- 1Univ. Lille, CNRS, INSERM, CHU Lille, Institut pour la Recherche contre le Cancer de Lille, UMR9020 – UMR-S 1277 – CANTHER - Cancer Heterogeneity, Plasticity and Resistance to Therapies, Lille, France
- 2Center for Hematology and Regenerative Medicine, Department of Medicine, Huddinge, Karolinska Institutet, Stockholm, Sweden
- 3Center for Cell Therapy and Allogeneic Stem Cell Transplantation, Karolinska University Hospital, Stockholm, Sweden
Natural killer (NK) cells are endowed with germline-encoded receptors that enable them to detect and kill malignant cells without prior priming. Over the years, overwhelming evidence has identified an essential role for NK cells in tumor immune surveillance. More recently, clinical trials have also highlighted their potential in therapeutic settings. Yet, data show that NK cells can be dysregulated within the tumor microenvironment (TME), rendering them ineffective in eradicating the cancer cells. This has been attributed to immune suppressive factors, including the tumor cells per se, stromal cells, regulatory T cells, and soluble factors such as reactive oxygen species and cytokines. However, the TME also hosts myeloid cells such as dendritic cells, macrophages, neutrophils, and myeloid-derived suppressor cells that influence NK cell function. Although the NK-myeloid cell crosstalk can promote anti-tumor responses, myeloid cells in the TME often dysregulate NK cells via direct cell-to-cell interactions down-regulating key NK cell receptors, depletion of nutrients and growth factors required for NK cell growth, and secretion of metabolites, chemokines and cytokines that ultimately alter NK cell trafficking, survival, and cytotoxicity. Here, we review the complex functions of myeloid-derived cytokines in both supporting and suppressing NK cells in the TME and how NK cell-derived cytokines can influence myeloid subsets. We discuss challenges related to these interactions in unleashing the full potential of endogenous and adoptively infused NK cells. Finally, we present strategies aiming at improving NK cell-based cancer immunotherapies via pathways that are involved in the NK-myeloid cell crosstalk in the TME.
Introduction
Natural Killer (NK) cells are cytotoxic lymphocytes that innately recognize their target cells based on signals from an array of germline-encoded inhibitory and activating cell surface receptors (1). While inhibition is mainly mediated by HLA class I-binding receptors such as KIR, LIR-1, and NKG2A, activation is triggered by the NKG2D, DNAM-1, NKp30, NKp46, and 2B4 receptors, among others (2). Experimental approaches delineating how NK cells target tumor cells have in more recent years been harmonized with studies evidencing their role in tumor immune surveillance (3) and clinical therapy to treat patients with cancer (4, 5). However, it has also become increasingly clear that NK cells are often dysfunctional in cancer patients (6, 7). This is most prominent in the tumor microenvironment (TME), although also observed in blood and other tissues in patients with advanced cancer (6–8). Factors suppressing endogenous or adoptively infused NK cells in the TME are likely limiting the full potential of NK cell-based cancer immunotherapies.
NK cells can be disarmed in the TME by both direct and indirect interactions with the tumor cells (6, 7, 9). However, several other cell types in the TME, such as stroma cells and immune cells, acting by direct interactions and via release of reactive oxygen species, growth factors, and cytokines, can also induce NK cell dysfunction (9–11). Both the degree and mode of NK cell suppression in the TME may dynamically vary from early to later stages of cancer development as well as between different tumor histotypes. Beyond reducing the anti-tumor cytotoxicity of NK cells per se, suppression of NK cells in the TME can also negatively impact their ability to recruit other immune cells (12–15), which is crucial for initiating and maintaining proper anti-tumor responses. In this regard, a pivotal interaction in the TME is the one between NK cells and myeloid cells, such as dendritic cells (DCs), macrophages, neutrophils, and myeloid-derived suppressor cells (MDSCs) (16–19). While NK cells positively promote DC infiltration and maturation via release of pro-inflammatory cytokines such as interferon (IFN)-γ (20), myeloid-derived cytokines, including interleukin (IL)-12, IL-15, and IL-18, critically promote NK cell maturation, proliferation, and anti-tumor functions (21). However, aggravation of the TME often observed in more advanced stages of cancer direct myeloid cells towards a suppressive phenotype that instead can impede NK cell functions via secretion of cytokines, such as transforming growth factor (TGF)-β, IL-1β, and IL-10 (22–24). Thus, the NK-myeloid crosstalk is intricate but critical for proper anti-tumor properties of NK cells in the TME.
Here we give an overview of the cytokines involved in the interplay between NK cells and myeloid cells in the general TME. We discuss how myeloid cells promote NK cell functions and vice versa, but foremost, how this interaction can hinder NK cell-mediated tumor rejection. We outline current methods and possible future approaches to enhance anti-tumor responses by NK cells via administration or manipulation of cytokines and cytokine signaling, as well as preventing myeloid cell infiltration into the TME. This review highlights that a better understanding of the crosstalk between myeloid cells and NK cells is likely critical to improve the efficacy of NK cell-based cancer immunotherapy.
Myeloid-Derived Cytokines Promoting Natural Killer Cell Responses to Cancer
Several myeloid cells, exemplified by macrophages and DCs, are characterized by a pro-inflammatory phenotype and release cytokines such as type-1 IFNs, IL-12, IL-15, IL-18, IL-21 (Table 1A) upon recognition of damage-associated molecular patterns (DAMPs) on the transformed cells (70, 71). This pro-inflammatory cytokine milieu, together with key chemokines, aid in the recruitment of NK cells to the tumor site while promoting their persistence and anti-tumor effector functions (70, 72). Several of these cytokines have overlapping functions but also possess specific functions in the regulation of NK cell responses in cancer (35, 73–79) (Figure 1 and Table 1A). In this section, we will present the key cytokines released by myeloid cells that promote anti-tumor cytotoxicity by NK cells but also give examples of how cytokines can have dual functions.
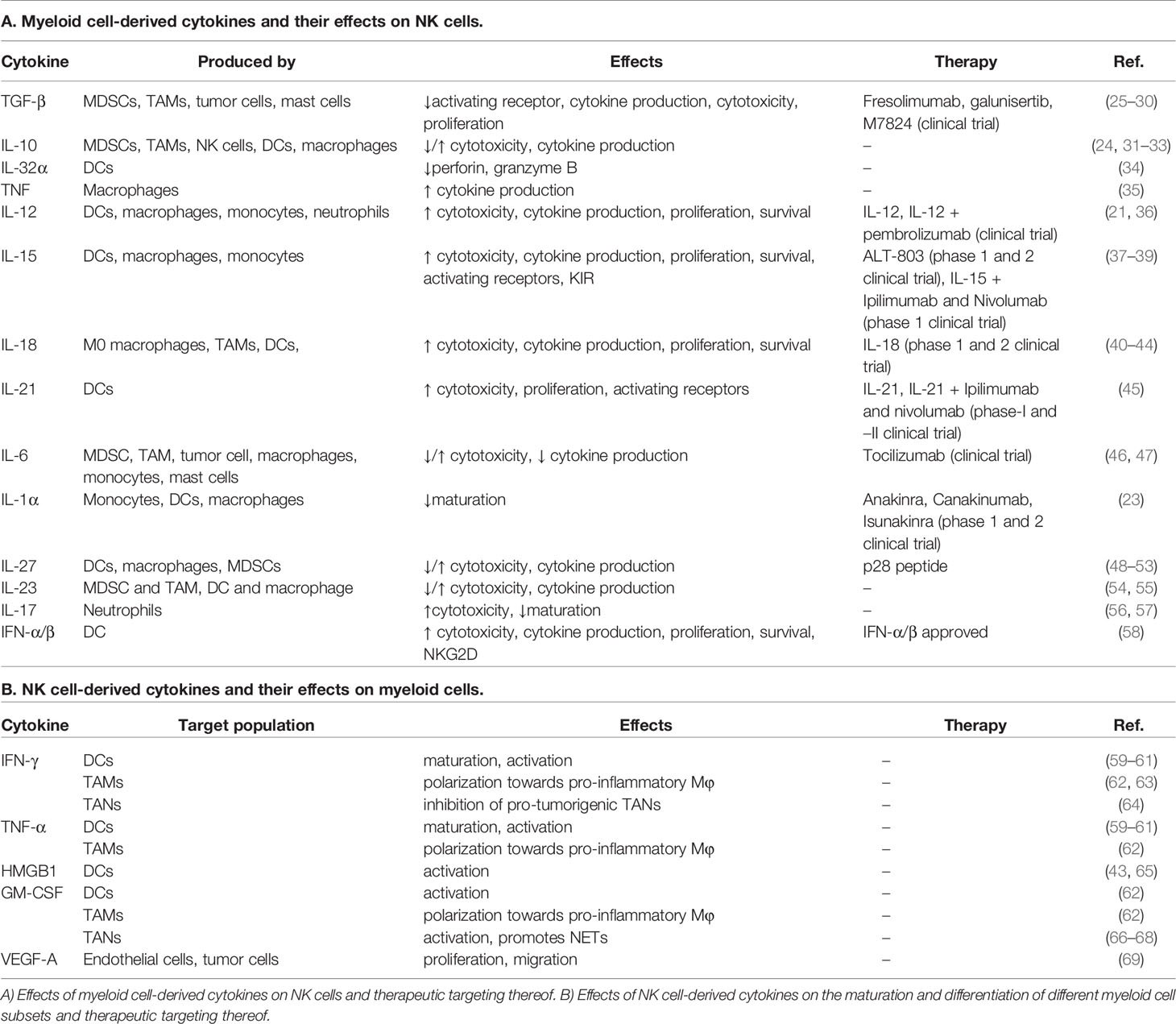
Table 1 Cytokines and chemokines involved in the NK-myeloid cell crosstalk and drugs directed to modulate these interactions.
