- 1Department of Laboratory Medicine, The Affiliated People’s Hospital, Jiangsu University, Zhenjiang, China
- 2Department of Immunology, Jiangsu Key Laboratory of Laboratory Medicine, School of Medicine, Jiangsu University, Zhenjiang, China
Primary Sjögren’s syndrome (pSS) is a chronic autoimmune disease associated with damage to multiple organs and glands. The most common clinical manifestations are dry eyes, dry mouth, and enlarged salivary glands. Currently, CD4+ T lymphocytes are considered to be key factors in the immunopathogenesis of pSS, but various studies have shown that CD8+ T lymphocytes contribute to acinar injury in the exocrine glands. Therefore, in this review, we discussed the classification and features of CD8+ T lymphocytes, specifically describing the role of CD8+ T lymphocytes in disease pathophysiology. Furthermore, we presented treatment strategies targeting CD8+ T cells to capitalize on the pathogenic and regulatory potential of CD8+ T lymphocytes in SS to provide promising new strategies for this inflammatory disease.
Introduction
Sjögren’s syndrome (SS) is defined as primary SS (pSS) or secondary SS (sSS) which is associated with other autoimmune diseases (usually rheumatoid arthritis (RA), lupus, or scleroderma). pSS may occur alone and is a chronic autoimmune disease characterized by dry mouth and eyes. pSS patients often have distinct clinical symptoms, such as slow salivary flow rates and decreased salivary mucin quality, accompanied by dry keratitis or conjunctivitis (1, 2). Glandular lesions in patients with pSS are characterized by the mass infiltration of inflammatory cells and formation of ectopic germinal centers (GCs), which are anatomically and functionally similar to the GCs found in the secondary lymphatic organs (3). These inflammatory cells include T lymphocytes, B lymphocytes, natural killer cells (NKs), dendritic cells (DCs) and macrophages (2, 4).
CD8+ T lymphocytes are a complex group of lymphocytes with different phenotypes which are known to display a critical role in tumor, viral infection, chronic inflammation, and autoimmune disease (5–8). Overactivity or abnormal proliferation of CD8+ T lymphocytes can be detected in the peripheral circulation and specific target tissues of SS patients (9, 10). In the damaged lacrimal and salivary glands of SS patients or non-obese diabetic (NOD) murine models, there are always some CD8+ T cells among the infiltrating T cells, even though the number of CD4+ T cells is higher. It has been observed that activated CD8+ T lymphocytes accumulate around apoptotic acinar epithelial cells, and the ascensive effector molecules of cytotoxic CD8+ T lymphocytes (CTLs) such as Granzyme B (GrB), perforin, interferon-γ (IFN-γ), and tumor necrosis factor-α (TNF-α) can be detected (11, 12). These results indicate the presence of a population of activated CD8+ T lymphocytes with cytotoxicity that are capable of killing the glandular epithelial cells and leading to the death or apoptosis of the cells. However, some researchers have found that CD8+ T cells located in the ocular surface of a murine model could regulate Th17 cells and prevent disease progression (13). Therefore, the pathogenicity and regulatory effects of CD8+ T cells still require further clarification.
The distinctive role of CD8+ T lymphocytes in SS is likely influenced by effector molecules and cellular distribution. Here, we discuss our current understanding of the involvement of CD8+ T lymphocytes in lacrimal and salivary gland injury during SS development and review possible targeted therapies for CD8+ T lymphocytes.
CD8+ T Lymphocytes as Effector T Cells: Pathogenicity
Traditionally, naïve CD8+ T lymphocytes expressing the surface markers CD27, CCR7, and CD45RA are located in the secondary T cell zone of the thymus gland. When professional antigen presenting cells (APCs), such as DCs and B cells, present specific antigens on major histocompatibility complex 1 (MHC-1) molecules to CD8+ T cells together with co-stimulatory molecules to release cytokine signaling, CD8+T cells are activated, express CD57, and become cytotoxic T lymphocytes (CTLs) (14). As previously described, there was a negative correlation between perforin and CD27 expression, and CD27low/−CD8+ T cell subsets showed high cytolytic activity, indicating that these subsets were mainly cytotoxic effector T cells. Thus, mature CTLs are CD27−CD28–CD45RA+ T cells (15).
Classification, Features, and Functions
Effector CD8+ T lymphocytes play a vital role in killing tumor cells and controlling pathogen-infected cells. CTLs directly affect target cells mainly through the release of GrB and perforin to clear target cells directly or Fas ligands (FasL), which is induced to bind to Fas death receptors on target cells, triggering apoptosis signalling (16). In addition to directly targeting infected cells, CTLs are likely to affect the occurrence and prognosis of diseases indirectly through the release of effector cytokines. This subset of CTLs expresses lower levels of GrB and perforin than other subsets but is more likely to participate in adaptive immune responses through a variety of proinflammatory cytokines, such as IFN-γ, IL-4, IL-17 and TNF-α. At present, it is believed that effector CD8+ T lymphocytes can be mainly divided into Tc1, Tc2, Tc9, Tc17, follicular cytotoxic T (Tfc), follicular helper T (CD8+ Tfh) and regulatory T (CD8+ Treg) cells in response to different stimuli, such as tumors, viral infections, allergies, autoimmune diseases and transplantation (17, 18) (Table 1).
According to the main effector factors and phenotype, CXCR5−CTLs are mostly known as Tc1 cells and kill target cells in the context of antitumor immunity. These cells secrete IFN-γ and TNF-α and have high cytotoxicity (40). Tc1 cells are pathogenic in insulin-dependent diabetes mellitus (IDDM) but protective in allergic airway inflammation (33). Compared with Tc1 cells, Tc2 cells have lower cytotoxic functions and produce IL-4 and IL-5 in response to specific allergens (33, 40). Tc2 cells can produce IL-13 to enhance inflammatory responses, but these cells are less cytotoxic in diabetes than Tc1 cells (41). In addition, Tc2 cells may be involved in the development of RA by selective enrichment of IL-4-producing CD8+ T cells (42). Tc9 cells promote airway inflammation through Th2 cells while inhibiting CD4+T cell-mediated colitis (59). Tc17 cells (also known as Tc3 cells) mainly secrete IL-17 without cytolysis (40). Tc17 cells are present in the cerebrospinal fluid (CSF) of patients with multiple sclerosis (MS) and are crucial for Th17-mediated experimental autoimmune encephalomyelitis (EAE) and Th1-mediated autoimmune diabetes (43) (Table 1). However, these CTL subtypes have not been determined in SS yet and need further exploration.
CXCR5+CTLs are characterized by the transcription factor Bcl6 and can be further divided into three subgroups. First, Tfc cells are the only subgroup with cytotoxic abilities in chronic viral infections. These cells are also known as the progenitors of exhausted T cells (TEX cells) and show higher proliferation and cytotoxicity than CXCR5-CTLs (40, 60). Tfc cells are particularly responsive to inhibitor checkpoint blockade, especially anti-PD-1 therapy and are excellent for treating cancer and chronic viral infections (19, 20, 34). Second, CD8+ Tfh cells interact with B cells and are recruited through CXCL13, producing high levels of IFN-γ and IL-21 and promoting autoimmune responses (21–24, 61, 62). The third subgroup is CD8+ Treg cells. QA-1-restricted CD8+ Treg cells inhibit Tfh- and Th17-mediated responses in diseases such as lupus-like syndrome, EAE and arthritis (25, 63, 64). Due to their special functions, Treg cells will be described in detail in the following section.
Distribution of CTLs in Peripheral Blood
The marked activation of T lymphocytes in the peripheral blood of patients with Sjögren’s syndrome shows increased HLA-DR expression. Yukinobu et al. showed that the percentage of peripheral HLA-DR+CD11−CTLs but not HLA-DR+CD11+CD8+T (T-suppressed) cells in SS patients was obviously high compared with that of the control (9). In addition, serum immunoglobulin levels were positively correlated with the percentage of HLA-DR+CTLs and T-suppressed cells (9). Surprisingly, only CD8+ T cells but not CD4+ T cells were associated with HLA-DR up-regulation in damaged glandular tissue (10). Moreover, the expression of HLA-DR in glandular CD8+ T lymphocytes was closely related to the clinical symptoms of SS, including the European League Against Rheumatism Sjögren’s syndrome Disease Activity Index (ESSDAI) scores, suggesting that effector CD8+ T lymphocytes have a pathogenic role (9, 15). The pathogenic factors of SS are not clear, but it is thought that viruses are important inducers. In general, CD8+ T cells play a key role in the clearance of viral infections. In addition, it showed that dysregulated SS gene signatures (SGS), including epigenomes, mRNAs and proteins in peripheral blood of SS patients, significantly overlapped with SS-causing genes which mainly involved in interferon signal and a disintegrin and metalloproteinase (ADAM) substrates (65). Most significantly, the SS pathogenic genes were specifically associated with activated CTLs, which may reflect disease characteristics. Remarkably, transcriptional module 1 (TR1) in CD8+T cells rather than in CD4+ T were present specifically in SS (65). The total percentage of CD27−CD57−/+CD45RA+CD8+T lymphocytes with a high level of perforin and killing activity in the peripheral blood of SS patients was lower than that of healthy subjects (15, 66). In contrast, Sudzius et al. proposed that the absolute number of effector CD27−CD8+ T cells was increased (67).
