- Institute of Reproductive Health, Tongji Medical College, Huazhong University of Science and Technology, Wuhan, China
Background: Varicocele (VC) is present in 35 - 40% of men with infertility. However, current surgical and antioxidant treatments are not completely effective. In addition to oxidative stress, it is likely that other factors such as testicular immune microenvironment disorder contribute to irreversible testicular. Evidence suggests that VC is associated with anti-sperm antibodies (ASAs), spermatogenesis and testosterone secretion abnormalities, and testicular cytokine production. Moreover, inhibition of inflammation can alleviate VC-mediated pathogenesis. The normal function of the testis depends on its immune tolerance mechanism. Testicular immune regulation is complex, and many infectious or non-infectious diseases may damage this precision system.
Results: The testicular immune microenvironment is composed of common immune cells and other cells involved in testicular immunity. The former includes testicular macrophages, T cells, dendritic cells (DCs), and mast cells, whereas the latter include Leydig cells and Sertoli cells (SCs). In animal models and in patients with VC, most studies have revealed an abnormal increase in the levels of ASAs and pro-inflammatory cytokines such as interleukin (IL)-1 and tumor necrosis factor (TNF)-alpha in the seminal plasma, testicular tissue, and even peripheral blood. It is also involved in the activation of potential inflammatory pathways, such as the nucleotide-binding oligomerization domain-like receptor family pyrin domain containing (NLRP)-3 pathway. Finally, the development of VC-mediated infertility (VMI) may be facilitated by abnormal permeability of proteins, such as claudin-11, that constitute the blood-testis barrier (BTB).
Conclusions: The testicular immune response, including the production of ASAs and inflammatory factors, activation of inflammatory pathways, and destruction of the BTB may be involved in the pathogenesis of VMI it is necessary to further explore how patient outcomes can be improved through immunotherapy.
Introduction
Approximately 15% of couples worldwide have infertility; half of these cases are attributable to male factors. Varicocele (VC), a vascular disease characterized by abnormal enlargement of the pampiniform plexus veins, is a highly treatable cause of infertility observed in 35–40% of men with infertility (1). VC is left-sided in at least 85% of cases (2); right-sided VCs are rare (3). Surgical ligation or embolization of the spermatic cord vein can improve semen quality, sperm DNA integrity, mitochondrial activity, and assisted reproductive cycle outcomes (4). It has been suggested that VC-mediated infertility (VMI) is not caused by a single factor but is the result of the synergy of genetic and other molecular factors, such as hypoxia, oxidative stress, and nutrient deprivation (5). However, some patients fail to regain fertility after surgery, antioxidant therapy, or other treatments. Interestingly, Mazdak Razi et al. (6) proposed that VMI is caused by endoplasmic reticulum stress and the unfolded protein response, which promote testicular cell apoptosis, and could be treated with antioxidants and anti-inflammatory molecules. In addition to the above factors, there are likely other factors that contribute to irreversible testicular damage. Evidence suggests that VC is associated with anti-sperm antibodies (ASAs) (7), spermatogenesis and testosterone secretion abnormalities (8), and cytokine production (9) in the testes. Moreover, inhibition of inflammation can alleviate VC-mediated pathogenesis (10). The normal function of the testis depends on its immune tolerance mechanism (11). The complex yet precise system of testicular immune regulation may be compromised by various infectious or non-infectious diseases.
The exact mechanism by which VC causes infertility remains unknown. It is generally accepted that the pathogenesis of VMI is complex and multifactorial (12, 13). Currently, immunotherapy is rarely applied to VMI. This review explores the mechanisms of VMI from the perspective of reproductive immunology. In the first half of this article, we review the composition of the testicular immune microenvironment under normal physiological conditions, and in the second half, we focus on the potential immunological pathogenesis of VMI, including the role of ASAs, the blood–testis barrier (BTB), cytokines, and inflammatory pathways. Rather than denying the important role of oxidative stress, this review explores the reproductive immune mechanism of VMI from an alternative perspective and provides ideas for future research and potential treatment of VMI.
Testicular Immunity
Mammalian testes create a unique immune environment; that is crucial for testicular function. The testis is a significant immune-sparing site that protects immunogenic germ cells from adverse effects of the immune response (14). Both local immunosuppression and systemic immune responses may be involved in maintaining testicular immunity (15). Pattern recognition receptors (PRRs) play a significant role in the testicular innate immune response. The BTB prevents harmful substances from entering the seminiferous epithelium and prevents sperm antigens from escaping into the seminiferous tubules and causing autoimmune reactions. Moreover, testicular cells secrete immunosuppressive factors and negative regulatory factors considered to be associated with several diverse immune cells (16), including testicular macrophages, lymphocytes, dendritic cells (DCs), and mast cells.
Common Immune Cells
Testicular Macrophages
Macrophages are tissue-specific immune cells that are widely present in various tissues; their functions differ depending on the site. Testicular macrophages are the major immune cells in the testes, accounting for approximately 20% of murine testicular stromal cells (17), which regulate the development of rat Leydig cells and steroidogenesis (18, 19). Specifically, testicular macrophages play a key role in fetal testicular organogenesis (20), testosterone synthesis (21), spermatogenesis (22), and testicular immunosuppression (23). Notably, the inflammatory response of testicular macrophages is lower than that of blood macrophages (24–26). Therefore, testicular macrophages are regarded as testicular guardians (27). In other words, testicular macrophages are characterized by a higher degree of immunosuppressive activity than macrophages from other tissues. Testicular macrophages are mainly polarized into pro-inflammatory (M1) and inhibitory-inflammatory (M2), the latter of which is characterized by high levels of anti-inflammatory cytokines, such as interleukin (IL)-10 and transforming growth factor-beta (TGF-β) (28). Furthermore, testicular macrophages in rats are less responsive to pathogen stimulation; and constitutively produce anti-inflammatory cytokines (29, 30), such as IL-10, which are essential for the prevention of organ-specific autoimmune inflammation (8). In contrast, under the influence of some negative regulatory factors, macrophages polarize to M1 and secrete high levels of pro-inflammatory cytokines, such as IL-1β and TNF-α (31).
Testicular Lymphocytes
Lymphocytes are cells with immune recognition functions. They can be classified as T cells, B cells, and natural killer (NK) cells according to their migration, surface molecules, and function. T cells are vital regulators of cellular and humoral immunity and can be distinguished as CD4+ T cells and CD8+ T cells based on their surface receptors. T cell receptors recognize antigenic proteins released by pathogens. Under normal physiological conditions, CD8+ T cells are the predominant lymphocytes in the testicular interstitial space (32). Regulatory T cells (T-regs) promote peripheral immune tolerance and exhibit immunosuppressive properties in the vasectomy model (33). Murine models of allogeneic islet cell transplantation have also exhibited the immunosuppressive effects of testicular T-regs characterized by CD4+ CD25+ (34, 35). T-regs inhibit the activation of effector T cells under normal physiological conditions (35) and increase T cells counts under inflammatory conditions (36). These observations suggest that T-regs contribute to testicular immunity. Induction of T cell apoptosis is one of the methods by which the testes suppress the immune response, and the Fas/Fas ligand (Fas/FasL) system is highly expressed in the testes (37, 38). Programmed deathligand-1(PDL-1) is significantly expressed in male germ cells. The Fas/FasL and the programmed death-1 (PD-1)/PDL-1 system can induce T cell apoptosis, thereby and protecting against islet allogeneic immune damage (39, 40). Testicular NK cells also have immunoregulatory properties, but there have been only a few studies on these cells. Previous studies have used methods such as flow cytometry to confirm that there are no B cells in the testes under physiologically normal conditions (41).
