- 1Department of Hematology, Hokkaido University Faculty of Medicine, Sapporo, Japan
- 2Clinical Research Division, Fred Hutchinson Cancer Research Center, Seattle, WA, United States
- 3Division of Medical Oncology, The University of Washington, Seattle, WA, United States
Allogeneic hematopoietic cell transplantation (HCT) is a curative treatment for hematologic malignancies, bone marrow failure syndromes, and inherited immunodeficiencies and metabolic diseases. Graft-versus-host disease (GVHD) is the major life-threatening complication after allogeneic HCT. New insights into the pathophysiology of GVHD garnered from our understanding of the immunological pathways within animal models have been pivotal in driving new therapeutic paradigms in the clinic. Successful clinical translations include histocompatibility matching, GVHD prophylaxis using cyclosporine and methotrexate, posttransplant cyclophosphamide, and the use of broad kinase inhibitors that inhibit cytokine signaling (e.g. ruxolitinib). New approaches focus on naïve T cell depletion, targeted cytokine modulation and the inhibition of co-stimulation. This review highlights the use of animal transplantation models to guide new therapeutic principles.
Early History
In 1956, two groups observed that mice exposed to lethal dose of total body irradiation (TBI) and administered allogeneic splenocytes survived for a shorter time than those transplanted with syngeneic splenocytes (1–3). The recipients of allogeneic cells exhibited diarrhea, weight loss, skin lesions, and died (1, 4). This syndrome was initially designated as “secondary disease”, which was later renamed as graft-versus-host disease (GVHD). In 1963, Mathé and colleagues reported the first case of a human allogeneic bone marrow transplantation (BMT) recipient that survived beyond a year. This patient had complete engraftment and the development of a lethal “secondary disease” was described (5). Subsequently, the clinical and pathological characteristics of GVHD was described (6). The outcomes for the initial 200 patients transplanted prior to 1967 were disparaging; all patients died of either graft failure, GVHD, infection, or leukemia relapse (7). These poor outcomes reflected a limited understanding of histocompatibility matching and the requirement for immune suppression after BMT to control GVHD (8).
Although many investigators lost their enthusiasm for BMT, several groups increasingly utilized animal models to gain a better understanding of the allogeneic barrier with regard to both GVHD and graft rejection. Studies of allogeneic BMT in Seattle using dog models in the 1980s provided the scientific groundwork for the field leading to the concepts of histocompatibility matching, conditioning regimens and pharmacological GVHD prophylaxis (9–13). These findings were soon translated to the clinic and successful clinical BMT was established (14), subsequently leading to E. Donnall Thomas being awarded the Nobel prize within Physiology or Medicine in 1990 (see Figure 1 for a timeline).
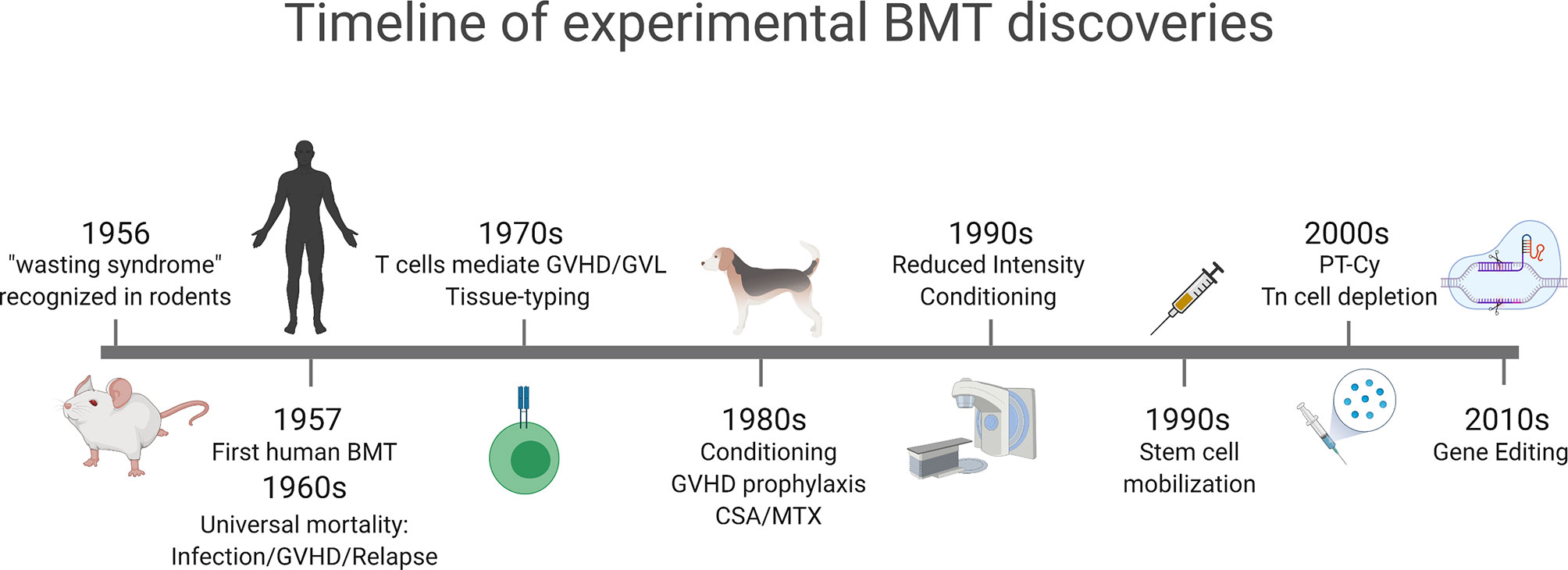
Figure 1 Timeline of major experimental concepts that have translated into clinical practice. GVHD was initially described as a wasting syndrome in transplanted mice in 1956. Early clinical bone marrow transplantation was associated with high mortality due to GVHD, infection and relapse. Recognition of T cells as the mediators of GVHD and GVL and initiating rudimentary tissue-typing. Conditioning and GVHD prophylaxis regimens were developed in dog models in the 1980s that led to reduced intensity and non-myeloablative conditioning in the 1990s. Stem cell mobilization following cytokine administration was developed in the early 1990s and gained widespread clinical translation. New approaches to GVHD prophylaxis, including post-transplant cyclophosphamide (PT-Cy) and naïve T (Tn) cell depletion developed in the 2000s and are increasingly utilized in the clinic. In the last decade the widespread use of gene editing, initially in T cells, has been widely translated to modulate GVHD and GVL. Figure generated with biorender.com.
For a detailed discussion of the various mouse models of GVHD currently available and the penetrance of disease therein, we refer the reader to some of the excellent reviews on this subject (15–17).
Acute GVHD
Donor T Cells
In the 1970’s and 1980’s, Korngold and Sprent performed extensive studies in a series of mouse models across MHC and/or minor histocompatibility antigen mismatches demonstrating the role of donor T cells in the etiology of GVHD. They showed that donor-derived T cells were causative of GVHD and identified T cell subsets (CD4 versus CD8) responsible for the induction of GVHD in each model (18–20). Following the observation that T cell depletion prevented GVHD in mice (18), clinical studies confirmed that GVHD also failed to develop following rigorous donor T cell depletion with CD34 positive selection of the donor inoculum and the administration of anti-thymocyte globulin (ATG); none developed GVHD even without posttransplant immunosuppression (21, 22).
Donor T cells exert graft-versus-leukemia (GVL) effects. In 1956, Barnes et al. reported that leukemia-bearing mice receiving allogeneic cells eventually died of GVHD without evidence of leukemia (2). Mathe et al. proposed a concept of GVL effect (23), which was soon demonstrated clinically (24–27). Importantly, pan T-cell depletion was shown to reduce GVHD at the expense of an increased risk of opportunistic infection and leukemia relapse (28). Shlomchik and colleagues refined our understanding of the subsets of mature T cells responsible for GVHD, demonstrating that naïve T cells rather than memory T cells played the major role in inducing GVHD in mice (29, 30). Early clinical trial data of naïve T cell-depleted PBSCT has shown promising results (31), but definitive randomized data is needed to confirm a role of naïve versus memory T cells in GVHD and GVL.
The predominant expansion of Th1/Tc1 and Th17/Tc17 cells in mice and the cytokines derived from these cells suggests that acute GVHD is primarily driven by Th1/Tc1- and Th17/Tc17-associated immune reactions (32–35). There is a crosstalk between GVHD and infection; GVHD-associated immunodeficiency, dysbiosis, and disruption of epithelial and mucosal barrier are risks for infections, while bacterial and viral infections are risks for GVHD by activating innate immunity (36). Neutrophils activated by translocation of intestinal bacteria can also accelerate GVHD early after BMT via tissue injury (34). Mechanistically, bacteria and virus-derived molecules behave as pathogen-associated molecular patterns (PAMPs) that accelerate allogeneic T cell responses. Candida colonization is a risk for acute GVHD and fluconazole prophylaxis is associated with reduction of severe acute GVHD (37, 38). In mouse models, fungal cell wall components such as sugar polymers, are recognized by macrophages and promote Th17 differentiation that exacerbate GVHD (39). These results highlight importance of infection prophylaxis in the control of GVHD.
Unfortunately, it is impossible to discern cause and effect from human microbiota studies which generate associations between bacterial taxa typically derived from 16s ribosomal sequencing and transplant outcomes (40). The use of shotgun sequencing allows for the imputation of various functional properties of bacterial species (e.g. the likely ability to generate various metabolites) which provide further granularity and allows hypothesis generation (41). Recently, gnotobiotic and/or antibiotic decolonized mice have allowed true cause and effect to be ascertained whilst permitting dissection of the mechanisms by which microbiota invoke GVHD, both at initiation and amplification phases of the disease (42, 43).
Regulatory T cells (Treg) defined by the transcription factor FoxP3 are pivotal for the maintenance of self-tolerance and the induction of tolerance after allogeneic hematopoietic stem cell transplantation (HCT). Depletion of CD25+ cells from the donor inoculum exacerbates acute GVHD and infusion of CD4+CD25+ Treg inhibits GVHD in mice (44–46). Mogamulizumab, anti-CCR4 antibody, eliminates Treg, in which CCR4 is highly expressed (47). Pretransplant administration of mogamulizumab is a risk for severe acute GVHD (48). Following the link between Treg and acute GVHD, early phase clinical studies of Treg infusion demonstrate safety of Treg infusion (49–54) but definitive data on efficacy is awaited. The use of epigenetic modifiers such as histone deacetylase inhibitors has been shown to attenuate acute GVHD and enhance regulatory T cell activity in preclinical systems (55). Promising activity in subsequent early phase clinical GVHD prophylaxis studies has also been seen (56). Additional immunomodulatory effects of these agents have recently been reviewed elsewhere (57).
