- 1Department of Epidemiology and Biostatistics, School of Public Health, Anhui Medical University, Hefei, China
- 2The Key Laboratory of Major Autoimmune Diseases of Anhui Province, Anhui Institute of Innovative Drugs, School of Pharmacy, Anhui Medical University, Hefei, China
- 3The Key Laboratory of Anti-inflammatory and Immune Medicines, Ministry of Education, Hefei, China
Acid-sensing ion channel 1a (ASIC1a) is a member of the extracellular H+-activated cation channel family. Emerging evidence has suggested that ASIC1a plays a crucial role in the pathogenesis of rheumatoid arthritis (RA). Specifically, ASIC1a could promote inflammation, synovial hyperplasia, articular cartilage, and bone destruction; these lead to the progression of RA, a chronic autoimmune disease characterized by chronic synovial inflammation and extra-articular lesions. In this review, we provided a brief overview of the molecular properties of ASIC1a, including the basic biological characteristics, tissue and cell distribution, channel blocker, and factors influencing the expression and function, and focused on the potential therapeutic targets of ASIC1a in RA and possible mechanisms of blocking ASIC1a to improve RA symptoms, such as regulation of apoptosis, autophagy, pyroptosis, and necroptosis of articular cartilage, and synovial inflammation and invasion of fibroblast-like cells in synovial tissue.
Introduction
As a chronic systemic autoimmune disease, the main pathological characteristics of rheumatoid arthritis (RA) include synovial cell proliferation, multiple inflammatory cell infiltration, pannus formation, and cartilage and bone tissue destruction, which thereby eventually lead to joint deformity and loss of function (1). Epidemiological data show that RA affects approximately 1% of the world’s population (2). Numerous studies have demonstrated that synovial inflammation and extracellular acidification play an important role in the occurrence and development of the RA-mediated destruction of articular cartilage and bone (3, 4). Currently, the most commonly used treatment for RA is the control of synovitis rather than its root causes (5). However, the use of long-term medication reportedly cannot cure arthritis and is usually accompanied by serious side effects (6). Therefore, the key approach in preventing the progression of RA is to elucidate the pathogenesis of synovial inflammation and destruction of articular cartilage and find new targets that would control or be used to control the development of RA.
Acid-sensitive ion channel 1a (ASIC1a) is a member of the degenerin/epithelial sodium channel protein superfamily that is transiently activated by extracellular H+, which plays a critical role in a variety of physiological and pathological processes, including RA (7). It has been shown that extracellular acidification is a common phenomenon that plays an important role in the physiological and pathological processes related to inflammation, including early wound healing, infectious diseases, bone remodeling, and tumorigenesis (8, 9). During inflammation, the drop in pH is a result of infiltration and activation of inflammatory cells in the tissue, which leads to increased energy and oxygen demand, accelerated glucose consumption via glycolysis and thus increased lactic acid secretion (10, 11). In a study, decreased pH was detected in the synovial fluid of RA patients (12–14) and adjuvant arthritis (AA) rats (4), which is an animal model widely used to explore the pathophysiological mechanism of RA. Our previous studies have demonstrated that ASIC1a is involved in the injury of articular chondrocytes that is caused by increased intracellular calcium ([Ca2+]i) induced by extracellular acidification; this can be significantly attenuated by the use of amiloride and the ASIC1a-specific blocker psalmotoxin 1 (PCTX-1) (15–17). Recently, it has been indicated that ASIC1a is highly expressed in RA synovial tissues and RA synovial fibroblasts (RASF); it also induces synovial inflammation and invasion, which are downregulated by ASIC1a-RNAi and PCTX-1 while they are increased by the overexpression of ASIC1a (18, 19). Thus, ASIC1a may be a potential therapeutic target for RA.
In the present review, we have provided a brief overview of the molecular properties of ASIC1a and discussed the therapeutic potential of ASIC1a and the possible mechanisms of blocking ASIC1a in articular chondrocytes and synovial fibroblasts to improve the disease symptoms of RA.
Basic Biological Characteristics of ASIC1a
ASICs are a class of extracellular H+-activated cation channels, also known as H+ non-voltage-gated cation channels, and belong to the superfamily of epithelial sodium channels (ENaC)/degradable proteins (DEG) (20). To date, seven ASIC subunits (ASIC1a, ASIC1b1, ASIC1b2, ASIC2a, ASIC2b, ASIC3, and ASIC4), which are encoded by four genes (ACCN1, ACCN2, ACCN3, and ACCN4), have been identified (21). ASICs, as acid receptors on the cell membrane, transmit the low-pH signal of the extracellular microenvironment to the cell such that the downstream signaling pathway is activated that would thereby cause a series of physiological and pathological changes (22). Compared to other ASIC subunits, ASIC1a not only has permeability to Na+ but also mediates the influx of extracellular Ca2+ (23). As a crucial secondary messenger, Ca2+ plays a pivotal role in the physiological and pathological processes of cells, including in RA (24). Increasing evidence indicates that ASIC1a contributes to acid-induced injury by increasing intracellular Ca2+ in rat articular chondrocytes and RASF.
Structure of ASIC1a
ASIC1a is composed of more than 500 amino acids, including two hydrophobic transmembrane domains (TM1 and TM2) and one large cysteine-rich extracellular ring (25). Its N- and C-terminals are both located in the cytoplasm (26). A crystal structure analysis has revealed that ASICs exist as trimers and that three subunits are necessary for the formation of functional channels (27).
Tissue and Cell Distribution of ASIC1a
ASIC1a is mainly distributed in the central cerebral cortex, hippocampus, cerebellum, pineal gland, amygdala, and spinal cord (28). It has been reported that ASIC1a is also expressed in isolated human monocytes and differentiated osteoclasts and is the most abundant in human chondrocytes (29). In a previous study, we found that ASIC1a, ASIC2a, and ASIC3 were expressed in the articular chondrocytes of rats with AA, and that the expression levels of ASIC1awere significantly higher than those of other subunits (4). More recently, it has been reported that the expression of ASIC1a is significantly increased in human RA synovial tissues, primary human RASF, and the ankle synovium of AA rats (18, 19). Table 1 summarizes the tissue and cell distribution of ASIC1a.
Channel Blocker of ASIC1a
Amiloride is a potassium-sparing diuretic that regulates K+ and Na+ balance in cells. The inhibition of the ENaCs of renal tubular cells is a classic therapeutic effect of amiloride (59). There is a general consensus that amiloride also blocks ASICs, which are members of the ENaC/DEG superfamily (60). As a non-selective ASIC blocker, amiloride is one of the main pharmacological tools that is used to study the function of ASICs, including ASIC1a (61). It has been shown that amiloride and its analogs inhibit ASIC1a currents expressed in Chinese hamster ovary cells and cortical neurons (62). Among the analogs, benzamil has been reported to be the most potent ASIC1a inhibitor (62).
A-317567, a nonspecific small molecule inhibitor of ASICs, is structurally unrelated to amiloride (60, 63). A previous study has indicated that A-317567 has the potential of treating ischemic stroke due to its potent inhibition of ASIC1a-like current (60), suggesting that A-317567 has promising therapeutic potential for the treatment of ASIC1a-related diseases.
PCTX1, a peptide purified from the venom of the southern spider tarantula Psalmopoeus cambridgei, can specifically and strongly inhibit the current of ASIC1a (64, 65). Moreover, studies have suggested that PCTX1 can block ASIC1a in articular chondrocytes and RASF in vitro (4, 18, 19, 66).
Non-steroidal anti-inflammatory drugs (NSAIDs) have also been reported to inhibit ASIC1a or ASIC1a-likecurrents. For example, an in vitro study showed that aspirin rapidly and reversibly inhibited 83.7% of the peak ASIC current in rat cortical neurons (67). Another study has demonstrated that both ibuprofen and flurbiprofen can inhibit ASIC1a current (68), thereby indicating that the mechanism of NSAIDs in improving RA symptoms may be related to ASIC1a blockade.
A common mechanism of many local anesthetics is to block the voltage-gated Na+ channel (69). It has been shown that lidocaine and tetracaine can reversibly inhibit ASIC1a current (60, 70); these drugs maybe potential candidates for the treatment of ASIC1a-related diseases.
Small interfering RNA (siRNA) is a powerful tool for functional genomics and gene therapy owing to its advantages of high efficiency, specificity, and easy operation (7). In a previous study, we designed and synthesized ASIC1a-specific siRNA and transiently transfected it into rat articular chondrocytes (72). ASIC1a-siRNA could be successfully transfected into rat articular chondrocytes; after transfection, the chondrocytes reduced the expression of ASIC1a mRNA and protein, suggesting that siRNA interference technology could successfully construct the ASIC1a gene silencing cell model.
Factors Influencing the Expression and Function of ASIC1a
As a membrane proton receptor, the number of ASIC1a present on the cell surface determines its physiological and pathological functions, and this number partially depends on protein synthesis, degradation, and membrane trafficking processes. Recently, several studies have shown that various factors affect these processes. Wang et al. summarized the factors regulating ASIC1a expression and activity in various conditions, including chemical regulation, metal ions, polypeptide toxins and small-molecule inhibitors, protein interactions, drugs, receptors (73). More recently, we further elucidated the major factors and underlying molecular mechanisms affecting ASIC1a protein expression and membrane trafficking (74). Specifically, acidosis, hypoxia, inflammatory cytokines, neurotrophins, hormones drugs, microRNAs, effector proteins, and chemicals have been reported to govern ASIC1a protein synthesis, degradation, and dynamic trafficking (74).
