- 1Priority Research Centre for Healthy Lungs, Hunter Medical Research Institute, University of Newcastle, Newcastle, NSW, Australia
- 2Priority Research Centre GrowUpWell, Hunter Medical Research Institute, University of Newcastle, Newcastle, NSW, Australia
- 3Department of Immunology and Pathology, Central Clinical School, Monash University, Melbourne, VIC, Australia
- 4Department of Respiratory and Sleep Medicine, John Hunter Hospital, Newcastle, NSW, Australia
Background: Asthma is the most frequent cause of hospitalisation among children; however, little is known regarding the effects of asthma on immune responses in children.
Objective: The present study aimed to evaluate cytokine responses of peripheral blood mononuclear cells (PBMCs), PBMC composition and lung function in children with and without asthma.
Methods: Using a case-control design, we compared 48 children with asthma aged 3-11 years with 14 age-matched healthy controls. PBMC composition and cytokine production including interferon (IFN)-γ, interleukin (IL)-1β, IL-5 and lL-6 following stimulation with rhinovirus-1B (RV1B), house dust mite (HDM) and lipopolysaccharide (LPS) were measured. Lung function was assessed using impulse oscillometry and nitrogen multiple breath washout.
Results: The frequency of group 2 innate lymphoid cells were significantly higher in asthmatics and PBMCs from asthmatics had deficient IFN-γ production in response to both RV1B and LPS compared with controls (P<0.01). RV1B-induced IL-1β response and HDM-stimulated IL-5 production was higher in asthmatics than controls (P<0.05). In contrast, IL-1β and IL-6 were significantly reduced in response to HDM and LPS in asthmatics compared to controls (P<0.05). Children with asthma also had reduced pulmonary function, indicated by lower respiratory reactance as well as higher area of-reactance and lung clearance index values compared with controls (P<0.05).
Conclusion: Our study indicates that children with asthma have a reduced lung function in concert with impaired immune responses and altered immune cell subsets. Improving our understanding of immune responses to viral and bacterial infection in childhood asthma can help to tailor management of the disease.
Introduction
Asthma is the most prevalent chronic childhood condition (1, 2). Children with asthma frequently experience exacerbations, and the majority of exacerbations in children are associated with viral infections (3). While rhinoviruses (RVs) are the most frequent precipitants of virus-associated exacerbations in children (4), asthma exacerbations may also be triggered by invasive bacterial infection (5). Indeed, there is evidence showing strong associations between levels of household lipopolysaccharide (LPS) and asthma exacerbations (6–8) and reduced lung function (7). However, in children with asthma, a greater understanding of the mechanisms underlying the immune response to different stimuli, and factors contributing to increased risk of infection are required.
There are numerous immune cells, including neutrophils, eosinophils, natural killer (NK) cells, dendritic cells (DCs), lymphocytes as well as structural cells such as epithelial cells, that may contribute to an altered immune response in childhood asthma. Recent studies have demonstrated that innate lymphoid cells (ILCs) also have a key role in the development of virus-induced asthma exacerbations (9). Group 2 ILC (ILC2) secrete interleukin (IL)-5, IL-9 and IL-13 in response to IL-25 and IL-33 stimulation (9, 10), and circulating ILC2 levels are increased in adults with asthma. However, the role of ILC2 in childhood asthma is less clear.
The aims of this study were to compare cytokine responses of peripheral blood mononuclear cells (PBMCs) (including interferon (IFN)-γ, IFN-λ, IL-1β, IL-5 and IL-6) stimulated with RV1B, LPS and house dust mite (HDM) in asthmatic children with healthy controls and to characterise immune cell subsets (ILCs, eosinophils, neutrophils, lymphocytes, NK cells and DCs) in whole blood. We further investigated the relationship between immune cell subset populations and PBMC cytokine responses. Additionally, we aimed to compare the lung function parameters between children with and without asthma.
Materials and Methods
Study Design and Participants
This was an observational, case-control study including children with asthma aged 3-11 years (n=48) and healthy, age-matched controls with no previous diagnosis of asthma or history of respiratory conditions (n=14). Children with asthma were recruited via attendance to the emergency department or admission to the John Hunter Children’s Hospital and Maitland Hospital, following an exacerbation of asthma. This study includes baseline data obtained from asthmatic children participating in a 26-week clinical trial evaluating the effects of a high fruit and vegetable diet on asthma exacerbation (ACTRN12615000851561). Healthy controls were recruited via flyers placed in community centres and at the University of Newcastle (UoN). The study was conducted at the Hunter Medical Research Institute (HMRI), Newcastle, Australia, between September 2015 and March 2019. All participants were screened for eligibility prior to enrolment.
Inclusion criteria for children with asthma were physician diagnosis of asthma; recent exacerbation/s (≥1 exacerbation in past 6 months or ≥2 in the past 12 months) and stable asthma at the visit. Exclusion criteria included other respiratory conditions, diagnosed intestinal disorders, or consumption of nutritional supplements (in previous 4 weeks). Inclusion and exclusion criteria were the same for the control group, with the exception that controls had no history of asthma or wheeze. All subjects were consuming a low fruit and vegetable diet (≤3 serves of fruit and vegetables per day (assessed over past week). The study was approved by the HNEH Ethics Committee (15/06/17/4.03) and registered with the UON Human Research Ethics Committee. Written informed parental consent and child assent (where applicable), was obtained prior to participation in the study.
Clinical Assessment
All participants fulfilling the inclusion criteria attended HMRI for clinical assessment and blood collection following a 12 hour overnight fast. Clinical assessments included anthropometry, nitrogen multi-breath washout (MBW), impulse oscillometry (IOS) and blood collection. For details, refer to supplement.
Laboratory Methods
Immunoglobulin E (IgE) specific antibodies against 4 allergens (dust mites, mixed moulds, mixed grasses, and mixed animal epithelial) were measured in plasma using an ImmunoCAP Fluorenzyme assay (Pathology North, Newcastle, NSW Australia).
PBMCs were isolated from whole blood by density gradient method (11) using Ficoll-PaqueTM PLUS (GE Healthcare, Sydney, Australia) and cultured with and without RV1B, LPS or HDM for 48 hours. The concentrations of IFN-γ, IL-1β and IL-6 in the culture supernatants were analysed using bead-based multiplex assay (BD Bioscience, Sydney, Australia) and IFN-λ and IL-5 concentrations in the culture supernatants were measured using high-sensitivity commercial ELISA assays (R&D Systems, Sydney, Australia) as per the manufacturer’s recommendations.
Quantification of major immune cell subsets in whole blood, including eosinophils and neutrophils, T lymphocytes, DCs, NK cells plus B cells and ILCs was performed by multi-parametric flow cytometry using the Fortessa LSR-X20 (BD Biosciences, Sydney, Australia).
For full laboratory analysis methods see supplement.
Statistical Analysis
Statistical analyses were performed using STATA 15 (StataCorp, College Station, Texas, USA). Data are reported as mean ± standard deviation or median (interquartile range). Significant differences between groups were determined using independent t-test (parametric data) or Wilcoxon Rank Sum tests (non-parametric data). Subgroup analyses on immune responses of PBMCs were performed on a subset of participants with negative RAST results (no history of allergy) as well as those with no history of OCS use. In adjusted comparisons, age, weight and height were adjusted using General Linear Model. Pearson’s Chi-squared test or Fisher’s exact test (expected cell sizes< 5) were used to test equality of proportions between groups. Exploratory analysis between immune cell subset populations and PBMC cytokine responses were assessed using Spearman’s correlations. Significance was accepted when P<0.05.
Results
Subject Characteristics
Demographic and clinical characteristics of the children with asthma and healthy controls enrolled in the study are listed in Table 1A. There were no significant differences in age, weight, height, BMI and BMI z score among the asthmatic children and healthy controls. The proportion of males was significantly higher in children with asthma compared to the healthy controls. Furthermore, the history of eczema (60.4% versus 0%, P<0.0001) or hayfever (60.4% versus 14.3%, P<0.01) was greater in the asthma group compared with the control group. Similarly, there were more children with history of food allergy in the asthmatics than in healthy controls, which appeared to be marginally significant (P=0.056). There were also more subjects with asthma that had a positive RAST result to dust mite (allergen specific IgE 15-50 kUA/L) (P<0.001).
Children with asthma were well-controlled at the time of sample collection [median asthma control score of 24 (21, 25)] (Table 1B). Of 48 subjects in the asthmatic group, 58.3% had ≥1 hospital admission for acute asthma exacerbation in the previous 12 months. Asthma medication use in the previous 12 months is presented in Table 1B.
