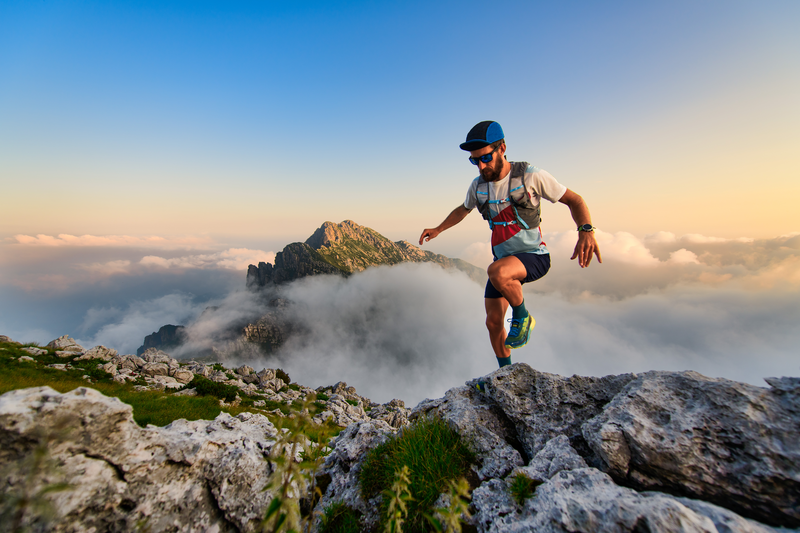
94% of researchers rate our articles as excellent or good
Learn more about the work of our research integrity team to safeguard the quality of each article we publish.
Find out more
ORIGINAL RESEARCH article
Front. Immunol. , 08 June 2021
Sec. Molecular Innate Immunity
Volume 12 - 2021 | https://doi.org/10.3389/fimmu.2021.625922
This article is part of the Research Topic Neutrophil Functions in Host Immunity, Inflammation and Tissue Repair View all 24 articles
Low density neutrophils (LDNs) are described in a number of inflammatory conditions, cancers and infections and associated with immunopathology, and a mechanistic role in disease. The role of LDNs at homeostasis in healthy individuals has not been investigated. We have developed an isolation protocol that generates high purity LDNs from healthy donors. Healthy LDNs were identical to healthy normal density neutrophils (NDNs), aside from reduced neutrophil extracellular trap formation. CD66b, CD16, CD15, CD10, CD54, CD62L, CXCR2, CD47 and CD11b were expressed at equivalent levels in healthy LDNs and NDNs and underwent apoptosis and ROS production interchangeably. Healthy LDNs had no differential effect on CD4+ or CD8+ T cell proliferation or IFNγ production compared with NDNs. LDNs were generated from healthy NDNs in vitro by activation with TNF, LPS or fMLF, suggesting a mechanism of LDN generation in disease however, we show neutrophilia in people with Cystic Fibrosis (CF) was not due to increased LDNs. LDNs are present in the neutrophil pool at homeostasis and have limited functional differences to NDNs. We conclude that increased LDN numbers in disease reflect the specific pathology or inflammatory environment and that neutrophil density alone is inadequate to classify discrete functional populations of neutrophils.
Heterogeneity is a characteristic of human neutrophil populations (1), yet whether this represents truly diverse neutrophil subsets, plasticity during the immune response or differences in maturation state is undetermined. Low density neutrophils (LDNs) are a suggested subpopulation of neutrophils, first described in humans with systemic lupus erythematosus (2), that may be separated from other granulocytes by discontinuous density gradient (2). LDNs have a similar density to peripheral blood mononuclear cells (PBMCs) and are isolated alongside these cells, in contrast to normal density neutrophils (NDNs) which segregate with other polymorphonuclear (PMN) cells during density exclusion (3, 4).
LDNs have been described in pregnancy (5), autoimmune disease (6, 7), cancer (8, 9), infection (10–13) and inflammation (14, 15), and speculated to contribute to pathophysiology. Across these studies, LDNs do not represent one discrete neutrophil sub-population, but rather a spectrum of multiple neutrophil phenotypes, which vary in morphology, maturation and activation dependent on the underlying disease process. In addition, previously characterised monocyte-derived suppressor cells (MDSCs) that suppress T cell function can also contribute to the LDN population (16, 17), further complicating the description of these cells between patient groups. Simply isolating LDN populations by density exclusion produces mixed populations of cells, and the lack of consistent LDN markers means that cell sorting techniques from whole blood are unsuitable.
Early studies counted LDNs based on density alone (2), and in some cases LDNs are identified by flow cytometry as neutrophils in the PBMC layer. These studies are limited to characterising neutrophil phenotype without assessing the function of pure LDNs (3, 5, 13, 14, 17–21). In addition, isolation of neutrophils within the PBMC and in the presence of monocytes can result in their activation (22). For functional assays, further isolation of LDNs from PBMC is performed by FACS (10, 12) which can also cause artefactual activation or by magnetic bead selection which is problematic if the NDNs do not undergo the same protocol (7, 23–27) and are instead subject to second density gradient or RBC lysis treatment. This is an important consideration as differences in isolation method can significantly alter granulocyte markers often measured in the context of LDN characterisation (28). Furthermore many functional studies of LDNs have compared LDNs isolated from patient groups to healthy control and patient NDNs (not healthy LDNs) as a control (15, 29, 30).
The described function of LDNs varies by the disease. In Systemic Lupus Erythematosus (SLE) and other autoimmune diseases, LDNs are highly inflammatory, produce type I interferon and activate T cells to produce TNFα and type II interferon (7, 25). A similar phenotype is also seen in some infections (10). Conversely in Mycobacterium tuberculosis infection or following surgical stress, LDNs suppress T cell proliferation and IFNγ production (26, 27). The ability of LDNs to generate neutrophil extracellular traps (NETs) also varies between conditions. In SLE and Psoriasis, LDNs produce increased NETs compared with NDNs from the same donor (14, 23), while in Rheumatoid Arthritis, NET formation did not appear to differ (30), but importantly healthy LDNs were not included as a comparator. These data suggest that low neutrophil density may represent ‘disease specific’ neutrophil populations rather than a distinct class of neutrophils common to all conditions.
To interrogate whether LDNs are a distinct class of neutrophil with unique functional characteristics, we first developed a robust method to isolate pure LDNs from healthy control blood. We then compared these to a disease control associated with infection and inflammation, namely Cystic Fibrosis, (CF). We have previously demonstrated a pro-survival phenotype and increased incidence of NET formation in CF neutrophils (31) but the contribution of LDNs to these findings has not been assessed.
