- 1Department of Pediatrics-Neonatal Intensive Care, V. Fazzi Hospital, ASL LE, Lecce, Italy
- 2Infectivology and Clinical Trials Research Department, Bambino Gesù Children's Hospital, IRCCS, Rome, Italy
- 3Section of Internal Medicine and Endocrinological and Metabolic Sciences, Department of Medicine, University of Perugia, Perugia, Italy
The autoimmune polyglandular syndrome type 1 (APS1) is caused by pathogenic variants of the autoimmune regulator (AIRE) gene, located in the chromosomal region 21q22.3. The related protein, AIRE, enhances thymic self-representation and immune self-tolerance by localization to chromatin and anchorage to multimolecular complexes involved in the initiation and post-initiation events of tissue-specific antigen-encoding gene transcription. Once synthesized, the self-antigens are presented to, and cause deletion of, the self-reactive thymocyte clones. The clinical diagnosis of APS1 is based on the classic triad idiopathic hypoparathyroidism (HPT)—chronic mucocutaneous candidiasis—autoimmune Addison's disease (AAD), though new criteria based on early non-endocrine manifestations have been proposed. HPT is in most cases the first endocrine component of the syndrome; however, APS1-associated AAD has received the most accurate biochemical, clinical, and immunological characterization. Here is a comprehensive review of the studies on APS1-associated AAD from initial case reports to the most recent scientific findings.
Introduction
The term autoimmune polyglandular syndrome (APS) designates a heterogeneous group of diseases sharing a common fundamental characteristic—damage to more than one organ, essentially but not exclusively endocrine—caused by pathological processes identifiable as autoimmune (1, 2). APSs were classified in the early 1980s (3–5). APS type 1 (APS1), often referred to as autoimmune polyendocrinopathy-candidiasis-ectodermal dystrophy (APECED) to summarize clinical features, is a monogenic disease with an autosomal recessive inheritance (6, 7). Traditionally, at least two of the major components—chronic mucocutaneous candidiasis (CMC), idiopathic hypoparathyroidism (HPT), and autoimmune Addison's disease (AAD)—are required for clinical diagnosis (8–10). The diagnostic pathway of APS1 cases with uncommon presentation and course may take advantage of early non-triad components, such as urticaria-like eruptions, gastrointestinal dysfunction, and enamel hypoplasia, along with type-1 interferon (IFN) antibody assay, pending molecular confirmation (11–16).
In APS type 2 (APS2), or Schmidt's syndrome, AAD is associated with autoimmune thyroid disease (ATD), type-1 diabetes (T1D), or both (17–19). In contrast, ATD along with other autoimmune diseases, HPT and AAD excluded, identifies APS type 3 (APS3) (20). Worldwide medical literature is closely aligned with this classification (21–28). However, it has been suggested to merge non-monogenic APSs into a single disease entity (29), as they recognize a multifactorial genetic predisposition, mostly related to the major histocompatibility complex class-I and class-II (MHCI and MHCII, respectively) genes and their regulators (30–35).
Since AAD is the bridge between APS1 and APS2, the revision of its principles implies the treatment of these subjects (36–41). This work aims to review the characteristics of APS1-associated AAD, providing a historical overview and looking at the most recent results deriving from studies on the animal models of the disease. Due to the common embryogenesis of the adrenal cortex and gonads (42), AAD intersects the autoimmune events involving the gonads, especially in the form of autoimmune primary ovarian insufficiency (APOI) of the female sex.
AIRE, APS1, and the Animal Models of Disease
AIRE: Basic Properties and Functions
Autoimmune regulator (AIRE), the gene responsible for APS1, is found in the chromosomal region 21q22.3 (6, 7, 43–45). The murine homolog (Aire) lies on chromosome 10 (46–48). The pool of tissues in which the gene is transcribed must be fully delineated (49–51), but undoubtedly the highest degree of expression is in medullary thymic epithelial cells (mTECs); here, the protein (AIRE/Aire) forms distinct nuclear speckles and co-localizes with the microtubular cytoskeleton (52, 53).
Earlier studies proved that bipotent thymic epithelial progenitor cells (TEPCs) give rise to mTEC and cortical TEC (cTEC) compartments in the embryonic and early neonatal thymus (54–58). It was then shown that selected TEPC clusters differentiate into mTEC sublineage derailing from a predefined cTEC development program (59–63). In the post-natal thymus, bipotent TEPCs become progressively quiescent, and the replenishment of TEC compartments is supported by sublineage-restricted precursors (64–69).
Committed mTECs descend from the apical layer of the thymic anlage, marked by tight-junction claudins 3 and 4 (70), while the initial stages of maturation require lymphostromal “crosstalk” with early T-cell subsets (71–73). MHCloCD80loAIRE− mTECs (mTECslo) include not only precursors of the mature MHChiCD80hiAIRE+ mTECs (mTECshi), but also cortico-medullary junctional TECs (jTECs) that recruit positively selected thymocytes into the medulla (74–78). In turn, mTECshi have a rapid turnover and give rise to various post-AIRE subsets (79–81); these include corneocyte-like mTECs and thymic tuft cells, which play a presumed role in addressing cytokine responses (82–87).
AIRE contains a caspase-activation and recruitment domain (CARD), a nuclear localization signal (NLS), a SAND (for Sp100-AIRE-NucP41/75-Deaf-1) domain, and two plant-homeodomain zinc fingers (PHD1 and PHD2, respectively) (88, 89). Amino-terminal and middle regions perform auxiliary functions, such as oligomerization, pro-apoptosis, nuclear shuttling, and DNA binding (90–93). At the carboxyl-terminal end, PHD1 binds to the tail of unmethylated histone H3 by electrostatic complementarity, and PHD2 activates gene transcription (94–97). To perform this function, AIRE interacts with enzymes, such as DNA-topoisomerases (DNA-TOPs) and DNA-activated protein kinase (DNA-PK), which belong to the multimolecular complex involved in DNA break and repair by non-homologous end joining (98–100).
As demonstrated in the murine thymus, Aire and co-actors localize to long stretches of chromatin known as super-enhancers, which enclose the transcription start sites of most Aire-dependent genes (101). Initiation of gene transcription is made effective by AIRE-induced recruitment of the positive transcriptional elongation factor b (P-TEFb), which enables elongation and pre-mRNA splicing into mature mRNA by phosphorylation and release of the stalled RNA-polymerase II (102, 103).
Due to the above properties, AIRE plays a crucial role in promiscuous gene expression within the thymus; in other words, AIRE drives the ectopic expression of genes that encode for enzymes, hormones, receptors, structural proteins and other molecules acting as self-antigens and normally synthesized in a few tissues (104–107). Their presentation to thymocytes induces apoptosis and deletion of self-reactive clones, which prevent their spreading as mature T cells (108–111). However, AIRE controls only a part of these genes; furthermore, the expression of any single AIRE-dependent gene affects a small percentage of mTECs and follows a stochastic pattern (112, 113), though co-expression pools of overlapping and complementary gene sets have been established (114–116).
AIRE also promotes the generation of regulatory T cells (111, 117, 118). The result of self-reactive thymocytes (i.e., negative selection or switch to tolerogenic function) depends on mTEC subsets and AIRE availability (119–122), division of labor and interplay between antigen-presenting cells (123–127), and degree of affinity between self-antigens and T-cell receptors (128, 129).
For a careful dissection of the above-mentioned arguments, the authors suggest readers to refer recent reviews that have thoroughly analyzed TEC dynamics and functions (130, 131), the molecular properties of AIRE, and its role in self-tolerance (132–135).
Clinical Picture of APS1 and the Animal Models of Disease
The clinical picture of APS1 is quite repetitive, although, as indicated, minor or uncommon entities may precede the main triad in a number of cases (15, 16). In other patients, severe forms of autoimmune hepatitis (136–139), lung disease (140, 141), and oral/esophageal carcinoma (142, 143) worsen the disease and progress into a life-threatening condition.
In vitro mutagenesis suggests that specific AIRE genotypes, particularly that of Iranian-Jewish patients with APS1, give rise to distinctive clinical features (144–147). Other peculiarities concern patients carrying mutated AIRE chains that co-localize with the wild-type protein and undermine the oligomeric structure in a dominant way. Incomplete penetrance, late-onset autoimmunity, and a milder phenotype characterize the clinical picture (148–151).
Additional genetic traits, with particular reference to the MHC alleles, can influence the APS1 phenotype so that in large APS1 patient cohorts most of the disease components mirror the HLA associations established for the general population (152). As an example, Finnish patients with APS1 have a high prevalence of T1D, while ATD is surprisingly common in those of Southern Italy (8, 153); actually, both observations reflect the MHC predispositions of the respective populations (154, 155).
Regarding APS1-associated AAD, no susceptibility related to MHCI and MHCII was initially reported, while HLA-DR3 and -DR4 were recognized to confer a significant risk for sporadic and APS2-associated forms (5, 156, 157). Subsequent analysis of larger patient cohorts contradicted the previous claim and revealed that patients with APS1 carrying the DRB1*03 allele have a significantly higher prevalence of AAD, while DRB1*04 appears to be more closely associated with alopecia (152). A number of other genes have been linked to non-APS1-associated AAD, but none of these play a role in APS1 phenotype (35). On the contrary, AIRE sequencing allows a correct classification of some AAD cases, which were previously framed within sporadic forms and actually being part of APS1 (158, 159).
The animal models of APS1 provide a formidable support for delineating AIRE-related self-tolerance mechanisms, but provide scant information, if any, on the characteristics of the disease components (108–111). This is because Aire-deficient (Aire−/−) mice exhibit different pictures than human APS1 (160, 161); typically, the exocrine glands are targets of autoimmunity, while the adrenal cortex, and other endocrine glands, shows little or no damage (108, 109, 111). Furthermore, the clinical picture of Aire−/− mice is modulated by the strain background, supporting the idea that gene modifiers control patterns of autoimmunity to each organ (162–164).
More recently, Aire−/− rats were engineered by zinc-finger nucleases; the animals exhibited various APS1-like ectodermal dystrophies, periportal lymphocyte infiltrates (with “piecemeal” necrosis) of the liver, and a broad spectrum of antibodies to self-antigens, type-1 IFNs included, but the endocrine glands were not significantly affected. In part contradicting the above findings, the reproductive capacity of the animals was impaired by the damage of testis Leydig cells (165).
Interestingly, the thymic expression of genes encoding for three enzymes of the cytochrome-P450 (CYP) family involved in the steroid pathway and electively targeted by adrenal autoimmunity in APS1 suggests differing and somewhat contrasting regulatory mechanisms between humans and mice. Murine mitochondrial cholesterol side-chain cleavage enzyme (CYP11A1, or CYPscc) and microsomal 21-hydroxylase (CYP21) have a strong and intermediate degree, respectively, of dependence on Aire, while the gene expression of another microsomal CYP enzyme, 17α-hydroxylase/17,20 lyase (CYP17), does not change significantly in murine Aire−/− mTECs (86, 109, 166–168). Unexpectedly, the expression of the human corresponding genes is unrelated to that of AIRE in thymoma and HEK293 cells, underlining that dependence on AIRE follows a species-specific pattern (169–171).
AAD and APOI as Parts of APS1
Prevalence of APS1-Associated AAD and APOI
The prevalence of AAD in APS2 and APS3 is, by definition, 100 and 0%, respectively. Regarding the prevalence of AAD in APS1, it should be noted that, prior to the APS classification (3–5), patients with APS1 are either described in case reports or grouped together with subjects suffering from other diseases. Consequently, a comprehensive overview of these APS1 cases is missing. Thanks to the collection of hundreds of scientific articles covering the whole relevant literature and considering the utmost care to avoid counting each patient more than once; the Authors reviewed 282 certain or highly probable APS1 cases reported till 1980. Personal data and clinical course of each patient and related references are listed in Supplementary Material. Any further information can be requested by e-mail to the corresponding author.
As shown in Table 1, AAD has a prevalence of ~57%, with no gender preponderance. Moreover, the mean age of the patients at disease onset was comparable: just under 12 years in males, and just over 11 years in female patients. AAD, in the form of acute adrenal failure neither readily recognized nor effectively treated, was a significant cause of death, starting with the little girl whose clinical course and autopsy findings were detailed by Ostertag (172).
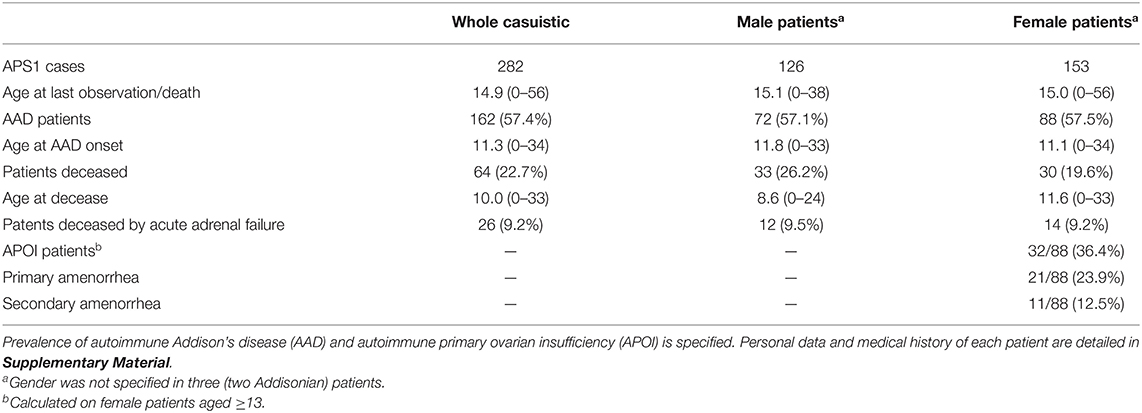
Table 1. Summary of data from 282 confirmed or highly probable cases of autoimmune polyglandular syndrome type 1 (APS1) reported up until 1980.
Among Finnish patients with APS1, who represent a reference cohort in terms of number, genetic homogeneity, data centralization, and serial updates (8, 173–180), AAD reaches an incidence of 84%. To retrieve the data, Finnish researchers estimate the onset of each APS1 component over fixed age ranges, assuming that all patients live up to 50 years old. As of the 2006 update, no AAD cases were reported up to a patient age of 2 years, while AAD rate increased to 8, 40, and 65% in the age ranges 2–5, 5–10, and 10–15, respectively (180).
Ferre et al. calculated the incidence of APS1 components in American (mostly the US) patients in the same way and obtained consistent percentages for AAD (16). Again, AAD had a prevalence of 73 and 67% in two major reviews of 41 and 112 patients with APS1 from Northern Italy and Russia, respectively (9, 10).
In Table 2, the prevalence of AAD and APOI in various APS1 patient cohorts is reported, with the specification of their ethnic backgrounds (15, 144, 153, 181–197). AAD prevalence can sometimes be influenced by the mean patient age, for example, in Saudi patients with APS1 described by Bin-Abbas et al. (193). Conversely, the extent of follow-up suggests that the low prevalence of AAD in Iranian-Jewish patients with APS1 does not recognize an age-related factor (144).