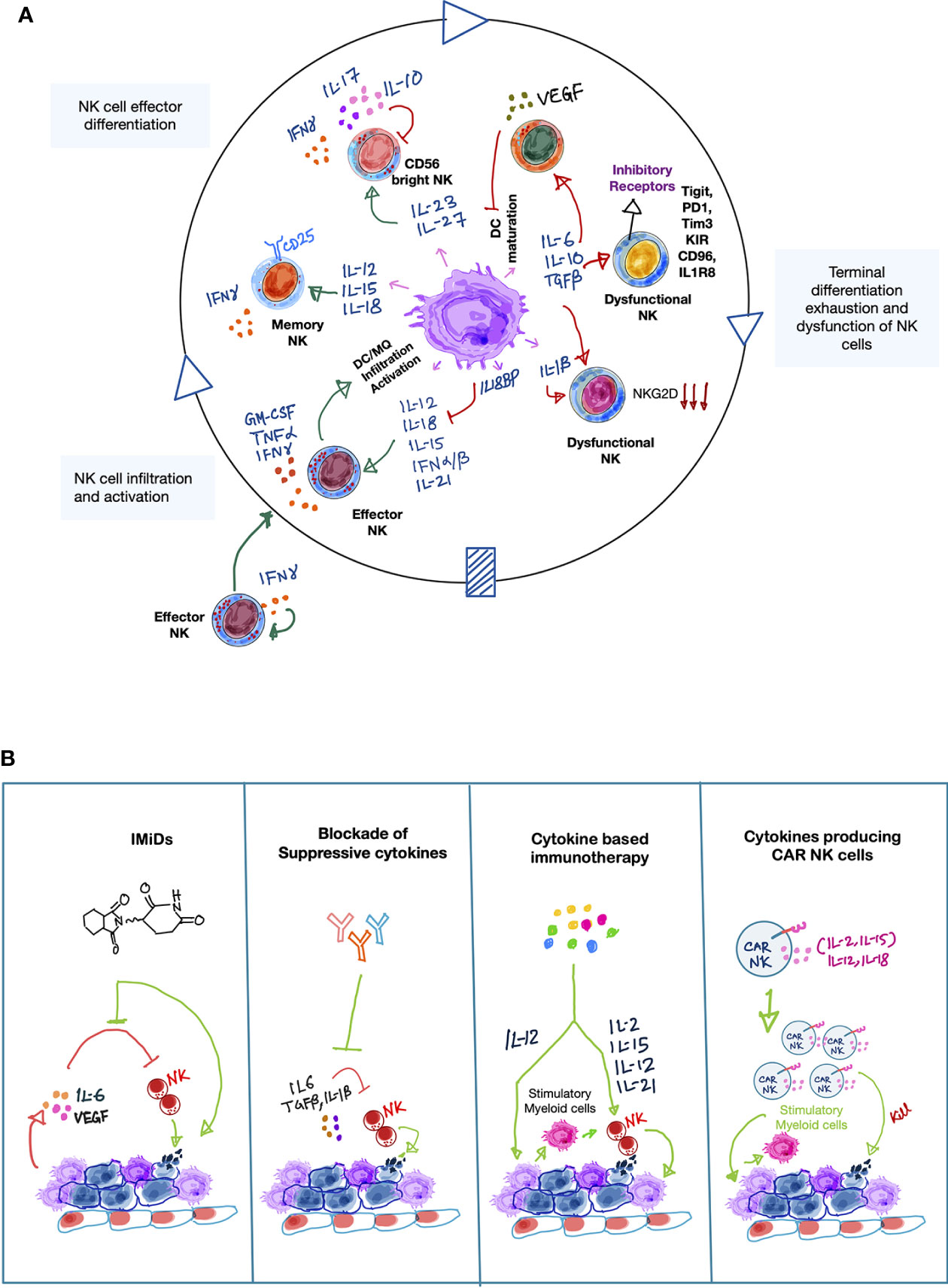
Figure 1 Cytokines involved in the NK-myeloid cell cross talk in the tumor microenvironment. (A) A simplified schematic illustration showing the orchestra of myeloid- and NK cell-derived cytokines involved in forming anti-tumor immune responses by NK cells in the tumor microenvironment (TME). Activated NK cells produce IFN-γ which indirectly promotes recruitment of other NK cells from peripheral blood to the tumor sites. Upon recognition of tumor antigens, myeloid cells, especially DCs and macrophages produce inflammatory cytokines such as type-1 IFNs, IL-12, IL-15, IL-18, IL-21. These cytokines either alone or cooperatively promote NK cell survival, proliferation, maturation and production of spectrum of pro-inflammatory cytokines, including IFN-γ, TNF-α, GM-CSF, which further boost anti-tumor immune-activating potential of myeloid cells while recruiting additional inflammatory myeloid cells [including M1 macrophages (MQ), mature dendritic cells (DCs)] to sustain the anti-tumor immune response. Furthermore, myeloid-derived IL-23 and IL-27 cytokines can also promote NK cell activity by inducing IFN-γ production, but they can also negatively influence the NK-myeloid cell anti-tumor crosstalk by secretion of tumor-promoting cytokines such as IL-17 and IL-10, respectively. This suggests a dual role of IL-23 and IL-27 in NK cell-mediated tumor immunity. In line with the dual roles of certain cytokines, myeloid cells can become immune suppressive (myeloid-derived suppressor cells; MDSCs). This is frequently occurring during cancer progression. MDSCs secrete a plethora of immune suppressive cytokines that negatively influence the anti-tumor potential of NK cells per se but also impair anti-tumor responses normally resulting from the NK-myeloid cell crosstalk. For example, suppressive cytokines promote NK cell exhaustion and directly impair NK cell-mediated cytolytic activity, while limiting the ability of myeloid cells to produce NK cell stimulatory cytokines such as IL-12, IL-15, and IL-18. Green arrows indicate positive interactions and red arrows indicate negative interactions. (B) Therapeutic approaches that directly or indirectly modulate cytokine mediators that enhance NK cell-mediated anti-cancer responses in the TME. Simplified illustrations showing validated therapeutic approaches that can either restore or reinforce a stimulatory cytokine environment to augment NK cell-mediated tumor killing activity. On one hand, immunomodulatory drugs (IMiDs) such as lenalidomide can indirectly augment NK cell anti-tumor activity by reducing the levels of pro-tumorigenic factors, such as IL-6 and VEGF, while stimulating other immune cells to secrete IL-2. Accordingly, targeted blockade of immune-suppressive cytokines, such as IL-6, TGF-β can also positively impact NK-myeloid anti-tumor cross-talk in a similar manner. On the other hand, recombinant or synthetic cytokines as well as cell-based therapies such as cytokine-secreting CAR-NK cells can directly influence NK cell-mediated cancer cell killing. Importantly, cytokine-activated NK cells can further edit myeloid cells to enhance anti-tumor response via the production of inflammatory cytokines, such as IFN-γ. Light green arrows show the mode of therapeutic action.
Direct and Indirect Contribution of Interferon-α and Interferon-β in Natural Killer Cell Activation
The type-1 IFNs, IFN-α, and IFN-β are secreted by activated myeloid cells and stimulate NK cell expansion while enhancing the effector functions upon stimulation of the IFN-α receptor (IFNAR) (80, 81). Inversely, as highlighted in experimental models using IFNAR-deficient NK cells and NK cells with defective downstream signaling molecule transducer and activator-1 (STAT1), impaired type-1 IFN signaling results in defective functional NK cell maturation and hampered anti-tumoricidal potential in sarcoma and lymphoma mouse models (82, 83). Importantly, while transient or intermittent type-1 IFN stimulation results in preferential phosphorylation of STAT4 than STAT1 and thereby increased IFN-γ production by NK cells promoting pro-inflammation, chronic stimulation coupled with increased levels of IFN-γ also bolster NK cell cytotoxicity (81, 84). This is due to increases basal levels of STAT1 protein expression following chronic IFN-γ stimulation that triggers preferential activation of STAT1 over STAT4 (81, 84). Additionally, autocrine type-1 IFN signaling in activated myeloid cells induces interleukin (IL)-15 production, which is a critical cytokine for NK cell development, proliferation and cytotoxic function (37, 85).
Regulation of Natural Killer Cell Activation and Effector Function by Interleukin-12 Family of Cytokines
The IL-12 family of heterodimeric cytokines, including IL-12, IL-23, and IL-27, critically regulate NK cell activation and effector functions (Figure 1A and Table 1A). Phagocytic macrophages and DCs produce these cytokines following the recognition of DAMPs on dying tumor cells. Despite sharing sequence similarly, these cytokines uniquely modulate NK cell function.
Interleukin-12
IL-12 (p35 and p40 complex) signals by engaging the heterodimeric receptor complex of IL-12Rβ1 and IL-12Rβ2 subunits that are readily expressed by mature activated but not immature NK cells. In NK cells, IL-12 signaling principally mediates STAT4 phosphorylation that is essential for both IFN-γ and perforin expression, as observed both in human NK cells in vitro and in murine NK cells in vivo (21, 86–89). Accordingly, blockade of IL-12 diminishes DC-induced IFN-γ production by NK cells, suggesting IL-12 is critical for optimal IFN-γ release by activated human NK cells (90). Additionally, IL-12 can work in concert with IL-15 and IL-18 to generate ‘memory-like’ NK cells, partly facilitated by epigenetic reprogramming of the CNS1 enhancer region of the Ifng locus in NK cells, which enables them to produce elevated levels of IFN-γ compared to conventional NK cells upon activation as shown by transferring IL-12/IL-15/IL-18-pretreated human NK cells in NSG mice (91).
Interleukin-23
IL-12p40 also interacts with p19 subunits forming the heterodimeric cytokine IL-23. Upon stimulation with IL-23, CD56bright NK cells release higher levels of IFN-γ as compared to CD56dim NK cells due to their higher expression levels of IL-23R. IL-23 also increases IL-18Rα expression, thus priming NK cells for IL-18-induced IFN-γ production. IL-23 stimulates human NK cell activation in vitro while inhibiting IL-15- and IL-18-induced NK cell proliferation (54). However, IL-23 reduces both cytotoxicity and IFN-γ production in vivo, indeed anti-IL-23 therapy synergizes with IL-2 or anti-erbB2 treatment in mammary and melanoma tumor models in an NK cell-dependent manner (55, 92).