Of note, T lymphocytes in the blood were negatively correlated with CD45+ immune cells infiltrating the glands and the frequency of activated HLA-DR+ cells in target tissues, indicating that the reduction in peripheral effector T cells is probably due to their accumulation in the glands. Furthermore, effector memory CD8highCD27+CD57− T cells with reduced cytotoxicity were significantly reduced in the peripheral blood of SS patients, which was shown to be due to increased spontaneous apoptosis or a sedentary response in the tissues (15, 68). We hypothesize, these cells migrate to local inflammatory sites during progressive disease and are responsible for destroying pathogens. According to some studies, CD27−CD57+CD8+T cells, a subset of CTLs, are potentially highly cytotoxic, but they are destined to migrate to non-lymphoid tissues without further circulation, which is consistent with the above hypothesis (68). At present, a number of studies have shown that mature effector CD8+ T cells no longer participate in peripheral circulation throughout disease progression. In advanced SS, CD8+ T cells are likely to reside in glandular tissues to exert immune effects. Data show that after 10 weeks of immunization, CD8+ T lymphocytes migrate and re-infiltrate with the accumulation of large amounts of the chemokine CCL22 and macrophages in the spleens or salivary glands of SS mice (69).
Distribution of CTLs in the Major Salivary Glands
The major salivary glands include the parotid, submandibular, and sublingual glands. Previous reports have shown an increase in IFN-γ, CXCL9 and CXCL10 in the salivary gland epithelial cells of SS patients, as well as constant infiltration of CTLs in inflammatory lesions in salivary glands in the female NOD murine model, which is representative of SS (35) IFN-γ derived from CTLs can alter tight junction integrity and function in parotid epithelial cells, leading to cell death (70, 71) (Figure 1).
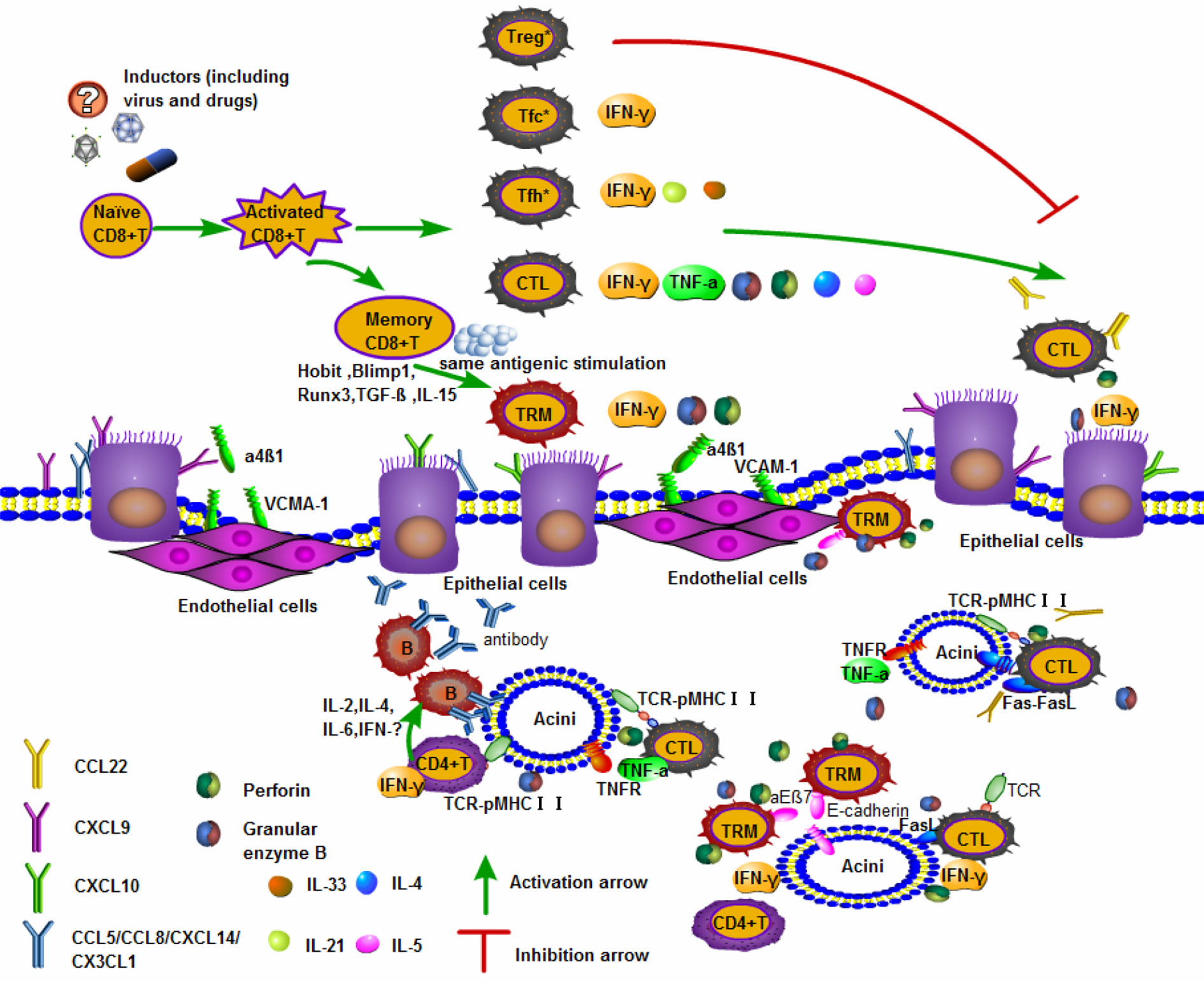
Figure 1 Activated CD8+T cells are involved in the mechanism of salivary gland injury. The upper part of the panel shows naïve CD8+ T differentiated into subgroups. CTLs and memory CD8+ T cells play important roles in the organization. The lower part of the panel highlights the tissues damage caused by activated CD8+ T entering the salivary glands. Pathological examination of apoptotic acinar cells in the salivary glands and lacrimal glands of patients with SS revealed the accumulation of CD8+ T cells and fewer CD4+ T cells expressing integrin αEβ7 (CD103) (12). A kind of cytotoxic memory T cell called CD8+ TRM cells and CTLs producing GrB/perforin can induce acinar cells death (53, 72). CTLs recognize pMHCI presented by the acinar cells, then specific T cell receptor (TCR) and co-receptors to contact parts, promoting the secretion of such as IFN-γ, TNF-α, GrB/perforin. In addition, CTLs can express FasL or secrete TNF-α, which bind to Fas and TNF receptors (TNFR) on the surface of acinar cells respectively and induce apoptosis mediated by caspase signaling pathways (73, 74). There are also a large number of inflammatory cells such as CD4+T cells and B cells in the glandular tissue, which cooperate with CD8+T cells to play an immune effect and cause tissues damage. Treg *: CXCR5+Foxp3+/−CD8+Treg; Tfc *: CXCR5+PD-1int-CD8+Tfc; Tfh *: CXCR5+PD-1highCD8+Tfh.
Recently, it was found that in aged Lgals1−/− mice (similar to SS, with decreased salivary and increased levels of anti-dsDNA, anti-nuclear and anti-SSA/Ro autoantibodies), the frequency of CD8+ T cells in submandibular glands was significantly increased. However, there was no significant difference in the frequency of CD4+ T and B220+ B cells. In particular, CD8+IFN-γ+ T and CD8+PD-1+ T cell infiltration was higher. Consistent with the higher expression of CXCL9 and CXCL10 in submandibular glands, CD8+CXCR3+ T cells’ infiltration increased as well, compared with WT mice (36). It suggested that CTLs could be actively recruited into this target organ. Kong et al. reported the expression of Fas and FasL in the duct lumen of salivary glands in SS patients (73). This finding suggested the occurrence of apoptosis and progressive damage to salivary glands through CTL-mediated cytotoxicity or the Fas-FasL pathway.
Furthermore, it has been well demonstrated that major salivary gland vascular endothelial cells express the cellular adhesion molecule VCAM-1, which is induced by systemic inflammation and binds with integrin α4β1 (the ligand of VCAM-1) (75). VCAM-1 promoted the migration of CTLs to exert immune effects in salivary glands, indicating that inflammation enhances T cell recruitment to the salivary glands. The salivary glands, particularly the parotid and submandibular glands, express many chemokines, such as CCL5, CXCL9, CCL28, CXCL14 and CX3CL1, while CD8+ T lymphocytes express chemokine receptors, such as CXCR4 and CXCR6, after stimulation (76) (Figure 1).
Therefore, we hypothesized that the destruction of salivary glands in advanced SS could be mediated by integrins, chemokines, and activated CD8+ T lymphocytes under sustained inflammatory stimulation, following the secretion of inflammatory factors.
Distribution of CTLs in the Lacrimal Glands
A large number of CTLs expressing IFN-γ surround apoptotic acinar epithelial cells of the lacrimal gland in SS patients (11). Moreover, the increased levels of CXCL9 and CXCL10 in the tears of SS patients are consistent with CTL-mediated IFN-γ induction (37). Subsequently, researchers demonstrated the presence of proliferating and activated CD11a+CD69+CD8+ T cells, which produced inflammatory molecules in the lacrimal glands of a NOD mouse model of SS (11). In particular, apoptosis mediated by the Fas-FasL interaction was observed in lacrimal acinar cells surrounded by CD107a+CD8+T cells, while FasL was only detected in SS patients (74, 77). Since CTLs expressing CD107a and GrB can kill the target cell directly, it has been suggested that the death of lacrimal epithelial cells could be independently mediated by CTLs (11). This finding is sufficient to show that CTLs are distributed in the lacrimal glands and play a pathogenic role in SS.
