- 1Department of Medicine, Case Western Reserve University, Cleveland, OH, United States
- 2Medical Affairs, Partner Therapeutics, Inc., Lexington, MA, United States
- 3Centre for Haematology, Department of Immunology and Inflammation, Imperial College London, London, United Kingdom
- 4Public Health Sciences and Clinical Research Divisions, Fred Hutchinson Cancer Research Center, Seattle, WA, United States
Background: Sargramostim [recombinant human granulocyte-macrophage colony-stimulating factor (rhu GM-CSF)] was approved by US FDA in 1991 to accelerate bone marrow recovery in diverse settings of bone marrow failure and is designated on the list of FDA Essential Medicines, Medical Countermeasures, and Critical Inputs. Other important biological activities including accelerating tissue repair and modulating host immunity to infection and cancer via the innate and adaptive immune systems are reported in pre-clinical models but incompletely studied in humans.
Objective: Assess safety and efficacy of sargramostim in cancer and other diverse experimental and clinical settings.
Methods and Results: We systematically reviewed PubMed, Cochrane and TRIP databases for clinical data on sargramostim in cancer. In a variety of settings, sargramostim after exposure to bone marrow-suppressing agents accelerated hematologic recovery resulting in fewer infections, less therapy-related toxicity and sometimes improved survival. As an immune modulator, sargramostim also enhanced anti-cancer responses in solid cancers when combined with conventional therapies, for example with immune checkpoint inhibitors and monoclonal antibodies.
Conclusions: Sargramostim accelerates hematologic recovery in diverse clinical settings and enhances anti-cancer responses with a favorable safety profile. Uses other than in hematologic recovery are less-well studied; more data are needed on immune-enhancing benefits. We envision significantly expanded use of sargramostim in varied immune settings. Sargramostim has the potential to reverse the immune suppression associated with sepsis, trauma, acute respiratory distress syndrome (ARDS) and COVID-19. Further, sargramostim therapy has been promising in the adjuvant setting with vaccines and for anti-microbial-resistant infections and treating autoimmune pulmonary alveolar proteinosis and gastrointestinal, peripheral arterial and neuro-inflammatory diseases. It also may be useful as an adjuvant in anti-cancer immunotherapy.
Highlights
● Sargramostim (yeast-derived rhu GM-CSF) accelerates bone marrow recovery after exposure to bone marrow damaging exposures. Safety and efficacy studies in other clinical settings warrant further study.
● Endogenous GM-CSF modulates the innate and adaptive immune systems and acts on multiple hematopoietic lineages.
● rhu GM-CSF and rhu G-CSF have distinct mechanisms of action with different clinical effects; they are not inter-changeable.
● Data in melanoma suggest the potential of adjuvant sargramostim to improve cancer outcomes and reduce the toxicity of immune checkpoint inhibitors.
● Sargramostim may have a role as an adjuvant to anti-fungal agents for resistant infections, improve immune suppression associated with sepsis and trauma, have benefit in lung disorders such as autoimmune pulmonary alveolar proteinosis and acute respiratory distress syndrome, and improve symptoms in neuro-degenerative disorders.
Introduction
Granulocyte-macrophage colony-stimulating factor (GM-CSF) is a protein central to regulation of hematopoiesis in mammals (1). Mouse GM-CSF was molecularly-cloned followed by molecular cloning of human GM-CSF (2, 3). Subsequently, recombinant human (rhu) GM-CSF was developed as a drug, the most common and only FDA-approved form of which is sargramostim (Leukine®, Partner Therapeutics, Inc., Lexington, MA), which is also now designated on the list of FDA Essential Medicines, Medical Countermeasures, and Critical Inputs (4). Other rhu GM-CSFs include molgramostim and regramostim.
The development timeline of sargramostim, yeast-derived rhu GM-CSF, is displayed in Figure 1. The first clinical use of rhu GM-CSF was in 1986 when Gale and Vorobiov, in the aftermath of the Chernobyl nuclear power facility accident tested safety and efficacy of rhu GM-CSF by injecting themselves with a 10-fold higher dose than previously given to sub-human primates (5). Subsequently, these scientists used rhu GM-CSF to treat 3 radiation accident victims. Clinical trials began in 1987 and sargramostim was approved by US FDA in 1991 to accelerate bone marrow recovery in allogeneic hematopoietic cell transplant recipients (5, 6). It was subsequently approved in persons receiving bone marrow-suppressive drugs and/or radiation and in persons with post-transplant graft-failure. Although safe and effective in these settings, concerns over fever and myalgia resulted in a clinical shift to recombinant human granulocyte colony-stimulating factor (rhu G-CSF) in many clinical settings (7–11). Some believe this shift also may reflect effective marketing. In contrast, some animal and human studies suggest recombinant G-CSF is likely to exacerbate lung injury in the setting of infection via neutrophil infiltration whereas mice and humans receiving recombinant GM-CSF therapy may be associated with less lung injury risk [see review; Lazarus and Gale (12)]. Although the few comparative studies of sargramostim and rhu G-CSF products (filgrastim, pegfilgrastim and their biosimilars) report similar safety and efficacy, rhu G-CSF products account for over 95 percent of myeloid hematopoietic growth factor use (12–14). A formal comparison of the clinical uses of rhu GM-CSF versus rhu G-CSF is beyond the scope of this review [reviewed in Lazarus and Gale (12)].
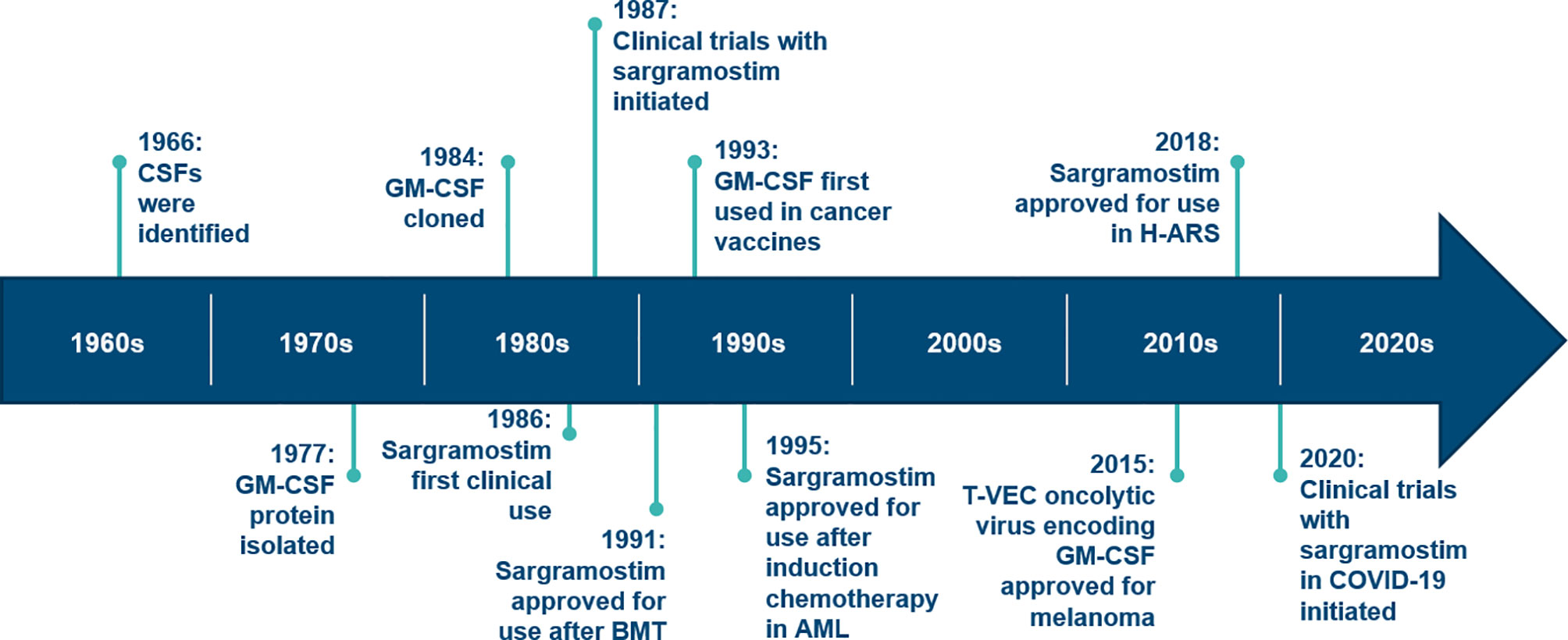
Figure 1 Timeline for discovery and development of GM-CSF. AML, acute myeloid leukemia; BMT, bone marrow transplant; CSF, colony-stimulating factor; GM-CSF, granulocyte-macrophage colony-stimulating factor; H-ARS, hematopoietic acute radiation syndrome; T-VEC, talimogene laherparepvec. (Modified from: Dougan M, Dranoff G, Dougan SK. GM-CSF, IL-3, and IL-5 family of cytokines: Regulators of Inflammation. Immunity. 2019;50(4):796‐811).
We conducted a systematic review of clinical uses of sargramostim in cancer. Because sargramostim is the only approved rhu GM-CSF available for clinical use, we focus on this drug. In addition to the effects of sargramostim on accelerating bone marrow recovery, we describe use as an adjunct in host immune modulation, anti-cancer therapy, and as an anti-cancer vaccine adjuvant. We discuss why there is also renewed interest in sargramostim because of its pleiotropic biologic and immune-enhancing activities. The sargramostim US FDA approved indications and emerging uses as an immune modulator are detailed in Table 1.
Methods
This study was conducted according to the Preferred Reporting Items for Systematic Review and Meta-Analyses (PRISMA) guidelines (15) and followed a prespecified protocol. A literature search was performed in PubMed, Cochrane and TRIP databases (search terms: “rhu-GM-CSF” OR “granulocyte-macrophage colony stimulating factor” OR “sargramostim” AND “cancer”) to identify clinical studies published in English between January 1, 1988 and May 1, 2020 that evaluated the efficacy and/or safety of sargramostim in patients with hematologic or solid tumors (Figure 2). Additional studies found on a detailed literature review were also included. A two-stage approach was used in which three independent reviewers screened titles and abstracts followed by full-text articles based on pre-defined inclusion and exclusion criteria. Discordances were adjudicated and confirmed by the authors. Inclusion criteria encompassed prospective interventional or observational studies of sargramostim given IV or subcutaneously (SC), alone or combined with other cytokines and/or treatments with ≥ 50 subjects with cancer. We excluded studies assessing sargramostim for blood cells mobilization, as an adjuvant to vaccines or GM-CSF–expressing vaccines, alternate rhu GM-CSFs (i.e., not sargramostim), those supporting current FDA-approved indications and all phase 1 studies. Data extracted included study-objective and -design, disease-type and, for clinical trials, safety and efficacy outcomes. All data were extracted and presented as reported in the original publications. Statistical significance, where specified, was based on the cut-off indicated in the original publication for determining significance (or p <.05 if not specified).
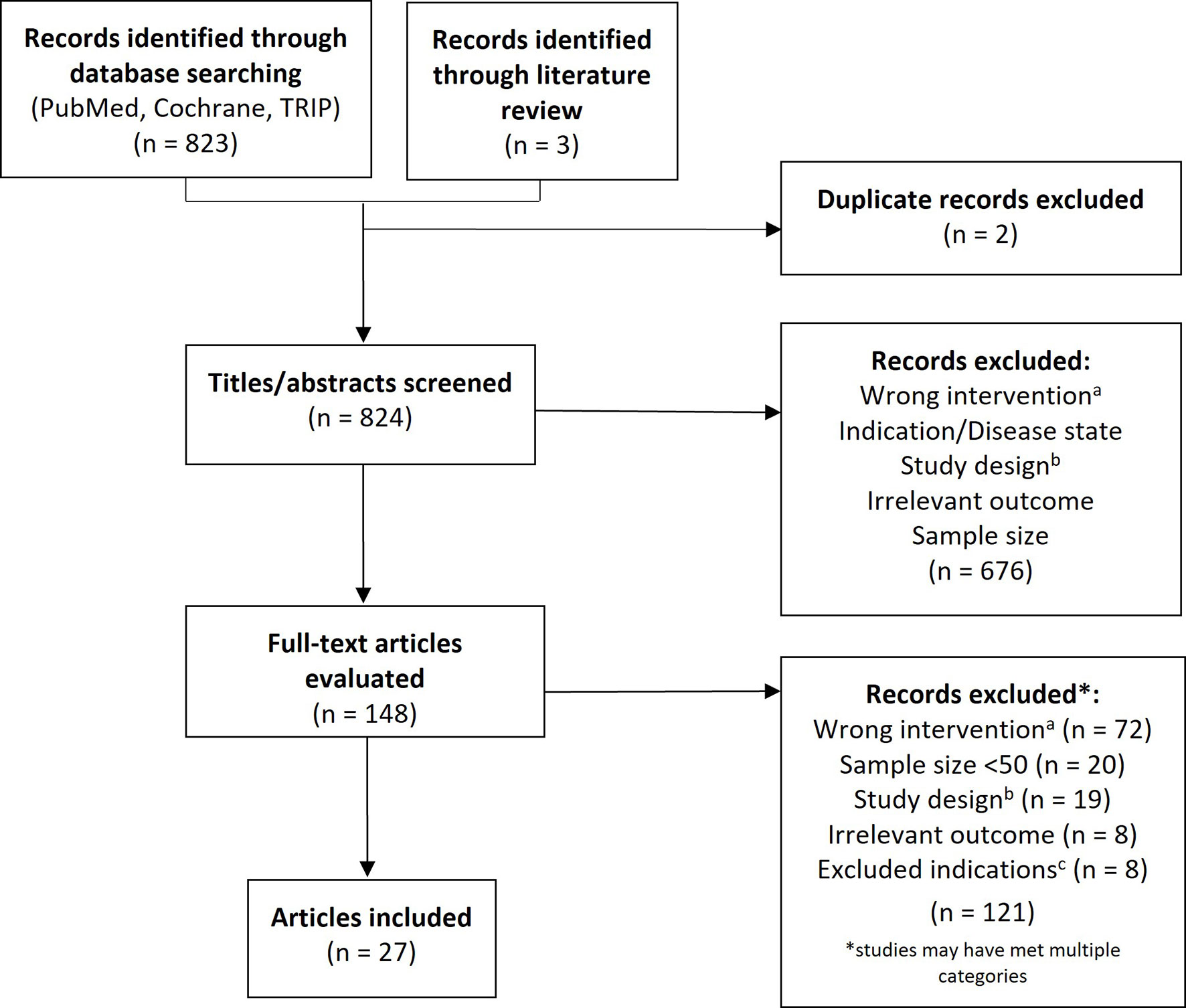
Figure 2 PRISMA flow diagram. PRISMA flow diagram. aIntervention not sargramostim (e.g., molgramostim) and/or not administered via intravenous or subcutaneous route; bExclusions comprised phase 1 trials and those that were not prospective, interventional, or observational studies (e.g., letter to the editor, retrospective studies, etc.); cExcluded studies were those assessing sargramostim for mobilization, as an adjuvant to vaccines or GM-CSF–expressing vaccines and studies supporting current FDA-approved indications.
