- 1Institute of Haematology, NSW Health Pathology, Royal Prince Alfred Hospital, Camperdown, NSW, Australia
- 2Faculty of Medicine and Health, The University of Sydney, Sydney, NSW, Australia
- 3School of Life Sciences, University of Technology Sydney, Ultimo, NSW, Australia
The classical paradigm of host-tumor interaction, i.e. elimination, equilibrium, and escape (EEE), is reflected in the clinical behavior of myeloma which progresses from the premalignant condition, Monoclonal Gammopathy of Unknown Significance (MGUS). Despite the role of other immune cells, CD4+ regulatory T cells (Treg) and cytotoxic CD8+ T cells have emerged as the dominant effectors of host control of the myeloma clone. Progression from MGUS to myeloma is associated with alterations in Tregs and terminal effector CD8+ T cells (TTE). These changes involve CD39 and CD69 expression, affecting the adenosine pathway and residency in the bone marrow (BM) microenvironment, together with oligoclonal expansion within CD8+ TTE cells. In this mini-review article, in the context of earlier data, we summarize our recent understanding of Treg involvement in the adenosine pathway, the significance of oligoclonal expansion within CD8+ TTE cells and BM-residency of CD8+ TTE cells in MGUS and newly diagnosed multiple myeloma patients.
Introduction
Multiple Myeloma (MM) is a malignancy of plasma cells which grow predominantly in the bone marrow (BM). The classical paradigm of host tumor interaction, i.e. elimination, equilibrium, and escape (EEE) is reflected in the clinical behavior of myeloma. The clinical counterparts of equilibrium include Monoclonal Gammopathy of Unknown Significance (MGUS), and plateau phase disease after therapy, implying that significant host-tumor interaction occurs. The clinical counterpart of escape is the progression from MGUS to MM or disease relapse after plateau phase (1).
Regulatory CD4+ T cells (Treg) and cytotoxic CD8+ T cells have emerged as the dominant effectors in host myeloma control in syngeneic transplantable murine myeloma models (2, 3) underpinning autologous graft versus myeloma as an important process in disease control (4). The dramatic success of Chimeric Antigen Receptor (CAR) T cell therapies in myeloma reinforces the vulnerability of the malignant plasma cell to T cell-mediated cytotoxicity, even though the durability of response remains an issue (5, 6). Changes in the BM microenvironment and endosteal niche are also crucial to malignant plasma cell dormancy and growth permissiveness. These changes involve not only Tregs and cytotoxic CD8+ T cells, but also NK cells, myeloid-derived suppressor cells (MDSC), and cytokine fluxes which promote inflammation and angiogenesis resulting in impaired tumor immunity and a favorable environment for tumor growth (7).
The clinical finding of an association between improved clinical outcome and reduced Treg/Th17 ratios (8) or Treg frequency (9, 10) and oligoclonal expansion of terminal effector CD8+ T cells (TTE) (11) suggests Treg and oligoclonal expansion of TTE as key players of immune surveillance in MM. This further suggests that T cells may recognize myeloma antigens and differentiate into TTE which are able to exert cytotoxicity against malignant plasma cells through perforin and granzyme expression. Until recently this possibility had not been demonstrated in an autologous setting, but the recent demonstration of cytotoxicity mediated by circulating oligoclonal expanded TTE against autologous malignant plasma cells provides proof for this concept and an opportunity to elucidate potential myeloma antigens as well as harnessing these cells for therapy (12). However, the crucial factor, or gatekeeper, which allows for BM-residency of Treg and TTE, and therefore immune surveillance at the site of malignant disease is yet to be determined. It is also unknown whether BM-residency, per se, is necessary to confer tumor control since the spatial and temporal relationship between circulating and BM-resident T cells and myeloma cells and other cells of the BM microenvironment is not yet elucidated. We postulate however that BM-residency of immune cells can be considered as the “Green Card” which allows for permanent myeloma surveillance at the site of disease initiation and progression.
Treg in Myeloma
The classical Treg is a subset of CD4+ T lymphocytes characterized by the surface CD25+CD127low phenotype and expression of transcription factor forkhead box P3 (FoxP3) (13). If the homeostatic balance between Treg-mediated suppression and T effector cell activation is unbalanced in favor of effector activation, autoimmune disease results. In the case of malignancy, excessive Treg activity leads to suppression or exhaustion of effector cells and a lack of tumor immune surveillance. Compared to MGUS, in MM there is skewing of the Treg and pro-inflammatory Th17 cell balance in favor of Tregs, and this balance may be more important than absolute numbers of Treg cells (8). In our study of long-term survivors of myeloma, normal or reduced Treg/Th17 ratios were seen (14). Thus, although this is merely a correlative finding, it is possible that in patients who have active myeloma the immune system tips in favor of myeloma cell growth due to immunosuppressive mechanisms mediated by regulatory immune cells (15). This normal or reduced Treg/Th17 ratio, however, must be interpreted with caution as this ratio could be due to the increased number of Th17 cells which contribute to myeloma pathology by sustaining malignant plasma cell proliferation even from the early stage of disease (16–20) and contribute to osteoclastogenesis (21). Other studies emphasized the relationship between increased Treg frequency and adverse clinical features such as hypercalcemia and IgA myeloma subtype (10) or inferior clinical outcomes in myeloma patients (9, 10). The increased frequency of Treg reported in MGUS and MM patients suggests that Treg are important players but increased frequency does not predict evolution of MGUS to MM (22, 23).
