- 1World Bank Africa Centre of Excellence in Public Health and Toxicological Research (PUTOR), University of Port Harcourt, Port Harcourt, Nigeria
- 2Department of Dental Health Sciences, Ogbia, Bayelsa State College of Health Technology, Otakeme, Nigeria
- 3Department of Experimental Pharmacology & Toxicology, Faculty of Pharmacy, University of Port Harcourtt, Port Harcourt, Nigeria
Hibiscus sabdariffa calyx (HS) water decoction extract is a commonly consumed beverage with various pharmacological properties. This systematic review examines the possible effect of HS intake on immune mediators. The Scopus and PUBMED databases were searched for all human and animal studies that investigated the effect of HS administration on immune related biomarkers. For each of the immune biomarkers, the mean, standard deviation and number of subjects were extracted for both the HS treated and untreated group. These values were used in the computation of standardized mean difference (SMD). Statistical analysis and forest plot were done with R statistical software (version 3.6.1). Twenty seven (27) studies met the eligibility criteria. Twenty two (22) of the studies were used for the meta-analysis which included a total of 1211 subjects. The meta-analysis showed that HS administration significantly lowered the levels of TNF-α (n=10; pooled SMD: -1.55; 95% CI: -2.43, -0.67; P < 0.01), IL-6 (n=11; pooled SMD:-1.09; 95% CI: -1.77, -0.40; P < 0.01), IL-1β (n=7; pooled SMD:-0.62; 95% CI: -1.25, 0.00; P = 0.05), Edema formation (n=4; pooled SMD: -2.29; 95% CI: -4.47, -0.11; P = 0.04), Monocyte Chemoattractant Protein -1 (n=4; pooled SMD: -1.17; 95% CI: -1.78, -0.57; P < 0.01) and Angiotensin converting enzyme cascade (n=6; pooled SMD: -0.91; 95% CI: -1.57, -0.25; P < 0.01). The levels of IL-10 (n=4; pooled SMD: -0.38; 95% CI: -1.67, 0.91; P = 0.56), Interleukin 8 (n=2; pooled SMD:-0.12; 95% CI: -0.76, 0.51; P = 0.71), iNOS (n=2; pooled SMD:-0.69; 95% CI: -1.60, 0.23 P = 0.14) and C- Reactive Protein (n=4; pooled SMD: 0.05; 95% CI: -0.26, 0.36; P = 0.75), were not significantly changed by HS administration. Some of the results had high statistical heterogeneity. HS may be promising in the management of disorders involving hyperactive immune system or chronic inflammation.
Introduction
Hibiscus sabdariffa (HS) is a shrub that belongs to the Malvaceae family (1). It is cultivated in many parts of the world such as in West Africa, South Asia (2), the West Indies, Jamaica, China, and the United States (3). It is also cultivated in Egypt, Saudi Arabia, and Sudan (1). It is popularly called Rosella, Mesta, Krajeab, Zobo, and Sorrel in Australia, India, Thailand, Nigeria, and Latin America, respectively (1). Water decoction from dried HS calyx is usually consumed as a beverage drink (4) and used in folk medicine for the management of diseases such as hypertension, liver disorders (5), pyrexia (1, 5), dyslipidemia, diabetes (6), high blood pressure, liver diseases, ulcers, abscesses, and anemia (1). The fleshy calyces of HS are consumed as vegetables (7) and used in making jellies, wine, cakes, syrup, and colorants (8).
The use of HS calyx extracts in traditional medicine has been justified by many studies. Such studies show that extracts from HS possess anti-oxidative (2, 9), radical scavenging (10, 11), angiotensin converting enzyme 1 (ACE1) inhibitory (12–14) activities. HS also possess antihypertensive, anti-bacterial (1), anti-proliferative, anti-glycemic (9, 15) anti-hyperlipidaemic (15), antiobesity (9, 16), anti-inflammatory, anti-carcinogenic (1, 9), antinociceptive, anti-diarrheal, hepatoprotective (1), and antiproliferative (17) activities.
Various phytochemicals such as organic acids (16) phenolic acids (6), flavones and anthocyanin flavonoids (5), essential oils (18), fatty acids (7), and polysaccharides (4) have been detected in extracts from HS. Major organic acids identified in HS include hydroxycitric acids, hibiscus acid, and its derivatives (16, 17, 19, 20). The predominant phenolic acids in extracts from HS include protocatechuic acid (PCA) (21), 3-O-caffeoylquinic acid (7), chlorogenic acid (6), neochlorogenic acid, methyl digallate, methyl chlorogenate, coumaroilquinic acid, dihyroferulic acid 4-o-glucoronide, 5-O-caffeoylshikimic acid, and ethyl chlorogenate, etc. (19). The aglycones, O, and 3-substituted derivatives of quercetin, myricetin, kaempferol, Gossypetin, and methyl epigallocatechin have also been identified from extracts of HS (7, 19, 20).
Anthocyanins are the major flavonoids present in HS; they are responsible for the color pigment. Anthocyanins are water soluble glycosides or acylglycosides of anthocyanidin which are derivates of the 2-phenylbenzopyrylium salt (5). Delphinidin 3-sambubioside forms about 85% of the anthocyanin contents of HS including cyanidin 3-sambubioside (major pigment), and delphinidin-3-glucoside and cyanidin 3-glucoside as minor pigments (5, 6, 22). Other derivatives of anthocyanins have also been reported (17). The carbohydrates identified from extracts of HS include glucose, galactose, xylose, arabinose, rhamnose, and pectin-like polysaccharides (4, 23). Fatty acids such as hexadecanoic, 14-Methyl-pentadecanoic acid, and methyl esters have also been identified in HS (18). Palmitic acid and alpha tocopherol are the most abundant lipophilic compounds in HS (7).
HS has recently gained attention because it has beneficial effects in the management of hypertension and other metabolic syndromes such as obesity and diabetes. Hypertensive subjects have elevated serum ACE1 activity (24, 25). Accumulating evidence demonstrates the inhibitory activity of HS extract on ACE1 activity (13, 25, 26). The catalytic conversion of angiotensin I (Ang I) to angiotensin II (Ang II) by ACE1 leads to the buildup of Ang II. Elevated levels of inflammatory mediators and the depletion of cellular antioxidant capacity have been observed in different models of hypertension (25, 27–29). Ang II-induced stimulation of the angiotensin II receptor type 1 (AT1 receptor) could lead to the activation of Nuclear Factor Kappa B (NF-κB), bringing about the expression of pro-inflammatory genes (29, 30). ACE1 inhibitors can lower Ang II levels thereby suppressing the Ang II-induced stimulation of AT1 receptors. The antioxidant (2, 21) and ACE1 inhibitory (13, 24) activities of constituents of HS extract, makes it potentially able to suppress inflammatory reactions. HS has been reported to suppress the levels of NF-κB in Lipopolysaccharide (LPS)-stimulated recombinant Human hepatoma cell line (HepG2) cells (31), metabolic syndrome, and Thioacetamide (TAA)-intoxicated rats (17). There is currently no systematic review or meta-analysis on the effect of HS extract supplementation on the expression of inflammatory cytokines in different disease and nondisease models. This review thus aimed to review the available evidence on the immunomodulatory potential of HS extracts and how such activity might be exploited in the management of pathological conditions linked to hyperactive immune responses.
Methods
This review was done according to the recommendations of the Preferred Reporting Items for Systematic Reviews and Meta-Analyses (PRISMA) (32).
Data Sources and Search Strategy
Online databases (Pubmed and Scopus) were systematically searched by two researchers reviewing independently (UF and NC) to find articles that documented the impact of HS on immune biomarkers. Date restriction was not applied in the search. The search was conducted between March 20, 2020, and June 5, 2020. The databases were searched with the keywords (“Hibiscus sabdariffa” OR roselle). The title and abstract of all the resultant search results were then screened for eligibility.
Eligibility Criteria
The following were inputted in the inclusion criteria; (1) original articles published in the English language. (2) Human and animal studies that used HS as an intervention agent in a healthy or disease model. (3) Studies that reported any immune or inflammatory related biomarkers such as Interleukins, Chemokines, Toll-like receptors (TLRs), Prostaglandins, Tumor Necrosis Factor-α (TNF-α), NF-κB, Monocyte chemoattractant protein-1 (MCP-1/CCL2), Inducible Nitric Oxide synthase (iNOS), components of the cyclooxygenase and lipoxygenases pathways, components of the ACE cascade (Ang I, Ang II, AT1 receptor, Angiotensin II receptor type 2 (AT2 receptor), ACE), inflammatory endpoints such as edema formation, and inflammatory diseases, etc. (4) Studies done using any extract from the calyces of HS. (5) Only studies that included both a control and an HS-intervention group were considered. Data obtained from in vitro studies, grey literature, meta-analysis, and reviews were excluded from the review. Studies done on extracts obtained from parts of HS (other than the calyx) or other species of Hibiscus were excluded.
Data Selection
Duplicate records were deleted, after which two authors (UF, NC) reviewed the collated articles to ascertain their suitability for inclusion. Where the two authors had different opinions on the inclusion of an article, the other researchers (EEBC, UJ, and BG) voted and a consensus was reached. Titles and abstracts of articles were first screened, and publications that did not meet the written criteria were removed. Five independent researchers (UF, BG, EEBC, UJ, NC) assessed the full texts of the remaining articles for eligibility. For each of the eligible studies, relevant data were extracted independently by each of the reviewers (UF, BG, EEBC, UJ, NC), into a predesigned data extraction table which consisted of the first author’s last name, year of study, experimental model, HS formulation, dosage/route of administration, analytical method, the inflammatory biomarker investigated, tissue investigated, study sample size and summary of major findings. The data extraction table generated by each reviewer was subsequently compared and collated.
Statistical Analysis
Missing or incomplete data was estimated with Cochrane’s review manager software (RevMan 5.3). Statistics and meta-analysis were done with R statistical software (version 3.6.1). A random effect model was assumed and meta-analysis was computed using the DerSimonian-Laird tau estimator. The forest plot was plotted with the “meta-forest” statistical package using R (33–35). The standardized mean difference (SMD) was used as an index of the effect size. SMD was used for the forest plot since varying units were showing a particular effect across studies (36). The random effect model was assumed to accommodate the different experimental models that generated the investigated effects (36).
The forest plot is essential for analyzing the pooled estimate of the mean differences between the control and the HS intervention group across different studies. The overall standardized mean difference and the pooled 95% confidence interval were presented for each biomarker analyzed. A forest plot was carried out on a particular immune-related biomarker only if more than one study reported complete data for that particular biomarker. The effect of HS on the levels of inflammatory biomarkers across healthy and diseased subgroups of the experimental models, as well as across human and rodent models were examined using subgroup analysis. Raw data for mean and standard deviation (SD) were extracted from chart images with the aid of a digital pixel ruler downloaded from www.arulerforwindows.com.
For studies that reported standard error of mean (SEM) values, the SD was calculated using SD = (SEM∗√n).
In estimating the pooled effect of HS administration on ACE signaling, data from different members of the pathways were combined in one analysis. For example the Ang II, ACE1, and Ang I receptors were all analyzed under ACE signaling. In studies where more than one dose of the HS extract was given, the dose that elicited the most effect was reported and used for the analyses. Some of the studies recorded some missing data. Such missing data (when possible), was estimated using RevMan 5.3. In some cases, the published data were not reported in forms that can be subjected to analysis with other data. Such data were transformed appropriately before analysis.
Results
Data Selection
The total search hits from both databases were 3718 papers (1943 from Scopus and 1775 from PubMed). A total of 78 relevant articles were downloaded after screening the title and abstract of the search results against the eligibility criteria. Duplicates articles (n=19) were removed and the remaining unique articles (n=59) were subjected to full-text screening. One article was excluded on account of being inaccessible. Twenty seven (27) articles were eventually selected for the review study, 22 of which were used for the meta-analysis (Figure 1). The meta-analysis consists of three pre-post clinical trials, three randomized double blind clinical trials, one randomized single blind clinical trial, 15 animal studies, and 1211 subjects in all. The details of the included and excluded study are shown in Table 1.
Effect of HS Extracts on Inflammatory and Immune Mediators
Administration of HS calyces extract significantly decreased the levels of different pro-inflammatory biomarkers such as TNF-α (9, 11, 16, 31, 40–43), Interleukin 6 (IL-6) (11, 16, 17, 40, 42–44), iNOS (31, 45), Toll-like receptor 4 (TLR4) (16), Interleukin 1β (IL-1β) (9, 16, 42), MCP-1 (11, 16, 42), Ang I (46), Ang II (26), ACE1 (24–26, 47), NF-κB (17), and Cyclooxygenase-2 (COX-2) (38, 48). It also significantly decreased the levels of inflammatory manifestations of ear edema (1, 49) and paw edema (11). HS treatments do not seem to have any significant effect on the levels of C-reactive protein (CRP) (42, 50, 51). There are conflicting reports on the effect of HS extracts on the levels of interleukin 10 (IL-10). While Fakeye et al. (41) and Villalpando-Arteaga et al. (9) did not detect any significant difference, Lubis et al. (40), and Nurkhasanah (39) reported a significant increase and Ali et al. (44), reported a significant decrease in IL-10 levels. Some studies did not detect any significant difference in the levels of ACE, IL-6 and IL-1β following treatments with HS calyx extract (9, 14, 42, 52).
Meta-Analysis of Effects Across Studies
Figures 2–11 show the meta-analysis of the effect of HS extract administration on the levels of Ang II cascade (ACE1, Ang II, Ang I, AT1 receptors), CRP, Edema formation, iNOS, IL-1β, IL-6, Interleukin 8 (IL-8), IL-10, MCP-1, and TNF-α across different studies.
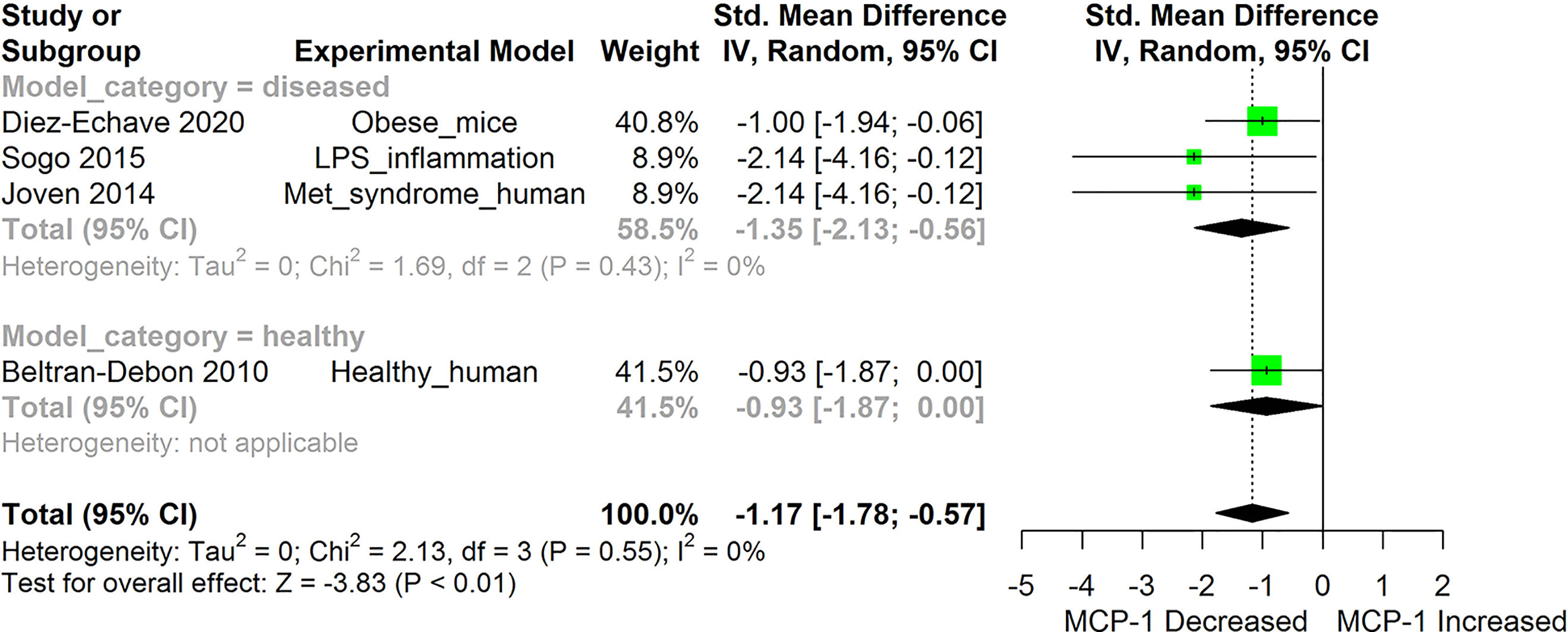
Figure 2 Meta-analysis and forest plot of the effect of HS administration on Monocyte Chemoattractant Protein -1b (MCP-1/CCL2). The meta-analysis was sub-grouped by health status (i.e diseased or healthy state). The standardized mean difference (SMD), which is an estimate of the effect size, is presented as a square on the forest plot, for each study and as a diamond for the pooled estimate of each subgroup or overall studies. The overall pooled SMD for all included studies is shown in the bottom section of the forest plot. SMD data points that are lower than zero signify that treatments with HS lowered the level of the biomarker while those greater than zero indicate that HS treatment causes an increase in the levels of the biomarker. The statistical significance of the pooled estimate is indicated by the Z test of significance. LPS, Lipopolysaccharide; Met, Metabolic.
