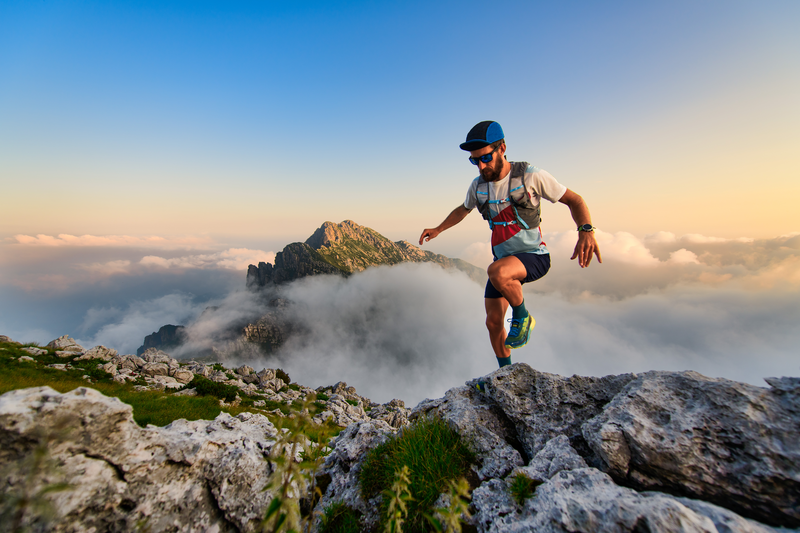
94% of researchers rate our articles as excellent or good
Learn more about the work of our research integrity team to safeguard the quality of each article we publish.
Find out more
MINI REVIEW article
Front. Immunol. , 12 July 2021
Sec. Inflammation
Volume 12 - 2021 | https://doi.org/10.3389/fimmu.2021.714042
This article is part of the Research Topic Molecular and Cellular Effectors in the Resolution of Inflammation View all 33 articles
Bioactive lipid mediators play a major role in regulating inflammatory processes. Herein, early pro-inflammatory phases are characterized and regulated by prostanoids and leukotrienes, whereas specialized pro-resolving mediators (SPM), including lipoxins, resolvins, protectins, and maresins, dominate during the resolution phase. While pro-inflammatory properties of prostanoids have been studied extensively, their impact on later phases of the inflammatory process has been attributed mainly to their ability to initiate the lipid-mediator class switch towards SPM. Yet, there is accumulating evidence that prostanoids directly contribute to the resolution of inflammation and return to homeostasis. In this mini review, we summarize the current knowledge of the resolution-regulatory properties of prostanoids and discuss potential implications for anti-inflammatory, prostanoid-targeted therapeutic interventions.
Inflammation is an integral part of protective host responses against pathogens or injury (1). Herein, inflammatory processes usually follow a defined sequence of reactions characterized by the rapid induction of a pro-inflammatory response, which is closely followed by an anti-inflammatory response. To regain homeostasis resolution of inflammation represents an integral and crucial part of acute inflammation. In fact, failure to completely resolve inflammatory processes is associated with the emergence of chronic inflammatory conditions. While resolution was considered to merely represent the downregulation or inactivation of inflammatory mediators for a long time, it is now appreciated to be active and complex, involving the formation of pro-resolving mediators (2). Moreover, it is widely accepted that resolution processes are initiated early during inflammation, and classical pro-inflammatory mediators have been shown to directly impact on resolution as well (3). Therefore, pro-resolving therapeutic approaches are increasingly being considered rather than anti-inflammatory ones as the latter might diminish the host response against pathogenic challenges (4, 5). Since lipid mediators play a crucial, yet often ambivalent role during the inflammatory process, and prostanoid synthesis represents a major target for anti-inflammatory therapies, the present mini review aims to recapitulate the current understanding of the role of prostanoids in the context of resolution of inflammation.
Upon an insult (e.g., pathogen contact, injury) local resident immune cells are the first responders. Among these, tissue resident macrophages (MΦ) represent not only an important first line of defense but even more importantly they establish an environment prone to recruit neutrophils and monocytes from the circulation. Neutrophils are main executers in the acute inflammatory environment, i.e. they produce high amounts of reactive oxygen and nitrogen species to eliminate pathogens, and secrete a plethora of inflammatory mediators (6). Within the inflammatory environment neutrophils rapidly die by apoptotic processes and are phagocytosed by MΦ in a process termed efferocytosis (7, 8). Importantly, uptake of apoptotic cells (AC) induces a shift in MΦ polarization from a pro-inflammatory to an anti-inflammatory and immunomodulatory phenotype (9–14). MΦ and other antigen-presenting cells such as dendritic cells (DC) eventually activate adaptive immune responses, which ensures complete removal of the invading pathogens and enables accelerated responses upon anew contact to the triggering stimulus (15). The return to cellular homeostasis further requires successful emigration of infiltrated immune cells from the cleared site of inflammation (16). In contrast to the concept of a rapid return to homeostasis, recent reports provided evidence that resolution is followed by a longer lasting, immune-suppressive post-resolution phase characterized by infiltrating regulatory T cells (Treg) and myeloid-derived suppressor cells (MDSC), but also MΦ, which might be decisive for a successful adaptation vs. chronic inflammation and/or autoimmunity (17–19).
Regulation and execution of inflammatory reactions is mediated by soluble mediators, such as cytokines and chemokines. In addition, bioactive lipids emerged as crucial factors during all phases of the inflammatory process (20, 21). Specifically, while leukotrienes and prostaglandins appear early during the onset of inflammation, specialized pro-resolving mediators (SPM), such as lipoxins, resolvins, and maresins, are produced later on, facilitating the resolution of inflammation (22). Prostanoids, like leukotrienes, belong to the eicosanoid family of lipid mediators (23). Both classes are synthesized from arachidonic acid (AA) after the latter is released from membrane phospholipids by phospholipase A2 (24). While leukotrienes are synthesized by the lipoxygenases, prostanoid formation initially requires conversion of AA to the unstable prostaglandin H2 (PGH2) via the bifunctional cyclooxygenases 1 (Cox-1) and 2 (25, 26). In line with the established pro-inflammatory function of the prostanoids, the cyclooxgenases became an important target in the therapy of inflammatory diseases, and as of today, non-steroidal anti-inflammatory drugs (NSAIDs) rank amongst the most important anti-inflammatory drugs (27, 28). Of note, while Cox-1 is constitutively expressed in most cells, Cox-2 often is inducible and emerged as the more important cyclooxygenase in the context of inflammation. Consequently Cox inhibitors (Coxibs) selectively targeting Cox-2 emerged as promising novel anti-inflammatory therapeutics (29, 30). The short-lived Cox product PGH2 is then substrate to specific synthases, which produce potent prostanoids including PGD2, PGE2, PGF2α, and PGI2, as well as thromboxane A2 (TXA2) (Figure 1).
Figure 1 Prostanoid synthesis. Arachidonic acid, liberated from membrane phospholipids by phospholipase A2, is converted to prostaglandin H2 (PGH2) by the dual peroxidase/cyclooxygenase activity of the cyclooxygenases (Cox-1, Cox-2). PGH2 serves as the substrate for the terminal synthases to produce PGD2, PGE2, PGF2α, PGI2, and thromboxane A2 (TXA2). PGD2 is further dehydrated and isomerized spontaneously to 15-deoxy−Δ12,14-PGJ2 (15d-PGJ2). Alternatively, arachidonic acid can be converted to lipoxins, i.e. SPM, via the activity of the lipoxygenases (LO). L-PGDS, lipocalin-type PGD synthase; H-PGDS, hematopoietic-type PGDS; mPGES, microsomal PGES; cPGES, cytosolic PGES; TXAS, TXA synthase.
Interestingly, the production of the different lipid mediators appears to be tightly connected across the course of inflammation. For example, the presumably pro-inflammatory PGE2 was shown to attenuate the synthesis of leukotrienes and to induce the production of SPM, thus initiating the so-called lipid-mediator class switch (31). While the SPM-regulatory impact of prostanoids on the resolution of inflammation is rather well characterized, there is accumulating evidence that prostanoids also directly impinge on other aspects of the resolution process. In the following sections, we will therefore summarize the current understanding of the role of the most prominent prostanoids in resolution of inflammation, aside from the aforementioned lipid-mediator class switch, with a special focus on PGE2.
TXA2 is predominantly produced by platelets, but also at appreciable amounts by MΦ (32). Interestingly, while thromboxane A synthase (TXAS) appears to be coupled to the activity of Cox-1 and Cox-2 constitutes the dominant Cox in inflammatory conditions, the activity of TXA2 is largely pro-inflammatory (33, 34). In fact, there is little evidence that TXA2 might contribute to the resolution of inflammation. In line, Kupffer cell-derived TXA2 was shown to contribute to liver fibrosis, a common outcome of ineffective resolution (35). The observation that TXA2 synthesis is induced by pro-inflammatory stimuli, while it is suppressed upon inflammatory restimulation (36), further suggest that the prevention of TXA2, e.g. via redirection of Cox-1-provided PGH2 towards PGE2 synthesis, might be part of the immune-suppressive environment typical for the post-resolution phase (18).
