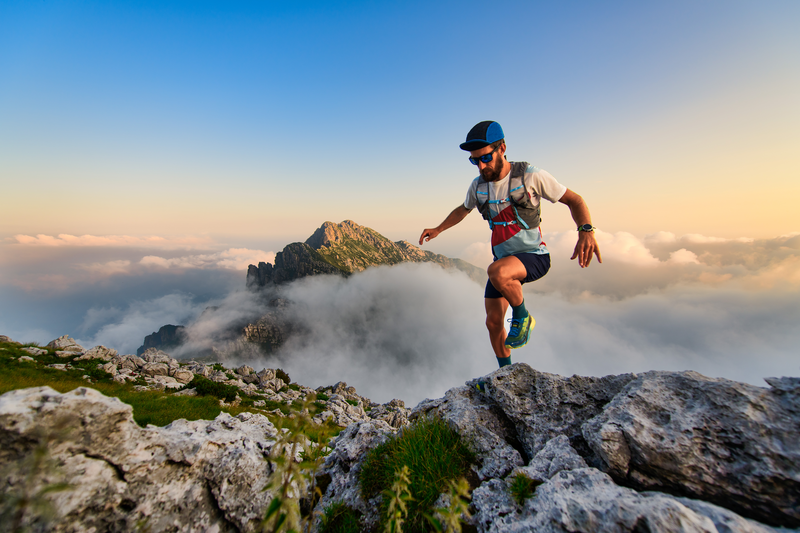
94% of researchers rate our articles as excellent or good
Learn more about the work of our research integrity team to safeguard the quality of each article we publish.
Find out more
REVIEW article
Front. Immunol. , 21 May 2021
Sec. Microbial Immunology
Volume 12 - 2021 | https://doi.org/10.3389/fimmu.2021.639834
This article is part of the Research Topic Recent Advances in Chlamydial Infection Immunology View all 4 articles
Chlamydial infection causes a number of clinically relevant diseases and induces significant morbidity in humans. Immune and inflammatory responses contribute to both the clearance of Chlamydia infection and pathology in host tissues. Chlamydia infection stimulates host cells to produce a large number of cytokines that trigger and regulate host immune responses against Chlamydia. However, inappropriate responses can occur with excessive production of cytokines, resulting in overreactive inflammatory responses and alterations in host or Chlamydia metabolism. As a result, Chlamydia persists and causes wound healing delays, leading to more severe tissue damage and triggering long-lasting fibrotic sequelae. Here, we summarize the roles of cytokines in Chlamydia infection and pathogenesis, thus advancing our understanding chlamydial infection biology and the pathogenic mechanisms involved.
Chlamydia are gram-negative prokaryotic organisms with obligate intracellular parasitism (1). Each species of Chlamydia is capable of infecting the host species—ranging from humans to amoebae—that they are adapted to (2). In animals, infections with Chlamydia can result in inflammatory pathologies at the sites of infection, including ocular, pulmonary, genital, articular, and intestinal tissues. Chlamydia infections and their transmission impose a significant medical and social burden, thus causing economic damage and representing a major public health challenge (3), and there is currently no optimal strategy to control chlamydial infections and stop their spread. Although chlamydial vaccine research dates to seventy years ago, an effective vaccine is not yet available for the limitations in the safety and protective immunity (4). Drug therapy is beneficial for temporary control of infection but unable to treat the irreversible lesions caused by reinfection and persistent asymptomatic infection (5). Therefore, it is crucial to deeply investigate the pathogenic mechanisms of Chlamydia to develop more effective strategies for the treatment and prevention of these diseases.
Chlamydia have a biphasic life cycle, alternating between the infectious elementary body (EB) and the replicative reticulate body (RB). Intracellular infection starts with the entry of EBs into a host cell. Then, the endocytosed EBs differentiates into noninfectious but metabolically active RBs (6), which replicates and converts into EBs again for transmission of the infection to a new host cell (1). Invasion of the host by Chlamydia and the ensuing chlamydial life cycle, involves series of poorly understood mechanisms that compromise and interfere with the function of the host cells, thus damaging host health. Instead, it is critical for the host to mount an immune response, including production of cytokines such as interleukin (IL)-1, IL-6, IL-8, and tumor necrosis factor alpha (TNF-α) that activate or recruit immune cells to trigger or amplify inflammation against Chlamydia (7, 8). These cytokines can be not only used by immune system to inhibit Chlamydia growth and control infection, which is helpful for preventing or slowing down the progression of chlamydial lesions (9, 10), but also used for microbial survival but not for clearance, and result in irreversible lesions and severe tissue damage (Table 1).
In vitro and in vivo studies on Chlamydia infection show that a variety of cytokines, including IL, interferon (IFN), and TNF are involved in the inflammatory response (Figure 1) and immune regulation in Chlamydia-induced diseases. Here, we attempt to summarize the roles of cytokines involved in Chlamydia infection and pathogenesis.
IFN was originally shown to induce an antiviral state in host cells, and was later found to be a cytokine with several effects on the immune system (26, 27). Type I interferon (IFN-α/β) mainly functions as an antiviral factor and immune regulator (26, 28). Type II interferon (IFN-γ) is involved in immunoregulation, and has anti-bacterial, anti-parasitic and anti-tumor functions (26, 27).
The level of IFN is elevated in the cell culture supernatants, serum, and cervical secretions after Chlamydia infection (20, 29, 30). During chlamydial infection, IFN-α/β activates macrophages, enhances the cytotoxic activity of natural killer (NK) cells, and promotes IFN-γ production or Th1 cell differentiation through the activator of transcription (STAT) signal pathway (31). However, the precise role of IFN-α/β in chlamydial infection is not very clear (32). IFN-γ plays an anti-Chlamydia role in the innate immune system and adaptive immune system. The secretion of IFN-γ is not only regulated by IL-12, IL-18, IL-10, and other cytokines after chlamydial infection, but is also enhanced through a positive feedback mechanism (33–35). The importance of IFN-γ in the host during chlamydial infection is evidenced by the elevated chlamydial load in IFN-γ -/-, IFN-γR -/- mice or mice treated with anti-IFN-γ antibody compared with that in the wild/control group (20–22).
IFN-γ inhibits the normal metabolism and replication of Chlamydia by affecting availability of essential nutrients for Chlamydia growth. IFN-γ not only strongly reduces C. trachomatis metabolic growth via cellular tryptophan depletion and glucose starvation (36), but also interferes with the iron metabolism of the host (37). In addition, IFN-γ has immune-defensive functions in the host. Severe combined immunodeficiency (SCID) mice treated with neutralized anti-IFN-γ antibody, or RAG-1-/-/IFN-γR-/- mice exhibit increased susceptibility to C. trachomatis compared with RAG-1-/- mice, suggesting that IFN-γ exerts beneficial effects on host innate immunity for controlling Chlamydia infection (38). Furthermore, the role of IFN-γ against Chlamydia in adaptive immune protection can be demonstrated by transfer of Chlamydia-specific CD4+ or CD8+ T cells, which induce IFN-γ production after infection and provide complementary mechanisms for maintaining protective levels of IFN-γ (39). Chlamydia-specific CD8+ T cells, derived from IFN-γ-deficient mice, failed to provide protection under conditions whereas the wild-type CD8+ T cells did (40, 41). IFN-γ also has a regulatory impact on anti-Chlamydia defense by altering the Th1/Th2 balance, which is modulated by STAT1 phosphorylation and subsequent activation of the Th1/Th2 cell differentiation-specific transcription factor T-bet (42, 43). However, low-level IFN-γ induces the formation of smaller atypical inclusions that contain large RBs and non-replicating aberrant bodies with no newly generated EBs, which are associated with the persistent infection of Chlamydia (32, 44).
IFN-γ not only has an anti-Chlamydia function, but also influences the outcome of Chlamydia infection. Under normal conditions, IFN-γ can accelerate the clearance of Chlamydia, control infection effectively, and alleviate the lesions that form. However, a high concentration of IFN-γ is related to excessive inflammatory reaction and infectious sequelae (45, 46). Thus, the diverse effects of IFN-γ on Chlamydia infection-induced immune response are related to its concentration, the immune microenvironment, and the stage of infection (32, 33). Anti-Chlamydia strategies seek to take advantage of the functions of IFN-γ: for example, cell-specific IFN-γ/IFN-γR gene knockout (KO) mice may be established using the Cre/loxP recombinant system, defining where IFN-γ exerts its anti-infective effects. Furthermore, magnifying the effects of cell-targeting IFN-γ in that particular area can be used to improve the sensitivity of IFN-γ treatment. Genital and intestinal epithelial cells should be the main focus in strategies against C. trachomatis infection.
TNF, an important inflammatory factor mainly produced by activated monocytes/macrophages (47), is divided into the categories of TNF-α and TNF-β (48). TNF-α is chiefly secreted in response to inflammatory stimuli and is well known for its killing effect against intracellular microbes (23). Chlamydia or its pathogenic substances such as pORF5 and lipopolysaccharide (LPS) induce TNF-α production in host serum, bronchial lavage fluid, vaginal secretions, and supernatants of cultured cells cells (49–51), through activating mitogen-activated protein kinases (MAPK) or other signaling pathways (52, 53). Toll-like receptor (TLRs) regulate TNF-α expression. Deficiency of TLR2 or TLR4 in macrophages significantly reduces TNF-α levels during chlamydial infection (53, 54),while deficiency of TLR3 in epithelial cells increases its levels at the early stage of chlamydial infection (55).
Elevated TNF-α levels have certain effects on Chlamydia infection. First, TNF-α works with IFN-γ to inhibits the metabolism of host cells by increasing the activity of indoleamine 2, 3-dioxygenase (IDO), and restricts the growth of Chlamydia (36, 56). Secondly, TNF-α influences the survival of Chlamydia in vivo by inducing apoptosis of host cells that provide suitable conditions for the growth of the organism (57–59). Furthermore, the adoptive transfer of TNF-α reduces the lung bacterial load of newborn mice infected with Chlamydia. It is possible that TNF-α strongly enhances the phagocytic activity of neutrophils and macrophages (60). However, deficiency of TNF-α or application of TNF-α inhibitors has no significant effect on Chlamydia clearance in vivo, suggesting that TNF-α may not be necessary for Chlamydia clearance (23).
It has been documented that TNF-α is related to the formation of Chlamydia-induced lesions. TNF-α not only up-regulates adhesion molecules, assisting the exudation of leukocytes to the site of inflammation, but also facilitates the expression of cytokines such as IL-6 and IL-8, which are connected with tissue fibrosis and scar formation (61, 62). Second, TNF-α accelerates the release of collagenase by stimulating the proliferation of tissue fibroblasts, which leads to histopathological damage (63). Third, deficiency of TNF-αR, the specific receptor of TNF-α that is essential for mediating its biological function; injection of TNF-α antibody; or knockout of the TNF-α gene significantly reduces the severity of mouse fallopian tube lesions due to chlamydial infection, illustrating that TNF-α play an important role in immune injury induced by Chlamydia (24, 25). Therefore, although TNF-α is not necessary for Chlamydia clearance, it can mediate immunopathological damage caused by Chlamydia.