DCs and Mast Cells
DCs are the most functional, professional antigen-presenting cells in the body and are highly efficient at ingesting, processing, and presenting antigens. DCs occur in the interstitial spaces of the testes and are immature under normal physiological conditions. The number of DCs increases significantly and they express maturation markers in the inflammatory state (42, 43), indicating that DCs play an important role in testicular autoimmunity (44). DCs minimize autoimmune responses by tolerating T cell auto-antigens under normal physiological conditions (45). However, the mechanism underlying their effects in the testes requires further study. According to Banchereau’s study (46), the maturation of testicular immunosuppressive T cells requires the assistance of mast cells.
Mast cells secrete a variety of cytokines and are involved in immune regulation and immediate hypersensitivity (type I allergy). The number of mast cells in the testes is small under normal physiological conditions. However, mast cells are heavily differentiated during inflammatory activation (47). Abnormal spermatogenesis is associated with an increased number of mast cells (48). A randomized controlled trial has demonstrated that Zaditen, a mast cell blocker, improves semen parameters, chromatin integrity, and pregnancy rates after VC surgery (49). Research on mast cells is limited, and the function of mast cells in testicular immunity is not completely understood. However, this review demonstrates, their important role in testicular immune disorders and their effects on sperm quality parameters.
Other Cells Involved in Testicular Immunity
Leydig Cells
In addition to regulating male sex differentiation and fertility by producing testosterone, Leydig cells can also indirectly contribute to the testicular immune microenvironment. Representing the majority of mesenchymal cells, Leydig cells have congenital antiviral infection function in rats (50, 51). Furthermore, testosterone inhibits systemic immune responses to autoantigens (52, 53). Androgen receptor-deficient murine Sertoli cells (SCs) impair immune exemption in the seminiferous tubules, suggesting that testosterone also plays a local role in maintaining testicular immune privilege (54). Leydig cells also play an important role in the innate immune defense of the testes. In microbial invasion, Leydig cells synthesize and release antiviral factors, such as TNF-α and interferon (IFN)- α, or anti-inflammatory factors, such as IL-6 and IL-1β (55, 56).
SCs
As part of the BTB, SCs are crucial for testicular immune privilege. Tight junctions form between adjacent SCs in the testicle; this is the basis of the BTB, blocking contact between systemic immune cells and spermatogenic cells in the stroma, thereby producing immune tolerance (57, 58). Once the tight junctions are destroyed, the BTB is weakened. More seriously, as demonstrated in mice, sperm cell differentiation is altered with significant germ cells loss, suggesting that the BTB/SC barrier effectively prevents humoral immune responses to late germ cells in normal testes (56).
Various cytokines secreted by SCs also form a part of the testicular immune microenvironment. TGF-β is mainly produced by testicular SCs in testis for immune suppression (59). SCs also express large amounts of activin A and B (60). The former is similar in structure to TGF-β, which inhibits the expression of pro-inflammatory cytokines (including IL-1 and IL-6), thereby inhibiting testicular inflammation. Once Toll-like receptors (TLRs) expressed on SCs are activated, a large number of pro-inflammatory factors are released (39). Gas6 is a functional ligand of the Tyro 3 receptor tyrosine kinase (RTK) subfamily, and its tyrosine kinase receptors TAM are constitutively expressed in murine testes (61). It mediates the ability of SCs to phagocytose apoptotic germ cells and plays a key role in testicular immunity (40, 62).
The Immune Mechanism of VC
The current consensus is that VMI is mainly caused by hypoxia and oxidative stress, as clarified in a review by Jensen (12), but some phenomena cannot be fully explained by these factors alone. Numerous studies have suggested that inflammation and immunity play a role as mediators, and any abnormal changes in the immune links of the testes may have a negative impact. Testicular macrophages are involved in the pathogenesis of various inflammatory diseases. Experimental autoimmune orchitis models have shown substantial macrophage infiltration and secretion of numerous inflammatory factors (25, 63). Some researchers have found that the pathological changes in the testes of experimental autoimmune orchitis models are similar to those in VC, both are associated with abnormalities of permeability and structure of the BTB structure (64). Our previous study showed that the inflammasome, NLRP3, is activated in testicular macrophages, producing a large amount of IL-1β and inhibiting the normal synthesis of testosterone (65).
ASAs
ASAs are present in 1-2% of fertile men and 5-15% of men with infertility (66). Sperm immunoglobulin (Ig) levels in the seminal plasma of men with infertility and VC were higher than those in men with infertility but without VC (67, 68). The positive rate of mixed antiglobulin reaction (MAR)-IgG in the seminal plasma of patients with VMI was more than three times that of normal seminal plasma (66). In patients with improved semen quality after surgery, the level of seminal ASAs decreased accordingly (69, 70). Animal experiments also showed similar results; i.e. the ASA of the VC rats model increased (71). The incidence of anti-sperm immune responses is associated with increased chromosomal abnormalities in the gametes. There was a significant positive correlation between the MAR-IgG percentage and sperm DNA fragmentation rate. ASAs can agglutinate sperm and bind to apoptosis-related proteins (e.g., caspase 3 and hsp70), leading to apoptosis in sperm cells (72).
ASAs affect male fertility through different mechanisms, and largely correlates with sperm autoimmunity and ASA antigen specificity (Figure 1A). The main role of the testicular autoimmune response is to reduce sperm movement and agglutination (73). An anti-spermatic immune response is associated with decreased sperm function and sperm quality parameters, such as DNA breaks. One study found that sperm quality in patients with ASA (+) VC is significantly reduced and correlates with the VC grade (66). Simultaneously, sperm damage is more severe in patients with ASA positive VC. The spermatozoa count in grade 2 ASA-positive VCs patients was less than half of that in ASA negative patients, whereas the reactive oxygen species (ROS) level in ASA (+)patients with infertility was 2.8 and 3.5 times higher than that of ASA (-) patients, respectively (5). However, the results of ASA testing in patients with infertility were similar regardless of whether the patient had been diagnosed with VC (2). In other words, VC may be an important cofactor for ASA production. VC increases the likelihood of immune infertility; after testicular trauma, the probability of immune infertility in patients with VC increases by a factor of 2 (66). Therefore, VC may not be the direct cause of ASA production, but VC is a synergistic factor that leads to immune sterility. However, Bozhemov et al. (66) suggested that VC is not a direct cause of the sperm autoimmune response; but a cofactor that increases the risk of ASA production. In VC patients, the odds ratio for immune infertility tripled after testicular trauma.
