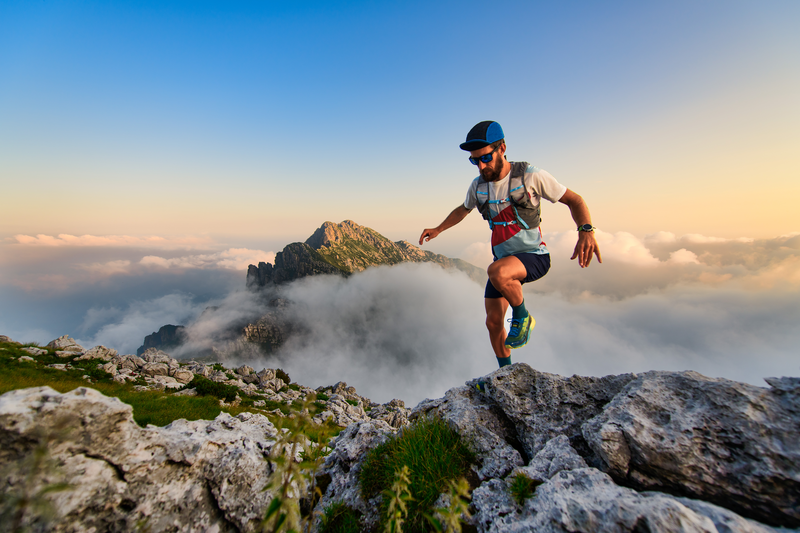
94% of researchers rate our articles as excellent or good
Learn more about the work of our research integrity team to safeguard the quality of each article we publish.
Find out more
ORIGINAL RESEARCH article
Front. Immunol. , 03 February 2021
Sec. Vaccines and Molecular Therapeutics
Volume 11 - 2020 | https://doi.org/10.3389/fimmu.2020.621537
Dendrimeric peptide constructs based on a lysine core that comprises both B- and T-cell epitopes of foot-and-mouth disease virus (FMDV) have proven a successful strategy for the development of FMD vaccines. Specifically, B2T dendrimers displaying two copies of the major type O FMDV antigenic B-cell epitope located on the virus capsid [VP1 (140–158)], covalently linked to a heterotypic T-cell epitope from either non-structural protein 3A [3A (21–35)] or 3D [3D (56–70)], named B2T-3A and B2T-3D, respectively, elicit high levels of neutralizing antibodies (nAbs) and IFN-γ-producing cells in pigs. To assess whether the inclusion and orientation of T-3A and T-3D T-cell epitopes in a single molecule could modulate immunogenicity, dendrimers with T epitopes juxtaposed in both possible orientations, i.e., constructs B2TT-3A3D and B2TT-3D3A, were made and tested in pigs. Both dendrimers elicited high nAbs titers that broadly neutralized type O FMDVs, although B2TT-3D3A did not respond to boosting, and induced lower IgGs titers, in particular IgG2, than B2TT-3A3D. Pigs immunized with B2, a control dendrimer displaying two B-cell epitope copies and no T-cell epitope, gave no nABs, confirming T-3A and T-3D as T helper epitopes. The T-3D peptide was found to be an immunodominant, as it produced more IFN-γ expressing cells than T-3A in the in vitro recall assay. Besides, in pigs immunized with the different dendrimeric peptides, CD4+ T-cells were the major subset contributing to IFN-γ expression upon in vitro recall, and depletion of CD4+ cells from PBMCs abolished the production of this cytokine. Most CD4+IFN-γ+ cells showed a memory (CD4+2E3−) and a multifunctional phenotype, as they expressed both IFN-γ and TNF-α, suggesting that the peptides induced a potent Th1 pro-inflammatory response. Furthermore, not only the presence, but also the orientation of T-cell epitopes influenced the T-cell response, as B2TT-3D3A and B2 groups had fewer cells expressing both cytokines. These results help understand how B2T-type dendrimers triggers T-cell populations, highlighting their potential as next-generation FMD vaccines.
Foot-and-mouth disease (FMD), an acute and systemic vesicular disease that affects cloven-hoofed animals, is caused by FMD virus (FMDV) from the Aphthovirus genus within the Picornaviridae family (1). FMD is included in the list of notifiable terrestrial and aquatic animal diseases of the World Organization for Animal Health (OIE), as the fatal impact of recurring FMD outbreaks causes huge economic losses in affected countries (2–4). Vaccination remains the most effective method to control FMD (5, 6), with the current OIE-approved vaccine types consisting of chemically inactivated whole viruses emulsified with different adjuvants (7). Although these conventional vaccines have demonstrated their success in eliciting protective immunity against the disease in endemic countries, they have shortcomings such the need for a cold-chain to preserve antigenicity, high-containment biosafety facilities, and difficulties to distinguish infected from vaccinated animals (DIVA capability), among others. These drawbacks underlie non-vaccination policies in some countries (8).
In the face of these limitations, alternative strategies, for instance peptide-based subunit vaccines targeting FMDV protein VP1 have been successfully used to induce anti-FMDV neutralizing antibodies (9). Advantages of such peptide vaccines include: (i) safety, as a non-infectious material is required, and no reversion to virulence is possible; (ii) DIVA condition; (iii) easy handling and storage, with no cold chain needed; (iv) chemical stability, and (v) efficient, affordable large scale production. However, early reports of livestock immunization with linear peptides showed modest levels of protection in livestock, lower than required for use as commercial vaccines (10, 11) and interest in peptide-based vaccines temporarily waned. However, with the advent of so-called multiple antigenic peptides (MAPs) pioneered by Tam (12), an effective approach to increase peptide immunogenicity was demonstrated, and peptide vaccines staged a comeback. In the context of FMD, our own research has focused on dendrimeric constructions, generically termed BnT, where several copies of a FMDV B-cell epitope from the G-H loop of VP1 protein in the FMDV capsid (13, 14) are covalently linked through a Lys core matrix to a FMDV T-cell epitope from a non-structural protein (i.e., originally 3A protein, residues 21–35) (15). The selected B-cell epitope shows amino acid variations among different serotypes while the T-cell epitope is highly conserved and therefore can evoke heterologous responses in swine. Interestingly, two doses of a dendrimeric peptide named B4T-3A, displaying four copies of a B cell epitope from type C FMDV linked to T-cell epitope 3A (21–35), was able to protect pigs against homologous FMDV challenge (16). Subsequently, a downsized version, i.e. B2T-3A, bearing only two copies of a type O FMDV B-cell epitope and being stable in serum for several hours (17), afforded full protection in swine, even upon a single dose (18–20). These protective responses of B2T dendrimers are correlated with the induction of high and long-lasting titers of nAbs and the activation of specific lymphocytes providing T-cell help (19, 21). Besides, such T-cell epitopes can also stimulate T-cell subsets leading to the expression of IFN-γ, a cytokine with a relevant role in the antiviral response (22). In another endeavor, a T-cell epitope at the 3D FMDV protein [3D (56-70)], previously shown to be promiscuous and heterotypic in swine (23), displayed as a B2T-3D construct, elicited nAbs titers and IFN-γ-producing cells in similar levels to B2T-3A (24). As previous results in our groups with linear FMDV peptides had shown that not only the presence but also the orientation of the T-cell epitope influenced the immune response (25), in this work the T-3A and T-3D T-cell epitopes have been juxtaposed in B2TT constructs with the two possible orientations, to assess how tandem display and orientation modulate immunogenicity, specifically the T-cell response, a crucial information for improving vaccine design. Also, the requirement of T-cell epitope inclusion in the peptide vaccine was again confirmed, by comparison with ineffective immunizations with construct B2, lacking a T-cell epitope.
The B-cell epitope from FMDV O UKG/11/2001, VP1 (residues 140–158), and the T-cell epitopes 3A (residues 21–35) and 3D (residues 56–70) were synthesized by Fmoc-solid phase synthesis. Bivalent branched dendrimers were prepared by conjugation in solution of two B-cell peptides containing an extra C-terminal Cys (free thiol form) to (one or tandem of two) T-cell epitope moieties N-terminally elongated with Lys residues defining a branching point further derivatized by maleimide units (Table 1). A Lys-Lys motif, defining a putative cleavage site for cathepsin D, a protease involved in in vivo antigen processing for presentation to the MHC class II molecules, was included in the constructs at one (N-terminal of the T-cell epitope in B2T, before the branching) or two positions (as above, plus between the T-cell epitope sequences in B2TT tandems). The end products were obtained via thiol–maleimide ligation at pH 6.0, purified by reverse-phase liquid chromatography and characterized by mass spectrometry (16–18). Final immunogenic peptide dendrimers were water-soluble and stable either lyophilized or in freeze-dried form. Formulation of peptide vaccines was done at the moment of administration.
