- 1Department of Gastrointestinal and Hepatology, Beijing You’An Hospital, Capital Medical University, Beijing, China
- 2Institute for Research in Biomedicine, Università della Svizzera italiana, Bellinzona, Switzerland
- 3Institute of Microbiology, Eidgenössische Technische Hochschule (ETH) Zürich, Zurich, Switzerland
- 4Beijing Institute of Hepatology, Beijing You’An Hospital, Capital Medical University, Beijing, China
Primary liver cancer (PLC) is one of the most common malignancies in China, where it ranks second in mortality and fifth in morbidity. Currently, liver transplantation, hepatic tumor resection, radiofrequency ablation, and molecular-targeted agents are the major treatments for hepatocellular carcinoma (HCC). Overall, HCC has a poor survival rate and a high recurrence rate. Tumor-infiltrating lymphocytes (TILs) have been discovered to play essential roles in the development, prognosis, and immunotherapy treatment of HCC. As the major component cells of TILs, T cells are also proved to show antitumor and protumor effects in HCC. Foxp3+, CD8+, CD3+, and CD4+ T lymphocytes are the broadly studied subgroups of TILs. This article reviews the roles and mechanisms of different tumor-infiltrating T lymphocyte subtypes in HCC.
Introduction
According to the International Agency of Research on Cancer of World Health Organization report, primary liver cancer (PLC) posed a severe threat to people’s life and health, with an estimated 906,000 new cases and 830,000 deaths globally in 2020 (1). In China, liver cancer ranked second in mortality and fifth in morbidity (2). There are three main pathological types of PLC, including hepatocellular carcinoma (HCC), intrahepatic cholangiocarcinoma (CC), and combined HCC/CC. They vary in pathogenesis, biological behavior, histological morphology, treatment, and prognosis. HCC accounts for 85%-90% among them (3). In this article, liver cancer as follows refers to HCC. For HCC, currently available therapeutic options include liver transplantation, tumor resection, radiofrequency ablation, and molecular-targeted agents. However, the survival rate of HCC is low, and the recurrence rate is high, due to the fact that when patients are first diagnosed with HCC, they often have intermediate-stage or advanced-stage HCC, or micro-metastasis tumors (4). The 1-year median survival rate of patients with end-stage HCC was 11% (3), whereas the 1-year recurrence rate following surgical resection was 70% (5), suggesting that these treatment options for HCC were limited. As a result, novel therapies for HCC are still needed. In the past few years, tumor immunotherapy has emerged as a new therapeutic procedure for HCC. Tumor immunotherapy works by generating and strengthening patients’ existing tumor-specific immune responses, which have high efficiency and strong selectivity when compared to traditional tumor therapies. The immunotherapy regimen that each patient should follow is determined by the host’s immunological specificity. In addition, the quantities and distributions of tumor-infiltrating immune cells also assist us in predicting the response of each patient to immunotherapy prior to treatment. The compositions of immune cells, on the other hand, significantly differ between HCC patients and healthy populations (6). A sufficient understanding of the tumor microenvironment (TME) is the key to successful immunotherapy. TME for HCC is a dynamic system that enriches multiple immune cells, such as regulatory T cells (Tregs), tissue-resident memory CD8+ T (Trm) cells, resident natural killer (NK) cells, tumor-associated macrophages (TAMs) (7). Through interacting with each other and the surrounding stromal cells, they form a complex network and play essential roles in the proliferation, migration, invasion, and angiogenesis of HCC (8).
Tumor-infiltrating lymphocytes (TILs) were formerly thought to host immune responses to tumors. With extensive study in this subject, people discover that TILs consist of a variety of lymphocyte subgroups, including innate immune and adaptive immune cells, such as mast cells, macrophages, NK cells, and T lymphocytes. There are many specific antigens on the surfaces of TILs, for example, Foxp3, CD3, CD4, CD8, CD16, CD20, CD56, CD57, CD68, and CD169 (9). It is widely thought that Foxp3, CD3, CD4, and CD8 are linked with T lymphocytes, CD16 with monocytes, CD20 with B lymphocytes, CD56 and CD57 with NK cells, as well as CD68 and CD169 with macrophages. TILs play significant roles in the development, treatment, and prognosis of HCC. Besides, the antitumor or protumor effects of TILs are related to the component of lymphocyte subsets in TME (10). T lymphocytes are the primary cells of TILs in HCC (11). In addition, Foxp3+, CD3+, CD4+, and CD8+ T lymphocytes are the broadly discussed subgroups of TILs (12). However, the results of TILs in HCC remain controversial. Here, the roles and mechanisms of common tumor-infiltrating T lymphocyte subsets in HCC are reviewed.
Foxp3+ T Lymphocytes
Forkhead box protein P3 (Foxp3), consisting of 12 exons, belongs to the forkhead family of transcriptional factors and regulates the expression of multiple genes. Foxp3 was revealed to be a reliable marker of Tregs as well as a key regulator of their growth and function (13, 14). Tregs were usually divided into two different subgroups: “natural Treg” cells (nTregs) and “induced Treg” cells (iTregs). It was commonly thought that nTregs differentiated from CD4+ CD25+ Foxp3+ T cells in the thymus, whereas iTregs developed from naive CD4+ Foxp3- T cells in the peripheral tissues, such as lymph node and spleen, and universally gained Foxp3 expression outside of the thymus after antigenic stimulation (15). Both two types of Tregs shared the common ability to suppress immune responses, but they used distinct ways to do so. nTregs played roles through cell contact-dependent ways via membrane-bound molecules, yet iTregs exerted their functions through cell contact independent ways via cytokines including interleukin (IL)-10 and transforming growth factor-β (TGF-β) (16). Now, there’s no reliable biomarker for distinguishing nTregs from iTregs. In recent years, Foxp3+ T cells have been found in many tumors, such as HCC (17), pancreatic ductal adenocarc (18), colorectal cancer (19), gastric cancer (20), and esophageal cancer (21), suggesting that Foxp3+ T cells participated in the tumor progression. However, the subsets of infiltrating Foxp3+ T cells in tumors remained unclear. With immunohistochemical staining analysis, Wang et al. (22) found that Foxp3 was detected in 48% of HCC tumor tissue but not in healthy liver tissue and para-tumor tissue, indicating that the recruitment of Foxp3+ T cells played an important effect in the development of HCC. Foxp3 was mainly present in the nucleus and cytoplasm of tumor cells (22). A synthetic peptide named P60 could bind to Foxp3, restrict its nuclear translocation, and reverse the biological activity for HCC (23), which indicated that Foxp3 was required to enter the nucleus to display its function. However, the expression and regulation mechanisms of Foxp3 are unknown. In tumor cells from patients with HBV-associated HCC, PreS2 overexpression could trigger the Foxp3 overexpression. In contrast, PreS2 knockdown could significantly reduce the expression of Foxp3. This was the first time a direct conclusion that PreS2 triggered Foxp3 expression in HCC was reached (24).
According to several studies, nTregs promoted tumor growth by limiting antitumor immune responses. iTregs, on the other hand, were shown to favor cancer progression by sustaining peripheral tolerance and controlling cancer-related inflammation (25). The exact roles of nTregs and iTregs in cancer are yet unknown. For this reason, we only talk about total Foxp3+ T cells in this review. Currently, the role of Foxp3+ T cells for HCC prognosis remains disputable. The majority of studies showed that Foxp3+ T cells were related to HCC migration, and high Foxp3 expression predicted a poor prognosis for HCC (17, 26–32) (Figure 1). A meta-analysis on HCC, which was conducted by Huang et al. (17), demonstrated that the high Foxp3+ T cells invading group had worse 1, 3, and 5-year survival than the low Foxp3+ T cells infiltrating group. At the same time, the high Foxp3+ T cells infiltrating group had better 1, 3, and 5 year-recurrence than the low Foxp3+ T cells infiltrating group. According to the above conclusion, high Foxp3+ T cells infiltrating was a poor prognostic factor for HCC. The mechanisms in which Foxp3+ T cells promoted HCC progression might include fostering angiogenesis (27), decreasing CD8+ T cells (32), progressively reducing CD4+ CTLs (31), and boosting the formation of portal vein tumor thrombi (PVTT) (29) and so on. Conversely, new data suggested that high Foxp3+ T cells in HCC improved the clinical outcome by suppressing proliferation, migration, and invasion of tumor cells (33) (Figure 1). Gong and colleagues first reported that those with high Foxp3 protein expression had better overall survival than those with low Foxp3 expression in 84 HCC patients. Based on those finds, they demonstrated that Foxp3 suppressed tumor growth in mouse tumor models by directly or indirectly inhibiting oncogene c-Myc (33).