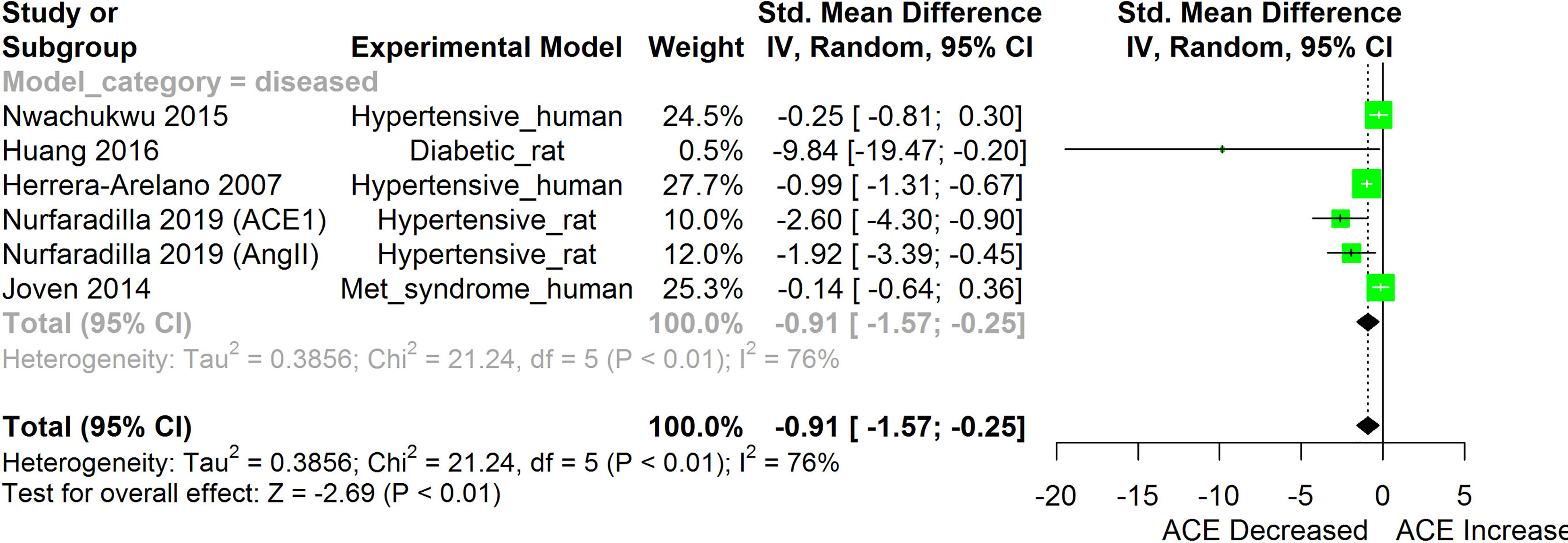
Figure 3 Meta-analysis and forest plot of the effect of HS administration on Angiotensin converting enzyme cascade. The meta-analysis was sub-grouped by health status (i.e diseased or healthy state). The standardized mean difference (SMD), which is an estimate of the effect size, is presented as a square on the forest plot, for each study and as a diamond for the pooled estimate of each subgroup or overall studies. The overall pooled SMD for all included studies is shown in the bottom section of the forest plot. SMD data points that are lower than zero signify that treatments with HS lower the level of the biomarker while those greater than zero signify that HS treatment causes an increase in the levels of the biomarker. The statistical significance of the pooled estimate is indicated by the Z test of significance. Met, Metabolic.
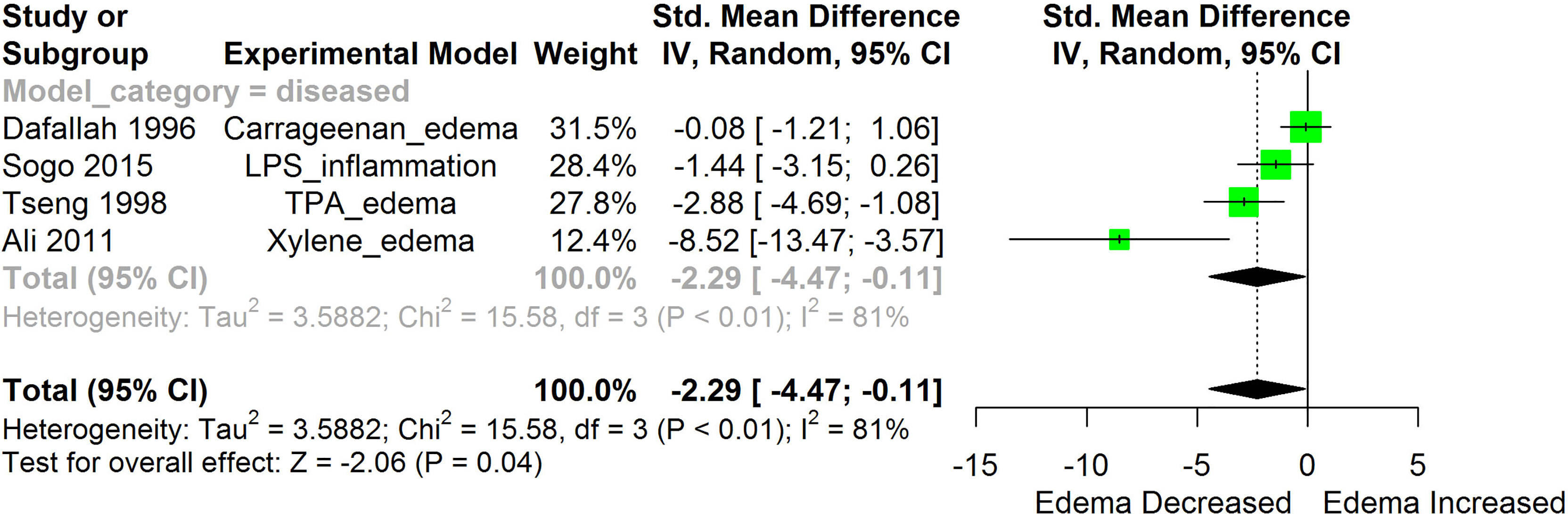
Figure 4 Meta-analysis and forest plot of the effect of HS administration on Edema formation. The meta-analysis was sub-grouped by health status (i.e diseased or healthy state). The standardized mean difference (SMD), which is an estimate of the effect size, is presented as a square on the forest plot, for each study and as a diamond for the pooled estimate of each subgroup or overall studies. The overall pooled SMD for all included studies is shown in the bottom section of the forest plot. SMD data points that are lower than zero signify that treatments with HS lowered the level of the biomarker while those greater than zero signify that HS treatment causes an increase in the levels of the biomarker. The statistical significance of the pooled estimate is indicated by the Z test of significance. LPS, Lipopolysaccharide; TPA, 12-O-tetradecanoylphorbol-13-acetate.
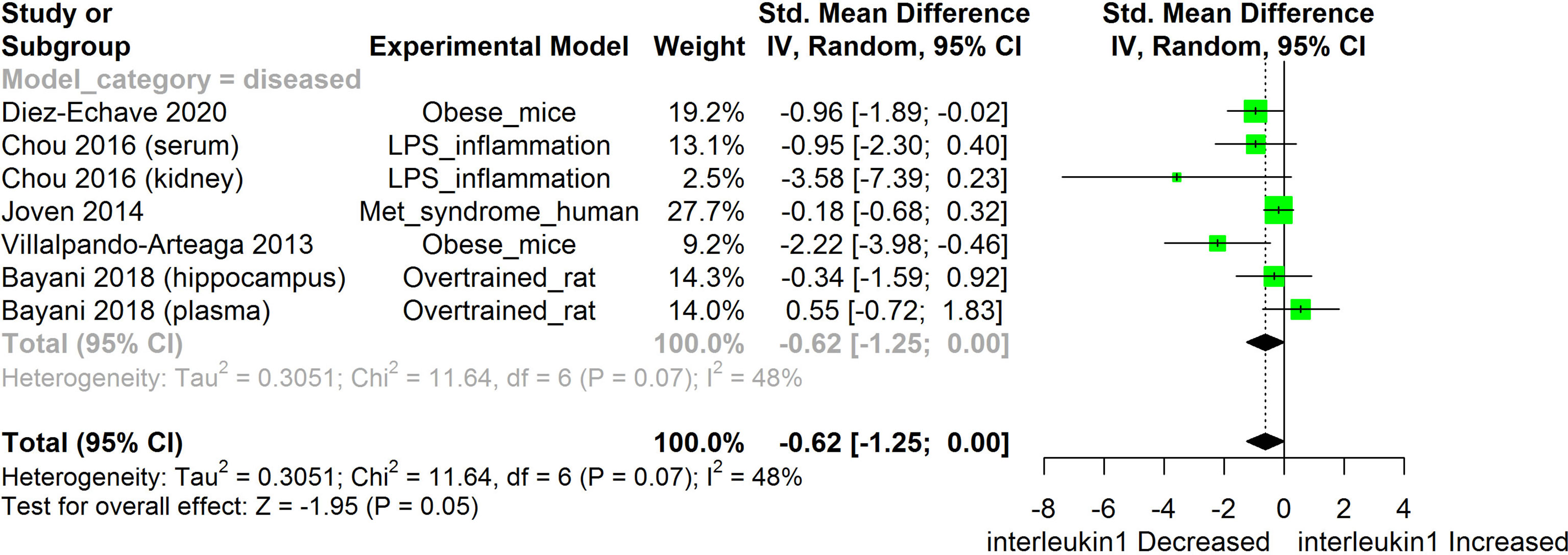
Figure 5 Meta-analysis and forest plot of the effect of HS administration on IL-1β. The meta-analysis was sub-grouped by health status (i.e diseased or healthy state). The standardized mean difference (SMD), which is an estimate of the effect size, is presented as a square on the forest plot, for each study and as a diamond for the pooled estimate of each subgroup or overall studies. The overall pooled SMD for all included studies is shown in the bottom section of the forest plot. SMD data points that are lower than zero signify treatments with HS lowered the level of the biomarker while those greater than zero signifies that HS treatment causes an increase in the levels of the biomarker. The statistical significance of the pooled estimate is indicated by the Z test of significance. LPS, Lipopolysaccharide; Met, Metabolic.
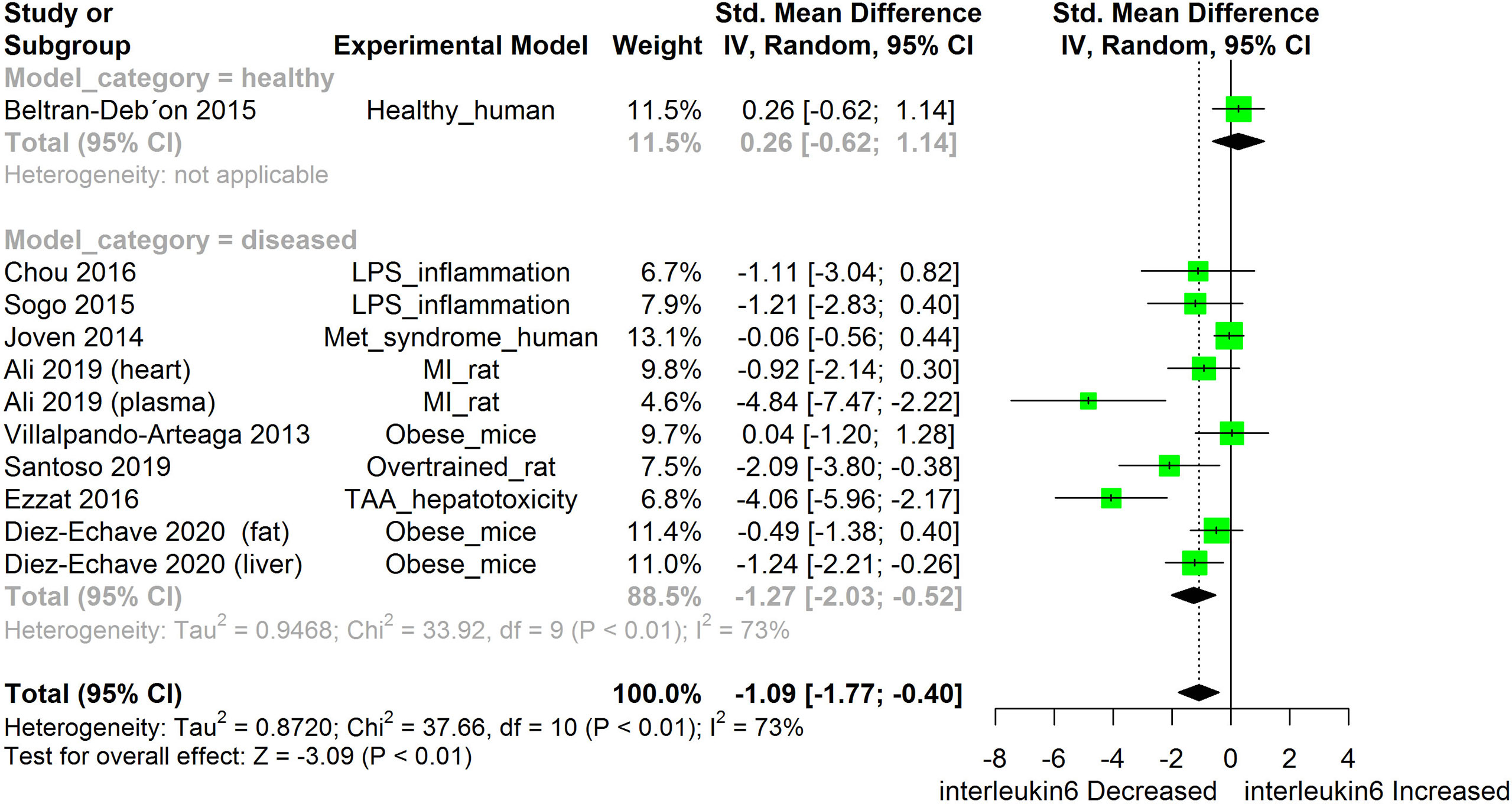
Figure 6 Meta-analysis and forest plot of the effect of HS administration on IL-6. The meta-analysis was sub-grouped by health status (i.e diseased or healthy state). The standardized mean difference (SMD) which is an estimate of the effect size, is presented as a square on the forest plot, for each study and as a diamond for the pooled estimate of each subgroup or overall studies. The overall pooled SMD for all included studies is shown in the bottom section of the forest plot. SMD data points that are lower than zero signify that treatments with HS lowered the level of the biomarker while those greater than zero signify that HS treatment caused an increase in the levels of the biomarker. The statistical significance of the pooled estimate is indicated by the Z test of significance. LPS, Lipopolysaccharide; Met, Metabolic; TAA, Thioacetamide; MI, Myocardiac infarction.