GVHD prophylaxis using calcineurin inhibitors (cyclosporin or tacrolimus) reduce the expansion of effector T cells (Teff) by blocking IL-2 and prevent acute GVHD, but fail to reduce chronic GVHD (58–60). Calcineurin inhibitors regulate Teff at the expense of Treg inhibition and the major challenge of GVHD prophylaxis is to selectively control Teff, while preserving Treg. In addition, calcineurin inhibitors are not sufficient in isolation to control GVHD in HLA-mismatched HCT (61, 62). Alternative approaches have been explored in preclinical systems. A study in the early 1960’s showed that high-dose cyclophosphamide (Cy) prolonged murine skin allograft survival only when given shortly after transplant (63). Mayumi and Nomoto then continued studies to elucidate mechanisms of tolerance induction by post-transplant Cy (PTCy) in mice (64). Tolerogenic effects of PTCy were exerted through selective elimination of alloreactive T cells, while preserving bystander T cells and Treg (65–67). Subsequently, the Johns Hopkins group translated PTCy to the clinic and confirmed a low incidence of both acute and chronic GVHD, even after haploidentical HCT (68, 69). GVHD prophylaxis using PTCy is a standard of care in haploidentical HCT and also potentially in HLA-matched related and unrelated donor transplantation, either with bone marrow or peripheral blood stem cell sources (70, 71).
Role of the Conditioning Regimen
In the setting of BMT, donor T cells are infused into recipients that have potentially experienced tissue injury by prior treatments of the underlying malignancy, infections, and more immediately, pre-transplant conditioning. The inflammatory environment invoked by these therapies predispose to a state of enhanced alloantigen-presentation. Johnson and Truitt demonstrated that delayed infusion of donor T cells induced less severe GVHD (72). The Ferrara group demonstrated that the conditioning regimen, particularly total body irradiation (TBI), induced proinflammatory cytokine secretion (e.g. IL-1 and TNF) and increased the severity of acute GVHD in animal models (73, 74). These studies demonstrated that GI tract injury and associated pathogen-derived danger signals are critical to the propagation of the ‘‘cytokine storm’’ characteristic of acute GVHD (75). In humans, clinical studies clearly show that myeloablative conditioning, particularly TBI is a risk for acute GVHD (76, 77). Given this link between conditioning intensity and acute GVHD, non-myeloablative and reduced intensity conditioning regimens were developed by Storb and colleagues in dog models (78). The translation of these to humans were associated with reduced incidence of acute GVHD although later-onset acute GVHD, occurring after day 100 was noted (79).
Antigen Presentation
In 1999, Shlomchik et al. demonstrated in preclinical mouse systems that recipient antigen presenting cells (APCs) were responsible for donor T cell activation and the induction of acute GVHD (80). They subsequently showed that although host APCs were much more potent, reconstituting donor hematopoietic APCs were necessary to invoke the full spectrum and severity of acute GVHD (81). They also demonstrated that these donor APCs could cross-present host antigens to induce chronic GVHD (82, 83). These hematopoietic (or professional) recipient or donor APCs were predominantly dendritic cells (DCs) (84, 85). Unexpectedly, Koyama et al. showed that non-hematopoietic recipient APCs exhibited a potent capacity to induce lethal acute GVHD (86) and consistent with this, depletion of recipient professional CD11c+ or CD11b+ APCs do not prevent GVHD (86, 87).
Subsequent mouse studies have demonstrated that intestine is a critical site for alloreactive T cell activation by APCs (86, 88, 89). Importantly, the pathogenic donor APCs in the colon are GM-CSF dependent, providing a potential therapeutic target [reviewed in (90)]. Intestinal epithelial cells highly express MHC class II and thereby regulate tolerance to intestinal commensals while inducing immunity against pathogens (91). Koyama et al. have demonstrated that prior to HCT, intestinal epithelial cells (IEC) express MHC class II in the ileum and can stimulate donor T cells and initiate acute GVHD, defining the lineage of the non-hematopoietic APC that initiates lethal GVHD (43). Both microbiota and conditioning invoke IL-12p40 dependent generation of interferon (IFN)-γ to mediate these effects by IEC (43). A translational clinical study has now commenced blocking IL-12p40 prior to conditioning in an attempt to prevent the initiation of MHC class II dependent GVHD within the GI tract (NCT04572815) (see also below).
Other mouse studies have been shown that intestine is a critical site for alloreactive T cell activation by APCs (86, 88). The α4β7 integrin-MAdCAM (mucosal addressin cell adhesion molecule) -1 pathway is critical for T cell homing to the intestine (88). Such a pathway found in mice has clinical potential for translation. Maraviroc is a small-molecule drug that block CCR5. However, addition of Maraviroc to standard GVHD prophylaxis composed of tacrolimus and methotrexate failed to reduce incidence of acute GVHD (92). Vedolizumab and Natalizumab, a humanized monoclonal antibody specifically target α4β7 integrin showed potentially promising results in phase I/II studies (93, 94), and these agents are currently tested in larger studies.
Co-Stimulation
The activation, proliferation and differentiation of donor T cells requires recognition of alloantigen presented within MHC in the context of additional signals, usually a cognate costimulatory signal (characteristically CD40L – CD40 and CD28 – CD80/86 on the T cell and APC, respectively) and a differentiation signal in the form of cytokine as defined above. The recognition of the importance of cognate costimulatory signals has led to the investigation of the relative ability of inhibitory antibodies that block these pathways in preclinical models. Thus CTLA-4-Ig that inhibits CD80 and CD86, anti-CD28 and anti-CD40 have all been shown to attenuate GVHD in preclinical models (95–97). The clinical reagent abatacept (CTLA-4-Ig) has also shown promise in preventing acute GVHD in early phase clinical studies (98, 99). Additional pathways such as OX40L and ICOSL may also be clinically tractable [reviewed in (100)].
Cytokines
Cytokines play a pivotal role in the pathogenesis of GVHD. Many inflammatory cytokines (e.g. IL-1, IL-6, TNF, HMGB1) are generated in response to chemoradiotherapy during conditioning and promote the activation of APCs. Other cytokines act in a costimulatory role to promote pathogenic T cell differentiation (e.g. IL-12, IL-6). Finally pivotal T cell derived cytokines (e.g. IFNγ, GM-CSF, IL-17) can in turn invoke target tissue apoptosis and secondary myeloid cell migration to amplify GVHD [reviewed in (100)]. Initial studies in experimental GVHD models suggested that cytotoxicity mediated by cytotoxic T lymphocytes (CTLs) have a central role in GVHD target tissue injury through the Fas/Fas ligand pathway and perforin/granzyme pathways (101–104). Subsequent studies demonstrated that inhibition of inflammatory cytokines such as TNF, IL-1 and IL-6 also ameliorated GVHD (74, 105–109). In 2002, Teshima et al. formally demonstrated that cytokines alone could generate the typical acute GVHD pathology in the absence of cognate cell-to-cell dependent cytotoxic mechanisms (110). These studies facilitated clinical trials of cytokine blockade for acute GVHD. However, individual cytokine blockade (e.g. TNF-α, IL-1, and IL-2) did not demonstrate significant benefits in randomized trials, suggesting considerable redundancy in these pathways and a likely requirement to inhibit multiple cytokines to gain clinical efficacy (111, 112). With this concept in mind, Ruxolitinib inhibits the signaling of multiple cytokines involved in the pathogenesis of experimental GVHD (113, 114) and a recent randomized study has demonstrated the efficacy of this agent in the treatment corticosteroid-refractory acute GVHD (115). This represents the first successful randomized study for the treatment of acute GVHD and highlights the successful translation of our understanding of the role of cytokines in GVHD from preclinical models. Ruxolitinib also targets Jak2, which relays signals for growth and differentiation of hematopoietic cells, in addition to Jak1, which relays inflammatory cytokines. Although it remains to be determined which pathway is critical for GVHD mitigation, animal studies suggest that neutrophils recruited to GI tract in response to bacterial translocation enhance intestinal GVHD via tissue damage (34). α1-Antitrypsin (ATT) inactivates serine proteases produced from neutrophils and macrophages and protect tissues from proteolytic degradation. Administration of AAT ameliorates murine acute GVHD via multiple mechanisms (116, 117). A phase 2 study of ATT shows promising results (118) and ATT is currently tested in larger studies.
Tissue Homeostasis in GVHD
The pathophysiology of GVHD beyond donor effector T cells is now better understood. Damage to the intestine plays a central role in propagating a proinflammatory cytokine milieu and amplifying systemic GVHD. Indeed, intestinal GVHD is the major cause of non-relapse mortality after allogeneic HCT (75). Intestinal GVHD is characterized by severe villous atrophy and crypt degeneration; the latter has long been thought of as the cardinal and pathognomonic feature of intestinal GVHD (119–121). Recent data indicate that intestinal stem cells (ISCs) and their Paneth cell niche are targeted in GVHD, resulting in dysregulation of intestinal homeostasis and associated microbial ecology (122–124). Goblet cells are also significantly reduced in GVHD, resulting in disruption of inner mucus layer of the colon and increased bacterial translocation into colonic mucosa (125). In humans, reduced Paneth-cell numbers in duodenal biopsies and Goblet-cell numbers in colon biopsies correlate with the severity of GI-GVHD and transplant outcome (125, 126). Patients who undergo allogeneic HCT exhibit dysbiosis characterized by loss of diversity and expansion of potentially pathogenic bacteria (127–129). The microbiota and their metabolites shape the immune system and intestinal homeostasis (130). Lower microbial diversity and Enterococcus domination are associated with increased GVHD and poor survival across diverse ethnicity (40, 42). In addition, recent studies suggest an unexpected association between fungal and viral colonization and GVHD (39, 131). However, there are many open questions to be addressed in this field (132). What are the most important microbes that control transplant outcomes? Should we consider microbiota stewardship in addition to antibiotic stewardship in our transplant teams? Can we use interventions that modify the microbiome to improve transplant outcomes? What is the role of skin microbes in skin GVHD?
