- Department of Internal Medicine and Infectious Diseases, University Medical Centre Utrecht, Utrecht, Netherlands
Infection with the human immunodeficiency virus (HIV) is characterized by progressive depletion of CD4+ lymphocytes cells as a result of chronic immune activation. Next to the decreases in the number of CD4+ cells which leads to opportunistic infections, HIV-related immune activation is associated with several prevalent comorbidities in the HIV-positive population such as cardiovascular and bone disease. Traditionally, combination antiretroviral therapy (cART) consists of three drugs with activity against HIV and is highly effective in diminishing the degree of immune activation. Over the years, questions were raised whether virological suppression could also be achieved with fewer antiretroviral drugs, i.e., dual- or even monotherapy. This is an intriguing question considering the fact that antiretroviral drugs should be used lifelong and their use could also induce cardiovascular and bone disease. Therefore, the equilibrium between drug-induced toxicity and immune activation related comorbidity is delicate. Recently, two large clinical trials evaluating two-drug cART showed non-inferiority with respect to virological outcomes when compared to triple-drug regimens. This led to adoption of dual antiretroviral therapy in current HIV treatment guidelines. However, it is largely unknown whether dual therapy is also able to suppress immune activation to the same degree as triple therapy. This poses a risk for an imbalance in the delicate equilibrium. This mini review gives an overview of the current available evidence concerning immune activation in the setting of cART with less than three antiretroviral drugs.
Introduction
In 1983, a group of French virologists identified a T-lymphotropic retrovirus—now called the human immunodeficiency virus (HIV)—as causative agent of the acquired immunodeficiency syndrome (AIDS) (1). The clinical picture of AIDS is characterized by opportunistic infections such as pneumocystis jirovecii pneumonia and candida esophagitis (2). These opportunistic infections are the result of a severe depletion of CD4+ lymphocytes, which are central mediators of immune response, coordinating both cellular and humoral responses against infections (3).
Although HIV uses the CD4 receptor to gain access to target cells, the depletion of CD4+ lymphocytes is only partly due to a direct cytolytic effect of HIV (4). The current leading hypothesis states that chronic HIV infection is accompanied by a hyperactive inflammatory state in which there is an increased turnover of activated naïve T-cells, eventually leading to T-cell depletion by means of apoptosis (5, 6). Immune activation is driven by both the HIV viremia and bacterial translocation from the gut (7, 8) and is associated with numerous comorbidities in HIV-positive patients (9–11). Therefore, immune activation is not only considered to be a predictor for the risk for progression to AIDS but also an important cause of HIV-related comorbidity (12, 13).
Till the end of 1995, the nucleoside reverse transcriptase inhibitors (NRTIs) were the only available antiretroviral agents – targeting reverse transcriptase, an enzyme essential for HIV replication (14). Unfortunately, NRTI mono- or dual therapy had only temporary effects due to rapid resistance development and virological failure (15). However, the perspective for people living with HIV changed dramatically as result of the introduction of a new class of drugs: the protease inhibitors (PIs) combined with a pharmacological booster (16). Combination antiretroviral therapy (cART)—drug regimens consisting of multiple antiretroviral classes—diminished the risk of resistance development and led to an spectacular increase in life expectancy (17). Over the years, the development of antiretroviral drugs took off and several other third drug (“anchors”) classes—such as the non-nucleoside reverse transcriptase inhibitors (NNRTIs) and integrase strand transfer inhibitors (INSTIs) —were introduced (18, 19). Nowadays, triple antiretroviral therapy is highly successful with most patients reaching the main treatment goal of an “undetectable” viral load—defined as <50 copies/ml of HIV RNA when measured by polymerase chain reaction—and with the mortality risk declining (20, 21). Current immunoassays however, due to improvement of sensitivity, are able to detect viral loads that are below 50 copies/ml but can still be quantified: so-called “residual viremia.” A small group of patients—“elite controllers”—are able to maintain an undetectable viral load in absence of antiretroviral drugs (22). However, these patients display significant immune activation when compared to HIV-negative controls (23, 24) and this is linked to an increased risk for cardiovascular disease in these patients (25). These findings emphasize the importance of immune activation in the pathophysiology of HIV-related comorbidity. In the modern antiretroviral era, there is no role for in depth monitoring of immune activation as these markers are generally considered to reduce simultaneously with the viral load, albeit they do not show complete normalization (26).
In the recent years, questions were raised whether there is a need to hold on to the mantra that cART should always consist of three antiretroviral drugs (27). Indeed, the current available agents have high genetic barriers for resistance and the life-long use of multiple drugs could lead to long-term toxicity. Numerous studies evaluated the efficacy of mono- or dual antiretroviral therapy (28–36) and some of these two-drug regimens gained ground in the current treatment guidelines (37, 38). However, there are concerns as to whether the two-drug regimens suppress the degree of HIV-related immune activation enough (39). A rebound in immune activation which occurs beneath the surface despite virological suppression could be harmful. In the end, the development of comorbidity in HIV is the net result of potential harmful effects of antiretroviral drugs vs. the degree in which these drugs suppress the virus and the related immune activation (Figure 1). Therefore, any change in the current standard of care might lead to disruption of this equilibrium. In this mini review, we will discuss the best current available data on immune activation in non-traditional cART regimens.
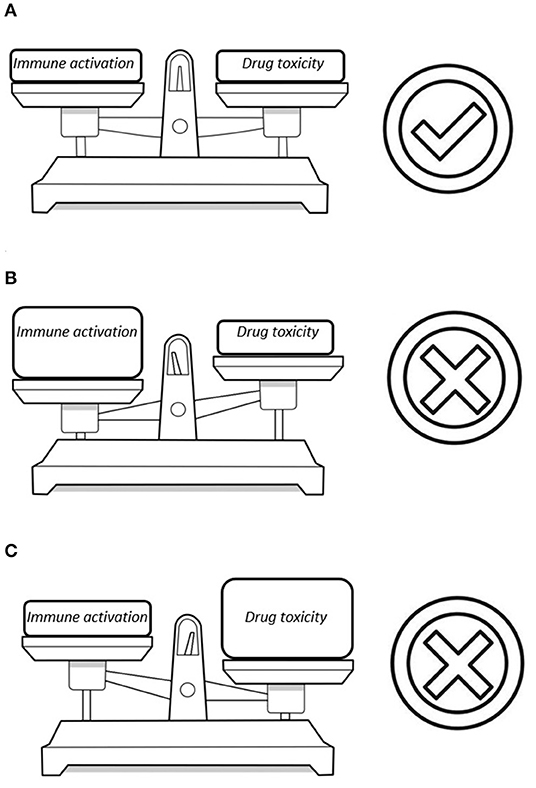
Figure 1. Three possible scenarios in the equilibrium between drug toxicity and damage from HIV-related immune activation: (A) A perfect balance between these factors with the smallest possible risk for comorbidity. (B) The reduction in the number of antiretroviral drugs diminishes the risk for drug toxicity but a flare in immune activation could lead to HIV-associated comorbidity. (C) Multiple antiretroviral drugs are able to fully suppress the virus but this poses a significant risk for cART-associated toxicity.