Interleukin-27
The IL-27 heterodimer is composed of p28 and EBI3 and promotes pro- and anti-inflammatory functions mainly through STAT1 and STAT3 (93). Like IL-23, IL-27 shows differential activity on human CD56bright and CD56dim subsets, maybe related to the higher expression of IL-27Rα in the CD56bright subset (48). While CD56dim NK cells are not affected, CD56bright NK cells acquire a regulatory phenotype. Yet, in vivo studies on a murine squamous cell carcinoma model and in vitro analysis on human NK cells also demonstrated that IL-27 primes NK cells to respond to IL-18, while inducing perforin, granzyme B, NKp46, and NKG2D expression, as well as promoting antibody-dependent cellular cytotoxicity (ADCC) (49–52, 94, 95).
Critical Role of Gamma-Chain Cytokines Released by Myeloid Cells in Promoting Natural Killer Cell Survival, Proliferation, and Functions
The gamma-chain family consists of cytokines such as IL-2, IL-4, IL-7, IL-9, IL-15, and IL-21 that all bind the common gamma-chain receptor. Below we will focus the discussion on IL-15 and IL-21 that are commonly derived from myeloid cells.
Interleukin-15
IL-15/IL-5Rα induces NK cell survival and proliferation by acting as a soluble form or presented at the DC membrane. As mentioned above, it is also an essential driver of NK cell development and activation (37–39, 96). IL-15 expression correlates with NK cell infiltration in human tumor samples (97), and data from a murine melanoma model indicate CD11b+Ly6ChiLy6G- monocytic cells are the major source of this cytokine (78). IL-15, together with IL-12, also indirectly regulates NK cell functions by controlling metabolism via mTORC1 activation, which stimulates nutrient uptake, glycolysis, and OXPHOS, thereby providing the energy for NK cell proliferation, proper functions and enhanced persistance (36, 98). Interestingly, chronic IL-15 stimulation of NK cells results in exhaustion by reducing the mitochondrial respiratory capacity (99).
Interleukin-21
In addition to IL-15, DCs also release IL-21, which following STAT3 and STAT1 signaling in NK cells, promotes NK cell cytotoxicity via increased granzyme B and perforin expression. Moreover, STAT1 and PI3K pathways are essential for IL-21-mediated reversal of NK cell exhaustion in mice and for intratumoral human NK cells cultured in vitro (45). Interestingly, IL-21 differentially regulates the expression of activating receptors by inducing NKp30 levels while reducing NKG2D/DAP10 expression in human NK cell (100). However, IL-21 contributes to tumor rejection in an NKG2D-dependent manner in multiple mouse tumor models (101).
Interleukin-17 Can Promote Natural Killer Cell Cytotoxicity While Limiting Terminal Differentiation of Natural Killer Cells
Neutrophils are the major producers of IL-17, which binds to a dimeric receptor and mainly signals through the NF-kB and ERK pathways (102). IL-17 has been shown to enhance NK cell recruitment in human esophageal cancer through tumor-derived chemokines and NK cell cytotoxicity through the increased expression of activating receptors, perforin, granzyme B, TNF-α, and IFN-γ (103). However, IL-17 has also been reported to limit IL-15-mediated terminal murine NK cell differentiation via upregulation of suppressor of cytokine signaling (SOCS), which inhibits STAT5 phosphorylation, and reduces NK cell killing in the presence of IFN-γ (56).
Context-Dependent Role of Interleukin-18 in Cancer
Upon interaction with its heterodimeric receptor and the activation of the MyD88 signaling pathway, IL-18 primes NK cells to produce IFN-γ (104). In vitro data also show that IL-18 can favor the differentiation of human CD56dim CCR7+ CD25+ CD83+ helper NK cells, which control tumor dissemination and CD8+ T cell activation through the crosstalk with DCs in the lymph nodes (40, 41, 105). Similar to other activating cytokines, such as IL-17 above, also IL-18 can display immunosuppressive features by boosting TGF-β-mediated immunosuppression (106), formation of MDSCs, and induction of PD1 expression on NK cells in mouse models (42, 107, 108). Notably, the upregulation of IL-18 binding protein (IL-18BP), which sequesters IL-18 as a physiological negative feedback mechanism, has been reported as an immune escape strategy (42, 109).
Natural Killer Cell Dysfunction in the Tumor Microenvironment Triggered by Myeloid-Derived Cytokines
Although the NK-myeloid crosstalk stimulates anti-tumor immunity by NK cells in the early tumor development, immunosuppressive cytokines promoting NK cell dysfunction predominate in the aggravated TME of more advanced tumors. Tumor-associated macrophages (TAMs) and MDSCs are usually the main myeloid cell populations in such TME and represent the major producers of NK cell suppressive TGF-β and IL-10 (Figure 1A and Table 1A) (110).
Transforming Growth Factor-β
As mentioned above, TGF-β is a key suppressor of NK cell migration, cytotoxicity, and cytokine production via transcriptional and post-transcriptional control of receptor and effector molecule expression (111). As an example, TGF-β-mediated downregulation of CX3CR1 can limit NK cell migration towards the tumor site (25); similarly, the downregulation of activating receptors including NKG2D and NKp30 as well as the adaptor proteins DAP10 and DAP12 triggered by TGF-β diminishes human NK cell cytotoxicity in vitro (26–28, 111). TGF-β-mediated NK cell conversion into Eomes- ILC1 with increased expression levels of inhibitory receptors may represent an additional mechanism to reduce NK cell cytotoxicity in mouse tumor models (29), while a third mechanism is the inhibition of signaling pathways downstream of pro-inflammatory cytokines (30, 36, 111, 112). TGF-β-induced miRNA targets STAT1, which is essential for perforin expression (111), whereas blockade of IL-15-mediated mTOR activity dampens NK cell metabolism. Beyond this, TGF-β also reduces NK cell-mediated IFN-γ and TNF-α production in both human and mouse (29, 30, 36, 111, 112).
Interleukin-10
Like TGF-β, IL-10 directly inhibits IFN-γ and TNF-α production by NK cells in vitro. This effect is also indirectly mediated via inhibition of IL-12, IL-15, and IL-18 production in myeloid cells (24, 58). Yet, in the presence of IL-12 and IL-18, IL-10 has also been shown to stimulate NK cell proliferation, cytotoxicity, and IFN-γ production in vitro via the STAT3 signaling pathway (24, 31, 113, 114). More studies are needed to determine this complex relationship between NK cell suppressive and possibly NK cell promoting properties of IL-10.
Interleukin-32α
Another myeloid-derived immunosuppressive cytokine that can characterize the NK cell suppressive TME is IL-32α. IL-32α, which is often found highly expressed in the TME (115), inhibits IL-15-induced upregulation of perforin and granzyme B in vitro (34). Interestingly, dysregulated levels of IL-32α impairs human NK cell functions in chronic myelomonocytic leukemia and myelodysplastic syndrome (116).
Interleukin-1β
IL-1β is released by monocytes, DCs, and macrophages and stimulates the expansion of CD11b+Gr-1+Ly6C- MDSCs, which are potent inhibitors of murine NK cells in vivo (117). This cytokine has also been reported to maintain human NK cells in an immature state in the presence of IL-15 in secondary lymphoid tissues (23). Similarily to several cytokines above, IL-1β can also have NK cell promoting effects. One example is by indirectly promoting NK cell IFN-γ release by inducing IL-21 production in Th9 cells in mice (118).
Natural Killer Cell-Derived Cytokines Regulating Myeloid Cells in the Tumor Microenvironment
Upon cytokine stimulation and target cell encounter, NK cells themselves produce a range of cytokines such as IFN-γ, TNF-α, granulocyte-macrophage colony-stimulating factor (GM-CSF), and in some cases IL-10, that in turn modulate myeloid cells (119–121) (Figure 1A and Table 1B). In this section, we will summarize how cytokines released by NK cells affect the NK-myeloid cell crosstalk.
Dendritic Cells
DCs are central in triggering immune responses by T and NK cells. However, NK cells are also important for the DC function. In addition to cell-to-cell contact, NK cell-derived IFN-γ, TNF-α, and GM-CSF play a key role in the maturation and activation of human antigen-presenting DCs in the TME and lymphoid organs (59–61, 122). Further, activated human DCs maintain the IFN-γ production and induce high mobility group box 1 (HMGB1) production in NK cells via secretion of IL-12 and IL-18, resulting in further DC activation and maturation (43, 65). Hence, NK cells can be central in promoting DC maturation and activation, thereby feeding the positive loop of NK-myeloid crosstalk involving DCs in the TME.
Tumor-Associated Macrophages
TAMs are often immunosuppressive and known drivers of tumor progression (123). However, macrophages in the TME have a high degree of plasticity and can even display a pro-inflammatory phenotype (M1) and release IFN-γ and TNF-α (124). In vitro studies have shown that IFN-γ is the main cytokine that drives classical activation of macrophages and polarizes them towards an M1-like phenotype (62). Indeed, as highlighted in a murine sarcoma model, NK cell-derived IFN-γ promotes M1 polarization of macrophages (63). Additionally, TNF-α, and GM-CSF also support an inflammatory phenotype in macrophages (62). Hence, NK cells can also have a critical role in maintaining a pro-inflammatory phenotype of TAMs.
Tumor-Associated Neutrophils
Similar to TAMs, tumor-associated neutrophils (TANs) can be both tumor-promoting or pro-inflammatory and thereby counteract tumor growth. Recently, the first studies have investigated the interaction of NK cells with TANs in mouse models. NK cell-derived IFN-γ inhibits the tumor-promoting function of TANs in murine sarcoma and lung cancer models (64). NK cell-derived GM-CSF appears to promote Neutrophils Extracellular Trap formation by neutrophils, which can support tumor metastasis (66, 67).
