- 1Paediatric Immunology and Vaccinology Unit, Division of General Paediatrics, Department of Paediatrics, Gynaecology and Obstetrics, Geneva University Hospitals and University of Geneva, Geneva, Switzerland
- 2Centre for Vaccinology and Neonatal Immunology, Department of Paediatrics and Pathology-Immunology, Medical Faculty and University Hospitals of Geneva, Geneva, Switzerland
Children with autoimmune disorders are especially at risk of vaccine-preventable diseases due to their underlying disease and the immunosuppressive treatment often required for a long period. In addition, vaccine coverage remains too low in this vulnerable population. This can be explained by a fear of possible adverse effects of vaccines under immunosuppression, but also a lack of data and clear recommendations, particularly with regard to vaccination with live vaccines. In this review, the latest literature and recommendations on vaccination in immunosuppressed children are discussed in detail, with the aim to provide a set of practical guidelines on vaccination for specialists caring for children suffering from different autoimmune disorders and treated with various immunosuppressive regimens.
Introduction
Autoimmune disorders include children with systemic autoimmune diseases and those with autoimmune diseases specific to a single organ, such as the digestive tract (inflammatory bowel diseases), eyes (uveitis), skin (psoriasis) or the central nervous system (multiple sclerosis). These children are treated with similar immunosuppressive therapy to control the disease, which includes traditional immunomodulatory drugs, such as glucocorticoids (GCs), disease-modifying antirheumatic drugs (DMARDs), and biologics. Currently, DMARDs are separated into conventional synthetic (csDMARDs), biological (bDMARDs), and targeted synthetic (tsDMARDs) (1) as shown in Table 1.
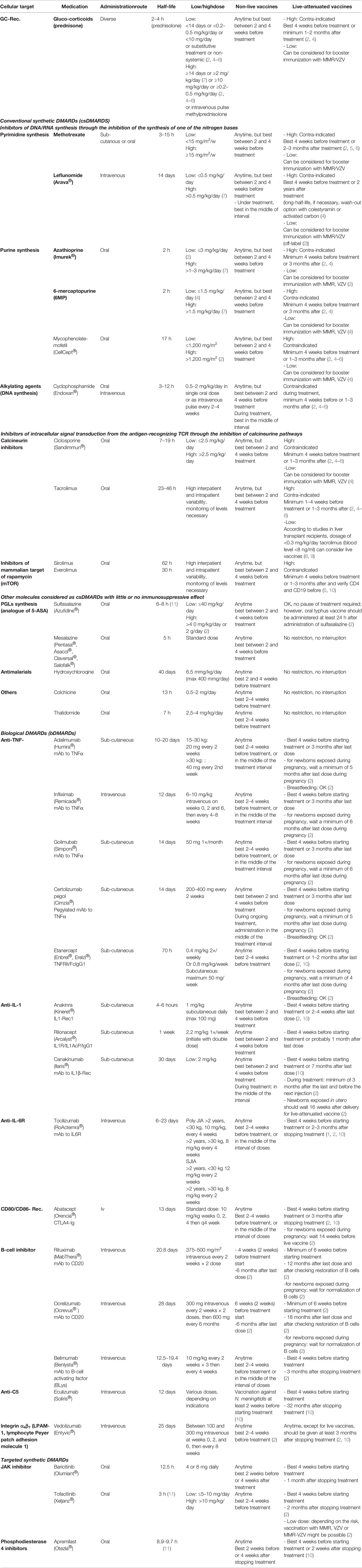
Table 1 List of immunosuppressive agents and current recommendations concerning vaccination [adapted from (2, 3)].
Children with autoimmune disorders are at an increased risk of infection due to their underlying disease and to their immunosuppressive treatment. A study assessing the risk of infection every 2 months for one year in juvenile idiopathic arthritis-children treated with bDMARDs reported that 57% (n = 175) of patients developed an infection. Upper respiratory tract infections were among the most frequent infections and mostly treated in ambulatory care (12).
Several studies have reported similar rates of serious infection in children with JIA receiving bDMARDs or csDMARDs (12, 13). However, other studies have reported that the highest rates of infection were in children treated with bDMARDs, especially anti-TNFα (such as etanercept and infliximab) (12, 14–16), or a combined treatment of csDMARDs (such as MTX) and bDMARDs (17). Upper respiratory tract infections (including severe influenza) were among the most frequent infections, together with complicated varicella (12, 14–19).
As most patients received a combined treatment rather than a single molecule, it is particularly challenging to design a clinical study to assess the effect of various immunosuppressive regimens on the risk of infection and to understand the biology behind the infectious risk.
Infectious diseases for which a vaccine is available for children include influenza virus, S. pneumoniae, H. influenzae, meningococcus, polioviruses, VZV, measles, mumps, rubella, HPV, hepatitis A (HAV) and B virus (HBV), tick-borne encephalitis, and travel vaccines (such as the yellow fever vaccine, rabies vaccine, and typhoid vaccine).
In this review, the challenges in vaccinating children with autoimmune and autoinflammatory disorders is discussed and how the immune response develops following vaccination and how it is affected by various immunosuppressive drugs is explained. Then, the review focuses on live vaccines, discusses the existing data, the controversies and existing recommendations, and does the same for non-live vaccine. At the end, a practical approach for vaccinating children with immune-mediated disorders is proposed. In this review, vaccination in function of the immunosuppressive regimen rather that in function of the various autoimmune and autoinflammatory disorders is discussed. Further, it should be noted that the majority of studies that we have in this field come from studies mainly done in children with JIA, SLE, and autoinflammatory disorders, and few studies in children with IBD, while there are almost no studies for other autoimmune diseases.
Challenges in the Vaccination of Children With Autoimmune Disorders
One of the major achievements in medicine is the development of vaccines, which allow protection against many potentially fatal infectious diseases, thus decreasing mortality worldwide. However, recent outbreaks of vaccine-preventable diseases, such as measles, show that reaching a sufficient vaccine coverage of the international population remains a challenge (20, 21).
Completion of vaccination series is even more important in children with autoimmune disorders. First, they are more susceptible to infections because of the underlying conditions that affect their immune system and influence their natural defense mechanisms against various infectious agents. Furthermore, these children often require a rapid start of immunosuppressive treatment after diagnosis, usually lasting for many months/years until the treatment can be reduced or interrupted, which renders vaccination challenging in this population. Indeed, it is expected that most immunosuppressive drugs will affect the immune capacity of the child to a different degree depending on the agent, thereby reducing their capacity to respond to many vaccines. In addition, only non-live vaccines are recommended during immunosuppressive treatment and the use of live-attenuated vaccines should be carefully assessed on a case-by-case basis.
In the specific population of children with immune-mediated disorders treated with various immunosuppressive agents, the indication for each vaccine can be complicated, and it becomes very challenging for the specialists who care for these children to decide upon the best vaccination scheme for their patients. Moreover, several concerns, misconceptions, and unanswered questions have led to decreased vaccination rates in children with chronic autoimmune diseases (22, 23). For example, in Ljublijana, Slovenia, only 65% of 18-year-old young adults with rheumatic diseases were up to date with their vaccines, with the most frequently omitted being hepatitis B (HBV) and a second dose of measles–mumps–rubella (MMR) (24). In addition, only 10% had received the seasonal influenza vaccine and 4% the pneumococcal 13-valent conjugate vaccine (PCV13) (24). Similarly, 40% of children with JIA in Canada have an incomplete vaccination record for their age (23).
The reasons described for these decreased rates were that medical specialists caring for patients with autoimmune diseases did not feel responsible for monitoring the vaccination schedules of their patients (25). Additionally, parents—and even specialists—remained uncertain about the safety of some vaccines in the context of children with autoimmune diseases and under immunosuppressive treatment (23). Safety aspects in terms of the potential interferences of vaccination on the underlying disease, as well as the question of whether vaccination under immunosuppressive treatment is sufficiently immunogenic/protective, are repeatedly subjects of discussion and debate (7, 26, 27). Specialists very much worry about the potential danger of administering a live-attenuated vaccine in a child with an autoimmune disease, even under low-immunosuppression, because of the risk of causing vaccine virus associated infections. In addition, current vaccine recommendations for pediatric populations with immune-mediated disorders are often based on small sample sizes with low levels of evidence, especially for the use of live-attenuated vaccines (7, 26, 27).
Immune Responses To Vaccination
During a first encounter with an antigen, only a small number of naïve B cells and T cells are able to recognize a given antigen. After around 2 weeks, clones of T and B cells are selected, expand, and give rise to a small pool of memory B and T cells, which are often too small and last for a too short period to offer protection against a given pathogen. Following subsequent encounters with the given antigen, memory B and T cells proliferate and expand. These cells respond more rapidly and more strongly following a smaller amount of antigen. This explains the principle of vaccines, which allows for producing a pool of memory B and T cells able to respond rapidly to a given antigen after infection and also giving rise to long-lived plasma cells that persist in the bone marrow (28) (Figure 1).
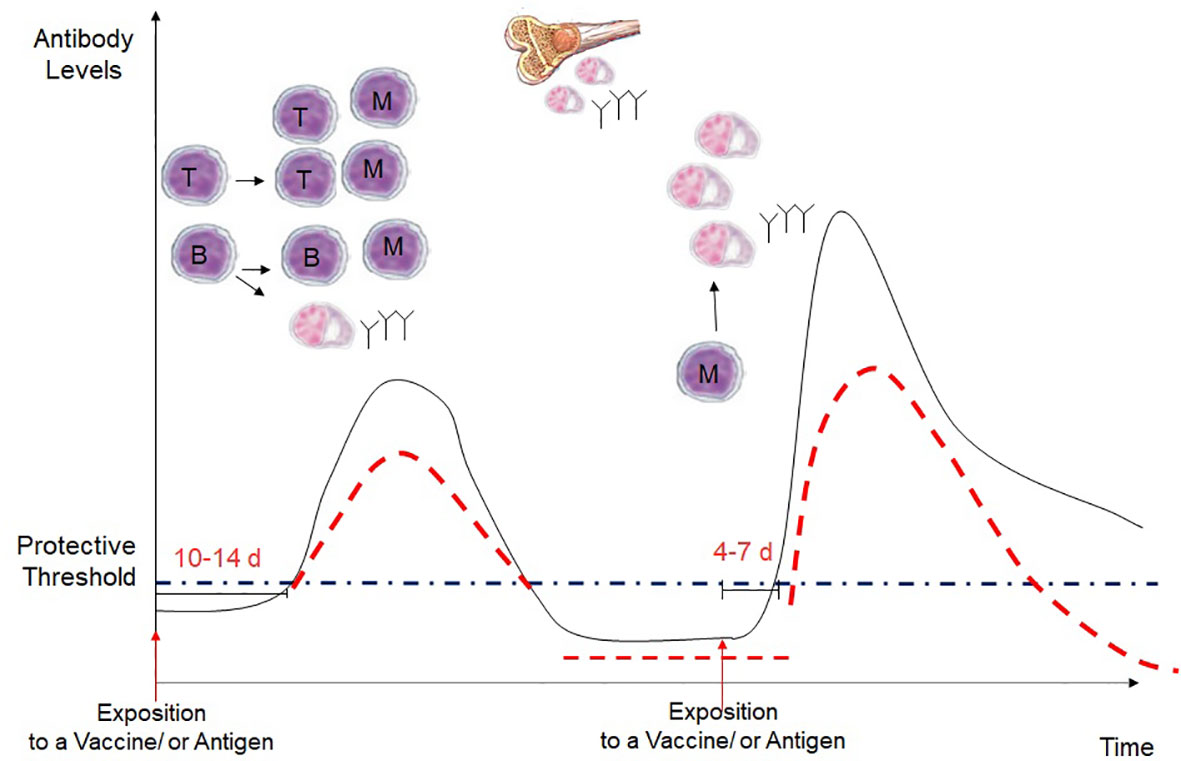
Figure 1 Kinetics of the antibody response in a primary and secondary immune response. Following a first exposure with an antigen, there is an interval of 10-14 days before the production of IgG antibodies, which occurs after the interaction of naive B and T cells, which become effector cells and develop into gentianée-specific memory B cells, short-lived plasma cells and long-lived plsame cells. Then, long-lived plasma cells in migrate in the bone marrow and continue to secrete IgG antibodies. However, with the time the antibodies decrease often under the protective levels. During a secondary exposure with the vaccine or the antigen, memory B and T cells are rapidly reactivated, with a more rapid and higher IgG increase that usually last longer. However, in immunosuppressed children, the peak of the antibody response is expected to be lower, and the antibodies are expected to decrease more rapidly after vaccination.
