- 1Institute of Animal Husbandry, Heilongjiang Academy of Agricultural Sciences, Harbin, China
- 2Information Department for Epidemic Prevention, Heilongjiang Provincial Animal Epidemic Prevention and Control Center, Harbin, China
- 3Hunan Co-Innovation Center of Animal Production Safety, CICAPS, College of Animal Science and Technology, Hunan Agricultural University, Changsha, China
- 4College of Life Sciences, Northeast Agricultural University, Harbin, China
Background: Ulva prolifera extract contains a variety of functional active substances. Whether these substances had any beneficial effects on the small intestine of weaned piglets under oxidative stress remained unknown.
Method: We explored the effects of U. prolifera extract on oxidative stress and related mechanisms in weaned piglets and intestinal porcine epithelial cells (IPEC-J2) challenged with hydrogen peroxide.
Results: U. prolifera extract was found to mainly consist of polyphenols and unsaturated fatty acids. U. prolifera extract increased total antioxidant capacity and superoxide dismutase (SOD) activity, while it decreased malondialdehyde content, in the serum of weaned piglets challenged with hydrogen peroxide. Moreover, U. prolifera extract increased mRNA expression of SOD and catalase, as well as the intestinal expression of nuclear NF-E2-related factor 2 (Nrf2), both in vitro and in vivo. Furthermore, U. prolifera extract decreased reactive oxygen species and improved mitochondrial respiration in IPEC-J2 cells treated with hydrogen peroxide. However, AMPK inhibition did not affect nuclear Nrf2 expression and only partially affected the effects of U. prolifera extract on oxidative stress.
Conclusion: We suggest that U. prolifera extract alleviates oxidative stress via Nrf2 signaling, but independent of AMPK pathway in weaned piglets challenged with hydrogen peroxide. These results shed new insight into the potential applications of U. prolifera extract as a therapeutic agent for the prevention and treatment of oxidative stress-induced intestinal diseases.
Introduction
Ulva prolifera is a common green algae that blooms in the Bohai and the Yellow Seas of China (1). In ancient China, U. prolifera is not only a food but also a pharmaceutical product for the treatment of diseases (2). In recent years, many components such as polyphenols, flavonoids, and polysaccharides have been extracted from U. prolifera (3–5). These components have been proven to widely exert antioxidant, anti-inflammatory, and hypolipidemic properties (1, 2, 6). Consequently, U. prolifera extract has the potential to be used for the prevention and treatment of diseases such as diabetes and non-alcoholic fatty liver diseases (4, 7). However, it remains unknown whether U. prolifera extract has any effects on oxidative damage in the intestine.
Redox homeostasis is a key factor in the maintenance of normal cellular and organic functions (8). The gastrointestinal tract is prone to oxidative damage as it faces a complicated luminal environment of ingested materials and microbial pathogens (9). The loss of redox homeostasis in the gastrointestinal tract due to oxidation-induced disruption can contribute to the development of various intestinal diseases such as ulcers, malignancies, and colitis (10). Hydrogen peroxide, a highly reactive oxygen species (ROS), causes the imbalance of intestinal cellular redox in vivo and in vitro (11). Previous studies have shown that hydrogen peroxide decreased the activity of antioxidant enzymes, increased ROS content and apoptosis level, and caused mitochondrial dysfunction in intestinal epithelial cells of weaned piglets (8, 11). These results suggested that weaned piglets challenged with hydrogen peroxide could provide appropriate dysfunctional intestine model for redox balance disruption. Additionally, many researchers suggested that the pig is a valuable resource for biomedical research, and experiments in pigs are much likely to be predictive of therapeutic treatments in humans (12). Because the pig is very similar to humans in terms of anatomy, genetics and physiology (13, 14).
Consequently, the present study was conducted to determine the effects of U. prolifera extract on oxidative stress and mitochondrial dysfunction, as well as its related mechanisms, in the intestine of hydrogen peroxide-challenged piglets. We expected that the results would shed new insight into the potential applications of U. prolifera extract as a therapeutic agent for the prevention and treatment of oxidative stress-induced intestinal diseases.
Materials and Methods
Sample Extraction
U. prolifera was collected in May 2018 from the coast of Beidaihe in the Bohai Sea. The collected U. prolifera was rinsed with running water and dried in the sun. One kilogram of dried U. prolifera was mixed with 5 L distilled water and extracted for 3 h at 90°C in an ultrasonic bath (200 W, 45 kHz). The supernatant was collected by filtration through siliceous earth and then run through an adsorption chromatography column (120 cm L × 150 mm ID—Huamei Experiment Instrument Plant, Shanghai, China) packed with AB-8 macroporous adsorption resin. After initial elution with distilled water, the final extract (UE) was obtained by elution with 70% methanol, followed by freeze-drying. For sample preparation, 10 mg of UE powder was dissolved in 200 ml of distilled water. Then a 1 ml aliquot of the upper layer was filtered through a 0.22 µm nylon membrane prior to UHPLC/Q-TOF-MS analysis.
UHPLC-Q-TOF-MS/MS Analysis
An Agilent 1290 Series UHPLC (Agilent, Palo Alto, CA, USA) was used and chromatography was performed in a ZORBAX Eclipse Plus C18 column (2.1 × 100 mm, 1.8 μm) (Agilent). The mobile phases consisted of water containing 0.1% formic acid (A) and acetonitrile (B). Gradient elution was performed with 5% B (0–1 min), 55% B (1–6 min), 95% B (6–20 min), 95% B (20–26 min), and 5% (1 min). Finally, the column was conditioned with 5% B for 8 min. The total run time was 35 min, with a flow rate of 0.25 ml/min, and an injection volume of 5 µl. The column temperature was 30°C.
MS was performed using an Agilent 6545 ESI-Q-TOF (Agilent). The optimum MS conditions consisted of a capillary voltage of 3500 V in negative ionization mode, a skimmer voltage of 65 V, and a fragmentor at 135 V. The gas temperature was 320°C, the drying gas flow rate was 8 L/min, and the nebulizer pressure was 35 psi. The sheath gas temperature was 350°C, and the sheath gas flow was 11 L/min. MS spectra were acquired at 100–1,700 m/z using an extended dynamic range at a scan rate of 2.0 spectra/s. A reference mass solution that contained reference ions (m/z 112.985587 and m/z 1033.988109) was used to maintain mass accuracy during the run. The MassHunter Workstation software (Agilent) was used to control the UHPLC/Q-TOF-MS system and to process recorded data, while the MassHunter Profiling software (Agilent) was used to screen the characteristic compound (15). The IM-MS Browser software (Agilent) and Traditional Chinese Medicine (TCM) database (Agilent) were used to identify the compound’s identity.
Experimental Design
Thirty-two Landrace × Large White piglets (mean body weight of 6.81 ± 0.21 kg) were weaned at the age of 21 days, and randomly assigned to one of four treatment groups (n = 8/group): (1) Piglets fed a basal diet (CONT); (2) Piglets fed a basal diet supplemented with 0.1% extract of U. prolifera (UE group); (3) Piglets fed a basal diet and intraperitoneal injection of 10% hydrogen peroxide (1 ml/kg body weight) (HP); (4) Piglets fed a basal diet supplemented with 0.1% extract of U. prolifera and intraperitoneal injection of 10% hydrogen peroxide (UEH). The experiment lasted for 14 days and all piglets had free access to feed and drinking water. The diets for the UE and UEH groups were supplemented with U. prolifera extract daily. Hydrogen peroxide were administered intraperitoneally in the HP and UEH groups on days 8 and 11 as previously did (8). The composition and nutrient levels of the basal diet met the nutrient requirements listed in Nutritional Requirements of Swine (NRC, 2012) (Supplementary Table 1). Piglets were weighed on days 1 and 14, and their feed intake was recorded daily. Average daily feed intake, average daily weight gain, and the ratio of feed intake to weight gain, were calculated. The experimental protocol was approved by the Protocol Management and Review Committee of the Institute of Animal Husbandry, Heilongjiang Academy of Agricultural Sciences. Pigs were cared for and slaughtered according to the guidelines of Heilongjiang Academy of Agricultural Sciences (Harbin, China).