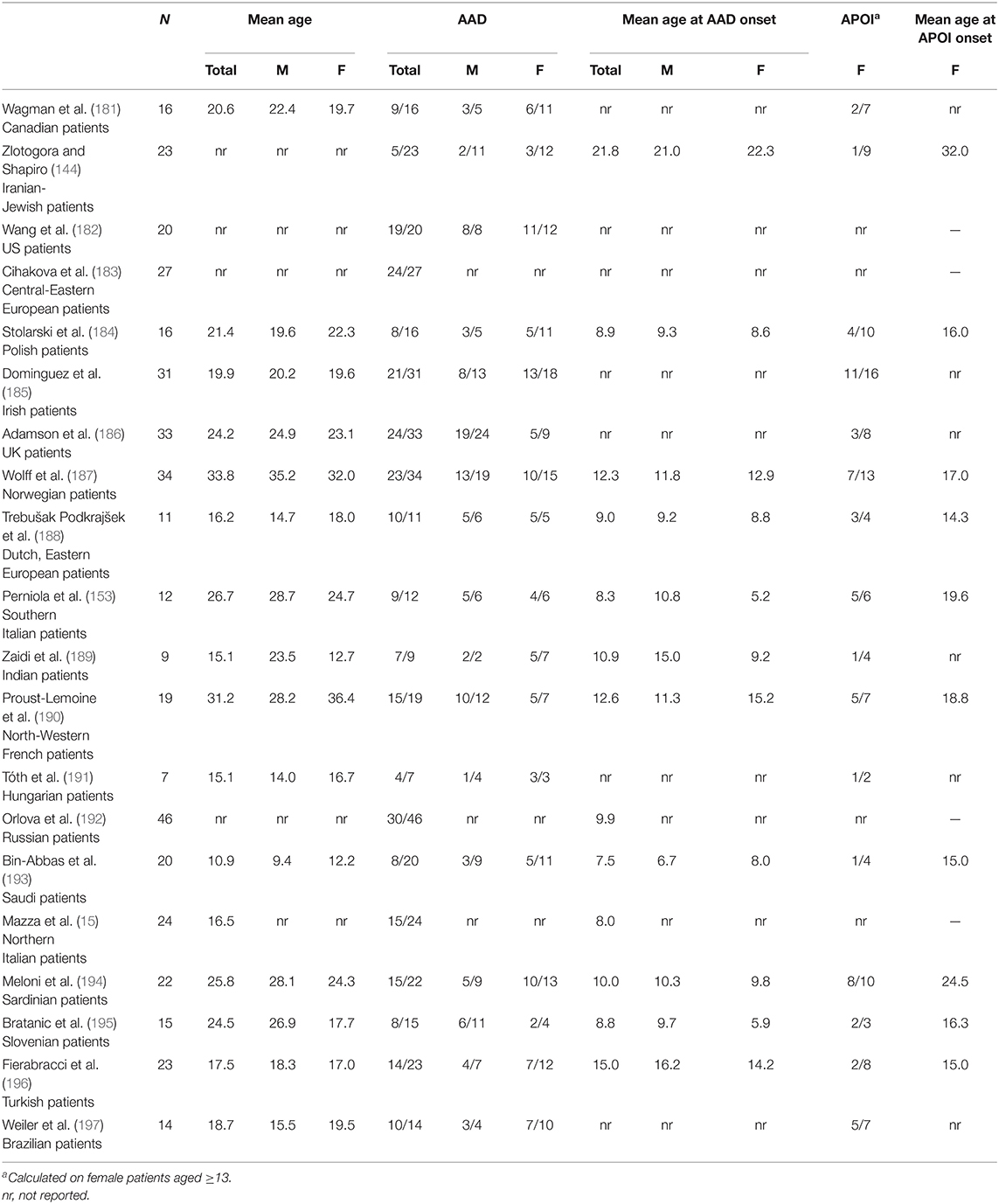
Table 2. Prevalence of autoimmune Addison's disease (AAD) and autoimmune primary ovarian insufficiency (APOI) in cohorts of autoimmune polyglandular syndrome type-1 (APS1) patients, with specification of their ethnic background.
Closely related to the mechanisms involved in AAD, a large percentage of patients with APS1 suffer from primary hypogonadism, with a privileged gender association. APS1 women have been included in APOI studies (198, 199), and APS1 has become a well-known condition causing this disease (200–218). Recently, the clinical and immunological features of APS1-associated APOI have been detailed in the Finnish cohort of APS1 women (219).
As shown in Table 2, APOI approaches or exceeds AAD prevalence in some APS1 patient cohorts. Surprisingly, Adamson et al. reported that 6 out of 19 UK APS1 male patients had gonadal insufficiency, but the criteria adopted to satisfy this diagnosis were not reported (186). Similar findings were outlined in Slovenian patients with APS1, but again the diagnostic criteria were not specified (195).
Clinical History and Particular Issues
Irvine and Barnes observed a bimodal age distribution at AAD onset; the first peak emerged at the end of the first decade and involved the majority of HPT patients. A later peak (fifth decade) mainly affected women with T1D, ATD, or both (220). In subsequent years, long-term observation of large AAD cohorts confirmed that the disease onset occurs at an early age only in patients with APS1 (221–227). Conversely, more recent nationwide AAD studies have purposely excluded patients with APS1, emphasizing the difference between monogenic and multifactorial pathogenesis (228, 229).
Furthermore, the prediction of AAD onset in patients with APS1 with related humoral autoimmunity has contributed to characterize the biochemical and clinical stages of the disease. Damage typically begins in the zona glomerulosa and causes impaired mineralocorticoid secretion and increased plasma renin activity. The subsequent involvement of the zona fasciculata has been divided into three stages of hypocortisolism: subnormal cortisol response after adrenocorticotropic-hormone (ACTH) stimulation test, persistent ACTH increase, and decrease in basal cortisol level, respectively (230–235).
Replacement therapy is unable to reproduce the natural hormone pulse, so the treatment of AAD is a challenge in itself and carries risks of suboptimal or excessive dosage (236). In APS1, the problem is accentuated by the coexistence of other hormonal deficits, with particular reference to HPT and ATD. Untreated AAD masks the early stages of HPT, as the hypercalciuric and hypocalcemic effects of glucocorticoids wear off. By the same principle, AAD replacement therapy can induce hypocalcemic seizures in patients with APS1 with subclinical HPT (237, 238). Fortunately, HPT treatment mitigates the negative impact of glucocorticoids on bone health (239).
Again, it is important to remember that, regardless of the underlying disease, untreated AAD can cause reversible thyroid dysfunction (240–242). Co-occurrence of AAD and ATD should caution when initiating thyroid replacement therapy due to the risk of raising the basal metabolic rate and precipitating an adrenal crisis.
Patients with APS1 have been included in AAD therapeutic trials (243–245), and special attention is paid to them in consensus statements on diagnosis, treatment, and follow-up (246).
APS1-Associated AAD and APOI: Humoral Immunity
Although in statistical terms AAD is the third component of APS1 triad, it has received the best immunological characterization; it is probably accepted that APS1 studies have made a substantial contribution to the delineation of the pathogenetic and immunological aspects of AAD.
The Founding Studies
Antibodies to adrenal cortex (AC-Abs) were first demonstrated by complement fixation (CF) in sera from patients with sporadic or APS2-associated AAD (247). Sera from unselected patients with APS1 were included in subsequent studies using both CF and indirect immunofluorescence (IIF) (248–255). The cell cytoplasm of the adrenal layers was stained in positive samples (248); the mitochondrial and microsomal fractions of the tissue extracts retained the antigenic properties, since pre-absorption with them inhibited the reaction (249, 253). In addition, AAD sera from the patients with associated HPT, CMC, or both contained other antibody specificities, such as those to thyroid (248, 249, 252, 254), salivary gland ducts (249), gastric parietal cells and intrinsic factor (250, 252, 254), parathyroid glands (251, 255), and liver (252).
In parallel studies, the same methods were used to test for antibodies to steroid-producing cells (StC-Abs). Five out of 77 AAD sera reacted against granulosa and theca interna cells of Graafian follicles, luteal cells, ovarian interstitial cells, Leydig cells, and placental syncytiotrophoblasts; three of these sera belonged to APS1 women with coexistent APOI (256, 257). A similar result was obtained with serum from a male patient with APS1 (258). Moreover, StC-Ab-positive sera from patients with APS1 showed in vitro cytotoxicity on granulosa cells of the Graafian follicle (259). The above-mentioned results were confirmed by subsequent studies (260–262).
Pre-absorption with adrenal and gonadal extracts reduced or abolished the antibody titer, leading researchers to infer that the self-antigens shared by the adrenal cortex and steroid-producing extra-adrenal tissues were related to steroid pathway enzymes (256–258, 262).
Antibodies to germline cells were also detected in some patients with APS1 (256, 257, 260, 263), following the studies of Vallotton and Forbes, who found them in patients with gonadal dysgenesis (264–266).
Unlike Anglo-American patients in the 1970s, Finnish patients with APS1 had already been grouped into a distinct disease entity called moniliasis-polyendocrinopathy syndrome; precipitating AC-Abs were detected in the serum of these patients by gel diffusion (267, 268). The association appeared to be restricted to APS1-associated AAD (269). Two specific adrenal antigens were targeted, one named P (particulate) and precipitating in the mitochondrial fraction, and the other named S (soluble) since it was present in all subcellular fractions; the latter contained a variety of determinants, partly common to human sera and sera from other species (270, 271).
Antibodies to CYP Enzymes
In the mid-1980s, genes encoding for the above-mentioned CYP enzymes were identified and cloned (272–274). CYP21 was soon identified as the major self-antigen target of adult-onset, either sporadic or APS2-associated AAD; generally, AC-Abs were searched for by IIF; the assay was followed by Western blot (WB) on tissue fractions separated by gel electrophoresis (275–277). Data were confirmed by immunoprecipitation (IP) of 35S-labeled-cell lysates from human adrenal cells (275) and by the reaction of human antibodies to recombinant CYP21 (CYP21-Abs) expressed in Saccharomyces cerevisiae (276, 277).
In contrast, the definition of adrenal autoimmunity in APS1 was delayed by contradicting data. For the first time, CYP17 was identified as the self-antigen recognized by precipitating AC-Abs of APS1 sera (278). In another study, while CYP21-Abs were found in sera from non-APS1 AAD patients as indicated, the serum from the only patient with APS1 stained testis Leydig cells and targeted CYPscc (275). Further analysis of APS1 sera confirmed these results (279, 280). Thus, APS1 sera appeared to react against either CYP17 or CYPscc, while CYP21 was thought to represent an exclusive target of autoimmunity in sporadic and APS2-associated AAD (281, 282).
Finally, Uibo et al., using WB on Escherichia coli lysates, which expressed recombinant CYP fragments, stated that all three enzymes are self-antigens in APS1 (283). Furthermore, the absorption studies were congruent with tissue distribution: since CYP21 is restricted to the adrenal cortex, CYP17 is also represented in the gonads, and the ubiquitous CYPscc is also found in the placenta, only incubation with adrenal homogenates could completely abolish reactivity. Ovarian and testis homogenates absorbed reactivity against CYPScc and CYP17, while placental homogenates selectively absorbed that against CYPScc (281). Several subsequent studies have confirmed the strong association (60–100% of cases) of adrenal autoimmunity, any form of AAD included, with AC-Abs and CYP21-Abs (284–293).
In contrast, StC-Abs and antibodies to CYP17 and CYPscc (CYP17-Abs and CYPscc-Abs, respectively) can be found in a limited number (5–42%) of sporadic or APS2-associated AAD cases and mainly identified in APOI women (286, 287, 292–295). As shown in Table 3, their prevalence is significantly higher in patients with APS1 (16, 152, 183, 187, 194, 283, 287, 296–298).
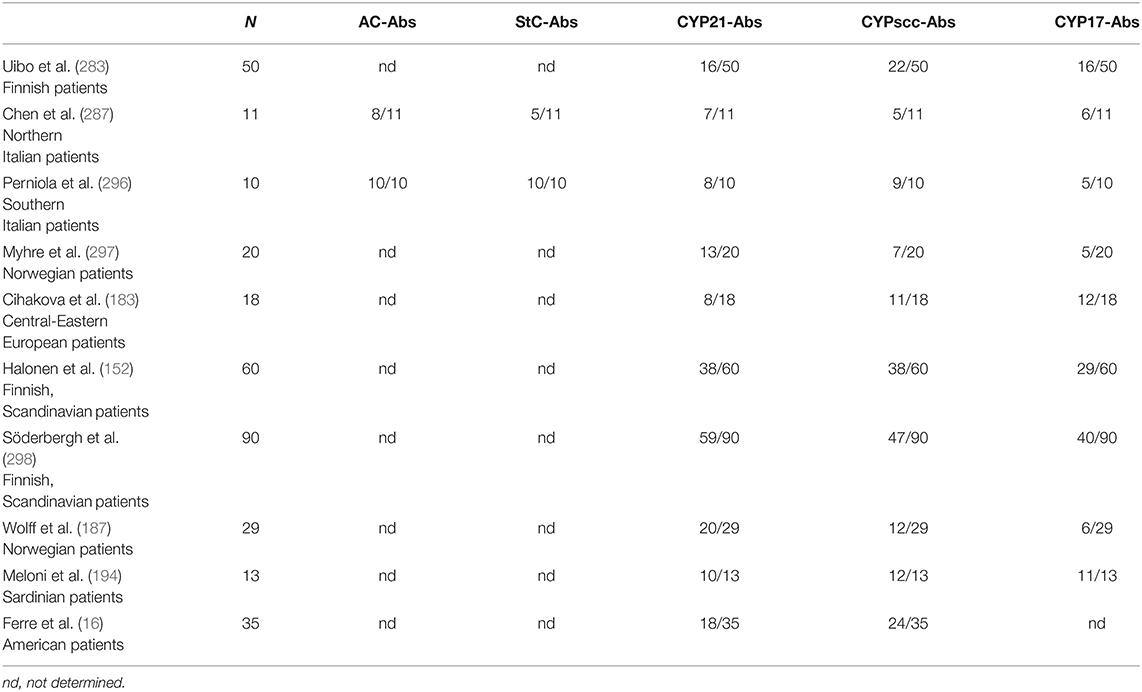
Table 3. Fraction of sera positive for antibodies to adrenal cortex (AC-Abs), steroid-producing cells (StC-Abs), cytochrome-P450 21-hydroxylase (CYP21-Abs), cytochrome-P450 cholesterol side-chain cleavage enzyme (CYPscc-Abs), and cytochrome-P450 17α-hydroxylase/17,20 lyase (CYP17-Abs) in cohorts of autoimmune polyglandular syndrome type-1 (APS1) patients, with specification of their ethnic background.
The same results have been achieved starting from the opposite point of view, i.e., extrapolating from patients with various autoimmune diseases, precisely those with gonadal insufficiency. Furthermore, gonadal autoimmunity markers prevail in APS1 and APS2 APOI women, while the percentage of sera positive for StC-Abs and CYPscc-Abs/CYP17-Abs falls in women with non-AAD-associated APOI, and in patients with other autoimmune diseases (299–302).
Lastly, in a minority of APS1 sera, it is possible to find antibodies against members of other steroid pathways; this is the case of antibodies to an enzyme belonging to the hydroxysteroid dehydrogenase (HSD) family, namely 3βHSD (303).
Concordance Between IIF and Antibodies to CYP Enzymes
Comparing IIF, a tool normally available in laboratory medicine, and the assays used to detect antibodies to CYP enzymes, which are generally reserved for experimental settings, presumably contains the most useful information for clinical purposes. Due to the selective synthesis of CYP21 in the adrenal cortex, AC-Abs and CYP21-Abs have a close relationship that produces very high correlation coefficients, regardless of the analytical methods (284, 285, 289, 290, 292, 293).
Progressive depletion of self-antigen source causes antibody titers to show an inverse correlation with disease duration (285, 291). However, the sensitivity of the analytical methods makes CYP21-Abs more reliable than AC-Abs in long-lasting AAD (more than 15–20 years of duration) (288). In other studies, CYP21-Abs neither correlate with AAD duration (286) nor are they prevalent over AC-Abs (293).
In relation to the tissue distribution of CYPs, StC-Abs, routinely tested on ovary and testis specimens, are associated with positivity for CYPscc-Abs, CYP17-Abs, or both; again, this relationship does not differ among the subcohorts of AAD patients (287, 293).
Predictive Role of AC-Abs, StC-Abs, and Antibodies to CYP Enzymes
In most cases, AC-Abs and CYP21-Abs anticipate the onset of AAD, and their predictive role is higher in patients with APS1 (233, 304). The same is true for StC-Abs, though the overlap of AC-Abs and StC-Abs creates a phenomenon of statistical redundancy in univariate analyses (233).
The above-mentioned results are more relevant when adding patients with other diseases. An Italian research group followed adult and pediatric patients suffering from various autoimmune diseases and divided according to AC-Ab positivity: while only three patients with APS1 were included in the group of adult subjects (one only being AC-Ab-positive and acquiring overt AAD at follow-up), all but one AC-Ab-positive children enrolled in the latter group were patients with APS1 (305, 306).
In a reappraisal of these studies, the same research group observed that, among the parameters at highest risk of acquiring overt AAD in AC-Ab-positive patients, was an impaired adrenal function at enrollment, the simultaneous presence of idiopathic HPT, CMC, or both, pediatric age, and a high titer of AC-Abs and CYP21-Abs. In particular, the cumulative risk of developing AAD at 11 years of age was 100% in patients with APS1, a much higher percentage than in patients with other autoimmune and non-autoimmune diseases (307, 308).
On the other hand, StC-Abs play a prominent role in predicting APOI in APS1 women, but the statistical power is slightly lower than that achieved by AC-Abs (and StC-Abs themselves) in predicting AAD (231). Interestingly, measurement of AC-Abs and CYP21-Abs in women with sporadic APOI may be important in identifying patients at risk of acquiring overt AAD (299).
Epitope Targeting
A first determination of the epitopes recognized by CYP21-Abs tested only sporadic and APS2-associated AAD sera (309). A subsequent study showed that the middle region of CYP21 (CYP21164−356) retained antigenic sites, and sera from patients with APS1 reacted not unlike those from patients with other AAD forms (310). The use of CYP21 fragments and mouse monoclonal antibodies emphasized the antigenic relevance of the middle and carboxyl-terminal regions of CYP21 and resulted in the detection of two short amino-acid stretches (CYP21335−339 and CYP21406−411) as the main epitopes independently from patient disease (sporadic AAD, APS1, APS2, and isolated CYP21-Ab positivity) (311, 312). The same is true for CYPscc epitopes (313).
