- 1Research Service, Veteran Administration Greater Los Angeles Healthcare System, Los Angeles, CA, United States
- 2David Geffen School of Medicine, University of California, Los Angeles, Los Angeles, CA, United States
Regulatory T cells have been implicated in the regulation and maintenance of immune homeostasis. Whether gender and sex hormones differentially influence the expression and function of regulatory T cell phenotype and their influence on FoxP3 expression remains obscure. We provide evidence in this study that the number and percent of human regulatory T cells (Tregs) expressing CD4+ and CD8+ are significantly reduced in healthy females compared to healthy males. In addition, both CD4+CD25+hi and CD8+CD25+hi subsets in healthy males have a 2-3 fold increase in FoxP3 mRNA expression compared to healthy females. Female SLE patients, compared to healthy women, have elevated plasma levels of estradiol and decreased levels of testosterone. Higher levels of testosterone correlate with higher expression of FoxP3 in CD4+CD25hiCD127low putative Tregs in women with SLE. Incubation of CD4+ regulatory T cells with 17β-estradiol at physiological levels generally decreased FoxP3 expression in females with SLE. These data suggest that females may be more susceptible than males to SLE and other autoimmune diseases in part because they have fewer Tregs and reduced FoxP3 expression within those cells due to normal E2 levels which suppress FoxP3 expression. In addition, low levels of plasma testosterone in women may further reduce the ability of the Tregs to express FoxP3. These data suggest that gender and sex hormones can influence susceptibility to SLE via effects on regulatory T cells and FoxP3 expression.
Introduction
Many autoimmune diseases including lupus are gender biased, with females outnumbering males 9:1 (1–5). Emerging evidence shows that sex hormones influence the expression and function of regulatory cells in both mice and humans (6) and that regulatory T cells (Tregs), which play an important role in the regulation and maintenance of a normal immune responses, are impaired in numbers or in function in many autoimmune diseases including SLE (7–9). Both CD4+ and CD8+ Tregs in the peripheral immune system have important roles in suppressing autoimmune disease (10–15). Impaired development and function (16–19) or removal (20–23) of Tregs can also promote the development of autoimmunity. We have shown previously that Tregs suppress autoreactive T and B cells in lupus-prone mice and protect against disease (24–27). Some recent reports suggest a decreased percentage of CD4+CD25+ cells (28–30); whereas, other reports seem to indicate normal or increased numbers of circulating Tregs (31–35) in active SLE patients. These differences may be due to differences in phenotyping methods, analyses, disease status, or therapies. Our own research points to a significantly decreased percentage of Tregs in our SLE cohort. However, gender-based differences in the roles of Tregs, expression of FoxP3, and their regulatory capacities have not been thoroughly studied.
Recent studies have shown that FoxP3 plays a significant role in regulatory T cell differentiation, function, and the prevention of auto-reactivity (8, 36, 37). FoxP3 is a critical transcription factor essential for determining the phenotype, development, and function of Tregs. FoxP3 deficiency or mutation results in the “Scurfy” phenotype in mice (38) and in humans results in IPEX (Immune dysregulation, Polyendocrinopathy, X-linked) syndrome (8, 9, 39–41).
Sex hormones have been known to play an important role in regulating lupus both in human and animal models (42, 43). Estrogen has been shown to increase calcineurin in T cells of SLE patients but not in age and sex matched healthy controls (44, 45). These previous studies did not examine the role of Tregs in SLE patients. A recent study identified the expansion of CD4+CD25+ and FoxP3+ Tregs during the follicular phase of the menstrual cycle in healthy females and found that an increase in Tregs correlated with changes in serum estradiol levels (46). In healthy males, testosterone antagonists have been shown to cause significant decreases (~30%) in the percentage of CD4+CD25+ T cells in comparison with baseline and with subjects in the placebo group. This decline normalized with the return of natural hormone levels after the antagonists were discontinued (47). In males with SLE, imbalances in estrogens and androgens could contribute to susceptibility to the disease (48–52).
Testosterone suppresses both IgG anti-dsDNA antibody and total IgG production in PBMCs from SLE patients (53, 54). Low levels of plasma androgens was reported (55) and androgen administration has been demonstrated to improve disease activity (56) in women with active SLE disease. However, this study (56) did not evaluate Treg function or the expression of FoxP3.
In the present study, we provide evidence that sex hormones and gender influence both the number and phenotype of Tregs and the Treg expression of FoxP3 differentially in men and women and also in SLE patients and healthy controls. Notably, we have found that plasma levels of estradiol are increased and testosterone levels are decreased in SLE females compared to healthy females. Furthermore, we have found that Treg exposure to testosterone in vitro increases FoxP3 expression in SLE females. Finally, we have demonstrated that plasma concentrations of testosterone in SLE females positively correlates with levels of FoxP3 expression. These data suggest that sex hormones and gender play pivotal roles in the regulation and maintenance of immune responses and provide novel evidence for a modulatory role of 17β-estradiol and androgen (DHT) in the phenotype and regulation of immune responses in autoimmunity.
Materials and Methods
Subjects
We enrolled 27 subjects who were 18 years or older and fulfilled the American College of Rheumatology revised criteria for the classification of SLE (57, 58) and 23 healthy donors (19–70 years of age) with no history of autoimmune disease. Patients with comorbid conditions were excluded from the study. Disease activity was recorded based on the SLE disease activity index (SLEDAI) (59). For estradiol and testosterone measurement, we obtained control and SLE plasma samples from the UCLA Rheumatology biobank. The study was approved by the Institutional Review Board of the University of California Los Angeles. Written informed consent was obtained from each subject who participated in the study.
Cell Isolation and Preparation
T cell enriched peripheral blood mononuclear cells (PBMCs) were isolated on a density gradient (Histopaque-1077, Sigma-Aldrich, St. Louis, MO, USA) from blood samples of lupus patients and healthy volunteers. Lymphocytes were washed twice in serum free media. Red blood cells (RBC) were lysed with RBC lysing solution (Sigma-Aldrich, St. Louis, MO, USA). CD4+CD25+hiCD127low and CD8+CD25+hiCD127low Tregs were sorted after staining using a FACS Aria flow cytometer (BD Biosciences) for sex hormone experiments.
Immunophenotyping and Flow Cytometry
Peripheral blood mononuclear cells (PBMCs) from patients and healthy volunteers were stained with CD4-FITC, clone (RPA-T4); CD8-PerCP, clone (SK1); CD25-APC, clone (BC96); and CD127-PE, clone (hIL7R-M21) fluorochrome-conjugated monoclonal antibodies (mAb). Intracellular staining for FoxP3 (clone-PCH101) was performed after cell surface staining by fixation and permeabilization as per manufacturer protocol (eBiosciences, San Diego, CA). The antibodies for cell surface staining and isotype controls were from BD Biosciences, eBiosciences, and from BioLegend. (San Diego, CA). Data were collected using FACSCalibur (BD Biosciences) and analyzed by BD Cell Quest software (Becton-Dickinson, Mountain View, CA) or FCS De Nova software (Thornhill, Ontario, Canada).
Cell Culture
PBMCs and sorted CD4+CD25+hiCD127low Tregs (1-2x106 cells) were cultured with the sex hormones 17β-estradiol (30, 60–100, 500 pg/ml) or testosterone (30, 60, 100, 500 pg/ml), or with TGFβ (20 ng/ml) with and without fetal calf serum in complete media for 24–72 h. After culture, supernatants were obtained; cells were washed and stained for FACS analyses, and lysed for RNA and Western blot analyses.
ELISA
Estradiol and testosterone levels were measured in plasma and culture supernatants by commercial ELISA (Calbiotech, Inc., Spring Valley, CA) as per manufacturer instructions.
RNA Isolation and Real Time PCR
RNA was isolated from sorted and cultured Tregs with TRIzol (Invitrogen) as per the manufacturer's protocol. Real time PCR was used to analyze mRNA gene expression as described earlier (1, 26, 27). Human FoxP3 specific primers and probes were synthesized from Applied Biosystems. Human FoxP3 primer and probe sequences are as follows: FoxP3 forward, 5′-TCTTCTCGGTATAAAAGCAAAGTTGTT-3′; reverse, 5′-GTGAAGTGGACTGACAGAAAAGGAT-3′; probe, 6FAM-TGATACGTGACAGTTTCCCACAAGCCA-TAMRA. Human GAPDH primers and probes were obtained from Applied Biosystems. The amplification primers were used at 900 nM and probes at 200 nM. All samples were run in duplicate. Data was normalized with the house-keeping gene GAPDH.
Statistical Analyses
Data was analyzed using Prism 4.0 (GraphPad Software, San Diego, CA). Comparisons were performed using paired one- or two-tailed test. Results are expressed as mean ± SEM. p < 0.05 was considered significant.