FMDV stocks O UKG/11/2001, O/SKR, O1Manisa, O1BFS (The Pirbright Institute, UK), and O1Campos (OPS-PanAftosa) were amplified in IBRS-2 cells and type C CS8-c1 virus (26) was amplified in BHK-21 cells.
Landrace x Large White female pigs, 9 to 12-week-old (20 Kg), were maintained in a conventional farm facility at the Departamento de Reproducción Animal, INIA, Madrid. Groups of four pigs were immunized with the B2T-3A (pigs 49, 54, 65, and 74), B2T-3D (pigs 75, 76, 77, 78), B2TT-3A3D (pigs 79, 90, 91, and 92) or B2TT-3D3A (pigs 93, 94, 95, and 96) constructs at day 0, with 2 ml of Montanide ISA 50V2 emulsion containing 2 mg of the corresponding peptide, and boosted likewise at day 21 pi. In addition, two pigs were immunized and boosted with B2 (pigs 97 and 98) and another two PBS-inoculated pigs were maintained as controls (pigs 99 and 100). Because of animal welfare and space requirements, half of the animals (10) corresponding to the lower T-cell responders, were euthanized at day 70 pi. The remaining pigs 10 were housed until the end of the experiment at day 183 pi. Blood samples were collected at days 0, 7, 14, 21, 29, 36, 49, 121, 157, and 183 pi to obtain serum and peripheral blood mononuclear cells (PBMCs). The study was approved (CBS2014/015 and CEEA2014/018) by the INIA Committees on Ethics of Animal Experiments and Biosafety, and by the National Committee on Ethics and Animal Welfare (PROEX 218/14).
Neutralization assays were performed in 96-well culture plates. Serial 2-fold dilutions of each serum sample (in DMEM containing 2% fetal bovine serum) were incubated with 100 infection units—50% tissue culture infective doses (TCID50)—of FMDV O UKG/11/2001 for 1 h at 37°C. Then, a cell suspension of IBRS-2 cells in DMEM was added and plates were incubated for 72 h. Monolayers were controlled for development of cytopathic effect (cpe), fixed and stained. End-point titers were calculated as the reciprocal of the final serum dilution that neutralized 100 TCID50 of homologous FMDV in 50% of the wells (18). For cross neutralization assays, incubation of sera with the panel of FMD viruses (O/SKR, O1Manisa, O1BFS and O1Campos) that belonged to different type O topotypes was performed in parallel to that of the homologous isolate O UKG/11/2001 and the type C CS8-c1 virus as a negative control.
Total anti-FMDV antibodies were determined by means of an indirect ELISA (25). Briefly, 96-well plates coated with peptide B (1µg) were incubated with three-fold dilutions of serum and detected using HRP-conjugated protein A. Plates were read at 450 nm and titers were expressed as the reciprocal of the last serum dilution given an absorbance range of two standard deviations above the background (serum at day 0). Specific IgG1 and IgG2 titers to peptide B were measured and expressed as above using isotype-specific mAbs (Serotec) that were detected with HRP-labeled anti-mouse IgGs (Thermo Fisher). Antibodies against the non-structural protein precursor 3ABC were detected basically as described (18, 27).
Porcine PBMCs were isolated from blood samples collected in Vacutainer tubes EDTA-K2, diluted 1:1 in PBS and then used to obtain PBMC by density-gradient centrifugation with Histopaque 1077 (Sigma) and Leucosep tubes (Greiner Bio-One) as described (27). The number of live PMBCs was calculated using a Neubauer chamber (Immune Systems) and cell staining with 0.4% Trypan Blue (Sigma) in PBS. In general, fresh cells were used in the experiments and those remaining were cryo-preserved in 90% FBS and 10% DMSO in liquid nitrogen. The minimum number of cells used for freezing was 2x107/vial.
Quantification of IFN-γ secreting cells from immunized and control animals was performed using an ELISPOT assay. Briefly, 2.5 × 105 PBMCs were shed in triplicate wells of Immobilon-P plates (Merck Millipore) coated as reported (18) and in vitro stimulated with 50 µg/ml of their respective dendrimers or with T or B peptides. As positive or negative controls, cells were incubated with 10 µg/ml of phytohaemagglutinin (Sigma) or only with medium, respectively. After 48 h at 37°C and 5% CO2, plates were washed and incubated with a biotinylated mouse anti-pig IFN-γ antibody (clone P2C11, BD) followed by HRP-streptavidin (BD). The frequency of peptide-specific T-cells was expressed as the mean number of spot-forming cells/106 PBMCs, with background values (number of spots in negative control wells) subtracted from the respective counts of stimulated cells. These experiments were performed using outbred domestic pigs with different individual genetic backgrounds. In any case, the levels of animal-to-animal variation did not exceed those observed in other related studies (11).
The following mouse anti-pig monoclonal antibodies (mAbs) were used as primary immunoreagents: anti-CD4 (74-12-4, IgG2b) (28), anti-CD8β (PG164A, IgG2a, Eurovet Veterinaria), anti-2E3 (IgM) (29), and anti-CD172a (BL1H7, IgG1) (30). Goat polyclonal Abs (pAbs) specific for mouse Ig subclasses labeled with Alexa647, Alexa488 (Fisher Scientific) or FITC (Bionova) were used as secondary antibodies. For cytokine staining, PE-conjugated anti-pig IFN-γ (P2G10, IgG1) or APC-conjugated anti-human TNF-α (Mab11, IgG1, BD Biosciences) were used. All immunoreagents were titrated for optimal signal/noise ratios. Primary and secondary isotype-matched antibodies were used as negative controls. No background or false staining was observed.
To detect the phenotype of cytokine-producing cells, intracellular staining of purified PBMCs was performed using 5x106 PBMCs from each pig that were in vitro stimulated or not for 18 h at 37°C with their respective peptide at a final concentration of 25 µg/ml. During the last 10 h, brefeldin A (Sigma) was present in cultures at a final concentration of 5 µg/ml. Medium- or PHA-M-incubated cultures (25 µg/ml) served as negative (non-stimulated) or positive controls, respectively, in a non-immunized animal of each experiment. Cells were washed in PBS-0.05% EDTA-5% FBS and surface stained by incubation with cell-surface primary antibodies for 30 min at 4°C in PBS-0.05% EDTA- 5% swine serum. After three washes in PBS-0.05% EDTA-5% FBS, cells were incubated with the adequate secondary labeled antibodies for 30 min at 4°C. Cells were washed three times, fixed and permeabilized for 20 min at 4°C using Cytofix/Cytoperm buffer (BD). Then, samples were washed in PERM-WASH buffer (BD), free binding sites of secondary antibodies were blocked with whole mouse IgG molecules, and samples were incubated with PE- and APC- conjugated antibodies for the detection of intracellular IFN-γ or TNF-α, respectively, for 30 min at 4°C. Finally, cells were washed three times in PERM-WASH buffer and fixed using a solution containing 2% paraformaldehyde (Electron Microscopy Science). After a further wash, cells were analyzed in a flow cytometer FACS CantoA (BD Biosciences). Data was processed using FACSDiva software (BD Biosciences) or FlowJo software (www.flowjo.com/, Tree Star, Ashland, OR, USA), and transferred to Microsoft Excel for further calculations and preparation of graphs with GraphPad Prism Software 5.0.