Determination of Serum Antioxidative Enzymes and Malondialdehyde
Malondialdehyde (MDA) content, total antioxidant capacity (T-AOC), and superoxide dismutase (SOD) activities were analyzed in serum using commercial kits according to the manufacturer’s instructions (Beyotime Biotechnology, Shanghai, China).
Cell Culture and Treatments
Intestinal porcine epithelial cells (IPEC-J2) were cultured in Dulbecco’s Modified Eagles Medium/Nutrient Mixture F-12 (Gibco, Carlsbad, CA, USA). After being seeded in 6-well plates and grown at ∼60−70% confluence, cells cultured in fresh medium without fetal bovine serum (FBS) for 12 h were pretreated with U. prolifera extract (40 μg/ml) for 12 h, and then treated with 200 μM hydrogen peroxide for 6 h. For the inhibition of AMPK, 5 μM Compound C (Selleck Chemicals, Shanghai, China) was added to the medium for 6 h.
RT-qPCR Analysis
Total RNA was extracted from ileum samples and then reverse-transcribed into cDNA using reverse transcriptase (Takara Bio, Tokyo, Japan). Pig-specific primers were designed using Primer 5.0 software (Supplementary Table 2). The mRNA expression level of the target gene was normalized using housekeeping genes β-actin and GAPDH. RT-PCR was performed as described previously (16, 17). Relative expression of the target gene in the treatment groups was expressed as a ratio to the expression of the target gene in the control group.
Protein Qualification by the Wes Simple Western System
Protein qualification was performed using the Wes Simple Western System (ProteinSimple, San Jose, CA, USA), an automated process of capillary gel electrophoresis. Proteins were extracted from IPEC-J2 cells, mixed with Simple Western Sample Buffer, Master Mix, dithiothreitol, and fluorescent standards (ProteinSimple), and then loaded into Wes 25-well plates. Thereafter, primary and secondary antibodies, luminol-peroxide mixture, stacking gel matrix, and separation gel matrix were added to the appropriate wells. Primary antibodies used in the experiment included antibodies against Keap1, Nrf2, β-actin (Abcam, Cambridge, MA, USA); lamin B1, AMPK and phospho-AMPK (Bioss, Beijing, China). Results were obtained using the “gel view” function of the software (ProteinSimple). Total cellular protein expression was normalized to β-actin, while nuclear Nrf2 and Keap1 protein expression was normalized to lamin B1.
Measurement of ROS in Mitochondria
IPEC-J2 cells seeded into confocal dishes were treated with 5 μM MitoSOX™ reagent working solution (Invitrogen, Shanghai, China) and incubated for 10 min at 37°C. Cells were then treated with anti-fluorescence quenching agent and observed using a ZEISS LSM 880 confocal microscope (ZEISS, Shanghai, China).
Mitochondrial Membrane Potential Assay
Mitochondrial membrane potential was assayed by double fluorescence staining with JC-1 (Invitrogen, Shanghai, China). After being seeded into confocal dishes, the cells were incubated with 10 μg/ml JC-1 for 30 min, and then washed twice with PBS. Cells were then treated with anti-fluorescence quenching agent and observed using a ZEISS LSM 880 confocal microscope (ZEISS, Shanghai, China).
Mitochondrial Respiration
Mitochondrial respiration was measured using an XF-24 Extracellular Flux Analyzer and a Cell Mito Stress Test Kit (Agilent, Beijing, China) according to the manufacturer’s instructions. The baseline oxygen-consumption rate (basal OCR), spare respiratory capacity, non-ATP-linked oxygen consumption (proton leakage), maximal respiratory capacity (maximal respiration), ATP-linked mitochondrial oxygen consumption (ATP production), and non-mitochondrial respiration were determined as previously described (18). Total cellular protein was analyzed for normalizing mitochondrial respiration rates.
Immunofluorescent Assay
IPEC-J2 cells seeded into confocal dishes were fixed with 4% paraformaldehyde for 15 min, permeabilized with 0.3% Triton X-100 for 15 min. Cells were then blocked in 10% BSA for 20 min, and incubated with primary antibodies Keap1 and Nrf2 (Abcam, Cambridge, MA, USA) overnight at 4°C. After being washed three times with PBS, cells were incubated with secondary antibodies for 1 h. The nuclear DNA was labeled with 4’,6-Diamidino-2-phenylindole (Sigma-Aldrich, St. Louis, MO, USA) for 2 min, and cells were then treated with anti-fluorescence quenching agent and observed using a ZEISS LSM 880 confocal microscope (ZEISS, Shanghai, China).
AMPK Activity Determination
AMPK activity was determined using AMPK Kinase Assay Kit (CycLex, Tokyo, Japan) as previously described (19). Briefly, IPEC-J2 cells were collected and lysed. Then, supernatant was obtained by centrifugation at 12,000 g and 4°C for 15 min, for the determination of the relative AMPK activity.
Statistical Analysis
Statistical analysis was performed by one-way ANOVA followed by Student-Newman-Keuls post hoc test using data statistics software SPSS 18.0. All the measurement data was expressed as the means ± standard error (SEM). P < 0.05 was considered statistically significant.
Results
Characterization of Components of U. Prolifera Extract
By screening with Agilent’s TCM database, U. prolifera extract was found to mainly consist of polyphenols and unsaturated fatty acids (Table 1).
U. prolifera Extract Improved Growth Performance and Alleviated Oxidative Stress in Weaned Piglets Challenged With Hydrogen Peroxide
Piglets challenged with hydrogen peroxide showed decreased average daily weight gain and feed intake, while showing an increased ratio of feed intake to weight gain, indicating reduced growth performance (Figures 1A–C). When the piglets’ diet was supplemented with U. prolifera extract, the growth performance was unchanged compared with control piglets, even if the piglets were challenged with hydrogen peroxide. Compared with control piglets, piglets challenged with hydrogen peroxide had lower T-AOC and SOD activities, but higher MDA content in serum, while no such changes were observed in piglets supplemented with U. prolifera extract (Figures 1D–F).
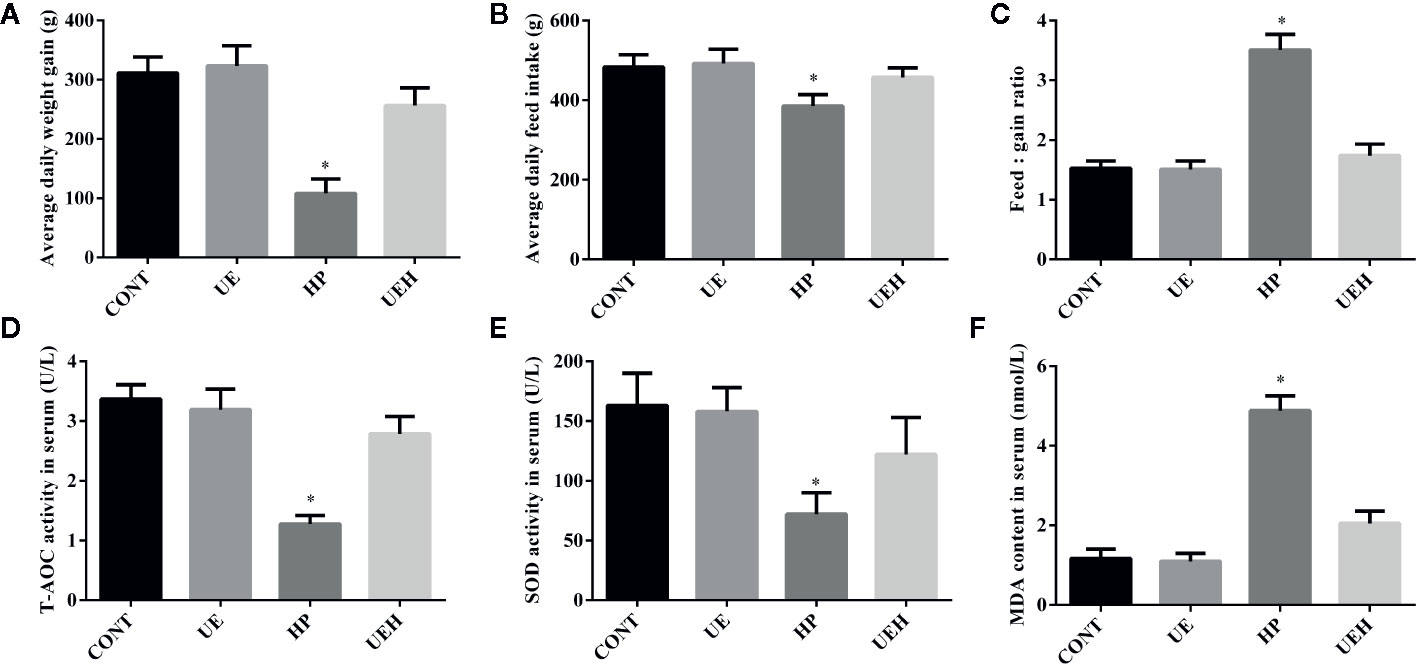
Figure 1 Ulva prolifera extract improved growth performance and alleviated oxidative stress in weaned piglets challenged with hydrogen peroxide. (A) Average daily weight gain; (B) Average daily feed intake; (C) Feed: gain ratio; (D) T-AOC activity in serum; (E) SOD activity in serum; (F) MDA content in serum. T-AOC, total antioxidant ability; SOD, superoxide dismutase; MDA, Malondialdehyde. CONT, piglets fed a basal diet and administrated intraperitoneally with saline (1 ml/kg BW); UE, piglets fed a basal diet and supplemented with 0.1% U. prolifera extract; HP, piglets fed a basal diet and administrated intraperitoneally with 10% hydrogen peroxide (1 ml/kg body weight); UEH, piglets fed a basal diet supplemented with 0.1% U. prolifera extract, and administrated intraperitoneally with 10% hydrogen peroxide. Values are expressed as mean ± SEM, n = 8; *p < 0.05.