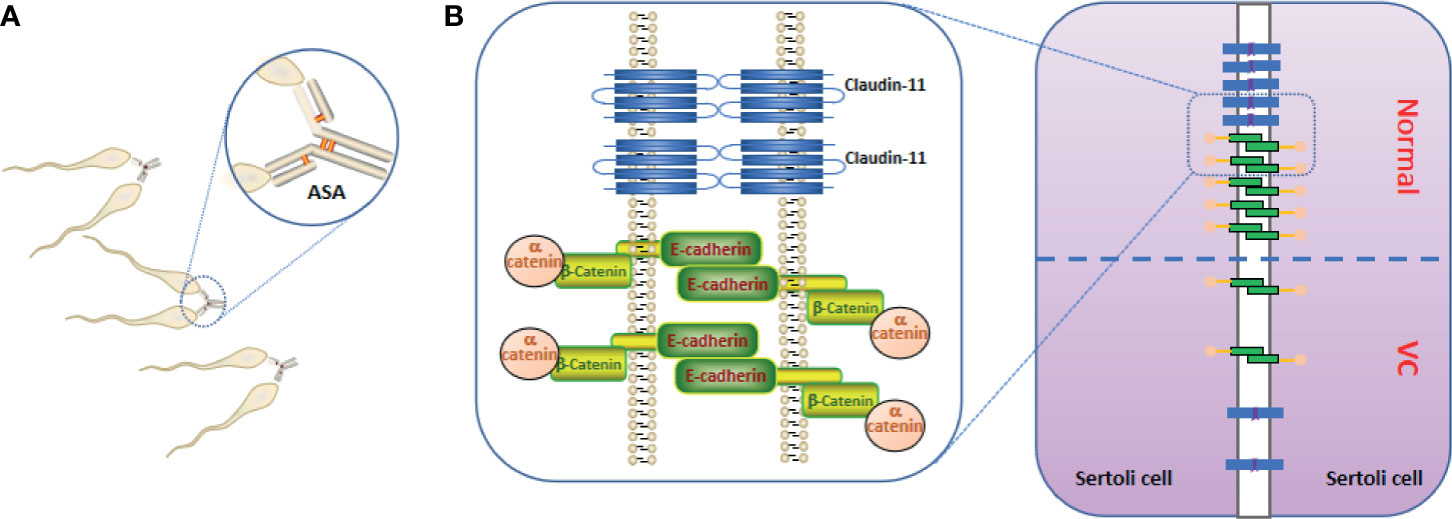
Figure 1 Anti-sperm antibodies (ASAs) and blood testes barrier (BTB). (A) ASAs bind to sperm, resulting in sperm agglutination and sperm motility reducing. (B) the important constituent proteins (claudin-11, E-cadherin, and α-catenin) that constitute the BTB are reduced in the testes of Varicocele (VC), resulting in increased BTB permeability.
Permeability of the BTB
In the VC animal model, Pan et al. (74) and Raĭtsina et al. (64) reported a significant disruption of the BTB, and the latter also found lymphocytes sensitized to spermatozoa antigens in the lymphatic organs of experimental rats. Soares et al. (75) suggested that the receptor for activated C kinase 1 up-regulation, induced by VC, may also be involved in the phosphorylation of focal adhesion kinase, thus affecting the dynamics of the BTB and apical ectoplasmic specialization. Immune factors in VC act on the adherent tight junction molecule of the BTB, increasing the permeability of the BTB. The immune factor subsequently enters the seminiferous tubule to destroy spermatogenic cells (73, 76–79). Simultaneously, sperm antigens may be exposed to the circulating blood. Claudin-11, a tight junction protein expressed by SCs, E-cadherin, and α-catenin have been shown to participate in cell adhesion, and all molecules are involved in the formation of the BTB but the levels in the seminiferous tubules were significantly down-regulated (Figure 1B) (8, 74, 80, 81).
Inflammatory Factors and Pathways
IL
Multiple studies have demonstrated the central role of IL-1α and IL-1β in a number of autoinflammatory diseases (82–85). In the testes, IL-1 regulates spermatogenesis through autocrine and paracrine expression, and overexpression of IL-1 is critical for improving VMI. Studies have shown that IL-1 expression in the testes has increased in VC animal models (86, 87). Kim’s study (88) and Sahin et al. (89) found that the expression of both IL-1α and IL-β increased in the testes of the VC model, which could be reversed by treatment with herbs. However, case-control studies found that, compared with that in the control group, the level of IL-1β in the seminal plasma of patients with VC did not change significantly (9, 55, 90). (Table 1) This contrasts with the increased IL-1 levels typically observed in the testicular tissues of patients with VMI. A potential explanation for this is that the liquid components of the semen originate from multiple sites, including the seminal vesicle gland and prostate, and may dilute the levels of the original substances in the testicles. Therefore, IL-1 is most likely a factor that causes infertility in patients with VC.
IL-6 is produced rapidly and briefly in response to infection and tissue damage, but the persistent dysregulation of IL-6 synthesis plays a pathological role in chronic inflammation and autoimmunity (99). Most studies (8, 55, 88, 91–94) support the view that the level of IL-6 is increased in VC animal models or patients with VMI (Table 1). The concentration of IL-6 in the seminal plasma of patients with VC is much higher than that in fertile men (55, 93, 94), but lower in Finelli’s study (9). However, the study conducted by Finelli et al. included only patients with VC, excluding those with VMI the focus of our review. This may be one of the main reasons why the levels of IL-1β, IL-6, IL-8, TNF-α, and IFN-γ found in that study are inconsistent with those found in other studies. In patients with VMI, it has been reported that levels of IL-6 and ROS are elevated and that total oxidative binding energy is decreased. In vitro, IL-6 reduces sperm motility, possibly attributed to excessive production of nitric oxide (NO) (100). IL-1 and IL-6 have inhibitory effects on the acrosomal reaction similar to those of TNFα (93). In short, IL-6, like IL-1, may be an important immunoregulatory factor affecting fertility in patients with VC.
The number of white blood cells in seminal plasma is significantly correlated with IL-8, also known as C-X-C motif chemokine ligand (CXCL) -8 (55, 73, 101). Compared with those in the control group, the levels of IL-8 in the seminal plasma of patients with VC was found to be higher (55, 95). The mechanism related to IL-6 and IL-8 has not yet been reported. Similarly, IL-17A and IL-18, as well as anti-inflammatory factors IL-10 and IL-37, have also been reported in a series of studies (9, 96–98) (Table 1). In general, the current research on IL-8, IL-10, IL-17A, IL-18 and IL-37 is limited, and it is difficult to draw definite conclusions on the role of ILs in VC. Further investigation on this topic is required, is needed in the future, particularly through high-quality human studies.
TNF-α, IFN-γ, and TGF-β
Multiple studies have reported an increase in TNF-α levels in both patients with VC and in animal models (Table 1). For other two studies (9, 98) did not find significant changes in TNF-α levels because they included patients different from those in the other studies, as described above. TNF-α alters mitochondrial function, increases NO production, and is inversely related to sperm motility. It also increases the production of malondialdehyde and inhibits spontaneous and induced acrosomal reactions (73). The role of pro-apoptotic TNF-α in the pathogenesis of VC-mediated dysfunction is currently under intense study (93). Animal studies demonstrated that IFN-α levels increased in the testes or serum, but human studies did not support this finding, possible reasons have been explained in IL-1. (Table 1) Habibi et al. (91) found that IFN-γ levels in serum and testicular tissue were increased in rat VC models, suggesting that VC has a detrimental, time-dependent effect on cytokine levels and decreases the number of SCs, and spermatogonia, as well as the seminiferous tubules diameter and sperm indices. TGF-β balances pro-inflammatory and anti-inflammatory effects by reducing cell growth of immune precursors and plays a key role in immune tolerance (102). Similar to those of most cytokines, TGF-β levels were also reported to be increased in the testes of VC model (Table 1) (71). Together, these results suggest that TNF-α and IFN-γ are potential markers of testicular damage in patients with VC.
Overall, most of the available data from patients with VC or animal VC models support the observation of elevated levels of pro-inflammatory factors in the testicular tissues and seminal plasma, to varying degrees. In particular, IL-1, IL-6, TNF-α, and IFN-γ may be key immunoregulatory factors for testicular injury in patients with VC. Simultaneously, some anti-inflammatory factors, such as IL-10, IL-37, and TGF-β, tend to be upregulated; this may be the result of the normal feedback mechanism of the body. These anti-inflammatory factors may also be of consequence to future treatment strategies, but their authenticity must first be confirmed by further studies.
