- 1Department of Radiation Oncology, Shandong Cancer Hospital and Institute, Shandong First Medical University and Shandong Academy of Medical Sciences, Jinan, China
- 2Department of Cardiology, Qilu Hospital Affiliated to Shandong University, Jinan, China
Recently, the overall survival (OS) and progression-free survival (PFS) of patients with advanced cancer has been significantly improved due to the application of immune checkpoint inhibitors (ICIs). Low response rate and high occurrence of immune-related adverse events (irAEs) make urgently need for ideal predictive biomarkers to identity efficient population and guide treatment strategies. Cytokines are small soluble proteins with a wide range of biological activity that are secreted by activated immune cells or tumor cells and act as a bridge between innate immunity, infection, inflammation and cancer. Cytokines can be detected in peripheral blood and suitable for dynamic detection. During the era of ICIs, many studies investigated the role of cytokines in prediction of the efficiency and toxicity of ICIs. Herein, we review the relevant studies on TNF-α, IFN-γ, IL-6, IL-8, TGF-β and other cytokines as biomarkers for predicting ICI-related reactions and adverse events, and explore the immunomodulatory mechanisms. Finally, the most important purpose of this review is to help identify predictors of ICI to screen patients who are most likely to benefit from immunotherapy.
Introduction
Immune checkpoint inhibitors (ICIs) therapy has shown improved clinical responses and significant survival benefits in patients with locally advanced or advanced solid tumor types. Specifically, programmed cell death 1 (PD-1), programmed cell death ligand 1 (PD-L1), and monoclonal antibodies against cytotoxic T lymphocyte antigen 4 (CTLA-4) have been approved in the first-line and second-line treatment of various malignant neoplasms, including non-small cell lung cancer (NSCLC), melanoma, renal cell carcinoma (RCC), etc. (1–4). To date, there are six Food and Drug Administration (FDA)-approved ICIs for PD-1/PD-L1/CTLA-4, including pembrolizumab and nivolumab (anti-PD-1) and atezolizumab, durvalumab and avelumab (anti-PD-L1). However, the first-line objective response rate (ORR) of NSCLC treated by ICIs plus chemotherapy was approximately 48-58% (5, 6), and ICI monotherapy was only approximately 27-46% (7, 8). Thus, a large number of patients did not receive beneficial effects. Additionally, immune-related adverse events (irAEs), which occurs mostly in derma, digestive system, endocrine organs and lungs. The response rate of any grade of irAEs was 30% and ≥grade 3 was 5-10%, even 1% death rate (9, 10). Hence, biomarkers are pressing needed for identification patients who will benefit most from ICIs and avoid over treatment.
Predictive biomarkers for prognosis and adverse reactions of ICI treatment received more attention and have been widely explored in recent years. According to the simple being test, current biomarkers are mainly including tumor tissue biomarkers [e.g. PD-L1 expression (11, 12), tumor mutation burden (TMB) (13), MHC molecule expression (14, 15)], circulating immune cells biomarkers [e.g. CD4+T cells (16, 17), myeloid-derived suppressive cells (MDSCs) (18)] and soluble systemic immune/inflammatory biomarkers [e.g. lactate dehydrogenase (LDH) (19, 20), cytokines (21, 22)]. However, definite conclusions have not been reached. So far, PD-L1 expression based on assays on tumor cells is the only biomarker that is approved and extensively used for selecting patients for PD1/PD-L1 immunotherapy (23, 24). However, response was observed in PD-L1 negative patients and not all PD-L1 positive patients benefit (25). In addition, PD-L1 detection requires tissue samples obtained by an invasive means and local tissue may provide an incomplete insight of TME. Circulating biomarkers based on plasma offer an alternative non-invasive solution to address these weaknesses.
Cytokines (CK) are a series of low molecular weight soluble proteins, including interleukin (IL), interferon (IFN), tumor necrosis factor (TNF) superfamily, colony stimulating factors (CSF), chemokines, and growth factors (GF), secreted by immune cells (such as monocytes, macrophages, T cells, B cells, and NK cells) and some nonimmune cells (endothelial cells, epidermal cells, and fibroblasts) after induction by immunogens, mitogens, or other stimulants (26). Autocrine, paracrine, or endocrine cytokines bind to the receptors on the target cell membrane to trigger intracellular signals and change specific cellular functions (27, 28). Changes of cytokines levels regulate tumor microenvironment, change the proliferation and differentiation of immune cells, and even influence the metastasis of cancer cells (29, 30).
A series of studies have evaluated the level of baseline and changes of cytokines in various tumors patients treated with ICIs. Several preclinical model studies have shown that a combination of certain cytokine drugs and ICIs can improve the prognosis (31, 32). In this review, we retrospect the potential value of cytokines in predicting the efficacy and adverse reactions of immune checkpoint therapy (ICT) and intends to identify patients who will benefit from ICIs.
TNF-α
Tumor necrosis factor (TNF) was named in 1975 by Carswell because TNF caused tumor bleeding and necrosis (33). TNF-α is mainly produced by monocytes and macrophages. Additionally, TNF-α is detected in tumor tissue and secreted by malignant tumor tissues or interstitial tumor cells. Soluble TNF-α (sTNF) and transmembrane TNF-α (tmTNF) are two forms of TNF-α. TNF-α binds to two receptors including TNFR1 and TNFR2. TNFR1 is ubiquitously expressed, and TNFR2 is expressed only in immune cells, neurons, and endothelial cells (34, 35). After binding to the receptors, TNF-α recruits death domain (DD) proteins, such as Fas-associated death domain (FADD) and TNFR associated DD (TRADD), activates apoptotic signal transduction pathways, and recruits TRAF family proteins, such as NF-kappa B and JNK, which accelerate cell proliferation and differentiation (36, 37). Moreover, TNF-α plays a key role in a number of systemic inflammatory diseases. Anti-TNF-α drugs are effective in the treatment of inflammation associated with a variety of autoimmune diseases, such as rheumatoid arthritis (RA), inflammatory bowel disease (IBD) (38), ankylosing spondylitis, and Behcet’s disease (39).
The associations of TNF-α with a response to ICIs was investigated in several studies. Tanaka et al. (40) showed serum levels of TNF-α were decreased in 6/9 malignant melanoma (MM) patients with complete remission (CR), partial remission (PR) or long-term stable disease (long SD), and elevated in six patients with progressive disease (PD) (P<0.05). It agrees with the preclinical study results that TNF induced resistance of immunotherapy (41). Several studies demonstrated the mechanism that TNF-α may acted as a negative biomarker. TNF-α upregulates the expression of PD-L1 in tumor cells and T cell immunoglobulin and mucin domain 3 (TIM-3) in CD8+ TIL (41, 42). TIM-3 has been reported as secondary immune checkpoints which limit the function of tumor reactive T cells (31, 43) and trigger TIL exhaustion (44). Additionally, TNF was shown to trigger CD8+ T cell activation-induced cell death (AICD) (45, 46). Blocking TNF and PD-1 increased the number and activity of tumor-infiltrating CD8+ TIL (31, 47) and enabled to achieve 75% survival versus 20% survival in the case of treatment with anti-PD-1 alone. In addition, Lim et al. found that TNF-α upregulates the expression of ubiquitin enzyme CSN5, which reduces the ubiquitination of PD-L1 and stabilizes its expression (41).
Different results have been reported in studies. Boutsikou et al. (21) reported increased TNF-α levels at the time before and 3 months after anti-PD-1 was correlated with improved response and prolonged survival (P=0.009) in 26 NSCLC patients treated with PD-1 inhibitors, while not association with PFS. Additionally, Ozawa et al. (45) showed no significant difference of TNF-α levels at time of before and 7 days anti-PD-1 in 10 NSCLC patients. However, no serious adverse effects were observed in patients with normal TNF-α levels. Baseline TNF-α may not act as an ideal biomarker for ICI treatment. Correlation between survival and non-synonymous TNF pathway mutations was not discovered in any cancer type (48). Changed TNF-α levels and suitable time for detecting plasma was needed further explored. A brief summary of clinical studies of the association of ICT response and irAEs with cytokines is respectively shown in Tables 1, 2.
Preclinical studies targeted the combination of TNF and ICIs have received improved prognosis. For instance, compared to anti-PD-1 alone, the combination of anti-PD-1 and a TNF/TNFR1 gene defect or TNF blockade had better therapeutic benefit (75% survival versus <20% survival) in melanoma and lung cancer mouse models (31). Moreover, TNF inhibitor enalapril or anti-TNF monoclonal antibody decreased the risk of hepatitis and colitis induced by double checkpoint inhibition (44, 46). Anti-TNF antibodies, such as etanercept and infliximab, have been used in clinic to treat serious irAEs (65, 66).
IFN-γ
Interferon-γ (IFN-γ) is a soluble cytokine dimer named for its ability to interfere with the growth of live viruses (67). IFN-γ is mainly produced by NK cells (68), activated T cells (69, 70), B cells (71, 72), and antigen-presenting cells [macrophages (73), monocytes (74), and dendritic cells (75)]. JAK/STAT is the main signaling pathway that mediates interferon-induced gene expression. IFN-γ binds to the cell surface receptors and triggers phosphorylation of JANUS family kinases JAK1 and JAK2. This phosphorylation activates signal transducer and transcriptional activator (STAT) protein. Response genes (e.g., IRF1) induced by STAT1 signaling enhance the transcription of the secondary response genes (76). IFN-γ is a multipotent cytokine with antiviral, proinflammatory, and immunomodulatory functions (77–79).
