- 1ISGlobal, Hospital Clínic - Universitat de Barcelona, Barcelona, Spain
- 2Centro de Investigação em Saúde de Manhiça (CISM), Maputo, Mozambique
- 3U.S. Military Malaria Vaccine Program, Walter Reed Army Institute of Research (WRAIR), Silver Spring, MD, United States
- 4Department of Physiologic Science, Clinical Pharmacology, Faculty of Medicine, Eduardo Mondlane University, Maputo, Mozambique
Transplacental transfer of antibodies is essential for conferring protection in newborns against infectious diseases. We assessed the impact of different factors, including gestational age and maternal infections such as HIV and malaria, on the efficiency of cord blood levels and placental transfer of IgG subclasses. We measured total IgG and IgG subclasses by quantitative suspension array technology against 14 pathogens and vaccine antigens, including targets of maternal immunization, in 341 delivering HIV-uninfected and HIV-infected mother-infant pairs from southern Mozambique. We analyzed the association of maternal HIV infection, Plasmodium falciparum exposure, maternal variables and pregnancy outcomes on cord antibody levels and transplacental transfer. Our results show that maternal antibody levels were the main determinant of cord antibody levels. Univariable and multivariable analysis showed that HIV reduced the placental transfer and cord levels of IgG and IgG1 principally, but also IgG2 to half of the antigens tested. P. falciparum exposure and prematurity were negatively associated with cord antibody levels and placental transfer, but this was antigen-subclass dependent. Our findings suggest that lower maternally transferred antibodies may underlie increased susceptibility to infections of HIV-exposed infants. This could affect efficacy of maternal vaccination, especially in sub-Saharan Africa, where there is a high prevalence of HIV, malaria and unfavorable environmental factors.
Introduction
Each year, 2.6 million deaths occur during the neonatal period, with infectious diseases being the leading cause of mortality, particularly in low-income countries (1, 2). Newborns are highly vulnerable to pathogens due to their functional immunological differences from adults as a result of living in a semi-allogeneic sterile environment, where exposure to microbial antigens is limited (3–6). For example, microorganisms such as respiratory syncytial virus (RSV) cause no or mild disease in adults but induce acute bronchiolitis, viral pneumoniae, and croup in infants, with those between 2 and 6 months of age at the highest risk, especially in low-income countries (7, 8).
Vaccination is among the most cost-effective public health measures worldwide (9), and regions with high rates of infant morbidity and mortality like sub-Saharan Africa benefit from the implementation of the Expanded Program of Immunization (EPI) (10). Nevertheless, acquisition of immunity from vaccination is not immediate and vaccines are not available for all infectious diseases. At present, only three vaccines are being administered at birth in some countries: Bacillus Calmette-Guérin (BCG), hepatitis B virus (HBV), and oral polio vaccine (OPV) (11–13). Therefore, newborns mostly rely on the protection elicited by maternal antibodies transferred across the placenta, which provide passive immunity against common pathogens (14).
Transplacental transfer of antibodies occurs in utero and it is facilitated by neonatal fragment crystallizable (Fc) region receptor (FcRn), expressed in the human syncytiotrophoblast (15, 16). Only IgG is transferred across the placenta with the highest rate occurring during the third trimester of pregnancy (17), although some studies suggest that maternal IgE may also be transferred to the fetus as IgG/IgE complexes (18). IgG subclasses have different affinities for the FcRn receptor leading to differences in the efficiency of transfer (19); classically it was stated that the greatest transport occurs for IgG1, followed by IgG4, IgG3, and finally IgG2 (20), although a recent update on transplacental transfer of IgG subclasses showed that it is different depending on the antigen and study populations (21).
To be effective, the transferred IgG must reach protective levels after birth. Maternal immunization is a valuable strategy to prevent newborn infections, ensuring a sufficient transfer of protective antibodies to the neonate (22, 23). Maternal vaccination against tetanus, pertussis, and influenza has been implemented in many populations and has been effective at protecting young infants from these pathogens (24–26), and could be used to protect newborns from RSV (27). However, it has been reported that IgG placental transfer and cord levels could be affected by some factors such as maternal antibody concentrations, gestational age, placental integrity, maternal infections, Fc binding strength, and the antigen specificity (16, 28–32), although the effects are not consistent among studies. Placental malaria (PM) has been shown to reduce transplacental transfer of antibodies against tetanus, measles, Streptococcus pneumoniae (S. pneumoniae), herpes simplex virus type 1 (HSV-1), RSV and varicella-zoster virus (VZV) (28, 33–35). However, other studies have shown no impact of PM on transplacental transfer of tetanus, S. pneumoniae, Haemophilus influenzae type b (Hib), diphtheria, measles, or RSV antibodies (16, 33, 35–38). The effect of maternal HIV infection is also controversial. Some studies demonstrated that HIV infection leads to a reduction of the transplacental transfer of Hib, diphtheria, pertussis, pneumococcus, measles, tetanus, and Plasmodium falciparum (P. falciparum) specific antibodies (28, 29, 39–45), but others have shown no effect (29, 33, 38, 41, 42, 46). Those studies had several limitations, as the numbers of antigens tested and HIV-infected women included in the analyses were low. Moreover, the information regarding IgG subclasses is scarce (41, 45, 46). Therefore, the effect of HIV and malaria on transplacental transfer of antibodies, particularly IgG subclasses, is still not clear.
In our study, we aimed to assess the impact of different factors, including maternal HIV infection and malaria in pregnancy on the placental transfer and cord levels of IgG and IgG subclasses to a broad range of highly prevalent microbial and vaccine antigens in a sub-Saharan African country, including: Corynebacterium diphtheriae (C. diphtheriae), Clostridium tetani (C. tetani), Bordetella pertussis (B. pertussis), Hib, S. pneumoniae, Shigella dysenteriae (S. dystenteriae), Vibrio cholerae (V. cholerae), hepatitis B virus (HBV), measles, RSV, Cryptosporidium parvum (C. parvum), Giardia intestinalis (G. intestinalis), and P. falciparum. A better understanding of factors affecting cord IgG levels will help with designing better preventive measures and strategies for maternal and child health.
Materials and Methods
Study Design and Sample Collection
A total of 197 HIV-uninfected and 144 HIV-infected women were recruited among those participating in two clinical trials of antimalarial intermittent preventive treatment in pregnancy (IPTp, ClinicalTrialGov NCT00811421 for both) (Supplementary Figure 1) in the Manhiça District, Southern Mozambique (47, 48), between May 2011 and September 2012, to perform an immunology ancillary study. The first clinical trial evaluated mefloquine (MQ) as an alternative IPTp drug to sulfadoxine-pyrimethamine (SP) in HIV-uninfected pregnant women. The study arms were (1) SP, (2) single dose MQ (MQ full), and (3) split dose over 2 days MQ (MQ split). The second trial evaluated MQ as IPTp drug in HIV-infected pregnant women in whom SP is contraindicated and who received daily cotrimoxazole (CTX), and women received either three monthly doses of MQ or placebo. All women received bed nets treated with long-lasting insecticide and supplements of folic acid and ferrous sulfate. All women also received tetanus toxoid vaccination during pregnancy. At the time of the study, the intensity of malaria transmission was low/moderate (49). Antiretroviral therapy (ART) with daily monotherapy with zidovudine (AZT) was recommended when CD4+ T cell count was below <350 cells/μL and/or when women were in WHO HIV clinical stage III or IV (50). The number of women on ART regime was 116, of which 81 started before pregnancy and 35 at the start of the study recruitment. A total of 24 women were not in an ART regime.
At delivery, peripheral and cord blood samples from women were collected into sodium heparin and EDTA vacutainers to perform the antibody assays. Plasma samples from peripheral blood and cord blood were available for this study from 332 (195 HIV-uninfected and 137 HIV-infected) and 303 women (178 HIV-uninfected and 125 HIV-infected), respectively. There were 294 mother-cord paired samples. The extraction of the maternal blood was done before delivery, when women were admitted to the hospital.
For the detection of P. falciparum species, thick and thin blood smears were assessed according to standard procedures (47, 48). Fifty microliter of maternal peripheral, placental, and cord blood samples were collected on Whatman 903™ filter paper during two visits before delivery (one during second trimester and the other during third trimester) and at delivery. Real-time quantitative polymerase-chain-reaction (qPCR) assay targeting the 18S ribosomal RNA was performed (51). Tissue samples from the maternal side of the placenta (decidua) were also collected for the assessment of placental malaria. Microscopy data of peripheral and placental blood smears at delivery were available for 308 and 340 women, respectively. Peripheral and placental blood qPCR data were available for 242 and 236 women, respectively.
Antibody Assays
Quantitative suspension array technology (qSAT) assays applying the xMAP™ technology (Luminex Corp., TX) were used to measure antigen-specific IgG, IgG1, IgG2, IgG3, and IgG4 responses to vaccine and pathogen antigens. A total of 16 recombinant proteins were selected for the analysis: diphtheria toxoid (Corynebacterium diphtheriae, Alpha Diagnostic DTOX15-N-500), tetanus toxin (Clostridium tetani, Santa Cruz SC222347), pertussis toxin (Bordetella pertussis, Santa Cruz SC200837), Hib Oligosaccharide (BEI Resources NR12268), pneumococcal surface protein A (PspA, Streptococcus pneumoniae, BEI Resources NR33179), shiga toxin (Shigella dysenteriae, BEI Resources NR4676), anti-O-specific polysaccharide (OSP, Vibrio cholerae, Massachusetts General Hospital, MA, USA) (52), hepatitis B surface antigen (HBsAg, Abcam ab91276), hemagglutinin (measles, Alpha Diagnostic RP655), viral protein 6 (VP6, rotavirus, Friedzgerald 80-1389), F protein (respiratory syncytial virus, BEI Resources NR31097), 17-kDA surface antigen (Cp17, Cryptosporidium parvum, Centers for Disease Control and Prevention, GA, USA) (53), variant-specific surface protein 5 (VSP5, Giardia intestinalis) (53), 42 kDA fragment of merozoite surface protein 1 (MSP142, P. falciparum, WRAIR) (54), merozoite surface protein 2 (MSP2, P. falciparum, University of Edinburgh) (55), and exported protein 1 (EXP1, P. falciparum, Sanaria) (56). MSP142 antigen was selected for representing P. falciparum. Eight recombinant proteins represented the most prevalent pathogens circulating in the study area (57–59) and six were from the vaccines administrated to the infants through the EPI in Mozambique (60).
qSAT assays were previously standardized and optimized to control for sources of variability (61–63). Briefly, antigens covalently coupled to MagPlex beads were added to a 96-well μClear® flat bottom plate (Greiner Bio-One) in multiplex resuspended in 50 μL of PBS, 1% BSA, 0.05% Azide pH 7.4 (PBS-BN). Fifty microliter of test samples, negative or positive controls (64) were added to multiplex wells and incubated overnight at 4°C protected from light. After incubation, plates were washed three times with PBS-Tween 20 0.05%, and 100 μL of anti-human IgG (Sigma B1140), anti-human IgG1 (Abcam ab99775), anti-human IgG2 (Invitrogen MA1-34755), anti-human IgG3 (Sigma B3523) or anti-human IgG4 (Invitrogen MA5-16716), each at their corresponding dilution, were added and incubated for 45 min. Then, plates were washed three times more and 100 μL of streptavidin-R-phycoerythrin (Sigma 42250) at the appropriate dilution were added to all wells and incubated 30 min for IgG, IgG1, and IgG3. For IgG2 and IgG4, 100 μL of anti-mouse IgG (Fc-specific)–biotin (Merck B7401) were added and incubated for 45 min, followed by another washing cycle and the incubation with streptavidin-R-phycoerythrin for 30 min. Finally, plates were washed and beads resuspended in 100 μL/well of PBS-BN. Plates were read using the Luminex 100/200 analyzer, and at least 20 microspheres per analyte were acquired per sample. Antibody levels were measured as median fluorescence intensity (MFI). Data were captured using xPonent software.
Test samples were assayed at 2 dilutions for IgG (1/250 and 1/10,000), and IgG1 and IgG3 (1/100 and1/2,500). For IgG2 and IgG4 only 1 dilution was tested (1/50) because of their usual low levels. Twelve serial dilutions (1:3, starting at 1/25) of a positive control (WHO Reference Reagent for anti-malaria P. falciparum human serum, NIBSC code: 10/198) were used for QA/QC and to select an optimal sample dilution for data analysis. Two blanks were also added to each plate for quality control purposes. Sample distribution across plates was designed to ensure a balanced distribution of groups. Single replicates of the assay were performed.