Granulocyte-Macrophage Colony-Stimulating Factor
Expression Systems
Sargramostim, produced in Saccharomyces cervisae, is a single-chain, glycosylated polypeptide (5, 6). It differs from human GM-CSF by substitution of leucine for arginine at position 23 rhu GM-CSFs derived in other expression systems [i.e., molgramostim (bacterial-derived) and regramostim (mammalian-derived)] have been studied but are not commercially available. These rhu GM-CSFs are not inter-changeable because the expression system determines the amino acid structure and degree of glycosylation which in turn influences pharmacokinetics (distribution and clearance), biologic activity and safety (16, 17). Compared with other rhu GM-CSFs the intermediate level of glycosylation of sargramostim results in biologic activity like that of native GM-CSF, greater stability, resistance to degradation, improved tolerability and less immunogenicity. Several studies reported a lower frequency and severity of fevers, chills, myalgias, bone pain, edema, dyspnea and local erythema with sargramostim compared with E. coli-derived, non-glycosylated molgramostim (16, 18).
Biology
GM-CSF causes rapid, sustained down-regulation and internalization of cell membrane GM-CSF receptor α subunit (GM-CSFRα) with the receptor playing a key role in ligand clearance (19, 20). Mice lacking a GM-CSF receptor develop high blood concentrations of GM-CSF after endotoxin challenge (21). Similarly, loss-of-function mutations in CSFRA is associated with markedly increased blood GM-CSF concentrations and pulmonary alveolar proteinosis because of the inability of alveolar macrophages to clear surfactant (22). Additionally, around birth there is a surge in alveolar GM-CSF causing immature alveolar macrophages to develop into functionally self-sustaining mature alveolar macrophages (23).
Wessendarp et al. recently reported the use of a GM-CSF receptor-β-chain deficient (Csf2rb–/–) murine model suggesting GM-CSF is critical for mitochondrial turnover, function and integrity (24). GM-CSF stimulation is required to maintain mitochondrial mass and function in macrophages, as well as for several other critical metabolic functions including self-renewal. Consequently, giving exogeneous GM-CSF may have profound effects on several metabolic pathways relevant to cellular proliferation including cytosolic and mitochondrial function.
Actions
Considerable data indicate GM-CSF stimulates proliferation, differentiation, activation and survival of granulocytes, monocytes and macrophages and stimulates growth and maturation of myeloid-derived dendritic cells (Figure 3) (1, 25–28). GM-CSF stimulates the innate immune response by activating macrophages and dendritic cells. By driving the immune function of dendritic cells, GM-CSF promotes development of antigen-specific T-cells and regulatory T-cells (Tregs), linking the innate and adaptive immune systems to increase host defenses (19). Additionally, cells associated with epithelial barriers, e.g. alveolar macrophages and gastrointestinal tract immune cells, are repaired and their functionality is restored by GM-CSF (Figure 4) (29, 30). Effects of GM-CSF on macrophages are thought to contribute to its ability to support epithelial cell function and repair of mucosal surfaces in the lung and gastrointestinal tract following direct injury or from infection (30, 31). Figure 3 displays actions of GM-CSF and how it differs from granulocyte colony-stimulating factor (G-CSF) (12). Hence, GM-CSF increases immunity via the innate and adaptive immune systems.
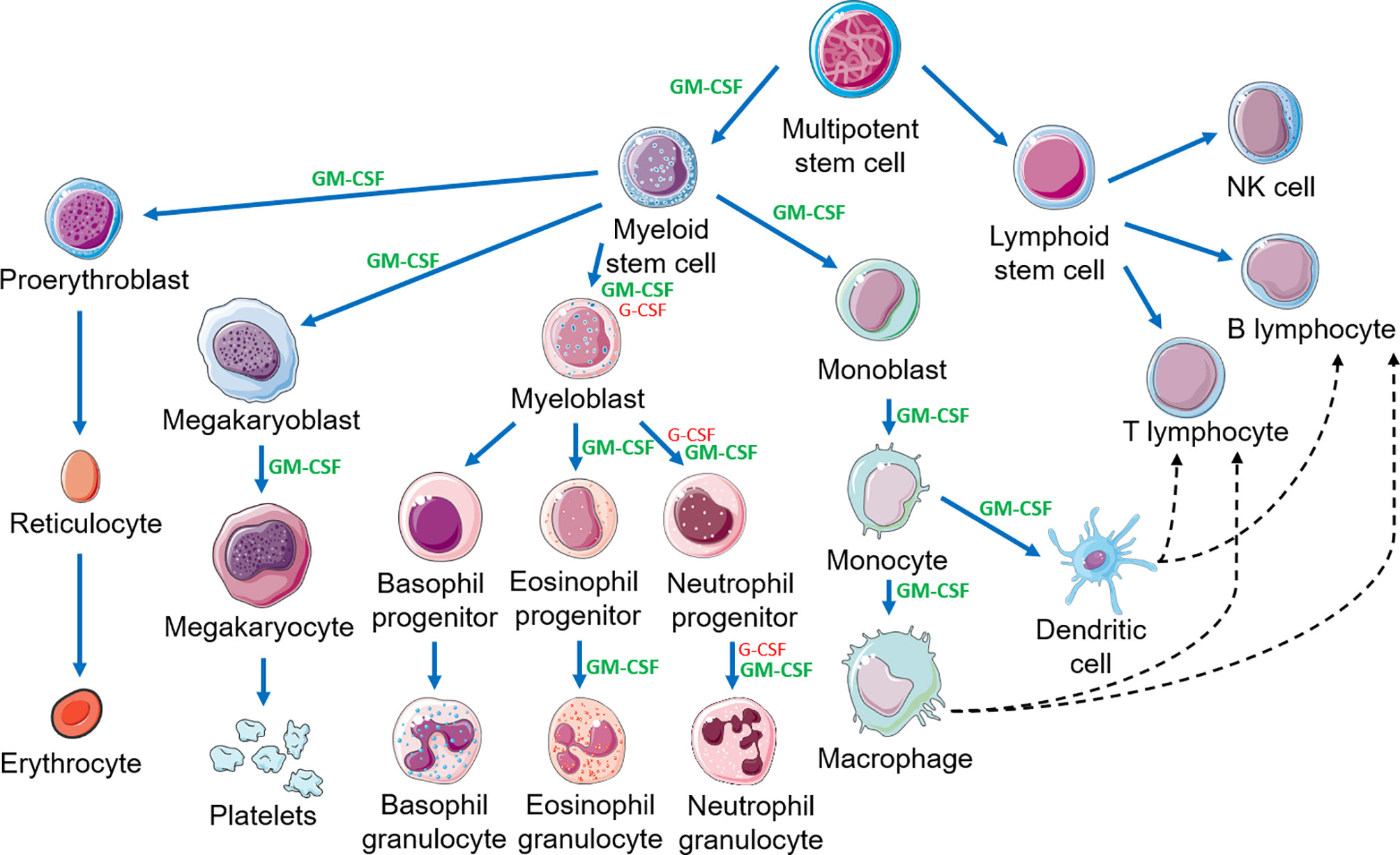
Figure 3 Hematopoietic cascade demonstrating the action of GM-CSF and G-CSF and the link between the innate and adaptive immune systems. This figure is a partial representation of the hematopoietic cascade. NK, natural killer. This figure was created using Servier Medical Art templates, which are licensed under a Creative Commons Attribution 3.0 Unported License; https://smart.servier.com.
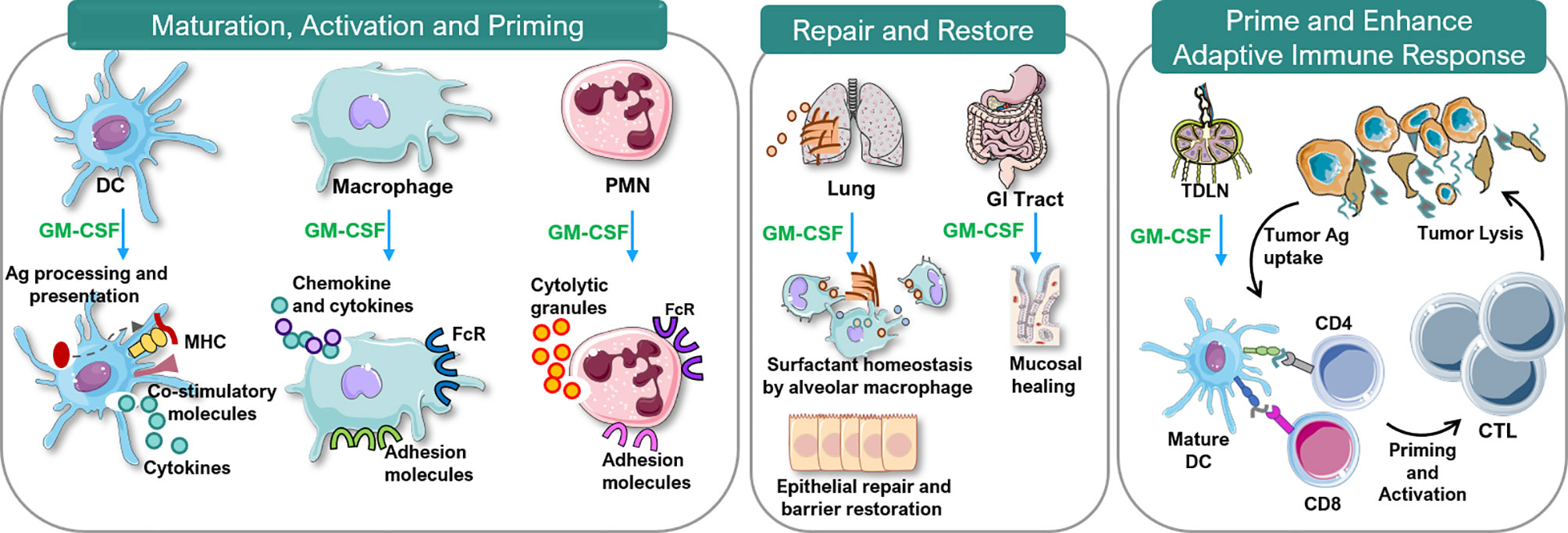
Figure 4 Immunobiology of GM-CSF. Ag, antigen; CTL, cytotoxic T lymphocytes; DC, dendritic cell; FcR, Fc receptor; GM-CSF, granulocyte-macrophage colony-stimulating factor; MHC, major histocompatibility complex; PMN, polymorphonuclear neutrophils; TDLN, tumor-draining lymph node. This figure was created using Servier Medical Art templates, which are licensed under a Creative Commons Attribution 3.0 Unported License; https://smart.servier.com.
Immune Modulation
GM-CSF increases monocyte killing of S. aureus and C. albicans in vitro in persons with solid cancers receiving chemotherapy (32). Sargramostim may reverse the immune suppression associated with severe illnesses such as sepsis, multiple organ dysfunction syndrome (MODS) and trauma (33–36). In persons with sepsis, sargramostim significantly increased the resolution rate of infections compared with placebo in a meta-analysis (37). In a randomized controlled trial of persons with severe sepsis or septic shock with sepsis-associated immune suppression, sargramostim increased monocyte HLA-DR levels, a marker of monocyte immune competence (38). Sargramostim-treated subjects had reduced need for mechanical ventilation and shorter intensive care unit and hospital stays. Hall et al. reported sargramostim prevented nosocomial infections in children with MODS (33). Considerable data indicate sargramostim stimulates immune function in persons with refractory bacterial and fungal infections including those with CARD9 deficiency (39–47). Recombinant GM-CSF is also reported to improve protection against and recovery from viral infections in mouse models (42, 48–50). Recent data indicate some cases of coronavirus disease-2019 (COVID-19)–associated acute respiratory distress syndrome (ARDS) results from cytokine release syndrome (CRS) (51–54). Sargramostim supports epithelial cell repair and reverses the immune suppression associated with CRS (31, 38, 55). Inhaled sargramostim could potentially reverse ARDS (56). Randomized trials testing this hypothesis in persons with COVID-19 are in progress (NCT04707664, NCT04326920, NCT04411680, NCT04642950) (57–61). Figure 5 illustrates restoration of immune competence with GM-CSF from dysfunctional CD14+ HLA-DRlo/neg monocytes to activated HLA-DRhi monocytes (62, 63).
GM-CSF is typically characterized as a pro-inflammatory cytokine but some data suggest anti-inflammatory effects. Recent data indicate roles regulating the immune response (19). Sargramostim may be effective in several inflammatory, autoimmune and neuro-inflammatory diseases but convincing data from large randomized controlled trials are not reported (discussed in Pulmonary and Gastrointestinal Repair; Emerging Uses in Neuro-degenerative Disorders; Other Emerging Uses).
Direct Anti-Tumor Properties of GM-CSF
GM-CSF stimulates production and proliferation of dendritic cells that may confer anti-tumor effect. Kurbacher et al. treated 19 subjects who had advanced cancers with sargramostim, 125-250 µg per day, subcutaneously (64). Subjects received a median of 4 prior drug regimens for metastatic breast, recurrent ovary, metastatic endometrial and recurrent cervix cancers. Sargramostim therapy was continued until progression or withdrawal of consent. Of the 19 subjects, one had a complete response and 6 had partial responses. Median response duration was 6 months. The authors attribute the anti-tumor response to dendritic cell activation by GM-CSF as well as GM-CSF-induced tumor growth arrest via stimulation of intratumoral GM-CSF receptors. The immune modulation driven by GM-CSF and the major role in the generation of dendritic cells from mononuclear precursors may be a signal as an important adjuvant in therapeutic vaccine approaches, which we discuss later (64).
