- 1Section of Immunology and Joint Immunology Program, Guangdong Provincial Academy of Chinese Medical Sciences, Guangzhou, China
- 2The Second Affiliated Hospital of Guangzhou University of Chinese Medicine, Guangzhou, China
Emerging evidence has linked the gut microbiota dysbiosis to transplant rejection while memory T-cells pose a threat to long-term transplant survival. However, it's unclear if the gut microbiome alters the formation and function of alloreactive memory T-cells. Here we studied the effects of berberine, a narrow-spectrum antibiotic that is barely absorbed when orally administered, on the gut microbiota, memory T-cells, and allograft survival. In this study, C57BL/6 mice transplanted with islets or a heart from BALB/c mice were treated orally with berberine. Allograft survival was observed, while spleen, and lymph node T-cells from recipient mice were analyzed using a flow cytometer. High-throughput sequencing and qPCR were performed to analyze the gut microbiota. CD8+ T-cells from recipients were cultured with the bacteria to determine potential T-cell memory cross-reactivity to a specific pathogen. We found that berberine suppressed islet allograft rejection, reduced effector CD8+CD44highCD62Llow and central memory CD8+CD44highCD62Lhigh T-cells (TCM), altered the gut microbiota composition and specifically lowered Bacillus cereus abundance. Further, berberine promoted long-term islet allograft survival induced by conventional costimulatory blockade and induced cardiac allograft tolerance as well. Re-colonization of B. cereus upregulated CD8+ TCM cells and reversed long-term islet allograft survival induced by berberine plus the conventional costimulatory blockade. Finally, alloantigen-experienced memory CD8+ T-cells from transplanted recipients rapidly responded to B. cereus in vitro. Thus, berberine prolonged allograft survival by repressing CD8+ TCM through regulating the gut microbiota. We have provided the first evidence that donor-specific memory T-cell generation is linked to a specific microbe and uncovered a novel mechanism underlying the therapeutic effects of berberine. This study may be implicated for suppressing human transplant rejection since berberine is already used in clinic to treat intestinal infections.
Introduction
The gut microbiota not only is involved in host physiological processes, including vitamin synthesis, metabolism and immune defense (1, 2), but also plays an important role in many diseases, including multiple sclerosis (3) and autoimmune arthritis (4). Recently, a growing number of studies revealed the relationship between the gut microbiota and allograft rejection and GVHD (5–13). Changes in urinary microbiota correlated with renal transplant outcomes (14, 15). It was shown that microbiota dysbiosis was obviously associated with post-operative complications, such as infection and graft rejection (16–18). Meanwhile, pretreatment of recipient mice with broad-spectrum antibiotics (ABX) also prolonged survival of minor antigen-mismatched skin transplants (19) while differences in gut microbes in mice derived from different vendors resulted in a different graft outcome (20). Acute infection could temporarily interrupt tolerance (21) while antibiotic pretreatment attenuated hepatic transplant injury (22). Although it remains not fully understood how microbiota regulates immunity, recent advances in the field showed that a short-chain fatty acid, butyrate, regulated energy metabolism and inflammation (23, 24). Interaction of microbiota and bile acids could also alter host metabolism (25). Furthermore, three major tryptophan metabolites that affect T cell function, including serotonin, kynurenine, and indole derivatives, were controlled by the gut microbiota (26). Thus, the gut microbiota could be a therapeutic target for preventing transplant rejection.
Berberine, a natural ingredient originally derived from the plant goldthread, has been widely used to treat bacteria-associated diarrhea as an antibiotic in China (27, 28). Berberine monomer is poorly absorbed by the gastrointestinal tract (29–32), and thus its modulation of gut microbiota has been hypothesized as one of the main mechanisms underlying its beneficial effects on diabetes (33). Moreover, some studies demonstrated that berberine ameliorated autoimmune diseases, including colitis, arthritis and experimental autoimmune encephalomyelitis (EAE), by inhibiting Th1 and/or Th17 responses in rodents (34–42). Nevertheless, the direct effects of berberine on transplant rejection remain undefined. This study was undertaken to investigate the effects of berberine on allograft rejection and its underlying mechanisms in a murine model. We found that berberine promoted long-term islet allograft survival and cardiac allograft tolerance induced by brief costimulatory blockade via shrinking alloreactive CD8+ TCM pool through altering the gut microbiota. This study may be implicated for the treatment of transplant rejection in humans.
Materials and Methods
Animals
BALB/c and C57BL/6 male mice (6 to 8 weeks-old) were obtained from Guangdong Medical Laboratory Animal Center (Guangdong, China), while C3H/HeJ mice (6 to 8 weeks-old) were purchased from Jiangsu ALF Biotechnology company (Nanjing, China). Mice were bred and maintained under a specific pathogen-free condition. Animal protocols involved in this study were approved by the Animal Ethics Committee of Guangdong Provincial Academy of Chinese Medical Sciences.
Treatment of Mice
Recipient mice were randomly divided into control groups and groups treated with berberine (Ber: 200 mg/kg body weight daily) or ABX via oral gavage. Both Ber (Alfa Biotechnology, China, purity >99%) and ABX (Solarbio, China) were prepared with sterilized water. Mice in ABX group received 200 μl of mixed antibiotics, including gentamycin (0.35 mg/ml), kanamycin (5.25 mg/ml), colistin (8,500 U), metronidazole (2.15 mg/ml), and vancomycin (0.5 mg/ml), as described previously (19). Mice were treated with Ber or ABX for 3 weeks after transplantation or until graft rejection, whichever came earlier. In some experiments, mice were pretreated with ABX for 6 days prior to transplantation and rested for 1 day before further treatment. To induce long-term allograft survival, some recipients received brief treatment with low-doses of anti-CD154 Ab (MR1) or CTLA4-Ig (Bio X Cell, West Lebanon, USA) at 0.2 mg on days 0 and 4.
Murine Islet and Heart Transplantation
Islet transplantation was performed as described in our previous studies (43, 44). Briefly, 2 ml of collagenase V (1 mg/mL, Sigma) was injected into common bile duct of BALB/c donors. The pancreas was removed and incubated in a 37°C water bath for 16–18 min and the crude preparation was filtered through a 100-μm nylon cell-strainer. Islets were counted and ~400 islets were injected into the renal subcapsular space of C57BL/6 recipients. Recipient mice were rendered diabetic by a single injection of streptozotocin (180 mg/kg, Sigma) 10–14 days before transplantation. Primary graft function was characterized by a blood glucose level of <200 mg/dL for 48 h after transplantation. Graft rejection was defined as a rise in blood glucose to >300 mg/dL for 2 consecutive days after primary function. Cardiac donors were 7 to 8-week-old BALB/c (H-2d) mice while recipients were 7 to 8-week-old C57BL/6 mice (H-2b). Fully vascularized heterotopic heart transplantation was performed as described previously (45, 46).
Flow Cytometry
Draining lymph node (dLN) and spleen cells were harvested and stained with anti-CD4-FITC, CD8-FITC, CD44-V450, CD62L-PE-Cy7, FoxP3-APC, EOMES-PE-Cy7, Bcl-6-PE, and anti-Thy1.1 (CD90.1)-PE Abs (eBioscience or BD Biosciences). To determine intracellular expression of FoxP3, EOMES, and Bcl-6, cells were fixed and permeated according to the protocol of Foxp3/Transcription Factor Fixation/Permeabilization Concentrate and Diluent Kit (eBioscience). Then, cells were stained with anti-FoxP3, EOMES, or Bcl-6 Abs and finally analyzed using FACSAria III (BD Biosciences). To purify CD8+ TCM cells, cells were stained with anti-CD8-FITC, CD44-V450, and anti-CD62L-PE-Cy7 Abs and CD8+CD44highCD62Lhigh T cells were sorted out via FACSAria III (BD Biosciences). To purify CD3+ T cells, splenocytes were stained with anti-CD3-PE Ab and CD3+ cells then were sorted out.
16S rRNA Gene Amplification and Sequencing
Caecum microbiota from recipient mice were collected and used for DNA extraction. Then V4 region of 16S rRNA genes was amplified from purified DNA using specific PCR primers (F515/R806). Replicate PCR products were quantified and pooled. Finally, all determinant libraries were sequenced on an Illumina MiSeq platform.
Sequencing Data Analysis
Sequencing data were analyzed via FLASH (Fast Length Adjustment of Short reads, v1.2.11) for generating Tags, which were subsequently clustered to OTU (Operational Taxonomic Unit) at 97% similarity by scripts of software USEARCH (v7.0.1090). Then OTU representative sequences were taxonomically classified using Ribosomal Database Project (RDP) Classifier v.2.2 (47) trained on the Greengenes database (48), utilizing 0.6 confidence value as cutoff.