Results
CD4+CD25+hiFoxP3+ and CD8+CD25+hiFoxP3+ Tregs Are Reduced in SLE Patients
In order to determine the number, phenotype, and homeostatic regulation and effects of sex hormones on Tregs in lupus patients compared to healthy controls, we performed an extensive immunophenotyping of PBMCs from each group (see gating scheme Figure 1A). Treg cells were identified as those cells expressing FoxP3 and CD25+high. We found that lupus patients exhibited significantly reduced percentages of CD4+FoxP3+ (p < 0.0041) and CD8+FoxP3+ T cells (p < 0.0102) when compared to healthy controls (Figures 1A–E). We also characterized levels of CD4+CD25+ (Figure 1F) and CD8+CD25+ T cells (Figure 1G) between healthy control and SLE patient and found that the percentage of CD4+CD25+hiFoxP3+ Tregs in peripheral blood was also significantly reduced in patients with SLE relative to healthy controls (Figure 1H; p < 0.0005). Collectively these data demonstrate that lupus patients have reduced percentages of both CD4+ and CD8+ Tregs.
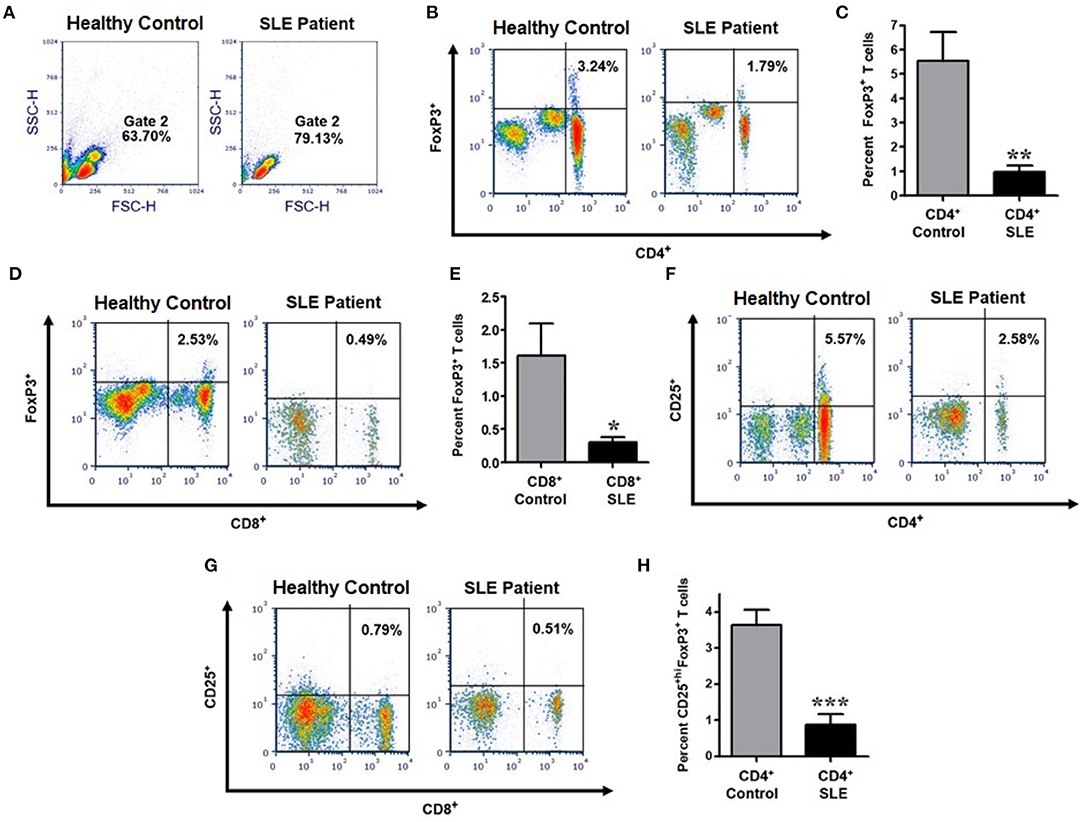
Figure 1. The percentage of CD4+ and CD8+ expressing characteristics of regulatory T cells are significantly reduced in SLE patients compared to gender and age matched healthy controls. Representative FACS analysis from PBMC of active female SLE patients compared with healthy controls (A,B,D,F,G). Peripheral blood mononuclear cells (PBMC) were isolated from 20 to 30 ml of blood obtained from SLE patients and healthy controls. 10,000 cells were gated and analyzed by FACS. Representative FACS analysis of FoxP3+ T cells (B) (with percent of positive cells in the Upper-Right quadrant indicated) were analyzed after gating of CD4+ T cells and CD8+ T cells from PBMC. (C) Cumulative data of CD4+FoxP3+ T cells in healthy controls (n = 14) and SLE patients (n = 25). (D) Representative FACS analysis of CD8+FoxP3+ T cells from PBMC of SLE patient vs healthy control. (E) Cumulative data of CD8+FoxP3+ T cells in healthy controls (n = 13) and SLE patients (n = 16). (F) Representative FACS analysis of CD4+CD25+ T cells from SLE patient vs. healthy control. (G) Representative FACS analysis of CD8+CD25+ T cells from SLE patient vs. healthy control. (H) Cumulative data of CD4+CD25+hiFoxP3+ T cells in healthy controls (n = 10) and SLE patients (n = 12). p values indicating significant differences are shown in each panel (C,E,F). *p < 0.05, **p < 0.01, ***p < 0.001.
CD4+CD25+hi and CD8+CD25+hi T Cells From Healthy Males Have Higher FoxP3 mRNA Levels Than Healthy Females
Healthy males had significantly higher percentages of CD4+CD25+hi and CD8+CD25+hi Tregs relative to healthy females (Figures 2A,B; p < 0.007 and p < 0.02). Since lupus is a gender-biased disease with a female to male ratio of 9:1, we determined whether FoxP3 expression in healthy male and female individuals varies in their regulatory T cell compartments. FoxP3 expression in CD4+ and CD8+ regulatory subsets, CD4+CD25+hi and CD8+CD25+hi T cell subsets were sorted by FACS. The sorted cells were subjected to RNA isolation from both healthy male and female subjects. We found that CD4+CD25+hi and CD8+CD25+hi subsets of healthy males had 2-3 times higher FoxP3 mRNA compared to healthy females (Figures 2C,D). Overall, we found that circulating CD4+CD25+high and CD8+CD25+high T cells are higher in healthy males than healthy females and, although, FoxP3 expression is decreased in both CD4+ and CD8+ T cells, it was only significantly decreased within the CD4+ T cell compartment.
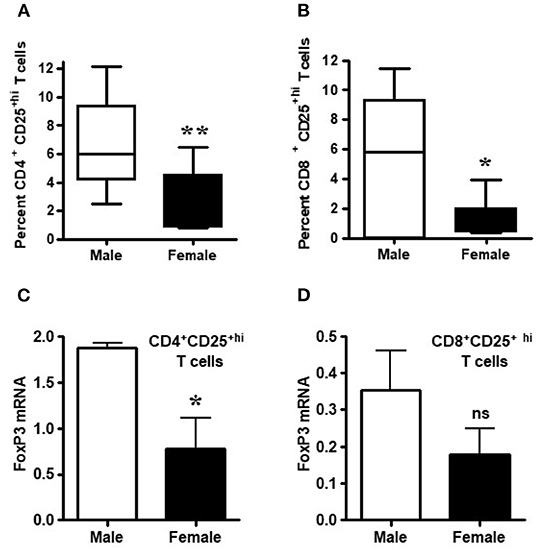
Figure 2. Circulating Tregs of healthy males have higher FoxP3 mRNA than cells from healthy females. Numbers of circulating CD4+ and CD8+ regulatory T cells are decreased in healthy females. (A) CD4+ regulatory T cell numbers were measured after FACS staining by CD4, CD8, and CD25 monoclonal antibodies from 6 healthy males and 10 healthy females, **p < 0.007 by Mann Whitney two-tailed t test. (B) CD8+ Tregs from 8 healthy males and 10 healthy age matched females, *p < 0.02 by paired two-tailed t test. Percent positive CD4+ Treg and CD8+ Tregs were determined from total CD4 and CD8 cells from PBMCs. T cells from male and female healthy subjects were sorted by FACS and total RNA isolated from CD4+CD25+hi (C) and CD8+CD25+hi (D) cells. 100 ng of RNA from each male and female was used for real-time PCR to quantitate FoxP3 mRNA levels, and data were normalized with GAPDH. Data shown are from 3 males and 5 females. *p < 0.05, **p < 0.001, ns, not significant.
Females Have Less Total FoxP3 Message in PBMCs Relative to Males; Evidence Suggests That TGFβ Promotes FoxP3 Expression in Both Sexes
Having examined the expression of FoxP3 in CD4+ and CD8+ Tregs of healthy males and females, we were interested to see whether transforming growth factor-β (TGFβ) promotes FoxP3 expression differently in whole peripheral blood mononuclear cells (PBMCs) of healthy males and females. Transforming growth factor-β (TGFβ) is a multifunctional cytokine regulating T cell biology (60, 61). It has been shown to induce FoxP3+ T regulatory cells from CD4+CD25− precursors (12, 62, 63). To address TGFβ effects on FoxP3 expression in healthy males and females, PBMCs were obtained, total RNA isolated, and real-time PCR performed to analyze FoxP3 expression. We found that female have less FoxP3 expression compared to age matched male healthy subject (Figure 3A). Overall, TGFβ increased FoxP3 expression in both healthy male and healthy female PBMCs (Figure 3B) however, the fold increase of FoxP3 expression was less in healthy females than in healthy males. These findings of a lower response to TGFβ in terms of expression of FoxP3—a classical marker of Tregs–in healthy females compared to healthy males might be one explanation of why SLE and other autoimmune disorders are predominantly expressed in females.