Potential Therapeutic Targets of ASIC1a in Rheumatoid Arthritis
Overexpression of ASIC1a in Rheumatoid Arthritis
Extracellular acidification, which promotes the defense of inflammatory cells against pathogens by regulating migration and phagocytosis, is a condition commonly associated with a variety of physiological and pathological situations (75). It has been shown that extracellular acidification is involved in the pathogenesis and development of RA (76). The pH of synovial fluid in RA patients may fall below 6.0 during active RA (12, 13, 77). Moreover, the acidification of synovial fluid has been associated with radiological joint destruction in patients with RA (14). Based on the above-mentioned studies and the fact that chondrocytes, the only cell type present in articular cartilage, are important in the pathogenesis of arthritis and are profoundly affected by local pH (78), acid-stimulated articular chondrocytes were selected as a cell model to examine the pathogenesis of RA in vitro (4, 66, 72, 79, 80). In previous studies, we have demonstrated that ASIC1a is expressed in rat articular chondrocytes and is increased in acid-induced chondrocytes in vitro (16, 81). There is growing evidence that activated RASF, a critical component of the synovial tissue to investigate the mechanism of synovial inflammation, plays a crucial role in the pathogenesis of joint destruction and RA (82). Moreover, membrane ASIC1a has been reported to be highly expressed in the RASF of three donors compared to the normal synovial fluid (NSF) of a donor (18).
Rat AA is an experimental animal model of polyarthritis and is induced by using complete Freund’s adjuvant, which is widely used as a tool to explore the pathophysiology of RA (83). In a study, a reduction in pH was detected in the synovial fluid of AA rats, and ASIC1a was upregulated in AA rat articular chondrocytes in vivo (4). Further studies have indicated that the expression level of ASIC1a is increased in the synovial tissues of AA rats than in normal rats, suggesting that ASIC1a may be involved in the pathological process of RA (18, 19).
Consistent with the results of studies wherein animal experiments were conducted, markedly increased expression level of ASIC1a in human RA synovial tissues with reference to those in normal synovial tissue was observed (18, 19).
Blocking ASIC1a to Relieve Rheumatoid Arthritis Symptoms
Articular cartilage is a connective tissue located at the ends of long bones, which consists of chondrocytes and extracellular matrix (ECM) (84). Chondrocytes account for 3% of the volume of cartilage tissue and play an important role in the formation, maintenance of normal metabolism, and repair of articular cartilage (85). The ECM is mainly composed of tissue fluid, type II collagen (COII), and proteoglycan (PG) (85). The interaction between chondrocytes and the extracellular matrix maintains the normal physiological characteristics of articular cartilage (85). The degeneration of articular cartilage and the destruction of COII and PG are the most evident symptoms among arthritis-related diseases (86). Furthermore, it has been reported that AA rats treated with the ASIC1a inhibitor amiloride improved prognoses in terms of pathological articular changes (including hyperplasia of the synovium, thickening of the lining layer, formation of pannus, infiltration of a variety of inflammatory cells), and upregulation of articular cartilage matrix COII and PG (87). Moreover, the HE staining and toluidine blue staining results of pertinent cells of AA rats that received ASIC1a-specific inhibitor PCTX-1 joint injection showed that synovial invasion and cartilage destruction were ameliorated, the swelling of joint was relieved, and arthritic severity was significantly alleviated compared to those in untreated AA rats (18, 19); this indicates that ASIC1a may be a master regulator of synovial invasion and joint inflammation.
Possible Mechanism of Blocking ASIC1a to Improve Rheumatoid Arthritis Symptoms
ASIC1a is expressed in human and rat articular cartilage and synovium; it is also upregulated during acid induction and inflammatory state, which are the two main initiating factors in the occurrence and development of RA (4, 18, 19). In this section, we explore the mechanism by which ASIC1a improves RA symptoms and leads to the alleviation of the destruction of articular cartilage (Figure 1) and synovial inflammation and invasion (Figure 2).
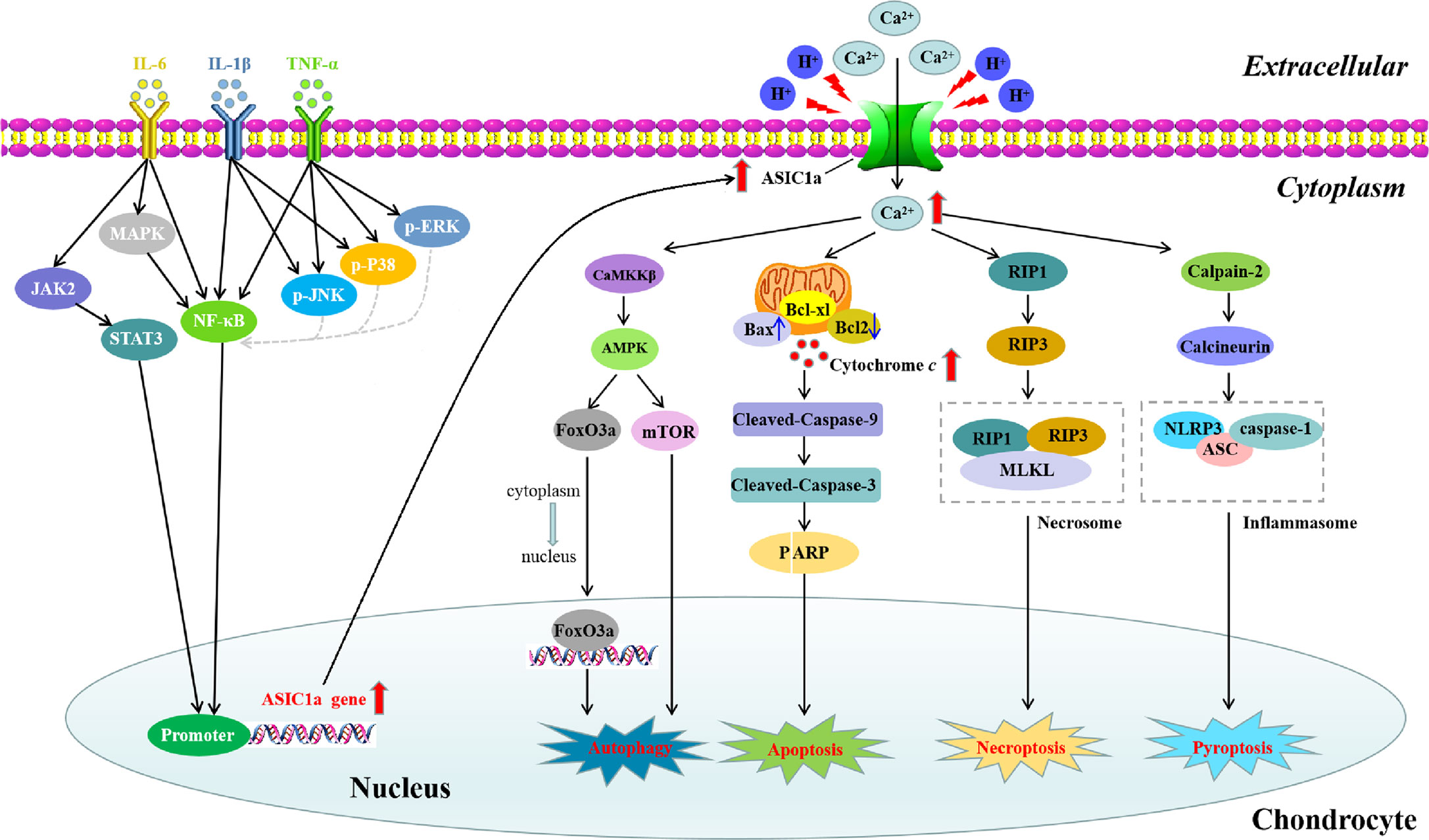
Figure 1 Signaling pathways involved in IL-6-, IL-1β-, and TNF-α-induced ASIC1a expression and the possible molecular mechanisms of ASC1a-mediated apoptosis, autophagy, pyroptosis, and necroptosis induced by extracellular acidification in articular chondrocytes. IL-6, IL-1β, and TNF-α activate STAT3 or/and NF-κB signaling pathways by binding to their respective receptors and lead to the upregulation of ASIC1a expression. Extracellular acidification activates ASIC1a and increases intracellular Ca2+ influx, which ultimately lead to the apoptosis, autophagy, pyroptosis, and necroptosis of articular chondrocytes.