Due to the similarity between the antigenic targets, it was suggested that CYP17-Abs and CYP21-Abs have immunological cross-reactivity (314), but subsequent studies in sera from patients with APS1 disproved this hypothesis (315, 316).
Finally, it has been shown that antibodies to CYP enzymes belong mainly to the IgG1 subclass in patients with APS1, indicating a predominant Th1 response (317). However, IgG4 isotype specificity identifies a small subset of patients with Th2-oriented response (318).
Other Antibody Specificities and Proteomics
Adrenal antibody specificities other than those related to steroid pathways have been occasionally described in patients with APS1: Kendall-Taylor et al. found IgG-class antibodies that antagonized ACTH action in an APS1 girl with AAD (319).
The view is somewhat richer if we look at the gonadal self-antigens; testis-specific protein 10 (TSGA10) was identified by immunoscreening of testis and pituitary cDNA expression library with sera from patients with APS1, but no clinical phenotype correlated with antibody positivity (320, 321).
In turn, based on the observation that the maternal antigen that the embryo requires (Mater) acts as an ovarian target of autoimmunity in an early thymectomy mouse model (322, 323), Brozzetti et al. searched for antibodies to the leucine-rich repeat protein 5 (NALP5), which represents the corresponding human self-antigen. Interestingly, antibody positivity involved the majority of patients with APS1 and included a number of patients with non-APS1-associated AAD, APOI, or both (324).
Currently, proteomics appears to be capable of capturing the enormous biodiversity of potential targets and offers an undisputable advantage over previous candidate-based approaches (325). Landegren et al. used sera from patients with APS1 to probe proteome arrays containing thousands of full-length human proteins. Together with established autoimmunity targets, they detected melanoma-associated antigen B2 (MAGEB2) and disulfide isomerase-like protein of the testis (PDILT) as gonadal self-antigens (326).
More recently, Vazquez et al., employing a high-throughput, proteome-wide phage display method, identified some novel self-antigens targeted by APS1 sera. No adrenal targets were included but, in turn, antibodies to the ovarian KH-domain-containing 3-like protein (KHDC3L), which form an oocyte-specific critical complex with NALP5, were involved in APS1-associated APOI (327).
APS1-Associated AAD: Cellular Immunity
Although antibodies to CYP enzymes play a prominent role in indicating the nature, antigenic targets and, especially in the APS1-associated form, the time interval before the clinical onset of AAD, it is well-known that cellular immunity is responsible for organ damage (328). Even circulating T-lymphocytes show clear signs of activation in the early stage of the disease (16, 185, 329–333). Further evidence is provided by the (rare) animal models of autoimmune adrenalitis (334).
Findings from patients with APS1 strongly support this belief—starting from the cited study of Ostertag (172), autopsy samples of patients with Addisonian APS1 show massive infiltration of the adrenal cortex by mononuclear cells; with time, gross atrophy of the gland is evident, characterized by the replacement of the adrenal layers with connective tissue. The medulla is usually spared (335–340). As expected, a variable degree of adrenal inflammation is frequently found in patients with APS1 who had not yet reached a symptomatic stage, an uncommon feature of autopsy, if present, in the general population (341).
Interestingly, the delineation of CYP21 epitopes benefited from interferon-γ (IFNγ, a type-2 IFN) assay in cultures of peripheral blood mononuclear cells challenged with several CYP21 fragments: some patients with APS1 carrying the MHCI HLA-B35 allele were included in these studies. A CYP21431−450 fragment stimulated lymphocytes from non-APS1 AAD patients, while those from two APS1 siblings reacted against CYP131−150. Lymphocytes from the third patient with APS1 did not proliferate, presumably due to long-lasting AAD (342, 343).
Conclusions
Several key issues need to be clarified in the relationship between the loss of self-tolerance resulting from AIRE/Aire deficiency and related organ failures. In particular, the non-dependence on AIRE of the main adrenal self-antigens in the human thymus raises questions about the intrinsic mechanisms that trigger autoimmunity in APS1. Dissection of these aspects can help plan suitable strategies for causal therapy.
Meanwhile, a look at the history of APS1 and APS1-associated AAD confirms the challenge that such disease implies—interpolation between AAD and other endocrine failures, mainly HPT and ATD, amplifies the risks and benefits of replacement therapies and highlights the need for proper use of mineralo- and glucocorticoids.
Above all, correct management of specific laboratory tests can identify early markers of adrenal impairment and avoid pitfalls and dangers deriving from the onset and stabilization of one of the most insidious APS1 components.
Author Contributions
RP revised APS1 case reports and cohort studies, detailed AIRE properties and drew up the manuscript. AFi described APS1 clinical picture and examined the genotype/phenotype relationship. AFa dissected the immunological aspects of APS1. All authors contributed to the article and approved the submitted version.
Conflict of Interest
The authors declare that the research was conducted in the absence of any commercial or financial relationships that could be construed as a potential conflict of interest.
Supplementary Material
The Supplementary Material for this article can be found online at: https://www.frontiersin.org/articles/10.3389/fimmu.2021.606860/full#supplementary-material
Abbreviations
AAD, autoimmune Addison's disease; AC-Abs, adrenal-cortex antibodies; ACTH, adrenocorticotropic hormone; AIRE/Aire, autoimmune regulator; APECED, autoimmune polyendocrinopathy-candidiasis-ectodermal dystrophy; APOI, autoimmune primary ovarian insufficiency; APS, autoimmune polyglandular syndrome; ATD, autoimmune thyroid disease; CARD, caspase-activation and recruitment domain; CMC, chronic mucocutaneous candidiasis; CF, complement fixation; CYP, cytochrome P450; CYP17, CYP 17α-hydroxylase/17,20 lyase; CYP17-Abs, antibodies to CYP17; CYP21, CYP 21-hydroxylase; CYP21-Abs, antibodies to CYP21; CYP11A1/CYPscc, CYP cholesterol side-chain cleavage enzyme; CYPscc-Abs, antibodies to CYPscc; DNA-PK, DNA-activated protein kinase; DNA-TOP, DNA topoisomerase; HPT, hypoparathyroidism; HSD, hydroxysteroid dehydrogenase; IFN, interferon; IIF, indirect immunofluorescence; IP, immunoprecipitation; KHDC3L, KH-domain-containing 3-like protein; MAGEB2, melanoma-associated antigen B2; Mater, maternal antigen that embryo requires; MHC, major histocompatibility complex; NALP5, leucine-rich-repeat protein 5; NLS, nuclear localization signal; PDILT, disulfide isomerase-like protein of the testis; PHD, plant-homeodomain (zinc finger); P-TEFb, positive transcriptional elongation factor b; SAND, Sp100-AIRE-NucP41/75-Deaf-1; StC-Abs, steroid-cell antibodies; T1D, type-1 diabetes; TEC, thymic epithelial cell; TEPC, thymic epithelial progenitor cell; TSGA10, testis-specific protein 10; WB, Western blot.
References
1. Witebsky E, Rose NR, Terplan K, Paine JR, Egan RW. Chronic thyroiditis and autoimmunization. J Am Med Assoc. (1957) 164:1439–47. doi: 10.1001/jama.1957.02980130015004
2. Rose NR, Bona C. Defining criteria for autoimmune diseases (Witebsky's postulates revisited). Immunol Today. (1993) 14:426–30. doi: 10.1016/0167-5699(93)90244-F
3. Neufeld M, Maclaren N, Blizzard R. Autoimmune polyglandular syndromes. Pediatr Ann. (1980) 9:154–62.
5. Neufeld M, Maclaren NK, Blizzard RM. Two types of autoimmune Addison's disease associated with different polyglandular autoimmune (PGA) syndromes. Medicine (Baltimore). (1981) 60:355–62. doi: 10.1097/00005792-198109000-00003
6. Nagamine K, Peterson P, Scott HS, Kudoh J, Minoshima S, Heino M, et al. Positional cloning of the APECED gene. Nat Genet. (1997) 17:393–8. doi: 10.1038/ng1297-393
7. Finnish-German APECED Consortium. An autoimmune disease, APECED, caused by mutations in a novel gene featuring two PHD-type zinc-finger domains. Nat Genet. (1997) 17:399–403. doi: 10.1038/ng1297-399
8. Ahonen P, Myllärniemi S, Sipilä I, Perheentupa J. Clinical variation of autoimmune polyendocrinopathy-candidiasis-ectodermal dystrophy (APECED) in a series of 68 patients. N Engl J Med. (1990) 322:1829–36. doi: 10.1056/NEJM199006283222601
9. Betterle C, Greggio NA, Volpato M. Autoimmune polyglandular syndrome type 1. J Clin Endocrinol Metab. (1998) 83:1049–55. doi: 10.1210/jcem.83.4.4682
10. Orlova EM, Sozaeva LS, Kareva MA, Oftedal BE, Wolff ASB, Breivik L, et al. Expanding the phenotypic and genotypic landscape of autoimmune polyendocrine syndrome type 1. J Clin Endocrinol Metab. (2017) 102:3546–56. doi: 10.1210/jc.2017-00139
11. Buzi F, Badolato R, Mazza C, Giliani S, Notarangelo LD, Radetti G, et al. Autoimmune polyendocrinopathy-candidiasis-ectodermal dystrophy syndrome: time to review diagnostic criteria? J Clin Endocrinol Metab. (2003) 88:3146–8. doi: 10.1210/jc.2002-021495
12. Meager A, Visvalingam K, Peterson P, Möll K, Murumägi A, Krohn K, et al. Anti-interferon autoantibodies in autoimmune polyendocrinopathy syndrome type 1. PLoS Med. (2006) 3:1152–64. doi: 10.1371/journal.pmed.0030289
13. Oftedal BE, Bøe Wolff AS, Bratland E, Kämpe O, Perheentupa J, Myhre AG, et al. Radioimmunoassay for autoantibodies against interferon omega; its use in the diagnosis of autoimmune polyendocrine syndrome type I. Clin Immunol. (2008) 129:163–9. doi: 10.1016/j.clim.2008.07.002
14. Meloni A, Furcas M, Cetani F, Marcocci C, Falorni A, Perniola R, et al. Autoantibodies against type I interferons as an additional diagnostic criterion for autoimmune polyendocrine syndrome type I. J Clin Endocrinol Metab. (2008) 93:4389–97. doi: 10.1210/jc.2008-0935
15. Mazza C, Buzi F, Ortolani F, Vitali A, Natarangelo LD, Weber G, et al. Clinical heterogeneity and diagnostic delay of autoimmune polyendocrinopathy-candidiasis-ectodermal dystrophy syndrome. Clin Immunol. (2011) 139:6–11. doi: 10.1016/j.clim.2010.12.021
16. Ferre EMN, Rose SR, Rosenzweig SD, Burbelo PD, Romito KR, Niemela JE, et al. Redefined clinical features and diagnostic criteria in autoimmune polyendocrinopathy-candidiasis-ectodermal dystrophy. JCI Insight. (2016) 1:e88782. doi: 10.1172/jci.insight.88782
17. Bennett IL Jr, Harvey AM, Carpenter CCJ, Solomon N, Silberberg SG, Bledsoe T, et al. Schmidt's syndrome (thyroid and adrenal insufficiency): a review of the literature and a report of fifteen new cases including ten instances of coexistent diabetes mellitus. Trans Am Clin Climatol Assoc. (1964) 75:27–36.
18. Lechuga-Gomez EE, Meyerson J, Bigazzi PE, Walfish PG. Polyglandular autoimmune syndrome type II. CMAJ. (1988) 138:632–4.
19. Betterle C, Volpato M, Greggio AN, Presotto F. Type 2 polyglandular autoimmune disease (Schmidt's syndrome). J Pediatr Endocrinol Metab. (1996) 9(S1):113–23. doi: 10.1515/jpem.1996.9.s1.113
20. Betterle C, Zanchetta R. Update on autoimmune polyendocrine syndromes (APS). Acta Biomed. (2003) 74:9–33.
21. Trence DL, Morley JE, Handwerger BS. Polyglandular autoimmune syndromes. Am J Med. (1984) 77:107–16. doi: 10.1016/0002-9343(84)90444-3
22. Meyerson J, Lechuga-Gomez EE, Bigazzi PE, Walfish PG. Polyglandular autoimmune syndrome: current concepts. CMAJ. (1988) 138:605–12.
24. Obermayer-Straub P, Manns MP. Autoimmune polyglandular syndromes. Baillieres Clin Gastroenterol. (1998) 12:293–315. doi: 10.1016/s0950-3528(98)90136-1
25. Eisenbarth GS, Gottlieb PA. Autoimmune Polyendocrine Syndromes. N Engl J Med. (2004) 350:2068–79. doi: 10.1056/NEJMra030158
26. Kahaly GJ. Polyglandular autoimmune syndromes. Eur J Endocrinol. (2009) 161:11–20. doi: 10.1530/EJE-09-0044
27. Michels AW, Gottlieb PA. Autoimmune polyglandular syndromes. Nat Rev Endocrinol. (2010) 6:270–7. doi: 10.1038/nrendo.2010.40
28. Frommer L, Kahaly GJ. Autoimmune polyendocrinopathy. J Clin Endocrinol Metab. (2019) 104:4769–82. doi: 10.1210/jc.2019-00602
29. Husebye ES, Anderson MS, Kämpe O. Autoimmune polyendocrine syndromes. N Engl J Med. (2018) 378:2543–4. doi: 10.1056/NEJMc1805308
30. Eisenbarth G, Wilson P, Ward F, Lebovitz HE. HLA type and occurrence of disease in familial polyglandular failure. N Engl J Med. (1978) 298:92–4. doi: 10.1056/NEJM197801122980209
31. Farid NR, Larsen B, Payne R, Noel EP, Sampson L. Polyglandular autoimmune disease and HLA. Tissue Antigens. (1980) 16:23–9. doi: 10.1111/j.1399-0039.1980.tb00284.x
32. Muir A, She JX. Advances in the genetics and immunology of autoimmune polyglandular syndrome II/III and their clinical applications. Ann Med Interne (Paris). (1999) 150:301–12.
33. Robles DT, Fain PR, Gottlieb PA, Eisenbarth GS. The genetics of autoimmune polyendocrine syndrome type II. Endocrinol Metab Clin North Am. (2002) 31:353–68. doi: 10.1016/s0889-8529(01)00015-9
34. Dittmar M, Kahaly GJ. Polyglandular autoimmune syndromes: immunogenetics and long-term follow-up. J Clin Endocrinol Metab. (2003) 88:2983–92. doi: 10.1210/jc.2002-021845
35. Falorni A, Brozzetti A, Perniola R. From genetic predisposition to molecular mechanisms of autoimmune primary adrenal insufficiency. Front Horm Res. (2016) 46:115–32. doi: 10.1159/000443871
36. Riley WJ, Maclaren NK. Autoimmune adrenal disease. In: Laron Z, editor. Pediatric and Adolescent Endocrinology. Vol. 13: Adrenal Diseases in Childhood. Basel: Karger (1984). p. 162–72.
37. Muir A, Schatz DA, Maclaren NK. Autoimmune Addison's disease. Springer Semin Immunopathol. (1993) 14:275–84. doi: 10.1007/BF00195978
38. Vaidya B, Pearce S, Kendall-Taylor P. Recent advances in the molecular genetics of congenital and acquired primary adrenocortical failure. Clin Endocrinol (Oxf). (2000) 53:403–18. doi: 10.1046/j.1365-2265.2000.01116.x
39. Peterson P, Uibo R, Krohn KJE. Adrenal autoimmunity: results and developments. Trends Endocrinol Metab. (2000) 11:285–90. doi: 10.1016/s1043-2760(00)00283-6
40. Betterle C, Dal Pra C, Mantero F, Zanchetta R. Autoimmune adrenal insufficiency and autoimmune polyendocrine syndromes: autoantibodies, autoantigens, and their applicability in diagnosis and disease prediction. Endocr Rev. (2002) 23:327–64. doi: 10.1210/edrv.23.3.0466
41. Arlt W, Allolio B. Adrenal insufficiency. Lancet. (2003) 361:1881–93. doi: 10.1016/S0140-6736(03)13492-7
42. Hatano O, Takakusu A, Nomura M, Morohashi K. Identical origin of adrenal cortex and gonad revealed by expression profiles of Ad4BP/SF-1. Genes Cells. (1996) 1:663–71. doi: 10.1046/j.1365-2443.1996.00254.x
43. Aaltonen J, Björses P, Sandkuijl L, Perheentupa J, Peltonen L. An autosomal locus causing autoimmune disease: autoimmune polyglandular disease type I assigned to chromosome 21. Nat Genet. (1994) 8:83–7. doi: 10.1038/ng0994-83
44. Björses P, Aaltonen J, Vikman A, Perheentupa J, Ben-Zion G, Chiumello G, et al. Genetic homogeneity of autoimmune polyglandular disease type I. Am J Hum Genet. (1996) 59:879–86.