Immunological Markers in HIV-Infection
The test battery for HIV-related immune activation is extending ever since the recognition of the hyperactive inflammatory status. The available markers can be divided into soluble and cellular markers for inflammation and immune activation, with some being more readily available than others (40) (Table 1).
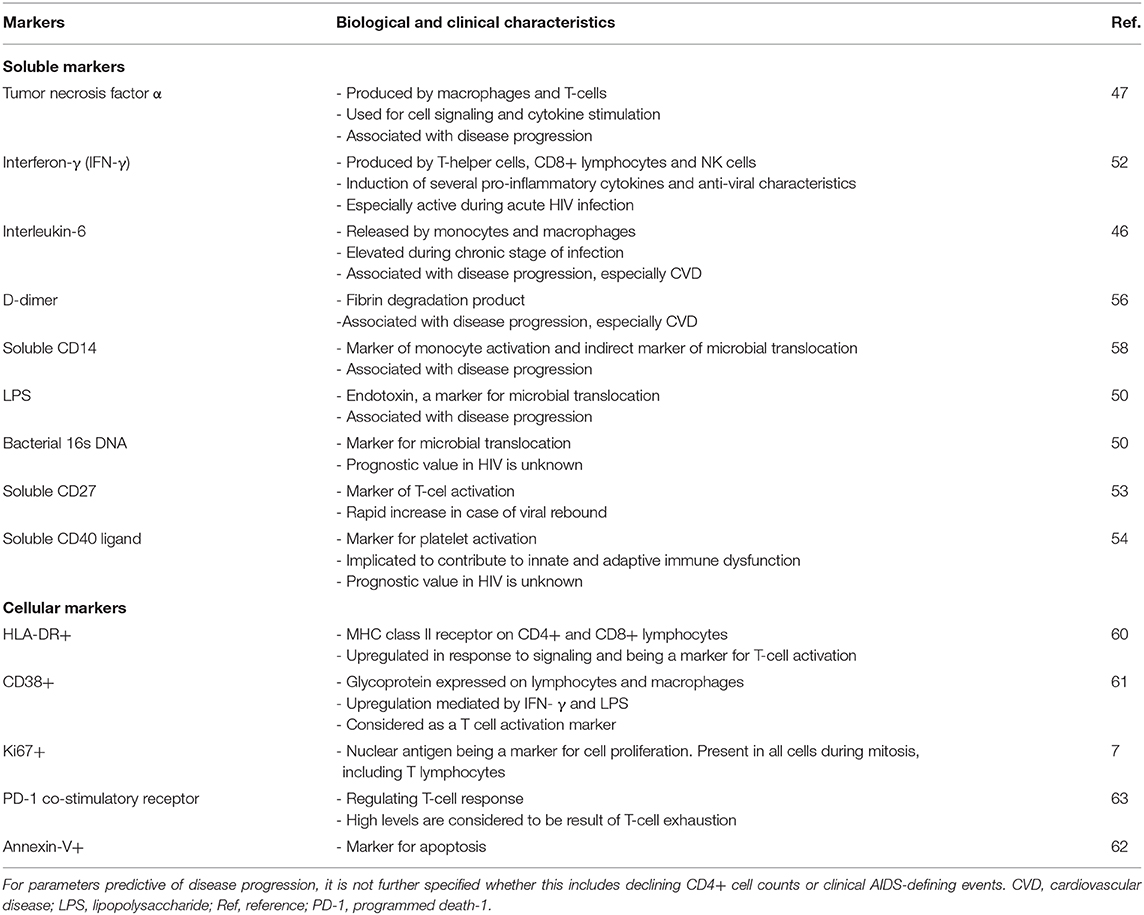
Table 1. An overview of the most important soluble and cellular markers for HIV-associated immune activation that are reported in current literature.
The soluble markers are easy to measure in a large number of test facilities and can subdivided into markers of inflammation, coagulation and microbial translocation. The most commonly used inflammation markers include high-sensitivity c-reactive protein (hs-CRP) and plasma interleukin-6 (IL-6), both considered to be extremely sensitive for systemic inflammation (41, 42) and associated with HIV-related mortality (43–46). Other soluble markers include tumor necrosis factor alpha (TNF-α), interferon-γ, neopterin, mitochondrial DNA (mtDNA), β2-microglobulin, soluble CD27, and soluble CD40 ligand (47–54). The latter two are markers of T-cell activation. The main example for coagulation markers is D-dimer, which levels increase in several pro-inflammatory states and high levels being associated with cardiovascular disease (55, 56). The last group of soluble markers are surrogates of microbial translocation. These include bacterial lipopolysaccharide (LPS)—present in gram-negative bacteria—and bacterial DNA (16s ribosomal RNA subunit) (57). In addition, plasma soluble CD14 (sCD14) and soluble CD163 (sCD163)—products of monocyte activation—are also considered to be a markers for impaired mucosal integrity (58). None of these markers are exclusively found in the setting of HIV-infection (59).
Although the soluble markers can be assessed relatively easy, their reflection of inflammation and immune activation is considered to be less specific than the cellular activation markers in the setting of HIV (40). Assessing cellular markers is more labor-intensive, requiring the isolation of peripheral blood mononuclear cells and performing flow cytometry. For the cellular activity, some well-defined markers are available: CD38+/HLA-DR+ expression on lymphocytes for T-cell activation (60, 61), Ki-67 positivity for proliferation (7), annexin-V for apoptosis (62) and programmed-death-1 co-stimulatory receptor for T-cell exhaustion (63). The CD4+ lymphocyte counts and CD4/CD8 ratio are more readily available but these changes occur more slowly and are therefore kept out of this review (64).
Residual Immune Activation During Triple-Drug Therapy
The initiation of cART results in fast virological suppression and significant reduction in immune activation in most patients, subsequently leading to CD4+ cell recovery (65). However, antiretroviral therapy does not normalize the HIV-induced inflammatory response with some residual immune activation persisting (66). Studies describing the effect of cART on the soluble markers report inconsistent outcomes (43, 67, 68), but especially the degree of T-cell activation rarely normalizes (69).
