- 1Department of Biology, American University of Beirut, Beirut, Lebanon
- 2Department of Pharmacology and Therapeutics, Beirut Arab University, Beirut, Lebanon
- 3Biomedical Research Center, Qatar University, Doha, Qatar
- 4Department of Pharmacology and Toxicology, American University of Beirut, Beirut, Lebanon
- 5Department of Pharmacology and Toxicology, Faculty of Pharmacy, Alexandria University, Alexandria, Egypt
- 6Department of Basic Medical Sciences, College of Medicine, Qatar University Health, Qatar University, Doha, Qatar
- 7Biomedical and Pharmaceutical Research Unit, Qatar University Health, Qatar University, Doha, Qatar
Globally, over two million people have perished due to the recent pandemic caused by SARS-CoV-2. The available epidemiological global data for SARS-CoV-2 portrays a higher rate of severity and mortality in males. Analyzing gender differences in the host mechanisms involved in SARS-CoV-2 infection and progression may offer insight into the more detrimental disease prognosis and clinical outcome in males. Therefore, we outline sexual dimorphisms which exist in particular host factors and elaborate on how they may contribute to the pronounced severity in male COVID-19 patients. This includes disparities detected in comorbidities, the ACE2 receptor, renin-angiotensin system (RAS), signaling molecules involved in SARS-CoV-2 replication, proteases which prime viral S protein, the immune response, and behavioral considerations. Moreover, we discuss sexual disparities associated with other viruses and a possible gender-dependent response to SARS-CoV-2 vaccines. By specifically highlighting these immune-endocrine processes as well as behavioral factors that differentially exist between the genders, we aim to offer a better understanding in the variations of SARS-CoV-2 pathogenicity.
Introduction
As of March 2021, the World Health Organization (WHO) reported over 100 million confirmed cases of coronavirus disease-2019 (COVID-19) and approximately 2.7 million deaths (1). The available data demonstrates a higher rate of male severity and mortality associated with COVID-19 (2). Even when female infection rates supersede that of males, men commonly present with higher intensive care unit (ICU) admissions and death rates (3). In fact, while females predominated mild and serious cases, there were three times as many male severe pneumonia cases compared to females (4). In addition, the male case fatality rate upon COVID-19 infection, has been consistently greater in countries throughout the world, including England (approximately 27% male: 15% female), Italy (17%: 8%), Spain (10%: 6%) and China (5%:3%) (5). Males generally constituted over 50% of COVID-19 related deaths, as indicated in China (64%), South Korea (53%) and the United Kingdom (UK) (61.7%) (3, 6, 7).
Significant gender-based differences appear to be a common theme in viral infections. Indeed, studies have demonstrated higher incidence and increased fatality rates in males compared to females upon infection with human coronaviruses, SARS-CoV-1 and Middle Eastern Respiratory Syndrome coronavirus (MERS-CoV) (8, 9). However, only a few studies have investigated causes for the discrepancy, which primarily cite an increased pro-inflammatory state in males (10, 11). On the other hand, young females emerged as the most vulnerable group to both the influenza and avian H5N1 virus (12). Moreover, during the 1957 H2N2 pandemic in the United States, the mortality rate of females was higher than males in the same age group of 1-44 years (13). Conversely, hepatitis B virus (HBV) and hepatitis C virus (HCV) are more prevalent in males and this been attributed to the sex hormones (14–16). Some studies linked the relatively higher HBV titer in male serum to testosterone and androgen receptors used to activate HBV genome transcription (16, 17). Testosterone can also increase scavenger receptors required for HCV viral entry (18, 19). By contrast, estrogen downregulates HBV transcription via estrogen receptor alpha (ERα), while selective estrogen receptor modulators (SERMs) inhibit the production of mature HCV (19, 20).
These studies highlight that, depending on the virus, an interplay of different factors like sex hormones and inflammatory processes, may allow the symptoms to manifest more severely in one of the genders. Therefore, it is important to characterize unique host factors involved in the pathogenicity of a specific virus, and how these components differ between males and females. Because the reasons for the apparent gender bias in COVID-19 patients are not completely elucidated, we explore the contribution of several relevant host factors that may drive the increased severity of symptoms detected in males. Many of these components include underlying comorbidities, immuno-endocrine processes, signaling molecules, and host proteases, among many others. Understanding how these may render more protection for females would provide more effective measures for managing COVID-19.
Gender Differences in Comorbidities and the Link to Creatinine
Comorbidities are highly prevalent among COVID-19 fatalities, especially hypertension, diabetes, cardiovascular disease (CVD), and chronic lung diseases (21, 22). Given that the severity and death rates are more pronounced in males, it would be plausible to assume that underlying conditions drive this bias. However, at first glance the relationship between comorbidities, gender and COVID-19 severity is not straightforward. In older individuals, more women (45%) than men (32%) have multiple morbidities (23). Type 2 diabetes (T2D), on the other hand, is equally prevalent in older males and females (24). In addition, deaths due to chronic obstructive pulmonary disease are more prominent in females (25, 26). Moreover, it was demonstrated in a particular study that a higher prevalence of CVDs is associated with postmenopausal women and that females are more prone to ischemic heart disease (27). To further corroborate the obscure link between comorbidities, gender, and COVID-19 severity, it was shown that there was no gender difference in the prevalence of comorbidities in those who perished, including the most common diseases of hypertension, diabetes, CVDs, and chronic lung diseases (4).
Although prevalence of comorbidities was common between the two genders, one of the most striking differences that did exist was the exceptionally elevated levels of creatinine in blood samples of males compared to females (4). High creatinine levels were detected in individuals with severe cases of COVID-19 who were transitioning to multi-organ failure (28). Elevated creatinine serum levels are often corollary to comorbid diseases including diabetes, cancer, chronic renal failure, and some viruses, which can eventually lead to acute kidney injury (AKI) in severely ill and hospitalized patients, including COVID-19 patients (29, 30). The development of AKI in patients with comorbidities is positively correlated with increased mortality. Up to 7% of hospitalized patients and 20%-50% of ICU patients develop AKI, of which 5% require renal replacement therapy (29). Stimulation of the inflammatory response, either to an underlying disease or infection, results in abundant expression of cytokines, like tumor necrosis factor alpha (TNFα) and interleukin 6 (IL6), which lead to endothelial activation of adhesion molecules and further chemokine production (28). Ultimately, leukocytes from the blood infiltrate the interstitial tissue of the kidney, disrupting its processes. The cytokine storm that arises in COVID-19 patients can further exacerbate this state (28). Importantly, it has been shown, that for the same disease, women develop lower levels of serum creatinine than males, like in the case of chronic kidney disease (31). Because comorbid diseases are shown to be equally prevalent in those who have perished from COVID-19, their physiological response- as demonstrated by a highly significant creatinine increase in males compared to females- may be one of the differential contributors to increased disease severity in men (4).
Gender Differences in Cardiac Repair Mechanisms
Notably, patients with underlying cardiovascular related pathologies such as hypertension and coronary heart disease (CHD) develop more detrimental COVID-19 symptoms (32–34). Additionally, CVD is one of the highest risk factors associated with COVID-19 severity and mortality (33–35). Therefore, it may be expected that severely infected males would have a higher CVD prevalence and severity. However, like other morbidities, CVD epidemiological data does not fully support this assumption. For example, while age-adjusted men have a higher incidence of CVD, it is the leading cause of mortality and hospitalizations in females (36). In fact, after the age of 50, women show higher CVD events (37). On the other hand, hypertension is more likely to lead to heart failure in males, rather than females (38). Given the more pronounced CVD symptoms in females and that it is one of the most common underlying co-morbidities in severe COVID-19 patients, it somewhat counter-intuitive that males would predominate the deaths resulting from SARS-CoV-2 (33). An alternative perspective would involve examining gender differences in cardiac repair mechanisms, following SARS-CoV-2 induced cardiac injury.
Direct cardiac injury due to the interaction between SARS-CoV-2 and cardiomyocytes, in addition to the concomitant systemic inflammation, is the primary mechanism by which the virus induces myocardial injury (39). Therefore, it is important to highlight sexual dimorphism which also may exist in cardiac restoration post-injury. Indeed, it was shown that the repair response after injury differs between males and females (38). In animal models, females displayed improved survival in the event of acute heart failure (38). This is associated with limited cardiac remodeling and more efficient functional recovery. Uniquely, females produced amplified levels of reparative leukocytes and epoxy-eicosatrienoic acids, which exert anti-hypertensive and anti-inflammatory effects on blood vessels (38). Collectively, such a female response is associated with a more adequate regulation of inflammation and survival post-cardiac injury. As such, although older females tend to have a higher incidence of CVD compared to age-matched males, it is possible that the reduced female mortality related to COVID-19, may partially be attributed to an enhanced reparative response following cardiovascular injury.
Gender Differences in Host Molecular Components Used by SARS-CoV-2
Cell Receptors
Angiotensin I-Converting Enzyme 2 (ACE2) Expression
One potential contributor to the apparent gender disparity in COVID-19 may be ACE2 expression. This enzyme has been established as the route of entry for SARS-CoV-1 and SARS-CoV-2, upon binding of its spike (S) protein, and it is abundantly expressed in the lungs and heart (40). SARS-CoVs reduce bioavailable ACE2 levels by competitive inhibition and by subsequent cleavage of the active site ectodomain (41–43). The loss of ACE2 is associated with increased lung edema, reduced lung function and diminished protection against acute lung injury and, sometimes, severe acute lung failure (42, 43). In addition, it has been suggested that higher levels of ACE2, ultimately lead to an increase in soluble ACE2 ectodomains in circulation (44). Subsequently, these enzymes would serve as circulating scavengers for SARS-CoV-2, thus limiting their interaction with cell-bound ACE2.
The ace2 gene locus lies on the X chromosome (45). Some scientists suggested that the presence of two alleles from the two X chromosomes in females, compared to males with only one X chromosome, may exert a protective effect (45). However, given that one of the X chromosomes is permanently inactivated in females, it is not likely that heterozygous alleles for ACE2 would emerge in a female. In addition, polymorphism analysis of ACE2 indicated that there are no significant sex-related differences (46). Therefore, while it may be an obvious contender for gender disparities in COVID-19, the effect is likely not associated with sex-related single nucleotide polymorphisms (SNPs).
ACE2 expression in rat lungs is significantly diminished in both genders upon aging (47). However, the reduction is more pronounced in older male rats, which display a 78% reduction in ACE2 expression, in contrast to older females which demonstrated a 67% decrease (47). This has been partially linked to the reduction of sex hormones in aging females and males (48). ACE2 is also reduced in individuals with chronic diseases (48).
According to this data, the individual with the lowest ACE2 expression would be an older male with comorbidities. The added burden of a COVID-19 infection would further jeopardize their already depressed ACE2 levels. This may partially explain their relatively higher severity (as reflected by ICU admissions) and mortality due to COVID-19 (33). However, it is important to also evaluate the change, if any, in ACE2 expression upon SARS-CoV-2 infection, and if the response differs between genders.