For isolation of CD4+ T cells, PBMC were incubated with mAb 74-12-4 for 30 min and then washed 2 times with PBS containing 2% FBS and 2.5 mM EDTA and 0.1% sodium azide (MACS buffer). Thereafter, cells were incubated with anti-mouse IgG magnetic microbeads (Miltenyi Biotec, Germany) for 15 min. After a further wash in MACS buffer, the cell suspension was passed through the autoMACS cell sorter and the labeled (CD4+) and non-labeled (CD4−) fractions were collected. The purity of each fraction was determined by flow cytometry. Cell staining with anti-CD172a antibody revealed the presence of around 4% of APCs in the CD4+ T-cell fraction (Supplemental Figure 1). Finally, cells were processed for IFN-γ detection by ELISPOT as described before.
We first addressed the effect on peptide immunogenicity of including both 3A and 3D T-cell epitopes and their orientation in the same molecule. To this end, T-3A and T-3D T-cell epitopes were combined in a B2T-type platform in the two possible orientations, to render peptides B2TT-3A3D and B2TT-3D3A (Table 1). Groups of four pigs were immunized and boosted at day 21 pi, with B2T-3A, B2T-3D, B2TT-3A3D, or B2TT-3D3A. Although previous studies with linear peptides had shown that the inclusion of a T-cell epitope was required for inducing optimal immune responses in pigs (31), this requirement for a T-cell epitope in the context of more complex platforms such as our dendrimeric peptides remained to be clarified. Thus, two additional pigs were immunized and boosted with a B2 peptide that harbored only two copies of the B-cell epitope without any additional porcine T-cell epitope. Two PBS-immunized animals were included as negative controls.
Since in previous work T-cell responses to B2T decreased over time, being more short-lived than antibody responses (24), the frequency of IFN-γ-secreting cells was only analyzed at early times pi (days 7, 14, and 21) and post-boost (days 28 and 35), and by the end of the experiment at day 183 (6 months pi) for those animals that remained alive.
Total IgGs in sera from peptide-immunized pigs were determined by ELISA. All the dendrimer-immunized animals elicited similar levels of specific antibodies, first detected at day 14 pi (about 3.6 log10) and slightly increased at day 21 pi (about 4 log10). Upon boosting, an increase in IgG titers was observed in the B2T-immunized groups at day 28 pi (about 5 log10) that remained stable up to day 49 pi, except in pigs immunized with B2TT-3D3A, whose titers showed a slight decrease. Interestingly, at day 121 (4 months pi) a sustained antibody response was still observed, with average titers about 4.5 log10. This long-lasting antibody response was consistently observed up to the end of the experiment at day 183 (6 months pi) (about 4 log10). No antibodies were detected in B2 and PBS-immunized groups (Figure 1).
Figure 1 Time course of specific IgG antibodies elicited by B2T constructions combining different T-cell epitopes. Each point represents the mean of sera from the indicated group at different days pi (n = 4; except for B2 and PBS groups in which n = 2) ± SD. Data on day 121 pi corresponds to two pigs per group. Arrow points the day of peptide boost.
Neutralizing activity against FMDV was tested in sera from pigs at different times pi (Figure 2); nAbs were not detected until day 14 pi (Figure 2B). At this time, slightly higher average VNTs were observed for constructions encompassing two T-cell epitopes in tandem (B2TT-3A3D: 1.6 ± 0.5; B2TT-3D3A: 1.5 ± 0.5 log10) in comparison with those with a single T-cell motif (B2T-3A: 1.3 ± 0.6; B2T-3D: 1 ± 0.4) (Figure 2B). At day 21 pi, an average increment was observed in all dendrimer-immunized groups, reaching similar average titers (B2T-3A: 1.9 ± 0.3; B2T-3D: 1.9 ± 0.4; B2TT-3A3D: 1.6 ± 0.7; B2TT-3D3A: 1.7 ± 0.5 log10) (Figure 2C). After the boost, at day 28 pi, an increment in VNTs was noticed in pigs immunized with B2T-3A (2.5 ± 0.6 log10), B2T-3D (2.5 ± 0.4 log10), and B2TT-3A3D (2.6 ± 0.3 log10), but not in animals from the B2TT-3D3A group (2 ± 0.6 log10) (Figure 2D). Titers remained similar over time with minor differences among groups in subsequent days (B2T-3A: 2.4 ± 0.5; B2T-3D: 2.5 ± 0.5; B2TT-3A3D: 2.5 ± 0.3; B2TT-3D3A: 1.9 ± 0.5 log10, at day 36 pi; B2T-3A: 2.3 ± 0.4; B2T-3D: 2.1 ± 0.6; B2TT-3A3D: 2.2 ± 0.3; B2TT-3D3A: 1.6 ± 0.3 log10, at day 49 pi) (Figures 2E, F). These differences were not statistically significant.
Figure 2 VNTs are influenced by the presence and orientation of the T-cell epitopes in the dendrimer. Sera samples were collected after one dose of each dendrimeric peptide (pre-boost) panels (A–C) and after peptide boost [panels (D–F). Each point represents the VNT of one single pig and the mean of each group is shown with a horizontal bar. Dotted line depicts the detection limit.
These results suggested that the presence of T-cell epitopes and their orientation within the dendrimer has an impact in the duration of neutralizing antibody responses against the FMDV G-H loop (B-cell epitope). Indeed, animals that were not euthanized—pigs 49 and 65 (B2T-3A), 76, 77 and 78 (B2T-3D), 91 and 92 (B2TT-3A3D), 93 and 94 (B2TT-3D3A)—showed at days 121, 157 and 183 pi (4, 5, and 6 months pi, respectively) VNTs similar to those observed at day 49 pi (data not shown). This result is consistent with previous observations on the long-lasting neutralizing responses elicited by B2T-dendrimers (19).
Remarkably, no nAbs were detected at any time point in pigs immunized with the B2 peptide, with the branched B-cell epitopes but lacking a T-cell motif. This lack of response confirms the functional role of T-3A and T-3D as T helper epitopes, i.e., inclusion of at least one of them is essential for nAbs to be elicited in pigs.
Since type O FMDVs are responsible for many current FMD outbreaks in endemic countries, a broad-spectrum response is necessary for effective vaccines against this serotype (32). Therefore, we were interested in assessing the neutralization range afforded by B2T-dendrimers. Recently, we observed that sera from pigs immunized with B2T-3A or B2T-3D were able to neutralize a panel of type O FMDVs belonging to various spatiotemporal locations (24). We decided to extend the study to animals vaccinated with B2T-dendrimers harboring two different T-cell epitopes in tandem, as well as with B2, to assess again the relevance of T-cell epitopes in the induction of neutralizing activity. As shown in Figure 3, both constructions with two T-cell epitopes induced nAbs against the panel of FMDVs, showing similar titers before boosting (Figure 3A), while only B2TT-3A3D had a VNT increase afterwards (Figure 3B). Neutralizing activity against viruses such as O1Campos and O/SKR appeared to be significantly higher, in some cases, than that against the homologous virus O UKG/11/2001; factors inherent to the assay, such as differential thermal stability among viral isolates, might contribute to explain these observations. As expected, no nAbs were detected in B2-immunized animals, confirming our previous results with homologous O UKG/11/2001 virus, nor in all animals tested against isolate CS8-c1 that belongs to a different serotype (Figure 3). Thus, B2TT-3A3D and B2TT-3D3A behave just like previously tested B2T-3A and B2T-3D, being able to induce a broad anti-FMDV immunity within a serotype.
Figure 3 Sera from pigs immunized with dendrimers can neutralize a panel of different FMDVs type O topotypes. Sera recovered from animals immunized with the different peptides after the first (day 21 pi or pre-boost) (A) and the second immunization (day 28 pi or post-boost) (B), were tested to neutralize a panel of different type O FMDVs. Individual columns represent the mean of each group (n = 4) ± SD. Values are expressed as the reciprocal log10 of the last serum dilution that neutralized 100 TCID50 of each FMDV. Statistically significant differences are indicated by one (*) or two (**) asterisks for p < 0.05 or p < 0.005, respectively.