Bacteria Strains, Culture, and Preparation of Bacterial Suspension
Bacteroides ovatus (Bacteroides ov.) was purchased from ATCC (ATCC 8483), and cultured in anaerobically sterilized ATCC medium 260 containing tryptone, soytone, NaCl, and sheep blood (defibrinated) under strict anaerobic conditions overnight. Bacillus ce. was bought from BeNa Culture Collection (BNCC103930) and cultured in anaerobically sterilized medium 260. Briefly, cultures were centrifuged at 9,000 rpm for 10 min and re-suspended in PBS containing 10% glycerol to 5 × 1010 colony-forming units (CFUs)/ml.
Quantification of the Abundance of Bacteria Via qPCR
Bacterial relative abundance was determined by qPCR using the ABI ViiA 7 detection system (Thermo Fisher Scientific) and THUNDERBIRD SYBR qPCR Mix (TOYOBO). The relative quantity of each bacteria was calculated by the ΔCt method and was normalized to the amount of total bacteria (16S). The primers for 16S rRNA gene were used as the following: Forward, 5′-ACTCCTACGGGAGGCAGCAG-3′, Reverse, 5′-ATTACCGCGGCTGCTGG-3′. For taxa assays, the following primers were used: Bacteroides ov., Forward, 5′-AAGTCGAGGGGCAGCATTTT-3′, Reverse, 5′- CACAACTGACTTAACAATCC-3′; and Bacillus ce., Forward, 5′-TTCAAATTCAAAAGAATGTTGAAGAAGG-3′, Reverse, 5′-GATTTGTTTGCTTATTCATTTCATCAC-3′.
Analysis of T Cell Apoptosis and Proliferation in vitro
FACS-sorted CD3+ T cells derived from Thy1.1+ C57BL/6 mice were sorted out, labeled with CFSE and then cultured with BALB/c splenocytes in the presence of berberine or CsA. Briefly, cells were stained for Annexin V-APC according to the protocol of Annexin V Apoptosis Detection Kit (BD Biosciences). For proliferation assays, cells were labeled with 1 μM CFSE (Invitrogen, Germany) at room temperature for 15 min. Then, cells (2 × 105 cells/well) were cultured with mitomycin C-treated BALB/c splenocytes (4 × 105 /well) in 96-well plates in complete RPMI-1640 medium in the presence of IL-2 (10 ng/ml, Peprotech) at 37°C for 4 days. These cells were treated with berberine (0.1 and 0.5μM) or CsA (100 mM). To measure memory T-cell response to Bacillus ce., CD8+ T-cells from previously transplanted mice were purified, labeled with CFSE and cultured with self-APCs in the presence of inactivated Bacillus ce. (1 × 105 CFU/well), for 24 h. Bacillus ce. was inactivated by incubating at 65°C for 1 h (49), while self-APCs (50) were obtained from C57BL/6 splenocytes depleted of CD3+ T cells using magnetic micro-beads. Finally, cells were harvested and their proliferation was measured after gating on Thy1.1+ populations using FACS.
Measurement of IFN-γ and IL-17A via ELISA
The protein levels of IFN-γ and IL-17A were measured using ELISA kits according to the manufacturer's instructions (Boster, China), and the absorbance was read at 450 nm in a microplate spectrophotometer (Thermo Fisher Scientific, USA).
Statistical Analysis
Comparisons of the means were performed using one-way ANOVA or Student t-test. Data analyzed using GraphPad Prism 7 (GraphPad Software, La Jolla, CA, USA). The analysis of graft survival was performed using Kaplan–Meier method (log-rank test). A value of P < 0.05 was considered statistically significant.
Results
Berberine Inhibits Murine Islet Allograft Rejection
To investigate the effects of berberine on allograft survival, C57BL/6 mice were transplanted with islets from BALB/c mice and treated with berberine (Ber) or broad-spectrum antibiotics (ABX). As shown in Table 1, berberine extended islet allograft survival in immune competent wild-type mice compared to the control, with a statistical significance (median survival time or MST = 33 vs. 14 days, P < 0.05), while ABX did not (MST = 18 vs. 14, P > 0.05), suggesting that berberine can regulate alloimmunity.
Berberine Diminishes Effector and Memory CD8+ T Cells
To determine whether berberine would regulate effector CD8+ T cells (CD44highCD62Llow, Teff) and central memory CD8+ T cells (CD44highCD62Lhigh, TCM), dLN and spleen cells from recipient mice treated with berberine or ABX were isolated 4 weeks after transplantation. As shown in Figures 1A,B, either berberine or ABX decreased CD8+ Teff number in dLNs while berberine, but not ABX, also reduced their number even in the spleen. However, it was berberine but not ABX that lowered CD8+ TCM number in dLNs and spleen of recipient mice (Figure 1B). Interestingly, berberine did not significantly alter CD4+FoxP3+ Tregs (Supplementary Figure 1).
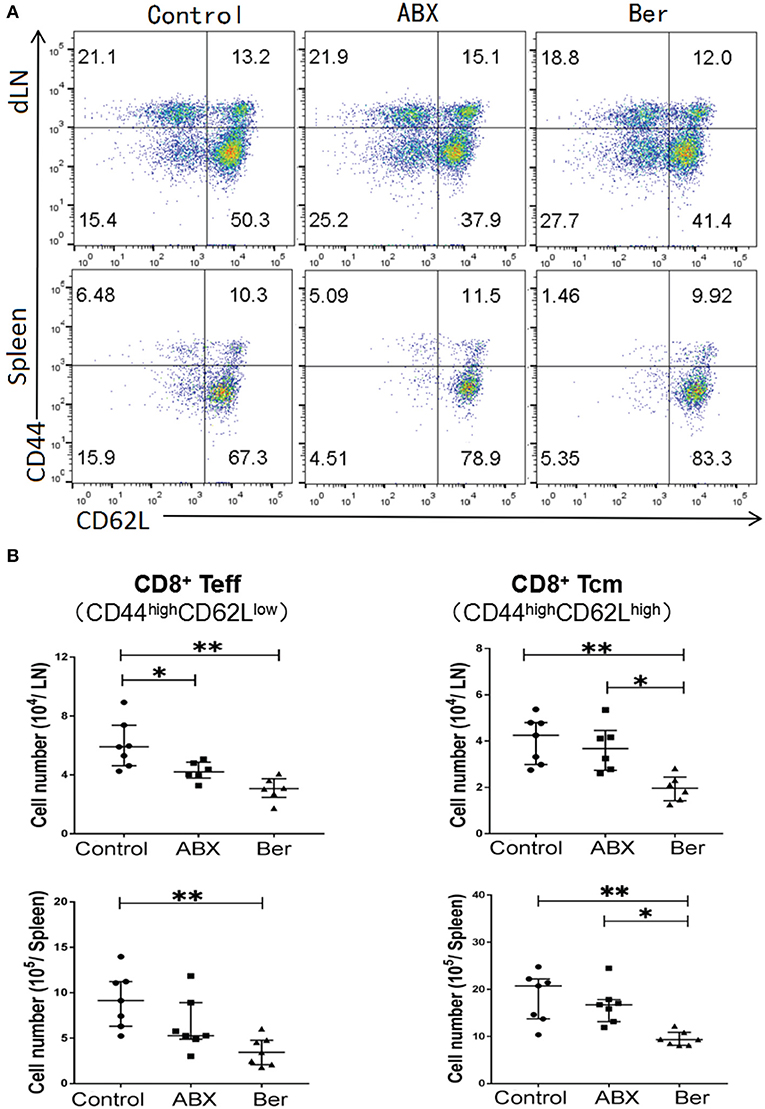
Figure 1. Berberine reduces effector and memory CD8+ T cells. Cells from dLN and spleen of recipient mice were harvested 4 weeks after islet transplantation and treatment with berberine. The percentages of CD8+CD44highCD62Llow (Teff) and CD8+CD44highCD62Lhigh (Tcm) cells were measured via a flow cytometer (A), while their absolute numbers were also calculated (B). Data of individual values (b) are presented as median ± interquartile range (*P < 0.05 and **P < 0.01, n = 6–7 mice/group).
Four weeks after transplantation, berberine also significantly reduced CD8+ TCM cell number in naïve mice without transplantation (Supplementary Figure 2). Moreover, we tracked CD8+/CD4+ Thy1.1+ TCM cell formation in transplanted mice adoptively transferred with naïve Thy1.1+ T cells. CD44highCD62LhighCD8+/CD4+TCM numbers within Thy1.1+ population were determined via FACS. We found that berberine, but not ABX, reduced the number of Thy1.1+CD8+, but not Thy1.1+CD4+, TCM cells in both dLNs and spleen (Supplementary Figure 3), indicating that it downregulates CD8+, but not CD4+, T-cell memory. To further track alloreactive CD8+ TCM cells that are still non-artificial or non-transgenic, CD3+ T cells isolated from naïve Thy1.1+ B6 mice were stained with CFSE and stimulated with BALB/c splenocytes in an MLR for 4 days. Thy1.1+CD8+ T cells that underwent at least two divisions were sorted and injected into C57BL/6 mice that were then transplanted with BALB/c islets and treated with berberine. We found that alloreactive Thy1.1+CD8+ TCM cell number was decreased by berberine, but not ABX (Supplementary Figure 4). Finally, berberine did not promote the apoptosis of CD4+ T, CD8+ T, CD19+, and CD11c+ cells (Supplementary Figure 5), suggesting that orally administered berberine is non-cytotoxic.