IL, which are produced by lymphocytes, macrophages, and monocytes, and direct certain immune cells to divide and differentiate, consists of several pro- or anti-inflammatory proteins, with pro-inflammatory IL-1β, IL-17A, IL-18 being its best characterized members. The main pro-inflammatory ILs include the IL-1 family, IL-17A, IL-8 (64). While, Major anti-inflammatory cytokines include IL-1 receptor antagonist, IL-4, IL-6, IL-10, IL-11, and IL-13 (65).
The IL-1 family consists of 12 members, including IL-1, IL-18, and IL-33 (66). These ILs are expressed and secreted by different cells, such as mast cells, eosinophils, macrophages, and may play an important role in biological activities such as regulation of immune responses; induction of the inflammatory response, cell proliferation, and differentiation; and promoting the secretion of other cytokines (67). The IL-1 family members are also the central mediators of innate immunity and inflammation (68). These multi-effect cytokines exert a variety of local or systemic responses to viral and bacterial infections and are involved in the pathogenesis of chronic inflammatory diseases (67, 68). This has also been observed in chlamydial infections (69, 70) (Table 2).
The increase in IL-8 and IL-1 levels can be detected in the blood and synovial tissue of patients infected with C. trachomatis (9). Further, alveolar macrophages and peripheral blood mononuclear cells (PBMCs) obtained from chronic obstructive pulmonary disease (COPD) patients with C. pneumoniae infection produce significantly higher amounts of IL-1β and lower amounts of IL-1R-antagonist (10) than cells from COPD patients without C. pneumoniae infection produce. In addition, elevated levels of IL-1, IL-6, and IL-8 can be detected in the female cervix with C. trachomatis infection (81). Based on these phenomena, IL-1 family might have vital functions in the immune response and acute or chronic inflammation following chlamydial infection (71, 82). Current evidence shows that IL-1α can affect the secretion and maturation of IL-8 during chlamydial infection in two ways. On the one hand, IL-1α combined with DNA of host cells enhances the expression of IL-8, mediating host inflammation in the early stage of chlamydial infection. On the other hand, IL-1α can be released from ruptured cells to bind to IL-1R, promoting the secretion of IL-8 in the later stage of infection (11). Through IL-8 activity, IL-1α might play a role in inflammation and tissue lesions induced by Chlamydia (83, 84). IL-1β evolved to assist host defense against chlamydial infection by inducing a wide spectrum of inflammatory cytokines and chemokines, such as IL-6 and IL-8 (71, 74). However, it was found that high expression of IL-1β could drive foam cell formation and accelerate atherosclerosis during C. pneumoniae infection (78). Furthermore, IL-1β is believed to be associated with exacerbation of upper genital tract pathology during infection with C. muridarum (75). IL-33 can activate ST2-mediated signaling pathways, which mainly promotes Th2 cytokine production (85). Because Th2 cytokines can suppress Th1 responses and inhibit IFN-γ production, it is not likely that IL-33 functions in blocking chlamydial infection (86). It is reported that IL-18 is the only member of the IL-1 family that can induce T, B and NK cells to produce IFN-γ, which is responsible to controlling chlamydial infection (80). However, the clearance of Chlamydia did not have significant difference on mouse pneumonitis (MoPn)-infected IL-18 KO mice and wild-type mice (12). Thus, it is speculated that IL-18 may not be necessary for the clearance of chlamydial infection.
The roles of IL-1 members are varied and complex, and their activities and interactions will change as the chlamydial infection progresses. Pathogenic molecules produced by Chlamydia, such as pORF5 and Hsp60, can induce the activation of caspase-1 and stimulate secretion of IL-1β, IL-18, and IL-33 through TLR2/myeloid differentiation primary response 88 (MyD88) or NLRP3/ASC/caspase-1 signaling pathways to promote local inflammatory responses to Chlamydia (74, 87, 88). Additionally, these proteins may also be related to the pathological mechanism of tubal edema and tissue fibrosis caused by Chlamydia (71, 82). It is important to define the role of IL-1β in this context: while this IL protects the host in the defense against chlamydial infection (77, 81), we still believe that it is a promoting disease-related factor in Chlamydia infection. IL-18 and IL-33 participate in immunologic injury induced by chlamydial infection, although the precise mechanisms in removing chlamydial are still unknown (11, 72, 89). In short, the IL-1 family not only includes members that regulate inflammation but also includes those are involved in the immunopathological damage caused by Chlamydia (72, 77, 87). Doubts still persist regarding the function of some IL-1 cytokines such as IL-37 and IL-38, and further studies are needed to identify their precise roles in chlamydiosis.
Like IL-1, IL-6 has a wide variety of activities related to immune cell functions. IL-6 promotes the terminal differentiation of B cells (90) and T cell survival (91) and helps T cells to overcome suppression by Tregs (92); its most noticeable role is in the defense against infection (90, 93). IL-6 is overexpressed in mice with Chlamydia-related tubal factor infertility or humans who suffered from Chlamydia-related disease (94, 95). IL-6 can also be detected in the semen and serum of asymptomatic patients with Chlamydia infection (96, 97). Elevated IL-6 levels were found to play a protective role in controlling Chlamydia infection, given that IL-6 deficient mice were significantly more susceptible to Chlamydia infection through the airway than wild-type mice (98, 99). Patients who received anti-IL-6 treatment were also found to be at increased risk of Chlamydia infection (13, 100). These results suggest that the role of IL-6 should not be ignored in the inhibition of and therapy for Chlamydia infection. There are a few potential explanations for the resistance of IL-6 to Chlamydia. IL-8, IL-1β, and other cytokines act as initiators of IL-6 production (101). The MAPK/extracellular regulated protein kinases (ERK) pathway or the Janus kinase (JAK)/signal transducer and STAT pathway mediate the expression and secretion of IL-6 at certain mucosal surfaces or in cell culture supernatants (94, 102). From the perspective of the innate immune response, IL-6 induces the recruitment of white blood cells and promotes apoptosis of neutrophils, mediating inflammation to control infection (93, 103). From the perspective of the adaptive immune response, high expression of IL-6 activates the Th1-like response to clear pathogens by regulating the production of IFN-γ and thus decrease susceptibility to Chlamydia infection (94). In addition, TNF-α, which is relevant to the degree of Chlamydia-induced fallopian tube obstruction and maintenance of the continuous state of disease, is inhibited by IL-6 in a dose-dependent manner (62, 104). Although IL-6 plays critical roles in controlling Chlamydia infections, the effects may vary. Some of the most interesting discoveries are relevant to the varying role of IL-6 in Chlamydia infection at different inoculating doses. IL-6 is required for controlling chlamydial infection by limiting replication and colonization by the pathogen, at either high dose or low dose of Chlamydia muridarum (C. muridarum) (94). In contrast, IL-6 is not essential for induced hydrosalpinx at a high dose of C. muridarum, but is required for exacerbating infection-induced hydrosalpinx with low dose of C. muridarum (94). The reason might be that IL-6 increases inflammatory infiltration in certain tissues and the specific CD4+ and CD8+ T cells that produce TNF-α under low-dose chlamydial inoculation (101, 104). These findings also show that host inflammatory responses to IL-6 do not match the extent of the infection.
Besides its role in host defense, IL-6 also mediates the inflammatory pathology. Regardless of infectious dose, the phenomenon correlating with IL-6-induced pathology has been reported mainly in C. pneumonia infections and C. muridarum mice models (94, 102). Patients with C. pneumonia-related COPD were shown to have high levels of IL-6, which exacerbates the disease state and has been proposed to be useful in evaluating the severity of COPD (62, 105). Anti-IL-6 therapy has been used to treat arthritis patients with C. trachomatis and C. pneumonia infection (14, 100). The possible reason why use anti-IL-6 treatment might be IL-6 participation in the mechanism of chronic inflammatory pathology or the resulting fibrosis (102, 106, 107).
The role of IL-6 in chlamydial infection and the pathological effects thus induced has been controversial. IL-6 mediates inflammation, in terms of both pro-inflammatory and anti-inflammatory functions, in bacterial infection, which is consistent with previous reports (81, 108). Furthermore, while IL-6 promotes host defense against chlamydial infection by balancing inflammatory and immune responses, this IL also exacerbates chlamydial pathogenicity through its involvement in inflammatory pathology. However, the mechanism involved has not yet been fully elucidated and further research is needed (94, 109, 110).
IL-8, a pro-inflammatory chemokine, participates in host defense by recruiting and regulating the activity of immune cells such as leukocytes, basophils, and T lymphocytes (111). IL-8 was found to be increased in the serum of patients suffering from Chlamydia-induced pneumonia (112), and has been found to be elevated in the culture supernatant of cervical and colon epithelial cells infected with Chlamydia (113). Thus, IL-8 might be related to acute or chronic inflammation after chlamydial infection. Further studies have provided a comprehensive understanding of the function and mechanism of IL-8 in chlamydial infection. Firstly, Chlamydia and its pathogenic substances can induce IL-8 secretion by activating the nuclear factor-κB (NF-κB) and MAPK/ERK signaling pathways (114, 115), by activating the IL-10-mediated JAK/STAT signaling pathway (116), or through an IL-1α-mediated IL-1RI-independent mechanism (11). Second, the local hypoxic environment, formed in the process of C. pneumoniae infection, also facilitates the secretion of IL-8 in another way (15). These processes are particularly important for continuing the induction of IL-8 at later phases of infection (84). Furthermore, IL-8 is also necessary for chlamydial replication and its synthesis of its components. The trend in up-regulation of IL-8 is consistent with the unique development cycle of Chlamydia (113). It is possible that IL-8 promotes the lipid metabolism of host cells, thus enabling the provision of nutrients for chlamydial growth and development (117). C. pneumoniae relies on the activation of the IL-8-mediated phosphatidylinositol 3-kinase (PI3K)/protein kinase B (Akt) signaling pathway to stabilize myeloid cell leukemia-1 (MCL-1) and inhibit the spontaneous apoptosis of neutrophils, which act as a transport vehicle and are beneficial for C. pneumoniae to establish a productive infection during the initial phase of infection (118). In addition, the IL-8 produced during chlamydial infection in turn reduces the sensitivity of Chlamydia to azithromycin and other therapeutic drugs (15). Thus, chlamydial infection and IL-8 form a positive feedback cycle. However, although it is inefficient in resolving chlamydial infection, IL-8 plays a certain role in preventing chlamydial invasion (119). Further research is needed to elucidate the contradictory roles of IL-8 in chlamydial infection. Studies under different infectious conditions may prove valuable.
IL-17, a hallmark cytokine of Th17 cells, performs a pro-inflammatory function and exerts a host-defensive role in many infectious diseases (120, 121). IL-17 is present in inflammatory tissues or the internal environment of almost all patients infected with Chlamydia (122, 123). Furthermore, the replicative ability of Chlamydia is enhanced in IL-17 KO mice or mice treated with IL-17 inhibitors (17, 124). Thus, IL-17 plays an anti-infective role against Chlamydia (125–127). This function is achieved not only by up-regulating inducible nitric oxide synthase (iNOS) production and the cooperative interaction between nitric oxide (NO) and IFN-γ (128), but also via the induction of type 1 T cell immunity by DCs (129). Furthermore, the IL-17 response in the early stage was found to be central to amplifying inflammation and initiating host defense against Chlamydia through synergy with other cytokines such as IL-6 and macrophage inflammatory protein-2 (MIP-2) (98).