To better understand the effect of each immunosuppressive drug on the immune response to vaccination, we should look at what happens specifically at the cellular level. Following a first exposure with the antigen, naïve T cells in lymph nodes recognize a peptide antigen presented on the surface of antigen-presenting cells (APCs) in the MHC molecule via the binding of their T-cell receptor (TCR) and co-stimulatory signals given by the CD80 and CD86 on the surface of DCs and CD28 on T cells. The extent and quality of antigen-presenting cell activations condition the T-cell responses. T-cell responses are often dependent on the inflammatory milieu (innate immune responses) created at the time of vaccination and these could be impacted by the various immunosuppressive drugs. This activates various signal transduction pathways in T cells, which activate the transcription of various factors that induce the expression of several molecules and, most importantly, IL-2, which binds to the CD25 receptor on the surface of activated T cells and induces its survival and proliferation. After 4–5 days of division, the activated T cells differentiate into effector and memory T cells (29, 30).
Naïve B cells that have bound antigen to their surface Ig receptors require co-stimulatory signals from CD4 helper T cells that are specific for the same antigen. This allows initiating a germinal center reaction in secondary lymph nodes and proliferating and mutating their antibody genes through somatic hypermutation to achieve higher affinity and then differentiate into antibody-producing plasma cells and memory B cells (Figure 2).
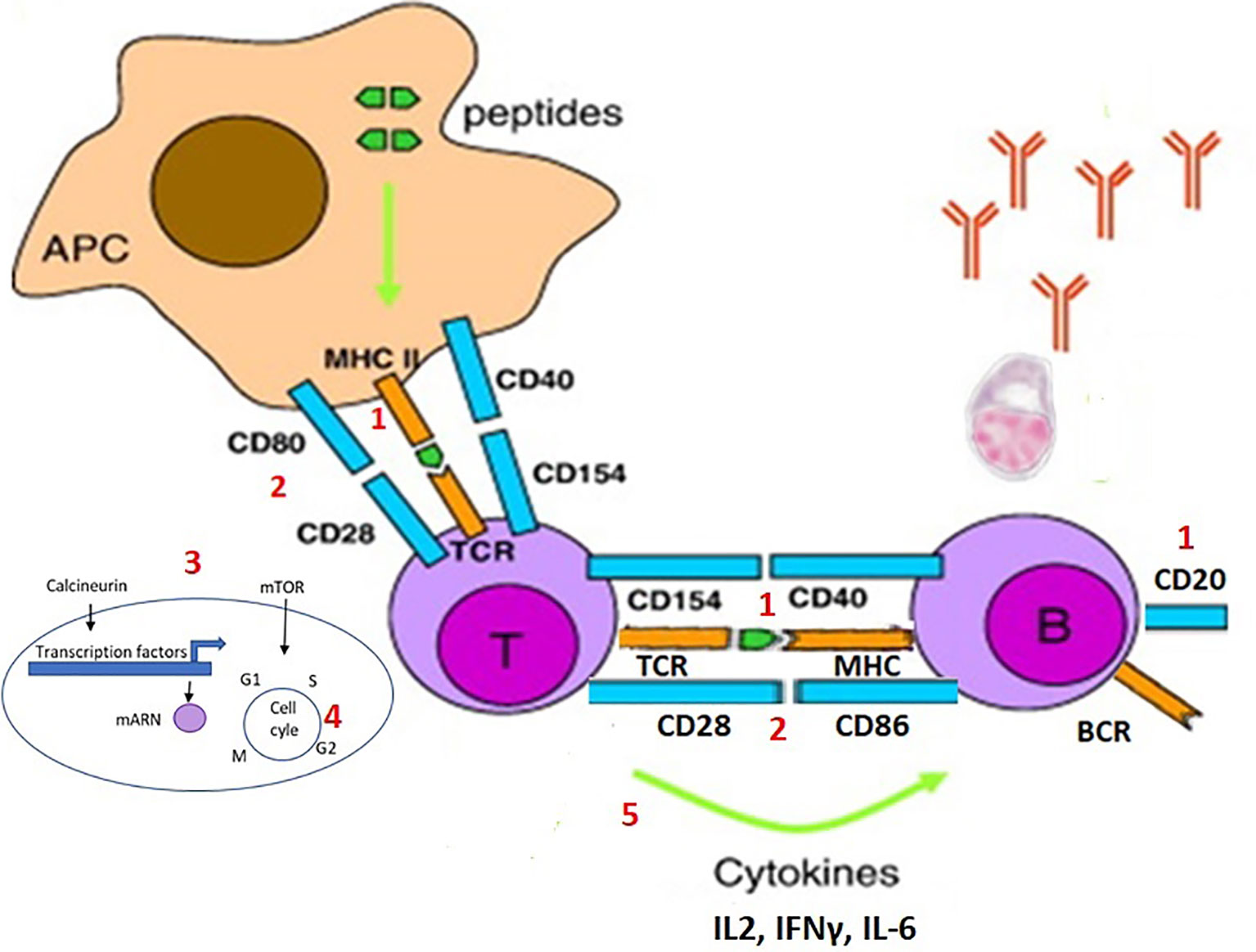
Figure 2 Steps of the activation and proliferation of T cells and B cells during an immune response, and possible targets of the immunosuppresive drugs. During an immune response, naïve T cells recognize a peptide antigen presented on the surface of antigen-presenting cells (APCs) in the MHC molecule via the binding of their T-cell receptor (TCR) and co-stimulatory signals given by the CD80 and CD86 on the surface of DCs and CD28 on T cells. This activates various signal transduction pathways in T cells, which activate the transcription of various factors that induce the expression of several molecules, such as IL-2. Naïve B cells that have bound antigen to their surface Ig receptors require co-stimulatory signals from T cells that are specific for the same antigen. This allows initiating a germinal center reaction with proliferation and mutation of the antibody genes and then differentiate into antibody-producing plasma cells and memory B cells. Each of the steps of the immune response can be the target of an immunosuppressive drug: 1) the depletion of the specific or cognate T and/or B cells (e.g. anti-CD20); 2) interference with the co-stimulatory signals (e.g. CTLA4-analog); 3) blockade of the intracellular signal (e.g. calcineurin inhibitor or mTOR inhibitor); 4) inhibition of DNA synthesis and cell proliferation (e.g. purine analog, or alkylating agents); 5) modulation of the effector T or B cell responses (various anticytokine monoclonal antibodies), included blocking inflammation reducing antigen presentation (anti-IL6, TNF, JAK, etc.).
General Principles of the Effect of Immunosuppressive Drugs on the Immune Response to Vaccination
Each of the steps of the immune response can be the target of an immunosuppressive drug (Figure 2) (29): 1) the depletion of the specific or cognate T and/or B cells (e.g. anti-CD20); 2) interference with the co-stimulatory signals (e.g. CTLA4-analog); 3) blockade of the intracellular signal of the antigen-recognizing TCR (e.g. calcineurin inhibitor or mTOR inhibitor); 4) inhibition of DNA synthesis and cell proliferation (e.g. purine analog, or alkylating agents); 5) modulation of the effector T or B cell responses (various anticytokine monoclonal antibodies), included blocking inflammation reducing antigen presentation (anti-IL6, TNF, JAK, etc.).
Overall, the result is that the various immunosuppressors block the clonal expansion of specific T and B cells. In general, the primary immune response to a novel antigen is typically more severely affected than a secondary immune response, as the threshold for activation of memory B and T cells is lower. However, some drugs may affect directly on T/B cell activation/trafficking. In addition, the clonal expansion of the memory cells is reduced, and the antibodies are produced in a lower amount and of reduced quality and therefore resulting in a shorter duration of protection.
Classical vaccines can be subdivided into two groups including the live-attenuated vaccines and the inactivated-subunit-killed vaccines (commonly named “non-live” vaccines). These groups differ in the way they stimulate the immune system. Inactivated vaccines are used against bacteria and viruses that cannot be attenuated. Their advantage is that the product is chemically defined, stable, safe and contains only B cell and T-cell specific epitopes. They can be administered without any risk in any patient, including those who are immunosuppressed. However, they require frequent booster immunizations.
Live-attenuated viral vaccines can be created with less virulent (attenuated) mutants of the wild-type virus or with viruses from other species that share antigenic determinants. Virus can be attenuated via passage through a foreign host, such as embryonated eggs or tissue culture, where they acquire mutations to infect the new host. The new virus population will be significantly different from the initial population and will not grow well in the original host. The disadvantages of these vaccines are that they require refrigeration and they cannot usually be administered to immunocompromised patients because of the risk of disease caused by the vaccine strains.
There is also the possibility to create vaccines with viruses that lack virulence properties using genetic engineering. Molecular techniques are now being used to develop new vaccines. By genetic engineering, new live-attenuated vaccines can be generated by the induction of mutations to delete or inactivate genes encoding virulence factors. These new techniques appear to be more reliable than random attenuation of the virus via passage through tissue culture. Hybrid virus vaccines can be formed when genes from infectious agents that cannot be easily attenuated are inserted into safe viruses. A defective, infectious, single-cycle (DISC) virus vaccine is formed by a virus with a deletion of an essential gene that is grown in a tissue culture cell that expresses the defective gene. In DNA vaccines, the genes coding for a protein that expresses an important B- and T-cell-specific viral or bacterial epitopes are inserted into a plasmid vector, thus permitting the protein to be expressed in eukaryotic cells. Plasmid DNA is injected into muscle or skin, and then taken up by DCs where the cDNA is transcribed and the immunogenic protein expressed, permitting the induction of a cell-mediated and humoral immune response. Attenuated viruses or bacteria, such as Escherichia coli, may be used as vectors containing the plasmid. Reverse vaccinology, which utilizes genomic sequence data, is a new approach for the development of vaccines. These new technologies are exploding in the context of the current severe acute respiratory syndrome coronavirus 2 (SARS-CoV-2) pandemic and it is anticipated that they will also influence the development of new vaccines for different populations such as the “too young, too old, or too immunocompromised”. Hopefully, these new vaccines and also the development of new adjuvant will help in developing vaccines that are more adapted to immunosuppressed patients.