The sensitivity of target tissues to GVHD may be modulated by tissue-intrinsic resilience and homeostasis. Thus, integration of both immune tolerance and tissue tolerance could optimize GVHD treatment (133). In the 1990’s, Ferrara’s group proposed a concept of using cytokine shields to prevent target tissue damage in the GI tract. IL-11 and keratinocyte growth factor (KGF) protect gut injury from TBI and have anti-inflammatory properties. In mice, IL-11 and KGF ameliorated GVHD (134–136). However, a clinical trial of IL-11 was halted by unexpected severe side effects (137). This trial highlighted that clinical toxicity cannot always be estimated in mice and subsequent studies have also utilized primate models to study efficacy and toxicity (138). Protection of the ISC-niche and modification of the intestinal microbiota and metabolome to restore intestinal homeostasis may also represent a novel approach to modulate GVHD and also infection. In mice, IL-22 and R-Spondin are growth factors for ISCs that ameliorate GVHD in mice (122, 123, 139, 140). Glucagon-like-peptide-2 (GLP-2) is an enteroendocrine tissue hormone. Administration of GLP-2 promoted regeneration of ISCs and Paneth cells and restored intestinal homeostasis, resulting in amelioration of GVHD (141). IL-25 protects Goblet cells and also could improve transplant outcome (125). ISCs and Paneth cells express IFN-γ receptors. IFN-γ secreted by donor T cells induces ISC and Paneth cell death in vitro (142, 143). Ruxolotinib inhibits IFN-γ signaling and protects ISCs and Paneth cells (142, 143), which may be an additional mechanism of ruxolitinib’s action in intestinal GVHD (113, 144). Despite the promising mouse data, it remains to be elucidated whether modification of GVHD target tissue sensitivity can attenuate clinical GVHD.
Although most studies of tissue stem cell injury in GVHD have focused on the intestine, a recent study demonstrated that skin stem cells are injured in GVHD in association with impaired skin homeostasis (145). Topical corticosteroids suppressed skin inflammation but failed to protect skin stem cells and restore skin homeostasis. In contrast, topical ruxolitinib protected skin stem cells, resulting in restoration of hair regeneration and wound healing (145). These results in animals deserve further clinical scrutiny but will promote to open a new avenue for improved tissue homeostasis in GVHD beyond the GI tract.
Chronic GVHD
Chronic GVHD, a pleiomorphic syndrome, is the major cause of nonrelapse mortality and severely impairs the quality of life in long-term survivors of allogeneic HCT. The highly variable clinical manifestations of chronic GVHD frequently involve the skin, liver, eyes, mouth, upper respiratory tract, esophagus, and less frequently serosal surfaces, lower gastrointestinal tract, female genitalia, and fascia (146). The biological mechanisms leading to chronic GVHD are not yet as well understood as those leading to acute GVHD, complicated by the fact that chronic GVHD can present with more heterogenous phenotypes than acute GVHD. Individual mouse models have dominant disease manifestations that typically involve a limited number of organs. The B6 into B10.BR model induces chronic GVHD primarily presenting as bronchiolitis obliterans (147). The B10.D2 into BALB/c model induces scleroderma as the primary manifestation (148). G-CSF-mobilized SCT (bothB6 into B6D2F1 and Balb/c to B6 mouse models) generate scleroderma, liver disease and Sjogren’s features (149). Using these preclinical models, significant progress has been made in the last decade and mouse models have demonstrated a critical role for donor Treg defects, germinal center B cell expansion and alloantibody secretion, and dysregulated Th17/Tc17 and T follicular helper (Tfh) differentiation in the development of chronic GVHD (150–157). Ibrutinib, an inhibitor of Bruton’s tyrosine kinase, has showed clinical efficacy in a phase II clinical trial and was approved for chronic GVHD, representing the first such agent (158). Treg are numerically decreased and dysfunctional in patients with chronic GVHD (159, 160). Low-dose IL-2 preferentially stimulates proliferation, function, and survival of Treg; low-dose IL-2 administration to patients has been shown expands Treg and ameliorates chronic GVHD in a proportion of patients (161, 162). Efavakeukin-α, IL-2 mutein, is currently tested in a clinical trial. Ruxolitinib suppresses dysregulated inflammatory cytokine responses in chronic GVHD and is effective in patients with chronic GVHD (144); results of a prospective phase 3 trial of ruxolitinib for steroid refractory chronic GVHD are expected soon. Tfh and germinal center B cells (GCB) play a role in the development of chronic GVHD and bronchiolitis obliterans syndrome (152, 153, 163). The rho-associated coiled-coil kinase 2 (ROCK2) inhibitor, belumosudil (KD025), inhibits the differentiation of Th17 and Tfh together with GCB, and alloantibody generation (164). Syk inhibition induces apoptosis of activated B cells and ameliorates chronic GVHD (165, 166). Belumosudil and the Syk inhibitor Fostamatinib are currently being tested in clinical trials. Tissue fibrosis, the main manifestation of chronic GVHD, is characterized by increased deposition of collagen fibers secreted from activated fibroblasts in response to profibrotic cytokines such as TGF-β and PDGF-α secreted by CSF-1R-dependent macrophages (157, 167–172) [reviewed in (173)]. This pathological cascade of fibrosis defined in mice, has given rise to a number of new potential targets, including TGF-β, PDGF-α and CSF-1R; CSF-1R antibody axatilimab, which inhibits signaling through CSF-1 and IL-34, is currently undergoing assessment in clinical trials (NCT04710576).
Conclusions
Mouse models of GVHD faithfully recapitulate the pathological lesions seen in clinical disease and allow the dissection of pathogenic versus protective immunological mechanisms of action and tissue resistance. While the ability to tightly control MHC and minor antigen barriers is a strength, the inbred nature of these systems may overlook variables present in outbred human populations (e.g. microbiota, age, obesity, prior therapy, comorbidities, conditioning, immune suppression). Some of these limitations can be overcome by more thorough study of these variables in mice (e.g. age, obesity, conditioning, concurrent immune suppression) and/or the use of non-human primates or dog models (especially pharmacological immune suppression). Additional limitations include the widespread use of cell lines to study graft-versus-leukemia effects and the lack of relevant models to study pathogen-specific immunity in the context of new therapies, at least until recently. Nevertheless, to date almost all effective therapeutic paradigms that are now established in humans have their genesis in animal models, suggesting that these systems will continue to provide valuable insights and therapeutic advances to the field. Importantly, it would seem critical that well-established preclinical systems are utilized to analyze the effects of various therapeutic interventions before they are translated into early phase clinical trials.
Author Contributions
TT and GH, wrote the manuscript. All authors contributed to the article and approved the submitted version.
Funding
TT is supported by JSPS KAKENHI (21H02944, 20K21610). GRH is supported by NIH R01 HL148164. The content is solely the responsibility of the authors and does not necessarily represent the official views of the NIH.
Conflict of Interest
TT: Grants from Kyowa Kirin, Chugai, Sanofi, Astellas, TEIJIN PHARMA, Fuji Pharma, NIPPON SHINYAKU, Personal Fees from Novartis, Merck, Kyowa Kirin, Takeda, Pfizer, Bristol-Myers Squibb, Non-Financial Support from Janssen, Novartis.
GRH has consulted for Generon Corporation, NapaJen Pharma, Neoleukin Therapeutics, iTeos Therapeutics and has received research funding from Roche Pharmaceuticals, Compass Therapeutics, Syndax Pharmaceuticals, Applied Molecular Transport and iTeos Therapeutics.
Publisher’s Note
All claims expressed in this article are solely those of the authors and do not necessarily represent those of their affiliated organizations, or those of the publisher, the editors and the reviewers. Any product that may be evaluated in this article, or claim that may be made by its manufacturer, is not guaranteed or endorsed by the publisher.
References
1. Vos O, Davids JA, Weyzen WW, Van Bekkum DW. Evidence for the Cellular Hypothesis in Radiation Protection by Bone Marrow Cells. Acta Physiol Pharmacol Neerl (1956) 4:482–6.
2. Barnes DW, Corp MJ, Loutit JF, Neal FE. Treatment of Murine Leukaemia With X Rays and Homologous Bone Marrow; Preliminary Communication. Br Med J (1956) 2:626–7. doi: 10.1136/bmj.2.4993.626
3. Barnes DW, Loutit JF. Treatment of Murine Leukaemia With X-Rays and Homologous Bone Marrow. II. Br J Haematol (1957) 3:241–52. doi: 10.1111/j.1365-2141.1957.tb05793.x
5. Mathe G, Amiel JL, Schwarzenberg L, Cattan A, Schneider M. Haematopoietic Chimera in Man After Allogenic (Homologous) Bone-Marrow Transplantation. (Control of the Secondary Syndrome. Specific Tolerance Due to the Chimerism). Br Med J (1963) 2:1633–5. doi: 10.1136/bmj.2.5373.1633
6. Mathe G, Schwarzenberg L, Amiel JL, Schneider M, Cattan A, Schlumberger JR, et al. Immunogenetic and Immunological Problems of Allogeneic Haemopoietic Radio-Chimaeras in Man. Scand J Haematol (1967) 4:193–216. doi: 10.1111/j.1600-0609.1967.tb01621.x
7. Bortin MM. A Compendium of Reported Human Bone Marrow Transplants. Transplantation (1970) 9:571–87. doi: 10.1097/00007890-197006000-00006
9. Santos GW. Preparative Regimens: Chemotherapy Versus Chemoradiotherapy. A Historical Perspective. Ann N Y Acad Sci (1995) 770:1–7. doi: 10.1111/j.1749-6632.1995.tb31039.x
10. Storb R, Epstein RB, Bryant J, Ragde H, Thomas ED. Marrow Grafts by Combined Marrow and Leukocyte Infusions in Unrelated Dogs Selected by Histocompatibility Typing. Transplantation (1968) 6:587–93. doi: 10.1097/00007890-196807000-00011
11. Storb R, Epstein RB, Graham TC, Thomas ED. Methotrexate Regimens for Control of Graft-Versus-Host Disease in Dogs With Allogeneic Marrow Grafts. Transplantation (1970) 9:240–6. doi: 10.1097/00007890-197003000-00007
12. Storb R, Graham TC, Shiurba R, Thomas ED. Treatment of Canine Graft-Versus-Host Disease With Methotrexate and Cyclo-Phosphamide Following Bone Marrow Transplantation From Histoincompatible Donors. Transplantation (1970) 10:165–72. doi: 10.1097/00007890-197008000-00003
13. Epstein RB, Storb R, Ragde H, Thomas ED. Cytotoxic Typing Antisera for Marrow Grafting in Littermate Dogs. Transplantation (1968) 6:45–58. doi: 10.1097/00007890-196801000-00005
14. Thomas E, Storb R, Clift RA, Fefer A, Johnson FL, Neiman PE, et al. Bone-Marrow Transplantation (First of Two Parts). N Engl J Med (1975) 292:832–43. doi: 10.1056/NEJM197504172921605
15. Reddy P, Negrin R, Hill GR. Mouse Models of Bone Marrow Transplantation. Biol Blood Marrow Transplant (2008) 14:129–35. doi: 10.1016/j.bbmt.2007.10.021
16. Arber C, Brenner MK, Reddy P. Mouse Models in Bone Marrow Transplantation and Adoptive Cellular Therapy. Semin Hematol (2013) 50:131–44. doi: 10.1053/j.seminhematol.2013.03.026
17. Markey KA, MacDonald KP, Hill GR. The Biology of Graft-Versus-Host Disease: Experimental Systems Instructing Clinical Practice. Blood (2014) 124:354–62. doi: 10.1182/blood-2014-02-514745
18. Korngold R, Sprent J. Lethal Graft-Versus-Host Disease After Bone Marrow Transplantation Across Minor Histocompatibility Barriers in Mice. Prevention by Removing Mature T Cells From Marrow. J Exp Med (1978) 148:1687–98. doi: 10.1084/jem.148.6.1687
19. Korngold R, Sprent J. Features of T Cells Causing H-2-Restricted Lethal Graft-vs.-Host Disease Across Minor Histocompatibility Barriers. J Exp Med (1982) 155:872–83. doi: 10.1084/jem.155.3.872
20. Korngold R, Sprent J. Surface Markers of T Cells Causing Lethal Graft-vs-Host Disease to Class I vs Class II H-2 Differences. J Immunol (1985) 135:3004–10.
