- 1College of Standardization, China Jiliang University, Hangzhou, China
- 2Key Laboratory of Applied Technology on Green-Eco-Healthy Animal Husbandry of Zhejiang Province, The Zhejiang Provincial Engineering Laboratory for Animal Health and Internet Technology, College of Animal Science and Technology, Zhejiang A & F University, Hangzhou, China
- 3Zhejiang Vegamax Biotechnology Co., Ltd., Anji, China
Grape seed is rich in vitamin E, flavonoids, and proanthocyanidins and has the potential to be used as an antibiotic substitute in broilers. We investigated the effects of grape seed proanthocyanidin extract (GSPE) on growth performance, immune responses, cecal microflora, and serum metabolism in early stage broilers. Data indicated that GSPE improved broiler growth performance by strengthening antioxidant capacity, enhancing immune responses, and increasing cecal short chain fatty acids. 16S rRNA sequencing indicated that GSPE changed the predominant cecal microflora and induced the metabolism of amino acids, lipids, and carbohydrates. An UPLC-Q-TOF/MS-based metabolomics analysis identified 23 serum metabolites (mainly related to lipid, amino acid, and alkaloid) were extremely changed by GSPE treatment. The correlations between the changes of cecal microflora and serum metabolites in birds fed with GSPE were analyzed. Hence, GSPE potentially provides active ingredients that may be used as antibiotic substitute and reduces environmental pollution by grape by-products.
Introduction
Recently, along the implementation of antibiotic ban in a growing number of countries, plenty of studies have been conducted to seek the antibiotic substitutions in livestock industry. Grape seed is rich in polyphenols (especially proanthocyanidins) and is a natural agricultural by-product with powerful antioxidant and anti-inflammatory effects. Grape seed is an outstanding antioxidative substance, with anti-inflammatory and anti-cancer effects (1, 2). Containing 60–70% of extractable polyphenols, the phenolic compounds from grape seeds comprise flavonoids, as well as catechins and their polymers (3, 4). The valorization of grape seed extract (GSE) could reduce the environmental pollution caused by wine production. It also potentially provides active ingredients for antibiotic substitution. Previous experiments have already revealed the potential applications of GSE in animal nutrition to enhance animal health status and product quality (5, 6).
Grape seed proanthocyanidin extract (GSPE) is enriched with polyphenolic flavonoids, oligomeric proanthocyanidins, and polymerized oligomers (7). Remarkably, previous studies revealed that GSPE has the wide positive effects, including anti-inflammatory, cardioprotective, and neuroprotective (8, 9). In addition to antioxidant function, GSPE shows beneficial effects on inflammatory processes (10). Moreover, proanthocyanidins are potential antibacterial compounds with the ability to inhibit the colonization of pathogenic intestinal bacteria (11, 12). Dietary polyphenol-rich grape products were also effective in boosting the development of extraordinary beneficial intestinal microbiota, competitively inhibiting particular harmful bacteria (6, 12).
In fact, increased efforts are now being directed toward a broader revaluation of polyphenol-rich plants for obtaining high-value grape seed by-products. In the mouse model induced by aflatoxin B1 (AFB1), GSPE significantly improved body weight gain, reduced oxidative damage to the spleen, and alleviated immune injury (13). A study by Cardona et al. (14). revealed that a dietary intake of red wine polyphenol increased the intestinal concentrations of healthy gut microbiota (including Enterococcus, Prevotella, Bacteroides, Bifidobacterium, and Blautiacoccoides). However, it did not change Lactobacillus spp. but significantly constrained the development of pathogenic bacteria (such as Clostridium difficile and Clostridium perfringens) (14). We suspected that there might be a relationship between intestinal microflora and metabolites in broilers supplemented with GSPE. Therefore, the present study was conducted to investigate whether GSPE influences the growth performance, immune response, cecal microflora, and serum metabolites in early stage broilers.
Materials and Methods
Test Substances Grape Seed Extracts
Grape (Vitis vinifera var. Bobal) seeds were purchased from Champagne (France). The GSE was extracted with water, concentrated, and spray dried. Its components were analyzed using the Agilent 1290 UPLC system (Ultra-Performance Liquid Chromatography) (Agilent, CA, USA) equipped with a binary pump, an auto-sampler, a photodiode array detector, and a column heater. The total polyphenol concentration (expressed as gallic acid equivalents) was determined following the methodology of Chamorro et al. (15). The resultant dry material was 80.5 ± 3.2 g/100 g. The major proanthocyanidins constituents of GSE were analyzed using high-performance liquid chromatography (HPLC). The major constituents were catechin (5.52 ± 0.21 g/100 g), epicatechin (3.14 ± 0.11 g/100 g), procyanidin B1 (1.48 ± 0.06 g/100 g), procyanidin B2 (1.16 ± 0.06 g/100 g), and procyanidin C1 (0.68 ± 0.04 g/100 g). In addition, the GSPE consisted of 4.80% water and 0.435% ash.
Animal Treatments and Design
A total of 960 AA+ one-day-old broilers were randomly divided into four treatment groups (8 replicates, 30 birds per pen). The birds in the control group were fed the basal diet (Control). The birds in the antibiotic group were fed the basal diet with added 20 mg bacitracin/kg (Anti). The birds in the low dose GSPE group were fed the basal diet with 200 mg GSPE/kg (GseL) added. The birds in the high dose GSPE group were fed the basal diet with an addition of 400 mg GSPE/kg (GseH). Throughout the 21-day experiment, all birds were cultivated on floor pens and provided the free access to food and water, with a 23-h photoperiod. In the first week, the room temperature was 35°C and was decreased 2°C each week. The composition and nutrients of basal diet aligned to meet the NRC Nutrient Requirement (2012) (Table 1). This study was performed in strict accordance with the Animal Management Rules of the Ministry of Health of the People’s Republic of China and approved by the Animal Care and Use Committee of Zhejiang A&F University (Hangzhou, China).
Representative sample birds were euthanized at day 21. Blood was collected from the jugular vein of the sample birds, 64 birds (4 treatment groups, with 8 replicate pens and 2 birds sampled per pen). Coagulation-promoting tubes were used to obtain the serum; the blood was allowed to stand at room temperature for 4 h and centrifuged at 3,000 g for 15 min. The cecal contents were collected in an aseptic cryopreservation tube and stored below −80° for the detection of SCFAs and microbial sequencing. After gently washing the intestinal content using a sterile PBS, the jejunal and ileal mucosa were wiped on sterile slides, and stored below −80°C for immune factors detecting.
Growth Performance
All birds per pen were weighted at the beginning and end of every week to calculate the average daily gain (ADG). Moreover, residue feeding and number of deaths were recorded to calculate the average daily feed intake (ADFI) and mortality. The feed:gain ratio (F:G) was calculated using the ADFI and ADG.
Serum Antioxidant Indexes
The serum antioxidant indexes were tested using commercial kits (Jiancheng Bioengineering Institute, Nanjing, China). The total antioxidant capacity (T-AOC), superoxide dismutase (SOD), glutathione peroxidase (GSH-Px), catalase (CAT), and malondialdehyde (MDA) were determined in accordance with the manufacturer’s instructions.
Immune Indexes
The serum contents of immunoglobulin A (IgA), immunoglobulin Y (IgY), and immunoglobulin M (IgM), and serum and mucosal interleukin-1β (IL-1β), interleukin-6 (IL-6), and interleukin-8 (IL-8) were detected using specific ELISA kits purchased from Cusabio (Wuhan, China). All methodology followed the manufacturer’s instructions.
Cecal SCFAs
The concentrations of cecal SCFAs were detected by gas chromatography. Briefly, the SCFA external standards (acetic acid, butyrate, propionic acid, isovalerate, isobutyric acid, and valerate) were obtained from Sigma-Aldrich (Shanghai, China). One gram of cecal content was mixed with 6% phosphorous acid (m/v, 1:3). After vibration and centrifugation, the supernatant was injected into the Agilent Technologies GC7890 Network System (Agilent Technologies, USA), with a column (30 m × 0.25 mm × 0.25 μm, Agilent Technologies) and flame ionization.
16S rRNA High Throughput Sequencing
The genomic DNA of the cecal content bacteria was extracted by DNeasy Power Soil Kit (QIAGEN, USA) following the manufacturer’s instructions. The content from both birds sampled in each replicate was mixed into one biological sample. The quality and quantity were determined for the extracted DNA prior to PCR amplification of 16S rRNA genes using the diluted DNA as template. Moreover, due to the bacterial diversity analysis, the V3–V4 variable regions were amplified with normal primers 343F (5’-TACGGRAGGCAGCAG-3’) and 798R (5’-AGGGTATCTAATCCT-3’). The sequencing was conducted at the Illumina Miseq platform from Shanghai OE Biotech Co., Ltd (Shanghai, China)..