Myeloid-Derived Suppressor Cells
MDSCs include a heterogenous group of myeloid cells that have a markedly strong immunosuppressive ability. While NK cell-derived GM-CSF results in DC activation and macrophage polarization towards an anti-tumorigenic phenotype, GM-CSF expands MDSCs in human and murine tumors (62, 125, 126). The exact role of NK cell-derived GM-CSF in promoting MDSC expansion in the TME remains unclear but should likely not be neglected. Importantly, it is clear that NK cells can promote MDSCs in the TME also by other mechanisms. A CD73+ IL-10-secreting subset of NK cells was recently identified in human sarcomas (127). While IL-10 promotes regulatory T cells (Tregs), it has also been shown to maintain the immune-suppressive functions of MDSCs in ovarian cancer (128).
Therapeutic Approaches to Directly Stimulate Natural Killer Cells or to Revert the Tumor Microenvironment to Favor Natural Killer Cell Anti-Tumor Responses
As highlighted in the previous sections, controlling the cytokine milieu in the TME is likely key to unleash the full potential of NK cell-based immunotherapies for several malignancies. Below, we will discuss ongoing and future approaches to enhance NK cell cytotoxicity in the TME (Figure 1B).
Administration of Cytokines
Administration of cytokines to boost NK cell anti-tumor cytotoxicity has been widely explored in the recent years. IL-2, IL-12, IL-15, and IL-21 represent the most promising cytokines under investigation. While IL-2 acts on several immune cell populations, the effects of IL-15 is mainly limited to NK cells and CD8+ T cells. Promising results have been obtained in phase I clinical trials for melanoma and hematologic malignancies using ALT-803 (an IL-15 mutein/IL-15Rα complex fused with the IgG1 Fc) (129, 130). However, side-effects observed following high-dose administration of both IL-2 and IL-15 represents a major challenge along with mobilization of Tregs (131, 132). An alternative strategy to reduce the risk of side-effects while stimulating NK cells more specifically is the use of IL-2 mutants, such as IL-2 F42K and IL-2 H9 that compared to wildtype IL-2 preferentially bind IL-2Rα and thereby increases NK cell activation without inducing Treg expansion (133–135). Likewise, although the use of IL-18 therapy did not show toxicity and was ineffective in several clinical trials, a novel IL-18 mutant has been recently shown to induce NK cell anti-tumor activity (136).
To avoid the toxicities of high-dose cytokines, investigators have also addressed the use of lower doses following adoptive NK cell transfer or combined with checkpoint blockade or fused to anti-tumor antibodies. Intermediate doses of IL-2 have been explored to support adoptively infused NK cells (5). In this context, IL-2 is intended to promote persistence and expansion of the infused donor NK cells, however, data also show IL-2 mobilizes Tregs which likely counteract the effect of the transferred NK cells. As clinical protocols on adoptive NK cell transfer where post-infusion IL-2 has been omitted report similar outcomes as those with IL-2 (137), it remains unclear as to whether post-NK cell infusion IL-2 is of benefit or not. Administration of IL-15 has also been used to support adoptively infused NK cells. However, although in initial trials report this cytokine may trigger better responses, intermediate doses of IL-15 were associated with cytokine-release syndrome (CRS) when administrated subcutaneously (138). Additional investigations are needed to identify which cytokines and the window in which they promote the effect of adoptively infused NK cells.
IL-15, IL-12, and IL-21 are under clinical investigation combined with anti-CTLA4, anti-TIM3, or anti-PD-1 (139, 140). Alternative approaches that are also currently explored are based on the generation of anti-tumor antibody-cytokine fusion molecules. Trispecific killer engagers (TriKEs) fused with IL-15 have demonstrated their ability to boost NK cell functions and persistence (141, 142), whereas IL-21 fused to anti-CD20 increases mouse survival in a lymphoma model (143). Future studies have to address the clinical efficacy of these approaches.
Modulation and Prevention of Cytokine Signaling
In addition to the more direct cytokine-based treatment approaches discussed above, there are also indirect strategies explored. Targeting pathways downstream of cytokine receptors may represent an alternative or complementary approach. Inhibition of SOCS proteins is promising since blockade of the STAT5 inhibitor CIS increases NK cell-mediated anti-tumor activity (144). In preclinical models, agonists of the stimulator of IFN genes (STING) pathway induce tumor regression by stimulating IL-15 production by infiltrating myeloid cells (145). Data also support the potential of GSK3 inhibitors in promoting maturation and cytotoxicity of NK cells following expansion ex vivo with IL-15 (146) GSK3 inhibition increased NK cell production of TNF and IFN-γ as well as bolstered the NK cytotoxicity per se and via ADCC, which translated into better tumor control of human ovarian cancer in a mouse model. An alterantive strategy is to alter the cytokine environment in the TME by neutralizing immunosuppressive cytokines. Ongoing trials with anti-TGF-β (Fresolimumab) and inhibitors of TGF-β signaling (140) will show if such approach has potential for the future. Depletion and/or prevention of the infiltration of suppressive cytokine-producing myeloid cells in the TME per se represents a tempting and yet incompletely explored alternative that indirectly would bolster the anti-tumor properties of NK cells. This approach needs further attention in future studies.
Concluding Remarks
The network of cells and signals in the TME is complex and not yet fully understood. However, ample evidence show that this environment most often is NK cell suppressive, especially in more advanced disease. Yet, the NK-myeloid cell crosstalk is central in shaping NK cell anti-tumor responses and that a better understanding of this crosstalk is required to improve outcomes of NK cell-based cancer immunotherapies. While strategies directed towards boosting NK cell cytotoxicity per se using cytokines or drugs that modulate cytokine signaling, other complementory approaches directed towards reverting the TME to favor anti-tumor immunity is likely required to promote long-term responses.
As pointed out in this mini review, challenges hindering prompt progress in the field include the multitude of different cell types in the TME along with the context-dependent functions of several cytokines derived from both the myeloid cells and the NK cells. We predict that recent developments related to genetic engineering of NK cells along with the arsenal of new cytokine mutants as well as targeted and immunomodulatory drugs, alone or combination, can facilitate progress in the field. We foresee the use of genetically engineered NK cells to help improve their efficacy per se but also to resist and persist in the TME and thereby have the chance to revert it to a more pro-inflammatory milieu optimal for initating potent durable anti-tumor immune reponses. CAR-NK cells equipped with cytokine signaling elements or dominant negative cytokine receptors are examples that hold promise. We are positive that this along with further insights in the basic biology of cytokines and cytokine signaling will help improve NK cell-based cancer immunotherapy.
Author Contributions
SG, KW, MC, and SM have together outlined and written the manuscript. All authors contributed to the article and approved the submitted version.
Funding
This work was supported by funding from Jeanssons Stiftelser (MC), Swedish Society for Medical Research (MC), Wallenberg Clinical Fellow (MC), Cancerfonden (MC) and the Swedish Research Council (MC) as well as from Fondation Arc Pour La Recherche Sur Le Cancer (SM), Ligue Nationale Contre Le Cancer (SM), Cancéropôle Nord-Ouest (SM), and CPER (SM).
Conflict of Interest
The authors declare that the research was conducted in the absence of any commercial or financial relationships that could be construed as a potential conflict of interest.
Acknowledgments
We would like to thank the funding agencies supporting our work.
