- 1Programa de Biología Celular y Molecular, Instituto de Ciencias Biomédicas, Facultad de Medicina, Universidad de Chile, Santiago, Chile
- 2Departamento de Tecnología Médica, Facultad de Medicina, Universidad de Chile, Santiago, Chile
- 3Facultad de Odontología, Universidad San Sebastián, Santiago, Chile
- 4Clínica INDISA, Santiago, Chile
- 5Escuela de Odontología, Facultad de Ciencias, Universidad Mayor, Santiago, Chile
- 6Programa de Inmunología, Instituto de Ciencias Biomédicas, Facultad de Medicina, Universidad de Chile, Santiago, Chile
Sjögren’s syndrome (SS) is an autoimmune disease that mainly affects salivary glands (SG) and is characterized by overactivation of the type I interferon (IFN) pathway. Type I IFNs can decrease the levels of hsa-miR-145-5p, a miRNA with anti-inflammatory roles that is downregulated in SG from SS-patients. Two relevant targets of hsa-miR-145-5p, mucin 1 (MUC1) and toll-like receptor 4 (TLR4) are overexpressed in SS-patients and contribute to SG inflammation and dysfunction. This study aimed to evaluate if hsa-miR-145-5p modulates MUC1 and TLR4 overexpression in SG from SS-patients in a type I IFN dependent manner. Labial SG (LSG) biopsies from 9 SS-patients and 6 controls were analyzed. We determined hsa-miR-145-5p levels by TaqMan assays and the mRNA levels of MUC1, TLR4, IFN-α, IFN-β, and IFN-stimulated genes (MX1, IFIT1, IFI44, and IFI44L) by real time-PCR. We also performed in vitro assays using type I IFNs and chemically synthesized hsa-miR-145-5p mimics and inhibitors. We validated the decreased hsa-miR-145-5p levels in LSG from SS-patients, which inversely correlated with the type I IFN score, mRNA levels of IFN-β, MUC1, TLR4, and clinical parameters of SS-patients (Ro/La autoantibodies and focus score). IFN-α or IFN-β stimulation downregulated hsa-miR-145-5p and increased MUC1 and TLR4 mRNA levels. Hsa-miR-145-5p overexpression decreased MUC1 and TLR4 mRNA levels, while transfection with a hsa-miR-145-5p inhibitor increased mRNA levels. Our findings show that type I IFNs decrease hsa-miR-145-5p expression leading to upregulation of MUC1 and TLR4. Together, this suggests that type I interferon-dependent hsa-miR-145-5p downregulation contributes to the perpetuation of inflammation in LSG from SS-patients.
Introduction
Sjögren’s syndrome (SS) is a chronic autoimmune disease that primarily affects exocrine glands (mainly salivary and lachrymal), causing signs and symptoms of secretory dysfunction (1). SS-patients have increased systemic and glandular levels of pro-inflammatory cytokines, synthesized by inflammatory and epithelial cells (2–4). Accumulating evidence shows the involvement of interferons (IFNs) in both the initiation and progression of SS (5, 6).
IFNs are a family of cytokines originally defined by their anti-viral activity and are divided into three classes: type I, type II, and type III. The type I IFN class comprises 13 IFN-α subtypes, as well as IFN-β, IFN-ε, IFN-κ and IFN-ω. The type II IFN class comprises IFN-γ, while the type III IFN class consists of IFN-λ1, IFN-λ2 and IFN-λ3 (IL-29, IL-28A, and IL-28B, respectively) (6). Type I IFNs mainly signal through IFNα/β receptor (IFNAR), consisting of two subunits IFNAR1 and IFNAR2. Type I IFNs binds to IFNAR and transactivate the associated Janus protein kinases (JAKs) Tyk2 and Jak1, which are autophosphorylated. Activated Tyk2 and Jak1 then phosphorylate tyrosines on the intracellular receptor domains which recruit the Signal Transducer and Activator of Transcription (STAT) 1 and STAT2 heterodimers (6–8). The binding of interferon-regulatory factor 9 (IRF-9) to STAT1/STAT2 leads to the formation of a complex known as IFN-stimulated gene factor 3 (ISGF3). This complex induces the expression of IFN-stimulated genes (ISGs) by binding to IFN-stimulated response elements (ISRE) (6–8). Thus, type I IFN activation is commonly measured by evaluating the expression of ISGs, also called the “type I IFN signature” (6).
The ISG proteins have a wide variety of anti-viral/antineoplastic effector functions (9). However, type I IFNs also have several immunomodulatory roles, including isotype switching (10), induction of B cell-activating factor (BAFF) (11), and cytotoxicity mediated by T-cells and natural killers (12). Thus, tight regulation of type I IFN is required to avoid collateral tissue damage induced by excessive host defense responses (8).
Upregulated ISGs are present in labial salivary gland (LSG) biopsies and ocular epithelial cells (13) of SS patients using global gene expression profiling and real-time PCR (13–15). Among the most studied ISGs to determine type I IFN signature are Myxovirus (Influenza Virus) resistance 1, interferon-inducible (MX1); Interferon Induced Protein with Tetratricopeptide Repeats 1 (IFIT1); Interferon Induced Protein 44 (IFI44); and Interferon Induced Protein 44 Like (IFI44L) (16). In a microarray analysis using enriched epithelial cell fractions obtained from SS-patient LSG, MX1 and IFIT1 were upregulated (17). ISG overexpression was found in peripheral blood mononuclear cells (PBMCs), isolated monocytes, plasmacytoid dendritic cells, and B cells of SS-patients (13, 14, 18–21). Together, these observations indicate overactivation of the type I IFN pathway in SS. Systemic upregulation of type I IFN was associated with higher serum IgG, lower complement C3 levels, lower lymphocyte and neutrophil counts, and the presence of anti-Ro/SSA and anti-La/SSB autoantibodies (7, 22). Additionally, several studies show increased EULAR Sjögren’s syndrome disease activity index (ESSDAI) scores in type I IFN positive SS-patients (22–24). Local upregulation of type I IFN in the LSG of SS-patients was also associated with higher prevalence of abnormal findings on sialometry, leukopenia, hyperglobulinemia, high-titer antinuclear antibody, anti-SSA, and a high focus score of the LSG biopsy (25): emphasizing the key role of these cytokines in the pathogenesis of SS.
While the regulation and role of protein-coding genes involved in type I IFNs responses are well characterized, the involvement of non-coding microRNAs (miRNAs) is less studied (26). MiRNAs are single-stranded, non-coding small RNAs whose sizes range from 18 to 25 nucleotides. They play indispensable roles in regulating gene or protein expression by enhancing mRNA degradation or translational repression by binding to the 3’-untranslational region (3’-UTR) of target mRNAs (27, 28). Through their role in regulating gene expression, miRNAs participate in immune homeostasis and inflammatory responses, and their expression is altered in various autoimmune diseases, including SS (27). Interestingly, microRNAs themselves may be induced or repressed directly through type I IFN signaling (26). Type I IFNs inhibit miR-145 gene transcription, leading to decreased hsa-miR-145-5p levels (28), a miRNA with anti-inflammatory roles, and is downregulated in the LSG of SS-patients (29). The hsa-miR-145-5p inhibits IL-6 secretion in airway smooth muscle cells, and its absence leads to an induction of pro-inflammatory signals of the innate immune response (30, 31). Hsa-miR-145-5p levels decrease in T cells from systemic lupus erythematosus patients (32), as well as in skin biopsies and fibroblasts from systemic sclerosis patients (33), in the damaged renal vessels of patients with lupus nephritis (34, 35), and in PBMCs from patients with myasthenia gravis (36). Interestingly, two relevant hsa-miR-145-5p targets are overexpressed in LSG from SS-patients: mucin 1 (MUC1) (37, 38) and toll-like receptor 4 (TLR4) (39, 40), which have important roles in innate immunity, inflammation and glandular dysfunction.
MUC1 transcripts generate three types of isoforms: transmembrane proteins with or without variable numbers of tandem repeats (VNTR) and secreted proteins (37, 41–44). MUC1/SEC corresponds to secreted MUC1 isoform and does not contain the cytoplasmic and transmembrane domains (43, 45). Also this isoform is the only one that contains a unique 11 amino-acid peptide at the COOH terminus (43, 45). This sequence is known as immuno-enhancing peptide (IEP) and can enhance the immune response (46, 47). Interestingly, IEP modulates both the innate and adaptive immune responses (46, 48). Overexpression of cytokines may be induced by MUC1/SEC, mediated by IEP, and/or via the formation of complexes with MUC1/Y (44). The production of cytokines and modulation of the immune response could be triggered by the binding of MUC1/Y and MUC1/SEC (37, 49). MUC1/Y induces the transcription of pro-inflammatory cytokines via NF-kB (50). mRNA and protein levels of both isoforms are significantly increased in LSG from SS-patients (37). Moreover, MUC1 is overexpressed and accumulates in the endoplasmic reticulum (ER) of LSG from these patients. Pro-inflammatory cytokines induced aberrant MUC1 accumulation, suggesting that chronic inflammation alters the secretory process of MUC1, causing ER stress and could affect the quality of saliva in SS patients (38).
The Toll-like receptors (TLR) belong to a conserved family of type I transmembrane receptors, and each member recognizes distinct elements of bacteria, fungi, or viruses known as pathogen-associated molecular patterns (PAMPs) (51). TLR can also recognize damage-associated molecular patterns (DAMPs) such as histones, S100 proteins, heat shock proteins, and some extracellular proteins (52). TLR activation primes the adaptive immune system and initiates inflammatory responses by inducing proinflammatory cytokines, chemokines, co-stimulatory and adhesion molecules (53, 54). Furthermore, the development and progression of organ-specific autoimmune lesions in various experimental animal models suggest a role for TLR triggering in the pathogenesis of autoimmune disorders (55–57). This study specifically aimed to evaluate if hsa-miR-145-5p modulates the increased MUC1 and TLR4 expression in LSG from SS-patients in a type I IFN dependent manner.
Materials and Methods
Patients With SS and Controls
The study group included 9 patients diagnosed with primary SS, based on the 2016 American College of Rheumatology/European League against Rheumatism Classification Criteria (58). The control group included 6 subjects, who did not fulfill the primary SS classification criteria, did not suffer systemic diseases, and whose LSG biopsy analysis was normal or revealed mild diffuse chronic sialadenitis. Table 1 summarizes the demographic, serological, and histological characteristics of SS-patient and control subjects. All individuals signed an informed consent according to the Declaration of Helsinki and the study was approved by the Ethics Committee of the Facultad de Medicina, Universidad de Chile (registration code CEISH 010-2016).
Biopsies
The LSG biopsies were obtained as described by Daniels et al. (59). Collected samples were immediately frozen in liquid nitrogen.
Taqman Assays
Total RNA extraction enriched in small RNAs was performed using the miRNeasy mini kit (QIAGEN Sciences, Maryland, USA). 40 ng of total RNA were reverse transcribed into cDNA using Taqman™ MicroRNA Reverse Transcription Kit (Applied Biosystems, CA, USA) and Taqman™ MicroRNA assays (Applied Biosystems, CA, USA) that include specific RT probes for hsa-miR-145-5p. To determine the expression levels of the miR-145-5p, Taqman™ Universal Master Mix II, no UNG (Applied Biosystems, CA, USA), and Taqman™ MicroRNA assays that include specific PCR probes for this miRNA were used. qPCR reactions for the hsa-miR-145-5p miRNA (20 μL reaction volume) were performed (at least in triplicate) using an MxPro 3000 termocycler (Stratagene). hsa-miR145-5p levels were normalized to those of the U48, and for calculating relative expression levels the efficiency-calibrated model was used (60).
Real Time-PCR
Total RNA was extracted using the miRNeasy mini kit (QIAGEN Sciences, Maryland, USA). 1 µg of total RNA was reverse transcribed with oligo (dT), random primers, and the Superscript II enzyme (Invitrogen by Thermo Fisher Scientific, USA). Specific primers for the MUC1, TLR4, IFN-α, IFN-β, MX1, IFIT1, IFI44, IFI44L, and h18S genes were designed with the AmplifiX 1.4 software (Supplementary Table 1). For real-time PCR reactions, the 5X Hot FirePol EvaGreen qPCR Mix Plus (Solis BioDyne, Estonia) was used. The relative expression ratio of a target gene was compared to h18S. Relative quantification of target mRNAs was accomplished by comparative Ct, using the efficiency-calibrated model (60).