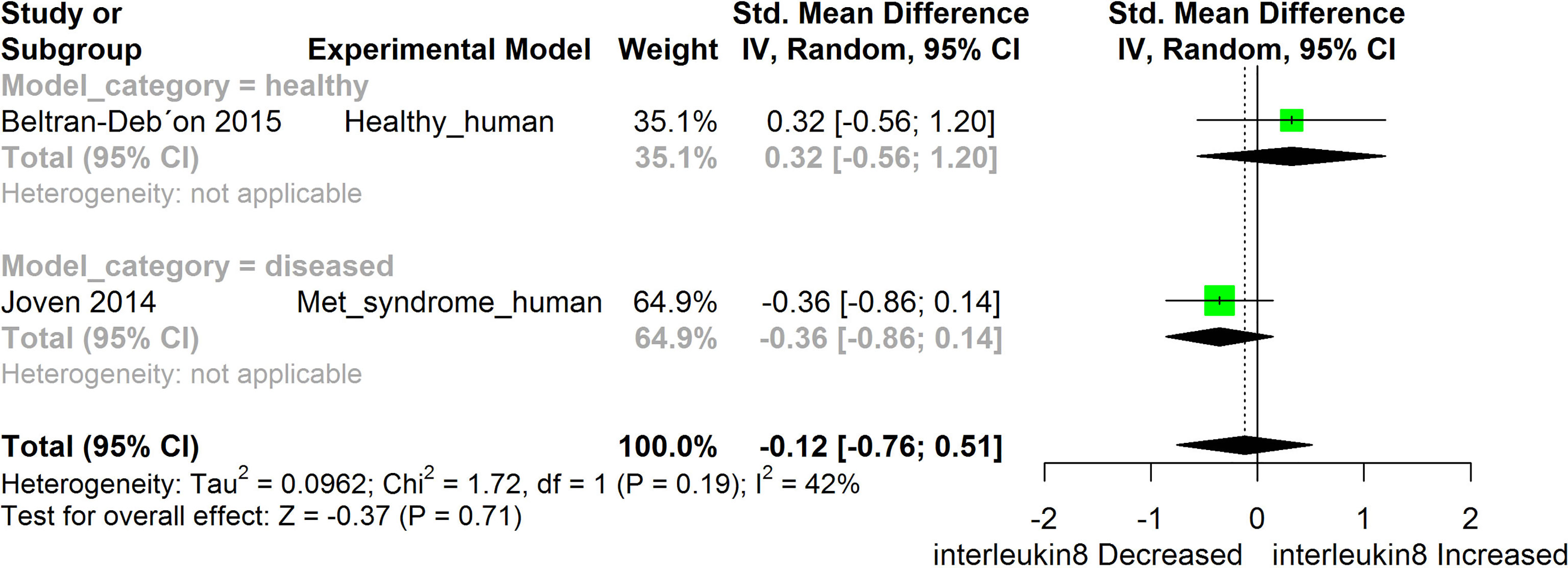
Figure 7 Meta-analysis and forest plot of the effect of HS administration on IL- 8. The meta-analysis was sub-grouped by health status (i.e diseased or healthy state). The standardized mean difference (SMD), which is an estimate of the effect size, is presented as a square on the forest plot, for each study and as a diamond for the pooled estimate of each subgroup or overall studies. The overall pooled SMD for all included studies is shown in the bottom section of the forest plot. SMD data points that are lower than zero signify that treatments with HS lowered the level of the biomarker while those greater than zero signify that HS treatment causes an increase in the levels of the biomarker. The statistical significance of the pooled estimate is indicated by the Z test of significance. Met, Metabolic.
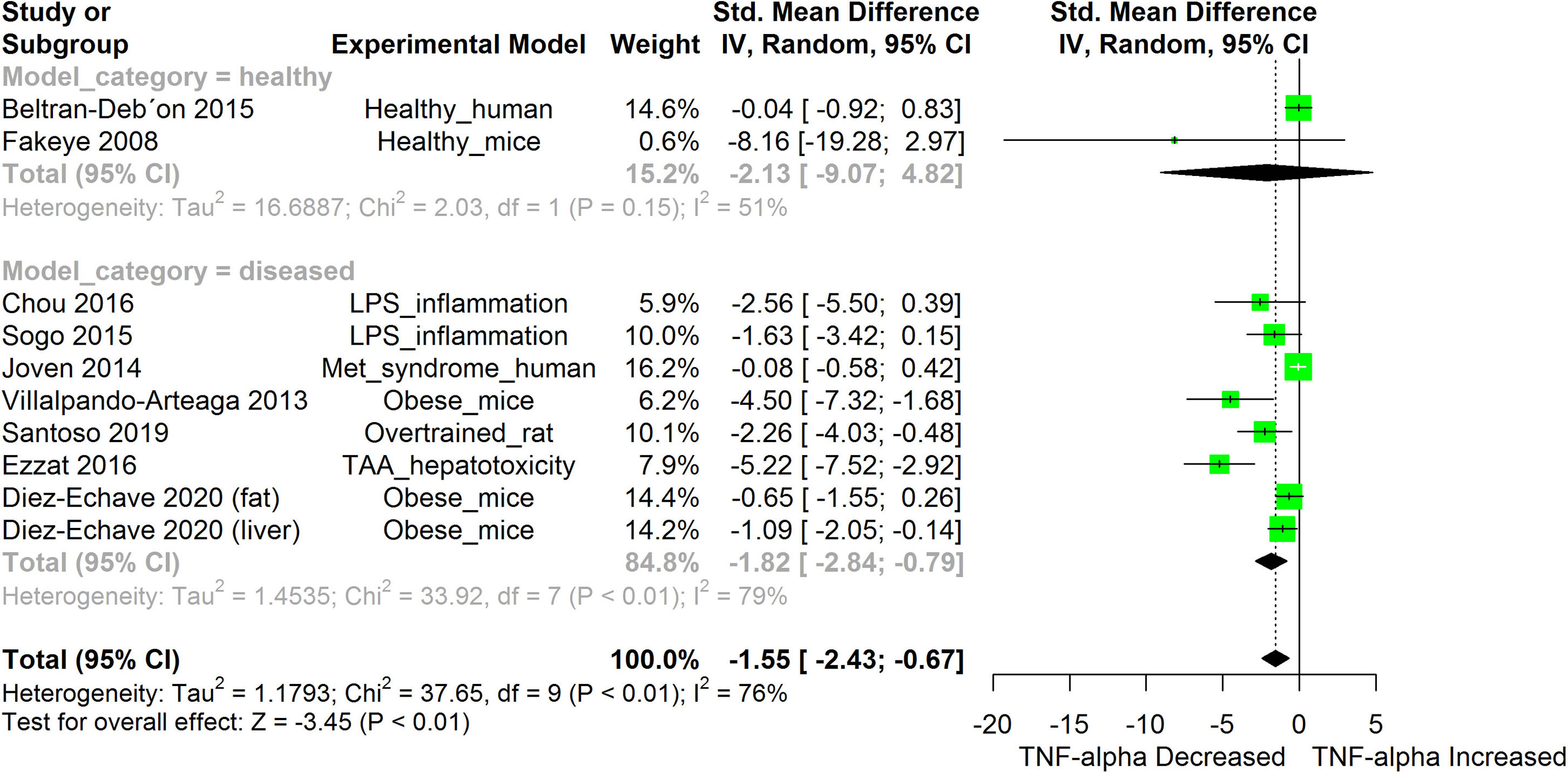
Figure 8 Meta-analysis and forest plot of the effect of HS administration on TNF-α. The meta-analysis was sub-grouped by health status (i.e diseased or healthy state). The standardized mean difference (SMD), which is an estimate of the effect size, is presented as a square on the forest plot, for each study and as a diamond for the pooled estimate of each subgroup or overall studies. The overall pooled SMD for all included studies is shown in the bottom section of the forest plot. SMD data points that are lower than zero signify that treatments with HS lowered the level of the biomarker while those greater than zero signify that HS treatment caused an increase in the levels of the biomarker. The statistical significance of the pooled estimate is indicated by the Z test of significance. LPS, Lipopolysaccharide; Met, Metabolic; TAA, Thioacetamide.
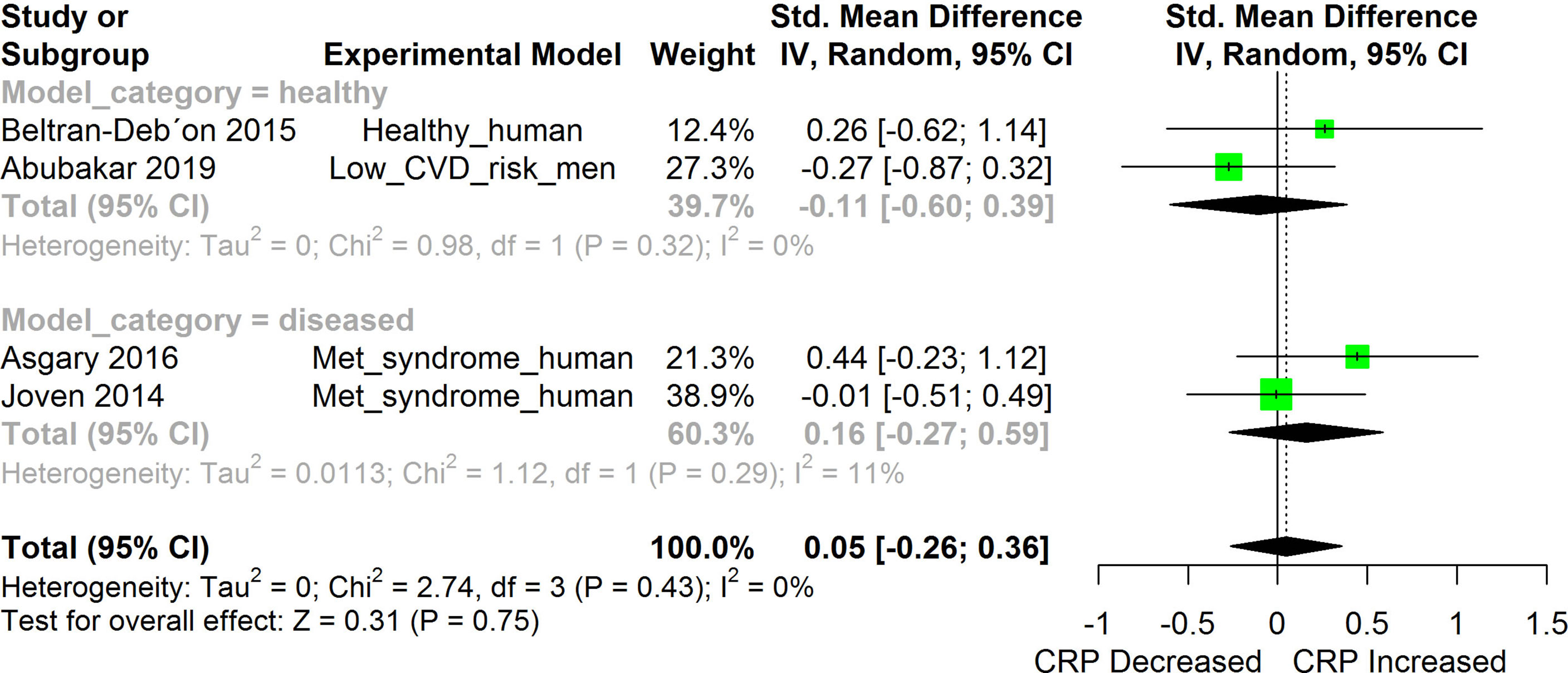
Figure 9 Meta-analysis and forest plot of the effect of HS administration on CRP. The meta-analysis was sub-grouped by health status (i.e diseased or healthy state). The standardized mean difference (SMD), which is an estimate of the effect size, is presented as a square on the forest plot, for each study and as a diamond for the pooled estimate of each subgroup or overall studies. The overall pooled SMD for all included studies is shown in the bottom section of the forest plot. SMD data points that are lower than zero signify that treatments with HS lowered the level of the biomarker while those greater than zero signify that HS treatment causes an increase in the levels of the biomarker. The statistical significance of the pooled estimate is indicated by the Z test of significance. Met, Metabolic.
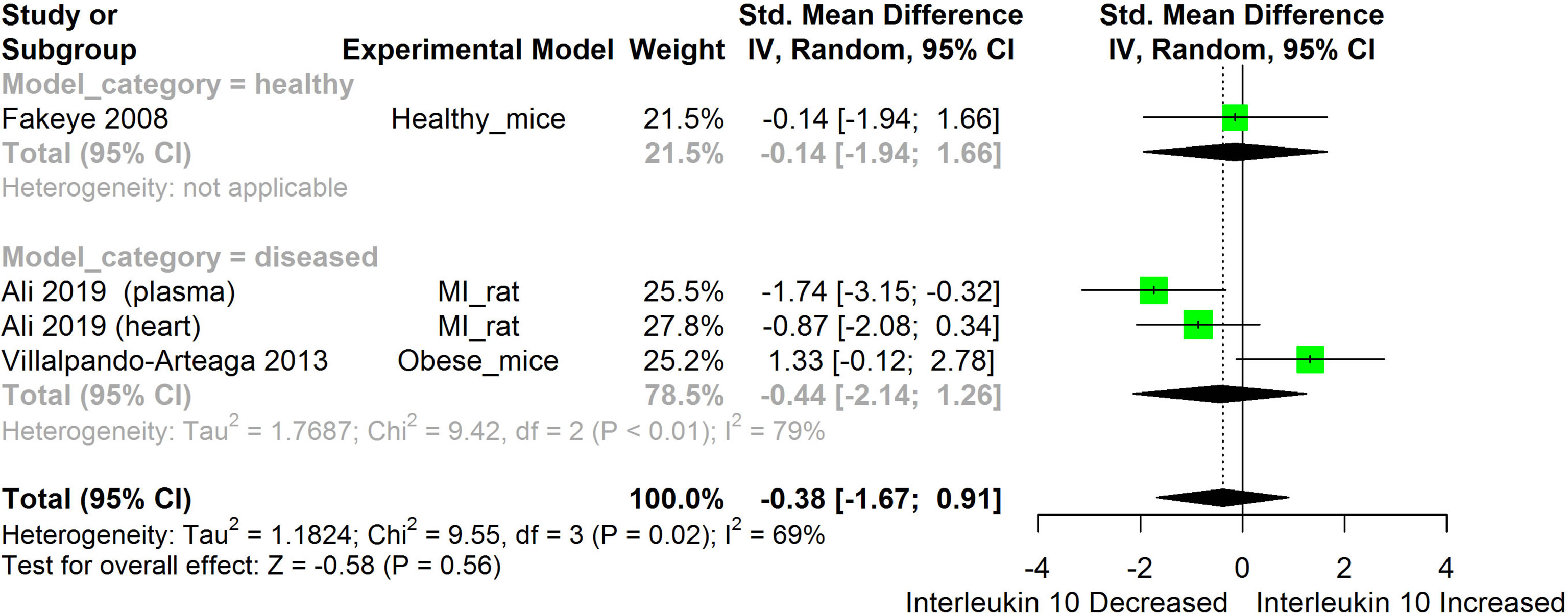
Figure 10 Meta-analysis and forest plot of the effect of HS administration on IL-10. The meta-analysis was sub-grouped by health status (i.e diseased or healthy state). The standardized mean difference (SMD) which is an estimate of the effect size, is presented as a square on the forest plot, for each study and as a diamond for the pooled estimate of each subgroup or overall studies. The overall pooled SMD for all included studies is shown in the bottom section of the forest plot. SMD data points that are lower than zero signify that treatments with HS lowered the level of the biomarker while those greater than zero signify that HS treatment caused an increase in the levels of the biomarker. The statistical significance of the pooled estimate is indicated by the Z test of significance. MI, Myocardiac infarction.