References
1. Chiossone L, Dumas P-Y, Vienne M, Vivier E. Natural killer cells and other innate lymphoid cells in cancer. Nat Rev Immunol (2018) 18:671–88. doi: 10.1038/s41577-018-0061-z
2. Long EO, Sik Kim H, Liu D, Peterson ME, Rajagopalan S. Controlling Natural Killer Cell Responses: Integration of Signals for Activation and Inhibition. Annu Rev Immunol (2013) 31:227–58. doi: 10.1146/annurev-immunol-020711-075005
3. Malmberg K-J, Carlsten M, Björklund A, Sohlberg E, Bryceson YT, Ljunggren H-G. Natural killer cell-mediated immunosurveillance of human cancer. Semin Immunol (2017) 31:20–9. doi: 10.1016/j.smim.2017.08.002
4. Ruggeri L, Capanni M, Urbani E, Perruccio K, Shlomchik WD, Tosti A, et al. Effectiveness of donor natural killer cell alloreactivity in mismatched hematopoietic transplants. Science (2002) 295:2097–100. doi: 10.1126/science.1068440
5. Miller JS, Soignier Y, Panoskaltsis-Mortari A, McNearney SA, Yun GH, Fautsch SK, et al. Successful adoptive transfer and in vivo expansion of human haploidentical NK cells in patients with cancer. Blood (2005) 105:3051–7. doi: 10.1182/blood-2004-07-2974
6. Carlsten M, Norell H, Bryceson YT, Poschke I, Schedvins K, Ljunggren H-G, et al. Primary human tumor cells expressing CD155 impair tumor targeting by down-regulating DNAM-1 on NK cells. J Immunol (2009) 183:4921–30. doi: 10.4049/jimmunol.0901226
7. Carlsten M, Baumann BC, Simonsson M, Jädersten M, Forsblom A-M, Hammarstedt C, et al. Reduced DNAM-1 expression on bone marrow NK cells associated with impaired killing of CD34+ blasts in myelodysplastic syndrome. Leukemia (2010) 24:1607–16. doi: 10.1038/leu.2010.149
8. Devillier R, Chrétien A-S, Pagliardini T, Salem N, Blaise D, Olive D. Mechanisms of NK cell dysfunction in the tumor microenvironment and current clinical approaches to harness NK cell potential for immunotherapy. J Leukocyte Biol (2020). doi: 10.1002/JLB.5MR0920-198RR
9. Melaiu O, Lucarini V, Cifaldi L, Fruci D. Influence of the Tumor Microenvironment on NK Cell Function in Solid Tumors. Front Immunol (2019) 10:3038. doi: 10.3389/fimmu.2019.03038
10. Sarhan D, Wang J, Sunil Arvindam U, Hallstrom C, Verneris MR, Grzywacz B, et al. Mesenchymal stromal cells shape the MDS microenvironment by inducing suppressive monocytes that dampen NK cell function. JCI Insight (2020) 5(5):e130155. doi: 10.1172/jci.insight.130155
11. Romero AI, Thorén FB, Brune M, Hellstrand K. NKp46 and NKG2D receptor expression in NK cells with CD56dim and CD56bright phenotype: regulation by histamine and reactive oxygen species. Br J Haematol (2006) 132:91–8. doi: 10.1111/j.1365-2141.2005.05842.x
12. Iyori M, Zhang T, Pantel H, Gagne BA, Sentman CL. TRAIL/DR5 plays a critical role in NK cell-mediated negative regulation of dendritic cell cross-priming of T cells. J Immunol (2011) 187:3087–95. doi: 10.4049/jimmunol.1003879
13. Pallmer K, Oxenius A. Recognition and Regulation of T Cells by NK Cells. Front Immunol (2016) 7:251. doi: 10.3389/fimmu.2016.00251
14. Raulet DH. Interplay of natural killer cells and their receptors with the adaptive immune response. Nat Immunol (2004) 5:996–1002. doi: 10.1038/ni1114
15. Salagianni M, Lekka E, Moustaki A, Iliopoulou EG, Baxevanis CN, Papamichail M, et al. NK cell adoptive transfer combined with Ontak-mediated regulatory T cell elimination induces effective adaptive antitumor immune responses. J Immunol (2011) 186:3327–35. doi: 10.4049/jimmunol.1000652
16. Kumar V, Patel S, Tcyganov E, Gabrilovich DI. The Nature of Myeloid-Derived Suppressor Cells in the Tumor Microenvironment. Trends Immunol (2016) 37:208–20. doi: 10.1016/j.it.2016.01.004
17. Veglia F, Gabrilovich DI. Dendritic cells in cancer: the role revisited. Curr Opin Immunol (2017) 45:43–51. doi: 10.1016/j.coi.2017.01.002
18. Kiss M, Van Gassen S, Movahedi K, Saeys Y, Laoui D. Myeloid cell heterogeneity in cancer: not a single cell alike. Cell Immunol (2018) 330:188–201. doi: 10.1016/j.cellimm.2018.02.008
19. Vivier E, Raulet DH, Moretta A, Caligiuri MA, Zitvogel L, Lanier LL, et al. Innate or adaptive immunity? The example of natural killer cells. Science (2011) 331:44–9. doi: 10.1126/science.1198687
20. Böttcher JP, Bonavita E, Chakravarty P, Blees H, Cabeza-Cabrerizo M, Sammicheli S, et al. NK Cells Stimulate Recruitment of cDC1 into the Tumor Microenvironment Promoting Cancer Immune Control. Cell (2018) 172:1022–1037.e14. doi: 10.1016/j.cell.2018.01.004
21. Walzer T, Dalod M, Robbins SH, Zitvogel L, Vivier E. Natural-killer cells and dendritic cells: “l’union fait la force.” Blood (2005) 106:2252–8. doi: 10.1182/blood-2005-03-1154
22. Yang L, Pang Y, Moses HL. TGF-beta and immune cells: an important regulatory axis in the tumor microenvironment and progression. Trends Immunol (2010) 31:220–7. doi: 10.1016/j.it.2010.04.002
23. Hughes T, Becknell B, Freud AG, McClory S, Briercheck E, Yu J, et al. Interleukin-1β Selectively Expands and Sustains Interleukin-22+ Immature Human Natural Killer Cells in Secondary Lymphoid Tissue. Immunity (2010) 32:803–14. doi: 10.1016/j.immuni.2010.06.007
24. Mannino MH, Zhu Z, Xiao H, Bai Q, Wakefield MR, Fang Y. The paradoxical role of IL-10 in immunity and cancer. Cancer Lett (2015) 367:103–7. doi: 10.1016/j.canlet.2015.07.009
25. Regis S, Caliendo F, Dondero A, Casu B, Romano F, Loiacono F, et al. TGF-β1 Downregulates the Expression of CX3CR1 by Inducing miR-27a-5p in Primary Human NK Cells. Front Immunol (2017) 8:868:868. doi: 10.3389/fimmu.2017.00868
26. Castriconi R, Cantoni C, Chiesa MD, Vitale M, Marcenaro E, Conte R, et al. Transforming growth factor β1 inhibits expression of NKp30 and NKG2D receptors: Consequences for the NK-mediated killing of dendritic cells. PNAS (2003) 100:4120–5. doi: 10.1073/pnas.0730640100
27. Espinoza JL, Takami A, Yoshioka K, Nakata K, Sato T, Kasahara Y, et al. Human microRNA-1245 down-regulates the NKG2D receptor in natural killer cells and impairs NKG2D-mediated functions. Haematologica (2012) 97:1295–303. doi: 10.3324/haematol.2011.058529
28. Park YP, Choi S-C, Kiesler P, Gil-Krzewska A, Borrego F, Weck J, et al. Complex regulation of human NKG2D-DAP10 cell surface expression: opposing roles of the γc cytokines and TGF-β1. Blood (2011) 118:3019–27. doi: 10.1182/blood-2011-04-346825
29. Gao Y, Souza-Fonseca-Guimaraes F, Bald T, Ng SS, Young A, Ngiow SF, et al. Tumor immunoevasion by the conversion of effector NK cells into type 1 innate lymphoid cells. Nat Immunol (2017) 18:1004–15. doi: 10.1038/ni.3800
30. Viel S, Marçais A, Guimaraes FS-F, Loftus R, Rabilloud J, Grau M, et al. TGF-β inhibits the activation and functions of NK cells by repressing the mTOR pathway. Sci Signal (2016) 9:ra19. doi: 10.1126/scisignal.aad1884
31. Lauw FN, Pajkrt D, Hack CE, Kurimoto M, van Deventer SJ, van der Poll T. Proinflammatory effects of IL-10 during human endotoxemia. J Immunol (2000) 165:2783–9. doi: 10.4049/jimmunol.165.5.2783
32. Cai G, Kastelein RA, Hunter CA. IL-10 enhances NK cell proliferation, cytotoxicity and production of IFN-γ when combined with IL-18. Eur J Immunol (1999) 29:2658–65. doi: 10.1002/(SICI)1521-4141(199909)29:09<2658::AID-IMMU2658>3.0.CO;2-G
33. Parato KG, Kumar A, Badley AD, Sanchez-Dardon JL, Chambers KA, Young CD, et al. Normalization of natural killer cell function and phenotype with effective anti-HIV therapy and the role of IL-10. AIDS (2002) 16:1251–6. doi: 10.1097/00002030-200206140-00007
34. Gorvel L, Korenfeld D, Tung T, Klechevsky E. Dendritic Cell–Derived IL-32α: A Novel Inhibitory Cytokine of NK Cell Function. J Immunol (2017) 199:1290–300. doi: 10.4049/jimmunol.1601477
35. Almishri W, Santodomingo-Garzon T, Le T, Stack D, Mody CH, Swain MG. TNFα Augments Cytokine-Induced NK Cell IFNγ Production through TNFR2. JIN (2016) 8:617–29. doi: 10.1159/000448077
36. O’Brien KL, Finlay DK. Immunometabolism and natural killer cell responses. Nat Rev Immunol (2019) 19:282–90. doi: 10.1038/s41577-019-0139-2
37. Lucas M, Schachterle W, Oberle K, Aichele P, Diefenbach A. Dendritic Cells Prime Natural Killer Cells by trans-Presenting Interleukin 15. Immunity (2007) 26:503–17. doi: 10.1016/j.immuni.2007.03.006
38. Koka R, Burkett P, Chien M, Chai S, Boone DL, Ma A. Cutting Edge: Murine Dendritic Cells Require IL-15Rα to Prime NK Cells. J Immunol (2004) 173:3594–8. doi: 10.4049/jimmunol.173.6.3594