Ex Vivo Stimulation of Cytokine Production by PBMCs
Cytokine responses of PBMCs to Human Rhinovirus-1B stimulation
PBMCs from children with asthma stimulated with RV1B produced significantly less IFN-γ compared with PBMCs from healthy controls (P<0.01) (Figure 1 and Table 2). A similar trend was observed for IFN-λ, although the difference did not reach statistical significance. In contrast, the concentrations of IL-1β in the supernatants of PBMCs infected with RV1B were significantly higher in children with asthma than in the healthy controls (P<0.05) (Figure 1 and Table 2). The RV1B induced IL-6 response was also higher in asthmatics than healthy controls; however, this was not significant.
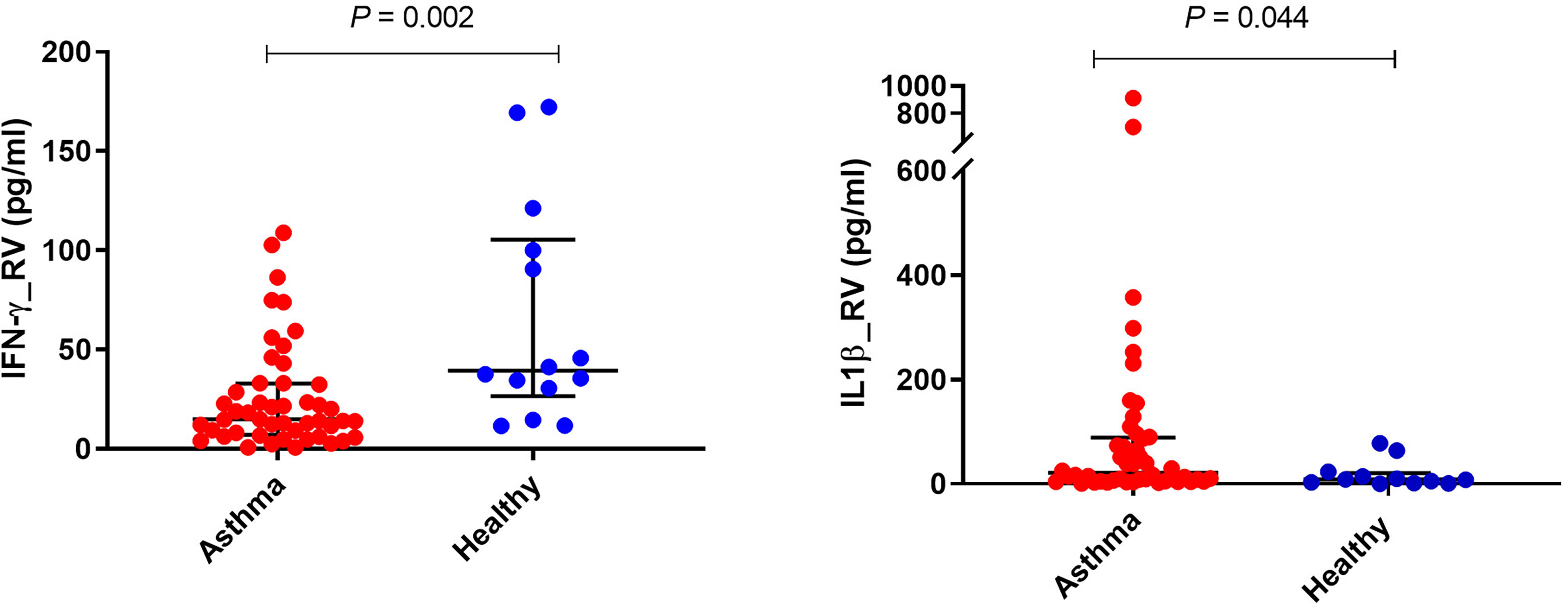
Figure 1 Effects of RV1B stimulation on cytokine responses of PBMCs. PBMCs from children with asthma (n=48) and healthy controls (n=14) were exposed to media or RV1B (MOI=20) for 48h. Bars represent median (interquartile range). Data adjusted for the levels in uninfected PBMCs (control). Plot represents median with interquartile ranges and individual’s values are represented by dots. PBMC, peripheral blood mononuclear cells; RV, rhinovirus; IFN, interferon.
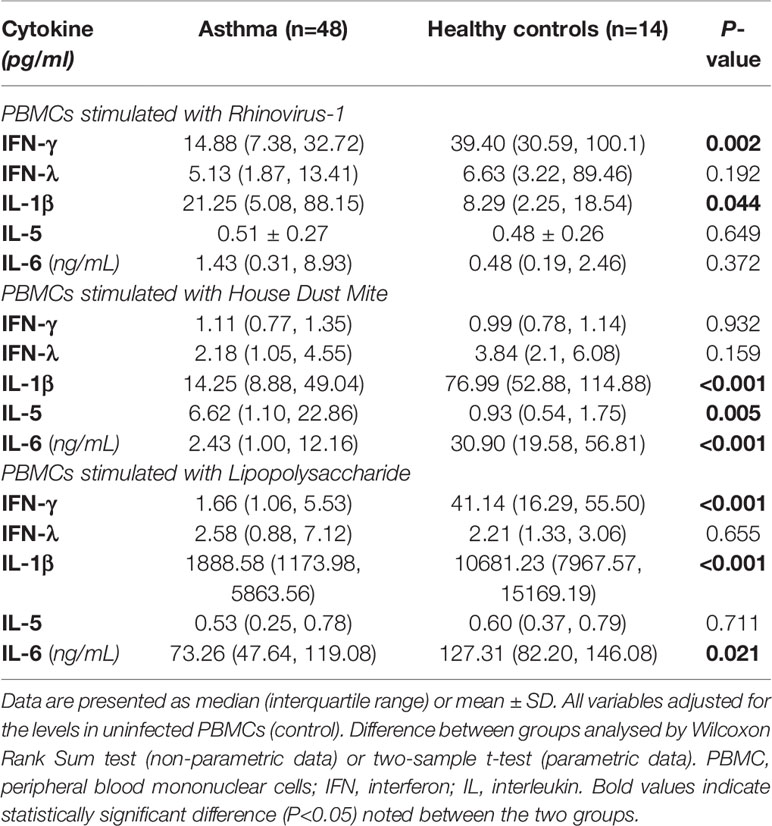
Table 2 Cell supernatant cytokine secretion of PBMCs in response to different stimuli in children with asthma and age-matched non-asthmatic group.
Cytokine responses of PBMCs to House Dust Mite stimulation
HDM-induced IL-1β and IL-6 production were significantly lower in children with asthma than healthy controls (P<0.001) (Figure 2 and Table 2). In contrast, the production of type 2 cytokine, IL-5, following HDM exposure was significantly higher in asthmatic children than in age-matched healthy controls (P<0.01) (Figure 2 and Table 2). However, there were no significant differences in HDM-induced IFN-γ, and IFN-λ production in PBMCs obtained from asthmatic children compared with those from healthy controls (P>0.05).