Anti-Cancer Immune Modulation
Many cancers inhibit host immune responses facilitating cancer growth and metastasis, sometimes by inducing myeloid-derived suppressor cells (MDSCs), a heterogeneous population of immature myeloid cells which suppress innate and adaptive immunity (63). Monocytic MDSCs are monocytes with little or no HLA-DR expression and reduced antigen-presenting capability (CD14+ HLA-DRlo/neg monocytes). In cancer MDSCs are important inhibitors of innate and adaptive anti-cancer immunity and decrease the efficacy of immune checkpoint inhibitors, CAR-T-cells and anti-cancer vaccines (65, 66). Some data suggest sargramostim can reverse this immune suppression by up-regulating HLA-DR expression and reversing effects of MDSCs and Tregs (33, 34, 38, 67–70). Up-regulation of monocyte HLA-DR expression and ex vivo lipopolysaccharide (LPS)-induced tumor necrosis factor-alpha responses are proposed biomarkers of immune suppression and could be useful to monitor responses to sargramostim therapy in persons with cancer (33, 34).
Anti-Cancer Vaccine Adjuvant
Sargramostim has been tested to enhance the efficacy of anti-cancer vaccines in several diseases. GM-CSF functions by recruiting and activating antigen-presenting cells at the injection site (71, 72). Cuzzubbo et al. reported sargramostim is the most common adjuvant in cancer vaccine trials (73). Results of these trials are mixed. Low doses of sargramostim, 40-80 μg for 1-5 days, may increase a vaccine-induced immune response, while higher doses may promote expansion of MDSCs (74). Petrina et al. reported adjuvant sargramostim in prostate cancer increased T-cell infiltration to the cancer microenvironment (75). The increased T cell infiltration into the tumor microenvironment may be promising for tumor suppression.
Pulmonary and Gastrointestinal Repair
GM-CSF facilitates repair of lung and gastrointestinal tract mucosa. In both tissues GM-CSF increases recruitment, differentiation and activation of dendritic cells and their interactions with T-cells to promote bacterial killing (30). GM-CSF also stimulates alveolar macrophage opsonization and phagocytosis of pathogens and enhances their clearance (31). GM-CSF promotes epithelial cell repair and prevents surfactant accumulation thereby supporting normal lung function. These observations prompted use of GM-CSF for pulmonary alveolar proteinosis (PAP).
PAP is a rare respiratory syndrome characterized by accumulation of surfactant in alveoli and terminal airways resulting in respiratory failure (76). In autoimmune PAP (aPAP), auto-antibodies to GM-CSF are found in broncho-alveolar lavage fluid and serum. PAP therapy with inhaled sargramostim appears to restore lung function and continues to be studied (77–80).
Paneth cells, highly specialized secretory epithelial cells located at the base of small intestinal crypts, express GM-CSF (81). Paneth and non-Paneth cells of the small intestine express the GM-CSFR β-chain. GM-CSF is also a key cytokine in the differentiation of intestinal dendritic cells and innate immune homeostasis (82). GM-CSF stimulates recruitment and activation of gut-derived immune cells, increases resistance to bacterial translocation and augments clearance of bacteria and viruses. In a murine model of Crohn disease GM-CSF activates monocytes and reduces disease severity (83). In humans, decreased bioavailability of GM-CSF is associated with more severe Crohn disease (84). Although most clinical data do not support a role for rhu GM-CSF in Crohn disease more studies appear needed (85).
Comparison of GM-CSF and G-CSF
GM-CSF and G-CSF are the two most common hematopoietic growth factors. At times, they are thought to be interchangeable; however this is not true (12). GM-CSF is produced by B- and T-cells, granulocytes, monocytes, macrophages, fibroblasts, epithelial cells, endothelial cells and microglia (42, 86). The G-CSF receptor (G-CSFR) is primarily on granulocytes and myeloid bone marrow precursor cells whereas the GM-CSFR (CD116) is on granulocytes, monocytes, eosinophils, basophils, dendritic cells and possibly B-cells (19, 42, 87). GM-CSF binding to these receptors results in rapid internalization and activation of intra-cellular signaling. Consequently, GM-CSF stimulates production of granulocytes and macrophages from precursor cells in the hematopoietic cascade whereas G-CSF only stimulates production and activity of granulocytes (1, 88–90) (Figure 3). Different receptor distributions explain many differences in the biological activities of G-CSF and GM-CSF. In one study, rhu GM-CSF accelerated granulocyte recovery earlier compared to control but slower than rhu G-CSF in persons receiving bone marrow damaging drugs (13). Other reports indicate rhu GM-CSF stimulates immune responses in persons unresponsive to G-CSF (39, 43, 44, 91, 92). A retrospective analysis of persons with leukemia receiving a hematopoietic cell transplantation after high-dose pretransplant conditioning receiving colony stimulating factors suggested that receipt of rhu GM-CSF + rhu G-CSF was associated with greater cognitive improvement than rhu G-CSF alone (93). Randomized controlled trials of the effects of rhu GM-CSF on cognitive functioning in humans are warranted and underway to confirm these preliminary findings. As discussed, a critical comparison of clinical uses of rhu GM-CSF versus rhu G-CSF is beyond the scope of this review (reviewed in Lazarus and Gale, 2020) (12).
Systematic Review
A total of 823 citations were screened by title and abstract of which 676 were excluded (Figure 2). Of the 148 articles included in the first-pass screening, 27 met all criteria for final inclusion following full-text screening and are detailed in Tables 2 through 5 and Supplementary Table 1.
Accelerating Hematopoietic Recovery
Supplementary Table 2 displays the studies resulting in US FDA approval of sargramostim to accelerate bone marrow recovery in persons receiving bone marrow suppressing drugs and exposed to ionizing radiations (100–106). In persons with delayed post-transplant bone marrow function, sargramostim use led to neutrophil recovery within 14 days in a majority of patients and provided a survival advantage compared with historical controls (100). Giving sargramostim post-transplant accelerated the interval to neutrophil recovery and shortened hospital stay without increased toxicities compared with placebo (101, 103). In a 3-year follow-up study, long-term toxicities such as delay in bone marrow recovery, increased leukemia risk, or death were not increased in persons receiving sargramostim compared with placebo (102).
Sargramostim given to older adults with de novo acute myeloid leukemia (AML) during intensive induction and consolidation chemotherapy decreased treatment-related morbidity and mortality primarily related to fewer infections (104, 105). Lastly, sargramostim use improved survival of non-human primates following exposure to high doses of acute whole body ionizing radiations not receiving blood transfusions (106–108). Based on these data sargramostim is approved in several settings including: 1. shortening interval to neutrophil recovery and reducing incidence of severe and life-threatening infection following induction chemotherapy in AML; 2. accelerating myeloid reconstitution after autologous transplantation; 3. accelerating myeloid recovery following allogeneic hematopoietic cell transplantation; 4. therapy for post-transplant delayed neutrophil recovery or graft-failure; and 5. increasing survival after exposure to acute, high-dose ionizing radiations.
Supplementary Table 1 displays data on effects of sargramostim on hematopoietic recovery after bone marrow suppressing exposures (7, 8, 10, 11, 13, 14, 109–113). Sargramostim was effective for enhancing neutrophil recovery in persons receiving cytotoxic therapy for non-Hodgkin lymphoma (NHL), plasma cell myeloma and breast cancer (7, 10, 110, 111).
Several studies compared efficacy of sargramostim and rhu G-CSF on neutrophil recovery in persons receiving bone marrow suppressive drugs. Beveridge et al. found no difference whereas Fields et al. reported delayed neutrophil recovery with sargramostim compared to rhu G-CSF therapy, but faster recovery compared to control (13, 14). Sargramostim versus no intervention was compared in two studies in subjects with small cell lung cancer (SCLC). Bunn et al. reported sargramostim was associated with less neutropenia but delayed platelet recovery and more non-hematologic adverse events and deaths (8). Steward and co-workers reported sargramostim failed to accelerate hematopoietic recovery nor decrease chemotherapy-related adverse events compared with placebo (112).
Overall, in a variety of settings, sargramostim administration after exposures to bone marrow suppressing agents usually accelerated hematologic recovery resulting in fewer infections, less therapy-related toxicity and sometimes improved survival.
Anti-Cancer Immune Modulation
Studies of sargramostim in persons with cancer are displayed in Tables 2 through 5. Anti-cancer effects of sargramostim were evaluated in 13 studies in solid cancers including melanoma (94–99) (Table 2), neuroblastoma (114–117) (Table 3) and prostate, colorectal and ovarian cancers (118–120) (Table 4). Anti-cancer effects of sargramostim were also evaluated in 3 studies in AML, chronic myelogenous (CML) and chronic lymphocytic leukemias (CLL) (121–123) (Table 5).
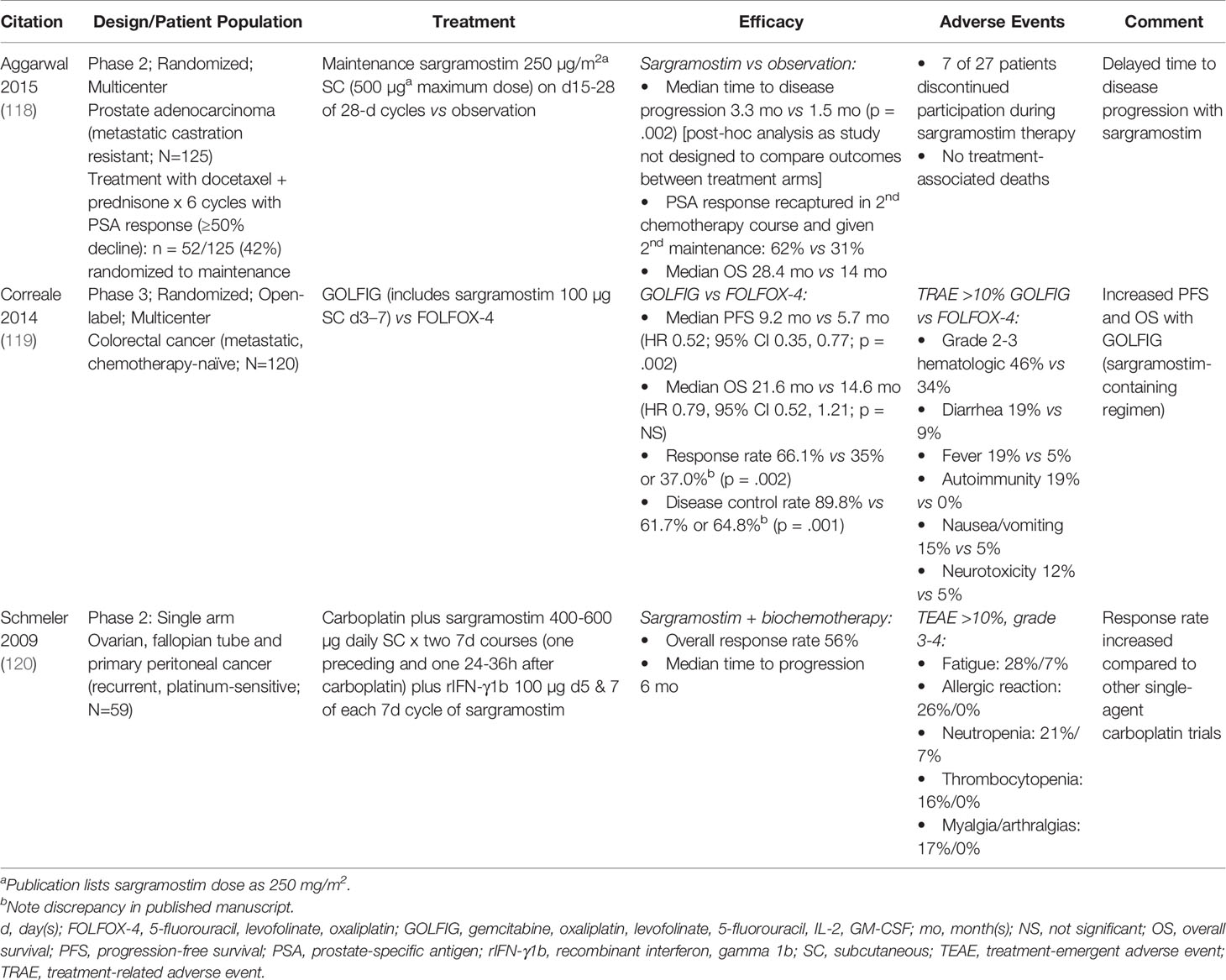
Table 4 Clinical studies evaluating immune-enhancing effects of sargramostim in prostate, colorectal and ovarian cancers.