In summary, we demonstrate a novel protocol to isolate highly pure populations of NDNs and LDNs from healthy donors. We define and comprehensively phenotype the characteristics of LDNs in the circulating neutrophil population of healthy donors and clinically stable CF patients, and found many similarities. We show that LDN nuclear morphology differs to autologous NDNs, and have reduced capacity for NET formation, despite similar levels of ROS generation and cell death by apoptosis. Finally, we demonstrate that NDNs can become low-density following activation with a number of inflammatory mediators. This research identifies an LDN population within healthy donors that have no significantly different function to circulating NDNs, aside from a perturbation of NET formation. In addition, it highlights the limitations of defining neutrophils by density alone and implies that neutrophils with low density are generated during inflammation or as a developmental step in maturation and are not a unique subset of cells with defined function.
Healthy blood was collected in accordance with The Centre for Inflammation Research Blood Resource (AMREC, 148 15/HV/013). Healthy donors were defined as age and sexed matched, non-CF donors with no evidence of any illness at the time of recruitment to the study. Clinically stable CF patients with one F508del mutation were recruited according to NRS Bioresource, East of Scotland research ethics committee 15/ES/0094. 12 ml of blood was collected into a tube containing 3.4% sodium citrate to prevent coagulation. Serum was isolated by centrifugation at 350 x g for 20 minutes at RT.
Total neutrophils were isolated from 12ml healthy or CF patient blood by negative selection using an EasySep™ Direct Human Neutrophil Isolation Kit (Stem Cell). LDN and NDNs were then isolated by discontinuous Percoll density gradient. Briefly, 27ml Percoll (GE Healthcare) was added to 3ml 10x PBS-/- (Gibco) to create a 100% solution. Then, 3ml 81% Percoll diluted in 1x PBS-/- (Gibco) was added to a 15ml tube (Falcon) carefully followed by a 3ml layer of 70% Percoll and finally, total neutrophils, resuspended in 3ml 55% Percoll. Centrifugation at 720xg without brake was performed at RT for 30 minutes. NDNs were recovered from the 71%/55% interface and LDNs from 70%/81% interface (see Figure 1A). Cell counts were performed on a NucleoCounter® NC-100 (Chemometec). Cytospins were performed in 1% BSA (Sigma) in PBS-/- in a Cytospin 4 (Thermo) and stained with Kwik-Diff stains (Shandon) according to manufacturer’s instructions. Neutrophil purity was determined by microscopic analysis of the cytospin. For identification of neutrophils in peripheral blood mononuclear cells (PBMC) and polymorphonuclear cells (PMN), 12 mls blood was centrifuged at 720 x g for 20 min, the plasma was removed, and the blood cells were resuspended in 50mls 0.9% saline containing 6mls 6% dextran. After sedimentation for 30 minutes at room temperature, the enriched white blood cell layer was aspirated, centrifuged and resuspended in 55% Percoll. A gradient was formed as above and after centrifugation, PBMC were recovered from the 71%/55% interface while PMN were recovered from the 81%/70% interface. Each were stained with CD66b and CD16 antibodies (Biolegend) for 30 mins at 4°C. Fc block was not used. Samples were then analysed on an Attune NxT Flow cytometer (Thermo).
Figure 1 Isolation of a high purity population of low density neutrophils from healthy donors (A) Overview of traditional isolation protocol PBMC, peripheral blood mononuclear cells PMN, polymorphonuclear cells; RBC, red blood cells. (B) Representative images of cells isolated by traditional protocol. Black arrows show neutrophils in PBMC layer. (C) Overview of magnetic, negative isolation protocol, LDN, low density neutrophils; NDN, normal density neutrophils. (D) Representative images of enriched LDNs and NDNs isolated by magnetic, negative selection protocol (E) Quantification of neutrophils isolated by each protocol, (N=5) (F) Total number of neutrophils (CD66b+ CD16+) identified within the PBMC population isolated from 12 mls blood by flow cytometry (N=5) (G) Total number of neutrophils isolated from 12mls blood by magnetic isolation (N=10). Statistical testing with two tailed unpaired T test where **p ≤ 0.01 Data shows means ± SEM error bars.
1x106 LDN or NDN were suspended in PBS-/- + 2% foetal calf serum (Gibco) and stained with CD66b, CD16, CD15, CD10, CD11b, CD54, CD47, CXCR2 and CD62L antibodies (Biolegend) for 30 minutes at 4°C. Fc block was not used. Samples were then analysed on an Aurora Spectral Flow cytometer (Cytek). Gating strategy shown in Figure 2A. Auto-fluorescence was measured by mean fluorescence intensity in each detector on unstained cells to produce a spectral map.
Figure 2 Expression of neutrophil markers on LDN and NDNs (A) Gating strategy to identify neutrophils (CD66b+ CD49d-) and remove any remaining eosinophils (CD66b+ CD49d+) after magnetic isolation. (B) Markers of neutrophil maturation measured by flow cytometry, shown as median fluorescence intensity (N=5). Linked samples show LDN and NDN from the same donor. (C) Markers of neutrophil activation measured by flow cytometry, shown as median fluorescence intensity (N=5). (D) NDN overlaid with LDN from a representative healthy donor to demonstrate combinations of markers previously reported to identify low density neutrophils, CD16HI, CD66bHI and CD62LLO,CD66b+. (E) Spectral intensity recorded on unstained LDN and NDN cells (N=5, Mean ± SEM error bars).
5x105 LDN or NDN were suspended in 200μl PBS-/- +2% donor serum and incubated at 37°C, 5% CO2 for 24 hrs. Neutrophils were then stained with Annexin-V-FLUOS Staining Kit (Sigma) following manufacturer’s instructions and analysed by flow cytometry on an LSR Fortessa (BD) and FlowJo software (BD) based on previously described methods (32).