PGI2 (prostacyclin) has been characterized as the counterpart of TXA2 as it inhibits platelet aggregation and acts as a potent vaso- and bronchodilator (37). It is produced primarily by vascular endothelial and smooth muscle cells, yet other cells such as fibroblasts and dendritic cells also synthesize PGI2 (38). In the context of inflammation, PGI2 was shown to inhibit LPS-induced expression of pro-inflammatory cytokines in MΦ, DC, CD4+ T cells, and endothelial cells (39–42). PGI2 further synergizes with anti-inflammatory cytokines interleukin-4 (IL-4) and IL-13 to suppress pro-inflammatory cytokines (43). Along the same lines, PGI2 receptor (IP) deficient mice displayed stronger allergic inflammation, which was attributable to enhanced Th2 cell function (44). Thus, PGI2 emerges as a predominant anti-inflammatory mediator, positioning it also as a counterpart of TXA2 in the context of inflammation.
PGD2 is produced by numerous immune cells including activated MΦ, DC, Th2 cells, eosinophils, platelets, but also endothelial cells (45). However, since its main source are mast cells (46, 47), PGD2 has been characterized extensively in the context of allergic reactions (48). PGD2 can further be metabolized to the cyclopentenone, PGJ2-type prostanoids, including PGJ2 and 15d-PGJ2, which also display biological activity in the context of resolution of inflammation (49). PGD2 binds to the PGD2 receptors 1 (DP1) and DP2 [also known as chemoattractant receptor-homologous molecule expressed on Th2 cells (CRTH2)] (50). Activation of DP2 is of specific importance in allergic inflammation, where mast cell-derived PGD2 stimulates the recruitment of innate lymphoid type 2 cells (51) and Th2 cells (52), and causes activation of these as well as of basophils and eosinophils (53). While PGD2 was shown to contribute to allergic inflammation, PGD2 synthase (PGDS) decreases during the acute inflammatory phase, while it rises again at later stages corresponding to the resolution phase in animal models (54). PGD2 and 15d-PGJ2 both exert pro-inflammatory functions via CRTH2 (55). In contrast, PGD2-dependent activation of DP1 as well as 15d-PGJ2-mediated activation of peroxisome proliferator-activated receptor γ (PPARγ) inhibit the production of inflammatory cytokines and chemokines by antigen-presenting cells including DC or MΦ by interfering with inflammatory transcription factors nuclear factor kappa B (NFκB), activator protein 1 (AP1), and signal transducer and activator of transcription 3 (STAT3) (56–58) and additionally by enhancing the activity of anti-inflammatory nuclear factor erythroid 2-like 2 (Nrf2) (59, 60). Consequently, PGD2 and 15d-PGJ2 support emigration of MΦ to the draining lymph nodes and attenuate the recruitment of leukocytes (54). They further inhibit effector functions of and induce apoptosis in T lymphocytes (57). Thus, PGD2 contributes to normalize the local environment, an important aspect of the resolution of inflammation (61).
PGF2α is produced by the aldo-keto reductase (AKR) 1C3, also known as PGF2α synthase (PGFS) using PGH2 or PGD2 (62). Alternatively, PGE2 can be converted to PGF2α by AKR1C1 or AKR1C2 (63). While PGF2α is synthesized in most tissues (64), its prime site of production is the female reproductive system (65), where PGF2α has been shown to be of functional importance (66, 67). Nevertheless, PGF2α also appears to be involved in inflammatory processes (64, 68). PGF2α is elevated in rheumatoid arthritis (69) and was shown to contribute to and correlate with fibrosis (70, 71), which is characteristic for insufficient resolution. Interestingly though, PGFS expression and concomitantly PGF2α levels decrease similar to PGD2 during acute inflammation, only to increase again in the resolution phase (72), which might be indicative for an active role of PGF2α in the resolution of inflammation. Yet, the exact role of PGF2α in inflammation-resolving processes remains to be determined.
The best characterized and presumably most important prostanoid in the context of inflammation is PGE2. It can be synthesized by all cell types. In the context of inflammation the prime producers are fibroblasts, epithelial cells, and immune cells (73). PGE2 exerts its functions via four G-protein-coupled PGE2 receptors (EP1-4) (74). While EP2 and 4 represent Gαs-coupled receptors increasing cAMP levels upon activation (75), the Gαi-coupled EP3 variants inhibit adenylate cyclase, thus reducing cAMP (76), and Gαq EP1 enhances intracellular Ca2+ levels (74). The distinct downstream signals as well as the cell type-specific distribution of the receptors, and their differential sensitivity for PGE2 account for the diverse functions of PGE2 also in inflammation (77).
Upon inflammatory stimulation, both the expression of Cox-2 and microsomal PGE2 synthase 1 (mPGES-1) are induced in MΦ (78), skewing the prostanoid spectrum towards PGE2 production in the acute inflammatory phase (73). PGE2 signals towards enhanced recruitment of neutrophils, MΦ, and mast cells (79–81) and enhances the expression and secretion of pro-inflammatory cytokines in DC, MΦ, and T cells (82–84). Its NFκB-activating properties further support neutrophil survival, thereby extending the pro-inflammatory impact of neutrophils in the inflammatory niche (85, 86). Yet, this initial increase in PGE2 is only moderate and transient in character, and PGE2 levels rise again during the resolution phase, eventually increasing to much higher levels in the post-resolution phase (18, 87, 88). This seemingly biphasic regulation of PGE2 might at least in part be due to a shift from transcriptional to post-transcriptional programs governing not only PGE2 production, but more generally the course of inflammation (89). With respect to the regulation of PGE2 synthesis, Cox-2 rapidly accumulates during early inflammation. In a negative feedback loop, elevated PGE2 induces the expression of dual specificity phosphatase 1 (DUSP1), thereby enhancing the activity of the RNA-binding protein tristetraprolin (TTP), which destabilizes the mRNA of Cox-2, but also of pro-inflammatory tumor necrosis factor (TNF) (90). The second wave of PGE2 production by MΦ appears to be facilitated by another increase of Cox-2 expression induced by sphingosine-1-phosphate released from apoptotic cells, which activates the RNA-stabilizing protein human antigen R (HuR) in MΦ to increase Cox-2 expression (91). As a side note, while PGE2 is predominantly produced in a Cox-2/mPGES-1 dependent manner during the inflammatory and early resolution phase, PGE2 levels during the post-resolution phase are approx. 3-fold higher, which is due to enhanced Cox-1/mPGES-1 expression in MΦ (18). While these findings might explain the kinetics of PGE2 production and even some of the inhibitory effects of PGE2 on pro-inflammatory mediators, further evidence for an immunosuppressive function of MΦ-derived PGE2 emerged in the tumor context, where PGE2 inhibits CD80 expression via EP2, thereby attenuating activation of cytotoxic T cells (92, 93). Similarly, PGE2 suppresses cytolytic functions of NK cells (94, 95) and inhibits phagocytic and bacteria killing activities of MΦ, thus preventing the establishment of appropriate inflammatory, anti-microbial responses largely via EP2-dependent cAMP induction (96–98). In line, EP2- and EP4-signaling limits secretion of TNF and IL-1β, and enhances expression of anti-inflammatory IL-10 in response to LPS by microglia (99), i.e. resident MΦ of the central nervous system (100). In general, EP2- and EP4-activation by PGE2 appears to be crucial to establish an anti-inflammatory and resolving MΦ phenotype (101, 102), which is characterized by further immune-modulatory factors such as transforming growth factor β (TGFβ) (103). Nevertheless, there are contradictory reports regarding the exact impact of PGE2 on T cell functions. On the one hand, PGE2 appears to inhibit IL-2 production by T cells, thereby attenuating both T cell activation and activation-dependent apoptosis (104–106). On the other hand, while PGE2 appears to contribute to sustained inflammation by differentiation and activation of Th1 and γδ T cells, considered to support inflammation (107–109), other findings indicate that PGE2 selectively inhibits Th1 cytokine production leaving Th2 cytokines, such as IL-4 and IL-5, unaffected (110, 111), thus provoking a PGE2-dependent shift towards Th2 responses, which are associated with repair mechanisms instead (112–114). This notion is substantiated by the high levels of PGE2 observed in Th2-driven diseases such as atopic allergy (115). Indeed, the intricate impact of PGE2 on the balance between different T cell subtypes might be one of the key mechanisms how PGE2 affects a self-limiting inflammation throughout resolution and post-resolution phases. Elevated PGE2 impairs interferon γ (IFN γ) synthesis, thereby directly attenuating Th1 responses, while leaving Th2 responses unaltered (110, 111). PGE2 further favors Th17 responses via EP2 and EP4 by shifting the IL-12/IL-23 balance towards Th17-supportive IL-23 (116–118). While Th17 cells are largely inflammatory in nature contributing to severe inflammatory diseases (107, 119), they have been shown to be highly plastic, being able to trans-differentiate into Treg thereby contributing to resolution of inflammation (120). Yet, PGE2 not only promotes differentiation of naïve T cells or Th17 cells towards Tregs (121, 122), it also supports further expansion of differentiated Tregs (123). Conclusively, the concentration, source, and timing of PGE2 appear critical to determine the exact T cell response in the context of inflammatory responses. Moreover, while the pro-resolving activity of cyclooxygenase metabolites has long been attributed predominantly to PGD2 and 15d-PGJ2 (124), PGE2 emerged as an important facilitator of the lipid-mediator class switch inducing not only the production of PGD2 and its derivatives, but also of the so-called specialized pro-resolving mediators (SPM) (20). SPM, synthesized by 15-lipoxygenase (ALOX15) (31, 125–127), are key players in the resolution of inflammation (114, 128). PGE2 induces the expression of the relevant lipoxygenases, thereby skewing the balance towards a pro-resolving lipid mediator profile (129, 130). While SPM levels are mostly considered to reciprocally reflect PGE2 levels during the course of inflammation, they in fact coexist (131). The exact temporal and spatial distribution of both PGE2 and SPM might eventually determine the course of the resolution of inflammation. Of note, SPM also have been shown to affect the T cell balance (132–134). Yet, there is mounting evidence that PGE2 also directly supports further resolution-associated functions. Along these lines, PGE2 has been shown to inhibit pro-inflammatory cytokine production (99, 135) and to stimulate the expression of anti-inflammatory cytokines (136, 137), thereby contributing to the early steps of the resolution process (138). Furthermore, blocking PGE2 synthesis attenuated efficient resolution in a peritonitis model by preventing the emigration of MΦ from the site of inflammation in a CX3CL1-dependent manner (87). Extending beyond its impact on the resolution phase, PGE2 contributes to the immune suppressive post-resolution phase, where PGE2 suppresses innate immune responses, inhibits lymphocyte functions, and contributes to the generation and activity of Treg (121, 139) and MDSC (140). While these immune suppressive effects appear negative in the context of novel infections, they lower the risk of autoimmune responses (18, 19) (Figure 2). The complexity of the resolution of inflammation both at cellular as well as (lipid) mediator level, highlights the need for further studies unraveling details of the resolution phase to allow for the development of refined therapeutic intervention strategies, especially for chronic inflammatory diseases lacking proper resolution mechanisms.
Figure 2 PGE2 in the context of inflammation. An inflammatory insult is recognized by resident immune cells such as resident MΦ. Upon inflammatory stimulation Cox-2 and mPGES-1 are induced in MΦ resulting in increased PGE2 synthesis. PGE2 then contributes to the MΦ-mediated recruitment of neutrophils, which act as a first line of defense to eliminate the pathogenic stimulus and incite further inflammatory responses. Already at this early stage of the inflammatory process PGE2 initiates the lipid mediator class switch towards the production of specialized pro-resolving mediators (SPM) including lipoxins, resolvins, maresins, and protectins e.g. in neutrophils. Neutrophils are rapidly followed by monocytes again facilitated by PGE2, which upon infiltration into the affected tissue differentiate into pro-inflammatorily activated MΦ and release soluble mediators including cytokines and chemokines, as well as further PGE2. PGE2-triggered transcriptional programs eventually induce post-transcriptional feedback circuits, which reduce Cox-2 mRNA stability and thus protein expression to eventually inhibit PGE2 production, and also attenuate the expression of pro-inflammatory cytokines such as TNF. In addition, anti-inflammatory mediators (including IL-10) are induced restricting the intensity of the inflammatory reactions. Within the inflammatory niche, activated neutrophils rapidly undergo apoptotic cell death and are phagocytosed by MΦ, which causes a shift in MΦ polarization towards an alternatively activated, immune-modulatory, resolution phenotype characterized by the secretion of e.g. TGFβ. Apoptotic cells further release sphingosine-1-phosphate (S1P), which enhances the mRNA stability of Cox-2 again, resulting in increasing PGE2 production. Within the resolving environment PGE2 supports a shift from Th1 T cells to the repair-associated Th2-type further supporting tissue normalization. In addition, PGE2 attenuates expression of CX3CL1 in MΦ, thereby eventually allowing their emigration from the resolving tissue. PGE2 further supports the recruitment of T cells and myeloid cells, which differentiate into regulatory T cells and myeloid-derived suppressor cells, respectively, thereby establishing an immune-suppressive post-resolution environment. Lower part: During inflammation, PGE2 levels transiently increase in the acute inflammatory phase. After a decrease during the anti-inflammatory and the early resolution phase, PGE2 tends to increase again during the progress of resolution, reaching highest levels in the post-resolution phase. AC, apoptotic cells; MΦ, macrophages (gray – naïve, resident; red – pro-inflammatory; blue – anti-inflammatory; green – resolution phase); MDSC, myeloid-derived suppressor cells; MO, monocytes; PMN, neutrophils (gray – naïve, red – inflammatory); T cells (red – Th1; blue – Th2; green – Treg); ⇨, infiltration/emigration; →, development within the inflammatory niche.
Cox inhibitors are amongst the most widely used over the counter anti-inflammatory drugs worldwide (141). Despite the undisputed beneficial effects of the broad spectrum Cox inhibitors, specific Cox-2 inhibitors (Coxibs) were developed to more selectively interfere with the production of inflammation-associated prostanoids (142). Due to the prominent role of PGE2 in the establishment of inflammatory processes, recent therapeutic approaches aimed at inhibiting the inflammation-associated terminal PGE2 synthase mPGES-1 to selectively block the production of PGE2 only (73, 143, 144). Yet, considering the major impact of PGE2 on successful resolution of inflammation, therapeutic approaches targeting PGE2 synthesis should be critically revisited as continued PGE2 inhibition in inflammatory diseases might be expected to lead to chronic diseases due to insufficient resolution. In fact, even attenuating inflammation itself might interfere with successful resolution, since the process of resolution is initiated already very early during the inflammatory process (3). Thus, it might be warranted to focus on therapeutic approaches promoting resolution rather than on anti-inflammatory ones in the future (4, 145). Indeed, there are numerous efforts to target SPM production or receptors (146). Considering promising effects of PGE2 receptor antagonists (e.g. EP4) in the context of chronic inflammatory diseases (147), it will be interesting to see combinatorial approaches in the future.
TS and BB wrote and edited the manuscript. All authors contributed to the article and approved the submitted version.
This work was supported by grants of the DFG (GRK 2336 TP6, SCHM2663/7, SFB1039 B04).
The authors declare that the research was conducted in the absence of any commercial or financial relationships that could be construed as a potential conflict of interest.
1. Medzhitov R. Origin and Physiological Roles of Inflammation. Nature (2008) 454(7203):428–35. doi: 10.1038/nature07201
2. Buckley CD, Gilroy DW, Serhan CN, Stockinger B, Tak PP. The Resolution of Inflammation. Nat Rev Immunol (2013) 13(1):59–66. doi: 10.1038/nri3362
3. Serhan CN, Savill J. Resolution of Inflammation: The Beginning Programs the End. Nat Immunol (2005) 6(12):1191–7. doi: 10.1038/ni1276
4. Fullerton JN, Gilroy DW. Resolution of Inflammation: A New Therapeutic Frontier. Nat Rev Drug Discov (2016) 15(8):551–67. doi: 10.1038/nrd.2016.39
5. Dalli J. Does Promoting Resolution Instead of Inhibiting Inflammation Represent the New Paradigm in Treating Infections? Mol Aspects Med (2017) 58:12–20. doi: 10.1016/j.mam.2017.03.007
6. Smith JA. Neutrophils, Host Defense, and Inflammation: A Double-Edged Sword. J Leukoc Biol (1994) 56(6):672–86. doi: 10.1002/jlb.56.6.672
7. Savill JS, Wyllie AH, Henson JE, Walport MJ, Henson PM, Haslett C. Macrophage Phagocytosis of Aging Neutrophils in Inflammation. Programmed Cell Death in the Neutrophil Leads to its Recognition by Macrophages. J Clin Invest (1989) 83(3):865–75. doi: 10.1172/JCI113970
8. Fadok VA, Voelker DR, Campbell PA, Cohen JJ, Bratton DL, Henson PM. Exposure of Phosphatidylserine on the Surface of Apoptotic Lymphocytes Triggers Specific Recognition and Removal by Macrophages. J Immunol (Baltimore Md: 1950) (1992) 148(7):2207–16.