Recently, the role of IFN-γ in the prediction of the efficacy of immunotherapy was evaluated. A phase II clinical trial (49) of MM patients treated with nivolumab indicated that serum IFN levels in patients with responders (CR/PR were significantly higher than those in non-responders (PD) (P<0.0001). And a study reported increased levels of IFN-γ after 3 months of anti-PD-1 inhibitors treatment in 26 NSCLC patients was correlated with improved response and prolonged survival(P=0.002) (21). Another study of NSCLC patients who received ICIs showed low baseline IFN-γ level (<10 IU/ml)and decreased IFN-γ level after ICI treatment was associated with progression disease and immunotherapy-induced pneumonitis (50). Above all, increased levels of IFN-γ may acted as a positive biomarker for ICI therapy response. In fact, IFN-γ modulated the tumor micro-environment of ICI therapy (80). Damage of IFN-γ stimulus response is correlated with both primary and acquired resistance to ICI therapy (81, 82). In a mouse model, the ability of IFN-γ production by peripheral blood lymphocyte was significantly was a potent biomarker for survival of double PD-1/CTLA-4 blockade (51). However, Costantini et al. reported no correlation between baseline or variation IFN-γ level with 43 advanced NSCLC patients treated with nivolumab (52).
In addition, several clinical studies have shown that IFN-γ gene signature is related to prognosis. Brandon et al. showed that in advanced NSCLC, patients with four genetic IFN-γ+ signatures (interferon γ, CD274, LAG3, and CXCL9) had longer median OS (18.1-22.7m vs 6.5-7.7m) and six-fold higher objective remission rate (ORR) regardless of the immunohistochemical (IHC) PD-L1 status (83). In Keynote-012 clinical trial (84, 85), the average levels of six interferon-gamma-related genes (CXCL9, CXCL10, IDO1, IFNG, HLA-DRA, and STAT1) were associated with OS (p=0.0047) and PFS (p=0.0009) in patients with head and neck squamous cell carcinoma and gastric cancer treated with pembrolizumab. Moreover, the results of a phase II randomized controlled trial (86) showed high levels of the expression of IFN-γ-associated genes had longer survival in patients treated with atezolizumab. Thus, high expression of IFN-γ gene may predict a good prognosis of ICIs.
The potential mechanism of the relationship between IFN-γ and the response to ICIs has been demonstrated. ICIs promote the production of IFN-γ; then, IFN-γ increases tumor immunogenicity, inhibits tumor cell proliferation, and enhances the cytotoxic function of NK cells and CTL (87–90). Additionally, IFN-γ induces the secretion of CXCL9 and CXCL10 chemokines that recruit additional tumor-reactive T cells (83, 90). Moreover, IFN-γ released by T cells stimulates neighboring dendritic cells (DCs) to produce IL-12, which in turn promotes the production of IFN-γ and forms a positive feedback loop (91). Moreover, IFN-γ induces the expression of inhibitory receptor LAG3 (lymphocyte-activation gene 3), which mainly expressed on dysfunctional or depleted T cells and induces immunosuppression. Regulatory T cells (Tregs) are a functional subset of suppressor T cells that suppress anti-tumor immunity. IFN-γ produced by Nrp1-deficient (Nrp1-/-) Tregs decreases the stability of surrounding wild-type Tregs and enhances anti-tumor immunity (92) (Figure 1).
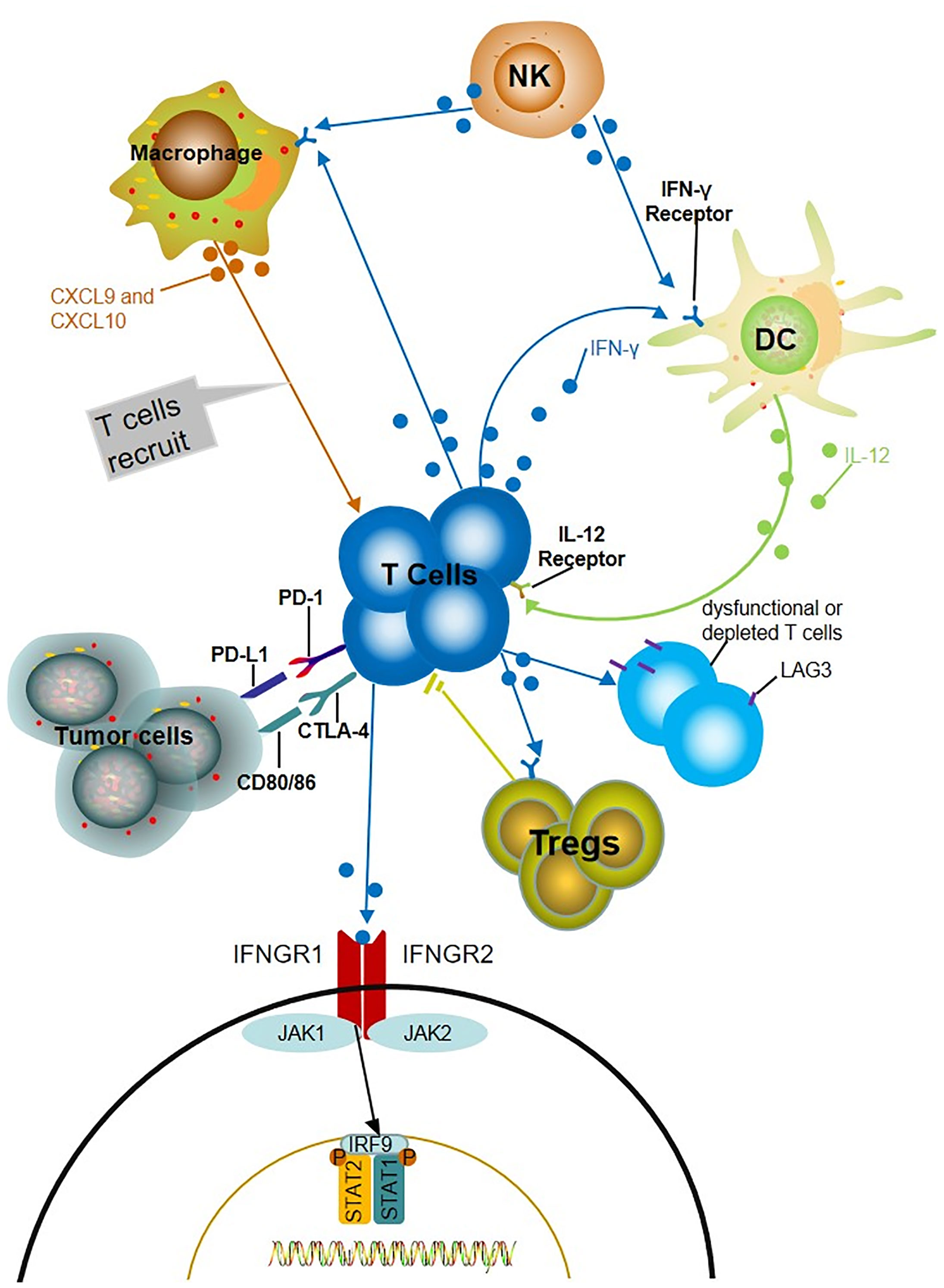
Figure 1 Potential mechanisms of IFN-γ predicting the prognosis of ICIs. The production of IFN-γ induces the increase of chemokine CXCL9 and CXCL10, which recruits more tumor reactive T cells and increased the level of IFN-γ. IFN-γ released by T cells stimulates neighboring dendritic cells (DCs) to produce IL-12, which in turn promotes the production of IFN-γ and forms a positive feedback loop. IFN- γ induces LAG3 which mainly express on dysfunctional or depleted T cells and induce immunosuppression. The fragility of Treg driven by IFN-γ produced by intratumoral Nrp1-/- treg limits the activity of CD4+Treg cells.
IL-6
Interleukin-6 (IL-6) is produced by fibroblasts, monocytes/macrophages, T lymphocytes, B lymphocytes, epithelial cells, and a variety of tumor cells (93, 94). IL-6 is involved in cell survival, growth, immune regulation, and inflammation via the JAK/STAT signaling pathway (95). Moreover, IL-6 signaling plays a key role in carcinogenesis, inhibition of antitumor immunity, and promotion of tumor transmission in tumor environment (96–100). An increase in IL-6 is detected earlier than that in other cytokines and lasts for a long time during inflammatory reactions. Therefore, IL-6 can be used in early diagnosis of acute infection to evaluate the severity of an infection and prognosis. Drugs targeting IL-6, IL-6 receptors, or JAK have been approved by the FDA for the treatment of multicentric Casterman disease (siltuximab), arthritis (tocilizumab), and CART-induced CRS.
Several studies demonstrated associations between baseline IL-6 level and ICI response. Yamazaki et al. showed the IL-6 level pretreatment in responders (CR/PR) was remarkably higher than those in non-responders (PD) in 35 advanced MM patients treated with nivolumab (P=0.0007) (49). However, a study by Hardy-Werbin et al. reported the baseline IL-6 level lower than cut-off (3.65pg/ml) was significantly associated with higher OS compared with those with lower level (18.5m vs 9.5m) in patients treated with ipilimumab and chemotherapy (P=0.026) (53). And another study showed a similar result in which higher baseline IL-6 levels was associated with shorter survival (54). Overall, baseline IL-6 level was a strong prognostic marker of ICI treatment. In view of the limitations of small number patients and different types of ICI, the relationship between them needed further prospective clinical exploration. Furthermore, association between changes of IL-6 were also studied. Ozawa et al. (45) investigated the early changes in the cytokines (0-7 days before the treatment and after the treatment) with response in NSCLC patients treated with nivolumab. The response rate in patients with elevated IL-6 or CRP was 46%, which was significantly higher than that in patients with no increase (0%). A study by Tsuka-moto et al. reported that increased IL-6 levels were associated with tumor progression in melanoma patients treated with nivolumab (55). These findings suggest that increased level of IL-6 was a negative biomarker of prognosis with ICI treatment. Notably, combined anti-IL-6 and ICI treatment showed synergistic anti-tumor activity and improved prognosis, which helps to confirm the negative role of IL-6 in immunotherapy (55, 101, 102). And studies reported that IL-6 induced production of myeloid-derived suppressor cells (MDSCs) and resulted in immunosuppressive, which may explain the above phenomenon (103).
