- 1Department of Colorectal Surgery, The First Affiliated Hospital of Zhengzhou University, Zhengzhou, China
- 2Academy of Medical Sciences, Zhengzhou University, Zhengzhou, China
RNA methylation is a kind of RNA modification that exists widely in eukaryotes and prokaryotes. RNA methylation occurs not only in mRNA but also in ncRNA. According to the different sites of methylation, RNA methylation includes m6A, m5C, m7G, and 2-O-methylation modifications. Modifications affect the splicing, nucleation, stability and immunogenicity of RNA. RNA methylation is involved in many physiological and pathological processes. In the immune system, especially for tumor immunity, RNA methylation affects the maturation and response function of immune cells. Through the influence of RNA immunogenicity and innate immune components, modifications regulate the innate immunity of the body. Some recent studies verified that RNA methylation can regulate tumor immunity, which also provides a new idea for the future of treating immunological diseases and tumor immunotherapy.
Introduction
More than 160 chemical modifications have been found in RNA (1, 2). Of these modifications, RNA methylation is a post-transcriptional modification that exists widely in eukaryotes and prokaryotes (3, 4). Methylation is the transfer of a methyl group from an active methyl compound, to another compound. As a post-transcriptional modification, RNA methylation regulates RNA splicing (5, 6), nuclear export (7), stability (8–10), translation (11–13), DNA damage repair (14), initiation of miRNA biogenesis (15) and immunogenicity (16) and then affects cellular differentiation (17), embryonic development (18), spermatogenesis (19), sex determination (20), learning and memory (12), immune response (21) and the occurrence and development of cancer (22–24). Since its discovery in the 1970s, m6A methylation has been considered to be the most abundant methylation modification in the mRNA of eukaryotes (25). With the discovery of methylases and demethylases, the view that m6A methylation is dynamic and reversible has also been confirmed (26, 27). The discovery of recognition factors provides a deeper understanding of RNA methylation (7, 8, 13).
In recent years, RNA methylation has been proven to be related to a variety of human physiologies and diseases, especially tumor immunity (24, 28–30). RNA methylation plays an important role in regulating normal physiological processes, and abnormal methylation will lead to diseases and cancers (28, 31–33). This provides new insights for us to understand diseases from the perspective of RNA methylation and find new treatment methods. In this review, we will focus on the relationship between RNA methylation and tumor immunity. When methylation acts on different RNAs, it will directly or indirectly affect immunity especially tumor immunity (21, 34), which means that RNA methylation has the potential to be applied to the treatment of a variety of diseases in the future, including autoimmune diseases and cancers.
Biological Features of RNA Methylation
Types of RNA Methylation
m6A
N6-methyladenosine (m6A) is generated by the methylation of adenosine at the N6 position. M6A methylation was discovered in the 1970s and is the most abundant modification in mammalian mRNA (25). In addition to being abundant in mammals, m6A modification is enriched in eukaryotes such as plants (35) and yeasts (36). In addition, m6A modification was also found in prokaryotes such as bacteria (37). M6A is enriched at exons, stop codons and 3′ UTRs of RNA and mainly on adenine in the RRACH sequence, where R is guanine or adenine and H is uracil, adenine or cytosine (38). M6A modification is a reversible process. Methyltransferase complexes, including METTL3, METTL14 and WTAP, methylate RNA (39). Demethylases, such as FTO and ALKBH5, demethylate RNA (27, 40). M6A binding proteins, such as YTHDC1/2 and YTHDF1/2/3, recognize and bind methylated RNA to perform functions (5, 8, 12, 13, 19). M6A regulates the processing (15), translation (11–13) and stability of RNA (9, 10) and controls a variety of biological functions, including spermatogenesis (19) and stem cell differentiation (17).
m5C
5-Methylcytidine (m5C) is a methylation modification of the fifth N of cytosine. M5C methylation is found in a variety of RNAs, including tRNAs (41), rRNAs (42), mRNAs (43), and sRNAs (42). M5C methylation affects the structural and metabolic stability of tRNAs (41) and plays an important role in the translation of tRNAs (44). M5C modification of mRNAs regulates their export (45). Common m5C methylases include DNMT2 (46) and Nsun2 (47). M5C can regulate cell division (48) and protein synthesis (49).
m7G
N7-methylguanosine (m7G) is a modification of the seventh N of RNA guanine with a methyl group. M7G modification improves the stability of mRNA (50, 51). In addition, m7G regulates cell differentiation (52). In addition to being present on mRNAs, m7G modification also exists on tRNAs, rRNAs and miRNAs (53–55). M7G methylation complexes include METTL1 and WDR4 (52).
2-O-Methylation
2-O-Methylation occurs in the methylation of RNA2′-OH and is widely found on tRNAs, rRNAs, and mRNAs in mammalian cells (1). 2-O-methylation regulates the splicing of pre-mRNAs and stability of small RNAs (56, 57).
RNA Types With Methylation Modification
RNA methylation exists in all types of RNAs, including mRNAs, tRNAs, rRNAs and some other ncRNAs.
mRNA
Methylation modifications in mRNA include m6A, m5C, and m7G. M6A methylation is the most common in mRNA. Methylation modification regulates mRNA transcription, nucleation, stability and translation and thus controls the expression of proteins (6, 11, 12, 40). Methylation in mRNA also plays a role in immune regulation. For example, N6-methyladenosine modification of an mRNA encoding a lysosomal protease increases its translation, which inhibits the presentation of tumor antigens (58).
Non-coding RNA
In addition to mRNAs that encode proteins being methylated, some ncRNAs can also be methylated. It has been shown that RNA methylation modification plays a role in the initiation of miRNA biogenesis (15). After being methylated, some immunogenic ncRNAs lose their immunogenicity and no longer have the ability to activate the immune response. N6-methyladenosine-modified self-circRNA suppresses circRNA immunity (59). A study confirmed that m6A methylation of lnc-Dpf3 increases CCR7-mediated DC migration (34).
Regulatory Mechanism of RNA Methylation
RNA methylation is a dynamic and reversible biological process that is regulated by methyltransferases and demethylases. The methylated RNA is recognized and bound by specific binding proteins and plays a corresponding role. Methyltransferases, demethylases and binding proteins are known as writers, erasers and readers, respectively.
Writers
RNA methylation is catalyzed by RNA methyltransferase complexes called “writers.” METTL3 was the first component identified in such a complex. Then, WTAP and METTL14 were confirmed to be components of RNA methyltransferase complexes. METTL14 reacts with METTL3 at a ratio of 1:1 to form a dimer. WTAP does not have the ability to catalyze methylation. It acts as a scaffold in the methylase complex and regulates the recruitment of the m6A methyltransferase complex to mRNA targets (39, 60, 61). KIAA1429, RNA binding motif protein 15/15B (RBM15/15B), ZC3H13, ZCCHC4 and METTL16 are also involved in mediating the methylation of RNA (62–66).
Erasers
Methylated RNA is demethylated by demethylases called “erasers,” which make reversible methylation of RNA possible. The main demethylases include FTO and ALKBH5, which have been shown to affect mRNA export and RNA metabolism (27, 40).
Readers
Methylated RNA binds to specific RNA-binding proteins to perform corresponding functions. These binding proteins are called “readers” and mainly include the YTH family members YTHDC1/2 and YTHDF1/2/3 and insulin-like growth factor 2 mRNA binding proteins (IGF2BP1/2/3). YTHDF1 binds to the translation initiator and ribosome to promote the translation of mRNA (13). YTHDF2 selectively recognizes and regulates the degradation of mRNA (8, 9). YTHDF3 cooperates with YTHDF1 and YTHDF2 to accelerate the translation and degradation of mRNA, respectively (67, 68). Mainly located in the nucleus, YTHDC1 recruits splicing factors and regulates the shearing and nucleation of mRNA (5, 7, 69). YTHDC2, as a methylation-binding protein, promotes translation and regulates spermatogenesis (19, 70). IGF2BPs, as methylation-binding proteins, regulate the stability and translation of RNA (71).
Different Immunoregulation Types Mediated by RNA Methylation
T Cells
T cells play an important role in immune regulation. T cells participate in adaptive immunity and secrete cytokines. The imbalance of T cell homeostasis is associated with some autoimmune diseases (72). T cells are also involved in the process of tumor immunity (73).
One study proved that RNA methylation played an essential role in maintaining T cell homeostasis. The absence of METTL3 makes T cells stay in the naive T cell stage for a longer time via METTL3-mediated m6A methylation targeting the IL-7/STAT5/SOCS pathway (Figure 1) (21). In addition to maintaining T cell homeostasis and differentiation, RNA methylation also promotes the expression of immune factors in T cells. IL-17 participates in the immune response and is related to some immune diseases. Nsun2 mediated the methylation of IL-17 mRNA in T cells, promoted the translation of IL-17 mRNA and increased the expression of IL-17 (74). Furthermore, in tumor immunity, the initiation of the immune response of T cells in YTHDF1-deficient mice is enhanced, featuring a stronger antitumour CD8+ T cell response (58).
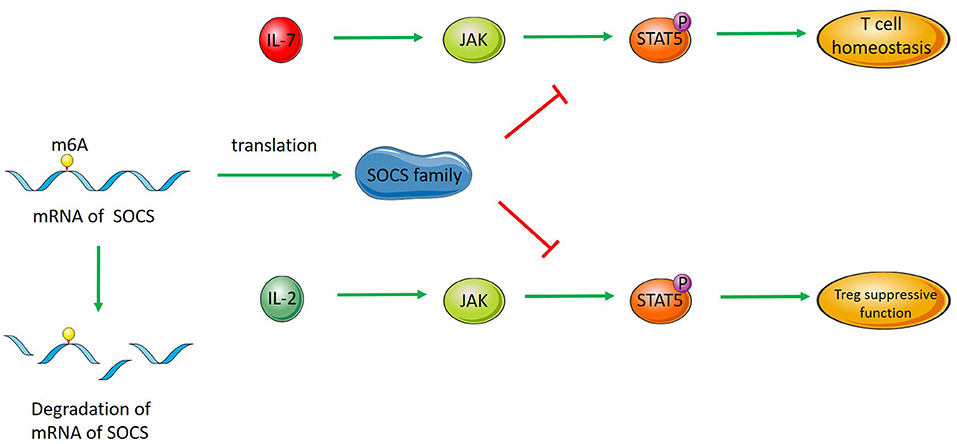
Figure 1. RNA methylation regulates T cells and Tregs. SOCS RNA modified by m6A methylation is degraded, which decreases the level of SOCS family members. SOCS proteins inhibit the activation of STAT5 in the IL-7/STAT5 pathway and regulate T cell homeostasis. SOCS proteins inhibit the activation of STAT5 in the IL-2/STAT5 pathway, thus regulating the inhibitory function of Tregs.