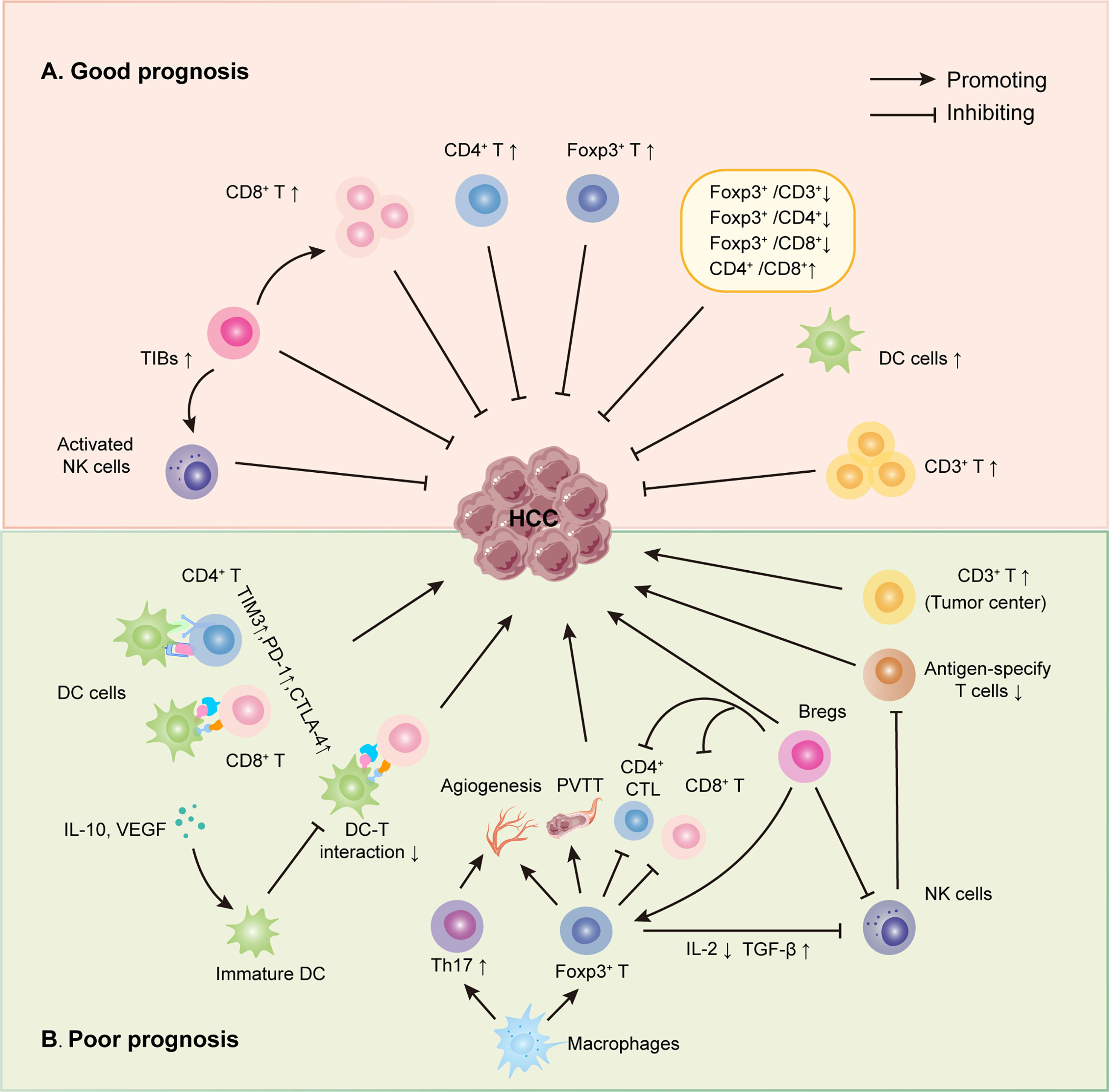
Figure 1 Roles of infiltrating immune cells in HCC. (A) The suppressing role of TILs on HCC. Initially, several infiltrating T lymphocytes, including CD3+, CD8+, activated NKs and Foxp3+ TILs, have been discovered to inhibit HCC directly. Secondly, some TILs could interact with other immune cells to suppress HCC, such as TIBs prohibited HCC by increasing CD8+ T cells and activating NK cells. Last, different TIL ratios also were proved to hinder HCC progress, just as low Foxp3+/CD3+ TIL ratio, high CD4+/CD8+ TIL ratio, et al. (B) The promoting role of TILs on HCC. Some infiltrating T lymphocytes have been shown to promote HCC directly, like Foxp3+, CD3+ TILs. Also, infiltrating T lymphocytes could interact with other immune cells to boost HCC progress via many mechanisms, including reduced DC-T interaction, weakening the function of CD4+ and CD8+ TILs et al.
In conclusion, most studies have found that a high frequency of infiltrating Foxp3+ T cells was associated with a poor prognosis in HCC. However, further study is needed to reach a consensus and explore the functions of nTregs and iTregs.
CD8+ T Lymphocytes
Cytotoxic T lymphocytes (CTLs) always have CD8 surface antigen and play a function in the antitumor immune response. In current studies, CD8+ T lymphocyte is the most prevalent T cell subset. It has been shown that high expression of CD8+ TILs linked to a good prognosis in multiple tumors, including colorectal cancer (19), esophageal cancer (34), gastric cancer (35). In HCC, CD8+ T cells are also widely concerned. Several recent investigations have stated that CD8+ TILs expression decreased in the tumor tissue with HCC. Based on immunohistochemistry staining for CD8+ TILs from 29 cases with HCC, Yarchoan’s team found that the expression of CD8+T cells in the tumor tissue was remarkably lower than that in the liver background, and the expression of CD8+ T cells in the tumor side was lower than that in the nontumor side of tumor interface (36). Furthermore, the distribution of CD8+ T lymphocytes in HCC significantly varied among different areas and etiologies of the tumor. In non-alcoholic steatohepatitis (NASH) patients and animal models, CD8+ T cells were accumulated with strong programmed cell death protein 1 (PD-1) expression (37). Compared to the invasive margin region of the tumor in HCC, the central tumor region had a lower expression and a smaller area of CD8+ T lymphocytes (38). This phenomenon was also considered to happen because CD8+ T lymphocytes usually migrated from the peritumor region towards the central region of the tumor, and Tregs contributed to suppressing their migration and proliferation (32). While due to the perspective that HCC is a solid human tumor with abundant neovascularization and high perivascular infiltration of CD8+ T cells, the infiltration of immune cells was also believed to originate from intratumor vessel extravasation, not the peritumor tissue migration (39).
As in other tumors, many experts have demonstrated that CD8+ T lymphocyte in HCC was a protective factor (38–42) (Figure 1). According to research, high expression of CD8+TILs in the invasive margin, intratumoral, and perivascular regions has been linked to improved survival (39, 41). In a cohort analysis of 446 HCC cases, the densities of CD8+ TILs both in the intratumor and margin area positively correlated with overall survival and disease-free survival, and a higher density CD8+ TILs indicated a reduced recurrence rate (38). In addition, Sideras et al. reported that patients with a low density of CD8+ TILs survived poorly after analyzing stored formalin-fixed paraffin-embedded HCC tissue samples from 154 patients using immunohistochemical analysis, which was also confirmed in their validation cohort (42). This result suggested that CD8+ TILs with high expression had a positive prognostic role. Yet, whether CD8+ TILs could exert antitumor activity normally was still determined by the expression level of inhibitory receptors on their surface. Another study on NASH-related HCC discovered that the elimination of enriched CD8+ PD-1 T cells, which represent exhausted effector cells, could reduce liver damage and HCC incidence. In comparison to virus-related HCC, NASH-related HCC responded to immunotherapy treatment worse due to the obviously increased expression of CD8+ PD-1 T cells (37). Interestingly, a few studies came to an opposite view that high infiltrating CD8+ T lymphocytes predicted high recurrence and poor prognosis (28, 43, 44), which contradicted the antitumor role of CTLs.
Overall, CD8+TIL acting as an antitumor effector decreased in the tumor tissue, which has been proved to link with poor prognosis in HCC in the majority of studies. In NASH-related HCC, however, accumulating CD8+ T cells with elevated PD-1 expression accelerated tumor growth and reduced immunotherapy response.
CD3+ T Lymphocytes
CD3 also is the typical surface antigen of T lymphocytes. Compared with HCV-associated cirrhosis, CD3+ TILs expression in HCV-associated HCC was significantly higher (43). In addition, the CD3+ TILs density of the intratumoral region was higher than that of the peritumoral area (45). Now the role of CD3+ TILs in HCC remains uncertain. Most studies showed that high CD3+ TILs expression was a protective factor against HCC (12, 38, 39, 46) (Figure 1). Patients having more CD3+ TILs in the intratumor tissue lived longer in HCC, according to Sun’s findings. This result, however, was not seen in the peritumor tissue. They came to the conclusion that CD3+ TILs in the central zone tumor was an independent indicator for HCC prognosis while the distribution of CD3+ TILs in peritumor had little impact on the prognostic value (38). In another research of 65 patients with I to IV stage HCC, high CD3+ TILs densities both in the center and margin of the tumors were found to predict low recurrence and prolonged disease-free survival (46). Yao’s group also came to the same conclusion in a meta-analysis that included 23 relevant papers with 3173 HCC patients (12). The value of CD3+ TILs in the perivascular area was likewise proved. A recent study found that patients with a high amount of CD3+ TILs at the perivascular region had long disease-free survival, implying that CD3+ TILs in the perivascular location could also be used as an independent predictor of HCC (39). Despite the above, the following paper showed that high CD3+ TILs was a poor prognostic factor for HCC (41). A meta-analysis of seven articles on TILs involving 1274 HCC patients revealed that high infiltration of CD3+ T lymphocytes at the tumor center was correlated with poor overall survival (Figure 1), whereas there was no correlation between CD3+ TILs density at the margin tumor and overall survival (41).
In summary, even though there were disputes, most studies found that a high concentration of CD3+ TILs in the tumor or perivascular region predicted a favorable outcome in HCC.
CD4+ T Lymphocytes
CD4+ T lymphocytes took on activities in the immune response against tumor by secreting cytokines and activating CD8+ T lymphocytes (31, 47). Naive CD4+ T cells can differentiate into many subsets following antigens stimulation, including T helper 1 (Th1), Th2, Th9, Th17, Th22, follicular helper T (Tfh) cells, and Tregs. In recent years, CD4+ T cells with cytotoxic activity, which produce granzyme A, granzyme B, and perforin, have been termed CD4+ CTLs. It has been shown that CD4+ CTLs could exert antiviral and antitumor effects (48). CD4+ T cells have been extensively discovered in many cancers including HCC. It has been found that the numbers of circulating CD4+T lymphocytes were significantly higher in HBV-associated HCC patients than that in chronic hepatitis B (CHB), HBV-related cirrhosis patients, and healthy individuals (31). Furthermore, HCV-related HCC had higher CD4+ TILs than HCV-related cirrhosis (43). When patients progressed to HCC, CD4+ T lymphocytes were redistributed in tumor tissue, with CD4+ TILs expression in the peritumoral area being greater than that in the intratumoral area (28). CD4+ TILs might be decreasing as HCC progressed. Compared with the early-stage HCC, the quantity of CD4+ TILs was lower in the advanced-stage HCC, suggesting that the gradual reduction of CD4+ TILs might correlate with HCC progression (31). In order to better understand the mechanism of CD4+ TILs decline in late-stage HCC, Chaoul’s group observed the subsets of CD4+ TILs. When compared to the circulating blood of HCC patients, they found that terminally differentiated effectors (TEFF) strongly reduced and exhausted in both peritumor and central tumor, indicating that CD4+ TILs were selectively recruited into the tumor to escape the immune system (49).