9. Fadok VA, Bratton DL, Konowal A, Freed PW, Westcott JY, Henson PM. Macrophages That Have Ingested Apoptotic Cells In Vitro Inhibit Proinflammatory Cytokine Production Through Autocrine/Paracrine Mechanisms Involving TGF-Beta, PGE2, and PAF. J Clin Invest (1998) 101(4):890–8. doi: 10.1172/JCI1112
10. Johann AM, Knethen Av, Lindemann D, Brüne B. Recognition of Apoptotic Cells by Macrophages Activates the Peroxisome Proliferator-Activated Receptor-Gamma and Attenuates the Oxidative Burst. Cell Death Differ (2006) 13(9):1533–40. doi: 10.1038/sj.cdd.4401832
11. Weigert A, Johann AM, Knethen Av, Schmidt H, Geisslinger G, Brüne B. Apoptotic Cells Promote Macrophage Survival by Releasing the Antiapoptotic Mediator Sphingosine-1-Phosphate. Blood (2006) 108(5):1635–42. doi: 10.1182/blood-2006-04-014852
12. Mora J, Schlemmer A, Wittig I, Richter F, Putyrski M, Frank A-C. Et alInterleukin-38 Is Released From Apoptotic Cells to Limit Inflammatory Macrophage Responses. J Mol Cell Biol (2016) 8(5):426–38. doi: 10.1093/jmcb/mjw006
13. Kourtzelis I, Li X, Mitroulis I, Grosser D, Kajikawa T, Wang B, et al. DEL-1 Promotes Macrophage Efferocytosis and Clearance of Inflammation. Nat Immunol (2019) 20(1):40–9. doi: 10.1038/s41590-018-0249-1
14. Watanabe S, Alexander M, Misharin AV, Budinger GRS. The Role of Macrophages in the Resolution of Inflammation. J Clin Invest (2019) 129(7):2619–28. doi: 10.1172/JCI124615
15. Newson J, Stables M, Karra E, Arce-Vargas F, Quezada S, Motwani M, et al. Resolution of Acute Inflammation Bridges the Gap Between Innate and Adaptive Immunity. Blood (2014) 124(11):1748–64. doi: 10.1182/blood-2014-03-562710
16. Sugimoto MA, Sousa LP, Pinho V, Perretti M, Teixeira MM. Resolution of Inflammation: What Controls Its Onset? Front Immunol (2016) 7:160. doi: 10.3389/fimmu.2016.00160
17. Rajakariar R, Lawrence T, Bystrom J, Hilliard M, Colville-Nash P, Bellingan G, et al. Novel Biphasic Role for Lymphocytes Revealed During Resolving Inflammation. Blood (2008) 111(8):4184–92. doi: 10.1182/blood-2007-08-108936
18. Newson J, Motwani MP, Kendall AC, Nicolaou A, Muccioli GG, Alhouayek M, et al. Inflammatory Resolution Triggers a Prolonged Phase of Immune Suppression Through COX-1/mPGES-1-Derived Prostaglandin E2. Cell Rep (2017) 20(13):3162–75. doi: 10.1016/j.celrep.2017.08.098
19. Motwani MP, Newson J, Kwong S, Richard-Loendt A, Colas R, Dalli J, et al. Prolonged Immune Alteration Following Resolution of Acute Inflammation in Humans. PLoS One (2017) 12(10):e0186964. doi: 10.1371/journal.pone.0186964
20. Buckley CD, Gilroy DW, Serhan CN. Proresolving Lipid Mediators and Mechanisms in the Resolution of Acute Inflammation. Immunity (2014) 40(3):315–27. doi: 10.1016/j.immuni.2014.02.009
21. Serhan CN, Haeggström JZ, Leslie CC. Lipid Mediator Networks in Cell Signaling: Update and Impact of Cytokines. FASEB J (1996) 10(10):1147–58. doi: 10.1096/fasebj.10.10.8751717
22. Serhan CN, Chiang N, Dalli J, Levy BD. Lipid Mediators in the Resolution of Inflammation. Cold Spring Harb Perspect Biol (2015) 7(2):a016311. doi: 10.1101/cshperspect.a016311
23. Korotkova M, Jakobsson P-J. Persisting Eicosanoid Pathways in Rheumatic Diseases. Nat Rev Rheumatol (2014) 10(4):229–41. doi: 10.1038/nrrheum.2014.1
24. Dennis EA. Phospholipase A2 in Eicosanoid Generation. Am J Respir Crit Care Med (2000) 161(2 Pt 2):S32–5. doi: 10.1164/ajrccm.161.supplement_1.ltta-7
25. Samuelsson B, Granström E, Green K, Hamberg M, Hammarström S. Prostaglandins. Annu Rev Biochem (1975) 44:669–95. doi: 10.1146/annurev.bi.44.070175.003321
26. Dubois RN, Abramson SB, Crofford L, Gupta RA, Simon LS, van de Putte LB, et al. Cyclooxygenase in Biology and Disease. FASEB J (1998) 12(12):1063–73. doi: 10.1096/fasebj.12.12.1063
27. Rainsford KD. Anti-Inflammatory Drugs in the 21st Century. Subcell Biochem (2007) 42:3–27. doi: 10.1007/1-4020-5688-5_1
28. Bacchi S, Palumbo P, Sponta A, Coppolino MF. Clinical Pharmacology of non-Steroidal Anti-Inflammatory Drugs: A Review. Antiinflamm Antiallergy Agents Med Chem (2012) 11(1):52–64. doi: 10.2174/187152312803476255
29. Mazaleuskaya LL, Ricciotti E. Druggable Prostanoid Pathway. Adv Exp Med Biol (2020) 1274:29–54. doi: 10.1007/978-3-030-50621-6_3
30. Ferrer MD, Busquets-Cortés C, Capó X, Tejada S, Tur JA, Pons A, et al. Cyclooxygenase-2 Inhibitors as a Therapeutic Target in Inflammatory Diseases. Curr Med Chem (2019) 26(18):3225–41. doi: 10.2174/0929867325666180514112124
31. Levy BD, Clish CB, Schmidt B, Gronert K, Serhan CN. Lipid Mediator Class Switching During Acute Inflammation: Signals in Resolution. Nat Immunol (2001) 2(7):612–9. doi: 10.1038/89759
32. Ricciotti E, FitzGerald GA. Prostaglandins and Inflammation. Arterioscler Thromb Vasc Biol (2011) 31(5):986–1000. doi: 10.1161/ATVBAHA.110.207449
33. Kobayashi T, Tahara Y, Matsumoto M, Iguchi M, Sano H, Murayama T, et al. Roles of Thromboxane A2 and Prostacyclin in the Development of Atherosclerosis in apoE-Deficient Mice. J Clin Invest (2004) 114(6):784–94. doi: 10.1172/JCI200421446
34. Nakahata N. Thromboxane A2: Physiology/Pathophysiology, Cellular Signal Transduction and Pharmacology. Pharmacol Ther (2008) 118(1):18–35. doi: 10.1016/j.pharmthera.2008.01.001
35. Miller AM, Masrorpour M, Klaus C, Zhang JX. LPS Exacerbates Endothelin-1 Induced Activation of Cytosolic Phospholipase A2 and Thromboxane A2 Production From Kupffer Cells of the Prefibrotic Rat Liver. J Hepatol (2007) 46(2):276–85. doi: 10.1016/j.jhep.2006.08.026
36. Naraba H, Murakami M, Matsumoto H, Shimbara S, Ueno A, Kudo I, et al. Segregated Coupling of Phospholipases A2, Cyclooxygenases, and Terminal Prostanoid Synthases in Different Phases of Prostanoid Biosynthesis in Rat Peritoneal Macrophages. J Immunol (Baltimore Md: 1950) (1998) 160(6):2974–82.
