- 1Department of Chemical and Systems Biology, Stanford University School of Medicine, Stanford, CA, United States
- 2School of Life Sciences, University of Technology, Sydney, NSW, Australia
- 3SPARK at Stanford, Stanford, CA, United States
Antiviral, antibacterial, and antiparasitic drugs and vaccines are essential to maintaining the health of humans and animals. Yet, their production can be slow and expensive, and efficacy lost once pathogens mount resistance. Chicken immunoglobulin Y (IgY) is a highly conserved homolog of human immunoglobulin G (IgG) that has shown benefits and a favorable safety profile, primarily in animal models of human infectious diseases. IgY is fast-acting, easy to produce, and low cost. IgY antibodies can readily be generated in large quantities with minimal environmental harm or infrastructure investment by using egg-laying hens. We summarize a variety of IgY uses, focusing on their potential for the detection, prevention, and treatment of human and animal infections.
Introduction
Immunoglobulin Y (IgY) is the main class of serum antibodies found in birds, amphibians, and reptiles (1). IgY, the avian homolog of immunoglobulin G (IgG) in humans (2), is the evolutionary ancestor of IgG (1) and shows significant sequence conservation (3). IgG and IgY are composed of two heavy and two light chains, each with constant and variable regions. The variable regions are critical for antigen recognition, whereas the constant regions provide the effector function. IgG and IgY are responsible for similar immune response functions (Figure 1) (1). Compared with IgG, IgY has a higher molecular weight (180 kDa vs. 150 kDa) and contains a larger heavy chain (65-68 kDa vs. 50 kDa) (1). In addition, IgY contains more carbohydrate sidechains (2 vs. 1), is more hydrophobic especially in the longer Fc portion of the molecule (3 constant domains in IgY vs. 2 in IgG), and is more resistant to proteolysis than its mammalian counterparts (4). IgY also retains 40% of its activity after incubation with trypsin or chymotrypsin for 8 hours (5).
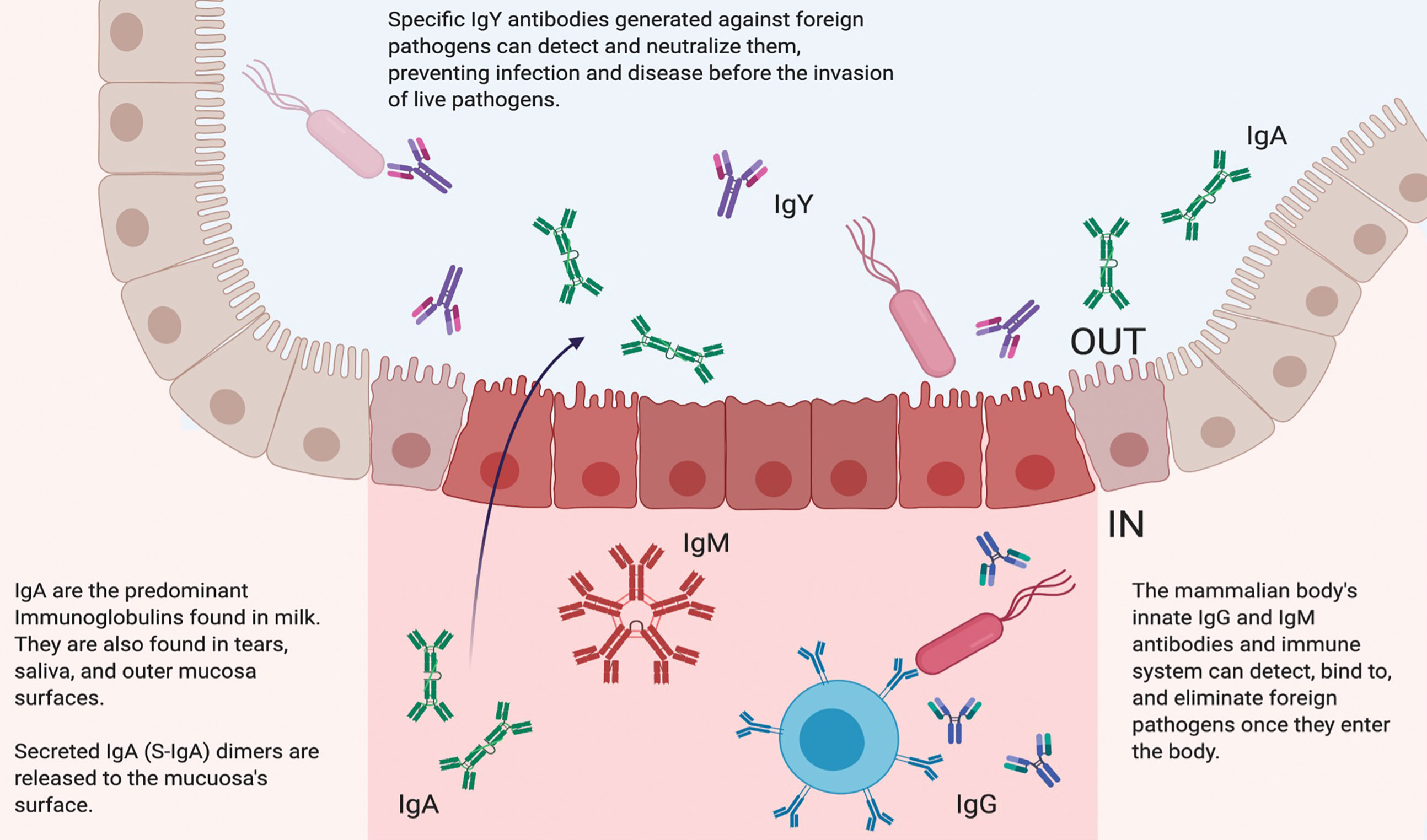
Figure 1 Biological Function of Different Immunoglobulins. In mammals, immunoglobulin G (IgG) is the main antibody in the circulating bloodstream. IgG antibodies are characterized by two antigen recognition sites that act as crucial components of the immune system. These sites are involved in the detection and subsequent elimination of foreign invaders that may also be circulating in the bloodstream. Mammalians also produce immunoglobulin M (IgM) antibodies, which are found in blood and lymph fluid and the first antibodies to appear in response to antigen exposure. Immunoglobulin A (IgA) antibodies are the main immunoglobulins found in milk, tears, saliva, and mucosa. IgA is characterized by a unique dimer structure and released to the outer mucosa surface. Immunoglobulin Y (IgY) is the main antibody found in birds, reptiles, and amphibians. Because of their unique structures, IgY antibodies are particularly resistant to low pH, high temperatures, and proteolysis. IgY can be effectively administered prophylactically and therapeutically to detect and neutralize pathogens without activating the host’s native immune system.
In healthy humans, serum half-life is approximately 23 days for IgG, 5 days for IgM, and 6 days for IgA (6). Although the precise in vivo half-life of IgY is not well characterized in humans, active IgY antibodies were still present in the saliva of patients with cystic fibrosis in the morning after gargling with an anti-P. aeruginosa IgY solution the previous evening (7). In suckling pigs, IgY had a half-life of 1.85 days in the sera and 1.73 hours in the gastrointestinal tract (8). Similar to IgG, IgY is involved in opsonization, complement system activation, and most effector functions in the chicken (9). However, IgY is unable to bind to or activate the mammalian complement system, Fc receptors, and rheumatoid factors (10, 11).
IgY may provide a low cost (only a few cents to produce an oral or nasal dose), safe (due to its molecular structure, see below), and fast approach (produced in 5-8 weeks, including the time required for immunogen preparation) for rapid prophylaxis and therapeutic development against a variety of pathogens. In particular, passive immunoprophylaxis by surface treatment with IgY (e.g. via nasal spray, lozenges, etc.) may be an effective method of capturing pathogens before they enter the body. This approach may be especially attractive in limited-resource countries where
vaccination is unavailable, where new viral variants may evade immunity produced by vaccines or previous infection, or where immediate immunity is required (such as an outbreak in a nursing home).
Antiviral drug and vaccine development is lengthy and costly. For example, the estimated mean duration of clinical development was 6.4 years for 48 new antiviral drugs licensed for use in the United Kingdom between 1981 and 2014 (12). Because of the accelerated research, development, and Emergency Use Authorization during the coronavirus disease 2019 (COVID-19) pandemic, pharmaceutical companies have been able to obtain approval for therapeutics and vaccines within a dramatically shorter time and at a lower cost. Typically, scaling up the production of antiviral therapeutics is difficult, even for costs to manufacture new COVID-19 treatments (13). This is especially challenging in resource-limited-income countries due to the costly infrastructure required to develop, distribute, and store antiviral agents and vaccines. In contrast, IgY production may take as little as 2 months and occur on a community level through the inoculation of laying hens. These hens can produce eggs containing protective IgY antibodies that can be easily processed into a long-lasting and easy-to-store lyophilized product.
IgY used as prophylaxis can also be tailored to be highly specific and is not dependent on the host immune response (Figure 1). This latter feature may enable its clinical application for protecting a wide range of especially vulnerable patients, including the elderly, immunocompromised, and young children (14). In addition, in animals raised for conventional antibody production, IgG is the main serum antibody collected. In contrast, IgY antibodies can be easily harvested and used in diagnostic assays, with lower background and cross-reactivity compared with current industry standards using IgG in animals raised for conventional antibody production (Figure 2).
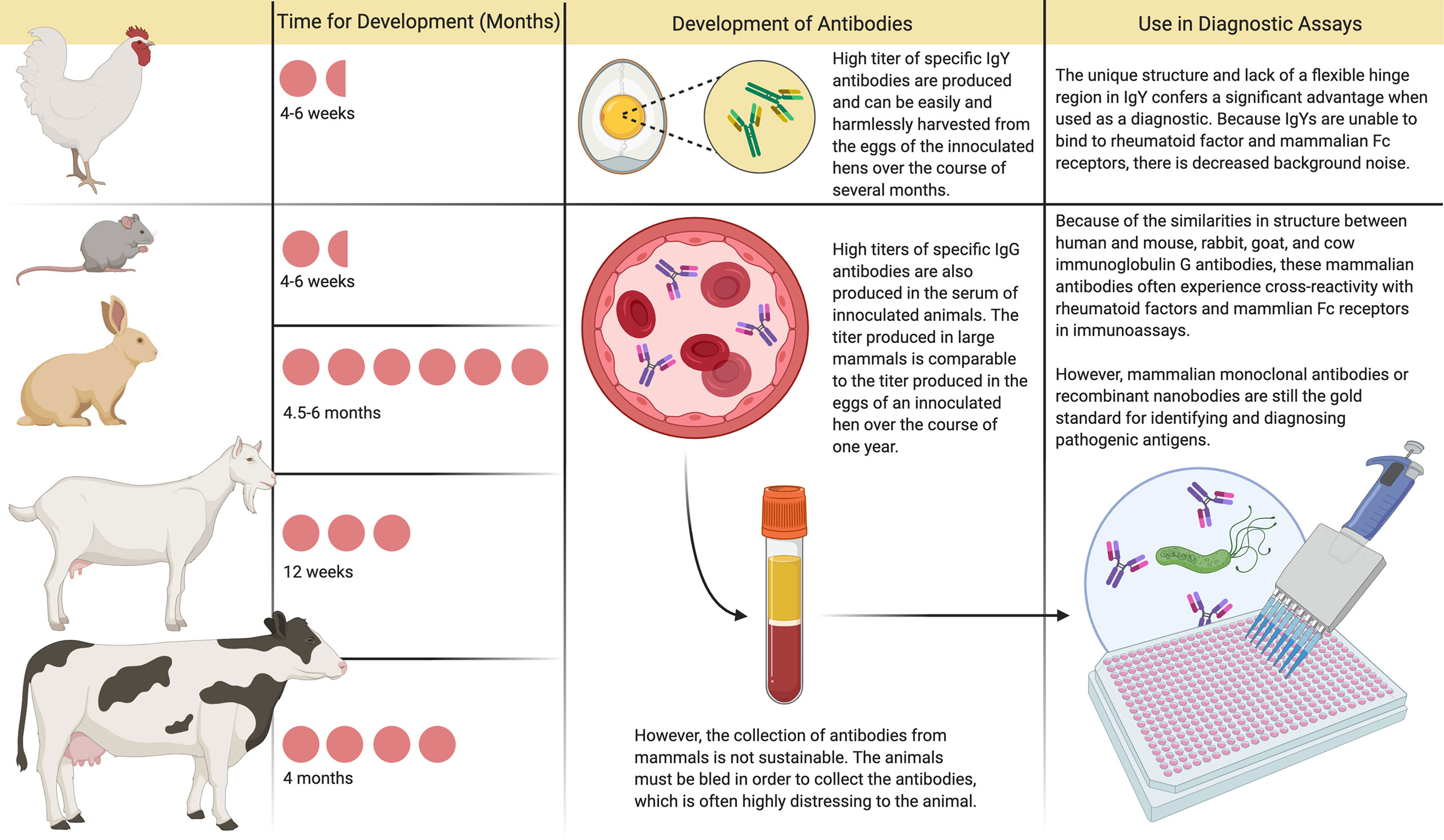
Figure 2 Immunoglobulin Y and Immunoglobulin G Antibody Development and Use in Diagnostic Assays. In animals raised for conventional antibody production, immunoglobulin G (IgG) is the main serum antibody collected. The amount of antibody that can be harvested from the blood in one setting is comparable to the amount of immunoglobulin Y (IgY) produced in the eggs of a commercial laying hen over a year. However, production of these conventional antibodies usually requires 2-6 months, and harvesting through bleeding is distressing to the animal. Currently, mammalian monoclonal antibodies are considered the gold standard for use in diagnostic assays, although they are prone to exhibit cross-reactivity and are costly to produce. In contrast, after inoculation with the desired antigen, hens produce specific antibodies in their eggs within 4-6 weeks. These IgY antibodies can be easily harvested and used in diagnostic assays, with lower background and cross-reactivity compared with current industry standards.
Here we provide a comprehensive overview of previous clinical applications of IgY in both humans and animals, including viral, bacterial, fungal, and parasitic infections.
Diagnosis and Treatment of Viral Infections
Detection and Neutralization of Coronaviruses: Severe Acute Respiratory Syndrome (SARS) Coronavirus and Middle East Respiratory Syndrome (MERS) Coronavirus
Severe Acute Respiratory Syndrome (SARS) is caused by SARS-associated coronavirus (SARS-CoV) and characterized by fever, chills, cough, pneumonia, and high infectivity. SARS was first identified in southern China in 2003 but quickly spread to over 30 countries, resulting in greater than 8000 cases and an estimated mortality rate of 15% (15). Middle East Respiratory Syndrome (MERS) is also caused by a coronavirus (MERS-CoV) and was first identified in humans in the Middle East in 2012; individuals infected with MERS showed similar clinical features to those infected with SARS (16). Because of the high infectivity and mortality caused by coronaviruses, prophylaxis to capture and neutralize the virus before it enters the nasopharynx using IgY against SARS-CoV or MERS-CoV could provide a means to curb the transmission and severity of disease. IgY against SARS-CoV or its spike protein neutralized the virus in infected Vero E6 cells (17, 18). Similarly, anti-MERS-CoV IgY neutralized MERS-CoV in infected Vero E6 cells, inhibited viral replication in vitro, and conferred protection when injected intraperitoneally to mice challenged with MERS-CoV (19). IgY against the nucleocapsid protein of SARS-CoV, which is shed in high amounts during the first week after infection, was found to be highly sensitive and capable of detecting the virus in serum and nasopharyngeal aspirate (20), indicating the potential for IgY-based diagnostic tools. When faced with highly contagious infectious diseases, such as those caused by coronaviruses, there may be several advantages to using IgY as a diagnostic tool (Figure 2) in addition to combating community transmission or providing passive immunization to exposed individuals.
IgY Against SARS-CoV-2
Besides regulatory authorizations or approvals of COVID-19 vaccines, antivirals, and neutralizing antibodies in various countries, there remains a global need to develop additional safe, effective, easy-to-produce, and inexpensive treatments to prevent or reduce the risk of acquiring severe acute respiratory syndrome coronavirus 2 (SARS-CoV-2) infection (21, 22). This need is of heightened importance for health care and other at-risk service workers in regions where new variants of SARS-CoV-2 may increase contagiousness or evade immunity produced by vaccines or previous infection (23, 24) or where COVID-19 vaccination is unavailable. Despite the use of personal protective equipment and recommended practices, these groups are also vulnerable during the immediate period after active vaccination due to continued close contact with both asymptomatic and symptomatic individuals infected with SARS-CoV-2 (25).
Active vaccination requires the induction of an immune response that takes time to develop and varies depending on the vaccine and recipient. In contrast, passive antibody administration may provide immediate immunity to susceptible persons. However, passive immunization via parenteral administration with sera from recovered patients infected with SARS-CoV-2 requires the availability of a population of donors who have recovered from the disease and can donate convalescent serum, blood banking facilities to process the serum, assays to detect SARS-CoV-2 in serum, assays to measure viral neutralization, and virology laboratory support to perform these assays (26). Passive immunization with anti-SARS-CoV-2 IgY given topically may offer another passive immunization with several advantages: the known safety profile of IgY, applicability to a wide array of individuals, high yield per egg, and rapid mass production at a low cost given the large production of eggs for human consumption (27, 28) (Figure 3). In addition, anti-SARS-CoV-2 IgY applied superficially would not be expected to elicit antibody-dependent enhancement of infection due to its topical application.
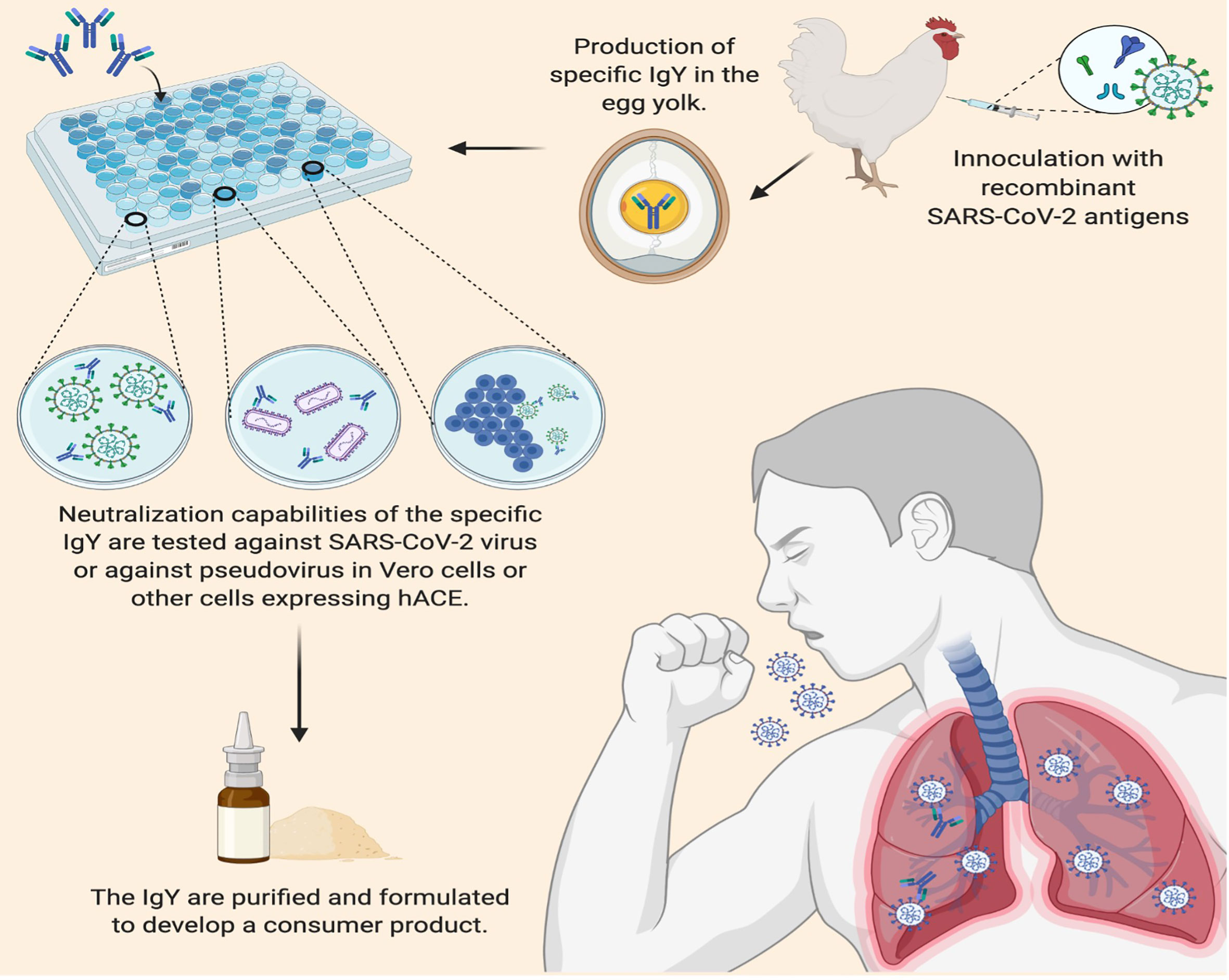
Figure 3 Applications of Immunoglobulin Y against SARS-CoV-2 to Inhibit COVID-19. IgY can be easily produced by inoculating egg-laying hens with recombinant SARS-CoV-2 antigens such as the spike protein on the viral envelope. Following a second inoculation, the yolk of the eggs laid by commercial hens contains 50-100 mg IgY per egg; up to 500 mg IgY per egg can be obtained from naïve, specific-pathogen-free hens. After harvesting the IgY from the egg yolks, the efficacy of IgY antibodies can be tested for neutralization activity before purification and processing into a lyophilized product to be evaluated in controlled trials as a potential (prophylactic) COVID-19 passive immunization.
