- 1INRAE, Université de Tours, ISP, Nouzilly, France
- 2Institut de l’Elevage, Paris, France
Neutrophils that reside in the bone marrow are swiftly recruited from circulating blood to fight infections. For a long time, these first line defenders were considered as microbe killers. However their role is far more complex as cross talk with T cells or dendritic cells have been described for human or mouse neutrophils. In cattle, these new roles are not documented yet. We identified a new subset of regulatory neutrophils that is present in the mouse bone marrow or circulate in cattle blood under steady state conditions. These regulatory neutrophils that display MHC-II on the surface are morphologically indistinguishable from classical MHC-IIneg neutrophils. However MHC-IIpos and MHC-IIneg neutrophils display distinct transcriptomic profiles. While MHC-IIneg and MHC-IIpos neutrophils display similar bacterial phagocytosis or killing activity, MHC-IIpos only are able to suppress T cell proliferation under contact-dependent mechanisms. Regulatory neutrophils are highly enriched in lymphoid organs as compared to their MHC-IIneg counterparts and in the mouse they express PDL-1, an immune checkpoint involved in T-cell blockade. Our results emphasize neutrophils as true partners of the adaptive immune response, including in domestic species. They open the way for discovery of new biomarkers and therapeutic interventions to better control cattle diseases.
Introduction
Neutrophils are major partners of the innate immune system and are considered the first line of defense against microbes (1). They originate from the bone marrow (BM) and circulate in large numbers in the blood from where they are swiftly recruited to sites of inflammation or infection to fight danger. They are functionally equipped to rapidly phagocytose invading microbes via a variety of receptors (2) and rapidly deliver large amounts of reactive oxygen species (ROS) into phagocytic vacuoles to kill microbes (3). If not tightly controlled these dangerous weapons lead to tissue damage and neutrophils are often a signature of uncontrolled inflammation or infection.
Neutrophils are the dominant cell population circulating in blood in a wide range of animal species and humans (4). However, in cattle or mouse blood they are less numerous than lymphocytes (5, 6). Compared to human or mouse neutrophils, bovine neutrophils present peculiarities such as unique receptors or the lack of N-formylated chemotactic peptide receptor (5). Neutrophil counts are an important signature of cattle condition. In lactating cows, they represent the most part of an abnormal somatic cell count in milk from mastitic cows. Indeed, their swift recruitment into the mammary gland is of critical importance in the fight against invasive pathogens (7). For a long time, neutrophils were thought to only travel from their birth place, the BM, to blood and tissues in response to inflammatory signals or infections. However, this has recently been revisited by a number of authors who have identified neutrophils residing in spleen (8, 9), lymph nodes (10), or lung vasculature (11). These new locations and features of resident neutrophils have been discovered in mice and humans but are not well documented in other animal species, including cattle, under normal conditions.
Long considered as “suicide bombers,” neutrophils were recently upgraded as global players of the immune response along with the discovery of new functions (12). They fully participate in shaping adaptive immunity by suppressing T cell proliferation and activity (13, 14) or by promoting IgM and IgG production by splenic B cells (9). This broad range of phenotypes and functions was recently linked to discrete populations of neutrophils characterized by unbiased single cell analyses (15). Like macrophages and dendritic cells, neutrophils are now recognized as plastic cells able to respond and adapt to their environment. Again, although neutrophil diversity and plasticity are now well documented for mouse and human neutrophils, they remain unknown for domestic species including cattle.
Here we conducted a parallel thorough characterization of neutrophils present in mouse BM or circulating in cattle blood at steady state. We define this term as animals without any apparent sign of disease or infection. In other words, animals where the immune system is at homeostasis. We discovered a new population of regulatory neutrophils that displayed suppressive activity on T-cells, at steady state.
Materials and Methods
Animal Protocols and Sampling
Experimental protocols complied with French law (Décret: 2001–464 29/05/01) and European directive 2010/63/UE for the care and use of laboratory animals and were carried out under Authorization for Experimentation on Laboratory Animals Number D-37-175-3 (Animal facility UE-PFIE, INRA Centre Val de Loire for mice) and E 37-175-2 (UE-PAO, INRAE Centre Val de Loire for bovine). Animal protocols were approved by the “Val de Loire” Ethics Committee for Animal Experimentation and were registered to the French National Committee for Animal Experimentation under N°2016091610026164.V3 (mice) or N°2016040410531486 (cattle). Cattle remained in their environment (UE-PAO) and no animal was sacrificed for this work. Six- to eight-week-old C57BL/6 mice and OT-II transgenic mice for the OVA MHC class II complex-specific TCR (16) on a C57BL/6 genetic background were bred at the resident PFIE animal facility before use.
Bovine blood was collected from Holstein Friesian cows at the jugular vein into vacutainer K2 EDTA tubes. Broncho-tracheal lymph nodes, spleen biopsy, and sternum BM were collected post-mortem at a commercial abattoir from Blonde d’Aquitaine, Limousine, Charolaise and Highlands cows. Mice were euthanized by CO2 inhalation. Blood (from heart), spleen, inguinal lymph nodes, and femurs were collected.
Preparation of Cells
Tubes containing blood were centrifuged at 1000xg for 10 min at 20°C before removal of the plasma layer and buffy coat. In indicated experiments, buffy coat was used in proliferation assay (see below). Red blood lysis buffer (Sigma 94 R7757) (4 vol/1 vol of blood) was added for 5 min at room temperature to lyse red blood cells. Cells were washed twice in D-PBS with 2mM EDTA. Bovine blood neutrophils were labeled with primary anti-G1 and anti-MHC-II Ab, followed by secondary fluorescent-conjugated Ab before purification by FACS (see next paragraph for labeling protocol). Mouse neutrophils were generally purified from the BM by positive magnetic selection with anti–Ly-6G PE-conjugated Ab (1A8; BD Biosciences) and anti-PE microbeads (Miltenyi Biotec) as described previously (17) except for cell sorting, where mouse neutrophils were enriched from BM by positive magnetic selection with anti–CD11b microbeads (Miltenyi Biotec) before labeling with antibodies. Bovine BM cells were collected post-mortem at local commercial abattoirs, by scratching an open rib (directly on the carcass) with a spatula, cells were diluted in PBS. Spleen biopsies, the broncho-tracheal lympho nodes (LN) and BM cells were transported at 4°C to our laboratory. Bovine and murine LN and spleen biopsies were disrupted mechanically and all cells were filtered through 100μm nylon cell strainer (BD Falcon), and washed twice. Cells were suspended in RPMI-1640 supplemented with 2mM L-glutamine, 10mM HEPES, and 1mg/ml of BSA with extremely low endotoxin level (≤1.0 EU/mg) (hereafter referred to as RPMI complete medium). Cell counts were determined after staining with Türk solution and numerated with a Malassez’s chamber.
Flow Cytometry
Bovine cells were suspended in PBS with 10% of horse serum (Gibco), 2mM EDTA, and labeled for 30 min with primary antibodies (see Supplementary Table S1). Mouse cells were suspended in PBS 2mM EDTA. After saturation with anti-CD32/CD16, murine cells were incubated 30min with fluorescent mAb. After washes in D-PBS (300xg, 10 min, 4°C), both murine and bovine cells were labeled 30min with the corresponding fluorescent-conjugated secondary antibodies. Cells were washed and fixed with BD cell fix diluted four times in PBS. Data were acquired with a LSR Fortessa™ X-20 Flow cytometer (Becton Dickinson) and results analyzed with Kaluza software (Beckman Coulter).
For neutrophil subsets purification, cell concentrations were adjusted to 107cells/ml and sorted with a MoFlo AstriosEQ high speed cell sorter (Beckman Coulter) according to our previously published protocol (18). Sorted cells were spread on microscope slides (Superfrost, Thermo) by cytocentrifugation (3 min, 700xg) and stained with May-Grünwald and Giemsa with the RAL 555 kit (RAL Diagnostics).
Neutrophils Functional Assays
Sorted neutrophils viability was evaluated after 1 h stimulation with 100ng/ml of LPS (Escherichia coli 0111:B4, Sigma). Neutrophils were then incubated 15min at room temperature with anti-annexin V antibodies in binding buffer (BD Bioscience). Cells were washed and incubated 15min at room temperature with streptavidin-APC-Cy7 (BD Bioscience). Cells were washed and incubated 5 min with 20µg/ml of propidium iodide (BD Bioscience) and then directly analyzed with LSR Fortessa X-20 flow cytometer. Phagocytosis was measured using pHrodo™ Red E. coli BioParticles® Conjugated (MolecularProbes®) following the manufacturer’s instructions. Briefly, purified neutrophils were first incubated in a 96 wells microplate for 30 min at 37°C in RPMI complete medium with or without 2µg/well of cytochalasin D (Sigma), and then for 1 h with 20µg/wells of pHrodo E. coli BioParticles. Fluorescence was directly measured with the LSR Fortessa™ X-20 Flow cytometer. ROS produced by neutrophils were quantified using the CellROX® Orange Flow Cytometry Assay Kits (MolecularProbes®, C10493) following the manufacturer’s instructions. Briefly, purified neutrophils were first incubated for 1 h at 37°C in RPMI complete medium with or without 400µM of TBHP in a 96-wells black microplate and then for 30 min with 100nM CellROX®. Fluorescence was measured with the LSR Fortessa™ X-20 Flow Cytometer. To test the bacterial killing capacity of neutrophils, we used the Escherichia coli P4 strain, isolated from a clinical case of bovine mastitis which causes severe infections in mice (19). Escherichia coli P4 bacteria were grown in 10 ml BHI medium overnight at 37°C without agitation. Bacteria were then diluted in BHI medium (1 vol/100 vol) and incubated for 6 h at 37°C without agitation. Bacterial concentration was determined by the optical density at 600 nm and adjusted at 4.105 CFU/ml in RPMI complete medium. Purified neutrophils were infected at a MOI of 0.2 in RPMI complete medium in 1.5ml Eppendorf tubes for 90 min at 37°C under agitation on a Rotator SB3 (Stuart equipment). Bacteria in RPMI complete medium without neutrophils were used as the reference to asses killing. Bacterial dilutions (in PBS 0.25% SDS) were plated on TSA agar plates and incubated for 16 h at 37°C before numeration of CFUs.