The clinical impact of this residual immune activation is largely unknown but, for example, the higher incidence of cardiovascular disease among HIV-positive individuals despite cART and the elite controllers implies clinical significance. The reason for residual immune activation in the setting of virological suppression has not been fully elucidated, but it is suggested that low-grade HIV replication in certain anatomical or cellular compartments is the main driver (70). These “sanctuary sites” are compartments, such as the central nervous system (CNS), gastrointestinal tract and lymph nodes, where cART reaches insufficient drug levels to completely suppress local viral replication and subsequent low-grade inflammation. The variable—and often suboptimal—drug penetration in lymph nodes (71), mucosal tissues (72) and the CNS (73) have been demonstrated in several papers. Besides these sites, persisting microbial translocation and the presence of viral coinfections are associated with persistent immune activation (74, 75). There is no consistent evidence that favors one anchor over another with respect to the degree of immune activation (76–78). Studies evaluating whether therapy intensification with additional anchors results in further suppression of immune activation, are conflicting (79–81).
Immune Activation and Virological Efficacy in Monotherapy
After the introduction of cART in the mid-nineties, monotherapy for HIV-infection was abandoned because of virological inferiority. However, the idea of antiretroviral monotherapy made a comeback after the introduction of agents with a high antiviral potency and a high genetic barrier for resistance. Such a mono-drug regimen have significant advantages, including less side-effects and pill burden. The hypothesis that one powerful antiretroviral drug would be sufficient to maintain virological suppression, led to several trials comparing the virological efficacy of PI or INSTI monotherapy to traditional three-drug regimens (28, 30–33). Unfortunately, monotherapy with these drugs seem to result in higher rates of virological rebound when compared to cART. Therefore, current guidelines recommend against monotherapy as maintenance therapy in treatment-experienced patients with a undetectable viral load (37, 38). However, from a pathophysiological viewpoint it is interesting to have a closer look at the impact of monotherapy on immune activation markers.
One study that provides an insight in the mechanisms of immune activation rebound was published by BenMarzoek-Hidalgo et al. (82). In their paper, the authors describe the relationship between microbial translocation and viremia with immune activation in 71 patients receiving boosted darunavir monotherapy. In this cohort, only 26% of the patients maintained a viral load below 20 copies/ml, while 16 patients displayed virological failure (2 consecutive HIV-RNA levels exceeding 200 copies/mL). The remaining patients had (transitory) episodes of a detectable viral load during follow-up yet without meeting the criteria for virological failure. Although separate analysis per outcome group found that only patients with virological failure showed an increase in T-cell activation, it became clear that time with viral suppression was inversely correlated with T-cell activation (percentage HLA-DR+-CD38+ lymphocytes in both CD8+ and CD4+ lymphocyte subsets) at a follow-up of 24 months. In this study, there was a clear correlation between the viral load and the percentage of activated CD4+ and CD8+ lymphocytes. In addition, another study showed that intensification with INSTI (raltegravir) to PI monotherapy (either darunavir/ritonavir or lopinavir/ritonavir), resulted in a decline in the degree of residual viremia and a decrease in the percentage of activated CD8+ lymphocytes (83).
There are several studies that evaluated the non-specific soluble markers in highly selected populations (84–86), while other studies evaluated the cellular markers. The smallest study of Merlini et al. did not find a difference in T-cell activation between baseline and after 96 weeks for both patients receiving PI monotherapy with atazanavir (n = 18) and those receiving atazanavir-based cART [n = 22 (87)]. However, patients on monotherapy were more likely to display increased T-cell apoptosis than patients receiving three drugs. Torres et al. evaluated the markers for monocyte activation in 40 patients receiving PI monotherapy (either lopinavir/ritonavir or darunavir/ritonavir) and 20 patients on PI-based cART for at least 48 weeks and an undetectable viral load (88). This cross-sectional analysis showed that patients on monotherapy display higher levels of monocyte activation—CD14+CD16-CD163+ cells and sCD14 levels—when compared to those receiving standard therapy. The last, most well-designed, study of Petrara et al. described the dynamics of the HIV-1 viral reservoir and T- and B-cell activation markers at 48 and 96 weeks of therapy in patients switched to PI mono-therapy (n = 32) and patients continuing PI-based triple therapy (n = 32) (89). It should be noted that ten percent of the patients in the monotherapy group experienced virological failure compared to zero patients receiving cART. Furthermore, the authors observed a significant increase of T- and B-cell activation in patients receiving one drug, while these markers remained low in patients on cART.
So the best available evidence suggests that a switch to monotherapy is associated with an increase of T-cell activation and apoptosis markers, while soluble markers data are more inconsistent. These observations seem to be the result of (low-grade) viral rebound. The increased risk for virological failure and the suggestion of a rebound in immune activation, disqualify monotherapy as maintenance therapy.
Immune Activation in Dual Therapy
Antiretroviral monotherapy is not likely to play a role in the near future, so the current focus is on the effectiveness of dual therapy. In fact, two-drug regimens have already gained a position in current HIV treatment guidelines; in 2018 a single-tablet regimen (STR) consisting of dolutegravir (INSTI) and rilpivirine (NNRTI) was introduced and in 2020 a STR with dolutegravir and lamivudine (NRTI) was registered as a first-line treatment option. Currently, there are several large trials that support the use of these two STRs in clinical practice: SWORD-1&2 (36), GEMINI-1&2 (35), and TANGO study (29).
The SWORD-1&2 studies evaluated the efficacy, safety and tolerability of dolutegravir/rilpivirine as maintenance therapy in patients with an undetectable viral load. Patients were randomized to either dual therapy (n = 512) vs. continuing triple-drug therapy (n = 516). After 148 weeks, the data showed that dolutegravir/rilpivirine was non-inferior with respect to virological outcomes to triple therapy (90). In the first paper evaluating this regimen, there was a brief mention on the dynamics of the inflammatory and cardiovascular markers in both groups. The authors state there was no consistent pattern of change from baseline to week 48 or differentiation between both groups in the following markers: IL-6, CRP, sCD14, sCD163, and D-dimer. Exact data were not shown and specific T-cell markers were not evaluated. The use of STR dolutegravir/lamivudine for treatment-experienced patients is supported by the TANGO study (29). In this study, 743 patients with an undetectable viral load were enrolled and were randomized to either dolutegravir/lamivudine or a triple drug regimen (two NRTIs as backbone and an anchor from one of major groups). In this study, dual therapy was also found to be non-inferior in maintaining virological suppression compared to triple therapy. In the study cohort, the authors describe a significantly smaller decrease in serum IL-6 levels in patients on dual therapy, but for sCD14 there was an exact opposite trend. The dynamics of D-dimer, hs-CRP and sCD163 were comparable for both groups. In the GEMINI-1&2 studies, it was shown that dolutegravir/lamivudine was virologically non-inferior to INSTI-based cART in treatment-naïve patients, but there were no data on immune activation (35).