21. Aversa F, Tabilio A, Velardi A, Cunningham I, Terenzi A, Falzetti F, et al. Treatment of High-Risk Acute Leukemia With T-Cell-Depleted Stem Cells From Related Donors With One Fully Mismatched HLA Haplotype. N Engl J Med (1998) 339:1186–93. doi: 10.1056/NEJM199810223391702
22. Aversa F, Tabilio A, Terenzi A, Velardi A, Falzetti F, Giannoni C, et al. Successful Engraftment of T-Cell Depleted Haploidentical “Three-Loci” Incompatible Transplants in Leukaemia Patients by Addition of Recombinant Human G-CSF Mobilised Peripheral Blood Progenitor Cells to Bone Marrow Inoculum. Blood (1994) 84:3948–55. doi: 10.1182/blood.V84.11.3948.bloodjournal84113948
23. Mathe G, Amiel JL, Schwarzenberg L, Cattan A, Schneider M. Adoptive Immunotherapy of Acute Leukemia: Experimental and Clinical Results. Cancer Res (1965) 25:1525–31.
24. Bortin MM, Rimm AA, Saltzstein E. Grfat-Versus-Leukemia: Quantification of Adoptive Immunotherapy in Murine Leukemia. Science (1973) 173:811–3. doi: 10.1126/science.179.4075.811
25. Weiden PL, Flournoy N, Thomas ED, Prentice R, Fefer A, Buckner CD, et al. Antileukemic Effect of Graft-Versus-Host Disease in Human Recipients of Allogeneic-Marrow Grafts. N Engl J Med (1979) 300:1068–73. doi: 10.1056/NEJM197905103001902
26. Horowitz MM, Gale RP, Sondel PM, Goldman JM, Kersey J, Kolb HJ, et al. Graft-Versus-Leukemia Reactions After Bone Marrow Transplantation. Blood (1990) 75:555–62. doi: 10.1182/blood.V75.3.555.bloodjournal753555
27. Kolb H, Mittermuller J, Clemm C, Holler E, Ledderose G, Brehm G, et al. Donor Leukocyte Transfusions for Treatment of Recurrent Chronic Myelogenousleukemia in Marrow Transplant Patients. Blood (1990) 76:2462–5. doi: 10.1182/blood.V76.12.2462.bloodjournal76122462
28. Marmont AM, Horowitz MM, Gale RP, Sobocinski K, Ash RC, van Bekkum DW, et al. T-Cell Depletion of HLA-Identical Transplants in Leukemia. Blood (1991) 78:2120–30.
29. Anderson BE, McNiff J, Yan J, Doyle H, Mamula M, Shlomchik MJ, et al. Memory CD4+ T Cells do Not Induce Graft-Versus-Host Disease. J Clin Invest (2003) 112:101–8. doi: 10.1172/JCI17601
30. Anderson BE, Taylor PA, McNiff JM, Jain D, Demetris AJ, Panoskaltsis-Mortari A, et al. Effects of Donor T-Cell Trafficking and Priming Site on Graft-Versus-Host Disease Induction by Naive and Memory Phenotype CD4 T Cells. Blood (2008) 111:5242–51. doi: 10.1182/blood-2007-09-107953
31. Bleakley M, Heimfeld S, Loeb KR, Jones LA, Chaney C, Seropian S, et al. Outcomes of Acute Leukemia Patients Transplanted With Naive T Cell-Depleted Stem Cell Grafts. J Clin Invest (2015) 125:2677–89. doi: 10.1172/JCI81229
32. Jung U, Foley JE, Erdmann AA, Eckhaus MA, Fowler DH. CD3/CD28-Costimulated T1 and T2 Subsets: Differential In Vivo Allosensitization Generates Distinct GVT and GVHD Effects. Blood (2003) 102:3439–46. doi: 10.1182/blood-2002-12-3936
33. Gartlan KH, Markey KA, Varelias A, Bunting MD, Koyama M, Kuns RD, et al. Tc17 Cells Are a Proinflammatory, Plastic Lineage of Pathogenic CD8+ T Cells That Induce GVHD Without Antileukemic Effects. Blood (2015) 126:1609–20. doi: 10.1182/blood-2015-01-622662
34. Schwab L, Goroncy L, Palaniyandi S, Gautam S, Triantafyllopoulou A, Mocsai A, et al. Neutrophil Granulocytes Recruited Upon Translocation of Intestinal Bacteria Enhance Graft-Versus-Host Disease via Tissue Damage. Nat Med (2014) 20:648–54. doi: 10.1038/nm.3517
35. Carlson MJ, West ML, Coghill JM, Panoskaltsis-Mortari A, Blazar BR, Serody JS. In Vitro-Differentiated TH17 Cells Mediate Lethal Acute Graft-Versus-Host Disease With Severe Cutaneous and Pulmonary Pathologic Manifestations. Blood (2009) 113:1365–74. doi: 10.1182/blood-2008-06-162420
36. Cantoni N, Hirsch HH, Khanna N, Gerull S, Buser A, Bucher C, et al. Evidence for a Bidirectional Relationship Between Cytomegalovirus Replication and Acute Graft-Versus-Host Disease. Biol Blood marrow Transplant (2010) 16:1309–14. doi: 10.1016/j.bbmt.2010.03.020
37. van der Velden WJ, Plantinga TS, Feuth T, Donnelly JP, Netea MG, Blijlevens NM. The Incidence of Acute Graft-Versus-Host Disease Increases With Candida Colonization Depending the Dectin-1 Gene Status. Clin Immunol (2010) 136:302–6. doi: 10.1016/j.clim.2010.04.007
38. Marr KA, Seidel K, Slavin MA, Bowden RA, Schoch HG, Flowers ME, et al. Prolonged Fluconazole Prophylaxis Is Associated With Persistent Protection Against Candidiasis-Related Death in Allogeneic Marrow Transplant Recipients: Long-Term Follow-Up of a Randomized, Placebo-Controlled Trial. Blood (2000) 96:2055–61. doi: 10.1182/blood.V96.6.2055.h8002055_2055_2061
39. Uryu H, Hashimoto D, Kato K, Hayase E, Matsuoka S, Ogasawara R, et al. Alpha-Mannan Induces Th17-Mediated Pulmonary Graft-Versus-Host Disease in Mice. Blood (2015) 125:3014–23. doi: 10.1182/blood-2014-12-615781
40. Peled JU, Gomes ALC, Devlin SM, Littmann ER, Taur Y, Sung AD, et al. Microbiota as Predictor of Mortality in Allogeneic Hematopoietic-Cell Transplantation. N Engl J Med (2020) 382:822–34. doi: 10.1056/NEJMoa1900623
41. Markey KA, Schluter J, Gomes ALC, Littmann ER, Pickard AJ, Taylor BP, et al. The Microbe-Derived Short-Chain Fatty Acids Butyrate and Propionate Are Associated With Protection From Chronic GVHD. Blood (2020) 136:130–6. doi: 10.1182/blood.2019003369
42. Stein-Thoeringer CK, Nichols KB, Lazrak A, Docampo MD, Slingerland AE, Slingerland JB, et al. Lactose Drives Enterococcus Expansion to Promote Graft-Versus-Host Disease. Science (2019) 366:1143–9. doi: 10.1126/science.aax3760
43. Koyama M, Mukhopadhyay P, Schuster IS, Henden AS, Hulsdunker J, Varelias A, et al. MHC Class II Antigen Presentation by the Intestinal Epithelium Initiates Graft-Versus-Host Disease and Is Influenced by the Microbiota. Immunity (2019) 51:885–98.e7. doi: 10.1016/j.immuni.2019.08.011
44. Taylor PA, Lees CJ, Blazar BR. The Infusion of Ex Vivo Activated and Expanded CD4(+)CD25(+) Immune Regulatory Cells Inhibits Graft-Versus-Host Disease Lethality. Blood (2002) 99:3493–9. doi: 10.1182/blood.V99.10.3493
45. Cohen JL, Trenado A, Vasey D, Klatzmann D, Salomon BL. CD4(+)CD25(+) Immunoregulatory T Cells: New Therapeutics for Graft-Versus-Host Disease. J Exp Med (2002) 196:401–6. doi: 10.1084/jem.20020090
46. Hoffmann P, Ermann J, Edinger M, Fathman CG, Strober S. Donor-Type CD4(+)CD25(+) Regulatory T Cells Suppress Lethal Acute Graft-Versus-Host Disease After Allogeneic Bone Marrow Transplantation. J Exp Med (2002) 196:389–99. doi: 10.1084/jem.20020399
47. Yamamoto K, Utsunomiya A, Tobinai K, Tsukasaki K, Uike N, Uozumi K, et al. Phase I Study of KW-0761, a Defucosylated Humanized Anti-CCR4 Antibody, in Relapsed Patients With Adult T-Cell Leukemia-Lymphoma and Peripheral T-Cell Lymphoma. J Clin Oncol (2010) 28:1591–8. doi: 10.1200/JCO.2009.25.3575
48. Fuji S, Inoue Y, Utsunomiya A, Moriuchi Y, Uchimaru K, Choi I, et al. Pretransplantation Anti-CCR4 Antibody Mogamulizumab Against Adult T-Cell Leukemia/Lymphoma Is Associated With Significantly Increased Risks of Severe and Corticosteroid-Refractory Graft-Versus-Host Disease, Nonrelapse Mortality, and Overall Mortality. J Clin Oncol (2016) 34:3426–33. doi: 10.1200/JCO.2016.67.8250
49. Brunstein CG, Miller JS, Cao Q, McKenna DH, Hippen KL, Curtsinger J, et al. Infusion of Ex Vivo Expanded T Regulatory Cells in Adults Transplanted With Umbilical Cord Blood: Safety Profile and Detection Kinetics. Blood (2011) 117:1061–70. doi: 10.1182/blood-2010-07-293795
50. Brunstein CG, Miller JS, McKenna DH, Hippen KL, DeFor TE, Sumstad D, et al. Umbilical Cord Blood-Derived T Regulatory Cells to Prevent GVHD: Kinetics, Toxicity Profile, and Clinical Effect. Blood (2016) 127:1044–51. doi: 10.1182/blood-2015-06-653667
51. Di Ianni M, Falzetti F, Carotti A, Terenzi A, Castellino F, Bonifacio E, et al. Tregs prevent GVHD And Promote Immune Reconstitution in HLA-Haploidentical Transplantation. Blood (2011) 117:3921–8. doi: 10.1182/blood-2010-10-311894
52. Martelli MF, Di Ianni M, Ruggeri L, Falzetti F, Carotti A, Terenzi A, et al. HLA-Haploidentical Transplantation With Regulatory and Conventional T-Cell Adoptive Immunotherapy Prevents Acute Leukemia Relapse. Blood (2014) 124:638–44. doi: 10.1182/blood-2014-03-564401
53. Kellner JN, Delemarre EM, Yvon E, Nierkens S, Boelens JJ, McNiece I, et al. Third Party, Umbilical Cord Blood Derived Regulatory T-Cells for Prevention of Graft Versus Host Disease in Allogeneic Hematopoietic Stem Cell Transplantation: Feasibility, Safety and Immune Reconstitution. Oncotarget (2018) 9:35611–22. doi: 10.18632/oncotarget.26242