It was found that CD4+ TILs served as a protective factor in HCC (31, 50) (Figure 1). The progressive decrease of CD4+ CTLs was related to a poor prognosis and a high recurrence of HCC (31). Another study performed in the NASH mice model revealed that inducing apoptosis of CD4+ T lymphocytes could promote HCC development while rescuing apoptosis of CD4+ T lymphocytes could prevent HCC development (50). Nevertheless, the mechanism of HCC progression mediated by a reduction of CD4+ TILs remains unclear. On the one hand, it was directly responsible for the reduced and exhausted TEFF of CD4+ TILs. On the other hand, insufficient stimulation of CD8+ TILs without the assistance of CD4 +TILs might play a potent role in the poor prognosis of HCC (51). However, several researches argued that there was no correlation between CD4+ TILs and HCC progression (12, 45). Even though positively correlated with elevated AFP and poor tumor differentiation, CD4+ TILs were discovered to be associated with neither overall survival nor disease-free survival (45).
Collectively, the decreased and impaired CD4+ TILs were observed in tumor tissue, which triggered the impaired activation of CD8+ TILs, and correlated with poor prognosis in HCC.
Foxp3+/CD3+, Foxp3+/CD4+, Foxp3+/CD8+, CD8+/CD3+, CD4+/CD8+T Lymphocytes Ratio
As stated previously, tumor-infiltrating T lymphocytes with multiple subgroups exhibited antitumor and protumor effects at the same time, showing significant predictive value for HCC. So it is necessary to discover their prognostic value of the different ratios between TIL subsets for HCC. Low Foxp3+/CD3+, Foxp3+/CD4+, Foxp3+/CD8+TIL ratio, and high CD4+/CD8 +TIL ratio were shown to correlate with good prognosis (Figure 1), while CD8+/CD3+TIL ratio showed no correlation with survival in HCC (12, 52–54). Yao and co-workers reported that patients with lower Foxp3+/CD4+ and Foxp3+/CD8+TIL ratios had better overall survival and disease-free survival (12). A recent study found that the mortality rate of the high Foxp3 +/CD4+ TIL ratio group was 3.5 times higher than that of the low ratio group after observing the distribution of immune cells of 57 HCC patients in tumor tissue and peritumor tissue and the correlation of immune cells with clinical outcome. Then it came to a conclusion that the Foxp3+/CD4+ TIL ratio in tumor tissue was an independent prognostic factor for HCC (54). Additionally, Mathai’s group discovered that a high Foxp3 +/CD8+TIL ratio was correlated with poor tumor differentiation, high recurrence, poor overall survival, and disease-free survival in post-surgery HCC patients (52). Finally, in 2006, a report including 69 HCC patients who accepted liver transplantation from 1998 to 2001 pointed out that patients with a high CD4 +/CD8+TIL ratio had low recurrence risk after treatment (53).
Given the above, the ratio between TIL subtypes might be a useful prognostic indicator in HCC. Low Foxp3+/CD3+, Foxp3+/CD4+, and Foxp3+/CD8+TIL ratio, as well as high CD4+/CD8+TIL ratio were found to predict a good prognosis in recent studies.
Interactions Between Tumor-Infiltrating T Lymphocytes and Other Immune Cells in HCC
B Lymphocytes
B cells infiltrating in tumor tissue are defined as tumor-infiltrating B cells (TIBs). Under the stimulation of different factors and cells in TME, TIBs can differentiate into different subtypes and then play a dual role in tumors by secreting antibodies, acting as antigen-presenting cells (APCs), and secreting cytokines (55). TIBs have been widely focused on many different types of tumors, such as liver cancer (56), breast cancer (57), oropharyngeal squamous cell carcinoma (58), cervical cancer (59). High levels of TIBs, which have been proved to be a protective factor against HCC, were linked to smaller tumors and the lack of vascular invasion (60) (Figure 1). Multiple subsets of TIBs, including CD20+ B cells, naive B cells (Bn), IgM+ memory B cells (Bm), CD27− isotype-switched memory B cells (CD27− Sw Bm), as well as plasma cells (PCs), co-existed in HCC. In HCC tumors, all five subtypes were reduced and impaired as compared to non-tumor tissue. High Bn and CD27− Sw Bm densities might be utilized as independent good predictor factors, possibly because of their cytokines secreting activity, such as interferon (IFN)- γ (61). Furthermore, B cells might boost the humoral response to eliminate tumor cells by secreting antitumor antibodies (62). Last, it has been shown that TIBs were able to influence HCC progression through interacting with other immune cells (63). TIBs could increase the infiltration of CD8+ T cells via IFN-γ, IL-12p40, granzyme B to inhibit HCC progression (60) (Figure 1). TIBs secreting IL-12 and IFN-γ also stimulated NK cells, which had an anticancer impact (64) (Figure 1). Regulating B cells (Bregs), a novel subset of B cells, could promote HCC by interacting with many immune cells. When compared to healthy liver tissue, the amount of Bregs was elevated while the level of CD4+ CTLs expressing granzyme and perforin was downregulated in HBV tumor tissue, according to Xue’s group (65) (Figure 1). Finally, they concluded that the interactions between Bregs and CD4+CTLs might be the mechanism for HCC progression. Bregs also promoted HCC by blocking CD8+ T cells and NK cells while increasing Foxp3+ T cells (66) (Figure 1).
The roles of TIBs, as outlined, are still open for debate in HCC. The dual roles of TIB were determined by its subtypes infiltrating in HCC.
Macrophages
There are two prominent macrophages in liver tissue: tissue-resident macrophages, such as Kupffer cells, and monocyte-derived macrophages (MDMs) (67). MDMs could differentiate into two different functional subtypes in TME, including M1 induced by lipopolysaccharide (LPS), IFN-γ, and M2 or tumor-associated macrophages (TAMs) induced by IL-4 and IL-10 (68). M1 macrophages mainly exert pro-inflammatory function, while M2 macrophages have an anti-inflammatory effect (69). Meanwhile, recent evidence suggested that M2 macrophages contributed to the progression of liver cancer by promoting pathogenic angiogenesis (70), tumor cell invasion and migration (71), epithelial-mesenchymal transition (EMT), and cancer stem cell-like characters (72, 73), as well as mediating drug resistance in HCC (74). According to research published in 2019 (74), not only did M2 macrophages prompt the progression and metastasis of liver cancer but also maintained the growth and metastasis of tumor cells by secreting hepatocyte growth factor (HGF), which significantly enhanced the resistance to sorafenib in liver cancer. Moreover, it was proposed that the interactions between macrophages and infiltrating T lymphocytes also contributed to liver cancer progression (Figure 1). Kuang et al. (75) reported that the distribution of CD68+TAMs in liver cancer tissue was positively correlated with that of Th17, and TAMs could induce the expansion of Th17, playing a role in fostering angiogenesis of cancer through secreting inflammatory factors, which promoted liver cancer finally. In addition, several studies have demonstrated that Tregs in tumor tissue were increased and activated by intratumoral macrophages, and their interactions promoted HCC progression at last (76, 77).
Finally, macrophages were proved to aid HCC progression through interacting with infiltrating T lymphocytes, such as Th17 and Tregs.
Dendritic Cells
In the healthy liver, DCs mainly captured antigens as APCs, presented antigens to T cells with the help of major histocompatibility complex (MHC), and then activated antigen-specific T lymphocytes, which linked innate and adaptive immunity together. The precise role of infiltrating DCs in HCC remains uncertain. It was widely thought that high infiltration of DCs was associated with a favorable prognosis and could predict recurrence and metastasis independently in individuals following surgery (78) (Figure 1). Their antitumor function was generated due to the mechanism that DCs could activate T cells responses to inhibit HCC progression (79). Other studies, on the other hand, revealed that DCs could be suppressed, leading to the development of HCC in patients with high AFP levels (80). Cross-talks between DCs and other cells, such as tumor cells and immune cells, have also been discussed in some studies. It has been shown that tumor cells could induce the immature differentiation of DCs by reducing adhesion molecules or secreting immune inhibition cytokines, such as IL-10 and vascular endothelial growth factor (VEGF) (81, 82). Compared with mature DCs, immature DCs could recognize and process antigens well while present antigens poorly (83). As a result, DC-T cell interactions were reduced, and T cells could not fully play the role of antitumor effector cells, which might be one of the mechanisms of immune resistance to tumors (84) (Figure 1). In an ex vivo study, DCs with low IL-12 production caused the functional impairment of T lymphocytes, confirming the interaction between them (85). In addition, another recent evidence (86) confirmed that the expression of inhibitory receptors on the surface of CD4+ and CD8+ T cells, such as PD-1, T-cell immunoglobulin and mucin-domain containing-3 (TIM3), cytotoxic T-lymphocyte-associated protein 4 (CTLA4), was higher in the tumor tissue than that in the non-tumor tissue and peripheral blood. Those inhibitory receptors could combine with corresponding inhibitory ligands of DCs and tumor cells, induce the disability of CD4+ TILs or suppress the cytotoxic reaction of CD8+ TILs, which finally mediated the immune tolerance to the tumor (Figure 1). The latest immune check-point treatment was founded on those theory bases and had been used in many types of tumors, including liver cancer. Compared with traditional tumor therapies, immune checkpoint treatment could recover the response of impaired TILs to tumor antigens and prolong the survival of HCC (84, 86).