Calculation of the Type I IFN Scores
The mean and standard deviation of each ISGs (MX1, IFIT1, IFI44, and IFI44L) obtained by real-time PCR in the control group LSGs were used to standardize the expression levels of each of these genes for each SS-patient, and IFN scores were calculated as described in previous studies (16, 22, 61, 62). Standardized expression levels were subsequently summed for each SS-patient to provide a type I IFN expression score, where i = each of the 4 IFN-inducible genes, Gene iSS= the gene expression level in each SS-patient, and Gene iCtr = the gene expression in controls:
Type I IFN Stimulation and Functional Assays
Human submandibular gland (HSG) cells were cultured as previously described (40, 63) and incubated with or without 10 ng/mL human recombinant IFN-α (Biolegend, CA, USA) or IFN-β (R&D systems, MC, USA) in serum-free medium for 24 h and subsequently lysed to isolate RNA. For functional assays, HSG cells were transfected with 100 nM of specific hsa-miR-145-5p mimic and inhibitor (Applied Biosystems, CA, USA) at 24 h. Transient transfections were performed using HiPerFect transfection reagent (QIAGEN Sciences, Maryland, USA). Transfected HSG cells were lysed, and extraction of total RNA enriched in small RNAs was performed as described above.
Protein Extraction and Western Blotting
HSG cells incubated with or without 10 ng/mL human recombinant IFN-α (Biolegend, CA, USA) or IFN-β (R&D systems, MC, USA) were homogenized using RIPA buffer and the Complete™ Protease Inhibitor Cocktail Mini Tablets (Roche, Mannheim, Germany). Proteins were quantified using the Bradford method (64) and separated according to their molecular weights by SDS-PAGE on 8% gels under reducing conditions. Separated proteins were transferred to nitrocellulose membranes (Bio-Rad Laboratories, Hercules, CA, USA) for 15 h at 4°C. Membranes were blocked for 1 h at room temperature (RT) in 5% skimmed-milk prepared in TBS-T buffer (10 mM Tris HCl [pH 7.5], 150 mM NaCl, 0.1% Tween 20). Blots were then separately incubated with primary antibodies against MUC1 or TLR4 (Supplementary Table 2) prepared in TBS-T buffer overnight at 4°C. After washes in TBS-T buffer, membranes were incubated with goat anti-mouse or anti-rabbit HRP-conjugated secondary antibodies (Supplementary Table 2) for 1 h at RT (Pierce® by Thermo Scientific, IL, USA). Target proteins were detected by chemiluminescence (Pierce, IL, USA). Protein bands were quantified by densitometry. Protein levels were normalized to β-actin.
Statistical Analysis
Mean values in SS-patients and controls groups or between different culture cell conditions were compared using the Mann-Whitney test. Spearman rank correlation analysis was also performed with P values less than 0.05 considered significant.
Results
hsa-miR-145-5p Is Downregulated in LSG From SS-Patients and Inversely Correlates With IFN-β and Type I IFN Score
Using TaqMan miRNA assays in LSG from 9 SS-patients and 6 controls, we validated hsa-miR-145-5p level. We observed decreased expression in SS-patients compared to controls (p=0.0004) (Figure 1A). Then, we assessed IFN-α and IFN-β transcript levels and their association with hsa-miR-145-5p. IFN-α transcript levels were near the limit of detection and no significant differences were found between groups (p=0.25) (Figure 1B), while IFNβ transcript levels were significantly increased in LSG from SS-patients compared to controls (p=0.0014) (Figure 1D). Spearman’s analysis showed a negative correlation between hsa-miR-145-5p and IFN-β (Figure 1E). However, there was no correlation between miR-145-5p and IFN-α (Figure 1C).
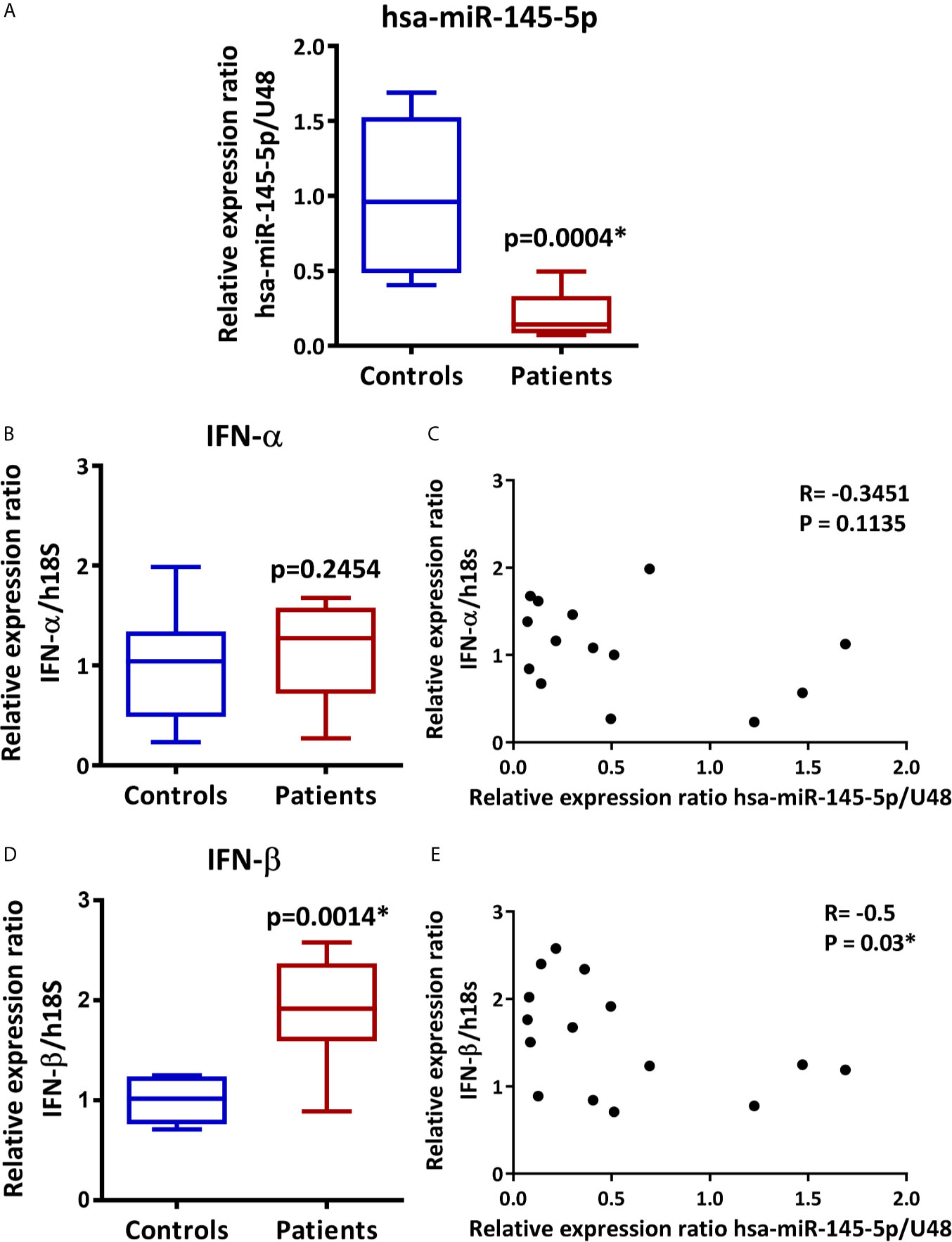
Figure 1 hsa-miR-145-5p is downregulated in LSG from SS-patients and inversely correlates with IFN-β mRNA levels. (A) hsa-miR-145-5p levels in SS-patients (n=9) and control subjects (n=6). U48 snRNA was used as a housekeeping. (B) IFN-α transcript levels in SS-patients and control subjects. h18S was used as a housekeeping gene. (C) Spearman correlation between hsa-miR-145-5p and IFN-α mRNA levels in SS-patients and controls. (D) IFN-β transcript levels in SS-patients and control subjects. h18S was used as a housekeeping gene. (E) Spearman correlation between hsa-miR-145-5p and IFN-β mRNA levels in SS-patients and controls. Data are representative of at least three independent measurements. (*) p-value ≤ 0.05 was considered significant.
The assessment of type I IFN score by real-time PCR analysis of multiple genes preferentially induced by type I IFNs or ISGs is a useful technique to determine the activation of the type I IFN pathway or type I IFN signature (16, 62). Therefore, the relative expression of MX1, IFIT1, IFI44, and IFI44L and the type I IFN score in LSG from SS-patients and controls were determined. As shown in Figure 2, mRNA levels of MX1 (p=0.0002), IFIT1 (p=0.0024), IFI44 (p=0.0002), and IFI44L (p=0.0002), were significantly higher in LSG from SS-patients compared to controls (Figures 2A–D). We calculated the type I IFN score and the results showed that the mean IFN score in SS patients was 34.5, with a range of 11.2 to 70.6, and in controls, the mean IFN score was -0.3, with a range of -3.5 to 3.6. Thus, the type I IFN score was significantly higher in SS-patients (p=0.0002) (Figure 2E), and there was a negative correlation between hsa-miR-145-5p levels and type I IFN score (p=0.0002) (Figure 2F).
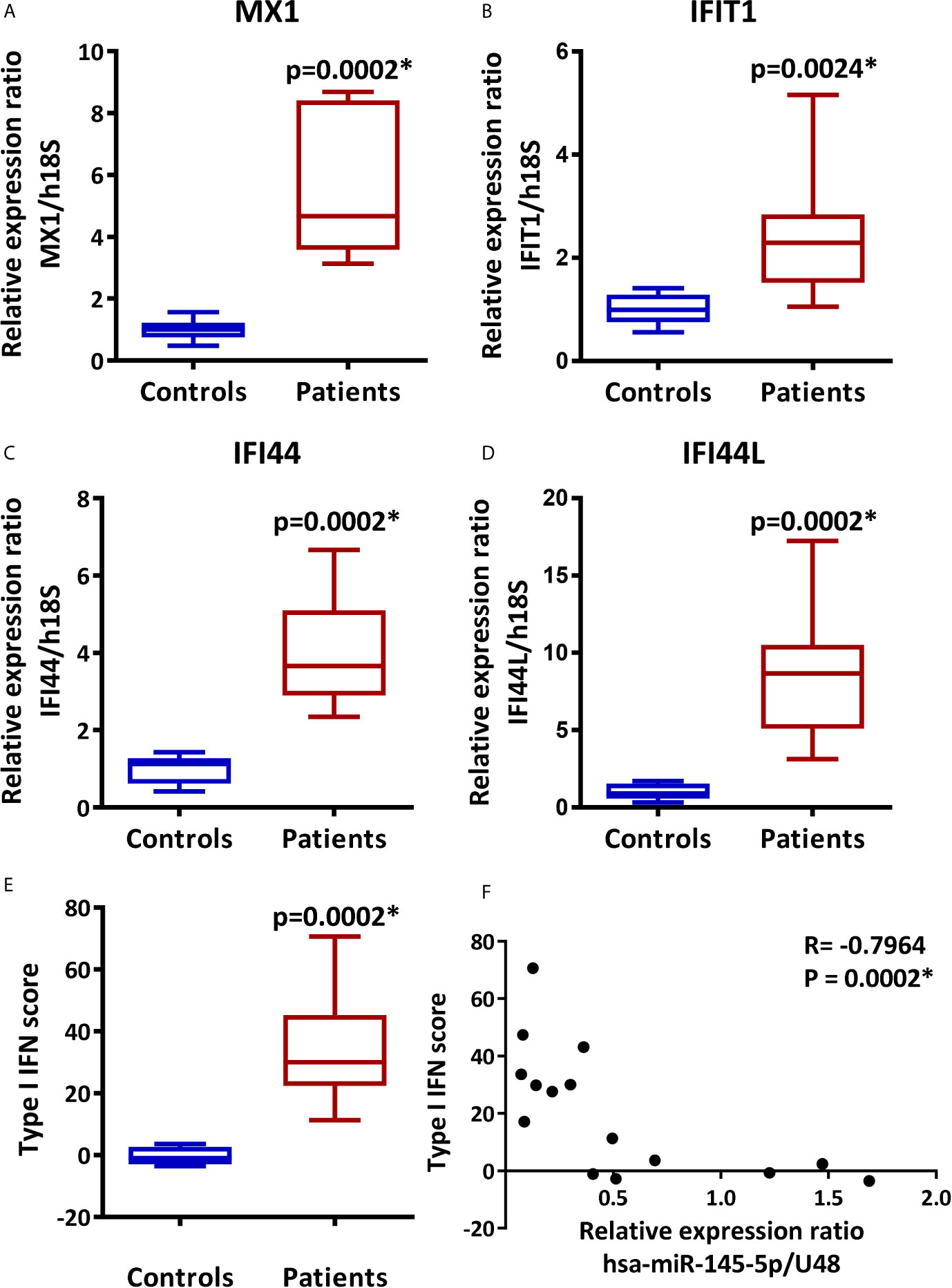
Figure 2 Overexpression of MX1, IFIT1, IFI44, and IFI44L and increased type I IFN score in LSG from SS-patients. Relative expression ratios of MX1 (A), IFIT1 (B), IFI44 (C) and IFI44L (D) in 9 SS-patients and 6 controls. h18S was used as a housekeeping gene. (E) Type I IFN score was calculated by summing the MX1, IFIT1, IFI44, and IFI44L standarized expression levels. (F) Spearman correlation between hsa-miR-145-5p levels and type I IFN score in SS-patients and controls. Data are representative of at least three independent measurements. (*) p-value ≤ 0.05 was considered significant.
