immunological Features of Severe and Moderate Coronavirus Disease 2019. J Clin Invest (2020) 130(5):2620–9. doi: 10.1172/JCI137244
10. Braun J, Loyal L, Frentsch M, Wendisch D, Georg P, Kurth F, et al. SARS-CoV-2-Reactive T Cells in Healthy Donors and Patients With COVID-19. Nature (2020) 587(7833):270–4. doi: 10.1038/s41586-020-2598-9
11. Fathi N, Rezaei N. Lymphopenia in COVID-19: Therapeutic Opportunities. Cell Biol Int (2020) 44(9):1792–7. doi: 10.1002/cbin.11403
12. Sekine T, Perez-Potti A, Rivera-Ballesteros O, Strålin K, Gorin JB, Olsson A, et al. Robust T Cell Immunity in Convalescent Individuals With Asymptomatic or Mild COVID-19. Cell (2020) 183(1):158–68.e14. doi: 10.1016/j.cell.2020.08.017
13. Schultheiß C, Paschold L, Simnica D, Mohme M, Willscher E, von Wenserski L, et al. Next-Generation Sequencing of T and B Cell Receptor Repertoires From COVID-19 Patients Showed Signatures Associated With Severity of Disease. Immunity (2020) 53(2):442–55.e4. doi: 10.1016/j.immuni.2020.06.024
14. Wilk AJ, Rustagi A, Zhao NQ, Roque J, Martínez-Colón GJ, McKechnie JL, et al. A Single-Cell Atlas of the Peripheral Immune Response in Patients With Severe COVID-19. Nat Med (2020) 26(7):1070–6. doi: 10.1038/s41591-020-0944-y
15. Agrati C, Castilletti C, Casetti R, Sacchi A, Falasca L, Turchi F, et al. Longitudinal Characterization of Dysfunctional T Cell-Activation During Human Acute Ebola Infection. Cell Death Dis (2016) 7(3):e2164. doi: 10.1038/cddis.2016.55
16. Wang Z, Zhu L, Nguyen T, Wan Y, Sant S, Quiñones-Parra SM, et al. Clonally Diverse CD38(+)HLA-DR(+)CD8(+) T Cells Persist During Fatal H7N9 Disease. Nat Commun (2018) 9(1):824. doi: 10.1038/s41467-018-03243-7
17. Thom R, Tipton T, Strecker T, Hall Y, Akoi Bore J, Maes P, et al. Longitudinal Antibody and T Cell Responses in Ebola Virus Disease Survivors and Contacts: An Observational Cohort Study. Lancet Infect Dis (2021) 21(4):507–16. doi: 10.1016/S1473-3099(20)30736-2
18. McElroy AK, Akondy RS, Davis CW, Ellebedy AH, Mehta AK, Kraft CS, et al. Human Ebola Virus Infection Results in Substantial Immune Activation. Proc Natl Acad Sci U S A (2015) 112(15):4719–24. doi: 10.1073/pnas.1502619112
19. Koutsakos M, Illing PT, Nguyen T, Mifsud NA, Crawford JC, Rizzetto S, et al. Human CD8(+) T Cell Cross-Reactivity Across Influenza A, B and C Viruses. Nat Immunol (2019) 20(5):613–25. doi: 10.1038/s41590-019-0320-6
20. Song JW, Zhang C, Fan X, Meng FP, Xu Z, Xia P, et al. Immunological and Inflammatory Profiles in Mild and Severe Cases of COVID-19. Nat Commun (2020) 11(1):3410. doi: 10.1038/s41467-020-17240-2
21. Habel JR, Nguyen T, van de Sandt CE, Juno JA, Chaurasia P, Wragg K, et al. Suboptimal SARS-CoV-2-Specific CD8(+) T Cell Response Associated With the Prominent HLA-A*02:01 Phenotype. Proc Natl Acad Sci U S A (2020) 117(39):24384–91. doi: 10.1073/pnas.2015486117
22. Mathew D, Giles JR, Baxter AE, Oldridge DA, Greenplate AR, Wu JE, et al. Deep Immune Profiling of COVID-19 Patients Reveals Distinct Immunotypes With Therapeutic Implications. Science (2020) 369(6508). doi: 10.1126/science.abc8511
23. Wang Z, Yang X, Zhou Y, Sun J, Liu X, Zhang J, et al. COVID-19 Severity Correlates With Weaker T-Cell Immunity, Hypercytokinemia, and Lung Epithelium Injury. Am J Respir Crit Care Med (2020) 202(4):606–10. doi: 10.1164/rccm.202005-1701LE
24. Nienhold R, Ciani Y, Koelzer VH, Tzankov A, Haslbauer JD, Menter T, et al. Two Distinct Immunopathological Profiles in Autopsy Lungs of COVID-19. Nat Commun (2020) 11(1):5086. doi: 10.1038/s41467-020-18854-2