5x104 LDN or NDN were suspended in 200μl PBS-/- +2% donor serum. NET formation was stimulated with 100nM PMA and detected with SYTOX™ Green nucleic acid stain (Thermo) at 528nm during 6 hr incubation at 37°C in a Synergy HT (BioTek) plate reader (as previously described) (31). NET formation was confirmed by fluorescence microscopy. For immunocytochemistry assay, 5x104 LDN or NDN were suspended in 200μl PBS-/- +2% donor serum and added to a 24 well plate containing a circular cover slip. Neutrophils were allowed to adhere for 30 minutes at 37°C. NET formation was stimulated with 10nM PMA and after 4 hours, samples were fixed in 4% PFA for 30minutes and stored overnight at 4°C. Samples were washed in PBS-/-, blocked in 25% goat serum in PBS-/- for 1 hour at room temperature, before staining with rabbit anti-human myeloperoxidase (Dako) and mouse anti-human neutrophil elastase (Dako) in 10% goat serum for 1 hour at room temperature. No permeabilisation step was performed. Fluorescently labelled secondary antibodies (goat anti-mouse AF488 and goat anti-rabbit AF647, Thermo) were added at room temperature for 1 hour. Finally, DNA was stained with the cell permeable dye Hoecsht for 5 minutes at room temperature. Samples were washed in PBS-/- between each step. Slides were then mounted in ProLong™ Diamond Antifade Mountant (Thermo) and analysed with a Thermo Scientific™ Invitrogen™ EVOS™ FL Auto 2 Imaging System. NETs (Hoecsht+, MPO+ and NE+ structures) are reported as a proportion of total neutrophils analysed.
2.5x105 LDN or NDN were suspended in 500μl HBSS+/+ (Gibco) and stained with Dihydrorhodamine 123 (Thermo) as previously described (33) followed by stimulation with 10nM fMLF for 20 minutes at 37°C. The assay was stopped by transference to ice for 10 minutes before fixation with 4% PFA. Cells were analysed by flow cytometry. DHR 123 is measured by 488 laser excitation with a 525/50 filter.
1x105 T cells were stained with CFSE (Thermo) and cultured in 200μl RPMI (Gibco) + 10% HI-FCS (Gibco) + 1% Peniciliin/Streptomycin (100x, Gibco) + 1% L –glutamine (100x, Gibco) with 2x104 Human T-Activator CD3/CD28 Dynabeads (Thermo) alone or with 2x105 LDNs or NDNs isolated form the same donor for 96 hours at 37°C 5% CO2. Activation cocktail containing protein transport inhibitors (500x eBioscience) was added for 4 hours then stained with Live/Dead Yellow (Thermo), followed by antibodies for CD4, CD8, CD66b (Biolegend) and intracellular IFNγ (Biolegend) following fixation and permeabilisation with 1x Fix/Perm solution (BD). Cells were then run on an LSR Fortessa flow cytometer (BD) and analysed with FlowJo Software (BD). Co-culture conditions were adapted from previously described methods (34).
5x106 PMN were suspended in 1ml HBSS-/- (Gibco) alone or with either 100ng/ml LPS (Invivogen), 100 ng/ml TNF (R&D) for 2 hours at 37°C, 5% CO2, with gentle agitation at 1 hour. For fMLF treatment, 10nM fMLF (Sigma) was added after 90 minutes for the remaining 30 minutes of incubation. Neutrophils were then washed x2 in HBSS-/- and resuspended in 55% Percoll for repeat density gradient.
Where two groups were analysed a two tailed, unpaired T test was used, or for non-parametric data, a Wilcoxon matched-pairs rank analysis. Where 3 or more groups were analysed, One-way analysis of variance was used with multiple comparisons. All graphs show mean ± standard error of the mean as average and error bars. Data was analysed in GraphPad Prism 9.
First, we compared LDN separation using the traditional method of a Percoll density gradient to a new strategy employing negative magnetic selection with the EasySep™ Direct Human Neutrophil Isolation Kit (STEMCELL), followed by discontinuous Percoll density gradient (Figures 1A, C). LDNs were distributed within a mixed population of lymphocytes and monocytes by the traditional isolation method (Figure 1B). Our new isolation strategy resulted in a highly pure (>95% by cytospin count) population of LDNs from healthy donors with no requirement for any further purification steps such as cell sorting (Figures 1D, E). The median number of LDNs identified by flow cytometry following traditional Percoll gradient of 12mls blood (N=5) was 794,000 which accounted for 2.95% of total neutrophils isolated on average (Figure 1F). The median number of LDNs isolated by the new protocol from (N=10) healthy donors was 630,000 from 12 mls whole blood, which accounted for 3.62% of total neutrophils isolated on average (Figure 1G). This number is sufficient to perform functional assays.
The markers used to identify LDNs differ between studies and between disease groups (literature reviewed in Table 1). These reports are frequently conflicting and a definitive set of LDN markers have yet to be resolved – in addition, very few studies have used healthy donor LDNs as the control group. We have generated high purity LDNs and NDNs from healthy individuals in a method that minimises artefactual activation. We therefore used flow cytometry to characterise the LDN and NDNs isolated from healthy donors in an attempt to identify key markers of LDNs that may be used to identify these neutrophils without density separation, in a clinical setting for example. First, we measured granulocyte maturation marker expression based on previously published observations. There was equal expression of CD16, CD15 and CD10 on LDNs and NDNs isolated from healthy individuals by the new protocol, despite variation between donors (Figure 2B). CD66b expression was modestly increased in NDNs compared to autologous LDNs, however, this did not achieve statistical significance. Following this we measured markers of neutrophil activation, which have also been used to speculate on LDN function. Unlike previous studies in disease populations, L-selectin (CD62L) and integrin alpha M (CD11b) did not differentiate LDNs from NDNs (Figure 2C) in the same healthy individuals. We hypothesized that neutrophil migration markers may be different on LDNs. CD54 (I-CAM1) and CXCR2 expression also failed to differentiate between the two neutrophil populations, as did integrin associated protein (CD47) (Figure 2C). Furthermore, we were unable to show that LDNs were significantly phenotypically different to NDNs from the same donor, for any previously reported combinations of markers (Figure 2D). Finally, as different immune cells have characteristic auto-fluorescence and absorption of different wavelengths of light, a factor exploited by flow cytometry, we used a spectral flow cytometer to generate an auto-fluorescence spectrum for the isolated LDNs and NDNs and found no change in auto-fluorescence across all detectors (Figure 2E).
As marker expression is unaltered, we next asked if neutrophil function was different between LDNs and NDNs isolated from healthy donors. In both Systemic Lupus Erythematosus (SLE) and Idiopathic inflammatory myopathy, neutrophil extracellular trap (NET) formation is increased in LDNs when compared to disease group NDNs and healthy control neutrophils (15, 19). In these and other studies ‘control neutrophils’ comprise neutrophils found in the PMN layer, isolated by Ficoll/Percoll gradient NET formation by healthy donor LDNs is therefore poorly described (Reviewed in Table 2). Healthy LDNs produced fewer NETs following PMA stimulation than NDNs over a 6-hour time course (Figure 3A) although this did not reach statistical significance. We confirmed the presence of NETs in both LDN and NDN cultures and identified both ‘cloud-like’ DNA structures (solid arrows) characteristic of NETs generated by PMA stimulation and some ‘stretched’ NETs (dashed arrows) more commonly seen in vivo (Figure 3B). We further quantified NET formation, by immunofluorescence microscopy for markers of granule proteins myeloperoxidase and neutrophil elastase (Figure 3C). The proportion of DNA+ MPO+NE+ NETs in LDNs and NDNs undergoing NETing at 4 hours post PMA stimulation was not significantly different (Figure 3D).