RNA Extraction and Gene Expression Analysis
Total RNAs were extracted from cell-sorter purified cells using NucleoSpin RNA kit with a DNase treatment (Macherey Nagel) and reverse transcribed with iScript™ Reverse Transcriptase mix (Bio-Rad) according to the manufacturer’s instructions. Primers (Eurogentec) are listed in Supplementary Table S2. Primers validation was performed on a serial diluted pool of cDNA (a mix of cDNA from spleen, lung, LN, blood, and BM cells for both species) with a LightCycler® 480 Real-Time PCR System (Roche). Gene expression was then assessed with the Biomark HD (Fluidigm) in a 48x48 wells plate, according to the manufacturer’s instructions. The annealing temperature was 60 and 62°C for bovine and mouse samples respectively. Data were analyzed with Fluidigm Real-Time PCR software to determine the cycle threshold (Ct) values. Messenger RNA (mRNA) expression was normalized to the mean expression of three housekeeping genes for each animal species to obtain the ΔCt value. Principal component analysis (PCA) and hierarchical clustering were performed with ΔCt values in R studio (Version 1.3.959, © 2009-2020 RStudio, PBC), using respectively the FactoMineR and pheatmap packages. Ward’s minimum variance method was applied for clustering, with dissimilarities squared before clustering (ward.D2). ΔCt values were centered to the median for clustering.
Measure of T-Cell Suppressive Activity of Neutrophils
For the mouse, splenocytes from OT-II mice were collected, homogenized to single-cell suspensions through nylon screens and resuspended in RPMI medium (Gibco) supplemented with 10% decomplemented fetal bovine serum (Gibco), 2 mM L-glutamine (Gibco), 100 U penicillin, and 100 μg/ml streptomycin (Gibco). 105 cells/well were distributed in a 96-wells round bottom plate (BD Falcon). OT-II splenocytes proliferation was induced by addition of 2 μg/ml of the OVA peptide 323-339 (PolyPeptide Group). As indicated, purified neutrophils were added to the culture at a ratio of 1 neutrophil:10 splenocytes in a final volume of 200µl. Wells without neutrophils were used as reference for maximal proliferation.
As indicated, neutrophils were separated from splenocytes by placing them in a HTS Transwell-96 permeable device with 0.4 μm pore and polycarbonate membrane and adapted receiver plate (Corning, reference CLS3381). To test the role of MHC-II and CD11b molecules in the suppression mechanism, neutrophils were also treated 1 h before incubation with splenocytes with 15µg/ml anti-CD11b mAb (clone M1/70) or rat IgG2bκ as isotype control; anti MHC-II mAb (clone 2G9) or rat IgG2aκ as isotype control. Plates were incubated at 37°C with 5%CO2. Cell proliferation was quantified after 3 days of culture using CyQUANT Cell Proliferation Assay tests (Thermo Fisher) according to the manufacturers’ instructions.
For the bovine, a mixed lymphocyte reaction assay was set up by mixing blood cells from two genetically distant cows with cow N°1 as the responding animal and cow N°2 as the stimulating animal. Briefly, blood was centrifuged at 1000xg, 15min, 20°C, and buffy coats were collected and diluted four times in PBS. PBMCs were collected at the interface of 1.077 density Percoll gradient (GE Healthcare) after centrifugation at 400g, 15min, 20°C, without brake. PBMCs from cow n°1 (responding) remained untreated while PBMCs from cow n°2 (stimulating) were incubated for 30 min at 38.5°C in 5% CO2 with 50µg/ml of mitomycin C from Streptomyces caespitosus (Sigma, M4287), to block their proliferation. After three washes, 105 stimulating cells were mixed with 105 responding cells in 96 wells plates (ratio 1:1) in a total volume of 150µl in RPMI complete medium supplemented with 100U/ml penicillin and 100μg/ml streptomycin. The negative control was responding cells alone and the reference maximal proliferation was responding and simulating cells together at ratio 1:1. Proliferation that started at day 6 was stopped at day 9. As indicated, at day 4, 105 of purified syngenic neutrophils from the responding animal were added to the well in a final volume of 200 µl. In one experiment, neutrophils were placed in a HTS Transwell-96 permeable device as for the mouse system. To quantify proliferation, plates were centrifuged at day 6 and day 9 for 5 min at 300xg, supernatants were discarded and cells were frozen at −80°C before addition of reagents from the CyQUANT Cell Proliferation Assay Kit (Thermo Fisher) as indicated by the manufacturer.
Statistical Analysis
Individual data and the median were presented in the figures. Statistical analyses were performed with Prism 6.0 software (GraphPad). Analyzes were performed on data from two to six independent experiments, Mann Whitney non-parametric tests, or two-way ANOVA test were used. Represented p-values were: ∗p < 0.05; ∗∗p < 0.01, and ∗∗∗p < 0.001.
Supplementary Information
Supplemental information can be found with this article online. Should the reader need additional details, please email a request to corresponding authors.
Results
Neutrophils Represent Discrete Populations in Mouse and Cattle
In cattle, neutrophils are often isolated by simple centrifugation of freshly collected blood to separate them from the buffy coat. They segregate at the bottom of the tube with red blood cells (20, 21). In mice, because of very small volumes, blood sampling is less practical. Therefore, high numbers of neutrophils are directly extracted from the BM by magnetic selection following labeling with antibody against Ly-6G which is highly expressed by mouse neutrophils (22). These methods, that lead to a fair level of purity for neutrophils, are convenient for most assays because they are quick and preserve these fragile cells. We prepared cattle and mouse neutrophils using these rapid procedures and analyzed them by flow cytometry (Figure 1). Following mouse bone-marrow cell preparation and labeling with anti-Ly-6G and magnetic separation (Figure 1A), banded-cells of apparent homogeneity were observed after May-Grümwald-Giemsa (MGG) staining (Figure 1B). However, the side scatter (SSC) and forward scatter (FSC) profile by flow cytometry displayed two discrete populations of heterogeneous size. Moreover, double labeling of these “pure” neutrophils with anti-Ly-6G and anti-CD11b distinguished CD11bhi from CD11bmed cell populations (Figure 1C). In cattle, after elimination of the buffy coat containing PBMCs, cells were collected from lower 2/3 of the tube (Figure 1D) and analyzed after centrifugation and MGG staining. This revealed heterogeneity of this cell fraction with the presence of eosinophils in variable proportions (Figure 1E). After labeling with anti G1, a marker that is highly expressed on the surface of bovine neutrophils (23, 24) and anti CD11b, cells were analyzed by flow cytometry. After SSC and FSC gating on the granulocytes populations (Figure 1F), we distinguished CD11bpos G1low eosinophils (representing between 2 and 8% of the SSC x FSC granulocyte gate depending on the animal) from G1hi neutrophils. Among G1hi neutrophils CD11b labeling segregated two populations: a main population of CD11bmed neutrophils (around 87% of the granulocyte gate) and a minor population (around 1.5%) of CD11bhi neutrophils (Figure 1F).
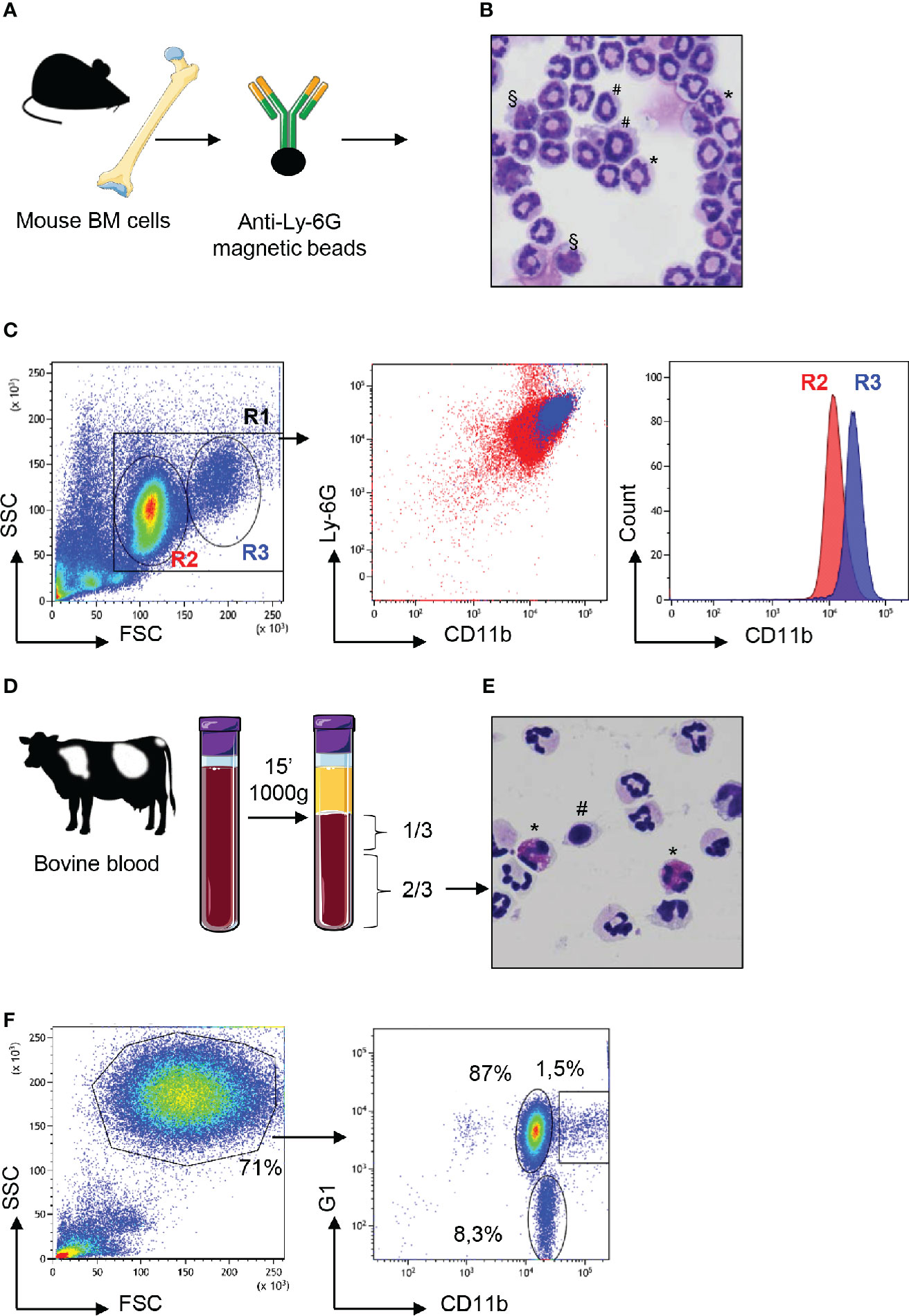
Figure 1 Neutrophils from mouse bone marrow or cattle blood display heterogeneous profiles. (A) Bone marrow (BM) cells from C57BL/6 adult mice were magnetically purified with anti-Ly6G beads. (B) These cells stained with May Grünwald Giemsa after cytocentrifugation on glass slides displayed heterogenous profiles with segmented nuclei (*), banded nuclei (#) as well as some nuclei with a mononuclear kidney shape (§). (C) After anti-Ly-6G magnetic purification, mouse cells were analyzed by flow cytometry using the LSR Fortessa™ X-20 apparatus and in the “granulocytes” R1 gate, a bimodal distribution of FSCmed (R2) and FSChigh (R3) cells was observed on a linear scale (first panel). Labeling with anti-Ly6-G and anti-CD11b confirmed this heterogeneity after dot plot analysis (middle panel) with bimodal distribution of CD11bmed (R2) and CD11bhi (R3) cells as displayed on the histogram analysis (right panel). (D) Blood from Hostein Friesian cows was centrifuged and the lower 2/3 of the tube enriched in neutrophils were collected. (E) These cells, as observed after cytocentrifugation and staining by May Grünwald Giemsa, were mainly neutrophils, characterized by their polylobed nucleus but monocytes (#) and eosinophils (*) were also observed in various proportions depending on the animal. (F) These cells were analyzed by flow cytometry and FSC x SSC dot plot showed granulocytes in a gate that represented 71% of analyzed cells (left panel). After labeling with anti-G1 and anti CD11b, dot-plot analysis of the “granulocytes” gate (right panel) revealed three subsets: eosinophils that were negative for the G1 marker (around 8% of granulocytes) and two subsets of G1pos neutrophils that were CD11bmed (87%) or CD11bhi (1.5%). The most representative animal is shown (n=4 for mouse, n=6 for bovine), although in cattle the proportion of eosinophils and CD11bhi neutrophils circulating in blood varied between animals.
