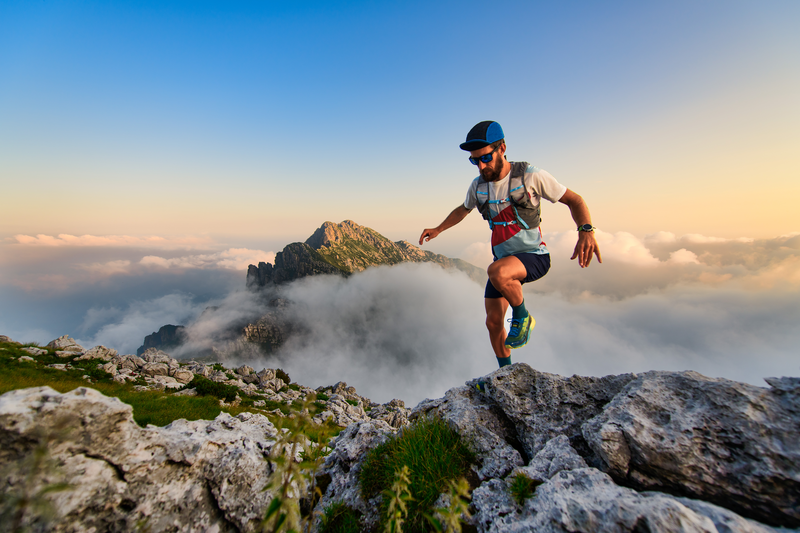
94% of researchers rate our articles as excellent or good
Learn more about the work of our research integrity team to safeguard the quality of each article we publish.
Find out more
ORIGINAL RESEARCH article
Front. Immunol. , 19 April 2021
Sec. Molecular Innate Immunity
Volume 12 - 2021 | https://doi.org/10.3389/fimmu.2021.581799
Forkhead box O 3 (FOXO3) is a transcription factor involved in cell metabolism, inflammation and longevity. Here, we investigated if metformin can activate FOXO3 in human immune cells and affects the subsequent level of reactive oxygen/nitrogen species (ROS/RNS) in immune cells. AMP-activated protein kinase (AMPK) and FOXO3 activation were investigated by immunoblot or flow cytometry (FC) analysis, respectively. FOXO3 target gene expression was quantified by real-time PCR. ROS/RNS measurement using dichlorodihydrofluorescein diacetate (DCFH-DA) dye was investigated by FC. The role of the FOXO3 single nucleotide polymorphisms (SNPs) rs12212067, rs2802292 and rs12206094 on ROS/RNS production was studied using allelic discrimination PCR. Metformin induced activation of AMPK (pT172) and FOXO3 (pS413). ROS/RNS level was reduced in immune cells after metformin stimulation accompanied by induction of the FOXO3 targets mitochondrial superoxide dismutase and cytochrome c. Studies in Foxo3 deficient (Foxo3-/-) mouse splenocytes confirmed that metformin mediates its effects via Foxo3 as it attenuates ROS/RNS in myeloid cells of wildtype (WT) but not of Foxo3-/- mice. Our results suggest that FOXO3 can be activated by metformin leading to reduced ROS/RNS level in immune cells. This may add to the beneficial clinical effects of metformin observed in large cohort studies on longevity, cardiovascular and cancer risk.
Forkhead box O 3 (FOXO3) belongs to the family of FoxO transcription factors and is crucial in the regulation of cellular processes such as cell cycle regulation, apoptosis, cell metabolism, stress resistance and immunity (1). Single-nucleotide polymorphisms (SNPs) for FOXO3 have been found to be associated with longevity (2, 3) and favorable outcomes in inflammatory disease (4). A role of FOXO3 in dampening immune reactions was discussed in several studies (5, 6). We showed that Foxo3 plays an important role in acute viral infection as its activation attenuated natural killer (NK) cell responses in a viral myocarditis model (7). Thus, FOXO3 modulation would have therapeutic potential in chronic and autoimmune diseases.
FOXO3 is phosphorylated by various kinases. Growth factor induced phosphorylation via phosphatidylinositol-4,5-bisphosphate 3-kinase (PI3K)/protein kinase B (Akt) pathway results in an increased binding to the regulator protein 14-3-3 and consequently in nuclear exclusion. This results in reduction of its transcriptional activity (1). The energy-sensing AMP-activated protein kinase (AMPK) mediated phosphorylation induces activation of FOXO3 (8). Moreover, acetylation/deacetylation and methylation are implicated in FOXO3 regulation (1).
