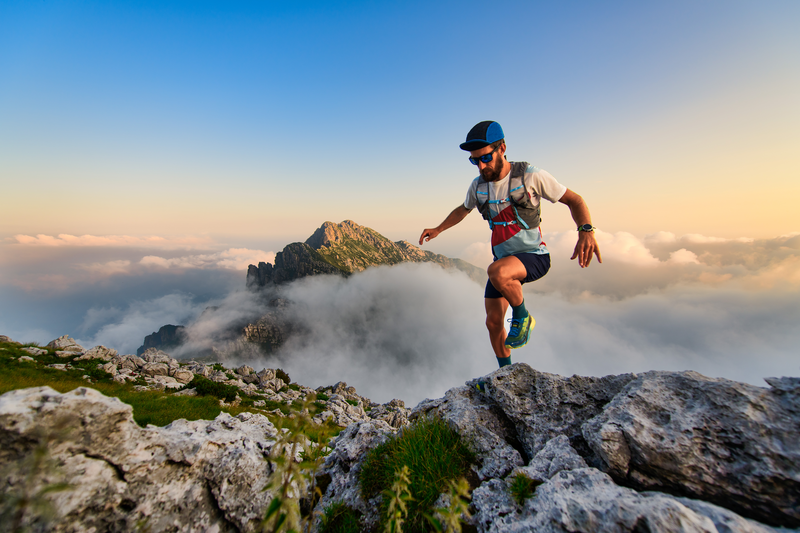
94% of researchers rate our articles as excellent or good
Learn more about the work of our research integrity team to safeguard the quality of each article we publish.
Find out more
MINI REVIEW article
Front. Immunol. , 19 March 2021
Sec. Viral Immunology
Volume 12 - 2021 | https://doi.org/10.3389/fimmu.2021.657679
This article is part of the Research Topic CD4 T cells in HIV: A Friend or Foe? View all 16 articles
Human Immunodeficiency Virus (HIV) and Simian Immunodeficiency Virus (SIV) are associated with severe perturbations in the gut mucosal environment characterized by massive viral replication and depletion of CD4 T cells leading to dysbiosis, breakdown of the epithelial barrier, microbial translocation, immune activation and disease progression. Multiple mechanisms play a role in maintaining homeostasis in the gut mucosa and protecting the integrity of the epithelial barrier. Among these are the secretory IgA (sIgA) that are produced daily in vast quantities throughout the mucosa and play a pivotal role in preventing commensal microbes from breaching the epithelial barrier. These microbe specific, high affinity IgA are produced by IgA+ plasma cells that are present within the Peyer’s Patches, mesenteric lymph nodes and the isolated lymphoid follicles that are prevalent in the lamina propria of the gastrointestinal tract (GIT). Differentiation, maturation and class switching to IgA producing plasma cells requires help from T follicular helper (Tfh) cells that are present within these lymphoid tissues. HIV replication and CD4 T cell depletion is accompanied by severe dysregulation of Tfh cell responses that compromises the generation of mucosal IgA that in turn alters barrier integrity leading to commensal bacteria readily breaching the epithelial barrier and causing mucosal pathology. Here we review the effect of HIV infection on Tfh cells and mucosal IgA responses in the GIT and the consequences these have for gut dysbiosis and mucosal immunopathogenesis.
Human and Simian Immunodeficiency Virus (HIV, SIV) infections are associated with dramatic changes in mucosal tissues such as the gastrointestinal tract (GIT) with massive replication and depletion of CD4 T cells during the acute stages of infection (1–17). Viral replication and associated inflammation persists in these tissues over the course of infection that is accompanied by damage to intestinal epithelial barrier, translocation of microbial products, immune activation and progressive loss of CD4 T cells (18–22). Though peripheral tissues experience significant levels of restoration after antiretroviral therapy, viral replication in mucosal tissues continues to persist with incomplete restoration of the immune system to a homeostatic state (23–38). As such, the inflammatory microenvironment in the mucosa remains in a state of high activation that is driven by microbial antigens that traverse the leaky epithelial barrier damaged during infection.
The GIT hosts the largest collection of microbes in the human body with bacteria accounting for a majority of the gut microflora. Studies have estimated that there are over 35,000 bacterial species in the human gut (39), a diversity that is only matched by the sheer magnitude of bacteria colonizing the GIT with estimates ranging from 1011 – 1013 bacteria (40). Within the GIT, the large intestine accounts for over 70% of the microbes that colonize the human body (41).
The resilience of the gastrointestinal mucosa to withstand continuous exposure to foreign antigens alongside the vast magnitude of microbes and their byproducts is exemplified by the homeostatic state that is maintained throughout life that ensures both intestinal and overall health. This homeostatic balance is maintained by a complex interplay between the host immune system and the gut microbiota that is characterized by a combination of tolerant and antigen specific immune responses that is constantly shaped and modulated by the microbes that reside in the GIT. This symbiotic relationship allows for physiological functions to proceed normally in a tightly controlled immunoregulatory environment. This regulation is essential to prevent any immunopathology that may develop due to responses to continuous exposure to both dietary and microbial antigens.
How the mucosal immune system regulates the mucosal microenvironment and at the same time keeps microbes from crossing the intestinal barrier has been an area of extensive research. In addition to innate mechanisms such as the thick mucus lining the mucosal surface, anti-microbial proteins like defensins, and lectins such as RegIIIγ (42–47), numerous studies have delineated the critical role for antibody responses such as microbe specific secretory IgA (sIgA) in preventing invasive microbes from breaching the epithelial barrier and gaining entry into the submucosa and systemic circulation (48–51).
Secretory IgA is the primary immunoglobulin found on mucosal surfaces and exists as a dimer that is bound to each other by the J chain, 15 kD polypeptide (52–55). The J chain binds to cysteine residues in the Fc regions of the heavy chain via disulphide bonds (55). Dimeric IgA is transported across the epithelium lining the gut by the polymeric immunoglobulin receptor that binds to IgA on the basolateral side of the epithelium and plays a role in transporting the sIgA across to the apical side and into the gut lumen (56–58). sIgA has significant antimicrobial activity and binds and neutralizes microbes, enhances agglutination of bacteria, and blocks cell adhesion thereby preventing access to the mucosal epithelium (56, 57).
Secretory IgA is the most abundant antibody (~ 50 mg/Kg/day) found in the lumen of the GIT with the gut associated lymphoid tissue (GALT) hosting ~ 80% of IgA producing plasma cells in the body (59, 60). Microbe specific sIgA are induced in the Peyer’s Patches and the lymphoid follicles in the GALT following antigen presentation by mucosal dendritic cells (DC) that sample and present microbial antigens to T and B cells. Following differentiation and class switching sIgA is transcytosed across the gut epithelium into the lumen of the GIT (61). sIgA are primarily generated in the Peyer’s patches, isolated lymphoid follicles, and mesenteric lymph nodes that drain the GIT; mice lacking Peyer’s patches have little or no IgA+ plasma cells and sIgA in the gut (62, 63). Isolated lymphoid follicles that develop in the lamina propria are significantly influenced by the microbiota colonizing the gut (64); in the absence of microbiota, germ free mice harbor low numbers of IgA producing plasma cells that secrete IgA that have low affinity to antigens and had failed to undergo somatic hypermutation. In contrast, in the presence of gut microbiota, B cells undergo class switch recombination and somatic hypermutation to produce antigen specific high affinity IgA (65, 66).
sIgA is abundant in mucosa associated lymphoid tissues (MALT) found in other mucosal sites such as the female genital tract (67), the LN that drain the upper and lower respiratory tracts (68). Unlike other MALT, however, Peyer’s patches are unique and specialized for production of isotype switched IgA, while other MALT produce a mix of both IgA and IgG (68–70).
Unlike the isolated lymphoid follicles in the lamina propria that can generate IgA responses in a T cell independent manner, most of the T cell dependent induction of IgA responses occurs within the Peyer’s patches and mesenteric lymph nodes (71–73). T cell dependent induction and differentiation of B cell responses relies on CD4 T cell help (74–78). Though different subsets of CD4 T cells such as Treg (79) and Th-17 (80, 81) have been shown to play a role in the generation of IgA responses in the GIT, T - follicular helper (Tfh) cells are essential for the generation of high affinity IgA responses (82).
Tfh cells are a subset of CD4 T cells that mainly reside in the germinal centers of lymph nodes and provide help to differentiating B cells during the germinal center reaction (83). Tfh cells express CXCR5, PD-1 and ICOS along with BCL6 (83–87), and are a primary source of IL-21, a key cytokine that drives the induction and differentiation of B cell responses (83, 86, 88, 89); mice lacking the IL-21 receptor was found to have defective antibody responses, class-switching, and germinal center (GC) formation (90). A number of other studies have established the central role of Tfh in the induction of high affinity B cell responses (83, 91).
HIV and SIV infections are characterized by loss of CD4 T cells that compromises the immune system with viral reservoirs persisting throughout the life of an infected subject (5, 92–104). Tfh cells residing within the lymph node GC have since been identified as a major source of the persistent latent and productive HIV reservoir (105) due to the inaccessibility of cytotoxic CD8 T cells into B cell follicles within the GC that creates a privileged environment for HIV replication and infection of Tfh cells, the predominant population of CD4 T cells in the follicles (106, 107). Numerous studies have explored the role of lymph nodes in HIV persistence and pathogenesis (108, 109). Perreau et al. (110) reported that HIV infected nonprogressors with low levels of viremia (<1000 HIV RNA copies) harbored high levels of HIV+ Tfh cells as compared to other CD4 T cells subsets. Similarly, SIV infection was associated with significantly high levels of infection in Tfh cells (111–113).