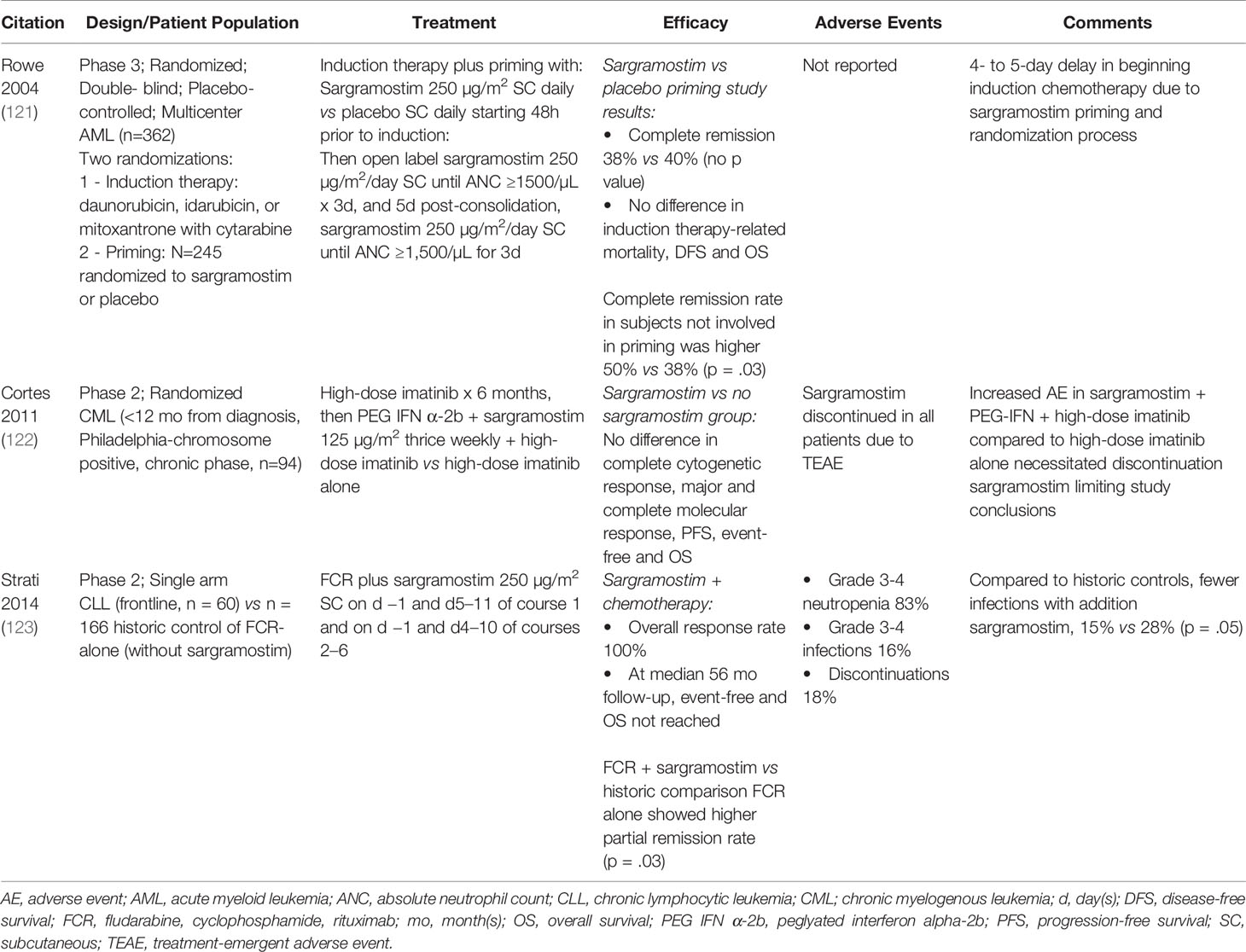
Table 5 Clinical studies evaluating immune-enhancing effects of sargramostim in hematologic malignancies (AML, CML, CLL).
Melanoma
Adding sargramostim to conventional melanoma therapies improved outcomes in some studies (Table 2). Hodi et al. (98) reported increased survival when sargramostim was added to immune checkpoint inhibitor (ipilimumab 10 mg/kg) treatment in a randomized phase 2 trial. Overall grade 3-5 treatment-related adverse effects were reduced including fewer gastrointestinal and no grade 3-5 pulmonary toxicities. Spitler and co-workers (94) reported increased disease-free and overall survival in subjects receiving sargramostim compared to matched historical controls. A study by Andtbacka et al. reported subcutaneous sargramostim and intra-lesional T-VEC therapy (a herpes simplex virus type 1–derived oncolytic immune therapy designed to selectively replicate within cancers and produce GM-CSF to enhance systemic antitumor immune responses) had similar survival rates in a phase 3 trial, ultimately leading to FDA approval for the use of T-VEC in melanoma (99). In a phase 2 study Spitler et al. (96) gave GM-CSF maintenance therapy to subjects with high risk of recurrence. Melanoma-specific survival was not reached after a median of 5.3 years follow-up, longer than observed in previous melanoma trials (Table 2). Further encouraging results are reported with the addition of sargramostim to other chemo- and bio-therapies (95, 97). In most of these studies treatment-related adverse effects of sargramostim were mild, the most common being injection-site erythema and fatigue (94–96, 98, 99), with the potential for a reduction in adverse effects with the addition sargramostim to therapy (98).
Several studies with sargramostim in persons with metastatic melanoma did not meet the literature search inclusion criteria. Si et al. and Elias et al. reported that intra-lesional injection of sargramostim in persons with metastatic melanoma resulted in regression of both injected and non-injected lesions suggesting systemic immune effects (124, 125). Additionally, reduced adverse events with the combination of ipilimumab 3 mg/kg (126) and 10 mg/kg (127) and sargramostim were reported in two real-world studies that corroborate the data presented here.
ICOS Ligand
Inducible co-stimulatory molecule ligand (ICOS-L) is a protein expressed on antigen-presenting cells, B- and T-memory and -effector cells, macrophages and dendritic cells (128) and on cells in the cancer micro-environment. Inhibition of the ICOS/ICOS-L signaling pathway on melanoma cells could improve treatment outcomes (129). Ipilimumab, a CTLA-4 inhibitor, increases CD4+ and CD8+ T-cell numbers and ICOS expression (130). Hodi and co-workers reported sargramostim added to ipilimumab increased ICOS expression on CD4+ and CD8+ T-cells compared with ipilimumab only (p = .11 and p = .01, respectively) (98) suggesting potential synergism.
Neuroblastoma
Sargramostim combined with an anti-GD2 monoclonal antibody was evaluated predominately in children with neuroblastoma (Table 3) (114–117). Yu and colleagues and Cheung and colleagues reported improved progression-free survival (PFS) and survival in subjects receiving sargramostim-containing regimens compared to standard chemotherapy and historical controls, respectively (114, 116). Adverse events were manageable. Based on these data dinutuximab (anti-GD2 monoclonal antibody, Unituxin®, United Therapeutics, Corp.) received FDA approval for the treatment of some children with high-risk neuroblastoma combined with rhu GM-CSF (i.e., sargramostim), IL-2 and isotretinoin (131). Naxitamab-gqgk (Danyelza®, Y-mAbs Therapeutics, Inc.), a humanized GD2-binding monoclonal antibody, also received FDA approval in combination with rhu GM-CSF (i.e., sargramostim) in certain patients with high-risk neuroblastoma (132).
Neutrophil-Driven Antibody-Dependent Cell-Mediated Cytotoxicity in Neuroblastoma
Sargramostim enhances neutrophil-driven antibody-dependent cell-mediated cytotoxicity (ADCC) in neuroblastoma when given with anti-GD2 antibodies (133). Patients with neuroblastoma undergoing high-dose chemotherapy have significantly attenuated lymphocyte anti-tumor responses, but neutrophils and macrophages are only transiently suppressed and can exert cell-mediated cytotoxicity and ADCC for marked tumor cell destruction (133, 134). Furthermore, the relative lack of complement-inhibitory proteins on the neuroblastoma cell surface renders these cancer cells more susceptible to cell-mediated cytotoxicity and antibody dependent cell cytotoxicity (135). Kushner and colleagues reported a 31 subject phase 1 dose-escalation study of anti-GD2 antibody therapy with sargramostim in treatment-resistant neuroblastoma (136). Fourteen (45%) subjects had complete or partial remission.
Other Solid Cancers
Adding sargramostim to conventional therapies was studied in other cancers including prostate, colorectal and ovary cancers (Table 4) (118–120). Investigators reported higher response rates with sargramostim and sargramostim-containing regimens compared with other similar trials. None of these studies were adequately controlled making a definitive conclusion impossible. These data suggest sargramostim may enhance anti-cancer responses in solid cancers when combined with conventional therapies but needs confirmation.
Hematologic Cancers
A few studies on the use of sargramostim in hematologic malignancies were included in this systematic review (Table 5). Some data suggest sargramostim might improve outcomes and reduce infections in persons with CLL receiving rituximab (123). In a study in subjects with CML adding sargramostim was no better than conventional therapy (122). Rowe et al. reported a phase 3 study in previously untreated persons with AML (121). Subjects received sargramostim before induction chemotherapy to stimulate leukemia cells to divide and thereby increasing their sensitivity to cell-cycle active drugs. No benefit was reported (121). In a recent report, Rong and associates (137) used an animal model to explore why some persons with extra-nodal natural killer/T cell lymphoma have rapid disease progression with GM-CSF treatment. They reported GM-CSF facilitated immune evasion by up-regulating PD-L1 expression. This effect could paradoxically increase immune checkpoint inhibitor activity similar to the use of prednisolone to increase CD20 cell expression and increase sensitivity to rituximab in children with B-cell precursor acute lymphoblastic leukemia during induction chemotherapy (138).
Attenuation of Adverse Events
Table 6 summarizes the evidence for sargramostim attenuation of adverse events when given with concurrent treatments in trials involving melanoma, AML and hematopoietic cell transplants. Reductions in infections and fatal infection are reported (100, 101, 103, 104). Decreases in other adverse events included less grade 3-5 AEs, grade 3-5 gastrointestinal (GI) and pulmonary AEs and mucositis (98, 103).
Sargramostim Enhancement of Abscopal Response
Radiation therapy has not been thought to elicit an immunologic effect, but there are several reports of inducing a clinical immune response in a cancer site non-contiguous with the radiation field. This phenomenon is referred to as abscopal effect. Leary and colleagues reviewed clinical trials and case reports where sargramostim was added to radiation therapy (139). They discuss enhancement of the abscopal effect and postulate that sargramostim enables the presentation of tumor-associated antigens to generate a T-cell response. Golden and co-workers reported a phase 2 trial in 41 subjects with diverse cancers (140). Abscopal responses were observed in 4 of 18 patients with lung cancer, 5 of 14 with breast cancer and 2 of 2 with a thymoma. These responses were associated with improved overall survival. Leary et al. also discuss abscopal effects with sargramostim and radiation therapy in 2 persons with pancreatic and lung cancers (139).
Discussion and Future Considerations
The sargramostim studies spanning 30 years of use highlight the extensive knowledge of sargramostim as a myeloid hematopoietic growth factor in accelerating bone marrow recovery after insult from bone marrow damaging exposures. The data presented in this systematic review suggest the innate and adaptive immune activity of sargramostim may improve cancer outcomes and reduce toxicity of chemo- and other immune therapies, including immune checkpoint inhibitors and GD2-binding monoclonal antibodies. Safety and efficacy in oncologic clinical settings warrants further study. Sargramostim may be less efficacious in hematologic malignancies, although data are limited.
The use of sargramostim in combination with immune checkpoint inhibitors in melanoma merits further study, as demonstrated by significantly mitigating immune checkpoint inhibitor-related adverse events. In melanoma, data indicate sargramostim improves survival and reduces toxicity associated with ipilimumab 10 mg/kg therapy (98). Furthermore, sargramostim is actively being studied in combination with the immune checkpoint inhibitor combination ipilimumab and nivolumab in patients with advanced melanoma; the ECOG phase 2-3 study achieved the phase 2 metric allowing the continuation into phase 3 (141). Also, sargramostim in combination with pembrolizumab is being studied in advanced melanoma (142). Other solid tumor investigations include sargramostim and pembrolizumab in biliary cancer (143, 144). As noted above, two GD2-binding monoclonal antibodies, dinutuximab and naxitamab-gqgk, are approved for use in combination with sargramostim in neuroblastoma (131, 132, 145).
Diverse activities of sargramostim are being evaluated (Table 1). These studies focus on increasing host defenses, reversing immune suppression, as a vaccine adjuvant and as combination therapy with immune checkpoint inhibitors. Other studies are evaluating different routes of administration such as intra- and peri-lesional injections for skin cancers. Many clinical trials evaluating the potential of sargramostim as an adjuvant for anti-cancer vaccines in diverse settings and improving efficacy of anti-bacterial and -virus vaccines are discussed above (146, 147).
Emerging Uses in Neuro-Degenerative Disorders
GM-CSF treatment is protective in animal models of neuro-degenerative disorders (148–150). Increasing Treg activity is postulated to improve signs and symptoms of disorders such as Alzheimer and Parkinson diseases, amyotrophic lateral sclerosis and stroke (151). Sargramostim is being evaluated in Parkinson disease (152) based on in vitro and recent pre-clinical studies reporting a positive influence on innate and/or adaptive cell-mediated immunity. The focus has been on regulatory T-cells (Tregs), a T-cell subset which modulates the immune system, maintains tolerance to self-antigens, prevents autoimmunity and downregulates induction and proliferation of effector T-cells. GM-CSF increases the frequency of Tregs suggesting increased immune regulation and efficacy in Parkinson disease (153, 154). The anti-inflammatory activity of sargramostim appears mediated by inducing tolerogenic dendritic cells and thereby preventing T-cell activation (84, 155, 156). Olson and colleagues (157) reported sargramostim, 3 μg/kg/day, given for one year was well-tolerated and increased numbers and function of Tregs in persons with Parkinson disease. Sargramostim supported a neuro-protective biomarker phenotype associated with stable Parkinson disease.
Potter and associates followed their preclinical murine model (158) by a randomized, double-blind, placebo-controlled clinical trial in patients with mild-to-moderate Alzheimer disease (AD) (159). Forty patients were given either placebo or sargramostim 250 µg/m2/day subcutaneous injection five days/week for 3 weeks; compared to placebo and baseline, sargramostim recipients showed significant improvement in Mini-Mental State Examination (MMSE) scores, along with a decrease in plasma markers of neurodegeneration, tau and plasma ubiquitin C-terminal hydrolase L1 (UCH-L1), and an increase in amyloid beta (Aβ40), an amyloid marker that is decreased in AD (159). Clinical studies with sargramostim therapy in neuro-inflammatory disease continue.
Other Emerging Uses
Other potential uses of sargramostim include as an adjunctive therapy in refractory bacterial and fungal infections and to reverse immune deficiency associated with sepsis and other acute illnesses (42, 47). Sargramostim mobilizes progenitor cells, particularly those of endothelial origin which are involved in vascular repair and regeneration into the circulation. These cells contribute to neo-vascularization in persons with symptomatic peripheral arterial disease (claudication) in whom sargramostim may improve walking performance (160, 161).
Lastly, as discussed above, sargramostim may be effective in respiratory diseases including autoimmune PAP (77–80) and the immune suppression associated with ARDS (38). There are ongoing trials of whether inhaled sargramostim might be effective in lung diseases such as COVID-19 (NCT0470766, NCT04326920, NCT04411680, NCT04642950) (57–60). The sum of these studies suggests a much wider potential role for sargramostim therapy than simply accelerating bone marrow recovery.
Conclusions
GM-CSF accelerates hematopoietic recovery after exposure to bone marrow-suppressing agents and reverses post-transplant graft-failure. In this systematic review we consider use of sargramostim in other settings including therapy of solid cancers as an immune modulating drug. The favorable clinical outcomes observed in patients treated with sargramostim, combined with its acceptable safety profile and diverse biological effects, warrant continued evaluation of its role as immunotherapy. Furthermore, we describe use as an adjunct to therapy for resistant infections, immune suppression associated with sepsis and trauma, and respiratory and neuro-inflammatory diseases. We emphasize the pleiotropic biologic activities of GM-CSF including effects on innate and adaptive immune responses. These considerations and encouraging results of exploratory clinical trials suggest continued evaluation of this agent in diverse conditions.