CD8+ T Lymphocytes as Memory T Cells
Memory CD8+ T lymphocytes differentiate from naïve CD8+ T cells and express CD45RO rather than CD45RA after antigen activation. Initially, memory CD8+ T lymphocytes predominantly circulate in the peripheral system and are divided into two classic subgroups: central memory (TCM) and effector memory (TEM) cells (78, 79). TCM cells are similar to naïve CD8+ T cells, express CCR5 and are present in secondary lymphoid organs, while TEM cells express CD45RA and exhibit cytotoxicity similar to that of CTLs in peripheral tissues (54, 80) (Table 1). Recently, a new subset of memory CD8+ T cells called tissue-resident memory CD8+ T lymphocytes (TRM cells) has been widely studied and expresses four classic surface markers: CD44, CD49a, CD69 and CD103 (53). This group is actively involved in disease progression, resides in peripheral tissues and is different from circulating memory CD8+ T cells, which will be investigated in detail.
CD8+ TRM cells circulate continuously in peripheral tissues and play a leading role in fighting peripheral infections, sometimes mediating stronger protection than any other memory T cells (81). CD44 could not be used to distinguish TRM cells from other CD8+T cells, but it could distinguish naïve, effector and memory cells according to the degree of expression. CD44 can bind to vascular endothelial cells to promote peripheral tissue cell migration during inflammation. CD49a is involved in TRM cells production of IFN-γ, GrB, and perforin, and it also interferes with TRM cell apoptosis in combination with collagenase type IV (82). In addition to CD8+ TRM cells, CD69 is also expressed in other killer cells, such as NKs, and antagonizes SIPR1 to promote tissue retention (83).
Furthermore, there is increasing evidence of TRM cells in autoimmune diseases and inflammatory responses. Recent pathological biopsies of patients with type 1 diabetes mellitus (T1D) showed that there were CD8+ TRM cells in the pathological islets, which were prone to producing inflammatory cytokines, including IFN-γ, IL-18 and IL-22 (84). Considering that CD103 on the surface of CD8+ TRM cells can bind E-cadherin on epithelial cells, CD8+ TRM cells are mainly present in mucosal tissues such as the respiratory tract, digestive or urogenital tract, secretory glands and skin (12, 53). In particular, the labial and submandibular glands, as exocrine glands, contain a large number of IFN-γ- and GrB-producing CD8+ TRM cells (11, 75). In murine SS, the salivary glands are typically damaged, which could be explained by the infiltration of pathogenic CD103+CD8+TRM cells in glandular tissues (55). It is worth noting that CD103+CD8+ T cells expressing IFN-γ appear to be controversial since they are regulatory cells.
Distribution of TRM Cells in the Minor Salivary Glands
Minor salivary glands (MSGs) are widely distributed in the lips, tongue, and palate. Although previous reports on the pathogenesis of SS indicated that CD4+ T cells were dominant in T lymphocyte-infiltrated lesions, in the labial salivary glands of SS patients, it was noted that there was a group of CD8+ T lymphocytes that outnumbered CD4+ T cells (55, 85). CD8+ T cells with a TRM phenotype secrete high levels of IFN-γ and are mainly localized near the duct epithelial cells and acinar cells of the MSGs (55). This finding could be logically explained by CD103 binding to E-cadherin on MSGs epithelial cells (12) (Figure 1).
Distribution of TRM Cells in the Major Salivary Glands
The striking thing is that the majority of infiltrating T lymphocytes in the submandibular glands of p40−/−CD25−/− mice, which recapitulate the main characteristics of human SS, are CD8+ T lymphocytes. They exhibit a TRM phenotype instead of CD4+ T cells, accompanied by significantly increased IFN-γ (55). CD8+ T cells are also a source of IFN-γ and cause direct destruction of glandular tissues. IFN-γ is closely connected to the death of parotid epithelial cells in the salivary glands (70, 71) IFN-γ significantly recruits the chemokines CXCL9 and CXCL10 on the surface of submandibular epithelial cells, then immune cells home to lesions, and exacerbate the progression of SS (35, 86) (Figure 1).
As CXCR3 expression is increased in T cells in draining lymph nodes, IFN-γ may promote CD8+T cell migration from draining lymph nodes to submandibular glands and exert effects in tissues, especially in the case of consistent inflammation and infection (55, 86). Therefore, the positive feedback pathway induced through the CD8+T-IFN-γ-CXCL9/10-CXCR3 axis further exacerbates tissue damage.
Moreover, researchers discovered that acinar atrophy, ductal injury and fibrosis were completely eliminated by knocking out CD8a in p40−/−CD25−/− mice, while CD4 knockout provided only mild relief. Moreover, only depletion of CD8a but not CD4 alleviated the lymphocyte lesions in the submandibular glands and restored salivary secretion (55). Tissue damage occurred despite inhibition of the germinal center responses and antibody production in p40−/vCD25v/−CD4−/− or IFN-γ−/− mice (55). In summary, CD8+T lymphocytes, especially CD8+ TRM cells, lead to salivary gland tissue damage in mice and may play a role that cannot be ignored in the occurrence and development of SS.
CD8+ T Lymphocytes as Regulatory T Cells
Regulatory CD8+ T cells (CD8+ Treg cells) are considered to be essential regulators and should be discussed. CD8+ Treg cells prevent the progression of autoimmune diseases by secreting a variety of cytokines to inhibit lymphocyte function (87–90). CD8+ Treg cells are mostly characterized by CD45RO expression and lack CD28, CD62L or CD122 expression (91, 92). CD8+ Treg cells can be divided into natural and induced subgroups according to their origins. Natural CD8+ Treg cells are generated and mature in the thymus and express human leukocyte antigen-G (HLA-G) or CD122 and CD28. The inhibitory effects of these cells are mediated by soluble factors such as HLA-G or IL-10 (93, 94). In contrast, induced Treg cells (including effector and memory CD8+ Treg cells) are derived from peripheral naïve T lymphocytes, which are stimulated by antigens and exert functions through cell–cell contact or the release of soluble factors (95, 96).
There is no real consensus on the phenotypic and molecular characteristics of regulatory CD8+ T cells, which appear to make up a heterogeneous group expressing Foxp3 (26). The phenotypic expression of CD8+ Treg cells, including CD122, CD28, CD45RC, CD103, and PD-1, is mainly associated with the differentiation status of CD8+ T lymphocytes among central or effector memory cells (97, 98). The inhibitory ability of induced CD8+ Treg cells is much stronger than that of naïve CD8+ Treg cells (99). Effector CD8+ Treg cells are present in the blood and secondary lymphoid organs, while memory CD8+ Treg cells are mainly distributed in peripheral tissues. A subset of induced Treg cells expressing CD8+CD28− can inhibit the production of high-affinity antibodies and autoantibodies to regulate humoral responses (40, 100). A reduction in the number of CD8+CD28− T lymphocytes with inhibitory effects has been described in animal models of autoimmune diabetes and experimental autoimmune encephalomyelitis (EAE) (100–103). The subset CD8+CD28−Foxp3+ T lymphocytes, which are similar to classic CD4hiCD25+Treg cells, inhibit the development of autoimmune diseases. Previously, it has been shown that CD8+CD28−Foxp3+T downregulate the expression of co-stimulatory molecules in DCs, reducing the efficiency of antigen presentation (104–106), regulating the activity of IDO+ plasmacytoid dendritic cells (pDCs), and mediating immune tolerance (104). In addition, CD8+ Treg cells could eliminate CD4+ Tfh cells by IL-21 and enhance perforin and IL-15 (27).
In addition, this subset includes a specific population called antibody-suppressor (CD8+ TAb-supp) cells that produce IFN-γ, which directly kills or inhibits IgG and IL-4 secretion by allogeneic B and CD4+ T cells (28) (Table 1). Reinforcing the notion that the key mediators of CD8+ Tregs inhibitory activity are IFN-γ and TGF-β, IFN-γ also participates in the pathogenicity of CTLs (107–110). An advantage is that CD8+ Treg cells’ activation is stimulated by almost all cell types, while CD4+ Tregs’ activation occurs only through cell expressing MHC-II molecules. CD8+ Tregs are useful in organ transplantation or graft-versus-host disease (GVHD) and are critical in autoimmune diseases (98, 111, 112).
Distribution of CD8+Tregs in the Blood
A recent study found that circulating regulatory CD8+CD28− T cells were negatively correlated with systemic disease activity in patients with SS (95, 113). Sudzius et al. demonstrated that CD8+Foxp3+ Treg cells showed a decreasing trend in the peripheral blood of pSS patients, even though it was not significantly different (67). Compared with that of healthy controls, the percentage of CD8+Foxp3+ Treg cells in the peripheral blood of pSS patients without clinical disease activity but with serological activity was significantly reduced, while there were no obvious changes in the frequency of CD4+ Treg cells (114). However, with the progression of the disease, advanced SS tissue lesions are exacerbated, and increased Treg cells are detected in the peripheral blood (115). The proportion of CD8+CD28-T cells in the peripheral blood of SS patients was significantly higher than that of healthy controls, as the level of soluble CD28 was also increased (113, 116). The migration of pathogenic or regulatory CD8+ T lymphocytes between the periphery and local disease-affected tissues is thought to occur, as CTLs have been detected in lacrimal and salivary glands (117).
Distribution of CD8+ Tregs at the Ocular Surface
Previous studies demonstrated that the number of infiltrating CD4+ Treg cells in the MSGs of SS patients was positively correlated with the gland biopsy lesion score but negatively correlated with the number of cells in the peripheral blood. Therefore, it can be posited that CD8+ Tregs exhibit opposing regulation in the peripheral blood and affected tissues, even though this regulation is reversible. Furuzawa-Carballeda et al. showed that IL-10+CD8+ Tregs migrated to lacrimal and salivary glands and appeared to directly contact target cells to mediate immune regulation (114). In addition to salivary glands and lacrimal glands, the ocular surface is another key site of inflammatory damage in SS. However, other researchers found that instead of causing disease, CD8+ T lymphocytes regulate the ocular surface in desiccating stress (DS)-induced SS mice, and so further research is needed. Although natural regulatory CD122+CD8+ T cells exist in the ocular surface, they seem to be independent of the development of SS (13, 73, 118). Zhang X demonstrated for the first time that CD103+CD8+ Tregs could significantly reduce Th17-mediated corneal barrier dysfunction in in a murine model of SS by inhibiting DCs activation (13). CD8+ T lymphocytes’ depletion increased DCs’ accumulation and activation and promoted DCs’ migration from the ocular surface to cervical lymph nodes (13). While we previously discussed CD103+CD8+ T cells with pathogenic effects on salivary glands, whether pathogenicity or regulation is related to the presence of these cells in different tissues or to the stage of SS development remains to be further clarified.
