- Department of Molecular Biology, Council of Scientific and Industrial Research, Institute of Microbial Technology, Chandigarh, India
The escalation in living standards and adoption of ‘Western lifestyle’ has an allied effect on the increased allergy and asthma burden in both developed and developing countries. Current scientific reports bespeak an association between allergic diseases and metabolic dysfunction; hinting toward the critical requirement of organized lifestyle and dietary habits. The ubiquitous nuclear receptors (NRs) translate metabolic stimuli into gene regulatory signals, integrating diet inflences to overall developmental and physiological processes. As a consequence of such promising attributes, nuclear receptors have historically been at the cutting edge of pharmacy world. This review discusses the recent findings that feature the cardinal importance of nuclear receptors and how they can be instrumental in modulating current asthma pharmacology. Further, it highlights a possible future employment of therapy involving dietary supplements and synthetic ligands that would engage NRs and aid in eliminating both asthma and linked comorbidities. Therefore, uncovering new and evolving roles through analysis of genomic changes would represent a feasible approach in both prevention and alleviation of asthma.
Introduction
The advancement of science has caused a reduction in both mortality and morbidity associated with infectious diseases. However, the modern lifestyle has constituted a world where cases of metabolic and allergic diseases are prolific. The incidences of allergic diseases constitute the most chronic maladies globally, with asthma leading the class. Asthma equally affects people from different socioeconomic status or age groups and accounts for 339 million affected people worldwide (1). An increase in allergic disorders has also been observed with the adoption of sedentary lifestyle and higher living standards. The condition further deteriorates due to the prevalence of comorbidities such as stress, obesity and viral infections (2–4). Despite the healthcare advancements, asthma is still under-diagnosed and under-treated creating huge healthcare burden and social implications to individuals and concerned families (5). Also surprisingly, even after taking the existent therapy, a significant portion of the affected population continues to experience a recalcitrant form of asthma (6). This difficult-to-treat asthma is an attribute of either insensitivity to corticosteroids or poor therapeutic adherence due to various associated side-effects. Albeit the fraction of poorly controlled asthma subjects is relatively small, they form a major portion of mortality, morbidity and the associated cost.
The impetus behind this review is to accentuate the unmet dearth of newer therapy targets and to emphasize the need for customized therapeutics to bestir the challenges of current asthma therapy. The present article elaborates on asthma and the associated allies, a newer arena of fascinating drug targets – nuclear receptors (NRs) and how these could be instrumental in combating asthma and comorbidities.
Asthma: Tale of an Atypical Ailment
The word ‘Asthma’ has Greek roots and its meaning translates to short of breath. However, it was in late 19th century when the term was refined with the publication of a treatise entitled “On Asthma and its Treatment”. Thus, from that time, asthma came to be recognized as a distinct ailment which gets triggered by a specific set of stimuli and possesses clinical consequences (7). The modern definition as provided by Global Initiative of Asthma (GINA) describes asthma as a complex disease of lower airways characterized by bronchoconstriction, excessive mucus production, breathlessness and wheezing illness (8). The disease is a mixed outcome of genetic susceptibility and environmental influence resulting in a lifelong ailment (9). A consistent variability is also seen in disease expression in terms of provoking elements, age, gender, various forms of airway inflammation and index of severity. The most commonly observed form is of allergic asthma and its physiology involves T-cells, mast cells and eosinophils majorly along with histamine, cytokines and leukotrienes as inflammatory mediators (10). Asthma symptoms can range from mild to severe and hence, has been classified into various ‘phenotypes’ based on observable symptoms or environmental triggers. However, various phenotypes give no comprehension about the underlying molecular mechanisms and therefore, classification of asthma subtypes on the basis of involved cellular mechanisms led to the recognition of different ‘endotypes’ (11). They consist of distinctive pathophysiological features like T helper type 2 (Th2) associated allergic asthma, severe eosinophilic, allergic bronchopulmonary mycosis, aspirin-sensitive asthma, obesity-related asthma (lifestyle-linked) and non-Th2 linked neutrophilic asthma (12). However, the genetics behind most of these endotypes are not thoroughly deciphered and the variations in disease progression and severity remains largely unexplained.
Asthma exists with numerous comorbidities that mutually influence its clinical expression, disease management and control. Comorbid conditions like sinusitis, rhinitis, microbial infections, obstructive sleep apnea, psychopathologies, hormonal disturbances and gastroesophageal reflux disease (GERD) have been found to be highly prevalent (13). Also, demographic observations suggest of a possible hormonal angle and this has been considerably documented that women suffer more severe asthma and subsequent hospitalisations than men (14–17) (Figure 1A). The adult form of asthma witness significantly more comorbidities than the general asthmatic population with a ratio of one being found in every four adults. Although children had a lower comorbidity burden, yet 12.6% harbored a linked chronic medical condition (18). Further, asthmatics are shown to be more prone to arthritis, heart disease, diabetes, cancer, stroke and osteoporosis (19).
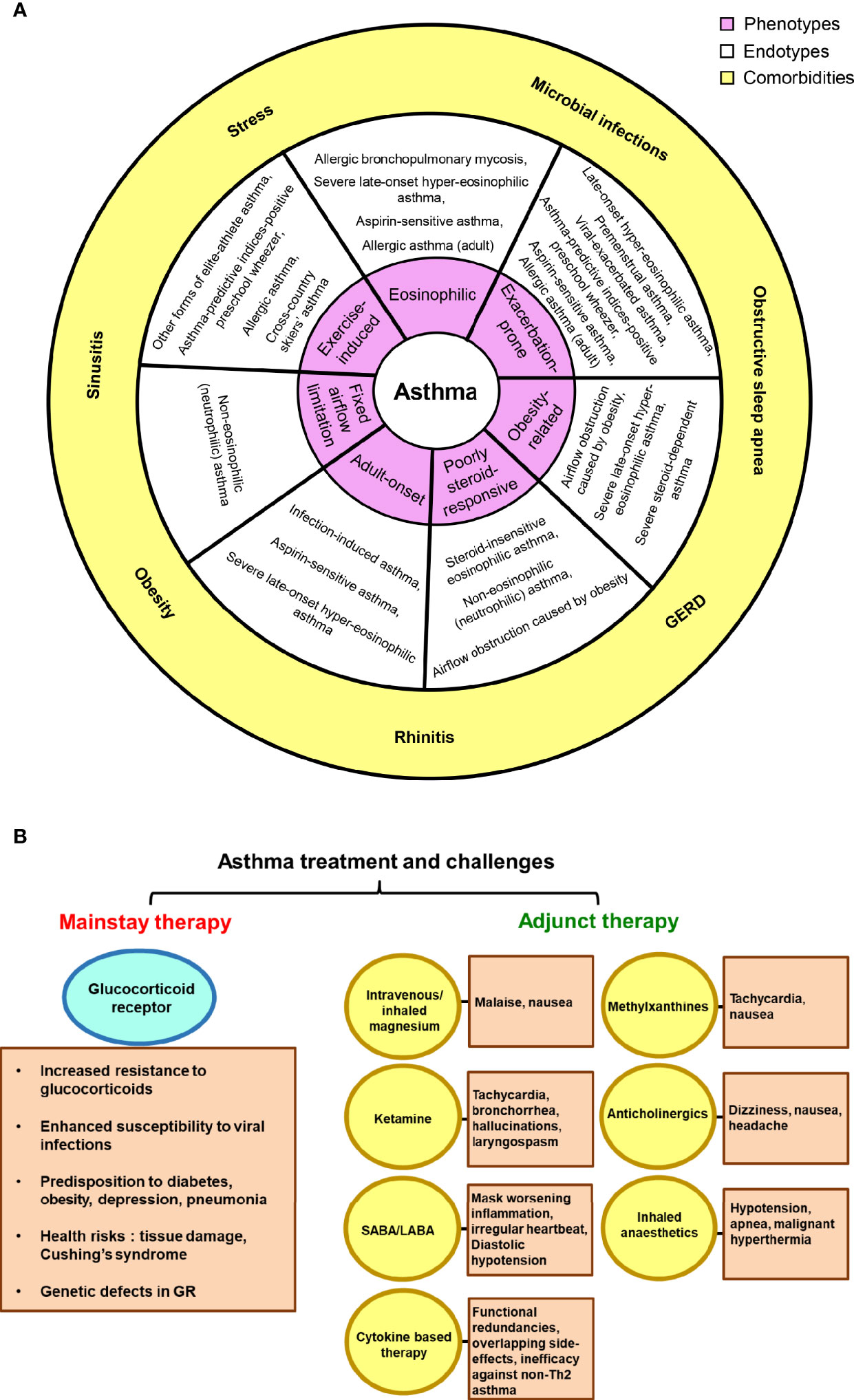
Figure 1 Asthma, comorbidities and treatment challenges. (A) The link between asthma phenotypes and endotypes is depicted; suggesting possible existence of multiple endotypes for a single phenotype and vice-versa. The outer sphere portrays the commonly observed comorbid conditions in asthma patients. (B) The reported side-effects and shortcomings of glucocorticoid receptor and other adjunct therapies in asthma have been highlighted.
As recommended by the disease-management guidelines, inhaled corticosteroids and short-/long- acting β2-adrenoceptor agonists (SABAs and LABAs) form the cornerstone of current asthma treatment. Salbutamol and terbutaline hail to the SABAs category and are the most effective asthma relievers available till today. The two LABAs, salmeterol and formoterol are used as a supplementary therapy where patients do not experience relief with inhaled corticosteroids. These successfully induce bronchodilation for 12 h. However, LABA has the potential to mask worsening inflammation and hence, a monotherapy with LABA is not prescribed (20–22). Mounting evidence suggests that a significant fraction of asthmatics respond poorly to inhaled and systemic steroid therapy (23, 24). Treatment of glucocorticoid-resistant patients with GCs produces major health risks, ranging from irreversible tissue damage to the development of Cushing’s syndrome, thereby increasing the overall morbidity and mortality rates (25). Moreover, along with osteoporosis and pneumonia, oral corticosteroids may sway our body toward glucose intolerance/diabetes and also enhance susceptibility to viral infections (26, 27). The general non-compliance to GC treatment, genetic defects in GR and abnormal steroid pharmacokinetics further add to the issue (28, 29). Several other adjunct therapies are prevalent along with the mainstay that provide relief to severe asthmatic cases, such as methylxanthines, magnesium, anticholinergic drugs, ketamine or other inhaled anesthetics. However, these too, are gloomed by the associated dire effects (30–32). Furthermore, new and emerging biologic therapies for severe asthma are cytokine-based (anti-IL-4, IL-5, or IL-13) and mostly target Th2-mediated pathways. Nonetheless, they suffer from issues like functional redundancies and overlapping side-effects; thereby failing in clinical efficacy (33) (Figure 1B). At present, FDA has approved Omalizumab, a recombinant humanized anti-IgE monoclonal antibody for severe asthma, which down regulates the high-affinity IgE receptor FcϵR1 on mast cells and basophils (34). Since there is an absence of unifying markers for non-Th2 asthmatics, novel strategies to target this section of patients have yielded poorer success ratios than therapies for Th2 high asthma. Hence, the lack of an alternative effective therapy leads to high healthcare costs.
Nuclear Receptors: A Clan of Idiosyncratic Transcription Factors
NRs hail from a large 48-membered ligand-dependent superfamily of transcription factors that are associated with the overall vertebrate development and are connected to a plethora of human diseases (35). The appeal of these receptors is that their activity can be regulated by binding to small lipophilic molecules called ligands (36). Ligands cross the lipid bilayer and carry out both intra- and extra-cellular physiological activities. The superfamily of NRs is typically grouped into three classes based on their ligand selectivity: class I, class II, and class III. The classical endocrine nuclear receptors catalog themselves to class I and comprise of estrogen (ER), glucocorticoid (GR), mineralocorticoid (MR), progesterone (PR), and androgen receptors (AR) as its members. Steroid hormones synthesized by endocrine glands serve as their ligands and these NRs bind to their DNA sequences in homodimeric fashion. NRs which get activated by dietary vitamins, importantly vitamin D receptor (VDR) and retinoic acid receptor (RAR) are also clubbed into this category, although they bind to DNA by forming heterodimers with other NRs. The ligand binding affinity is very high in such receptors due to a smaller ligand binding pocket. Class II corresponds to orphan NRs for which respective ligands have not been discovered so far. They bind DNA usually as monomer or homodimer and examples of such NRs include retinoid-related orphan receptors (RORs) and nerve growth factor IB (Nurr77). Class III represents adopted orphan NRs and comprise of those NRs which earlier belonged to orphan category but with recent discovery of their ligands, have constituted a new class. On comparison with endocrine NRs, these have larger ligand binding pockets and lower affinity for ligands. Peroxisome proliferator-activated receptors (PPARs), farnesoid X receptor (FXR), liver X receptor (LXR) and retinoid X receptor (RXR) are all examples of adopted orphan receptor (37, 38). The NRs largely share a conserved structure consisting of a DNA-binding domain (DBD), hinge region, ligand-binding domain (LBD) along with variable N-terminal and C-terminal domains. N-terminal is known for carrying out transactivation functions via activation function-1 (AF-1); independent of the ligand binding to the receptor. The DBD binds to the target DNA by recognizing certain conserved sequences known as response elements while hinge is a flexible region that connects DBD and LBD. The LBD is responsible for ligand based interaction and recruits coregulators through the activation function-2 (AF-2), which is followed by a C-terminal extension. NRs can bind to response elements of their target genes as homodimers, heterodimers and monomers and classically act as transcriptional repressors in the absence of a ligand. However, upon ligand binding, NRs undergo conformational changes that lead to release of corepressors, recruitment of coactivators and subsequent target gene activation (39). In addition to this, the class of adopted orphan receptors are more desirable as drug targets than endocrine receptors. Since adopted orphan receptors do not command the hormonal system, the adverse effects of immune and metabolic imbalance are averted. Moreover, orphan receptors also hold certain charm and curiosity because discovery of their ligands hold the potential of being used as new ‘modulatory switches’ of the human system.
In current years, NRs have been accentuated as strategic therapeutic targets due to their ability to translate nutritional and metabolic signals into gene regulation; significantly impacting human health and disease progression (40). Interestingly, the market share for drug targets also reveals the dominance of G-protein-coupled receptors (GPCRs), NRs and voltage gated ion channels, underlying their immense clinical importance (41, 42). Since NRs communicate directly through DNA response elements of their target genes and can also crosstalk with various other signalling pathways, the spectrum of advantages is considerably more. NRs are deep-seated inside the cells, have cleaner effector functions and exhibit specificity both at the DNA binding and ligand binding levels. As these ligand-dependent transcription factors glean their ligands from a varied set of fat-soluble hormones, vitamins and diet stemmed components, NRs provide us with a considerably better opportunity for functional modifications (35). In addition to this, modulation by synthetic ligands makes them the ideal target for ‘small molecule drugs’ family (43). As small molecules can be easily manipulated, drugs can be designed that are even more potent than the endogenous ligand, for example, dexamethasone which brings a greater biological response than cortisol (44). Due to the immense success of NRs as drug targets, discovering newer roles of NRs in diseases and designing agonist/antagonist for them appear as a promising research initiative. Therefore, it wouldn’t be a mendacious statement to say that NRs are the future of genomic medicine and would soon compete with GPCRs, ion-channels and kinases as ‘next-generation targets’.
Nuclear Receptors in Asthma
With the recognition of NRs significance in physiology, they are now being extensively researched in numerous human diseases. In reference to asthma too, the accumulated data from scientific world stipulates the involvement of NRs in the pathology of asthma and its progression. Both kinds of NRs; those that abate asthma (Figure 2) and the ones that tend to promote the disease severity (Figure 3) are documented.