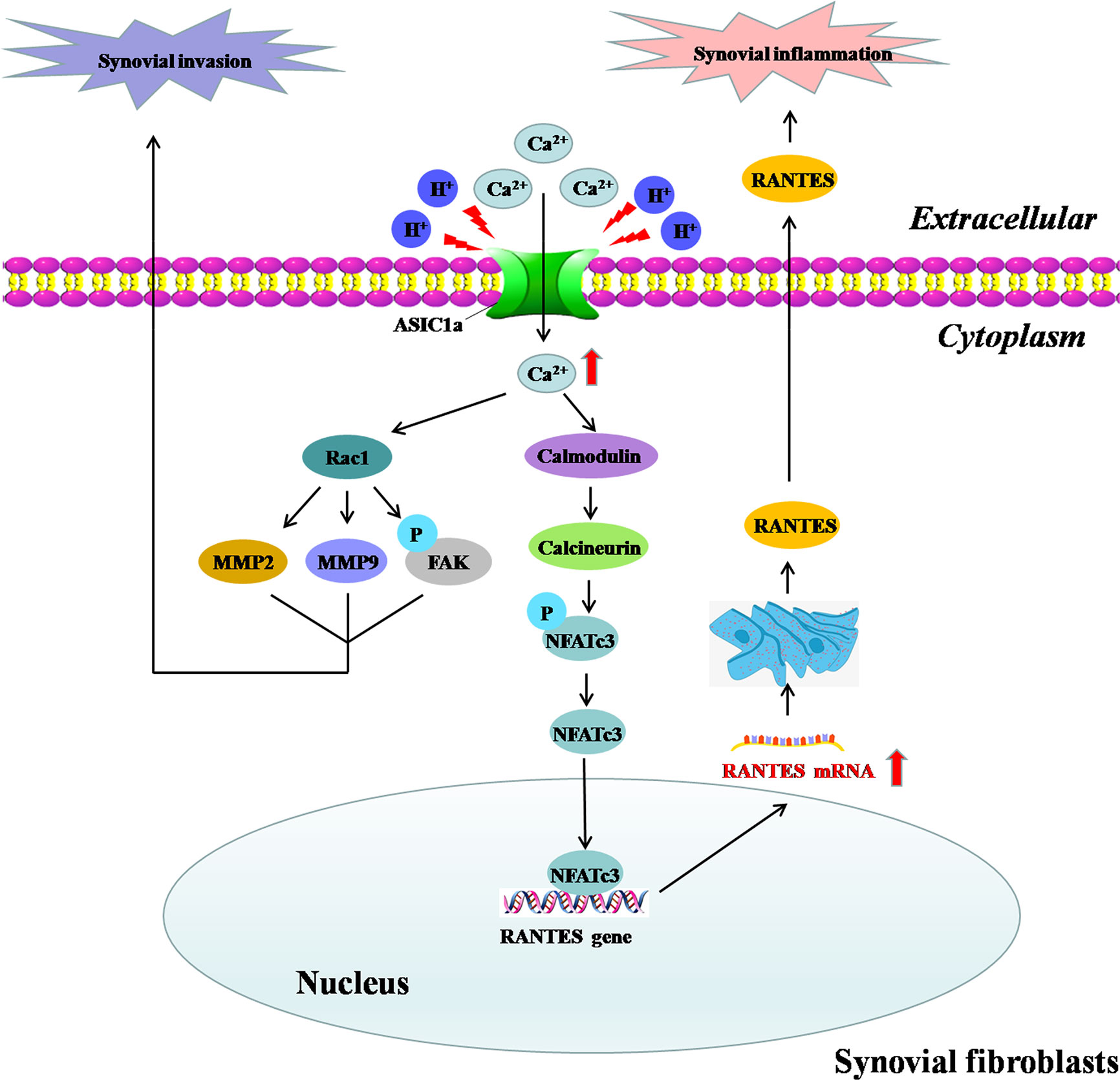
Figure 2 Molecular mechanism of ASIC1a-mediated synovial inflammation and invasion in RASF. Extracellular acidification activates ASIC1a and increases intracellular Ca2+ influx to mediate the nuclear translocation of NFATc3,which binds to the RANTES promoter to directly regulate RANTES transcription and enhance its expression in RASF; this is involved in the induction of synovial inflammation. On the other hand, ASIC1a may partially mediate the acid-induced migration and invasion of RASF through [Ca2+]i-Rac1 signaling, thereby contributing to the synovial invasive destruction of cartilage.
ASIC1a Is Involved in the Regulation of Apoptosis in Articular Cartilage in RA
Apoptosis, which is an autonomic ordered programmed cell death, plays a significant role in normal homeostasis, physiologic cell removal, and inflammatory joint diseases occurring in RA (88). Current evidence indicates that the early and late stages of apoptosis of primary human chondrocytes contribute to the joint damage observed in the pathogenesis of RA (89). In a previous study, we showed that more than half of the chondrocytes underwent apoptosis after extracellular acidification (15). Treatment with amiloride in acid-induced chondrocytes resulted in a dose-dependent decline in apoptosis (15). More specifically, amiloride partly restored mitochondrial membrane potential by regulating the mRNA expression of apoptosis-related Bcl-2 family genes and caspase 3/9 activity in chondrocytes induced by extracellular acid (15). Another study also demonstrated that blocking ASIC1a by amiloride could protect articular chondrocytes from acid-induced apoptosis through the downregulation of Ca2+-dependent signaling pathways such as calpain and calcineurin and the inhibition of caspase-3 activity (17); these findings indicated that ASIC1a mediated the apoptosis of acid-induced articular chondrocyte by causing [Ca2+]i overload.
Proinflammatory cytokines such as interleukin-1β (IL-1β) and tumor necrosis factor-α (TNF-α) lead to the progressive destruction of articular structures in RA by stimulating synovial hyperplasia and bone destruction (90). A recent study reports that extracellular acidosis activates ASIC1a and reduces cell viability by triggering the apoptosis of rat articular chondrocytes and that pretreatment with TNF-α and IL-1β can enhance this process (4). Furthermore, IL-1β and TNF-α upregulated the expression of ASIC1a in primary rat articular chondrocytes in a time- and dose-dependent manner. Moreover, pretreatment with IL-1β and TNF-α significantly reduced cell viability and increased LDH release, [Ca2+]i elevation, and apoptosis by promoting the depolarization of the mitochondrial membrane and by upregulating the expression levels of cleaved PARP, cleaved caspase-3, and cleaved caspase-9 in acid-induced articular chondrocytes; the blockade of ASIC1a with PcTX1 or ASIC1a-shRNA dramatically decreased the expression of pro-apoptotic proteins (4). These results indicated that the pro-apoptotic effects of IL-1β and TNF-α in acid-stimulated articular chondrocytes are at least partially due to their involvement in regulating the expression and function of ASIC1a.
ASIC1a Is Involved in the Regulation of Autophagy in Articular Cartilage in RA
As an intracellular degradation system, autophagy mainly promotes the degradation of long-lived proteins and provides nutrition for the survival of cells during starvation (91). Increasing evidence shows that autophagy may lead to the degradation of damaged or organelles in excess, including mitochondria and endoplasmic reticulum (92). Recently, autophagy has been found to not only play a role in cell protection but also in the promotion of cell death in many types of cells. The overactivation of autophagy can lead to type II programmed cell death (93). In a study, it has been demonstrated that the level of autophagy in the synovial tissue of patients with active RA is increased and is related to the severity of the disease, thereby indicating that autophagy may play a crucial role in the regulation of RA (94). Intracellular Ca2+ is a regulator of autophagy (95). In a previous study, we showed that acidified activated ASIC1a promoted the autophagy of articular chondrocytes by mediating extracellular Ca2+ influx (96). Extracellular acidification could increase the levels of ASIC1a and autophagy-related markers (including LC3B-II and Beclin1) in articular chondrocytes, which was inhibited by the ASIC1a-specific blocker PcTx1 and the calcium-chelating agent BAPTA-AM (96). Moreover, the AMPK/FOXO3a signaling pathway has been considered to be involved in ASIC1a mediated autophagy. Extracellular acidification could activate AMPK and increase the levels of total FOXO3a and intranuclear FOXO3a, which could be reversed by the blockage of ASIC1a with PcTx1 or the blockage of Ca2+ with BAPTA-AM (96). The gene silencing of AMPK and FOXO3a can reduce the expression of LC3B-II and other autophagy-related markers in acid-induced articular chondrocytes (96).
Ca2+/CaMKKβ/AMPK/mTOR pathway has been reported to be involved in the progression of autophagy (97). Thus, the CaMKKβ/AMPK/mTOR signaling pathway was also evaluated to examine the mechanisms of ASIC1a in autophagy. It has been shown that the downregulated protein levels of p-mTOR/mTOR and the upregulated protein levels of CaMKKβ/β-actin and p-AMPK/AMPK in acid-induced activated articular chondrocytes were reversed by the inhibition of ASIC1a or BAPTA-AM; this suggests that the CaMKKβ/AMPK/mTOR signaling pathway may be related to the role of ASIC1a in autophagy (72).
Altogether, these results suggest that Ca2+ is an important factor in the acid-induced autophagy of articular chondrocytes and that ASIC1amayact as an upstream regulator of autophagy by inhibiting the influx of intracellular Ca2+.