Associations of IL-6 with irAEs were also been studied extensively. Two studies reported increased level of IL-6 after nivolumab treatment was associated with psoriasiform dermatitis in patients with malanoma (40, 62). Tanaka et al. reported increased level of IL-6 was associated with higher incidence of psoriasis (p=0.018) in melanoma patients treated with nivolumab (40). SAEs rate in NSCLC patients treated with PD-1 inhibitors was 43% vs 0% in the group with increased IL-6 level compared to the normal IL-6 level group (45). Notably, a case report by Yoshino et al. showed decreased level of IL-6 and CRP accompanied with the clinical remission of colitis after corticosteroid treatment (104). In summary, the increased IL-6 level after ICIs treatment was an efficient biomarker for irAEs. Additionally, low baseline IL-6 level may use as a predictor for irAEs. Chaput et al. reported low baseline IL-6, IL-8, and sCD25 was associated with colitis related to ipilimumab treatment in melanoma patients (63). And another study by Valpione et al. showed low baseline IL-6 level act as independent predicted factor for irAEs (P=0.007) (64). In summary, high baseline level of IL-6 was a risk marker for irAEs, but the cut-off of high level needed further definition by more clinical trials. The elevation of IL-6 after ICI application may indicate the immune-sensitive individuals and play a predictive role before the occurrence of irAEs.
Interestingly, the association of irAEs and response was investigated by several studies (105–108). ORR in NSCLC patients with irAEs was reported higher than that in patients without irAEs (63.6% versus 7.4%, p <0.01) (109). Another study showed that patients with AE after nivolumab treatment had better ORR (37% versus 17%, p=0.17) and longer median PFS (6.4 versus 1.5 months, p=0.01) than those with no irAEs (108). The mechanism of associations of irAEs with response are unclear and need further investigation. Similar to cytokine release syndrome (CRS) after adoptive T cell therapy, ICIs may lead to hyper-physiological levels of proinflammatory cytokines, especially IL-6, resulting in irAEs.
IL-8
Interleukin-8 (IL-8), also known as CXCL8, is a proinflammatory chemokine whose function was mediated by binding to two cell-surface G protein coupled receptors, termed CXCR1 and CXCR2 (110, 111). lL-8 was produced by immune cells (including macrophages, neutrophils and T cells) and non-immune cells (including epithelial and endothelial cells) (112, 113). IL-8 is identified as a neutrophil-activating cytokine and stimulated by hypoxia/anoxia, death receptors (Fas, DR5), and a variety of cellular stresses (114). Literatures reported IL-8 expression was higher in various tumor than healthy tissues (115). In addition, IL-8-CXCR1/2 pathway play an important role in tumor progression and metastasis (116), and activating proliferation of endothelial cells in tumor vasculature and induced vascular building was mainly mechanism (117).
Boutsikou et al. reported that increased level of IL-8 (3 months after immunotherapy) was correlated with prolonged OS (P=0.015) in 26 NSCLC patients treated with first or second line anti-PD-1 (nivolumab or pembrolizumab) (21). With controversial result, A study showed the increased level of IL-8 (2 months after immunotherapy) was associated with poor OS (P=0.025) (56). Sanmamed et al. analyzed early increased IL-8 level(2-4 weeks after anti-PD-1) was associated with lower response rate in patients with MM (P<0.001) and NSCLC (P=0.001) with high sensitivity and specificity (57). Significantly, IL-8 level maintains a level below the baseline when tumor with pseudo-progression, but progressively increased when tumor with a real progression. These findings consist with the conclusion that IL-8 level reflect the tumor burden (118).
The relationship between baseline IL-8 levels have also been discussed. Higher baseline levels of IL-8 were associated with poor OS in SCLC patients whatever treated with chemotherapy alone (n=47) or chemotherapy plus ipilimumab (n=37). In ipilimumab cohort, patients with high level of IL-8 (≥13.82pg/mL) had a worse median OS (5.3m vs 17m) (53). In the study by Yuen et al. demonstrated that high baseline levels of IL-8 were correlated with poor efficiency by evaluating 1445 patients in metastatic urothelial carcinoma (mUC) and metastatic renal cell carcinoma (mRCC) from three large atezolizumab trails (58). Similar results were acted by assessing 1344 patients (NSCLC and RCC) treated with nivolumab or nivolumab plus ipilimumab and determined cut-off (23pg/mL) may guide clinical application of ICIs (59). High baseline and early increased IL-8 levels may act as a strong negative biomarker for ICI therapy.
TGF-β
Transforming growth factor-β (TGF-β) induces transformation and growth of certain fibroblasts in combination with epidermal growth factor (EGF) (119). In addition to TGF-β, the TGF-β superfamily includes activin, inhibin, bone morphogenetic protein (BMP), and growth and differentiation factor (GDF). Cells with active differentiation often contain high levels of TGF-β, including osteoblasts, kidney, bone marrow, and fetal liver hematopoietic cells. Activated T cells, B cells, and numerous types of tumor cells can secrete TGF-β. TGF-β receptors (TGF-βR), including types I, II, and III, are involved in signal transduction. Typical TGF-β signal transduction includes TGF-β binding to TGF-βRII, which recruits and phosphorylates TGF-βRI. Then, these receptors phosphorylate smad2, smad3, and smad4 to induce gene transcription and expression (120, 121). TGF-β signal can be transduced by the MAPK, PI3K, and Rho-GTP pathways (122, 123). The TGF-β pathway plays an important role in the regulation of cell proliferation, growth, differentiation, apoptosis, and autoimmunity (124). Due to the diversity of TGF-β functions, dysfunctions of the TGF-β signal transduction are associated with many diseases, including systemic sclerosis, fibrosis, hereditary diseases, and cancer (125–129).
TGF-β has been shown to be associated with ICI response. A prospective phase II study showed mean TGF-β levels was higher in non-responders than responders (1071.8 pg/mL vs 141.9 pg/mL) in 24 patients with advanced liver cancer treated with pembrolizumab. And TGF-β≥200pg/mL was assigned as a poor indicator of response with pembrolizumab (60). The median PFS in patients with TGF-β levels <200 pg/mL compared with TGF-β level ≥200 pg/mL was 2 months versus >25 months. Mariathasan et al. reported increased TGF-β ligand1 (TGF-β1) and TGF-β receptor2 (TGF-βR2) levels was correlated with non-response (P=0.00011) and OS (P=0.0096) in patients with metastatic urothelial cancer (MUC) treated with atezolizumab (61). To sum up, increased TGF-β level acted as a negative biomarker of response of ICIs treatment. In general, TGF-β plays an inhibitory role in normal cells and early cancer cells, including cell cycle arrest and apoptosis (122). However, in advanced cancer, TGF-β can be used as a cancer-promoting factor to enhance tumorigenesis, including immunosuppression, tumor metastasis, and drug resistance (128, 129). Several studies have shown that the abnormal TGF-β signaling pathway may affect immune regulation, including antigen presentation, differentiation of CD4 Th1 cells, infiltration and proliferation of CD8+ T cells, and production of long-term memory T cells (130–134). These effects may result in low response and poor prognosis of ICT.
A combination of anti-PD-L1 and TGF-β antibody was studied to determine the negative effects of TGF-β on ICT in several studies. In a mouse model of urothelial cancer (UC) (61), combined application of anti-PD-L1 and TGF-β antibody reduced TGF-β signal transduction in stromal cells and promoted T cell into the tumor. An increased number of infiltrating CD8+ T cells resulted in tumor suppression. In addition, ICI monotherapy produce unsatisfactory results in phase II and III trials (135, 136). However, Jiao et al. (137) demonstrated that anti-CTLA-4 combined with anti-TGF-β therapy enhances the response to ICI therapy in a bone metastasis model of CRPC. Similarly, ICI combined with TGF-β antibody resulted in improved prognosis compared to that in ICI monotherapy in the experiments (22, 138). Interestingly, Lan et al. (139) designed a novel bifunctional drug M7824 which composed of PD-L1 monoclonal antibody and the extracellular domain of human TGF-βRII. M7824 is anti-PD-L1 and promote the activation of CD8+T cells and NK cells resulting in a higher OS and PFS (139, 140).
Other IL Family Members
The relationships between IL family members, including IL-1β, IL-2, IL-4 and IL-10, and ICT response were evaluated in a number of studies. Boutsikou et al. assayed cytokines by flow cytometry in patients with NSCLC receiving pembrolizumab or nivolumab before treatment and 3 months after the treatment. The results showed that an increase in the levels of IL-1β (p=0.038), IL-2 (p=0.011), IL-4 (p=0.018), IL-6 (p=0.014), IFN-γ (p=0.00), and TNF-α (p=0.006) was associated with increased response rate (RR) (21). In another study, 65 cytokines were profiled in patients with unresectable stage III or IV melanoma treated with anti-PD-1 monotherapy (cohort 1) or anti-PD-1 plus anti-CTLA-4 (cohort 2). The results showed that the expression levels of IL-2 (p=0.041) in cohort 1 and TNF-α (p=0.0189) in cohort 2 were associated with OS (22). Additionally, several studied validated that high baseline levels of IL-2 and IL-4 are correlated with higher OS (53, 57). Studies summary is in Table 3. Increased cytokines mentioned after ICT related to better response and OS. Generally elevated levels of cytokines may reveal extensive immune activity, therefore, predicting good prognosis. Moreover, depletion of TILs is one of the possibly reason for patients with ICIs ineffectiveness (142). A study reported that IL-10–Fc enhanced immunotherapies by accelerating oxidative phosphorylation (OXPHOS) to reactive T cells and directly expand terminally exhausted CD8+ TILs (143). And in microsatellite stable (MSS) colorectal cancer (CRC) mouse models, IL-17A blocking combined with ICIs showed improved efficiency (144).