Although IL-17 elicits protection against chlamydial infections via its pro-inflammatory function, it can promote inflammatory pathology and participate in the pathogenesis of chlamydial diseases. IL-17 increases local neutrophil infiltration by regulating the expression of chemokines and adhesion molecules in early stage of Chlamydia infection (130, 131). It can also drive the secretion of a series of cytokines that cause excessive tissue damage and fibrosis repair (132). The C-Fos/IL-17C signal pathway mediates vascular smooth muscle cell (VSMC) migration and accelerates atherosclerosis resulted from C. pneumonia infection (133). Taken together, IL-17 exerts anti-infective effects but is inadequate to clear Chlamydia infection. More importantly, it unarguably contributes to the inflammatory pathology of Chlamydia infection (134).
IL-4 is crucial for the function of T and B lymphocytes. It can elicit many responses, in particular the humoral immune response that is associated with antibody production (135, 136). IL-4 can be detected in the culture supernatant of PBMCs isolated from patients with Chlamydia infection (137, 138). Therefore, IL-4 is considered to play a role in Chlamydia infections. It enhances the antigen-presenting ability of B cells by boosting the expression of MHC II, FcϵRII/CD23, and CD40 molecules, thus magnifying immune responses beneficial for eliminating Chlamydia (139). This IL-4-mediated enhancement of immune responses also triggers delayed type hypersensitivity (DTH) during Chlamydia infection, which might be associated with asthma due to Chlamydia (140). Furthermore, IL-4 reduces the secretion of inflammatory cytokines from mononuclear macrophages, inhibiting local tissue damage resulting from excessive Th1 immune responses (141, 142). This explains the previous finding that IL-4 can effectively prevent endometrial injury caused by C. trachomatis (18). Based on these data, strongly expressed IL-4 could inhibit Chlamydia infection to a certain extent, and also effectively prevent tissue damage. However, the role of IL-4 in Chlamydia infection has not been adequately investigated and further elucidation is necessary.
IL-13 has an amino acid homology of 20%-25% with IL-4, thus sharing some, but not all functional properties (143). IL-13 can enhance the host’s resistance to intracellular parasites by enhancing Th2-type cell response (144). However, this appears to differ for Chlamydia. The elevated IL-13 levels observed in vaginal secretions and serum after Chlamydia infection have been frequently linked to the function of promoting infection and aggravating lesions (145). In vivo and in vitro experiments indicate, to a certain extent, that IL-13 may enhance Chlamydia replication in cell or animal models of infection (16). Together with TNF-α, IL-13 induces certain cells to produce cytokines related to scar formation, such as transforming growth factor-β (TGF-β) (146). In comparison with wild-type mice, C. muridarum-infected IL-13 KO mice have faster pathogen clearance, and milder tissue lesions (145). Therefore, it is hypothesized that IL-13 mediates Chlamydia-related immunopathology and reflects disease severity after Chlamydia infection (16). IL-13 thus has the potential to develop as an evaluation index of Chlamydia-induced disease severity. Interestingly, in a mouse model of C. trachomatis genital tract infection, two studies have found that a subset of CD4 or CD8 cell populations can produce both IFN-γ and IL-13 (termed CD4γ13 or CD8γ13 T cells, respectively); adoptive transfer of either Chlamydia-specific CD4γ13 or CD8γ13 T cells protects oviducts from immunopathology, suggesting a protective role of IL-13 during Chlamydia infection (146, 147). The reason for the contradictory result of IL-13 remains unclear. Perhaps IL-13 secreted by innate immune cells in the early stage of infection is mainly associated with pathogenicity, while in the late stage of infection, IL-13 produced by specific T cells, especially those have immune memory, exert protective effect in anti-infection immunity. IL-13 from specific CD4γ13 or CD8γ13 T cells probably is a very small proportion of the total IL-13 produced, so protective IL-13 doesn’t work well in the context of pathogenic IL-13. Knockout of IL-13 gene, but not adoptive transfer of CD4γ13 or CD8γ13 T cells, may affect important immune cells in anti-infection immunity. Anyway, targeted blocking of IL-13 action in Chlamydia infection through clarification of cell specific IL-13 mechanisms is a critical goal to aim for in related research.
IL-10 is synthesized by a wide range of cell types such as macrophages, monocytes, Th2, and Treg cells (148). As a potent anti‐inflammatory cytokine, IL-10 not only limits and terminates inflammatory responses, but also plays a crucial role in the control of diseases caused by infectious pathogens; its levels are inversely correlated with disease incidence and severity (149).
The functions of IL-10 in chlamydial infection are however more complex. Chlamydial factors such as heat shock proteins 60 (Hsp60) and LPS interact with TLRs to induce high expression of IL-10 from host cells (83, 150). IL-10 can be also secreted from PBMCs infected with C. trachomatis, which is dependent on the ERK and p38 signaling pathways (116). It has also been found that the inhibition of TLR2, MyD88, and NF-κB in C. psittaci-infected HD11 macrophages significantly reduces IL-10 cytokine production (151). Thus, elevated IL-10 levels can be detected not only in serum, scar tissue homogenates, bronchoalveolar lavage fluid, and cervical secretions from Chlamydia-infected hosts (152–154), but also in the supernatant of Chlamydia-infected HeLa cells, DCs, and PBMCs (137, 155, 156).
In addition to findings about changes in expression and the possible roles of IL-10 in chlamydial infection, some deeper insights into the roles of IL-10 were gained. Yang and his co-workers revealed that C57BL/6 mice with lower levels of IL-10 had faster clearance of the organism from the lungs after infection with MoPn than BALB/c mice (35). Furthermore, IL-10 KO mice also presented faster clearance of MoPn in lung and genital tract than wild-type mice (157, 158). It’s possible that IL-10 inhibits pro-inflammatory cytokine production for chlamydial clearance by regulating the activation of certain signaling pathways (150, 159). Further, IL-10 down-regulates the expression of MHC I molecules, impairing the presentation of MHC-bacterial epitopes and eliminating activity of CD8+ T cells against infected cells, thus reducing the clearance of Chlamydia (19). In addition, IL-10 enhances the survival of Chlamydia by inhibiting apoptosis of DCs or controlling its antigen presentation function and weakening the immune response (160, 161). Therefore, IL-10 can suppress inflammation-related immune responses against Chlamydia species.
Inflammatory processes are responsible for complications induced by Chlamydia infections, and IL-10 is hypothesized to be involved in the processes. IL-10 levels in cervical secretions were higher in C. trachomatis-infected infertile women than in fertile women (162). The reason could be that IL-10, as an anti-inflammatory factor, reduces local inflammatory infiltration and attenuates the pathological damage such as mice tubal edema due to C. trachomatis infection (71). It also decreases the activity of CD8+ T cells against infected cells and thereby reduces tissue damage (19). IL-10 is however also responsible for the severe damage, such as tubal infertility and ectopic pregnancy, induced by Chlamydia infection (71, 140, 162). These findings are in contrast to the observed mild pathologies induced by IL-10 in Chlamydia infection, which may be due to the differences in pathogenesis of Chlamydia species in infectious models.
IL-10 is an important factor for balancing the immune system after Chlamydia infection, and the modulation of its expression in Chlamydia-infected hosts is cautiously performed. Although it can eliminate or promote infection with differing intensity and duration at different infectious phases, the presence of IL-10 decreases Chlamydia eradication via anti-inflammatory action, and is helpful for controlling and minimizing Chlamydia-induced diseases and complications. Moreover, genetic variations in IL-10 gene may be associated with its different expression and the polymorphisms within IL-10 gene may explain interindividual variation in host immune responses to Chlamydia infection (150). However, researchers have different opinions on the association between IL-10 polymorphisms and the outcome of Chlamydia infections (163). Future investigations regarding the role of IL-10 polymorphisms in Chlamydia infections are required.
Members of the IL family play important roles in Chlamydia infection, either promoting infection and accelerating the disease, or suppressing Chlamydia and alleviating tissue injures. One of our main tasks now is to make the best use of information on ILs for the prevention and treatment of Chlamydia infection. A potential method is to identify the function of a certain IL in Chlamydia infection by using KO mice, siRNA/chemical inhibition or antibody blockade: this work has already been done for some ILs; second, to generate cells/tissue-specific IL gene-KO mice, and determine the main environmental conditions in which the IL functions optimally. Based on the former two steps, aiming at specific cells/tissues, the relevant IL or antibody/inhibitor of the harmful IL may be used to control Chlamydia infection. It might be possible to extend this technique to other diseases in the future.
In addition to the above cytokines, other cytokines such as IL-12, IL-5, and GM-CSF have also been reported in studies on chlamydial infection, although sufficient data are not available. IL-12 coordinates its functions with IFN-γ in Chlamydia infection to collectively prevent neonatal pneumonia caused by C. pneumonia (164). If either IL-12 or IFN-γ is deficient, the other correspondingly decreases and Chlamydia clearance is affected (12, 164). A positive feedback loop exists between the effects of IL-12 and IFN-γ, which strengthens the host’s ability to resist infection. However, excessive IL-12 has a negative impact on the development of CD8+ T memory cells, which is not conducive to the host’s resistance to Chlamydia reinfection (33). In addition, high levels of IL-5 are closely related to C. pneumonia-caused asthma (165). It has also been reported that Th2 type DTH-related immunity and pathology to Chlamydia infection is associated with high levels of IL-5 and IL-4 (140). Elevated GM-CSF expression is also observed during Chlamydia infection, although the mechanism is not quite clear (166).
Here, we summarized the effects and interactions of important cytokines involved in Chlamydia infection (Figure 2), and offered some valuable insights into the potential mechanisms and proposed countermeasures. A range of factors contribute to cytokine production during Chlamydia infection (Supplementary Table 1). First, Chlamydia species (with different components), the number of Chlamydia (or dose of their products) and the route of infection are implicated in cytokine production (167). A certain chlamydial strain and the corresponding products exert effects on their cytokine production, which related to the varied toxicity in host (168). For example, plasmid might partially explain the different pathological features and cytokine production in plasmid-deficient and plasmid-competent Chlamydia (169). In C. trachomatis D/UW-3/CX strain, MOMP, CPAF and HSP60 are all important cytokine inducers, while CPAF and MOMP are more potent in triggering IL-1β, as compared to HSP60 (109). Second, major factors influencing cytokine variation in hosts include host genetics, non-heritable factors and the microbiome (167). Host genetic variation accounts for a significant part of variability in cytokine production by different strains of mice. For example, 27 oviduct cytokines were significantly higher in highly susceptible (hydrosalpinx) CBA/J than those of resistant A/J mice (170). 16 oviduct cytokines were significantly higher in C5-competent than those of C5-deficient mice (171). Some cytokine coding genes are known to be highly polymorphic, which are also regarded as a possible cause for difference in the cytokine production and contribute to various pathological conditions (172). In addition, non-heritable factors, including age, body weight and gender, and gut microbiome may also engage in variation in cytokine production during Chlamydia infection (167). The variability of cytokine production makes their biological function to be systemic or tissue-specific, complementary (several cytokines work together) or pleiotropic (a cytokine with different functions). Further identifying the causes and consequences of variation in cytokine production is a crucial step to better understand the pathogenesis of Chlamydia infection.