Statistical Analysis
To stabilize the variance, the analysis was done on log10-transformed values of the MFI measurements. A positive control curve was used to select the sample dilution for each antigen-isotype/subclass-plate. The dilution nearest to the midpoint between the two positive control curve serial dilutions ranging the maximum slope was chosen. Plates were normalized using the positive control curve in each plate and the average positive control curve from all plates. The MFI values of samples were multiplied by the corresponding normalization factor (MFI value of the chosen dilution from the average positive control curve divided by the MFI value of same dilution in the plate curve).
The Shapiro-Wilk test of normality confirmed that most of the antibody data were not normally distributed. The Chi-square and the non-parametric Wilcoxon-Mann-Whitney tests were used to compare categorical and continuous variables, respectively, between HIV-infected and HIV-uninfected women. Comparisons of crude Ig levels across antigens and subclasses between HIV exposure groups were assessed by Wilcoxon-Mann-Whitney tests, and between ART groups, were assessed by Kruskal-Wallis and Dunn's tests. Kruskal-Wallis and Dunn's tests were also performed in order to compare IgG subclass placental transfer for each antigen, represented as the cord/mother ratios. Univariable linear regression models were fit to determine the effect of variables on the cord blood antibody levels (log10) or the cord blood/mother ratio (log10) (Supplementary Material 1). The variables considered in this analysis were log10 maternal antibody levels, maternal HIV infection, P. falciparum exposure, PM (acute, defined by the presence of parasites on sections without malaria pigment; chronic, by presence of parasites and pigment; or past, by the presence of pigment alone), age, gravidity (defined as primigravidae and multigravidae), maternal anemia (defined as hemoglobin level <11 g/dL), low birth weight (defined as <2,500 g at birth), prematurity (defined as delivery before 37 weeks of gestational age), gestational age [measured by Ballard score (65)], treatment (defined as MQ or placebo in the HIV-infected women ancillary study and MQ full, MQ split or SP in HIV-uninfected women ancillary study), ART received before pregnancy or at recruitment, CD4+ T cell counts (<350 or ≥350 cells/μL), HIV viral load (<400, 400–999, 1,000–9,999, and >9,999 copies/mL), and seasonality (dry or rainy). Exposure to P. falciparum was computed as the sum of the maternal IgG antibody levels (MFI) for the following immunogenic P. falciparum antigens: MSP142, MSP2, and EXP1, as antibody levels to these antigens have been shown to reflect exposure to malaria (66, 67). Seasonality was computed for each woman based on the pregnancy period—if at least four of the pregnancy months fell under the category of rainy period (November through April), the season was defined as such. In any other case, the season was defined as dry. A base multivariable model including maternal antibody levels, maternal HIV infection and P. falciparum exposure was established for each antigen and IgG or IgG subclass. Base model for MSP142 did not include P. falciparum exposure as this variable includes antibodies to this antigen. We performed additional regression models testing exhaustively all possible combinations of predictor variables (added to our base model) and selected the models based on the Akaike information criterion (AIC), Bayesian information criterion (BIC) and adjusted r-square parameters. All p-values were considered statistically significant when <0.05 after adjusting for multiple testing through Benjamini-Hochberg. All data collected were pre-processed, managed, and analyzed using the R software version 3.6.3 and its package devtools (68). The ggplot2 package was used to perform boxplot graphs (69). The FactoMineR and factoextra packages were used to perform Principal Component Analysis (PCA) (70, 71).
Results
Description of Participants
A total of 341 women (197 HIV-uninfected and 144 HIV-infected) participated in the study (Table 1). HIV-infected women were older than the HIV-uninfected and there were more primigravidae among the HIV-uninfected. HIV-infected women had significantly more anemia than the HIV-uninfected. There were no significant differences in birth weight or prematurity between infants born to HIV-infected and those born to HIV-uninfected women. Among the 155 infants born from HIV-infected women, eight tested HIV-positive at 6 weeks of age by polymerase chain reaction (PCR) analysis performed following national guidelines. Placental histology was performed on 307 samples from study participants, of which three had acute PM and eight past PM. In total, 20 women had PM (positive in placental blood, by microscopy or PCR at delivery, or acute or past PM by histology), but there were no differences by HIV infection. Peripheral malaria (positive in peripheral blood by microscopy or PCR at any of the visits during pregnancy) was detected in 51 women, but there were no differences by HIV infection. Finally, P. falciparum exposure was lower among HIV-infected women.
Profile of Antibody Levels in Cord Blood
PCA of the cord antibody levels and maternal antibody levels separately, including IgG, IgG1, IgG2, IgG3, and IgG4 to all antigens tested, were performed to reduce the dimensionality of the data and get insights into the overall antibody patterns. Cord and maternal PCA looked very similar (data not shown). Cord antibody responses clearly clustered by IgG subclasses (Figure 1A). The distribution of the IgG subclass clusters across the PCA dimensions was influenced by the antigens. Specifically, IgG and IgG1 clusters were closer, showing similar responses, whereas IgG4 and IgG3 were the most distant. Cord antibody responses also clustered by antigens (Figure 1B). Hib cluster was clearly separated from the rest, indicating a different antibody profile (Figure 1B). Overall, PCA results suggested different antibody profiles depending on the antigen specificity. Consistently, median IgG and IgG1 levels were higher than the rest of IgG subclasses and were both similar between them for most of the antigens with the exception of Hib (Figure 1C). IgG2 had lower median levels than IgG1, followed by IgG3 and the lowest levels were shown for IgG4.
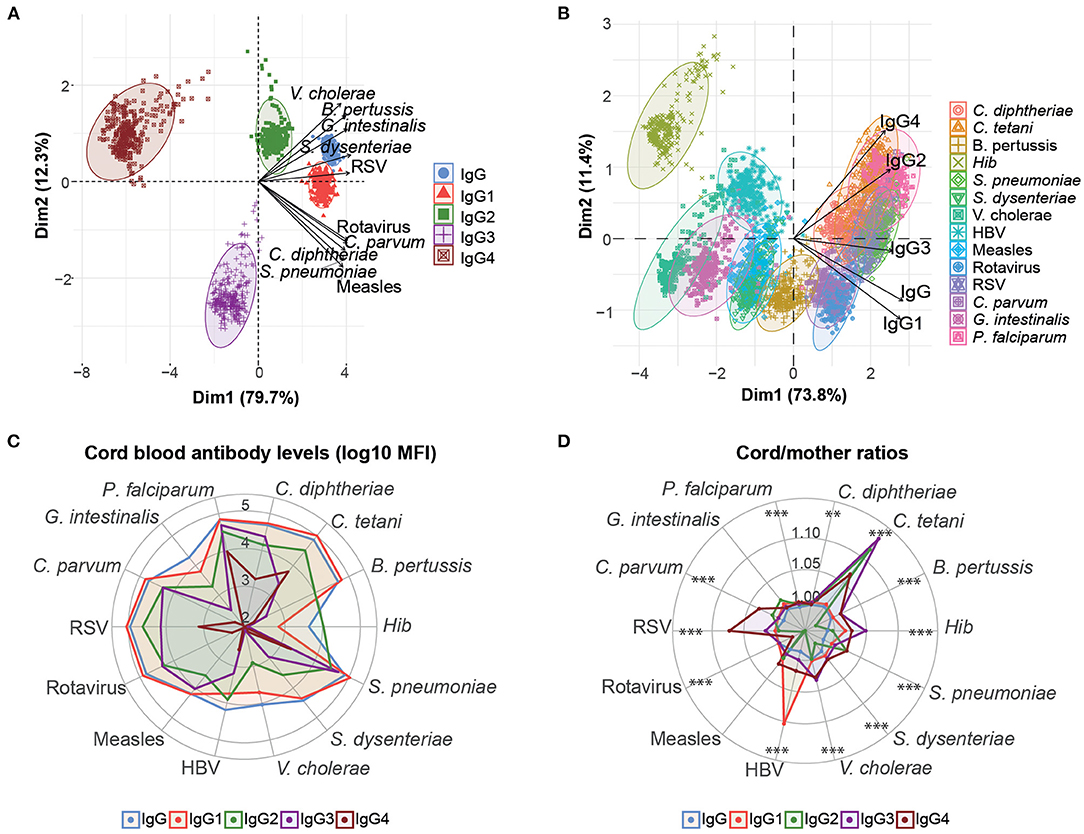
Figure 1. Overview of cord blood levels of IgG and IgG subclasses for all women. Principal component analysis (PCA) plots of cord IgG and IgG subclass levels against all antigens clustered by subclass type (A) and antigen type (B). The two principal components (Dim 1, Dim2) that explained the highest percentage of the variance of the data (percentage in parenthesis) were chosen for representation. The arrows in (A) and (B) represent how the variables contribute to each of the two principal components. (C) Medians of IgG and IgG subclass levels (log10 MFI) in cord blood for each antigen. (D) Medians of IgG and IgG subclass placental transfer for each antigen, represented as the cord/mother ratios. Medians of IgG subclass placental transfer for each antigen were compared by Kruskal-Wallis test and p-values were adjusted for multiple testing by the Benjamini-Hochberg approach (False Discovery Rate 5%). ***p < 0.001, **p < 0.01.
We also determined the placental transfer of antibodies, measured as the ratio of cord blood levels to the maternal levels. The efficiency of placental transfer was different depending on the IgG subclass in all antigens with the exception of measles and G. intestinalis (Figure 1D). The transfer efficiency was greatest for IgG1, IgG3, or IgG4 depending on the antigen. IgG1 placental transfer was significantly higher for C. diphtheriae, P. falciparum, HBV, and rotavirus. IgG3 placental transfer was significantly higher for B. pertussis, C. tetani, Hib, and V. cholerae. IgG4 placental transfer was significantly greater for C. parvum, S. dysenteriae, and RSV. The least efficiently transferred subclass was IgG2 for most of the antigens, with the exception of S. pneumoniae for which IgG2 and IgG4 were the greatest.
Altered Maternal and Cord Blood Antibody Levels in HIV-Infected Women
We first compared total IgG levels and IgG subclasses in maternal and cord blood from HIV-infected and HIV-uninfected women. Significant differences in maternal antibody levels were only detected for C. tetani (IgG and IgG1), S. pneunomiae and RSV (IgG2), and C. diphtheriae and P. falciparum (IgG4), with lower antibody levels in HIV-infected compared to HIV-uninfected women (Figure 2). In contrast, higher G. intestinalis and HBV IgG levels were found in HIV-infected women (Figure 2A).
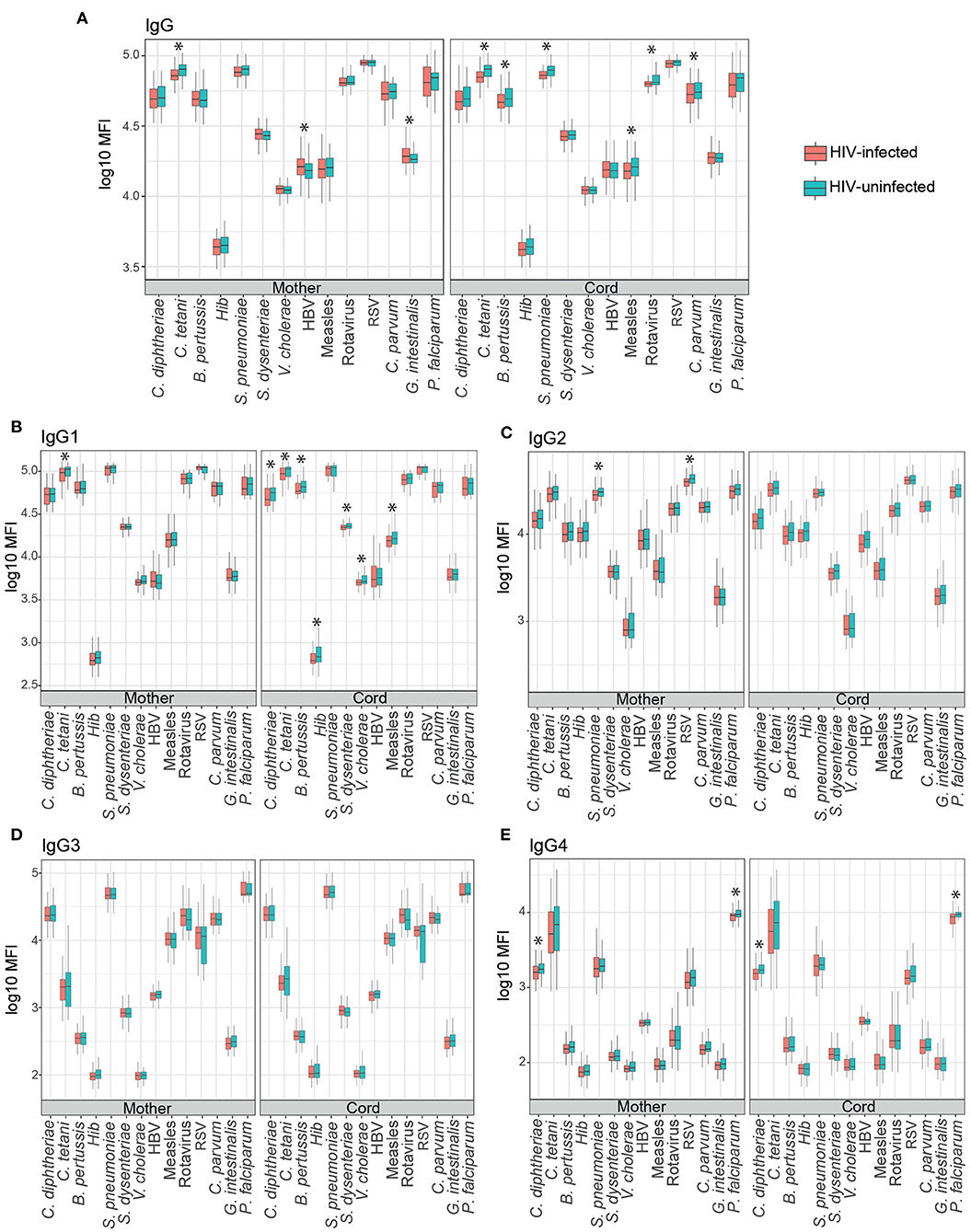
Figure 2. Mother and cord blood antibody levels (log10 MFI) in HIV-positive and HIV-negative women. Boxplots illustrate the medians and the interquartile range for IgG (A), IgG1 (B), IgG2 (C), IgG3 (D), and IgG4 (E) subclasses in HIV-positive (red) and HIV-negative women (blue). Levels between groups were compared by Wilcoxon-Mann–Whitney test and p-values were adjusted for multiple testing by the Benjamini-Hochberg approach (False Discovery Rate 5%). Statistically significant differences are highlighted with an asterisk.