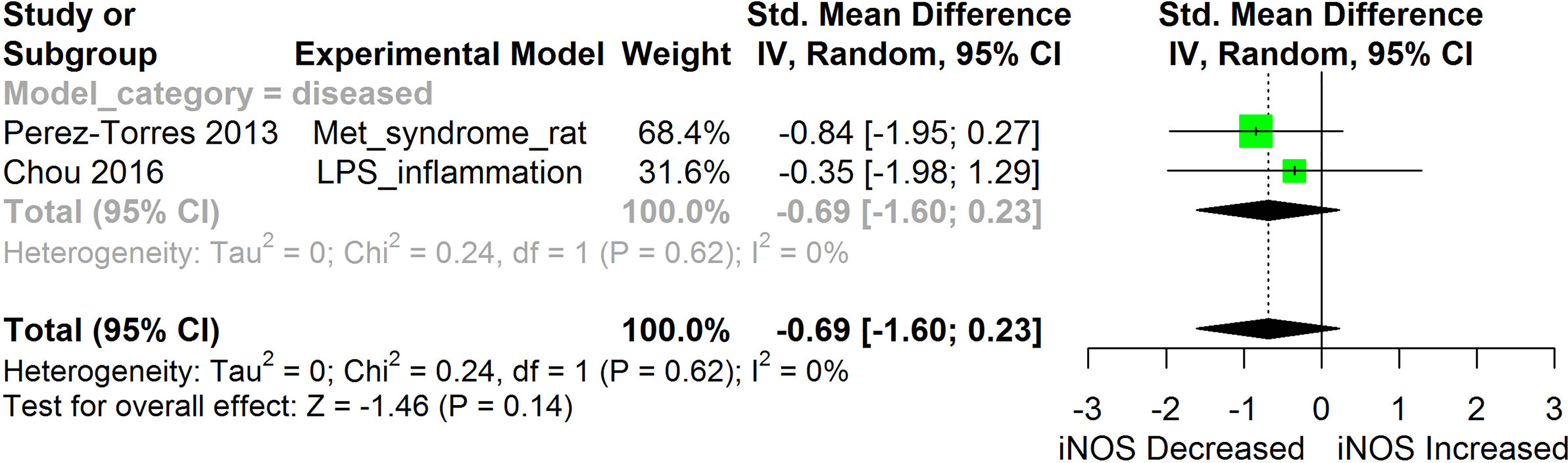
Figure 11 Meta-analysis and forest plot of the effect of HS administration on iNOS. The meta-analysis was sub-grouped by health status (i.e diseased or healthy state). The standardized mean difference (SMD), which is an estimate of the effect size, is presented as a square on the forest plot, for each study and as a diamond for the pooled estimate of each subgroup or overall studies. The overall pooled SMD for all included studies is shown in the bottom section of the forest plot. SMD data points that are lower than zero signify that treatments with HS lowered the level of the biomarker while those greater than zero signify that HS treatment causes an increase in the levels of the biomarker. The statistical significance of the pooled estimate is indicated by the Z test of significance. LPS, Lipopolysaccharide; Met, Metabolic.
HS administration lowered the MCP-1 levels when compared to untreated groups in the studies examined. The pooled SMD showed a significant decrease in MCP-1 (Figure 2) following treatments with HS extract (n=4; pooled SMD:-1.17; 95% CI: -1.78, -0.57; P < 0.01). Components of the ACE cascade (Figure 3) were lowered significantly following HS administration (n=6; pooled SMD:-0.91; 95% CI: -1.57, -0.25; P < 0.01). Edema formation (Figure 4) was significantly reduced following HS treatment (n=4; pooled SMD:-2.29; 95% CI: -4.47, -0.11; P = 0.04). IL-1β (Figure 5) was significantly lowered by HS treatment (n=7; pooled SMD:-0.62; 95% CI: -1.25, 0.00; P = 0.05). IL-6 (Figure 6) was also lowered significantly (n=11; pooled SMD:-1.09; 95% CI: -1.77, -0.40; P < 0.01). IL-8 was not significantly altered by HS administration (Figure 7; n=2; pooled SMD:-0.12; 95% CI: -0.76, 0.51; P = 0.71). TNF-α was significantly reduced following treatments with HS (Figure 8: n=10; pooled SMD:-1.55; 95% CI: -2.43, -0.67; P < 0.01). The levels of CRP (Figure 9: n=4; pooled SMD: 0.05; 95% CI: -0.26, 0.36; P = 0.75), IL-10 (Figure 10: n=4; pooled SMD:-0.38; 95% CI: -1.67, 0.91; P = 0.56), and iNOS (Figure 11: n=2; pooled SMD:-0.69; 95% CI: -1.60, 0.23 P = 0.14) were not significantly affected by HS treatment. A high heterogeneity was observed for TNF, IL-6, ACE, and edema formation. The diverse experimental models used in most of the included studies could partly explain the observed heterogeneity. The observed heterogeneity could affect the extrapolation of this meta-analysis to other similar populations (36).
The meta-analysis (Figures 2–11) showed that the levels of TNF-β, IL-6, IL-1β, MCP-1, and ACE were significantly lowered by HS. An attempt was made to compare the efficacy of HS extract in lowering the levels of TNF-a, IL-6, IL-1β, MCP-1, and ACE, in human and animal disease models. This was done by running a subgroup meta-analysis using the biomarkers TNF- α, IL-6, IL-1β, MCP-1, and ACE on diseased models of humans and rodents. The result is shown in Figure 12. The result shows that HS extract is more active in suppressing the inflammatory biomarkers in rodents (n=27; pooled SMD:-1.53; 95% CI: -1.98, -1.07; p < 0.01) than in human subjects (n=7; pooled SMD:-0.36; 95% CI: -0.74, 0.01; p=0.06). This result may be due to the comparatively low number of human studies. It may also be due to the limited experimental models reported in available human studies.
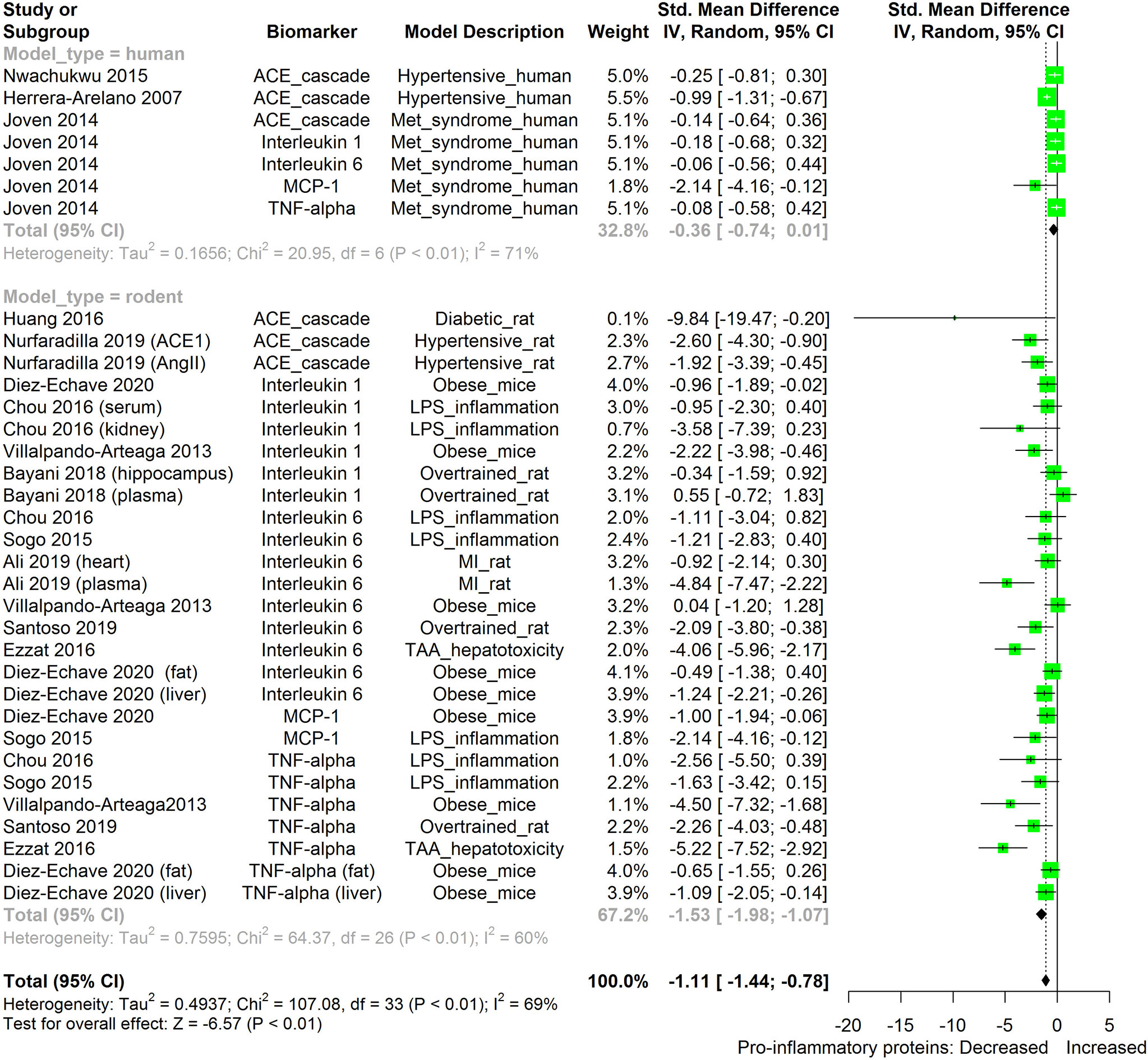
Figure 12 Meta-analysis and forest plot of the effect of HS administration on selected pro-inflammatory biomarkers (ACE, TNF-α, IL-6, IL-1β, MCP-1) from diseased human and rodent models. The meta-analysis was sub-grouped by model organism (i.e diseased human or diseased rodents models). The standardized mean difference (SMD), which is an estimate of the effect size, is presented as a square on the forest plot, for each study and as a diamond for the pooled estimate of each subgroup or overall studies. The overall pooled SMD for all included studies is shown in the bottom section of the forest plot. SMD data points that are lower than zero signify that treatments with HS lowered the level of the biomarker while those greater than zero signify that HS treatment causes an increase in the levels of biomarker. The statistical significance of the pooled estimate is indicated by the Z test of significance. MI, Myocardiac infarction; LPS, Lipopolysaccharide; TAA, Thioacetamide; Met, Metabolic; TNF-alpha, Tumour necrosis factor-alpha; MCP-1, Monocyte chemoattractant protein-1; ACE, Angiotensin-converting enzyme.
We also attempted to compare the immunosuppressive potential of HS in different animal disease models using TNF-α, IL-6, IL-1β, MCP-1, and ACE as biomarkers (Figure 13). The levels of the inflammatory biomarkers TNF-α, IL-6, IL-1β, MCP-1, and ACE appeared to be more readily suppressed by HS in some disease models than in other disease models. HS appears to have the lowest effect in obese mice; however, the low number of studies on the subject limits the strength of this line of reasoning.
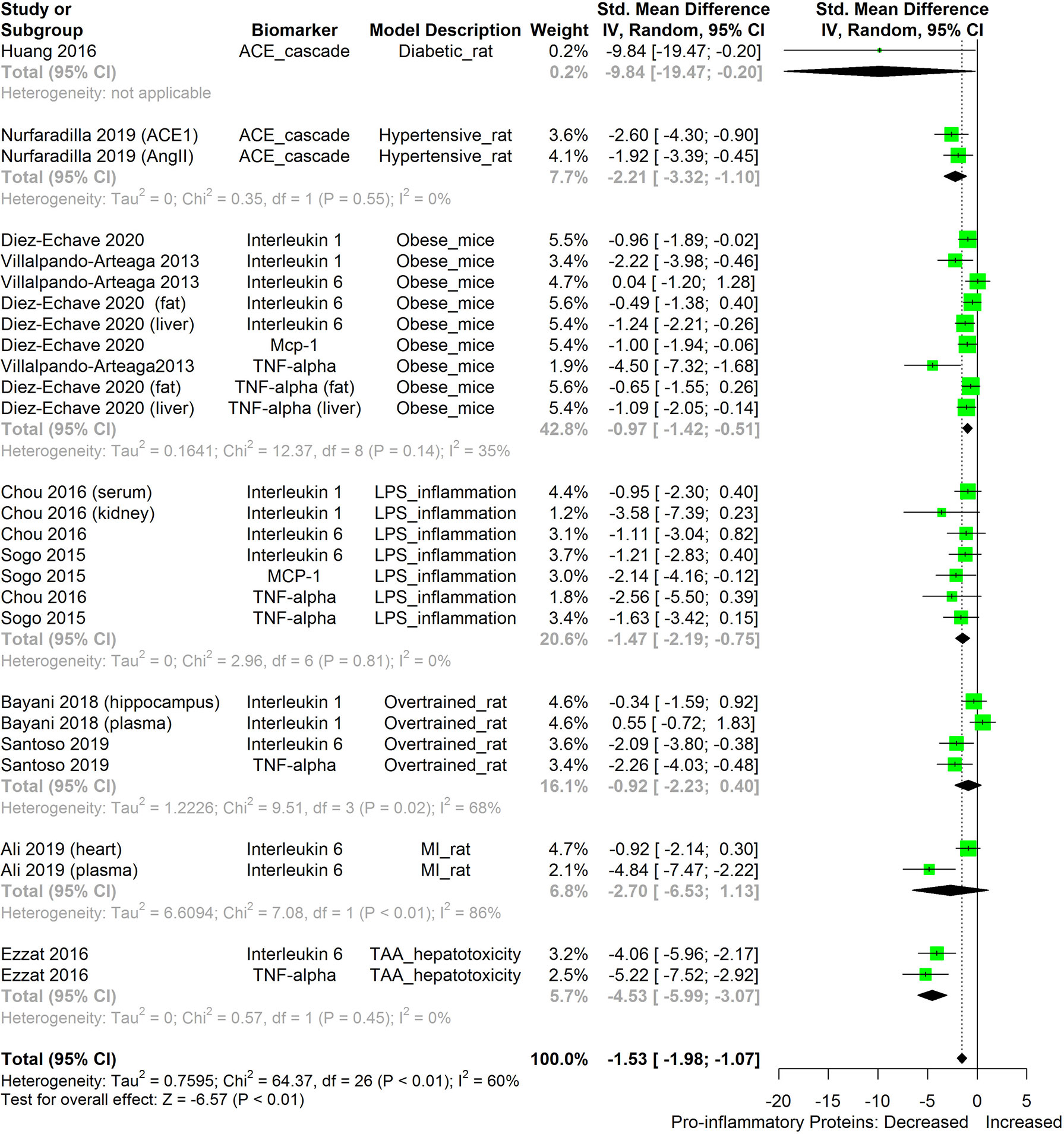
Figure 13 Meta-analysis and forest plot of the effect of HS administration on selected pro-inflammatory biomarkers (ACE, TNF-α, IL-6, IL-1β, MCP-1) across different diseased-rodent models. The meta-analysis was sub-grouped by rodent’s disease models (i.e diabetic rats, hypertensive rats, LPS-inflammation model, over-trained rats, MI-rats, TAA-hepatotoxicity models). The standardized mean difference (SMD), which is an estimate of the effect size, is presented as a square on the forest plot, for each study and as a diamond for the pooled estimate of each subgroup or overall studies. The overall pooled SMD for all included studies is shown in the bottom section of the forest plot. SMD data points that are lower than zero signify that treatments with HS lowered the level of the biomarker while those greater than zero signify that HS treatment causes an increase in the levels of the biomarker. The statistical significance of the pooled estimate is indicated by the Z test of significance. MI, Myocardiac infarction; LPS, Lipopolysaccharide; TAA, Thioacetamide; TNF-alpha, Tumor necrosis factor-alpha; MCP-1, Monocyte chemoattractant protein-1; ACE, Angiotensin-converting enzyme.
Effects Across Different Experimental Models
The anti-inflammatory effects of HS calyx extract were observed in healthy mice (41), healthy humans (20, 47), and in different disease models such as dextran sodium sulfate-induced colitis mice (40), xylene-induced ear edema mice (1), sucrose induced metabolic syndrome rat (45), hypertensive rat (two-kidney-one-clip (2K1C) model of hypertension (26), men with low risk of cardiovascular-disease (51), human subjects with metabolic syndrome (42, 50), Dimethylbenz(A)Anthracene (DMBA) treated rats (39), high fat diet (HFD) induced obese mice (9, 16, 46), STZ-induced Diabetic rats (37, 46), LPS-induced inflammation in rats (38), hypertensive humans (24), myocardial infarction rat (44), LPS-induced inflammation in mice (11, 48), over trained rats (43, 52), N(G)-nito-L-arginine-methyl ester (L-NAME)- induced hypertensive rats (25), Thioacetamide (TAA)-induced hepatotoxic rats (17) and 12-O-tetradecanoylphorbol-13-acetate (TPA) induced mouse ear edema (49) as shown in Table 2.