Follicular helper T cell (Tfh) is a unique CD4+T cell, which can promote the response of B cells. Inducible costimulator (ICOS) plays an important role in the initiation and development of Tfh cells. A recent study found that Von Hippel–Lindau (VHL) promotes the initiation of Tfh cells through glycolysis mediated by HIF-1 α. In this process, HIF-1 α induced GAPDH to promote ICOS mRNA degradation through m6A modification, thus regulating the expression of ICOS. Mechanistically, enhanced glycolysis induced the gene expression of METTL3 and Mettl14 methyltransferase complex, and catalyzed the m6A modification of ICOS mRNA, thus inhibiting the expression of ICOS (75).
DCs
Dendritic cells (DCs) are antigen-presenting cells that ingest, process and present antigens. Immature DCs have strong migration ability, and mature DCs stimulate and activate T cells. DCs regulate T cell differentiation and play an important role in both innate immunity and adaptive immunity. Abnormalities in the quantity and function of DCs cause colitis, among other diseases (76–79).
Methylation of mRNA affects protein expression in dendritic cells (80). N6-methyladenosine modification mediated by the methyltransferase METTL3 promotes the activation of DCs and enhances the ability of DCs to stimulate T cell activation (81). Demethylation of Lnc-Dpf3 mRNA increases the level of Lnc-Dpf3, which in turn enhances the inhibition of Lnc-Dpf3 on DC migration (34). DCs are stimulated after being exposed to non-self components. RNA transcribed in vitro, such as in mammalian necrotic cells, stimulates DC activation (82). RNAs with methylation modifications, including m6A, m5C, and 2-O- methylation, attenuate or eliminate this stimulation. The higher the level of modification, the stronger the inhibition of stimulation (16).
In tumor immunity, deletion of YTHDF1 enhances the tumor antigen cross-presentation ability of DCs and promotes the activation of T cells. In mice, an RNA encoding a lysosomal protease can be methylated. The methylated RNA interacts with YTHDF1, which promotes the translation of RNA. In YTHDF1−/− mice, the translation of methylated RNA is not promoted, causing a low level of lysosomal protein. Limiting the degradation ability of lysosomal proteases can prevent the destruction of tumor antigens, which enhances the ability of DCs to present tumor antigens and promotes the activation of T cells (58).
Tregs
Regulatory T cells (Tregs) play an important role in maintaining immunological self-tolerance and autoimmunity (83, 84). N6-methyladenosine modification has been shown to maintain the inhibitory function of Tregs. Tong reported that mice with m6A-deficient Tregs showed severe autoimmune diseases and died 8–9 weeks later. Naive T cells cocultured with Tregs without m6A modification showed increased proliferation, which indicates that Tregs without m6A modification lose their ability to inhibit T cell proliferation. In Tregs with METTL3 defects, SOCS family mRNAs reduce m6A methylation and increase stability, which increases the level of SOCS protein. The SOCS protein regulates the inhibitory function of Tregs by the IL2-STAT5 signaling pathway. Previous studies have found that the IL2-STAT5 pathway can regulate the function of Tregs. Methylation modifications regulate the function of Tregs by affecting those RNAs that encode inhibitor proteins of this pathway (Figure 1) (85).
Innate Immunity
In innate immunity, macrophages are involved in regulating tissue dynamic balance, defending against viral infection and inflammation. Under different conditions, it can be polarized to M1 and M2. M1 has anti-tumor activity. M2 macrophages can inhibit inflammation, promote angiogenesis and tissue repair, and also participate in tumor metastasis (86–89).
A recent study found that silent FTO can inhibit the polarization of M1 and M2. NF-κB signal pathway is the main activation pathway of M1 polarization. It has been found that knockout of FTO can inhibit the phosphorylation of NF- κ B signal pathway to inhibit M1 polarization. However, it has not been proved whether RNA methylation is involved in this process. In addition, STAT1 promotes M1 polarization, while PPAR- γ promotes M2 polarization. Knockout of FTO reduces the stability of STAT1 and PPAR- γ mRNA through YTHDF2-mediated degradation, which results in the inhibition of polarization of M1 and M2 (90). In an earlier study, METTL3 promoted the methylation of STAT1 and enhanced the stability of STAT1mRNA, up-regulated the expression of STAT1 and promoted the polarization of M1. However, in this process, the reader that recognizes the methylation and enhances the stability of mRNA has not been found (91). RNA-binding motif 4 (RBM4) interacts with YTHDF2 to degrade M6A modified STAT1mRNA to regulate glycolysis and M1 macrophage polarization (92). In a recent study on acute coronary syndrome, it was found that IRF-1 promoted the expression of methyltransferase METTL3 in macrophages and inhibited expression of circ_0029589 by regulating its m6A modification, thus promoting the pyroptosis of macrophages (93).
In innate immunity, the immune system initiates a series of immune responses by recognizing foreign components. These foreign components are recognized by receptors located in the cell membrane or cytoplasm, such as Toll-like receptors and RIG-I-like receptors. After the receptor recognizes the foreign component, it stimulates the immune response and produces cytokines.
Toll-like receptors (TLRs) recognize foreign components and induce the immune response. Foreign RNAs recognized by TLRs stimulate the immune system and trigger immune responses with the production of type I interferon (82, 94). RNAs containing nucleotide modifications, including RNA methylation, suppress or avoid the activation of innate immunity (95, 96). Previous research has proven that methylation modifications inhibit the ability of RNAs to stimulate TLR3 and block the stimulation of TLR7 and TLR8 (16). Similarly, protein kinase R (PKR) is an innate immune sensor that recognizes foreign RNA and initiates innate immunity. Retinoic acid-inducible gene I (RIG-I) is a cytoplasmic innate immune receptor that recognizes RNAs of invasive pathogens (97). RNA modification, including methylation, eliminates or suppresses stimulation of PKR and RIG (98, 99).
As non-coding RNAs, foreign circRNAs have immunogenicity and act as adjuvants to induce T cell activation and antibody production (100). M6A modification can significantly inhibit the immune activation of circRNAs. RIG-I binds to foreign circRNAs, and with the participation of K63-polyubiquitin, it stimulates mitochondrial antiviral signaling protein (MAVS) filamentation and ultimately stimulates IRF-3 dimerization. Foreign circRNAs modified by m6A cannot stimulate Mavs filamentation and IRF-3 dimerization after being bound by RIG-I, and YTHDF2 binding to methylated circRNA inhibits circRNA immunity. In vivo, the immunogenicity of circRNAs as adjuvants is also inhibited by m6A modification (59).
In addition to affecting the immunogenicity of RNA itself, methylation modification also affects innate immunity by regulating innate immune components. Type I interferon is key for antiviral immunity (101). M6A regulates the production of type I interferon, which is stimulated by viruses. The deletion of METTL14 increases the level of type I interferon mRNA, and the deletion of ALKBH5 decreases the level of type I interferon mRNA, which regulates the level of type I interferon. Without the m6A modification, the type I interferon mRNA is more stable, which means a stronger immune response (102).
In vesicular stomatitis virus infection, knockout of the eraser ALKBH5 increases the level of interferon induced by the virus, while overexpression of ALKBH5 decreases the level of interferon. ALKBH5, as a demethylase, demethylates the transcripts of some antiviral proteins, such as Mavs, Traf3 and Traf6. These demethylated transcripts remain in the nucleus, and translation is inhibited, thus inhibiting the production of interferon (103).
Methylation modification can regulate innate immunity not only by affecting the molecules involved in the immune response but also by directly affecting antigens. However, the function of methylation is different between the two mechanisms. The former mainly affects the protein translated by RNA, while the latter directly affects the immunogenicity of RNAs (Figure 2).
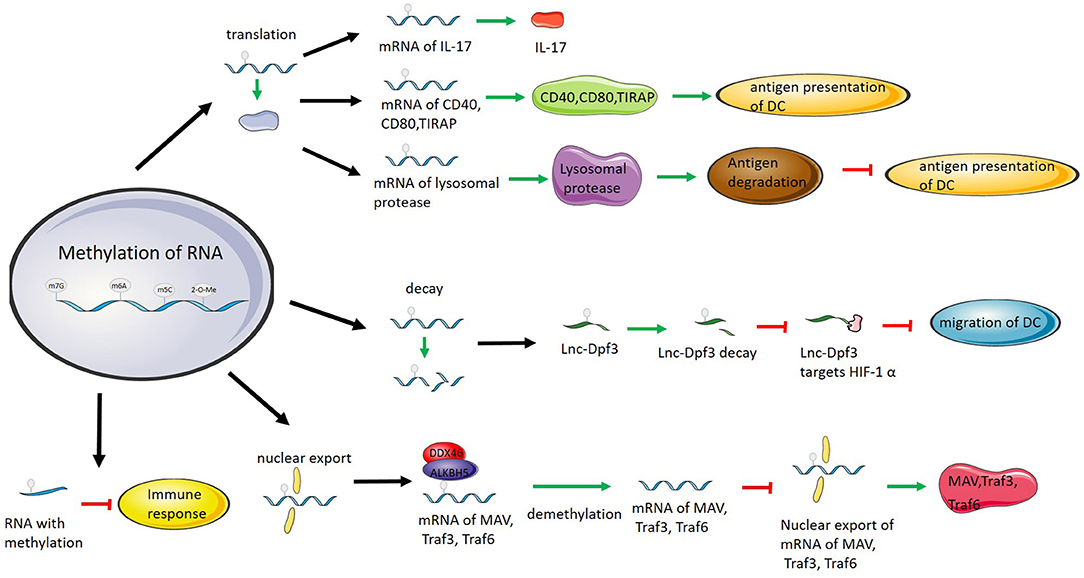
Figure 2. RNA methylation regulates immunity by affecting RNA translation, degradation, and nuclear export and directly inhibiting RNA immunogenicity. RNA methylation promotes IL-17 translation. RNA methylation promotes the translation of CD40, CD80, and TIRAP and improves antigen presentation by DCs. RNA methylation promotes the translation of lysosomal proteases, degrades antigens and inhibits antigen presentation by DCs. RNA methylation promotes Lnc-Dpf3 degradation to prevent Lnc-Dpf3 from inhibiting DC migration by targeting HIF-1α. DDX46 recruits ALKBH5 to demethylate MAV, Traf3, and Traf6 mRNAs, inhibit the enucleation of mRNAs, and thus inhibit the immune function of MAV, Traf3, and Traf6. Methylation of RNAs suppresses the immunogenicity of RNAs and eliminates or weakens the ability of RNAs to activate the immune response.