Total IgG cord blood levels were lower in HIV-infected than HIV-uninfected women for C. tetani, B. pertussis, S. pneumoniae, measles, rotavirus, and C. parvum. Similarly, HIV-infected women had lower IgG1 cord levels for C. diphtheriae, C. tetani, B. pertussis, Hib, S. dysenteriae, V. cholerae, and measles for IgG1 (Figure 2B). No differences were observed between groups for IgG2 and IgG3 (Figures 2C,D), whereas lower C. diphtheriae and P. falciparum IgG4 levels were also found in cord blood of HIV-infected than HIV-uninfected women (Figure 2E).
We also compared cord blood levels of IgG and IgG subclasses between HIV-infected women taking ART before pregnancy, at recruitment or without ART regime during pregnancy. The only significant differences were observed for S. dysenteriae and RSV IgG4 (Supplementary Figure 2E). HIV-infected women taking ART before pregnancy had higher cord blood levels of IgG4 against S. dysenteriae and RSV than those women taking no ART (p < 0.05 in Dunn's test adjusted by Benjamini-Hochberg, False Discovery Rate 5%), but no differences were found among women taking ART at recruitment than those not taking ART. Similarly, no differences were found between women taking ART before pregnancy or at recruitment.
Multivariable models were also performed to assess the factors associated with cord blood levels of total IgG and IgG subclasses. Maternal antibodies, HIV infection and P. falciparum exposure were included in the models. Maternal antibody levels had the strongest positive correlation with cord antibody levels for all the antigens and subclasses (Figure 3A). However, the effect of maternal antibody levels was more variable for IgG3-4 than for total IgG and IgG1 subclass. A 10% increase in maternal total IgG levels was associated with increases from 8.1 to 9.7% in total IgG cord blood levels, depending on the antigen. For IgG subclasses, a 10% increase in maternal antibody levels was associated with increases of cord blood levels from 7.6 to 10.9% for IgG1, 5.4 to 9.6% for IgG2, 5 to 9.9% for IgG3, and 5.3 to 9.3% for IgG4.
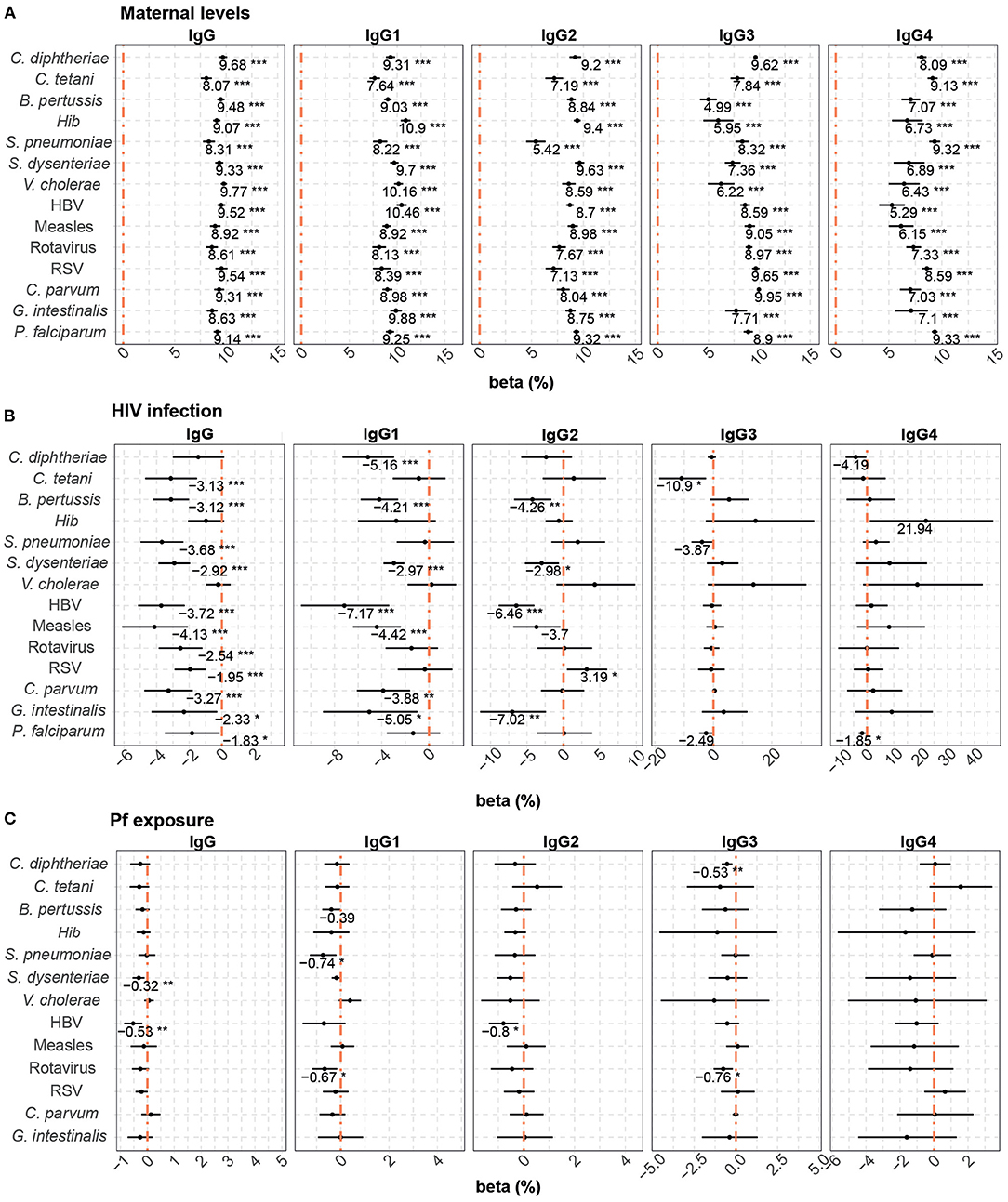
Figure 3. Difference of IgG and IgG subclass levels in cord blood by study factors. Forest plots show the effect (in percentage) of maternal antibody levels (A), HIV infection (B) and P. falciparum exposure (Pf exposure) (C) on cord blood levels of IgG and IgG subclasses for all the antigens tested. The differences in percentage correspond to beta-transformed values (%) calculated from the beta values obtained in the multivariable models. Beta transformed values (%) are displayed when raw p-values are significant. Asterisks are shown when adjusted p-values by Benjamini-Hochberg are significant (False Discovery Rate 5%). ***p < 0.001, **p < 0.01, *p < 0.05.
Maternal HIV infection (Figure 3B) had a negative effect on total IgG cord blood levels to all antigens, except for C. diphtheriae, Hib, and V. cholerae. HIV infection was associated with a reduction ranging from 1.83 to 4.13% in the IgG cord blood levels. For IgG1, HIV infection negatively impacted cord blood levels against C. diphtheriae, B. pertussis, S. dysenteriae, HBV, measles, C. parvum, and G. intestinalis (from 2.97 to 7.17% reduction). For IgG2, a reduction was observed against B. pertussis, S. dysenteriae, HBV, and G. intestinalis (from 2.98 to 7.02% reduction), whereas HIV was associated with an increase of 3.19% of IgG2 to RSV. Finally, we only detected a negative effect of HIV infection on IgG3 levels to C. tetani (10.90% reduction) and IgG4 to P. falciparum (1.85% reduction).
P. falciparum exposure was negatively associated with cord blood IgG levels against S. dysenteriae and HBV, IgG1 against S. pneumoniae and rotavirus, IgG2 against HBV and IgG3 against C. diphtheriae and rotavirus (Figure 3C). Depending on the IgG sublcass and antigen, 10% increase in P. falciparum exposure reduced the cord blood levels ranging from 0.32 to 0.80%.
Previous studies suggest that PM rather than peripheral malaria may affect cord blood levels and transplacental transfer of antibodies and lead to adverse outcomes due to the damaged placental tissue (37, 72, 73). Therefore, we explored the effect of PM on cord blood levels in multivariable models without P. falciparum exposure despite the low number of women with any evidence of PM. PM had no significant associations with antibody cord blood levels (data not shown). When analyzing HIV-infected women only, PM was associated with lower B. pertussis IgG1, C. diphtheriae IgG2 and HBV IgG3 levels in cord blood (Supplementary Figure 3).
Prematurity, previously shown to have a detrimental effect on placental transfer of antibodies (74), was added to the multivariable model, which included the variables maternal antibody levels, HIV infection and P. falciparum exposure, as it increased the quality (AIC) of some of the models. Prematurity (Figure 4) was associated with lower cord blood total IgG levels against Hib (4.36% reduction compared with term cord blood levels), V. cholerae (2.37% reduction), measles (5.83% reduction), and C. parvum (3.53% reduction without statistical significance after adjusting for multiple testing).
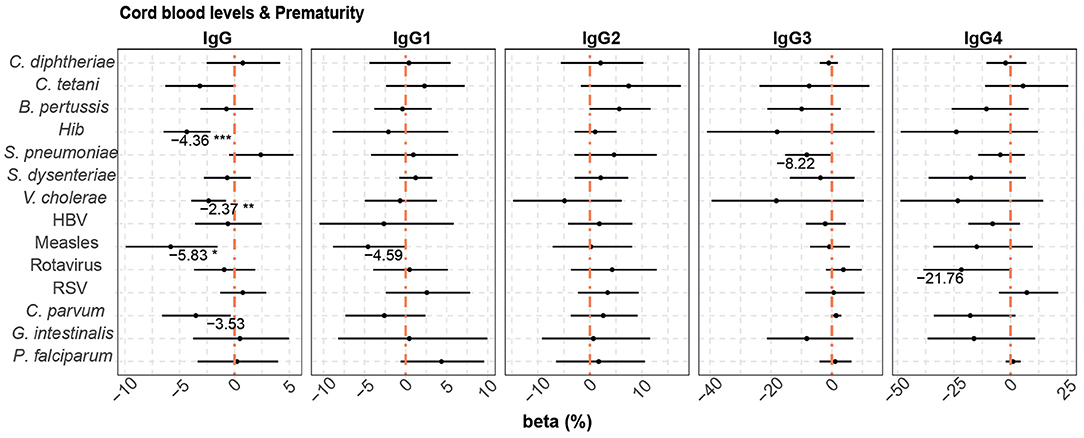
Figure 4. Difference of IgG and IgG subclass levels in cord blood by prematurity. Forest plots show the effect (in percentage) of prematurity on cord blood levels of IgG and IgG subclasses, for all the antigens tested. The differences in percentage correspond to beta-transformed values (%) that were calculated from the beta values in the multivariable models. This beta value was obtained when prematurity was included in multivariable models with maternal antibody levels, HIV infection and P. falciparum exposure. Beta transformed values (%) are displayed when raw p-values are significant. Asterisks are shown when adjusted p-values by Benjamini-Hochberg are significant (False Discovery Rate 5%). ***p < 0.001, **p < 0.01, *p < 0.05.
The rest of the variables (age, maternal anemia, gravidity, low birth weight, IPTp treatment, seasonality; and CD4+ T cell counts, ART and viral load for HIV-infected women) did not provide any added value to the multivariable models and were not included.