Figure 3 LDNs form fewer NETs than NDNs in healthy individuals. (A) NET formation by 5x104 LDNs or NDNs, stimulated with PMA measured by SYTOX green fluorescence over 6 hours (N=4-6). (B) Representative images of NETs formed by LDNs and NDNs after 3 hour stimulation with PMA. Solid arrows show ‘cloud-like’ NETs and dashed arrows show ‘stretched’ NETs. (C) Representative NET formation, stained with Hoecsht for DNA (blue) and myeloperoxidase (red) and neutrophil elastase (green) antibodies 4 hours post PMA stimulation. Solid arrows show NETing neutrophils, dashed arrow shows non-NETing neutrophils. All images are from a single donor. (D) Quantification of DNA+ MPO+ NE+ NETs in culture after 4 hours stimulation with PMA, presented as percentage of total neutrophils (N=4). (E) Apoptotic neutrophils measured by Annexin V and PI expression after 20 hours in culture (N=4-5) (F) Percentage of LDNs and NDNs positive for ROS before and after FMLF stimulation, measured by DHR123+ fluorescence. (N=5-6) (G) ROS generated by LDNs and NDNs in response to FMLF stimulation, expressed as DHR123 mean fluorescence intensity (N=5-6).
Neutrophils would normally undergo apoptotic cell death before clearance from the site of inflammation. We have previously shown in patients with CF that extended neutrophil life span contributes to increased NET formation (31). In Rheumatoid Arthritis (RA), LDNs have reduced apoptosis compared with RA NDNs, even when treated with GM-CSF (30). We therefore cultured isolated LDNs and NDNs for 20 hours in vitro then stained with Annexin V and Propidium Iodide to determine the levels of apoptosis. There was no significant difference in apoptosis rates between the neutrophil densities, with ~70% of LDNs and NDNs undergoing apoptosis at the 20 hour time point (Figure 3E). In SLE, LDNs produce similar amounts of ROS to NDNs (25) yet production of reactive oxygen species (ROS) is required for some types of NET formation (36) and we have shown differences in NET formation in healthy LDNs. We therefore questioned if ROS production is impaired in healthy LDNs. LDNs isolated from healthy donors are capable of producing ROS (Figure 3F) and the extent of ROS production is the same as that in NDNs upon FMLF stimulation (Figure 3G).
Neutrophil - T cell interaction is now well described as a mechanism of innate and adaptive immune cross talk. Sub-populations of neutrophils have been shown to suppress proliferation of T cells (37) and LDN responses are often linked to activation or suppression of T cell responses, particularly in the tumour microenvironment (34, 38). Neutrophils in circulation suppress T cell proliferation and T cells stimulated with BCG, have decreased proliferative responses and IFN-γ production in the presence of LDNs (26). Progenitor cells from the bone marrow however are incapable of T cell suppression (39) and while LDNs from SLE patients activate T cell IFN-γ and TNF production they do not affect proliferation (7). To determine how the LDNs found in healthy controls compare with these phenotypes, we co-cultured LDNs and NDNs from healthy donors for 96 hours with T cells. Beads coated with anti-CD3/anti-CD28 antibodies were used to activate the T cells. T cells were analysed by flow cytometry (Figures 4A). Total CD4+ or CD8+ T cell numbers were unaltered by co-culture with neutrophils for 96 hours (Figures 4B, F). The proportion of CD4+ and CD8+ T cells producing IFN-γ after 96 hour co-culture with LDNs or NDNs was also unaltered compared with T cell only controls (Figures 4C, G) and amount of IFN-γ was unchanged (Figures 4D, H). Proliferation was measured by dilution of CFSE labelled T cells and quantified by flow cytometry. T cells cultured alone showed significant proliferation following 96 hour stimulation (Figures 4E, I). Co-culture with either LDN or NDNs resulted in decreased numbers of proliferating CD4+ and CD8+ T cells, but this response was not significantly different between the two neutrophil densities. (Figures 4E, I respectively).
Figure 4 Co-culture of T cells with either LDNs or NDNs reduces T cell proliferation (A) Gating strategy to identify T cells after 96 hour co-culture with neutrophils. (B) Percentage of CD4+ T cells in culture after 96 hours (N=3). (C) Percentage of CD4+ T cells producing intracellular IFN-γ after 96 hour co-culture with neutrophils then 4 hour stimulation with T cell activation cocktail (N=3). (D) Amount of intracellular IFN-γ production in CD4+ T cells, shown as mean fluorescence intensity (N=3). (E) CD4+ T cell proliferation, measured by reduction in peaks of CFSE fluorescence (N=3). (F) Percentage of CD8+ T cells in culture after 96 hours (N=3). (G) Percentage of CD8+ T cells producing intracellular IFN-γ after 96 hour co-culture with neutrophils then 4 hour stimulation with T cell activation cocktail (N=3). (H) Amount of intracellular IFN-γ production in CD8+ T cells, shown as mean fluorescence intensity (N-3). (I) CD8+ T cell proliferation, measured by reduction in peaks of CFSE fluorescence (N=3). All data represented as individual values with mean ± SEM). Statistical testing by one way ANOVA with multiple comparisons. Statistical testing by one way ANOVA with multiple comparisons, where *p<0.05 and **p<0.01.
The generation of LDNs is poorly understood, yet increased numbers are observed in many inflammatory environments (12, 15). We therefore hypothesised that activation during various disease states would affect the production of LDNs from already circulating NDNs. As the aim of this experiment was to generate LDNs from the NDN population, neutrophils were isolated from healthy donor whole blood by dextran sedimentation followed by traditional discontinuous Percoll density gradient (55%/70%/81%). Five million neutrophils recovered in the ‘PMN layer’ (NDNs) were then cultured either untreated or in the presence of three different inflammatory stimuli (TNF or LPS for 2 hours, or FMLF for 30 minutes), or left untreated. Following stimulation, the neutrophils were again separated by discontinuous density gradient as before (Figure 5A). NDNs which were untreated with stimuli and re-run through the Percoll gradients were largely recovered in the NDN layer as before, indicating that the isolation was reproducible and that two hours’ incubation at 37°C had not altered their density. However, NDNs activated with any of the inflammatory stimuli were now predominantly recovered from the LDN phase (Figures 5B shows NDN recovery and 5C shows LDN recovery). Accompanying cytospins of LDNs from each treatment conditions demonstrate the varied neutrophil morphologies (Figure 5D). The nuclear structure in the primed and activated cells retains its mature, segmented phenotype, but the cytosol in fMLF treated cells became vacuolated and LPS treated neutrophils demonstrated membrane ruffling and protrusions from the cell surface. Control cells that were isolated as LDNs after 2 hour culture had a similar appearance to NDNs before culture.