Neuropilin-1 (NRP1)
The S protein in SARS-CoV-2 contains a furin cleavage site which is absent in the S protein of other coronaviruses (49). This added site may contribute to enhanced viral binding and host cell invasion upon its cleavage (50). While ACE2 binds to the receptor binding domain (RBD) on the S protein, another cell receptor, neuropilin-1 (NRP1), has recently been shown to bind to the furin-cleavage site on the S1 segment of the S protein (51). Incidentally, endothelial and respiratory epithelial cells express the highest level of NRP1 in the body, which may explain the excessive damage exerted upon lung and myocardial tissue particularly in COVID-19 patients (50). Indeed, blocking NRP1 with monoclonal antibodies greatly reduced SARS-CoV-2 infection of cells in in vitro conditions (51).
NRP1 is often studied in the context of tumorigenesis as it is a receptor for vascular endothelial growth factor (VEGF) and contributes to the cancer hallmarks of angiogenesis, invasion and metastasis (52). In fact, it is often upregulated in several cancers, including stomach adenocarcinoma, squamous cell carcinoma and melanoma (52–55). Although NRP1 expression increases according to the size of these tumors and degree of metastasis, there is no significant difference in expression between males and females (55). Studies comparing baseline NRP1 tissue expression of men and women without cancer or those with various diseases, however, are still lacking. Elucidating this component would establish whether or not NRP1 partially drives the gender disparity associated with COVID-19 severity.
CD147
Cluster of differentiation 147 (CD147), also known as basic immunoglobulin (basigin) or extracellular matrix metalloproteinase inducer (EMMPRIN) is a membrane receptor shown to interact with SARS-CoV-2 (56). In fact, inhibition of this receptor by meplazumab was shown to reduce SARS-CoV-2 amplification (56). It is present in several tissues in the body, and it is especially overexpressed in inflamed tissues. As its name suggests, it induces extracellular matrix proteases, which may contribute to the development of fibrosis. Incidentally, it is relatively abundant in fibrotic lung tissue compared to normal lung tissue (57).
A CD147 expression study has demonstrated that it is not differentially expressed between males and females in primary bronchial epithelial cells or in blood cells (58). However, gender differences were detected in gastric cancer cells, in which males had significantly higher expression of CD147 and this was correlated to a worse prognosis (59). It has yet to be examined whether or not there is differential CD147 expression between men and women at homeostatic conditions or in the presence of other underlying diseases.
Renin-Angiotensin System
The renin-angiotensin system (RAS) has been characterized as the main homeostatic regulator of extracellular fluid via renal, cardiovascular, and cerebral interactions (60). Alterations in fluid balance trigger a series of enzymatic cleavages beginning with renin and culminating in the production of angiotensin II (AngII) by angiotensin-converting enzyme 1 (ACE1) (61). Upon binding with high affinity to angiotensin receptor 1 (AT1), AngII initiates a variety of responses that include vasoconstriction and renal sodium retention (61). On the other hand, the metabolism of AngII to angiotensin 1-7 (Ang1-7) by ACE2 can counteract these activities (62, 63). After identifying RAS components on a variety of other tissues, other roles became evident (62). For example, the ACE1/AngII arm also results in pro-inflammatory, pro-fibrotic and pro-oxidative effects (64–66), while the ACE2/Ang1-7 arm counteracts these processes (64, 67). As such, the ACE2/Ang1-7 axis is mainly characterized as the protective arm of RAS.
Studies have indicated that females have significantly higher blood levels of Ang1-7 compared to males, but similar levels of AngII (68, 69). Correspondingly, these women also had a lower incidence of hypertension compared to males with the same body mass index (BMI) and waist circumference (68, 69). The higher levels of Ang1-7 may enable females to counteract inflammation, such as that induced by COVID-19, more effectively. In fact, supplementation with Ang1-7 is currently being suggested as treatment against SARS-CoV-2 (70).
Signaling Molecules
ERK
Mitogen activated protein kinase (MAPK) pathways involve the sequential phosphorylation of three MAP kinases and culminate in the activation of a downstream effector involved in cellular growth, proliferation, differential, or death (71). An extracellular signal-regulated kinase (ERK) is a major MAPK and is significantly upregulated in most comorbidities present in COVID-19 patients like ischemic heart, CVD, hypertension, diabetes, and cancer (71–77).
It has been shown that several viruses, including some coronaviruses like SARS-CoV-1, Middle East respiratory syndrome (MERS) and likely SARS-CoV-2 also induce ERK for viral replication, and its inhibition results in depressed viral progeny (78, 79). Moreover, cells infected with SARS-CoV-1 display upregulation of ERK and its downstream pro-inflammatory cytokine and monocyte chemoattractant protein-1 (MCP-1) (80). Other cytokines are likely to be potentiated via the same mediator, thus contributing to the detrimental cytokine storm induced by SARS-CoV-2 (80). As such, enhanced ERK activation in chronic inflammatory diseases may amplify SARS-CoV-2 replication and pathogenicity.
Animal studies have demonstrated that ERK is differentially activated in males and females during inflammation (81). Specifically, male- and female-derived rat aortic smooth muscle cells (RASMCs) were induced with conditions of abdominal aortic aneurysm (AAA) (81). As a result, activated (phosphorylated) ERK (p-ERK) expression was significantly higher in male derived cells compared to female cells. Moreover, the downstream target, matrix metalloprotease 2 (MMP-2), which is involved in tissue remodeling, was also correspondingly increased in males (81).
A relatively higher expression of ERK in males compared to females has also been corroborated in human neutrophils, particularly in the context of leukotriene (LT) synthesis by 5-lipoxygenase (5-LO) (82). Production of LTs is preceded by 5-LO translocation from the cytoplasm to the nuclear envelope. Trafficking is more pronounced in activated female neutrophils and highly lacking in males. Translocation of 5-LO is not required in male neutrophils because it already resides in the peri-nuclear region, consequently limiting LT production (82). This phenomenon is due higher levels of dihydrotestosterone (5α-DHT), which activate ERK, thus maintaining a constant localization of 5-LO near the nuclear envelope. These findings indicate that male sex hormones can rapidly induce and sustain enhanced expression of ERK, possibly via 5α-DHT receptors like those that may exist on neutrophils (82).
These studies demonstrated in both inflammatory states (AAA) and normal physiological states (neutrophils) that ERK activation is relatively higher in males. The apparent male bias in COVID-19 severity could partially be attributed to higher basal levels of ERK expression, which are further exacerbated in chronic diseases, thus enhancing viral replication and associated tissue damage.
STAT1
One of the most rapid anti-viral responses in the host involves the secretion of type I interferons α and β (IFNα/β) (83). Type I IFN receptor is present throughout most host cells and activates the downstream JAK1/STAT1 (Janus kinase 1/signal transducer and activator of transcription 1) signaling pathway (83). STAT1 predominantly induces the expression of genes that hinder viral replication (83). Incidentally, coronaviruses like SARS-CoV-1 are sensitive to the activity of type I IFNs, and their growth can be hindered by the addition of IFNα/β. However, SARS-CoVs do not endogenously induce IFNα/β in infected host cells, which contributes to their severe and prolonged pathogenicity due to their ability to evade the initial immune response (83).
In animal models, healthy females have presented with higher baseline expression of IFNα-induced genes in immune cells, such as B lymphocytes (84). This phenomenon was linked to 17-β-estradiol in females, which further augments JAK1/STAT1 signaling (84). Moreover, estrogen signaling via ERα has been shown to promote type I IFN synthesis (85).
Moreover, in certain chronic diseases, STAT1 in females is more upregulated compared to age-matched males (84). These findings suggest that higher STAT1 levels in females, especially in immune cells, may equip them with a more ‘prepared’ initial anti-viral response. It may also offer insight into why females, who have a higher prevalence of the underlying chronic diseases, present with less COVID-19 severity and mortality compared to males.
Host Proteases Associated With SARS-Cov-2 Entry
ADAM17
Efficient cellular entry of SARS-CoVs involves adhesion of the outer spike protein (S) to the membrane bound ACE2 (86, 87). Thereafter, the membrane-bound enzyme, a disintegrin and metalloproteinase 17 (ADAM17), cleaves the active site ectodomain of ACE2, shedding it into the plasma (88). Inhibition of ADAM17 is associated with lower coronavirus entry into a cell, indicating its relevant, yet covert, role in viral uptake (89). ADAM17 also cleaves and activates a number of immunomodulatory membrane-associated proteins like tumor necrosis factor α (TNFα) (90, 91). In fact, concomitant to ACE2 cleavage, is increased activation of TNFα, which is thought to initiate the tissue damage associated with viral invasion (89).
Gender differences in ADAM17 activity and expression are generally lacking. However, sex disparities in ADAM17 have been detected in relation to AAA (92). A particular SNP in the ADAM17 gene was linked to a higher expression and activity of ADAM17, and an increased risk of AAA in males compared to females (92). It may be worth examining if pulmonary ADAM17 activity varies between males and females to better understand the gender gap in SARS-CoV-2 severity.
TMPRSS2
Indispensable for SARS-CoV-2 entry into host cells is the transmembrane serine protease 2 (TMPRSS2) (87). Upon cleaving the S protein at S1 and S2’, TMPRSS2 facilitates the initial partitioning of this protein into the host cell membrane (93). TMPRSS2 has previously been established as a gender biased protein as it is regulated by an androgen-sensitive promoter and is most abundantly expressed in prostate cells (94). Four androgen receptor (AR) binding sites (ARBs) are located in the promoter region of TMPRSS2, two of which respond to androgen stimulation and require the transcription factors GATA Zinc Finger Domain-Containing Protein 2A and 2B (GATA2A and GATA2B, respectively) for efficient transcription (94).
In animal studies, it was previously shown that GATA transcription factors, including GATA2, are differentially expressed in male and female tissues, particularly at different stages of development (95). It is not yet known if SARS-CoV-2 enhances GATA expression and if there is gender disparity in its expression. Moreover, because TMPRSS2 is androgen-responsive, it would therefore be tempting to expect TMPRSS2 expression to be elevated in males. However, a study examining lung TMPRSS2 expression in the lungs indicated that there is no significant difference between males and females regardless of the age group (46). Nevertheless, this does not necessarily rule out differential expression in other tissues.
Although expression levels did not differ, several distinct SNPs are prevalent in the TMPRSS2 gene. In fact, one of the most prominent variant haplotypes, termed the “European” haplotype was completely absent in Asians (46). Importantly, this haplotype is suggested to have an enhanced androgen-response for TMPRSS2 expression (46). It is not yet known if this haplotype is also more prevalent in males. Because TMPRSS2 plays an integral role in viral invasion, a thorough examination of its expression in various tissues among males and females – in both homeostatic and pathological conditions- would further clarify the male bias in COVID-19.
Furin
Another important membrane protease associated with SARS-CoV-2 viral entry is furin (50). The furin cleavage site (FCS) is located upstream of the S1 cleavage site in the S protein. The FCS is not present in SARS-CoV-1 and it is thought to lend a greater capacity for SARS-CoV-2 to infect cells- however this is still disputed (49). It has been demonstrated, though, that deletion of the FCS within the S protein is associated with reduced replication in respiratory cells and pathogenesis in vivo (50). Gender disparity in furin expression has been previously demonstrated in asthmatic patients. Specifically, bronchial cell samples obtained from asthmatic patients revealed that furin is positively associated with the male gender and this was speculated as a possible factor contributing to COVID-19 severity (96). Further analysis of genetic variations at the FCS and any associated gender differences may offer insight to the more pronounced COVID-19 severity and mortality in males.