U. prolifera Extract Alleviated Oxidative Stress and Activated AMPK/Nrf2 Signaling in the Ileum of Weaned Piglets Challenged With Hydrogen Peroxide
Compared with those in control piglets, piglets challenged with hydrogen peroxide had higher SOD1, SOD2, and CAT mRNA expression in the ileum, while no changes were observed in piglets supplemented with U. prolifera extract (Figures 2A–C). No change in Gpx1 mRNA expression in the piglet ileum was observed across the four treatments (Figure 2D). Hydrogen peroxide challenge caused significant decreases in protein expression of AMPK and phosphorylated AMPK, as well as nuclear Nrf2 and Keap1, in the weaned piglet ileum, while U. prolifera extract significantly prevented these changes (Figure 3).
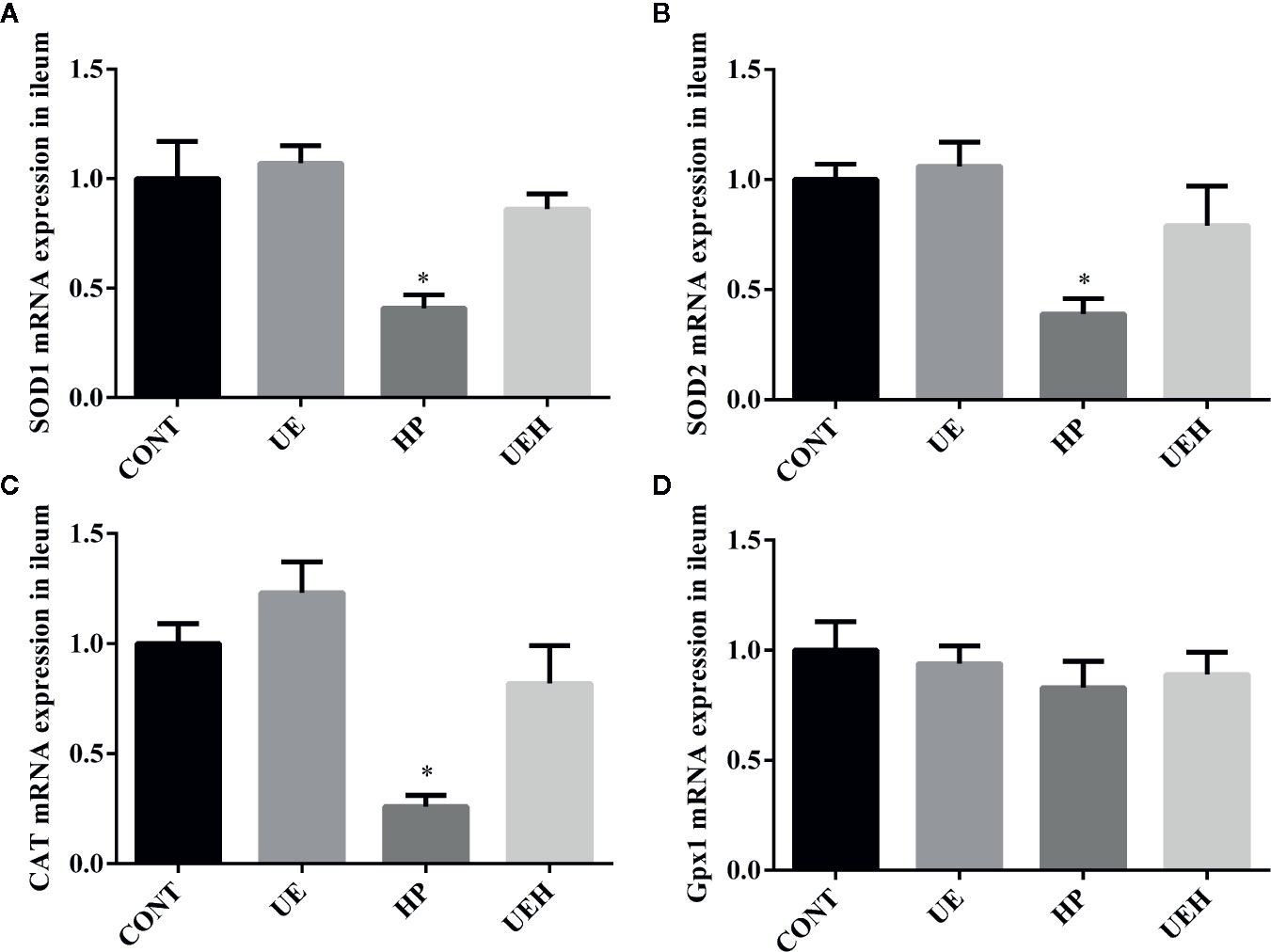
Figure 2 Ulva prolifera extract promoted expression of the antioxidant genes in the ileum of weaned piglets challenged with hydrogen peroxide. Gene expression of SOD1 (A), SOD2 (B), CAT (C), and Gpx1 (D) in ileum. SOD, superoxide dismutase; CAT, catalase; Gpx1, glutathione peroxidase 1. CONT, piglets fed a basal diet and administrated intraperitoneally with saline (1 ml/kg BW); UE, piglets fed a basal diet and supplemented with 0.1% U. prolifera extract; HP, piglets fed a basal diet and administrated intraperitoneally with 10% hydrogen peroxide (1 ml/kg body weight); UEH, piglets fed a basal diet supplemented with 0.1% U. prolifera extract, and administrated intraperitoneally with 10% hydrogen peroxide. Values are expressed as mean ± SEM, n = 8; *p < 0.05.