As mentioned above, the registration trials briefly addressed the concerns regarding HIV-related immune activation in dual therapy. In general, the results were inconsistent and focused on soluble markers. Fortunately, a few other studies described this issue more extensively although not for the registered treatment regimens. In the study of Concepción Romero-Sánchez et al. 58 patients, having an undetectable viral load for at least 6 months, were switched to a two-drug regimen consisting of a boosted PI and Maraviroc, a HIV entry inhibitor; there was no control group in this study (91). The authors observed no change in β2-microglobuline, sCD40L, sCD14, hsCRP, D-dimer, and mtDNA at 24 (±12) weeks of follow-up when compared to baseline. However, for patients with high baseline levels of β2-microglobuline, sCD40L and hsCRP there was marked decrease at final follow-up. Two other papers evaluated the differences between patients on dual antiretroviral therapy vs. those on triple therapy. Belmonti et al. describe the dynamics of IL-6, CRP, sCD14 and D-dimer from baseline to 48 weeks (92). A switch to dual therapy (n = 70 boosted atazanavir plus lamivudine) did not result in a significant changes in the markers mentioned above and did not differ from the markers in patients continuing triple therapy (n = 69). In addition, Vallejo et al. published a cross-sectional pilot study evaluating a broad spectrum of inflammation and immune activation biomarkers (interferon-gamma-induced protein 10, hs-CRP, sCD14, D-dimer, interferon-γ, TNF-α and IL-4) in patients on dual therapy vs. those continuing triple therapy (93). The dual therapy group consisted of 13 patients that were evaluated at 24 weeks after switch and 36 patients at 48 weeks, the control group included 26 patients. The authors found the lowest IL-6 and sCD14 levels in the patients on dual therapy for 48 weeks; the other markers were not different from the triple-therapy groups. Other studies worth to mention were performed by Quiros-Roldan et al. and Mussini et al. but these papers reported less commonly used parameters such as CD4/CD8 ratio, platelet-to-lymphocyte and neutrophil-to-lymphocyte ratio (94, 95).
In the studies presented above, the switch from triple to dual therapy is not accompanied with a consistent increase in the soluble inflammatory markers. However, in contrast to the monotherapy studies none of the papers assessed T-cell activation, proliferation or apoptosis markers. At this moment, there is sufficient evidence to support certain two drug regimens as treatment options for HIV in terms of virological efficacy but robust data on effects on immune activation are lacking.
Conclusions
In this review we presented the current best available evidence on the dynamics in immune activation in non-traditional antiretroviral therapy. We found that the most well-designed studies show that monotherapy is associated with insufficient suppression of T-cell activation when compared to traditional triple therapy; there might be an association with a detectable viral load. Furthermore, we observed that the dynamics of T-cell activation, proliferation and apoptosis do not necessarily follow the trends observed in the soluble markers, confirming earlier observations.
Especially the last finding is of great importance when we have a look at the data presented for the two-drug regimens, which now have become a reasonable option in modern antiretroviral therapy. The fact that the large registration trials for treatment-experienced patients included inflammatory makers as secondary outcomes is laudable; it emphasizes the recognition of the importance of this outcome. In contrast, the founders of these studies missed an excellent opportunity for a thorough assessment of the immune activation markers in dual therapy. In SWORD-1&2 and TANGO, the soluble markers are only briefly mentioned or the authors stay away from firm statements. Furthermore, the studies only included soluble markers but there are no data on T-cell activation. As we learned from the monotherapy data, especially those markers might display abnormalities. The fact that T-cell activation is correlated with a detectable viremia and that the two-drug regimens show virological non-inferiority with the 50 copies/ml threshold, is reassuring. However, as we are not aware of the degree of residual viremia in the two-drug regimens, a negative impact of dual drug therapy cannot be excluded at this moment.
Based on the presented studies, we believe there is insufficient evidence that mono- and dual therapy are non-inferior to triple therapy when it comes to the suppression of HIV-related immune activation. Although dual therapy is an attractive option as it diminishes the life-time exposure to antiretroviral drugs with potential toxicity, the impact of a rebound in immune activation are currently unknown. We need to keep the potential negative impact of cART in an equilibrium with the degree of immune activation, as a misbalance could lead to HIV or cART-related comorbidity. There is a need for well-designed, longitudinal studies with a proper, unbiased patients selection evaluating both the soluble and the cellular immune activation markers. Only such studies can tell us whether everything is quiet beneath the surface in dual therapy.
Author Contributions
BW and PO: draft of the manuscript and editing. AH: critical review and editing. All authors contributed to the article and approved the submitted version.
Conflict of Interest
The authors declare that the research was conducted in the absence of any commercial or financial relationships that could be construed as a potential conflict of interest.
References
1. Barre-Sinoussi F, Chermann JC, Rey F, Nugeyre MT, Chamaret S, Gruest J, et al. Isolation of a T-lymphotropic retrovirus from a patient at risk for acquired immune deficiency syndrome (AIDS). Science. (1983) 220:868–71. doi: 10.1126/science.6189183
2. Deeks SG, Overbaugh J, Phillips A, Buchbinder S. HIV infection. Nat Rev Dis Prim. (2015) 1:15035. doi: 10.1038/nrdp.2015.35
3. Swain SL, McKinstry KK, Strutt TM. Expanding roles for CD4+ T cells in immunity to viruses. Nat Rev Immunol. (2012) 12:136–48. doi: 10.1038/nri3152
4. Vidya Vijayan KK, Karthigeyan KP, Tripathi SP, Hanna LE. Pathophysiology of CD4+ T-cell depletion in HIV-1 and HIV-2 infections. Front Immunol. (2017) 8:580. doi: 10.3389/fimmu.2017.00580
5. Sodora DL, Silvestri G. Immune activation and AIDS pathogenesis. AIDS. (2008) 22:439–46. doi: 10.1097/QAD.0b013e3282f2dbe7
6. Yates A, Stark J, Klein N, Antia R, Callard R. Understanding the slow depletion of memory CD4+ T cells in HIV infection. PLoS Med. (2007) 4:e177. doi: 10.1371/journal.pmed.0040177
7. Orendi JM, Bloem AC, Borleffs JC, Wijnholds FJ, de Vos NM, Nottet HS, et al. Activation and cell cycle antigens in CD4+ and CD8+ T cells correlate with plasma human immunodeficiency virus (HIV-1) RNA level in HIV-1 infection. J Infect Dis. (1998) 178:1279–87. doi: 10.1086/314451
8. Brenchley JM, Price DA, Schacker TW, Asher TE, Silvestri G, Rao S, et al. Microbial translocation is a cause of systemic immune activation in chronic HIV infection. Nat Med. (2006) 12:1365–71. doi: 10.1038/nm1511
9. Teer E, Joseph DE, Driescher N, Nell TA, Dominick L, Midgley N, et al. HIV and cardiovascular diseases risk: exploring the interplay between T-cell activation, coagulation, monocyte subsets, and lipid subclass alterations. Am J Physiol Heart Circ Physiol. (2019) 316:H1146–57. doi: 10.1152/ajpheart.00797.2018
10. Goh SSL, Lai PSM, Tan ATB, Ponnampalavanar S. Reduced bone mineral density in human immunodeficiency virus-infected individuals: a meta-analysis of its prevalence and risk factors. Osteoporos Int. (2018) 29:595–613. doi: 10.1007/s00198-017-4305-8
11. van BJ, Mudrikova Welzen T, El Idrissi A, Hoepelman AIM, Arends JE. A review of non-alcoholic fatty liver disease in HIV-infected patients: the next big thing? Infect Dis Ther. (2019) 8:33–50. doi: 10.1007/s40121-018-0229-7
12. Giorgi JV, Liu Z, Hultin LE, Cumberland WG, Hennessey K, Detels R. Elevated levels of CD38+ CD8+ T cells in HIV infection add to the prognostic value of low CD4+ T cell levels: results of 6 years of follow-up. The Los Angeles Center, Multicenter AIDS Cohort Study. J Acquir Immune Defic Syndr. (1993) 6:904–12.