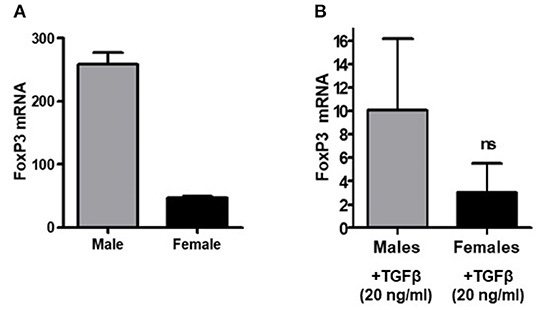
Figure 3. TGFβ promotes FoxP3 expression in human PBMCs in vitro. PBMCs (2–4 × 106) were isolated from healthy males (n = 5) and healthy females (n = 5) and cultured with TGFβ (20 ng/ml) for 24–48 h range in complete media. RNA was isolated and real time PCR was performed for FoxP3 mRNA expression. 100 ng of RNA was used for FoxP3 mRNA expression with a specific primer and probe for the human FoxP3 gene. (A) PBMCs were isolated from a 21-year-old male and 22-year-old female, RNA was isolated, and real-time PCR was performed. Experiment was performed in triplicate and error bar shows the values from the triplicates. A deficiency of FoxP3 mRNA levels was noted in the female subject as compared to male. (B) PBMCs from healthy males (n = 5) had a larger magnitude of response to TGFβ treatment (20 ng/ml) than female cells (n = 5).
Female SLE Patients Have Altered Sex Hormone Levels—Increased Plasma Estradiol Levels and Decreased Testosterone Levels
Since chronic exposure to estradiol leads to activation of pro-inflammatory cells and genes, we measured 17β-estradiol levels in SLE patients and age- and sex-matched controls. We found that female SLE patients have significantly increased plasma estradiol levels compared to healthy controls (Figure 4A). We also found that testosterone levels were decreased in female SLE patients compared to healthy female controls (Figure 4B).
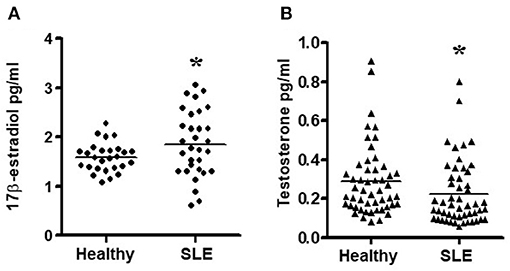
Figure 4. Estradiol and testosterone levels in plasma of SLE patients and healthy controls. (A) Female SLE patients (n = 31) have increased plasma estradiol levels compared to female healthy controls. (n = 27) and (B) Female SLE patients (n = 51) have decreased testosterone levels compared to healthy female controls (n = 51). Plasma estradiol and testosterone levels were measured by ELISA. *p < 0.05.
Sex Hormones Influence CD4, CD25, and FoxP3 Expression Differentially in Healthy Males and Females and in SLE Female PBMCs
It is not clear whether sex hormones influence CD4, CD25 and FoxP3 expression differentially in humans, both healthy and in SLE patient cells. To address this, we isolated PBMCs from healthy male and female volunteers and cultured their PBMCs with different concentrations of 17β-estradiol (E2). In preliminary in vitro experiments using different concentrations of E2 (30–150 pg/ml), we determined that maximal responses in both healthy and SLE individuals occurred at the 30–60 pg/mL range (data not shown). Of significance, we found that incubation with E2 at physiologic range (60 pg/ml) significantly increased CD4, CD25, and FoxP3 (mean fluorescence intensity) expression in PBMCs from a healthy female (Figures 5A–C) but not in PBMCs from a healthy male (Figures 5D–F). In contrast, in SLE patients (both males and females) PBMCs treated with E2 at a physiological dose (60 pg/ml) was associated with significantly reduced FoxP3 mRNA expression (Figures 6A,B). These differences in response to E2 in SLE patients' immune cells vs. healthy cells suggest that the disease-inflammatory microenvironment may play a significant role. Next, we wanted to see how CD4+CD25− T cells from SLE patients would response to E2. We found that CD4+CD25− T cells treated with E2 were unable to drive FoxP3 protein expression (Figure 6C) and that the mean fluorescence intensity of FoxP3 was significantly (p < 0.04) decreased in E2-treated CD4+CD25− T cells (Figure 6D). These data suggest that in healthy females E2 promotes an increase in regulatory T cell numbers and FoxP3 expression; whereas, females with SLE have defective regulatory T cell responses to E2 at physiologic levels. Therefore, females with SLE do not expand Tregs normally in response to estradiol stimulation.
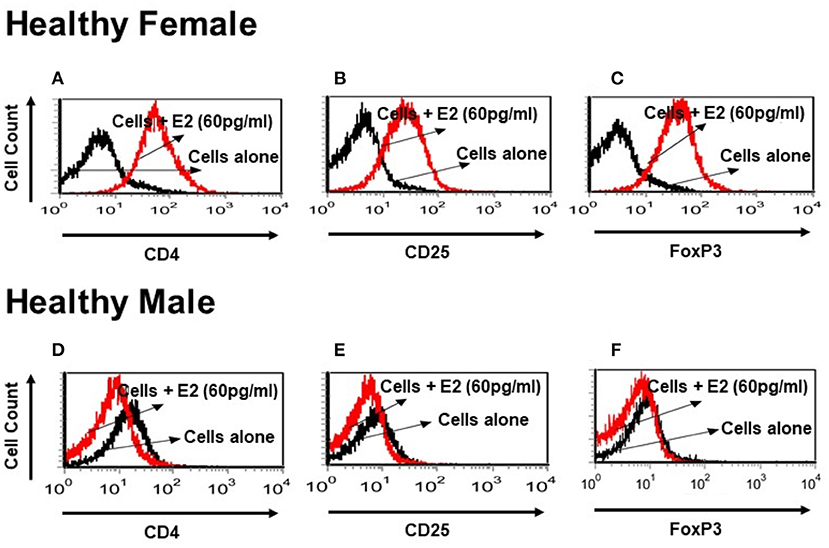
Figure 5. Estrogen increases expression of CD4, CD25, and FoxP3 in vitro in a healthy female subject but not in a healthy male subject. PBMCs (2–4 × 106) were isolated from a healthy female and a healthy male subject and cultured for 24–48 h range with physiological concentrations of E2 (60 pg/ml). Estrogen increases CD4 and CD25 cell surface expression and intracellular FoxP3 expression in a healthy female PBMCs (A–C). These effects were not seen in a healthy male PBMCs cells (D–F).
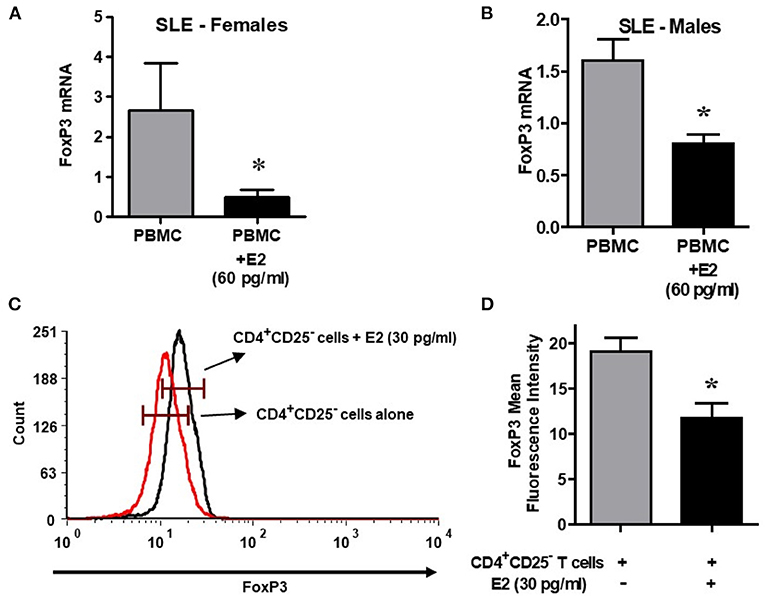
Figure 6. Lupus patient immune cells respond to E2 with a decrease in FoxP3 expression. Estrogen decreases expression of FoxP3 in both female and male SLE patient PBMCs. Lupus patient PBMCs (2–4 × 106 cells) were isolated and cultured (24–48 h range) with E2 at physiological concentrations (60 pg/ml). RNA was isolated, and real time PCR was performed with FoxP3 primers and probes. GAPDH was used as the house keeping gene. FoxP3 mRNA expression was reduced in female (n = 5) and male (n = 3) SLE pts (A,B). (C) Estrogen decreases FoxP3 protein in CD4+CD25− T cells from SLE patients. CD4+CD25− T cells were isolated from female SLE patient cells (n = 3) and cultured for in the 24–48 h range with E2 at 30 pg/ml concentration. FoxP3 intracellular protein was measured by FACS. (D) Mean fluorescence intensity (MFI) decreases in CD4+CD25− T cells from SLE patients treated with E2. *p < 0.05.