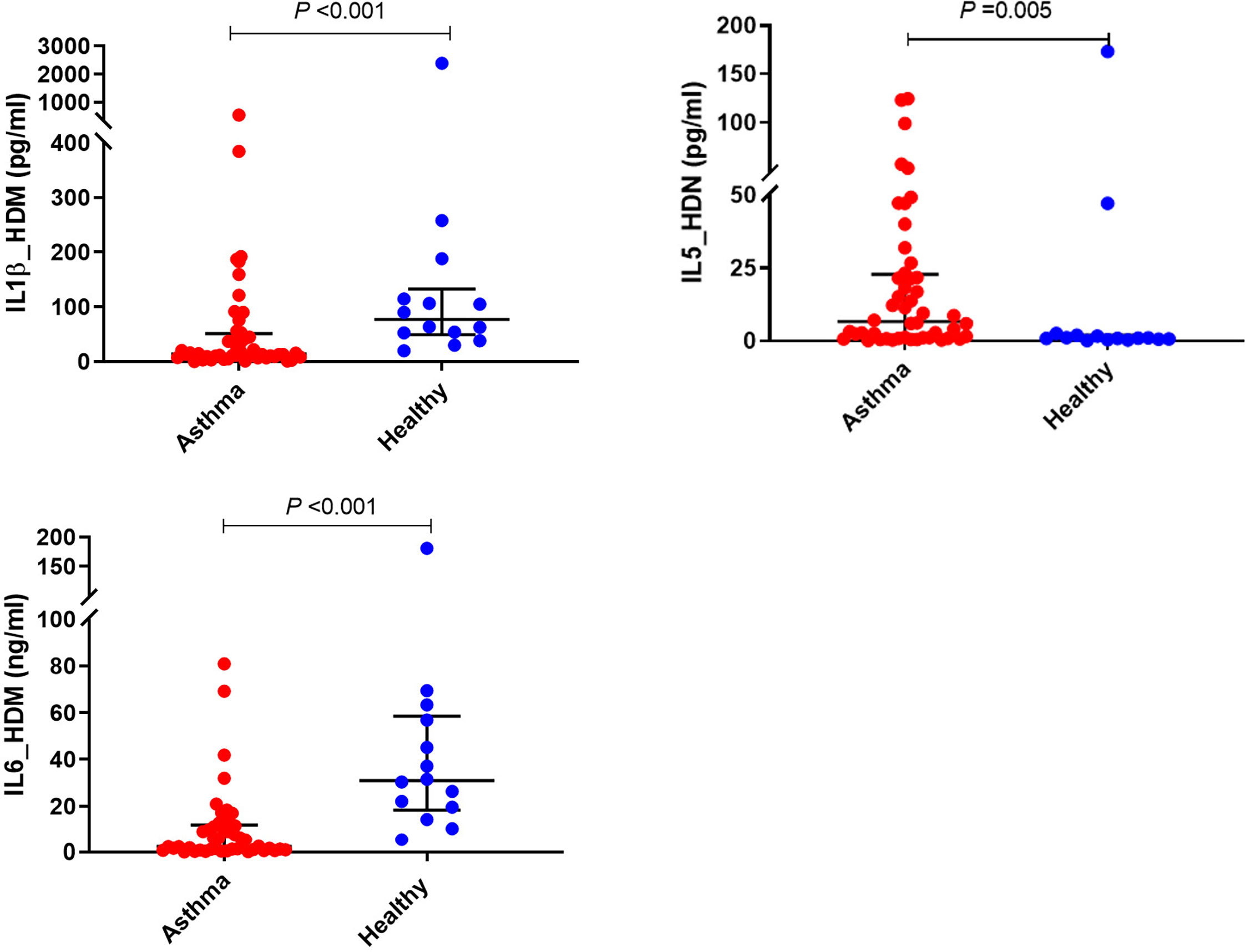
Figure 2 Effects of HDM stimulation on cytokine responses of PBMCs. PBMCs from children with asthma (n=48) and healthy controls (n=14) were exposed to media or HDM for 48h. Bars represent median (interquartile range). Data adjusted for the levels in uninfected PBMCs (control). Plot represents median with interquartile ranges and individual’s values are represented by dots. PBMC, peripheral blood mononuclear cells; HDM, house dust mite; IL, interleukin.
Cytokine responses of PBMCs to Lipopolysaccharide stimulation
There was significantly less IFN-γ produced by LPS stimulated PBMCs from children with asthma compared with those from the controls (P<0.001) (Figure 3 and Table 2); however, no significant difference was found in LPS- induced IFN-λ production in PBMCs obtained from children with asthma compared with PBMCs from controls. Furthermore, PBMCs stimulated with LPS produced the same pattern of IL-1β and IL-6 response observed for HDM with PBMCs from asthmatic children producing significantly lower IL-1β and IL-6 compared with PBMCs from healthy controls (P<0.001 and P<0.05, respectively) (Figure 3 and Table 2).
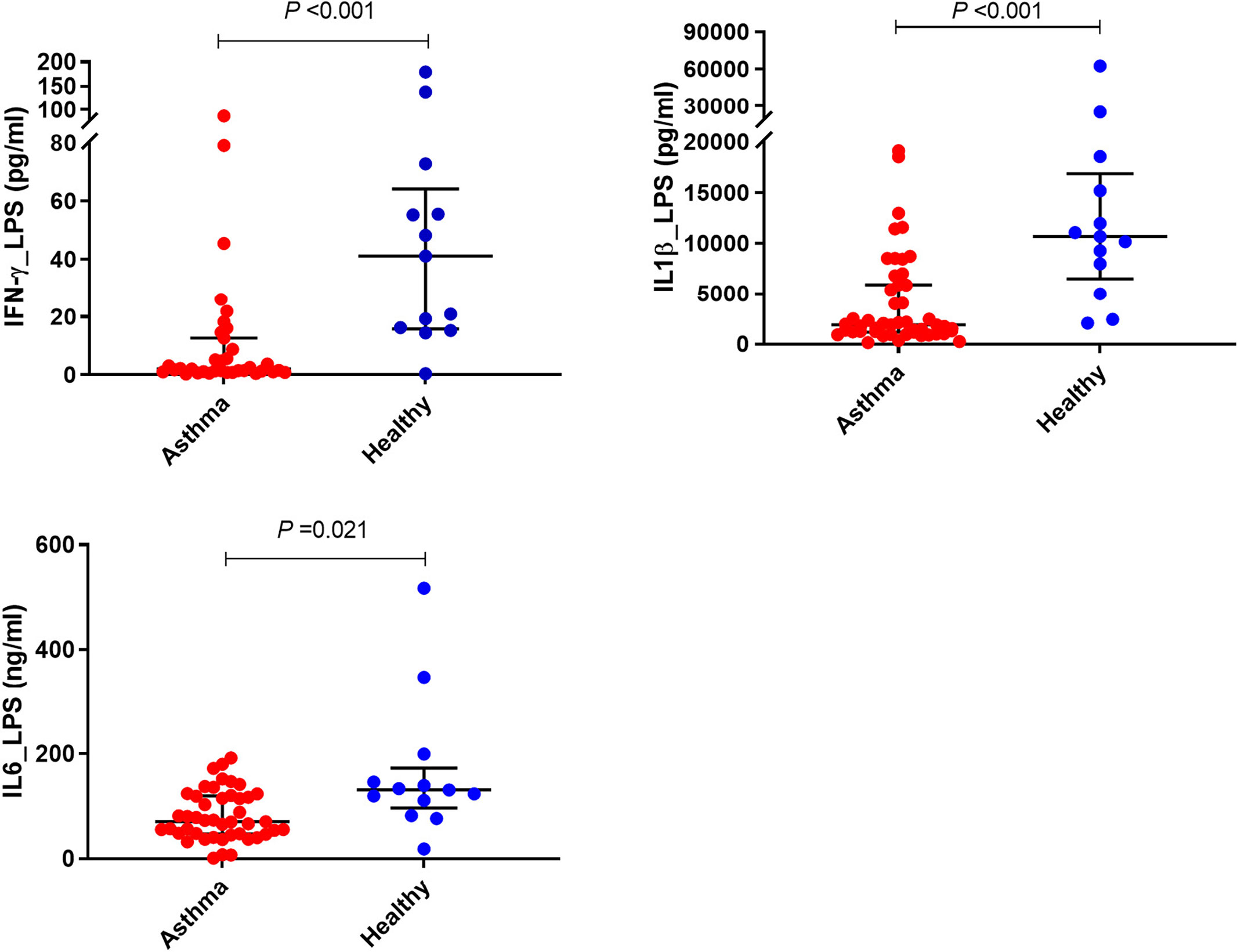
Figure 3 Effects of LPS stimulation on cytokine responses of PBMCs. PBMCs from children with asthma (n=48) and healthy controls (n=14) were exposed to media or LPS for 48h. Bars represent median (interquartile range). Data adjusted for the levels in uninfected PBMCs (control). Plot represents median with interquartile ranges and individual’s values are represented by dots. PBMC, peripheral blood mononuclear cells; LPS, lipopolysaccharide; IFN, interferon.
Subgroup analyses
A subgroup analysis on children with negative RAST results was performed on 19 children with asthma and 13 healthy controls. Production of IFN-γ in response to both RV1B and LPS was lower in asthmatic children than healthy controls (P= 0.018 and P= 0.009, respectively). Additionally, HDM stimulated IL-1β and IL-6 production (P=0.002 and P= 0.001, respectively) and as well as LPS induced IL-1β release were found to be lower in asthmatics compared to the healthy controls (P=0.005) (Table S3).
A subgroup analysis was also carried out on a subset of participants with no history of OCS use (asthmatics=27, healthy controls= 14). Children with asthma had deficient IFN-γ production in response to both RV1B and LPS compared with healthy controls (P=0.003 and P<0.0001, respectively). Moreover, IL-1β and IL-6 production in response to both HDM (P <0.001 and P<0.001, respectively) and LPS (P <0.001, P=0.029, respectively) were found to be lower in asthmatic children than in their age-matched counterparts (Table S4).