Various Immunoregulation Mechanism of RNA Methylation
RNA methylation regulates immunity through a variety of mechanisms.
The transcripts encoding lysosomal proteases are recognized by YTHDF1 after methylation, and YTHDF1 combines with the methylated transcripts to promote the translation of the transcripts and increase the level of lysosomal proteases. More lysosomal proteases degrade phagocytosed tumor antigens, and DCs inhibit the presentation of antigens, resulting in immune escape (58).
In DCs, the transcripts of the immune components CD40, CD80, and Tirap are methylated by METTL3. Methylated CD40 and CD80 mRNAs are recognized by YTHDF1, and the translation of CD40, CD80 and Tirap is enhanced. When DCs express more CD40, CD80, and Tirap, the antigen presentation function of DCs is enhanced (81). Methylation of lnc-Dpf3 affects DC migration. The methylated lnc-Dpf3 is recognized and bound by YTHDF2, which enhances the degradation of lnc-Dpf3. CCR7 stimulation decreases the methylation level of lnc-Dpf3. When the methylation level of Lnc-Dpf3 decreases, the level of Lnc-Dpf3 increases due to decreased degradation. Rapid glycolytic reprogramming is triggered when DCs are stimulated to meet metabolic requirements. Increased Lnc-Dpf3 targets HIF-1α and inhibits HIF-1α-dependent transcription of the glycolysis gene LDHA. Glycolysis and migration of DCs are inhibited (34).
M6A methylation regulates the proliferation and differentiation of immature T cells by affecting the degradation of SOCS family mRNAs in the IL7/STAT5/SOCS pathway. In METTL3-deficient T cells, the degradation of SOCS family member mRNA transcription is decreased. The expression of STAT5 inhibitory protein is increased, which blocks the stimulation of T cell proliferation and differentiation by IL-7 and makes T cells exist for a longer time in the immature T cell stage (21).
Similar to its regulation of T cell proliferation and differentiation, m6A regulates the inhibitory function of Tregs through the IL2-STAT5 signaling pathway. In Tregs with METTL3 conditional knockout, the mRNA stability of SOCS family members with decreased m6A methylation increased, and the level of SOCS protein increased. The increased SOCS proteins inhibited the phosphorylation of STAT5 stimulated by IL-2, which in turn affected the inhibitory function of Tregs (85).
DDX46 belongs to the DEAD-box family. Most of the family members are located in the nucleus and participate in the metabolism of RNA, which plays an important role in antiviral immunity. DDX46 is located in the nucleus and recruits ALKBH5 in antiviral immunity. ALKBH5, as an eraser, can demethylate the mRNA of antiviral substances such as Mavs, Traf3, and Traf6. After demethylation, the nucleation of mRNA is inhibited, translation of mRNA is blocked, and the expression of antiviral molecules is reduced. Knockout of DDX46 in vivo can significantly improve the antiviral response of the body (103).
In terms of effects on immunity, on the one hand, RNA methylation affects the stability, translation and nuclear export of RNAs after modification and thus affects the levels of RNAs and proteins, which are involved in the immune process. In addition, it also affects immunity by directly affecting the immunogenicity of RNA and reduces the immune stimulation by RNA.
Immunoregulation of RNA Methylation in Various Cancers
RNA methylation plays an essential role in tumorigenesis and development. Abnormal RNA methylation has been confirmed to be associated with human cancer (104, 105). In addition to directly affecting the occurrence and development of tumors, RNA methylation affects tumors by regulating tumor immunity. The immune system plays an important role in the development, growth, invasion and metastasis of tumors. On the one hand, immune cells attack tumor cells and inhibit the development of tumors; on the other hand, immune escape by tumors promotes their development, growth, invasion and metastasis (106). RNA methylation affects tumors through the regulation of related components in the immune system (Table 1 and Figure 3).
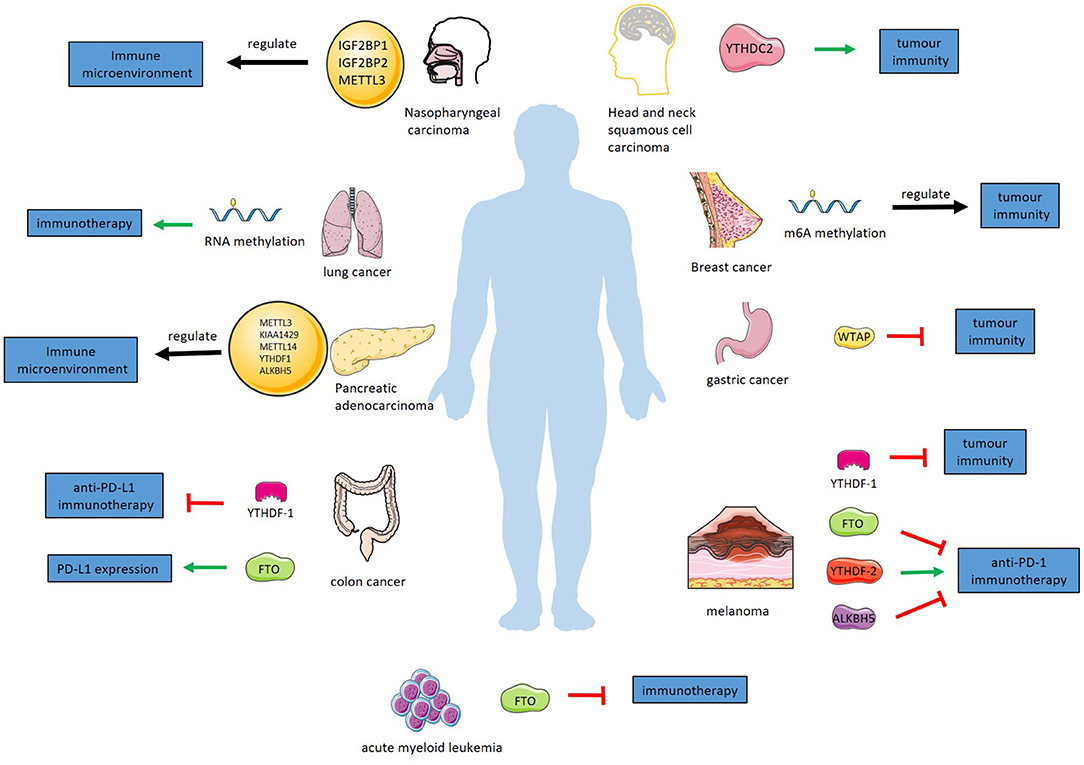
Figure 3. RNA methylation regulates tumor immunity in various cancers. In melanoma, YTHDF-1 inhibits tumor immunity. In anti-PD-1 immunotherapy of melanoma, YTHDF-2 has a promoting effect and FTO and alkbh5 have inhibitory effect. YTHDF-1 suppress anti-PD-L1 immunotherapy for colon cancer. FTO promotes PD-L1 expression in colon cancer. WTAP suppress tumor immunity of gastric cancer. RNA methylation regulates immunotherapy for lung cancer. FTO inhibits immunotherapy of acute myeloid leukemia. IGF2BP1, IGF2BP2, and METTL3 regulate immune microenvironment of nasopharyngeal carcinoma. YTHDC-2 has a promoting effect in tumor immunity of HNSCC. M6A methylation regulates tumor immunity of breast cancer. METTL3, KIAA1429, METTL14, YTHDF1, ALKBH5 regulate immune microenvironment of pancreatic adenocarcinoma.
Melanoma
Tumor neoantigens play an important role in tumor immunotherapy (121). M6A methylation regulates the long-lasting specific immunity of tumor neoantigens through m6A-binding proteins. In a melanoma model in mice, YTHDF1-deficient mice showed slower tumor growth and longer survival than wild-type mice. By restricting the lysosomal protease expression of DCs cross-presentation is enhanced so that CD8+ T cells can better exert antitumour immune effects (58).
M6A modification inhibits the growth, metastasis, invasion and viability of melanoma cells by regulating tumor immunity. As a demethylase, FTO has been shown to promote the development of melanoma. PD-1 mRNA, as a target of FTO, is demethylated. M6A-methylated PD-1 mRNA is recognized by YTHDF2, which promotes its degradation. Therefore, the knockout of FTO decreases the level of PD-1 mRNA, which means lower expression of PD-1. In a melanoma mouse model treated with anti-PD-1 immunotherapy, knockout of FTO significantly inhibited tumor growth (107). Knockout of YTHDF-1 or blocking methylation of lysosomal protein RNA in DC inhibits tumor immune escape in melanoma patients. This provides a method of immunotherapy. The overexpression of YTHDF-2 in immune cells or the enhancement of PD-1 RNA methylation reduces the expression of PD-1 in immune cells, which enhances the effect of anti-PD-1 immunotherapy. These provide help in the clinical treatment of melanoma.
A recent study found that in anti-PD-1 therapy for melanoma, the deletion of ALKBH5 decreased the levels of infiltrating Tregs and polymorphonuclear myeloid derived suppressor cells (PMN-MDSCs), while the level of dendritic cells increased. In the process of this regulation, the m6A modification of the target gene Mct4/Slc16a3 increased, which means a higher stability and level. Mct4 is the key enzyme that catalyzes the rapid transport of lactic acid across the plasma membrane. And extracellular lactic acid directly affects the recruitment of Tregs and MDSC in the tumor microenvironment. A small-molecule ALKBH5 inhibitor, which can enhance the effect of anti-PD-1 therapy for melanoma, was found in this study (108).
Colon Carcinoma
Similarly, immunoregulation of RNA methylation is essential in colon cancer therapy. In a colon cancer tumor-bearing mouse model, YTHDF1-deficient mice show tumor growth inhibition and longer survival than control mice. Early activation of T cells in YTHDF1-deficient mice was enhanced, and the immune infiltration levels of CD8+ cytotoxic T cells and natural killer cells were higher, while the infiltration of bone marrow-derived suppressor cells was lower. DCs showed a stronger ability to cross-present tumor antigens in this process. YTHDF1-deficient mice showed a higher cure rate with anti-PD-L1 treatment than control mice (58). Similar to the melanoma mentioned above, limiting the expression of YTHDF-1 is also used in the treatment of colon cancer and shows a higher survival rate in anti-PD-L1 immunotherapy for colon cancer. These results provide a new idea for immunotherapy of colon cancer. In addition, the detection of YTHDF-1 is used to evaluate the prognosis of patients with colon cancer.