The mechanism of Treg interactions with the myeloma microenvironment is unclear. It has been postulated that Treg are implicated in myeloma progression based on their contribution to the complex immunosuppressive environment via secretion of the cytokines interleukin (IL)-10 and transforming growth factor β (TGF-β) by APRIL/TACI dependent mechanisms (24), the CD39/CD73 adenosine pathway (25) and direct inhibition of effector T cell responses (26). Myeloma cells can also induce Treg expansion and activation by secreting type 1 interferon (2), or acquired Treg by transferring membrane proteins to Treg by trogocytosis (27, 28).
Polychromatic fluorescence-based flow cytometry, the benchmark technology to study T-cell diversity, has divided Treg based on CD25, CD45RA, and FoxP3 expression into three subsets; resting Treg (CD25+CD45RA+FoxP3lo), activated Treg (CD25+CD45RA−FoxP3hi) and non-suppressive Treg (CD25+CD45RA−FoxP3lo) (13). Expression of ectonucleotidase CD39 defines a subset of Tregs involved in the canonical CD39/CD73 adenosine pathway (29), a key immunosuppressive mechanism operating in tumor microenvironments (30, 31). Restrictions in the number of available fluorophores, and the complexity associated with spectral overlap, however, currently limits flow cytometry technology beyond 34 parameters (32). Mass cytometry time-of-flight (CyTOF) technology provides an alternative methodology, allowing for more than 50 different parameters without the limitations associated with spectral analysis and represents an important advantage in the field of T-cell biology (33). Recently by using the advantages afforded by mass cytometry and the unsupervised clustering flow self-organizing map (FlowSOM) algorithm, we interrogated the distribution of multiple subsets within the Treg compartment in the matched BM and peripheral blood (PB) samples of MGUS and Newly Diagnosed (ND)MM patients and demonstrated some novel findings (34).
In contrast to the established role of CD39+ Treg in suppressive tumor microenvironments (including colorectal, pancreatic, head, and neck cancer) (30, 31, 35), we found a trend toward increased CD39− Treg within the Treg compartment in BM and PB of NDMM patients compared to MGUS patients (34). Treg expressing the ectonucleotidase CD39 in conjunction with CD73-expressing cells hydrolyze extracellular ATP and generate adenosine (29). Following the engagement with its cognate receptors extracellularly produced adenosine suppresses effector T-cell responses and induces MDSC (36). In humans, CD39+ Treg are defined as activated memory cells and are implicated in the suppression of Th17 responses and the control of autoimmunity (37). Thus, it may be speculated that although increased Tregs in myeloma may outweigh the number of myeloma permissive Th17 cells, the detrimental effect on Th17 cells may be compensated by reduced ability of Treg to suppress IL-17 production due to in increased proportion of CD39- Treg.
In addition to CD39− Treg frequency, their subset composition also distinguishes between MGUS and NDMM patients (34). The FlowSOM clustering algorithm identified two subsets that emerged within CD39− Treg of NDMM patients which were negligible or absent in CD39− Treg of MGUS patients. One subset was found in both BM and PB which phenotypically resembled activated Treg based on CD45RO, CD49d, and CD62L expression; another subset resembled BM-resident Treg based on CD69 expression, a key marker of tissue residency, and restricted location within the BM. Based on their low/lack of CD38 expression these two subsets of CD39- Treg are likely resistant to anti-CD38 monoclonal antibody therapy and may limit its effectiveness in MM patients (38).
Our data provide additional evidence for divergence between Treg in elimination/equilibrium (MGUS) and escape stage (myeloma). This divergence has now been shown to be more than a skewing of balance between Treg and pro-inflammatory Th17 in favor of Treg, but also an active Treg differentiation process involving regulation of ectonucleotidase CD39 expression, activation and BM-residency (Figure 1). These changes in the Treg compartment have real potential to improve our understanding of the clinical stability in MGUS and disease progression into MM, and to further advance the clinical diagnosis, prognosis, and therapeutic implications for MM.