Berberine Suppresses EOMES Expression in CD8+ T Cells in vivo
EOMES, a transcription factor, is considered to be crucial for the maintenance of TCM cells (51, 52). Bcl-6 is another transcription factor that is also important for the generation of TCM cells (53). We found that berberine downregulated EOMES expression in CD8+ T cells from recipient mice, but did not significantly reduce Bcl-6 expression (Figure 2). However, there was no significant difference in their expression between ABX and control groups.
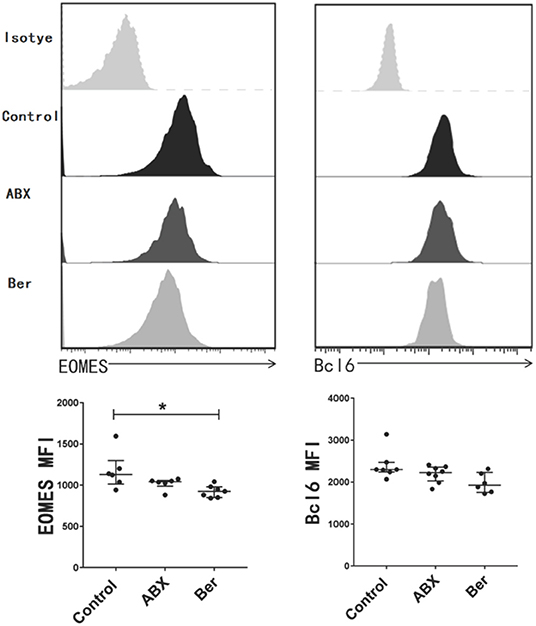
Figure 2. Berberine inhibits the expression of EOMES in CD8+ T cells. dLN cells were harvested 14 days after allotransplantation with various treatments. The expression of EOMES and Bcl-6 in CD8+ T cells was detected via a flow cytometer and quantified as mean fluorescence intensity (MFI). Histogram is shown after gating on CD8+ population. Plots of individual values are presented as median ± interquartile range (*P < 0.05, n = 6–8 mice/group).
Berberine Alters the Composition of the Gut Microbiota in Recipient Mice
To characterize the potential changes in gut flora 14 days after transplantation, bacterial DNA was extracted from caecal samples of recipients and 16S rRNA sequencing was performed. Berberine did not significantly decrease overall bacterial richness, as determined by analysis of OTUs (operational taxonomic units), which contrasted with a reduction in bacterial richness in ABX-treated mice (data not shown). Therefore, berberine treatment did not alter general bacterial richness. Furthermore, we analyzed the microbial abundance to identify berberine-sensitive microorganism. Among the top 26 genus-level taxa, berberine-treated recipients had much lower relative abundance of Bacillus in their microbiota and higher abundance of Bacteroides and Turicibacter (Figure 3A). Quantitative PCR also was performed to validate the sequencing results, and the expansion of Bacteroides ov. and contraction of Bacillus ce. were indeed observed in the cecum microbiota of berberine-treated mice (Figure 3B).
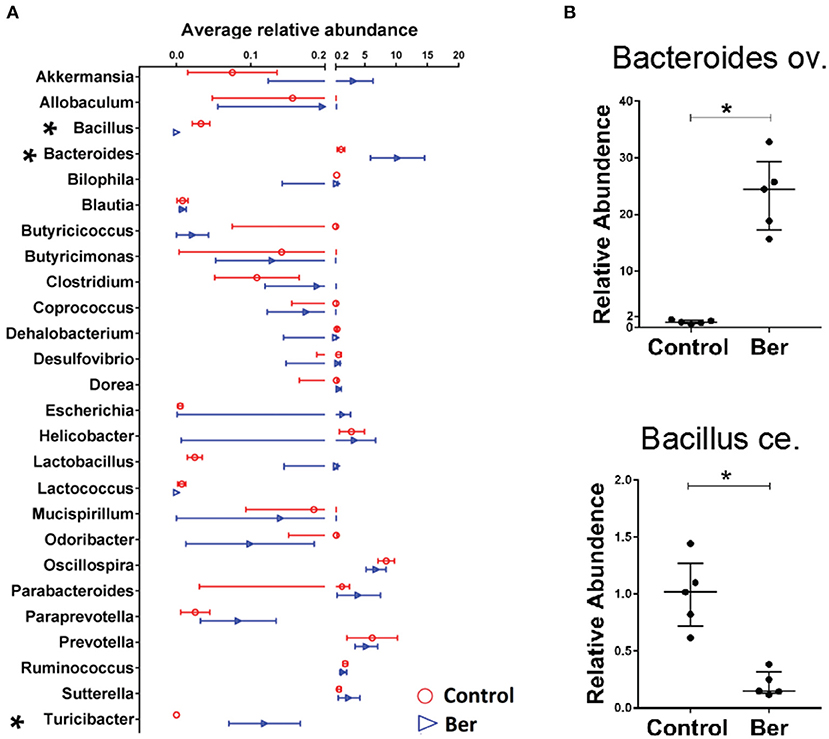
Figure 3. Berberine alters the composition of gut microbiota in recipient mice. Bacterial DNA was isolated from the cecum of B6 recipient mice transplanted with BALB/c islets 14 days after berberine treatment, and was analyzed via high-throughput sequencing. (A) The distribution of the top 26 bacterial taxa in berberine-treated and control groups at genus level (n = 4/group, shown as mean ± SEM, *P < 0.05). (B) Quantitative PCR was performed for validation of relative abundance of Bacteroides ov. and Bacillus ce. in caecum of control and berberine-treated mice (n = 5 mice/group, shown as median ± interquartile range with control group being set as 1.0).
Berberine Promotes Long-Term Islet Allograft Survival Under the Cover of Costimulatory Blockade While Addition of Exogenous CD8+ TCM Cells or Bacillus ce. Reverses It
Since berberine reduced CD8+ TCM formation, we then asked if berberine would help induce long-term islet allograft survival. We found that treatment with berberine plus either anti-CD154 Ab (MR1) (Figure 4A) or CTLA4-Ig (Figure 4B) induced long-term islet allograft survival (>90 days) in some recipients although berberine, MR1 or CTLA4-Ig alone extended islet allograft survival. On day 28, some groups of transplanted mice that were treated with berberine plus MR1 or CTLA4-Ig also received a single dose of bacterial strain (Bacillus ce. or Bacteroides ov.: 1 × 107 CFU) or CD8+ TCM cells derived from C57BL/6 recipients that were previously transplanted/primed with either BALB/c or C3H/HeJ skin (3rd-Party). As shown in Figure 4, administration of alloreactive, but not 3rd -party, CD8+ TCM cells reversed the long-term islet allograft survival, with all recipients rejecting their allografts within 60 days, and so did Bacillus ce. transplantation. However, addition of Bacteroides ov. failed to alter long-term islet survival induced by Ber + MR1 (Figure 4A), while treatment with ABX + CTLA4-Ig also did not further extend the islet allograft survival compared to CTLA4-Ig alone (Figure 4B). Thus, although berberine increased the abundance of Bacteroides ov., the latter did not prolong allograft survival.