Metformin (1,1-dimethylbiguanide hydrochloride) is the first-line oral drug for treatment of type 2 diabetes. It has been shown to reduce cardiac events in diabetic patients and improve the overall outcome and prognosis (9). In vitro metformin activates AMPK, which is upstream of FOXO3 (10). Further, a reduction of reactive oxygen/nitrogen species (ROS/RNS) in primary hepatocytes (11) and human monocytes and macrophages by metformin has been described (12, 13). Based on the favorable outcome of diabetic patients taking metformin, several studies are now investigating the influence of metformin on aging (targeting/taming aging with metformin, TAME study (14) and metformin in Longevity Study, MILES study, ClinicalTrials.gov Identifier: NCT02432287). We hypothesized that metformin is a drug that can activate FOXO3 in immune cells. Hence, the effect of metformin on FOXO3 activation, downstream targets and ROS/RNS level in immune cells was investigated in the present study.
The study was approved and performed according to the ethical guidelines and regulations by the Institutional Ethics Committee (Charité Berlin). Informed consent was obtained from all subjects. Fresh PBMCs from healthy subjects were isolated from heparinized whole blood by density gradient centrifugation.
Splenocytes of FVB/N wildtype (WT) and FVB/N Foxo3 deficient (Foxo3-/-) littermates were used. Experiments were conducted conform to the NIH Guide for the care and use of laboratory animals and were approved by regional authorities for provisions on labor, health, and technical safety, Berlin, Germany. WT and Foxo3-/- mice were sacrificed and spleens were taken. Single cell suspensions were made by passing the spleen through a cell strainer (BD) and diluting the suspension in cold PBS (4°C). The cells were cultured in RPMI 1640 containing l-glutamine and supplemented with 10% FCS, and 1% each of penicillin and streptomycin at 37°C, 5% CO2, or used immediately.
Freshly isolated human PBMCs were incubated for 3 h with metformin [0.01 - 10 mM]. The cells were then washed twice in ice cold PBS and solubilized in lysis buffer for 30 min on ice. After centrifugation for 10 min at 12000 x g and 4°C the supernatant was harvested. 20 µg of the protein lysate was separated on a gradient gel (7.5 -12.5%) and transferred to a nitrocellulose membrane. After blocking in 5% BSA for 1h, the membranes were incubated with the primary antibodies mouse-α-GAPDH (FF26A/F9) (1:10000, BioLegend), rabbit-α-AMPK (Thr172) 40H9 (1:500; Cell Signaling), mouse-α-AMPKalpha (F6) (1:500; Cell Signaling). The primary antibody was incubated overnight at 4°C in 5% BSA (in 0,05% TBS-T) and the secondary antibody goat-α-rabbit or α -mouse Ig-HRP 1:2000 (#7074/7076 Cell Signaling) was incubated for 1 h. Detection was achieved by using an enhanced chemiluminescence system (Pierce™ ECL Western Blotting Substrate) according to the manufacturer’s instructions. Quantification was performed by ImageJ software.
CytoFLEX S, CytoFLEX LX (Backman Coulter), or LSR Fortessa (BD) was used. Data was analysed with FlowJo software 10.0.08. All gating strategies are shown in the Supplementary Figure 1. PBMCs were stained after stimulation with metformin [1 and 10 mM] or Compound C [CC, 10 µM] for 30 min at 37°C. 2% formalin fixed and 90% methanol permeabilized cells are blocked with 2% Flebo-γ. FOXO3 activation is visualized with an unlabeled monoclonal rabbit α -phospho-FOXO3 (Ser413) (D77C9) antibody (Cell Signaling) for 1 h. Secondary AF700-labelled goat-α-rabbit antibody (Invitrogen) was incubated for 30 min. Cell surface molecules CD3-PB (UCHT1; BioLegend), CD56-PE (HCD56; BioLegend), and CD14-FITC (HCD14; Biolegend) are stained afterwards for 15 min at 4°C.
Intracellular ROS/RNS staining was performed following 3 h metformin stimulation [0.1 - 20 mM]. For this, cells were stained with CD3-Per.CP-Cy5.5 (SK7); CD14-APC (M5E2); CD19-PE-Cy7 (HIB19) and CD56-PE (HCD56) purchased from BioLegend. After 10 min stimulation with phorbol 12‐myristate 13‐acetate (PMA) (Sigma Aldrich) [100 ng/ml] as positive control, cells were stained with viable and dead cells LIVE/DEAD™ Fixable Aqua dead cell stain kit (Life Technologies) followed by dichlorodihydrofluorescein diacetate (DCFH-DA) [5 µM; Sigma-Aldrich] incubation for 30 min. Mouse ROS/RNS measurement was performed according to the same protocol using staining antibodies (CD3-AF700 (17A2; Invitrogen); CD19-APC (6D5; BioLegend); Nkp46-APC (29A1.4; BioLegend); CD11b-PE (M1/70; eBioscience); CD45-PE-Cy7 (30-F11; eBioscience); Ly6C-PerCPCy5.5 (HK1.4; Invitrogen).
Total RNA was extracted (RNeasy® Mini Kit QIAGEN®) according to the manufacturer’s instructions. cDNA was prepared by reverse transcription and real-time PCR was performed using TaqMan® Universal PCR Master Mix (Applied Biosystems) and TaqMan® Gene Expression Assays for SOD2, HMOX1, CYCS and 18S rRNA (Applied Biosystems). All analyses were performed with the ABI7200 and software Step One Plus as absolute quantification according to manufacturer’s instruction. Relative expression was analysed using the ΔΔCT method and normalized against 18S rRNA.