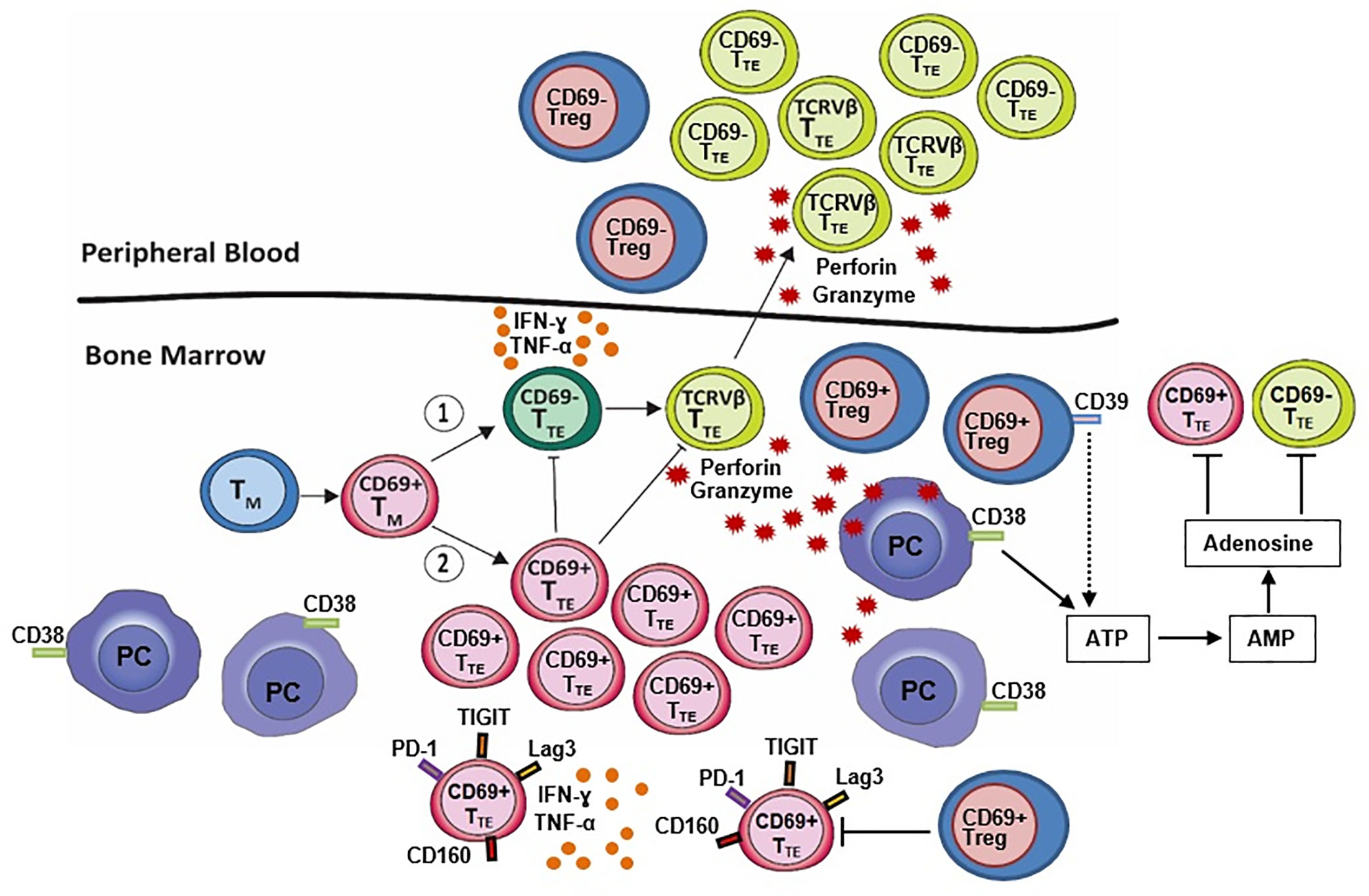
Figure 1 BM-resident CD69+ regulatory T cells (CD69+ Treg) decrease expression of CD39 which is required for the canonical CD39/CD73 adenosine pathway. This suggests that an alternative adenosine pathway involving ectoenzyme CD38, expressed on malignant plasma cells (PC), is active in the BM niches of NDMM patients. CD8+CD57+ terminal effector T cells (TTE) originate from memory T cells (TM) and may (1) undergo oligoclonal expansion into cytotoxic CD69- TTE (TCRVβ TTE) with high expression of perforin and granzyme B, the ability to eliminate autologous PC in vitro and circulate between BM and PB or, (2) remain within the BM as non-cytotoxic BM-resident CD69+ TTE with high inhibitory checkpoint expression (PD-1, TIGIT, Lag3, CD160) possibly promoted by BM-resident CD69+ Treg. Both CD69- TTE and BM-resident CD69+ TTE produce large amounts of the inflammatory cytokines interferon-γ (IFN-γ) and tumor necrosis factor α (TNF-α). CD69− TTE and CD69+ TTE establish an inverse relationship within the BM-resident T cell compartment of NDMM patients. Changes in Treg and CD69− TTE and CD69+ TTE within the BM-resident T cell compartment of NDMM patients may be critical for myeloma surveillance at the site of disease initiation and progression.
Significance of Oligoclonal Expansion of CD8+TTE Cells in Myeloma
In addition to Treg, oligoclonal expansion of CD8+CD57+ TTE cells in myeloma patients have remained an attractive research topic and matter of debate for decades (11, 39–42). Although the clinical relevance of this phenomenon is well established, the biological explanation remain elusive, two mutually opposite concepts have been proposed. The first proposes that oligoclonal expanded TTE represent exhausted and senescent T effector cells and are detrimental in myeloma immunity (39). The second proposes that oligoclonal expanded TTE are key to disease control in myeloma as they are prognostically significant and correlate with improved survival (11).
Our recent research provides novel evidence supporting the idea that oligoclonal expanded TTE are key players in myeloma disease control (12). We demonstrated that oligoclonal expansions not limited to PB CD8+ TTE also occurred in CD8+ TTE which belong to the pool of marrow infiltrating lymphocytes (MILs) (12). The mechanism underlining oligoclonal expansions of TTE cells is unclear but infers reactivity against putative myeloma antigens. Such oligoclonal expansions of TTE in MM patients may result from persistent stimulation of CD8+ T cells in the BM in the absence of effective clearance of the malignant clone and then oligoclonal expanded TTE exit BM and circulate between BM and PB. Expanded oligoclonal CD8+ TTE in BM and PB share same phenotype indicative of strong cytotoxic function with high expression of perforin and granzyme B and a TbethiEomeslo/neg signature (Figure 1). Low expression of the inhibitory checkpoints CD279 (PD-1), TIGIT, CD223 (Lag3) and CD160, suggests that these cells may not be exhausted and thus not an appropriate or optimal target for checkpoint blockade immunotherapy (12, 42, 43).