45. Aaltonen J, Horelli-Kuitunen N, Fan JB, Björses P, Perheentupa J, Myers R, et al. High-resolution physical and trascriptional mapping of the autoimmune polyendocrinopathy-candidiasis-ectodermal dystrophy locus on chromosome 21q22.3 by FISH. Genome Res. (1997) 7:820–9. doi: 10.1101/gr.7.8.820
46. Mittaz L, Rossier C, Heino M, Peterson P, Krohn KJE, Gos A, et al. Isolations and characterization of the mouse Aire gene. Biochem Biophys Res Commun. (1999) 255:483–90. doi: 10.1006/bbrc.1999.0223
47. Blechschmidt K, Schweiger M, Wertz K, Poulson R, Christensen HM, Rosenthal A, et al. The mouse Aire gene: comparative genomic sequencing, gene organization, and expression. Genome Res. (1999) 9:158–66. doi: 10.1101/gr.9.2.158
48. Wang CY, Shi JD, Davoodi-Semiromi A, She JX. Cloning of Aire, the mouse homologue of the autoimmune regulator (AIRE) gene responsible for autoimmune polyglandular syndrome type 1 (APS1). Genomics. (1999) 55:322–6. doi: 10.1006/geno.1998.5656
49. Eldershaw SA, Sansom DM, Narendran P. Expression and function of the autoimmune regulator (Aire) gene in non-thymic tissue. Clin Exp Immunol. (2011) 163:296–308. doi: 10.1111/j.1365-2249.2010.04316.x
50. Perniola R. Expression of the autoimmune regulator gene and its relevance to the mechanisms of central and peripheral tolerance. Clin Dev Immunol. (2012) 2012:e207403. doi: 10.1155/2012/207403
51. Zhao B, Chang L, Fu H, Sun G, Yang W. The role of autoimmune regulator (AIRE) in peripheral tolerance. J Immunol Res. (2018) 2018:e3930750. doi: 10.1155/2018/3930750
52. Heino M, Peterson P, Kudoh J, Nagamine K, Lagerstedt A, Ovod V, et al. Autoimmune regulator is expressed in the cells regulating immune tolerance in thymus medulla. Biochem Biophys Res Commun. (1999) 257:821–5. doi: 10.1006/bbrc.1999.0308
53. Heino M, Peterson P, Sillanpää N, Guérin S, Wu L, Anderson G, et al. RNA and protein expression of the murine autoimmune regulator gene (Aire) in normal, RelB-deficient and in NOD mouse. Eur J Immunol. (2000) 30:1884–93. doi: 10.1002/1521-4141(200007)30:7<1884::AID-IMMU1884>3.0.CO;2-P
54. Bennett AR, Farley A, Blair NF, Gordon J, Sharp L, Blackburn CC. Identification and characterization of thymic epithelial progenitor cells. Immunity. (2002) 16:803–14. doi: 10.1016/S1074-7613(02)00321-728
55. Gill J, Malin M, Holländer GA, Boyd R. Generation of a complete thymic micro-environment by MTS24+ epithelial cells. Nat Immunol. (2002) 3:635–42. doi: 10.1038/ni812
56. Rossi SW, Jenkinson WE, Anderson G, Jenkinson EJ. Clonal analysis reveals a common progenitor for thymic cortical and medullary epithelium. Nature. (2006) 441:988–91. doi: 10.1038/nature04813
57. Bleul CC, Corbeaux T, Reuter A, Fisch P, Mönting JS, Boehm T. Formation of a functional thymus initiated by a postnatal epithelial progenitor cell. Nature. (2006) 441:992–6. doi: 10.1038/nature04850
58. Rossi SW, Chidgey AP, Parnell SM, Jenkinson WE, Scott HS, Boyd RL, et al. Redefining epithelial progenitor potential in the developing thymus. Eur J Immunol. (2007) 37:2411–8. doi: 10.1002/eji.200737275
59. Shakib S, Desanti GE, Jenkinson WE, Parnell SM, Jenkinson EJ, Anderson G. Checkpoints in the development of thymic cortical epithelial cells. J Immunol. (2009) 182:130–7. doi: 10.4049/jimmunol.182.1.130
60. Baik S, Jenkinson EJ, Lane PJL, Anderson G, Jenkinson WE. Generation of both cortical and Aire+ medullary thymic epithelial compartments from CD205+ progenitors. Eur J Immunol. (2013) 43:589–94. doi: 10.1002/eji.201243209
61. Ohigashi I, Zuklys S, Sakata M, Mayer CE, Zhanybekova S, Murata S, et al. Aire-expressing thymic medullary epithelial cells originate from β5t-expressing progenitor cells. Proc Natl Acad Sci USA. (2013) 110:9885–90. doi: 10.1073/pnas.1301799110
62. Mayer CE, Žuklys S, Zhanybekova S, Ohigashi I, Teh HY, Sansom SN, et al. Dynamic spatio-temporal contribution of single β5t+ cortical epithelial precursors to the thymus medulla. Eur J Immunol. (2016) 46:846–56. doi: 10.1002/eji.201545995
63. Alves NL, Takahama Y, Ohigashi I, Ribeiro AR, Baik S, Anderson G, et al. Serial progression of cortical and medullary thymic epithelial microenvironments. Eur J Immunol. (2014) 44:16–22. doi: 10.1002/eji.201344110
64. Wong K, Lister NL, Barsanti M, Lim JMC, Hammett MV, Khong DM, et al. Multilineage potential and self-renewal define an epithelial progenitor cell population in the adult thymus. Cell Rep. (2014) 8:1198–209. doi: 10.1016/j.celrep.2014.07.029
65. Ucar A, Ucar O, Klug P, Matt S, Brunk F, Hofmann TG, et al. Adult thymus contains FoxN1− epithelial stem cells that are bipotent for medullary and cortical thymic epithelial lineages. Immunity. (2014) 41:257–69. doi: 10.1016/j.immuni.2014.07.005
66. Ohigashi I, Zuklys S, Sakata M, Mayer CE, Hamazaki Y, Minato N, et al. Adult thymic medullary epithelium is maintained and regenerated by lineage-restricted cells rather than bipotent progenitors. Cell Rep. (2015) 13:1432–43. doi: 10.1016/j.celrep.2015.10.012
67. Ulyanchenko S, O'Neill KE, Medley T, Farley AM, Vaidya HJ, Cook AM, et al. Identification of a bipotent epithelial progenitor population in the adult thymus. Cell Rep. (2016) 14:2819–32. doi: 10.1016/j.celrep.2016.02.080
68. Dumont-Lagacé M, Gerbe H, Daouda T, Laverdure JP, Brochu S, Lemieux S, et al. Detection of quiescent radioresistant epithelial progenitors in the adult thymus. Front Immunol. (2017) 8:e1717. doi: 10.3389/fimmu.2017.01717
69. Lepletier A, Hun ML, Hammett MV, Wong K, Naeem H, Hedger M, et al. Interplay between Follistatin, Activin A, and BMP4 signaling regulates postnatal thymic epithelial progenitor cell differentiation during aging. Cell Rep. (2019) 27:3887–901. doi: 10.1016/j.celrep.2019.05.045
70. Hamazaki Y, Fujita H, Kobayashi T, Choi Y, Scott HS, Matsumoto M, et al. Medullary thymic epithelial cells expressing Aire represent a unique lineage derived from cells expressing claudin. Nat Immunol. (2007) 8:304–11. doi: 10.1038/ni1438
71. Rossi SW, Kim MY, Leibbrandt A, Parnell SM, Jenkinson WE, Glanville SH, et al. RANK signals from CD4+3− inducer cells regulate development of Aire-expressing epithelial cells in the thymic medulla. J Exp Med. (2007) 204:1267–72. doi: 10.1084/jem.20062497
72. Roberts NA, White AJ, Jenkinson WE, Turchinovich G, Nakamura K, Withers DR, et al. Rank signaling links the development of invariant γδ T cell progenitors and Aire+ medullary epithelium. Immunity. (2012) 36:427–37. doi: 10.1016/j.immuni.2012.01.016
73. Lopes N, Sergé A, Ferrier P, Irla M. Thymic crosstalk coordinates medulla organization and T-cell tolerance induction. Front Immunol. (2015) 6:e365. doi: 10.3389/fimmu.2015.00365
74. Kurobe H, Liu C, Ueno T, Saito F, Ohigashi I, Seach N, et al. CCR7-dependent cortex-to-medulla migration of positively selected thymocytes is essential for establishing central tolerance. Immunity. (2006) 24:165–77. doi: 10.1016/j.immuni.2005.12.011
75. Lkhagvasuren E, Sakata M, Ohigashi I, Takahama Y. Lymphotoxin β receptor regulates the development of CCL21-expressing subset of postnatal medullary thymic epithelial cells. J Immunol. (2013) 190:5110–7. doi: 10.4049/jimmunol.120320
76. Onder L, Nindl V, Scandella E, Chai Q, Cheng HW, Caviezel-Firner S, et al. Alternative NF-κB signaling regulates mTEC differentiation from podoplanin-expressing precursors in the cortico-medullary junction. Eur J Immunol. (2015) 45:2218–31. doi: 10.1002/eji.201545677
77. Kozai M, Kubo Y, Katakai T, Kondo H, Kiyonari H, Schaeuble K, et al. Essential role of CCL21 in establishment of central self-tolerance in T cells. J Exp Med. (2017) 214:1925–35. doi: 10.1084/jem.20161864
78. Miragaia RJ, Zhang X, Gomes T, Svensson V, Ilicic T, Henriksson J, et al. Single-cell RNA-sequencing resolves self-antigen expression during mTEC development. Sci Rep. (2018) 8:e685. doi: 10.1038/s41598-017-19100-4
79. Gray DHD, Seach N, Ueno T, Milton MK, Liston A, Lew AM, et al. Developmental kinetics, turnover, and stimulatory capacity of thymic epithelial cells. Blood. (2006) 108:3777–85. doi: 10.1182/blood-2006-02-004531
80. Gray D, Abramson J, Benoist C, Mathis D. Proliferative arrest and rapid turnover of thymic epithelial cells expressing Aire. J Exp Med. (2007) 204:2521–8. doi: 10.1084/jem.20070795
81. Colomé N, Collado J, Bech-Serra JJ, Liiv I, Antón LC, Peterson P, et al. Increased apoptosis after autoimmune regulator expression in epithelial cells revealed by a combined quantitative proteomics approach. J Proteome Res. (2010) 9:2600–9. doi: 10.1021/pr100044d
82. Yano M, Kuroda N, Han H, Meguro-Horike M, Nishikawa Y, Kiyonari H, et al. Aire controls the differentiation program of thymic epithelial cells in the medulla for the establishment of self-tolerance. J Exp Med. (2008) 205:2827–38. doi: 10.1084/jem.20080046
83. White AJ, Nakamura K, Jenkinson WE, Saini M, Sinclair C, Seddon B, et al. Lymphotoxin signals from positively selected thymocytes regulate the terminal differentiation of medullary thymic epithelial cells. J Immunol. (2010) 185:4769–76. doi: 10.4049/jimmunol.1002151
84. Wang X, Laan M, Bichele R, Kisand K, Scott HS, Peterson P. Post-Aire maturation of thymic medullary epithelial cells involves selective expression of keratinocyte-specific autoantigens. Front Immunol. (2012) 3:e19. doi: 10.3389/fimmu.2012.00019
85. Michel C, Miller CN, Küchler R, Brors B, Anderson MS, Kyewski B, et al. Revisiting the road map of medullary thymic epithelial cell differentiation. J Immunol. (2017) 199:3488–503. doi: 10.4049/jimmunol.1700203
86. Bornstein C, Nevo S, Giladi A, Kadouri N, Pouzolles M, Gerbe F, et al. Single-cell mapping of the thymic stroma identifies IL-25-producing tuft epithelial cells. Nature. (2018) 559:622–6. doi: 10.1038/s41586-018-0346-1
87. Miller CN, Proekt I, von Moltke J, Wells KL, Rajpurkar AR, Wang H, et al. Thymic tuft cells promote an IL-4-enriched medulla and shape thymocyte development. Nature. (2018) 559:627–31. doi: 10.1038/s41586-018-0345-2
88. Björses P, Aaltonen J, Horelli-Kuitunen N, Yaspo ML, Peltonen L. Gene defect behind APECED: a new clue to autoimmunity. Hum Mol Genet. (1998) 7:1547–53. doi: 10.1093/hmg/7.10.1547
89. Peterson P, Nagamine K, Scott H, Heino M, Kudoh J, Shimizu N, et al. APECED: a monogenic autoimmune disease providing new clues to self-tolerance. Immunol Today. (1998) 19:384–6. doi: 10.1016/S0167-5699(98)01293-6
90. Pitkänen J, Doucas V, Sternsdorf T, Nakajima T, Aratani S, Jensen K, et al. The autoimmune regulator protein has transcriptional transactivating properties and interacts with the common coactivator CREB-binding protein. J Biol Chem. (2000) 275:16802–9. doi: 10.1074/jbc.M908944199
91. Ferguson BJ, Alexander C, Rossi SW, Liiv I, Rebane A, Worth CL, et al. AIRE's CARD revealed, a new structure for central tolerance provokes transcriptional plasticity. J Biol Chem. (2008) 283:1723–31. doi: 10.1074/jbc.M707211200
92. Ilmarinen T, Melén K, Kangas H, Julkunen I, Ulmanen I, Eskelin P. The monopartite nuclear localization signal of autoimmune regulator mediates its nuclear import and interaction with multiple importin α molecules. FEBS J. (2006) 273:315–24. doi: 10.1111/j.1742-4658.2005.05065.x
93. Gibson TJ, Ramu C, Gemünd C, Aasland R. The APECED polyglandular autoimmune syndrome protein, AIRE-1, contains the SAND domain and is probably a transcription factor. Trends Biochem Sci. (1998) 23:242–4. doi: 10.1016/S0968-0004(98)01231-6
94. Org T, Chignola F, Hetényi C, Gaetani M, Rebane A, Liiv I, et al. The autoimmune regulator PHD finger binds to non-methylated histone H3K4 to activate gene expression. EMBO Rep. (2008) 9:370–6. doi: 10.1038/sj.embor.2008.11
95. Koh AS, Kuo AJ, Park SY, Cheung P, Abramson J, Bua D, et al. Aire employs a histone-binding module to mediate immunological tolerance, linking chromatin regulation with organ-specific autoimmunity. Proc Natl Acad Sci USA. (2008) 105:15878–83. doi: 10.1073/pnas.0808470105
96. Gaetani M, Matafora V, Saare M, Spiliotopoulos D, Mollica L, Quilici G, et al. AIRE-PHD fingers are structural hubs to maintain the integrity of chromatin-associated interactome. Nucleic Acids Res. (2012) 40:11756–68. doi: 10.1093/nar/gks933
97. Yang S, Bansal K, Lopes J, Benoist C, Mathis D. Aire's plant homeodomain(PHD)-2 is critical for induction of immunological tolerance. Proc Natl Acad Sci USA. (2013) 110:1833–8. doi: 10.1073/pnas.1222023110
98. Liiv I, Rebane A, Org T, Saare M, Maslovskaja J, Kisand K, et al. DNA-PK contributes to the phosphorylation of AIRE: importance in transcriptional activity. Biochim Biophys Acta. (2008) 1783:74–83. doi: 10.1016/j.bbamcr.2007.09.003
99. Abramson J, Giraud M, Benoist C, Mathis D. Aire's partners in the molecular control of immunological tolerance. Cell. (2010) 140:123–35. doi: 10.1016/j.cell.2009.12.030