Inflammatory Pathways
PRRs can be divided into five categories, including TLRs, located on the cell membrane, and NLRs, located in the cytoplasm (103). They occur mainly on immunocytes such as macrophages, DCs, and rarely non-immunized cells. Thus, once PRRs are activated by pathogen-associated molecular patterns (PAMPs) or danger-associated molecular patterns (DAMPs), the signal will eventually be transmitted to the downstream target gene, which regulates immunity and inflammation (104). PRRs and their specific ligands are listed in detail in a review by Wang et al. (103).
TLR2, one of the TLRs, can also recognize PAMPs and/or DAMPs (105), the latter including heat shock proteins (HSP60, HSP70, Gp96), advanced glycation end-products, high mobility group box 1, and serum amyloid A (103, 106). The average relative expression of TLR2 in the semen of men with infertility and VC was twice as high as that in fertile men, but the difference was not statistically significant (107).
NLRP3 is a subtype of the NLR family, that can be directly triggered by cytoplasmic DAMPS, such as peptides, DNA, and RNA (103). NLRP3 inflammasomes are composed of cytoplasmic sensor molecules, such as pyrin domain-containing protein 3, adaptor proteins (e.g., caspase-recruiting domain, ASC, and apoptosis-associated speck-like proteins), and effector proteins (e.g., pro-caspase-1). NLRP3 and ASC promote the cleavage of pro-caspase-1 and form an active complex, which triggers the cleavage of pro-IL-1β into mature IL-1β (65). The VC model showed a significant increase in NLRP3 gene expression after partial ligation of the left renal vein (108). Interestingly, this trend was reversed by the administration of Resveratrol (3,5,4′-trihydoxy-trans-stilbene), which is an anti-inflammatory, anti-apoptotic compound in some plants (109). We previously found a significant up-regulation of prokineticin 2 (PK2) in the VC animal model (110), whereas in the orchitis model PK2 promoted IL-1β secretion via the NLRP3 pathway (111). We speculate that this process is also involved in the onset of VMI, although this hypothesis has not been validated with rigorous experiments.
Future Perspective
The current treatment approach of VC is mainly surgical, but a certain proportion of patients find it difficult to regain natural fertility even after surgery. Whether normal fertility can be restored in these patients through immunotherapy is worth further exploration. Although the studies reviewed here have reported various abnormalities of immune-related cells or active molecules in the testicular tissue or seminal plasma of patients with VC, little is known about how these factors interact or function. However, there are currently at least three strategies to protect the testicular immune microenvironment in cases of VMI reduction of the production of ASAs and removal of ASAs from the body, reduction of BTB permeability, i.e., reduction of sperm antigen exposure; maintenance of the balance of pro-inflammatory and anti-inflammatory molecules and cells in testes, as well as inhibition of related inflammatory pathways. It should be emphasized that the molecular targets of these three strategies are not clearly defined and need further exploration.
Conclusions
The mechanism by which VC induces male infertility is complex and multi-factorial. However, at its core, it is a disorder of the testicular immune microenvironment caused by various injury factors. Where testicular immunity and inflammation are concerned, it mainly involves the production of ASAs, an increased permeability of the BTB caused by abnormal proteins, the release of a series of inflammatory factors, and the activation of inflammatory pathways. Although some protective molecules, such as anti-inflammatory cytokines, are up-regulated, this is not enough to combat the negative regulatory of damaging factors. Given that immune mechanisms are involved in the pathogenesis of VMI, it is necessary to investigate the use of immunotherapy to improve patient outcomes in the future.
Author Contributions
YF, YS, and HZ designed the work. YF, YS, JX, and ZH collected and analyzed the relevant reports. YF, YS, and JX wrote the paper. KZ and CL provided substantial contributions to improve the content of the article. All authors contributed to the article and approved the submitted version.
Funding
This work was funded by National Key R&D Program of China (Grant numbers: 2018YFC1004300, 2018YFC1004304), National Natural Foundation of China (Grant numbers: 81701539).
Conflict of Interest
The authors declare that the research was conducted in the absence of any commercial or financial relationships that could be construed as a potential conflict of interest.
Publisher’s Note
All claims expressed in this article are solely those of the authors and do not necessarily represent those of their affiliated organizations, or those of the publisher, the editors and the reviewers. Any product that may be evaluated in this article, or claim that may be made by its manufacturer, is not guaranteed or endorsed by the publisher.
References
1. Masson P, Brannigan RE. The Varicocele. Urol Clin North Am (2014) 41(1):129–44. doi: 10.1016/j.ucl.2013.08.001
2. Damsgaard J, Joensen UN, Carlsen E, Erenpreiss J, Jensen MB, Matulevicius V, et al. Varicocele Is Associated With Impaired Semen Quality and Reproductive Hormone Levels: A Study of 7035 Healthy Young Men From Six European Countries. Eur Urol (2016) 70(6):1019–29. doi: 10.1016/j.eururo.2016.06.044
3. Dubin L, Amelar RD. Varicocelectomy: 986 Cases in a Twelve-Year Study. Urology (1977) 10(5):446–9. doi: 10.1016/0090-4295(77)90132-7
4. Baazeem A, Belzile E, Ciampi A, Dohle G, Jarvi K, Salonia A, et al. Varicocele and Male Factor Infertility Treatment: A New Meta-Analysis and Review of the Role of Varicocele Repair. Eur Urol (2011) 60(4):796–808. doi: 10.1016/j.eururo.2011.06.018
5. Eisenberg ML, Lipshultz LI. Varicocele-Induced Infertility: Newer Insights Into Its Pathophysiology. Indian J Urol (2011) 27(1):58–64. doi: 10.4103/0970-1591.78428
6. Hosseini M, Shaygannia E, Rahmani M, Eskandari A, Golsefid AA, Tavalaee M, et al. Endoplasmic Reticulum Stress (ER Stress) and Unfolded Protein Response (UPR) Occur in a Rat Varicocele Testis Model. Oxid Med Cell Longev (2020) 2020:5909306. doi: 10.1155/2020/5909306
7. Tchiokadze S, Galdava G. Humoral Immunity Status If Infertile Men Antisperm Antibodies and Various Pathologies of Reproductive Organs. Georgian Med News (2015) 241):58–62.