Research Agenda
● Evaluate the ability of sargramostim to restore immune function.
● Evaluate sargramostim in the setting of immune checkpoint inhibitor therapy.
● Develop new, targeted ways to administer sargramostim including delivery as an inhalation.
● Evaluate sargramostim in infection and as a vaccine adjuvant.
● Explore sargramostim in the treatment of peripheral artery disease and neuro-inflammatory disease.
Data Availability Statement
The original contributions presented in the study are included in the article/Supplementary Material. Further inquiries can be directed to the corresponding author.
Author Contributions
GL and CR designed the systematic review. CR, along with two other data abstractors, conducted the systematic review. HL, CR, RG, and GL summarized the data and prepared the typescript. All authors contributed to the article and approved the submitted version.
Funding
Publication of this manuscript was supported, in part, by Partner Therapeutics, Inc.
Conflict of Interest
HL is a paid consultant for Partner Therapeutics, Inc., including in relation to this manuscript. HL has stock options and participated on an advisory board for Partner Therapeutics, Inc. outside of the current work. CR is an employee of and has stock options for Partner Therapeutics, Inc. RG and GL received funds from Partner Therapeutics for consulting but none in relation to this manuscript. Additionally, RG is a consultant to BeiGene Ltd., Kite Pharma, Inc., FFF Enterprises, Inc., CStone Pharmaceuticals; advisor to LaJolla NanoMedical, Inc, Mindsight Pharma, Antegene Biotech LLC; partner, AZAC Inc.; Board of Directors for Russian Foundation for Cancer Research Support; and Scientific Advisory Board for StemRad Ltd. Additionally, GL is PI on research grant from Amgen to the Fred Hutchinson Cancer Research Center and has consulted for G1 Therapeutics, BeyondSpring, Sandoz, ER Squibb, Seattle Genetics, Jazz Pharmaceuticals, TEVA, Samsung, and Merck outside of the current work.
The authors declare that this study received funding from Partner Therapeutics, Inc. The funder had the following involvement with the study: Partner Therapeutics funded the professional writer, Jill Luer (Secundum Artem) who contributed to study design and data abstraction, and another data abstractor.
Publisher’s Note
All claims expressed in this article are solely those of the authors and do not necessarily represent those of their affiliated organizations, or those of the publisher, the editors and the reviewers. Any product that may be evaluated in this article, or claim that may be made by its manufacturer, is not guaranteed or endorsed by the publisher.
Acknowledgments
We thank Jill Luer (Secundum Artem) for support in study design and data abstraction and Ila Joshi (Partner Therapeutics) for constructing Figure 4.
Supplementary Material
The Supplementary Material for this article can be found online at: https://www.frontiersin.org/articles/10.3389/fimmu.2021.706186/full#supplementary-material
References
1. Burgess AW, Metcalf D. The Nature and Action of Granulocyte-Macrophage Colony Stimulating Factors. Blood (1980) 56(6):947–58. doi: 10.1182/blood.V56.6.947.947
2. Metcalf D. Hematopoietic Cytokines. Blood (2008) 111(2):485–91. doi: 10.1182/blood-2007-03-079681
3. Hamilton JA, Anderson GP. GM-CSF Biology. Growth Factors (2004) 22(4):225–31. doi: 10.1080/08977190412331279881
4. United States Food and Drug Administration. Executive Order 13944 List of Essential Medicines, Medical Countermeasures, and Critical Inputs. (2021). Available at: https://www.fda.gov/about-fda/reports/executive-order-13944-list-essential-medicines-medical-countermeasures-and-critical-inputs (Accessed April 12, 2021).
5. Gale RP, Vorobiov A. First Use of Myeloid Colony-Stimulating Factors in Humans. Bone Marrow Transplant (2013) 48(10):1358. doi: 10.1038/bmt.2013.57
6. Partner Therapeutics, Inc. LEUKINE®(sargramostim) for Injection, for Subcuteous or Intravenous Use Prescribing Information. (2018).
7. O’Day SJ, Rabinowe SN, Neuberg D, Freedman AS, Soiffer RJ, Spector NA, et al. A Phase II Study of Continuous Infusion Recombinant Human Granulocyte-Macrophage Colony-Stimulating Factor as an Adjunct to Autologous Bone Marrow Transplantation for Patients With Non-Hodgkin’s Lymphoma in First Remission. Blood (1994) 83(9):2707–14. doi: 10.1182/blood.V83.9.2707.2707
8. Bunn PA Jr, Crowley J, Kelly K, Hazuka MB, Beasley K, Upchurch C, et al. Chemoradiotherapy With or Without Granulocyte-Macrophage Colony-Stimulating Factor in the Treatment of Limited-Stage Small-Cell Lung Cancer: A Prospective Phase III Randomized Study of the Southwest Oncology Group. J Clin Oncol (1995) 13(7):1632–41. doi: 10.1200/JCO.1995.13.7.1632
9. Neidhart JA, Mangalik A, Stidley CA, Tebich SL, Sarmiento LE, Pfile JE, et al. Dosing Regimen of Granulocyte-Macrophage Colony-Stimulating Factor to Support Dose-Intensive Chemotherapy. J Clin Oncol (1992) 10(9):1460–9. doi: 10.1200/JCO.1992.10.9.1460
10. Yau JC, Neidhart JA, Triozzi P, Verma S, Nemunaitis J, Quick DP, et al. Randomized Placebo-Controlled Trial of Granulocyte-Macrophage Colony-Stimulating-Factor Support for Dose-Intensive Cyclophosphamide, Etoposide, and Cisplatin. Am J Hematol (1996) 51(4):289–95. doi: 10.1002/(SICI)1096-8652(199604)51:4<289::AID-AJH7>3.0.CO;2-S
11. Beveridge RA, Miller JA, Kales AN, Binder RA, Robert NJ, Heisrath-Evans J, et al. Randomized Trial Comparing the Tolerability of Sargramostim (Yeast-Derived RhuGM-CSF) and Filgrastim (Bacteria-Derived RhuG-CSF) in Cancer Patients Receiving Myelosuppressive Chemotherapy. Support Care Cancer (1997) 5(4):289–98. doi: 10.1007/PL00009894
12. Lazarus HM, Gale RP. G-CSF and GM-CSF Are Different. Which One Is Better for COVID-19? Acta Haematol (2021) 144(4):355–9. doi: 10.1159/000510352
13. Fields KK, Elfenbein GJ, Perkins JB, Janssen WE, Ballester OF, Hiemenz JW, et al. High-Dose Ifosfamide/Carboplatin/Etoposide: Maximum Tolerable Doses, Toxicities, and Hematopoietic Recovery After Autologous Stem Cell Reinfusion. Semin Oncol (1994) 21(5 Suppl 12):86–92.
14. Beveridge RA, Miller JA, Kales AN, Binder RA, Robert NJ, Harvey JH, et al. A Comparison of Efficacy of Sargramostim (Yeast-Derived RhuGM-CSF) and Filgrastim (Bacteria-Derived RhuG-CSF) in the Therapeutic Setting of Chemotherapy-Induced Myelosuppression. Cancer Invest (1998) 16(6):366–73. doi: 10.3109/07357909809115775
15. Liberati A, Altman DG, Tetzlaff J, Mulrow C, Gotzsche PC, Ioannidis JP, et al. The PRISMA Statement for Reporting Systematic Reviews and Meta-Analyses of Studies That Evaluate Healthcare Interventions: Explanation and Elaboration. BMJ (2009) 339:b2700. doi: 10.1136/bmj.b2700
16. Dorr RT. Clinical Properties of Yeast-Derived Versus Escherichia Coli-Derived Granulocyte-Macrophage Colony-Stimulating Factor. Clin Ther (1993) 15(1):19–29, discussion 18.
17. Sola RJ, Griebenow K. Effects of Glycosylation on the Stability of Protein Pharmaceuticals. J Pharm Sci (2009) 98(4):1223–45. doi: 10.1002/jps.21504
18. Bennett CL, George SL, Vose JM, Nemunaitis JJ, Armitage JL, Armitage JO, et al. Granulocyte-Macrophage Colony-Stimulating Factor as Adjunct Therapy in Relapsed Lymphoid Malignancy: Implications for Economic Analyses of Phase III Clinical Trials. Stem Cells (1995) 13(4):414–20. doi: 10.1002/stem.5530130412
19. Bhattacharya P, Thiruppathi M, Elshabrawy HA, Alharshawi K, Kumar P, Prabhakar BS. GM-CSF: An Immune Modulatory Cytokine That can Suppress Autoimmunity. Cytokine (2015) 75(2):261–71. doi: 10.1016/j.cyto.2015.05.030
20. De Alessandris S, Ferguson GJ, Dodd AJ, Juss JK, Devaprasad A, Piper S, et al. Neutrophil GM-CSF Receptor Dynamics in Acute Lung Injury. J Leukoc Biol (2019) 105(6):1183–94. doi: 10.1002/JLB.3MA0918-347R
21. Metcalf D, Nicola NA, Mifsud S, Di Rago L. Receptor Clearance Obscures the Magnitude of Granulocyte-Macrophage Colony-Stimulating Factor Responses in Mice to Endotoxin or Local Infections. Blood (1999) 93(5):1579–85. doi: 10.1182/blood.V93.5.1579.405k01_1579_1585
22. Suzuki T, Sakagami T, Young LR, Carey BC, Wood RE, Luisetti M, et al. Hereditary Pulmonary Alveolar Proteinosis: Pathogenesis, Presentation, Diagnosis, and Therapy. Am J Respir Crit Care Med (2010) 182(10):1292–304. doi: 10.1164/rccm.201002-0271OC
23. Guilliams M, De Kleer I, Henri S, Post S, Vanhoutte L, De Prijck S, et al. Alveolar Macrophages Develop From Fetal Monocytes That Differentiate Into Long-Lived Cells in the First Week of Life Via GM-CSF. J Exp Med (2013) 210(10):1977–92. doi: 10.1084/jem.20131199
24. Wessendarp M, Watanabe M, Liu S, Stankiewicz T, Ma Y, Shima K, et al. Role of GM-CSF in Regulating Metabolism and Mitochondrial Functions Critical to Macrophage Proliferation. bioRxiv (2021) 02.10.430444. doi: 10.1101/2021.02.10.430444
25. Inaba K, Inaba M, Romani N, Aya H, Deguchi M, Ikehara S, et al. Generation of Large Numbers of Dendritic Cells From Mouse Bone Marrow Cultures Supplemented With Granulocyte/Macrophage Colony-Stimulating Factor. J Exp Med (1992) 176(6):1693–702. doi: 10.1084/jem.176.6.1693
26. Hercus TR, Thomas D, Guthridge MA, Ekert PG, King-Scott J, Parker MW, et al. The Granulocyte-Macrophage Colony-Stimulating Factor Receptor: Linking Its Structure to Cell Signaling and Its Role in Disease. Blood (2009) 114(7):1289–98. doi: 10.1182/blood-2008-12-164004
27. Larrivee B, Pollet I, Karsan A. Activation of Vascular Endothelial Growth Factor Receptor-2 in Bone Marrow Leads to Accumulation of Myeloid Cells: Role of Granulocyte-Macrophage Colony-Stimulating Factor. J Immunol (2005) 175(5):3015–24. doi: 10.4049/jimmunol.175.5.3015
28. Sudo T, Motomura Y, Okuzaki D, Hasegawa T, Yokota T, Kikuta J, et al. Group 2 Innate Lymphoid Cells Support Hematopoietic Recovery Under Stress Conditions. J Exp Med (2021) 218(5):e20200817. doi: 10.1084/jem.20200817
29. Steinman RM. The Dendritic Cell System and Its Role in Immunogenicity. Annu Rev Immunol (1991) 9:271–96. doi: 10.1146/annurev.iy.09.040191.001415
30. Dabritz J. Granulocyte Macrophage Colony-Stimulating Factor and the Intestinal Innate Immune Cell Homeostasis in Crohn’s Disease. Am J Physiol Gastrointest Liver Physiol (2014) 306(6):G455–65. doi: 10.1152/ajpgi.00409.2013
31. Rosler B, Herold S. Lung Epithelial GM-CSF Improves Host Defense Function and Epithelial Repair in Influenza Virus Pneumonia-A New Therapeutic Strategy? Mol Cell Pediatr (2016) 3(1):29. doi: 10.1186/s40348-016-0055-5
32. Nemunaitis J, Cox J, Meyer W, Courtney A, Hanson T, Green-Weaver C, et al. Comparison of Neutrophil and Monocyte Function by Microbicidal Cell-Kill Assay in Patients With Cancer Receiving Granulocyte Colony-Stimulating Factor, Granulocyte-Macrophage Colony-Stimulating Factor, or No Cytokine After Cytotoxic Chemotherapy: A Phase II Trial. Am J Clin Oncol (1998) 21(3):308–12. doi: 10.1097/00000421-199806000-00023
33. Hall MW, Knatz NL, Vetterly C, Tomarello S, Wewers MD, Volk HD, et al. Immunoparalysis and Nosocomial Infection in Children With Multiple Organ Dysfunction Syndrome. Intensive Care Med (2011) 37(3):525–32. doi: 10.1007/s00134-010-2088-x
34. Hall MW. Immune Modulation in Pediatric Sepsis. J Pediatr Intensive Care (2019) 8(1):42–50. doi: 10.1055/s-0038-1676607
35. Mathias B, Szpila BE, Moore FA, Efron PA, Moldawer LL. A Review of GM-CSF Therapy in Sepsis. Med (Baltimore) (2015) 94(50):e2044. doi: 10.1097/MD.0000000000002044
36. ClinicalTrials.gov. GM-CSF for Immunomodulation Following Trauma (GIFT) Study. (2021). Available at: https://www.clinicaltrials.gov/ct2/show/NCT01495637 (Accessed April 21, 2021).