As we were intrigued by the presence of different neutrophil populations in mouse BM and cattle blood at steady state, we performed a more thorough characterization by flow cytometry using a panel of markers (Figure 2). In mouse, CD11b was used to gate myeloid cells (Figure 2A) and a combination of anti-Ly-6C and anti-Ly-6G allowed us to distinguish bona-fide neutrophils (Ly-6Ghi) from monocytes (Ly-6Gneg) (25). Among Ly-6C+ Ly-6Ghi cells, a minor subset (1.2%) of MHC-IIpos Ly-6C+ Ly-6Ghi neutrophils was clearly distinguished from the main population (92.5%) of the MHC-IIneg neutrophils. In cattle, among the neutrophils that highly expressed the G1 marker, MHC-IIneg neutrophils represented the main population (93%). However, a minor population of G1hi neutrophils (around 1.5%) expressed MHC-II antigens on the surface as observed in the mouse (Figure 2A). The two MHCneg et MHCpos subsets were sorted by flow cytometry in both species. After centrifugation onto glass slides and MGG staining, they were indistinguishable under a microscope (Figure 2B). Their banded or segmented nuclei morphology, for mouse bone marrow or bovine blood neutrophils respectively, probably reflected their different maturation stage. Sorted MHC-IIneg and MHC-IIpos neutrophils (purity >99%) were then labeled with a panel of antibodies (Figure 2C). In both bovine and mouse, in comparison with classical MCH-IIneg neutrophils, the MHC-IIpos subset overexpressed CD11b on the surface as well as L-selectin CD62L. The CD14 LPS coreceptor was not detected on MHC-IIneg neutrophils. By contrast around 50% of MHC-IIpos neutrophils displayed this receptor on their surface in both the mouse and the bovine. In mouse, we also observed that the MHC-IIpos subset expressed high levels of CD44 as well as CD274 (PDL-1) the ligand for PD-1 involved in T cell exhaustion (26). Since neutrophils are fragile cells which could be exacerbated by flow cytometry sorting, we next asked if the two subsets displayed different ex-vivo survival times. The two subsets were incubated with LPS for 1 hour and stained with annexin V and propidium iodide (Figure 2D). The large majority of neutrophils remained alive after this treatment and no significant difference was observed between classical MHC-IIneg neutrophils (97% alive in mouse or bovine) and MHC-IIpos neutrophils (95% alive in mouse or bovine).
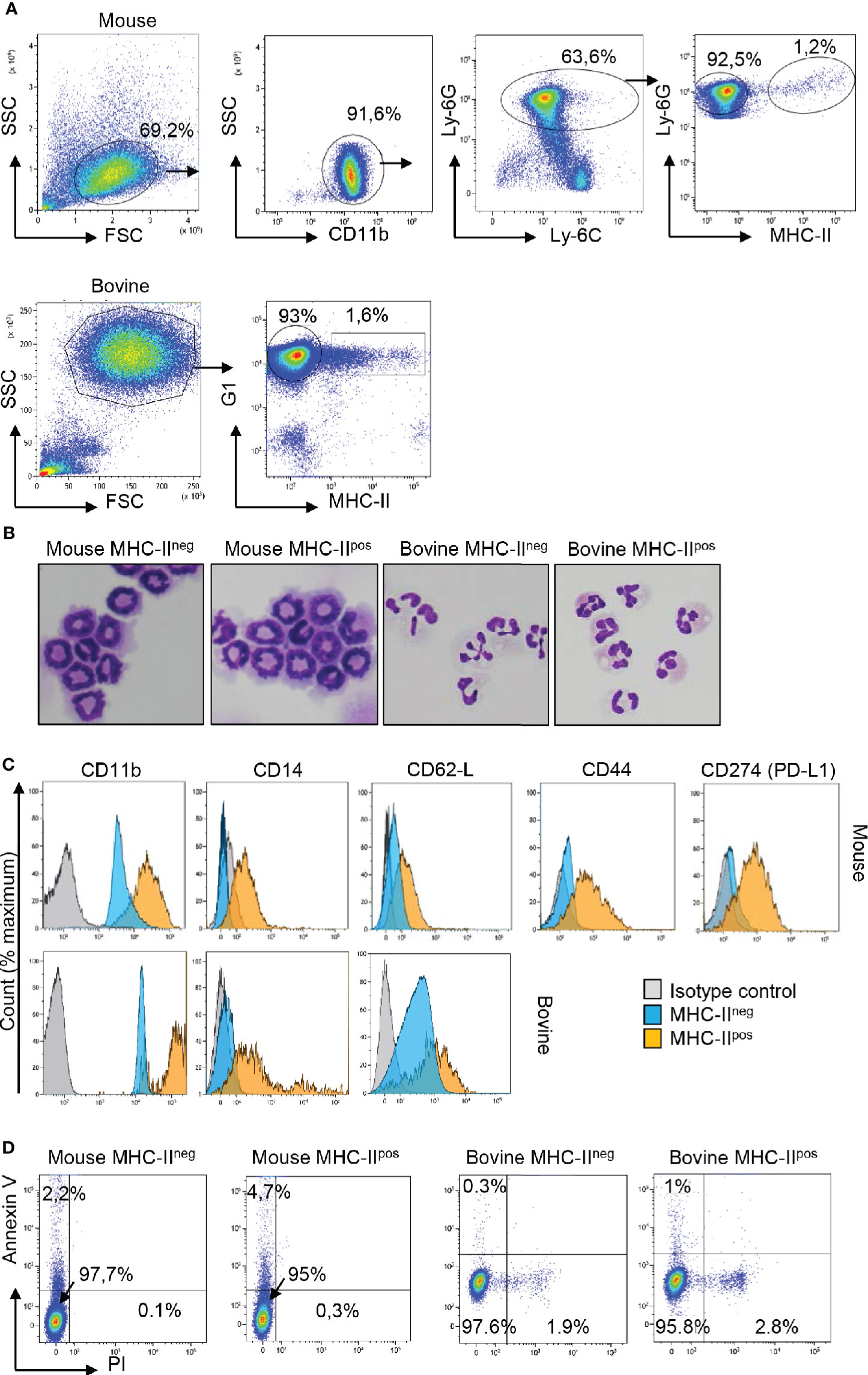
Figure 2 Two populations of neutrophils can be distinguished on the basis of specific surface makers, including MHC-II, in the mouse, and the bovine. (A) Cells were prepared from mouse bone marrow (BM) or cattle blood as described in Figure 1 and neutrophils were sorted with a MoFlo AstriosEQ apparatus after labeling with eFluor viability dye 780, anti-CD11b, anti-Ly-6C, anti-Ly-6G, and anti MHC-II in the mouse or eFluor viability dye 780, anti-G1, and anti-MHC-II in the bovine. Representative plots that show the major proportion of MHC-IIneg and the minor proportion of MHC-IIpos neutrophils in both species are depicted. (B) The two MHC-IIneg and MHC-IIpos neutrophil populations among total CD11bpos Ly6-Gpos Ly6-Cpos mouse neutrophils, or G1pos bovine neutrophils, were sorted by flow cytometry (purity >99%), cytocentrifuged and were indistinguishable after May Grünwald Giemsa staining. (C) Histograms represent expression of CD11b, CD14, CD62-L for both species and CD44 and CD274 (PD-L1) for the mouse samples on the surface of sorted MHC-IIneg and MHC-IIpos neutrophils in comparison to isotype controls. (A–C) Results from one representative animal are depicted (n=6 for mouse, n=6 for bovine). (D) After purification by flow cytometry, MHC-IIneg and MHC-IIpos neutrophils from the two species were incubated with LPS for 1 h and labeled with annexin-V and propidium iodide (PI) to analyze apoptotic (annexin V+; PI−) and dead cells (annexin V+; PI+). Dot plots from one representative animal are depicted (n=4 for mouse, n=3 for bovine).
Therefore, in mouse BM or in cattle blood, two subsets of neutrophils could be distinguished at steady state by MHC-II labeling. Both subsets displayed similar survival profiles after short-term ex-vivo LPS stimulation.
MHC-IIpos Neutrophils Are Enriched in Lymphoid Organs
Although neutrophils are mostly present in the BM reservoir and in circulating blood, recent data in the mouse model and in humans highlighted their wider distribution in tissues (27) including lymphoid organs. We next examined the distribution of MHC-IIneg versus MHC-IIpos neutrophils in different compartments in both mouse and cattle. Cells were extracted and prepared from BM, lymph nodes (LN, inguinal in the mouse and tracheobronchial in bovine), and spleen. For analysis of circulating neutrophils, total blood leukocytes were analyzed by flow cytometry (see Supplementary Figure S1 for isotype controls and Supplementary Figure S2 for gating strategy) after lysis of red cells. In the BM, neutrophils represented around 30% of total leukocytes in both species (Figure 3A). This was expected since BM is the principal compartment hosting neutrophils. In blood, neutrophils represented 30 or 20% of total cells in mouse and cattle, respectively. In both the mouse and the bovine LN, even though neutrophils represented less than 1% of cells at steady state, they were consistently detected. Similarly, between 2 and 3% of cells collected from mouse or bovine spleen after lysis of red blood cells were neutrophils (Figure 3A). In all analyzed compartments classical neutrophils as well as MHC-IIpos neutrophils were detected (Figure 3B). Interestingly, whereas MHC-IIpos neutrophils represented only 2.4 ± 0.6 or 4.6 ± 1.4% of total neutrophils in the mouse bone-marrow or cattle blood, respectively, they represented around 40 or 60% of total neutrophils in mouse or bovine lymph nodes, respectively. In spleen, around 20% of total mouse neutrophils and 30% of bovine were MHC-IIpos (Figure 3B). Therefore MHC-IIpos neutrophils were highly enriched in lymphoid organs in both species, at steady state.