Collectively, three kinds of CD8+ T lymphocytes have been summarized in this section (Table 1). We discussed the different CD8+ T subsets that contribute to SS in depth. CD8+ T lymphocyte subsets are widely distributed in the peripheral blood and tissues with complex classifications and diverse phenotypes. However, subsets of the same phenotype may exhibit pathogenicity or regulation through different distributions in SS patients. Therefore, because CD8+ T lymphocytes have not been extensively reported in detail in SS, we need to further explore the role and influence of CD8+ T lymphocytes.
Targeting CD8+ T Lymphocytes: A Promising Treatment for SS
At present, it has been reported in the literature that the pathogenesis of Sjögren’s syndrome is probably related to the up-regulation of type I interferon. Consistent with this, SIGLEC1 (known as sialoadhesin and CD169, responsive protein of IFN-α) on mononuclear cells of SS patients was also significantly up-regulated, which has been proven to be related to the disease activity (119). Besides, B-cell aggregating in ectopic GCs is also pathogenic, producing antibodies and forming immune complexes with autoantigens. It can be deposited in tissues, promoting inflammation (120). However, the pathogenesis of SS is diverse without a complete understanding of the underlying pathogenic factors, and there is currently no specific cure. SS is characterized by systemic dryness, especially dry mouth and dry eyes, which are also observed in SICCA syndrome (1, 4). Therefore, it is difficult to diagnose SS through clinical symptoms, which urges us to look for characteristic indicators such as changes in autoantibodies, cytokines, effector molecules or immune cell subsets for diagnosis. The subsets of CD8+ T lymphocytes in SS are a controversial issue because the retention of CD8+ T cells in tissues contributes to both pathogenicity and regulation during disease development.
Given the key role of CD8+ T lymphocytes in the pathogenesis of SS, CD8+ T cell-targeted therapy has great promise. Unfortunately, no satisfactory results have been achieved. However, treatment of the disease with damaged glandular cells may be effective. For example, the proteasome inhibitor lactacystin suppresses the formation of immunoproteasomes in human-SG (HSG) cells by binding to the N-terminal of the β1i subunit (121, 122). IFN-γ is overexpressed during the progression of SS and up-regulates the immunoproteasome in SG cells, promoting the expression of MHC-I-associated peptides (123). MHC class I may be recognized and targeted by autoreactive CD8+T cells and then destroy SG cells in SS patients. Hence, the use of proteasome inhibitors to inhibit the immunoproteasome or targeting peptide epitopes on HSG cells is a promising strategy for the treatment of SS. Moreover, the direct use of MHC class I and self-peptide complexes can selectively induce autoreactive CD8+T cells apoptosis (124). Furthermore, blocking effector molecules associated with CD8+T cells might also be used to reduce the cytotoxicity and apoptosis of glandular epithelial cells or acinar cells.
On the other hand, improving SS may occur through targeting CD8+ T lymphocytes directly. Barr et al. demonstrated that NOD mice had lacrimal inflammation and cytotoxic CD8+ T cell infiltration, and the lack of CD8+ T cells reduced disease severity. In fact, cyclosporine inhibits the proliferation of CTLs in vitro (125). Douglas A. treated MRL/lpr mice with cyclosporine at the early stage and found that cyclosporine was effective in controlling autoimmune diseases, especially in alleviating lacrimal glands and intraocular inflammatory lesions (126). Cytotoxic T cell antigen 4 (CTLA-4), which can block the binding of B7 to CD28, leading to immune tolerance. CTLA-4Ig is a fusion protein composed of CTLA-4 extracellular functional genes and Fc segments of IgG1, which can destroy T cell activation. This may be a potential treatment for inhibiting the progression of pSS through suppressing CD8+ T activated in tissues. Meiners PM et al. found that abatacept treatment was effective, safe, and well tolerated in early pSS patients, because it resulted in a significant reduction level of ESSDAI and EULAR Sjögren’s syndrome Patient Reported Index (ESSPRI) (127). However, in the single-center abatacept Sjögren Active Patients phase III (ASAP-III) study, there was no difference between pSS patients treated with abatacept and those treated with placebo measured by ESSDAI or ESSPRI (128). Baer AN et al. also suggested that abatacept did not produce significant clinical efficacy compared with placebo in patients with moderate or severe pSS. Limitations in this study may be the heterogeneity of patients and missing CD8+ T cell population detection. While they detected improvements in some disease-relevant biomarkers and pathogenic cell populations such as CD4+T, it is difficult to determine whether it is effective against CD8+ T. Therefore, further studies are needed to assess the effect of abatacept on specific disease-relevant CD8+ T cells changes of pSS (129). In addition, the number of CD8+ Treg cells was negatively correlated with disease activity, regulating SS development (95, 113, 115). Thus, CTL depletion and powerful CD8+Tregs amplification may be achieved in early SS when certain drugs like abatacept and cytokines are applied.
Currently, new targets under investigation, including several approaches discussed in this review, which may intervene in the involvement of CD8+ T lymphocytes in SS, are shown in Table 2. Advances in our understanding of the pathogenic or regulatory mechanism of activated CD8+ T subsets are expected to provide effective treatments for SS patients in the future.
Conclusion
The exocrine glands of SS patients have different degrees of inflammatory cell infiltration or ectopic germinal centers. In particular, activated CD8+ T lymphocytes in the tissues in SS are characterized as cytotoxic, promoting apoptosis or even inhibiting the disease, and these findings have been shown in current studies and support the idea that these cells are actively involved in the development of SS. The targets of this disease, salivary gland and lacrimal gland epithelial cells, maintain cross-talk with activated CD8+ T subsets. Environmental triggers occur through heredity, viral invasion and estrogen imbalance, leading to the activation of specific CD8+T cell subsets, especially TRM cells that reside in tissues and exhibit high cytotoxicity, which mediate glandular cell apoptosis through the Fas death pathway or releases toxic particles to target cell destruction (81, 85). Whether CD8+ T lymphocytes can induce or exacerbate the destruction of lacrimal and salivary glands remains to be further explored. Furthermore, the evidence for SS discussed in this review suggests that targeting CD8+ T cells may be a complementary strategy for disease immunotherapy. In summary, we explored evidence for the participation of CD8+ T lymphocyte subsets in the pathogenesis or regulation of SS with the hope of gaining insights to enhance CD8+T cell-targeted therapies.
Author Contributions
HZ drafted the manuscript. JY and JT discussed and revised the manuscript. SW designed the study and revised the manuscript. All authors contributed to the article and approved the submitted version.
Funding
This work was supported by the National Natural Science Foundation of China (Grant Nos. 81971542, 81771759) and Jiangsu Province’s Key Medical Talents Program (Grant No. ZDRCB2016018).
Conflict of Interest
The authors declare that the research was conducted in the absence of any commercial or financial relationships that could be construed as a potential conflict of interest.