Genomic DNA was isolated (QIAmp® DNA Blood Mini Kit QIAGEN®) according to the manufacturer’s protocol. Analysis of the FOXO3 SNP rs12212067 (ID: C:30780203_10), SNP rs2802292 (ID: C:16097219_10) and SNP rs12206094 (ID: C:30780173_10) was performed by allelic discrimination PCR according to the manufacturer’s instruction using Applied Biosystems 7500 Fast Real-Time PCR system. 10 ng of genomic DNA were used in a PCR reaction. TaqMan® SNP Genotyping Assays were purchased from Applied Biosystems. SNP genotypes are shown in the Supplementary Table.
Statistical data analysis was carried out using GraphPad Prism software, version 6.0. and 7.0 Continuous variables were expressed as median and interquartile range, if not indicated otherwise. Univariate comparison of two independent groups were done using Mann-Whitney U test (Mann Whitney test) and of dependent groups with the Wilcoxon matched-pairs signed rank test (Wilcoxon test). P values were calculated in a two-tailed manner. In all cases, the significance level was set to *P value < 0.05, **P < 0.01.
To investigate the activation of FOXO3, we first studied the effect of metformin on the AMPK kinase upstream of FOXO3 by immunoblot. We observed a dose dependent increase of the activating phosphorylation of AMPK (pT172) after incubation with metformin in human blood immune cells (Figure 1).
Figure 1 AMPK is activated in a dose dependent manner by metformin. (A) Representative image of the protein amount of pAMPK (pT172), AMPKalpha and GAPDH analysed in lysates of PBMCs stimulated for 3 h with metformin using specific antibodies by immunoblot analysis. pAMPK (pT172) and AMPKalpha are run on two separate gels in parallel each with GAPDH as control. (B) Activating phosphorylation of AMPK was quantified using Image J (NIH). The median stimulation index (SI, treated/untreated) is shown for the pAMPK/AMPKalpha ratio normalized to unstimulated control. Significance was calculated with the Mann-Whitney test between indicated groups. **P ≤ 0.01.
Next, the effect of metformin on the activating phosphorylation of FOXO3 (pS413) was studied in blood immune cells by FC. We observed an activation of FOXO3 by upregulation of pS413 in all human immune cell subpopulations including T cells, B cells, monocytes and both CD56bright and CD56dim NK cells after metformin incubation (Figure 2A). To elucidate the involvement of AMPK in metformin induced FOXO3 activation, AMPK was additionally inhibited by Compound C. The inhibition of AMPK resulted in a significant reduction of metformin induced phosphorylation of FOXO3 in all immune cells (Figure 2B).
Figure 2 FOXO3 is activated by metformin in an AMPK dependent manner. (A) FOXO3 (pS413) expression in T cells, B cells, monocytes and NK cells (total, CD56dim and CD56bright) after 30 min stimulation analysed by flow cytometry. (B) Addition of the AMPK inhibitor Compound C (CC) [10 µM] abrogated the effect of 10 mM metformin. SI: The MFI (AF700) of FOXO3 (pS413) expression subtracted by background signal (GAR) is depicted in relation to the unstimulated control. Median with interquartile range is shown. Wilcoxon test is used for statistical analysis in relation to unstimulated control or between indicated groups. *P ≤ 0.05, **P ≤ 0.01.
Next, the effect of metformin on ROS/RNS level was studied in immune cells under similar conditions. PMA-induced ROS/RNS level was diminished by metformin in CD14+ monocytes, CD3+ T cells, CD19+ B cells and CD56+ NK cells in a dose-dependent manner (Figures 3A–D). In unstimulated CD14+ monocytes ROS/RNS level was already diminished when incubated with 10 mM metformin (Figure 3C).
Figure 3 Metformin reduces ROS/RNS level in human immune cells in a dose-dependent manner. (A–D) ROS/RNS level was measured in blood immune cells, cultured with metformin for 3 h (n=6; met alone n=2). Cells were either unstimulated or stimulated with PMA [100 ng/ml] for 10 min and ROS/RNS generation was measured by FC in (A) T cells, (B) B cells, (C) monocytes and (D) NK cells using DCFH-DA. Median with interquartile range is depicted and Wilcoxon test is used for statistical analysis. *P ≤ 0.05.
