- 1Research Center of Avian Disease, College of Veterinary Medicine of Sichuan Agricultural University, Chengdu, China
- 2Institute of Preventive Veterinary Medicine, Sichuan Agricultural University, Chengdu, China
- 3Key Laboratory of Animal Disease and Human Health of Sichuan Province, Sichuan Agricultural University, Chengdu, China
Interleukin-7 (IL-7) is produced by stromal cells, keratinocytes, and epithelial cells in host tissues or tumors and exerts a wide range of immune effects mediated by the IL-7 receptor (IL-7R). IL-7 is primarily involved in regulating the development of B cells, T cells, natural killer cells, and dendritic cells via the JAK-STAT, PI3K-Akt, and MAPK pathways. This cytokine participates in the early generation of lymphocyte subsets and maintain the survival of all lymphocyte subsets; in particular, IL-7 is essential for orchestrating the rearrangement of immunoglobulin genes and T-cell receptor genes in precursor B and T cells, respectively. In addition, IL-7 can aid the activation of immune cells in anti-virus and anti-tumor immunity and plays important roles in the restoration of immune function. These biological functions of IL-7 make it an important molecular adjuvant to improve vaccine efficacy as it can promote and extend systemic immune responses against pathogens by prolonging lymphocyte survival, enhancing effector cell activity, and increasing antigen-specific memory cell production. This review focuses on the biological function and mechanism of IL-7 and summarizes its contribution towards improved vaccine efficacy. We hope to provide a thorough overview of this cytokine and provide strategies for the development of the future vaccines.
Introduction
In 1987, Hunt et al. discovered a cytokine that promoted the development of pro- and pre-B cells while exploring the potential role of bone marrow stromal cells in pre-B cell subset growth (1). The following year, Namen et al. isolated and purified a cytokine from the culture supernatant of mouse bone marrow stromal cells and named it lymphocyte growth factor-1 (lymphopoetin-1, LP-1) (2). This cytokine was officially named interleukin-7 (IL-7) at the 6th International Lymphokine Conference, France (2). Subsequently, it has been confirmed that IL-7 is mainly produced by thymus and bone marrow stromal cells (3), but can also be secreted by lymphoid organs (spleen, tonsil), non-lymphoid tissues (liver, lung, intestine, and skin), and tumors (colorectal cancer, prostate cancer) (4–11).
B and T lymphocytes develop from hematopoietic stem cells (HSCs) and play critical roles in regulating immune responses. IL-7 is a highly pleiotropic cytokine that is required for the efficient generation of lymphocytes from HSCs (12) and maintains the survival of B and T cells by regulating B-cell lymphoma-2 (Bcl-2) family proteins and providing proliferation signals to these lymphocytes (13–15). Gene rearrangements allow lymphocytes to recognize various antigens. For instance, IL-7 regulates the rearrangement of immunoglobulin (Ig) genes in immature B cell subsets and T cell receptor (TCR) genes in precursor T cell subsets via the IL-7 receptor (IL-7R) signaling pathway to ensure primary antibody repertoire and T cell diversity (16, 17). In T cells, IL-7 can also restore T cell homeostasis by enhancing thymic output (18) and trigger the expression of chemokines (CCL4, CCL25, CCL28) and integrins (α4β7, α2β1), leading to T cell homing in local organs (19–21).
IL-7 quantitatively and qualitatively modulates the responses of immune cells, such as natural killer (NK) cells, dendritic cells (DCs), B cells, and T cells, against pathogens (22–28). Indeed, IL-7 treatment promotes Th2 cell immune responses, increases the production of neutralizing antibodies, and increases the cytotoxicity of antigen-specific cytotoxic T lymphocytes (CTL) (29). Furthermore, it controls the survival of mature and memory T cells by upregulating the expression of anti-apoptotic Bcl-2 family proteins and the glycerol channel aquaporin 9, which promotes long-term immunity (30–32), as well as being an important regulator of immune balance (33). Besides, IL-7 exerts significant effects on anti-virus and anti-tumor activities, as demonstrated multiple times in vitro and in vivo. The administration of IL-7 has been found to significantly boost mouse anti-cynomolgus-monkey anti-simian immunodeficiency virus (SIV) infection (34), mouse anti-4T1 breast tumor invasion (35), lymphocytic choriomeningitis virus (LCMV) infection (36), and mouse anti-E.G7-OVA tumor invasion (37). Moreover, IL-7 has been tried to apply in patient against human diseases (Table 1).
Given the powerful biological functions of IL-7, several groups have successfully used IL-7 as a molecular adjuvant to enhance immunogenicity and prolong the protection period of vaccines against human, avian, and canine-related pathogens (46–48). In this review, we summarize and update our understanding of the biological function, mechanism, and adjuvanticity of IL-7 and highlight this cytokine as a promising molecule for future vaccine research.
IL-7 Is Essential for Immune Cell Development
Lymphocytes such as B cells, T cells, and NK cells differentiate from common lymphoid progenitors (CLPs) derived from HSCs. CLPs differentiate into lymphocytes with the help of several cytokines, including IL-7, which plays important roles in cell differentiation, proliferation, survival, and activation. In particular, IL-7 is the predominant cytokine associated with B and T cell development, as demonstrated by Von et al. who reported that mice lacking IL-7 or IL-7Rα are unable to maintain normal numbers of B and T cells (49). NK cells were one of the earliest reported lymphocytes and are the main effector cells of the innate immune system, with essential roles in early host defenses against intracellular pathogen infection (50). In addition, IL-7 contributes towards maintaining and enhancing NK cell-based cytotoxicity (24). DCs are considered to be the most powerful antigen-presenting cells (APCs) and play central regulatory roles in immunity, such as in antigen recognition, processing, and peptide presentation to naive T cells (Tn) via the major histocompatibility complex (MHC), which effectively activates adaptive immune responses (51). Since IL-7 is critical for bone marrow-derived and peripheral blood monocyte-differentiated DCs (25), it may play crucial roles in host immune system development and immune response regulation. Taking these facts into account, the review next expands on the role of IL-7 on the development of immune cells.
IL-7 in B Cell Development
Cytokine action causes CLPs to sequentially progress from pro-B cells (Igα/Igβ expressed and B cell receptor (BCR) gene rearrangement) to pre-B cells (pre-BCR complex expression) and finally develop into immature B cells (membrane IgM molecule engagement). Immature B cells then migrate into secondary lymphoid tissues, where they differentiate into mature B cells (membrane IgM and IgD expression). When mature B cells respond to antigens in peripheral lymphoid organs, they transiently form germinal centers (GCs) where Ig genes are hypermutated and selected, leading to the formation of plasma cells and memory B cells (52, 53).
Previous studies have shown that the cytokines IL-4, IL-7, IL-9, IL-13, and IL-21 play important roles in the development of B cells. In particular, IL-7 is essential for B cell development since it can enhance and maintain B lymphopoiesis-specific transcription factor early B cell factor 1 (EBF1) expression to induce B cell production and to cause early B cell differentiation at the CLP stage (54, 55). IL-7 also critically assists the transformation of pre-pro-B cells into pro-B cells, with pre-pro-B cells from IL-7-/- mice losing their ability to differentiate into pro-B cells (56). In this process, pre-pro-B cell growth stimulating factor (PPBSF) is an important cofactor of IL-7, which up-regulates the expression of IL-7Rα, making pro-B cells better respond to IL-7 (57). In addition, IL-7 is essential for pre-B cell formation from pro-B cells in the bone marrow: when IL-7 is neutralized using a monoclonal antibody, pro-B cells are unable to develop into pre-B cells (58), but their differentiation is rescued by IL-7 supplementation (59). However, the function of IL-7 varies between different early B cell subsets. For instance, recombinant IL-7 administration was found to increase the number of pro-B and pre-B cells in wildtype mice and renal adenocarcinoma mice through transducing replicative signals for cell proliferation by inducing signal transducer and activator of transcription-5 (STAT5) phosphorylation (60–62), whereas other experiments have shown that it provides survival signals instead of proliferation signals for CD19- B-cell progenitors (63).
The Ig loci of B cells recombine in a stepwise and reproducible manner. Ig heavy chain (HC) genes are rearranged in pro-B cells, whereas Ig light chain (LC) genes are rearranged in pre-B cells (64). IL-7 is essential for orchestrating the rearrangement of both the Ig HC and LC loci in early B cell development (65). In addition, IL-7 co-ordinates with the transcription factor paired box protein 5 (PAX5) to regulate Ig HC variable-region (VH) gene rearrangement that generates antibody repertoire during B lymphocyte development (66). In particular, IL-7 influences the distal VH locus by activating H3K36me2 histone modification, whereas PAX5 controls the proximal VH locus by increasing H3K27me3 histone modification (65). In IL-7-/- mice, splenic B cell structure is absent or aberrant and the IgM xenoantibody (IgMXAb)-producing function of marginal zone B cells is blocked (67). During B cell development, IL-7 regulates the expression of anti-apoptotic genes (Bcl-2, Bcl-xL, Mcl-1) and pro-apoptotic genes (Bax, Bad, Bim) to affect the survival of precursor B cell subsets via IL-7R-mediated signals (68, 69). A previous study showed that mature B cells accumulate in IL-7-/- mice, indicating that immature B cells do not require IL-7 for survival or further development once they have left the bone marrow (69). Although the conclusions of recent studies have been controversial, IL-7 has been harnessed as a molecular adjuvant in vaccines to significantly promote the production of neutralizing antibodies and GC B cells (70, 71), suggesting that IL-7 may assist mature B cell development in the formation of plasma and memory B cells. This function may be indirectly regulated by IL-7 through T follicular helper (Tfh) cells, which assist B cells to produce pathogen neutralizing antibodies and to form memory B cells (72, 73). They prove that IL-7 plays a directly or indirectly role in the development of B cells (Figure 1). Although IL-7 is a key cytokine in B cell progenitors development in the mouse, it has less critical function in human (74).
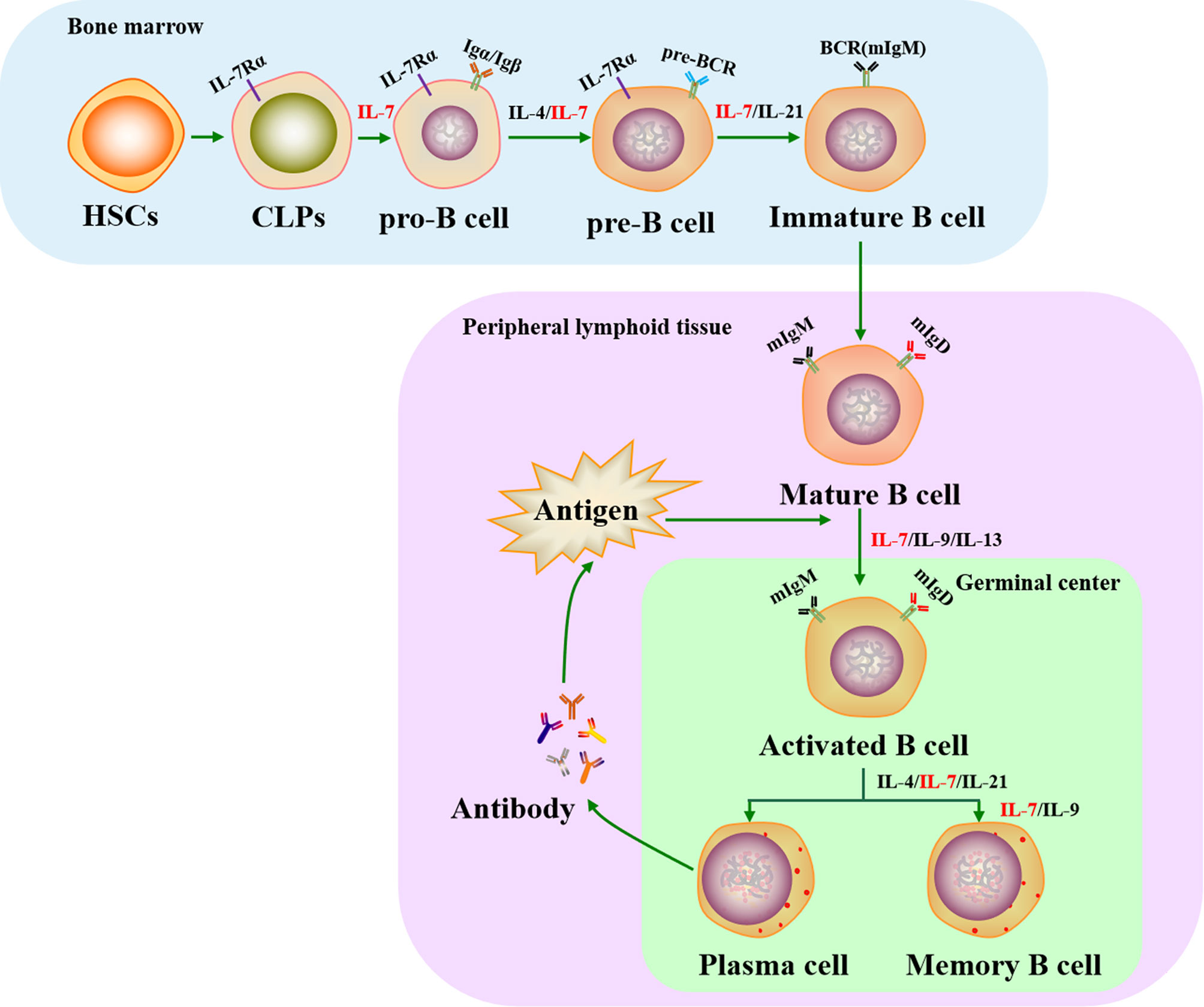
Figure 1 Role of IL-7 in the life cycle of B cells. IL-7 plays an essential role in B cell development in both bone marrow and peripheral lymphoid tissues. It is involved in regulating the production of pro-B, pre-B, immature B, plasma, and memory B cells. HSCs, hematopoietic stem cells; CLPs, common lymphoid progenitors; IL-7Rα, IL-7 receptor α chain; BCR, B cell receptor; mIgM, membrane IgM; mIgD, membrane IgD.