As can be observed, DCs had a role in the development of HCC due to inadequate DC-T interactions and an increased inhibitory function on effector T cells.
Natural Killer Cells
NK cells, which belong to innate immune cells, account for 5-20% of circulating lymphocytes and over 50% of intrahepatic lymphocytes. They mainly recognize abnormal cells with low expression of MHC I, such as tumor cells and infected cells. Then, activating receptors on NK cells will be activated, which causes an unbalance towards activated NK cells, and NK cells can eradicate target cells directly or indirectly, such as NK cell-mediated cytotoxicity and secreting pro-inflammatory cytokines (87) (Figure 1). Compared to healthy liver tissue, the quantity of infiltrating NK cells changed, and their function did in HCC tissue. Wu’s group (88) reported that the number of infiltrating NK cells in HCC tissue significantly reduced, and their functions were also impaired, which might trigger immune evasion of the tumor and finally cause the progression of HCC. This suggested that NK cells had the antitumor role. Consistent with that, another study discovered that higher frequency of NK cells of intratumor tissues was related to longer recurrence-free and overall survival in HCC patients treated with sorafinib (89). Aside from the reduced expression and dysfunction of NKs, the following mechanisms involved in NKs might promote HCC progression. First, the final result of HCC patients was determined by the balance between infiltrating NKs, which could destroy tumors, and other immune cells, such as Tregs, which might inhibit immune response. Wang and co-workers (90) found that, compared with non-tumor tissue, both Tregs and the activated NK cells significantly increased in liver cancer tissue. Now, the increased Tregs were shown to be associated with poor prognosis in HCC (91). Second, the state of NK cells would be influenced by tumor cells. On the surface of NK cells, there were activating receptors that could activate NKs to exert immunity surveillance and inhibitory receptors which could inhibit NKs to trigger immune evasion, and their imbalance would determine the function of NKs in HCC (92). NKG2D was one of the most well discussed activating receptors in HCC. Although, tumor cells might still manage to escape the immune surveillance by down-regulating NKG2D via increased TGF-β secretion (93) or decreased expression of its ligands, such as major histocompatibility chain-related protein A (MICA) (94). Third, NKs might promote HCC progression via interacting with infiltrating T cells. An earlier review (95) discovered that antigen-specify T lymphocytes regulated by NK cells would boost as the depletion of NK cells. Hence, it concluded that NK cells finally promoted HCC progression by lowering the immune activity against tumors via diminishing T cells (Figure 1). It has been found that Treg cells could directly inhibit the function of NK cells by expressing membrane-bound TGF-β (96), or reduce the sensitivity of NK cells to tumor cells via diminishing IL-2 (95) (Figure 1).
Ultimately, NKs failed to exert the antitumor effect for the sake of their frequency decreasing, function impairment, and interaction with surrounding tumor cells or other types of infiltrating immune cells in HCC.
Critical Signaling Pathways Related to TILs in HCC
TGF-β Signaling
The TGF-β family comprises TGF-βs, activins, inhibins, bone morphogenetic proteins (BMPs), as well as growth and differentiation factors (GDFs). The activation of this signaling pathway starts with the binding of ligands to the extracellular region of TGF-β type I and type II receptors (TβRI and TβRII), followed by activating SMAD-dependent and SMAD-independent pathways to trigger downstream cascades. In HCC, TGF-β signaling is involved in almost each stage of tumor formation (97). TME had a large amount of TGF-β, which was overexpressed by tumor cells and other immune cells. During the early stage of liver cancer, TGF-β suppressed the proliferation of premalignant hepatocytes. But in the advanced HCC phase, it contributed to tumor progression through regulating immune cells, such as Tregs, CTLs, TAMs, NKs (98). First, TGF-β could induce the expression of Foxp3 on CD4+CD25- naive T cells through activating SMAD-dependent pathways, mediating the formation of Tregs (99) (Figure 2). Tregs in periphery blood also infiltrated into HCC tumor tissues to suppress the antitumor effect of CTLs (100) (Figure 2). In addition, tumor cells of HCC increasingly expressed TGF-β, which was capable of upregulating the expression of PD-1 on CD8+ CTLs. CTLs then bound to the PD-L1 on tumor cells and APC to induce CTLs exhaustion (101). At last, TGF-β signaling could participate in the regulation of the innate immune system by directly suppressing NK cells and promoting the differentiation of TAM towards M2 to induce immune escape (102) (Figure 2). Taken together, TGF-β signaling pathways were broadly involved in the regulation of TILs, and finally exerted promotion function in HCC.
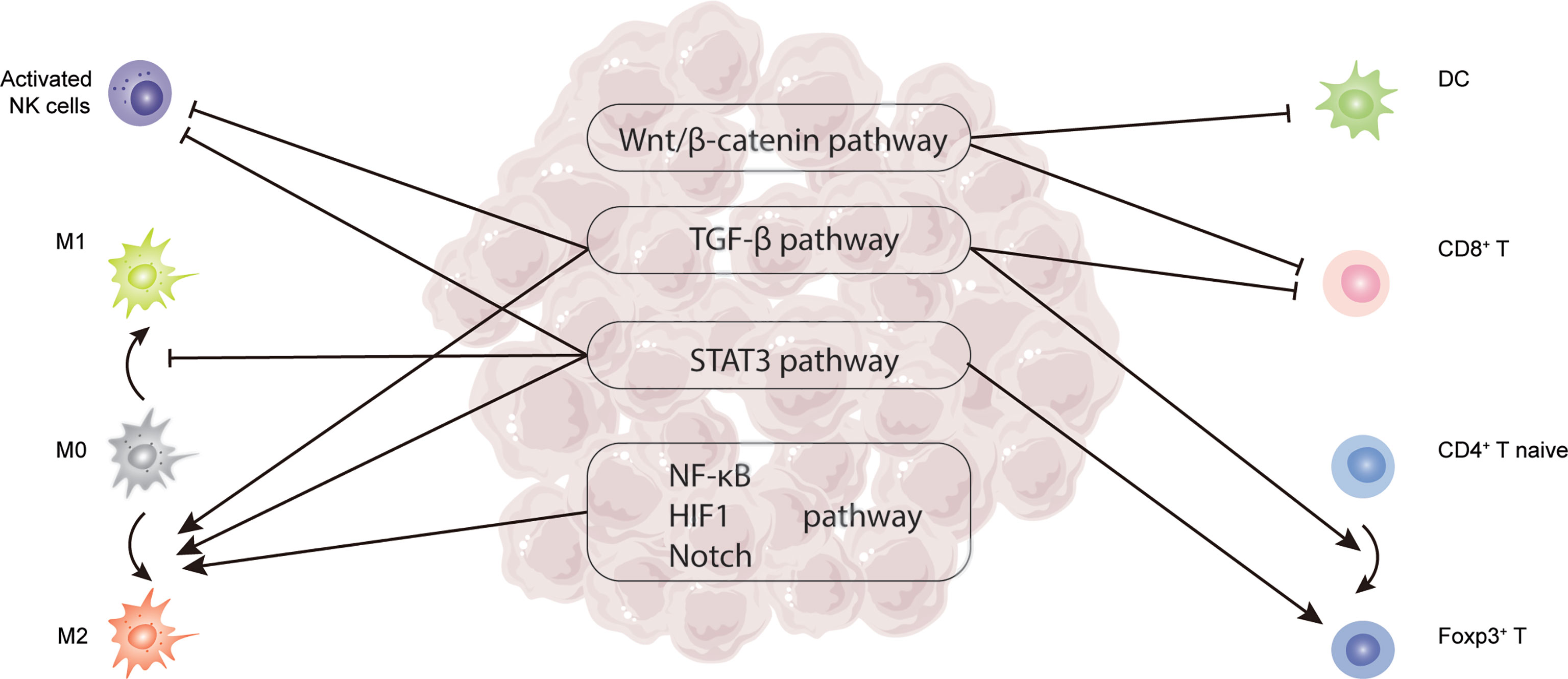
Figure 2 Critical signaling pathways related to TILs in HCC, including TGF-β, STAT3, Wnt/β-catenin, NF-κB, HIF-1, and Notch pathway.
Signal Transducer and Activator of Transcription 3 Signaling
The human signal transducer and activator of transcription (STAT) protein family consist of 7 members, including STAT1, STAT2, STAT3, STAT4, STAT5A, STAT5B, and STAT6. Among them, STAT3 has been focused by many studies on cancer regulation. STAT3 was thought to be activated rapidly and transiently in healthy cells by several cytokines, such as IL-6, IL-10, IFNs, and growth factors, such as VEGF. Nevertheless, it was stimulated persistently and abnormally in tumor cells. According to a recent study, phosphorylated STAT3 could be detected in around 60% of liver cancer samples, which was related to poor prognosis (103). STAT3 signaling pathway mainly exerted protumor function through regulating DCs, differentiation of TAMs, NKs, and Tregs. In DCs, IL-6 secreted by liver tumor cells and hepatic carcinoma-associated fibroblasts (hCAFs) could bind to the corresponding IL-6R on DCs, activate janus kinase (JAK), and then start the downstream phosphorylation cascade of STAT3 signaling pathway to inhibit T cells proliferation and increase Tregs production (104) (Figure 2). Moreover, STAT3 signaling was proved to inhibit the expression of activation receptor NKD2G on NKs and its corresponding ligands MICA/B on tumor cells, which could block the activation of NKs and result in failing immune surveillance in HCC (Figure 2). Suppressing STAT3 signaling in HCC could reactive NKs to exert antitumor function through altering cytokines in TME, such as reducing the level of IL-10 (105). Apart from that, IL‐6/STAT3 signaling facilitated tumor development by reducing M1 polarization while increasing M2 differentiation of TAM (106) (Figure 2). As can be seen, STAT3 signaling influenced multiple immune cells, which aided in the development of HCC.