Revaccination with non-live vaccine is therefore expected to induce a reduced immune response in immunosuppressed compared to healthy children. In contrast, during a primary vaccination with a novel antigen, additional doses may be necessary to reach the protective antibody threshold. For this reason, when possible, it is very important to verify the antibody titer post-vaccination to decide on whether additional doses must be given. In addition, following immunization with live-attenuated vaccines, there is the risk that the inhibition of the clonal expansion of T and B cells may lead to the inability to clear the replicating attenuated vaccine-strain virus, leading to the possibility of severe vaccine-associated disease and adverse events.
The introduction of bDMARDs in 1995 has revolutionized the treatment of autoimmune diseases, as these new treatments target specific pathways that are involved in immune function, inflammation and autoimmunity, rather than broadly suppress cell activation/proliferation as csDMARDs. The advantage is that bDMARDs are more targeted and effective in treating autoimmune diseases. Of note, there is a continuous introduction of new monoclonal antibodies, but only those currently used in children are listed in Table 1, classified according to their mode of action. The current recommendations of vaccination with non-live and live-attenuated vaccines are summarized for each type of immunosuppressive drug. There will be a need to revise and adapt vaccination guidelines regularly due to constant advances in this field.
Vaccination With Live-Attenuated Vaccines
Vaccination schedules for MMR and VZV vaccines differ among countries. While the first MMR vaccine dose is given around 9–15 months of age in all countries, the timing of the second dose varies greatly. It is recommended before the age of 2 years in Switzerland and Australia, or between 4 and 6 years in countries such as France, Spain, the United Kingdom, USA and Canada, or even as late as at 9 years old in Hungary, The Netherlands, Estonia, Norway, Poland and the Slovak Republic (17, 31–33). Most European countries do not vaccinate against varicella, while VZV vaccine is part of the routine vaccination schedule in Australia, Canada, and the USA. Hence, depending on the age at onset of the autoimmune disease, the child might not be immune against measles and varicella at the time of diagnosis. In healthy children, VZV vaccine is 82% effective at preventing any form of varicella after one dose and 98% after 2 doses, but 100% effective at preventing severe varicella after one dose (34). MMR vaccine is very safe and effective in healthy people. One dose of the vaccine in 93% effective, while two doses are 97% effective at preventing measles (34).
The risk of measles and varicella infections in immunocompromised children is even more important at the current time of increasing vaccine hesitancy and measle outbreaks worldwide. Therefore, assuring a protective immunity against measles and varicella in immunocompromised children can be very challenging. Once the immunosuppressive treatment has been introduced, it is no longer possible to vaccinate against these diseases, as only live-attenuated vaccines are available. Furthermore, vaccinating children during the acute phase of disease with a live-attenuated vaccine is often difficult as a time interval of minimum 4 weeks is necessary between vaccination and the beginning of the immunosuppression, and even more if two doses are needed.
Previous studies that have assessed the safety and immunogenicity of live-attenuated vaccines in children on immunosuppressive treatment are summarized in Table 2. There are almost no data on primary vaccination with MMR in immunosuppressed children as the first dose of this vaccine is typically given before the onset of most autoimmune disorders.
Evidence for Safety and Immunogenicity of Live-Attenuated Vaccines
Measles, Mumps, Rubella
In a prospective, nested, case–control study, the immune response following a booster dose of MMR was comparable in both healthy controls and 15 children with JIA treated with low-dose MTX + or anti-TNFα (etanercept) (36). A Dutch randomized, multicenter, open-label clinical equivalence trial assessed the effect of a MMR booster dose in 137 JIA patients aged between 4 and 9 years (60, MTX; 15, bDMARDs) in which patients were randomly assigned to receive MMR booster or placebo. Among patients taking bDMARDs, treatments were interrupted five times their half-lives prior to vaccination. The authors observed a good immunogenicity of the booster dose of MMR in JIA patients and no increase in disease flares in the year following vaccination (39). A retrospective, single-center Dutch study compared the long-term persistence of antibody to MMR, diphtheria, and tetanus toxoids in 400 JIA patients compared to 2,176 healthy controls. They reported lower levels of antigen-specific antibodies in JIA patients for all antigens, except measles, although seroprotection rates were similar in JIA patients and controls. Furthermore, the use of MTX and GCs had no effect on antibody persistence (38). Other studies have reported that revaccination with MMR in patients treated with various immunosuppressive treatments was safe and immunogenic, although the antibody response was lower in the short and longer term (35, 37, 40, 42) (Table 2).
Varicella (Chickenpox) Vaccine
In a prospective controlled study, 25 children with various rheumatic diseases (seventeen JIA, four juvenile dermatomyositis, three juvenile scleroderma, one vasculitis), treated with MTX alone or with prednisone (maximum 10 mg/day) or other csDMARDs, received a single primary dose of VZV vaccine. Three patients with JIA presented a mild self-limited varicella-like rash in the first 2 weeks post-vaccination, without any other symptoms, and the rash spontaneously resolved after 5 to 7 days. More importantly, the number of active joints in JIA patients significantly decreased at month 3 after vaccination (43). In another prospective controlled study, 54 children with SLE treated with various csDMARDs and immune for varicella were randomly assigned to receive a single booster dose of VZV vaccine or placebo. There was no difference in the rates of adverse events or frequency of SLE flares between the vaccinated and non-vaccinated children (45). A case series reported the administration of a first dose of VZV vaccine in 4/6 children with JIA treated with bDMARDs. They reported that the vaccine was safe, but not efficacious in all children as one patient did not respond and presented a mild varicella infection 4 months later. Although it was a very small sample size, it appears that patients treated with anti-TNFα (etanercept) responded less well (46). Another case–control study assessed the immune response to a booster dose of VZV vaccine in 49 children with diverse rheumatic diseases (three of whom were treated with bDMARDs) compared to 18 healthy controls. They reported good safety data and similar humoral responses in patients compared to healthy controls (47). Similarly, another prospective study assessed the immune response to primary and booster doses of VZV vaccine in children on immunosuppressive treatments, nine of whom were on bDMARDs. They used a pre-vaccination checklist with basic laboratory tests: white blood cell count ≥3,000/mm3, lymphocytes ≥1,200/mm3, serum IgG ≥500 mg/dl, IgM ≥20 mg/dl, tetanus toxoid antibody ≥0.1 IU/ml. In the case of high immunosuppression, additional specifications included a CD4+ lymphocyte count ≥200/mm3 and a positive T-cell function (via the positive control of a standard tuberculosis interferon-gamma-release-assay indicating mitogen-induced T-cell proliferation). Patients who met the criteria of the pre-vaccination checklist received the first and/or second VZV vaccination, with good safety and immunogenicity results (48).
A retrospective multicenter survey in which physicians treating children with autoinflammatory diseases on anti-IL-1 and anti-IL-6 were contacted and asked to report safety data concerning the vaccination with live-attenuated vaccines. Good safety data were reported concerning 17 children (seven with sJIA and ten with periodic fever syndromes), apart from two serious adverse effects: a VZV infection after a VZV booster in a child on anti-IL-1 (anakinra), low GCs and several csDMARDs and a pneumonia after a MMR booster in a child on anti-IL-1 (canakinumab), low GCs and MTX (49). Finally, a retrospective study from the Paediatric Rheumatology European Society (PRES) Vaccinations Working Group reported good safety data of 234 patients with various rheumatic diseases receiving booster doses of MMR/MMRV while treated with various immunosuppressive treatments (40).
Other Vaccines
There are a few studies that have shown that the yellow fever vaccine was relatively safe in immunocompromised patients (solid organ transplant recipients, patients with immunosuppressive treatment like corticosteroids, mesalazine, MTX, and bDMARDs) and was not associated with infections by the attenuated vaccine strain (50–52). One case series has also assessed the immune response to a booster dose of yellow fever vaccine in 15 adults with various rheumatic diseases treated with MTX and anti-TNFα reported a similar antibody response to healthy controls and no adverse events, although there was a trend towards a lower immune response in patients, but due to the small sample size, no formal statistics could be performed (53).
What Do We Know and What Are the Controversies
Overall, these studies show that children treated with low-dose csDMARDs and GCs who received booster doses of MMR, VZV, or primary vaccination against VZV, had no severe adverse reactions and no cases of vaccine-derived viral infections or worsening of disease activity (35, 36, 43, 53). Therefore, even if larger studies are necessary, it appears that booster vaccinations with live-attenuated vaccines can be considered in patients with autoimmune disorders treated with various csDMARDs at low dose or GCs, or even some bDMARDs (54) However, more data are needed for these new treatments as they are more specific and, therefore, they could impact on a similar pathway needed for vaccine responses. An immunology work-up could also be done before vaccination with live-attenuated vaccines by looking at total lymphocyte count, IgG levels, vaccine antibody levels, including lymphocyte immunophenotyping looking at CD4 and CD8 counts and a T-cell stimulation test (4, 48).
Concerning immunogenicity, all these results show that live-attenuated vaccines induce a good immune response in the short term in children with various immune-mediated disorders on GCs, csDMARDs or bDMARDs (anti-TNF, anti-IL-1, anti-IL-6) (36, 38–40, 43, 45–48) as summarized in Table 2. However, a rapid loss of the antibodies can be expected in the longer term under immunosuppression, although persistence may be maintained with some csDMARDs. Results also suggest that the responses are lower in children on bDMARDs. These results are very important in the context of measle outbreaks occurring worldwide as immunosuppressed children not up to date with their vaccines are particularly at risk of infection. Booster doses may be needed, but it is difficult to establish common guidelines as to when booster should be given as the long-term effect may depend on the complexity of therapy.
Recommendations
The recommendations of the PRES concerning live-attenuated vaccines in children with rheumatic disease were published in 2011 (7) and updated in 2015 (54). According to PRES, live-attenuated vaccines against MMR and VZV can be given safely in children with rheumatic disease without immunosuppression according to national guidelines (7, 54). As soon as an immune-mediated disorder is suspected, screening for VZV and measles should be done systematically through infection and vaccine history and, if possible, confirmation by vaccine serology (4). If the surrogate marker is below the threshold considered protective, seronegative patients for VZV and measles should be vaccinated before the start of immunosuppressive/immunomodulatory therapy. Two vaccine doses, at least 1 month apart, should be administered and the last dose should be given ≥1 month before the start of immunosuppressive therapy (4, 7, 55, 56).
Under immunosuppression, it is recommended to first give a non-live vaccine (preferably following a novel antigen, such as hepatitis A) and assess the antibody response one month after vaccination, as well as to measure the number of CD4/CD8 cells. If the antibody response is good, including the T-cell numbers, a live-attenuated vaccine can be considered (4).
In general, live-attenuated vaccines are contraindicated under immunosuppressive therapy. However, as the replication potential of varicella vaccine is low and antivirals are available, varicella vaccine can be considered in any stable child under low-dose therapy with MTX, AZA, or 6-MP (4, 57), while MMR and yellow fever vaccinations can be considered in clinically stable patients during low-dosage GCs and MTX therapy ≤15 mg/m2/week (7, 54). According to other recommendations, booster vaccinations against VZV, MMR, and yellow fever, can also be considered in patients on low-dose csDMARDs (2, 4) (Table 3).
Live-attenuated vaccines should be avoided in children on high-dose immunosuppression (7, 54). Indeed, the replication of the live-attenuated vaccine may not be sufficiently controlled under strong immunosuppression and attenuated vaccines have the theoretical risk of a reversion to the virulent form, thereby inducing overt disease (49, 58). In the healthy population, this presentation is extremely rare, generally mild, and self-limited (23).