37. Camacho M, Rodríguez C, Guadall A, Alcolea S, Orriols M, Escudero J-R, et al. Hypoxia Upregulates PGI-Synthase and Increases PGI2 Release in Human Vascular Cells Exposed to Inflammatory Stimuli. J Lipid Res (2011) 52(4):720–31. doi: 10.1194/jlr.M011007
38. Dorris SL, Peebles RS. PGI2 as a Regulator of Inflammatory Diseases. Mediators Inflamm (2012) 2012:926968. doi: 10.1155/2012/926968
39. Luttmann W, Herzog V, Virchow JC, Matthys H, Thierauch KH, Kroegel C. Prostacyclin Modulates Granulocyte/Macrophage Colony-Stimulating Factor Release by Human Blood Mononuclear Cells. Pulm Pharmacol (1996) 9(1):43–8. doi: 10.1006/pulp.1996.0005
40. Zhou W, Blackwell TS, Goleniewska K, O’Neal JF, FitzGerald GA, Lucitt M, et al. Prostaglandin I2 Analogs Inhibit Th1 and Th2 Effector Cytokine Production by CD4 T Cells. J Leukoc Biol (2007) 81(3):809–17. doi: 10.1189/jlb.0606375
41. Zhou W, Hashimoto K, Goleniewska K, O’Neal JF, Ji S, Blackwell TS, et al. Prostaglandin I2 Analogs Inhibit Proinflammatory Cytokine Production and T Cell Stimulatory Function of Dendritic Cells. J Immunol (Baltimore Md: 1950) (2007) 178(2):702–10. doi: 10.4049/jimmunol.178.2.702
42. Di Francesco L, Totani L, Dovizio M, Piccoli A, Di Francesco A, Salvatore T, et al. Induction of Prostacyclin by Steady Laminar Shear Stress Suppresses Tumor Necrosis Factor-Alpha Biosynthesis via Heme Oxygenase-1 in Human Endothelial Cells. Circ Res (2009) 104(4):506–13. doi: 10.1161/CIRCRESAHA.108.191114
43. Luttmann W, Herzog V, Matthys H, Thierauch KH, Virchow JC, Kroegel C. Modulation of Cytokine Release From Mononuclear Cells by Prostacyclin, IL-4 and IL-13. Cytokine (1999) 11(2):127–33. doi: 10.1006/cyto.1998.0410
44. Takahashi Y, Tokuoka S, Masuda T, Hirano Y, Nagao M, Tanaka H, et al. Augmentation of Allergic Inflammation in Prostanoid IP Receptor Deficient Mice. Br J Pharmacol (2002) 137(3):315–22. doi: 10.1038/sj.bjp.0704872
45. Marone G, Galdiero MR, Pecoraro A, Pucino V, Criscuolo G, Triassi M, et al. Prostaglandin D2 Receptor Antagonists in Allergic Disorders: Safety, Efficacy, and Future Perspectives. Expert Opin Investig Drugs (2019) 28(1):73–84. doi: 10.1080/13543784.2019.1555237
46. Lewis RA, Soter NA, Diamond PT, Austen KF, Oates JA, Roberts LJ. Prostaglandin D2 Generation After Activation of Rat and Human Mast Cells With Anti-IgE. J Immunol (Baltimore Md: 1950) (1982) 129(4):1627–31.
47. Urade Y, Ujihara M, Horiguchi Y, Igarashi M, Nagata A, Ikai K, et al. Mast Cells Contain Spleen-Type Prostaglandin D Synthetase. J Biol Chem (1990) 265(1):371–5. doi: 10.1016/S0021-9258(19)40239-1
48. Kupczyk M, Kuna P. Targeting the PGD2/CRTH2/DP1 Signaling Pathway in Asthma and Allergic Disease: Current Status and Future Perspectives. Drugs (2017) 77(12):1281–94. doi: 10.1007/s40265-017-0777-2
49. Surh Y-J, Na H-K, Park J-M, Lee H-N, Kim W, Yoon I-S, et al. 15-Deoxy-Δ1214;-Prostaglandin J2, an Electrophilic Lipid Mediator of Anti-Inflammatory and Pro-Resolving Signaling. Biochem Pharmacol (2011) 82(10):1335–51. doi: 10.1016/j.bcp.2011.07.100
50. Pettipher R, Hansel TT, Armer R. Antagonism of the Prostaglandin D2 Receptors DP1 and CRTH2 as an Approach to Treat Allergic Diseases. Nat Rev Drug Discov (2007) 6(4):313–25. doi: 10.1038/nrd2266
51. Wojno EDT, Monticelli LA, Tran SV, Alenghat T, Osborne LC, Thome JJ, et al. The Prostaglandin D2 Receptor CRTH2 Regulates Accumulation of Group 2 Innate Lymphoid Cells in the Inflamed Lung. Mucosal Immunol (2015) 8(6):1313–23. doi: 10.1038/mi.2015.21
52. Hirai H, Tanaka K, Yoshie O, Ogawa K, Kenmotsu K, Takamori Y, et al. Prostaglandin D2 Selectively Induces Chemotaxis in T Helper Type 2 Cells, Eosinophils, and Basophils via Seven-Transmembrane Receptor CRTH2. J Exp Med (2001) 193(2):255–61. doi: 10.1084/jem.193.2.255
53. Nagata K, Hirai H, Tanaka K, Ogawa K, Aso T, Sugamura K, et al. CRTH2, an Orphan Receptor of T-Helper-2-Cells, is Expressed on Basophils and Eosinophils and Responds to Mast Cell-Derived Factor(s). FEBS Lett (1999) 459(2):195–9. doi: 10.1016/S0014-5793(99)01251-X
54. Rajakariar R, Hilliard M, Lawrence T, Trivedi S, Colville-Nash P, Bellingan G, et al. Hematopoietic Prostaglandin D2 Synthase Controls the Onset and Resolution of Acute Inflammation Through PGD2 and 15-Deoxydelta12 14 PGJ2. Proc Natl Acad Sci U S A (2007) 104(52):20979–84. doi: 10.1073/pnas.0707394104
55. Xue L, Gyles SL, Wettey FR, Gazi L, Townsend E, Hunter MG, et al. Prostaglandin D2 Causes Preferential Induction of Proinflammatory Th2 Cytokine Production Through an Action on Chemoattractant Receptor-Like Molecule Expressed on Th2 Cells. J Immunol (Baltimore Md: 1950) (2005) 175(10):6531–6. doi: 10.4049/jimmunol.175.10.6531
56. Herlong JL, Scott TR. Positioning Prostanoids of the D and J Series in the Immunopathogenic Scheme. Immunol Lett (2006) 102(2):121–31. doi: 10.1016/j.imlet.2005.10.004
57. Appel S, Mirakaj V, Bringmann A, Weck MM, Grünebach F, Brossart P. PPAR-Gamma Agonists Inhibit Toll-Like Receptor-Mediated Activation of Dendritic Cells via the MAP Kinase and NF-kappaB Pathways. Blood (2005) 106(12):3888–94. doi: 10.1182/blood-2004-12-4709
58. Jennewein C, Kuhn A-M, Schmidt MV, Meilladec-Jullig V, Knethen Av, Gonzalez FJ, et al. Sumoylation of Peroxisome Proliferator-Activated Receptor Gamma by Apoptotic Cells Prevents Lipopolysaccharide-Induced NCoR Removal From kappaB Binding Sites Mediating Transrepression of Proinflammatory Cytokines. J Immunol (Baltimore Md: 1950) (2008) 181(8):5646–52. doi: 10.4049/jimmunol.181.8.5646
59. Itoh K, Mochizuki M, Ishii Y, Ishii T, Shibata T, Kawamoto Y, et al. Transcription Factor Nrf2 Regulates Inflammation by Mediating the Effect of 15-Deoxy-Delta(12,14)-Prostaglandin J(2). Mol Cell Biol (2004) 24(1):36–45. doi: 10.1128/MCB.24.1.36-45.2004
60. Kuhn A-M, Tzieply N, Schmidt MV, Knethen Av, Namgaladze D, Yamamoto M, et al. Antioxidant Signaling via Nrf2 Counteracts Lipopolysaccharide-Mediated Inflammatory Responses in Foam Cell Macrophages. Free Radic Biol Med (2011) 50(10):1382–91. doi: 10.1016/j.freeradbiomed.2011.02.036
61. Burstein SH. The Chemistry, Biology and Pharmacology of the Cyclopentenone Prostaglandins. Prostaglandins other Lipid Mediators (2020) 148:106408. doi: 10.1016/j.prostaglandins.2020.106408
62. Suzuki-Yamamoto T, Nishizawa M, Fukui M, Okuda-Ashitaka E, Nakajima T, Ito S, et al. cDNA Cloning, Expression and Characterization of Human Prostaglandin F Synthase. FEBS Lett (1999) 462(3):335–40. doi: 10.1016/S0014-5793(99)01551-3
63. Nishizawa M, Nakajima T, Yasuda K, Kanzaki H, Sasaguri Y, Watanabe K, et al. Close Kinship of Human 20alpha-Hydroxysteroid Dehydrogenase Gene With Three Aldo-Keto Reductase Genes. Genes Cells (2000) 5(2):111–25. doi: 10.1046/j.1365-2443.2000.00310.x
64. Basu S. Novel Cyclooxygenase-Catalyzed Bioactive Prostaglandin F2alpha From Physiology to New Principles in Inflammation. Med Res Rev (2007) 27(4):435–68. doi: 10.1002/med.20098