Trimmomatic software was used to detect and dissect ambiguous bases, the raw sequencing data were presented in FASTQ format. FLASH software was used to assemble the paired-end reads. Noise reduction of the sequences and removal of reads with chimera were performed utilizing QIIME software (version 1.8.0). The clustering of operational taxonomic units (OTUs) was generated from clean reads using VSEARCH software (version 2.4.2) with a 97% similarity requirement. The entire representative reads selected by the QIIME package were annotated with the SILVA database (Version 123) using a RDP classifier (with a 70% confidence threshold). According to the OTU clustering, a flower plot analysis identified the common and particular OTUs among all treatment samples. The alpha-indexes were used to describe the degree of cecal microfloral diversity within each group; the beta-diversity was analyzed for the difference among all the treatments (using principal component analysis, PCA; Principal Coordinates Analysis, PCoA). Moreover, the linear discriminant analysis (LDA) was coupled with effect size measurements (LEfSe) to distinguish the bacteria between all the treatments, the LDA score was set at 3.5. PICRUSt software (based on the Greengenes data) was applied to predict the composition of the known microbial gene functions. A Kruskal-Wallis test was used to calculate the functional differences between all samples.
UPLC-Q-TOF/MS Analysis for Serum Metabolomics
The detection of the serum metabolites in the birds utilized the methodology provided in Li et al. (16). A total of 42 serum samples were analyzed to detect the variation in the bird metabolites, including 12 Control samples and 10 samples of each treatment (GseL, GseH and Anti). An Agilent 6545 Q-TOF/MS system (Agilent Technologies) was used for the chromatographic separation of the serum extract. The UPLC system was equipped with an Agilent Zorbox Eclipse Plus C18 (2.1 × 100 mm, 1.8 μm; Waters Corp., MA, USA). Mobile phases A and B were 95% ACN (containing 10 mM ammonium formate and 1 μl of formic acid) and 50% ACN (containing 10 mM ammonium formate and 1 μl of formic acid), respectively. In the negative ionization mode, mobile phases A and B were 95% ACN (containing 10 mM ammonium acetate, with an adjusted pH to 9 using ammonium hydroxide solution) and 50% ACN (containing 10 mM ammonium acetate, pH 9.0), respectively. The solvent gradient elution in the positive ionization mode involved: 0−2 min, 5% B; 2−20.0 min 5−100% B; 20−25 min, 100% B. The injection volume was 2 μl, and flow rate was set to 0.3 ml/min.
The UPLC system was connected to an Agilent 6545 ESI-Q-TOF high-resolution accurate-mass spectrometer (Agilent Technologies). Both the positive and negative ionization modes were performed. The MS conditions involved: 3.5 kV for positive, capillary voltage; 325°C, gas temperature; 11 L/min, drying gas flow rate; 35 psi, nebulizer pressure; 370°C, sheath gas temperature; 11 L/min, sheath gas flow rate; m/z 100−1,700, mass range. Metabolites were identified using Agilent Mass Hunter Profinder (Agilent Technologies) and the METLIN database (DB).
Statistical Analysis
SPSS version 24 (IBM Corp.) was used to perform a t test and one-way ANOVA analysis to determine significant differences among groups, differences were considered statistically significant at P < 0.05, tendency changes but no significant difference were considered at 0.1 < P < 0.05. The KEGG data were analyzed using SIMCA-P version 13.0 (Sartorius Stedim Biotech Ltd., Umea, Sweden) for the PCA analysis, and the heat map analysis utilized Multi Experiment Viewer version 4.8 (Boston, MA, USA). The metabolomic data were further analysis by screening the fold change > 2 and P value < 0.05 using a t test. The metabolism pathway analysis was conducted using MetaboAnalyst 4.0 (http://www.metaboanalyst.ca) and KEGG (http://www.kegg.jp) based on the screened serum metabolites with significant differences (P < 0.05). GraphPad Prism 7.0 (GraphPad Software Inc., San Diego, CA, USA) was used to produce the figures.
Results
Effects of GSPE on Growth Performance
There were no significant differences in the ADG of birds among all the treatments from d 1 to 7 and d 8 to 14 (Figure 1A). Compared with the Control birds, the Anti and GseL birds had significant higher (P < 0.05) ADG from d 15 to 21. From d 1 to 21, the ADG of the GseL and Anti birds were higher (P < 0.05) than the Control birds. Moreover, the Anti birds had significant higher ADG than the GseL and GseH birds. Furthermore, the supplementation of GSPE significantly decreased (P < 0.05) the ADFI of the birds from d 1 to 21 (Figure 1B). Both the supplementation of GSPE and antibiotic significantly decreased (P < 0.001) the F:G of birds (Figure 1C). There was no significant difference between the treatments in bird mortality (Figure 1D).
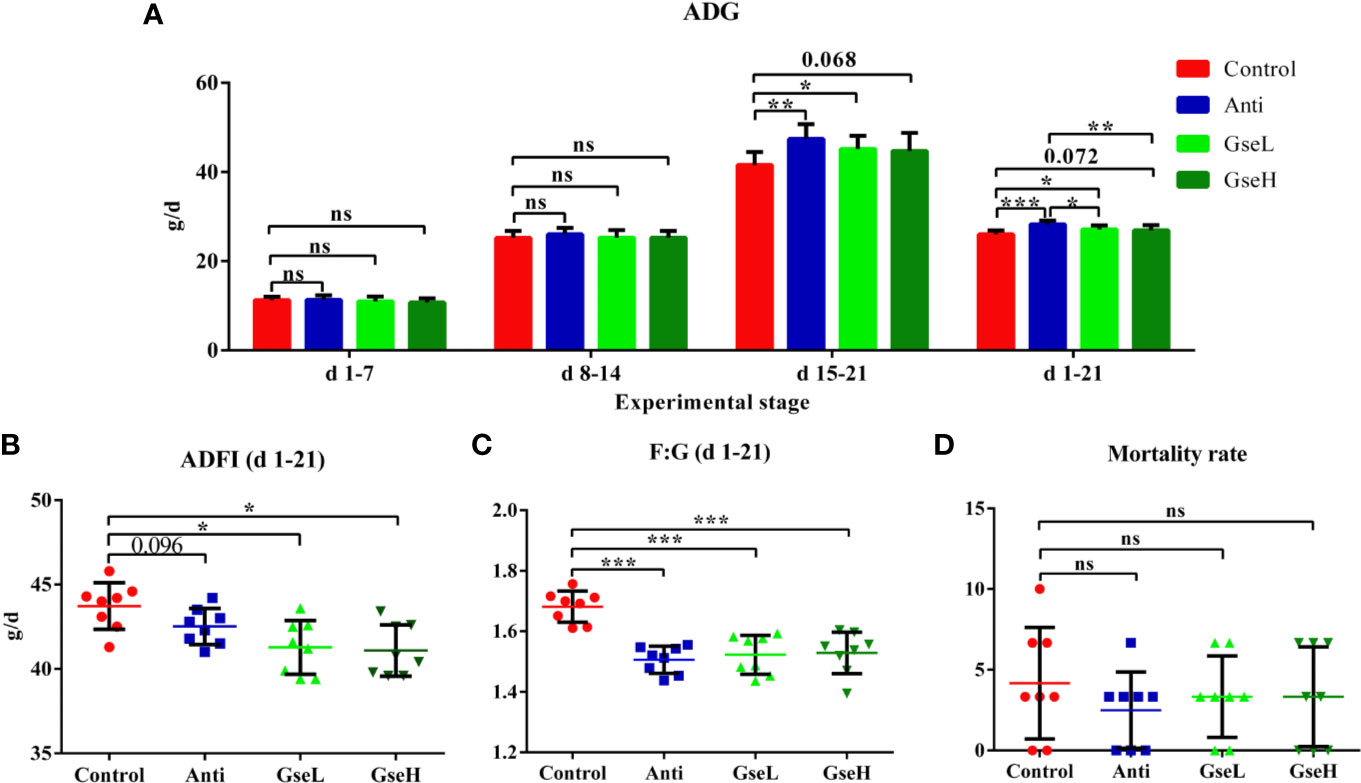
Figure 1 Effects of Anti, GseL, and GseH on (A) ADG (B), ADFI (C), F:G, and (D) Mortality in broilers from d 1 to 21. *P < 0.05 **P < 0.01, ***P < 0.001, tendency changes but no significant difference were considered at 0.1 < P < 0.05, when compared to the Control treatment. N = 8 per treatment group. ns, not significant.
Effects of GSPE on Serum Antioxidant Indexes
The effects of GSPE on the serum antioxidant parameters in early stage broilers are shown in Figure 2. The dietary supplementation of the antibiotic and GSPE significantly increased the serum concentration of T-AOC (P < 0.01). Supplementation with GSPE significantly (P < 0.01) increased the content of serum T-AOC to a higher level when compared to the antibiotic treatment (Figure 2A). The level of serum SOD in GseL birds was significant (P < 0.05) higher than that in the Control birds (Figure 2B). Compared with the Control birds, the GseH-treated birds exhibited significant higher content of serum GSH-Px (Figure 2C). Moreover, the supplementation of both the antibiotic and GSPE significantly increased (P < 0.01) the content of serum CAT and decreased the MDA of birds (Figures 2D, E).