Figure 5 NDNs become LDNs upon activation with inflammatory stimuli. (A) Diagram of method. PMN isolated by traditional methods are activated in vitro with inflammatory stimuli and then undergo repeat Percoll gradient. (B) Number of neutrophils recovered from the ‘PMN/NDN’ layer after second Percoll gradient. (N=4) (C) Number of neutrophils recovered from the ‘PBMC/LDN’ layer after second Percoll gradient. (N=4) (D) Representative cytospin images of neutrophils recovered in each condition. Statistical analysis by One-way ANOVA with multiple comparisons where ***p ≤ 0.001.
We have shown that inflammation or activation of healthy NDNs can generate LDNs. We therefore asked if we could isolate LDNs with our new protocol from a patient cohort with a well characterised neutrophil phenotype. Our lab has previously demonstrated a pro-survival, apoptosis resistant response in neutrophils from people with Cystic Fibrosis that results in increased production of NETs (31). We asked if LDNs accounted for the significant increase in neutrophils seen in this patient group. The median number of LDNs isolated from people with CF was 230,000 (N=5) and accounted for 4.64% of total neutrophils (Figures 6A). This is not significantly different to the proportion found in healthy individuals (described in Figure 1). The total number of NDNs however, was significantly higher in people with CF when compared to healthy controls (p=0.0047, by two tailed T test, Figures 1D and 6A). In addition, the pro-survival ‘anti-apoptotic’ phenotype previously described in CF neutrophils was present in both CF NDNs and LDNs (Figure 6B). The mean number of live cells after 20 hour culture was 45.23% ± 10.43% (Mean ± SEM) for CF NDNs and 53.18% ± 10.26% for CF LDNs compared with 36.03% ± 5.31% for healthy LDNs and 36.42% ± 4.81% for healthy NDNs. NET formation is reduced in CF LDNs compared with CF NDNs, mirroring the phenotype observed in healthy individuals (Figure 6C).
Figure 6 LDNs are not elevated in people with Cystic Fibrosis. (A) Total numbers of LDNs isolated from 12 mls blood by magnetic separation and Percoll gradient. (N=5) (B) Rate of apoptosis after in vitro culture for 20 hrs, measured by Annexin V and Propidium Iodide staining. (N=4-6) (C) NET formation, measured by SYTOX green fluorescence over 6 hours after stimulation with 100nM PMA (N=5-6) Data shown as mean ± SEM error bars.
We have developed a modified protocol to isolate LDNs from whole blood that can be used to study LDN phenotype and function in healthy controls and disease populations. We show that healthy LDNs are present in circulation, and, in contrast to LDNs observed in SLE, have marginally lower capacity to form NETs - yet lack any distinguishing markers of activation or maturation characteristic of LDNs in disease. Healthy LDNs have unaltered ROS production, undergo apoptosis at the same rate as autologous NDNs, and furthermore do not alter T cell proliferation or IFNγ production in comparison to NDNs.
Our simple protocol, of magnetic negative selection of total neutrophils from small volumes (12 mls) of blood followed by Percoll gradient, isolates highly enriched populations of low and normal density neutrophils from healthy donors. This is rapid, less likely to activate neutrophils than methods such as FACS, effectively nullifies any chance of activation by monocytes, and leads to >95% pure populations of LDN. Healthy control LDNs are usually not quantified in studies of LDN function during disease or infection owing to the perception that they cannot be readily harvested (Table 3). Previous studies therefore lack the crucial control of healthy LDNs.
Phenotypic markers used to identify LDNs differ between studies and disease groups and as such a definitive set of LDN markers has yet to be resolved (Table 1). Granulocyte markers, CD66b, CD15 and CD16 (FcγRIII) are frequently used to identify LDNs within the PBMC cell population (see Table 1). In Rheumatoid Arthritis and ANCA positive Vasculitis, CD16 expression is reduced on LDNs (30, 40) while in lung adenocarcinoma and HIV infection, CD15 is elevated on LDNs (3, 13). Furthermore LDNs in gastric cancers have high CD66b expression (9). CD10 (Neprilysin, CALLA) expression is increased on mature neutrophils. These are discernible from CD10LOW immature neutrophils which suppress T cell activation (42). In severely infected patients, CD10LOW neutrophils increase with infection severity and this is correlated with increased numbers of LDNs (11). Surprisingly, we found that none of these markers were effective at differentiating between healthy LDNs from healthy NDNs, despite markers such as CD66b being modestly upregulated on LDNs when compared to autologous NDNs. This suggests that the differences in many published studies are not intrinsic features of less dense neutrophils or are insufficient for identification or isolation of LDNs when all neutrophils are isolated as a single population.
We therefore turned our attention to the function of LDNs. Healthy LDNs had no differences in rate of apoptosis or oxidative burst compared to NDNs. LDNs from healthy individuals appeared to produce fewer NETs than NDN, in direct contrast to LDNs isolated from chronic inflammatory conditions such as psoriasis and idiopathic inflammatory myopathy, where LDNs produce increased numbers of NETs (14, 15). The proportion of NETing LDNs and NDNs was however not statistically different when measured by fluorescence microscopy for granule proteins neutrophil elastase and myeloperoxidase. It is difficult to reconcile many of the features of LDNs as healthy LDNs have normal expression of CD10 and CD16 characteristic of mature neutrophils and no reduction in ROS production.