The associations of the levels of IL and irAEs were also investigated. Tarhini et al. demonstrated that elevated baseline IL-17 levels were significantly associated with high risk of grade 3 diarrhea or colitis (p=0.02) in patients with advanced melanoma receiving neoadjuvant ipilimumab therapy (145). In another study, increased 11 cytokines (G-CSF, GM-CSF, fractalkine, FGF-2, IFNα2, IL-12p70, IL-1a, IL-1β, IL-1RA, IL-2, and IL-13) were all associated with severe toxicity and they integrated a CYTOX (cytokine toxicity) score which with AUC 0.68 at PRE (95% CI, 0.51-0.84, p=0.037) and 0.70 at EDT (95% CI, 0.55-0.85, p=0.017) (141). Additionally, a study reported that decreased IL-10 level in patients after anti-CTLA-4 treatment was associated with pancreatitis and uveitis, but the level of IFN-γ was not associated with irAEs (146). Table 4 lists these studies. To sum up, these studies demonstrated lower baseline and increased levels of extensively cytokines after ICI treatment were associated with irAEs.
Conclusion
Low efficiency and high irAEs occurrence urgently require biomarkers to identify patients that benefit from the immune checkpoint inhibitors therapy. Cytokines produced by immune cells and tumor cells in the tumor microenvironment may acted as a suitable candidate biomarker due to its no-invasive obtaining, easy for dynamic monitoring and cost-effective. The investigations of TNF-α, IFN-γ, IL-6, IL-8, TGF-β and other cytokines as predictors of the responses and adverse events of ICT produced encouraging results. Increased level of IFN-γ and IFN-γ pathway genes always acted as positive biomarkers for response and irAEs, while high baseline and increased level of IL-8, increased level of IL-6 and TGF-β was negative biomarkers. TNF-αwas generally regarded as an negative biomarker, but its predictive function is needed further exploration. Negative cytokines in the tumor microenvironment induce immunosuppression and even adverse reactions in ICT by regulating T cells, B cells, and related immune checkpoints. Thus, the combinations of cytokine drugs and ICIs improved prognosis and resulted in a number of ongoing clinical trials. However, it is unlikely a single cytokine will be sufficient to predict immunotherapy response and irAEs for the complexity of TME and interaction between cytokines. In future, development of multifactorial synergistic predictive markers is necessary to achieve individualized treatment and minimize adverse reactions.
Author Contributions
HZ designed the study. MW collected literatures together with XZ and SX and drafted the manuscript. JG and JL coordinated. All authors contributed to the article and approved the submitted version.
Funding
This work was supported by Innovation Project of Shandong Academy of Medical Sciences (2019-04) and the Academic Promotion Program of Shandong First Medical University [grant number: 2019ZL002]; the National Natural Science Foundation of China [grant number: 81972862]; and CSCO-Pilot Cancer Research Fund [grant number: Y-2019AZZD-0352]; and Key Research and Development Program of Shandong Province: Study on the role and mechanism of myocardial -AR in cardiac radiation injury [grant number: 2018GSF118067].
Conflict of Interest
The authors declare that the research was conducted in the absence of any commercial or financial relationships that could be construed as a potential conflict of interest.
References
1. Robert C, Schachter J, Long GV, Arance A, Grob JJ, Mortier L, et al. Pembrolizumab Versus Ipilimumab in Advanced Melanoma. N Engl J Med (2015) 372:2521–32. doi: 10.1056/NEJMoa1503093
2. Motzer RJ, Escudier B, McDermott DF, George S, Hammers HJ, Srinivas S, et al. Nivolumab Versus Everolimus in Advanced Renal-Cell Carcinoma. N Engl J Med (2015) 373:1803–13. doi: 10.1056/NEJMoa1510665
3. Bellmunt J, de Wit R, Vaughn DJ, Fradet Y, Lee JL, Fong L, et al. Pembrolizumab as Second-Line Therapy for Advanced Urothelial Carcinoma. N Engl J Med (2017) 376:1015–26. doi: 10.1056/NEJMoa1613683
4. Reck M, Rodriguez-Abreu D, Robinson AG, Hui R, Csoszi T, Fulop A, et al. Pembrolizumab Versus Chemotherapy for PD-L1-Positive Non-Small-Cell Lung Cancer. N Engl J Med (2016) 375:1823–33. doi: 10.1056/NEJMoa1606774
5. Gadgeel S, Rodriguez-Abreu D, Speranza G, Esteban E, Felip E, Domine M, et al. Updated Analysis From KEYNOTE-189: Pembrolizumab or Placebo Plus Pemetrexed and Platinum for Previously Untreated Metastatic Nonsquamous Non-Small-Cell Lung Cancer. J Clin Oncol (2020) 38:1505–17. doi: 10.1200/JCO.19.03136
6. Paz-Ares L, Luft A, Vicente D, Tafreshi A, Gumus M, Mazieres J, et al. Pembrolizumab Plus Chemotherapy for Squamous Non-Small-Cell Lung Cancer. N Engl J Med (2018) 379:2040–51. doi: 10.1056/NEJMoa1810865
7. Mok TSK, Wu YL, Kudaba I, Kowalski DM, Cho BC, Turna HZ, et al. Pembrolizumab Versus Chemotherapy for Previously Untreated, PD-L1-Expressing, Locally Advanced or Metastatic Non-Small-Cell Lung Cancer (KEYNOTE-042): A Randomised, Open-Label, Controlled, Phase 3 Trial. Lancet (2019) 393:1819–30. doi: 10.1016/S0140-6736(18)32409-7
8. Reck M, Rodriguez-Abreu D, Robinson AG, Hui R, Csoszi T, Fulop A, et al. Updated Analysis of KEYNOTE-024: Pembrolizumab Versus Platinum-Based Chemotherapy for Advanced Non-Small-Cell Lung Cancer With PD-L1 Tumor Proportion Score of 50% or Greater. J Clin Oncol (2019) 37:537–46. doi: 10.1200/JCO.18.00149
9. Fujii T, Colen RR, Bilen MA, Hess KR, Hajjar J, Suarez-Almazor ME, et al. Incidence of Immune-Related Adverse Events and Its Association With Treatment Outcomes: The MD Anderson Cancer Center Experience. Invest New Drugs (2018) 36:638–46. doi: 10.1007/s10637-017-0534-0
10. Wang PF, Chen Y, Song SY, Wang TJ, Ji WJ, Li SW, et al. Immune-Related Adverse Events Associated With Anti-PD-1/PD-L1 Treatment for Malignancies: A Meta-Analysis. Front Pharmacol (2017) 8:730. doi: 10.3389/fphar.2017.00730
11. Taube JM, Klein A, Brahmer JR, Xu H, Pan X, Kim JH, et al. Association of PD-1, PD-1 Ligands, and Other Features of the Tumor Immune Microenvironment With Response to Anti-PD-1 Therapy. Clin Cancer Res (2014) 20:5064–74. doi: 10.1158/1078-0432.ccr-13-3271
12. Daud AI, Wolchok JD, Robert C, Hwu WJ, Weber JS, Ribas A, et al. Programmed Death-Ligand 1 Expression and Response to the Anti-Programmed Death 1 Antibody Pembrolizumab in Melanoma. J Clin Oncol (2016) 34:4102–9. doi: 10.1200/jco.2016.67.2477
13. Goodman AM, Kato S, Bazhenova L, Patel SP, Frampton GM, Miller V, et al. Tumor Mutational Burden as an Independent Predictor of Response to Immunotherapy in Diverse Cancers. Mol Cancer Ther (2017) 16:2598–608. doi: 10.1158/1535-7163.mct-17-0386
14. Johnson DB, Estrada MV, Salgado R, Sanchez V, Doxie DB, Opalenik SR, et al. Melanoma-Specific MHC-II Expression Represents A Tumour-Autonomous Phenotype and Predicts Response to Anti-PD-1/PD-L1 Therapy. Nat Commun (2016) 7:10582. doi: 10.1038/ncomms10582
15. Wang X, Schoenhals JE, Li A, Valdecanas DR, Ye H, Zang F, et al. Suppression of Type I IFN Signaling in Tumors Mediates Resistance to Anti-PD-1 Treatment That Can Be Overcome by Radiotherapy. Cancer Res (2017) 77:839–50. doi: 10.1158/0008-5472.can-15-3142
16. Zheng H, Liu X, Zhang J, Rice SJ, Wagman M, Kong Y, et al. Expression of PD-1 on CD4+ T Cells in Peripheral Blood Associates With Poor Clinical Outcome in Non-Small Cell Lung Cancer. Oncotarget (2016) 7:56233–40. doi: 10.18632/oncotarget.9316
17. Arrieta O, Montes-Servin E, Hernandez-Martinez JM, Cardona AF, Casas-Ruiz E, Crispin JC, et al. Expression of PD-1/PD-L1 and PD-L2 in Peripheral T-Cells From Non-Small Cell Lung Cancer Patients. Oncotarget (2017) 8:101994–2005. doi: 10.18632/oncotarget.22025