To test whether the inclusion of two consecutive T-cell epitopes in the dendrimer and their orientation could favor expression of a specific IgG isotype and influence the class switching, the anti B-peptide IgG1 and IgG2 profile was analyzed by ELISA in serum samples from pigs immunized with the different constructs at day 21 pi. All dendrimers induced specific IgG1 and IgG2 albeit to different extents (Figure 4). Three animals from the B2T-3A group showed high titers of both IgG1 and IgG2 (about 5 log10) and only one pig did not develop high IgG2 titers (< 3 log10) (Figure 4A). In the same day, all animals immunized with B2T-3D reached similar IgG1 and IgG2 titers (about 4 log10) that were, in average, lower than those of B2T-3A group (Figure 4B). Moreover, pigs immunized with B2TT-3A3D reached isotype titers similar to those of the B2T-3D group suggesting that T-3D was modulating the isotype switching (Figure 4C). On the other hand, the construction B2TT-3D3A induced low (titers < 3 log10) IgG2 titers, in two of the four animals (Figure 4D).
Figure 4 IgG1 and IgG2 profiles elicited by B2T constructions combining different T-cell epitopes. Anti-B peptide IgG1 and IgG2 profiles in sera from pigs collected at 21 days pi with: B2T-3A (A), B2T-3D (B), B2TT-3A3D (C), and B2TT-3D3A (D). Each point represents the value of a duplicate of an individual animal. Endpoint titers are expressed as the reciprocal of serum dilution (log10) giving the absorbance recorded in control wells (sera collected at day 0) + 2 x SD. Each symbol represents the IgG1 and IgG2 titers (X and Y values respectively) for an individual pig. In the lower right quadrant, the percentage of pigs with titers above 3 log10 is shown. No individual spontaneous reactivity was observed in the titers determined at day 0.
The T-cell responses elicited by dendrimers B2TT-3A3D, B2TT-3D3A, B2T-3A, B2T-3D, and B2 were analyzed using PBMCs collected at different days pi. Cells were in vitro stimulated with the homologous peptide as well as with the heterologous one (B2TT-3A3D/B2TT-3D3A or B2T-3A/B2T-3D) and with the B- and the 3A and 3D T-cell peptides, in an attempt to further understand the antigenic specificity of the elicited responses (Figure 5).
Figure 5 T-cell responses elicited by B2TT-3A3D and B2TT-3D3A unveil 3D peptide as an immunodominant T-cell epitope. PBMCs were isolated from individual animals of each group, at different days pi. Cells were in vitro stimulated for 48 h with dendrimers and the frequency of IFN-γ producing cells was determined by ELISPOT. (A) Frequency of IFN-γ secreting cells in the groups of pigs immunized as indicated, in response to the homologous dendrimer and the different constructions indicated. Each line corresponds to an individual animal and arrows show the day of the boost. (B) IFN-γ production was stimuli-specific and sequence-dependent. The values corresponded to PBMC recovered at day 183 and are presented as IFN-γ spots per 106 cells). Each column represents the mean (n = 4) ± SD.
When T-3D and T-3A were joined together in dendrimers B2TT-3A3D and B2TT-3D3A, a consistent T-cell response was observed in pigs immunized with these constructions. Similar high levels of IFN-γ producing cells were found upon in vitro stimulation with the homologous or the heterologous dendrimeric peptides that only differed in the T-cell epitope orientation. Interestingly, the response was higher when cells were in vitro stimulated with peptide T-3D than with peptide T-3A, suggesting an immunodominance of T-3D T-cell epitope over T-3A. Low levels of IFN-γ secreting cells were noticed when the B-cell peptide was used as stimulus, unlike what observed in the responses induced in pigs from the B2T-3A and B2T-3D groups (Figure 5A).
Significant frequencies of IFN-γ expressing cells were detected in the B2T-3A group upon in vitro recall with the homologous dendrimer. In general, responses were high in all the animals. The number of IFN-γ secreting cells detected was stimulus-dependent, the highest values being induced by the homologous dendrimer B2T-3A. Responses to T-3A were also significant, confirming that this peptide was recognized as a T-cell epitope. The specificity of the T-cell response was confirmed as no IFN-γ spots were detected upon stimulation with the heterologous T-cell epitope T-3D. However, a heterologous response against B2T-3D peptide was also noticed, similar to that elicited against B-cell epitope. These results point to a contribution of the B-cell epitope present in the B2T dendrimers to the effector cellular responses (Figure 5A), although we cannot rule out that the overall configuration of the dendrimer platform itself may also play a role in T-cell activation.
Peptide B2T-3D was also able to induce similar frequencies of IFN-γ expressing cells in response to peptide T-3D in all the animals. This result confirms that T-3D can be considered a T-cell epitope able to induce an IFN-γ effector immune response. As observed in animals immunized with B2T-3A, the B-cell peptide was also able to induce IFN-γ secretion, suggesting, again, that this peptide may be recognized as a T-cell epitope (Figure 5A).
Low levels of IFN-γ expressing cells were also detected in animals immunized with peptide B2. This poor recognition of B2 as a T-cell epitope highlights the decisive contribution of 3A and 3D epitopes to the cellular immune response elicited by the dendrimer peptides. As expected, non-immunized pigs from the control group did not show specific T-cell responses against any of the peptides (Figure 5A).
In order to rule out whether the induction of T-cell responses could be influenced by non-specific mechanisms, a dendrimer with a scrambled amino acid sequence (src) in both the B- and the T-3A epitopes (B2scr-Tscr) was used to stimulate PBMCs from day 183. No IFN-γ secreting cells were detected neither in response to the whole scrambled dendrimeric peptide (B2scr- Tscr) nor to the scrambled T-cell peptide alone (Tscr) (Figure 5B), showing that a branched architecture is not enough to induce an effector T-cell response, and that specific T-cell epitope sequences are required.
Flow cytometry assays, including both surface marker staining and intracellular cytokine staining (ICS), were performed in dendrimer-stimulated PBMCs from immunized pigs at day 21 pi, in order to evaluate the specific T-cell populations induced upon immunization with B2T peptides. To make the assay feasible, the two best responders from each group, in terms of levels of IFN-γ producing cells determined by ELISPOT assays, were selected: pigs 49 and 65 (B2T-3A), pigs 76 and 77 (B2T-3D), pigs 91 and 92 (B2TT-3A3D), pigs 93 and 94 (B2TT-3D3A), pig 98 (B2) and pig 100 (PBS).
PMBCs were stained for CD4 or CD8β expression, followed by intracellular cytokine staining for IFN-γ in CD4+ (helper) or CD8β+ (cytotoxic) T-cells. The percentages of CD4+ and CD8β+ populations differed among pigs (17.4 ± 3.7% and 10.1 ± 3.2%, respectively) (Figure 6A). Upon specific stimulation, IFN-γ was preferentially expressed by CD4+ T-cells in all immunized animals (n = 2). The percentage of CD4+IFN-γ+ cells was variable, being higher in B2T-3D group (5.2 ± 0.3%), followed by B2TT-3A3D (3.6 ± 1.9%), B2TT-3D3A (3 ± 1.1%), and B2T-3A (2.8 ± 2%) groups. Notably, the B2-immunized animal (pig 98) hardly induced CD4+IFN-γ+ T-cells (0.4%), further supporting the need for a T-cell epitope to induce a cellular response, as observed by ELISPOT (Figure 5). IFN-γ production was less evident in CD8β+ T-cells, where the highest response was found in the B2TT-3D3A group (1.7 ± 0.1%), followed by B2T-3D (1 ± 0.1%), B2TT-3A3D (0.9 ± 0.1), B2 [pig 98 (0.8%)] and B2T-3A (0.7 ± 0.4%) groups. These results point to CD4+ T-cells as the main population involved in dendrimer-induced T-cell immunity (Figure 6B).
Figure 6 CD4+ and CD8β+ specific T-cell responses elicited by different dendrimeric peptides in PBMCs from immunized animals. Intracellular cytokine staining was performed in overnight dendrimer stimulated PBMCs from immunized animals at day 21 pi. (A) PBMCs were stained and analyzed for surface CD4 or CD8β expression (representative plot from pig #49 is shown). (B) CD4+ or CD8β+ populations from each pig were gated and further analyzed for IFN-γ expression. IFN-γ-producing cells are shown as percent of total CD4+ or CD8β+ T cells.