Androgen/Testosterone Increases the Expression of FoxP3 mRNA and Protein in Regulatory T Cells of SLE Patients
In the present study, we also tested the androgen effect on isolated CD4+CD25+CD127low Tregs in female SLE patients. Isolated cells were cultured with testosterone (100 ng/ml) and then lysed, RNA isolated, and RT-PCR performed. We found that treatment with testosterone/DHT (100 ng/mL) significantly increased FoxP3 mRNA expression in SLE patient' CD4+ Tregs (Figure 7A). The positive effect of testosterone on FoxP3 expression is further suggested by the data shown in Figure 7B, which indicates that plasma concentrations of testosterone in females with SLE correlates significantly with the expression of FoxP3 in their CD4+CD25+CD127low T cells. Furthermore, we found that incubation with androgen increased the expression of total FoxP3 protein in the PBMCs of female SLE patients (Figure 7C). These data suggest that androgens positively regulate FoxP3 expression in SLE patients.
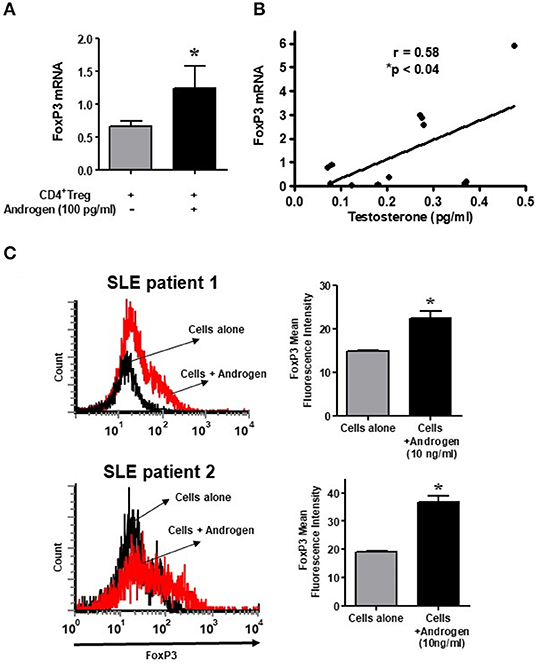
Figure 7. Testosterone increases the expression of FoxP3 mRNA in female SLE patient Tregs in vitro, and the plasma level of testosterone correlates with FoxP3 mRNA in SLE patients. (A) Androgen/testosterone treatment (100 pg/ml) increases FoxP3 expression significantly in female SLE patient' Tregs. CD4+CD25+highCD127low Tregs were isolated from female SLE patients (n = 7-10) cells after cultured for 24–48 h range with androgen. RNA was isolated, and real time PCR was performed with FoxP3 primers and probes. GAPDH was used to normalize data. (B) Positive correlation between plasma levels of testosterone and expression of FoxP3 in female SLE patients (n = 12) immune cells. *p < 0.05. (C) Female PBMCs were isolated from SLE patients and cultured at a density of 2-3 × 106 cells per well with and without androgen (10 ng/ml) for 24–48 h range. Cells were washed, stained with CD4 and FoxP3 antibodies, and analyzed with FACS. A minimum of 10,000 cells were gated. Representative FACS analysis from two female SLE patients is shown. Mean fluorescence intensity (MFI). *p < 0.05.
Discussion
Environmental factors, genetic defects, and hormones can regulate immune responses and therefore may influence SLE susceptibility (64–67). Regulatory T cells (Tregs) play a key role in maintaining immune homeostasis including the prevention of autoimmunity, maintenance of self-tolerance, and regulation of immune responses against infection (68–70). The functional failure of Tregs can result in development of autoimmune diseases including SLE (18, 71–73). Regulatory B cells (Breg) and myeloid-derived suppressor cells (MDSC) (74–79) and type1 regulatory T cells (CD4+IL-10+FoxP3−) (80– 85) have an immunosuppression role and promote immune tolerance. Genetic polymorphisms in the FoxP3 gene and imbalances of regulatory T cells and autoimmunity have also been reported (86) as-well-as polymorphisms of genes involved in Tregs activation and function (87). Gender effects have also been reported.
Women are more prone than men to the development of autoimmune diseases including SLE (3, 4) and the role of sex hormones (17β-estradiol and androgen) has been demonstrated in SLE (88). The female sex hormone (estrogen) contributes to the pathogenesis of SLE by activating T cells and by modulating the function of regulatory T cells (89–91). However, it is not clear whether sex hormones and/or gender regulate the function of these regulatory cells or expression of markers including FoxP3 differentially in humans.
The role of sex hormones (17β-estradiol and androgen) has been demonstrated in SLE (88). The female sex hormone (estrogen) contributes to the pathogenesis of SLE by activating T cells and by modulating the function of regulatory T cells (89–91). In addition, an association of single-nucleotide polymorphisms in the FoxP3 gene have been correlated with SLE susceptibility (92). However, it is not clear whether sex hormones and/or gender regulate the function of these regulatory cells or expression of markers including FoxP3 differentially in humans. In the present study, we did not address the issue of gene polymorphisms or the possibility that individual SNPs may play a significant role in healthy Treg populations which have low FoxP3 expression. Future study will be needed to address these possibilities. Previous studies have shown that estradiol treatment of PBMCs affects T cells, B cells, and monocytes (54, 93, 94); and gender differences in estrogen receptor (ER) expression were documented (95). However, the effect of gender and sex hormones on the phenotype and function of Tregs and expression of FoxP3 in SLE patients compared to healthy controls is less well studied. Recent evidence in a mouse model of autoimmune diseases indicates that female sex hormone (17β-estradiol-E2) influences the expression of FoxP3 and Treg number and function. Further, estradiol has been shown to influence the activation and function of many immune cells (74, 93) including CD4+ (Th1, Th2, Th17, and Treg) and CD8+ T cells (96–98). It has been reported that estrogen exposure directly activated T cells through the cell membrane estrogen receptor (99) and that 17β-estradiol receptors are differential expressed in women with SLE (100).
Estrogen binds to nuclear receptors (ERα and ERβ) on various cells, including CD4+ T cells, thus altering the rate of gene transcription (48-51). It also acts independently of ER (estrogen receptor) through alterations in the plasma membrane. Exposure of T cells to 17β-estradiol stimulates kinase activation and calcium flux. In normal mice, administration of physiologic doses of 17β-estradiol to ovariectomized females increased 2-to-3-fold the numbers of CD4+CD25+ and CD4+FoxP3+ T cells in PBL, spleens and lymph nodes, suggesting that 17β-estradiol in the absence of other ovarian hormones strongly influences expansion of Tregs (101). In vivo or in vitro exposure to 17β-estradiol increases CD4+CD25+ T cell numbers and FoxP3 expression in Experimental Autoimmune Encephalomyelitis (EAE) (102).
Male hormones (androgens) also influence the immune response in many diseases including SLE (47). In males with SLE, imbalances in both estrogens and androgens could contribute to increased susceptibility to the active disease (48–52). Earlier studies indicated that testosterone suppressed both IgG anti-dsDNA antibody and total IgG production in PBMCs from SLE patients (53, 54). Androgen administration (of prasterone, which can be metabolized to testosterone) has recently been demonstrated to improve disease activity in females with SLE (56). The effect of testosterone on CD4+ Tregs and FoxP3 expression, stability, and plasticity in SLE patients is not clear and has not been studied extensively. Although recent evidence suggests that FoxP3 protein stability is controlled by several proteins including cyclin dependent kinase−2 (CDK-2) (103), Pim-2 kinase (104), Nemo-like kinase, and CNS2 (non–coding sequence 2 demethylation by TET (ten-eleven translocation) protein (105, 106). However, the molecular mechanisms that control FoxP3 stability and Treg plasticity remains to be identified in SLE.
In the present study, we demonstrate that the percentage of CD4+ and CD8+ Tregs are significantly reduced in SLE patients compared to gender and age matched healthy controls (Figure 1); and that the percentage of both CD4+ and CD8+ Tregs is reduced in healthy females compared to healthy males. Our data is the first report that healthy male cells express higher FoxP3 mRNA levels than healthy female cells (Figure 2). We show that female SLE patients have increased plasma levels of estradiol (Figure 4) and that incubation of CD4+ Tregs with 17β-estradiol (at physiological levels) either maintains or decreases FoxP3 expression in females with SLE, in contrast to inducing a significant increase in CD4+ Treg in healthy females (Figures 5, 6). We further show that TGFβ treatment induces FoxP3 expression in PBMC of both healthy males and females, but at a larger degree in males than healthy females (Figure 3). At the mechanistic level, we demonstrate that estrogen increases expression of CD4, CD25, and FoxP3 in a healthy female but not in a healthy male subject (Figure 5). Finally, at the clinical and translational significance level, we showed for the first time that in female SLE patients (1) testosterone levels are reduced (Figure 4); (2) testosterone exposure increases the expression of FoxP3 mRNA in Tregs (Figure 7); and (3) that the plasma levels of testosterone positively correlates with FoxP3 mRNA (Figure 7). Thus, our data suggest that sex hormones and gender influences the expression of FoxP3 and regulatory T cells differentially in SLE.