Other studies have reported that HIV specific Tfh significantly expand during chronic HIV infection (114). Similar findings have been reported in a subset of SIV infected macaques where an accumulation of Tfh cells in the lymph nodes that are latently infected is accompanied by higher frequencies of activated GC B cells and SIV specific antibody responses (112, 115–117). Mylvaganam et al. (118) demonstrated that SIV infection was associated with an increase in the frequency of aberrant Tfh in the lymphoid follicles of the rectal mucosa that correlated with lack of viral control. Loss of IL-21+ CD4 T cells was associated with the depletion of other T cell subsets such as Th17 cells and disease progression (119). Numerous studies have documented the depletion of Th17 cells in the mucosa and correlated them with microbial translocation and immune activation (5, 120, 121). A detailed phenotype of Tfh cells that is unique to the GIT is still under investigation. Tfh cells in other specialized lymphoid organs such as the spleen, however express typical Tfh markers, and were shown to decline early during SIV infection (122).
In contrast to the accumulation of Tfh in a subset of infected subjects, numerous studies have reported that HIV and SIV infection were characterized by a depletion of Tfh cells that was associated with altered B cell responses. Mesenteric lymph node Tfh cells were lost very early during SIV infection that was accompanied by a loss of GC and memory B cells (116, 122, 123). Others have reported that the development of both GC reaction and Tfh within these GCs were significantly impaired during HIV and SIV infections that likely contributed to the dysregulation of B cell responses (124–126). Rabezanahary et al. (127) reported a significant decline in Tfh cells in MLN and spleen of SIV infected animals, whereas McGray et al. (128) described a similar decline in the GIT of SIV infected animals during the acute stages of infection. Interestingly, Velu et al. (129) reported that Tfh that expand in the GC during chronic infection harbor a Th-1 bias that differed from conventional Tfh cells raising the possibility that accumulation or depletion of Tfh cells may depend on factors that drive their differentiation.
Despite early antiretroviral therapy, Tfh cells remain major reservoirs in lymphoid tissues and peripheral blood (127, 130, 131). Studies have shown that Tfh were a major source of replication competent HIV and persistent HIV-1 transcription in treated aviremic patients on long term HAART (132). Yu et al. reported that Tfh were enriched for replication competent X4 tropic HIV virus and correlated with progression of disease (133). HIV specific Tfh levels have been shown to significantly correlate with plasma HIV specific antibody levels during HAART (134). Early initiation of HAART maintained HIV-1-reactive memory B cells and Tfh in the gut (135). Rabezanahary et al. (127) reported that Tfh CD4+ T cells in the mesenteric lymph nodes harbor significant levels of viral DNA and RNA in SIV infected rhesus macaques treated with antiretroviral therapy suggesting that Tfh niches that reside in the lymph node GCs were major sites of viral persistence and like other sites are dysregulated during infection. Thornhill et al. (136) demonstrated that CD32 high doublets in the GALT of HIV infected subjects undergoing antiretroviral therapy were primarily composed of Tfh cells that correlated with high levels of rectal HIV DNA.
Mucosal IgA plays a critical role in protecting the mucosa from invasive bacteria that colonize the GIT (137). IgA are primarily produced in a T- cell dependent manner within the Peyer’s Patches and mesenteric lymph node GC with Tfh driving the differentiation, maturation and class switching on B cells (90, 138–142). Chaoul et al. (143) examined T cell dependent IgA responses in the Peyer’s Patches and isolated lymphoid follicles in SIV infected rhesus macaques and detected no IgA+ plasma cells in the GC at these sites during acute infection that was accompanied by a significant decrease in the amount of IgA detectable in the duodenum and ileum. Rather surprisingly, both GC and Tfh within the Peyer’s Patches and isolated lymphoid follicles were functional and intact. Schäfer et al. (144) reported that the amount of total intestinal IgA decreased and remained low during the first few months after SIV infection. Likewise, numerous studies have reported low levels of IgA in mucosal secretions of HIV infected subjects (145–151) whereas Scamurra et al. (152) showed that low levels of IgA in intestinal secretions during HIV infection was associated with lower frequencies of IgA plasma cells in the GIT. It is highly likely that HIV infection significantly impairs B cell differentiation and IgA class switching in the GALT as reported by Xu et al. (153). Levesque et al. (154) reported that GALT GC display significant levels of apoptosis during HIV infection that do not effectively reconstitute after antiretroviral therapy (155). Given the central role of Tfh cells in this process, the loss or dysregulation of Tfh cells during HIV infection likely contributes to B cell dysfunction in the gut that in turn affects the protective mechanisms at the mucosal epithelial barrier. In line with this argument, Hel et al. (123) demonstrated that HIV infected subjects display lower gut microbiota specific IgA and IgG responses in the GIT that likely contributes to microbial translocation and chronic immune activation. Numerous studies have reported the breakdown of gut epithelial barrier during HIV and SIV infections (25, 121, 156–161).
The decrease in intestinal IgA is consequential given its central importance in preventing gut microbes from accessing the intestinal epithelium. Gut commensal bacteria and their metabolites play a role in inducing effector T cells and maintaining immune homeostasis in the GIT (162–167). Hegazy et al. (168) reported that gut microbiota reactive CD4 T cells in intestinal mucosa are readily detectable in healthy humans and were a normal feature of the repertoire of CD4 T cells. Likewise, the development of IgA responses is significantly influenced by the microbiota that colonize the gut (65). Large numbers of Tfh cells reside in the GC of Peyer’s patches where gut microbes and their byproducts continuously shape the GC reaction and production of mucosal IgA (169, 170) that in turn regulates gut microbiome interactions with the host. Maruya et al. (171) reported that PD-1 deficiency alters the phenotype of Tfh cells leading to the induction of IgA with reduced capacity to bind gut bacteria that in turn leads to alterations in the composition of the commensal bacteria in the GIT. Loss of PD-1 was also associated with an increase in dysfunctional Tfh cells in the Peyer’s patches that is characterized by reduced production of IL-21, increased secretion of IFNγ, and production of IgA with poor affinity maturation (172). Low affinity IgA responses were accompanied by a profound loss of “healthy bacteria” such as Bifidobacterium and Bacteroides, and an increase in Enterobacteriaceae (172). Others (173) have shown that Tfh cells within the Peyer’s Patches play an essential role in systemic arthritis induced by segmented filamentous bacteria by inhibiting IL-2 signaling with DCs contributing to SFB-mediated Tfh cell induction and IL-2 receptor α (IL-2Rα) suppression. IL-2 signaling pathway has been shown to inhibit Tfh cell differentiation by altering Bcl-6 expression (174, 175). Impairment of Tfh cells, either due to lack of inhibitory co-receptor PD-1 or ATP-gated ionotropic P2RX7 receptors, was found to alter the gut commensal bacteria (172, 176). Micci et al. (119) reported that depletion of IL-21 producing CD4 T cells was associated with progression of SIV disease. Taken together, these findings suggest that compromise of mucosal Tfh cells and high affinity IgA responses probably play a role in altering the composition of gut microbiota during HIV infection.
HIV infection is associated with changes in the composition of the gut microbiota (22, 177, 178) characterized by a decrease in Bacteroides and Firmicutes and an increase in the prevalence Proteobacteria (179–181). Others have reported alterations in microbial richness (182) and Prevotella during chronic HIV infection (181, 183–186). Gut dysbiosis has been reported in SIV infected rhesus macaques that was partially restored with combination antiretroviral therapy (187). SIV infected macaques were found to display an expansion of enteropathogens during advanced stages of disease (188), and macaques with severe disease displayed changes in their bacterial diversity that was characterized by an altered abundance of Enterobacteriaceae and Moraxellaceae similar to that of HIV infected patients who have low CD4 T cell counts. Lower microbial richness and diversity was associated with poor CD4 T cell reconstitution in HIV infected subjects undergoing treatment with HAART (189, 190). Numerous studies have suggested that some level of gut dysbiosis persists during HAART. Likewise, Tfh cells dysregulated during HIV infection are not fully restored to their healthy state during HART suggesting that B cell dysfunction is likely to persist during therapy that in turn could lead to inadequate protection of the epithelial barrier.
In conclusion, the dysregulation of Tfh cells that compromises B cell responses especially the secretion of microbe specific IgA is one of the likely drivers of gut microbial dysbiosis during HIV infection. This dysregulation that accompanies high levels of HIV replication, loss of mucosal CD4 T cells, breakdown of the integrity of epithelial barrier significantly alters mucosal immune homeostasis leading to microbial translocation, immune activation and progression of disease. Strategies that can preserve and maintain Tfh responses in the mucosa could potentially restore high affinity mucosal IgA that could aid in protecting the mucosal epithelial barrier from invasive dysbiotic bacteria.
OO and JM wrote the manuscript. All authors contributed to the article and approved the submitted version.