IL-7 in T Cell Development
T cells play a key role in cellular immunity against intracellular pathogens. Pro-T cells differentiate and mature from HSCs that have migrated into the thymus, a primary lymphoid organ, where they transition through several developmental stages, including double-negative T cells (DN, pre-T cells), double positive T cells (DP, immature T cells), and single positive T cells (SP, Tn cells). Tn cells migrate into secondary lymphoid tissues where they can encounter antigens, leading to their activation, proliferation, and differentiation into effector T cells. Approximately 90–95% of effector T cells die after pathogen clearance and the remaining cells become memory T cells (75, 76).
Previous studies have shown that T cell development is regulated by several cytokines, including IL-2, IL-7, IL-15, and IL-21. As a pluripotent cytokine, IL-7 is important for regulating T cell survival, proliferation, differentiation, and activation (Figure 2). In addition, IL-7-/- mice display approximately 10-20-fold fewer total T cells, indicating that IL-7 plays a critical role in T cell development (77). However, the demand for IL-7 in T cell development varies at different stages and the dynamic interplay between IL-7 requirement and the T cell subset is regulated by IL-7R. Pro-T, pre-T, Tn, and memory T cell subsets display relatively high IL-7R expression, whereas IL-7R expression is low or absent in immature T and activated T cell subsets. Therefore, the effect of intrinsic IL-7 may be greater during the pro-T, pre-T, Tn, and memory T cell stages than in immature and activated T cells (78). Overall, several studies have assisted us to understand the important function of IL-7 in the development and activation of T cells in vitro and in vivo.
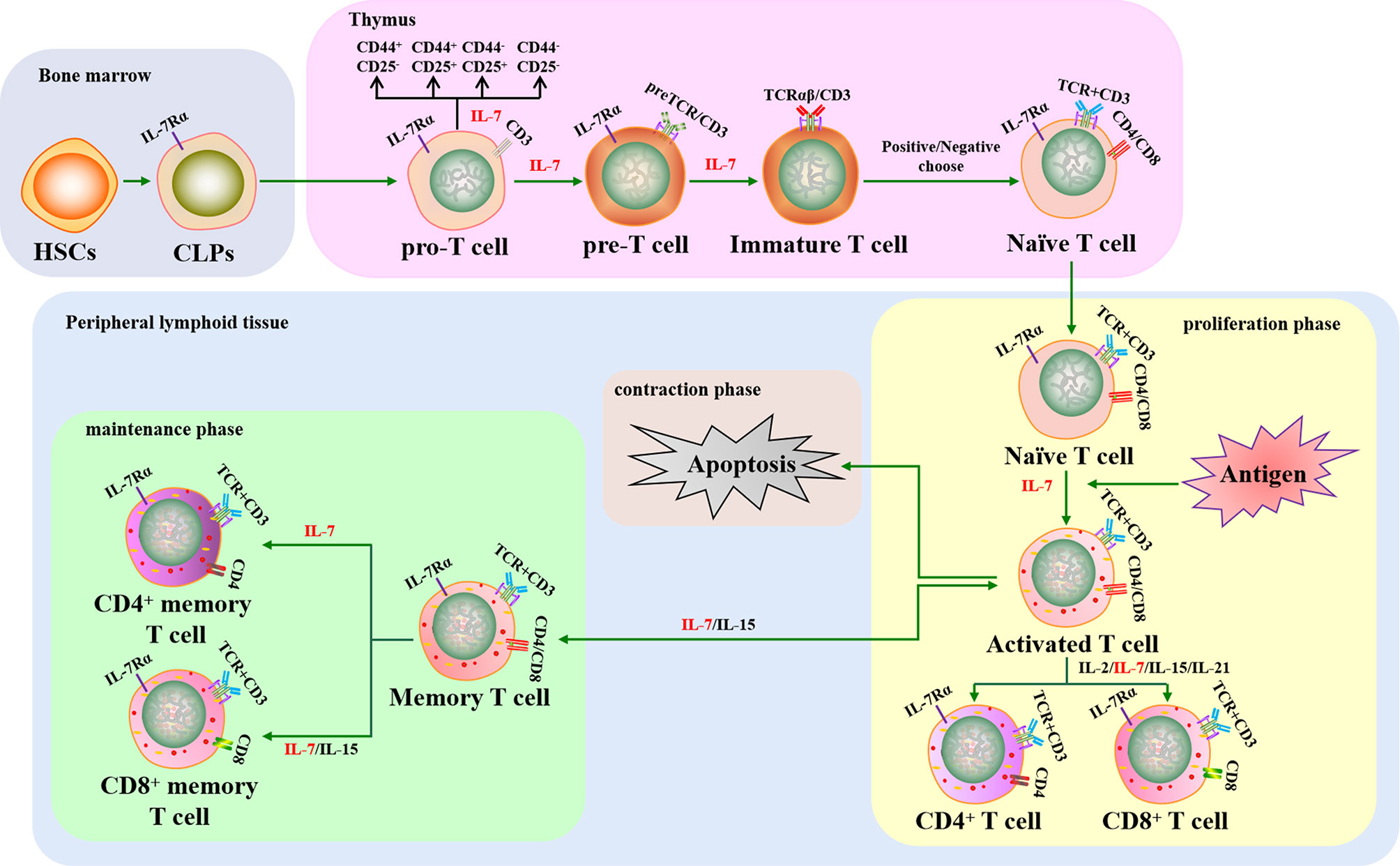
Figure 2 The crucial role of IL-7 in T cell development and activation. IL-7 contributes to T cell lymphopoiesis and survival. When T cells encounter antigens, IL-7 can boost T cell immune responses to fight against pathogens, including rescuing T cells from apoptosis, promoting naive T cells to differentiate into effector T cells, and improving memory T cell production. HSCs, hematopoietic stem cells; CLPs, common lymphoid progenitors; IL-7Rα, IL-7 receptor α chain; TCR, T cell receptor.
Thymocytes in IL-7-/- mice have been shown to arrest in G0/G1 phase and lose Bcl-2 expression, leading to defects in T lymphopoiesis that can be rescued when the thymocytes are cultured with IL-7 (79). Subsequent studies have demonstrated that IL-7 contributes towards T lymphopoiesis by enhancing the expression of Bcl-2, Cdk2, Rb, GSK-3, and Mcl-1 and downregulating p27, Bax, Bad, and Bim (80–85). IL-7 can also rescue thymic CD4+CD8+ T cell subsets from apoptosis and is required to sustain the metabolism of Tn cells to inhibit cell atrophy (86). Long-term IL-7 monoclonal antibody treatment was found to interrupt the proliferation of CD44+CD25+ T cells in mice, causing the sharp depletion of the CD4+CD8+ T cell subset (87). In addition, studies have shown that IL-7 tightly regulates Tn cell proliferation to maintain T cell homeostasis in peripheral lymphoid tissues (33, 35, 88). However, the role of IL-7 in early T cell differentiation in vitro remains controversial. One study showed that IL-7 crucially controls the development of CD4-CD8- DN T cells into CD4+CD8+ DP T cells and the differentiation of DP T cells into CD4+CD8-/CD4-CD8+ SP T cells when HSCs were cultured in vitro (89). Conversely, the transition of DN T cells into DP T cells was reduced when CD4-CD8- DN thymocytes were cultured with IL-7 in vitro, indicating that IL-7 mainly provides survival signaling rather than DN T cell subset differentiation signaling (90). Later research demonstrated that IL-7 signaling was required for DN-DP differentiation, but it could delay DN T cells differentiation into DP T cells for maintaining DN self-renewal in vitro and in vivo (91). Additionally, IL-7 could induce the expression of CXCR4, a chemokine receptor, to support CD4+ and CD8+ SP T cell survival and recruitment in secondary lymphoid tissues (92, 93). Therefore, IL-7 is a crucial cytokine in regulating T cell production, survival, proliferation, and differentiation.
IL-7 can also boost T cell immune responses against pathogens by promoting the differentiation of Tn cells into effector T cells and then memory T cells (18, 94, 95). IL-7 has been used as an activator to augment mature T cell immune responses against chronic viruses and cancers. Recent reports have shown that IL-7 can stimulate CD8+ T cell proliferation and promote their cytotoxicity by upregulating perforin, granzyme B, and IFN-γ expression in patients with viral infection and tumors (96–99). The cytokine promotes the activation of both CD4+ and CD8+ T cells by increasing CXCR3 expression to control Lewis lung tumor growth (100). Ubiquitin ligases are crucial factors in protein degradation that also participate in both the innate and adaptive immune responses (101) and are involved in IL-7-mediated T cell immune responses. This notion is supported by the fact that IL-7 represses Casitas B lymphoma-b (Cbl-b) expression and enhances SMAD-specific E3 ubiquitin protein ligase-2 (Smurf2) expression to augment T cell activation during antitumor immune responses (102). Increased IL-7 usage can also enhance pathogen-specific memory T cell responses against cancer and chronic viral infections (32, 103). In addition, IL-7 serves as a key factor in promoting T-cell reconstitution, with IL-7 treatment increasing CD4+ T-cell circulation to maintain CD4+ T cell homeostasis in idiopathic CD4 lymphopenia (ICL) and human immunodeficiency virus (HIV) infections (104–106). Furthermore, it has been confirmed that the homeostatic proliferation and survival of memory CD4+ T cells rely on IL-7, whereas these aspects of memory CD8+ T cells require a combination of IL-7 and IL-15 (12, 107–110). Hence, IL-7 can enhance cell-mediated immune responses, including antigen-specific and -non-specific cellular immune responses, by promoting the production and function of effector T cells and memory T cells.
IL-7 in NK Cell and DC Development
NK cells are a fundamental component of bodily frontline defense systems that participate in both innate and adaptive immune responses against tumors and viruses (111). IL-7Rα is expressed in thymus-dependent and -independent NK cell progenitors (NKPs) in mouse lymph nodes, indicating that IL-7 signaling may contribute towards their development (112). IL-7 not only plays important roles in NK cell maturation, but also in their survival via the anti-apoptotic protein Bcl-2 (113). In addition, IL-7 is strictly responsible for maintaining NK cell homeostasis (114) and is related to their activation. Dadmarz et al. showed that IL-7 could stimulate the generation of lymphokine-activated killer cells from mouse CD56bright NK cells and enhance the cytotoxicity of mature NK cells (115). These findings are consistent with the ability of IL-7 to promote the cytolytic activity of mature CD56bright NK cells against K562 cells by increasing CD69 expression, IFN-γ production, and CD107α expression (116). However, compared with IL-2, IL-15 and IL-21, IL-7 plays a minor role in initiating and sustaining the proliferation of NK cell (117).
DCs are a major type of antigen-presenting cell derived from bone marrow that recognize, process, and present antigens to T cells and then induce T cell-dependent immune responses. Mature DCs further differentiate into conventional DCs (cDCs) and plasmacytoid DCs (pDCs), which regulate the activation of adaptive immunity (118, 119). When DC progenitors isolated from the thymus were cultured together with GM-CSF, IL-3, and IL-7 in vitro, efficient DC differentiation was observed compared to stimulation with GM-CSF alone (120), suggesting that IL-7 is involved in DC differentiation. Moreover, DCs derived from both bone marrow and lymph nodes express IL-7R, while reduced proportions of cDCs and pDCs have been observed without IL-7 (25). Thus, it has been clarified that IL-7 participates in DC development.
Taken together, IL-7 is an essential cytokine in regulating both innate and adaptive immune responses through participation in B cell, T cell, NK cell and DC development to fight against pathogen infection. Although some controversial issues regarding immune cells development require further study, the feature of IL-7 lays the foundation for its application in vaccine research.