ASIC1a Is Involved in the Regulation of Pyroptosis in Articular Cartilage in RA
Pyroptosis, which is a proinflammatory programmed cell death, is characterized by caspase-1 activation and the secretion of the proinflammatory cytokines IL-1β and IL-18 (98). Pyroptosis is inherently inflammatory and involves the activation of a caspase-activating complex known as the inflammasome (99). The inflammasome is mainly composed of three parts: receptor proteins, apoptosis-associated speck-like protein (ASC), and effect molecule pro-caspase-1 (100). According to different receptor proteins, inflammasomes can be divided into NLRP1 (101), NLRP3 (102), NLRC4 (103), and AIM2 inflammasome (104). Recent studies have found that pyroptosis is related to the occurrence and development of some autoimmune diseases, such as RA (105, 106). In cells exposed to Ca2+, bacterial toxin, and H+, the classical signaling pathway of the pyroptosis mediated by caspase-1 is activated (107, 108). Thus, in our previous study, we aimed to observe the pyroptosis of articular chondrocytes during the occurrence of RA in vivo and in vitro and to explore the role of ASIC1a in the pyroptosis of articular chondrocytes and its possible mechanism (87). The results of the in vivo experiments showed that ASC, NLRP3, caspase-1, IL-1β, and IL-18 were upregulated in the joints of AA rats compared to those in normal rats, whereas the expression of Col2a in cartilage was decreased. These changes were reversed by the ASIC1a inhibitor amiloride (87). Consistently, the results of in vitro experiments showed that the expression levels of ASC, NLRP3, caspase-1, IL-1β, and IL-18 were increased by extracellular acidosis and were reversed by PCTX-1 or BAPTA-AM (87). These results indicate that ASIC1a mediates pyroptosis in chondrocytes from AA rats, a mechanism which may be associated with the ability of ASIC1a to promote [Ca2+]i and upregulate the expression of the NLRP3 inflammasome.
ASIC1a Is Involved in the Regulation of Necroptosis in Articular Cartilage in RA
Necroptosis, a necrotic cell death pathway regulated by receptor interacting protein (RIP) 1 and 3, plays a critical role in the pathogenesis of several human inflammatory diseases (109). A recent study has shown that leptin can protect chondrocytes from necrosis, thereby indicating that necrosis may be related to the death of chondrocytes (110). The serine/threonine kinase activity of RIP1 is essential for necroptosis (111). Moreover, it has been reported that acid stimulation recruits RIP1 to the C-terminus of the ASIC1a in the cytoplasm; this causes the phosphorylation of RIP1 and subsequent neuronal death (112). Moreover, the deletion of ASIC1a gene significantly prevented the phosphorylation of RIP1 and brain injury, thereby indicating that the activation of RIP1 mediated by ASIC1a played an important role in ischemic neuronal injury (112). In a study, ASIC1a and RIP1 immunostaining signals were found to be highly co-expressed in articular cartilage tissues of AA rats than in control rats by using double-labeling immunofluorescence (79). Necrostatin-1 is a potent inhibitor of RIP1 kinase activity (113). In a previous study, we demonstrated that necrostatin-1 could reduce articular cartilage damage and necroinflammation in AA rats (79). Additionally, the ASIC1a-specific blockers PcTX-1 or ASIC1a short hairpin RNA inhibited the increase in the acid-induced necrosis markers RIP1 and RIP3, respectively, suggesting that acid-induced chondrocyte necrosis was mediated by ASIC1a (79). These findings indicate that blocking the ASIC1a-mediated necrosis of chondrocytes may provide a potential therapeutic strategy for the treatment of RA.
ASIC1a Promotes Synovial Inflammation and Invasion of Fibroblast-Like Cells in RA
Several studies have shown that fibroblast-like cells (FLSs) contribute significantly to the initiation and perpetuation of RA (114). Specifically, it has been reported that stable activated FLSs of RA can escape the growth limit by contact inhibition and gain the ability to migrate and invade, thereby leading to RA progression and cartilage destruction (115). Therefore, inhibiting the migration and invasion of RA-FLSs maybe a therapeutic strategy for the destructive progression of RA (116). Previous studies have suggested that ASIC1amaybe related to the proliferation and migration of tumors (117, 118). We explored the role of ASIC1a in the migration and invasion of RA-FLSs and whether blocking ASIC1a could reduce the migration and invasion of RA-FLSs and control the progression of RA (19). The results of HE and toluidine blue staining showed that synovial invasion was inhibited by the ASIC1a-specific inhibitor PcTX-1 in AA rats (19), thereby indicating that the inhibition of ASIC1a protected articular cartilage from synovial invasion and destruction in RA. Furthermore, the possible mechanism of ASIC1a involvement in synovial invasion was explored. As the key members of the MMP family, MMP-2, and MMP-9 have the ability to cleave gelatin, collagen type I, IV, and V, elastin, and vitronectin, which provide the conditions for cell migration and invasion (119, 120). Focal adhesion kinase (FAK), a non-receptor protein tyrosine kinase, plays a key role in integrin-mediated signaling pathways that are relevant to cell adhesion, migration, and invasion (121). ASIC1a-RNAi and PCTX-1 have been reported to decrease the extracellular acidification-induced invasion and migration of RA-FLSs and the expression of MMP2, MMP9, and p-FAK, which are upregulated by ASIC1a (19), suggesting that these proteins may be downstream signaling molecules of ASIC1a that are involved in synovial migration and invasion. Additionally, Ras-related C3 botulinum toxin substrate 1 (Rac1), a member of the Rho family of Ras-like small GTPases, interacts with a series of effectors and thus mediates various biological functions (122). Ca2+/Rac1 signaling has been reported to regulate the migration and invasion of RA-FLSs (123, 124). In a study, we demonstrated that the migration, invasion, and expression of MMP2, MMP9, and p-FAKin RA-FLSs were decreased by the intracellular calcium chelating agent BAPTA-AM or Rac1 specific blocker NSC23766 (19).Thus, we conclude that ASIC1a maybe a master regulator of synovial invasion via the Ca2+/Rac1 pathway and that inhibition of synovium invasion maybe one of the mechanisms forASIC1a in the treatment of RA.
Synovial inflammation is the main pathogenic factor of RA and plays an important role in the occurrence and development of RA-mediated articular cartilage and destruction of the bone (125, 126). RASF, as a key cell component of synovial tissues, plays an important role in synovial inflammation and joint structure destruction (76). The acidification of synovial fluid may be a key factor in synovial inflammation during the pathogenesis of RA (127). In a study, we demonstrated that extracellular acidification induced cartilage destruction by activating ASIC1a (87). For synovial tissues, it has been shown that the expression of ASIC1a in human RA synovial tissue, primary human RASF, and ankle synovium of AA rats was significantly increased (18). Consistently, in vitro experiments showed that extracellular acidification upregulated the expression of ASIC1a in RASF. Moreover, the expression of the inflammatory cytokines “regulated on activation, normal T cell expressed and secreted” (RANTES), sTNF RI, MIP-1a, IL-8, sTNFRII, and ICAM-1 were increased in RASF by extracellular acidification, which was significantly attenuated by ASIC1a-RNAi and PCTX-1. This indicated that ASIC1a induced synovial inflammation. Nuclear factor of activated T cells (NFATs), as a group of calcium-dependent transcription factors, regulate the transcription of inflammation-related genes, which drive the inflammatory process (128). Emerging evidence has indicated that ASIC1a induces the nuclear translocation of NFAT3 by mediating Ca2+ into pulmonary artery smooth muscle cells (129). Our research showed that extracellular acidification activated ASIC1a for the mediation of [Ca2+]i and the nuclear translocation of NFATc3. RANTES, also known as chemokine C-C motif ligand 5 (CCL5), is an important inflammatory cytokine that promotes the progression of RA. Recent studies have shown that the levels of RANTES in the plasma, synovial fluid and synovial tissue of RA patients are increased (130). NFATc3 binds to the RANTES promoter, directly regulates the transcription of RANTES, and enhances its expression; thus, RANTES is involved in the induction of synovial inflammation. These findings suggest that ASIC1a may be a potential therapeutic target for preventing synovial inflammation and controlling the progression of RA.
Acid-base balance is an important condition for maintaining normal physiological activities. Many diseases, such as ischemia, inflammation, hypoxia, and cancer, cause pH changes. ASICs, a member of the extracellular H+-activated cation channel family, affects the pathological and physiological changes of tissues. Numerous evidences have shown that ASIC1a promotes inflammation, synovial hyperplasia, articular cartilage, and bone destruction, which together lead to the progression of RA; these pathologic events maybe significantly attenuated by blocking ASIC1a, thereby indicating that ASIC1a may represent a novel target for the treatment of RA. The mechanism of ASIC1a blockade to improve symptoms of RA may be related to reduced apoptosis, autophagy, pyroptosis, and necroptosis of articular chondrocytes and inflammation and invasion of synovium. Further studies are required, especially in humans, to comprehensively explore the therapeutic potential of ASIC1a in RA.
Author Contributions
YX and FC designed this work and revised the manuscript. YX wrote the manuscript. All authors contributed to the article and approved the submitted version.
Funding
This work was supported by the National Natural Science Foundation of China (grant number 81873986).
Conflict of Interest
The authors declare that the research was conducted in the absence of any commercial or financial relationships that could be construed as a potential conflict of interest.