Altered Placental Transfer of Antibodies in HIV-Infected Women
Placental transfer of total IgG and IgG1 was significantly lower in HIV-infected women for all antigens except for Hib and V. cholerae (total IgG) and C. tetani, S. pneumoniae, V. cholera, and RSV (IgG1) (Figures 5A,B). For IgG2, only G. intestinalis, B. pertussis, and HBV had significantly lower transfer in HIV-infected women, while S. pneumoniae and RSV had higher transfer in HIV-infected women (Figure 5C). For IgG3, only C. tetani had a significantly lower transfer in HIV-infected compared to HIV-uninfected women (Figure 5D). No significant differences in placental transfer between the two groups were found for IgG4 (Figure 5E).
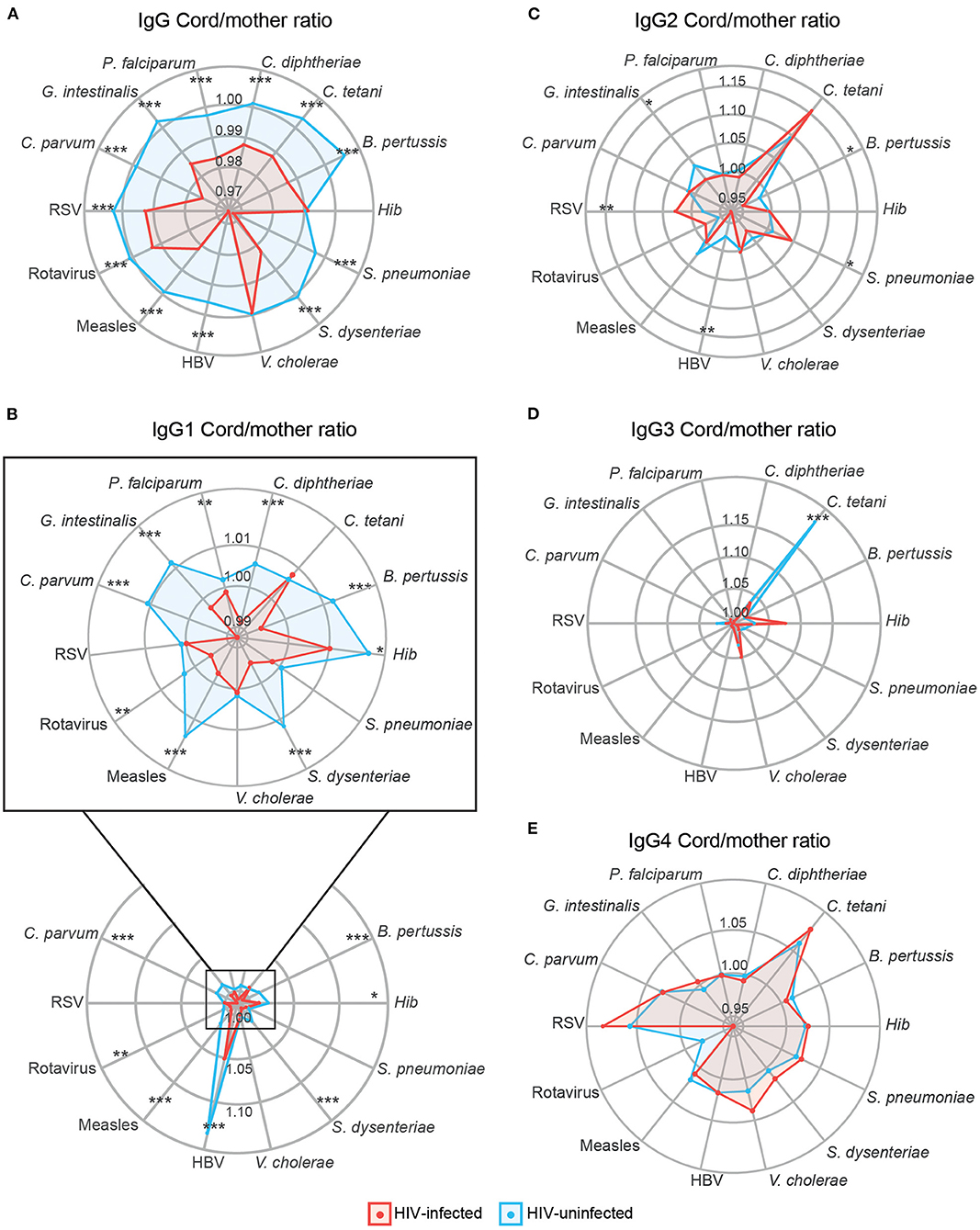
Figure 5. Antibody placental transfer in HIV-positive and HIV-negative women. Radar charts representing the medians of each antigen antibody cord/mother ratio in HIV-positive and HIV-negative women for IgG (A), IgG1 subclass (B), IgG2 (C), IgG3 (D), and IgG4 (E). Ratios between HIV-positive and negative women were compared by Wilcoxon-Mann-Whitney test and p-values were adjusted for multiple testing by the Benjamini-Hochberg approach (False Discovery Rate 5%). Statistically significant differences between HIV-positive and negative women ratios are highlighted with an asterisk. ***p < 0.001, **p < 0.01, *p < 0.05. HIV-positive women are represented in red and HIV-negative women in blue.
We compared placental transfer of IgG and IgG subclasses between HIV-infected women taking ART before pregnancy, at recruitment or without ART regime. No significant differences were found, with the exception of C. parvum IgG4 (Supplementary Figure 4E), for which HIV-infected women taking ART before pregnancy or at recruitment had higher placental transfer than those not taking ART (p < 0.05 in Dunn's test adjusted by Benjamini-Hochberg, False Discovery Rate 5%). There were no differences between taking ART before pregnancy or at recruitment in the C. parvum IgG4 placental transfer.
In multivariable models including HIV infection and P. falciparum exposure, HIV infection (Figure 6A) in general was associated with reduced placental transfer of total IgG and IgG1 (from 1.99 to 6.75% reduction depending on the antigen). HIV infection was also associated with a reduced transfer of IgG2 against B. pertussis, HBV, and G. intestinalis, but an increase in IgG2 RSV transfer (5.42% increase). Although adjusted p-values were not significant, a similar trend of positive correlation was found for S. pneumoniae IgG2 and Hib and V. cholerae IgG3 and IgG4.
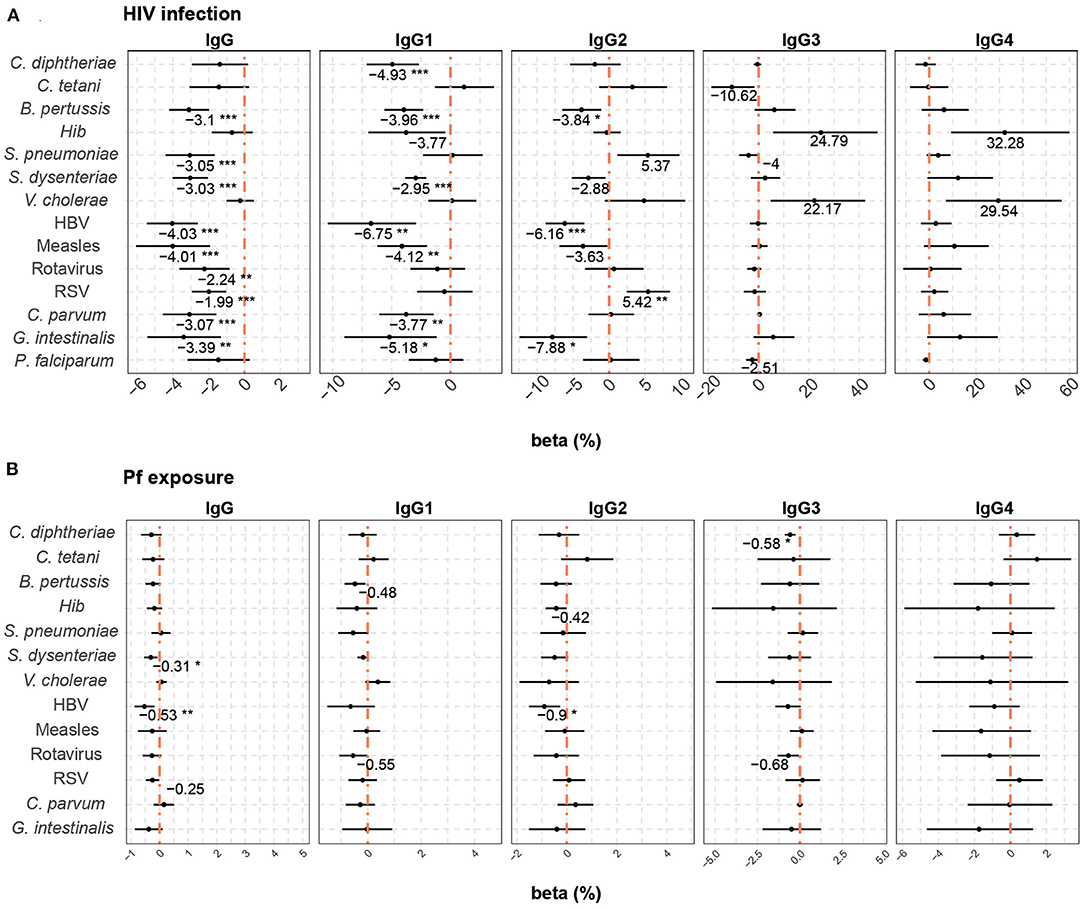
Figure 6. Difference of IgG and IgG subclass placental transfer by study factors. Forest plots show the effect (in percentage) of HIV infection (A) and P. falciparum exposure (Pf exposure) (B) on transplacental transfer of IgG and IgG subclasses for all the antigens tested. The differences in percentage correspond to beta-transformed values (%) that were calculated from the beta values obtained in the multivariable models. Beta transformed values (%) are displayed when raw p-values are significant. Asterisks are shown when adjusted p-values by Benjamini-Hochberg are significant (False Discovery Rate 5%). ***p < 0.001, **p < 0.01, *p < 0.05.
P. falciparum exposure (Figure 6B) had a negative effect on the placental transfer of antibodies for some antigens. An increase of 10% in P. falciparum exposure reduced the placental transfer of total IgG against S. dysenteriae and HBV by 0.31 and 0.53%, respectively, IgG2 against HBV by 0.90% and IgG3 against C. diphtheriae by 0.58%.
PM, in contrast to P. falciparum exposure, did not have any impact on transplacental transfer of antibodies in exploratory analyses and did not improve any of the models, although it had similar correlation trends for total IgG. Nevertheless, PM was associated with a diminished placental transfer of IgG1 B. pertussis in HIV-infected women (Supplementary Figure 5).
When prematurity was added to the multivariable models that included HIV infection and P. falciparum exposure, this additional covariable had a negative effect on placental transfer of only Hib and V. cholerae total IgG antibodies (4.43 and 2.38% reduction in premature vs. term newborns, respectively) (Figure 7).
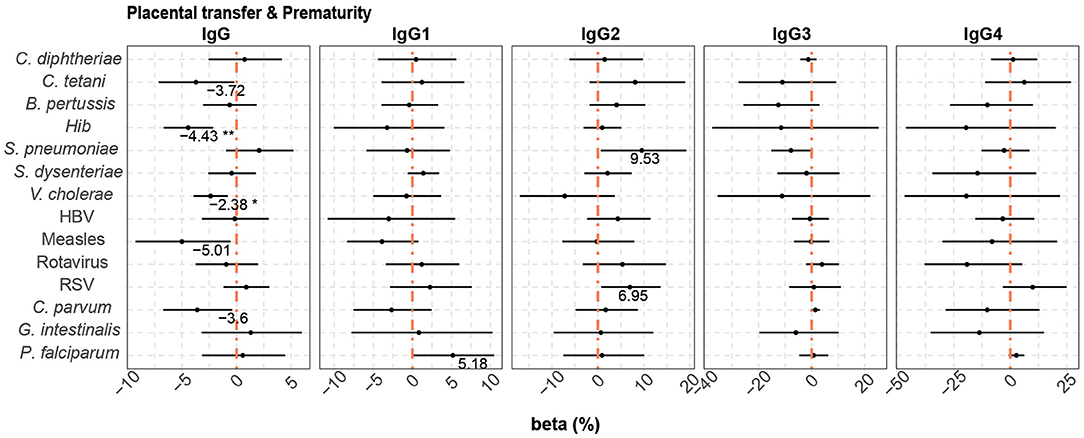
Figure 7. Difference of IgG and IgG subclass placental transfer by prematurity. Forest plots show the effect (in percentage) of prematurity on transplacental transfer of IgG and IgG subclasses, for all the antigens tested. The differences in percentage correspond to beta transformed values (%) that were calculated from the beta values in the multivariable models. This beta value was obtained when prematurity was included in multivariable models with HIV infection and P. falciparum exposure. Beta transformed values (%) are displayed when raw p-values are significant. Asterisks are shown when adjusted p-values by Benjamini-Hochberg are significant (False Discovery Rate 5%). **p < 0.01, *p < 0.05.