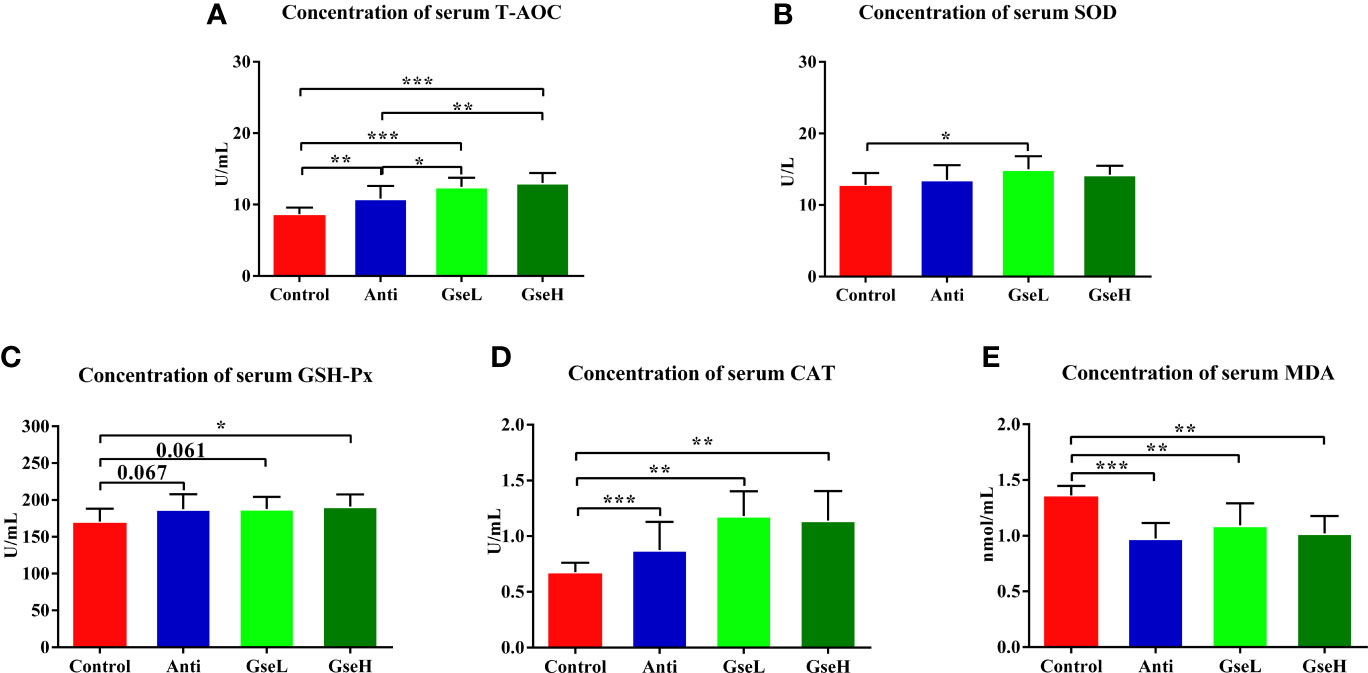
Figure 2 Effects of Anti, GseL, and GseH on the serum contents of (A) T-AOC (B), SOD (C), GSH-Px (D), CAT, and (E) MDA in broilers on d 21. *P < 0.05 **P < 0.01, ***P < 0.001, tendency changes but no significant difference were considered at 0.1 < P < 0.05, when compared to the Control treatment. N = 16 per treatment group.
Effects of GSPE on Serum and Mucosal Immune Indexes
As demonstrated in Figure 3A, the levels of serum IgA in the GseL and Anti treated broilers were significantly higher than in the Control birds, while no significant difference was found in the serum IgM regardless of treatments. In addition, the level of serum IgY in the GseH birds was higher (P = 0.071) than that in the Control birds, while no significant difference was found within other groups. Compared with the Control birds, the GSPE birds had significantly (P < 0.05) fewer IL-6 and IL-1β in their serum (Figure 3B). Additionally, the supplementation of Anti (P = 0.063) and GseH (P = 0.081) decreased the level of IL-6 when compared with the Control group, which there was no treatment effects. Moreover, the contents of ileal and jejunal mucosal IL-1β in birds supplemented with Anti or GSPE were significant lower (P < 0.05) than those in the Control birds (Figures 3C, D). Compared with the Control birds, although there was no significant difference, the supplementation of GseL (P = 0.091) and GseH (P = 0.070) decreased the jejunal mucosal IL-6, while only GseH decreased (P = 0.073) the ileal mucosal IL-8.
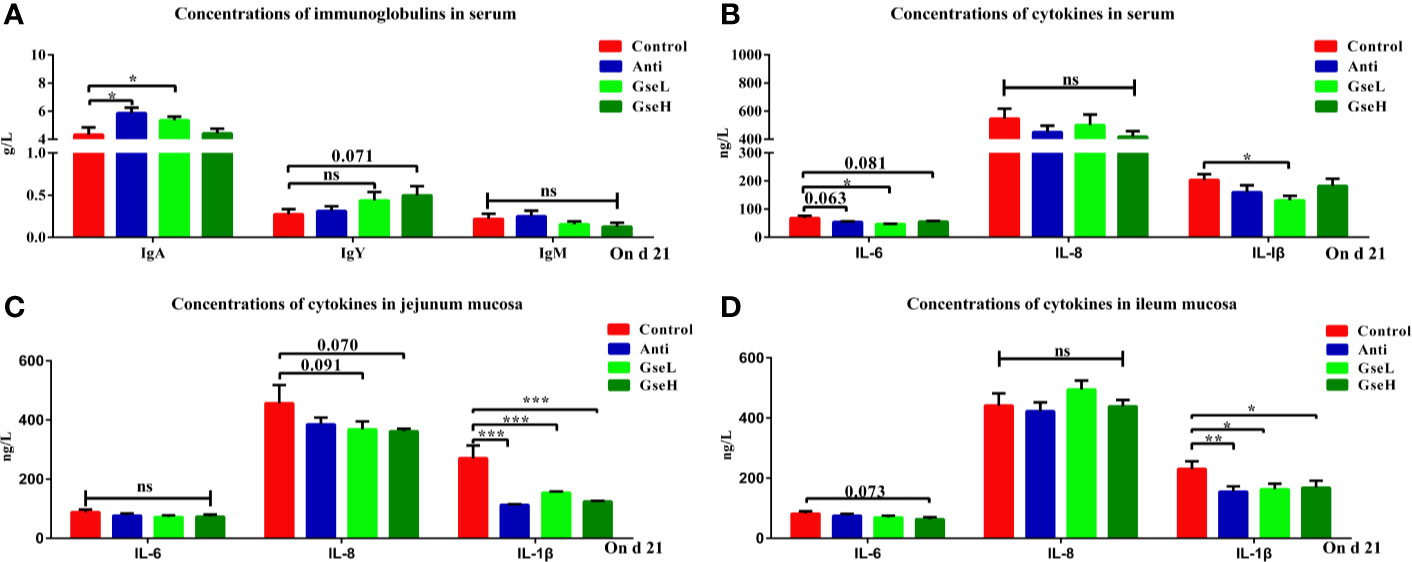
Figure 3 Effects of Anti, GseL, and GseH on (A) levels of serum immunoglobulins (B), levels of serum cytokines (C), levels of jejunum mucosal cytokines, and (D) levels of ileum mucosal cytokines in broilers on d 21. *P < 0.05 **P < 0.01, ***P < 0.001, tendency changes but no significant difference were considered at 0.1 < P < 0.05, when compared to the Control treatment. N = 16 per treatment group. ns, not significant.
Effects of GSPE on Cecal SCFAs
The effects of GSPE on the concentrations of cecal SCFAs are shown in Figure 4. Both the supplementation of antibiotic and GSPE significantly increased (P < 0.05) the concentrations of butyrate and valerate in the colonic content of birds (Figures 4A, B). The GseL birds had a significant higher (P < 0.05) concentration of isobutyrate than the Anti and GseH birds. In addition, the supplementation of GseH increased (P = 0.096) the concentration of propionate to a higher level when compared to the Control treatment, although there was no significant difference. The GseL birds had a higher (P = 0.068) concentration of isovalerate when compared to the Anti birds, while there was no significant difference.