37. Bo L, Wang F, Zhu J, Li J, Deng X. Granulocyte-Colony Stimulating Factor (G-CSF) and Granulocyte-Macrophage Colony Stimulating Factor (GM-CSF) for Sepsis: A Meta-Analysis. Crit Care (2011) 15(1):R58. doi: 10.1186/cc10031
38. Meisel C, Schefold JC, Pschowski R, Baumann T, Hetzger K, Gregor J, et al. Granulocyte-Macrophage Colony-Stimulating Factor to Reverse Sepsis-Associated Immunosuppression: A Double-Blind, Randomized, Placebo-Controlled Multicenter Trial. Am J Respir Crit Care Med (2009) 180(7):640–8. doi: 10.1164/rccm.200903-0363OC
39. Abzug MJ, Walsh TJ. Interferon-Gamma and Colony-Stimulating Factors as Adjuvant Therapy for Refractory Fungal Infections in Children. Pediatr Infect Dis J (2004) 23(8):769–73. doi: 10.1097/01.inf.0000134314.65398.bf
40. Baltch AL, Bopp LH, Smith RP, Ritz WJ, Carlyn CJ, Michelsen PB. Effects of Voriconazole, Granulocyte-Macrophage Colony-Stimulating Factor, and Interferon Gamma on Intracellular Fluconazole-Resistant Candida Glabrata and Candida Krusei in Human Monocyte-Derived Macrophages. Diagn Microbiol Infect Dis (2005) 52(4):299–304. doi: 10.1016/j.diagmicrobio.2005.02.017
41. Goldman C, Akiyama MJ, Torres J, Louie E, Meehan SA. Scedosporium Apiospermum Infections and the Role of Combination Antifungal Therapy and GM-CSF: A Case Report and Review of the Literature. Med Mycol Case Rep (2016) 11:40–3. doi: 10.1016/j.mmcr.2016.04.005
42. Damiani G, McCormick TS, Leal LO, Ghannoum MA. Recombinant Human Granulocyte Macrophage-Colony Stimulating Factor Expressed in Yeast (Sargramostim): A Potential Ally to Combat Serious Infections. Clin Immunol (2020) 210:108292. doi: 10.1016/j.clim.2019.108292
43. Hariadi NI, Blackwood RA. Disseminated Mycobacterium Avium Complex in an Adolescent With Perinatally-Acquired HIV Infection. Infect Dis Rep (2017) 9(2):6884. doi: 10.4081/idr.2017.6884
44. Mir N, Edmonson R, Yeghen T, Rashid H. Gastrointestinal Mucormycosis Complicated by Arterio-Enteric Fistula in a Patient With Non-Hodgkin’s Lymphoma. Clin Lab Haematol (2000) 22(1):41–4. doi: 10.1046/j.1365-2257.2000.00264.x
45. Gavino C, Cotter A, Lichtenstein D, Lejtenyi D, Fortin C, Legault C, et al. CARD9 Deficiency and Spontaneous Central Nervous System Candidiasis: Complete Clinical Remission With GM-CSF Therapy. Clin Infect Dis (2014) 59(1):81–4. doi: 10.1093/cid/ciu215
46. Gavino C, Hamel N, Zeng JB, Legault C, Guiot MC, Chankowsky J, et al. Impaired RASGRF1/ERK-Mediated GM-CSF Response Characterizes CARD9 Deficiency in French-Canadians. J Allergy Clin Immunol (2016) 137(4):1178–88.e7. doi: 10.1016/j.jaci.2015.09.016
47. Chu S, McCormick TS, Lazarus HM, Leal LO, Ghannoum MA. Invasive Fungal Disease and the Immunocompromised Host Including Allogeneic Hematopoietic Cell Transplant Recipients: Improved Understanding and New Strategic Approach With Sargramostim. Clin Immunol (2021) 228:108731. doi: 10.1016/j.clim.2021.108731
48. Subramaniam R, Hillberry Z, Chen H, Feng Y, Fletcher K, Neuenschwander P, et al. Delivery of GM-CSF to Protect Against Influenza Pneumonia. PloS One (2015) 10(4):e0124593. doi: 10.1371/journal.pone.0124593
49. Huang FF, Barnes PF, Feng Y, Donis R, Chroneos ZC, Idell S, et al. GM-CSF in the Lung Protects Against Lethal Influenza Infection. Am J Respir Crit Care Med (2011) 184(2):259–68. doi: 10.1164/rccm.201012-2036OC
50. Huang H, Li H, Zhou P, Ju D. Protective Effects of Recombinant Human Granulocyte Macrophage Colony Stimulating Factor on H1N1 Influenza Virus-Induced Pneumonia in Mice. Cytokine (2010) 51(2):151–7. doi: 10.1016/j.cyto.2010.04.001
51. England JT, Abdulla A, Biggs CM, Lee AYY, Hay KA, Hoiland RL, et al. Weathering the COVID-19 Storm: Lessons From Hematologic Cytokine Syndromes. Blood Rev (2021) 45:100707. doi: 10.1016/j.blre.2020.100707
52. Qin C, Zhou L, Hu Z, Zhang S, Yang S, Tao Y, et al. Dysregulation of Immune Response in Patients With Coronavirus 2019 (COVID-19) in Wuhan, China. Clin Infect Dis (2020) 71(15):762–8. doi: 10.1093/cid/ciaa248
53. Huang C, Wang Y, Li X, Ren L, Zhao J, Hu Y, et al. Clinical Features of Patients Infected With 2019 Novel Coronavirus in Wuhan, China. Lancet (2020) 395(10223):497–506. doi: 10.1016/S0140-6736(20)30183-5
54. Wang W, Liu X, Wu S, Chen S, Li Y, Nong L, et al. Definition and Risks of Cytokine Release Syndrome in 11 Critically Ill COVID-19 Patients With Pneumonia: Analysis of Disease Characteristics. J Infect Dis (2020) 222(9):1444–51. doi: 10.1093/infdis/jiaa387
55. Hall MW, Joshi I, Leal L, Ooi EE. Immune Modulation in COVID-19: Strategic Considerations for Personalized Therapeutic Intervention. Clin Infect Dis (2020) ciaa904. doi: 10.1093/cid/ciaa904
56. Herold S, Hoegner K, Vadasz I, Gessler T, Wilhelm J, Mayer K, et al. Inhaled Granulocyte/Macrophage Colony-Stimulating Factor as Treatment of Pneumonia-Associated Acute Respiratory Distress Syndrome. Am J Respir Crit Care Med (2014) 189(5):609–11. doi: 10.1164/rccm.201311-2041LE
57. ClinicalTrials.gov. Sargramostim Use in COVID-19 to Recover Patient Health (SCOPE). (2021). Available at: https://clinicaltrials.gov/ct2/show/NCT04707664 (Accessed April 21, 2021).
58. ClinicalTrials.gov. Study of Sargramostim in Patients With COVID-19 (Ileukpulm). (2021). Available at: https://www.clinicaltrials.gov/ct2/show/NCT04411680 (Accessed April 21, 2021).
59. ClinicalTrials.gov. A Phase II/III Study of Sargramostim. (2021). Available at: https://www.clinicaltrials.gov/ct2/show/NCT04642950 (Accessed April 21, 2021).
60. Bosteels C, Maes B, Van Damme K, De Leeuw E, Declercq J, Delporte A, et al. Correction to: Sargramostim to Treat Patients With Acute Hypoxic Respiratory Failure Due to COVID-19 (SARPAC): A Structured Summary of a Study Protocol for a Randomised Controlled Trial. Trials (2020) 21(1):554. doi: 10.1186/s13063-020-04520-x
61. Sargramostim in Patients With Acute Hypoxic Respiratory Failure Due to COVID-19 (SARPAC). Available at: https://clinicaltrials.gov/ct2/show/NCT04326920 (Accessed on April 21, 2021).
62. Schulte-Schrepping J, Reusch N, Paclik D, Bassler K, Schlickeiser S, Zhang B, et al. Severe COVID-19 Is Marked by a Dysregulated Myeloid Cell Compartment. Cell (2020) 182(6):1419–40.e23. doi: 10.1016/j.cell.2020.08.001
63. Mengos AE, Gastineau DA, Gustafson MP. The CD14(+)HLA-DR(lo/neg) Monocyte: An Immunosuppressive Phenotype That Restrains Responses to Cancer Immunotherapy. Front Immunol (2019) 10:1147. doi: 10.3389/fimmu.2019.01147
64. Kurbacher CM, Kurbacher JA, Cramer EM, Rhiem K, Mallman PK, Reichelt R, et al. Continuous Low-Dose GM-CSF as Salvage Therapy in Refractory Recurrent Breast or Female Genital Tract Carcinoma. Oncol (Williston Park) (2005) 19(4 Suppl 2):23–6.
65. Innamarato P, Kodumudi K, Asby S, Schachner B, Hall M, Mackay A, et al. Reactive Myelopoiesis Triggered by Lymphodepleting Chemotherapy Limits the Efficacy of Adoptive T Cell Therapy. Mol Ther (2020) 28(10):2252–70. doi: 10.1016/j.ymthe.2020.06.025
66. Gunther P, Schultze JL. Mind the Map: Technology Shapes the Myeloid Cell Space. Front Immunol (2019) 10:2287. doi: 10.3389/fimmu.2019.02287
67. Le DT, Wang-Gillam A, Picozzi V, Greten TF, Crocenzi T, Springett G, et al. Safety and Survival With GVAX Pancreas Prime and Listeria Monocytogenes-Expressing Mesothelin (CRS-207) Boost Vaccines for Metastatic Pancreatic Cancer. J Clin Oncol (2015) 33(12):1325–33. doi: 10.1200/JCO.2014.57.4244
68. Lawson DH, Lee S, Zhao F, Tarhini AA, Margolin KA, Ernstoff MS, et al. Randomized, Placebo-Controlled, Phase III Trial of Yeast-Derived Granulocyte-Macrophage Colony-Stimulating Factor (GM-CSF) Versus Peptide Vaccination Versus GM-CSF Plus Peptide Vaccination Versus Placebo in Patients With No Evidence of Disease After Complete Surgical Resection of Locally Advanced and/or Stage IV Melanoma: A Trial of the Eastern Cooperative Oncology Group-American College of Radiology Imaging Network Cancer Research Group (E4697). J Clin Oncol (2015) 33(34):4066–76. doi: 10.1200/JCO.2015.62.0500
69. Spies C, Luetz A, Lachmann G, Renius M, von Haefen C, Wernecke KD, et al. Influence of Granulocyte-Macrophage Colony-Stimulating Factor or Influenza Vaccination on HLA-DR, Infection and Delirium Days in Immunosuppressed Surgical Patients: Double Blind, Randomised Controlled Trial. PloS One (2015) 10(12):e0144003. doi: 10.1371/journal.pone.0144003
70. Sloot S, Rashid OM, Sarnaik AA, Zager JS. Developments in Intralesional Therapy for Metastatic Melanoma. Cancer Control (2016) 23(1):12–20. doi: 10.1177/107327481602300104
71. Basta S, Alatery A. The Cross-Priming Pathway: A Portrait of an Intricate Immune System. Scand J Immunol (2007) 65(4):311–9. doi: 10.1111/j.1365-3083.2007.01909.x
72. Dranoff G, Jaffee E, Lazenby A, Golumbek P, Levitsky H, Brose K, et al. Vaccination With Irradiated Tumor Cells Engineered to Secrete Murine Granulocyte-Macrophage Colony-Stimulating Factor Stimulates Potent, Specific, and Long-Lasting Anti-Tumor Immunity. Proc Natl Acad Sci USA (1993) 90(8):3539–43. doi: 10.1073/pnas.90.8.3539
73. Cuzzubbo S, Mangsbo S, Nagarajan D, Habra K, Pockley AG, McArdle SEB. Cancer Vaccines: Adjuvant Potency, Importance of Age, Lifestyle, and Treatments. Front Immunol (2020) 11:615240. doi: 10.3389/fimmu.2020.615240
74. Parmiani G, Castelli C, Pilla L, Santinami M, Colombo MP, Rivoltini L. Opposite Immune Functions of GM-CSF Administered as Vaccine Adjuvant in Cancer Patients. Ann Oncol (2007) 18(2):226–32. doi: 10.1093/annonc/mdl158
75. Petrina M, Martin J, Basta S. Granulocyte Macrophage Colony-Stimulating Factor Has Come of Age: From a Vaccine Adjuvant to Antiviral Immunotherapy. Cytokine Growth Factor Rev (2021) 59:101–10. doi: 10.1016/j.cytogfr.2021.01.001
76. Kitamura T, Tanaka N, Watanabe J, Uchida, Kanegasaki S, Yamada Y, et al. Idiopathic Pulmonary Alveolar Proteinosis as an Autoimmune Disease With Neutralizing Antibody Against Granulocyte/Macrophage Colony-Stimulating Factor. J Exp Med (1999) 190(6):875–80. doi: 10.1084/jem.190.6.875
77. Tazawa R, Trapnell BC, Inoue Y, Arai T, Takada T, Nasuhara Y, et al. Inhaled Granulocyte/Macrophage-Colony Stimulating Factor as Therapy for Pulmonary Alveolar Proteinosis. Am J Respir Crit Care Med (2010) 181(12):1345–54. doi: 10.1164/rccm.200906-0978OC
78. Tazawa R, Ueda T, Abe M, Tatsumi K, Eda R, Kondoh S, et al. Inhaled GM-CSF for Pulmonary Alveolar Proteinosis. N Engl J Med (2019) 381(10):923–32. doi: 10.1056/NEJMoa1816216
79. Sheng G, Chen P, Wei Y, Chu J, Cao X, Zhang HL. Better Approach for Autoimmune Pulmonary Alveolar Proteinosis Treatment: Inhaled or Subcutaneous Granulocyte-Macrophage Colony-Stimulating Factor: A Meta-Analyses. Respir Res (2018) 19(1):163. doi: 10.1186/s12931-018-0862-4
80. Ohashi K, Sato A, Takada T, Arai T, Nei T, Kasahara Y, et al. Direct Evidence That GM-CSF Inhalation Improves Lung Clearance in Pulmonary Alveolar Proteinosis. Respir Med (2012) 106(2):284–93. doi: 10.1016/j.rmed.2011.10.019
81. Fukuzawa H, Sawada M, Kayahara T, Morita-Fujisawa Y, Suzuki K, Seno H, et al. Identification of GM-CSF in Paneth Cells Using Single-Cell RT-PCR. Biochem Biophys Res Commun (2003) 312(4):897–902. doi: 10.1016/j.bbrc.2003.11.009
82. Dabritz J. GM-CSF and the Role of Myeloid Regulatory Cells in the Pathogenesis and Treatment of Crohn’s Disease. Mol Cell Pediatr (2015) 2(1):12. doi: 10.1186/s40348-015-0024-4
83. Sainathan SK, Hanna EM, Gong Q, Bishnupuri KS, Luo Q, Colonna M, et al. Granulocyte Macrophage Colony-Stimulating Factor Ameliorates DSS-Induced Experimental Colitis. Inflamm Bowel Dis (2008) 14(1):88–99. doi: 10.1002/ibd.20279
84. Lotfi N, Thome R, Rezaei N, Zhang GX, Rezaei A, Rostami A, et al. Roles of GM-CSF in the Pathogenesis of Autoimmune Diseases: An Update. Front Immunol (2019) 10:1265. doi: 10.3389/fimmu.2019.01265
85. Roth L, MacDonald JK, McDonald JW, Chande N. Sargramostim (GM-CSF) for Induction of Remission in Crohn’s Disease: A Cochrane Inflammatory Bowel Disease and Functional Bowel Disorders Systematic Review of Randomized Trials. Inflamm Bowel Dis (2012) 18(7):1333–9. doi: 10.1002/ibd.22973
86. Malone DG, Pierce JH, Falko JP, Metcalfe DD. Production of Granulocyte-Macrophage Colony-Stimulating Factor by Primary Cultures of Unstimulated Rat Microvascular Endothelial Cells. Blood (1988) 71(3):684–9. doi: 10.1182/blood.V71.3.684.684
87. Dabusti M, Castagnari B, Moretti S, Ferrari L, Tieghi A, Lanza F. CD116 (Granulocyte-Macrophage Colony Stimulating Factor Receptor). J Biol Regul Homeost Agents (2001) 15(1):86–9.