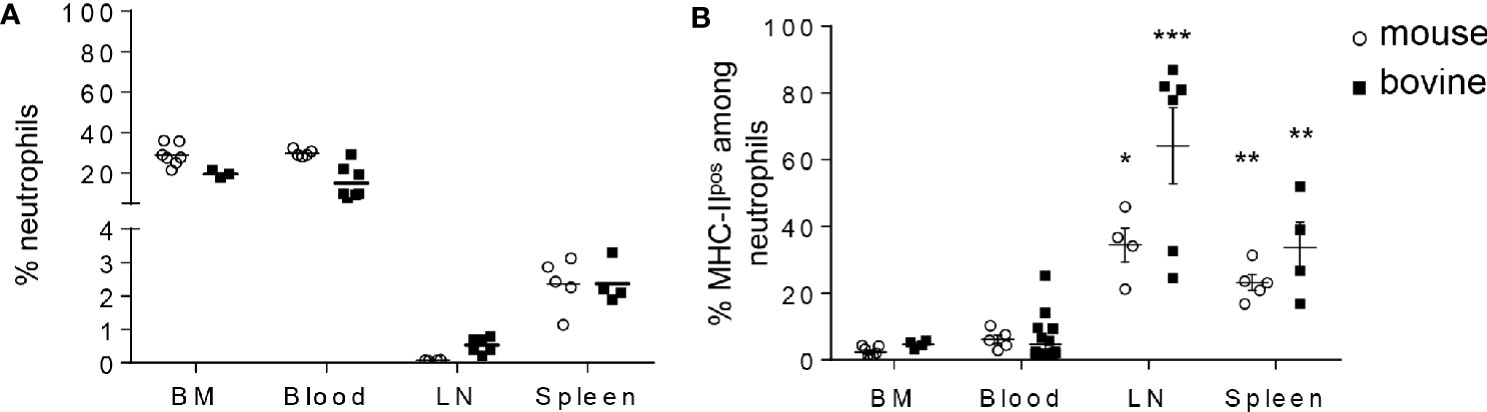
Figure 3 MHC-IIpos neutrophils are enriched in lymphoid organs in both species. (A, B) Cells were prepared from bone marrow (BM) or blood from the two species and neutrophils analyzed by flow cytometry as in Figure 2. Neutrophils present in the lymphoid organs were also analyzed after collecting post mortem the inguinal (mouse) or tracheobronchial (cattle) lymph nodes (LN) and spleen. (A) The percentage of Ly6-Gpos, Ly6-Cpos mouse neutrophils or G1pos cattle neutrophils among total leukocytes and (B) The percentage of the MHC-IIpos subset among total neutrophils, was analyzed in each compartment. Individual data and the median in each group are presented. This percentage was significantly higher in lymphoid organs than in mouse BM or cattle blood. *P<0.05; **P<0.01; ***P<0.001 (Mann Whitney non-parametric test).
RNA Profiling Distinguishes Classical From MHC-IIpos Neutrophils in Both the Mouse and the Bovine
To analyze the transcriptional profile of MHC-IIpos and MHC-IIneg neutrophils, we sorted the two populations from either BM (mouse) or blood (cattle) to more than 99% purity and performed a transcriptomic profiling using 48 validated primer pairs (Supplementary Table S1) designed to cover a large set of neutrophils functions such as synthesis of cytokines or chemokines, enzymes stored in granules, surface receptors, as well as transcription factors. Some weakly expressed genes were removed from the panel (highlighted in Supplementary Table S1). We then performed unsupervised principal component analysis (PCA) and observed that the different subsets were significantly discriminated (Figure 4A). The first axis of the PCA (explaining 26.4% of the variance) separated bovine and mouse samples, as expected. The second axis (explaining 19.1% of the variance) clearly separated the MHC-IIpos from the MHC-IIneg neutrophils in each species (Figure 4A), indicating that they belonged to different subsets. As expected, in the bovine, gene expression profiles were more dispersed than in the inbred mouse, due to inter-individual variability. In both species, the two neutrophil subsets were also segregated by hierarchical clustering based on ΔCT values (Figure 4B). Most of the genes were significantly less expressed in MHC-IIpos neutrophils as compared to MHC-IIneg. However, expression of the following genes was significantly higher in the MHC-IIpos subset: elane, proteinase3, PD-L1 in murine and MHC-II; AHR in bovine cells. A trend for higher expression was observed in MHC-IIpos neutrophils for CD14 (both species) and proteinase3 (bovine). Therefore, in both species the transcriptomic signature significantly differed between the two neutrophil subsets which suggested different biological roles.
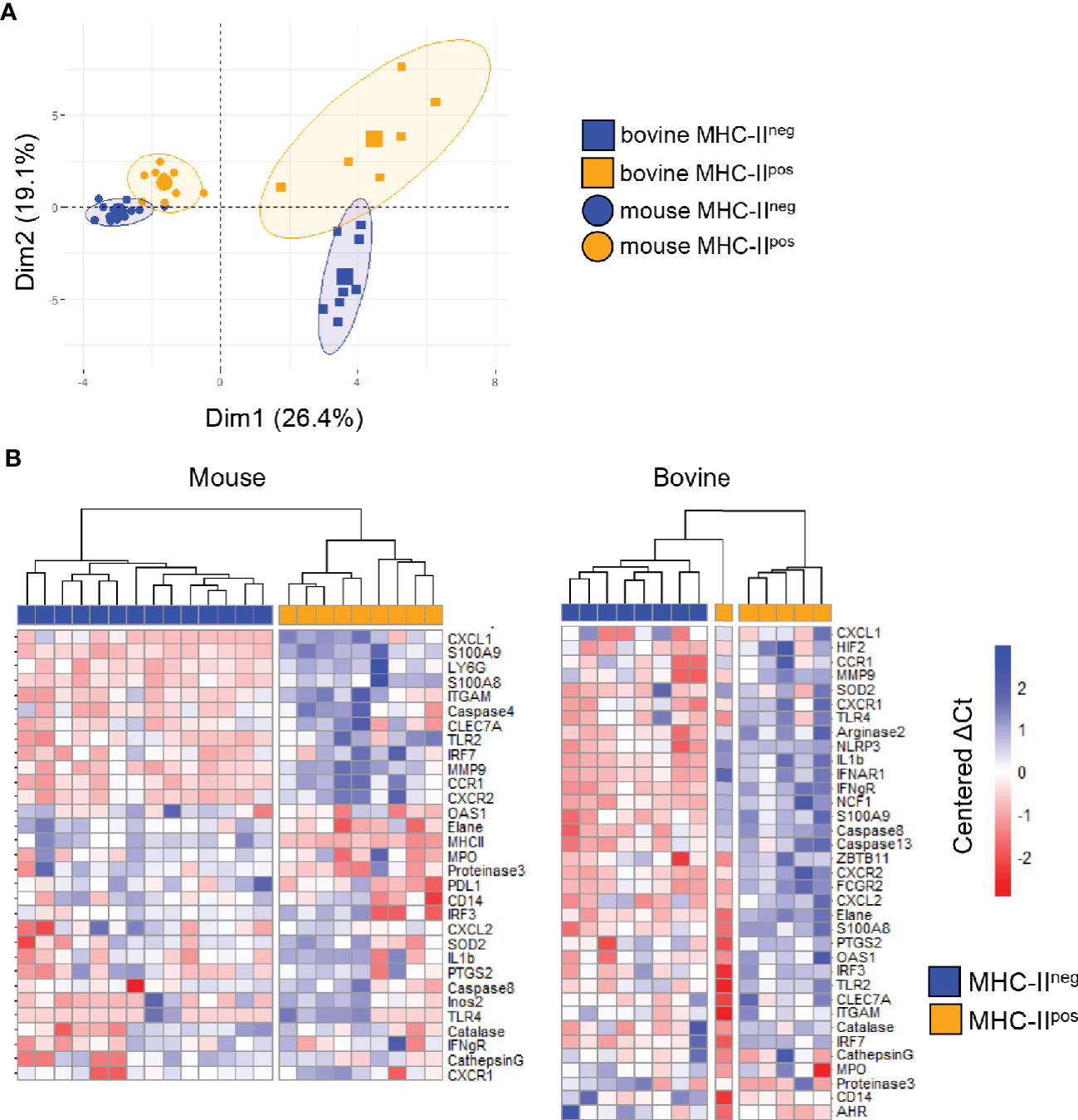
Figure 4 MHC-IIpos and MHC-IIneg neutrophils display distinct transcriptomic signatures. Gene expression of the two purified neutrophil subsets (more than 99% purity) from the two species was assessed by quantitative Real Time PCR using sets of primer pairs designed to cover a large range of neutrophils functions. mRNA expression was normalized to the expression of three housekeeping genes for both species to calculate the ΔCt values (A). principal component analysis (PCA) was performed on ΔCt values and the two first dimensions of the PCA plot are depicted. Inclusion in groups of sets of samples are delineated in the colored areas on the graph with a confidence level of 90% (B) Hierarchical clustering of gene expression was performed on median centered ΔCt values for mouse and bovine data sets, using the ward.D2 method. For each gene, ΔCt values were centered to the median ΔCt value. Higher or lower ΔCt expression compared to the median value were represented respectively in deep to light red or blue. Expression of a selected set of genes indicated on the figure was clearly distinct in MHC-IIpos neutrophils or MHC-IIneg neutrophils. Data represent individual samples (mouse: n= 14 for MHC-IIneg, n=9 for MHC-IIpos Bovine: n=8 for MHC-IIneg n=6 for bovine MHC-IIpos).
MHC-IIpos Neutrophils Produce Higher Levels of ROS Than MHC-IIneg but Similarly Phagocytose Bioparticles and Kill Bacteria
In order to gain further insight into the functions of the two neutrophil populations, we first compared their phagocytic ability using conjugated pHrodo™ Red E. coli BioParticles™ that only fluoresce once inside the phagosome or endosome of the cell (28). Neutrophils were then analyzed by flow cytometry and the percentage of phagocytosis was directly correlated with the mean fluorescence intensity (see Supplementary Figure S3). Phagocytosis by MHC-IIneg or MHC-IIpos neutrophils was compared in individual animals from each species. Both subsets actively phagocytosed bioparticles which was dramatically reduced by treatment with cytochalasin D (Figure 5A). We did not detect a significant difference in phagocytosis between MHC-IIneg and MHC-IIpos neutrophils neither in the mouse nor the bovine (Figure 5A). We next measured their potential for total ROS production after incubation with the non-specific chemical inducer tert-butyl hydroperoxide (TBHP) using the fluorescent probe CellROX that fluoresces when oxidized (see Supplementary Figure S3). Mouse bone-marrow neutrophils incubated with medium produced very low levels of ROS whereas bovine blood neutrophils produced higher levels (Figure 5B). As expected, TBHP treatment dramatically increased ROS production by both MHC-IIneg and MHC-IIpos neutrophils in both species. However, in both conditions and for both species, MHC-IIpos neutrophils produced significantly more ROS than MHC-IIneg cells (Figure 5B). We next analyzed the killing activity, under non-opsonic conditions, of the two subsets against the Escherichia coli P4 strain isolated from a case of bovine clinical mastitis (19). Both types of neutrophils efficiently killed E. coli and no significant difference was observed between MHC-IIneg or MHC-IIpos neutrophils (Figure 5C).