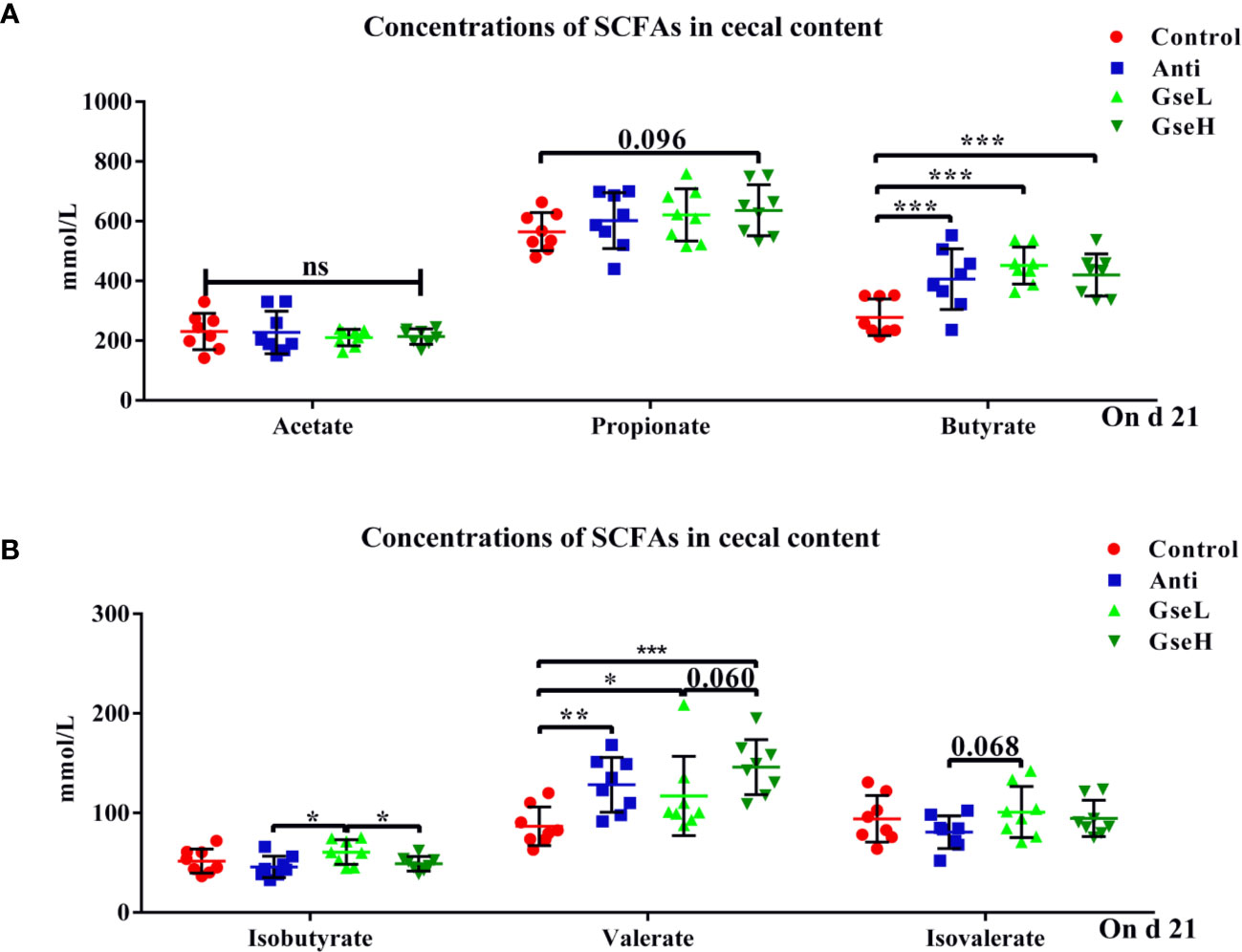
Figure 4 Effects of Anti, GseL, and GseH on the cecal concentrations of (A) acetate, propionate, and butyrate (B), isobutyrate, valerate, and isovalerate in broilers on d 21. *P < 0.05 **P < 0.01, ***P < 0.001, tendency changes but no significant difference were considered at 0.1 < P < 0.05, when compared to the Control treatment. N = 8 per treatment group.
Effects of GSPE on Cecal Microflora
Birds in all treatment groups shared 567 OTUs of cecal microflora in the Venn diagram (Figure 5A). The Control, Anti, GseL, and GseH birds had 145, 101, 74, and 150 particular OTUs, respectively. Through the relative abundance of top bacterial strains in genus level (Figure 5B), Bacteroides, Escherichia-Shigella, Alistipes, Ruminococcaceae_UCG-014, Faecalibacterium, Ruminococcaceae_UCG-005, Parasutterella, Butyricicoccus, Lachnospiraceae_NK4A136_group, and Lactobacillus predominated in all birds. There was no significant difference in the alpha-diversity index (only the Shannon index is provided) within all the treatments (Figure 5C). Conversely, the beta-diversity analysis using principal component analysis (PCA) showed that the Control samples were the most different to other groups (Figure 5D). The PCoA 3D diagram shows samples were clustered within their group, the four treatment groups were well separated from each other (Figure 5E).
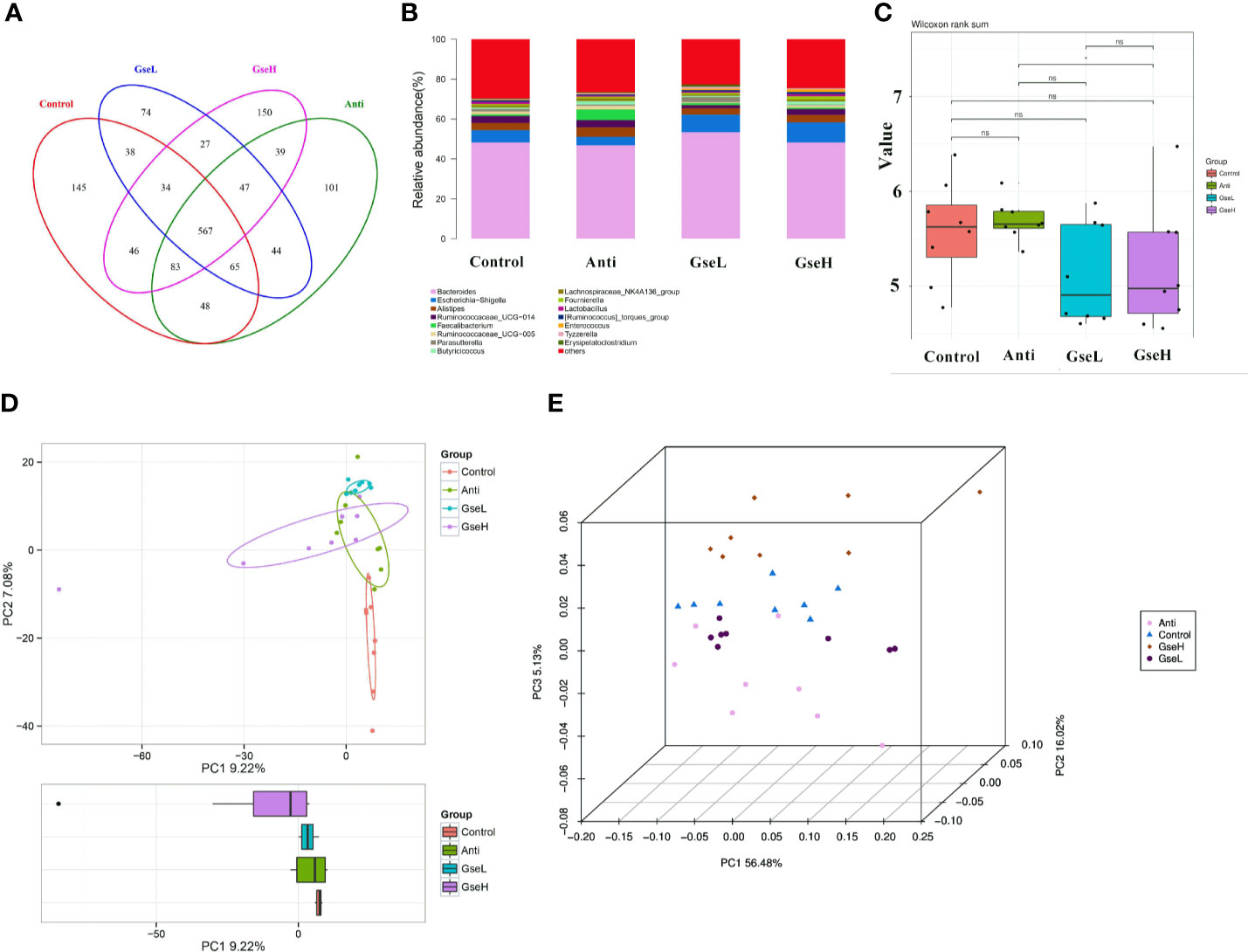
Figure 5 Summary of the microbial community in the cecal contents of broilers on d 21 (A). is a Venn diagram summarizing the numbers of common and unique observed taxonomic units (OTUs) in the microflora (B). is the top 15 relative abundances of bacterial strains (at the genus level) between groups (C). is the Shannon Index reflecting species diversity within and between groups (D). is the PCA plot (E). is the PCoA analysis. N = 8 per treatment group.