Intranasal antibody prophylaxis is an especially effective means against multiple viral pathogens (29–32). Further, key characteristics of the mechanism of action of anti-SARS- CoV-2 IgY may be ideal for effective transient immunization while awaiting global COVID-19 vaccination: a) anti-spike-S1 IgY can neutralize or bind SARS-CoV-2 in vitro (33–35), b) superficially administered anti-SARS-CoV-2 IgY may bind to the spike protein on the surface of the virus, competing with the binding of the viral spike protein to the human angiotensin-converting enzyme 2 receptor to prevent cell entry and infection, c) anti-SARS-CoV-2 IgY may also agglutinate SARS-CoV-2 on the surface of the mucosa, thus preventing viral lateral motility and entry across the mucosa, and d) intranasal administration can deliver anti-SARS-CoV-2 IgY to the nasal passage and throat mucosa (through mucociliary clearance), the main routes of viral entry and replication. This approach may be especially valuable for immediate and short-lived protection in health care and other at-risk service workers in regions where new variants of SARS-CoV-2 may evade immunity produced by vaccines or where COVID-19 vaccination is unavailable.
Detection and Treatment of Influenza Viruses
Influenza is a highly contagious infection of the respiratory tract that is caused by the influenza family of viruses. There are four types of influenza viruses, but types A and B most commonly cause seasonal influenza pandemics. Influenza is generally a self-limited illness but is a significant global concern due to complications such as pneumonia and organ failure that can lead to death, especially in vulnerable patients (36). Although novel seasonal influenza vaccines are developed annually, influenza viruses are extremely prone to antigenic drift and effective vaccines reduce the risk of flu illness by only about 50% (37, 38). Because of the limitations of vaccination and antiviral drugs, anti-influenza IgY may be an inexpensive and rapid prophylaxis that can be given via intranasal spray or drops to bind to numerous epitopes on the virus and thus prevent viral entry into the body (39). This approach may be practical for those where vaccination is unavailable or as a temporary measure (such as during travel) before or to supplement vaccination.
IgY developed against various influenza strains was effective in neutralizing the virus and protecting against infection. In vitro, IgY anti-H1N1 inhibited hemagglutination (39, 40) and infection of Madin-Darby canine kidney cells by binding specifically to virus neuraminidase and hemagglutinin (39, 41). In a mouse model of H1N1 infection, intranasally administered anti-H1N1 IgY reduced the viral titer and minimized damage to the lung tissue while conferring significant protection comparable to oseltamivir (39), a neuraminidase inhibitor approved to treat acute uncomplicated influenza and for prophylaxis of influenza. Similar results were also observed using IgY developed against inactivated H3N2, H5N1, and influenza B viruses; these neutralized the virus in vitro and protected challenged mice when administered intranasally at (42–44). In addition, anti-H1N1 and anti-H5N1 IgY exhibited cross-reactivity to other influenza A strains (40, 41, 43).
IgY has also been developed against specific influenza proteins. For example, IgY has been designed to target the influenza matrix 2 protein, which is secreted in high concentrations during infection and can be used in the diagnosis and treatment of influenza. In immunoassays, IgY anti- matrix 2 protein were specifically bound to the influenza virus and caused aggregation when coupled to a latex nanoparticle (45). IgY generated against the highly conserved nucleoprotein of influenza A strains bound specifically to the viral protein and virus in infected Madin-Darby canine kidney cells (46). Therefore, IgY may prove useful for diagnosis and prophylaxis against various circulating or novel strains of human, avian, or swine influenza viruses.
Treatment of Hepatitis B
Hepatitis B viruses have a narrow range of host specificity (47). Similar to the human hepatitis B virus (HBV), duck HBV infection can lead to chronic hepatitis, fibrosis, cirrhosis, and hepatocellular carcinoma (48). In eggs laid by ducks that were immunized against duck HBV, significant titers of specific IgY were found in the yolk (49, 50). Also, uninfected treated ducks were protected against the virus, and treated carriers had decreased serum and liver levels of duck HBV (51, 52). Despite the availability of an effective vaccine, rates of vaccination in humans are poor and immunotherapies may be unavailable in resource-limited settings, or not tolerated. IgY may also warrant evaluation as a therapeutic for the treatment of postexposure HBV infection in humans where standard treatments are not readily available.
Treatment of Rotavirus Group A
Rotaviruses cause gastrointestinal and diarrheal diseases, resulting in high rates of mortality in various animal species and severe watery diarrhea and vomiting in infants and young children (53). Although vaccines and symptomatic treatments are available, there is a special need for effective control strategies to eliminate rotavirus infections in animal settings to protect herds (54); IgY against the bovine Group A Rotavirus VP8 capsid protein may be one such avenue (55, 56). In challenged calves, specific IgY against bovine rotavirus, combined with milk formula or distilled water, increased the response of the mucosal antibody-secreting cells, reduced viral shedding, decreased the severity and duration of diarrhea, and minimized body weight loss (44, 57–60). Furthermore, an orally administered solution of specific IgY against the MO strain conferred complete or significant protection against strains of rotavirus in later infected BALB/c mice (61, 62). With combination IgY treatment against the G1, G4, and G9 serotypes, protection was also observed in infected mice administered feed containing specific IgY, as well as a marked reduction in viral load, histopathological damage, and diarrhea (63). Decreases in duration and extent of diarrhea were observed in infected mice treated with an orally administered solution of specific IgY (4), a Rotamix IgY treatment (64), or feed containing IgY in phosphate-buffered saline (65). In a neonatal gnotobiotic piglet disease model, a 4.8% milk solution supplemented with anti-human rotavirus IgY increased IgG and IgM antibody response against the virus, decreased viral shedding, and protected against diarrhea (66).
Rotavirus is the most common cause of severe diarrhea in children and outbreaks can occur in both vaccinated and unvaccinated children (67). When administered to pediatric patients who tested positive for rotavirus infection, orally administered treatments of 20% IgY sachets (64) or 4 doses of 10 g of IgY powder (68) significantly reduced the time for viral clearance in the feces, volume and duration of oral rehydration, intravenous fluid administration, duration of diarrhea, and recovery time. Wang et al. (69) conducted a meta-analysis involving 2626 infants with rotavirus diarrhea from 17 randomized clinical trials. Among these infants, 1347 received anti-rotavirus IgY taken orally and 1279 received conventional treatment. Anti-rotavirus IgY treatment was significantly more effective than conventional treatment. These preliminary data demonstrate the utility of IgY as an antiviral therapy for infantile rotavirus enteritis.
Treatment of Zika Virus
In 2016, a global health emergency was declared by the World Health Organization after the observation of hundreds of thousands of infections by the Zika virus. Zika virus is transmitted by Aedes mosquitoes (70). The illness is characterized by fever, rash, arthritis (71), and less commonly, Guillain-Barré syndrome (72). In pregnant women, Zika virus infection may cause severe birth defects including microcephaly (73, 74). There is no effective drug or vaccine for Zika virus infection in part because of antibody-dependent enhancement, a phenomenon in which prior infection results in virus-specific antibodies enhancing replication of virus into monocytes/macrophages and granulocytic cells through interaction with Fc or complement receptors (75). IgY against the Zika virus was able to neutralize the virus in vitro at a concentration of 25 µg/mL (76). Furthermore, intraperitoneal injection with 1 mg of specific IgY protected 3-week-old IFNAR−/− mice that received a lethal challenge of Zika virus without inducing antibody-dependent enhancement (76). Zika virus-specific IgY may warrant further evaluation as passive immunotherapy, with caution for the potential to elicit an allergic response.
Detection and Treatment of Dengue Fever
Dengue fever, dengue hemorrhagic fever, and dengue shock syndrome are tropical, mosquito-borne diseases that are caused by infection with one of four dengue virus serotypes (77). In the last 50 years, dengue virus-associated diseases have re-emerged, causing millions of infected cases and tens of thousands of deaths annually (78). Although there is a commercially available vaccine to treat dengue fever, the World Health Organization recommends only vaccinating seropositive individuals who have a history of dengue virus infection, or by age 9 years in areas where the infection is prevalent (79). Currently, IgG-coupled enzyme-linked immunosorbent assays (ELISAs) are used for serological testing for dengue virus infection (79), suggesting that IgY can also be used in a diagnostic test for this disease.
Specific IgY has been developed against nonstructural protein 1, which is secreted by the dengue virus during infection and is detectable for up to 9 days after infection (80, 81). IgY anti-nonstructural protein 1 that is highly specific can neutralize the virus in immunoassays (82–84). Specific IgY generated against dengue virus serotype 2 also demonstrated a similar ability to neutralize the virus (85). In vivo, IgY antibodies given at a dose of 150 μg (82) or 1 mg through intraperitoneal injection (85) protected mice lethally challenged with dengue virus, suggesting that specific IgY may be used as a treatment of dengue virus-associated diseases in humans provided that a severe immune response (e.g., anaphylactic shock) to the IgY will not be generated (86). IgY antibodies are not likely to lead to immune amplification of dengue virus infection, unlike IgG where enhanced viral uptake via IgG-bound dengue virus enters into monocytes/macrophages via Fc receptors (87).
Treatment of Hantavirus Pulmonary Syndrome
Hantavirus Pulmonary Syndrome (HPS) is a rare, severe, and potentially fatal respiratory disease caused by infection with hantaviruses (most commonly, Andes virus) (88, 89). Infection is believed to occur primarily through inhalation or ingestion of rodent feces, urine, and saliva, or by rodent bites. Person-to-person transmission is also recognized. The mortality rate is estimated at 38% (90). There are no current immunotherapies, antiviral treatments, or vaccines to treat HPS (91). Specific IgY developed after immunization of ducks with Andes virus neutralized the virus in vitro (92, 93) and protected Syrian hamsters administered a dose of 12,000 neutralizing antibody units/kg through intranasal delivery (92) or 20,000 neutralizing antibody units/kg through subcutaneous injection (93) after receiving intramuscular and intranasal challenge. This suggests that IgY may warrant evaluation as a treatment following infection with the Andes virus to prevent the onset of HPS, especially in settings of clustering of cases.
Treatment of Ebola Virus Infection
Ebola virus infection is rare but results in high mortality rates in humans and nonhuman primates (94). Ebola virus also can persist in survivors and relapse has been documented. In addition to supported care, two monoclonal antibody treatments and a vaccine have been approved (94).
Anti-Ebola virus IgY was harvested from hens immunized with a recombinant vesicular stomatitis virus vector encoding Ebola virus glycoproteins. Anti-Ebola virus IgY was then evaluated in newborn Balb/c mice challenged with a lethal dose of Ebola pseudovirus 2 or 24 hours after infection (95). Animals receiving a high dose of anti-Ebola virus IgY showed complete protection, while the low dose group showed partial protection. All mice receiving naïve IgY (i.e., from hens not immunized with Ebola glycoproteins) died. Zhang et al. note that because Ebola epidemics typically occur in impoverished hot African areas where electricity and cold-chain storage may be limited, advantages of low-cost mass production and avoidance of antibody-dependent enhancement may make anti-Ebola virus IgY an especially novel approach that warrants further investigation (95).
Viruses of Specific Interest in Diseases of Livestock
Treatment of Infectious Bursal Disease
Infectious bursal disease is a highly contagious avian immunosuppressive illness that is caused by the infectious bursal disease virus (IBDV). IBDV infection in bursal B cells of lymphoid organs can lead to cell destruction, suppression of the immune system, secondary infection, and death (96). Despite the development of various diagnostic methods as well as a recombinant DNA-IBDV vaccine to treat this disease (97), IBD remains a significant problem in the poultry industry due to the emergence of new variants (98). Anti-IBDV IgY can protect against infection, with decreased mortality, postmortem lesions, bursal tissue, and body weight loss in young broiler chicks lethally challenged with IBDV (99–101). This protective effect was most significant when specific IgY was combined with the live intermediate IBDV vaccine (D78) (99).
Detection and Treatment of Reovirus
In poultry, the most frequent reovirus-associated disease is arthritis with malabsorption syndrome, immunosuppression, pericarditis, myocarditis, and osteoporosis as other common features (102, 103). Although several commercial vaccines have been developed against avian reovirus, it is difficult to detect and treat infected flocks with multiple or novel strains of circulating reovirus (104). Specific IgY against avian reovirus in infected birds displayed a high sensitivity to the virus, detected the presence of the virus in contaminated tissue, and neutralized the virus in BHK-21 cells without binding to heterologous viral strains (105). Reovirus is also a growing problem in aquaculture, causing high mortality rates of swimming crabs. Use of anti-swimming crab reovirus IgY effectively detected the virus in contaminated samples with high consistency, suggesting potential benefit in reovirus-associated disease outbreaks in aquaculture (106).
Treatment of Bovine Respiratory Syncytial Virus
Bovine respiratory syncytial virus is a pneumovirus in the Paramyxovirus family that afflicts young calves and is difficult to diagnose and treat due to its lability and poor growth in cell culture (107). However, IgY against bovine respiratory syncytial virus specifically recognized and neutralized the virus in vitro when analyzed in dot blot and virus neutralization assays (108). Because of the success of IgY production and activity in vitro, IgY may be a novel prophylactic treatment to combat bovine respiratory disease in infected calves.
Treatment of Bovine Leukemia Virus
Bovine leukemia virus (BLV) is a retrovirus that causes enzootic bovine leukosis, a chronic and slow-developing disease in cattle (109). While most infected animals remain asymptomatic, others can develop lymphosarcoma or persistent lymphocytosis.
BLV can easily be transmitted through birth and contaminated colostrum, milk, blood, exudates, and tissue (110). Currently, methods to minimize BLV transmission include careful herd management (110) given the unavailability of effective antiviral drugs and vaccines (111). IgY antibodies generated against the whole virus or the p24 core protein specifically bound to BLV particles (in an infected cell line), purified p24, and supernatants from ex vivo cultures of peripheral blood mononuclear cells from naturally infected animals (111). IgY against BLV may warrant evaluation as a passive immunization against this virus for enzootic bovine leukosis.
Treatment of Bacterial Infections
Besides use as therapeutics, IgY used prophylactically may provide passive immunization to the treated host through neutralization of bacterial toxins, prevention of adhesion to host cells, and inhibition or destruction of bacterial enzymes (112). Here we review the use of IgY as treatments focused on bacteria mainly of the pulmonary, gastrointestinal, and skin systems.
Lung Infections
Treatment of Pseudomonas aeruginosa in Cystic Fibrosis
Cystic fibrosis is a common, hereditary, and life-threatening disease associated with damage to the lungs, pancreas, and male sex organs (113, 114). Patients with cystic fibrosis are especially prone to debilitating chronic lung infections caused by bacteria such as Pseudomonas aeruginosa (115). Due to a fear of developing antibiotic-resistant strains, alternative treatments to chronic antibiotic therapy have been studied, including the use of IgY against P. aeruginosa as a method of passive immunization. Anti-P. aeruginosa IgY significantly increased the neutrophil-mediated respiratory burst and subsequent bacterial killing of P. aeruginosa in vitro (116, 117). Anti-P. aeruginosa IgY also inhibited murine pneumonia when administered intranasally as evidenced by reduced bacterial burden, inflammatory cytokines, inflammation of the lung tissue, and clinical symptoms, an effect enhanced by pretreatment with azithromycin (118). The benefit of specific IgY anti-P. aeruginosa is believed to be against the flagellin protein implicated in the motility, adhesion, and inflammation of P. aeruginosa (119).
In 17 patients with cystic fibrosis, prophylactic continuous oral treatment (solution gargled for 2 minutes and swallowed in the evening) with specific IgY against P. aeruginosa to prevent pulmonary infections for up to 12 years (114 patient-years) showed significant reduction in P. aeruginosa infections compared with 23 cystic fibrosis control patients, with no adverse events (120).
A randomized, double-blind, placebo-controlled Phase 3 trial of 164 patients age 5 and older with cystic fibrosis was conducted at 47 European sites from 2011 to 2015 to evaluate treatment with oral anti-P. aeruginosa IgY gargling solution (n=83) vs. placebo (n=81). Study drug was to be gargled and then swallowed once daily in the evening. Study duration (and primary outcome measure) was until the next P. aeruginosa infection was diagnosed or 2 years, whichever came first. There was no significant difference between treatment groups in time to first recurrence of P. aeruginosa infection (median, 26.3 months for IgY-treated group) or secondary endpoints of number of exacerbations, number of days of illness, and use of antibiotics (121). Despite findings of no efficacy, this study provided an excellent safety database. A total of 1972 adverse events (AEs), mostly mild in severity, were reported, 989 of which were in the placebo group and 980 in the IgY group. The incidence of AEs was also similar between the two groups. The most commonly reported AEs were abdominal pain, vomiting, pyrexia, nasopharyngitis, and upper respiratory tract infection. No deaths occurred. Only 5 AEs in the IgY-treated group and 20 AEs in the placebo group (none serious) were judged to be related to study drug (121).
Treatment of Mycobacterium Tuberculosis
Mycobacterium tuberculosis (MBTC) is responsible for the development of tuberculosis, a potentially fatal respiratory disease that also can cause extrapulmonary disease (e.g., of the urinary system). MBTC is increasingly becoming more difficult to treat due to antibiotic resistance (122). In a rat peripheral blood mononuclear cell model, administration of high concentrations of IgY anti-MBTC increased interleukin-2 and interferon expression (123). IgY against MBTC may warrant evaluation for use in combination with other immunotherapeutic treatments of tuberculosis (124). In addition, IgY may also be of interest as a novel treatment of pulmonary nontuberculous mycobacteria, regarded as more challenging to treat because of frequent antimicrobial intolerance, toxicities, resistance, and drug-drug interactions (125).
Treatment of Acinetobacter baumannii
Acinetobacter baumannii, a gram-negative bacterium, is the cause of nosocomial infections and outbreaks in hospitals worldwide, such as sepsis, urinary tract infections, pneumonia, or surgical wound infections. Due to its resistance to desiccation and antimicrobial agents, A. baumannii is associated with significant mortality, costs, and hospital stays, particularly in vulnerable patients (126). In a mouse model of A. baumannii-associated pneumonia, intraperitoneal anti-A. baumannii IgY specific to pan-drug-resistant strains reduced levels of inflammatory cytokines, lung inflammation, and mortality (127). Similar results were also seen with intraperitoneal injections of 40 μg of IgY developed against the inactivated whole-cell or outer membrane proteins of A. baumannii, which protected against nasally challenged mice, possibly by inhibiting bacterial adhesion (128).
Oral and Gastrointestinal Infections
Treatment of Vibrio
The genus Vibrio is responsible for seaborne diseases that can cause illness in aquatic animals raised for consumption, as well as foodborne infections and gastroenteritis in humans who consume raw or undercooked seafood (129). IgY may offer promise for diagnosing and treating virulent Vibrio-associated diseases in aquaculture. In the past, Vibrio treatment relied on vaccination and antibiotics, which may cause unintentional side effects, antibiotic resistance, and the buildup of toxins (130, 131).
IgY has been tested against Vibrio alginolyticus, which causes infections and high rates of mortality in abalone (132–134). When incorporated into the feed, IgY anti-V. alginolyticus significantly increased the survival of small abalones challenged by V. alginolyticus (135). Similar results were obtained using IgY against Vibrio anguillarum in rainbow trout (136), ayu fish (137, 138), half-smooth tongue sole (139), shrimp (140), and carp (141).
Vibrio parahaemolyticus, which causes acute hepatopancreatic necrosis and death in white shrimp in aquacultures (142), can cause serious foodborne illness in humans. Anti-V. parahaemolyticus IgY inhibited bacterial growth in liquid media (143, 144), decreased bacterial load, and increased survival rates in V. parahaemolyticus-challenged white pacific shrimp (144), suggesting its potential use to prevent foodborne illness in humans. Another strain, V. harveyi, which decreases health and survival in white shrimp (145), is inhibited through agglutinating IgY antibodies (146). Anti-V. harveyi IgY, incorporated into feed before being challenged with virulent V. harveyi, also increased levels of jejunioxyhemocyanin, prophenoloxidase, lysozyme, phagocytosis, and bacterial agglutinin, as well as augmented coagulase activity and intracellular superoxide anion production in treated Indian white shrimp (147). The potential value of anti-V. paraphaemolyticus IgY to prevent foodborne illness was tested in mice; 300 µg/ml IgY administered by oral gavage decreased the levels of bacteria in the feces and increased survival compared to vehicle-treated infected mice or mice treated with non-specific IgY (148).
Much research has been done to address Vibrio cholerae, which causes cholera in humans. Cholera is a global and life-threatening gastrointestinal disease characterized by severe diarrhea (149). Through the consumption of V. cholerae-contaminated food and water, hundreds of thousands of infections occur every year, especially in developing countries (150). Current treatments of V. cholerae include rehydration and vaccines, although their efficacy is limited (151). The finding that orally administered anti-V. cholerae IgY can prevent and treat cholera in suckling mice (151) and that only 2.5 micrograms protected against cholera in these animals (152) is encouraging.
Treatment of Helicobacter pylori
Helicobacter pylori (H. pylori) is a bacterial cause of gastritis, gastric ulcers, and gastric cancer (153). Because of the development of resistance to antibiotics, treatment of H. pylori requires the incorporation of multiple antibiotics. H. pylori exhibits resistance to metronidazole (78%), levofloxacin (56%), multidrug treatments (53%), and clarithromycin (31%) (154). Thus, orally administered immunoglobulins, and particularly IgY, have been suggested as an alternative approach to treat H. pylori-related infections because they limit the development of antibiotic resistance. IgY has been developed against H. pylori urease (HPU), a protein likely required for bacterial adhesion to mucin or the surface of epithelial cells in the gastric mucosa (155).