No additional variables were included in the multivariable analysis as they did not provide any added value to the model.
Discussion
Our comprehensive analysis of maternal and cord plasma total IgG and IgG subclasses against a wide range of microbial and vaccine antigens allowed a deep immunoprofiling, that is essential to decipher the mechanisms affecting antibody placental transfer and maternal and newborn immunity in women chronically exposed to pathogens. We confirmed that the main determinant of cord total IgG and IgG subclass levels are the corresponding maternal antibody levels, and that maternal HIV infection is associated with a reduction of total IgG levels in the cord due to low maternal levels, but also to a reduction of IgG and IgG1 placental transfer.
Maternal and cord blood antibody levels are usually correlated in many studies, suggesting that maternal levels are the main determinant for transfer efficiency (14, 75, 76). However, the effect of HIV infection on placental transfer is not consistent among studies and prior analyses of the effect of HIV on maternal and cord blood levels have mainly focused on total IgG (16, 28, 29, 33, 39, 41–43, 46). Our results showed that HIV infection reduced the total IgG maternal levels for some antigens, the cord blood levels overall, and also had a negative effect on transplacental transfer of total IgG antibodies. It is interesting that although we found higher maternal HBV and G. intestinalis IgG levels among HIV-infected women, cord blood levels and transplacental transfer were lower than in HIV-uninfected women. Higher maternal antibody levels against these pathogens in HIV-infected women may be due to an increased susceptibility to co-infections with these pathogens, as described before (77–79), but it seems that they are not being transferred as efficiently as in HIV-uninfected women. This could be due to hypergammaglobulinemia, demonstrated to be common among HIV-infected individuals (80) and previously shown to impair transplacental transfer of antibodies (14, 16, 35), or due to an impairment of the Fc receptors caused by maternal HIV infection (16).
Our results are consistent with previous studies reporting that HIV infection led to a reduction of the cord blood levels and transplacental transfer of total IgG against B. pertussis (29, 42), C. tetani (28, 29, 40, 42), S. pneumoniae (33, 40, 42, 44, 81), RSV (82, 83), and measles (39, 40). Some studies also found a negative effect on Hib (29, 81) that is not appreciated in our study, although we found reduced IgG1 levels in cord in univariable analyses. However, our results differ from other studies that did not find any effect of HIV status on IgG levels against C. diphtheriae (38), C. tetani (33, 38), S. pneumoniae (46, 83), HBV (38), and measles (33, 38). These discrepancies could be due to the different geographical areas, statistical methods used, small sample sizes in the previous studies, type of antigen tested and the serological method applied.
IgG subclasses may be elicited differently depending on the pathogen, the antigen or the epitope (84), and the differences in Fc region between IgG subclasses, which mediates effector functions, confers them different roles during infection and pathogen clearance (84–86). The efficiency of the antibody placental transfer varies for each subclass due to differential affinity of the receptors FcRn (19). We found that the efficacy of IgG placental transfer also depended on the antigen. IgG1, IgG3, or IgG4 transferred better than IgG2, except for S. pneumoniae, for which IgG2 transfer was significantly higher. This was unexpected as classically it has been described that the greatest transport occurs for IgG1, followed by IgG4, IgG3, and finally IgG2 (20), although a recent report showed a different hierarchy of subclass transfer and identified a number of other studies that also observed different transfer efficiencies, depending on the antigen tested (21). However, IgG1 levels were the highest for almost all antigens in cord blood, probably because of the overall higher levels of this IgG subclass in maternal blood. One exception was Hib that presented higher IgG2 cord levels than IgG1, although IgG1 transplacental transfer was higher than IgG2 consistently with previous studies (87). The mothers had an IgG2-predominant response to Hib, and consequently higher IgG2 than IgG1 levels were found in cord blood as previously described (88, 89).
In our multivariable models, we observed that HIV infection reduced mainly IgG1 cord levels due to a diminished transplacental transfer, similarly to IgG. Interestingly, maternal HIV infection increased the placental transfer of IgG2 to RSV and had a positive effect on RSV IgG2 cord blood levels, although IgG2 maternal levels were lower in HIV-infected women. To our knowledge, an increased placental transfer by HIV infection has not been described before. This may have implications for maternal immunization with RSV vaccines under development. Natural RSV infection seems to elicit an age-dependent IgG1, IgG2, and IgG3 response against the F protein, the major target of the host's immune response (90, 91), and of some RSV vaccines (92). Antibodies binding to the F protein are protective (93), and IgG1 is the IgG subclass mainly produced in response to RSV infection (94, 95) and an IgG1 monoclonal antibody against RSV F protein with neutralizing function, has shown to be effective (96), suggesting that the protective response is predominantly IgG1. Here, we found that total IgG and IgG1 against RSV F protein had the highest levels in cord blood compared to other subclasses, but HIV infection reduced total IgG cord blood levels and placental transfer. Therefore, HIV infection could compromise the levels of RSV neutralizing antibodies transferred to the newborn and, consequently, diminish the effectivity of an RSV vaccine.
Regarding other variables, our data did not show any significant association between CD4+ T cell counts or HIV viral load on cord blood levels and transplacental transfer of antibodies. Even though these results agree with previous studies that did not find any associations (29, 81, 97), other reports describe that lower CD4+ T cell counts and higher HIV viral load lead to a reduction of the transfer of some pathogen-specific antibodies and vaccines such as measles and S. pneumoniae (37, 43, 98). Some studies describe that HIV-infected women receiving ART transferred higher pathogen-specific antibodies than those who were not under ART (97) or who initiated it during pregnancy (99). However, in our cohort we did not find any significant associations in regards to ART. The discrepancies between studies could be explained by the different ART drug regime type.
At the time of the study, malaria transmission intensity was very low in the area and only a few women had active malaria during pregnancy. Nonetheless, we found a negative correlation between P. falciparum exposure and both placental transfer and cord blood antibody levels for some antigens and IgG subclasses. Previous studies are contradictory, as some found that PM led to a reduction of the transplacental transfer of some pathogen-specific IgG to C. tetani (34), measles (35, 39), RSV (37) and S. pneumoniae (33), but others did not find any effect for IgG against C. diphtheriae (37, 38), C. tetani (28, 35, 38), Hib (37), HBV (38), measles (38) RSV (36), and S. pneumoniae (37). Discrepancies between studies could be due to differences in study sites, prevalence of malaria, sample sizes, type of antigens, sensitivities of serological methods, exposure to the pathogens tested, and other co-infections. We also found that P. falciparum exposure was lower among HIV-infected women. Although all HIV-infected women were on prophylactic treatment with CTX, a broad-spectrum antibiotic that has been suggested to be protective against malaria (100, 101), HIV-negative women were also on IPTp with SP or MQ that has been demonstrated to be effective (47). Thus, this lower exposure could be more related to the fact that exposure to P. falciparum was computed as the sum of the maternal IgG antibody levels against three immunogenic P. falciparum antigens, and maternal HIV infection could reduce the IgG levels against them.
We found prematurity to be associated with lower cord blood IgG levels and placental transfer for some antigens, as previous studies have shown (74, 102, 103), although the effect was not consistent among subclasses. It has already been reported that the greatest transport occurs in the third trimester of gestation (17), and due to this fact, preterm infants may have lower amounts of transplacental IgG than term infants (74, 103, 104).
Our results are important for maternal immunization implementation in settings with a high prevalence of HIV infection. Here, the only vaccine given during pregnancy was tetanus. Although HIV infection was associated with lower maternal and cord blood tetanus toxoid IgG and IgG1 levels in univariable models, HIV did not affect cord blood IgG1 levels in multivariable models adjusted by maternal levels. Systemic tetanus vaccination during pregnancy has been implemented in Africa and has demonstrated a high efficacy (105). Pertussis vaccination in pregnancy has also been implemented in some countries such as Canada, US, and UK (106, 107), but not in Africa. Acellular pertussis vaccine induces mainly IgG and IgG1 responses that are thought to confer protection (108–110). We found lower cord blood levels and a reduced placental transfer of IgG and IgG1 against B. pertussis among HIV-infected women and those exposed to P. falciparum. These results highlight the need for further studies assessing the impact of these infections on pertussis vaccine efficacy and antibody placental transfer when implemented in pregnant women from African countries.
As study limitations, we could not establish the threshold of protection of antibody levels, therefore it is difficult to infer the clinical relevance of the reductions in antibodies detected in cord blood from the HIV-infected women. We could not measure hypergammaglobulinemia, which has been associated with a reduced transplacental transfer of antibodies (35–37), and demonstrated to be induced by chronic infections such as HIV, malaria or helminthiasis (80, 111, 112). Also, diabetes may have an impact on placental transfer of antibodies (31), but this diagnosis was not available in our cohort. Another limitation is that this study was only performed in a Mozambican cohort, therefore results obtained may not be representative of other populations. Specifically, IgG allotype diversity, or ethnic/geographic differences in FcRn, may impact the effect of HIV and malaria on placental transfer. As HIV-infected mothers also receive CTX, it could potentially limit antigen exposure and, therefore, antibody responses. Finally, we had to assess malaria exposure instead of PM due to the low number of PM cases and consequently our results are not comparable with those assessing PM.
In conclusion, our results demonstrate that maternal HIV infection was associated with reduced levels of antibodies against a broad range of pathogens and vaccine antigens in cord blood. Part of this reduction in antibody levels was due to altered antibody levels in the mother, which are the main determinants of cord blood levels, but HIV-infection also diminished transplacental transfer of antibodies. Importantly, IgG1 was the most affected by maternal HIV infection but, depending on the pathogen, other subclasses were also affected. P. falciparum exposure also reduced the levels and transfer of some antibodies, although overall the effect was lower than HIV infection. In future studies, the clinical impact of the reduced placental transfer of antibodies caused by HIV infection and P. falciparum exposure will be assessed. Our findings are important for effective maternal immunization strategies and for newborn and infant's health.
Data Availability Statement
The raw data supporting the conclusions of this article will be made available by the authors upon request.
Ethics Statement
The studies involving human participants were reviewed and approved by Comitè Ètic d'Investigació Clínica (CEIC, Hospital Clínic, UB), Spain, Comité Nacional de Bioética (CNBS), Mozambique. Written informed consent to participate in this study was provided by the participants' legal guardian/next of kin.
Author Contributions
SA, CD, and GM wrote the first draft of the manuscript, conceived the immunological study, the experimental design, and interpreted the data. CD, GM, RA, and SA designed the analysis and selection of the antigens. SA and MV performed the antibody Luminex assay. SA and MC produced antigens. EA provided the MSP142 protein. GR-O and MV-S performed the statistical analysis. AM, CD, and GM designed the immunology study ancillary to the clinical trials. MM, RB, and CJ processed the samples. PC and LF-S performed the PCR. RG, MR, JA, EM, AV, ES, and CM designed and enrolled participants in the clinical trials. AN was the clinical trial data manager. JA was the clinical trial statistician. RA, MC, RG, CM, GR-O, and MV-S contributed to the write up of the manuscript. All authors reviewed and approved the manuscript.
Funding
This study was supported by the European and Developing Countries Clinical Trials Partnership (EDCTP; IP.2007.31080.002), the Malaria in Pregnancy Consortium, which was funded by a grant from the Bill and Melinda Gates Foundation to the Liverpool School of Tropical Medicine, and the Instituto de Salud Carlos III (PI08/0564). The Centro de Investigacao em Saude da Manhica receives core support from the Spanish Agency for International Cooperation and Development (AECID). The study received funds from MINECO (SAF2008-00743, RYC-2008-02631 to CD), from Instituto de Salud Carlos III (PS09/01113, PI13/01478 to AM) and the Department d'Universitats I Recerca de la Generalitat de Catalunya (AGAUR; 2017SGR664 to AM, 2014SGR991 to CD). GM had the support of the Department of Health, Catalan Government (SLT006/17/00109). This research was part of the ISGlobal's Program on the Molecular Mechanisms of Malaria which is partially supported by the Fundación Ramón Areces and we acknowledge support from the Spanish Ministry of Science and Innovation through the Centro de Excelencia Severo Ochoa 2019–2023 Program (CEX2018-000806-S), and support from the Generalitat de Catalunya through the CERCA Program.
Conflict of Interest
The authors declare that the research was conducted in the absence of any commercial or financial relationships that could be construed as a potential conflict of interest.