To confirm the phenotype of the T-cell subset responsible for IFN-γ expression, CD4+ T-cells were depleted of PBMCs isolated from a B2T-3D immunized pig (pig #77), which was selected for being a high IFN-γ producer when tested in ELISPOT and ICS assays (Figures 5 and 6). The magnetically isolated positive (CD4+) and negative (CD4−) fractions were in vitro stimulated with B2T-3D or T-3D peptides and IFN-γ expression was determined by ELISPOT. Unfractioned PBMCs and PBMCs reconstituted with the CD4+ and CD4- fractions (CD4+/CD4−) were included as controls. The efficiency of the isolation process was verified by flow cytometry. As shown in Figure 7, 21.1% of initial PBMCs were CD4+. After the sorting, a drastic reduction in the number of CD4+ cells (0.1%) was found in the eluted negative fraction (CD4−) while the positive fraction (CD4+) showed a notable enrichment in CD4+ (95.6%) (Figure 7A). No fluorescence was detected in cells incubated with secondary antibodies alone (data not shown). Both unsorted PBMCs and magnetically sorted fractions were stimulated with B2T-3D and T-3D peptides to quantify IFN-γ expression by ELISPOT. As shown in Figure 7B only the CD4+ cells produce IFN-γ upon stimulation with either the dendrimer or the T cell epitope, while no IFN-γ spots were detected in the CD4− fraction. As expected, total PBMCs and the reconstituted fraction CD4+/CD4− showed similar levels of IFN-γ secreting cells. These results confirm that CD4+ T-cells are the main population responsible for specific IFN-γ release.
Figure 7 Depletion of CD4+ T-cells abolishes IFN-γ production of peptide-stimulated PBMCs. Cells from a B2T-3D immunized pig (pig #77) were magnetically sorted using an anti-CD4 mAb. (A) FACS analysis was performed to determine the CD4 staining quality of initial pre-sorted PBMCs and each purified fraction after MACS fractioning. (B) Pre-sorted PBMCs and fractioned positive fraction (CD4+), negative fraction (CD4−) and reconstituted fraction (CD4+/CD4−) were plated in triplicates and stimulated either with B2T-3D or T-3D peptides for 48 h and IFN-γ production was determined by ELISPOT. The values are presented as IFN-γ spots per 106 cells (spots from mock-stimulated cells are subtracted). Each column represents the mean of a triplicate ± SD. Statistically significant differences between the positive fraction (CD4+) and the rest of the groups are indicated by three asterisks (***) for p < 0.001.
2E3 expression has been used in swine immunological studies to differentiate naïve and memory CD4+ T-cell subpopulations. This marker is expressed on the surface of naïve CD4+ cells in swine PBMCs but not in memory CD4+ cells (29, 33). Dendrimer-stimulated PBMCs from immunized pigs were stained for the expression of CD4 in combination with 2E3, and ICS for IFN-γ was performed in both gated CD4+ T cell subpopulations (i.e., CD4+2E3+ and CD4+2E3−). The percentage of CD4+2E3+ and CD4+2E3− cells in the pigs varied, being higher for the latter subset (3.3 ± 1.3% and 8.9 ± 3.2%, respectively) (Figure 8A). CD4+2E3+ subset showed a low percentage of IFN-γ-expressing cells, with higher levels in the B2T-3A group (1.5 ± 0.6%), followed by B2TT-3A3D (0.9 ± 0.6%), B2T-3D (0.4 ± 0.3%) and B2TT-3D3A and B2 (0.2 ± 0.2%) groups. A clear induction of IFN-γ was observed in the CD4+2E3− subset from all immunized groups, which was higher in B2T-3D (4.6 ± 0.8%), B2TT-3A3D (4.2 ± 1.4%), and B2T-3A (3.6 ± 0.5%) groups than in B2TT-3D3A (1.2 ± 0%) and B2 (1.3%) groups (Figure 8B). No fluorescence signal was detected in non-stimulated cells (data not shown).
Figure 8 CD4+2E3- T-cells are the major subset producing IFN-γ. Intracellular cytokine staining was performed in overnight dendrimer stimulated-PBMCs isolated from immunized pigs at day 14 pi. (A) PBMCs were stained and analyzed for surface CD4 and 2E3 expression (representative plot from pig #49 is shown). (B) CD4+ 2E3+ and CD4+2E3− populations were gated and further analyzed for IFN-γ expression. IFN-γ-producing cells are shown as percent of total CD4+2E3+ or CD4+2E3− T cells.
These results suggest that memory CD4+ T-cells are the major subset involved in IFN-γ production and in the T-cell response elicited by B2T dendrimers.
Studies on multifunctional T-cells, i.e., T-cells performing several functions like cytokine production or degranulation, have revealed their central role in protective immune responses (34). IFN-γ is the main effector cytokine expressed in Th1 T-cells, but other pro-inflammatory cytokines are also involved in Th1 effector responses and are important in T-cell immunity. Therefore, in order to evaluate whether CD4+ T-cells activated by the B2T dendrimers were producing effector cytokines other than IFN-γ, ICS was performed for IFN-γ and TNF-α expression (Figure 9A; a representative plot from pig #91 is shown). When the expression of IFN-γ and TNF-α was analyzed in the gated CD4+ T-cell population, a strong fluorescence signal was detected in PBMCs stimulated with homologous peptides (Figure 9B).
Figure 9 Multifunctionality of CD4 T cells from immunized pigs upon stimulation with homologous dendrimer. Intracellular cytokine staining of PBMCs was performed following overnight in vitro stimulation with homologous dendrimers. (A) CD4 staining and expression was analyzed (representative plot of animal #49 is shown). (B) CD4+ T cells were gated and analyzed for production of a single cytokine (IFN-γ−TNF-α+ and IFN-γ+TNF-α− subsets, in upper left and bottom right quadrants) or of both cytokines (IFN-γ+TNF-α+ subset in upper right quadrant). Cytokine-producing cells are shown as percent of total CD4+ T cells.
Most of the activated CD4+ T-cells expressed both cytokines, which is consistent with a multifunctional CD4+IFN-γ+TNF-α+ phenotype. This was particularly evident in pigs immunized with B2T-3A (2.7 ± 1.9%), B2T-3D (2 ± 0.6%), and B2TT-3A3D (1.5 ± 0.8%). In contrast, in B2TT-3D3A (0.8 ± 0.1%) and B2 [pig 98 (0.5%)] groups showed lower levels of cells expressing both cytokines (Figure 9B). A lower percentage of CD4+ T cells producing a single cytokine CD4+IFN-γ+TNF-α− and CD4+ IFN-γ−TNF-α+ was also detected with average values of 0.4 and 0.7%, respectively.
In summary, these data indicate that a considerable proportion of dendrimer-responding CD4+ T cells is multifunctional in terms of IFN-γ and TNF-α production, and that not only the presence, but also the orientation of T-cell epitopes influences the T-cell response evoked by these peptides.
The protective responses elicited by B2T-3A and other related dendrimeric constructs are associated with the induction of high titers of nAb and lymphocytes activation providing a T-cell helper response (16, 18). Such T-helper epitopes can also stimulate T-cell subsets expressing IFN-γ, a cytokine relevant to antiviral response (22). Thus, characterization of the functional role of the T-cell epitope(s) recognized by swine lymphocytes in our B2T-type molecules is relevant for optimizing our vaccine constructions. MHC restriction can pose a bottleneck in subunit vaccines development, as T-cell epitope recognition may vary within species with MHC repertoires (35). For this reason, expanding T-cell epitope diversity in dendrimeric vaccines may improve recognition and presentation to genetically different individuals.