Gender Differences in the Immune Response
Immune Response Background
An effective immune response is essential for viral elimination, yet, upon over-activation, as in some chronic conditions, it can induce cellular injury and lead to aberrant antigenic response with immunological pathology (97). Activation of host cell pattern recognition receptors (PRRs) in response to viral pathogen-associated molecular patterns (PAMPs) in innate immune cells, including dendritic cells and macrophages, initiates the response of the innate immune system. Specifically, PRRs which include toll-like receptors (TLRs) and retinoic acid-inducible gene I-like receptors (RLRs), lead to downstream activation of the JAK/STAT pathway, resulting in the production and secretion of pro-inflammatory cytokines particularly type I and III interferons (IFNs) (98, 99). Other pro-inflammatory molecules secreted include interleukin-12 (IL-12), TNFα, and chemokine ligand 2 (CCL2), and upon uncontrolled expression, are collectively responsible for the associated pulmonary injury (98, 100). As a consequence, the degree to which the virus activates PRR influences the magnitude of immune cell recruitment and inflammatory cytokine production.
Gender Disparities in the Innate and Adaptive Anti-Viral Immune Response
Sexual dimorphism in the susceptibility and response to viral pathogens has been previously documented in both clinical and pre-clinical studies. In general, females seem to be less susceptible to viral infections. Indeed, females mount higher innate immune responses than males, resulting in a faster viral recognition and production of IFN and inflammatory cytokines, inducing more rapid viral clearance (101, 102). In fact, the activity and number of innate active immune cells, including macrophages, monocytes, and dendritic cells (DCs), are higher in females than males (103–105). In addition, the innate activity of PRRs in detecting viral nucleic acids is more effective in females (104). For example, during viral infection, TLR7 – a PRR that detects single stranded viral RNA- stimulates higher production of IFNβ in females (97). These findings suggest that upon infection with SARS-CoV-2, females may be better equipped to initially respond, and attenuate viral invasion and pathogenicity compared to males.
Gender differences have also been detected in the adaptive immune response, where females exhibit a higher humoral and cell-mediated immune responses than males (106). In females, antigen-presenting cells (APC) are more efficient at presenting peptides than males (107). Thus, communication between the innate and adaptive cells may occur more rapidly in females once SARS-CoV-2 is detected. In fact, following the activation of the adaptive immune cells by APC, the levels of antibodies (106, 108), as well as immunoglobulins, are greater in females than males in both human and experimental murine models (109, 110). Specifically, upon viral infection, females exhibit higher levels of cluster of differentiation 4 (CD4+) T cells, thus activating a greater number of effector T cells (111, 112). Additionally, a more robust inflammatory/cytotoxic T-cell response along with an upregulation of the pro-inflammatory genes is reported in females, indicating that viral clearance is also more adequate (113) (Figure 1).
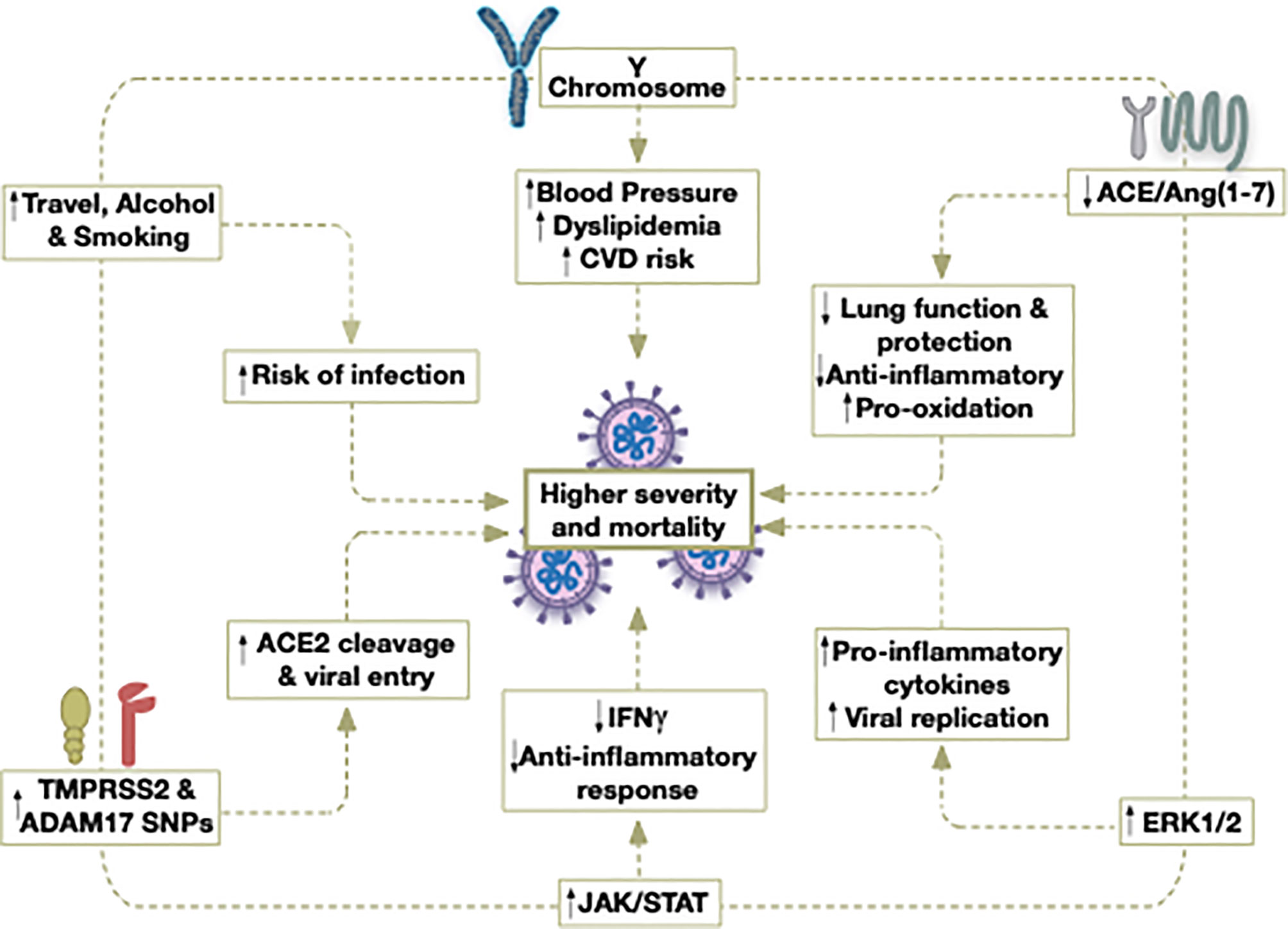
Figure 1 Potential contributors to the higher severity and mortality caused by COVID-19 in older males.
Based on this, one can deduce that females, and due to their stronger, more effective anti-viral immunity beginning from initial viral invasion and culminating with viral clearance, are less prone to develop severe viral infections than males do. These findings may also be extended to the response of females after infection with SARS-CoV-2. However, it should be noted that, following viral clearance and return to homeostasis, a prolonged immune system activation in women may contribute to increased development of immunopathology associated with viral infections (101, 108). On the other hand, the lower immune response to the viral infection at homeostasis is responsible for the persistent viral infections seen in males (101, 108). The seemingly more robust immune response present in females may be partially attributed to the role of sex hormones.
As for SARS-CoV-2, what is known till now is that once the virus is recognized by alveolar macrophages, it will trigger the generation of pro-inflammatory cytokines and chemokines (114–116). Studies have shown that patients with severe symptoms, a dysfunctional immune response occurs caused by hyperactivation of macrophages and monocytes, which triggers a cytokine storm mediating a widespread inflammation and thus multi-organ damage (114, 116). Cytotoxic T cells are thereafter recruited and Th1/Th7 cells stimulation could lead to aggravated inflammatory response (117). B cells recognize viral proteins and are activated to produce SARS-CoV-2 specific antibodies (118). The detected humoral response directed against SARS-CoV-2 has been found to be of great similarity with other coronavirus infections, initiating the production of specific immunoglobulin G and M (IgG and IgM) (119, 120). These specific antibodies produced aid in viral deactivation as well as providing systemic immunity in different organs (117). Though studies are now compiling, it seems that SARS-CoV-2 possess similar cellular invasion mechanisms as SARS-CoV-1 and as such a similar trend in gender differences could be observed.
Estrogen Commonly Enhances the Innate and Adaptive Immune Response
Apart from factors merely related to discrepancies in the immune and inflammatory responses, other factors, including sex hormones, act as key modulators of immune responses (121). Receptors for sex hormones have been identified on immune cells, suggesting a direct effect of those hormones on the immune response (122). The binding of hormones to their respective receptors directly alters cell signaling pathways, including nuclear factor kappa-light-chain-enhancer of activated B cells (NF-kB), the transcription factor cJun, and IFN regulatory factor (IRF), resulting in variable production of cytokines and chemokines, in addition to regulating the development and maturation of immune cells (122).
Estrogen receptor (ER) subtypes ERα and ERβ, are expressed in various immune cells such as lymphocytes, macrophages, and dendritic cells (85). ERα appears to be the key regulator in cellular differentiation of hematopoietic progenitor cells (123). ERs induce activation of surface receptors (as insulin growth factor), ERK/MAPK, protein kinase C (PKC) and cyclic adenosine monophosphate (cAMP) signaling (124, 125). Additionally, ERs expressed on leukocytes modulate their function: low levels of estrogen tend to increase the synthesis of pro-inflammatory cytokines and high levels decrease their production (Figure 2) (108, 126). Moreover, estrogen promotes adaptive T cell response against viral infection through increasing neutrophil accumulation (127). It also induces monocyte differentiation into inflammatory DC, inducing greater production of cytokines and IFNs and activates T helper 1 cells (Th1) (108). Additionally, estrogen inhibits TLR4-mediated NFκB activation in macrophages via suppression of micro-RNAs and increases anti-inflammatory type-1 IFN via TLR (10, 11). Estrogen also regulates STAT activity by increasing the expression of cytokine inhibitor proteins in T cells and macrophages (128). ER activation suppresses the expression of CC-motif ligand-2 (CCL2) in leukocytes, therefore decreasing their migration (128). Estrogen has a crucial role in modulating zinc finger proteins that regulate various metabolic functions, cell cycle, and lipid metabolism pathways involved in the progression of influenza A infection (129).