To get further evidence that metformin attenuates ROS via FOXO3 we comparatively analysed WT and Foxo3-/- mice. As observed in human monocytes, incubation of splenocytes with metformin diminished ROS/RNS level in WT CD11b+ monocytes. In contrast metformin had no effect on monocytes in ROS/RNS in Foxo3-/- mice, providing further evidence that Foxo3 is involved in this pathway (Figure 4A). ROS/RNS level in CD3+ T cells of WT mice were not reduced by metformin (Figure 4B). Stimulation of splenocytes with PMA leads to an increased ROS/RNS production in 3 of 5 Foxo3-/- mice in CD11b+ (not significant) and in all 5 Foxo3-/- mice in T cells (p=0.03) (Figures 4C, D). Interestingly, the rather short incubation time of 10 min with PMA chosen to avoid toxicity in Foxo3-/- mice was not sufficient to induce the ROS/RNS production in WT mice.
Figure 4 ROS/RNS production is reduced by metformin in WT and induced by PMA in Foxo3-/- mice. ROS/RNS level was analysed in WT vs. Foxo3-/- splenocytes after 3 h of metformin [0.1 mM] (A, B) or 10 min of PMA [100 ng/ml] treatment (C, D). Mouse splenocytes were labeled with DCFH-DA and ROS/RNS level was analysed by FC (n=6) in (A, C) CD11b+ myeloid cells and (B, D) CD3+ T cells. Significance was evaluated using the Wilcoxon test. *P ≤ 0.05; ns, not significant.
We further studied the expression of FOXO3 regulated antioxidative enzymes SOD2, CYCS and HMOX1. Metformin stimulated the gene expression of SOD2 and CYCS but not of HMOX1 (Figure 5A). The enhanced gene expression was abolished by coincubation with the AMPK inhibitor Compound C (Figure 5B).
Figure 5 Gene expression of antioxidative enzymes is induced by metformin. (A) RNA expression analysis of SOD2, CYCS and HMOX1, three FOXO3 target genes after 3 h incubation with different dosages of metformin (n=4-7). (B) Antioxidative gene expression by metformin is AMPK dependent. Gene expression analysis of SOD2, CYCS and HMOX1 after 3 h incubation with metformin [10 mM] alone or in combination with CC [10 µM] (n=7). Gene expression was normalized to 18S rRNA and is depicted as fold induction to unstimulated control. Median with interquartile range is shown and significance was evaluated between unnormalized data and refers to unstimulated control using the Wilcoxon test. *P ≤ 0.05; ns, not significant.
In addition, we studied the gene expression of MAP1LC3A coding for the autophagic marker LC3. The gene expression was induced in PBMCs treated with 10 mM metformin compared to untreated control (p=0.047) (Figure S2). However, this increase was not observed in cells co-treated with Compound C, implicating AMPK in FOXO3 dependent autophagic gene regulation by metformin.
Finally, the three different gain-of-function FOXO3 SNPs rs12212067, rs2802292 and rs12206094 associated with aging (2, 3) and inflammation (4) were analysed for their potential influence on ROS/RNS level in immune cells of healthy controls. We observed neither differences in the ROS/RNS level in monocytes at baseline (data not shown) nor after 10 min of PMA stimulation in carriers of the longevity/immune dampening-associated alleles of the SNPs (Figure 6).
Figure 6 FOXO3 gain-of-function SNPs have no influence on ROS/RNS level. ROS/RNS level in CD14+ monocytes were investigated following stimulation with PMA [100 ng/ml] for 10 min by DCFH-DA staining using FC. (A) rs12212067 SNP carrier minor/heterozygous n=12 and major n=30. Minor allele carrier is depicted with an open circle (○). (B) rs2802292 SNP carrier minor n=10, heterozygous n=21 and major n=11 and (C) rs12206094 SNP carrier: minor n=4, heterozygous n=21 and major n=17. Median with interquartile range is depicted and Mann-Whitney test is used for statistical analysis between independent cohorts.
FOXO3 is a gene associated with healthy aging in centenarians, a lower prevalence of cardiovascular events and a better outcome in inflammatory disorders (2–4, 15). Therefore, it is of great interest to find drugs that activate FOXO3 and target downstream ROS and inflammatory cytokine responses. Here, we provide evidence that metformin activates FOXO3 via the AMPK signalling pathway which subsequently leads to the induction of FOXO3 antioxidative target genes SOD2 and CYCS as well as the induction of autophagic gene expression. Further we show that triggering this pathway by metformin is associated with reduced ROS/RNS level. To our knowledge this is the first study providing evidence that FOXO3 can be activated by metformin in immune cells.