References
1. Aletaha D, Smolen JS. Diagnosis and Management of Rheumatoid Arthritis: A Review. JAMA (2018) 320(13):1360–72. doi: 10.1001/jama.2018.13103
2. Gibofsky A. Epidemiology, pathophysiology, and diagnosis of rheumatoid arthritis: A Synopsis. Am J Manag Care (2014) 20(7 Suppl):S128–35.
3. Falconer J, Murphy AN, Young SP, Clark AR, Tiziani S, Guma M, et al. Review: Synovial Cell Metabolism and Chronic Inflammation in Rheumatoid Arthritis. Arthritis Rheumatol (2018) 70(7):984–99. doi: 10.1002/art.40504
4. Zhou RP, Dai BB, Xie YY, Wu XS, Wang ZS, Li Y, et al. Interleukin-1beta and tumor necrosis factor-alpha augment acidosis-induced rat articular chondrocyte apoptosis via nuclear factor-kappaB-dependent upregulation of ASIC1a channel. Biochim Biophys Acta Mol Basis Dis (2018) 1864(1):162–77. doi: 10.1016/j.bbadis.2017.10.004
5. Mahajan TD, Mikuls TR. Recent advances in the treatment of rheumatoid arthritis. Curr Opin Rheumatol (2018) 30(3):231–7. doi: 10.1097/BOR.0000000000000496
6. Wang W, Zhou H, Liu L. Side effects of methotrexate therapy for rheumatoid arthritis: A systematic review. Eur J Med Chem (2018) 158:502–16. doi: 10.1016/j.ejmech.2018.09.027
7. Wemmie JA, Taugher RJ, Kreple CJ. Acid-sensing ion channels in pain and disease. Nat Rev Neurosci (2013) 14(7):461–71. doi: 10.1038/nrn3529
8. Riemann A, Ihling A, Thomas J, Schneider B, Thews O, Gekle M. Acidic environment activates inflammatory programs in fibroblasts via a cAMP-MAPK pathway. Biochim Biophys Acta (2015) 1853(2):299–307. doi: 10.1016/j.bbamcr.2014.11.022
9. Riemann A, Rauschner M, Giesselmann M, Reime S, Haupt V, Thews O. Extracellular Acidosis Modulates the Expression of Epithelial-Mesenchymal Transition (EMT) Markers and Adhesion of Epithelial and Tumor Cells. Neoplasia (2019) 21(5):450–8. doi: 10.1016/j.neo.2019.03.004
10. Rajamäki K, Nordström T, Nurmi K, Åkerman K, Kovanen P, Öörni K, et al. Extracellular acidosis is a novel danger signal alerting innate immunity via the NLRP3 inflammasome. J Biol Chem (2013) 288(19):13410–9. doi: 10.1074/jbc.M112.426254
11. Tannahill G, O’Neill L. The emerging role of metabolic regulation in the functioning of Toll-like receptors and the NOD-like receptor Nlrp3. FEBS Lett (2011) 585(11):1568–72. doi: 10.1016/j.febslet.2011.05.008
12. Cummings NA, Nordby GL. Measurement of synovial fluid pH in normal and arthritic knees. Arthritis Rheum (1966) 9(1):47–56. doi: 10.1002/art.1780090106
13. Farr M, Garvey K, Bold AM, Kendall MJ, Bacon PA. Significance of the hydrogen ion concentration in synovial fluid in rheumatoid arthritis. Clin Exp Rheumatol (1985) 3(2):99–104.
14. Geborek P, Saxne T, Pettersson H, Wollheim FA. Synovial fluid acidosis correlates with radiological joint destruction in rheumatoid arthritis knee joints. J Rheumatol (1989) 16(4):468–72.
15. Rong C, Chen FH, Jiang S, Hu W, Wu FR, Chen TY, et al. Inhibition of acid-sensing ion channels by amiloride protects rat articular chondrocytes from acid-induced apoptosis via a mitochondrial-mediated pathway. Cell Biol Int (2012) 36(7):635–41. doi: 10.1042/CBI20110432
16. Yuan FL, Chen FH, Lu WG, Li X, Wu FR, Li JP, et al. Acid-sensing ion channel 1a mediates acid-induced increases in intracellular calcium in rat articular chondrocytes. Mol Cell Biochem (2010) 340(1-2):153–9. doi: 10.1007/s11010-010-0412-y
17. Hu W, Chen FH, Yuan FL, Zhang TY, Wu FR, Rong C, et al. Blockade of acid-sensing ion channels protects articular chondrocytes from acid-induced apoptotic injury. Inflammation Res (2012) 61(4):327–35. doi: 10.1007/s00011-011-0414-6
18. Zhang Y, Qian X, Yang X, Niu R, Song S, Zhu F, et al. ASIC1a induces synovial inflammation via the Ca(2+)/NFATc3/ RANTES pathway. Theranostics (2020) 10(1):247–64. doi: 10.7150/thno.37200
19. Niu R, Hang X, Feng Y, Zhang Y, Qian X, Song S, et al. ASIC1a promotes synovial invasion of rheumatoid arthritis via Ca(2+)/Rac1 pathway. Int Immunopharmacol (2020) 79:106089. doi: 10.1016/j.intimp.2019.106089
20. Waldmann R, Champigny G, Bassilana F, Heurteaux C, Lazdunski M. A proton-gated cation channel involved in acid-sensing. Nature (1997) 386(6621):173–7. doi: 10.1038/386173a0
21. Lingueglia E. Acid-sensing ion channels in sensory perception. J Biol Chem (2007) 282(24):17325–9. doi: 10.1074/jbc.R700011200
22. Yoder N, Yoshioka C, Gouaux E. Gating mechanisms of acid-sensing ion channels. Nature (2018) 555(7696):397–401. doi: 10.1038/nature25782
23. Xu Y, Jiang YQ, Li C, He M, Rusyniak WG, Annamdevula N, et al. Human ASIC1a mediates stronger acid-induced responses as compared with mouse ASIC1a. FASEB J (2018) 32(7):3832–43. doi: 10.1096/fj.201701367R
24. Pathak T, Trebak M. Mitochondrial Ca(2+) signaling. Pharmacol Ther (2018) 192:112–23. doi: 10.1016/j.pharmthera.2018.07.001
25. Vullo S, Kellenberger S. A molecular view of the function and pharmacology of acid-sensing ion channels. Pharmacol Res (2020) 154:104166. doi: 10.1016/j.phrs.2019.02.005
26. Rook ML, Musgaard M, MacLean DM. Coupling structure with function in acid-sensing ion channels: Challenges in pursuit of proton sensors. J Physiol (2020). doi: 10.1113/JP278707
27. Jasti J, Furukawa H, Gonzales EB, Gouaux E. Structure of acid-sensing ion channel 1 at 1.9 A resolution and low pH. Nature (2007) 449(7160):316–23. doi: 10.1038/nature06163
28. Deval E, Lingueglia E. Acid-Sensing Ion Channels and nociception in the peripheral and central nervous systems. Neuropharmacology (2015) 94:49–57. doi: 10.1016/j.neuropharm.2015.02.009
29. Jahr H, van Driel M, van Osch GJ, Weinans H, van Leeuwen JP. Identification of acid-sensing ion channels in bone. Biochem Biophys Res Commun (2005) 337(1):349–54. doi: 10.1016/j.bbrc.2005.09.054
30. Duan B, Wu L, Yu Y, Ding Y, Jing L, Xu L, et al. Upregulation of acid-sensing ion channel ASIC1a in spinal dorsal horn neurons contributes to inflammatory pain hypersensitivity. J Neurosci Off J Soc Neurosci (2007) 27(41):11139–48. doi: 10.1523/jneurosci.3364-07.2007
31. Dusenkova S, Ru F, Surdenikova L, Nassenstein C, Hatok J, Dusenka R, et al. The expression profile of acid-sensing ion channel (ASIC) subunits ASIC1a, ASIC1b, ASIC2a, ASIC2b, and ASIC3 in the esophageal vagal afferent nerve subtypes. Am J Physiol Gastrointest Liver Physiol (2014) 307(9):G922–30. doi: 10.1152/ajpgi.00129.2014
32. Hughes P, Brierley S, Young R, Blackshaw L. Localization and comparative analysis of acid-sensing ion channel (ASIC1, 2, and 3) mRNA expression in mouse colonic sensory neurons within thoracolumbar dorsal root ganglia. J Comp Neurol (2007) 500(5):863–75. doi: 10.1002/cne.21204
33. Page A, Brierley S, Martin C, Price M, Symonds E, Butler R, et al. Different contributions of ASIC channels 1a, 2, and 3 in gastrointestinal mechanosensory function. Gut (2005) 54(10):1408–15. doi: 10.1136/gut.2005.071084
34. Huang C, Hu Z, Wu W, Yu D, Xiong Q, Song J, et al. Existence and distinction of acid-evoked currents in rat astrocytes. Glia (2010) 58(12):1415–24. doi: 10.1002/glia.21017
35. Yu XW, Hu ZL, Ni M, Fang P, Zhang PW, Shu Q, et al. Acid-sensing ion channels promote the inflammation and migration of cultured rat microglia. Glia (2015) 63(3):483–96. doi: 10.1002/glia.22766
36. Berdiev BK, Xia J, McLean LA, Markert JM, Gillespie GY, Mapstone TB, et al. Acid-sensing ion channels in malignant gliomas. J Biol Chem (2003) 278(17):15023–34. doi: 10.1074/jbc.M300991200
37. Richter TA, Dvoryanchikov GA, Roper SD, Chaudhari N. Acid-sensing ion channel-2 is not necessary for sour taste in mice. J Neurosci (2004) 24(16):4088–91. doi: 10.1523/JNEUROSCI.0653-04.2004
38. Chai S, Li M, Branigan D, Xiong ZG, Simon RP. Activation of acid-sensing ion channel 1a (ASIC1a) by surface trafficking. J Biol Chem (2010) 285(17):13002–11. doi: 10.1074/jbc.M109.086041
39. Zhou R, Leng T, Yang T, Chen F, Hu W, Xiong ZG. beta-Estradiol Protects Against Acidosis-Mediated and Ischemic Neuronal Injury by Promoting ASIC1a (Acid-Sensing Ion Channel 1a) Protein Degradation. Stroke (2019) 50(10):2902–11. doi: 10.1161/STROKEAHA.119.025940
40. Sun X, Jin J, Zhang JG, Qi L, Braun FK, Zhang XD, et al. Expression of acid-sensing ion channels in nucleus pulposus cells of the human intervertebral disk is regulated by non-steroid anti-inflammatory drugs. Acta Biochim Biophys Sin (Shanghai) (2014) 46(9):774–81. doi: 10.1093/abbs/gmu067