Significant alterations were observed in the levels of most of the inflammatory biomarkers investigated following induction of each disease condition when compared to their respective un-induced controls. HS extract exerted significant anti-inflammatory effects against most of the investigated biomarkers of inflammation. With a few exceptions, the levels of most of the investigated inflammatory cytokines in healthy models were generally low, sometimes undetectable (20), and often without significant alterations after treatment with HS. Some exceptions included the suppression of TNF-α in healthy mice (41), suppression of MCP-1, and ACE1 in healthy humans (20, 47). Significant changes were not observed in the levels of CRP following treatments with HS in healthy humans (20), human subjects with metabolic syndrome (50), and men with low risk of cardiovascular disease (51).
Extract Formulations
A description of the extract formulation of each included study is presented in Table 2. Anti-inflammatory activities were observed in different formulations of HS calyx such as ethanol extract (1, 25, 40, 41, 52), hot or cold aqueous extract (9, 16, 20, 24, 31, 37, 45, 51), methanol extract (38, 43), trifluoroacetic acid (TFA) extract (17), Polyphenol Extract (42), and protocatechuic acid extracts (49) of HS calyces. A study done with the encapsulated whole calyx powder did not yield a significant difference in the level of CRP when compared with the untreated group.
Affected Organs
The tissues/organs that were analyzed in each study are presented in Table 2. HS calyx extract elicited significant anti-inflammatory effects in different tissues and organs including blood (11, 17, 24, 25, 41, 47); spleen (39); liver (9, 37, 38, 45); heart (42–44, 50); kidney (31, 46), liver Adipose (16); ear (1, 49); colon (40), and paw (11). Significant alteration was not detected in IL-1β levels in the hippocampus of rats following treatment with HS calyx extract (52).
The route of administration, the applied doses of HS extract, and the duration of treatment in the included studies are shown in Table 2. All but two studies administered HS extract formulation orally. Sogo et al. (11), administered pure anthocyanin from HS intraperitoneally while Tseng et al. (49) applied protocatechuic acid extract of HS topically. In most of the studies (with few exceptions), the administration of HS calyx extract on experimental subjects lasted up to (or more than) three weeks. However, HS was administered for three hours in the works of Beltrán-Debón et al. (20), Beltrán-Debón et al. (47), and Dafallah et al. (53). HS administration lasted between four to 7 days in the works of Sogo et al. (11), Tseng et al. (49), Kao et al. (38), Chou et al. (31), and Ali et al. (44). HS treatment lasted for two weeks in the study of Nurfaradilla (26). One time administration of HS was reported in the work of Abubakar et al. (51) and Ali (1).
Discussion
Chemical Constituents and Bioactivity of Different HS Extract Formulations
The anti-inflammatory effect of HS extracts has been reported in many studies using various formulations of HS. Each of these formulations contains different proportions of bioactive agents which contribute to the observed bioactivities. The aqueous extracts of HS have comparatively higher contents of cyanidin-3-sambubioside and delphinidin-3-sambubioside as well as a higher antioxidant activity than methanol, ethylacetate, and formic acid extract (2). Flavonoids are more enriched in extracts obtained using less polar solvents (6). Hydroxycitric, hibiscus, and chlorogenic acid are the most abundant phenolic acids detected in aqueous extracts of HS (50). Delphinidin 3-sambubioside and Cyanidin 3-sambubioside are the predominant anthocyanins present in aqueous extract of HS (9, 26, 31, 50).
The mechanisms involved in the anti-inflammatory activities of HS extract appear to be multifunctional, involving different bioactive agents which can interact with different biological targets to elicit the observed anti-inflammatory effects. Immunomodulatory activities have been reported for pectin-rich polysaccharide obtained from water soluble fractions of HS (4). HS extracts containing PCA (7%), catechins (9.97%), epigallocatechin (10.23%), epigallocatechin-3-gallate (20%), and caffeic acid (18.%) have been shown to possess in-vitro antioxidant, nitric oxide inhibitory and Prostaglandin E2 inhibitory activities (38). The antioxidant capability was improved in diabetic rats after the intake of PCA and gallic acids extracted from HS (21). Metabolites of Delphinidin-3-glucoside have strong radical scavenging activities (11). Essential oils obtained from HS (containing fatty acids such as hexadecanoic and 14-methyl-pentadecanoic acid methyl ester) were reported to elicit anti-inflammatory activity against LPS-stimulated RAW264.7 cells (18). Anthocyanin-rich water soluble extracts of HS inhibited ACE1 enzyme in-vitro (12). Delphinidin-3-sambubioside containing extract of HS (obtained using ethylacetate and methanol) has been reported to possess cyclooxygenase 1 inhibitory activity in-vitro (8).
Bioavailability and Pharmacokinetics of HS Extracts Formulation
HS is most commonly consumed as a decoction of the dried HS calyx. Many in-vivo and in-vitro studies have shown the immunosuppressive effect of aqueous HS extract. To fully elucidate the mechanism of the anti-inflammatory activities of HS, the bioavailability of the bioactive constituents of HS need to be considered. Within 1 hour after ingesting aqueous extract of HS, a small percentage (1%) of its flavonoids and anthocyanin contents are rapidly absorbed and metabolized before appearing in the blood plasma as flavonoid conjugates and hippuric acid respectively (10, 51). Anthocyanin glycosides are more readily absorbed than the aglycone equivalent (11). Flavonoid O-glucosides are more readily absorbed than their aglycone form or derivatives with rhamnose glycosylation (54). Urinary levels of the metabolites of anthocyanin peak around 2 hours following the intake of HS extract (5). Unabsorbed anthocyanin is further metabolized by intestinal microbiota which hydrolyses and stimulates the absorption of the aglycones of anthocyanin (11). Unabsorbed anthocyanin is excreted through the feces (10). PCA is the main metabolite found in rat’s plasma after the consumption of cyanidin 3-O-glucoside (11, 55). Less than 1% of the ingested phenols were detected in the plasma, with plasma levels peaking within 90 minutes of HS intake (51). The hibiscus acid content of HS extract is metabolized into hydroxycitric acid, which attains its peak plasma level in about 2 hours following ingestion (54). The presence of chlorogenic acid, methyl digallates, and feruloyltyramine in the plasma within 1 hr of HS ingestion suggests the possibility of direct absorption of the phenolic acids into the bloodstream in un-metabolized forms (54). Abubakar et al. (51), also reported the detection of 3-O-methylgallic acid, hippuric acid, gallic acid, and 4-O-methylgallic acid in blood plasma following the intake of HS extract. Fernandez-Arroyo et al. (54), report that about 5 µM of flavonols were detected in blood plasma within 2 hours of intake of 1200 mg/kg of HS polyphenol extract. The foregoing suggests that many components of HS have low bioavailability and are metabolically transformed and eliminated through urine within about 2 hours of ingestion.
Potential Mechanisms of the Immunosuppressive Activity of HS Extract
As discussed previously, the presence of different bioactive agents in extracts of HS, suggests the possible involvement of several mechanisms in the suppression of immune and inflammatory responses. Suggested mechanisms include the suppression of cellular stress, inhibition of enzymes that can trigger inflammatory reactions, and down-regulation of pro-inflammatory genes.
Suppression of Cellular Stress
An important mechanism involved in the anti-inflammatory activities of HS is its ability to suppress the generation of oxidative stress and cellular damage in cells. Several pathological conditions are associated with oxidative stress. Increased oxidative stress can cause cellular damages leading to the release of stress factors such as heat shock protein, reactive oxygen species (ROS), necrotic, and apoptotic factors, etc. These factors are capable of activating TLR proteins in immune cells leading to downstream expression of several inflammatory genes (56). Oxidative stress is reported in most of the different experimental disease models used in the included studies. For instance LPS treatment depleted the anti-oxidant status of cells (38), over-training elevated the levels of malondialdehyde (57), models of hypertension had elevated malondialdehyde levels as well as depleted glutathione (25), mechanism of Isoprenaline -induced myocardiac infarction involves free radical generation, increased necrosis, inflammation and cardiac fibrosis (44), TAA-intoxication causes an increase in reactive oxygen species (ROS) which may result in centrilobular necrosis (17), metabolic syndrome and non-communicable diseases such as diabetes and obesity can be caused by excess oxidative stress arising from disrupted cellular redox control (58).
Several components of HS extract have been demonstrated to possess high radical scavenging potentials in in-vivo and in-vitro studies. Anthocyanins can reduce oxidative stress through the direct scavenging of free radicals or the induction of anti-oxidant enzymes (10). HS possesses potent in-vitro anti-oxidant capability (2). Anti-oxidant capacity was improved in diabetic rats after the administration of PCA and gallic acids extracted from HS (21). The phenolic acid constituents of HS, though short-lived in the plasma, can improve the anti-oxidant capability of the cells in a mechanism that involves iron homeostasis (54, 59). In mechanisms that involve the enhancement of antioxidant capabilities, anthocyanin has been reported to trigger the activation of protein kinase B signaling, inhibition of inhibitor of κB phosphorylation, and the suppression of NF-κB in hydrogen peroxide (H2O2)-stimulated rat dermal fibroblast (60). The conjugated forms of quercetin and kaempferol often detected in the plasma after intake of HS could indicate long-lasting cellular antioxidant effects because of their long plasma half life (54). Some of the anti-inflammatory properties of anthocyanin may be explained by the antioxidant effects they exhibit (10). Beltrán-Debón et al. (47) suggest the possible involvement of the Heme-oxygenase (HO)-biliverdin reductase system in the in-vivo antioxidant and anti-inflammatory effect of HS. When the anti-oxidant capacity of cells are improved following HS treatments, the levels of oxidative stress-induced damages would be suppressed and TLR stress related agonist will not be generated.
Inhibition of Enzymes Capable of Triggering Immune Responses
ACE
Direct Inhibition of enzyme systems that are capable of stimulating inflammatory response can explain some of the anti-inflammatory activities of HS. One of such enzyme systems is the ACE1. ACE1 is a component of the Renin-Angiotensin-System which functions in the homeostatic control of pressure in arteries, perfusion of tissues, and regulation of extracellular cell volume. The Renin-Angiotensin-System system consists of two ACE enzymes, ACE1 and angiotensin converting enzyme -2 (ACE2), which functions in an opposing manner to both increase and decrease vasoconstriction respectively (61). ACE1 catalyzes the conversion of Ang I into Ang II which then activates the AT1 receptor to trigger several downstream processes including vasoconstriction, sodium/water re-absorption, proliferation and hypertrophy, fibrosis, oxidative stress, and arrhythmogenesis (61). Ang II can promote vascular permeability, leukocyte infiltration, activate the Nf-κB signaling pathway, and inadvertently produces inflammatory mediators (25). HS administration has been reported to suppress both Ang II and AT 1 receptors in diabetic and hypertensive rat models (26, 46). In-vitro studies have also demonstrated the ACE1 inhibitory effects of Anthocyanin-rich extracts of HS (12). The inhibition of ACE1, Ang II, and AT 1 receptor by HS extract could potentially inhibit Ang II-mediated activation of the AT1 receptor. This would subsequently suppress the downstream activation of Nf-κB as well as ROS production (30).
ACE2, a homologue and regulatory arm of ACE 1, diverts some of the Ang II generated by ACE1 into the production of a potent vasodilatory growth inhibitory peptide, angiotensin (1–7), which activates the Mas AT2 receptor and receptor Mas (61). Activation of the AT2 receptor is thought to enhance ACE2 activities and subsequently leads to an ACE2-mediated inhibition of NF-kB pro-inflammatory signaling as well as the downstream inhibition of TNF-α-mediated ICAM-1 expression (62). ACE2 knockout mice were observed to suffer from more pulmonary inflammation following exposure to cigarette smoke (63), probably as a result of unregulated ACE1 activity. Conditions that suppress the level of ACE2, for instance as observed in coronavirus binding to ACE2 (64, 65) would interrupt the immunomodulatory activities of ACE2 thereby bringing about an increase in AT1 receptor-mediated pro-inflammatory responses (66). Agents capable of inhibiting ACE1 activities hold promise in the management of pulmonary inflammatory disorders (66).
Inhibition of components of the angiotensin converting enzyme (ACE) cascades by HS extract have been reported in both human and animal studies (24, 25). The result obtained following the meta-analysis of the effects of HS on components of the ACE cascade, as shown in this review (Figure 3), showed that HS administration had an overall inhibitory effect on the ACE cascade. Possible mechanisms of the HS-induced inhibition of components of the ACE cascade may involve both direct and indirect mechanisms. Delphinidin-3-O-sambubioside and cyanidin-3-O-sambubioside from HS have been reported to competitively inhibit ACE activity in-vitro (13). Polyphenols from HS were observed to suppress the expression of AT1 and AT2 receptors in HK-2 cells that were exposed to high glucose but did not have a significant effect on the expression of the AT1 and AT2 receptors in HK-2 cells that were not exposed to high glucose (15). The inhibition of the ACE cascade would possibly suppress the conversion of Ang 1 to Ang II thereby halting the downstream consequences of AT1 receptor activation and the consequent production of oxidative stress and inflammatory responses (25).
Lipoxygenase and Cyclooxygenase
HS has also been reported to inhibit lipoxygenase and cyclooxygenase enzyme systems (10, 59). This enzyme functions in the conversion of arachidonic acid to prostaglandins. The over expression of the enzymes of prostaglandin synthetic pathways (COX-2 and lipooxygenase), can lead to different pathologies such as renal disorders and nephritis (31). HS treatments significantly lowered the activities of COX-2 in-vivo studies (31). Lipoxygenase was inhibited in-vitro by PCA from HS extract (59). This implies that treatment with HS could lower the level of prostaglandin and suppress prostaglandin-mediated inflammatory responses. Prostaglandin E2 has been reported to contribute to the formation of pulmonary edema (67). The observed reduction in edema formation (Figure 4) following treatment with HS may be explained in part by the inhibition of the enzymes of the prostaglandin synthesis by HS.
Down-Regulation of Pro-Inflammatory Genes
Down-regulation of pro-inflammatory genes and factors is another potential mechanism involved in HS anti-inflammatory activities. Different in-vivo and in-vitro studies have demonstrated the ability of HS or known components of HS to downregulate the expression of pro-inflammatory genes such as NF-κB, TNF-α, interleukins, iNOS (17, 20, 31, 44). The exact mechanism involved in the down-regulation of pro-inflammatory genes are unknown but accumulating evidence suggests that HS may affect one or more upstream component of the pro-inflammatory gene expression cascade. An instance is the HS-induced suppression of the levels of Ang II and AT1 receptors (26, 46) which prevents the downstream NF-κB mediated expression of the pro-inflammatory gene. There is also the possibility that there is post transcriptional modulation of inflammatory gene products. Ali et al. (44) reported that IL-6 protein levels were significantly reduced by HS whereas IL-6 (mRNA) was not significantly affected in a myocardiac infarction model. There is also the possibility of direct interaction between components of HS extract and the surface receptors of immune cells that can lead to modulation in gene expression. For instance, Zheng et al. (23), reported that polysaccharide extract of HS increased proliferation and NF-κB expression of RAW264.7 cells in a mechanism that was likely due to the enhancement of macrophage proliferation or the activation of specific surface receptors on macrophages. The anti-inflammatory activity of Delphinidin 3-sambubioside and Delphinidin against LPS-induced cells were reported to be independent of direct TLR4 binding interaction (11).
Microarray gene profiling studies by Chou et al. (31), showed that 26 genes including interleukins, interferons chemokines, tumor necrosis family, as well as COX and iNOS genes were significantly altered following LPS-intoxication and subsequent HS treatment in mice kidney (31). Polyphenol extracts from HS inhibited the activation of c-Jun N-terminal kinase (JNK) and p38 mitogen-activated protein kinase (MAPK or MAP kinase) in LPS-challenged rats hereby suppressing NF-κB activation and the downstream expression of COX-2 proteins (38). The metabolites of protocatechuic acid and cyaninin can suppress COX-2 and iNOS expression (10).
NF-κB
Treatment with HS extract has been reported to down-regulate the activity of NF-κB (p65) subunit in different animal and cell line models (17, 18, 31). NF-κB is a family of inducible transcription factors that regulate several genes which function in inflammation and immune responses (68). NF-κB is ubiquitously expressed and comprises several subunits such as NF-κB1 (p50), NF-κB2(p52), RelA(p65), RelB, and c-Rel (69). NF-κB is commonly associated with an inhibitory subunit, IκB. NF-κB can be activated in a pathway that is either dependent or independent on the IκB complex degradation. NF-κB is typically activated through the degradation of its associated IκB-α upon phosphorylation by the IKK complex. The IKK itself is activated by several upstream signals including cytokines, growth factors, antigenic factors (66, 70). On activation, NF-κB can migrate into the nucleus to activate gene expression (71). Dysfunctional NF-κB signaling has been implicated in several inflammatory disorders (68). Suppression of NF-κB activity will reduce the downstream expression of pro-inflammatory genes thereby effectively attenuating immune responses (18).