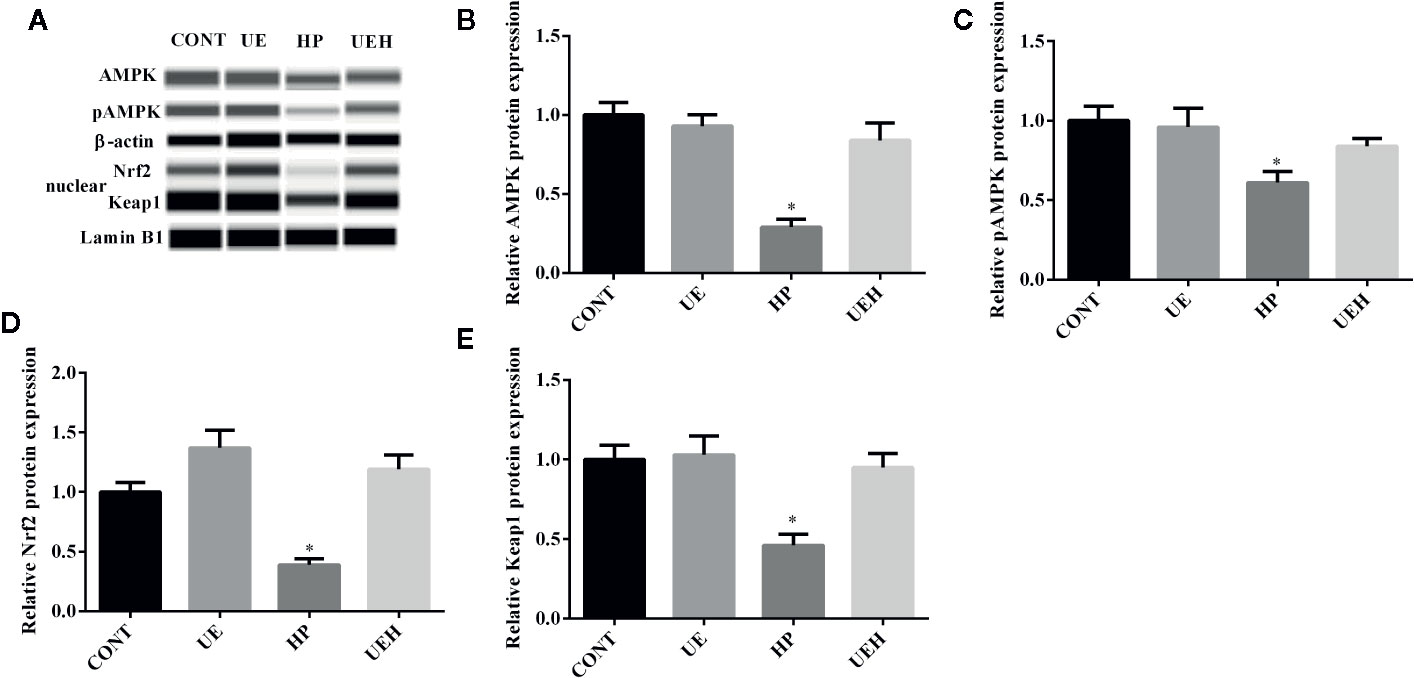
Figure 3 Ulva prolifera extract activated the AMPK/Nrf2 signaling pathway in the ileum of weaned piglets challenged with hydrogen peroxide. (A) Western blotting results; (B) AMPK abundance relative to β-actin; (C) pAMPK abundance relative to AMPK; (D) Nuclear Nrf2 abundance relative to Lamin B1; (E) Nuclear Keap1 abundance relative to Lamin B1. CONT, piglets fed a basal diet and administrated intraperitoneally with saline (1 ml/kg BW); UE, piglets fed a basal diet and supplemented with 0.1% U. prolifera extract; HP, piglets fed a basal diet and administrated intraperitoneally with 10% hydrogen peroxide (1 ml/kg body weight); UEH, piglets fed a basal diet supplemented with 0.1% U. prolifera extract, and administrated intraperitoneally with 10% hydrogen peroxide. AMPK, AMP-activated protein kinase; Nrf2, NF-E2-related factor 2; Keap1, inhibitor of Nrf2. Values are expressed as mean ± SEM, n = 3; *p < 0.05.
U. prolifera Extract Alleviated Oxidative Stress in the IPEC-J2 Cells Treated With Hydrogen Peroxide
Hydrogen peroxide treatment caused significant decreases in mRNA expression of SOD1, SOD2, and CAT in IPEC-J2 cells, while U. prolifera extract significantly prevented these changes (Figures 4A–C). Moreover, cells further treated with compound C, an inhibitor of AMPK, showed significantly less effect of U. prolifera extract, even though mRNA expression of SOD1 and SOD2 remained significantly higher than observed in hydrogen peroxide-treated cells. No changes in Gpx1 mRNA expression were observed in IPEC-J2 cells across the five treatments (Figure 4D). ROS content was significantly higher in cells treated with hydrogen peroxide, while no difference in ROS content was observed when the cells were treated with U. prolifera extract (Figures 5A, B). ROS content in hydrogen peroxide-induced cells treated with both U. prolifera extract and compound C was significantly higher compared with control cells, but ROS content was lower when compared with cells treated only with hydrogen peroxide.
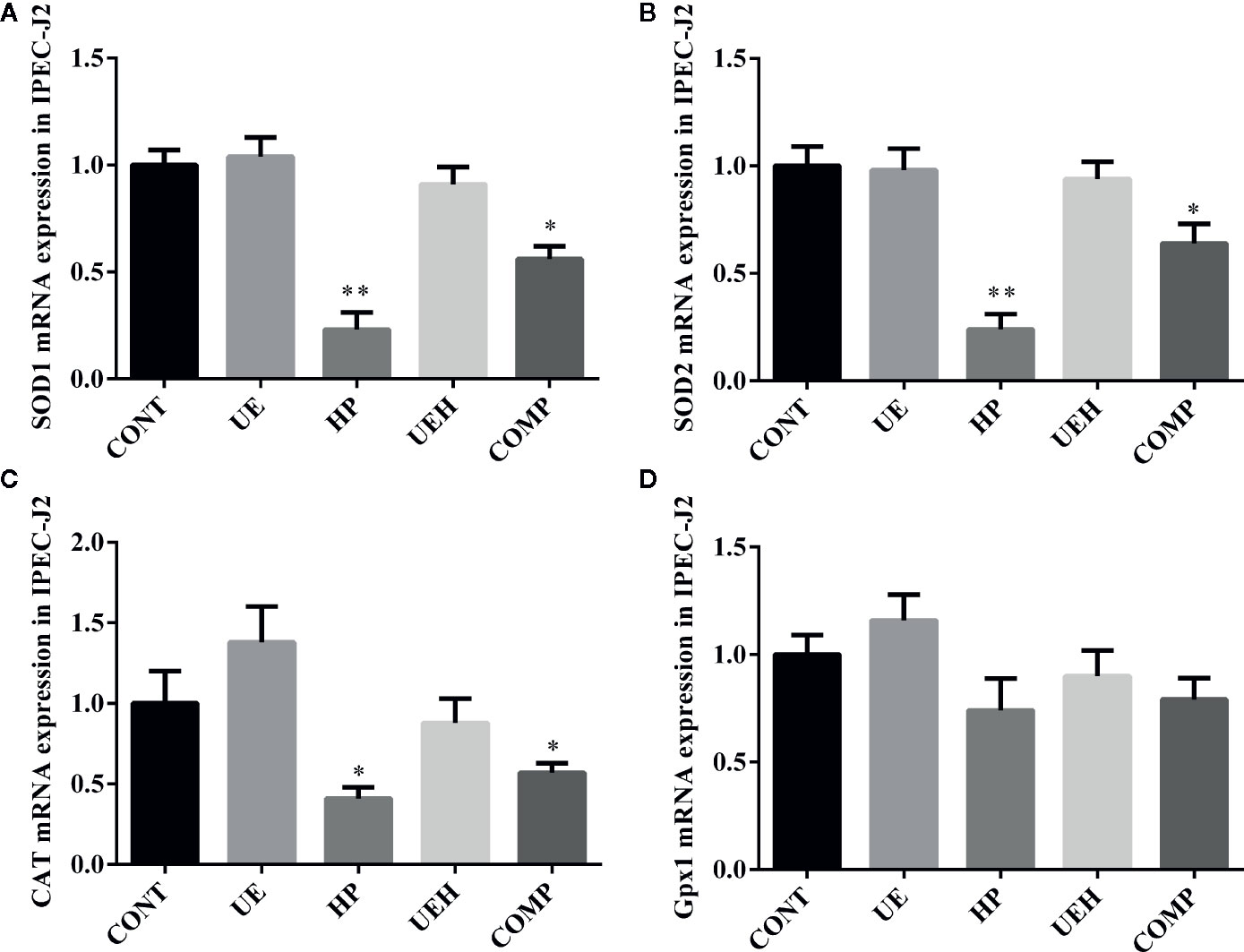
Figure 4 Ulva prolifera extract promoted expression of the antioxidant genes in IPEC-J2 cells treated with hydrogen peroxide. Gene expression of SOD1 (A), SOD2 (B), CAT (C), and Gpx1 (D) in IPEC-J2 cells. SOD, superoxide dismutase; CAT, catalase; Gpx1, glutathione peroxidase 1. CONT, control cells; UE, cells treated with 40 μg/ml U. prolifera extract; HP, cells treated with 200 μM hydrogen peroxide; UEH, cells treated with 40 μg/ml U. prolifera extract and 200 μM hydrogen peroxide; COMP, cells treated with 40 μg/ml U. prolifera extract, 200 μM hydrogen peroxide, and 5 μM compound C. Values are expressed as mean ± SEM, n = 6; *p < 0.05, **p < 0.01.