8. Oh YS, Jo NH, Park JK, Gye MC. Changes in Inflammatory Cytokines Accompany Deregulation of Claudin-11, Resulting in Inter-Sertoli Tight Junctions in Varicocele Rat Testes. J Urol (2016) 196(4):1303–12. doi: 10.1016/j.juro.2016.05.004
9. Finelli R, Pallotti F, Cargnelutti F, Faja F, Carlini T, Rizzo F, et al. Sperm DNA Damage and Cytokines in Varicocele: A Case-Control Study. Andrologia (2021) 53(5):e14023. doi: 10.1111/and.14023
10. Mazhari S, Razi M, Sadrkhanlou R. Silymarin and Celecoxib Ameliorate Experimental Varicocele-Induced Pathogenesis: Evidences for Oxidative Stress and Inflammation Inhibition. Int Urol Nephrol (2018) 50(6):1039–52. doi: 10.1007/s11255-018-1862-5
11. Iosub R, Klug J, Fijak M, Schneider E, Frohlich S, Blumbach K, et al. Development of Testicular Inflammation in the Rat Involves Activation of Proteinase-Activated Receptor-2. J Pathol (2006) 208(5):686–98. doi: 10.1002/path.1938
12. Jensen CFS, Ostergren P, Dupree JM, Ohl DA, Sonksen J, Fode M. Varicocele and Male Infertility. Nat Rev Urol (2017) 14(9):523–33. doi: 10.1038/nrurol.2017.98
13. Razi M, Tavalaee M, Sarrafzadeh-Rezaei F, Moazamian A, Gharagozloo P, Drevet JR, et al. Varicocoele and Oxidative Stress: New Perspectives From Animal and Human Studies. Andrology (2021) 9(2):546–58. doi: 10.1111/andr.12940
14. Chen Q, Deng T, Han D. Testicular Immunoregulation and Spermatogenesis. Semin Cell Dev Biol (2016) 59:157–65. doi: 10.1016/j.semcdb.2016.01.019
15. Gu X, Li SY, DeFalco T. Immune and Vascular Contributions to Organogenesis of the Testis and Ovary. FEBS J (2021). doi: 10.1111/febs.15848
16. Yu LL, Li CL, Zhang HP. Updated Immunoregulation Mechanism of the Testis. Zhonghua Nan Ke Xue (2013) 19(5):456–9. doi: 10.13263/j.cnki.nja.2013.05.019
17. Hedger MP. Macrophages and the Immune Responsiveness of the Testis. J Reprod Immunol (2002) 57(1-2):19–34. doi: 10.1016/s0165-0378(02)00016-5
18. Hutson JC. Physiologic Interactions Between Macrophages and Leydig Cells. Exp Biol Med (2006) 231(1):1–7. doi: 10.1177/153537020623100101
19. Guo JT, Grow EJ, Mlcochova H, Maher GJ, Lindskog C, Nie XC, et al. The Adult Human Testis Transcriptional Cell Atlas. Cell Res (2018) 28(12):1141–57. doi: 10.1038/s41422-018-0099-2
20. DeFalco T, Bhattacharya I, Williams AV, Sams DM, Capel B. Yolk-Sac-Derived Macrophages Regulate Fetal Testis Vascularization and Morphogenesis. Proc Natl Acad Sci USA (2014) 111(23):E2384–93. doi: 10.1073/pnas.1400057111
21. Smith LB, Walker WH. The Regulation of Spermatogenesis by Androgens. Semin Cell Dev Biol (2014) 30:2–13. doi: 10.1016/j.semcdb.2014.02.012
22. Oatley JM, Brinster RL. Regulation of Spermatogonial Stem Cell Self-Renewal in Mammals. Annu Rev Cell Dev Biol (2008) 24:263–86. doi: 10.1146/annurev.cellbio.24.110707.175355
23. Mossadegh-Keller N, Gentek R, Gimenez G, Bigot S, Mailfert S, Sieweke MH. Developmental Origin and Maintenance of Distinct Testicular Macrophage Populations. J Exp Med (2017) 214(10):2829–41. doi: 10.1084/jem.20170829
24. Kern S, Robertson SA, Mau VJ, Maddocks S. Cytokine Secretion by Macrophages in the Rat Testis. Biol Reprod (1995) 53(6):1407–16. doi: 10.1095/biolreprod53.6.1407
25. Rival C, Theas MS, Suescun MO, Jacobo P, Guazzone V, van Rooijen N, et al. Functional and Phenotypic Characteristics of Testicular Macrophages in Experimental Autoimmune Orchitis. J Pathol (2008) 215(2):108–17. doi: 10.1002/path.2328
26. Theas MS, Rival C, Jarazo-Dietrich S, Jacobo P, Guazzone VA, Lustig L. Tumour Necrosis Factor-Alpha Released by Testicular Macrophages Induces Apoptosis of Germ Cells in Autoimmune Orchitis. Hum Reprod (2008) 23(8):1865–72. doi: 10.1093/humrep/den240
27. Mossadegh-Keller N, Sieweke MH. Testicular Macrophages: Guardians of Fertility. Cell Immunol (2018) 330:120–5. doi: 10.1016/j.cellimm.2018.03.009
28. Xue J, Schmidt SV, Sander J, Draffehn A, Krebs W, Quester I, et al. Transcriptome-Based Network Analysis Reveals a Spectrum Model of Human Macrophage Activation. Immunity (2014) 40(2):274–88. doi: 10.1016/j.immuni.2014.01.006
29. Bhushan S, Hossain H, Lu Y, Geisler A, Tchatalbachev S, Mikulski Z, et al. Uropathogenic E. Coli Induce Different Immune Response in Testicular and Peritoneal Macrophages: Implications for Testicular Immune Privilege. PLoS One (2011) 6(12):e28452. doi: 10.1371/journal.pone.0028452
30. Winnall WR, Muir JA, Hedger MP. Rat Resident Testicular Macrophages Have an Alternatively Activated Phenotype and Constitutively Produce Interleukin-10 In Vitro. J Leukoc Biol (2011) 90(1):133–43. doi: 10.1189/jlb.1010557
31. O'Bryan MK, Gerdprasert O, Nikolic-Paterson DJ, Meinhardt A, Muir JA, Foulds LM, et al. Cytokine Profiles in the Testes of Rats Treated With Lipopolysaccharide Reveal Localized Suppression of Inflammatory Responses. Am J Physiol Regul Integr Comp Physiol (2005) 288(6):R1744–55. doi: 10.1152/ajpregu.00651.2004
32. Hedger MP, Meinhardt A. Local Regulation of T Cell Numbers and Lymphocyte-Inhibiting Activity in the Interstitial Tissue of the Adult Rat Testis. J Reprod Immunol (2000) 48(2):69–80. doi: 10.1016/s0165-0378(00)00071-1
33. Wheeler K, Tardif S, Rival C, Luu B, Bui E, Del Rio R, et al. Regulatory T Cells Control Tolerogenic Versus Autoimmune Response to Sperm in Vasectomy. Proc Natl Acad Sci USA (2011) 108(18):7511–6. doi: 10.1073/pnas.1017615108
34. Dai Z, Nasr IW, Reel M, Deng S, Diggs L, Larsen CP, et al. Impaired Recall of CD8 Memory T Cells in Immunologically Privileged Tissue. J Immunol (2005) 174(3):1165–70. doi: 10.4049/jimmunol.174.3.1165
35. Nasr IW, Wang Y, Gao G, Deng S, Diggs L, Rothstein DM, et al. Testicular Immune Privilege Promotes Transplantation Tolerance by Altering the Balance Between Memory and Regulatory T Cells. J Immunol (2005) 174(10):6161–8. doi: 10.4049/jimmunol.174.10.6161
36. Jacobo P, Guazzone VA, Jarazo-Dietrich S, Theas MS, Lustig L. Differential Changes in CD4+ and CD8+ Effector and Regulatory T Lymphocyte Subsets in the Testis of Rats Undergoing Autoimmune Orchitis. J Reprod Immunol (2009) 81(1):44–54. doi: 10.1016/j.jri.2009.04.005
37. Sun B, Qi N, Shang T, Wu H, Deng TT, Han DS. Sertoli Cell-Initiated Testicular Innate Immune Response Through Toll-Like Receptor-3 Activation Is Negatively Regulated by Tyro3, Axl, and Mer Receptors. Endocrinology (2010) 151(6):2886–97. doi: 10.1210/en.2009-1498
38. Zhang XY, Wang T, Deng TT, Xiong WP, Lui P, Li N, et al. Damaged Spermatogenic Cells Induce Inflammatory Gene Expression in Mouse Sertoli Cells Through the Activation of Toll-Like Receptors 2 and 4. Mol Cell Endocrinol (2013) 365(2):162–73. doi: 10.1016/j.mce.2012.10.016