Replacement of T-3A or its combination with other T-cell peptides was a reasonable approach to explore alternative recognition of B2T-type constructions by T- cells. We previously demonstrated that a B2T dendrimer with a T-3D epitope elicits high levels of neutralizing antibodies and a potent IFN-γ response (24). In this work, we have confirmed that B2T-3D and B2T-3A induce similar nAb titers in pigs, and shown that when juxtaposed in the same molecule, T-3A/T-3D orientation influences the response, as B2TT-3A3D, unlike B2TT-3D3A, elicited optimal nAb titers, especially after boost. These results evidence differences in the immunomodulation of the antibody response. When compared with the response elicited by a commercial FMD vaccine, the magnitude of the neutralization titers elicited by the dendrimer constructs studied is about 1 log10 lower than that evoked by type O (O Campos) commercial vaccine (27), and similar to those elicited by B2TA that result in protection against virus challenge (19). Experiments are being arranged to confirm such correlation in the new constructions analyzed. In addition, the failure of a B2 construct lacking the T-cell motif to induce antibodies is consistent with the need of specific T-cell help (36). Nevertheless, the influence of other factors such as possible non-functional B-cell epitope conformations in B2 resulting in impaired presentation to B- and/or T-cells, cannot be excluded. Interestingly, B2T-3A also induced IFN-γ expressing T-cells that were recalled with similar time courses by T-3A and, to a lesser extent, by B peptide suggesting that both sequences were recognized as T-cell epitopes. Conversely, the IFN-γ expressing cells elicited by B2T-3D preferentially recognized the T-3D peptide, supporting its dominance. When the two epitopes were combined in a single molecule, B2TT-3A3D and B2TT-3D3A induced a clear activation of specific T-cells. In fact, when peptides with each of the T-cell epitopes (T-3A and T-3D) were used as stimuli, a marked hierarchy of T-cell responses were observed, peptide T-3D being the main inducer of IFN-γ. The mechanisms underlying the immunodominance of T-3D peptide over the other epitopes (T-3A and B) remain to be clarified.
Regarding the isotype profile of the antibody response, B2TT-3A3D induced high IgG1 and IgG2 titers similar to those of the animals immunized with B2T-3D and B2T-3A, suggesting the ability of T-cell peptides T-3A and T-3D to induce the IgG class-switching, as observed in IFN-γ inductions. Thus, when individually included in a B2T-dendrimer, T-3A and T-3D can provide similar T-cell help for the production of antibodies, including nAbs. However, B2TT-3D3A induced, in two out of four tested animals, lower titers of both IgG isotypes than B2TT-3A3D, indicating that the T-cell epitopes orientation in the dendrimer is important for the IgG class-switching. This difference in immunomodulation is also observed in the lack of boost effect in the nAbs levels induced by B2TT-3D3A.
In humans and mice, IFN-γ is mainly produced by T lymphocytes and NK cells (37, 38). The subject is less clear in the case of pigs, reflecting the complexity of the cell subsets involved and the limited availability of specific reagents for the characterization of swine antigens (39, 40). Thus, a wide variety of IFN-γ producing cells are known to be induced by specific stimuli or cytokines, including Th-cells, Tc-cells, NK cells and γδ T-cells, the latter being highly abundant in swine (39, 41, 42). Flow cytometry analyses of PBMCs from peptide-immunized pigs showed a percentage of CD4+ and CD8β+ positive populations that agreed with those described for outbred 3–5 month-old pig populations (43). Indeed, a strong IFN-γ production was noticed in the CD4+ subset derived from vaccinated animals upon homologous stimulation, ranging from 3–5%, depending on the peptide. Although a limited number of animals was used for the analysis (n = 2), B2T-3D induced the highest IFN-γ secretion (Figure 6). These results correlated with those of the IFN-γ ELISPOT and point to the epitope T-3D as a potent IFN-γ inductor as well as mainly recognized by CD4+ cells. In contrast, a limited activation of the cytolytic CD8β+ subset was noticed in these experiments (ranging from <1–1.5% depending on the peptide), suggesting a minor contribution of CD8β+ cells in the response to the dendrimers. These results are consistent with the kind of T-cell epitopes included in the dendrimers, as they were previously identified as swine T helper epitopes (15, 23). The possibility of eliciting heterotypic CD8 responses against highly conserved FMDV epitopes can interestingly contribute to potentiate the protective responses against this highly antigenic variable virus. Induction of CD8 responses has been described upon vaccination of pigs (44, 45) and cattle (46, 47). Therefore, further understanding of the role that CD8 responses can play in the protection conferred by the B2T peptides as well as the development of strategies aimed at increasing activation of CD8 T-cells, are among the goals for improving dendrimeric peptide vaccines.
The importance of CD4+ T-cells upon FMDV vaccination is supported by the observation that depletion of this subset in vivo ablated antigen-dependent lymphoproliferative responses (48). On the other hand, a CD4+ T-cell-independent nAb response has been reported using in vivo depletion of this cell subset in a different species (36). The major role of the CD4+ cell subset on the response to dendrimer B2T-3D was confirmed when this population was depleted from PBMCs. The sorted CD4- subset failed to produce IFN-γ whereas the CD4+ enriched fraction substantially produced IFN-γ upon dendrimer and T-peptide stimulation (Figure 7).
A fraction of primed T-cells can persist in the absence of specific antigen (memory T-cells) being necessary for a rapid response upon antigen recall (38, 49, 50). Considering that the PBMCs used for the enrichment studies were collected at 3.5 months pi, the presence in them of a substantial proportion of memory T-cells was expected. Indeed, the mild expression of IFN-γ in the CD4+2E3+ (naïve T cells) in contrast with the strong cytokine detection in the effector/memory CD4+2E3- subset, suggests that effector/memory T-cells were responsible of responses in the two animals analyzed from each peptide group (Figure 8). This effect was more prominent for B2T-3D immunized animals and correlated with ELISPOT results (Figure 5), further supporting the capacity of T-3D in eliciting potent Th1 responses.
Not only IFN-γ but other pro-inflammatory cytokines such as TNF-α can drive T-cells towards a Th1 pathway, the main effector cells for viral clearance. Indeed, T cells producing several cytokines, also called multifunctional T cells, are considered to be functionally superior to their single-producing counterparts, and have been associated/correlated with protection in several virus infections (51, 52). Our results showed that in dendrimer-immunized animals the majority of IFN-γ producing CD4+ T cells also produced TNF-α, although there were also minor subsets of CD4+ IFN-γ+TNF-α- and IFN-γ-TNF-α+ single producers (Figure 9). Interestingly, IFN-γ+ and/or TNF-α+ producing CD4+T-cells were less frequently observed in the B2TT-3D3A immunized pigs, as in the B2-immunized group/animals, indicating again that the T-cell epitope orientation in dendrimer B2TT-3D3A is less efficient in T-cell activation. To our knowledge, this is the first study that addresses in detail the T-cell subsets stimulated upon FMDV immunization with either the whole virus or its subunits. Furthermore, B2 peptide was unable to induce IFN-γ in CD4+ subsets, strengthening the importance of the incorporation of a specific T-cell epitope for the dendrimers to elicit IFN-γ secreting T cells.
Altogether, our results point to Th1 responses as the main effector mechanism of the T-cell activation elicited by the dendrimeric constructs. The overall results presented support the use of the dendrimer platforms, such as those studied here, as potential FMDV heterotypic next-generation vaccines.
The original contributions presented in the study are included in the article/Supplementary Material. Further inquiries can be directed to the corresponding authors.
The animal study was reviewed and approved by CSIC Committees on Ethical and Animal Welfare (CBS2014/015 and CEEA2014/018) by the INIA Committees on Ethics of Animal Experiments and Biosafety, and by the National Committee on Ethics and Animal Welfare (PROEX 218/14).
FS, EB, DA, PL, RC-A, and SD conceived and designed the experiments. PL, RC-A, ET, SD, MF, MB, and CR performed the experiments. PL, RC-A, JD, EB, DA, and FS analyzed the data and wrote the manuscript. All authors contributed to the article and approved the submitted version.
The research was supported by the Spanish Ministry of Science, Innovation and Universities grants AGL2014-48923-C2 and AGL2017-89097-C2-2-R (to FS and DA) and AGL2016-349 76445-R to EB), as well as by Comunidad de Madrid co-financed with ECFEDER funds (S2013/ABI-350 2906-PLATESA and P2018/BAA-4370 to FS and EB) and by Generalitat de Catalunya (2009SGR492 to DA). Work at Centro de Biología Molecular “Severo Ochoa” and at UPF was supported by Fundación Ramón Areces and by the Maria de Maeztu Program of the Spanish Ministry of Science, Innovation and Universities, respectively. RC-A and MF were holders of a PhD fellowship from the Spanish Ministry of Science, Innovation and University (FPI programme).
The authors declare that the research was conducted in the absence of any commercial or financial relationships that could be construed as a potential conflict of interest.
We thank the staff of the CBMSO and the INIA animal facilities for their assistance.