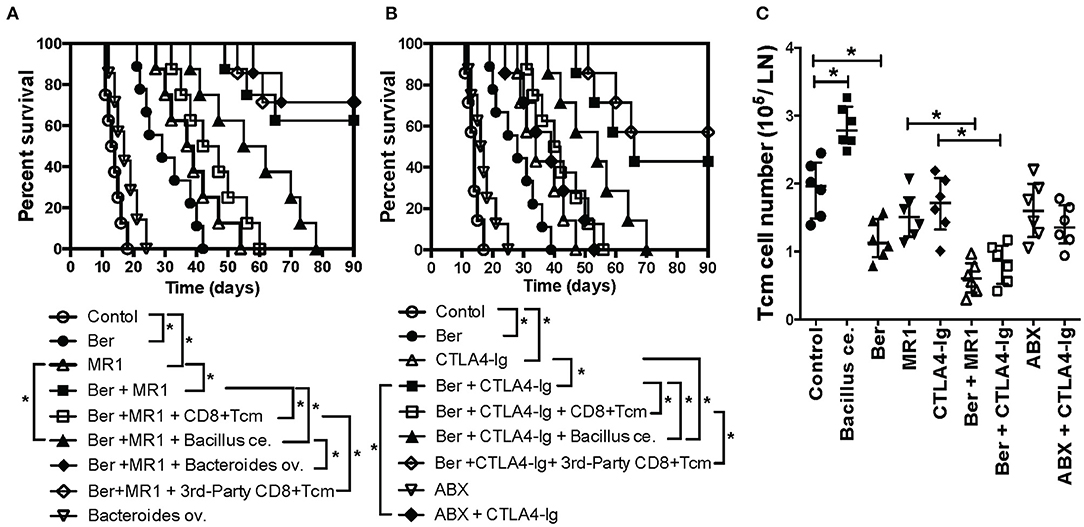
Figure 4. Berberine promotes long-term islet allograft survival induced by costimulatory blockade while addition of exogenous CD8+ TCM cells or Bacillus ce. reverses the long-term islet survival. C57BL/6 mice were transplanted with islets from BALB/c mice and treated with berberine for 3 weeks and/or MR1/CTLA4-Ig on days 0 and 4. On day 28, some of transplanted mice that were treated with berberine plus MR1 (A) or plus CTLA4-Ig (B) received a single dose of bacterial strain (Bacillus ce. or Bacteroides ov.: 1 × 107 CFU) or 1 × 106 CD8+ TCM cells isolated from an untreated B6 recipient that was previously transplanted/primed with BALB/c or C3H/HeJ skin (3rd-Party). Islet allograft survival was observed (n = 7–9 mice, *P < 0.05). In some groups, transplanted mice were also treated with ABX and/or CTLA4-Ig. (C) Draining dLN cells were harvested and assessed using a flow cytometer 4 weeks after transplantation with various treatments. The absolute cell numbers of CD8+CD44highCD62Lhigh (TCM) cells were measured via FACS. Data are presented as median ± interquartile range (*P < 0.05, n = 6 mice/group).
Bacillus ce. Transplantation, but Not Costimulatory Blockade, Increases CD8+ TCM Cells
To examine direct effects of Bacillus ce. on CD8+ TCM generation, we performed re-colonization of Bacillus ce. in some islet recipients. CD8+ TCM cells were quantified via FACS analyses. As shown in Figure 4C, Bacillus ce. colonization increased CD8+ TCM cells. However, colonization of Bacteroides ov. did not alter their number (data not shown). Interestingly, either ABX or costimulatory blockade alone failed to significantly change their number, whereas treatment with Ber + MR1 or Ber + CTLA4-Ig further reduced their number compared to MR1 or CTLA4-Ig alone (Figure 4C). These results suggest that Bacillus ce. upregulates CD8+ TCM cells while berberine does the opposite, with the costimulatory blockade of CD154 or CD28 alone failing to alter CD8+ TCM formation.
Berberine Induces Cardiac Allograft Tolerance Under the Cover of Costimulatory Blockade
To further confirm if berberine would help induce long-term allograft survival or tolerance in solid organ transplantation, C57BL/6 mice received a heart derived from BALB/c mice and were treated with berberine and/or MR1. As shown in Figure 5A, we found that berberine alone indeed delayed cardiac allograft rejection (MST = 32 vs. 6 days) while berberine plus MR1 induced long-term cardiac allograft survival (>90 days) in 6 of 9 recipients. Among the 6 recipients with an accepted cardiac allograft, 3 of them rejected a new skin allograft from C3H/HeJ donor while the rest of the recipients accepted a new one from BALB/c donor (Figure 5B), suggesting that berberine induces allospecific cardiac transplant tolerance.
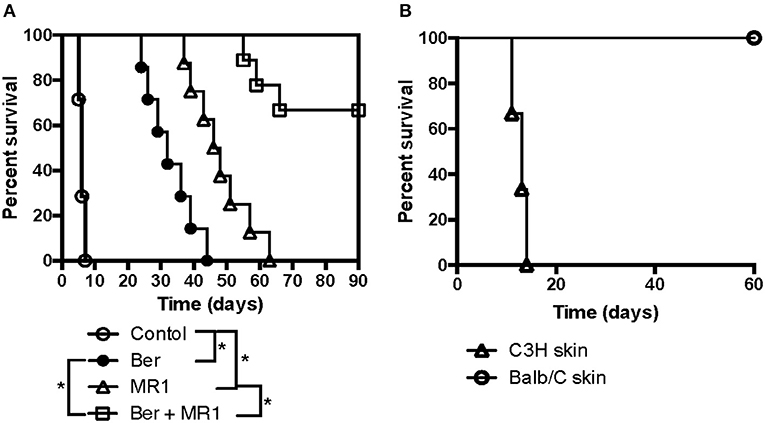
Figure 5. Berberine induces cardiac allograft tolerance under the cover of costimulatory blockade. To further confirm if berberine would help induce long-term allograft survival or tolerance in solid organ transplantation, C57BL/6 mice received a heart derived from a BALB/c mouse and were treated with berberine and/or MR1. Cardiac allograft rejection was observed (n = 7–9 mice/group, *p < 0.05) (A). Moreover, C57BL/6 recipients with an accepted cardiac allograft (BALB/c) for 90 days were transplanted with a skin graft from a BALB/c or C3H/HeJ (third-party) donor (n = 3 mice/group), and skin allograft rejection was observed (B).
Berberine, at Low Concentrations Close to Those Achieved in Blood in vivo, Does Not Suppress T Cell Proliferation and Differentiation in vitro
Orally administered berberine is barely absorbed in the intestinal tract, with a maximal concentration of <0.1 μM achieved in the blood (29–32). To determine if berberine directly inhibits T cell activation in vitro, FACS-sorted CD3+ T cells from Thy1.1+ C57BL/6 mice were stimulated with mitomycin C-treated BALB/c splenocytes for 4 days. As shown in Figure 6A, T cell apoptosis started to increase when berberine was used at 2.5 μM and reached 46.4% when used at 12.5 μM. Importantly, berberine, at either 0.1 or 0.5 μM, which did not yet promote T cell death, did not suppress T cell proliferation whereas CsA did so (Figure 6B). Similarly, berberine failed to reduce IFNγ (Figure 6C) or IL-17A (Figure 6D) level in the supernatant. These findings indicate that berberine, at relatively low concentrations that can be maximally achieved in the blood following oral administration, does not inhibit T cell response in an MLR, a setting of alloreactivity.
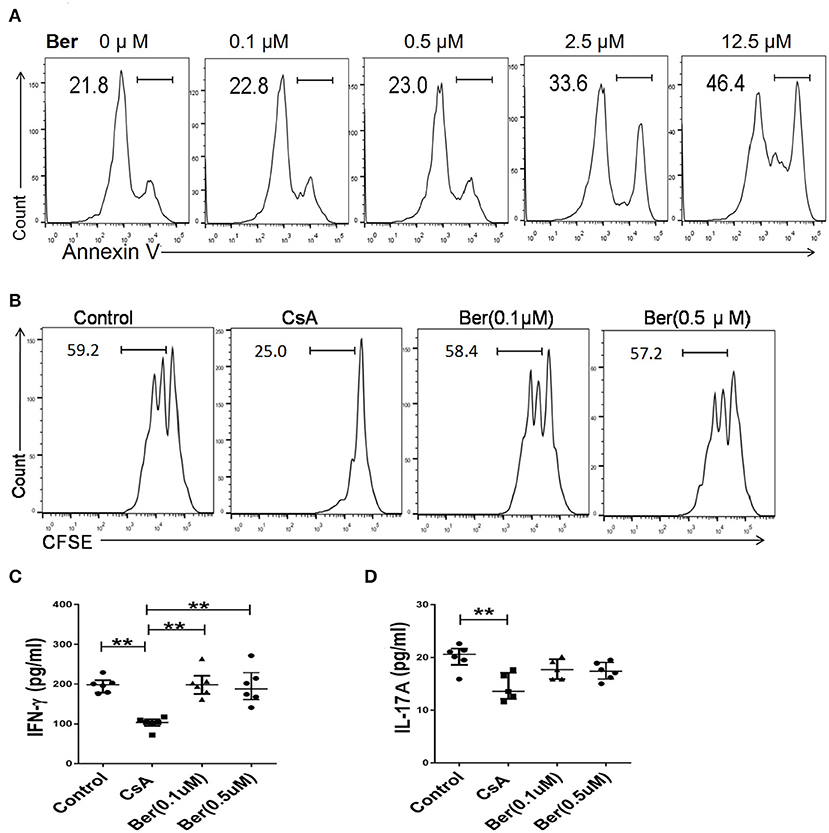
Figure 6. Berberine, at maximal concentrations achieved in the blood upon oral administration, does not inhibit T cell response in vitro. (A) FACS-sorted CD3+ T cells derived from Thy1.1+ C57BL/6 mice were sorted out and cultured with mitomycin-C-treated BALB/c splenocytes in the presence of various concentrations of berberine or CsA for 4 days, as detailed in the supporting information (item 2.3). Cells were then stained with Annexin V to detect their apoptosis. (B) CD3+ T cells, as described above, were labeled with CFSE before their culture. Four days later, they were stained for surface Thy1.1 and analyzed via FACS. Histograms were gated on Thy1.1+ T cells and one of the three sets was shown. Moreover, the culture medium was collected to determine the level of IFN-γ (C) and IL-17A (D) via ELISA. Plots of individual values are presented as median ± interquartile range (*P < 0.05, **P < 0.01, n = 5–6 mice/group from three separate experiments).