Peripheral Whole Blood Immune Cell Profiles
Immune cell subset frequency was assessed in PBMCs from 17 asthmatics and 11 healthy children. Asthmatic children showed a significantly higher number of ILC1 and ILC2 compared to healthy controls [(51.09 (39.04, 100.62) versus 21.91 (13.04, 67.60), P<0.05 and 151.80 (81.16, 335.17) versus 8.40 (6.52, 11.46), P<0.001, respectively]. Moreover, while there were no significant differences in numbers of ILC3 with natural cytotoxicity receptor (NCR+) between the two groups, the number of circulating ILC3 NCR- was significantly higher in children with asthma compared to healthy controls [25.00 (2.52, 70.99) versus 0.0 (0, 0.53), P<0.001] (Figure 4 and Table 3). The frequency of granulocytes, DCs, NK cells and lymphocytes were similar in the two groups (Table 3).
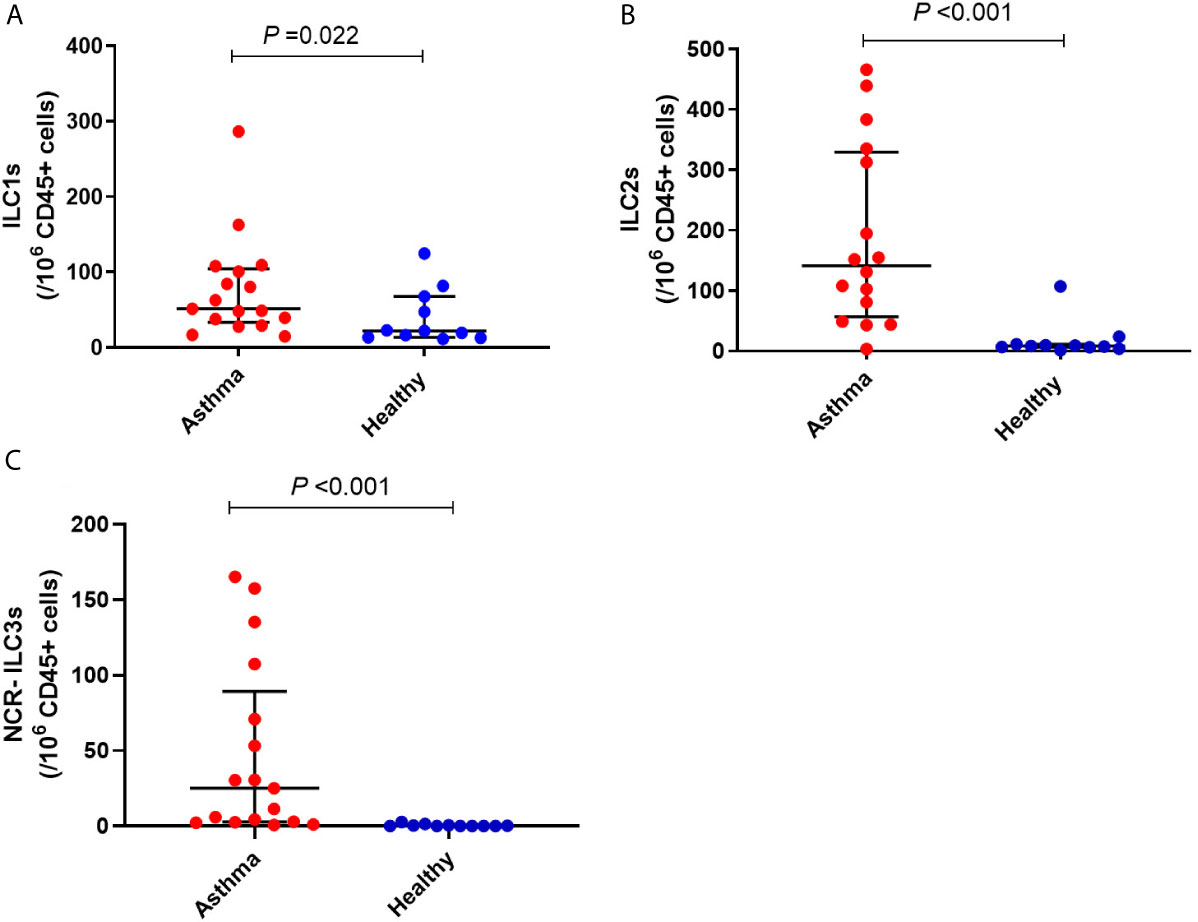
Figure 4 Frequency of (A) ILC1, (B) ILC2 and (C) NCR- ILC3 in children with asthma and healthy controls. Bars represent median (interquartile range). Immune cell phenotyping was performed in whole blood using a lyse-wash procedure. Cells are per 106 CD45+ cells. Plot represents median with interquartile ranges and individual’s values are represented by dots. ILCs, innate lymphoid cells; NCR, natural cytotoxicity receptor.
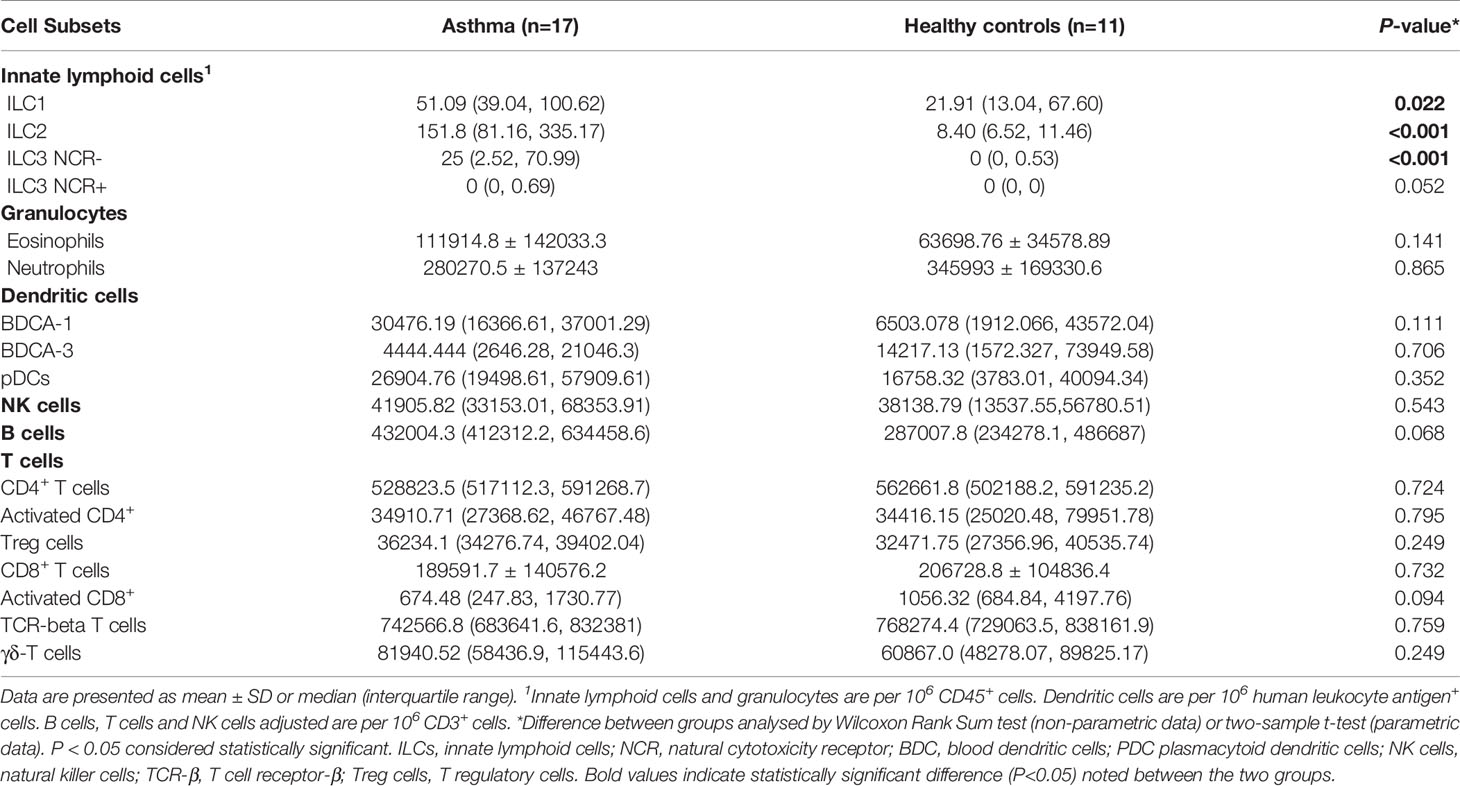
Table 3 Frequency of circulating immune cell subsets in children with asthma and age-matched non-asthmatic group.