The authors declare that the research was conducted in the absence of any commercial or financial relationships that could be construed as a potential conflict of interest.
The opinions or assertions contained herein are the private ones of the authors and are not to be construed as official or reflecting the views of the Department of the Navy, Department of Defense, the Uniformed Services University of the Health Sciences, National Institutes of Health or any other agency of the U.S. Government.
1. Allers K, Kunkel D, Hofmann J, Stahl-Hennig C, Moos V, Schneider T. Cell-Associated Simian Immunodeficiency Virus Accelerates Initial Virus Spread and CD4+ T-Cell Depletion in the Intestinal Mucosa. J Infect Dis (2018) 217:1421–5. doi: 10.1093/infdis/jiy055
2. Brenchley JM, Douek DC. HIV infection and the gastrointestinal immune system. Mucosal Immunol (2008) 1:23–30. doi: 10.1038/mi.2007.1
3. Eberly MD, Kader M, Hassan W, Rogers KA, Zhou J, Mueller YM, et al. Increased IL-15 production is associated with higher susceptibility of memory CD4 T cells to simian immunodeficiency virus during acute infection. J Immunol (2009) 182:1439–48. doi: 10.4049/jimmunol.182.3.1439
4. George J, Johnson RC, Mattapallil MJ, Renn L, Rabin R, Merrell DS, et al. Gender differences in innate responses and gene expression profiles in memory CD4 T cells are apparent very early during acute simian immunodeficiency virus infection. PloS One (2019) 14:e0221159. doi: 10.1371/journal.pone.0221159
5. Kader M, Wang X, Piatak M, Lifson J, Roederer M, Veazey R, et al. Alpha4(+)beta7(hi)CD4(+) memory T cells harbor most Th-17 cells and are preferentially infected during acute SIV infection. Mucosal Immunol (2009) 2:439–49. doi: 10.1038/mi.2009.90
6. Kovacs SB, Sheikh V, Thompson WL, Morcock DR, Perez-Diez A, Yao MD, et al. T-Cell Depletion in the Colonic Mucosa of Patients With Idiopathic CD4+ Lymphopenia. J Infect Dis (2015) 212:1579–87. doi: 10.1093/infdis/jiv282
7. Li Q, Duan L, Estes JD, Ma ZM, Rourke T, Wang Y, et al. Peak SIV replication in resting memory CD4+ T cells depletes gut lamina propria CD4+ T cells. Nature (2005) 434:1148–52. doi: 10.1038/nature03513
8. Mattapallil JJ, Douek DC, Hill B, Nishimura Y, Martin M, Roederer M. Massive infection and loss of memory CD4+ T cells in multiple tissues during acute SIV infection. Nature (2005) 434:1093–7. doi: 10.1038/nature03501
9. Mattapallil JJ, Letvin NL, Roederer M. T-cell dynamics during acute SIV infection. AIDS (2004) 18:13–23. doi: 10.1097/00002030-200401020-00002
10. Mattapallil JJ, Roederer M. Acute HIV infection: it takes more than guts. Curr Opin HIV AIDS (2006) 1:10–5. doi: 10.1097/01.COH.0000191896.70685.74
11. Moore AC, Bixler SL, Lewis MG, Verthelyi D, Mattapallil JJ. Mucosal and peripheral Lin- HLA-DR+ CD11c/123- CD13+ CD14- mononuclear cells are preferentially infected during acute simian immunodeficiency virus infection. J Virol (2012) 86:1069–78. doi: 10.1128/JVI.06372-11
12. Nishimura Y, Brown CR, Mattapallil JJ, Igarashi T, Buckler-White A, Lafont BA, et al. Resting naive CD4+ T cells are massively infected and eliminated by X4-tropic simian-human immunodeficiency viruses in macaques. Proc Natl Acad Sci U.S.A. (2005) 102:8000–5. doi: 10.1073/pnas.0503233102
13. Smit-McBride Z, Mattapallil JJ, McChesney M, Ferrick D, Dandekar S. Gastrointestinal T lymphocytes retain high potential for cytokine responses but have severe CD4(+) T-cell depletion at all stages of simian immunodeficiency virus infection compared to peripheral lymphocytes. J Virol (1998) 72:6646–56. doi: 10.1128/JVI.72.8.6646-6656.1998
14. Song K, Rabin RL, Hill BJ, De Rosa SC, Perfetto SP, Zhang HH, et al. Characterization of subsets of CD4+ memory T cells reveals early branched pathways of T cell differentiation in humans. Proc Natl Acad Sci U.S.A. (2005) 102:7916–21. doi: 10.1073/pnas.0409720102
15. Tokarev A, McKinnon LR, Pagliuzza A, Sivro A, Omole TE, Kroon E, et al. Preferential Infection of alpha4beta7+ Memory CD4+ T Cells During Early Acute Human Immunodeficiency Virus Type 1 Infection. Clin Infect Dis (2020) 71:e735–e43. doi: 10.1093/cid/ciaa497
16. Veazey RS. Intestinal CD4 Depletion in HIV / SIV Infection. Curr Immunol Rev (2019) 15:76–91. doi: 10.2174/1573395514666180605083448
17. Veazey RS, DeMaria M, Chalifoux LV, Shvetz DE, Pauley DR, Knight HL, et al. Gastrointestinal tract as a major site of CD4+ T cell depletion and viral replication in SIV infection. Science (1998) 280:427–31. doi: 10.1126/science.280.5362.427
18. Brenchley JM, Price DA, Schacker TW, Asher TE, Silvestri G, Rao S, et al. Microbial translocation is a cause of systemic immune activation in chronic HIV infection. Nat Med (2006) 12:1365–71. doi: 10.1038/nm1511
19. Epple HJ, Zeitz M. HIV infection and the intestinal mucosal barrier. Ann N Y Acad Sci (2012) 1258:19–24. doi: 10.1111/j.1749-6632.2012.06512.x
20. Gordon SN, Cervasi B, Odorizzi P, Silverman R, Aberra F, Ginsberg G, et al. Disruption of intestinal CD4+ T cell homeostasis is a key marker of systemic CD4+ T cell activation in HIV-infected individuals. J Immunol (2010) 185:5169–79. doi: 10.4049/jimmunol.1001801
21. Marchetti G, Tincati C, Silvestri G. Microbial translocation in the pathogenesis of HIV infection and AIDS. Clin Microbiol Rev (2013) 26:2–18. doi: 10.1128/CMR.00050-12
22. Mudd JC, Brenchley JM. Gut Mucosal Barrier Dysfunction, Microbial Dysbiosis, and Their Role in HIV-1 Disease Progression. J Infect Dis (2016) 214 Suppl 2:S58–66. doi: 10.1093/infdis/jiw258
23. Brown D, Mattapallil JJ. Gastrointestinal tract and the mucosal macrophage reservoir in HIV infection. Clin Vaccine Immunol (2014) 21:1469–73. doi: 10.1128/CVI.00518-14
24. Cassol E, Malfeld S, Mahasha P, Bond R, Slavik T, Seebregts C, et al. Impaired CD4+ T-cell restoration in the small versus large intestine of HIV-1-positive South Africans receiving combination antiretroviral therapy. J Infect Dis (2013) 208:1113–22. doi: 10.1093/infdis/jit249
25. Estes JD, Harris LD, Klatt NR, Tabb B, Pittaluga S, Paiardini M, et al. Damaged intestinal epithelial integrity linked to microbial translocation in pathogenic simian immunodeficiency virus infections. PloS Pathog (2010) 6:e1001052. doi: 10.1371/journal.ppat.1001052
26. George J, Wagner W, Lewis MG, Mattapallil JJ. Significant Depletion of CD4(+) T Cells Occurs in the Oral Mucosa during Simian Immunodeficiency Virus Infection with the Infected CD4(+) T Cell Reservoir Continuing to Persist in the Oral Mucosa during Antiretroviral Therapy. J Immunol Res (2015) 2015:673815. doi: 10.1155/2015/673815
27. Kader M, Bixler S, Roederer M, Veazey R, Mattapallil JJ. CD4 T cell subsets in the mucosa are CD28+Ki-67-HLA-DR-CD69+ but show differential infection based on alpha4beta7 receptor expression during acute SIV infection. J Med Primatol (2009) 38 Suppl 1:24–31. doi: 10.1111/j.1600-0684.2009.00372.x
28. Kader M, Hassan WM, Eberly M, Piatak M, Lifson JD, Roederer M, et al. Antiretroviral therapy prior to acute viral replication preserves CD4 T cells in the periphery but not in rectal mucosa during acute simian immunodeficiency virus infection. J Virol (2008) 82:11467–71. doi: 10.1128/JVI.01143-08
29. Klatt NR, Canary LA, Vanderford TH, Vinton CL, Engram JC, Dunham RM, et al. Dynamics of simian immunodeficiency virus SIVmac239 infection in pigtail macaques. J Virol (2012) 86:1203–13. doi: 10.1128/JVI.06033-11
30. Klatt NR, Chomont N, Douek DC, Deeks SG. Immune activation and HIV persistence: implications for curative approaches to HIV infection. Immunol Rev (2013) 254:326–42. doi: 10.1111/imr.12065