A major function attributed to LDNs is their ability to either activate T cells as in SLE (7), or to suppress T cell proliferation as described in cancer (27). Our data show that in healthy donors, LDNs and NDNs did not affect intracellular IFNγ at the 96 hour time point or the frequency of CD4+ and CD8+ T cells, but both populations significantly impaired T cell proliferation. T cell suppression assays are not without limitations, as neutrophils may prevent αCD3/αCD28 bead stimulation of T cells in some part by phagocytosing beads (43). Furthermore, after 96 hours in culture, ‘suppression’ of T cell division may be due to the presence of apoptotic cells. We demonstrated no significant differences between LDNs and NDNs on T cell responses in vitro in spite of these limitations, however it is important to note that the outcome of T cell and neutrophil interactions are altered by the activation state of both cell types (44). Absence of CD10 expression is a marker used in characterisation of immature LDNs that can suppress T cells (11) yet the relationship between CD10 and LDNs is complex. LDNs in autoimmune disease are CD10+ (25) and LDNs identified in cardiovascular risk associated with psoriasis are identified by CD10hi expression (14). As CD10 expression was equal in LDNs and NDNs from healthy individuals and T cell suppression was seen in both neutrophil densities, we would propose that the T cell suppression is disease specific, and perhaps CD10- LDNs seen in disease may be a result of emergency granulopoiesis (42) and are therefore not present in healthy individuals.
Mycobacterium tuberculosis (Mtb) infection of healthy blood samples induces additional numbers of LDNs (12), suggesting that LDNs may be directly generated by inflammation or infection. To assess if we could induce LDNs from NDNs, we isolated NDN and then stimulated cells with either LPS, TNF or FMLF before separating once again by Percoll gradient (Figure 5). Activated, previously normal density neutrophils now segregated to the PBMC/LDN layer. While this does not explain the population of LDNs isolated from healthy donors at baseline, this experiment suggests that neutrophil priming and stimulation in disease could be an important driver of increased LDN populations. We show that a corresponding change in density is linked to multiple, well documented methods of activation that cause diverse transcriptional responses (32, 45), suggesting that multiple neutrophil populations with assorted functions are present within the LDN pool. This understanding may change our interpretation of LDNs as a single population found in many different diseases or the suggestion of density as a defining neutrophil characteristic.
CF is a progressive disease associated with neutrophilic lung pathology. We have previously demonstrated that neutrophils in CF have a pro-survival phenotype (31) but it is unknown whether LDNs contribute to this observation. Interestingly, people with CF had significantly increased levels of NDNs in comparison to healthy donors, but a similar proportion of LDNs, suggesting they are not a major feature of the neutrophilia seen in CF. Notably, LDNs in our CF population were also resistant to apoptosis mirroring the phenotype seen in CF NDNs, yet were less capable of NET formation than CF NDNs (Figure 6C). These data may be explained by the compartmentalised nature of inflammation in the CF lung while systemic inflammation is less prominent in times of disease stability.
Low density neutrophils have been given significance in diseases ranging from cancer to autoimmune disease. Our data demonstrate that LDNs are part of the normal spectrum of neutrophils and that in health, aside from a reduction in NET formation capacity, they do not display a divergent phenotype. Furthermore, known activators of neutrophils can induce NDNs to become LDNs. We propose that standardised density protocols for neutrophil and mononuclear cell isolation has incorrectly led to the characterisation of LDNs a highly unique sub-population of neutrophils with defined function. Low neutrophil density could actually be a reflection of maturation or activation status. It may also be suggested that in health and disease that neutrophils range in sizes and densities, and the application of arbitrary cut-offs particularly in states of inflammation may explain the variable functions of LDNs.
The raw data supporting the conclusions of this article will be made available by the authors, without undue reservation.
Healthy blood was collected in accordance with The Centre for Inflammation Research Blood Resource (AMREC, 148 15/HV/013). Clinically stable CF patients with one F508del mutation were recruited according to NRS Bioresource, East of Scotland research ethics committee 15/ES/0094. The patients/participants provided their written informed consent to participate in this study.
GH, DD, EF, and RG designed the experiments. GH, FL, DM, and JG collected the data. GH, EF and RG analysed the data. Manuscript was drafted and edited by GH, DD, EF, and RG. All authors contributed to the article and approved the submitted version.
This work was funded by a Chief Scientist Office Senior Clinical Academic Fellowship (SCAF/16/02) awarded to RG and Medical Research Council Senior Fellowship (G1002046) awarded to DD.
The authors declare that the research was conducted in the absence of any commercial or financial relationships that could be construed as a potential conflict of interest.
We would like to acknowledge the contributions of Professor Julia Dorin, University of Edinburgh to the discussion and development of this work. Flow cytometry data was generated with support from the QMRI Flow Cytometry and cell sorting facility, University of Edinburgh.
1. Grieshaber-Bouyer R, Nigrovic PA. Neutrophil Heterogeneity as Therapeutic Opportunity in Immune-Mediated Disease. Front Immunol (2019) 10:346. doi: 10.3389/fimmu.2019.00346