MUC1 and TLR4 Are Overexpressed in LSG From SS-Patients and Inversely Correlate With hsa-miR-145-5p
We measured MUC1 and TLR4 transcript levels by real-time PCR in LSG samples and evaluated their association with hsa-miR-145-5p. Results showed an increase in MUC1 and TLR4 transcripts levels in SS-patients (p=0.044 and 0.0014, respectively) (Figures 3A, C). Spearman’s analysis showed a negative correlation between hsa-miR-145-5p and MUC1 transcript levels (Figure 3B) and between hsa-miR-145-5p and TLR4 (Figure 3D) transcript levels.
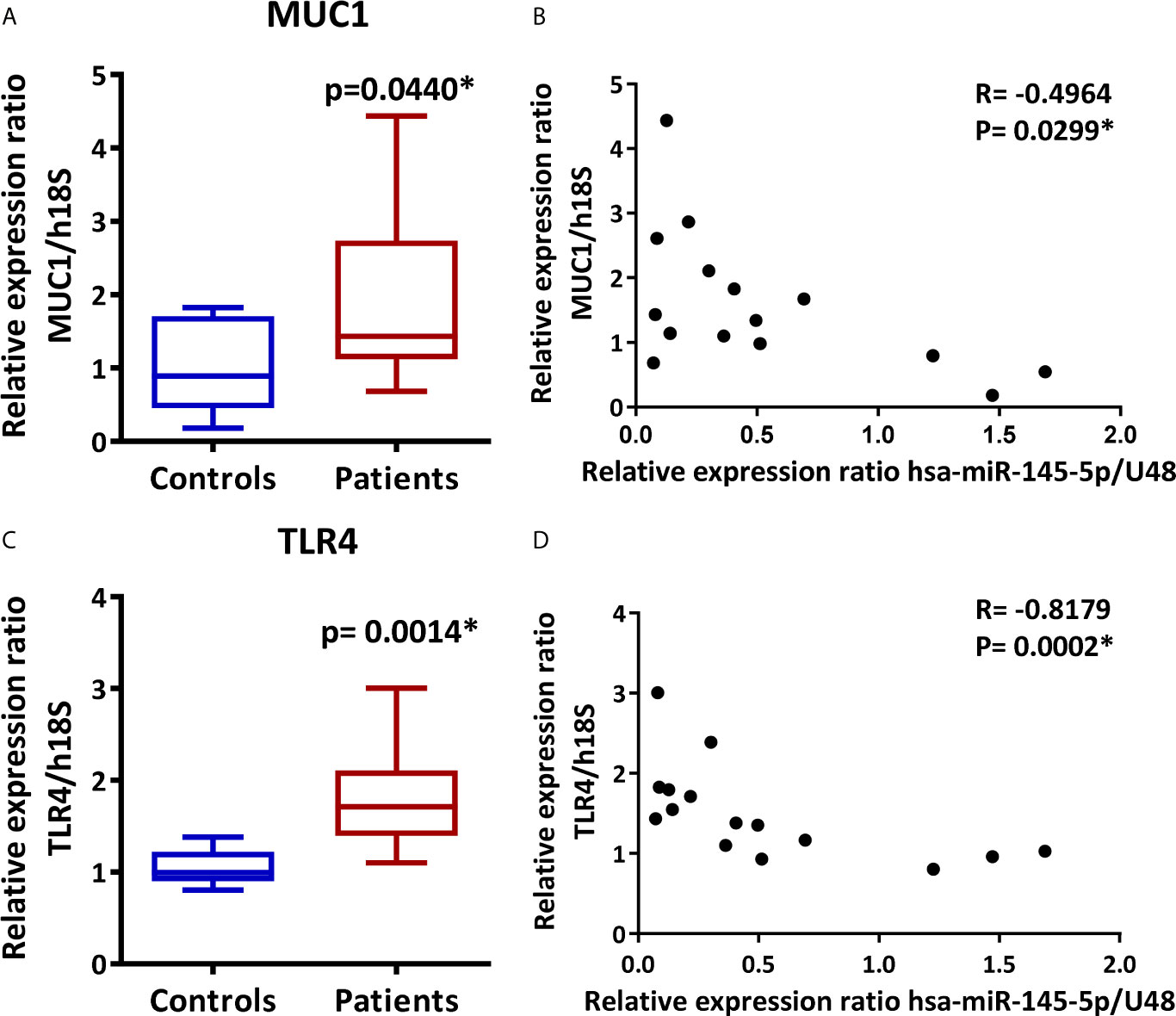
Figure 3 MUC1 and TLR4 are overexpressed in LSG from SS-patients and inversely correlate with hsa-miR-145-5p. (A) MUC1 transcript levels in SS-patients and control subjects. h18S was used as a housekeeping gene. (B) Spearman correlation between hsa-miR-145-5p and MUC1 mRNA levels in SS-patients and controls. (C) TLR4 transcript levels in SS-patients and control subjects. h18S was used as a housekeeping gene. (D) Spearman correlation between hsa-miR-145-5p and TLR4 mRNA levels in SS-patients and controls. Data are representative of at least three independent measurements. (*) p-value ≤ 0.05 was considered significant.
hsa-miR-145-5p Levels Are Inversely Correlated With Glandular Inflammation
According to its anti-inflammatory role, we found a negative correlation between hsa-miR-145-5p levels and clinical parameters of SS-patients: Ro autoantibodies (r= -0.82, p<0.001); La autoantibodies (r= - 0.59, p= 0.021), and focus score (r= -0.81, p<0.001) (Table 2). Additionally, we observed a positive correlation between these clinical parameters and mRNA levels of TLR4, ISGs, and type I IFN score (Table 2). ISGs negatively correlated with hsa-miR-145-5p levels, while ISGs and mRNA levels positively correlated with IFN-β and TLR4 (Table 3).
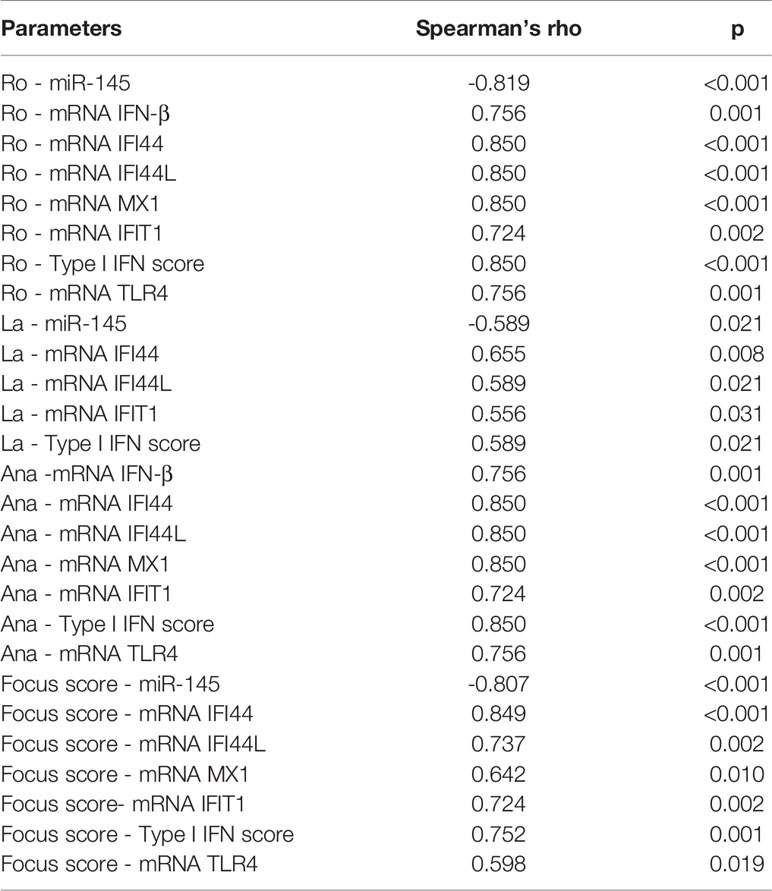
Table 2 Spearman’s rank correlation coefficients between hsa-miR-145-5p levels, ISGs mRNA levels and clinical parameters of SS-patients and control subjects.
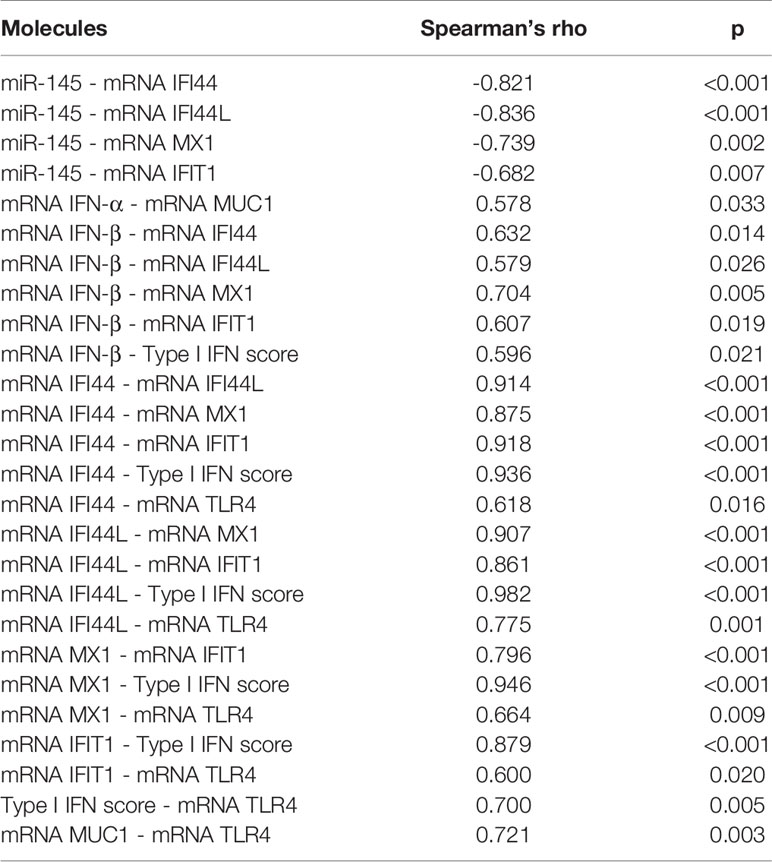
Table 3 Spearman’s rank correlation coefficients between hsa-miR-145-5p levels and mRNA levels of each analyzed gene in LSG from SS-patients and control subjects.