As a demethylase, FTO is thought to regulate the methylation of PD-L1mRNA to affect the expression of PD-L1 in colon cancer cells. The detailed regulation mechanism of this process is not clear, but with the further research, it will provide new ideas for immunotherapy of colon cancer (109). In addition, in mismatch-repair-proficient or microsatellite instability-low (pMMR-MSI-L) colorectal cancer and melanoma, the deletion of METTL3 and METTL14 increased the infiltration of CD8+T cells and the secretion of IFN- γ, Cxcl9 and Cxcl10, and enhanced the anti-PD-1 response. The absence of METTL3 and METTL14 reduced the m6A modification of STAT1 and IRF1 mRNA and the YTHDF2-mediated mRNA degradation to increase the levels of STAT1 and IRF1 which participate in IFN- γ-Stat1-Irf1 signal axis and play an important role in anti-PD-1 response (110).
Gastric Cancer
WTAP is an important component of the RNA methylase complex and recruits m6A methyltransferases. WTAP is present at a high level in the tumor tissues of patients with gastric cancer and is significantly related to the prognosis of patients. A study found that patients with high expression of WTAP had lower levels of Tregs and CD4 memory-activated T cells. High infiltration of Tregs and CD4 memory-activated T cells can improve the prognosis of patients with gastric cancer. This means that high expression of WTAP affects the prognosis of patients with gastric cancer by suppressing tumor immunity. Therefore, detecting the level of WTAP could evaluate the prognosis of patients with gastric cancer and provide a direction for immunotherapy of patients with gastric cancer (111).
By analyzing 1,938 cases of gastric cancer, Zhang et al. concluded that m6A modification is significantly related to tumor immune microenvironment and tumor immunotherapy. A recent study by Mo et al. build an m6A risk score: FTO × 0.127+YTHDF1 × 0.004+KIAA1429 × 0.044+YTHDC2 × 0.112-RBM15 × 0.135-ALKBH5 × 0.019-YTHDF2 × 0.028. It is considered that the risk score can be used as an independent prognostic index of gastric adenocarcinoma. Low risk score is associated with high expression of immune checkpoints, such as PD-1, PD-L1, and CTLA-4, which means that the risk factor can be used to evaluate the efficacy of immunotherapy for gastric cancer. The above view has not been clinically verified, but it suggests that the methylation of RNA can be used as an independent marker for predicting prognosis and guiding immunotherapy in patients with gastric cancer. With further understanding of the relationship between RNA methylation and immunity, altering methylation modification may provide help for personalized and accurate treatment of gastric cancer (112, 113).
Lung Cancer
Immunotherapy plays an important role in the treatment of lung cancer by affecting RNA methylation. In a study on the prognosis of lung cancer, it was found that high-risk patients who responded better to immunotherapy had more methyltransferases than high-risk patients who did not respond well to immunotherapy. This may suggest that RNA methylation has the capacity to predict prognosis and guide the use of drugs in immunotherapy for lung cancer (114).
Acute Myeloid Leukemia
A recent study has found that some FTO inhibitors have been identified to play a role in tumor immunotherapy by interfering with RNA methylation. In addition to the common immune checkpoints such as PD-L1, LILRB4 also plays an important role in immunotherapy in AML. By inhibiting the methylation of LILRB4 regulated by FTO, it can affect the expression of LILRB4, make AML cells more sensitive to T cell toxicity, and play a synergistic role in the immunotherapy of AML. Previous studies have found FTO inhibitors suitable for clinical use, which will be of great help to the immunotherapy of AML (115).
Nasopharyngeal Carcinoma
In a recently study, Lu build a risk model of nasopharyngeal carcinoma with m6A-related genes, the risk score = IGF2BP1 × 0.161557 + IGF2BP2 × 0.01313 + METTL3 × (−0.0624), which is considered an independent prognostic factor. In nasopharyngeal carcinoma patients, the infiltration of immune cells (B naïve cells, B memory cells, CD8T, CD4 memory T activated, T gamma delta, Mast cells resting, and activated Mast cells) is significantly increased and the infiltration of immune cells (CD4 memory T resting, Macrophages M0, and Macrophages M2) is significantly decreased in the high risk score group compared to low risk score group. This finding revealed the potential role of m6A modification in nasopharyngeal carcinoma, providing novel insight into nasopharyngeal carcinoma prognosis and therapeutic strategies (116).
Breast Cancer
A recent study analyzed the RNA sequence data of 24 m6A regulators in 775 breast cancer patients in TCGA. Patients with performing consensus clustering of 24 commonly reported m6A regulators based on the RNA expression data from TCGA, were divided into two groups. The two groups were significantly correlated with the number of tumor infiltrating CD8+T cells, helper T cells, activated NK cells, macrophage M2 and regulatory T cells. It was also significantly correlated with the expression of PD-L1, TIM3, LAG3, and CCR4, which are well-known T-cell exhaustion markers and important targets in immunotherapy. Compared with the low methylation group, the number of tumor infiltrating CD8+T cells, helper T cells and activated NK cells increased significantly, while the expression of PD-L1, PD-L2, TIM3, and CCR4 decreased significantly in the high methylation group. These results suggest that m6A regulators are closely related to the anti-tumor immunity of breast cancer. However, these analyses come from bioinformatics tools, and there are no further experiments to verify them. However, this provides comprehensive evidence for the further study of m6A regulators in breast cancer, and provides new clues for the epigenetic regulation of anti-tumor immune response (117).
Another study based on bioinformatics suggests that the low expression of METTL14 and ZC3H13 mRNA indicates a poor prognosis of breast cancer. The expression of METTL14 and ZC3H13 in breast cancer was positively correlated with the infiltration of CD4+T cells, CD8+T cells, neutrophils, macrophages and DC, and negatively correlated with Treg cells. The study puts forward a scientific hypothesis: the low expression of METTL14 and ZC3H13 leads to the decrease of Adenomatous polyposis coli (APC) mRNA methylation, which in turn reduces the stability of APC mRNA. The decrease of APC level leads to the abnormal activation of Wnt signal pathway which plays an important role in the regulation of tumor immune microenvironment and regulate the infiltration and activity of various types of T cells in tumor (118).
Pancreatic Adenocarcinoma
Based on the analysis of the expression of m6A methylation regulatory gene in pancreatic Adenocarcinoma and normal tissue data from TCGA and GEO database, a recent study suggested that m6A methylation regulatory gene is related to the prognosis and immune microenvironment of pancreatic Adenocarcinoma, and constructed a m6A-related risk signature: risk score= −0.087 × METTL3 + 0.233 × KIAA1429-0.132 × METTL14-0.035 × YTHDF1-0.0286 × ALKBH5. In the high risk group, the infiltration of macrophage M0 and macrophage M2 increased, while the levels of B cells, CD8+T cells and regulatory T cells decreased. These results suggest that m6A regulatory gene regulates the immune microenvironment of pancreatic Adenocarcinoma through a comprehensive and complex process. Similarly, these results have not been verified by experiments, and the specific regulation mechanism has not been clarified (119).
Head and Neck Squamous Cell Carcinoma
YTHDC2 is a reader of N6-methyladenosine. Recent studies have found that YTHDC2 is associated with the level of immune infiltration of B cells, CD8+T cells, CD4+T cells, neutrophils and dendritic cells in head and neck squamous cell carcinoma (HNSCC). Through the analysis of gene expression and prognosis of HNSCC patients in GEO, Oncolnc, Kaplan-Meier plotter, TIMER and other databases, it was found that YTHDC2 could be an independent tumor suppressor gene to judge the prognosis of HNSCC, and the expression level of YTHDC2 was positively correlated with the infiltration level of CD4+T cell subsets. However, the correlation between YTHDC2 and CD4+T cell subsets and its specific mechanism need further experimental study (120).
Dual Roles of RNA Methylation in Immunity
As mentioned above, RNA methylation has dual effects on tumor regulation of immunity. In different types of tumors, RNA methylation can have a promoting or inhibitory role. The existing data suggest that RNA methylation also has dual roles in immunity.
The absence of m6A inhibited the differentiation and proliferation of T cells, which could prevent colitis in experimental mice. However, in Tregs, the absence of m6A inhibited their inhibitory function, which could lead to autoimmune diseases in experimental mice (21, 85).
On the one hand, methylation promotes immunity, while demethylation suppresses immunity. After methylation, CD40, CD80, and TIRAP on DCs promote the activation of DCs and enhance immunity. During the migration of DCs stimulated by CCR7, lnc-Dpf3 methylation promotes the migration and maturation of DCs, leading to abnormal inflammation. In melanoma cells, the inhibition of demethylation enhances tumor immunity. After the methylation of antiviral transcripts in cells is erased by demethylases, innate immunity is suppressed (34, 81, 107). On the other hand, methylation weakens the antigenicity of RNA and suppresses the immune response. After methylation modification, the immunogenicity of RNA is weakened or disappears, and innate immunity is no longer triggered. The methylation of mRNAs encoding lysosomal proteases inhibits the presentation of tumor antigens, resulting in tumor immune escape. After methylation of type I interferon mRNA, mRNA degradation increased, interferon production decreased, and innate immunity was suppressed (58, 102).
Clinical Implications and Future Directions
The immune system is the host defense system against infection and disease. As a kind of genetic post-modification, RNA methylation not only participates in the immune response, but also participates in the occurrence and development of tumor by affecting splicing, nucleation, stability and immunogenicity of RNA. In addition to eliminating the immunogenicity of therapeutic RNA and treating immune diseases, RNA methylation provides a broader prospect for tumor immunotherapy.