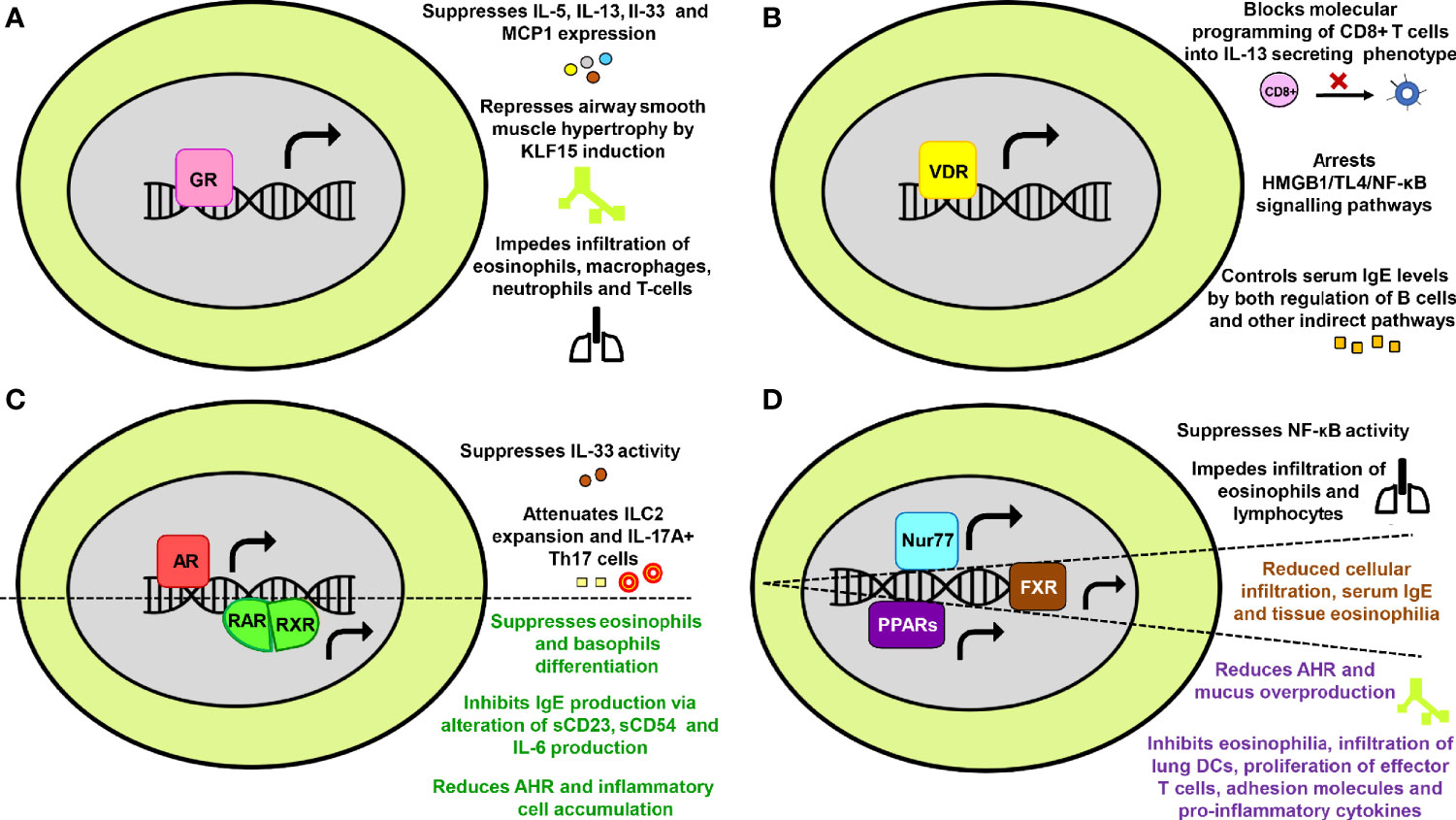
Figure 2 The protective effects of nuclear receptors (NRs) in asthma. Key mechanisms through which (A) Glucocorticoid receptor (B) Vitamin D receptor (C) Androgen receptor and Retinoic acid receptor-Retinoic X receptor (D) Nurr77, Farnesoid X receptor and Peroxisome proliferator-activated receptors provide protection against asthma have been summarized in a schematic form.
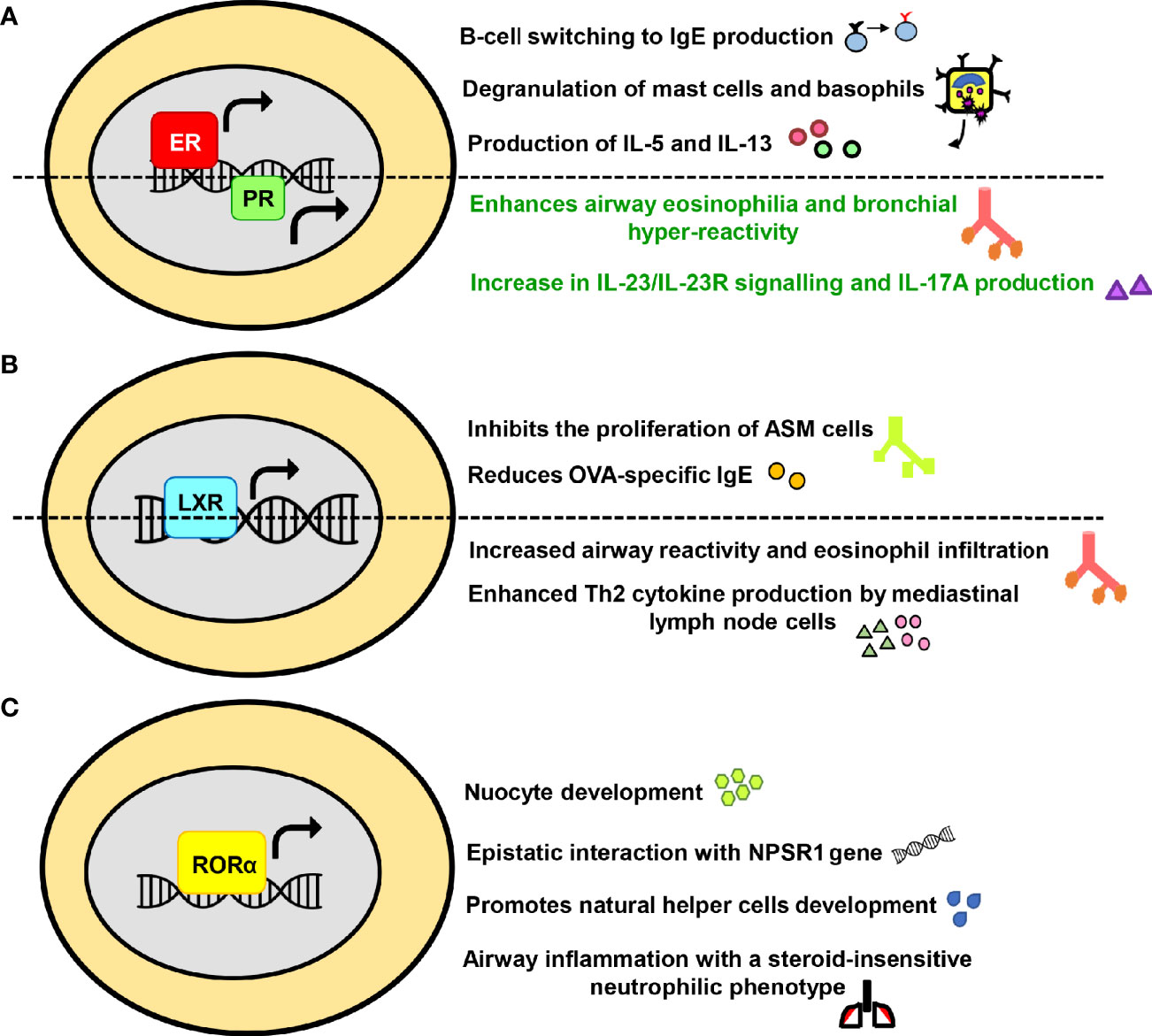
Figure 3 The exacerbating or promiscuous effects of nuclear receptors (NRs) in asthma. The influence of (A) Estrogen receptor and Progesterone receptor (B) Liver-X-receptor (C) Retinoic acid receptor-related orphan receptor alpha on major immunological pathways in asthma have been summarized in a schematic form.
Endocrine Receptors
Glucocorticoid Receptor
Glucocorticoids are among the most ubiquitous hormones present in mammals that carry out majority of their action through GRs. The GRs are involved in gluconeogenesis, postnatal insulin-like growth factor-1 production, behavioral abnormalities and physiological control of inflammation (45–51). In the context of asthma, GR is the most extensively studied NR. Glucocorticoids are known to have a suppressive effect on IL-5, IL-13, IL-33, and MCP1 expression, thereby impeding infiltration of eosinophils, macrophages, neutrophils and T cells into the lungs of asthmatic mice (52). GR also represses airway smooth muscle (ASM) hypertrophy in human ASM cells by inducing kruppel-like factor 15 (KLF15) (53). Furthermore, the GR expression levels or its subsequent phosphorylation are disrupted by multiple factors such as exposure to Aspergillus fumigatus (54), increased protein phosphatase 5 (PP5) activity (55), enhanced nuclear factor interleukin-3 (NFIL3) expression levels (56) and activation of H1R by histamine (57) (Figure 2A). Moreover, some recent reports have also linked gene polymorphism associated with GR as one of the plausible causes behind insensitivity to corticosteroid therapy (58, 59). Limited GR nuclear bioavailability (60, 61) and secondary effects of fetal sex and maternal asthma on placental GR expression are some of the other rationalities behind increased asthma susceptibility and exacerbations (62).
Vitamin D Receptor
Vitamin D earlier had its roots of significance among skeletal disorders such as osteoporosis, fractures, muscle strength, calcium, phosphorus and bone metabolism (63–66). However, research conducted in the past decade have established non-skeletal roles of vitamin D in chronic maladies including cardiovascular, infectious, metabolic, autoimmune diseases, cancer, and mortality (67–72). Vitamin D has been reported to arrest HMGB1/TLR4/NF-κB signaling pathway (73), inhibit the conversion of CD8+ T-cell to an IL-13 secreting phenotype through VDR recruitment to Cyp11a1 promoter (74) and keep a check on serum IgE levels in B cells through both direct and indirect mechanisms (75) in asthmatic mice. In human B cells, IgE production, B cell proliferation and subsequent differentiation into IgE, IgG and IgA producing plasma cells are also inhibited by active form of vitamin D (1α,25-dihydroxyvitamin D(3)) via limiting the population of immunoglobulin‐secreting cells, reducing the activation of NF-κB and impeding switch recombination (76, 77) (Figure 2B). Moreover, factors like vitamin D deficiency and VDR gene polymorphism prevalent in human population have also been included in the ‘high susceptibility to asthma’ list (78–80).
Androgen Receptor
Primarily, AR plays a pivotal role in the development and maintenance of male phenotype (81). Along with sex-differentiation, fertility and male-specific pathology, AR is shown to modulate hair growth, immune functions, female reproductive growth, metabolic disorders and certain cancers (82–87). Since there is a visible sex bias in asthma, possible role of testosterone and other androgens in its immunopathogenesis have been studied. Gonadectomized male mice exhibited an increase in level of IL-13 protein expression, lymphocytes and eosinophils in comparison to the hormonally intact, sham-operated male mice (88). Another study has documented androgen-mediated inhibition of IL-33 driven lung inflammation and impaired expansion of group 2 innate lymphoid cells (ILC2) (89). Furthermore, stimulation of female mice with extract of Alternaria alternata, house dust mite (HDM) or ovalbumin (OVA) leads to increased production of IL-5 and IL-13 by ILC2 when compared with stimulated male mice (88–90). AR signalling has also been reported to directly attenuate IL-17A+ Th17 cells in the mice lung and indirectly reduces IL-13+ Th2 cells through impairment of HDM-induced IL-4 production (91) (Figure 2C). Since androgens also modulate airway hyperresponsiveness (AHR) and smooth muscle contractility (92, 93), AR signalling appears to play an important role in the regulation of allergic airway inflammation. However, a distinct role of AR in promoting M2 macrophage polarization has recently been revealed in a murine model of asthma (94). This finding perhaps suggests of a different role of AR in macrophages in contrast to other immune cells and therefore, requires further research.
Retinoic Acid Receptor and Retinoid X Receptor
The retinoid receptors comprise of two distinct subgroups, namely RAR and RXR that are known to modulate embryonic development, wound healing, neuronal differentiation, carcinogenesis, immunity and inflammation (95–98). Vitamin A serves as a ligand for both RAR and RXR, and provides shield against allergies by inhibiting IgE synthesis via alteration of sCD23, sCD54 and IL-6 production in anti-CD40 plus IL-4 stimulated human B cells (99). It also suppresses the differentiation of eosinophils and basophils at early stages of lineage commitment in HL-60 cells (human cell line) (100). Moreover, administration of RXR partial agonist NEt-4IB in mice has been found to significantly reduce AHR and inflammatory cell accumulation along with suppressing NF-κB expression (101) (Figure 2C).
Estrogen and Progesterone Receptors
Besides the effect on female reproductive system, estrogen and progesterone also significantly impact skeletal homeostasis, glucose and lipid metabolism, central nervous system and various cancers (102–107). Over the past decade, the role of female hormones in asthma, particularly estrogen, has been extensively recognized. The allergic responses are favored by estrogen in numerous ways such as fostering Th2 polarization, promoting B-cell switching to IgE production, enhancing mucus synthesis, increasing M2 polarization and assisting degranulation of basophils and mast cells (108–111). A couple of initial studies have reported that interaction of mast cells with estrogen enhances the release of histamines (112, 113). Other than histamine, estrogen mediated production of IL-5 and IL-13 from mediastinal lymph nodes has also been observed in an animal model of asthma (114). Moreover, it must be noted that exogenous compounds with estrogenic activity, such as xeno- and phytoestrogens interact well with ERs and are known to potentially influence histamine release and allergic responses (115–117). Additionally, an expression analysis has reported the expression of ER and PR in mast cells of human upper airways where they have been known to activate mast cells (118, 119). Differential expression of ER in asthmatics and receptor polymorphism have also been held accountable for elevating the asthma pathogenesis (120, 121). Interestingly, the role of ERβ differs from that of ERα and few recent studies have demonstrated its protective role in asthma via inhibition of PDGF induced proliferation, suppression of the NF-κB pathway, downregulation of AHR and airway remodeling (122–126). Furthermore, the impact of female hormone progesterone has been investigated in a murine model of asthma where it enhances airway eosinophilia and bronchial hyper-reactivity (127). The exposure to a combination of 17β-estradiol (E2) and progesterone (P4) also leads to an increase in IL-23/IL-23R signalling and IL-17A production in patients with severe form of asthma (128) (Figure 3A). Conceivably, the cardinal involvement of female hormones in asthma immunopathogenesis accounts for the observed gender disparity.
Adopted Orphan/Orphan Receptors
Peroxisome Proliferator-Activated Receptors
The members of PPAR family are major regulators of energy homeostasis and metabolic function. However, broader roles have now been discovered and they are being increasingly recognized as key players involved in inflammatory, metabolic and neurodegenerative disorders (129). Experimental evidences have suggested that activation of PPARs produces anti-inflammatory effects in lung diseases (130). Deficiency of PPARα enhanced AHR and eosinophilia in a murine model of asthma while its agonist alleviated the disease (131–133). It is also reported that administration of PPARγ agonists or overexpression through adeno-PPARγ provides protection against asthma in mice (134–136). Several beneficial effects of PPARγ agonists in reducing multiple asthma features, such as AHR, leukocyte infiltration, mucus overproduction, infiltration of lung DCs, proliferation of effector T cells, adhesion molecules and pro-inflammatory cytokines have been observed in various murine asthma models (137–144). Troglitazone, another PPARγ agonist, impeded eosinophilia through inhibition of their IL-5 directed survival and eotaxin mediated chemotaxis (145) (Figure 2D). Collectively, the protective role of PPARs in lung disorders is affirmed by both experimental and clinical data (146–150). However, few recent animal based studies have instituted that PPARγ promotes type 2 effector responses in DCs and T cells (151, 152). Furthermore, therapy with PPARγ agonist (pioglitazone and rosiglitazone) showed modest or no improvement in subjects with asthma, thereby implying insufficient intervention (153, 154). Thus, rigorous studies and clinical research are required where a larger sample size and distinct endotypes are considered in order to translate PPARγ agonists into asthma drugs.
Nur77
The orphan nuclear receptor Nur77, also known as the nerve growth factor IB (NGFIB) is highly expressed in eosinophils, tolerant T cells and lung epithelium. It plays a cardinal role in mediating inflammatory responses in macrophages (155) and is also involved in cell growth, apoptosis, T cells function, neuronal regulation, muscle homeostasis and energy metabolism (156–164). Nur77 has been found to provide protection against asthma by suppressing nuclear factor kappa-light-chain-enhancer of activated B cells (NF-κB) activity. The knockdown of Nur77 led to enhanced inhibitor kappa B alpha (IκBα) phosphorylation and Nur77 knock-out mice had an increased infiltration of lymphocytes and eosinophils with aggravated mucus production. Moreover, bronchial hyper-responsiveness is also determined by single nucleotide polymorphism (SNP) present in Nur77 gene (165) (Figure 2D).
Farnesoid X Receptor
The bile acid sensing receptor FXR largely controls hepatic triglyceride and glucose homeostasis (166). In addition to the above, its non-hepatic roles in fluid homeostasis, atherosclerosis, cancer, cardiovascular, renal, neuronal and other inflammatory diseases have also been documented (167–170). FXR is shown to have an immunomodulatory role in asthma and predominantly reduces the disease severity. Treatment with natural FXR agonists (chenodeoxycholic acid and ursodeoxycholic acid) significantly reduced cellular infiltration in peri-bronchial areas, mucus production, serum IgE and tissue eosinophilia in mice (171, 172) (Figure 2D).
Liver X Receptor
LXR finds its expression in a large number of cell types and is responsible for maintaining whole-body lipid and cholesterol metabolism (173). Newly discovered roles of LXR, linking lipid metabolism and inflammation, have also emerged; highlighting its importance in immune disorders such as infection, atherosclerosis and Alzheimer disease (174). There are several reports for the role of LXR in airway diseases and one of the earliest studies states that LXR agonist T1317 (T0901317) inhibited the proliferation of ASM cells (175). Similarly, agonist T0901317 provided protection in asthma via attenuation of OVA-specific IgE and reduction in collagen deposition and ASM thickness (176). However, LXR agonist GW3965 mediated enhanced airway reactivity that increased the growth of ASM in pre-clinical models of asthma is also documented (177). In addition to this, a recent study involving LXR-knockout mice have shown that eosinophilic airway inflammation and production of Th2 cytokines by mediastinal lymph node cells was abolished in the LXRα−/−β−/− mice in both the OVA and HDM-induced asthma models (178). Furthermore, administration of LXR agonist GW3965 exhibited an increase in the eosinophilic airway disease and production of type 2 cytokines (178) (Figure 3B). Given the immunomodulatory role of LXR in asthma, these differential effects of ligands need to be adequately addressed.