Type I IFN Stimulation Downregulates hsa-miR-145-5p and Induces MUC1 and TLR4 Overexpression in HSG Cells
To evaluate if the decreased levels of hsa-miR-145-5p observed in SS-patients are associated with type I IFNs and the activation of this specific pathway, we assessed the effect of IFN-α or IFN-β stimulation on hsa-miR-145-5p levels and its targets MUC1 and TLR4 in HSG cells. First, to assess type I IFN pathway activation, we measured ISG expression in HSG cells stimulated with IFN-α or IFN-β. Stimulation with 10 ng/mL IFN-α induced an increase of MX1 (p<0.0001) (Figure 4A), IFIT1 (p<0.0001) (Figure 4B), IFI44 (p<0.0001) (Figure 4C), and IFI44L (p<0.0001) (Figure 4D) mRNA levels. In addition, stimulation of HSG cells with 10 ng/mL IFN-β induced the expression of MX1 (p<0.0001) (Figure 4E), IFIT1 (p<0.0001) (Figure 4F), IFI44 (p<0.0001) (Figure 4G), and IFI44L (p<0.0001) (Figure 4H) transcripts. A significant decrease of hsa-miR-145-5p levels were observed in HSG cells stimulated with 10 ng/mL IFN-α (p<0.0001) (Figure 5A). IFN-α stimulation also induces the expression of MUC1 (p<0.0001) (Figure 5B) and TLR4 (p=0.0012) (Figure 5C) transcript levels, which inversely correlated with hsa-miR-145-5p levels (Figures 5D, E). Stimulation with 10 ng/mL IFN-β also reduced hsa-miR-145-5p levels (p<0.0001) (Figure 5F), and increased MUC1 (p<0.0001) (Figure 5G) and TLR4 (p<0.0001) (Figure 5H) transcript levels. MUC1 and TLR4 mRNA levels negatively correlated with hsa-miR-145-5p levels in IFN-β stimulated HSG cells (Figures 5I, J). In addition, MUC1 and TLR4 protein levels were significantly increased after 10 ng/mL IFN-α or IFN-β stimulation (Figures 6A–D).
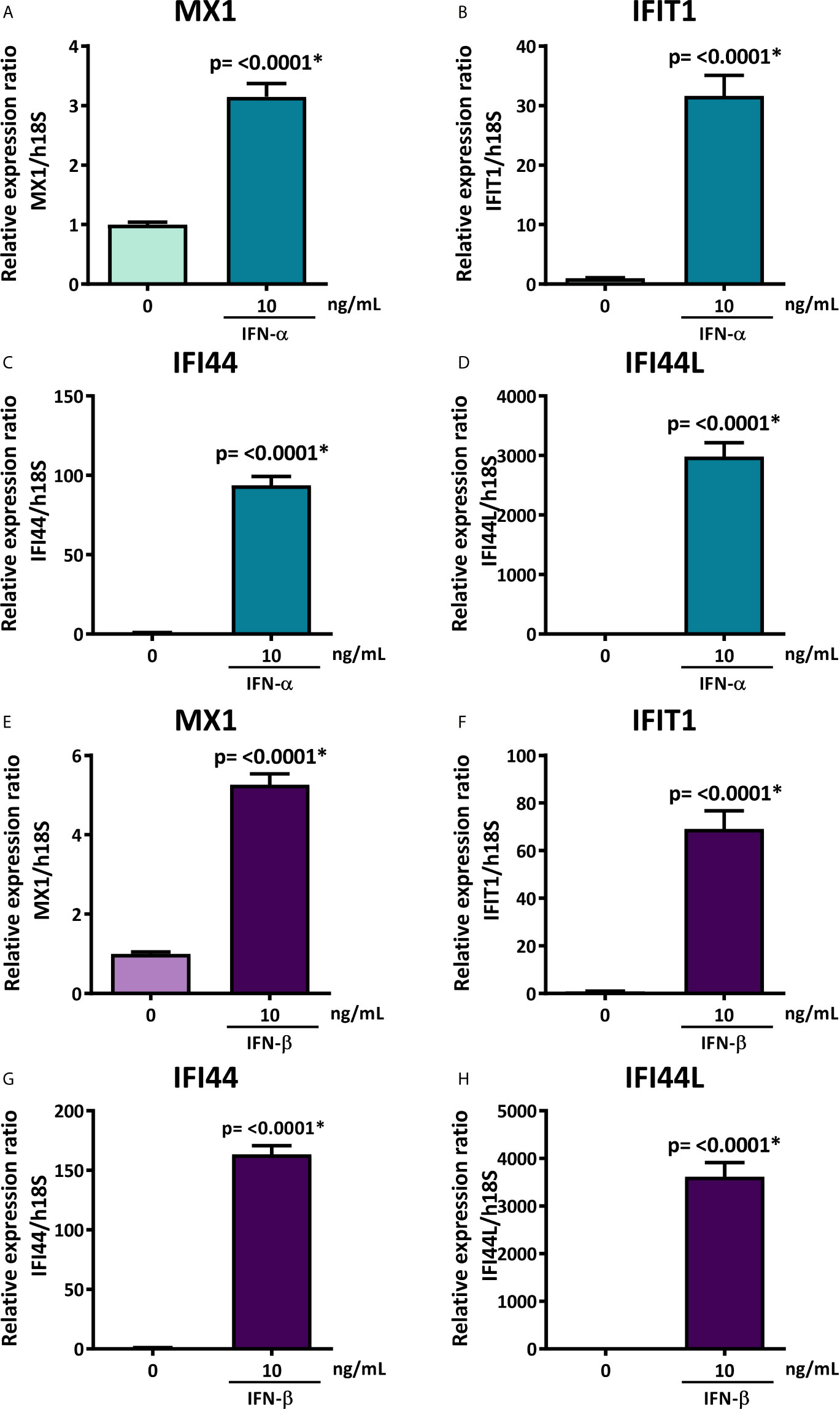
Figure 4 Overexpression of MX1, IFIT1, IFI44, and IFI44L in IFN-α or IFN-β-stimulated HSG cells. Relative expression ratios of MX1 (A), IFIT1 (B), IFI44 (C) and IFI44L (D) in HSG cells stimulated with or without 10 ng/mL human recombinant IFN-α for 24 h. h18S was used as a housekeeping gene. Relative expression ratios of MX1 (E), IFIT1 (F), IFI44 (G) and IFI44L (H) in HSG cells stimulated with or without 10 ng/mL human recombinant IFN-β for 24 h. h18S was used as a housekeeping gene. Data are representative of at least three independent experiments. (*) p-value ≤ 0.05 was considered significant.
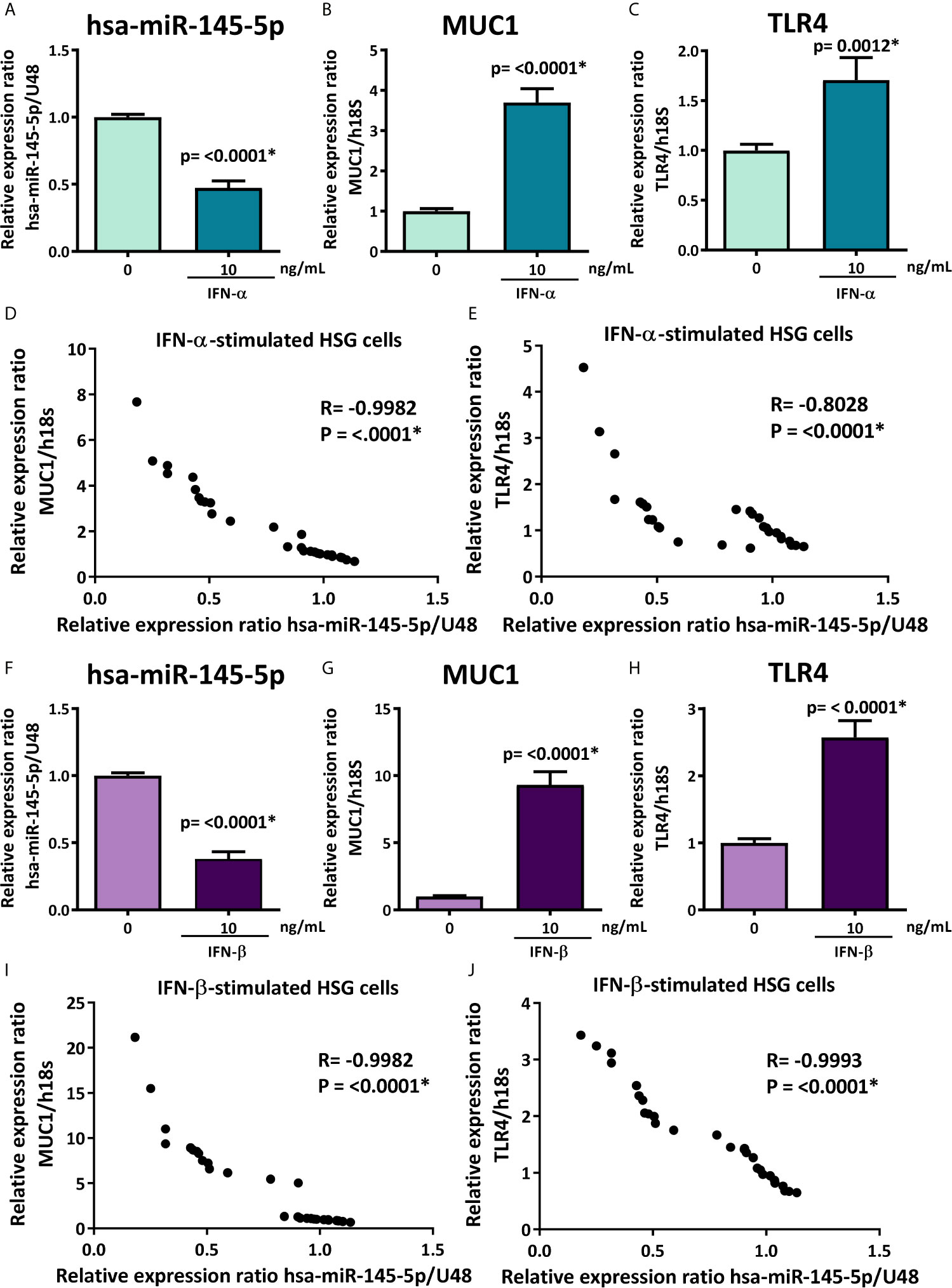
Figure 5 hsa-miR-145-5p is downregulated and MUC1 and TLR4 are overexpressed in type I IFNs-stimulated HSG cells. (A) hsa-miR-145-5p levels in HSG cells stimulated with or without 10 ng/mL human recombinant IFN-α for 24 h. U48 was used as a housekeeping gene. Transcript levels of MUC1 (B) and TLR4 (C) in HSG cells stimulated with IFN-α. Spearman correlation between hsa-miR-145-5p and MUC1 (D) or TLR4 (E) transcript levels in IFN-α stimulated HSG cells. (F) hsa-miR-145-5p levels in HSG cells stimulated with or without 10 ng/mL human recombinant IFN-β for 24 h. U48 was used as a housekeeping gene. Transcript levels of MUC1 (G) and TLR4 (H) in HSG cells stimulated with IFN-β. Spearman correlation between hsa-miR-145-5p and MUC1 (I) or TLR4 (J) transcript levels in IFN-β stimulated HSG cells. Data are representative of at least three independent experiments. (*) p-value ≤ 0.05 was considered significant.