31. Klatt NR, Funderburg NT, Brenchley JM. Microbial translocation, immune activation, and HIV disease. Trends Microbiol (2013) 21:6–13. doi: 10.1016/j.tim.2012.09.001
32. Klatt NR, Vinton CL, Lynch RM, Canary LA, Ho J, Darrah PA, et al. SIV infection of rhesus macaques results in dysfunctional T- and B-cell responses to neo and recall Leishmania major vaccination. Blood (2011) 118:5803–12. doi: 10.1182/blood-2011-07-365874
33. Mattapallil JJ, Hill B, Douek DC, Roederer M. Systemic vaccination prevents the total destruction of mucosal CD4 T cells during acute SIV challenge. J Med Primatol (2006) 35:217–24. doi: 10.1111/j.1600-0684.2006.00170.x
34. Mattapallil JJ, Reay E, Dandekar S. An early expansion of CD8alphabeta T cells, but depletion of resident CD8alphaalpha T cells, occurs in the intestinal epithelium during primary simian immunodeficiency virus infection. AIDS (2000) 14:637–46. doi: 10.1097/00002030-200004140-00002
35. Mavigner M, Cazabat M, Dubois M, L’Faqihi FE, Requena M, Pasquier C, et al. Altered CD4+ T cell homing to the gut impairs mucosal immune reconstitution in treated HIV-infected individuals. J Clin Invest (2012) 122:62–9. doi: 10.1172/JCI59011
36. Merriam D, Chen C, Mendez-Lagares G, Rogers KA, Michaels AJ, Yan J, et al. Depletion of Gut-Resident CCR5(+) Cells for HIV Cure Strategies. AIDS Res Hum Retroviruses (2017) 33:S70–80. doi: 10.1089/aid.2017.0159
37. Petravic J, Ribeiro RM, Casimiro DR, Mattapallil JJ, Roederer M, Shiver JW, et al. Estimating the impact of vaccination on acute simian-human immunodeficiency virus/simian immunodeficiency virus infections. J Virol (2008) 82:11589–98. doi: 10.1128/JVI.01596-08
38. Sainz T, Serrano-Villar S, Mann S, Ma ZM, Utay NS, Thompson CG, et al. Delayed gastrointestinal-associated lymphoid tissue reconstitution in duodenum compared with rectum in HIV-infected patients initiating antiretroviral therapy. AIDS (2019) 33:2289–98. doi: 10.1097/QAD.0000000000002361
39. Frank DN, St Amand AL, Feldman RA, Boedeker EC, Harpaz N, Pace NR. Molecular-phylogenetic characterization of microbial community imbalances in human inflammatory bowel diseases. Proc Natl Acad Sci U.S.A. (2007) 104:13780–5. doi: 10.1073/pnas.0706625104
40. Sender R, Fuchs S, Milo R. Are We Really Vastly Outnumbered? Revisiting the Ratio of Bacterial to Host Cells in Humans. Cell (2016) 164:337–40. doi: 10.1016/j.cell.2016.01.013
41. Jandhyala SM, Talukdar R, Subramanyam C, Vuyyuru H, Sasikala M, Nageshwar Reddy D. Role of the normal gut microbiota. World J Gastroenterol (2015) 21:8787–803. doi: 10.3748/wjg.v21.i29.8787
42. Brandl K, Plitas G, Schnabl B, DeMatteo RP, Pamer EG. MyD88-mediated signals induce the bactericidal lectin RegIII gamma and protect mice against intestinal Listeria monocytogenes infection. J Exp Med (2007) 204:1891–900. doi: 10.1084/jem.20070563
43. Cash HL, Whitham CV, Behrendt CL, Hooper LV. Symbiotic bacteria direct expression of an intestinal bactericidal lectin. Science (2006) 313:1126–30. doi: 10.1126/science.1127119
44. Johansson ME, Larsson JM, Hansson GC. The two mucus layers of colon are organized by the MUC2 mucin, whereas the outer layer is a legislator of host-microbial interactions. Proc Natl Acad Sci U.S.A. (2011) 108 Suppl 1:4659–65. doi: 10.1073/pnas.1006451107
45. Johansson ME, Phillipson M, Petersson J, Velcich A, Holm L, Hansson GC. The inner of the two Muc2 mucin-dependent mucus layers in colon is devoid of bacteria. Proc Natl Acad Sci U.S.A. (2008) 105:15064–9. doi: 10.1073/pnas.0803124105
46. Vaishnava S, Behrendt CL, Ismail AS, Eckmann L, Hooper LV. Paneth cells directly sense gut commensals and maintain homeostasis at the intestinal host-microbial interface. Proc Natl Acad Sci U.S.A. (2008) 105:20858–63. doi: 10.1073/pnas.0808723105
47. Vaishnava S, Yamamoto M, Severson KM, Ruhn KA, Yu X, Koren O, et al. The antibacterial lectin RegIIIgamma promotes the spatial segregation of microbiota and host in the intestine. Science (2011) 334:255–8. doi: 10.1126/science.1209791
48. Apter FM, Lencer WI, Finkelstein RA, Mekalanos JJ, Neutra MR. Monoclonal immunoglobulin A antibodies directed against cholera toxin prevent the toxin-induced chloride secretory response and block toxin binding to intestinal epithelial cells in vitro. Infect Immun (1993) 61:5271–8. doi: 10.1128/IAI.61.12.5271-5278.1993
49. Hutchings AB, Helander A, Silvey KJ, Chandran K, Lucas WT, Nibert ML, et al. Secretory immunoglobulin A antibodies against the sigma1 outer capsid protein of reovirus type 1 Lang prevent infection of mouse Peyer’s patches. J Virol (2004) 78:947–57. doi: 10.1128/jvi.78.2.947-957.2004
50. Macpherson AJ, Gatto D, Sainsbury E, Harriman GR, Hengartner H, Zinkernagel RM. A primitive T cell-independent mechanism of intestinal mucosal IgA responses to commensal bacteria. Science (2000) 288:2222–6. doi: 10.1126/science.288.5474.2222
51. Macpherson AJ, Uhr T. Induction of protective IgA by intestinal dendritic cells carrying commensal bacteria. Science (2004) 303:1662–5. doi: 10.1126/science.1091334
52. Senior BW, Woof JM. The influences of hinge length and composition on the susceptibility of human IgA to cleavage by diverse bacterial IgA1 proteases. J Immunol (2005) 174:7792–9. doi: 10.4049/jimmunol.174.12.7792
53. Underdown BJ, Schiff JM. Immunoglobulin A: strategic defense initiative at the mucosal surface. Annu Rev Immunol (1986) 4:389–417. doi: 10.1146/annurev.iy.04.040186.002133
54. Woof JM, Mestecky J. Mucosal immunoglobulins. Immunol Rev (2005) 206:64–82. doi: 10.1111/j.0105-2896.2005.00290.x
55. Woof JM, Russell MW. Structure and function relationships in IgA. Mucosal Immunol (2011) 4:590–7. doi: 10.1038/mi.2011.39
56. Brandtzaeg P. Secretory IgA: Designed for Anti-Microbial Defense. Front Immunol (2013) 4:222. doi: 10.3389/fimmu.2013.00222
57. Brandtzaeg P. Gate-keeper function of the intestinal epithelium. Benef Microbes (2013) 4:67–82. doi: 10.3920/BM2012.0024
58. Kaetzel CS, Robinson JK, Chintalacharuvu KR, Vaerman JP, Lamm ME. The polymeric immunoglobulin receptor (secretory component) mediates transport of immune complexes across epithelial cells: a local defense function for IgA. Proc Natl Acad Sci U.S.A. (1991) 88:8796–800. doi: 10.1073/pnas.88.19.8796
59. Brandtzaeg P, Johansen FE. Mucosal B cells: phenotypic characteristics, transcriptional regulation, and homing properties. Immunol Rev (2005) 206:32–63. doi: 10.1111/j.0105-2896.2005.00283.x
60. Fritz JH, Rojas OL, Simard N, McCarthy DD, Hapfelmeier S. Acquisition of a multifunctional IgA+ plasma cell phenotype in the gut. Nature (2011) 481:199–203. doi: 10.1038/nature10698
61. Kaetzel CS. The polymeric immunoglobulin receptor: bridging innate and adaptive immune responses at mucosal surfaces. Immunol Rev (2005) 206:83–99. doi: 10.1111/j.0105-2896.2005.00278.x
62. Cherrier M, Eberl G. The development of LTi cells. Curr Opin Immunol (2012) 24:178–83. doi: 10.1016/j.coi.2012.02.003
63. Kruglov AA, Grivennikov SI, Kuprash DV, Winsauer C, Prepens S, Seleznik GM, et al. Nonredundant function of soluble LTalpha3 produced by innate lymphoid cells in intestinal homeostasis. Science (2013) 342:1243–6. doi: 10.1126/science.1243364
64. Pabst O, Herbrand H, Worbs T, Friedrichsen M, Yan S, Hoffmann MW, et al. Cryptopatches and isolated lymphoid follicles: dynamic lymphoid tissues dispensable for the generation of intraepithelial lymphocytes. Eur J Immunol (2005) 35:98–107. doi: 10.1002/eji.200425432
65. Hapfelmeier S, Lawson MA, Slack E, Kirundi JK, Stoel M. Reversible microbial colonization of germ-free mice reveals the dynamics of IgA immune responses. Science (2010) 328:1705–9. doi: 10.1126/science.1188454