CD8+ T Cells From Mice Previously Primed With BALB/c Skin Rapidly Respond to Bacillus ce. in vitro
To determine whether alloreactive memory CD8+ T cells developed in transplanted mice would rapidly cross-react to Bacillus ce., FACS-sorted CD8+ T cells derived from C57BL/6 recipients previously transplanted with BALB/c or C3H/HeJ (third-party) skin were stained with CFSE and cultured with inactivated Bacillus ce. and self APCs. CD8+ T cell division was measured via FACS while IFNγ expression was measured using ELISA. As shown in Figure 7A, 17.7% of CD8+ T cells, which were derived from C57BL/6 recipients previously transplanted with BALB/c skin, proliferated compared with only 2% of the cells from untransplanted naïve mice or 3.6% of the cells from those transplanted with skin of C3H/HeJ mice. Few control cells without Bacillus ce. stimulation proliferated. Furthermore, CD8+ T cells derived from C57BL/6 recipients previously transplanted with BALB/c skin also produced more IFNγ than control cells or those from recipients previously transplanted with C3H/HeJ skin (a third-party) (Figure 7B). These findings indicate that CD8+ T cells from transplanted/primed mice contain BALB/c-specific memory T cells rapidly cross-reacting to Bacillus ce, but do not necessarily imply that this cross-reactivity is limited to BALB/c donor antigens.
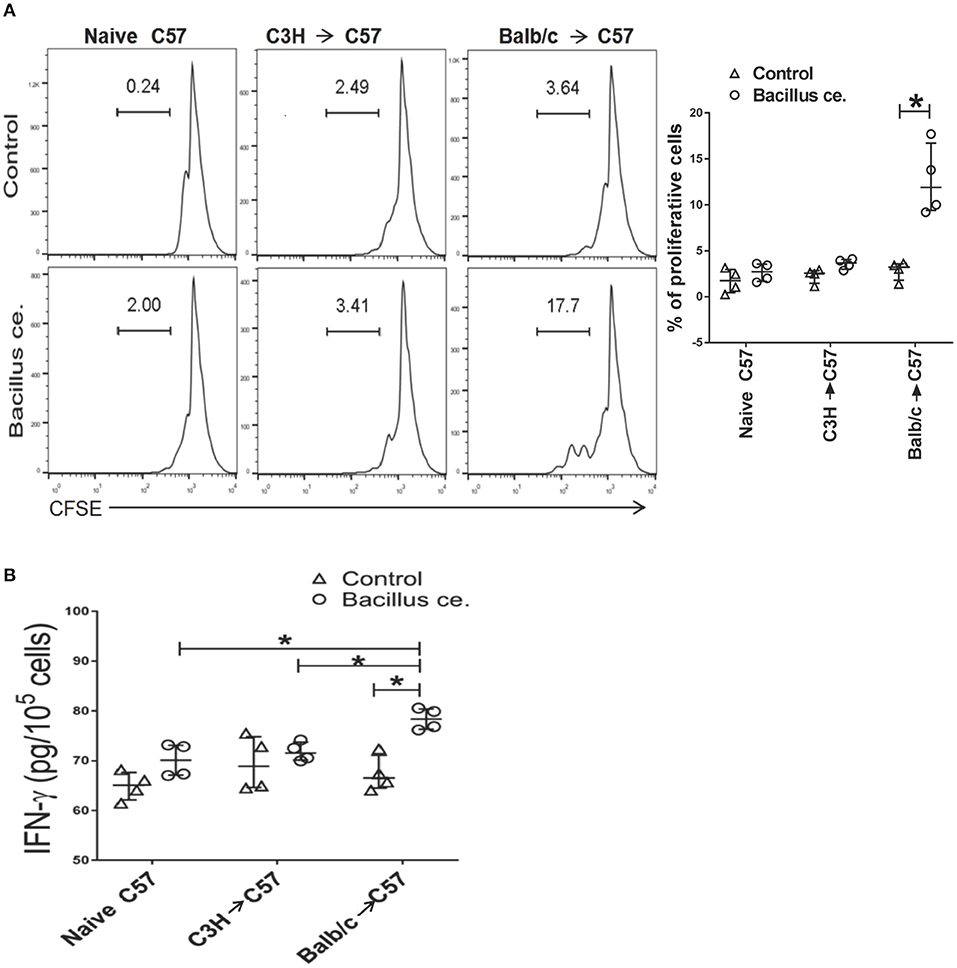
Figure 7. Bacillus ce. induces in vitro proliferation and IFN-γ expression by CD8+ T cells derived from recipient mice that were primed with BALB/c skin. FACS-sorted CD8+ T cells derived from naïve or C57BL/6 recipients transplanted with BALB/c or C3H/HeJ skin 4 weeks earlier were stained with CFSE and cultured in the presence of inactivated Bacillus ce. plus inactivated self APCs obtained from C57BL/6 splenocytes depleted of CD3+ T cells via magnetic micro-beads detachment. Twenty-four hours later, CD8+ T cell division was measured via FACS analysis after gating on CD8+ population (A), while one representative of three separate sets of histograms is shown. Besides, IFNγ expression by the lyzed cells was also measured via ELISA (B). Data of individual values are presented as median ± interquartile range (*P < 0.05, n = 4 mice/group).
Discussion
Using murine models of islet and cardiac allotransplantation, we found that berberine, a natural compound used to treat bacteria-associated diarrhea, delayed acute allograft rejection, and induced long-term allograft survival or tolerance under the cover of brief costimulatory blockade. In contrast, broad-spectrum ABX alone did not significantly delay acute rejection of a fully MHC-mismatched allograft, which is consistent with a previous study (54). Instead, Lei et al. demonstrated that ABX alone mainly extended minor antigen-mismatched skin graft survival (19). Collectively, previous studies and ours indicated that ABX did not dramatically suppress fully MHC-mismatched allograft rejection. Further, we found that berberine repressed CD8+ TCM without a confounding effect on CD4+Foxp3+ Tregs. In contrast, ABX resulted in a reduction in Treg frequency in recipient mice, which was consistent with a recent study by Guo et al. (12). This phenomenon may have restricted otherwise beneficiary effects of ABX on allograft survival.
Previous studies have shown that berberine attenuates autoimmunity in animal models by inhibiting Th1/Th2 responses (34–42). For those studies in vitro, berberine was used at a concentration of ≥5 μM. However, it's well-known that the concentrations of berberine can not reach more than 0.1 μM upon the oral administration because it is barely absorbed by the intestine (29–32). We found that berberine, at a concentration of up to 0.5 μM, did not inhibit T cell proliferation and Th1/Th17 responses in an MLR, an in vitro setting of alloimmunity, indicating that berberine in our model does not directly suppress alloreactive Th1/Th17 responses. In contrast, we demonstrated that a higher concentration of berberine, at 12.5 μM, caused significant T cell apoptosis, indicating its cytotoxicity in vivo if it is directly injected. Moreover, previously studies were concerning animal models of autoimmunity, but not alloimmunity. It is ruled out that berberine, even with ultra-low concentrations in the blood, may still moderately suppress autoimmunity, directly or through altering the gut microbiota in vivo. At higher concentrations, such as 50–100 μM, berberine might also have suppressed Th1/Th17 cells by killing T cells in those studies. Therefore, it's very likely that berberine helps promote long-term allograft survival induced by costimulatory blockade via indirectly altering the gut microbiota, instead of directly inhibiting Th1/Th17 responses, given that berberine simply could not reach 0.1 μM in the blood after oral administration (29–32) and that, at up to 0.5 μM, it still did not suppress alloreactive T cell responses in our study in vitro. Importantly, our findings that treatment with berberine plus ABX failed to suppress allograft rejection also indicate that berberine does not directly inhibit alloreactive T cells in the presence of ABX.
Donor-specific memory T cells pose a significant challenge to long-term allograft survival. The presence of memory T cells in the blood of kidney graft recipients has been associated with poor outcomes in clinical transplantation (55, 56). Studies in animal models also revealed that memory T cells triggered rapid allograft rejection (57–59). Moreover, endogenous memory CD8+ T cells could still respond to alloantigens despite costimulatory blockade of CD28-CD80/86 or CD40-CD154 (60) while CD122 as a component of high-affinity IL-15 receptor was critical for memory CD8+ T cell recall response that was also independent of CD28 signaling (61). Our study revealed that berberine downregulated both CD8+ Teff and CD8+ TCM cells, implying that it is a potential agent to be used for induction of transplant tolerance. Interestingly, a previous study demonstrated that costimulatory blockade-mediated lung allograft acceptance was dependent on the intragraft infiltration of CD8+ TCM cells (62), suggesting that a regulatory subset of CD8+ TCM cells may be induced in that model. Importantly, it's likely that not all CD44high T cells in our model are alloreactive. Thus, there is a limitation in defining alloreactive CD8+ TCM cells using CD44 marker. To further determine an effect of berberine on donor-specific CD8+ TCM generation, we therefore used an adoptive transfer model of pre-activated Thy1.1+CD8+ T cells with at least two divisions in an MLR in vitro, we found that berberine indeed reduced alloreactive Thy1.1+CD8+ TCM cells in recipient mice. It remains to be defined if berberine can also hinder the formation of CD8+ TCM cells in humans.