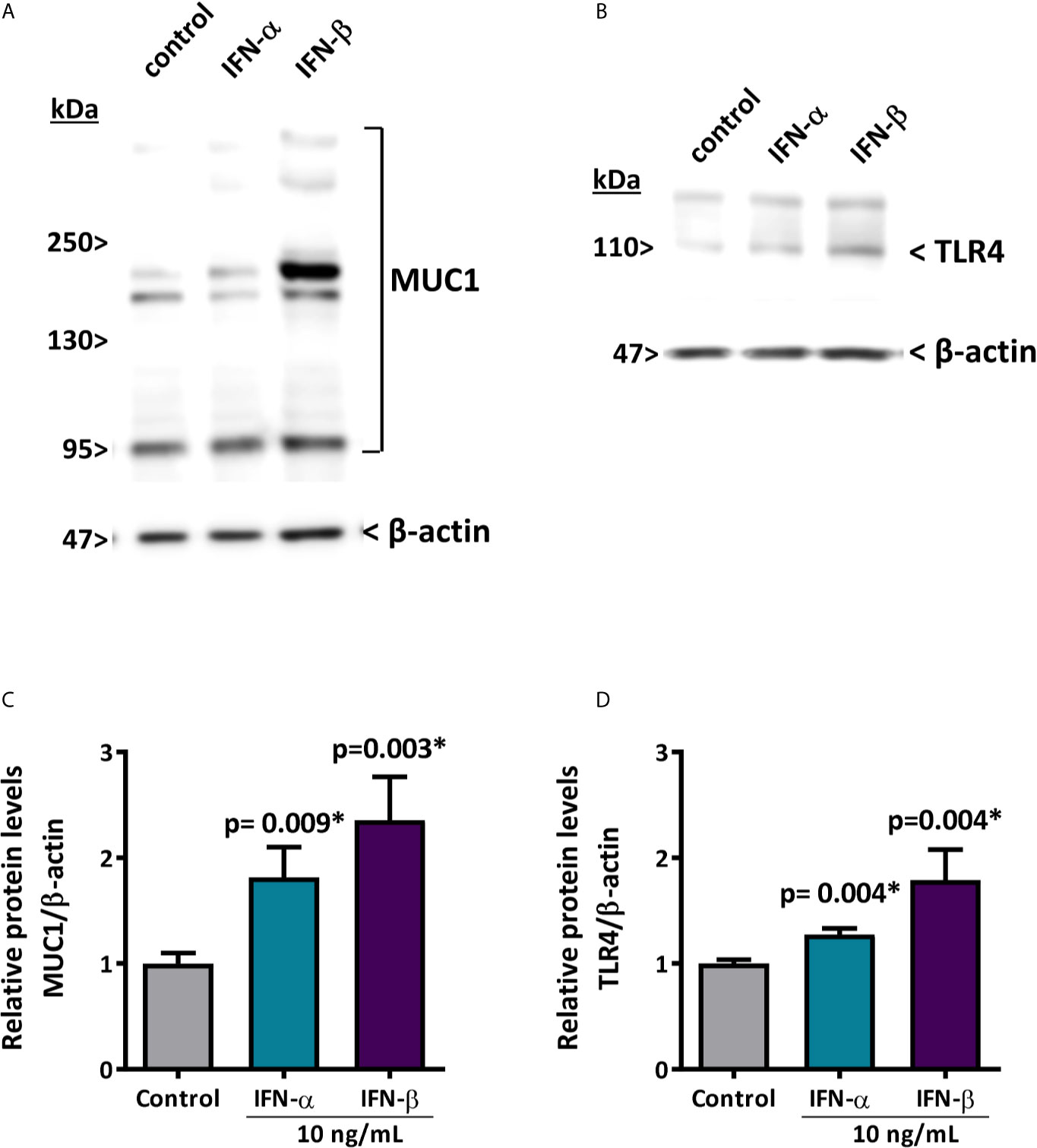
Figure 6 IFN-α and IFN-β increase MUC1 and TLR4 protein levels in HSG cells. (A, C) representative MUC1 western blot (90-300 kDa) in HSG cells stimulated with 10 ng/mL IFN-α or IFN-β for 24 h. Relative protein levels were normalized to the control condition. β-actin was used as a loading control. (B, D) representative TLR4 western blot (95 kDa) in HSG cells stimulated with 10 ng/mL IFN-α or IFN-β for 24 h. Relative protein levels were normalized to the control condition. β-actin was used as a loading control. Data are representative of at least three independent experiments. (*) p-value ≤ 0.05 was considered significant.
hsa-miR-145-5p Overexpression Downregulates MUC1 and TLR4
Upregulation and downregulation assays identify target genes regulated by specific miRNAs. When evaluating whether hsa-miR-145-5p modulates MUC1 and TLR4 expression in HSG cells, we used mimic and inhibitor miRNAs which are chemically synthesized double-stranded RNA molecules imitating mature miRNA duplexes. HSG cells transfected with 100 nM of mimic hsa-miR-145-5p significantly increased miRNA levels (p<0.0001) (Figure 7A), while 100 nM of the hsa-miR-145-5p inhibitor decreased miRNA levels (p=0.05) (Figure 7B). MUC1 transcript levels were decreased in HSG cells transfected with mimic hsa-miR-145-5p (p= 0.0369) and increased in HSG cells transfected with the miRNA inhibitor (p=0.0003) (Figure 7C). Transfection with the mimic hsa-miR-145-5p significantly decreased TLR4 transcript levels (p=0.0062), and transfection with the miRNA inhibitor increased them (p=0.0345) (Figure 7D).
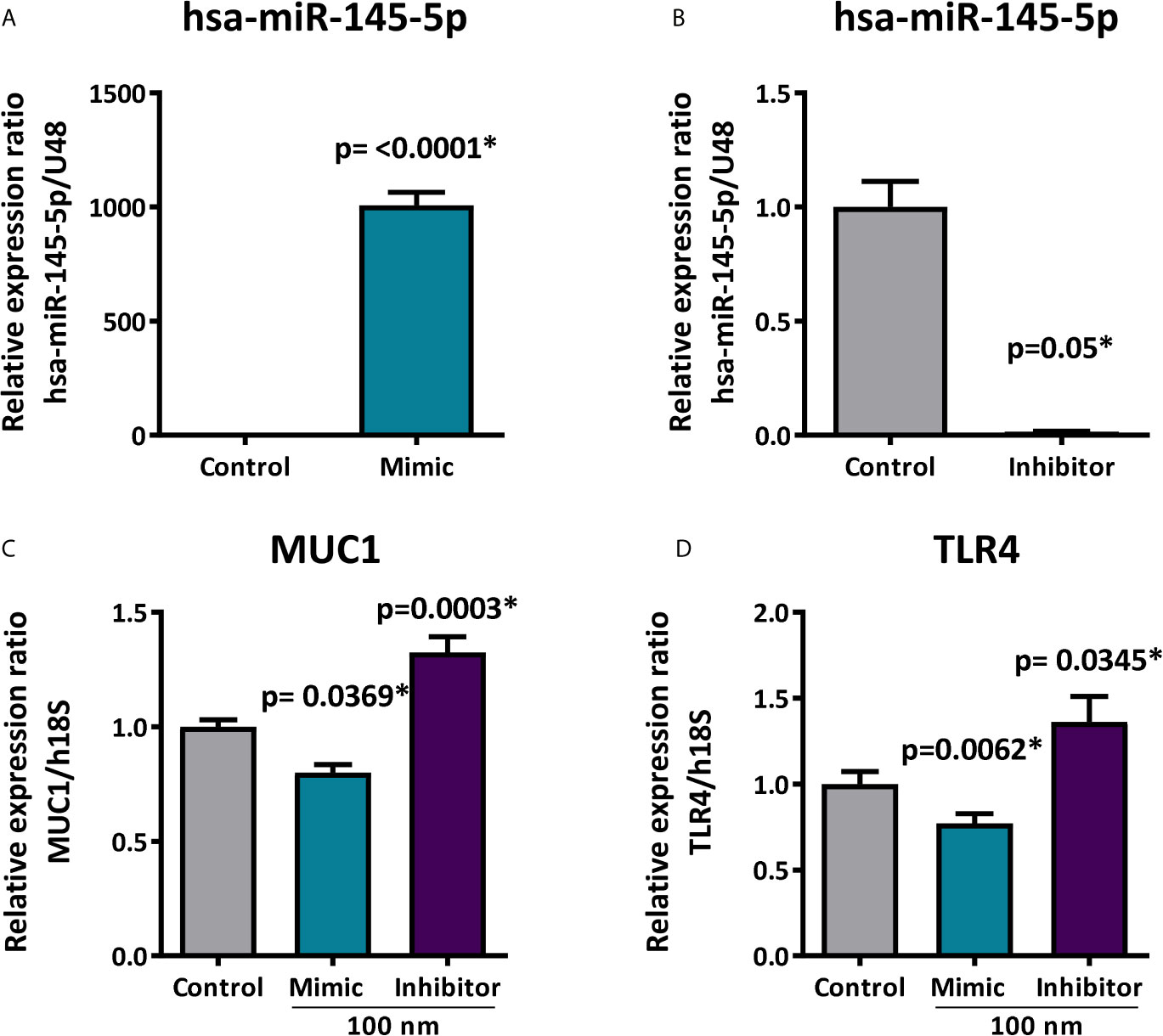
Figure 7 Expression of MUC1 and TLR4 after hsa-miR-145-5p overexpression or inhibition. (A) Increased hsa-miR-145-5p levels in HSG cells transfected with the mimic miRNA. (B) Decreased hsa-miR-145-5p levels in HSG cells transfected with inhibitor miRNA. (C) Relative expression ratio of MUC1 transcripts in HSG cells transfected with mimic hsa-miR-145-5p or inhibitor (D) Relative expression ratio of TLR4 transcripts in HSG cells transfected with mimic hsa-miR-145-5p or inhibitor. Data are representative of at least three independent experiments. (*) p-value ≤ 0.05 was considered significant.
Discussion
This study aimed to evaluate if miR-145-5p modulates MUC1 and TLR4 expression in LSG from SS-patients in a type I IFNs dependent manner. To our knowledge, this is the first study validating hsa-miR-145-5p levels in LSG from SS-patients by real-time PCR. Our results confirm the observations from a previous study showing a significant decrease of hsa-miR-145-5p in a miRNA expression profile generated by miRNA microarrays in LSG from SS-patients compared with control subjects (29). We observed a negative correlation between hsa-miR-145-5p and type I IFN score in LSG together with our in vitro results showing decreased hsa-miR-145-5p levels after IFN-α or IFN-β stimulation. These results suggest that type I IFNs and the activation of its pathway could downregulate hsa-miR-145-5p in LSG from SS-patients. Hsa-miR-145-5p is downregulated in LPS-stimulated macrophages, which depends on type I IFN production and the downstream IFNAR-JAK1-STAT1 signal cascade (28). Functional analyses in LPS-triggered macrophages have demonstrated that hsa-miR-145-5p functions as an anti-inflammatory molecule, promoting IL-10 expression by directly targeting the epigenetic Il10 gene silencer HDAC11 (histone deacetylase 11). In these cells, type I IFNs decrease hsa-miR-145-5p expression, thus alleviating HDAC11 repression, resulting in IL-10 production and precise coordination of innate immune responses (28). Therefore, hsa-miR-145-5p could help avoid overactivation of immune responses and subsequent inflammatory damage to host tissue (28).