65. Hao C-M, Breyer MD. Physiological Regulation of Prostaglandins in the Kidney. Annu Rev Physiol (2008) 70:357–77. doi: 10.1146/annurev.physiol.70.113006.100614
66. Poyser NL. The Control of Prostaglandin Production by the Endometrium in Relation to Luteolysis and Menstruation. Prostaglandins Leukot Essential Fatty Acids (1995) 53(3):147–95. doi: 10.1016/0952-3278(95)90115-9
67. Sugimoto Y, Yamasaki A, Segi E, Tsuboi K, Aze Y, Nishimura T, et al. Failure of Parturition in Mice Lacking the Prostaglandin F Receptor. Science (New York N Y) (1997) 277(5326):681–3. doi: 10.1126/science.277.5326.681
68. Basu S. Bioactive Eicosanoids: Role of Prostaglandin F(2α) and F2-Isoprostanes in Inflammation and Oxidative Stress Related Pathology. Mol Cells (2010) 30(5):383–91. doi: 10.1007/s10059-010-0157-1
69. Basu S, Whiteman M, Mattey DL, Halliwell B. Raised Levels of F(2)-Isoprostanes and Prostaglandin F(2alpha) in Different Rheumatic Diseases. Ann Rheum Dis (2001) 60(6):627–31. doi: 10.1136/ard.60.6.627
70. Oga T, Matsuoka T, Yao C, Nonomura K, Kitaoka S, Sakata D, et al. Prostaglandin F(2alpha) Receptor Signaling Facilitates Bleomycin-Induced Pulmonary Fibrosis Independently of Transforming Growth Factor-Beta. Nat Med (2009) 15(12):1426–30. doi: 10.1038/nm.2066
71. Aihara K, Handa T, Oga T, Watanabe K, Tanizawa K, Ikezoe K, et al. Clinical Relevance of Plasma Prostaglandin F2α Metabolite Concentrations in Patients With Idiopathic Pulmonary Fibrosis. PLoS One (2013) 8(6):e66017. doi: 10.1371/journal.pone.0066017
72. Colville-Nash PR, Gilroy DW, Willis D, Paul-Clark MJ, Moore AR, Willoughby DA. Prostaglandin F2alpha Produced by Inducible Cyclooxygenase may Contribute to the Resolution of Inflammation. Inflammopharmacology (2005) 12(5-6):473–6; discussion 477-80. doi: 10.1163/156856005774382616
73. Park JY, Pillinger MH, Abramson SB. Prostaglandin E2 Synthesis and Secretion: The Role of PGE2 Synthases. Clin Immunol (Orlando Fla) (2006) 119(3):229–40. doi: 10.1016/j.clim.2006.01.016
74. Hata AN, Breyer RM. Pharmacology and Signaling of Prostaglandin Receptors: Multiple Roles in Inflammation and Immune Modulation. Pharmacol Ther (2004) 103(2):147–66. doi: 10.1016/j.pharmthera.2004.06.003
75. Fujino H, Salvi S, Regan JW. Differential Regulation of Phosphorylation of the cAMP Response Element-Binding Protein After Activation of EP2 and EP4 Prostanoid Receptors by Prostaglandin E2. Mol Pharmacol (2005) 68(1):251–9. doi: 10.1124/mol.105.011833
76. Yang J, Xia M, Goetzl EJ, An S. Cloning and Expression of the EP3-Subtype of Human Receptors for Prostaglandin E2. Biochem Biophys Res Commun (1994) 198(3):999–1006. doi: 10.1006/bbrc.1994.1142
77. Tsuge K, Inazumi T, Shimamoto A, Sugimoto Y. Molecular Mechanisms Underlying Prostaglandin E2-Exacerbated Inflammation and Immune Diseases. Int Immunol (2019) 31(9):597–606. doi: 10.1093/intimm/dxz021
78. Claveau D, Sirinyan M, Guay J, Gordon R, Chan C-C, Bureau Y, et al. Microsomal Prostaglandin E Synthase-1 is a Major Terminal Synthase That is Selectively Up-Regulated During Cyclooxygenase-2-Dependent Prostaglandin E2 Production in the Rat Adjuvant-Induced Arthritis Model. J Immunol (Baltimore Md: 1950) (2003) 170(9):4738–44. doi: 10.4049/jimmunol.170.9.4738
79. Yu Y, Chadee K. Prostaglandin E2 Stimulates IL-8 Gene Expression in Human Colonic Epithelial Cells by a Posttranscriptional Mechanism. J Immunol (Baltimore Md: 1950) (1998) 161(7):3746–52.
80. Weller CL, Collington SJ, Hartnell A, Conroy DM, Kaise T, Barker JE, et al. Chemotactic Action of Prostaglandin E2 on Mouse Mast Cells Acting via the PGE2 Receptor 3. Proc Natl Acad Sci U S A (2007) 104(28):11712–7. doi: 10.1073/pnas.0701700104
81. Treutlein E-M, Kern K, Weigert A, Tarighi N, Schuh C-D, Nüsing RM, et al. The Prostaglandin E2 Receptor EP3 Controls CC-Chemokine Ligand 2-Mediated Neuropathic Pain Induced by Mechanical Nerve Damage. J Biol Chem (2018) 293(25):9685–95. doi: 10.1074/jbc.RA118.002492
82. Sheibanie AF, Yen J-H, Khayrullina T, Emig F, Zhang M, Tuma R, et al. The Proinflammatory Effect of Prostaglandin E2 in Experimental Inflammatory Bowel Disease is Mediated Through the IL-23–IL-17 Axis. J Immunol (Baltimore Md: 1950) (2007) 178(12):8138–47. doi: 10.4049/jimmunol.178.12.8138
83. Raucci F, Saviano A, Casillo GM, Guerra-Rodriguez M, Mansour AA, Piccolo M, et al. IL-17-Induced Inflammation Modulates the mPGES-1/PPAR-γ Pathway in Monocytes/Macrophages. Br J Pharmacol (2021). doi: 10.1111/bph.15413
84. Schiffmann S, Weigert A, Männich J, Eberle M, Birod K, Häussler A, et al. PGE2/EP4 Signaling in Peripheral Immune Cells Promotes Development of Experimental Autoimmune Encephalomyelitis. Biochem Pharmacol (2014) 87(4):625–35. doi: 10.1016/j.bcp.2013.12.006
85. Poligone B, Baldwin AS. Positive and Negative Regulation of NF-kappaB by COX-2: Roles of Different Prostaglandins. J Biol Chem (2001) 276(42):38658–64. doi: 10.1074/jbc.M106599200
86. Ward C, Walker A, Dransfield I, Haslett C, Rossi AG. Regulation of Granulocyte Apoptosis by NF-Kappab. Biochem Soc Trans (2004) 32(Pt3):465–7. doi: 10.1042/bst0320465
87. Rappl P, Rösser S, Maul P, Bauer R, Huard A, Schreiber Y, et al. Inhibition of mPGES-1 Attenuates Efficient Resolution of Acute Inflammation by Enhancing CX3CL1 Expression. Cell Death Dis (2021) 12(2):135. doi: 10.1038/s41419-021-03423-2
88. Gilroy DW, Colville-Nash PR, Willis D, Chivers J, Paul-Clark MJ, Willoughby DA. Inducible Cyclooxygenase may Have Anti-Inflammatory Properties. Nat Med (1999) 5(6):698–701. doi: 10.1038/9550
89. Rappl P, Brüne B, Schmid T. Role of Tristetraprolin in the Resolution of Inflammation. Biology (Basel) (2021) 10(1):66. doi: 10.3390/biology10010066
90. Tang T, Scambler TE, Smallie T, Cunliffe HE, Ross EA, Rosner DR, et al. Macrophage Responses to Lipopolysaccharide are Modulated by a Feedback Loop Involving Prostaglandin E2, Dual Specificity Phosphatase 1 and Tristetraprolin. Sci Rep (2017) 7(1):4350. doi: 10.1038/s41598-017-04100-1
91. Johann AM, Weigert A, Eberhardt W, Kuhn A-M, Barra V, Knethen Av, et al. Apoptotic Cell-Derived Sphingosine-1-Phosphate Promotes HuR-Dependent Cyclooxygenase-2 mRNA Stabilization and Protein Expression. J Immunol (Baltimore Md: 1950) (2008) 180(2):1239–48. doi: 10.4049/jimmunol.180.2.1239
92. Sha W, Olesch C, Hanaka H, Rådmark O, Weigert A, Brüne B. Necrosis in DU145 Prostate Cancer Spheroids Induces COX-2/mPGES-1-Derived PGE2 to Promote Tumor Growth and to Inhibit T Cell Activation. Int J Cancer (2013) 133(7):1578–88. doi: 10.1002/ijc.28181
93. Olesch C, Sha W, Angioni C, Sha LK, Açaf E, Patrignani P, et al. MPGES-1-Derived PGE2 Suppresses CD80 Expression on Tumor-Associated Phagocytes to Inhibit Anti-Tumor Immune Responses in Breast Cancer. Oncotarget (2015) 6(12):10284–96. doi: 10.18632/oncotarget.3581