IL-1β
The results from the meta-analysis suggest that HS administration suppressed the levels of IL-1β across different experimental models (Figure 6). IL-1β and TLRs are membrane bound proteins that are commonly expressed on immune cells. TLRs are activated by the binding of antigenic substances from bacteria and viruses or stress signals from the host (72). IL-1β is a component of the innate immune response that is rapidly produced following stimulation of TLR or interleukin-1 receptor (IL-1R) by microbial antigenic substances or stress signals from the host (Martin2002, Thobakgale2017). IL-1β also facilitates the maturation, proliferation, and differentiation of immune cells (73). IL-1R is activated by IL-1β. Following the binding of its agonist, IL-1R triggers the release of inflammasomes which matures into active IL-1β and then mediates inflammatory reactions (74). Activation of IL-1R and TLR triggers a cascade of signaling which involves the interleukin-1 receptor associated kinase (IRAK)/MAPK/JNK2/extracellular signal-regulated kinases (ERK) and eventually culminates in the activation of NF-κB and other transcription factors that are involved in the expression of inflammatory mediators such as MCP-1, IL-8, and IL-6 (74). Hyper-activation or dysfunctional regulation of the activities of the IL-1R and TLR can cause tissue damage and auto-immune diseases (74).
TNF-α
The result from the meta-analysis indicates that HS supplementation significantly suppressed the levels of TNF-α across the different studies (Figure 8). TNF-α are mainly produced by immune cells such as activated macrophages, lymphocytes, endothelial, epithelia cells, smooth muscle cells, and cardiac myocytes (71). It participates in pro-inflammatory reactions. TNF-α, on binding to its receptors (Tumor necrosis factor receptor (TNFR) 1 and 2) triggers the activation of NF-κB, MAPK/c-Jun, and caspase apoptotic signaling in a process mediated by the TNF receptor associated factor 2 (TRAF2) (71). The activated Mitogen Activated Protein (MAP) kinase kinase kinase (MAP3K)/JNK system generates c-Jun and Activation protein 1 (AP-1) factors that activate the expression of pro-inflammatory genes (71). The persistent overproduction of TNF-α could be deleterious to tissues (71). The ability of HS to inhibit TNF-α-induced activation of NF-κB presents an interesting prospect for the use of HS in managing inflammatory disorders. Inhibition of TNF-α reduces the upstream signals that can potentially stimulate the NF-κB induced expression of inflammatory cytokines.
MCP-1
Evidence from this meta-analysis shows that HS treatment can significantly suppress the levels of MCP-1 (Figure 2). Furthermore, the low heterogeneity (0%) observed following the meta-analysis of the evidence gathered from both human and animal studies, suggests that the MCP-1-lowering effect of HS is independent of the type and health state of the model. MCP-1 are inflammatory chemokines that regulate the migration and infiltration of macrophages and monocytes to sites of inflammation (75). The levels of MCP-1/CCL2 and its receptor C-C chemokine receptor type 2 (CCR2), are increased in different inflammatory related diseases such as atherosclerosis, inflammatory bowel disease, allergic asthma, rheumatoid arthritis, and Type 2 diabetes, etc. (75). The increased expression of MCP-1 in the kidney and vasculatures in hypertensive subjects results in increased infiltration of monocytes expressing CCL2 receptors (CCR2) into the kidney (76). The inhibitors of MCP-1 could lower excessive renal accumulation of monocytes and macrophages (77). While many inflammatory cytokines are either undetectable or very low in a healthy subject, It is interesting to note that MCP-1 was present in healthy subjects and was significantly lowered after HS treatment (20). The mechanism involved in HS-induced reduction in MCP-1 levels does not involve the enhancement of the antioxidant capability of the plasma (20). The ability to suppress MCP-1 levels is one of the prominent anti-inflammatory potentials of HS. Since most of the inflammatory cytokines are secreted by specialized immune cells, suppression of MCP-1 activity would reduce the aggregation of circulating monocytes at inflammation sites. This would reduce the numbers of circulating monocytes that are able to develop into specialized cytokine-releasing immune cells and thus reduce the consequent inflammation to body tissues.
IL-6
The meta-analysis showed that HS administration significantly suppressed the cellular levels of IL-6 in different disease models (Figure 6). IL-6 are pro-inflammatory cytokines that are produced in response to the presence of inflammatory stimuli such as IL-1, LPS, TNF-α, and IL-4 (78). IL-6 acts by binding to soluble or bound IL-6 receptors which are expressed on many endothelial cells. The downstream effect of IL-6 includes increased expression of the AT1 receptor (78), adhesion molecules [including ICAM-1, vascular cell adhesion molecule 1 (VCAM-1)], vascular endothelial growth factor (VEGF), and other inflammatory cytokines such as MCP, IL-8, and IL-6 (79, 80). Dysfunctional IL-6 signaling has been implicated in autoimmune diseases, chronic inflammation endothelial. dysfunction, and fibrogenesis. Suppressing IL-6 signaling could be beneficial to patients with these disease conditions (81). In many disease models, inhibition of IL-6 expression has been shown to prevent, heal or suppress disease progression (82). The IL-6 suppressing effect of HS across different disease models suggests a role for HS in the management of chronic inflammatory diseases.
IL-10
IL-10 is an anti-inflammatory cytokine produced by virtually all immune cells. Its main function is to prevent immunopathology during inflammatory responses (83). The result obtained from the pooled meta-analysis suggests that IL-10 was not significantly altered by HS treatment (Figure 10). This could, however, be due to the small number of studies used for the meta-analysis.
iNOS
Inducible nitric oxide synthase (iNOS) synthesizes nitric oxide a regulator of immune responses. Overexpression of iNOS and the consequent over production of nitric oxide can lead to cellular injury including DNA damage (31). Findings from studies conducted by Chou et al. (31) and Perez-Torres et al. (45) suggest that HS can lower iNOS. This makes HS potentially useful in protecting against nitric oxide-induced cellular damage in the conditions of chronic inflammatory diseases. Our meta-analysis of the available evidence (Figure 11) showed that HS lowered iNOS, however, the result was not statistically significant (p=0.14).
The suggested mechanism of HS anti-inflammatory activities is outlined in Figure 14. The pathway leading to the buildup of inflammatory and immune responses includes: (1) build up of oxidative stress, which leads to cellular damage and the consequent release of endogenous stress signal molecules, i.e, damage-associated molecular patterns (DAMP). Stimulation by pathogens also triggers the release of pathogen-associated molecular pattern molecules (PAMP). PAMP and DAMP binding to Toll-like receptor proteins (eg TLR4) leads to the downstream expression of inflammatory mediators including MCP-1, TNF-α, IL-1β, IL-6, etc. (2) MCP-1, IL-1β, IL-6, and other cytokines trigger the accumulation and infiltration of circulating monocytes into sites of inflammation, resulting in the differentiation and maturation of the monocytes to specialized immune cells. (3) Cytokine receptors (eg TNFR, IL-6R, IL-1R) on the surface of immune or epithelial cells are activated by the binding of their respective ligands, leading to the expression of more inflammatory cytokines in an NF-κB dependent and independent pathway. (4) The activities of ACE1 lead to the buildup of Ang II. This increases the stimulation of the AT1 receptors and its associated downstream expression of inflammatory cytokines. IL-6 further enhances the expression of AT1 receptors, leading to more downstream effects.
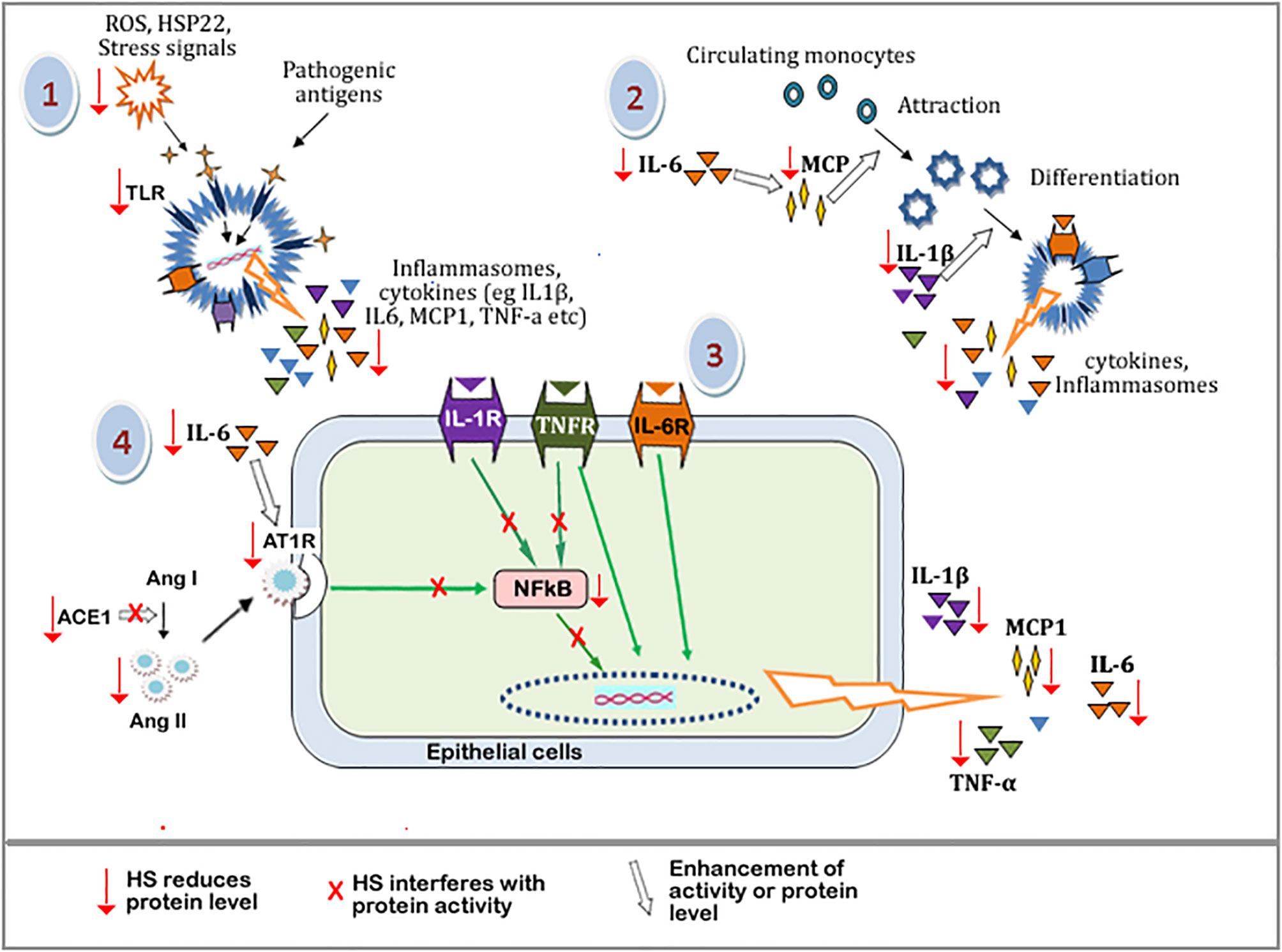
Figure 14 Schematic diagram showing the tentative mechanism of HS anti-inflammatory activities. Oxidative stress and other cellular damage cause the activation of TLRs, which leads to the expression of inflammatory mediators. These mediators further perpetuate immune responses causing the release of more inflammatory mediators. HS interferes with various steps along these pathways (shown by red arrow). Steps with a red arrow indicate that HS reduced the level of the component of that step. Steps with a red “X” means that HS inhibited or interfered with the protein activity along that pathway. The white arrow means that the activity or level of a component is enhanced.\ TLRs, Toll-like receptors; ROS, Reactive oxygen species; HSP22, heat shock protein 22; IL-6, Interleukin 6; IL-1b, interleukin 1b; MCP-1, Monocyte Chemoattractant Protein-1; NFkB, Nuclear factor kappa B; IL-1R, Interleukin 1 receptor; TNFR, tumor necrosis factor receptor; IL-6R, Interleukin 6 receptor; Ang I, Angiotensin I; Ang II, Angiotensin II; ACE-1, Angiotensin converting enzyme 1; AT-1R, Angiotensin II type 1 receptor.
Components of HS extracts can suppress TLR mediated expression of inflammatory mediators by reducing the levels of DAMP molecules (through their radical scavenging activities) and suppressing the expression of TLR. HS extract also suppresses the levels of MCP-1, TNF-α, IL-1β, IL-6 resulting in a reduction in the overall inflammatory/immune responses. HS extract can inhibit ACE1 activity as well as reduce the levels of ACE1 and Ang II proteins hereby suppressing downstream activation of AT1 receptor-mediated expression of inflammatory cytokines. HS can also interfere with components of NF-κB cascade thereby further suppressing the expression of inflammatory genes.
The mechanism involved in the anti-inflammatory effect of HS extract in different disease models is multifaceted and involves the interaction of different constituents of HS with different cellular targets. It appears that the mechanism that predominates depends on the causative factors of each disease model. Diseases linked to dysfunctional oxidative stress most likely could involve the antioxidant capacity enhancement potentials of HS to restore normal antioxidant levels, bringing about the cessation of the observed inflammation. In pathological conditions caused by localized inflammation, the MCP-1, COX2, and lipoxygenase suppressing properties of HS might come into play to reduce inflammation. Reduced expression of MCP-1 will suppress the infiltration of circulating monocytes into inflammatory sites, reducing the extent of the innate immune response at such sites. The ability to inhibit ACE suppresses the possibility of having an enhanced Ang II/AT1 receptor-mediated downstream oxidative dysfunction and inflammatory response. A combination of all these effects could be beneficial for suppressing immune responses in conditions of chronic systemic inflammatory disorders.
Apart from anti-inflammatory effects, other beneficial health effects have also been observed following HS supplementation. Using a combination of transcriptomics and metabolomics, Beltrán-Debón et al. (47) showed that ingestion of aqueous extract of HS triggered several components of mitochondrial functions, energy metabolism, and cardiovascular protection. Improvement of glucose tolerance, insulin sensitivity, gut integrity, and restoration of gastrointestinal microbiota was observed in experimental models of diet-induced obesity which received oral doses of HS polyphenol extracts (16). HS has been recommended for use as adjuvant therapy in the management of metabolic syndrome diseases (16), Inflammatory bowel disease (40), diabetic nephropathy (15), and wounds (60). The use of HS as an adjuvant needs to be done cautiously since HS can interact with conventional drugs such as hydrochlorothiazide, chloroquine, ciprofloxacin, and acetaminophen, as such affecting the efficacy and kinetics of the primary drug (26, 84). HS did not improve nor affect the antihypertensive potency of captopril when both were co-administered in a hypertensive rat model (26). In terms of toxicological safety, HS is reported to have a lethal dose of 50 that is greater than 5000 mg/kg weight of experimental animals (85, 86).
Limitations
This systematic review is affected by the various limitations that are characteristics of systematic reviews. Some of such limitations include the fact that only published data that were identified using the search strategies and databases specified in our method section were accounted for in the data synthesis. The possible omission of other relevant studies either because they were not published or because they not were indexed in the databases we consulted cannot be ruled out. The data used in this review were obtained from in-vivo (animal and human) studies in a limited disease model. Furthermore, there were few studies per disease model and in some cases, conclusions were drawn using models from just two studies. Only two disease models were reported for humans (i.e hypertensive and metabolic syndrome), as such, the possible impact of the human disease model on HS efficacy could not be reliably ascertained from this study. The small number of studies (per each biomarker) made it impossible to identify the possible causes of the heterogeneity observed in some of the pooled estimates. The number of studies used to generate the pooled estimate might not be sufficient to entirely reflect the real situation. Finally, most of the data used for this systematic review and meta-analysis were obtained from studies that exposed experimental models to chronic or sub-chronic levels of HS. The instantaneous efficacy of HS administration could not be thus ascertained from this systematic review.
Conclusion
This systematic review and meta-analysis revealed that HS extracts possess immunosuppressive capabilities. This finding suggests that HS extracts might be beneficial in the treatment/management of pathological conditions associated with a hyperactive immune system. Further studies should verify the efficacy of HS in suppressing immune responses in autoimmune or chronic inflammatory disease conditions.