In this study, hsa-miR-145-5p inversely correlated with MUC1 and TLR4 mRNA levels, two targets overexpressed in LSG from SS-patients (37–40). Our functional results show reduced MUC1 mRNA levels after transfection with mimic hsa-miR-145-5p, while MUC1 mRNA levels increased after hsa-miR-145-5p inhibition. These results complement those from a previous report showing that MUC1 is a direct target for hsa-miR-145, and suppression of MUC1 is dependent on the 3′-UTR in metastatic breast cancer cell lines, demonstrated by luciferase reporter assays (65). MUC1 has several splicing variants that share the same 3′-UTR: therefore, hsa-miR-145-5p is expected to suppress all variants. This was supported by Western blot results showing decreased levels of large and small isoforms of MUC1 after expression of hsa-miR-145-5p in breast cancer cell lines (65). Hsa-miR-145-5p was downregulated in freshly frozen ovarian carcinoma samples and ovarian carcinoma cell line (SKOV3) based on Northern blot and microarray analysis (66). MUC1 was overexpressed in ovarian (66), breast, colon, pancreas, and bladder tumors and was often associated with the epithelial-mesenchymal transition of different cancer cells, being considered an important metastasis gene (67, 68). Hsa-miR-145-5p overexpression inhibits SKOV3 cell migration and invasion and remarkably reduced the protein but not mRNA expression of MUC1 (69). To further explore the mechanism by which hsa-miR-145-5p inhibits invasion and metastasis, Wang et al. cloned the wild type and mutant miR-145 target sequences of the MUC1 3-’UTR into luciferase reporter vectors and performed the luciferase reporter gene assay, demonstrating that hsa-miR-145-5p negatively regulates MUC1 expression by directly targeting the MUC1 3-’UTR (69). Moreover, MUC1 overexpression alleviated the hsa-miR-145-5p-mediated inhibition of cell invasion, suggesting that miR-145 regulates cell growth and invasion, functioning as a tumor suppressor by directly targeting MUC1 (66). Interestingly, several MUC1 variants are overexpressed and aberrantly localized in LSG from SS-patients (37, 38). Under physiological conditions, salivary mucins are only secreted towards the mouth and are efficient mucosal moisturizers that prevent desiccation (70). One serious problem that SS-patients suffer daily is the dryness of their oral mucosa. We previously demonstrated that this dryness is not just a consequence of the reduced saliva volume (70, 71), but also that mucins play a leading role in mucosa hydration and protection, with hydrophilic groups essentially retaining water molecules in the epithelial surface (70). Previous evidence from our laboratory revealed that MUC1 accumulates in the ER, co-localizing and co-precipitating with GRP78, a chaperone that binds to unfolded proteins in the ER lumen in LSG from SS-patients (38). Also, changes indicative of ER stress and altered unfolded protein response in LSG from SS-patients, such as ER cistern dilation (72), decreased IRE1α/XBP1 pathway activation (73), and ATF6α pathway activation promoting increased ER-associated protein degradation (ERAD) (63) have been observed. In the present study, increased MUC1 mRNA induced by IFN-α and IFN-β together with functional assays using mimic hsa-miR-145-5p and inhibitors suggest that the type I IFN signaling increases MUC1 synthesis mediated by hsa-miR-145-5p inhibition in LSG of SS-patients. This mechanism could contribute to the MUC1 overexpression leading to ER synthesis machinery overload, MUC1 accumulation, and ER stress observed in LSG from SS-patients. MUC1 also induces pro-inflammatory cytokines (46, 49, 50), therefore, MUC1 overexpression mediated through type I dependent hsa-miR-145-5p downregulation could contribute to perpetuating glandular inflammation in LSG from SS-patients.
LSG from SS-patients present significantly increased TLR4 expression compared to controls (39, 40). In the present study, we confirmed TLR4 overexpression in LSG from a different cohort of SS-patients. Also, TLR4 mRNA levels significantly increased after IFN-α and IFN-β stimulation and were repressed by hsa-miR-145-5p overexpression. Hsa-miR-145-5p was down-regulated in high glucose-treated retinal endothelial cells (a cellular model of diabetic retinopathy), and hsa-miR-145-5p overexpression significantly reduced TLR4 at both the protein and mRNA levels (74). Additionally, several studies using luciferase reporter assays provided evidence that the 3’-UTR of TLR4 mRNA is a direct target of hsa-miR-145-5p (74–77). High glucose levels increased TNF-α and IL-1β expression in retinal endothelial cells and were substantially suppressed by hsa-miR-145-5p overexpression, with consequent TLR4 downregulation (74). Furthermore, hsa-miR-145-5p overexpression attenuated the oxidative stress and inflammation induced by high glucose levels, suggesting that hsa-miR-145-5p might exert both anti-oxidative and anti-inflammatory roles in diabetic retinopathy (74). TLR4 expression was decreased in osteoporotic samples compared with nonosteoporotic samples, while hsa-miR-145-5p levels were higher in osteoporotic samples, revealing a significantly negative correlation (75). The luciferase activity of HEK293T cells cotransfected with mimic hsa-miR-145-5p and TLR4‐WT was significantly reduced, while the luciferase activity of cells cotransfected with hsa-miR-145-5p and TLR4‐mutant was similar to control conditions (75). In other study, hsa-miR-145-5p was significantly down-regulated both in mice with acute lung injury and LPS-induced type II alveolar epithelial cells (77). Hsa-miR-145-5p overexpression decreased IL-1β, IL-6, and TNF-α expression levels. Also, it blocked LPS-induced activation of nuclear factor kappa B (NF-κB) pathway and reactive oxygen species (ROS) accumulation in LPS-induced type II alveolar epithelial cells. In these cells, it was confirmed that TLR4 mRNA is a direct target of hsa-miR-145-5p by dual luciferase assays (77). Additionally, hsa-miR-145-5p overexpression alleviated lung tissue injury, decreased the expression levels of IL-1β, IL-6, and TNF-α and reduced myeloperoxidase activity in acute lung injury mouse model, demonstrating that miR-145-5p participated in the progression and development of acute lung injury by decreasing the production of pro-inflammatory cytokines (77). Also, hsa-miR-145-5p inhibits tumor occurrence and metastasis through the NF‐κB signaling pathway by targeting TLR4 in malignant melanoma (76). This miRNA was downregulated in melanoma tissues and cells and suppressed TLR4 expression by binding to its 3’-UTR in melanoma cells (76). Moreover, TLR4 overexpression abolished the inhibition of cell proliferation, colony formation, migration, and invasion abilities induced by hsa-miR-145-5p in melanoma cells (76). Meanwhile, hsa-miR-145-5p restrained melanoma tumor growth in vivo by targeting TLR4. Furthermore, hsa-miR-145-5p overexpression inactivated the NF-κB pathway in melanoma in vitro and in vivo, which was reversed by TLR4 overexpression, suggesting that miR-145-5p hindered the occurrence and metastasis of melanoma cells (76). Also, alterations in cell polarity lead to loss of the innate epithelial barrier function in LSG from SS-patients, triggering a series of changes that result in mucin release to the extracellular matrix (78, 79). Epithelial cell TLR4 recognizes ectopic mucins (mucin obtained from bovine submaxillary glands; MUC5B isolated from human whole saliva; and synthetic sulpho-Lewis (SO3-3Galβ1-3GlcNAc) antigen, and initiates a pro-inflammatory response through transcription of IFN-α, IFN-β, TNF-α, IL-6, IL-1β, and CXCL8 in HSG cells (40). These signals, produced initially by epithelial cells, could attract inflammatory cells, perpetuating inflammation and developing a chronic disease (40).
Finally, TLR4 is an N-glycoprotein that is synthesized in the ER and consequently can cause ER stress. The crosstalk between TLR4-mediated signaling and ER stress promotes the production of pro-inflammatory cytokines (80). TLR4 activation can trigger the IRE1α/XBP-1s axis of the UPR and promote pro-inflammatory cytokine expression, such as IL-6 (81). Moreover, TLR4 activation and ER stress inducers synergize the production of pro-inflammatory cytokines, mainly mediated by upregulated p38 expression via ATF6 (82): a UPR transcriptional factor increased in SG from SS-patients (63). Since SS-patients exhibit ER stress and ER stress can synergize pro-inflammatory cytokine production mediated by TLR4, overexpression of TLR4 could further favor an inflammatory response in the SG of SS-patients.
In conclusion, our findings suggest that type I IFNs could downregulate hsa-miR-145-5p leading to MUC1 and TLR4 overexpression in LSG from SS-patients. Both MUC1 and TLR4 contribute to inflammation and glandular dysfunction in LSG from SS-patients; thus, these results suggest the anti-inflammatory role of hsa-miR-145-5p and unveil the contribution of type I IFNs in the perpetuation of inflammation.
Data Availability Statement
The raw data supporting the conclusions of this article will be made available by the authors, without undue reservation.
Ethics Statement
The studies involving human participants were reviewed and approved by the Ethics Committee of the Facultad de Medicina, Universidad de Chile. The patients/participants provided their written informed consent to participate in this study.
Author Contributions
DJ, PC, and M-JG conceived the study. DJ and PC conceived and designed the experiments while DJ, PC, IC, M-JB, SA, SG, CM, and MH performed them. SA, SG, and CM were involved in clinical data collection. IC, DJ, PC, M-JB, SA, SG, CM, MH, and MJG wrote the manuscript. All authors contributed to the article and approved the submitted version.
Funding
This work was supported by Fondecyt-Chile [1210055 to M-JG, SA, IC, CM, SG, M-JB); Fondecyt-Chile [1160015 to M-JG, SA, IC, CM, SG); Enlace-VID Universidad de Chile [ENL04/20 to MJG]; Fondecyt-Iniciación [11170049 to IC]; Fondecyt-Iniciación [11201058 to MJB], and PhD fellowship Conicyt-Chile to DJ and PC.
Conflict of Interest
The authors declare that the research was conducted in the absence of any commercial or financial relationships that could be construed as a potential conflict of interest.
Acknowledgments
We thank all the patients who participated in this study. We also thank Amber Philp for her editing contribution.
Supplementary Material
The Supplementary Material for this article can be found online at: https://www.frontiersin.org/articles/10.3389/fimmu.2021.685837/full#supplementary-material
References
1. Mavragani CP, Moutsopoulos HM. Sjögren’s Syndrome: Old and New Therapeutic Targets. J Autoimmun (2020) 110:102364. doi: 10.1016/j.jaut.2019.102364
2. Fox RI, Kang HI, Ando D, Abrams J, Pisa E. Cytokine mRNA Expression in Salivary Gland Biopsies of Sjögren’s Syndrome. J Immunol (1994) 152(11):5532–9.
3. Fox PC, Brennan M, Di Sun P. Cytokine Expression in Human Labial Minor Salivary Gland Epithelial Cells in Health and Disease. Arch Oral Biol (1999) 44 Suppl 1:S49–52. doi: 10.1016/S0003-9969(99)90018-3
4. Roescher N, Tak PP, Illei GG. Cytokines in Sjögren’s Syndrome. Oral Dis (2009) 15(8):519–26. doi: 10.1111/j.1601-0825.2009.01582.x
5. Yao Y, Liu Z, Jallal B, Shen N, Rönnblom L. Type I Interferons in Sjögren’s Syndrome. Autoimmun Rev (2013) 12(5):558–66. doi: 10.1016/j.autrev.2012.10.006
6. Bodewes ILA, Björk A, Versnel MA, Wahren-Herlenius M. Innate Immunity and Interferons in the Pathogenesis of Sjögren’s Syndrome. Rheumatol (Oxford) (2019). doi: 10.1093/rheumatology/key360
7. Bodewes ILA, Al-Ali S, van Helden-Meeuwsen CG, Maria NI, Tarn J, Lendrem DW, et al. Systemic Interferon Type I and Type II Signatures in Primary Sjögren’s Syndrome Reveal Differences in Biological Disease Activity. Rheumatol (Oxford) (2018) 57(5):921–30. doi: 10.1093/rheumatology/kex490
8. Marketos N, Cinoku I, Rapti A, Mavragani CP. Type I Interferon Signature in Sjögren’s Syndrome: Pathophysiological and Clinical Implications. Clin Exp Rheumatol (2019) 37 Suppl 118(3):185–91.