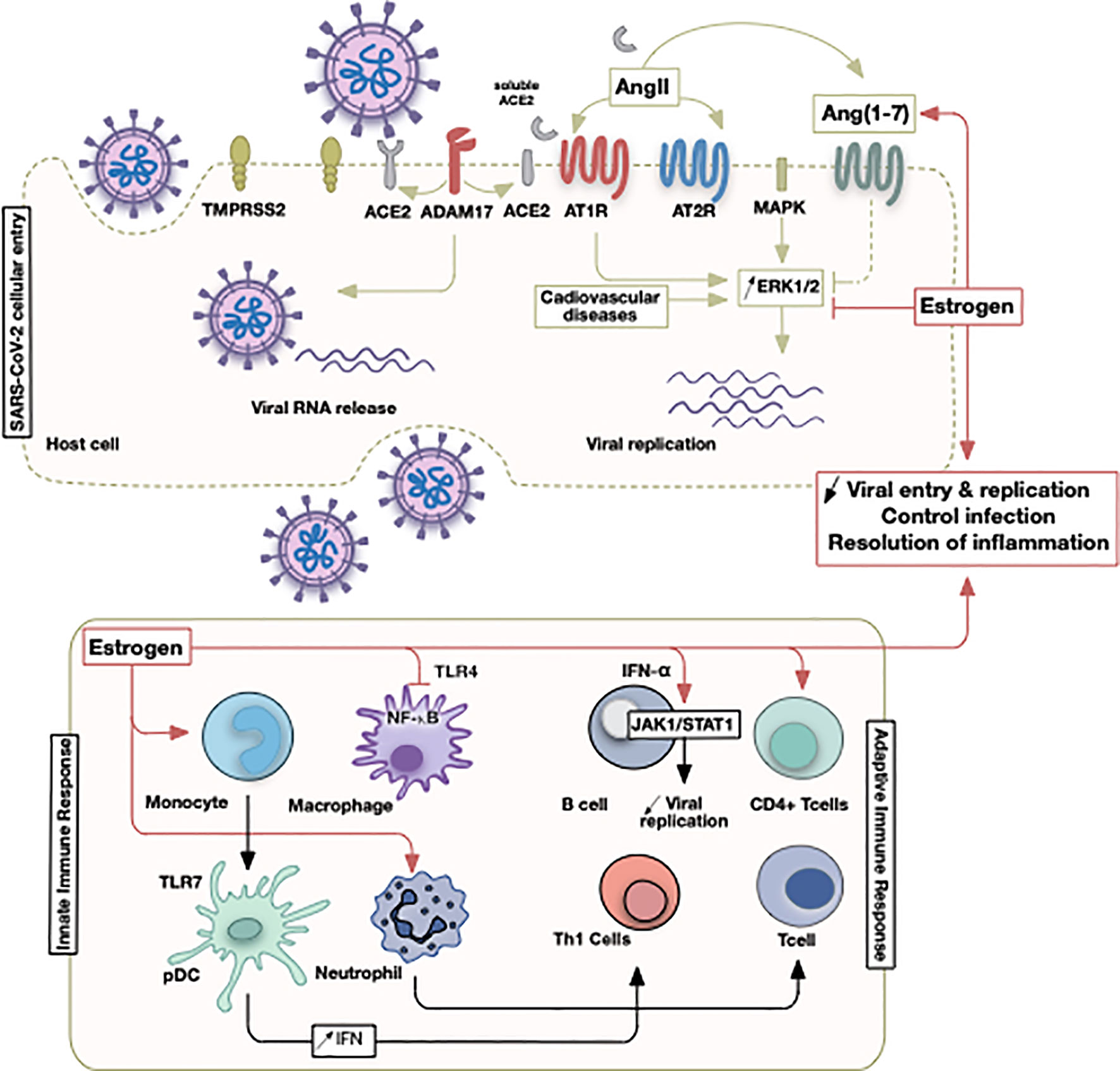
Figure 2 Illustrative summary of events involving estrogen in COVID-19. The initial SARS-CoV-2 viral entry and recruitment of innate and adaptive immune cells culminate in a pathological state following viral infection or successful control of infection. Sex discrepancies could be pertinent due to estrogen hormone that operates at different points as indicated.
While estrogen often activates target immune cells, testosterone and its active metabolite suppress immune cell activity as well as the production of inflammatory cytokines (130, 131). Specifically, stimulation of androgen receptors reduces the production of pro-inflammatory cytokines (132). Indeed, androgen deficiency in males is associated with high levels of inflammatory cytokines and increased CD4+/CD8+ T-cells ratio (132, 133).
Despite these findings, it is essential to highlight that age-related changes to innate immunity should not be overseen, which have previously been described (134). Primarily, levels of hormones in both males and females are not always stable. Estrogen levels in females vary during the menstrual cycle, pregnancy and fall after menopause; however, in males, testosterone levels remain stable until 60 years of age. More importantly, high estrogen levels decrease innate immunity, increase Th2-induced responses, and enhance humoral immune activity (135). Therefore, women, based on their age and estrogen and receptor levels, might present multiple reactions to viral infections. Nevertheless, these overall findings suggest that the commonly pronounced levels of estrogen in females are driving a more robust response against the infection with SARS-CoV-2.
Selective Estrogen Receptor Modulators as a Treatment Against COVID-19?
As presented earlier, over expression of ERs was found to suppress viral replication (136). Repurposing selective estrogen receptor modulators (SERMs) as anti-viral drugs had led to their use against Ebola virus, human immunodeficiency virus (HIV), and HCV infections (137). SERMs were found to interfere at the post-viral entry step and suppress Th1 cells while inducing Th2 cytokine expression (138). A particular SERM, tamoxifen, has been repurposed for anti-viral activity in cases of vesicular stomatitis as well as HCV infections. It inhibits vesicular stomatitis viral replication by enhancing type I IFN response and macrophage stimulation (139). Moreover, Tamoxifen targets several steps in HCV infection via ERα signaling (20). HCV replication, post replication, viral attachment on the cell surface as well as viral entry were inhibited by Tamoxifen (20).
Another SERM drug, Raloxifene, was found to reduce influenza A virus titer in human female nasal epithelial cells (129). Viral infections by MERS-CoV and SARS-CoV in established cell lines were inhibited by Toremifene, a nonsteroidal SERM (140). The drug’s mechanism of action involves interaction with CoV membrane glycoproteins, thereafter destabilizing it and inhibiting its replication (140).
Importantly, ERs are expressed in the upper and lower respiratory tract and targeting these receptors in respiratory viral infections is of great importance (141). The presence of ER implies an important role of estrogen in pulmonary cellular physiology and offers a possible route for protecting against lung diseases using SERMs. Importantly, many women currently taking SERMs as estrogen replacement therapy or as a treatment for other diseases may benefit from their mitigating effects on spontaneous viral infections (127). Altogether, SERMs (like Toremifene and Raloxifene) could offer a possible add on treatment for COVID-19.
Behavioral and Social Gender Differences
Gender discrepancies in the exposure to viral infections are due to evident disparities in social roles, activities, and behaviors (142). Proper assessment of differences in disease exposure is warranted when studying gender discrepancies in the incidence of infections. Males tend to have more social contacts, travel more frequently, and spend more time in settings that could be conducive to viral transmission (143). In addition, gender roles influence how and where males and females spend their time and the quality of healthcare they receive. Moreover, gender differences in access to healthcare facilities in specific countries may lead to further variability in disease progression. For example, boys in India usually have better follow up from healthcare professionals and medical care than girls (144).
Additionally, behavioral factors are involved in gender discrepancies affecting the burden of viral infections (145–147). Alcohol consumption and cigarette smoking exert complex and detrimental effects on systemic and pulmonary immunity. Both factors suppress a wide range of immune responses, therefore, predispose individuals to the acquisition of further infections (145, 146, 148). Alcohol drinking and cigarette smoking habit are higher in males than females, which would therefore render men more prone to alterations in the immune responses (145, 146, 149–151). For example, the risk of tuberculosis infection is seen to be higher in men than in women (147). Furthermore, high alcohol consumption is linked to liver cirrhosis as well as increased mortality and morbidity rates. Recent studies have documented the association of liver diseases as well as smoking with a higher risk for severe COVID-19 and mortality; therefore, smoking and alcohol cessation should be encouraged (152, 153).
Gender Differences in Vaccine Response
COVID-19 vaccine development is expected to be the most effective way to control the pandemic and combat this novel virus. It is quite interesting that besides the role of sexual differences in infection immunity, sexual differences are documented to be important in the immune response to vaccines (154, 155). Females do mount greater immune response to vaccinations than men thus facilitating vaccine efficacy, yet they more commonly experience severe side effects (154, 156, 157). Unfortunately, there is serious lack of attention to sexual dimorphism in vaccine clinical trials.
There are currently several vaccines against SARS-CoV-2 that have been rolled out (158). Early reports of clinical trials for SARS-CoV-2 vaccination have not addressed sexual differences (159–161). Studies reported promising immunogenicity and safety outcomes. In addition, local and general adverse effects are being addressed yet not segregated by gender (160, 161). However, a clinical trial of the adenovirus-vector vaccine candidate measured adverse effects outcomes and reported that females experienced side effects such as fever more commonly than males (162).
Concluding Remarks
To date, COVID-19 still represents a worldwide health concern. Culminating evidence demonstrates more favorable COVID-19 outcomes in adult females, especially in the mortality rate. Among the possible factors discussed are those related to females’ molecular factors associated with comorbidities, factors affecting SARS-CoV-2 cellular entry, RAS, specific signaling pathways affecting viral replication, and tissue damage as well as those related to immune-endocrine processes. The data presented above provide potential mechanisms, including the role of sex hormones in the different susceptibility and outcomes of COVID-19 infection (Table 1). However, because virus severity is not always lower in females (as demonstrated by some influenza viruses), it is important to recognize unique host cell components exploited by individual viruses and to characterize any associated sexual dimorphism. In our opinion, further epidemiological and molecular studies are needed to identify the key-players in gender bias in response to illness, disease outcomes and more importantly in response to anti-viral treatment modalities.
Author Contributions
AE conceived of the idea. ZW, SH, MF, and HY wrote the first draft. HY critically edited the first draft. AE-Y and AE did the critical edit of the next four drafts. AE made the final edits on the last draft. All authors contributed to the article and approved the submitted version.
Conflict of Interest
The authors declare that the research was conducted in the absence of any commercial or financial relationships that could be construed as a potential conflict of interest.
References
1. World Health Organization. (2021). Available at: https://www.who.int/emergencies/diseases/novel-coronavirus-2019.
2. Abduljalil JM, Abduljalil BM. Epidemiology, Genome, and Clinical Features of the Pandemic SARS-CoV-2: A Recent View. New Microbes New Infect (2020) 35:100672. doi: 10.1016/j.nmni.2020.100672
3. Shim E, Tariq A, Choi W, Lee Y, Chowell G. Transmission Potential and Severity of COVID-19 in South Korea. Int J Infect Dis (2020) 93:339–44. doi: 10.1016/j.ijid.2020.03.031
4. Jin JM, Bai P, He W, Wu F, Liu XF, Han DM, et al. Gender Differences in Patients With COVID-19: Focus on Severity and Mortality. Front Public Health (2020) 8:152. doi: 10.3389/fpubh.2020.00152
5. Scully EP, Haverfield J, Ursin RL, Tannenbaum C, Klein SL. Considering How Biological Sex Impacts Immune Responses and COVID-19 Outcomes. Nat Rev Immunol (2020) 20(7):442–7. doi: 10.1038/s41577-020-0348-8
6. Wu Z, McGoogan JM. Characteristics of and Important Lessons From the Coronavirus Disease 2019 (Covid-19) Outbreak in China: Summary of a Report of 72314 Cases From the Chinese Center for Disease Control and Prevention. Jama (2020) 323:1239–42. doi: 10.1001/jama.2020.2648
7. Mohamed MO, Gale CP, Kontopantelis E, Doran T, de Belder M, Asaria M, et al. Sex Differences in Mortality Rates and Underlying Conditions for COVID-19 Deaths in England and Wales. Mayo Clin Proc (2020) 95(10):2110–24. doi: 10.1016/j.mayocp.2020.07.009
8. Karlberg J, Chong DS, Lai WY. Do Men Have a Higher Case Fatality Rate of Severe Acute Respiratory Syndrome Than Women do? Am J Epidemiol (2004) 159(3):229–31. doi: 10.1093/aje/kwh056
9. Leong HN, Earnest A, Lim HH, Chin CF, Tan C, Puhaindran ME, et al. SARS in Singapore–predictors of Disease Severity. Ann Acad Med Singapore (2006) 35(5):326–31.