100. Guha M, Saare M, Maslovskaja J, Kisand K, Liiv I, Haljasorg U, et al. DNA breaks and chromatin structural changes enhance the transcription of autoimmune regulator target genes. J Biol Chem. (2017) 292:6542–54. doi: 10.1074/jbc.M116.764704
101. Bansal K, Yoshida H, Benoist C, Mathis D. The transcriptional regulator Aire binds to and activates super-enhancers. Nat Immunol. (2017) 18:263–73. doi: 10.1038/ni.3675
102. Oven I, Brdičková N, Kohoutec J, Vaupotič T, Narat M, Peterlin BM. AIRE recruits P-TEFb for transcriptional elongation of target genes in medullary thymic epithelial cells. Mol Cell Biol. (2007) 27:8815–23. doi: 10.1128/MCB.01085-07
103. Giraud M, Yoshida H, Abramson J, Rahl PB, Young RA, Mathis D, et al. Aire unleashes stalled mRNA polymerase to induce ectopic gene expression in thymic epithelial cells. Proc Natl Acad Sci USA. (2012) 109:535–40. doi: 10.1073/pnas.1119351109
104. Derbinski J, Schulte A, Kyewski B, Klein L. Promiscuous gene expression in medullary thymic epithelial cells mirrors the peripheral self. Nat Immunol. (2001) 2:1032–9. doi: 10.1038/ni723
105. Kyewski B, Derbinski J, Gotter J, Klein L. Promiscuous gene expression and central T-cell tolerance: more than meets the eye. Trends Immunol. (2002) 23:364–71. doi: 10.1016/S1471-4906(02)02248-2
106. Kyewski B, Derbinski J. Self-representation in the thymus: an extended view. Nat Rev Immunol. (2004) 4:688–98. doi: 10.1038/nri1436
107. Kyewski B, Klein L. A central role for central tolerance. Annu Rev Immunol. (2006) 24:571–606. doi: 10.1146/annurev.immunol.23.021704.115601
108. Ramsey C, Winqvist O, Puhakka L, Halonen M, Moro A, Kämpe O, et al. Aire deficient mice develop multiple features of APECED phenotype and show altered immune response. Hum Mol Genet. (2002) 11:397–409. doi: 10.1093/hmg/11.4.397
109. Anderson MS, Venanzi ES, Klein L, Chen Z, Berzins SP, Turley SJ, et al. Projection of an immunological self shadow within the thymus by the Aire protein. Science. (2002) 298:1395–401. doi: 10.1126/science.1075958
110. Liston A, Lesage S, Wilson J, Peltonen L, Goodnow CC. Aire regulates negative selection of organ-specific T cells. Nat Immunol. (2003) 4:350–4. doi: 10.1038/ni906
111. Kuroda N, Mitani T, Takeda N, Ishimaru N, Arakaki R, Hayashi Y, et al. Development of autoimmunity against transcriptionally unrepressed target antigen in the thymus of Aire-deficient mice. J Immunol. (2005) 174:1862–70. doi: 10.4049/jimmunol.174.4.1862
112. Derbinski J, Pinto S, Rösch S, Hexel K, Kyewski B. Promiscuous gene expression patterns in single medullary thymic epithelial cells argue for a stochastic mechanism. Proc Natl Acad Sci USA. (2008) 105:657–62. doi: 10.1073/pnas.0707486105
113. Villaseñor J, Besse W, Benoist C, Mathis D. Ectopic expression of peripheral-tissue antigens in the thymic epithelium: probabilistic, monoallelic, misinitiated. Proc Natl Acad Sci USA. (2008) 105:15854–9. doi: 10.1073/pnas.0808069105
114. Pinto S, Michel C, Schmidt-Glenewinkel H, Harder N, Rohr K, Wild S, et al. Overlapping gene coexpression patterns in human medullary thymic epithelial cells generate self-antigen diversity. Proc Natl Acad Sci USA. (2013) 110:E3497–505. doi: 10.1073/pnas.1308311110
115. Brennecke P, Reyes A, Pinto S, Rattay K, Nguyen M, Küchler R, et al. Single-cell transcriptome analysis reveals coordinated ectopic gene-expression patterns in medullary thymic epithelial cells. Nat Immunol. (2015) 16:933–41. doi: 10.1038/ni.3246
116. Meredith M, Zemmour D, Mathis D, Benoist C. Aire controls gene expression in the thymic epithelium with ordered stochasticity. Nat Immunol. (2015) 16:942–9. doi: 10.1038/ni.3247
117. Anderson MS, Venanzi ES, Chen Z, Berzins SP, Benoist C, Mathis D. The cellular mechanism of Aire control of T cell tolerance. Immunity. (2005) 23:227–39. doi: 10.1016/j.immuni.2005.07.005
118. Chen Z, Benoist C, Mathis D. How defects in central tolerance impinge on a deficiency in regulatory T cells. Proc Natl Acad Sci USA. (2005) 102:14735–40. doi: 10.1073/pnas.0507014102
119. Aschenbrenner K, D'Cruz LM, Vollmann EH, Hinterberger M, Emmerich J, Swee LK, et al. Selection of Foxp3+ regulatory T cells specific for self antigen expressed and presented by Aire+ medullary thymic epithelial cells. Nat Immunol. (2007) 8:351–8. doi: 10.1038/ni1444
120. Aichinger M, Wu C, Nedjic J, Klein L. Macroautophagy substrates are loaded onto MHC class II of medullary thymic epithelial cells for central tolerance. J Exp Med. (2013) 210:287–300. doi: 10.1084/jem.20122149
121. Mouri Y, Ueda Y, Yamano T, Matsumoto M, Tsuneyama K, Kinashi T, et al. Mode of tolerance induction and requirement for Aire are governed by the cell types that express self-antigen and those that present antigen. J Immunol. (2017) 199:3959–71. doi: 10.4049/jimmunol.1700892
122. Lebel MÈ, Coutelier M, Galipeau M, Kleinman CL, Moon JJ, Melichar HJ. Differential expression of tissue-restricted antigens among mTEC is associated with distinct autoreactive T cell fates. Nat Commun. (2020) 11:e3734. doi: 10.1038/s41467-020-17544-3
123. Hubert FX, Kinkel SA, Davey GM, Phipson B, Mueller SN, Liston A, et al. Aire regulates the transfer of antigen from mTECs to dendritic cells for induction of thymic tolerance. Blood. (2011) 118:2462–72. doi: 10.1182/blood-2010-06-286393
124. Taniguchi RT, DeVoss JJ, Moon JJ, Sidney J, Sette A, Jenkins MK, et al. Detection of an autoreactive T-cell population within the polyclonal repertoire that undergoes distinct autoimmune regulator (Aire)-mediated selection. Proc Natl Acad Sci USA. (2012) 109:7847–52. doi: 10.1073/pnas.1120607109
125. Perry JSA, Lio CWJ, Kau AL, Nutsch K, Yang Z, Gordon JI, et al. Distinct contributions of Aire and antigen-presenting-cell subsets to the generation of self-tolerance in the thymus. Immunity. (2014) 41:414–26. doi: 10.1016/j.immuni.2014.08.007
126. Skogberg G, Lundberg V, Berglund M, Gudmundsdottir J, Telemo E, Lindgren S, et al. Human thymic epithelial primary cells produce exosomes carrying tissue-restricted antigens. Immunol Cell Biol. (2015) 93:727–34. doi: 10.1038/icb.2015.33
127. Lancaster JN, Thyagarajan HM, Srinivasan J, Li Y, Hu Z, Ehrlich LIR. Live-cell imaging reveals the relative contributions of antigen-presenting cell subsets to thymic central tolerance. Nat Commun. (2019) 10:e2220. doi: 10.1038/s41467-019-09727-4
128. Wirnsberger G, Mair F, Klein L. Regulatory T cell differentiation of thymocytes does not require a dedicated antigen-presenting cell but is under T cell-intrinsic developmental control. Proc Natl Acad Sci USA. (2009) 106:10278–83. doi: 10.1073/pnas.0901877106
129. Hinterberger M, Aichinger M, Prazeres da Costa O, Voehringer D, Hoffmann R, Klein L. Autonomous role of medullary thymic epithelial cells in central CD4+ T cell tolerance. Nat Immunol. (2010) 11:512–20. doi: 10.1038/ni.1874
130. Abramson J, Anderson G. Thymic epithelial cells. Annu Rev Immunol. (2017) 26:85–118. doi: 10.1146/annurev-immunol-051116-052320
131. Kadouri N, Nevo S, Goldfarb Y, Abramson J. Thymic epithelial cell heterogeneity: TEC by TEC. Nat Rev Immunol. (2020) 20:239–53. doi: 10.1038/s41577-019-0238-0
132. Perniola R, Musco G. The biophysical and biochemical properties of the autoimmune regulator (AIRE) protein. Biochim Biophys Acta. (2014) 1842:326–37. doi: 10.1016/j.bbadis.2013.11.020
133. Proekt I, Miller CN, Lionakis MS, Anderson MS. Insights into immune tolerance from AIRE deficiency. Curr Opin Immunol. (2017) 49:71–8. doi: 10.1016/j.coi.2017.10.003
135. Passos GA, Speck-Hernandez CA, Assis AF, Mendes-da-Cruz DA. Update on Aire and thymic negative selection. Immunology. (2018) 153:10–20. doi: 10.1111/imm.12831
136. Michele TM, Fleckenstein J, Sgrignoli AR, Thuluvath PJ. Chronic active hepatitis in the type I polyglandular autoimmune syndrome. Postgrad Med J. (1994) 70:128–31. doi: 10.1136/pgmj.70.820.128
137. Gebre-Medhin G, Husebye ES, Gustafsson J, Winqvist O, Goksøyr A, Rorsman F, et al. Cytochrome P450IA2 and aromatic l-amino acid decarboxylase are hepatic autoantigens in autoimmune polyendocrine syndrome type I. FEBS Lett. (1997) 412:439–45. doi: 10.1016/s0014-5793(97)00797-7
138. Clemente MG, Obermayer-Straub P, Meloni A, Strassburg CP, Arangino V, Tukey RH, et al. Cytochrome P450 1A2 is a hepatic autoantigen in autoimmune polyglandular syndrome type 1. J Clin Endocrinol Metab. (1997) 82:1353–61. doi: 10.1210/jcem.82.5.3913
139. Clemente MG, Meloni A, Obermayer-Straub P, Frau F, Manns MP, De Virgiliis S. Two cytochromes P450 are major hepatocellular autoantigens in autoimmune polyglandular syndrome type 1. Gastroenterology. (1998) 114:324–8. doi: 10.1016/s0016-5085(98)70484-6
140. Alimohammadi M, Dubois N, Sköldberg F, Hallgren Å, Tardivel I, Hedstrand H, et al. Pulmonary autoimmunity as a feature of autoimmune polyendocrine syndrome type 1 and identification of KCNRG as a bronchial autoantigen. Proc Natl Acad Sci USA. (2009) 106:4396–401. doi: 10.1073/pnas.0809986106
141. Shum AK, Alimohammadi M, Tan CL, Cheng MH, Metzger TC, Law CS, et al. BPIFB1 is a lung-specific autoantigen associated with interstitial lung disease. Sci Transl Med. (2013) 5:e206ra139. doi: 10.1126/scitranslmed.3006998
142. Rautemaa R, Hietanen J, Niissalo S, Pirinen S, Perheentupa J. Oral and oesophageal squamous cell carcinoma – a complication or component of autoimmune polyendocrinopathy-candidiasis-ectodermal dystrophy (APECED, APS-I). Oral Oncol. (2007) 43:607–13. doi: 10.1016/j.oraloncology.2006.07.005
143. Böckle BC, Wilhelm M, Müller H, Götsch C, Sepp NT. Oral mucous squamous cell carcinoma – an anticipated consequence of autoimmune polyendocrinopathy-candidiasis-ectodermal dystrophy (APECED). J Am Acad Dermatol. (2010) 62:864–8. doi: 10.1016/j.jaad.2009.06.061
144. Zlotogora J, Shapiro MS. Polyglandular autoimmune syndrome type I among Iranian Jews. J Med Genet. (1992) 29:824–6. doi: 10.1136/jmg.29.11.824
145. Björses P, Halonen M, Palvimo JJ, Kolmer M, Aaltonen J, Ellonen P, et al. Mutations in the AIRE gene: effects on subcellular location and transactivation function of the autoimmune polyendocrinopathy-candidiasis-ectodermal dystrophy protein. Am J Hum Genet. (2000) 66:378–92. doi: 10.1086/302765
146. Ramsey C, Bukrinsky A, Peltonen L. Systematic mutagenesis of the functional domains of AIRE reveals their role in intracellular targeting. Hum Mol Genet. (2002) 11:3299–308. doi: 10.1093/hmg/11.26.3299
147. Halonen M, Kangas H, Rüppell T, Ilmarinen T, Ollila J, Kolmer M, et al. APECED-causing mutations in AIRE reveal the functional domains of the protein. Hum Mutat. (2004) 23:245–57. doi: 10.1002/humu.20003
148. Cetani F, Barbesino G, Borsari S, Pardi E, Cianferotti L, Pinchera A, et al. A novel mutation of the autoimmune regulator gene in an Italian kindred with autoimmune polyendocrinopathy-candidiasis-ectodermal dystrophy, acting in a dominant fashion and strongly cosegregating with hypothyroid autoimmune thyroiditis. J Clin Endocrinol Metab. (2001) 86:4747–52. doi: 10.1210/jcem.86.10.7884
149. Bellacchio E, Palma A, Corrente C, Di Girolamo F, Kemp EH, Di Matteo G, et al. The possible implication of the S250C variant of the autoimmune regulator protein in a patient with autoimmunity and immunodeficiency: in silico analysis suggests a molecular pathogenic mechanism for the variant. Gene. (2014) 549:286–94. doi: 10.1016/j.gene.2014.07.064
150. Oftedal BE, Hellesen A, Erichsen MM, Bratland E, Vardi A, Perheentupa J, et al. Dominant mutations in the autoimmune regulator AIRE are associated with common organ-specific autoimmune diseases. Immunity. (2015) 42:1185–96. doi: 10.1016/j.immuni.2015.04.021
151. Abbott JK, Huoh YS, Reynolds PR, Yu L, Rewers M, Reddy M, et al. Dominant-negative loss of function arises from a second, more frequent variant within the SAND domain of autoimmune regulator (AIRE). J Autoimmun. (2018) 88:114–20. doi: 10.1016/j.jaut.2017.10.010
152. Halonen M, Eskelin P, Myhre AG, Perheentupa J, Husebye ES, Kämpe O, et al. AIRE mutations and human leukocyte antigen genotypes as determinants of the autoimmune polyendocrinopathy-candidiasis-ectodermal dystrophy phenotype. J Clin Endocrinol Metab. (2002) 87:2568–74. doi: 10.1210/jcem.87.6.8564
153. Perniola R, Filograna O, Greco G, Pellegrino V. High prevalence of thyroid autoimmunity in Apulian patients with autoimmune polyglandular syndrome type 1. Thyroid. (2008) 18:1027–9. doi: 10.1089/thy.2008.0027
154. Tuomilehto J, Karvonen M, Pitkäniemi J, Virtala E, Kohtamäki K, Toivanen L, et al. Record-high incidence of Type I (insulin-dependent) diabetes mellitus in Finnish children. Diabetologia. (1999) 42:655–60. doi: 10.1007/s001250051212
155. Svejgaard A, Morling N, Platz P, Ryder LP, Thomsen M. HLA and disease associations with special reference to mechanisms. Transplant Proc. (1981) 13:913–7.