39. Shang T, Zhang XY, Wang T, Sun B, Deng TT, Han DS. Toll-Like Receptor-Initiated Testicular Innate Immune Responses in Mouse Leydig Cells. Endocrinology (2011) 152(7):2827–36. doi: 10.1210/en.2011-0031
40. Zhang Y, Li N, Chen QY, Yan KQ, Liu ZH, Zhang XY, et al. Breakdown of Immune Homeostasis in the Testis of Mice Lacking Tyro3, Axl and Mer Receptor Tyrosine Kinases. Immunol Cell Biol (2013) 91(6):416–26. doi: 10.1038/icb.2013.22
41. Zhao S, Zhu W, Xue S, Han D. Testicular Defense Systems: Immune Privilege and Innate Immunity. Cell Mol Immunol (2014) 11(5):428–37. doi: 10.1038/cmi.2014.38
42. Keir ME, Butte MJ, Freeman GJ, Sharpe AH. PD-1 and Its Ligands in Tolerance and Immunity. Annu Rev Immunol (2008) 26:677–704. doi: 10.1146/annurev.immunol.26.021607.090331
43. Cheng XY, Dai HH, Wan N, Moore Y, Vankayalapati R, Dai ZH. Interaction of Programmed Death-1 and Programmed Death-1 Ligand-1 Contributes to Testicular Immune Privilege. Transplantation (2009) 87(12):1778–86. doi: 10.1097/TP.0b013e3181a75633
44. Ju ST, Panka DJ, Cui H, Ettinger R, el-Khatib M, Sherr DH, et al. Fas(CD95)/FasL Interactions Required for Programmed Cell Death After T-Cell Activation. Nature (1995) 373(6513):444–8. doi: 10.1038/373444a0
45. Suda T, Takahashi T, Golstein P, Nagata S. Molecular Cloning and Expression of the Fas Ligand, a Novel Member of the Tumor Necrosis Factor Family. Cell (1993) 75(6):1169–78. doi: 10.1016/0092-8674(93)90326-l
46. Banchereau J, Steinman RM. Dendritic Cells and the Control of Immunity. Nature (1998) 392(6673):245–52. doi: 10.1038/32588
47. Rival C, Lustig L, Iosub R, Guazzone VA, Schneider E, Meinhardt A, et al. Identification of a Dendritic Cell Population in Normal Testis and in Chronically Inflamed Testis of Rats With Autoimmune Orchitis. Cell Tissue Res (2006) 324(2):311–8. doi: 10.1007/s00441-005-0129-5
48. Hussein MR, Abou-Deif ES, Bedaiwy MA, Said TM, Mustafa MG, Nada E, et al. Phenotypic Characterization of the Immune and Mast Cell Infiltrates in the Human Testis Shows Normal and Abnormal Spermatogenesis. Fertil Steril (2005) 83(5):1447–53. doi: 10.1016/j.fertnstert.2004.11.062
49. Azadi L, Abbasi H, Deemeh MR, Tavalaee M, Arbabian M, Pilevarian AA, et al. Zaditen (Ketotifen), as Mast Cell Blocker, Improves Sperm Quality, Chromatin Integrity and Pregnancy Rate After Varicocelectomy. Int J Androl (2011) 34(5 Pt 1):446–52. doi: 10.1111/j.1365-2605.2010.01112.x
50. Le Tortorec A, Denis H, Satie AP, Patard JJ, Ruffault A, Jegou B, et al. Antiviral Responses of Human Leydig Cells to Mumps Virus Infection or Poly I:C Stimulation. Hum Reprod (2008) 23(9):2095–103. doi: 10.1093/humrep/den207
51. Guazzone VA, Hollwegs S, Mardirosian M, Jacobo P, Hackstein H, Wygrecka M, et al. Characterization of Dendritic Cells in Testicular Draining Lymph Nodes in a Rat Model of Experimental Autoimmune Orchitis. Int J Androl (2011) 34(3):276–89. doi: 10.1111/j.1365-2605.2010.01082.x
52. Dulin JN, Moore ML, Gates KW, Queen JH, Grill RJ. Spinal Cord Injury Causes Sustained Disruption of the Blood-Testis Barrier in the Rat. PLoS One (2011) 6(1):e16456. doi: 10.1371/journal.pone.0016456
53. Cui D, Han GW, Shang YG, Liu CC, Xia LB, Li LK, et al. Antisperm Antibodies in Infertile Men and Their Effect on Semen Parameters: A Systematic Review and Meta-Analysis. Clin Chim Acta (2015) 444:29–36. doi: 10.1016/j.cca.2015.01.033
54. La Vignera S, Condorelli RA, Vicari E, Tumino D, Morgia G, Favilla V, et al. Markers of Semen Inflammation: Supplementary Semen Analysis? J Reprod Immunol (2013) 100(1):2–10. doi: 10.1016/j.jri.2013.05.001
55. Moretti E, Cosci I, Spreafico A, Serchi T, Cuppone AM, Collodel G. Semen Characteristics and Inflammatory Mediators in Infertile Men With Different Clinical Diagnoses. Int J Androl (2009) 32(6):637–46. doi: 10.1111/j.1365-2605.2008.00911.x
56. Meng J, Greenlee AR, Taub CJ, Braun RE. Sertoli Cell-Specific Deletion of the Androgen Receptor Compromises Testicular Immune Privilege in Mice. Biol Reprod (2011) 85(2):254–60. doi: 10.1095/biolreprod.110.090621
57. Lu LF, Lind EF, Gondek DC, Bennett KA, Gleeson MW, Pino-Lagos K, et al. Mast Cells Are Essential Intermediaries in Regulatory T-Cell Tolerance. Nature (2006) 442(7106):997–1002. doi: 10.1038/nature05010
58. Melaine N, Lienard MO, Guillaume E, Ruffault A, Dejucq-Rainsford N, Jegou B. Production of the Antiviral Proteins 2'5'oligoadenylate Synthetase, PKR and Mx in Interstitial Cells and Spermatogonia. J Reprod Immunol (2003) 59(1):53–60. doi: 10.1016/s0165-0378(02)00061-x
59. Gartner R, Schopohl D, Schaefer S, Dugrillon A, Erdmann A, Toda S, et al. Regulation of Transforming Growth Factor Beta(1) Messenger Ribonucleic Acid Expression in Porcine Thyroid Follicles In Vitro by Growth Factors, Iodine, or Delta-Iodolactone. Thyroid (1997) 7(4):633–40. doi: 10.1089/thy.1997.7.633
60. Buzzard JJ, Loveland KL, O'Bryan MK, O'Connor AE, Bakker M, Hayashi T, et al. Changes in Circulating and Testicular Levels of Inhibin A and B and Activin A During Postnatal Development in the Rat. Endocrinology (2004) 145(7):3532–41. doi: 10.1210/en.2003-1036
61. Rothlin CV, Ghosh S, Zuniga EI, Oldstone MBA, Lemke G. TAM Receptors Are Pleiotropic Inhibitors of the Innate Immune Response. Cell (2007) 131(6):1124–36. doi: 10.1016/j.cell.2007.10.034
62. Chen YM, Wang HZ, Qi N, Wu H, Xiong WP, Ma J, et al. Functions of TAM RTKs in Regulating Spermatogenesis and Male Fertility in Mice. Reproduction (2009) 138(4):655–66. doi: 10.1530/Rep-09-0101
63. Gerdprasert O, O'Bryan MK, Muir JA, Caldwell AM, Schlatt S, de Kretser DM, et al. The Response of Testicular Leukocytes to Lipopolysaccharide-Induced Inflammation: Further Evidence for Heterogeneity of the Testicular Macrophage Population. Cell Tissue Res (2002) 308(2):277–85. doi: 10.1007/s00441-002-0547-6
64. Raitsina SS, Kurnosov AV, Iarovaia IM, Gladkova NS, Davydova AI. Autoimmune Nature of Spermatogenesis Disorders in Varicocele Models in Rats. Biull Eksp Biol Med (1979) 87(1):44–8. doi: 10.1007/BF00802352
65. Su Y, Zhang Y, Hu Z, He L, Wang W, Xu J, et al. Prokineticin 2 via Calcium-Sensing Receptor Activated NLRP3 Inflammasome Pathway in the Testicular Macrophages of Uropathogenic Escherichia Coli-Induced Orchitis. Front Immunol (2020) 11:570872. doi: 10.3389/fimmu.2020.570872
66. Bozhedomov VA, Lipatova NA, Rokhlikov IM, Alexeev RA, Ushakova IV, Sukhikh GT. Male Fertility and Varicocoele: Role of Immune Factors. Andrology (2014) 2(1):51–8. doi: 10.1111/j.2047-2927.2013.00160.x