We validated the alteration of two microbial species, including Bacteroides ov. and Bacillus ce., by quantitative qPCR. Bacteroides ov., a species member of Bacteroidaceae family that is a predominant group in gut bacteria of almost all mammals (63), was previously reported to alleviate LPS-induced inflammation in mice (64) and was increased in berberine-treated recipient mice in our study. Nevertheless, we found that colonization of Bacteroides ov. alone was insufficient to ameliorate acute allograft rejection. On the other hand, we demonstrated that berberine reduced overall levels of Bacillus ce., the toxin-producing bacteria capable of causing human diarrhea, while recolonization of Bacillus ce. reversed long-term islet allograft survival induced by berberine plus brief costimulatory blockade, implying that Bacillus ce. is a pathogen precipitating allograft rejection. More importantly, our mechanistic studies revealed that colonization of Bacillus ce. increased CD8+ Teff and TCM cells whereas addition of Bacteroides ov. did not. This finding underscores differences in their immunomodulation and suggests that berberine can eliminate bacterial species that would otherwise promote alloreactive CD8+ TCM generation. Indeed, we found that Bacillus ce. contained a cross-reactive antigen that stimulated the formation of alloreactive CD8+ TCM cells, because Bacillus ce. rapidly stimulated in vitro proliferation and IFN-γ expression by alloreactive CD8+ TCM cells derived from recipient mice that were previously transplanted or primed with specific donor organs. It is possible that endotoxins released by Bacillus ce. could also stimulate CD8+ T cells in vitro. Fortunately, the response to endotoxins, released possibly by the bacteria, would not be so fast, such as 24 h, because this response was an alloreactive memory recall.
Taken together, berberine promotes the induction of allograft tolerance via downregulating memory CD8+ T cells through altering the gut microbiota because: (1) Berberine promoted long-term allograft survival, downregulated CD8+ Tcm and lowered Bacillus cereus abundance; (2) Addition of CD8+ Tcm or B. cereus reversed allograft survival induced by berberine plus costimulatory blockade; and (3) Furthermore, CD8+ Tcm cells recovered once B. cereus was added back to the recipients. Our findings could be implicated for clinical transplantation since berberine has already been widely used to treat gastroenteritis and diarrhea in China (65, 66). Berberine can support a healthy balance of the gut microbiota (67). Furthermore, previous studies have reported that berberine has several pharmacological properties, including improvement of insulin resistance and cholesterol- or glucose-lowering activities (29, 68–72). A recent clinical trial in individuals with ulcerative colitis also showed that berberine reduced the Geboes grade in colonic tissue of patients (73). Thus, berberine can be used to treat multiple diseases in clinic.
Data Availability Statement
The datasets presented in this study can be found in online repositories. The names of the repository/repositories and accession number(s) can be found at: NCBI Sequence Read Archive, PRJNA693134.
Ethics Statement
This animal study was reviewed and approved by the Animal Ethics Committee of Guangdong Provincial Academy of Chinese Medical Sciences.
Author Contributions
FQ performed experiments and wrote the original manuscript. WL provided critical experimental design and some reagents. SY, HL, and QZe performed some experiments. HH, C-LL, and YC worked on some animal experiments. FZ and QZh analyzed the data. C-JL provided main ideas and vital reagents. ZD designed the study and edited the manuscript. All authors contributed to the article and approved the submitted version.
Funding
This work was supported by Natural Science Foundation of Guangdong Province (2017A030310127, 2018A030310530, and 2019A1515110741) and the Specific Research Fund for TCM Science and Technology of Guangdong Provincial Hospital of Chinese Medicine (YN2016ZD01, YN2019MJ03, YN2019QJ07, and YN2019QJ02).
Conflict of Interest
The authors declare that the research was conducted in the absence of any commercial or financial relationships that could be construed as a potential conflict of interest.
Supplementary Material
The Supplementary Material for this article can be found online at: https://www.frontiersin.org/articles/10.3389/fimmu.2021.646831/full#supplementary-material
Abbreviations
ABX, broad-spectrum antibiotics; Ber, berberine; dLN, draining lymph node; EOMES, eomesodermin; Treg, regulatory T cell; TCM, central memory T cell; TEM, effector memory T cell; Teff, effector T cell.
References
1. Shreiner AB, Kao JY, Young VB. The gut microbiome in health and in disease. Curr Opin Gastroen. (2015) 31:69–75. doi: 10.1097/MOG.0000000000000139
2. Eckburg PB, Bik EM, Bernstein CN, Purdom E, Dethlefsen L, Sargent M, et al. Diversity of the human intestinal microbial flora. Science. (2005) 308:1635–8. doi: 10.1126/science.1110591
3. Ochoa-Reparaz J, Mielcarz DW, Wang Y, Begum-Haque S, Dasgupta S, Kasper DL, et al. A polysaccharide from the human commensal Bacteroides fragilis protects against CNS demyelinating disease. Mucosal Immunol. (2010) 3:487–95. doi: 10.1038/mi.2010.29
4. Wu HJ, Ivanov II, Darce J, Hattori K, Shima T, Umesaki Y, et al. Gut-residing segmented filamentous bacteria drive autoimmune arthritis via T helper 17 cells. Immunity. (2010) 32:815–27. doi: 10.1016/j.immuni.2010.06.001
5. Bromberg JS, Fricke WF, Brinkman CC, Simon T, Mongodin EF. Microbiota-implications for immunity and transplantation. Nat Rev Nephrol. (2015) 11:342–53. doi: 10.1038/nrneph.2015.70
6. Oh PL, Martinez I, Sun Y, Walter J, Peterson DA, Mercer DF. Characterization of the ileal microbiota in rejecting and nonrejecting recipients of small bowel transplants. Am J Transplant. (2012) 12:753–62. doi: 10.1111/j.1600-6143.2011.03860.x
7. Riwes M, Reddy P. Microbial metabolites and graft versus host disease. Am J Transplant. (2018) 18:23–9. doi: 10.1111/ajt.14443
8. Alegre ML, Mannon RB, Mannon PJ. The microbiota, the immune system and the allograft. Am J Transplant. (2014) 14:1236–48. doi: 10.1111/ajt.12760
9. Fricke WF, Maddox C, Song Y, Bromberg JS. Human microbiota characterization in the course of renal transplantation. Am J Transplant. (2014) 14:416–27. doi: 10.1111/ajt.12588
10. Fishman JA, Thomson AW. Clinical implications of basic science discoveries: immune homeostasis and the microbiome-dietary and therapeutic modulation and implications for transplantation. Am J Transplant. (2015) 15:1755–8. doi: 10.1111/ajt.13236
11. Zhang Z, Liu L, Tang H, Jiao W, Zeng S, Xu Y, et al. Immunosuppressive effect of the gut microbiome altered by high-dose tacrolimus in mice. Am J Transplant. (2018) 18:1646–56. doi: 10.1111/ajt.14661
12. Guo Y, Wang Q, Li D, Onyema OO, Mei Z, Manafi A, et al. Vendor-specific microbiome controls both acute and chronic murine lung allograft rejection by altering CD4(+) Foxp3(+) regulatory T cell levels. Am J Transplant. (2019) 19:2705–18. doi: 10.1111/ajt.15523
13. Han L, Zhao K, Li Y, Han H, Zhou L, Ma P, et al. A gut microbiota score predicting acute graft-versus-host disease following myeloablative allogeneic hematopoietic stem cell transplantation. Am J Transplant. (2020) 20:1014–27. doi: 10.1111/ajt.15654
14. Modena BD, Milam R, Harrison F, Cheeseman JA, Abecassis MM, Friedewald JJ, et al. Changes in urinary microbiome populations correlate in kidney transplants with interstitial fibrosis and tubular atrophy documented in early surveillance biopsies. Am J Transplant. (2017) 17:712–23. doi: 10.1111/ajt.14038
15. Colas L, Mongodin EF, Montassier E, Chesneau M, Guerif P, Hittle L, et al. Unique and specific proteobacteria diversity in urinary microbiota of tolerant kidney transplanted recipients. Am J Transplant. (2020) 20:145–58. doi: 10.1111/ajt.15549