The interval after which a live vaccine can safely be given after interruption of the immunosuppressive drug is dependent on the pharmacokinetics and pharmacodynamics of the molecule. In principle, it is considered that five times the specific half-life of a drug corresponds to the time needed to clear the drug from the body, but the immunosuppressive effect can last longer. For anti-cytokine drugs, the immunosuppressive effects are expected to be of shorter duration than for drugs inhibiting cell division or cell function. Therefore, some guidelines recommend “5 × t1/2 elimination + immunosuppressive effect” (which is 2 weeks for anti-cytokines and 4 weeks for other drugs) (6). In general, it is recommended to wait for at least 4 weeks after discontinuation of high-dose GCs, at least 3 months after discontinuation of csDMARDs, and at least 3 months after discontinuation of a bDMARDs (56).
Table 1 summarizes the effects of each immunosuppressive drug and the delay necessary before vaccination with a live-attenuated vaccine. These tables should be taken as indicative and not as strict guidelines according to expert consensus (2–5, 10, 56). Delays were calculated according to the half-lives of the drugs (usually five half-lives) and the expected duration of the immunosuppressive effect after interruption. The various delays can be followed before planning any live-attenuated vaccines in children on immunosuppressive treatments while considering the risk and benefit of vaccination in each situation.
It is also recommended to verify the vaccination status of the household and other close contacts and vaccinate them if indicated so as to minimize the risk for immunocompromised children through a “cocooning strategy” (56).
In addition, if there is no time to administer live-attenuated attenuated vaccines before starting immunosuppression, patients should be informed of their risk in the case of known exposure and advised to consult rapidly to receive prophylactic treatment antivirals/Igs (4).
Concerning other live-attenuated vaccines, they are usually contraindicated in patients on immunosuppression. The same recommendations should be followed for VZV and MMR vaccines. If travel is planned to an endemic country for yellow fever soon after the diagnosis, this vaccine should be administered before starting immunosuppression. In general, families should be discouraged from traveling to countries endemic for yellow fever, and other diseases for which only live-attenuated vaccines are available, these are contraindicated. Yellow fever vaccination can be given in clinically stable patients during low dosage MTX (4). If yellow fever vaccine has been already administered previously, an antibody measurement should be performed. Seropositivity indicates past immunity and enables travel to yellow fever endemic areas, regardless of the time that elapsed since immunization. As a precaution, oral typhoid vaccination (Vivotif®) and BCG vaccine should generally be avoided in all patients under immunosuppression (4).
Vaccination With Non-Live Vaccines
Several studies have shown that non-live vaccines in children with autoimmune disorders treated with different immunosuppressive drugs do not worsen the disease or cause serious adverse events compared with healthy subjects as reviewed in detail in (7) and (54).
For children with rheumatic diseases, EULAR recommends adhering to national vaccination guidelines for diphtheria, Hib, hepatitis A and B, pertussis, pneumococci, poliomyelitis, meningococci, rabies, tetanus, and tick-borne encephalitis. Vaccination schedules differ among countries (31–33, 59). Table 4 summarizes the recommendations for the various non-live vaccines.
When a disease that potentially requires an immunosuppressive treatment is diagnosed, the vaccine status of the child should be verified. All non-live vaccines can be given without restriction, but they should be given 2 weeks before treatment starts to increase immunogenicity. When possible, the vaccine-specific antibody responses should be verified, especially following primary immunization and for children treated with high-dose immunosuppression and bDMARDs (7, 54, 55). Table 5 shows whether a meaningful serological test is available. Table 4 summarizes the general recommendations for each non-live vaccines.
Influenza
Evidence for Immunogenicity
The response to influenza vaccine of children with rheumatic disease on immunosuppressive treatment has been widely studied, especially during the influenza A H1N1/2009 pandemic. It was observed in a case–control study of 95 patients with JIA compared to 91 healthy controls that the immune response was generally good, but sometimes associated with a reduced immune response in children with polyarticular JIA (60). In addition, another case–control study assessed the antibody response in 118 SLE patients and 102 healthy controls and reported that high disease activity was associated with a decrease in the antibody response to influenza A H1N1/2009 (61). Two other case–control studies of children with various rheumatic diseases (JIA, SLE, JDM) compared to healthy children reported a decreased immune response in those treated with high-dose GCs (62, 63) or combination treatment with GCs, MTX, and cyclosporin (63).
Therefore, the recommendation to vaccinate all children under the age of 9 years receiving the seasonal influenza vaccine for the first time with two doses at 1 month apart should perhaps be extended also to older immunosuppressed children. In a prospective case–control study, Aikawa et al. assessed the immunogenicity of two doses of the non-adjuvanted influenza A H1N1/2009 vaccine in children younger than 9 years with rheumatic disease compared to healthy controls and reported it to be safe and immunogenic in this patient population (64).
Recommendations
Seasonal influenza vaccination is recommended annually to all children with immune-mediated disorders treated or not with immunosuppressive drugs as influenza can be very severe and increase the risk of secondary bacterial infections (7). The first year, two doses are recommended in patients <9 years who have never been vaccinated against influenza or for whom the vaccination history is unknown (31–33, 59). The vaccination status of the household and other close contacts should be verified, and they should be encouraged to receive the current seasonal influenza vaccine.
Hepatitis A
Evidence for Immunogenicity
Previous studies have reported a good immunogenicity of the hepatitis A vaccine (HAV) in children on immunosuppressive treatment, except in some conditions. Indeed, a case–control study assessing the antibody response to HAV in JIA and healthy controls reported a decrease of the antibody response in children with active systemic JIA on anti-TNFα (65). However, other case–control studies have reported high seroconversion rates following HAV vaccine in patients with IBD on infliximab (66), 6-MP, or AZA (67) compared to healthy controls.
Recommendations
HAV should be offered to seronegative children with autoimmune disorders who travel frequently to endemic countries. The schedule should follow national guidelines (31–33, 59). A control of the response to HAV is recommended in immunosuppressed children by serology (4). If short-term protection is necessary, a serology can be performed 1 month after the first dose and, if necessary, a second dose can be administered at a short interval. For long-term protection, a serology should be performed 1 month after the last dose (6 months after the first dose) and, if necessary, further vaccine doses should be administered (4).
Hepatitis B
Evidence for Immunogenicity
Several case–control studies in children suffering from various immune-mediated disorders (autoimmune hepatitis, IBD, and JIA) have observed a reduced immune response following HBV vaccine in children with immune-mediated disorders compared to healthy children and a decreased long-term antibody persistence, particularly in those treated with GCs, AZA, and anti-TNFα (68–71). In another case–control study, 14 children with IBD non-responders to three doses of hepatitis B vaccine (20 mcg) received a booster dose of hepatitis B vaccine. After this additional dose, 7/14 (50%) seroconverted. Overall, seroprotection was 85% after a full vaccination scheme and a booster dose (72), even if an adjuvant was used (aluminium), suggesting that this may not be sufficient.
Recommendations
Hepatitis B vaccination is recommended for children with autoimmune disorders because of potential severe disease during immunosuppression. All children should be screened by serology soon after the diagnosis. Hepatitis B should be administered to children seronegative (without anti-HBs antibodies) according to national guidelines (31–33, 59), which is three doses at 0, 1 and 6 months for most countries (and in some countries two doses at 0 and 6 months for children 11–15 years). If protection is needed more rapidly, the accelerated scheme (1, 7, 21 days, 6–12 months) is indicated, e.g. patients who need to start rapid immunosuppression. In the case that the family travels extensively and no natural immunity against hepatitis A has been acquired yet, the combined hepatitis A and B vaccines (Twinrix®) should be chosen, as it is known to be more immunogenic than the monovalent HBV. It is recommended to verify the antibody titer one month after the 3rd vaccination (scheme 0, 1, and 6 months) and after the 4th dose if the scheme is 0, 7, 21 days and 6–12 months. Levels of anti-HBs >100 mIU/ml should be achieved. If necessary, booster doses should be administered. There are no data on the maximal doses to be given in the case of an absence of response, but usually up to six doses are given. In addition, Twinrix® can be given in immunosuppressed children in the case of an absence of response (usually three doses at 0, 1, and 2 months) according to a recent study (73).
Maintenance of HBs antibody should be monitored on a regular basis in immunosuppressed children. A booster dose of hepatitis B vaccine should be given if anti-HBs fall below 10 IU/l (4, 6).
HPV
Evidence for Immunogenicity
Heijstek et al. assessed the immunogenicity and safety of the bivalent HPV in young females with JIA, SLE, and JDM in a case–control study and reported lower antibody and memory B cell concentrations in patients compared to healthy controls, although not statistically significant. There was no significant effect of the various immunosuppressive treatments (MTX and anti-TNFα) on the immune response to HPV vaccine. However, it has been reported in two case–control studies of patients with JIA or JDM that the antibody concentrations tended to be lower in patients than in healthy controls. This was even observed in adolescent girls who were not receiving any immunosuppression, due to an unclear mechanism that remains to be elucidated (74, 75), despite the fact that the vaccine was adjuvanted with aluminium. Similarly, an adult case–control study reported a reduced immunogenicity of the quadrivalent HPV vaccine in adult patients with SLE compared to healthy controls (76).
Recommendations
HPV vaccination is recommended for young adults aged 11–26 years with autoimmune disorders according to the national vaccine schedule (31–33, 59). The immunogenicity results of previous studies suggest that assessing the vaccine response and antibody persistence following HPV vaccination in this population may be useful, although there is still no recognized seroprotection cut-off for this age group.
Pneumococcal Vaccines
Evidence for Immunogenicity
In a case–control study of JIA children and healthy controls, Farmaki et al. observed that following the pneumococcal protein 7-valent conjugate vaccine (PCV7), JIA-children had a normal antibody response when treated with MTX or cyclosporine, either with or without GCs, but a lower antibody response if treated with anti-TNFα (77). Other studies have reported severe local and systemic reactions following the plain polysaccharide pneumococcal vaccine in children and young adults with an autoinflammatory disease, such as cryopyrin-associated-periodic syndromes (78, 79), but probably due to the disease itself and not due to the therapy (inflammasome trigger). Therefore, for this population of children, the PCV may be better.
Recommendations
Both the PCV13 and PPV23 pneumococcal vaccines are still recommended in many countries, such as the USA, Canada, Cyprus, Greece, France, and Spain (31–33, 59). For example, the US Advisory Committee on Immunization Practices recommends the following vaccination plan for children with chronic diseases: four doses of PCV13 at 2, 4, 6 and 12–15 months of age, followed by two additional doses of PPV23 at 5 years’ interval between the ages of 2 and 18 years (32). The rationale being that the PPV23 adds protection against a larger number of serotypes than the PCV13. However, because of its T-independent characteristic, it only induces short-term immunity and weaker immune responses than the PCV13, which is a T-dependent antigen (57). For this reason, only PCV13 is recommended in Switzerland. In general, conjugate pneumococcal vaccine should be preferred over polysaccharide vaccine as conjugate vaccines produce higher affinity antibody responses, longer lasting immune responses, as well as the production of memory B cells. Booster vaccinations after conjugate vaccines permit an amplification of the pool of memory B and T cells. In contrast, booster vaccination with plain polysaccharide vaccines may deplete the pool of memory B cells due to a lack of induction of memory cells (80). The fact that some countries still include the polysaccharide vaccine in their recommendations depends on the pneumococcal serotype distribution circulating in the country.
Vaccination against pneumococcal disease is recommended for all children with autoimmune disorders according to national immunization guidelines (31–33, 59). Ideally, the vaccination should be administered prior to the start of immunosuppressive therapy. If immunosuppressive therapy has already been started, the vaccination should be administered at a time point when the level of immunosuppression is lowest. Whether and when booster vaccination may be needed following PCV13 priming remains to be defined. Immunogenicity may be reduced under some immunosuppressive treatments and, if possible, verification of the immune response should be performed 1 month after vaccination and regularly in children remaining under immunosuppression (4).