IL-7 Signaling Pathway
The biological effects of IL-7 are mediated by binding to its receptor, IL-7R, which is a transmembrane heterodimer of the IL-7Rα (CD127) and γ (CD132) chains. The α chain is used by IL-7R and thymic stromal lymphopoietin receptor (TSLPR), also termed cytokine receptor-like factor 2 (CRLF2), while the γ chain (or common γ (γc) chain) is shared by IL-7R, IL-2R, IL-4R, IL-9R, IL-15R, and IL-21R (121). IL-7Rα is mainly found in T, B, and NK cells as well as DCs, innate lymphoid cells (ILCs), and lymphoid tissue inducer (Lti) cells (122), whereas γc is expressed in all HSC-derived cell types (84). Both IL-7Rα and γc are high-affinity IL-7 receptors; however, non-receptor kinases and adaptors are employed to transduce IL-7 signals via IL-7R since IL-7Rα and γc both lack intrinsic tyrosine kinase activity. When IL-7 binds to IL-7Rα, the α and γc chains dimerize, triggering kinase activation (Figure 3). Subsequent IL-7/IL-7R signal transduction is mainly dependent on the Janus kinase (JAK)-STAT and phosphatidylinositol 3-kinase (PI3K)-Akt pathways.
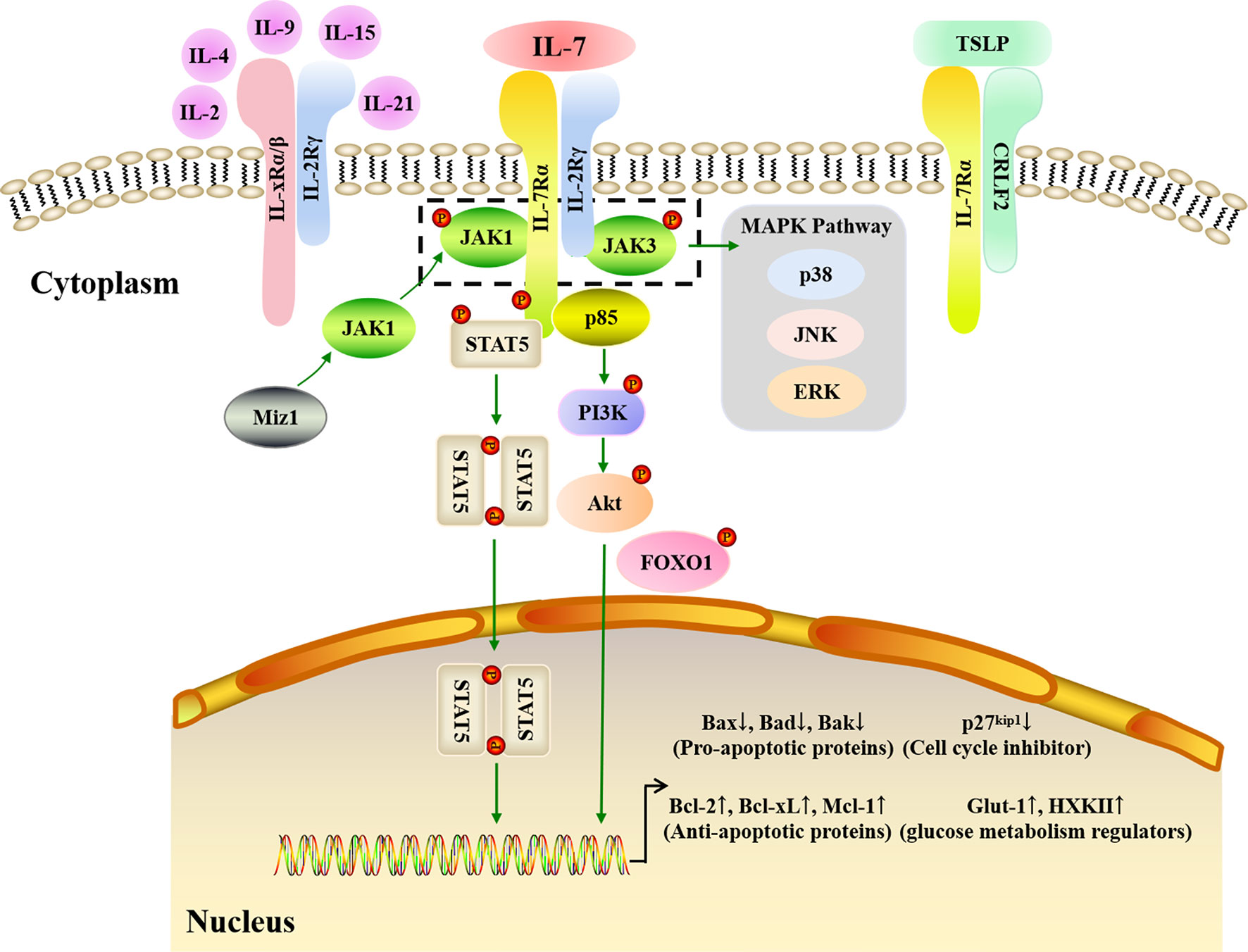
Figure 3 IL-7 signaling pathways. IL-7 must combine with the IL-7R to carry out its biological function. IL-7R is a transmembrane heterodimer consisting of the α chain and γ chain (common γ chain, γc chain). IL-7Rα is used by IL-7R and the thymic stromal lymphopoietin receptor (TSLPR), while γc is shared by IL-7R, IL-2R, IL-4R, IL-9R, IL-15R, and IL-21R. JAK-STAT, PI3K-Akt, and MAPK pathways are involved in IL-7 signaling transduction. When IL-7 binds to IL-7Rα, the α and γc chains dimerize. JAK1 and JAK3 are major kinases that respond to IL-7/IL-7R signaling in the JAK-STAT pathway. Myc interacting zinc finger protein 1 (Miz1) recruits JAK1 to IL-7Rα. The phosphorylation of JAK1 and JAK3 triggers STAT5 phosphorylation and dimerization to upregulate anti-apoptotic gene expression and downregulate pro-apoptotic gene expression. In addition, the MAPK pathway is also activated by the phosphorylated JAK1 and JAK3. When p85 tethers to IL-7Rα, the PI3K-Akt pathway is activated. Subsequently, the Akt is phosphorylated, which then induces glucose metabolism regulator gene expression, and inhibits p27 kinase inhibitor protein 1 (p27kip1) expression with phosphorylated Forkhead box protein 1 (FOXO1).
The JAK family consists of JAK1, JAK2, JAK3, and tyrosine kinase-2 (TYK-2), while the STAT family has seven members: STAT1, STAT2, STAT3, STAT4, STAT5α, STAT5β, and STAT6. JAK1, JAK3, and STAT5 are the predominant proteins that respond to IL-7/IL-7R signaling: when IL-7 combines with IL-7R, JAK1 and JAK3 tether to IL-7Rα and γc, respectively, alongside IL-7Rα C-terminal phosphorylation. Next, STAT1, STAT3, and STAT5 are recruited and phosphorylated by JAK1 and JAK3 (123), with phosphorylated STAT5 production occurring in an IL-7 dose-dependent manner (124). Following its phosphorylation, STAT5 dimerizes and is translocated into the nucleus, which in turn triggers the expression of downstream genes involved in cell survival and proliferation (125–127). Via the JAK-STAT pathway, IL-7 upregulates the expression of anti-apoptotic genes mainly belonging to the Bcl-2 family, including Bcl-2, Mcl-1, and Bcl-xL, while also downregulating pro-apoptotic genes, including Bax, Bad, and Bak, to promote the survival of cells in vitro and in vivo (128). Lu et al. quantified B cell apoptosis and examined the expression of Bcl-2 family proteins in IL-7-/- mice and IL-7 transgenic mice, finding that IL-7 strongly increased Bcl-2 levels and decreased Bax levels to promote precursor B cell survival (128). Myc interacting zinc finger protein 1 (Miz1) is a crucial activator that also participates in the JAK-STAT signaling transduction pathway by recruiting JAK1, activating STAT5, and upregulating Bcl-2 expression when IL-7 triggers early B and T cell survival and proliferation (129, 130). Therefore, the lymphocyte survival signaling provided by IL-7 is predominantly dependent on the JAK-STAT pathway.
The PI3K-Akt signaling transduction pathway is an important intracellular signaling pathway that regulates cell growth, survival, and glucose metabolism (131). Indeed, PI3K inhibitors have been found to attenuate the proliferation and activation of murine T cells in response to IL-7 (132), while Akt phosphorylation increased when IL-7 was used to improve adipose-derived stem cell differentiation (133). The pathway starts with the recruitment of p85, a regulatory subunit of PI3K, when IL-7 interacts with IL-7R. P85 then associates with IL-7Rα and induces the phosphorylation of tyrosine in the IL-7Rα cytoplasmic tail (134). Subsequently, Akt is activated to induce downstream gene expression (135). p27 kinase inhibitor protein 1 (p27kip1) is a crucial molecule in this pathway and its expression is downregulated by PI3K-Akt signaling via Forkhead box protein 1 (FOXO1) phosphorylation (136). p27kip1 degradation can induce cyclin D1 expression and promote the G1 to S phase transition for cell proliferation during IL-7-dependent cell cycle progression (80, 137). Furthermore, PI3K-Akt signaling can drive the cytoplasmic localization of p27kip1 and thereby allow cell proliferation (138, 139). Glucose is the main nutrient for cell development and activation, and studies have shown that IL-7 can upregulate the expression of the glycolytic enzymes hexokinase II (HXK II) and glucose transporter-1 (Glut-1), thereby augmenting glycolysis by increasing glucose uptake (140, 141). Furthermore, it has been clarified that IL-7-mediated glucose utilization occurs in a PI3K-Akt-dependent fashion (83).These results suggest that the PI3K-Akt pathway is necessary for effective IL-7 signaling during cell cycle progression.
In addition to the JAK-STAT and PI3K-Akt pathways, mitogen-activated protein kinases (MAPK), including p38 kinase, extracellular signal regulated kinase (ERK), and c-Jun N-terminal kinase (JNK), may also contribute towards IL-7/IL-7R signaling. Importantly, the MAPK pathway allows the transduction of signals from the cell membrane to the nucleus. The p38 MAPK pathway may play a critical role in IL-7 signaling since specific p38 inhibitors can suppress T cell proliferation driven by IL-7 (142). Moreover, IL-7 withdrawal results in IL-7-dependent thymocyte death caused by blocking the activation of p38 and JNK kinases (143). In addition to JAK3 activation, the phosphorylation of p44, an ERK isoform, was found to significantly increase after IL-7 stimulation in murine T helper cells (144). Furthermore, IL-7 signaling may affect the migration of peripheral blood monocytes in rheumatoid arthritis (RA), a chronic autoimmune disorder caused by ERK (145). Therefore, the ERK MAPK pathway appears to play a role in IL-7-mediated signal transduction to regulate cell development and activation.
IL-7 Applications in Vaccines
An ideal vaccine would permanently protect the host from related pathogens after only one immunization; therefore, it is important to develop efficient vaccines with broad, robust, and long-lasting immunogenicity. Cytokines are commonly employed as adjuvants to enhance and extend the vaccine effect, with early studies revealing that IL-7 can improve vaccine protection by driving T lymphocyte responses as a molecular adjuvant (29, 146, 147). Recent studies have also shown that IL-7 can not only enhance cellular immune responses, but also improves the humoral immune responses induced by vaccines (71, 148–150). As an adjuvant, IL-7 has been shown to stimulate antigen-specific CD4+ T cell, CD8+ T cell, and B cell responses to boost vaccine potency, as well as increasing the production of memory T and B cells to prolong the protective effects of vaccines (108, 151–154) (Table 2).
The IL-7 protein possesses strong adjuvant activity to improve and extend vaccine protection. Indeed, costimulation of the male HY antigen with recombinant human IL-7 (hIL-7) protein may augment HY specific-CD8+ effector T cell generation and improve the survival of HY specific-CD8+ memory T cells (155). In addition, administering recombinant hIL-7 protein with a trivalent inactivated influenza vaccine (TIV; H1N1 A/New Caledonia/20/99, H3N2 A/Fujian/411/2002, and B/Shanghai/361/2002) could facilitate the generation of GC B cells and increase neutralizing antibodies against homologous and heterologous (PR8/H1N1) influenza viruses (70). Nanjappa et al. found that the codelivery of hIL-7 protein with a DNA vaccine encoding LCMV nucleoprotein (NP) in mice during the contraction phase of the T cell response could increase the number of specific mouse anti-LCMV memory CD8+ T cells and enhance T cell proliferation and cytotoxicity to improve viral control (155). Using hIL-7 protein as an adjuvant may also improve mouse anti-tumor immune responses by increasing pro-inflammatory cytokine production [IL-6, IL-1α, IL-1β, IL-12, TNF-α, C-C chemokine ligand-5 (CCL-5), macrophage inflammatory protein 1α (MIP-1α)], augmenting Th17 cell differentiation, repressing Cbl-b expression, and enhancing SMAD-specific E3 ubiquitin protein ligase 2 (Smurf2) expression (98). Furthermore, recombinant chicken IL-7 (chIL-7) protein expressed in E. coli and administered with the inactivated infectious bursal disease virus (IBDV) vaccine was found to significantly upregulate chicken anti-IBDV antibody titers, lymphocyte proliferation, and IFN-γ and IL-4 production (163). This increased the survival rate of chickens to 91–97% compared to 78% when vaccinated with the inactivated IBDV vaccine alone (163). Alfredo et al. also assessed the adjuvanticity of chIL-7 protein with the Eimeria tenella elongation factor 1α (EF-1α) DNA vaccine, finding that chIL-7 boosted humoral and cellular immunity against live E. acervulina challenge in broiler chickens that received the EF-1α DNA vaccine (157).