94. Walker W, Rotondo D. Prostaglandin E2 Is a Potent Regulator of Interleukin-12- and Interleukin-18-Induced Natural Killer Cell Interferon-Gamma Synthesis. Immunology (2004) 111(3):298–305. doi: 10.1111/j.1365-2567.2004.01810.x
95. Joshi PC, Zhou X, Cuchens M, Jones Q. Prostaglandin E2 Suppressed IL-15-Mediated Human NK Cell Function Through Down-Regulation of Common Gamma-Chain. J Immunol (Baltimore Md: 1950) (2001) 166(2):885–91. doi: 10.4049/jimmunol.166.2.885
96. Serezani CH, Chung J, Ballinger MN, Moore BB, Aronoff DM, Peters-Golden M. Prostaglandin E2 Suppresses Bacterial Killing in Alveolar Macrophages by Inhibiting NADPH Oxidase. Am J Respir Cell Mol Biol (2007) 37(5):562–70. doi: 10.1165/rcmb.2007-0153OC
97. Aronoff DM, Canetti C, Peters-Golden M. Prostaglandin E2 Inhibits Alveolar Macrophage Phagocytosis Through an E-Prostanoid 2 Receptor-Mediated Increase in Intracellular Cyclic AMP. J Immunol (Baltimore Md: 1950) (2004) 173(1):559–65. doi: 10.4049/jimmunol.173.1.559
98. O’Brien AJ, Fullerton JN, Massey KA, Auld G, Sewell G, James S, et al. Immunosuppression in Acutely Decompensated Cirrhosis is Mediated by Prostaglandin E2. Nat Med (2014) 20(5):518–23. doi: 10.1038/nm.3516
99. Brenneis C, Coste O, Altenrath K, Angioni C, Schmidt H, Schuh C-D, et al. Anti-Inflammatory Role of Microsomal Prostaglandin E Synthase-1 in a Model of Neuroinflammation. J Biol Chem (2011) 286(3):2331–42. doi: 10.1074/jbc.M110.157362
100. Ginhoux F, Prinz M. Origin of Microglia: Current Concepts and Past Controversies. Cold Spring Harb Perspect Biol (2015) 7(8):a020537. doi: 10.1101/cshperspect.a020537
101. Takayama K, García-Cardena G, Sukhova GK, Comander J, Gimbrone MA, Libby P. Prostaglandin E2 Suppresses Chemokine Production in Human Macrophages Through the EP4 Receptor. J Biol Chem (2002) 277(46):44147–54. doi: 10.1074/jbc.M204810200
102. Bystrom J, Evans I, Newson J, Stables M, Toor I, van Rooijen N, et al. Resolution-Phase Macrophages Possess a Unique Inflammatory Phenotype That is Controlled by cAMP. Blood (2008) 112(10):4117–27. doi: 10.1182/blood-2007-12-129767
103. Korns D, Frasch SC, Fernandez-Boyanapalli R, Henson PM, Bratton DL. Modulation of Macrophage Efferocytosis in Inflammation. Front Immunol (2011) 2:57. doi: 10.3389/fimmu.2011.00057
104. Walker C, Kristensen F, Bettens F, deWeck AL. Lymphokine Regulation of Activated (G1) Lymphocytes. I. Prostaglandin E2-Induced Inhibition of Interleukin 2 Production. J Immunol (Baltimore Md: 1950) (1983) 130(4):1770–3.
105. Kolenko V, Rayman P, Roy B, Cathcart MK, O’Shea J, Tubbs R, et al. Downregulation of JAK3 Protein Levels in T Lymphocytes by Prostaglandin E2 and Other Cyclic Adenosine Monophosphate-Elevating Agents: Impact on Interleukin-2 Receptor Signaling Pathway. Blood (1999) 93(7):2308–18. doi: 10.1182/blood.V93.7.2308.407k16_2308_2318
106. Porter BO, Malek TR. Prostaglandin E2 Inhibits T Cell Activation-Induced Apoptosis and Fas-Mediated Cellular Cytotoxicity by Blockade of Fas-Ligand Induction. Eur J Immunol (1999) 29(7):2360–5. doi: 10.1002/(SICI)1521-4141(199907)29:07<2360::AID-IMMU2360>3.0.CO;2-A
107. Yao C, Sakata D, Esaki Y, Li Y, Matsuoka T, Kuroiwa K, et al. Prostaglandin E2-EP4 Signaling Promotes Immune Inflammation Through Th1 Cell Differentiation and Th17 Cell Expansion. Nat Med (2009) 15(6):633–40. doi: 10.1038/nm.1968
108. Yao C, Hirata T, Soontrapa K, Ma X, Takemori H, Narumiya S. Prostaglandin E2 Promotes Th1 Differentiation via Synergistic Amplification of IL-12 Signalling by cAMP and PI3-Kinase. Nat Commun (2013) 4:1685. doi: 10.1038/ncomms3315
109. Du B, Zhu M, Li Y, Li G, Xi X. The Prostaglandin E2 Increases the Production of IL-17 and the Expression of Costimulatory Molecules on γδ T Cells in Rheumatoid Arthritis. Scand J Immunol (2020) 91(5):e12872. doi: 10.1111/sji.12872
110. Betz M, Fox BS. Prostaglandin E2 Inhibits Production of Th1 Lymphokines But Not of Th2 Lymphokines. J Immunol (Baltimore Md: 1950) (1991) 146(1):108–13.
111. Snijdewint FG, Kaliński P, Wierenga EA, Bos JD, Kapsenberg ML. Prostaglandin E2 Differentially Modulates Cytokine Secretion Profiles of Human T Helper Lymphocytes. J Immunol (Baltimore Md: 1950) (1993) 150(12):5321–9.
112. Kalinski P. Regulation of Immune Responses by Prostaglandin E2. J Immunol (Baltimore Md: 1950) (2012) 188(1):21–8. doi: 10.4049/jimmunol.1101029
113. Placek K, Schultze JL, Aschenbrenner AC. Epigenetic Reprogramming of Immune Cells in Injury, Repair, and Resolution. J Clin Invest (2019) 129(8):2994–3005. doi: 10.1172/JCI124619
114. Snodgrass RG, Benatzy Y, Schmid T, Namgaladze D, Mainka M, Schebb NH, et al. Efferocytosis Potentiates the Expression of Arachidonate 15-Lipoxygenase (ALOX15) in Alternatively Activated Human Macrophages Through LXR Activation. Cell Death Differ (2021) 28(4):1301–16. doi: 10.1038/s41418-020-00652-4
115. Kapsenberg ML, Hilkens CM, Wierenga EA, Kalinski P. The Paradigm of Type 1 and Type 2 Antigen-Presenting Cells. Implications for Atopic Allergy. Clin Exp Allergy (1999) 29(Suppl 2):33–6. doi: 10.1046/j.1365-2222.1999.00006.x-i2
116. Khayrullina T, Yen J-H, Jing H, Ganea D. In Vitro Differentiation of Dendritic Cells in the Presence of Prostaglandin E2 Alters the IL-12/IL-23 Balance and Promotes Differentiation of Th17 Cells. J Immunol (Baltimore Md: 1950) (2008) 181(1):721–35. doi: 10.4049/jimmunol.181.1.721
117. Boniface K, Bak-Jensen KS, Li Y, Blumenschein WM, McGeachy MJ, McClanahan TK, et al. Prostaglandin E2 Regulates Th17 Cell Differentiation and Function Through Cyclic AMP and EP2/EP4 Receptor Signaling. J Exp Med (2009) 206(3):535–48. doi: 10.1084/jem.20082293
118. Maseda D, Banerjee A, Johnson EM, Washington MK, Kim H, Lau KS, et al. mPGES-1-Mediated Production of PGE2 and EP4 Receptor Sensing Regulate T Cell Colonic Inflammation. Front Immunol (2018) 9:2954. doi: 10.3389/fimmu.2018.02954
119. Maseda D, Johnson EM, Nyhoff LE, Baron B, Kojima F, Wilhelm AJ, et al. Mpges1-Dependent Prostaglandin E2 (PGE2) Controls Antigen-Specific Th17 and Th1 Responses by Regulating T Autocrine and Paracrine PGE2 Production. J Immunol (Baltimore Md: 1950) (2018) 200(2):725–36. doi: 10.4049/jimmunol.1601808
120. Gagliani N, Amezcua Vesely MC, Iseppon A, Brockmann L, Xu H, Palm NW, et al. Th17 Cells Transdifferentiate Into Regulatory T Cells During Resolution of Inflammation. Nature (2015) 523(7559):221–5. doi: 10.1038/nature14452
121. Baratelli F, Lin Y, Zhu L, Yang S-C, Heuzé-Vourc’h N, Zeng G, et al. Prostaglandin E2 Induces FOXP3 Gene Expression and T Regulatory Cell Function in Human CD4+ T Cells. J Immunol (Baltimore Md: 1950) (2005) 175(3):1483–90. doi: 10.4049/jimmunol.175.3.1483
122. Soontrapa K, Honda T, Sakata D, Yao C, Hirata T, Hori S, et al. Prostaglandin E2-Prostaglandin E Receptor Subtype 4 (EP4) Signaling Mediates UV Irradiation-Induced Systemic Immunosuppression. Proc Natl Acad Sci U S A (2011) 108(16):6668–73. doi: 10.1073/pnas.1018625108