156. Maclaren NK, Riley WJ. Inherited susceptibility to autoimmune Addison's disease is linked to human leukocyte antigens-DR3 and/or DR4, except when associated with type I autoimmune polyglandular syndrome. J Clin Endocrinol Metab. (1986) 62:455–9. doi: 10.1210/jcem-62-3-455
157. Huang W, Connor E, Dela Rosa T, Muir A, Schatz D, Silverstein J, et al. Although DR3-DQB1*0201 may be associated with multiple component diseases of the autoimmune polyglandular syndromes, the human leukocyte antigen DR4-DQB1*0302 haplotype is implicated only in β-cell autoimmunity. J Clin Endocrinol Metab. (1996) 81:2559–63. doi: 10.1210/jcem.81.7.8675578
158. Bøe AS, Knappskog PM, Myhre AG, Sørheim JI, Husebye ES. Mutational analysis of the autoimmune regulator (AIRE) gene in sporadic autoimmune Addison's disease can reveal patients with unidentified autoimmune polyendocrine syndrome type I. Eur J Endocrinol. (2002) 146:519–22. doi: 10.1530/eje.0.1460519
159. Eriksson D, Bianchi M, Landegren N, Dalin F, Skov J, Hultin-Rosenberg L, et al. Common genetic variation in the autoimmune regulator (AIRE) locus is associated with autoimmune Addison's disease in Sweden. Sci Rep. (2018) 8:e8395. doi: 10.1038/s41598-018-26842-2
160. Kekäläinen E, Miettinen A, Arstila TP. Does the deficiency of Aire in mice really resemble human APECED? Nat Rev Immunol. (2007) 7:1. doi: 10.1038/nri2136-c1
161. Hubert FX, Kinkel SA, Crewther PE, Cannon PZF, Webster KE, Link M, et al. Aire-deficient C57BL/6 mice mimicking the common human 13-base pair deletion mutant present with only a mild autoimmune phenotype. J Immunol. (2009) 182:3902–18. doi: 10.4049/jimmunol.0802124
162. Jiang W, Anderson MA, Bronson R, Mathis D, Benoist C. Modifier loci condition autoimmunity provoked by Aire deficiency. J Exp Med. (2005) 202:805–15. doi: 10.1084/jem.20050693
163. Niki S, Oshikawa K, Mouri Y, Hirota F, Matsushima A, Yano M, et al. Alteration of intra-pancreatic target-organ specificity by abrogation of Aire in NOD mice. J Clin Invest. (2006) 116:1292–301. doi: 10.1172/JCI26971
164. Han H. Target-organ specificity of autoimmunity is modified by thymic stroma and bone marrow-derived cells. J Med Invest. (2007) 54:54–64. doi: 10.2152/jmi.54.54
165. Ossart J, Moreau A, Autrusseau E, Ménoret S, Martin JC, Besnard M, et al. Breakdown of immune tolerance in AIRE-deficient rats induces a severe autoimmune polyendocrinopathy-candidiasis-ectodermal dystrophy-like autoimmune disease. J Immunol. (2018) 201:874–87. doi: 10.4049/jimmunol.1701318
166. Derbinski J, Gäbler J, Brors B, Tierling S, Jonnakuty S, Hergenhahn M, et al. Promiscuous gene expression in thymic epithelial cells is regulated at multiple levels. J Exp Med. (2005) 202:33–45. doi: 10.1084/jem.20050471
167. Shikama N, Nusspaumer G, Holländer GA. Clearing the AIRE: on the pathophysiological basis of the autoimmune polyendocrinopathy syndrome type-1. Endocrinol Metab Clin North Am. (2009) 38:273–88. doi: 10.1016/j.ecl.2009.01.011
168. Sansom SN, Shikama-Dorn N, Zhanybekova S, Nusspaumer G, Macaulay IC, Deadman ME, et al. Population and single-cell genomics reveal the Aire dependency, relief from Polycomb silencing, and distribution of self-antigen expression in thymic epithelia. Genome Res. (2014) 24:1918–31. doi: 10.1101/gr.171645.113
169. Ströbel P, Murumägi A, Klein R, Luster M, Lahti M, Krohn K, et al. Deficiency of the autoimmune regulator AIRE in thymomas is insufficient to elicit autoimmune polyendocrinopathy syndrome type I (APS-I). J Pathol. (2007) 211:563–71. doi: 10.1002/path.2141
170. Org T, Rebane A, Kisand K, Laan M, Haljasorg U, Andreson R, et al. AIRE activated tissue specific genes have histone modifications associated with inactive chromatin. Hum Mol Genet. (2009) 18:4699–710. doi: 10.1093/hmg/ddp433
171. Wolff AS, Kärner J, Owe JF, Oftedal BE, Gilhus NE, Erichsen MM, et al. Clinical and serologic parallels to APS-I in patients with thymomas and autoantigen transcripts in their tumors. J Immunol. (2014) 193:3880–90. doi: 10.4049/jimmunol.1401068
172. Ostertag B. Die an bestimmte Lokalisation gebundenen Konkremente des Zentralnervensystems und ihre Beziehung zur “Verkalkung intracelebraler Getäße” beigewissen endokrinen Erkrankungen. Virchows Arch Pathol Anat Physiol Klin Med. (1930) 275:828–59. doi: 10.1007/BF01947423
173. Perheentupa J. Kaksi ryhmää lisämunuaiskuoren periytyviä vajaatoimintatiloja. Duodecim. (1972) 88:119–27.
174. Perheentupa J, Hiekkala H. Twenty cases of the syndrome of autoimmune endocrinopathy and candidiasis. Acta Paediatr Scand. (1973) 62:110–1. doi: 10.1111/j.1651-2227.1973.tb08074.x
175. Myllärniemi S, Perheentupa J. Oral findings in the autoimmune polyendocrinopathy-candidosis syndrome (APECS) and other forms of hypoparathyroidism. Oral Surg Oral Med Oral Pathol. (1978) 45:721–9. doi: 10.1016/0030-4220(78)90147-0
176. Perheentupa J, Tiilikainen A, Lokki ML. Autoimmune polyendocrinopathy-candidosis syndrome (APECS): clinical variation, inheritance and HLA association in 40 Finnish patients. Pediatr Res. (1978) 12:1087. doi: 10.1203/00006450-197811000-00038
177. Perheentupa J. Autoimmune Polyendocrinopathy – Candidosis – Ectodermal Dystrophy (APECED). In: Eriksson AW, Forsius HR, Nevanlinna HR, Workman PL, Norio RK, editors. Population Structure and Genetic Disorders. London: Academic Press. (1980). p. 583–7.
178. Perheentupa J. Autoimmune polyendocrinopathy-candidiasis-ectodermal dystrophy (APECED). Horm Metab Res. (1996) 28:353–6. doi: 10.1055/s-2007-979814
179. Perheentupa J. APS-I/APECED: the clinical disease and therapy. Endocrinol Metab Clin North Am. (2002) 31:295–320. doi: 10.1016/s0889-8529(01)00013-5
180. Perheentupa J. Autoimmune polyendocrinopathy-candidiasis-ectodermal dystrophy. J Clin Endocrinol Metab. (2006) 91:2843–50. doi: 10.1210/jc.2005-2611
181. Wagman RD, Kazdan JJ, Kooh SW, Fraser D. Keratitis associated with the multiple endocrine deficiency, autoimmune disease, and candidiasis syndrome. Am J Ophthalmol. (1987) 103:569–75. doi: 10.1016/s0002-9394(14)74281-3
182. Wang CY, Davoodi-Semiromi A, Huang W, Connor E, Shi JD, She JX. Characterization of mutations in patients with autoimmune polyglandular syndrome type 1 (APS1). Hum Genet. (1998) 103:681–5. doi: 10.1007/s004390050891
183. Cihakova D, Trebusak K, Heino M, Fadeyev V, Tiulpakov A, Battelino T, et al. Novel AIRE mutations and P450 cytochrome autoantibodies in Central and Eastern European patients with APECED. Hum Mutat. (2001) 18:225–32. doi: 10.1002/humu.1178
184. Stolarski B, Pronicka E, Korniszewski L, Pollak A, Kostrzewa G, Rowińska E, et al. Molecular background of polyendocrinopathy-candidiasis-ectodermal dystrophy syndrome in a Polish population: novel AIRE mutations and an estimate of disease prevalence. Clin Genet. (2006) 70:348–54. doi: 10.1111/j.1399-0004.2006.00690.x
185. Dominguez M, Crushell E, Ilmarinen T, McGovern E, Collins S, Chang B, et al. Autoimmune polyendocrinopathy-candidiasis-ectodermal dystrophy (APECED) in the Irish population. J Pediatr Endocrinol Metab. (2006) 19:1343–52. doi: 10.1515/jpem.2006.19.11.1343
186. Adamson KA, Cheetham TD, Kendall-Taylor P, Seckl JR, Pearce SHS. The role of the IDDM2 locus in the susceptibility of UK APS1 subjects to type 1 diabetes mellitus. Int J Immunogenet. (2007) 34:17–21. doi: 10.1111/j.1744-313X.2006.00643.x
187. Wolff ASB, Erichsen MM, Meager A, Magitta NF, Myhre AG, Bollerslev J, et al. Autoimmune polyendocrine syndrome type 1 in Norway: phenotypic variation, autoantibodies, and novel mutations in the autoimmune regulator gene. J Clin Endocrinol Metab. (2007) 92:595–603. doi: 10.1210/jc.2006-1873
188. Trebušak Podkrajšek K, Milenković T, Odink RJ, Claasen-van der Grinten HL, Bratanić N, Hovnik T, et al. Detection of a complete autoimmune regulator gene deletion and two additional novel mutations in a cohort of patients with atypical phenotypic variants of autoimmune polyglandular syndrome type 1. Eur J Endocrinol. 2008; 159:633–9. doi: 10.1530/EJE-08-0328
189. Zaidi G, Sahu RP, Zhang L, George G, Bhavani N, Shah N, et al. Two novel Aire mutations in autoimmune polyendocrinopathy-candidiasis-ectodermal dystrophy (APECED) among Indians. Clin Genet. (2009) 76:441–8. doi: 10.1111/j.1399-0004.2009.01280.x
190. Proust-Lemoine E, Saugier-Véber P, Lefranc D, Dubucquoi S, Ryndak A, Buob D, et al. Autoimmune polyendocrine syndrome type 1 in North-Western France: AIRE gene mutation specificities and severe forms needing immunosuppressive therapies. Horm Res Paediatr. (2010) 74:275–84. doi: 10.1159/000297714
191. Tóth B, Wolff ASB, Halász Z, Tar A, Szüts P, Ilyés I, et al. Novel sequence variation of AIRE and detection of interferon-ω antibodies in early infancy. Clin Endocrinol (Oxf). (2010) 72:641–7. doi: 10.1111/j.1365-2265.2009.03740.x
192. Orlova EM, Bukina AM, Kuznetsova ES, Kareva MA, Zakharova EU, Peterkova VA, et al. Autoimmune polyglandular syndrome type 1 in Russian patients: clinical variants and autoimmune regulator mutations. Horm Res Paediatr. (2010) 73:449–57. doi: 10.1159/000313585
193. Bin-Abbas BS, Faiyaz-Ul-Haque M, Al-Fares AH, Al-Gazlan SS, Bhuiyan JA, Al-Muhsen SZ. Autoimmune polyglandular syndrome type 1 in Saudi children. Saudi Med J. (2010) 31:788–92.
194. Meloni A, Willcox N, Meager A, Atzeni M, Wolff ASB, Husebye ES, et al. Autoimmune polyendocrine syndrome type 1: an extensive longitudinal study in Sardinian patients. J Clin Endocrinol Metab. (2012) 97:1114–24. doi: 10.1210/jc.2011-2461
195. Bratanic N, Kisand K, Avbelj Stefanija M, Battelino T, Trebusak Podkrajsek K. Clinical, genetic and immunological characteristics of paediatric autoimmune polyglandular syndrome type 1 patients in Slovenia. Zdrav Var. (2015) 54:112–8. doi: 10.1515/sjph-2015-0017
196. Fierabracci A, Pellegrino M, Frasca F, Kilic SS, Betterle C. APECED in Turkey: a case report and insights on genetic and phenotypic variability. Clin Immunol. (2018) 194:60–6. doi: 10.1016/j.clim.2018.06.012
197. Weiler FG, Peterson P, Costa-Carvalho BT, de Barros Dorna M, Correia-Deur JE, Sader SL, et al. The heterogeneity of autoimmune polyendocrine syndrome type 1: clinical features, new mutations and cytokine autoantibodies in a Brazilian cohort from tertiary care centers. Clin Immunol. (2018) 197:231–8. doi: 10.1016/j.clim.2018.09.012
198. de Moraes Ruehsen M, Blizzard RM, Garcia-Bunuel R, Jones GS. Autoimmunity and ovarian failure. Am J Obstet Gynecol. (1972) 112:693–703. doi: 10.1016/0002-9378(72)90797-1
199. Fénichel P, Sosset C, Barbarino-Monnier P, Gobert B, Hiéronimus S, Béné MC, et al. Prevalence, specificity and significance of ovarian antibodies during spontaneous premature ovarian failure. Hum Reprod. (1997) 12:2623–8. doi: 10.1093/humrep/12.12.2623
200. Moncayo R, Moncayo HE. The association of autoantibodies directed against ovarian antigens in human disease: a clinical review. J Intern Med. (1993) 234:371–8. doi: 10.1111/j.1365-2796.1993.tb00758.x
201. Hoek A, Schoemaker J, Drexhage HA. Premature ovarian failure and ovarian autoimmunity. Endocr Rev. (1997) 18:107–34. doi: 10.1210/edrv.18.1.0291
202. Kalantaridou SN, Davs SR, Nelson LM. Premature ovarian failure. Endocrinol Metab Clin North Am. (1998) 27:989–1006. doi: 10.1016/s0889-8529(05)70051-7
203. Anasti JN. Premature ovarian failure: an update. Fertil Steril. (1998) 70:1–15. doi: 10.1016/s0015-0282(98)00099-5
204. Christin-Maitre S, Vasseur C, Portnoï MF, Bouchard P. Genes and premature ovarian failure. Mol Cell Endocrinol. (1998) 145:75–80. doi: 10.1016/s0303-7207(98)00172-5
205. Maclaren N, Chen QY, Kukreja A, Marker J, Zhang CH, Sun ZS. Autoimmune hypogonadism as part of an autoimmune polyglandular syndrome. J Soc Gynecol Investig. (2001) 8:S52–4. doi: 10.1016/s1071-5576(00)00109-x
206. Laml T, Preyer O, Umek W, Hengstschläger M, Hanzal E. Genetic disorders in premature ovarian failure. Hum Reprod Update. (2002) 8:483–91. doi: 10.1093/humupd/8.5.483
207. Layman LC. Human gene mutations causing infertility. J Med Genet. (2002) 39:153–61. doi: 10.1136/jmg.39.3.153
208. Practice Committee of the American Society for Reproductive Medicine. Current evaluation of amenorrhea. Fertil Steril. (2004) 82:266–72. doi: 10.1016/j.fertnstert.2004.02.098
209. Forges T, Monnier-Barbarino P, Faure GC, Béné MC. Autoimmunity and antigenic targets in ovarian pathology. Hum Reprod Update. (2004) 10:163–75. doi: 10.1093/humupd/dmh014
210. Goswami D, Conway GS. Premature ovarian failure. Hum Reprod Update. (2005) 11:391–410. doi: 10.1093/humupd/dmi012
211. Welt CK. Autoimmune oophoritis in the adolescent. Ann N Y Acad Sci. (2008) 1135:118–22. doi: 10.1196/annals.1429.006
212. Broekmans FJ, Soules MR, Fauser BC. Ovarian aging: mechanisms and clinical consequences. Endocr Rev. (2009) 30:465–93. doi: 10.1210/er.2009-0006
213. Nelson LM. Primary ovarian insufficiency. N Engl J Med. (2009) 360:606–14. doi: 10.1056/NEJMcp0808697
214. Kokcu A. Premature ovarian failure from current perspective. Gynecol Endocrinol. (2010) 26:555–62. doi: 10.3109/09513590.2010.488773
215. Persani L, Rossetti R, Cacciatore C. Genes involved in human premature ovarian failure. J Mol Endocrinol. (2010) 45:257–79. doi: 10.1677/JME-10-0070
216. Warren BD, Kinsey WK, McGinnis LK, Christenson LK, Jasti S, Stevens AM, et al. Ovarian autoimmune disease: clinical concepts and animal models. Cell Mol Immunol. (2014) 11:510–21. doi: 10.1038/cmi.2014.97
217. Greene AD, Patounakis G, Segars JH. Genetic associations with diminished ovarian reserve: a systematic review of the literature. J Assist Reprod Genet. (2014) 31:935–46. doi: 10.1007/s10815-014-0257-5
218. Kirshenbaum M, Orvieto R. Premature ovarian insufficiency (POI) and autoimmunity–an update appraisal. J Assist Reprod Genet. (2019) 36:2207–15. doi: 10.1007/s10815-019-01572-0
219. Saari V, Holopainen E, Mäkitie O, Laakso S. Pubertal development and premature ovarian insufficiency in patients with APECED. Eur J Endocrinol. (2020) 183:513–20. doi: 10.1530/EJE-20-0516
220. Irvine WJ, Barnes EW. Addison's disease, ovarian failure and hypoparathyroidism. Clin Endocrinol Metab. (1975) 4:379–434. doi: 10.1016/S0300-595X(75)80027-2
221. Muir A, Maclaren NK. Autoimmune diseases of the adrenal glands, parathyroid glands, gonads, and hypothalamic-pituitary axis. Endocrinol Metab Clin North Am. (1991) 20:619–44. doi: 10.1016/S0889-8529(18)30261-5