9. Schoggins JW, Rice CM. Interferon-Stimulated Genes and Their Antiviral Effector Functions. Curr Opin Virol (2011) 1(6):519–25. doi: 10.1016/j.coviro.2011.10.008
10. Le Bon A, Schiavoni G, D’Agostino G, Gresser I, Belardelli F, Tough DF. Type I Interferons Potently Enhance Humoral Immunity and can Promote Isotype Switching by Stimulating Dendritic Cells In Vivo. Immunity (2001) 14(4):461–70. doi: 10.1016/S1074-7613(01)00126-1
11. Mavragani CP, Niewold TB, Moutsopoulos NM, Pillemer SR, Wahl SM, Crow MK. Augmented Interferon-Alpha Pathway Activation in Patients With Sjögren’s Syndrome Treated With Etanercept. Arthritis Rheum (2007) 56(12):3995–4004. doi: 10.1002/art.23062
12. Hervas-Stubbs S, Perez-Gracia JL, Rouzaut A, Sanmamed MF, Le Bon A, Melero I. Direct Effects of Type I Interferons on Cells of the Immune System. Clin Cancer Res (2011) 17(9):2619–27. doi: 10.1158/1078-0432.CCR-10-1114
13. Gottenberg JE, Cagnard N, Lucchesi C, Letourneur F, Mistou S, Lazure T, et al. Activation of IFN Pathways and Plasmacytoid Dendritic Cell Recruitment in Target Organs of Primary Sjögren’s Syndrome. Proc Natl Acad Sci U S A (2006) 103(8):2770–5. doi: 10.1073/pnas.0510837103
14. Hjelmervik TO, Petersen K, Jonassen I, Jonsson R, Bolstad AI. Gene Expression Profiling of Minor Salivary Glands Clearly Distinguishes Primary Sjögren’s Syndrome Patients From Healthy Control Subjects. Arthritis Rheum (2005) 52(5):1534–44. doi: 10.1002/art.21006
15. Wakamatsu E, Nakamura Y, Matsumoto I, Goto D, Ito S, Tsutsumi A, et al. DNA Microarray Analysis of Labial Salivary Glands of Patients With Sjogren’s Syndrome. Ann Rheum Dis (2007) 66(6):844–5. doi: 10.1136/ard.2006.063370
16. Nezos A, Gravani F, Tassidou A, Kapsogeorgou EK, Voulgarelis M, Koutsilieris M, et al. Type I and II Interferon Signatures in Sjogren’s Syndrome Pathogenesis: Contributions in Distinct Clinical Phenotypes and Sjogren’s Related Lymphomagenesis. J Autoimmun (2015) 63:47–58. doi: 10.1016/j.jaut.2015.07.002
17. Pérez P, Anaya JM, Aguilera S, Urzúa U, Munroe D, Molina C, et al. Gene Expression and Chromosomal Location for Susceptibility to Sjögren’s Syndrome. J Autoimmun (2009) 33(2):99–108. doi: 10.1016/j.jaut.2009.05.001
18. Wildenberg ME, van Helden-Meeuwsen CG, van de Merwe JP, Drexhage HA, Versnel MA. Systemic Increase in Type I Interferon Activity in Sjögren’s Syndrome: A Putative Role for Plasmacytoid Dendritic Cells. Eur J Immunol (2008) 38(7):2024–33. doi: 10.1002/eji.200738008
19. Emamian ES, Leon JM, Lessard CJ, Grandits M, Baechler EC, Gaffney PM, et al. Peripheral Blood Gene Expression Profiling in Sjögren’s Syndrome. Genes Immun (2009) 10(4):285–96. doi: 10.1038/gene.2009.20
20. Imgenberg-Kreuz J, Sandling JK, Almlöf JC, Nordlund J, Signér L, Norheim KB, et al. Genome-Wide DNA Methylation Analysis in Multiple Tissues in Primary Sjögren’s Syndrome Reveals Regulatory Effects at Interferon-Induced Genes. Ann Rheum Dis (2016) 75(11):2029–36. doi: 10.1136/annrheumdis-2015-208659
21. Maria NI, Steenwijk EC, IJpma AS, van Helden-Meeuwsen CG, Vogelsang P, Beumer W, et al. Contrasting Expression Pattern of RNA-sensing Receptors TLR7, RIG-I and MDA5 in Interferon-Positive and Interferon-Negative Patients With Primary Sjögren’s Syndrome. Ann Rheum Dis (2017) 76(4):721–30. doi: 10.1136/annrheumdis-2016-209589
22. Brkic Z, Maria NI, van Helden-Meeuwsen CG, van de Merwe JP, van Daele PL, Dalm VA, et al. Prevalence of Interferon Type I Signature in CD14 Monocytes of Patients With Sjogren’s Syndrome and Association With Disease Activity and BAFF Gene Expression. Ann Rheum Dis (2013) 72(5):728–35. doi: 10.1136/annrheumdis-2012-201381
23. Maria NI, Brkic Z, Waris M, van Helden-Meeuwsen CG, Heezen K, van de Merwe JP, et al. Mxa as a Clinically Applicable Biomarker for Identifying Systemic Interferon Type I in Primary Sjogren’s Syndrome. Ann Rheum Dis (2014) 73(6):1052–9. doi: 10.1136/annrheumdis-2012-202552
24. Maria NI, van Helden-Meeuwsen CG, Brkic Z, Paulissen SM, Steenwijk EC, Dalm VA, et al. Association of Increased Treg Cell Levels With Elevated Indoleamine 2,3-Dioxygenase Activity and an Imbalanced Kynurenine Pathway in Interferon-Positive Primary Sjögren’s Syndrome. Arthritis Rheumatol (2016) 68(7):1688–99. doi: 10.1002/art.39629
25. Hall JC, Baer AN, Shah AA, Criswell LA, Shiboski CH, Rosen A, et al. Molecular Subsetting of Interferon Pathways in Sjögren’s Syndrome. Arthritis Rheumatol (2015) 67(9):2437–46. doi: 10.1002/art.39204
26. Forster SC, Tate MD, Hertzog PJ. MicroRNA as Type I Interferon-Regulated Transcripts and Modulators of the Innate Immune Response. Front Immunol (2015) 6:334. doi: 10.3389/fimmu.2015.00334
27. Cha S, Mona M, Lee KE, Kim DH, Han K. MicroRNAs in Autoimmune Sjögren’s Syndrome. Genomics Inform (2018) 16(4):e19. doi: 10.5808/GI.2018.16.4.e19
28. Lin L, Hou J, Ma F, Wang P, Liu X, Li N, et al. Type I IFN Inhibits Innate IL-10 Production in Macrophages Through Histone Deacetylase 11 by Downregulating Microrna-145. J Immunol (2013) 191(7):3896–904. doi: 10.4049/jimmunol.1203450
29. Alevizos I, Alexander S, Turner RJ, Illei GG. MicroRNA Expression Profiles as Biomarkers of Minor Salivary Gland Inflammation and Dysfunction in Sjögren’s Syndrome. Arthritis Rheum (2011) 63(2):535–44. doi: 10.1002/art.30131
30. Pekow JR, Dougherty U, Mustafi R, Zhu H, Kocherginsky M, Rubin DT, et al. miR-143 and miR-145 are Downregulated in Ulcerative Colitis: Putative Regulators of Inflammation and Protooncogenes. Inflammation Bowel Dis (2012) 18(1):94–100. doi: 10.1002/ibd.21742
31. O’Leary L, Sevinç K, Papazoglou IM, Tildy B, Detillieux K, Halayko AJ, et al. Airway Smooth Muscle Inflammation is Regulated by microRNA-145 in COPD. FEBS Lett (2016) 590(9):1324–34. doi: 10.1002/1873-3468.12168
32. Lu MC, Lai NS, Chen HC, Yu HC, Huang KY, Tung CH, et al. Decreased microRNA(miR)-145 and Increased miR-224 Expression in T Cells From Patients With Systemic Lupus Erythematosus Involved in Lupus Immunopathogenesis. Clin Exp Immunol (2013) 171(1):91–9. doi: 10.1111/j.1365-2249.2012.04676.x
33. Zhu H, Li Y, Qu S, Luo H, Zhou Y, Wang Y, et al. MicroRNA Expression Abnormalities in Limited Cutaneous Scleroderma and Diffuse Cutaneous Scleroderma. J Clin Immunol (2012) 32(3):514–22. doi: 10.1007/s10875-011-9647-y
34. Cai Z, Xiang W, Peng X, Ding Y, Liao W, He X. Microrna-145 Involves in the Pathogenesis of Renal Vascular Lesions and May Become a Potential Therapeutic Target in Patients With Juvenile Lupus Nephritis. Kidney Blood Press Res (2019) 44(4):643–55. doi: 10.1159/000500923
35. Ding Y, Liao W, Yi Z, Xiang W, He X. Association of miRNA-145 Expression in Vascular Smooth Muscle Cells With Vascular Damages in Patients With Lupus Nephritis. Int J Clin Exp Pathol (2015) 8(10):12646–56.
36. Wang J, Zheng S, Xin N, Dou C, Fu L, Zhang X, et al. Identification of Novel MicroRNA Signatures Linked to Experimental Autoimmune Myasthenia Gravis Pathogenesis: Down-Regulated miR-145 Promotes Pathogenetic Th17 Cell Response. J Neuroimmune Pharmacol (2013) 8(5):1287–302. doi: 10.1007/s11481-013-9498-9
37. Sung HH, Castro I, González S, Aguilera S, Smorodinsky NI, Quest A, et al. MUC1/SEC and MUC1/Y Overexpression is Associated With Inflammation in Sjögren’s Syndrome. Oral Dis (2015) 21(6):730–8. doi: 10.1111/odi.12339
38. Castro I, Albornoz N, Aguilera S, Barrera MJ, González S, Núñez M, et al. Aberrant MUC1 Accumulation in Salivary Glands of Sjögren’s Syndrome Patients is Reversed by TUDCA In Vitro. Rheumatol (Oxford) (2020) 59(4):742–53. doi: 10.1093/rheumatology/kez316
39. Spachidou MP, Bourazopoulou E, Maratheftis CI, Kapsogeorgou EK, Moutsopoulos HM, Tzioufas AG, et al. Expression of Functional Toll-like Receptors by Salivary Gland Epithelial Cells: Increased mRNA Expression in Cells Derived From Patients With Primary Sjögren’s Syndrome. Clin Exp Immunol (2007) 147(3):497–503. doi: 10.1111/j.1365-2249.2006.03311.x
40. Barrera MJ, Aguilera S, Veerman E, Quest AF, Díaz-Jiménez D, Urzúa U, et al. Salivary Mucins Induce a Toll-like Receptor 4-Mediated Pro-Inflammatory Response in Human Submandibular Salivary Cells: Are Mucins Involved in Sjögren’s Syndrome? Rheumatol (Oxford) (2015) 54(8):1518–27. doi: 10.1093/rheumatology/kev026
41. Zhang L, Vlad A, Milcarek C, Finn OJ. Human Mucin MUC1 RNA Undergoes Different Types of Alternative Splicing Resulting in Multiple Isoforms. Cancer Immunol Immunother (2013) 62(3):423–35. doi: 10.1007/s00262-012-1325-2
42. Ligtenberg MJ, Vos HL, Gennissen AM, Hilkens J. Episialin, a Carcinoma-Associated Mucin, is Generated by a Polymorphic Gene Encoding Splice Variants With Alternative Amino Termini. J Biol Chem (1990) 265(10):5573–8. doi: 10.1016/S0021-9258(19)39399-8
43. Wreschner DH, Hareuveni M, Tsarfaty I, Smorodinsky N, Horev J, Zaretsky J, et al. Human Epithelial Tumor Antigen cDNA Sequences. Differential Splicing may Generate Multiple Protein Forms. Eur J Biochem (1990) 189(3):463–73. doi: 10.1111/j.1432-1033.1990.tb15511.x
44. Zrihan-Licht S, Vos HL, Baruch A, Elroy-Stein O, Sagiv D, Keydar I, et al. Characterization and Molecular Cloning of a Novel MUC1 Protein, Devoid of Tandem Repeats, Expressed in Human Breast Cancer Tissue. Eur J Biochem (1994) 224(2):787–95. doi: 10.1111/j.1432-1033.1994.00787.x
45. Smorodinsky N, Weiss M, Hartmann ML, Baruch A, Harness E, Yaakobovitz M, et al. Detection of a Secreted MUC1/SEC Protein by MUC1 Isoform Specific Monoclonal Antibodies. Biochem Biophys Res Commun (1996) 228(1):115–21. doi: 10.1006/bbrc.1996.1625
46. Herbert LM, Grosso JF, Dorsey M, Fu T, Keydar I, Cejas MA, et al. A Unique Mucin Immunoenhancing Peptide With Antitumor Properties. Cancer Res (2004) 64(21):8077–84. doi: 10.1158/0008-5472.CAN-04-0853
47. Ilkovitch D, Handel-Fernandez ME, Herbert LM, Lopez DM. Antitumor Effects of Mucin 1/Sec Involves the Modulation of Urokinase-Type Plasminogen Activator and Signal Transducer and Activator of Transcription 1 Expression in Tumor Cells. Cancer Res (2008) 68(7):2427–35. doi: 10.1158/0008-5472.CAN-07-5651
48. Grosso JF, Herbert LM, Owen JL, Lopez DM. MUC1/Sec-Expressing Tumors are Rejected In Vivo by a T Cell-Dependent Mechanism and Secrete High Levels of CCL2. J Immunol (2004) 173(3):1721–30. doi: 10.4049/jimmunol.173.3.1721
49. Baruch A, Hartmann M, Yoeli M, Adereth Y, Greenstein S, Stadler Y, et al. The Breast Cancer-Associated MUC1 Gene Generates Both a Receptor and its Cognate Binding Protein. Cancer Res (1999) 59(7):1552–61.