Wnt/β-Catenin Signaling
When Wnt ligands bind to their corresponding receptors on the surface of cells, the Wnt signaling cascade is activated. β-catenin, usually located in the adherent junctions and cytoplasm of cells, is driven to accumulate in the cytoplasm and translocate into the nucleus, where it exerts regulation function (107). Wnt/β-catenin signaling was frequently stimulated in HCC (108), which mainly reduced the frequency of TILs and impaired their function (109). The activation of Wnt/-catenin signaling in HCC was considered to impair the innate immune system by lowering DC infiltration (Figure 2), which then impaired the adaptive immune response through reducing antigen-specific CD8+ T cell migration (110). Apart from that, Wnt/-catenin signaling also interfered with the effect of CTLs, causing them to become exhausted (111) (Figure 2). In summary, Wnt/β-catenin signaling was frequently activated and promoted HCC through interacting with TILs, such as CD8+ T cells and DCs.
Other Signaling Pathways
NF-κB signaling pathway including canonical and non-canonical plays significant roles in many kinds of liver disease, such as hepatitis, liver cirrhosis, and liver cancer (112). In HCC, hepatoma-derived factors expressed by tumor cells could bind to hepatoma-derived toll-like receptor 2 (TLR2) to active canonical NF-κB signaling pathway. Subsequently, TAMs differentiation towards M2 was enhanced and acted as protumor function (113) (Figure 2). Moreover, another two signaling pathways, named HIF-1 and Notch signaling, have been proved to be involved in the recruitment and activation of TAM in HCC (114, 115) (Figure 2). Ultimately, the formation and development of HCC are complicated. The signaling pathways related to TILs in HCC played potent roles in this process. And further research is still needed to clarify the mechanisms of HCC progression deeply.
Conclusion
Overall, TILs have been shown to exhibit both antitumor and protumor properties in HCC. They cross-talked to create a complex map with multiple signaling pathways connecting them in the development of HCC. Future investigations are necessary to reveal the undiscovered TIL domains, such as the specific functions and mechanisms through which TILs function. In the long term, studying on TILs in the roles and mechanisms of HCC progression might help us best understand the law of HCC progression, and identify innovative immunotherapy strategies.
Author Contributions
XZ prepared and wrote the manuscript. WJ drafted and corrected the figures. SW contributed to design the manuscript of the second edition. HD critically revised and edited the manuscript content. All authors contributed to the article and approved the submitted version.
Funding
This study was supported by National Natural Science Foundation (81672725) and Sino-German Cooperation Group (GZ1517).
Conflict of Interest
The authors declare that the research was conducted in the absence of any commercial or financial relationships that could be construed as a potential conflict of interest.
Publisher’s Note
All claims expressed in this article are solely those of the authors and do not necessarily represent those of their affiliated organizations, or those of the publisher, the editors and the reviewers. Any product that may be evaluated in this article, or claim that may be made by its manufacturer, is not guaranteed or endorsed by the publisher.
References
1. Sung H, Ferlay J, Siegel RL, Laversanne M, Soerjomataram I, Jemal A, et al. Global Cancer Statistics 2020: GLOBOCAN Estimates of Incidence and Mortality Worldwide for 36 Cancers in 185 Countries. CA: Cancer J Clin (2021) 71(3):209–49. doi: 10.3322/caac.21660
2. Zhang S, Sun K, Zheng R, Zeng H, Wang S, Chen R, et al. Cancer Incidence and Mortality in China, 2015. J Natl Cancer Center (2021) 1(1):2–11. doi: 10.1016/j.jncc.2020.12.001
3. Liver EAFT. EASL Clinical Practice Guidelines: Management of Hepatocellular Carcinoma. J Hepatol (2018) 69(1):182–236. doi: 10.1016/j.jhep.2018.03.019
4. Yang JD, Hainaut P, Gores GJ, Amadou A, Plymoth A, Roberts LR, et al. A Global View of Hepatocellular Carcinoma: Trends, Risk, Prevention and Management. Nat Rev Gastroenterol Hepatol (2019) 16(10):589–604. doi: 10.1038/s41575-019-0186-y
5. Tabrizian P, Jibara G, Shrager B, Schwartz M, Roayaie S. Recurrence of Hepatocellular Cancer After Resection: Patterns, Treatments, and Prognosis. Ann Surg (2015) 261(5):947–55. doi: 10.1097/SLA.0000000000000710
6. Rohr-Udilova N, Klinglmuller F, Schulte-Hermann R, Stift J, Herac M, Salzmann M, et al. Deviations of the Immune Cell Landscape Between Healthy Liver and Hepatocellular Carcinoma. Sci Rep (2018) 8(1):6220. doi: 10.1038/s41598-018-24437-5
7. Chew V, Lai L, Pan L, Lim CJ, Li J, Ong R, et al. Delineation of an Immunosuppressive Gradient in Hepatocellular Carcinoma Using High-Dimensional Proteomic and Transcriptomic Analyses. Proc Natl Acad Sci USA (2017) 114(29):E5900–9. doi: 10.1073/pnas.1706559114
8. Hernandez Gea V, Toffanin S, Friedman SL, Llovet JM, et al. Role of the Microenvironment in the Pathogenesis and Treatment of Hepatocellular Carcinoma. Gastroenterology (2013) 144(3):512–27. doi: 10.1053/j.gastro.2013.01.002
9. Sprinzl MF, Galle PR. Immune Control in Hepatocellular Carcinoma Development and Progression: Role of Stromal Cells. Semin Liver Dis (2014) 34(4):376–88. doi: 10.1055/s-0034-1394138
10. Hiraoka N. Tumor-Infiltrating Lymphocytes and Hepatocellular Carcinoma: Molecular Biology. Int J Clin Oncol (2010) 15(6):544–51. doi: 10.1007/s10147-010-0130-1
11. Shirabe K, Motomura T, Muto J, Toshima T, Matono R, Mano Y, et al. Tumor-Infiltrating Lymphocytes and Hepatocellular Carcinoma: Pathology and Clinical Management. Int J Clin Oncol (2010) 15(6):552–8. doi: 10.1007/s10147-010-0131-0
12. Yao W, He JC, Yang Y, Wang JM, Qian YW, Yang T, et al. The Prognostic Value of Tumor-Infiltrating Lymphocytes in Hepatocellular Carcinoma: A Systematic Review and Meta-Analysis. Sci Rep (2017) 7(1):7525. doi: 10.1038/s41598-017-08128-1
13. Fontenot JD, Gavin MA, Rudensky AY. Foxp3 Programs the Development and Function of CD4+CD25+ Regulatory T Cells. Nat Immunol (2003) 4(4):330–6. doi: 10.1038/ni904
14. Yagi H, Nomura T, Nakamura K, Yamazaki S, Kitawaki T, Hori S, et al. Crucial Role of FOXP3 in the Development and Function of Human CD25+CD4+ Regulatory T Cells. Int Immunol (2004) 16(11):1643–56. doi: 10.1093/intimm/dxh165
15. Curotto De Lafaille MA, Lafaille JJ. Natural and Adaptive Foxp3+ Regulatory T Cells: More of the Same or a Division of Labor? Immunity (2009) 30(5):626–35. doi: 10.1016/j.immuni.2009.05.002
16. Jonuleit H, Schmitt E. The Regulatory T Cell Family: Distinct Subsets and Their Interrelations. J Immunol (2003) 171(12):6323–7. doi: 10.4049/jimmunol.171.12.6323
17. Huang Y, Liao H, Zhang Y, Yuan R, Wang F, Gao Y, et al. Prognostic Value of Tumor-Infiltrating FoxP3+ T Cells in Gastrointestinal Cancers: A Meta Analysis. PloS One (2014) 9(5):e94376. doi: 10.1371/journal.pone.0094376
18. Wang X, Lang M, Zhao T, Feng X, Zheng C, Huang C, et al. Cancer-FOXP3 Directly Activated CCL5 to Recruit FOXP3(+)Treg Cells in Pancreatic Ductal Adenocarcinoma. Oncogene (2017) 36(21):3048–58. doi: 10.1038/onc.2016.458
19. Zhao Y, Ge X, He J, Cheng Y, Wang Z, Wang J, et al. The Prognostic Value of Tumor-Infiltrating Lymphocytes in Colorectal Cancer Differs by Anatomical Subsite: A Systematic Review and Meta-Analysis. World J Surg Oncol (2019) 17(1):85. doi: 10.1186/s12957-019-1621-9
20. Mansuri N, Birkman EM, Heuser VD, Lintunen M, Algars A, Sundstrom J, et al. Association of Tumor-Infiltrating T Lymphocytes With Intestinal-Type Gastric Cancer Molecular Subtypes and Outcome. Virchows Arch (2020) 478:717–17. doi: 10.1007/s00428-020-02932-3