123. Banerjee DK, Dhodapkar MV, Matayeva E, Steinman RM, Dhodapkar KM. Expansion of FOXP3high Regulatory T Cells by Human Dendritic Cells (DCs) In Vitro and After Injection of Cytokine-Matured DCs in Myeloma Patients. Blood (2006) 108(8):2655–61. doi: 10.1182/blood-2006-03-011353
124. Willoughby DA, Moore AR, Colville-Nash PR, Gilroy D. Resolution of Inflammation. Int J Immunopharmacol (2000) 22(12):1131–5. doi: 10.1016/S0192-0561(00)00064-3
125. Serhan CN, Chiang N, van Dyke TE. Resolving Inflammation: Dual Anti-Inflammatory and Pro-Resolution Lipid Mediators. Nat Rev Immunol (2008) 8(5):349–61. doi: 10.1038/nri2294
126. Bannenberg G, Serhan CN. Specialized Pro-Resolving Lipid Mediators in the Inflammatory Response: An Update. Biochim Biophys Acta (2010) 1801(12):1260–73. doi: 10.1016/j.bbalip.2010.08.002
127. Snodgrass RG, Brüne B. Regulation and Functions of 15-Lipoxygenases in Human Macrophages. Front Pharmacol (2019) 10:719. doi: 10.3389/fphar.2019.00719
128. Serhan CN, Gupta SK, Perretti M, Godson C, Brennan E, Li Y, et al. The Atlas of Inflammation Resolution (AIR). Mol Aspects Med (2020) 74:100894. doi: 10.1101/2020.01.27.921882
129. Mancini AD, Di Battista JA. The Cardinal Role of the Phospholipase A(2)/cyclooxygenase-2/Prostaglandin E Synthase/Prostaglandin E(2) (PCPP) Axis in Inflammostasis. Inflammation Res (2011) 60(12):1083–92. doi: 10.1007/s00011-011-0385-7
130. Loynes CA, Lee JA, Robertson AL, Steel MJ, Ellett F, Feng Y, et al. PGE2 Production at Sites of Tissue Injury Promotes an Anti-Inflammatory Neutrophil Phenotype and Determines the Outcome of Inflammation Resolution In Vivo. Sci Adv (2018) 4(9):eaar8320. doi: 10.1126/sciadv.aar8320
131. Chiurchiù V, Leuti A, Maccarrone M. Bioactive Lipids and Chronic Inflammation: Managing the Fire Within. Front Immunol (2018) 9:38. doi: 10.3389/fimmu.2018.00038
132. Chiurchiù V, Leuti A, Dalli J, Jacobsson A, Battistini L, Maccarrone M, et al. Proresolving Lipid Mediators Resolvin D1, Resolvin D2, and Maresin 1 are Critical in Modulating T Cell Responses. Sci Transl Med (2016) 8(353):353ra111. doi: 10.1126/scitranslmed.aaf7483
133. Jin S, Chen H, Li Y, Zhong H, Sun W, Wang J, et al. Maresin 1 Improves the Treg/Th17 Imbalance in Rheumatoid Arthritis Through miR-21. Ann Rheum Dis (2018) 77(11):1644–52. doi: 10.1136/annrheumdis-2018-213511
134. Cheng T, Ding S, Liu S, Li X, Tang X, Sun L. Resolvin D1 Improves the Treg/Th17 Imbalance in Systemic Lupus Erythematosus Through miR-30e-5p. Front Immunol (2021) 12:668760. doi: 10.3389/fimmu.2021.668760
135. Ratcliffe MJ, Walding A, Shelton PA, Flaherty A, Dougall IG. Activation of E-Prostanoid4 and E-Prostanoid2 Receptors Inhibits TNF-Alpha Release From Human Alveolar Macrophages. Eur Respir J (2007) 29(5):986–94. doi: 10.1183/09031936.00131606
136. Strassmann G, Patil-Koota V, Finkelman F, Fong M, Kambayashi T. Evidence for the Involvement of Interleukin 10 in the Differential Deactivation of Murine Peritoneal Macrophages by Prostaglandin E2. J Exp Med (1994) 180(6):2365–70. doi: 10.1084/jem.180.6.2365
137. Takayama K, Sukhova GK, Chin MT, Libby P. A Novel Prostaglandin E Receptor 4-Associated Protein Participates in Antiinflammatory Signaling. Circ Res (2006) 98(4):499–504. doi: 10.1161/01.RES.0000204451.88147.96
138. Serhan CN, Brain SD, Buckley CD, Gilroy DW, Haslett C, O’Neill LAJ, et al. Resolution of Inflammation: State of the Art, Definitions and Terms. FASEB J (2007) 21(2):325–32. doi: 10.1096/fj.06-7227rev
139. Mahic M, Yaqub S, Johansson CC, Taskén K, Aandahl EM. FOXP3+CD4+CD25+ Adaptive Regulatory T Cells Express Cyclooxygenase-2 and Suppress Effector T Cells by a Prostaglandin E2-Dependent Mechanism. J Immunol (Baltimore Md: 1950) (2006) 177(1):246–54. doi: 10.4049/jimmunol.177.1.246
140. Obermajer N, Muthuswamy R, Lesnock J, Edwards RP, Kalinski P. Positive Feedback Between PGE2 and COX2 Redirects the Differentiation of Human Dendritic Cells Toward Stable Myeloid-Derived Suppressor Cells. Blood (2011) 118(20):5498–505. doi: 10.1182/blood-2011-07-365825
141. Fuentes AV, Pineda MD, Venkata KCN. Nagulapalli. Comprehension of Top 200 Prescribed Drugs in the US as a Resource for Pharmacy Teaching, Training and Practice. Pharm (Basel Switzerland) (2018) 6(2):43. doi: 10.3390/pharmacy6020043
142. Hinz B, Brune K. Cyclooxygenase-2–10 Years Later. J Pharmacol Exp Ther (2002) 300(2):367–75. doi: 10.1124/jpet.300.2.367
143. Hara S. Prostaglandin Terminal Synthases as Novel Therapeutic Targets. Proc Japan Academy Ser B Phys Biol Sci (2017) 93(9):703–23. doi: 10.2183/pjab.93.044
144. Bergqvist F, Morgenstern R, Jakobsson P-J. A Review on mPGES-1 Inhibitors: From Preclinical Studies to Clinical Applications. Prostaglandins other Lipid Mediators (2020) 147:106383. doi: 10.1016/j.prostaglandins.2019.106383
145. Werner M, Jordan PM, Romp E, Czapka A, Rao Z, Kretzer C, et al. Targeting Biosynthetic Networks of the Proinflammatory and Proresolving Lipid Metabolome. FASEB J (2019) 33(5):6140–53. doi: 10.1096/fj.201802509R
146. Park J, Langmead CJ, Riddy DM. New Advances in Targeting the Resolution of Inflammation: Implications for Specialized Pro-Resolving Mediator GPCR Drug Discovery. ACS Pharmacol Transl Sci (2020) 3(1):88–106. doi: 10.1021/acsptsci.9b00075
Keywords: prostaglandin, prostacyclin, thromboxane, specialized pro-resolving mediator, inflammation, resolution, macrophage
Citation: Schmid T and Brüne B (2021) Prostanoids and Resolution of Inflammation – Beyond the Lipid-Mediator Class Switch. Front. Immunol. 12:714042. doi: 10.3389/fimmu.2021.714042
Received: 24 May 2021; Accepted: 30 June 2021;
Published: 12 July 2021.
Edited by:
Amiram Ariel, University of Haifa, IsraelReviewed by:
Eoin Brennan, University College Dublin, IrelandCopyright © 2021 Schmid and Brüne. This is an open-access article distributed under the terms of the Creative Commons Attribution License (CC BY). The use, distribution or reproduction in other forums is permitted, provided the original author(s) and the copyright owner(s) are credited and that the original publication in this journal is cited, in accordance with accepted academic practice. No use, distribution or reproduction is permitted which does not comply with these terms.
*Correspondence: Tobias Schmid, dC5zY2htaWRAYmlvY2hlbS51bmktZnJhbmtmdXJ0LmRl
Disclaimer: All claims expressed in this article are solely those of the authors and do not necessarily represent those of their affiliated organizations, or those of the publisher, the editors and the reviewers. Any product that may be evaluated in this article or claim that may be made by its manufacturer is not guaranteed or endorsed by the publisher.
Research integrity at Frontiers
Learn more about the work of our research integrity team to safeguard the quality of each article we publish.