Associations
Correlation analysis between peripheral blood immune cell numbers and cytokine responses of PBMCs revealed an inverse association between the frequency of ILC1 and LPS-induced IL-1β (rs=-0.45, P=0.030). Furthermore, the frequency of ILC2 was inversely correlated with LPS-induced IL-1β and IL-6 (rs=-0.43, P=0.035; and rs=-0.45, P=0.030, respectively), and HDM-induced IL-6 (rs=-0.54, P=0.006). Inverse associations were also found between the prevalence of ILC3 NCR- and RV1B-induced IFN-γ (rs=-0.48, P=0.019), HDM-induced IL-6 (rs=-0.77, P<0.0001), and LPS-induced IL-1β (rs=-0.48, P=0.018). Moreover, the frequency of B cells was inversely associated with RV1B-induced IFN-λ (rs=-0.45, P=0.035). In addition, the frequency of TCR- β cells was positively associated with LPS-induced IL-1β (rs=0.44, P=0.031).
Lung Function Assessments
Impulse Oscillometry
IOS variables included in the analysis were airway reactance (Xrs), respiratory resistance (Rrs) (all measured at 5, 10, 15 and 20 Hz), as well as respiratory impedance measured at 5 Hz (Z5), and reactance area (Ax). Children with asthma demonstrated lower reactance at 5, 10, 15 and 20 Hz compared with healthy controls (Table 4). Whereas, Ax values were greater in asthmatics than in healthy controls (Table 4). No difference was found in respiratory resistance and respiratory impedance between the two groups. After adjusting for age, weight and height, the difference between airway resistance at 5Hz and 20Hz (R5-R20) was significantly higher in asthmatics than in healthy controls (P<0.05). Additionally, the differences in airway reactance remained significant after adjusting for age, weight and height, while Ax value was no longer different between the groups.
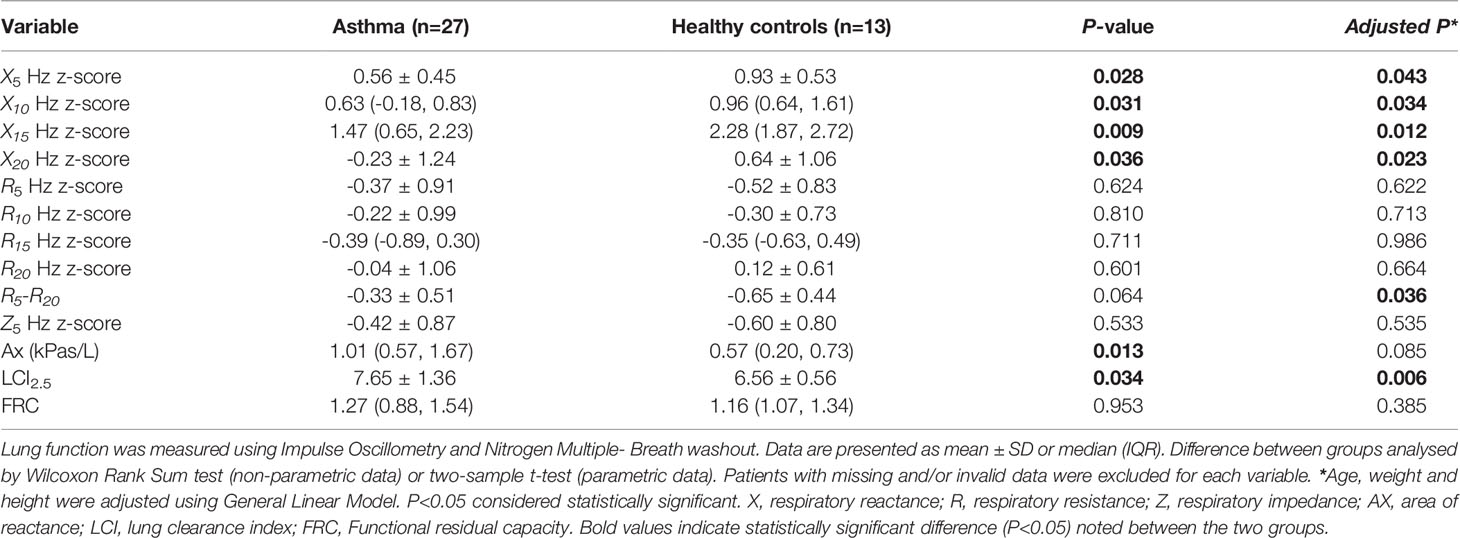
Table 4 Comparison of lung function parameters between children with asthma and age matched non-asthmatic group.
Nitrogen Multiple-Breath Washout
Lung clearance index (LCI)2.5 values were on average 1.09 units (95% confidence interval: 0.09- 2.09, P< 0.05) higher in children with asthma compared with healthy controls (Table 4). No significant difference was detected in functional residual capacity between the groups. Adjusting for age, weight and height did not alter the results.
Discussion
For the first time, this study has demonstrated that children with asthma had deficient IFN-γ production in response to both RV1B and LPS infection, compared with the healthy controls. RV1B induced IL-1β response was higher in asthmatics than healthy controls. HDM-stimulated IL-5 production was also significantly greater in asthmatic children than in healthy controls. In contrast, both IL-1β and IL-6 production were significantly lower in response to HDM and LPS in children with asthma. Furthermore, the frequency of ILC1, ILC2, and ILC3 NCR- were significantly higher in children with asthma compared to healthy controls, while other immune cells such as granulocytes, DCs, B cells and T-cells were present in whole blood in similar numbers. These results indicate that innate immune dysfunction in asthma is not limited to adults and may explain the increased susceptibility of asthmatic children to viral and bacterial respiratory infections.
In the present study, we observed that ex vivo RV1B infection of PBMCs from asthmatic children resulted in >2.6 times less IFN-γ production compared with PBMCs from healthy controls. Whereas, RV1B-induced IL-1β production was 2.5-fold higher in asthmatic cells than in healthy controls, which suggests inflammasome induction (12). Our results suggest that diminished IFN-γ production, as well as overproduction of inflammatory cytokines (IL-1β) in children with asthma, may be involved in their high susceptibility to lower airway symptoms caused by RV infection. These findings are in concordance with another study in pre-school children (13) that showed reduced serum IFN-α levels in children with asthma compared to healthy controls. In line with our findings, numerous studies in adults reported that following RV exposure PBMCs from adult patients with asthma produced lower levels of IFN-γ compared with cells from healthy controls (14, 15).
IFNs can decrease susceptibility of host cells to viral infection, and an inverse association between IFN-γ production and viral load has been shown previously (3, 16). The antiviral activities of IFNs are mediated directly through the inhibition of viral replication in cells and indirectly through the induction of cytokines and chemokines, which results in recruitment of NK cells as well as CD4 and CD8 T cells (17). Diminished antiviral IFN responses in asthma could be the main mechanism for enhanced susceptibility to respiratory viral infection (18). This mechanism could be an explanation for asthmatic patients having more severe lower respiratory tract symptoms and declines in lung function of greater duration and severity following RV infection (18).
IFN-γ also appears to be a key mediator of LPS-induced immune responses (19). We observed a deficient IFN-γ response to LPS in asthmatic children compared with age-matched healthy controls. Similarly, Contoli et al. showed primary bronchial epithelial cells and alveolar macrophages from adult asthmatic patients produced lower levels of IFN-λ following LPS stimulation than healthy controls (5). IL-6 and IL-1β production by PBMCs in response to HDM and LPS were impaired in children with asthma in our study. There is apparent controversy about the immune responses to LPS in patients with asthma. A previous study showed that following LPS exposure, PBMCs from adult asthmatic patients produced more IL-1β than cells from healthy controls (20), while, another study in adults reported that asthmatic patients have defective innate immune responses to LPS demonstrated by lower LPS-induced IL-1B response in asthmatics compared to healthy participants (21). Recent studies have highlighted the role of toll-like receptors (TLRs) in the innate and adaptive immune responses. TLRs are involved in the initial immune response to pathogens or environmental stimuli and are broadly expressed by a variety of tissues and cell types (22, 23). Previous studies have demonstrated an association between TLRs and the pathogenesis of asthma (22). One of the well-characterised TLRs that can recognise ligands such as LPS and HDM is TLR4. It has been shown that the expression of TLR4 on PBMCs is diminished in asthmatic patients (22). Our finding of a significantly lower level of IL-6 and IL-1B in response to HDM and LPS in the asthmatic group is in concordance with the previous study that reported lower expression of TLR4 in asthma (22).
This study also demonstrated that HDM-stimulated IL-5 release was significantly higher in children with asthma than in healthy controls. This is in consistent with previous studies that reported children and adolescents with asthma had enhanced TH2 cytokines responses to HDM in comparison with healthy controls (24–26). Previous research suggested that HDM can trigger a TH2-type response in the T cells that specifically recognize the allergen but that allergen-responsive T cells might be lower in healthy subjects than in patients with asthma. It can be suggested that the impaired inflammatory response observed in patients with asthma is due to an imbalance between type I and type II cytokines.