10. Murphy AJ, Guyre PM, Pioli PA. Estradiol Suppresses NF-Kappa B Activation Through Coordinated Regulation of let-7a and miR-125b in Primary Human Macrophages. J Immunol (2010) 184(9):5029–37. doi: 10.4049/jimmunol.0903463
11. Channappanavar R, Fett C, Mack M, Ten Eyck PP, Meyerholz DK, Perlman S. Sex-Based Differences in Susceptibility to Severe Acute Respiratory Syndrome Coronavirus Infection. J Immunol (2017) 198(10):4046–53. doi: 10.4049/jimmunol.1601896
12. Klein SL, Pekosz APC, Anker M. P. O. Sex, Gender and Influenza. Geneva: World Health Organization (2010). p. 1–58.
13. Serfling RE, Sherman IL, Houseworth WJ. Excess Pneumonia-Influenza Mortality by Age and Sex in Three Major Influenza A2 Epidemics, United States, 1957-58, 1960 and 1963. Am J Epidemiol (1967) 86(2):433–41. doi: 10.1093/oxfordjournals.aje.a120753
14. Ruggieri A, Barbati C, Malorni W. Cellular and Molecular Mechanisms Involved in Hepatocellular Carcinoma Gender Disparity. Int J Cancer (2010) 127(3):499–504. doi: 10.1002/ijc.25298
15. Tsay PK, Tai DI, Chen YM, Yu CP, Wan SY, Shen YJ, et al. Impact of Gender, Viral Transmission and Aging in the Prevalence of Hepatitis B Surface Antigen. Chang Gung Med J (2009) 32(2):155–64.
16. Breidbart S, Burk RD, Saenger P. Hormonal Regulation of Hepatitis B Virus Gene Expression: Influence of Androgen Receptor. Pediatr Res (1993) 34(3):300–2. doi: 10.1203/00006450-199309000-00012
17. Ruggieri A, Gagliardi MC, Anticoli S. Sex-Dependent Outcome of Hepatitis B and C Viruses Infections: Synergy of Sex Hormones and Immune Responses? Front Immunol (2018) 9:2302. doi: 10.3389/fimmu.2018.02302
18. Scarselli E, Ansuini H, Cerino R, Roccasecca RM, Acali S, Filocamo G, et al. The Human Scavenger Receptor Class B Type I is a Novel Candidate Receptor for the Hepatitis C Virus. EMBO J (2002) 21(19):5017–25. doi: 10.1093/emboj/cdf529
19. Hayashida K, Shoji I, Deng L, Jiang DP, Ide YH, Hotta H. 17β-Estradiol Inhibits the Production of Infectious Particles of Hepatitis C Virus. Microbiol Immunol (2010) 54(11):684–90. doi: 10.1111/j.1348-0421.2010.00268.x
20. Murakami Y, Fukasawa M, Kaneko Y, Suzuki T, Wakita T, Fukazawa H. Selective Estrogen Receptor Modulators Inhibit Hepatitis C Virus Infection At Multiple Steps of the Virus Life Cycle. Microbes Infect (2013) 15(1):45–55. doi: 10.1016/j.micinf.2012.10.003
21. Feng Y, Ling Y, Bai T, Xie Y, Huang J, Li J, et al. Covid-19 With Different Severity: A Multi-Center Study of Clinical Features. Am J Respir Crit Care Med (2020) 201:1380–8. doi: 10.1164/rccm.202002-0445OC
22. Garg S, Kim L, Whitaker M, O’Halloran A, Cummings C, Holstein R, et al. Hospitalization Rates and Characteristics of Patients Hospitalized With Laboratory-Confirmed Coronavirus Disease 2019 - COVID-NET, 14 States, March 1-30, 2020. MMWR Morb Mortal Wkly Rep (2020) 69(15):458–64. doi: 10.15585/mmwr.mm6915e3
23. Abad-Díez JM, Calderón-Larrañaga A, Poncel-Falcó A, Poblador-Plou B, Calderón-Meza JM, Sicras-Mainar A, et al. Age and Gender Differences in the Prevalence and Patterns of Multimorbidity in the Older Population. BMC Geriatr (2014) 14:75. doi: 10.1186/1471-2318-14-75
24. Gale EA, Gillespie KM. Diabetes and Gender. Diabetologia (2001) 44(1):3–15. doi: 10.1007/s001250051573
25. Han MK, Postma D, Mannino DM, Giardino ND, Buist S, Curtis JL, et al. Gender and Chronic Obstructive Pulmonary Disease: Why it Matters. Am J Respir Crit Care Med (2007) 176(12):1179–84. doi: 10.1164/rccm.200704-553CC
26. Podcasy JL, Epperson CN. Considering Sex and Gender in Alzheimer Disease and Other Dementias. Dialog Clin Neurosci (2016) 18(4):437–46. doi: 10.31887/DCNS.2016.18.4/cepperson
27. Li J, Chen X, McClusky R, Ruiz-Sundstrom M, Itoh Y, Umar S, et al. The Number of X Chromosomes Influences Protection From Cardiac Ischaemia/Reperfusion Injury in Mice: One X is Better Than Two. Cardiovasc Res (2014) 102(3):375–84. doi: 10.1093/cvr/cvu064
28. Ragab D, Salah Eldin H, Taeimah M, Khattab R, Salem R. The COVID-19 Cytokine Storm; What We Know So Far. Front Immunol (2020) 11:1446. doi: 10.3389/fimmu.2020.01446
29. Farooqi S, Dickhout JG. Major Comorbid Disease Processes Associated With Increased Incidence of Acute Kidney Injury. World J Nephrol (2016) 5(2):139–46. doi: 10.5527/wjn.v5.i2.139
30. Raza A, Estepa A, Chan V, Jafar MS. Acute Renal Failure in Critically Ill COVID-19 Patients With a Focus on the Role of Renal Replacement Therapy: A Review of What We Know So Far. Cureus (2020) 12(6):e8429. doi: 10.7759/cureus.8429
31. Coggins CH, Breyer Lewis J, Caggiula AW, Castaldo LS, Klahr S, Wang SR. Differences Between Women and Men With Chronic Renal Disease. Nephrol Dial Transplant (1998) 13(6):1430–7. doi: 10.1093/ndt/13.6.1430
32. Guan WJ, Ni ZY, Hu Y, Liang WH, Ou CQ, He JX, et al. Clinical Characteristics of Coronavirus Disease 2019 in China. N Engl J Med (2020) 58:711–2. doi: 10.1056/NEJMoa2002032
33. Huang C, Wang Y, Li X, Ren L, Zhao J, Hu Y, et al. Clinical Features of Patients Infected With 2019 Novel Coronavirus in Wuhan, China. Lancet (2020) 395(10223):497–506. doi: 10.1016/S0140-6736(20)30183-5
34. Wehbe Z, Hammoud S, Soudani N, Zaraket H, El-Yazbi A, Eid AH. Molecular Insights Into SARS Cov-2 Interaction With Cardiovascular Disease: Role of RAAS and MAPK Signaling. Front Pharmacol (2020) 11:836. doi: 10.3389/fphar.2020.00836
35. Zheng YY, Ma YT, Zhang JY, Xie X. Covid-19 and the Cardiovascular System. Nat Rev Cardiol (2020) 17:259–60. doi: 10.1038/s41569-020-0360-5
36. Woodward M. Cardiovascular Disease and the Female Disadvantage. Int J Environ Res Public Health (2019) 16(7):1165. doi: 10.3390/ijerph16071165
37. Möller-Leimkühler AM. Gender Differences in Cardiovascular Disease and Comorbid Depression. Dialog Clin Neurosci (2007) 9(1):71. doi: 10.31887/DCNS.2007.9.1/ammoeller
38. Pullen AB, Kain V, Serhan CN, Halade GV. Molecular and Cellular Differences in Cardiac Repair of Male and Female Mice. J Am Heart Assoc (2020) 9(8):e015672. doi: 10.1161/JAHA.119.015672
39. Bansal M. Cardiovascular Disease and COVID-19. Diabetes Metab Syndr (2020) 14(3):247–50. doi: 10.1016/j.dsx.2020.03.013
40. Chen Y, Guo Y, Pan Y, Zhao ZJ. Structure Analysis of the Receptor Binding of 2019-nCoV. Biochem Biophys Res Commun (2020) 525:135–40. doi: 10.1016/j.bbrc.2020.02.071
41. Fu Y, Cheng Y, Wu Y. Understanding SARS-CoV-2-Mediated Inflammatory Responses: From Mechanisms to Potential Therapeutic Tools. Virol Sin (2020) 35:266–71. doi: 10.1007/s12250-020-00207-4
42. Kuba K, Imai Y, Rao S, Gao H, Guo F, Guan B, et al. A Crucial Role of Angiotensin Converting Enzyme 2 (ACE2) in SARS Coronavirus-Induced Lung Injury. Nat Med (2005) 11(8):875–9. doi: 10.1038/nm1267
43. Imai Y, Kuba K, Rao S, Huan Y, Guo F, Guan B, et al. Angiotensin-Converting Enzyme 2 Protects From Severe Acute Lung Failure. Nature (2005) 436(7047):112–6. doi: 10.1038/nature03712
44. Brojakowska A, Narula J, Shimony R, Bander J. Clinical Implications of SARS-CoV-2 Interaction With Renin Angiotensin System: Jacc Review Topic of the Week. J Am Coll Cardiol (2020) 75(24):3085–95. doi: 10.1016/j.jacc.2020.04.028
45. Gemmati D, Bramanti B, Serino ML, Secchiero P, Zauli G, Tisato V. Covid-19 and Individual Genetic Susceptibility/Receptivity: Role of ACE1/ACE2 Genes, Immunity, Inflammation and Coagulation. Might the Double X-Chromosome in Females be Protective Against SARS-CoV-2 Compared to the Single X-Chromosome in Males? Int J Mol Sci (2020) 21(10):3474. doi: 10.3390/ijms21103474
46. Asselta R, Paraboschi EM, Mantovani A, Duga S. ACE2 and TMPRSS2 variants and expression as candidates to sex and country differences in COVID-19 severity in Italy. Aging (Albany NY) (2020) 12(11):10087–98. doi: 10.18632/aging.103415
47. Xie X, Xudong X, Chen J, Junzhu C, Wang X, Xingxiang W, et al. Age- and Gender-Related Difference of ACE2 Expression in Rat Lung. Life Sci (2006) 78(19):2166–71. doi: 10.1016/j.lfs.2005.09.038
48. Cristiani L, Mancino E, Matera L, Nenna R, Pierangeli A, Scagnolari C, et al. Will Children Reveal Their Secret? The Coronavirus Dilemma. Eur Respir J (2020) 55(4). doi: 10.1183/13993003.00749-2020
49. Coutard B, Valle C, de Lamballerie X, Canard B, Seidah NG, Decroly E. The Spike Glycoprotein of the New Coronavirus 2019-nCoV Contains a Furin-Like Cleavage Site Absent in CoV of the Same Clade. Antiviral Res (2020) 176:104742. doi: 10.1016/j.antiviral.2020.104742