In animal models, a chow diet containing 25 mg anti-HPU IgY/g and 0.16 mg famotidine/g reduced H. pylori activity in infected Mongolian gerbils and prevented colonization of H. pylori in the gastrointestinal tracts of uninfected controls (156). In the Mongolian gerbil model, anti- H. pylori IgY reduced inflammation, neutrophil and leukocyte infiltration, and gastric mucosal injury by interfering with the adhesion of H. pylori via its urease (157). A similar anti-inflammatory effect and reduction of H. pylori in the gastric mucosa were also observed in C57BL6/j mice treated with 60 mg of anti-H. pylori urease C IgY in either powder form or dissolved in phosphate-buffered saline (158). Importantly, orally administered IgY (100- 500 mg) in male C57BL/6 mice were more efficacious in eliminating H. pylori compared to treatment with the commonly used proton pump inhibitor pantoprazole (159). IgY against other H. Pylori antigens with good efficacy includes IgY against the outer inflammatory protein (160, 161), the neutrophil-activating protein (162), and the native or recombinant VacA protein l (163, 164).
An egg yolk powder dietary supplement containing IgY anti-HPU administered to a cohort of asymptomatic H. pylori-positive patients reduced the levels of H. pylori and aided the treatment of H. pylori-associated gastritis, with no side effects reported (165). In a study of 42
H. pylori-positive subjects, drinkable yogurt fortified with anti-HPU IgY significantly suppressed H. pylori infection and was well tolerated with no adverse effects (166).
Treatment of Porphyromonas gingivalis
Porphyromonas gingivalis (P. gingivalis), which causes biofilm on teeth (167), is quite resistant to the host immune response and leads to inflammation and bone loss associated with periodontitis (168). Anti-P. gingivalis IgY against gingipains, a protein family released by P. gingivalis, inhibited attachment of the bacteria in cultured human epithelial cells (169). In addition, IgY against a 40 kDa outer membrane protein prevented the aggregation of P.gingivalis with Streptococcus gordonii, another bacterial strain implicated in periodontitis (170). Furthermore, IgY-anti-P. gingivalis in dry feed or administered as an ointment in a dog model reduced biofilm formation and inflammation (171). Sublingual application of IgY anti-P. gingivalis in five periodontitis patients reduced the levels of the bacteria and gum bleeding (172).
Treatment of Prevotella intermedia
Like Porphyromonas gingivalis, Prevotella intermedia also causes gingivitis and other periodontal diseases (173) and can be associated in humans with systemic diseases such as diabetes mellitus (174), respiratory illnesses (175), cardiovascular disease (176), ischemic stroke (177), osteoporosis (178), and risk of low birthweight preterm pregnancies (179). In rat models challenged with P. intermedia on gingivae, IgY anti-P. intermedia treatment protected against gingivitis by decreasing gingival index, plaque index, bleeding on probing, white blood cell counts, and local inflammation typically associated with periodontal disease (180). Because of the success of anti-P. intermedia IgY in rat models, as well as the general challenge due to increased resistance to antibiotics, IgY treatment may provide an alternative in humans.
Treatment of Solobacterium moorei
Solobacterium moorei causes oral halitosis, periodontitis, and gingivitis (181, 182). S. moorei is susceptible to common antibiotics such as penicillin, vancomycin, and moxifloxacin (183). Specific IgY inhibited bacterial growth in liquid media and biofilm formation in vitro (184). In a mouse model challenged with S. moorei, 20 to 40 mg/ml of specific IgY decreased bacterial counts in the oral cavities of treated animals (184). Benefit in humans has not been determined.
Treatment of Fusobacterium nucleatum
Fusobacterium nucleatum is one of many pathogenic bacterial strains that contributes to halitosis and periodontitis (185). Although available treatments include chemical antiseptics, antimicrobials, and mechanical therapy (186), efficacy is limited by poor compliance and the development of antibiotic resistance. IgY anti-F. nucleatum has been suggested as an immunotherapeutic alternative to mediating the development of F. nucleatum and other bacteria in the oral cavity. In a periodontitis rat model, IgY anti-F. nucleatum inhibited the development of volatile sulfurous and odorous compounds and decreased the malodor index, levels of anti-inflammatory cytokines, and alveolar bone loss, while aiding periodontal restoration (186).
Treatment of Streptococcus mutans
Streptococcus mutans (S. mutans) is the main odontopathogen implicated in the development of dental carries in humans (187). Although most antibiotic treatments of S. mutans are effective, resistance to penicillin, erythromycin, amoxicillin, clindamycin, and lincomycin is common (188). IgY developed against S. mutans is highly stable and cross-reacts with other serotypes, including S. salivarum (189–191). IgY against S. mutans inhibited in vitro bacterial growth, biofilm development, and binding to bacterial adhesion proteins, and inducing agglutination of S. mutans (189, 191, 192). Rats exposed to S. mutans and fed a caries-inducing diet had reduced dental caries when lyophilized anti-S. mutans IgY was incorporated into their feed (193, 194), topical gel (195), or chitosan-enriched soy milk (192).
The efficacy of anti-S. mutans IgY in humans has also been evaluated. IgY anti-S. mutans incorporated into a mouth rinse significantly reduced levels of S. mutans in saliva within 4 hours, and in plaque within 7 days (189). In comparison to commercial toothpaste, anti-S. mutans IgY toothpaste reduced levels of S. mutans more quickly and colonization was suppressed as long as 2 weeks after discontinuation (196). Similar results were also observed with the administration of lozenges containing IgY against S. mutans glucosyltransferase, which significantly decreased salivary levels of S. mutans after 5 days (197).
Treatment of Salmonella
The Salmonella species, particularly S. typhimurium and S. enteritidis, are human and chicken pathogens (198, 199). Salmonella-specific IgY inhibited bacterial cell growth (200) by binding to and structurally altering antigens on the surface of the bacterium (200) or by causing bacterial agglutination (201). In a human epithelial Caco2 cell model, IgY anti-Salmonella antibodies also prevented the adhesion of the bacterium to cells (202).
IgY anti-S. typhimurium and IgY anti-S. enteritidis exhibited significant cross-reactivity (200) and agglutination (201, 203), which indicates that IgY against a specific Salmonella serovar may be useful in treating a broad range of different Salmonella strains. IgY anti-S. typhimurium reduced immune cell recruitment and cytokine release in a mouse model infected with these bacteria (204). A combination treatment of a probiotic with IgY anti-S. enteritidis also decreased colonization and fecal shedding in young, market-aged broiler chicks challenged with S. enteritidis (205), indicating the additional potential benefit of using IgY anti-Salmonella antibodies in animals consumed as food.
Treatment of Clostridium difficile
Clostridium difficile (CD) is a cause of morbidity due to diarrhea and mortality due to inflammation of the colon, especially in the elderly, immunosuppressed, and after chronic antibiotic use. This serious condition has been increasing in incidence. IgY anti-CD has shown promise for patients based on animal studies (206). For example, 0.5 mg of IgY against the CD’s FliD colonization-associated factor administered by gavage prevented the adhesion of CD and significantly enhanced survival rates in CD-challenged Syrian hamsters (207). Oral gavage treatments of 0.6 mg of anti-CD spore IgY delayed diarrhea onset and reduced spore adhesion to intestinal cells in mouse models, especially when coupled with an existing antibiotics treatment such as vancomycin (208). IgY anti-CD toxin A and B neutralized toxins and prevented recurrent infections in a hamster model (209). Delivery of IgY anti-CD toxins to the colon instead of the upper gastrointestinal tract was enhanced when IgY was coated on microbeads (210) or encapsulated in chitosan-Ca pectinate microbeads (211) in a rat model.
Detection and Treatment of Campylobacter jejuni
The Campylobacter species, in particular Campylobacter jejuni, is the most common cause of gastroenteritis in humans worldwide (212). Most human C. jejuni infections are caused by the consumption of contaminated poultry. However, C. jejuni seems to have a commensal relationship with chickens while acting as a pathogen in humans (213). Because of the use of growth-promoting antibiotics in the meat-producing industry, there is a rise in antibiotic-resistant Campylobacter strains (214). IgY against C. jejuni may provide an alternative to antibiotic use (215, 216). Because of its high specificity and limited cross-reactivity, IgY anti-C. jejuni also can provide a highly accurate method to detect food contamination with C. jejuni (217, 218).
To reduce the spread of C. jejuni from poultry to humans, IgY has been administered as a passive vaccine to chickens. IgY against C. jejuni has prophylactic and therapeutic effects through its ability to decrease overall and fecal bacterial levels in C. jejuni-challenged chickens (219–221). Similar results were also observed using IgY against C. jejuni adhesins and flagellins, which significantly reduced caecal colonization by C. jejuni and initiated the production of C. jejuni specific antibodies, although these may not play a role in protection (222, 223). Administration of anti-C. jejuni IgY also resulted in a significant reduction in transmission of C. jejuni to non-inoculated birds (220), without altering the microflora of the intestinal tract (221).
Sepsis
Detection and Treatment of Escherichia coli
Escherichia coli is an integral constituent of the mammalian microflora, with several pathotypes of E. coli implicated in the development of enteric and extraintestinal diseases such as diarrhea, sepsis, meningitis, and urinary tract infections (224). Among the enteric E. coli-associated diseases, there are at least six different categories: enterotoxigenic E.coli, enteropathogenic E. coli, enterohemorrhagic E. coli, enteroaggregative E. coli, enteroinvasive E. coli, and diffusely adherent E. coli (225). Due to widespread antibiotic resistance of E.coli (226), IgY may serve as an alternative method to neutralize virulent E. coli in food, animals, and humans.
The major factor in the pathogenicity of E. coli is production of Shiga-like toxin. Numerous assays using IgY have been developed to detect the presence of Shiga-toxin-producing enterohemorrhagic E.coli. For instance, an ELISA assay involving IgY against E.coli O157:H7 was able to detect as little as 40 CFU/ml of E. coli O157:H7, suggesting that such assays can be used for detecting foodborne pathogens (227). Furthermore, because Shiga-toxin is uniformly expressed by all enterohemorrhagic E. coli, IgY can be used to detect different serotypes and variants of Shiga-toxin-producing E. coli (228). Similarly, toxin-specific IgY has also been used to detect and neutralize heat-labile toxin produced by enterotoxigenic E. coli (229).
Chicken or ostrich IgY against E. coli O157:H7 and O78:K80 inhibited bacterial growth in liquid medium (227, 230–232). The benefit of IgY given by intramuscular injection as prophylaxis against E. coli-associated respiratory, enteric, and septicemic diseases has been demonstrated in young broiler chicks (233). In piglets infected with enterotoxigenic E. coli, specific IgY decreased the severity of diarrhea (234) when encapsulated in chitosan-alginate microcapsules or hydrogel-carbon nanotube composites (235, 236), suggesting that this approach may be especially useful to neutralize E. coli IgY in food and veterinary settings.
A randomized, double-blind, placebo-controlled trial evaluated 301 Guatemalan children (154 intervention and 147 placebo) with acute non-bloody diarrhea who received PTM202 (combined IgY specifically targets rotavirus, enterotoxigenic E. coli, Shiga toxin-positive E. coli, and salmonella) or placebo for 3 days (237). PTM202 led to a reduction in duration of diarrhea among children whose diarrheal stool at enrollment contained one or more PTM202-targeted organisms. No adverse events were reported.
Detection and Treatment of Staphylococcus aureus
Staphylococcus aureus is a pathogenic bacterial strain that causes food poisoning, toxic shock syndrome, endocarditis, sepsis, soft tissue infections, and in-hospital infections (238, 239). Although S. aureus is normally found in mammals and 30-50% of humans, it remains a dangerous pathogen due to its endotoxicity, virulence, invasiveness, and antibiotic resistance (240). S. aureus has increasingly displayed resistance to common antibiotic treatments such as methicillin and vancomycin (241, 242) and antimicrobial-resistant strains are now detected in the community, not just in healthcare settings (243, 244). Stronger measures to contain S. aureus infections, as well as alternative and combination treatments, are now promoted to combat increasing antibiotic resistance.
S. aureus is commonly found in food samples. IgY has been employed as a method to detect the bacterium, as well as its associated proteins and toxins to prevent S. aureus-induced food poisoning. IgY against S. aureus or its endotoxins has been used in sandwich ELISAs, immuncapture polymerase chain reaction ELISAs, lateral flow devices, immunopillar chips, immunochromatography, and fluorescence resonance energy transfer assays (245–252). The success of these approaches is considered due to IgY’s lack of reactivity with protein A of S.aureus, which often results in false positives results in other assays (253). In vitro, specific IgY had high binding specificity and inhibited bacterial growth in culture, possibly by interrupting interactions with surface antigens (254). IgY generated against S.aureus also caused agglutination of the bacterium, and did not show cross-reactivity with other bacterial strains such as Streptococcus epidermidis, Escherichia coli, and Pseudomonas aeruginosa that are commonly found in milk (255).
S. aureus is also implicated in ruminant mastitis, which affects the quality and quantity of milk produced, as well as the health of infected animals (256). Although there are antibiotic treatments and vaccines available, they are not fully effective and S. aureus-associated infections often recur (257). IgY produced against S. aureus was highly specific to mastitis-causing strains, enhanced the phagocytic activity of milk macrophages (258), and reduced bacterial growth in culture (259). In addition, specific IgY blocked the internalization and infection of bovine mammary epithelial cells by S.aureus in vitro (260). At a concentration of 20 mg/ml, IgY anti-S.aureus infused by insertion into the teat canal decreased somatic cell and bacterial counts, while curing most experimentally challenged lactating cows (258). Similar cure rates were also observed in challenged buffaloes with mastitis, and specific IgY administered through intramammary infusions at a dose rate of 20 mg/ml improved milk yield (261).
The anterior nare of the nose is the most frequent carriage site for S. aureus (262). When the nares are treated topically to eliminate nasal carriage, in most cases the organism also disappears from other body areas (262). Anti-S. aureus IgY given intranasally may be of special interest in the treatment of this pathogen, including for the growing threat of methicillin-resistant S. aureus (263).
Detection and Treatment of Aeromonas
Aeromonas is commonly found in aquatic environments and the microflora of animals and humans. However, certain strains of Aeromonas have been implicated in the development of sepsis and gastroenteritis in humans (264), as well as fish, other animals, and environmental reservoirs (265). Notably, almost all subspecies of Aeromonas express strong resistance to beta-lactam antibiotics such as penicillin, ampicillin, and carbenicillin, which has led to the pursuit of alternative and combination antimicrobial therapies (266).
IgY-specific antibodies have been investigated as an alternative method to diagnose Aeromonas-diseased aquatic animals. IgY produced against Aeromonas hydrophila detected A. hydrophila in tissues and phagocytes in infected Nile tilapia (267), neutralized bacterial adhesins and toxins released by the bacteria, promoted agglutination, inhibited bacterial growth, and enhanced the phagocytic activity of infected Nile tilapia, blunt snout bream (268, 269), and polyploid gibel carp (269, 270). When added to rearing water, specific IgY eliminated the development of skin ulcers, as well as transmission of the infection between different fish (271).
Skin-Related Infections
Treatment of Propionibacterium
Acne vulgaris is a skin condition that affects most humans at some time and is thought to be caused by multiple factors, including increased sebaceous gland sebum production, hormones, cytokines, nutrition, and bacteria such as Propionibacterium acnes (272). Because of rising antibiotic resistance, IgY has also been proposed as a cost-effective alternative to antimicrobial treatments of acne. IgY anti-P. acnes inhibited growth of P. acnes colonies as well as biofilm development by preventing bacterial adhesion (273).
Diagnosis and Treatment of Fungal Infections
Treatment of Candida albicans
Typically, Candida albicans is a normal, commensal member of the human microbiome (274). However, C. albicans can also express virulence factors that can cause candidiasis infections of the mucosal membranes of the oral cavity, esophagus, gastrointestinal system, vagina, vascular system, and skin, as well as serious and life-threatening pneumonia and systemic infections (275). Because of limited antifungal therapeutics, virulence, and the development of resistance to existing drugs (276), novel approaches to treatment, such as the use of IgY antibodies, have been investigated. IgY generated against C. albicans inhibited biofilm formation and growth in liquid media (277), as well as adhesion of C. albicans to denture-based material (272), human oral epithelial cells (277, 278), and human pharyngeal carcinoma cells (274). In addition, cross-reactivity to other Candida subspecies such as C. glabrata was observed, suggesting that anti-C. albicans IgY may also be used to prevent the dissemination of different Candida strains (277, 279). In mouse models, orally administered treatments of a gel containing 0.5 g of IgY against C. albicans (280) or a 20 mg specific IgY/ml phosphate-buffered saline solution (276) protected challenged animals and prevented C. albicans colonization and the subsequent development of kidney and tongue lesions. Because there was no correlation observed between drug-resistant strains of C. albicans and the growth-inhibition ability of anti-C. albicans IgY (278), specific IgY may warrant evaluation as a novel therapeutic for fungal infections in humans.
Diagnosis and Treatment of Parasitic Diseases
Treatment of Trypanosoma
Infection by the protozoan parasite Trypanosoma cruzi results in Chagas disease, which is transmitted to animals and humans by insect vectors that are found only in the Americas. Chronic infection can lead to heart and gastrointestinal disease that can be life-threatening (281). Vaccines developed against T. cruzi are only partially protective since defined antigens must be used to prevent the occurrence of cryptic infections (282). Furthermore, drugs approved for the treatment of Chagas disease can have toxic, mutagenic, and other adverse side effects (283). Since no cytotoxic or proliferative effects were observed on mononuclear and VERO cells in vitro when treated with IgY against T. cruzi, specific IgY has been considered as a possible therapeutic for Chagas disease (284). In a mouse model, anti-T. cruzi IgY administered prophylactically at 50 mg/kg reduced parasitemia post-challenge and prevented the development of cardiac lesions by amastigotes. These same effects were also observed with the therapeutic administration of 50 mg/kg of IgY, which also improved the immune response by preventing an increase in activity of E-NTPDase and E- ADA activities in the splenic lymphocytes of the animals (285).
Another member of the Trypanosoma family, T. evansi, infects a wide range of domesticated livestock worldwide (286), causing anemia (287). One case of T. evansi infection in humans has also been documented, possibly by blood transmission from an infected animal (288). Current strategies for controlling the dissemination of T. evansi include herd culling (289) and chemical therapy of infected animals, although this latter approach has limited use due to high toxicity and the development of drug-resistant strains (290). In contrast, no significant cytotoxic or genotypic toxicity was observed when IgY anti-T. evansi was used to treat peripheral blood samples, although an increase in cell viability and lymphocyte proliferation was observed when a concentration of 10 mg/ml of specific IgY was used (291). In vivo, specific IgY against T. evansi administered intraperitoneally at a dose of 10 mg/kg increased the longevity and survival of infected animals (292).
Detection and Treatment of Cryptosporidiosis
Several members of the Cryptosporidium family are implicated in the development of cryptosporidiosis, an intestinal infection that causes diarrhea and, less commonly, pneumonia in humans (293). There are no therapies to fully treat cryptosporidiosis or prevent the infection in humans and animals (294) although hydration and passive immunization through the administration of monoclonal antibodies (295), nitazoxanide, or hyperimmune bovine colostrum have limited efficacy (296, 297). IgY has been explored as a treatment against Cryptosporidium infection. In vitro, IgY antibodies generated against C. parvum oocyst antigens were highly specific (298), decreased binding of the parasite to Caco-2 cells, and blocked the vitality of C. parvum (299). However, in a severe combined immunodeficiency mouse model, treatment using feed containing 25% specific IgY powder and a 20% specific IgY solution was only capable of partially reducing oocyst shedding in challenged animals, and was unable to eliminate infection (299). Similarly, IgY against the P23 protein in C. parvum also has high specificity for the parasite (300, 301). Using a mouse model, the anti-P23 IgY reduced oocyst shedding by 70% (300). Specific IgY against the GP60 glycoprotein in Cryptosporidium hominis, another strain implicated in cryptosporidiosis, was also found to specifically bind to the antigen, as well as the parasite (302). The high specificity and protective effectiveness of these antibodies suggest that IgY warrants evaluation as a novel diagnostic test for cryptosporidiosis and a passive immunization treatment in immunocompromised individuals.
Treatment of Eimeria
The Eimeria family of parasites is responsible for the development of avian coccidiosis, a severe intestinal disease with varying rates of morbidity and mortality (303). Because of the significant economic effect that coccidiosis has on the poultry industry, coccidiostat drugs have been undertaken. Unfortunately, numerous Eimeria strains bear multiple resistance to these anticoccidials (304). Thus, other measures such as live attenuated vaccines (303) and IgY antibodies against Eimeria have been explored (305).
Using maternal immunization against the three major species of Eimeria that cause coccidiosis (E. tenella, E. acervulina, and E. maxima), Wallach et al. demonstrated that IgY protected offspring chicks up to 3 weeks of age (306). By strongly blocking the exponential rise in oocyst numbers, this was expected to also protect against the pathogenic effects of the disease for their entire 5-7-week growth period. Studies have occurred on a very large commercial scale where millions of hens and their offspring chicks were vaccinated on 4 continents (307). Maternal immunity can also lead to results similar or even better than that achieved using the best anticoccidial drugs (307).
In young broiler chicks challenged with E. acervulina oocysts, specific IgY against multiple strains of Eimeria decreased the number of oocysts in fecal matter and increased the body weight of treated animals (308). Similar improvements, as well as decreased intestinal lesions, were also observed in chicks challenged with E. tenella or E. maxima that were treated with Supracox, a commercially available egg yolk powder containing specific IgY against oocysts of three different Eimeria strains (309). With an IgY treatment generated against five different species of Eimeria, chicks challenged with E. tenella had reduced mortality, increased body weight gain, reduced oocyst shedding, reduced caecal lesion score, and increased anticoccidial index (310). IgY generated against a recombinant protein (3-1E) from E. acervulina merozoites also improved body weight gain and decreased oocyst production in chickens infected with E. acervulina or E. tenella (311). The Fc fragments of IgY have also been coupled to stable transgenic Eimeria mitis, which decreased oocyst output in challenged animals (312) and suggests that the method of expressing Fc-fused exogenous antigens on transgenic Eimeria might be explored as a more efficient vaccine.