67. Bozhedomov VA, Teodorovich OV. Epidemiology and Causes of Autoimmune Male Infertility. Urologiia (2005) 1(1):35–44.
68. Solis EA, Gatti VN, Brufman AS, Bouvet BR, Conforti CB, Papparella CV, et al. Immunology and Deterioration of Seminal Parameters in Varicocele. Arch Esp Urol (2001) 54(8):797–800.
69. Djaladat H, Mehrsai A, Rezazade M, Djaladat Y, Pourmand G. Varicocele and Antisperm Antibody: Fact or Fiction? South Med J (2006) 99(1):44–7. doi: 10.1097/01.smj.0000197036.08282.70
70. Bozhedomov VA, Lipatova NA, Alexeev RA, Alexandrova LM, Nikolaeva MA, Sukhikh GT. The Role of the Antisperm Antibodies in Male Infertility Assessment After Microsurgical Varicocelectomy. Andrology (2014) 2(6):847–55. doi: 10.1111/j.2047-2927.2014.00254.x
71. Zhang L, Zhao X, Wang F, Lin Q, Wang W. Effects of Morinda Officinalis Polysaccharide on Experimental Varicocele Rats. Evid Based Complement Alternat Med (2016) 2016:5365291. doi: 10.1155/2016/5365291
72. Bohring C, Krause E, Habermann B, Krause W. Isolation and Identification of Sperm Membrane Antigens Recognized by Antisperm Antibodies, and Their Possible Role in Immunological Infertility Disease. Mol Hum Reprod (2001) 7(2):113–8. doi: 10.1093/molehr/7.2.113
73. Zhang H, Yin Y, Wang G, Liu Z, Liu L, Sun F. Interleukin-6 Disrupts Blood-Testis Barrier Through Inhibiting Protein Degradation or Activating Phosphorylated ERK in Sertoli Cells. Sci Rep (2014) 4:4260. doi: 10.1038/srep04260
74. Pan J, Zhu Z, Xu G, Niu L, Yu L, Luo Z, et al. Expression of Claudin11 in a Rat Model of Varicocele and Its Effects on the Bloodtestis Barrier. Mol Med Rep (2018) 18(6):5647–51. doi: 10.3892/mmr.2018.9603
75. Soares TS, Fernandes SAF, Lima ML, Stumpp T, Schoorlemmer GH, Lazari MFM, et al. Experimental Varicocoele in Rats Affects Mechanisms That Control Expression and Function of the Androgen Receptor. Andrology (2013) 1(5):670–81. doi: 10.1111/j.2047-2927.2013.00103.x
76. Aljabari B, Calogero AE, Perdichizzi A, Vicari E, Karaki R, Lahloub T, et al. Imbalance in Seminal Fluid MIF Indicates Male Infertility. Mol Med (2007) 13(3-4):199–202. doi: 10.2119/2006-00114.Aljabari
77. Bian SL, Jin HB, Wang SZ, Zhang HF, Gu CR, Zhang W, et al. Effects of Interferon-Gamma and Tumor Necrosis Factor-Alpha on the Fertilizing Capacity of Human Sperm and Their Mechanisms. Zhonghua Nan Ke Xue (2007) 13(8):681–4. doi: 10.13263/j.cnki.nja.2007.08.003
78. Carli C, Leclerc P, Metz CN, Akoum A. Direct Effect of Macrophage Migration Inhibitory Factor on Sperm Function: Possible Involvement in Endometriosis-Associated Infertility. Fertil Steril (2007) 88(4 Suppl):1240–7. doi: 10.1016/j.fertnstert.2007.04.002
79. Sarkar O, Mathur PP, Cheng CY, Mruk DD. Interleukin 1 Alpha (IL1A) Is a Novel Regulator of the Blood-Testis Barrier in the Rat. Biol Reprod (2008) 78(3):445–54. doi: 10.1095/biolreprod.107.064501
80. Ha HK, Park HJ, Park NC. Expression of E-Cadherin and Alpha-Catenin in a Varicocele-Induced Infertility Rat Model. Asian J Androl (2011) 13(3):470–5. doi: 10.1038/aja.2010.94
81. Koksal IT, Ishak Y, Usta M, Danisman A, Guntekin E, Bassorgun IC, et al. Varicocele-Induced Testicular Dysfunction may be Associated With Disruption of Blood-Testis Barrier. Arch Androl (2007) 53(1):43–8. doi: 10.1080/01485010600822606
82. Martinon F, Mayor A, Tschopp J. The Inflammasomes: Guardians of the Body. Annu Rev Immunol (2009) 27:229–65. doi: 10.1146/annurev.immunol.021908.132715
83. Farasat S, Aksentijevich I, Toro JR. Autoinflammatory Diseases: Clinical and Genetic Advances. Arch Dermatol (2008) 144(3):392–402. doi: 10.1001/archderm.144.3.392
84. Dinarello CA. Biologic Basis for Interleukin-1 in Disease. Blood (1996) 87(6):2095–147. doi: 10.1182/blood.V87.6.2095.bloodjournal8762095
85. Weber A, Wasiliew P, Kracht M. Interleukin-1 (IL-1) Pathway. Sci Signal (2010) 3(105):cm1. doi: 10.1126/scisignal.3105cm1
86. Chen LP, Wang SQ, Zhao YZ, Liu L, Wang SX, Lu YZ. The Changes of IL-1 and NO Levels in the Testes of Rats With Experimental Varicocele. Zhonghua Nan Ke Xue (2002) 8(2):125–6. doi: 10.13263/j.cnki.nja.2002.02.016
87. Xu F, Chen Y, Chen H, Xu ZP, Han YF, Yu W, et al. Impact of Varicocele and Varicocelectomy on the Apoptosis of Spermatogenic Cells and the Levels of Nitrogen Monoxidum and Interleukin 1 in the Rat Testis. Zhonghua Nan Ke Xue (2016) 22(3):200–4. doi: 10.13263/j.cnki.nja.2016.03.002
88. Kim SJ, Jeon SH, Kwon EB, Jeong HC, Choi SW, Bae WJ, et al. Early and Synergistic Recovery Effect of Herbal Combination on Surgically Corrected Varicocele. Altern Ther Health Med (2020) 26(3):24–31.