18. Kim HR, Park SM, Seo SU, Jung I, Yoon HI, Gabrilovich DI, et al. The Ratio of Peripheral Regulatory T Cells to Lox-1(+) Polymorphonuclear Myeloid-Derived Suppressor Cells Predicts the Early Response to Anti-PD-1 Therapy in Patients With Non-Small Cell Lung Cancer. Am J Respir Crit Care Med (2019) 199:243–6. doi: 10.1164/rccm.201808-1502LE
19. Zhang J, Yao YH, Li BG, Yang Q, Zhang PY, Wang HT. Prognostic Value of Pretreatment Serum Lactate Dehydrogenase Level in Patients With Solid Tumors: A Systematic Review and Meta-Analysis. Sci Rep (2015) 5:9800. doi: 10.1038/srep09800
20. Deng T, Zhang J, Meng Y, Zhou Y, Li W. Higher Pretreatment Lactate Dehydrogenase Concentration Predicts Worse Overall Survival in Patients With Lung Cancer. Med (Baltimore) (2018) 97:e12524. doi: 10.1097/MD.0000000000012524
21. Boutsikou E, Domvri K, Hardavella G, Tsiouda D, Zarogoulidis K, Kontakiotis T. Tumour Necrosis Factor, Interferon-Gamma and Interleukins As Predictive Markers of Antiprogrammed Cell-Death Protein-1 Treatment in Advanced Non-Small Cell Lung Cancer: A Pragmatic Approach in Clinical Practice. Ther Adv Med Oncol (2018) 10:1758835918768238. doi: 10.1177/1758835918768238
22. Vanpouille-Box C, Formenti S. Dual Transforming Growth Factor-β and Programmed Death-1 Blockade: A Strategy for Immune-Excluded Tumors? Trends Immunol (2018) 39:435–7. doi: 10.1016/j.it.2018.03.002
23. Aguilar EJ, Ricciuti B, Gainor JF, Kehl KL, Kravets S, Dahlberg S, et al. Outcomes to First-Line Pembrolizumab in Patients With Non-Small-Cell Lung Cancer and Very High PD-L1 Expression. Ann Oncol (2019) 30:1653–9. doi: 10.1093/annonc/mdz288
24. Topalian SL, Hodi FS, Brahmer JR, Gettinger SN, Smith DC, McDermott DF, et al. Safety, Activity, and Immune Correlates of Anti-PD-1 Antibody in Cancer. N Engl J Med (2012) 366:2443–54. doi: 10.1056/NEJMoa1200690
25. Sharma P, Hu-Lieskovan S, Wargo JA, Ribas A. Primary, Adaptive, and Acquired Resistance to Cancer Immunotherapy. Cell (2017) 168:707–23. doi: 10.1016/j.cell.2017.01.017
26. Oppenheim JJ. Cytokines: Past, Present, and Future. Int J Hematol (2001) 74:3–8. doi: 10.1007/BF02982543
27. Kelso A. Cytokines and Their Receptors: An Overview. Ther Drug Monit (2000) 22:40–3. doi: 10.1097/00007691-200002000-00008
28. Barrett KE. Cytokines: Sources, Receptors and Signalling. Baillieres Clin Gastroenterol (1996) 10:1–15. doi: 10.1016/s0950-3528(96)90036-6
29. Conlon KC, Miljkovic MD, Waldmann TA. Cytokines in the Treatment of Cancer. J Interferon Cytokine Res (2019) 39:6–21. doi: 10.1089/jir.2018.0019
30. Yoshimura A, Ito M, Chikuma S, Akanuma T, Nakatsukasa H. Negative Regulation of Cytokine Signaling in Immunity. Cold Spring Harb Perspect Biol (2018) 10(7):a028571. doi: 10.1101/cshperspect.a028571
31. Bertrand F, Montfort A, Marcheteau E, Imbert C, Gilhodes J, Filleron T, et al. TNFα Blockade Overcomes Resistance to Anti-PD-1 in Experimental Melanoma. Nat Commun (2017) 8:2256. doi: 10.1038/s41467-017-02358-7
32. Tauriello DVF, Palomo-Ponce S, Stork D, Berenguer-Llergo A, Badia-Ramentol J, Iglesias M, et al. TGFβ Drives Immune Evasion in Genetically Reconstituted Colon Cancer Metastasis. Nature (2018) 554:538–43. doi: 10.1038/nature25492
33. Carswell EA, Old LJ, Kassel RL, Green S, Fiore N, Williamson B. An Endotoxin-Induced Serum Factor That Causes Necrosis of Tumors. Proc Natl Acad Sci USA (1975) 72:3666–70. doi: 10.1073/pnas.72.9.3666
34. Faustman D, Davis M. TNF Receptor 2 Pathway: Drug Target for Autoimmune Diseases. Nat Rev Drug Discov (2010) 9:482–93. doi: 10.1038/nrd3030
35. Probert L. TNF and Its Receptors in the CNS: The Essential, the Desirable and the Deleterious Effects. Neuroscience (2015) 302:2–22. doi: 10.1016/j.neuroscience.2015.06.038
36. Brenner D, Blaser H, Mak TW. Regulation of Tumour Necrosis Factor Signalling: Live or Let Die. Nat Rev Immunol (2015) 15:362–74. doi: 10.1038/nri3834
37. Dostert C, Grusdat M, Letellier E, Brenner D. The TNF Family of Ligands and Receptors: Communication Modules in the Immune System and Beyond. Physiol Rev (2019) 99:115–60. doi: 10.1152/physrev.00045.2017
38. Croft M, Benedict CA, Ware CF. Clinical Targeting of the TNF and TNFR Superfamilies. Nat Rev Drug Discov (2013) 12:147–68. doi: 10.1038/nrd3930
39. Arida A, Fragiadaki K, Giavri E, Sfikakis PP. Anti-TNF Agents for Behçet’s Disease: Analysis of Published Data on 369 Patients. Semin Arthritis rheumatism (2011) 41:61–70. doi: 10.1016/j.semarthrit.2010.09.002
40. Tanaka R, Okiyama N, Okune M, Ishitsuka Y, Watanabe R, Furuta J, et al. Serum Level of Interleukin-6 Is Increased in Nivolumab-Associated Psoriasiform Dermatitis and Tumor Necrosis Factor-α Is a Biomarker of Nivolumab Recativity. J Dermatol Sci (2017) 86:71–3. doi: 10.1016/j.jdermsci.2016.12.019
41. Lim SO, Li CW, Xia W, Cha JH, Chan LC, Wu Y, et al. Deubiquitination and Stabilization of PD-L1 by CSN5. Cancer Cell (2016) 30:925–39. doi: 10.1016/j.ccell.2016.10.010
42. Bertrand F, Montfort A, Marcheteau E, Imbert C, Gilhodes J, Filleron T, et al. TNFalpha Blockade Overcomes Resistance to Anti-PD-1 in Experimental Melanoma. Nat Commun (2017) 8:2256. doi: 10.1038/s41467-017-02358-7
43. Koyama S, Akbay EA, Li YY, Herter-Sprie GS, Buczkowski KA, Richards WG, et al. Adaptive Resistance to Therapeutic PD-1 Blockade Is Associated With Upregulation of Alternative Immune Checkpoints. Nat Commun (2016) 7:10501. doi: 10.1038/ncomms10501
44. Sakuishi K, Apetoh L, Sullivan JM, Blazar BR, Kuchroo VK, Anderson AC. Targeting Tim-3 and PD-1 Pathways to Reverse T Cell Exhaustion and Restore Anti-Tumor Immunity. J Exp Med (2010) 207:2187–94. doi: 10.1084/jem.20100643
45. Ozawa Y, Amano Y, Kanata K, Hasegwa H, Matsui T, Kakutani T, et al. Impact of Early Inflammatory Cytokine Elevation After Commencement of PD-1 Inhibitors to Predict Efficacy in Patients With Non-Small Cell Lung Cancer. Med Oncol (Northwood Lond Engl) (2019) 36:33. doi: 10.1007/s12032-019-1255-3
46. Perez-Ruiz E, Minute L, Otano I, Alvarez M, Ochoa MC, Belsue V, et al. Prophylactic TNF Blockade Uncouples Efficacy and Toxicity in Dual CTLA-4 and PD-1 Immunotherapy. Nature (2019) 569:428–32. doi: 10.1038/s41586-019-1162-y
47. Zheng L, Fisher G, Miller RE, Peschon J, Lynch DH, Lenardo MJ. Induction of Apoptosis in Mature T Cells by Tumour Necrosis Factor. Nature (1995) 377:348–51. doi: 10.1038/377348a0
48. Vredevoogd DW, Kuilman T, Ligtenberg MA, Boshuizen J, Stecker KE, de Bruijn B, et al. Augmenting Immunotherapy Impact by Lowering Tumor TNF Cytotoxicity Threshold. Cell (2019) 178(3):585–99.e15. doi: 10.1016/j.cell.2019.06.014
49. Yamazaki N, Kiyohara Y, Uhara H, Iizuka H, Uehara J, Otsuka F, et al. Cytokine Biomarkers to Predict Antitumor Responses to Nivolumab Suggested in a Phase 2 Study for Advanced Melanoma. Cancer Sci (2017) 108:1022–31. doi: 10.1111/cas.13226
50. Hirashima T, Kanai T, Suzuki H, Yoshida H, Matsushita A, Kawasumi H, et al. The Levels of Interferon-Gamma Release as a Biomarker for Non-Small-Cell Lung Cancer Patients Receiving Immune Checkpoint Inhibitors. Anticancer Res (2019) 39:6231–40. doi: 10.21873/anticanres.13832
51. McNamara MJ, Hilgart-Martiszus I, Barragan Echenique DM, Linch SN, Kasiewicz MJ, Redmond WL. Interferon-Gamma Production by Peripheral Lymphocytes Predicts Survival of Tumor-Bearing Mice Receiving Dual PD-1/CTLA-4 Blockade. Cancer Immunol Res (2016) 4:650–7. doi: 10.1158/2326-6066.CIR-16-0022
52. Costantini A, Julie C, Dumenil C, Helias-Rodzewicz Z, Tisserand J, Dumoulin J, et al. Predictive Role of Plasmatic Biomarkers in Advanced Non-Small Cell Lung Cancer Treated by Nivolumab. Oncoimmunology (2018) 7:e1452581. doi: 10.1080/2162402X.2018.1452581
53. Hardy-Werbin M, Rocha P, Arpi O, Taus A, Nonell L, Duran X, et al. Serum Cytokine Levels as Predictive Biomarkers of Benefit From Ipilimumab in Small Cell Lung Cancer. Oncoimmunology (2019) 8:e1593810. doi: 10.1080/2162402X.2019.1593810