50. Johnson BA, Xie X, Kalveram B, Lokugamage KG, Muruato A, Zou J, et al. Furin Cleavage Site Is Key to SARS-CoV-2 Pathogenesis. (2020). doi: 10.1101/2020.08.26.268854
51. Cantuti-Castelvetri L, Ojha R, Pedro LD, Djannatian M, Franz J, Kuivanen S, et al. Neuropilin-1 Facilitates SARS-CoV-2 Cell Entry and Infectivity. Science (2020) 370(6518):856–60. doi: 10.1126/science.abd2985
52. Lu J, Cheng Y, Zhang G, Tang Y, Dong Z, McElwee KJ, et al. Increased Expression of Neuropilin 1 in Melanoma Progression and its Prognostic Significance in Patients With Melanoma. Mol Med Rep (2015) 12(2):2668–76. doi: 10.3892/mmr.2015.3752
53. Kang JY, Gil M, Kim KE. Neuropilin1 Expression Acts as a Prognostic Marker in Stomach Adenocarcinoma by Predicting the Infiltration of Treg Cells and M2 Macrophages. J Clin Med (2020) 9(5):1430. doi: 10.3390/jcm9051430
54. Cao H, Li Y, Huang L, Bai B, Xu Z. Clinicopathological Significance of Neuropilin 1 Expression in Gastric Cancer: A Meta-Analysis. Dis Markers (2020) 2020:4763492. doi: 10.1155/2020/4763492
55. Alattar M, Omo A, Elsharawy M, Li J. Neuropilin-1 Expression in Squamous Cell Carcinoma of the Oesophagus. Eur J Cardiothorac Surg (2014) 45(3):514–20. doi: 10.1093/ejcts/ezt380
56. Wang K, Chen W, Zhang Z, Deng Y, Lian JQ, Du P, et al. CD147-Spike Protein is a Novel Route for SARS-CoV-2 Infection to Host Cells. Signal Transd Target Ther (2020) 5(1):283. doi: 10.1038/s41392-020-00426-x
57. Masre SF, Jufri NF, Ibrahim FW, Abdul Raub SH. Classical and Alternative Receptors for SARS-CoV-2 Therapeutic Strategy. Rev Med Virol (2020) 27:e2207. doi: 10.1002/rmv.2207
58. Radzikowska U, Ding M, Tan G, Zhakparov D, Peng Y, Wawrzyniak P, et al. Distribution of ACE2, Cd147, CD26, and Other SARS-CoV-2 Associated Molecules in Tissues and Immune Cells in Health and in Asthma, COPD, Obesity, Hypertension, and COVID-19 Risk Factors. Allergy (2020) 75(11):2829–45. doi: 10.1111/all.14429
59. Zheng HC, Gong BC. CD147 Expression was Positively Linked to Aggressiveness and Worse Prognosis of Gastric Cancer: A Meta and Bioinformatics Analysis. Oncotarget (2017) 8(52):90358–70. doi: 10.18632/oncotarget.20089
60. Almeida LF, Tofteng SS, Madsen K, Jensen BL. Role of the Renin-Angiotensin System in Kidney Development and Programming of Adult Blood Pressure. Clin Sci (Lond) (2020) 134(6):641–56. doi: 10.1042/CS20190765
61. Sparks MA, Crowley SD, Gurley SB, Mirotsou M, Coffman TM. Classical Renin-Angiotensin System in Kidney Physiology. Compr Physiol (2014) 4(3):1201–28. doi: 10.1002/cphy.c130040
62. Hamming I, Timens W, Bulthuis ML, Lely AT, Navis G, van Goor H. Tissue Distribution of ACE2 Protein, the Functional Receptor for SARS Coronavirus. A First Step Understanding SARS Pathogen J Pathol (2004) 203(2):631–7. doi: 10.1002/path.1570
63. Simões e Silva AC, Silveira KD, Ferreira AJ, Teixeira MM. ACE2, Angiotensin-(1-7) and Mas Receptor Axis in Inflammation and Fibrosis. Br J Pharmacol (2013) 169(3):477–92. doi: 10.1111/bph.12159
64. Paz Ocaranza M, Riquelme JA, García L, Jalil JE, Chiong M, Santos RAS, et al. Counter-Regulatory Renin-Angiotensin System in Cardiovascular Disease. Nat Rev Cardiol (2020) 17(2):116–29. doi: 10.1038/s41569-019-0244-8
65. Rocha NP, Simoes E Silva AC, Prestes TRR, Feracin V, Machado CA, Ferreira RN, et al. RAS in the Central Nervous System: Potential Role in Neuropsychiatric Disorders. Curr Med Chem (2018) 25(28):3333–52. doi: 10.2174/0929867325666180226102358
66. Kangussu LM, Marzano LAS, Souza CF, Dantas CC, Miranda AS, Simões E Silva AC. The Renin-Angiotensin System and the Cerebrovascular Diseases: Experimental and Clinical Evidence. Protein Pept Lett (2019) 27:463–75. doi: 10.2174/0929866527666191218091823
67. Tikellis C, Thomas MC. Angiotensin-Converting Enzyme 2 (Ace2) Is a Key Modulator of the Renin Angiotensin System in Health and Disease. Int J Pept (2012) 2012:256294. doi: 10.1155/2012/256294
68. Cohall DH, Scantlebury-Manning T, James S, Hall K, Ferrario CM. Renin-Angiotensin-Aldosterone System Gender Differences in an Afro-Caribbean Population. J Renin Angiotensin Aldosterone Syst (2015) 16(3):539–46. doi: 10.1177/1470320314523659
69. Sullivan JC, Rodriguez-Miguelez P, Zimmerman MA, Harris RA. Differences in Angiotensin (1-7) Between Men and Women. Am J Physiol Heart Circ Physiol (2015) 308(9):H1171–6. doi: 10.1152/ajpheart.00897.2014
70. Imanpour H, Rezaee H, Nouri-Vaskeh M. Angiotensin 1-7: A Novel Strategy in COVID-19 Treatment. Adv Pharm Bull (2020) 10(4):488–9. doi: 10.34172/apb.2020.068
71. Muslin AJ. MAPK Signalling in Cardiovascular Health and Disease: Molecular Mechanisms and Therapeutic Targets. Clin Sci (Lond) (2008) 115(7):203–18. doi: 10.1042/CS20070430
72. Bueno OF, De Windt LJ, Tymitz KM, Witt SA, Kimball TR, Klevitsky R, et al. The MEK1-ERK1/2 Signaling Pathway Promotes Compensated Cardiac Hypertrophy in Transgenic Mice. EMBO J (2000) 19(23):6341–50. doi: 10.1093/emboj/19.23.6341
73. Haq S, Choukroun G, Lim H, Tymitz KM, del Monte F, Gwathmey J, et al. Differential Activation of Signal Transduction Pathways in Human Hearts With Hypertrophy Versus Advanced Heart Failure. Circulation (2001) 103(5):670–7. doi: 10.1161/01.cir.103.5.670
74. Esposito G, Prasad SV, Rapacciuolo A, Mao L, Koch WJ, Rockman HA. Cardiac Overexpression of a G(q) Inhibitor Blocks Induction of Extracellular Signal-Regulated Kinase and C-Jun NH(2)-terminal Kinase Activity in In Vivo Pressure Overload. Circulation (2001) 103(10):1453–8. doi: 10.1161/01.cir.103.10.1453
75. Gao JH, Wang CH, Tong H, Wen SL, Huang ZY, Tang CW. Targeting Inhibition of Extracellular Signal-Regulated Kinase Kinase Pathway With AZD6244 (Arry-142886) Suppresses Growth and Angiogenesis of Gastric Cancer. Sci Rep (2015) 5:16382. doi: 10.1038/srep16382
76. Mohammad G, Mairaj Siddiquei M, Imtiaz Nawaz M, Abu El-Asrar AM. The ERK1/2 Inhibitor U0126 Attenuates Diabetes-Induced Upregulation of MMP-9 and Biomarkers of Inflammation in the Retina. J Diabetes Res (2013) 2013:658548. doi: 10.1155/2013/658548
77. Cao Z, Tong X, Xia W, Chen L, Zhang X, Yu B, et al. CXCR7/P-ERK-Signaling Is a Novel Target for Therapeutic Vasculogenesis in Patients With Coronary Artery Disease. PloS One (2016) 11(9):e0161255. doi: 10.1371/journal.pone.0161255
78. Cai Y, Liu Y, Zhang X. Suppression of Coronavirus Replication by Inhibition of the MEK Signaling Pathway. J Virol (2007) 81(2):446–56. doi: 10.1128/JVI.01705-06
79. Icard P, Lincet H, Wu Z, Coquerel A, Forgez P, Alifano M, et al. The Key Role of Warburg Effect in SARS-CoV-2 Replication and Associated Inflammatory Response. Biochimie (2021) 180:169–77. doi: 10.1016/j.biochi.2020.11.010
80. Varshney B, Lal SK. Sars-CoV Accessory Protein 3b Induces AP-1 Transcriptional Activity Through Activation of JNK and ERK Pathways. Biochemistry (2011) 50(24):5419–25. doi: 10.1021/bi200303r
81. Ehrlichman LK, Ford JW, Roelofs KJ, Tedeschi-Filho W, Futchko JS, Ramacciotti E, et al. Gender-Dependent Differential Phosphorylation in the ERK Signaling Pathway is Associated With Increased MMP2 Activity in Rat Aortic Smooth Muscle Cells. J Surg Res (2010) 160(1):18–24. doi: 10.1016/j.jss.2009.03.095
82. Pergola C, Dodt G, Rossi A, Neunhoeffer E, Lawrenz B, Northoff H, et al. ERK-Mediated Regulation of Leukotriene Biosynthesis by Androgens: A Molecular Basis for Gender Differences in Inflammation and Asthma. Proc Natl Acad Sci USA (2008) 105(50):19881–6. doi: 10.1073/pnas.0809120105
83. Thiel V, Weber F. Interferon and Cytokine Responses to SARS-coronavirus Infection. Cytokine Growth Factor Rev (2008) 19(2):121–32. doi: 10.1016/j.cytogfr.2008.01.001
84. Dong G, Fan H, Yang Y, Zhao G, You M, Wang T, et al. 17β-Estradiol Enhances the Activation of IFN-α Signaling in B Cells by Down-Regulating the Expression of let-7e-5p, miR-98-5p and miR-145a-5p That Target Ikkϵ. Biochim Biophys Acta (2015) 1852(8):1585–98. doi: 10.1016/j.bbadis.2015.04.019
85. Kovats S. Estrogen Receptors Regulate Innate Immune Cells and Signaling Pathways. Cell Immunol (2015) 294(2):63–9. doi: 10.1016/j.cellimm.2015.01.018
86. Jia HP, Look DC, Shi L, Hickey M, Pewe L, Netland J, et al. ACE2 Receptor Expression and Severe Acute Respiratory Syndrome Coronavirus Infection Depend on Differentiation of Human Airway Epithelia. J Virol (2005) 79(23):14614–21. doi: 10.1128/JVI.79.23.14614-14621.2005