Figure 2 The regulatory network and function of different cytokines upon Chlamydia infection. The host cells produce a variety of cytokines after chlamydial infection. Cytokines induce cell immunity response and produce more cytokines. As descripted in text, the impact of all the cytokines on the host chlamydial infection can be divided into two aspects: favorable and unfavorable.
In chlamydial infection, the behavior of some cytokines is a double-edged sword in inflammation and immune-mediated pathogenesis (Figures 1, 2; Table 2). Although the role of cytokines at different stages of Chlamydia infection has been extensively studied, still some important questions warrant further exploration. For instance, how do different cytokines coordinate their roles in chlamydial pathogenesis? Why do cytokines from different cells play different roles in chlamydial infection (32, 173)? The intestinal tract, which is colonized by more virulent Chlamydia strains and a higher chlamydial burden, might also take part in the pathogenesis and development of chlamydial diseases. Whether cytokines from innate lymphoid cells (ILCs), especially ILC3 play a role in Chlamydia caused diseases. How does gut microbiota affect the host cytokine network during chlamydial infection? How to dynamic remodeling cytokine network for clearing Chlamydia infection or promoting body recovery? With the solution of these important questions, we believe that manipulation of key cytokines in chlamydial infection will represent a novel strategy to treat chlamydial diseases.
WX conceived of the presented idea and drafted the manuscript. ZZ, NY, XL and ST gave some idea to draft the manuscript. ZZ, AL, and LH critically revised the manuscript. All authors contributed to the article and approved the submitted version.
This work was supported by the National Natural Science Foundation of China (No. 31570179), the Hunan Natural Science Foundation (No. 2020JJ4084), the Hunan Provincial Key Laboratory for Prevention and Control of Special Pathogens (No. 2014-5), the Hunan Province Cooperative Innovation Center for Molecular Target New Drug Study (2015–351), and the Hunan Provincial Key Discipline Project (No. 2011-76).
LH was employed by Huang Nanyue Biopharmaceutical Co. Ltd.
The remaining authors declare that the research was conducted in the absence of any commercial or financial relationships that could be construed as a potential conflict of interest.
The Supplementary Material for this article can be found online at: https://www.frontiersin.org/articles/10.3389/fimmu.2021.639834/full#supplementary-material
1. Elwell C, Mirrashidi K, Engel J. Chlamydia Cell Biology and Pathogenesis. Nat Rev Microbiol (2016) 14(6):385–400. doi: 10.1038/nrmicro.2016.30
2. Bachmann NL, Polkinghorne A, Timms P. Chlamydia Genomics: Providing Novel Insights Into Chlamydial Biology. Trends Microbiol (2014) 22(8):464–72. doi: 10.1016/j.tim.2014.04.013
3. Pultorak E, Wong W, Rabins C, Mehta SD. Economic Burden of Sexually Transmitted Infections: Incidence and Direct Medical Cost of Chlamydia, Gonorrhea, and Syphilis Among Illinois Adolescents and Young Adults, 2005-2006. Sex Transm Dis (2009) 36(10):629–36. doi: 10.1097/OLQ.0b013e3181a96d23
4. Phillips S, Quigley BL, Timms P. Seventy Years of Chlamydia Vaccine Research - Limitations of the Past and Directions for the Future. Front Microbiol (2019) 10:70. doi: 10.3389/fmicb.2019.00070
5. Stagg AJ. Vaccines Against Chlamydia: Approaches and Progress. Mol Med Today (1998) 4(4):166–73. doi: 10.1016/s1357-4310(98)01232-5
6. Moulder JW. Interaction of Chlamydiae and Host Cells In Vitro. Microbiol Rev (1991) 55(1):143–90. doi: 10.1128/MR.55.1.143-190.1991
7. Faris R, Andersen SE, McCullough A, Gourronc F, Klingelhutz AJ, Weber MM. Chlamydia Trachomatis Serovars Drive Differential Production of Proinflammatory Cytokines and Chemokines Depending on the Type of Cell Infected. Front Cell Infect Microbiol (2019) 9:399. doi: 10.3389/fcimb.2019.00399
8. Mpiga P, Mansour S, Morisset R, Beaulieu R, Ravaoarinoro M. Sustained Interleukin-6 and Interleukin-8 Expression Following Infection With Chlamydia Trachomatis Serovar L2 in a Hela/THP-1 Cell Co-Culture Model. Scand J Immunol (2006) 63(3):199–207. doi: 10.1111/j.1365-3083.2006.01734.x
9. Jendro MC, Raum E, Schnarr S, Kohler L, Zeidler H, Kuipers JG, et al. Cytokine Profile in Serum and Synovial Fluid of Arthritis Patients With Chlamydia Trachomatis Infection. Rheumatol Int (2005) 25(1):37–41. doi: 10.1007/s00296-003-0393-1
10. Rupp J, Kothe H, Mueller A, Maass M, Dalhoff K. Imbalanced Secretion of IL-1beta and IL-1RA in Chlamydia Pneumoniae-Infected Mononuclear Cells From COPD Patients. Eur Respir J (2003) 22(2):274–9. doi: 10.1183/09031936.03.00007303
11. Cheng W, Shivshankar P, Zhong Y, Chen D, Li Z, Zhong G. Intracellular Interleukin-1alpha Mediates Interleukin-8 Production Induced by Chlamydia Trachomatis Infection Via a Mechanism Independent of Type I Interleukin-1 Receptor. Infect Immun (2008) 76(3):942–51. doi: 10.1128/IAI.01313-07
12. Lu H, Yang X, Takeda K, Zhang D, Fan Y, Luo M, et al. Chlamydia Trachomatis Mouse Pneumonitis Lung Infection in IL-18 and IL-12 Knockout Mice: IL-12 is Dominant Over IL-18 for Protective Immunity. Mol Med (2000) 6(7):604–12. doi: 10.1007/BF03401798
13. Edwards CJ. IL-6 Inhibition and Infection: Treating Patients With Tocilizumab is There a Greater Risk of Infections? Rheumatology (2012) 51(5):769–70. doi: 10.1093/rheumatology/ker311
14. Edwards CJ. IL-6 Inhibition and Infection: Treating Patients With Tocilizumab. Rheumatol (Oxford) (2012) 51(5):769–70. doi: 10.1093/rheumatology/ker311
15. Matsuo J, Sakai K, Okubo T, Yamaguchi H. Chlamydia Pneumoniae Enhances Interleukin 8 (Il-8) Production With Reduced Azithromycin Sensitivity Under Hypoxia. APMIS (2019) 127(3):131–8. doi: 10.1111/apm.12924
16. Zhong G. Chlamydia Spreading From the Genital Tract to the Gastrointestinal Tract - a Two-Hit Hypothesis. Trends Microbiol (2018) 26(7):611–23. doi: 10.1016/j.tim.2017.12.002
17. Andrew DW, Cochrane M, Schripsema JH, Ramsey KH, Dando SJ, O’Meara CP, et al. The Duration of Chlamydia Muridarum Genital Tract Infection and Associated Chronic Pathological Changes are Reduced in IL-17 Knockout Mice But Protection is Not Increased Further by Immunization. PloS One (2013) 8(9):e76664. doi: 10.1371/journal.pone.0076664
18. Vicetti Miguel RD, Quispe Calla NE, Dixon D, Foster RA, Gambotto A, Pavelko SD, et al. IL-4-Secreting Eosinophils Promote Endometrial Stromal Cell Proliferation and Prevent Chlamydia-Induced Upper Genital Tract Damage. Proc Natl Acad Sci USA (2017) 114(33):E6892–901. doi: 10.1073/pnas.1621253114
19. Caspar-Bauguil S, Puissant B, Nazzal D, Lefevre JC, Thomsen M, Salvayre R, et al. Chlamydia Pneumoniae Induces Interleukin-10 Production That Down-Regulates Major Histocompatibility Complex Class I Expression. J Infect Dis (2000) 182(5):1394–401. doi: 10.1086/315856
20. Williams DM, Byrne GI, Grubbs B, Marshal TJ, Schachter J. Role in Vivo for Gamma Interferon in Control of Pneumonia Caused by Chlamydia Trachomatis in Mice. Infect Immun (1988) 56(11):3004–6. doi: 10.1128/IAI.56.11.3004-3006.1988
21. Cotter TW, Ramsey KH, Miranpuri GS, Poulsen CE, Byrne GI. Dissemination of Chlamydia Trachomatis Chronic Genital Tract Infection in Gamma Interferon Gene Knockout Mice. Infect Immun (1997) 65(6):2145–52. doi: 10.1128/IAI.65.6.2145-2152.1997
22. Ito JI, Lyons JM. Role of Gamma Interferon in Controlling Murine Chlamydial Genital Tract Infection. Infect Immun (1999) 67(10):5518–21. doi: 10.1128/IAI.67.10.5518-5521.1999
23. Kamalakaran S, Chaganty BK, Gupta R, Guentzel MN, Chambers JP, Murthy AK, et al. Vaginal Chlamydial Clearance Following Primary or Secondary Infection in Mice Occurs Independently of TNF-Alpha. Front Cell Infect Microbiol (2013) 3:11. doi: 10.3389/fcimb.2013.00011
24. Manam S, Thomas JD, Li W, Maladore A, Schripsema JH, Ramsey KH, et al. Tumor Necrosis Factor (TNF) Receptor Superfamily Member 1b on CD8+ T Cells and TNF Receptor Superfamily Member 1a on Non-CD8+ T Cells Contribute Significantly to Upper Genital Tract Pathology Following Chlamydial Infection. J Infect Dis (2015) 211(12):2014–22. doi: 10.1093/infdis/jiu839
25. Zafiratos MT, Cottrell JT, Manam S, Henderson KK, Ramsey KH, Murthy AK. Tumor Necrosis Factor Receptor Superfamily Members 1a and 1b Contribute to Exacerbation of Atherosclerosis by Chlamydia Pneumoniae in Mice. Microbes Infect (2019) 21(2):104–8. doi: 10.1016/j.micinf.2018.09.003
26. Schroder K, Hertzog PJ, Ravasi T, Hume DA. Interferon-Gamma: An Overview of Signals, Mechanisms and Functions. J Leukoc Biol (2004) 75(2):163–89. doi: 10.1189/jlb.0603252
27. Kak G, Raza M, Tiwari BK. Interferon-Gamma (IFN-Gamma): Exploring Its Implications in Infectious Diseases. Biomol Concepts (2018) 9(1):64–79. doi: 10.1515/bmc-2018-0007
28. Baird NL, Bowlin JL, Hotz TJ, Cohrs RJ, Gilden D. Interferon Gamma Prolongs Survival of Varicella-Zoster Virus-Infected Human Neurons In Vitro. J Virol (2015) 89(14):7425–7. doi: 10.1128/Jvi.00594-15
29. Cohen CR, Nguti R, Bukusi EA, Lu H, Shen C, Luo M, et al. Human Immunodeficiency Virus Type 1-Infected Women Exhibit Reduced Interferon-Gamma Secretion After Chlamydia Trachomatis Stimulation of Peripheral Blood Lymphocytes. J Infect Dis (2000) 182(6):1672–7. doi: 10.1086/317616
30. Morrison RP. Differential Sensitivities of Chlamydia Trachomatis Strains to Inhibitory Effects of Gamma Interferon. Infect Immun (2000) 68(10):6038–40. doi: 10.1128/iai.68.10.6038-6040.2000
31. Burian K, Endresz V, Deak J, Kormanyos Z, Pal A, Nelson D, et al. Transcriptome Analysis Indicates an Enhanced Activation of Adaptive and Innate Immunity by Chlamydia-Infected Murine Epithelial Cells Treated With Interferon Gamma. J Infect Dis (2010) 202(9):1405–14. doi: 10.1086/656526
32. Rottenberg ME, Gigliotti-Rothfuchs A, Wigzell H. The Role of IFN-Gamma in the Outcome of Chlamydial Infection. Curr Opin Immunol (2002) 14(4):444–51. doi: 10.1016/s0952-7915(02)00361-8
33. Zhang X, Starnbach MN. An Excess of the Proinflammatory Cytokines IFN-Gamma and IL-12 Impairs the Development of the Memory CD8+ T Cell Response to Chlamydia Trachomatis. J Immunol (2015) 195(4):1665–75. doi: 10.4049/jimmunol.1500457
34. Netea MG, Kullberg BJ, Jacobs LE, Verver-Jansen TJ, van der Ven-Jongekrijg J, Galama JM, et al. Chlamydia Pneumoniae Stimulates IFN-Gamma Synthesis Through Myd88-Dependent, TLR2- and TLR4-Independent Induction of IL-18 Release. J Immunol (2004) 173(2):1477–82. doi: 10.4049/jimmunol.173.2.1477
35. Yang X, HayGlass KT, Brunham RC. Genetically Determined Differences in IL-10 and IFN-Gamma Responses Correlate With Clearance of Chlamydia Trachomatis Mouse Pneumonitis Infection. J Immunol (1996) 156(11):4338–44.