Principles Regarding the Clinical Use of IgY
Physicochemical Properties of Chicken IgY Contribute to Safety
IgY consumed orally is considered to be GRAS (“Generally Recognized as Safe”) by the U.S. Food and Drug Administration. At a biochemical level, this may be because IgY, unlike IgG, does not have a hinge region but contains a short region between the Fa and Fc segments of the antibody’s stock (313). This short region is rich in proline and glycine residues, allowing only limited flexibility of its Fab. Due to this difference, IgY is unable to bind to mammalian Fc receptors and therefore does not readily cause T cell activation (314). In addition, because of its unique Fc characteristics, IgY does not cause the false-positive results or aggregation in immunoassays often seen with use of monoclonal and polyclonal mammalian antibodies (5). When used as a therapeutic, IgY - unlike mammalian antibodies - also cannot activate the mammalian complement cascade that may cause cell lysis (314), even after repetitive subcutaneous injections in vivo. However, IgY used as a repetitive parenteral product is unlikely due to risk of an adverse immune response.
In humans, controlled clinical trials have shown that IgY has a favorable safety profile with its known nonparenteral administration. Continuous prophylactic treatment by a daily mouth rinse with specific IgY against P. aeruginosa in 17 patients with cystic fibrosis for up to 12 years (114 patient-years) significantly reduced pulmonary P. aeruginosa infections compared with 23 cystic fibrosis control patients, with no adverse events (119, 315). Use of oral anti-P. aeruginosa IgY gargling solution by 83 patients with cystic fibrosis for a median of 26.3 months resulted in a similar number and incidence of adverse events compared with 81 patients receiving placebo (120).
Additional clinical studies showing favorable safety of IgY given orally include treatment with a gel containing anti-Candida IgY (316), treatment with lozenges containing anti-P. gingivalis IgY (317), ingestion of anti-rotavirus IgY in pediatric patients with rotavirus diarrhea (63, 67), and ingesting yogurt containing anti-H. pylori IgY (167). Parenteral administration of IgY has not been evaluated but may be of value as a single injection in situations such as the need for antivenom treatment. IgY-based antivenom given parenterally resulted in complete protection in animal models of lethal venomous bites and stings (318–321).
Stability
Due to its physical and biochemical properties, IgY is protected from inactivation down to pH 3 (8), heat up to 60°C (156), and proteolytic enzymes (313). At a pH lower than 3, such as in the gastrointestinal tract, inactivation of IgY is similar to other immunoglobulins but can be minimized by encapsulation (322). IgY can also be degraded by stomach protease and pepsin, thereby reducing IgY’s efficacy in the treatment of gastrointestinal diseases (4). However, a variety of formulation ingredients can increase IgY stability. For example, a 50% solution of sorbitol is a polyol that is commonly used to improve protein stability. Sorbitol at this concentration is sufficient to prevent the pH-dependent inactivation of IgY by strengthening the hydrophobic interactions and by surrounding and preventing the exposure of its aromatic or carboxylic amino acids, the sites of pepsin proteolysis (323).
Coupling of polyethylene-glycol improves the heat stability of IgG, and therefore may also increase the heat resistance of IgY (324). High concentrations of sucrose also had a stabilizing effect on IgY: in a 50% sucrose solution, the activity of IgY was completely retained even when heated to 80°C (325). Furthermore, the encapsulation of IgY in liposomes renders IgY resistant to acidic conditions and pepsin (326, 327). Orally administered chitosan-alginate microcapsules also protected premature degradation and improved bioactivity and targeting of IgY to microorganisms in the lower intestinal tract of neonatal and early-weaned piglets (235).
Production Process
IgY may provide a low-cost and fast method for the development of prophylactic immunization strategies. The phylogenetic relationship between birds and mammals not only permits the adaptation of IgY as an alternative to mammalian antibodies for clinical use, but also increases sensitivity of IgY to human antigens for diagnostics. Thus, immunization of laying hens twice with the antigen of interest is sufficient to produce a humoral response that results in the production of large amounts of specific IgY molecules in their eggs for several months. Typically, older hens produce a higher titer compared with younger chickens (328). As mentioned previously, IgY collection does not require bleeding of the immunized laying hens or their progeny, thus reducing animal distress and other ethical considerations.
A typical egg of an immunized hen contains about 100 mg of IgY and a single immunized hen lays approximately 325 eggs per year, resulting in a total production yield of up to 40 g of IgY per hen per year (329). We estimate that each egg can produce approximately 10 doses of treatment/person, varying with the indication. Two to 10% of the IgY found in eggs of immunized commercial hens is specific to the antigen of interest (330). A much higher amount, 500 mg of IgY per egg, is produced in specific pathogen-free hens, hence the percentage of specific antibodies is likely higher; specific IgY levels can be maintained without further inoculation of the laying hen for up to half a year (230). IgY can be purified via a variety of nontoxic methods, including water dilution and low pH-induced precipitation, as well as polyethylene glycol-, dextran sulfate- and xanthan gum-induced precipitations (331) or NaCl extraction (332). Therefore, in terms of environmental impact, IgY can be efficiently collected and processed on an industrial scale without the usage of harsh chemicals and the production of toxic byproducts (8). Very large quantities of IgY antibodies can be harvested from the eggs laid by a single immunized hen, and the overall yield is comparable to the amount that can be collected from large mammals such as goats and cows, and 18-times higher than what is collected from rabbits (9).
Concluding Remarks and Future Perspectives
Use of avian IgY antibodies has important implications for a wide range of human and veterinary clinical applications. As a diagnostic tool, the inflexibility of IgY’s structure prevents binding to rheumatoid factor, which is found in mammalian samples. Because of this advantage conferred by IgY’s structure, the use of IgY antibodies in immunoassays may result in less background noise, fewer false positives, and decreased aggregation of antibodies, which are common issues observed with both monoclonal and polyclonal mammalian antibodies. Furthermore, IgY antibodies have also shown high binding specificity and low cross-reactivity with other antigens comparable to current industry standards and may have value in a variety of applications to detect pathogens.
IgY has been explored as a prophylactic agent with the potential to neutralize pathogens in vivo. IgY antibodies can be developed into highly stable and concentrated products that can be evaluated under controlled settings in human and veterinary subjects, with the route depending on the intended target of prophylaxis. Nonparenterally administered IgY products, such as oral ingestibles, nasal sprays, and nasal drops, may provide widespread protection against pathogens that can colonize, infect, or damage the gastrointestinal and respiratory tracts. Neutralizing IgY antibodies may also warrant evaluation to target specific pathogens circulating in the bloodstream or localized in a specific area. Because IgY administration before infection has been demonstrated to have significant protective effects in vivo in animal models, evaluation of its use as prophylactic therapy in humans may be of special interest, including for SARS-CoV-2 in context of the current COVID-19 pandemic. Besides use in passive immunotherapy, IgY antibodies have also shown promise as a potential therapeutic agent for a wide spectrum of clinical applications. Specific IgY has detected and neutralized both surface and internal pathogenic antigens when administered after infection or consumption in multiple preclinical models. In most of these applications, IgY is not curative and has a greater therapeutic benefit with greater protection when used as prophylactic treatment or in conjunction to supplement existing standard treatments.
Limitations of IgY include possible host anti-IgY antibody responses. Parenteral administration has potential for serum sickness, an immune-complex-mediated hypersensitivity reaction. IgY immunogenicity has been tested in a pig model, which revealed that an anti-IgY antibody response was induced upon both local and systemic routes of administration of IgY (333, 334). Further safety studies are necessary to explore the potential of an antigenic or allergic response in both human and veterinary applications of IgY. Because of the phylogenetic distance between IgY and mammalian immunoglobulins, IgY is unable to bind to and activate Fc receptors, rheumatoid factors, and the mammalian complement system. Acute responses through these mechanisms would be unexpected.
Lack of intellectual property for IgY has hampered the commercial development of therapeutics by industry. Despite this, the potential of IgY to address a wide range of common and fatal diseases in both farm animals and humans is gaining interest (335–338) and warrants further exploration against infectious agents, including those associated with pandemics. For example, the distribution of COVID-19 vaccines with outstanding safety and efficacy profiles remains limited, with an estimated 5.5% of the world population fully vaccinated as of June 1, 2121 (339). A key bottleneck in mRNA (COVID-19) vaccine manufacturing is a global shortage of essential components (such as nucleotides, enzymes, and lipids) (340), which has lessened production of the estimated 11 billion doses needed to fully vaccinate 70% of the world’s population – the figure assumed needed to reach herd immunity. This shortage especially impacts low- and lower-middle-income countries (340). In contrast, within 6 weeks of identifying an epidemic or pandemic-causing virus, millions of egg-laying hens immunized throughout the world can provide billions of doses of drug substance, as each egg can produce many doses.
Author Contributions
Wrote or contributed to the writing of the manuscript: LL, KS, MW, LF, and DM-R. All authors contributed to the article and approved the submitted version.
Funding
The authors would like to thank SPARK at Stanford and the Stanford ChEM-H and the Innovative Medicines Accelerator Award, May 2020 COVID-19 Response: Drug and Vaccine Prototyping Seed Grant Program for support of the project.
Conflict of Interest
The authors declare that the research was conducted in the absence of any commercial or financial relationships that could be construed as a potential conflict of interest.
Acknowledgments
We also thank Drs. David Goodkin, W. Conrad Liles, and Tom St. John for helpful advice and critical review.
Abbreviations
AE, adverse event; BLV, bovine leukemia virus; CD, Clostridium difficile; COVID-19, coronavirus disease 2019; ELISA, enzyme-linked immunosorbent assay; IgG, immunoglobulin G; IgY, immunoglobulin Y; HBV, hepatitis B virus; HPS, hantavirus pulmonary syndrome; HPU, H. pylori urease; IBDV, infectious bursal disease virus; MERS, Middle East Respiratory Syndrome; MERS-CoV, Middle East respiratory syndrome coronavirus; MBTC, Mycobacterium tuberculosis; SARS, Severe Acute Respiratory Syndrome; SARS-CoV, SARS-associated coronavirus; SARS-CoV-2, severe acute respiratory syndrome coronavirus 2.
References
1. Warr GW, Magor KE, Higgins DA. Igy: Clues to the Origins of Modern Antibodies. Immunol Today (1995) 16:392–98. doi: 10.1016/0167-5699(95)80008-5
2. Leslie GA, Clem LW. Phylogeny of Immunoglobulin Structure and Function: Iii. Immunoglobulins of the Chicken. J Exp Med (1969) 130:1337–52. doi: 10.1084/jem.130.6.1337
3. Taylor AI, Fabiane SM, Sutton BJ, Calvert RA. The Crystal Structure of an Avian Igy- Fc Fragment Reveals Conservation With Both Mammalian Igg and Ige. Biochemistry (2009) 48:558–62. doi: 10.1021/bi8019993
4. Hatta H, Tsuda K, Akachi S, Kim M, Yamamoto T, Ebina T. Oral Passive Immunization Effect of Anti-Human Rotavirus Igy and its Behavior Against Proteolytic Enzymes. Biosci Biotechnol Biochem (1993) 57:1077–81. doi: 10.1271/bbb.57.1077
5. Dávalos-Pantoja L, Ortega-Vinuesa JL, Bastos-González D, Hidalgo-Álvarez R. A Comparative Study Between the Adsorption of Igy and Igg on Latex Particles. J Biomater Sci Polym Ed (2000) 11:657–73. doi: 10.1163/156856200743931
6. Frazer JK, Capra JD. “Immunoglobulins: Structure and Function.” In: Paul WE, editor. Fundamental Immunology, 4th edition. Philadelphia: Lippincott-Raven (1999). p. 37–74.
7. Carlander D, Sundstrom J, Berglund A, Larsson A, Wretlind B, Kollberg H. Immunoglobulin Y (Igy)—a New Tool for the Prophylaxis Against Pseudomonas Aeruginosa in Cystic Fibrosis Patients. Pediatr Pulmonol [Suppl] (1999) 28:241.
8. Yokoyama H, Peralta RC, Sendo S, Ikemori Y, Kodama Y. Detection of Passage and Absorption of Chicken Egg Yolk Immunoglobulins in the Gastrointestinal Tract of Pigs by Use of Enzyme-Linked Immunosorbent Assay and Fluorescent Antibody Testing. Am J Vet Res (1993) 54:867–72.
9. Karlsson M, Kollberg H, Larsson A. Chicken Igy: Utilizing the Evolutionary Advantage. Worlds Poult Sci J (2004) 60:341–8. doi: 10.1079/WPS200422
10. Larsson A, Karlsson-Parra A, Sjöquist J. Use of Chicken Antibodies in Enzyme Immunoassays to Avoid Interference by Rheumatoid Factors. Clin Chem (1991) 37:411–4. doi: 10.1093/clinchem/37.3.411
11. Larsson A, Wejaker P-E, Forsberg P-O, Lindahl T. Chicken Antibodies: A Tool to Avoid Interference by Complement Activation in ELISA. J Immunol Methods (1992) 156:79–83. doi: 10.1016/0022-1759(92)90013-J
12. Ward DJ, Hammond E, Linden-Phillips L, Stevens AJ. Trends in Clinical Development Timeframes for Antiviral Drugs Launched in the UK, 1981–2014: A Retrospective Observational Study. BMJ Open (2015) 5:e009333. doi: 10.1136/bmjopen-2015-009333
13. Hill A, Wang J, Levi J, Heath K, Fortunak J. Minimum Costs to Manufacture New Treatments for COVID-19. J Virus Erad (2020) 6:61–9. doi: 10.1016/S2055-6640(20)30018-2
14. Rahman S, Van Nguyen S, Icatlo FC Jr., Umeda K, Kodama Y. Oral Passive Igy-Based Immunotherapeutics: A Novel Solution for Prevention and Treatment of Alimentary Tract Diseases. Hum Vaccin Immunother (2013) 9:1039–48. doi: 10.4161/hv.23383
15. Chang S-C. Clinical Findings, Treatment and Prognosis in Patients With Severe Acute Respiratory Syndrome (Sars). J Chin Med Assoc (2005) 68:106–7. doi: 10.1016/S1726-4901(09)70229-1
16. de Groot RJ, Baker SC, Baric RS, Brown CS, Drosten C, Enjuanes L, et al. Middle East Respiratory Syndrome Coronavirus (Mers-Cov): Announcement of the Coronavirus Study Group. J Virol (2013) 87:7790–2. doi: 10.1128/JVI.01244-13
17. Hu G, Nie R, Zhang J, Wu D, Yang H, Wen Z, et al. Study on the Effect of Specific Egg Yolk Immunoglobulins (Igy) Inhibiting Sars-Cov. Zhonghua Min Guo Wei Sheng Wu Ji Mian Yi Xue Za Zhi (2004) 24:123–5. (article in Chinese).
18. Lee Y-C, Leu S-JC, Hung H-C, Wu H-H, Huang IJ, Hsieh W-S, et al. A Dominant Antigenic Epitope on SARS-Cov Spike Protein Identified by an Avian Single-Chain Variable Fragment (Scfv)-Expressing Phage. Vet Immunol Immunopathol (2007) 117:75–85. doi: 10.1016/j.vetimm.2007.02.001
19. Abbas AT, El-Kafrawy SA, Sohrab SS, Tabll AA, Hassan AM, Iwata-Yoshikawa N, et al. Anti-S1 MERS-Cov Igy Specific Antibodies Decreases Lung Inflammation and Viral Antigen Positive Cells in the Human Transgenic Mouse Model. Vaccines (Basel) (2020) 8:634. doi: 10.3390/vaccines8040634
20. Kammila S, Das D, Bhatnagar PK, Sunwoo HH, Zayas-Zamora G, King M, et al. A Rapid Point of Care Immunoswab Assay for SARS-Cov Detection. J Virol Methods (2008) 152:77–84. doi: 10.1016/j.jviromet.2008.05.023
21. Koff WC, Schenkelberg T, Williams T, Baric RS, McDermott A, Cameron CM, et al. Development and Deployment of COVID-19 Vaccines for Those Most Vulnerable. Sci Transl Med (2021) 13:eabd1525. doi: 10.1126/scitranslmed.abd1525
22. Pardi N, Weissman D. Development of Vaccines and Antivirals for Combating Viral Pandemics. Nat BioMed Eng (2020) 4:1128–33. doi: 10.1038/s41551-020-00658-w
23. Callaway E, Ledford H. How to Redesign Covid Vaccines So They Protect Against Variants. Nature (2021) 590:15–6. doi: 10.1038/d41586-021-00241-6
24. Lauring AS, Hodcroft EB. Genetic Variants of SARS-Cov-2-What do They Mean? JAMA (2021) 325:529–31. doi: 10.1001/jama.2020.27124
25. Centers for Diseases Control and Prevention. Information for Healthcare Professionals About Coronavirus (Covid-19) (2021). Available at: https://www.cdc.gov/coronavirus/2019-ncov/hcp/index.html (Accessed April 15, 2021).
26. Casadevall A, Pirofski LA. The Convalescent Sera Option for Containing Covid-19. J Clin Invest (2020) 130:1545–8. doi: 10.1172/JCI138003
27. Constantin C, Neagu M, Supeanu TD, Chiurciu V, Spandidos A. Igy - Turning the Page Toward Passive Immunization in COVID-19 Infection (Review). Exp Ther Med (2020) 20:151– 8. doi: 10.3892/etm.2020.8704
28. Somasundaram R, Choraria A, Antonysamy M. An Approach Towards Development of Monoclonal Igy Antibodies Against SARS Cov-2 Spike Protein (s) Using Phage Display Method: A Review. Int Immunopharmacol (2020) 85:106654. doi: 10.1016/j.intimp.2020.106654
29. Hemmingsson P, Hammarström L. Nasal Administration of Immunoglobulin as Effective Prophylaxis Against Infections in Elite Cross-Country Skiers. Scand J Infect Dis (1993) 25:783–5. doi: 10.3109/00365549309008580
30. Giraudi V, Riganti C, Torales MR, Sédola H, Gaddi E. Upper Respiratory Infections in Children: Response to Endonasal Administration of IGA. Int J Pediatr Otorhinolaryngol (1997) 39:103–10. doi: 10.1016/S0165-5876(96)01472-3
31. Heikkinen TA, Ruohola A, Ruuskanen O, Waris M, Uhari M, Hammarström L. Intranasally Administered Immunoglobulin for the Prevention of Rhinitis in Children. Pediatr Infect Dis J (1998) 17:367–72. doi: 10.1097/00006454-199805000-00004
32. Weltzin R, Monath TP. Intranasal Antibody Prophylaxis for Protection Against Viral Disease. Clin Microbiol Rev (1999) 12:383–93. doi: 10.1128/CMR.12.3.383
33. Wei S, Duan S, Liu X, Wang H, Ding S, Chen Y, et al. Chicken Egg Yolk Antibodies (Igys) Block the Binding of Multiple Sars-Cov-2 Spike Protein Variants to Human Ace2. Int Immunopharmacol (2021) 90:107172. doi: 10.1016/j.intimp.2020.107172
34. Lu Y, Wang Y, Zhang Z, Huang J, Yao M, Huang G, et al. Generation of Chicken Igy Against Sars-Cov-2 Spike Protein and Epitope Mapping. J Immunol Res (2020) 2020:9465398. doi: 10.1155/2020/9465398
35. Shen H, Cai Y, Zhang H, Wu J, Ye L, Yang P, et al. Igy Isolated From Egg Yolks of Hens Immunized With Inactivated Sars-Cov-2 for Immunoprophylaxis of COVID-19. Virol Sin (2021) 1–3. doi: 10.1007/s12250-021-00371-1. Online ahead of print.
36. World Health Organization. Influenza (2021). Available at: https://www.who.int/influenza/en/ (Accessed April 15, 2021).