Acknowledgments
We are grateful to the volunteers and their families; the clinical, field, and lab teams at the Manhiça Health Research Centre, particularly the lab personnel Bendita Zavale, Lázaro Quimice, Elias Matusse, Eugenio Mussa, and Edmundo José. Special thanks to Laura Puyol from ISGlobal for her logistic support and Robert Andrew Mitchell for proofreading and reviewing the manuscript. We thank Luis Izquierdo for the assessment of the protein expression protocol. We are grateful to Jeffrey Priest from CDC for the Cp17 and VSP5 plasmid proteins, Edward Ryan from Massachusetts General Hospital for the OSP antigen, and David Cavanagh from University of Edinburgh for the MSP2. This manuscript has been released as a pre-print at bioRxiv (113).
Supplementary Material
The Supplementary Material for this article can be found online at: https://www.frontiersin.org/articles/10.3389/fimmu.2021.614246/full#supplementary-material
Supplementary Figure 1. IPTp trial profile.
Supplementary Figure 2. Cord blood antibody levels in HIV-positive women taking ART. Radar charts representing the medians of each analyte antibody cord levels in HIV-positive women who started ART before pregnancy, at recruitment or were not taking ART for IgG (A), IgG1 subclass (B), IgG2 (C), IgG3 (D), and IgG4 (E). Cord blood levels were compared by Kruskal-Wallis test and p-values were adjusted for multiple testing by the Benjamini-Hochberg approach (False Discovery Rate 5%). Statistically significant differences between ART before pregnancy, ART at recruitment and no ART are highlighted with an asterisk. **p < 0.01, *p < 0.05. ART at recruitment is represented in green, ART before pregnancy is represented in blue and no ART is represented in red.
Supplementary Figure 3. Difference of IgG and IgG subclass levels in cord blood levels of HIV-infected women. Forest plots show the effect (in percentage) of placental malaria on cord blood levels of IgG and IgG subclasses, for all the antigens tested, when placental malaria was included in multivariable models with maternal antibody levels. The differences in percentage correspond to beta-transformed values (%) that were calculated from the beta values obtained in the multivariable models. Beta transformed values (%) are displayed when raw p-values are significant. Asterisks are shown when adjusted p-values by Benjamini-Hochberg are significant (False Discovery Rate 5%). **p < 0.01, *p < 0.05.
Supplementary Figure 4. Antibody placental transfer in HIV-positive women taking ART. Radar charts representing the medians of each analyte antibody cord/mother ratio in HIV-positive who started ART before pregnancy, at recruitment or were not taking ART for IgG (A), IgG1 subclass (B), IgG2 (C), IgG3 (D), and IgG4 (E). Ratios were compared by Kruskal-Wallis test and p-values were adjusted for multiple testing by the Benjamini-Hochberg approach (False Discovery Rate 5%). Statistically significant differences between ART before pregnancy, ART at recruitment and no ART are highlighted with an asterisk. *p < 0.05. ART at recruitment is represented in green, ART before pregnancy is represented in blue and no ART is represented in red.
Supplementary Figure 5. Difference of IgG and IgG subclass placental transfer in HIV-infected women. Forest plots show the effect (in percentage) of placental malaria on transplacental transfer of IgG and IgG subclasses, for all the antigens tested, when placental malaria was included in multivariable models. The differences in percentage correspond to beta-transformed values (%) that were calculated from the beta values obtained in the multivariable models. Beta transformed values (%) are displayed when raw p-values are significant. Asterisks are shown when adjusted p-values by Benjamini-Hochberg are significant (False Discovery Rate 5%). *p < 0.05.
Supplementary Material 1. Cord blood levels and placental transfer of antibodies univariable models.
References
1. World Health Organization. World Health Statistics 2018: Monitoring Health for the SDGs, Geneva (2018).
2. WHO. Infant Mortality. WHO (2018). Available online at: https://www.who.int/gho/child_health/mortality/neonatal_infant_text/en/ (accessed April 10, 2019).
3. Kollmann TR, Kampmann B, Mazmanian SK, Marchant A, Levy O. Protecting the newborn and young infant from infectious diseases: lessons from immune ontogeny. Immunity. (2017) 46:350–63. doi: 10.1016/j.immuni.2017.03.009
4. Basha S, Surendran N, Pichichero M. Immune responses in neonates. Expert Rev Clin Immunol. (2014) 10:1171–84. doi: 10.1586/1744666X.2014.942288
5. Prabhudas M, Adkins B, Gans H, King C, Levy O, Ramilo O, et al. Challenges in infant immunity: implications for responses to infection and vaccines. Nat Immunol. (2011) 12:189–94. doi: 10.1038/ni0311-189
6. Zhang X, Zhivaki D, Lo-Man R. Unique aspects of the perinatal immune system. Nat Rev Immunol. (2017) 17:495–507. doi: 10.1038/nri.2017.54
7. O'Brien KL, Baggett HC, Brooks WA, Feikin DR, Hammitt LL, Higdon MM, et al. Causes of severe pneumonia requiring hospital admission in children without HIV infection from Africa and Asia: the PERCH multi-country case-control study. Lancet. (2019) 394:757–79. doi: 10.1016/S0140-6736(19)30721-4
8. Kabego L, Balol'Ebwami S, Kasengi JB, Miyanga S, Bahati YL, Kambale R, et al. Human respiratory syncytial virus: prevalence, viral co-infections and risk factors for lower respiratory tract infections in children under 5 years of age at a general hospital in the democratic republic of Congo. J Med Microbiol. (2018) 67:514–22. doi: 10.1099/jmm.0.000713
9. Deogaonkar R, Hutubessy R, van der Putten I, Evers S, Jit M. Systematic review of studies evaluating the broader economic impact of vaccination in low and middle income countries. BMC Public Health. (2012) 12:878. doi: 10.1186/1471-2458-12-878
10. Clemens J, Holmgren J, Kaufmann SHE, Mantovani A. Ten years of the global alliance for vaccines and immunization: challenges and progress. Nat Immunol. (2010) 11:1069–72. doi: 10.1038/ni1210-1069
11. Trunz BB, Fine P, Dye C. Effect of BCG vaccination on childhood tuberculous meningitis and miliary tuberculosis worldwide: a meta-analysis and assessment of cost-effectiveness. Lancet. (2006) 367:1173–80. doi: 10.1016/S0140-6736(06)68507-3
12. World Health Organization. Hepatitis B Control Through Immunization: A Reference Guide. Geneva (2014) 5:13–62.
13. Lund N, Andersen A, Hansen ASK, Jepsen FS, Barbosa A, Biering-Sørensen S, et al. The effect of oral polio vaccine at birth on infant mortality: a randomized trial. Clin Infect Dis. (2015) 61:1504–11. doi: 10.1093/cid/civ617
14. Palmeira P, Quinello C, Silveira-Lessa AL, Zago CA, Carneiro-Sampaio M. IgG placental transfer in healthy and pathological pregnancies. Clin Dev Immunol. (2012) 2012:985646. doi: 10.1155/2012/985646
15. Simister NE, Story CM, Chen H-LL, Hunt JS. An IgG-transporting Fc receptor expressed in the syncytiotrophoblast of human placenta. Eur J Immunol. (1996) 26:1527–31. doi: 10.1002/eji.1830260718
16. Martinez DR, Fong Y, Li SH, Yang F, Jennewein MF, Weiner JA, et al. Fc characteristics mediate selective placental transfer of IgG in HIV-infected women. Cell. (2019) 178:190–201.e11. doi: 10.1016/j.cell.2019.05.046
17. Malek A, Sager R, Kuhn P, Nicolaides KH, Schneider H. Evolution of maternofetal transport of immunoglobulins during human pregnancy. Am J Reprod Immunol. (1996) 36:248–55. doi: 10.1111/j.1600-0897.1996.tb00172.x
18. Bundhoo A, Paveglio S, Rafti E, Dhongade A, Blumberg RS, Matson AP. Evidence that FcRn mediates the transplacental passage of maternal IgE in the form of IgG anti-IgE/IgE immune complexes. Clin Exp Allergy. (2015) 45:1085–98. doi: 10.1111/cea.12508
19. Abdiche YN, Yeung YA, Chaparro-Riggers J, Barman I, Strop P, Chin SM, et al. The neonatal Fc receptor (FcRn) binds independently to both sites of the IgG homodimer with identical affinity. MAbs. (2015) 7:331–43. doi: 10.1080/19420862.2015.1008353
20. Costa-Carvalho BT, Vieira HM, Dimantas RBR, Arslanian C, Naspitz CK, Solé D, et al. Transfer of IgG subclasses across placenta in term and preterm newborns. Brazil J Med Biol Res. (1996) 29:201–4.
21. Clements T, Rice TF, Vamvakas G, Barnett S, Barnes M, Donaldson B, et al. Update on transplacental transfer of IgG subclasses: impact of maternal and fetal factors. Front Immunol. (2020) 11:1920. doi: 10.3389/fimmu.2020.01920
22. Marchant A, Sadarangani M, Garand M, Dauby N, Verhasselt V, Pereira L, et al. Maternal immunisation: collaborating with mother nature. Lancet Infect Dis. (2017) 17:e197–208. doi: 10.1016/S1473-3099(17)30229-3
23. Fouda GG, Martinez DR, Swamy GK, Permar SR. The impact of IgG transplacental transfer on early life immunity. ImmunoHorizons. (2018) 2:14–25. doi: 10.4049/immunohorizons.1700057
24. Buchy P, Badur S, Kassianos G, Preiss S, Tam JS. Vaccinating pregnant women against influenza needs to be a priority for all countries: an expert commentary. Int J Infect Dis. (2020) 92:1–12. doi: 10.1016/j.ijid.2019.12.019
25. Thwaites CL, Beeching NJ, Newton CR. Maternal and neonatal tetanus. Lancet. 385:362–70. doi: 10.1016/S0140-6736(14)60236-1
26. Gkentzi D, Katsakiori P, Marangos M, Hsia Y, Amirthalingam G, Heath PT, et al. Maternal vaccination against pertussis: a systematic review of the recent literature. Arch Dis Child Fetal Neonatal Ed. (2017) 102:F456–63. doi: 10.1136/archdischild-2016-312341
27. Heath PT, Culley FJ, Jones CE, Kampmann B, Le Doare K, Nunes MC, et al. Group B streptococcus and respiratory syncytial virus immunisation during pregnancy: a landscape analysis. Lancet Infect Dis. (2017) 17:e223–34. doi: 10.1016/S1473-3099(17)30232-3
28. Cumberland P, Shulman CE, Maple PA, Bulmer JN, Dorman EK, Kawuondo K, et al. Maternal HIV infection and placental malaria reduce transplacental antibody transfer and tetanus antibody levels in newborns in Kenya. J Infect Dis. (2007) 196:550–7. doi: 10.1086/519845
29. Jones CE, Naidoo S, De Beer C, Esser M, Kampmann B, Hesseling AC. Maternal HIV infection and antibody responses against vaccine-preventable diseases in uninfected infants. J Am Med Assoc. (2011) 305:576–84. doi: 10.1001/jama.2011.100
30. Caceres VM, Strebel PM, Sutter RW. Factors determining prevalence of maternal antibody to measles virus throughout infancy: a review. Clin Infect Dis. (2000) 31:110–9. doi: 10.1086/313926
31. Wilcox CR, Holder B, Jones CE. Factors affecting the FcRn-mediated transplacental transfer of antibodies and implications for vaccination in pregnancy. Front Immunol. (2017) 8:1294. doi: 10.3389/fimmu.2017.01294
32. Van Den Berg JP, Westerbeek EAM, Berbers GAM, Van Gageldonk PGM, Van Der Klis FRM, Van Elburg RM. Transplacental transport of IgG antibodies specific for pertussis, diphtheria, tetanus, haemophilus influenzae type b, and neisseria meningitidis serogroup C is lower in preterm compared with term infants. Pediatr Infect Dis J. (2010) 29:801–5. doi: 10.1097/INF.0b013e3181dc4f77
33. De Moraes-Pinto MI, Verhoeff F, Chimsuku L, Milligan PJM, Wesumperuma L, Broadhead RL, et al. Placental antibody transfer: influence of maternal HIV infection and placental malaria. Arch Dis Child Fetal Neonatal Ed. (1998) 79:F202–5. doi: 10.1136/fn.79.3.F202
34. Brair M-E, Brabin B, Milligan P, Maxwell S, Hart CA. Reduced transfer of tetanus antibodies with placental malaria. Lancet. (1994) 343:208–9. doi: 10.1016/S0140-6736(94)90991-1