We found that FOXO3 is activated by metformin stimulation in immune cells. Using the AMPK inhibitor Compound C we provide evidence that metformin-triggered FOXO3 activation is AMPK-dependent. However, Compound C has been shown to inhibit several other kinases, including the FOXO3 regulator Akt (16). We cannot thus exclude the possibility that other kinases might have been affected by Compound C in our experiments. However, in line with our findings a metformin-triggered, AMPK-dependent activation of FOXO3 was shown previously by Chou et al. in cancer cells by targeting the Akt-MDM2-FOXO3 signalling axis (17). Further, we provide evidence in our study that FOXO3 is phosphorylated at an AMPK specific phosphorylation site, Serine 413, following metformin incubation. Furthermore, metformin activates gene expression of FOXO3 targets SOD2 and CYCS. The upregulation was diminished by co-treatment with the AMPK inhibitor Compound C indicating an involvement of AMPK function. In addition, metformin incubation resulted in reduced PMA-stimulated ROS/RNS production in human immune cells. Mitochondrial SOD2 is important for neutralizing ROS. The enzyme binds to superoxide byproducts of oxidative phosphorylation and converts them to hydrogen peroxide and diatomic oxygen (18). CYCS is a small hemeprotein found loosely associated with the inner membrane of the mitochondrion. It belongs to the cytochrome c family of proteins and can remove superoxide (O2–) and H2O2 from mitochondria (19). Reduction of ROS/RNS levels in human cells by metformin has already been shown by other groups for other cell types including primary hepatocytes and vestibular cells (11, 20). The study of Hou et al. indicates that metformin reduces ROS by inducing Trx expression via AMPK-FOXO3 signalling in aortic endothelial cells (21). In addition, we observed an increased gene expression of LC3, an autophagic marker, in metformin treated, but not in Compound C co-treated cells. These data suggest, that metformin might induce autophagy in PBMCs probably in an AMPK-dependent manner. Autophagy is an essential mechanism in response to oxidative stress and associated mitochondrial dysfunction (22). It enables the clearance of damaged proteins and cell organelles. Interestingly, the LC3 gene is described as target gene for FOXO3 (23) and it was shown in several studies that autophagy can be induced via AMPK-FOXO3 signalling (24–26). These data corroborate our results about the effects of metformin-AMPK-FOXO-signalling in immune cells. In a recent study by Gillespie et al. it was shown that metformin stimulation results in decreased fibroblast proliferation and increased FOXO3 promotor occupancy. However, pathway analysis of FOXO3 activation and downstream effects were not studied (27).
Using Foxo3-/- mice we provide further evidence that the reduction of ROS/RNS levels by metformin at least partly depends on Foxo3 as we observed that metformin reduced basal intracellular ROS/RNS level in WT but not Foxo3-/- mice. A metformin dependent reduction of the mitochondrial ROS production by direct inhibition of mitochondrial complex I was discussed in several studies (28). We cannot exclude a partial direct effect of metformin on the mitochondrial ROS production in our experiments. As metformin-induced antioxidative gene expression was observed in our study we provide evidence about an AMPK-FOXO3-axis dependent cellular stress response also involved in the decline in ROS level. In addition, our experiments using Foxo3-/- mice further confirm the antioxidative properties of Foxo3, as PMA enhanced ROS/RNS in CD11b+ and T cells of Foxo3-/- mice but not WT mice. This is in line with the study of Joseph et al. Here the ROS production, induced by a chronic Salmonella typhimurium infection, was more prominent in splenocytes of Foxo3a−/− when compared to WT mice (29). Furthermore, they observed a decreased expression of antioxidative genes in infected Foxo3a−/− mice. In general, the important role of FOXO3 in the intracellular oxidative stress response and damage repair via gene expression of antioxidative as well as autophagy-related genes has been determined in several studies (1, 23).
SNPs in FOXO3 were shown in previous studies to be associated with ameliorated clinical course of rheumatoid arthritis (30), Crohn´s disease (4) and viral myocarditis (7). In the study by Lee et al. monocytes from healthy subjects carrying the SNP rs12212067 had lower TNF-α response upon LPS stimulation (4). In our previous study, healthy subjects with the FOXO3 SNP rs12212067 had lower IFN-γ production in peripheral NK cells after 18 h of R848 stimulation (7). Furthermore two of the here analysed SNPs (rs2802292, rs12206094) are associated with longevity (2, 3). In the present study we therefore wanted to investigate if these three SNPs, located within different intronic regions with a low linkage disequilibrium, have an influence on ROS/RNS level in human immune cells and if so may influence the response to metformin. Grossi et al. showed that the SNP rs2802292 is associated with diminished ROS content, measured with DCFH-DA in human primary dermal fibroblasts after stressing the cells with H2O2. They found that the SNP, located in the intron 2 of FOXO3 influences enhancer function creating a novel binding site for HSF1, so inducing FOXO3 expression along with stress response via upregulation of target genes (SOD2, CAT, GADD45A, HSPA1A) (31). In contrast we could not observe an association of any of the 3 SNPs with levels of ROS/RNS in immune cells.
We have evidence from our previous study that FOXO3 plays a role in attenuating cytokine responses. We could show that NK cells of Foxo3-/- mice had an increased IFN-γ response to Il-2. Furthermore, healthy carrier of the immune dampening-associated FOXO3 SNP rs12212067 had reduced IFN-γ producing CD56bright NK cells after 18 h of R848 stimulation (7). Diminished levels of ROS itself may play a role in attenuation of cytokine levels as it was shown that ROS activates NF-κB and TH1 cytokine production (32). Thus, FOXO3 may affect cytokine production by reducing intracellular ROS. However, other studies showed that metformin can inhibit NF-κB directly (33). Further, it was shown that FOXO3 antagonizes signalling intermediates downstream of the Toll‐like receptor (TLR) 4, such as NF‐κB and IRFs, resulting in decreased IFN‐β expression in human monocyte‐derived DCs (34). Buldak et al. showed that metformin in a dose of 0.02 and 2 mM reduces the LPS-induced TNF-α response in human monocyte derived macrophages after 24 h (13). We could not observe an influence of metformin on LPS induced TNF-α production in human monocytes under similar conditions (data not shown).