References
1. Brito-Zeron P, Baldini C, Bootsma H, Bowman SJ, Jonsson R, Mariette X, et al. Sjogren syndrome. Nat Rev Dis Primers (2016) 2:16047. doi: 10.1038/nrdp.2016.47
2. Mavragani CP. Mechanisms and New Strategies for Primary Sjogren’s Syndrome. Annu Rev Med (2017) 68:331–43. doi: 10.1146/annurev-med-043015-123313
3. Nocturne G, Mariette X. B cells in the pathogenesis of primary Sjogren syndrome. Nat Rev Rheumatol (2018) 14(3):133–45. doi: 10.1038/nrrheum.2018.1
4. Baldini C, Ferro F, Elefante E, Bombardieri S. Biomarkers for Sjögren’s syndrome. Biomark Med (2018) 12(3):275–86. doi: 10.2217/bmm-2017-0297
5. Farhood B, Najafi M, Mortezaee K. CD8(+) cytotoxic T lymphocytes in cancer immunotherapy: A review. J Cell Physiol (2019) 234(6):8509–21. doi: 10.1002/jcp.27782
6. Lang PA, Crome SQ, Xu HC, Lang KS, Chapatte L, Deenick EK, et al. NK Cells Regulate CD8+ T Cell Mediated Autoimmunity. Front Cell Infect Microbiol (2020) 10:36. doi: 10.3389/fcimb.2020.00036
7. Chen T, Chen L, Song H, Chen X, Xie R, Xia Q, et al. Clinical relevance of T lymphocyte subsets in pediatric Graves’ disease. J Pediatr Endocrinol Metab (2020) 33(11):1425–30. doi: 10.1515/jpem-2020-0158
8. Goplen NP, Wu Y, Son YM, Li C, Wang Z, Cheon IS, et al. Tissue-resident CD8+T cells drive age-associated chronic lung sequelae after viral pneumonia. Sci Immunol (2020) 5(53):eabc4557. doi: 10.1126/sciimmunol.abc4557
9. Youinou P, Pennec YL, Blaschek MA, Gentric A, Jouquan J, Lamour A, et al. Activation of peripheral blood lymphocytes in patients with primary Sjögren’s syndrome. Rheumatol Int (1988) 8(3):125–30. doi: 10.1007/BF00272434
10. Mingueneau M, Boudaoud S, Haskett S, Reynolds TL, Nocturne G, Norton E, et al. Cytometry by time-of-flight immunophenotyping identifies a blood Sjogren’s signature correlating with disease activity and glandular inflammation. J Allergy Clin Immunol (2016) 137(6):1809–21.e1812. doi: 10.1016/j.jaci.2016.01.024
11. Barr JY, Wang X, Meyerholz DK, Lieberman SM. CD8 T cells contribute to lacrimal gland pathology in the nonobese diabetic mouse model of Sjogren syndrome. Immunol Cell Biol (2017) 95(8):684–94. doi: 10.1038/icb.2017.38
12. Fujihara T, Fujita H, Tsubota K, Saito K, Tsuzaka K, Abe T, et al. Preferential localization of CD8+αEβ7+T cells around acinar epithelial cells with apoptosis in patients with Sjögren’s syndrome. J Immunol (1999) 163(4):2226–35. doi: 10.1007/s00170-015-7241-9
13. Zhang X, Schaumburg CS, Coursey TG, Siemasko KF, Volpe EA, Gandhi NB, et al. CD8(+) cells regulate the T helper-17 response in an experimental murine model of Sjogren syndrome. Mucosal Immunol (2014) 7(2):417–27. doi: 10.1038/mi.2013.61
14. Mittrucker HW, Visekruna A, Huber M. Heterogeneity in the differentiation and function of CD8(+) T cells. Arch Immunol Ther Exp (Warsz) (2014) 62(6):449–58. doi: 10.1007/s00005-014-0293-y
15. Narkeviciute I, Sudzius G, Mieliauskaite D, Mackiewicz Z, Butrimiene I, Viliene R, et al. Are cytotoxic effector cells changes in peripheral blood of patients with Sjogren’s syndrome related to persistent virus infection: Suggestions and conundrums. Cell Immunol (2016) 310:123–30. doi: 10.1016/j.cellimm.2016.08.013
16. Gravano DM, Hoyer KK. Promotion and prevention of autoimmune disease by CD8+ T cells. J Autoimmun (2013) 45:68–79. doi: 10.1016/j.jaut.2013.06.004
17. Buchholz VR, Graf P, Busch DH. The origin of diversity: studying the evolution of multi-faceted CD8+ T cell responses. Cell Mol Life Sci (2012) 69(10):1585–95. doi: 10.1007/s00018-012-0967-8
18. Yu D, Ye L. A Portrait of CXCR5(+) Follicular Cytotoxic CD8(+) T cells. Trends Immunol (2018) 39(12):965–79. doi: 10.1016/j.it.2018.10.002
19. Collins DR, Gaiha GD, Walker BD. CD8(+) T cells in HIV control, cure and prevention. Nat Rev Immunol (2020) 20(8):471–82. doi: 10.1038/s41577-020-0274-9
20. Leong YA, Chen Y, Ong HS, Wu D, Man K, Deleage C, et al. CXCR5(+) follicular cytotoxic T cells control viral infection in B cell follicles. Nat Immunol (2016) 17(10):1187–96. doi: 10.1038/ni.3543
21. Valentine KM, Davini D, Lawrence TJ, Mullins GN, Manansala M, Al-Kuhlani M, et al. CD8 Follicular T Cells Promote B Cell Antibody Class Switch in Autoimmune Disease. J Immunol (2018) 201(1):31–40. doi: 10.4049/jimmunol.1701079
22. Chen Y, Yu M, Zheng Y, Fu G, Xin G, Zhu W, et al. CXCR5(+)PD-1(+) follicular helper CD8 T cells control B cell tolerance. Nat Commun (2019) 10(1):4415. doi: 10.1038/s41467-019-12446-5
23. Kang YM, Zhang X, Wagner UG, Yang H, Beckenbaugh RD, Kurtin PJ, et al. CD8 T cells are required for the formation of ectopic germinal centers in rheumatoid synovitis. J Exp Med (2002) 195(10):1325–36. doi: 10.1084/jem.20011565
24. Shen J, Luo X, Wu Q, Huang J, Xiao G, Wang L, et al. A Subset of CXCR5(+)CD8(+) T Cells in the Germinal Centers From Human Tonsils and Lymph Nodes Help B Cells Produce Immunoglobulins. Front Immunol (2018) 9:2287. doi: 10.3389/fimmu.2018.02287
25. Kim HJ, Cantor H. Regulation of self-tolerance by Qa-1-restricted CD8(+) regulatory T cells. Semin Immunol (2011) 23(6):446–52. doi: 10.1016/j.smim.2011.06.001
26. Flippe L, Bezie S, Anegon I, Guillonneau C. Future prospects for CD8(+) regulatory T cells in immune tolerance. Immunol Rev (2019) 292(1):209–24. doi: 10.1111/imr.12812
27. Kim HJ, Verbinnen B, Tang X, Lu L, Cantor H. Inhibition of follicular T-helper cells by CD8(+) regulatory T cells is essential for self tolerance. Nature (2010) 467(7313):328–32. doi: 10.1038/nature09370
28. Zimmerer JM, Ringwald BA, Elzein SM, Avila CL, Warren RT, Abdel-Rasoul M, et al. Antibody-suppressor CD8+ T Cells Require CXCR5. Transplantation (2019) 103(9):1809–20. doi: 10.1097/TP.0000000000002683
29. Kim H-J, Wang X, Radfar S, Sproule TJ, Roopenian DC, Cantor H. CD8+T regulatory cells express the Ly49 Class I MHC receptor and are defective in autoimmune prone B6-Yaa mice. Proc Natl Acad Sci (2011) 108(5):2010–5. doi: 10.1073/pnas.1018974108
30. Lu L, Kim HJ, Werneck MB, Cantor H. Regulation of CD8+ regulatory T cells: Interruption of the NKG2A-Qa-1 interaction allows robust suppressive activity and resolution of autoimmune disease. Proc Natl Acad Sci U S A (2008) 105(49):19420–5. doi: 10.1073/pnas.0810383105
31. Saligrama N, Zhao F, Sikora MJ, Serratelli WS, Fernandes RA, Louis DM, et al. Opposing T cell responses in experimental autoimmune encephalomyelitis. Nature (2019) 572(7770):481–7. doi: 10.1038/s41586-019-1467-x
32. Bezie S, Anegon I, Guillonneau C. Advances on CD8+ Treg Cells and Their Potential in Transplantation. Transplantation (2018) 102(9):1467–78. doi: 10.1097/TP.0000000000002258
33. Vizler C, Bercovici N, Heurtier A, Pardigon N, Goude K, Bailly K, et al. Relative diabetogenic properties of islet-specific Tc1 and Tc2 cells in immunocompetent hosts. J Immunol (2000) 165(11):6314–21. doi: 10.4049/jimmunol.165.11.6314
34. He R, Hou S, Liu C, Zhang A, Bai Q, Han M, et al. Erratum: Follicular CXCR5-expressing CD8+ T cells curtail chronic viral infection. Nature (2016) 540(7633):470. doi: 10.1038/nature20107
35. Ogawa N, Ping L, Zhenjun L, Takada Y, Sugai S. Involvement of the interferon-gamma-induced T cell-attracting chemokines, interferon-gamma-inducible 10-kd protein (CXCL10) and monokine induced by interferon-gamma (CXCL9), in the salivary gland lesions of patients with Sjogren’s syndrome. Arthritis Rheumatol (2002) 46(10):2730–41. doi: 10.1002/art.10577
36. Martinez Allo VC, Hauk V, Sarbia N, Pinto NA, Croci DO, Dalotto-Moreno T, et al. Suppression of age-related salivary gland autoimmunity by glycosylation-dependent galectin-1-driven immune inhibitory circuits. Proc Natl Acad Sci USA (2020) 117(12):6630–9. doi: 10.1073/pnas.1922778117
37. Yoon KC, Park CS, You IC, Choi H-J, Lee K-H, Im S-K, et al. Expression of CXCL9, -10, -11, and CXCR3 in the tear film and ocular surface of patients with dry eye syndrome. Invest Ophthalmol Vis Sci (2010) 51(2):643–50. doi: 10.1167/iovs.09-3425