We postulate that women are predisposed to SLE and to the maintenance of disease activity in part because of their relatively high levels of estradiol (E2), and their low levels of testosterone and related active metabolites. We also suggest that the sex hormone (estradiol that has higher concentrations in females) work in part by suppressing the numbers and functional activity of CD4+ Tregs, with resultant inadequate suppression of CD4+CD25− effector T cells and of autoantibody-producing B cells. Our data suggest that CD4+CD25+hi and CD4+FoxP3+ T cells are lower in numbers in women with SLE than in matched healthy controls, and that these cells are relatively insensitive to E2 stimulation at the levels that increase FoxP3 expression in normal Tregs but not in SLE. Our results are in-line with other investigators who found that estradiol treatment enhances increased expression of CD25+ T cells and increased FoxP3 expression in mice treated with estradiol (102). Furthermore, estradiol treatment increased Treg numbers and functions and induces FoxP3 expression both in vitro and in vivo (6). These data indicate that lupus patient' immune cells (PBMCs) behave differently than those from healthy subjects when they are cultured in vitro with 17β-estradiol (Figure 6).
Androgen-induced immunosuppression was reviewed recently (107) in both sexes and shown to affect the differentiation and function of Tregs differently in men and women. In vivo administration of androgens to women with adrenal insufficiency and rats with experimental autoimmune orchitis has been shown to increase Treg numbers (108, 109). Further it was suggested that, androgens are capable of directly converting peripheral T cells into Tregs in women. However, in a contradicting report it was suggested that androgens interfere with Treg function in men, as occurs in a mouse model of Sjögren's syndrome which predominantly affects male mice (110). Further it has been shown that administration of 5-dehydroepiandrosterone (DHEA), which is metabolized to testosterone, reduces disease activity in women with SLE (56). However, this DHEA study did not evaluate the expression of FoxP3 nor regulatory T cell numbers or function. In our current study, testosterone significantly increases FoxP3 expression in CD4+CD25+hi cells from females with SLE in vitro. Furthermore, we showed that testosterone increases the expression of FoxP3 mRNA in female SLE patient' Tregs (Figure 7A). In addition, we showed that plasma concentrations of testosterone positively correlated in those females with the expression of FoxP3 in their CD4+CD25+hi T cells (Figure 7B) suggesting that this response to testosterone may be normal in women with SLE, in contrast to their response to estradiol.
Our data is in agreement with a previous study which showed that androgen causes expansion of Tregs and a significant androgen-dependent increase of FoxP3 expression in human T-cells from women; however, this response was not seen in males. The study also identified a functional androgen response element (ARE) within the FoxP3 locus (111) and showed that binding of the androgen receptor (AR) to the ARE leads to epigenetic changes. The authors were able to show that the FoxP3 gene is more responsive to androgen treatment in T cells isolated from women than in men, indicating gender-specific androgen signaling. These studies, together with our data, demonstrate that healthy females may be more susceptible than males to SLE and other autoimmune diseases in part because females have fewer Tregs with reduced FoxP3 expression within those cells. In addition, females with SLE have less ability to generate CD4+ Tregs in response to physiologic concentrations of 17β-estradiol in comparison to healthy females; whereas, an active testosterone metabolite can increase the generation of CD4+ Tregs in SLE females. The responsiveness of female T cells to induce or transform into Tregs, under the effect of androgen, may provide a mechanistic basis to control excessive and damaging immune responses (autoimmunity). Future studies will be required addressing the exact mechanism of this immune homeostasis.
In summary, our results provide novel evidence for a functional modulatory role of sex hormones (estradiol and androgen) in the differentiation of Treg cells in SLE. Further, we provided evidence that androgen effects the regulation of the FoxP3 expression on T regulatory cells differentially in women and men.
Data Availability Statement
The raw data supporting the conclusions of this article will be made available by the authors, without undue reservation.
Ethics Statement
The studies involving human participants were reviewed and approved by UCLA IRB. The patients/participants provided their written informed consent to participate in this study.
Author Contributions
RPS conceived the idea, designed the experiments, performed the experiments, obtained data, analyzed, wrote the manuscript, and obtained funding. DSB analyzed the data, figure drawing and edited the manuscript. Both authors contributed to the article and approved the submitted version.
Funding
This study was supported by NIH grants AR54034, AI 083894, AI65645 to RPS, UCLA Senate Core Grant to Bevra Hahn and RPS, UCLA Oppenheimer Clinical Seed Grant and American Autoimmune Related Disease Association grant to RPS.
Conflict of Interest
The authors declare that the research was conducted in the absence of any commercial or financial relationships that could be construed as a potential conflict of interest.
Acknowledgments
We would like to thank all the patients, volunteers, and the UCLA Rheumatology clinicians who participated in the study by providing blood samples for our analysis. We also thank the UCLA Flow Cytometry Core Facility for cell sorting and FACS experiments, Ravi Dinesh for technical help and Drs. Antonio La Cava and Bevra Hahn for intellectual insights.
Abbreviations
E2, 17β-estradiol; Tregs, regulatory T cells; RT-PCR, real-time PCR; SLE, systemic lupus erythematosus; PBMC, peripheral blood mononuclear cells; TGF-β, transforming growth factor beta; CD25, IL-2 receptor α chain; FoxP3, Forkhead box protein-3; FACS, fluorescence-activated cell sorting.
References
1. Dinesh R, Hahn BH, Singh RP. Gender and sex hormones influence CD4+ regulatory T cells and their expression of FoxP3 in healthy people and in SLE. Arthr Rheum Abstr. (2010) 62:1257.
2. Dinesh RK, Skaggs BJ, La Cava A, Hahn BH, Singh RP. CD8+ Tregs in lupus, autoimmunity, and beyond. Autoimm Rev. (2010) 9:560–8. doi: 10.1016/j.autrev.2010.03.006
3. Kotzin BL. Systemic lupus erythematosus. Cell. (1996) 85:303–6. doi: 10.1016/S0092-8674(00)81108-3
4. Whitacre CC. Sex differences in autoimmune disease. Nat Immunol. (2001) 2:777–80. doi: 10.1038/ni0901-777
5. Ngo ST, Steyn FJ, McCombe PA. Gender differences in autoimmune disease. Front Neuroendocrinol. (2014) 35:347–69. doi: 10.1016/j.yfrne.2014.04.004
6. Nie J, Li YY, Zheng SG, Tsun A, Li B. FOXP3(+) Treg cells and gender bias in autoimmune diseases. Front Immunol. (2015) 6:493. doi: 10.3389/fimmu.2015.00493
7. Yagi H, Nomura T, Nakamura K, Yamazaki S, Kitawaki T, Hori S, et al. Crucial role of FOXP3 in the development and function of human CD25+CD4+ regulatory T cells. International immunology. (2004) 16:1643–56. doi: 10.1093/intimm/dxh165
8. Fontenot JD, Gavin MA, Rudensky AY. Foxp3 programs the development and function of CD4+CD25+ regulatory T cells. Nat Immunol. (2003) 4:330–6. doi: 10.1038/ni904
9. Khattri R, Cox T, Yasayko SA, Ramsdell F. An essential role for Scurfin in CD4+CD25+ T regulatory cells. Nat Immunol. (2003) 4:337–42. doi: 10.1038/ni909
10. Chen W, Jin W, Hardegen N, Lei KJ, Li L, Marinos N, et al. Conversion of peripheral CD4+CD25- naive T cells to CD4+CD25+ regulatory T cells by TGF-beta induction of transcription factor Foxp3. J Exp Med. (2003) 198:1875–86. doi: 10.1084/jem.20030152
11. Zheng SG, Wang JH, Koss MN, Quismorio FJr, Gray JD, Horwitz DA. CD4+ and CD8+ regulatory T cells generated ex vivo with IL-2 and TGF-beta suppress a stimulatory graft-versus-host disease with a lupus-like syndrome. J Immunol. (2004) 172:1531–9. doi: 10.4049/jimmunol.172.3.1531
12. Fu S, Zhang N, Yopp AC, Chen D, Mao M, Zhang H, et al. TGF-beta induces Foxp3 + T-regulatory cells from CD4 + CD25 - precursors. Am J Transplant. (2004) 4:1614–27. doi: 10.1111/j.1600-6143.2004.00566.x
13. Rao PE, Petrone AL, Ponath PD. Differentiation and expansion of T cells with regulatory function from human peripheral lymphocytes by stimulation in the presence of TGF-{beta}. J Immunol. (2005) 174:1446–55. doi: 10.4049/jimmunol.174.3.1446
14. Park HB, Paik DJ, Jang E, Hong S, Youn J. Acquisition of anergic and suppressive activities in transforming growth factor-beta-costimulated CD4+CD25- T cells. Int Immunol. (2004) 16:1203–13. doi: 10.1093/intimm/dxh123
15. Fantini MC, Becker C, Monteleone G, Pallone F, Galle PR, Neurath MF. Cutting edge: TGF-beta induces a regulatory phenotype in CD4+CD25- T cells through Foxp3 induction and down-regulation of Smad7. J Immunol. (2004) 172:5149–53. doi: 10.4049/jimmunol.172.9.5149
16. Miyara M, Amoura Z, Parizot C, Badoual C, Dorgham K, Trad S, et al. Global natural regulatory T cell depletion in active systemic lupus erythematosus. J Immunol. (2005) 175:8392–400. doi: 10.4049/jimmunol.175.12.8392
17. Lee JH, Wang LC, Lin YT, Yang YH, Lin DT, Chiang BL. Inverse correlation between CD4+ regulatory T-cell population and autoantibody levels in paediatric patients with systemic lupus erythematosus. Immunology. (2006) 117:280–6. doi: 10.1111/j.1365-2567.2005.02306.x
18. Franz B, Fritzsching B, Riehl A, Oberle N, Klemke CD, Sykora J, et al. Low number of regulatory T cells in skin lesions of patients with cutaneous lupus erythematosus. Arthritis Rheum. (2007) 56:1910–20. doi: 10.1002/art.22699
19. Valencia X, Yarboro C, Illei G, Lipsky PE. Deficient CD4+CD25high T regulatory cell function in patients with active systemic lupus erythematosus. J Immunol. (2007) 178:2579–88. doi: 10.4049/jimmunol.178.4.2579
20. Suri-Payer E, Amar AZ, Thornton AM, Shevach EM. CD4+CD25+ T cells inhibit both the induction and effector function of autoreactive T cells and represent a unique lineage of immunoregulatory cells. J Immunol. (1998) 160:1212–8.