37. Ferguson NM, Galvani AP, Bush RM. Ecological and Immunological Determinants of Influenza Evolution. Nature (2003) 422:428–33. doi: 10.1038/nature01509
38. Demicheli V, Jefferson T, Ferroni E, Rivetti A, Pietrantonj CD. Vaccines for Preventing Influenza in Healthy Adults. Cochrane Database Syst Rev (2018) 2:CD001269. doi: 10.1002/14651858.CD001269.pub6
39. Yang Y-e, Wen J, Zhao S, Zhang K, Zhou Y. Prophylaxis and Therapy of Pandemic H1n1 Virus Infection Using Egg Yolk Antibody. J Virol Methods (2014) 206:19–26. doi: 10.1016/j.jviromet.2014.05.016
40. Adachi K, Takama K, Tsukamoto M, Inai M, Handharyani E, Hiroi S, et al. Ostrich Produce Cross-Reactive Neutralization Antibodies Against Pandemic Influenza Virus a/H1N1 Following Immunization With a Seasonal Influenza Vaccine. Exp Ther Med (2011) 2:41–5. doi: 10.3892/etm.2010.180
41. Tsukamoto M, Hiroi S, Adachi K, Kato H, Inai M, Konishi I, et al. Antibodies Against Swine Influenza Virus Neutralize the Pandemic Influenza Virus a/H1N1. Mol Med Rep (2011) 4:209–14. doi: 10.3892/mmr.2011.410
42. Nguyen HH, Tumpey TM, Park H-J, Byun Y-H, Tran LD, Nguyen VD, et al. Prophylactic and Therapeutic Efficacy of Avian Antibodies Against Influenza Virus H5N1 and H1N1 in Mice. PloS One (2010) 5:e10152. doi: 10.1371/journal.pone.0010152
43. Wallach MG, Webby RJ, Islam F, Walkden-Brown S, Emmoth E, Feinstein R, et al. Cross-Protection of Chicken Immunoglobulin Y Antibodies Against H5N1 and H1N1 Viruses Passively Administered in Mice. Clin Vaccine Immunol (2011) 18:1083–90. doi: 10.1128/CVI.05075-11
44. Wen J, Zhao S, He D, Yang Y, Li Y, Zhu S. Preparation and Characterization of Egg Yolk Immunoglobulin Y Specific to Influenza B Virus. Antiviral Res (2012) 93:154–9. doi: 10.1016/j.antiviral.2011.11.005
45. Budama-Kilinc Y, Cakir-Koc R, Ozdemir B, Kaya Z, Badur S. Production and Characterization of a Conserved M2e Peptide-Based Specific Igy Antibody: Evaluation of the Diagnostic Potential Via Conjugation With Latex Nanoparticles. Prep Biochem Biotech (2018) 48:930–9. doi: 10.1080/10826068.2018.1525564
46. da Silva MC, Schaefer R, Gava D, Souza CK, da Silva Vaz I, Bastos AP, et al. Production and Application of Anti-Nucleoprotein Igy Antibodies for Influenza a Virus Detection in Swine. J Immunol Methods (2018) 461:100–5. doi: 10.1016/j.jim.2018.06.023
47. Maenz C, Chang S-F, Iwanski A, Burns M. Entry of Duck Hepatitis B Into Primary Duck Liver and Kidney Cells After Discovery of a Fusogenic Region Within the Large Surface Protein. J Virol (2007) 81:5014–23. doi: 10.1128/JVI.02290-06
48. Omata M, Uchiumi K, Ito Y, Yokosuka O, Mori J, Terao K, et al. Duck Hepatitis B Virus and Liver Diseases. Gastroenterology (1983) 85:260–7. doi: 10.1016/0016-5085(83)90309-8
49. Abouzid K, Ndeboko B, Durantel S, Jamard C, Zoulim F, Buronfosse T, et al. Genetic Vaccination for Production of DNA-Designed Antibodies Specific to Hepadnavirus Envelope Proteins. Vaccine (2006) 24:4615–7. doi: 10.1016/j.vaccine.2005.08.085
50. Rollier C, Charollois C, Jamard C, Trepo C, Cova L. Early Life Humoral Response of Ducks to DNA Immunization Against Hepadnavirus Large Envelope Protein. Vaccine (2000) 18:3091–6. doi: 10.1016/S0264-410X(00)00130-4
51. Rollier C, Sunyach C, Barraud L, Madani N, Jamard C, Trepo C, et al. Protective and Therapeutic Effect of DNA-Based Immunization Against Hepadnavirus Large Envelope Protein. Gastroenterology (1999) 116:658–65. doi: 10.1016/S0016-5085(99)70188-5
52. Thermet A, Robaczewska M, Rollier C, Hantz O, Trepo C, Deleage G, et al. Identification of Antigenic Regions of Duck Hepatitis B Virus Core Protein With Antibodies Elicited by DNA Immunization and Chronic Infection. J Virol (2004) 78:1945–53. doi: 10.1128/JVI.78.4.1945-1953.2004
54. Vega C, Bok M, Saif L, Fernandez F, Parreño V. Egg Yolk Igy Antibodies: A Therapeutic Intervention Against Group a Rotavirus in Calves. Res Vet Sci (2015) 103:1–10. doi: 10.1016/j.rvsc.2015.09.005
55. Dai Y-C, Zhang X-F, Tan M, Huang P, Lei W, Fang H, et al. A Dual Chicken Igy Against Rotavirus and Norovirus. Antiviral Res (2013) 97:293–300. doi: 10.1016/j.antiviral.2012.12.011
56. Kim HS, Lee B, Han SY, Jung YT. Expression of Bovine Rotavirus VP8 and Preparation of Igy Antibodies Against Recombinant Vp8. Acta Virol (2017) 61:143–9. doi: 10.4149/av_2017_02_03
57. Kuroki M, Ohta M, Ikemori Y, Icatlo FC, Kobayashi C, Yokoyama H, et al. Field Evaluation of Chicken Egg Yolk Immunoglobulins Specific for Bovine Rotavirus in Neonatal Calves. Arch Virol (1997) 142:843–51. doi: 10.1007/s007050050123
58. Masahiko K, Yutaka I, Hideaki Y, Peralta RC, Icatlo FC, Yoshikatsu K. Passive Protection Against Bovine Rotavirus-Induced Diarrhea in Murine Model by Specific Immunoglobulins From Chicken Egg Yolk. Vet Microbiol (1993) 37:135–46. doi: 10.1016/0378-1135(93)90188-D
59. Thu HM, Myat TW, Win MM, Thant KZ, Rahman S, Umeda K, et al. Chicken Egg Yolk Antibodies (Igy) for Prophylaxis and Treatment of Rotavirus Diarrhea in Human and Animal Neonates: A Concise Review. Korean J Food Sci Anim Resour (2017) 37:1– 9. doi: 10.5851/kosfa.2017.37.1.1
60. Vega C, Bok M, Chacana P, Saif L, Fernandez F, Parreño V. Egg Yolk Igy: Protection Against Rotavirus Induced Diarrhea and Modulatory Effect on the Systemic and Mucosal Antibody Responses in Newborn Calves. Veterinary Immunol Immunopathol (2011) 142:156–69. doi: 10.1016/j.vetimm.2011.05.003
61. Ebina T. Prophylaxis of Rotavirus Gastroenteritis Using Immunoglobulin. Arch Virol Suppl (1996) 12:217–23. doi: 10.1007/978-3-7091-6553-9_23
62. Ebina T, Tsukada K, Umezu K, Nose M, Tsuda K, Hatta H, et al. Gastroenteritis in Suckling Mice Caused by Human Rotavirus can be Prevented With Egg Yolk Immunoglobulin (Igy) and Treated With a Protein-Bound Polysaccharide Preparation (Psk). Microbiol Immunol (1990) 34:617–29. doi: 10.1111/j.1348-0421.1990.tb01037.x
63. Buragohain M S, Dhale G, Ghalsasi G R, Chitambar SD. Evaluation of Hyperimmune Hen Egg Yolk Derived Anti-Human Rotavirus Antibodies (Anti-Hrv Igy) Against Rotavirus Infection. World J Vaccines (2012) 2:73–84. doi: 10.4236/wjv.2012.22010
64. Rahman S, Higo-Moriguchi K, Htun KW, Taniguchi K, Icatlo FC, Tsuji T, et al. Randomized Placebo-Controlled Clinical Trial of Immunoglobulin Y as Adjunct to Standard Supportive Therapy for Rotavirus-Associated Diarrhea Among Pediatric Patients. Vaccine (2012) 30:4661–9. doi: 10.1016/j.vaccine.2012.04.091
65. Sarker SA, Pant N, Juneja LR, Hammarström L. Successful Treatment of Rotavirus- Induced Diarrhoea in Suckling Mice With Egg Yolk Immunoglobulin. J Health Popul Nutr (2007) 25:465–8.
66. Vega CG, Bok M, Vlasova AN, Chattha KS, Fernández FM, Wigdorovitz A, et al. Igy Antibodies Protect Against Human Rotavirus Induced Diarrhea in the Neonatal Gnotobiotic Piglet Disease Model. PloS One (2012) 7:e42788. doi: 10.1371/journal.pone.0042788
67. Burke RM, Tate JE, Barin N. Three Rotavirus Outbreaks in the Postvaccine Era - California, 2017. MMWR Morb Mortal Wkly Rep (2018) 67:470–2. doi: 10.15585/mmwr.mm6716a3
68. Sarker SA, Casswall TH, Juneja LR, Hoq E, Hossain I, Fuchs GJ, et al. Randomized, Placebo-Controlled, Clinical Trial of Hyperimmunized Chicken Egg Yolk Immunoglobulin in Children With Rotavirus Diarrhea. J Pediatr Gastroenterol Nutr (2001) 32:19–25. doi: 10.1097/00005176-200101000-00009
69. Wang X, Song L, Tan W, Zhao W. Clinical Efficacy of Oral Immunoglobulin Y in Infant Rotavirus Enteritis: Systematic Review and Meta-Analysis. Med (Baltimore) (2019) 98:e16100. doi: 10.1097/MD.0000000000016100
70. Dick GWA. Zika Virus (Ii). Pathogenicity and Physical Properties. Trans R Soc Trop Med Hyg (1952) 46:521–34. doi: 10.1016/0035-9203(52)90043-6
71. Duffy MR, Chen T-H, Hancock WT, Powers AM, Kool JL, Lanciotti RS, et al. Zika Virus Outbreak on Yap Island, Federated States of Micronesia. N Engl J Med (2009) 360:2536–43. doi: 10.1056/NEJMoa0805715
72. Cao-Lormeau V-M, Blake A, Mons S, Lastère S, Roche C, Vanhomwegen J, et al. Guillain-Barré Syndrome Outbreak Associated With Zika Virus Infection in French Polynesia: A Case-Control Study. Lancet (2016) 387:1531–9. doi: 10.1016/S0140-6736(16)00562-6
73. Brasil P, Pereira JP, Moreira ME, Ribeiro Nogueira RM, Damasceno L, Wakimoto M, et al. Zika Virus Infection in Pregnant Women in Rio De Janeiro. N Engl J Med (2016) 375:2321–34. doi: 10.1056/NEJMoa1602412
74. Rasmussen SA, Jamieson DJ, Honein MA, Petersen LR. Zika Virus and Birth Defects-Reviewing the Evidence for Causality. N Engl J Med (2016) 374:1981–7. doi: 10.1056/NEJMsr1604338
75. Tirado SMC, Yoon KJ. Antibody-Dependent Enhancement of Virus Infection and Disease. Viral Immunol (2003) 16:69–86. doi: 10.1089/088282403763635465
76. O’Donnell KL, Meberg B, Schiltz J, Nilles M,L, Bradley DS. Zika Virus-Specific Igy Results are Therapeutic Following a Lethal Zika Virus Challenge Without Inducing Antibody-Dependent Enhancement. Viruses (2019) 11:301. doi: 10.3390/v11030301
77. Gubler DJ, Clark GG. Dengue/Dengue Hemorrhagic Fever: The Emergence of a Global Health Problem. Emerg Infect Dis (1995) 1:55–7. doi: 10.3201/eid0102.952004
78. Wilder-Smith A, Lindsay SW, Scott TW, Ooi EE, Gubler DJ, Das P. The Lancet Commission on Dengue and Other Aedes-Transmitted Viral Diseases. Lancet (2020) 395:1890–1. doi: 10.1016/S0140-6736(20)31375-1
79. World Health Organization. Dengue Vaccine: Who Position Paper, September 2018 – Recommendations. Vaccine (2019) 37:4848–9. doi: 10.1016/j.vaccine.2018.09.063
80. Dussart P, Labeau B, Lagathu G, Louis P, Nunes MRT, Rodrigues SG, et al. Evaluation of an Enzyme Immunoassay for Detection of Dengue Virus NS1 Antigen in Human Serum. Clin Vaccine Immunol (2006) 13:1185–9. doi: 10.1128/CVI.00229-06
81. Lapphra K, Sangcharaswichai A, Chokephaibulkit K, Tiengrim S, Piriyakarnsakul W, Chakorn T, et al. Evaluation of an NS1 Antigen Detection for Diagnosis of Acute Dengue Infection in Patients With Acute Febrile Illness. Diagn Microbiol Infect Dis (2008) 60:387–91. doi: 10.1016/j.diagmicrobio.2007.11.010
82. Figueiredo A, Vieira NCS, dos Santos JF, Janegitz BC, Aoki SM, Junior PP, et al. Electrical Detection of Dengue Biomarker Using Egg Yolk Immunoglobulin as the Biological Recognition Element. Sci Rep (2015) 5:7865. doi: 10.1038/srep07865
83. O’Donnell KL, Fink A, Nilles ML, Bradley DS. Dengue NS1-Specific Igy Antibodies Neutralizes Dengue Infection Without Inducing Antibody Dependent Enhancement. J Immunol (2017) 198(1 Supplement):225.223.
84. O’Donnell KL, Espinosa DA, Puerta-Guardo H, Biering SB, Warnes CM, Schiltz J, et al. Avian Anti-NS1 Igy Antibodies Neutralize Dengue Virus Infection and Protect Against Lethal Dengue Virus Challenge. Antiviral Res (2020) 183:104923. doi: 10.1016/j.antiviral.2020.104923
85. Fink AL, Williams KL, Harris E, Alvine TD, Henderson T, Schiltz J, et al. Dengue Virus Specific Igy Provides Protection Following Lethal Dengue Virus Challenge and is Neutralizing in the Absence of Inducing Antibody Dependent Enhancement. PloS Negl Trop Dis (2017) 11:e0005721. doi: 10.1371/journal.pntd.0005721
86. Yao L, Zhao H, Tang H, Song J, Dong H, Zou F, et al. Chicken Igy Facilitates Allergic Airway Inflammation in a Chemical-Induced Murine Asthma Model by Potentiating Il-4 Release. Toxicol Lett (2015) 239:22–31. doi: 10.1016/j.toxlet.2015.08.1108
87. Goncalvez AP, Engle RE, Claire MS, Purcell RH, Lai C-J. Monoclonal Antibody-Mediated Enhancement of Dengue Virus Infection in Vitro and In Vivo and Strategies for Prevention. Proc Natl Acad Sci USA (2007) 104:9422–7. doi: 10.1073/pnas.0703498104
88. Padula PJ, Edelstein A, Miguel SDL, López NM, Rossi CM, Rabinovich RD. Hantavirus Pulmonary Syndrome Outbreak in Argentina: Molecular Evidence for Person- to-Person Transmission of Andes Virus. Virology (1998) 241:323–30. doi: 10.1006/viro.1997.8976
89. Zaki SR, Greer PW, Coffield LM, Goldsmith CS, Nolte KB, Foucar K, et al. Hantavirus Pulmonary Syndrome. Pathogenesis of an Emerging Infectious Disease. Am J Pathol (1995) 146:552–9.
90. Centers for Disease Control and Prevention. (2021). Available at: https://www.cdc.gov/hantavirus/hps/symptoms.html (Accessed April 15, 2021).
91. Custer DM, Thompson E, Schmaljohn CS, Ksiazek TG, Hooper JW. Active and Passive Vaccination Against Hantavirus Pulmonary Syndrome With Andes Virus M Genome Segment- Based DNA Vaccine. J Virol (2003) 77:9894–905. doi: 10.1128/JVI.77.18.9894-9905.2003
92. Brocato R, Josleyn M, Ballantyne J, Vial P, Hooper JW. Dna Vaccine-Generated Duck Polyclonal Antibodies as a Postexposure Prophylactic to Prevent Hantavirus Pulmonary Syndrome (Hps). PloS One (2012) 7:e35996. doi: 10.1371/journal.pone.0035996
93. Haese N, Brocato RL, Henderson T, Nilles ML, Kwilas SA, Josleyn MD, et al. Antiviral Biologic Produced in DNA Vaccine/Goose Platform Protects Hamsters Against Hantavirus Pulmonary Syndrome When Administered Post-Exposure. PloS Negl Trop Dis (2015) 9:e0003803. doi: 10.1371/journal.pntd.0003803
94. World Health Organization. Ebola (2021). Available at: https://www.who.int/health-topics/ebola (Accessed April 15, 2021).
95. Zhang Y, Wei Y, Li Y, Wang X, Liu Y, Tian D, et al. Igy Antibodies Against Ebola Virus Possess Post-Exposure Protection in a Murine Pseudovirus Challenge Model and Excellent Thermostability. PloS Negl Trop Dis (2021) 15:e0008403. doi: 10.1371/journal.pntd.0008403
96. Lukert PD, Saif YM. Infectious Bursal Disease. In: Saif YM, Glisson JR, Fadhy AM, McDougal LR, Swayne DE, eds. Diseases of Poultry, 11th ed. AMES: Iowa State University Press (2003). p. 161–80.
97. Schijns VEJC, Sharma J. “IAN Practical Aspects of Poultry Vaccination”. In: Davison F, Kaspers B, Schat KA, editors. Avian Immunology, London: Academic Press (2008). p. 373–93.
98. Mahgoub HA. An Overview of Infectious Bursal Disease. Arch Virol (2012) 157:2047–57. doi: 10.1007/s00705-012-1377-9
99. El-Ghany WAA. Comparison Between Immunoglobulins Igy and the Vaccine for Prevention of Infectious Bursal Disease in Chickens. Glob Vet (2011) 6:16–24.
100. Farooq A, Rabbani M, Muhammad K, Akram Z, Ahad A, Fatima Z, et al. Passive Immunization in Infectious Bursal Disease Virus Infected Birds Using Chemically Purified Immune Yolk Immunoglobulins (Igy). Afr J Microbiol Res (2012) 6:2993–8. doi: 10.5897/AJMR12.049
101. Yousif AA, Mohammad WA, Khodeir MH, Zeid AZAA, El-Sanousi AA, MS S, et al. Oral Administration of Hyperimmune Igy: An Immunoecological. Approach to Curbing Acute Infectious Bursal Disease Virus Infection. Egypt J Immunol (2006) 13:85–94.
102. Fahey JE, Crawley JF. Studies on Chronic Respiratory Disease of Chickens Ii. Isolation of a Virus. Can J Comp Med Vet Sci (1954) 18:13–21.
103. Jones RC. “Reovirus Infections”. In: Swayne DE, editor. Diseases of Poultry, 13th Edition. Hoboken: Wiley-Blackwell (2013). p. 351–73.
104. Sellers HS. Current Limitations in Control of Viral Arthritis and Tenosynovitis Caused by Avian Reoviruses in Commercial Poultry. Vet Microbiol (2017) 206:152–6. doi: 10.1016/j.vetmic.2016.12.014
105. Jung KM, Bae EH, Jung YT, Kim JW. Use of Igy Antibody to Recombinant Avian Reovirus ςc Protein in the Virus Diagnostics. Acta Virol (2014) 58:108–13. doi: 10.4149/av_2014_02_108
106. Zhang L, Li D, Liu L, Zhang G. Rapid Immunochromatographic Test Strip to Detect Swimming Crab Portunus Trituberculatus Reovirus. Dis Aquat Organ (2015) 117:21–9. doi: 10.3354/dao02921
107. Larsen LE. Bovine Respiratory Syncytial Virus (Brsv): A Review. Acta Vet Scand (2000) 41:1–24. doi: 10.1186/BF03549652
108. Ferella A, Bellido D, Chacana P, Wigdorovitz A, Santos MJD, Mozgovoj MV. Chicken Egg Yolk Antibodies Against Bovine Respiratory Syncytial Virus Neutralize the Virus In Vitro. Proc Vaccinol (2012) 6:33–8. doi: 10.1016/j.provac.2012.04.006
109. Ghysdael J, Bruck C, Kettmann R, Burny A. Bovine Leukemia Virus. Curr Top Microbiol Immunol (1984) 112:1–19. doi: 10.1007/978-3-642-69677-0_1
110. Hopkins SG, DiGiacomo RF. Natural Transmission of Bovine Leukemia Virus in Dairy and Beef Cattle. Vet Clin North Am Food Anim (1997) 13:107–28. doi: 10.1016/S0749-0720(15)30367-4
111. Martínez C, Gutiérrez G, Alvarez I, Porta N, Lomónaco M, Wigdorovitz A, et al. Egg Yolk Antibodies (Igy) Against Bovine Leukemia Virus. Retrovirology (2014) 11:P46. doi: 10.1186/1742-4690-11-S1-P46
112. Müller S, Schubert A, Zajac J, Dyck T, Oelkrug C IgY antibodies in human nutrition for disease prevention. Nutr J. (2015) 14:109.
113. Collins FS. Cystic Fibrosis: Molecular Biology and Therapeutic Implications. Science (1992) 256:774–9. doi: 10.1126/science.1375392
114. Govan JR, Deretic V. Microbial Pathogenesis in Cystic Fibrosis: Mucoid Pseudomonas Aeruginosa and Burkholderia Cepacia. Microbiol Rev (1996) 60:539–74. doi: 10.1128/MR.60.3.539-574.1996
115. Ulrich M, Worlitzsch D, Viglio S, Siegmann N, Iadarola P, Shute JK, et al. Alveolar Inflammation in Cystic Fibrosis. J Cyst Fibros (2010) 9:217–27. doi: 10.1016/j.jcf.2010.03.001
116. Thomsen K, Christophersen L, Bjarnsholt T, Jensen PØ, Moser C, Høiby N. Anti- Pseudomonas Aeruginosa Igy Antibodies Induce Specific Bacterial Aggregation and Internalization in Human Polymorphonuclear Neutrophils. Infect Immun (2015) 83:2686–93. doi: 10.1128/IAI.02970-14
117. Thomsen K, Christophersen L, Jensen PØ, Bjarnsholt T, Moser C, Høiby N. Anti- Pseudomonas Aeruginosa Igy Antibodies Promote Bacterial Opsonization and Augment the Phagocytic Activity of Polymorphonuclear Neutrophils. Hum Vaccin Immunother (2016) 12:1690–9. doi: 10.1080/21645515.2016.1145848
118. Thomsen K, Christophersen L, Lerche CJ, Holmgaard DB, Calum H, Høiby N, et al. Azithromycin Potentiates Avian Igy Effect Against Pseudomonas Aeruginosa in a Murine Pulmonary Infection Model. Int J Antimicrob Agents (2021) 57:106213. doi: 10.1016/j.ijantimicag.2020.106213
119. Nilsson E, Amini A, Wretlind B, Larsson A. Pseudomonas Aeruginosa Infections are Prevented in Cystic Fibrosis Patients by Avian Antibodies Binding Pseudomonas Aeruginosa Flagellin. J Chromatogr B (2007) 856:75–80. doi: 10.1016/j.jchromb.2007.05.029
120. Nilsson E, Larsson A, Olesen HV, Wejåker P-E, Kollberg H. Good Effect of Igy Against Pseudomonas Aeruginosa Infections in Cystic Fibrosis Patients. Pediatr Pulmonol (2008) 43:892–9. doi: 10.1002/ppul.20875
121. EU Clinical Trials Register. Clinical Trial Results: Prospective Randomized, Placebo- Controlled, Double Blind, Multicenter Study (Phase III) to Evaluate Clinical Efficacy and Safety of Avian Polyclonal Anti-Pseudomonas Antibodies (Igy), in: Prevention of Recurrence of Pseudomonas Aeruginosa Infection in Cystic Fibrosis Patients . Available at: https://www.clinicaltrialsregister.eu/ctr-search/trial/2011-000801-39/results (Accessed April 15, 2021).