2. Hacbarth E, Kajdacsy-Balla A. Low Density Neutrophils in Patients With Systemic Lupus Erythematosus, Rheumatoid Arthritis, and Acute Rheumatic Fever. Arthritis Rheum (1986) 29:1334–42. doi: 10.1002/art.1780291105
3. Liu Y, Hu Y, Gu F, Liang J, Zeng Y, Hong X, et al. Phenotypic and Clinical Characterization of Low Density Neutrophils in Patients With Advanced Lung Adenocarcinoma. Oncotarget (2017) 8:90969–78. doi: 10.18632/oncotarget.18771
4. Zipursky A, Bow E, Seshadri RS, Brown EJ. Leukocyte Density and Volume in Normal Subjects and in Patients With Acute Lymphoblastic Leukemia. Blood (1976) 48:361–71. doi: 10.1182/blood.V48.3.361.bloodjournal483361
5. Ssemaganda A, Kindinger L, Bergin P, Nielsen L, Mpendo J, Ssetaala A, et al. Characterization of Neutrophil Subsets in Healthy Human Pregnancies. PloS One (2014) 9:e85696. doi: 10.1371/journal.pone.0085696
6. Carmona-Rivera C, Kaplan MJ. Low-Density Granulocytes: A Distinct Class of Neutrophils in Systemic Autoimmunity. Semin Immunopathol (2013) 35:455–63. doi: 10.1007/s00281-013-0375-7
7. Rahman S, Sagar D, Hanna RN, Lightfoot YL, Mistry P, Smith CK, et al. Low-Density Granulocytes Activate T Cells and Demonstrate a Non-Suppressive Role in Systemic Lupus Erythematosus. Ann Rheum Dis (2019) 78:957–66. doi: 10.1136/annrheumdis-2018-214620
8. Sagiv JY, Voels S, Granot Z. Isolation and Characterization of Low- vs. High-Density Neutrophils in Cancer. Methods Mol Biol (2016) 1458:179–93. doi: 10.1007/978-1-4939-3801-8_13
9. Kanamaru R, Ohzawa H, Miyato H, Matsumoto S, Haruta H, Kurashina K, et al. Low Density Neutrophils (LDN) in Postoperative Abdominal Cavity Assist the Peritoneal Recurrence Through the Production of Neutrophil Extracellular Traps (NETs). Sci Rep (2018) 8(1):632. doi: 10.1038/s41598-017-19091-2
10. Li Y, Li H, Wang H, Pan H, Zhao H, Jin H, et al. The Proportion, Origin and Pro-Inflammation Roles of Low Density Neutrophils in SFTS Disease. BMC Infect Dis (2019) 19(1):109. doi: 10.1186/s12879-019-3701-4
11. Morisaki T, Goya T, Ishimitsu T, Torisu M. The Increase of Low Density Subpopulations and CD10 (CALLA) Negative Neutrophils in Severely Infected Patients. Surg Today (1992) 22:322–7. doi: 10.1007/BF00308740
12. Deng Y, Ye J, Luo Q, Huang Z, Peng Y, Xiong G, et al. Low-Density Granulocytes Are Elevated in Mycobacterial Infection and Associated With the Severity of Tuberculosis. PloS One (2016) 11:e0153567. doi: 10.1371/journal.pone.0153567
13. Cloke T, Munder M, Taylor G, Müller I, Kropf P. Characterization of a Novel Population of Low-Density Granulocytes Associated With Disease Severity in HIV-1 Infection. PloS One (2012) 7:e48939. doi: 10.1371/journal.pone.0048939
14. Teague HL, Varghese NJ, Tsoi LC, Dey AK, Garshick MS, Silverman JI, et al. Neutrophil Subsets, Platelets, and Vascular Disease in Psoriasis. JACC Basic Transl Sci (2019) 4:1–14. doi: 10.1016/j.jacbts.2018.10.008
15. Seto N, Torres-Ruiz JJ, Carmona-Rivera C, Pinal-Fernandez I, Pak K, Purmalek MM, et al. Neutrophil Dysregulation Is Pathogenic in Idiopathic Inflammatory Myopathies. JCI Insight (2020) 5(3):e134189. doi: 10.1172/jci.insight.134189
16. Jayaraman P, Parikh F, Newton JM, Hanoteau A, Rivas C, Krupar R, et al. TGF-β1 Programmed Myeloid-Derived Suppressor Cells (MDSC) Acquire Immune-Stimulating and Tumor Killing Activity Capable of Rejecting Established Tumors in Combination With Radiotherapy. OncoImmunology (2018) 7:e1490853. doi: 10.1080/2162402X.2018.1490853
17. Sagiv JY, Michaeli J, Assi S, Mishalian I, Kisos H, Levy L, et al. Phenotypic Diversity and Plasticity in Circulating Neutrophil Subpopulations in Cancer. Cell Rep (2015) 10:562–73. doi: 10.1016/j.celrep.2014.12.039
18. Rocha BC, Marques PE, Leoratti FM de S, Junqueira C, Pereira DB, Antonelli LR do V, et al. Type I Interferon Transcriptional Signature in Neutrophils and Low-Density Granulocytes Are Associated With Tissue Damage in Malaria. Cell Rep (2015) 13:2829–41. doi: 10.1016/j.celrep.2015.11.055
19. van den Hoogen LL, van der Linden M, Meyaard L, Fritsch-Stork RDE, van Roon JA, Radstake TR. Neutrophil Extracellular Traps and Low-Density Granulocytes Are Associated With the Interferon Signature in Systemic Lupus Erythematosus, But Not in Antiphospholipid Syndrome. Ann Rheum Dis (2020) 79(10):e135. doi: 10.1136/annrheumdis-2019-215781
20. Torres-Ruiz J, Carrillo-Vázquez DA, Tapia-Rodríguez M, Garcia-Galicia JA, Alcocer-Varela J, Gómez-Martín D. The Role of Low Density Granulocytes and NETosis in the Pathogenesis of Adult-Onset Still’s Disease. Clin Exp Rheumatol (2019) 37 Suppl 121:74–82.
21. Ramanathan K, Glaser A, Lythgoe H, Ong J, Beresford MW, Midgley A, et al. Neutrophil Activation Signature in Juvenile Idiopathic Arthritis Indicates the Presence of Low-Density Granulocytes. Rheumatology (2018) 57:488–98. doi: 10.1093/rheumatology/kex441
22. Quach A, Ferrante A. The Application of Dextran Sedimentation as an Initial Step in Neutrophil Purification Promotes Their Stimulation, Due to the Presence of Monocytes. J Immunol Res (2017) 2017:1–10. doi: 10.1155/2017/1254792
23. Villanueva E, Yalavarthi S, Berthier CC, Hodgin JB, Khandpur R, Lin AM, et al. Netting Neutrophils Induce Endothelial Damage, Infiltrate Tissues, and Expose Immunostimulatory Molecules in Systemic Lupus Erythematosus. J Immunol (2011) 187:538–52. doi: 10.4049/jimmunol.1100450
24. Su R, Peng Y, Deng Z, Deng Y, Ye J, Guo Y, et al. Mycobacterium Tuberculosis Infection Induces Low-Density Granulocyte Generation by Promoting Neutrophil Extracellular Trap Formation Via ROS Pathway. Front Microbiol (2019) 10:1468. doi: 10.3389/fmicb.2019.01468
25. Denny MF, Yalavarthi S, Zhao W, Thacker SG, Anderson M, Sandy AR, et al. A Distinct Subset of Proinflammatory Neutrophils Isolated From Patients With Systemic Lupus Erythematosus Induces Vascular Damage and Synthesizes Type I IFNs. J Immunol (2010) 184:3284–97. doi: 10.4049/jimmunol.0902199