Flexner et al. were the first to report the use of a cytokine gene as a biological adjuvant, having inserted mIl-2 into the vaccinia virus genome to construct a recombinant virus as a novel vaccine. They found that mIl-2 was able to improve the safety and immunogenicity of the vaccinia virus (164), providing a foundation for the application of cytokine genes in vaccines. Subsequently, numerous studies have attempted to co-administer cytokine genes with vaccines to improve their efficiency. IL-7 has been confirmed to exhibit efficient adjuvanticity to boost vaccine immunogenicity, with the codelivery of hIL-7-encoding plasmids with hepatitis C virus (HCV) NS2-E1E2-NS3-NS4 DNA vaccine increasing anti-HCV antibody levels and widening the T cell response compared to the DNA vaccine alone (158). A DNA vaccine co-expressing IBDV VP2366 and chIL-7 via an internal ribosome entry site (IRES) connection was constructed and then used to intramuscularly immunize SPF chickens, with significantly strengthened immunogenicity compared to the IBDV VP2366 DNA vaccine (156). In particular, the VP2366-chIL-7 DNA vaccine increased the titer of neutralizing antibodies as well as IFN-γ and IL-4 production, while chIL-7 enhanced IBDV VP2366 DNA vaccine protection by approximately 25% (156). The murine Il-7 (mIl-7) gene has also been inserted into the genome of the nonlytic Newcastle disease virus (NDV) LX strain, which is an autologous tumor vaccine modified using the reverse genetic method that has been used against murine tumors (HCC, lymphoma, and melanoma) (160). Interestingly, the NDV LX-mIL-7 vaccine displayed strong anti-tumor activity, with mIL-7 expression by the NDV LX strain inducing higher IFN-γ production, greater levels of tumor-infiltrating CD4+ and CD8+ T cells, and stronger cytotoxicity of tumor-specific CD8+ T cells than the NDV LX vaccine (160). Similarly, when mIl-7 was inserted into the recombinant canine distemper virus (rCDV) genome, it significantly promoted the generation of GC B cells and plasma cells in draining lymph nodes (LNs) (71). The number of antigen-specific memory B cells was also highly increased and the presence of virus-neutralizing antibodies was prolonged (up to 360 days) when mice were injected with a recombinant attenuated rabies virus (rLBNSE) expressing mIL-7 (150). These results suggest that the Il-7 gene can be directly used in vaccine development. Although IL-7 has strong adjuvanticity, its immunomodulatory properties may be different in certain diseases. The vaccine efficacy of Friend Virus was no significant changed when with or without adenoviral vectors encoding hIL-7 (165). Therefore, further research is needed to pinpoint the benefits of IL-7 in different pathogen vaccines, and the adjuvanticity of IL-7 in different vector-based vaccines.
Another strategy to improve the immunogenicity of vaccines is to combine IL-7 with other cytokines. When mIL-7 or mIL-33 was subcloned into pVAX1 vector, both mIL-7 and mIL-33 could enhance the immunogenicity of varicella-zoster virus (VZV) glycoprotein E (gE) DNA vaccine. However, compared with the coadministration of mIL-7 and mIL-33, the DNA vaccine with mIL-7 alone enhanced the weaker VZV-specific T cell immune responses and protection (153). Coadministering mice with bacille Calmette-Guerin (BCG) vaccine and mIL-7/mIL-15 proteins promoted T cell proliferation, cytokine production, and both CD4+ and CD8+ memory T cell responses compared to the BCG vaccine alone (162), indicating that mIL-7 and mIL-15 proteins can induce long-lasting T cell immune responses against Mycobacterium tuberculosis. Similarly, recombinant NDV expressing mIL-7/mIL-15 also improved the anti-tumor activity of the NDV LX strain (166), while the delivery of mIL-7/mIL-15 co-expression plasmids as molecular adjuvants with the Toxoplasma gondii calcium-dependent protein kinase 1 (TgCDPK1) DNA vaccine enhanced Toxoplasma-specific IgG2a levels and CD8+/CD4+IFN-γ+ T cell frequencies induced by the vaccine and prolonged the survival of mice post lethal infection by approximately 7 days (149). Furthermore, mIl-7/mIl-15 genes subcloned into an adenovirus were able to extend the protective efficacy of the M. tuberculosis subunit vaccine (LT70 and MH fusion protein) by augmenting M. tuberculosis-specific central memory-like T cell responses (167). When mIl-7/mIl-2 were co-administered with the ovalbumin (OVA) DNA vaccine, mIL-7 and mIL-2 mutually improved its immunogenicity by up-regulating the specific antibody titer, IFN-γ production, and T cell proliferation (168). This may have occurred via a mechanism wherein mIL-2 induced the generation of mIL-7Rα-expressing lymphocytes, which were increased in number by mIL-7 (168). These findings show that other adjuvants are needed to enhance the adjuvanticity of IL-7 in some vaccines. As a result, further studies about the form and mechanism of IL-7 in the development of specific vaccines should be conducted.
The mucosal immune system plays a fundamental role in defending against infection through food, water, pathogens, and direct contact with mucosal surfaces. The Fc fragment is always employed to enhance the mucosal delivery of a target protein. When mice were intranasally treated with Fc-fused mouse IL-7 (mIL-7-mFc) not native IL-7 protein before lethal influenza A virus (IAV) infection, the percentage of mice that survived was significantly increased (169). In particular, mIL-7-mFc recruited both T cells and pDCs from circulation into the lungs, with T cells transitioning into lung-specific memory-like T cells (TRM-like) and pDCs strengthening lung-specific anti-IAV CTL responses (169, 170), thereby providing long-lasting immune responses against lethal AIV infection by altering the pulmonary immune environment. Since IL-7 is locally produced by intestinal epithelial cells, particularly epithelial goblet cells, it can regulate the phenotype and function of intraepithelial lymphocytes (IELs) and lamina propria lymphocytes (LPLs) (171, 172). Indeed, mIL-7 prevented approximately 50% of IELs from undergoing spontaneous apoptosis via both caspase-dependent and Bcl-2-dependent pathways in vitro (173). Exogenous mIL-7 was also able to reverse the mucosal damage induced by parenteral nutrition (PN) and increase the levels of secretory IgA in the intestine and bronchoalveolae, meaning that mice with PN could resist lethal Pseudomonas aeruginosa infection (174). In addition, the hIL-7-hFc protein not hIL-7 was shown to enhance genital mucosal CD8+ T cell immune responses to the human papillomavirus (HPV) GX188 DNA vaccine and promote the anti-tumor activity of the vaccine (159). The vaccine has entered preclinical trials and demonstrated that hIL-7-hFc can be used with safety (175). Together, these studies indicate that IL-7 could be used as a promising molecular adjuvant to improve mucosal immune responses to vaccines. Moreover, the formation of IL-7 with Fc fragment should be considered in future mucosal vaccine development.
Conclusion
Vaccines play fundamental roles in helping the body to resist pathogenic infections; however, antigens alone are unable to stimulate persistent protective immune responses. To solve this problem, cytokines have been employed to improve vaccine-specific immunity and promote vaccine-specific memory immune responses. In the past three decades, numerous trials have confirmed that IL-7 can alter immune responses and promote immune reconstitution by inducing the development and activation of B cells, T cells, NK cells, and DCs. Moreover, IL-7 exhibits adjuvant properties, enhancing effector and memory cell immune responses, and has been used in preventive and therapeutic vaccines.
As mucosal vaccines always present poor immunogenicity, an appropriate adjuvant to enhance mucosal immunity is necessary. In view of the biological activity and tissue distribution of IL-7, it has been used in vaccines to enhance mucosal immune responses. It has been proved that IL-7 can not only promote the humoral and cellular immune responses of vaccines, but also improve their mucosal immunity. It can recruit DCs, NK cells, B cells, and T cells to infiltrate in local mucosal tissues. IL-7 has been employed in the development of IAV (169), HPV (159), DT (176), and other mucosal vaccines. This strategy has been demonstrated to be toxicity-free.
These findings have made IL-7 an important adjuvant in vaccine development. Here, we reviewed the biological function and mechanism of IL-7, and summarized its applications in vaccines to provide a new perspective on clinical vaccine design.
Author Contributions
JH and ZL wrote the manuscript. RJ, MW, DZ, ML, SC, XZ, QY, YW, SZ, BT, SM, XO, DS, QG, and AC revised the review. All authors contributed to the article and approved the submitted version.
Funding
This work was supported by the National Natural Science Foundation of China (grant number 31902286), the Program Sichuan Veterinary Medicine and Drug Innovation Group of China Agricultural Research System (SCCXTD-2021-18) and China Agriculture Research System of MOF and MARA.
Conflict of Interest
The authors declare that the research was conducted in the absence of any commercial or financial relationships that could be construed as a potential conflict of interest.
Publisher’s Note
All claims expressed in this article are solely those of the authors and do not necessarily represent those of their affiliated organizations, or those of the publisher, the editors and the reviewers. Any product that may be evaluated in this article, or claim that may be made by its manufacturer, is not guaranteed or endorsed by the publisher.
Acknowledgments
We thank all our colleagues who were not included as authors of this review.