21. Salomon B, Lenschow DJ, Rhee L, Ashourian N, Singh B, Sharpe A, et al. B7/CD28 costimulation is essential for the homeostasis of the CD4+CD25+ immunoregulatory T cells that control autoimmune diabetes. Immunity. (2000) 12:431–40. doi: 10.1016/S1074-7613(00)80195-8
22. Sakaguchi S. Naturally arising CD4+ regulatory t cells for immunologic self-tolerance and negative control of immune responses. Annu Rev Immunol. (2004) 22:531–62. doi: 10.1146/annurev.immunol.21.120601.141122
23. Paust S, Cantor H. Regulatory T cells and autoimmune disease. Immunol Rev. (2005) 204:195–207. doi: 10.1111/j.0105-2896.2005.00247.x
24. La Cava A, Ebling FM, Hahn BH. Ig-reactive CD4+CD25+ T cells from tolerized (New Zealand Black x New Zealand White)F1 mice suppress in vitro production of antibodies to DNA. J Immunol. (2004) 173:3542–8. doi: 10.4049/jimmunol.173.5.3542
25. Hahn BH, Singh RP, La Cava A, Ebling FM. Tolerogenic treatment of lupus mice with consensus peptide induces Foxp3-expressing, apoptosis-resistant, TGFbeta-secreting CD8+ T cell suppressors. J Immunol. (2005) 175:7728–37. doi: 10.4049/jimmunol.175.11.7728
26. Singh RP, La Cava A, Wong M, Ebling F, Hahn BH. CD8+ T cell-mediated suppression of autoimmunity in a murine lupus model of peptide-induced immune tolerance depends on Foxp3 expression. J Immunol. (2007) 178:7649–57. doi: 10.4049/jimmunol.178.12.7649
27. Singh RP, La Cava A, Hahn BH. pConsensus peptide induces tolerogenic CD8+ T cells in lupus-prone (NZB x NZW)F1 mice by differentially regulating Foxp3 and PD1 molecules. J Immunol. (2008) 180:2069–80. doi: 10.4049/jimmunol.180.4.2069
28. Crispin JC, Martinez A, Alcocer-Varela J. Quantification of regulatory T cells in patients with systemic lupus erythematosus. J Autoimm. (2003) 21:273–6. doi: 10.1016/S0896-8411(03)00121-5
29. Liu MF, Wang CR, Fung LL, Wu CR. Decreased CD4+CD25+ T cells in peripheral blood of patients with systemic lupus erythematosus. Scand J Immunol. (2004) 59:198–202. doi: 10.1111/j.0300-9475.2004.01370.x
30. Lyssuk EY, Torgashina AV, Soloviev SK, Nassonov EL, Bykovskaia SN. Reduced number and function of CD4+CD25highFoxP3+ regulatory T cells in patients with systemic lupus erythematosus. Adv Exp Med Biol. (2007) 601:113–9. doi: 10.1007/978-0-387-72005-0_12
31. Azab NA, Bassyouni IH, Emad Y, Abd El-Wahab GA, Hamdy G, Mashahit MA. CD4+CD25+ regulatory T cells (TREG) in systemic lupus erythematosus (SLE) patients: the possible influence of treatment with corticosteroids. Clin Immun. (2008) 127:151–7. doi: 10.1016/j.clim.2007.12.010
32. Bonelli M, von Dalwigk K, Savitskaya A, Smolen JS, Scheinecker C. Foxp3 expression in CD4+ T cells of patients with systemic lupus erythematosus: a comparative phenotypic analysis. Ann Rheum Dis. (2008) 67:664–71. doi: 10.1136/ard.2007.074690
33. Lin SC, Chen KH, Lin CH, Kuo CC, Ling QD, Chan CH. The quantitative analysis of peripheral blood FOXP3-expressing T cells in systemic lupus erythematosus and rheumatoid arthritis patients. Eur J Clin Invest. (2007) 37:987–96. doi: 10.1111/j.1365-2362.2007.01882.x
34. Yates J, Whittington A, Mitchell P, Lechler RI, Lightstone L, Lombardi G. Natural regulatory T cells: number and function are normal in the majority of patients with lupus nephritis. Clin Exp Immun. (2008) 153:44–55. doi: 10.1111/j.1365-2249.2008.03665.x
35. Rifa'i M, Shi Z, Zhang SY, Lee YH, Shiku H, Isobe K, et al. CD8+CD122+ regulatory T cells recognize activated T cells via conventional MHC class I-alphabetaTCR interaction and become IL-10-producing active regulatory cells. Int Immunol. (2008) 20:937–47. doi: 10.1093/intimm/dxn052
36. Bonelli M, Smolen JS, Scheinecker C. Treg and lupus. Ann Rheum Dis. (2010) 69 Suppl. 1:i65–66. doi: 10.1136/ard.2009.117135
37. Horwitz DA. Regulatory T cells in systemic lupus erythematosus: past, present and future. Arthritis Res Ther. (2008) 10:227. doi: 10.1186/ar2511
38. Brunkow ME, Jeffery EW, Hjerrild KA, Paeper B, Clark LB, Yasayko SA, et al. Disruption of a new forkhead/winged-helix protein, scurfin, results in the fatal lymphoproliferative disorder of the scurfy mouse. Nat Gene. (2001) 27:68–73. doi: 10.1038/83784
39. Chatila TA, Blaeser F, Ho N, Lederman HM, Voulgaropoulos C, Helms C, et al. JM2, encoding a fork head-related protein, is mutated in X-linked autoimmunity-allergic disregulation syndrome. J Clin Invest. (2000) 106:R75–81. doi: 10.1172/JCI11679
40. Hori S, Nomura T, Sakaguchi S. Control of regulatory T cell development by the transcription factor Foxp3. Science. (2003) 299:1057–61. doi: 10.1126/science.1079490
41. Ziegler SF. FOXP3: of mice and men. Annu Rev Immunol. (2006) 24:209–26. doi: 10.1146/annurev.immunol.24.021605.090547
42. McMurray RW. Sex hormones in the pathogenesis of systemic lupus erythematosus. Front Biosci. (2001) 6:E193–206. doi: 10.2741/A708
43. McMurray RW, May W. Sex hormones and systemic lupus erythematosus: review and meta-analysis. Arthritis Rheum. (2003) 48:2100–10. doi: 10.1002/art.11105
44. Rider V, Foster RT, Evans M, Suenaga R, Abdou NI. Gender differences in autoimmune diseases: estrogen increases calcineurin expression in systemic lupus erythematosus. Clin Immunol Immunopathol. (1998) 89:171–80. doi: 10.1006/clin.1998.4604
45. Rider V, Jones SR, Evans M, Abdou NI. Molecular mechanisms involved in the estrogen-dependent regulation of calcineurin in systemic lupus erythematosus T cells. Clin Immunol. (2000) 95:124–34. doi: 10.1006/clim.2000.4844
46. Arruvito L, Sanz M, Banham AH, Fainboim L. Expansion of CD4+CD25+and FOXP3+ regulatory T cells during the follicular phase of the menstrual cycle: implications for human reproduction. J Immunol. (2007) 178:2572–8. doi: 10.4049/jimmunol.178.4.2572
47. Page ST, Plymate SR, Bremner WJ, Matsumoto AM, Hess DL, Lin DW, et al. Effect of medical castration on CD4+ CD25+ T cells, CD8+ T cell IFN-gamma expression, and NK cells: a physiological role for testosterone and/or its metabolites. Am J Physiol Endocrinol Metab. (2006) 290:E856–63. doi: 10.1152/ajpendo.00484.2005
48. Inman RD, Jovanovic L, Markenson JA, Longcope C, Dawood MY, Lockshin MD. Systemic lupus erythematosus in men. Genetic and endocrine features. Arch Intern Med. (1982) 142:1813–5. doi: 10.1001/archinte.142.10.1813
49. Miller MH, Urowitz MB, Gladman DD, Killinger DW. Systemic lupus erythematosus in males. Medicine. (1983) 62:327–34. doi: 10.1097/00005792-198309000-00005
50. Lavalle C, Loyo E, Paniagua R, Bermudez JA, Herrera J, Graef A, et al. Correlation study between prolactin and androgens in male patients with systemic lupus erythematosus. J Rheumatol. (1987) 14:268–72.