In recent years, emerging tumor immunotherapy strategies have brought new hope to tumor patients. Some immunotherapeutic drugs, such as PD-1/PD-L1 inhibitors, have been approved for the clinical treatment of melanoma, lung cancer and colorectal cancer (122, 123). In melanoma, the deletion of YTHDF-1 enhances the anti-tumor immune response. Knock out FTO or ALKBH-5 enhances anti-PD-1 immunotherapy. In colon cancer, knockout YTHDF-1 enhances PD-L1 immunotherapy. The deletion of METTL3 and METTL14 enhances the anti-PD-1 response. In gastric cancer, high expression of WTAP suppresses tumor immunity and affects prognosis. In AML, suppression of FTO expression enhances immunotherapy. By targeting these methylation regulatory molecules, it can directly enhance tumor immunotherapy. In nasopharyngeal carcinoma, breast cancer, Pancreatic adenocarcinoma and HNSCC, using bioinformatics tool to analyze the database, it is found that many modulators have a strong correlation with tumor immunity and immunotherapy. Most of these conclusions have not been confirmed by experiments, but they provide a lot of directions for tumor immunotherapy. For example, these modulators can be used as biomarkers for biological prediction in immunotherapy and as targets to improve the response to immunotherapy. In addition, it can also be used alone or in combination with other drugs to treat tumor. For example, as a broad-spectrum antiviral drug, Ribavirin has been found in recent years to be used as an antitumour drug to participate in the treatment of a variety of tumors. It can not only be effective as a monotherapy, but also has been shown to enhance the effect of radiotherapy or chemotherapy (124). Previous study has found that Ribavirin competes with eIF4E to combine with m7G to block the translation of mRNA and play a role in the treatment of tumors. With the progress of RNA methylation research, the combination of Ribavirin and drugs regulating m7G in the treatment of tumor is also worth looking forward to (125).
Compared with RNA methylation, DNA methylation has become the focus of cancer research earlier. Some DNA methylation drugs have been widely used clinically, such as Azacytidine and Decitabine. Previous studies have found that the DNA demethylation drug Azacytidine inhibits the RNA transferase DNMT2 to regulate tRNA methylation (126). In a recent study, RNA methylation modification affected resistance in leukemia treated with the DNA demethylation drug 5-Aza (127). In the future, the combined application of DNA demethylation drugs and RNA methylation drugs may provide new treatments for tumors. In addition, RNA methylation modulators can also be used as new markers for predicting the sensitivity of DNA methylation drugs. Those DNA methylation drugs that can regulate RNA methylation also have the potential to be applied to RNA methylation therapy or immunotherapy.
Conclusion
RNA methylation, which regulates not only mRNAs but also ncRNAs, has been proven to be able to regulate a variety of biological functions, especially tumor immunity. RNA methylation can regulate a variety of immune cells and has a variety of regulatory modes and mechanisms, such as regulating the differentiation of T cells, the state of Treg cells, and the maturation of DCs. In addition, modifications can also regulate a variety of immune cytokines, such as IL-17. RNA methylation regulates human immune status and tumor immunity. RNA methylation also shows dual roles in regulating immunity, which affects immune function. In a variety of tumors, RNA methylation affects the effectiveness of tumor immunotherapy by regulating immune function. At present, research on the relationship between RNA methylation and tumor immunity is still in its infancy, and more in-depth research is needed to explore the mechanism. RNA methylation has the potential to provide new ideas for the identification of tumor patients who will be sensitive to a given drug, the determination of prognostic indicators, and research and development of targeted drugs.
Author Contributions
ZS and JS provided direction and guidance throughout the preparation of this manuscript. MZ wrote and edited the manuscript. WY reviewed and made significant revisions to the manuscript. WZ collected and prepared the related papers. All authors read and approved the final manuscript.
Funding
This study was supported by The National Natural Science Foundation of China (81972663, 81560385), Key Scientific Research Projects of Institutions of Higher Education in Henan Province (19A310024), The Medical Scientific and Technological Research Project of Henan Province (201702027), Youth Innovation Fund Project of The First Affiliated Hospital of Zhengzhou University (YNQN2017035), The China Postdoctoral Science Foundation (2019T120648, 2017M610462), The National Natural Science Foundation of Henan Province (182300410342), and The Health Commission Technology Talents Overseas Training Project of Henan Province (2018140).
Conflict of Interest
The authors declare that the research was conducted in the absence of any commercial or financial relationships that could be construed as a potential conflict of interest.
Abbreviations
ALKBH5, alkylation repair homing protein 5; CCR7, chemokine-C receptor 7; DCs, Dendritic cells; DDX46, DEAD-box 46; DNMT, DNA methyltransferase; ERK, extracellular regulated protein kinases; FTO, fat mass and obesity-associated protein; HIF-1α, hypoxia inducible factor-1α; IGF2BP1/2/3, insulin-like growth factor 2 mRNA binding protein 1/2/3; IL-17, interleukin 17; IL-2, interleukin 2; IL-7, interleukin 7; IRF3, Interferon regulatory Factor 3; LDHA, lactate dehydrogenase A; Mavs, mitochondrial antiviral signaling protein; METTL1, methyltransferase-like 1; METTL14, methyltransferase-like 14; METTL16, methyltransferase-like 16; METTL3, methyltransferase-like 3; MSI, microsatellite instability; m6A, N6-methyladenosine; m5C, 5-methylcytosine; m7G, N7-methylguanosine; ncRNAs, non-coding RNAs; Nsun2, NOP2/Sun RNA methyltransferase 2; PD-L1, Programmed cell death 1 ligand 1; PD-1, programmed cell death protein 1; PKR, protein kinase R; RBM15/15B, RNA binding motif protein 15/15B; RIG-I, retinoic acid-inducible gene I; snoRNA, small nucleolar RNA; snRNA, small nuclear RNA; SOCS, Suppressor of cytokine signaling; STAT5, Signal transducers and activators of transcription 5; TIRAP, Toll-interleukin1 receptor domain containing adaptor protein; TLRs, Toll-like receptors; TMB, tumor mutational burden; Traf3, TNF receptor associated factor 6; Traf6, TNF receptor associated factor 6; Tregs, Regulatory T cells; WDR4, WD repeat domain 4; WTAP, Wilms tumor 1-associated protein; YTHDC1/2, YTH domain-containing 1/2; YTHDF1/2/3, YTH N6-methyladenosine RNA binding protein 1/2/3; ZCCHC4, CCHC zinc finger-containing protein 4; ZC3H13, Zinc finger CCCH domaincontaining protein 13.
References
1. Boccaletto P, Machnicka MA, Purta E, Piatkowski P, Baginski B, Wirecki TK, et al. MODOMICS: a database of RNA modification pathways. 2017 update. Nucleic Acids Res. (2018) 46:D303–d7. doi: 10.1093/nar/gkx1030
2. Mathlin J, Le Pera L, Colombo T. A census and categorization method of epitranscriptomic marks. Int J Mol Sci. (2020) 21:4684. doi: 10.3390/ijms21134684
3. Chen Y, Wang J, Xu D, Xiang Z, Ding J, Yang X, et al. m(6)A mRNA methylation regulates testosterone synthesis through modulating autophagy in Leydig cells. Autophagy. (2020) 1–19. doi: 10.1080/15548627.2020.1720431. [Epub ahead of print].
4. Courtney DG, Tsai K, Bogerd HP, Kennedy EM, Law BA, Emery A, et al. Epitranscriptomic addition of m(5)C to HIV-1 transcripts regulates viral gene expression. Cell Host Microbe. (2019) 26:217–27.e6. doi: 10.1016/j.chom.2019.07.005
5. Xiao W, Adhikari S, Dahal U, Chen YS, Hao YJ, Sun BF, et al. Nuclear m(6)A reader YTHDC1 regulates mRNA splicing. Mol Cell. (2016) 61:507–19. doi: 10.1016/j.molcel.2016.01.012
6. Zhao X, Yang Y, Sun BF, Shi Y, Yang X, Xiao W, et al. FTO-dependent demethylation of N6-methyladenosine regulates mRNA splicing and is required for adipogenesis. Cell Res. (2014) 24:1403–19. doi: 10.1038/cr.2014.151
7. Roundtree IA, Luo GZ, Zhang Z, Wang X, Zhou T, Cui Y, et al. YTHDC1 mediates nuclear export of N(6)-methyladenosine methylated mRNAs. eLife. (2017) 6:e31311. doi: 10.7554/eLife.31311
8. Wang X, Lu Z, Gomez A, Hon GC, Yue Y, Han D, et al. N6-methyladenosine-dependent regulation of messenger RNA stability. Nature. (2014) 505:117–20. doi: 10.1038/nature12730