122. Chauhan A, Kumar M, Kumar A, Kanchan K. Comprehensive Review on Mechanism of Action, Resistance and Evolution of Antimycobacterial Drugs. Life Sci (2021) 274:119301. doi: 10.1016/j.lfs.2021.119301
123. Sudjarwo SA, Eraiko K, Sudjarwo GW, Koerniasari E. The Activity of Immunoglobulin Y Anti-Mycobacterium Tuberculosis on Proliferation and Cytokine Expression of Rat Peripheral Blood Mononuclear Cells. Pharmacognosy (2017) 9:S5–8. doi: 10.4103/pr.pr_66_17
124. Sudjarwo SA, Eraiko K, Sudjarwo GW, Koerniasari E. The Potency of Chicken Egg Yolk Immunoglobulin (Igy) Specific as Immunotherapy to Mycobacterium Tuberculosis Infection. J Adv Pharm Technol Res (2017) 8:91–6. doi: 10.4103/japtrJAPTR_167_16
125. Shulha JA, Escalante P, Wilson JW. Pharmacotherapy Approaches in Nontuberculous Mycobacteria Infections. Mayo Clin Proc (2019) 94:1567–81. doi: 10.1016/j.mayocp.2018.12.011
126. Fournier PE, Richet H, Weinstein RA. The Epidemiology and Control of Acinetobacter Baumannii in Health Care Facilities. Clin Infect Dis (2006) 42:692–9. doi: 10.1086/500202
127. Shi H, Zhu J, Zou B, Shi L, Du L, Long Y, et al. Effects of Specific Egg Yolk Immunoglobulin on Pan-Drug-Resistant Acinetobacter Baumannii. BioMed Pharmacother (2017) 95:1734–42. doi: 10.1016/j.biopha.2017.09.112
128. Jahangiri A, Owlia P, Rasooli I, Salimian J, Derakhshanifar E, Naghipour Erami A, et al. Specific Egg Yolk Antibodies (Igy) Confer Protection Against Acinetobacter Baumannii in a Murine Pneumonia Model. J Appl Microbiol (2019) 126:624–32. doi: 10.1111/jam.14135
129. Oliver J, Pruzzo C, Vezzulli L, Kaper JB. “Vibrio Species”. In: Doyle MP, Buchanan RL, editors. Food Microbiology: Fundamentals and Frontiers, 4th Edition. Hoboken: Wiley-Blackwell (2012). p. 263–300.
130. Coleman M. “Using Egg Antibodies to Treat Diseases”. In: Sim JS, Nakai S, Guenter W, editors. Egg Nutrition Biotechnology. Wallingford: CABI International (1999). p. 351.
131. Smith PD. “Vaccination Against Vibriosis”. In: Ellis AE, editor. Fish Vaccination. London: Academic Press (1988).
132. Elston R, Lockwood GS. Pathogenesis of Vibriosis in Cultured Juvenile Red Abalone, Haliotis Rufescens Swainson. J Fish Dis (1983) 6:111–28. doi: 10.1111/j.1365-2761.1983.tb00059.x
133. Casandra AB, Searcy-Bernal R, Lizárraga-Partida ML. Pathogenic Effects of Vibrio Alginolyticus on Larvae and Postlarvae of the Red Abalone Haliotis Rufescens. Dis Aquat Organ (1998) 33:119–22. doi: 10.3354/dao033119
134. Liu PC, Chen YC, Huang CY, Lee KK. Virulence of Vibrio Parahaemolyticus Isolated From Cultured Small Abalone, Haliotis Diversicolor Supertexta, With Withering Syndrome. Lett Appl Microbiol (2000) 31:433–7. doi: 10.1046/j.1365-2672.2000.00843.x
135. Wu C-J, Wang H, Chan Y-L, Li T-L. Passive Immune-Protection of Small Abalone Against Vibrio Alginolyticus Infection by Anti-Vibrio Igy-Encapsulated Feed. Fish Shellfish Immunol (2011) 30:1042–8. doi: 10.1016/j.fsi.2011.01.026
136. Arasteh N, Aminirissehei AH, Yousif AN, Albright LJ, Durance TD. Passive Immunization of Rainbow Trout (Oncorhynchus Mykiss) With Chicken Egg Yolk Immunoglobulins (Igy). Aquaculture (2004) 231:23–36. doi: 10.1016/j.aquaculture.2003.11.004
137. Kanno T, Nakai T, Muroga K. Mode of Transmission of Vibriosis Among Ayu Plecoglossus Altivelis. J Aquat Anim Health (1989) 1:2–6. doi: 10.1577/1548-8667(1989)001<0002:MOTOVA>2.3.CO;2
138. Li X, Jing K, Wang X, Li Y, Zhang M, Li Z, et al. Protective Effects of Chicken Egg Yolk Antibody (Igy) Against Experimental Vibrio Splendidus Infection in the Sea Cucumber (Apostichopus Japonicus). Fish Shellfish Immunol (2016) 48:105–11. doi: 10.1016/j.fsi.2015.11.024
139. Gao X, Zhang X, Sun J, Du X, Li X, Zhang Y, et al. Passive Protection Effect of Anti- Vibrio Anguillarum Igy-Encapsulated Feed on Half-Smooth Tongue Sole (Cynoglossus Semilaevi) Against V. Anguillarum. Fish Shellfish Immunol (2016) 56:483–8. doi: 10.1016/j.fsi.2016.07.041
140. Keetanon A, Chuchird N, Bae H-D, Won M-K, Kim S-Y, Elahi F. Effects of Igy Antibody on Growth, Survival, Immune Responses and Protection Against Vibrio Parahaemolyticus in Pacific White Shrimp. J Fish Environ (2021) 45:1–6.
141. Gao X, Chen N, Zhang Y, Zhang X, Bing X. Non-O1 Vibrio Cholerae Pathogen From Cyprinus Carpio and Control With Anti-non-O1 V. Cholerae Egg Yolk Powder (Igy). Aquaculture (2017) 479:69–74. doi: 10.1016/j.aquaculture.2017.05.015
142. Soto-Rodriguez SA, Gomez-Gil B, Lozano-Olvera R, Betancourt-Lozano M, Morales- Covarrubias MS. Field and Experimental Evidence of Vibrio Parahaemolyticus as the Causative Agent of Acute Hepatopancreatic Necrosis Disease of Cultured Shrimp (Litopenaeus Vannamei) in Northwestern Mexico. Appl Environ Microbiol (2015) 81:1689–99. doi: 10.1128/AEM.03610-14
143. Kassim N, Mtenga AB, Shim W-B, Chung DH. The In Vitro and In Vivo Efficacy of Hen Igy Against Vibrio Parahaemolyticus and Vibrio Vulnificus. J Microbiol Biotechnol (2012) 22:1423–31. doi: 10.4014/jmb.1204.04006
144. Hu B, Yang X, Guo E, Zhou P, Xu D, Qi Z, et al. The Preparation and Antibacterial Effect of Egg Yolk Immunoglobulin (Igy) Against the Outer Membrane Proteins of Vibrio Parahaemolyticus. J Sci Food Agric (2019) 99:2565–71. doi: 10.1002/jsfa.9470
145. Soto-Rodriguez SA, Gomez-Gil B, Lozano R, del Rio-Rodríguez R, Diéguez AL, Romalde JL. Virulence of Vibrio Harveyi Responsible for the “Bright-Red” Syndrome in the Pacific White Shrimp Litopenaeus Vannamei. J Invertebr Pathol (2012) 109:307–17. doi: 10.1016/j.jip.2012.01.006
146. Punyokun K, Hongprayoon R, Srisapoome P, Sirinarumitr T. The Production of Anti- Vibrio Harveyi Egg Yolk Immunoglobulin and Evaluation of its Stability and Neutralisation Efficacy. Food Agr Immunol (2013) 24:279–94. doi: 10.1080/09540105.2012.684203
147. Kumaran T, Thirumalaikumar E, Lelin C, Palanikumar P, Michaelbabu M, Citarasu T. Physicochemical Properties of Anti Vibrio Harveyi Egg Yolk Antibody (Igy) and its Immunological Influence in Indian White Shrimp Fenneropenaeus Indicus. Fish Shellfish Immunol (2018) 74:349–62. doi: 10.1016/j.fsi.2017.12.062
148. Kassim N, Mtenga AB, Lee W-G, Kim J-S, Shim W-B, Chung D-H. Production of Coturnix Quail Immunoglobulins Y (Igys) Against Vibrio Parahaemolyticus and Vibrio Vulnificus. Food Sci Biotechnol (2011) 20:1577–83. doi: 10.1007/s10068-011-0218-z
149. Clemens JD, Nair GB, Ahmed T, Qadri F, Holmgren J. Cholera. Lancet (2017) 390:1539– 49. doi: 10.1016/S0140-6736(17)30559-7
150. Faruque SM, Albert MJ, Mekalanos JJ. Epidemiology, Genetics, and Ecology of Toxigenic Vibrio Cholerae. Microbiol Mol Biol Rev (1998) 62:1301–14. doi: 10.1128/MMBR.62.4.1301-1314.1998
151. Hirai K, Arimitsu H, Umeda K, Yokota K, Shen L, Ayada K, et al. Passive Oral Immunization by Egg Yolk Immunoglobulin (Igy) to Vibrio Cholerae Effectively Prevents Cholera. Acta Med Okayama (2010) 64:163–70.
152. Akbari MR, Ahmadi A, Mirkalantari S, Salimian J. Anti-Vibrio Cholerae Igy Antibody Inhibits Mortality in Suckling Mice Model. J Natl Med Assoc (2018) 110:84–7. doi: 10.1016/j.jnma.2017.04.001
153. Suerbaum S, Michetti P. Helicobacter Pylori Infection. N Engl J Med (2002) 347:1175–86. doi: 10.1056/NEJMra020542
154. Wang D, Guo Q, Yuan Y, Gong Y. The Antibiotic Resistance of Helicobacter Pylori to Five Antibiotics and Influencing Factors in an Area of China With a High Risk of Gastric Cancer. BMC Microbiol (2019) 19:152. doi: 10.1186/s12866-019-1517-4
155. Marcus EA, Scott DR. Cell Lysis is Responsible for the Appearance of Extracellular Urease in Helicobacter Pylori. Helicobacter (2001) 6:93–9. doi: 10.1046/j.1523-5378.2001.00014.x
156. Nomura S, Suzuki H, Masaoka T, Kurabayashi K, Ishii H, Kitajima M, et al. Effect of Dietary Anti-Urease Immunoglobulin Y on Helicobacter Pylori Infection in Mongolian Gerbils. Helicobacter (2005) 10:43–52. doi: 10.1111/j.1523-5378.2005.00290.x
157. Shin JH, Yang M, Nam SW, Kim JT, Myung NH, Bang W-G, et al. Use of Egg Yolk- Derived Immunoglobulin as an Alternative to Antibiotic Treatment for Control of Helicobacter Pylori Infection. Clin Diagn Lab Immunol (2002) 9:1061–6. doi: 10.1128/CDLI.9.5.1061-1066.2002
158. Malekshahi ZV, Gargari SLM, Rasooli I, Ebrahimizadeh W. Treatment of Helicobacter Pylori Infection in Mice With Oral Administration of Egg Yolk-Driven Anti-Urec Immunoglobulin. Microb Pathog (2011) 51:366–72. doi: 10.1016/j.micpath.2011.06.002
159. Yang Y-H, Park D, Yang G, Lee SH, Bae DK, Kyung J, et al. Anti-Helicobacter Pylori Effects of Igy From Egg York of Immunized Hens. Lab Anim Res (2012) 28:55–60. doi: 10.5625/lar.2012.28.1.55
160. Dossumbekova A, Prinz C, Mages J, Lang R, Kusters JG, Van Vliet AHM, et al. Helicobacter Pylori Hoph (Oipa) and Bacterial Pathogenicity: Genetic and Functional Genomic Analysis of Hoph Gene Polymorphisms. J Infect Dis (2006) 194:1346–55. doi: 10.1086/508426
161. Franco AT, Johnston E, Krishna U, Yamaoka Y, Israel DA, Nagy TA, et al. Regulation of Gastric Carcinogenesis by Helicobacter Pylori Virulence Factors. Cancer Res (2008) 68:379–87. doi: 10.1158/0008-5472.CAN-07-0824
162. Evans DJ Jr, Evans DG, Takemura T, Nakano H, Lampert HC, Graham DY, et al. Characterization of a Helicobacter Pylori Neutrophil-Activating Protein. Infect Immunol (1995) 63:2213–20. doi: 10.1128/IAI.63.6.2213-2220.1995
163. Xun Z, Li-yuan G, Zhibang Y, Xiaoping C. Protective Effects of Sucralfate on Anti-H.Pylori Vaca Igy in Vivo and In Vitro. Afr J Microbiol Res (2010) 4:1091–9.
164. Xia L-J, Yang Z-B, Huang W, Zhang R-F, Jiang R-J, Jiang Y. Preventive Effect of Hpaa- Vaca Igy on Intragastric Infection With Helicobacter Pylori in Mice. Chin J Biol (2011) 24:34–36+40. (article in Chinese).
165. Suzuki H, Nomura S, Masaoka T, Goshima H, Kamata N, Kodama Y, et al. Effect of Dietary Anti-Helicobacter Pylori-Urease Immunoglobulin Y on Helicobacter Pylori Infection. Aliment Pharmacol Ther (2004) 20:185–92. doi: 10.1111/j.1365-2036.2004.02027.x
166. Horie K, Horie N, Abdou AM, Yang JO, Yun SS, Chun HN, et al. Suppressive Effect of Functional Drinking Yogurt Containing Specific Egg Yolk Immunoglobulin on Helicobacter Pylori in Humans. J Dairy Sci (2004) 87:4073–9. doi: 10.3168/jds.S0022-0302(04)73549-3
167. Costalonga M, Herzberg MC. The Oral Microbiome and the Immunobiology of Periodontal Disease and Caries. Immunol Lett (2014) 162:22–38. doi: 10.1016/j.imlet.2014.08.017
168. Hajishengallis G, Lamont RJ. Beyond the Red Complex and Into More Complexity: The Polymicrobial Synergy and Dysbiosis (Psd) Model of Periodontal Disease Etiology. Mol Oral Microbiol (2012) 27:409–19. doi: 10.1111/j.2041-1014.2012.00663.x
169. Yokoyama K, Sugano N, Rahman AKMS, Oshikawa M, Ito K. Activity of Anti- Porphyromonas Gingivalis Egg Yolk Antibody Against Gingipains In Vitro. Oral Microbiol Immunol (2007) 22:352–5. doi: 10.1111/j.1399-302X.2007.00358.x
170. Hamajima S, Maruyama M, Hijiya T, Hatta H, Abiko Y. Egg Yolk-Derived Immunoglobulin (Igy) Against Porphyromonas Gingivalis 40-Kda Outer Membrane Protein Inhibits Coaggregation Activity. Arch Oral Biol (2007) 52:697–704. doi: 10.1016/j.archoralbio.2006.12.013
171. Rahman S, Galila E, Isoda R, Umeda K, Nguyen V, Kodama Y. Effect of Passive Immunization by Anti-Gingipain Igy on Periodontal Health of Dogs. Vet Sci Dev (2011) 1:35–9. doi: 10.4081/vsd.2011.2204
172. Yokoyama K, Sugano N, Shimada T, Shofiqur RAKM, Ibrahim E-SM, Isoda R, et al. Effects of Egg Yolk Antibody Against Porphyromonas Gingivalis Gingipains in Periodontitis Patients. J Oral Sci (2007) 49:201–6. doi: 10.2334/josnusd.49.201
173. Maeda N, Okamoto M, Kondo K, Ishikawa H, Osada R, Tsurumoto A, et al. Incidence of Prevotella Intermedia and Prevotella Nigrescens in Periodontal Health and Disease. Microbiol Immunol (1998) 42:583–9. doi: 10.1111/j.1348-0421.1998.tb02328.x
174. Schara R, Skaleric E, Seme K, Skaleric U. Prevalence of Periodontal Pathogens and Metabolic Control of Type 1 Diabetes Patients. J Int Acad Periodontol (2013) 15:29–34.
175. Widmer RP. Oral Health of Children With Respiratory Diseases. Paediatr Respir Rev (2010) 11:226–32. doi: 10.1016/j.prrv.2010.07.006
176. Beck J, Garcia R, Heiss G, Vokonas PS, Offenbacher S. Periodontal Disease and Cardiovascular Disease. J Periodontol (1996) 67:1123–37. doi: 10.1902/jop.1996.67.10s.1123
177. Joshipura Kaumudi J, Hung H-C, Rimm Eric B, Willett Walter C, Ascherio A. Periodontal Disease, Tooth Loss, and Incidence of Ischemic Stroke. Stroke (2003) 34:47–52. doi: 10.1161/01.STR.0000052974.79428.0C
178. von Wowern N, Klausen B, Kollerup G. Osteoporosis: A Risk Factor in Periodontal Disease. J Periodontol (1994) 65:1134–8. doi: 10.1902/jop.1994.65.12.1134
179. Offenbacher S, Katz V, Fertik G, Collins J, Boyd D, Maynor G, et al. Periodontal Infection as a Possible Risk Factor for Preterm Low Birth Weight. J Periodontol (1996) 67:1103–13. doi: 10.1902/jop.1996.67.10s.1103
180. Hou YY, Zhen YH, Wang D, Zhu J, Sun DX, Liu XT, et al. Protective Effect of an Egg Yolk-Derived Immunoglobulin (Igy) Against Prevotella Intermedia-Mediated Gingivitis. J Appl Microbiol (2014) 116:1020–7. doi: 10.1111/jam.12419
181. Haraszthy VI, Gerber D, Clark B, Moses P, Parker C, Sreenivasan PK, et al. Characterization and Prevalence of Solobacterium Moorei Associated With Oral Halitosis. J Breath Res (2008) 2:017002. doi: 10.1088/1752-7155/2/1/017002
182. Vancauwenberghe F, Dadamio J, Laleman I, Tornout MV, Teughels W, Coucke W, et al. The Role of Solobacterium Moorei in Oral Malodour. J Breath Res (2013) 7:046006. doi: 10.1088/1752-7155/7/4/046006
183. Pedersen RM, Holt HM, Justesen US. Solobacterium Moorei Bacteremia: Identification, Antimicrobial Susceptibility, and Clinical Characteristics. J Clin Microbiol (2011) 49:2766–8. doi: 10.1128/JCM.02525-10
184. Li X, Liu H, Xu Y, Xu F, Wang L, You J, et al. Chicken Egg Yolk Antibody (Igy) Controls Solobacterium Moorei Under In Vitro and In Vivo Conditions. Appl Biochem Biotechnol (2012) 168:1448–58. doi: 10.1007/s12010-012-9869-3
185. Pianotti R, Lachette S, Dills S. Desulfuration of Cysteine and Methionine by Fusobacterium Nucleatum. J Dent Res (1986) 65:213–7. doi: 10.1177/00220345860650061101
186. Wang F, Qiao W, Bao B, Wang S, Regenstein J, Shi Y, et al. Effect of Igy on Periodontitis and Halitosis Induced by Fusobacterium Nucleatum. J Microbiol Biotechnol (2019) 29:311–20. doi: 10.4014/jmb.1810.10044
187. Loesche WJ. Role of Streptococcus Mutans in Human Dental Decay. Microbiol Rev (1986) 50:353– 80. doi: 10.1128/MR.50.4.353-380.1986
188. Al-Shami I, Al-Shamahy H, Lutf A. Efficacy of Some Antibiotics Against Streptococcus Mutans Associated With Tooth Decay in Children and Their Mothers. Online J Dentistry Oral Health (2019) 2:1–4. doi: 10.33552/OJDOH.2019.02.000530
189. Hatta H, Tsuda K, Ozeki M, Kim M, Yamamoto T, Otake S, et al. Passive Immunization Against Dental Plaque Formation in Humans: Effect of a Mouth Rinse Containing Egg Yolk Antibodies (Igy) Specific to Streptococcus Mutans. Caries Res (1997) 31:268–74. doi: 10.1159/000262410
190. Chang HM, Ouyang R, Chen Y, Chen C. Productivity and Some Properties of Immunoglobulin Specific Against Streptococcus Mutans Serotype C in Chicken Egg Yolk (Igy). J Agric Food Chem (1999) 47:61–6. doi: 10.1021/jf980153u
191. Moreno L, Moreno C, Bilbao V, Acevedo A, Felizzola O, Zerpa N, et al. Producción Y Evaluación In Vitro De Igy Contra Streptococcus Mutans. Rev la Sociedad Venezolana Microbiología (2011) 31:118–23. (article in Spanish).
192. Bachtiar EW, Soejoedono RD, Bachtiar BM, Henrietta A, Farhana N, Yuniastuti M. Effects of Soybean Milk, Chitosan, and Anti-Streptococcus Mutans Igy in Malnourished Rats’ Dental Biofilm and the Igy Persistency in Saliva. Interv Med Appl Sci (2015) 7:118–23. doi: 10.1556/1646.7.2015.3.6
193. Otake S, Nishihara Y, Makimura M, Hatta H, Kim M, Yamamoto T, et al. Protection of Rats Against Dental Caries by Passive Immunization With Hen-Egg-Yolk Antibody (Igy). J Dent Res (1991) 70:162–6. doi: 10.1177/00220345910700030101
194. Fan M, Jiang Q, Bian Z. [the Study on Specific Anti-Streptococcus Mutans Igy Against Dental Caries in Rats]. Hua Xi Kou Qiang Yi Xue Za Zhi (2003) 21:339–41. (article in Chinese).