There are several additional points that warrant consideration. The number of children with sensitization to dust mite was significantly higher in children with asthma compared with healthy controls (61.7% vs. 0%, respectively). Increased IgE antibodies to allergens was found to be correlated with increased risk for lower respiratory tract symptoms with viral infections such as rhinovirus in patients with asthma (16). To examine whether allergy status is one of the causes of the observed significant differences in immune responses of PBMCs, we performed a subgroup analysis on children with negative RAST results (n= 32) and differences in the following variables remained statistically significant between the two groups: RV1B induced IFN-γ (P= 0.018), HDM stimulated IL-1β and IL-6 production (P=0.002, P= 0.001, respectively) and as well as LPS induced IFN-γ and IL-1β release (P= 0.009 and P=0.005, respectively) (Table S3). These findings confirm that the deficient IFN responses observed in children with asthma is not related to their allergy status. However, it should also be noted that no significant difference was observed in HDM-specific IL-5 responses between the two groups after adjusting for allergy status. Our results indicate that HDM-induced IL-5 production can be considered as a predictor for the presence of atopy in children with asthma, as has been suggested by others (27). Additional questions of concern relate to the effects that corticosteroids may have on the observed differences in immune responses of PBMCs. Prior studies have reported immune-suppressive characteristics of steroids (28, 29). Twenty-one children in asthma group (43.7%) used intermittent oral corticosteroids, while none of the healthy controls used corticosteroids for any health conditions. Our subgroup analysis of subjects with no history of OCS use (n=41) revealed the following: IFN-γ production in response to both RV1B and LPS remained to be significantly lower in children with asthma compared with healthy controls (P=0.003 and P<0.0001). Additionally, IL-1β and IL-6 production in response to both HDM and LPS were remained lower in asthmatic children than in their age-matched counterparts (P <0.001) (Table S4). These findings are in line with previous in vitro studies that reported corticosteroids inhibit viral-induced cytokines but do not inhibit interferons (30). However, the interaction between corticosteroids and virus infection is controversial and some studies demonstrated that treatment with corticosteroids induced viral replication in the epithelial cells by suppressing type I and type III IFN production (31). It is also worth noting that in our study there was an apparent increase in RV1B induced IFN-λ production in subjects with no history of OCS; however, this was not significantly different between the two groups. These data indicate the need to further in vivo and in vitro studies in the role of corticosteroids in innate immune responses and activation of immune cells in asthma.
We also measured the frequency of whole blood immune cell subsets and found a higher prevalence of ILC1, ILC2, and ILC3 NCR- in children with asthma compared to healthy controls. These findings are consistent with previous studies that also reported higher prevalence of ILC2 in blood from adult asthmatic patients than healthy controls (9, 10, 32), which was associated with worse asthma control (10). However, in another study, ILC2 were on average 50% lower in the blood of children with acute asthma compared with healthy controls (33). Compelling evidence indicates that ILCs have significant roles in asthma development, specifically virus-induced asthma (9), as they link the innate and the adaptive immune responses within the hypersensitive airway (10). The airway epithelial cells, as the first natural barrier, protect the body from external antigens. In response to pathogen recognition, allergen exposure or viral infection, epithelial cell-derived cytokines such as IL-25 and IL-33 are released. Following secretion, IL-25 and IL-33 can bind to their receptors on the surface of ILC2 and affect the growth and proliferation of these cells (9, 34). Activated ILC2 cells are potent secretors of Th2 cytokines (e.g., IL-5, IL-13) (9, 34), which results in airway hyperactivity, mucus overproduction, airway smooth muscle constriction as well as airway remodelling (35).
In an exploratory analysis, we examined the relationship between peripheral blood immune cell numbers and cytokine responses of PBMCs to RV1B, HDM, and LPS stimulation. We found that the number of ILC2 and ILC3s NCR- was inversely correlated with HDM-induced IL-1β. LPS-induced IL-1β was found to be inversely correlated with ILC1s, ILC2 and ILC3s NCR-, while, positively associated with TCR+ cells. Inverse correlations were also found between ILC2 and IL-6 from LPS-stimulated PBMCs, as well as between ILC3s NCR- and IFN-γ production in response to RV1B stimulation. These findings suggest that increased circulating ILCs, in particular, ILC2 might be involved in dysregulated innate immune responses in asthmatic children. Additionally, we found that the frequency of B cells was inversely associated with RV1B-induced IFN-λ. This inverse correlation confirms the previous observation that virus-induced IFN-λ can reduce proliferation of B cells in a dose-dependent manner (36). To our knowledge, this is the first study to suggest a relationship between whole blood immunophenotype and innate immune responses of PBMCs. Previous research has explored the association between cord blood immune cell subsets and airway immune mediators and reported a positive association between activated CD4 and CD8 T cells and TNF-α, while regulatory T cells and CD4 T cells were reported to be inversely correlated with IL-1β (37).
Our study also revealed that compared with healthy controls, asthmatic children had lower lung reactance (Xrs) values, while area of reactance was found to be significantly higher in asthmatics than in healthy controls. Moreover, after adjusting for age, weight and height, asthmatic children had a greater R5-R20 value compared with controls. This is consistent with previous studies that reported peripheral airway IOS indices (including Ax and R5-R20) were correlated with asthma control in both children and adults (38–41). Our findings are also in line with previous research showing that Xrs predicted values were significantly lower in asthmatics than in controls (42). In a cohort of 162 children aged 2-5 years, lower z-scores of reactance X5 were observed in persistent asthmatics compared with children with intermittent asthma (43). Our results showed that functional impairment of the airways is present, even in young children with stable asthma. The ability to predict loss of asthma control in children can decrease asthma related mobility and mortality. Thus, IOS can be used to identify paediatric patients with stable asthma who are at risk of losing asthma control.
The measurements from nitrogen MBW showed that, as expected, LCI2.5 was higher in asthmatic children than in healthy controls. These results are in agreement with previous research (44, 45). Baseline LCI was found to be significantly higher in school-aged children with asthma compared with healthy age-matched controls (40). Similarly, another study demonstrated that clinically stable paediatric patients with asthma had a significantly greater LCI value compared to healthy controls, which persisted after salbutamol use (41). These findings suggest that ventilation inhomogeneity is present in the airways of asthmatic children even in those with stable asthma. LCI has been found be useful in the recognition of early lung disease as well as in the prediction of lung function in children (46–49). Further studies are needed to assess the role of LCI in tracking the progression of early airway remodelling in children with asthma.
There are several limitations to our study, including the relatively small sample size of the control group. The rate of recruitment was lower than we had anticipated, with objections to the venous blood draw being the primary reason for parental refusal. Although, the sample size in this study was comparable to similar studies conducted in adults (50, 51) and children (52). Nonetheless, the study was adequately powered to detect important differences in circulating ILC subsets in children with asthma compared with healthy controls, and associations between immune cell subsets and responses to virus, HDM and LPS exposure, which are important observations.
A key strength of the present study is that the children with and without asthma were matched for age, which is known to affect immune responses (53). Additionally, asthma was defined by a doctors diagnosis (54). Another strength of this study is the exploration of the associations between whole blood immunophenotype and innate immune responses of PBMCs for the first time in children with asthma.
In summary, our study indicates that children with asthma have impaired innate immune responses, which may explain the high frequency of viral-induced acute exacerbations in this population. Furthermore, asthma was associated with increased frequency of ILC subsets, which could contribute to airway inflammation and tissue remodelling. Increased understanding of innate immune responses may facilitate the development of therapeutic strategies to prevent acute asthma exacerbations in young children with asthma.
Data Availability Statement
The datasets presented in this study can be found in online repositories. The names of the repository/repositories and accession number(s) can be found below: https://ogma.newcastle.edu.au/vital/access/%20/manager/Repository/uon:37141.
Ethics Statement
The studies involving human participants were reviewed and approved by HNEH Ethics Committee (15/06/17/4.03). Written informed consent to participate in this study was provided by the participants’ legal guardian/next of kin.
Author Contribution
LW, BB, MS, AC, MJ, and PW were involved in design of the study. BB, BH, and RM conducted the study. KN was involved in developing the laboratory methods for PBMC isolating and culture. MS, AC, and BH were involved in developing laboratory methods for cell quantification. BH and KN performed the experiments. BH performed the analysis and drafted the manuscript with input from BB, MS, AC, and LW. BB and LW verified the analytical methods. All authors discussed the results and commented on the manuscript. All authors contributed to the article and approved the submitted version.
Funding
This study has been funded by Hunter Medical Research Institute Gastronomic Lunch project grant (G1500957). Funding sources had no role in the study design, collection, analysis, and interpretation of data or in the decision to submit or writing the manuscript.