51. Peeva E, Michael D, Cleary J, Rice J, Chen X, Diamond B. Prolactin modulates the naive B cell repertoire. J Clin Invest. (2003) 111:275–83. doi: 10.1172/JCI200316530
52. Lahita RG, Bradlow L, Fishman J, Kunkel HG. Estrogen metabolism in systemic lupus erythematosus: patients and family members. Arthritis Rheum. (1982) 25:843–6. doi: 10.1002/art.1780250726
53. Kanda N, Tsuchida T, Tamaki K. Estrogen enhancement of anti-double-stranded DNA antibody and immunoglobulin G production in peripheral blood mononuclear cells from patients with systemic lupus erythematosus. Arthritis Rheum. (1999) 42:328–37. doi: 10.1002/1529-0131(199902)42:2<328::AID-ANR16>3.0.CO;2-#
54. Kanda N, Tamaki K. Estrogen enhances immunoglobulin production by human PBMCs. J Allergy Clin Immunol. (1999) 103:282–8. doi: 10.1016/S0091-6749(99)70503-8
55. Lahita RG, Bradlow HL, Ginzler E, Pang S, New M. Low plasma androgens in women with systemic lupus erythematosus. Arthritis Rheum. (1987) 30:241–8. doi: 10.1002/art.1780300301
56. Petri MA, Mease PJ, Merrill JT, Lahita RG, Iannini MJ, Yocum DE, et al. Effects of prasterone on disease activity and symptoms in women with active systemic lupus erythematosus. Arthritis Rheum. (2004) 50:2858–68. doi: 10.1002/art.20427
57. Hochberg MC. Updating the American College of Rheumatology revised criteria for the classification of systemic lupus erythematosus. Arthritis Rheum. (1997) 40:1725. doi: 10.1002/art.1780400928
58. Tan EM, Cohen AS, Fries JF, Masi AT, McShane DJ, Rothfield NF, et al. The 1982 revised criteria for the classification of systemic lupus erythematosus. Arthritis Rheum. (1982) 25:1271–7. doi: 10.1002/art.1780251101
59. Bombardier C, Gladman DD, Urowitz MB, Caron D, Chang CH. Derivation of the SLEDAI. A disease activity index for lupus patients. The Committee on Prognosis Studies in SLE. Arthritis Rheum. (1992) 35:630–40. doi: 10.1002/art.1780350606
60. Gorelik L, Flavell RA. Transforming growth factor-beta in T-cell biology. Nat Rev. (2002) 2:46–53. doi: 10.1038/nri704
61. Wahl SM. Transforming growth factor beta: the good, the bad, the ugly. J Exp Med. (1994) 180:1587–90. doi: 10.1084/jem.180.5.1587
62. Xu A, Liu Y, Chen W, Wang J, Xue Y, Huang F, et al. TGF-beta-induced regulatory T cells directly suppress B cell responses through a noncytotoxic mechanism. J Immunol. (2016) 196:3631–41. doi: 10.4049/jimmunol.1501740
63. Peng Y, Laouar Y, Li MO, Green EA, Flavell RA. TGF-beta regulates in vivo expansion of Foxp3-expressing CD4+CD25+ regulatory T cells responsible for protection against diabetes. Proc Natl Acad Sci USA. (2004) 101:4572–7. doi: 10.1073/pnas.0400810101
64. Bertsias GK, Salmon JE, Boumpas DT. Therapeutic opportunities in systemic lupus erythematosus: state of the art and prospects for the new decade. Ann Rheum Dis. (2010) 69:1603–11. doi: 10.1136/ard.2010.135186
65. Kamen DL. Environmental influences on systemic lupus erythematosus expression. Rheum Dis Clin. (2014) 40:401–12. doi: 10.1016/j.rdc.2014.05.003
66. Parks CG, de Souza Espindola Santos A, Barbhaiya M, Costenbader KH. Understanding the role of environmental factors in the development of systemic lupus erythematosus. Best Pract Res Clin Rheumatol. (2017) 31:306–20. doi: 10.1016/j.berh.2017.09.005
67. Tsokos GC. Systemic lupus erythematosus. N Engl J Med. (2011) 365:2110–21. doi: 10.1056/NEJMra1100359
68. Okeke EB, Uzonna JE. The pivotal role of regulatory T cells in the regulation of innate immune cells. Front Immunol. (2019) 10:680. doi: 10.3389/fimmu.2019.00680
69. Sakaguchi S, Sakaguchi N, Asano M, Itoh M, Toda M. Immunologic self-tolerance maintained by activated T cells expressing IL-2 receptor alpha-chains (CD25). Breakdown of a single mechanism of self-tolerance causes various autoimmune diseases. J Immunol. (1995) 155:1151–64.
70. Sakaguchi S, Yamaguchi T, Nomura T, Ono M. Regulatory T cells and immune tolerance. Cell. (2008) 133:775–87. doi: 10.1016/j.cell.2008.05.009
71. Bonelli M, Savitskaya A, Steiner CW, Rath E, Smolen JS, Scheinecker C. Phenotypic and functional analysis of CD4+ CD25- Foxp3+ T cells in patients with systemic lupus erythematosus. J Immunol. (2009) 182:1689–95. doi: 10.4049/jimmunol.182.3.1689
72. Bonelli M, Savitskaya A, von Dalwigk K, Steiner CW, Aletaha D, Smolen JS, et al. Quantitative and qualitative deficiencies of regulatory T cells in patients with systemic lupus erythematosus (SLE). Int Immunol. (2008) 20:861–8. doi: 10.1093/intimm/dxn044
73. Scheinecker C, Bonelli M, Smolen JS. Pathogenetic aspects of systemic lupus erythematosus with an emphasis on regulatory T cells. J Autoimmun. (2010) 35:269–75. doi: 10.1016/j.jaut.2010.06.018
74. Veglia F, Perego M, Gabrilovich D. Myeloid-derived suppressor cells coming of age. Nat Immunol. (2018) 19:108–119. doi: 10.1038/s41590-017-0022-x
75. Ji J, Xu J, Zhao S, Liu F, Qi J, Song Y, et al. Myeloid-derived suppressor cells contribute to systemic lupus erythaematosus by regulating differentiation of Th17 cells and Tregs. Clin Sci. (2016) 130:1453–67. doi: 10.1042/CS20160311
76. Park MJ, Lee SH, Kim EK, Lee EJ, Park SH, Kwok SK, et al. Myeloid-derived suppressor cells induce the expansion of regulatory B cells and ameliorate autoimmunity in the sanroque mouse model of systemic lupus erythematosus. Arthritis Rheumatol. (2016) 68:2717–27. doi: 10.1002/art.39767
77. Ma H, Xia CQ. Phenotypic and functional diversities of myeloid-derived suppressor cells in autoimmune diseases. Mediators Inflamm. (2018) 2018:4316584. doi: 10.1155/2018/4316584
78. Mauri C, Menon M. Human regulatory B cells in health and disease: therapeutic potential. J Clin Invest. (2017) 127:772–9. doi: 10.1172/JCI85113
79. Gao N, Dresel J, Eckstein V, Gellert R, Storch H, Venigalla RK, et al. Impaired suppressive capacity of activation-induced regulatory B cells in systemic lupus erythematosus. Arthritis Rheumatol. (2014) 66:2849–61. doi: 10.1002/art.38742
80. Gagliani N, Magnani CF, Huber S, Gianolini ME, Pala M, Licona-Limon P, et al. Coexpression of CD49b and LAG-3 identifies human and mouse T regulatory type 1 cells. Nat Med. (2013) 19:739–46. doi: 10.1038/nm.3179
81. Mascanfroni ID, Takenaka MC, Yeste A, Patel B, Wu Y, Kenison JE, et al. Metabolic control of type 1 regulatory T cell differentiation by AHR and HIF1-alpha. Nat Med. (2015) 21:638–46. doi: 10.1038/nm.3868
82. Geem D, Harusato A, Flannigan K, Denning TL. Harnessing regulatory T cells for the treatment of inflammatory bowel disease. Inflamm Bowel Dis. (2015) 21:1409–18. doi: 10.1097/MIB.0000000000000343
83. Pot C, Apetoh L, Kuchroo VK. Type 1 regulatory T cells (Tr1) in autoimmunity. Semin Immunol. (2011) 23:202–8. doi: 10.1016/j.smim.2011.07.005
84. Pot C, Apetoh L, Awasthi A, Kuchroo VK. Induction of regulatory Tr1 cells and inhibition of T(H)17 cells by IL-27. Semin Immunol. (2011) 23:438–45. doi: 10.1016/j.smim.2011.08.003
85. Chen Y, Kuchroo VK, Inobe J, Hafler DA, Weiner HL. Regulatory T cell clones induced by oral tolerance: suppression of autoimmune encephalomyelitis. Science. (1994) 265:1237–40. doi: 10.1126/science.7520605