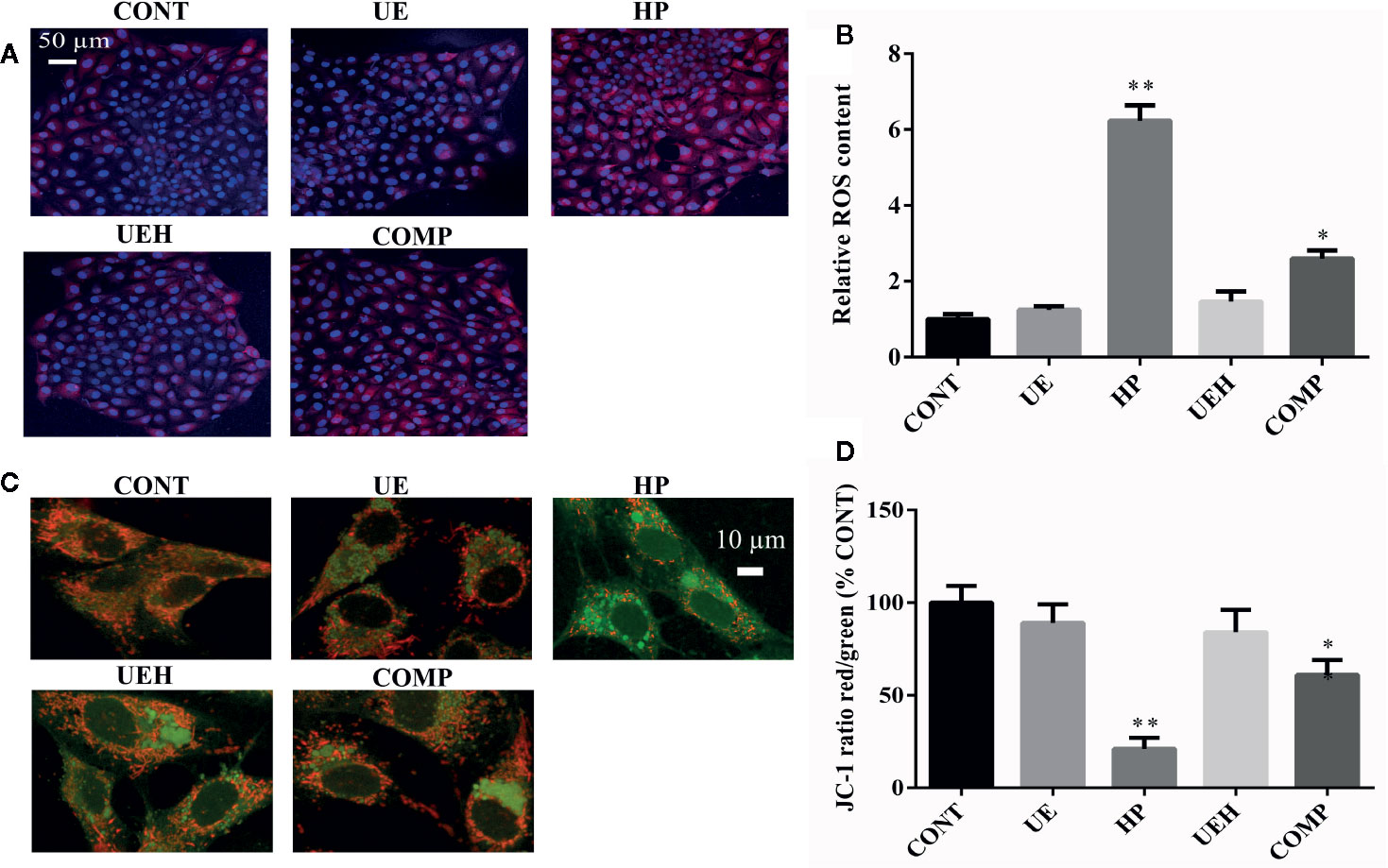
Figure 5 Ulva prolifera extract decreased ROS content and alleviated oxidative stress in IPEC-J2 cells treated with hydrogen peroxide. (A) ROS staining (red, ROS; blue, DAPI); (B) Relative ROS content; (C) JC-1 staining (red, aggregate; green, monomer); (D) JC-1 ratio (red to green). ROS, reactive oxygen species. CONT, control cells; UE, cells treated with 40 μg/ml U. prolifera extract; HP, cells treated with 200 μM hydrogen peroxide; UEH, cells treated with 40 μg/ml U. prolifera extract and 200 μM hydrogen peroxide; COMP, cells treated with 40 μg/ml U. prolifera extract, 200 μM hydrogen peroxide and 5 μM compound C. Values are expressed as mean ± SEM, n = 3; *p < 0.05, **p < 0.01.
JC-1 staining, as a marker of mitochondrial membrane potential, was further used to assess mitochondrial stress. The formation of JC-1 aggregates (red fluorescence) reflects lower stress while JC-1 monomers (green fluorescence) suggest higher stress. Hydrogen peroxide induced a decrease in the ratio of red to green fluorescence, while U. prolifera extract diminished this ratio decrease (Figures 5C, D). The ratio of red to green fluorescence in cells treated with compound C was higher than the ratio observed in control cells and U. prolifera extract-treated cells, but lower than observed in hydrogen peroxide-treated cells.
U. prolifera Extract Promoted Mitochondrial Respiration in IPEC-J2 Cells Treated With Hydrogen Peroxide
The results of mitochondrial respiration determination are shown in Figure 6A. Challenge with hydrogen peroxide significantly decreased basal OCR, maximal respiration, spare respiration capacity, non-mitochondrial respiration, and ATP production, and increased proton leak, while U. prolifera extract alleviated these changes in IPEC-J2 cells (Figures 6B–G). Cells further treated with compound C did not show any change in these parameters, except that spare respiration capacity was not altered between cells treated with compound C and cells treated with hydrogen peroxide only.