54. MacMillan ML, Hippen KL, McKenna DH, Kadidlo D, Sumstad D, DeFor TE, et al. First-in-Human Phase 1 Trial of Induced Regulatory T Cells for Graft-Versus-Host Disease Prophylaxis in HLA-Matched Siblings. Blood Adv (2021) 5:1425–36. doi: 10.1182/bloodadvances.2020003219
55. Reddy P, Sun Y, Toubai T, Duran-Struuck R, Clouthier SG, Weisiger E, et al. Histone Deacetylase Inhibition Modulates Indoleamine 2,3-Dioxygenase-Dependent DC Functions and Regulates Experimental Graft-Versus-Host Disease in Mice. J Clin Invest (2008) 118:2562–73. doi: 10.1172/JCI34712
56. Choi SW, Braun T, Henig I, Gatza E, Magenau J, Parkin B, et al. Vorinostat Plus Tacrolimus/Methotrexate to Prevent GVHD After Myeloablative Conditioning, Unrelated Donor HCT. Blood (2017) 130:1760–7. doi: 10.1182/blood-2017-06-790469
57. Xu X, Li X, Zhao Y, Huang H. Immunomodulatory Effects of Histone Deacetylation Inhibitors in Graft-vs.-Host Disease After Allogeneic Stem Cell Transplantation. Front Immunol (2021) 12:641910. doi: 10.3389/fimmu.2021.641910
58. Deeg HJ, Lin D, Leisenring W, Boeckh M, Anasetti C, Appelbaum FR, et al. Cyclosporine or Cyclosporine Plus Methylprednisolone for Prophylaxis of Graft-Versus-Host Disease: A Prospective, Randomized Trial. Blood (1997) 89:3880–7. doi: 10.1182/blood.V89.10.3880.3880_3880_3887
59. Storb R, Deeg HJ, Pepe M, Doney K, Appelbaum F, Beatty P, et al. Graft-Versus-Host Disease Prevention by Methotrexate Combined With Cyclosporin Compared to Methotrexate Alone in Patients Given Marrow Grafts for Severe Aplastic Anaemia: Long-Term Follow-Up of a Controlled Trial. Br J Haematol (1989) 72:567–72. doi: 10.1111/j.1365-2141.1989.tb04325.x
60. Kansu E, Gooley T, Flowers ME, Anasetti C, Deeg HJ, Nash RA, et al. Administration of Cyclosporine for 24 Months Compared With 6 Months for Prevention of Chronic Graft-Versus-Host Disease: A Prospective Randomized Clinical Trial. Blood (2001) 98:3868–70. doi: 10.1182/blood.V98.13.3868
61. Powles RL, Morgenstern GR, Kay HE, McElwain TJ, Clink HM, Dady PJ, et al. Mismatched Family Donors for Bone-Marrow Transplantation as Treatment for Acute Leukaemia. Lancet (1983) 1:612–5. doi: 10.1016/S0140-6736(83)91793-2
62. Beatty PG, Clift RA, Mickelson EM, Nisperos BB, Flournoy N, Martin PJ, et al. Marrow Transplantation From Related Donors Other Than HLA-Identical Siblings. N Engl J Med (1985) 313:765–71. doi: 10.1056/NEJM198509263131301
63. Berenbaum MC, Brown IN. Prolongation of Homograft Survival in Mice With Single Doses of Cyclophosphamide. Nature (1963) 200:84. doi: 10.1038/200084a0
64. Mayumi H, Himeno K, Shin T, Nomoto K. Drug-Induced Tolerance to Allografts in Mice. IV. Mechanisms and Kinetics of Cyclophosphamide-Induced Tolerance. Transplantation (1985) 39:209–15. doi: 10.1097/00007890-198502000-00019
65. Eto M, Mayumi H, Tomita Y, Yoshikai Y, Nishimura Y, Nomoto K. Sequential Mechanisms of Cyclophosphamide-Induced Skin Allograft Tolerance Including the Intrathymic Clonal Deletion Followed by Late Breakdown of the Clonal Deletion. J Immunol (1990) 145:1303–10.
66. Ross D, Jones M, Komanduri K, Levy RB. Antigen and Lymphopenia-Driven Donor T Cells Are Differentially Diminished by Post-Transplantation Administration of Cyclophosphamide After Hematopoietic Cell Transplantation. Biol Blood marrow Transplant (2013) 19:1430–8. doi: 10.1016/j.bbmt.2013.06.019
67. Kanakry CG, Ganguly S, Zahurak M, Bolaños-Meade J, Thoburn C, Perkins B, et al. Aldehyde Dehydrogenase Expression Drives Human Regulatory T Cell Resistance to Posttransplantation Cyclophosphamide. Sci Trans Med (2013) 5:211ra157. doi: 10.1126/scitranslmed.3006960
68. O’Donnell PV, Luznik L, Jones RJ, Vogelsang GB, Leffell MS, Phelps M, et al. Nonmyeloablative Bone Marrow Transplantation From Partially HLA-Mismatched Related Donors Using Posttransplantation Cyclophosphamide. Biol Blood Marrow Transplant (2002) 8:377–86. doi: 10.1053/bbmt.2002.v8.pm12171484
69. Luznik L, O’Donnell PV, Symons HJ, Chen AR, Leffell MS, Zahurak M, et al. HLA-Haploidentical Bone Marrow Transplantation for Hematologic Malignancies Using Nonmyeloablative Conditioning and High-Dose, Posttransplantation Cyclophosphamide. Biol Blood Marrow Transplant (2008) 14:641–50. doi: 10.1016/j.bbmt.2008.03.005
70. Kwon M, Bailen R, Pascual-Cascon MJ, Gallardo-Morillo AI, Garcia Sola A, Balsalobre P, et al. Posttransplant Cyclophosphamide vs Cyclosporin a and Methotrexate as GVHD Prophylaxis in Matched Sibling Transplantation. Blood Adv (2019) 3:3351–9. doi: 10.1182/bloodadvances.2019000236
71. Gagelmann N, Bacigalupo A, Rambaldi A, Hoelzer D, Halter J, Sanz J, et al. Haploidentical Stem Cell Transplantation With Posttransplant Cyclophosphamide Therapy vs Other Donor Transplantations in Adults With Hematologic Cancers: A Systematic Review and Meta-Analysis. JAMA Oncol (2019) 5:1739–48. doi: 10.1001/jamaoncol.2019.3541
72. Johnson BD, Truitt RL. Delayed Infusion of Immunocompetent Donor Cells After Bone-Marrow Transplantation Breaks Graft-Host Tolerance and Allows for Persistent Antileukemic Reactivity Without Severe Graft-Versus-Host Disease. Blood (1995) 85:3302–12. doi: 10.1182/blood.V85.11.3302.bloodjournal85113302
73. Hill GR, Crawford JM, Cooke KR, Brinson YS, Pan L, Ferrara JL. Total Body Irradiation and Acute Graft-Versus-Host Disease: The Role of Gastrointestinal Damage and Inflammatory Cytokines. Blood (1997) 90:3204–13. doi: 10.1182/blood.V90.8.3204
74. Cooke KR, Hill GR, Crawford JM, Bungard D, Brinson Y, Delmonte J, et al. Tnfa Production to LPS Stimulation by Donor Cells Predicts the Severity of Experimental Acute Graft-Versus-Host Disease. J Clin Invest (1998) 102:1882–91. doi: 10.1172/JCI4285
75. Hill GR, Ferrara JLM. The Primacy of the Gastrointestinal Tract as a Target Organ of Acute Graft-Versus-Host Disease: Rationale for the Use of Cytokine Shields in Allogeneic Bone Marrow Transplantation. Blood (2000) 95:2754–9. doi: 10.1182/blood.V95.9.2754.009k25_2754_2759
76. Jagasia M, Arora M, Flowers ME, Chao NJ, McCarthy PL, Cutler CS, et al. Risk Factors for Acute GVHD and Survival After Hematopoietic Cell Transplantation. Blood (2012) 119:296–307. doi: 10.1182/blood-2011-06-364265
77. Nakasone H, Fukuda T, Kanda J, Mori T, Yano S, Kobayashi T, et al. Transplantation, Impact of Conditioning Intensity and TBI on Acute GVHD After Hematopoietic Cell Transplantation. Bone Marrow Transplant (2015) 50:559–65. doi: 10.1038/bmt.2014.293
78. Storb RF, Champlin R, Riddell SR, Murata M, Bryant S, Warren EH. Non-Myeloablative Transplants for Malignant Disease. Hematol Am Soc Hematol Educ Program (2001) p. 375–91. doi: 10.1182/asheducation-2001.1.375
79. Mielcarek M, Martin PJ, Leisenring W, Flowers ME, Maloney DG, Sandmaier BM, et al. Graft-Versus-Host Disease After Nonmyeloablative Versus Conventional Hematopoietic Stem Cell Transplantation. Blood (2003) 102:756–62. doi: 10.1182/blood-2002-08-2628
80. Shlomchik WD, Couzens MS, Tang CB, McNiff J, Robert ME, Liu J, et al. Prevention of Graft Versus Host Disease by Inactivation of Host Antigen-Presenting Cells. Science (1999) 285:412–5. doi: 10.1126/science.285.5426.412
81. Matte CC, Liu J, Cormier J, Anderson BE, Athanasiadis I, Jain D, et al. Donor APCs Are Required for Maximal GVHD But Not for GVL. Nat Med (2004) 10:987–92. doi: 10.1038/nm1089
82. Anderson BE, McNiff JM, Jain D, Blazar BR, Shlomchik WD, Shlomchik MJ. Distinct Roles for Donor- and Host-Derived Antigen-Presenting Cells and Costimulatory Molecules in Murine Chronic Graft-Versus-Host Disease: Requirements Depend on Target Organ. Blood (2005) 105:2227–34. doi: 10.1182/blood-2004-08-3032
83. Wang X, Li H, Matte-Martone C, Cui W, Li N, Tan HS, et al. Mechanisms of Antigen Presentation to T Cells in Murine Graft-Versus-Host Disease: Cross-Presentation and the Appearance of Cross-Presentation. Blood (2011) 118:6426–37. doi: 10.1182/blood-2011-06-358747
84. Duffner UA, Maeda Y, Cooke KR, Reddy P, Ordemann R, Liu C, et al. Host Dendritic Cells Alone Are Sufficient to Initiate Acute Graft-Versus-Host Disease. J Immunol (2004) 172:7393–8. doi: 10.4049/jimmunol.172.12.7393
85. Markey KA, Banovic T, Kuns R, Olver S, Don A, Raffelt N, et al. Conventional Dendritic Cells Are the Critical Donor APC Presenting Alloantigen After Experimental BMT. Blood (2009) 113:5644–9.