86. Oda JM, Hirata BK, Guembarovski RL, Watanabe MA. Genetic polymorphism in FOXP3 gene: imbalance in regulatory T-cell role and development of human diseases. J Genet. (2013) 92:163–71. doi: 10.1007/s12041-013-0213-7
87. Nedoszytko B, Sokolowska-Wojdylo M, Renke J, Lange M, Trzonkowski P, Sobjanek M, et al. The role of regulatory T cells and genes involved in their differentiation in pathogenesis of selected inflammatory and neoplastic skin diseases. Part III: Polymorphisms of genes involved in Tregs' activation and function. Postepy Dermatol Alergol. (2017) 34:517–25. doi: 10.5114/pdia.2017.67053
88. Rider V, Abdou NI, Kimler BF, Lu N, Brown S, Fridley BL. Gender bias in human systemic lupus erythematosus: a problem of steroid receptor action? Front Immunol. (2018) 9:611. doi: 10.3389/fimmu.2018.00611
89. Khan D, Dai R, Ansar Ahmed S. Sex differences and estrogen regulation of miRNAs in lupus, a prototypical autoimmune disease. Cell Immunol. (2015) 294:70–9. doi: 10.1016/j.cellimm.2015.01.004
90. Moulton VR. Sex hormones in acquired immunity and autoimmune disease. Front Immunol. (2018) 9:2279. doi: 10.3389/fimmu.2018.02279
91. Mohammad I, Starskaia I, Nagy T, Guo J, Yatkin E, Vaananen K, et al. Estrogen receptor alpha contributes to T cell-mediated autoimmune inflammation by promoting T cell activation and proliferation. Sci Signal. (2018) 11:9415. doi: 10.1126/scisignal.aap9415
92. Lin YC, Lee JH, Wu AS, Tsai CY, Yu HH, Wang LC, et al. Association of single-nucleotide polymorphisms in FOXP3 gene with systemic lupus erythematosus susceptibility: a case-control study. Lupus. (2011) 20:137–43. doi: 10.1177/0961203310382428
93. Ahmed SA, Hissong BD, Verthelyi D, Donner K, Becker K, Karpuzoglu-Sahin E. Gender and risk of autoimmune diseases: possible role of estrogenic compounds. Environ Health Persp. (1999) 107 Suppl. 5:681–6. doi: 10.1289/ehp.99107s5681
94. Grimaldi CM, Jeganathan V, Diamond B. Hormonal regulation of B cell development: 17 beta-estradiol impairs negative selection of high-affinity DNA-reactive B cells at more than one developmental checkpoint. J Immunol. (2006) 176:2703–10. doi: 10.4049/jimmunol.176.5.2703
95. Phiel KL, Henderson RA, Adelman SJ, Elloso MM. Differential estrogen receptor gene expression in human peripheral blood mononuclear cell populations. Immunol Lett. (2005) 97:107–13. doi: 10.1016/j.imlet.2004.10.007
96. Lelu K, Laffont S, Delpy L, Paulet PE, Perinat T, Tschanz SA, et al. Estrogen receptor alpha signaling in T lymphocytes is required for estradiol-mediated inhibition of Th1 and Th17 cell differentiation and protection against experimental autoimmune encephalomyelitis. J Immunol. (2011) 187:2386–93. doi: 10.4049/jimmunol.1101578
97. Priyanka HP, Krishnan HC, Singh RV, Hima L, Thyagarajan S. Estrogen modulates in vitro T cell responses in a concentration- and receptor-dependent manner: effects on intracellular molecular targets and antioxidant enzymes. Mol Immunol. (2013) 56:328–39. doi: 10.1016/j.molimm.2013.05.226
98. Robinson DP, Hall OJ, Nilles TL, Bream JH, Klein S. 17beta-estradiol protects females against influenza by recruiting neutrophils and increasing virus-specific CD8 T cell responses in the lungs. J Virol. (2014) 88:4711–20. doi: 10.1128/JVI.02081-13
99. Benten WP, Lieberherr M, Giese G, Wunderlich F. Estradiol binding to cell surface raises cytosolic free calcium in T cells. FEBS Lett. (1998) 422:349–53. doi: 10.1016/S0014-5793(98)00039-8
100. Rider V, Li X, Peterson G, Dawson J, Kimler BF, Abdou NI. Differential expression of estrogen receptors in women with systemic lupus erythematosus. J Rheum. (2006) 33:1093–101.
101. Tai P, Wang J, Jin H, Song X, Yan J, Kang Y, et al. Induction of regulatory T cells by physiological level estrogen. J Cell Physiol. (2008) 214:456–64. doi: 10.1002/jcp.21221
102. Polanczyk MJ, Carson BD, Subramanian S, Afentoulis M, Vandenbark AA, Ziegler SF, et al. Cutting edge: estrogen drives expansion of the CD4+CD25+ regulatory T cell compartment. J Immunol. (2004) 173:2227–30. doi: 10.4049/jimmunol.173.4.2227
103. Morawski PA, Mehra P, Chen C, Bhatti T, Wells AD. Foxp3 protein stability is regulated by cyclin-dependent kinase 2. J Biol Chem. (2013) 288:24494–502. doi: 10.1074/jbc.M113.467704
104. Deng G, Nagai Y, Xiao Y, Li Z, Dai S, Ohtani T, et al. Pim-2 kinase influences regulatory T cell function and stability by mediating Foxp3 protein N-terminal phosphorylation. J Biol Chem. (2015) 290:20211–20. doi: 10.1074/jbc.M115.638221
105. Yue X, Trifari S, Aijo T, Tsagaratou A, Pastor WA, Zepeda-Martinez JA, et al. Control of Foxp3 stability through modulation of TET activity. J Exp Med. (2016) 213:377–97. doi: 10.1084/jem.20151438
106. Someya K, Nakatsukasa H, Ito M, Kondo T, Tateda KI, Akanuma T, et al. Improvement of Foxp3 stability through CNS2 demethylation by TET enzyme induction and activation. Int Immunol. (2017) 29:365–75. doi: 10.1093/intimm/dxx049
107. Gubbels Bupp MR, Jorgensen TN. Androgen-induced immunosuppression. Front Immunol. (2018) 9:794. doi: 10.3389/fimmu.2018.00794
108. Fijak M, Schneider E, Klug J, Bhushan S, Hackstein H, Schuler G, et al. Testosterone replacement effectively inhibits the development of experimental autoimmune orchitis in rats: evidence for a direct role of testosterone on regulatory T cell expansion. J Immunol. (2011) 186:5162–72. doi: 10.4049/jimmunol.1001958
109. Rutkowski K, Sowa P, Rutkowska-Talipska J, Kuryliszyn-Moskal A, Rutkowski R. Dehydroepiandrosterone (DHEA): hypes and hopes. Drugs. (2014) 74:1195–207. doi: 10.1007/s40265-014-0259-8
110. Lieberman SM, Kreiger PA, Koretzky GA. Reversible lacrimal gland-protective regulatory T-cell dysfunction underlies male-specific autoimmune dacryoadenitis in the non-obese diabetic mouse model of Sjogren syndrome. Immunology. (2015) 145:232–41. doi: 10.1111/imm.12439
Keywords: sex hormones, gender, regulatory T cells, systemic lupus erythematosus, 17β-estradiol
Citation: Singh RP and Bischoff DS (2021) Sex Hormones and Gender Influence the Expression of Markers of Regulatory T Cells in SLE Patients. Front. Immunol. 12:619268. doi: 10.3389/fimmu.2021.619268
Received: 19 October 2020; Accepted: 01 February 2021;
Published: 03 March 2021.
Edited by:
James A. Lederer, Brigham and Women's Hospital and Harvard Medical School, United StatesReviewed by:
Maria G. Petrillo, National Institute of Environmental Health Sciences (NIEHS), United StatesRaza Naqvi, University of Illinois at Chicago, United States
Carlo Riccardi, University of Perugia, Italy
Copyright © 2021 Singh and Bischoff. This is an open-access article distributed under the terms of the Creative Commons Attribution License (CC BY). The use, distribution or reproduction in other forums is permitted, provided the original author(s) and the copyright owner(s) are credited and that the original publication in this journal is cited, in accordance with accepted academic practice. No use, distribution or reproduction is permitted which does not comply with these terms.
*Correspondence: Ram P. Singh, cmFtLnNpbmdoQHZhLmdvdg==; cnBzaW5naDExQGhvdG1haWwuY29t