26. La Manna MP, Orlando V, Paraboschi EM, Tamburini B, Di Carlo P, Cascio A, et al. Mycobacterium Tuberculosis Drives Expansion of Low-Density Neutrophils Equipped With Regulatory Activities. Front Immunol (2019) 10:2761. doi: 10.3389/fimmu.2019.02761
27. Kumagai Y, Ohzawa H, Miyato H, Horie H, Hosoya Y, Lefor AK, et al. Surgical Stress Increases Circulating Low-Density Neutrophils Which May Promote Tumor Recurrence. J Surg Res (2020) 246:52–61. doi: 10.1016/j.jss.2019.08.022
28. Zhou L, Somasundaram R, Nederhof RF, Dijkstra G, Faber KN, Peppelenbosch MP, et al. Impact of Human Granulocyte and Monocyte Isolation Procedures on Functional Studies. Clin Vaccine Immunol (2012) 19:1065–74. doi: 10.1128/CVI.05715-11
29. Shaul ME, Eyal O, Guglietta S, Aloni P, Zlotnik A, Forkosh E, et al. Circulating Neutrophil Subsets in Advanced Lung Cancer Patients Exhibit Unique Immune Signature and Relate to Prognosis. FASEB J (2020) 34:4204–18. doi: 10.1096/fj.201902467R
30. Wright HL, Makki FA, Moots RJ, Edwards SW. Low-Density Granulocytes: Functionally Distinct, Immature Neutrophils in Rheumatoid Arthritis With Altered Properties and Defective TNF Signalling. J Leukoc Biol (2017) 101:599–611. doi: 10.1189/jlb.5A0116-022R
31. Gray RD, Hardisty G, Regan KH, Smith M, Robb CT, Duffin R, et al. Delayed Neutrophil Apoptosis Enhances NET Formation in Cystic Fibrosis. Thorax (2018) 73:134–44. doi: 10.1136/thoraxjnl-2017-210134
32. Wright HL, Thomas HB, Moots RJ, Edwards SW. RNA-Seq Reveals Activation of Both Common and Cytokine-Specific Pathways Following Neutrophil Priming. PloS One (2013) 8:e58598. doi: 10.1371/journal.pone.0058598
33. Fu J, Tobin MC, Thomas LL. Neutrophil-Like Low-Density Granulocytes Are Elevated in Patients With Moderate to Severe Persistent Asthma. Ann Allergy Asthma Immunol (2014) 113:635–40.e2. doi: 10.1016/j.anai.2014.08.024
34. Mackey JBG, Coffelt SB, Carlin LM. Neutrophil Maturity in Cancer. Front Immunol (2019) 10:1912. doi: 10.3389/fimmu.2019.01912
35. Herteman N, Vargas A, Lavoie J-P. Characterization of Circulating Low-Density Neutrophils Intrinsic Properties in Healthy and Asthmatic Horses. Sci Rep (2017) 7(1):7743. doi: 10.1038/s41598-017-08089-5
36. Remijsen Q, Berghe TV, Wirawan E, Asselbergh B, Parthoens E, De Rycke R, et al. Neutrophil Extracellular Trap Cell Death Requires Both Autophagy and Superoxide Generation. Cell Res (2011) 21:290–304. doi: 10.1038/cr.2010.150
37. Pillay J, Kamp VM, van Hoffen E, Visser T, Tak T, Lammers J-W, et al. A Subset of Neutrophils in Human Systemic Inflammation Inhibits T Cell Responses Through Mac-1. J Clin Invest (2012) 122:327–36. doi: 10.1172/JCI57990
38. Hsu BE, Tabariès S, Johnson RM, Andrzejewski S, Senecal J, Lehuédé C, et al. Immature Low-Density Neutrophils Exhibit Metabolic Flexibility That Facilitates Breast Cancer Liver Metastasis. Cell Rep (2019) 27:3902–15.e6. doi: 10.1016/j.celrep.2019.05.091
39. Aarts CEM, Hiemstra IH, Tool ATJ, van den Berg TK, Mul E, van Bruggen R, et al. Neutrophils as Suppressors of T Cell Proliferation: Does Age Matter? Front Immunol (2019) 10:2144. doi: 10.3389/fimmu.2019.02144
40. Ui Mhaonaigh A, Coughlan AM, Dwivedi A, Hartnett J, Cabral J, Moran B, et al. Low Density Granulocytes in ANCA Vasculitis Are Heterogenous and Hypo-Responsive to Anti-Myeloperoxidase Antibodies. Front Immunol (2019) 10:2603. doi: 10.3389/fimmu.2019.02603
41. Ronchezel MV, Hacbarth ET, Len CA, Terreri MT, Andrade LE, Hilário MO. Low Density Neutrophils in Patients With Juvenile Idiopathic Arthritis. J Investig Allergol Clin Immunol (2003) 13(2):103–7.
42. Marini O, Costa S, Bevilacqua D, Calzetti F, Tamassia N, Spina C, et al. Mature CD10 + and Immature CD10 – Neutrophils Present in G-CSF–Treated Donors Display Opposite Effects on T Cells. Blood (2017) 129:1343–56. doi: 10.1182/blood-2016-04-713206
43. Negorev D, Beier UH, Zhang T, Quatromoni JG, Bhojnagarwala P, Albelda SM, et al. Human Neutrophils can Mimic Myeloid-Derived Suppressor Cells (PMN-MDSC) and Suppress Microbead or Lectin-Induced T Cell Proliferation Through Artefactual Mechanisms. Sci Rep (2018) 8(1):3135. doi: 10.1038/s41598-018-21450-6
44. Minns D, Smith KJ, Hardisty G, Rossi AG, Gwyer Findlay E. The Outcome of Neutrophil-T Cell Contact Differs Depending on Activation Status of Both Cell Types. Front Immunol (2021) 12:633486. doi: 10.3389/fimmu.2021.633486
Keywords: neutrophils, low-density neutrophils, inflammation, cystic fibrosis, neutrophil T cell interactions, neutrophil extracellular traps
Citation: Hardisty GR, Llanwarne F, Minns D, Gillan JL, Davidson DJ, Gwyer Findlay E and Gray RD (2021) High Purity Isolation of Low Density Neutrophils Casts Doubt on Their Exceptionality in Health and Disease. Front. Immunol. 12:625922. doi: 10.3389/fimmu.2021.625922
Received: 04 November 2020; Accepted: 13 May 2021;
Published: 08 June 2021.
Edited by:
Felix Ellett, Massachusetts General Hospital and Harvard Medical School, United StatesReviewed by:
Zdenek Hel, University of Alabama at Birmingham, United StatesCopyright © 2021 Hardisty, Llanwarne, Minns, Gillan, Davidson, Gwyer Findlay and Gray. This is an open-access article distributed under the terms of the Creative Commons Attribution License (CC BY). The use, distribution or reproduction in other forums is permitted, provided the original author(s) and the copyright owner(s) are credited and that the original publication in this journal is cited, in accordance with accepted academic practice. No use, distribution or reproduction is permitted which does not comply with these terms.
*Correspondence: Robert D. Gray, Ui5ELkdyYXlAZWQuYWMudWs=
Disclaimer: All claims expressed in this article are solely those of the authors and do not necessarily represent those of their affiliated organizations, or those of the publisher, the editors and the reviewers. Any product that may be evaluated in this article or claim that may be made by its manufacturer is not guaranteed or endorsed by the publisher.
Research integrity at Frontiers
Learn more about the work of our research integrity team to safeguard the quality of each article we publish.