Data Availability Statement
The original contributions presented in the study are included in the article/supplementary material. Further inquiries can be directed to the corresponding author.
Author Contributions
FU conducted the search, participated in data extraction, ran the statistical and forest plot analyses, designed the graphic abstract, and contributed to the introduction and discussion sections. JU and GB wrote the introduction and materials and methods and undertook data extraction. BE-E participated in data extraction, contributed to the discussion section, and collated the work. NC searched the databases and participated in data extraction. OO mentored the authors, reviewed the draft manuscript, and certified the final manuscript. All authors contributed to the article and approved the submitted version.
Conflict of Interest
The authors declare that the research was conducted in the absence of any commercial or financial relationships that could be construed as a potential conflict of interest.
Abbreviations
ACE1, Angiotensin converting enzyme -1; ACE2, Angiotensin converting enzyme -2; Ang I, Angiotensin I; Ang II , Angiotensin II; AT1 receptor, Angiotensin II receptor type 1; AT2 receptor, Angiotensin II receptor type 2; CCR2, C-C chemokine receptor type 2; COX-2, Cyclooxygenase-2; CRP, C-reactive protein; DAMP, damage-associated molecular patterns; DMBA, Dimethylbenz(A)Anthracene; ERK, Extracellular signal-regulated kinases; HepG2, Human hepatoma cell line; HFD, High fat diet; HS, Hibiscus sabdariffa calyx; ICAM-1, Intercellular Adhesion Molecule 1; IL-10, Interleukin 10; IL-1R, Interleukin-1 receptor; IL-1β, Interleukin 1β; IL-6, Interleukin 6; IL-8, Interleukin 8; iNOS, Inducible nitric oxide synthase; JNK, c-Jun N-terminal kinase; L-NAME, N(G)-nito-L-arginine-methyl ester; LPS , Lipopolysaccharide; MAP3K, Mitogen Activated Protein (MAP) kinase kinase kinase; MAPK, mitogen-activated protein kinase; MCP-1 or CCL2, Monocyte Chemoattractant Protein-1; NF-κB, Nuclear Factor Kappa B; PAMP, pathogen-associated molecular pattern molecules; PCA, protocatechuic acid; PRISMA, Preferred Reporting Items for Systematic reviews and Meta-Analysis; ROS, Reactive oxygen species; SD, standard deviation; SEM, standard error of mean; SMD, standardized mean difference; TAA, Thioacetamide; TFA, Trifluoroacetic acid; TLR, Toll like receptor; TLR4, Toll like receptor 4; TNFR, Tumor necrosis factor receptor; TNF-α, tumor necrosis factor-α; TPA, 12-O-tetradecanoylphorbol-13-acetate; TRAF2, TNF receptor associated factor 2; VCAM-1, vascular cell adhesion molecule 1; VEGF, vascular endothelial growth factor.
References
1. Ali MK, Ashraf A, Biswas NN, Karmakar UK, Afroz S. Antinociceptive, Anti-Inflammatory and Antidiarrheal Activities of Ethanolic Calyx Extract of Hibiscus Sabdariffa Linn.(Malvaceae) in Mice. Zhong xi yi jie he xue bao= J Chin Integr Med (2011) 9(6):626–31. doi: 10.3736/jcim20110608
2. Sindi HA, Marshall LJ, Morgan MRA. Comparative Chemical and Biochemical Analysis of Extracts of Hibiscus Sabdariffa. Food Chem (2014) 164:23–9. doi: 10.1016/j.foodchem.2014.04.097
3. Gerald CL, McClendon CJ, Ranabhat RS, Waterman JT, Kloc LL, Conklin DR, et al. Sorrel Extract Reduces Oxidant Production in Airway Epithelial Cells Exposed to Swine Barn Dust Extract In Vitro. Mediators Inflammation (2019) 2019:7420468. doi: 10.1155/2019/7420468
4. Müller BM, Franz G. Chemical Structure and Biological Activity of Polysaccharides From Hibiscus Sabdariffa. Planta Med (1992) 58(01):60–7. doi: 10.1055/s-2006-961391
5. Frank T, Janßen M, Netzel M, Straß G, Kler A, Kriesl E, et al. Pharmacokinetics of anthocyanidin-3-glycosides Following Consumption of Hibiscus Sabdariffa L. extract. J Clin Pharmacol (2005) 45(2):203–10. doi: 10.1177/0091270004270561
6. Jiménez-Ferrer E, Alarcón-Alonso J, Aguilar-Rojas A, Zamilpa A, Tortoriello J, Herrera-Ruiz M, et al. Diuretic Effect of Compounds From Hibiscus Sabdariffa by Modulation of the Aldosterone Activity. Planta Med (2012) 78(18):1893–8. doi: 10.1055/s-0032-1327864
7. Jabeur I, Pereira E, Caleja C, Calhelha RC, Soković M, Catarino L, et al. Exploring the Chemical and Bioactive Properties of Hibiscus Sabdariffa L. Calyces From Guinea-Bissau (West Africa). Food Funct (2019) 10(4):2234–43. doi: 10.1039/C9FO00287A
8. Christian KR, Nair MG, Jackson JC. Antioxidant and Cyclooxygenase Inhibitory Activity of Sorrel (Hibiscus Sabdariffa). J Food Compos Anal (2006) 19(8):778–83. doi: 10.1016/j.jfca.2006.04.004
9. Villalpando-Arteaga EV, Mendieta-Condado E, Esquivel-Solís H, Canales-Aguirre AA, Gálvez-Gastélum FJ, Mateos-Díaz JC, et al. Hibiscus Sabdariffa L. Aqueous Extract Attenuates Hepatic Steatosis Through Down-Regulation of PPAR-$γ$ and SREBP-1c in Diet-Induced Obese Mice. Food Funct (2013) 4(4):618–26. doi: 10.1039/c3fo30270a
10. Li S, Wu B, Fu W, Reddivari L. The Anti-Inflammatory Effects of Dietary Anthocyanins Against Ulcerative Colitis. Int J Mol Sci (2019) 20(10):2588. doi: 10.3390/ijms20102588
11. Sogo T, Terahara N, Hisanaga A, Kumamoto T, Yamashiro T, Wu S, et al. Anti-Inflammatory Activity and Molecular Mechanism of Delphinidin 3-Sambubioside, a Hibiscus Anthocyanin. BioFactors (2015) 41(1):58–65. doi: 10.1002/biof.1201
12. Vargas-León EA, Díaz-Batalla L, González-Cruz L, Bernardino-Nicanor A, Castro-Rosas J, Reynoso-Camacho R, et al. Effects of Acid Hydrolysis on the Free Radical Scavenging Capacity and Inhibitory Activity of the Angiotensin Converting Enzyme of Phenolic Compounds of Two Varieties of Jamaica (Hibiscus Sabdariffa). Ind Crops Prod (2018) 116(October 2017):201–8. doi: 10.1016/j.indcrop.2018.02.044
13. Ojeda D, Jiménez-Ferrer E, Zamilpa A, Herrera-Arellano A, Tortoriello J, Alvarez L. Inhibition of Angiotensin Convertin Enzyme (ACE) Activity by the Anthocyanins Delphinidin-and Cyanidin-3-O-sambubiosides From Hibiscus Sabdariffa. J Ethnopharmacol (2010) 127(1):7–10. doi: 10.1016/j.jep.2009.09.059
14. Nwachukwu DC, Aneke EI, Obika LF, Nwachukwu NZ. Effects of Aqueous Extract of Hibiscus Sabdariffa on the Renin-Angiotensin-Aldosterone System of Nigerians With Mild to Moderate Essential Hypertension: A Comparative Study With Lisinopril. Indian J Pharmacol (2015) 47(5):540. doi: 10.4103/0253-7613.165194
15. Yang Y-S, Wang C-J, Huang C-N, Chen M-L, Chen M-J, Peng C-H. Polyphenols of Hibiscus Sabdariffa Improved Diabetic Nephropathy Via Attenuating Renal Epithelial Mesenchymal Transition. J Agric Food Chem (2013) 61(31):7545–51. doi: 10.1021/jf4020735
16. Diez-Echave P, Vezza T, Rodríguez-Nogales A, Ruiz-Malagón AJ, Hidalgo-García L, Garrido-Mesa J, et al. The Prebiotic Properties of Hibiscus Sabdariffa Extract Contribute to the Beneficial Effects in Diet-Induced Obesity in Mice. Food Res Int (2020) 127:108722. doi: 10.1016/j.foodres.2019.108722
17. Ezzat SM, Salama MM, Seif el-Din SH, Saleh S, El-Lakkany NM, Hammam OA, et al. Metabolic Profile and Hepatoprotective Activity of the Anthocyanin-Rich Extract of Hibiscus Sabdariffa Calyces. Pharm Biol (2016) 54(12):3172–81. doi: 10.1080/13880209.2016.1214739
18. Shen C-Y, Zhang T-T, Zhang W-L, Jiang J-G. Anti-Inflammatory Activities of Essential Oil Isolated From the Calyx of Hibiscus Sabdariffa L. Food Funct (2016) 7(10):4451–9. doi: 10.1039/C6FO00795C
19. Pimentel-Moral S, Borrás-Linares I, Lozano-Sánchez J, Arráez-Román D, Martínez-Férez A, Segura-Carretero A. Microwave-Assisted Extraction for Hibiscus Sabdariffa Bioactive Compounds. J Pharm BioMed Anal (2018) 156:313–22. doi: 10.1016/j.jpba.2018.04.050
20. Beltrán-Debón R, Alonso-Villaverde C, Aragones G, Rodriguez-Medina I, Rull A, Micol V, et al. The Aqueous Extract of Hibiscus Sabdariffa Calices Modulates the Production of Monocyte Chemoattractant Protein-1 in Humans. Phytomedicine (2010) 17(3–4):186–91. doi: 10.1016/j.phymed.2009.08.006
21. Alegbe EO, Teralí K, Olofinsan KA, Surgun S, Ogbaga CC, Ajiboye TO. Antidiabetic Activity-Guided Isolation of Gallic and Protocatechuic Acids From Hibiscus Sabdariffa Calyxes. J Food Biochem (2019) 43(7):e12927. doi: 10.1111/jfbc.12927
22. Maciel LG, do Carmo MAV, Azevedo L, Daguer H, Molognoni L, de Almeida MM, et al. Hibiscus Sabdariffa Anthocyanins-Rich Extract: Chemical Stability, In Vitro Antioxidant and Antiproliferative Activities. Food Chem Toxicol (2018) 113:187–97. doi: 10.1016/j.fct.2018.01.053
23. Zheng D, Zou Y, Cobbina SJ, Wang W, Li Q, Chen Y, et al. Purification, Characterization and Immunoregulatory Activity of a Polysaccharide Isolated From Hibiscus Sabdariffa L. J Sci Food Agric (2017) 97(5):1599–606. doi: 10.1002/jsfa.7908
24. Herrera-Arellano A, Miranda-Sánchez J, Ávila-Castro P, Herrera-Álvarez S, Jiménez-Ferrer JE, Zamilpa A, et al. Clinical Effects Produced by a Standardized Herbal Medicinal Product of Hibiscus Sabdariffa on Patients With Hypertension. A Randomized, Double-Blind, Lisinopril-Controlled Clinical Trial. Planta Med (2007) 73(01):6–12. doi: 10.1055/s-2006-957065
25. Abdel-Rahman RF, Hessin AF, Abdelbaset M, Ogaly HA, Abd-Elsalam RM, Hassan SM. Antihypertensive Effects of Roselle-Olive Combination in L-NAME-Induced Hypertensive Rats. Oxid Med Cell Longev (2017) 2017:9460653. doi: 10.1155/2017/9460653
26. Nurfaradilla SA, Saputri FC, Harahap Y. Effects of Hibiscus Sabdariffa Calyces Aqueous Extract on the Antihypertensive Potency of Captopril in the Two-Kidney-One-Clip Rat Hypertension Model. Evidence-Based Complement Altern Med (2019) 2019:9694212. doi: 10.1155/2019/9694212
27. Abdel-Zaher AO, Farghaly HSM, El-Refaiy AEM, Abd-Eldayem AM. Protective Effect of the Standardized Leaf Extract of G Inkgo Biloba (Egb761) Against Hypertension-Induced Renal Injury in Rats. Clin Exp Hypertens (2018) 40(8):703–14. doi: 10.1080/10641963.2018.1425421
28. Li Y, Wang Y, Ding X, Duan B, Li L, Wang X. Serum Levels of TNF-$α$ and IL-6 are Associated With Pregnancy-Induced Hypertension. Reprod Sci (2016) 23(10):1402–8. doi: 10.1177/1933719116641760
29. Dange RB, Agarwal D, Masson GS, Vila J, Wilson B, Nair A, et al. Central Blockade of TLR4 Improves Cardiac Function and Attenuates Myocardial Inflammation in Angiotensin II-induced Hypertension. Cardiovasc Res (2014) 103(1):17–27. doi: 10.1093/cvr/cvu067
30. Mezzano S, Aros C, Droguett A, Burgos ME, Ardiles L, Flores C, et al. Nf-κb Activation and Overexpression of Regulated Genes in Human Diabetic Nephropathy. Nephrol Dial Transplant (2004) 19(10):2505–12. doi: 10.1093/ndt/gfh207
31. Chou ST, Lo HY, Li CC, Cheng LC, Chou PC, Lee YC, et al. Exploring the Effect and Mechanism of Hibiscus Sabdariffa on Urinary Tract Infection and Experimental Renal Inflammation. J Ethnopharmacol (2016) 194(October):617–25. doi: 10.1016/j.jep.2016.10.059
32. Moher D, Liberati A, Tetzlaff J, Altman DG, Group P, others. Preferred Reporting Items for Systematic Reviews and Meta-Analyses: The PRISMA Statement. PloS Med (2009) 6(7):e1000097. doi: 10.1371/journal.pmed.1000097
33. Team RC, others. R: A Language and Environment for Statistical Computing. Vienna: R Foundation for Statistical Computing (2013). Available at: https://www.R-project.org.
34. Higgins JPT, Thompson SG. Spiegelhalter Dj. A Re-Evaluation of Random-Effects Meta-Analysis. J R Stat Soc Ser A (Statistics Soc (2009) 172(1):137–59. doi: 10.1111/j.1467-985X.2008.00552.x
35. Guddat C, Grouven U, Bender R, Skipka G. A Note on the Graphical Presentation of Prediction Intervals in Random-Effects Meta-Analyses. Syst Rev (2012) 1(1):34. doi: 10.1186/2046-4053-1-34
36. Takeshima N, Sozu T, Tajika A, Ogawa Y, Hayasaka Y, Furukawa TA. Which is More Generalizable, Powerful and Interpretable in Meta-Analyses, Mean Difference or Standardized Mean Difference? BMC Med Res Methodol (2014) 14(1):30. doi: 10.1186/1471-2288-14-30
37. Wang S-C, Lee S-F, Wang C-J, Lee C-H, Lee W-C, Lee H-J. Aqueous Extract From Hibiscus Sabdariffa Linnaeus Ameliorate Diabetic Nephropathy Via Regulating Oxidative Status and Akt/Bad/14-3-3$γ$ in an Experimental Animal Model. Evidence-Based Complement Altern Med (2011) 2011:938126. doi: 10.1093/ecam/nep181
38. Kao E-S, Hsu J-D, Wang C-J, Yang S-H, Cheng S-Y, Lee H-J. Polyphenols Extracted From Hibiscus Sabdariffa L. Inhibited Lipopolysaccharide-Induced Inflammation by Improving Antioxidative Conditions and Regulating Cyclooxygenase-2 Expression. Biosci Biotechnol Biochem (2009) 73(2):385–90. doi: 10.1271/bbb.80639
39. Nurkhasanah N. The EFFECT of ROSELLA (Hibiscus Sabdariffa L) Treatment ON Il-10 AND Il-14 SECRETION on DIMETHYLBENZ (a) ANTHRACENE (Dmba) INDUCED Rat. Int J Pharm Pharm Sci (2015) 7(4):402–4.