41. Li M, Zhou P, Chen X, Xu H, Wang J, Chen L, et al. NO in the dPAG modulates panic-like responses and ASIC1a expression in the prefrontal cortex and hippocampus in mice. Biochem Biophys Res Commun (2019) 511(2):274–9. doi: 10.1016/j.bbrc.2019.02.020
42. Brockway L, Zhou Z, Bubien J, Jovov B, Benos D, Keyser K. Rabbit retinal neurons and glia express a variety of ENaC/DEG subunits. Am J Physiol Cell Physiol (2002) 283(1):C126–34. doi: 10.1152/ajpcell.00457.2001
43. Ettaiche M, Deval E, Cougnon M, Lazdunski M, Voilley N. Silencing acid-sensing ion channel 1a alters cone-mediated retinal function. J Neurosci Off J Soc Neurosci (2006) 26(21):5800–9. doi: 10.1523/jneurosci.0344-06.2006
44. Lilley S, LeTissier P, Robbins J. The discovery and characterization of a proton-gated sodium current in rat retinal ganglion cells. J Neurosci Off J Soc Neurosci (2004) 24(5):1013–22. doi: 10.1523/jneurosci.3191-03.2004
45. Su X, Li Q, Shrestha K, Cormet-Boyaka E, Chen L, Smith P, et al. Interregulation of proton-gated Na(+) channel 3 and cystic fibrosis transmembrane conductance regulator. J Biol Chem (2006) 281(48):36960–8. doi: 10.1074/jbc.M608002200
46. Grifoni S, Jernigan N, Hamilton G, Drummond H. ASIC proteins regulate smooth muscle cell migration. Microvasc Res (2008) 75(2):202–10. doi: 10.1016/j.mvr.2007.08.003
47. Friese MA, Craner MJ, Etzensperger R, Vergo S, Wemmie JA, Welsh MJ, et al. Acid-sensing ion channel-1 contributes to axonal degeneration in autoimmune inflammation of the central nervous system. Nat Med (2007) 13(12):1483–9. doi: 10.1038/nm1668
48. Li X, Ye J, Xu M, Zhao M, Yuan F. Evidence that activation of ASIC1a by acidosis increases osteoclast migration and adhesion by modulating integrin/Pyk2/Src signaling pathway. Osteoporos Int J Established as Result Coop Between Eur Found Osteoporos Natl Osteoporos Found USA (2017) 28(7):2221–31. doi: 10.1007/s00198-017-4017-0
49. Zhang Y, Qian X, Yang X, Niu R, Song S, Zhu F, et al. ASIC1a induces synovial inflammation via the Ca/NFATc3/ RANTES pathway. Theranostics (2020) 10(1):247–64. doi: 10.7150/thno.37200
50. Kong X, Tang X, Du W, Tong J, Yan Y, Zheng F, et al. Extracellular acidosis modulates the endocytosis and maturation of macrophages. Cell Immunol (2013) 281(1):44–50. doi: 10.1016/j.cellimm.2012.12.009
51. Ni L, Fang P, Hu ZL, Zhou HY, Chen JG, Wang F, et al. Identification and Function of Acid-sensing Ion Channels in RAW 264.7 Macrophage Cells. Curr Med Sci (2018) 38(3):436–42. doi: 10.1007/s11596-018-1897-y
52. Dong X, Ko K, Chow J, Tuo B, Barrett K, Dong H. Expression of acid-sensing ion channels in intestinal epithelial cells and their role in the regulation of duodenal mucosal bicarbonate secretion. Acta Physiol (Oxford England) (2011) 201(1):97–107. doi: 10.1111/j.1748-1716.2010.02207.x
53. Thongon N, Ketkeaw P, Nuekchob C. The roles of acid-sensing ion channel 1a and ovarian cancer G protein-coupled receptor 1 on passive Mg2+ transport across intestinal epithelium-like Caco-2 monolayers. J Physiol Sci (2014) 64(2):129–39. doi: 10.1007/s12576-013-0301-8
54. Corrow K, Girard B, Vizzard M. Expression and response of acid-sensing ion channels in urinary bladder to cyclophosphamide-induced cystitis. Am J Physiol Renal Physiol (2010) 298(5):F1130–9. doi: 10.1152/ajprenal.00618.2009
55. Tong J, Wu W, Kong X, Wu P, Tian L, Du W, et al. Acid-sensing ion channels contribute to the effect of acidosis on the function of dendritic cells. J Immunol (Baltimore Md. 1950) (2011) 186(6):3686–92. doi: 10.4049/jimmunol.1001346
56. Zuo L, Zhu Y, Hu L, Liu Y, Wang Y, Hu Y, et al. PI3-kinase/Akt pathway-regulated membrane transportation of acid-sensing ion channel 1a/Calcium ion influx/endoplasmic reticulum stress activation on PDGF-induced HSC Activation. J Cell Mol Med (2019) 23(6):3940–50. doi: 10.1111/jcmm.14275
57. Jiang Y, Zha X. miR-149 reduces while let-7 elevates ASIC1a expression in vitro. Int J Physiol Pathophysiol Pharmacol (2017) 9(5):147–52.
58. Herbert LM, Resta TC, Jernigan NL. RhoA increases ASIC1a plasma membrane localization and calcium influx in pulmonary arterial smooth muscle cells following chronic hypoxia. Am J Physiol Cell Physiol (2018) 314(2):C166–76. doi: 10.1152/ajpcell.00159.2017
59. Frelin C, Barbry P, Vigne P, Chassande O, Cragoe EJ Jr, Lazdunski M. Amiloride and its analogs as tools to inhibit Na+ transport via the Na+ channel, the Na+/H+ antiport and the Na+/Ca2+ exchanger. Biochimie (1988) 70(9):1285–90. doi: 10.1016/0300-9084(88)90196-4
60. Leng TD, Xiong ZG. The pharmacology and therapeutic potential of small molecule inhibitors of acid-sensing ion channels in stroke intervention. Acta Pharmacol Sin (2013) 34(1):33–8. doi: 10.1038/aps.2012.81
61. Boscardin E, Alijevic O, Hummler E, Frateschi S, Kellenberger S. The function and regulation of acid-sensing ion channels (ASICs) and the epithelial Na(+) channel (ENaC): IUPHAR Review 19. Br J Pharmacol (2016) 173(18):2671–701. doi: 10.1111/bph.13533
62. Leng TD, Si HF, Li J, Yang T, Zhu M, Wang B, et al. Amiloride Analogs as ASIC1a Inhibitors. CNS Neurosci Ther (2016) 22(6):468–76. doi: 10.1111/cns.12524
63. Dube GR, Lehto SG, Breese NM, Baker SJ, Wang X. M. A. Matulenko, J. D. Brioni, et al. Electrophysiological and in vivo characterization of A-317567, a novel blocker of acid sensing ion channels. Pain (2005) 117(1-2):88–96. doi: 10.1016/j.pain.2005.05.021
64. Escoubas P, De Weille JR, Lecoq A, Diochot S, Waldmann R, Champigny G, et al. Isolation of a tarantula toxin specific for a class of proton-gated Na+ channels. J Biol Chem (2000) 275(33):25116–21. doi: 10.1074/jbc.M003643200
65. Chen X, Kalbacher H, Grunder S. The tarantula toxin psalmotoxin 1 inhibits acid-sensing ion channel (ASIC) 1a by increasing its apparent H+ affinity. J Gen Physiol (2005) 126(1):71–9. doi: 10.1085/jgp.200509303
66. Zhou R, Wu X, Wang Z, Ge J, Chen F. Interleukin-6 enhances acid-induced apoptosis via upregulating acid-sensing ion channel 1a expression and function in rat articular chondrocytes. Int Immunopharmacol (2015) 29(2):748–60. doi: 10.1016/j.intimp.2015.08.044
67. Wang W, Ye SD, Zhou KQ, Wu LM, Huang YN. High doses of salicylate and aspirin are inhibitory on acid-sensing ion channels and protective against acidosis-induced neuronal injury in the rat cortical neuron. J Neurosci Res (2012) 90(1):267–77. doi: 10.1002/jnr.22742
68. Voilley N, de Weille J, Mamet J, Lazdunski M. Nonsteroid anti-inflammatory drugs inhibit both the activity and the inflammation-induced expression of acid-sensing ion channels in nociceptors. J Neurosci (2001) 21(20):8026–33. doi: 10.1523/JNEUROSCI.21-20-08026.2001
69. Ragsdale DS, McPhee JC, Scheuer T, Catterall WA. Molecular determinants of state-dependent block of Na+ channels by local anesthetics. Science (1994) 265(5179):1724–8. doi: 10.1126/science.8085162
70. Lin J, Chu X, Maysami S, Li M, Si H, Cottrell JE, et al. Inhibition of acid sensing ion channel currents by lidocaine in cultured mouse cortical neurons. Anesth Analg (2011) 112(4):977–81. doi: 10.1213/ANE.0b013e31820a511c
71. Morris KV, Chan SW, Jacobsen SE, Looney DJ. Small interfering RNA-induced transcriptional gene silencing in human cells. Science (2004) 305(5688):1289–92. doi: 10.1126/science.1101372
72. Gao WF, Xu YY, Ge JF, Chen FH. Inhibition of acidsensing ion channel 1a attenuates acidinduced activation of autophagy via a calcium signaling pathway in articular chondrocytes. Int J Mol Med (2019) 43(4):1778–88. doi: 10.3892/ijmm.2019.4085
73. Wang Y, O’Bryant Z, Wang H, Huang Y. Regulating Factors in Acid-Sensing Ion Channel 1a Function. Neurochem Res (2016) 41(4):631–45. doi: 10.1007/s11064-015-1768-x
74. Xu Y, Chen F. Factors and Molecular Mechanisms Influencing the Protein Synthesis, Degradation and Membrane Trafficking of ASIC1a. Front Cell Dev Biol (2020) 8:596304:596304. doi: 10.3389/fcell.2020.596304
75. Trevani AS, Andonegui G, Giordano M, Lopez DH, Gamberale R, Minucci F, et al. Extracellular acidification induces human neutrophil activation. J Immunol (1999) 162(8):4849–57.