Supplementary Material
The Supplementary Material for this article can be found online at: https://www.frontiersin.org/articles/10.3389/fimmu.2021.680442/full#supplementary-material
References
1. Hunt P, Robertson D, Weiss D, Rennick D, Lee F, Witte ON. A Single Bone Marrow-Derived Stromal Cell Type Supports the In Vitro Growth of Early Lymphoid and Myeloid Cells. Cell (1987) 48(6):997–1007. doi: 10.1016/0092-8674(87)90708-2
2. Namen AE, Lupton S, Hjerrild K, Wignall J, Mochizuki DY, Schmierer A, et al. Stimulation of B-Cell Progenitors by Cloned Murine Interleukin-7. Nature (1988) 333(6173):571–3. doi: 10.1038/333571a0
3. Mazzucchelli RI, Warming S, Lawrence SM, Ishii M, Abshari M, Washington AV, et al. Visualization and Identification of IL-7 Producing Cells in Reporter Mice. PloS One (2009) 4(11):e7637. doi: 10.1371/journal.pone.0007637
4. Heufler C, Topar G, Grasseger A, Stanzl U, Koch F, Romani N, et al. Interleukin 7 Is Produced by Murine and Human Keratinocytes. J Exp Med (1993) 178(3):1109–14. doi: 10.1084/jem.178.3.1109
5. Maeurer MJ, Walter W, Martin D, Zitvogel L, Elder E, Storkus W, et al. Interleukin-7 (IL-7) in Colorectal Cancer: Il-7 Is Produced by Tissues From Colorectal Cancer and Promotes Preferential Expansion of Tumour Infiltrating Lymphocytes. Scand J Immunol (1997) 45(2):182–92. doi: 10.1046/j.1365-3083.1997.d01-384.x
6. Link A, Vogt TK, Favre S, Britschgi MR, Acha-Orbea H, Hinz B, et al. Fibroblastic Reticular Cells in Lymph Nodes Regulate the Homeostasis of Naive T Cells. Nat Immunol (2007) 8(11):1255–65. doi: 10.1038/ni1513
7. Sawa Y, Arima Y, Ogura H, Kitabayashi C, Jiang JJ, Fukushima T, et al. Hepatic Interleukin-7 Expression Regulates T Cell Responses. Immunity (2009) 30(3):447–57. doi: 10.1016/j.immuni.2009.01.007
8. Rane L, Rahman S, Magalhaes I, Ahmed R, Spångberg M, Kondova I, et al. Increased (6 Exon) Interleukin-7 Production After M. Tuberculosis Infection and Soluble Interleukin-7 Receptor Expression in Lung Tissue. Genes Immun (2011) 12(7):513–22. doi: 10.1038/gene.2011.29
9. Shitara S, Hara T, Liang B, Wagatsuma K, Zuklys S, Holländer GA, et al. IL-7 Produced by Thymic Epithelial Cells Plays a Major Role in the Development of Thymocytes and Tcrγδ+ Intraepithelial Lymphocytes. J Immunol (2013) 190(12):6173–9. doi: 10.4049/jimmunol.1202573
10. Zhang W, Du JY, Yu Q, Jin JO. Interleukin-7 Produced by Intestinal Epithelial Cells in Response to Citrobacter Rodentium Infection Plays a Major Role in Innate Immunity Against This Pathogen. Infect Immun (2015) 83(8):3213–23. doi: 10.1128/IAI.00320-15
11. Qu H, Zou Z, Pan Z, Zhang T, Deng N, Chen G, et al. IL-7/IL-7 Receptor Axis Stimulates Prostate Cancer Cell Invasion and Migration via AKT/NF-κb Pathway. Int Immunopharmacol (2016) 40:203–10. doi: 10.1016/j.intimp.2016.08.017
12. Bradley LM, Haynes L, Swain SL. IL-7: Maintaining T-Cell Memory and Achieving Homeostasis. Trends Immunol (2005) 26(3):172–6. doi: 10.1016/j.it.2005.01.004
13. Fry TJ, Mackall CL. Interleukin-7: From Bench to Clinic. Blood (2002) 99(11):3892–904. doi: 10.1182/blood.v99.11.3892
14. Fistonich C, Zehentmeier S, Bednarski JJ, Miao R, Schjerven H, Sleckman BP, et al. Cell Circuits Between B Cell Progenitors and IL-7+ Mesenchymal Progenitor Cells Control B Cell Development. J Exp Med (2018) 215(10):2586–99. doi: 10.1084/jem.20180778
15. Ribeiro D, Melão A, Van Boxtel R, Santos CI, Silva A, Silva MC, et al. STAT5 Is Essential for IL-7-Mediated Viability, Growth, and Proliferation of T-Cell Acute Lymphoblastic Leukemia Cells. Blood Adv (2018) 2(17):2199–213. doi: 10.1182/bloodadvances.2018021063
16. Corcoran AE, Riddell A, Krooshoop D, Venkitaraman AR. Impaired Immunoglobulin Gene Rearrangement in Mice Lacking the IL-7 Receptor. Nature (1998) 391(6670):904–7. doi: 10.1038/36122
17. Kang J, Coles M, Raulet DH. Defective Development of Gamma/Delta T Cells in Interleukin 7 Receptor-Deficient Mice Is Due to Impaired Expression of T Cell Receptor Gamma Genes. J Exp Med (1999) 190(7):973–82. doi: 10.1084/jem.190.7.973
18. Terrazzini N, Mantegani P, Kern F, Fortis C, Mondino A, Caserta S. Interleukin-7 Unveils Pathogen-Specific T Cells by Enhancing Antigen-Recall Responses. J Infect Dis (2018) 217(12):1997–2007. doi: 10.1093/infdis/jiy096
19. Cimbro R, Vassena L, Arthos J, Cicala C, Kehrl JH, Park C, et al. IL-7 Induces Expression and Activation of Integrin α4β7 Promoting Naive T-Cell Homing to the Intestinal Mucosa. Blood (2012) 120(13):2610–9. doi: 10.1182/blood-2012-06-434779
20. El Azreq MA, Arseneault C, Boisvert M, Pagé N, Allaeys I, Poubelle PE, et al. Cooperation Between IL-7 Receptor and Integrin α2β1 (CD49b) Drives Th17-Mediated Bone Loss. J Immunol (2015) 195(9):4198–209. doi: 10.4049/jimmunol.1500437
21. Ponte R, Rancez M, Figueiredo-Morgado S, Dutrieux J, Fabre-Mersseman V, Charmeteau-De-Muylder B, et al. Acute Simian Immunodeficiency Virus Infection Triggers Early and Transient Interleukin-7 Production in the Gut, Leading to Enhanced Local Chemokine Expression and Intestinal Immune Cell Homing. Front Immunol (2017) 8:588. doi: 10.3389/fimmu.2017.00588
22. Morrissey PJ, Goodwin RG, Nordan RP, Anderson D, Grabstein KH, Cosman D, et al. Recombinant Interleukin 7, Pre-B Cell Growth Factor, has Costimulatory Activity on Purified Mature T Cells. J Exp Med (1989) 169(3):707–16. doi: 10.1084/jem.169.3.707
23. Murray R, Suda T, Wrighton N, Lee F, Zlotnik A. IL-7 Is a Growth and Maintenance Factor for Mature and Immature Thymocyte Subsets. Int Immunol (1989) 1(5):526–31. doi: 10.1093/intimm/1.5.526
24. Alderson MR, Sassenfeld HM, Widmer MB. Interleukin 7 Enhances Cytolytic T Lymphocyte Generation and Induces Lymphokine-Activated Killer Cells From Human Peripheral Blood. J Exp Med (1990) 172(2):577–87. doi: 10.1084/jem.172.2.577
25. Vogt TK, Link A, Perrin J, Finke D, Luther SA. Novel Function for Interleukin-7 in Dendritic Cell Development. Blood (2009) 113(17):3961–8. doi: 10.1182/blood-2008-08-176321
26. Meazza R, Azzarone B, Orengo AM, Ferrini S. Role of Common-Gamma Chain Cytokines in NK Cell Development and Function: Perspectives for Immunotherapy. J BioMed Biotechnol (2011) 2011:861920. doi: 10.1155/2011/861920
27. Lin J, Zhu Z, Xiao H, Wakefield MR, Ding VA, Bai Q, et al. The Role of IL-7 in Immunity and Cancer. Anticancer Res (2017) 37(3):963–7. doi: 10.21873/anticanres.11405
28. Kulkarni U, Herrmenau C, Win SJ, Bauer M, Kamradt T. IL-7 Treatment Augments and Prolongs Sepsis-Induced Expansion of IL-10-Producing B Lymphocytes and Myeloid-Derived Suppressor Cells. PloS One (2018) 13(2):e0192304. doi: 10.1371/journal.pone.0192304
29. Sin JI, Kim J, Pachuk C, Weiner DB. Interleukin 7 can Enhance Antigen-Specific Cytotoxic-T-Lymphocyte and/or Th2-Type Immune Responses In Vivo. Clin Diagn Lab Immunol (2000) 7(5):751–8. doi: 10.1128/cdli.7.5.751-758.2000
30. Ponchel F, Cuthbert RJ, Goëb V. IL-7 and Lymphopenia. Clin Chim Acta (2011) 412(1–2):7–16. doi: 10.1016/j.cca.2010.09.002
31. Cui G, Staron MM, Gray SM, Ho PC, Amezquita RA, Wu J, et al. IL-7-Induced Glycerol Transport and TAG Synthesis Promotes Memory CD8+ T Cell Longevity. Cell (2015) 161(4):750–61. doi: 10.1016/j.cell.2015.03.021
32. Raeber ME, Zurbuchen Y, Impellizzieri D, Boyman O. The Role of Cytokines in T-Cell Memory in Health and Disease. Immunol Rev (2018) 283(1):176–93. doi: 10.1111/imr.12644
33. Sportès C, Hakim FT, Memon SA, Zhang H, Chua KS, Brown MR, et al. Administration of rhIL-7 in Humans Increases In Vivo TCR Repertoire Diversity by Preferential Expansion of Naive T Cell Subsets. J Exp Med (2008) 205(7):1701–14. doi: 10.1084/jem.20071681
34. Fry TJ, Moniuszko M, Creekmore S, Donohue SJ, Douek DC, Giardina S, et al. IL-7 Therapy Dramatically Alters Peripheral T-Cell Homeostasis in Normal and SIV-Infected Nonhuman Primates. Blood (2003) 101(6):2294–9. doi: 10.1182/blood-2002-07-2297
35. Cha E, Graham L, Manjili MH, Bear HD. IL-7 + IL-15 Are Superior to IL-2 for the Ex Vivo Expansion of 4T1 Mammary Carcinoma-Specific T Cells With Greater Efficacy Against Tumors In Vivo. Breast Cancer Res Treat (2010) 122(2):359–69. doi: 10.1007/s10549-009-0573-0
36. Nanjappa SG, Kim EH, Suresh M. Immunotherapeutic Effects of IL-7 During a Chronic Viral Infection in Mice. Blood (2011) 117(19):5123–32. doi: 10.1182/blood-2010-12-323154
37. Toyota H, Yanase N, Yoshimoto T, Harada M, Kato Y, Mizuguchi J. Vaccination With OVA-Bound Nanoparticles Encapsulating IL-7 Inhibits the Growth of OVA-Expressing E.G7 Tumor Cells In Vivo. Oncol Rep (2015) 33(1):292–6. doi: 10.3892/or.2014.3603
38. Sportès C, Babb RR, Krumlauf MC, Hakim FT, Steinberg SM, Chow CK, et al. Phase I Study of Recombinant Human Interleukin-7 Administration in Subjects With Refractory Malignancy. Clin Cancer Res (2010) 16(2):727–35. doi: 10.1158/1078-0432.CCR-09-1303
39. Sereti I, Dunham RM, Spritzler J, Aga E, Proschan MA, Medvik K, et al. IL-7 Administration Drives T Cell-Cycle Entry and Expansion in HIV-1 Infection. Blood (2009) 113(25):6304–14. doi: 10.1182/blood-2008-10-186601
40. Levy Y, Lacabaratz C, Weiss L, Viard J-P, Goujard C, Lelièvre J-D. Enhanced T Cell Recovery in HIV-1-Infected Adults Through IL-7 Treatment. J Clin Invest (2009) 119(4):997–1007. doi: 10.1172/JCI38052
41. Venet F, Foray A-P, Villars-Méchin A, Malcus C, Poitevin-Later F, Lepape A, et al. IL-7 Restores Lymphocyte Functions in Septic Patients. J Immunol (2012) 189(10):5073–81. doi: 10.4049/jimmunol.1202062
42. Sheikh V, Porter BO, DerSimonian R, Kovacs SB, Thompson WL, Perez-Diez A, et al. Administration of Interleukin-7 Increases CD4 T Cells in Idiopathic CD4 Lymphocytopenia. Blood (2016) 127(8):977–88. doi: 10.1182/blood-2015-05-645077
43. Thampy LK, Remy KE, Walton AH, Hong Z, Liu K, Liu R, et al. Restoration of T Cell Function in Multi-Drug Resistant Bacterial Sepsis After Interleukin-7, Anti-PD-L1, and OX-40 Administration. PloS One (2018) 13(6):e0199497. doi: 10.1371/journal.pone.0199497.
44. Perales M-A, Goldberg JD, Yuan J, Koehne G, Lechner L, Papadopoulos EB, et al. Recombinant Human Interleukin-7 (CYT107) Promotes T-Cell Recovery After Allogeneic Stem Cell Transplantation. Blood (2012) 120(24):4882–91. doi: 10.1182/blood-2012-06-437236
45. Pachynski RK, Morishima C, Szmulewitz R, Harshman L, Appleman L, Monk P, et al. IL-7 Expands Lymphocyte Populations and Enhances Immune Responses to Sipuleucel-T in Patients With Metastatic Castration-Resistant Prostate Cancer (mCRPC). J Immunother Cancer (2021) 9(8):e002903. doi: 10.1136/jitc-2021-002903
46. Colombetti S, Lévy F, Chapatte L. IL-7 Adjuvant Treatment Enhances Long-Term Tumor-Antigen-Specific CD8+ T-Cell Responses After Immunization With Recombinant Lentivector. Blood (2009) 113(26):6629–37. doi: 10.1182/blood-2008-05-155309
47. Li Y, Zhou M, Luo Z, Zhang Y, Cui M, Chen H, et al. Overexpression of Interleukin-7 Extends the Humoral Immune Response Induced by Rabies Vaccination. J Virol (2017) 91(7):e02324–16. doi: 10.1128/JVI.02324-16
48. Cui D, Zhang J, Zuo Y, Huo S, Zhang Y, Wang L, et al. Recombinant Chicken Interleukin-7 as a Potent Adjuvant Increases the Immunogenicity and Protection of Inactivated Infectious Bursal Disease Vaccine. Vet Res (2018) 49(1):10. doi: 10.1186/s13567-017-0497-3
49. Von Freeden-Jeffry U, Vieira P, Lucian LA, Mcneil T, Burdach SE, Murray R. Lymphopenia in Interleukin (IL)-7 Gene-Deleted Mice Identifies IL-7 as a Nonredundant Cytokine. J Exp Med (1995) 181(4):1519–26. doi: 10.1084/jem.181.4.1519
50. Kumar V. Natural Killer Cells in Sepsis: Underprivileged Innate Immune Cells. Eur J Cell Biol (2019) 98(2-4):81–93. doi: 10.1016/j.ejcb.2018.12.003
51. Gardner A, Ruffell B. Dendritic Cells and Cancer Immunity. Trends Immunol (2016) 37(12):855–65. doi: 10.1016/j.it.2016.09.006
52. Good-Jacobson KL, Shlomchik MJ. Plasticity and Heterogeneity in the Generation of Memory B Cells and Long-Lived Plasma Cells: The Influence of Germinal Center Interactions and Dynamics. J Immunol (2010) 185(6):3117–25. doi: 10.4049/jimmunol.1001155
53. Zhang Y, Garcia-Ibanez L, Toellner KM. Regulation of Germinal Center B-Cell Differentiation. Immunol Rev (2016) 270(1):8–19. doi: 10.1111/imr.12396
54. Boller S, Grosschedl R. The Regulatory Network of B-Cell Differentiation: A Focused View of Early B-Cell Factor 1 Function. Immunol Rev (2014) 261(1):102–15. doi: 10.1111/imr.12206
55. Wang Y, Liu J, Burrows PD, Wang JY. B Cell Development and Maturation. Adv Exp Med Biol (2020) 1254:1–22. doi: 10.1007/978-981-15-3532-1_1
56. Kikuchi K, Kasai H, Watanabe A, Lai AY, Kondo M. IL-7 Specifies B Cell Fate at the Common Lymphoid Progenitor to pre-proB Transition Stage by Maintaining Early B Cell Factor Expression. J Immunol (2008) 181(1):383–92. doi: 10.4049/jimmunol.181.1.383
57. Chiju W, Lai L, Goldschneider I. Pre-Pro-B Cell Growth-Stimulating Factor (PPBSF) Upregulates IL-7Ralpha Chain Expression and Enables Pro-B Cells to Respond to Monomeric IL-7. J Interf Cytokine Res (2002) 22(7):823–32. doi: 10.1089/107999002320271422
58. Grabstein KH, Waldschmidt TJ, Finkelman FD, Hess BW, Alpert AR, Boiani NE, et al. Inhibition of Murine B and T Lymphopoiesis In Vivo by an Anti-Interleukin 7 Monoclonal Antibody. J Exp Med (1993) 178(1):257–64. doi: 10.1084/jem.178.1.257
59. Namikawa R, Muench MO, de Vries JE, Roncarolo MG. The FLK2/FLT3 Ligand Synergizes With Interleukin-7 in Promoting Stromal-Cell-Independent Expansion and Differentiation of Human Fetal Pro-B Cells In Vitro. Blood (1996) 87(5):1881–90. doi: 10.1182/blood.V87.5.1881.1881
60. Morrissey PJ, Conlon P, Braddy S, Williams DE, Namen AE, Mochizuki DY. Administration of IL-7 to Mice With Cyclophosphamide-Induced Lymphopenia Accelerates Lymphocyte Repopulation. J Immunol (1991) 146(5):1547–52.