The Supplementary Material for this article can be found online at: https://www.frontiersin.org/articles/10.3389/fimmu.2020.621537/full#supplementary-material
1. Nfon C, Lung O, Embury-Hyatt C, Alexandersen S. Clinical Signs and Pathology of Foot-and-Mouth Disease. In: Sobrino F, Domingo E, editors. Foot-and-mouth Disease Virus Current Research and Emerging Trends. Norfolk, UK: Caister Academic Press (2017). p. 171–8.
2. Knight-Jones TJ, Robinson L, Charleston B, Rodriguez LL, Gay CG, Sumption KJ, et al. Global Foot-and-Mouth Disease Research Update and Gap Analysis: 1 - Overview of Global Status and Research Needs. Transbound Emerg Dis (2016) 63(Suppl 1):3–13. doi: 10.1111/tbed.12528
4. Sobrino F, Domingo E. Foot-and-mouth disease in Europe. FMD is economically the most important disease of farm animals. Its re-emergence in Europe is likely to have consequences that go beyond severe alterations of livestock production and trade. EMBO Rep. (2001) 2(6):459–61. doi: 10.1093/embo-reports/kve122
5. Parida S. Vaccination against foot-and-mouth disease virus: strategies and effectiveness. Expert Rev Vaccines (2009) 8:347–65. doi: 10.1586/14760584.8.3.347
6. Robinson L, Knight-Jones TJ, Charleston B, Rodriguez LL, Gay CG, Sumption KJ, et al. Global Foot-and-Mouth Disease Research Update and Gap Analysis: 6 - Immunology. Transbound Emerg Dis (2016) 63(Suppl 1):56–62. doi: 10.1111/tbed.12518
7. Smitsaart E, Bergmann I. Quality Attributes of Current Inactivated Foot-and-mouth Disease Vaccines and their Effects on the Success of Vaccination. In: Sobrino F, Domingo E, editors. Foot-and-mouth Disease Virus Current Research and Emerging Trends. Norfolk, UK: Caister Academic Press (2017). p. 287–316.
8. Grubman MJ, Baxt B. Foot-and-mouth disease. Clin Microbiol Rev (2004) 17:465–93. doi: 10.1128/CMR.17.2.465-493.2004
9. de Los Santos T, Diaz-San Segundo F, Rodriguez LL. The need for improved vaccines against foot-and-mouth disease. Curr Opin Virol (2018) 29:16–25. doi: 10.1016/j.coviro.2018.02.005
10. Rodriguez LL, Barrera J, Kramer E, Lubroth J, Brown F, Golde WT. A synthetic peptide containing the consensus sequence of the G-H loop region of foot-and-mouth disease virus type-O VP1 and a promiscuous T-helper epitope induces peptide-specific antibodies but fails to protect cattle against viral challenge. Vaccine (2003) 21:3751–6. doi: 10.1016/S0264-410X(03)00364-5
11. Taboga O, Tami C, Carrillo E, Nunez JI, Rodriguez A, Saiz JC, et al. A large-scale evaluation of peptide vaccines against foot-and-mouth disease: lack of solid protection in cattle and isolation of escape mutants. J Virol (1997) 71:2606–14. doi: 10.1128/JVI.71.4.2606-2614.1997
12. Tam JP. Synthetic peptide vaccine design: synthesis and properties of a high-density multiple antigenic peptide system. Proc Natl Acad Sci USA (1988) 85:5409–13. doi: 10.1073/pnas.85.15.5409
13. Acharya R, Fry E, Stuart D, Fox G, Rowlands D, Brown F. The three-dimensional structure of foot-and-mouth disease virus at 2.9 A resolution. Nature (1989) 337:709–16. doi: 10.1038/337709a0
14. Pfaff E, Mussgay M, Bohm HO, Schulz GE, Schaller H. Antibodies against a preselected peptide recognize and neutralize foot and mouth disease virus. EMBO J (1982) 1:869–74. doi: 10.1002/j.1460-2075.1982.tb01262.x
15. Blanco E, Garcia-Briones M, Sanz-Parra A, Gomes P, De Oliveira E, Valero ML, et al. Identification of T-cell epitopes in nonstructural proteins of foot-and-mouth disease virus. J Virol (2001) 75:3164–74. doi: 10.1128/JVI.75.7.3164-3174.2001
16. Cubillos C, de la Torre BG, Jakab A, Clementi G, Borras E, Barcena J, et al. Enhanced mucosal immunoglobulin A response and solid protection against foot-and-mouth disease virus challenge induced by a novel dendrimeric peptide. J Virol (2008) 82:7223–30. doi: 10.1128/JVI.00401-08
17. Monso M, de la Torre BG, Blanco E, Moreno N, Andreu D. Influence of conjugation chemistry and B epitope orientation on the immune response of branched peptide antigens. Bioconjug Chem (2013) 24:578–85. doi: 10.1021/bc300515t
18. Blanco E, Guerra B, de la Torre BG, Defaus S, Dekker A, Andreu D, et al. Full protection of swine against foot-and-mouth disease by a bivalent B-cell epitope dendrimer peptide. Antiviral Res (2016) 129:74–80. doi: 10.1016/j.antiviral.2016.03.005
19. Cañas-Arranz R, Forner M, Defaus S, de León P, Bustos M, Torres E, et al. A single Dose of Dendrimer B2T Peptide Vaccine Partially Protects Pigs against Foot-and-Mouth Disease Virus Infection. Vaccines (2020) 8:406. doi: 10.3390/vaccines8010019
20. Defaus S, Forner M, Canas-Arranz R, de Leon P, Bustos MJ, Rodriguez-Pulido M, et al. Designing Functionally Versatile, Highly Immunogenic Peptide-Based Multiepitopic Vaccines against Foot-and-Mouth Disease Virus. Vaccines (Basel) (2020) 8(3):406. doi: 10.3390/vaccines8030406
21. Canas-Arranz R, Forner M, Defaus S, Rodriguez-Pulido M, de Leon P, Torres E, et al. A bivalent B-cell epitope dendrimer peptide can confer long-lasting immunity in swine against foot-and-mouth disease. Transbound Emerg Dis (2020b) 67:1614–22. doi: 10.1111/tbed.13497
22. McCullough K, Sobrino F. Immunology of Foot-and-Mouth Disease. In: Sobrino F, Domingo E, editors. Foot-and-Mouth Disease Current Perspectives. Norfolk, UK: Horizon Bioscience (2004). p. 173–222.
23. Garcia-Briones MM, Blanco E, Chiva C, Andreu D, Ley V, Sobrino F. Immunogenicity and T cell recognition in swine of foot-and-mouth disease virus polymerase 3D. Virology (2004) 322:264–75. doi: 10.1016/j.virol.2004.01.027
24. Canas-Arranz R, de Leon P, Forner M, Defaus S, Bustos MJ, Torres E, et al. Immunogenicity of a Dendrimer B2T Peptide Harboring a T-Cell Epitope From FMDV Non-structural Protein 3D. Front Vet Sci (2020a) 7:498. doi: 10.3389/fvets.2020.00498
25. Blanco E, Cubillos C, Moreno N, Barcena J, de la Torre BG, Andreu D, et al. B epitope multiplicity and B/T epitope orientation influence immunogenicity of foot-and-mouth disease peptide vaccines. Clin Dev Immunol (2013) 2013:475960. doi: 10.1155/2013/475960
26. Sobrino F, Davila M, Ortin J, Domingo E. Multiple genetic variants arise in the course of replication of foot-and-mouth disease virus in cell culture. Virology (1983) 128:310–8. doi: 10.1016/0042-6822(83)90258-1
27. Borrego B, Blanco E, Rodriguez Pulido M, Mateos F, Lorenzo G, Cardillo S, et al. Combined administration of synthetic RNA and a conventional vaccine improves immune responses and protection against foot-and-mouth disease virus in swine. Antiviral Res (2017) 142:30–6. doi: 10.1016/j.antiviral.2017.03.009
28. Pescovitz MD, Lunney JK, Sachs DH. Preparation and characterization of monoclonal antibodies reactive with porcine PBL. J Immunol (1984) 133:368–75.