Significantly, oligoclonal expanded TTE from the PB of NDMM patients are capable of eliminating autologous CD38+ plasma cells in-vitro, attributable to their perforin and granzyme B production (12). Direct testing of oligoclonal expanded TTE residing in the BM for their myeloma reactivity remains challenging due to their limited numbers and small volume of diagnostic BM samples available for research. However, based on their cytotoxic function they likely resemble myeloma-reactive T cells reported in the BM of MM patients (44) and may be pivotal to favorable clinical outcome in MM patients receiving MILs as adoptive T-cell therapy (45). These cells also adopt a secretory phenotype and produce large amounts of the inflammatory cytokines interferon-γ (IFN-γ) and tumor necrosis factor α (TNF-α) (Figure 1) (12) aligning with the previously described senescence-associate secretory phenotype (SASP) (46–49).
These findings raise the possibility of the development of novel personalized therapies based on expanded clonal TTE and a means of identifying new, previously occult, myeloma antigens.
CD69: the Gatekeeper or “Green Card” Allowing BM-Residency of CD8+ TTE
It is likely that capacity of CD8+ TTE to maintain immune surveillance over time at the site of malignant disease in MGUS and MM patients is linked to their retention within BM-resident T cell compartment, namely their BM-residency. Although understanding regulation of human BM-resident T cell compartment is in infancy, signals derived from endothelial cells (50) and attrition of memory stem-like TCF1/7hi T cells (51) are considered important regulators factors in MGUS and MM patients.
Our recent investigations determined CD69 as a gatekeeper, or “green card”, allowing residence of CD8+ TTE in the BM of MGUS and NDMM patients (12). CD69+ TTE, being a constituent of MILs have many characteristics in common with resident memory T cells (TRM) including expression of CD69, a key marker of tissue-residency, cytokine production, and inhibitory receptor expression (Figure 1) (52). Only a minority of CD69+ TTE express another marker of residency, CD103 (52). Non-circulating CD69+ TRM lacking CD103 expression can be found in secondary lymphoid tissue following virus exposure and the expression of CD103 is a requirement for epithelial tissue-residency and adhesion to E-cadherin (53). CD69+ TTE are not characterized by oligoclonal expansions, possess low perforin and granzyme expression and TbetloEomeshi transcriptional signature (12). Enhanced inhibitory receptor expression on BM-resident CD69+ TTE marked them as exhausted and thus a suitable target for checkpoint inhibition immunotherapy.
BM-resident CD69+ TTE are not restricted to myeloma, comprise approximately half of CD8+ TTE in the BM of healthy controls, and a small proportion of CD8+ TTE in the BM of MGUS patients. However, an inverse relationship between BM-resident CD69+ TTE and their CD69- TTE counterpart encompassing oligoclonal expanded TTE discriminates NDMM from MGUS patients and healthy controls (12). BM-resident CD69+ TTE also maintain an inverse relationship with oligoclonal expanded CD69- TTE found in the PB of NDMM patients. The documentation of a unique relationship between BM-resident CD69+ TTE and circulating cytotoxic CD8+ TTE in myeloma is of great interest, as the cardinal feature of TRM cells is a lack of equilibration with the circulating memory T-cell pool (54). It remains unclear whether CD69+ TTE can be replenished from circulating CD69- T cells or whether the self-renewal of CD69+ T is sufficient to maintain population stability.
Our data established that CD69+ TTE independent of disease reside within the BM-resident T cell compartment, however, regulatory mechanism develop between CD69+ TTE and CD69- TTE may impact immune surveillance in MGUS and NDMM patients.
Discussion
In this mini-review, in the context of earlier published data, we discussed our recent data which suggest that the host-myeloma escape stage clinically defined by progression from MGUS to MM is characterized by lessening involvement of Treg in suppressive CD39/CD73 adenosine pathway and development of the regulatory mechanism between BM-resident CD69+ TTE and their circulating CD69- TTE counterpart encompassing oligoclonal expanded TTE. We also discussed breaking discovery that clonally expanded TTE are capable of eliminating autologous CD38+ plasma cells in-vitro and such play a key role in myeloma immunity.
It is surprising that Treg during myeloma escape decrease CD39 expression and become less involved in a key suppressive CD39/CD73 adenosine pathway operating in tumor microenvironment (30, 31, 35). Pertinent to their immunosuppressive fate, Treg must be able to adjust for this reduced involvement in the immunosuppressive pathway. Plausible adjustments are: reduced ability to suppress IL-17 production due to low CD39 expression and capacity of CD39- Treg to produce IL-17 (37) a growth factor for myeloma (3). In particular BM-resident CD39-CD69+ Treg subset may be an important source of IL-17 at the site of disease initiation and progression. Lessening involvement of Treg in suppressive CD39/CD73 adenosine pathway is likely compensated by alternative adenosine pathway involving NAD(+)-consuming enzyme CD38 which is active in the BM niches of myeloma (55) and correlates with progression of myeloma (Figure 1) (56).