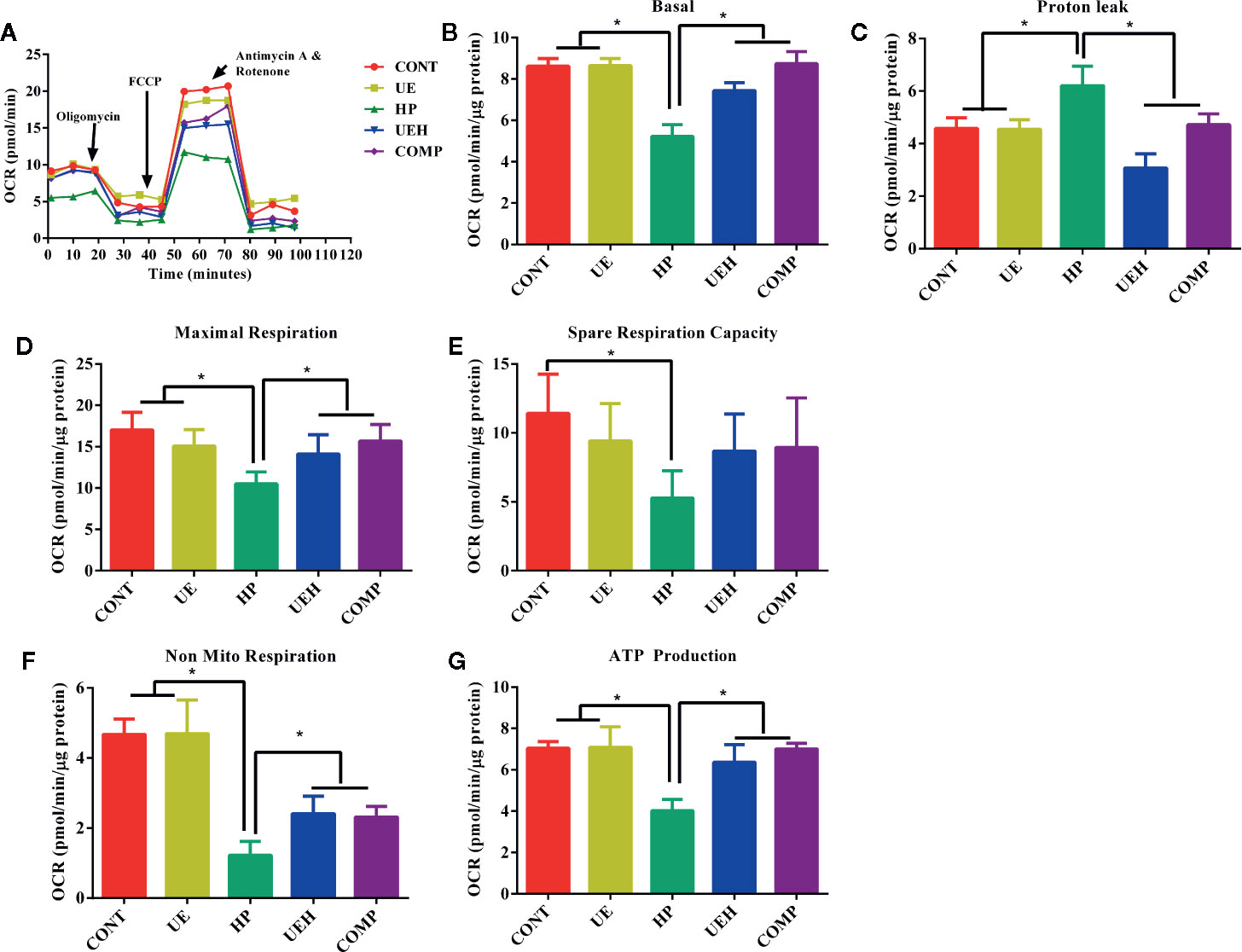
Figure 6 Ulva prolifera extract promoted mitochondrial respiration in IPEC-J2 cells treated with hydrogen peroxide. (A) mitochondrial respiration curve; (B) Basal respiration; (C) Proton leak; (D) Maximal respiration; (E) Spare respiration capacity; (F) Non mitochondrial respiration; (G) ATP production. CONT, control cells; UE, cells treated with 40 μg/ml U. prolifera extract; HP, cells treated with 200 μM hydrogen peroxide; UEH, cells treated with 40 μg/ml U. prolifera extract and 200 μM hydrogen peroxide; COMP, cells treated with 40 μg/ml U. prolifera extract, 200 μM hydrogen peroxide, and 5 μM compound C. Values are expressed as mean ± SEM, n = 3; *p < 0.05.
U. prolifera Extract Activated AMPK/Nrf2 Signaling in the IPEC-J2 Cells Treated With Hydrogen Peroxide
The results of immunofluorescent assay show that nuclear Nrf2 and Keap1 expression was decreased by hydrogen peroxide, while U. prolifera extract alleviated these changes in IPEC-J2 cells (Figures 7 and 8). Compound C treatment did not alter the effects of U. prolifera extract on Nrf2 and Keap1 expression. We determined the activity of AMPK to further investigate any changes to AMPK signaling. The results showed that hydrogen peroxide caused significant decrease of AMPK activity, and this decrease was alleviated by U. prolifera extract (Figure 8).
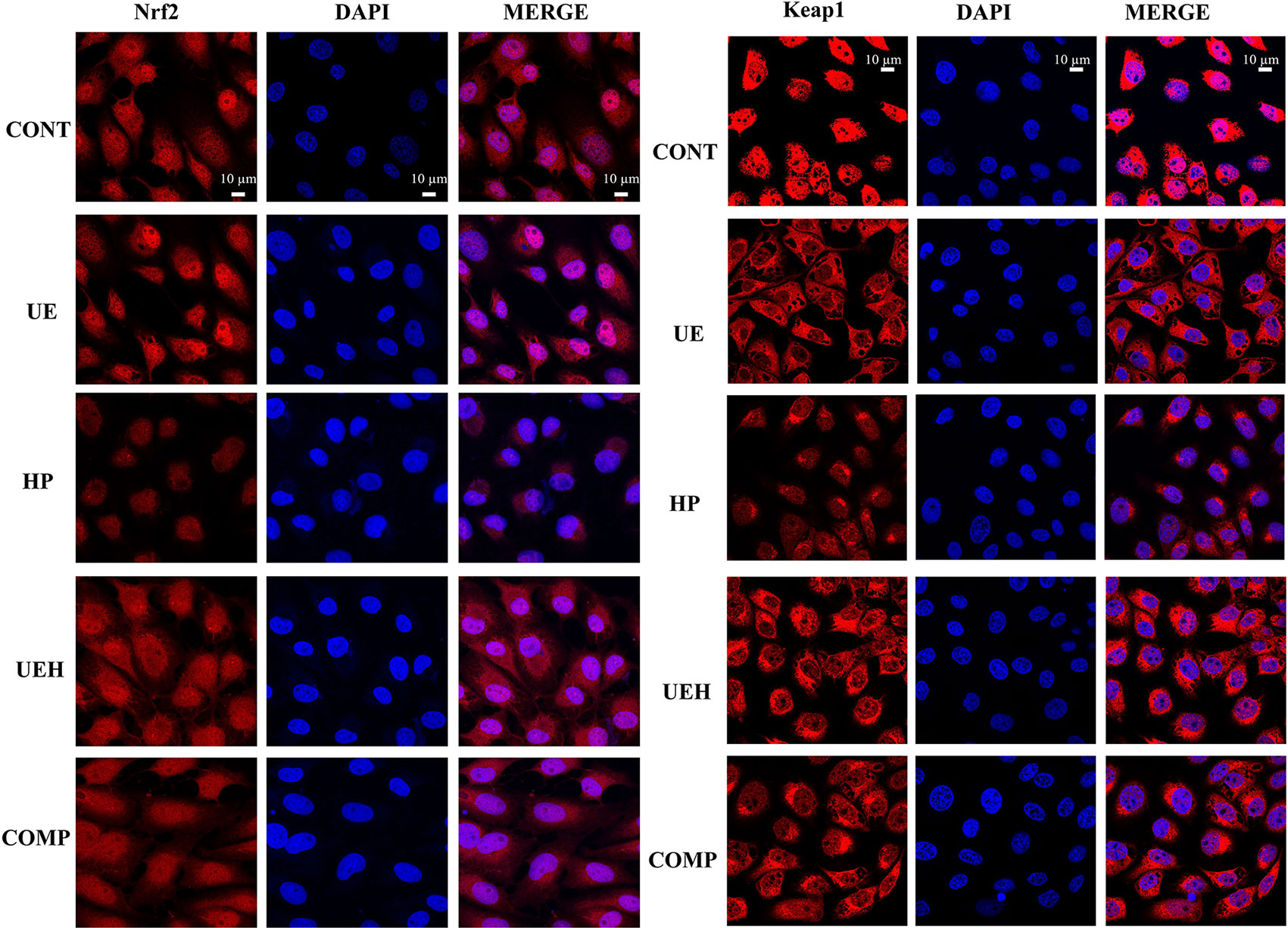
Figure 7 Ulva prolifera extract increased expression of Nrf2 and Keap1 in IPEC-J2 cells treated with hydrogen peroxide. CONT, control cells; UE, cells treated with 40 μg/ml U. prolifera extract; HP, cells treated with 200 μM hydrogen peroxide; UEH, cells treated with 40 μg/ml U. prolifera extract and 200 μM hydrogen peroxide; COMP, cells treated with 40 μg/ml U. prolifera extract, 200 μM hydrogen peroxide, and 5 μM compound C. Nrf2, NF-E2-related factor 2; Keap1, inhibitor of Nrf2.