In conclusion, our data provide evidence that metformin activates FOXO3 in immune cells resulting in reductions of ROS/RNS stress. This effect may be beneficial in chronic inflammation and atherosclerosis and may play a role in the improved morbidity and mortality of diabetes patients taking metformin (9). Ongoing trials (TAME, MILES) are examining the effect of metformin on morbidity and mortality in healthy older people (ClinicalTrials.gov Identifier: NCT02432287 (14). Based on our findings it would be very interesting to study the effect of metformin on FOXO3 activation in clinical trials and the potential influence of FOXO3 SNPs.
The raw data supporting the conclusions of this article will be made available by the authors, without undue reservation.
The studies involving human participants were reviewed and approved by Institutional Ethics Committee (Charité Berlin). The patients/participants provided their written informed consent to participate in this study. The animal study was reviewed and approved by Landesamt für Gesundheit und Soziales (LAGeSo).
Funding Acquisition: ML, CSc and CSk. Conceptualization: JH, FS, ML and CSc. Investigation: JH carried out the experiments with the exception that SS performed DCFH-DA staining in healthy controls. Writing-Original Draft Preparation: JH, CSc and FS. Resources: SB contributed to materials and reagents, ZK and CR helped designing the mice study and provided samples. Formal Analysis: JH, FS and CSc. Writing-Review and Editing: FS, ML, ZK, CR, CSc and CSk. All authors contributed to the article and approved the submitted version.
This study was supported by a Grant of the German Research Council (DFG) (SCHE 478/9-1) to CSc and CSk.
The authors declare that the research was conducted in the absence of any commercial or financial relationships that could be construed as a potential conflict of interest.
The content of this manuscript has been published as part of the thesis of JH (35). Available at: http://dx.doi.org/10.14279/depositonce-8723 (Accessed October 23, 2019).
The Supplementary Material for this article can be found online at: https://www.frontiersin.org/articles/10.3389/fimmu.2021.581799/full#supplementary-material
1. Morris BJ, Willcox DC, Donlon TA, Willcox BJ. FOXO3: A Major Gene for Human Longevity–a Mini-Review. Gerontology (2015) 61(6):515–25. doi: 10.1159/000375235
2. Flachsbart F, Caliebe A, Kleindorp R, Blanche H, von Eller-Eberstein H, Nikolaus S, et al. Association of FOXO3A Variation With Human Longevity Confirmed in German Centenarians. Proc Natl Acad Sci USA (2009) 106(8):2700–5. doi: 10.1073/pnas.0809594106
3. Flachsbart F, Dose J, Gentschew L, Geismann C, Caliebe A, Knecht C, et al. Identification and Characterization of Two Functional Variants in the Human Longevity Gene FOXO3. Nat Commun (2017) 8(1):2063. doi: 10.1038/s41467-017-02183-y
4. Lee JC, Espeli M, Anderson CA, Linterman MA, Pocock JM, Williams NJ, et al. Human SNP Links Differential Outcomes in Inflammatory and Infectious Disease to a FOXO3-Regulated Pathway. Cell (2013) 155(1):57–69. doi: 10.1016/j.cell.2013.08.034
5. Brandstetter B, Dalwigk K, Platzer A, Niederreiter B, Kartnig F, Fischer A, et al. FOXO3 is Involved in the Tumor Necrosis Factor-Driven Inflammatory Response in Fibroblast-Like Synoviocytes. Lab Invest (2019) 99(5):648–58. doi: 10.1038/s41374-018-0184-7
6. Penrose HM, Cable C, Heller S, Ungerleider N, Nakhoul H, Baddoo M, et al. Loss of Forkhead Box O3 Facilitates Inflammatory Colon Cancer: Transcriptome Profiling of the Immune Landscape and Novel Targets. Cell Mol Gastroenterol Hepatol (2019) 7(2):391–408. doi: 10.1016/j.jcmgh.2018.10.003
7. Loebel M, Holzhauser L, Hartwig JA, Shukla PC, Savvatis K, Jenke A, et al. The Forkhead Transcription Factor Foxo3 Negatively Regulates Natural Killer Cell Function and Viral Clearance in Myocarditis. Eur Heart J (2018) 39(10):876–87. doi: 10.1093/eurheartj/ehx624