Retinoid Acid Receptor-Related Orphan Receptor
RORα is functionally linked to have roles in circadian rhythm, immune regulation, cancer progression, neural development, cellular metabolism and autoimmune diseases (179, 180). Recently, RORα has been associated with allergic diseases as its expression level was found to be on the higher side in patients with therapy-refractory asthma along with RORα rs11071559C>T gene polymorphism accounting for elevated susceptibility to asthma (181–183). This finding is further affirmed by other reports that discuss the link of RORα SNPs with increased childhood asthma and exhibits an epistatic interaction with neuropeptide S receptor 1 (NPSR1), leading to the modification of joint risk effects (184). RORα has also been identified as a key factor required for ILC2 differentiation and is critically required for nuocyte development (185, 186). Furthermore, this NR is necessary for natural helper (NH) cells development and allergic inflammation (187). Few other animal studies have shown that RORαsg/sg and RORγ-/- mice exhibit a severely impaired allergic response to OVA (188, 189). Another member of the ROR family, RORγt, is reported to drive the allergic airway inflammation toward a steroid-insensitive neutrophilic phenotype (190). Inhibition of RORγt also led to suppression of allergic airway hyperresponsiveness and pulmonary neutrophilia in a murine model of asthma (191, 192) (Figure 3C).
Nuclear Receptors at the Crossroads of Diet and Asthma: Key Molecules for Nutritional Targeting of Disease
Diet is considered as a major contributor to education and regulation of the immune system, particularly impacting systemic inflammation and generation of oral tolerance. Epidemiological cohort studies have showcased that diets which are rich in fruits and vegetables (e.g. Mediterranean diet) had lower allergic index while high maternal consumption of oily and processed foods enhanced the risk for allergies (193). In relation to asthma, high fat meals led to an increase in sputum neutrophils and several other genes regulating airway inflammation (194–196). On the other hand, reduction in airway neutrophil influx and IL-8 protein in nasal lavage was observed upon intake of fruits and vegetables (197, 198). The components of diet not only impact adult life but also influence the health of child during pregnancy. There are considerable evidences linking low maternal intakes of vitamins and fiber in pregnancy with increased risk of asthma development in children (199–202). Moreover, development of allergic airway diseases was also found to be inhibited by diet through significant regulation of the gut microflora composition (202–204).
Food components alter gene expression through NRs and epigenetic modifications; contributing profoundly to phenotypic plasticity and susceptibility to chronic diseases (205–207). Along with hormones, bile acid and sterols, several NRs are activated by components directly originating from food or drugs. Examples include vitamin A (RAR, RXR), vitamin D (VDR), fatty acids (PPAR), plant steroids (ER), and xenobiotic drugs (CAR, PXR) (208). Moreover, dietary components like curcumin are also known to cause histone modifications (acetylation/deacetylation) (209). Couple of studies have revealed high fat diet induced alterations in NRs expression and activity (210–212). Flavonoids, a category of plant secondary metabolites, also exert some of their effects through NRs and play a key role in dietary modulation of metabolism and linked diseases (213). Thus, NRs are cardinal players that influence both the genome and epigenome of an organism, thereby bridging this gap between genotype and phenotype. Since NRs effect on gene expression is sequence specific, identification of spatial arrangement present on binding sequence in food-bound NRs would provide a greater understanding of epigenetic modulation associated with a particular disease.
Expert Opinion: Scientific Challenges and Future Perspectives
>The field of biology is marked by an extraordinary spatiotemporal interplay. NRs are exemplary in the same context as each NR regulates vast array of gene networks, distinct to cell type and origin. The breakthrough in the field of genetics, molecular and structural biology has edified our knowledge about the functional regulation of NRs. However, there are still innumerable unmet queries regarding the understanding of how dynamically these NRs govern transcriptional processes. One of the future goals could be: comprehending their interaction abilities with the genome, how they peddle chromatin remodeling and corresponding interactions with binding partners like other NRs or coregulators (enhancers/repressors). The physiological complexity that we see is perhaps, a reflection of the genomic complexity. Therefore, another key area that can be chased would be discovering factors that collaborate with NRs and aid them in carrying out tissue-specific roles. An understanding of their functional aspects would provide insights into various human physiological pathways and lead to the genesis of ‘customized and tailored’ therapeutics.
In the context of asthma too, scientific findings have pointed toward the existence of NRs that tend to promote or abate asthma. These discoveries fortify the importance of NRs in maintaining immune homeostasis. The enormous success of GR in inflammatory diseases is a well-acclaimed example of NR serving as an excellent druggable target. However, an important aspect arising out of the several studies is that the NRs like ER, AR or GR must be kept out of druggable zones. This is because they dictate the sensitive hormonal arm and if tinkered, would have a substantial impact on immune system, systemic inflammation and body homeostasis. Conceivably, this could be one of the reasons behind GR-linked dire effects and hence, it would be a sagacious policy to focus on the family of orphan NRs. Presently, the role of six orphan NRs; Nurr77, FXR, LXR, PPAR, ROR, and RXR have been reviewed in the context of asthma biology. Howbeit, the role of other members of this family is still encrypted and leaves an open canvas for future research. The complete landscaping of orphan NRs in immunopathogenesis of asthma would increase our understanding of how these NRs impact the complex gene networks behind various disease endotypes.
It is noteworthy to mention that many food ligands as well as drugs interact with NRs and activate them functionally. ERα ligand genistein, a food isoflavone, has been found to elevate the therapeutic efficacy of ERα antagonist tamoxifen in breast cancer (214). On the other hand, glucocorticoid-induced hyperinsulinemia was inhibited by PPARα agonist fenofibrate in mice fed on high-fat diet while enhancing the anti-inflammatory effects of GR on transrepression of NF-κB (215). Therefore, future efforts must be directed toward in-depth study of how these food-driven synergies and antagonisms influence NRs and the response to asthma therapy. In addition to this, role of gut microbiota in asthma and their regulation through healthy diet has gained much scientific attention. So, another interesting question to chase would be to decipher how the gut microbiota crosstalk through NRs and its impact on the gut microbiota-diet axis. However, before giving it a translational outlook, a comprehensive study elucidating the role of all 48 NRs in asthma and allergies needs to be done. Post the explication of their roles in current disease biology, alternative adjunct-therapies could be developed and chosen NRs could be targeted either via drugs (small-molecules) or dietary supplements. A combinatorial therapy of the FDA approved ligands; agonists for defender-NRs that possess anti-inflammatory attributes along with antagonist for disease-exacerbating NRs could be employed to relieve asthma in a synergistic way (43) (Table 1).
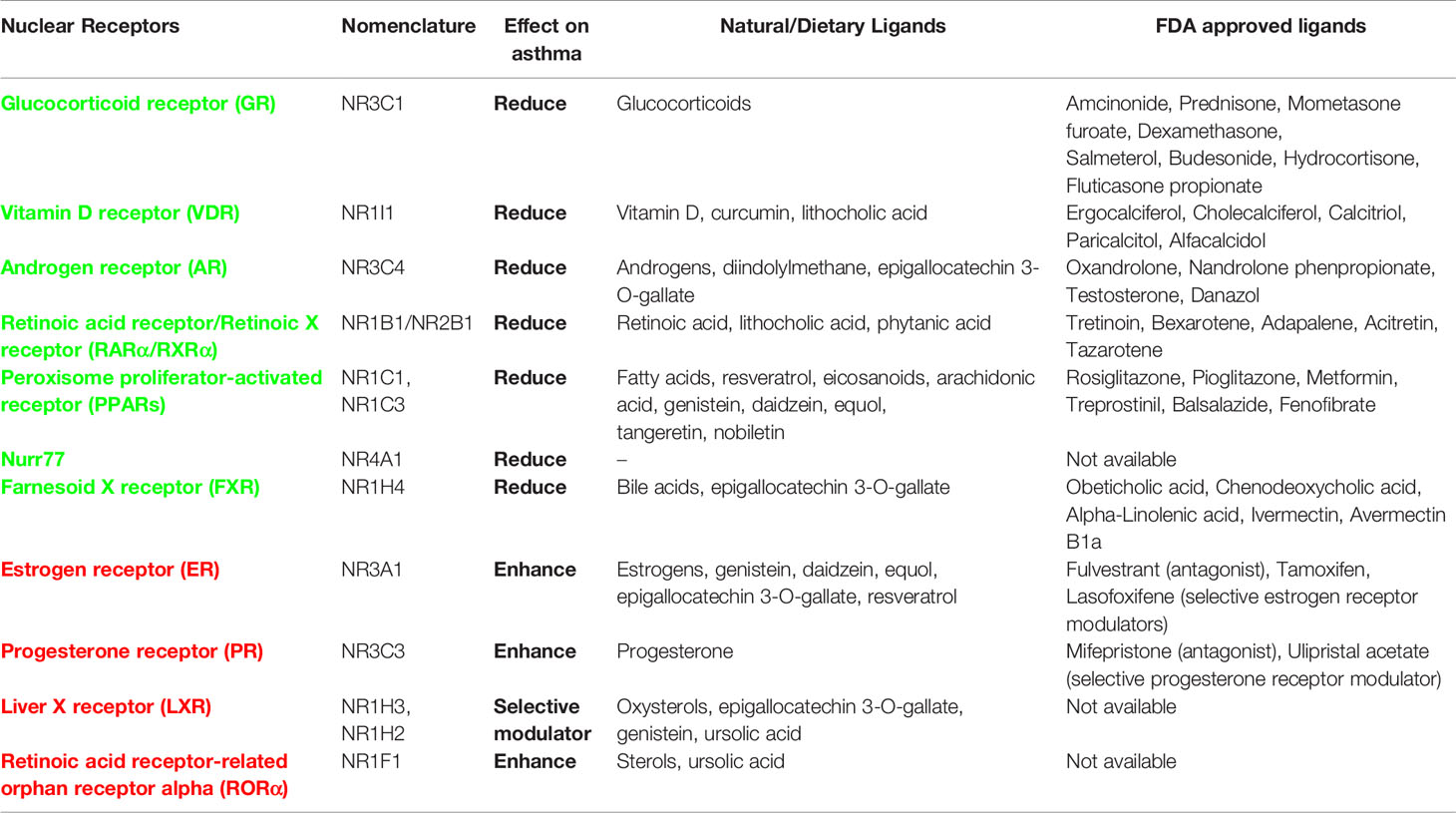
Table 1 List of nuclear receptors (NRs) and their effect on asthma pathogenesis along with information about natural/dietary and FDA approved ligands (agonists for protective NRs: green colored and antagonists for exacerbating NRs: red colored).
The NRs annotated so far in asthma biology have their impacts on comorbidities as well. Whether its metabolic syndromes, microbial infections, cardiovascular or inflammatory disorders, all of these biological province corroborate either healing or augmenting roles of various NRs (216–220). With the cognition of available literature, it becomes apparent that GR is an odious target for the treatment of refractory asthma and comorbid conditions as both aberrant GR expression and gene polymorphism is found to be associated with several metabolic, inflammatory and psychiatric illnesses. The GR has been reported to promote adipogenesis, obesity, musculoskeletal disorders, and psychosocial stress along with reduction in body’s ability to clear infection (27, 221–229). Another NR, RORα has similar attributes and it exacerbates both asthma and associated comorbidities (230–235). On the other hand, NRs like VDR and Nurr77 seem to be promising in their action and allay asthma, rhinitis, obesity, infection, stress or other co-existing conditions (236–245). Although Nurr77 is a desirable target but is considerably less explored and requires more translational studies. However, PPARγ, FXR, RXR, RAR, and LXR have multifarious effects where they mitigate few while exacerbates other comorbidities (101, 246–287). However, they can be critically chosen for creating a therapeutic cocktail in context of subjects exhibiting a particular comorbid condition along with asthma, thereby opening customized therapy avenues. Also, for a broader effect on clinical symptoms, there is a necessity to empower defender NRs as well as to countervail the disease advocating NRs. Therefore, a better remedy option would comprise of respective agonists of VDR and Nurr77 along with antagonist of RORα for its neutralization. Before advancing toward designing therapeutic cocktails, there is an inevitable need to evaluate the role of all 48 NRs in both asthma and comorbid conditions. Since asthma manifestation requires a genetic pre-disposition and fomenting environment, a better comprehension of the genetic basis would certainly speed-up the journey of finding new targets and subsequent drug development. These efforts would aid in the discovery of a cleaner target that might soothe both asthma and associated comorbidities (Figures 4 and 5).
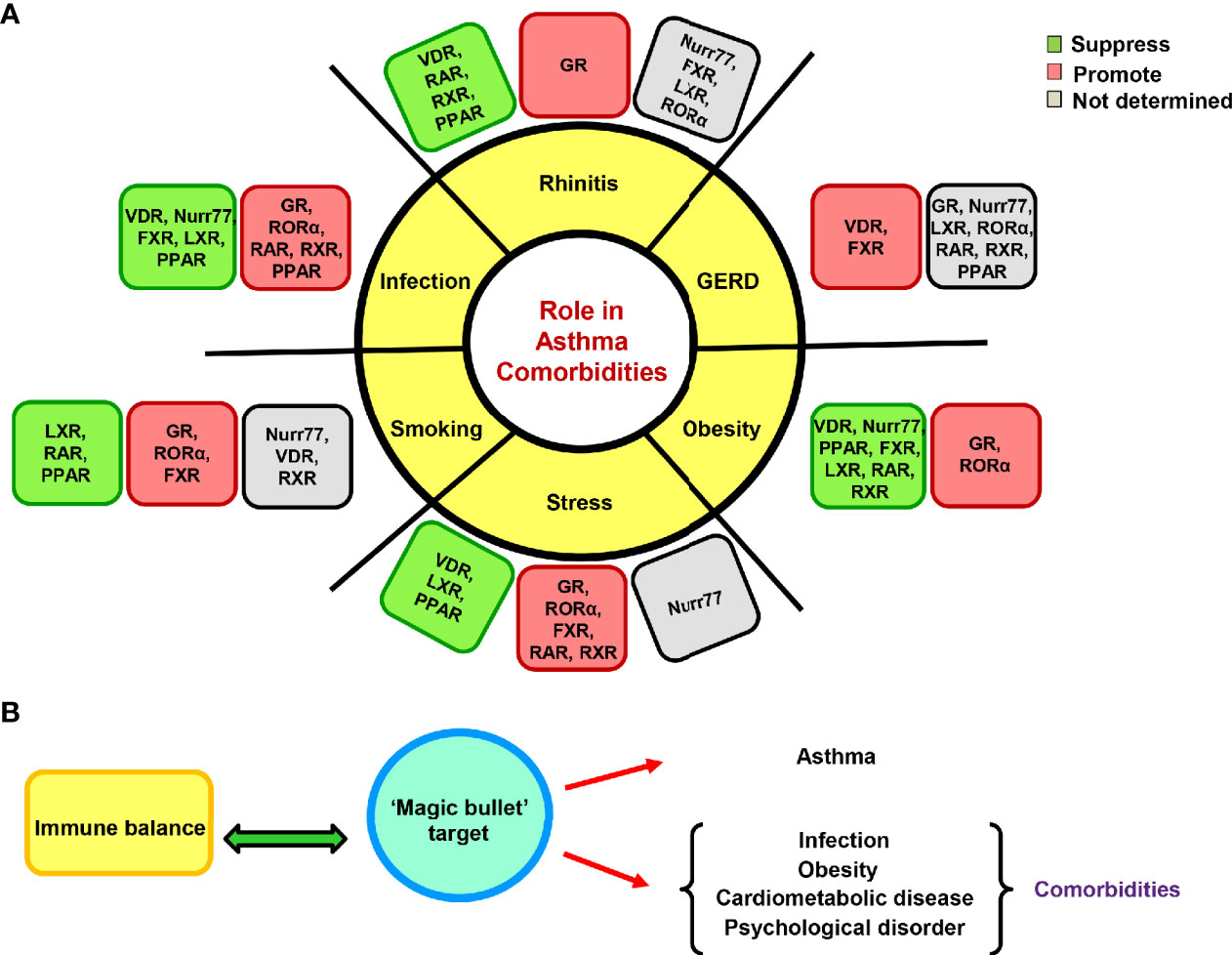
Figure 4 Effects of nuclear receptors (NRs) on asthma comorbidities. The NRs are also known to impact asthma comorbidities; both protective and exacerbating roles have been documented. (A) The illustration depicts the reported roles of GR and adopted orphan/orphan receptors in various comorbid conditions. (B) A proposed hypothetical model of a cleaner therapeutic target as a remedy for asthma and associated comorbidities that can also maintain immune homeostasis.