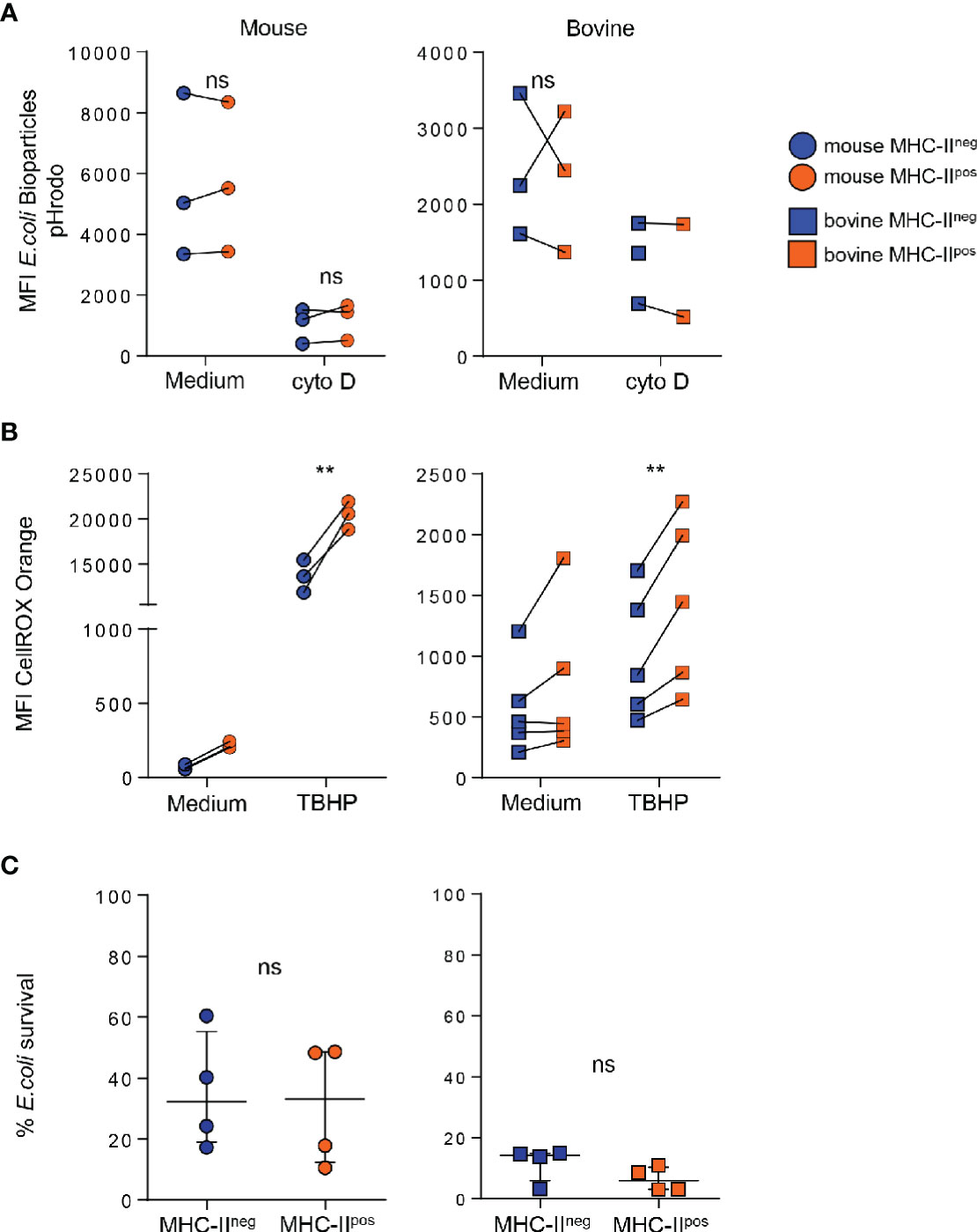
Figure 5 MHC-IIpos phagocytose bioparticles and kill Escherichia coli similarly to MHC-IIneg neutrophils but produce higher levels of reactive oxygen species (ROS). (A) After purification by cell sorting from the bone marrow (BM) (mouse) or blood (bovine), phagocytosis by MHC-IIpos or MHC-IIneg neutrophils was assessed using pHrodo E. coli bioparticles. Mean fluorescence intensity was directly correlated to ingested particles. Cells were treated with cytochalasin D as indicated. (B) Oxidative stress was measured in MHC-IIpos and MHC-IIneg neutrophils using the CellROX Orange probe that reacts with all ROS species. Cells were activated with TBHP or incubated with medium alone and levels of ROS were measured by flow cytometry. (A, B) Mean fluorescence intensity in each sample is depicted and paired MHC-IIpos and MHC-IIneg samples were analyzed for each animal. (C) Purified MHC-IIpos and MHC-IIneg neutrophils were infected with the E. coli P4 strain and bacterial survival was calculated by determining the ratio of bacteria incubated alone or in presence of neutrophils. Data represent n=4 independent experiments with neutrophils pooled from independent lots of 10 mice, or prepared from blood of n=4 independent cows. (A–C) **P<0.01; (2way ANOVA). ns, non significant.
MHC-IIpos but Not MHC-IIneg Neutrophils Exert Contact-Dependent Suppression of T Cells at Steady State
We wondered if surface proteins such as MHC-II (mouse and cattle) or PDL-1 (mouse) or enrichment of MHC-IIpos neutrophils in lymphoid organs could be linked to regulatory functions on T cells. To address this, we set up in vitro assays that were either antigen specific in the mouse (Figure 6A) or polyclonal in cattle (Figure 6C). Using the OT-II transgenic mice that bear the OVA peptide 323-339—MHC class II complex-specific TCR (16) we observed strong proliferation of splenocytes when stimulated with OVA peptide for 72 hours. Taking this condition as the maximum proliferation (100%) we compared the impact of adding MHC-IIneg and MHC-IIpos neutrophils to the proliferating cells (ratio 10 splenocytes:1 neutrophil). The classical MHC-IIneg neutrophils had no measurable effect on OT-II splenocytes proliferation (Figure 6B). By contrast, addition of MHC-IIpos neutrophils purified either from mouse BM or blood, decreased the capacity of CD4 OT-II cells to proliferate by 66 ± 3% (Figure 6B). The suppressive activity of mouse MHC-IIpos neutrophils depended on contact with the proliferating T-cells as no effect was observed when cells were separated by a Transwell device (Figure 6B). We then used blocking antibodies in order to define if MHC-II or CD11b, that were used to distinguish the two subsets as both are highly expressed on the surface of MHC-IIpos neutrophils, were involved in this suppressive activity. Both anti-MHC-II and anti-CD11b partially relieved suppression by MHC-IIpos neutrophils that reached 70 ± 7 and 75 ± 1% of total proliferation respectively (Figure 6B). This indicated that both molecules were involved in the suppression mechanism although they were obviously not the only ones.
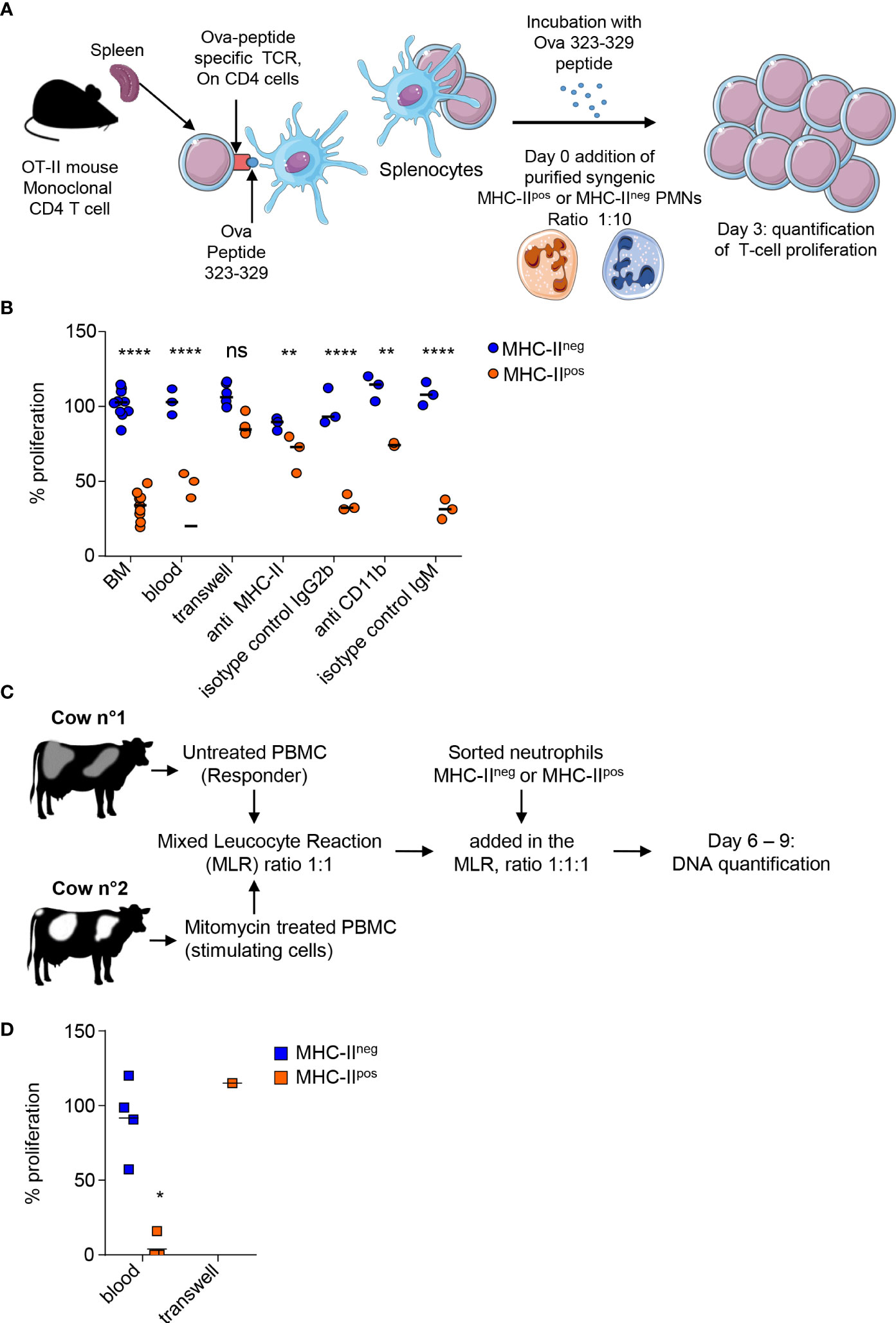
Figure 6 MHC-IIpos but not MHC-IIneg neutrophils exert suppression of T cells at steady state. (A) In the mouse an antigen -specific proliferation assay was set up with OT-II transgenic mice that carry the monoclonal population of T-cells specific for the Ova 323-329 peptide. Proliferation of splenocytes from OT-II mice activated with the Ova 323-329 peptide was set up as the maximum and compared to conditions where MHC-IIpos or MHC-IIneg neutrophils purified from syngenic C57BL/6 mice after cell sorting (99% purity) were added with a ratio of 1:10. (B) The percentage of T cell proliferation after addition of MHC-IIpos or MHC-IIneg neutrophils was calculated based on OT-II splenocytes proliferation with ova peptide only. Neutrophils were prepared from the BM of syngenic mice except for the “blood” sample as indicated on the graph. Different conditions for the assay are also indicated, with cells separated by a transwell device, or neutrophils incubated with anti-MHC-II, anti-CD11b, or isotype controls before addition to the proliferating splenocytes. (C) For the bovine, a mixed leucocyte reaction (MLR) was set up to assess polyclonal T cell proliferation. PBMCs from the responder animal were isolated and left untreated, while PBMCs from the stimulating animal were incubated with mitomycin C. PBMCs from the two cows were incubated at ratio of 1:1. To assess the impact of neutrophils on cattle T cell proliferation, 105 of sorted MHC-IIpos or MHC-IIneg neutrophils from the responder animal were added to the reaction at day 4. DNA was quantified at day 6 and 9. (D) The proliferation was calculated by subtracting DNA values at day 9 from day 6 values. Proliferation measured in the MLR without neutrophils was defined as the reference 100% proliferation. The proliferation observed in the presence of sorted MHC-IIpos or MHC-IIneg neutrophils was calculated according to the DNA content (day 9–day 6) and expressed as percentage of the reference value. In one experiment, MHC-IIpos neutrophils were separated from proliferating cells by a Transwell device. (B, D) Individual data and the median in each group are represented (3 independent experiments, n≥3 pool of 10 mice, n=4 cows, n=1 cow for transwell experiment; each represented value is the mean of technical triplicates). *P<0.05; **P<0.01; ****P<0.0001 (Mann Whitney non-parametric test).