36. Brunham RC, Rey-Ladino J. Immunology of Chlamydia Infection: Implications for a Chlamydia Trachomatis Vaccine. Nat Rev Immunol (2005) 5(2):149–61. doi: 10.1038/nri1551
37. Jerchel S, Kaufhold I, Schuchardt L, Shima K, Rupp J. Host Immune Responses After Hypoxic Reactivation of IFN-Gamma Induced Persistent Chlamydia Trachomatis Infection. Front Cell Infect Microbiol (2014) 4:43. doi: 10.3389/fcimb.2014.00043
38. Wang S, Fan Y, Brunham RC, Yang X. IFN-Gamma Knockout Mice Show Th2-Associated Delayed-Type Hypersensitivity and the Inflammatory Cells Fail to Localize and Control Chlamydial Infection. Eur J Immunol (1999) 29(11):3782–92. doi: 10.1002/(SICI)1521-4141(199911)29:11<3782::AID-IMMU3782>3.0.CO;2-B
39. Loomis WP, Starnbach MN. T Cell Responses to Chlamydia Trachomatis. Curr Opin Microbiol (2002) 5(1):87–91. doi: 10.1016/s1369-5274(02)00291-6
40. Zhang DJ, Yang X, Shen C, Brunham RC. Characterization of Immune Responses Following Intramuscular DNA Immunization With the MOMP Gene of Chlamydia Trachomatis Mouse Pneumonitis Strain. Immunology (1999) 96(2):314–21. doi: 10.1046/j.1365-2567.1999.00682.x
41. Lampe MF, Wilson CB, Bevan MJ, Starnbach MN. Gamma Interferon Production by Cytotoxic T Lymphocytes is Required for Resolution of Chlamydia Trachomatis Infection. Infect Immun (1998) 66(11):5457–61. doi: 10.1128/IAI.66.11.5457-5461.1998
42. Gondek DC, Roan NR, Starnbach MN. T Cell Responses in the Absence of IFN-Gamma Exacerbate Uterine Infection With Chlamydia Trachomatis. J Immunol (2009) 183(2):1313–9. doi: 10.4049/jimmunol.0900295
43. Bakshi RK, Gupta K, Jordan SJ, Chi X, Lensing SY, Press CG, et al. An Adaptive Chlamydia Trachomatis-Specific IFN-Gamma-Producing CD4(+) T Cell Response is Associated With Protection Against Chlamydia Reinfection in Women. Front Immunol (2018) 9:1981. doi: 10.3389/fimmu.2018.01981
44. Mannonen L, Kamping E, Penttila T, Puolakkainen M. IFN-Gamma Induced Persistent Chlamydia Pneumoniae Infection in HL and Mono Mac 6 Cells: Characterization by Real-Time Quantitative PCR and Culture. Microb Pathog (2004) 36(1):41–50. doi: 10.1016/j.micpath.2003.09.001
45. Ishihara T, Aga M, Hino K, Ushio C, Taniguchi M, Iwaki K, et al. Inhibition of Chlamydia Trachomatis Growth by Human Interferon-Alpha: Mechanisms and Synergistic Effect With Interferon-Gamma and Tumor Necrosis Factor-Alpha. BioMed Res (2005) 26(4):179–85. doi: 10.2220/biomedres.26.179
46. Mascellino MT, Boccia P, Oliva A. Immunopathogenesis in Chlamydia Trachomatis Infected Women. ISRN Obstet Gynecol (2011) 2011:436936. doi: 10.5402/2011/436936
47. Idriss HT, Naismith JH. TNF Alpha and the TNF Receptor Superfamily: Structure-Function Relationship(S). Microsc Res Tech (2000) 50(3):184–95. doi: 10.1002/1097-0029(20000801)50:3<184::AID-JEMT2>3.0.CO;2-H
48. Horiuchi T, Mitoma H, Harashima S, Tsukamoto H, Shimoda T. Transmembrane TNF-Alpha: Structure, Function and Interaction With Anti-TNF Agents. Rheumatol (Oxford) (2010) 49(7):1215–28. doi: 10.1093/rheumatology/keq031
49. Kaukoranta-Tolvanen SS, Teppo AM, Laitinen K, Saikku P, Linnavuori K, Leinonen M. Growth of Chlamydia Pneumoniae in Cultured Human Peripheral Blood Mononuclear Cells and Induction of a Cytokine Response. Microb Pathog (1996) 21(3):215–21. doi: 10.1006/mpat.1996.0056
50. Abu el-Asrar AM, Geboes K, Tabbara KF, Missotten L, Desmet V. Immunopathogenesis of Conjunctival Scarring in Trachoma. Eye (Lond) (1998) 12( Pt 3a):453–60. doi: 10.1038/eye.1998.104
51. Kragsbjerg P, Vikerfors T, Holmberg H. Cytokine Responses in Patients With Pneumonia Caused by Chlamydia or Mycoplasma. Respiration (1998) 65(4):299–303. doi: 10.1159/000029280
52. Wajant H, Pfizenmaier K, Scheurich P. Tumor Necrosis Factor Signaling. Cell Death Differ (2003) 10(1):45–65. doi: 10.1038/sj.cdd.4401189
53. Jiang SJ, Kuo CC, Berry MW, Lee AW, Campbell LA. Identification and Characterization of Chlamydia Pneumoniae-Specific Proteins That Activate Tumor Necrosis Factor Alpha Production in RAW 264.7 Murine Macrophages. Infect Immun (2008) 76(4):1558–64. doi: 10.1128/IAI.01331-07
54. Nagarajan UM, Sikes J, Prantner D, Andrews CW,Jr., Frazer L, Goodwin A, et al. Myd88 Deficiency Leads to Decreased NK Cell Gamma Interferon Production and T Cell Recruitment During Chlamydia Muridarum Genital Tract Infection, But a Predominant Th1 Response and Enhanced Monocytic Inflammation are Associated With Infection Resolution. Infect Immun (2011) 79(1):486–98. doi: 10.1128/IAI.00843-10
55. Carrasco SE, Hu S, Imai DM, Kumar R, Sandusky GE, Yang XF, et al. Toll-Like Receptor 3 (TLR3) Promotes the Resolution of Chlamydia Muridarum Genital Tract Infection in Congenic C57BL/6N Mice. PloS One (2018) 13(4):e0195165. doi: 10.1371/journal.pone.0195165
56. Njau F, Geffers R, Thalmann J, Haller H, Wagner AD. Restriction of Chlamydia Pneumoniae Replication in Human Dendritic Cell by Activation of Indoleamine 2,3-Dioxygenase. Microbes Infect (2009) 11(13):1002–10. doi: 10.1016/j.micinf.2009.07.006
57. Darville T, Andrews CW, Jr., Rank RG. Does Inhibition of Tumor Necrosis Factor Alpha Affect Chlamydial Genital Tract Infection in Mice and Guinea Pigs? Infect Immun (2000) 68(9):5299–305. doi: 10.1128/iai.68.9.5299-5305.2000
58. Perfettini JL, Darville T, Gachelin G, Souque P, Huerre M, Dautry-Varsat A, et al. Effect of Chlamydia Trachomatis Infection and Subsequent Tumor Necrosis Factor Alpha Secretion on Apoptosis in the Murine Genital Tract. Infect Immun (2000) 68(4):2237–44. doi: 10.1128/iai.68.4.2237-2244.2000
59. Radomski N, Karger A, Franzke K, Liebler-Tenorio E, Jahnke R, Matthiesen S, et al. Chlamydia Psittaci-Infected Dendritic Cells Communicate With NK Cells Via Exosomes to Activate Antibacterial Immunity. Infect Immun (2019) 88(1). doi: 10.1128/IAI.00541-19
60. Pal S, de la Maza LM. Mechanism of T-Cell Mediated Protection in Newborn Mice Against a Chlamydia Infection. Microbes Infect (2013) 15(8-9):607–14. doi: 10.1016/j.micinf.2013.04.010
61. Shen F, Verma AH, Volk A, Jones B, Coleman BM, Loza MJ, et al. Combined Blockade of TNF-Alpha and IL-17A Alleviates Progression of Collagen-Induced Arthritis Without Causing Serious Infections in Mice. J Immunol (2019) 202(7):2017–26. doi: 10.4049/jimmunol.1801436
62. Zhao XM, Zhu DY, Ye JB, Li XQ, Wang ZB, Zhang LF, et al. The Potential Protective Role of the Combination of IL-22 and TNF-Alpha Against Genital Tract Chlamydia Trachomatis Infection. Cytokine (2015) 73(1):66–73. doi: 10.1016/j.cyto.2015.01.027
63. Igietseme JU, Omosun Y, Stuchlik O, Reed MS, Partin J, He Q, et al. Role of Epithelial-Mesenchyme Transition in Chlamydia Pathogenesis. PloS One (2015) 10(12):e0145198. doi: 10.1371/journal.pone.0145198
64. Palomo J, Dietrich D, Martin P, Palmer G, Gabay C. The Interleukin (IL)-1 Cytokine Family - Balance Between Agonists and Antagonists in Inflammatory Diseases. Cytokine (2015) 76(1):25–37. doi: 10.1016/j.cyto.2015.06.017
65. Opal SM, DePalo VA. Anti-Inflammatory Cytokines. Chest (2000) 117(4):1162–72. doi: 10.1378/chest.117.4.1162
66. Yazdi AS, Ghoreschi K. The Interleukin-1 Family. Adv Exp Med Biol (2016) 941:21–9. doi: 10.1007/978-94-024-0921-5_2
67. Dinarello CA. Biologic Basis for Interleukin-1 in Disease. Blood (1996) 87(6):2095–147. doi: 10.1182/blood.V87.6.2095.bloodjournal8762095
68. Dinarello CA. Overview of the IL-1 Family in Innate Inflammation and Acquired Immunity. Immunol Rev (2018) 281(1):8–27. doi: 10.1111/imr.12621
69. Rothermel CD, Schachter J, Lavrich P, Lipsitz EC, Francus T. Chlamydia Trachomatis-Induced Production of Interleukin-1 by Human Monocytes. Infect Immun (1989) 57(9):2705–11. doi: 10.1128/IAI.57.9.2705-2711.1989
70. Gervassi A, Alderson MR, Suchland R, Maisonneuve JF, Grabstein KH, Probst P. Differential Regulation of Inflammatory Cytokine Secretion by Human Dendritic Cells Upon Chlamydia Trachomatis Infection. Infect Immun (2004) 72(12):7231–9. doi: 10.1128/IAI.72.12.7231-7239.2004