Meningococcal Vaccines
Evidence for Immunogenicity
Stoof et al. conducted a retrospective cohort study of the kinetics of specific antibody responses following the meningococcal serogroup C-conjugate vaccine over time in children with JIA. They observed a similar antibody response and waning of meningococcus-specific IgG titers over time in patients and healthy controls. However, the loss of antibodies was more rapid in patients on bDMARDs than on csDMARDs (81).
Recommendations
Monovalent (capsular groups A and C) or quadrivalent (MenACWY) meningococcal conjugate vaccine, as well as the vaccine against serogroup B (MenB), is recommended in several European countries and in the USA and Canada, depending on the endemicity of the various meningococcal serogroups in the different locations worldwide (31–33, 59). Patients with acquired complement deficiency, such as patients receiving the monoclonal antibody eculizumab, and other children who are receiving an immunosuppressive treatment are also at risk of hyposplenism and should be up to date with their meningococcal immunization (10).
Tetanus-Diphtheria-Acellular Pertussis-Polio Vaccines
Evidence for Immunogenicity
In general, the antibody response to tetanus–diphtheria vaccination is similar in patients with immune-mediated disorders and healthy controls. However, case–control studies assessing measles and tetanus antibodies in children with SLE observed that the antibody titers tended to decrease more rapidly in patients treated with immunosuppressive drugs (37, 38). Another retrospective, cross-sectional study in children with various rheumatic diseases and healthy controls also reported a decrease in antibody in children with rheumatic disease (37, 38).
Recommendations
Tetanus, diphtheria, acellular pertussis, and poliomyelitis vaccinations are recommended for all children with immune-mediated disorders, according to the national immunization guidelines specific to the country (31–33, 59). The timing and number of doses depend on the number of previous doses received and the interval since their last dose of vaccination. In young adults, after primary vaccination, booster doses of diphtheria/tetanus vaccine should probably be given more frequently than in healthy persons, i.e. every 10 years (4).
Haemophilus Influenzae Type B
Recommendations
Hib vaccination should be administered according to national immunization guidelines (31–33, 59). Based on the current epidemiology, Hib immunization is not recommended after the age of 5 years, even in immunosuppressed patients, except in the Czech Republic, Greece, the USA, and Canada (31–33, 59).
Other Vaccines: Rabies, Japanese Encephalitis, Parenteral Typhoid Vaccines, Tick-Borne Encephalitis
Evidence for Immunogenicity
In the literature, no data were found on the safety and immunogenicity of the inactivated vaccinations against rabies, Japanese encephalitis, typhoid fever, or tick-borne encephalitis in children with autoimmune diseases.
Recommendations
Vaccinations against rabies, Japanese encephalitis, or typhoid fever are indicated for specific risk situations according to national immunization guidelines before traveling to endemic areas (31–33, 59). The indications should be discussed individually with specialists before planning international travel.
A vaccination against tick-borne encephalitis is recommended for children with an increased risk of exposure according to the national immunization guidelines for each country (32, 59). The usual course of vaccination should be followed (three dose-scheme with a booster dose every 10 years). In immunosuppressed patients, a serology should be performed 1 month after the last dose.
Particular Situations
Children Treated With Intravenous Immunoglobulin
In the case of treatment with IVIg, the immune response to live-attenuated vaccines may be reduced if the vaccine is administered immediately before or 1–4 weeks (or more) after IVIg. Live vaccines should be given either 2 weeks before or should be delayed for 3–11 months after IVIg, depending on the dose. In the case of treatment with IVIG within 14 days of a live vaccine, the vaccine should be verified after 3–11 months after IVIg treatment, and the vaccine re-administered if necessary.
Children Treated With B-Cell Depleting Drugs
There are no data on the immune response post-vaccination in children treated with anti-CD20. In a prospective controlled study, Oren et al. assessed the humoral immune response to the seasonal influenza vaccine in three groups, i.e. 29 adults with rheumatoid arthritis, 14 rheumatoid arthritis adults treated with rituximab in the previous 18 months, and 21 healthy adults. They observed that patients treated with rituximab responded less well compared to the two other groups but still developed a partial immune response to the seasonal influenza vaccine (82). Nagel et al. showed in a prospective controlled study that belimumab given in addition to csDMARDs did not decrease the antibody response to PCV13 in SLE patients (83). In a phase 4, open-label study among patients randomized to receive the PPV23 either 4 weeks prior to belimumab or 24 weeks after starting 4-weekly belimumab treatment, Chatham et al. observed that both groups responded similarly to the PPV23 (41). Other groups have shown that there is very little antibody induced, but T cells may be preserved (unpublished).
During 6–9 months following treatment with anti-CD20 monoclonal antibodies or anti-BLys, immune responses to vaccination are severely impaired as many antibody-producing plasma cells are short-lived and require replacement from CD20+ precursors. In addition, the number of memory B cells in the bone marrow is also reduced (84), and B cells returning from the bone marrow to the peripheral blood have an immature phenotype (CD27−IgD−) or naïve (CD27−IgD+) rather than memory B cells. The development of new memory B cells appears to be delayed for many years. However, it appears that long-lived plasma cells may not be affected by B cell depleting drugs. Therefore, it is recommended to administer primary immunization before anti-CD20-depleting antibodies. Secondary immunization can be administered 6 months after these treatments for non-live vaccines, but only after 12 months for live-attenuated vaccines (5, 6, 29). Prolonged hypogammaglobulinemia and B-cell depletion have been reported following rituximab. Since there are recommendations to document prior to therapy and then monitor Ig and B-cell levels during therapy, it may be reasonable to ensure that these levels have normalized prior to any immunizations.
Although the immune response is expected to be diminished in individuals under B cell-depleting drugs, the seasonal influenza vaccine is still recommended, as we expect to induce a T-cell immune response (4).
Infants Born to Mothers Who Received Immunosuppressive Treatment During Pregnancy
As some immunosuppressive drugs pass the placental barrier, they can be found in new-borns for 6–8 months, especially if they are taken at the end of pregnancy by mothers. These drugs can affect the development of the immune system of the new-borns and also affect the response to vaccination. For example, a case of fatal “BCGitis” has been reported in a 3-month-old infant whose mother had been treated with infliximab during pregnancy (85). Drugs such as MTX, MMF, leflunomide, and cyclophosphamide are teratogenous and contraindicated during pregnancy (86). Other medications such as antimalarials, sulfasalazine, AZT, cyclosporine, tacrolimus, and colchicine are not immunosuppressive and can be administered during pregnancy (86). COX2 selective non-steroidal anti-inflammatory drugs (NSAIDs) and corticosteroids can be given until 28 gestational weeks (86). In severe refractory maternal disease during pregnancy, pulses of methylprednisolone and IVIg can also be given until the end of pregnancy if necessary. It should be noted that biological monoclonal antibodies are transferred through the placenta, like other Igs, from week 13 until the end of pregnancy, with a peak during the last 4 weeks of pregnancy, resulting in a blood level 120–130% higher than the blood levels of the mother. Then, it appears that the half-life of the biological molecules is prolonged in new-borns (infliximab can be measured for up to 6–12 months in babies, adalimumab for 3–6 months). Concerning anti-TNFα, they can be given during the two first trimesters and it seems that etanercept and certolizumab can also be given until the end of pregnancy due to a low rate of transplacental passage. Other bDMARDs should not be used during pregnancy (86).
EULAR recommends vaccinating infants according to the normal schedule if biological agents have been discontinued before week 22 of gestation. However, if immunosuppressive treatment is continued past 22 weeks in the mother, live-attenuated vaccines (including BCG, rotavirus, oral polio, MMR and VZV) should be given after the age of 6 months. It is also possible to measure the metabolite levels in the blood of the infant. In contrast, inactivated vaccines can be given according to the normal schedule (86).
Most csDMARDs, bDMARDs, and tsDMARDs are contraindicated during breastfeeding, except for antimalarials, sulfasalazine, AZT, cyclosporine, tacrolimus, colchicine, prednisone, Ig, and anti-TNF because of a low transfer to breast milk. Therefore, children who are only exposed to those immunosuppressive drugs during breastfeeding can be vaccinated normally (86).
Proposed Practical Approach Concerning the Vaccination of Children With Immune-Mediated Disorders Who Should Start Immunosuppression or Already Under Immunosuppressive Treatment
In A Child Newly Diagnosed With an Immune-Mediated Disorder
- Check the immune status of the child through natural infection and vaccine history and serology.
Administer missing vaccines according to the age and condition if possible and prior to initiation of immunosuppressive treatment.
- For non-live vaccine, 2 weeks are generally required for the development of the immune response following primary immunization and around 1 week following booster immunization.
Verify when possible the vaccine response 1 month later and if vaccine antibodies remain below protective threshold as defined in Table 5, administer additional doses of vaccine.
- For live-attenuated vaccine, if time is sufficient (at least 4 weeks), administer one or two doses of the live-attenuated vaccine at 4 weeks interval. Verify the response after the first or second dose.
- However, in a case of good epidemiological situation, it is better not to vaccinate during an active disease, and to wait that the disease is stable on therapy to vaccinate, at least with non-live vaccines.
In Children Already Under Immunosuppression
- Define the effect of the underlying disease and/or immunosuppressive treatment on the immune response to vaccination.
- Using Table 1, determine how the immunosuppressive treatment will affect the cellular and humoral immune response to vaccines and whether any precautions are required before administering non-live or live-attenuated vaccines.
- For non-live vaccine, all vaccines are allowed; however, the immune response may be diminished with high-dose immunosuppression. Therefore, it is recommended to check the antibody response 1 month after vaccination when possible.
If a Live-Attenuated Vaccine Is Required Despite Immunosuppression
- Live-attenuated vaccines are usually contraindicated during immunosuppressive treatment. They can be considered in some circumstances if the personal risk of exposure to a given disease is high.
If the child has already received in the past the vaccine antigen for which he has no longer protective antibody levels, revaccination can be considered under certain conditions (low-dose immunosuppression.
- If primary immunization with a live-attenuated vaccine is needed, depending on the planned duration of the treatment and risk of exposure to the pathogen, it may be possible to interrupt temporarily the immunosuppressive treatment. In this case, follow Table 1 showing the minimal time interval between interruption of a certain immunosuppressive treatment and the administration of live-attenuated vaccines. However, the risk of exacerbation of the autoimmune disorders should also be considered.
When the Vaccine Antibodies Should Be Verified
- Under immunosuppression, the antibody response to primary immunization should be verified at 1 month post-vaccination when possible as the immune response may be decreased depending on the treatment, and additional doses may be necessary.
- Secondary immunization is expected to give rise to protective antibody levels, although at lower levels and for a shorter duration than in healthy children. Therefore, it is advisable to regularly assess vaccine antibody in a short period after vaccination (few month) and in the long-term (years) because children treated with immunosuppressive therapy might need booster doses more often than healthy children might. The timing of revaccination should be decided according to vaccine serology. This is particularly important for those pathogens that present a significant risk for community acquisition because of poor vaccine uptake (measles, varicella) and/or pathogens that present a significant lifelong risk and for which regular boosters are recommended (tetanus, pneumococci, hepatitis B).
Patients Treated With IVIG
- These treatments are not considered as immunosuppressive. However, they affect the immune responses to vaccinations.