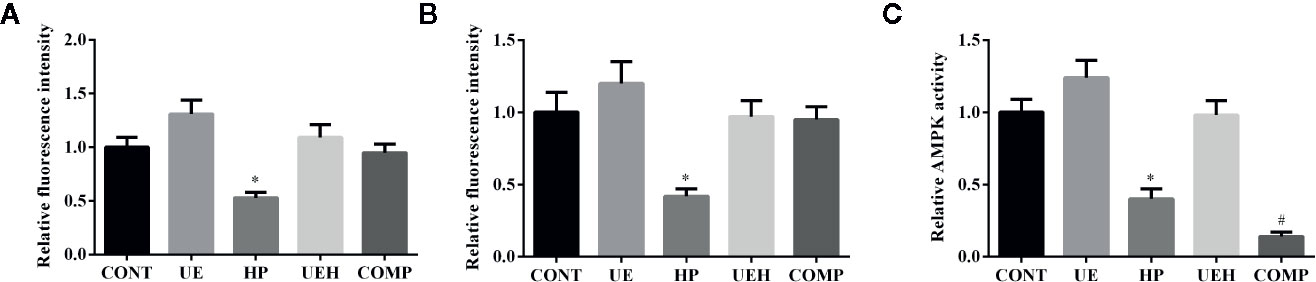
Figure 8 Ulva prolifera extract activated AMPK/Nrf2 signaling pathway in IPEC-J2 cells treated with hydrogen peroxide. Relative fluorescence intensity of Nrf2 (A) and Keap1 (B) based on the results of Figure 7; (C) Relative AMPK activity. CONT, control cells; UE, cells treated with 40 μg/ml U. prolifera extract; HP, cells treated with 200 μM hydrogen peroxide; UEH, cells treated with 40 μg/ml U. prolifera extract and 200 μM hydrogen peroxide; COMP, cells treated with 40 μg/ml U._ prolifera extract, 200 μM hydrogen peroxide, and 5 μM compound C. AMPK, AMP-activated protein kinase; Nrf2, NF-E2-related factor 2; Keap1, inhibitor of Nrf2. Values are expressed as mean ± SEM, n = 3; *p < 0.05 relative to CONT, UE, and UEH; #p < 0.05 relative to HP.
Discussion
U. prolifera, the major causative species of green tides, has bloomed in the Yellow Sea since 2008 and bloomed in the Bohai Sea in 2015, leading to marine ecological disasters as well as great losses to tourism and aquaculture (1). However, many bioactive materials in U. prolifera are considered nutritious and have functional activities. In the present study, we extracted bioactive constituents from U. prolifera using hot water extraction, analyzed the components by UHPLC-Q-TOF-MS/MS analysis, and screened the components using Agilent’s TCM database. Except that other researchers who extracted polysaccharides and flavonoids in the green algae, U. prolifera extract was found to contain polyphenols and unsaturated fatty acids in our study (2, 4). By supplementation with U. prolifera extract in hydrogen peroxide-challenged piglets, interestingly, we found that U. prolifera extract protected the intestine from oxidative damage. U. prolifera extract also improved mitochondrial function and activated the AMPK/Nrf2 signaling pathway in intestinal porcine epithelial cells.
Hydrogen peroxide can diffuse across the cell membrane and penetrate throughout the mitochondria (20). Overaccumulation of hydrogen peroxide and its metabolites, such as hydroxyl radicals, oxidizes carbohydrates, lipids, nucleic acids, and proteins, resulting in cellular injury that includes mitochondrial dysfunction. This state of oxidative stress further impairs organ function. Previous studies have shown that hydrogen peroxide not only inhibited SOD and T-AOC activities but also increased MDA content, indicating an oxidative imbalance in weaned piglets challenged with hydrogen peroxide (8, 20–22). Additionally, hydrogen peroxide treatment caused morphological injury and mitochondrial dysfunction, and promoted apoptosis in intestinal porcine epithelial cells (11). These results suggested that weaned piglets challenged with hydrogen peroxide would be a suitable model for studying intestinal oxidative damage and ROS-induced intestinal diseases.
In the present study, we used the described piglet model and our results suggested that the weaned piglets were under oxidative stress after peritoneal injections with hydrogen peroxide, which is in agreement with previous studies (8, 11). Interestingly, U. prolifera extract showed a strong protective effect against hydrogen peroxide-induced oxidative responses in weaned piglets. Importantly, U. prolifera extract exerted beneficial effects on mitochondrial function via preventing the impairment of ATP-linked respiration and maximal respiratory capacity from hydrogen peroxide challenge, according to the results of the mitochondrial respiration assay (23). Mitochondria play critical roles in generating, sensing, and scavenging ROS (24). We proposed that U. prolifera extract may help maintain mitochondrial function by improving mitochondrial metabolism of ROS when the intestinal porcine epithelial cells were challenged with hydrogen peroxide. This was evidenced by lower mitochondrial ROS content and higher JC-1 red to green fluorescence ratios in cells treated with hydrogen peroxide and U. prolifera extract, which suggested lower mitochondrial stress.
AMP-activated protein kinase (AMPK), a cellular sensor of redox balance, is involved in the regulation of cellular antioxidant response. It has been suggested that AMPK is an upstream regulator of Nrf2 and its activation causes nuclear accumulation of Nrf2 (25). However, our results suggested that inhibition of AMPK did not affect nuclear Nrf2 expression, although the effects of U. prolifera extract on oxidative stress was partially affected. Based on these results, we suggest that U. prolifera extract may exert direct influence on the Nrf2 pathway or has activated other upstream regulators of Nrf2. The Nrf2/Keap1 pathway is considered the cell’s most important antioxidant system (26). The initial effect of ROS on the Nrf2/Keap1 pathway is to promote the dissociation of Nrf2 from the Nrf2/Keap1 complex, which then translocates into the nucleus to promote the expression of its downstream antioxidant enzyme genes. However, continuously increased ROS further exerts accumulative effects that lead to the degradation of nuclear Nrf2, switching off the target gene expression activation. Hydrogen peroxide was hypothesized to influence the formation of the Nrf2/Keap1 complex and translocation of Nrf2 by promoting the production of ROS (18, 27). We observed the accumulative negative effects of hydrogen peroxide in the intestine of weaned piglets. Importantly, U. prolifera extract protected the intestinal porcine epithelial cells from a dysfunctional Nrf2/Keap1 signaling pathway. We suggest that U. prolifera extract exerted these protective effects via the elimination of ROS overproduction.
In conclusion, our results suggested that U. prolifera extract alleviated oxidative stress and improved mitochondrial function in the small intestine of weaned piglets challenged with hydrogen peroxide. Moreover, the Nrf2 signaling pathway played a critical role in mediating these effects. Our results provide potential applications of U. prolifera in animal feed and for medical purposes. These alternative uses indicate effective methods to bring the environmental damage under control and to profit from green tide events.
Data Availability Statement
The raw data supporting the conclusion of this article will be made available by the authors, without undue reservation.
Ethics Statement
The animal study was reviewed and approved by Ethics of Animal Experiments of Heilongjiang Academy of Agricultural Sciences.
Author Contributions
YF, FL, and DL designed the experiment and drafted the manuscript. HC, XH, WW, XL, and HZ carried out the animal trials and sample analysis. XL did data analysis work. FL and DL were responsible for the integrity of the work as a whole. All authors contributed to the article and approved the submitted version.
Funding
This work was supported by the Cooperative Innovation and Popularization System of Modern Agricultural Technology of Heilongjiang Provincial Pig Production and the Earmarked Fund for China Agriculture Research System (CARS-36).
Conflict of Interest
The authors declare that the research was conducted in the absence of any commercial or financial relationships that could be construed as a potential conflict of interest.