71. Hvid M, Baczynska A, Deleuran B, Fedder J, Knudsen HJ, Christiansen G, et al. Interleukin-1 is the Initiator of Fallopian Tube Destruction During Chlamydia Trachomatis Infection. Cell Microbiol (2007) 9(12):2795–803. doi: 10.1111/j.1462-5822.2007.00996.x
72. Nagarajan UM, Sikes JD, Yeruva L, Prantner D. Significant Role of IL-1 Signaling, But Limited Role of Inflammasome Activation, in Oviduct Pathology During Chlamydia Muridarum Genital Infection. J Immunol (2012) 188(6):2866–75. doi: 10.4049/jimmunol.1103461
73. Rank RG, Lacy HM, Goodwin A, Sikes J, Whittimore J, Wyrick PB, et al. Host Chemokine and Cytokine Response in the Endocervix Within the First Developmental Cycle of Chlamydia Muridarum. Infect Immun (2010) 78(1):536–44. doi: 10.1128/IAI.00772-09
74. Shimada K, Crother TR, Karlin J, Chen S, Chiba N, Ramanujan VK, et al. Caspase-1 Dependent IL-1beta Secretion is Critical for Host Defense in a Mouse Model of Chlamydia Pneumoniae Lung Infection. PloS One (2011) 6(6):e21477. doi: 10.1371/journal.pone.0021477
75. Prantner D, Darville T, Sikes JD, Andrews CW,Jr., Brade H, Rank RG, et al. Critical Role for Interleukin-1beta (IL-1beta) During Chlamydia Muridarum Genital Infection and Bacterial Replication-Independent Secretion of IL-1beta in Mouse Macrophages. Infect Immun (2009) 77(12):5334–46. doi: 10.1128/IAI.00883-09
76. Carlin JM, Weller JB. Potentiation of Interferon-Mediated Inhibition of Chlamydia Infection by Interleukin-1 in Human Macrophage Cultures. Infect Immun (1995) 63(5):1870–5. doi: 10.1128/IAI.63.5.1870-1875.1995
77. Poston TB, Lee DE, Darville T, Zhong W, Dong L, O’Connell CM, et al. Cervical Cytokines Associated With Chlamydia Trachomatis Susceptibility and Protection. J Infect Dis (2019) 220(2):330–9. doi: 10.1093/infdis/jiz087
78. Tumurkhuu G, Dagvadorj J, Porritt RA, Crother TR, Shimada K, Tarling EJ, et al. Chlamydia Pneumoniae Hijacks a Host Autoregulatory IL-1beta Loop to Drive Foam Cell Formation and Accelerate Atherosclerosis. Cell Metab (2018) 28(3):432–48 e4. doi: 10.1016/j.cmet.2018.05.027
79. Carter JD, Inman RD, Whittum-Hudson J, Hudson AP. Chlamydia and Chronic Arthritis. Ann Med (2012) 44(8):784–92. doi: 10.3109/07853890.2011.606830
80. Lu H, Shen C, Brunham RC. Chlamydia Trachomatis Infection of Epithelial Cells Induces the Activation of Caspase-1 and Release of Mature IL-18. J Immunol (2000) 165(3):1463–9. doi: 10.4049/jimmunol.165.3.1463
81. Marconi C, Santos-Greatti MM, Parada CM, Pontes A, Pontes AG, Giraldo PC, et al. Cervicovaginal Levels of Proinflammatory Cytokines are Increased During Chlamydial Infection in Bacterial Vaginosis But Not in Lactobacilli-Dominated Flora. J Low Genit Tract Dis (2014) 18(3):261–5. doi: 10.1097/LGT.0000000000000003
82. He X, Mekasha S, Mavrogiorgos N, Fitzgerald KA, Lien E, Ingalls RR. Inflammation and Fibrosis During Chlamydia Pneumoniae Infection is Regulated by IL-1 and the NLRP3/ASC Inflammasome. J Immunol (2010) 184(10):5743–54. doi: 10.4049/jimmunol.0903937
83. Jha HC, Srivastava P, Prasad J, Mittal A. Chlamydia Pneumoniae Heat Shock Protein 60 Enhances Expression of ERK, TLR-4 and IL-8 in Atheromatous Plaques of Coronary Artery Disease Patients. Immunol Invest (2011) 40(2):206–22. doi: 10.3109/08820139.2010.534217
84. Buchholz KR, Stephens RS. Activation of the Host Cell Proinflammatory Interleukin-8 Response by Chlamydia Trachomatis. Cell Microbiol (2006) 8(11):1768–79. doi: 10.1111/j.1462-5822.2006.00747.x
85. Schmitz J, Owyang A, Oldham E, Song Y, Murphy E, McClanahan TK, et al. IL-33, an Interleukin-1-Like Cytokine That Signals Via the IL-1 Receptor-Related Protein ST2 and Induces T Helper Type 2-Associated Cytokines. Immunity (2005) 23(5):479–90. doi: 10.1016/j.immuni.2005.09.015
86. Igietseme JU, He Q, Joseph K, Eko FO, Lyn D, Ananaba G, et al. Role of T Lymphocytes in the Pathogenesis of Chlamydia Disease. J Infect Dis (2009) 200(6):926–34. doi: 10.1086/605411
87. Abu el-Asrar AM, Geboes K, Missotten L. Immunology of Trachomatous Conjunctivitis. Bull Soc Belge Ophtalmol (2001) 280):73–96.
88. Cheok YY, Lee CYQ, Cheong HC, Looi CY, Wong WF. Chronic Inflammatory Diseases At Secondary Sites Ensuing Urogenital or Pulmonary Chlamydia Infections. Microorganisms (2020) 8(1):127. doi: 10.3390/microorganisms8010127
89. Vardhan H, Dutta R, Vats V, Gupta R, Jha R, Jha HC, et al. Persistently Elevated Level of IL-8 in Chlamydia Trachomatis Infected Hela 229 Cells is Dependent on Intracellular Available Iron. Mediators Inflammation (2009) 2009:417658. doi: 10.1155/2009/417658
90. Heinrich PC, Behrmann I, Haan S, Hermanns HM, Muller-Newen G, Schaper F. Principles of Interleukin (IL)-6-Type Cytokine Signalling and Its Regulation. Biochem J (2003) 374(Pt 1):1–20. doi: 10.1042/BJ20030407
91. Tanaka T, Narazaki M, Kishimoto T. IL-6 in Inflammation, Immunity, and Disease. Cold Spring Harb Perspect Biol (2014) 6(10):a016295. doi: 10.1101/cshperspect.a016295
92. Weissenbach M, Clahsen T, Weber C, Spitzer D, Wirth D, Vestweber D, et al. Interleukin-6 is a Direct Mediator of T Cell Migration. Eur J Immunol (2004) 34(10):2895–906. doi: 10.1002/eji.200425237
93. Rose-John S. Interleukin-6 Family Cytokines. Cold Spring Harb Perspect Biol (2018) 10(2). doi: 10.1101/cshperspect.a028415
94. Sun X, Tian Q, Wang L, Xue M, Zhong G. IL-6-Mediated Signaling Pathways Limit Chlamydia Muridarum Infection and Exacerbate Its Pathogenicity in the Mouse Genital Tract. Microbes Infect (2017) 19(11):536–45. doi: 10.1016/j.micinf.2017.08.007
95. Kishimoto T. IL-6: From Its Discovery to Clinical Applications. Int Immunol (2010) 22(5):347–52. doi: 10.1093/intimm/dxq030
96. Bua A, Cannas S, Zanetti S, Molicotti P. Levels of Different Cytokines in Women and Men With Asymptomatic Genital Infection Caused by Chlamydia. J Infect Dev Countr (2019) 13(9):847–50. doi: 10.3855/jidc.9810
97. Martinez-Prado E, Camejo Bermudez MI. Expression of IL-6, IL-8, TNF-Alpha, IL-10, HSP-60, Anti-HSP-60 Antibodies, and Anti-Sperm Antibodies, in Semen of Men With Leukocytes and/or Bacteria. Am J Reprod Immunol (2010) 63(3):233–43. doi: 10.1111/j.1600-0897.2009.00786.x
98. Zhang XY, Li JL, Dong MG, Gao LF, Zhong GM. [Early Production of Interleukin-17 in Airway Upon Chlamydia Trachomatis Infection Increases the Local Secretion of IL-6 and MIP-2]. Beijing Da Xue Xue Bao Yi Xue Ban (2010) 42(5):509–13.
99. Williams DM, Grubbs BG, Darville T, Kelly K, Rank RG. A Role for Interleukin-6 in Host Defense Against Murine Chlamydia Trachomatis Infection. Infect Immun (1998) 66(9):4564–7. doi: 10.1128/IAI.66.9.4564-4567.1998
100. Bouwman JJ, Visseren FL, Bouter PK, Diepersloot RJ. Azithromycin Inhibits Interleukin-6 But Not Fibrinogen Production in Hepatocytes Infected With Cytomegalovirus and Chlamydia Pneumoniae. J Lab Clin Med (2004) 144(1):18–26. doi: 10.1016/j.lab.2004.03.012
101. Refaat B, Ashshi AM, Batwa SA, Ahmad J, Idris S, Kutbi SY, et al. The Prevalence of Chlamydia Trachomatis and Mycoplasma Genitalium Tubal Infections and Their Effects on the Expression of IL-6 and Leukaemia Inhibitory Factor in Fallopian Tubes With and Without an Ectopic Pregnancy. Innate Immun-London (2016) 22(7):534–45. doi: 10.1177/1753425916662326
102. Magee DM, Smith JG, Bleicker CA, Carter CJ, Bonewald LF, Schachter J, et al. Chlamydia Trachomatis Pneumonia Induces in Vivo Production of Interleukin-1 and -6. Infect Immun (1992) 60(3):1217–20. doi: 10.1128/IAI.60.3.1217-1220.1992
103. Cunningham K, Stansfield SH, Patel P, Menon S, Kienzle V, Allan JA, et al. The IL-6 Response to Chlamydia From Primary Reproductive Epithelial Cells is Highly Variable and May Be Involved in Differential Susceptibility to the Immunopathological Consequences of Chlamydial Infection. BMC Immunol (2013) 14. doi: 10.1186/1471-2172-14-50
104. Ilumets H, Rytila P, Demedts I, Brusselle GG, Sovijarvi A, Myllarniemi M, et al. Matrix Metalloproteinases -8, -9 and -12 in Smokers and Patients With Stage 0 COPD. Int J Chron Obstruct Pulmon Dis (2007) 2(3):369–79.