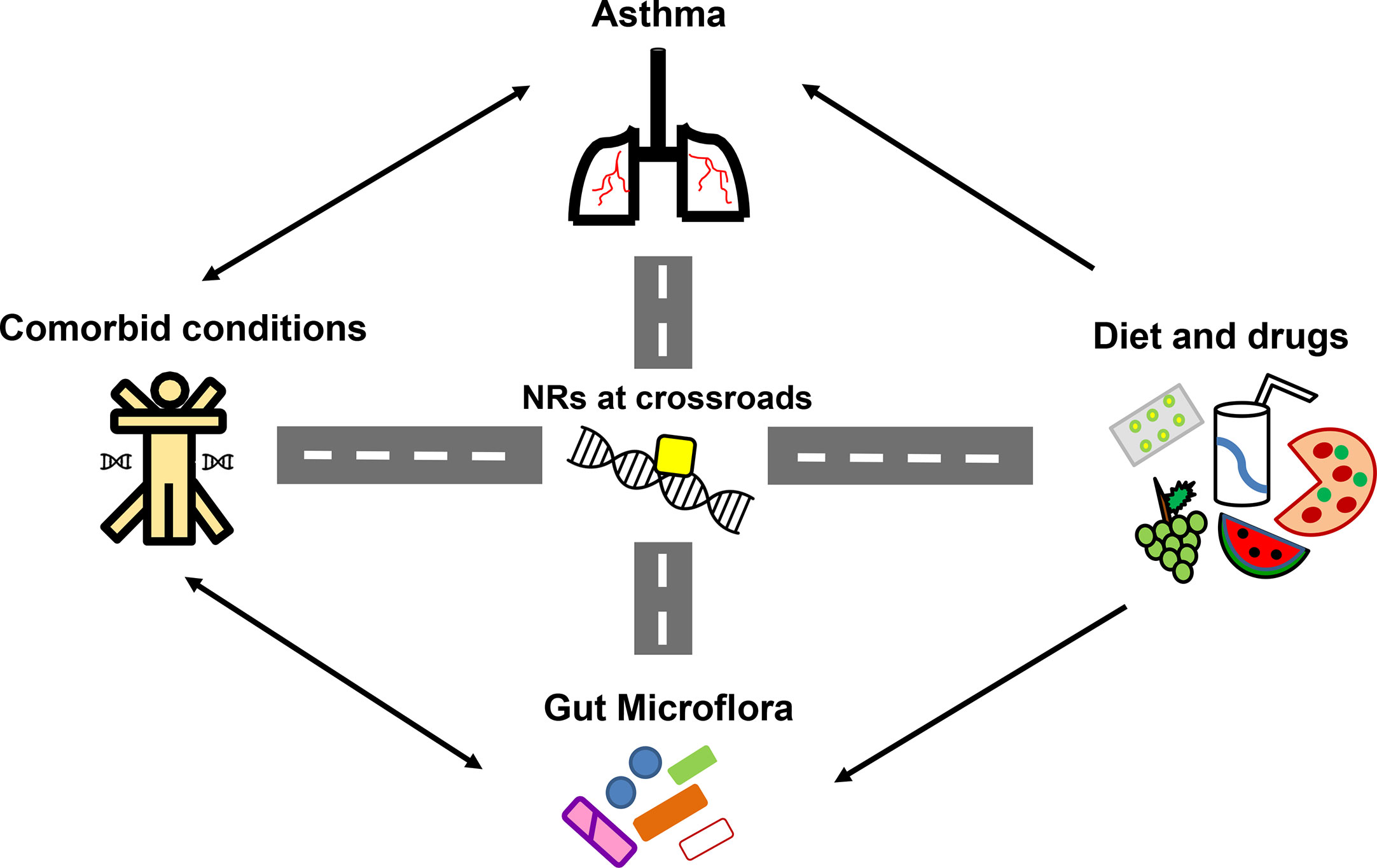
Figure 5 Nuclear receptors (NRs) at the crossroads of asthma, comorbidities, diet and gut microflora. Numerous studies have highlighted the significant influence of diet, gut microflora and existing comorbidities in asthma pathogenesis and therapy outcomes. With reported roles of NRs in asthma and comorbidities along with its ability to crosstalk with diet, drugs and microflora, NRs become the strategic molecules for therapeutic intervention in asthma.
Conclusion
● There is no skepticism associated with the intake of a balanced diet and proper lifestyle patterns leading to a healthier immune system. Hustle and bustle of earning the daily bread and raised living standards have led to poor health quality and increased allergy burden. This review highlights the unmet dearth of newer therapy targets and elaborates on asthma therapy challenges like GR-associated side-effects and resistance issues along with a therapy need for linked comorbidities. It also discusses the cardinal importance of NRs in maintaining immune homeostasis and provides a knowledge window of how they serve as excellent druggable targets. Additionally, the advantages of adopted orphan NRs over endocrine NRs and why they should be the cynosure of all pharmacological efforts is discussed.
● The diets rich in fibers and micronutrients involving essential vitamins like A or D provide a natural defense against allergies while those that are calorie rich raise the vulnerability index. Taken together, these discoveries propose a possible future employment of patient-friendly therapy involving a combination of dietary supplements and synthetic ligands that would trigger the activation of defender NRs.
● The extent to which this perception of comorbid conditions worsening asthma holds true, the similar amount of veracity retains in the fact that asthma too can induce or even worsen comorbidities. They either share similar pathophysiological pathway (e.g. rhinitis) or alter asthma phenotypes (e.g. obesity, smoking, respiratory infections). Therefore, the apprehension and medication of comorbidities must be considered essential during the course of asthma evaluation. Considering the NRs significance in both asthma and comorbidities, there is a requisite to engage defender NRs alongside the negation of exacerbating NRs for a better cure. Moreover, mysteries to the underlying molecular link connecting asthma to these specific comorbid ailments still remain unresolved and finding answers to them would bring about a cut above understanding of disorders like asthma that display complicated etiology. The application of systems biology and findings from holistic studies would help in uncovering cleaner targets that are more desirable and possess some kind of ‘magic-bullet’ properties.
Author Contributions
PG conceived the idea. DT and PG wrote the review manuscript. All authors contributed to the article and approved the submitted version.
Funding
This work was supported by the Council of Scientific and Industrial Research (CSIR) 12th Plan Network project Bugs to Drugs (BSC0211) and OLP (0704) to PG.
Conflict of Interest
The authors declare that the research was conducted in the absence of any commercial or financial relationships that could be construed as a potential conflict of interest.
Acknowledgments
We thank IMTECH (a constituent laboratory of the Council of Scientific and Industrial Research) for facilities and financial support.
Abbreviations
AR, androgen receptor; ASM, airway smooth muscle; ER, estrogen receptor; FXR, farnesoid X receptor; GR, glucocorticoid receptor; LXR, liver X receptor NR, nuclear receptor; PPAR, peroxisome proliferator-activated receptor; PR, progesterone receptor; RAR, retinoic acid receptor; ROR, retinoid-related orphan receptor; RXR, retinoid X receptor; Th2, T helper cells type 2; VDR, vitamin D receptor.
References
1. Global Asthma Network. The Global Asthma Report. (2018). www.globalasthmanetwork.org.
2. Gern JE. The Urban Environment and Childhood Asthma Study. J Allergy Clin Immunol (2010) 125:545–9. doi: 10.1016/j.jaci.2010.01.037
3. Oliver BGG, Robinson P, Peters M, Black J. Viral infections and asthma: an inflammatory interface? Eur Respir J (2014) 44:1666–81. doi: 10.1183/09031936.00047714
4. Moreira A, Bonini M, Garcia-Larsen V, Bonini S, Del Giacco SR, Agache I, et al. Weight loss interventions in asthma: EAACI Evidence-Based Clinical Practice Guideline (Part I). Allergy (2013) 68:425–39. doi: 10.1111/all.12106
5. Nunes C, Pereira AM, Morais-Almeida M. Asthma costs and social impact. Asthma Res Pract (2017) 3:1. doi: 10.1186/s40733-016-0029-3
6. Chung KF, Wenzel SE, Brozek JL, Bush A, Castro M, Sterk PJ, et al. International ERS/ATS guidelines on definition, evaluation and treatment of severe asthma. Eur Respir J (2014) 43:343–73. doi: 10.1183/09031936.00202013
7. Holgate ST. A look at the pathogenesis of asthma: the need for a change in direction. Discovery Med (2010) 9:439–47.
8. Global Initiative for Asthma. Global Strategy for Asthma Management and Prevention. (2019). www.ginasthma.org.
9. Arruda LK, Solé D, Baena-Cagnani CE, Naspitz CK. Risk factors for asthma and atopy. Curr Opin Allergy Clin Immunol (2005) 5:153–9. doi: 10.1097/01.all.0000162308.89857.6c
10. Kontakioti E, Domvri K, Papakosta D, Daniilidis M. HLA and asthma phenotypes/endotypes: A review. Hum Immunol (2014) 75:930–9. doi: 10.1016/j.humimm.2014.06.022
11. Meyer N, Dallinga JW, Nuss SJ, Moonen EJ, van Berkel JJ, Akdis C, et al. Defining adult asthma endotypes by clinical features and patterns of volatile organic compounds in exhaled air. Respir Res (2014) 15:136. doi: 10.1186/s12931-014-0136-8
12. Lötvall J, Akdis CA, Bacharier LB, Bjermer L, Casale TB, Custovic A, et al. Asthma endotypes: A new approach to classification of disease entities within the asthma syndrome. J Allergy Clin Immunol (2011) 127:355–60. doi: 10.1016/j.jaci.2010.11.037
13. Boulet L-P, Boulay M-È. Asthma-related comorbidities. Expert Rev Respir Med (2011) 5:377–93. doi: 10.1586/ers.11.34
14. Sears MR. Epidemiology of asthma exacerbations. J Allergy Clin Immunol (2008) 122:662–8. doi: 10.1016/j.jaci.2008.08.003
15. Macsali F, Real FG, Plana E, Sunyer J, Anto J, Dratva J, et al. Early Age at Menarche, Lung Function, and Adult Asthma. Am J Respir Crit Care Med (2011) 183:8–14. doi: 10.1164/rccm.200912-1886OC
16. Brenner BE, Holmes TM, Mazal B, Camargo CA. Relation between phase of the menstrual cycle and asthma presentations in the emergency department. Thorax (2005) 60:806–9. doi: 10.1136/thx.2004.033928
17. Postma DS. Gender differences in asthma development and progression. Gender Med (2007) 4(Suppl B):S133–46. doi: 10.1016/s1550-8579(07)80054-4
18. Prosser R, Carleton B, Smith A. The comorbidity burden of the treated asthma patient population in British Columbia. Chronic Dis Canada (2010) 30:46–55.
19. Adams RJ, Wilson DH, Taylor AW, Daly A, Tursan d’Espaignet E, Dal Grande E, et al. Coexistent Chronic Conditions and Asthma Quality of Life. Chest (2006) 129:285–91. doi: 10.1378/chest.129.2.285
20. Holgate ST, Polosa R. Treatment strategies for allergy and asthma. Nat Rev Immunol (2008) 8:218–30. doi: 10.1038/nri2262
21. Johnston SL, Edwards MR. Mechanisms of adverse effects of {beta}-agonists in asthma. Thorax (2009) 64:739–41. doi: 10.1136/thx.2009.119230
22. Wraight JM, Smith AD, Cowan JO, Flannery EM, Herbison GP, Taylor DR. Adverse effects of short-acting beta-agonists: Potential impact when anti-inflammatory therapy is inadequate. Respirology (2004) 9:215–21. doi: 10.1111/j.1440-1843.2004.00557.x
23. Wenzel SE, Busse WW. National Heart, Lung, and Blood Institute’s Severe Asthma Research Program. Severe asthma: Lessons from the Severe Asthma Research Program. J Allergy Clin Immunol (2007) 119:14–21. doi: 10.1016/j.jaci.2006.10.025
24. Kupczyk M, Wenzel S. US and European severe asthma cohorts: what can they teach us about severe asthma? J Internal Med (2012) 272:121–32. doi: 10.1111/j.1365-2796.2012.02558.x
25. Newell-Price J, Bertagna X, Grossman AB, Nieman LK. Cushing’s syndrome. Lancet (2006) 367:1605–17. doi: 10.1016/S0140-6736(06)68699-6
26. Coutinho AE, Chapman KE. The anti-inflammatory and immunosuppressive effects of glucocorticoids, recent developments and mechanistic insights. Mol Cell Endocrinol (2011) 335:2–13. doi: 10.1016/j.mce.2010.04.005
27. Ng SSM, Li A, Pavlakis GN, Ozato K, Kino T. Viral Infection Increases Glucocorticoid-Induced Interleukin-10 Production through ERK-Mediated Phosphorylation of the Glucocorticoid Receptor in Dendritic Cells: Potential Clinical Implications. PloS One (2013) 8:e63587. doi: 10.1371/journal.pone.0063587
28. Adcock IM, Lane SJ, Brown CR, Peters MJ, Lee TH, Barnes PJ. Differences in binding of glucocorticoid receptor to DNA in steroid-resistant asthma. J Immunol (Baltimore Md : 1950) (1995) 154:3500–5.