First, vaccine serology is not reliable.
- The responses to live-attenuated vaccines are altered as the Igs will inhibit the replication of the live-attenuated virus. Therefore, a delay of 3–11 months is recommended between the end of the IVIG and the administration of live-attenuated vaccine, depending on the IVIG dose. It is also recommended to verify that vaccine antibodies (passively transferred through the IVIG) have disappeared before administering live-attenuated vaccines.
There is no delay necessary between non-live vaccines and IVIG perfusion.
Discussion
Overall, there are many studies concerning the vaccination of children with autoimmune disorders; however, there are still many open research questions (Table 6).
All published studies are very reassuring from a safety point of view, and most vaccines appear to be safe in children with autoimmune disorders on immunosuppressive treatment. They do not frequently cause serious adverse events and do not increase disease activity. However, there are only a few studies that have assessed vaccination with live-attenuated vaccines in this patient population.
It appears to be safe to vaccinate children treated with low dose csDMARDs and GCs, including booster doses of MMR and VZV or primary vaccination against VZV, as there has been no report of severe adverse reactions or cases of vaccine-derived viral infections or worsening of the disease activity under these conditions. However, larger studies are necessary to define the exact conditions under which live-attenuated vaccines can be given in children on high-dose DMARDs, bDMARDs. Indeed, live-attenuated vaccines can be considered on a case-by-case basis for children with autoimmune disorders treated with higher immunosuppression. For these patients, it is important to have a systematic approach to assess vaccine status and to plan the vaccinations at a specific time of the disease.
Concerning immunogenicity, most immunosuppressive treatments at low dose induce a normal antibody response in the short term. However, immunogenicity of some vaccines under higher immunosuppression is less clear. Although all non-live vaccines can be given even under high immunosuppression, it is not always very clear how the child will respond to the vaccination. Therefore, when possible, it is important to assess the antibody response 1 month after vaccination, as it might be necessary to give a supplementary dose of vaccine for some children.
Most studies have analyzed the short-term responses post-vaccination in children with immune-mediated disorders. However, long-term protection depends on persisting antibody levels above the threshold of protection until we know if the immunological memory can act rapidly enough to induce protective antibody levels in case of infection. Of note, this threshold of protection has been only established in healthy children and may be different in immunocompromised children. Therefore, a correlate of protection needs to be defined for this specific population to ensure that long-term protection is maintained. Therefore, it is very important to verify that children treated continuously with immunosuppressive treatment or suffering from various immune-mediated disorders that can affect their response to vaccination maintain protective antibody in the long-term. Indeed, it has been observed that the specific antibodies post-vaccination wane more rapidly in immunocompromised children than in healthy children. The speed of decline of the specific antibodies post-immunization in this population of children with immune-mediated disorders may depend on various parameters, such as the type and dose of immunosuppressive treatment, previous vaccinations, time since last vaccines, age, and the activity of the disease, but more studies are needed to define the exact factors that affect the rapidity of this decline. It is important to recommend how frequent the vaccine serology should be assessed in this population and how often vaccine booster doses should be given. For the moment, antibody persistence should be assessed more systematically in all children on immunosuppressive treatments, especially those on bDMARDs and against diseases for which the risk of exposure is continuous, such as pneumococci, influenza, tetanus, hepatitis B, VZV, and measles. There is also a need to develop laboratory tests, which are more widely available to help in monitoring long-term immunity to all vaccine-preventable diseases in high-risk children.
Assessment of immunity is largely restricted to antibody responses because of the difficulty measuring B- and T- cell-specific responses outside of specialized laboratories. However, long-term protection is more complex than just measuring the level of neutralizing antibodies. The quality of antibody and B cells (function, repertoire) is also important. There are only few data on recall responses in immunocompromised children and no data on B cell memory functions. It is well known that antibody levels can be under the protective threshold, although the individual may still be protected by the memory immunity, which can be re-activated very rapidly for some antigens, at least in healthy individuals. Studying the antibody and cellular immune responses in the short- and longer-term post-vaccination in this vulnerable population is crucial for the improved development of vaccine strategies (such as the use of new adjuvants or the use of DNA vaccines) to increase vaccine protection among these children.
Conclusion
Vaccination of immunocompromised children is safe with non-live vaccines and in specific situations also with live-attenuated vaccines, and should be a priority and a concern of every doctor in charge of these patients. As soon as an autoimmune disorder is suspected, vaccine status should be verified and all missing vaccines administered if time is sufficient. During immunosuppression, immune responses may be decreased, especially in children treated with high-dose csDMARDs or bDMARDs. In addition, antibody titers decrease more rapidly in the long term than in healthy children. Therefore, the vaccine response should be assessed not only at one month post-immunization when possible, but also on a regular basis for some antigens like tetanus, pneumococci, and hepatitis B, in order to administer booster doses when necessary. Of note, it is important to determine who has the responsibility for these assessments among the health care providers of these children. Specialists are often the primary care providers of those complex patients and should be more pro-active in verifying the vaccine status of their patients and making recommendations as to when to administer particular vaccines.
Even if larger studies are needed, live-attenuated vaccines appear to be safe under low-dose immunosuppression or after temporarily interrupting immunosuppressive treatment. It would be helpful to have studies that test a specific protocol to assess immune competence before vaccinating with live-attenuated vaccine, such as giving first the hepatitis A vaccine, assess the antibody titer after one month, and vaccinate with live-attenuated vaccines if the response to hepatitis A is good and if the total lymphocyte count is good enough. Indeed, clear guidelines are thus needed in order to define in which situations live-attenuated vaccines can be used.
Finally, new vaccine technologies are exploding in the context of the SARS-CoV-2 pandemic, based mostly on gene analysis to indicate targets and then combining this information with new ways to target and “trick” the immune system into responding appropriately. Similarly, a better understanding of the pathology of immune-mediated disorders has led to the development of more targeted approaches. It is hoped that the current pandemic will also trigger the development of an array of vaccines to be used in different populations for the same pathogen, with special vaccines for immunocompromised children.
Author Contributions
The author confirms being the sole contributor of this work and has approved it for publication.
Conflict of Interest
The author declares that the research was conducted in the absence of any commercial or financial relationships that could be construed as a potential conflict of interest.
Publisher’s Note
All claims expressed in this article are solely those of the authors and do not necessarily represent those of their affiliated organizations, or those of the publisher, the editors and the reviewers. Any product that may be evaluated in this article, or claim that may be made by its manufacturer, is not guaranteed or endorsed by the publisher.
Acknowledgments
I am very grateful to Dr Christiane Eberhardt, Prof Arnaud Didierlaurent and Prof Christoph Berger for carefully reading the first draft of the manuscript and for their very useful comments.
References
1. Furer V, Rondaan C, Heijstek MW, Agmon-Levin N, van Assen S, Bijl M, et al. 2019 Update of EULAR Recommendations for Vaccination in Adult Patients With Autoimmune Inflammatory Rheumatic Diseases. Ann Rheum Dis (2020) 79(1):39–52.
2. Wagner N, Assmus F, Arendt G, Baum E, Baumann U, Bogdan C, et al. Bundesgesundheitsblatt Gesundheitsforschung Gesundheitsschutz, Vol. 62. (2019). pp. 494–515.
3. Bühler S, Eperon G, Ribi C, Kyburz D, van Gompel F, Visser LG, et al. Vaccination Recommendations for Adult Patients With Autoimmune Inflammatory Rheumatic Diseases. Swiss Med Wkly (2015) 145:w14159.
4. Bühler S HC. Background Document on Immune-Mediated Inflammatory Diseases (IMID), Module 2: Vaccination in Patients With Inflammatory Bowel Diseases and Other Gastroenterological (Auto)Immune Conditions, S.F.A.C.o. Immunisation. (2017).
5. Wiedermann U, Sitte HH, Burgmann H, Eser A, Falb P, Holzmann H, et al. Guidelines for Vaccination of Immunocompromised Individuals. Wien Klin Wochenschr (2016) 128 Suppl 4:337–76. doi: 10.1007/s00508-016-1033-6
6. Belgium, S.H.C.o. Vaccination of Immunocompromised or Chronically Ill Children and/or Adults, S.H.C. Food Chain Safety and Environment. Belgium (2019).
7. Heijstek MW, Ott de Bruin LM, Bijl M, Borrow R, van der Klis F, Kone-Paut I, et al. EULAR Recommendations for Vaccination in Paediatric Patients With Rheumatic Diseases. Ann Rheum Dis (2011) 70(10):1704–12. doi: 10.1136/ard.2011.150193
8. Verolet CM, Pittet LF, Wildhaber BE, McLin VA, Rodriguez M, Grillet S, et al. Long-Term Seroprotection of Varicella-Zoster Immunization in Pediatric Liver Transplant Recipients. Transplantation (2019) 103(11):e355–64. doi: 10.1097/TP.0000000000002866
9. Posfay-Barbe KM, Pittet LF, Sottas C, Grillet S, Wildhaber BE, Rodriquez M, et al. Varicella-Zoster Immunization in Pediatric Liver Transplant Recipients: Safe and Immunogenic. Am J Transplant (2012) 12(11):2974–85. doi: 10.1111/j.1600-6143.2012.04273.x
10. Eperon G, Buhler S, Enriquez N, Vaudaux B. The Immunosuppressed Traveler: Vaccination Guidelines. Rev Med Suisse (2018) 14(605):922–33.
11. Papp KA, et al. Vaccination Guidelines for Patients With Immune-Mediated Disorders on Immunosuppressive Therapies. J Cutan Med Surg (2019) 23(1):50–74. doi: 10.1177/1203475418811335
12. Aygun D, Sahin S, Adrovic A, Barut K, Cokugras H, Camcioglu Y, et al. The Frequency of Infections in Patients With Juvenile Idiopathic Arthritis on Biologic Agents: 1-Year Prospective Study. Clin Rheumatol (2019) 38(4):1025–30. doi: 10.1007/s10067-018-4367-9
13. Aeschlimann FA, Chong SL, Lyons TW, Beinvogl BC, Goez-Mogollon LM, Tan S, et al. Risk of Serious Infections Associated With Biologic Agents in Juvenile Idiopathic Arthritis: A Systematic Review and Meta-Analyses. J Pediatr (2019) 204:162–71.e3. doi: 10.1016/j.jpeds.2018.08.065
14. Toussi SS, Pan N, Walters HM, Walsh TJ. Infections in Children and Adolescents With Juvenile Idiopathic Arthritis and Inflammatory Bowel Disease Treated With Tumor Necrosis Factor-Alpha Inhibitors: Systematic Review of the Literature. Clin Infect Dis (2013) 57(9):1318–30. doi: 10.1093/cid/cit489
15. Nagy A, Matrai P, Hegyi P, Alizadeh H, Bajor J, Czopf L, et al. The Effects of TNF-Alpha Inhibitor Therapy on the Incidence of Infection in JIA Children: A Meta-Analysis. Pediatr Rheumatol Online J (2019) 17(1):4. doi: 10.1186/s12969-019-0305-x
16. Lee WJ, Lee TA, Suda KJ, Calip GS, Briars L, Schumock GT, et al. Risk of Serious Bacterial Infection Associated With Tumour Necrosis Factor-Alpha Inhibitors in Children With Juvenile Idiopathic Arthritis. Rheumatol (Oxford) (2018) 57(2):273–82. doi: 10.1093/rheumatology/kex049
17. Toplak N, Uziel Y. Vaccination for Children on Biologics. Curr Rheumatol Rep (2020) 22(7):26. doi: 10.1007/s11926-020-00905-8
18. Gluck T, Kiefmann B, Grohmann M, Falk W, Straub RH, Scholmerich J, et al. Immune Status and Risk for Infection in Patients Receiving Chronic Immunosuppressive Therapy. J Rheumatol (2005) 32(8):1473–80.