29. Revilla C, Rodriguez-Carreno MP, Alvarez B, Chamorro S, Alonso LM, Ezquerra A, et al. 2E3, a new marker that selectively identifies porcine CD4+ naive T cells. Dev Comp Immunol (2004) 28:239–50. doi: 10.1016/S0145-305X(03)00137-X
30. Alvarez B, Sanchez C, Bullido R, Marina A, Lunney J, Alonso F, et al. A porcine cell surface receptor identified by monoclonal antibodies to SWC3 is a member of the signal regulatory protein family and associates with protein-tyrosine phosphatase SHP-1. Tissue Antigens (2000) 55:342–51. doi: 10.1034/j.1399-0039.2000.550408.x
31. Cubillos C, de la Torre BG, Barcena J, Andreu D, Sobrino F, Blanco E. Inclusion of a specific T cell epitope increases the protection conferred against foot-and-mouth disease virus in pigs by a linear peptide containing an immunodominant B cell site. Virol J (2012) 9:66. doi: 10.1186/1743-422X-9-66
32. Mahapatra M, Parida S. Foot and mouth disease vaccine strain selection: current approaches and future perspectives. Expert Rev Vaccines (2018) 17:577–91. doi: 10.1080/14760584.2018.1492378
33. Moreno S, Alvarez B, Martinez P, Uenishi H, Revilla C, Ezquerra A, et al. Analysis of chemokine receptor CCR7 expression on porcine blood T lymphocytes using a CCL19-Fc fusion protein. Dev Comp Immunol (2013) 39:207–13. doi: 10.1016/j.dci.2012.11.010
34. Sallusto F, Lanzavecchia A. Heterogeneity of CD4+ memory T cells: functional modules for tailored immunity. Eur J Immunol (2009) 39:2076–82. doi: 10.1002/eji.200939722
35. Hammer SE, Ho CS, Ando A, Rogel-Gaillard C, Charles M, Tector M, et al. Importance of the Major Histocompatibility Complex (Swine Leukocyte Antigen) in Swine Health and Biomedical Research. Annu Rev Anim Biosci (2020) 8:171–98. doi: 10.1146/annurev-animal-020518-115014
36. Juleff N, Windsor M, Lefevre EA, Gubbins S, Hamblin P, Reid E, et al. Foot-and-mouth disease virus can induce a specific and rapid CD4+ T-cell-independent neutralizing and isotype class-switched antibody response in naive cattle. J Virol (2009) 83:3626–36. doi: 10.1128/JVI.02613-08
37. Bonilla FA, Oettgen HC. Adaptive immunity. J Allergy Clin Immunol (2010) 125:S33–40. doi: 10.1016/j.jaci.2009.09.017
38. Sallusto F, Lanzavecchia A, Araki K, Ahmed R. From vaccines to memory and back. Immunity (2010) 33:451–63. doi: 10.1016/j.immuni.2010.10.008
39. Gerner W, Hammer SE, Wiesmuller KH, Saalmuller A. Identification of major histocompatibility complex restriction and anchor residues of foot-and-mouth disease virus-derived bovine T-cell epitopes. J Virol (2009) 83:4039–50. doi: 10.1128/JVI.01534-08
40. Talker SC, Kaser T, Reutner K, Sedlak C, Mair KH, Koinig H, et al. Phenotypic maturation of porcine NK- and T-cell subsets. Dev Comp Immunol (2013) 40:51–68. doi: 10.1016/j.dci.2013.01.003
41. Pintaric M, Gerner W, Saalmuller A. Synergistic effects of IL-2, IL-12 and IL-18 on cytolytic activity, perforin expression and IFN-gamma production of porcine natural killer cells. Vet Immunol Immunopathol (2008) 121:68–82. doi: 10.1016/j.vetimm.2007.08.009
42. Rodriguez-Carreno MP, Lopez-Fuertes L, Revilla C, Ezquerra A, Alonso F, Dominguez J. Phenotypic characterization of porcine IFN-gamma-producing lymphocytes by flow cytometry. J Immunol Methods (2002) 259:171–9. doi: 10.1016/S0022-1759(01)00511-7
43. Zuckermann FA, Husmann RJ. Functional and phenotypic analysis of porcine peripheral blood CD4/CD8 double-positive T cells. Immunology (1996) 87:500–12.
44. Fernandez-Sainz I, Gavitt TD, Koster M, Ramirez-Medina E, Rodriguez YY, Wu P, et al. The VP1 G-H loop hypervariable epitope contributes to protective immunity against Foot and Mouth Disease Virus in swine. Vaccine (2019) 37:3435–42. doi: 10.1016/j.vaccine.2019.05.019
45. Patch JR, Pedersen LE, Toka FN, Moraes M, Grubman MJ, Nielsen M, et al. Induction of foot-and-mouth disease virus-specific cytotoxic T cell killing by vaccination. Clin Vaccine Immunol (2011) 18:280–8. doi: 10.1128/CVI.00417-10
46. Guzman E, Taylor G, Charleston B, Ellis SA. Induction of a cross-reactive CD8(+) T cell response following foot-and-mouth disease virus vaccination. J Virol (2010) 84:12375–84. doi: 10.1128/JVI.01545-10
47. Guzman E, Taylor G, Charleston B, Skinner MA, Ellis SA. An MHC-restricted CD8+ T-cell response is induced in cattle by foot-and-mouth disease virus (FMDV) infection and also following vaccination with inactivated FMDV. J Gen Virol (2008) 89:667–75. doi: 10.1099/vir.0.83417-0
48. Naessens J, Scheerlinck JP, De Buysscher EV, Kennedy D, Sileghem M. Effective in vivo depletion of T cell subpopulations and loss of memory cells in cattle using mouse monoclonal antibodies. Vet Immunol Immunopathol (1998) 64:219–34. doi: 10.1016/S0165-2427(98)00138-X
49. Gray D. A role for antigen in the maintenance of immunological memory. Nat Rev Immunol (2002) 2:60–5. doi: 10.1038/nri706
50. Zinkernagel RM. On natural and artificial vaccinations. Annu Rev Immunol (2003) 21:515–46. doi: 10.1146/annurev.immunol.21.120601.141045
51. Kannanganat S, Ibegbu C, Chennareddi L, Robinson HL, Amara RR. Multiple-cytokine-producing antiviral CD4 T cells are functionally superior to single-cytokine-producing cells. J Virol (2007) 81:8468–76. doi: 10.1128/JVI.00228-07
Keywords: foot-and-mouth disease virus, vaccines, dendrimer peptide, T cell phenotype, cytokine
Citation: de León P, Cañas-Arranz R, Defaus S, Torres E, Forner M, Bustos MJ, Revilla C, Dominguez J, Andreu D, Blanco E and Sobrino F (2021) Swine T-Cells and Specific Antibodies Evoked by Peptide Dendrimers Displaying Different FMDV T-Cell Epitopes. Front. Immunol. 11:621537. doi: 10.3389/fimmu.2020.621537
Received: 26 October 2020; Accepted: 18 December 2020;
Published: 03 February 2021.
Edited by:
Pedro A. Reche, Complutense University of Madrid, SpainReviewed by:
Rupsa Basu, TechnoVax Inc, United StatesCopyright © 2021 de León, Cañas-Arranz, Defaus, Torres, Forner, Bustos, Revilla, Dominguez, Andreu, Blanco and Sobrino. This is an open-access article distributed under the terms of the Creative Commons Attribution License (CC BY). The use, distribution or reproduction in other forums is permitted, provided the original author(s) and the copyright owner(s) are credited and that the original publication in this journal is cited, in accordance with accepted academic practice. No use, distribution or reproduction is permitted which does not comply with these terms.
*Correspondence: Esther Blanco, YmxhbmNvQGluaWEuZXM=; Francisco Sobrino, ZnNvYnJpbm9AY2JtLmNzaWMuZXM=
†These authors have contributed equally to this work
Disclaimer: All claims expressed in this article are solely those of the authors and do not necessarily represent those of their affiliated organizations, or those of the publisher, the editors and the reviewers. Any product that may be evaluated in this article or claim that may be made by its manufacturer is not guaranteed or endorsed by the publisher.
Research integrity at Frontiers
Learn more about the work of our research integrity team to safeguard the quality of each article we publish.