In cattle, we set up a mixed-leukocyte-reaction (MLR) by mixing PBMCs from a responder animal with mitomycin-C treated PBMCs from a genetically unmatched animal as stimulating cells (ratio 1:1) for a total of 9 days. After initial decline of total DNA content during 6 days due to cells dying in the wells, proliferation of T-cells from the responder animal was measured by an increase of the DNA content at day 9 in the control wells, correlating with polyclonal activation of T-cells (Supplementary Figure S4). Level of proliferation obtained under these control conditions was set as 100% (Figure 6D). Addition of classical MHC-IIneg bovine neutrophils to the PBMCs (ratio 1:1) from the responder animal did not change the proliferative capacity. By contrast, addition of MHC-IIpos neutrophils from the responder animal to PBMCs (ratio 1:1) strongly suppressed T-cells as the proliferation was completely inhibited for 3 animals, and only 16% of proliferation remained for 1 animal, as compared to control wells (Figure 6D). Decreasing the MHC-IIpos neutrophils concentration, alleviated the suppressive effect (Supplementary Figure S5) showing the dose-response effect of MHC-IIpos neutrophils on T-cells. In one experiment we could separate MHC-IIpos neutrophils from the proliferating PBMCs in a transwell device and observed that suppression was abolished, indicating that the suppressive activity of MHC-IIpos neutrophils was contact-dependent as in the mouse (Figure 6D). Therefore, both in the mouse and the bovine, a subset of MHC-IIpos neutrophils can be distinguished from classical neutrophils as displaying suppressive activity on T-cells at steady state.
Discussion
We reported here that a population of MHC-IIpos neutrophils was present in the BM reservoir, circulated in blood and was enriched in lymphoid organs, in the apparently healthy bovine and mouse. Both MHC-IIpos and MHC-IIneg neutrophils displayed the polylobed nucleus, and were undistinguishable by this gold standard of neutrophils characterization (14). Similar to classical MHC-IIneg neutrophils, the MHC-IIpos subset displayed important functions of neutrophils such as ROS production, phagocytosis, and bacterial killing. However, unlike classical MHC-IIneg neutrophils, the MHC-IIpos neutrophils were able to suppress T-cells, a function that is reported here for the first time for bovine neutrophils. Heterogeneity or plasticity of neutrophils has largely emerged in the literature in humans or mice and new models of neutrophil differential development are proposed (15). Here, mouse and bovine MHC-IIpos suppressive neutrophils were detected in the BM reservoir. They were the only ones to exert suppressive activity on T cells. They displayed a clearly distinct transcriptomic profile as compared to MHC-IIneg neutrophils. They were also highly enriched in lymphoid organs. Thus, they may represent a distinct subset, produced in the BM for rapid mobilization and regulation of T cells. On the other hand, CD11b upregulation that was observed on these neutrophils could also sign an activated state (29). Recently, CD11bhi primed neutrophils were also reported to circulate in blood in healthy mice and humans to quickly respond to danger (30). Neutrophils from healthy humans could be induced in vitro to exert ROS, CD11b and contact-dependent suppressive activity on T cells upon activation with specific stimuli (31). We detected MHC-IIpos neutrophils in the BM and, at least in the mouse, they were able to suppress T-cell proliferation, suggesting that they were already present as regulatory cells in the reservoir. Moreover, the regulatory neutrophils from the two species did not undergo higher apoptosis or death as compared to their classical neutrophils counterparts upon short-term ex-vivo stimulation with LPS, indicating they were not “older” or hyperactivated (32). In addition, MHC-II expression by MHC-IIneg neutrophils could not be induced in vitro by incubation with LPS (data not shown). Whether heterogenous neutrophils are released from the bone-marrow as distinct subsets under steady state conditions or correspond to activation or polarization states in situ remains an open debate (33) but what we observed here is that MHC-IIpos neutrophils able to regulate T cells display a specific neutrophil “phenotype” (34).
Suppressive neutrophils that may be included within a broader category of cells termed “myeloid-derived suppressor cells (MDSCs)” (35) accumulate mostly under pathological conditions. Two main branches of MDSCs have been described: the monocytic MDSCs and the granulocytic MDSCs (G-MDSCs) (35). In cancer, G-MDSCs are associated with tumor progression and escape to the immune surveillance (36). In chronic infections such as tuberculosis (37, 38) or AIDS (39), or acute inflammatory syndrome such as sepsis (40) MDSCs are targeted for new host-directed therapies. However, MDSCs, including regulatory neutrophils, are also beneficial to the host under some circumstances. Because they sustain the generation of regulatory T-cells (41) they may help avoiding graft rejection (42). They can positively regulate autoimmune disorders (43) or protect the lung from destructive inflammation during Pseudomonas aeruginosa or Klebsiella pneumoniae infections (44). They accumulate during pregnancy where they are important regulators of fetal-maternal tolerance (45) and in neonates where they could prevent overwhelming inflammation following microbial colonization (46). Recently, Aarts and colleagues demonstrated that neutrophils from healthy humans could become T-cell suppressive under specific stimulation (31). Along the same line, Fine et al. recently described that neutrophils circulate in healthy donors under several states included as “primed” cells that are first recruited to the injury site to combat infection (30). In both these studies, high levels of CD11b signed the phenotype of these neutrophils, alike the MHC-IIpos regulatory neutrophil subset that we describe here. In cattle, there is no description to date of suppressive neutrophils or G-MDSCs. In a recent report, Li and colleagues (47) identified a subset of MHC-IIpos neutrophils that accumulated in spleen during Ostertagia ostertagi parasitic infection in cattle and produced the suppressive cytokine IL-10. This subset was the master regulator of immune suppression in parasitized animals. We did not observe il10 transcription in any neutrophil subset nor IL-10 production in the supernatant of neutrophil-T-cells cocultures in our study (data not shown). Whether MHC-IIpos regulatory neutrophils that we identified are related to the subset identified by Li et al. (47) remains an open question.
Regulatory neutrophils, or G-MDSCs, block T-cell proliferation through different mechanisms (14) including ROS production (48). We observed in both species that direct contact between MHC-IIpos neutrophils and T-cells was necessary to induce suppression, as was also reported for G-MDSCs. Formation of a synapse is believed to greatly enhance suppression especially when the delivery of short-lived molecules such as H2O2 is instrumental (35). Interestingly, we observed here a significantly higher production of total ROS upon chemical stimulation by MHC-IIpos neutrophils as compared to their MHC-IIneg counterparts which did not translate into higher killing of E. coli. Pliyev and colleagues demonstrated that NADPH dependent ROS were required for the expression of MHC-II in human neutrophils activated by GM-CSF and IFNγ (49). Whether the higher ROS production recorded in our study is linked to the suppression mechanism remains to be investigated. In both the mouse and the bovine (data not shown), MHC-II was also involved in the mechanism, as blocking this molecule partially relieved the suppression of T-cell proliferation, emphasizing the need for synapse formation between the T-cell and the regulatory neutrophil. We established in the past the important role of CD11b expressed by neutrophils in phagocytic synapse and crosstalk with other cells, as well as specific signaling pathways (50, 51). In the present study, we observed that CD11b was also involved in the suppression mechanism in mice, as reported for human neutrophils acquiring suppressive activity upon in vitro stimulation. Direct CD11b-dependent interactions between neutrophils and T cells was required for suppression but additional molecules such as ICAM-3 on T-cells may also function as ligands for CD11b (31). Similarly, our results in the mouse model suggested that a synapse with T-cells, involving CD11b and MHC-II on the surface of neutrophils, was necessary to exert suppression. However, suppression by MHC-IIpos neutrophils clearly involved other mechanisms that we will investigate in the near future. In that respect, surface expression and up-regulated gene transcription of PD-L1 by mouse regulatory neutrophils is an interesting avenue. This molecule is an important immune check-point and a favorite target for cancer treatment. MDSCs express high levels of CD274 (52) which is involved in T-cell suppression. Of note, CD14pos monocytes expressing CD274 accumulate in blood of cattle infected by Mycoplasma bovis (53), a strongly immunosuppressive pathogen causing antibiotic resistant mastitis ant other diseases. CD274 is now a target for host-directed therapies of cattle infected by this pathogen (54). We could not investigate CD274 expression by cattle MHC-IIpos regulatory neutrophils due to a lack of available Ab. We did not observe differences at the mRNA level between the two subsets (data not shown) but whether such differences exist at the protein level remains to be investigated.