88. Kaushansky K. Lineage-Specific Hematopoietic Growth Factors. N Engl J Med (2006) 354(19):2034–45. doi: 10.1056/NEJMra052706
89. Fleetwood AJ, Cook AD, Hamilton JA. Functions of Granulocyte-Macrophage Colony-Stimulating Factor. Crit Rev Immunol (2005) 25(5):405–28. doi: 10.1615/critrevimmunol.v25.i5.50
90. Amgen Inc. NEUPOGEN®(filgrastim) Injection, for Subcuteous or Intravenous Use Prescribing Information. (2018).
91. Ma B, Seymour JF, Januszewicz H, Slavin MA. Cure of Pulmonary Rhizomucor Pusillus Infection in a Patient With Hairy-Cell Leukemia: Role of Liposomal Amphotericin B and GM-CSF. Leuk Lymphoma (2001) 42(6):1393–9. doi: 10.3109/10428190109097768
92. Lewis R, Hogan H, Howell A, Safdar A. Progressive Fusariosis: Unpredictable Posaconazole Bioavailability, and Feasibility of Recombinant Interferon-Gamma Plus Granulocyte Macrophage-Colony Stimulating Factor for Refractory Disseminated Infection. Leuk Lymphoma (2008) 49(1):163–5. doi: 10.1080/10428190701724819
93. Jim HS, Boyd TD, Booth-Jones M, Pidala J, Potter H. Granulocyte Macrophage Colony Stimulating Factor Treatment Is Associated With Improved Cognition in Cancer Patients. Brain Disord Ther (2012) 1(1):1000101. doi: 10.4172/bdt.1000101
94. Spitler LE, Grossbard ML, Ernstoff MS, Silver G, Jacobs M, Hayes FA, et al. Adjuvant Therapy of Stage III and IV Malignant Melanoma Using Granulocyte-Macrophage Colony-Stimulating Factor. J Clin Oncol (2000) 18(8):1614–21. doi: 10.1200/JCO.2000.18.8.1614
95. O’Day SJ, Atkins MB, Boasberg P, Wang HJ, Thompson JA, Anderson CM, et al. Phase II Multicenter Trial of Maintenance Biotherapy After Induction Concurrent Biochemotherapy for Patients With Metastatic Melanoma. J Clin Oncol (2009) 27(36):6207–12. doi: 10.1200/JCO.2008.20.3075
96. Spitler LE, Weber RW, Allen RE, Meyer J, Cruickshank S, Garbe E, et al. Recombinant Human Granulocyte-Macrophage Colony-Stimulating Factor (GM-CSF, Sargramostim) Administered for 3 Years as Adjuvant Therapy of Stages II(T4), III, and IV Melanoma. J Immunother (2009) 32(6):632–7. doi: 10.1097/CJI.0b013e3181a7d60d
97. Eroglu Z, Kong KM, Jakowatz JG, Samlowski W, Fruehauf JP. Phase II Clinical Trial Evaluating Docetaxel, Vinorelbine and GM-CSF in Stage IV Melanoma. Cancer Chemother Pharmacol (2011) 68(4):1081–7. doi: 10.1007/s00280-011-1703-z
98. Hodi FS, Lee S, McDermott DF, Rao UN, Butterfield LH, Tarhini AA, et al. Ipilimumab Plus Sargramostim vs Ipilimumab Alone for Treatment of Metastatic Melanoma: A Randomized Clinical Trial. JAMA (2014) 312(17):1744–53. doi: 10.1001/jama.2014.13943
99. Andtbacka RH, Kaufman HL, Collichio F, Amatruda T, Senzer N, Chesney J, et al. Talimogene Laherparepvec Improves Durable Response Rate in Patients With Advanced Melanoma. J Clin Oncol (2015) 33(25):2780–8. doi: 10.1200/JCO.2014.58.3377
100. Nemunaitis J, Singer JW, Buckner CD, Durnam D, Epstein C, Hill R, et al. Use of Recombinant Human Granulocyte-Macrophage Colony-Stimulating Factor in Graft Failure After Bone Marrow Transplantation. Blood (1990) 76(1):245–53. doi: 10.1182/blood.V76.1.245.245
101. Nemunaitis J, Rabinowe SN, Singer JW, Bierman PJ, Vose JM, Freedman AS, et al. Recombinant Granulocyte-Macrophage Colony-Stimulating Factor After Autologous Bone Marrow Transplantation for Lymphoid Cancer. N Engl J Med (1991) 324(25):1773–8. doi: 10.1056/NEJM199106203242504
102. Rabinowe SN, Neuberg D, Bierman PJ, Vose JM, Nemunaitis J, Singer JW, et al. Long-Term Follow-Up of a Phase III Study of Recombinant Human Granulocyte-Macrophage Colony-Stimulating Factor After Autologous Bone Marrow Transplantation for Lymphoid Malignancies. Blood (1993) 81(7):1903–8. doi: 10.1182/blood.V81.7.1903.1903
103. Nemunaitis J, Rosenfeld CS, Ash R, Freedman MH, Deeg HJ, Appelbaum F, et al. Phase III Randomized, Double-Blind Placebo-Controlled Trial of rhGM-CSF Following Allogeneic Bone Marrow Transplantation. Bone Marrow Transplant (1995) 15(6):949–54. doi: 10.1182/blood.V87.6.2205.bloodjournal8762205
104. Rowe JM, Andersen JW, Mazza JJ, Bennett JM, Paietta E, Hayes FA, et al. A Randomized Placebo-Controlled Phase III Study of Granulocyte-Macrophage Colony-Stimulating Factor in Adult Patients (> 55 to 70 Years of Age) With Acute Myelogenous Leukemia: A Study of the Eastern Cooperative Oncology Group (E1490). Blood (1995) 86(2):457–62. doi: 10.1182/blood.V86.2.457.bloodjournal862457
105. Rowe JM, Rubin A, Mazza JJ, Bennett JM, Paietta E, Anderson JW, et al. Incidence of Infections in Adult Patients (>55 Years) With Acute Myeloid Leukemia Treated With Yeast-Derived GM-CSF (Sargramostim): Results of a Double-Blind Prospective Study by the Eastern Cooperative Oncology Group. In: Hiddeman W, Buchner T, Wormann B, Schellong L, Ritter J, Creutzig U, editors. Acute Leukemias V: Experimental Approaches and Management of Refractory Disease. Berlin, Germany: Springer-Verlag (1996). p. 178–84.
106. Clayton NP, Khan-Malek RC, Dangler CA, Zhang D, Ascah A, Gains M, et al. Sargramostim (Rhu GM-CSF) Improves Survival of Non-Human Primates With Severe Bone Marrow Suppression After Acute, High-Dose, Whole-Body Irradiation. Radiat Res (2021) 195(2):191–9. doi: 10.1667/RADE-20-00131.1
107. Gale RP, Armitage JO. Use of Molecularly-Cloned Haematopoietic Growth Factors in Persons Exposed to Acute High-Dose, High-Dose Rate Whole-Body Ionizing Radiations. Blood Rev (2021) 45:100690. doi: 10.1016/j.blre.2020.100690
108. Zhong Y, Pouliot M, Downey AM, Mockbee C, Roychowdhury D, Wierzbicki W, et al. Efficacy of Delayed Administration of Sargramostim Up to 120 Hours Post Exposure in a Nonhuman Primate Total Body Radiation Model. Int J Radiat Biol (2020) 1–17. doi: 10.1080/09553002.2019.1673499
109. O’Shaughnessy JA, Tolcher A, Riseberg D, Venzon D, Zujewski J, Noone M, et al. Prospective, Randomized Trial of 5-Fluorouracil, Leucovorin, Doxorubicin, and Cyclophosphamide Chemotherapy in Combination With the Interleukin-3/Granulocyte-Macrophage Colony-Stimulating Factor (GM-CSF) Fusion Protein (PIXY321) Versus GM-CSF in Patients With Advanced Breast Cancer. Blood (1996) 87(6):2205–11. doi: 10.1182/blood.V87.6.2205.bloodjournal8762205
110. Schiller G, Vescio R, Freytes C, Spitzer G, Lee M, Wu CH, et al. Autologous CD34-Selected Blood Progenitor Cell Transplants for Patients With Advanced Multiple Myeloma. Bone Marrow Transplant (1998) 21(2):141–5. doi: 10.1038/sj.bmt.1701055
111. Jones SE, Schottstaedt MW, Duncan LA, Kirby RL, Good RH, Mennel RG, et al. Randomized Double-Blind Prospective Trial to Evaluate the Effects of Sargramostim Versus Placebo in a Moderate-Dose Fluorouracil, Doxorubicin, and Cyclophosphamide Adjuvant Chemotherapy Program for Stage II and III Breast Cancer. J Clin Oncol (1996) 14(11):2976–83. doi: 10.1200/JCO.1996.14.11.2976
112. Steward WP, von Pawel J, Gatzemeier U, Woll P, Thatcher N, Koschel G, et al. Effects of Granulocyte-Macrophage Colony-Stimulating Factor and Dose Intensification of V-ICE Chemotherapy in Small-Cell Lung Cancer: A Prospective Randomized Study of 300 Patients. J Clin Oncol (1998) 16(2):642–50. doi: 10.1200/JCO.1998.16.2.642
113. Le Cesne A, Judson I, Crowther D, Rodenhuis S, Keizer HJ, Van Hoesel Q, et al. Randomized Phase III Study Comparing Conventional-Dose Doxorubicin Plus Ifosfamide Versus High-Dose Doxorubicin Plus Ifosfamide Plus Recombinant Human Granulocyte-Macrophage Colony-Stimulating Factor in Advanced Soft Tissue Sarcomas: A Trial of the European Organization for Research and Treatment of Cancer/Soft Tissue and Bone Sarcoma Group. J Clin Oncol (2000) 18(14):2676–84. doi: 10.1200/JCO.2000.18.14.2676
114. Yu AL, Gilman AL, Ozkaynak MF, London WB, Kreissman SG, Chen HX, et al. Anti-GD2 Antibody With GM-CSF, Interleukin-2, and Isotretinoin for Neuroblastoma. N Engl J Med (2010) 363(14):1324–34. doi: 10.1056/NEJMoa0911123
115. Cheung IY, Hsu K, Cheung NK. Activation of Peripheral-Blood Granulocytes Is Strongly Correlated With Patient Outcome After Immunotherapy With Anti-GD2 Monoclonal Antibody and Granulocyte-Macrophage Colony-Stimulating Factor. J Clin Oncol (2012) 30(4):426–32. doi: 10.1200/JCO.2011.37.6236
116. Cheung NK, Cheung IY, Kramer K, Modak S, Kuk D, Pandit-Taskar N, et al. Key Role for Myeloid Cells: Phase II Results of Anti-G(D2) Antibody 3F8 Plus Granulocyte-Macrophage Colony-Stimulating Factor for Chemoresistant Osteomedullary Neuroblastoma. Int J Cancer (2014) 135(9):2199–205. doi: 10.1002/ijc.28851
117. Ozkaynak MF, Gilman AL, London WB, Naranjo A, Diccianni MB, Tenney SC, et al. A Comprehensive Safety Trial of Chimeric Antibody 14.18 With GM-CSF, IL-2, and Isotretinoin in High-Risk Neuroblastoma Patients Following Myeloablative Therapy: Children’s Oncology Group Study ANBL0931. Front Immunol (2018) 9:1355. doi: 10.3389/fimmu.2018.01355
118. Aggarwal RR, Beer TM, Weinberg VK, Higano C, Taplin ME, Ryan CJ, et al. Intermittent Chemotherapy as a Platform for Testing Novel Agents in Patients With Metastatic Castration-Resistant Prostate Cancer: A Department of Defense Prostate Cancer Clinical Trials Consortium Randomized Phase II Trial of Intermittent Docetaxel With Prednisone With or Without Maintenance GM-CSF. Clin Genitourin Cancer (2015) 13(3):e191–8. doi: 10.1016/j.clgc.2014.12.004
119. Correale P, Botta C, Rotundo MS, Guglielmo A, Conca R, Licchetta A, et al. Gemcitabine, Oxaliplatin, Levofolinate, 5-Fluorouracil, Granulocyte-Macrophage Colony-Stimulating Factor, and Interleukin-2 (GOLFIG) Versus FOLFOX Chemotherapy in Metastatic Colorectal Cancer Patients: The GOLFIG-2 Multicentric Open-Label Randomized Phase III Trial. J Immunother (2014) 37(1):26–35. doi: 10.1097/CJI.0000000000000004
120. Schmeler KM, Vadhan-Raj S, Ramirez PT, Apte SM, Cohen L, Bassett RL, et al. A Phase II Study of GM-CSF and rIFN-Gamma1b Plus Carboplatin for the Treatment of Recurrent, Platinum-Sensitive Ovarian, Fallopian Tube and Primary Peritoneal Cancer. Gynecol Oncol (2009) 113(2):210–5. doi: 10.1016/j.ygyno.2009.02.007
121. Rowe JM, Neuberg D, Friedenberg W, Bennett JM, Paietta E, Makary AZ, et al. A Phase 3 Study of Three Induction Regimens and of Priming With GM-CSF in Older Adults With Acute Myeloid Leukemia: A Trial by the Eastern Cooperative Oncology Group. Blood (2004) 103(2):479–85. doi: 10.1182/blood-2003-05-1686
122. Cortes J, Quintas-Cardama A, Jones D, Ravandi F, Garcia-Manero G, Verstovsek S, et al. Immune Modulation of Minimal Residual Disease in Early Chronic Phase Chronic Myelogenous Leukemia: A Randomized Trial of Frontline High-Dose Imatinib Mesylate With or Without Pegylated Interferon Alpha-2b and Granulocyte-Macrophage Colony-Stimulating Factor. Cancer-Am Cancer Soc (2011) 117(3):572–80. doi: 10.1002/cncr.25438
123. Strati P, Ferrajoli A, Lerner S, O’Brien S, Wierda W, Keating MJ, et al. Fludarabine, Cyclophosphamide and Rituximab Plus Granulocyte Macrophage Colony-Stimulating Factor as Frontline Treatment for Patients With Chronic Lymphocytic Leukemia. Leuk Lymphoma (2014) 55(4):828–33. doi: 10.3109/10428194.2013.819574
124. Si Z, Hersey P, Coates AS. Clinical Responses and Lymphoid Infiltrates in Metastatic Melanoma Following Treatment With Intralesional GM-CSF. Melanoma Res (1996) 6(3):247–55. doi: 10.1097/00008390-199606000-00008
125. Elias EG, Sharma BK. Targeting Melanoma Sites In Vivo can Induce Complete Tumor Ablation and Prolong Patient Survival: An Exploratory Study. J Cancer Sci Ther (2013) 5(7):244–48. doi: 10.4172/1948-5956.1000212
126. Luke JJ, Donahue H, Nishino M, Giobbie-Hurder A, Davis M, Bailey N, et al. Single Institution Experience of Ipilimumab 3 Mg/Kg With Sargramostim (GM-CSF) in Metastatic Melanoma. Cancer Immunol Res (2015) 3(9):986–91. doi: 10.1158/2326-6066.CIR-15-0066
127. Kwek SS, Kahn J, Greaney SK, Lewis J, Cha E, Zhang L, et al. GM-CSF and Ipilimumab Therapy in Metastatic Melanoma: Clinical Outcomes and Immunologic Responses. Oncoimmunology (2016) 5(4):e1101204. doi: 10.1080/2162402X.2015.1101204
128. Marinelli O, Nabissi M, Morelli MB, Torquati L, Amantini C, Santoni G. ICOS-L as a Potential Therapeutic Target for Cancer Immunotherapy. Curr Protein Pept Sci (2018) 19(11):1107–13. doi: 10.2174/1389203719666180608093913
129. Martin-Orozco N, Li Y, Wang Y, Liu S, Hwu P, Liu YJ, et al. Melanoma Cells Express ICOS Ligand to Promote the Activation and Expansion of T-Regulatory Cells. Cancer Res (2010) 70(23):9581–90. doi: 10.1158/0008-5472.CAN-10-1379
130. Fu T, He Q, Sharma P. The ICOS/ICOSL Pathway Is Required for Optimal Antitumor Responses Mediated by Anti-CTLA-4 Therapy. Cancer Res (2011) 71(16):5445–54. doi: 10.1158/0008-5472.CAN-11-1138