66. Slack E, Balmer ML, Fritz JH, Hapfelmeier S. Functional flexibility of intestinal IgA - broadening the fine line. Front Immunol (2012) 3:100:100. doi: 10.3389/fimmu.2012.00100
67. Hickey DK, Patel MV, Fahey JV, Wira CR. Innate and adaptive immunity at mucosal surfaces of the female reproductive tract: stratification and integration of immune protection against the transmission of sexually transmitted infections. J Reprod Immunol (2011) 88:185–94. doi: 10.1016/j.jri.2011.01.005
68. Randall TD, Kern JA. Tertiary lymphoid structures target the antitumor immune response to lung cancer. Am J Respir Crit Care Med (2014) 189:767–9. doi: 10.1164/rccm.201402-0317ED
69. Craig SW, Cebra JJ. Peyer’s patches: an enriched source of precursors for IgA-producing immunocytes in the rabbit. J Exp Med (1971) 134:188–200. doi: 10.1084/jem.134.1.188
70. Fagarasan S, Kinoshita K, Muramatsu M, Ikuta K, Honjo T. In situ class switching and differentiation to IgA-producing cells in the gut lamina propria. Nature (2001) 413:639–43. doi: 10.1038/35098100
71. Brandtzaeg P. Mucosal immunity: induction, dissemination, and effector functions. Scand J Immunol (2009) 70:505–15. doi: 10.1111/j.1365-3083.2009.02319.x
72. Cerutti A, Chen K, Chorny A. Immunoglobulin responses at the mucosal interface. Annu Rev Immunol (2011) 29:273–93. doi: 10.1146/annurev-immunol-031210-101317
73. Fagarasan S, Kawamoto S, Kanagawa O, Suzuki K. Adaptive immune regulation in the gut: T cell-dependent and T cell-independent IgA synthesis. Annu Rev Immunol (2010) 28:243–73. doi: 10.1146/annurev-immunol-030409-101314
74. Katz DH, Benacerraf B. The regulatory influence of activated T cells on B cell responses to antigen. Adv Immunol (1972) 15:1–94. doi: 10.1016/s0065-2776(08)60683-5
75. Miller JF, Mitchell GF. Cell to cell interaction in the immune response. I. Hemolysin-forming cells in neonatally thymectomized mice reconstituted with thymus or thoracic duct lymphocytes. J Exp Med (1968) 128:801–20. doi: 10.1084/jem.128.4.801
76. Mitchell GF, Miller JF. Cell to cell interaction in the immune response. II. The source of hemolysin-forming cells in irradiated mice given bone marrow and thymus or thoracic duct lymphocytes. J Exp Med (1968) 128:821–37. doi: 10.1084/jem.128.4.821
77. Nossal GJ, Cunningham A, Mitchell GF, Miller JF. Cell to cell interaction in the immune response. 3. Chromosomal marker analysis of single antibody-forming cells in reconstituted, irradiated, or thymectomized mice. J Exp Med (1968) 128:839–53. doi: 10.1084/jem.128.4.839
78. Raff MC. Role of thymus-derived lymphocytes in the secondary humoral immune response in mice. Nature (1970) 226:1257–8. doi: 10.1038/2261257a0
79. Cong Y, Feng T, Fujihashi K, Schoeb TR, Elson CO. A dominant, coordinated T regulatory cell-IgA response to the intestinal microbiota. Proc Natl Acad Sci U.S.A. (2009) 106:19256–61. doi: 10.1073/pnas.0812681106
80. Cao AT, Yao S, Gong B, Elson CO, Cong Y. Th17 cells upregulate polymeric Ig receptor and intestinal IgA and contribute to intestinal homeostasis. J Immunol (2012) 189:4666–73. doi: 10.4049/jimmunol.1200955
81. Hirota K, Turner JE, Villa M, Duarte JH, Demengeot J, Steinmetz OM, et al. Plasticity of Th17 cells in Peyer’s patches is responsible for the induction of T cell-dependent IgA responses. Nat Immunol (2013) 14:372–9. doi: 10.1038/ni.2552
82. Tsuji M, Komatsu N, Kawamoto S, Suzuki K, Kanagawa O. Preferential generation of follicular B helper T cells from Foxp3+ T cells in gut Peyer’s patches. Science (2009) 323:1488–92. doi: 10.1126/science.1169152
83. Crotty S. Follicular helper CD4 T cells (TFH). Annu Rev Immunol (2011) 29:621–63. doi: 10.1146/annurev-immunol-031210-101400
84. Johnston RJ, Poholek AC, DiToro D, Yusuf I, Eto D, Barnett B, et al. Bcl6 and Blimp-1 are reciprocal and antagonistic regulators of T follicular helper cell differentiation. Science (2009) 325:1006–10. doi: 10.1126/science.1175870
85. Nurieva RI, Chung Y, Martinez GJ, Yang XO, Tanaka S, Matskevitch TD, et al. Bcl6 mediates the development of T follicular helper cells. Science (2009) 325:1001–5. doi: 10.1126/science.1176676
86. Onabajo OO, George J, Lewis MG, Mattapallil JJ. Rhesus macaque lymph node PD-1(hi)CD4+ T cells express high levels of CXCR5 and IL-21 and display a CCR7(lo)ICOS+Bcl6+ T-follicular helper (Tfh) cell phenotype. PloS One (2013) 8:e59758. doi: 10.1371/journal.pone.0059758
87. Schaerli P, Willimann K, Lang AB, Lipp M, Loetscher P, Moser B. CXC chemokine receptor 5 expression defines follicular homing T cells with B cell helper function. J Exp Med (2000) 192:1553–62. doi: 10.1084/jem.192.11.1553
88. Linterman MA, Beaton L, Yu D, Ramiscal RR, Srivastava M, Hogan JJ, et al. IL-21 acts directly on B cells to regulate Bcl-6 expression and germinal center responses. J Exp Med (2010) 207:353–63. doi: 10.1084/jem.20091738
89. Nurieva RI, Chung Y, Hwang D, Yang XO, Kang HS, Ma L, et al. Generation of T follicular helper cells is mediated by interleukin-21 but independent of T helper 1, 2, or 17 cell lineages. Immunity (2008) 29:138–49. doi: 10.1016/j.immuni.2008.05.009
90. Ozaki K, Spolski R, Feng CG, Qi CF, Cheng J, Sher A, et al. A critical role for IL-21 in regulating immunoglobulin production. Science (2002) 298:1630–4. doi: 10.1126/science.1077002
91. Crotty S. T follicular helper cell differentiation, function, and roles in disease. Immunity (2014) 41:529–42. doi: 10.1016/j.immuni.2014.10.004
92. Churchill MJ, Deeks SG, Margolis DM, Siliciano RF, Swanstrom R. HIV reservoirs: what, where and how to target them. Nat Rev Microbiol (2016) 14:55–60. doi: 10.1038/nrmicro.2015.5
93. Cohn LB, Chomont N, Deeks SG. The Biology of the HIV-1 Latent Reservoir and Implications for Cure Strategies. Cell Host Microbe (2020) 27:519–30. doi: 10.1016/j.chom.2020.03.014
94. George J, Cofano EB, Lybarger E, Louder M, Lafont BA, Mascola JR, et al. Early short-term antiretroviral therapy is associated with a reduced prevalence of CD8(+)FoxP3(+) T cells in simian immunodeficiency virus-infected controller rhesus macaques. AIDS Res Hum Retroviruses (2011) 27:763–75. doi: 10.1089/AID.2010.0251
95. George J, Renn L, Verthelyi D, Roederer M, Rabin RL, Mattapallil JJ. Early treatment with reverse transcriptase inhibitors significantly suppresses peak plasma IFNalpha in vivo during acute simian immunodeficiency virus infection. Cell Immunol (2016) 310:156–64. doi: 10.1016/j.cellimm.2016.09.003
96. Kuwata T, Dehghani H, Brown CR, Plishka R, Buckler-White A, Igarashi T, et al. Infectious molecular clones from a simian immunodeficiency virus-infected rapid-progressor (RP) macaque: evidence of differential selection of RP-specific envelope mutations in vitro and in vivo. J Virol (2006) 80:1463–75. doi: 10.1128/JVI.80.3.1463-1475.2006
97. Mattapallil JJ, Smit-McBride Z, Dailey P, Dandekar S. Activated memory CD4(+) T helper cells repopulate the intestine early following antiretroviral therapy of simian immunodeficiency virus-infected rhesus macaques but exhibit a decreased potential to produce interleukin-2. J Virol (1999) 73:6661–9. doi: 10.1128/JVI.73.8.6661-6669.1999
98. Mattapallil JJ, Smit-McBride Z, McChesney M, Dandekar S. Intestinal intraepithelial lymphocytes are primed for gamma interferon and MIP-1beta expression and display antiviral cytotoxic activity despite severe CD4(+) T-cell depletion in primary simian immunodeficiency virus infection. J Virol (1998) 72:6421–9. doi: 10.1128/JVI.72.8.6421-6429.1998