29. Ito K, Chung KF, Adcock IM. Update on glucocorticoid action and resistance. J Allergy Clin Immunol (2006) 117:522–43. doi: 10.1016/j.jaci.2006.01.032
30. Iwaki H, Nomoto M. [The adverse effects of anticholinergic drugs]. Brain Nerve = Shinkei kenkyu no shinpo (2014) 66:551–60. doi: 10.11477/mf.1416101793
31. Wong JJ, Lee JH, Turner DA, Rehder KJ. A review of the use of adjunctive therapies in severe acute asthma exacerbation in critically ill children. Expert Rev Respir Med (2014) 8:423–41. doi: 10.1586/17476348.2014.915752
32. Rehder KJ. Adjunct Therapies for Refractory Status Asthmaticus in Children. Respir Care (2017) 62:849–65. doi: 10.4187/respcare.05174
33. Baldo BA. Side Effects of Cytokines Approved for Therapy. Drug Saf (2014) 37:921–43. doi: 10.1007/s40264-014-0226-z
34. Strunk RC, Bloomberg GR. Omalizumab for asthma. New Engl J Med (2006) 354:2689–95. doi: 10.1056/NEJMct055184
35. Mangelsdorf DJ, Thummel C, Beato M, Herrlich P, Schütz G, Umesono K, et al. The nuclear receptor superfamily: the second decade. Cell (1995) 83:835–9. doi: 10.1016/0092-8674(95)90199-x
36. Burris TP, Solt LA, Wang Y, Crumbley C, Banerjee S, Griffett K, et al. Nuclear Receptors and Their Selective Pharmacologic Modulators. Pharmacol Rev (2013) 65:710–78. doi: 10.1124/pr.112.006833
37. Chawla A, Repa JJ, Evans RM, Mangelsdorf DJ. Nuclear receptors and lipid physiology: opening the X-files. Sci (New York NY) (2001) 294:1866–70. doi: 10.1126/science.294.5548.1866
38. Shi Y. Orphan nuclear receptors in drug discovery. Drug Discovery Today (2007) 12:440–5. doi: 10.1016/j.drudis.2007.04.006
39. Huang P, Chandra V, Rastinejad F. Structural Overview of the Nuclear Receptor Superfamily: Insights into Physiology and Therapeutics. Annu Rev Physiol (2010) 72:247–72. doi: 10.1146/annurev-physiol-021909-135917
40. Pearen MA, Muscat GEO. Orphan Nuclear Receptors and the Regulation of Nutrient Metabolism: Understanding Obesity. Physiology (2012) 27:156–66. doi: 10.1152/physiol.00007.2012
41. Overington JP, Al-Lazikani B, Hopkins AL. How many drug targets are there? Nat Rev Drug Discovery (2006) 5:993–6. doi: 10.1038/nrd2199
42. Santos R, Ursu O, Gaulton A, Bento AP, Donadi RS, Bologa CG, et al. A comprehensive map of molecular drug targets. Nat Rev Drug Discovery (2016) 16:19–34. doi: 10.1038/nrd.2016.230
43. Nanduri R, Bhutani I, Somavarapu AK, Mahajan S, Parkesh R, Gupta P. ONRLDB—manually curated database of experimentally validated ligands for orphan nuclear receptors: insights into new drug discovery. Database (2015) 2015:bav112. doi: 10.1093/database/bav112
44. Yen PM. Classical nuclear hormone receptor activity as a mediator of complex biological responses: A look at health and disease. Best Pract Research: Clin Endocrinol Metab (2015) 29:517–28. doi: 10.1016/j.beem.2015.07.005
45. Opherk C, Tronche F, Kellendonk C, Kohlmüller D, Schulze A, Schmid W, et al. Inactivation of the glucocorticoid receptor in hepatocytes leads to fasting hypoglycemia and ameliorates hyperglycemia in streptozotocin-induced diabetes mellitus. Mol Endocrinol (2004) 18:1346–53. doi: 10.1210/me.2003-0283
46. Tronche F, Opherk C, Moriggl R, Kellendonk C, Reimann A, Schwake L, et al. Glucocorticoid receptor function in hepatocytes is essential to promote postnatal body growth. Genes Dev (2004) 18:492–7. doi: 10.1101/gad.284704
47. Boyle MP, Kolber BJ, Vogt SK, Wozniak DF, Muglia LJ. Forebrain glucocorticoid receptors modulate anxiety-associated locomotor activation and adrenal responsiveness. J Neurosci (2006) 26:1971–8. doi: 10.1523/JNEUROSCI.2173-05.2006
48. Bhattacharyya S, Brown DE, Brewer JA, Vogt SK, Muglia LJ. Macrophage glucocorticoid receptors regulate Toll-like receptor 4-mediated inflammatory responses by selective inhibition of p38 MAP kinase. Blood (2007) 109:4313–9. doi: 10.1182/blood-2006-10-048215
49. Kleiman A, Tuckermann JP. Glucocorticoid receptor action in beneficial and side effects of steroid therapy: Lessons from conditional knockout mice. Mol Cell Endocrinol (2007) 275:98–108. doi: 10.1016/j.mce.2007.05.009
50. Tuckermann JP, Kleiman A, Moriggl R, Spanbroek R, Neumann A, Illing A, et al. Macrophages and neutrophils are the targets for immune suppression by glucocorticoids in contact allergy. J Clin Invest (2007) 117:1381–90. doi: 10.1172/JCI28034
51. Goodwin JE, Feng Y, Velazquez H, Sessa WC. Endothelial glucocorticoid receptor is required for protection against sepsis. Proc Natl Acad Sci United States America (2013) 110:306–11. doi: 10.1073/pnas.1210200110
52. Klaßen C, Karabinskaya A, Dejager L, Vettorazzi S, Van Moorleghem J, Lühder F, et al. Airway Epithelial Cells Are Crucial Targets of Glucocorticoids in a Mouse Model of Allergic Asthma. J Immunol (2017) 199:48–61. doi: 10.4049/jimmunol.1601691
53. Sasse SK, Kadiyala V, Danhorn T, Panettieri RA, Phang TL, Gerber AN. Glucocorticoid Receptor ChIP-Seq Identifies PLCD1 as a KLF15 Target that Represses Airway Smooth Muscle Hypertrophy. Am J Respir Cell Mol Biol (2017) 57:226–37. doi: 10.1165/rcmb.2016-0357OC
54. Zhou X, Dang Y-J, Wang G-F, Jin X-Q. Effects of Aspergillus fumigatus on glucocorticoid receptor and β2-adrenergic receptor expression in a rat model of asthma. Exp Lung Res (2017) 43:197–207. doi: 10.1080/01902148.2017.1339142
55. Pazdrak K, Straub C, Maroto R, Stafford S, White WI, Calhoun WJ, et al. Cytokine-Induced Glucocorticoid Resistance from Eosinophil Activation: Protein Phosphatase 5 Modulation of Glucocorticoid Receptor Phosphorylation and Signaling. J Immunol (2016) 197:3782–91. doi: 10.4049/jimmunol.1601029
56. Pazdrak K, Moon Y, Straub C, Stafford S, Kurosky A. Eosinophil resistance to glucocorticoid-induced apoptosis is mediated by the transcription factor NFIL3. Apoptosis: an Int J Programmed Cell Death (2016) 21:421–31. doi: 10.1007/s10495-016-1226-5
57. Zappia CD, Granja-Galeano G, Fernández N, Shayo C, Davio C, Fitzsimons CP, et al. Effects of histamine H1 receptor signaling on glucocorticoid receptor activity. Role of canonical and non-canonical pathways. Sci Rep (2015) 5:17476. doi: 10.1038/srep17476
58. Kmyta V, Orlovskyi V, Prystupa L, Prystupa E. Bcl1 polymorphism of glucocorticoids receptor gene and bronchial asthma. Georgian Med News (2015), 51–5. doi: 10.3103/S0095452716030063
59. Panek M, Pietras T, Antczak A, Górski P, Kuna P, Szemraj J. The role of functional single nucleotide polymorphisms of the human glucocorticoid receptor gene NR3C1 in Polish patients with bronchial asthma. Mol Biol Rep (2012) 39:4749–57. doi: 10.1007/s11033-011-1267-3
60. Chang P-J, Michaeloudes C, Zhu J, Shaikh N, Baker J, Chung KF, et al. Impaired Nuclear Translocation of the Glucocorticoid Receptor in Corticosteroid-Insensitive Airway Smooth Muscle in Severe Asthma. Am J Respir Crit Care Med (2015) 191:54–62. doi: 10.1164/rccm.201402-0314OC
61. Cornejo S, Tantisira K, Raby BA, Weiss ST, Kaplan F. Nuclear bioavailability of the glucocorticoid receptor in a pediatric asthma cohort with variable corticosteroid responsiveness. Pediatr Res (2015) 78:505–12. doi: 10.1038/pr.2015.148
62. Saif Z, Hodyl NA, Hobbs E, Tuck AR, Butler MS, Osei-Kumah A, et al. The human placenta expresses multiple glucocorticoid receptor isoforms that are altered by fetal sex, growth restriction and maternal asthma. Placenta (2014) 35:260–8. doi: 10.1016/j.placenta.2014.01.012
63. Lips P. Vitamin D physiology. Prog Biophys Mol Biol (2006) 92:4–8. doi: 10.1016/j.pbiomolbio.2006.02.016
64. Visser M, Deeg DJH, Lips P. Low Vitamin D and High Parathyroid Hormone Levels as Determinants of Loss of Muscle Strength and Muscle Mass (Sarcopenia): The Longitudinal Aging Study Amsterdam. J Clin Endocrinol Metab (2003) 88:5766–72. doi: 10.1210/jc.2003-030604
65. Tang BM, Eslick GD, Nowson C, Smith C, Bensoussan A. Use of calcium or calcium in combination with vitamin D supplementation to prevent fractures and bone loss in people aged 50 years and older: a meta-analysis. Lancet (2007) 370:657–66. doi: 10.1016/S0140-6736(07)61342-7
66. Bischoff-Ferrari HA, Willett WC, Wong JB, Stuck AE, Staehelin HB, Orav EJ, et al. Prevention of nonvertebral fractures with oral vitamin D and dose dependency: A meta-analysis of randomized controlled trials. Arch Internal Med (2009) 169:551–61. doi: 10.1001/archinternmed.2008.600
67. Rai V, Agrawal DK. Role of Vitamin D in Cardiovascular Diseases. Endocrinol Metab Clinics North America (2017) 46:1039–59. doi: 10.1016/j.ecl.2017.07.009
68. Pittas AG, Lau J, Hu FB, Dawson-Hughes B. The Role of Vitamin D and Calcium in Type 2 Diabetes. A Systematic Review and Meta-Analysis. J Clin Endocrinol Metab (2007) 92:2017–29. doi: 10.1210/jc.2007-0298
69. Yamshchikov AV, Desai NS, Blumberg HM, Ziegler TR, Tangpricha V. Vitamin D for treatment and prevention of infectious diseases: A systematic review of randomized controlled trials. Endocr Pract (2009) 15:438–49. doi: 10.4158/EP09101.ORR
70. Cutolo M, Otsa K, Uprus M, Paolino S, Seriolo B. Vitamin D in rheumatoid arthritis. Autoimmun Rev (2007) 7:59–64. doi: 10.1016/j.autrev.2007.07.001
71. Myhr K-M. Vitamin D treatment in multiple sclerosis. J Neurological Sci (2009) 286:104–8. doi: 10.1016/j.jns.2009.05.002
72. Lappe JM, Travers-Gustafson D, Davies KM, Recker RR, Heaney RP. Vitamin D and calcium supplementation reduces cancer risk: results of a randomized trial. Am J Clin Nutr (2007) 85:1586–91. doi: 10.1093/ajcn/85.6.1586
73. Qiao J-Y, Song L, Zhang Y-L, Luan B. [HMGB1/TLR4/NF-κB signaling pathway and role of vitamin D in asthmatic mice]. Zhongguo dang dai er ke za zhi = Chin J Contemp Pediatr (2017) 19:95–103. doi: 10.7499/j.issn.1008-8830.2017.01.016
74. Schedel M, Jia Y, Michel S, Takeda K, Domenico J, Joetham A, et al. 1,25D3 prevents CD8(+)Tc2 skewing and asthma development through VDR binding changes to the Cyp11a1 promoter. Nat Commun (2016) 7:10213. doi: 10.1038/ncomms10213
75. James J, Weaver V, Cantorna MT. Control of Circulating IgE by the Vitamin D Receptor In Vivo Involves B Cell Intrinsic and Extrinsic Mechanisms. J Immunol (Baltimore Md: 1950) (2017) 198:1164–71. doi: 10.4049/jimmunol.1601213
76. Hartmann B, Heine G, Babina M, Steinmeyer A, Zügel U, Radbruch A, et al. Targeting the vitamin D receptor inhibits the B cell-dependent allergic immune response. Allergy: Eur J Allergy Clin Immunol (2011) 66:540–8. doi: 10.1111/j.1398-9995.2010.02513.x
77. Heine G, Anton K, Henz BM, Worm M. 1alpha,25-dihydroxyvitamin D3 inhibits anti-CD40 plus IL-4-mediated IgE production in vitro. Eur J Immunol (2002) 32:3395–404. doi: 10.1002/1521-4141(200212)32:12<3395::AID-IMMU3395>3.0.CO;2-
78. Einisman H, Reyes ML, Angulo J, Cerda J, López-Lastra M, Castro-Rodriguez JA. Vitamin D levels and vitamin D receptor gene polymorphisms in asthmatic children: a case-control study. Pediatr Allergy Immunol (2015) 26:545–50. doi: 10.1111/pai.12409
79. Zhao D-D, Yu D-D, Ren Q-Q, Dong B, Zhao F, Sun Y-H. Association of vitamin D receptor gene polymorphisms with susceptibility to childhood asthma: A meta-analysis. Pediatr Pulmonol (2017) 52:423–9. doi: 10.1002/ppul.23548
80. Han J-C, Du J, Zhang Y-J, Qi G-B, Li H-B, Zhang YJ, et al. Vitamin D receptor polymorphisms may contribute to asthma risk. J Asthma (2016) 53:790–800. doi: 10.3109/02770903.2016.1158267
81. Davey RA, Grossmann M. Androgen Receptor Structure, Function and Biology: From Bench to Bedside. Clin biochemist Rev (2016) 37:3–15.
82. Obana N, Chang C, Uno H. Inhibition of hair growth by testosterone in the presence of dermal papilla cells from the frontal bald scalp of the postpubertal stumptailed macaque. Endocrinology (1997) 138:356–61. doi: 10.1210/endo.138.1.4890
83. Mantalaris A, Panoskaltsis N, Sakai Y, Bourne P, Chang C, Messing EM, et al. Localization of androgen receptor expression in human bone marrow. J Pathol (2001) 193:361–6. doi: 10.1002/1096-9896(0000)9999:9999<::AID-PATH803>3.0.CO;2-W
84. Olsen NJ, Kovacs WJ. Effects of androgens on T and B lymphocyte development. Immunologic Res (2001) 23:281–8. doi: 10.1385/IR:23:2-3:281
85. Chang C, Lee SO, Wang RS, Yeh S, Chang TM. Androgen receptor (AR) physiological roles in male and female reproductive systems: Lessons learned from AR-Knockout mice lacking AR in selective cells. Biol Reprod (2013) 89:21. doi: 10.1095/biolreprod.113.109132
86. Navarro G, Allard C, Xu W, Mauvais-Jarvis F. The role of androgens in metabolism, obesity, and diabetes in males and females. Obesity (2015) 23:713–9. doi: 10.1002/oby.21033
87. Hu C, Fang D, Xu H, Wang Q, Xia H. The androgen receptor expression and association with patient’s survival in different cancers. Genomics (2020) 112:1926–40. doi: 10.1016/j.ygeno.2019.11.005
88. Cephus JY, Stier MT, Fuseini H, Yung JA, Toki S, Bloodworth MH, et al. Testosterone Attenuates Group 2 Innate Lymphoid Cell-Mediated Airway Inflammation. Cell Rep (2017) 21:2487–99. doi: 10.1016/j.celrep.2017.10.110
89. Laffont S, Blanquart E, Guéry J-C. Sex Differences in Asthma: A Key Role of Androgen-Signaling in Group 2 Innate Lymphoid Cells. Front Immunol (2017) 8:1069. doi: 10.3389/fimmu.2017.01069
90. Warren KJ, Sweeter JM, Pavlik JA, Nelson AJ, Devasure JM, Dickinson JD, et al. Sex differences in activation of lung-related type 2 innate lymphoid cells in experimental asthma. Ann Allergy Asthma Immunol (2017) 118:233–4. doi: 10.1016/j.anai.2016.11.011
91. Fuseini H, Yung JA, Cephus JY, Zhang J, Goleniewska K, Polosukhin VV, et al. Testosterone Decreases House Dust Mite–Induced Type 2 and IL-17A–Mediated Airway Inflammation. J Immunol (2018) 201:1843–54. doi: 10.4049/jimmunol.1800293
92. Card JW, Carey MA, Bradbury JA, DeGraff LM, Morgan DL, Moorman MP, et al. Gender Differences in Murine Airway Responsiveness and Lipopolysaccharide-Induced Inflammation. J Immunol (2006) 177:621–30. doi: 10.4049/jimmunol.177.1.621
93. Card JW, Voltz JW, Ferguson CD, Carey MA, DeGraff LM, Peddada SD, et al. Male sex hormones promote vagally mediated reflex airway responsiveness to cholinergic stimulation. Am J Physiol Lung Cell Mol Physiol (2007) 292:L908–14. doi: 10.1152/ajplung.00407.2006
94. Becerra-Díaz M, Strickland AB, Keselman A, Heller NM. Androgen and Androgen Receptor as Enhancers of M2 Macrophage Polarization in Allergic Lung Inflammation. J Immunol (2018) 201:2923–33. doi: 10.4049/jimmunol.1800352
95. Polcz ME, Barbul A. The Role of Vitamin A in Wound Healing. Nutr Clin Pract (2019) 34:695–700. doi: 10.1002/ncp.10376
96. Janesick A, Wu SC, Blumberg B. Retinoic acid signaling and neuronal differentiation. Cell Mol Life Sci (2015) 72:1559–76. doi: 10.1007/s00018-014-1815-9
97. Siddikuzzaman, Guruvayoorappan C, Berlin Grace VM. All trans retinoic acid and cancer. Immunopharmacol Immunotoxicol (2011) 33:241–9. doi: 10.3109/08923973.2010.521507
98. Conserva MR, Anelli L, Zagaria A, Specchia G, Albano F. The pleiotropic role of retinoic acid/retinoic acid receptors signaling: From vitamin A metabolism to gene rearrangements in acute promyelocytic Leukemia. Int J Mol Sci (2019) 20:2921. doi: 10.3390/ijms20122921
99. Scheffel F, Heine G, Henz BM, Worm M. Retinoic acid inhibits CD40 plus IL-4 mediated IgE production through alterations of sCD23, sCD54 and IL-6 production. Inflammation Res (2005) 54:113–8. doi: 10.1007/s00011-004-1331-8
100. Leber BF, Denburg JA. Retinoic acid modulation of induced basophil differentiation. Allergy (1997) 52:1201–6. doi: 10.1111/j.1398-9995.1997.tb02524.x
101. Fujii U, Miyahara N, Taniguchi A, Oda N, Morichika D, Murakami E, et al. Effect of a retinoid X receptor partial agonist on airway inflammation and hyperresponsiveness in a murine model of asthma. Respir Res (2017) 18:23. doi: 10.1186/s12931-017-0507-z
102. Jia M, Dahlman-Wright K, Gustafsson JÅ. Estrogen receptor alpha and beta in health and disease. Best Pract Research: Clin Endocrinol Metab (2015) 29:557–68. doi: 10.1016/j.beem.2015.04.008
103. Nilsson S, Gustafsson JÅ. Estrogen receptors: Therapies targeted to receptor subtypes. Clin Pharmacol Ther (2011) 89:44–55. doi: 10.1038/clpt.2010.226
104. Compston JE. Sex steroids and bone. Physiol Rev (2001) 81:419–47. doi: 10.1152/physrev.2001.81.1.419
105. Flock GB, Cao X, Maziarz M, Drucker DJ. Activation of enteroendocrine membrane progesterone receptors promotes incretin secretion and improves glucose tolerance in mice. Diabetes (2013) 62:283–90. doi: 10.2337/db12-0601
106. Theis V, Theiss C. Progesterone Effects in the Nervous System. Anatomical Record (2019) 302:1276–86. doi: 10.1002/ar.24121
107. Valadez-Cosmes P, Vázquez-Martínez ER, Cerbón M, Camacho-Arroyo I. Membrane progesterone receptors in reproduction and cancer. Mol Cell Endocrinol (2016) 434:166–75. doi: 10.1016/j.mce.2016.06.027
108. Bonds RS, Midoro-Horiuti T. Estrogen effects in allergy and asthma. Curr Opin Allergy Clin Immunol (2013) 13:92–9. doi: 10.1097/ACI.0b013e32835a6dd6
109. Tam A, Wadsworth S, Dorscheid D, Man SFP, Sin DD. Estradiol increases mucus synthesis in bronchial epithelial cells. PloS One (2014) 9:e100633. doi: 10.1371/journal.pone.0100633
110. Keselman A, Fang X, White PB, Heller NM. Estrogen Signaling Contributes to Sex Differences in Macrophage Polarization during Asthma. J Immunol (2017) 199:1573–83. doi: 10.4049/jimmunol.1601975
111. Yung JA, Fuseini H, Newcomb DC. Hormones, sex, and asthma. Ann Allergy Asthma Immunol (2018) 120:488–94. doi: 10.1016/j.anai.2018.01.016
112. Cocchiara R, Albeggiani G, Di Trapani G, Azzolina A, Lampias N, Rizzo F, et al. Modulation of rat peritoneal mast cell and human basophil histamine release by estrogens. Int Arch Allergy Immunol (1990) 93:192–7. doi: 10.1159/000235300
113. Cocchiara R, Albeggiani G, Di Trapani G, Azzolina A, Lampiasi N, Rizzo F, et al. Oestradiol enhances in vitro the histamine release induced by embryonic histamine-releasing factor (EHRF) from uterine mast cells. Hum Reprod (1992) 7:1036–41. doi: 10.1093/oxfordjournals.humrep.a137790
114. Cai Y, Zhou J, Webb DC. Estrogen stimulates Th2 cytokine production and regulates the compartmentalisation of eosinophils during allergen challenge in a mouse model of asthma. Int Arch Allergy Immunol (2012) 158:252–60. doi: 10.1159/000331437
115. Narita SI, Goldblum RM, Watson CS, Brooks EG, Estes DM, Curran EM, et al. Environmental estrogens induce mast cell degranulation and enhance IgE-mediated release of allergic mediators. Environ Health Perspect (2007) 115:48–52. doi: 10.1289/ehp.9378
116. Maranghi F, Rescia M, Macrì C, Di Consiglio E, De Angelis G, Testai E, et al. Lindane may modulate the female reproductive development through the interaction with ER-β: an in vivo-in vitro approach. Chemico Biol Interact (2007) 169:1–14. doi: 10.1016/j.cbi.2007.04.008
117. British Dietetic Association. Paediatric group position statement on the use of soya protein for infants. J Fam Health Care (2003) 13:93.