195. Endang WB, Anggraeni A, Ratna M, Retno DS, Erni P. Effect of Topical Anti- Streptococcus Mutans Igy Gel on Quantity of s. Mutans on Rats’ Tooth Surface. Acta Microbio Imm Hung (2016) 63:159–69. doi: 10.1556/030.63.2016.2.2
196. Chi Z-B, Gao Y-X, Pan Y, Zhang B, Feng X-P. The Inhibitive Effect of Igy Toothpaste Against Oral Streptococcus Mutans. Shanghai Kou Qiang Yi Xue (2004) 13:256–8.
197. Nguyen SV, Icatlo FC, Nakano T, Isogai E, Hirose K, Mizugai H, et al. Anti-Cell-Associated Glucosyltransferase Immunoglobulin Y Suppression of Salivary Mutans Streptococci in Healthy Young Adults. J Am Dent Assoc (2011) 142:943–9. doi: 10.14219/jada.archive.2011.0301
198. Haselbeck AH, Panzner U, Im J, Baker S, Meyer CG, Marks F. Current Perspectives on Invasive Nontyphoidal Salmonella Disease. Curr Opin Infect Dis (2017) 30:498–503. doi: 10.1097/QCO.0000000000000398
199. Broom LJ, Kogut MH. Deciphering Desirable Immune Responses From Disease Models With Resistant and Susceptible Chickens. Poult Sci (2019) 98:1634–42. doi: 10.3382/ps/pey535
200. Lee EN, Sunwoo HH, Menninen K, Sim JS. In Vitro Studies of Chicken Egg Yolk Antibody (Igy) Against Salmonella Enteritidis and Salmonella Typhimurium. Poult Sci (2002) 81:632–41. doi: 10.1093/ps/81.5.632
201. Mine Y. Separation of Salmonella Enteritidis From Experimentally Contaminated Liquid Eggs Using a Hen Igy Immobilized Immunomagnetic Separation System. J Agric Food Chem (1997) 45:3723–37. doi: 10.1021/jf9701998
202. Chalghoumi R, Thewis A, Beckers Y, Marcq C, Portetelle D, Schneider Y. Adhesion and Growth Inhibitory Effect of Chicken Egg Yolk Antibody (Igy) on Salmonella Enterica Serovars Enteritidis and Typhimurium In Vitro. Foodborne Pathog Dis (2009) 6:593–604. doi: 10.1089/fpd.2008.0258
203. Terzolo HR, Sandoval VE, Caffer MI, Terragno R, Alcain A. Agglutination of Hen Egg-Yolk Immunoglobulins (Igy) Against Salmonella Enterica, Serovar Enteritidis. Rev Argen Microbiol (1998) 30:84–92. (article in Spanish).
204. Li X, Yao Y, Wang X, Zhen Y, Thacker PA, Wang L, et al. Chicken Egg Yolk Antibodies (Igy) Modulate the Intestinal Mucosal Immune Response in a Mouse Model of Salmonella Typhimurium Infection. Int Immunopharmacol (2016) 36:305–14. doi: 10.1016/j.intimp.2016.04.036
205. Rahimi S, Shiraz Z, Salehi T, Karimi Torshizi MA, Grimes J. Prevention of Salmonella Infection in Poultry by Specific Egg-Derived Antibody. Int J Poult Sci (2007) 6:230–5. doi: 10.3923/ijps.2007.230.235
206. Khanna S, Pardi DS. Clinical Implications of Antibiotic Impact on Gastrointestinal Microbiota and Clostridium Difficile Infection. Expert Rev Gastroenterol Hepatol (2016) 10:1145–52. doi: 10.1586/17474124.2016.1158097
207. Mulvey G, Dingle T, Fang L, Strecker J, Armstrong G. Therapeutic Potential of Egg Yolk Antibodies for Treating Clostridium Difficile Infection. J Med Microbiol (2011) 60:1181–7. doi: 10.1099/jmm.0.029835-0
208. Pizarro-Guajardo M, Díaz-González F, Álvarez-Lobos M, Paredes-Sabja D. Characterization of Chicken Igy Specific to Clostridium Difficile R20291 Spores and the Effect of Oral Administration in Mouse Models of Initiation and Recurrent Disease. Front Cell Infect Microbiol (2017) 7:365. doi: 10.3389/fcimb.2017.00365
209. Kink JA, Williams JA. Antibodies to Recombinant Clostridium Difficile Toxins a and B are an Effective Treatment and Prevent Relapse of C. Difficile-Associated Disease in a Hamster Model of Infection. Infect Immun (1998) 66:2018–25. doi: 10.1128/IAI.66.5.2018-2025.1998
210. Zhang S, Xing P, Guo G, Liu H, Lin D, Dong C, et al. Development of Microbeads of Chicken Yolk Antibodies Against Clostridium Difficile Toxin a for Colonic- Specific Delivery. Drug Delivery (2016) 23:1940–7. doi: 10.3109/10717544.2015.1022836
211. Xing P, Shi Y, Dong C, Liu H, Cheng Y, Sun J, et al. Colon-Targeted Delivery of Igy Against Clostridium Difficile Toxin a and B by Encapsulation in Chitosan-Ca Pectinate Microbeads. AAPS PharmSciTech (2017) 18:1095–103. doi: 10.1208/s12249-016-0656-2
212. World Health Organization. Campylobacter (2021). Available at: https://www.who.int/news-room/fact-sheets/detail/campylobacter (Accessed April 15, 2021).
213. Young KT, Davis LM, DiRita VJ. Campylobacter Jejuni: Molecular Biology and Pathogenesis. Nat Rev Microbiol (2007) 5:665–79. doi: 10.1038/nrmicro1718
214. Alfredson DA, Korolik V. Antibiotic Resistance and Resistance Mechanisms in Campylobacter Jejuni and Campylobacter Coli. FEMS Microbiol Lett (2007) 277:123–32. doi: 10.1111/j.1574-6968.2007.00935.x
215. Al-Adwani SR, Crespo R, Shah DH. Production and Evaluation of Chicken Egg-Yolk- Derived Antibodies Against Campylobacter Jejuni Colonization-Associated Proteins. Foodborne Pathog Dis (2013) 10:624–31. doi: 10.1089/fpd.2012.1313
216. Thibodeau A, Fravalo P, Perron A, Laurent-Lewandowski S, Letellier A. Production and Characterization of Anti-Campylobacter Jejuni Igy Derived From Egg Yolks. Acta Vet Scand (2017) 59:80. doi: 10.1186/s13028-017-0346-4
217. Hochel I, Viochna D, Škvor J, Musil M. Development of an Indirect Competitive ELISA for Detection of Campylobacter Jejuni Subsp. Jejuni O: 23 in Foods. Folia Microbiol (2004) 49:579– 86. doi: 10.1007/BF02931537
218. Horák D, Hochel I. Magnetic Poly(Glycidyl Methacrylate) Microspheres for Campylobacter Jejuni Detection in Food. e-Polymers (2005) 5:1–12. doi: 10.1515/epoly.2005.5.1.643
219. Tsubokura K, Berndtson E, Bogstedt A, Kaijser B, Kim M, Ozeki M, et al. Oral Administration of Antibodies as Prophylaxis and Therapy in Campylobacter Jejuni- Infected Chickens. Clin Exp Immunol (1997) 108:451–5. doi: 10.1046/j.1365-2249.1997.3901288.x
220. Hermans D, Van Steendam K, Verbrugghe E, Verlinden M, Martel A, Seliwiorstow T, et al. Passive Immunization to Reduce Campylobacter Jejuni Colonization and Transmission in Broiler Chickens. Vet Res (2014) 45:27. doi: 10.1186/1297-9716-45-27
221. Nothaft H, Davis B, Lock YY, Perez-Munoz ME, Vinogradov E, Walter J, et al. Engineering the Campylobacter Jejuni N-Glycan to Create an Effective Chicken Vaccine. Sci Rep (2016) 6:1–12. doi: 10.1038/srep26511
222. Chintoan-Uta C, Cassady-Cain R, Stevens M. Evaluation of Flagellum-Related Proteins Flid and Fspa as Subunit Vaccines Against Campylobacter Jejuni Colonisation in Chickens. Vaccine (2016) 34:1739–43. doi: 10.1016/j.vaccine.2016.02.052
223. Neal-McKinney JM, Samuelson DR, Eucker TP, Nissen MS, Crespo R. Konkel ME Reducing Campylobacter Jejuni Colonization of Poultry Via Vaccination. PloS One (2014) 9:e114254. doi: 10.1371/journal.pone.0114254
224. Kaper JB, Nataro JP, Mobley HL. Pathogenic Escherichia Coli. Nat Rev Microbiol (2004) 2:123–40. doi: 10.1038/nrmicro818
225. Nataro JP, Kaper JB. Diarrheagenic Escherichia Coli. Clin Microbiol Rev (1998) 11:142–201. doi: 10.1128/CMR.11.1.142
226. Sáenz Y, Briñas L, Domínguez E, Ruiz J, Zarazaga M, Vila J, et al. Mechanisms of Resistance in Multiple-Antibiotic-Resistant Escherichia Coli Strains of Human, Animal, and Food Origins. Antimicrob Agents Chemother (2004) 48:3996–4001. doi: 10.1128/AAC.48.10.3996-4001.2004
227. Sunwoo HH, Wang WW, Sim JS. Detection of Escherichia Coli O157: H7 Using Chicken Immunoglobulin Y. Immunol Lett (2006) 106:191–3. doi: 10.1016/j.imlet.2006.05.005
228. Parma YR, Chacana PA, Lucchesi PMA, Roge A, Granobles Velandia CV, Krüger A, et al. Detection of Shiga Toxin-Producing Escherichia Coli by Sandwich Enzyme-Linked Immunosorbent Assay Using Chicken Egg Yolk Igy Antibodies. Front Cell Infect Microbiol (2012) 2:84. doi: 10.3389/fcimb.2012.00084
229. Akita EM, Li-Chan ECY, Nakai S. Neutralization of Enterotoxigenic Escherichia Coli Heat-Labile Toxin by Chicken Egg Yolk Immunoglobulin Y and its Antigen-Binding Fragments. Food Agr Immunol (1998) 10:161–72. doi: 10.1080/09540109809354979
230. Mahdavi A, Rahmani H, Nili N, Samie A, Soleimania S. Chicken Egg Yolk Antibody (Igy) Powder Against Escherichia Coli O78:K80. J Anim Vet Adv (2010) 9:366–73. doi: 10.3923/javaa.2010.366.373
231. Meenatchisundaram S, Shanmugam V, Anjali V. Development of Chicken Egg Yolk Antibodies Against Streptococcus Mitis–Purification and Neutralizing Efficacy. J Basic Clin Pharm (2011) 2:109–14.
232. Tobias FL, Garcia LNN, Kanashiro MM, Medina-Acosta E, Brom-de-Luna JG, Costa de Almeida CM, et al. Growth Inhibition of Staphylococcus Aureus and Escherichia Coli Strains by Neutralizing Igy Antibodies From Ostrich Egg Yolk. Braz J Microbiol (2012) 43:544–51. doi: 10.1590/S1517-83822012000200015
233. Kariyawasam S, Wilkie BN, Gyles CL. Resistance of Broiler Chickens to Escherichia Coli Respiratory Tract Infection Induced by Passively Transferred Egg-Yolk Antibodies. Vet Microbiol (2004) 98:273–84. doi: 10.1016/j.vetmic.2003.10.022
234. Wang Z, Li J, Li J, Li Y, Wang W, Wang Q, et al. Protective Effect of Chicken Egg Yolk Immunoglobulins (Igy) Against Enterotoxigenic Escherichia Coli K88 Adhesion in Weaned Piglets. BMC Vet Res (2019) 15:234. doi: 10.1186/s12917-019-1958-x
235. Li X-Y, Jin L-J, Uzonna JE, Li S-Y, Liu J-J, Li H-Q, et al. Chitosan–Alginate Microcapsules for Oral Delivery of Egg Yolk Immunoglobulin (Igy): In Vivo Evaluation in a Pig Model of Enteric Colibacillosis. Vet Immunol Immunopathol (2009) 29:132–6. doi: 10.1016/j.vetimm.2008.12.016
236. Alustiza F, Bellingeri R, Picco N, Motta C, Grosso MC, Barbero CA, et al. Igy Against Enterotoxigenic Escherichia Coli Administered by Hydrogel-Carbon Nanotubes Composites to Prevent Neonatal Diarrhoea in Experimentally Challenged Piglets. Vaccine (2016) 34:3291–7. doi: 10.1016/j.vaccine.2016.05.004
237. Gaensbauer JT, Melgar MA, Calvimontes DM, Lamb MM, Asturias EJ, Contreras-Roldan IL, et al. Efficacy of a Bovine Colostrum and Egg-Based Intervention in Acute Childhood Diarrhoea in Guatemala: A Randomised, Double- Blind, Placebo-Controlled Trial. BMJ Glob Health (2017) 2:e000452. doi: 10.1136/bmjgh-2017-000452
238. Lowy FD. Staphylococcus Aureus Infections. N Engl J Med (1998) 339:520–32. doi: 10.1056/NEJM199808203390806
239. Henderson A, Nimmo GR. Control of Healthcare- and Community-Associated Mrsa: Recent Progress and Persisting Challenges. Br Med Bull (2018) 125:25–41. doi: 10.1093/bmb/ldx046
240. Le Loir Y, Baron F, Gautier M. Staphylococcus Aureus and Food Poisoning. Genet Mol Res (2003) 2:63–76.
241. Smith TL, Pearson ML, Wilcox KR, Cruz C, Lancaster MV, Robinson-Dunn B, et al. Emergence of Vancomycin Resistance in Staphylococcus Aureus. Glycopeptide-Intermediate Staphylococcus Aureus Working Group. N Engl J Med (1999) 340:493–501. doi: 10.1056/NEJM199902183400701
242. Klevens RM, Morrison MA, Nadle J, Petit S, Gershman K, Ray S, et al. Invasive Methicillin-Resistant Staphylococcus Aureus Infections in the United States. JAMA (2007) 298:1763–71. doi: 10.1001/jama.298.15.1763
243. Chambers HF. The Changing Epidemiology of Staphylococcus Aureus? Emerg Infect Dis (2001) 7:178–82. doi: 10.3201/eid0702.010204
244. Saveli CC, Belknap RW, Morgan SJ, Price CS. The Role of Prophylactic Antibiotics in Open Fractures in an Era of Community-Acquired Methicillin-Resistant Staphylococcus Aureus. Orthopedics (2011) 34:611–6. doi: 10.3928/01477447-20110627-25
245. Mizutani N, Sugita-Konishi Y, Omoe K, Shinagawa K, Kawakami H, Kanno S, et al. Advantages of Immunoglobulin Y for the Detection of Staphylococcal Enterotoxin a in a Double-Antibody Sandwich Enzyme-Linked Immunosorbent Assay. Int J Food Sci Technol (2012) 47:155–9. doi: 10.1111/j.1365-2621.2011.02821.x
246. Jin W, Yamada K, Ikami M, Kaji N, Tokeshi M, Atsumi Y, et al. Application of Igy to Sandwich Enzyme-Linked Immunosorbent Assays, Lateral Flow Devices, and Immunopillar Chips for Detecting Staphylococcal Enterotoxins in Milk and Dairy Products. J Microbiol Methods (2013) 92:323–31. doi: 10.1016/j.mimet.2013.01.001
247. Reddy PN, Nagaraj S, Sripathy MH, Batra HV. Use of Biotin-Labeled Igy Overcomes Protein a Interference in Immunoassays Involving Staphylococcus Aureus Antigens. Ann Microbiol (2015) 65:1915–22. doi: 10.1007/s13213-014-1029-2
248. Reddy P, Ramlal S, Sripathy MH, Batra HV. Development and Evaluation of Igy Immunocapture PCR ELISA for Detection of Staphylococcus Aureus Enterotoxin a Devoid of Protein a Interference. J Immunol Methods (2014) 408:114–22. doi: 10.1016/j.jim.2014.05.012
249. Reddy PK, Shekar A, Kingston JJ, Sripathy MH, Batra H. Evaluation of Igy Capture ELISA for Sensitive Detection of Alpha Hemolysin of Staphylococcus Aureus Without Staphylococcal Protein a Interference. J Immunol Methods (2013) 391:31–8. doi: 10.1016/j.jim.2013.02.004
250. Vinayaka AC, Muthukumar SP, Thakur MS. Avian Antibodies for Staphylococcal Enterotoxin B as an Efficient Tool for FRET-Based Fluoroimmunosensor. Bionanoscience (2013) 3:232–40. doi: 10.1007/s12668-013-0101-x
251. Yamada K, Wanchun J, Ohkura T, Murai A, Hayakawa R, Kinoshita K, et al. Detection of Methicillin-Resistant Staphylococcus Aureus Using a Specific Anti-Pbp2a Chicken Igy Antibody. Jpn J Infect Dis (2013) 66:103–8. doi: 10.7883/yoken.66.103
252. Nagaraj S, Ramlal S, Kingston J, Batra H. Development of Igy Based Sandwich ELISA for the Detection of Staphylococcal Enterotoxin G (SEG), an Egc Toxin. Int J Food Microbiol (2016) 237:136–41. doi: 10.1016/j.ijfoodmicro.2016.08.009
253. Kota RK, Srirama K, Reddy PN. Igy Antibodies of Chicken do Not Bind Staphylococcal Binder of Immunoglobulin (Sbi) From Staphylococcus Aureus. Ann Microbiol (2019) 69:531–40. doi: 10.1007/s13213-019-1441-8
254. Guimarães MCC, Amaral LG, Rangel LBA, Silva IV, Matta CGF, de Matta MFR. Growth Inhibition of Staphylococcus Aureus by Chicken Egg Yolk Antibodies. Arch Immunol Ther Exp (Warsz) (2009) 57:377–82. doi: 10.1007/s00005-009-0041-x
255. Jagadeeswari M, Dhanabalan R, Saranya G. Detection of Staphylococcus Aureus From Clinical and Sub Clinical Bovine Mastitis Milk Samples Using Chicken Egg Yolk Antibodies (Igy). Int J Appl Pure Sci Agric (2015) 7:1–6.
256. Baselga R, Albizu I. Amorena B Staphylococcus Aureus Capsule and Slime as Virulence Factors in Ruminant Mastitis. A Review. Vet Microbiol (1994) 39:195–204. doi: 10.1016/0378-1135(94)90157-0
257. Hwang CY, Pak SI, Han HR. Effects of Autogenous Toxoid-Bacterin in Lactating Cows With Staphylococcus Aureus Subclinical Mastitis. J Vet Sci (2000) 62:875–80. doi: 10.1292/jvms.62.875
258. Zhen YH, Jin LJ, Guo J, Li XY, Li Z, Fang R, et al. Characterization of Specific Egg Yolk Immunoglobulin (Igy) Against Mastitis-Causing Staphylococcus Aureus. J Appl Microbiol (2008) 105:1529–35. doi: 10.1111/j.1365-2672.2008.03920.x
259. Mahenthiran R, Naveen S, Diraviyam T, Kumar KR, Michael A. Generation Purification and Neutralization Potential of Chicken Egg Yolk Antibodies Igy Against Mastitis Causing Escherichia Coli and Staphylococcus Aureus. Int J Pharma Bio Sci (2013) 4:687–96.
260. Wang L-H, Li X-Y, Jin L-J, You J-S, Zhou Y, Li S-Y, et al. Characterization of Chicken Egg Yolk Immunoglobulins (Igys) Specific for the Most Prevalent Capsular Serotypes of Mastitis-Causing Staphylococcus Aureus. Vet Microbiol (2011) 149:415–21. doi: 10.1016/j.vetmic.2010.11.029
261. Iqbal M, Ahmad T, Yousaf A, Sajjad-ur R, Saqib M, et al. In Vivo Comparison of Specific Activity of Egg Yolk Immunoglobulins (Igy) and Antibiotic Against Staphylococcus Aureus Causing Mastitis in Buffaloes (Bubalus Bubalis). Buffalo Bull (2013) 32:1017–20.