Conflict of Interest
The authors declare that the research was conducted in the absence of any commercial or financial relationships that could be construed as a potential conflict of interest.
Supplementary Material
The Supplementary Material for this article can be found online at: https://www.frontiersin.org/articles/10.3389/fimmu.2021.664668/full#supplementary-material
References
1. Masoli M, Fabian D, Holt S, Beasley R. Global Initiative for Asthma (GINA) Program. The Global Burden of Asthma: Executive Summary of the GINA Dissemination Committee Report. Allergy (2004) 59:469–78. doi: 10.1111/j.1398-9995.2004.00526.x
2. Schmudde I, Laumonnier Y, Kohl J. Anaphylatoxins Coordinate Innate and Adaptive Immune Responses in Allergic Asthma. Semin Immunol (2013) 25:2–11. doi: 10.1016/j.smim.2013.04.009
3. Xepapadaki P, Papadopoulos NG. Childhood Asthma and Infection: Virus-Induced Exacerbations as Determinants and Modifiers. Eur Respir J (2010) 36:438–45. doi: 10.1183/09031936.00149009
4. Steinke JW, Borish L. Immune Responses in Rhinovirus-Induced Asthma Exacerbations. Curr Allergy Asthma Rep (2016) 16:78. doi: 10.1007/s11882-016-0661-2
5. Contoli M, Message SD, Laza-Stanca V, Edwards MR, Wark PA, Bartlett NW, et al. Role of Deficient Type III Interferon-Lambda Production in Asthma Exacerbations. Nat Med (2006) 12:1023–6. doi: 10.1038/nm1462
6. Kumari A, Dash D, Singh R. Lipopolysaccharide (LPS) Exposure Differently Affects Allergic Asthma Exacerbations and its Amelioration by Intranasal Curcumin in Mice. Cytokine (2015) 76:334–42. doi: 10.1016/j.cyto.2015.07.022
7. Rabinovitch N, Liu AH, Zhang L, Rodes CE, Foarde K, Dutton SJ, et al. Importance of the Personal Endotoxin Cloud in School-Age Children With Asthma. J Allergy Clin Immunol (2005) 116:1053–7. doi: 10.1016/j.jaci.2005.08.045
8. Thorne PS, Kulhankova K, Yin M, Cohn R, Arbes SJ Jr., Zeldin DC. Endotoxin Exposure is a Risk Factor for Asthma: The National Survey of Endotoxin in United States Housing. Am J Respir Crit Care Med (2005) 172:1371–7. doi: 10.1164/rccm.200505-758OC
9. Kim HY, Umetsu DT, Dekruyff RH. Innate Lymphoid Cells in Asthma: Will They Take Your Breath Away? Eur J Immunol (2016) 46:795–806. doi: 10.1002/eji.201444557
10. Jia Y, Fang X, Zhu X, Bai C, Zhu L, Jin M, et al. IL-13+ Type 2 Innate Lymphoid Cells Correlate With Asthma Control Status and Treatment Response. Am J Respir Cell Mol Biol (2016) 55:675–83. doi: 10.1165/rcmb.2016-0099OC
11. Gunawardhana LP, Gibson PG, Simpson JL, Powell H, Baines KJ. Activity and Expression of Histone Acetylases and Deacetylases in Inflammatory Phenotypes of Asthma. Clin Exp Allergy (2014) 44:47–57. doi: 10.1111/cea.12168
12. Han M, Ishikawa T, Bermick JR, Rajput C, Lei J, Goldsmith AM, et al. IL-1β Prevents ILC2 Expansion, Type 2 Cytokine Secretion, and Mucus Metaplasia in Response to Early-Life Rhinovirus Infection in Mice. Allergy (2020) 75:2005–19. doi: 10.1111/all.14241
13. Bergauer A, Sopel N, Kroß B, Vuorinen T, Xepapadaki P, Weiss ST, et al. IFN-α/Ifn-λ Responses to Respiratory Viruses in Paediatric Asthma. Eur Respir J (2017) 49:1600969. doi: 10.1183/13993003.00969-2016
14. Papadopoulos NG, Stanciu LA, Papi A, Holgate ST, Johnston SL. A Defective Type 1 Response to Rhinovirus in Atopic Asthma. Thorax (2002) 57:328–32. doi: 10.1136/thorax.57.4.328
15. Jurak LM, Xi Y, Landgraf M, Carroll ML, Murray L, Upham JW. Interleukin 33 Selectively Augments Rhinovirus-Induced Type 2 Immune Responses in Asthmatic But Not Healthy People. Front Immunol (2018) 9:1895. doi: 10.3389/fimmu.2018.01895
16. Kelly JT, Busse WW. Host Immune Responses to Rhinovirus: Mechanisms in Asthma. J Allergy Clin Immunol (2008) 122:671–82. doi: 10.1016/j.jaci.2008.08.013
17. Ritchie AI, Jackson DJ, Edwards MR, Johnston SL. Airway Epithelial Orchestration of Innate Immune Function in Response to Virus Infection. A Focus Asthma Ann Am Thorac Soc (2016) 13(Suppl 1):S55–63. doi: 10.1513/AnnalsATS.201507-421MG
18. Gill MA, Bajwa G, George TA, Dong CC, Dougherty II, Jiang N, et al. Counterregulation Between the FcepsilonRI Pathway and Antiviral Responses in Human Plasmacytoid Dendritic Cells. J Immunol (2010) 184:5999–6006. doi: 10.4049/jimmunol.0901194
19. Varma TK, Lin CY, Toliver-Kinsky TE, Sherwood ER. Endotoxin-Induced Gamma Interferon Production: Contributing Cell Types and Key Regulatory Factors. Clin Diagn Lab Immunol (2002) 9:530–43. doi: 10.1128/CDLI.9.3.530-543.2002
20. Hallsworth MP, Soh CP, Lane SJ, Arm JP, Lee TH. Selective Enhancement of GM-CSF, TNF-Alpha, IL-1 Beta and IL-8 Production by Monocytes and Macrophages of Asthmatic Subjects. Eur Respir J (1994) 7:1096–102.