54. Laino AS, Woods D, Vassallo M, Qian X, Tang H, Wind-Rotolo M, et al. Serum Interleukin-6 and C-Reactive Protein Are Associated With Survival in Melanoma Patients Receiving Immune Checkpoint Inhibition. J Immunother Cancer (2020) 8(1):e000842. doi: 10.1136/jitc-2020-000842
55. Tsukamoto H, Fujieda K, Miyashita A, Fukushima S, Ikeda T, Kubo Y, et al. Combined Blockade of IL6 and PD-1/PD-L1 Signaling Abrogates Mutual Regulation of Their Immunosuppressive Effects in the Tumor Microenvironment. Cancer Res (2018) 78:5011–22. doi: 10.1158/0008-5472.CAN-18-0118
56. Agullo-Ortuno MT, Gomez-Martin O, Ponce S, Iglesias L, Ojeda L, Ferrer I, et al. Blood Predictive Biomarkers for Patients With Non-Small-Cell Lung Cancer Associated With Clinical Response to Nivolumab. Clin Lung Cancer (2020) 21:75–85. doi: 10.1016/j.cllc.2019.08.006
57. Sanmamed M, Perez-Gracia J, Schalper K, Fusco J, Gonzalez A, Rodriguez-Ruiz M, et al. Changes in Serum Interleukin-8 (IL-8) Levels Reflect and Predict Response to Anti-PD-1 Treatment in Melanoma and Non-Small-Cell Lung Cancer Patients. Ann Oncol (2017) 28:1988–95. doi: 10.1093/annonc/mdx190
58. Yuen KC, Liu LF, Gupta V, Madireddi S, Keerthivasan S, Li C, et al. High Systemic and Tumor-Associated IL-8 Correlates With Reduced Clinical Benefit of PD-L1 Blockade. Nat Med (2020) 26:693–8. doi: 10.1038/s41591-020-0860-1
59. Schalper KA, Carleton M, Zhou M, Chen T, Feng Y, Huang SP, et al. Elevated Serum Interleukin-8 Is Associated With Enhanced Intratumor Neutrophils and Reduced Clinical Benefit of Immune-Checkpoint Inhibitors. Nat Med (2020) 26:688–92. doi: 10.1038/s41591-020-0856-x
60. Feun L, Li Y, Wu C, Wangpaichitr M, Jones P, Richman S, et al. Phase 2 Study of Pembrolizumab and Circulating Biomarkers to Predict Anticancer Response in Advanced, Unresectable Hepatocellular Carcinoma. Cancer (2019) 125:3603–14. doi: 10.1002/cncr.32339
61. Mariathasan S, Turley SJ, Nickles D, Castiglioni A, Yuen K, Wang Y, et al. TGFbeta Attenuates Tumour Response to PD-L1 Blockade by Contributing to Exclusion of T Cells. Nature (2018) 554:544–8. doi: 10.1038/nature25501
62. Okiyama N, Tanaka R. Varied Immuno-Related Adverse Events Induced by Immune-Check Point Inhibitors - Nivolumab-Associated Psoriasiform Dermatitis Related With Increased Serum Level of Interleukin-6. Nihon Rinsho Meneki Gakkai Kaishi (2017) 40:95–101. doi: 10.2177/jsci.40.95
63. Chaput N, Lepage P, Coutzac C, Soularue E, Le Roux K, Monot C, et al. Baseline Gut Microbiota Predicts Clinical Response and Colitis in Metastatic Melanoma Patients Treated With Ipilimumab. Ann Oncol (2017) 28:1368–79. doi: 10.1093/annonc/mdx108
64. Valpione S, Pasquali S, Campana LG, Piccin L, Mocellin S, Pigozzo J, et al. Sex and Interleukin-6 Are Prognostic Factors for Autoimmune Toxicity Following Treatment With Anti-CTLA4 Blockade. J Transl Med (2018) 16:94. doi: 10.1186/s12967-018-1467-x
65. Minor DR, Chin K, Kashani-Sabet M. Infliximab in the Treatment of Anti-CTLA4 Antibody (Ipilimumab) Induced Immune-Related Colitis. Cancer Biother Radiopharm (2009) 24:321–5. doi: 10.1089/cbr.2008.0607
66. Merrill SP, Reynolds P, Kalra A, Biehl J, Vandivier RW, Mueller SW. Early Administration of Infliximab for Severe Ipilimumab-Related Diarrhea in a Critically Ill Patient. Ann Pharmacother (2014) 48:806–10. doi: 10.1177/1060028014528152
67. Isaacs A, Lindenmann J. Biological Cciences. Virus Interference. I. The Interferon. Proc R Soc Lond B Biol Sci (1957) 147:258–67. doi: 10.1098/rspb.1957.0048
68. Kasahara T, Hooks JJ, Dougherty SF, Oppenheim JJ. Interleukin 2-Mediated Immune Interferon (IFN-Gamma) Production by Human T Cells and T Cell Subsets. J Immunol (1983) 130:1784–9.
69. Yu J, Wei M, Becknell B, Trotta R, Liu S, Boyd Z, et al. Pro- and Antiinflammatory Cytokine Signaling: Reciprocal Antagonism Regulates Interferon-Gamma Production by Human Natural Killer Cells. Immunity (2006) 24:575–90. doi: 10.1016/j.immuni.2006.03.016
70. Cooper MA, Fehniger TA, Turner SC, Chen KS, Ghaheri BA, Ghayur T, et al. Human Natural Killer Cells: A Unique Innate Immunoregulatory Role for the CD56(bright) Subset. Blood (2001) 97:3146–51. doi: 10.1182/blood.v97.10.3146
71. Harris DP, Goodrich S, Gerth AJ, Peng SL, Lund FE. Regulation of IFN Gamma Production by B Effector 1 Cells: Essential Roles for T-Bet and the IFN Gamma Receptor. J Immunol (2005) 174:6781–90. doi: 10.4049/jimmunol.174.11.6781
72. Olalekan S, Cao Y, Hamel K, Finnegan A. B Cells Expressing IFN-γ Suppress Treg-Cell Differentiation and Promote Autoimmune Experimental Arthritis. Eur J Immunol (2015) 45:988–98. doi: 10.1002/eji.201445036
73. Robinson C, O’Dee D, Hamilton T, Nau G. Cytokines involved in interferon-gamma production by human macrophages. J Innate Immun (2010) 2:56–65. doi: 10.1159/000247156
74. Kraaij M, Vereyken E, Leenen P, van den Bosch T, Rezaee F, Betjes M, et al. Human Monocytes Produce Interferon-Gamma Upon Stimulation With LPS. Cytokine (2014) 67:7–12. doi: 10.1016/j.cyto.2014.02.001
75. Ohteki T, Fukao T, Suzue K, Maki C, Ito M, Nakamura M, et al. Interleukin 12-Dependent Interferon Gamma Production by CD8alpha+ Lymphoid Dendritic Cells. J Exp Med (1999) 189:1981–6. doi: 10.1084/jem.189.12.1981
76. Decker T, Lew DJ, Mirkovitch J, Darnell JE Jr. Cytoplasmic Activation of GAF, an IFN-Gamma-Regulated DNA-Binding Factor. EMBO J (1991) 10:927–32. doi: 10.1002/j.1460-2075.1991.tb08026.x
77. Enzler T, Gillessen S, Manis JP, Ferguson D, Fleming J, Alt FW, et al. Deficiencies of GM-CSF and Interferon Gamma Link Inflammation and Cancer. J Exp Med (2003) 197:1213–9. doi: 10.1084/jem.20021258
78. Kaplan DH, Shankaran V, Dighe AS, Stockert E, Aguet M, Old LJ, et al. Demonstration of an Interferon Gamma-Dependent Tumor Surveillance System in Immunocompetent Mice. Proc Natl Acad Sci USA (1998) 95:7556–61. doi: 10.1073/pnas.95.13.7556
79. Shankaran V, Ikeda H, Bruce AT, White JM, Swanson PE, Old LJ, et al. IFNgamma and Lymphocytes Prevent Primary Tumour Development and Shape Tumour Immunogenicity. Nature (2001) 410:1107–11. doi: 10.1038/35074122
80. Teng F, Meng X, Kong L, Yu J. Progress and Challenges of Predictive Biomarkers of Anti PD-1/PD-L1 Immunotherapy: A Systematic Review. Cancer Lett (2018) 414:166–73. doi: 10.1016/j.canlet.2017.11.014
81. Zaretsky JM, Garcia-Diaz A, Shin DS, Escuin-Ordinas H, Hugo W, Hu-Lieskovan S, et al. Mutations Associated With Acquired Resistance to PD-1 Blockade in Melanoma. N Engl J Med (2016) 375:819–29. doi: 10.1056/NEJMoa1604958
82. Gao J, Shi LZ, Zhao H, Chen J, Xiong L, He Q, et al. Loss of IFN-Gamma Pathway Genes in Tumor Cells as a Mechanism of Resistance to Anti-CTLA-4 Therapy. Cell (2016) 167:397–404.e399. doi: 10.1016/j.cell.2016.08.069
83. Higgs BW, Morehouse CA, Streicher K, Brohawn PZ, Pilataxi F, Gupta A, et al. Interferon Gamma Messenger RNA Signature in Tumor Biopsies Predicts Outcomes in Patients With Non-Small Cell Lung Carcinoma or Urothelial Cancer Treated With Durvalumab. Clin Cancer Res (2018) 24:3857–66. doi: 10.1158/1078-0432.CCR-17-3451
84. Seiwert TY, Burtness B, Mehra R, Weiss J, Berger R, Eder JP, et al. Safety and Clinical Activity of Pembrolizumab for Treatment of Recurrent or Metastatic Squamous Cell Carcinoma of the Head and Neck (KEYNOTE-012): An Open-Label, Multicentre, Phase 1b Trial. Lancet Oncol (2016) 17:956–65. doi: 10.1016/S1470-2045(16)30066-3
85. Muro K, Chung HC, Shankaran V, Geva R, Catenacci D, Gupta S, et al. Pembrolizumab for Patients With PD-L1-Positive Advanced Gastric Cancer (KEYNOTE-012): A Multicentre, Open-Label, Phase 1b Trial. Lancet Oncol (2016) 17:717–26. doi: 10.1016/S1470-2045(16)00175-3
86. Fehrenbacher L, Spira A, Ballinger M, Kowanetz M, Vansteenkiste J, Mazieres J, et al. Atezolizumab Versus Docetaxel for Patients With Previously Treated Non-Small-Cell Lung Cancer (POPLAR): A Multicentre, Open-Label, Phase 2 Randomised Controlled Trial. Lancet (2016) 387:1837–46. doi: 10.1016/S0140-6736(16)00587-0