86. Koyama M, Kuns RD, Olver SD, Raffelt NC, Wilson YA, Don AL, et al. Recipient Nonhematopoietic Antigen-Presenting Cells Are Sufficient to Induce Lethal Acute Graft-Versus-Host Disease. Nat Med (2011) 18:135–42. doi: 10.1038/nm.2597
87. Li H, Demetris AJ, McNiff J, Matte-Martone C, Tan HS, Rothstein DM, et al. Profound Depletion of Host Conventional Dendritic Cells, Plasmacytoid Dendritic Cells, and B Cells Does Not Prevent Graft-Versus-Host Disease Induction. J Immunol (2012) 188:3804–11. doi: 10.4049/jimmunol.1102795
88. Murai M, Yoneyama H, Ezaki T, Suematsu M, Terashima Y, Harada A, et al. Peyer’s Patch Is the Essential Site in Initiating Murine Acute and Lethal Graft-Versus-Host Reaction. Nat Immunol (2003) 4:154–60. doi: 10.1038/ni879
89. Koyama M, Cheong M, Markey KA, Gartlan KH, Kuns RD, Locke KR, et al. Donor Colonic CD103+ Dendritic Cells Determine the Severity of Acute Graft-Versus-Host Disease. J Exp Med (2015) 212:1303–21. doi: 10.1084/jem.20150329
90. Koyama M, Hill GR. The Primacy of Gastrointestinal Tract Antigen-Presenting Cells in Lethal Graft-Versus-Host Disease. Blood (2019) 134:2139–48. doi: 10.1182/blood.2019000823
91. Wosen JE, Mukhopadhyay D, Macaubas C, Mellins ED. Epithelial MHC Class II Expression and Its Role in Antigen Presentation in the Gastrointestinal and Respiratory Tracts. Front Immunol (2018) 9:2144. doi: 10.3389/fimmu.2018.02144
92. Bolanos-Meade J, Reshef R, Fraser R, Fei M, Abhyankar S, Al-Kadhimi Z, et al. Three Prophylaxis Regimens (Tacrolimus, Mycophenolate Mofetil, and Cyclophosphamide; Tacrolimus, Methotrexate, and Bortezomib; or Tacrolimus, Methotrexate, and Maraviroc) Versus Tacrolimus and Methotrexate for Prevention of Graft-Versus-Host Disease With Haemopoietic Cell Transplantation With Reduced-Intensity Conditioning: A Randomised Phase 2 Trial With a Non-Randomised Contemporaneous Control Group (BMT CTN 1203). Lancet Haematol (2019) 6:e132–43. doi: 10.1016/S2352-3026(18)30221-7
93. Chen YB, Shah NN, Renteria AS, Cutler C, Jansson J, Akbari M, et al. Vedolizumab for Prevention of Graft-Versus-Host Disease After Allogeneic Hematopoietic Stem Cell Transplantation. Blood Adv (2019) 3:4136–46. doi: 10.1182/bloodadvances.2019000893
94. Kekre N, Kim HT, Hofer J, Ho VT, Koreth J, Armand P, et al. Phase II Trial of Natalizumab With Corticosteroids as Initial Treatment of Gastrointestinal Acute Graft-Versus-Host Disease. Bone Marrow Transplant (2020) 56:1006–12. doi: 10.1038/s41409-020-01083-y
95. Blazar BR, Taylor PA, Linsley PS, Vallera DA. In Vivo Blockade of CD28/CTLA4: B7/BB1 Interaction With CTLA4-Ig Reduces Lethal Murine Graft-Versus-Host Disease Across the Major Histocompatibility Complex Barrier in Mice. Blood (1994) 83:3815–25. doi: 10.1182/blood.V83.12.3815.bloodjournal83123815
96. Miller WP, Srinivasan S, Panoskaltsis-Mortari A, Singh K, Sen S, Hamby K, et al. GVHD After Haploidentical Transplantation: A Novel, MHC-Defined Rhesus Macaque Model Identifies CD28- CD8+ T Cells as a Reservoir of Breakthrough T-Cell Proliferation During Costimulation Blockade and Sirolimus-Based Immunosuppression. Blood (2010) 116:5403–18. doi: 10.1182/blood.V116.21.2550.2550
97. Watkins BK, Tkachev V, Furlan SN, Hunt DJ, Betz K, Yu A, et al. CD28 Blockade Controls T Cell Activation to Prevent Graft-Versus-Host Disease in Primates. J Clin Invest (2018) 128:3991–4007. doi: 10.1172/JCI98793
98. Koura DT, Horan JT, Langston AA, Qayed M, Mehta A, Khoury HJ, et al. In Vivo T Cell Costimulation Blockade With Abatacept for Acute Graft-Versus-Host Disease Prevention: A First-in-Disease Trial. Biol Blood Marrow Transplant (2013) 19:1638–49. doi: 10.1016/j.bbmt.2013.09.003
99. Watkins BK, Qayed M, Bratrude B, NBetz K, Sinclair S, Suessmuth Y, et al. T Cell Costimulation Blockade with CTLA4-Ig (Abatacept) for Acute Gvhd Prevention in HLA Matched and Mismatched Unrelated Donor Transplantation: Results of the First Phase 2 Trial. 2019 TCT | Transplantation & Cellular Therapy Meetings of ASBMT and CIBMTR. (2019). doi: 10.1016/j.bbmt.2018.12.129
100. Hill GR, Koyama M. Cytokines and Costimulation in Acute Graft-Versus-Host Disease. Blood (2020) 136:418–28. doi: 10.1182/blood.2019000952
101. Baker MB, Altman NH, Podack ER, Levy RB. The Role of Cell-Mediated Cytotoxicity in Acute GVHD After MHC-Matched Allogeneic Bone Marrow Transplantation in Mice. J Exp Med (1996) 183:2645–56. doi: 10.1084/jem.183.6.2645
102. Braun MY, Lowin B, French L, AchaOrbea H, Tschopp J. Cytotoxic T Cells Deficient in Both Functional Fas Ligand and Perforin Show Residual Cytolytic Activity Yet Lose Their Capacity to Induce Lethal Acute Graft-Versus-Host Disease. J Exp Med (1996) 183:657–61. doi: 10.1084/jem.183.2.657
103. Graubert TA, DiPersio JF, Russell JH, Ley TJ. Perforin/Granzyme-Dependent and Independent Mechanisms Are Both Important for the Development of Graft-Versus-Host Disease After Murine Bone Marrow Transplantation. J Clin Invest (1997) 100:904–11. doi: 10.1172/JCI119606
104. Blazar BR, Taylor PA, Vallera DA. CD4(+) and CD8(+) T Cells Each Can Utilize a Perforin-Dependent Pathway to Mediate Lethal Graft-Versus-Host Disease in Major Histocompatibility Complex Disparate Recipients. Transplantation (1997) 64:571–6. doi: 10.1097/00007890-199708270-00004
105. Nestel FP, Price KS, Seemayer TA, Lapp WS. Macrophage Priming and Lipopolysaccharide-Triggered Release of Tumor Necrosis Factor Alpha During Graft-Versus-Host Disease. J Exp Med (1992) 175:405–13. doi: 10.1084/jem.175.2.405
106. Hattori K, Hirano T, Miyajima H, Yamakawa N, Tateno M, Oshimi K, et al. Differential Effects of Anti-Fas Ligand and Anti-Tumor Necrosis Factor-α Antibodies on Acute Graft-Versus-Host Disease Pathologies. Blood (1998) 91:4051–5. doi: 10.1182/blood.V91.11.4051.411k16_4051_4055
107. Hill GR, Teshima T, Gerbitz A, Pan L, Cooke KR, Brinson YS, et al. Differential Roles of IL-1 and TNF-Alpha on Graft-Versus-Host Disease and Graft Versus Leukemia. J Clin Invest (1999) 104:459–67. doi: 10.1172/JCI6896
108. Cooke KR, Gerbitz A, Crawford JM, Teshima T, Hill GR, Tesolin A, et al. LPS Antagonism Reduces Graft-Versus-Host Disease and Preserves Graft-Versus-Leukemia Activity After Experimental Bone Marrow Transplantation. J Clin Invest (2001) 107:1581–9. doi: 10.1172/JCI12156
109. Wilkinson AN, Chang K, Kuns RD, Henden AS, Minnie SA, Ensbey KS, et al. IL-6 Dysregulation Originates in Dendritic Cells and Initiates Graft-Versus-Host Disease via Classical Signaling. Blood (2019) 134:2092–106. doi: 10.1182/blood.2019000396
110. Teshima T, Ordemann R, Reddy P, Gagin S, Liu C, Cooke KR, et al. Acute Graft-Versus-Host Disease Does Not Require Alloantigen Expression on Host Epithelium. Nat Med (2002) 8:575–81. doi: 10.1038/nm0602-575
111. Antin JH, Chen AR, Couriel DR, Ho VT, Nash RA, Weisdorf D. Novel Approaches to the Therapy of Steroid-Resistant Acute Graft-Versus-Host Disease. Biol Blood Marrow Transplant (2004) 10:655–68. doi: 10.1016/j.bbmt.2004.07.007
112. Lee SJ, Zahrieh D, Agura E, MacMillan ML, Maziarz RT, McCarthy PL Jr., et al. Effect of Up-Front Daclizumab When Combined With Steroids for the Treatment of Acute Graft-Versus-Host Disease: Results of a Randomized Trial. Blood (2004) 104:1559–64. doi: 10.1182/blood-2004-03-0854
113. Spoerl S, Mathew NR, Bscheider M, Schmitt-Graeff A, Chen S, Mueller T, et al. Activity of Therapeutic JAK 1/2 Blockade in Graft-Versus-Host Disease. Blood (2014) 123:3832–42. doi: 10.1182/blood-2013-12-543736
114. Teshima T. JAK Inhibitors: A Home Run for GVHD Patients? Blood (2014) 123:3691–3. doi: 10.1182/blood-2014-04-570325