39. Wagner JA, Rosario M, Romee R, Berrien-Elliott MM, Schneider SE, Leong JW, et al. CD56bright NK cells exhibit potent antitumor responses following IL-15 priming. J Clin Invest (2017) 127:4042–58. doi: 10.1172/JCI90387
40. Mailliard RB, Alber SM, Shen H, Watkins SC, Kirkwood JM, Herberman RB, et al. IL-18–induced CD83+CCR7+ NK helper cells. J Exp Med (2005) 202:941–53. doi: 10.1084/jem.20050128
41. Wong JL, Mailliard RB, Moschos SJ, Edington H, Lotze MT, Kirkwood JM, et al. Helper Activity of Natural Killer Cells During the Dendritic Cell-mediated Induction of Melanoma-specific Cytotoxic T Cells. J Immunother (2011) 34:270–8. doi: 10.1097/CJI.0b013e31820b370b
42. Fabbi M, Carbotti G, Ferrini S. Context-dependent role of IL-18 in cancer biology and counter-regulation by IL-18BP. J Leukocyte Biol (2015) 97:665–75. doi: 10.1189/jlb.5RU0714-360RR
43. Semino C, Angelini G, Poggi A, Rubartelli A. NK/iDC interaction results in IL-18 secretion by DCs at the synaptic cleft followed by NK cell activation and release of the DC maturation factor HMGB1. Blood (2005) 106:609–16. doi: 10.1182/blood-2004-10-3906
44. Bellora F, Castriconi R, Dondero A, Pessino A, Nencioni A, Liggieri G, et al. TLR activation of tumor-associated macrophages from ovarian cancer patients triggers cytolytic activity of NK cells. Eur J Immunol (2014) 44:1814–22. doi: 10.1002/eji.201344130
45. Seo H, Jeon I, Kim B-S, Park M, Bae E-A, Song B, et al. IL-21-mediated reversal of NK cell exhaustion facilitates anti-tumour immunity in MHC class I-deficient tumours. Nat Commun (2017) 8:15776. doi: 10.1038/ncomms15776
46. Jones SA, Jenkins BJ. Recent insights into targeting the IL-6 cytokine family in inflammatory diseases and cancer. Nat Rev Immunol (2018) 18:773–89. doi: 10.1038/s41577-018-0066-7
47. Cifaldi L, Prencipe G, Caiello I, Bracaglia C, Locatelli F, De Benedetti F, et al. Inhibition of Natural Killer Cell Cytotoxicity by Interleukin-6: Implications for the Pathogenesis of Macrophage Activation Syndrome. Arthritis Rheumatol (2015) 67:3037–46. doi: 10.1002/art.39295
48. Laroni A, Gandhi R, Beynon V, Weiner HL. IL-27 Imparts Immunoregulatory Function to Human NK Cell Subsets. PloS One (2011) 6:e26173. doi: 10.1371/journal.pone.0026173
49. Ziblat A, Domaica CI, Spallanzani RG, Iraolagoitia XLR, Rossi LE, Avila DE, et al. IL-27 stimulates human NK-cell effector functions and primes NK cells for IL-18 responsiveness. Eur J Immunol (2015) 45:192–202. doi: 10.1002/eji.201444699
50. Matsui M, Kishida T, Nakano H, Yoshimoto K, Shin-Ya M, Shimada T, et al. Interleukin-27 Activates Natural Killer Cells and Suppresses NK-Resistant Head and Neck Squamous Cell Carcinoma through Inducing Antibody-Dependent Cellular Cytotoxicity. Cancer Res (2009) 69:2523–30. doi: 10.1158/0008-5472.CAN-08-2793
51. Hemati M, Rasouli Nejad Z, Shokri M-R, Ghahremanfard F, Mir Mohammadkhani M, Kokhaei P. IL-27 impact on NK cells activity: Implication for a robust anti-tumor response in chronic lymphocytic leukemia. Int Immunopharmacol (2020) 82:106350. doi: 10.1016/j.intimp.2020.106350
52. Dondero A, Casu B, Bellora F, Vacca A, Luisi AD, Frassanito MA, et al. NK cells and multiple myeloma-associated endothelial cells: molecular interactions and influence of IL-27. Oncotarget (2017) 8(21):35088–102. doi: 10.18632/oncotarget.17070
53. Wei J, Xia S, Sun H, Zhang S, Wang J, Zhao H, et al. Critical Role of Dendritic Cell–Derived IL-27 in Antitumor Immunity through Regulating the Recruitment and Activation of NK and NKT Cells. J Immunol (2013) 191:500–8. doi: 10.4049/jimmunol.1300328
54. Ziblat A, Nuñez SY, Raffo Iraolagoitia XL, Spallanzani RG, Torres NI, Sierra JM, et al. Interleukin (IL)-23 Stimulates IFN-γ Secretion by CD56bright Natural Killer Cells and Enhances IL-18-Driven Dendritic Cells Activation. Front Immunol (2018) 8:1959. doi: 10.3389/fimmu.2017.01959
55. Teng MWL, Andrews DM, McLaughlin N, von Scheidt B, Ngiow SF, Möller A, et al. IL-23 suppresses innate immune response independently of IL-17A during carcinogenesis and metastasis. PNAS (2010) 107:8328–33. doi: 10.1073/pnas.1003251107
56. Wang X, Sun R, Hao X, Lian Z-X, Wei H, Tian Z. IL-17 constrains natural killer cell activity by restraining IL-15–driven cell maturation via SOCS3. PNAS (2019) 116:17409–18. doi: 10.1073/pnas.1904125116
57. Qian X, Chen H, Wu X, Hu L, Huang Q, Jin Y. Interleukin-17 acts as double-edged sword in anti-tumor immunity and tumorigenesis. Cytokine (2017) 89:34–44. doi: 10.1016/j.cyto.2015.09.011
58. Konjević GM, Vuletić AM, Mirjačić Martinović KM, Larsen AK, Jurišić VB. The role of cytokines in the regulation of NK cells in the tumor environment. Cytokine (2019) 117:30–40. doi: 10.1016/j.cyto.2019.02.001
59. Piccioli D, Sbrana S, Melandri E, Valiante NM. Contact-dependent stimulation and inhibition of dendritic cells by natural killer cells. J Exp Med (2002) 195:335–41. doi: 10.1084/jem.20010934
60. Gerosa F, Baldani-Guerra B, Nisii C, Marchesini V, Carra G, Trinchieri G. Reciprocal activating interaction between natural killer cells and dendritic cells. J Exp Med (2002) 195:327–33. doi: 10.1084/jem.20010938
61. Vitale M, Della Chiesa M, Carlomagno S, Pende D, Aricò M, Moretta L, et al. NK-dependent DC maturation is mediated by TNFalpha and IFNgamma released upon engagement of the NKp30 triggering receptor. Blood (2005) 106:566–71. doi: 10.1182/blood-2004-10-4035
62. Sica A, Mantovani A. Macrophage plasticity and polarization: in vivo veritas. J Clin Invest (2012) 122:787–95. doi: 10.1172/JCI59643
63. O’Sullivan T, Saddawi-Konefka R, Vermi W, Koebel CM, Arthur C, White JM, et al. Cancer immunoediting by the innate immune system in the absence of adaptive immunity. J Exp Med (2012) 209:1869–82. doi: 10.1084/jem.20112738
64. Ogura K, Sato-Matsushita M, Yamamoto S, Hori T, Sasahara M, Iwakura Y, et al. NK Cells Control Tumor-Promoting Function of Neutrophils in Mice. Cancer Immunol Res (2018) 6:348–57. doi: 10.1158/2326-6066.CIR-17-0204
65. Mailliard RB, Son Y-I, Redlinger R, Coates PT, Giermasz A, Morel PA, et al. Dendritic cells mediate NK cell help for Th1 and CTL responses: two-signal requirement for the induction of NK cell helper function. J Immunol (2003) 171:2366–73. doi: 10.4049/jimmunol.171.5.2366
66. Bertin F-R, Rys RN, Mathieu C, Laurance S, Lemarié CA, Blostein MD. Natural killer cells induce neutrophil extracellular trap formation in venous thrombosis. J Thromb Haemost (2019) 17:403–14. doi: 10.1111/jth.14339
67. Yang L, Liu Q, Zhang X, Liu X, Zhou B, Chen J, et al. DNA of neutrophil extracellular traps promotes cancer metastasis via CCDC25. Nature (2020) 583(7814):133–8. doi: 10.1038/s41586-020-2394-6
68. Louis C, Souza-Fonseca-Guimaraes F, Yang Y, D’Silva D, Kratina T, Dagley L, et al. NK cell-derived GM-CSF potentiates inflammatory arthritis and is negatively regulated by CIS. J Exp Med (2020) 217(5):e20191421. doi: 10.1084/jem.20191421
69. Gotthardt D, Putz EM, Grundschober E, Prchal-Murphy M, Straka E, Kudweis P, et al. STAT5 Is a Key Regulator in NK Cells and Acts as a Molecular Switch from Tumor Surveillance to Tumor Promotion. Cancer Discovery (2016) 6:414–29. doi: 10.1158/2159-8290.CD-15-0732
70. Huntington ND, Cursons J, Rautela J. The cancer-natural killer cell immunity cycle. Nat Rev Cancer (2020) 20(8):437–54. doi: 10.1038/s41568-020-0272-z
71. Galluzzi L, Buqué A, Kepp O, Zitvogel L, Kroemer G. Immunogenic cell death in cancer and infectious disease. Nat Rev Immunol (2017) 17:97–111. doi: 10.1038/nri.2016.107
72. Castriconi R, Carrega P, Dondero A, Bellora F, Casu B, Regis S, et al. Molecular Mechanisms Directing Migration and Retention of Natural Killer Cells in Human Tissues. Front Immunol (2018) 9:2324. doi: 10.3389/fimmu.2018.02324
73. O’Sullivan TE, Sun JC, Lanier LL. Natural Killer Cell Memory. Immunity (2015) 43:634–45. doi: 10.1016/j.immuni.2015.09.013
74. Fehniger TA, Shah MH, Turner MJ, VanDeusen JB, Whitman SP, Cooper MA, et al. Differential Cytokine and Chemokine Gene Expression by Human NK Cells Following Activation with IL-18 or IL-15 in Combination with IL-12: Implications for the Innate Immune Response. J Immunol (1999) 162:4511–20.