21. Gao Y, Guo W, Geng X, Zhang Y, Zhang G, Qiu B, et al. Prognostic Value of Tumor-Infiltrating Lymphocytes in Esophageal Cancer: An Updated Meta-Analysis of 30 Studies With 5,122 Patients. Ann Transl Med (2020) 8(13):822. doi: 10.21037/atm-20-151
22. Wang W, Jiang C, Yan W, Zhang Y, Yang J, Zhang C, et al. FOXP3 Expression and Clinical Characteristics of Hepatocellular Carcinoma. World J Gastroenterol WJG (2010) 16(43):5502–9. doi: 10.3748/wjg.v16.i43.5502
23. Casares N, Rudilla F, Arribillaga L, Llopiz D, Riezu-Boj JI, Lozano T, et al. A Peptide Inhibitor of FOXP3 Impairs Regulatory T Cell Activity and Improves Vaccine Efficacy in Mice. J Immunol (2010) 185(9):5150–9. doi: 10.4049/jimmunol.1001114
24. Zhang X, Gao L, Liang X, Guo M, Wang R, Pan Y, et al. HBV Pres2 Transactivates FOXP3 Expression in Malignant Hepatocytes. Liver Int (2015) 35(3):1087–94. doi: 10.1111/liv.12642
25. Whiteside TL, Schuler P, Schilling B. Induced and Natural Regulatory T Cells in Human Cancer. Expert Opin Biol Ther (2012) 12(10):1383–97. doi: 10.1517/14712598.2012.707184
26. Lin SZ, Chen KJ, Xu ZY, Chen H, Zhou L, Xie HY, et al. Prediction of Recurrence and Survival in Hepatocellular Carcinoma Based on Two Cox Models Mainly Determined by FoxP3+ Regulatory T Cells. Cancer Prev Res (Phila) (2013) 6(6):594–602. doi: 10.1158/1940-6207.CAPR-12-0379
27. Huang Y, Wang FM, Wang T, Wang YJ, Zhu ZY, Gao YT, et al. Tumor-Infiltrating FoxP3+ Tregs and CD8+ T Cells Affect the Prognosis of Hepatocellular Carcinoma Patients. Digestion (2012) 86(4):329–37. doi: 10.1159/000342801
28. Chen K, Zhou L, Xie H, Ahmed T, Feng X, Zheng S. Intratumoral Regulatory T Cells Alone or in Combination With Cytotoxic T Cells Predict Prognosis of Hepatocellular Carcinoma After Resection. Med Oncol (2012) 29(3):1817–26. doi: 10.1007/s12032-011-0006-x
29. Shen SL, Liang LJ, Peng BG, He Q, Kuang M, Lai JM. Foxp3+ Regulatory T Cells and the Formation of Portal Vein Tumour Thrombus in Patients With Hepatocellular Carcinoma. Can J Surg (2011) 54(2):89–94. doi: 10.1503/cjs.028009
30. Yang Z, Yang Z, Zhang L, Ping-Bie, Wang S, Ma K, et al. Increased Liver-Infiltrating CD8+FoxP3+ Regulatory T Cells are Associated With Tumor Stage in Hepatocellular Carcinoma Patients. Hum Immunol (2010) 71(12):1180–6. doi: 10.1016/j.humimm.2010.09.011
31. Fu J, Zhang Z, Zhou L, Qi Z, Xing S, Lv J, et al. Impairment of CD4+ Cytotoxic T Cells Predicts Poor Survival and High Recurrence Rates in Patients With Hepatocellular Carcinoma. Hepatology (2013) 58(1):139–49. doi: 10.1002/hep.26054
32. Fu J, Xu D, Liu Z, Shi M, Zhao P, Fu B, et al. Increased Regulatory T Cells Correlate With CD8 T-Cell Impairment and Poor Survival in Hepatocellular Carcinoma Patients. Gastroenterology (2007) 132(7):2328–39. doi: 10.1053/j.gastro.2007.03.102
33. Gong Z, Jia H, Yu J, Liu Y, Ren J, Yang S, et al. Nuclear FOXP3 Inhibits Tumor Growth and Induced Apoptosis in Hepatocellular Carcinoma by Targeting C-Myc. Oncogenesis (2020) 9(10):97. doi: 10.1038/s41389-020-00283-x
34. Hao J, Li M, Zhang T, Yu H, Liu Y, Xue Y, et al. Prognostic Value of Tumor-Infiltrating Lymphocytes Differs Depending on Lymphocyte Subsets in Esophageal Squamous Cell Carcinoma: An Updated Meta-Analysis. Front Oncol (2020) 10:614. doi: 10.3389/fonc.2020.00614
35. Lee JS, Won HS, Sun DS, Hong JH, Ko YH. Prognostic Role of Tumor-Infiltrating Lymphocytes in Gastric Cancer. Medicine (2018) 97(32):e11769. doi: 10.1097/MD.0000000000011769
36. Yarchoan M, Xing D, Luan L, Xu H, Sharma RB, Popovic A, et al. Characterization of the Immune Microenvironment in Hepatocellular Carcinoma. Clin Cancer Res (2017) 23(23):7333–9. doi: 10.1158/1078-0432.CCR-17-0950
37. O'Leary K. T Cell Drivers in NASH-HCC. Nat Rev Cancer (2021) 21(6):341. doi: 10.1038/s41568-021-00362-0
38. Sun C, Xu J, Song J, Liu C, Wang J, Weng C, et al. The Predictive Value of Centre Tumour CD8(+) T Cells in Patients With Hepatocellular Carcinoma: Comparison With Immunoscore. Oncotarget (2015) 6(34):35602–15. doi: 10.18632/oncotarget.5801
39. Hao J, Schoenberg MB, Bucher JN, Miksch RC, Wolfgang HJ, Anger B, et al. Perivascular Tumor-Infiltrating Leukocyte Scoring for Prognosis of Resected Hepatocellular Carcinoma Patients. HPB (2019) 21:S225. doi: 10.1016/j.hpb.2019.10.1628
40. Xu X, Tan Y, Qian Y, Xue W, Wang Y, Du J, et al. Clinicopathologic and Prognostic Significance of Tumor-Infiltrating CD8+ T Cells In Patients With Hepatocellular Carcinoma: A Meta-Analysis. Med (Baltimore) (2019) 98(2):e13923. doi: 10.1097/MD.0000000000013923
41. Ding W, Xu X, Qian Y, Xue W, Wang Y, Du J, et al. Prognostic Value of Tumor-Infiltrating Lymphocytes in Hepatocellular Carcinoma: A Meta-Analysis. Med (Baltimore) (2018) 97(50):e13301. doi: 10.1097/MD.0000000000013301
42. Sideras K, Biermann K, Verheij J, Takkenberg BR, Mancham S, Hansen BE, et al. PD-L1, Galectin-9 and CD8(+) Tumor-Infiltrating Lymphocytes Are Associated With Survival in Hepatocellular Carcinoma. Oncoimmunology (2017) 6(2):e1273309. doi: 10.1080/2162402X.2016.1273309
43. Ramzan M, Sturm N, Decaens T, Bioulac-Sage P, Bancel B, Merle P, et al. Liver-Infiltrating CD8+ Lymphocytes as Prognostic Factor for Tumour Recurrence In Hepatitis C Virus-Related Hepatocellular Carcinoma. Liver Int (2016) 36(3):434–44. doi: 10.1111/liv.12927
44. Ozgur HH, Ercetin AP, Eliyatkin N, Seren A, Kupelioglu A, Ortac R, et al. Regulatory T Cells and Their Prognostic Value in Hepatopancreatobiliary Tumours. Hepato-gastroenterology (2014) 61(135):1847–51. doi: 10.5754/hge14619
45. Gao Q, Qiu SJ, Fan J, Zhou J, Wang XY, Xiao YS, et al. Intratumoral Balance of Regulatory and Cytotoxic T Cells is Associated With Prognosis of Hepatocellular Carcinoma After Resection. J Clin Oncol (2007) 25(18):2586–93. doi: 10.1200/JCO.2006.09.4565
46. Gabrielson A, Wu Y, Wang H, Jiang J, Kallakury B, Gatalica Z, et al. Intratumoral CD3 and CD8 T-Cell Densities Associated With Relapse-Free Survival in HCC. Cancer Immunol Res (2016) 4(5):419–30. doi: 10.1158/2326-6066.CIR-15-0110
47. Antony PA, Piccirillo CA, Akpinarli A, Finkelstein SE, Speiss PJ, Surman DR, et al. CD8+ T Cell Immunity Against a Tumor/Self-Antigen Is Augmented by CD4+ T Helper Cells and Hindered by Naturally Occurring T Regulatory Cells. J Immunol (2005) 174(5):2591–601. doi: 10.4049/jimmunol.174.5.2591
48. Takeuchi A, Saito T. CD4 CTL, a Cytotoxic Subset of CD4 + T Cells, Their Differentiation and Function. Front Immunol (2017) 8:194. doi: 10.3389/fimmu.2017.00194
49. Chaoul N, Mancarella S, Lupo L, Giannelli G, Dituri F, et al. Impaired Anti-Tumor T Cell Response in Hepatocellular Carcinoma. Cancers (2020) 12(3):627. doi: 10.3390/cancers12030627
50. Brown ZJ, Fu Q, Ma C, Kruhlak M, Zhang H, Luo J, et al. Carnitine Palmitoyltransferase Gene Upregulation by Linoleic Acid Induces CD4+ T Cell Apoptosis Promoting HCC Development. Cell Death Dis (2018) 9(6):614–20. doi: 10.1038/s41419-018-0687-6
51. Witkowski M, Spangenberg HC, Neumann-Haefelin C, Buttner N, Breous E, et al. Lack of Ex Vivo Peripheral and Intrahepatic Alpha-Fetoprotein-Specific CD4+ Responses in Hepatocellular Carcinoma. Int J Cancer (2011) 129(9):2171–82. doi: 10.1002/ijc.25866
52. Mathai AM, Kapadia MJ, Alexander J, Kernochan LE, Swanson PE, Yeh MM, et al. Role of Foxp3-Positive Tumor-Infiltrating Lymphocytes in the Histologic Features and Clinical Outcomes of Hepatocellular Carcinoma. Am J Surg Pathol (2012) 36(7):980–6. doi: 10.1097/PAS.0b013e31824e9b7c