16. Lee JR, Muthukumar T, Dadhania D, Toussaint NC, Ling LL, Pamer E, et al. Gut microbial community structure and complications after kidney transplantation: a pilot study. Transplantation. (2014) 98:697–705. doi: 10.1097/TP.0000000000000370
17. Lee JR, Magruder M, Zhang L, Westblade LF, Satlin MJ, Robertson A, et al. Gut microbiota dysbiosis and diarrhea in kidney transplant recipients. Am J Transplant. (2019) 19:488–500. doi: 10.1111/ajt.14974
18. Ingham AC, Kielsen K, Cilieborg MS, Lund O, Holmes S, Aarestrup FM, et al. Specific gut microbiome members are associated with distinct immune markers in pediatric allogeneic hematopoietic stem cell transplantation. Microbiome. (2019) 7:131. doi: 10.1186/s40168-019-0745-z
19. Lei YM, Chen LQ, Wang Y, Stefka AT, Molinero LL, Theriault B, et al. The composition of the microbiota modulates allograft rejection. J Clin Invest. (2016) 126:2736–44. doi: 10.1172/JCI85295
20. McIntosh CM, Chen L, Shaiber A, Eren AM, Alegre ML. Gut microbes contribute to variation in solid organ transplant outcomes in mice. Microbiome. (2018) 6:96. doi: 10.1186/s40168-018-0474-8
21. Miller ML, Daniels MD, Wang T, Chen J, Young J, Xu J, et al. Spontaneous restoration of transplantation tolerance after acute rejection. Nat Commun. (2015) 6:7566. doi: 10.1038/ncomms8566
22. Nakamura K, Kageyama S, Ito T, Hirao H, Kadono K, Aziz A, et al. Antibiotic pretreatment alleviates liver transplant damage in mice and humans. J Clin Invest. (2019) 129:3420–34. doi: 10.1172/JCI127550
23. Donohoe DR, Garge N, Zhang X, Sun W, O'Connell TM, Bunger MK, et al. The microbiome and butyrate regulate energy metabolism and autophagy in the mammalian colon. Cell Metab. (2011) 13:517–26. doi: 10.1016/j.cmet.2011.02.018
24. Usami M, Kishimoto K, Ohata A, Miyoshi M, Aoyama M, Fueda Y, et al. Butyrate and trichostatin A attenuate nuclear factor kappaB activation and tumor necrosis factor alpha secretion and increase prostaglandin E2 secretion in human peripheral blood mononuclear cells. Nutr Res. (2008) 28:321–8. doi: 10.1016/j.nutres.2008.02.012
25. Wahlstrom A, Sayin SI, Marschall HU, Backhed F. Intestinal crosstalk between bile acids and microbiota and its impact on host metabolism. Cell Metab. (2016) 24:41–50. doi: 10.1016/j.cmet.2016.05.005
26. Agus A, Planchais J, Sokol H. Gut microbiota regulation of tryptophan metabolism in health and disease. Cell Host Microbe. (2018) 23:716–24. doi: 10.1016/j.chom.2018.05.003
27. Bandyopadhyay S, Patra PH, Mahanti A, Mondal DK, Dandapat P, Bandyopadhyay S, et al. Potential antibacterial activity of berberine against multi drug resistant enterovirulent Escherichia coli isolated from yaks (Poephagus grunniens) with haemorrhagic diarrhoea. Asian Pac J Trop Med. (2013) 6:315–9. doi: 10.1016/S1995-7645(13)60063-2
28. Rabbani GH, Butler T, Knight J, Sanyal SC, Alam K. Randomized controlled trial of berberine sulfate therapy for diarrhea due to enterotoxigenic Escherichia coli and Vibrio cholerae. J Infect Dis. (1987) 155:979–84. doi: 10.1093/infdis/155.5.979
29. Kong WJ, Wei J, Abidi P, Lin MH, Inaba S, Li C, et al. Berberine is a novel cholesterol-lowering drug working through a unique mechanism distinct from statins. Nat Med. (2004) 10:1344–51. doi: 10.1038/nm1135
30. Chen W, Miao YQ, Fan DJ, Yang SS, Lin X, Meng LK, et al. Bioavailability study of berberine and the enhancing effects of TPGS on intestinal absorption in rats. Aaps Pharmscitech. (2011) 12:705–11. doi: 10.1208/s12249-011-9632-z
31. Fan D, Wu X, Dong W, Sun W, Li J, Tang X. Enhancement by sodium caprate and sodium deoxycholate of the gastrointestinal absorption of berberine chloride in rats. Drug Dev Ind Pharm. (2013) 39:1447–56. doi: 10.3109/03639045.2012.723219
32. Tan XS, Ma JY, Feng R, Ma C, Chen WJ, Sun YP, et al. Tissue distribution of berberine and its metabolites after oral administration in rats. PLoS ONE. (2013) 8:e77969. doi: 10.1371/journal.pone.0077969
33. Zhang X, Zhao Y, Zhang M, Pang X, Xu J, Kang C, et al. Structural changes of gut microbiota during berberine-mediated prevention of obesity and insulin resistance in high-fat diet-fed rats. PLoS ONE. (2012) 7:e42529. doi: 10.1371/journal.pone.0042529
34. Takahara M, Takaki A, Hiraoka S, Adachi T, Shimomura Y, Matsushita H, et al. Berberine improved experimental chronic colitis by regulating interferon-gamma- and IL-17A-producing lamina propria CD4(+) T cells through AMPK activation. Sci Rep. (2019) 9:11934. doi: 10.1038/s41598-019-48331-w
35. Zhou J, Yu Y, Yang X, Wang Y, Song Y, Wang Q, et al. Berberine attenuates arthritis in adjuvant-induced arthritic rats associated with regulating polarization of macrophages through AMPK/NF-small ka, CyrillicB pathway. Eur J Pharmacol. (2019) 852:179–88. doi: 10.1016/j.ejphar.2019.02.036
36. Wu X, Li X, Dang Z, Jia Y. Berberine demonstrates anti-inflammatory properties in Helicobacter pylori-infected mice with chronic gastritis by attenuating the Th17 response triggered by the B cell-activating factor. J Cell Biochem. (2018) 119:5373–81. doi: 10.1002/jcb.26681
37. Yue M, Xia Y, Shi C, Guan C, Li Y, Liu R, et al. Berberine ameliorates collagen-induced arthritis in rats by suppressing Th17 cell responses via inducing cortistatin in the gut. FEBS J. (2017) 284:2786–801. doi: 10.1111/febs.14147
38. Liu X, Zhang X, Ye L, Yuan H. Protective mechanisms of berberine against experimental autoimmune myocarditis in a rat model. Biomed Pharmacother. (2016) 79:222–30. doi: 10.1016/j.biopha.2016.02.015
39. Li YH, Xiao HT, Hu DD, Fatima S, Lin CY, Mu HX, et al. Berberine ameliorates chronic relapsing dextran sulfate sodium-induced colitis in C57BL/6 mice by suppressing Th17 responses. Pharmacol Res. (2016) 110:227–39. doi: 10.1016/j.phrs.2016.02.010
40. Li C, Xi Y, Li S, Zhao Q, Cheng W, Wang Z, et al. Berberine ameliorates TNBS induced colitis by inhibiting inflammatory responses and Th1/Th17 differentiation. Mol Immunol. (2015) 67(2 Pt. B):444–54. doi: 10.1016/j.molimm.2015.07.013
41. Qin X, Guo BT, Wan B, Fang L, Lu L, Wu L, et al. Regulation of Th1 and Th17 cell differentiation and amelioration of experimental autoimmune encephalomyelitis by natural product compound berberine. J Immunol. (2010) 185:1855–63. doi: 10.4049/jimmunol.0903853
42. Cui G, Qin X, Zhang Y, Gong Z, Ge B, Zang YQ. Berberine differentially modulates the activities of ERK, p38 MAPK, and JNK to suppress Th17 and Th1 T cell differentiation in type 1 diabetic mice. J Biol Chem. (2009) 284:28420–9. doi: 10.1074/jbc.M109.012674
43. Zeng YQ, Liu XS, Wu S, Zou C, Xie Q, Xu SM, et al. Kaempferol promotes transplant tolerance by sustaining CD4+FoxP3+regulatory T cells in the presence of calcineurin inhibitor. Am J Transplant. (2015) 15:1782–92. doi: 10.1111/ajt.13261
44. Dai Z, Zhang S, Xie Q, Wu S, Su J, Li S, et al. Natural CD8+CD122+T cells are more potent in suppression of allograft rejection than CD4+CD25+regulatory T cells. Am J Transplant. (2014) 14:39–48. doi: 10.1111/ajt.12515
45. Corry RJ, Winn HJ, Russell PS. Primarily vascularized allografts of hearts in mice. The role of H-2D, H-2K, and non-H-2 antigens in rejection. Transplantation. (1973) 16:343–50. doi: 10.1097/00007890-197310000-00010
46. Dai Z, Li Q, Wang Y, Gao G, Diggs LS, Tellides G, et al. CD4+CD25+regulatory T cells suppress allograft rejection mediated by memory CD8+T cells via a CD30-dependent mechanism. J Clin Invest. (2004) 113:310–7. doi: 10.1172/JCI19727
47. Cole JR, Wang Q, Fish JA, Chai BL, McGarrell DM, Sun YN, et al. Ribosomal database project: data and tools for high throughput rRNA analysis. Nucleic Acids Res. (2014) 42:D633-42. doi: 10.1093/nar/gkt1244
48. DeSantis TZ, Hugenholtz P, Larsen N, Rojas M, Brodie EL, Keller K, et al. Greengenes, a chimera-checked 16S rRNA gene database and workbench compatible with ARB. Appl Environ Microb. (2006) 72:5069–72. doi: 10.1128/AEM.03006-05
49. Cekanaviciute E, Yoo BB, Runia TF, Debelius JW, Singh S, Nelson CA, et al. Gut bacteria from multiple sclerosis patients modulate human T cells and exacerbate symptoms in mouse models. Proc Natl Acad Sci USA. (2017) 114:10713–8.