There was no significant difference in the relative abundance ratio of Firmicutes : Bacteroidetes in the cecal microflora within all the groups (Figure 6A). The LEfSe analysis indicated that the predominant bacterial strains of GseL were Gammaproteobacteria, Parasutterella, Betaproteobacteriales, and Erysipelatoclostridium. The predominant bacterial strains of GseH were Lactobacillales, Lewinalla, Lactobacillus, and Candidatus-Stoquefichus. The predominant bacterial strains in the Control group were Muribaculaceae, Mollicutes, Tenericutes, and Anaeroplasmataceae. The predominant bacterial strains in the Anti group were Clostridiales, Ruminococcaceae, Faecalibacterium, Ruminococcaceae-UCG-005, Ruminococcaceae-UCG-014, and Lachnospiraceae-NK4A136 (Figure 6B). A combined analysis of contents of cecal SCFAs and the top 15 cecal bacteria strains in genus level in the differential dietary treatments, the redundancy analysis (RDA) revealed that the GSPE treatment played a more valuable role in the cecal microfloral variation in birds when compared with antibiotic treatment. The correlations between the GSPE and Anti treatments were negative. Moreover, Lactobacillus and the Ruminococcaceae_torques_group had the highest correlation with the production of propionate. Meanwhile, the content of isobutyrate and valerate played a positive role in distinguishing the change of cecal microfloral structure (Figure 6C). Based on the Greengenes data, PICRUSt analysis showed that the dietary GSPE treatment induced higher heatmap scores in the known functional genes for amino acid metabolism, lipid metabolism, carbohydrate metabolism, cell communication, cellular processes and signaling, energy metabolism, metabolism of cofactors and vitamins, and xenobiotics biodegradation and metabolism when compared to the Control treatment (Figure 6D).
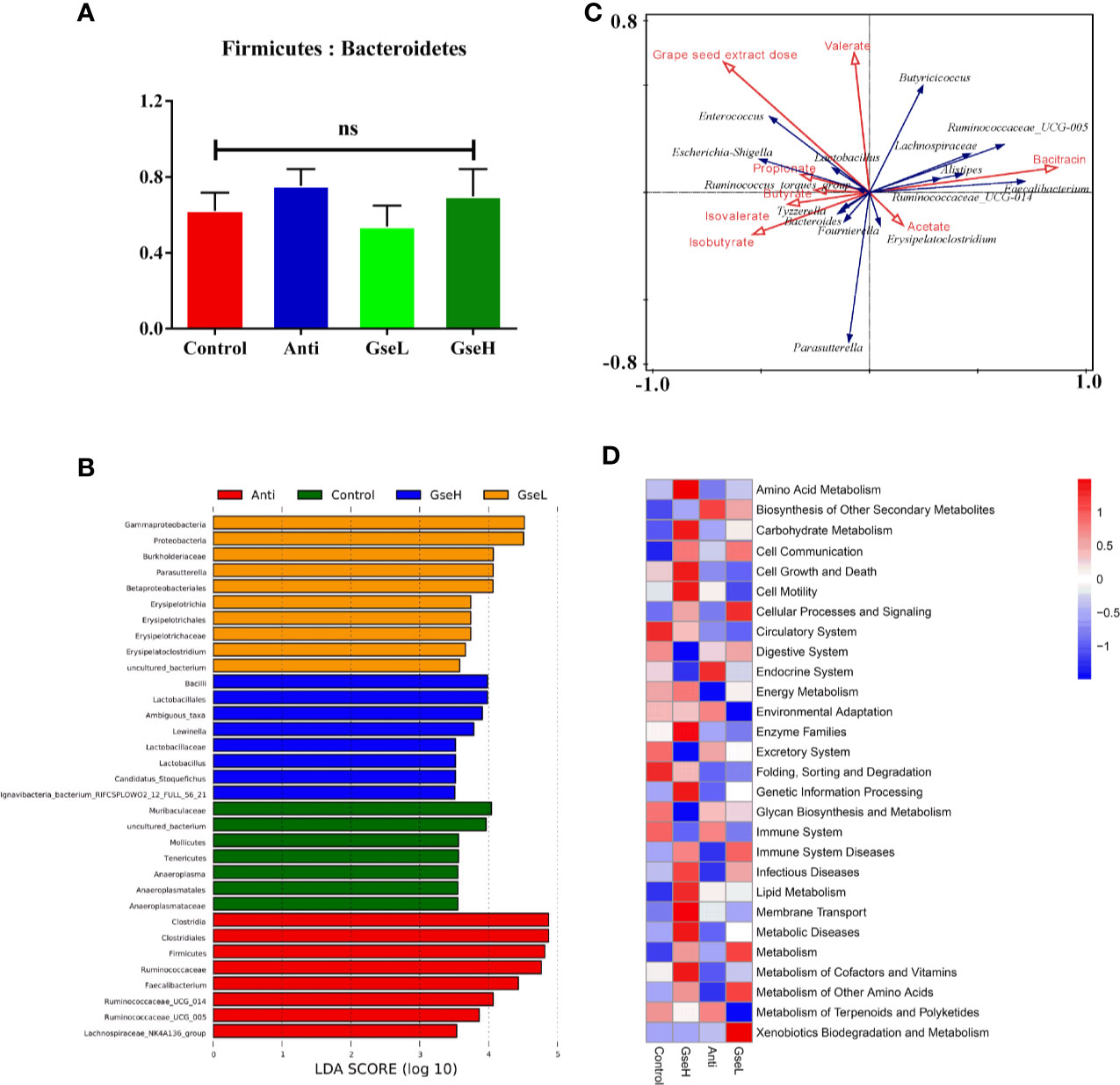
Figure 6 Summary of microbial communities in the cecal contents of broilers on day 21 (A). is the Firmicutes/Bacteroidetes (B). represents the LEfSe analysis (C). is the RDA (D). provides the PICRUSt analysis. N = 8 per treatment group. ns, not significant.
Effects of GSPE on Serum Metabolome
To investigate the metabolic regulation in GSPE treated birds, the serum metabolites were analyzed using UPLC-Q-TOF/MS. Data showed that 369 identified metabolites were shared among all the treatment groups. There were 394, 64, 135, and 246 metabolites identified in the serum of birds in Control, Anti, GseL, and GseH groups respectively (Figure 7A). The Control and Anti samples were well distinguished with those of the GSPE treatment groups using the PCA analysis. There was no definite separation between the GseL and GseH groups (Figure 7B). Hence, we hypothesized that the GSE dosage (i.e., 400 mg/kg) was not enough to cause marked changes in the serum metabolites within the GSPE treatment groups.
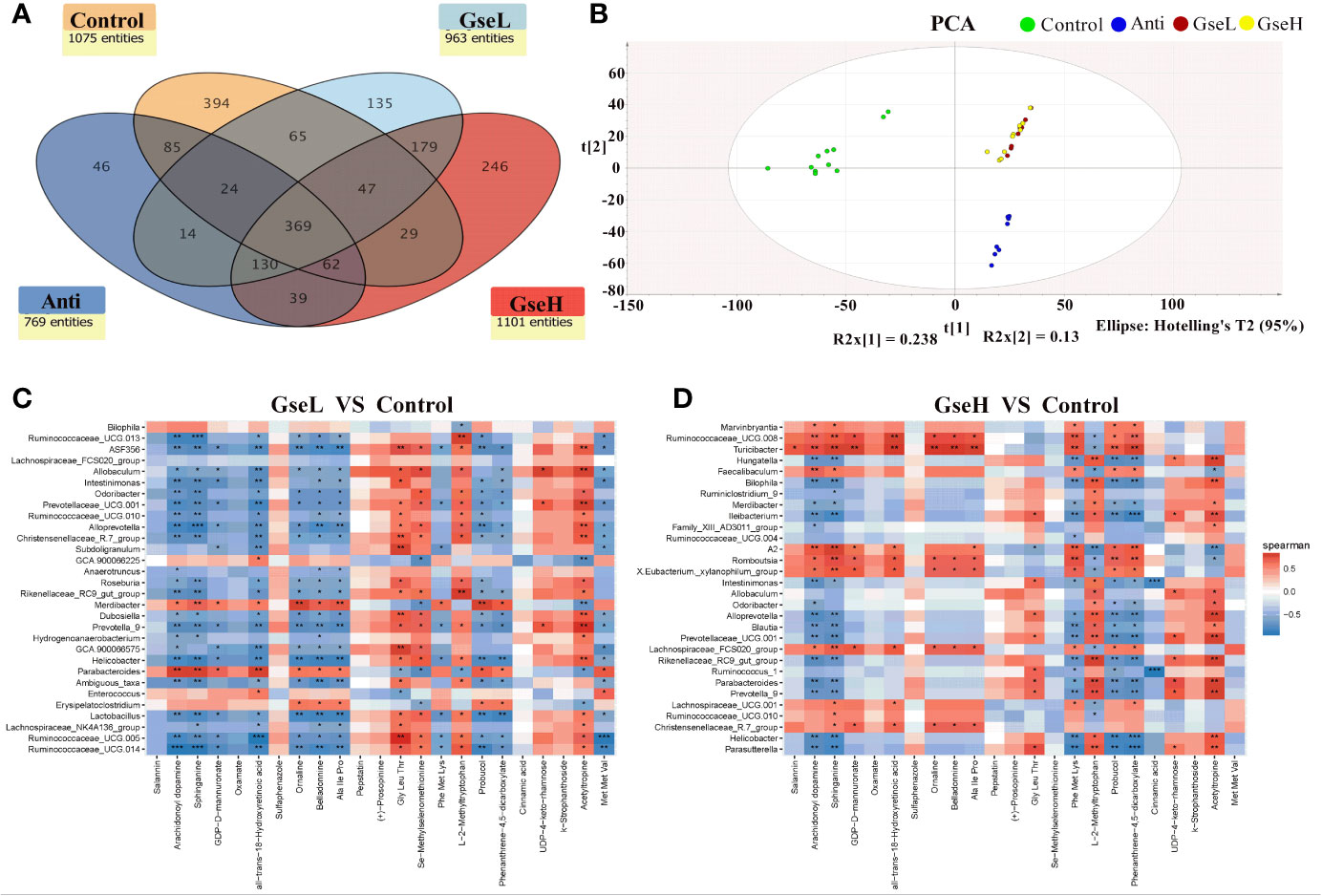
Figure 7 (A) is a Venn diagram summarizing the numbers of common and unique observed entities in serum metabolome. (B). represents the PCA plot of the broilers’ serum metabolome. (C, D). represent the correlation between significantly changed serum metabolites and the top 30 distinguished cecal bacteria strains (at the genus level) between the GSPE-treated and Control groups. *P < 0.05 **P < 0.01, ***P < 0.001, compared to the Control treatment. N (Control) = 12; N (Anti, GseL, GseH) = 10.