53. Unitt E, Marshall A, Gelson W, Rushbrook SM, Davies S, Vowler SL, et al. Tumour Lymphocytic Infiltrate and Recurrence of Hepatocellular Carcinoma Following Liver Transplantation. J Hepatol (2006) 45(2):246–53. doi: 10.1016/j.jhep.2005.12.027
54. Tu JF, Ding YH, Ying XH, Wu FZ, Zhou XM, Zhang DK, et al. Regulatory T Cells, Especially ICOS(+) FOXP3(+) Regulatory T Cells, are Increased in the Hepatocellular Carcinoma Microenvironment and Predict Reduced Survival. Sci Rep (2016) 6:35056. doi: 10.1038/srep35056
55. Martin F, Chan AC. B Cell Immunobiology in Disease: Evolving Concepts From the Clinic. Annu Rev Immunol (2006) 24:467–96. doi: 10.1146/annurev.immunol.24.021605.090517
56. Garnelo M, Tan A, Her Z, Yeong J, Lim CJ, Chen J, et al. Interaction Between Tumour-Infiltrating B Cells and T Cells Controls the Progression of Hepatocellular Carcinoma. Gut (2017) 66(2):342–51. doi: 10.1136/gutjnl-2015-310814
57. Shen M, Wang J, Ren X. New Insights Into Tumor-Infiltrating B Lymphocytes in Breast Cancer: Clinical Impacts and Regulatory Mechanisms. Front Immunol (2018) 9:470. doi: 10.3389/fimmu.2018.00470
58. Hladikova K, Koucky V, Boucek J, Laco J, Grega M, Hodek M, et al. Tumor-Infiltrating B Cells Affect the Progression of Oropharyngeal Squamous Cell Carcinoma via Cell-to-Cell Interactions With CD8(+) T Cells. J Immunother Cancer (2019) 7(1):261. doi: 10.1186/s40425-019-0726-6
59. Rossetti R, Lorenzi N, Yokochi K, Rosa M, Benevides L, Margarido P, et al. B Lymphocytes can be Activated to Act as Antigen Presenting Cells to Promote Anti-Tumor Responses. PloS One (2018) 13(7):e199034. doi: 10.1371/journal.pone.0199034
60. Shi J, Gao Q, Wang Z, Zhou J, Wang X, Min Z, et al. Margin-Infiltrating CD20+ B Cells Display an Atypical Memory Phenotype and Correlate With Favorable Prognosis in Hepatocellular Carcinoma. Clin Cancer Res (2013) 19(21):5994–6005. doi: 10.1158/1078-0432.CCR-12-3497
61. Zhang Z, Ma L, Goswami S, Ma J, Zheng B, Duan M, et al. Landscape of Infiltrating B Cells and Their Clinical Significance in Human Hepatocellular Carcinoma. Oncoimmunology (2019) 8(4):e1571388. doi: 10.1080/2162402X.2019.1571388
62. Fridman WH, Zitvogel L, Sautes-Fridman C, Kroemer G. The Immune Contexture in Cancer Prognosis and Treatment. Nat Rev Clin Oncol (2017) 14(12):717–34. doi: 10.1038/nrclinonc.2017.101
63. Guo FF, Cui JW, Riccardo M, Masetti R. The Role of Tumor-Infiltrating B Cells in Tumor Immunity. J Oncol (2019) 2019:1–9. doi: 10.1155/2019/2592419
64. Sharonov GV, Serebrovskaya EO, Yuzhakova DV, Britanova OV, Chudakov DM. B Cells, Plasma Cells and Antibody Repertoires in the Tumour Microenvironment. Nat Rev Immunol (2020) 20(5):294–307. doi: 10.1038/s41577-019-0257-x
65. Xue H, Lin F, Tan H, Zhu ZQ, Zhang ZY, Zhao L, et al. Overrepresentation of IL-10-Expressing B Cells Suppresses Cytotoxic CD4+ T Cell Activity in HBV-Induced Hepatocellular Carcinoma. PloS One (2016) 11(5):e154815. doi: 10.1371/journal.pone.0154815
66. Yuen GJ, Demissie E, Pillai S. B Lymphocytes and Cancer: A Love-Hate Relationship. Trends Cancer (2016) 2(12):747–57. doi: 10.1016/j.trecan.2016.10.010
67. Li Y, Li S, Duan X, Yang C, Xu M, Chen L. Macrophage Phenotypes and Hepatitis B Virus Infection. J Clin Trans Hepatol (2020) 8(4):1–8. doi: 10.14218/JCTH.2020.00046
68. Huang Y, Ge W, Zhou J, Gao B, Qian X, Wang W. The Role of Tumor Associated Macrophages in Hepatocellular Carcinoma. J Cancer (2021) 12(5):1284–94. doi: 10.7150/jca.51346
69. Yunna C, Mengru H, Lei W, Weidong C. Macrophage M1/M2 Polarization. Eur J Pharmacol (2020) 877:173090. doi: 10.1016/j.ejphar.2020.173090
70. Bartneck M, Schrammen PL, Möckel D, Govaere O, Liepelt A, Krenkel O, et al. The CCR2+ Macrophage Subset Promotes Pathogenic Angiogenesis for Tumor Vascularization in Fibrotic Livers. Cell Mol Gastroenterol Hepatol (2019) 7(2):371–90. doi: 10.1016/j.jcmgh.2018.10.007
71. Liu G, Yin L, Ouyang X, Zeng K, Xiao Y, Li Y. M2 Macrophages Promote HCC Cells Invasion and Migration via miR-149-5p/MMP9 Signaling. J Cancer (2020) 11(5):1277–87. doi: 10.7150/jca.35444
72. Yao RR, Li JH, Zhang R, Chen RX, Wang YH. M2-Polarized Tumor-Associated Macrophages Facilitated Migration and Epithelial-Mesenchymal Transition of HCC Cells via the TLR4/STAT3 Signaling Pathway. World J Surg Oncol (2018) 16(1):9. doi: 10.1186/s12957-018-1312-y
73. Fan QM, Jing YY, Yu GF, Kou XR, Ye F, Gao L, et al. Tumor-Associated Macrophages Promote Cancer Stem Cell-Like Properties via Transforming Growth Factor-Beta1-Induced Epithelial-Mesenchymal Transition in Hepatocellular Carcinoma. Cancer Lett (2014) 352(2):160–8. doi: 10.1016/j.canlet.2014.05.008
74. Dong N, Shi X, Wang S, Gao Y, Kuang Z, Xie Q, et al. M2 Macrophages Mediate Sorafenib Resistance by Secreting HGF in a Feed-Forward Manner in Hepatocellular Carcinoma. Br J Cancer (2019) 121(1):22–33. doi: 10.1038/s41416-019-0482-x
75. Kuang DM, Peng C, Zhao Q, Wu Y, Chen MS, Zheng L. Activated Monocytes in Peritumoral Stroma of Hepatocellular Carcinoma Promote Expansion of Memory T Helper 17 Cells. Hepatology (2010) 51(1):154–64. doi: 10.1002/hep.23291
76. Zhou J, Ding T, Pan W, Zhu LY, Li L, Zheng L. Increased Intratumoral Regulatory T Cells Are Related to Intratumoral Macrophages and Poor Prognosis in Hepatocellular Carcinoma Patients. Int J Cancer (2009) 125(7):1640–8. doi: 10.1002/ijc.24556
77. Yang J, Zhang JX, Wang H, Wang GL, Hu QG, Zheng QC. Hepatocellular Carcinoma and Macrophage Interaction Induced Tumor Immunosuppression via Treg Requires TLR4 Signaling. World J Gastroenterol (2012) 18(23):2938–47. doi: 10.3748/wjg.v18.i23.2938
78. Cai X, Gao Q, Qiu S, Ye S, Wu Z, Fan J, et al. Dendritic Cell Infiltration and Prognosis of Human Hepatocellular Carcinoma. J Cancer Res Clin Oncol (2006) 132(5):293–301. doi: 10.1007/s00432-006-0075-y
79. Shibolet O, Alper R, Zlotogarov L, Thalenfeld B, Engelhardt D, Rabbani E, et al. NKT and CD8 Lymphocytes Mediate Suppression of Hepatocellular Carcinoma Growth via Tumor Antigen-Pulsed Dendritic Cells. Int J Cancer (2003) 106(2):236–43. doi: 10.1002/ijc.11201
80. Santos PM, Menk AV, Shi J, Tsung A, Delgoffe GM, Butterfield LH. Tumor-Derived Alpha-Fetoprotein Suppresses Fatty Acid Metabolism and Oxidative Phosphorylation in Dendritic Cells. Cancer Immunol Res (2019) 7(6):1001–12. doi: 10.1158/2326-6066.CIR-18-0513
81. Dudek AM, Martin S, Garg AD, Agostinis P. Immature, Semi-Mature, and Fully Mature Dendritic Cells: Toward a DC-Cancer Cells Interface That Augments Anticancer Immunity. Front Immunol (2013) 4:438. doi: 10.3389/fimmu.2013.00438
82. Vicari AP, Caux C, Trinchieri G. Tumour Escape From Immune Surveillance Through Dendritic Cell Inactivation. Semin Cancer Biol (2002) 12(1):33–42. doi: 10.1006/scbi.2001.0400
83. Chen C, Ma Y, Zhang Y, Zhang F, Zhou N, Wang X, et al. Effect of Dendritic Cell–Based Immunotherapy on Hepatocellular Carcinoma: A Systematic Review and Meta-Analysis. Cytother (Oxf Engl) (2018) 20(8):975–89. doi: 10.1016/j.jcyt.2018.06.002
84. Lurje I, Hammerich L, Tacke F. Dendritic Cell and T Cell Crosstalk in Liver Fibrogenesis and Hepatocarcinogenesis: Implications for Prevention and Therapy of Liver Cancer. Int J Mol Sci (2020) 21(19):1. doi: 10.3390/ijms21197378
85. Ormandy LA, Farber A, Cantz T, Petrykowska S, Wedemeyer H, Horning M, et al. Direct Ex Vivo Analysis of Dendritic Cells in Patients With Hepatocellular Carcinoma. World J Gastroenterol (2006) 12(20):3275–82. doi: 10.3748/wjg.v12.i20.3275
86. Zhou G, Sprengers D, Boor PPC, Doukas M, Schutz H, Mancham S, et al. Antibodies Against Immune Checkpoint Molecules Restore Functions of Tumor-Infiltrating T Cells in Hepatocellular Carcinomas. Gastroenterology (2017) 153(4):1107–19. doi: 10.1053/j.gastro.2017.06.017