40. Lubis M, Siregar GA, Bangun H, Ilyas S. The Effect of Roselle Flower Petals Extract (Hibiscus Sabdariffa Linn.) on Reducing Inflammation in Dextran Sodium Sulfateinduced Colitis. Med Glas Off Publ Med Assoc Zenica-doboj Canton Bosnia Herzegovina (2020) 17(2):395–401. doi: 10.17392/1095-20
41. Fakeye TO, Pal A, Bawankule DU, Khanuja SPS. Immunomodulatory Effect of Extracts of Hibiscus Sabdariffa L. (Family Malvaceae) Mouse Model Phyther Res Int J Devoted to Pharmacol Toxicol Eval Nat Prod Deriv (2008) 22(5):664–8. doi: 10.1002/ptr.2370
42. Joven J, March I, Espinel E, Fernández-Arroyo S, Rodríguez-Gallego E, Aragonès G, et al. Hibiscus Sabdariffa Extract Lowers Blood Pressure and Improves Endothelial Function. Mol Nutr Food Res (2014) 58(6):1374–8. doi: 10.1002/mnfr.201300774
43. Santoso DIS, Yunita S, Paramita N, Andraini T, Kartinah NT, El Bayani GF, et al. Effect of Hibiscus Sabdariffa Linn on IL-6 and TNF-α Levels in Overtrained Rat Heart. Int J Appl Pharm (2019) 11(Special Issue 6):42–5. doi: 10.22159/ijap.2019.v11s6.33535
44. Ali SS, Mohamed SFA, Rozalei NH, Boon YW, Zainalabidin S. Anti-Fibrotic Actions of Roselle Extract in Rat Model of Myocardial Infarction. Cardiovasc Toxicol (2019) 19(1):72–81. doi: 10.1007/s12012-018-9478-7
45. Pérez-Torres I, Zuniga Munoz A, Beltrán-Rodríguez U, Díaz-Díaz E, Martínez-Memije R, Guarner Lans V. Modification of the Liver Fatty Acids by Hibiscus Sabdariffa Linnaeus (Malvaceae) Infusion, its Possible Effect on Vascular Reactivity in a Metabolic Syndrome Model. Clin Exp Hypertens (2014) 36(3):123–31. doi: 10.3109/10641963.2013.789046
46. Huang C-N, Wang C-J, Yang Y-S, Lin C-L, Peng C-H. Hibiscus Sabdariffa Polyphenols Prevent Palmitate-Induced Renal Epithelial Mesenchymal Transition by Alleviating Dipeptidyl peptidase-4-mediated Insulin Resistance. Food Funct (2016) 7(1):475–82. doi: 10.1039/C5FO00464K
47. Beltrán-Debón R, Rodr’iguez-Gallego E, Fernández-Arroyo S, Senan-Campos O, Massucci FA, Hernández-Aguilera A, et al. The Acute Impact of Polyphenols From Hibiscus Sabdariffa in Metabolic Homeostasis: An Approach Combining Metabolomics and Gene-Expression Analyses. Food Funct (2015) 6(9):2957–66. doi: 10.1039/C5FO00696A
48. Chou C-C, Wang C-P, Chen J-H, Lin H-H. Anti-Atherosclerotic Effect of Hibiscus Leaf Polyphenols Against Tumor Necrosis Factor-alpha-Induced Abnormal Vascular Smooth Muscle Cell Migration and Proliferation. Antioxidants (2019) 8(12):620. doi: 10.3390/antiox8120620
49. Tseng T-H, Hsu J-D, Lo M-H, Chu C-Y, Chou F-P, Huang C-L, et al. Inhibitory Effect of Hibiscus Protocatechuic Acid on Tumor Promotion in Mouse Skin. Cancer Lett (1998) 126(2):199–207. doi: 10.1016/S0304-3835(98)00010-X
50. Asgary S, Soltani R, Zolghadr M, Keshvari M, Sarrafzadegan N. Evaluation of the Effects of Roselle (Hibiscus Sabdariffa L.) on Oxidative Stress and Serum Levels of Lipids, Insulin and hs-CRP in Adult Patients With Metabolic Syndrome: A Double-Blind Placebo-Controlled Clinical Trial. J Complement Integr Med (2016) 13(2):175–80. doi: 10.1515/jcim-2015-0030
51. Abubakar SM, Ukeyima MT, Spencer JPE, Lovegrove JA. Acute Effects of Hibiscus Sabdariffa Calyces on Postprandial Blood Pressure, Vascular Function, Blood Lipids, Biomarkers of Insulin Resistance and Inflammation in Humans. Nutrients (2019) 11(2):1–21. doi: 10.3390/nu11020341
52. El Bayani GF, Marpaung NLE, Simorangkir DAS, IR S, Ibrahim N, NT K, et al. Anti-Inflammatory Effects of Hibiscus Sabdariffa Linn. on the IL-1β/IL-1ra Ratio in Plasma and Hippocampus of Overtrained Rats and Correlation With Spatial Memory. Kobe J Med Sci (2018) 64(2):E73–83.
53. Dafallah AA, Al-Mustafa Z. Investigation of the Anti-Inflammatory Activity of Acacia Nilotica and Hibiscus Sabdariffa. Am J Chin Med (1996) 24(03n04):263–9. doi: 10.1142/S0192415X96000323
54. Fernández-Arroyo S, Herranz-López M, Beltrán-Debón R, Borrás-Linares I, Barrajón-Catalán E, Joven J, et al. Bioavailability Study of a Polyphenol-Enriched Extract From H Ibiscus Sabdariffa in Rats and Associated Antioxidant Status. Mol Nutr Food Res (2012) 56(10):1590–5. doi: 10.1002/mnfr.201200091
55. Tsuda T, Horio F, Osawa T. Absorption and Metabolism of Cyanidin 3-O-$β$-D-glucoside in Rats. FEBS Lett (1999) 449(2–3):179–82. doi: 10.1016/S0014-5793(99)00407-X
56. Piccinini AM, Midwood KS. Dampening Inflammation by Modulating TLR Signalling. Mediators Inflammation (2010) 2010:672395. doi: 10.1155/2010/672395
57. Hurst RD, Lyall KA, Wells RW, Sawyer GM, Lomiwes D, Ngametua N, et al. Daily Consumption of an Anthocyanin-Rich Extract Made From New Zealand Blackcurrants for 5 Weeks Supports Exercise Recovery Through the Management of Oxidative Stress and Inflammation: A Randomized Placebo Controlled Pilot Study. Front Nutr (2020) 7:16. doi: 10.3389/fnut.2020.00016
58. Carrier A. Metabolic Syndrome and Oxidative Stress: A Complex Relationship. 140 Huguenot Street, 3rd Floor New Rochelle, NY 10801 USA: Mary Ann Liebert, Inc (2017).
59. Liu C-L, Wang J-M, Chu C-Y, Cheng M-T, Tseng T-H. In Vivo Protective Effect of Protocatechuic Acid on Tert-Butyl Hydroperoxide-Induced Rat Hepatotoxicity. Food Chem Toxicol (2002) 40(5):635–41. doi: 10.1016/S0278-6915(02)00002-9
60. Palungwachira P, Tancharoen S, Phruksaniyom C, Klungsaeng S, Srichan R, Kikuchi K, et al. Antioxidant and Anti-Inflammatory Properties of Anthocyanins Extracted From Oryza Sativa L. @ in Primary Dermal Fibroblasts. Oxid Med Cell Longev (2019) 2019:2089817. doi: 10.1155/2019/2089817
61. Chamsi-Pasha MAR, Shao Z, Tang WHW. Angiotensin-Converting Enzyme 2 as a Therapeutic Target for Heart Failure. Curr Heart Fail Rep (2014) 11(1):58–63. doi: 10.1007/s11897-013-0178-0
62. Zhu L, Carretero OA, Xu J, Harding P, Ramadurai N, Gu X, et al. Activation of Angiotensin II Type 2 Receptor Suppresses TNF-$α$-Induced ICAM-1 Via NF-кB: Possible Role of ACE2. Am J Physiol Circ Physiol (2015) 309(5):H827–34. doi: 10.1152/ajpheart.00814.2014
63. Hung Y-H, Hsieh W-Y, Hsieh J-S, Liu C, Tsai C-H, Lu L-C, et al. Alternative Roles of STAT3 and MAPK Signaling Pathways in the MMPs Activation and Progression of Lung Injury Induced by Cigarette Smoke Exposure in ACE2 Knockout Mice. Int J Biol Sci (2016) 12(4):454. doi: 10.7150/ijbs.13379
64. Kuba K, Imai Y, Ohto-Nakanishi T, Penninger JM. Trilogy of ACE2: A Peptidase in the Renin–Angiotensin System, a SARS Receptor, and a Partner for Amino Acid Transporters. Pharmacol Ther (2010) 128(1):119–28. doi: 10.1016/j.pharmthera.2010.06.003
65. South AM, Diz D, Chappell MC. COVID-19, ACE2, and the cardiovascular consequences. Am J Physiol Heart Circ Physiol (2020) 318(5):H1084–90. doi: 10.1152/ajpheart.00217.2020
66. Sun ML, Yang JM, Sun YP, Su GH. Inhibitors of RAS Might be a Good Choice for the Therapy of COVID-19 Pneumonia. Zhonghua jie he he hu xi za zhi= Zhonghua jiehe he huxi zazhi= Chin J Tuberc Respir Dis (2020) 43:E014. doi: 10.3760/cma.j.issn.1001-0939.2020.0014
67. Göggel R, Hoffman S, Nüsing R, Narumiya S, Uhlig S. Platelet-Activating Factor–induced Pulmonary Edema is Partly Mediated by Prostaglandin E2, E-Prostanoid 3-Receptors, and Potassium Channels. Am J Respir Crit Care Med (2002) 166(5):657–62. doi: 10.1164/rccm.200111-071OC
68. Liu T, Zhang L, Joo D, Sun S-C. Nf-$κ$B Signaling in Inflammation. Signal Transduct Target Ther (2017) 2(1):1–9. doi: 10.1038/sigtrans.2017.23
69. Locke M, Anderson J. Nf-$κ$B Activation in Organs From STZ-treated Rats. Appl Physiol Nutr Metab (2011) 36(1):121–7. doi: 10.1139/H10-094
70. Israël A. The IKK Complex, a Central Regulator of NF-kappaB Activation. Cold Spring Harb Perspect Biol (2010) 2:a000158. doi: 10.1101/cshperspect.a000158
71. Urschel K, Cicha I. Tnf-$α$ in the Cardiovascular System: From Physiology to Therapy. Intern J Interf Cytokine Med Res (2015) 7:9–25. doi: 10.2147/IJICMR.S64894
72. Azam S, Jakaria M, Kim I-S, Kim J, Haque ME, Choi D-K. Regulation of Toll-Like Receptor (TLR) Signaling Pathway by Polyphenols in the Treatment of Age-Linked Neurodegenerative Diseases: Focus on TLR4 Signaling. Front Immunol (2019) 10:1000. doi: 10.3389/fimmu.2019.01000
73. Martin MU, Wesche H. Summary and Comparison of the Signaling Mechanisms of the Toll/interleukin-1 Receptor Family. Biochim Biophys Acta (BBA)-Molecular Cell Res (2002) 1592(3):265–80. doi: 10.1016/S0167-4889(02)00320-8
75. Deshmane SL, Kremlev S, Amini S, Sawaya BE. Monocyte Chemoattractant Protein-1 (MCP-1): An Overview. J Interf Cytokine Res (2009) 29(6):313–26. doi: 10.1089/jir.2008.0027
76. Rudemiller NP, Patel MB, Zhang J, Jeffs AD, Karlovich NS, Griffiths R, et al. Cc Motif Chemokine 5 Attenuates Angiotensin II–dependent Kidney Injury by Limiting Renal Macrophage Infiltration. Am J Pathol (2016) 186(11):2846–56. doi: 10.1016/j.ajpath.2016.07.015
77. Zoja C, Corna D, Locatelli M, Rottoli D, Pezzotta A, Morigi M, et al. Effects of MCP-1 Inhibition by Bindarit Therapy in a Rat Model of Polycystic Kidney Disease. Nephron (2015) 129(1):52–61. doi: 10.1159/000369149
78. Su H, Lei C-T, Zhang C. Interleukin-6 Signaling Pathway and its Role in Kidney Disease: An Update. Front Immunol (2017) 8:405. doi: 10.3389/fimmu.2017.00405
79. Kaplanski G, Marin V, Montero-Julian F, Mantovani A, Farnarier C. Il-6: A Regulator of the Transition From Neutrophil to Monocyte Recruitment During Inflammation. Trends Immunol (2003) 24(1):25–9. doi: 10.1016/S1471-4906(02)00013-3
80. Nakahara H, Song J, Sugimoto M, Hagihara K, Kishimoto T, Yoshizaki K, et al. Anti–Interleukin-6 Receptor Antibody Therapy Reduces Vascular Endothelial Growth Factor Production in Rheumatoid Arthritis. Arthritis Rheum Off J Am Coll Rheumatol (2003) 48(6):1521–9. doi: 10.1002/art.11143
81. Barnes TC, Anderson ME, Moots RJ. The Many Faces of interleukin-6: The Role of IL-6 in Inflammation, Vasculopathy, and Fibrosis in Systemic Sclerosis. Int J Rheumatol (2011) 2011:721608. doi: 10.1155/2011/721608
82. Tanaka T, Narazaki M, Kishimoto T. IL-6 in Inflammation, Immunity, and Disease. Cold Spring Harb Perspect Biol (2014) 6(10):a016295. doi: 10.1101/cshperspect.a016295
83. Rojas J, Avia M, Martin V, Sevilla N. Il-10: A Multifunctional Cytokine in Viral Infections. J Immunol Res (2017) 2017:6104054. doi: 10.1155/2017/6104054
84. Ahad A, Raish M, Bin Jardan YA, Alam MA, Al-Mohizea AM, Al-Jenoobi FI. Effect of Hibiscus Sabdariffa and Zingiber Officinale on the Antihypertensive Activity and Pharmacokinetic of Losartan in Hypertensive Rats. Xenobiotica 50(7):847–57. doi: 10.1080/00498254.2020.1729446
85. Ozkol HU, Koyuncu I, Tuluce Y, Dilsiz N, Soral S, Ozkol H. Anthocyanin-Rich Extract From Hibiscus Sabdariffa Calyx Counteracts UVC-caused Impairments in Rats. Pharm Biol (2015) 53(10):1435–41. doi: 10.3109/13880209.2014.980586
86. Onyenekwe PC, Ajani EO, Ameh DA, Gamaniel KS. Antihypertensive Effect of Roselle (Hibiscus Sabdariffa) Calyx Infusion in Spontaneously Hypertensive Rats and a Comparison of its Toxicity With That in Wistar Rats. Cell Biochem Funct Cell Biochem its Modul by Act Agents Dis (1999) 17(3):199–206. doi: 10.1002/(SICI)1099-0844(199909)17:3<199::AID-CBF829>3.0.CO;2-2
Keywords: Hibiscus sabdariffa, functional food, polyphenols, Anthocyanin, immunomodulatory, Anti-inflammatory, cytokines
Citation: Umeoguaju FU, Ephraim-Emmanuel BC, Uba JO, Bekibele GE, Chigozie N and Orisakwe OE (2021) Immunomodulatory and Mechanistic Considerations of Hibiscus sabdariffa (HS) in Dysfunctional Immune Responses: A Systematic Review. Front. Immunol. 12:550670. doi: 10.3389/fimmu.2021.550670
Received: 10 April 2020; Accepted: 08 April 2021;
Published: 10 May 2021.
Edited by:
Wilson Savino, Oswaldo Cruz Foundation (Fiocruz), BrazilReviewed by:
Sonia G. Sáyago-Ayerdi, Instituto Tecnológico de Tepic, MexicoEsmeralda Rangel Vargas, Autonomous University of the State of Hidalgo, Mexico
Copyright © 2021 Umeoguaju, Ephraim-Emmanuel, Uba, Bekibele, Chigozie and Orisakwe. This is an open-access article distributed under the terms of the Creative Commons Attribution License (CC BY). The use, distribution or reproduction in other forums is permitted, provided the original author(s) and the copyright owner(s) are credited and that the original publication in this journal is cited, in accordance with accepted academic practice. No use, distribution or reproduction is permitted which does not comply with these terms.
*Correspondence: Orish Ebere Orisakwe, b3Jpc2hlYmVyZUBnbWFpbC5jb20=; b3Jpc2gub3Jpc2Frd2VAdW5pcG9ydC5lZHUubmc=