Moreover, 23 serum metabolites were significantly changed (up-regulated and down-regulated) and detected within all the differential groups after screening with log2 fold change > 2 and P-value < 0.05 (Table 2). It can be seen that both the dietary GSPE and Anti significantly increased the serum concentration of all-trans-18-hydroxyretinoic acid, arachidonoyl dopamine, sphinganine, and probucol (mainly belonging to prenol lipids and sphingolipid) to higher levels when compared with the Control treatment. The dietary GSPE significantly down-regulated the k-strophanthoside related with sterol lipids. The dietary supplementation with both GseL and the antibiotic substantially increased ornaline and oxamate to higher levels when compared with the Control treatment. The GSPE treatment significantly down-regulated the L-2-methyltryptophan and pepstatin. The dietary GSE treatment up-regulated the GDP-D-mannuronate and UDP-4-keto-rhamnose (related with amino sugar and nucleotide sugar metabolism). Moreover, three kinds of alkaloids (acetyltropine, belladonnine, and salannin) were significantly increased by the GseL treatment (P < 0.05). One alkaloid [(+)-prosopinine] was significantly decreased by the GSPE and the antibiotic treatment (P < 0.05). The GSPE supplementation significantly increased small peptides (Ala Ile Pro and Met Met Val) and decreased Gly Leu Thr and Phe Met Lys. In addition, the dietary supplementation of GSPE and the antibiotic extremely increased the concentration of Se-methylselenomethionine and decreased that of phenanthrene-4,5-dicarboxylate and sulfaphenazole.
The correlation between the significantly changed serum metabolites and the top 30 distinguished cecal bacteria strains at the genus level is provided in a visual model (as shown in Figures 7C, D). We found that sphinganine, all-trans-18-hydroxyretinoic acid, and belladonnine were negatively correlated with the relative abundance of Ruminococcaceae_UCG.14, Ruminococcaceae_UCG.005, Lactobacillus, Helicobacter, Alloprevotella, Ruminococcaceae_UCG.013, and Intestinimonas. Conversely, they positively correlated to the relative abundance of Parabacteroides and Merdibacter in birds that received GseL supplementation. Interestingly, sphinganine, all-trans-18-hydroxyretinoic acid, and belladonnine were positively correlated with the relative abundance of Ruminococcaceae_UCG.010, Christensenellaceae_R.7_group, Lachnospiraceae_UCG.001, Lachnospiraceae_FCS020_group, Romboutsia, X.Eubacterium._xylanophilum_group, and Ruminococcaceae_UCG.008 in birds that received the GseH supplementation. Sphinganine was negatively correlated with Rikenellaceae_RC9_gut_group, Prevotellaceae_UCG.001, Blautia, Alloprevotella, Ileibacterium, Bilophila, and Hungatella in birds pretreated with GseH.
Discussion
Currently, our results suggest that dietary supplements of phenolic compounds are likely to improve the health status without affecting productive performance. Proanthocyanidins are natural compounds in plant-based foods (i.e., GSE),. A couple of recently studies also have been conducted to investigate the applications and effects of GSPE in poultry industry (17, 18). To date, adequate evidence exists to support the antioxidation, anti-inflammatory, and antimicrobial activity of polyphenols. A previous study conducted by Abu Hafsa and Ibrahim (19) confirmed that grape seed could be treated as an herbal additive agent in the diet of broilers to improve the body weight gain and final body weight (19). GSPE supplementation eliminated the toxic effect of AFB1 on the growth performance of broilers by improving ADFI, ADG, and FCR (13, 19). The present study aligned with previous studies, the addition of GSPE increased the ADG of birds. In addition, the treatment with GSPE decreased plasma ghrelin which normal functions to inhibit food intake and animal weight loss (20, 21). Our results also revealed the supplementation of GSPE markedly decreased the ADFI of birds. Similar to antibiotic treatment, GSPE treatments caused an extreme reduction in F:G. Viveros et al. (12). observed that the supplementation of polyphenol-rich grape pomace extract improved the F:G ratio and nutrition digestibility by increasing the absorptive surface in the intestine of broilers (12). The growth performance of the birds was not influenced by the higher GSPE dose in the present study.
Previous studies revealed that the addition of dietary GSPE could improve the oxidative stress resistance by activating the Nrf2 signaling pathway in broilers (13, 22). Diets containing GSPE (250 and 500 mg/kg) significantly increased the total protein, albumin, and globulin. It decreased the levels of aspartate aminotransferase, alanine aminotransferase, and alkaline phosphatase in the serum of broilers infected with AFB1 (7). We found that the dietary supplementation of GSPE improved the antioxidant levels in birds in the early developmental stage, including increased serum T-AOC, SOD, and GSH-Px and decreased MDA. In addition, the supplementation of commercial grape extracts in broiler chicken diets improved the oxidative stability of the meat (23). It is well known that antioxidative substances derived from plant can greatly reduce damage according to the oxidants by neutralizing free radicals before they attack host cells, and then prevent injuring to proteins, enzymes, and lipids. Dietary grape products might cause an increment in circulating phenolic metabolites by increasing plasmatic α- and γ-tocopherol concentrations, which improves the antioxidant status of broilers (5).
GSPE supplementation significantly ameliorated the serum IL-1β, IL-6, IL-10, and TNF-α. It down-regulated the mRNA expression of serum pro-inflammatory cytokines IL-1β, IL-6, and TNF-α in the spleens of broilers exposed in AFB1 (7). A study by Liu et al. (24). also confirmed that GSE ameliorated host inflammation in mice fed a high-fat diet by markedly decreased the concentrations of serum pro-inflammatory cytokines such as TNF-α, IL-6, and MCP-1 (24). Our results show the supplementation of GSPE to basal diets increased the content of serum IgA and IgY and decreased the content of intestinal IL-1β, and serum IL-6 and IL-1β. GSPE may have changed the mucosal immune system at the jejunum and ileum surfaces by regulating gut microbiota, as suggested by Koren et al. (25). Salinas-Sánchez et al. (26). also speculated that proanthocyanidin derived from GSPE plays an anti-inflammatory role by modulating the microbiota (26). The links between the anti-inflammatory effect and the regulation of intestinal microbiota of GSPE are yet to be elucidated.
The digestive tract contains an abundance of microbial-derived metabolites, which can be easily absorbed through the intestine and play a valuable role in the maintenance of intestinal health (27). Studies confirmed that the SCFAs derived from intestinal microbiota may directly or indirectly affect the unabsorbed nutrients in the intestinal tract (28, 29). As suggested by previous studies, dietary proanthocyanidins can be degraded by intestinal microorganisms into simple phenolic compounds (24, 30). The ingested GSPE was relatively stable in the colon and may affect gastrointestinal tract health (31). GSPE significantly reduced cecal butyrate concentration and modulated the ratio of acetate:propionate:butyrate in mice (32). These studies were in accordance with our findings that the dietary GSPE treatment led to the increment of major colonic SCFAs (butyrate, propionate, and isobutyrate) in present study.
Grape by-products (are extensively debated as antibacterial and feed additives) change the gastrointestinal microflora of broilers (29, 33). Research has confirmed that the flavonoids existing in grape by-products are able to increase the growth of beneficial intestinal bacteria and also restrict certain pathogenic organisms, such as E. coli, Candida albicans, and Staphylococcus aureus (4, 6). Casanova-Martí et al. (32). identified that GSPE changes the diversity of cecal microbiota. This result aligns with the general opinion that polyphenols increase Bacteroidetes and decrease Firmicutes phyla (32). In the present study, the ratio of Firmicutes : Bacteroidetes populations in the GSPE group was not substantially changed; the ratio is normally treated as a marker for intestinal inflammation. Therefore, we undertook a LEfSe analysis and found Betaproteobacteriales, Erysipelatoclostridium, Parasutterella, Lewinalla, Lactobacillus, and Candidatus-Stoquefichus dominated the GSPE treatment. Obviously, the dosage of GSPE influenced the dominant microflora. Other studies produced similar results, dietary polyphenols can help to maintain bowel health by promoting the development of beneficial bacteria (i.e., Lactobacilli and Bifidobacteria), thus demonstrating the positive prebiotic effect of polyphenols (34). Anhê et al. (35). also confirmed that polyphenols extracted from different plants played a beneficial role in modulating the gut microbiota composition, which increased the abundance of Bifidobactrium spp., Clostridiales, Faecalibacterium spp., Lactobacillus spp., and especially the growth of Akkermansia muciniphila (35). Some micro-organisms, such as Lactobacilli, have been confirmed to use polyphenols compounds in GSE as nutritional substrates to metabolize phenolic compounds for providing energy to cells and effectively affecting bacterial metabolism (12, 36).