38. Mylvaganam GH, Rios D, Abdelaal HM, Iyer S, Tharp G, Mavigner M, et al. Dynamics of SIV-specific CXCR5+ CD8 T cells during chronic SIV infection. Proc Natl Acad Sci USA (2017) 114(16):E3366. doi: 10.1073/pnas.1703867114
39. Vinuesa CG, Linterman MA, Yu D, MacLennan IC. Follicular Helper T Cells. Annu Rev Immunol (2016) 34:335–68. doi: 10.1146/annurev-immunol-041015-055605
40. Fousteri G, Kuka M. The elusive identity of CXCR5(+) CD8 T cells in viral infection and autoimmunity: Cytotoxic, regulatory, or helper cells? Mol Immunol (2020) 119:101–5. doi: 10.1016/j.molimm.2020.01.007
41. Tang Y, Guan SP, Chua BY, Zhou Q, Ho AW, Wong KH, et al. Antigen-specific effector CD8 T cells regulate allergic responses via IFN-gamma and dendritic cell function. J Allergy Clin Immunol (2012) 129(6):1611–20 e1614. doi: 10.1016/j.jaci.2011.12.976
42. Cho BA, Sim JH, Park JA, Kim HW, Yoo W-H, Lee S-H, et al. Characterization of effector memory CD8+ T cells in the synovial fluid of rheumatoid arthritis. J Clin Immunol (2012) 32(4):709–20. doi: 10.1007/s10875-012-9674-3
43. Huber M, Heink S, Pagenstecher A, Reinhard K, Ritter J, Visekruna A, et al. IL-17A secretion by CD8+ T cells supports Th17-mediated autoimmune encephalomyelitis. J Clin Invest (2012) 123(1):247–60. doi: 10.1172/JCI63681
44. Goedhart M, Cornelissen AS, Kuijk C, Geerman S, Kleijer M, van Buul JD, et al. Interferon-Gamma Impairs Maintenance and Alters Hematopoietic Support of Bone Marrow Mesenchymal Stromal Cells. Stem Cells Dev (2018) 27(9):579–89. doi: 10.1089/scd.2017.0196
45. Becker TC, Wherry EJ, Boone D, Murali-Krishna K, Antia R, Ma A, et al. Interleukin 15 Is Required for Proliferative Renewal of Virus-specific Memory CD8 T Cells. J Exp Med (2002) 195(12):1541–8. doi: 10.1084/jem.20020369
46. Goldrath AW, Sivakumar PV, Glaccum M, Kennedy MK, Bevan MJ, Benoist C, et al. Cytokine requirements for acute and Basal homeostatic proliferation of naïve and memory CD8+ T cells. J Exp Med (2002) 195(12):1515–22. doi: 10.1084/jem.20020033
47. Almeida JR, Price DA, Papagno L, Arkoub ZA, Sauce D, Bornstein E, et al. Superior control of HIV-1 replication by CD8+ T cells is reflected by their avidity, polyfunctionality, and clonal turnover. J Exp Med (2007) 204(10):2473–85. doi: 10.1084/jem.20070784
48. Wimmers F, Aarntzen EH, Duiveman-deBoer T, Figdor CG, Jacobs JFM, Tel J, et al. Long-lasting multifunctional CD8(+) T cell responses in end-stage melanoma patients can be induced by dendritic cell vaccination. Oncoimmunology (2016) 5(1):e1067745. doi: 10.1080/2162402X.2015.1067745
49. Kupz A, Guarda G, Gebhardt T, Sander LE, Short KR, Diavatopoulos DA, et al. NLRC4 inflammasomes in dendritic cells regulate noncognate effector function by memory CD8+ T cells. Nat Immunol (2012) 13(2):162–9. doi: 10.1038/ni.2195
50. Kohlmeier JE, Cookenham T, Roberts AD, Miller SC, Woodland DL. Type I interferons regulate cytolytic activity of memory CD8(+) T cells in the lung airways during respiratory virus challenge. Immunity (2010) 33(1):96–105. doi: 10.1016/j.immuni.2010.06.016
51. Freeman BE, Hammarlund E, Raué HP, Slifka MK. Regulation of innate CD8+ T-cell activation mediated by cytokines. Proc Natl Acad Sci U S A (2012) 109(25):9971–6. doi: 10.1073/pnas.1203543109
52. Salerno F, Guislain A, Cansever D, Wolkers MC. TLR-Mediated Innate Production of IFN-gamma by CD8+ T Cells Is Independent of Glycolysis. J Immunol (2016) 196(9):3695–705. doi: 10.4049/jimmunol.1501997
53. Topham DJ, Reilly EC. Tissue-Resident Memory CD8(+) T Cells: From Phenotype to Function. Front Immunol (2018) 9:515. doi: 10.3389/fimmu.2018.00515
54. Romero P, Zippelius A, Kurth I, Pittet MJ, Touvrey C, Iancu EM, et al. Four functionally distinct populations of human effector-memory CD8+ T lymphocytes. J Immunol (2007) 178(7):4112–9. doi: 10.4049/jimmunol.178.7.4112
55. Gao CY, Yao Y, Li L, Yang SH, Chu H, Tsuneyama K, et al. Tissue-Resident Memory CD8+ T Cells Acting as Mediators of Salivary Gland Damage in a Murine Model of Sjogren’s Syndrome. Arthritis Rheumatol (2019) 71(1):121–32. doi: 10.1002/art.40676
56. Ely KH, Cookenham T, Roberts AD, Woodland DL. Memory T cell populations in the lung airways are maintained by continual recruitment. J Immunol (2006) 176(1):537–43. doi: 10.4049/jimmunol.176.1.537
57. Ray SJ, Franki SN, Pierce RH, Dimitrova S, Koteliansky V, Sprague AG, et al. The collagen binding alpha1beta1 integrin VLA-1 regulates CD8 T cell-mediated immune protection against heterologous influenza infection. Immunity (2004) 20(2):167–79. doi: 10.1016/S1074-7613(04)00021-4
58. Yang R, Masters AR, Fortner KA, Champagne DP, Yanguas-Casás N, Silberger DJ, et al. IL-6 promotes the differentiation of a subset of naïve CD8+ T cells into IL-21-producing B helper CD8+ T cells. J Exp Med (2016) 213(11):2281–91. doi: 10.1084/jem.20160417
59. Visekruna A, Ritter J, Scholz T, Campos L, Guralnik A, Poncette L, et al. Tc9 cells, a new subset of CD8(+) T cells, support Th2-mediated airway inflammation. Eur J Immunol (2013) 43(3):606–18. doi: 10.1002/eji.201242825
60. Quigley MF, Gonzalez VD, Granath A, Andersson J, Sandberg JK. CXCR5+ CCR7- CD8 T cells are early effector memory cells that infiltrate tonsil B cell follicles. Eur J Immunol (2007) 37(12):3352–62. doi: 10.1002/eji.200636746
61. Li Y, Tang L, Guo L, Chen C, Gu S, Zhou Y, et al. CXCL13-mediated recruitment of intrahepatic CXCR5(+)CD8(+) T cells favors viral control in chronic HBV infection. J Hepatol (2020) 72(3):420–30. doi: 10.1016/j.jhep.2019.09.031
62. Sage PT, Sharpe AH. T follicular regulatory cells. Immunol Rev (2016) 271(1):246–59. doi: 10.1111/imr.12411
63. Hu D, Ikizawa K, Lu L, Sanchirico ME, Shinohara ML, Cantor H. Analysis of regulatory CD8 T cells in Qa-1-deficient mice. Nat Immunol (2004) 5(5):516–23. doi: 10.1038/ni1063
64. Leavenworth JW, Tang X, Kim HJ, Wang X, Cantor H. Amelioration of arthritis through mobilization of peptide-specific CD8+ regulatory T cells. J Clin Invest (2013) 123(3):1382–9. doi: 10.1172/JCI66938
65. Tasaki S, Suzuki K, Nishikawa A, Kassai Y, Takiguchi M, Kurisu R, et al. Multiomic disease signatures converge to cytotoxic CD8 T cells in primary Sjogren’s syndrome. Ann Rheum Dis (2017) 76(8):1458–66. doi: 10.1136/annrheumdis-2016-210788
66. Wu RC, Hwu P, Radvanyi LG. New insights on the role of CD8(+)CD57(+) T-cells in cancer. Oncoimmunology (2012) 1(6):954–6. doi: 10.4161/onci.20307
67. Sudzius G, Mieliauskaite D, Siaurys A, Viliene R, Butrimiene I, Characiejus D, et al. Distribution of Peripheral Lymphocyte Populations in Primary Sjogren’s Syndrome Patients. J Immunol Res (2015) 2015:854706. doi: 10.1155/2015/854706
68. Le Priol Y, Puthier D, Lecureuil C, Combadière C, Debrè P, Nguyen C, et al. High cytotoxic and specific migratory potencies of senescent CD8+ CD57+ cells in HIV-infected and uninfected individuals. J Immunol (2006) 177(8):5145–54. doi: 10.4049/jimmunol.177.8.5145
69. Ushio A, Arakaki R, Otsuka K, et al. CCL22-Producing Resident Macrophages Enhance T Cell Response in Sjogren’s Syndrome. Front Immunol (2018) 9:2594. doi: 10.3389/fimmu.2018.02594
70. Baker OJ, Camden JM, Redman RS, Jones JE, Seye CI, Erb L, et al. Proinflammatory cytokines tumor necrosis factor-alpha and interferon-gamma alter tight junction structure and function in the rat parotid gland Par-C10 cell line. Am J Physiol Cell Physiol (2008) 295(5):C1191–1201. doi: 10.1152/ajpcell.00144.2008
71. Matsumura R, Umemiya K, Goto T, Nakazawa T, Ochiai K, Kagami M, et al. Interferon gamma and tumor necrosis factor alpha induce Fas expression and anti-Fas mediated apoptosis in a salivary ductal cell line. Clin Exp Rheumatol (2000) 18(3):311–8. doi: 10.1002/1529-0131(200005)43:5<1190::AID-ANR37>3.0.CO;2-C
72. Lowin B, Hahne M, Mattmann C, Tschopp J. Cytolytic T-cell cytotoxicity is mediated through perforin and Fas lytic pathways. Nature (1994) 370(6491):650–2. doi: 10.1038/370650a0