9. Du H, Zhao Y, He J, Zhang Y, Xi H, Liu M, et al. YTHDF2 destabilizes m(6)A-containing RNA through direct recruitment of the CCR4-NOT deadenylase complex. Nat Commun. (2016) 7:12626. doi: 10.1038/ncomms12626
10. Lee Y, Choe J, Park OH, Kim YK. Molecular mechanisms driving mRNA degradation by m(6)A modification. Trends Genet TIG. (2020) 36:177–88. doi: 10.1016/j.tig.2019.12.007
11. Meyer KD, Patil DP, Zhou J, Zinoviev A, Skabkin MA, Elemento O, et al. 5' UTR m(6)A promotes cap-independent translation. Cell. (2015) 163:999–1010. doi: 10.1016/j.cell.2015.10.012
12. Shi H, Zhang X, Weng YL, Lu Z, Liu Y, Lu Z, et al. m(6)A facilitates hippocampus-dependent learning and memory through YTHDF1. Nature. (2018) 563:249–53. doi: 10.1038/s41586-018-0666-1
13. Wang X, Zhao BS, Roundtree IA, Lu Z, Han D, Ma H, et al. N(6)-methyladenosine modulates messenger RNA translation efficiency. Cell. (2015) 161:1388–99. doi: 10.1016/j.cell.2015.05.014
14. Xiang Y, Laurent B, Hsu CH, Nachtergaele S, Lu Z, Sheng W, et al. RNA m(6)A methylation regulates the ultraviolet-induced DNA damage response. Nature. (2017) 543:573–6. doi: 10.1038/nature21671
15. Alarcon CR, Lee H, Goodarzi H, Halberg N, Tavazoie SF. N6-methyladenosine marks primary microRNAs for processing. Nature. (2015) 519:482–5. doi: 10.1038/nature14281
16. Kariko K, Buckstein M, Ni H, Weissman D. Suppression of RNA recognition by Toll-like receptors: the impact of nucleoside modification and the evolutionary origin of RNA. Immunity. (2005) 23:165–75. doi: 10.1016/j.immuni.2005.06.008
17. Batista PJ, Molinie B, Wang J, Qu K, Zhang J, Li L, et al. m(6)A RNA modification controls cell fate transition in mammalian embryonic stem cells. Cell Stem Cell. (2014) 15:707–19. doi: 10.1016/j.stem.2014.09.019
18. Wang Y, Li Y, Yue M, Wang J, Kumar S, Wechsler-Reya RJ, et al. N(6)-methyladenosine RNA modification regulates embryonic neural stem cell self-renewal through histone modifications. Nat Neurosci. (2018) 21:195–206. doi: 10.1038/s41593-017-0057-1
19. Hsu PJ, Zhu Y, Ma H, Guo Y, Shi X, Liu Y, et al. Ythdc2 is an N(6)-methyladenosine binding protein that regulates mammalian spermatogenesis. Cell Res. (2017) 27:1115–27. doi: 10.1038/cr.2017.99
20. Haussmann IU, Bodi Z, Sanchez-Moran E, Mongan NP, Archer N, Fray RG, et al. m(6)A potentiates Sxl alternative pre-mRNA splicing for robust Drosophila sex determination. Nature. (2016) 540:301–4. doi: 10.1038/nature20577
21. Li HB, Tong J, Zhu S, Batista PJ, Duffy EE, Zhao J, et al. m(6)A mRNA methylation controls T cell homeostasis by targeting the IL-7/STAT5/SOCS pathways. Nature. (2017) 548:338–42. doi: 10.1038/nature23450
22. Li Z, Weng H, Su R, Weng X, Zuo Z, Li C, et al. FTO plays an oncogenic role in acute myeloid leukemia as a N(6)-methyladenosine RNA demethylase. Cancer Cell. (2017) 31:127–41. doi: 10.1016/j.ccell.2016.11.017
23. Zhang S, Zhao BS, Zhou A, Lin K, Zheng S, Lu Z, et al. m(6)A demethylase ALKBH5 maintains tumorigenicity of glioblastoma stem-like cells by sustaining FOXM1 expression and cell proliferation program. Cancer Cell. (2017) 31:591–606.e6. doi: 10.1016/j.ccell.2017.02.013
24. Wang Q, Chen C, Ding Q, Zhao Y, Wang Z, Chen J, et al. METTL3-mediated m(6)A modification of HDGF mRNA promotes gastric cancer progression and has prognostic significance. Gut. (2020) 69:1193–1205. doi: 10.1136/gutjnl-2019-319639
25. Desrosiers R, Friderici K, Rottman F. Identification of methylated nucleosides in messenger RNA from Novikoff hepatoma cells. Proc Natl Acad Sci USA. (1974) 71:3971–5. doi: 10.1073/pnas.71.10.3971
26. Bokar JA, Rath-Shambaugh ME, Ludwiczak R, Narayan P, Rottman F. Characterization and partial purification of mRNA N6-adenosine methyltransferase from HeLa cell nuclei. Internal mRNA methylation requires a multisubunit complex. J Biol Chem. (1994) 269:17697–704. doi: 10.1016/S0021-9258(17)32497-3
27. Jia G, Fu Y, Zhao X, Dai Q, Zheng G, Yang Y, et al. N6-methyladenosine in nuclear RNA is a major substrate of the obesity-associated FTO. Nature chemical biology. 2011;7(12):885-7. doi: 10.1038/nchembio.687
28. Berulava T, Buchholz E, Elerdashvili V, Pena T, Islam MR, Lbik D, et al. Changes in m6A RNA methylation contribute to heart failure progression by modulating translation. Eur J Heart Fail. (2020) 22:54–66. doi: 10.1002/ejhf.1672
29. Dorn LE, Lasman L, Chen J, Xu X, Hund TJ, Medvedovic M, et al. The N(6)-methyladenosine mRNA methylase METTL3 controls cardiac homeostasis and hypertrophy. Circulation. (2019) 139:533–45. doi: 10.1161/CIRCULATIONAHA.118.036146
30. Lan Q, Liu PY, Haase J, Bell JL, Hüttelmaier S, Liu T. The critical role of RNA m(6)A methylation in cancer. Cancer Res. (2019) 79:1285–92. doi: 10.1158/0008-5472.CAN-18-2965
31. Xu H, Dzhashiashvili Y, Shah A, Kunjamma RB, Weng YL, Elbaz B, et al. m(6)A mRNA methylation is essential for oligodendrocyte maturation and CNS myelination. Neuron. (2020) 105:293–309.e5. doi: 10.1016/j.neuron.2019.12.013
32. Huang H, Weng H, Chen J. m(6)A modification in coding and non-coding RNAs: roles and therapeutic implications in cancer. Cancer Cell. (2020) 37:270–88. doi: 10.1016/j.ccell.2020.02.004
33. Han X, Wang M, Zhao YL, Yang Y, Yang YG. RNA methylations in human cancers. Semin Cancer Biol. (2020). doi: 10.1016/j.semcancer.2020.11.007
34. Liu J, Zhang X, Chen K, Cheng Y, Liu S, Xia M, et al. CCR7 chemokine receptor-inducible lnc-Dpf3 restrains dendritic cell migration by inhibiting HIF-1alpha-mediated glycolysis. Immunity. (2019) 50:600–15.e15. doi: 10.1016/j.immuni.2019.01.021
35. Luo GZ, MacQueen A, Zheng G, Duan H, Dore LC, Lu Z, et al. Unique features of the m6A methylome in Arabidopsis thaliana. Nat Commun. (2014) 5:5630. doi: 10.1038/ncomms6630
36. Bushkin GG, Pincus D, Morgan JT, Richardson K, Lewis C, Chan SH, et al. m(6)A modification of a 3' UTR site reduces RME1 mRNA levels to promote meiosis. Nat Commun. (2019) 10:3414. doi: 10.1038/s41467-019-11232-7
37. Deng X, Chen K, Luo GZ, Weng X, Ji Q, Zhou T, et al. Widespread occurrence of N6-methyladenosine in bacterial mRNA. Nucleic Acids Res. (2015) 43:6557–67. doi: 10.1093/nar/gkv596
38. Meyer KD, Saletore Y, Zumbo P, Elemento O, Mason CE, Jaffrey SR. Comprehensive analysis of mRNA methylation reveals enrichment in 3' UTRs and near stop codons. Cell. (2012) 149:1635–46. doi: 10.1016/j.cell.2012.05.003
39. Liu J, Yue Y, Han D, Wang X, Fu Y, Zhang L, et al. A METTL3-METTL14 complex mediates mammalian nuclear RNA N6-adenosine methylation. Nat Chem Biol. (2014) 10:93–5. doi: 10.1038/nchembio.1432
40. Zheng G, Dahl JA, Niu Y, Fedorcsak P, Huang CM, Li CJ, et al. ALKBH5 is a mammalian RNA demethylase that impacts RNA metabolism and mouse fertility. Mol Cell. (2013) 49:18–29. doi: 10.1016/j.molcel.2012.10.015
41. David R, Burgess A, Parker B, Li J, Pulsford K, Sibbritt T, et al. Transcriptome-wide mapping of RNA 5-methylcytosine in arabidopsis mRNAs and noncoding RNAs. Plant Cell. (2017) 29:445–60. doi: 10.1105/tpc.16.00751
42. Huang W, Lan MD, Qi CB, Zheng SJ, Wei SZ, Yuan BF, et al. Formation and determination of the oxidation products of 5-methylcytosine in RNA. Chem Sci. (2016) 7:5495–502. doi: 10.1039/C6SC01589A
43. Cui X, Liang Z, Shen L, Zhang Q, Bao S, Geng Y, et al. 5-Methylcytosine RNA methylation in Arabidopsis thaliana. Mol Plant. (2017) 10:1387–99. doi: 10.1016/j.molp.2017.09.013
44. Shanmugam R, Fierer J, Kaiser S, Helm M, Jurkowski TP, Jeltsch A. Cytosine methylation of tRNA-Asp by DNMT2 has a role in translation of proteins containing poly-Asp sequences. Cell Discov. (2015) 1:15010. doi: 10.1038/celldisc.2015.10
45. Yang X, Yang Y, Sun BF, Chen YS, Xu JW, Lai WY, et al. 5-methylcytosine promotes mRNA export—NSUN2 as the methyltransferase and ALYREF as an m(5)C reader. Cell Res. (2017) 27:606–25. doi: 10.1038/cr.2017.55
46. Goll MG, Kirpekar F, Maggert KA, Yoder JA, Hsieh CL, Zhang X, et al. Methylation of tRNAAsp by the DNA methyltransferase homolog Dnmt2. Science (New York, NY). (2006) 311:395–8. doi: 10.1126/science.1120976
47. Moon HJ, Redman KL. Trm4 and Nsun2 RNA:m5C methyltransferases form metabolite-dependent, covalent adducts with previously methylated RNA. Biochemistry. (2014) 53:7132–44. doi: 10.1021/bi500882b
48. Xing J, Yi J, Cai X, Tang H, Liu Z, Zhang X, et al. NSun2 promotes cell growth via elevating cyclin-dependent kinase 1 translation. Mol Cell Biol. (2015) 35:4043–52. doi: 10.1128/MCB.00742-15
49. Tuorto F, Liebers R, Musch T, Schaefer M, Hofmann S, Kellner S, et al. RNA cytosine methylation by Dnmt2 and NSun2 promotes tRNA stability and protein synthesis. Nat Struct Mol Biol. (2012) 19:900–5. doi: 10.1038/nsmb.2357
50. Furuichi Y, LaFiandra A, Shatkin AJ. 5'-Terminal structure and mRNA stability. Nature. (1977) 266:235–9. doi: 10.1038/266235a0