131. United Therapeutics, Corp. UNITUXIN®(dinutuximab) Injection, for Intravenous Use Prescribing Information. (2020).
132. Y-mabs Therapeutics, Inc. DANYELZA®(naxitamab-Gqgk) Injection, for Intravenous Use Prescribing Information. (2020).
133. Sait S, Modak S. Anti-GD2 Immunotherapy for Neuroblastoma. Expert Rev Anticancer Ther (2017) 17(10):889–904. doi: 10.1080/14737140.2017.1364995
134. Kushner BH, Cheung NK. Clinically Effective Monoclonal Antibody 3F8 Mediates Nonoxidative Lysis of Human Neuroectodermal Tumor Cells by Polymorphonuclear Leukocytes. Cancer Res (1991) 51(18):4865–70.
135. Imai M, Landen C, Ohta R, Cheung NK, Tomlinson S. Complement-Mediated Mechanisms in Anti-GD2 Monoclonal Antibody Therapy of Murine Metastatic Cancer. Cancer Res (2005) 65(22):10562–8. doi: 10.1158/0008-5472.CAN-05-1894
136. Kushner BH, Cheung IY, Modak S, Basu EM, Roberts SS, Cheung NK. Humanized 3F8 Anti-GD2 Monoclonal Antibody Dosing With Granulocyte-Macrophage Colony-Stimulating Factor in Patients With Resistant Neuroblastoma: A Phase 1 Clinical Trial. JAMA Oncol (2018) 4(12):1729–35. doi: 10.1001/jamaoncol.2018.4005
137. Rong QX, Wang F, Guo ZX, Hu Y, An SN, Luo M, et al. GM-CSF Mediates Immune Evasion via Upregulation of PD-L1 Expression in Extranodal Natural Killer/T Cell Lymphoma. Mol Cancer (2021) 20(1):80. doi: 10.1186/s12943-021-01374-y
138. Dworzak MN, Schumich A, Printz D, Potschger U, Husak Z, Attarbaschi A, et al. CD20 Up-Regulation in Pediatric B-Cell Precursor Acute Lymphoblastic Leukemia During Induction Treatment: Setting the Stage for Anti-CD20 Directed Immunotherapy. Blood (2008) 112(10):3982–8. doi: 10.1182/blood-2008-06-164129
139. Leary R, Gardner RB, Mockbee C, Roychowdhury DF. Boosting Abscopal Response to Radiotherapy With Sargramostim: A Review of Data and Ongoing Studies. Cureus (2019) 11(3):e4276. doi: 10.7759/cureus.4276
140. Golden EB, Chhabra A, Chachoua A, Adams S, Donach M, Fenton-Kerimian M, et al. Local Radiotherapy and Granulocyte-Macrophage Colony-Stimulating Factor to Generate Abscopal Responses in Patients With Metastatic Solid Tumours: A Proof-of-Principle Trial. Lancet Oncol (2015) 16(7):795–803. doi: 10.1016/S1470-2045(15)00054-6
141. ClinicalTrials.gov. A Phase II/III Trial of Nivolumab, Ipilimumab, and GM-CSF in Patients With Advanced Melanoma. (2021). Available at: https://www.clinicaltrials.gov/ct2/show/NCT02339571 (Accessed April 21, 2021).
142. ClinicalTrials.gov. Sargramostim (GM-CSF) + PD-1. (2021). Available at: https://www.clinicaltrials.gov/ct2/show/NCT04703426 (Accessed April 21, 2021).
143. Kelley RK ME, Behr S, Hwang J, Keenan B, Cheung A, Gordan JD, et al. Phase II Trial of Pembrolizumab (PEM) Plus Granulocyte Macrophage Colony Stimulating Factor (GM-CSF) in Advanced Biliary Cancers (ABC). J Clin Oncol (2018) 36(4):386. doi: 10.1200/JCO.2018.36.4_suppl.386
144. ClinicalTrials.gov. Pembrolizumab and GM-CSF in Biliary Cancer (2021). Available at: https://www.clinicaltrials.gov/ct2/show/NCT02703714 (Accessed April 21, 2021).
145. Yu AL, Gilman AL, Ozkaynak MF, Naranjo A, Diccianni MB, Gan J, et al. Long-Term Follow-Up of a Phase III Study of Ch14.18 (Dinutuximab) + Cytokine Immunotherapy in Children With High-Risk Neuroblastoma: COG Study ANBL0032. Clin Cancer Res (2021) 27(8):2179–89. doi: 10.1158/1078-0432.CCR-20-3909
146. Nahas MR, Rosenblatt J, Lazarus HM, Avigan D. Anti-Cancer Vaccine Therapy for Hematologic Malignancies: An Evolving Era. Blood Rev (2018) 32(4):312–25. doi: 10.1016/j.blre.2018.02.002
147. McMasters M, Blair BM, Lazarus HM, Alonso CD. Casting a Wider Protective Net: Anti-Infective Vaccine Strategies for Patients With Hematologic Malignancy and Blood and Marrow Transplantation. Blood Rev (2021) 47:100779. doi: 10.1016/j.blre.2020.100779
148. Olson KE, Namminga KL, Schwab AD, Thurston MJ, Lu Y, Woods A, et al. Neuroprotective Activities of Long-Acting Granulocyte-Macrophage Colony-Stimulating Factor (Mpdm608) in 1-Methyl-4-Phenyl-1,2,3,6-Tetrahydropyridine-Intoxicated Mice. Neurotherapeutics (2020) 17(4):1861–77. doi: 10.1007/s13311-020-00877-8
149. Kiyota T, Machhi J, Lu Y, Dyavarshetty B, Nemati M, Yokoyama I, et al. Granulocyte-Macrophage Colony-Stimulating Factor Neuroprotective Activities in Alzheimer’s Disease Mice. J Neuroimmunol (2018) 319:80–92. doi: 10.1016/j.jneuroim.2018.03.009
150. Olson KE, Namminga KL, Lu Y, Thurston MJ, Schwab AD, de Picciotto S, et al. Granulocyte-Macrophage Colony-Stimulating Factor mRNA and Neuroprotective Immunity in Parkinson’s Disease. Biomaterials (2021) 272:120786. doi: 10.1016/j.biomaterials.2021.120786
151. Machhi J, Kevadiya BD, Muhammad IK, Herskovitz J, Olson KE, Mosley RL, et al. Harnessing Regulatory T Cell Neuroprotective Activities for Treatment of Neurodegenerative Disorders. Mol Neurodegener (2020) 15(1):32. doi: 10.1186/s13024-020-00375-7
152. Schwab AD, Thurston MJ, Machhi J, Olson KE, Namminga KL, Gendelman HE, et al. Immunotherapy for Parkinson’s Disease. Neurobiol Dis (2020) 137:104760. doi: 10.1016/j.nbd.2020.104760
153. Saunders JA, Estes KA, Kosloski LM, Allen HE, Dempsey KM, Torres-Russotto DR, et al. CD4+ Regulatory and Effector/Memory T Cell Subsets Profile Motor Dysfunction in Parkinson’s Disease. J Neuroimmune Pharmacol (2012) 7(4):927–38. doi: 10.1007/s11481-012-9402-z
154. Gendelman HE, Zhang Y, Santamaria P, Olson KE, Schutt CR, Bhatti D, et al. Evaluation of the Safety and Immunomodulatory Effects of Sargramostim in a Randomized, Double-Blind Phase 1 Clinical Parkinson’s Disease Trial. NPJ Parkinsons Dis (2017) 3:10. doi: 10.1038/s41531-017-0013-5
155. Bhattacharya P, Budnick I, Singh M, Thiruppathi M, Alharshawi K, Elshabrawy H, et al. Dual Role of GM-CSF as a Pro-Inflammatory and a Regulatory Cytokine: Implications for Immune Therapy. J Interferon Cytokine Res (2015) 35(8):585–99. doi: 10.1089/jir.2014.0149
156. Schutt CR, Gendelman HE, Mosley RL. Tolerogenic Bone Marrow-Derived Dendritic Cells Induce Neuroprotective Regulatory T Cells in a Model of Parkinson’s Disease. Mol Neurodegener (2018) 13(1):26. doi: 10.1186/s13024-018-0255-7
157. Olson KE, Namminga KL, Lu Y, Schwab AD, Thurston MJ, Abdelmoaty MM, et al. Safety, Tolerability, and Immune-Biomarker Profiling for Year-Long Sargramostim Treatment of Parkinson’s Disease. EBioMedicine (2021) 67:103380. doi: 10.1016/j.ebiom.2021.103380
158. Boyd TD, Bennett SP, Mori T, Governatori N, Runfeldt M, Norden M, et al. GM-CSF Upregulated in Rheumatoid Arthritis Reverses Cognitive Impairment and Amyloidosis in Alzheimer Mice. J Alzheimers Dis (2010) 21(2):507–18. doi: 10.3233/JAD-2010-091471
159. Potter H, Woodcock JH, Boyd TD, Coughlan CM, O’Shaughnessy JR, Borges MT, et al. Safety and Efficacy of Sargramostim (GM-CSF) in the Treatment of Alzheimer’s Disease. Alzheimers Dement (NY) (2021) 7(1):e12158. doi: 10.1002/trc2.12158
160. Subramaniyam V, Waller EK, Murrow JR, Manatunga A, Lonial S, Kasirajan K, et al. Bone Marrow Mobilization With Granulocyte Macrophage Colony-Stimulating Factor Improves Endothelial Dysfunction and Exercise Capacity in Patients With Peripheral Arterial Disease. Am Heart J (2009) 158(1):53–60.e1. doi: 10.1016/j.ahj.2009.04.014
Keywords: GM-CSF, sargramostim, immune modulation, innate immune response, adaptive immune response, granulocyte-macrophage colony-stimulating factor, immune therapy, cancer
Citation: Lazarus HM, Ragsdale CE, Gale RP and Lyman GH (2021) Sargramostim (rhu GM-CSF) as Cancer Therapy (Systematic Review) and An Immunomodulator. A Drug Before Its Time? Front. Immunol. 12:706186. doi: 10.3389/fimmu.2021.706186
Received: 06 May 2021; Accepted: 26 July 2021;
Published: 17 August 2021.
Edited by:
Peter Brossart, University of Bonn, GermanyReviewed by:
Yang Liang, Sun Yat-sen University Cancer Center (SYSUCC), ChinaHoward Eliot Gendelman, University of Nebraska Medical Center, United States
Copyright © 2021 Lazarus, Ragsdale, Gale and Lyman. This is an open-access article distributed under the terms of the Creative Commons Attribution License (CC BY). The use, distribution or reproduction in other forums is permitted, provided the original author(s) and the copyright owner(s) are credited and that the original publication in this journal is cited, in accordance with accepted academic practice. No use, distribution or reproduction is permitted which does not comply with these terms.
*Correspondence: Hillard M. Lazarus, hillard.lazarus@case.edu