73. Kong L, Ogawa N, Nakabayashi T, Liu GT, D’Souza E, McGuff HS, et al. Fas and Fas ligand expression in the salivary glands of patients with primary Sjögren’s syndrome. Arthritis Rheumatol (1997) 40(1):87–97. doi: 10.1002/art.1780400113
74. Nakamura H, Horai Y, Shimizu T, Kawakami A. Modulation of Apoptosis by Cytotoxic Mediators and Cell-Survival Molecules in Sjogren’s Syndrome. Int J Mol Sci (2018) 19(8):2369. doi: 10.3390/ijms19082369
75. Woyciechowski S, Hofmann M, Pircher H. alpha4 beta1 integrin promotes accumulation of tissue-resident memory CD8(+) T cells in salivary glands. Eur J Immunol (2017) 47(2):244–50. doi: 10.1002/eji.201646722
76. Sato T, Thorlacius H, Johnston B, Staton TL, Xiang W, Littman DR, et al. Role for CXCR6 in recruitment of activated CD8+ lymphocytes to inflamed liver. J Immunol (2005) 174(1):277–83. doi: 10.4049/jimmunol.174.1.277
77. Nakamura H, Koji T, Tominaga M, Kawakami A, Migita K, Kawabe Y, et al. Apoptosis in labial salivary glands from Sjögren’s syndrome (SS) patients: comparison with human T lymphotropic virus-I (HTLV-I)-seronegative and -seropositive SS patients. Clin Exp Immunol (1998) 114(1):106–12. doi: 10.1046/j.1365-2249.1998.00692.x
78. Usherwood EJ, Hogan RJ, Crowther G, Surman SL, Hogg TL, Altman JD, et al. Functionally heterogeneous CD8(+) T-cell memory is induced by Sendai virus infection of mice. J Virol (1999) 73(9):7278–86. doi: 10.1128/JVI.73.9.7278-7286.1999
79. Klonowski KD, Williams KJ, Marzo AL, Blair DA, Lingenheld EG, Lefrançois L. Dynamics of blood-borne CD8 memory T cell migration in vivo. Immunity (2004) 20(5):551–62. doi: 10.1016/S1074-7613(04)00103-7
80. Sallusto F, Geginat J, Lanzavecchia A. Central memory and effector memory T cell subsets: function, generation, and maintenance. Annu Rev Immunol (2004) 22:745–63. doi: 10.1146/annurev.immunol.22.012703.104702
81. Jiang X, Clark RA, Liu L, Wagers AJ, Fuhlbrigge RC, Kupper TS. Skin infection generates non-migratory memory CD8+ T(RM) cells providing global skin immunity. Nature (2012) 483(7388):227–31. doi: 10.1038/nature10851
82. Roberts AI, Brolin RE, Ebert EC. Integrin alpha1beta1 (VLA-1) mediates adhesion of activated intraepithelial lymphocytes to collagen. Immunology (1999) 97(4):679–85. doi: 10.1046/j.1365-2567.1999.00812.x
83. Mackay LK, Braun A, Macleod BL, Collins N, Tebartz C, Bedoui S, et al. Cutting edge: CD69 interference with sphingosine-1-phosphate receptor function regulates peripheral T cell retention. J Immunol (2015) 194(5):2059–63. doi: 10.4049/jimmunol.1402256
84. Kuric E, Seiron P, Krogvold L, Edwin B, Buanes T, Hanssen KF, et al. Demonstration of Tissue Resident Memory CD8 T Cells in Insulitic Lesions in Adult Patients with Recent-Onset Type 1 Diabetes. Am J Pathol (2017) 187(3):581–8. doi: 10.1016/j.ajpath.2016.11.002
85. Christodoulou MI, Kapsogeorgou EK, Moutsopoulos HM. Characteristics of the minor salivary gland infiltrates in Sjögren’s syndrome. J Autoimmun (2010) 34(4):400–7. doi: 10.1016/j.jaut.2009.10.004
86. Groom JR, Luster AD. CXCR3 in T cell function. Exp Cell Res (2011) 317(5):620–31. doi: 10.1016/j.yexcr.2010.12.017
87. Tang XL, Smith TR, Kumar V. Specific control of immunity by regulatory CD8 T cells. Cell Mol Immunol (2005) 2(1):11–9.
88. Dinesh RK, Skaggs BJ, La Cava A, Hahn BH, Singh RP. CD8+ Tregs in lupus, autoimmunity, and beyond. Autoimmun Rev (2010) 9(8):560–8. doi: 10.1016/j.autrev.2010.03.006
89. Suzuki M, Konya C, Goronzy JJ, Weyand CM. Inhibitory CD8+ T cells in autoimmune disease. Hum Immunol (2008) 69(11):781–9. doi: 10.1016/j.humimm.2008.08.283
90. Konya C, Goronzy JJ, Weyand CM. Treating autoimmune disease by targeting CD8(+) T suppressor cells. Expert Opin Biol Ther (2009) 9(8):951–65. doi: 10.1517/14712590903020759
91. Bezie S, Meistermann D, Boucault L, Kilens S, Zoppi J, Autrusseau E, et al. Ex Vivo Expanded Human Non-Cytotoxic CD8(+)CD45RC(low/-) Tregs Efficiently Delay Skin Graft Rejection and GVHD in Humanized Mice. Front Immunol (2017) 8:2014. doi: 10.3389/fimmu.2017.02014
92. Liu Q, Zheng H, Chen X, Peng Y, Huang W, Li X, et al. Human mesenchymal stromal cells enhance the immunomodulatory function of CD8(+)CD28(-) regulatory T cells. Cell Mol Immunol (2015) 12(6):708–18. doi: 10.1038/cmi.2014.118
93. Feger U, Tolosa E, Huang YH, Waschbisch A, Biedermann T, Melms A, et al. HLA-G expression defines a novel regulatory T-cell subset present in human peripheral blood and sites of inflammation. Blood (2007) 110(2):568–77. doi: 10.1182/blood-2006-11-057125
94. Gilliet M, Liu YJ. Generation of human CD8 T regulatory cells by CD40 ligand-activated plasmacytoid dendritic cells. J Exp Med (2002) 195(6):695–704. doi: 10.1084/jem.20011603
95. Filaci G, Fenoglio D, Indiveri F. CD8+T regulatory/suppressor cells and their relationships with autoreactivity and autoimmunity. Autoimmunity (2010) 44(1):51–7. doi: 10.3109/08916931003782171
96. Mills KH. Regulatory T cells: friend or foe in immunity to infection? Nat Rev Immunol (2004) 4(11):841–55. doi: 10.1038/nri1485
97. Sallusto F, Lenig D, Förster R, Lipp M, Lanzavecchia A. Two subsets of memory T lymphocytes with distinct homing potentials and effector functions. Nature (1999) 401(6754):708–12. doi: 10.1038/44385
98. Robb RJ, Lineburg KE, Kuns RD, Wilson YA, Raffelt NC, Olver SD, et al. Identification and expansion of highly suppressive CD8(+)FoxP3(+) regulatory T cells after experimental allogeneic bone marrow transplantation. Blood (2012) 119(24):5898–908. doi: 10.1182/blood-2011-12-396119
99. Lei H, Kuchenbecker L, Streitz M, Sawitzki B, Vogt K, Landwehr-Kenzel S, et al. Human CD45RA(-) FoxP3(hi) Memory-Type Regulatory T Cells Show Distinct TCR Repertoires With Conventional T Cells and Play an Important Role in Controlling Early Immune Activation. Am J Transplant (2015) 15(10):2625–35. doi: 10.1111/ajt.13315
100. Tsai S, Shameli A, Yamanouchi J, Clemente-Casares X, Wang J, Serra P, et al. Reversal of autoimmunity by boosting memory-like autoregulatory T cells. Immunity (2010) 32(4):568–80. doi: 10.1016/j.immuni.2010.03.015
101. Najafian N, Chitnis T, Salama AD, Zhu B, Benou C, Yuan X, et al. Regulatory functions of CD8+CD28– T cells in an autoimmune disease model. J Clin Invest (2003) 112(7):1037–48. doi: 10.1172/JCI17935
102. Abdul-Majid K-B, Wefer J, Stadelmann C, Stefferl A, Lassmann H, Olsson T, et al. Comparing the pathogenesis of experimental autoimmune encephalomyelitis in CD4–/– and CD8–/– DBA/1 mice defines qualitative roles of different T cell subsets. J Neuroimmunol (2003) 141(1-2):10–9. doi: 10.1016/S0165-5728(03)00210-8
103. York NR, Mendoza JP, Ortega SB, Benagh A, Tyler AF, Firan M, et al. Immune regulatory CNS-reactive CD8+T cells in experimental autoimmune encephalomyelitis. J Autoimmun (2010) 35(1):33–44. doi: 10.1016/j.jaut.2010.01.003
104. Correale J, Villa A. Role of CD8+ CD25+ Foxp3+ regulatory T cells in multiple sclerosis. Ann Neurol (2010) 67(5):625–38. doi: 10.1002/ana.21944
105. Yang ZQ, Yang ZY, Zhang LD, Ping-Bie, Wang SG, Ma KS, et al. Increased liver-infiltrating CD8+FoxP3+ regulatory T cells are associated with tumor stage in hepatocellular carcinoma patients. Hum Immunol (2010) 71(12):1180–6. doi: 10.1016/j.humimm.2010.09.011
106. Peterson RA. Regulatory T-cells: diverse phenotypes integral to immune homeostasis and suppression. Toxicol Pathol (2012) 40(2):186–204. doi: 10.1177/0192623311430693
107. Guillonneau C, Hill M, Hubert FX, Chiffoleau E, Hervé C, Li XL, et al. CD40Ig treatment results in allograft acceptance mediated by CD8CD45RC T cells, IFN-gamma, and indoleamine 2,3-dioxygenase. J Clin Invest (2007) 117(4):1096–106. doi: 10.1172/JCI28801
108. Zhang J. Yin and yang interplay of IFN-γ in inflammation and autoimmune disease. J Clin Invest (2007) 117(4):871–3. doi: 10.1172/JCI31860