13. Hazenberg MD, Otto SA, van Benthem BHB, Roos MTL, Coutinho RA, Lange JMA, et al. Persistent immune activation in HIV-1 infection is associated with progression to AIDS. AIDS. (2003) 17:1881–8. doi: 10.1097/00002030-200309050-00006
14. Fischl MA, Richman DD, Grieco MH, Gottlieb MS, Volberding PA, Laskin OL, et al. The efficacy of azidothymidine (AZT) in the treatment of patients with AIDS and AIDS-related complex. A double-blind, placebo-controlled trial. N Engl J Med. (1987) 317:185–91. doi: 10.1056/NEJM198707233170401
15. Lorenzi P, Opravil M, Hirschel B, Chave JP, Furrer HJ, Sax H, et al. Impact of drug resistance mutations on virologic response to salvage therapy. Swiss HIV Cohort Study. AIDS. (1999) 13:F17–21. doi: 10.1097/00002030-199902040-00001
16. Kitchen VS, Skinner C, Ariyoshi K, Lane EA, Duncan IB, Burckhardt J, et al. Safety and activity of saquinavir in HIV infection. Lancet. (1995) 345:952–5. doi: 10.1016/S0140-6736(95)90699-1
17. Gulick RM, Mellors JW, Havlir D, Eron JJ, Gonzalez C, McMahon D, et al. Treatment with indinavir, zidovudine, and lamivudine in adults with human immunodeficiency virus infection and prior antiretroviral therapy. N Engl J Med. (1997) 337:734–9. doi: 10.1056/NEJM199709113371102
18. Vazquez E. Nevirapine approval expands combo possibilities–and HIV suppression. Posit Aware. (1996) 7:9.
19. Markowitz M, Nguyen B-Y, Gotuzzo E, Mendo F, Ratanasuwan W, Kovacs C, et al. Rapid and durable antiretroviral effect of the HIV-1 integrase inhibitor raltegravir as part of combination therapy in treatment-naive patients with HIV-1 infection: results of a 48-week controlled study. J Acquir Immune Defic Syndr. (2007) 46:125–33. doi: 10.1097/QAI.0b013e318157131c
20. van Sighem AI, Wit F, Boyd A, Smit C, Matser A, Reiss P. Monitoring Report 2019. Human Immunodeficiency Virus (HIV) Infection in the Netherlands. Amsterdam: Stichting HIV Monitoring (2019).
21. Smith CJ, Ryom L, Weber R, Morlat P, Pradier C, Reiss P, et al. Trends in underlying causes of death in people with HIV from 1999 to 2011 (D:A:D): a multicohort collaboration. Lancet. (2014) 384:241–8. doi: 10.1016/S0140-6736(14)60604-8
22. Gebara NY, El Kamari V, Rizk N. HIV-1 elite controllers: an immunovirological review and clinical perspectives. J Virus Erad. (2019) 5:163–6. doi: 10.1016/S2055-6640(20)30046-7
23. Hunt PW, Brenchley J, Sinclair E, McCune JM, Roland M, Page-Shafer K, et al. Relationship between T cell activation and CD4+ T cell count in HIV-seropositive individuals with undetectable plasma HIV RNA levels in the absence of therapy. J Infect Dis. (2008) 197:126–33. doi: 10.1086/524143
24. Prabhu VM, Singh AK, Padwal V, Nagar V, Patil P, Patel V. Monocyte based correlates of immune activation and viremia in HIV-Infected long-term non-progressors. Front Immunol. (2019) 10:2849. doi: 10.3389/fimmu.2019.02849
25. Crowell TA, Hatano H. Clinical outcomes and antiretroviral therapy in “elite” controllers: a review of the literature. J Virus Erad. (2015) 1:72–7. doi: 10.1016/S2055-6640(20)30488-X
26. Gandhi RT, McMahon DK, Bosch RJ, Lalama CM, Cyktor JC, Macatangay BJ, et al. Levels of HIV-1 persistence on antiretroviral therapy are not associated with markers of inflammation or activation. PLoS Pathog. (2017) 13:e1006285. doi: 10.1371/journal.ppat.1006285
27. Moreno S, Perno CF, Mallon PW, Behrens G, Corbeau P, Routy J-P, et al. Two-drug vs. three-drug combinations for HIV-1: do we have enough data to make the switch? HIV Med. (2019) 20 (Suppl. 4):2–12. doi: 10.1111/hiv.12716
28. Wijting I, Rokx C, Boucher C, van Kampen J, Pas S, de Vries-Sluijs T, et al. Dolutegravir as maintenance monotherapy for HIV (DOMONO): a phase 2, randomised non-inferiority trial. Lancet HIV. (2017) 4:e547–54. doi: 10.1016/S2352-3018(17)30152-2
29. van Wyk J. Switching to DTG/3TC Fixed-Dose Combination (FDC) is Non-inferior to Continuing a TAF-Based Regimen in Maintaining Virological Suppression Through 48 Weeks (TANGO Study). (2019). Mexico City: IAS.