35. Okoko BJ, Wesuperuma LH, Ota MO, Banya WA, Pinder M, Gomez FS, et al. Influence of placental malaria infection and maternal hypergammaglobulinaemia on materno-foetal transfer of measles and tetanus antibodies in a rural west African population. J Health Popul Nutr. (2001) 19:59–65. doi: 10.3329/jhpn.v19i2.77
36. Atwell JE, Thumar B, Robinson LJ, Tobby R, Yambo P, Ome-Kaius M, et al. Impact of placental malaria and hypergammaglobulinemia on transplacental transfer of respiratory syncytial virus antibody in Papua New Guinea. J Infect Dis. (2016) 213:423–31. doi: 10.1093/infdis/jiv401
37. Okoko BJ, Wesumperuma LH, Ota MO, Pinder M, Banya W, Gomez SF, et al. The influence of placental malaria infection and maternal hypergammaglobulinemia on transplacental transfer of antibodies and IgG subclasses in a rural West African population. J Infect Dis. (2001) 184:627–32. doi: 10.1086/322808
38. Ray JE, Dobbs KR, Ogolla SO, Daud II, Vulule J, Sumba PO, et al. Reduced transplacental transfer of antimalarial antibodies in kenyan HIV-exposed uninfected infants. Open Forum Infect Dis. (2019) 6:ofz237. doi: 10.1093/ofid/ofz237
39. Scott S, Cumberland P, Shulman CE, Cousens S, Cohen BJ, Brown DWG, et al. Neonatal measles immunity in rural Kenya: the Influence of HIV and placental malaria infections on placental transfer of antibodies and levels of antibody in maternal and cord serum samples. J Infect Dis. (2005) 191:1854–60. doi: 10.1086/429963
40. de Moraes-Pinto MI, Almeida AC, Kenj G, Filgueiras TE, Tobias W, Santos AM, et al. Placental transfer and maternally acquired neonatal IgG immunity in human immunodeficiency virus infection. J Infect Dis. (1996) 173:1077–84. doi: 10.1093/infdis/173.5.1077
41. Moro L, Bardaji A, Nhampossa T, Mandomando I, Serra-Casas E, Sigauque B, et al. Malaria and HIV infection in mozambican pregnant women are associated with reduced transfer of antimalarial antibodies to their newborns. J Infect Dis. (2015) 211:1004–14. doi: 10.1093/infdis/jiu547
42. Jones C, Pollock L, Barnett SM, Battersby A, Kampmann B. Specific antibodies against vaccine-preventable infections: a mother-infant cohort study. BMJ Open. (2013) 3:e002473. doi: 10.1136/bmjopen-2012-002473
43. Farquhar C, Nduati R, Haigwood N, Sutton W, Mbori-Ngacha D, Richardson B, et al. High maternal HIV-1 viral load during pregnancy is associated with reduced placental transfer of measles IgG antibody. J Acquir Immune Defic Syndr. (2005) 40:494–7. doi: 10.1097/01.qai.0000168179.68781.95
44. Dangor Z, Kwatra G, Izu A, Adrian P, Van Niekerk N, Cutland CL, et al. HIV-1 is associated with lower group B streptococcus capsular and surface-protein IgG antibody levels and reduced transplacental antibody transfer in pregnant women. J Infect Dis. (2015) 212:453–62. doi: 10.1093/infdis/jiv064
45. Babakhanyan A, Ekali GL, Dent A, Kazura J, Nguasong JT, Fodjo BAY, et al. Maternal human immunodeficiency virus-associated hypergammaglobulinemia reduces transplacental transfer of immunoglobulin G to Plasmodium falciparum antigens in Cameroonian neonates. Open Forum Infect Dis. (2016) 3:ofw092. doi: 10.1093/ofid/ofw092
46. Le Doare K, Taylor S, Allen L, Gorringe A, Heath PT, Kampmann B, et al. Placental transfer of anti-group B Streptococcus immunoglobulin G antibody subclasses from HIV-infected and uninfected women to their uninfected infants. AIDS. (2016) 30:471–5. doi: 10.1097/QAD.0000000000000923
47. González R, Mombo-Ngoma G, Ouédraogo S, Kakolwa MA, Abdulla S, Accrombessi M, et al. Intermittent preventive treatment of malaria in pregnancy with mefloquine in HIV-negative women: a multicentre randomized controlled trial. PLoS Med. (2014) 11:e1001733. doi: 10.1371/journal.pmed.1001733
48. González R, Desai M, Macete E, Ouma P, Kakolwa MA, Abdulla S, et al. Intermittent preventive treatment of malaria in pregnancy with mefloquine in HIV-infected women receiving cotrimoxazole prophylaxis: a multicenter randomized placebo-controlled trial. PLoS Med. (2014) 11:e1001735. doi: 10.1371/journal.pmed.1001735
49. Mayor A, Bardají A, Macete E, Nhampossa T, Fonseca AM, González R, et al. Changing Trends in P. falciparum burden, immunity, and disease in pregnancy. N Engl J Med. (2015) 373:1607–17. doi: 10.1056/NEJMoa1406459
50. WHO. Interim WHO Clinical Staging of HIV/AIDS and HIV/AIDS Case Definitions for Surveillance. African Region, Geneva (2005).
51. Mayor A, Serra-Casas E, Bardají A, Sanz S, Puyol L, Cisteró P, et al. Sub-microscopic infections and long-term recrudescence of Plasmodium falciparum in Mozambican pregnant women. Malar J. (2009) 8:1–10. doi: 10.1186/1475-2875-8-9
52. Sayeed MA, Bufano MK, Xu P, Eckhoff G, Charles RC, Alam MM, et al. A cholera conjugate vaccine containing ospecific polysaccharide (OSP) of V. cholera o1 inaba and recombinant fragment of tetanus toxin heavy chain (OSP:rTTHC) induces serum, memory and lamina proprial responses against OSP and is protective in mice. PLoS Negl Trop Dis. (2015) 9:e0003881. doi: 10.1371/journal.pntd.0003881
53. Priest JW, Moss DM, Visvesvara GS, Jones CC, Li A, Isaac-Renton JL. Multiplex assay detection of immunoglobulin G antibodies that recognize Giardia intestinalis and Cryptosporidium parvum antigens. Clin Vaccine Immunol. (2010) 17:1695–707. doi: 10.1128/CVI.00160-10
54. Angov E, Aufiero BM, Turgeon AM, Van Handenhove M, Ockenhouse CF, Kester KE, et al. Development and pre-clinical analysis of a Plasmodium falciparum merozoite surface protein-142 malaria vaccine. Mol Biochem Parasitol. (2003) 128:195–204. doi: 10.1016/S0166-6851(03)00077-X
55. Metzger WG, Okenu DMN, Cavanagh DR, Robinson JV, Bojang KA, Weiss HA, et al. Serum IgG3 to the Plasmodium falciparum merozoite surface protein 2 is strongly associated with a reduced prospective risk of malaria. Parasite Immunol. (2003) 25:307–12. doi: 10.1046/j.1365-3024.2003.00636.x
56. Doolan DL, Hedstrom RC, Rogers WO, Charoenvit Y, Rogers M, De La Vegar P, et al. Identification and characterization of the protective hepatocyte erythrocyte protein 17 dDa gene of Plasmodium yoelii, homolog of Plasmodium falciparum exported protein 1. J Biol Chem. (1996) 271:17861–8. doi: 10.1074/jbc.271.30.17861
57. Dgedge M, Novoa A, Macassa G, Sacarlal J, Black J, Michaud C, et al. The burden of disease in Maputo City, Mozambique: registered and autopsied deaths in 1994. Bull World Health Organ. (2001) 79:546–52. doi: 10.1590/S0042-96862001000600011
58. Kotloff KL, Nataro JP, Blackwelder WC, Nasrin D, Farag TH, Panchalingam S, et al. Burden and aetiology of diarrhoeal disease in infants and young children in developing countries (the Global Enteric Multicenter Study, GEMS): a prospective, case-control study. Lancet. (2013) 382:209–22. doi: 10.1016/S0140-6736(13)60844-2
59. Troeger C, Blacker B, Khalil IA, Rao PC, Cao J, Zimsen SRM, et al. Estimates of the global, regional, and national morbidity, mortality, and aetiologies of lower respiratory infections in 195 countries, 1990–2016: a systematic analysis for the Global Burden of Disease Study 2016. Lancet Infect Dis. (2018) 18:1191–210. doi: 10.1016/S1473-3099(18)30310-4
60. Lanaspa M, Balcells R, Sacoor C, Nhama A, Aponte JJ, Bassat Q. The performance of the expanded programme on immunization in a rural area of Mozambique. Acta Trop. (2015) 149:262–6. doi: 10.1016/j.actatropica.2015.06.007
61. Vidal M, Aguilar R, Campo JJ, Dobaño C. Development of quantitative suspension array assays for six immunoglobulin isotypes and subclasses to multiple Plasmodium falciparum antigens. J Immunol Methods. (2018) 455:41–54. doi: 10.1016/j.jim.2018.01.009
62. Ubillos I, Jiménez A, Vidal M, Bowyer PW, Gaur D, Dutta S, et al. Optimization of incubation conditions of Plasmodium falciparum antibody multiplex assays to measure IgG, IgG1-4, IgM and IgE using standard and customized reference pools for sero-epidemiological and vaccine studies. Malar J. (2018) 17:216. doi: 10.1186/s12936-018-2369-3
63. Ubillos I, Aguilar R, Sanz H, Jiménez A, Vidal M, Valmaseda A, et al. Analysis of factors affecting the variability of a quantitative suspension bead array assay measuring IgG to multiple Plasmodium antigens. PLoS ONE. (2018) 13:0199278. doi: 10.1371/journal.pone.0199278
64. Bryan D, Silva N, Rigsby P, Dougall T, Corran P, Bowyer PW, et al. The establishment of a WHO Reference Reagent for anti-malaria (Plasmodium falciparum) human serum. Malar J. (2017) 16:314. doi: 10.1186/s12936-017-1958-x
65. Ballard JL, Khoury JC, Wedig K, Wang L, Eilers-Walsman BL, Lipp R. New Ballard Score, expanded to include extremely premature infants. J Pediatr. (1991) 119:417–23. doi: 10.1016/S0022-3476(05)82056-6
66. Weiss GE, Traore B, Kayentao K, Ongoiba A, Doumbo S, Doumtabe D, et al. The Plasmodium falciparum-specific human memory b cell compartment expands gradually with repeated malaria infections. PLoS Pathog. (2010) 6:e1000912. doi: 10.1371/journal.ppat.1000912
67. Dobaño C, Ubillos I, Jairoce C, Gyan B, Vidal M, Jiménez A, et al. RTS,S/AS01E immunization increases antibody responses to vaccine-unrelated Plasmodium falciparum antigens associated with protection against clinical malaria in African children: a case-control study. BMC Med. (2019) 17:157. doi: 10.1186/s12916-019-1378-6
68. Wickham H, Hester J, Chang W. devtools: TOOLS to Make Developing R Packages Easier. R Package Devtools Version 2.3.0. (2020). Available online at: https://cran.r-project.org/package=devtools (accessed July 7, 2020).
70. Lê S, Josse J, Husson F. FactoMineR: an R package for multivariate analysis. J Stat Softw. (2008) 25:1–18. doi: 10.18637/jss.v025.i01
71. Kassambara A, Mundt F. factoextra: Extract and Visualize the Results of Multivariate Data Analyses. R Package Version 1.0.7. (2020). Available online at: https://cran.r-project.org/package=factoextra
72. Dechavanne C, Cottrell G, Garcia A, Migot-Nabias F. Placental malaria: decreased transfer of maternal antibodies directed to Plasmodium falciparum and impact on the incidence of febrile infections in infants. PLoS ONE. (2015) 10:0145464. doi: 10.1371/journal.pone.0145464
73. Boudová S, Divala T, Mungwira R, Mawindo P, Tomoka T, Laufer MK. Placental but not peripheral Plasmodium falciparum infection during pregnancy is associated with increased risk of malaria in infancy. J. Infect. Dis. (2017) 216:732–5. doi: 10.1093/infdis/jix372
74. van den Berg JP, Westerbeek EAM, van der Klis FRM, Berbers GAM, Van Elburg RM. Transplacental transport of IgG antibodies to preterm infants: a review of the literature. Early Hum Dev. (2011) 87:67–72. doi: 10.1016/j.earlhumdev.2010.11.003
75. Fu C, Lu L, Wu H, Shaman J, Cao Y, Fang F, et al. Placental antibody transfer efficiency and maternal levels: specific for measles, coxsackievirus A16, enterovirus 71, poliomyelitis I-III and HIV-1 antibodies. Sci Rep. (2016) 6:1–6. doi: 10.1038/srep38874
76. Pou C, Nkulikiyimfura D, Henckel E, Olin A, Lakshmikanth T, Mikes J, et al. The repertoire of maternal anti-viral antibodies in human newborns. Nat Med. (2019) 25:591–6. doi: 10.1038/s41591-019-0392-8
77. Askari A, Hakimi H, Nasiri Ahmadabadi B, Hassanshahi G, Kazemi Arababadi M. Prevalence of hepatitis B co-infection among HIV positive patients: narrative review article. Iran J Public Health. (2014) 43:705–12.