222. Kasperlik-Zaluska AA, Migdalska B, Czarnocka B, Drac-Kaniewska J, Niegowska E, Czech W. Association of Addison's disease with autoimmune disorders – a long term observation of 180 patients. Postgrad Med J. (1991) 67:984–7. doi: 10.1136/pgmj.67.793.984
223. Kong MF, Jeffcoate W. Eighty-six cases of Addison's disease. Clin Endocrinol (Oxf). (1994) 41:757–61. doi: 10.1111/j.1365-2265.1994.tb02790.x
224. Betterle C, Dal Pra C, Greggio N, Volpato M, Zanchetta R. Autoimmunity in isolated Addison's disease and in polyglandular autoimmune diseases type 1, 2 and 4. Ann Endocrinol (Paris). (2001) 62:193–201. doi: 10.1210/AE-04-2001-62-2-0003-4266-101019-ART2
225. Falorni A, Laureti S, De Bellis A, Zanchetta R, Tiberti C, Arnaldi G, et al. Italian Addison Network Study: update of diagnostic criteria for the etiological classification of primary adrenal insufficiency. J Clin Endocrinol Metab. (2004) 89:1598–604. doi: 10.1210/jc.2003-030954
226. Perry R, Kecha O, Paquette J, Huot C, Van Vliet G, Deal C. Primary adrenal insufficiency in children: twenty years experience at the Sainte-Justine Hospital, Montreal. J Clin Endocrinol Metab. (2005) 90:3243–50. doi: 10.1210/jc.2004-0016
227. Kasperlik-Zaluska AA, Czarnocka B, Jeske W, Papierska L. Addison's disease revisited in Poland: year 2008 versus year 1990. Autoimmune Dis. (2010) 2010:e731834. doi: 10.4061/2010/731834
228. Dalin F, Nordling Eriksson G, Dahlqvist P, Hallgren Å, Wahlberg J, Ekwall O, et al. Clinical and immunological characteristics of autoimmune Addison Disease: a nationwide Swedish multicenter study. J Clin Endocrinol Metab. (2017) 102:379–89. doi: 10.1210/jc.2016-2522
229. Regan EA, Vaidya A, Margulies PL, Make BJ, Lowe KE, Crapo JD. Primary adrenal insufficiency in the United States: diagnostic error and patient satisfaction with treatment. Diagnosis (Berl). (2019) 6:343–50. doi: 10.1515/dx-2019-0013
230. Saenger P, Levine LS, Irvine WJ, Gottesdiener K, Rauh W, Sonino N, et al. Progressive adrenal failure in polyglandular autoimmune disease. J Clin Endocrinol Metab. (1982) 54:863–8. doi: 10.1210/jcem-54-4-863
231. Leisti S, Ahonen P, Perheentupa J. The diagnosis and staging of hypocortisolism in progressing autoimmune adrenalitis. Pediatr Res. (1983) 17:861–7. doi: 10.1203/00006450-198311000-00005
232. Ketchum CH, Riley WJ, Maclaren NK. Adrenal dysfunction in asymptomatic patients with adrenocortical autoantibodies. J Clin Endocrinol Metab. (1984) 58:1166–70. doi: 10.1210/jcem-58-6-1166
233. Ahonen P, Miettinen A, Perheentupa J. Adrenal and steroidal cell antibodies in patients with autoimmune polyglandular disease type I and risk of adrenocortical and ovarian failure. J Clin Endocrinol Metab. (1987) 64:494–500. doi: 10.1210/jcem-64-3-494
234. Betterle C, Scalici C, Presotto F, Pedini B, Moro L, Rigon F, et al. The natural history of adrenal function in autoimmune patients with adrenal autoantibodies. J Endocrinol. (1988) 117:467–75. doi: 10.1677/joe.0.1170467
235. Barat P, Joussein M, Carel JC. À propos de deux cas de déficit en minéralo-corticoïdes dû à une première manifestation endocrinienne du syndrome APECED. Arch Pediatr. (2003) 10:137–9. doi: 10.1016/s0929-693x(03)00311-7
236. Løvås K, Husebye ES. Replacement therapy in Addison's disease. Expert Opin Pharmacother. (2003) 4:2145–9. doi: 10.1517/14656566.4.12.2145
237. Jeffcoate WJ, Hosking DJ, Jones RM. Hypoparathyroidism and Addison's disease: a potentially lethal combination. J R Soc Med. (1987) 80:709–10. doi: 10.1177/014107688708001119
238. Husebye ES, Perheentupa J, Rautemaa R, Kämpe O. Clinical manifestations and management of patients with autoimmune polyendocrine syndrome type I. J Intern Med. (2009) 265:514–29. doi: 10.1111/j.1365-2796.2009.02090.x
239. Mäkitie O, Sochett EB, Bondestam S, Sipilä I, Perheentupa J. Bone health in autoimmune polyendocrinopathy-candidiasis-ectodermal dystrophy (APECED): findings in 25 adults. Clin Endocrinol (Oxf). (2006) 64:489–94. doi: 10.1111/j.1365-2265.2006.02495.x
240. Gharib H, Gastineau CF, Hodgson SF, Scholz DA, Smith LA. Reversible hypothyroidism in Addison's disease. Lancet. (1972) 300:734–6. doi: 10.1016/s0140-6736(72)92024-7
241. Topliss DJ, White EL, Stockigt JR. Significance of thyrotropin excess in untreated primary adrenal insufficiency. J Clin Endocrinol Metab. (1980) 50:52–6. doi: 10.1210/jcem-50-1-52
242. Ismail AAA, Burr WA, Walker PL. Acute changes in serum thyrotrophin in treated Addison's disease. Clin Endocrinol (Oxf). (1989) 30:225–30. doi: 10.1111/j.1365-2265.1989.tb02230.x
243. Løvås K, Loge JH, Husebye ES. Subjective health status in Norwegian patients with Addison's disease. Clin Endocrinol (Oxf). (2002) 56:581–8. doi: 10.1046/j.1365-2265.2002.01466.x
244. Løvas K, Husebye ES, Holsten F, Bjorvatn B. Sleep disturbances in patients with Addison's disease. Eur J Endocrinol. (2003) 148:449–56. doi: 10.1530/eje.0.1480449
245. Løvås K, Gjesdal CG, Christensen M, Wolff AB, Almås B, Svartberg J, et al. Glucocorticoid replacement therapy and pharmacogenetics in Addison's disease: effects on bone. Eur J Endocrinol. (2009) 160:993–1002. doi: 10.1530/EJE-08-0880
246. Husebye ES, Allolio B, Arlt W, Badenhoop K, Bensing S, Betterle C, et al. Consensus statement on the diagnosis, treatment and follow-up of patients with primary adrenal insufficiency. J Intern Med. (2014) 275:104–15. doi: 10.1111/joim.12162
247. Anderson JR, Goudie RB, Gray KG, Timbury GC. Auto-antibodies in Addison's disease. Lancet. (1957) 269:1123–4. doi: 10.1016/s0140-6736(57)91687-2
248. Blizzard RM, Kyle MA, Chandler RW, Hung W. Adrenal antibodies in Addison's disease. Lancet. (1962) 280:901–3. doi: 10.1016/s0140-6736(62)90681-5
249. Blizzard RM, Kyle M. Studies of the adrenal antigens and antibodies in Addison's disease. J Clin Invest. (1963) 42:1653–60. doi: 10.1172/JCI104851
250. Goudie RB, Anderson JR, Gray KK, Whyte WG. Autoantibodies in Addison's disease. Lancet. (1966) 287:1173–6. doi: 10.1016/S0140-6736(66)91070-1
251. Blizzard RM, Chee D, Davis W. The incidence of adrenal and other antibodies in the sera of patients with idiopathic adrenal insufficiency (Addison's disease). Clin Exp Immunol. (1967) 2:19–30.
252. Irvine WJ, Stewart AG, Scarth L. A clinical and immunological study of adrenocortical insufficiency (Addison's disease). Clin Exp Immunol. (1967) 2:31–69.
253. Goudie RB, McDonald E, Anderson JR, Gray K. Immunological features of idiopathic Addison's disease: characterization of the adrenocortical antigens. Clin Exp Immunol. (1968) 3:119–31.
254. Irvine WJ. Clinical and immunological associations in adrenal disorders. Proc R Soc Med. (1968) 61:271–5.
255. Irvine WJ, Scarth L. Antibody to the oxyphil cells of the human parathyroid in idiopathic hypoparathyroidism. Clin Exp Immunol. (1969) 4:505–10.
256. Irvine WJ, Chan MMW, Scarth L, Kolb FO, Hartog M, Bayliss RIS, et al. Immunological aspects of premature ovarian failure associated with idiopathic Addison's disease. Lancet. (1968) 292:883–7. doi: 10.1016/s0140-6736(68)91053-2
257. Irvine WJ, Chan MMW, Scarth L. The further characterization of autoantibodies reactive with extra-adrenal steroid-producing cells in patients with adrenal disorders. Clin Exp Immunol. (1969) 4:489–503.
258. Anderson JR, Goudie RB, Gray K, Stuart-Smith DA. Immunological features of idiopathic Addison's disease: an antibody to cells producing steroid hormones. Clin Exp Immunol. (1968) 3:107–17.
259. McNatty KP, Short RV, Barnes EW, Irvine WJ. The cytotoxic effect of serum from patients with Addison's disease and autoimmune ovarian failure of human granulosa cells in culture. Clin Exp Immunol. (1975) 22:378–84.
260. Sotsiou F, Bottazzo GF, Doniach D. Immunofluorescence studies on autoantibodies to steroid-producing cells, and to germline cells in endocrine disease and infertility. Clin Exp Immunol. (1980) 39:97–111.
261. Khoury EL, Hammond L, Bottazzo GF, Doniach D. Surface-reactive antibodies to human adrenal cells in Addison's disease. Clin Exp Immunol. (1981) 45:48–55.
262. Elder M, Maclaren N, Riley W. Gonadal autoantibodies in patients with hypogonadism and/or Addison's disease. J Clin Endocrinol Metab. (1981) 52:1137–42. doi: 10.1210/jcem-52-6-1137
263. Irvine WJ. Premature menopause in autoimmune diseases. Lancet. (1969) 293:264. doi: 10.1016/s0140-6736(69)91280-x
264. Vallotton MB, Forbes AP. Antibodies to cytoplasm of ova. Lancet. (1966) 288:264–5. doi: 10.1016/s0140-6736(66)92546-3
265. Vallotton MB, Forbes AP. Autoimmunity in gonadal dysgenesis and Klinefelter syndrome. Lancet. (1967) 289:648–51. doi: 10.1016/s0140-6736(67)92543-3
266. Vallotton MB, Forbes AP. Premature menopause in autoimmune diseases. Lancet. (1969) 293:156–7. doi: 10.1016/s0140-6736(69)91171-4
267. Krohn K, Heinonen E, Pelkonen R, Perheentupa J. Precipitating antiadrenal antibodies in Addison's disease. Scand J Immunol. (1973) 2:450. doi: 10.1016/0090-1229(74)90023-3
268. Krohn K, Perheentupa J, Heinonen E. Precipitating anti-adrenal antibodies in Addison's disease. Clin Immunol Immunopathol. (1974) 3:59–68.
269. Heinonen E, Krohn K, Perheentupa J, Aro A, Pelkonen R. Association of precipitating anti-adrenal antibodies with moniliasis-polyendocrinopathy syndrome. Ann Clin Res. (1976) 8:262–5.
270. Heinonen E. Variety of determinants in an adrenal antigen common to man and some animals. Med Biol. (1976) 54:341–6.
271. Heinonen E, Krohn K. Studies on an adrenal antigen common to man and different animals. Med Biol. (1977) 55:48–53.
272. Matteson KJ, Picado-Leonard J, Chung BC, Mohandas TK, Miller WL. Assignment of the gene for adrenal P450c17 (steroid 17α-hydroxylase/17,20 lyase) to human chromosome 10. J Clin Endocrinol Metab. (1986) 63:789–91. doi: 10.1210/jcem-63-3-789
273. White PC, New MI, Dupont B. Structure of human steroid 21-hydroxylase genes. Proc Natl Acad Sci USA. (1986) 83:5111–5. doi: 10.1073/pnas.83.14.5111
274. Chung B, Matteson KJ, Voutilainen R, Mohandas TK, Miller WL. Human cholesterol side-chain cleavage enzyme, P450scc: cDNA cloning, assignment of the gene to chromosome 15, and expression in the placenta. Proc Natl Acad Sci USA. (1986) 83:8962–6. doi: 10.1073/pnas.83.23.8962
275. Winqvist O, Karlsson FA, Kämpe O. 21-hydroxylase, a major autoantigen in idiopathic Addison's disease. Lancet. (1992) 339:1559–62. doi: 10.1016/0140-6736(92)91829-w
276. Bednarek J, Furmaniak J, Wedlock N, Kiso Y, Baumann-Antczak A, Fowler S, et al. Steroid 21-hydroxylase is a major autoantigen involved in adult onset autoimmune Addison's disease. FEBS Lett. (1992) 309:51–5. doi: 10.1016/0014-5793(92)80737-2
277. Baumann-Antczak A, Wedlock N, Bednarek J, Kiso Y, Krishnan H, Fowler S, et al. Autoimmune Addison's disease and 21-hydroxylase. Lancet. (1992) 340:429–30. doi: 10.1016/0140-6736(92)91513-8
278. Krohn K, Uibo R, Aavik E, Peterson P, Savilahti K. Identification by molecular cloning of an autoantigen associated with Addison's disease as steroid 17α-hydroxylase. Lancet. (1992) 339:770–3. doi: 10.1016/0140-6736(92)91894-e
279. Winqvist O, Gustafsson J, Rorsman F, Karlsson FA, Kämpe O. Two different cytochrome P450 enzymes are the adrenal antigens in autoimmune polyendocrine syndrome type I and Addison's disease. J Clin Invest. (1993) 92:2377–85. doi: 10.1172/JCI116843
280. Karlsson FA, Kämpe O, Winqvist O, Burman P. Autoimmune disease of the adrenal cortex, pituitary, parathyroid glands and gastric mucosa. J Intern Med. (1993) 234:379–86. doi: 10.1111/j.1365-2796.1993.tb00759.x
281. Uibo R, Perheentupa J, Ovod V, Krohn KJE. Characterization of adrenal autoantigens recognized by sera from patients with autoimmune polyglandular syndrome (APS) type I. J Autoimmun. (1994) 7:399–411. doi: 10.1006/jaut.1994.1029
282. Weetman AP. Autoimmunity to steroid-producing cells and familial polyendocrine autoimmunity. Baillieres Clin Endocrinol Metab. (1995) 9:157–74. doi: 10.1016/s0950-351x(95)80899-x
283. Uibo R, Aavik E, Peterson P, Perheentupa J, Aranko S, Pelkonen R, et al. Autoantibodies to cytochrome P450 enzymes P450scc, P450c17, and P450c21 in autoimmune polyglandular disease types I and II and in isolated Addison's disease. J Clin Endocrinol Metab. (1994) 78:323–8. doi: 10.1210/jcem.78.2.8106620
284. Colls J, Betterle C, Volpato M, Prentice L, Rees Smith B, Furmaniak J. Immunoprecipitation assay for autoantibodies to steroid 21-hydroxylase in autoimmune adrenal diseases. Clin Chem. (1995) 41:375–80.
285. Falorni A, Nikoshkov A, Laureti S, Grenbäck E, Hulting AL, Casucci G, et al. High diagnostic accuracy for idiopathic Addison's disease with a sensitive radiobinding assay for autoantibodies against recombinant human 21-hydroxylase. J Clin Endocrinol Metab. (1995) 80:2752–5. doi: 10.1210/jcem.80.9.7673419
286. Söderbergh A, Winqvist O, Norheim I, Rorsman F, Husebye ES, Dolva Ø, et al. Adrenal autoantibodies and organ-specific autoimmunity in patients with Addison's disease. Clin Endocrinol (Oxf). (1996) 45:453–60. doi: 10.1046/j.1365-2265.1996.8040813.x
287. Chen S, Sawicka J, Betterle C, Powell M, Prentice L, Volpato M, et al. Autoantibodies to steroidogenic enzymes in autoimmune polyglandular syndrome, Addison's disease, and premature ovarian failure. J Clin Endocrinol Metab. (1996) 81:1871–6. doi: 10.1210/jcem.81.5.8626850
288. Falorni A, Laureti S, Nikoshkov A, Picchio ML, Hallengren B, Vandewalle CL, et al. 21-hydroxylase autoantibodies in adult patients with endocrine autoimmune diseases are highly specific for Addison's disease. Clin Exp Immunol. (1997) 107:341–6. doi: 10.1111/j.1365-2249.1997.262-ce1153.x
289. Tanaka H, Perez MS, Powell M, Sanders JF, Sawicka J, Chen S, et al. Steroid 21-hydroxylase autoantibodies: measurements with a new immunoprecipitation assay. J Clin Endocrinol Metab. (1997) 82:1440–6. doi: 10.1210/jcem.82.5.3929
290. Betterle C, Volpato M, Pedini B, Chen S, Furmaniak J, Rees Smith B. Adrenal-cortex and 21-hydroxylase autoantibodies in autoimmune Addison's disease. J Endocrinol Invest. (1997) 20(S4):91.
291. Degros V, Pons L, Ghulam A, Racadot A. Intérêt du dosage des anticorps anti-21 hydroxylase comme marqueur de l'atteinte surrénale dans les endocrinopathies auto-immunes. Ann Biol Clin. (1999) 57:705–9.