21. Hernandez ML, Herbst M, Lay JC, Alexis NE, Brickey WJ, Ting JPY, et al. Atopic Asthmatic Patients Have Reduced Airway Inflammatory Cell Recruitment After Inhaled Endotoxin Challenge Compared With Healthy Volunteers. J Allergy Clin Immunol (2012) 130:869–76.e2. doi: 10.1016/j.jaci.2012.05.026
22. Lun SW, Wong CK, Ko FW, Hui DS, Lam CW. Expression and Functional Analysis of Toll-Like Receptors of Peripheral Blood Cells in Asthmatic Patients: Implication for Immunopathological Mechanism in Asthma. J Clin Immunol (2009) 29:330–42. doi: 10.1007/s10875-008-9269-1
23. Zhu L, Lee PK, Lee WM, Zhao Y, Yu D, Chen Y. Rhinovirus-Induced Major Airway Mucin Production Involves a Novel TLR3-EGFR-dependent Pathway. Am J Respir Cell Mol Biol (2009) 40:610–9. doi: 10.1165/rcmb.2008-0223OC
24. Nurse B, Puterman AS, Haus M, Berman D, Weinberg EG, Potter PC. Pbmcs From Both Atopic Asthmatic and Nonatopic Children Show a TH2 Cytokine Response to House Dust Mite Allergen. J Allergy Clin Immunol (2000) 106:84–91. doi: 10.1067/mai.2000.107397
25. Noma T, Hayashi M, Kawano Y, Yoshizawa I, Ishikawa Y, Saeki T, et al. Functional Interleukin-5 Activity in Peripheral Blood Mononuclear Cells From Adolescents With Mite Antigen Asthma in Remission. Clin Exp Allergy (1999) 29:780–5. doi: 10.1046/j.1365-2222.1999.00584.x
26. Boberg E, Johansson K, Malmhäll C, Weidner J, Rådinger M. House Dust Mite Induces Bone Marrow IL-33-Responsive ILC2s and T(H) Cells. Int J Mol Sci (2020) 21(11):3751. doi: 10.3390/ijms21113751
27. Weber-Chrysochoou C, Crisafulli D, Kemp AS, Britton WJ, Marks GB, Investigators C. Allergen-Specific IL-5 Responses in Early Childhood Predict Asthma at Age Eight. PloS One (2014) 9:e97995–e. doi: 10.1371/journal.pone.0097995
28. Della Corte CM, Morgillo F. Early Use of Steroids Affects Immune Cells and Impairs Immunotherapy Efficacy. ESMO Open (2019) 4:e000477. doi: 10.1136/esmoopen-2018-000477
29. Mahata B, Zhang X, Kolodziejczyk AA, Proserpio V, Haim-Vilmovsky L, Taylor AE, et al. Single-Cell RNA Sequencing Reveals T Helper Cells Synthesizing Steroids De Novo to Contribute to Immune Homeostasis. Cell Rep (2014) 7:1130–42. doi: 10.1016/j.celrep.2014.04.011
30. Oliver BGG, Robinson P, Peters M, Black J. Viral Infections and Asthma: An Inflammatory Interface? Eur Respir J (2014) 44:1666. doi: 10.1183/09031936.00047714
31. Thomas BJ, Porritt RA, Hertzog PJ, Bardin PG, Tate MD. Glucocorticosteroids Enhance Replication of Respiratory Viruses: Effect of Adjuvant Interferon. Sci Rep (2014) 4:7176. doi: 10.1038/srep07176
32. Bartemes KR, Kephart GM, Fox SJ, Kita H. Enhanced Innate Type 2 Immune Response in Peripheral Blood From Patients With Asthma. J Allergy Clin Immunol (2014) 134:671–8.e4. doi: 10.1016/j.jaci.2014.06.024
33. Barraclough B, Sen A, Hussain S, Gaillard E, Cousins D, McCarthy J. Type Two Innate Lymphoid Cells (ILC2s) in the Blood of Children With Acute Asthma. Eur Respir J (2017) 50:PA1336. doi: 10.1183/1393003.congress-2017.PA1336
34. Deckers J, Branco Madeira F, Hammad H. Innate Immune Cells in Asthma. Trends Immunol (2013) 34:540–7. doi: 10.1016/j.it.2013.08.004
35. Loh Z, Simpson J, Ullah A, Zhang V, Gan WJ, Lynch JP, et al. HMGB1 Amplifies ILC2-induced Type-2 Inflammation and Airway Smooth Muscle Remodelling. PloS Pathog (2020) 16:e1008651–e. doi: 10.1371/journal.ppat.1008651
36. Egli A, Santer DM, O’Shea D, Barakat K, Syedbasha M, Vollmer M, et al. IL-28B is a Key Regulator of B- and T-cell Vaccine Responses Against Influenza. PloS Pathog (2014) 10:e1004556. doi: 10.1371/journal.ppat.1004556
37. Thysen AH, Rasmussen MA, Kreiner-Moller E, Larsen JM, Folsgaard NV, Bonnelykke K, et al. Season of Birth Shapes Neonatal Immune Function. J Allergy Clin Immunol (2016) 137:1238–46.e1–13. doi: 10.1016/j.jaci.2015.08.041
38. Gonem S, Natarajan S, Desai D, Desai D, Desai D, Desai D, et al. Clinical Significance of Small Airway Obstruction Markers in Patients With Asthma. Clin Exp Allergy (2014) 44:499–507. doi: 10.1111/cea.12257
39. Shi Y, Aledia AS, Galant SP, George SC. Peripheral Airway Impairment Measured by Oscillometry Predicts Loss of Asthma Control in Children. J Allergy Clin Immunol (2013) 131:718–23. doi: 10.1016/j.jaci.2012.09.022
40. Marotta A, Klinnert MD, Price MR, Larsen GL, Liu AH. Impulse Oscillometry Provides an Effective Measure of Lung Dysfunction in 4-Year-Old Children at Risk for Persistent Asthma. J Allergy Clin Immunol (2003) 112:317–22. doi: 10.1067/mai.2003.1627
41. Schulze J, Biedebach S, Christmann M, Herrmann E, Voss S, Zielen S. Impulse Oscillometry as a Predictor of Asthma Exacerbations in Young Children. Respiration (2016) 91:107–14. doi: 10.1159/000442448
42. Kim HY, Shin YH, Jung DW, Jee HM, Park HW, Han MY. Resistance and Reactance in Oscillation Lung Function Reflect Basal Lung Function and Bronchial Hyperresponsiveness Respectively. Respirology (2009) 14:1035–41. doi: 10.1111/j.1440-1843.2009.01605.x
43. Kim HY, Shin YH, Jung DW, Jee HM, Park HW, Han MY. Use of Impulse Oscillometry System in Assessment of Asthma Severity for Preschool Children. J Asthma (2013) 50:198–203. doi: 10.3109/02770903.2012.751996
44. Macleod KA, Horsley AR, Bell NJ, Greening AP, Innes JA, Cunningham S. Ventilation Heterogeneity in Children With Well Controlled Asthma With Normal Spirometry Indicates Residual Airways Disease. Thorax (2009) 64:33. doi: 10.1136/thx.2007.095018
45. Zwitserloot A, Fuchs SI, Müller C, Bisdorf K, Gappa M. Clinical Application of Inert Gas Multiple Breath Washout in Children and Adolescents With Asthma. Respir Med (2014) 108:1254–9. doi: 10.1016/j.rmed.2014.07.003
46. Ramsey KA, Foong RE, Grdosic J, Harper A, Skoric B, Clem C, et al. Multiple-Breath Washout Outcomes Are Sensitive to Inflammation and Infection in Children With Cystic Fibrosis. Ann Am Thorac Soc (2017) 14:1436–42. doi: 10.1513/AnnalsATS.201611-935OC
47. Nenna R, Midulla F, Lambiase C, De Castro G, Zicari AM, Indinnimeo L, et al. Effects of Inhaled Hypertonic (7%) Saline on Lung Function Test in Preschool Children With Cystic Fibrosis: Results of a Crossover, Randomized Clinical Trial. Ital J Pediatr (2017) 43:60. doi: 10.1186/2Fs13052-017-0376-6
48. Fainardi V, Lombardi E. Lung Function Tests to Monitor Respiratory Disease in Preschool Children. Acta BioMed (2018) 89:148–56. doi: 10.23750/abm.v89i2.7155
49. Nuttall AGL, Velásquez W, Beardsmore CS, Gaillard EA. Lung Clearance Index: Assessment and Utility in Children With Asthma. Eur Respir Rev (2019) 28:154. doi: 10.1183/16000617.0046-2019
50. Wark PA, Johnston SL, Bucchieri F, Powell R, Puddicombe S, Laza-Stanca V, et al. Asthmatic Bronchial Epithelial Cells Have a Deficient Innate Immune Response to Infection With Rhinovirus. J Exp Med (2005) 201:937–47. doi: 10.1084/jem.20041901
51. Forbes RL, Gibson PG, Murphy VE, Wark PA. Impaired Type I and III Interferon Response to Rhinovirus Infection During Pregnancy and Asthma. Thorax (2012) 67:209–14. doi: 10.1136/thoraxjnl-2011-200708
52. Iikura K, Katsunuma T, Saika S, Saito S, Ichinohe S, Ida H, et al. Peripheral Blood Mononuclear Cells From Patients With Bronchial Asthma Show Impaired Innate Immune Responses to Rhinovirus In Vitro. Int Arch Allergy Immunol (2011) 155 Suppl 1:27–33. doi: 10.1159/000327262
53. Simon AK, Hollander GA, McMichael A. Evolution of the Immune System in Humans From Infancy to Old Age. Proc Biol Sci (2015) 282:20143085. doi: 10.1098/rspb.2014.3085
54. Australian Asthma Handbook – Quick Reference Guide, Version 1.3 (2017). National Asthma Council. Available at: http://www.asthmahandbook.org.au (Accessed 15/01/2018).
Keywords: asthma, children, innate immune response, innate lymphoid cells, lung function
Citation: Hosseini B, Berthon BS, Starkey MR, Collison A, McLoughlin RF, Williams EJ, Nichol K, Wark PAB, Jensen ME, Da Silva Sena CR, Baines KJ, Mattes J and Wood LG (2021) Children With Asthma Have Impaired Innate Immunity and Increased Numbers of Type 2 Innate Lymphoid Cells Compared With Healthy Controls. Front. Immunol. 12:664668. doi: 10.3389/fimmu.2021.664668
Received: 05 February 2021; Accepted: 17 May 2021;
Published: 17 June 2021.
Edited by:
Lena Uller, Lund University, SwedenCopyright © 2021 Hosseini, Berthon, Starkey, Collison, McLoughlin, Williams, Nichol, Wark, Jensen, Da Silva Sena, Baines, Mattes and Wood. This is an open-access article distributed under the terms of the Creative Commons Attribution License (CC BY). The use, distribution or reproduction in other forums is permitted, provided the original author(s) and the copyright owner(s) are credited and that the original publication in this journal is cited, in accordance with accepted academic practice. No use, distribution or reproduction is permitted which does not comply with these terms.
*Correspondence: Lisa G. Wood, lisa.wood@newcastle.edu.au