19. Singh JA, Wells GA, Christensen R, Tanjong E, Ghogomu L, Maxwell JK, et al. Adverse Effects of Biologics: A Network Meta-Analysis and Cochrane Overview. Cochrane Database Syst Rev (2011) 2):CD008794. doi: 10.1002/14651858.CD008794
20. Nelson R. US Measles Outbreak Concentrated Among Unvaccinated Children. Lancet Infect Dis (2019) 19(3):248. doi: 10.1016/S1473-3099(19)30074-X
21. Porter A, Goldfarb J. Measles: A Dangerous Vaccine-Preventable Disease Returns. Cleve Clin J Med (2019) 86(6):393–8. doi: 10.3949/ccjm.86a.19065
22. Dell’Era L, Corona F, Daleno C, Scala A, Principi N, Esposito S, et al. Immunogenicity, Safety and Tolerability of MF59-Adjuvanted Seasonal Influenza Vaccine in Children With Juvenile Idiopathic Arthritis. Vaccine (2012) 30(5):936–40. doi: 10.1016/j.vaccine.2011.11.083
23. Morin MP, Quach C, Fortin E, Chedeville G. Vaccination Coverage in Children With Juvenile Idiopathic Arthritis Followed at a Paediatric Tertiary Care Centre. Rheumatol (Oxford) (2012) 51(11):2046–50. doi: 10.1093/rheumatology/kes175
24. Bizjak M, Blazina S, Zajc Avramovic M, Markelj G, Avcin T, Toplak N, et al. Vaccination Coverage in Children With Rheumatic Diseases. Clin Exp Rheumatol (2020) 38(1):164–70.
25. McCarthy EM, Azeez MA, Fitzpatrick FM, Donnelly S. Knowledge, Attitudes, and Clinical Practice of Rheumatologists in Vaccination of the at-Risk Rheumatology Patient Population. J Clin Rheumatol (2012) 18(5):237–41. doi: 10.1097/RHU.0b013e3182611547
26. Bijl M, Agmon-Levin N, Dayer JM, Israeli E, Gatto M, Shoenfeld Y, et al. Vaccination of Patients With Autoimmune Inflammatory Rheumatic Diseases Requires Careful Benefit-Risk Assessment. Autoimmun Rev (2012) 11(8):572–6. doi: 10.1016/j.autrev.2011.10.015
27. Heijstek MW, Ott de Bruin LM, Borrow R, van der Klis F, Kone-Paut I, Fasth A, et al. Vaccination in Paediatric Patients With Autoimmune Rheumatic Diseases: A Systemic Literature Review for the European League Against Rheumatism Evidence-Based Recommendations. Autoimmun Rev (2011) 11(2):112–22. doi: 10.1016/j.autrev.2011.08.010
28. McHeyzer-Williams LJ, McHeyzer-Williams MG. Antigen-Specific Memory B Cell Development. Annu Rev Immunol (2005) 23:487–513. doi: 10.1146/annurev.immunol.23.021704.115732
29. Visser LG. The Immunosuppressed Traveler. Infect Dis Clin North Am (2012) 26(3):609–24. doi: 10.1016/j.idc.2012.06.003
30. Halloran PF. Immunosuppressive Drugs for Kidney Transplantation. N Engl J Med (2004) 351(26):2715–29. doi: 10.1056/NEJMra033540
31. Control, E.C.f.D.P.a. Vaccine Schedules in European Countries. Available at: https://vaccine-schedule.ecdc.europa.eu/Scheduler/ByDisease?SelectedDiseaseId (Accessed August 11th 2020).
32. Prevention, C.C.f.D.C.a. US Immunization Guide. Available at: https://www.cdc.gov/vaccines/schedules/hcp/imz/child-adolescent.html.
33. Government C. Canadian Immunization Guide. Available at: https://www.canada.ca/en/public-health/services/publications/healthy-living/canadian-immunization-guide-part-1-key-immunization-information/page-13-recommended-immunization-schedules.html (Accessed August 11th 2020).
34. Prevention, C.C.f.D.C.a. Vaccines and Preventable Diseases (2021). Available at: https://www.cdc.gov/vaccines/vpd/measles/index.html.
35. Heijstek MW, Pileggi GC, Zonneveld-Huijssoon E, Armbrust W, Hoppenreijs EP, Uiterwaal CS, et al. Safety of Measles, Mumps and Rubella Vaccination in Juvenile Idiopathic Arthritis. Ann Rheum Dis (2007) 66(10):1384–7. doi: 10.1136/ard.2006.063586
36. Borte S, Liebert UG, Borte M, Sack U. Efficacy of Measles, Mumps and Rubella Revaccination in Children With Juvenile Idiopathic Arthritis Treated With Methotrexate and Etanercept. Rheumatol (Oxford) (2009) 48(2):144–8. doi: 10.1093/rheumatology/ken436
37. Miyamoto M, Ono E, Barbosa C, Terreri M, Hilario M, Salomao R, et al. Vaccine Antibodies and T- and B-Cell Interaction in Juvenile Systemic Lupus Erythematosus. Lupus (2011) 20(7):736–44. doi: 10.1177/0961203310397409
38. Heijstek MW, van Gageldonk PG, Berbers GA, Wulffraat NM. Differences in Persistence of Measles, Mumps, Rubella, Diphtheria and Tetanus Antibodies Between Children With Rheumatic Disease and Healthy Controls: A Retrospective Cross-Sectional Study. Ann Rheum Dis (2012) 71(6):948–54. doi: 10.1136/annrheumdis-2011-200637
39. Heijstek MW, Kamphuis S, Armbrust W, Swart J, Gorter S, de Vries LD, et al. Effects of the Live Attenuated Measles-Mumps-Rubella Booster Vaccination on Disease Activity in Patients With Juvenile Idiopathic Arthritis: A Randomized Trial. JAMA (2013) 309(23):2449–56. doi: 10.1001/jama.2013.6768
40. Uziel Y, Moshe V, Onozo B, Kulcsar A, Trobert-Sipos D, Akikusa JD, et al. Live Attenuated MMR/V Booster Vaccines in Children With Rheumatic Diseases on Immunosuppressive Therapy are Safe: Multicenter, Retrospective Data Collection. Vaccine (2020) 38(9):2198–201. doi: 10.1016/j.vaccine.2020.01.037
41. Chatham W, Chadha A, Fettiplace J, Kleoudis C, Bass D, Roth D, et al. A Randomized, Open-Label Study to Investigate the Effect of Belimumab on Pneumococcal Vaccination in Patients With Active, Autoantibody-Positive Systemic Lupus Erythematosus. Lupus (2017) 26(14):1483–90. doi: 10.1177/0961203317703495
42. Maritsi DN, Kopsidas I, Vartzelis G, Spyridis N, Tsolia MN, et al. Long-Term Preservation of Measles and Rubella Specific-IgG Antibodies in Children With Enthesitis Related Arthritis on Anti-TNFalpha Treatment: A Prospective Controlled Study. Rheumatol (Oxford) (2019) 58(9):1686–8. doi: 10.1093/rheumatology/kez096
43. Pileggi GS, de Souza CB, Ferriani VP. Safety and Immunogenicity of Varicella Vaccine in Patients With Juvenile Rheumatic Diseases Receiving Methotrexate and Corticosteroids. Arthritis Care Res (Hoboken) (2010) 62(7):1034–9. doi: 10.1002/acr.20183
44. Lu Y, Bousvaros A. Varicella Vaccination in Children With Inflammatory Bowel Disease Receiving Immunosuppressive Therapy. J Pediatr Gastroenterol Nutr (2010) 50(5):562–5. doi: 10.1097/MPG.0b013e3181bab351
45. Barbosa CM, Terreri MT, Rosario PO, de Moraes-Pinto MI, Silva CA, Hilario MO, et al. Immune Response and Tolerability of Varicella Vaccine in Children and Adolescents With Systemic Lupus Erythematosus Previously Exposed to Varicella-Zoster Virus. Clin Exp Rheumatol (2012) 30(5):791–8.
46. Toplak N, Avcin T. Long-Term Safety and Efficacy of Varicella Vaccination in Children With Juvenile Idiopathic Arthritis Treated With Biologic Therapy. Vaccine (2015) 33(33):4056–9. doi: 10.1016/j.vaccine.2015.06.086
47. Groot N, Pileggi G, Sandoval CB, Grein I, Berbers G, Ferriani VPL, et al. Varicella Vaccination Elicits a Humoral and Cellular Response in Children With Rheumatic Diseases Using Immune Suppressive Treatment. Vaccine (2017) 35(21):2818–22. doi: 10.1016/j.vaccine.2017.04.015
48. Speth F, Hinze CH, Andel S, Mertens T, Haas JP, et al. Varicella-Zoster-Virus Vaccination in Immunosuppressed Children With Rheumatic Diseases Using a Pre-Vaccination Check List. Pediatr Rheumatol Online J (2018) 16(1):15. doi: 10.1186/s12969-018-0231-3
49. Jeyaratnam J, Ter Haar NM, Lachmann HJ, Kasapcopur O, Ombrello AK, Rigante D, et al. The Safety of Live-Attenuated Vaccines in Patients Using IL-1 or IL-6 Blockade: An International Survey. Pediatr Rheumatol Online J (2018) 16(1):19. doi: 10.1186/s12969-018-0235-z
50. Lara AN, Miyaji KT, Ibrahim KY, Lopes MH, Sartori AMC, et al. Adverse Events Following Yellow Fever Vaccination in Immunocompromised Persons. Rev Inst Med Trop Sao Paulo (2021) 63:e13. doi: 10.1590/s1678-9946202163013
51. Huber R, Schlodder D, Effertz C, Rieger S, Troger W. Safety of Intravenously Applied Mistletoe Extract - Results From a Phase I Dose Escalation Study in Patients With Advanced Cancer. BMC Complement Altern Med (2017) 17(1):465. doi: 10.1186/s12906-017-1971-1
52. Azevedo LS, Lasmar EP, Contieri FL, Boin I, Percegona L, Saber LT, et al. Yellow Fever Vaccination in Organ Transplanted Patients: Is it Safe? A Multicenter Study. Transpl Infect Dis (2012) 14(3):237–41. doi: 10.1111/j.1399-3062.2011.00686.x
53. Scheinberg M, Guedes-Barbosa LS, Mangueira C, Rosseto EA, Mota L, Oliveira AC, et al. Yellow Fever Revaccination During Infliximab Therapy. Arthritis Care Res (Hoboken) (2010) 62(6):896–8. doi: 10.1002/acr.20045
54. Groot N, Heijstek MW, Wulffraat NM. Vaccinations in Paediatric Rheumatology: An Update on Current Developments. Curr Rheumatol Rep (2015) 17(7):46. doi: 10.1007/s11926-015-0519-y
55. Akikusa JD, Crawford NW. Vaccination in Paediatric Rheumatology. Curr Rheumatol Rep (2014) 16(8):432. doi: 10.1007/s11926-014-0432-9
56. Rheumatology, B.S.a.H.C.o.b.o.t.F.A.C.o.I.a.e.b.t.S.S.f.A.a.I.a.t.S.S.f. Background Document on Immune-Mediated Inflammatory Diseases (IMID) Module 1: Vaccination in Patients With Autoimmune Inflammatory Rheumatic Diseases (AIIRD). (2013).