Supplementary Material
The Supplementary Material for this article can be found online at: https://www.frontiersin.org/articles/10.3389/fimmu.2020.599735/full#supplementary-material
References
1. Song W, Wang Z, Zhang X, Li Y. Ethanol extract from Ulva prolifera prevents high-fat diet-induced insulin resistance, oxidative stress, and inflammation response in mice. BioMed Res Int (2018) 2018:1374565. doi: 10.1155/2018/1374565
2. Teng Z, Qian L, Zhou Y. Hypolipidemic activity of the polysaccharides from Enteromorpha prolifera. Int J Biol Macromol (2013) 62:254–6. doi: 10.1016/j.ijbiomac.2013.09.010
3. Shao LL, Xu J, Shi MJ, Wang XL, Li YT, Kong LM, et al. Preparation, antioxidant and antimicrobial evaluation of hydroxamated degraded polysaccharides from Enteromorpha prolifera. Food Chem (2017) 237:481–7. doi: 10.1016/j.foodchem.2017.05.119
4. Yan X, Yang C, Lin G, Chen Y, Miao S, Liu B, et al. Antidiabetic potential of green seaweed enteromorpha prolifera flavonoids regulating insulin signaling pathway and gut microbiota in Type 2 diabetic mice. J Food Sci (2019) 84(1):165–73. doi: 10.1111/1750-3841.14415
5. Lin G, Liu X, Yan X, Liu D, Yang C, Liu B, et al. Role of green macroalgae enteromorpha prolifera polyphenols in the modulation of gene expression and intestinal microflora profiles in Type 2 diabetic mice. Int J Mol Sci (2018) 20(1):25. doi: 10.3390/ijms20010025
6. Cho M, Lee HS, Kang IJ, Won MH, You S. Antioxidant properties of extract and fractions from Enteromorpha prolifera, a type of green seaweed. Food Chem (2011) 127(3):999–1006. doi: 10.1016/j.foodchem.2011.01.072
7. Ren R, Gong J, Zhao Y, Zhuang X, Ye Y, Lin W. Sulfated polysaccharides from Enteromorpha prolifera suppress SREBP-2 and HMG-CoA reductase expression and attenuate non-alcoholic fatty liver disease induced by a high-fat diet. Food Funct (2017) 8(5):1899–904. doi: 10.1039/c7fo00103g
8. Duan J, Yin J, Ren W, Liu T, Cui Z, Huang X, et al. Dietary supplementation with L-glutamate and L-aspartate alleviates oxidative stress in weaned piglets challenged with hydrogen peroxide. Amino Acids (2016) 48(1):53–64. doi: 10.1007/s00726-015-2065-3
9. Circu ML, Aw TY. Intestinal redox biology and oxidative stress. Semin Cell Dev Biol (2012) 23(7):729–37. doi: 10.1016/j.semcdb.2012.03.014
10. Bhattacharyya A, Chattopadhyay R, Mitra S, Crowe SE. Oxidative stress: an essential factor in the pathogenesis of gastrointestinal mucosal diseases. Physiol Rev (2014) 94(2):329–54. doi: 10.1152/physrev.00040.2012
11. Yin J, Wu M, Li Y, Ren W, Xiao H, Chen S, et al. Toxicity assessment of hydrogen peroxide on Toll-like receptor system, apoptosis, and mitochondrial respiration in piglets and IPEC-J2 cells. Oncotarget (2017) 8(2):3124–31. doi: 10.18632/oncotarget.13844
12. Nossol C, Barta-Boszormenyi A, Kahlert S, Zuschratter W, Faber-Zuschratter H, Reinhardt N, et al. Comparing two intestinal porcine epithelial cell lines (IPECs): morphological differentiation, function and metabolism. PLoS One (2015) 10(7):e0132323. doi: 10.1371/journal.pone.0132323
13. Flisikowska T, Kind A, Schnieke A. Genetically modified pigs to model human diseases. J Appl Genet (2014) 55(1):53–64. doi: 10.1007/s13353-013-0182-9
14. Meurens F, Summerfield A, Nauwynck H, Saif L, Gerdts V. The pig: a model for human infectious diseases. Trends Microbiol (2012) 20(1):50–7. doi: 10.1016/j.tim.2011.11.002
15. Jin XL, Wang K, Li QQ, Tian WL, Xue XF, Wu LM, et al. Antioxidant and anti-inflammatory effects of Chinese propolis during palmitic acid-induced lipotoxicity in cultured hepatocytes. J Funct Foods (2017) 34:216–23. doi: 10.1016/j.jff.2017.04.039
16. Zhou X, He L, Zuo S, Zhang Y, Wan D, Long C, et al. Serine prevented high-fat diet-induced oxidative stress by activating AMPK and epigenetically modulating the expression of glutathione synthesis-related genes. Biochim Biophys Acta Mol Basis Dis (2018) 1864(2):488–98. doi: 10.1016/j.bbadis.2017.11.009
17. Zhou X, He L, Wu C, Zhang Y, Wu X, Yin Y. Serine alleviates oxidative stress via supporting glutathione synthesis and methionine cycle in mice. Mol Nutr Food Res (2017) 61(11):1700262. doi: 10.1002/mnfr.201700262
18. He L, Wu J, Tang W, Zhou X, Lin Q, Luo F, et al. Prevention of Oxidative Stress by alpha-Ketoglutarate via Activation of CAR Signaling and Modulation of the Expression of Key Antioxidant-Associated Targets in Vivo and in Vitro. J Agric Food Chem (2018) 66(43):11273–83. doi: 10.1021/acs.jafc.8b04470
19. He L, Long J, Zhou X, Liu Y, Li T, Wu X. Serine is required for the maintenance of redox balance and proliferation in the intestine under oxidative stress. FASEB J (2020) 34(3):4702–17. doi: 10.1096/fj.201902690R
20. Yin J, Duan JL, Cui ZJ, Ren WK, Li TJ, Yin YL. Hydrogen peroxide-induced oxidative stress activates NF-kappa B and Nrf2/Keap1 signals and triggers autophagy in piglets. Rsc Adv (2015) 5(20):15479–86. doi: 10.1039/c4ra13557a
21. Duan JL, Yin J, Ren WK, Wu MM, Chen S, Cui ZJ, et al. Pyrrolidine dithiocarbamate restores gastric damages and suppressive autophagy induced by hydrogen peroxide. Free Radic Res (2015) 49(2):210–8. doi: 10.3109/10715762.2014.993627
22. Wang K, Jin X, Li Q, Sawaya A, Le Leu RK, Conlon MA, et al. Propolis from different geographic origins decreases intestinal inflammation and Bacteroides spp. populations in a model of DSS-induced colitis. Mol Nutr Food Res (2018) 62(17):e1800080. doi: 10.1002/mnfr.201800080
23. Ferramosca A, Pinto Provenzano S, Montagna DD, Coppola L, Zara V. Oxidative stress negatively affects human sperm mitochondrial respiration. Urology (2013) 82(1):78–83. doi: 10.1016/j.urology.2013.03.058
24. Starkov AA, Andreyev AY, Zhang SF, Starkova NN, Korneeva M, Syromyatnikov M, et al. Scavenging of H2O2 by mouse brain mitochondria. J Bioenerg Biomembr (2014) 46(6):471–7. doi: 10.1007/s10863-014-9581-9
25. Joo MS, Kim WD, Lee KY, Kim JH, Koo JH, Kim SG. AMPK facilitates nuclear accumulation of Nrf2 by phosphorylating at serine 550. Mol Cell Biol (2016) 36(14):1931–42. doi: 10.1128/Mcb.00118-16
26. Kaspar JW, Niture SK, Jaiswal AK. Nrf2:INrf2 (Keap1) signaling in oxidative stress. Free Radic Biol Med (2009) 47(9):1304–9. doi: 10.1016/j.freeradbiomed.2009.07.035
Keywords: hydrogen peroxide, oxidative stress, piglet, Ulva prolifera, intestine
Citation: Feng Y, An Z, Chen H, He X, Wang W, Li X, Zhang H, Li F and Liu D (2020) Ulva prolifera Extract Alleviates Intestinal Oxidative Stress via Nrf2 Signaling in Weaned Piglets Challenged With Hydrogen Peroxide. Front. Immunol. 11:599735. doi: 10.3389/fimmu.2020.599735
Received: 28 August 2020; Accepted: 12 October 2020;
Published: 30 October 2020.
Edited by:
Kai Wang, Chinese Academy of Agricultural Sciences (CAAS), ChinaCopyright © 2020 Feng, An, Chen, He, Wang, Li, Zhang, Li and Liu. This is an open-access article distributed under the terms of the Creative Commons Attribution License (CC BY). The use, distribution or reproduction in other forums is permitted, provided the original author(s) and the copyright owner(s) are credited and that the original publication in this journal is cited, in accordance with accepted academic practice. No use, distribution or reproduction is permitted which does not comply with these terms.
*Correspondence: Fenglan Li, bGlmZW5nbGFuMTAwQDE2My5jb20=; Di Liu, bGxpdWRpMTk2M0AxNjMuY29t
†These authors have contributed equally to this work