30. Hocqueloux L, Raffi F, Prazuck T, Bernard L, Sunder S, Esnault J-L, et al. Dolutegravir monotherapy vs. dolutegravir/abacavir/lamivudine for virologically suppressed people living with chronic human immunodeficiency virus infection: the randomized noninferiority MONotherapy of TiviCAY trial. Clin Infect Dis. (2019) 69:1498–505. doi: 10.1093/cid/ciy1132
31. Braun DL, Turk T, Tschumi F, Grube C, Hampel B, Depmeier C, et al. Noninferiority of simplified dolutegravir monotherapy compared to continued combination antiretroviral therapy that was initiated during primary human immunodeficiency virus infection: a randomized, controlled, multisite, open-label, noninferiority trial. Clin Infect Dis. (2019) 69:1489–97. doi: 10.1093/cid/ciy1131
32. Seang S, Schneider L, Nguyen T, Lê MP, Soulie C, Calin R, et al. Darunavir/ritonavir monotherapy at a low dose (600/100 mg/day) in HIV-1-infected individuals with suppressed HIV viraemia. J Antimicrob Chemother. (2018) 73:490–3. doi: 10.1093/jac/dkx417
33. Galli L, Spagnuolo V, Bigoloni A, D'Arminio Monforte A, Montella F, Antinori A, et al. Atazanavir/ritonavir monotherapy: 96 week efficacy, safety and bone mineral density from the MODAt randomized trial. J Antimicrob Chemother. (2016) 71:1637–42. doi: 10.1093/jac/dkw031
34. Swindells S, Andrade-Villanueva J-F, Richmond GJ, Rizzardini G, Baumgarten A, Masiá M, et al. Long-acting cabotegravir and rilpivirine for maintenance of HIV-1 suppression. N Engl J Med. (2020) 382:1112–23. doi: 10.1056/NEJMoa1904398
35. Cahn P, Madero JS, Arribas JR, Antinori A, Ortiz R, Clarke AE, et al. Durable efficacy of dolutegravir plus lamivudine in antiretroviral treatment-naive adults with HIV-1 infection: 96-week results from the GEMINI-1 and GEMINI-2 randomized clinical trials. J Acquir Immune Defic Syndr. (2020) 83:310–8. doi: 10.1097/QAI.0000000000002275
36. Aboud M, Orkin C, Podzamczer D, Bogner JR, Baker D, Khuong-Josses M-A, et al. Efficacy and safety of dolutegravir-rilpivirine for maintenance of virological suppression in adults with HIV-1: 100-week data from the randomised, open-label, phase 3 SWORD-1 and SWORD-2 studies. Lancet HIV. (2019) 6:e576–87. doi: 10.1016/S2352-3018(19)30149-3
37. European AIDS Clinical Society (EACS). Guidelines. Version 10.0 November 2019. (2019). Available online at: https://www.eacsociety.org/files/2019_guidelines-10.0_final.pdf (accessed December 1, 2020).
38. Panel on Antiretroviral Guidelines for Adults Adolescents. Guidelines for the Use of Antiretroviral Agents in Adults Adolescents With HIV. Department of Health and Human Services. Available online at: http://aidsinfo.nih.gov/contentfiles/lvguidelines/ (accessed December 1, 2020).
39. Serrano-Villar S, Moreno S. Changes in inflammatory biomarkers in SWORD-1 and SWORD-2 studies. Lancet HIV. (2020) 7:e158. doi: 10.1016/S2352-3018(20)30028-X
40. Nixon DE, Landay AL. Biomarkers of immune dysfunction in HIV. Curr Opin HIV AIDS. (2010) 5:498–503. doi: 10.1097/COH.0b013e32833ed6f4
41. Kaur S, Bansal Y, Kumar R, Bansal G. A panoramic review of IL-6: structure, pathophysiological roles and inhibitors. Bioorg Med Chem. (2020) 28:115327. doi: 10.1016/j.bmc.2020.115327
42. Pepys MB, Baltz ML. Acute phase proteins with special reference to C-reactive protein and related proteins (pentaxins) and serum amyloid A protein. Adv Immunol. (1983) 34:141–212. doi: 10.1016/S0065-2776(08)60379-X
43. Baker JV, Neuhaus J, Duprez D, Kuller LH, Tracy R, Belloso WH, et al. Changes in inflammatory and coagulation biomarkers: a randomized comparison of immediate vs. deferred antiretroviral therapy in patients with HIV infection. J Acquir Immune Defic Syndr. (2011) 56:36–43. doi: 10.1097/QAI.0b013e3181f7f61a
44. Neuhaus J, Jacobs DRJ, Baker JV, Calmy A, Duprez D, La Rosa A, et al. Markers of inflammation, coagulation, and renal function are elevated in adults with HIV infection. J Infect Dis. (2010) 201:1788–95. doi: 10.1086/652749
45. Kalayjian RC, Machekano RN, Rizk N, Robbins GK, Gandhi RT, Rodriguez BA, et al. Pretreatment levels of soluble cellular receptors and interleukin-6 are associated with HIV disease progression in subjects treated with highly active antiretroviral therapy. J Infect Dis. (2010) 201:1796–805. doi: 10.1086/652750
46. Kedzierska K, Crowe SM. Cytokines and HIV-1: interactions and clinical implications. Antivir Chem Chemother. (2001) 12:133–50. doi: 10.1177/095632020101200301
47. Vaidya SA, Korner C, Sirignano MN, Amero M, Bazner S, Rychert J, et al. Tumor necrosis factor α is associated with viral control and early disease progression in patients with HIV type 1 infection. J Infect Dis. (2014) 210:1042–6. doi: 10.1093/infdis/jiu206
48. Hunt PW. HIV and inflammation: mechanisms and consequences. Curr HIV AIDS Rep. (2012) 9:139–47. doi: 10.1007/s11904-012-0118-8
49. Cobos Jiménez V, Wit F, Joerink M, Maurer I, Harskamp AM, Schouten J, et al. T-cell activation independently associates with immune senescence in HIV-infected recipients of long-term antiretroviral treatment. J Infect Dis. (2016) 214:216–25. doi: 10.1093/infdis/jiw146
50. Nasi M, Pecorini S, De Biasi S, Digaetano M, Chester J, Aramini B, et al. Short communication: circulating mitochondrial DNA and lipopolysaccharide-binding protein but not bacterial DNA are increased in acute human immunodeficiency virus infection. AIDS Res Hum Retroviruses. (2020) 36:817–20. doi: 10.1089/aid.2020.0098
51. Uysal HK, Sohrabi P, Habip Z, Saribas S, Kocazeybek E, Seyhan F, et al. Neopterin and soluble CD14 levels as indicators of immune activation in cases with indeterminate pattern and true positive HIV-1 infection. PLoS ONE. (2016) 11:e0152258. doi: 10.1371/journal.pone.0152258
52. Roff SR, Noon-Song EN, Yamamoto JK. The significance of interferon-γ in HIV-1 pathogenesis, therapy, and prophylaxis. Front Immunol. (2014) 4:498. doi: 10.3389/fimmu.2013.00498