8. Greer EL, Oskoui PR, Banko MR, Maniar JM, Gygi MP, Gygi SP, et al. The Energy Sensor AMP-Activated Protein Kinase Directly Regulates the Mammalian FOXO3 Transcription Factor. J Biol Chem (2007) 282(41):30107–19. doi: 10.1074/jbc.M705325200
9. Group* UPDSU. Effect of Intensive Blood-Glucose Control With Metformin on Complications in Overweight Patients With Type 2 Diabetes (UKPDS 34). Lancet (1998) 352(9131):854–65. doi: 10.1016/s0140-6736(98)07037-8
10. Zhou G, Myers R, Li Y, Chen Y, Shen X, Fenyk-Melody J, et al. Role of AMP-Activated Protein Kinase in Mechanism of Metformin Action. J Clin Invest (2001) 108(8):1167–74. doi: 10.1172/jci13505
11. Ota S, Horigome K, Ishii T, Nakai M, Hayashi K, Kawamura T, et al. Metformin Suppresses Glucose-6-Phosphatase Expression by a Complex I Inhibition and AMPK Activation-Independent Mechanism. Biochem Biophys Res Commun (2009) 388(2):311–6. doi: 10.1016/j.bbrc.2009.07.164
12. Kajiwara C, Kusaka Y, Kimura S, Yamaguchi T, Nanjo Y, Ishii Y, et al. Metformin Mediates Protection Against Legionella Pneumonia Through Activation of AMPK and Mitochondrial Reactive Oxygen Species. J Immunol (2018) 200(2):623–31. doi: 10.4049/jimmunol.1700474
13. Buldak L, Labuzek K, Buldak RJ, Kozlowski M, Machnik G, Liber S, et al. Metformin Affects Macrophages’ Phenotype and Improves the Activity of Glutathione Peroxidase, Superoxide Dismutase, Catalase and Decreases Malondialdehyde Concentration in a Partially AMPK-Independent Manner in LPS-Stimulated Human Monocytes/Macrophages. Pharmacol Rep PR (2014) 66(3):418–29. doi: 10.1016/j.pharep.2013.11.008
14. Barzilai N, Crandall JP, Kritchevsky SB, Espeland MA. Metformin as a Tool to Target Aging. Cell Metab (2016) 23(6):1060–5. doi: 10.1016/j.cmet.2016.05.011
15. Xin Z, Ma Z, Jiang S, Wang D, Fan C, Di S, et al. Foxos in the Impaired Heart: New Therapeutic Targets for Cardiac Diseases. Biochim Biophys Acta Mol Basis Dis (2017) 1863(2):486–98. doi: 10.1016/j.bbadis.2016.11.023
16. Bain J, Plater L, Elliott M, Shpiro N, Hastie CJ, Mclauchlan H, et al. The Selectivity of Protein Kinase Inhibitors: A Further Update. Biochem J (2007) 408(3):297–315. doi: 10.1042/bj20070797
17. Chou CC, Lee KH, Lai IL, Wang D, Mo X, Kulp SK, et al. AMPK Reverses the Mesenchymal Phenotype of Cancer Cells by Targeting the Akt-MDM2-Foxo3a Signaling Axis. Cancer Res (2014) 74(17):4783–95. doi: 10.1158/0008-5472.CAN-14-0135
18. Fridovich I. The Trail to Superoxide Dismutase. Protein Sci Publ Protein Soc (1998) 7(12):2688–90. doi: 10.1002/pro.5560071225
19. Zhao Y, Wang ZB, Xu JX. Effect of Cytochrome C on the Generation and Elimination of O2*- and H2O2 in Mitochondria. J Biol Chem (2003) 278(4):2356–60. doi: 10.1074/jbc.M209681200
20. Lee JY, Lee SH, Chang JW, Song JJ, Jung HH, Im GJ. Protective Effect of Metformin on Gentamicin-Induced Vestibulotoxicity in Rat Primary Cell Culture. Clin Exp Otorhinolaryngol (2014) 7(4):286–94. doi: 10.3342/ceo.2014.7.4.286
21. Hou X, Song J, Li XN, Zhang L, Wang X, Chen L, et al. Metformin Reduces Intracellular Reactive Oxygen Species Levels by Upregulating Expression of the Antioxidant Thioredoxin Via the AMPK-FOXO3 Pathway. Biochem Biophys Res Commun (2010) 396(2):199–205. doi: 10.1016/j.bbrc.2010.04.017
22. Lee J, Giordano S, Zhang J. Autophagy, Mitochondria and Oxidative Stress: Cross-Talk and Redox Signalling. Biochem J (2012) 441(2):523–40. doi: 10.1042/BJ20111451
23. Eijkelenboom A, Burgering BM. Foxos: Signalling Integrators for Homeostasis Maintenance. Nat Rev Mol Cell Biol (2013) 14(2):83–97. doi: 10.1038/nrm3507
24. Sanchez AM, Csibi A, Raibon A, Cornille K, Gay S, Bernardi H, et al. AMPK Promotes Skeletal Muscle Autophagy Through Activation of Forkhead Foxo3a and Interaction With Ulk1. J Cell Biochem (2012) 113(2):695–710. doi: 10.1002/jcb.23399