87. Hoffmann M, Kleine-Weber H, Schroeder S, Krüger N, Herrler T, Erichsen S, et al. SARS-Cov-2 Cell Entry Depends on ACE2 and TMPRSS2 and Is Blocked by a Clinically Proven Protease Inhibitor. Cell (2020) 181:271–80. doi: 10.1016/j.cell.2020.02.052
88. Jia HP, Look DC, Tan P, Shi L, Hickey M, Gakhar L, et al. Ectodomain Shedding of Angiotensin Converting Enzyme 2 in Human Airway Epithelia. Am J Physiol Lung Cell Mol Physiol (2009) 297(1):L84–96. doi: 10.1152/ajplung.00071.2009
89. Haga S, Yamamoto N, Nakai-Murakami C, Osawa Y, Tokunaga K, Sata T, et al. Modulation of TNF-alpha-converting Enzyme by the Spike Protein of SARS-CoV and ACE2 Induces TNF-alpha Production and Facilitates Viral Entry. Proc Natl Acad Sci USA (2008) 105(22):7809–14. doi: 10.1073/pnas.0711241105
90. Menghini R, Fiorentino L, Casagrande V, Lauro R, Federici M. The Role of ADAM17 in Metabolic Inflammation. Atherosclerosis (2013) 228(1):12–7. doi: 10.1016/j.atherosclerosis.2013.01.024
91. Düsterhöft S, Lokau J, Garbers C. The Metalloprotease ADAM17 in Inflammation and Cancer. Pathol Res Pract (2019) 215(6):152410. doi: 10.1016/j.prp.2019.04.002
92. Li Y, Yang C, Ma G, Cui L, Gu X, Chen Y, et al. Analysis of ADAM17 Polymorphisms and Susceptibility to Sporadic Abdominal Aortic Aneurysm. Cell Physiol Biochem (2014) 33(5):1426–38. doi: 10.1159/000358708
93. Mollica V, Rizzo A, Massari F. The Pivotal Role of TMPRSS2 in Coronavirus Disease 2019 and Prostate Cancer. Future Oncol (2020) 16(27):2029–33. doi: 10.2217/fon-2020-0571
94. Clinckemalie L, Spans L, Dubois V, Laurent M, Helsen C, Joniau S, et al. Androgen Regulation of the TMPRSS2 Gene and the Effect of a SNP in an Androgen Response Element. Mol Endocrinol (2013) 27(12):2028–40. doi: 10.1210/me.2013-1098
95. Piprek RP, Damulewicz M, Tassan JP, Kloc M, Kubiak JZ. Transcriptome Profiling Reveals Male- and Female-Specific Gene Expression Pattern and Novel Gene Candidates for the Control of Sex Determination and Gonad Development in Xenopus Laevis. Dev Genes Evol (2019) 229(2-3):53–72. doi: 10.1007/s00427-019-00630-y
96. Kermani NZ, Song WJ, Badi Y, Versi A, Guo Y, Sun K, et al. Sputum ACE2, TMPRSS2 and FURIN Gene Expression in Severe Neutrophilic Asthma. Respir Res (2021) 22(1):10. doi: 10.1186/s12931-020-01605-8
97. Ghosh S, Klein RS. Sex Drives Dimorphic Immune Responses to Viral Infections. J Immunol (2017) 198(5):1782–90. doi: 10.4049/jimmunol.1601166
98. Newton AH, Cardani A, Braciale TJ. The Host Immune Response in Respiratory Virus Infection: Balancing Virus Clearance and Immunopathology. Semin Immunopathol (2016) 38(4):471–82. doi: 10.1007/s00281-016-0558-0
99. Horvath CM. The Jak-STAT Pathway Stimulated by Interferon Alpha or Interferon Beta. Sci STKE (2004) 2004(260). doi: 10.1126/stke.2602004tr10
100. Yoo JK, Kim TS, Hufford MM, Braciale TJ. Viral Infection of the Lung: Host Response and Sequelae. J Allergy Clin Immunol (2013) 132(6):1263–76. doi: 10.1016/j.jaci.2013.06.006
101. Klein SL. Sex Influences Immune Responses to Viruses, and Efficacy of Prophylaxis and Treatments for Viral Diseases. Bioessays (2012) 34(12):1050–9. doi: 10.1002/bies.201200099
102. Melgert BN, Oriss TB, Qi Z, Dixon-McCarthy B, Geerlings M, Hylkema MN, et al. Macrophages: Regulators of Sex Differences in Asthma? Am J Respir Cell Mol Biol (2010) 42(5):595–603. doi: 10.1165/rcmb.2009-0016OC
103. Pisitkun P, Deane JA, Difilippantonio MJ, Tarasenko T, Satterthwaite AB, Bolland S. Autoreactive B Cell Responses to RNA-related Antigens Due to TLR7 Gene Duplication. Science (2006) 312(5780):1669–72. doi: 10.1126/science.1124978
104. Berghöfer B, Frommer T, Haley G, Fink L, Bein G, Hackstein H. TLR7 Ligands Induce Higher IFN-alpha Production in Females. J Immunol (2006) 177(4):2088–96. doi: 10.4049/jimmunol.177.4.2088
105. Fish EN. The X-files in Immunity: Sex-Based Differences Predispose Immune Responses. Nat Rev Immunol (2008) 8(9):737–44. doi: 10.1038/nri2394
106. Klein SL, Jedlicka A Fau - Pekosz A, Pekosz A. The Xs and Y of Immune Responses to Viral Vaccines. Lancet Infect Dis (2010) 10:1474–4457. doi: 10.1016/S1473-3099(10)70049-9
107. Weinstein Y, Ran S, Segal S. Sex-Associated Differences in the Regulation of Immune Responses Controlled by the MHC of the Mouse. J Immunol (1984) 132(2):656–61.
108. Klein SL, Flanagan KL. Sex Differences in Immune Responses. Nat Rev Immunol (2016) 16(10):626–38. doi: 10.1038/nri.2016.90
109. Butterworth M, McClellan B, Allansmith M. Influence of Sex in Immunoglobulin Levels. Nature (1967) 214(5094):1224–5. doi: 10.1038/2141224a0
110. Klein SL, Marriott I, Fish EN. Sex-Based Differences in Immune Function and Responses to Vaccination. Trans R Soc Trop Med Hyg (2015) 109(1):9–15. doi: 10.1093/trstmh/tru167
111. Donnelly CA, Bartley LM, Ghani AC, Le Fevre AM, Kwong GP, Cowling BJ, et al. Gender Difference in HIV-1 RNA Viral Loads. HIV Med (2005) 6(3):170–8. doi: 10.1111/j.1468-1293.2005.00285.x
112. Hsieh AR, Fann CS, Yeh CT, Lin HC, Wan SY, Chen YC, et al. Effects of Sex and Generation on Hepatitis B Viral Load in Families With Hepatocellular Carcinoma. World J Gastroenterol (2017) 23(5):876–84. doi: 10.3748/wjg.v23.i5.876
113. Hewagama A, Patel D, Yarlagadda S, Strickland FM, Richardson BC. Stronger Inflammatory/Cytotoxic T-cell Response in Women Identified by Microarray Analysis. Genes Immun (2009) 10(5):509–16. doi: 10.1038/gene.2009.12
114. Tay MZ, Poh CM, Rénia L, MacAry PA, Ng LFP. The Trinity of COVID-19: Immunity, Inflammation and Intervention. Nat Rev Immunol (2020) 20:1–12. doi: 10.1038/s41577-020-0311-8
115. di Mauro G, Cristina S, Concetta R, Francesco R, Annalisa C. Sars-Cov-2 Infection: Response of Human Immune System and Possible Implications for the Rapid Test and Treatment. Int Immunopharmacol (2020) 84:106519. doi: 10.1016/j.intimp.2020.106519
116. Nikolich-Zugich J, Knox KS, Rios CT, Natt B, Bhattacharya D, Fain MJ. Sars-CoV-2 and COVID-19 in Older Adults: What We may Expect Regarding Pathogenesis, Immune Responses, and Outcomes. GeroScience (2020) 42:1–10. doi: 10.1007/s11357-020-00186-0
117. Shah VK, Firmal P, Alam A, Ganguly D, Chattopadhyay S. Overview of Immune Response During SARS-Cov-2 Infection: Lessons From the Past. Front Immunol (2020) 11:1949. doi: 10.3389/fimmu.2020.01949
118. Zhang Y, Xu J, Jia R, Yi C, Gu W, Liu P, et al. Protective Humoral Immunity in SARS-CoV-2 Infected Pediatric Patients. Cell Mol Immunol (2020) 17(7):768–70. doi: 10.1038/s41423-020-0438-3
119. Li G, Chen X, Xu A. Profile of Specific Antibodies to the SARS-associated Coronavirus. N Engl J Med (2003) 349(5):508–9. doi: 10.1056/nejm200307313490520
120. Wu HS, Hsieh YC, Su IJ, Lin TH, Chiu SC, Hsu YF, et al. Early Detection of Antibodies Against Various Structural Proteins of the SARS-associated Coronavirus in SARS Patients. J BioMed Sci (2004) 11(1):117–26. doi: 10.1007/bf02256554
121. Roved J, Westerdahl H, Hasselquist D. Sex Differences in Immune Responses: Hormonal Effects, Antagonistic Selection, and Evolutionary Consequences. Horm Behav (2017) 88:95–105. doi: 10.1016/j.yhbeh.2016.11.017
122. Bhatia A, Sekhon HK, Kaur G. Sex Hormones and Immune Dimorphism. ScientificWorldJournal (2014) 2014:159150. doi: 10.1155/2014/159150
123. Nakada D, Oguro H, Levi BP, Ryan N, Kitano A, Saitoh Y, et al. Oestrogen Increases Haematopoietic Stem-Cell Self-Renewal in Females and During Pregnancy. Nature (2014) 505(7484):555–8. doi: 10.1038/nature12932
124. Zhou T, Yang Z, Chen Y, Chen Y, Huang Z, You B, et al. Estrogen Accelerates Cutaneous Wound Healing by Promoting Proliferation of Epidermal Keratinocytes Via Erk/Akt Signaling Pathway. Cell Physiol Biochem (2016) 38(3):959–68. doi: 10.1159/000443048
125. Xie H, Hua C, Sun L, Zhao X, Fan H, Dou H, et al. 17β-Estradiol Induces CD40 Expression in Dendritic Cells Via MAPK Signaling Pathways in a Minichromosome Maintenance Protein 6-Dependent Manner. Arthritis Rheum (2011) 63(8):2425–35. doi: 10.1002/art.30420
126. Xing D, Miller A, Novak L, Rocha R, Chen YF, Oparil S. Estradiol and Progestins Differentially Modulate Leukocyte Infiltration After Vascular Injury. Circulation (2004) 109(2):234–41. doi: 10.1161/01.Cir.0000105700.95607.49
127. Robinson DP, Hall OJ, Nilles TL, Bream JH, Klein SL. 17β-estradiol protects females against influenza by recruiting neutrophils and increasing virus-specific cd8 t cell responses in the lungs. J Virol (2014) 88(9):4711–20. doi: 10.1128/jvi.02081-13