105. Prohl A, Ostermann CH, Rummel CD, Roth J, Reinhold P. Circulating and Broncho-Alveolar Interleukin-6 in Relation to Body Temperature in an Experimental Model of Bovine Chlamydia Psittaci Infection. PloS One (2017) 12(12):e0189321. doi: 10.1371/journal.pone.0189321
106. Kotas ME, Medzhitov R. Homeostasis, Inflammation, and Disease Susceptibility. Cell (2015) 160(5):816–27. doi: 10.1016/j.cell.2015.02.010
107. Shapouri-Moghaddam A, Mohammadian S, Vazini H, Taghadosi M, Esmaeili SA, Mardani F, et al. Macrophage Plasticity, Polarization, and Function in Health and Disease. J Cell Physiol (2018) 233(9):6425–40. doi: 10.1002/jcp.26429
108. Hunter CA, Jones SA. IL-6 as a Keystone Cytokine in Health and Disease. Nat Immunol (2015) 16(5):448–57. doi: 10.1038/ni.3153
109. Cheong HC, Lee CYQ, Cheok YY, Shankar EM, Sabet NS, Tan GMY, et al. CPAF, HSP60 and MOMP Antigens Elicit Pro-Inflammatory Cytokines Production in the Peripheral Blood Mononuclear Cells From Genital Chlamydia Trachomatis-Infected Patients. Immunobiology (2019) 224(1):34–41. doi: 10.1016/j.imbio.2018.10.010
110. Strowig T, Henao-Mejia J, Elinav E, Flavell R. Inflammasomes in Health and Disease. Nature (2012) 481(7381):278–86. doi: 10.1038/nature10759
111. Remick DG. Interleukin-8. Crit Care Med (2005) 33(12 Suppl):S466–7. doi: 10.1097/01.ccm.0000186783.34908.18
112. Kragsbjerg P, Jones I, Vikerfors T, Holmberg H. Diagnostic Value of Blood Cytokine Concentrations in Acute Pneumonia. Thorax (1995) 50(12):1253–7. doi: 10.1136/thx.50.12.1253
113. Rasmussen SJ, Eckmann L, Quayle AJ, Shen L, Zhang YX, Anderson DJ, et al. Secretion of Proinflammatory Cytokines by Epithelial Cells in Response to Chlamydia Infection Suggests a Central Role for Epithelial Cells in Chlamydial Pathogenesis. J Clin Invest (1997) 99(1):77–87. doi: 10.1172/JCI119136
114. Zhou H, Huang Q, Li Z, Wu Y, Xie X, Ma K, et al. PORF5 Plasmid Protein of Chlamydia Trachomatis Induces MAPK-Mediated Pro-Inflammatory Cytokines Via TLR2 Activation in THP-1 Cells. Sci China Life Sci (2013) 56(5):460–6. doi: 10.1007/s11427-013-4470-8
115. Dehghan Marvast L, Aflatoonian A, Talebi AR, Ghasemzadeh J, Pacey AA. Semen Inflammatory Markers and Chlamydia Trachomatis Infection in Male Partners of Infertile Couples. Andrologia (2016) 48(7):729–36. doi: 10.1111/and.12501
116. Du K, Zhou M, Li Q, Liu XZ. Chlamydia Trachomatis Inhibits the Production of Pro-Inflammatory Cytokines in Human Pbmcs Through Induction of IL-10. J Med Microbiol (2018) 67(2):240–8. doi: 10.1099/jmm.0.000672
117. Bas S, James RW, Gabay C. Serum Lipoproteins Attenuate Macrophage Activation and Toll-Like Receptor Stimulation by Bacterial Lipoproteins. BMC Immunol (2010) 11:46. doi: 10.1186/1471-2172-11-46
118. Sarkar A, Moller S, Bhattacharyya A, Behnen M, Rupp J, van Zandbergen G, et al. Mechanisms of Apoptosis Inhibition in Chlamydia Pneumoniae-Infected Neutrophils. Int J Med Microbiol (2015) 305(6):493–500. doi: 10.1016/j.ijmm.2015.04.006
119. Rajeeve K, Das S, Prusty BK, Rudel T. Chlamydia Trachomatis Paralyses Neutrophils to Evade the Host Innate Immune Response. Nat Microbiol (2018) 3(7):824–35. doi: 10.1038/s41564-018-0182-y
120. Khader SA, Gopal R. IL-17 in Protective Immunity to Intracellular Pathogens. Virulence (2010) 1(5):423–7. doi: 10.4161/viru.1.5.12862
121. Amatya N, Garg AV, Gaffen SL. IL-17 Signaling: The Yin and the Yang. Trends Immunol (2017) 38(5):310–22. doi: 10.1016/j.it.2017.01.006
122. Rizzo A, Di Domenico M, Carratelli CR, Mazzola N, Paolillo R. Induction of Proinflammatory Cytokines in Human Osteoblastic Cells by Chlamydia Pneumoniae. Cytokine (2011) 56(2):450–7. doi: 10.1016/j.cyto.2011.06.027
123. Hakimi H, Akhondi MM, Sadeghi MR, Chamani L, Arababadi MK, Ahmadabadi BN, et al. Seminal Levels of IL-10, IL-12, and IL-17 in Men With Asymptomatic Chlamydia Infection. Inflammation (2014) 37(1):122–6. doi: 10.1007/s10753-013-9719-7
124. Bai H, Gao X, Zhao L, Peng Y, Yang J, Qiao S, et al. Respective IL-17A Production by Gammadelta T and Th17 Cells and Its Implication in Host Defense Against Chlamydial Lung Infection. Cell Mol Immunol (2017) 14(10):850–61. doi: 10.1038/cmi.2016.53
125. Lu C, Zeng H, Li Z, Lei L, Yeh IT, Wu Y, et al. Protective Immunity Against Mouse Upper Genital Tract Pathology Correlates With High Ifngamma But Low IL-17 T Cell and Anti-Secretion Protein Antibody Responses Induced by Replicating Chlamydial Organisms in the Airway. Vaccine (2012) 30(2):475–85. doi: 10.1016/j.vaccine.2011.10.059
126. Zhang X, Gao L, Lei L, Zhong Y, Dube P, Berton MT, et al. A Myd88-Dependent Early IL-17 Production Protects Mice Against Airway Infection With the Obligate Intracellular Pathogen Chlamydia Muridarum. J Immunol (2009) 183(2):1291–300. doi: 10.4049/jimmunol.0803075
127. Vicetti Miguel RD, Quispe Calla NE, Pavelko SD, Cherpes TL. Intravaginal Chlamydia Trachomatis Challenge Infection Elicits TH1 and TH17 Immune Responses in Mice That Promote Pathogen Clearance and Genital Tract Damage. PloS One (2016) 11(9):e0162445. doi: 10.1371/journal.pone.0162445
128. Zhang Y, Wang H, Ren J, Tang X, Jing Y, Xing D, et al. IL-17A Synergizes With IFN-Gamma to Upregulate Inos and NO Production and Inhibit Chlamydial Growth. PloS One (2012) 7(6):e39214. doi: 10.1371/journal.pone.0039214
129. Bai H, Cheng J, Gao X, Joyee AG, Fan Y, Wang S, et al. Il-17/Th17 Promotes Type 1 T Cell Immunity Against Pulmonary Intracellular Bacterial Infection Through Modulating Dendritic Cell Function. J Immunol (2009) 183(9):5886–95. doi: 10.4049/jimmunol.0901584
130. Arkatkar T, Gupta R, Li W, Yu JJ, Wali S, Neal Guentzel M, et al. Murine Microrna-214 Regulates Intracellular Adhesion Molecule (ICAM1) Gene Expression in Genital Chlamydia Muridarum Infection. Immunology (2015) 145(4):534–42. doi: 10.1111/imm.12470
131. Qiao S, Zhang H, Zha X, Niu W, Liang J, Pang G, et al. Endogenous IL-17A Mediated Neutrophil Infiltration by Promoting Chemokines Expression During Chlamydial Lung Infection. Microb Pathog (2019) 129:106–11. doi: 10.1016/j.micpath.2019.01.039
132. Burton MJ, Ramadhani A, Weiss HA, Hu V, Massae P, Burr SE, et al. Active Trachoma is Associated With Increased Conjunctival Expression of IL17A and Profibrotic Cytokines. Infection Immun (2011) 79(12):4977–83. doi: 10.1128/Iai.05718-11
133. Zheng NB, Zhang LJ, Wang BB, Wang GY, Liu JY, Miao GL, et al. Chlamydia Pneumoniae Infection Promotes Vascular Smooth Muscle Cell Migration Via C-Fos/Interleukin-17c Signaling. Int J Med Microbiol (2019) 309(8):151340. doi: 10.1016/j.ijmm.2019.151340
134. Scurlock AM, Frazer LC, Andrews CW, Jr, O’Connell CM, Foote IP, Bailey SL, et al. Interleukin-17 Contributes to Generation of Th1 Immunity and Neutrophil Recruitment During Chlamydia Muridarum Genital Tract Infection But is Not Required for Macrophage Influx or Normal Resolution of Infection. Infect Immun (2011) 79(3):1349–62. doi: 10.1128/IAI.00984-10
135. Choi P, Reiser H. IL-4: Role in Disease and Regulation of Production. Clin Exp Immunol (1998) 113(3):317–9. doi: 10.1046/j.1365-2249.1998.00690.x
136. Ho IC, Miaw SC. Regulation of IL-4 Expression in Immunity and Diseases. Regul Cytokine Gene Expression Immun Dis (2016) 941:31–77. doi: 10.1007/978-94-024-0921-5_3
137. Holland MJ, Bailey RL, Conway DJ, Culley F, Miranpuri G, Byrne GI, et al. T Helper Type-1 (Th1)/Th2 Profiles of Peripheral Blood Mononuclear Cells (PBMC); Responses to Antigens of Chlamydia Trachomatis in Subjects With Severe Trachomatous Scarring. Clin Exp Immunol (1996) 105(3):429–35. doi: 10.1046/j.1365-2249.1996.d01-792.x
138. Smith-Norowitz TA, Huang Y, Loeffler J, Klein E, Norowitz YM, Hammerschlag MR, et al. Azithromycin Decreases Chlamydia Pneumoniae-Mediated Interleukin-4 Responses But Not Immunoglobulin E Responses. PloS One (2020) 15(6):e0234413. doi: 10.1371/journal.pone.0234413
139. Williams DM, Grubbs BG, Pack E, Kelly K, Rank RG. Humoral and Cellular Immunity in Secondary Infection Due to Murine Chlamydia Trachomatis. Infect Immun (1997) 65(7):2876–82. doi: 10.1128/IAI.65.7.2876-2882.1997
140. Yang X. Role of Cytokines in Chlamydia Trachomatis Protective Immunity and Immunopathology. Curr Pharm Des (2003) 9(1):67–73. doi: 10.2174/1381612033392486
141. Vicetti Miguel RD, Harvey SA, LaFramboise WA, Reighard SD, Matthews DB, Cherpes TL. Human Female Genital Tract Infection by the Obligate Intracellular Bacterium Chlamydia Trachomatis Elicits Robust Type 2 Immunity. PloS One (2013) 8(3):e58565. doi: 10.1371/journal.pone.0058565
142. Clancy R, Ren Z, Pang G, Fletcher P, D’Este C. Chronic Chlamydia Pneumoniae Infection May Promote Coronary Artery Disease in Humans Through Enhancing Secretion of Interleukin-4. Clin Exp Immunol (2006) 146(2):197–202. doi: 10.1111/j.1365-2249.2006.03185.x