115. Zeiser R, von Bubnoff N, Butler J, Mohty M, Niederwieser D, Or R, et al. Ruxolitinib for Glucocorticoid-Refractory Acute Graft-Versus-Host Disease. N Engl J Med (2020) 382:1800–10. doi: 10.1056/NEJMoa1917635
116. Tawara I, Sun Y, Lewis EC, Toubai T, Evers R, Nieves E, et al. Alpha-1-Antitrypsin Monotherapy Reduces Graft-Versus-Host Disease After Experimental Allogeneic Bone Marrow Transplantation. Proc Natl Acad Sci U.S.A. (2012) 109:564–9. doi: 10.1073/pnas.1117665109
117. Marcondes AM, Karoopongse E, Lesnikova M, Margineantu D, Welte T, Dinarello CA, et al. Alpha-1-Antitrypsin (AAT)-Modified Donor Cells Suppress GVHD But Enhance the GVL Effect: A Role for Mitochondrial Bioenergetics. Blood (2014) 124:2881–91. doi: 10.1182/blood-2014-04-570440
118. Magenau JM, Goldstein SC, Peltier D, Soiffer RJ, Braun T, Pawarode A, et al. Alpha1-Antitrypsin Infusion for Treatment of Steroid-Resistant Acute Graft-Versus-Host Disease. Blood (2018) 131:1372–9. doi: 10.1182/blood-2017-11-815746
119. Epstein RJ, McDonald GB, Sale GE, Shulman HM, Thomas ED. The Diagnostic Accuracy of the Rectal Biopsy in Acute Graft-Versus-Host Disease: A Prospective Study of Thirteen Patients. Gastroenterology (1980) 78:764–71. doi: 10.1016/0016-5085(80)90681-2
120. Sale GE, Shulman HM, McDonald GB, Thomas ED. Gastrointestinal Graft-Versus-Host Disease in Man. A Clinicopathologic Study of the Rectal Biopsy. Am J Surg Pathol (1979) 3:291–9. doi: 10.1097/00000478-197908000-00001
121. Wall AJ, Rosenberg JL, Reilly RW. Small Intestinal Injury in the Immunologically Runted Mouse. Morphologic and Autoradiographic Studies. J Lab Clin Med (1971) 78:833–4.
122. Hanash AM, Dudakov JA, Hua G, O’Connor MH, Young LF, Singer NV, et al. Interleukin-22 Protects Intestinal Stem Cells From Immune-Mediated Tissue Damage and Regulates Sensitivity to Graft Versus Host Disease. Immunity (2012) 37:339–50. doi: 10.1016/j.immuni.2012.05.028
123. Takashima S, Kadowaki M, Aoyama K, Koyama M, Oshima T, Tomizuka K, et al. The Wnt Agonist R-Spondin1 Regulates Systemic Graft-Versus-Host Disease by Protecting Intestinal Stem Cells. J Exp Med (2011) 208:285–94. doi: 10.1084/jem.20101559
124. Eriguchi Y, Takashima S, Oka H, Shimoji S, Nakamura K, Uryu H, et al. Graft-Versus-Host Disease Disrupts Intestinal Microbial Ecology by Inhibiting Paneth Cell Production of Alpha-Defensins. Blood (2012) 120:223–31. doi: 10.1182/blood-2011-12-401166
125. Ara T, Hashimoto D, Hayase E, Noizat C, Kikuchi R, Hasegawa Y, et al. Intestinal Goblet Cells Protect Against GVHD After Allogeneic Stem Cell Transplantation via Lypd8. Sci Trans Med (2020) 12(550):eaaw0720. doi: 10.1126/scitranslmed.aaw0720
126. Levine JE, Huber E, Hammer ST, Harris AC, Greenson JK, Braun TM, et al. Low Paneth Cell Numbers at Onset of Gastrointestinal Graft-Versus-Host Disease Identify Patients at High Risk for Nonrelapse Mortality. Blood (2013) 122:1505–9. doi: 10.1182/blood-2013-02-485813
127. Jenq RR, Ubeda C, Taur Y, Menezes CC, Khanin R, Dudakov JA, et al. Regulation of Intestinal Inflammation by Microbiota Following Allogeneic Bone Marrow Transplantation. J Exp Med (2012) 209:903–11. doi: 10.1084/jem.20112408
128. Taur Y, Jenq RR, Perales MA, Littmann ER, Morjaria S, Ling L, et al. The Effects of Intestinal Tract Bacterial Diversity on Mortality Following Allogeneic Hematopoietic Stem Cell Transplantation. Blood (2014) 124:1174–82. doi: 10.1182/blood-2014-02-554725
129. Holler E, Butzhammer P, Schmid K, Hundsrucker C, Koestler J, Peter K, et al. Metagenomic Analysis of the Stool Microbiome in Patients Receiving Allogeneic Stem Cell Transplantation: Loss of Diversity Is Associated With Use of Systemic Antibiotics and More Pronounced in Gastrointestinal Graft-Versus-Host Disease. Biol Blood Marrow Transplant (2014) 20:640–5. doi: 10.1016/j.bbmt.2014.01.030
130. Fujiwara H, Docampo MD, Riwes M, Peltier D, Toubai T, Henig I, et al. Microbial Metabolite Sensor GPR43 Controls Severity of Experimental GVHD. Nat Commun (2018) 9:3674. doi: 10.1038/s41467-018-06048-w
131. Legoff J, Resche-Rigon M, Bouquet J, Robin M, Naccache SN, Mercier-Delarue S, et al. The Eukaryotic Gut Virome in Hematopoietic Stem Cell Transplantation: New Clues in Enteric Graft-Versus-Host Disease. Nat Med (2017) 23:1080–5. doi: 10.1038/nm.4380
132. Andermann TM, Peled JU, Ho C, Reddy P, Riches M, Storb R, et al. Blood and N. Marrow Transplant Clinical Trials, the Microbiome and Hematopoietic Cell Transplantation: Past, Present, and Future. Biol Blood Marrow Transplant (2018) 24:1322–40. doi: 10.1016/j.bbmt.2018.02.009
133. Wu SR, Reddy P. Tissue Tolerance: A Distinct Concept to Control Acute GVHD Severity. Blood (2017) 129:1747–52. doi: 10.1182/blood-2016-09-740431
134. Hill GR, Cooke KR, Teshima T, Crawford JM, Keith JC Jr., Brinson YS, et al. Interleukin-11 Promotes T Cell Polarization and Prevents Acute Graft-Versus-Host Disease After Allogeneic Bone Marrow Transplantation. J Clin Invest (1998) 102:115–23. doi: 10.1172/JCI3132
135. Teshima T, Hill GR, Pan L, Brinson YS, van den Brink MR, Cooke KR, et al. IL-11 Separates Graft-Versus-Leukemia Effects From Graft-Versus-Host Disease After Bone Marrow Transplantation. J Clin Invest (1999) 104:317–25. doi: 10.1172/JCI7111
136. Krijanovski OI, Hill GR, Cooke KR, Teshima T, Crawford JM, Brinson YS, et al. Keratinocyte Growth Factor Separates Graft-Versus-Leukemia Effects From Graft-Versus-Host Disease. Blood (1999) 94:825–31. doi: 10.1182/blood.V94.2.825
137. Antin JH, Lee SJ, Neuberg D, Alyea E, Soiffer RJ, Sonis S, et al. A Phase I/II Double-Blind, Placebo-Controlled Study of Recombinant Human Interleukin-11 for Mucositis and Acute GVHD Prevention in Allogeneic Stem Cell Transplantation. Bone Marrow Transplant (2002) 29:373–7. doi: 10.1038/sj.bmt.1703394
138. Furlan SN, Watkins B, Tkachev V, Flynn R, Cooley S, Ramakrishnan S, et al. Transcriptome Analysis of GVHD Reveals Aurora Kinase a as a Targetable Pathway for Disease Prevention. Sci Trans Med (2015) 7:315ra191. doi: 10.1126/scitranslmed.aad3231
139. Hayase E, Hashimoto D, Nakamura K, Noizat C, Ogasawara R, Takahashi S, et al. R-Spondin1 Expands Paneth Cells and Prevents Dysbiosis Induced by Graft-Versus-Host Disease. J Exp Med (2017) 214:3507–18. doi: 10.1084/jem.20170418
140. Lindemans CA, Calafiore M, Mertelsmann AM, O’Connor MH, Dudakov JA, Jenq RR, et al. Interleukin-22 Promotes Intestinal-Stem-Cell-Mediated Epithelial Regeneration. Nature (2015) 528:560–4. doi: 10.1038/nature16460
141. Norona J, Apostolova P, Schmidt D, Ihlemann R, Reischmann N, Taylor G, et al. Glucagon-Like Peptide 2 for Intestinal Stem Cell and Paneth Cell Repair During Graft-Versus-Host Disease in Mice and Humans. Blood (2020) 136:1442–55. doi: 10.1182/blood.2020005957
142. Eriguchi Y, Nakamura K, Yokoi Y, Sugimoto R, Takahashi S, Hashimoto D, et al. Essential Role of IFN-Gamma in T Cell-Associated Intestinal Inflammation. JCI Insight (2018) 3(18):e121886. doi: 10.1172/jci.insight.121886
143. Takashima S, Martin ML, Jansen SA, Fu Y, Bos J, Chandra D, et al. T Cell-Derived Interferon-Gamma Programs Stem Cell Death in Immune-Mediated Intestinal Damage. Sci Immunol (2019) 4(42):eaay8556. doi: 10.1126/sciimmunol.aay8556
144. Zeiser R, Burchert A, Lengerke C, Verbeek M, Maas-Bauer K, Metzelder SK, et al. Ruxolitinib in Corticosteroid-Refractory Graft-Versus-Host Disease After Allogeneic Stem Cell Transplantation: A Multicenter Survey. Leukemia (2015) 29:2062–8. doi: 10.1038/leu.2015.212
145. Takahashi S, Hashimoto D, Hayase E, Ogasawara R, Ohigashi H, Ara T, et al. Ruxolitinib Protects Skin Stem Cells and Maintains Skin Homeostasis in Murine Graft-Versus-Host Disease. Blood (2018) 131:2074–85. doi: 10.1182/blood-2017-06-792614