87. Gaczynska M, Rock KL, Spies T, Goldberg AL. Peptidase Activities of Proteasomes are Differentially Regulated by the Major Histocompatibility Complex-Encoded Genes for LMP2 and LMP7. Proc Natl Acad Sci USA (1994) 91:9213–7. doi: 10.1073/pnas.91.20.9213
88. Harvat BL, Seth P, Jetten AM. The Role of p27Kip1 in Gamma Interferon-Mediated Growth Arrest of Mammary Epithelial Cells and Related Defects in Mammary Carcinoma Cells. Oncogene (1997) 14:2111–22. doi: 10.1038/sj.onc.1201055
89. Peng W, Liu C, Xu C, Lou Y, Chen J, Yang Y, et al. PD-1 Blockade Enhances T-Cell Migration to Tumors by Elevating IFN-Gamma Inducible Chemokines. Cancer Res (2012) 72:5209–18. doi: 10.1158/0008-5472.CAN-12-1187
90. Dulos J, Carven GJ, van Boxtel SJ, Evers S, Driessen-Engels LJ, Hobo W, et al. PD-1 Blockade Augments Th1 and Th17 and Suppresses Th2 Responses in Peripheral Blood From Patients With Prostate and Advanced Melanoma Cancer. J Immunother (2012) 35:169–78. doi: 10.1097/CJI.0b013e318247a4e7
91. Garris CS, Arlauckas SP, Kohler RH, Trefny MP, Garren S, Piot C, et al. Successful Anti-PD-1 Cancer Immunotherapy Requires T Cell-Dendritic Cell Crosstalk Involving the Cytokines IFN-Gamma and IL-12. Immunity (2018) 49:1148–61.e1147. doi: 10.1016/j.immuni.2018.09.024
92. Overacre-Delgoffe AE, Chikina M, Dadey RE, Yano H, Brunazzi EA, Shayan G, et al. Interferon-Gamma Drives Treg Fragility to Promote Anti-Tumor Immunity. Cell (2017) 169:1130–41.e1111. doi: 10.1016/j.cell.2017.05.005
93. Walter M, Liang S, Ghosh S, Hornsby PJ, Li R. Interleukin 6 Secreted From Adipose Stromal Cells Promotes Migration and Invasion of Breast Cancer Cells. Oncogene (2009) 28:2745–55. doi: 10.1038/onc.2009.130
94. Kumari N, Dwarakanath BS, Das A, Bhatt AN. Role of Interleukin-6 in Cancer Progression and Therapeutic Resistance. Tumour Biol (2016) 37:11553–72. doi: 10.1007/s13277-016-5098-7
95. Jones SA, Scheller J, Rose-John S. Therapeutic Strategies for the Clinical Blockade of IL-6/Gp130 Signaling. J Clin Invest (2011) 121:3375–83. doi: 10.1172/JCI57158
96. Bromberg J, Wang TC. Inflammation and Cancer: IL-6 and STAT3 Complete the Link. Cancer Cell (2009) 15:79–80. doi: 10.1016/j.ccr.2009.01.009
97. Yu H, Kortylewski M, Pardoll D. Crosstalk Between Cancer and Immune Cells: Role of STAT3 in the Tumour Microenvironment. Nat Rev Immunol (2007) 7:41–51. doi: 10.1038/nri1995
98. Siegel AM, Heimall J, Freeman AF, Hsu AP, Brittain E, Brenchley JM, et al. A Critical Role for STAT3 Transcription Factor Signaling in the Development and Maintenance of Human T Cell Memory. Immunity (2011) 35:806–18. doi: 10.1016/j.immuni.2011.09.016
99. Kortylewski M, Yu H. Role of Stat3 in Suppressing Anti-Tumor Immunity. Curr Opin Immunol (2008) 20:228–33. doi: 10.1016/j.coi.2008.03.010
100. Lee H, Pal SK, Reckamp K, Figlin RA, Yu H. STAT3: A Target to Enhance Antitumor Immune Response. Curr Top Microbiol Immunol (2011) 344:41–59. doi: 10.1007/82_2010_51
101. Mace TA, Shakya R, Pitarresi JR, Swanson B, McQuinn CW, Loftus S, et al. IL-6 and PD-L1 Antibody Blockade Combination Therapy Reduces Tumour Progression in Murine Models of Pancreatic Cancer. Gut (2018) 67:320–32. doi: 10.1136/gutjnl-2016-311585
102. Liu H, Shen J, Lu K. IL-6 and PD-L1 Blockade Combination Inhibits Hepatocellular Carcinoma Cancer Development in Mouse Model. Biochem Biophys Res Commun (2017) 486:239–44. doi: 10.1016/j.bbrc.2017.02.128
103. Sosa A, Lopez Cadena E, Simon Olive C, Karachaliou N, Rosell R. Clinical Assessment of Immune-Related Adverse Events. Ther Adv Med Oncol (2018) 10:1758835918764628. doi: 10.1177/1758835918764628
104. Yoshino K, Nakayama T, Ito A, Sato E, Kitano S. Severe Colitis After PD-1 Blockade With Nivolumab in Advanced Melanoma Patients: Potential Role of Th1-Dominant Immune Response in Immune-Related Adverse Events: Two Case Reports. BMC Cancer (2019) 19:1019. doi: 10.1186/s12885-019-6138-7
105. Weber JS, Hodi FS, Wolchok JD, Topalian SL, Schadendorf D, Larkin J, et al. Safety Profile of Nivolumab Monotherapy: A Pooled Analysis of Patients With Advanced Melanoma. J Clin Oncol (2017) 35:785–92. doi: 10.1200/JCO.2015.66.1389
106. Sanlorenzo M, Vujic I, Daud A, Algazi A, Gubens M, Luna S, et al. Pembrolizumab Cutaneous Adverse Events and Their Association With Disease Progression. JAMA Dermatol (2015) 151:1206–12. doi: 10.1001/jamadermatol.2015.1916
107. Haratani K, Hayashi H, Chiba Y, Kudo K, Yonesaka K, Kato R, et al. Association of Immune-Related Adverse Events With Nivolumab Efficacy in Non-Small-Cell Lung Cancer. JAMA Oncol (2018) 4:374–8. doi: 10.1001/jamaoncol.2017.2925
108. Teraoka S, Fujimoto D, Morimoto T, Kawachi H, Ito M, Sato Y, et al. Early Immune-Related Adverse Events and Association With Outcome in Advanced Non-Small Cell Lung Cancer Patients Treated With Nivolumab: A Prospective Cohort Study. J Thorac Oncol (2017) 12:1798–805. doi: 10.1016/j.jtho.2017.08.022
109. Sato K, Akamatsu H, Murakami E, Sasaki S, Kanai K, Hayata A, et al. Correlation Between Immune-Related Adverse Events and Efficacy in Non-Small Cell Lung Cancer Treated With Nivolumab. Lung Cancer (Am Neth) (2018) 115:71–4. doi: 10.1016/j.lungcan.2017.11.019
110. Holmes WE, Lee J, Kuang WJ, Rice GC, Wood WI. Structure and Functional Expression of a Human Interleukin-8 Receptor. Science (1991) 253:1278–80. doi: 10.1126/science.1840701
111. Murphy PM, Tiffany HL. Cloning of Complementary DNA Encoding a Functional Human Interleukin-8 Receptor. Science (1991) 253:1280–3. doi: 10.1126/science.1891716
112. Kufareva I. Chemokines and Their Receptors: Insights From Molecular Modeling and Crystallography. Curr Opin Pharmacol (2016) 30:27–37. doi: 10.1016/j.coph.2016.07.006
113. Atretkhany KN, Drutskaya MS, Nedospasov SA, Grivennikov SI, Kuprash DV. Chemokines, Cytokines and Exosomes Help Tumors to Shape Inflammatory Microenvironment. Pharmacol Ther (2016) 168:98–112. doi: 10.1016/j.pharmthera.2016.09.011
114. Brat DJ, Bellail AC, Van Meir EG. The Role of Interleukin-8 and Its Receptors in Gliomagenesis and Tumoral Angiogenesis. Neuro Oncol (2005) 7:122–33. doi: 10.1215/S1152851704001061
115. David JM, Dominguez C, Hamilton DH, Palena C. The IL-8/IL-8r Axis: A Double Agent in Tumor Immune Resistance. Vaccines (Basel) (2016) 4(3):22. doi: 10.3390/vaccines4030022
116. Gales D, Clark C, Manne U, Samuel T. The Chemokine CXCL8 in Carcinogenesis and Drug Response. ISRN Oncol (2013) 2013:859154. doi: 10.1155/2013/859154
117. Li A, Dubey S, Varney ML, Dave BJ, Singh RK. IL-8 Directly Enhanced Endothelial Cell Survival, Proliferation, and Matrix Metalloproteinases Production and Regulated Angiogenesis. J Immunol (2003) 170:3369–76. doi: 10.4049/jimmunol.170.6.3369
118. Sanmamed MF, Carranza-Rua O, Alfaro C, Onate C, Martin-Algarra S, Perez G, et al. Serum Interleukin-8 Reflects Tumor Burden and Treatment Response Across Malignancies of Multiple Tissue Origins. Clin Cancer Res (2014) 20:5697–707. doi: 10.1158/1078-0432.CCR-13-3203
119. Roberts AB, Anzano MA, Lamb LC, Smith JM, Sporn MB. New Class of Transforming Growth Factors Potentiated by Epidermal Growth Factor: Isolation From Non-Neoplastic Tissues. Proc Natl Acad Sci USA (1981) 78:5339–43. doi: 10.1073/pnas.78.9.5339
120. Hata A, Chen YG. TGF-Beta Signaling From Receptors to Smads. Cold Spring Harb Perspect Biol (2016) 8(9):a022061. doi: 10.1101/cshperspect.a022061
121. Miyazono K. TGF-Beta Signaling by Smad Proteins. Cytokine Growth Factor Rev (2000) 11:15–22. doi: 10.1016/s1359-6101(99)00025-8
122. Xie F, Ling L, van Dam H, Zhou F, Zhang L. TGF-Beta Signaling in Cancer Metastasis. Acta Biochim Biophys Sin (Shanghai) (2018) 50:121–32. doi: 10.1093/abbs/gmx123
123. Xiao Y, Song YJ, Song B, Huang CB, Ling Q, Yu X. TGF-Beta/MAPK Signaling Mediates the Effects of Bone Marrow Mesenchymal Stem Cells on Urinary Control and Interstitial Cystitis After Urinary Bladder Transplantation. Am J Transl Res (2017) 9:1193–202.