143. Minty A, Chalon P, Derocq JM, Dumont X, Guillemot JC, Kaghad M, et al. Interleukin-13 is a New Human Lymphokine Regulating Inflammatory and Immune Responses. Nature (6417) 1993) 362:248–50. doi: 10.1038/362248a0
144. Wynn TA. Il-13 Effector Functions. Annu Rev Immunol (2003) 21:425–56. doi: 10.1146/annurev.immunol.21.120601.141142
145. Asquith KL, Horvat JC, Kaiko GE, Carey AJ, Beagley KW, Hansbro PM, et al. Interleukin-13 Promotes Susceptibility to Chlamydial Infection of the Respiratory and Genital Tracts. PloS Pathog (2011) 7(5):e1001339. doi: 10.1371/journal.ppat.1001339
146. Johnson RM, Kerr MS, Slaven JE. An Atypical CD8 T-Cell Response to Chlamydia Muridarum Genital Tract Infections Includes T Cells That Produce Interleukin-13. Immunology (2014) 142(2):248–57. doi: 10.1111/imm.12248
147. Johnson RM, Olivares-Strank N. Peng G. a Class II-Restricted CD8gamma13 T-Cell Clone Protects During Chlamydia Muridarum Genital Tract Infection. J Infect Dis (2020) 221(11):1895–906. doi: 10.1093/infdis/jiz685
148. Saraiva M, O’Garra A. The Regulation of IL-10 Production by Immune Cells. Nat Rev Immunol (2010) 10(3):170–81. doi: 10.1038/nri2711
149. Ouyang W, Rutz S, Crellin NK, Valdez PA, Hymowitz SG. Regulation and Functions of the IL-10 Family of Cytokines in Inflammation and Disease. Annu Rev Immunol (2011) 29:71–109. doi: 10.1146/annurev-immunol-031210-101312
150. Hakimi H, Zare-Bidaki M, Zainodini N, Assar S, Arababadi MK. Significant Roles Played by IL-10 in Chlamydia Infections. Inflammation (2014) 37(3):818–23. doi: 10.1007/s10753-013-9801-1
151. Chu J, Li X, Qu G, Wang Y, Li Q, Guo Y, et al. Chlamydia Psittaci Pmpd-N Exacerbated Chicken Macrophage Function by Triggering Th2 Polarization and the TLR2/Myd88/NF-Kappab Signaling Pathway. Int J Mol Sci (2020) 21(6):2003. doi: 10.3390/ijms21062003
152. Faal N, Bailey RL, Jeffries D, Joof H, Sarr I, Laye M, et al. Conjunctival FOXP3 Expression in Trachoma: Do Regulatory T Cells Have a Role in Human Ocular Chlamydia Trachomatis Infection? PloS Med (2006) 3(8):e266. doi: 10.1371/journal.pmed.0030266
153. Agrawal T, Vats V, Wallace PK, Salhan S, Mittal A. Cervical Cytokine Responses in Women With Primary or Recurrent Chlamydial Infection. J Interferon Cytokine Res (2007) 27(3):221–6. doi: 10.1089/jir.2006.0132
154. Kaiko GE, Phipps S, Hickey DK, Lam CE, Hansbro PM, Foster PS, et al. Chlamydia Muridarum Infection Subverts Dendritic Cell Function to Promote Th2 Immunity and Airways Hyperreactivity. J Immunol (2008) 180(4):2225–32. doi: 10.4049/jimmunol.180.4.2225
155. Yu JL, Yu P, Li LX. [Hela Cells Secrete Interleukin-8 and Interleukin-10 Response to Chlamydia Trachomatis Entry]. Hunan Yi Ke Da Xue Xue Bao (2003) 28(2):174–6.
156. Han X, Wang S, Fan Y, Yang J, Jiao L, Qiu H, et al. Chlamydia Infection Induces ICOS Ligand-Expressing and IL-10-Producing Dendritic Cells That Can Inhibit Airway Inflammation and Mucus Overproduction Elicited by Allergen Challenge in BALB/C Mice. J Immunol (2006) 176(9):5232–9. doi: 10.4049/jimmunol.176.9.5232
157. Yang X, Gartner J, Zhu L, Wang S, Brunham RC. IL-10 Gene Knockout Mice Show Enhanced Th1-Like Protective Immunity and Absent Granuloma Formation Following Chlamydia Trachomatis Lung Infection. J Immunol (1999) 162(2):1010–7.
158. Penttila T, Haveri A, Tammiruusu A, Vuola JM, Lahesmaa R, Puolakkainen M. Chlamydia Pneumoniae Infection in IL-10 Knock Out Mice: Accelerated Clearance But Severe Pulmonary Inflammatory Response. Microb Pathog (2008) 45(1):25–9. doi: 10.1016/j.micpath.2008.02.004
159. Yilma AN, Singh SR, Fairley SJ, Taha MA, Dennis VA. The Anti-Inflammatory Cytokine, Interleukin-10, Inhibits Inflammatory Mediators in Human Epithelial Cells and Mouse Macrophages Exposed to Live and UV-Inactivated Chlamydia Trachomatis. Mediators Inflamm (2012) 2012:520174. doi: 10.1155/2012/520174
160. Joyee AG, Yang X. Plasmacytoid Dendritic Cells Mediate the Regulation of Inflammatory Type T Cell Response for Optimal Immunity Against Respiratory Chlamydia Pneumoniae Infection. PloS One (2013) 8(12):e83463. doi: 10.1371/journal.pone.0083463
161. Omosun Y, McKeithen D, Ryans K, Kibakaya C, Blas-Machado U, Li D, et al. Interleukin-10 Modulates Antigen Presentation by Dendritic Cells Through Regulation of NLRP3 Inflammasome Assembly During Chlamydia Infection. Infect Immun (2015) 83(12):4662–72. doi: 10.1128/IAI.00993-15
162. Nsonwu-Anyanwu AC, Charles-Davies MA, Bello FA, Taiwo VO, Bin L, Oni AA. Cytokine Profile in Nigerians With Tubal Infertility. Cent Eur J Immunol (2016) 41(1):101–6. doi: 10.5114/ceji.2015.56969
163. Almeida NCC, Queiroz MAF, Lima SS, Brasil Costa I, Ayin Fossa MA, Vallinoto ACR, et al. Association of Chlamydia Trachomatis, C. Pneumoniae, and IL-6 and IL-8 Gene Alterations With Heart Diseases. Front Immunol (2019) 10:87. doi: 10.3389/fimmu.2019.00087
164. Jupelli M, Selby DM, Guentzel MN, Chambers JP, Forsthuber TG, Zhong G, et al. The Contribution of Interleukin-12/Interferon-Gamma Axis in Protection Against Neonatal Pulmonary Chlamydia Muridarum Challenge. J Interferon Cytokine Res (2010) 30(6):407–15. doi: 10.1089/jir.2009.0083
165. Kraft M, Cassell GH, Pak J, Martin RJ. Mycoplasma Pneumoniae and Chlamydia Pneumoniae in Asthma: Effect of Clarithromycin. Chest (2002) 121(6):1782–8. doi: 10.1378/chest.121.6.1782
166. Krull M, Bockstaller P, Wuppermann FN, Klucken AC, Muhling J, Schmeck B, et al. Mechanisms of Chlamydophila Pneumoniae-Mediated GM-CSF Release in Human Bronchial Epithelial Cells. Am J Respir Cell Mol Biol (2006) 34(3):375–82. doi: 10.1165/rcmb.2004-0157OC
167. Schirmer M, Kumar V, Netea MG, Xavier RJ. The Causes and Consequences of Variation in Human Cytokine Production in Health. Curr Opin Immunol (2018) 54:50–8. doi: 10.1016/j.coi.2018.05.012
168. Chen C, Zhou Z, Conrad T, Yang Z, Dai J, Li Z, et al. In Vitro Passage Selects for Chlamydia Muridarum With Enhanced Infectivity in Cultured Cells But Attenuated Pathogenicity in Mouse Upper Genital Tract. Infect Immun (2015) 83(5):1881–92. doi: 10.1128/IAI.03158-14
169. Chen J, Yang Z, Sun X, Tang L, Ding Y, Xue M, et al. Intrauterine Infection With Plasmid-Free Chlamydia Muridarum Reveals a Critical Role of the Plasmid in Chlamydial Ascension and Establishes a Model for Evaluating Plasmid-Independent Pathogenicity. Infect Immun (2015) 83(6):2583–92. doi: 10.1128/IAI.00353-15
170. Chen J, Zhang H, Zhou Z, Yang Z, Ding Y, Zhou Z, et al. Chlamydial Induction of Hydrosalpinx in 11 Strains of Mice Reveals Multiple Host Mechanisms for Preventing Upper Genital Tract Pathology. PloS One (2014) 9(4):e95076. doi: 10.1371/journal.pone.0095076
171. Yang Z, Conrad T, Zhou Z, Chen J, Dutow P, Klos A, et al. Complement Factor C5 But Not C3 Contributes Significantly to Hydrosalpinx Development in Mice Infected With Chlamydia Muridarum. Infect Immun (2014) 82(8):3154–63. doi: 10.1128/IAI.01833-14
172. Trenova AG, Slavov GS, Manova MG, Miteva LD. Stanilova SA. a Role of Cytokine Gene Polymorphisms in Cognitive Functioning. Folia Med (Plovdiv) (2018) 60(2):191–9. doi: 10.1515/folmed-2017-0094
Keywords: Chlamydia, infection, cytokines, inflammation, pathology
Citation: Xiang W, Yu N, Lei A, Li X, Tan S, Huang L and Zhou Z (2021) Insights Into Host Cell Cytokines in Chlamydia Infection. Front. Immunol. 12:639834. doi: 10.3389/fimmu.2021.639834
Received: 10 December 2020; Accepted: 05 May 2021;
Published: 21 May 2021.
Edited by:
Esaki M. SHANKAR, Central University of Tamil Nadu, IndiaReviewed by:
Stephen James Jordan, Indiana University, United StatesCopyright © 2021 Xiang, Yu, Lei, Li, Tan, Huang and Zhou. This is an open-access article distributed under the terms of the Creative Commons Attribution License (CC BY). The use, distribution or reproduction in other forums is permitted, provided the original author(s) and the copyright owner(s) are credited and that the original publication in this journal is cited, in accordance with accepted academic practice. No use, distribution or reproduction is permitted which does not comply with these terms.
*Correspondence: Zhou Zhou, U3VzaWV6aG91OTk1MDNAMTYzLmNvbQ==
Disclaimer: All claims expressed in this article are solely those of the authors and do not necessarily represent those of their affiliated organizations, or those of the publisher, the editors and the reviewers. Any product that may be evaluated in this article or claim that may be made by its manufacturer is not guaranteed or endorsed by the publisher.
Research integrity at Frontiers
Learn more about the work of our research integrity team to safeguard the quality of each article we publish.