262. Kluytmans J, van Belkum A, Verbrugh H. Nasal Carriage of Staphylococcus Aureus: Epidemiology, Underlying Mechanisms, and Associated Risks. Clin Microbiol Rev (1997) 10:505–20. doi: 10.1128/CMR.10.3.505
263. Jernigan JA, Hatfield KM, Wolford H, Nelson RE, Olubajo B, Reddy SC, et al. Multidrug-Resistant Bacterial Infections in U.s. Hospitalized Patients, 2012-2017. N Engl J Med (2020) 382:1309–19. doi: 10.1056/NEJM0Q1914433
264. Merino S, Rubires X, Knøchel S, Tomás JM. Emerging Pathogens: Aeromonas Spp. Int J Food Microbiol (1995) 28:157–68. doi: 10.1016/0168-1605(95)00054-2
265. Parker JL, Shaw JG. Aeromonas Spp. Clinical Microbiology and Disease. J Infect (2011) 62:109–18. doi: 10.1016/j.jinf.2010.12.003
266. Altwegg M, Geiss HK, Freij BJ. Aeromonas as a Human Pathogen. Crit Rev Microbiol (1989) 16:253–86. doi: 10.3109/10408418909105478
267. Fernandes DC, Eto SF, Funnicelli MIG, Fernandes CC, Charlie-Silva I, Belo MAA, et al. Immunoglobulin Y in the Diagnosis of Aeromonas Hydrophila Infection in Nile Tilapia (Oreochromis Niloticus). Aquaculture (2019) 500:576–85. doi: 10.1016/j.aquaculture.2018.10.045
268. Fernandes DC, Eto SF, Moraes AC, Prado EJR, Medeiros ASR, Belo MAA, et al. Phagolysosomal Activity of Macrophages in Nile Tilapia (Oreochromis Niloticus) Infected In Vitro by Aeromonas Hydrophila: Infection and Immunotherapy. Fish Shellfish Immunol (2019) 87:51–61. doi: 10.1016/j.fsi.2018.12.074
269. Qin Z, Sarath Babu V, Li N, Fu T, Li J, Yi L, et al. Protective Effects of Chicken Egg Yolk Immunoglobulins (Igy) Against Experimental Aeromonas Hydrophila Infection in Blunt Snout Bream (Megalobrama Amblycephala). Fish Shellfish Immunol (2018) 78:26–34. doi: 10.1016/j.fsi.2018.04.001
270. Li X-l, Shuai J-b, Fang W-h. Protection of Carassius Auratus Gibelio Against Infection by Aeromonas Hydrophila Using Specific Immunoglobulins From Hen Egg Yolk. J Zhejiang Univ Sci (2006) 7:922–8. doi: 10.1631/jzus.2006.B0922
271. Gan H, He H, Sato A, Hatta H, Nakao M, Somamoto T. Ulcer Disease Prophylaxis in Koi Carp by Bath Immersion With Chicken Egg Yolk Containing Anti-Aeromonas Salmonicida Igy. Res Vet Sci (2015) 99:82–6. doi: 10.1016/j.rvsc.2015.01.016
272. Kurokawa I, Danby FW, Ju Q, Wang X, Xiang LF, Xia L, et al. New Developments in Our Understanding of Acne Pathogenesis and Treatment. Exp Dermatol (2009) 18:821–32. doi: 10.1111/j.1600-0625.2009.00890.x
273. Revathy J, Karthika S, Sentila R, Michael A. In Vitro Evaluation of the Efficacy of Chicken Egg Yolk Antibodies (Igy) Generated Against Propionibacterium Acnes. Int J Cosmet Sci (2014) 36:68–73. doi: 10.1111/ics.12097
274. Ruhnke M. “Skin and Mucous Membrane Infections”. Candida candidiasis Washington DC: ASM Press (2002), 307–25.
275. Calderone RA, Fonzi WA. Virulence Factors of Candida Albicans. Trends Microbiol (2001) 9:327–35. doi: 10.1016/S0966-842X(01)02094-7
276. Abdelnoor AM, Rahal E, Zeidan JA, Halas YA, Sleiman F. Preparation of Anti-Candida Albicans Antibodies in an Egg-Laying Hen and Their Protective Efficacy in Mice. J Appl Res (2006) 6:62–8.
277. Fujibayashi T, Nakamura M, Tominaga A, Satoh N, Kawarai T, Narisawa N, et al. Effects of Igy Against Candida Albicans and Candida Spp. Adherence and Biofilm Formation. Jpn J Infect Dis (2009) 62:337–42.
278. Wang X, Fan B, Liu L, Hu X, Li R, Wei Y, et al. In Vitro Inhibition of OralCandida Albicans by Chicken Egg Yolk Antibody (Igy). Mycopathologia (2008) 165:381. doi: 10.1007/s11046-008-9097-0
279. Kamikawa Y, Fujisaki J, Nagayama T, Kawasaki K, Hirabayashi D, Hamada T, et al. Use of Candida-Specific Chicken Egg Yolk Antibodies to Inhibit the Adhering of Candida to Denture Base Materials: Prevention of Denture Stomatitis. Gerodontology (2016) 33:342–7. doi: 10.1111/ger.12163
280. Ibrahim E-SM, Rahman AS, Isoda R, Umeda K, Van Sa N, Kodama Y. In Vitro and In Vivo Effectiveness of Egg Yolk Antibody Against Candida Albicans (Anti-CA Igy). Vaccine (2008) 26:2073–80. doi: 10.1016/j.vaccine.2008.02.046
281. Bern C, Kjos S, Yabsley MJ, Montgomery SP. Trypanosoma Cruzi and Chagas’ Disease in the United States. Clin Microbiol Rev (2011) 24:655–81. doi: 10.1128/CMR.00005-11
282. Brener Z. Biology of Trypanosoma Cruzi. Annu Rev Microbiol (1973) 27:347–82. doi: 10.1146/annurev.mi.27.100173.002023
283. Castro JA, deMecca MM, Bartel LC. Toxic Side Effects of Drugs Used to Treat Chagas’ Disease (American Trypanosomiasis). Hum Exp Toxicol (2006) 25:471–9. doi: 10.1191/0960327106het653oa
284. Grando TH, Baldissera MD, de Sá MF, do Carmo GM, Porto BCZ, Aguirre GS, et al. Avian Antibodies (Igy) Against Trypanosoma Cruzi: Purification and Characterization Studies. J Immunol Methods (2017) 449:56– 61. doi: 10.1016/j.jim.2017.07.002
285. Grando TH, Baldissera MD, Do Carmo G, Oliveira CB, Santi ET, Doleski PH, et al. Ecto-Enzymes Activities in Splenic Lymphocytes of Mice Experimentally Infected by Trypanosoma Cruzi and Treated With Specific Avian Immunoglobulins: An Attempt to Improve the Immune Response. Mol Cell Biochem (2018) 448:9–15. doi: 10.1007/s11010-018-3308-x
286. Luckins A. Trypanosoma Evansi in Asia. Parasitol Today (1988) 4:137–42. doi: 10.1016/0169-4758(88)90188-3
287. Trail J, d’Ieteren G, Feron A, Kakiese O, Mulungo M, Pelo M. Effect of Trypanosome Infection, Control of Parasitaemia and Control of Anaemia Development on Productivity of N’dama Cattle. Acta Trop (1990) 48:37–45. doi: 10.1016/0001-706X(90)90063-6
288. Joshi PP, Shegokar VR, Powar RM, Herder S, Katti R, Salkar HR, et al. Human Trypanosomiasis Caused by Trypanosoma Evansi in India: The First Case Report. Am J Trop Med (2005) 73:491–5. doi: 10.4269/ajtmh.2005.73.491
289. Herrera HM, Dávila A, Norek A, Abreu U, Souza S, D’Andrea PS, et al. Enzootiology of Trypanosoma Evansi in Pantanal, Brazil. Vet Parasitol (2004) 125:263–75. doi: 10.1016/j.vetpar.2004.07.013
290. Silva R, Rivera Davila AM, Seidl A, Ramirez L. Trypanosoma Evansi E Trypanosoma Vivax: Biologia, Diagnóstico E Controle. Corumbá: Embrapa Pantanal-Livro Científico (ALICE) (2002). (book in Portuguese).
291. Sampaio LCL, Baldissera MD, Sagrilo M, Heres T, Oliveira CB, Stainki DR, et al. In Vitro Cytotoxicity and Genotoxicity of Chicken Egg Yolk Antibodies (Igy) Against Trypanosoma Evansi in Human Lymphocytes. Int J Pharm Pharm Sci (2014) 6:167–70.
292. Sampaio LCL, Baldissera MD, Grando TH, Gressler LT, de Menezes Capeleto D, de Sa MF, et al. Production, Purification and Therapeutic Potential of Egg Yolk Antibodies for Treating Trypanosoma Evansi Infection. Vet Parasitol (2014) 204:96–103. doi: 10.1016/j.vetpar.2014.05.032
294. Pinto DJ, Vinayak S. Cryptosporidium: Host-Parasite Interactions and Pathogenesis. Curr Clin Microbiol Rep (2021) 8:62–7. doi: 10.1007/s40588-021-00159-7
295. Perryman LE, Kegerris K, Mason PH. Effect of Orally Administered Monoclonal Antibody on Persistent Cryptosporidium Parvum Infection in Scid Mice. Infect Immun (1993) 61:4906–8. doi: 10.1128/IAI.61.11.4906-4908.1993
296. Nord J, Ma P, Dijohn D, Tzipori S, Tacket CO. Treatment With Bovine Hyperimmune Colostrum of Cryptosporidial Diarrhea in AIDS Patients. AIDS (1990) 4:581–4. doi: 10.1097/00002030-199006000-00015
297. Ungar BL, Ward DJ, Fayer R, Quinn CA. Cessation of Cryptosporidium-Associated Diarrhea in an Acquired Immunodeficiency Syndrome Patient After Treatment With Hyperimmune Bovine Colostrum. Gastroenterology (1990) 98:486–9. doi: 10.1016/0016-5085(90)90842-O
298. Hashemzadeh Farhang H, Shahbazi P. Production of Specific Egg Yolk Antibody (Igy) Against Cryptosporidium Parvum Oocysts. Med Lab J (2016) 10:38–42.
299. Kobayashi C, Yokoyama H, Van Nguyen S, Kodama Y, Kimata T, Izeki M. Effect of Egg Yolk Antibody on Experimental Cryptosporidium Parvum Infection in Scid Mice. Vaccine (2004) 23:232–5. doi: 10.1016/j.vaccine.2004.05.034
300. Omidian Z, Ebrahimzadeh E, Shahbazi P, Asghari Z, Shayan P. Application of Recombinant Cryptosporidium Parvum P23 for Isolation and Prevention. Parasitol Res (2014) 113:229–37. doi: 10.1007/s00436-013-3648-0
301. Shahbazi P, Shayan P, Ebrahimzadeh E, Rahbari S. Specific Egg Yolk Antibody Against Recombinant Cryptosporidium Parvum P23 Protein. Iran J Parasitol (2009) 4:15–24. doi: 10.1007/S00436-013-3648-0
302. Miura VC, Aoki SM, Peitl Junior P, Pires LC, Dalmagro P, Nakamura AA, et al. Evaluation of Recombinant Cryptosporidium Hominis Gp60 Protein and Anti-GP60 Chicken Polyclonal Igy for Research and Diagnostic Purposes. Rev Bras Parasitol V (2017) 26:205–10. doi: 10.1590/s1984-29612017032
303. McDonald V, Shirley M. Past and Future: Vaccination Against Eimeria. Parasitology (2009) 136:1477. doi: 10.1017/S0031182009006349
304. Stephan B, Rommel M, Daugschies A, Haberkorn A. Studies of Resistance to Anticoccidials in Eimeria Field Isolates and Pure Eimeria Strains. Vet Parasitol (1997) 69:19–29. doi: 10.1016/S0304-4017(96)01096-5
305. Danforth H. Use of Live Oocyst Vaccines in the Control of Avian Coccidiosis: Experimental Studies and Field Trials. Int J Parasitol (1998) 28:1099–109. doi: 10.1016/S0020-7519(98)00078-2
306. Wallach M, Smith NC, Petracca M, Miller CM, Eckert J, Braun R. Eimeria Maxima Gametocyte Antigens: Potential Use in a Subunit Maternal Vaccine Against Coccidiosis in Chickens. Vaccine (1995) 13:347–54. doi: 10.1016/0264-410X(95)98255-9
307. Wallach MG, Ashash U, Michael A, Smith NC. Field Application of a Subunit Vaccine Against an Enteric Protozoan Disease. PloS One (2008) 3:e3948. doi: 10.1371/journal.pone.0003948
308. Lillehoj H, Lee S, Park D, Jang S, Lillehoj E, Morales A, et al. Induction of Passive Immunity in Broiler Chickens Against Eimeria Acervulina by Hyperimmune Egg Yolk Igy. Poult Sci (2010) 88:562–66. doi: 10.3382/ps.2008-00340
309. Lee S, Lillehoj H, Park D, Jang S, Morales A, Garcia D, et al. Protective Effect of Hyperimmune Egg Yolk Igy Antibodies Against Eimeria Tenella and Eimeria Maxima Infections. Vet Parasitol (2009) 163:123–6. doi: 10.1016/j.vetpar.2009.04.020
310. Xu J, Ren C, Wang S, Liu D, Cao L, Tao J. Protection Efficacy of Multivalent Egg Yolk Immunoglobulin Against Eimeria Tenella Infection in Chickens. Iran J Parasitol (2013) 8:449–58.
311. Lillehoj H, Ngyyen S, Donahue J, Yokohama A, Kodama Y. Passive Protection Against Two Eimeria Species in Chickens by Orally Administered Antibodies Specific for a Single Eimeria Protein. Am Assoc Veterinary Parasitologists Proc (2004) 21.
312. Qin M, Tang X, Yin G, Liu X, Suo J, Tao G, et al. Chicken Igy Fc Expressed by Eimeria Mitis Enhances the Immunogenicity of E. Mitis. Parasit Vectors (2016) 9:164. doi: 10.1186/s13071-016-1451-3
313. Zhang X, Calvert RA, Sutton BJ, Doré KA. Igy: A Key Isotype in Antibody Evolution. Biol Rev (2017) 92:2144–56. doi: 10.1111/brv.12325
314. Carlander D, Kollberg H, Wejåker P-E, Larsson A. Peroral Immunotheraphy With Yolk Antibodies for the Prevention and Treatment of Enteric Infections. Immunol Res (2000) 21:1–6. doi: 10.1385/IR:21:1:1
315. Kollberg H, Carlander D, Olesen H, Wejåker PE, Johannesson M, Larsson A. Oral Administration of Specific Yolk Antibodies (Igy) may Prevent Pseudomonas Aeruginosa Infections in Patients With Cystic Fibrosis: A Phase I Feasibility Study. Pediatr Pulmonol (2003) 35:433–40. doi: 10.1002/ppul.10290
316. Takeuchi S, Motohashi J, Kimori H, Nakagawa Y, Tsurumoto A. Effects of Oral Moisturising Gel Containing Egg Yolk Antibodies Against Candida Albicans in Older People. Gerodontology (2016) 33:128–34. doi: 10.1111/ger.12139
317. Nguyen SV, Nguyen MTH, Tran BC, Ho MTQ, Umeda K, Rahman S. Evaluation of Lozenges Containing Egg Yolk Antibody Against Porphyromonas Gingivalis Gingipains as an Adjunct to Conventional non-Surgical Therapy in Periodontitis Patients: A Randomized Controlled Clinical Trial. J Periodontol (2018) 89:1334–9. doi: 10.1002/JPER.18-0037
318. Chiou VY. The Development of Igy(Deltafc) Antibody Based Neuro Toxin Antivenoms and the Study on Their Neutralization Efficacies. Clin Toxicol (Phila) (2008) 46:539–44. doi: 10.1080/15563650701771973
319. da Rocha DG, Fernandez JH, de Almeida CMC, da Silva CL, Magnoli FC, da Silva OÉ, et al. Development Of Igy Antibodies Against Anti-Snake Toxins Endowed With Highly Lethal Neutralizing Activity. Eur J Pharm Sci (2017) 106:404–12. doi: 10.1016/j.ejps.2017.05.069
320. Sifi A, Adi-Bessalem S, Laraba-Djebari F. Development of a New Approach of Immunotherapy Against Scorpion Envenoming: Avian Igys an Alternative to Equine Iggs. Int Immunopharmacol (2018) 61:256–65. doi: 10.1016/j.intimp.2018.06.013
321. Leiva CL, Geoghegan P, Lammer M, Cangelosi A, Mariconda V, Celi AB, et al. In Vivo Neutralization of Bee Venom Lethality by Igy Antibodies. Mol Immunol (2021) 135:183–90. doi: 10.1016/j.molimm.2021.04.015
322. Lee J, Kang H-E, Woo H-J. Stability of Orally Administered Immunoglobulin in the Gastrointestinal Tract. J Immunol Methods (2012) 384:143–7. doi: 10.1016/j.jim.2012.06.001
323. Lee KA, Chang SK, Lee YJ, Lee JH, Koo NS. Acid Stability of Anti-Helicobacter Pyroli Igy in Aqueous Polyol Solution. J Biochem Mol Biol (2002) 35:488–93. doi: 10.5483/BMBRep.2002.35.5.488
324. Suzuki T, Kanbara N, Tomono T, Hayashi N, Shinohara I. Physicochemical and Biological Properties of Poly (Ethylene Glycol)-Coupled Immunoglobulin G. Biochim Biophys Acta (1984) 788:248–55. doi: 10.1016/0167-4838(84)90268-1
325. Shimizu M, Nagashima H, Hashimoto K, Suzuki T. Egg Yolk Antibody (Ig Y) Stability in Aqueous Solution With High Sugar Concentrations. J Food Sci (1994) 59:763–5. doi: 10.1111/j.1365-2621.1994.tb08122.x
326. Shimizu M, Miwa Y, Hashimoto K, Goto A. Encapsulation of Chicken Egg Yolk Immunoglobulin G (Igy) by Liposomes. Biosci Biotechnol Biochem (1993) 57:1445–9. doi: 10.1271/bbb.57.1445
327. Chang HM, Lee YC, Chen C, Tu YY. Microencapsulation Protects Immunoglobulin in Yolk (Igy) Specific Against Helicobacter Pylori Urease. J Food Sci (2002) 67:15–20. doi: 10.1111/j.1365-2621.2002.tb11351.x
328. Trott D, Yang M, Utterback P, Utterback C, Koelkeback K, Cook M. Utility of Spent Single Comb White Leghorn Hens for Production of Polyclonal Egg Yolk Antibody. J Appl Poult Res (2009) 18:679–89. doi: 10.3382/japr.2008-00142
329. Pauly D, Dorner M, Zhang X, Hlinak A, Dorner B, Schade R. Monitoring of Laying Capacity, Immunoglobulin Y Concentration, and Antibody Titer Development in Chickens Immunized With Ricin and Botulinum Toxins Over a Two-Year Period. Poult Sci (2009) 88:281–90. doi: 10.3382/ps.2008-00323
330. Schade R, Bürger W, Schöneberg T, Schniering A, Schwarzkopf C, Hlinak A, et al. Avian Egg Yolk Antibodies. The Egg Laying Capacity of Hens Following Immunisation With Antigens of Different Kind and Origin and the Efficiency of Egg Yolk Antibodies in Comparison to Mammalian Antibodies. ALTEX (1994) 11:75–84.
331. Akita E, Nakai S. Production and Purification of Fab′ Fragments From Chicken Egg Yolk Immunoglobulin Y (Igy). J Immunol Methods (1993) 162:155–64. doi: 10.1016/0022-1759(93)90380-P
332. Kubickova B, Majerova B, Hadrabova J, Noskova L, Stiborova M, Hodek P. Effect of Chicken Antibodies on Inflammation in Human Lung Epithelial Cell Lines. Neuro Endocrinol Lett (2014) 35:99–104.
333. Torché A-M, Dimna ML, Le Corre P, Mesplède A, Le Gal S, Cariolet R, et al. Immune Responses After Local Administration of Igy Loaded-Plga Microspheres in Gut-Associated Lymphoid Tissue in Pigs. Vet Immunol Immunopathol (2006) 109:209–17. doi: 10.1016/j.vetimm.2005.08.016
334. Vega CG, Bok M, Vlasova AN, Chattha KS, Fernández FM, Wigdorovitz A, et al. Igy Antibodies Protect Against Human Rotavirus Induced Diarrhea in the Neonatal Gnotobiotic Piglet Disease Model. PloS One (2012) 7:e42788. doi: 10.1371/journal.pone.0042788
335. Pereira EPV, van Tilburg MF, Florean EOPT, Guedes MIF. Egg Yolk Antibodies (Igy) and Their Applications in Human and Veterinary Health: A Review. Int Immunopharmacol (2019) 73:293–303. doi: 10.1016/j.intimp.2019.05.015
336. Thirumalai D, Ambi SV, Vieira-Pires RS, Xiaoying Z, Sekaran S, Krishnan U. Chicken Egg Yolk Antibody (Igy) as Diagnostics and Therapeutics in Parasitic Infections - a Review. Int J Biol Macromol (2019) 136:755–63. doi: 10.1016/j.ijbiomac.2019.06.118
337. Leiva CL, Gallardo MJ, Casanova N, Terzolo H, Chacana P. Igy-Technology (Egg Yolk Antibodies) in Human Medicine: A Review of Patents and Clinical Trials. Int Immunopharmacol (2020) 81:106269. doi: 10.1016/j.intimp.2020.106269
338. Hakalehto E. Chicken Igy Antibodies Provide Mucosal Barrier Against SARS-Cov-2 Virus and Other Pathogens. Isr Med Assoc J (2021) 4:208–11.
339. University of Oxford. Our World in Data. Vaccinations by Location . Available at: https://www.google.com/search?q=how+many+percentage+vaccinated+covid+worldwide&ie=UTF-8&oe=UTF-8&hl=en-us&client=safari (Accessed June 1, 2021).
Keywords: immunoglobulin Y, IgY, chicken immunoglobulin, pathogen, viral (or virus)
Citation: Lee L, Samardzic K, Wallach M, Frumkin LR and Mochly-Rosen D (2021) Immunoglobulin Y for Potential Diagnostic and Therapeutic Applications in Infectious Diseases. Front. Immunol. 12:696003. doi: 10.3389/fimmu.2021.696003
Received: 15 April 2021; Accepted: 26 May 2021;
Published: 09 June 2021.
Edited by:
Gee W. Lau, University of Illinois at Urbana-Champaign, United StatesReviewed by:
Wei Luo, Purdue University Indianapolis, United StatesSunil Joshi, University of Miami, United States
Copyright © 2021 Lee, Samardzic, Wallach, Frumkin and Mochly-Rosen. This is an open-access article distributed under the terms of the Creative Commons Attribution License (CC BY). The use, distribution or reproduction in other forums is permitted, provided the original author(s) and the copyright owner(s) are credited and that the original publication in this journal is cited, in accordance with accepted academic practice. No use, distribution or reproduction is permitted which does not comply with these terms.
*Correspondence: Daria Mochly-Rosen, bW9jaGx5QHN0YW5mb3JkLmVkdQ==