99. Mueller YM, Do DH, Boyer JD, Kader M, Mattapallil JJ, Lewis MG, et al. CD8+ cell depletion of SHIV89.6P-infected macaques induces CD4+ T cell proliferation that contributes to increased viral loads. J Immunol (2009) 183:5006–12. doi: 10.4049/jimmunol.0900141
100. Nishimura Y, Sadjadpour R, Mattapallil JJ, Igarashi T, Lee W, Buckler-White A, et al. High frequencies of resting CD4+ T cells containing integrated viral DNA are found in rhesus macaques during acute lentivirus infections. Proc Natl Acad Sci U.S.A. (2009) 106:8015–20. doi: 10.1073/pnas.0903022106
101. Petrovas C, Price DA, Mattapallil J, Ambrozak DR, Geldmacher C, Cecchinato V, et al. SIV-specific CD8+ T cells express high levels of PD1 and cytokines but have impaired proliferative capacity in acute and chronic SIVmac251 infection. Blood (2007) 110:928–36. doi: 10.1182/blood-2007-01-069112
102. Sengupta S, Siliciano RF. Targeting the Latent Reservoir for HIV-1. Immunity (2018) 48:872–95. doi: 10.1016/j.immuni.2018.04.030
103. Vaccari M, Mattapallil J, Song K, Tsai WP, Hryniewicz A, Venzon D, et al. Reduced protection from simian immunodeficiency virus SIVmac251 infection afforded by memory CD8+ T cells induced by vaccination during CD4+ T-cell deficiency. J Virol (2008) 82:9629–38. doi: 10.1128/JVI.00893-08
104. Wilson DP, Mattapallil JJ, Lay MD, Zhang L, Roederer M, Davenport MP. Estimating the infectivity of CCR5-tropic simian immunodeficiency virus SIV(mac251) in the gut. J Virol (2007) 81:8025–9. doi: 10.1128/JVI.01771-06
105. Fukazawa Y, Lum R, Okoye AA, Park H, Matsuda K, Bae JY, et al. B cell follicle sanctuary permits persistent productive simian immunodeficiency virus infection in elite controllers. Nat Med (2015) 21:132–9. doi: 10.1038/nm.3781
106. Connick E, Mattila T, Folkvord JM, Schlichtemeier R, Meditz AL, Ray MG, et al. CTL fail to accumulate at sites of HIV-1 replication in lymphoid tissue. J Immunol (2007) 178:6975–83. doi: 10.4049/jimmunol.178.11.6975
107. Sasikala-Appukuttan AK, Kim HO, Kinzel NJ, Hong JJ, Smith AJ, Wagstaff R, et al. Location and dynamics of the immunodominant CD8 T cell response to SIVDeltanef immunization and SIVmac251 vaginal challenge. PloS One (2013) 8:e81623. doi: 10.1371/journal.pone.0081623
108. Dimopoulos Y, Moysi E, Petrovas C. The Lymph Node in HIV Pathogenesis. Curr HIV/AIDS Rep (2017) 14:133–40. doi: 10.1007/s11904-017-0359-7
109. Hong JJ, Chang KT, Villinger F. The Dynamics of T and B Cells in Lymph Node during Chronic HIV Infection: TFH and HIV, Unhappy Dance Partners? Front Immunol (2016) 7:522:522. doi: 10.3389/fimmu.2016.00522
110. Perreau M, Savoye AL, De Crignis E, Corpataux JM, Cubas R, Haddad EK, et al. Follicular helper T cells serve as the major CD4 T cell compartment for HIV-1 infection, replication, and production. J Exp Med (2013) 210:143–56. doi: 10.1084/jem.20121932
111. Hong JJ, Amancha PK, Rogers K, Ansari AA, Villinger F. Spatial alterations between CD4(+) T follicular helper, B, and CD8(+) T cells during simian immunodeficiency virus infection: T/B cell homeostasis, activation, and potential mechanism for viral escape. J Immunol (2012) 188:3247–56. doi: 10.4049/jimmunol.1103138
112. Petrovas C, Yamamoto T, Gerner MY, Boswell KL, Wloka K, Smith EC, et al. CD4 T follicular helper cell dynamics during SIV infection. J Clin Invest (2012) 122:3281–94. doi: 10.1172/JCI63039
113. Xu Y, Weatherall C, Bailey M, Alcantara S, De Rose R, Estaquier J, et al. Simian immunodeficiency virus infects follicular helper CD4 T cells in lymphoid tissues during pathogenic infection of pigtail macaques. J Virol (2013) 87:3760–73. doi: 10.1128/JVI.02497-12
114. Lindqvist M, van Lunzen J, Soghoian DZ, Kuhl BD, Ranasinghe S, Kranias G, et al. Expansion of HIV-specific T follicular helper cells in chronic HIV infection. J Clin Invest (2012) 122:3271–80. doi: 10.1172/JCI64314
115. Onabajo OO, Lewis MG, Mattapallil JJ. Chronic simian immunodeficiency virus infection is associated with contrasting phenotypes of dysfunctional Bcl6(+) germinal center B cells or Bcl6(-) Bcl2(+) non-germinal center B cells. J Cell Mol Med (2018) 22:5682–7. doi: 10.1111/jcmm.13844
116. Onabajo OO, Mattapallil JJ. Expansion or depletion of T follicular helper cells during HIV infection: consequences for B cell responses. Curr HIV Res (2013) 11:595–600. doi: 10.2174/1570162x12666140225153552
117. Xu H, Wang X, Malam N, Aye PP, Alvarez X, Lackner AA, et al. Persistent Simian Immunodeficiency Virus Infection Drives Differentiation, Aberrant Accumulation, and Latent Infection of Germinal Center Follicular T Helper Cells. J Virol (2016) 90:1578–87. doi: 10.1128/JVI.02471-15
118. Mylvaganam GH, Velu V, Hong JJ, Sadagopal S, Kwa S, Basu R, et al. Diminished viral control during simian immunodeficiency virus infection is associated with aberrant PD-1hi CD4 T cell enrichment in the lymphoid follicles of the rectal mucosa. J Immunol (2014) 193:4527–36. doi: 10.4049/jimmunol.1401222
119. Micci L, Cervasi B, Ende ZS, Iriele RI, Reyes-Aviles E, Vinton C, et al. Paucity of IL-21-producing CD4(+) T cells is associated with Th17 cell depletion in SIV infection of rhesus macaques. Blood (2012) 120:3925–35. doi: 10.1182/blood-2012-04-420240
120. Bixler SL, Mattapallil JJ. Loss and dysregulation of Th17 cells during HIV infection. Clin Dev Immunol (2013) 2013:852418. doi: 10.1155/2013/852418
121. Bixler SL, Sandler NG, Douek DC, Mattapallil JJ. Suppressed Th17 levels correlate with elevated PIAS3, SHP2, and SOCS3 expression in CD4 T cells during acute simian immunodeficiency virus infection. J Virol (2013) 87:7093–101. doi: 10.1128/JVI.00600-13
122. Moukambi F, Rabezanahary H, Fortier Y, Rodrigues V, Clain J, Benmadid-Laktout G, et al. Mucosal T follicular helper cells in SIV-infected rhesus macaques: contributing role of IL-27. Mucosal Immunol (2019) 12:1038–54. doi: 10.1038/s41385-019-0174-0
123. Hel Z, Xu J, Denning WL, Helton ES, Huijbregts RP, Heath SL, et al. Dysregulation of Systemic and Mucosal Humoral Responses to Microbial and Food Antigens as a Factor Contributing to Microbial Translocation and Chronic Inflammation in HIV-1 Infection. PloS Pathog (2017) 13:e1006087. doi: 10.1371/journal.ppat.1006087
124. Cubas RA, Mudd JC, Savoye AL, Perreau M, van Grevenynghe J, Metcalf T, et al. Inadequate T follicular cell help impairs B cell immunity during HIV infection. Nat Med (2013) 19:494–9. doi: 10.1038/nm.3109
125. Pallikkuth S, de Armas L, Rinaldi S, Pahwa ST. T Follicular Helper Cells and B Cell Dysfunction in Aging and HIV-1 Infection. Front Immunol (2017) 8:1380:1380. doi: 10.3389/fimmu.2017.01380
126. Xu H, Ziani W, Shao J, Doyle-Meyers LA, Russell-Lodrigue KE, Ratterree MS, et al. Impaired Development and Expansion of Germinal Center Follicular Th Cells in Simian Immunodeficiency Virus-Infected Neonatal Macaques. J Immunol (2018) 201:1994–2003. doi: 10.4049/jimmunol.1800235
127. Rabezanahary H, Moukambi F, Palesch D, Clain J, Racine G, Andreani G, et al. Despite early antiretroviral therapy effector memory and follicular helper CD4 T cells are major reservoirs in visceral lymphoid tissues of SIV-infected macaques. Mucosal Immunol (2020) 13:149–60. doi: 10.1038/s41385-019-0221-x
128. McGary CS, Deleage C, Harper J, Micci L, Ribeiro SP, Paganini S, et al. CTLA-4(+)PD-1(-) Memory CD4(+) T Cells Critically Contribute to Viral Persistence in Antiretroviral Therapy-Suppressed, SIV-Infected Rhesus Macaques. Immunity (2017) 47:776–88 e5. doi: 10.1016/j.immuni.2017.09.018