61. Komschlies KL, Gregorio TA, Gruys ME, Back TC, Faltynek CR, Wiltrout RH. Administration of Recombinant Human IL-7 to Mice Alters the Composition of B-Lineage Cells and T Cell Subsets, Enhances T Cell Function, and Induces Regression of Established Metastases. J Immunol (1994) 152(12):5776–84.
62. Johnson SE, Shah N, Panoskaltsis-Mortari A, Lebien TW. Murine and Human IL-7 Activate STAT5 and Induce Proliferation of Normal Human Pro-B Cells. J Immunol (2005) 175(11):7325–31. doi: 10.4049/jimmunol.175.11.7325
63. Wu TH, Bolt AM, Chou H, Plourde D, De Jay N, Guilbert C, et al. Tungsten Blocks Murine B Lymphocyte Differentiation and Proliferation Through Downregulation of IL-7 Receptor/Pax5 Signaling. Toxicol Sci (2019) 170(1):45–56. doi: 10.1093/toxsci/kfz080
64. Corfe SA, Paige CJ. The Many Roles of IL-7 in B Cell Development; Mediator of Survival, Proliferation and Differentiation. Semin Immunol (2012) 24(3):198–208. doi: 10.1016/j.smim.2012.02.001
65. Petkau G, Turner M. Signalling Circuits That Direct Early B-Cell Development. Biochem J (2019) 476(5):769–78. doi: 10.1042/BCJ20180565
66. Xu CR, Schaffer L, Head SR, Feeney AJ. Reciprocal Patterns of Methylation of H3K36 and H3K27 on Proximal vs. Distal IgVH Genes Are Modulated by IL-7 and Pax5. Proc Natl Acad Sci USA (2008) 105(25):8685–90. doi: 10.1073/pnas.0711758105
67. Willems L, Li S, Rutgeerts O, Lenaerts C, Waer M, Billiau AD. IL-7 Is Required for the Development of the Intrinsic Function of Marginal Zone B Cells and the Marginal Zone Microenvironment. J Immunol (2011) 187(7):3587–94. doi: 10.4049/jimmunol.1004012
68. Wei C, Zeff R, Goldschneider I. Murine Pro-B Cells Require IL-7 and Its Receptor Complex to Up-Regulate IL-7R Alpha, Terminal Deoxynucleotidyltransferase, and C Mu Expression. J Immunol (2000) 164(4):1961–70. doi: 10.4049/jimmunol.164.4.1961
69. Oliver PM, Wang M, Zhu Y, White J, Kappler J, Marrack P. Loss of Bim Allows Precursor B Cell Survival But Not Precursor B Cell Differentiation in the Absence of Interleukin 7. J Exp Med (2004) 200(9):1179–87. doi: 10.1084/jem.20041129
70. Seo YB, Im SJ, Namkoong H, Kim SW, Choi YW, Kang MC, et al. Crucial Roles of Interleukin-7 in the Development of T Follicular Helper Cells and in the Induction of Humoral Immunity. J Virol (2014) 88(16):8998–9009. doi: 10.1128/JVI.00534-14
71. Chen C, Zhou M, Yan XG, Chen YX, Cui M, Chen HC, et al. A Recombinant Canine Distemper Virus Expressing Interleukin-7 Enhances Humoral Immunity. J Gen Virol (2019) 100(4):602–15. doi: 10.1099/jgv.0.001247
72. McDonald PW, Read KA, Baker CE, Anderson AE, Powell MD, Ballesteros-Tato A, et al. IL-7 Signalling Represses Bcl-6 and the TFH Gene Program. Nat Commun (2016) 7:10285. doi: 10.1038/ncomms10285
73. Shaan Lakshmanappa Y, Elizaldi SR, Roh JW, Schmidt BA, Carroll TD, Weaver KD, et al. SARS-CoV-2 Induces Robust Germinal Center CD4 T Follicular Helper Cell Responses in Rhesus Macaques. Nat Commun (2021) 12(1):541. doi: 10.1038/s41467-020-20642-x
74. Puel A, Ziegler SF, Buckley RH, Leonard WJ. Defective IL7R Expression in T(-)B(+)NK(+) Severe Combined Immunodeficiency. Nat Genet (1998) 20(4):394–7. doi: 10.1038/3877
75. Williams MA, Bevan MJ. Effector and Memory CTL Differentiation. Annu Rev Immunol (2007) 25:171–92. doi: 10.1146/annurev.immunol.25.022106.141548
76. Gerlach C, Rohr JC, Perié L, Van Rooij N, Van Heijst JW, Velds A, et al. Heterogeneous Differentiation Patterns of Individual CD8+ T Cells. Science (2013) 340(6132):635–9. doi: 10.1126/science.1235487
77. Moore TA, Von Freeden-Jeffry U, Murray R, Zlotnik A. Inhibition of Gamma Delta T Cell Development and Early Thymocyte Maturation in IL-7 -/- Mice. J Immunol (1996) 157(6):2366–73.
78. Mazzucchelli R, Durum SK. Interleukin-7 Receptor Expression: Intelligent Design. Nat Rev Immunol (2007) 7(2):144–54. doi: 10.1038/nri2023
79. Von Freeden-Jeffry U, Solvason N, Howard M, Murray R. The Earliest T Lineage-Committed Cells Depend on IL-7 for Bcl-2 Expression and Normal Cell Cycle Progression. Immunity (1997) 7(1):147–54. doi: 10.1016/s1074-7613(00)80517-8
80. Barata JT, Cardoso AA, Nadler LM, Boussiotis VA. Interleukin-7 Promotes Survival and Cell Cycle Progression of T-Cell Acute Lymphoblastic Leukemia Cells by Down-Regulating the Cyclin-Dependent Kinase Inhibitor P27(Kip1). Blood (2001) 98(5):1524–31. doi: 10.1182/blood.v98.5.1524
81. Khaled AR, Li WQ, Huang J, Fry TJ, Khaled AS, Mackall CL, et al. Bax Deficiency Partially Corrects Interleukin-7 Receptor Alpha Deficiency. Immunity (2002) 17(5):561–73. doi: 10.1016/s1074-7613(02)00450-8
82. Opferman JT, Letai A, Beard C, Sorcinelli MD, Ong CC, Korsmeyer SJ. Development and Maintenance of B and T Lymphocytes Requires Antiapoptotic MCL-1. Nature (2003) 426(6967):671–6. doi: 10.1038/nature02067
83. Barata JT, Silva A, Brandao JG, Nadler LM, Cardoso AA, Boussiotis VA. Activation of PI3K Is Indispensable for Interleukin 7-Mediated Viability, Proliferation, Glucose Use, and Growth of T Cell Acute Lymphoblastic Leukemia Cells. J Exp Med (2004) 200(5):659–69. doi: 10.1084/jem.20040789
84. Jiang Q, Li WQ, Aiello FB, Mazzucchelli R, Asefa B, Khaled AR, et al. Cell Biology of IL-7, a Key Lymphotrophin. Cytokine Growth Factor Rev (2005) 16(4–5):513–33. doi: 10.1016/j.cytogfr.2005.05.004
85. Silva A, Laranjeira AB, Martins LR, Cardoso BA, Demengeot J, Yunes JA, et al. IL-7 Contributes to the Progression of Human T-Cell Acute Lymphoblastic Leukemias. Cancer Res (2011) 71(14):4780–9. doi: 10.1158/0008-5472.CAN-10-3606
86. Jacobs SR, Michalek RD, Rathmell JC. IL-7 Is Essential for Homeostatic Control of T Cell Metabolism In Vivo. J Immunol (2010) 184(7):3461–9. doi: 10.4049/jimmunol.0902593
87. Bhatia SK, Tygrett LT, Grabstein KH, Waldschmidt TJ. The Effect of In Vivo IL-7 Deprivation on T Cell Maturation. J Exp Med (1995) 181(4):1399–409. doi: 10.1084/jem.181.4.1399
88. Tan JT, Dudl E, Leroy E, Murray R, Sprent J, Weinberg KI, et al. IL-7 Is Critical for Homeostatic Proliferation and Survival of Naive T Cells. Proc Natl Acad Sci USA (2001) 98(15):8732–7. doi: 10.1073/pnas.161126098
89. Wang H, Pierce LJ, Spangrude GJ. Distinct Roles of IL-7 and Stem Cell Factor in the OP9-DL1 T-Cell Differentiation Culture System. Exp Hematol (2006) 34(12):1730–40. doi: 10.1016/j.exphem.2006.08.001
90. Xiong J, Parker BL, Dalheimer SL, Yankee TM. Interleukin-7 Supports Survival of T-Cell Receptor-β-Expressing CD4(-) CD8(-) Double-Negative Thymocytes. Immunology (2013) 138(4):382–91. doi: 10.1111/imm.12050
91. Boudil A, Matei IR, Shih H-Y, Bogdanoski G, Yuan JS, Chang SG, et al. IL-7 Coordinates Proliferation, Differentiation and Tcra Recombination During Thymocyte β-Selection. Nat Immune (2015) 16(4):397–405. doi: 10.1038/ni.3122
92. Jourdan P, Vendrell JP, Huguet MF, Segondy M, Bousquet J, Pène J, et al. Cytokines and Cell Surface Molecules Independently Induce CXCR4 Expression on CD4+ CCR7+ Human Memory T Cells. J Immunol (2000) 165(2):716–24. doi: 10.4049/jimmunol.165.2.716
93. Shimba A, Cui G, Tani-ichi S, Ogawa M, Abe S, Okazaki F, et al. Glucocorticoids Drive Diurnal Oscillations in T Cell Distribution and Responses by Inducing Interleukin-7 Receptor and CXCR4. Immunity (2018) 48(2):286–98.e6. doi: 10.1016/j.immuni.2018.01.004
94. Colpitts SL, Dalton NM, Scott P. IL-7 Receptor Expression Provides the Potential for Long-Term Survival of Both CD62Lhigh Central Memory T Cells and Th1 Effector Cells During Leishmania Major Infection. J Immunol (2009) 182(9):5702–11. doi: 10.4049/jimmunol.0803450
95. Le Campion A, Pommier A, Delpoux A, Stouvenel L, Auffray C, Martin B, et al. IL-2 and IL-7 Determine the Homeostatic Balance Between the Regulatory and Conventional CD4+ T Cell Compartments During Peripheral T Cell Reconstitution. J Immunol (2012) 189(7):3339–46. doi: 10.4049/jimmunol.1103152
96. Mackall CL, Fry TJ, Gress RE. Harnessing the Biology of IL-7 for Therapeutic Application. Nat Rev Immunol (2011) 11(5):330–42. doi: 10.1038/nri2970
97. Hou H, Kang Y, Zeng Y, Li Y, Shang J. Interleukin-7 Augments CD8+ T Cells Function and Promotes Viral Clearance in Chronic Hepatitis C Virus Infection. Cytokine (2018) 102:26–33. doi: 10.1016/j.cyto.2017.12.014
98. Teng D, Ding L, Cai B, Luo Q, Wang H. Interleukin-7 Enhances Anti-Tumor Activity of CD8+ T Cells in Patients With Hepatocellular Carcinoma. Cytokine (2019) 118:115–23. doi: 10.1016/j.cyto.2018.04.003
99. Tian X, Su Z, Guo S, Wang C, Zhang X, Du J. Interleukin-7 Promotes CD8+ T Cell Activity in Patients With Enterovirus 71 Associated Encephalitis. Int Immunopharmacol (2019) 75:105773. doi: 10.1016/j.intimp.2019.105773
100. Andersson A, Yang SC, Huang M, Zhu L, Kar UK, Batra RK, et al. IL-7 Promotes CXCR3 Ligand-Dependent T Cell Antitumor Reactivity in Lung Cancer. J Immunol (2009) 182(11):6951–8. doi: 10.4049/jimmunol.0803340
101. Hu H, Sun SC. Ubiquitin Signaling in Immune Responses. Cell Res (2016) 26(4):457–83. doi: 10.1038/cr.2016.40
102. Pellegrini M, Calzascia T, Elford AR, Shahinian A, Lin AE, Dissanayake D, et al. Adjuvant IL-7 Antagonizes Multiple Cellular and Molecular Inhibitory Networks to Enhance Immunotherapies. Nat Med (2009) 15(5):528–36. doi: 10.1038/nm.1953
103. Fry TJ, Mackall CL. The Many Faces of IL-7: From Lymphopoiesis to Peripheral T Cell Maintenance. J Immunol (2005) 174(11):6571–6. doi: 10.4049/jimmunol.174.11.6571