89. Sahin Z, Celik-Ozenci C, Akkoyunlu G, Korgun ET, Acar N, Erdogru T, et al. Increased Expression of Interieukin-1 Alpha and Interleukin-1 Beta Is Associated With Experimental Varicocele. Fertil Steril (2006) 85:1265–75. doi: 10.1016/j.fertnstert.2005.10.025
90. Papadimas J, Goulis DG, Sotiriades A, Daniilidis M, Fleva A, Bontis JN, et al. Interleukin-1 Beta and Tumor Necrosis Factor-Alpha in Normal/Infertile Men. Arch Androl (2002) 48(2):107–13. doi: 10.1080/014850102317267418
91. Habibi B, Seifi B, Mougahi SM, Ojaghi M, Sadeghipour HR. Increases in Interleukin-6 and Interferon-Gamma Levels Is Progressive in Immature Rats With Varicocele. Ir J Med Sci (2015) 184(2):531–7. doi: 10.1007/s11845-014-1167-3
92. Hassani-Bafrani H, Najaran H, Razi M, Rashtbari H. Berberine Ameliorates Experimental Varicocele-Induced Damages at Testis and Sperm Levels; Evidences for Oxidative Stress and Inflammation. Andrologia (2019) 51(2):e13179. doi: 10.1111/and.13179
93. Nallella KP, Allamaneni SS, Pasqualotto FF, Sharma RK, Thomas AJ Jr., Agarwal A. Relationship of Interleukin-6 With Semen Characteristics and Oxidative Stress in Patients With Varicocele. Urology (2004) 64(5):1010–3. doi: 10.1016/j.urology.2004.05.045
94. Micheli L, Collodel G, Cerretani D, Menchiari A, Noto D, Signorini C, et al. Relationships Between Ghrelin and Obestatin With MDA, Proinflammatory Cytokines, GSH/GSSG Ratio, Catalase Activity, and Semen Parameters in Infertile Patients With Leukocytospermia and Varicocele. Oxid Med Cell Longev (2019) 2019:7261842. doi: 10.1155/2019/7261842
95. Lotti F, Corona G, Mancini M, Biagini C, Colpi GM, Innocenti SD, et al. The Association Between Varicocele, Premature Ejaculation and Prostatitis Symptoms: Possible Mechanisms. J Sex Med (2009) 6(10):2878–87. doi: 10.1111/j.1743-6109.2009.01417.x
96. Matalliotakis IM, Cakmak H, Fragouli Y, Kourtis A, Arici A, Huszar G. Increased IL-18 Levels in Seminal Plasma of Infertile Men With Genital Tract Infections. Am J Reprod Immunol (2006) 55(6):428–33. doi: 10.1111/j.1600-0897.2006.00380.x
97. Zeinali M, Hadian Amree A, Khorramdelazad H, Karami H, Abedinzadeh M. Inflammatory and Anti-Inflammatory Cytokines in the Seminal Plasma of Infertile Men Suffering From Varicocele. Andrologia (2017) 49(6):e12685. doi: 10.1111/and.12685
98. Wang H, Lv Y, Hu K, Feng T, Jin Y, Wang Y, et al. Seminal Plasma Leptin and Spermatozoon Apoptosis in Patients With Varicocele and Leucocytospermia. Andrologia (2015) 47(6):655–61. doi: 10.1111/and.12313
99. Tanaka T, Narazaki M, Kishimoto T. IL-6 in Inflammation, Immunity, and Disease. Cold Spring Harb Perspect Biol (2014) 6(10):a016295. doi: 10.1101/cshperspect.a016295
100. Sakamoto Y, Ishikawa T, Kondo Y, Yamaguchi K, Fujisawa M. The Assessment of Oxidative Stress in Infertile Patients With Varicocele. BJU Int (2008) 101(12):1547–52. doi: 10.1111/j.1464-410X.2008.07517.x
101. Bezzerri V, Borgatti M, Finotti A, Tamanini A, Gambari R, Cabrini G. Mapping the Transcriptional Machinery of the IL-8 Gene in Human Bronchial Epithelial Cells. J Immunol (2011) 187(11):6069–81. doi: 10.4049/jimmunol.1100821
102. Akdis M, Aab A, Altunbulakli C, Azkur K, Costa RA, Crameri R, et al. Interleukins (From IL-1 to IL-38), Interferons, Transforming Growth Factor Beta, and TNF-Alpha: Receptors, Functions, and Roles in Diseases. J Allergy Clin Immunol (2016) 138(4):984–1010. doi: 10.1016/j.jaci.2016.06.033
103. Wang X, Antony V, Wang Y, Wu G, Liang G. Pattern Recognition Receptor-Mediated Inflammation in Diabetic Vascular Complications. Med Res Rev (2020) 40(6):2466–84. doi: 10.1002/med.21711
104. Takeuchi O, Akira S. Pattern Recognition Receptors and Inflammation. Cell (2010) 140(6):805–20. doi: 10.1016/j.cell.2010.01.022
105. Guo M, Suo Y, Gao Q, Du H, Zeng W, Wang Y, et al. The Protective Mechanism of Ginkgolides and Ginkgo Flavonoids on the TNF-Alpha Induced Apoptosis of Rat Hippocampal Neurons and Its Mechanisms In Vitro. Heliyon (2015) 1(1):e00020. doi: 10.1016/j.heliyon.2015.e00020
106. Lin M, Tang SC. Toll-Like Receptors: Sensing and Reacting to Diabetic Injury in the Kidney. Nephrol Dial Transplant (2014) 29(4):746–54. doi: 10.1093/ndt/gft446
107. Ghandehari-Alavijeh R, Tavalaee M, Zohrabi D, Foroozan-Broojeni S, Abbasi H, Nasr-Esfahani MH. Hypoxia Pathway has More Impact Than Inflammation Pathway on Etiology of Infertile Men With Varicocele. Andrologia (2019) 51(2):e13189. doi: 10.1111/and.13189
108. Baazm M, Ghafarizadeh AA, Noshad Kamran AR, Beyer C, Zendedel A. Presence of The NLRP3 Inflammasome Components in Semen of Varicocele Patients. Int J Fertil Steril (2020) 14(1):46–50. doi: 10.22074/ijfs.2020.5734
109. Hajipour E, Mashayekhi FJ, Mosayebi G, Baazm M, Zendedel A. Resveratrol Decreases Apoptosis and NLRP3 Complex Expressions in Experimental Varicocele Rat Model. Iran J Basic Med Sci (2018) 21(2):225–9. doi: 10.22038/IJBMS.2018.21943.5625
110. Li Y, Zhou T, Su YF, Hu ZY, Wei JJ, Wang W, et al. Prokineticin 2 Overexpression Induces Spermatocyte Apoptosis in Varicocele in Rats. Asian J Androl (2020) 22(5):500–6. doi: 10.4103/aja.aja_109_19
Keywords: varicocele, male infertility, sperm, testicular immunity, inflammation
Citation: Fang Y, Su Y, Xu J, Hu Z, Zhao K, Liu C and Zhang H (2021) Varicocele-Mediated Male Infertility: From the Perspective of Testicular Immunity and Inflammation. Front. Immunol. 12:729539. doi: 10.3389/fimmu.2021.729539
Received: 23 June 2021; Accepted: 16 August 2021;
Published: 31 August 2021.
Edited by:
Remo Castro Russo, Federal University of Minas Gerais, BrazilReviewed by:
Paulo Henrique Campos-Junior, Universidade Federal de São João del-Rei, BrazilDavid Diamond, Boston Children’s Hospital, United States
Mohammad Hossein Nasr-Esfahani, Royan Institute, Iran
Copyright © 2021 Fang, Su, Xu, Hu, Zhao, Liu and Zhang. This is an open-access article distributed under the terms of the Creative Commons Attribution License (CC BY). The use, distribution or reproduction in other forums is permitted, provided the original author(s) and the copyright owner(s) are credited and that the original publication in this journal is cited, in accordance with accepted academic practice. No use, distribution or reproduction is permitted which does not comply with these terms.
*Correspondence: Huiping Zhang, emhwbWVkQDEyNi5jb20=
†These authors have contributed equally to this work