129. Velu V, Mylvaganam GH, Gangadhara S, Hong JJ, Iyer SS, Gumber S, et al. Induction of Th1-Biased T Follicular Helper (Tfh) Cells in Lymphoid Tissues during Chronic Simian Immunodeficiency Virus Infection Defines Functionally Distinct Germinal Center Tfh Cells. J Immunol (2016) 197:1832–42. doi: 10.4049/jimmunol.1600143
130. Morou A, Brunet-Ratnasingham E, Dube M, Charlebois R, Mercier E, Darko S, et al. Altered differentiation is central to HIV-specific CD4(+) T cell dysfunction in progressive disease. Nat Immunol (2019) 20:1059–70. doi: 10.1038/s41590-019-0418-x
131. Pallikkuth S, Sharkey M, Babic DZ, Gupta S, Stone GW, Fischl MA, et al. Peripheral T Follicular Helper Cells Are the Major HIV Reservoir within Central Memory CD4 T Cells in Peripheral Blood from Chronically HIV-Infected Individuals on Combination Antiretroviral Therapy. J Virol (2015) 90:2718–28. doi: 10.1128/JVI.02883-15
132. Banga R, Procopio FA, Noto A, Pollakis G, Cavassini M, Ohmiti K, et al. PD-1(+) and follicular helper T cells are responsible for persistent HIV-1 transcription in treated aviremic individuals. Nat Med (2016) 22:754–61. doi: 10.1038/nm.4113
133. Yu F, Li Q, Chen X, Liu J, Li L, Li B, et al. X4-Tropic Latent HIV-1 Is Enriched in Peripheral Follicular Helper T Cells and Is Correlated with Disease Progression. J Virol (2020) 94:e01219–19. doi: 10.1128/JVI.01219-19
134. Niessl J, Baxter AE, Morou A, Brunet-Ratnasingham E, Sannier G, Gendron-Lepage G, et al. Persistent expansion and Th1-like skewing of HIV-specific circulating T follicular helper cells during antiretroviral therapy. EBioMedicine (2020) 54:102727. doi: 10.1016/j.ebiom.2020.102727
135. Planchais C, Hocqueloux L, Ibanez C, Gallien S, Copie C, Surenaud M, et al. Early Antiretroviral Therapy Preserves Functional Follicular Helper T and HIV-Specific B Cells in the Gut Mucosa of HIV-1-Infected Individuals. J Immunol (2018) 200:3519–29. doi: 10.4049/jimmunol.1701615
136. Thornhill JP, Pace M, Martin GE, Hoare J, Peake S, Herrera C, et al. CD32 expressing doublets in HIV-infected gut-associated lymphoid tissue are associated with a T follicular helper cell phenotype. Mucosal Immunol (2019) 12:1212–9. doi: 10.1038/s41385-019-0180-2
137. Suzuki K, Fagarasan S. How host-bacterial interactions lead to IgA synthesis in the gut. Trends Immunol (2008) 29:523–31. doi: 10.1016/j.it.2008.08.001
138. Cazac BB, Roes J. TGF-beta receptor controls B cell responsiveness and induction of IgA in vivo. Immunity (2000) 13:443–51. doi: 10.1016/s1074-7613(00)00044-3
139. Cerutti A. The regulation of IgA class switching. Nat Rev Immunol (2008) 8:421–34. doi: 10.1038/nri2322
140. Cerutti A, Rescigno M. The biology of intestinal immunoglobulin A responses. Immunity (2008) 28:740–50. doi: 10.1016/j.immuni.2008.05.001
141. Fagarasan S, Honjo T. Intestinal IgA synthesis: regulation of front-line body defences. Nat Rev Immunol (2003) 3:63–72. doi: 10.1038/nri982
142. Macpherson AJ, McCoy KD, Johansen FE, Brandtzaeg P. The immune geography of IgA induction and function. Mucosal Immunol (2008) 1:11–22. doi: 10.1038/mi.2007.6
143. Chaoul N, Burelout C, Peruchon S, van Buu BN, Laurent P, Proust A, et al. Default in plasma and intestinal IgA responses during acute infection by simian immunodeficiency virus. Retrovirology (2012) 9:43. doi: 10.1186/1742-4690-9-43
144. Schafer F, Kewenig S, Stolte N, Stahl-Hennig C, Stallmach A, Kaup FJ, et al. Lack of simian immunodeficiency virus (SIV) specific IgA response in the intestine of SIV infected rhesus macaques. Gut (2002) 50:608–14. doi: 10.1136/gut.50.5.608
145. Barassi C, Lazzarin A, Lopalco L. CCR5-specific mucosal IgA in saliva and genital fluids of HIV-exposed seronegative subjects. Blood (2004) 104:2205–6. doi: 10.1182/blood-2004-06-2134
146. Bishop PE, McMillan A, Gilmour HM. Immunological study of the rectal mucosa of men with and without human immunodeficiency virus infection. Gut (1987) 28:1619–24. doi: 10.1136/gut.28.12.1619
147. Kotler DP, Scholes JV, Tierney AR. Intestinal plasma cell alterations in acquired immunodeficiency syndrome. Dig Dis Sci (1987) 32:129–38. doi: 10.1007/BF01297100
148. Mestecky J, Wright PF, Lopalco L, Staats HF, Kozlowski PA, Moldoveanu Z, et al. Scarcity or absence of humoral immune responses in the plasma and cervicovaginal lavage fluids of heavily HIV-1-exposed but persistently seronegative women. AIDS Res Hum Retroviruses (2011) 27:469–86. doi: 10.1089/aid.2010.0169
149. Nilssen DE, Oktedalen O, Brandtzaeg P. Intestinal B cell hyperactivity in AIDS is controlled by highly active antiretroviral therapy. Gut (2004) 53:487–93. doi: 10.1136/gut.2003.027854
150. Raux M, Finkielsztejn L, Salmon-Ceron D, Bouchez H, Excler JL, Dulioust E, et al. Comparison of the distribution of IgG and IgA antibodies in serum and various mucosal fluids of HIV type 1-infected subjects. AIDS Res Hum Retroviruses (1999) 15:1365–76. doi: 10.1089/088922299310070
151. Schneider T, Zippel T, Schmidt W, Pauli G, Wahnschaffe U, Chakravarti S, et al. Increased immunoglobulin G production by short term cultured duodenal biopsy samples from HIV infected patients. Gut (1998) 42:357–61. doi: 10.1136/gut.42.3.357
152. Scamurra RW, Nelson DB, Lin XM, Miller DJ, Silverman GJ, Kappel T, et al. Mucosal plasma cell repertoire during HIV-1 infection. J Immunol (2002) 169:4008–16. doi: 10.4049/jimmunol.169.7.4008
153. Xu W, Santini PA, Sullivan JS, He B, Shan M, Ball SC, et al. HIV-1 evades virus-specific IgG2 and IgA responses by targeting systemic and intestinal B cells via long-range intercellular conduits. Nat Immunol (2009) 10:1008–17. doi: 10.1038/ni.1753
154. Levesque MC, Moody MA, Hwang KK, Marshall DJ, Whitesides JF, Amos JD, et al. Polyclonal B cell differentiation and loss of gastrointestinal tract germinal centers in the earliest stages of HIV-1 infection. PloS Med (2009) 6:e1000107. doi: 10.1371/journal.pmed.1000107
155. Kok A, Hocqueloux L, Hocini H, Carriere M, Lefrou L, Guguin A, et al. Early initiation of combined antiretroviral therapy preserves immune function in the gut of HIV-infected patients. Mucosal Immunol (2015) 8:127–40. doi: 10.1038/mi.2014.50
156. Burgener A, McGowan I, Klatt NR. HIV and mucosal barrier interactions: consequences for transmission and pathogenesis. Curr Opin Immunol (2015) 36:22–30. doi: 10.1016/j.coi.2015.06.004
157. Dandekar S, George MD, Baumler AJ. Th17 cells, HIV and the gut mucosal barrier. Curr Opin HIV AIDS (2010) 5:173–8. doi: 10.1097/COH.0b013e328335eda3
158. Hirao LA, Grishina I, Bourry O, Hu WK, Somrit M, Sankaran-Walters S, et al. Early mucosal sensing of SIV infection by paneth cells induces IL-1beta production and initiates gut epithelial disruption. PloS Pathog (2014) 10:e1004311. doi: 10.1371/journal.ppat.1004311
159. Hunt PW, Sinclair E, Rodriguez B, Shive C, Clagett B, Funderburg N, et al. Gut epithelial barrier dysfunction and innate immune activation predict mortality in treated HIV infection. J Infect Dis (2014) 210:1228–38. doi: 10.1093/infdis/jiu238
160. Ponte R, Mehraj V, Ghali P, Couedel-Courteille A, Cheynier R, Routy JP. Reversing Gut Damage in HIV Infection: Using Non-Human Primate Models to Instruct Clinical Research. EBioMedicine (2016) 4:40–9. doi: 10.1016/j.ebiom.2016.01.028
161. Raehtz KD, Barrenas F, Xu C, Busman-Sahay K, Valentine A, Law L. African green monkeys avoid SIV disease progression by preventing intestinal dysfunction and maintaining mucosal barrier integrity. PloS Pathog (2020) 16:e1008333. doi: 10.1371/journal.ppat.1008333
162. Atarashi K, Tanoue T, Shima T, Imaoka A, Kuwahara T, Momose Y, et al. Induction of colonic regulatory T cells by indigenous Clostridium species. Science (2011) 331:337–41. doi: 10.1126/science.1198469