25. Kong Y, Wang Y, Wu X, Han J, Li G, Hua M, et al. Storm of Soluble Immune Checkpoints Associated With Disease Severity of COVID-19. Signal Transduct Target Ther (2020) 5(1):192. doi: 10.1038/s41392-020-00308-2
26. Kong Y, Han J, Wu X, Zeng H, Liu J, Zhang H. VEGF-D: A Novel Biomarker for Detection of COVID-19 Progression. Crit Care (2020) 24(1):373. doi: 10.1186/s13054-020-03079-y
27. De Biasi S, Meschiari M, Gibellini L, Bellinazzi C, Borella R, Fidanza L, et al. Marked T Cell Activation, Senescence, Exhaustion and Skewing Towards TH17 in Patients With COVID-19 Pneumonia. Nat Commun (2020) 11(1):3434. doi: 10.1038/s41467-020-17292-4
28. Paces J, Strizova Z, Smrz D, Cerny J. COVID-19 and the Immune System. Physiol Res (2020) 69(3):379–88. doi: 10.33549/physiolres.934492
29. Kratzer B, Trapin D, Ettel P, Körmöczi U, Rottal A, Tuppy F, et al. Immunological Imprint of COVID-19 on Human Peripheral Blood Leukocyte Populations. Allergy (2021) 76(3):751–65. doi: 10.1111/all.14647
30. Ruibal P, Oestereich L, Lüdtke A, Becker-Ziaja B, Wozniak DM, Kerber R, et al. Unique Human Immune Signature of Ebola Virus Disease in Guinea. Nature (2016) 533(7601):100–4. doi: 10.1038/nature17949
31. Radziewicz H, Ibegbu CC, Hon H, Osborn MK, Obideen K, Wehbi M, et al. Impaired Hepatitis C Virus (HCV)-Specific Effector CD8+ T Cells Undergo Massive Apoptosis in the Peripheral Blood During Acute HCV Infection and in the Liver During the Chronic Phase of Infection. J Virol (2008) 82(20):9808–22. doi: 10.1128/JVI.01075-08
32. Hua S, Lécuroux C, Sáez-Cirión A, Pancino G, Girault I, Versmisse P, et al. Potential Role for HIV-Specific CD38-/HLA-DR+ CD8+ T Cells in Viral Suppression and Cytotoxicity in HIV Controllers. PloS One (2014) 9(7):e101920. doi: 10.1371/journal.pone.0101920
33. Murray SM, Down CM, Boulware DR, Stauffer WM, Cavert WP, Schacker TW, et al. Reduction of Immune Activation With Chloroquine Therapy During Chronic HIV Infection. J Virol (2010) 84(22):12082–6. doi: 10.1128/JVI.01466-10
34. Chandele A, Sewatanon J, Gunisetty S, Singla M, Onlamoon N, Akondy RS, et al. Characterization of Human CD8 T Cell Responses in Dengue Virus-Infected Patients From India. J Virol (2016) 90(24):11259–78. doi: 10.1128/JVI.01424-16
35. Fox A, Le NM, Horby P, van Doorn HR, Nguyen VT, Nguyen HH, et al. Severe Pandemic H1N1 2009 Infection Is Associated With Transient NK and T Deficiency and Aberrant CD8 Responses. PloS One (2012) 7(2):e31535. doi: 10.1371/journal.pone.0031535
36. Zhou R, To KK, Wong YC, Liu L, Zhou B, Li X, et al. Acute SARS-CoV-2 Infection Impairs Dendritic Cell and T Cell Responses. Immunity (2020) 53:864–877.e5. doi: 10.1016/j.immuni.2020.07.026
37. Zeng Q, Li YZ, Dong SY, Chen ZT, Gao XY, Zhang H, et al. Dynamic SARS-CoV-2-Specific Immunity in Critically Ill Patients With Hypertension. Front Immunol (2020) 11:596684. doi: 10.3389/fimmu.2020.596684
38. Gu D, Ao X, Yang Y, Chen Z, Xu X. Soluble Immune Checkpoints in Cancer: Production, Function and Biological Significance. J Immunother Cancer (2018) 6:132. doi: 10.1186/s40425-018-0449-0
39. Jalali S, Price-Troska T, Paludo J, Villasboas J, Kim HJ, Yang ZZ, et al. Soluble PD-1 Ligands Regulate T-Cell Function in Waldenstrom Macroglobulinemia. Blood Adv (2018) 2:1985–97. doi: 10.1182/bloodadvances.2018021113
40. Moore JB, June CH. Cytokine Release Syndrome in Severe COVID-19. Science (2020) 368:473–4. doi: 10.1126/science.abb8925