118. Zhao XJ, McKerr G, Dong Z, Higgins CA, Carson J, Yang ZQ, et al. Expression of oestrogen and progesterone receptors by mast cells alone, but not lymphocytes, macrophages or other immune cells in human upper airways. Thorax (2001) 56:205–11. doi: 10.1136/thorax.56.3.205
119. Jain R, Ray JM, Pan JH, Brody SL. Sex hormone-dependent regulation of cilia beat frequency in airway epithelium. Am J Respir Cell Mol Biol (2012) 46:446–53. doi: 10.1165/rcmb.2011-0107OC
120. Aravamudan B, Goorhouse KJ, Unnikrishnan G, Thompson MA, Pabelick CM, Hawse JR, et al. Differential Expression of Estrogen Receptor Variants in Response to Inflammation Signals in Human Airway Smooth Muscle. J Cell Physiol (2017) 232:1754–60. doi: 10.1002/jcp.25674
121. Bloodworth MH, Rusznak M, Bastarache L, Wang J, Newcomb DC. Association of estrogen receptor α polymorphism rs1999805 with asthma. Ann Allergy Asthma Immunol (2019) 122:208–10. doi: 10.1016/j.anai.2018.11.005
122. Ambhore NS, Kalidhindi RSR, Loganathan J, Sathish V. Role of differential estrogen receptor activation in airway hyperreactivity and remodeling in a murine model of asthma. Am J Respir Cell Mol Biol (2019) 61:469–80. doi: 10.1165/rcmb.2018-0321OC
123. Ambhore NS, Katragadda R, Raju Kalidhindi RS, Thompson MA, Pabelick CM, Prakash YS, et al. Estrogen receptor beta signaling inhibits PDGF induced human airway smooth muscle proliferation. Mol Cell Endocrinol (2018) 476:37–47. doi: 10.1016/j.mce.2018.04.007
124. Ambhore NS, Kalidhindi RSR, Pabelick CM, Hawse JR, Prakash YS, Sathish V. Differential estrogen-receptor activation regulates extracellular matrix deposition in human airway smooth muscle remodeling via NF-κB pathway. FASEB J : Off Publ Fed Am Societies Exp Biol (2019) 33:13935–50. doi: 10.1096/fj.201901340R
125. Kalidhindi RSR, Ambhore NS, Bhallamudi S, Loganathan J, Sathish V. Role of estrogen receptors a and b in a murine model of asthma: Exacerbated airway hyperresponsiveness and remodeling in erβ knockout mice. Front Pharmacol (2020) 10:1499. doi: 10.3389/fphar.2019.01499
126. Bhallamudi S, Connell J, Pabelick CM, Prakash YS, Sathish V. Estrogen receptors differentially regulate intracellular calcium handling in human nonasthmatic and asthmatic airway smooth muscle cells. Am J Physiol - Lung Cell Mol Physiol (2020) 318:L112–24. doi: 10.1152/ajplung.00206.2019
127. Hellings PW, Vandekerckhove P, Claeys R, Billen J, Kasran A, Ceuppens JL. Progesterone increases airway eosinophilia and hyper-responsiveness in a murine model of allergic asthma. Clin Exp Allergy (2003) 33:1457–63. doi: 10.1046/j.1365-2222.2003.01743.x
128. Newcomb DC, Cephus JY, Boswell MG, Fahrenholz JM, Langley EW, Feldman AS, et al. Estrogen and progesterone decrease let-7f microRNA expression and increase IL-23/IL-23 receptor signaling and IL-17A production in patients with severe asthma. J Allergy Clin Immunol (2015) 136:1025–1034.e11. doi: 10.1016/j.jaci.2015.05.046
129. Tyagi S, Gupta P, Saini A, Kaushal C, Sharma S. The peroxisome proliferator-activated receptor: A family of nuclear receptors role in various diseases. J Adv Pharm Technol Res (2011) 2:236–40. doi: 10.4103/2231-4040.90879
130. Becker J, Delayre-Orthez C, Frossard N, Pons F. Regulation of inflammation by PPARs: A future approach to treat lung inflammatory diseases? Fundam Clin Pharmacol (2006) 20:429–47. doi: 10.1111/j.1472-8206.2006.00425.x
131. Woerly G, Honda K, Loyens M, Papin JP, Auwerx J, Staels B, et al. Peroxisome proliferator-activated receptors α and γ down-regulate allergic inflammation and eosinophil activation. J Exp Med (2003) 198:411–21. doi: 10.1084/jem.20021384
132. Trifilieff A, Bench A, Hanley M, Bayley D, Campbell E, Whittaker P. PPAR-α and -γ but not -δ agonists inhibit airway inflammation in a murine model of asthma: In vitro evidence for an NF-κB-independent effect. Br J Pharmacol (2003) 139:163–71. doi: 10.1038/sj.bjp.0705232
133. Delayre-Orthez C, Becker J, Auwerx J, Frossard N, Pons F. Suppression of allergen-induced airway inflammation and immune response by the peroxisome proliferator-activated receptor-alpha agonist fenofibrate. Eur J Pharmacol (2008) 581:177–84. doi: 10.1016/j.ejphar.2007.11.040
134. Lee KS, Park SJ, Hwang PH, Yi HK, Song CH, Chai OH, et al. PPAR-gamma modulates allergic inflammation through up-regulation of PTEN. FASEB J (2005) 19:1033–5. doi: 10.1096/fj.04-3309fje
135. So RK, Kyung SL, Hee SP, Seoung JP, Kyung HM, Sun MJ, et al. Involvement of IL-10 in peroxisome proliferator-activated receptor γ-mediated anti-inflammatory response in asthma. Mol Pharmacol (2005) 68:1568–75. doi: 10.1124/mol.105.017160
136. Honda K, Marquillies P, Capron M, Dombrowicz D. Peroxisome proliferator-activated receptor γ is expressed in airways and inhibits features of airway remodeling in a mouse asthma model. J Allergy Clin Immunol (2004) 113:882–8. doi: 10.1016/j.jaci.2004.02.036
137. Ward JE, Fernandes DJ, Taylor CC, Bonacci JV, Quan L, Stewart AG. The PPARγ ligand, rosiglitazone, reduces airways hyperresponsiveness in a murine model of allergen-induced inflammation. Pulmon Pharmacol Ther (2006) 19:39–46. doi: 10.1016/j.pupt.2005.02.005
138. Narala VR, Ranga R, Smith MR, Berlin AA, Standiford TJ, Lukacs NW, et al. Pioglitazone is as effective as dexamethasone in a cockroach allergen-induced murine model of asthma. Respir Res (2007) 8:90. doi: 10.1186/1465-9921-8-90
139. Mueller C, Weaver V, Vanden Heuvel JP, August A, Cantorna MT. Peroxisome proliferator-activated receptor γ ligands attenuate immunological symptoms of experimental allergic asthma. Arch Biochem Biophys (2003) 418:186–96. doi: 10.1016/j.abb.2003.08.006
140. Hammad H, De Heer HJ, Soullié T, Angeli V, Trottein F, Hoogsteden HC, et al. Activation of Peroxisome Proliferator-Activated Receptor-γ in Dendritic Cells Inhibits the Development of Eosinophilic Airway Inflammation in a Mouse Model of Asthma. Am J Pathol (2004) 164:263–71. doi: 10.1016/S0002-9440(10)63116-1
141. Lee KS, Park SJ, Kim SR, Min KH, Jin SM, Lee HK, et al. Modulation of Airway Remodeling and Airway Inflammation by Peroxisome Proliferator-Activated Receptor γ in a Murine Model of Toluene Diisocyanate-Induced Asthma. J Immunol (2006) 177:5248–57. doi: 10.4049/jimmunol.177.8.5248
142. Zhang G, Yang J, Li P, Cao J, Nie H. Rosiglitazone inhibits HMC-1 cell migration and adhesion through a peroxisome proliferator-activated receptor gamma-dependent mechanism. Iranian J allergy asthma Immunol (2014) 13:11–8.
143. Spears M, McSharry C, Thomson NC. Peroxisome proliferator-activated receptor-? agonists as potential anti-inflammatory agents in asthma and chronic obstructive pulmonary disease. Clin Exp Allergy (2006) 36:1494–504. doi: 10.1111/j.1365-2222.2006.02604.x
144. Zhao Y, Huang Y, He J, Li C, Deng W, Ran X, et al. Rosiglitazone, a peroxisome proliferator-activated receptor-γ agonist, attenuates airway inflammation by inhibiting the proliferation of effector T cells in a murine model of neutrophilic asthma. Immunol Lett (2014) 157:9–15. doi: 10.1016/j.imlet.2013.11.004
145. Ueki S, Matsuwaki Y, Kayaba H, Oyamada H, Kanda A, Usami A, et al. Peroxisome proliferator-activated receptor γ regulates eosinophil functions: A new therapeutic target for allergic airway inflammation. Int Arch Allergy Immunol (2004) 134 Suppl 1):30–6. doi: 10.1159/000077790
146. Banno AT, Reddy AP, Lakshmi SC, Reddy R. PPARs: Key Regulators of Airway Inflammation and Potential Therapeutic Targets in Asthma. Nuclear Receptor Res (2018) 5:101306. doi: 10.11131/2018/101306
147. Hashimoto Y, Nakahara K. Improvement of asthma after administration of pioglitazone. Diabetes Care (2002) 25:401. doi: 10.2337/diacare.25.2.401
148. Sandhu MS, Dimov V, Sandhu AK, Walters RW, Wichman T, Casale T. The use of the peroxisome proliferator-activated receptors γ agonist rosiglitazone to treat airway hyperreactivity. Ann Allergy Asthma Immunol (2012) 109:75–7. doi: 10.1016/j.anai.2012.05.001
149. Nobs SP, Kopf M. PPAR-γ in innate and adaptive lung immunity. J Leukocyte Biol (2018) 104:737–41. doi: 10.1002/JLB.3MR0118-034R
150. Belvisi MG, Mitchell JA. Targeting PPAR receptors in the airway for the treatment of inflammatory lung disease. Br J Pharmacol (2009) 158:994–1003. doi: 10.1111/j.1476-5381.2009.00373.x
151. Nobs SP, Natali S, Pohlmeier L, Okreglicka K, Schneider C, Kurrer M, et al. PPARγ in dendritic cells and T cells drives pathogenic type-2 effector responses in lung inflammation. J Exp Med (2017) 214:3015–35. doi: 10.1084/jem.20162069
152. Chen T, Tibbitt CA, Feng X, Stark JM, Rohrbeck L, Rausch L, et al. PPAR-γ promotes type 2 immune responses in allergy and nematode infection. Sci Immunol (2017) 2:eaal5196. doi: 10.1126/sciimmunol.aal5196
153. Anderson JR, Mortimer K, Pang L, Smith KM, Bailey H, Hodgson DB, et al. Evaluation of the PPAR-γ Agonist Pioglitazone in Mild Asthma: A Double-Blind Randomized Controlled Trial. PloS One (2016) 11:e0160257. doi: 10.1371/journal.pone.0160257
154. Richards DB, Bareille P, Lindo EL, Quinn D, Farrow SN. Treatment with a peroxisomal proliferator activated receptor gamma agonist has a modest effect in the allergen challenge model in asthma: A randomised controlled trial. Respir Med (2010) 104:668–74. doi: 10.1016/j.rmed.2009.11.006
155. Pei L, Castrillo A, Tontonoz P. Regulation of macrophage inflammatory gene expression by the orphan nuclear receptor Nur77. Mol Endocrinol (2006) 20:786–94. doi: 10.1210/me.2005-0331
156. Brás A, Albar JP, Leonardo E, González De Buitrago G, Martínez-A C. Ceramide-induced cell death is independent of the Fas/Fas ligand pathway and is prevented by Nur77 overexpression in A20 B cells. Cell Death Differ (2000) 7:262–71. doi: 10.1038/sj.cdd.4400653
157. Li QX, Ke N, Sundaram R, Wong-Staal F. NR4A1, 2, 3 - An orphan nuclear hormone receptor family involved in cell apoptosis and carcinogenesis. Histol Histopathol (2006) 21:533–40. doi: 10.14670/HH-21.533
158. Lin B, Kolluri SK, Lin F, Liu W, Han YH, Cao X, et al. Conversion of Bcl-2 from Protector to Killer by Interaction with Nuclear Orphan Receptor Nur77/TR3. Cell (2004) 116:527–40. doi: 10.1016/S0092-8674(04)00162-X
159. Pearen MA, Ryall JG, Maxwell MA, Ohkura N, Lynch GS, Muscat GEO. The orphan nuclear receptor, NOR-1, is a target of β-adrenergic signaling in skeletal muscle. Endocrinology (2006) 147:5217–27. doi: 10.1210/en.2006-0447
160. Maxwell MA, Cleasby ME, Harding A, Stark A, Cooney GJ, Muscat GEO. Nur77 regulates lipolysis in skeletal muscle cells: Evidence for cross-talk between the β-adrenergic and an orphan nuclear hormone receptor pathway. J Biol Chem (2005) 280:12573–84. doi: 10.1074/jbc.M409580200
161. Chao LC, Zhang Z, Pei L, Saito T, Tontonoz P, Pilch PF. Nur77 coordinately regulates expression of genes linked to glucose metabolism in skeletal muscle. Mol Endocrinol (2007) 21:2152–63. doi: 10.1210/me.2007-0169
162. Kanzleiter T, Schneider T, Walter I, Bolze F, Eickhorst C, Heldmaier G, et al. Evidence for Nr4a1 as a cold-induced effector of brown fat thermogenesis. Physiol Genomics (2005) 24:37–44. doi: 10.1152/physiolgenomics.00204.2005
163. Gilbert F, Morissette M, St-Hilaire M, Paquet B, Rouillard C, Di Paolo T, et al. Nur77 Gene Knockout Alters Dopamine Neuron Biochemical Activity and Dopamine Turnover. Biol Psychiatry (2006) 60:538–47. doi: 10.1016/j.biopsych.2006.04.023
164. Liu X, Wang Y, Lu H, Li J, Yan X, Xiao M, et al. Genome-wide analysis identifies NR4A1 as a key mediator of T cell dysfunction. Nature (2019) 567:525–9. doi: 10.1038/s41586-019-0979-8
165. Kurakula K, Vos M, Logiantara A, Roelofs JJ, Nieuwenhuis MA, Koppelman GH, et al. Nuclear Receptor Nur77 Attenuates Airway Inflammation in Mice by Suppressing NF-κB Activity in Lung Epithelial Cells. J Immunol (2015) 195:1388–98. doi: 10.4049/jimmunol.1401714
166. Jiao Y, Lu Y, Li XY. Farnesoid X receptor: A master regulator of hepatic triglyceride and glucose homeostasis. Acta Pharmacol Sin (2015) 36:44–50. doi: 10.1038/aps.2014.116
167. Mroz MS, Keating N, Ward JB, Sarker R, Amu S, Aviello G, et al. Farnesoid X receptor agonists attenuate colonic epithelial secretory function and prevent experimental diarrhoea in vivo. Gut (2014) 63:808–17. doi: 10.1136/gutjnl-2013-305088
168. Mencarelli A, Renga B, Distrutti E, Fiorucci S. Antiatherosclerotic effect of farnesoid X receptor. Am J Physiol - Heart Circulatory Physiol (2009) 296:H272–81. doi: 10.1152/ajpheart.01075.2008
169. Shaik FB, Prasad DVR, Narala VR. Role of farnesoid X receptor in inflammation and resolution. Inflammation Res (2014) 64:9–20. doi: 10.1007/s00011-014-0780-y
170. Han CY. Update on FXR biology: Promising therapeutic target? Int J Mol Sci (2018) 19:2069. doi: 10.3390/ijms19072069
171. Shaik FB, Panati K, Narasimha VR, Narala VR. Chenodeoxycholic acid attenuates ovalbumin-induced airway inflammation in murine model of asthma by inhibiting the T(H)2 cytokines. Biochem Biophys Res Commun (2015) 463:600–5. doi: 10.1016/j.bbrc.2015.05.104
172. Willart MAM, Van Nimwegen M, Grefhorst A, Hammad H, Moons L, Hoogsteden HC, et al. Ursodeoxycholic acid suppresses eosinophilic airway inflammation by inhibiting the function of dendritic cells through the nuclear farnesoid X receptor. Allergy (2012) 67:1501–10. doi: 10.1111/all.12019