87. Sharrock J. Natural Killer Cells and Their Role in Immunity. EMJ Allergy Immunol (2019) 4(1):108–16.
88. Wu Y, Kuang DM, Pan WD, Wan YL, Lao XM, Wang D, et al. Monocyte/Macrophage-Elicited Natural Killer Cell Dysfunction in Hepatocellular Carcinoma Is Mediated by CD48/2B4 Interactions. Hepatology (2013) 57(3):1107–16. doi: 10.1002/hep.26192
89. Wu M, Mei F, Liu W, Jiang J. Comprehensive Characterization of Tumor Infiltrating Natural Killer Cells and Clinical Significance in Hepatocellular Carcinoma Based on Gene Expression Profiles. BioMed Pharmacother (2020) 121:109637. doi: 10.1016/j.biopha.2019.109637
90. Wang L, Yang Z, Cao Y. Regulatory T Cell and Activated Natural Killer Cell Infiltration in Hepatocellular Carcinoma: Immune Cell Profiling Using the CIBERSORT. Ann Transl Med (2020) 8(22):1483. doi: 10.21037/atm-20-5830
91. Yu S, Wang Y, Hou J, Li W, Wang X, Xiang L, et al. Tumor-Infiltrating Immune Cells in Hepatocellular Carcinoma: Tregs Is Correlated With Poor Overall Survival. PloS One (2020) 15(4):e231003. doi: 10.1371/journal.pone.0231003
92. Bozward AG, Warricker F, Oo YH, Khakoo SI. Natural Killer Cells and Regulatory T Cells Cross Talk in Hepatocellular Carcinoma: Exploring Therapeutic Options for the Next Decade. Front Immunol (2021) 12:643310. doi: 10.3389/fimmu.2021.643310
93. Lazarova M, Steinle A. Impairment of NKG2D-Mediated Tumor Immunity by TGF-Beta. Front Immunol (2019) 10:2689. doi: 10.3389/fimmu.2019.02689
94. Zhang J, Xu Z, Zhou X, Zhang H, Yang N, Wu Y, et al. Loss of Expression of MHC Class I-Related Chain A (MICA) Is a Frequent Event and Predicts Poor Survival in Patients With Hepatocellular Carcinoma. Int J Clin Exp Pathol (2014) 7(6):3123–31.
95. Juengpanich S, Shi L, Iranmanesh Y, Chen J, Cheng Z, Khoo AK, et al. The Role of Natural Killer Cells in Hepatocellular Carcinoma Development and Treatment: A Narrative Review. Transl Oncol (2019) 12(8):1092–107. doi: 10.1016/j.tranon.2019.04.021
96. Ghiringhelli F, Ménard C, Terme M, Flament C, Taieb J, Chaput N, et al. CD4+CD25+ Regulatory T Cells Inhibit Natural Killer Cell Functions in a Transforming Growth Factor-Beta-Dependent Manner. J Exp Med (2005) 202(8):1075–85. doi: 10.1084/jem.20051511
97. Katz LH, Likhter M, Jogunoori W, Belkin M, Ohshiro K, Mishra L. TGF-β Signaling in Liver and Gastrointestinal Cancers. Cancer Lett (2016) 379(2):166–72. doi: 10.1016/j.canlet.2016.03.033
98. Yamazaki K, Masugi Y, Sakamoto M. Molecular Pathogenesis of Hepatocellular Carcinoma: Altering Transforming Growth Factor-Beta Signaling in Hepatocarcinogenesis. Dig Dis (2011) 29(3):284–8. doi: 10.1159/000327560
99. Chen W, Jin W, Hardegen N, Lei KJ, Li L, Marinos N, et al. Conversion of Peripheral CD4+CD25- Naive T Cells to CD4+CD25+ Regulatory T Cells by TGF-Beta Induction of Transcription Factor Foxp3. J Exp Med (2003) 198(12):1875–86. doi: 10.1084/jem.20030152
100. Flecken T, Schmidt N, Hild S, Gostick E, Drognitz O, Zeiser R, et al. Immunodominance and Functional Alterations of Tumor-Associated Antigen-Specific CD8+ T-Cell Responses in Hepatocellular Carcinoma. Hepatology (2014) 59(4):1415–26. doi: 10.1002/hep.26731
101. Park BV, Freeman ZT, Ghasemzadeh A, Chattergoon MA, Rutebemberwa A, Steigner J, et al. TGFbeta1-Mediated SMAD3 Enhances PD-1 Expression on Antigen-Specific T Cells in Cancer. Cancer Discovery (2016) 6(12):1366–81. doi: 10.1158/2159-8290.CD-15-1347
102. Marvin DL, Heijboer R, Ten Dijke P, Ritsma L. TGF-β Signaling in Liver Metastasis. Clin Trans Med (2020) 10(7):e160. doi: 10.1002/ctm2.160
103. He G, Yu GY, Temkin V, Ogata H, Kuntzen C, Sakurai T, et al. Hepatocyte IKKbeta/NF-kappaB Inhibits Tumor Promotion and Progression by Preventing Oxidative Stress-Driven STAT3 Activation. Cancer Cell (2010) 17(3):286–97. doi: 10.1016/j.ccr.2009.12.048
104. Cheng JT, Deng YN, Yi HM, Wang GY, Fu BS, Chen WJ, et al. Hepatic Carcinoma-Associated Fibroblasts Induce IDO-Producing Regulatory Dendritic Cells Through IL-6-Mediated STAT3 Activation. Oncogenesis (2016) 5:e198. doi: 10.1038/oncsis.2016.7
105. Sun X, Sui Q, Zhang C, Tian Z, Zhang J. Targeting Blockage of STAT3 in Hepatocellular Carcinoma Cells Augments NK Cell Functions via Reverse Hepatocellular Carcinoma-Induced Immune Suppression. Mol Cancer Ther (2013) 12(12):2885–96. doi: 10.1158/1535-7163.MCT-12-1087
106. Yin Z, Ma T, Lin Y, Lu X, Zhang C, Chen S, et al. IL-6/STAT3 Pathway Intermediates M1/M2 Macrophage Polarization During the Development of Hepatocellular Carcinoma. J Cell Biochem (2018) 119(11):9419–32. doi: 10.1002/jcb.27259
107. Perugorria MJ, Olaizola P, Labiano I, Esparza-Baquer A, Marzioni M, Marin JJG, et al. Wnt-β-Catenin Signalling in Liver Development, Health and Disease. Nat Rev Gastroenterol Hepatol (2019) 16(2):121–36. doi: 10.1038/s41575-018-0075-9
108. Suarez MI, Uribe D, Jaramillo CM, Osorio G, Perez JC, Lopez R, et al. Wnt/beta-Catenin Signaling Pathway in Hepatocellular Carcinomas Cases From Colombia. Ann Hepatol (2015) 14(1):64–74. doi: 10.1016/S1665-2681(19)30802-6
109. Nishida N. Role of Oncogenic Pathways on the Cancer Immunosuppressive Microenvironment and Its Clinical Implications in Hepatocellular Carcinoma. Cancers (Basel) (2021) 13(15). doi: 10.3390/cancers13153666
110. Ruiz DGM, Bresnahan E, Molina-Sanchez P, Lindblad KE, Maier B, Sia D, et al. Beta-Catenin Activation Promotes Immune Escape and Resistance to Anti-PD-1 Therapy in Hepatocellular Carcinoma. Cancer Discovery (2019) 9(8):1124–41. doi: 10.1158/2159-8290.CD-19-0074
111. Schinzari V, Timperi E, Pecora G, Palmucci F, Gallerano D, Grimaldi A, et al. Wnt3a/beta-Catenin Signaling Conditions Differentiation of Partially Exhausted T-Effector Cells in Human Cancers. Cancer Immunol Res (2018) 6(8):941–52. doi: 10.1158/2326-6066.CIR-17-0712
112. Sun B, Karin M. NF-kappaB Signaling, Liver Disease and Hepatoprotective Agents. Oncogene (2008) 27(48):6228–44. doi: 10.1038/onc.2008.300
113. Chang CP, Su YC, Lee PH, Lei HY. Targeting NFKB by Autophagy to Polarize Hepatoma-Associated Macrophage Differentiation. Autophagy (2013) 9(4):619–21. doi: 10.4161/auto.23546
114. Chen J, Gingold JA, Su X. Immunomodulatory TGF-Beta Signaling in Hepatocellular Carcinoma. Trends Mol Med (2019) 25(11):1010–23. doi: 10.1016/j.molmed.2019.06.007
Keywords: hepatocellular carcinoma, tumor-infiltrating lymphocyte, T lymphocyte, interaction, signaling pathway
Citation: Zheng X, Jin W, Wang S and Ding H (2021) Progression on the Roles and Mechanisms of Tumor-Infiltrating T Lymphocytes in Patients With Hepatocellular Carcinoma. Front. Immunol. 12:729705. doi: 10.3389/fimmu.2021.729705
Received: 23 June 2021; Accepted: 17 August 2021;
Published: 03 September 2021.
Edited by:
David L. Wiest, Fox Chase Cancer Center, United StatesCopyright © 2021 Zheng, Jin, Wang and Ding. This is an open-access article distributed under the terms of the Creative Commons Attribution License (CC BY). The use, distribution or reproduction in other forums is permitted, provided the original author(s) and the copyright owner(s) are credited and that the original publication in this journal is cited, in accordance with accepted academic practice. No use, distribution or reproduction is permitted which does not comply with these terms.
*Correspondence: Huiguo Ding, dinghuiguo@ccmu.edu.cn