53. De Milito A, Aleman S, Marenzi R, Sonnerborg A, Fuchs D, Zazzi M, et al. Plasma levels of soluble CD27: a simple marker to monitor immune activation during potent antiretroviral therapy in HIV-1-infected subjects. Clin Exp Immunol. (2002) 127:486–94. doi: 10.1046/j.1365-2249.2002.01786.x
54. Miller EA, Gopal R, Valdes V, Berger JS, Bhardwaj N, O'Brien MP. Soluble CD40 ligand contributes to dendritic cell-mediated T-cell dysfunction in HIV-1 infection. AIDS. (2015) 29:1287–96. doi: 10.1097/QAD.0000000000000698
55. Vos AG, Idris NS, Barth RE, Klipstein-Grobusch K, Grobbee DE. Pro-Inflammatory markers in relation to cardiovascular disease in HIV infection. A systematic review. PLoS ONE. (2016) 11:e0147484. doi: 10.1371/journal.pone.0147484
56. Borges AH, O'Connor JL, Phillips AN, Baker JV, Vjecha MJ, Losso MH, et al. Factors associated with D-dimer levels in HIV-infected individuals. PLoS ONE. (2014) 9:e90978. doi: 10.1371/journal.pone.0090978
57. Sandler NG, Douek DC. Microbial translocation in HIV infection: causes, consequences and treatment opportunities. Nat Rev Microbiol. (2012) 10:655–66. doi: 10.1038/nrmicro2848
58. Sandler NG, Wand H, Roque A, Law M, Nason MC, Nixon DE, et al. Plasma levels of soluble CD14 independently predict mortality in HIV infection. J Infect Dis. (2011) 203:780–90. doi: 10.1093/infdis/jiq118
59. Dayer E, Dayer JM, Roux-Lombard P. Primer: the practical use of biological markers of rheumatic and systemic inflammatory diseases. Nat Clin Pract Rheumatol. (2007) 3:512–20. doi: 10.1038/ncprheum0572
60. Kestens L, Vanham G, Gigase P, Young G, Hannet I, Vanlangendonck F, et al. Expression of activation antigens, HLA-DR and CD38, on CD8 lymphocytes during HIV-1 infection. AIDS. (1992) 6:793–7. doi: 10.1097/00002030-199208000-00004
61. Savarino A, Bottarel F, Malavasi F, Dianzani U. Role of CD38 in HIV-1 infection: an epiphenomenon of T-cell activation or an active player in virus/host interactions? AIDS. (2000) 14:1079–89. doi: 10.1097/00002030-200006160-00004
62. Niehues T, McCloskey TW, Ndagijimana J, Horneff G, Wahn V, Pahwa S. Apoptosis in T-lymphocyte subsets in human immunodeficiency virus-infected children measured immediately ex vivo and following in vitro activation. Clin Diagn Lab Immunol. (2001) 8:74–8. doi: 10.1128/CDLI.8.1.74-78.2001
63. Velu V, Shetty RD, Larsson M, Shankar EM. Role of PD-1 co-inhibitory pathway in HIV infection and potential therapeutic options. Retrovirology. (2015) 12:14. doi: 10.1186/s12977-015-0144-x
64. Mussini C, Lorenzini P, Cozzi-Lepri A, Lapadula G, Marchetti G, Nicastri E, et al. CD4/CD8 ratio normalisation and non-AIDS-related events in individuals with HIV who achieve viral load suppression with antiretroviral therapy: an observational cohort study. Lancet HIV. (2015) 2:e98–106. doi: 10.1016/S2352-3018(15)00006-5
65. Sereti I, Krebs SJ, Phanuphak N, Fletcher JL, Slike B, Pinyakorn S, et al. Persistent, albeit reduced, chronic inflammation in persons starting antiretroviral therapy in acute HIV infection. Clin Infect Dis. (2017) 64:124–31. doi: 10.1093/cid/ciw683
66. Bloch M, John M, Smith D, Rasmussen TA, Wright E. Managing HIV-associated inflammation and ageing in the era of modern ART. HIV Med. (2020) 21 (Suppl. 3):2–16. doi: 10.1111/hiv.12952
67. McComsey GA, Kitch D, Daar ES, Tierney C, Jahed NC, Melbourne K, et al. Inflammation markers after randomization to abacavir/lamivudine or tenofovir/emtricitabine with efavirenz or atazanavir/ritonavir. AIDS. (2012) 26:1371–85. doi: 10.1097/QAD.0b013e328354f4fb
68. Eastburn A, Scherzer R, Zolopa AR, Benson C, Tracy R, Do T, et al. Association of low level viremia with inflammation and mortality in HIV-infected adults. PLoS ONE. (2011) 6:e26320. doi: 10.1371/journal.pone.0026320
69. Robbins GK, Spritzler JG, Chan ES, Asmuth DM, Gandhi RT, Rodriguez BA, et al. Incomplete reconstitution of T cell subsets on combination antiretroviral therapy in the AIDS Clinical Trials Group protocol 384. Clin Infect Dis. (2009) 48:350–361. doi: 10.1086/595888
70. Massanella M, Fromentin R, Chomont N. Residual inflammation and viral reservoirs: alliance against an HIV cure. Curr Opin HIV AIDS. (2016) 11:234–41. doi: 10.1097/COH.0000000000000230
71. Dyavar SR, Gautam N, Podany AT, Winchester LC, Weinhold JA, Mykris TM, et al. Assessing the lymphoid tissue bioavailability of antiretrovirals in human primary lymphoid endothelial cells and in mice. J Antimicrob Chemother. (2019) 74:2974–8. doi: 10.1093/jac/dkz273
72. Thompson CG, Cohen MS, Kashuba ADM. Antiretroviral pharmacology in mucosal tissues. J Acquir Immune Defic Syndr. (2013) 63 (Suppl. 2):S240–7. doi: 10.1097/QAI.0b013e3182986ff8
73. Caniglia EC, Cain LE, Justice A, Tate J, Logan R, Sabin C, et al. Antiretroviral penetration into the CNS and incidence of AIDS-defining neurologic conditions. Neurology. (2014) 83:134–41. doi: 10.1212/WNL.0000000000000564
74. Cassol E, Malfeld S, Mahasha P, van der Merwe S, Cassol S, Seebregts C, et al. Persistent microbial translocation and immune activation in HIV-1-infected South Africans receiving combination antiretroviral therapy. J Infect Dis. (2010) 202:723–33. doi: 10.1086/655229
75. Boulougoura A, Sereti I. HIV infection and immune activation: the role of coinfections. Curr Opin HIV AIDS. (2016) 11:191–200. doi: 10.1097/COH.0000000000000241
76. Hileman CO, Kinley B, Scharen-Guivel V, Melbourne K, Szwarcberg J, Robinson J, et al. Differential reduction in monocyte activation and vascular inflammation with integrase inhibitor-based initial antiretroviral therapy among HIV-infected individuals. J Infect Dis. (2015) 212:345–54. doi: 10.1093/infdis/jiv004
77. Kelesidis T, Tran TTT, Stein JH, Brown TT, Moser C, Ribaudo HJ, et al. Changes in inflammation and immune activation with atazanavir-, raltegravir-, darunavir-based initial antiviral therapy: ACTG 5260s. Clin Infect Dis. (2015) 61:651–60. doi: 10.1093/cid/civ327
78. Martínez E, D'Albuquerque PM, Llibre JM, Gutierrez F, Podzamczer D, Antela A, et al. Changes in cardiovascular biomarkers in HIV-infected patients switching from ritonavir-boosted protease inhibitors to raltegravir. AIDS. (2012) 26:2315–26. doi: 10.1097/QAD.0b013e328359f29c