78. Feitosa G, Bandeira AC, Sampaio DP, Badaró R, Brites C. High prevalence of giardiasis and stronglyloidiasis among HIV-infected patients in Bahia, Brazil. Braz J Infect Dis. (2001) 5:339–44. doi: 10.1590/S1413-86702001000600008
79. Angarano G, Maggi P, Di Bari MA, Larocca AMV, Congedo P, Di Bari C, et al. Giardiasis in HIV: a possible role in patients with severe immune deficiency. Eur J Epidemiol. (1997) 13:485–7. doi: 10.1023/A:1007375421908
80. De Milito A, Nilsson A, Titanji K, Thorstensson R, Reizenstein E, Narita M, et al. Mechanisms of hypergammaglobulinemia and impaired antigen-specific humoral immunity in HIV-1 infection. Blood. (2004) 103:2180–6. doi: 10.1182/blood-2003-07-2375
81. Gupta A, Mathad JS, Yang WT, Singh HK, Gupte N, Mave V, et al. Maternal pneumococcal capsular IgG antibodies and transplacental transfer are low in South Asian HIV-infected mother-infant pairs. Vaccine. (2014) 32:1466–72. doi: 10.1016/j.vaccine.2014.01.033
82. Jallow S, Agosti Y, Kgagudi P, Vandecar M, Cutland CL, Simões EAF, et al. Impaired transplacental transfer of respiratory syncytial virus–neutralizing antibodies in human immunodeficiency virus–infected versus –uninfected pregnant women. Clin Infect Dis. (2019) 69:151–4. doi: 10.1093/cid/ciy1071
83. Weinberg A, Mussi-Pinhata MM, Yu Q, Cohen RA, Almeida VC, Amaral F, et al. Excess respiratory viral infections and low antibody responses among HIV-exposed, uninfected infants. AIDS. (2017) 31:669–79. doi: 10.1097/QAD.0000000000001393
84. Vidarsson G, Dekkers G, Rispens T. IgG subclasses and allotypes: from structure to effector functions. Front Immunol. (2014) 5:520. doi: 10.3389/fimmu.2014.00520
85. Irani V, Guy AJ, Andrew D, Beeson JG, Ramsland PA, Richards JS. Molecular properties of human IgG subclasses and their implications for designing therapeutic monoclonal antibodies against infectious diseases. Mol Immunol. (2015) 67:171–82. doi: 10.1016/j.molimm.2015.03.255
86. Hjelholt A, Christiansen G, Sørensen US, Birkelund S. IgG subclass profiles in normal human sera of antibodies specific to five kinds of microbial antigens. Pathog Dis. (2013) 67:206–13. doi: 10.1111/2049-632X.12034
87. Einhorn MS, Granoff DM, Nahm MH, Quinn A, Shackelford PG. Concentrations of antibodies in paired maternal and infant sera: relationship to IgG subclass. J Pediatr. (1987) 111:783–8. doi: 10.1016/S0022-3476(87)80268-8
88. Seppälä I, Sarvas H, Mäkelä O, Mattila P, Eskola J, Käyhty H. Human antibody responses to two conjugate vaccines of haemophilus influenzae type B saccharides and diphtheria toxin. Scand J Immunol. (1988) 28:471–9. doi: 10.1111/j.1365-3083.1988.tb01478.x
89. Parkkali T, Käyhty H, Anttila M, Ruutu T, Wuorimaa T, Soininen A, et al. IgG subclasses and avidity of antibodies to polysaccharide antigens in allogeneic BMT recipients after vaccination with pneumococcal polysaccharide and Haemophilus influenzae type b conjugate vaccines. Bone Marrow Transplant. (1999) 24:671–8. doi: 10.1038/sj.bmt.1701967
90. Wagner DK, Muelenaer P, Henderson FW, Snyder MH, Reimer CB, Walsh EE, et al. Serum immunoglobulin G antibody subclass response to respiratory syncytial virus F and G glycoproteins after first, second, and third infections. J Clin Microbiol. (1989) 27:589–92. doi: 10.1128/JCM.27.3.589-592.1989
91. Jounai N, Yoshioka M, Tozuka M, Inoue K, Oka T, Miyaji K, et al. Age-specific profiles of antibody responses against respiratory syncytial virus infection. EBioMedicine. (2017) 16:124–35. doi: 10.1016/j.ebiom.2017.01.014
92. Higgins D, Trujillo C, Keech C. Advances in RSV vaccine research and development - a global agenda. Vaccine. (2016) 34:2870–5. doi: 10.1016/j.vaccine.2016.03.109
93. Piedra PA, Jewell AM, Cron SG, Atmar RL, Paul Glezen W. Correlates of immunity to respiratory syncytial virus (RSV) associated-hospitalization: establishment of minimum protective threshold levels of serum neutralizing antibodies. Vaccine. (2003) 21:3479–82. doi: 10.1016/S0264-410X(03)00355-4
94. Queiróz DAO, Durigon EL, Botosso VF, Ejzemberg B, Vieira SE, Mineo JR, et al. Immune response to respiratory syncytial virus in young Brazilian children. Brazil J Med Biol Res. (2002) 35:1183–93. doi: 10.1590/S0100-879X2002001000011
95. Freitas GRO, Silva DAO, Yokosawa J, Paula NT, Costa LF, Carneiro BM, et al. Antibody response and avidity of respiratory syncytial virus-specific total IgG, IgG1, and IgG3 in young children. J Med Virol. (2011) 83:1826–33. doi: 10.1002/jmv.22134
96. Connor EM. Palivizumab, a humanized respiratory syncytial virus monoclonal antibody, reduces hospitalization from respiratory syncytial virus infection in high-risk infants. Pediatrics. (1998) 102:531–7. doi: 10.1542/peds.102.3.531
97. Bosire R, Farquhar C, Nduati R, Broliden K, Luchters S, Van De Perre P, et al. Higher transplacental pathogen-specific antibody transfer among pregnant women randomized to triple antiretroviral treatment versus short course zidovudine. Pediatr Infect Dis J. (2018) 37:246–52. doi: 10.1097/INF.0000000000001749
98. Baroncelli S, Galluzzo CM, Mancinelli S, Andreotti M, Jere H, Amici R, et al. Antibodies against pneumococcal capsular polysaccharide in Malawian HIV-positive mothers and their HIV-exposed uninfected children. Infect Dis. (2016) 48:317–21. doi: 10.3109/23744235.2015.1115895
99. Goetghebuer T, Smolen KK, Adler C, Das J, Mcbride T, Smits G, et al. Initiation of antiretroviral therapy before pregnancy reduces the risk of infection-related hospitalization in human immunodeficiency virus-exposed uninfected infants born in a high-income country. Clin Infect Dis. (2019) 68:1193–203. doi: 10.1093/cid/ciy673
100. Kasirye R, Baisley K, Munderi P, Grosskurth H. Effect of cotrimoxazole prophylaxis on malaria occurrence in HIV-infected patients on antiretroviral therapy in sub-Saharan Africa. Trop Med Int Heal. (2015) 20:569–80. doi: 10.1111/tmi.12463
101. Davis NL, Barnett EJ, Miller WC, Dow A, Chasela CS, Hudgens MG, et al. Impact of daily cotrimoxazole on clinical malaria and asymptomatic parasitemias in HIV-exposed, uninfected infants. Clin Infect Dis. (2015) 61:368–74. doi: 10.1093/cid/civ309
102. Wesumperuma HL, Perera AJ, Pharoah POD, Hart CA. The influence of prematurity and low birthweight on transplacental antibody transfer in Sri Lanka. Ann Trop Med Parasitol. (1999) 93:169–77. doi: 10.1080/00034989958654
103. Okoko BJ, Wesumperuma LH, Hart AC. Materno-foetal transfer of H. influenzae and pneumococcal antibodies is influenced by prematurity and low birth weight: implications for conjugate vaccine trials. Vaccine. (2001) 20:647–50. doi: 10.1016/S0264-410X(01)00418-2
104. Linder N, Tallen-Gozani E, German B, Duvdevani P, Ferber A, Sirota L. Placental transfer of measles antibodies: effect of gestational age and maternal vaccination status. Vaccine. (2004) 22:1509–14. doi: 10.1016/j.vaccine.2003.10.009
105. Messeret ES, Masresha B, Yakubu A, Daniel F, Mihigo R, Nshimirimana D, et al. Maternal and neonatal tetanus elimination (MNTE) in the WHO African region. J Immunol Sci. (2018) 15:103–7. doi: 10.29245/2578-3009/2018/si.1115
106. Furuta M, Sin J, Ng ESW, Wang K. Efficacy and safety of pertussis vaccination for pregnant women – a systematic review of randomised controlled trials and observational studies. BMC Pregnancy Childbirth. (2017) 17:390. doi: 10.1186/s12884-017-1559-2
107. Brousseau N, Gagnon D, Vivion M, Poliquin V, Boucoiran I, Tapiéro B, et al. Expected challenges of implementing universal pertussis vaccination during pregnancy in Quebec: a cross-sectional survey. C Open. (2018) 6:E391–7. doi: 10.9778/cmajo.20180040
108. Zackrisson G, Lagergård T, Trollfors B. Subclass compositions of immunoglobulin G to pertussis toxin in patients with whooping cough, in healthy individuals, and in recipients of a pertussis toxoid vaccine. J Clin Microbiol. (1989) 27:1567–71. doi: 10.1128/JCM.27.7.1567-1571.1989
109. Hendrikx LH, Schure RM, Öztürk K, de Rond LGH, de Greeff SC, Sanders EAM, et al. Different IgG-subclass distributions after whole-cell and acellular pertussis infant primary vaccinations in healthy and pertussis infected children. Vaccine. (2011) 29:6874–80. doi: 10.1016/j.vaccine.2011.07.055
110. Giammanco A, Taormina S, Chiarini A, Dardanoni G, Stefanelli P, Salmaso S, et al. Analogous IgG subclass response to pertussis toxin in vaccinated children, healthy or affected by whooping cough. Vaccine. (2003) 21:1924–31. doi: 10.1016/S0264-410X(02)00823-X
111. Silveira ELV, Dominguez MR, Soares IS. To B or not to B: understanding B cell responses in the development of malaria infection. Front Immunol. (2018) 9:2961. doi: 10.3389/fimmu.2018.02961
112. Pritchard DI, Williams DJ, Behnke JM, Lee TD. The role of IgG1 hypergammaglobulinaemia in immunity to the gastrointestinal nematode Nematospiroides dubius. The immunochemical purification, antigen-specificity and in vivo anti-parasite effect of IgG1 from immune serum. Immunology. (1983) 49:353–65.
Keywords: antibody, maternal antibodies, placental transfer, HIV, pathogens, malaria, IgG, IgG subclasses
Citation: Alonso S, Vidal M, Ruiz-Olalla G, González R, Manaca MN, Jairoce C, Vázquez-Santiago M, Balcells R, Vala A, Rupérez M, Cisteró P, Fuente-Soro L, Cova M, Angov E, Nhacolo A, Sevene E, Aponte JJ, Macete E, Aguilar R, Mayor A, Menéndez C, Dobaño C and Moncunill G (2021) Reduced Placental Transfer of Antibodies Against a Wide Range of Microbial and Vaccine Antigens in HIV-Infected Women in Mozambique. Front. Immunol. 12:614246. doi: 10.3389/fimmu.2021.614246
Received: 05 October 2020; Accepted: 08 February 2021;
Published: 03 March 2021.
Edited by:
Remi Cheynier, INSERM U1016 Institut Cochin, FranceReviewed by:
Justin Pollara, Duke University, United StatesMangai Asokan, Independent Researcher, Bengaluru, India
Copyright © 2021 Alonso, Vidal, Ruiz-Olalla, González, Manaca, Jairoce, Vázquez-Santiago, Balcells, Vala, Rupérez, Cisteró, Fuente-Soro, Cova, Angov, Nhacolo, Sevene, Aponte, Macete, Aguilar, Mayor, Menéndez, Dobaño and Moncunill. This is an open-access article distributed under the terms of the Creative Commons Attribution License (CC BY). The use, distribution or reproduction in other forums is permitted, provided the original author(s) and the copyright owner(s) are credited and that the original publication in this journal is cited, in accordance with accepted academic practice. No use, distribution or reproduction is permitted which does not comply with these terms.
*Correspondence: Gemma Moncunill, gemma.moncunill@isglobal.org; Carlota Dobaño, carlota.dobano@isglobal.org
†These authors have contributed equally to this work
‡These authors have contributed equally to this work and share senior authorship
§Present address: María Rupérez, London School of Hygiene and Tropical Medicine (LSHTM), London, United Kingdom