124. Chen W, Ten Dijke P. Immunoregulation by Members of the TGFbeta Superfamily. Nat Rev Immunol (2016) 16:723–40. doi: 10.1038/nri.2016.112
125. Lafyatis R. Transforming Growth Factor Beta–at the Centre of Systemic Sclerosis. Nat Rev Rheumatol (2014) 10:706–19. doi: 10.1038/nrrheum.2014.137
126. Meng XM, Nikolic-Paterson DJ, Lan HY. TGF-Beta: The Master Regulator of Fibrosis. Nat Rev Nephrol (2016) 12:325–38. doi: 10.1038/nrneph.2016.48
127. McDonald J, Wooderchak-Donahue W, VanSant Webb C, Whitehead K, Stevenson DA, Bayrak-Toydemir P. Hereditary Hemorrhagic Telangiectasia: Genetics and Molecular Diagnostics in a New Era. Front Genet (2015) 6:1. doi: 10.3389/fgene.2015.00001
129. Wakefield LM, Hill CS. Beyond TGFbeta: Roles of Other TGFbeta Superfamily Members in Cancer. Nat Rev Cancer (2013) 13:328–41. doi: 10.1038/nrc3500
130. Papaspyridonos M, Matei I, Huang Y, do Rosario Andre M, Brazier-Mitouart H, Waite JC, et al. Id1 Suppresses Anti-Tumour Immune Responses and Promotes Tumour Progression by Impairing Myeloid Cell Maturation. Nat Commun (2015) 6:6840. doi: 10.1038/ncomms7840
131. Ito M, Minamiya Y, Kawai H, Saito S, Saito H, Nakagawa T, et al. Tumor-Derived TGFbeta-1 Induces Dendritic Cell Apoptosis in the Sentinel Lymph Node. J Immunol (2006) 176:5637–43. doi: 10.4049/jimmunol.176.9.5637
132. Chen H, Liakou C, Kamat A, Pettaway C, Ward J, Tang D, et al. Anti-CTLA-4 Therapy Results in Higher CD4+ICOShi T Cell Frequency and IFN-Gamma Levels in Both Nonmalignant and Malignant Prostate Tissues. Proc Natl Acad Sci USA (2009) 106:2729–34. doi: 10.1073/pnas.0813175106
133. Liakou C, Kamat A, Tang D, Chen H, Sun J, Troncoso P, et al. CTLA-4 Blockade Increases IFNgamma-Producing CD4+ICOShi Cells to Shift the Ratio of Effector to Regulatory T Cells in Cancer Patients. Proc Natl Acad Sci USA (2008) 105:14987–92. doi: 10.1073/pnas.0806075105
134. Wei SC, Levine JH, Cogdill AP, Zhao Y, Anang NAS, Andrews MC, et al. Distinct Cellular Mechanisms Underlie Anti-CTLA-4 and Anti-PD-1 Checkpoint Blockade. Cell (2017) 170:1120–33.e1117. doi: 10.1016/j.cell.2017.07.024
135. Beer TM, Kwon ED, Drake CG, Fizazi K, Logothetis C, Gravis G, et al. Randomized, Double-Blind, Phase III Trial of Ipilimumab Versus Placebo in Asymptomatic or Minimally Symptomatic Patients With Metastatic Chemotherapy-Naive Castration-Resistant Prostate Cancer. J Clin Oncol (2017) 35:40–7. doi: 10.1200/JCO.2016.69.1584
136. Berish RB, Ali AN, Telmer PG, Ronald JA, Leong HS. Translational Models of Prostate Cancer Bone Metastasis. Nat Rev Urol (2018) 15:403–21. doi: 10.1038/s41585-018-0020-2
137. Jiao S, Subudhi SK, Aparicio A, Ge Z, Guan B, Miura Y, et al. Differences in Tumor Microenvironment Dictate T Helper Lineage Polarization and Response to Immune Checkpoint Therapy. Cell (2019) 179:1177–90.e1113. doi: 10.1016/j.cell.2019.10.029
138. Terabe M, Robertson F, Clark K, De Ravin E, Bloom A, Venzon D, et al. Blockade of Only TGF-β 1 and 2 Is Sufficient to Enhance the Efficacy of Vaccine and PD-1 Checkpoint Blockade Immunotherapy. Oncoimmunology (2017) 6:e1308616. doi: 10.1080/2162402x.2017.1308616
139. Knudson K, Hicks K, Luo X, Chen J, Schlom J, Gameiro SJO. M7824, a Novel Bifunctional Anti-PD-L1/TGFβ Trap Fusion Protein, Promotes Anti-Tumor Efficacy as Monotherapy and in Combination With Vaccine. Oncoimmunology (2018) 7:e1426519. doi: 10.1080/2162402x.2018.1426519
140. David J, Dominguez C, McCampbell K, Gulley J, Schlom J, Palena CJO. A Novel Bifunctional Anti-PD-L1/TGF-β Trap Fusion Protein (M7824) Efficiently Reverts Mesenchymalization of Human Lung Cancer Cells. Oncoimmunology (2017) 6:e1349589. doi: 10.1080/2162402x.2017.1349589
141. Lim S, Lee J, Gide T, Menzies A, Guminski A, Carlino M, et al. Circulating Cytokines Predict Immune-Related Toxicity in Melanoma Patients Receiving Anti-PD-1-Based Immunotherapy. Clin Cancer Res (2019) 25:1557–63. doi: 10.1158/1078-0432.Ccr-18-2795
142. Miller BC, Sen DR, Al Abosy R, Bi K, Virkud YV, LaFleur MW, et al. Subsets of Exhausted CD8(+) T Cells Differentially Mediate Tumor Control and Respond to Checkpoint Blockade. Nat Immunol (2019) 20:326–36. doi: 10.1038/s41590-019-0312-6
143. Guo Y, Xie YQ, Gao M, Zhao Y, Franco F, Wenes M, et al. Metabolic Reprogramming of Terminally Exhausted CD8(+) T Cells by IL-10 Enhances Anti-Tumor Immunity. Nat Immunol (2021) 22:746–56. doi: 10.1038/s41590-021-00940-2
144. Liu C, Liu R, Wang B, Lian J, Yao Y, Sun H, et al. Blocking IL-17A Enhances Tumor Response to Anti-PD-1 Immunotherapy in Microsatellite Stable Colorectal Cancer. J Immunother Cancer (2021) 9(1):e001895. doi: 10.1136/jitc-2020-001895
145. Tarhini A, Zahoor H, Lin Y, Malhotra U, Sander C, Butterfield L, et al. Baseline Circulating IL-17 Predicts Toxicity While TGF-β1 and IL-10 Are Prognostic of Relapse in Ipilimumab Neoadjuvant Therapy of Melanoma. J Immunother Cancer (2015) 3:39. doi: 10.1186/s40425-015-0081-1
Keywords: cytokines, immune checkpoint inhibitors, predictive factors, adverse events, response
Citation: Wang M, Zhai X, Li J, Guan J, Xu S, Li Y and Zhu H (2021) The Role of Cytokines in Predicting the Response and Adverse Events Related to Immune Checkpoint Inhibitors. Front. Immunol. 12:670391. doi: 10.3389/fimmu.2021.670391
Received: 21 February 2021; Accepted: 05 July 2021;
Published: 22 July 2021.
Edited by:
Jonathan Pol, Institut National de la Santé et de la Recherche Médicale (INSERM), FranceReviewed by:
Marcia Antoniazi Michelin, Universidade Federal do Triângulo Mineiro, BrazilJamshid Hadjati, Tehran University of Medical Sciences, Iran
Copyright © 2021 Wang, Zhai, Li, Guan, Xu, Li and Zhu. This is an open-access article distributed under the terms of the Creative Commons Attribution License (CC BY). The use, distribution or reproduction in other forums is permitted, provided the original author(s) and the copyright owner(s) are credited and that the original publication in this journal is cited, in accordance with accepted academic practice. No use, distribution or reproduction is permitted which does not comply with these terms.
*Correspondence: Hui Zhu, ZHJ6aHVoQDEyNi5jb20=