We discovered that CD69 acts as a gatekeeper, or “green card”, allowing residence of CD8+ TTE in the BM (12). Our preliminary data suggest that the development of BM-resident CD69+ TTE may be more closely related to the transition from TM cells expressing CD69 rather than from CD69+ TTE and that regulation of this transition may differ between NDMM and MGUS patients (12). Low perforin and granzyme expression by BM-resident CD69+ TTE rule out their contribution to cytotoxic activity against myeloma cells. Cytotoxic activity against myeloma cells is limited to CD69- TTE encompassing oligoclonal expanded TTE which circulate between BM and PB in NDMM patients (Figure 1). One of the possible functions of BM-resident CD69+ TTE in myeloma is the recruitment of other immune cells from the circulation to the site of disease, as has been demonstrated with resident and circulating memory T cells in a murine vitiligo model (57). BM-resident CD69+ TTE may potentially have a beneficial effect against myeloma by recruiting oligoclonal expanded cytotoxic CD69- TTE and maintaining dormancy of the malignant clone. Alternatively, the accumulation of CD69+ TTE within MILs may contribute to local inflammation through the production of the pro-inflammatory cytokines IFN-γ and TNF-α and promote myeloma growth (58). Tracing BM-resident CD69+ TTE within MILs and correlating their numbers with clinical outcome in MM patients receiving MILs as adoptive T-cell therapy (45) could provide essential insights into the role of BM-resident CD69+ TTE in myeloma immunity.
It is possible that BM-resident CD69+ TTE and circulating CD69- TTE cooperate in anti-tumor immunity based on the inverse relationship between CD69+ TTE and CD69- TTE observed within the BM-resident T cell compartment in NDMM patients (12). We furthermore suggest that a competitive flux, or equilibrium, between BM-resident CD69+ TTE and circulating CD69- TTE regulates the egress of myeloma-reactive CD69- TTE from the BM to PB and contributes to senescence (CD69-PD-1lo TTE) or exhaustion (CD69+PD-1hi TTE) of CD8+ TTE. This is also applies to the effectiveness of CAR T cells which are likely impacted by the ability to CAR T cells to reach disease sites and to avoid the development of senescence and exhaustion while remaining in the BM.
Further studies are required to determine the role of BM-resident cells including CD69+ TTE and Treg, and circulating oligoclonal expanded CD69- TTE in the healthy and disease-affected BM microenvironments. These will require further functional studies of these subsets and their fluxes in the evolution of myeloma activity.
Author Contributions
DJ wrote the manuscript with assistance from SV and JF and was reviewed by all other authors. All authors contributed to the article and approved the submitted version.
Funding
This work and open access publication fees were funded by Sydney Blood Cancer Research and The Institute of Haematology, Royal Prince Alfred Hospital.
Conflict of Interest
The authors declare that the research was conducted in the absence of any commercial or financial relationships that could be construed as a potential conflict of interest.
References
1. Joshua D, Suen H, Brown R, Bryant C, Ho PJ, Hart D, et al. The T Cell in Myeloma. Clin Lymphoma Myeloma Leuk (2016) 16(10):537–42. doi: 10.1016/j.clml.2016.08.003
2. Kawano Y, Zavidij O, Park J, Moschetta M, Kokubun K, Mouhieddine TH, et al. Blocking IFNAR1 inhibits multiple myeloma-driven Treg expansion and immunosuppression. J Clin Invest (2018) 128(6):2487–99. doi: 10.1172/JCI88169
3. Vuckovic S, Minnie SA, Smith D, Gartlan KH, Watkins TS, Markey KA, et al. Bone marrow transplantation generates T cell-dependent control of myeloma in mice. J Clin Invest (2019) 129(1):106–21. doi: 10.1172/JCI98888
4. Dong S, Ghobrial IM. Autologous graft versus myeloma: it’s not a myth. J Clin Invest (2019) 129(1):48–50. doi: 10.1172/JCI125431
5. D’Agostino M, Raje N. Anti-BCMA CAR T-cell therapy in multiple myeloma: can we do better? Leukemia (2020) 34(1):21–34. doi: 10.1038/s41375-019-0669-4
6. Raje N, Berdeja J, Lin Y, Siegel D, Jagannath S, Madduri D, et al. Anti-BCMA CAR T-Cell Therapy bb2121 in Relapsed or Refractory Multiple Myeloma. N Engl J Med (2019) 380(18):1726–37. doi: 10.1056/NEJMoa1817226
7. Lomas OC, Tahri S, Ghobrial IM. The microenvironment in myeloma. Curr Opin Oncol (2020) 32(2):170–5. doi: 10.1097/CCO.0000000000000615