25. Nepal S, Park PH. Activation of Autophagy by Globular Adiponectin Attenuates Ethanol-Induced Apoptosis in Hepg2 Cells: Involvement of AMPK/Foxo3a Axis. Biochim Biophys Acta (2013) 1833(10):2111–25. doi: 10.1016/j.bbamcr.2013.05.013
26. Chi Y, Shi C, Zhao Y, Guo C, Forkhead box O. (FOXO) 3 Modulates Hypoxia-Induced Autophagy Through AMPK Signalling Pathway in Cardiomyocytes. Biosci Rep (2016) 36(3):e00345. doi: 10.1042/BSR20160091
27. Gillespie ZE, Wang C, Vadan F, Yu TY, Ausio J, Kusalik A, et al. Metformin Induces the AP-1 Transcription Factor Network in Normal Dermal Fibroblasts. Sci Rep (2019) 9(1):5369. doi: 10.1038/s41598-019-41839-1
28. Vial G, Detaille D, Guigas B. Role of Mitochondria in the Mechanism(S) of Action of Metformin. Front Endocrinol (Lausanne) (2019) 10:294. doi: 10.3389/fendo.2019.00294
29. Joseph J, Ametepe ES, Haribabu N, Agbayani G, Krishnan L, Blais A, et al. Inhibition of ROS and Upregulation of Inflammatory Cytokines by Foxo3a Promotes Survival Against Salmonella Typhimurium. Nat Commun (2016) 7:12748. doi: 10.1038/ncomms12748
30. Viatte S, Lee JC, Fu B, Espeli M, Lunt M, De Wolf JN, et al. Association Between Genetic Variation in FOXO3 and Reductions in Inflammation and Disease Activity in Inflammatory Polyarthritis. Arthritis Rheumatol (2016) 68(11):2629–36. doi: 10.1002/art.39760
31. Grossi V, Forte G, Sanese P, Peserico A, Tezil T, Lepore Signorile M, et al. The Longevity SNP Rs2802292 Uncovered: HSF1 Activates Stress-Dependent Expression of FOXO3 Through an Intronic Enhancer. Nucleic Acids Res (2018) 46(11):5587–600. doi: 10.1093/nar/gky331
32. Kesarwani P, Murali AK, Al-Khami AA, Mehrotra S. Redox Regulation of T-Cell Function: From Molecular Mechanisms to Significance in Human Health and Disease. Antioxid Redox Signaling (2013) 18(12):1497–534. doi: 10.1089/ars.2011.4073
33. Hattori Y, Suzuki K, Hattori S, Kasai K. Metformin Inhibits Cytokine-Induced Nuclear Factor Kappab Activation Via AMP-Activated Protein Kinase Activation in Vascular Endothelial Cells. Hypertension (2006) 47(6):1183–8. doi: 10.1161/01.HYP.0000221429.94591.72
34. Luron L, Saliba D, Blazek K, Lanfrancotti A, Udalova IA. FOXO3 as a New IKK-Epsilon-Controlled Check-Point of Regulation of IFN-Beta Expression. Eur J Immunol (2012) 42(4):1030–7. doi: 10.1002/eji.201141969
Keywords: metformin, FOXO3, AMPK, reactive oxygen species, immune cells
Citation: Hartwig J, Loebel M, Steiner S, Bauer S, Karadeniz Z, Roeger C, Skurk C, Scheibenbogen C and Sotzny F (2021) Metformin Attenuates ROS via FOXO3 Activation in Immune Cells. Front. Immunol. 12:581799. doi: 10.3389/fimmu.2021.581799
Received: 18 August 2020; Accepted: 31 March 2021;
Published: 19 April 2021.
Edited by:
Janos G. Filep, Université de Montréal, CanadaReviewed by:
Brian James Morris, The University of Sydney, AustraliaCopyright © 2021 Hartwig, Loebel, Steiner, Bauer, Karadeniz, Roeger, Skurk, Scheibenbogen and Sotzny. This is an open-access article distributed under the terms of the Creative Commons Attribution License (CC BY). The use, distribution or reproduction in other forums is permitted, provided the original author(s) and the copyright owner(s) are credited and that the original publication in this journal is cited, in accordance with accepted academic practice. No use, distribution or reproduction is permitted which does not comply with these terms.
*Correspondence: Franziska Sotzny, ZnJhbnppc2thLnNvdHpueUBjaGFyaXRlLmRl
Disclaimer: All claims expressed in this article are solely those of the authors and do not necessarily represent those of their affiliated organizations, or those of the publisher, the editors and the reviewers. Any product that may be evaluated in this article or claim that may be made by its manufacturer is not guaranteed or endorsed by the publisher.
Research integrity at Frontiers
Learn more about the work of our research integrity team to safeguard the quality of each article we publish.