104. Leone A, Rohankhedkar M, Okoye A, Legasse A, Axthelm MK, Villinger F, et al. Increased CD4+ T Cell Levels During IL-7 Administration of Antiretroviral Therapy-Treated Simian Immunodeficiency Virus-Positive Macaques Are Not Dependent on Strong Proliferative Responses. J Immunol (2010) 185(3):1650–9. doi: 10.4049/jimmunol.0902626
105. Rajasuriar R, Booth D, Solomon A, Chua K, Spelman T, Gouillou M, et al. Biological Determinants of Immune Reconstitution in HIV-Infected Patients Receiving Antiretroviral Therapy: The Role of Interleukin 7 and Interleukin 7 Receptor α and Microbial Translocation. J Infect Dis (2010) 202(8):1254–64. doi: 10.1086/656369
106. Sheikh V, Porter BO, Dersimonian R, Kovacs SB, Thompson WL, Perez-Diez A, et al. Administration of Interleukin-7 Increases CD4 T Cells in Idiopathic CD4 Lymphocytopenia. Blood (2016) 127(8):977–88. doi: 10.1182/blood-2015-05-645077
107. Schluns KS, Kieper WC, Jameson SC, Lefrançois L. Interleukin-7 Mediates the Homeostasis of Naïve and Memory CD8 T Cells In Vivo. Nat Immunol (2000) 1(5):426–32. doi: 10.1038/80868
108. Tan JT, Ernst B, Kieper WC, Leroy E, Sprent J, Surh CD. Interleukin (IL)-15 and IL-7 Jointly Regulate Homeostatic Proliferation of Memory Phenotype CD8+ Cells But Are Not Required for Memory Phenotype CD4+ Cells. J Exp Med (2002) 195(12):1523–32. doi: 10.1084/jem.20020066
109. Schluns KS, Lefrançois L. Cytokine Control of Memory T-Cell Development and Survival. Nat Rev Immunol (2003) 3(4):269–79. doi: 10.1038/nri1052
110. Chetoui N, Boisvert M, Gendron S, Aoudjit F. Interleukin-7 Promotes the Survival of Human CD4+ Effector/Memory T Cells by Up-Regulating Bcl-2 Proteins and Activating the JAK/STAT Signalling Pathway. Immunology (2010) 130(3):418–26. doi: 10.1111/j.1365-2567.2009.03244.x
111. O’Brien KL, Finlay DK. Immunometabolism and Natural Killer Cell Responses. Nat Rev Immunol (2019) 19(5):282–90. doi: 10.1038/s41577-019-0139-2
112. Luther C, Warner K, Takei F. Unique Progenitors in Mouse Lymph Node Develop Into CD127+ NK Cells: Thymus-Dependent and Thymus-Independent Pathways. Blood (2011) 117(15):4012–21. doi: 10.1182/blood-2010-07-298901
113. Michaud A, Dardari R, Charrier E, Cordeiro P, Herblot S, Duval M. IL-7 Enhances Survival of Human CD56bright NK Cells. J Immunother (2010) 33(4):382–90. doi: 10.1097/CJI.0b013e3181cd872d
114. Vosshenrich CA, García-Ojeda ME, Samson-Villéger SI, Pasqualetto V, Enault L, Richard-Le Goff O, et al. A Thymic Pathway of Mouse Natural Killer Cell Development Characterized by Expression of GATA-3 and CD127. Nat Immunol (2006) 7(11):1217–24. doi: 10.1038/ni1395
115. Dadmarz R, Bockstoce DC, Golub SH. Interleukin-7 Selectively Enhances Natural Kill Cytotoxicity Mediated by the CD56bright Natural Killer Subpopulation. Lymphokine Cytokine Res (1994) 13(6):349–57.
116. Judge CJ, Kostadinova L, Sherman KE, Butt AA, Falck-Ytter Y, Funderburg NT, et al. CD56bright NK IL-7rα Expression Negatively Associates With HCV Level, and IL-7-Induced NK Function Is Impaired During HCV and HIV Infections. J Leukoc Biol (2017) 102(1):171–84. doi: 10.1189/jlb.5A1116-456R
117. Abel AM, Yang C, Thakar MS, Malarkannan S. Natural Killer Cells: Development, Maturation, and Clinical Utilization. Front Immunol (2018) 9:1869. doi: 10.3389/fimmu.2018.01869
118. Shortman K, Naik SH. Steady-State and Inflammatory Dendritic-Cell Development. Nat Rev Immunol (2007) 7(1):19–30. doi: 10.1038/nri1996
119. Collin M, Bigley V. Human Dendritic Cell Subsets: An Update. Immunology (2018) 154(1):3–20. doi: 10.1111/imm.12888
120. Saunders D, Lucas K, Ismaili J, Wu L, Maraskovsky E, Dunn A, et al. Dendritic Cell Development in Culture From Thymic Precursor Cells in the Absence of Granulocyte/Macrophage Colony-Stimulating Factor. J Exp Med (1996) 184(6):2185–96. doi: 10.1084/jem.184.6.2185
121. Bikker A, Hack CE, Lafeber FP, Van Roon JA. Interleukin-7: A Key Mediator in T Cell-Driven Autoimmunity, Inflammation, and Tissue Destruction. Curr Pharm Des (2012) 18(16):2347–56. doi: 10.2174/138161212800165979
122. Barata JT, Durum SK, Seddon B. Flip the Coin: IL-7 and IL-7R in Health and Disease. Nat Immunol (2019) 20(12):1584–93. doi: 10.1038/s41590-019-0479-x
123. Leonard WJ, Imada K, Nakajima H, Puel A, Soldaini E, John S. Signaling via the IL-2 and IL-7 Receptors From the Membrane to the Nucleus. Cold Spring Harb Symp Quant Biol (1999) 64:417–24. doi: 10.1101/sqb.1999.64.417
124. Mohamed MF, Beck D, Camp HS, Othman AA. Preferential Inhibition of JAK1 Relative to JAK3 by Upadacitinib: Exposure–Response Analyses of Ex Vivo Data From 2 Phase 1 Clinical Trials and Comparison to Tofacitinib. J Clin Pharmacol (2020) 60(2):188–97. doi: 10.1002/jcph.1513
125. Gao J, Zhao L, Wan YY, Zhu B. Mechanism of Action of IL-7 and Its Potential Applications and Limitations in Cancer Immunotherapy. Int J Mol Sci (2015) 16(5):10267–80. doi: 10.3390/ijms160510267
126. Leonard WJ, Imada K, Nakajima H, Puel A, Soldaini E, John S. Signaling via the IL-2 and IL-7 Receptors From the Membrane to the Nucleus. Cold Spring Harb Symp Quant Biol (1999) 64:417–24. doi: 10.1101/sqb.1999.64.417
127. Tamarit B, Bugault F, Pillet AH, Lavergne V, Bochet P, Garin N, et al. Correction: Membrane Microdomains and Cytoskeleton Organization Shape and Regulate the IL-7 Receptor Signalosome in Human CD4 T-Cells. J Biol Chem (2019) 294(13):5212–3. doi: 10.1074/jbc.AAC119.008288
128. Lu L, Chaudhury P, Osmond DG. Regulation of Cell Survival During B Lymphopoiesis: Apoptosis and Bcl-2/Bax Content of Precursor B Cells in Bone Marrow of Mice With Altered Expression of IL-7 and Recombinase-Activating Gene-2. J Immunol (1999) 162(4):1931–40.
129. Kosan C, Saba I, Godmann M, Herold S, Herkert B, Eilers M, et al. Transcription Factor Miz-1 Is Required to Regulate Interleukin-7 Receptor Signaling at Early Commitment Stages of B Cell Differentiation. Immunity (2010) 33(6):917–28. doi: 10.1016/j.immuni.2010.11.028
130. Möröy T, Saba I, Kosan C. The Role of the Transcription Factor Miz-1 in Lymphocyte Development and Lymphomagenesis-Binding Myc Makes the Difference. Semin Immunol (2011) 23(5):379–87. doi: 10.1016/j.smim.2011.09.001
131. Xie Y, Shi X, Sheng K, Han G, Li W, Zhao Q, et al. PI3K/Akt Signaling Transduction Pathway, Erythropoiesis and Glycolysis in Hypoxia [Review]. Mol Med Rep (2019) 19(2):783–91. doi: 10.3892/mmr.2018.9713
132. Lali FV, Crawley J, Mcculloch DA, Foxwell BM. A Late, Prolonged Activation of the Phosphatidylinositol 3-Kinase Pathway Is Required for T Cell Proliferation. J Immunol (2004) 172(6):3527–34. doi: 10.4049/jimmunol.172.6.3527
133. Sun Y, Lu B, Deng J, Jiang Z, Cao W, Dai T, et al. IL-7 Enhances the Differentiation of Adipose-Derived Stem Cells Toward Lymphatic Endothelial Cells Through AKT Signaling. Cell Biol Int (2019) 43(4):394–401. doi: 10.1002/cbin.11093
134. Venkitaraman AR, Cowling RJ. Interleukin-7 Induces the Association of Phosphatidylinositol 3-Kinase With the Alpha Chain of the Interleukin-7 Receptor. Eur J Immunol (1994) 24(9):2168–74. doi: 10.1002/eji.1830240935
135. Oliveira ML, Akkapeddi P, Ribeiro D, Melão A, Barata JT. IL-7R-Mediated Signaling in T-Cell Acute Lymphoblastic Leukemia: An Update. Adv Biol Regul (2019) 71:88–96. doi: 10.1016/j.jbior.2018.09.012
136. Yang F, Chen E, Yang Y, Han F, Han S, Wu G, et al. The Akt/FoxO/p27Kip1 Axis Contributes to the Anti-Proliferation of Pentoxifylline in Hypertrophic Scars. J Cell Mol Med (2019) 23(9):6164–72. doi: 10.1111/jcmm.14498
137. Li WQ, Jiang Q, Aleem E, Kaldis P, Khaled AR, Durum SK. IL-7 Promotes T Cell Proliferation Through Destabilization of p27Kip1. J Exp Med (2006) 203(3):573–82. doi: 10.1084/jem.20051520
138. Fujita N, Sato S, Katayama K, Tsuruo T. Akt-Dependent Phosphorylation of p27Kip1 Promotes Binding to 14-3-3 and Cytoplasmic Localization. J Biol Chem (2002) 277(32):28706–13. doi: 10.1074/jbc.M203668200
139. Nam S, Gupta VK, Lee HP, Lee JY, Wisdom KM, Varma S, et al. Cell Cycle Progression in Confining Microenvironments Is Regulated by a Growth-Responsive TRPV4-PI3K/Akt-p27Kip1 Signaling Axis. Sci Adv (2019) 5(8):eaaw6171. doi: 10.1126/sciadv.aaw6171
140. Wofford JA, Wieman HL, Jacobs SR, Zhao Y, Rathmell JC. IL-7 Promotes Glut1 Trafficking and Glucose Uptake via STAT5-Mediated Activation of Akt to Support T-Cell Survival. Blood (2008) 111(4):2101–11. doi: 10.1182/blood-2007-06-096297
141. Chehtane M, Khaled AR. Interleukin-7 Mediates Glucose Utilization in Lymphocytes Through Transcriptional Regulation of the Hexokinase II Gene. Am J Physiol Cell Physiol (2010) 298(6):C1560–71. doi: 10.1152/ajpcell.00506.2009
142. Crawley JB, Rawlinson L, Lali FV, Page TH, Saklatvala J, Foxwell BM. T Cell Proliferation in Response to Interleukins 2 and 7 Requires P38map Kinase Activation. J Biol Chem (1997) 272(23):15023–7. doi: 10.1074/jbc.272.23.15023
143. Rajnavolgyi E, Benbernou N, Rethi B, Reynolds D, Young HA, Magocsi M, et al. IL-7 Withdrawal Induces a Stress Pathway Activating P38 and Jun N-Terminal Kinases. Cell Signal (2002) 14(9):761–9. doi: 10.1016/s0898-6568(02)00026-8
144. Zeng YX, Takahashi H, Shibata M, Hirokawa K. JAK3 Janus Kinase Is Involved in Interleukin 7 Signal Pathway. FEBS Lett (1994) 353(3):289–93. doi: 10.1016/0014-5793(94)01065-x