50. Cascio S, Zhang L, Finn OJ. MUC1 Protein Expression in Tumor Cells Regulates Transcription of Proinflammatory Cytokines by Forming a Complex With Nuclear Factor-κb p65 and Binding to Cytokine Promoters: Importance of Extracellular Domain. J Biol Chem (2011) 286(49):42248–56. doi: 10.1074/jbc.M111.297630
51. Takeda K, Kaisho T, Akira S. Toll-Like Receptors. Annu Rev Immunol (2003) 21:335–76. doi: 10.1146/annurev.immunol.21.120601.141126
52. Roh JS, Sohn DH. Damage-Associated Molecular Patterns in Inflammatory Diseases. Immune Netw (2018) 18(4):e27. doi: 10.4110/in.2018.18.e27
53. Han DC, Huang GT, Lin LM, Warner NA, Gim JS, Jewett A. Expression of MHC Class II, Cd70, CD80, CD86 and Pro-Inflammatory Cytokines is Differentially Regulated in Oral Epithelial Cells Following Bacterial Challenge. Oral Microbiol Immunol (2003) 18(6):350–8. doi: 10.1046/j.0902-0055.2003.00094.x
54. Kawai T, Takeuchi O, Fujita T, Inoue J, Mühlradt PF, Sato S, et al. Lipopolysaccharide Stimulates the MyD88-independent Pathway and Results in Activation of IFN-regulatory Factor 3 and the Expression of a Subset of Lipopolysaccharide-Inducible Genes. J Immunol (2001) 167(10):5887–94. doi: 10.4049/jimmunol.167.10.5887
55. Anders HJ, Vielhauer V, Eis V, Linde Y, Kretzler M, Perez de Lema G, et al. Activation of Toll-Like Receptor-9 Induces Progression of Renal Disease in MRL-Fas(lpr) Mice. FASEB J (2004) 18(3):534–6. doi: 10.1096/fj.03-0646fje
56. Kobayashi Y, Murakami H, Akbar SM, Matsui H, Onji M. A Novel and Effective Approach of Developing Aggressive Experimental Autoimmune Gastritis in Neonatal Thymectomized BALB/c Mouse by Polyinosinic:Polycytidylic Acid. Clin Exp Immunol (2004) 136(3):423–31. doi: 10.1111/j.1365-2249.2004.02467.x
57. Qu WM, Miyazaki T, Terada M, Okada K, Mori S, Kanno H, et al. A Novel Autoimmune Pancreatitis Model in MRL Mice Treated With Polyinosinic:Polycytidylic Acid. Clin Exp Immunol (2002) 129(1):27–34. doi: 10.1046/j.1365-2249.2002.01881.x
58. Shiboski CH, Shiboski SC, Seror R, Criswell LA, Labetoulle M, Lietman TM, et al. 2016 American College of Rheumatology/European League Against Rheumatism Classification Criteria for Primary Sjögren’s Syndrome: A Consensus and Data-Driven Methodology Involving Three International Patient Cohorts. Ann Rheum Dis (2017) 76(1):9–16. doi: 10.1136/annrheumdis-2016-210571
59. Daniels TE. Labial Salivary Gland Biopsy in Sjögren’s Syndrome. Assessment as a Diagnostic Criterion in 362 Suspected Cases. Arthritis Rheum (1984) 27(2):147–56. doi: 10.1002/art.1780270205
60. Pfaffl MW, Horgan GW, Dempfle L. Relative Expression Software Tool (REST) for Group-Wise Comparison and Statistical Analysis of Relative Expression Results in Real-Time PCR. Nucleic Acids Res (2002) 30(9):e36. doi: 10.1093/nar/30.9.e36
61. Kirou KA, Lee C, George S, Louca K, Papagiannis IG, Peterson MG, et al. Coordinate Overexpression of Interferon-Alpha-Induced Genes in Systemic Lupus Erythematosus. Arthritis Rheum (2004) 50(12):3958–67. doi: 10.1002/art.20798
62. Feng X, Wu H, Grossman JM, Hanvivadhanakul P, FitzGerald JD, Park GS, et al. Association of Increased Interferon-Inducible Gene Expression With Disease Activity and Lupus Nephritis in Patients With Systemic Lupus Erythematosus. Arthritis Rheum (2006) 54(9):2951–62. doi: 10.1002/art.22044
63. Barrera MJ, Aguilera S, Castro I, Cortés J, Bahamondes V, Quest AF, et al. Pro-Inflammatory Cytokines Enhance ERAD and ATF6α Pathway Activity in Salivary Glands of Sjögren’s Syndrome Patients. J Autoimmun (2016) 75:68–81. doi: 10.1016/j.jaut.2016.07.006
64. Bradford MM. A Rapid and Sensitive Method for the Quantitation of Microgram Quantities of Protein Utilizing the Principle of Protein-Dye Binding. Anal Biochem (1976) 72:248–54. doi: 10.1016/0003-2697(76)90527-3
65. Sachdeva M, Mo YY. MicroRNA-145 Suppresses Cell Invasion and Metastasis by Directly Targeting Mucin 1. Cancer Res (2010) 70(1):378–87. doi: 10.1158/0008-5472.CAN-09-2021
66. Wu H, Xiao Z, Wang K, Liu W, Hao Q. MiR-145 is Downregulated in Human Ovarian Cancer and Modulates Cell Growth and Invasion by Targeting p70S6K1 and MUC1. Biochem Biophys Res Commun (2013) 441(4):693–700. doi: 10.1016/j.bbrc.2013.10.053
67. Roy LD, Sahraei M, Subramani DB, Besmer D, Nath S, Tinder TL, et al. MUC1 Enhances Invasiveness of Pancreatic Cancer Cells by Inducing Epithelial to Mesenchymal Transition. Oncogene (2011) 30(12):1449–59. doi: 10.1038/onc.2010.526
68. Rahn JJ, Chow JW, Horne GJ, Mah BK, Emerman JT, Hoffman P, et al. MUC1 Mediates Transendothelial Migration In Vitro by Ligating Endothelial Cell ICAM-1. Clin Exp Metastasis (2005) 22(6):475–83. doi: 10.1007/s10585-005-3098-x
69. Wang L, Wu X, Wang B, Wang Q, Han L. Mechanisms of miR-145 Regulating Invasion and Metastasis of Ovarian Carcinoma. Am J Transl Res (2017) 9(7):3443–51.
70. Castro I, Sepúlveda D, Cortés J, Quest AF, Barrera MJ, Bahamondes V, et al. Oral Dryness in Sjögren’s Syndrome Patients. Not Just a Question of Water. Autoimmun Rev (2013) 12(5):567–74. doi: 10.1016/j.autrev.2012.10.018
71. Alliende C, Kwon YJ, Brito M, Molina C, Aguilera S, Pérez P, et al. Reduced Sulfation of muc5b is Linked to Xerostomia in Patients With Sjögren Syndrome. Ann Rheum Dis (2008) 67(10):1480–7. doi: 10.1136/ard.2007.078246
72. Goicovich E, Molina C, Pérez P, Aguilera S, Fernández J, Olea N, et al. Enhanced Degradation of Proteins of the Basal Lamina and Stroma by Matrix Metalloproteinases From the Salivary Glands of Sjögren’s Syndrome Patients: Correlation With Reduced Structural Integrity of Acini and Ducts. Arthritis Rheum (2003) 48(9):2573–84. doi: 10.1002/art.11178
73. Sepúlveda D, Barrera MJ, Castro I, Aguilera S, Carvajal P, Lagos C, et al. Impaired IRE1α/XBP-1 Pathway Associated to DNA Methylation Might Contribute to Salivary Gland Dysfunction in Sjögren’s Syndrome Patients. Rheumatol (Oxford) (2018) 57(6):1021–32. doi: 10.1093/rheumatology/key021
74. Hui Y, Yin Y. MicroRNA-145 Attenuates High Glucose-Induced Oxidative Stress and Inflammation in Retinal Endothelial Cells Through Regulating TLR4/NF-κb Signaling. Life Sci (2018) 207:212–8. doi: 10.1016/j.lfs.2018.06.005
75. Yu L, Qu H, Yu Y, Li W, Zhao Y, Qiu G. LncRNA-PCAT1 Targeting miR-145-5p Promotes TLR4-associated Osteogenic Differentiation of Adipose-Derived Stem Cells. J Cell Mol Med (2018) 22(12):6134–47. doi: 10.1111/jcmm.13892
76. Jin C, Wang A, Liu L, Wang G, Li G, Han Z. miR-145-5p Inhibits Tumor Occurrence and Metastasis Through the NF-κb Signaling Pathway by Targeting TLR4 in Malignant Melanoma. J Cell Biochem (2019) 120(7):11115–26. doi: 10.1002/jcb.28388
77. Yu YL, Yu G, Ding ZY, Li SJ, Fang QZ. Overexpression of miR-145-5p Alleviated LPS-induced Acute Lung Injury. J Biol Regul Homeost Agents (2019) 33(4):1063–72.
78. Barrera MJ, Sánchez M, Aguilera S, Alliende C, Bahamondes V, Molina C, et al. Aberrant Localization of Fusion Receptors Involved in Regulated Exocytosis in Salivary Glands of Sjögren’s Syndrome Patients is Linked to Ectopic Mucin Secretion. J Autoimmun (2012) 39(1-2):83–92. doi: 10.1016/j.jaut.2012.01.011
79. Bahamondes V, Albornoz A, Aguilera S, Alliende C, Molina C, Castro I, et al. Changes in Rab3D Expression and Distribution in the Acini of Sjögren’s Syndrome Patients are Associated With Loss of Cell Polarity and Secretory Dysfunction. Arthritis Rheum (2011) 63(10):3126–35. doi: 10.1002/art.30500
80. Kim S, Joe Y, Surh YJ, Chung HT. Differential Regulation of Toll-Like Receptor-Mediated Cytokine Production by Unfolded Protein Response. Oxid Med Cell Longev (2018) 2018:9827312. doi: 10.1155/2018/9827312
81. Martinon F, Chen X, Lee AH, Glimcher LH. TLR Activation of the Transcription Factor XBP1 Regulates Innate Immune Responses in Macrophages. Nat Immunol (2010) 11(5):411–8. doi: 10.1038/ni.1857
Keywords: Sjögren’s syndrome, Type I interferons, hsa-miR-145-5p, Mucin 1, Toll-like receptor 4
Citation: Jara D, Carvajal P, Castro I, Barrera M-J, Aguilera S, González S, Molina C, Hermoso M and González M-J (2021) Type I Interferon Dependent hsa-miR-145-5p Downregulation Modulates MUC1 and TLR4 Overexpression in Salivary Glands From Sjögren’s Syndrome Patients. Front. Immunol. 12:685837. doi: 10.3389/fimmu.2021.685837
Received: 25 March 2021; Accepted: 12 May 2021;
Published: 02 June 2021.
Edited by:
Kristi A. Koelsch, University of Oklahoma Health Sciences Center, United StatesReviewed by:
John Chiorini, National Institutes of Health Clinical Center (NIH), United StatesFloris Bikker, VU University Amsterdam, Netherlands
Copyright © 2021 Jara, Carvajal, Castro, Barrera, Aguilera, González, Molina, Hermoso and González. This is an open-access article distributed under the terms of the Creative Commons Attribution License (CC BY). The use, distribution or reproduction in other forums is permitted, provided the original author(s) and the copyright owner(s) are credited and that the original publication in this journal is cited, in accordance with accepted academic practice. No use, distribution or reproduction is permitted which does not comply with these terms.
*Correspondence: María-Julieta González, jgonzale@med.uchile.cl
†These authors have contributed equally to this work and share first authorship