50. Storni T, Bachmann MF. On the role of APC-activation for in vitro versus in vivo T cell priming. Cell Immunol. (2003) 225:1–11. doi: 10.1016/j.cellimm.2003.09.001
51. Paley MA, Gordon SM, Bikoff EK, Robertson EJ, Wherry EJ, Reiner SL. Technical advance: fluorescent reporter reveals insights into eomesodermin biology in cytotoxic lymphocytes. J Leukocyte Biol. (2013) 93:307–15. doi: 10.1189/jlb.0812400
52. Intlekofer AM, Takemoto N, Wherry EJ, Longworth SA, Northrup JT, Palanivel VR, et al. Effector and memory CD8(+) T cell fate coupled by T-bet and eomesodermin. Nat Immunol. (2005) 6:1236–44. doi: 10.1038/ni1268
53. Ichii H, Sakamoto A, Hatano M, Okada S, Toyama H, Taki S, et al. Role for Bcl-6 in the generation and maintenance of memory CD8(+) T cells. Nat Immunol. (2002) 3:558–63. doi: 10.1038/ni802
54. Bromberg JS, Hittle L, Xiong Y, Saxena V, Smyth EM, Li L, et al. Gut microbiota-dependent modulation of innate immunity and lymph node remodeling affects cardiac allograft outcomes. JCI Insight. (2018) 3:e121045. doi: 10.1172/jci.insight.121045
55. Heeger PS, Greenspan NS, Kuhlenschmidt S, Dejelo C, Hricik DE, Schulak JA, et al. Pretransplant frequency of donor-specific, IFN-gamma-producing lymphocytes is a manifestation of immunologic memory and correlates with the risk of posttransplant rejection episodes. J Immunol. (1999) 163:2267–75
56. Augustine JJ, Siu DS, Clemente MJ, Schulak JA, Heeger PS, Hricik DE. Pre-transplant IFN-gamma ELISPOTs are associated with post-transplant renal function in African American renal transplant recipients. Am J Transplant. (2005) 5:1971–5. doi: 10.1111/j.1600-6143.2005.00958.x
57. Schenk AD, Nozaki T, Rabant M, VaIujskikh A, Fairchild RL. Donor-reactive CD8 memory T cells infiltrate cardiac allografts within 24-h posttransplant in naive recipients. Am J Transplant. (2008) 8:1652–61. doi: 10.1111/j.1600-6143.2008.02302.x
58. Su CA, Iida S, Abe T, Fairchild RL. Endogenous memory CD8 T cells directly mediate cardiac allograft rejection. Am J Transplant. (2014) 14:568–79. doi: 10.1111/ajt.12605
59. Zhang QW, Chen YF, Fairchild RL, Heeger PS, Valujskikh A. Lymphoid sequestration of alloreactive memory CD4 T cells promotes cardiac allograft survival. J Immunol. (2006) 176:770–7. doi: 10.4049/jimmunol.176.2.770
60. Benichou G, Gonzalez B, Marino J, Ayasoufi K, Valujskikh A. Role of memory T Cells in allograft rejection and tolerance. Front Immunol. (2017) 8:170. doi: 10.3389/fimmu.2017.00170
61. Mathews DV, Dong Y, Higginbotham LB, Kim SC, Breeden CP, Stobert EA, et al. CD122 signaling in CD8+ memory T cells drives costimulation-independent rejection. J Clin Invest. (2018) 128:4557–72. doi: 10.1172/JCI95914
62. Krupnick AS, Lin X, Li W, Higashikubo R, Zinselmeyer BH, Hartzler H, et al. Central memory CD8+ T lymphocytes mediate lung allograft acceptance. J Clin Invest. (2014) 124:1130–43. doi: 10.1172/JCI71359
63. Miyamoto Y, Watanabe K, Tanaka R, Itoh K. Distribution analysis of six predominant bacteroides species in normal human feces using 16S rDNA-targeted species-specific primers. Microb Ecol Health Dis. (2002) 14:133–6. doi: 10.1080/089106002320644302
64. Tan HZ, Zhao JX, Zhang H, Zhai QX, Chen W. Novel strains of Bacteroides fragilis and Bacteroides ovatus alleviate the LPS-induced inflammation in mice. Appl Microbiol Biot. (2019) 103:2353–65. doi: 10.1007/s00253-019-09617-1
65. Rabbani GH. Mechanism and treatment of diarrhoea due to Vibrio cholerae and Escherichia coli: roles of drugs and prostaglandins. Dan Med Bull. (1996) 43:173–85.
66. Hu Q, Peng Z, Li L, Zou X, Xu L, Gong J, et al. The efficacy of berberine-containing quadruple therapy on helicobacter pylori eradication in China: a systematic review and meta-analysis of randomized clinical trials. Front Pharmacol. (2019) 10:1694. doi: 10.3389/fphar.2019.01694
67. Zhang W, Xu JH, Yu T, Chen QK. Effects of berberine and metformin on intestinal inflammation and gut microbiome composition in db/db mice. Biomed Pharmacother. (2019) 118:109131. doi: 10.1016/j.biopha.2019.109131
68. Cicero AFG, Fogacci F, Bove M, Giovannini M, Veronesi M, Borghi C. Short-term effects of dry extracts of artichokeand berberis in hypercholesterolemic patients without cardiovascular disease. Am J Cardiol. (2019) 123:588–91.
69. Liu D, Zhang Y, Liu Y, Hou L, Li S, Tian H, et al. Berberine modulates gut microbiota and reduces insulin resistance via the TLR4 signaling pathway. Exp Clin Endocrinol Diabetes. (2018) 126:513–20. doi: 10.1055/s-0043-125066
70. Lan J, Zhao Y, Dong F, Yan Z, Zheng W, Fan J, et al. Meta-analysis of the effect and safety of berberine in the treatment of type 2 diabetes mellitus, hyperlipemia and hypertension. J Ethnopharmacol. (2015) 161:69–81. doi: 10.1016/j.jep.2014.09.049
71. Zhang Y, Gu Y, Ren H, Wang S, Zhong H, Zhao X, et al. Gut microbiome-related effects of berberine and probiotics on type 2 diabetes (the PREMOTE study). Nat Commun. (2020) 11:5015. doi: 10.1038/s41467-020-18414-8
72. Zhang Y, Li X, Zou D, Liu W, Yang J, Zhu N, et al. Treatment of type 2 diabetes and dyslipidemia with the natural plant alkaloid berberine. J Clin Endocrinol Metab. (2008) 93:2559–65. doi: 10.1210/jc.2007-2404
Keywords: immunological tolerance, berberine, allograft rejection, memory T cell, gut microbiota
Citation: Qiu F, Lu W, Ye S, Liu H, Zeng Q, Huang H, Liang C-L, Chen Y, Zheng F, Zhang Q, Lu C-J and Dai Z (2021) Berberine Promotes Induction of Immunological Tolerance to an Allograft via Downregulating Memory CD8+ T-Cells Through Altering the Gut Microbiota. Front. Immunol. 12:646831. doi: 10.3389/fimmu.2021.646831
Received: 28 December 2020; Accepted: 26 January 2021;
Published: 12 February 2021.
Edited by:
Yong Zhao, Institute of Zoology (CAS), ChinaReviewed by:
Zhongquan Qi, Guangxi University, ChinaQing Ding, University of Pittsburgh, United States
Copyright © 2021 Qiu, Lu, Ye, Liu, Zeng, Huang, Liang, Chen, Zheng, Zhang, Lu and Dai. This is an open-access article distributed under the terms of the Creative Commons Attribution License (CC BY). The use, distribution or reproduction in other forums is permitted, provided the original author(s) and the copyright owner(s) are credited and that the original publication in this journal is cited, in accordance with accepted academic practice. No use, distribution or reproduction is permitted which does not comply with these terms.
*Correspondence: Zhenhua Dai, emRhaTIwMDkmI3gwMDA0MDtvdXRsb29rLmNvbQ==; Chuan-Jian Lu, bGNqJiN4MDAwNDA7Z3p1Y20uZWR1LmNu
†These authors have contributed equally to this work