173. Baranowski M. Biological role of liver X receptors. J Physiol Pharmacol (2008) 59(Suppl 7):31–55.
174. Wang B, Tontonoz P. Liver X receptors in lipid signalling and membrane homeostasis. Nat Rev Endocrinol (2018) 14:452–63. doi: 10.1038/s41574-018-0037-x
175. Delvecchio CJ, Bilan P, Radford K, Stephen J, Trigatti BL, Cox G, et al. Liver X Receptor Stimulates Cholesterol Efflux and Inhibits Expression of Proinflammatory Mediators in Human Airway Smooth Muscle Cells. Mol Endocrinol (2007) 21:1324–34. doi: 10.1210/me.2007-0017
176. Shi Y, Xu X, Tan Y, Mao S, Fang S, Gu W. A liver-X-receptor ligand, T0901317, attenuates IgE production and airway remodeling in chronic asthma model of mice. PloS One (2014) 9:92668. doi: 10.1371/journal.pone.0092668
177. Birrell MA, De Alba J, Catley MC, Hardaker E, Wong S, Collins M, et al. Liver X Receptor Agonists Increase Airway Reactivity in a Model of Asthma via Increasing Airway Smooth Muscle Growth. J Immunol (2008) 181:4265–71. doi: 10.4049/jimmunol.181.6.4265
178. Smet M, Van Hoecke L, De Beuckelaer A, Vander Beken S, Naessens T, Vergote K, et al. Cholesterol-sensing liver X receptors stimulate Th2-driven allergic eosinophilic asthma in mice. Immunity Inflammation Dis (2016) 4:350–61. doi: 10.1002/iid3.118
179. Jetten AM. Retinoid-related orphan receptors (RORs): critical roles in development, immunity, circadian rhythm, and cellular metabolism. Nuclear Receptor Signaling (2009) 7:e003. doi: 10.1621/nrs.07003
180. Eftekharian MM, Noroozi R, Sayad A, Sarrafzadeh S, Toghi M, Azimi T, et al. RAR-related orphan receptor A (RORA): A new susceptibility gene for multiple sclerosis. J Neurological Sci (2016) 369:259–62. doi: 10.1016/j.jns.2016.08.045
181. Persson H, Kwon AT, Ramilowski JA, Silberberg G, Söderhäll C, Orsmark-Pietras C, et al. Transcriptome analysis of controlled and therapy-resistant childhood asthma reveals distinct gene expression profiles. J Allergy Clin Immunol (2015) 136:638–48. doi: 10.1016/j.jaci.2015.02.026
182. Cai X, Lin M, Cao S, Liu Y, Lin N. The association of RAR-related orphan receptor A (RORA ) gene polymorphisms with the risk of asthma. Ann Hum Genet (2018) 82:158–64. doi: 10.1111/ahg.12238
183. Ramasamy A, Kuokkanen M, Vedantam S, Gajdos ZK, Couto Alves A, Lyon HN, et al. Genome-Wide Association Studies of Asthma in Population-Based Cohorts Confirm Known and Suggested Loci and Identify an Additional Association near HLA. PloS One (2012) 7:e44008. doi: 10.1371/journal.pone.0044008
184. Acevedo N, Sääf A, Söderhäll C, Melén E, Mandelin J, Pietras CO, et al. Interaction between Retinoid Acid Receptor-Related Orphan Receptor Alpha (RORA) and Neuropeptide S Receptor 1 (NPSR1) in Asthma. PloS One (2013) 8:e60111. doi: 10.1371/journal.pone.0060111
185. McKenzie ANJ, Spits H, Eberl G. Innate Lymphoid Cells in Inflammation and Immunity. Immunity (2014) 41:366–74. doi: 10.1016/J.IMMUNI.2014.09.006
186. Wong SH, Walker JA, Jolin HE, Drynan LF, Hams E, Camelo A, et al. Transcription factor RORα is critical for nuocyte development. Nat Immunol (2012) 13:229–36. doi: 10.1038/ni.2208
187. Halim TYF, MacLaren A, Romanish MT, Gold MJ, McNagny KM, Takei F. Retinoic-Acid-Receptor-Related Orphan Nuclear Receptor Alpha Is Required for Natural Helper Cell Development and Allergic Inflammation. Immunity (2012) 37:463–74. doi: 10.1016/j.immuni.2012.06.012
188. Jaradat M, Stapleton C, Tilley SL, Dixon D, Erikson CJ, McCaskill JG, et al. Modulatory role for retinoid-related orphan receptor α in allergen-induced lung inflammation. Am J Respir Crit Care Med (2006) 174:1299–309. doi: 10.1164/rccm.200510-1672OC
189. Tilley SL, Jaradat M, Stapleton C, Dixon D, Hua X, Erikson CJ, et al. Retinoid-Related Orphan Receptor γ Controls Immunoglobulin Production and Th1/Th2 Cytokine Balance in the Adaptive Immune Response to Allergen. J Immunol (2007) 178:3208–18. doi: 10.4049/jimmunol.178.5.3208
190. Ano S, Morishima Y, Ishii Y, Yoh K, Yageta Y, Ohtsuka S, et al. Transcription Factors GATA-3 and RORγt Are Important for Determining the Phenotype of Allergic Airway Inflammation in a Murine Model of Asthma. J Immunol (2013) 190:1056–65. doi: 10.4049/jimmunol.1202386
191. Whitehead GS, Kang HS, Thomas SY, Medvedev A, Karcz TP, Izumi G, et al. Therapeutic suppression of pulmonary neutrophilia and allergic airway hyperresponsiveness by an RORγt inverse agonist. JCI Insight (2019) 5:e125528. doi: 10.1172/jci.insight.125528
192. Na H, Lim H, Choi G, Kim BK, Kim SH, Chang YS, et al. Concomitant suppression of TH2 and TH17 cell responses in allergic asthma by targeting retinoic acid receptor–related orphan receptor γt. J Allergy Clin Immunol (2018) 141:2061–73.e5. doi: 10.1016/j.jaci.2017.07.050
193. Netting MJ, Middleton PF, Makrides M. Does maternal diet during pregnancy and lactation affect outcomes in offspring? A systematic review of food-based approaches. Nutrition (2014) 30:1225–41. doi: 10.1016/j.nut.2014.02.015
194. Li Q, Baines KJ, Gibson PG, Wood LG. Changes in expression of genes regulating airway inflammation following a high-fat mixed meal in asthmatics. Nutrients (2016) 8:30. doi: 10.3390/nu8010030
195. Wood LG, Garg ML, Gibson PG. A high-fat challenge increases airway inflammation and impairs bronchodilator recovery in asthma. J Allergy Clin Immunol (2011) 127:1133–40. doi: 10.1016/j.jaci.2011.01.036
196. Berthon BS, MacDonald-Wicks LK, Gibson PG, Wood LG. Investigation of the association between dietary intake, disease severity and airway inflammation in asthma. Respirology (2013) 18:447–54. doi: 10.1111/resp.12015
197. Wood LG, Garg ML, Powell H, Gibson PG. Lycopene-rich treatments modify noneosinophilic airway inflammation in asthma: Proof of concept. Free Radical Res (2008) 42:94–102. doi: 10.1080/10715760701767307
198. Romieu I, Barraza-Villarreal A, Escamilla-Núñez C, Texcalac-Sangrador JL, Hernandez-Cadena L, Díaz-Sánchez D, et al. Dietary intake, lung function and airway inflammation in Mexico City school children exposed to air pollutants. Respir Res (2009) 10:122. doi: 10.1186/1465-9921-10-122
199. Allan KM, Prabhu N, Craig LCA, McNeill G, Kirby B, McLay J, et al. Maternal vitamin D and E intakes during pregnancy are associated with asthma in children. Eur Respir J (2015) 45:1027–36. doi: 10.1183/09031936.00102214
200. Gray LEK, O’Hely M, Ranganathan S, Sly PD, Vuillermin P. The maternal diet, gut bacteria, and bacterial metabolites during pregnancy influence offspring asthma. Front Immunol (2017) 8:365. doi: 10.3389/fimmu.2017.00365
201. Baïz N, Just J, Chastang J, Forhan A, De Lauzon-Guillain B, Magnier AM, et al. Maternal diet before and during pregnancy and risk of asthma and allergic rhinitis in children. Allergy Asthma Clin Immunol (2019) 15:40. doi: 10.1186/s13223-019-0353-2
202. Thorburn AN, McKenzie CI, Shen S, Stanley D, MacIa L, Mason LJ, et al. Evidence that asthma is a developmental origin disease influenced by maternal diet and bacterial metabolites. Nat Commun (2015) 6:7320. doi: 10.1038/ncomms8320
203. Zhang Z, Shi L, Pang W, Liu W, Li J, Wang H, et al. Dietary fiber intake regulates intestinal microflora and inhibits ovalbumin-induced allergic airway inflammation in a mouse model. PloS One (2016) 11:e0147778. doi: 10.1371/journal.pone.0147778
204. Anand S, Mande SS. Diet, microbiota and gut-lung connection. Front Microbiol (2018) 9:2147. doi: 10.3389/fmicb.2018.02147
205. Greathouse KL, Bredfeldt T, Everitt JI, Lin K, Berry T, Kannan K, et al. Environmental estrogens differentially engage the histone methyltransferase EZH2 to increase risk of uterine tumorigenesis. Mol Cancer Res (2012) 10:546–57. doi: 10.1158/1541-7786.MCR-11-0605
206. Hong T, Nakagawa T, Pan WJ, Kim MY, Kraus WL, Ikehara T, et al. Isoflavones stimulate estrogen receptor-mediated core histone acetylation. Biochem Biophys Res Commun (2004) 317:259–64. doi: 10.1016/j.bbrc.2004.03.041
207. Jalili M, Pati S, Rath B, Bjørklund G, Singh RB. Effect of Diet and Nutrients on Molecular Mechanism of Gene Expression Mediated by Nuclear Receptor and Epigenetic Modulation. Open Nutraceuticals J (2013) 6:27–34. doi: 10.2174/1876396020130419002
208. Enmark E, Gustafsson J-Å, Ke Gustafsson J-AÊ. Nutrients and environmental factors as regulators of gene expression. Scand J Nutr (2002) 46:13–9. doi: 10.1080/110264802753704082
209. Hassan FU, Rehman MSU, Khan MS, Ali MA, Javed A, Nawaz A, et al. Curcumin as an alternative epigenetic modulator: Mechanism of action and potential effects. Front Genet (2019) 10:514. doi: 10.3389/fgene.2019.00514
210. Delage B, Bairras C, Buaud B, Pallet V, Cassand P. A high-fat diet generates alterations in nuclear receptor expression: Prevention by vitamin A and links with cyclooxygenase-2 and β-catenin. Int J Cancer (2005) 116:839–46. doi: 10.1002/ijc.21108
211. Starling S. Diet reprogrammes glucocorticoid rhythms. Nat Rev Endocrinol (2020) 16:5. doi: 10.1038/s41574-019-0297-0
212. Kohsaka A, Laposky AD, Ramsey KM, Estrada C, Joshu C, Kobayashi Y, et al. High-Fat Diet Disrupts Behavioral and Molecular Circadian Rhythms in Mice. Cell Metab (2007) 6:414–21. doi: 10.1016/j.cmet.2007.09.006
213. Avior Y, Bomze D, Ramon O, Nahmias Y. Flavonoids as dietary regulators of nuclear receptor activity. Food Funct (2013) 4:831–44. doi: 10.1039/c3fo60063g
214. Mai Z, Blackburn GL, Zhou JR. Genistein sensitizes inhibitory effect of tamoxifen on the growth of estrogen receptor-positive and HER2-overexpressing human breast cancer cells. Mol Carcinogenesis (2007) 46:534–42. doi: 10.1002/mc.20300
215. Bougarne N, Paumelle R, Caron S, Hennuyer N, Mansouri R, Gervois P, et al. PPARα blocks glucocorticoid receptor α-mediated transactivation but cooperates with the activated glucocorticoid receptor α for transrepression on NF-κB. Proc Natl Acad Sci USA (2009) 106:7397–402. doi: 10.1073/pnas.0806742106
216. Marciano DP, Chang MR, Corzo CA, Goswami D, Lam VQ, Pascal BD, et al. The Therapeutic Potential of Nuclear Receptor Modulators for Treatment of Metabolic Disorders: PPARγ, RORs, and Rev-erbs. Cell Metab (2014) 19:193–208. doi: 10.1016/j.cmet.2013.12.009
217. Mahajan S, Saini A, Kalra R, Gupta P. Frienemies of infection: A chronic case of host nuclear receptors acting as cohorts or combatants of infection. Crit Rev Microbiol (2014) 42:1–9. doi: 10.3109/1040841X.2014.970122
218. Staels B. Introduction on the ATVB Review Series “Nuclear Receptors in Metabolism and Cardiovascular Disease”. Arterioscler Thromb Vasc Biol (2010) 30:1504–5. doi: 10.1161/ATVBAHA.110.210823
219. Pascual G, Glass CK. Nuclear receptors versus inflammation: mechanisms of transrepression. Trends Endocrinol Metab (2006) 17:321–7. doi: 10.1016/j.tem.2006.08.005
220. Huang W, Glass CK. Nuclear Receptors and Inflammation Control: Molecular Mechanisms and Pathophysiological Relevance. Arteriosclerosis Thrombosis Vasc Biol (2010) 30:1542–9. doi: 10.1161/ATVBAHA.109.191189
221. Ishida A, Ohta N, Koike S, Aoyagi M, Yamakawa M. Overexpression of glucocorticoid receptor-β in severe allergic rhinitis. Auris Nasus Larynx (2010) 37:584–8. doi: 10.1016/j.anl.2009.12.002
222. Moraitis AG, Block T, Nguyen D, Belanoff JK. The role of glucocorticoid receptors in metabolic syndrome and psychiatric illness. J Steroid Biochem Mol Biol (2017) 165:114–20. doi: 10.1016/j.jsbmb.2016.03.023
223. Lee M-J, Fried SK. The glucocorticoid receptor, not the mineralocorticoid receptor, plays the dominant role in adipogenesis and adipokine production in human adipocytes. Int J Obes (2014) 38:1228–33. doi: 10.1038/ijo.2014.6
224. Majer-Łobodzińska A, Adamiec-Mroczek J. Glucocorticoid receptor polymorphism in obesity and glucose homeostasis. Adv Clin Exp medicine : Off Organ Wroclaw Med Univ (2017) 26:143–8. doi: 10.17219/acem/41231
225. Yan Y-X, Dong J, Liu Y-Q, Zhang J, Song M-S, He Y, et al. Association of suboptimal health status with psychosocial stress, plasma cortisol and mRNA expression of glucocorticoid receptor α / β in lymphocyte. Stress (2015) 18:29–34. doi: 10.3109/10253890.2014.999233
226. Barik J, Marti F, Morel C, Fernandez SP, Lanteri C, Godeheu G, et al. Chronic Stress Triggers Social Aversion via Glucocorticoid Receptor in Dopaminoceptive Neurons. Science (2013) 339:332–5. doi: 10.1126/science.1226767
227. Kadmiel M, Cidlowski JA. Glucocorticoid receptor signaling in health and disease. Trends Pharmacol Sci (2013) 34:518–30. doi: 10.1016/j.tips.2013.07.003
228. Deobagkar-Lele M, Chacko SK, Victor ES, Kadthur JC, Nandi D. Interferon-γ- and glucocorticoid-mediated pathways synergize to enhance death of CD4(+) CD8(+) thymocytes during Salmonella enterica serovar Typhimurium infection. Immunology (2013) 138:307–21. doi: 10.1111/imm.12047
229. Stolberg VR, McCubbrey AL, Freeman CM, Brown JP, Crudgington SW, Taitano SH, et al. Glucocorticoid-Augmented Efferocytosis Inhibits Pulmonary Pneumococcal Clearance in Mice by Reducing Alveolar Macrophage Bactericidal Function. J Immunol (2015) 195:174–84. doi: 10.4049/jimmunol.1402217
230. Kang HS, Okamoto K, Takeda Y, Beak JY, Gerrish K, Bortner CD, et al. Transcriptional profiling reveals a role for RORalpha in regulating gene expression in obesity-associated inflammation and hepatic steatosis. Physiol Genomics (2011) 43:818–28. doi: 10.1152/physiolgenomics.00206.2010
231. Liu Y, Chen Y, Zhang J, Liu Y, Zhang Y, Su Z. Retinoic acid receptor-related orphan receptor alpha stimulates adipose tissue inflammation by modulating endoplasmic reticulum stress. J Biol Chem (2017) 292:13959–69. doi: 10.1074/jbc.M117.782391
232. Saini A, Mahajan S, Ahuja N, Bhagyaraj E, Kalra R, Janmeja AK, et al. An Accord of Nuclear Receptor Expression in M. tuberculosis Infected Macrophages and Dendritic Cells. Sci Rep (2018) 8:2296. doi: 10.1038/s41598-018-20769-4
233. Lo BC, Gold MJ, Hughes MR, Antignano F, Valdez Y, Zaph C, et al. The orphan nuclear receptor RORα and group 3 innate lymphoid cells drive fibrosis in a mouse model of Crohn’s disease. Sci Immunol (2016) 1:eaaf8864. doi: 10.1126/sciimmunol.aaf8864
234. Hennings JM, Uhr M, Klengel T, Weber P, Pütz B, Touma C, et al. RNA expression profiling in depressed patients suggests retinoid-related orphan receptor alpha as a biomarker for antidepressant response. Trans Psychiatry (2015) 5:e538. doi: 10.1038/tp.2015.9
235. Shi Y, Cao J, Gao J, Zheng L, Goodwin A, An CH, et al. Retinoic acid-related orphan receptor-α is induced in the setting of DNA damage and promotes pulmonary emphysema. Am J Respir Crit Care Med (2012) 186:412–9. doi: 10.1164/rccm.201111-2023OC
236. Liu Z-Q, Li M-G, Geng X-R, Liu J, Yang G, Qiu S-Q, et al. Vitamin D regulates immunoglobulin mucin domain molecule-4 expression in dendritic cells. Clin Exp Allergy (2017) 47:656–64. doi: 10.1111/cea.12894
237. Christensen JM, Cheng J, Earls P, Gunton J, Sewell W, Sacks R, et al. Vitamin D pathway regulatory genes encoding 1α-hydroxylase and 24-hydroxylase are dysregulated in sinonasal tissue during chronic rhinosinusitis. Int Forum Allergy Rhinol (2017) 7:169–76. doi: 10.1002/alr.21852
238. Slusher AL, McAllister MJ, Huang C-J. A therapeutic role for vitamin D on obesity-associated inflammation and weight-loss intervention. Inflammation Res (2015) 64:565–75. doi: 10.1007/s00011-015-0847-4
239. Wu S, Sun J. Vitamin D, vitamin D receptor, and macroautophagy in inflammation and infection. Discovery Med (2011) 11:325–35.