109. Zhang S, Ke X, Zeng S, Wu M, Lou J, Wu L, et al. Analysis of CD8+ Treg cells in patients with ovarian cancer: a possible mechanism for immune impairment. Cell Mol Immunol (2015) 12(5):580–91. doi: 10.1038/cmi.2015.57
110. Chen ML, Yan BS, Kozoriz D, Weiner HL. Novel CD8+ Treg suppress EAE by TGF-beta- and IFN-gamma-dependent mechanisms. Eur J Immunol (2009) 39(12):3423–35. doi: 10.1002/eji.200939441
111. Dai Z, Zhang S, Xie Q, Wu S, Su J, Li S, et al. Natural CD8+CD122+ T Cells Are More Potent in Suppression of Allograft Rejection Than CD4+CD25+ Regulatory T Cells. Am J Transplant (2014) 14(1):39–48. doi: 10.1111/ajt.12515
112. Tsai YG, Yang KD, Wen YS, Hung CH, Chien JW, Lin CY. Allergen-specific immunotherapy enhances CD8(+) CD25(+) CD137(+) regulatory T cells and decreases nasal nitric oxide. Pediatr Allergy Immunol (2019) 30(5):531–9. doi: 10.1111/pai.13061
113. Smoleńska Ż, Pawłowska J, Zdrojewski Z, Daca A, Bryl E. Increased percentage of CD8+CD28– T cells correlates with clinical activity in primary Sjögren’s syndrome. Cell Immunol (2012) 278(1-2):143–51. doi: 10.1016/j.cellimm.2012.08.001
114. Furuzawa-Carballeda J, Hernandez-Molina G, Lima G, Rivera-Vicencio Y, Ferez-Blando K, Llorente L. Peripheral regulatory cells immunophenotyping in primary Sjogren’s syndrome: a cross-sectional study. Arthritis Res Ther (2013) 15(3):R68. doi: 10.1186/ar4245
115. Christodoulou MI, Kapsogeorgou EK, Moutsopoulos NM, Moutsopoulos HM. Foxp3+ T-regulatory cells in Sjogren’s syndrome: correlation with the grade of the autoimmune lesion and certain adverse prognostic factors. Am J Pathol (2008) 173(5):1389–96. doi: 10.2353/ajpath.2008.080246
116. Hebbar M, Jeannin P, Magistrelli G, Hatron PY, Hachulla E, Devulder B, et al. Detection of circulating soluble CD28 in patients with systemic lupus erythematosus, primary Sjogren’s syndrome and systemic sclerosis. Clin Exp Immunol (2004) 136(2):388–92. doi: 10.1111/j.1365-2249.2004.02427.x
117. Matsumoto I, Okada S, Kuroda K, Iwamoto I, Saito Y, Tokuhisa T, et al. Single cell analysis of T cells infiltrating labial salivary glands from patients with Sjögren’s syndrome. Int J Mol Med (1999) 4(5):519–27. doi: 10.3892/ijmm.4.5.519
118. Rifa’i M, Kawamoto Y, Nakashima I, Suzuki H. Essential roles of CD8+CD122+ regulatory T cells in the maintenance of T cell homeostasis. J Exp Med (2004) 200(9):1123–34. doi: 10.1084/jem.20040395
119. Lisney AR, Szelinski F, Reiter K, Burmester GR, Rose T, Dorner T. High maternal expression of SIGLEC1 on monocytes as a surrogate marker of a type I interferon signature is a risk factor for the development of autoimmune congenital heart block. Ann Rheum Dis (2017) 76(8):1476–80. doi: 10.1136/annrheumdis-2016-210927
120. Hoyne GF, Elliott H, Mutsaers SE, Prele CM. Idiopathic pulmonary fibrosis and a role for autoimmunity. Immunol Cell Biol (2017) 95(7):577–83. doi: 10.1038/icb.2017.22
121. Craiu A, Gaczynska M, Akopian T, Gramm CF, Fenteany G, Goldberg AL, et al. Lactacystin and clasto-lactacystin beta-lactone modify multiple proteasome beta-subunits and inhibit intracellular protein degradation and major histocompatibility complex class I antigen presentation. J Biol Chem (1997) 272(20):13437–45. doi: 10.1074/jbc.272.20.13437
122. Fenteany G, Schreiber SL. Lactacystin, proteasome function, and cell fate. J Biol Chem (1998) 273(15):8545–8. doi: 10.1074/jbc.273.15.8545
123. Arellano-Garcia ME, Misuno K, Tran SD, Hu S. Interferon-gamma induces immunoproteasomes and the presentation of MHC I-associated peptides on human salivary gland cells. PloS One (2014) 9(8):e102878. doi: 10.1371/journal.pone.0102878
124. Tran SD, Kodama S, Lodde BM, Szalayova I, Key S, Khalili S, et al. Reversal of Sjogren’s-like syndrome in non-obese diabetic mice. Ann Rheum Dis (2007) 66(6):812–4. doi: 10.1136/ard.2006.064030
125. Hess AD. Mechanisms of action of cyclosporine: considerations for the treatment of autoimmune diseases. Clin Immunol Immunopathol (1993) 68(2):220–8. doi: 10.1006/clin.1993.1122
126. Jabs DA, Lee B, Burek CL, Saboori AM, Prendergast RA. Cyclosporine therapy suppresses ocular and lacrimal gland disease in MRL/Mp-lpr/lpr mice. Invest Ophthalmol Vis Sci (1996) 37(2):377–83.
127. Meiners PM, Vissink A, Kroese FG, Spijkervet FK, Smitt-Kamminga NS, Abdulahad WH, et al. Abatacept treatment reduces disease activity in early primary Sjogren’s syndrome (open-label proof of concept ASAP study). Ann Rheum Dis (2014) 73(7):1393–6. doi: 10.1136/annrheumdis-2013-204653
128. Onuora S. Abatacept no better than placebo for pSS. Nat Rev Rheumatol (2020) 16(4):186. doi: 10.1038/s41584-020-0390-8
129. Baer AN, Gottenberg JE, St Clair EW, Sumida T, Takeuchi T, Seror R, et al. Efficacy and safety of abatacept in active primary Sjogren’s syndrome: results of a phase III, randomised, placebo-controlled trial. Ann Rheum Dis (2020). doi: 10.1136/annrheumdis-2020-218599
130. Santana-de Anda K, Gomez-Martin D, Soto-Solis R, Alcocer-Varela J. Plasmacytoid dendritic cells: key players in viral infections and autoimmune diseases. Semin Arthritis Rheumatol (2013) 43(1):131–6. doi: 10.1016/j.semarthrit.2012.12.026
131. Narain S, Berman N, Furie R. Biologics in the treatment of Sjogren’s syndrome, systemic lupus erythematosus, and lupus nephritis. Curr Opin Rheumatol (2020) 32(6):609–16. doi: 10.1097/BOR.0000000000000754
132. Ping L, Ogawa N, Sugai S. Novel role of CD40 in Fas-dependent apoptosis of cultured salivary epithelial cells from patients with Sjögren’s syndrome. Arthritis Rheum (2005) 52(2):573–81. doi: 10.1002/art.20789
133. Wieczorek G, Bigaud M, Pfister S, Ceci M, McMichael K, Afatsawo C, et al. Blockade of CD40-CD154 pathway interactions suppresses ectopic lymphoid structures and inhibits pathology in the NOD/ShiLtJ mouse model of Sjogren’s syndrome. Ann Rheum Dis (2019) 78(7):974–8. doi: 10.1136/annrheumdis-2018-213929
134. Fisher BA, Szanto A, Ng W-F, Bombardieri M, Posch MG, Papas AS, et al. Assessment of the anti-CD40 antibody iscalimab in patients with primary Sjögren’s syndrome: a multicentre, randomised, double-blind, placebo-controlled, proof-of-concept study. Lancet Rheumatol (2020) 2(3):e142–52. doi: 10.1016/S2665-9913(19)30135-3
135. Sun J, Yang Y, Huo X, Zhu B, Li Z, Jiang X, et al. Efficient Therapeutic Function and Mechanisms of Human Polyclonal CD8(+)CD103(+)Foxp3(+) Regulatory T Cells on Collagen-Induced Arthritis in Mice. J Immunol Res (2019) 2019:8575407. doi: 10.1155/2019/8575407
136. Stocks BT, Wilson CS, Marshall AF, Hoopes EM, Moore DJ. Regulation of Diabetogenic Immunity by IL-15-Activated Regulatory CD8 T Cells in Type 1 Diabetes. J Immunol (2019) 203(1):158–66. doi: 10.4049/jimmunol.1800976
137. Bezie S, Picarda E, Ossart J, Tesson L, Usal C, Renaudin K, et al. IL-34 is a Treg-specific cytokine and mediates transplant tolerance. J Clin Invest (2015) 125(10):3952–64. doi: 10.1172/JCI81227
Keywords: Sjögren’s syndrome, CD8+ T lymphocyte, pathophysiology, tissue resident lymphocyte, immune regulation
Citation: Zhou H, Yang J, Tian J and Wang S (2021) CD8+ T Lymphocytes: Crucial Players in Sjögren’s Syndrome. Front. Immunol. 11:602823. doi: 10.3389/fimmu.2020.602823
Received: 04 September 2020; Accepted: 10 December 2020;
Published: 28 January 2021.
Edited by:
Kristi A. Koelsch, University of Oklahoma Health Sciences Center, United StatesReviewed by:
Tsutomu Takeuchi, Keio University, JapanRoberto Giacomelli, University of L’Aquila, Italy
Copyright © 2021 Zhou, Yang, Tian and Wang. This is an open-access article distributed under the terms of the Creative Commons Attribution License (CC BY). The use, distribution or reproduction in other forums is permitted, provided the original author(s) and the copyright owner(s) are credited and that the original publication in this journal is cited, in accordance with accepted academic practice. No use, distribution or reproduction is permitted which does not comply with these terms.
*Correspondence: Shengjun Wang, sjwjs@ujs.edu.cn; Jie Tian, 1000004415@ujs.edu.cn