76. McInnes IB, Schett G. The pathogenesis of rheumatoid arthritis. N Engl J Med (2011) 365(23):2205–19. doi: 10.1056/NEJMra1004965
77. Goldie I, Nachemson A. Synovial pH in rheumatoid knee-joints. I. The effect of synovectomy. Acta Orthop Scand (1969) 40(5):634–41. doi: 10.3109/17453676908989529
78. Chang J, Wang W, Zhang H, Hu Y, Wang M, Yin Z. The dual role of autophagy in chondrocyte responses in the pathogenesis of articular cartilage degeneration in osteoarthritis. Int J Mol Med (2013) 32(6):1311–8. doi: 10.3892/ijmm.2013.1520
79. Chen Y, Zhu CJ, Zhu F, Dai BB, Song SJ, Wang ZQ, et al. Necrostatin-1 ameliorates adjuvant arthritis rat articular chondrocyte injury via inhibiting ASIC1a-mediated necroptosis. Biochem Biophys Res Commun (2018) 504(4):843–50. doi: 10.1016/j.bbrc.2018.09.031
80. Zhou R, Zhu F, Wu X, Song S, Chen Y, Zhu C, et al. Effects of autophagy on apoptosis of articular chondrocytes in adjuvant arthritis rats. J Cell Mol Med (2019) 23(11):7879–84. doi: 10.1111/jcmm.14629
81. Yuan FL, Chen FH, Lu WG, Li X, Li JP, Li CW, et al. Inhibition of acid-sensing ion channels in articular chondrocytes by amiloride attenuates articular cartilage destruction in rats with adjuvant arthritis. Inflammation Res (2010) 59(11):939–47. doi: 10.1007/s00011-010-0206-4
82. Pap T, Muller-Ladner U, Gay RE, Gay S. Fibroblast biology. Role of synovial fibroblasts in the pathogenesis of rheumatoid arthritis. Arthritis Res (2000) 2(5):361–7. doi: 10.1186/ar113
83. Hu Y, Cheng W, Cai W, Yue Y, Li J, Zhang P. Advances in research on animal models of rheumatoid arthritis. Clin Rheumatol (2013) 32(2):161–5. doi: 10.1007/s10067-012-2041-1
84. Sophia Fox AJ, Bedi A, Rodeo SA. The basic science of articular cartilage: structure, composition, and function. Sports Health (2009) 1(6):461–8. doi: 10.1177/1941738109350438
85. Espanha MM. [Articular cartilage: structure and histochemical composition]. Acta Reumatol Port (2010) 35(5):424–33.
86. DeGroot J, Verzijl N, Wenting-Van Wijk MJ, Bank RA, Lafeber FP, Bijlsma JW, et al. Age-related decrease in susceptibility of human articular cartilage to matrix metalloproteinase-mediated degradation: the role of advanced glycation end products. Arthritis Rheum (2001) 44(11):2562–71. doi: 10.1002/1529-0131(200111)44:11<2562::aid-art437>3.0.co;2-1
87. Wu X, Ren G, Zhou R, Ge J, Chen FH. The role of Ca(2+) in acid-sensing ion channel 1a-mediated chondrocyte pyroptosis in rat adjuvant arthritis. Lab Invest (2019) 99(4):499–513. doi: 10.1038/s41374-018-0135-3
88. Nygaard G, Di Paolo JA, Hammaker D, Boyle DL, Budas G, Notte GT, et al. Regulation and function of apoptosis signal-regulating kinase 1 in rheumatoid arthritis. Biochem Pharmacol (2018) 151:282–90. doi: 10.1016/j.bcp.2018.01.041
89. Rohner E, Detert J, Kolar P, Hocke A, N’Guessan P, Matziolis G, et al. Induced apoptosis of chondrocytes by Porphyromonas gingivalis as a possible pathway for cartilage loss in rheumatoid arthritis. Calcif Tissue Int (2010) 87(4):333–40. doi: 10.1007/s00223-010-9389-5
91. Levine B, Kroemer G. Autophagy in the pathogenesis of disease. Cell (2008) 132(1):27–42. doi: 10.1016/j.cell.2007.12.018
92. Shintani T, Klionsky DJ. Autophagy in health and disease: a double-edged sword. Science (2004) 306(5698):990–5. doi: 10.1126/science.1099993
93. Ding WX, Yin XM. Mitophagy: mechanisms, pathophysiological roles, and analysis. Biol Chem (2012) 393(7):547–64. doi: 10.1515/hsz-2012-0119
94. Feng Y, Li B, Li XY, Wu ZB. The Role of Autophagy in Rheumatic Disease. Curr Drug Targets (2018) 19(9):1009–17. doi: 10.2174/1389450117666160819155028
95. Sun F, Xu X, Wang X, Zhang B. Regulation of autophagy by Ca(2). Tumour Biol (2016) 37(12):15467–76. doi: 10.1007/s13277-016-5353-y
96. Dai B, Zhu F, Chen Y, Zhou R, Wang Z, Xie Y, et al. ASIC1a Promotes Acid-Induced Autophagy in Rat Articular Chondrocytes through the AMPK/FoxO3a Pathway. Int J Mol Sci (2017) 18(10):2125. doi: 10.3390/ijms18102125
97. Sun B, Ou H, Ren F, Huan Y, Zhong T, Gao M, et al. Propofol inhibited autophagy through Ca(2+)/CaMKKbeta/AMPK/mTOR pathway in OGD/R-induced neuron injury. Mol Med (2018) 24(1):58. doi: 10.1186/s10020-018-0054-1
98. Bergsbaken T, Fink SL, Cookson BT. Pyroptosis: host cell death and inflammation. Nat Rev Microbiol (2009) 7(2):99–109. doi: 10.1038/nrmicro2070
99. Miao EA, Leaf IA, Treuting PM, Mao DP, Dors M, Sarkar A, et al. Caspase-1-induced pyroptosis is an innate immune effector mechanism against intracellular bacteria. Nat Immunol (2010) 11(12):1136–42. doi: 10.1038/ni.1960
100. Martinon F, Burns K, Tschopp J. The inflammasome: a molecular platform triggering activation of inflammatory caspases and processing of proIL-beta. Mol Cell (2002) 10(2):417–26. doi: 10.1016/s1097-2765(02)00599-3
101. Chavarria-Smith J, Mitchell PS, Ho AM, Daugherty MD, Vance RE. Functional and Evolutionary Analyses Identify Proteolysis as a General Mechanism for NLRP1 Inflammasome Activation. PloS Pathog (2016) 12(12):e1006052. doi: 10.1371/journal.ppat.1006052
102. Liu D, Zeng X, Li X, Mehta JL, Wang X. Role of NLRP3 inflammasome in the pathogenesis of cardiovascular diseases. Basic Res Cardiol (2018) 113(1):5. doi: 10.1007/s00395-017-0663-9
103. Ip WK, Medzhitov R. Macrophages monitor tissue osmolarity and induce inflammatory response through NLRP3 and NLRC4 inflammasome activation. Nat Commun (2015) 6:6931. doi: 10.1038/ncomms7931
104. Rathinam VA, Jiang Z, Waggoner SN, Sharma S, Cole LE, Waggoner L, et al. The AIM2 inflammasome is essential for host defense against cytosolic bacteria and DNA viruses. Nat Immunol (2010) 11(5):395–402. doi: 10.1038/ni.1864
105. Choulaki C, Papadaki G, Repa A, Kampouraki E, Kambas K, Ritis K, et al. Enhanced activity of NLRP3 inflammasome in peripheral blood cells of patients with active rheumatoid arthritis. Arthritis Res Ther (2015) 17:257. doi: 10.1186/s13075-015-0775-2
106. Kastbom A, Verma D, Eriksson P, Skogh T, Wingren G, Soderkvist P. Genetic variation in proteins of the cryopyrin inflammasome influences susceptibility and severity of rheumatoid arthritis (the Swedish TIRA project). Rheumatol (Oxford) (2008) 47(4):415–7. doi: 10.1093/rheumatology/kem372
107. Eldridge MJ, Shenoy AR. Antimicrobial inflammasomes: unified signalling against diverse bacterial pathogens. Curr Opin Microbiol (2015) 23:32–41. doi: 10.1016/j.mib.2014.10.008