The growing evidence reveals that dietary polyphenols from plants including those from grape, tea, and coffee influence the glucose, protein, amino acids, and lipid metabolism in vitro and in vivo studies (37), which is similar our results to some extent. An earlier study found that GSPE influenced the lipid metabolism by reducing the levels of circulating free fatty acids, limiting the dietary fat absorption and the accumulation of fat in adipose tissue (38). Dietary polyphenols may modulate the glucose metabolism by stimulating peripheral glucose uptake (39). In another study, El-Alfy et al. (40). found that orally administered GSPE decreased the lipid peroxidation and substantially inhibited the rise in blood glucose levels in rats injected with alloxan (40). A rare test was conducted to estimate the change in serum metabolites in GSPE supplemented broilers. Changes in metabolites for lipids, amino acids, alkaloids, and aspartate protease were consistent with previous results in the PICRUSt analysis. Hence, we used MetaboAnalyst 4.0 for analyzing the significantly changed serum metabolites in Gallus gallus to identify the related metabolism pathways. Both sphinganine (belonging to the sphingolipid metabolism pathway) and all-trans-18-Hydroxyretinoic acid (belonging to the retinol metabolism pathway) were up-regulated due to the GSPE treatment. Moreover, although no study on the effects of GSPE on serum alkaloids have been reported, the increment of acetyltropine, belladonnine, and salannin and the decrement of (+)-prosopinine were screened for the GSPE treatment. We considered them as the biomarkers for evaluating the influence of dietary GSPE treatment on bird serum metabolites.
GSPE (with their metabolites) reach the colon by enterohepatic recirculation. They were catabolized by colonic microbiota before absorption, generating metabolites in the serum (5). The dietary GSPE encouraged the prevention and treatment of metabolic disorders by targeting intestinal microbiota through a potential prebiotic agent (24). Interestingly, the acetyltropine was oppositely correlated with the bacterial strains related with sphinganine, all-trans-18-hydroxyretinoic acid, and belladonnine. Further study is required to provide a definite explanation. Additional tests should be conducted to investigate the relationship between the digestive microflora and serum metabolome in broilers supplemented with GSPE.
Conclusion
In summary, we found that GSPE significantly improved the growth performance, increased the serum IgA and IgY concentrations, decreased the mucosal IL-6 and IL-1β, and increased kinds of SCFAs in broilers. Data of 16S rRNA high throughput sequencing of cecal microflora revealed that Betaproteobacteriales, Erysipelatoclostridium, Parasutterella, Lewinalla, Lactobacillus, and Candidatus-Stoquefichus dominated the cecal microflora of birds with GSPE treatment, which higher heatmap scores in the known functional genes for amino acid, lipid, carbohydrate cofactors and vitamins metabolism, cell communication, cellular processes and signaling, and xenobiotics biodegradation and metabolism in GSPE-treated birds from PICRUSt analysis. Based on the non-targeted serum metabolomic method using UPLC-Q-TOF/MS, our statistical analysis found that 13 serum metabolites were significantly up-regulated, and 10 metabolites were significantly down-regulated by the GSPE-supplemented diets in broilers. Additionally, we also identified that all-trans-18-hydroxyretinoic acid and sphinganine may be used as potential biomarkers for evaluating the GSPE regulations. Our findings provide a scientific foundation for GSPE application in broiler feed in the future.
Data Availability Statement
The original contributions presented in the study are publicly available. This data can be found here: http://www.ncbi.nlm.nih.gov/bioproject/68270; PRJNA682703.
Ethics Statement
The animal study was reviewed and approved by the Animal Care and Use Committee of Zhejiang A&F University.
Author Contributions
GC and CY designed the study, conducted the experiments, and wrote the manuscript. FY and YW detected the samples. XZ, JL, ZX, and FT analyzed the results. All authors contributed to the article and approved the submitted version.
Funding
We are very grateful for the support of the Natural Science Foundation of Zhejiang Province (No. Q20C170006), the National Natural Science Foundation of China (No. 32002195, 31702153), the Natural Science Foundation of Zhejiang Province (No. LY17C170004), and Basic Technology Research Programs of Zhejiang Province (No. LGN18C200024).
Conflict of Interest
XZ, JL, and ZX were employed by Zhejiang Vegamax Biotechnology Co., Ltd.
The remaining authors declare that the research was conducted in the absence of any commercial or financial relationships that could be construed as a potential conflict of interest.
Acknowledgments
We acknowledge Zhejiang Vegamax Biotechnology Co., Ltd. and Zhejiang Skyherb Biotechnology Co., Ltd (Anji, Zhejiang, China) for providing the dietary GSPE.
References
1. Sayin VI, Ibrahim MX, Larsson E, Nilsson JA, Lindahl P, Bergo MO. Antioxidants accelerate lung cancer progression in mice. Sci Transl Med (2014) 6:221ra15. doi: 10.1126/scitranslmed.3007653
2. Ouédraogo M, Charles C, Ouédraogo M, Guissou IP, Stévigny C, Duez P. An overview of cancer chemopreventive potential and safety of proanthocyanidins. Nutr Cancer (2011) 63:1163–73. doi: 10.1080/01635581.2011.607549
3. Badet C, Preedy VR, Watson RR, Patel VB. Antibacterial Activity of Grape (Vitis vinifera, Vitis rotundifolia) Seeds. Nuts Seeds Health Dis Prev (2011) 65:545–52. doi: 10.1016/B978-0-12-375688-6.10065-9
4. Silvan JM, Mingo E, Hidalgo M, de Pascual-Teresa S, Carrascosa AV, Martinez-Rodriguez AJ. Antibacterial activity of a grape seed extract and its fractions against Campylobacter spp. Food Control (2013) 29:25–31. doi: 10.1016/j.foodcont.2012.05.063
5. Irene M, Chamorro S, Jara P, Patricia L, Inmaculada Á, Herrero AM, et al. Phenolic metabolites in plasma and thigh meat of chickens supplemented with grape byproducts. J Agric Food Chem (2019) 67:4463–71. doi: 10.1021/acs.jafc.9b00222
6. Brenes A, Viveros A, Chamorroa S, Arija I. Use of polyphenol-rich grape by-products in monogastric nutrition. Anim Feed Sci Technol (2016) 211:1–17. doi: 10.1016/j.anifeedsci.2015.09.016
7. Ali RS, Sun L, Zhang N, Mohamed Khalil M, Gao X, Ling Z, et al. Ameliorative effects of grape seed proanthocyanidin extract on growth performance, immune function, antioxidant capacity, biochemical constituents, liver histopathology and aflatoxin residues in broilers exposed to aflatoxin b1. Toxins (2017) 9:371. doi: 10.3390/toxins9110371
8. Demirkaya E, Avci A, Kesik V, Karslioglu Y, Oztas E, Kismet E, et al. Cardioprotective roles of aged garlic extract, grape seed proanthocyanidin, and hazelnut on doxorubicin-induced cardiotoxicity. Can J Physiol Pharmacol (2009) 87:633–40. doi: 10.1139/y09-051
9. Ahn SH, Kim HJ, Jeong I, Hong YJ, Kim MJ, Rhie DJ, et al. Grape seed proanthocyanidin extract inhibits glutamate-induced cell death through inhibition of calcium signals and nitric oxide formation in cultured rat hippocampal neurons. BMC Neurosci (2011) 12:78. doi: 10.1186/1471-2202-12-78
10. Wang CZ, Mehendale SR, Calway T, Yuan CS. Botanical flavonoids on coronary heart disease. Am J Chin Med (2011) 39:661–71. doi: 10.1142/S0192415X1100910X
11. Papadopoulou C, Soulti K, Roussis IG. Potential antimicrobial activity of red and white wine phenolic extracts against strains of S. aureus, E. coli and Candida albi-cans. J Food Technol Biotechnol (2005) 43:41–6. doi: 10.1080/08905430500316474
12. Viveros A, Chamorro S, Pizarro M, Arija I, Centeno C, Brenes A. Effects of dietary polyphenol-rich grape products on intestinal microflora and gut morphology in broiler chicks. Poult Sci (2011) 90:566–78. doi: 10.3382/ps.2010-00889