79. van Lelyveld SFL, Drylewicz J, Krikke M, Veel EM, Otto SA, Richter C, et al. Maraviroc intensification of cART in patients with suboptimal immunological recovery: a 48-week, placebo-controlled randomized trial. PLoS ONE. (2015) 10:e0132430. doi: 10.1371/journal.pone.0132430
80. Kim CJ, Rousseau R, Huibner S, Kovacs C, Benko E, Shahabi K, et al. Impact of intensified antiretroviral therapy during early HIV infection on gut immunology and inflammatory blood biomarkers. AIDS. (2017) 31:1529–34. doi: 10.1097/QAD.0000000000001515
81. Llibre JM, Buzón MJ, Massanella M, Esteve A, Dahl V, Puertas MC, et al. Treatment intensification with raltegravir in subjects with sustained HIV-1 viraemia suppression: a randomized 48-week study. Antivir Ther. (2012) 17:355–64. doi: 10.3851/IMP1917
82. BenMarzouk-Hidalgo OJ, Torres-Cornejo A, Gutiérrez-Valencia A, Ruiz-Valderas R, Viciana P, López-Cortés LF. Differential effects of viremia and microbial translocation on immune activation in HIV-infected patients throughout ritonavir-boosted darunavir monotherapy. Medicine. (2015) 94:e781. doi: 10.1097/MD.0000000000000781
83. Puertas MC, Gómez-Mora E, Santos JR, Moltó J, Urrea V, Morón-López S, et al. Impact of intensification with raltegravir on HIV-1-infected individuals receiving monotherapy with boosted PIs. J Antimicrob Chemother. (2018) 73:1940–8. doi: 10.1093/jac/dky106
84. Arenas-Pinto A, Milinkovic A, Peppa D, McKendry A, Maini M, Gilson R. Systemic inflammation and residual viraemia in HIV-positive adults on protease inhibitor monotherapy: a cross-sectional study. BMC Infect Dis. (2015) 15:138. doi: 10.1186/s12879-015-0889-9
85. Arribas J, Hill A, Xi N, van Delft Y, Moecklinghoff C. Interleukin-6 and C-reactive protein levels after 3 years of treatment with darunavir/ritonavir monotherapy or darunavir/ritonavir + two nucleoside reverse transcriptase inhibitors in the MONET trial. J Antimicrob Chemother. (2012) 67:1804–6. doi: 10.1093/jac/dks102
86. Estébanez M, Stella-Ascariz N, Mingorance J, Pérez-Valero I, Bernardino JI, Zamora FX, et al. Inflammatory, procoagulant markers and HIV residual viremia in patients receiving protease inhibitor monotherapy or triple drug therapy: a cross-sectional study. BMC Infect Dis. (2014) 14:379. doi: 10.1186/1471-2334-14-379
87. Merlini E, Galli L, Tincati C, Cannizzo ES, Galli A, Gianotti N, et al. Immune activation, inflammation and HIV DNA after 96 weeks of ATV/r monotherapy: a MODAt substudy. Antivir Ther. (2018) 23:633–7. doi: 10.3851/IMP3234
88. Torres B, Guardo AC, Leal L, Leon A, Lucero C, Alvarez-Martinez MJ, et al. Protease inhibitor monotherapy is associated with a higher level of monocyte activation, bacterial translocation and inflammation. J Int AIDS Soc. (2014) 17:19246. doi: 10.7448/IAS.17.1.19246
89. Petrara MR, Cattelan AM, Sasset L, Freguja R, Carmona F, Sanavia S, et al. Impact of monotherapy on HIV-1 reservoir, immune activation, and co-infection with Epstein-Barr virus. PLoS ONE. (2017) 12:e0185128. doi: 10.1371/journal.pone.0185128
90. van Wyk J, Orkin C, Rubio R, Bogner J, Baker D, Khuong-Josses M-A, et al. Brief report: durable suppression and low rate of virologic failure 3 years after switch to dolutegravir + rilpivirine 2-drug regimen: 148-week results from the SWORD-1 and SWORD-2 randomized clinical trials. J Acquir Immune Defic Syndr. (2020) 85:325–30. doi: 10.1097/QAI.0000000000002449
91. Romero-Sánchez MC, Alvarez-Ríos AI, Bernal-Morell E, Genebat M, Vera F, Benhnia MR-E-I, et al. Maintenance of virologic efficacy and decrease in levels of β2-microglobulin, soluble CD40L and soluble CD14 after switching previously treated HIV-infected patients to an NRTI-sparing dual therapy. Antiviral Res. (2014) 111:26–32. doi: 10.1016/j.antiviral.2014.08.011
92. Belmonti S, Lombardi F, Quiros-Roldan E, Latini A, Castagna A, Borghetti A, et al. Systemic inflammation markers after simplification to atazanavir/ritonavir plus lamivudine in virologically suppressed HIV-1-infected patients: ATLAS-M substudy. J Antimicrob Chemother. (2018) 73:1949–54. doi: 10.1093/jac/dky125
93. Vallejo A, Molano M, Monsalvo-Hernando M, Hernández-Walias F, Fontecha-Ortega M, Casado JL. Switching to dual antiretroviral regimens is associated with improvement or no changes in activation and inflammation markers in virologically suppressed HIV-1-infected patients: the TRILOBITHE pilot study. HIV Med. (2019) 20:555–60. doi: 10.1111/hiv.12749
94. Quiros-Roldan E, Magro P, Raffetti E, Izzo I, Borghetti A, Lombardi F, et al. Biochemical and inflammatory modifications after switching to dual antiretroviral therapy in HIV-infected patients in Italy: a multicenter retrospective cohort study from 2007 to 2015. BMC Infect Dis. (2018) 18:285. doi: 10.1186/s12879-018-3198-2
Keywords: HIV, antiretroviral therapy, immune activation, dual therapy, cART
Citation: van Welzen BJ, Oomen PGA and Hoepelman AIM (2021) Dual Antiretroviral Therapy—All Quiet Beneath the Surface? Front. Immunol. 12:637910. doi: 10.3389/fimmu.2021.637910
Received: 04 December 2020; Accepted: 22 January 2021;
Published: 12 February 2021.
Edited by:
Caroline Petitdemange, Institut Pasteur, FranceReviewed by:
Giulia Carla Marchetti, University of Milan, ItalyClaudia Cicala, National Institutes of Health (NIH), United States
Copyright © 2021 van Welzen, Oomen and Hoepelman. This is an open-access article distributed under the terms of the Creative Commons Attribution License (CC BY). The use, distribution or reproduction in other forums is permitted, provided the original author(s) and the copyright owner(s) are credited and that the original publication in this journal is cited, in accordance with accepted academic practice. No use, distribution or reproduction is permitted which does not comply with these terms.
*Correspondence: Berend J. van Welzen, b.j.vanwelzen@umcutrecht.nl