To conclude, comparative analysis of mouse and bovine species allowed us to characterize a new subset of regulatory neutrophils that are able to suppress T cells. In the near future, we will investigate how these cells behave during clinical conditions in cattle such as mastitis, which remains one of the most important issue in dairy farming. We believe that such studies are of utmost importance to better understand the physiopathology of this disease, especially during chronic infections that remain difficult to treat. Our findings could lead to the discovery of new biomarkers and development of innovative host-directed therapies (54) targeting regulatory neutrophils for more effective clearance of pathogens and better control of mammary gland inflammation and damage.
Data Availability Statement
The original contributions presented in the study are included in the article/Supplementary Material. Further inquiries can be directed to the corresponding authors.
Ethics Statement
The animal study was reviewed and approved by Val de Loire Ethics Committee for Animal Experimentation.
Author Contributions
MR designed and did most of the experiments for cattle neutrophils and ED-D for mouse neutrophils. They analyzed data and prepared all manuscript figures. YV performed the flow cytometry analysis and sorting of mouse and cattle neutrophils. PC helped with the mixed leukocyte reaction in cattle. PG helped with transcriptomic analysis, critically analyzed the data, and revised the manuscript. PR brought valuable expertise on cattle neutrophil purification methods, critically analyzed the data, and revised the manuscript. NW and AR obtained grants, supervised all aspects of the work, critically analyzed the data, and wrote the manuscript. AR also contributed to the important experiments in cattle. All authors contributed to the article and approved the submitted version.
Funding
This work was supported by a grant from the French Agence Nationale de la Recherche under the Carnot Program France Future Elevage (BoNeutro), the EGER program of APIS-GENE (MASTICELLS), and the Région Centre Val de Loire (grant N°32000584 “inflammation et infection”) and the EUROFERI project (FEDER-FSE Centre Val de Loire 2014-2020, N° EX 010233).
Conflict of Interest
The authors declare that the research was conducted in the absence of any commercial or financial relationships that could be construed as a potential conflict of interest.
Acknowledgments
MR conducts her doctoral project under a CIFRE agreement (industrial agreement of learning/training by research, CIFRE N° 2019/0776) signed with Institut de l’Elevage IDELE. We are grateful to Théodore Vinais for his early contribution during his internship to the characterization of the two neutrophil subsets in cattle. Corinne Beauge and her team (PFIE, INRAE, Nouzilly) are gratefully acknowledged for mice care. We warmly thank Eric Briant and his team (UE-PAO, INRAE, Nouzilly) for bovine blood sampling and animal care. We thank the staff of the Abattoir du Perche Vendômois for valuable access to and assistance for bovine post-mortem sampling. Bovine CD11b hybridoma was a kind gift from Dr. Dirk Werling (Royal Veterinary College of London).
Supplementary Material
The Supplementary Material for this article can be found online at: https://www.frontiersin.org/articles/10.3389/fimmu.2021.625244/full#supplementary-material
Supplementary Table 1 | Antibodies used in the study. The specificity, origin, clone number and commercial provenance of all antibodies used in the study are listed.
Supplementary Table 2 | Sequences of primers used in this study. Primers were designed using Geneious software, in intron-spanning regions when possible. The annealing temperature was set at 60 and 62°C for bovine and mouse samples respectively. Housekeeping genes used as the reference to calculate ΔCT for each species are indicated in the yellow boxes and weakly expressed genes that were removed from the Principal Component Analysis presented in Figure 4 are indicated in the grey boxes.
Supplementary Figure 1 | Isotype controls for neutrophil diversity analysis in mouse bone marrow and cattle blood. Mouse and bovine neutrophils were labelled as described in Figure 2. Appropriate isotype controls for all antibodies in each experiment were used to correctly set the analysis and sorting gates. Dot plots from one representative animal are depicted (3 independent experiments, n=4 mice, n=6 for bovine).
Supplementary Figure 2 | Gating strategy for neutrophil diversity analysis in all organs. Mouse and bovine neutrophils were labelled as described in Figure 2. Similar procedures were set up with isotype controls for all antibodies in each experiment to correctly set the analysis gates. Dot plots from one representative animal are depicted (3 independent experiments, n=4 mice, n=6 for bovine).
Supplementary Figure 3 | Gating strategy for analysis of phagocytosis and ROS production by neutrophils. Neutrophils were labelled and sorted as described in Figures 2 and S1. (A) After purification by cell sorting from the BM (mouse) or blood (bovine) phagocytosis by MHC-IIpos or MHC-IIneg neutrophils was assessed using pHrodo E.coli bioparticles with or without previous treatment with cytochalasin D. Dot plots from one representative animal are depicted (3 independent experiments, n=3 pool of 10 mice, n=3 for bovine). (B) Oxidative stress was measured in MHC-IIpos and MHC-IIneg sorted neutrophils using the CellROX Orange probe that reacts with all ROS species. Cells were activated with TBHP or incubated with medium alone and levels of ROS were measured by flow cytometry among the live cells (unstained with eFluor780 viability dye). Dot plots from one representative animal are depicted (3 and 4 independent experiments for mice and cattle respectively, n=3 pool of 10 mice, n=5 for bovine).
Supplementary Figure 4 | Kinetics of bovine Mixed Leukocyte Reaction and analysis of neutrophil suppressive activity. (A) PBMCs from the responder animal were isolated and left untreated, while PBMCs from the stimulating animal were incubated with mitomycin C to block their proliferation. PBMCs from the two cows were incubated at ratio of 1:1. Sorted MHC-IIpos or MHC-IIneg neutrophils from the responder animal were added to the reaction at day 4. (B) DNA was quantified at different time points with CyQUANT Cell Proliferation Assay tests according to manufacturer’s instruction and fluorescence was read at 530nm. DNA extracted from PBMCs cultivated separately decreased along the assay indicated the absence of proliferation (dotted lines). In the MLR reaction, while DNA content declined between day 1 and 6, PBMCs proliferation could be measured between day 6 and 9 (black). The effect of adding sorted MHC-IIneg neutrophils (blue) or MHC-IIpos neutrophils (orange) to the proliferating cells could then be measured. One representative experiment is shown and data represent the mean ± SEM of technical triplicates. Four independent experiments were conducted with different pairs of cows.
Supplementary Figure 5 | Bovine MHC-IIpos neutrophil suppressive activity is dose-dependent. The suppressive assay was performed as described in Figures 6 and S4. At day 4, 1x105, 5x104 or 1x104 purified MHC-IIpos neutrophils from the responder animal were added to the MLR reaction. One experiment was performed (technical duplicates are depicted).
References
1. Kolaczkowska E, Kubes P. Neutrophil recruitment and function in health and inflammation. Nat Rev Immunol (2013) 13:159–75. doi: 10.1038/nri3399
2. Futosi K, Fodor S, Mócsai A. Neutrophil cell surface receptors and their intracellular signal transduction pathways. Int Immunopharmacol (2013) 17:638–50. doi: 10.1016/j.intimp.2013.06.034
3. Nguyen GT, Green ER, Mecsas J. Neutrophils to the ROScue: Mechanisms of NADPH oxidase activation and bacterial resistance. Front Cell Infect Microbiol (2017) 7:373–3. doi: 10.3389/fcimb.2017.00373
4. Reagan WJ, Rovira ARI, DeNicola DB. Veterinary hematology: atlas of common domestic and non-domestic species. Chichester, UK. Wiley (2019).
5. Bassel LL, Caswell JL. Bovine neutrophils in health and disease. Cell Tissue ResS (2018) 371:617–37. doi: 10.1007/s00441-018-2789-y
6. Mestas J, Hughes CCW. Of mice and not men: differences between mouse and human immunology. J Immunol (2004) 172:2731–8. doi: 10.4049/jimmunol.172.5.2731
7. Rainard P, Riollet C. Mobilization of neutrophils and defense of the bovine mammary gland. Reprod Nutr Dev (2003) 43:439–57. doi: 10.1051/rnd:2003031
8. Deniset JF, Surewaard BG, Lee W-Y, Kubes P. Splenic Ly6Ghigh mature and Ly6Gint immature neutrophils contribute to eradication of S. Pneumoniae J Exp Med (2017) 214:1333–50. doi: 10.1084/jem.20161621
9. Puga I, Cols M, Barra CM, He B, Cassis L, Gentile M, et al. B cell-helper neutrophils stimulate the diversification and production of immunoglobulin in the marginal zone of the spleen. Nat Immunol (2011) 13:170–80. doi: 10.1038/ni.2194
10. Hampton HR, Chtanova T. The lymph node neutrophil. Semin Immunol (2016) 28:129–36. doi: 10.1016/j.smim.2016.03.008
11. Yipp BG, Kim JH, Lima R, Zbytnuik LD, Petri B, Swanlund N, et al. The lung is a host defense niche for immediate neutrophil-mediated vascular protection. Sci Immunol (2017) 2:eaam8929. doi: 10.1126/sciimmunol.aam8929
12. Mócsai A. Diverse novel functions of neutrophils in immunity, inflammation, and beyond. J Exp Med (2013) 210:1283–99. doi: 10.1084/jem.20122220
13. Mantovani A, Cassatella MA, Costantini C, Jaillon S. Neutrophils in the activation and regulation of innate and adaptive immunity. Nat Rev Immunol (2011) 11:519–31. doi: 10.1038/nri3024
14. Pillay J, Tak T, Kamp VM, Koenderman L. Immune suppression by neutrophils and granulocytic myeloid-derived suppressor cells: similarities and differences. Cell Mol Life Sci CMLS (2013) 70:3813–27. doi: 10.1007/s00018-013-1286-4