128. Pan T, Zhong L, Wu S, Cao Y, Yang Q, Cai Z, et al. 17β-Oestradiol Enhances the Expansion and Activation of Myeloid-Derived Suppressor Cells Via Signal Transducer and Activator of Transcription (STAT)-3 Signalling in Human Pregnancy. Clin Exp Immunol (2016) 185(1):86–97. doi: 10.1111/cei.12790
129. Peretz J, Pekosz A, Lane AP, Klein SL. Estrogenic Compounds Reduce Influenza A Virus Replication in Primary Human Nasal Epithelial Cells Derived From Female, But Not Male, Donors. Am J Physiol Lung Cell Mol Physiol (2016) 310(5):L415–25. doi: 10.1152/ajplung.00398.2015
130. Roberts CW, Walker W, Alexander J. Sex-Associated Hormones and Immunity to Protozoan Parasites. Clin Microbiol Rev (2001) 14(3):476–88. doi: 10.1128/cmr.14.3.476-488.2001
131. Maggio M, Basaria S, Ble A, Lauretani F, Bandinelli S, Ceda GP, et al. Correlation Between Testosterone and the Inflammatory Marker Soluble Interleukin-6 Receptor in Older Men. J Clin Endocrinol Metab (2006) 91(1):345–7. doi: 10.1210/jc.2005-1097
132. Malkin CJ, Pugh PJ, Jones RD, Kapoor D, Channer KS, Jones TH. The Effect of Testosterone Replacement on Endogenous Inflammatory Cytokines and Lipid Profiles in Hypogonadal Men. J Clin Endocrinol Metab (2004) 89(7):3313–8. doi: 10.1210/jc.2003-031069
133. Bobjer J, Katrinaki M, Tsatsanis C, Lundberg Giwercman Y, Giwercman A. Negative Association Between Testosterone Concentration and Inflammatory Markers in Young Men: A Nested Cross-Sectional Study. PloS One (2013) 8(4):e61466. doi: 10.1371/journal.pone.0061466
134. Gubbels Bupp MR, Potluri T, Fink AL, Klein SL. The Confluence of Sex Hormones and Aging on Immunity. Front Immunol (2018) 9:1269. doi: 10.3389/fimmu.2018.01269
135. Seillet C, Laffont S, Trémollières F, Rouquié N, Ribot C, Arnal JF, et al. The TLR-mediated Response of Plasmacytoid Dendritic Cells is Positively Regulated by Estradiol In Vivo Through Cell-Intrinsic Estrogen Receptor α Signaling. Blood (2012) 119(2):454–64. doi: 10.1182/blood-2011-08-371831
136. Lasso G, Mayer SV, Winkelmann ER, Chu T, Elliot O, Patino-Galindo JA, et al. A structure-informed atlas of human-virus interactions. Cell (2019) 178(6):1526–41.e1516. doi: 10.1016/j.cell.2019.08.005
137. Montoya MC, Krysan DJ. Repurposing Estrogen Receptor Antagonists for the Treatment of Infectious Disease. mBio (2018) 9(6). doi: 10.1128/mBio.02272-18
138. Johansen LM, Brannan JM, Delos SE, Shoemaker CJ, Stossel A, Lear C, et al. Fda-approved selective estrogen receptor modulators inhibit ebola virus infection. Sci Transl Med (2013) 5190:190ra179. doi: 10.1126/scitranslmed.3005471
139. Cham LB, Friedrich SK, Adomati T, Bhat H, Schiller M, Bergerhausen M, et al. Tamoxifen Protects From Vesicular Stomatitis Virus Infection. Pharmaceut (Basel) (2019) 12(4):142. doi: 10.3390/ph12040142
140. Dyall J, Coleman CM, Hart BJ, Venkataraman T, Holbrook MR, Kindrachuk J, et al. Repurposing of clinically developed drugs for treatment of middle east respiratory syndrome coronavirus infection. Antimicrob Agents Chemother (2014) 58(8):4885–93. doi: 10.1128/aac.03036-14
141. Ivanova MM, Mazhawidza W, Dougherty SM, Minna JD, Klinge CM. Activity and Intracellular Location of Estrogen Receptors Alpha and Beta in Human Bronchial Epithelial Cells. Mol Cell Endocrinol (2009) 305(1–2):12–21. doi: 10.1016/j.mce.2009.01.021
142. Nhamoyebonde S, Leslie A. Biological Differences Between the Sexes and Susceptibility to Tuberculosis. J Infect Dis (2014) 209 Suppl 3:S100–6. doi: 10.1093/infdis/jiu147
143. Oni T, Gideon HP, Bangani N, Tsekela R, Seldon R, Wood K, et al. Smoking, BCG and Employment and the Risk of Tuberculosis Infection in HIV-infected Persons in South Africa. PloS One (2012) 7(10):e47072. doi: 10.1371/journal.pone.0047072
144. Anker M. Addressing Sex and Gender in Epidemic-Prone Infectious Diseases. Geneva: World Health Organ Rep (2007).
145. Perumal R, Naidoo K, Padayatchi N. TB Epidemiology: Where are the Young Women? Know Your Tuberculosis Epidemic, Know Your Response. BMC Public Health (2018) 18(1):417. doi: 10.1186/s12889-018-5362-4
146. Qiu F, Liang CL, Liu H, Zeng YQ, Hou S, Huang S, et al. Impacts of Cigarette Smoking on Immune Responsiveness: Up and Down or Upside Down? Oncotarget (2017) 8(1):268–84. doi: 10.18632/oncotarget.13613
147. Yates TA, Atkinson SH. Ironing Out Sex Differences in Tuberculosis Prevalence. Int J Tuberc Lung Dis (2017) 21(5):483–4. doi: 10.5588/ijtld.17.0194
148. Romeo J, Wärnberg J, Marcos A. Drinking Pattern and Socio-Cultural Aspects on Immune Response: An Overview. Proc Nutr Soc (2010) 69(3):341–6. doi: 10.1017/s0029665110001904
149. Zeman MV, Hiraki L, Sellers EM. Gender Differences in Tobacco Smoking: Higher Relative Exposure to Smoke Than Nicotine in Women. J Womens Health Gend Based Med (2002) 11(2):147–53. doi: 10.1089/152460902753645281
150. Han J, Chen X. A Meta-Analysis of Cigarette Smoking Prevalence Among Adolescents in China: 1981-2010. Int J Environ Res Public Health (2015) 12(5):4617–30. doi: 10.3390/ijerph120504617
151. Ely M, Hardy R, Longford NT, Wadsworth ME. Gender Differences in the Relationship Between Alcohol Consumption and Drink Problems are Largely Accounted for by Body Water. Alcohol Alcohol (1999) 34(6):894–902. doi: 10.1093/alcalc/34.6.894
152. Vardavas CI, Nikitara K. Covid-19 and Smoking: A Systematic Review of the Evidence. Tob Induc Dis (2020) 18:20. doi: 10.18332/tid/119324
153. Guo YR, Cao QD, Hong ZS, Tan YY, Chen SD, Jin HJ, et al. The Origin, Transmission and Clinical Therapies on Coronavirus Disease 2019 (COVID-19) Outbreak - an Update on the Status. Mil Med Res (2020) 7(1):11. doi: 10.1186/s40779-020-00240-0
154. Fischinger S, Boudreau CM, Butler AL, Streeck H, Alter G. Sex Differences in Vaccine-Induced Humoral Immunity. Semin Immunopathol (2019) 41(2):239–49. doi: 10.1007/s00281-018-0726-5
155. Harper A, Flanagan KL. Effect of Sex on Vaccination Outcomes: Important But Frequently Overlooked. Curr Opin Pharmacol (2018) 41:122–7. doi: 10.1016/j.coph.2018.05.009
156. Fink AL, Klein SL. Sex and Gender Impact Immune Responses to Vaccines Among the Elderly. Physiol (Bethesda) (2015) 30(6):408–16. doi: 10.1152/physiol.00035.2015
157. Fink AL, Klein SL. The Evolution of Greater Humoral Immunity in Females Than Males: Implications for Vaccine Efficacy. Curr Opin Physiol (2018) 6:16–20. doi: 10.1016/j.cophys.2018.03.010
158. Krammer F. Sars-CoV-2 Vaccines in Development. Nature (2020) 586(7830):516–27. doi: 10.1038/s41586-020-2798-3
159. Brady E, Nielsen MW, Andersen JP, Oertelt-Prigione S. Lack of Consideration of Sex and Gender in Clinical Trials for COVID-19. (2020). doi: 10.1101/2020.09.13.20193680
160. Jackson LA, Anderson EJ, Rouphael NG, Roberts PC, Makhene M, Coler RN, et al. An Mrna Vaccine Against SARS-CoV-2 - Preliminary Report. N Engl J Med (2020) 383(20):1920–31. doi: 10.1056/NEJMoa2022483
161. Folegatti PM, Ewer KJ, Aley PK, Angus B, Becker S, Belij-Rammerstorfer S, et al. Safety and Immunogenicity of the ChAdOx1 nCoV-19 Vaccine Against SARS-CoV-2: A Preliminary Report of a Phase 1/2, Single-Blind, Randomised Controlled Trial. Lancet (2020) 396(10249):467–78. doi: 10.1016/s0140-6736(20)31604-4
Keywords: COVID-19, SARS-CoV-2, vaccine, ACE-2, gender difference
Citation: Wehbe Z, Hammoud SH, Yassine HM, Fardoun M, El-Yazbi AF and Eid AH (2021) Molecular and Biological Mechanisms Underlying Gender Differences in COVID-19 Severity and Mortality. Front. Immunol. 12:659339. doi: 10.3389/fimmu.2021.659339
Received: 27 January 2021; Accepted: 20 April 2021;
Published: 07 May 2021.
Edited by:
Anna Ruggieri, National Institute of Health (ISS), ItalyReviewed by:
Helena Nunes-Cabaço, University of Lisbon, PortugalZhenlong Liu, McGill University, Canada
Copyright © 2021 Wehbe, Hammoud, Yassine, Fardoun, El-Yazbi and Eid. This is an open-access article distributed under the terms of the Creative Commons Attribution License (CC BY). The use, distribution or reproduction in other forums is permitted, provided the original author(s) and the copyright owner(s) are credited and that the original publication in this journal is cited, in accordance with accepted academic practice. No use, distribution or reproduction is permitted which does not comply with these terms.
*Correspondence: Ali H. Eid, YWxpLmVpZEBxdS5lZHUucWE=
†These authors have contributed equally to this work