8. Favaloro J, Brown R, Aklilu E, Yang S, Suen H, Hart D, et al. Myeloma skews regulatory T and pro-inflammatory T helper 17 cell balance in favor of a suppressive state. Leuk Lymphoma (2014) 55(5):1090–8. doi: 10.3109/10428194.2013.825905
9. Alrasheed N, Lee L, Ghorani E, Henry JY, Conde L, Chin M, et al. Marrow-Infiltrating Regulatory T Cells Correlate with the Presence of Dysfunctional CD4(+)PD-1(+) Cells and Inferior Survival in Patients with Newly Diagnosed Multiple Myeloma. Clin Cancer Res (2020) 26(13):3443–54. doi: 10.1158/1078-0432.CCR-19-1714
10. Muthu Raja KR, Rihova L, Zahradova L, Klincova M, Penka M, Hajek R. Increased T regulatory cells are associated with adverse clinical features and predict progression in multiple myeloma. PloS One (2012) 7(10):e47077. doi: 10.1371/journal.pone.0047077
11. Brown RD, Spencer A, Ho PJ, Kennedy N, Kabani K, Yang S, et al. Prognostically significant cytotoxic T cell clones are stimulated after thalidomide therapy in patients with multiple myeloma. Leuk Lymphoma (2009) 50(11):1860–4. doi: 10.3109/10428190903216804
12. Vuckovic S, Bryant CE, Lau KHA, Yang S, Favaloro J, McGuire HM, et al. Inverse relationship between oligoclonal expanded CD69- TTE and CD69+ TTE cells in bone marrow of multiple myeloma patients. Blood Adv (2020) 4(19):4593–604. doi: 10.1182/bloodadvances.2020002237
13. Miyara M, Yoshioka Y, Kitoh A, Shima T, Wing K, Niwa A, et al. Functional delineation and differentiation dynamics of human CD4+ T cells expressing the FoxP3 transcription factor. Immunity (2009) 30(6):899–911. doi: 10.1016/j.immuni.2009.03.019
14. Bryant C, Suen H, Brown R, Yang S, Favaloro J, Aklilu E, et al. Long-term survival in multiple myeloma is associated with a distinct immunological profile, which includes proliferative cytotoxic T-cell clones and a favourable Treg/Th17 balance. Blood Cancer J (2013) 3(9):e148. doi: 10.1038/bcj.2013.34
15. Raja KR, Hajek R. Contribution of regulatory T cells to immunosuppression and disease progression in multiple myeloma patients. Oncoimmunology (2013) 2(9):e25619. doi: 10.4161/onci.25619
16. Calcinotto A, Brevi A, Chesi M, Ferrarese R, Garcia Perez L, Grioni M, et al. Microbiota-driven interleukin-17-producing cells and eosinophils synergize to accelerate multiple myeloma progression. Nat Commun (2018) 9(1):4832. doi: 10.1038/s41467-018-07305-8
17. Di Lullo G, Marcatti M, Protti MP. Non-redundant roles for Th17 and Th22 cells in multiple myeloma clinical correlates. Oncoimmunology (2016) 5(4):e1093278. doi: 10.1080/2162402X.2015.1093278
18. Dhodapkar KM, Barbuto S, Matthews P, Kukreja A, Mazumder A, Vesole D, et al. Dendritic cells mediate the induction of polyfunctional human IL17-producing cells (Th17-1 cells) enriched in the bone marrow of patients with myeloma. Blood (2008) 112(7):2878–85. doi: 10.1182/blood-2008-03-143222
19. Prabhala RH, Pelluru D, Fulciniti M, Prabhala HK, Nanjappa P, Song W, et al. Elevated IL-17 produced by TH17 cells promotes myeloma cell growth and inhibits immune function in multiple myeloma. Blood (2010) 115(26):5385–92. doi: 10.1182/blood-2009-10-246660
20. Prabhala RH, Fulciniti M, Pelluru D, Rashid N, Nigroiu A, Nanjappa P, et al. Targeting IL-17A in multiple myeloma: a potential novel therapeutic approach in myeloma. Leukemia (2016) 30(2):379–89. doi: 10.1038/leu.2015.228
21. Noonan K, Marchionni L, Anderson J, Pardoll D, Roodman GD, Borrello I. A novel role of IL-17-producing lymphocytes in mediating lytic bone disease in multiple myeloma. Blood (2010) 116(18):3554–63. doi: 10.1182/blood-2010-05-283895
22. Beyer M, Kochanek M, Giese T, Endl E, Weihrauch MR, Knolle PA, et al. In vivo peripheral expansion of naive CD4+CD25high FoxP3+ regulatory T cells in patients with multiple myeloma. Blood (2006) 107(10):3940–9. doi: 10.1182/blood-2005-09-3671
23. Lad D, Huang Q, Hoeppli R, Garcia R, Xu L, Levings M, et al. Evaluating the role of Tregs in the progression of multiple myeloma. Leuk Lymphoma (2019) 60(9):2134–42. doi: 10.1080/10428194.2019.1579324
24. Tai YT, Lin L, Xing L, Cho SF, Yu T, Acharya C, et al. APRIL signaling via TACI mediates immunosuppression by T regulatory cells in multiple myeloma: therapeutic implications. Leukemia (2019) 33(2):426–38. doi: 10.1038/s41375-018-0242-6
25. Chillemi A, Quarona V, Antonioli L, Ferrari D, Horenstein AL, Malavasi F. Roles and Modalities of Ectonucleotidases in Remodeling the Multiple Myeloma Niche. Front Immunol (2017) 8:305. doi: 10.3389/fimmu.2017.00305