51. Shimotohno K, Kodama Y, Hashimoto J, Miura KI. Importance of 5'-terminal blocking structure to stabilize mRNA in eukaryotic protein synthesis. Proc Natl Acad Sci USA. (1977) 74:2734–8. doi: 10.1073/pnas.74.7.2734
52. Lin S, Liu Q, Lelyveld VS, Choe J, Szostak JW, Gregory RI. Mettl1/Wdr4-mediated m(7)G tRNA methylome is required for normal mRNA translation and embryonic stem cell self-renewal and differentiation. Mol Cell. (2018) 71:244–55.e5. doi: 10.1016/j.molcel.2018.06.001
53. Pandolfini L, Barbieri I, Bannister AJ, Hendrick A, Andrews B, Webster N, et al. METTL1 promotes let-7 microRNA processing via m7G methylation. Mol Cell. (2019) 74:1278–90.e9. doi: 10.1016/j.molcel.2019.03.040
54. Guy MP, Phizicky EM. Two-subunit enzymes involved in eukaryotic post-transcriptional tRNA modification. RNA Biol. (2014) 11:1608–18. doi: 10.1080/15476286.2015.1008360
55. Sloan KE, Warda AS, Sharma S, Entian KD, Lafontaine DLJ, Bohnsack MT. Tuning the ribosome: the influence of rRNA modification on eukaryotic ribosome biogenesis and function. RNA Biol. (2017) 14:1138–52. doi: 10.1080/15476286.2016.1259781
56. Ge J, Liu H, Yu YT. Regulation of pre-mRNA splicing in Xenopus oocytes by targeted 2'-O-methylation. RNA (New York, NY). (2010) 16:1078–85. doi: 10.1261/rna.2060210
57. Ji L, Chen X. Regulation of small RNA stability: methylation and beyond. Cell Res. (2012) 22:624–36. doi: 10.1038/cr.2012.36
58. Han D, Liu J, Chen C, Dong L, Liu Y, Chang R, et al. Anti-tumour immunity controlled through mRNA m(6)A methylation and YTHDF1 in dendritic cells. Nature. (2019) 566:270–4. doi: 10.1038/s41586-019-0916-x
59. Chen YG, Chen R, Ahmad S, Verma R, Kasturi SP, Amaya L, et al. N6-Methyladenosine modification controls circular RNA immunity. Mol Cell. (2019) 76:96–109.e9. doi: 10.1016/j.molcel.2019.07.016
60. Wang P, Doxtader KA, Nam Y. Structural basis for cooperative function of Mettl3 and Mettl14 methyltransferases. Mol Cell. (2016) 63:306–17. doi: 10.1016/j.molcel.2016.05.041
61. Wang X, Feng J, Xue Y, Guan Z, Zhang D, Liu Z, et al. Structural basis of N(6)-adenosine methylation by the METTL3-METTL14 complex. Nature. (2016) 534:575–8. doi: 10.1038/nature18298
62. Schwartz S, Mumbach MR, Jovanovic M, Wang T, Maciag K, Bushkin GG, et al. Perturbation of m6A writers reveals two distinct classes of mRNA methylation at internal and 5' sites. Cell Rep. (2014) 8:284–96. doi: 10.1016/j.celrep.2014.05.048
63. Meyer KD, Jaffrey SR. Rethinking m(6)A readers, writers, and erasers. Ann Rev Cell Dev Biol. (2017) 33:319–42. doi: 10.1146/annurev-cellbio-100616-060758
64. Wen J, Lv R, Ma H, Shen H, He C, Wang J, et al. Zc3h13 regulates nuclear RNA m(6)A methylation and mouse embryonic stem cell self-renewal. Mol Cell. (2018) 69:1028–38.e6. doi: 10.1016/j.molcel.2018.02.015
65. Ma H, Wang X, Cai J, Dai Q, Natchiar SK, Lv R, et al. N(6-)Methyladenosine methyltransferase ZCCHC4 mediates ribosomal RNA methylation. Nat Chem Biol. (2019) 15:88–94. doi: 10.1038/s41589-018-0184-3
66. Warda AS, Kretschmer J, Hackert P, Lenz C, Urlaub H, Hobartner C, et al. Human METTL16 is a N(6)-methyladenosine (m(6)A) methyltransferase that targets pre-mRNAs and various non-coding RNAs. EMBO Rep. (2017) 18:2004–14. doi: 10.15252/embr.201744940
67. Li A, Chen YS, Ping XL, Yang X, Xiao W, Yang Y, et al. Cytoplasmic m(6)A reader YTHDF3 promotes mRNA translation. Cell Res. (2017) 27:444–7. doi: 10.1038/cr.2017.10
68. Shi H, Wang X, Lu Z, Zhao BS, Ma H, Hsu PJ, et al. YTHDF3 facilitates translation and decay of N(6)-methyladenosine-modified RNA. Cell Res. (2017) 27:315–28. doi: 10.1038/cr.2017.15
69. Xu C, Wang X, Liu K, Roundtree IA, Tempel W, Li Y, et al. Structural basis for selective binding of m6A RNA by the YTHDC1 YTH domain. Nat Chem Biol. (2014) 10:927–9. doi: 10.1038/nchembio.1654
70. Mao Y, Dong L, Liu XM, Guo J, Ma H, Shen B, et al. m(6)A in mRNA coding regions promotes translation via the RNA helicase-containing YTHDC2. Nat Commun. (2019) 10:5332. doi: 10.1038/s41467-019-13317-9
71. Huang H, Weng H, Sun W, Qin X, Shi H, Wu H, et al. Recognition of RNA N(6)-methyladenosine by IGF2BP proteins enhances mRNA stability and translation. Nat Cell Biol. (2018) 20:285–95. doi: 10.1038/s41556-018-0045-z
72. Sharabi A, Tsokos GC. T cell metabolism: new insights in systemic lupus erythematosus pathogenesis and therapy. Nat Rev Rheumatol. (2020) 16:100–12. doi: 10.1038/s41584-019-0356-x
73. Walsh SR, Simovic B, Chen L, Bastin D, Nguyen A, Stephenson K, et al. Endogenous T cells prevent tumor immune escape following adoptive T cell therapy. J Clin Investig. (2019) 129:5400–10. doi: 10.1172/JCI126199
74. Wang N, Tang H, Wang X, Wang W, Feng J. Homocysteine upregulates interleukin-17A expression via NSun2-mediated RNA methylation in T lymphocytes. Biochem Biophys Res Commun. (2017) 493:94–9. doi: 10.1016/j.bbrc.2017.09.069
75. Zhu Y, Zhao Y, Zou L, Zhang D, Aki D, Liu YC. The E3 ligase VHL promotes follicular helper T cell differentiation via glycolytic-epigenetic control. J Exp Med. (2019) 216:1664–81. doi: 10.1084/jem.20190337
76. Merad M, Sathe P, Helft J, Miller J, Mortha A. The dendritic cell lineage: ontogeny and function of dendritic cells and their subsets in the steady state and the inflamed setting. Ann Rev Immunol. (2013) 31:563–604. doi: 10.1146/annurev-immunol-020711-074950
77. Waisman A, Lukas D, Clausen BE, Yogev N. Dendritic cells as gatekeepers of tolerance. Semin Immunopathol. (2017) 39:153–63. doi: 10.1007/s00281-016-0583-z
78. Eisenbarth SC. Dendritic cell subsets in T cell programming: location dictates function. Nat Rev Immunol. (2019) 19:89–103. doi: 10.1038/s41577-018-0088-1
79. Ardouin L, Luche H, Chelbi R, Carpentier S, Shawket A, Montanana Sanchis F, et al. Broad and largely concordant molecular changes characterize tolerogenic and immunogenic dendritic cell maturation in thymus and periphery. Immunity. (2016) 45:305–18. doi: 10.1016/j.immuni.2016.07.019
80. Sikorski PJ, Warminski M, Kubacka D, Ratajczak T, Nowis D, Kowalska J, et al. The identity and methylation status of the first transcribed nucleotide in eukaryotic mRNA 5' cap modulates protein expression in living cells. Nucleic Acids Res. (2020) 48:1607–26. doi: 10.1093/nar/gkaa032
81. Wang H, Hu X, Huang M, Liu J, Gu Y, Ma L, et al. Mettl3-mediated mRNA m(6)A methylation promotes dendritic cell activation. Nat Commun. (2019) 10:1898. doi: 10.1038/s41467-019-09903-6
82. Kariko K, Ni H, Capodici J, Lamphier M, Weissman D. mRNA is an endogenous ligand for toll-like receptor 3. J Biol Chem. (2004) 279:12542–50. doi: 10.1074/jbc.M310175200
83. Kim JM, Rasmussen JP, Rudensky AY. Regulatory T cells prevent catastrophic autoimmunity throughout the lifespan of mice. Nat Immunol. (2007) 8:191–7. doi: 10.1038/ni1428
84. Josefowicz SZ, Lu LF, Rudensky AY. Regulatory T cells: mechanisms of differentiation and function. Ann Rev Immunol. (2012) 30:531–64. doi: 10.1146/annurev.immunol.25.022106.141623
85. Tong J, Cao G, Zhang T, Sefik E, Amezcua Vesely MC, Broughton JP, et al. m(6)A mRNA methylation sustains Treg suppressive functions. Cell Res. (2018) 28:253–6. doi: 10.1038/cr.2018.7
86. Sica A, Erreni M, Allavena P, Porta C. Macrophage polarization in pathology. Cell Mol Life Sci. (2015) 72:4111–26. doi: 10.1007/s00018-015-1995-y
87. Li C, Xu MM, Wang K, Adler AJ, Vella AT, Zhou B. Macrophage polarization and meta-inflammation. Transl Res. (2018) 191:29–44. doi: 10.1016/j.trsl.2017.10.004
88. Braga TT, Agudelo JS, Camara NO. Macrophages during the fibrotic process: M2 as friend and foe. Front Immunol. (2015) 6:602. doi: 10.3389/fimmu.2015.00602
89. Shrivastava R, Asif M, Singh V, Dubey P, Ahmad Malik S, Lone MU, et al. M2 polarization of macrophages by Oncostatin M in hypoxic tumor microenvironment is mediated by mTORC2 and promotes tumor growth and metastasis. Cytokine. (2019) 118:130–43. doi: 10.1016/j.cyto.2018.03.032
90. Gu X, Zhang Y, Li D, Cai H, Cai L, Xu Q. N6-methyladenosine demethylase FTO promotes M1 and M2 macrophage activation. Cell Signal. (2020) 69:109553. doi: 10.1016/j.cellsig.2020.109553
91. Liu Y, Liu Z, Tang H, Shen Y, Gong Z, Xie N, et al. The N(6)-methyladenosine (m(6)A)-forming enzyme METTL3 facilitates M1 macrophage polarization through the methylation of STAT1 mRNA. Am J Physiol Cell Physiol. (2019) 317:C762–75. doi: 10.1152/ajpcell.00212.2019
92. Huangfu N, Zheng W, Xu Z, Wang S, Wang Y, Cheng J, et al. RBM4 regulates M1 macrophages polarization through targeting STAT1-mediated glycolysis. Int Immunopharmacol. (2020) 83:106432. doi: 10.1016/j.intimp.2020.106432