41. Hu B, Huang S, Yin L. The Cytokine Storm and COVID-19. J Med Virol (2021) 93:250–6. doi: 10.1002/jmv.26232
42. Picchianti Diamanti A, Rosado MM, Pioli C, Sesti G, Laganà B. Cytokine Release Syndrome in COVID-19 Patients, A New Scenario for an Old Concern: The Fragile Balance Between Infections and Autoimmunity. Int J Mol Sci (2020) 21. doi: 10.3390/ijms21093330
43. Bidari Zerehpoosh F, Sabeti S, Bahrami-Motlagh H, Mokhtari M, Naghibi Irvani SS, Torabinavid P, et al. Post-Mortem Histopathologic Findings of Vital Organs in Critically Ill Patients With COVID-19. Arch Iran Med (2021) 24:144–51. doi: 10.34172/aim.2021.23
44. Ribeiro Dos Santos Miggiolaro ,AF, da Silva Motta Junior J, Busatta Vaz de Paula C, Nagashima S, Alessandra Scaranello Malaquias M, Baena Carstens L, et al. Covid-19 Cytokine Storm in Pulmonary Tissue: Anatomopathological and Immunohistochemical Findings. Respir Med Case Rep (2020) 31:101292. doi: 10.1016/j.rmcr.2020.101292
45. Gauchotte G, Venard V, Segondy M, Cadoz C, Esposito-Fava A, Barraud D, et al. SARS-Cov-2 Fulminant Myocarditis: An Autopsy and Histopathological Case Study. Int J Legal Med (2021) 135:577–81. doi: 10.1007/s00414-020-02500-z
46. Bournazos S, Corti D, Virgin HW, Ravetch JV. Fc-Optimized Antibodies Elicit CD8 Immunity to Viral Respiratory Infection. Nature (2020) 588:485–90. doi: 10.1038/s41586-020-2838-z
47. Sridhar S, Begom S, Bermingham A, Hoschler K, Adamson W, Carman W, et al. Cellular Immune Correlates of Protection Against Symptomatic Pandemic Influenza. Nat Med (2013) 19:1305–12. doi: 10.1038/nm.3350
48. Sakabe S, Sullivan BM, Hartnett JN, Robles-Sikisaka R, Gangavarapu K, Cubitt B, et al. Analysis of CD8(+) T Cell Response During the 2013-2016 Ebola Epidemic in West Africa. Proc Natl Acad Sci U S A (2018) 115:E7578–7578E7586. doi: 10.1073/pnas.1806200115
49. Zhao F, Yang Y, Wang Z, Li L, Liu L, Liu Y, et al. The Time Sequences of Respiratory and Rectal Viral Shedding in Patients With Coronavirus Disease 2019. Gastroenterology (2020) 159:1158–1160.e2. doi: 10.1053/j.gastro.2020.05.035
Keywords: COVID-19, HLA-DR, CD38, severity, immune disorder
Citation: Du J, Wei L, Li G, Hua M, Sun Y, Wang D, Han K, Yan Y, Song C, Song R, Zhang H, Han J, Liu J and Kong Y (2021) Persistent High Percentage of HLA-DR+CD38high CD8+ T Cells Associated With Immune Disorder and Disease Severity of COVID-19. Front. Immunol. 12:735125. doi: 10.3389/fimmu.2021.735125
Received: 02 July 2021; Accepted: 09 August 2021;
Published: 09 September 2021.
Edited by:
Giulia Carla Marchetti, University of Milan, ItalyReviewed by:
Gabriella d’Ettorre, Sapienza University of Rome, ItalySylvain Cardinaud, Institut National de la Santé et de la Recherche Médicale (INSERM), France
Copyright © 2021 Du, Wei, Li, Hua, Sun, Wang, Han, Yan, Song, Song, Zhang, Han, Liu and Kong. This is an open-access article distributed under the terms of the Creative Commons Attribution License (CC BY). The use, distribution or reproduction in other forums is permitted, provided the original author(s) and the copyright owner(s) are credited and that the original publication in this journal is cited, in accordance with accepted academic practice. No use, distribution or reproduction is permitted which does not comply with these terms.
*Correspondence: Yaxian Kong, a29uZ3lheGlhbkBjY211LmVkdS5jbg==; Jingyuan Liu, ZHR5eWljdUBjY211LmVkdS5jbg==; Junyan Han, aGFuanVueWFuQGNjbXUuZWR1LmNu
†These authors have contributed equally to this work