292. Seissler J, Schott M, Steinbrenner H, Peterson P, Scherbaum WA. Autoantibodies to adrenal cytochrome P450 antigens in isolated Addison's disease and autoimmune polyendocrine syndrome type II. Exp Clin Endocrinol Diabetes. (1999) 107:208–13. doi: 10.1055/s-0029-1212100
293. Betterle C, Volpato M, Pedini B, Chen S, Rees Smith B, Furmaniak J. Adrenal-cortex autoantibodies and steroid-producing cells autoantibodies in patients with Addison's disease: comparison of immunofluorescence and immunoprecipitation assays. J Clin Endocrinol Metab. (1999) 84:618–22. doi: 10.1210/jcem.84.2.5459
294. Reato G, Morlin L, Chen S, Furmaniak J, Rees Smith B, Masiero S, et al. Premature ovarian failure in patients with autoimmune Addison's disease: clinical, genetic, and immunological evaluation. J Clin Endocrinol Metab. (2011) 96:E1255–61. doi: 10.1210/jc.2011-0414
295. Dalla Costa M, Bonanni G, Masiero S, Faggian D, Chen S, Furmaniak J, et al. Gonadal function in males with autoimmune Addison's disease and autoantibodies to steroidogenic enzymes. Clin Exp Immunol. (2014) 176:373–9. doi: 10.1111/cei.12303
296. Perniola R, Falorni A, Clemente MG, Forini F, Accogli E, Lobreglio G. Organ-specific and non-organ-specific autoantibodies in children and young adults with autoimmune polyendocrinopathy-candidiasis-ectodermal dystrophy (APECED). Eur J Endocrinol. (2000) 143:497–503. doi: 10.1530/eje.0.1430497
297. Myhre AG, Halonen M, Eskelin P, Ekwall O, Hedstrand H, Rorsman F, et al. Autoimmune polyendocrine syndrome type 1 (APS I) in Norway. Clin Endocrinol (Oxf). (2001) 54:211–7. doi: 10.1046/j.1365-2265.2001.01201.x
298. Söderbergh A, Myhre AG, Ekwall O, Gebre-Medhin G, Hedstrand H, Landgren E, et al. Prevalence and clinical associations of 10 defined autoantibodies in autoimmune polyendocrine syndrome type I. J Clin Endocrinol Metab. (2004) 89:557–62. doi: 10.1210/jc.2003-030279
299. Betterle C, Rossi A, Dalla Pria S, Artifoni A, Pedini B, Gavasso S, et al. Premature ovarian failure: autoimmunity and natural history. Clin Endocrinol (Oxf). (1993) 39:35–43. doi: 10.1111/j.1365-2265.1993.tb01748.x
300. Winqvist O, Gebre-Medhin G, Gustafsson J, Ritzén EM, Lundkvist Ö, Karlsson FA, et al. Identification of the main gonadal autoantigens in patients with adrenal insufficiency and associated ovarian failure. J Clin Endocrinol Metab. (1995) 80:1717–23. doi: 10.1210/jcem.80.5.7745025
301. Falorni A, Laureti S, Candeloro P, Perrino S, Coronella C, Bizzarro A, et al. Steroid-cell autoantibodies are preferentially expressed in women with premature ovarian failure who have adrenal autoimmunity. Fertil Steril. (2002) 78:270–9. doi: 10.1016/s0015-0282(02)03205-3
302. Dal Pra C, Chen S, Furmaniak J, Rees Smith B, Pedini B, Moscon A, et al. Autoantibodies to steroidogenic enzymes in patients with premature ovarian failure with and without Addison's disease. Eur J Endocrinol. (2003) 148:565–70. doi: 10.1530/eje.0.1480565
303. Reimand K, Peterson P, Hyöti H, Uibo R, Cooke I, Weetman AP, et al. 3β-Hydroxysteroid dehydrogenase autoantibodies are rare in premature ovarian failure. J Clin Endocrinol Metab. (2000) 85:2324–6. doi: 10.1210/jcem.85.6.6630
304. Betterle C, Zanette F, Zanchetta R, Pedini B, Trevisan A, Mantero F, et al. Complement-fixing adrenal autoantibodies as a marker for predicting onset of idiopathic Addison's disease. Lancet. (1983) 321:1238–41. doi: 10.1016/s0140-6736(83)92695-8
305. Betterle C, Volpato M, Rees Smith B, Furmaniak J, Chen S, Greggio NA, et al. I. Adrenal cortex and steroid 21-hydroxylase autoantibodies in adult patients with organ-specific autoimmune diseases: markers of low progression to clinical Addison's disease. J Clin Endocrinol Metab. (1997) 82:932–8. doi: 10.1210/jcem.82.3.3819
306. Betterle C, Volpato M, Rees Smith B, Furmaniak J, Chen S, Zanchetta R, et al. II. Adrenal cortex and steroid 21-hydroxylase autoantibodies in children with organ-specific autoimmune diseases: markers of high progression to clinical Addison's disease. J Clin Endocrinol Metab. (1997) 82:939–42. doi: 10.1210/jcem.82.3.3849
307. Betterle C, Coco G, Zanchetta R. Adrenal cortex autoantibodies in subjects with normal adrenal function. Best Pract Res Clin Endocrinol Metab. (2005) 19:85–99. doi: 10.1016/j.beem.2004.11.008
308. Coco G, Dal Pra C, Presotto F, Albergoni MP, Canova C, Pedini B, et al. Estimated risk for developing autoimmune Addison's disease in patients with adrenal cortex autoantibodies. J Clin Endocrinol Metab. (2006) 91:1637–45. doi: 10.1210/jc.2005-0860
309. Wedlock N, Asawa T, Baumann-Antczak A, Rees Smith B, Furmaniak J. Autoimmune Addison's disease. Analysis of autoantibody binding sites on human steroid 21-hydroxylase. FEBS Lett. (1993) 332:123–6. doi: 10.1016/0014-5793(93)80497-i
310. Song YH, Connor EL, Muir A, She JX, Zorovich B, Derovanesian D, et al. Autoantibody epitope mapping of the 21-hydroxylase antigen in autoimmune Addison's disease. J Clin Endocrinol Metab. (1994) 78:1108–12. doi: 10.1210/jcem.78.5.7513715
311. Volpato M, Prentice L, Chen S, Betterle C, Rees Smith B, Furmaniak J. A study of the epitopes on steroid 21-hydroxylase recognized by autoantibodies in patients with or without Addison's disease. Clin Exp Immunol. (1998) 111:422–8. doi: 10.1046/j.1365-2249.1998.00475.x
312. Chen S, Sawicka J, Prentice L, Sanders JF, Tanaka H, Petersen V, et al. Analysis of autoantibody epitopes on steroid 21-hydroxylase using a panel of monoclonal antibodies. J Clin Endocrinol Metab. (1998) 83:2977–86. doi: 10.1210/jcem.83.8.5010
313. Liiv I, Teesalu K, Peterson P, Clemente MG, Perheentupa J, Uibo R. Epitope mapping of cytochrome P450 cholesterol side-chain cleavage enzyme by sera from patients with autoimmune polyglandular syndrome type 1. Eur J Endocrinol. (2002) 146:113–9. doi: 10.1530/eje.0.1460113
314. Peterson P, Krohn KJE, Mapping of B cell epitopes on steroid 17α-hydroxylase an autoantigen in autoimmune polyglandular syndrome type I. Clin Exp Immunol. (1994) 98:104–9. doi: 10.1111/j.1365-2249.1994.tb06614.x
315. Weetman AP. Autoantigens in Addison's disease and associated syndromes. Clin Exp Immunol. (1997) 107:227–9. doi: 10.1111/j.1365-2249.1997.1137a.x
316. Peterson P, Uibo R, Peränen J, Krohn K. Immunoprecipitation of steroidogenic enzyme autoantigens with autoimmune polyglandular syndrome type I (APS I) sera; further evidence for independent humoral immunity to P450c17 and P450c21. Clin Exp Immunol. (1997) 107:335–40. doi: 10.1111/j.1365-2249.1997.282-ce1175.x
317. Bøe AS, Bredholt G, Knappskog PM, Hjelmervik TO, Mellgren G, Winqvist O, et al. Autoantibodies against 21-hydroxylase and side-chain cleavage enzyme in autoimmune Addison's disease are mainly immunoglobulin G1. Eur J Endocrinol. (2004) 150:49–56. doi: 10.1530/eje.0.1500049
318. Brozzetti A, Marzotti S, La Torre D, Bacosi ML, Morelli S, Bini V, et al. Autoantibody responses in autoimmune ovarian insufficiency and in Addison's disease are IgG1 dominated and suggest a predominant, but not exclusive, Th1 type of response. Eur J Endocrinol. (2010) 163:309–17. doi: 10.1530/EJE-10-0257
319. Kendall-Taylor P, Lambert A, Mitchell R, Robertson WR. Antibody that blocks stimulation of cortisol secretion by adrenocorticotrophic hormone in Addison's disease. Br Med J (Clin Res Ed). (1988) 296:1489–91. doi: 10.1136/bmj.296.6635.1489
320. Reimand K, Perheentupa J, Link M, Krohn K, Peterson P, Uibo R. Testis-expressed protein TSGA10 – an auto-antigen in autoimmune polyendocrine syndrome type I. Int Immunol. (2008) 20:39–44. doi: 10.1093/intimm/dxm118
321. Smith CJA, Oscarson M, Rönnblom L, Alimohammadi M, Perheentupa J, Husebye ES, et al. TSGA10 – a target for autoantibodies in autoimmune polyendocrine syndrome type 1 and systemic lupus erythematosus. Scand J Immunol. (2011) 73:147–53. doi: 10.1111/j.1365-3083.2010.02486.x
322. Tong ZB, Nelson LM. A mouse gene encoding an oocyte antigen associated with premature ovarian failure. Endocrinology. (1999) 140:3720–6. doi: 10.1210/endo.140.8.6911
323. Otsuka N, Tong ZB, Vanevski K, Tu W, Cheng MH, Nelson LM. Autoimmune oophoritis with multiple molecular targets mitigated by transgenic expression of mater. Endocrinology. (2011) 152:2465–73. doi: 10.1210/en.2011-0022
324. Brozzetti A, Alimohammadi M, Morelli S, Minarelli V, Hallgren Å, Giordano R, et al. Autoantibody response against NALP5/MATER in primary ovarian insufficiency and in autoimmune Addison's disease. J Clin Endocrinol Metab. (2015) 100:1941–8. doi: 10.1210/jc.2014-3571
325. Moritz CP, Paul S, Stoevesandt O, Tholance Y, Camdessanché JP, Antoine JC. Autoantigenomics: holistic characterization of autoantigen repertoires for a better understanding of autoimmune diseases. Autoimmun Rev. (2020) 19:e102450. doi: 10.1016/j.autrev.2019.102450
326. Landegren N, Sharon D, Freyhult E, Hallgren Å, Eriksson D, Edqvist PH, et al. Proteome-wide survey of the autoimmune target repertoire in autoimmune polyendocrine syndrome type 1. Sci Rep. (2016) 6:e20104. doi: 10.1038/srep20104
327. Vazquez SE, Ferré EMN, Scheel DW, Sunshine S, Miao B, Mandel-Brehm C, et al. Identification of novel, clinically correlated autoantigens in the monogenic autoimmune syndrome APS1 by proteome-wide PhIP-Seq. eLife. (2020) 9:e55053. doi: 10.7554/eLife.55053
328. Winqvist O, Söderbergh A, Kämpe O. The autoimmune basis of adrenocortical destruction in Addison's disease. Mol Med Today. (1996) 2:282–9. doi: 10.1016/1357-4310(96)10024-1
329. Rabinowe SL, Jackson RA, Dluhy RG, Williams GH. Ia-positive T lymphocytes in recently diagnosed idiopathic Addison's disease. Am J Med. (1984) 77:597–601. doi: 10.1016/0002-9343(84)90348-6
330. Šedivá A, Ciháková D, Lebl J. Immunological findings in patients with autoimmune polyendocrinopathy-candidiasis-ectodermal dystrophy (APECED) and their family members: are heterozygotes subclinically affected? J Pediatr Endocrinol Metab. (2002) 15:1491–6. doi: 10.1515/jpem.2002.15.9.1491
331. Perniola R, Lobreglio G, Rosatelli MC, Pitotti E, Accogli E, De Rinaldis C. Immunophenotypic characterisation of peripheral blood lymphocytes in autoimmune polyglandular syndrome type 1: clinical study and review of the literature. J Pediatr Endocrinol Metab. (2005) 18:155–64. doi: 10.1515/jpem.2005.18.2.155
332. Przybylski P, Kurowska M, Glazer M, Plewik D, Radej S, Wiktor K, et al. Analysis of blood dendritic cells and lymphocytes in patients with autoimmune polyglandular syndromes (APS) and isolated autoimmune endocrine diseases – a pilot study. Ann Univ Mariae Curie Sklodowska Med. (2008) 63:83–7. doi: 10.2478/v10079-008-0055-6
333. Wolff ASB, Oftedal BEV, Kisand K, Ersvær E, Lima K, Husebye ES. Flow cytometry study of blood cell subtypes reflects autoimmune and inflammatory processes in autoimmune polyendocrine syndrome type I. Scand J Immunol. (2010) 71:459–67. doi: 10.1111/j.1365-3083.2010.02397.x
334. Fujii Y, Kato N, Kito J, Asai J, Yokochi T. Experimental autoimmune adrenalitis: a murine model for Addison's disease. Autoimmunity. (1992) 12:47–52. doi: 10.3109/08916939209146129
335. Dudley HR, Ritchie AC, Schilling A, Baker WH. Pathologic changes associated with the use of sodium ethylene diamine tetra-acetate in the treatment of hypercalcemia. Report of two cases with autopsy findings. N Engl J Med. (1955) 252:331–7. doi: 10.1056/NEJM195503032520901
336. Craig JM, Schiff LH, Boone JE. Chronic moniliasis associated with Addison's disease. AMA Am J Dis Child. (1955) 89:669–84. doi: 10.1001/archpedi.1955.02050110809003
337. Perlmutter M, Ellison RR, Norsa L, Kantrowitz AR. Idiopathic hypoparathyroidism and Addison's disease. Am J Med. (1956) 21:634–43. doi: 10.1016/0002-9343(56)90078-x
338. Whitaker J, Landing BH, Esselborn VM, Williams RR. The syndrome of familial juvenile hypoadrenocorticism, hypoparathyroidism and superficial moniliasis. J Clin Endocrinol Metab. (1956) 16:1374–87. doi: 10.1210/jcem-16-10-1374
339. McMahon FG, Cookson DU, Kabler JD, Inhorn SL. Idiopathic hypoparathyroidism and idiopathic adrenal cortical insufficiency occurring with cystic fibrosis of the pancreas. Ann Intern Med. (1959) 51:371–84. doi: 10.7326/0003-4819-51-2-371
340. Carter AC, Kaplan SA, DeMayo AP, Rosenblum DJ. An unusual case of idiopathic hypoparathyroidism, adrenal insufficiency, hypothyroidism and metastatic calcification. J Clin Endocrinol Metab. (1959) 19:1633–41. doi: 10.1210/jcem-19-12-1633
341. Petri M, Nerup J. Addison's adrenalitis. Studies on diffuse lymphocytic adrenalitis (idiopathic Addison's disease) and focal lymphocytic infiltration in a control material. Acta Pathol Microbiol Scand A. (1971) 79:381–8. doi: 10.1111/j.1699-0463.1971.tb01835.x
342. Rottembourg D, Le Deist F, Carel JC, Mallone R, Caillat-Zucman S, Deal C. Autoreactive T cells in patients with autoimmune adrenal diseases. Horm Res. (2009) 72(S3):217. doi: 10.1159/000239668
Keywords: Addison's disease, autoimmune polyendocrinopathies, cytochrome P450 enzyme system, history, transcription factors
Citation: Perniola R, Fierabracci A and Falorni A (2021) Autoimmune Addison's Disease as Part of the Autoimmune Polyglandular Syndrome Type 1: Historical Overview and Current Evidence. Front. Immunol. 12:606860. doi: 10.3389/fimmu.2021.606860
Received: 15 September 2020; Accepted: 25 January 2021;
Published: 26 February 2021.
Edited by:
Ger Rijkers, University College Roosevelt, NetherlandsCopyright © 2021 Perniola, Fierabracci and Falorni. This is an open-access article distributed under the terms of the Creative Commons Attribution License (CC BY). The use, distribution or reproduction in other forums is permitted, provided the original author(s) and the copyright owner(s) are credited and that the original publication in this journal is cited, in accordance with accepted academic practice. No use, distribution or reproduction is permitted which does not comply with these terms.
*Correspondence: Roberto Perniola, cnBlcm5pb2xhJiN4MDAwNDA7aG90bWFpbC5jb20=