93. Guo M, Yan R, Ji Q, Yao H, Sun M, Duan L, et al. IFN regulatory factor-1 induced macrophage pyroptosis by modulating m6A modification of circ_0029589 in patients with acute coronary syndrome. Int Immunopharmacol. (2020) 86:106800. doi: 10.1016/j.intimp.2020.106800
94. Schlee M, Hartmann G. Discriminating self from non-self in nucleic acid sensing. Nat Rev Immunol. (2016) 16:566–80. doi: 10.1038/nri.2016.78
95. Gehrig S, Eberle ME, Botschen F, Rimbach K, Eberle F, Eigenbrod T, et al. Identification of modifications in microbial, native tRNA that suppress immunostimulatory activity. J Exp Med. (2012) 209:225–33. doi: 10.1084/jem.20111044
96. Durbin AF, Wang C, Marcotrigiano J, Gehrke L. RNAs containing modified nucleotides fail to trigger RIG-I conformational changes for innate immune signaling. mBio. (2016) 7. doi: 10.1128/mBio.00833-16
97. Loo YM, Gale M, Jr. Immune signaling by RIG-I-like receptors. Immunity. (2011) 34:680–92. doi: 10.1016/j.immuni.2011.05.003
98. Hull CM, Bevilacqua PC. Discriminating self and non-self by RNA: roles for RNA structure, misfolding, and modification in regulating the innate immune sensor PKR. Acc Chem Res. (2016) 49:1242–9. doi: 10.1021/acs.accounts.6b00151
99. Devarkar SC, Wang C, Miller MT, Ramanathan A, Jiang F, Khan AG, et al. Structural basis for m7G recognition and 2'-O-methyl discrimination in capped RNAs by the innate immune receptor RIG-I. Proc Natl Acad Sci USA. (2016) 113:596–601. doi: 10.1073/pnas.1515152113
100. Chen YG, Kim MV, Chen X, Batista PJ, Aoyama S, Wilusz JE, et al. Sensing self and foreign circular RNAs by intron identity. Mol Cell. (2017) 67:228–38.e5. doi: 10.1016/j.molcel.2017.05.022
101. Gonzalez-Navajas JM, Lee J, David M, Raz E. Immunomodulatory functions of type I interferons. Nat Rev Immunol. (2012) 12:125–35. doi: 10.1038/nri3133
102. Winkler R, Gillis E, Lasman L, Safra M, Geula S, Soyris C, et al. m(6)A modification controls the innate immune response to infection by targeting type I interferons. Nat Immunol. (2019) 20:173–82. doi: 10.1038/s41590-018-0275-z
103. Zheng Q, Hou J, Zhou Y, Li Z, Cao X. The RNA helicase DDX46 inhibits innate immunity by entrapping m(6)A-demethylated antiviral transcripts in the nucleus. Nat Immunol. (2017) 18:1094–103. doi: 10.1038/ni.3830
104. Barbieri I, Tzelepis K, Pandolfini L, Shi J, Millan-Zambrano G, Robson SC, et al. Promoter-bound METTL3 maintains myeloid leukaemia by m(6)A-dependent translation control. Nature. (2017) 552:126–31. doi: 10.1038/nature24678
105. Liu J, Eckert MA, Harada BT, Liu SM, Lu Z, Yu K, et al. m(6)A mRNA methylation regulates AKT activity to promote the proliferation and tumorigenicity of endometrial cancer. Nat Cell Biol. (2018) 20:1074–83. doi: 10.1038/s41556-018-0174-4
106. Galon J, Bruni D. Tumor immunology and tumor evolution: intertwined histories. Immunity. (2020) 52:55–81. doi: 10.1016/j.immuni.2019.12.018
107. Yang S, Wei J, Cui YH, Park G, Shah P, Deng Y, et al. m(6)A mRNA demethylase FTO regulates melanoma tumorigenicity and response to anti-PD-1 blockade. Nat Commun. (2019) 10:2782. doi: 10.1038/s41467-019-10669-0
108. Li N, Kang Y, Wang L, Huff S, Tang R, Hui H, et al. ALKBH5 regulates anti-PD-1 therapy response by modulating lactate and suppressive immune cell accumulation in tumor microenvironment. Proc Natl Acad Sci USA. (2020) 117:20159–70. doi: 10.1073/pnas.1918986117
109. Tsuruta N, Tsuchihashi K, Ohmura H, Yamaguchi K, Ito M, Ariyama H, et al. RNA N6-methyladenosine demethylase FTO regulates PD-L1 expression in colon cancer cells. Biochem Biophys Res Commun. (2020) 530:235–9. doi: 10.1016/j.bbrc.2020.06.153
110. Wang L, Hui H, Agrawal K, Kang Y, Li N, Tang R, et al. m(6) A RNA methyltransferases METTL3/14 regulate immune responses to anti-PD-1 therapy. EMBO J. (2020) 39:e104514. doi: 10.15252/embj.2020104514
111. Li H, Su Q, Li B, Lan L, Wang C, Li W, et al. High expression of WTAP leads to poor prognosis of gastric cancer by influencing tumour-associated T lymphocyte infiltration. J Cell Mol Med. (2020) 24:4452–4465. doi: 10.1111/jcmm.15104
112. Zhang B, Wu Q, Li B, Wang D, Wang L, Zhou YL. m(6)A regulator-mediated methylation modification patterns and tumor microenvironment infiltration characterization in gastric cancer. Mol Cancer. (2020) 19:53. doi: 10.1186/s12943-020-01170-0
113. Mo P, Xie S, Cai W, Ruan J, Du Q, Ye J, et al. N(6)-methyladenosine (m(6)A) RNA methylation signature as a predictor of stomach adenocarcinoma outcomes and its association with immune checkpoint molecules. J Int Med Res. (2020) 48:300060520951405. doi: 10.1177/0300060520951405
114. Xu F, Zhang H, Chen J, Lin L, Chen Y. Immune signature of T follicular helper cells predicts clinical prognostic and therapeutic impact in lung squamous cell carcinoma. Int Immunopharmacol. (2019) 105932. doi: 10.1016/j.intimp.2019.105932
115. Su R, Dong L, Li Y, Gao M, Han L, Wunderlich M, et al. Targeting FTO suppresses cancer stem cell maintenance and immune evasion. Cancer Cell. (2020) 38:79–96.e11. doi: 10.1016/j.ccell.2020.04.017
116. Lu S, Yu Z, Xiao Z, Zhang Y. Gene signatures and prognostic values of m(6)A genes in nasopharyngeal carcinoma. Front Oncol. (2020) 10:875. doi: 10.3389/fonc.2020.00875
117. He X, Tan L, Ni J, Shen G. Expression pattern of m(6)A regulators is significantly correlated with malignancy and antitumor immune response of breast cancer. Cancer Gene Ther. (2020). doi: 10.1038/s41417-020-00208-1
118. Gong PJ, Shao YC, Yang Y, Song WJ, He X, Zeng YF, et al. Analysis of N6-methyladenosine methyltransferase reveals METTL14 and ZC3H13 as tumor suppressor genes in breast cancer. Front Oncol. (2020) 10:578963. doi: 10.3389/fonc.2020.578963
119. Xu F, Zhang Z, Yuan M, Zhao Y, Zhou Y, Pei H, et al. M6A Regulatory genes play an important role in the prognosis, progression and immune microenvironment of pancreatic adenocarcinoma. Cancer Investig. (2021) 39:39–54. doi: 10.1080/07357907.2020.1834576
120. Li Y, Zheng JN, Wang EH, Gong CJ, Lan KF, Ding X. The m6A reader protein YTHDC2 is a potential biomarker and associated with immune infiltration in head and neck squamous cell carcinoma. PeerJ. (2020) 8:e10385. doi: 10.7717/peerj.10385
121. Yarchoan M, Johnson BA, III, Lutz ER, Laheru DA, Jaffee EM. Targeting neoantigens to augment antitumour immunity. Nat Rev Cancer. (2017) 17:209–22. doi: 10.1038/nrc.2016.154
122. Brahmer JR, Tykodi SS, Chow LQ, Hwu WJ, Topalian SL, Hwu P, et al. Safety and activity of anti-PD-L1 antibody in patients with advanced cancer. N Engl J Med. (2012) 366:2455–65. doi: 10.1056/NEJMoa1200694
123. Ganesh K, Stadler ZK, Cercek A, Mendelsohn RB, Shia J, Segal NH, et al. Immunotherapy in colorectal cancer: rationale, challenges and potential. Nat Rev Gastroenterol Hepatol. (2019) 16:361–75. doi: 10.1038/s41575-019-0126-x
124. Casaos J, Gorelick NL, Huq S, Choi J, Xia Y, Serra R, et al. The use of ribavirin as an anticancer therapeutic: will it go viral? Mol Cancer Ther. (2019) 18:1185–94. doi: 10.1158/1535-7163.MCT-18-0666
125. Kentsis A, Topisirovic I, Culjkovic B, Shao L, Borden KL. Ribavirin suppresses eIF4E-mediated oncogenic transformation by physical mimicry of the 7-methyl guanosine mRNA cap. Proc Natl Acad Sci USA. (2004) 101:18105–10. doi: 10.1073/pnas.0406927102
126. Schaefer M, Hagemann S, Hanna K, Lyko F. Azacytidine inhibits RNA methylation at DNMT2 target sites in human cancer cell lines. Cancer Res. (2009) 69:8127–32. doi: 10.1158/0008-5472.CAN-09-0458
Keywords: RNA methylation, M6A, immunoregulation, tumor immunity, immunotherapy
Citation: Zhang M, Song J, Yuan W, Zhang W and Sun Z (2021) Roles of RNA Methylation on Tumor Immunity and Clinical Implications. Front. Immunol. 12:641507. doi: 10.3389/fimmu.2021.641507
Received: 14 December 2020; Accepted: 15 February 2021;
Published: 10 March 2021.
Edited by:
Khashayarsha Khazaie, Mayo Clinic College of Medicine and Science, United StatesReviewed by:
Claude Perreault, Université de Montréal, CanadaJulie Cunningham, Mayo Clinic, United States
Copyright © 2021 Zhang, Song, Yuan, Zhang and Sun. This is an open-access article distributed under the terms of the Creative Commons Attribution License (CC BY). The use, distribution or reproduction in other forums is permitted, provided the original author(s) and the copyright owner(s) are credited and that the original publication in this journal is cited, in accordance with accepted academic practice. No use, distribution or reproduction is permitted which does not comply with these terms.
*Correspondence: Junmin Song, zzusjm@163.com; Zhenqiang Sun, fccsunzq@zzu.edu.cn