146. Jagasia MH, Greinix HT, Arora M, Williams KM, Wolff D, Cowen EW, et al. National Institutes of Health Consensus Development Project on Criteria for Clinical Trials in Chronic Graft-Versus-Host Disease: I. The 2014 Diagnosis and Staging Working Group Report. Biol Blood marrow Transplant (2015) 21:389–401.e1. doi: 10.1016/j.bbmt.2015.02.025
147. Panoskaltsis-Mortari A, Tram KV, Price AP, Wendt CH, Blazar BR. A New Murine Model for Bronchiolitis Obliterans Post-Bone Marrow Transplant. Am J Respir Crit Care Med (2007) 176:713–23. doi: 10.1164/rccm.200702-335OC
148. Jaffee BD, Claman HN. Chronic Graft-Versus-Host Disease (GVHD) as a Model for Scleroderma. I. Description of Model Systems. Cell Immunol (1983) 77:1–12. doi: 10.1016/0008-8749(83)90001-1
149. Hill GR, Olver SD, Kuns RD, Varelias A, Raffelt NC, Don AL, et al. Stem Cell Mobilization With G-CSF Induces Type 17 Differentiation and Promotes Scleroderma. Blood (2010) 116:819–28. doi: 10.1182/blood-2009-11-256495
150. Zhang C, Todorov I, Zhang Z, Liu Y, Kandeel F, Forman S, et al. Donor CD4+ T and B Cells in Transplants Induce Chronic Graft-Versus-Host Disease With Autoimmune Manifestations. Blood (2006) 107:2993–3001. doi: 10.1182/blood-2005-09-3623
151. Young JS, Wu T, Chen Y, Zhao D, Liu H, Yi T, et al. Donor B Cells in Transplants Augment Clonal Expansion and Survival of Pathogenic CD4+ T Cells That Mediate Autoimmune-Like Chronic Graft-Versus-Host Disease. J Immunol (2012) 189:222–33. doi: 10.4049/jimmunol.1200677
152. Srinivasan M, Flynn R, Price A, Ranger A, Browning JL, Taylor PA, et al. Donor B-Cell Alloantibody Deposition and Germinal Center Formation Are Required for the Development of Murine Chronic GVHD and Bronchiolitis Obliterans. Blood (2012) 119:1570–80. doi: 10.1182/blood-2011-07-364414
153. Flynn R, Du J, Veenstra RG, Reichenbach DK, Panoskaltsis-Mortari A, Taylor PA, et al. Increased T Follicular Helper Cells and Germinal Center B Cells Are Required for Cgvhd and Bronchiolitis Obliterans. Blood (2014) 123:3988–98. doi: 10.1182/blood-2014-03-562231
154. McDonald-Hyman C, Flynn R, Panoskaltsis-Mortari A, Peterson N, MacDonald KP, Hill GR, et al. Therapeutic Regulatory T-Cell Adoptive Transfer Ameliorates Established Murine Chronic GVHD in a CXCR5-Dependent Manner. Blood (2016) 128:1013–7. doi: 10.1182/blood-2016-05-715896
155. Gartlan KH, Bommiasamy H, Paz K, Wilkinson AN, Owen M, Reichenbach DK, et al. A Critical Role for Donor-Derived IL-22 in Cutaneous Chronic GVHD. Am J Transplant (2018) 18:810–20. doi: 10.1111/ajt.14513
156. MacDonald KP, Blazar BR, Hill GR. Cytokine Mediators of Chronic Graft-Versus-Host Disease. J Clin Invest (2017) 127:2452–63. doi: 10.1172/JCI90593
157. Yamakawa T, Ohigashi H, Hashimoto D, Hayase E, Takahashi S, Miyazaki M, et al. Vitamin a-Coupled Liposomes Containing Sirna Against HSP47 Ameliorate Skin Fibrosis in Chronic Graft-Versus-Host Disease. Blood (2018) 131:1476–85. doi: 10.1182/blood-2017-04-779934
158. Miklos D, Cutler CS, Arora M, Waller EK, Jagasia M, Pusic I, et al. Ibrutinib for Chronic Graft-Versus-Host Disease After Failure of Prior Therapy. Blood (2017) 130:2243–50. doi: 10.1182/blood-2017-07-793786
159. Zorn E, Kim HT, Lee SJ, Floyd BH, Litsa D, Arumugarajah S, et al. Reduced Frequency of FOXP3+ CD4+CD25+ Regulatory T Cells in Patients With Chronic Graft-Versus-Host Disease. Blood (2005) 106:2903–11. doi: 10.1182/blood-2005-03-1257
160. Matsuoka K, Kim HT, McDonough S, Bascug G, Warshauer B, Koreth J, et al. Altered Regulatory T Cell Homeostasis in Patients With CD4+ Lymphopenia Following Allogeneic Hematopoietic Stem Cell Transplantation. J Clin Invest (2010) 120:1479–93. doi: 10.1172/JCI41072
161. Matsuoka K, Koreth J, Kim HT, Bascug G, McDonough S, Kawano Y, et al. Low-Dose Interleukin-2 Therapy Restores Regulatory T Cell Homeostasis in Patients With Chronic Graft-Versus-Host Disease. Sci Trans Med (2013) 5:179ra43. doi: 10.1126/scitranslmed.3005265
162. Koreth J, Matsuoka K, Kim HT, McDonough SM, Bindra B, Alyea EP 3rd. P. Armand, C. Cutler, V.T. Ho, N.s. Treister, D.C. Bienfang, s. Prasad, D. Tzachanis, R.M. Joyce, D.E. Avigan, J.H. Antin, J. Ritz, and R.J. Soiffer, Interleukin-2 and Regulatory T Cells in Graft-Versus-Host Disease. N Engl J Med (2011) 365:2055–66. doi: 10.1056/NEJMoa1108188
163. Deng R, Hurtz C, Song Q, Yue C, Xiao G, Yu H, et al. Extrafollicular CD4(+) T-B Interactions Are Sufficient for Inducing Autoimmune-Like Chronic Graft-Versus-Host Disease. Nat Commun (2017) 8:978. doi: 10.1038/s41467-017-00880-2
164. Flynn R, Paz K, Du J, Reichenbach DK, Taylor PA, Panoskaltsis-Mortari A, et al. Targeted Rho-Associated Kinase 2 Inhibition Suppresses Murine and Human Chronic GVHD Through a Stat3-Dependent Mechanism. Blood (2016) 127:2144–54. doi: 10.1182/blood-2015-10-678706
165. Flynn R, Allen JL, Luznik L, MacDonald KP, Paz K, Alexander KA, et al. Targeting Syk-Activated B Cells in Murine and Human Chronic Graft-Versus-Host Disease. Blood (2015) 125:4085–94. doi: 10.1182/blood-2014-08-595470
166. Leonhardt F, Zirlik K, Buchner M, Prinz G, Hechinger AK, Gerlach UV, et al. Spleen Tyrosine Kinase (Syk) Is a Potent Target for Gvhd Prevention at Different Cellular Levels. Leukemia (2012) 26:1617–29. doi: 10.1038/leu.2012.10
167. Hinz B, Phan SH, Thannickal VJ, Galli A, Bochaton-Piallat ML, Gabbiani G. The Myofibroblast: One Function, Multiple Origins. Am J Pathol (2007) 170:1807–16. doi: 10.2353/ajpath.2007.070112
168. Wynn TA, Ramalingam TR. Mechanisms of Fibrosis: Therapeutic Translation for Fibrotic Disease. Nat Med (2012) 18:1028–40. doi: 10.1038/nm.2807
169. Alexander KA, Flynn R, Lineburg KE, Kuns RD, Teal BE, Olver SD, et al. CSF-1-Dependant Donor-Derived Macrophages Mediate Chronic Graft-Versus-Host Disease. J Clin Invest (2014) 124:4266–80. doi: 10.1172/JCI75935
170. MacDonald KP, Hill GR, Blazar BR. Chronic Graft-Versus-Host Disease: Biological Insights From Preclinical and Clinical Studies. Blood (2017) 129:13–21. doi: 10.1182/blood-2016-06-686618
171. Du J, Paz K, Flynn R, Vulic A, Robinson TM, Lineburg KE, et al. Pirfenidone Ameliorates Murine Chronic GVHD Through Inhibition of Macrophage Infiltration and TGF-Beta Production. Blood (2017) 129:2570–80. doi: 10.1182/blood-2017-01-758854
172. Ohigashi H, Hashimoto D, Hayase E, Takahashi S, Ara T, Yamakawa T, et al. Ocular Instillation of Vitamin a-Coupled Liposomes Containing HSP47 Sirna Ameliorates Dry Eye Syndrome in Chronic GVHD. Blood Adv (2019) 3:1003–10. doi: 10.1182/bloodadvances.2018028431
Keywords: graft-versus-host disease, animal models, pathophysiology, history, treatment
Citation: Teshima T and Hill GR (2021) The Pathophysiology and Treatment of Graft-Versus-Host Disease: Lessons Learnt From Animal Models. Front. Immunol. 12:715424. doi: 10.3389/fimmu.2021.715424
Received: 26 May 2021; Accepted: 26 July 2021;
Published: 19 August 2021.
Edited by:
Xue-Zhong Yu, Medical University of South Carolina, United StatesReviewed by:
Daniel Wolff, University Hospital Regensburg, GermanyHaiyan Liu, National University of Singapore, Singapore
Yi Zhang, Temple University, United States
Copyright © 2021 Teshima and Hill. This is an open-access article distributed under the terms of the Creative Commons Attribution License (CC BY). The use, distribution or reproduction in other forums is permitted, provided the original author(s) and the copyright owner(s) are credited and that the original publication in this journal is cited, in accordance with accepted academic practice. No use, distribution or reproduction is permitted which does not comply with these terms.
*Correspondence: Geoffrey R. Hill, Z3JoaWxsQGZyZWRodXRjaC5vcmc=; Takanori Teshima, dGVzaGltYUBtZWQuaG9rdWRhaS5hYy5qcA==