240. Wu M, Yue M, Huang P, Zhang Y, Xie C, Yu R, et al. Vitamin D level and vitamin D receptor genetic variations contribute to HCV infection susceptibility and chronicity in a Chinese population. Infect Genet Evol (2016) 41:146–52. doi: 10.1016/j.meegid.2016.03.032
241. Salamon H, Bruiners N, Lakehal K, Shi L, Ravi J, Yamaguchi KD, et al. Cutting Edge: Vitamin D Regulates Lipid Metabolism in Mycobacterium tuberculosis Infection. J Immunol (2014) 193:30–4. doi: 10.4049/jimmunol.1400736
242. Cuomo A, Giordano N, Goracci A, Fagiolini A. Depression and Vitamin D Deficiency: Causality, Assessment, and Clinical Practice Implications. Neuropsychiatry (London) (2017) 7:606–14. doi: 10.4172/Neuropsychiatry.1000255
243. Perez-Sieira S, Martinez G, Porteiro B, Lopez M, Vidal A, Nogueiras R, et al. Female Nur77-Deficient Mice Show Increased Susceptibility to Diet-Induced Obesity. PloS One (2013) 8:e53836. doi: 10.1371/journal.pone.0053836
244. Egarnes B, Blanchet M-R, Gosselin J. Treatment with the NR4A1 agonist cytosporone B controls influenza virus infection and improves pulmonary function in infected mice. PloS One (2017) 12:e0186639. doi: 10.1371/journal.pone.0186639
245. Janmaat VT, Van De Winkel A, Peppelenbosch MP, Spaander MCW, Uitterlinden AG, Pourfarzad F, et al. Vitamin D Receptor Polymorphisms Are Associated with Reduced Esophageal Vitamin D Receptor Expression and Reduced Esophageal Adenocarcinoma Risk. Mol Med (Cambridge Mass) (2015) 21:346–54. doi: 10.2119/molmed.2012.00336
246. De Gottardi A, Dumonceau J-M, Bruttin F, Vonlaufen A, Morard I, Spahr L, et al. Expression of the bile acid receptor FXR in Barrett’s esophagus and enhancement of apoptosis by guggulsterone in vitro. Mol Cancer (2006) 5:48. doi: 10.1186/1476-4598-5-48
247. Chen W-G, Zheng J-X, Xu X, Hu Y-M, Ma Y-M. Hippocampal FXR plays a role in the pathogenesis of depression: A preliminary study based on lentiviral gene modulation. Psychiatry Res (2018) 264:374–9. doi: 10.1016/j.psychres.2018.04.025
248. Chen B, You W-J, Xue S, Qin H, Zhao X-J, Zhang M, et al. Overexpression of farnesoid X receptor in small airways contributes to epithelial to mesenchymal transition and COX-2 expression in chronic obstructive pulmonary disease. J thoracic Dis (2016) 8:3063–74. doi: 10.21037/jtd.2016.11.08
249. Grenningloh R, Gho A, di Lucia P, Klaus M, Bollag W, Ho I-C, et al. Cutting Edge: Inhibition of the retinoid X receptor (RXR) blocks T helper 2 differentiation and prevents allergic lung inflammation. J Immunol (Baltimore Md : 1950) (2006) 176:5161–6. doi: 10.4049/jimmunol.176.9.5161
250. Berry DC, Noy N. All-trans-retinoic acid represses obesity and insulin resistance by activating both peroxisome proliferation-activated receptor beta/delta and retinoic acid receptor. Mol Cell Biol (2009) 29:3286–96. doi: 10.1128/MCB.01742-08
251. Pinaire JA, Reifel-Miller A. Therapeutic potential of retinoid x receptor modulators for the treatment of the metabolic syndrome. PPAR Res (2007) 2007:94156. doi: 10.1155/2007/94156
252. Nunez V, Alameda D, Rico D, Mota R, Gonzalo P, Cedenilla M, et al. Retinoid X receptor controls innate inflammatory responses through the up-regulation of chemokine expression. Proc Natl Acad Sci (2010) 107:10626–31. doi: 10.1073/pnas.0913545107
253. Angulo A, Chandraratna RA, LeBlanc JF, Ghazal P. Ligand induction of retinoic acid receptors alters an acute infection by murine cytomegalovirus. J Virol (1998) 72:4589–600. doi: 10.1128/JVI.72.6.4589-4600.1998
254. Bremner JD, McCaffery P. The neurobiology of retinoic acid in affective disorders. Prog Neuropsychopharmacol Biol Psychiatry (2008) 32:315–31. doi: 10.1016/j.pnpbp.2007.07.001
255. Son HL, Park HR, Park YJ, Kim SW. Effect of Retinoic Acid in a Mouse Model of Allergic Rhinitis. Allergy asthma Immunol Res (2015) 7:590–8. doi: 10.4168/aair.2015.7.6.590
256. Gundra UM, Poles J, Loke P. Role of retinoic acid in diet induced obesity. J Immunol (2016) 196:124.53.
257. Guan B, Li H, Yang Z, Hoque A, Xu X. Inhibition of farnesoid X receptor controls esophageal cancer cell growth in vitro and in nude mouse xenografts. Cancer (2013) 119:1321–9. doi: 10.1002/cncr.27910
258. Jeyakumar SM, Vajreswari A. Vitamin A as a key regulator of obesity & its associated disorders: Evidences from an obese rat model. Indian J Med Res (2015) 141:275–84. doi: 10.4103/0971-5916.156554
259. Wang X-C, Zhao G-Q, Lin J, Li C, Jiang N, Zhang J. Effects of retinoic acid receptor-γ on the Aspergillus fumigatus induced innate immunity response in human corneal epithelial cells. Int J Ophthalmol (2016) 9:1713–8. doi: 10.18240/ijo.2016.12.02
260. Recio JA, Martínez de la Mata J, Martín-Nieto J, Aranda A. Retinoic acid stimulates HIV-1 transcription in human neuroblastoma SH-SY5Y cells. FEBS Lett (2000) 469:118–22. doi: 10.1016/s0014-5793(00)01249-7
261. Chen X-N, Meng Q-Y, Bao A-M, Swaab DF, Wang G-H, Zhou J-N. The Involvement of Retinoic Acid Receptor-α in Corticotropin-Releasing Hormone Gene Expression and Affective Disorders. Biol Psychiatry (2009) 66:832–9. doi: 10.1016/j.biopsych.2009.05.031
262. O’Reilly KC, Shumake J, Gonzalez-Lima F, Lane MA, Bailey SJ. Chronic Administration of 13-Cis-Retinoic Acid Increases Depression-Related Behavior in Mice. Neuropsychopharmacology (2006) 31:1919–27. doi: 10.1038/sj.npp.1300998
263. Chiang MY, Misner D, Kempermann G, Schikorski T, Giguère V, Sucov HM, et al. An essential role for retinoid receptors RARbeta and RXRgamma in long-term potentiation and depression. Neuron (1998) 21:1353–61. doi: 10.1016/s0896-6273(00)80654-6
264. Wang J, Liu W, Marion C, Singh R, Andrews N, Lee CG, et al. Regulation of Retinoic Acid Receptor Beta by Interleukin-15 in the Lung during Cigarette Smoking and Influenza Virus Infection. Am J Respir Cell Mol Biol (2015) 53:822–33. doi: 10.1165/rcmb.2014-0448OC
265. Hind M, Stinchcombe S. Palovarotene, a novel retinoic acid receptor gamma agonist for the treatment of emphysema. Curr Opin Invest Drugs (London England : 2000) (2009) 10:1243–50.
266. Kurie JM, Lotan R, Lee JJ, Lee JS, Morice RC, Liu DD, et al. Treatment of Former Smokers With 9-cis-Retinoic Acid Reverses Loss of Retinoic Acid Receptor- Expression in the Bronchial Epithelium: Results From a Randomized Placebo-Controlled Trial. JNCI J Natl Cancer Institute (2003) 95:206–14. doi: 10.1093/jnci/95.3.206
267. Dib L, Bugge A, Collins S. LXRα fuels fatty acid-stimulated oxygen consumption in white adipocytes. J Lipid Res (2014) 55:247–57. doi: 10.1194/jlr.M043422
268. De Magalhaes Filho CD, Downes M, Evans RM. Farnesoid X Receptor an Emerging Target to Combat Obesity. Digest Dis (2017) 35:185–90. doi: 10.1159/000450909
269. Wang S, Moustaid-Moussa N, Chen L, Mo H, Shastri A, Su R, et al. Novel insights of dietary polyphenols and obesity. J Nutr Biochem (2014) 25:1–18. doi: 10.1016/j.jnutbio.2013.09.001
270. Joseph SB, Bradley MN, Castrillo A, Bruhn KW, Mak PA, Pei L, et al. LXR-Dependent Gene Expression Is Important for Macrophage Survival and the Innate Immune Response. Cell (2004) 119:299–309. doi: 10.1016/j.cell.2004.09.032
271. Wang YY, Ryg U, Dahle MK, Steffensen KR, Thiemermann C, Chaudry IH, et al. Liver X Receptor Protects against Liver Injury in Sepsis Caused by Rodent Cecal Ligation and Puncture. Surg Infect (2011) 12:283–9. doi: 10.1089/sur.2010.066
272. Peng Z, Deng B, Jia J, Hou W, Hu S, Deng J, et al. Liver X receptor β in the hippocampus: A potential novel target for the treatment of major depressive disorder? Neuropharmacology (2018) 135:514–28. doi: 10.1016/j.neuropharm.2018.04.014
273. Kratzer A, Salys J, Zamora M, Taraseviciene-Stewart L. The nuclear liver X receptor and its role in smoke exposed rat lungs. Eur Respir J (2011) 38:p428.
274. Higham A, Lea S, Plumb J, Maschera B, Simpson K, Ray D, et al. The role of the liver X receptor in chronic obstructive pulmonary disease. Respir Res (2013) 14:106. doi: 10.1186/1465-9921-14-106
275. Stienstra R, Duval C, Müller M, Kersten S. PPARs, obesity, and inflammation. PPAR Res (2007) 2007. doi: 10.1155/2007/95974
276. Ulrich-Lai YM, Ryan KK. PPARγ and stress: Implications for aging. Exp Gerontol (2013) 48:671–6. doi: 10.1016/j.exger.2012.08.011
277. Guo M, Li C, Lei Y, Xu S, Zhao D, Lu XY. Role of the adipose PPARÎ 3-adiponectin axis in susceptibility to stress and depression/anxiety-related behaviors. Mol Psychiatry (2017) 22:1056–68. doi: 10.1038/mp.2016.225
278. Morissette MC, Shen P, Thayaparan D, Stämpfli MR. Impacts of peroxisome proliferatoractivated receptor-γ activation on cigarette smoke-induced exacerbated response to bacteria. Eur Respir J (2015) 45:191–200. doi: 10.1183/09031936.00004314
279. Fang S, Suh JM, Reilly SM, Yu E, Osborn O, Lackey D, et al. Intestinal FXR agonism promotes adipose tissue browning and reduces obesity and insulin resistance. Nat Med (2015) 21:159–65. doi: 10.1038/nm.3760
280. Wang L, Yin Y, Hou G, Kang J, Wang Q. Peroxisome proliferator-activated receptor (PPARγ) plays a protective role in cigarette smoking-induced inflammation via ampactivated protein kinase (AMPK) signaling. Med Sci Monit (2018) 24:5168–77. doi: 10.12659/MSM.909285
281. Huang S, Zhu B, Cheon IS, Goplen NP, Jiang L, Zhang R, et al. PPAR-γ in Macrophages Limits Pulmonary Inflammation and Promotes Host Recovery following Respiratory Viral Infection. J Virol (2019) 93:e00030–19. doi: 10.1128/jvi.00030-19
282. Reddy AT, Lakshmi SP, Reddy RC. PPAR γ in Bacterial Infections: A Friend or Foe? PPAR Res (2016) 2016:7963540. doi: 10.1155/2016/7963540
283. Ma H, Patti ME. Bile acids, obesity, and the metabolic syndrome. Best Pract Res Clin Gastroenterol (2014) 28:573–83. doi: 10.1016/j.bpg.2014.07.004
284. Tremblay S, Romain G, Roux M, Chen X-L, Brown K, Gibson DL, et al. Bile Acid Administration Elicits an Intestinal Antimicrobial Program and Reduces the Bacterial Burden in Two Mouse Models of Enteric Infection. Infect Immun (2017) 85:e00942–16. doi: 10.1128/IAI.00942-16
285. Honke N, Shaabani N, Hardt C, Krings C, Häussinger D, Lang PA, et al. Farnesoid X Receptor in Mice Prevents Severe Liver Immunopathology During Lymphocytic Choriomeningitis Virus Infection. Cell Physiol Biochem (2017) 41:323–38. doi: 10.1159/000456168
286. Inagaki T, Moschetta A, Lee Y-K, Peng L, Zhao G, Downes M, et al. Regulation of antibacterial defense in the small intestine by the nuclear bile acid receptor. Proc Natl Acad Sci (2006) 103:3920–5. doi: 10.1073/pnas.0509592103
Keywords: asthma, comorbidities, glucocorticoids, diet, nuclear receptors
Citation: Tiwari D and Gupta P (2021) Nuclear Receptors in Asthma: Empowering Classical Molecules Against a Contemporary Ailment. Front. Immunol. 11:594433. doi: 10.3389/fimmu.2020.594433
Received: 13 August 2020; Accepted: 09 December 2020;
Published: 26 January 2021.
Edited by:
Junji Yodoi, Kyoto University, JapanReviewed by:
Hiroshi Masutani, Tenri Health Care University, JapanAtsuhito Nakao, University of Yamanashi, Japan
Copyright © 2021 Tiwari and Gupta. This is an open-access article distributed under the terms of the Creative Commons Attribution License (CC BY). The use, distribution or reproduction in other forums is permitted, provided the original author(s) and the copyright owner(s) are credited and that the original publication in this journal is cited, in accordance with accepted academic practice. No use, distribution or reproduction is permitted which does not comply with these terms.
*Correspondence: Pawan Gupta, cGF3YW5AaW10ZWNoLnJlcy5pbg==