75. Leong JW, Chase JM, Romee R, Schneider SE, Sullivan RP, Cooper MA, et al. Preactivation with IL-12, IL-15, and IL-18 Induces CD25 and a Functional High-Affinity IL-2 Receptor on Human Cytokine-Induced Memory-like Natural Killer Cells. Biol Blood Marrow Transplant (2014) 20:463–73. doi: 10.1016/j.bbmt.2014.01.006
76. Burkholder B, Huang R-Y, Burgess R, Luo S, Jones VS, Zhang W, et al. Tumor-induced perturbations of cytokines and immune cell networks. Biochim Biophys Acta (BBA) Rev Cancer (2014) 1845:182–201. doi: 10.1016/j.bbcan.2014.01.004
77. Ewen E-M, Pahl JHW, Miller M, Watzl C, Cerwenka A. KIR downregulation by IL-12/15/18 unleashes human NK cells from KIR/HLA-I inhibition and enhances killing of tumor cells. Eur J Immunol (2018) 48:355–65. doi: 10.1002/eji.201747128
78. Carrero RMS, Beceren-Braun F, Rivas SC, Hegde SM, Gangadharan A, Plote D, et al. IL-15 is a component of the inflammatory milieu in the tumor microenvironment promoting antitumor responses. PNAS (2019) 116:599–608. doi: 10.1073/pnas.1814642116
79. Nguyen KB, Salazar-Mather TP, Dalod MY, Van Deusen JB, Wei X, Liew FY, et al. Coordinated and distinct roles for IFN-alpha beta, IL-12, and IL-15 regulation of NK cell responses to viral infection. J Immunol (2002) 169:4279–87. doi: 10.4049/jimmunol.169.8.4279
80. Müller L, Aigner P, Stoiber D. Type I Interferons and Natural Killer Cell Regulation in Cancer. Front Immunol (2017) 8:304. doi: 10.3389/fimmu.2017.00304
81. Paolini R, Bernardini G, Molfetta R, Santoni A. NK cells and interferons. Cytokine Growth Factor Rev (2015) 26:113–20. doi: 10.1016/j.cytogfr.2014.11.003
82. Dunn GP, Bruce AT, Sheehan KCF, Shankaran V, Uppaluri R, Bui JD, et al. A critical function for type I interferons in cancer immunoediting. Nat Immunol (2005) 6:722–9. doi: 10.1038/ni1213
83. Swann JB, Hayakawa Y, Zerafa N, Sheehan KCF, Scott B, Schreiber RD, et al. Type I IFN Contributes to NK Cell Homeostasis, Activation, and Antitumor Function. J Immunol (2007) 178:7540–9. doi: 10.4049/jimmunol.178.12.7540
84. Gough DJ, Messina NL, Clarke CJP, Johnstone RW, Levy DE. Constitutive type I interferon modulates homeostatic balance through tonic signaling. Immunity (2012) 36:166–74. doi: 10.1016/j.immuni.2012.01.011
85. Montoya M, Schiavoni G, Mattei F, Gresser I, Belardelli F, Borrow P, et al. Type I interferons produced by dendritic cells promote their phenotypic and functional activation. Blood (2002) 99:3263–71. doi: 10.1182/blood.v99.9.3263
86. Thierfelder WE, van Deursen JM, Yamamoto K, Tripp RA, Sarawar SR, Carson RT, et al. Requirement for Stat4 in interleukin-12-mediated responses of natural killer and T cells. Nature (1996) 382:171–4. doi: 10.1038/382171a0
87. Sun JC, Madera S, Bezman NA, Beilke JN, Kaplan MH, Lanier LL. Proinflammatory cytokine signaling required for the generation of natural killer cell memory. J Exp Med (2012) 209:947–54. doi: 10.1084/jem.20111760
88. Yamamoto K, Shibata F, Miyasaka N, Miura O. The human perforin gene is a direct target of STAT4 activated by IL-12 in NK cells. Biochem Biophys Res Commun (2002) 297:1245–52. doi: 10.1016/s0006-291x(02)02378-1
89. Trinchieri G. Interleukin-12 and the regulation of innate resistance and adaptive immunity. Nat Rev Immunol (2003) 3:133–46. doi: 10.1038/nri1001
90. Ferlazzo G, Pack M, Thomas D, Paludan C, Schmid D, Strowig T, et al. Distinct roles of IL-12 and IL-15 in human natural killer cell activation by dendritic cells from secondary lymphoid organs. Proc Natl Acad Sci USA (2004) 101:16606–11. doi: 10.1073/pnas.0407522101
91. Ni J, Hölsken O, Miller M, Hammer Q, Luetke-Eversloh M, Romagnani C, et al. Adoptively transferred natural killer cells maintain long-term antitumor activity by epigenetic imprinting and CD4+ T cell help. Oncoimmunology (2016) 5:e1219009. doi: 10.1080/2162402X.2016.1219009
92. Teng MWL, von Scheidt B, Duret H, Towne JE, Smyth MJ. Anti-IL-23 Monoclonal Antibody Synergizes in Combination with Targeted Therapies or IL-2 to Suppress Tumor Growth and Metastases. Cancer Res (2011) 71:2077–86. doi: 10.1158/0008-5472.CAN-10-3994
93. Yoshida H, Hunter CA. The immunobiology of interleukin-27. Annu Rev Immunol (2015) 33:417–43. doi: 10.1146/annurev-immunol-032414-112134
94. Murugaiyan G, Saha B. IL-27 in tumor immunity and immunotherapy. Trends Mol Med (2013) 19:108–16. doi: 10.1016/j.molmed.2012.12.002
95. Zwirner NW, Ziblat A. Regulation of NK Cell Activation and Effector Functions by the IL-12 Family of Cytokines: The Case of IL-27. Front Immunol (2017) 8:25. doi: 10.3389/fimmu.2017.00025
96. Anton OM, Peterson ME, Hollander MJ, Dorward DW, Arora G, Traba J, et al. Trans -endocytosis of intact IL-15Rα–IL-15 complex from presenting cells into NK cells favors signaling for proliferation. Proc Natl Acad Sci USA (2020) 117:522–31. doi: 10.1073/pnas.1911678117
97. Cursons J, Souza-Fonseca-Guimaraes F, Foroutan M, Anderson A, Hollande F, Hediyeh-Zadeh S, et al. A Gene Signature Predicting Natural Killer Cell Infiltration and Improved Survival in Melanoma Patients. Cancer Immunol Res (2019) 7:1162–74. doi: 10.1158/2326-6066.CIR-18-0500
98. Mao Y, van Hoef V, Zhang X, Wennerberg E, Lorent J, Witt K, et al. IL-15 activates mTOR and primes stress-activated gene expression leading to prolonged antitumor capacity of NK cells. Blood (2016) 128:1475–89. doi: 10.1182/blood-2016-02-698027
99. Felices M, Lenvik AJ, McElmurry R, Chu S, Hinderlie P, Bendzick L, et al. Continuous treatment with IL-15 exhausts human NK cells via a metabolic defect. JCI Insight (2018) 3(3):e96219. doi: 10.1172/jci.insight.96219
100. Burgess SJ, Marusina AI, Pathmanathan I, Borrego F, Coligan JE. IL-21 Down-Regulates NKG2D/DAP10 Expression on Human NK and CD8+ T Cells. J Immunol (2006) 176:1490–7. doi: 10.4049/jimmunol.176.3.1490
101. Takaki R, Hayakawa Y, Nelson A, Sivakumar PV, Hughes S, Smyth MJ, et al. IL-21 Enhances Tumor Rejection through a NKG2D-Dependent Mechanism. J Immunol (2005) 175:2167–73. doi: 10.4049/jimmunol.175.4.2167
102. Amatya N, Garg AV, Gaffen SL. IL-17 Signaling: The Yin and the Yang. Trends Immunol (2017) 38:310–22. doi: 10.1016/j.it.2017.01.006
103. Lu L, Pan K, Zheng H-X, Li J-J, Qiu H-J, Zhao J-J, et al. IL-17A Promotes Immune Cell Recruitment in Human Esophageal Cancers and the Infiltrating Dendritic Cells Represent a Positive Prognostic Marker for Patient Survival. J Immunother (2013) 36:451–8. doi: 10.1097/CJI.0b013e3182a802cf
104. Chaix J, Tessmer MS, Hoebe K, Fuséri N, Ryffel B, Dalod M, et al. Priming of Natural Killer cells by Interleukin-18. J Immunol (2008) 181:1627–31. doi: 10.4049/jimmunol.181.3.1627
105. Wong JL, Berk E, Edwards RP, Kalinski P. IL-18–Primed Helper NK Cells Collaborate with Dendritic Cells to Promote Recruitment of Effector CD8+ T Cells to the Tumor Microenvironment. Cancer Res (2013) 73:4653–62. doi: 10.1158/0008-5472.CAN-12-4366
106. Casu B, Dondero A, Regis S, Caliendo F, Petretto A, Bartolucci M, et al. Novel Immunoregulatory Functions of IL-18, an Accomplice of TGF-β1. Cancers (2019) 11:75. doi: 10.3390/cancers11010075
107. Terme M, Ullrich E, Aymeric L, Meinhardt K, Coudert JD, Desbois M, et al. Cancer-Induced Immunosuppression: IL-18–Elicited Immunoablative NK Cells. Cancer Res (2012) 72:2757–67. doi: 10.1158/0008-5472.CAN-11-3379
108. Lim HX, Hong H-J, Cho D, Kim TS. IL-18 Enhances Immunosuppressive Responses by Promoting Differentiation into Monocytic Myeloid-Derived Suppressor Cells. J Immunol (2014) 193:5453–60. doi: 10.4049/jimmunol.1401282
109. Carbotti G, Barisione G, Orengo AM, Brizzolara A, Airoldi I, Bagnoli M, et al. The IL-18 Antagonist IL-18–Binding Protein Is Produced in the Human Ovarian Cancer Microenvironment. Clin Cancer Res (2013) 19:4611–20. doi: 10.1158/1078-0432.CCR-13-0568
110. Grivennikov SI, Greten FR, Karin M. Immunity, Inflammation, and Cancer. Cell (2010) 140:883–99. doi: 10.1016/j.cell.2010.01.025
111. Regis S, Dondero A, Caliendo F, Bottino C, Castriconi R. NK Cell Function Regulation by TGF-β-Induced Epigenetic Mechanisms. Front Immunol (2020) 11:311. doi: 10.3389/fimmu.2020.00311
112. Zaiatz-Bittencourt V, Finlay DK, Gardiner CM. Canonical TGF-β Signaling Pathway Represses Human NK Cell Metabolism. J Immunol (2018) 200:3934–41. doi: 10.4049/jimmunol.1701461
113. Park JY, Lee SH, Yoon S-R, Park Y-J, Jung H, Kim T-D, et al. IL-15-Induced IL-10 Increases the Cytolytic Activity of Human Natural Killer Cells. Mol Cells (2011) 32:265–72. doi: 10.1007/s10059-011-1057-8
114. Shibata Y, Foster LA, Kurimoto M, Okamura H, Nakamura RM, Kawajiri K, et al. Immunoregulatory Roles of IL-10 in Innate Immunity: IL-10 Inhibits Macrophage Production of IFN-γ-Inducing Factors but Enhances NK Cell Production of IFN-γ. J Immunol (1998) 161:4283–8.