26. Strauss L, Bergmann C, Szczepanski M, Gooding W, Johnson JT, Whiteside TL. A unique subset of CD4+CD25highFoxp3+ T cells secreting interleukin-10 and transforming growth factor-beta1 mediates suppression in the tumor microenvironment. Clin Cancer Res (2007) 13(15 Pt 1):4345–54. doi: 10.1158/1078-0432.CCR-07-0472
27. Brown R, Kabani K, Favaloro J, Yang S, Ho PJ, Gibson J, et al. CD86+ or HLA-G+ can be transferred via trogocytosis from myeloma cells to T cells and are associated with poor prognosis. Blood (2012) 120(10):2055–63. doi: 10.1182/blood-2012-03-416792
28. Brown R, Suen H, Favaloro J, Yang S, Ho PJ, Gibson J, et al. Trogocytosis generates acquired regulatory T cells adding further complexity to the dysfunctional immune response in multiple myeloma. Oncoimmunology (2012) 1(9):1658–60. doi: 10.4161/onci.22032
29. Borsellino G, Kleinewietfeld M, Di Mitri D, Sternjak A, Diamantini A, Giometto R, et al. Expression of ectonucleotidase CD39 by Foxp3+ Treg cells: hydrolysis of extracellular ATP and immune suppression. Blood (2007) 110(4):1225–32. doi: 10.1182/blood-2006-12-064527
30. Bastid J, Cottalorda-Regairaz A, Alberici G, Bonnefoy N, Eliaou JF, Bensussan A. ENTPD1/CD39 is a promising therapeutic target in oncology. Oncogene (2013) 32(14):1743–51. doi: 10.1038/onc.2012.269
31. Tøndell A, Wahl SGF, Sponaas AM, Sørhaug S, Børset M, Haug M. Ectonucleotidase CD39 and Checkpoint Signalling Receptor Programmed Death 1 are Highly Elevated in Intratumoral Immune Cells in Non-small-cell Lung Cancer. Transl Oncol (2020) 13(1):17–24. doi: 10.1016/j.tranon.2019.09.003
32. Yekyung Seong DN, Thakur A, Harding FA, Nguyen A. Novel fluorochrome development enables 34-color high-content flow cytometry. J Immunol (2020) 204:1. doi: 10.1101/2020.06.25.165381
33. Bendall SC, Simonds EF, Qiu P, Amir el AD, Krutzik PO, Finck R, et al. Single-cell mass cytometry of differential immune and drug responses across a human hematopoietic continuum. Science (2011) 332(6030):687–96. doi: 10.1126/science.1198704
34. Marsh-Wakefield F, Kruzins A, McGuire HM, Yang S, Bryant C, Fazekas de St Groth B, et al. Mass Cytometry Discovers Two Discrete Subsets of CD39(-)Treg Which Discriminate MGUS From Multiple Myeloma. Front Immunol (2019) 10:1596. doi: 10.3389/fimmu.2019.01596
35. Bastid J, Regairaz A, Bonnefoy N, Déjou C, Giustiniani J, Laheurte C, et al. Inhibition of CD39 enzymatic function at the surface of tumor cells alleviates their immunosuppressive activity. Cancer Immunol Res (2015) 3(3):254–65. doi: 10.1158/2326-6066.CIR-14-0018
36. Antonioli L, Blandizzi C, Pacher P, Hasko G. Immunity, inflammation and cancer: a leading role for adenosine. Nat Rev Cancer (2013) 13(12):842–57. doi: 10.1038/nrc3613
37. Fletcher JM, Lonergan R, Costelloe L, Kinsella K, Moran B, O’Farrelly C, et al. CD39+Foxp3+ regulatory T Cells suppress pathogenic Th17 cells and are impaired in multiple sclerosis. J Immunol (2009) 183(11):7602–10. doi: 10.4049/jimmunol.0901881
38. Usmani SZ, Khan I, Chiu C, Foureau D, Druhan LJ, Rigby K, et al. Deep sustained response to daratumumab monotherapy associated with T-cell expansion in triple refractory myeloma. Exp Hematol Oncol (2018) 7:3. doi: 10.1186/s40164-018-0096-7
39. Zelle-Rieser C, Thangavadivel S, Biedermann R, Brunner A, Stoitzner P, Willenbacher E, et al. T cells in multiple myeloma display features of exhaustion and senescence at the tumor site. J Hematol Oncol (2016) 9(1):116. doi: 10.1186/s13045-016-0345-3
40. Sze DM, Brown RD, Yuen E, Gibson J, Ho J, Raitakari M, et al. Clonal cytotoxic T cells in myeloma. Leuk Lymphoma (2003) 44(10):1667–74. doi: 10.1080/1042819031000097438
41. Sze DM, Giesajtis G, Brown RD, Raitakari M, Gibson J, Ho J, et al. Clonal cytotoxic T cells are expanded in myeloma and reside in the CD8(+)CD57(+)CD28(-) compartment. Blood (2001) 98(9):2817–27. doi: 10.1182/blood.V98.9.2817
42. Suen H, Brown R, Yang S, Ho PJ, Gibson J, Joshua D. The failure of immune checkpoint blockade in multiple myeloma with PD-1 inhibitors in a phase 1 study. Leukemia (2015) 29(7):1621–2. doi: 10.1038/leu.2015.104
43. Badros A, Hyjek E, Ma N, Lesokhin A, Dogan A, Rapoport AP, et al. Pembrolizumab, pomalidomide, and low-dose dexamethasone for relapsed/refractory multiple myeloma. Blood (2017) 130(10):1189–97. doi: 10.1182/blood-2017-03-775122