145. Chen Z, Kim SJ, Chamberlain ND, Pickens SR, Volin MV, Volkov S, et al. The Novel Role of IL-7 Ligation to IL-7 Receptor in Myeloid Cells of Rheumatoid Arthritis and Collagen-Induced Arthritis. J Immunol (2013) 190(10):5256–66. doi: 10.4049/jimmunol.1201675
146. Kim JH, Loveland JE, Sitz KV, Ratto Kim S, Mclinden RJ, Tencer K, et al. Expansion of Restricted Cellular Immune Responses to HIV-1 Envelope by Vaccination: Il-7 and IL-12 Differentially Augment Cellular Proliferative Responses to HIV-1. Clin Exp Immunol (1997) 108(2):243–50. doi: 10.1046/j.1365-2249.1997.d01-1006.x
147. Kim HR, Hong MS, Dan JM, Kang I. Altered IL-7Ralpha Expression With Aging and the Potential Implications of IL-7 Therapy on CD8+ T-Cell Immune Responses. Blood (2006) 107(7):2855–62. doi: 10.1182/blood-2005-09-3560
148. Su B, Wang J, Zhao G, Wang X, Li J, Wang B. Sequential Administration of Cytokine Genes to Enhance Cellular Immune Responses and CD4 (+) T Memory Cells During DNA Vaccination. Hum Vaccin Immunother (2012) 8(11):1659–67. doi: 10.4161/hv.22105
149. Chen J, Li ZY, Petersen E, Liu WG, Zhu XQ. Co-Administration of Interleukins 7 and 15 With DNA Vaccine Improves Protective Immunity Against Toxoplasma Gondii. Exp Parasitol (2016) 162:18–23. doi: 10.1016/j.exppara.2015.12.013
150. Li Y, Zhou M, Luo Z, Zhang Y, Cui M, Chen H, et al. Overexpression of Interleukin-7 Extends the Humoral Immune Response Induced by Rabies Vaccination. J Virol (2017) 91(7):e02324–16. doi: 10.1128/JVI.02324-16
151. Melchionda F, Fry TJ, Milliron MJ, Mckirdy MA, Tagaya Y, Mackall CL. Adjuvant IL-7 or IL-15 Overcomes Immunodominance and Improves Survival of the CD8+ Memory Cell Pool. J Clin Invest (2005) 115(5):1177–87. doi: 10.1172/JCI23134
152. Ahn SS, Jeon BY, Park SJ, Choi DH, Ku SH, Cho SN, et al. Nonlytic Fc-Fused IL-7 Synergizes With Mtb32 DNA Vaccine to Enhance Antigen-Specific T Cell Responses in a Therapeutic Model of Tuberculosis. Vaccine (2013) 31(27):2884–90. doi: 10.1016/j.vaccine.2013.04.029
153. Kim AR, Park J, Kim JH, Kwak JE, Cho Y, Lee H, et al. Herpes Zoster DNA Vaccines With IL-7 and IL-33 Molecular Adjuvants Elicit Protective T Cell Immunity. Immune Netw (2018) 18(5):e38. doi: 10.4110/in.2018.18.e38
154. Huo S, Zhang J, Fan J, Wang X, Wu F, Zuo Y, et al. Co-Expression of Chicken IL-2 and IL-7 Enhances the Immunogenicity and Protective Efficacy of a VP2-Expressing DNA Vaccine Against IBDV in Chickens. Viruses (2019) 11(5):476. doi: 10.3390/v11050476
155. Nanjappa SG, Walent JH, Morre M, Suresh M. Effects of IL-7 on Memory CD8 T Cell Homeostasis Are Influenced by the Timing of Therapy in Mice. J Clin Invest (2008) 118(3):1027–39. doi: 10.1172/JCI32020
156. Huo S, Zuo Y, Li N, Li X, Zhang Y, Wang L, et al. Chicken IL-7 as a Potent Adjuvant Enhances IBDV VP2 DNA Vaccine Immunogenicity and Protective Efficacy. Vet Microbiol (2016) 193:145–55. doi: 10.1016/j.vetmic.2016.08.016
157. Panebra A, Lillehoj HS. Eimeria Tenella Elongation Factor-1α (EF-1α) Coadministered With Chicken IL-7 (chIL-7) DNA Vaccine Emulsified in Montanide Gel 01 Adjuvant Enhanced the Immune Response to E. Acervulina Infection in Broiler Chickens. Avian Dis (2019) 63(2):342–50. doi: 10.1637/11976-092418-Reg.1
158. Park SH, Song MY, Nam HJ, Im SJ, Sung YC. Codelivery of IL-7 Augments Multigenic HCV DNA Vaccine-Induced Antibody as Well as Broad T Cell Responses in Cynomolgus Monkeys. Immune Netw (2010) 10(6):198–205. doi: 10.4110/in.2010.10.6.198
159. Choi YW, Kang MC, Seo YB, Namkoong H, Park Y, Choi DH, et al. Intravaginal Administration of Fc-Fused IL7 Suppresses the Cervicovaginal Tumor by Recruiting HPV DNA Vaccine-Induced CD8 T Cells. Clin Cancer Res (2016) 22(23):5898–908. doi: 10.1158/1078-0432.CCR-16-0423
160. Zhao L, Mei Y, Sun Q, Guo L, Wu Y, Yu X, et al. Autologous Tumor Vaccine Modified With Recombinant New Castle Disease Virus Expressing IL-7 Promotes Antitumor Immune Response. J Immunol (2014) 193(2):735–45. doi: 10.4049/jimmunol.1400004
161. Shinya H, Toshiki T, Masayuki U, Goro M, Naoya O, Yasunobu Y. Recombinant Mycobacterium Bovis Bacillus Calmette-Guérin Expressing Ag85B-IL-7 Fusion Protein Enhances IL-17A-Producing Innate γδ T Cells. Vaccine (2016) 34(22):2490–5. doi: 10.1016/j.vaccine.2016.03.096
162. Singh V, Gowthaman U, Jain S, Parihar P, Banskar S, Gupta P, et al. Coadministration of Interleukins 7 and 15 With Bacille Calmette–Guérin Mounts Enduring T Cell Memory Response Against Mycobacterium Tuberculosis. J Infect Dis (2010) 202(3):480–9. doi: 10.1086/653827
163. Cui D, Zhang J, Zuo Y, Huo S, Zhang Y, Wang L, et al. Recombinant Chicken Interleukin-7 as a Potent Adjuvant Increases the Immunogenicity and Protection of Inactivated Infectious Bursal Disease Vaccine. Vet Res (2018) 49(1):10. doi: 10.1186/s13567-017-0497-3
164. Flexner C, Hügin A, Moss B. Prevention of Vaccinia Virus Infection in Immunodeficient Mice by Vector-Directed IL-2 Expression. Nature (1987) 330(6145):259–62. doi: 10.1038/330259a0
165. Bekele Y, Sui Y, Berzofsky JA. IL-7 in SARS-CoV-2 Infection and as a Potential Vaccine Adjuvant. Front Immunol (2021) 12:737406. doi: 10.3389/fimmu.2021.737406
166. Xu X, Sun Q, Mei Y, Liu Y, Zhao L. Newcastle Disease Virus Co-Expressing Interleukin 7 and Interleukin 15 Modified Tumor Cells as a Vaccine for Cancer Immunotherapy. Cancer Sci (2018) 109(2):279–88. doi: 10.1111/cas.13468
167. Bai C, Zhou L, Tang J, He J, Han J, Niu H, et al. Fusion Cytokines IL-7-Linker-IL-15 Promote Mycobacterium Tuberculosis Subunit Vaccine to Induce Central Memory Like T Cell-Mediated Immunity. Vaccines (Basel) (2020) 8(4):715. doi: 10.3390/vaccines8040715
168. Zhang Y, Liang S, Li X, Wang L, Zhang J, Xu J, et al. Mutual Enhancement of IL-2 and IL-7 on DNA Vaccine Immunogenicity Mainly Involves Regulations on Their Receptor Expression and Receptor-Expressing Lymphocyte Generation. Vaccine (2015) 33(30):3480–7. doi: 10.1016/j.vaccine.2015.05.068
169. Kang MC, Choi DH, Choi YW, Park SJ, Namkoong H, Park KS, et al. Intranasal Introduction of Fc-Fused Interleukin-7 Provides Long-Lasting Prophylaxis Against Lethal Influenza Virus Infection. J Virol (2015) 90(5):2273–84. doi: 10.1128/JVI.02768-15
170. Kang MC, Park HW, Choi DH, Choi YW, Park Y, Sung YC, et al. Plasmacytoid Dendritic Cells Contribute to the Protective Immunity Induced by Intranasal Treatment With Fc-Fused Interleukin-7 Against Lethal Influenza Virus Infection. Immune Netw (2017) 17(5):343–51. doi: 10.4110/in.2017.17.5.343
171. Watanabe M, Ueno Y, Yajima T, Iwao Y, Tsuchiya M, Ishikawa H, et al. Interleukin 7 Is Produced by Human Intestinal Epithelial Cells and Regulates the Proliferation of Intestinal Mucosal Lymphocytes. J Clin Invest (1995) 95(6):2945–53. doi: 10.1172/JCI118002
172. Yang H, Madison B, Gumucio DL, Teitelbaum DH. Specific Overexpression of IL-7 in the Intestinal Mucosa: The Role in Intestinal Intraepithelial Lymphocyte Development. Am J Physiol Gastrointest Liver Physiol (2008) 294(6):G1421–30. doi: 10.1152/ajpgi.00060.2008
173. Yada S, Nukina H, Kishihara K, Takamura N, Yoshida H, Inagaki-Ohara K, et al. IL-7 Prevents Both Caspase-Dependent and -Independent Pathways That Lead to the Spontaneous Apoptosis of I-IEL. Cell Immunol (2001) 208(2):88–95. doi: 10.1006/cimm.2001.1765
174. Fukatsu K, Moriya T, Murakoshi S, Yasuhara H. Interleukin-7 Treatment Reverses Parenteral Nutrition-Induced Impairment of Resistance to Bacterial Pneumonia With Increased Secretory Immunoglobulin A Levels. J Surg Res (2012) 174(2):334–8. doi: 10.1016/j.jss.2010.12.004
175. Han KH, Jang MS, Han HY, Im WJ, Jung KJ, Park KS, et al. Preclinical Safety Assessment of a Therapeutic Human Papillomavirus DNA Vaccine Combined With Intravaginal Interleukin-7 Fused With Hybrid Fc in Female Rats. Toxicol Appl Pharmacol (2021) 413:115406. doi: 10.1016/j.taap.2021.115406
Keywords: IL-7, biological function, mechanism, vaccine, molecular adjuvant
Citation: Huang J, Long Z, Jia R, Wang M, Zhu D, Liu M, Chen S, Zhao X, Yang Q, Wu Y, Zhang S, Tian B, Mao S, Ou X, Sun D, Gao Q and Cheng A (2021) The Broad Immunomodulatory Effects of IL-7 and Its Application In Vaccines. Front. Immunol. 12:680442. doi: 10.3389/fimmu.2021.680442
Received: 14 March 2021; Accepted: 24 November 2021;
Published: 10 December 2021.
Edited by:
Jochen Mattner, University of Erlangen Nuremberg, GermanyReviewed by:
Jose Alejandro Chabalgoity, University of the Republic, UruguayJana Trifinopoulos, Veterinärmedizinische Universität Wien, Austria
Stephen Brown, The University of Sheffield, United Kingdom
Copyright © 2021 Huang, Long, Jia, Wang, Zhu, Liu, Chen, Zhao, Yang, Wu, Zhang, Tian, Mao, Ou, Sun, Gao and Cheng. This is an open-access article distributed under the terms of the Creative Commons Attribution License (CC BY). The use, distribution or reproduction in other forums is permitted, provided the original author(s) and the copyright owner(s) are credited and that the original publication in this journal is cited, in accordance with accepted academic practice. No use, distribution or reproduction is permitted which does not comply with these terms.
*Correspondence: Juan Huang, aHVhbmdqdWFuNjEwQDE2My5jb20=; Anchun Cheng, Y2hlbmdhbmNodW5AdmlwLjE2My5jb20=
†These authors have contributed equally to this work