115. Sloot YJE, Smit JW, Joosten LAB, Netea-Maier RT. Insights into the role of IL-32 in cancer. Semin Immunol (2018) 38:24–32. doi: 10.1016/j.smim.2018.03.004
116. Marcondes AM, Mhyre AJ, Stirewalt DL, Kim S-H, Dinarello CA, Deeg HJ. Dysregulation of IL-32 in myelodysplastic syndrome and chronic myelomonocytic leukemia modulates apoptosis and impairs NK function. PNAS (2008) 105:2865–70. doi: 10.1073/pnas.0712391105
117. Elkabets M, Ribeiro VSG, Dinarello CA, Ostrand-Rosenberg S, Santo JPD, Apte RN, et al. IL-1β regulates a novel myeloid-derived suppressor cell subset that impairs NK cell development and function. Eur J Immunol (2010) 40:3347–57. doi: 10.1002/eji.201041037
118. Végran F, Berger H, Boidot R, Mignot G, Bruchard M, Dosset M, et al. The transcription factor IRF1 dictates the IL-21-dependent anticancer functions of TH9 cells. Nat Immunol (2014) 15:758–66. doi: 10.1038/ni.2925
119. Cooper MA, Fehniger TA, Turner SC, Chen KS, Ghaheri BA, Ghayur T, et al. Human natural killer cells: a unique innate immunoregulatory role for the CD56(bright) subset. Blood (2001) 97:3146–51. doi: 10.1182/blood.v97.10.3146
120. Fauriat C, Long EO, Ljunggren H-G, Bryceson YT. Regulation of human NK-cell cytokine and chemokine production by target cell recognition. Blood (2010) 115:2167–76. doi: 10.1182/blood-2009-08-238469
121. Delahaye NF, Rusakiewicz S, Martins I, Ménard C, Roux S, Lyonnet L, et al. Alternatively spliced NKp30 isoforms affect the prognosis of gastrointestinal stromal tumors. Nat Med (2011) 17:700–7. doi: 10.1038/nm.2366
122. Morrissey PJ, Bressler L, Park LS, Alpert A, Gillis S. Granulocyte-macrophage colony-stimulating factor augments the primary antibody response by enhancing the function of antigen-presenting cells. J Immunol (1987) 139:1113–9.
123. Jayasingam SD, Citartan M, Thang TH, Mat Zin AA, Ang KC, Ch’ng ES. Evaluating the Polarization of Tumor-Associated Macrophages Into M1 and M2 Phenotypes in Human Cancer Tissue: Technicalities and Challenges in Routine Clinical Practice. Front Oncol (2019) 9:1512. doi: 10.3389/fonc.2019.01512
124. Mantovani A, Marchesi F, Malesci A, Laghi L, Allavena P. Tumour-associated macrophages as treatment targets in oncology. Nat Rev Clin Oncol (2017) 14:399–416. doi: 10.1038/nrclinonc.2016.217
125. Becher B, Tugues S, Greter M. GM-CSF: From Growth Factor to Central Mediator of Tissue Inflammation. Immunity (2016) 45:963–73. doi: 10.1016/j.immuni.2016.10.026
126. Gabrilovich DI, Nagaraj S. Myeloid-derived suppressor cells as regulators of the immune system. Nat Rev Immunol (2009) 9:162–74. doi: 10.1038/nri2506
127. Neo SY, Yang Y, Record J, Ma R, Chen X, Chen Z, et al. CD73 immune checkpoint defines regulatory NK cells within the tumor microenvironment. J Clin Invest (2020) 130:1185–98. doi: 10.1172/JCI128895
128. Hart KM, Byrne KT, Molloy MJ, Usherwood EM, Berwin B. IL-10 immunomodulation of myeloid cells regulates a murine model of ovarian cancer. Front Immunol (2011) 2:29. doi: 10.3389/fimmu.2011.00029
129. Margolin K, Morishima C, Velcheti V, Miller JS, Lee SM, Silk AW, et al. Phase I Trial of ALT-803, A Novel Recombinant IL15 Complex, in Patients with Advanced Solid Tumors. Clin Cancer Res (2018) 24:5552–61. doi: 10.1158/1078-0432.CCR-18-0945
130. Romee R, Cooley S, Berrien-Elliott MM, Westervelt P, Verneris MR, Wagner JE, et al. First-in-human phase 1 clinical study of the IL-15 superagonist complex ALT-803 to treat relapse after transplantation. Blood (2018) 131:2515–27. doi: 10.1182/blood-2017-12-823757
131. Conlon KC, Lugli E, Welles HC, Rosenberg SA, Fojo AT, Morris JC, et al. Redistribution, Hyperproliferation, Activation of Natural Killer Cells and CD8 T Cells, and Cytokine Production During First-in-Human Clinical Trial of Recombinant Human Interleukin-15 in Patients With Cancer. J Clin Oncol (2015) 33:74–82. doi: 10.1200/JCO.2014.57.3329
132. Abbas AK, Trotta E, Simeonov DR, Marson A, Bluestone JA. Revisiting IL-2: Biology and therapeutic prospects. Sci Immunol (2018) 3:eaat1482. doi: 10.1126/sciimmunol.aat1482
133. Sim GC, Liu C, Wang E, Liu H, Creasy C, Dai Z, et al. IL2 Variant Circumvents ICOS+ Regulatory T-cell Expansion and Promotes NK Cell Activation. Cancer Immunol Res (2016) 4:983–94. doi: 10.1158/2326-6066.CIR-15-0195
134. Ardolino M, Azimi CS, Iannello A, Trevino TN, Horan L, Zhang L, et al. Cytokine therapy reverses NK cell anergy in MHC-deficient tumors. J Clin Invest (2014) 124:4781–94. doi: 10.1172/JCI74337
135. Mitra S, Leonard WJ. Biology of IL-2 and its therapeutic modulation: Mechanisms and strategies. J Leukoc Biol (2018) 103:643–55. doi: 10.1002/JLB.2RI0717-278R
136. Zhou T, Damsky W, Weizman O-E, McGeary MK, Hartmann KP, Rosen CE, et al. IL-18BP is a secreted immune checkpoint and barrier to IL-18 immunotherapy. Nature (2020) 583(7817):609–14. doi: 10.1038/s41586-020-2422-6
137. Björklund AT, Carlsten M, Sohlberg E, Liu LL, Clancy T, Karimi M, et al. Complete Remission with Reduction of High-Risk Clones following Haploidentical NK-Cell Therapy against MDS and AML. Clin Cancer Res (2018) 24:1834–44. doi: 10.1158/1078-0432.CCR-17-3196
138. Cooley S, He F, Bachanova V, Vercellotti GM, DeFor TE, Curtsinger JM, et al. First-in-human trial of rhIL-15 and haploidentical natural killer cell therapy for advanced acute myeloid leukemia. Blood Adv (2019) 3:1970–80. doi: 10.1182/bloodadvances.2018028332
139. Waldmann TA. Cytokines in Cancer Immunotherapy. Cold Spring Harb Perspect Biol (2018) 10:a028472. doi: 10.1101/cshperspect.a028472
140. Berraondo P, Sanmamed MF, Ochoa MC, Etxeberria I, Aznar MA, Pérez-Gracia JL, et al. Cytokines in clinical cancer immunotherapy. Br J Cancer (2019) 120:6–15. doi: 10.1038/s41416-018-0328-y
141. Vallera DA, Felices M, McElmurry R, McCullar V, Zhou X, Schmohl JU, et al. IL15 Trispecific Killer Engagers (TriKE) Make Natural Killer Cells Specific to CD33+ Targets While Also Inducing Persistence, In Vivo Expansion, and Enhanced Function. Clin Cancer Res (2016) 22:3440–50. doi: 10.1158/1078-0432.CCR-15-2710
142. Felices M, Kodal B, Hinderlie P, Kaminski MF, Cooley S, Weisdorf DJ, et al. Novel CD19-targeted TriKE restores NK cell function and proliferative capacity in CLL. Blood Adv (2019) 3:897–907. doi: 10.1182/bloodadvances.2018029371
143. Bhatt S, Parvin S, Zhang Y, Cho H-M, Kunkalla K, Vega F, et al. Anti-CD20-interleukin-21 fusokine targets malignant B cells via direct apoptosis and NK-cell–dependent cytotoxicity. Blood (2017) 129:2246–56. doi: 10.1182/blood-2016-09-738211
144. Scarno G, Pietropaolo G, Di Censo C, Gadina M, Santoni A, Sciumè G. Transcriptional, Epigenetic and Pharmacological Control of JAK/STAT Pathway in NK Cells. Front Immunol (2019) 10:2456. doi: 10.3389/fimmu.2019.02456
145. Carrero RMS, Beceren-Braun F, Rivas SC, Hegde SM, Gangadharan A, Plote D, et al. IL-15 is a component of the inflammatory milieu in the tumor microenvironment promoting antitumor responses. PNAS (2019) 116:599–608. doi: 10.1073/pnas.1814642116
Keywords: natural killer (NK) cells, myeloid cells, cytokines, tumor microenvironment, cancer immunotherapy, tumor immunity
Citation: Gaggero S, Witt K, Carlsten M and Mitra S (2021) Cytokines Orchestrating the Natural Killer-Myeloid Cell Crosstalk in the Tumor Microenvironment: Implications for Natural Killer Cell-Based Cancer Immunotherapy. Front. Immunol. 11:621225. doi: 10.3389/fimmu.2020.621225
Received: 25 October 2020; Accepted: 09 December 2020;
Published: 29 January 2021.
Edited by:
Erik Wennerberg, Weill Cornell Medicine, United StatesReviewed by:
Rachel Burga, George Washington University, United StatesAna Stojanovic, Heidelberg University, Germany
Copyright © 2021 Gaggero, Witt, Carlsten and Mitra. This is an open-access article distributed under the terms of the Creative Commons Attribution License (CC BY). The use, distribution or reproduction in other forums is permitted, provided the original author(s) and the copyright owner(s) are credited and that the original publication in this journal is cited, in accordance with accepted academic practice. No use, distribution or reproduction is permitted which does not comply with these terms.
*Correspondence: Mattias Carlsten, mattias.carlsten@ki.se
†These authors share first authorship
‡These authors share last authorship