44. Noonan K, Matsui W, Serafini P, Carbley R, Tan G, Khalili J, et al. Activated marrow-infiltrating lymphocytes effectively target plasma cells and their clonogenic precursors. Cancer Res (2005) 65(5):2026–34. doi: 10.1158/0008-5472.CAN-04-3337
45. Noonan KA, Huff CA, Davis J, Lemas MV, Fiorino S, Bitzan J, et al. Adoptive transfer of activated marrow-infiltrating lymphocytes induces measurable antitumor immunity in the bone marrow in multiple myeloma. Sci Transl Med (2015) 7(288):288ra78. doi: 10.1126/scitranslmed.aaa7014
46. Malaquin N, Tu V, Rodier F. Assessing Functional Roles of the Senescence-Associated Secretory Phenotype (SASP). Methods Mol Biol (2019) 1896:45–55. doi: 10.1007/978-1-4939-8931-7_6
47. Rao SG, Jackson JG. SASP: Tumor Suppressor or Promoter? Yes! Trends Cancer (2016) 2(11):676–87. doi: 10.1016/j.trecan.2016.10.001
48. Callender LA, Carroll EC, Beal RWJ, Chambers ES, Nourshargh S, Akbar AN, et al. Human CD8(+) EMRA T cells display a senescence-associated secretory phenotype regulated by p38 MAPK. Aging Cell (2018) 17(1):1–9. doi: 10.1111/acel.12675
49. Suen H, Brown R, Yang S, Weatherburn C, Ho PJ, Woodland N, et al. Multiple myeloma causes clonal T-cell immunosenescence: identification of potential novel targets for promoting tumour immunity and implications for checkpoint blockade. Leukemia (2016) 30(8):1716–24. doi: 10.1038/leu.2016.84
50. Leone P, Di Lernia G, Solimando AG, Cicco S, Saltarella I, Lamanuzzi A, et al. Bone marrow endothelial cells sustain a tumor-specific CD8(+) T cell subset with suppressive function in myeloma patients. Oncoimmunology (2019) 8(1):e1486949. doi: 10.1080/2162402X.2018.1486949
51. Bailur JK, McCachren SS, Doxie DB, Shrestha M, Pendleton K, Nooka AK, et al. Early alterations in stem-like/resident T cells, innate and myeloid cells in the bone marrow in preneoplastic gammopathy. JCI Insight (2019) 5:1–10. doi: 10.1172/jci.insight.127807
52. Kumar BV, Ma W, Miron M, Granot T, Guyer RS, Carpenter DJ, et al. Human Tissue-Resident Memory T Cells Are Defined by Core Transcriptional and Functional Signatures in Lymphoid and Mucosal Sites. Cell Rep (2017) 20(12):2921–34. doi: 10.1016/j.celrep.2017.08.078
53. Corgnac S, Boutet M, Kfoury M, Naltet C, Mami-Chouaib F. The Emerging Role of CD8(+) Tissue Resident Memory T (T(RM)) Cells in Antitumor Immunity: A Unique Functional Contribution of the CD103 Integrin. Front Immunol (2018) 9:1904. doi: 10.3389/fimmu.2018.01904
54. Schenkel JM, Masopust D. Tissue-resident memory T cells. Immunity (2014) 41(6):886–97. doi: 10.1016/j.immuni.2014.12.007
55. Costa F, Dalla Palma B, Giuliani N. CD38 Expression by Myeloma Cells and Its Role in the Context of Bone Marrow Microenvironment: Modulation by Therapeutic Agents. Cells (2019) 8(12):1–12. doi: 10.3390/cells8121632
56. Horenstein AL, Quarona V, Toscani D, Costa F, Chillemi A, Pistoia V, et al. Adenosine Generated in the Bone Marrow Niche Through a CD38-Mediated Pathway Correlates with Progression of Human Myeloma. Mol Med (2016) 22:694–704. doi: 10.2119/molmed.2016.00198
57. Richmond JM, Strassner JP, Rashighi M, Agarwal P, Garg M, Essien KI, et al. Resident Memory and Recirculating Memory T Cells Cooperate to Maintain Disease in a Mouse Model of Vitiligo. J Invest Dermatol (2019) 139(4):769–78. doi: 10.1016/j.jid.2018.10.032
Keywords: T cells, myeloma, oligoclonal expansions, CD69+TTE, CD39-Treg
Citation: Joshua DE, Vuckovic S, Favaloro J, Lau KHA, Yang S, Bryant CE, Gibson J and Ho PJ (2021) Treg and Oligoclonal Expansion of Terminal Effector CD8+ T Cell as Key Players in Multiple Myeloma. Front. Immunol. 12:620596. doi: 10.3389/fimmu.2021.620596
Received: 23 October 2020; Accepted: 12 January 2021;
Published: 23 February 2021.
Edited by:
Matteo Bellone, San Raffaele Hospital (IRCCS), ItalyReviewed by:
Michael Hundemer, Heidelberg University Hospital, GermanyKwee Lan Yong, University College London, United Kingdom
Copyright © 2021 Joshua, Vuckovic, Favaloro, Lau, Yang, Bryant, Gibson and Ho. This is an open-access article distributed under the terms of the Creative Commons Attribution License (CC BY). The use, distribution or reproduction in other forums is permitted, provided the original author(s) and the copyright owner(s) are credited and that the original publication in this journal is cited, in accordance with accepted academic practice. No use, distribution or reproduction is permitted which does not comply with these terms.
*Correspondence: Douglas E. Joshua, RG91Z2xhcy5qb3NodWFAc3lkbmV5LmVkdS5hdQ==