163. Furusawa Y, Obata Y, Fukuda S, Endo TA, Nakato G, Takahashi D, et al. Commensal microbe-derived butyrate induces the differentiation of colonic regulatory T cells. Nature (2013) 504:446–50. doi: 10.1038/nature12721
164. Hooper LV, Littman DR, Macpherson AJ. Interactions between the microbiota and the immune system. Science (2012) 336:1268–73. doi: 10.1126/science.1223490
165. Muranski P, Restifo NP. Essentials of Th17 cell commitment and plasticity. Blood (2013) 121:2402–14. doi: 10.1182/blood-2012-09-378653
166. Telesford KM, Yan W, Ochoa-Reparaz J, Pant A, Kircher C, Christy MA, et al. A commensal symbiotic factor derived from Bacteroides fragilis promotes human CD39(+)Foxp3(+) T cells and Treg function. Gut Microbes (2015) 6:234–42. doi: 10.1080/19490976.2015.1056973
167. Wu HJ, Wu E. The role of gut microbiota in immune homeostasis and autoimmunity. Gut Microbes (2012) 3:4–14. doi: 10.4161/gmic.19320
168. Hegazy AN, West NR, Stubbington MJT, Wendt E, Suijker KIM, Datsi A, et al. Circulating and Tissue-Resident CD4(+) T Cells With Reactivity to Intestinal Microbiota Are Abundant in Healthy Individuals and Function Is Altered During Inflammation. Gastroenterology (2017) 153:1320–37 e16. doi: 10.1053/j.gastro.2017.07.047
169. Gutzeit C, Magri G, Cerutti A. Intestinal IgA production and its role in host-microbe interaction. Immunol Rev (2014) 260:76–85. doi: 10.1111/imr.12189
170. Jones L, Ho WQ, Ying S, Ramakrishna L, Srinivasan KG, Yurieva M, et al. A subpopulation of high IL-21-producing CD4(+) T cells in Peyer’s Patches is induced by the microbiota and regulates germinal centers. Sci Rep (2016) 6:30784. doi: 10.1038/srep30784
171. Maruya M, Kawamoto S, Kato LM, Fagarasan S. Impaired selection of IgA and intestinal dysbiosis associated with PD-1-deficiency. Gut Microbes (2013) 4:165–71. doi: 10.4161/gmic.23595
172. Kawamoto S, Tran TH, Maruya M, Suzuki K, Doi Y, Tsutsui Y, et al. The inhibitory receptor PD-1 regulates IgA selection and bacterial composition in the gut. Science (2012) 336:485–9. doi: 10.1126/science.1217718
173. Teng F, Klinger CN, Felix KM, Bradley CP, Wu E, Tran NL, et al. Gut Microbiota Drive Autoimmune Arthritis by Promoting Differentiation and Migration of Peyer’s Patch T Follicular Helper Cells. Immunity (2016) 44:875–88. doi: 10.1016/j.immuni.2016.03.013
174. Ballesteros-Tato A, Leon B, Graf BA, Moquin A, Adams PS, Lund FE, et al. Interleukin-2 inhibits germinal center formation by limiting T follicular helper cell differentiation. Immunity (2012) 36:847–56. doi: 10.1016/j.immuni.2012.02.012
175. Johnston RJ, Choi YS, Diamond JA, Yang JA, Crotty S. STAT5 is a potent negative regulator of TFH cell differentiation. J Exp Med (2012) 209:243–50. doi: 10.1084/jem.20111174
176. Proietti M, Cornacchione V, Rezzonico Jost T, Romagnani A, Faliti CE, Perruzza L, et al. ATP-gated ionotropic P2X7 receptor controls follicular T helper cell numbers in Peyer’s patches to promote host-microbiota mutualism. Immunity (2014) 41:789–801. doi: 10.1016/j.immuni.2014.10.010
177. Dillon SM, Frank DN, Wilson CC. The gut microbiome and HIV-1 pathogenesis: a two-way street. AIDS (2016) 30:2737–51. doi: 10.1097/QAD.0000000000001289
178. Zevin AS, McKinnon L, Burgener A, Klatt NR. Microbial translocation and microbiome dysbiosis in HIV-associated immune activation. Curr Opin HIV AIDS (2016) 11:182–90. doi: 10.1097/COH.0000000000000234
179. Dillon SM, Lee EJ, Kotter CV, Austin GL, Dong Z, Hecht DK, et al. An altered intestinal mucosal microbiome in HIV-1 infection is associated with mucosal and systemic immune activation and endotoxemia. Mucosal Immunol (2014) 7:983–94. doi: 10.1038/mi.2013.116
180. Mutlu EA, Keshavarzian A, Losurdo J, Swanson G, Siewe B, Forsyth C, et al. A compositional look at the human gastrointestinal microbiome and immune activation parameters in HIV infected subjects. PloS Pathog (2014) 10:e1003829. doi: 10.1371/journal.ppat.1003829
181. Vujkovic-Cvijin I, Dunham RM, Iwai S, Maher MC, Albright RG, Broadhurst MJ, et al. Dysbiosis of the gut microbiota is associated with HIV disease progression and tryptophan catabolism. Sci Transl Med (2013) 5:193ra91. doi: 10.1126/scitranslmed.3006438
182. Le Chatelier E, Nielsen T, Qin J, Prifti E, Hildebrand F, Falony G, et al. Richness of human gut microbiome correlates with metabolic markers. Nature (2013) 500:541–6. doi: 10.1038/nature12506
183. Lozupone CA, Li M, Campbell TB, Flores SC, Linderman D, Gebert MJ, et al. Alterations in the gut microbiota associated with HIV-1 infection. Cell Host Microbe (2013) 14:329–39. doi: 10.1016/j.chom.2013.08.006
184. Noguera-Julian M, Rocafort M, Guillen Y, Rivera J, Casadella M, Nowak P, et al. Gut Microbiota Linked to Sexual Preference and HIV Infection. EBioMedicine (2016) 5:135–46. doi: 10.1016/j.ebiom.2016.01.032
185. Vazquez-Castellanos JF, Serrano-Villar S, Latorre A, Artacho A, Ferrus ML, Madrid N, et al. Altered metabolism of gut microbiota contributes to chronic immune activation in HIV-infected individuals. Mucosal Immunol (2015) 8:760–72. doi: 10.1038/mi.2014.107
186. Yu G, Fadrosh D, Ma B, Ravel J, Goedert JJ. Anal microbiota profiles in HIV-positive and HIV-negative MSM. AIDS (2014) 28:753–60. doi: 10.1097/QAD.0000000000000154
187. Blum FC, Hardy BL, Bishop-Lilly KA, Frey KG, Hamilton T, Whitney JB, et al. Microbial Dysbiosis During Simian Immunodeficiency Virus Infection is Partially Reverted with Combination Anti-retroviral Therapy. Sci Rep (2020) 10:6387. doi: 10.1038/s41598-020-63196-0
188. Handley SA, Thackray LB, Zhao G, Presti R, Miller AD, Droit L, et al. Pathogenic simian immunodeficiency virus infection is associated with expansion of the enteric virome. Cell (2012) 151:253–66. doi: 10.1016/j.cell.2012.09.024
189. Monaco CL, Gootenberg DB, Zhao G, Handley SA, Ghebremichael MS, Lim ES, et al. Altered Virome and Bacterial Microbiome in Human Immunodeficiency Virus-Associated Acquired Immunodeficiency Syndrome. Cell Host Microbe (2016) 19:311–22. doi: 10.1016/j.chom.2016.02.011
Keywords: HIV, SIV, microbiome, Tfh, IgA, mucosa, GALT, microbial translocation
Citation: Onabajo OO and Mattapallil JJ (2021) Gut Microbiome Homeostasis and the CD4 T- Follicular Helper Cell IgA Axis in Human Immunodeficiency Virus Infection. Front. Immunol. 12:657679. doi: 10.3389/fimmu.2021.657679
Received: 23 January 2021; Accepted: 01 March 2021;
Published: 19 March 2021.
Edited by:
Vijayakumar Velu, Emory University, United StatesReviewed by:
Huanbin Xu, Tulane University, United StatesCopyright © 2021 Onabajo and Mattapallil. This is an open-access article distributed under the terms of the Creative Commons Attribution License (CC BY). The use, distribution or reproduction in other forums is permitted, provided the original author(s) and the copyright owner(s) are credited and that the original publication in this journal is cited, in accordance with accepted academic practice. No use, distribution or reproduction is permitted which does not comply with these terms.
*Correspondence: Joseph J. Mattapallil, am9zZXBoLm1hdHRhcGFsbGlsQHVzdWhzLmVkdQ==
Disclaimer: All claims expressed in this article are solely those of the authors and do not necessarily represent those of their affiliated organizations, or those of the publisher, the editors and the reviewers. Any product that may be evaluated in this article or claim that may be made by its manufacturer is not guaranteed or endorsed by the publisher.
Research integrity at Frontiers
Learn more about the work of our research integrity team to safeguard the quality of each article we publish.