15. Ng LG, Ostuni R, Hidalgo A. Heterogeneity of neutrophils. Nat Rev Immunol (2019) 19:255–65. doi: 10.1038/s41577-019-0141-8
16. Barnden MJ, Allison J, Heath WR, Carbone FR. Defective TCR expression in transgenic mice constructed using cDNA-based alpha- and beta-chain genes under the control of heterologous regulatory elements. Immunol Cell Biol (1998) 76:34–40. doi: 10.1046/j.1440-1711.1998.00709.x
17. Abadie V, Badell E, Douillard P, Ensergueix D, Leenen PJ, Tanguy M, et al. Neutrophils rapidly migrate via lymphatics after Mycobacterium bovis BCG intradermal vaccination and shuttle live bacilli to the draining lymph nodes. Blood (2005) 106:1843–50. doi: 10.1182/blood-2005-03-1281
18. Rambault M, Borkute R, Doz-Deblauwe E, Le Vern Y, Winter N, Dorhoi A, et al. Isolation of Bovine Neutrophils by Fluorescence- and Magnetic-Activated Cell Sorting. Methods. Mol Biol (2021) 2236:203–17. doi: 10.1007/978-1-0716-1060-2_16
19. Roussel P, Porcherie A, Répérant-Ferter M, Cunha P, Gitton C, Rainard P, et al. Escherichia coli mastitis strains: In vitro phenotypes and severity of infection in vivo. PloS One (2017) 12:e0178285. doi: 10.1371/journal.pone.0178285
20. Carlson GP, Kaneko JJ. Isolation of leukocytes from bovine peripheral blood. Proceedings of the Society for Experimental Biology and Medicine. Soc Exp Biol Med (New York NY) (1973) 142:853–6. doi: 10.3181/00379727-142-37131
21. Rainard P, Riollet C, Poutrel B, Paape MJ. Phagocytosis and killing of Staphylococcus aureus by bovine neutrophils after priming by tumor necrosis factor-alpha and the des-arginine derivative of C5a. Am J Vet Res (2000) 61:951–9. doi: 10.2460/ajvr.2000.61.951
22. Daley JM, Thomay AA, Connolly MD, Reichner JS, Albina JE. Use of Ly6G-specific monoclonal antibody to deplete neutrophils in mice. J Leukoc Biol (2008) 83:64–70. doi: 10.1189/jlb.0407247
23. Davis WC, Marusic S, Lewin HA, Splitter GA, Perryman LE, McGuire TC, et al. The development and analysis of species specific and cross reactive monoclonal antibodies to leukocyte differentiation antigens and antigens of the major histocompatibility complex for use in the study of the immune system in cattle and other species. Vet Immunol Immunopathol (1987) 15:337–76. doi: 10.1016/0165-2427(87)90005-5
24. Grandoni F, Elnaggar MM, Abdellrazeq GS, Signorelli F, Fry LM, Marchitelli C, et al. Characterization of leukocyte subsets in buffalo (Bubalus bubalis) with cross-reactive monoclonal antibodies specific for bovine MHC class I and class II molecules and leukocyte differentiation molecules. Dev Comp Immunol (2017) 74:101–9. doi: 10.1016/j.dci.2017.04.013
25. Lombard R, Doz E, Carreras F, Epardaud M, Le Vern Y, Buzoni-Gatel D, et al. IL-17RA in non-hematopoietic cells controls CXCL-1 and 5 critical to recruit neutrophils to the lung of mycobacteria-infected mice during the adaptive immune response. PloS One (2016) 11:e0149455. doi: 10.1371/journal.pone.0149455
26. Pauken KE, Wherry EJ. Overcoming T cell exhaustion in infection and cancer. Trends Immunol (2015) 36:265–76. doi: 10.1016/j.it.2015.02.008
27. Hidalgo A, Chilvers ER, Summers C, Koenderman L. The neutrophil life cycle. Trends Immunol (2019) 40:584–97. doi: 10.1016/j.it.2019.04.013
28. Segal AW. How neutrophils kill microbes. Annu Rev Immunol (2005) 23:197–223. doi: 10.1146/annurev.immunol.23.021704.115653
29. Miralda I, Uriarte SM, McLeish KR. Multiple phenotypic changes define neutrophil priming. Front Cell Infect Microbiol (2017) 7:217–7. doi: 10.3389/fcimb.2017.00217
30. Fine N, Barzilay O, Sun C, Wellappuli N, Tanwir F, Chadwick JW, et al. Primed PMNs in healthy mouse and human circulation are first responders during acute inflammation. Blood Adv (2019) 3:1622–37. doi: 10.1182/bloodadvances.2018030585
31. Aarts CEM, Hiemstra IH, Béguin EP, Hoogendijk AJ, Bouchmal S, van Houdt M, et al. Activated neutrophils exert myeloid-derived suppressor cell activity damaging T cells beyond repair. Blood Adv (2019) 3:3562–74. doi: 10.1182/bloodadvances.2019031609
32. Lawrence SM, Corriden R, Nizet V. How neutrophils meet their end. Trends Immunol (2020) 41:531–44. doi: 10.1016/j.it.2020.03.008
33. Deniset JF, Kubes P. Neutrophil heterogeneity: Bona fide subsets or polarization states? J Leukoc Biol (2018) 103:829–38. doi: 10.1002/JLB.3RI0917-361R
34. Hellebrekers P, Vrisekoop N, Koenderman L. Neutrophil phenotypes in health and disease. Eur J Clin Invest (2018) 48:e12943. doi: 10.1111/eci.12943
35. Gabrilovich DI, Nagaraj S. Myeloid-derived suppressor cells as regulators of the immune system. Nat Rev Immunol (2009) 9:162–74. doi: 10.1038/nri2506
36. Lecot P, Sarabi M, Pereira Abrantes M, Mussard J, Koenderman L, Caux C, et al. Neutrophil heterogeneity in cancer: from biology to therapies. Front Immunol (2019) 10:2155. doi: 10.3389/fimmu.2019.02155
37. du Plessis N, Loebenberg L, Kriel M, von Groote-Bidlingmaier F, Ribechini E, Loxton AG, et al. Increased frequency of myeloid-derived suppressor cells during active tuberculosis and after recent mycobacterium tuberculosis infection suppresses T-cell function. Am J Respir Crit Care Med (2013) 188:724–32. doi: 10.1164/rccm.201302-0249OC
38. Knaul JK, Jörg S, Oberbeck-Mueller D, Heinemann E, Scheuermann L, Brinkmann V, et al. Lung-residing myeloid-derived suppressors display dual functionality in murine pulmonary tuberculosis. Am J Respir Crit Care Med (2014) 190:1053–66. doi: 10.1164/rccm.201405-0828OC
39. Dorhoi A, Kotzé LA, Berzofsky JA, Sui Y, Gabrilovich DI, Garg A, et al. Therapies for tuberculosis and AIDS: myeloid-derived suppressor cells in focus. J Clin Invest (2020) 130:2789–99. doi: 10.1172/JCI136288
40. Schrijver IT, Théroude C, Roger T. Myeloid-Derived Suppressor Cells in sepsis. Front Immunol (2019) 10:327. doi: 10.3389/fimmu.2019.00327
41. Amodio G, Cichy J, Conde P, Matteoli G, Moreau A, Ochando J, et al. Role of myeloid regulatory cells (MRCs) in maintaining tissue homeostasis and promoting tolerance in autoimmunity, inflammatory disease and transplantation. Cancer Immunol Immunother (2019) 68:661–72. doi: 10.1007/s00262-018-2264-3
42. Wu T, Zhao Y, Zhao Y. The roles of myeloid-derived suppressor cells in transplantation. Expert Rev Clin Immunol (2014) 10:1385–94. doi: 10.1586/1744666X.2014.948424
43. Boros P, Ochando J, Zeher M. Myeloid derived suppressor cells and autoimmunity. Hum Immunol (2016) 77:631–6. doi: 10.1016/j.humimm.2016.05.024
44. Poe SL, Arora M, Oriss TB, Yarlagadda M, Isse K, Khare A, et al. STAT1-regulated lung MDSC-like cells produce IL-10 and efferocytose apoptotic neutrophils with relevance in resolution of bacterial pneumonia. Mucosal Immunol (2013) 6:189–99. doi: 10.1038/mi.2012.62
45. Ahmadi M, Mohammadi M, Ali-Hassanzadeh M, Zare M, Gharesi-Fard B. MDSCs in pregnancy: Critical players for a balanced immune system at the feto-maternal interface. Cell Immunol (2019) 346:103990. doi: 10.1016/j.cellimm.2019.103990
46. He YM, Li X, Perego M, Nefedova Y, Kossenkov AV, Jensen EA, et al. Transitory presence of myeloid-derived suppressor cells in neonates is critical for control of inflammation. Nat Med (2018) 24:224–31. doi: 10.1038/nm.4467
47. Li L, Si H, Wu SW, Mendez JO, Zarlenga D, Tuo W, et al. Characterization of IL-10-producing neutrophils in cattle infected with Ostertagia ostertagi. Sci Rep (2019) 9:20292. doi: 10.1038/s41598-019-56824-x
48. Ohl K, Tenbrock K. Reactive Oxygen Species as regulators of MDSC-mediated immune suppression. Front Immunol (2018) 9:2499. doi: 10.3389/fimmu.2018.02499
49. Pliyev BK, Dimitrieva TV, Savchenko VG. Cytokine-mediated induction of MHC class II in human neutrophils is dependent on NADPH oxidase activity. Eur J Cell Biol (2015) 94:67–70. doi: 10.1016/j.ejcb.2014.11.001
50. Doz E, Lombard R, Carreras F, Buzoni-Gatel D, Winter N. Mycobacteria-infected dendritic cells attract neutrophils that produce IL-10 and specifically shut down Th17 CD4 T cells through their IL-10 receptor. J Immunol (2013) 191:3818–26. doi: 10.4049/jimmunol.1300527
51. Doz-Deblauwe É, Carreras F, Arbues A, Remot A, Epardaud M, Malaga W, et al. CR3 engaged by PGL-I triggers Syk-calcineurin-NFATc to rewire the innate immune response in leprosy. Front Immunol (2019) 10:2913. doi: 10.3389/fimmu.2019.02913
52. Weber R, Fleming V, Hu X, Nagibin V, Groth C, Altevogt P, et al. Myeloid-Derived Suppressor Cells hinder the anti-cancer activity of immune checkpoint inhibitors. Front Immunol (2018) 9:1310. doi: 10.3389/fimmu.2018.01310
53. Goto S, Konnai S, Okagawa T, Nishimori A, Maekawa N, Gondaira S, et al. Increase of cells expressing PD-1 and PD-L1 and enhancement of IFN-γ production via PD-1/PD-L1 blockade in bovine mycoplasmosis. Immun Inflammation Dis (2017) 5:355–63. doi: 10.1002/iid3.173
Keywords: neutrophil, regulation, cattle, T-cell, suppression, subset
Citation: Rambault M, Doz-Deblauwe É, Le Vern Y, Carreras F, Cunha P, Germon P, Rainard P, Winter N and Remot A (2021) Neutrophils Encompass a Regulatory Subset Suppressing T Cells in Apparently Healthy Cattle and Mice. Front. Immunol. 12:625244. doi: 10.3389/fimmu.2021.625244
Received: 02 November 2020; Accepted: 11 January 2021;
Published: 26 February 2021.
Edited by:
Humberto Lanz-Mendoza, National Institute of Public Health, MexicoReviewed by:
Dirk Werling, Royal Veterinary College (RVC), United KingdomJodi L. McGill, Iowa State University, United States
Copyright © 2021 Rambault, Doz-Deblauwe, Le Vern, Carreras, Cunha, Germon, Rainard, Winter and Remot. This is an open-access article distributed under the terms of the Creative Commons Attribution License (CC BY). The use, distribution or reproduction in other forums is permitted, provided the original author(s) and the copyright owner(s) are credited and that the original publication in this journal is cited, in accordance with accepted academic practice. No use, distribution or reproduction is permitted which does not comply with these terms.
*Correspondence: Nathalie Winter, nathalie.winter@inrae.fr; Aude Remot, aude.remot@inrae.fr
†These authors have contributed equally to this work