57. Rubin LG, Levin MJ, Ljungman P, Davies EG, Avery R, Tomblyn M, et al. 2013 IDSA Clinical Practice Guideline for Vaccination of the Immunocompromised Host. Clin Infect Dis (2014) 58(3):e44–100. doi: 10.1093/cid/cit684
58. Leung J, Siegel S, Jones JF, Schulte C, Blog D, Schmid DS, et al. Fatal Varicella Due to the Vaccine-Strain Varicella-Zoster Virus. Hum Vaccin Immunother (2014) 10(1):146–9. doi: 10.4161/hv.26200
59. OFSP. Plan De Vaccination Suisse 2020 (2020). Available at: https://www.bag.admin.ch/bag/fr/home/gesund-leben/gesundheitsfoerderung-und-praevention/impfungen-prophylaxe/schweizerischer-impfplan.html.
60. Aikawa NE, Campos LM, Goldenstein-Schainberg C, Saad CG, Ribeiro AC, Bueno C, et al. Effective Seroconversion and Safety Following the Pandemic Influenza Vaccination (Anti-H1N1) in Patients With Juvenile Idiopathic Arthritis. Scand J Rheumatol (2013) 42(1):34–40. doi: 10.3109/03009742.2012.709272
61. Campos LM, Silva CA, Aikawa NE, Jesus AA, Moraes JC, Miraglia J, et al. High Disease Activity: An Independent Factor for Reduced Immunogenicity of the Pandemic Influenza a Vaccine in Patients With Juvenile Systemic Lupus Erythematosus. Arthritis Care Res (Hoboken) (2013) 65(7):1121–7. doi: 10.1002/acr.21948
62. Aikawa NE, Campos LM, Silva CA, Carvalho JF, Saad CG, Trudes G, et al. Glucocorticoid: Major Factor for Reduced Immunogenicity of 2009 Influenza A (H1N1) Vaccine in Patients With Juvenile Autoimmune Rheumatic Disease. J Rheumatol (2012) 39(1):167–73. doi: 10.3899/jrheum.110721
63. Guissa VR, Pereira RM, Sallum AM, Aikawa NE, Campos LM, Silva CA, et al. Influenza A H1N1/2009 Vaccine in Juvenile Dermatomyositis: Reduced Immunogenicity in Patients Under Immunosuppressive Therapy. Clin Exp Rheumatol (2012) 30(4):583–8.
64. Aikawa NE, Trudes G, Campos LM, Pereira RM, Moraes JC, Ribeiro AC, et al. Immunogenicity and Safety of Two Doses of a non-Adjuvanted Influenza A H1N1/2009 Vaccine in Young Autoimmune Rheumatic Diseases Patients. Lupus (2013) 22(13):1394–8. doi: 10.1177/0961203313505926
65. Erguven M, Kaya B, Hamzah OY, Tufan F. Evaluation of Immune Response to Hepatitis A Vaccination and Vaccine Safety in Juvenile Idiopathic Arthritis. J Chin Med Assoc (2011) 74(5):205–8. doi: 10.1016/j.jcma.2011.03.004
66. Moses J, Alkhouri N, Shannon A, Feldstein A, Carter-Kent C. Response to Hepatitis A Vaccine in Children With Inflammatory Bowel Disease Receiving Infliximab. Inflammation Bowel Dis (2011) 17(12):E160. doi: 10.1002/ibd.21892
67. Radzikowski A, Banaszkiewicz A, Lazowska-Przeorek I, Grzybowska-Chlebowczyk U, Wos H, Pytrus T, et al. Immunogenecity of Hepatitis A Vaccine in Pediatric Patients With Inflammatory Bowel Disease. Inflammation Bowel Dis (2011) 17(5):1117–24. doi: 10.1002/ibd.21465
68. Maritsi D, Vartzelis G, Soldatou A, Garoufi A, Spyridis N. Markedly Decreased Antibody Titers Against Hepatitis B in Previously Immunised Children Presenting With Juvenile Idiopathic Arthritis. Clin Exp Rheumatol (2013) 31(6):969–73.
69. Moses J, Alkhouri N, Shannon A, Raig K, Lopez R, Danziger-Isakov L, et al. Hepatitis B Immunity and Response to Booster Vaccination in Children With Inflammatory Bowel Disease Treated With Infliximab. Am J Gastroenterol (2012) 107(1):133–8. doi: 10.1038/ajg.2011.295
70. Kasapcopur O, Cullu F, Kamburoglu-Goksel A, Cam H, Akdenizli E, Calykan S, et al. Hepatitis B Vaccination in Children With Juvenile Idiopathic Arthritis. Ann Rheum Dis (2004) 63(9):1128–30. doi: 10.1136/ard.2003.013201
71. Beran J, Dedek P, Stepanova V, Spliio M, Pozler O. Safety and Immunogenicity of a Combined Vaccine Against Hepatitis A and B in Patients With Autoimmune Hepatitis. Cent Eur J Public Health (2005) 13(1):20–3.
72. Urganci N, Kalyoncu D. Immunogenecity of Hepatitis A and B Vaccination in Pediatric Patients With Inflammatory Bowel Disease. J Pediatr Gastroenterol Nutr (2013) 56(4):412–15. doi: 10.1097/MPG.0b013e31827dd87d
73. Raven SFH, Hoebe C, Vossen A, Visser LG, Hautvast JLA, Roukens AHE, et al. Serological Response to Three Alternative Series of Hepatitis B Revaccination (Fendrix, Twinrix, and HBVaxPro-40) in Healthy non-Responders: A Multicentre, Open-Label, Randomised, Controlled, Superiority Trial. Lancet Infect Dis (2020) 20(1):92–101. doi: 10.1016/S1473-3099(19)30417-7
74. Heijstek MW, Scherpenisse M, Groot N, Wulffraat NM, Van Der Klis FR, et al. Immunogenicity of the Bivalent Human Papillomavirus Vaccine in Adolescents With Juvenile Systemic Lupus Erythematosus or Juvenile Dermatomyositis. J Rheumatol (2013) 40(9):1626–7. doi: 10.3899/jrheum.130246
75. Heijstek MW, Scherpenisse M, Groot N, Tacke N, Schepp C, Buisman RM, et al. Immunogenicity and Safety of the Bivalent HPV Vaccine in Female Patients With Juvenile Idiopathic Arthritis: A Prospective Controlled Observational Cohort Study. Ann Rheum Dis (2014) 73(8):1500–7. doi: 10.1136/annrheumdis-2013-203429
76. Mok CC, Ho LY, Fong LS, To CH. Immunogenicity and Safety of a Quadrivalent Human Papillomavirus Vaccine in Patients With Systemic Lupus Erythematosus: A Case-Control Study. Ann Rheum Dis (2013) 72(5):659–64. doi: 10.1136/annrheumdis-2012-201393
77. Farmaki E, Kanakoudi-Tsakalidou F, Spoulou V, Trachana M, Pratsidou-Gertsi P, Tritsoni M, et al. The Effect of Anti-TNF Treatment on the Immunogenicity and Safety of the 7-Valent Conjugate Pneumococcal Vaccine in Children With Juvenile Idiopathic Arthritis. Vaccine (2010) 28(31):5109–13. doi: 10.1016/j.vaccine.2010.03.080
78. Walker UA, Hoffman HM, Williams R, Kuemmerle-Deschner J, Hawkins PN, et al. Brief Report: Severe Inflammation Following Vaccination Against Streptococcus Pneumoniae in Patients With Cryopyrin-Associated Periodic Syndromes. Arthritis Rheumatol (2016) 68(2):516–20. doi: 10.1002/art.39482
79. Jaeger VK, Hoffman HM, van der Poll T, Tilson H, Seibert J, Speziale A, et al. Safety of Vaccinations in Patients With Cryopyrin-Associated Periodic Syndromes: A Prospective Registry Based Study. Rheumatol (Oxford) (2017) 56(9):1484–91. doi: 10.1093/rheumatology/kex185
80. Bjarnarson SP, Benonisson H, Del Giudice G, Jonsdottir I. Pneumococcal Polysaccharide Abrogates Conjugate-Induced Germinal Center Reaction and Depletes Antibody Secreting Cell Pool, Causing Hyporesponsiveness. PloS One (2013) 8(9):e72588. doi: 10.1371/journal.pone.0072588
81. Stoof SP, Heijstek MW, Sijssens KM, van der Klis F, Sanders EA, Teunis PF, et al. Kinetics of the Long-Term Antibody Response After Meningococcal C Vaccination in Patients With Juvenile Idiopathic Arthritis: A Retrospective Cohort Study. Ann Rheum Dis (2014) 73(4):728–34. doi: 10.1136/annrheumdis-2012-202561
82. Oren S, Mandelboim M, Braun-Moscovici Y, Paran D, Ablin J, Litinsky D, et al. Vaccination Against Influenza in Patients With Rheumatoid Arthritis: The Effect of Rituximab on the Humoral Response. Ann Rheum Dis (2008) 67(7):937–41. doi: 10.1136/ard.2007.077461
83. Nagel J, Saxne T, Geborek P, Bengtsson AA, Jacobsen S, Svaerke Joergensen C, et al. Treatment With Belimumab in Systemic Lupus Erythematosus Does Not Impair Antibody Response to 13-Valent Pneumococcal Conjugate Vaccine. Lupus (2017) 26(10):1072–81. doi: 10.1177/0961203317695465
84. Rehnberg M, Amu S, Tarkowski A, Bokarewa MI, Brisslert M. Short- and Long-Term Effects of Anti-CD20 Treatment on B Cell Ontogeny in Bone Marrow of Patients With Rheumatoid Arthritis. Arthritis Res Ther (2009) 11(4):R123. doi: 10.1186/ar2789
85. Cheent K, Nolan J, Shariq S, Kiho L, Pal A, Arnold J, et al. Case Report: Fatal Case of Disseminated BCG Infection in an Infant Born to a Mother Taking Infliximab for Crohn’s Disease. J Crohns Colitis (2010) 4(5):603–5. doi: 10.1016/j.crohns.2010.05.001
Keywords: auto-immune, vaccination, children, immunosuppressed, immunomodulatory drugs
Citation: Blanchard-Rohner G (2021) Vaccination in Children With Autoimmune Disorders and Treated With Various Immunosuppressive Regimens: A Comprehensive Review and Practical Guide. Front. Immunol. 12:711637. doi: 10.3389/fimmu.2021.711637
Received: 18 May 2021; Accepted: 12 July 2021;
Published: 02 August 2021.
Edited by:
Susu M. Zughaier, Qatar University, QatarReviewed by:
Natasa Toplak, Univerzitetnega Kliničnega Centra Ljubljana, SloveniaGecilmara Salviato Pileggi, Faculty of Health Sciences of Barretos Dr. Paulo Prata (FACISB), Brazil
Copyright © 2021 Blanchard-Rohner. This is an open-access article distributed under the terms of the Creative Commons Attribution License (CC BY). The use, distribution or reproduction in other forums is permitted, provided the original author(s) and the copyright owner(s) are credited and that the original publication in this journal is cited, in accordance with accepted academic practice. No use, distribution or reproduction is permitted which does not comply with these terms.
*Correspondence: Geraldine Blanchard-Rohner, R2VyYWxkaW5lLmJsYW5jaGFyZHJvaG5lckBoY3VnZS5jaA==