108. Dostert C, Petrilli V, Van Bruggen R, Steele C, Mossman BT, Tschopp J. Innate immune activation through Nalp3 inflammasome sensing of asbestos and silica. Science (2008) 320(5876):674–7. doi: 10.1126/science.1156995
109. Christofferson DE, Yuan J. Necroptosis as an alternative form of programmed cell death. Curr Opin Cell Biol (2010) 22(2):263–8. doi: 10.1016/j.ceb.2009.12.003
110. Lee SW, Rho JH, Lee SY, Kim JH, Cheong JH, Kim HY, et al. Leptin protects rat articular chondrocytes from cytotoxicity induced by TNF-alpha in the presence of cyclohexamide. Osteoarthr Cartil (2015) 23(12):2269–78. doi: 10.1016/j.joca.2015.06.005
111. Holler N, Zaru R, Micheau O, Thome M, Attinger A, Valitutti S, et al. Fas triggers an alternative, caspase-8-independent cell death pathway using the kinase RIP as effector molecule. Nat Immunol (2000) 1(6):489–95. doi: 10.1038/82732
112. Wang YZ, Wang JJ, Huang Y, Liu F, Zeng WZ, Li Y, et al. Tissue acidosis induces neuronal necroptosis via ASIC1a channel independent of its ionic conduction. Elife (2015) 4:e05682. doi: 10.7554/eLife.05682
113. Degterev A, Hitomi J, Germscheid M, Ch’en IL, Korkina O, Teng X, et al. Identification of RIP1 kinase as a specific cellular target of necrostatins. Nat Chem Biol (2008) 4(5):313–21. doi: 10.1038/nchembio.83
114. Huber LC, Distler O, Tarner I, Gay RE, Gay S, Pap T. Synovial fibroblasts: key players in rheumatoid arthritis. Rheumatol (Oxford) (2006) 45(6):669–75. doi: 10.1093/rheumatology/kel065
115. Liu Y, Pan YF, Xue YQ, Fang LK, Guo XH, Guo X, et al. uPAR promotes tumor-like biologic behaviors of fibroblast-like synoviocytes through PI3K/Akt signaling pathway in patients with rheumatoid arthritis. Cell Mol Immunol (2018) 15(2):171–81. doi: 10.1038/cmi.2016.60
116. Li N, Gong Z, Li X, Ma Q, Wu M, Liu D, et al. Betulinic acid inhibits the migration and invasion of fibroblast-like synoviocytes from patients with rheumatoid arthritis. Int Immunopharmacol (2019) 67:186–93. doi: 10.1016/j.intimp.2018.11.042
117. Chen X, Sun X, Wang Z, Zhou X, Xu L, Li F, et al. Involvement of acid-sensing ion channel 1a in gastric carcinoma cell migration and invasion. Acta Biochim Biophys Sin (Shanghai) (2018) 50(5):440–6. doi: 10.1093/abbs/gmy026
118. Wu Y, Gao B, Xiong QJ, Wang YC, Huang DK, Wu WN. Acid-sensing ion channels contribute to the effect of extracellular acidosis on proliferation and migration of A549 cells. Tumour Biol (2017) 39(6):1010428317705750. doi: 10.1177/1010428317705750
119. Babykutty S, S PP, J NR, Kumar MA, Nair MS, Srinivas P, et al. Nimbolide retards tumor cell migration, invasion, and angiogenesis by downregulating MMP-2/9 expression via inhibiting ERK1/2 and reducing DNA-binding activity of NF-kappaB in colon cancer cells. Mol Carcinog (2012) 51(6):475–90. doi: 10.1002/mc.20812
120. Zhou R, Xu L, Ye M, Liao M, Du H, Chen H. Formononetin inhibits migration and invasion of MDA-MB-231 and 4T1 breast cancer cells by suppressing MMP-2 and MMP-9 through PI3K/AKT signaling pathways. Horm Metab Res (2014) 46(11):753–60. doi: 10.1055/s-0034-1376977
121. Hauck CR, Hsia DA, Schlaepfer DD. The focal adhesion kinase–a regulator of cell migration and invasion. IUBMB Life (2002) 53(2):115–9. doi: 10.1080/15216540211470
122. Payapilly A, Malliri A. Compartmentalisation of RAC1 signalling. Curr Opin Cell Biol (2018) 54:50–6. doi: 10.1016/j.ceb.2018.04.009
123. Chan A, Akhtar M, Brenner M, Zheng Y, Gulko PS, Symons M. The GTPase Rac regulates the proliferation and invasion of fibroblast-like synoviocytes from rheumatoid arthritis patients. Mol Med (2007) 13(5-6):297–304. doi: 10.2119/2007-00025.Chan
124. Lao M, Shi M, Zou Y, Huang M, Ye Y, Qiu Q, et al. Protein Inhibitor of Activated STAT3 Regulates Migration, Invasion, and Activation of Fibroblast-like Synoviocytes in Rheumatoid Arthritis. J Immunol (2016) 196(2):596–606. doi: 10.4049/jimmunol.1403254
125. Ito H, Moritoshi F, Hashimoto M, Tanaka M, Matsuda S. Control of articular synovitis for bone and cartilage regeneration in rheumatoid arthritis. Inflammation Regener (2018) 38:7. doi: 10.1186/s41232-018-0064-y
126. Boissier MC, Semerano L, Challal S, Saidenberg-Kermanac’h N, Falgarone G. Rheumatoid arthritis: from autoimmunity to synovitis and joint destruction. J Autoimmun (2012) 39(3):222–8. doi: 10.1016/j.jaut.2012.05.021
127. Fujii W, Kawahito Y, Nagahara H, Kukida Y, Seno T, Yamamoto A, et al. Monocarboxylate transporter 4, associated with the acidification of synovial fluid, is a novel therapeutic target for inflammatory arthritis. Arthritis Rheumatol (2015) 67(11):2888–96. doi: 10.1002/art.39270
128. Klein-Hessling S, Muhammad K, Klein M, Pusch T, Rudolf R, Floter J, et al. NFATc1 controls the cytotoxicity of CD8(+) T cells. Nat Commun (2017) 8(1):511. doi: 10.1038/s41467-017-00612-6
129. Gonzalez Bosc LV, Plomaritas DR, Herbert LM, Giermakowska W, Browning C, Jernigan NL. ASIC1-mediated calcium entry stimulates NFATc3 nuclear translocation via PICK1 coupling in pulmonary arterial smooth muscle cells. Am J Physiol Lung Cell Mol Physiol (2016) 311(1):L48–58. doi: 10.1152/ajplung.00040.2016
Keywords: acid-sensing ion channel 1a, rheumatoid arthritis, articular chondrocyte, synovial fibroblast, therapeutic target
Citation: Xu Y and Chen F (2021) Acid-Sensing Ion Channel-1a in Articular Chondrocytes and Synovial Fibroblasts: A Novel Therapeutic Target for Rheumatoid Arthritis. Front. Immunol. 11:580936. doi: 10.3389/fimmu.2020.580936
Received: 07 July 2020; Accepted: 14 December 2020;
Published: 28 January 2021.
Edited by:
Dimitrios Petrou Bogdanos, University of Thessaly, GreeceReviewed by:
Åsa Andersson, Halmstad University, SwedenFeng Lai Yuan, Nanjing University of Chinese Medicine, China
Copyright © 2021 Xu and Chen. This is an open-access article distributed under the terms of the Creative Commons Attribution License (CC BY). The use, distribution or reproduction in other forums is permitted, provided the original author(s) and the copyright owner(s) are credited and that the original publication in this journal is cited, in accordance with accepted academic practice. No use, distribution or reproduction is permitted which does not comply with these terms.
*Correspondence: Feihu Chen, chenfeihu@ahmu.edu.cn