13. Long M, Zhang Y, Li P, Yang SH, Zhang WK, Han JX, et al. Intervention of grape seed proanthocyanidin extract on the subchronic immune injury in mice induced by aflatoxin B1. Int J Mol Sci (2016) 17:516. doi: 10.3390/ijms17040516
14. Cardona F, Andres-Lacueva C, Tulipani S, Tinahones FJ, Queipo-Ortuno MI. Benefits of polyphenols on gut microbiota and implications in human health. J Nutr Biochem (2013) 24:1415–22. doi: 10.1016/j.jnutbio.2013.05.001
15. Chamorro S, Goñi I, Viveros A, Hervert-Hernandez D, Brenes A. Changes in polyphenolic content and antioxidant activity after thermal treatments of grape seed extract and grape pomace. Eur Food Res Technol (2012) 234:147–55. doi: 10.1007/s00217-011-1621-7
16. Li Q, Sun M, Wan Z, Liang J, Wang K. Bee pollen extracts modulate serum metabolism in lipopolysaccharide-induced acute lung injury mice with anti-inflamm-atory effects. J Agric Food Chem (2019) 67:7855–68. doi: 10.1021/acs.jafc.9b03082
17. Ebrahimzadeh SK, Navidshad B, Farhoomand P, Mirzaei Aghjehgheshlagh F. The metabolizable energy content and effect of grape pomace with or without tannase enzyme treatment in broiler chickens. Iranian J Appl Anim Sci (2017) 7(3):479–86. doi: 10.5402/2011/780540
18. Azizi M, Seidavi AR, Ragni M, Laudadio V, Tufarelli V. Practical applications of agricultural wastes in poultry feeding in Mediterranean and Middle East regions. Part 1: citrus, grape, pomegranate and apple wastes. World Poult Sci J (2018) 74(3):489–98. doi: 10.1017/S0043933918000478
19. Abu Hafsa SH, Ibrahim SA. Effect of dietary polyphenol-rich grape seed on growth performance, antioxidant capacity and ileal microflora in broiler chicks. J Anim Physiol N (2018) 102:268–75. doi: 10.1111/jpn.12688
20. Serrano J, Casanova-Martí À, Gual A, PérezVendrell AM, Blay MT, Terra X, et al. A specific dose of grape seed-derived proanthocyanidins to inhibit body weight gain limits food intake and increases energy expenditure in rats. J Nutr (2017) 56:1629–36. doi: 10.1007/s00394-016-1209-x
21. Ginés I, Gil-Cardoso K, Terra X, Blay MT, Pérez-Vendrell AM, Pinent M, et al. Grape seed proanthocyanidins target the enteroendocrine system in cafeteria-diet-fed rats. Mol Nutr Food Res (2019) 63:e1800912. doi: 10.1002/mnfr.201800912
22. Ali Rajput S, Sun L, Zhang NY, Khalil M, Zhao L, Li C, et al. Grape seed proanthocyanidin extract alleviates aflatoxinB1-induced immunotoxicity and oxidative stress via modulation of NF-κB and Nrf2 signaling pathways in broilers. Toxins (2019) 11:23. doi: 10.3390/toxins11010023
23. Brenes A, Viveros A, Goñi I, Centeno C, Saura-Calixto F, Arija I. Effect of grape seed extract on growth performance, protein and polyphenol digestibilities, and antioxidant activity in chickens. Span J Agric Res (2010) 8:326–35. doi: 10.5424/sjar/2010082-1199
24. Liu W, Zhao S, Wang J, Shi J, Sun Y, Wang W, et al. Grape seed proanthocyanidin extract ameliorates inflammation and adiposity by modulating gut microbiota in high-fat diet mice. Mol Nutr Food Res (2017) 61:1601082. doi: 10.1002/mnfr
25. Koren O, Goodrich JK, Cullender TC, Spor A, Laitinen K, Backhed HK, et al. Host remodeling of the gut microbiome and metabolic changes during pregnancy. Cell (2012) 150:470–80. doi: 10.1016/j.cell.2012.07.008
26. Salinas-Sánchez DO, Jimenez-Ferrer E, Sanchez-Sanchez V, Zamilpa A, Gonzalez-Cortazar M, Tortoriello J, et al. Anti-inflammatory activity of a polymeric proanthocyanidin from Serjania schiedeana. Molecules (2017) 22:E863. doi: 10.3390/molecules22060863
27. Chamorro S, Romero C, Brenes A, Sanchez-Patan F, Bartolome B, Viveros A, et al. Impact of a sustained consumption of grape extract on digestion, gut microbial metabolism and intestinal barrier in broiler chickens. Food Funct (2019) 10:1444–54. doi: 10.1039/c8fo02465k
28. Hino S, Takemura N, Sonoyama K, Morita A, Kawagishi H, Aoe S, et al. Small intestinal goblet cell proliferation induced by ingestion of soluble and insoluble dietary fiber is characterized by an increase in sialylated mucins in rats. Nutr (2012) 142:1429–36. doi: 10.3945/jn.112.159731
29. Barcelo A, Claustre J, Moro F, Chayvialle J, Cuber J, Plaisancie P. Mucin secretion is modulated by luminal factors in the isolated vascularly perfused rat colon. Gut (2000) 46:218–24. doi: 10.1136/gut.46.2.218
30. Ou K, Sarnoski P, Schneider KR, Song K, Khoo C, Gu L. Microbial catabolism of procyanidins by human gut microbiota. Mol Nutr Food Res (2014) 58:2196–205. doi: 10.1002/mnfr.201400243
31. Choy YY, Jaggers GK, Oteiza PI, Waterhouse AL. Bioavailability of intact proanthocyanidins in the rat colon after ingestion of grape seed extract. J Agric Food Chem (2013) 61:121–7. doi: 10.1021/jf301939e
32. Casanova-Martí À, Serrano J, Portune KJ, Sanz Y, Blay MT, Terra X, et al. Grape seed proanthocyanidins influence gut microbiota and enteroendocrine secretions in female rats. Food Func (2018) 9:1672–82. doi: 10.1039/c7fo02028g
33. Xia EQ, Deng GF, Guo YJ, Li HB. Biological activities of polyphenols from grapes. Int J Mol Sci (2010) 11:622–46. doi: 10.3390/ijms11020622
34. Duenas M, Munoz-Gonzalez I, Cueva C, Jimenez-Giron A, Sanchez-Patan F, Santos-Buelga C, et al. A survey of modulation of gut microbiota by dietary polyphenols. BioMed Res Int (2015) 2015:850902. doi: 10.1155/2015/850902
35. Anhê FF, Varin TV, Le Barz M, Desjardins Y, Levy E, Roy D, et al. Gut microbiota dysbiosis in obesity-linked metabolic diseases and prebiotic potential of polyphenol-rich extracts. Curr Obes Rep (2015) 4:389–400. doi: 10.1007/s13679-015-0172-9
36. García-Ruíz A, Bartolomé B, Martínez-Rodríguez AJ, Puello E, Martín-Álvarez PJ, Moreno-Arribas MV. Potential of phenolic compounds for controlling lactic acid bacteria growth in wine. Food Control (2008) 19:835–41. doi: 10.1016/j.foodcont.2007.08.018
37. Goñi I, Brenes A, Centeno C, Viveros A, Saura-Calixto F, Rebolé A, et al. Effect of dietary grape pomace and vitamin E on growth performance, nutrient digestibility and susceptibility to meat lipid oxidation in chickens. Poult Sci (2007) 86:508–16. doi: 10.1093/ps/86.3.508
38. Diego AM, Nebojsa I, Alexander P, Dawn LB, Susan KF, Ilya R. Inhibitory effects of grape seed extract on lipases. Nutrition (2003) 19:876–9. doi: 10.1016/s0899-9007(03)00167-9
39. Hanhineva K, Törrönen R, Bondia-Pons I, Pekkinen J, Kolehmainen M, Mykkänen H, et al. Impact of dietary polyphenols on carbohydrate metabolism. Int J Mol Sci (2010) 11:1365–402. doi: 10.3390/ijms11041365
Keywords: anti-inflammatory effect, proanthocyanidins, grape seed extract, cecal microflora, serum metabolomics
Citation: Cao G, Zeng X, Liu J, Yan F, Xiang Z, Wang Y, Tao F and Yang C (2020) Change of Serum Metabolome and Cecal Microflora in Broiler Chickens Supplemented With Grape Seed Extracts. Front. Immunol. 11:610934. doi: 10.3389/fimmu.2020.610934
Received: 28 September 2020; Accepted: 02 November 2020;
Published: 08 December 2020.
Edited by:
Kai Wang, Chinese Academy of Agricultural Sciences (CAAS), ChinaReviewed by:
Qingbiao Xu, Huazhong Agricultural University, ChinaHeng-wei Cheng, Livestock Behavior Research Unit, USDA, United States
Alireza Seidavi, Islamic Azad University, Iran
Copyright © 2020 Cao, Zeng, Liu, Yan, Xiang, Wang, Tao and Yang. This is an open-access article distributed under the terms of the Creative Commons Attribution License (CC BY). The use, distribution or reproduction in other forums is permitted, provided the original author(s) and the copyright owner(s) are credited and that the original publication in this journal is cited, in accordance with accepted academic practice. No use, distribution or reproduction is permitted which does not comply with these terms.
*Correspondence: Caimei Yang, eWFuZ2NhaW1laTIwMTJAMTYzLmNvbQ==